- 1Yunnan Key Laboratory of Stomatology, School of Stomatology, Kunming Medical University, Kunming, China
- 2Department of Periodontology, Kunming Medical University School and Hospital of Stomatology, Kunming Medical University, Kunming, China
- 3Department of Oral Implantology, Kunming Medical University School and Hospital of Stomatology, Kunming Medical University, Kunming, China
Oral squamous cell carcinoma (OSCC) is a crucial public health problem, accounting for approximately 2% of all cancers globally and 90% of oral malignancies over the world. Unfortunately, despite the achievements in surgery, radiotherapy, and chemotherapy techniques over the past decades, OSCC patients still low 5-year survival rate. Cisplatin, a platinum-containing drug, serves as one of the first-line chemotherapeutic agents of OSCC. However, the resistance to cisplatin significantly limits the clinical practice and is a crucial factor in tumor recurrence and metastasis after conventional treatments. Ferroptosis is an iron-based form of cell death, which is initiated by the intracellular accumulation of lipid peroxidation and reactive oxygen species (ROS). Interestingly, cisplatin-resistant OSCC cells exhibit lower level of ROS and lipid peroxidation compared to sensitive cells. The reduced ferroptosis in cisplatin resistance cells indicates the potential relationship between cisplatin resistance and ferroptosis, which is proved by recent studies showing that in colorectal cancer cells. However, the modulation pathway of ferroptosis reversing cisplatin resistance in OSCC cells still remains unclear. This article aims to concisely summarize the molecular mechanisms and evaluate the relationship between ferroptosis and cisplatin resistance OSCC cells, thereby providing novel strategies for overcoming cisplatin resistance and developing new therapeutic approaches.
1 Introduction
Globally, head and neck cancer (HNC) ranks as the sixth most common type of cancer. OSCC is one of the most prevalent forms of the HNC, accounting for approximately 2% of all cancers globally and 90% of oral malignancies, with around 377,000 new cases diagnosed and 177,000 deaths annually (Sung et al., 2021). Worldwide, OSCC is a crucial public health problem, which ranks as the fourth most prevalent type of cancer in low/medium-income countries (Nagao and Warnakulasuriya, 2020). The conventional and standard treatment of OSCC is generally surgery followed by chemotherapy and radiotherapy. As a conventional treatment option for various types of cancer, including OSCC, chemotherapy is also a popular adjunct treatment for the advanced stages (Tan et al., 2023). However, due to the locally advanced stage because of a lack of awareness and a delay in initial diagnosis, OSCC patients still have a 50%–60% 5-year survival rate, despite the achievements in surgery, radiotherapy, and chemotherapy techniques over the past decades (Chinn and Myers, 2015).
Cisplatin, as a classical platinum-based chemotherapeutic agent, was discovered in 1845, which is widely used in solid cancer as a valid treatment choice (Ghosh, 2019). However, the resistance to chemotherapeutic reagents, such as cisplatin, leads to recurrence and metastasis after treatment. Plenty of basic and clinical studies aimed to reveal the mechanisms and overcome drug resistance.
As a novel form of programmed cell death, ferroptosis can be characterized by its morphological features, which was initially reported in 2012 (Dixon Scott et al., 2012). The main morphological appearance of ferroptosis is the alternations in mitochondria, for example, reduction or loss of mitochondrial cristae, rupture of the outer mitochondrial membrane, and increase in mitochondrial membrane density. Besides, neither the alternations in typical necrosis, such as the cellular swelling and the rupture of the cellular membrane, nor the characteristics of apoptosis, like the shrinkage of the nucleus and the condensation of chromatin, cannot be observed in ferroptosis cells. Researchers have discovered that the initiation of ferroptosis is triggered by the build-up of iron-dependent lipid peroxides in cells (Mou et al., 2019). Nowadays, ferroptosis has been discovered to correlate with chemoresistance, and inducing ferroptosis can reverse the drug resistance (Zhang et al., 2022). This review aims to concisely summarize the current molecular mechanisms of ferroptosis and cisplatin resistance in OSCC cells, then discusses how the inducing of ferroptosis can be an underlying strategy to overcome the cisplatin resistance in the realm of chemotherapy and expects that this review could stimulate some novel thoughts of developing ferroptosis-based therapies to reverse cisplatin-resistant in OSCC.
2 The mechanisms and pathways of ferroptosis
Ferroptosis can be promoted by iron ions and lipids, such as polyunsaturated fatty acids (PUFA), while can be inhibited by some products of amino acid metabolism, like glutathione (GSH) serving as a substrate of glutathione peroxidase 4 (GPX4), which can relieve the toxicity of lipid peroxides. When cells are unable to eliminate the excessive intracellular reactive oxygen species (ROS), the accumulated lipid peroxidation will induce ferroptosis (Louandre et al., 2013; Hassannia et al., 2019) (Figure 1).
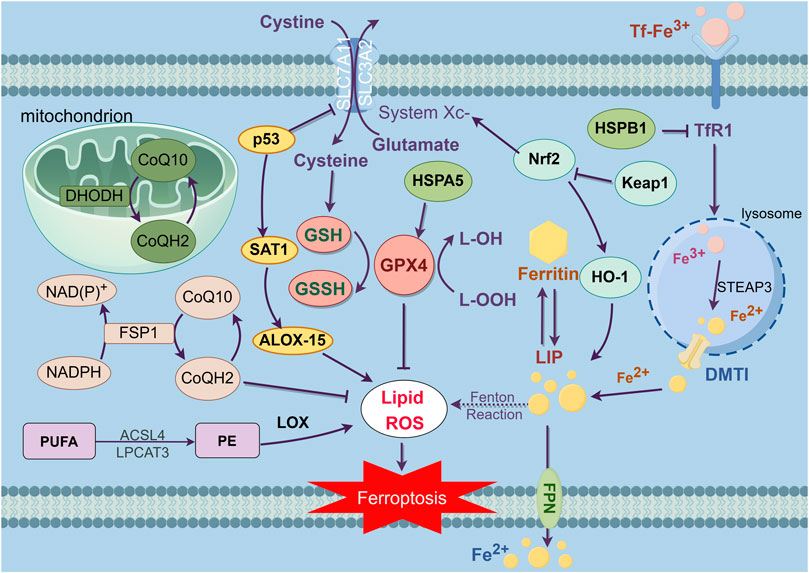
Figure 1. This figure exhibits the main regulation mechanisms of ferroptosis, which can be separated into three pathways. The first part is system XC-/GSH/GPX4 pathway. As an antitransporter, system XC- pumps cystine in the cell while pumping glutamate out, then synthesizes GSH which decreases ROS under the influence of GPX4, thereby inhibiting ferroptosis. Second, the iron metabolism, TfR1 inhales Tf-Fe3+ complex, then separating Fe3+ and reverting Fe3+ to Fe2+, which forms the reactive LIP. As the intracellular Fe2+ aggregates, resulting in the Fenton reaction, inducing LIP to produce large amounts of lipid peroxides and oxidative free radicals, which triggers ferroptosis. The third mechanism is related to the regulation of lipid metabolism. As a kind of PUFA, PE is synthesized and remodeled through two kinds of essential enzymes, ACSL4 and LPCAT3. The accumulation of PE is the symbol and the destination of ferroptosis. Furthermore, it has been revealed that the NADPH/FSP1/CoQ10 pathway and mitochondria defense system dihydroorotate dehydrogenase (DHODH) are independent parallel systems that cooperate with GPX4 pathway to inhibit ferroptosis.
2.1 Metabolism of iron ions
Iron ions are key materials in the synthesis of DNA and protein and in the process of cell growth. The lack of iron ions will result in the growth arrest and death of cells (Galaris et al., 2019). Physiologically, iron ions are in the form of Fe2+ and Fe3+, which can maintain relative homeostasis of redox states and ion concentrations. Extracellularly, Fe3+ binds into transferrin (Tf) to form the Tf- Fe3+ complex. Then, the Tf- Fe3+ complex will be transported to different tissues and organs through blood circulation. Transferrin receptor 1 (TfR1) in the cell membrane can recognize Tf- Fe3+ complex and inhale the complex into the cell by endocytosis, then separate Fe3+ from the complex in the lysosome. After that, the Fe3+ is reduced to Fe2+ in the lysosome by six transmembrane epithelial antigen of the prostate 3 (STEAP3). Subsequently, Fe2+ will be transferred to cytoplasm by divalent metal transporter 1 (DMT1) (Sendamarai et al., 2008; Wolff et al., 2018). The free Fe2+ in the cytoplasm will form the reactive labile iron pool (LIP). Fe2+ in LIP can be transferred to other organelles, and then to be participated in the activation of protein activity in these organelles (Mu et al., 2021). Ferroportin (FPN) can export the Fe2+ to the outside of the cells, thus avoiding the accumulation of excess iron ions (Yang et al., 2014). Normally, LIP would maintain the balance of iron ions intracellular, while pathologically, intracellular Fe2+ aggregates, resulting in the Fenton reaction, which can produce large amounts of lipid peroxides and oxidative free radicals. Failing to clear the excess free radicals will trigger ferroptosis (Song et al., 2019; Mu et al., 2021).
Nuclear factor erythroid 2-related factor 2 (Nrf2) is a major intracellular modulator of the antioxidant defense system, which is involved in signal transduction related to multiple intracellular defense mechanisms. Physiologically, Nrf2 acts as a compound with Kelch-like ECH-associated protein 1 (Keap1) in the cellular. When being stimulated by oxidative stress, excess ROS will dissociate Nrf2 from the compound and activate a series of antioxidant stress factors (Korytina et al., 2019). HO-1 is a target protein downstream of Nrf2, which can degrade heme to release biliverdin, CO, and Fe2+. On one hand, the activation of HO-1 would lead to the accumulation of Fe2+ which can induce ferroptosis. On the other hand, Fe2+ could be oxidized to Fe3+, then it will be stored by ferritin or exported outside the cell by FPN-1, which has been proven to contribute to inhibit ferroptosis (Fahrer et al., 2023), suggesting that the role of HO-1 in ferroptosis may depend on different conditions and need further research. In conclusion, the excess iron ions are necessary for the occurrence of ferroptosis. When intracellular iron-related proteins and iron homeostasis are dysregulated, intracellular iron ion concentration will increase, inducing the accumulation of an excess of ROS, and resulting in ferroptosis.
2.2 Metabolism of lipids
Ferroptosis is marked by the build-up of lipid peroxides that rely on iron for their formation. Lipid peroxides are a kind of intracellular ROS. PUFA and monounsaturated fatty acid (MUFA) are two kinds of the reaction substrates of lipid peroxidation. Between them, PUFA is more susceptible to be oxidized than MUFA (Yang et al., 2016). The free PUFA undergoes esterification to become membrane phospholipids, which can induce ferroptosis after further oxidation (Kagan et al., 2017). The symbol and the destination of ferroptosis is the accumulation of PUFA in cells (D Herde and Krysko, 2017). The difference in PUFA levels would cause the different rates of intracellular lipid peroxidation, and the varied intensity of ferroptosis. ROS is a general term for peroxides with oxygenated radicals in organisms, which can destroy intercellular structures such as RNA, DNA and lead to cell death (Lin et al., 2018). As a group of PUFA-related phospholipids, phosphatidyl ethanolamine (PE) is rich in arachidonic acid which has been proven to be the key material in activating ferroptosis (Kagan et al., 2017). Intercellular acyl-CoA synthetase long-chain family member 4 (ACSL4) and lysophosphatidylcholine acyltransferase 3 (LPCAT3) are essential enzymes in the process of lipid peroxidation. ACSL4 is involved in the synthesis of PE, while LPCAT3 contributes to the remodeling of PE. The lack of these two kinds of enzymes results in a PUFA decrease following the reduction in PE, which would then suppress the process of ferroptosis (Doll et al., 2017; Kagan et al., 2017). The peroxidation of PUFA is also catalyzed by lipoxygenase (LOX). When reducing the amount of intracellular LOX can inhibit the accumulation of PUFAs, which results in the inhibition of ferroptosis. In a word, dysregulation of lipid peroxide metabolism in cells is the premise for the occurrence of ferroptosis.
