- 1Department of Cardiology, Guang’anmen Hospital, China Academy of Chinese Medical Sciences, Beijing, China
- 2Department of Intensive Care Unit, Guang’anmen Hospital, China Academy of Chinese Medical Sciences, Beijing, China
- 3Department of Rehabilitation, Dongfang Hospital, Beijing University of Chinese Medicine, Beijing, China
- 4Graduate School, Henan University of Chinese Medicine, Zhengzhou, China
Cardiovascular diseases (CVD) remain the leading cause of death worldwide and represent a major public health challenge. YiyiFuzi Powder (YYFZ), composed of Coicis semen and Fuzi, is a classical traditional Chinese medicine prescription from the Synopsis of Golden Chamber dating back to the Han Dynasty. Historically, YYFZ has been used to treat various CVD, rooted in Chinese therapeutic principles. Network pharmacology analysis indicated that YYFZ may exhibit direct or indirect effects on mitochondria-endoplasmic reticulum (ER) interactions. This review, focusing on the cardiovascular protective effects of Coicis semen and Fuzi, summarizes the potential mechanisms by which YYFZ acts on mitochondria and the ER. The underlying mechanisms are associated with regulating cardiovascular risk factors (such as blood lipids and glucose), impacting mitochondrial structure and function, modulating ER stress, inhibiting oxidative stress, suppressing inflammatory responses, regulating cellular apoptosis, and maintaining calcium ion balance. The involved pathways include, but were not limited to, upregulating the IGF-1/PI3K/AKT, cAMP/PKA, eNOS/NO/cGMP/SIRT1, SIRT1/PGC-1α, Klotho/SIRT1, OXPHOS/ATP, PPARα/PGC-1α/SIRT3, AMPK/JNK, PTEN/PI3K/AKT, β2-AR/PI3K/AKT, and modified Q cycle signaling pathways. Meanwhile, the MCU, NF-κB, and JAK/STAT signaling pathways were downregulated. The PERK/eIF2α/ATF4/CHOP, PERK/SREBP-1c/FAS, IRE1, PINK1-dependent mitophagy, and AMPK/mTOR signaling pathways were bidirectionally regulated. High-quality experimental studies are needed to further elucidate the underlying mechanisms of YYFZ in CVD treatment.
1 Introduction
Cardiovascular diseases (CVD) remain the leading global cause of mortality, presenting a major public health challenge (Perk, 2017; Luo F. et al., 2018). In 2022, approximately 775 million individuals worldwide were affected by CVD, contributing to over 19.8 million deaths (Mensah et al., 2023). Currently, there is compelling evidence indicating that the occurrence and progression of CVD are frequently associated with aberrant interactions between mitochondria and endoplasmic reticulum (ER) (Gao et al., 2020; Wang et al., 2021e).
Mitochondria serve as the cellular powerhouses, generating energy in the form of adenosine triphosphate (ATP) and playing crucial roles in many cellular processes. Meanwhile, the ER is vital for protein synthesis, folding, translocation, and maintaining calcium (Ca2+) homeostasis (Zhou et al., 2021). In recent years, the focus on regulating mitochondrial quality control (MQC) has opened new avenues for treating CVD (Chang et al., 2022; Chang et al., 2023a; Chang et al., 2023b; Chang et al., 2023c). This autonomic system safeguards cardiomyocytes during stress responses by inhibiting mitochondrial apoptosis, mitochondrial-related ER stress, and the mitochondrial-related unfolded protein response (UPR). As a result, it mitigates ischemia and hypoxia injuries in sinoatrial node cells (Chang et al., 2023a; Chang et al., 2024b) and normal cardiomyocytes (Chang et al., 2021; Chang et al., 2022; Chang et al., 2023b; Chang et al., 2024a), ultimately protecting cardiovascular health. It is evident that the roles of mitochondria and ER in cardiovascular health are intertwined, indicating an interconnected relationship rather than independent functions. These two organelles are intricately connected both structurally and functionally, forming specialized domains known as mitochondria-associated endoplasmic reticulum membranes (MAM) (Marchi et al., 2014; Szymanski et al., 2017; Gao et al., 2020), which are integral to fundamental biological processes. Abnormal MAM structure and mitochondria-ER interactions can lead to disruptions in mitochondrial structure, function, DNA, and biosynthesis (Zhu L. et al., 2020; Glanz et al., 2020) as well as altered lipid metabolism, impaired Ca2+ handling, ER stress, oxidative stress, inflammation, and apoptosis (Gao et al., 2020; Wang et al., 2021e; Luan et al., 2021). Dysfunction in both mitochondria and ER can contribute to the development and progression of various CVD, including heart failure (HF) (Liu et al., 1992; Lu et al., 2017), cardiac hypertrophy (Dromparis et al., 2013; Koyama et al., 2014; Wu Y. et al., 2016), atherosclerosis (Glanz et al., 2020), coronary heart disease (CHD) (Liu and Wu, 2022), and myocardial infarction (MI) (Liu et al., 1992; Wang N. N. et al., 2021).
Traditional Chinese medicine (TCM), an essential complementary and alternative medicine, has been used for thousands of years to treat various diseases with multitarget effects and reported clinical benefits (Lu et al., 2004; Meng et al., 2021; Lv S. et al., 2022; Liu Y. J. et al., 2022). YiyiFuzi Powder (YYFZ), composed of Coicis semen and Fuzi (Figure 1), is a classical prescription from the Synopsis of Golden Chamber by Zhang Zhongjing in the Han Dynasty, which historically relieved cardiothoracic pain. In TCM theory, Coicis semen removes dampness and eliminates blockages (Han et al., 2017; Luo Y. Y. et al., 2018), while Fuzi warms and strengthens Yang, relieves pain, and disperses cold (Commission, 2020). Combining Coicis semen and Fuzi can remove the paralysis of cold and dampness contributing to cardiothoracic pain (Yang et al., 2016). Additional benefits have been noted in unstable angina patients, including improvements in their clinical symptoms, signs, and electrocardiogram ischemia, as well as reductions in homocysteine levels (Wang, 2018). The formula also treats other systemic conditions such as rheumatoid arthritis (Zhang F. F. et al., 2023; Wang Y. Y. et al., 2023) and bronchial asthma (Yu Z. X. et al., 2011; Yu et al., 2016).
Network pharmacology analysis suggests that YYFZ may impact mitochondria-ER interactions (Wang, 2019; Wang Y. et al., 2023). Our study reviews the cardiovascular protective effects of YYFZ by directly regulating mitochondrial and ER function and indirectly influencing cardiovascular risk factors (such as lipid and glucose metabolism), oxidative stress, inflammatory responses, cellular apoptosis, and Ca2+ balance to impact the mitochondria-ER interactions. These findings provide a theoretical basis for the rational clinical application of the TCM formula YYFZ.
2 The cardiovascular protective activity of YYFZ
Modern studies have demonstrated the effectiveness of YYFZ in therapeutic applications for CVD, including chronic heart failure (CHF) (Wang Y. Y. et al., 2023), CHD (Wang, 2018), myocardial ischemia (Li et al., 2023), and myocarditis (Liu, 1991), with reported analgesic, anti-anginal, and cardiotonic effects (Wang, 2019). Due to the lack of a boiling process, the pharmacological effects of the powdered formula YYFZ are simple a sum of the individual effects of its two component botanical drugs, Coicis semen and Fuzi. Hence, it is advisable to separately review the pharmacological effects and cardiovascular protective attributes of Coicis semen and Fuzi to understand the YYFZ’s pharmacological effects.
2.1 Coicis semen
Coicis semen, also known as Coix seed (Coix lacryma-jobi L. var. ma-yuen Stapf), is a TCM widely recognized for its rich nutritional and medicinal value. Fingerprinting of Coicis semen from dried tissues using the sequence-independent microarray (Niu et al., 2013) and the high-performance liquid chromatography (HPLC)-evaporative light scattering detector (Du et al., 2021) has been reported. It contains more than 80 active components including polysaccharides, fatty acids (FAs), phenolic acids, sterols, lipids, flavonoids, triterpenes, alkaloids, and adenosine (Zhu, 2017; Yu J. et al., 2022; Huang et al., 2022; Pan et al., 2023; Wei et al., 2023). The HPLC-atmospheric pressure chemical ionization-mass spectrometry (MS) method identified 12 triacylglycerols in coix oil (Xiang et al., 2005). Among them, the contents exceeding 3% include glycerol trioleate, glycerol dioleate, glycerol palmitate, and glycerol linoleate, comprising more than 90% of the triglycerides. The chromatographic fingerprint revealed the main pharmacologically active ingredients of Coicis semen, including 1,3-Dioleoyl-2-palmitoylglycerol, 1,2-dilinoleoyl-3-oleoyl-rac-glycerol, 1,3-Dipalmitoyl-2-Linolein, 1,2-Dilinoleoyl-3-palmitoyl-rac-glycerol, 1,2-Dioleoyl-3-linoleoyl-rac-glycerol, and glycerol trioleate (Du et al., 2021). These components are primarily responsible for improving lipid metabolism, antioxidation, and anti-inflammation, providing analgesic effects, and offering cardiovascular protection (Zhu R. et al., 2020; Chen et al., 2022; Yang et al., 2022d; Zeng et al., 2022; Pan et al., 2023), thereby supporting proper heart function and protecting against CVD (Xu et al., 2017; Chen et al., 2022). Studies have shown that Coicis semen has positive effects on CHDs (Yu F. et al., 2011; Wang et al., 2012), atherosclerosis (Check and K'Ombut, 1995; Yu F. et al., 2011), myocardial ischemia, MI(Yu J. et al., 2022), hypertension (Li et al., 2017; Chen et al., 2020), hypercholesterolemia (Wang et al., 2012), and hyperlipidemia (Chen et al., 2022).
2.2 Fuzi
Fuzi, also known as Aconiti Lateralis Radix Praeparata (Aconitum carmichaelii Debx.), is another TCM that contains more than 120 chemical components. HPLC-MS fingerprinting and Quadrupole Time-of-Flight (Q-TOF)-MS were used to analyze the chemical ingredients of Fuzi decoction sediments. As a result, 28 compounds were identified, with 25 of their structures confirmed (Zhang et al., 2012). The main components found in Fuzi include flavonoids, alkaloids such as aconitine (AC), salsolinol (SAL), fuziline (FZL), mesaconitine (MA), higenamine (HG), and hypaconitine (HA) (Zhao et al., 2012; Zhang et al., 2022d), polysaccharides, flavonoids, FAs, and ceramides (Zhang et al., 2022d; Zhang W. et al., 2023). Zhang et al. employed chromatographic fingerprinting to quantitatively analyze the diterpenoid alkaloids (DAs) of Fuzi, and utilized HPLC coupled with hybrid ion trap-time-of-flight mass spectrometry for qualitative assessment (Zhang et al., 2016). Among the samples, HA was the most abundant alkaloid in Fuzi, with a content range of 325.25–835.80 μg/g, followed by MA (96.41–541.62 μg/g), benzoylmesaconine (83.47–313.79 μg/g), AC (31.94–139.45 μg/g), benzoylhypaconine (22.39–96.93 μg/g), and benzoylaconine (BAC) (9.76–79.72 μg/g). In total, 99 DAs were identified and characterized, including 9 with clear identities, 77 with probable assignments, and 13 unknown compounds. However, HPLC fingerprinting demonstrated that the alkaloid content of Fuzi varies by geographical origin (Luo et al., 2019; Miao et al., 2019; Wang X. Y. et al., 2020; Ding et al., 2022; Qu et al., 2023). Luo et al. utilized HPLC coupled with an evaporative light-scattering detector to quantify the alkaloids. They observed average alkaloid contents in Fuzi as follows: HA at 1168.25 μg/g, MA at 1133.80 μg/g, Neoline at 766.62 μg/g, FZL at 750.32 μg/g, Songorine at 451. 89 μg/g, AC at 259.95 μg/g, and benzoylmeaconitine at 158.18 μg/g (Luo et al., 2019). Components of Fuzi, particularly alkaloids and polysaccharides, contribute to its antioxidant activities, anti-inflammatory properties, regulation of apoptosis, analgesic properties, and cardiotonic effects (Zhao et al., 2012; Yan et al., 2020; Yang et al., 2021b; Zhang et al., 2022d; Tian et al., 2022; Hacanli et al., 2023). However, HPLC-Q-TOF-MS and ultra-HPLC fingerprinting revealed distinct main activities among the different processed products of Fuzi (Zheng et al., 2014; Tong et al., 2021). In total, Fuzi helps protect against various CVD, particularly HF, and supports overall cardiovascular health (Yang et al., 2019; Tai et al., 2022). Long-term use of Fuzi is beneficial in preventing cardiovascular events (Tai et al., 2022).
3 The impact of YYFZ on mitochondria-ER interactions through data mining
Data-mining research has demonstrated that YYFZ can affect mitochondria-ER interactions by regulating mitochondrial function and ER function (Wang, 2019; Wang Y. et al., 2023; Wang Y. Y. et al., 2023). In addition, network pharmacology has shown that YYFZ can modulate the phosphoinositide 3-kinase (PI3K)/AKT, modulating mitogen-activated protein kinase (MAPK), and Ras signaling pathways (Wang Y. et al., 2023), all of which potentially affect mitochondria-ER interactions. Abnormal mitochondrial-ER interactions are thought to be key mechanisms in CVD such as CHD, HF, pulmonary hypertension, and atherosclerosis (Zhang et al., 2020). It can be speculated that the cardiovascular protective effect of YYFZ may be related to maintaining the homeostasis of mitochondria-ER interactions.
Studies have shown that the YYFZ may intervene in CHF through MAPK3(Wang Y. Y. et al., 2023). MAPK3 belongs to the MAPK family, which participates in diverse cellular functions such as inflammation, apoptosis, proliferation, and differentiation. Both in the in-vivo and in-vitro experiments, upregulation of MAPK3 attenuates ischemia/reperfusion (I/R) injury by reducing cardiomyocyte apoptosis (Wang H. et al., 2021). However, inhibiting MAPK phosphorylation levels in CHF model rats activates the peroxisome proliferator-activated receptor γ (PPAR-γ) pathway by participating in mitochondrial biogenesis, thereby promoting energy generation, improving cardiac energy metabolism, and slowing the pathological progression of CHF (Wang Y. W. et al., 2020).
Through metabolomics and network pharmacology analysis, it was found that YYFZ improves the efficiency of endoplasmic reticulum-associated protein degradation (ERAD), a process that removes misfolded proteins from the ER. By enhancing ERAD, it can reduce the accumulation of misfolded proteins and subsequently alleviate ER stress (Wang Y. et al., 2023). ER stress is a cellular condition that occurs when there is an accumulation of unfolded or misfolded proteins in the ER (Haeri and Knox, 2012), which triggers a cellular response known as the UPR (Hetz, 2012). It is speculated that YYFZ may also have the ability to modulate the UPR, therefore helping restore ER homeostasis and reducing the burden on the ER. Another clinical metabolomics combined with network pharmacology analysis showed that HG, β-sitosterol, and stigmasterol in YYFZ can bind to ER proteins and affect the sphingomyelin pathway, thereby reducing intravascular lipid accumulation, inhibiting inflammation, and exerting potential therapeutic effects on the cardiovascular system (Wang Y. et al., 2022). The study also suggests that ASM enzymes, a part of the sphingomyelin pathway, may be key targets for the treatment of CHD.
4 The potential impact of YYFZ on mitochondria-ER interactions
A mouse study found that YYFZ enhanced the biological activity of nitric oxide (NO), restored mitochondrial membrane potential (MMP), and reduced reactive oxygen species (ROS) generation, thereby alleviating mitochondrial and vascular endothelial dysfunction and improving the pathological state of myocardial ischemia (Li et al., 2023). However, direct laboratory evidence demonstrating YYFZ’s effects on mitochondria-ER interactions is still lacking. Further exploration is needed to elucidate the potential mechanism. Nevertheless, the constituent botanical drugs of YYFZ, Coicis semen and Fuzi, have been shown to play a significant role in regulating mitochondria-ER interactions and conferring cardiovascular protection individually.
4.1 Regulating cardiovascular risk factors
4.1.1 Regulating lipid metabolism
YYFZ potentially modulates mitochondria-ER interactions to regulate cardiometabolic risk factors such as lipids and glucose (Table 1; Figure 2), thereby exerting a cardiovascular protective effect. Dysregulated lipid metabolism is a major risk factor for CVD (Zeng et al., 2022). Coicis semen has shown effects on lipid metabolism by inhibiting fatty acid synthase (FAS), decreasing cholesterol synthesis, and supporting immune functions (Chen et al., 2022; Zhao et al., 2023). Studies found that Coicis semen decreases serum total cholesterol (TC), low-density lipoprotein cholesterol (LDL-C), and triglycerides (TG) in animal models (Lin and Tsai, 2008; Wang et al., 2012; Zhang et al., 2022c; Yang et al., 2022d). Coicis semen is rich in branched-chain amino acids (BCAAs), especially leucine (Liu et al., 2015). In mice, leucine decreased hepatic TG accumulation and atherosclerotic lesion area, exhibiting lipid-lowering and cardiovascular protective properties (Yokota et al., 2016; Zhao et al., 2016). Coix seed polysaccharides (CSP), active components of Coicis semen, have been found to alleviate type 2 diabetes mellitus in mouse models by increasing high-density lipoprotein cholesterol levels (Yu W. et al., 2022).
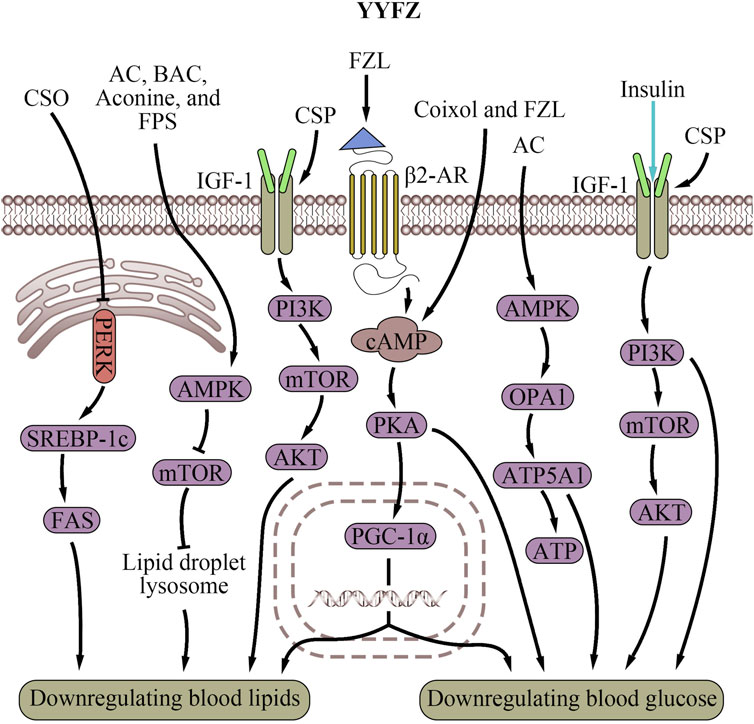
Figure 2. Cardiovascular risk factor regulatory effects of YiyiFuzi Powder (Abbreviations: AC, aconitine; AMPK, AMP-activated protein kinase; ATP, adenosine triphosphate; ATP5A1, adenosine triphosphate synthase, H+ transporting, mitochondrial F1 complex, alpha subunit 1; BAC, benzoylaconine; β2-AR, β2-adrenergic receptor; cAMP, cyclic adenosine monophosphate; CSO, Coix seed oil; CSP, Coix seed polysaccharides; FAS, fatty acid synthase; FPS, polysaccharides from Fuzi; FZL, fuziline; IGF-1, insulin-like Growth Factor 1; mTOR, mechanistic target of rapamycin; OPA1, optic atrophy 1; PERK, protein kinase RNA-like endoplasmic reticulum kinase; PGC-1α, peroxisome proliferator-activated receptor-γ coactivator-1α; PI3K, phosphoinositide 3-kinase; PKA, protein kinase A; SREBP-1c, sterol regulatory element-binding protein-1c; YYFZ, YiyiFuzi Powder).
