- 1Department of Pharmacy, Kunshan Hospital of Traditional Chinese Medicine, Kunshan, China
- 2Jiangsu Biodep Biotechnology, Jiangyin, China
- 3Department of Pharmacology, School of Pharmacy, Nantong University, Nantong, China
- 4Department of Pharmacy, Changzhou Geriatric Hospital Affiliated to Soochow University, Changzhou, China
- 5Department of Gastroenterology, Henan Provincial People’s Hospital, People’s Hospital of Zhengzhou University, Zhengzhou, Henan, China
Introduction: Probiotics have been recognized for their various biological activities, including antioxidant and anti-inflammatory properties. This study investigates the therapeutic effect of a novel probiotic formula, BLLL, consisting of Bifidobacterium breve, Lactobacillus plantarum, Lactobacillus paracasei, and Lactobacillus helveticus, on chronic stress-induced depression-like behaviors in mice.
Methods: The BLLL formula or phosphate-buffered saline (PBS) was given orally at a dose of 2, 4, or 8 × 1010 CFU/kg once daily for 10 days in mice treated with chronic unpredictable stress (CUS) treated or vehicle. Depression-like behaviors were assessed using the sucrose preference test (SPT), the forced swimming test (FST), and the tail suspension test (TST). The mRNA and/or protein expression of interleukin-1β (IL-1β), IL-6, tumor necrosis factor-α (TNF-α), IL-4, IL-10, and chitinase-3-like protein 3 (CHI3L1, also known as Ym-1), as well as the concentration of nitrite, malondialdehyde (MDA), glutathione (GSH), and brain-derived neurotrophic factor (BDNF) in the hippocampus and medial prefrontal cortex were examined.
Results: The BLLL formula treatment at a dose of 8 × 1010 CFU/kg, but not at a dose of 2 or 4 × 1010 CFU/kg, improved CUS-induced depression-like behaviors in mice, as shown by the decrease in immobility time in the TST and FST and the increase in sucrose intake in the SPT. Further analysis revealed that BLLL treatment suppressed the CUS-induced increase in IL-1β, IL-6, and TNF-α mRNA and protein levels, as well as the CUS-induced decrease in IL-4, IL-10, and Ym-1 mRNA and/or protein levels in the hippocampus and medial prefrontal cortex. In addition, treatment with the BLLL formula countered the CUS-induced increase in nitrite and MDA levels and the CUS-induced decrease in GSH content and BDNF concentration in the hippocampus and medial prefrontal cortex.
Conclusion: These results demonstrate that the novel probiotic formula BLLL ameliorates chronic stress-induced depression-like behavior in mice by suppressing neuroinflammation and oxido-nitrosative stress in the brain.
Introduction
Chronic harmful stress is a widespread phenomenon in modern society. It can cause various types of mental disorders, including depression (Smail et al., 2020). In the clinic, medications developed according to the monoamine imbalance hypothesis are used to treat depression (Kraus et al., 2017). However, these medications can have numerous harmful effects, such as sleep disturbances (Crawford et al., 2014) and an increased risk of suicide (Moller et al., 2008). Notably, only a small proportion of patients receiving antidepressants experience a significant therapeutic effect (Bollini et al., 1999; Furukawa et al., 2022). Therefore, there is an urgent need to search for new drugs to treat depression triggered by chronic stress.
In recent years, the brain-gut axis has attracted much attention. The gut microbiota has been shown to control brain development, function, and behavior (Sampson and Mazmanian, 2015). Many gastrointestinal diseases are linked to the development of various mental disorders, such as anxiety and depression (Barberio et al., 2021). Further, gut microbiota dysfunction plays a significant role in the progression of mental disorders, including depression (Dinan and Cryan, 2019; Cussotto et al., 2021). Compared to healthy individuals, depression patients tend to have more pro-inflammatory bacteria and fewer anti-inflammatory bacteria in their gut microbiota (Simpson et al., 2021; Liu et al., 2022). Since a systemic inflammatory state is associated with increased gut permeability and can affect the integrity of the brain-blood barrier, the balance of the gut microbiota is closely related to neuroinflammation (Reyes-Martinez et al., 2023).