2.3 Metabolism of amino acids
System Xc-, composed of SLC7A11 and SLC3A2, is a crucial intracellular antioxidant protein, which is widely dispersed in phospholipid bilayers. It can transport amino acids at a 1:1 ratio, pumping cystine into the cell while pumping glutamate out of the cell, thereby maintaining a dynamic balance between these two amino acids in cells (Dixon Scott et al., 2012). Intracellularly, cystine is converted to cysteine catalyzed by enzymes, then combining with glutamic acid and glycine to generate GSH (Koppula et al., 2020). As the predominant intracellular antioxidant, GSH plays a crucial role in reducing ROS under the influence of GPXs, thereby safeguarding cells against oxidative harm. In the GPX family, GPX4 inhibits the accumulation of lipid peroxides and is a pivotal inhibitor of ferroptosis. With a strong antioxidant capacity, GSH can be oxidize to glutathione disulfide (GSSG) by GPX4, and then reduce lipid peroxides to the corresponding alcohols. Normally, cancer cells have a higher level of ROS than non-cancer cells, while reducing the level of ROS inhibits the proliferation of cancer cells. However, as the level of ROS increased, cancer cells are facing a higher burden on antioxidant systems, which leading to an overexpression of GSH or Nrf2 in plenty of cancer cells (Kennedy et al., 2020). Nrf2-Keap1 can also upregulate system Xc- and increase the secretion of glutamate thereby inhibiting ferroptosis (Fan et al., 2017). RSL3 and erastin can respectively act on GPX4 and SLC7A11, then degrading the antioxidant ability of the cells and leading to the accumulation of ROS, eventually resulting in cell ferroptosis. As the latest reported ferroptosis agonist, Fin56 can exert synergy effects with the activation of cytogenesis autophagy to promote the degradation of GPX4, though its mechanism needs further research (Sun et al., 2021). System Xc-/GSH/GPX4 is a pivotal inhibitory pathway of ferroptosis (Dixon Scott et al., 2012).
2.4 Other factors
Furthermore, ferroptosis is also modulated by several factors, including ferroptosis suppressor protein 1 (FSP1), p53, heat shock protein (HSP), and mitochondria
p53 is an important antioncogene, which can regulate cancer cell proliferation, differentiation, and death. Recent studies have found that p53 can combine with the SLC7A11 promoter leading to inhibit the expression and the transport capacity of the system Xc-, which results in the decline in cellular antioxidant ability and the build-up of lipid peroxide, and finally induces ferroptosis (Jiang et al., 2015a; Jiang et al., 2015b). Additionally, abundant p53 can activate spermidine/spermine N1-acetyl-transferase 1 (SAT1), which can regulate the arachidonate 15-lipoxygenase (ALOX-15) resulting in the increase of intracellular lipid peroxidation, and will eventually induce ferroptosis (Ou et al., 2016).
FSP1 is a novel important ferroptosis-regulating protein that can restrain cells from ferroptosis when GPX4 pathway is blocked. As a kind of oxidoreductase of NADPH-dependent Coenzyme Q (CoQ), FSP1 can reduce coenzyme Q10 (CoQ10) to dihydro-ubiquinone (CoQH2). CoQH2 is a kind of antioxidant that traps lipophilic free radicals, which inhibits the build-up of lipid peroxides (Bersuker et al., 2019; Doll et al., 2019). As a parallel system, the NADPH/FSP1/CoQ10 pathway can not only work cooperate with the GPX4 pathway, but also in the absence of the GPX4 pathway to inhibit ferroptosis.
HSP is a kind of highly conserved molecular chaperone, which will be expressed under environmental pressure, to protect cells from different types of cell death. Heat shock protein beta-1 (HSPB1) can decrease cellular iron uptake, reducing intracellular ROS and inhibiting ferroptosis (Sun et al., 2015). Heat shock protein family A member 5 (HSPA5) can combine with GPX4, thereby keeping the stability of GPX4 from GPX4 protein degradation, thus inhibiting cell lipid peroxidation and ferroptosis (Zhu et al., 2017).
The defense system localized at mitochondria is mediated by dihydroorotate dehydrogenase (DHODH) which is an enzyme crucial for pyrimidine synthesis reducing CoQ10 to CoQH2 at the inner mitochondrial membrane, thereby limiting the buildup of lipid peroxides. Notably, when the expression of GPX4 is downregulated, flux through DHODH will increase significantly, leading to increased expression of CoQH2, thereby protecting cells from lipid peroxidation and inhibiting ferroptosis in mitochondria. Emerging proofs suggests that the potential role of targeting DHODH to induce mitochondria damage and the downregulation of both mitochondrial GPX4 and DHODH will lead to mitochondrial lipid peroxidation, ultimately triggering ferroptosis (Mao et al., 2021).
3 Relationship between ferroptosis and cancer cells
Cancer cells are cells that have lost normal physiological regulation and developed metabolic plasticity which has unlimited potential for growth. To meet the demands of their constant growth, malignant cells have a higher appetite for iron than normal cells (Hassannia et al., 2019). The specific metabolic reprogramming observed in cancer cells has been linked to an increased susceptibility to ferroptosis (Friedmann et al., 2019). As the iron ions accumulates in cancer cells, it will lead to an elevated level of lipid peroxides and ROS, while downregulating the level of ROS inhibits the proliferation of cancer cells. To cope with this characteristic, the expression of GSH or Nrf2 in malignant cells is upregulated to maintain a balanced level of ROS, which helps them to avoid ferroptosis, but it also results in the ferroptosis vulnerability of cancer cells will increase significantly when the GSH-GPX4 pathway is blocked (Kennedy et al., 2020).
3.1 The “iron addiction” of cancer cells
The high-speed proliferation characteristic of cancer cells results in a higher requirement of iron in these cells than in normal cells. As the most popular way for cancer cells to obtain iron, TfR1 is highly expressed in many cancer cells. Normally, the high expression of TfR1 indicates an advanced stage and poor prognosis of tumors (Candelaria et al., 2021).
A previous study has found that various cancer cells are sensitive to ferroptosis inducers (Wu Y. et al., 2023a; Feng et al., 2023; Shan et al., 2023; Zhang et al., 2023). Cancer stem cells (CSCs) are unique cancer cell subpopulations which have stem cell-like characteristics, the capacity for self-renewal and multidirectional differentiation allowing them to promote tumor growth and heterogeneity which is also a key reason for tumor recurrence and metastasis (Marquardt et al., 2018). However, CSCs can be induced death selectively through ferroptosis inducers by increasing sensitivity of cancer cells to chemotherapy, thereby eliminating malignant cells (Walcher et al., 2020). In tumor microenvironment, CSCs have a much higher efficiency of iron absorption than non-CSCs, with an increased expression level of Tf and TfR1, indicating that Tf and TfR1 are essential for the survival of CSCs, and emphasizing the critical importance of iron within these subgroups (Schonberg et al., 2015; Hamaï et al., 2017). The synthesis of polyunsaturated fatty acid-containing phospholipid (PUFA-PL) is significantly enhanced in CSCs, forcing CSCs to rely on GPX4 to detoxify lipid peroxides, indicating that CSCs are extremely vulnerable to ferroptosis when the pathway is being inhibited (Viswanathan et al., 2017; Bersuker et al., 2019; Doll et al., 2019).
Glioblastoma (GBM) is a highly malignant brain cancer with a poor treatment effect and prognosis. Researchers have found that GBM cells request more iron than normal brain cells, which upregulates the expression of TfR1 to intake more Tf- Fe3+ complexes to achieve more iron ions (Schonberg et al., 2015; Kawak et al., 2023). As a malignant cancer which occurs in the ovarian epithelium, the high-malignant serous ovarian cancer (SOC) has a higher expression of TfR1 and a lower one of FPN than the low-malignant SOC (Wu et al., 2022). All these studies manifest that cancer cells are addicted to iron.
3.2 The “vulnerability” of cancer cells to ferroptosis
The development of resistance of malignant cells to chemotherapy leads to recurrence and metastasis of tumor after treatment. Genes carried by these cancer cells may have been mutated which helps tumors to escape from cancer treatment (Shen et al., 2020). Moreover, metabolic and epigenetic plasticity can be altered by malignant cells to develop resistance to chemotherapy. This adaptability allows them to survive and continue to proliferate even after treatment, which brings heterogeneity to cancer cells, allowing them to be more resistant to conventional therapy strategies (Marine et al., 2020). As the research continues, the vulnerability of cancer cells to ferroptosis has been discovered. This vulnerability is mostly manifested in the dependence of cancer cells on iron, as well as the regulation of the epigenetic plasticity of iron in the drug-resistant status of cancer cells.
Studies have revealed that autophagic degradation of ferritin results in the release of unstable iron, which would lead to cellular ferroptosis (Mancias et al., 2014). In the pancreatic ductal adenocarcinoma mouse model, cysteine depletion also causes cellular ferroptosis (Badgley et al., 2020). The maintenance of resistance to chemotherapy of cancer cells highly depends on the pathway of lipid peroxidase, which reveals that the main characteristic of the mesenchymal status of cancer cells is the enrichment and esterification of PUFAs on their membranes (Hangauer et al., 2017). These PUFAs induce cellular ferroptosis when being catalyzed by LOX. Astrocytes are dependent on the presence of PUFA-rich membranes which can significantly alter cellular metabolic activity, manifesting that cells are forced to reprogram neurons, resulting in enhanced vulnerability of ferroptosis in cells (Gascon et al., 2016). As a kind of enzyme involved in the synthesis of PE, the level of ACSL4 is reduced in imatinib-resistant gastrointestinal stromal tumors cells, while upregulating the expression ACSL4 can induce ferroptosis and alleviate the resistance of imatinib (Cui et al., 2024). It is shown that the expression of Nrf2 is increased in plenty of cancer cell. Drug-resistant malignant cells can even inhibit ferroptosis through upregulating the level of Nrf2 (Adinolfi et al., 2023). Arenobufagin, a bioactive component extracted from toads, can reduce the expression of Nrf2 in colorectal cancer and gastric cancer, inducing ferroptosis, thereby inhibiting proliferation, migration and invasion of malignant cells. Nevertheless, activation of Nrf2 can inhibit ferroptosis and reverse these effects (Long et al., 2024; Wang et al., 2024).