In obese mice, Coix seed oil (CSO) protects mitochondrial function from lipid droplet-induced stress (Chen et al., 2022). Obesity increases oxidative stress, inflammation, and ER stress via the protein kinase RNA-like endoplasmic reticulum kinase (PERK)/sterol regulatory element-binding protein-1c (SREBP-1c)/FAS signaling pathway (Xue et al., 2005; Zhang and Kaufman, 2008; Han and Kaufman, 2016; Saltiel and Olefsky, 2017; Wang S. et al., 2021). PERK is an ER-resident transmembrane kinase that is a part of the UPR. PERK is activated when the ER is under stress, leading to the activation of SREBP-1c, which promotes lipogenesis (Han and Kaufman, 2016). CSO may exert cardiovascular protection by reducing oxidative and ER stress and protecting mitochondria through suppressing the PERK/SREBP-1c/FAS signaling pathway. Another study found that Coix seed extracts (CSEs) reduced chronic inflammation, insulin resistance, weight gain, fat content, and lipid levels in obese mice (Zhang et al., 2022c). Researchers speculated that this reduction was due to the CSE group’s reduction of liver fat weight and lipid control as a result of reduced ER stress.
Active components in Fuzi also contribute to YYFZ’s lipid-regulating effects. Studies show that Fuzi can lower LDL-C and TC, preventing atherosclerosis (Huang et al., 2010). The potential mechanism is that Fuzi upregulates the expression of fatty-acid-metabolism genes such as Acot1, Acot2, Acsl3, Acox3, and Acox2, which are involved in FA hydrolysis, beta-oxidation, and acyl-CoA production (Yu et al., 2012). Additionally, Fuzi was identified as improving energy metabolism, including lipid regulation, through the crucial AMP-activated protein kinase (AMPK) signaling pathway (Deng et al., 2019). This pathway ensures energy homeostasis and mitochondrial biogenesis (Hardie and Sakamoto, 2006; Jager et al., 2007). The diterpene alkaloid in Fuzi, FZL (Xiong et al., 2012; Fan et al., 2020), activates β-adrenergic receptors (β-AR), which then activate the downstream cyclic adenosine monophosphate (cAMP)/protein kinase A (PKA) signaling pathway. This enhances mitochondrial energy metabolism and stimulates liver lipolysis to improve lipid profiles (Gao et al., 2023). Overall, YYFZ helps maintain mitochondrial function, biogenesis, energy metabolism, and ER stress through its upregulation of the cAMP/PKA signaling pathways, and downregulation of the PERK/SREBP-1c/FAS signaling pathways, thereby modulating lipid metabolism and protecting the cardiovascular system.
4.1.2 Regulating blood glucose metabolism
Regulating blood glucose is another important way that YYFZ protects cardiovascular health. CSP, Coixol, FZL, and AC are the main constituents contributing to the glucose regulation effects of YYFZ. CSP increased serum insulin levels to alleviate type 2 diabetes mellitus in mouse models (Yu W. et al., 2022). CSP treatment also modulated gut microbiota composition via activation of the insulin-like Growth Factor 1 (IGF-1)/PI3K/AKT signaling pathway (Xia et al., 2021). The IGF-1/PI3K/AKT signaling pathway plays a significant role in regulating blood glucose, primarily involving maintaining normal insulin sensitivity, increasing glucose uptake and utilization, decreasing plasma triglyceride levels, and regulating cholesterol levels (Clemmons, 2004; Ng et al., 2008; Jensen-Cody and Potthoff, 2021). Additionally, this pathway is closely related to the structure and function of mitochondria (Radovic et al., 2019) and ER (Novosyadlyy et al., 2008). The IGF-1 signaling pathway supports mitochondrial biogenesis and function by maintaining MMP, increasing ATP production, reducing mitochondrial ROS production, and promoting mitochondrial protein complexes (Puche et al., 2008). IGF-1 also interacts with the ER (Paharkova-Vatchkova and Lee, 2010). Additionally, PI3K signaling regulates mitochondrial physiology at MAM through mTORC2 and AKT action, controlling mitochondrial metabolism, membrane integrity, and cell survival (Betz et al., 2013). The IGF-1/PI3K/AKT signaling pathway also regulates Ca2+ exchange between the ER and mitochondria (Lv Y. et al., 2022).
Coixol, an alkaloid extracted from Coicis semen, enhances glucose-stimulated insulin secretion via the cAMP/PKA signaling pathway (Hameed et al., 2019; Wang et al., 2021d). This pathway may interact with peroxisome proliferator-activated receptor γ coactivator 1α (PGC-1α) to modulate mitochondrial biogenesis, an important regulator of blood sugar (Wang et al., 2021d). It is plausible that Coixol increases cAMP levels to activate PGC-1α, resulting in more mitochondria formed in cells, thereby playing a significant role in sugar consumption and regulating blood sugar levels (Kim et al., 2008; Kwak et al., 2010; Wang et al., 2021d).
FZL increases mitochondrial function in muscle, enhancing glucose transport and lowering blood glucose (Gao et al., 2023). Interestingly, this process is associated with the rise in mitochondrial temperatures and MMP. Additionally, by enhancing mitochondrial energy metabolism, FZL also increases liver glycogenolysis via the cAMP/PKA signaling pathway (Gao et al., 2023). AC, another alkaloid found in Fuzi, improves energy metabolism disorders, resulting in the remodeling of mitochondrial function and mediating mitochondrial function via upregulating the AMPK/optic atrophy 1 (OPA1)/adenosine triphosphate synthase, H+ transporting, mitochondrial F1 complex, alpha subunit 1 (ATP5A1) signaling pathway (Qiu et al., 2021). AMPK also stimulates glucose metabolism in the ER (Long and Zierath, 2006). By activating AMPK, Fuzi may impact glucose handling and reverse diabetic cardiomyopathy (DCM), suppressing high glucose-induced effects on MAM formation, mitochondrial Ca2+ increase, and mitochondrial dysfunction (Wu S. et al., 2019). In summary, both botanical drugs in YYFZ likely work in coordination to regulate blood sugar levels through modulating the IGF-1/PI3K/AKT, cAMP/PKA, and AMPK/OPA1/ATP5A1 pathways which are involved in mitochondrial biogenesis, function, and interactions with the ER. This helps protect cardiovascular health by mitigating hyperglycemia-related risk.
4.2 Impact of YYFZ on mitochondria
4.2.1 Regulating mitochondrial biogenesis and structure
Mitochondrial biogenesis is the process of generating new mitochondria within cells. BCAAs in Coicis semen along with intermediate metabolites such as C3 and C5 acylcarnitine, acetyl-CoA, succinyl-CoA, and 3-hydroxyisobutyrate, directly induce mitochondrial biogenesis through protein acetylation and phosphorylation modifications, transcriptional regulation by promoting the expression of mitochondrial transcription factor A mRNA, and increasing mtDNA copy number (Ye et al., 2020). PGC-1α is another underlying pharmacological mechanism of BCAAs generating mitochondria, extensively described as a master regulator of mitochondrial biogenesis in skin and muscle tissues (Mootha et al., 2003; Nisoli et al., 2003). BCAAs promote the gene expression of PGC-1α through the endothelial nitric oxide synthase/NO/cGMP/sirtuin 1 (SIRT1) and SIRT1/Liver kinase B1 (LKB1)/AMPK signaling pathway (D'Antona et al., 2010; Hatazawa et al., 2014; Liang et al., 2014; Schnuck et al., 2016; Chen et al., 2019). Furthermore, leucine also directly activates SIRT1 and the mammalian target of rapamycin (mTOR) to promote PGC-1α expression (Liang et al., 2014; Schnuck et al., 2016; Chen et al., 2019).
Several active compounds in Fuzi, such as AC, BAC, and aconine, significantly increase mitochondrial mass (Park et al., 2016) by activating the AMPK signaling pathway (Wu J. et al., 2016; Deng et al., 2019), which induces mitochondrial biogenesis by regulating morphology and suppressing ROS-associated ER stress (Wu and Zou, 2020). Additionally, Fuzi formulations like Qili Qiangxin Capsule (QLQX) enhance cardiomyocyte metabolism, mitochondrial content, and mitochondrial biogenesis via PGC-1α activation (Lin et al., 2015). Fuzi also increases heart mitochondrial biogenesis when combined with Zingiberis Rhizoma (ZR), potentially via the SIRT1/PGC-1α signaling pathway (Lu et al., 2017). YYFZ botanical drugs support mitochondrial biogenesis (Table 2; Figure 3) by coordinating the effects of Coicis semen and Fuzi on mitochondrial quantity, quality, and gene expression networks.
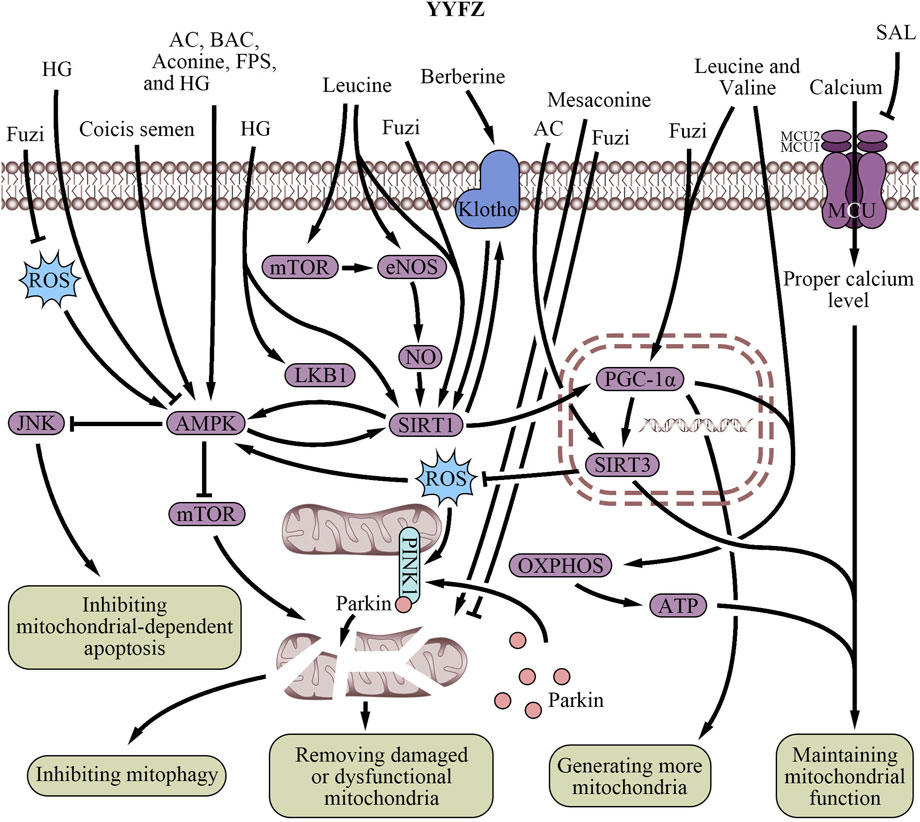
Figure 3. Regulatory effects of YiyiFuzi Powder’s major components on mitochondrial structure and function (Abbreviations: AC, aconitine; AMPK, AMP-activated protein kinase; ATP, adenosine triphosphate; BAC, benzoylaconine; eNOS, endothelial nitric oxide synthase; FPS, polysaccharides from Fuzi; HG, higenamine; JNK, c-Jun N-terminal kinase; LKB1, liver kinase B1; MCU, mitochondrial calcium uniporter; mTOR, mechanistic target of rapamycin; NO, nitric oxide; OXPHOS, oxidative phosphorylation; PGC-1α, peroxisome proliferator-activated receptor-γ coactivator-1α; PINK1, PTEN-induced putative kinase 1; ROS, reactive oxygen species; SAL, salsolinol; SIRT, sirtuin; YYFZ, YiyiFuzi Powder).
4.2.2 Regulating mitochondrial function and energy metabolism
Mitochondrial dysfunction plays a significant role in ischemic CVD caused by endothelial dysfunction (Song et al., 2013). Most compounds from Coicis semen primarily impact mitochondrial function rather than mitochondrial biogenesis or structure (Table 2; Figure 3). Berberine, a bioactive component of Coicis semen, may increase the Klotho/SIRT1 signaling pathway to alleviate mitochondrial dysfunction and cardiac senescence (Zhang T. et al., 2023). Klotho is an anti-aging protein that effectively reduces oxidative stress, inhibits apoptosis, and preserves mitochondrial function (Li et al., 2022; Donate-Correa et al., 2023). This protective effect was found in doxorubicin (DOX)-induced senescent H9c2 cells and naturally aged rats (Li et al., 2022).
Energy metabolism plays a crucial role in mitochondrial function. In recent years, the relationship between mitochondria and cardiomyocyte energy metabolism has gradually become a hot topic in clinical research. Efficient mitochondrial respiration is essential for ATP synthesis which preserves the energy supply (Aon et al., 2015; Viloria et al., 2022), reduces oxidative stress (Tsutsui et al., 2009; Kowalczyk et al., 2021), and prevents heart damage (Powers et al., 2014). CSEs have been shown to reduce the consumption of reducing sugars by the probiotic bacterium L. reuteri while increasing its growth (Yang et al., 2022c). This indicates that CSEs might be involved in energy supply and mitochondrial function. CSO enhances mitochondrial respiration and ATP production, thereby regulating blood sugar and improving overall energy metabolism (Chen et al., 2022). BCAAs like leucine and valine in Coicis semen are linked to lower CVD risk (Zheng et al., 2022), and they synergize with FAs to upregulate PGC-1α expression and enhance the mitochondrial oxidative phosphorylation (OXPHOS)/ATP signaling pathway (Ye et al., 2020). In coronary artery disease patients, a shift in mitochondrial metabolism from OXPHOS to glycolysis was observed (Ait-Aissa et al., 2019). The reduced OXPHOS leads to decreased mitochondrial respiration, mitochondrial damage, decreased ATP production, and a corresponding rise in glycolytic flux, all of which can contribute to the progression of heart disease (Ait-Aissa et al., 2019). Thus, BCAAs could help prevent this shift and mitochondrial damage by enhancing OXPHOS to support cardiovascular health.
Fuzi can be used in the treatment of CVD especially in HF, due to its valuable cardiotonic effect which helps improve heart function and enhance the contractility of the heart muscle (Zhao et al., 2012; Zhang J. et al., 2022). In addition, Fuzi has been studied for its potential anti-MI effects, including mitigating mitochondrial dysfunction caused by MI and restoring energy metabolism in the heart (Wu H. et al., 2019). This effect maintains mitochondrial health and function (Table 2; Figure 3), improves blood flow and oxygen delivery to the body’s tissues, and ensures an adequate supply of energy for the heart (Wang N. N. et al., 2021; Liu X. et al., 2022). SAL, a bioactive component of Fuzi, has been identified as a cardiotonic component (Zhou, 2011). Studies have shown that SAL improves mitochondrial function in H9c2 cardiomyocytes and attenuates DOX-induced CHF in rats (Wen et al., 2019b). This plant-based isoquinoline alkaloid ameliorates DOX-induced mitochondrial dysfunction and increases mitochondrial oxygen consumption rate (OCR) in H9c2 cardiomyocytes. It was suggested that SAL might improve mitochondrial respiratory function and energy metabolism by inhibiting excessive activation of the mitochondrial calcium uniporter (MCU) pathway in H9c2 cells (Wen et al., 2019b). Mesaconine, another bioactive component of Fuzi, activates the cardiac phosphatase and tensin homolog (PTEN)-induced putative kinase 1 (PINK1)-dependent mitophagy pathway (Zhou et al., 2023), which also helps remove damaged or dysfunctional mitochondria to maintain overall mitochondrial population health and function (Wallace et al., 2020; Zhou et al., 2023). Besides, AC activates SIRT3 activity in mitochondria, reducing the degree of Cyp-D acetylation, inhibiting the opening of the mitochondrial permeability transition pore, and improving mitochondrial energy metabolism (Wang et al., 2019). These biological processes protect myocardial tissue. Furthermore, water-soluble alkaloids in Fuzi also modulate mitochondria-mediated pathways to promote mitochondrial energy metabolism (Zhang J. et al., 2022).
HG, a natural benzylisoquinoline alkaloid found in Fuzi (Zhang et al., 2022d), was reported to upregulate mRNA and protein expression, such as LKB1, AMPKα1, and SIRT1, alleviating disturbances in myocardial mitochondrial energy metabolism when combined with 6-gingerol (Wen et al., 2020). The combination of Fuzi and Zingiber officinale (ZR) has also been found to promote mitochondrial energy metabolism via activating the peroxisome proliferator-activated receptor α (PPARα)/PGC-1α/SIRT3 signaling pathway (Wen et al., 2019a; Wen et al., 2019b). This is particularly relevant to HF, as energy metabolism is often impaired in this condition (Xing et al., 2023). Several TCM formulas containing Fuzi and other botanical drugs have been shown to improve mitochondrial energy metabolism and function. QLQX has been shown to enhance metabolism in cardiomyocytes, reducing cardiomyocyte apoptosis and improving heart function in infarcted hearts via suppression of the ROS/AMPK/mTOR signaling pathway (Fan et al., 2022) and PINK1/Parkin-mediated mitophagy (Zhou et al., 2020). This involves removing damaged mitochondria from cells, helping maintain their function and protect against cell death. A study showed that Sini decoction reduced lipid peroxidation incidence in myocardial mitochondria by increasing levels of antioxidant enzymes (copper-zinc superoxide dismutase and manganese superoxide dismutase, Mn-SOD (Zhao et al., 2005)) and decreasing malondialdehyde level, further protecting mitochondrial membrane integrity and reducing HF incidence (Zhao et al., 2010). Yang-xin-xue Keli, a TCM formula containing Fuzi, was found to improve cardiac function and reverse myocardial loss and fibrosis in rats with DOX-induced HF. This effect was attributed to the regulation of mitochondrial homeostasis and function (Long et al., 2022).
4.2.3 Regulating mitochondrial membrane potential
Regulating MMP is another critical aspect of regulating mitochondrial function (Table 2). The MMP is essential for various cellular processes, including ATP production, mitochondrial homeostasis, and the maintenance of mitochondrial quality (Vasan et al., 2022). Polysaccharides are beneficial to the cardiovascular system (Yin et al., 2018) and have been found to regulate MMP(Wang et al., 2014). Studies have identified that some polysaccharides exert a cardiovascular protective effect by maintaining MMP, increasing ATP generation, and increasing the OCR of cardiomyocytes (Zuo et al., 2018), which is crucial for energy production in cardiomyocytes. Both Coicis semen and Fuzi contain large amounts of polysaccharides, which are the main components of YYFZ that exert potential MMP regulation effects. Liao et al. demonstrated that polysaccharides from Fuzi (FPS) treatment attenuate starvation-induced cell viability reduction and MMP collapse in a concentration-dependent manner (Liao et al., 2013). Nevertheless, MMP collapse is a standard indicator of mitochondrial damage (Hussain et al., 2008). CSP and FPS may potentially protect mitochondria by regulating MMP collapse, thereby serving cardiovascular aims.