An enhanced neuroinflammatory response is a new hypothesis to explain the pathogenesis of depression (Ji et al., 2022). Previous studies have consistently observed elevated levels of pro-inflammatory cytokines such as interleukin-1β (IL-1β), IL-6, and tumor necrosis factor-α (TNF-α) in the blood of patients with depression [15–17]. Animal models of depression have confirmed that high concentrations of pro-inflammatory cytokines and low concentrations of anti-inflammatory mediators like IL-10 and IL-4 in the brain correlate with the progression of depression [18–20]. Central infusion of pro-inflammatory cytokines such as IL-1β and interferon-α (INF-α) can induce depression-like behaviors in animals (Anisman et al., 2008; Hayley et al., 2013). Clinically available antidepressants such as fluoxetine have been reported to improve depressive symptoms in part by inhibiting the production of pro-inflammatory cytokines in the brains of chronically stressed mice (Du et al., 2016) or in the blood of patients with depression (Wang et al., 2019). Mechanistic studies have shown that pro-inflammatory cytokines promote the progression of depression by increasing oxido-nitrosative stress (Sulakhiya et al., 2014; Yang et al., 2017) and impairing the expression of brain-derived neurotrophic factor (BDNF) (Calabrese et al., 2014). Therefore, regulating the gut microbiota and shifting the neuroinflammatory response to an anti-inflammatory state while also attenuating oxido-nitrosative stress could mitigate depressive symptoms triggered by harmful stress, which could be a promising strategy for the treatment of depression.
Probiotics are live, nonpathogenic microorganisms that can improve microbial balance and are widely used as dietary supplements and foods (Williams, 2010). Strategies that correct gut microbiota dysfunction with probiotics have been widely reported to alleviate depressive symptoms in animals (Park et al., 2018; Kosuge et al., 2021) and humans (Huang et al., 2016; Tian et al., 2022), which is likely achieved by targeting the nervous system via inhibiting neuroinflammation and oxido-nitrosative stress. However, there are still no clinically available probiotics for the treatment of depression, although numerous probiotics for this purpose are being developed. In the present study, we investigated the effects and mechanisms of a new formula of probiotics called the BLLL formula, consisting of Bifidobacterium breve, Lactobacillus plantarum, Lactobacillus paracasei, and Lactobacillus helveticus, provided by Biodep Biotechnology Company, on chronic stress-induced depression-like behaviors in mice. The effects of the individual components of the BLLL formula on depression have been described in prior studies. For example, Bifidobacterium has been reported to improve depression-like behaviors and microbiota dysbiosis in animals induced by chronic stress (Tian et al., 2019; Tian et al., 2020). Lactobacillus plantarum can alleviate depression-like behaviors in depressed animals by regulating the gut microbiota (Zhu R. et al., 2023) and improving the function of monoamine neurotransmitters and the CREB-BDNF signaling cascade in the hippocampus (Ma et al., 2024). Both L. paracasei (Xu et al., 2022) and extracellular vesicles released by Lactobacillus paracasei (Kwon et al., 2023) also have antidepressant-like effects in animal models of depression. The role of L. helveticus in the regulation of depression has also been described and discussed in numerous studies (Maehata et al., 2019; Partrick et al., 2021). We hypothesize that the BLLL formula can reverse depression-like behavior in chronically stressed mice by altering brain neuroinflammatory responses and oxido-nitrosative stress.