Lipid peroxides are unstable substrates, which can easily be converted to reactive oxygen radicals when being catalyzed by iron ions, then forming toxic lipid peroxidation catabolic products, such as phospholipid hydroperoxides, etc (Yang et al., 2016). If these lipid peroxidation catabolic products are not degraded by ferroptosis-inhibiting systems such as GPX4 or FSP1, they will eventually lead to cellular ferroptosis (Kagan et al., 2017). This vulnerability is manifested in different drug-resistant cancer cells, including ZEB1-driven cells which are susceptible to epithelial-mesenchymal transition (EMT) (Krebs et al., 2017). It is shown that the overexpression of ZEb1 increases the synthetic levels of PUFAs, which upregulates the levels of active lipid peroxides, enhancing the sensitivity of EMT cells to ferroptosis (Han et al., 2021). Through differentially expressed genes (DEGs), it is shown that palmitoyl protein thioesterase 1 (PPT1) is a core gene in OSCC. Overexpression of PPT1 enhances the proliferation of OSCC cells and associates with a poor prognosis, by upregulating the level of GPX4 and inhibiting ferroptosis, while knockdown PPT1 can reverse these effect and increase the sensitivity to ferroptosis inducers (Luo et al., 2024). As the inducers of ferroptosis, RSL3 and erastin have been proved to inhibit the system Xc-/GSH/GPX4 pathway, which provides novel ideas for treating drug-resistant cancer cells.
4 Mechanism of cisplatin resistance in OSCC cells
As the most commonly utilized chemotherapy medication, cisplatin has a high antitumor activity against many cancers and serves as the first-line treatment for locally advanced OSCC. The absorption of cisplatin is mediated by copper transporter protein 1 (CTR1). Those cells with higher expression of CTR1 have a higher level of cisplatin, which gives them a better expectation of chemotherapy (Ghosh, 2019). Once the cisplatin reaches the cytoplasm, it will be activated by replacing the chlorine atoms with water molecules (Dasari and Tchounwou, 2014). Then it will target to make lesions in DNA by producing oxidative stress such as ROS which leads to peroxidation of lipids and depletion of sulfhydryl groups. If the amount of damaged DNA is beyond the capacity of the DNA repair system, it will eventually lead to cell death through apoptosis (Galluzzi et al., 2012). However, cancer cells become resistant to chemotherapy medications such as cisplatin after being exposed to it several times, leading to recurrent and metastasis which greatly limits the clinical practice of it (Sha et al., 2021). Thus, it will be beneficial to provide potential therapeutic strategies by understanding the mechanism of cisplatin resistance in OSCC (Figure 2).
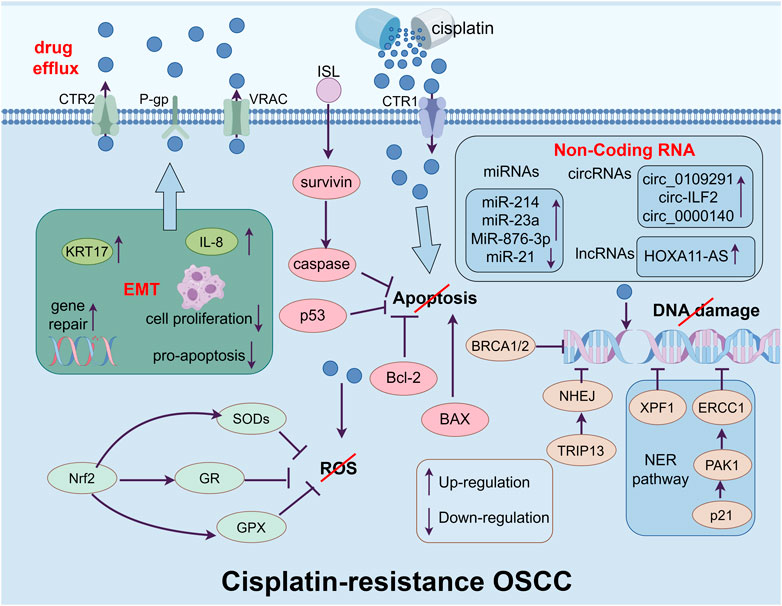
Figure 2. The figure shows the main mechanisms of cisplatin-resistance in OSCC cells, which include enhancing drug efflux ability form drug transporters, inhibiting apoptosis, repairing the damage of DNA causing by cisplatin, limiting the accumulation of ROS in cytoplasm, inducing EMT and regulating the level of non-coding RNA.
4.1 Exceeding export of cisplatin
ATP-binding cassette transporter (ABC transporter) is a kind of membrane protein which exports drugs and toxins from the cell (Fletcher et al., 2016). As the first found ABC transporter, in higher expressed OSCC cells, P-glycoprotein (P-gp) can export cisplatin thereby inhibiting its sensitivity (Kim et al., 2016a). Additionally, the export of cisplatin is also mediated by copper transporter protein 2 (CTR2). It is shown that the knockdown of CTR2 greatly increases the level of cisplatin in tumor cells, thereby significantly enhancing its drug effect (Blair et al., 2011). The patients with a higher level of CTR2 have a poorer prognosis in cisplatin-based chemotherapy. Moreover, volume-regulated anion channel (VRAC) is another kind of transmembrane protein which can export ions and other osmotic agents when the cell is swelling (Jentsch et al., 2016). The deletion of VRAC drastically enhances the resistance of cancer cells to cisplatin (Siemer et al., 2021).
4.2 Enhanced DNA repair mechanism
Once the cisplatin enters the cells, it will induce DNA damage and oxidative stress. In response to this damage, cells enhance the DNA repair system to maintain genetic integrity, which causes drug resistance. As an important way to repair DNA, the nucleotide excision repair pathway (NER pathway) can moderate the sensitivity of platinum-based chemotherapy drugs (Marteijn et al., 2014). Excision repair cross-complementing 1 (ERCC1) and Xeroderma pigmentosum complementation group F protein 1 (XPF1) are two kinds of pivotal endonucleases in the NER pathway. These two enzymes, acting as the step that controls the pace of the NER process, can associate with each other to form a compound that facilitates the elimination of platinum adducts (Sabatella et al., 2021). The overexpression of p21 protein activates kinase 1 (PAK1) to upregulate the level of ERCC1 in OSCC cells, which desensitizes the cancer cells to cisplatin (Lin et al., 2017). The overexpression of ERCC1 and XPF1 patients normally leads to a shorter progression-free survival and overall survival (Chiu et al., 2011; Zuo et al., 2023). The homologous recombination pathway (HR) which is mediated by BRCA1/2 will continue to repair them when the NER pathway is unable to repair the DNA lesions (Murai and Pommier, 2023). Patients with a lower level of BRCA1 have a longer overall survival (Burcher et al., 2021). As another DNA repair system, non-homologous end joining (NHEJ) can also induce cisplatin resistance through the overexpression of thyroid hormone receptor interactor 13 (TRIP13) (Banerjee et al., 2014).
4.3 Detoxification of reactive oxygen species
Over the past decades, cisplatin-based cell death was thought to be caused by DNA damage. However, it has been shown that the vital role of the accumulation of oxidative stress, such as ROS, in cisplatin-induced cell death (Kuo et al., 2022). Cisplatin can induce mitochondria to release ROS into the cytoplasm. As the ROS accumulates, the high level of oxidative stress contributes to lesions of lipids, proteins, and DNA which can induce cell death (Ma et al., 2016). When facing the overexpressed ROS, cells develop an intricate defending system consisting of superoxide dismutase (SOD), GPX, and glutathione reductase (GR), which can be induced by Nrf2, thereby reversing the accumulation of ROS and prevent cell death (Noman et al., 2020). Researchers have discovered that cisplatin-resistant OSCC cells have a high expression of Nrf2 (Zeng et al., 2021; Praharaj et al., 2023). As a kind of natural flavonoid, wogonin can selectively kill cancer cells by inducing the accumulation of intracellular ROS and decreasing the expression of Nrf2 to reverse the cisplatin resistance (Kim et al., 2016b). It is shown that in ovarian cancer, the expression of deoxycytidine triphosphate pyrophosphatase 1 (DCTPP1) increases after treating with cisplatin, which associates with a poor prognosis, especially in cisplatin-resistant cells. Downregulating the level of DCTPP1 suppresses the proliferation of cancer cells, leads to a higher level of intracellular ROS, and increases the sensitivity to cisplatin, indicating the crucial rule of ROS in cisplatin treatment (Wang et al., 2022).
4.4 Blocking of the apoptosis pathway
Platinum-based chemotherapy drugs can induce cell death through apoptosis. The cisplatin resistance is correlated with genes and proteins associated with apoptosis dysregulation (Siu et al., 2019; Zhang et al., 2019). B-cell lymphoma 2 (Bcl-2) is a kind of apoptosis-regulating proteins, which consists of anti-apoptosis protein and pro-apoptosis protein (Kaloni et al., 2023). Bcl-2 is overexpressed in drug-resistance OSCC cells and the knockdown of it can reverse the resistance to cisplatin (Xiong et al., 2016; Hsu et al., 2019; Qiao et al., 2021). Moreover, as a member of the inhibitor of apoptosis proteins (IAPs), survivin can regulate G2/M cell cycle transition and bind with caspase to inhibit cancer cells from apoptosis, resulting in resistance to chemotherapy, including cisplatin resistance (Ju et al., 2018; Kondapuram et al., 2023). Isoliquiritigenin (ISL) is a natural compound which can suppress survivin from phosphorylation and facilitate survivin for ubiquitination degradation. Combined with cisplatin, ISL can overcome cisplatin resistance caused by the overexpression of survivin in OSCC cells (Zhou et al., 2023). As an important antioncogene, p53 can also trigger apoptosis in cells (Aubrey et al., 2018). The acetylation and transactivation of p53 can significantly enhance the sensitivity of apoptosis induced by cisplatin in OSCC cells (Li et al., 2020), while the mutation of p53 leads to cisplatin resistance in OSCC cells (Alam et al., 2019).
4.5 Enhanced EMT capacity
As a cellular reprogramming event, EMT is a process that epithelial cells undergo transformation into mesenchymal cells, which allows cancer cells to invade, extravasate, and, eventually, form metastases (Wangmo et al., 2020). Researchers have found that EMT is likely to be a partial and flexible reprogramming progress in cancer cells that enables them to express mesenchymal and epithelial genes simultaneously (Yang et al., 2020). Recently, EMT has been deemed to be linked with CSC phenotype and drug resistance (Dongre and Weinberg, 2019). EMT cells have increased the expression of gene repairing mechanisms, drug efflux, inhibited cell proliferation, and pro-apoptotic pathways (Shibue and Weinberg, 2017). The level of genes alters based on the requirement of the needs of cancer cells (Pastushenko et al., 2018). It has been shown that the expression of transcription factors like Snail, Twist1 and Zeb1 are increased in EMT cell, which promote cells resistant to chemotherapy, making them crucial targeting points for determining sensitivity or resistance for cancer cells to chemotherapy (Zhang et al., 2024). Studies have found that the silence of keratin 17 (KRT17) results in significantly reduced expression of mesenchymal DNA in cancer cells leading to decreased migratory, invasive abilities, and reversing the resistance to cisplatin (Jang et al., 2022). The level of interleukin-8 (IL-8) is also found to be upregulated in a range of cancers and contribute to EMT and resistance to cisplatin in OSCC cells, while the blocking of it can reverse these effects (Joshi et al., 2023).