4.3 Regulating ER stress
The ER stress response and the UPR, triggered by ER stress, play crucial roles in maintaining cardiomyocyte homeostasis (Table 3; Figure 4), which are important for cardiovascular health (Zhang et al., 2017; Ren et al., 2021; Pietrafesa et al., 2023). Excessive ER stress disrupts secretory pathways and contributes to the progression of CVD (Zhang et al., 2017; Fu and Doroudgar, 2022). However, proper ER stress and early UPR response during cell injury may play a protective role in the cardiovascular system by maintaining cellular homeostasis, reducing tissue damage, and facilitating tissue repair (Glembotski, 2008; Kitakaze and Tsukamoto, 2010; Wang et al., 2018). Therefore, strategies to modulate ER stress could benefit CVD prevention and treatment. Coicis semen has demonstrated beneficial effects on obesity and dyslipidemia, which may involve regulating ER stress. In Chang liver cells, an ethanol extract of Coicis semen induced ER stress and stimulated the UPR via activation of the PERK and IRE1 pathways (Kim et al., 2018). When subjected to ER stressors, cells react rapidly to halt their translational machinery via PERK activation (Wong et al., 1993; Harding et al., 1999). The PERK pathway is necessary to protect the heart from pressure overload-induced congestive HF(Liu et al., 2014). Mice with cardiac-specific PERK knockout exhibited impaired cardiac function and increased damage, such as left ventricular fibrosis, enhanced cardiomyocyte apoptosis, and exacerbated lung remodeling, in response to chronic pressure overload (Liu et al., 2014). Likewise, IRE1 activation rapidly occurs during ER stress and protects against myocardial I/R injury (DuRose et al., 2006; Wu T. et al., 2019). The IRE1 pathway also prevents cell death by inhibiting Bax- and Bak-mediated ER membrane permeabilization in CVD, which prevents the leakage of ER contents, accumulation of Ca2+ in the mitochondria, oxidative stress, and ultimately cell death (Siwecka et al., 2021). In summary, Coicis semen shows the potential to enhance ER stress, and thus activation of the PERK and IRE1 pathways is associated with cardiovascular protective effects (Liu et al., 2014; Wu T. et al., 2019). However, further research is warranted to fully elucidate the mechanisms and determine optimal dosing for Coicis semen to properly regulate ER stress in CVD treatment.
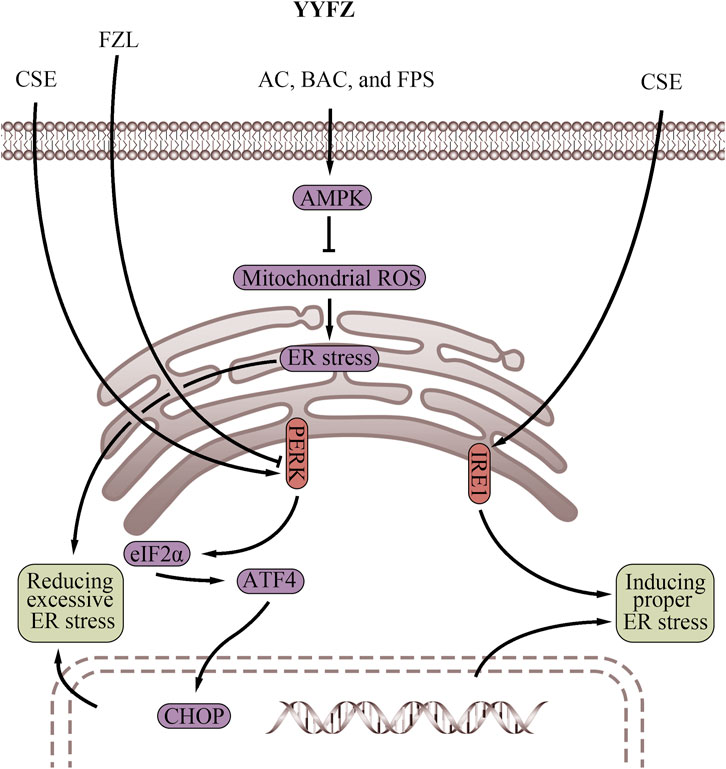
Figure 4. Endoplasmic reticulum regulatory effects of YiyiFuzi Powder (Abbreviations: AC, aconitine; AMPK, AMP-activated protein kinase; ATF4, activating transcription factor 4; BAC, benzoylaconine; CHOP, C/EBP homologous protein; CSE, Coix seed extract; eIF2α, eukaryotic initiation factor 2 alpha; ER, endoplasmic reticulum; FPS, polysaccharides from Fuzi; FZL, fuziline; IRE1, inositol-requiring enzyme 1; PERK, protein kinase RNA-like endoplasmic reticulum kinase; ROS, reactive oxygen species; YYFZ, YiyiFuzi Powder).
Both mitochondrial energy metabolism and apoptosis can be influenced by ER function which Fuzi has been shown to improve (Wu H. et al., 2019; Zhang J. et al., 2022). FZL was found to protect against isoproterenol (ISO)-induced myocardial injury in vivo by suppressing ER stress via downregulating the PERK/eukaryotic initiation factor 2 alpha (eIF2α)/activating transcription factor 4 (ATF4)/C/EBP homologous protein (CHOP) pathway (Fan et al., 2020). Western blot analysis further demonstrated that FZL reduced ER stress-induced apoptosis and effectively improved cardiac function in rats with ISO-induced myocardial injury (Fan et al., 2020). BAC, AC, and FPS have also been shown to activate the AMPK signaling pathway (Liao et al., 2013; Deng et al., 2019; Wang W. et al., 2022). Interestingly, activation of AMPK suppresses mitochondrial ROS-associated ER stress. Therefore, compounds in Fuzi may exert cardiovascular protection through AMPK activation, which serves to dampen mitochondrial ROS generation and subsequent ER stress induction (Wu and Zou, 2020). By alleviating aberrant mitochondria-ER crosstalk involved in CVD pathogenesis, the Fuzi components may help reduce mitochondrial dysfunction and protein misfolding in the ER. Further research is warranted to validate if AMPK stimulation represents a key mechanism through which Fuzi contributes to the cardiovascular effects of YYFZ. Properly controlling ER function through modulation of ER stress response pathways like PERK, IRE1, and AMPK could offer new opportunities for managing CVD. Further studies on YYFZ may provide valuable insights into developing improved strategies targeting this mechanism and fully elucidating potential drug interactions or side effects.
4.4 Reducing oxidative stress
The mitochondrial respiratory chain is the main inducer of oxidative stress by producing ROS(Petrosillo et al., 2003; Batandier et al., 2004; Paradies et al., 2004). Increased mitochondrial permeability can lead to elevated ROS production. This may contribute to the loss of myocardial contractility and increased myocardial stiffness, promoting cardiac remodeling processes (Gomez et al., 2007). Dysregulated ROS production and oxidative stress have been linked to cardiac hypertrophy, HF, cardiac I/R injury, and DCM (Peoples et al., 2019). A double-blind clinical trial using drugs targeting mitochondrial ROS may improve or even restore myocardial energetics, showing promise in limiting HF progression (Daubert et al., 2017). Amino acid metabolomics analysis has demonstrated that YYFZ regulates the glutathione and taurine synthesis pathways as well as phenylalanine and tyrosine metabolism (Wang Y. et al., 2023). Both glutathione and taurine are powerful antioxidant molecules that help protect cells from oxidative damage (Kerksick and Willoughby, 2005; Schaffer and Kim, 2018; Kwon et al., 2019; Surai et al., 2021), thereby safeguarding mitochondria from harm. YYFZ powder may enhance the synthesis of glutathione and taurine by regulating the metabolism of amino acids such as cysteine, glutamate, glycine, and methionine. Furthermore, phenylalanine and tyrosine serve as precursors for the synthesis of various antioxidant molecules (Szewczyk et al., 2021), including catecholamines and melanin. Metabolomic and network pharmacology analyses have also shown that YYFZ indirectly contributes to antioxidant effects by reducing lipid accumulation, increasing glucose utilization and ATP production, promoting the production of intermediates, donating electrons to the electron transport chain (ETC.), and driving ATP synthesis (Wang Y. et al., 2023).
Coicis semen contains high levels of antioxidants, including phenolic compounds, flavonoids, gamma-aminobutyric acid, CSP, and polyphenols (Xu et al., 2017; Zeng et al., 2021; Fang et al., 2023; Lee et al., 2023; Zhao et al., 2023). These antioxidants help prevent cellular oxidative stress and mitochondrial oxidative damage (Table 4; Figure 5), protecting mitochondrial integrity (Brand et al., 2004) and function (Chen et al., 2022). CSO also contains small amounts of free FAs, including arachidonic acid (AA) (Chen et al., 2022). However, AA exerts different effects on the mitochondrial energy-conserving system depending on its concentration. At sub-micromolar concentrations, AA can rescue proton pumping by the complex III (bc1 complex) of the mitochondrial, ETC., (Di Paola and Lorusso, 2006). Bc1 complex plays a key role in transferring electrons from ubiquinol to cytochrome c (Cyto-C) while pumping protons, which belongs to the modified Q cycle pathway and is necessary for ATP synthesis (Alberts et al., 2002; Springett, 2021). However, impaired, ETC., increases mitochondrial ROS production. Acute ROS exposure shuts down mitochondrial energy production, while chronic ROS causes oxidative damage to mitochondrial and cellular proteins, lipids, and nucleic acids (Chen and Zweier, 2014). Meanwhile, at micromolar concentrations, AA can increase inner mitochondrial membrane proton conductance, acting as a protonophore (Di Paola and Lorusso, 2006). Increasing inner membrane proton conductance can protect the cardiovascular system by reducing ROS production and limiting mitochondrial damage (Cheng et al., 2017). This process also belongs to the modified Q cycle pathway (Di Paola and Lorusso, 2006). Therefore, the small amount of AA in CSO may enhance mitochondrial electron transport and reduce ROS production via the modified Q cycle pathway, thus protecting mitochondria and the cardiovascular system.
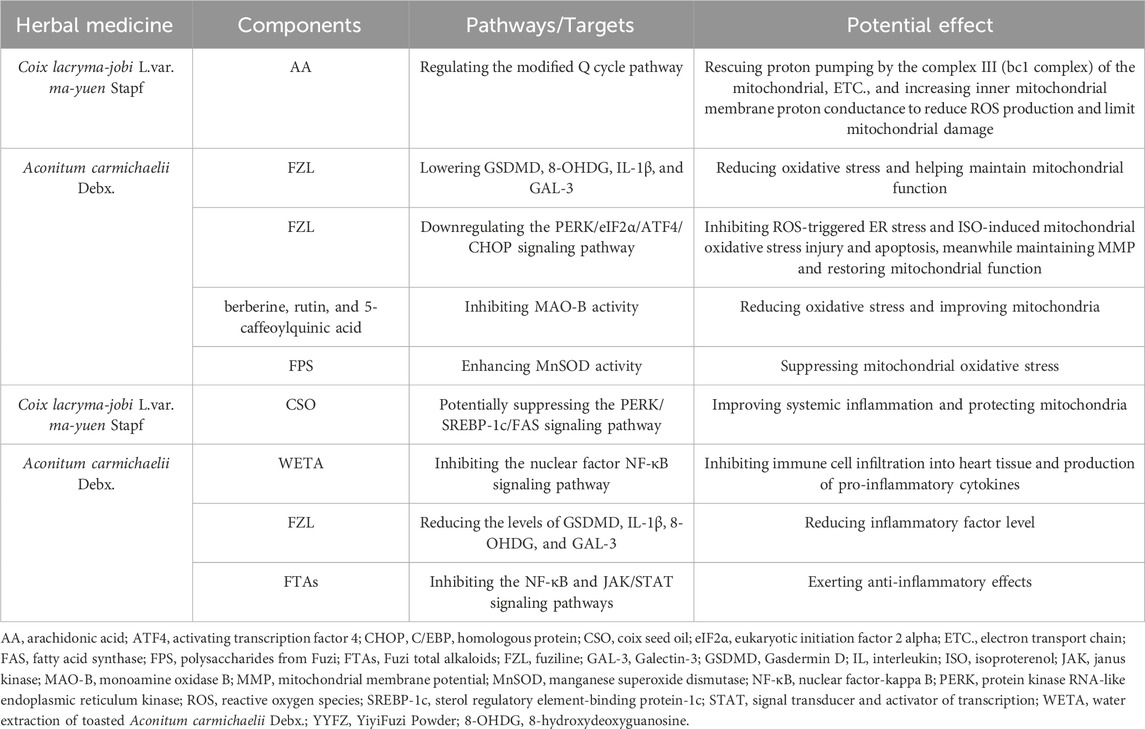
Table 4. Regulatory effects of YYFZ’s major components on oxidative stress and inflammatory response.
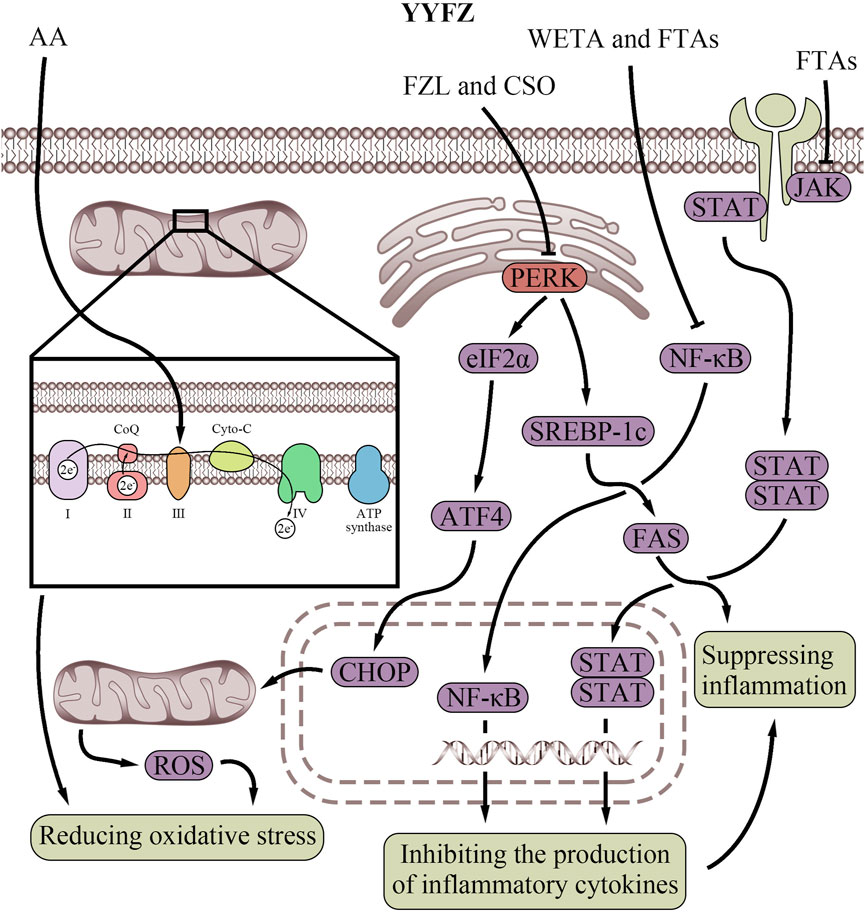
Figure 5. Antioxidant and anti-inflammatory effects of YiyiFuzi Powder’s major components (Abbreviations: AA, arachidonic acid; ATF4, activating transcription factor 4; ATP, adenosine triphosphate; CHOP, C/EBP homologous protein; Co Q, Coenzyme Q; CSO, Coix seed oil; Cyto-C, cytochrome C; eIF2α, eukaryotic initiation factor 2 alpha; FAS, fatty acid synthase; FPS, polysaccharides from Fuzi; FTAs, Fuzi total alkaloids; FZL, fuziline; JAK, Janus kinase; NF-κB, nuclear factor-kappa B; PERK, protein kinase RNA-like endoplasmic reticulum kinase; ROS, reactive oxygen species; SREBP-1c, sterol regulatory element-binding protein-1c; STAT, signal transducer and activator of transcription; WETA, water extraction of toasted Aconitum carmichaelii Debx.; YYFZ, YiyiFuzi Powder).
Antioxidants in Fuzi also help reduce oxidative stress (Table 4; Figure 5), protecting against mitochondrial and cardiovascular damage. A study found that FZL reduced dobutamine-induced cardiac damage in mice by lowering Gasdermin D (GSDMD), interleukin (IL)-1β, 8-hydroxydeoxyguanosine (8-OHDG), and Galectin-3 (GAL-3), and balancing oxidant and antioxidant levels, thereby reducing oxidative stress (Hacanli et al., 2023). Reducing oxidative stress helps maintain mitochondrial function and enhances left ventricular function (Lucas and Szweda, 1998). Research also showed that FZL can alleviate ISO-induced injury, both in vitro and in vivo, by inhibiting ROS-triggered ER stress via suppressing the PERK/eIF2α/ATF4/CHOP pathway (Fan et al., 2020). This pathway is a key ER stress response route, and its dysregulation can lead to cell death and cardiovascular disorders (Zhang et al., 2017). In this study, FZL also inhibited ISO-induced mitochondrial oxidative stress injury and apoptosis, maintained MMP, and restored mitochondrial function to play the cardiovascular protective role (Fan et al., 2020). Besides, Fuzi contains bioactive components (including berberine, rutin, and 5-caffeoylquinic acid) (Ayeni et al., 2023) that inhibit an enzyme, monoamine oxidase B, thereby reducing oxidative stress, improving mitochondria, and contributing to the enhancement of left ventricular function (Kaludercic et al., 2014). In addition, studies in SD rats have shown that FPS exerts mitochondrial and cardiomyocyte protective effects (Liu and Ji, 2011). FPS suppresses mitochondrial oxidative stress by enhancing the gene and activity expression of Mn-SOD, an important antioxidant enzyme. Additionally, FPS was demonstrated to inhibit cardiomyocyte apoptosis, reduce lipid peroxidation, and stabilize MMP function (Liu and Ji, 2011; Xing et al., 2023).
4.5 Inhibiting inflammatory responses
Inflammatory responses may lead to abnormal mitochondrial structure and function (López-Armada et al., 2013), as well as ER stress (Degechisa et al., 2022). Evidence also suggests that mitochondrial damage and ER stress can activate inflammation (Gao et al., 2020; Degechisa et al., 2022). However, reducing the inflammatory response has been linked to protecting both mitochondria and the ER, thereby contributing to cardiovascular protection (Liu et al., 2020).
The anti-inflammatory properties of YYFZ were demonstrated through clinical metabolomics, network pharmacology, molecular docking, and atomic force microscopy analysis (Wang, 2019; Wang Y. et al., 2023), via inhibiting the activation of the MAPK signaling pathways, reducing the production of TNF-α, and promoting the release of anti-inflammatory substances. This powder is commonly used in China to treat various inflammatory conditions such as arthritis and myocarditis (Liu, 1991; Zhang F. F. et al., 2023; Wang Y. Y. et al., 2023). Its anti-inflammatory effects are attributed to its active components, including flavonoids, stilbenes, and anthraquinones (Chung et al., 2010; Zhu, 2017; Feng et al., 2021; Sui and Xu, 2022).
Coicis semen possesses anti-inflammatory properties (Table 4; Figure 5) and helps protect against CVD, as chronic inflammation is a risk factor (Zeng et al., 2022). Coicis semen’s anti-inflammatory ingredients include polyphenols, coixol, polysaccharides, eriodictyol, ceramide, and p-coumaric acid. Eriodictyol, ceramide, and p-coumaric acid from the ethanol extraction of Coicis semen hull were demonstrated to exhibit anti-inflammatory properties by increasing NO and prostaglandin E2 production induced by lipopolysaccharides (Huang et al., 2009). Methanol extraction of Coicis semen also shows anti-inflammatory properties, including the inhibition of NO and superoxide production by activated macrophages (Seo et al., 2000). Furthermore, CSO significantly prevents obesity and improves systemic inflammation (Liu et al., 2019). CSO may exert cardiovascular protection by reducing obesity-induced inflammation and protecting mitochondria through the PERK/SREBP-1c/FAS signaling pathway, which regulates lipid metabolism and inflammation (Xue et al., 2005; Zhang and Kaufman, 2008; Han and Kaufman, 2016; Saltiel and Olefsky, 2017; Wang S. et al., 2021; Chen et al., 2022).