Materials and methods
Animals
Seven-week-old male C57BL/6J mice (body weight: 22–26 g) were purchased from Beijing Vital River Laboratory Animal Technology Co, Ltd (Beijing, China) and housed five per cage for 1 week before experimental procedures started. All mice were kept under conditions with a 12-h light-dark cycle (lights on from 07:00 to 19:00), 23°C ± 1°C ambient temperature, 55% ± 10% relative humidity, without any environmental enrichment, and free access to food and water. Animal experiments were approved by the Animal Ethics Committee of Nantong University (Permit Number: 2110836) and conducted according to internationally accepted guidelines for the use of animals in toxicology adopted by the Society of Toxicology in 1999.
Materials
The BLLL formulation containing B. breve (25%), L. plantarum (25%), L. paracasei (25%), and L. helveticus (25%) was provided by Biodep Biotechnology Company and stored at −20°C.
Procedure for drug treatment, behavioral tests, and biochemical measurements
Mice exposed or not to chronic unpredictable stress (CUS) were orally administered the BLLL formula at a dose of 2, 4, or 8 × 1010 CFU/kg once daily for 10 days. The control animals, exposed or not to CUS, received the same amount of phosphate-buffered saline (PBS). PBS solution is a neutral fluid with minimal chemical reactions with other substances, making it safe and effective. In addition, the concentration of PBS is similar to the cell fluid of mice; therefore, it does not lead to dehydration of the cells. To this end, PBS has been widely used in numerous previous studies as a control substance to explore the advantages of probiotics (Ducray et al., 2020; Mulaw et al., 2020; Sun et al., 2020; Xiao et al., 2022; Fitri et al., 2023). The BLLL formula was dissolved in PBS immediately before administration. Gavage tubes containing BLLL or PBS were administered 200 μL per mouse at 8 a.m. In experiments to assess depression-like behaviors, a separate cohort of stress-naïve and CUS mice was divided into eight groups administered either vehicle or 2, 4, or 8 × 1010 CFU/kg BLLL (10 in each group). Behavioral tests were carried out 24 h after the final probiotic treatment. These tests included the sucrose preference test (SPT), the tail suspension test (TST), the forced swimming test (FST), and the open field test (OFT). The OFT was performed 1 day before the TST, followed by the FST. The SPT was conducted on a separate group of mice. The dose of BLLL application was chosen according to previous studies (Gao et al., 2022; Ramalho et al., 2022). The schematic diagrams for the specific arrangement of the tests for depression-like behaviors are shown in Figure 1A. The mice in the control group were kept in the same room where the stressed mice were exposed. The researchers were blinded to group assignment during the experiments and data analysis. The behavioral tests were performed during the light phase; each group consisted of 10 mice. To avoid a possible influence of the behavioral tests on the molecular analysis, we chose a dose of 8 × 1010 CFU/kg BLLL, and divided the other animals into four groups (vehicle, BLLL, CUS + vehicle, and CUS + BLLL). Each group consisted of eight mice to prepare the hippocampus and medial prefrontal cortex for further real-time PCR experiments and biochemical measurements. For real-time PCR and biochemical assays, the hippocampal and mPFC tissues were manually dissected bilaterally using a scalpel on wet ice. The mPFC tissues were collected according to “The Mouse Brain Atlas in Stereotaxic Coordinates” by Keith Franklin and George Paxinos (2008, Academic Press) using a mouse brain matrix from Ted Pella, Inc. Since the sample size of the animals is relatively small, the mice were randomly assigned to the experimental groups using the stratified randomization method. All mice were transported in their home cage and temporarily kept in a new cage in the experimental room during the test. The environment in the experimental room was the same as that in the housing room.