4.6 Non-coding RNA-based mechanisms
About 98% of the human genome is transcribed into RNA without the ability to code protein, which is commonly referred to as non-coding RNA (ncRNA). These ncRNAs are significantly involved in various physiological and pathological processes (Adams et al., 2017). Increasing evidence also indicates that ncRNAs can lead to cisplatin resistance in OSCC cells (Meng et al., 2021).
As a group of small ncRNAs, microRNAs (miRNAs) act as regulatory molecules in cancer, which play dual roles as both oncogenes and tumor suppressors (Wang et al., 2020).It has been discovered that the levels of miR-214 and miR-23a were increased in cisplatin-resistant OSCC cells, while the level of miR-21 was reduced. Moreover, the silencing or overexpressing of the expression level can reverse the resistance (Yu et al., 2010). It has been shown that cancer-associated fibroblast-derived extracellular vesicle (EV) treats OSCC cells to acquire cisplatin resistance, by upregulating the expression of GATA-binding protein 1 (GATA1), a downstream factor of miR-876-3p. Silencing miR-876-3p can inhibit cisplatin resistance in OSCC cells (Kang et al., 2024).
Circular ncRNAs (circRNAs) are transcripts that exhibit a closed-loop structure, which contributes to oncogenic processes (Lei et al., 2020). Circ_0109291 and circ-ILF2 are highly expressed in cisplatin-resistant OSCC cells compared with sensitive ones. As a sponge of miR-188-3p, knockdown of circ_0109291 and overexpression of miR-188-3p suppressed the resistance to cisplatin by enhancing the apoptosis of the resistant tumor cells. As a negatively regulated downstream factor of miR-188-3p, suppressing the expression of ATP-binding cassette sub-family B member 1 (ABCB1) can reverse the silencing of circ_010929. The regulation of circ_0109291 is a potential strategy to alleviate the cisplatin resistance of OSCC cells (Gao et al., 2022). Circ-ILF2 serves as a sponge of miR-1252, downregulating the level of Krüppel-like factor 8 (KLF8) in OSCC cells. The upregulation of circ-ILF2 can greatly decrease cisplatin-induced apoptosis and induce the M2 polarization of macrophages through circ-ILF2/miR-1252/KLF8 axis (Wu et al., 2023b).
Plenty of long ncRNAs (lncRNAs) have also been found to play a crucial rule in chemoresistance in OSCC cells (Meng et al., 2021). Similar to circRNAs, lncRNAs can also act as sponges to impair the modulations of miRNAs on mRNAs (Thomson and Dinger, 2016). The level of lncRNA Homeobox A11 antisense RNA (HOXA11-AS) was discovered to be upregulated in OSCC cells compared to neighboring healthy human oral cells (Niu et al., 2020). The upregulation of HOXA11-AS can lower the level of miR-214-3p, thereby positively regulates the proto-oncogene serine/threonine-protein kinase (PIM1). The knockdown of HOXA11-AS and PIM1 or the upregulation of miR-214-3p can increased cisplatin-induced cytotoxicity and reverse cisplatin resistance of OSCC cells (Wang et al., 2019).
5 Reversing cisplatin resistance in OSCC cells by inducing ferroptosis
Chemoresistance is one of the most common reasons for the failure of traditional tumor treatments. Research has shown that melanoma cells exhibit a preference for metastasizing via the lymphatic system rather than the bloodstream, which attributes to the elevated levels of GSH and the reduced availability of free iron in the lymphatic environment (Ubellacker et al., 2020), indicating that ferroptosis plays a crucial role in tumor suppression and results in the improvement of prognosis. For example, ferroptosis can interfere with lipid metabolism and disrupt iron metabolic equilibrium in chemoresistance colorectal cancer cells, thereby reversing drug resistance (Wang et al., 2023). Moreover, ferroptosis reverses the sorafenib resistance in hepatocellular carcinoma cancer cells (Guo et al., 2023) (Figure 3).
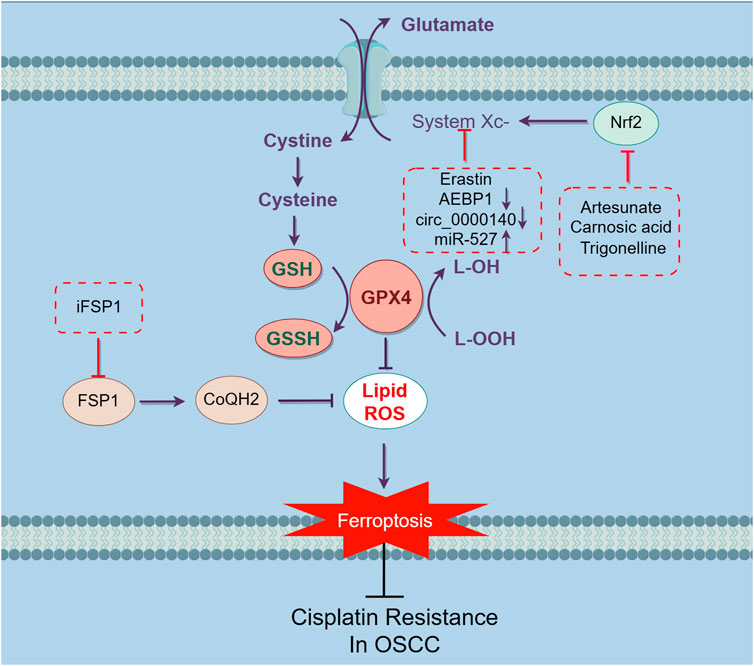
Figure 3. Mechanisms inducing ferroptosis and reversing cisplatin-resistance in OSCC cells. Targeting ferroptosis is an effective way to sensitize cisplatin-resistant OSCC cells. Ferroptosis is triggered by the accumulation of iron-based lipid peroxides and ROS. The main mechanism of inhibiting ferroptosis can be separated into three parts, inhibiting the build-up of Fe2+, suppressing the accumulation of iron dependent lipid peroxides and reducing ROS through intracellular antioxidant (Figure 1). The strategies have been discovered to overcome cisplatin-resistance in OSCC basically focused on SLC7A11/GSH/GPX4 pathway. Inhibit system Xc- by earstin or silence AEBP1 and circ_0000140, downregulate the upstream regulation factor Nrf2 through artesunate, carnosic acid and trigonelline, thereby inhibiting the SLC7A11/GSH/GPX4 pathway. Block FSP1 through iFSP1, reducing the level of CoQH2, impairing the capacity of cells to traps lipophilic free radicals. Altogether, through these ways can induce ferroptosis in cisplatin-resistant OSCC cells, thereby reversing the resistance to eliminate malignant cells.
Cisplatin-resistant HNSCC cells exhibit lower level of ROS and lipid peroxidation compared to sensitive ones after being exposed to cisplatin, which are also less sensitive to ferroptosis inducers. Treating with like N-acetyl cysteine (NAC), a free radical scavenger, reverses the effect of ferroptosis inducers and cisplatin (Table 1). Indication that cisplatin-resistant HNSCC cells are also resistant to ferroptosis. Bioinformatic analysis also shows that the development of cisplatin-resistance results in gene mutations, increased gene expression associated with EMT and β-catenin signaling, as well as decreased gene expression related to the G2/M checkpoint (Yu et al., 2020). Thereby, inducing ferroptosis in these resistant cells could be a potential strategy to reverse cisplatin-resistance.
5.1 Blocking GPX4 pathway by system Xc-inhibitors and SSZ
Studies also reveal that inducing ferroptosis might be a latent way to reverse cisplatin resistance in OSCCs. As mentioned before, system XC-/GSH/GPX4 is a pivotal ferroptosis-inhibiting pathway. Researchers have discovered that both cisplatin-sensitive and resistant OSCC cells can induce ferroptosis in a conditioned media without cystine or with excessive glutamine while the apoptosis markers remain. This effect can be blocked by ferroptosis inducer, but not necroptosis inhibitor. After being exposed to erastin, an inhibitor of system XC-, all resistant cells significantly increased their susceptibility to cisplatin and caused ferroptosis. Moreover, similar results were achieved on nude mice models (Roh et al., 2016).
As a kind of ferroptosis inducer, sulfasalazine (SSZ) can enhance the anti-cancer effects of cisplatin by inhibiting the system Xc-in colorectal cancer cells (Ma et al., 2015). As a potential oncogene, Adipocyte enhancer-binding protein 1 (AEBP1) is upregulated in various malignant cells (Majdalawieh et al., 2020). It is shown that cisplatin-resistant OSCC cells are also less sensitive to SSZ treatment. However, AEBP1 silencing significantly sensitizes cisplatin-resistant OSCC cells to SSZ, leading to an increasing the level of Fe2+ and ROS, with a decreasing one with GSH, which induces ferroptosis in both vivo and vitro, thereby reversing the resistance to cisplatin (Zhou et al., 2022).
5.2 Attenuating the expression of Nrf2 through inhibitor and carnosic acid
Carnosic acid (CA), a polyphenolic abietane diterpene found in rosemary and common sage, has been proven to exhibit potent activity against lipid peroxidation and carcinogen detoxification enzymes in suppressing oral carcinogenesis (Manoharan et al., 2010; Das et al., 2019). Treatment with CA increases sensitivity to cisplatin in cisplatin-resistant OSCC cells by inducing ferroptosis via the blocking of the Nrf2/system XC- pathway. The expression of GSH upregulates, while ROS and lipid peroxidation downregulate in the cells, and CA can reverse the expression. Furthermore, reactivating the Nrf2 signaling and utilizing ferroptosis antagonist can counteract the suppression, indicating that inducing ferroptosis can be a potential strategy to overcome cisplatin resistance (Han et al., 2022).
Artesunate (Arts) is a semi-synthetic derivative of artemisinin, which has been found to manifest anticancer effects through inducing oxidative DNA damage and ROS production (Yu et al., 2019). It is also shown to induce ferroptosis in glioblastoma cells (Song et al., 2022). However, this capacity is limited in cisplatin-resistant HNC due to the upregulation of Nrf2 in the cells (Shin et al., 2018). Trigonelline (Trig), a Nrf2 inhibitor contributes to attenuating this resistance. Cooperating with inhibitor, Arts can increase the ability to induce ferroptosis, indicating that this resistance is partly due to Nrf2 overexpression. Combined utilization of Nrf2 inhibitor and Arts can be a promising therapeutic strategy in overcoming the cisplatin resistance in HNC cells (Roh et al., 2017). It has been discovered that combined treatment with Arts, cisplatin and iron can greatly enhance the effect of cisplatin and induce a stronger apoptosis in HNSCC cells (Okamoto et al., 2023). Similar result is also shown in myeloma cells. Arts can increase the level of Fe2+, ROS and ACSL4, while the expression of GPX4 decreased, thereby inducing ferroptosis, which can be reversed by ferroptosis inhibitor (Liang et al., 2023).
5.3 Regulation of non-coding RNA and FSP1 pathway
Altered expression patterns of circRNAs have been discovered in OSCC (Paramasivam, 2022). It should be noted that certain circRNAs relate to cisplatin resistance in OSCC cells (Qiu et al., 2021; Gao et al., 2022). Moreover, the overexpression of circ_0000140 was discovered in cisplatin-resistant OSCC cell lines. Interestingly, knocking down this circRNA remarkably reverses the cisplatin resistance and promotes ferroptosis. The overexpression of circ_0000140 results in the dysregulation of the miR-527, thereby affecting the expression of SLC7A11 to inhibit ferroptosis, and the upregulation of miR-527 and the downregulation of SLC7A11 can reverse this effect (Ma et al., 2023).