Fuzi can protect against HF via its anti-inflammatory effects in vivo (Liu X. et al., 2022). Specifically, Water extraction of toasted Aconitum carmichaelii Debx. inhibits immune cell infiltration into heart tissue and the production of pro-inflammatory cytokines, which cause cardiovascular damage and contribute to HF progression by inhibiting the activation of the nuclear factor-kappa B (NF-κB) signaling pathway (Xing et al., 2023). FZL was also found to reduce the levels of inflammatory factors, such as GSDMD, IL-1β, 8-OHDG, and GAL-3, in mice with dobutamine-induced heart damage (Hacanli et al., 2023). Fuzi total alkaloids (FTAs) exert anti-inflammatory effects by promoting apoptosis, inhibiting hyperactivity of the NF-κB and Janus kinase/signal transducer and activator of transcription signaling pathways, and downregulating proliferation of tumor necrosis factor -α-induced MH7A cells (Wu et al., 2022). They also regulate related proteins of the mitochondrial apoptosis signaling pathway. This suggests that Fuzi’s anti-inflammatory properties are also mediated by mitochondria. Moreover, inflammation can affect Ca2+ flux between the ER and mitochondria, which is crucial for energy production and cell death. During inflammation, Ca2+ overload can cause mitochondrial damage, further activating the NLRP3 inflammasome (Giorgi et al., 2018). By mitigating inflammation, Fuzi safeguards mitochondrial and ER functions, ensuring balanced Ca2+ transport between these two organelles, and thereby exerting cardiovascular protection (Table 4; Figure 5).
4.6 Regulating cell autophagy and apoptosis
While the specific mechanism of action of YYFZ in regulating mitochondrial apoptosis is not fully understood, research suggests that it may involve multiple pathways (Table 5; Figure 6). One possible way is to modulate the balance of pro-apoptotic and anti-apoptotic protein expression. YYFZ may interact with Caspases, Bcl-2 family proteins, and the MAPK signaling pathways (Wang, 2019; Wang Y. et al., 2023; Wang Y. Y. et al., 2023). YYFZ can also indirectly protect cells from apoptosis-induced oxidative stress, for it scavenges ROS and reduces oxidative stress (Wang, 2019; Wang Y. et al., 2023; Wang Y. Y. et al., 2023). In addition to the above mechanisms, network pharmacology analysis showed that YYFZ acts through the PI3K/AKT, Ras(Wang Y. et al., 2023), and cAMP signaling pathway (Wang, 2019), with direct or indirect effects on interactions of mitochondria and ER.
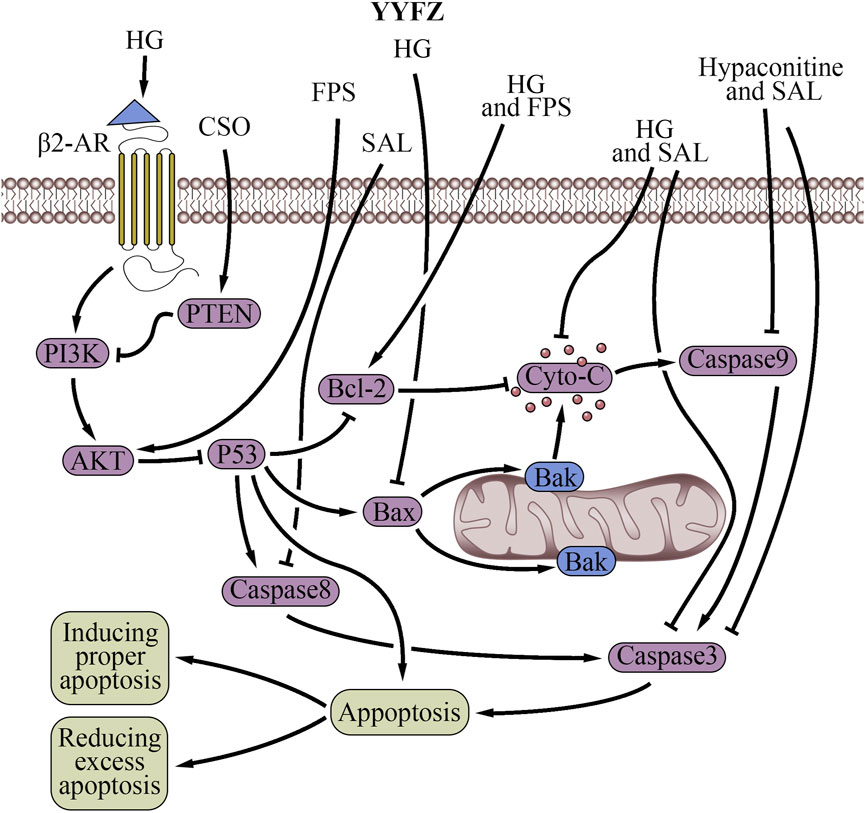
Figure 6. Cell apoptosis regulatory effects of YiyiFuzi Powder (Abbreviations: AMPK, AMP-activated protein kinase; β2-AR, β2-adrenergic receptors; CSO, Coix seed oil; Cyto-C, cytochrome C; FPS, polysaccharides from Fuzi; HG, higenamine; PI3K, phosphoinositide 3-kinase; PTEN, phosphatase and tensin homolog; SAL, salsolinol; YYFZ, YiyiFuzi Powder).
The mitochondria-mediated apoptotic pathway, known as the intrinsic pathway, is a major pathway that induces apoptosis (Ding et al., 2012), which is a critical mechanism for programmed cell death. Prolonged ER stress or adaptive response failure also leads to apoptotic cell death (Szegezdi et al., 2006). Both ER stress and mitochondria-induced apoptosis are involved in physiological and pathological processes. Nuanxin capsules containing Coicis semen had a protective effect on HF which enhanced cardiac function by inhibiting mitochondrial-dependent apoptosis through the AMPK/c-Jun N-terminal kinase signaling pathway under oxidative stress (Lou et al., 2021). FZL prevented cardiac myocyte necrosis (Hacanli et al., 2023). It reduced ER stress-induced apoptosis and improved cardiac function in rats with ISO-induced injury by suppressing the mitochondrial apoptosis pathway (Fan et al., 2020).
FPS inhibited apoptosis in a myocardial I/R injury cell model by promoting the expression of the anti-apoptotic gene Bcl-2 and scavenging excess mitochondrial oxygen radicals (Liu and Ji, 2011; Xing et al., 2023). Studies showed that Fuzi decreases Caspase three and Caspase nine expression to prevent unwanted activation of the mitochondrial apoptotic pathway in healthy cardiac cells (Wang and Youle, 2009). Caspase nine is an initiator caspase that activates the executioner caspase, Caspase 3, which then carries out the process of apoptosis (Mondello et al., 2021). The anti-apoptotic effects through the mitochondrial apoptosis signaling pathway are also associated with the decrease of Caspase 8, Cyto-C, Fas, and Bax expression, and the increase of Bcl-2 expression. Decreasing Caspase 8, Cyto-C, and Fas expression can potentially protect cardiac cells from apoptosis and mitigate various cardiac pathologies, including MI, cardiomyopathy, and HF (van Empel et al., 2005; Cao et al., 2023). Furthermore, Bax is a proapoptotic protein that promotes cell death, while Bcl-2 is an antiapoptotic protein that inhibits cell death. Fang, et al. reported that HA extracted from Fuzi at a concentration of 250 ng/mL reduces the H2O2-induced apoptosis of myocardial cells (Fang et al., 2010). They hypothesized that the mechanism probably involves the decrease in Caspase three and Caspase nine expression and the increase in cell proliferation activity (Fang et al., 2010). According to Wen et al., SAL in Fuzi could reduce adriamycin-induced myocardial injury and apoptosis in acute exhausted SD rats (Wen et al., 2019b). This study found that SAL decreased the expressions of Fas, Cyto-C, Caspase 3, Caspase 8, and Caspase 9, and increased the expression of Bcl-2. Earlier study found that the administration of HG significantly decreased Caspase three activity, Bax expression, and Cyto-C release, while upregulated Bcl-2 expression before rats underwent I/R injury (Lee et al., 2006). Furthermore, research has indicated that HG can attenuate DOX-induced cardiac remodeling and myocyte apoptosis by suppressing AMPK activation (Jin et al., 2022). Another in-depth study found that HG counteracted apoptosis and protected against I/R-induced MI in rat cardiomyocytes by activating β2-AR and the β2-AR/PI3K/AKT signaling pathway (Wu M. P. et al., 2016). Upon activation of the AKT pathway, antiapoptotic proteins such as Bcl-2 and Bcl-xL are upregulated, preventing the translocation of proapoptotic factors like Bad and Bax to mitochondria (Davidson et al., 2020). Previous studies have demonstrated that FPS treatment results in increased expression of the antiapoptotic protein Bcl-2 using an in-vitro model of myocardial I/R injury. This suggests FPS may promote cardiomyocyte survival by reducing apoptosis through Bcl-2-mediated mechanisms via the AKT pathway (Liu et al., 2012). The Mahuang Xixin Fuzi decoction inhibited apoptosis through the mitochondrial pathway by ameliorating MMP reduction, blocking mitochondrial Cyto-C release, reducing Bax level, increasing Bcl-2 level, and suppressing Caspase nine and Caspase three activation (Yang et al., 2022b).
However, the regulatory effect of YYFZ on apoptosis is bidirectional. Ethanol extract of Coicis semen was shown to stimulate the UPR or induce ER stress via activation of the IRE1 and PERK signaling pathways in normal liver cells while inducing apoptosis via increasing the synthesis of CHOP through the UPR pathway (Szegezdi et al., 2006; Oslowski and Urano, 2011; Kim et al., 2018). An in-vitro study demonstrated that CSO upregulates the PTEN protein while inhibiting the expression of p-AKT and p-PI3K proteins, thereby inducing apoptosis in human pancreatic cancer cells through the PTEN/PI3K/AKT signaling pathway (Yang et al., 2022a). Additionally, Coicis semen has been found to inhibit proliferation and promote the apoptosis of various cancer cell types, including cancer cells of the lung (Chang et al., 2003), colon (Lee et al., 2008), hepatic (Qu et al., 2016), mouth (Manosroi et al., 2019), breast (Fang et al., 2020; Chiang et al., 2022), uterine (Huang et al., 2021), cervical (Chen et al., 2018; Guo et al., 2019; Chiang et al., 2022), and pancreatic (Yang et al., 2022a). While not directly related to cardiovascular health, this could contribute to overall health and wellbeing (Zeng et al., 2022). Fuzi also possesses the ability to promote apoptosis. FTAs promoted apoptosis by regulating the mitochondrial apoptosis signaling pathway and its related proteins in vitro (Wu et al., 2022). Li et al. demonstrated that FPS induces autophagy and attenuates starvation-induced cardiomyocyte (H9c2 cells) death through the AMPK/mTOR pathway activation. AMPK helps restore energy supply and mitochondrial function by promoting ATP production in the failing heart (Liao et al., 2013). In summary, YYFZ has bidirectional effects on mitochondrial apoptosis regulation. The effect of YYFZ inhibits apoptosis mainly through suppressing the UPR and mitochondrial apoptosis signaling pathway, while the promotion of apoptosis occurs via the activation of the IRE1, PERK, UPR, and AMPK/mTOR signaling pathways.
4.7 Calcium homeostasis
Mitochondrial Ca2+ homeostasis plays a vital role in cardiomyocyte energy metabolism and function (Zhang D. et al., 2022). Ca2+ overload damages mitochondria and impairs mitochondrial function. Wang et al. demonstrated that YYFZ regulates Ca2+ by modulating the expression and activity of calcium channels and transporters in a network pharmacology analysis (Wang, 2019). Previous studies have shown that ethyl acetate CSEs decreased uterine contraction and intracellular Ca2+ concentrations by blocking extracellular Ca2+ influx in rats (Zeng et al., 2022). Naringenin and quercetin have been identified as major constituents present in these extracts. This suggests naringenin and quercetin may interact with calcium channels or other elements of the Ca2+ signaling pathway, thereby influencing Ca2+ homeostasis. In HF, abnormal Ca2+ handling is characterized by elevated end-diastolic cytosolic Ca2+ levels and prolonged calcium transients during diastole (Gorski et al., 2015). Therefore, blocking Ca2+ influx could help restore balance and improve cardiac function. Further research is still needed to determine whether Coicis semen regulates Ca2+ homeostasis to prevent CVD.
The MCU on the inner membrane is primarily responsible for mitochondrial Ca2+ uptake due to its high selectivity and low affinity for Ca2+(Kirichok et al., 2004). The MCU, along with mitochondrial calcium uptake 1 (MICU1) and MICU2, are key components of the MCU signaling pathway, regulating cardiomyocyte function by controlling mitochondrial Ca2+ uptake (Mammucari et al., 2018; Wen et al., 2019b). By inhibiting the overactivation of the MCU signaling pathway, SAL can reduce adriamycin-induced myocardial injury and apoptosis (Wen et al., 2019b). This improves mitochondrial energy metabolism and cardiac function by reducing MCU, MICU1, and MICU2 levels both in mRNA and protein levels, controlling Ca2+ transport, and maintaining intracellular Ca2+ homeostasis (Wen et al., 2019b). Shenfu injection (SFI), a TCM containing Fuzi, has been used in treating CVD for a long time in China (Committee, 2005). Appropriate doses of SFI decreased Ca2+ overload and protected myocardial mitochondria in an I/R rabbit model (Cao et al., 2005). Moreover, inflammation can impact Ca2+ flux between the ER and mitochondria, which is crucial for energy production and cell death. During inflammation, mitochondrial damage from Ca2+ overload can further enhance NLRP3 inflammasome activation (Giorgi et al., 2018). Fuzi has demonstrated anti-inflammatory effects that may safeguard against CVD by helping maintain balanced Ca2+ transport between mitochondria and ER to prevent mitochondrial damage (Table 5; Figure 3).
5 Toxicity
Coicis semen has been demonstrated to promote apoptosis of cancer cells to maintain homeostasis, and Fuzi also possesses this ability, as mentioned earlier. Therefore, indiscriminate use of YYFZ may also induce cardiomyocyte apoptosis, potentially damaging the myocardium. Besides, Coicis semen treatment has been found to promote angiogenesis in ischemic stroke models. This effect is mediated through the transforming growth factor-beta (TGF-β)/ALK1/Smad1/5 signaling pathway (Du et al., 2020). However, in the context of atherosclerosis, it has been suggested that the balance between Smad1/5- and Smad2/3-dependent signaling determines the outcome of the effect of TGF-β. Smad2/3 regulates the atheroprotective effects, whereas Smad1/5 is responsible for the proatherogenic effects of TGF-β(Yuan and Jing, 2010). Furthermore, in a study on mouse podocytes, TGF-β1 was found to increase mitochondrial OCR and ATP generation (Abe et al., 2013), which may exacerbate myocardial hypoxia.
The expression of PGC-1α and mitochondrial biogenesis can be suppressed by AC. This eventually leads to apoptosis in H9c2 cardiac cells, thereby demonstrating the toxic side effects of aconitum (Gao et al., 2018). PGC-1α plays a significant role in promoting mitochondrial biosynthesis (Fontecha-Barriuso et al., 2020; Abu Shelbayeh et al., 2023). A series of changes after PGC-1α expression reduction, such as a significant decrease in Bcl-2/Bax ratio and an increase in the levels of Cyto-C and Caspase 3, ultimately led to apoptosis in H9C2 cells (Gao et al., 2018).
Another in-vitro study found that AC treatment significantly reduced the phosphorylation of AMPK in SH-SY5Y cells. This suggests that certain doses of Fuzi may suppress the AMPK signaling pathway. Consequently, by inhibiting the AMPK signaling pathway, Fuzi could induce mitochondrial energetic and metabolic dysfunction with aberrant mitochondrial dynamics in cells (Yang et al., 2021a). This research highlights the toxicity of Fuzi, and overdosage in clinical practice warrants prudence. Nevertheless, Fuzi has been found to have a synergistic effect and reduce its toxicity when used in combination with other TCM(Liu X. et al., 2022). It can be inferred that the combination of Coicis semen and Fuzi may strengthen the therapeutic effect and reduce toxicity. However, due to their known toxicity, caution must be exercised when clinically employing YYFZ, and more importantly, under the guidance of a healthcare professional.
6 Conclusion
YYFZ, an effective TCM prescription for CVD, improves clinical symptoms, alleviates myocardial damage, enhances myocardial function, and reduces cardiovascular risk factors. Its mechanisms are associated with antioxidant, anti-inflammatory, immunomodulatory, regulation of cell proliferation and apoptosis, and modulation of mitochondrial and ER functions, reflecting the multicomponent, multitarget, and multi-pathway properties of TCM. However, due to the known toxicity of Coicis semen and Fuzi, caution should be exercised when using YYFZ, and it should be administered under the guidance of professional TCM practitioners. More rigorous experimental research and high-quality clinical studies are needed in the future to confirm these findings.
Author contributions
JD: Conceptualization, Data curation, Formal Analysis, Investigation, Methodology, Supervision, Visualization, Writing–original draft, Writing–review and editing. RJ: Conceptualization, Methodology, Visualization, Writing–original draft, Writing–review and editing. ZW: Data curation, Methodology, Supervision, Writing–review and editing. YJ: Data curation, Investigation, Methodology, Writing–review and editing. TM: Data curation, Investigation, Methodology, Writing–review and editing. XS: Data curation, Investigation, Validation, Writing–review and editing. JG: Data curation, Investigation, Validation, Writing–review and editing. QH: Conceptualization, Funding acquisition, Writing–review and editing.
Funding
The author(s) declare that financial support was received for the research, authorship, and/or publication of this article. This work was financially supported by the Traditional Chinese Medicine Ancient Book Documents and Characteristic Technology Inheritance Project of the National Administration of Traditional Chinese Medicine (GZY-KJS-2020-079) and Research and Transformation Application of Clinical Characteristic Diagnosis and Treatment Techniques in the Capital (Z221100007422081).
Acknowledgments
During the preparation of this study, we used ChatGPT in order to improve language and readability. We reviewed and edited the content and take full responsibility for the content of the publication.
Conflict of interest
The authors declare that the research was conducted in the absence of any commercial or financial relationships that could be construed as a potential conflict of interest.
Publisher’s note
All claims expressed in this article are solely those of the authors and do not necessarily represent those of their affiliated organizations, or those of the publisher, the editors and the reviewers. Any product that may be evaluated in this article, or claim that may be made by its manufacturer, is not guaranteed or endorsed by the publisher.
References
Abe, Y., Sakairi, T., Beeson, C., and Kopp, J. B. (2013). TGF-β1 stimulates mitochondrial oxidative phosphorylation and generation of reactive oxygen species in cultured mouse podocytes, mediated in part by the mTOR pathway. Am. J. Physiol. Ren. Physiol. 305 (10), F1477–F1490. doi:10.1152/ajprenal.00182.2013
Abu Shelbayeh, O., Arroum, T., Morris, S., and Busch, K. B. (2023). PGC-1α is a master regulator of mitochondrial lifecycle and ROS stress response. Antioxidants (Basel) 12 (5), 1075. doi:10.3390/antiox12051075
Ait-Aissa, K., Blaszak, S. C., Beutner, G., Tsaih, S. W., Morgan, G., Santos, J. H., et al. (2019). Mitochondrial oxidative phosphorylation defect in the heart of subjects with coronary artery disease. Sci. Rep. 9 (1), 7623. doi:10.1038/s41598-019-43761-y
Alberts, B., Johnson, A. J. L., Raff, M., Roberts, K., and Walter, P. (2002). Molecular biology of the cell. New York: Garland Science.