Drug-tolerant persister (DTP) is a prestate of drug-resistant cells, which enables malignant cells to endure the chemotherapy for a period, thereby allowing them to develop drug resistance through different methods (Cabanos and Hata, 2021). As a parallel system to the system Xc-/GSH/GPX4 pathway, FSP1 generates an antioxidant form of CoQH2 thereby inhibiting ferroptosis. Interestingly, FSP1 is highly expressed in HNC cells from cisplatin-resistant patients. The overexpression of FSP1 is also discovered in DTP cells which results in cisplatin resistance, enhanced migratory and invasive capacities. Not only can the FSP1 inhibitor, iFSP1, and shRNA reverse the capacity, but allow cisplatin to exhibit a more potent effect (Wu et al., 2024).
6 Conclusion
In brief, ferroptosis might be a potential strategy to overcome cisplatin resistance in OSCC cells. Combined treatment with ferroptosis inducers and chemotherapy could be a potent way to increase the 5-year survival rate and the prognosis of OSCC patients. However, the underlying mechanisms of how ferroptosis reverses cisplatin resistance still remains unclear, making it still far away from clinical practice of drug combination of cisplatin and ferroptosis inducers. Furthermore, ferroptosis can aggravate inflammation in a variety of disease. Treatment with ferroptosis inducers would be harmful to those cancer patients with underlying conditions. It might be benefit to combine with targeted treating, through directly targeting malignant cells, alleviating or avoiding unnecessary damage to healthy cells.
Nowadays, bioinformatic analysis has been widely used to predict the possible function of various genes and protein in cancers, thereby screening targeting points. Further research could focus on these bioinformatic analysis provided biomarkers, predicting the efficacy of ferroptosis inducers in OSCC, exploring new ferroptosis regulators, understanding the complicated molecule mechanisms, refining therapeutic procedures, and providing personalized medicine strategies. It is believed that in the near future, researchers will provide more combined treatment strategies to eliminate malignant cells.
Author contributions
RC: Conceptualization, Data curation, Formal Analysis, Investigation, Visualization, Writing–original draft, Writing–review and editing. SZ: Investigation, Writing–review and editing. RZ: Writing–review and editing. WL: Writing–review and editing. LJ: Writing–review and editing. XR: Methodology, Supervision, Writing–review and editing. HH: Methodology, Project administration, Supervision, Writing–review and editing.
Funding
The author(s) declare that financial support was received for the research, authorship, and/or publication of this article. This research was funded by the National Natural Science Foundation of China (Grant No. 81660184), the Natural Science Foundation of Yunnan, China (Grant nos 2019FE001-168 and 202001AY070001-085), the Yunnan Provincial Oral Disease Clinical Medical Research Center Scientific Research Fund (2022ZD001 and 2022YB001), and Technical Innovation Personnel Training Object Item (202405AD350005).
Conflict of interest
The authors declare that the research was conducted in the absence of any commercial or financial relationships that could be construed as a potential conflict of interest.
Publisher’s note
All claims expressed in this article are solely those of the authors and do not necessarily represent those of their affiliated organizations, or those of the publisher, the editors and the reviewers. Any product that may be evaluated in this article, or claim that may be made by its manufacturer, is not guaranteed or endorsed by the publisher.
References
Adams, B. D., Parsons, C., Walker, L., Zhang, W. C., and Slack, F. J. (2017). Targeting noncoding RNAs in disease. J. Clin. Investig. 127 (3), 761–771. doi:10.1172/JCI84424
Adinolfi, S., Patinen, T., Jawahar Deen, A., Pitkänen, S., Härkönen, J., Kansanen, E., et al. (2023). The KEAP1-NRF2 pathway: targets for therapy and role in cancer. Redox Biol. 63, 102726. doi:10.1016/j.redox.2023.102726
Alam, M., Kashyap, T., Mishra, P., Panda, A. K., Nagini, S., and Mishra, R. (2019). Role and regulation of proapoptotic Bax in oral squamous cell carcinoma and drug resistance. Head. Neck 41 (1), 185–197. doi:10.1002/hed.25471
Aubrey, B. J., Kelly, G. L., Janic, A., Herold, M. J., and Strasser, A. (2018). How does p53 induce apoptosis and how does this relate to p53-mediated tumour suppression? Cell. Death Differ. 25 (1), 104–113. doi:10.1038/cdd.2017.169
Badgley, M. A., Kremer, D. M., Maurer, H. C., DelGiorno, K. E., Lee, H. J., Purohit, V., et al. (2020). Cysteine depletion induces pancreatic tumor ferroptosis in mice. Science 368 (6486), 85–89. doi:10.1126/science.aaw9872
Banerjee, R., Russo, N., Liu, M., Basrur, V., Bellile, E., Palanisamy, N., et al. (2014). TRIP13 promotes error-prone nonhomologous end joining and induces chemoresistance in head and neck cancer. Nat. Commun. 5, 4527. doi:10.1038/ncomms5527
Bersuker, K., Hendricks, J. M., Li, Z., Magtanong, L., Ford, B., Tang, P. H., et al. (2019). The CoQ oxidoreductase FSP1 acts parallel to GPX4 to inhibit ferroptosis. Nature 575 (7784), 688–692. doi:10.1038/s41586-019-1705-2
Blair, B. G., Larson, C. A., Adams, P. L., Abada, P. B., Pesce, C. E., Safaei, R., et al. (2011). Copper transporter 2 regulates endocytosis and controls tumor growth and sensitivity to cisplatin in vivo. Mol. Pharmacol. 79 (1), 157–166. doi:10.1124/mol.110.068411
Burcher, K. M., Faucheux, A. T., Lantz, J. W., Wilson, H. L., Abreu, A., Salafian, K., et al. (2021). Prevalence of DNA repair gene mutations in blood and tumor tissue and impact on prognosis and treatment in HNSCC. Cancers (Basel) 13 (13), 3118. doi:10.3390/cancers13133118
Cabanos, H. F., and Hata, A. N. (2021). Emerging insights into targeted therapy-tolerant persister cells in cancer. Cancers (Basel) 13 (11), 2666. doi:10.3390/cancers13112666
Candelaria, P. V., Leoh, L. S., Penichet, M. L., and Daniels-Wells, T. R. (2021). Antibodies targeting the transferrin receptor 1 (TfR1) as direct anti-cancer agents. Front. Immunol. 12, 607692. doi:10.3389/fimmu.2021.607692
Chinn, S. B., and Myers, J. N. (2015). Oral cavity carcinoma: current management, controversies, and future directions. J. Clin. Oncol. 33 (29), 3269–3276. doi:10.1200/JCO.2015.61.2929
Chiu, T. J., Chen, C. H., Chien, C. Y., Li, S. H., Tsai, H. T., and Chen, Y. J. (2011). High ERCC1 expression predicts cisplatin-based chemotherapy resistance and poor outcome in unresectable squamous cell carcinoma of head and neck in a betel-chewing area. J. Transl. Med. 9, 31. doi:10.1186/1479-5876-9-31
Cui, Z., Sun, H., Gao, Z., Li, C., Xiao, T., Bian, Y., et al. (2024). TRIM21/USP15 balances ACSL4 stability and the imatinib resistance of gastrointestinal stromal tumors. Br. J. Cancer 130 (4), 526–541. doi:10.1038/s41416-023-02562-x
Dasari, S., and Tchounwou, P. B. (2014). Cisplatin in cancer therapy: molecular mechanisms of action. Eur. J. Pharmacol. 740, 364–378. doi:10.1016/j.ejphar.2014.07.025
Das, S., Dewanjee, S., Dua, T. K., Joardar, S., Chakraborty, P., Bhowmick, S., et al. (2019). Carnosic acid attenuates cadmium induced nephrotoxicity by inhibiting oxidative stress, promoting Nrf2/HO-1 signalling and impairing TGF-β1/smad/collagen IV signalling. Molecules 24 (22), 4176. doi:10.3390/molecules24224176
D Herde, K., and Krysko, D. V. (2017). Ferroptosis: oxidized PEs trigger death. Nat. Chem. Biol. 13 (1), 4–5. doi:10.1038/nchembio.2261
Dixon Scott, J., Lemberg Kathryn, M., Lamprecht, MICHAEL R., Skouta, R., Zaitsev, E. M., Gleason, C. E., et al. (2012). Ferroptosis: an iron-dependent form of nonapoptotic cell death. Cell. 149 (5), 1060–1072. doi:10.1016/j.cell.2012.03.042
Doll, S., Freitas, F. P., Shah, R., Aldrovandi, M., da Silva, M. C., Ingold, I., et al. (2019). FSP1 is a glutathione-independent ferroptosis suppressor. Nature 575 (7784), 693–698. doi:10.1038/s41586-019-1707-0
Doll, S., Proneth, B., Tyurina, Y. Y., Panzilius, E., Kobayashi, S., Ingold, I., et al. (2017). ACSL4 dictates ferroptosis sensitivity by shaping cellular lipid composition. Nat. Chem. Biol. 13 (1), 91–98. doi:10.1038/nchembio.2239
Dongre, A., and Weinberg, R. A. (2019). New insights into the mechanisms of epithelial-mesenchymal transition and implications for cancer. Nat. Rev. Mol. Cell. Biol. 20 (2), 69–84. doi:10.1038/s41580-018-0080-4
Fahrer, J., Wittmann, S., Wolf, A. C., and Kostka, T. (2023). Heme oxygenase-1 and its role in colorectal cancer. Antioxidants (Basel) 12 (11), 1989. doi:10.3390/antiox12111989
Fan, Z., Wirth, A. K., Chen, D., Wruck, C. J., Rauh, M., Buchfelder, M., et al. (2017). Nrf2-Keap1 pathway promotes cell proliferation and diminishes ferroptosis. Oncogenesis 6 (8), e371. doi:10.1038/oncsis.2017.65
Feng, S., Rao, Z., Zhang, J., She, X., Chen, Y., Wan, K., et al. (2023). Inhibition of CARM1-mediated methylation of ACSL4 promotes ferroptosis in colorectal cancer. Adv. Sci. (Weinh) 10, e2303484. doi:10.1002/advs.202303484