Aon, M. A., Tocchetti, C. G., Bhatt, N., Paolocci, N., and Cortassa, S. (2015). Protective mechanisms of mitochondria and heart function in diabetes. Antioxid. Redox Signal 22 (17), 1563–1586. doi:10.1089/ars.2014.6123
Ayeni, E. A., Ma, C., Zhang, Y. M., Fan, W. Q., and Liao, X. (2023). Chemical components and monoamine oxidase B inhibition activities from the tubers of Sauromatum giganteum (Engl.) Cusimano and Hett. Nat. Prod. Res. 37 (17), 2916–2923. doi:10.1080/14786419.2022.2137502
Batandier, C., Leverve, X., and Fontaine, E. (2004). Opening of the mitochondrial permeability transition pore induces reactive oxygen species production at the level of the respiratory chain complex I. J. Biol. Chem. 279 (17), 17197–17204. doi:10.1074/jbc.M310329200
Betz, C., Stracka, D., Prescianotto-Baschong, C., Frieden, M., Demaurex, N., and Hall, M. N. (2013). Feature Article: mTOR complex 2-Akt signaling at mitochondria-associated endoplasmic reticulum membranes (MAM) regulates mitochondrial physiology. Proc. Natl. Acad. Sci. U. S. A. 110 (31), 12526–12534. doi:10.1073/pnas.1302455110
Brand, M. D., Affourtit, C., Esteves, T. C., Green, K., Lambert, A. J., Miwa, S., et al. (2004). Mitochondrial superoxide: production, biological effects, and activation of uncoupling proteins. Free Radic. Biol. Med. 37 (6), 755–767. doi:10.1016/j.freeradbiomed.2004.05.034
Cao, J., Zheng, C. D., Zhang, G. X., Zhang, Y. J., and Min, S. (2005). Protective effect of Shenfu injection on myocardial mitochondria injured by ischemia-reperfusion in rabbits. Chin. Med. J. Engl. 118 (6), 505–507. doi:10.3760/j.issn:0366-6999.2005.06.012
Cao, X., Yao, F., Zhang, B., and Sun, X. (2023). Mitochondrial dysfunction in heart diseases: potential therapeutic effects of Panax ginseng. Front. Pharmacol. 14, 1218803. doi:10.3389/fphar.2023.1218803
Chang, H. C., Huang, Y. C., and Hung, W. C. (2003). Antiproliferative and chemopreventive effects of adlay seed on lung cancer in vitro and in vivo. J. Agric. Food Chem. 51 (12), 3656–3660. doi:10.1021/jf021142a
Chang, X., Li, Y., Liu, J., Wang, Y., Guan, X., Wu, Q., et al. (2023a). ß-tubulin contributes to Tongyang Huoxue decoction-induced protection against hypoxia/reoxygenation-induced injury of sinoatrial node cells through SIRT1-mediated regulation of mitochondrial quality surveillance. Phytomedicine 108, 154502. doi:10.1016/j.phymed.2022.154502
Chang, X., Liu, J., Wang, Y., Guan, X., and Liu, R. (2023b). Mitochondrial disorder and treatment of ischemic cardiomyopathy: potential and advantages of Chinese herbal medicine. Biomed. Pharmacother. 159, 114171. doi:10.1016/j.biopha.2022.114171
Chang, X., Liu, R., Li, R., Peng, Y., Zhu, P., and Zhou, H. (2023c). Molecular mechanisms of mitochondrial quality control in ischemic cardiomyopathy. Int. J. Biol. Sci. 19 (2), 426–448. doi:10.7150/ijbs.76223
Chang, X., Toan, S., Li, R., and Zhou, H. (2022). Therapeutic strategies in ischemic cardiomyopathy: focus on mitochondrial quality surveillance. EBioMedicine 84, 104260. doi:10.1016/j.ebiom.2022.104260
Chang, X., Zhang, Q., Huang, Y., Liu, J., Wang, Y., Guan, X., et al. (2024a). Quercetin inhibits necroptosis in cardiomyocytes after ischemia-reperfusion via DNA-PKcs-SIRT5-orchestrated mitochondrial quality control. Phytother. Res. 38 (5), 2496–2517. doi:10.1002/ptr.8177
Chang, X., Zhang, T., Meng, Q., Wang, S., Yan, P., Wang, X., et al. (2021). Quercetin improves cardiomyocyte vulnerability to hypoxia by regulating SIRT1/TMBIM6-related mitophagy and endoplasmic reticulum stress. Oxid. Med. Cell Longev. 2021, 5529913. doi:10.1155/2021/5529913
Chang, X., Zhou, S., Liu, J., Wang, Y., Guan, X., Wu, Q., et al. (2024b). Zishen Tongyang Huoxue decoction (TYHX) alleviates sinoatrial node cell ischemia/reperfusion injury by directing mitochondrial quality control via the VDAC1-β-tubulin signaling axis. J. Ethnopharmacol. 320, 117371. doi:10.1016/j.jep.2023.117371
Check, J. B., and K'Ombut, F. O. (1995). The effect on fibrinolytic system of blood plasma of Wister rats after feeding them with Coix mixed diet. East Afr. Med. J. 72 (1), 51–55.
Chen, L., Xue, S., Dai, B., and Zhao, H. (2022). Effects of coix seed oil on high fat diet-induced obesity and dyslipidemia. Foods 11 (20), 3267. doi:10.3390/foods11203267
Chen, P. Y., Li, L. L., Huo, X. Q., Qiao, L. S., Zhang, Y. L., Chen, Z. J., et al. (2020). New angiotensin-converting enzyme inhibitory peptide from Coix prolamin and its influence on the gene expression of renin-angiotensin system in vein endothelial cells. J. Cereal Sci. 96, 103099. doi:10.1016/j.jcs.2020.103099
Chen, X., Xiang, L., Jia, G., Liu, G., Zhao, H., and Huang, Z. (2019). Leucine regulates slow-twitch muscle fibers expression and mitochondrial function by Sirt1/AMPK signaling in porcine skeletal muscle satellite cells. Anim. Sci. J. 90 (2), 255–263. doi:10.1111/asj.13146
Chen, Y., Qu, D., Fu, R., Guo, M., Qin, Y., Guo, J., et al. (2018). A Tf-modified tripterine-loaded coix seed oil microemulsion enhances anti-cervical cancer treatment. Int. J. Nanomedicine 13, 7275–7287. doi:10.2147/IJN.S182475
Chen, Y. R., and Zweier, J. L. (2014). Cardiac mitochondria and reactive oxygen species generation. Circ. Res. 114 (3), 524–537. doi:10.1161/CIRCRESAHA.114.300559
Cheng, J., Nanayakkara, G., Shao, Y., Cueto, R., Wang, L., Yang, W. Y., et al. (2017). Mitochondrial proton leak plays a critical role in pathogenesis of cardiovascular diseases. Adv. Exp. Med. Biol. 982, 359–370. doi:10.1007/978-3-319-55330-6_20
Chiang, Y. F., Chung, C. P., Lin, J. H., Chiang, W., Chen, H. Y., Ali, M., et al. (2022). Adlay seed (coix lacryma-jobi L. Var. Ma-yuen Stapf.) ethanolic extract fractions and subfractions induce cell cycle arrest and apoptosis in human breast and cervical cancer cell lines. Molecules 27 (13), 3984. doi:10.3390/molecules27133984
Chung, C. P., Hsu, H. Y., Huang, D. W., Hsu, H. H., Lin, J. T., Shih, C. K., et al. (2010). Ethyl acetate fraction of adlay bran ethanolic extract inhibits oncogene expression and suppresses DMH-induced preneoplastic lesions of the colon in F344 rats through an anti-inflammatory pathway. J. Agric. Food Chem. 58 (13), 7616–7623. doi:10.1021/jf101084e
Clemmons, D. R. (2004). The relative roles of growth hormone and IGF-1 in controlling insulin sensitivity. J. Clin. Invest. 113 (1), 25–27. doi:10.1172/JCI20660
Commission, C.P. (2020). Pharmacopoeia of the People's Republic of China 2020. Beijing, China: China Medical Science Press.
Committee, C.P. (2005). Pharmacopoeia of the People's Republic of China. Beijing, China: Chemical Industry Press.
D'Antona, G., Ragni, M., Cardile, A., Tedesco, L., Dossena, M., Bruttini, F., et al. (2010). Branched-chain amino acid supplementation promotes survival and supports cardiac and skeletal muscle mitochondrial biogenesis in middle-aged mice. Cell Metab. 12 (4), 362–372. doi:10.1016/j.cmet.2010.08.016
Daubert, M. A., Yow, E., Dunn, G., Marchev, S., Barnhart, H., Douglas, P. S., et al. (2017). Novel mitochondria-targeting peptide in heart failure treatment: a randomized, placebo-controlled trial of elamipretide. Circ. Heart Fail 10 (12), e004389. doi:10.1161/CIRCHEARTFAILURE.117.004389
Davidson, S. M., Adameova, A., Barile, L., Cabrera-Fuentes, H. A., Lazou, A., Pagliaro, P., et al. (2020). Mitochondrial and mitochondrial-independent pathways of myocardial cell death during ischaemia and reperfusion injury. J. Cell Mol. Med. 24 (7), 3795–3806. doi:10.1111/jcmm.15127
Degechisa, S. T., Dabi, Y. T., and Gizaw, S. T. (2022). The mitochondrial associated endoplasmic reticulum membranes: a platform for the pathogenesis of inflammation-mediated metabolic diseases. Immun. Inflamm. Dis. 10 (7), e647. doi:10.1002/iid3.647
Deng, X. H., Liu, J. J., Sun, X. J., Dong, J. C., and Huang, J. H. (2019). Benzoylaconine induces mitochondrial biogenesis in mice via activating AMPK signaling cascade. Acta Pharmacol. Sin. 40 (5), 658–665. doi:10.1038/s41401-018-0174-8
Ding, F., Shao, Z. W., Yang, S. H., Wu, Q., Gao, F., and Xiong, L. M. (2012). Role of mitochondrial pathway in compression-induced apoptosis of nucleus pulposus cells. Apoptosis 17 (6), 579–590. doi:10.1007/s10495-012-0708-3
Ding, M. L., Cai, S. H., Zhang, D., Zhang, W., and Di, L. Q. (2022). Evaluation of Aconiti Lateralis Radix Praeparata water decoction by fingerprint and multivariable statistics. Chin. Arch. Tradit. Chin. Med. 37 (10), 5918–5922.
Di Paola, M., and Lorusso, M. (2006). Interaction of free fatty acids with mitochondria: coupling, uncoupling and permeability transition. Biochim. Biophys. Acta 1757 (9-10), 1330–1337. doi:10.1016/j.bbabio.2006.03.024
Donate-Correa, J., Martin-Carro, B., Cannata-Andia, J. B., Mora-Fernandez, C., and Navarro-Gonzalez, J. F. (2023). Klotho, oxidative stress, and mitochondrial damage in kidney disease. Antioxidants (Basel) 12 (2), 239. doi:10.3390/antiox12020239
Dromparis, P., Paulin, R., Stenson, T. H., Haromy, A., Sutendra, G., and Michelakis, E. D. (2013). Attenuating endoplasmic reticulum stress as a novel therapeutic strategy in pulmonary hypertension. Circulation 127 (1), 115–125. doi:10.1161/CIRCULATIONAHA.112.133413
Du, J., Yin, G., Hu, Y., Shi, S., Jiang, J., Song, X., et al. (2020). Coicis semen protects against focal cerebral ischemia-reperfusion injury by inhibiting oxidative stress and promoting angiogenesis via the TGFβ/ALK1/Smad1/5 signaling pathway. Aging (Albany NY) 13 (1), 877–893. doi:10.18632/aging.202194
Du, W., Zhu, W., Ge, W., and Li, C. (2021). Research on the effect of spleen-invigorating and anti-swelling active ingredients in crude and processed coix seed based on Spectrum - effects relationship combined with chemometrics. J. Pharm. Biomed. Anal. 205, 114350. doi:10.1016/j.jpba.2021.114350
DuRose, J. B., Tam, A. B., and Niwa, M. (2006). Intrinsic capacities of molecular sensors of the unfolded protein response to sense alternate forms of endoplasmic reticulum stress. Mol. Biol. Cell 17 (7), 3095–3107. doi:10.1091/mbc.e06-01-0055
Fan, C. L., Cai, W. J., Ye, M. N., Chen, M., and Dai, Y. (2022). Qili Qiangxin, a compound herbal medicine formula, alleviates hypoxia-reoxygenation-induced apoptotic and autophagic cell death via suppression of ROS/AMPK/mTOR pathway in vitro. J. Integr. Med. 20 (4), 365–375. doi:10.1016/j.joim.2022.04.005
Fan, C. L., Yao, Z. H., Ye, M. N., Fu, L. L., Zhu, G. N., Dai, Y., et al. (2020). Fuziline alleviates isoproterenol-induced myocardial injury by inhibiting ROS-triggered endoplasmic reticulum stress via PERK/eIF2α/ATF4/Chop pathway. J. Cell Mol. Med. 24 (2), 1332–1344. doi:10.1111/jcmm.14803
Fang, J., You, S., Sun, Q., Wang, Z., Wang, C., Wang, D., et al. (2023). Protection impacts of coix lachryma-jobi L. Seed lactobacillus reuteri fermentation broth on hydrogen peroxide-induced oxidative stress in human skin fibroblasts. Appl. Sci. 13 (1), 540. doi:10.3390/app13010540
Fang, K., Li, Z. H., Li, G. H., and Shao, X. T. (2010). Protection of hypaconitine on H2O2-induced myocardial apoptosis in rats. Chin. J. Tradit. Med. Sci. Technol. 17 (4), 315–318.
Fang, T., Jiang, Y. X., Chen, L., Huang, L., Tian, X. H., Zhou, Y. D., et al. (2020). Coix seed oil exerts an anti-triple-negative breast cancer effect by disrupting miR-205/S1PR1 Axis. Front. Pharmacol. 11, 529962. doi:10.3389/fphar.2020.529962
Feng, W., Liu, J., Zhang, D., Tan, Y., Cheng, H., and Peng, C. (2021). Revealing the efficacy-toxicity relationship of Fuzi in treating rheumatoid arthritis by systems pharmacology. Sci. Rep. 11 (1), 23083. doi:10.1038/s41598-021-02167-5
Fontecha-Barriuso, M., Martin-Sanchez, D., Martinez-Moreno, J. M., Monsalve, M., Ramos, A. M., Sanchez-Nino, M. D., et al. (2020). The role of PGC-1α and mitochondrial biogenesis in kidney diseases. Biomolecules 10 (2), 347. doi:10.3390/biom10020347
Fu, F., and Doroudgar, S. (2022). IRE1/XBP1 and endoplasmic reticulum signaling - from basic to translational research for cardiovascular disease. Curr. Opin. Physiol. 28, 100552. doi:10.1016/j.cophys.2022.100552
Gao, H., Li, Z., Cheng, C., Cui, J., Peng, J., Wang, X., et al. (2023). Fuziline ameliorates glucose and lipid metabolism by activating beta adrenergic receptors to stimulate thermogenesis. Int. J. Mol. Sci. 24 (9), 8362. doi:10.3390/ijms24098362
Gao, P., Yan, Z., and Zhu, Z. (2020). Mitochondria-associated endoplasmic reticulum membranes in cardiovascular diseases. Front. Cell Dev. Biol. 8, 604240. doi:10.3389/fcell.2020.604240
Gao, X., Zhang, X., Hu, J., Xu, X., Zuo, Y., Wang, Y., et al. (2018). Aconitine induces apoptosis in H9c2 cardiac cells via mitochondria-mediated pathway. Mol. Med. Rep. 17 (1), 284–292. doi:10.3892/mmr.2017.7894
Giorgi, C., Marchi, S., and Pinton, P. (2018). The machineries, regulation and cellular functions of mitochondrial calcium. Nat. Rev. Mol. Cell Biol. 19 (11), 713–730. doi:10.1038/s41580-018-0052-8
Glanz, V. Y., Sobenin, I. A., Grechko, A. V., Yet, S. F., and Orekhov, A. N. (2020). The role of mitochondria in cardiovascular diseases related to atherosclerosis. Front. Biosci. Elite Ed. 12 (1), 102–112. doi:10.2741/E860
Glembotski, C. C. (2008). The role of the unfolded protein response in the heart. J. Mol. Cell Cardiol. 44 (3), 453–459. doi:10.1016/j.yjmcc.2007.10.017
Gomez, L., Thibault, H., Gharib, A., Dumont, J. M., Vuagniaux, G., Scalfaro, P., et al. (2007). Inhibition of mitochondrial permeability transition improves functional recovery and reduces mortality following acute myocardial infarction in mice. Am. J. Physiol. Heart Circ. Physiol. 293 (3), H1654–H1661. doi:10.1152/ajpheart.01378.2006
Gorski, P. A., Ceholski, D. K., and Hajjar, R. J. (2015). Altered myocardial calcium cycling and energetics in heart failure--a rational approach for disease treatment. Cell Metab. 21 (2), 183–194. doi:10.1016/j.cmet.2015.01.005
Guo, M., Qu, D., Qin, Y., Chen, Y., Liu, Y., Huang, M., et al. (2019). Transferrin-functionalized microemulsions coloaded with coix seed oil and tripterine deeply penetrate to improve cervical cancer therapy. Mol. Pharm. 16 (12), 4826–4835. doi:10.1021/acs.molpharmaceut.9b00717
Hacanli, Y., Aydin, M. S., Ersoz, E. K., Kankilic, N., Koyuncu, I., Guldur, M. E., et al. (2023). Protective effects of fuziline on dobutamine-induced heart damage in mice. Braz J. Cardiovasc Surg. 38 (4), e20220251. doi:10.21470/1678-9741-2022-0251
Haeri, M., and Knox, B. E. (2012). Endoplasmic reticulum stress and unfolded protein response pathways: potential for treating age-related retinal degeneration. J. Ophthalmic Vis. Res. 7 (1), 45–59.