Fletcher, J. I., Williams, R. T., Henderson, M. J., Norris, M. D., and Haber, M. (2016). ABC transporters as mediators of drug resistance and contributors to cancer cell biology. Drug Resist Updat 26, 1–9. doi:10.1016/j.drup.2016.03.001
Friedmann, ANGELI J. P., Krysko, D. V., and Conrad, M. (2019). Ferroptosis at the crossroads of cancer-acquired drug resistance and immune evasion. Nat. Rev. Cancer 19 (7), 405–414. doi:10.1038/s41568-019-0149-1
Galaris, D., Barbouti, A., and Pantopoulos, K. (2019). Iron homeostasis and oxidative stress: an intimate relationship. Biochimica Biophysica Acta (BBA) - Mol. Cell. Res. 1866 (12), 118535. doi:10.1016/j.bbamcr.2019.118535
Galluzzi, L., Senovilla, L., Vitale, I., Michels, J., Martins, I., Kepp, O., et al. (2012). Molecular mechanisms of cisplatin resistance. Oncogene 31 (15), 1869–1883. doi:10.1038/onc.2011.384
Gao, F., Han, J., Wang, Y., Jia, L., Luo, W., and Zeng, Y. (2022). Circ_0109291 promotes cisplatin resistance of oral squamous cell carcinoma by sponging miR-188-3p to increase ABCB1 expression. Cancer Biother Radiopharm. 37 (4), 233–245. doi:10.1089/cbr.2020.3928
Gascon, S., Murenu, E., Masserdotti, G., Ortega, F., Russo, G. L., Petrik, D., et al. (2016). Identification and successful negotiation of a metabolic checkpoint in direct neuronal reprogramming. Cell. Stem Cell. 18 (3), 396–409. doi:10.1016/j.stem.2015.12.003
Ghosh, S. (2019). Cisplatin: the first metal based anticancer drug. Bioorg Chem. 88, 102925. doi:10.1016/j.bioorg.2019.102925
Guo, L., Hu, C., Yao, M., and Han, G. (2023). Mechanism of sorafenib resistance associated with ferroptosis in HCC. Front. Pharmacol. 14, 1207496. doi:10.3389/fphar.2023.1207496
Hamaï, A., CañEQUE, T., MüLLER, S., Mai, T. T., Hienzsch, A., Ginestier, C., et al. (2017). An iron hand over cancer stem cells. Autophagy 13 (8), 1465–1466. doi:10.1080/15548627.2017.1327104
Hangauer, M. J., Viswanathan, V. S., Ryan, M. J., Bole, D., Eaton, J. K., Matov, A., et al. (2017). Drug-tolerant persister cancer cells are vulnerable to GPX4 inhibition. Nature 551 (7679), 247–250. doi:10.1038/nature24297
Han, L., Li, L., and Wu, G. (2022). Induction of ferroptosis by carnosic acid-mediated inactivation of Nrf2/HO-1 potentiates cisplatin responsiveness in OSCC cells. Mol. Cell. Probes 64, 101821. doi:10.1016/j.mcp.2022.101821
Han, X., Duan, X., Liu, Z., Long, Y., Liu, C., Zhou, J., et al. (2021). ZEB1 directly inhibits GPX4 transcription contributing to ROS accumulation in breast cancer cells. Breast Cancer Res. Treat. 188 (2), 329–342. doi:10.1007/s10549-021-06301-9
Hassannia, B., Vandenabeele, P., and Vanden Berghe, T. (2019). Targeting ferroptosis to iron out cancer. Cancer Cell. 35 (6), 830–849. doi:10.1016/j.ccell.2019.04.002
Hsu, T. N., Huang, C. M., Huang, C. S., Yeh, C. T., Chao, T. Y., et al. (2019). Targeting FAT1 inhibits carcinogenesis, induces oxidative stress and enhances cisplatin sensitivity through deregulation of LRP5/WNT2/GSS signaling Axis in oral squamous cell carcinoma. Cancers (Basel) 11 (12), 1883. doi:10.3390/cancers11121883
Jang, T. H., Huang, W. C., Tung, S. L., Lin, S. C., Chen, P. M., Cho, C. Y., et al. (2022). MicroRNA-485-5p targets keratin 17 to regulate oral cancer stemness and chemoresistance via the integrin/FAK/Src/ERK/β-catenin pathway. J. Biomed. Sci. 29 (1), 42. doi:10.1186/s12929-022-00824-z
Jentsch, T. J., Lutter, D., Planells-Cases, R., Ullrich, F., and Voss, F. K. (2016). VRAC: molecular identification as LRRC8 heteromers with differential functions. Pflugers Arch. 468 (3), 385–393. doi:10.1007/s00424-015-1766-5
Jiang, L., Hickman, J. H., Wang, S. J., and Gu, W. (2015a). Dynamic roles of p53-mediated metabolic activities in ROS-induced stress responses. Cell. Cycle 14 (18), 2881–2885. doi:10.1080/15384101.2015.1068479
Jiang, L., Kon, N., Li, T., Wang, S. J., Su, T., Hibshoosh, H., et al. (2015b). Ferroptosis as a p53-mediated activity during tumour suppression. Nature 520 (7545), 57–62. doi:10.1038/nature14344
Joshi, S., Pandey, R., Kumar, A., Gupta, V., and Arya, N. (2023). Targeted blockade of interleukin-8 negates metastasis and chemoresistance via Akt/Erk-NFκB axis in oral cancer. Cytokine 166, 156155. doi:10.1016/j.cyto.2023.156155
Ju, X., Yu, H., Liang, D., Jiang, T., Liu, Y., Chen, L., et al. (2018). LDR reverses DDP resistance in ovarian cancer cells by affecting ERCC-1, Bcl-2, Survivin and Caspase-3 expressions. Biomed. Pharmacother. 102, 549–554. doi:10.1016/j.biopha.2018.03.092
Kagan, V. E., Mao, G., Qu, F., Angeli, J. P. F., Doll, S., Croix, C. S., et al. (2017). Oxidized arachidonic and adrenic PEs navigate cells to ferroptosis. Nat. Chem. Biol. 13 (1), 81–90. doi:10.1038/nchembio.2238
Kaloni, D., Diepstraten, S. T., Strasser, A., and Kelly, G. L. (2023). BCL-2 protein family: attractive targets for cancer therapy. Apoptosis 28 (1-2), 20–38. doi:10.1007/s10495-022-01780-7
Kang, S. H., Oh, S. Y., Lee, K. Y., Kim, M. S., Kwon, T. G., et al. (2024). Differential effect of cancer-associated fibroblast-derived extracellular vesicles on cisplatin resistance in oral squamous cell carcinoma via miR-876-3p. Theranostics 14 (2), 460–479. doi:10.7150/thno.87329
Kawak, P., Sawaftah, N. M. A., Pitt, W. G., and Husseini, G. A. (2023). Transferrin-targeted liposomes in glioblastoma therapy: a review. Int. J. Mol. Sci. 24 (17), 13262. doi:10.3390/ijms241713262
Kennedy, L., Sandhu, J. K., Harper, M.-E., and Cuperlovic-Culf, M. (2020). Role of glutathione in cancer: from mechanisms to therapies. Biomolecules 10 (10), 1429. doi:10.3390/biom10101429
Kim, E. H., Jang, H., Shin, D., Baek, S. H., and Roh, J. L. (2016b). Targeting Nrf2 with wogonin overcomes cisplatin resistance in head and neck cancer. Apoptosis 21 (11), 1265–1278. doi:10.1007/s10495-016-1284-8
Kim, J. W., Park, Y., Roh, J. L., Cho, K. J., Choi, S. H., Nam, S. Y., et al. (2016a). Prognostic value of glucosylceramide synthase and P-glycoprotein expression in oral cavity cancer. Int. J. Clin. Oncol. 21 (5), 883–889. doi:10.1007/s10147-016-0973-1
Kondapuram, S. K., Ramachandran, H. K., Arya, H., and Coumar, M. S. (2023). Targeting survivin for cancer therapy: strategies, small molecule inhibitors and vaccine based therapeutics in development. Life Sci. 335, 122260. doi:10.1016/j.lfs.2023.122260
Koppula, P., Zhuang, L., and Gan, B. (2020). Cystine transporter SLC7A11/xCT in cancer: ferroptosis, nutrient dependency, and cancer therapy. Protein and Cell. 12 (8), 599–620. doi:10.1007/s13238-020-00789-5
Korytina, G. F., Akhmadishina, L. Z., Aznabaeva, Y. G., Kochetova, O. V., Zagidullin, N. S., Kzhyshkowska, J. G., et al. (2019). Associations of the NRF2/KEAP1 pathway and antioxidant defense gene polymorphisms with chronic obstructive pulmonary disease. Gene 692, 102–112. doi:10.1016/j.gene.2018.12.061
Krebs, A. M., Mitschke, J., Lasierra Losada, M., Schmalhofer, O., Boerries, M., Busch, H., et al. (2017). The EMT-activator Zeb1 is a key factor for cell plasticity and promotes metastasis in pancreatic cancer. Nat. Cell. Biol. 19 (5), 518–529. doi:10.1038/ncb3513
Kuo, C. L., Ponneri Babuharisankar, A., Lin, Y. C., Lien, H. W., Lo, Y. K., Chou, H. Y., et al. (2022). Mitochondrial oxidative stress in the tumor microenvironment and cancer immunoescape: foe or friend? J. Biomed. Sci. 29 (1), 74. doi:10.1186/s12929-022-00859-2
Lei, M., Zheng, G., Ning, Q., Zheng, J., and Dong, D. (2020). Translation and functional roles of circular RNAs in human cancer. Mol. Cancer 19 (1), 30. doi:10.1186/s12943-020-1135-7
Li, X. H., Li, D., Liu, C., Zhang, M. M., Guan, X. J., and Fu, Y. P. (2020). p33ING1b regulates acetylation of p53 in oral squamous cell carcinoma via SIR2. Cancer Cell. Int. 20, 398. doi:10.1186/s12935-020-01489-0
Liang, L., Liu, Y., Wu, X., and Chen, Y. (2023). Artesunate induces ferroptosis by inhibiting the nuclear localization of SREBP2 in myeloma cells. Int. J. Med. Sci. 20 (12), 1535–1550. doi:10.7150/ijms.86409
Lin, L. S., Song, J., Song, L., Ke, K., Liu, Y., Zhou, Z., et al. (2018). Simultaneous fenton-like ion delivery and glutathione depletion by MnO(2) -based nanoagent to enhance chemodynamic therapy. Angew. Chem. Int. Ed. Engl. 57 (18), 4902–4906. doi:10.1002/anie.201712027
Lin, X. J., He, C. L., Sun, T., Duan, X. J., Sun, Y., and Xiong, S. J. (2017). hsa-miR-485-5p reverses epithelial to mesenchymal transition and promotes cisplatin-induced cell death by targeting PAK1 in oral tongue squamous cell carcinoma. Int. J. Mol. Med. 40 (1), 83–89. doi:10.3892/ijmm.2017.2992
Long, J., Wang, W., Chu, J., Li, Y., Wang, M., Su, J., et al. (2024). Overexpression of Nrf2 reverses ferroptosis induced by Arenobufagin in gastric cancer. Toxicol. Appl. Pharmacol. 484, 116842. doi:10.1016/j.taap.2024.116842
Louandre, C., Ezzoukhry, Z., Godin, C., Barbare, J. C., Mazière, J. C., Chauffert, B., et al. (2013). Iron-dependent cell death of hepatocellular carcinoma cells exposed to sorafenib. Int. J. Cancer 133 (7), 1732–1742. doi:10.1002/ijc.28159
Luo, Q., Hu, S., Tang, Y., Yang, D., and Chen, Q. (2024). PPT1 promotes growth and inhibits ferroptosis of oral squamous cell carcinoma cells. Curr. Cancer Drug Targets 24. doi:10.2174/0115680096294098240123104657