Hameed, A., Hafizur, R. M., Khan, M. I., Jawed, A., Wang, H., Zhao, M., et al. (2019). Coixol amplifies glucose-stimulated insulin secretion via cAMP mediated signaling pathway. Eur. J. Pharmacol. 858, 172514. doi:10.1016/j.ejphar.2019.172514
Han, J., and Kaufman, R. J. (2016). The role of ER stress in lipid metabolism and lipotoxicity. J. Lipid Res. 57 (8), 1329–1338. doi:10.1194/jlr.R067595
Han, X., Ji, X., Zhao, H., Zhang, Y., Liu, G., Wang, Y., et al. (2017). Mechanisms of coix seed compositions in the treatment of spleen deficiency and wet dampness zheng. Afr. J. Tradit. Complement. Altern. Med. 14 (4), 239–246. doi:10.21010/ajtcam.v14i4.26
Hardie, D. G., and Sakamoto, K. (2006). AMPK: a key sensor of fuel and energy status in skeletal muscle. Skelet. Muscle 21 (1), 48–60. doi:10.1152/physiol.00044.2005
Harding, H. P., Zhang, Y., and Ron, D. (1999). Protein translation and folding are coupled by an endoplasmic-reticulum-resident kinase. Nature 397 (6716), 271–274. doi:10.1038/16729
Hatazawa, Y., Tadaishi, M., Nagaike, Y., Morita, A., Ogawa, Y., Ezaki, O., et al. (2014). PGC-1α-mediated branched-chain amino acid metabolism in the skeletal muscle. PLoS One 9 (3), e91006. doi:10.1371/journal.pone.0091006
Hetz, C. (2012). The unfolded protein response: controlling cell fate decisions under ER stress and beyond. Nat. Rev. Mol. Cell Biol. 13 (2), 89–102. doi:10.1038/nrm3270
Huang, D. W., Chung, C. P., Kuo, Y. H., Lin, Y. L., and Chiang, W. (2009). Identification of compounds in adlay (Coix lachryma-jobi L. var. ma-yuen Stapf) seed hull extracts that inhibit lipopolysaccharide-induced inflammation in RAW 264.7 macrophages. J. Agric. Food Chem. 57 (22), 10651–10657. doi:10.1021/jf9028514
Huang, X., Tang, J., Zhou, Q., Lu, H., Wu, Y., and Wu, W. (2010). Polysaccharide from fuzi (FPS) prevents hypercholesterolemia in rats. Lipids Health Dis. 9, 9. doi:10.1186/1476-511X-9-9
Huang, Y. C., Yan, S. D., Su, Z. X., Xia, L., Xie, J. L., Zhang, F., et al. (2022). Aquaporins: a new target for traditional Chinese medicine in the treatment of digestive system diseases. Front. Pharmacol. 13, 1069310–1069322. doi:10.3389/fphar.2022.1069310
Huang, Y. J., Chang, C. C., Wang, Y. Y., Chiang, W. C., Shih, Y. H., Shieh, T. M., et al. (2021). Adlay testa (coix lachryma-jobi L. Var. Ma-yuen Stapf.) ethanolic extract and its active components exert anti-proliferative effects on endometrial cancer cells via cell cycle arrest. Molecules 26 (7), 1966. doi:10.3390/molecules26071966
Hussain, S. R., Lucas, D. M., Johnson, A. J., Lin, T. S., Bakaletz, A. P., Dang, V. X., et al. (2008). Flavopiridol causes early mitochondrial damage in chronic lymphocytic leukemia cells with impaired oxygen consumption and mobilization of intracellular calcium. Blood 111 (6), 3190–3199. doi:10.1182/blood-2007-10-115733
Jager, S., Handschin, C., St-Pierre, J., and Spiegelman, B. M. (2007). AMP-activated protein kinase (AMPK) action in skeletal muscle via direct phosphorylation of PGC-1alpha. Proc. Natl. Acad. Sci. U. S. A. 104 (29), 12017–12022. doi:10.1073/pnas.0705070104
Jensen-Cody, S. O., and Potthoff, M. J. (2021). Hepatokines and metabolism: deciphering communication from the liver. Mol. Metab. 44, 101138. doi:10.1016/j.molmet.2020.101138
Jin, C., Chai, Y., Hu, Z., Tian, W., Ling, W., Li, J., et al. (2022). Higenamine attenuates doxorubicin-induced cardiac remodeling and myocyte apoptosis by suppressing AMPK activation. Front. Cell Dev. Biol. 10, 809996. doi:10.3389/fcell.2022.809996
Kaludercic, N., Carpi, A., Nagayama, T., Sivakumaran, V., Zhu, G., Lai, E. W., et al. (2014). Monoamine oxidase B prompts mitochondrial and cardiac dysfunction in pressure overloaded hearts. Antioxid. Redox Signal 20 (2), 267–280. doi:10.1089/ars.2012.4616
Kerksick, C., and Willoughby, D. (2005). The antioxidant role of glutathione and N-acetyl-cysteine supplements and exercise-induced oxidative stress. J. Int. Soc. Sports Nutr. 2 (2), 38–44. doi:10.1186/1550-2783-2-2-38
Kim, H. Y., Song, H. N., Davaatseren, M., Chang, H. J., and Chun, H. S. (2018). Endoplasmic reticulum stress induced by an ethanol extract of Coicis semen in Chang liver cells. BMC Complement. Altern. Med. 18 (1), 100. doi:10.1186/s12906-018-2175-z
Kim, J. A., Wei, Y., and Sowers, J. R. (2008). Role of mitochondrial dysfunction in insulin resistance. Circ. Res. 102 (4), 401–414. doi:10.1161/CIRCRESAHA.107.165472
Kirichok, Y., Krapivinsky, G., and Clapham, D. E. (2004). The mitochondrial calcium uniporter is a highly selective ion channel. Nature 427 (6972), 360–364. doi:10.1038/nature02246
Kitakaze, M., and Tsukamoto, O. (2010). What is the role of ER stress in the heart? Introduction and series overview. Circ. Res. 107 (1), 15–18. doi:10.1161/CIRCRESAHA.110.222919
Kowalczyk, P., Sulejczak, D., Kleczkowska, P., Bukowska-Osko, I., Kucia, M., Popiel, M., et al. (2021). Mitochondrial oxidative stress-A causative factor and therapeutic target in many diseases. Int. J. Mol. Sci. 22 (24), 13384. doi:10.3390/ijms222413384
Koyama, M., Furuhashi, M., Ishimura, S., Mita, T., Fuseya, T., Okazaki, Y., et al. (2014). Reduction of endoplasmic reticulum stress by 4-phenylbutyric acid prevents the development of hypoxia-induced pulmonary arterial hypertension. Am. J. Physiol. Heart Circ. Physiol. 306 (9), H1314–H1323. doi:10.1152/ajpheart.00869.2013
Kwak, S. H., Park, K. S., Lee, K. U., and Lee, H. K. (2010). Mitochondrial metabolism and diabetes. J. Diabetes Investig. 1 (5), 161–169. doi:10.1111/j.2040-1124.2010.00047.x
Kwon, D. H., Cha, H. J., Lee, H., Hong, S. H., Park, C., Park, S. H., et al. (2019). Protective effect of glutathione against oxidative stress-induced cytotoxicity in RAW 264.7 macrophages through activating the nuclear factor erythroid 2-related factor-2/heme oxygenase-1 pathway. Antioxidants (Basel) 8 (4), 82. doi:10.3390/antiox8040082
Lee, E. S., Kim, Y. I., Lee, J. H., Kim, Y. G., Han, K. S., Yoon, Y. H., et al. (2023). Comparison of quality, antioxidant capacity, and anti-inflammatory activity of adlay [coix lacryma-jobi L. Var. ma-yuen (rom. Caill.) Stapf.] sprout at several harvest time. Plants (Basel) 12 (16), 2975. doi:10.3390/plants12162975
Lee, M. Y., Lin, H. Y., Cheng, F., Chiang, W., and Kuo, Y. H. (2008). Isolation and characterization of new lactam compounds that inhibit lung and colon cancer cells from adlay (Coix lachryma-jobi L. var. ma-yuen Stapf) bran. Food Chem. Toxicol. 46 (6), 1933–1939. doi:10.1016/j.fct.2008.01.033
Lee, Y. S., Kang, Y. J., Kim, H. J., Park, M. K., Seo, H. G., Lee, J. H., et al. (2006). Higenamine reduces apoptotic cell death by induction of heme oxygenase-1 in rat myocardial ischemia-reperfusion injury. Apoptosis 11 (7), 1091–1100. doi:10.1007/s10495-006-7110-y
Li, B., Qiao, L., Li, L., Zhang, Y., Li, K., Wang, L., et al. (2017). A novel antihypertensive derived from adlay (coix larchryma-jobi L. Var. ma-yuen Stapf) glutelin. Molecules 22 (1), 123. doi:10.3390/molecules22010123
Li, C., Jiang, S., Wang, H., Wang, Y., Han, Y., and Jiang, J. (2022). Berberine exerts protective effects on cardiac senescence by regulating the Klotho/SIRT1 signaling pathway. Biomed. Pharmacother. 151, 113097. doi:10.1016/j.biopha.2022.113097
Li, C., Ma, Z. S., Huang, S. M., Yin, B. Q., Chen, Z. F., Nie, S., et al. (2023). Protective effect of Yiyi Fuzisan on myocardium ischemia and vascular endothelial function injury in mice and its mechanism. J. Jilin Univ. Med. Ed. 49 (3), 580–589.
Liang, C., Curry, B. J., Brown, P. L., and Zemel, M. B. (2014). Leucine modulates mitochondrial biogenesis and SIRT1-AMPK signaling in C2C12 myotubes. J. Nutr. Metab. 2014, 239750. doi:10.1155/2014/239750
Liao, L. Z., Chen, Y. L., Lu, L. H., Zhao, Y. H., Guo, H. L., and Wu, W. K. (2013). Polysaccharide from Fuzi likely protects against starvation-induced cytotoxicity in H9c2 cells by increasing autophagy through activation of the AMPK/mTOR pathway. Am. J. Chin. Med. 41 (2), 353–367. doi:10.1142/S0192415X13500262
Lin, S., Wu, X., Tao, L., Bei, Y., Zhang, H., Zhou, Y., et al. (2015). The metabolic effects of traditional Chinese medication qiliqiangxin on H9C2 cardiomyocytes. Cell Physiol. Biochem. 37 (6), 2246–2256. doi:10.1159/000438580
Lin, Y., and Tsai, C. E. (2008). A study of adlay on lowering serum and liver lipids in hamsters. J. food lipids 15 (2), 176–189. doi:10.1111/j.1745-4522.2008.00110.x
Liu, H., Liu, X., Zhuang, H., Fan, H., Zhu, D., Xu, Y., et al. (2020). Mitochondrial contact sites in inflammation-induced cardiovascular disease. Front. Cell Dev. Biol. 8, 692. doi:10.3389/fcell.2020.00692
Liu, M., and Wu, Y. (2022). Role of mitophagy in coronary heart disease: targeting the mitochondrial dysfunction and inflammatory regulation. Front. Cardiovasc Med. 9, 819454. doi:10.3389/fcvm.2022.819454
Liu, S., Li, F., and Zhang, X. (2019). Structural modulation of gut microbiota reveals Coix seed contributes to weight loss in mice. Appl. Microbiol. Biotechnol. 103 (13), 5311–5321. doi:10.1007/s00253-019-09786-z
Liu, X., Engelman, R. M., Moraru, I. I., Rousou, J. A., Flack, J. E., Deaton, D. W., et al. (1992). Heat shock. A new approach for myocardial preservation in cardiac surgery. Circulation 86 (5 Suppl. l), II358–363.
Liu, X., Kwak, D., Lu, Z., Xu, X., Fassett, J., Wang, H., et al. (2014). Endoplasmic reticulum stress sensor protein kinase R-like endoplasmic reticulum kinase (PERK) protects against pressure overload-induced heart failure and lung remodeling. Hypertension 64 (4), 738–744. doi:10.1161/HYPERTENSIONAHA.114.03811
Liu, X., Xie, X., Luo, M., Zhao, Y., Li, M., Peng, F., et al. (2022a). The synergistic compatibility mechanisms of fuzi against chronic heart failure in animals: a systematic review and meta-analysis. Front. Pharmacol. 13, 954253. doi:10.3389/fphar.2022.954253
Liu, X., Zhang, X., Rong, Y. Z., Wu, J. H., Yang, Y. J., and Wang, Z. W. (2015). Rapid determination of fat, protein and amino acid content in coix seed using near-infrared spectroscopy technique. Food Anal. Methods 8 (2), 334–342. doi:10.1007/s12161-014-9897-4
Liu, Y., and Ji, C. (2011). Effects of fuzi polymccharide postconditioning on expression of manganese superoxide dismutase in neonatal rat cardiomyocytes with hypoxia-reoxygenation. Pharmacol. Clin. Chin. Mater. Medica 27 (5), 53–56.
Liu, Y., Ji, C., and Zheng, Q. L. (2012). Effects of bushen jiannao recipe on the content of acetylcholine and the hippocampal ERK1 and ERK2 protein expressions of vascular dementia rats. Tradit. Chin. Drug Res. Clin. Pharmacol. 23 (5), 504–509.
Liu, Y. J., Li, Z., Wang, X., Ni, T. Y., Ma, M., He, Y. Y., et al. (2022b). Effects of adjuvant Chinese patent medicine therapy on major adverse cardiovascular events in patients with coronary heart disease angina pectoris: a population-based retrospective cohort study. Acupunct. Herb. Med. 2 (2), 109–117. doi:10.1097/hm9.0000000000000028
Liu, Y. X. (1991). Treatment of myocarditis in critical cases by YiyiFuzi powder: examples. Hebei J. Tradit. Chin. Med. (06), 17–18.
Long, K., Zhao, Z., Chen, J., Zhi, L., Wang, C., Liao, D., et al. (2022). Yang-xin-xue keli exerts therapeutic effects via regulating mitochondrial homeostasis and function in doxorubicin-induced rat heart failure. Front. Pharmacol. 13, 931453. doi:10.3389/fphar.2022.931453
Long, Y. C., and Zierath, J. R. (2006). AMP-activated protein kinase signaling in metabolic regulation. J. Clin. Invest. 116 (7), 1776–1783. doi:10.1172/JCI29044
López-Armada, M. J., Riveiro-Naveira, R. R., Vaamonde-García, C., and Valcárcel-Ares, M. N. (2013). Mitochondrial dysfunction and the inflammatory response. Mitochondrion 13 (2), 106–118. doi:10.1016/j.mito.2013.01.003
Lou, T., Ma, J., Xie, Y., Yao, G., Fan, Y., Ma, S., et al. (2021). Nuanxin capsule enhances cardiac function by inhibiting oxidative stress-induced mitochondrial dependent apoptosis through AMPK/JNK signaling pathway. Biomed. Pharmacother. 135, 111188. doi:10.1016/j.biopha.2020.111188
Lu, A. P., Jia, H. W., Xiao, C., and Lu, Q. P. (2004). Theory of traditional Chinese medicine and therapeutic method of diseases. World J. Gastroenterol. 10 (13), 1854–1856. doi:10.3748/wjg.v10.i13.1854
Lu, X., Zhang, L., Li, P., Wang, J., Li, R., Huang, Y., et al. (2017). The protective effects of compatibility of Aconiti Lateralis Radix Praeparata and Zingiberis Rhizoma on rats with heart failure by enhancing mitochondrial biogenesis via Sirt1/PGC-1α pathway. Biomed. Pharmacother. 92, 651–660. doi:10.1016/j.biopha.2017.05.117
Luan, Y., Luan, Y., Yuan, R. X., Feng, Q., Chen, X., and Yang, Y. (2021). Structure and function of mitochondria-associated endoplasmic reticulum membranes (MAMs) and their role in cardiovascular diseases. Oxid. Med. Cell Longev. 2021, 4578809. doi:10.1155/2021/4578809
Lucas, D. T., and Szweda, L. I. (1998). Cardiac reperfusion injury: aging, lipid peroxidation, and mitochondrial dysfunction. Proc. Natl. Acad. Sci. U. S. A. 95 (2), 510–514. doi:10.1073/pnas.95.2.510
Luo, C., Yi, F., Xia, Y., Huang, Z., Zhou, X., Jin, X., et al. (2019). Comprehensive quality evaluation of the lateral root of Aconitum carmichaelii Debx. (Fuzi): simultaneous determination of nine alkaloids and chemical fingerprinting coupled with chemometric analysis. J. Sep. Sci. 42 (5), 980–990. doi:10.1002/jssc.201800937
Luo, F., Wang, T., Zeng, L., Zhu, S., Cao, W., Wu, W., et al. (2018a). Diagnostic potential of circulating LncRNAs in human cardiovascular disease: a meta-analysis. Biosci. Rep. 38 (6), BSR20181610. doi:10.1042/BSR20181610
Luo, Y. Y., Du, W. F., Kang, X. J., Zhao, J. K., Ying, Z. Q., Yang, L., et al. (2018b). Process of application history and modern research of coix seed. China J. Tradit. Chin. Med. Pharm. 33 (12), 5666–5673.