Ma, M. Z., Chen, G., Wang, P., Lu, W. H., Zhu, C. F., Song, M., et al. (2015). Xc-inhibitor sulfasalazine sensitizes colorectal cancer to cisplatin by a GSH-dependent mechanism. Cancer Lett. 368 (1), 88–96. doi:10.1016/j.canlet.2015.07.031
Majdalawieh, A. F., Massri, M., and Ro, H. S. (2020). AEBP1 is a novel oncogene: mechanisms of action and signaling pathways. J. Oncol. 2020, 8097872. doi:10.1155/2020/8097872
Ma, L., Wang, H., Wang, C., Su, J., Xie, Q., Xu, L., et al. (2016). Failure of elevating calcium induces oxidative stress tolerance and imparts cisplatin resistance in ovarian cancer cells. Aging Dis. 7 (3), 254–266. doi:10.14336/AD.2016.0118
Mancias, J. D., Wang, X., Gygi, S. P., Harper, J. W., and Kimmelman, A. C. (2014). Quantitative proteomics identifies NCOA4 as the cargo receptor mediating ferritinophagy. Nature 509 (7498), 105–109. doi:10.1038/nature13148
Manoharan, S., Vasanthaselvan, M., Silvan, S., Baskaran, N., Kumar Singh, A., and Vinoth Kumar, V. (2010). Carnosic acid: a potent chemopreventive agent against oral carcinogenesis. Chemico-Biological Interact. 188 (3), 616–622. doi:10.1016/j.cbi.2010.08.009
Mao, C., Liu, X., Zhang, Y., Lei, G., Yan, Y., Lee, H., et al. (2021). DHODH-mediated ferroptosis defence is a targetable vulnerability in cancer. Nature 593 (7860), 586–590. doi:10.1038/s41586-021-03539-7
Marine, J. C., Dawson, S. J., and Dawson, M. A. (2020). Non-genetic mechanisms of therapeutic resistance in cancer. Nat. Rev. Cancer 20 (12), 743–756. doi:10.1038/s41568-020-00302-4
Marquardt, S., Solanki, M., Spitschak, A., Vera, J., and Pützer, B. M. (2018). Emerging functional markers for cancer stem cell-based therapies: understanding signaling networks for targeting metastasis. Seminars Cancer Biol. 53, 90–109. doi:10.1016/j.semcancer.2018.06.006
Marteijn, J. A., Lans, H., Vermeulen, W., and Hoeijmakers, J. H. (2014). Understanding nucleotide excision repair and its roles in cancer and ageing. Nat. Rev. Mol. Cell. Biol. 15 (7), 465–481. doi:10.1038/nrm3822
Ma, Y., Gao, J., and Guo, H. (2023). Circ_0000140 alters miR-527/slc7a11-mediated ferroptosis to influence oral squamous cell carcinoma cell resistance to DDP. Pharmgenomics Pers. Med. 16, 1079–1089. doi:10.2147/PGPM.S426205
Meng, X., Lou, Q. Y., Yang, W. Y., Wang, Y. R., Chen, R., Wang, L., et al. (2021). The role of non-coding RNAs in drug resistance of oral squamous cell carcinoma and therapeutic potential. Cancer Commun. (Lond) 41 (10), 981–1006. doi:10.1002/cac2.12194
Mou, Y., Wang, J., Wu, J., He, D., Zhang, C., Duan, C., et al. (2019). Ferroptosis, a new form of cell death: opportunities and challenges in cancer. J. Hematol. Oncol. 12 (1), 34. doi:10.1186/s13045-019-0720-y
Mu, Q., Chen, L., Gao, X., Shen, S., Sheng, W., Min, J., et al. (2021). The role of iron homeostasis in remodeling immune function and regulating inflammatory disease. Sci. Bull. 66 (17), 1806–1816. doi:10.1016/j.scib.2021.02.010
Murai, J., and Pommier, Y. (2023). BRCAness, homologous recombination deficiencies, and synthetic lethality. Cancer Res. 83 (8), 1173–1174. doi:10.1158/0008-5472.CAN-23-0628
Nagao, T., and Warnakulasuriya, S. (2020). Screening for oral cancer: future prospects, research and policy development for Asia. Oral Oncol., 105. doi:10.1016/j.oraloncology.2020.104632
Niu, X., Yang, B., Liu, F., and Fang, Q. (2020). LncRNA HOXA11-AS promotes OSCC progression by sponging miR-98-5p to upregulate YBX2 expression. Biomed. Pharmacother. 121, 109623. doi:10.1016/j.biopha.2019.109623
Noman, A. S. M., Parag, R. R., Rashid, M. I., Islam, S., Rahman, M. Z., Chowdhury, A. A., et al. (2020). Chemotherapeutic resistance of head and neck squamous cell carcinoma is mediated by EpCAM induction driven by IL-6/p62 associated Nrf2-antioxidant pathway activation. Cell. Death Dis. 11 (8), 663. doi:10.1038/s41419-020-02907-x
Okamoto, H., Yoshikawa, K., Shimada, A., Sano, R., Inukai, D., Yamanaka, S., et al. (2023). Artesunate and cisplatin synergistically inhibit HNSCC cell growth and promote apoptosis with artesunate-induced decreases in Rb and phosphorylated Rb levels. Oncol. Rep. 50 (2), 154. doi:10.3892/or.2023.8591
Ou, Y., Wang, S. J., Li, D., Chu, B., and Gu, W. (2016). Activation of SAT1 engages polyamine metabolism with p53-mediated ferroptotic responses. Proc. Natl. Acad. Sci. U. S. A. 113 (44), E6806-E6812–E12. doi:10.1073/pnas.1607152113
Paramasivam, A. (2022). Circulating circular RNAs: novel potential biomarkers and therapeutic targets for oral cancer. Oral Oncol. 134, 106067. doi:10.1016/j.oraloncology.2022.106067
Pastushenko, I., Brisebarre, A., Sifrim, A., Fioramonti, M., Revenco, T., Boumahdi, S., et al. (2018). Identification of the tumour transition states occurring during EMT. Nature 556 (7702), 463–468. doi:10.1038/s41586-018-0040-3
Praharaj, P. P., Singh, A., Patra, S., and Bhutia, S. K. (2023). Co-targeting autophagy and NRF2 signaling triggers mitochondrial superoxide to sensitize oral cancer stem cells for cisplatin-induced apoptosis. Free Radic. Biol. Med. 207, 72–88. doi:10.1016/j.freeradbiomed.2023.07.008
Qiao, X., Liu, J., Zhu, L., Song, R., Zhong, M., and Guo, Y. (2021). Long noncoding RNA CEBPA-DT promotes cisplatin chemo-resistance through CEBPA/BCL2 mediated apoptosis in oral squamous cellular cancer. Int. J. Med. Sci. 18 (16), 3728–3737. doi:10.7150/ijms.64253
Qiu, F., Qiao, B., Zhang, N., Fang, Z., Feng, L., Zhang, S., et al. (2021). Blocking circ-SCMH1 (hsa_circ_0011946) suppresses acquired DDP resistance of oral squamous cell carcinoma (OSCC) cells both in vitro and in vivo by sponging miR-338-3p and regulating LIN28B. Cancer Cell. Int. 21 (1), 412. doi:10.1186/s12935-021-02110-8
Roh, J.-L., Kim, E. H., Jang, H. J., Park, J. Y., and Shin, D. (2016). Induction of ferroptotic cell death for overcoming cisplatin resistance of head and neck cancer. Cancer Lett. 381 (1), 96–103. doi:10.1016/j.canlet.2016.07.035
Roh, J. L., Kim, E. H., Jang, H., and Shin, D. (2017). Nrf2 inhibition reverses the resistance of cisplatin-resistant head and neck cancer cells to artesunate-induced ferroptosis. Redox Biol. 11, 254–262. doi:10.1016/j.redox.2016.12.010
Sabatella, M., Thijssen, K. L., Davo-Martinez, C., Vermeulen, W., and Lans, H. (2021). Tissue-specific DNA repair activity of ERCC-1/XPF-1. Cell. Rep. 34 (2), 108608. doi:10.1016/j.celrep.2020.108608
Schonberg, DAVID L., Miller Tyler, E., Wu, Q., Flavahan, W. A., Das, N. K., Hale, J. S., et al. (2015). Preferential iron trafficking characterizes glioblastoma stem-like cells. Cancer Cell. 28 (4), 441–455. doi:10.1016/j.ccell.2015.09.002
Sendamarai, A. K., Ohgami, R. S., Fleming, M. D., and Lawrence, C. M. (2008). Structure of the membrane proximal oxidoreductase domain of human Steap3, the dominant ferrireductase of the erythroid transferrin cycle. Proc. Natl. Acad. Sci. U. S. A. 105 (21), 7410–7415. doi:10.1073/pnas.0801318105
Sha, J., Bai, Y., Ngo, H. X., Okui, T., and Kanno, T. (2021). Overview of evidence-based chemotherapy for oral cancer: focus on drug resistance related to the epithelial-mesenchymal transition. Biomolecules 11 (6), 893. doi:10.3390/biom11060893
Shan, G., Bi, G., Zhao, G., Liang, J., Bian, Y., Zhang, H., et al. (2023). Inhibition of PKA/CREB1 pathway confers sensitivity to ferroptosis in non-small cell lung cancer. Respir. Res. 24 (1), 277. doi:10.1186/s12931-023-02567-3
Shen, S., Vagner, S., and Robert, C. (2020). Persistent cancer cells: the deadly survivors. Cell. 183 (4), 860–874. doi:10.1016/j.cell.2020.10.027
Shibue, T., and Weinberg, R. A. (2017). EMT, CSCs, and drug resistance: the mechanistic link and clinical implications. Nat. Rev. Clin. Oncol. 14 (10), 611–629. doi:10.1038/nrclinonc.2017.44
Shin, D., Kim, E. H., Lee, J., and Roh, J. L. (2018). Nrf2 inhibition reverses resistance to GPX4 inhibitor-induced ferroptosis in head and neck cancer. Free Radic. Biol. Med. 129, 454–462. doi:10.1016/j.freeradbiomed.2018.10.426
Siemer, S., Fauth, T., Scholz, P., Al-Zamel, Y., Khamis, A., Gül, D., et al. (2021). Profiling cisplatin resistance in head and neck cancer: a critical role of the VRAC ion channel for chemoresistance. Cancers (Basel) 13 (19), 4831. doi:10.3390/cancers13194831
Siu, K. T., Huang, C., Panaroni, C., Mukaihara, K., Fulzele, K., Soucy, R., et al. (2019). BCL2 blockade overcomes MCL1 resistance in multiple myeloma. Leukemia 33 (8), 2098–2102. doi:10.1038/s41375-019-0421-0
Song, Q., Peng, S., Che, F., and Zhu, X. (2022). Artesunate induces ferroptosis via modulation of p38 and ERK signaling pathway in glioblastoma cells. J. Pharmacol. Sci. 148 (3), 300–306. doi:10.1016/j.jphs.2022.01.007
Song, Y., Yang, H., Lin, R., Jiang, K., and Wang, B. M. (2019). The role of ferroptosis in digestive system cancer. Oncol. Lett. 18 (3), 2159–2164. doi:10.3892/ol.2019.10568
Sung, H., Ferlay, J., Siegel, R. L., Laversanne, M., Soerjomataram, I., Jemal, A., et al. (2021). Global cancer statistics 2020: GLOBOCAN estimates of incidence and mortality worldwide for 36 cancers in 185 countries. CA A Cancer J. Clin. 71 (3), 209–249. doi:10.3322/caac.21660