Lv, S., Zhao, Y., Wang, L., Yu, Y., Li, J., Huang, Y., et al. (2022a). Antidepressant active components of bupleurum chinense DC-paeonia lactiflora pall herb pair: pharmacological mechanisms. Biomed. Res. Int. 2022, 1024693. doi:10.1155/2022/1024693
Lv, Y., Cheng, L., and Peng, F. (2022b). Compositions and functions of mitochondria-associated endoplasmic reticulum membranes and their contribution to cardioprotection by exercise preconditioning. Front. Physiol. 13, 910452. doi:10.3389/fphys.2022.910452
Mammucari, C., Raffaello, A., Vecellio Reane, D., Gherardi, G., De Mario, A., and Rizzuto, R. (2018). Mitochondrial calcium uptake in organ physiology: from molecular mechanism to animal models. Pflugers Arch. 470 (8), 1165–1179. doi:10.1007/s00424-018-2123-2
Manosroi, J., Chankhampan, C., Kitdamrongtham, W., Manosroi, W., and Manosroi, A. (2019). Potent in vitro anti-mouth cancer (KB) and immunostimulating activities of the job's tears (coix lachryma-jobi linn.) seed semi-purified extract cocktails containing linoleic acid. J. Oleo Sci. 68 (4), 351–359. doi:10.5650/jos.ess18255
Marchi, S., Patergnani, S., and Pinton, P. (2014). The endoplasmic reticulum-mitochondria connection: one touch, multiple functions. Biochim. Biophys. Acta 1837 (4), 461–469. doi:10.1016/j.bbabio.2013.10.015
Meng, H., Du, Z., Lu, W., Wang, Q., Sun, X., Jiang, Y., et al. (2021). Baoyuan decoction (BYD) attenuates cardiac hypertrophy through ANKRD1-ERK/GATA4 pathway in heart failure after acute myocardial infarction. Phytomedicine 89, 153617. doi:10.1016/j.phymed.2021.153617
Mensah, G. A., Fuster, V., Murray, C. J. L., Roth, G. A., Global Burden of Cardiovascular, D., and Risks, C. (2023). Global burden of cardiovascular diseases and risks, 1990-2022. J. Am. Coll. Cardiol. 82 (25), 2350–2473. doi:10.1016/j.jacc.2023.11.007
Miao, L. L., Zhou, Q. M., Peng, C., Meng, C. W., Wang, X. Y., and Xiong, L. (2019). Discrimination of the geographical origin of the lateral roots of aconitum carmichaelii using the fingerprint, multicomponent quantification, and chemometric methods. Molecules 24 (22), 4124. doi:10.3390/molecules24224124
Mondello, C., Stassi, C., Minutoli, L., Baldino, G., Alibrandi, A., Spatola, G. F., et al. (2021). Caspase 9 and caspase 3 immunohistochemical pattern in skeletal and cardiac muscles at different times after death: an experimental study on PMI estimation. Diagn. (Basel) 11 (6), 1062. doi:10.3390/diagnostics11061062
Mootha, V. K., Lindgren, C. M., Eriksson, K. F., Subramanian, A., Sihag, S., Lehar, J., et al. (2003). PGC-1alpha-responsive genes involved in oxidative phosphorylation are coordinately downregulated in human diabetes. Nat. Genet. 34 (3), 267–273. doi:10.1038/ng1180
Ng, Y., Ramm, G., Lopez, J. A., and James, D. E. (2008). Rapid activation of Akt2 is sufficient to stimulate GLUT4 translocation in 3T3-L1 adipocytes. Cell Metab. 7 (4), 348–356. doi:10.1016/j.cmet.2008.02.008
Nisoli, E., Clementi, E., Paolucci, C., Cozzi, V., Tonello, C., Sciorati, C., et al. (2003). Mitochondrial biogenesis in mammals: the role of endogenous nitric oxide. Science 299 (5608), 896–899. doi:10.1126/science.1079368
Niu, L., Mantri, N., Wohlmuth, H., Li, C., Xue, C. C., and Pang, E. (2013). First report of fingerprinting dried herbal products using a subtractive diversity array. J. Complement. Integr. Med. 10, 2012–0002. doi:10.1515/jcim-2012-0002
Novosyadlyy, R., Kurshan, N., Lann, D., Vijayakumar, A., Yakar, S., and LeRoith, D. (2008). Insulin-like growth factor-I protects cells from ER stress-induced apoptosis via enhancement of the adaptive capacity of endoplasmic reticulum. Cell Death Differ. 15 (08), 1304–1317. doi:10.1038/cdd.2008.52
Oslowski, C. M., and Urano, F. (2011). Measuring ER stress and the unfolded protein response using mammalian tissue culture system. Methods Enzymol. 490, 71–92. doi:10.1016/B978-0-12-385114-7.00004-0
Paharkova-Vatchkova, V., and Lee, K. W. (2010). Nuclear export and mitochondrial and endoplasmic reticulum localization of IGF-binding protein 3 regulate its apoptotic properties. Endocr. Relat. Cancer 17 (2), 293–302. doi:10.1677/ERC-09-0106
Pan, X., Shen, Q., Zhang, C., Zhang, X., Li, Y., Chang, Z., et al. (2023). Coicis Semen for the treatment of malignant tumors of the female reproductive system: a review of traditional Chinese medicinal uses, phytochemistry, pharmacokinetics, and pharmacodynamics. Front. Pharmacol. 14, 1129874. doi:10.3389/fphar.2023.1129874
Paradies, G., Petrosillo, G., Pistolese, M., Di Venosa, N., Federici, A., and Ruggiero, F. M. (2004). Decrease in mitochondrial complex I activity in ischemic/reperfused rat heart: involvement of reactive oxygen species and cardiolipin. Circ. Res. 94 (1), 53–59. doi:10.1161/01.RES.0000109416.56608.64
Park, G., Kim, K. M., Choi, S., and Oh, D. S. (2016). Aconitum carmichaelii protects against acetaminophen-induced hepatotoxicity via B-cell lymphoma-2 protein-mediated inhibition of mitochondrial dysfunction. Environ. Toxicol. Pharmacol. 42, 218–225. doi:10.1016/j.etap.2016.01.012
Peoples, J. N., Saraf, A., Ghazal, N., Pham, T. T., and Kwong, J. Q. (2019). Mitochondrial dysfunction and oxidative stress in heart disease. Exp. Mol. Med. 51 (12), 1–13. doi:10.1038/s12276-019-0355-7
Perk, J. (2017). The 2016 version of the European guidelines on cardiovascular prevention. Eur. Heart J. Cardiovasc Pharmacother. 3 (1), 9–10. doi:10.1093/ehjcvp/pvw030
Petrosillo, G., Ruggiero, F. M., Di Venosa, N., and Paradies, G. (2003). Decreased complex III activity in mitochondria isolated from rat heart subjected to ischemia and reperfusion: role of reactive oxygen species and cardiolipin. FASEB J. 17 (6), 714–716. doi:10.1096/fj.02-0729fje
Pietrafesa, G., De Zio, R., Scorza, S. I., Armentano, M. F., Pepe, M., Forleo, C., et al. (2023). Targeting unfolded protein response reverts ER stress and ER Ca(2+) homeostasis in cardiomyocytes expressing the pathogenic variant of Lamin A/C R321X. J. Transl. Med. 21 (1), 340. doi:10.1186/s12967-023-04170-y
Powers, S. K., Sollanek, K. J., Wiggs, M. P., Demirel, H. A., and Smuder, A. J. (2014). Exercise-induced improvements in myocardial antioxidant capacity: the antioxidant players and cardioprotection. Free Radic. Res. 48 (1), 43–51. doi:10.3109/10715762.2013.825371
Puche, J. E., Garcia-Fernandez, M., Muntane, J., Rioja, J., Gonzalez-Baron, S., and Castilla Cortazar, I. (2008). Low doses of insulin-like growth factor-I induce mitochondrial protection in aging rats. Endocrinology 149 (5), 2620–2627. doi:10.1210/en.2007-1563
Qiu, L. Z., Zhou, W., Yue, L. X., Wang, Y. H., Hao, F. R., Li, P. Y., et al. (2021). Repeated aconitine treatment induced the remodeling of mitochondrial function via AMPK-OPA1-atp5a1 pathway. Front. Pharmacol. 12, 646121. doi:10.3389/fphar.2021.646121
Qu, D., Sun, W., Liu, M., Liu, Y., Zhou, J., and Chen, Y. (2016). Bitargeted microemulsions based on coix seed ingredients for enhanced hepatic tumor delivery and synergistic therapy. Int. J. Pharm. 503 (1-2), 90–101. doi:10.1016/j.ijpharm.2016.03.001
Qu, L. Y., Xue, R., Gong, J. W., Zhang, W. Y., Ji, D., Mao, C. Q., et al. (2023). Prediction of quality markers of heishun tablet based on fingerprint, network pharmacology and molecular docking. World J. Tradit. Chin. Med. 18 (19), 2719–2726. doi:10.3969/j.issn.1673-7202.2023.19.002
Radovic, S. M., Starovlah, I. M., Capo, I., Miljkovic, D., Nef, S., Kostic, T. S., et al. (2019). Insulin/IGF1 signaling regulates the mitochondrial biogenesis markers in steroidogenic cells of prepubertal testis, but not ovary†. Biol. Reprod. 100 (1), 253–267. doi:10.1093/biolre/ioy177
Ren, J., Bi, Y., Sowers, J. R., Hetz, C., and Zhang, Y. (2021). Endoplasmic reticulum stress and unfolded protein response in cardiovascular diseases. Nat. Rev. Cardiol. 18 (7), 499–521. doi:10.1038/s41569-021-00511-w
Saltiel, A. R., and Olefsky, J. M. (2017). Inflammatory mechanisms linking obesity and metabolic disease. J. Clin. Invest. 127 (1), 1–4. doi:10.1172/JCI92035
Schaffer, S., and Kim, H. W. (2018). Effects and mechanisms of taurine as a therapeutic agent. Biomol. Ther. Seoul. 26 (3), 225–241. doi:10.4062/biomolther.2017.251
Schnuck, J. K., Sunderland, K. L., Gannon, N. P., Kuennen, M. R., and Vaughan, R. A. (2016). Leucine stimulates PPARβ/δ-dependent mitochondrial biogenesis and oxidative metabolism with enhanced GLUT4 content and glucose uptake in myotubes. Biochimie 128-129, 1–7. doi:10.1016/j.biochi.2016.06.009
Seo, W. G., Pae, H. O., Chai, K. Y., Yun, Y. G., Kwon, T. H., and Chung, H. T. (2000). Inhibitory effects of methanol extract of seeds of Job's Tears (Coix lachryma-jobi L. var. ma-yuen) on nitric oxide and superoxide production in RAW 264.7 macrophages. Immunopharmacol. Immunotoxicol. 22 (3), 545–554. doi:10.3109/08923970009026011
Siwecka, N., Rozpedek-Kaminska, W., Wawrzynkiewicz, A., Pytel, D., Diehl, J. A., and Majsterek, I. (2021). The structure, activation and signaling of IRE1 and its role in determining cell fate. Biomedicines 9 (2), 156. doi:10.3390/biomedicines9020156
Song, C., Huang, Z., Chen, W., Wang, F., Cai, L., Zhao, F., et al. (2013). Empagliflozin alleviates cardiac microvascular ischemia/reperfusion injury by maintaining myocardial mitochondrial homeostasis. J. South Med. Univ. 43 (7), 1136–1144. doi:10.12122/j.issn.1673-4254.2023.07.10
Springett, R. (2021). The proton pumping mechanism of the bc(1) complex. Biochim. Biophys. Acta Bioenerg. 1862 (3), 148352. doi:10.1016/j.bbabio.2020.148352
Sui, Y., and Xu, D. (2022). Isolation and identification of anti-inflammatory and analgesic polysaccharides from Coix seed (Coix lacryma-jobi L.var. Ma-yuen (Roman.) Stapf). Nat. Prod. Res., 1–10. doi:10.1080/14786419.2022.2162896
Surai, P. F., Earle-Payne, K., and Kidd, M. T. (2021). Taurine as a natural antioxidant: from direct antioxidant effects to protective action in various toxicological models. Antioxidants (Basel) 10 (12), 1876. doi:10.3390/antiox10121876
Szegezdi, E., Logue, S. E., Gorman, A. M., and Samali, A. (2006). Mediators of endoplasmic reticulum stress-induced apoptosis. EMBO Rep. 7 (9), 880–885. doi:10.1038/sj.embor.7400779
Szewczyk, A., Kwiecien, I., Grabowski, M., Rajek, K., Cavo, E., Taviano, M. F., et al. (2021). Phenylalanine increases the production of antioxidant phenolic acids in ginkgo biloba cell cultures. Molecules 26 (16), 4965. doi:10.3390/molecules26164965
Szymanski, J., Janikiewicz, J., Michalska, B., Patalas-Krawczyk, P., Perrone, M., Ziolkowski, W., et al. (2017). Interaction of mitochondria with the endoplasmic reticulum and plasma membrane in calcium homeostasis, lipid trafficking and mitochondrial structure. Int. J. Mol. Sci. 18 (7), 1576. doi:10.3390/ijms18071576
Tai, C. J., El-Shazly, M., Yang, Y. H., Tsai, Y. H., Csupor, D., Hohmann, J., et al. (2022). The effectiveness of Fuzi in combination with routine heart failure treatment on chronic heart failure patients. J. Ethnopharmacol. 289, 115040. doi:10.1016/j.jep.2022.115040
Tian, M., Wang, L., Dong, Z., Wang, X., Qin, X., Wang, C., et al. (2022). Preparation, structural characterization, antioxidant activity and protection against cisplatin-induced acute kidney injury by polysaccharides from the lateral root of Aconitum carmichaelii. Front. Pharmacol. 13, 1002774. doi:10.3389/fphar.2022.1002774
Tong, H., Zhu, J., Gong, F., Zhong, L., and Xu, T. (2021). Relationship between cardiotonic activity of Fuzi (Radix Aconiti Lateralis Preparata) and its fingerprint determined by liquid chromatography-mass spectrometry. J. Tradit. Chin. Med. 41 (1), 140–149. doi:10.19852/j.cnki.jtcm.2021.01.016
Tsutsui, H., Kinugawa, S., and Matsushima, S. (2009). Mitochondrial oxidative stress and dysfunction in myocardial remodelling. Cardiovasc Res. 81 (3), 449–456. doi:10.1093/cvr/cvn280
van Empel, V. P., Bertrand, A. T., Hofstra, L., Crijns, H. J., Doevendans, P. A., and De Windt, L. J. (2005). Myocyte apoptosis in heart failure. Cardiovasc Res. 67 (1), 21–29. doi:10.1016/j.cardiores.2005.04.012
Vasan, K., Clutter, M., Fernandez Dunne, S., George, M. D., Luan, C. H., Chandel, N. S., et al. (2022). Genes involved in maintaining mitochondrial membrane potential upon electron transport chain disruption. Front. Cell Dev. Biol. 10, 781558. doi:10.3389/fcell.2022.781558
Viloria, M. A. D., Li, Q., Lu, W., Nhu, N. T., Liu, Y., Cui, Z. Y., et al. (2022). Effect of exercise training on cardiac mitochondrial respiration, biogenesis, dynamics, and mitophagy in ischemic heart disease. Front. Cardiovasc Med. 9, 949744. doi:10.3389/fcvm.2022.949744
Wallace, K. B., Sardao, V. A., and Oliveira, P. J. (2020). Mitochondrial determinants of doxorubicin-induced cardiomyopathy. Circ. Res. 126 (7), 926–941. doi:10.1161/CIRCRESAHA.119.314681
Wang, B. L. (2019). Research on network pharmacology of yiyi fuzi powder based on bioinformatic Technology. Tradit. Chin. Drug Res. Clin. Pharmacol. 30 (05), 564–570. doi:10.19378/j.issn.1003-9783.2019.05.010
Wang, C., and Youle, R. J. (2009). The role of mitochondria in apoptosis. Annu. Rev. Genet. 43, 95–118. doi:10.1146/annurev-genet-102108-134850
Wang, H., Pang, W., Xu, X., You, B., Zhang, C., and Li, D. (2021a). Cryptotanshinone attenuates ischemia/reperfusion-induced apoptosis in myocardium by upregulating MAPK3. J. Cardiovasc Pharmacol. 77 (3), 370–377. doi:10.1097/FJC.0000000000000971
Wang, L., Gao, S., Jiang, W., Luo, C., Xu, M., Bohlin, L., et al. (2014). Antioxidative dietary compounds modulate gene expression associated with apoptosis, DNA repair, inhibition of cell proliferation and migration. Int. J. Mol. Sci. 15 (9), 16226–16245. doi:10.3390/ijms150916226
Wang, L., Sun, J., Yi, Q., Wang, X., and Ju, X. (2012). Protective effect of polyphenols extract of adlay (Coix lachryma-jobi L. var. ma-yuen Stapf) on hypercholesterolemia-induced oxidative stress in rats. Molecules 17 (8), 8886–8897. doi:10.3390/molecules17088886
Wang, N. N., Xu, H. H., Zhou, W., Yang, H. X., Wang, J., Ma, Z. C., et al. (2021b). Aconitine attenuates mitochondrial dysfunction of cardiomyocytes via promoting deacetylation of cyclophilin-D mediated by sirtuin-3. J. Ethnopharmacol. 270, 113765. doi:10.1016/j.jep.2020.113765
Wang, S., Binder, P., Fang, Q., Wang, Z., Xiao, W., Liu, W., et al. (2018). Endoplasmic reticulum stress in the heart: insights into mechanisms and drug targets. Br. J. Pharmacol. 175 (8), 1293–1304. doi:10.1111/bph.13888
Wang, S., Tao, J., Chen, H., Kandadi, M. R., Sun, M., Xu, H., et al. (2021c). Ablation of Akt2 and AMPKα2 rescues high fat diet-induced obesity and hepatic steatosis through Parkin-mediated mitophagy. Acta Pharm. Sin. B 11 (11), 3508–3526. doi:10.1016/j.apsb.2021.07.006
Wang, W., Jiang, J., Huang, Y., Peng, F., Hu, T., Wu, J., et al. (2022a). Aconitine induces autophagy via activating oxidative DNA damage-mediated AMPK/ULK1 signaling pathway in H9c2 cells. J. Ethnopharmacol. 282, 114631. doi:10.1016/j.jep.2021.114631
Wang, X. L. (2018). Clinical Observation on the Treatment of Unstable angina Pectoris of coronary heart disease With yangoi Deficiency and Failure by yiyi fuzi power add-minus. M.M. Heilongjiang Univ. Tradit. Chin. Med.
Wang, X. Y., Xiong, L., Meng, C. W., Peng, C., Miao, L. L., Guo, L., et al. (2020a). Quality evaluation of heishunpian from different areas based on fingerprints and chemometrics. Tradit. Chin. Drug Res. Clin. Pharmacol. 31 (03), 353–358. doi:10.19378/j.issn.1003-9783.2020.03.017
Wang, Y., Geng, J., Jiang, M., Li, C., Han, Y., and Jiang, J. (2019). The cardiac electrophysiology effects of higenamine in Guinea pig heart. Biomed. Pharmacother. 109, 2348–2356. doi:10.1016/j.biopha.2018.10.022
Wang, Y., Li, X., Gu, K., Gou, J., Li, X., Dong, Y., et al. (2022b). Study on the potential mechanism of the active components in YiYiFuZi powder in homotherapy for hetropathy of coronary heart disease and rheumatoid arthritis. Front. Chem. 10, 926950. doi:10.3389/fchem.2022.926950
Wang, Y., Li, X., Qi, M., Li, X., Zhang, F., Wang, Y., et al. (2023a). Pharmacological effects and mechanisms of YiYiFuZi powder in chronic heart disease revealed by metabolomics and network pharmacology. Front. Mol. Biosci. 10, 1203208. doi:10.3389/fmolb.2023.1203208
Wang, Y., Liu, Q., Kang, S. G., Huang, K., and Tong, T. (2021d). Dietary bioactive ingredients modulating the cAMP signaling in diabetes treatment. Nutrients 13 (9), 3038. doi:10.3390/nu13093038
Wang, Y., Zhang, X., Wen, Y., Li, S., Lu, X., Xu, R., et al. (2021e). Endoplasmic reticulum-mitochondria contacts: a potential therapy target for cardiovascular remodeling-associated diseases. Front. Cell Dev. Biol. 9, 774989. doi:10.3389/fcell.2021.774989
Wang, Y. W., Ren, G. J., Wang, B., Wang, D. M., Liu, L. F., and Li, X. J. (2020b). Effect of Wenyang Buxin Decoction on energy metabolism of myocardial mitochondria and MAPK/PPAR- γ signaling pathway in rats with chronic heart failure. J. Emerg. Tradit. Chin. Med. 29 (2), 197–200.
Wang, Y. Y., Zhang, F. F., Li, X., Wang, Y. M., and Li, Y. B. (2023b). Mechanism of Yiyi Fuzi Powder in homotherapy for rheumatoid arthritis and chronic heart failure based on network pharmacology and molecular docking. Drug Eval. Res. 46 (2), 321–329.