Sun, X., Ou, Z., Xie, M., Kang, R., Fan, Y., Niu, X., et al. (2015). HSPB1 as a novel regulator of ferroptotic cancer cell death. Oncogene 34 (45), 5617–5625. doi:10.1038/onc.2015.32
Sun, Y., Berleth, N., Wu, W., Schlütermann, D., Deitersen, J., Stuhldreier, F., et al. (2021). Fin56-induced ferroptosis is supported by autophagy-mediated GPX4 degradation and functions synergistically with mTOR inhibition to kill bladder cancer cells. Cell. Death Dis. 12 (11), 1028. doi:10.1038/s41419-021-04306-2
Tan, Y., Wang, Z., Xu, M., Li, B., Huang, Z., Qin, S., et al. (2023). Oral squamous cell carcinomas: state of the field and emerging directions. Int. J. Oral Sci. 15 (1), 44. doi:10.1038/s41368-023-00249-w
Thomson, D. W., and Dinger, M. E. (2016). Endogenous microRNA sponges: evidence and controversy. Nat. Rev. Genet. 17 (5), 272–283. doi:10.1038/nrg.2016.20
Ubellacker, J. M., Tasdogan, A., Ramesh, V., Shen, B., Mitchell, E. C., Martin-Sandoval, M. S., et al. (2020). Lymph protects metastasizing melanoma cells from ferroptosis. Nature 585 (7823), 113–118. doi:10.1038/s41586-020-2623-z
Viswanathan, V. S., Ryan, M. J., Dhruv, H. D., Gill, S., Eichhoff, O. M., Seashore-Ludlow, B., et al. (2017). Dependency of a therapy-resistant state of cancer cells on a lipid peroxidase pathway. Nature 547 (7664), 453–457. doi:10.1038/nature23007
Walcher, L., Kistenmacher, A.-K., Suo, H., Kitte, R., Dluczek, S., Strauß, A., et al. (2020). Cancer stem cells—origins and biomarkers: perspectives for targeted personalized therapies. Front. Immunol. 11, 1280. doi:10.3389/fimmu.2020.01280
Wang, G., Wang, J. J., Zhi-Min, Z., Xu, X. N., Shi, F., and Fu, X. L. (2023). Targeting critical pathways in ferroptosis and enhancing antitumor therapy of Platinum drugs for colorectal cancer. Sci. Prog. 106 (1), 368504221147173. doi:10.1177/00368504221147173
Wang, M., Hu, S., Yang, J., Yuan, L., Han, L., Liang, F., et al. (2024). Arenobufagin inhibits lung metastasis of colorectal cancer by targeting c-MYC/Nrf2 axis. Phytomedicine 127, 155391. doi:10.1016/j.phymed.2024.155391
Wangmo, C., Charoen, N., Jantharapattana, K., Dechaphunkul, A., and Thongsuksai, P. (2020). Epithelial-mesenchymal transition predicts survival in oral squamous cell carcinoma. Pathol. Oncol. Res. 26 (3), 1511–1518. doi:10.1007/s12253-019-00731-z
Wang, S., Li, M. Y., Liu, Y., Vlantis, A. C., Chan, J. Y., Xue, L., et al. (2020). The role of microRNA in cisplatin resistance or sensitivity. Expert Opin. Ther. Targets 24 (9), 885–897. doi:10.1080/14728222.2020.1785431
Wang, X., Li, H., and Shi, J. (2019). LncRNA HOXA11-AS promotes proliferation and cisplatin resistance of oral squamous cell carcinoma by suppression of miR-214-3p expression. Biomed. Res. Int. 2019, 8645153. doi:10.1155/2019/8645153
Wang, Y., Chen, P., Chen, X., Gong, D., Wu, Y., Huang, L., et al. (2022). ROS-induced DCTPP1 upregulation contributes to cisplatin resistance in ovarian cancer. Front. Mol. Biosci. 9, 838006. doi:10.3389/fmolb.2022.838006
Wolff, N. A., Garrick, M. D., Zhao, L., Garrick, L. M., Ghio, A. J., and Thévenod, F. (2018). A role for divalent metal transporter (DMT1) in mitochondrial uptake of iron and manganese. Sci. Rep. 8 (1), 211. doi:10.1038/s41598-017-18584-4
Wu, Y. C., Huang, C. S., Hsieh, M. S., Setiawan, S. A., Yeh, C. T., et al. (2024). Targeting of FSP1 regulates iron homeostasis in drug-tolerant persister head and neck cancer cells via lipid-metabolism-driven ferroptosis. Aging (Albany NY) 16 (1), 627–647. doi:10.18632/aging.205409
Wu, H., He, H., Huang, J., Wang, C., Dong, Y., Lin, R., et al. (2022). Identification and validation of transferrin receptor protein 1 for predicting prognosis and immune infiltration in lower grade glioma. Front. Mol. Neurosci. 15, 972308. doi:10.3389/fnmol.2022.972308
Wu, S., Lv, X., Wei, H., Chen, W., Zheng, J., Li, X., et al. (2023b). Circ-ILF2 in oral squamous cell carcinoma promotes cisplatin resistance and induces M2 polarization of macrophages. J. Cell. Mol. Med. 27 (24), 4133–4144. doi:10.1111/jcmm.17998
Wu, Y., Franzmeier, S., Liesche-Starnecker, F., and Schlegel, J. (2023a). Enhanced sensitivity to aldh1a3-dependent ferroptosis in TMZ-resistant glioblastoma cells. Cells 12 (21), 2522. doi:10.3390/cells12212522
Xiong, L., Tang, Y., Liu, Z., Dai, J., and Wang, X. (2016). BCL-2 inhibition impairs mitochondrial function and targets oral tongue squamous cell carcinoma. SpringerPlus 5 (1), 1626. doi:10.1186/s40064-016-3310-2
Yang, W. S., Kim, K. J., Gaschler, M. M., Patel, M., Shchepinov, M. S., and Stockwell, B. R. (2016). Peroxidation of polyunsaturated fatty acids by lipoxygenases drives ferroptosis. Proc. Natl. Acad. Sci. U. S. A. 113 (34), E4966–E4975. doi:10.1073/pnas.1603244113
Yang, W. S., Sriramaratnam, R., Welsch, M. E., Shimada, K., Skouta, R., Viswanathan, V. S., et al. (2014). Regulation of ferroptotic cancer cell death by GPX4. Cell. 156 (1-2), 317–331. doi:10.1016/j.cell.2013.12.010
Yang, J., Antin, P., Berx, G., Blanpain, C., Brabletz, T., Bronner, M., et al. (2020). Guidelines and definitions for research on epithelial-mesenchymal transition. Nat. Rev. Mol. Cell. Biol. 21 (6), 341–352. doi:10.1038/s41580-020-0237-9
Yu, Z. W., Zhong, L. P., Ji, T., Zhang, P., Chen, W. t., and Zhang, C. p. (2010). MicroRNAs contribute to the chemoresistance of cisplatin in tongue squamous cell carcinoma lines. Oral Oncol. 46 (4), 317–322. doi:10.1016/j.oraloncology.2010.02.002
Yu, C., Sun, P., Zhou, Y., et al. (2019). Inhibition of AKT enhances the anti-cancer effects of Artemisinin in clear cell renal cell carcinoma. Biomed. Pharmacother., 118. doi:10.1016/j.biopha.2019.109383
Yu, W., Chen, Y., Putluri, N., Coarfa, C., Robertson, M. J., Putluri, V., et al. (2020). Acquisition of cisplatin resistance shifts head and neck squamous cell carcinoma metabolism toward neutralization of oxidative stress. Cancers (Basel) 12 (6), 1670. doi:10.3390/cancers12061670
Zeng, H., Zhao, X., and Tang, C. (2021). Downregulation of SELENBP1 enhances oral squamous cell carcinoma chemoresistance through KEAP1-NRF2 signaling. Cancer Chemother. Pharmacol. 88 (2), 223–233. doi:10.1007/s00280-021-04284-4
Zhang, C., Liu, X., Jin, S., Chen, Y., and Guo, R. (2022). Ferroptosis in cancer therapy: a novel approach to reversing drug resistance. Mol. Cancer 21 (1), 47. doi:10.1186/s12943-022-01530-y
Zhang, H., Chen, N., Ding, C., Liu, D., and Liu, S. (2024). Ferroptosis and EMT resistance in cancer: a comprehensive review of the interplay. Front. Oncol. 14, 1344290. doi:10.3389/fonc.2024.1344290
Zhang, X., Qi, Z., Yin, H., and Yang, G. (2019). Interaction between p53 and Ras signaling controls cisplatin resistance via HDAC4- and HIF-1α-mediated regulation of apoptosis and autophagy. Theranostics 9 (4), 1096–1114. doi:10.7150/thno.29673
Zhang, Y., Shen, G., Meng, T., Lv, Z., Li, X., Li, J., et al. (2023). Eicosapentaenoic acid enhances the sensitivity of osteosarcoma to cisplatin by inducing ferroptosis through the DNA-PKcs/AKT/NRF2 pathway and reducing PD-L1 expression to attenuate immune evasion. Int. Immunopharmacol. 125 (Pt B), 111181. doi:10.1016/j.intimp.2023.111181
Zhou, Q., Wang, X., Zhang, Y., Wang, L., and Chen, Z. (2022). Inhibition of AEBP1 predisposes cisplatin-resistant oral cancer cells to ferroptosis. BMC Oral Health 22 (1), 478. doi:10.1186/s12903-022-02503-9
Zhou, Z., Han, S., Liao, J., Wang, R., Yu, X., and Li, M. (2023). Isoliquiritigenin inhibits oral squamous cell carcinoma and overcomes chemoresistance by destruction of survivin. Am. J. Chin. Med. 51 (8), 2221–2241. doi:10.1142/S0192415X23500957
Zhu, S., Zhang, Q., Sun, X., Zeh, H. J., Lotze, M. T., Kang, R., et al. (2017). HSPA5 regulates ferroptotic cell death in cancer cells. Cancer Res. 77 (8), 2064–2077. doi:10.1158/0008-5472.CAN-16-1979
Keywords: ferroptosis, OSCC, HNSCC, cisplatin, chemoresistance, chemotherapy
Citation: Chen R, Zhu S, Zhao R, Liu W, Jin L, Ren X and He H (2024) Targeting ferroptosis as a potential strategy to overcome the resistance of cisplatin in oral squamous cell carcinoma. Front. Pharmacol. 15:1402514. doi: 10.3389/fphar.2024.1402514
Received: 17 March 2024; Accepted: 29 March 2024;
Published: 22 April 2024.
Edited by:
Qingbin Cui, University of Toledo College of Medicine and Life Sciences, United StatesReviewed by:
Xiaolin Qian, Southern Research Institute, United StatesYang Tian, University of Arkansas, United States
Yanning Hao, United States Food and Drug Administration, United States
Copyright © 2024 Chen, Zhu, Zhao, Liu, Jin, Ren and He. This is an open-access article distributed under the terms of the Creative Commons Attribution License (CC BY). The use, distribution or reproduction in other forums is permitted, provided the original author(s) and the copyright owner(s) are credited and that the original publication in this journal is cited, in accordance with accepted academic practice. No use, distribution or reproduction is permitted which does not comply with these terms.
*Correspondence: Xiaobin Ren, renxiaobin6688@163.com; Hongbing He, hehongbing@kmmu.edu.cn