Wei, X., Li, Y., Zhou, S., Guo, C., Dong, X., Li, Q., et al. (2023). The differences of nutrient components in edible and feeding coix seed at different developmental stages based on a combined analysis of metabolomics. Molecules 28 (9), 3759. doi:10.3390/molecules28093759
Wen, J., Wang, J., Li, P., Wang, R., Wang, J., Zhou, X., et al. (2019a). Protective effects of higenamine combined with [6]-gingerol against doxorubicin-induced mitochondrial dysfunction and toxicity in H9c2 cells and potential mechanisms. Biomed. Pharmacother. 115, 108881. doi:10.1016/j.biopha.2019.108881
Wen, J., Zhang, L., Liu, H., Wang, J., Li, J., Yang, Y., et al. (2019b). Salsolinol attenuates doxorubicin-induced chronic heart failure in rats and improves mitochondrial function in H9c2 cardiomyocytes. Front. Pharmacol. 10, 1135. doi:10.3389/fphar.2019.01135
Wen, J., Zhang, L., Wang, J., Wang, J., Wang, L., Wang, R., et al. (2020). Therapeutic effects of higenamine combined with [6]-gingerol on chronic heart failure induced by doxorubicin via ameliorating mitochondrial function. J. Cell Mol. Med. 24 (7), 4036–4050. doi:10.1111/jcmm.15041
Wong, W. L., Brostrom, M. A., Kuznetsov, G., Gmitter-Yellen, D., and Brostrom, C. O. (1993). Inhibition of protein synthesis and early protein processing by thapsigargin in cultured cells. Biochem. J. 289 (Pt 1), 71–79. doi:10.1042/bj2890071
Wu, H., Liu, X., Gao, Z. Y., Dai, Z. F., Lin, M., Tian, F., et al. (2019a). Anti-myocardial infarction effects of radix aconiti lateralis preparata extracts and their influence on small molecules in the heart using matrix-assisted laser desorption/ionization-mass spectrometry imaging. Int. J. Mol. Sci. 20 (19), 4837. doi:10.3390/ijms20194837
Wu, J., Lin, N., Li, F., Zhang, G., He, S., Zhu, Y., et al. (2016a). Induction of P-glycoprotein expression and activity by Aconitum alkaloids: implication for clinical drug-drug interactions. Sci. Rep. 6, 25343. doi:10.1038/srep25343
Wu, M. P., Zhang, Y. S., Zhou, Q. M., Xiong, J., Dong, Y. R., and Yan, C. (2016b). Higenamine protects ischemia/reperfusion induced cardiac injury and myocyte apoptosis through activation of β2-AR/PI3K/AKT signaling pathway. Pharmacol. Res. 104, 115–123. doi:10.1016/j.phrs.2015.12.032
Wu, S., Lu, Q., Ding, Y., Wu, Y., Qiu, Y., Wang, P., et al. (2019b). Hyperglycemia-driven inhibition of AMP-activated protein kinase α2 induces diabetic cardiomyopathy by promoting mitochondria-associated endoplasmic reticulum membranes in vivo. Circulation 139 (16), 1913–1936. doi:10.1161/CIRCULATIONAHA.118.033552
Wu, S., and Zou, M. H. (2020). AMPK, mitochondrial function, and cardiovascular disease. Int. J. Mol. Sci. 21 (14), 4987. doi:10.3390/ijms21144987
Wu, T., Jiang, N., Ji, Z., and Shi, G. (2019c). The IRE1 signaling pathway is involved in the protective effect of low-dose LPS on myocardial ischemia-reperfusion injury. Life Sci. 231, 116569. doi:10.1016/j.lfs.2019.116569
Wu, Y., Adi, D., Long, M., Wang, J., Liu, F., Gai, M. T., et al. (2016c). 4-Phenylbutyric acid induces protection against pulmonary arterial hypertension in rats. PLoS One 11 (6), e0157538. doi:10.1371/journal.pone.0157538
Wu, Y., Liu, Y., Zhang, L., Wen, L., and Xie, Y. (2022). Aconiti lateralis radix praeparata total alkaloids exert anti-RA effects by regulating NF-κB and JAK/STAT signaling pathways and promoting apoptosis. Front. Pharmacol. 13, 980229. doi:10.3389/fphar.2022.980229
Xia, T., Liu, C. S., Hu, Y. N., Luo, Z. Y., Chen, F. L., Yuan, L. X., et al. (2021). Coix seed polysaccharides alleviate type 2 diabetes mellitus via gut microbiota-derived short-chain fatty acids activation of IGF1/PI3K/AKT signaling. Food Res. Int. 150 (Pt A), 110717. doi:10.1016/j.foodres.2021.110717
Xiang, Z. M., Zhu, M., Chen, B. L., and Chen, Y. (2005). Identification of triacylglycerols in coix oil by high performance liquid chromatography-atmospheric pressure chemical ionization-mass spectrometry. China J. Chin. Mater Med. 30 (18), 1436–1438. doi:10.3321/j.issn:1001-5302.2005.18.014
Xing, Z., Chen, J., Yu, T., Li, X., Dong, W., Peng, C., et al. (2023). Aconitum carmichaelii Debx. Attenuates heart failure through inhibiting inflammation and abnormal vascular remodeling. Int. J. Mol. Sci. 24 (6), 5838. doi:10.3390/ijms24065838
Xiong, L., Peng, C., Xie, X. F., Guo, L., He, C. J., Geng, Z., et al. (2012). Alkaloids isolated from the lateral root of Aconitum carmichaelii. Molecules 17 (8), 9939–9946. doi:10.3390/molecules17089939
Xu, L., Chen, L., Ali, B., Yang, N., Chen, Y., Wu, F., et al. (2017). Impact of germination on nutritional and physicochemical properties of adlay seed (Coixlachryma-jobi L). Food Chem. 229, 312–318. doi:10.1016/j.foodchem.2017.02.096
Xue, X., Piao, J. H., Nakajima, A., Sakon-Komazawa, S., Kojima, Y., Mori, K., et al. (2005). Tumor necrosis factor alpha (TNFalpha) induces the unfolded protein response (UPR) in a reactive oxygen species (ROS)-dependent fashion, and the UPR counteracts ROS accumulation by TNFalpha. J. Biol. Chem. 280 (40), 33917–33925. doi:10.1074/jbc.M505818200
Yan, P., Mao, W., Jin, L., Fang, M., Liu, X., Lang, J., et al. (2020). Crude radix aconiti lateralis preparata (fuzi) with Glycyrrhiza reduces inflammation and ventricular remodeling in mice through the TLR4/NF-κB pathway. Mediat. Inflamm. 2020, 5270508. doi:10.1155/2020/5270508
Yang, J., Liu, Y., Lu, S., Sun, X., Yin, Y., Wang, K., et al. (2022a). Coix seed oil regulates mitochondrial functional damage to induce apoptosis of human pancreatic cancer cells via the PTEN/PI3K/AKT signaling pathway. Mol. Biol. Rep. 49 (7), 5897–5909. doi:10.1007/s11033-022-07371-8
Yang, J., Sun, Q., Ma, Q., Yu, Q., Liu, X., Liu, Y., et al. (2022b). Mahuang Xixin Fuzi decoction ameliorates apoptosis via the mitochondrial-mediated signaling pathway in MCM cells. J. Ethnopharmacol. 297, 115538. doi:10.1016/j.jep.2022.115538
Yang, L., Chen, Y., Zhou, J., Sun, J., Jiang, W., Liu, T., et al. (2021a). Aconitine induces mitochondrial energy metabolism dysfunction through inhibition of AMPK signaling and interference with mitochondrial dynamics in SH-SY5Y cells. Toxicol. Lett. 347, 36–44. doi:10.1016/j.toxlet.2021.04.020
Yang, L., Huang, G. Y., Wang, Y. G., Han, B. Q., Zheng, B., Zhu, J. M., et al. (2021b). Efficacy of renshen (radix ginseng) plus fuzi (radix aconiti lateralis preparata) on myocardial infarction by enhancing autophagy in rat. J. Tradit. Chin. Med. 41 (6), 909–918. doi:10.19852/j.cnki.jtcm.2021.06.009
Yang, M. Q., Song, Y. M., Gao, H. Y., and Xue, Y. T. (2019). Efficacy and safety of fuzi formulae on the treatment of heart failure as complementary therapy: a systematic review and meta-analysis of high-quality randomized controlled trials. Evid. Based Complement. Altern. Med. 2019, 9728957. doi:10.1155/2019/9728957
Yang, T., Zhong, X. X., and He, Q. Y. (2016). A preliminary study of Associate Professor He Qingyong's thoughts on the application of YiyiFuzi powder in the treatment of chest obstruction and heartache. J. Emerg. Tradit. Chin. Med. 25 (05), 821–822.
Yang, Z., Wen, A., Qin, L., and Zhu, Y. (2022c). Effect of coix seed extracts on growth and metabolism of limosilactobacillus reuteri. Foods 11 (2), 187. doi:10.3390/foods11020187
Yang, Z., Zhu, X., Wen, A., Ran, J., Qin, L., and Zhu, Y. (2022d). Coix seed-based milk fermented with limosilactobacillus reuteri improves lipid metabolism and gut microbiota in mice fed with a high-fat diet. Front. Nutr. 9, 921255. doi:10.3389/fnut.2022.921255
Ye, Z., Wang, S., Zhang, C., and Zhao, Y. (2020). Coordinated modulation of energy metabolism and inflammation by branched-chain amino acids and fatty acids. Front. Endocrinol. (Lausanne) 11, 617. doi:10.3389/fendo.2020.00617
Yin, H. M., Wang, S. N., Nie, S. P., and Xie, M. Y. (2018). Coix polysaccharides: gut microbiota regulation and immunomodulatory. Bioact. Carbohydr. Diet. Fibre 16, 53–61. doi:10.1016/j.bcdf.2018.04.002
Yokota, S. I., Ando, M., Aoyama, S., Nakamura, K., and Shibata, S. (2016). Leucine restores murine hepatic triglyceride accumulation induced by a low-protein diet by suppressing autophagy and excessive endoplasmic reticulum stress. Amino Acids 48 (4), 1013–1021. doi:10.1007/s00726-015-2149-0
Yu, F., Gao, J., Zeng, Y., and Liu, C. X. (2011a). Effects of adlay seed oil on blood lipids and antioxidant capacity in hyperlipidemic rats. J. Sci. Food Agric. 91 (10), 1843–1848. doi:10.1002/jsfa.4393
Yu, H. Y., Wang, S. J., Teng, J. L., Ji, X. M., Wu, Z. C., Ma, Q. C., et al. (2012). Effects of Radix aconiti lateralis preparata and Rhizoma zingiberis on energy metabolism and expression of the genes related to metabolism in rats. Chin. J. Integr. Med. 18 (1), 23–29. doi:10.1007/s11655-012-0964-7
Yu, J., Wang, X., Yao, X., and Wu, X. (2022a). Safety evaluation of heavy metal contamination and pesticide residues in coix seeds in guizhou province, China. Foods 11 (15), 2286. doi:10.3390/foods11152286
Yu, W., Zeng, D., Xiong, Y., Shan, S., Yang, X., Zhao, H., et al. (2022b). Health benefits of functional plant polysaccharides in metabolic syndrome: an overview. J. Funct. Foods 95, 105154. doi:10.1016/j.jff.2022.105154
Yu, Z. X., Hu, D. M., and Li, Q. (2011b). Clinical observation on the treatment of asthma exacerbation with YiyiFuzi powder. Guangming J. Chin. Med. 26 (11). doi:10.3969/j.issn.1003-8914.2011.011.031
Yu, Z. X., Li, Q., Sun, G. Q., and Hu, D. M. (2016). Effect of Yiyi Fuzi powder on the FEV1/FVC% and the quality of life of patients with bronchial asthma. Clin. J. Chin. Med. 8 (30), 13–15.
Yuan, S. M., and Jing, H. (2010). Cardiac pathologies in relation to Smad-dependent pathways. Interact. Cardiovasc Thorac. Surg. 11 (4), 455–460. doi:10.1510/icvts.2010.234773
Zeng, H., Qin, L., Liu, X., and Miao, S. (2021). Increases of lipophilic antioxidants and anticancer activity of coix seed fermented by Monascus purpureus. Foods 10 (3), 566. doi:10.3390/foods10030566
Zeng, Y., Yang, J., Chen, J., Pu, X., Li, X., Yang, X., et al. (2022). Actional mechanisms of active ingredients in functional food adlay for human health. Molecules 27 (15), 4808. doi:10.3390/molecules27154808
Zhang, C., Syed, T. W., Liu, R., and Yu, J. (2017). Role of endoplasmic reticulum stress, autophagy, and inflammation in cardiovascular disease. Front. Cardiovasc Med. 4, 29. doi:10.3389/fcvm.2017.00029
Zhang, D., Wang, F., Li, P., and Gao, Y. (2022a). Mitochondrial Ca(2+) homeostasis: emerging roles and clinical significance in cardiac remodeling. Int. J. Mol. Sci. 23 (6), 3025. doi:10.3390/ijms23063025
Zhang, F. F., Li, X. K., Li, X., Wang, Y. Y., Wu, J. K., Wang, Y. M., et al. (2023a). Study on the mechanism of Yiyi Fuzi powder in the treatment of rheumatoid arthritis based on metabolomics and network pharmacology. Acta Pharm. Sin. 58 (7), 1802–1811. doi:10.16438/j.0513-4870.2022-1146
Zhang, J., Li, D., Zhong, D., Zhou, Q., Yin, Y., Gao, J., et al. (2022b). Processed lateral root of Aconitum carmichaelii Debx.: a review of cardiotonic effects and cardiotoxicity on molecular mechanisms. Front. Pharmacol. 13, 1026219. doi:10.3389/fphar.2022.1026219
Zhang, J. M., Li, L., Gao, F., Li, Y., He, Y., and Fu, C. M. (2012). Chemical ingredient analysis of sediments from both single radix aconiti lateralis decoction and radix aconiti lateralis - radix glycyrrhizae decoction by HPLC-MS. Acta Pharm. Sin. 47 (11), 1527–1533.
Zhang, K., and Kaufman, R. J. (2008). From endoplasmic-reticulum stress to the inflammatory response. Nature 454 (7203), 455–462. doi:10.1038/nature07203
Zhang, N., Song, Y., Song, Q., Shi, S., Zhang, Q., Zhao, Y., et al. (2016). Qualitative and quantitative assessments of aconiti lateralis radix praeparata using high-performance liquid chromatography coupled with diode array detection and hybrid ion trap-time-of-flight mass spectrometry. J. Chromatogr. Sci. 54 (6), 888–901. doi:10.1093/chromsci/bmv245
Zhang, T., Xu, L., Guo, X., Tao, H., Liu, Y., Liu, X., et al. (2023b). The potential of herbal drugs to treat heart failure: the roles of Sirt1/AMPK. J. Pharm. Anal. 14 (2), 157–176. doi:10.1016/j.jpha.2023.09.001
Zhang, W., Cai, S., Qin, L., Ding, M., Luo, Z., Shan, J., et al. (2023c). Alkaloids of Aconiti Lateralis Radix Praeparata treated non-small cell lung cancer by regulating glycolysis via the PI3K/AKT-mTOR signaling pathway. Res. Square. doi:10.21203/rs.3.rs-3087978/v1
Zhang, W., Jia, X., Xu, Y., Xie, Q., Zhu, M., Zhang, H., et al. (2022c). Effects of coix seed extract, bifidobacterium BPL1, and their combination on the glycolipid metabolism in obese mice. Front. Nutr. 9, 939423. doi:10.3389/fnut.2022.939423
Zhang, W., Lu, C., Cai, S., Feng, Y., Shan, J., and Di, L. (2022d). Aconiti lateralis radix praeparata as potential anticancer herb: bioactive compounds and molecular mechanisms. Front. Pharmacol. 13, 870282. doi:10.3389/fphar.2022.870282
Zhang, Z. B., Jia, M., Wang, M., Zhang, P. F., Zhang, H. F., Gao, F., et al. (2020). Research advance in effects of endoplasmic reticulum-mitochondria interaction on cardiovascular diseases. Chin. Heart J. 32 (5), 522–527. doi:10.12125/j.chj.202006027
Zhao, D., Yan, M., Xu, H., Liang, H., Zhang, J., Li, M., et al. (2023). Antioxidant and antiaging activity of fermented coix seed polysaccharides on Caenorhabditis elegans. Nutrients 15 (11), 2474. doi:10.3390/nu15112474
Zhao, D. D., Wang, J., Cui, Y. J., and Wu, X. F. (2012). Pharmacological effects of Chinese herb aconite (fuzi) on cardiovascular system. J. Tradit. Chin. Med. 32 (3), 308–313. doi:10.1016/s0254-6272(13)60030-8
Zhao, M. Q., Wu, W. K., Zhao, D. Y., Duan, X. F., and Liu, Y. (2010). Effects of Sini decoction on mitochondrial mediated apoptosis in Adriamycin-induced heart failure rats. China Trop. Med. 10 (07), 844–845+852. doi:10.13604/j.cnki.46-1064/r.2010.07.014
Zhao, M. Q., Wu, W. K., Zhao, D. Y., Liu, Y., Liu, Y., Liang, T. W., et al. (2005). Protective effects of sini decoction on adriamycin-induced heart failure and its mechanism: role of superoxide dismutase. China J. Chin. Mater. Medica 30 (14), 1111–1114. doi:10.3321/j.issn:1001-5302.2005.14.017
Zhao, Y., Dai, X. Y., Zhou, Z., Zhao, G. X., Wang, X., and Xu, M. J. (2016). Leucine supplementation via drinking water reduces atherosclerotic lesions in apoE null mice. Acta Pharmacol. Sin. 37 (2), 196–203. doi:10.1038/aps.2015.88
Zheng, L., Cai, J., Feng, Y. H., Su, X., Chen, S. Y., Liu, J. Z., et al. (2022). The association between dietary branched-chain amino acids and the risk of cardiovascular diseases in Chinese patients with type 2 diabetes: a hospital-based case-control study. Front. Nutr. 9, 999189. doi:10.3389/fnut.2022.999189
Zheng, Q., Zhao, Y., Wang, J., Liu, T., Zhang, B., Gong, M., et al. (2014). Spectrum-effect relationships between UPLC fingerprints and bioactivities of crude secondary roots of Aconitum carmichaelii Debeaux (Fuzi) and its three processed products on mitochondrial growth coupled with canonical correlation analysis. J. Ethnopharmacol. 153 (3), 615–623. doi:10.1016/j.jep.2014.03.011
Zhou, J., Wang, Z., He, Y., Luo, X., Zhang, W., Yu, L., et al. (2020). Qiliqiangxin reduced cardiomyocytes apotosis and improved heart function in infarcted heart through Pink1/Parkin -mediated mitochondrial autophagy. BMC Complement. Med. Ther. 20 (1), 203. doi:10.1186/s12906-020-02992-7
Zhou, J. C., Jin, C. C., Wei, X. L., Xu, R. B., Wang, R. Y., Zhang, Z. M., et al. (2023). Mesaconine alleviates doxorubicin-triggered cardiotoxicity and heart failure by activating PINK1-dependent cardiac mitophagy. Front. Pharmacol. 14, 1118017. doi:10.3389/fphar.2023.1118017
Zhou, Y., Murugan, D. D., Khan, H., Huang, Y., and Cheang, W. S. (2021). Roles and therapeutic implications of endoplasmic reticulum stress and oxidative stress in cardiovascular diseases. Antioxidants (Basel) 10 (8), 1167. doi:10.3390/antiox10081167
Zhou, Y. P. (2011). Review and evaluation of water-soluble active ingredients of aconite in the cardiovascular system. Pharmacol. Clin. Chin. Mater Med. 27, 106–110.
Zhu, F. (2017). Coix: chemical composition and health effects. Trends Food Sci. Technol. 61, 160–175. doi:10.1016/j.tifs.2016.12.003
Zhu, L., Chen, Z., Han, K., Zhao, Y., Li, Y., Li, D., et al. (2020a). Correlation between mitochondrial dysfunction, cardiovascular diseases, and traditional Chinese medicine. Evid. Based Complement. Altern. Med. 2020, 2902136. doi:10.1155/2020/2902136
Zhu, R., Xu, X., Shan, Q., Wang, K., Cao, G., and Wu, X. (2020b). Determination of differentiating markers in Coicis semen from multi-sources based on structural similarity classification coupled with UPCC-xevo G2-XS QTOF. Front. Pharmacol. 11, 549181. doi:10.3389/fphar.2020.549181
Zuo, Y. H., Han, Q. B., Dong, G. T., Yue, R. Q., Ren, X. C., Liu, J. X., et al. (2018). Panax ginseng polysaccharide protected H9c2 cardiomyocyte from hypoxia/reoxygenation injury through regulating mitochondrial metabolism and RISK pathway. Front. Physiol. 9, 699. doi:10.3389/fphys.2018.00699
Glossary
Keywords: cardiovascular disease, mitochondria, endoplasmic reticulum, Chinese herbal medicine, YiYiFuZi powder
Citation: Ding J, Ji R, Wang Z, Jia Y, Meng T, Song X, Gao J and He Q (2024) Cardiovascular protection of YiyiFuzi powder and the potential mechanisms through modulating mitochondria-endoplasmic reticulum interactions. Front. Pharmacol. 15:1405545. doi: 10.3389/fphar.2024.1405545
Received: 23 March 2024; Accepted: 28 May 2024;
Published: 24 June 2024.
Edited by:
Kroekkiat Chinda, Naresuan University, ThailandReviewed by:
Kai Yang, Shandong University of Traditional Chinese Medicine, ChinaPeizheng Yan, Shandong University of Traditional Chinese Medicine, China
Pingjun Zhu, People’s Liberation Army General Hospital, China
Copyright © 2024 Ding, Ji, Wang, Jia, Meng, Song, Gao and He. This is an open-access article distributed under the terms of the Creative Commons Attribution License (CC BY). The use, distribution or reproduction in other forums is permitted, provided the original author(s) and the copyright owner(s) are credited and that the original publication in this journal is cited, in accordance with accepted academic practice. No use, distribution or reproduction is permitted which does not comply with these terms.
*Correspondence: Qingyong He, heqingyongg@163.com
†These authors have contributed equally to this work and share first authorship