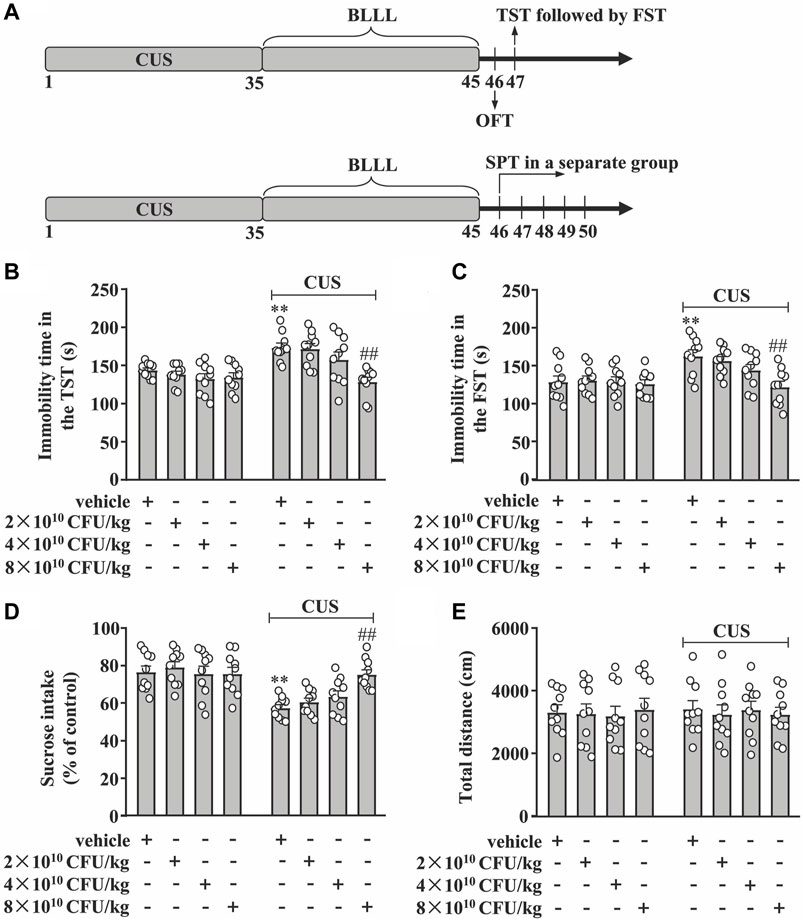
Figure 1. Dose-dependent effect of BLLL formula on CUS-induced depression-like behavior. (A) A schematic diagram showing the timeline for the evaluation of the dose-dependent effect of BLLL formula on CUS-induced depression-like behaviors in mice. (B, C) Quantitative analysis showing the effect of different doses of BLLL formula treatment (2×1010, 4×1010, and 8 × 1010 CFU/kg; 10 days) on CUS-induced increases in the immobility time in TST (B) and FST (C) in mice (n = 10, **p < 0.01 vs vehicle; ##p < 0.01 vs vehicle + CUS). (D) Quantitative analysis showing the effect of different doses of BLLL formula treatment (2×1010, 4×1010, and 8 × 1010 CFU/kg; 10 days) on CUS-induced decrease in sucrose intake in SPT (n = 10, **p < 0.01 vs vehicle; ##p < 0.01 vs vehicle + CUS). (E) Quantitative analysis showing the effect of different doses of BLLL formula treatment (2×1010, 4×1010, and 8 × 1010 CFU/kg; 10 days) on the total distance of mice treated with vehicle, BLLL formula, and/or CUS (n = 10). Data are shown as mean ± SEM.
Establishment of the CUS model
The CUS model was established according to previous studies [48–53]. In brief, mice were exposed daily (35 days) over a period of 5 weeks to two of the following stressors in random order: shaking the cage for 1 h, light throughout the night (12 h), housing in a 4°C cold room for 1 h, light restraint (by housing in small cages) for 2 h, tilting the cage 45° for 14 h, no light for 3 h during the daylight phase, wet cage for 14 h, flashing light for 6 h, noise in the room for 3 h, and water deprivation for 12 h during the dark phase. This method is well described as a model for depression and was performed according to a standard protocol.
Behavioral tests
The TST and the FST were performed following previous studies (Tong et al., 2017; Liu et al., 2021; Wang et al., 2021; Wang Y. et al., 2022; Tang et al., 2022; Wu et al., 2022). Mice were habituated to the test room for 20 min before the session began. In the TST, mice were suspended 50 cm above the floor for 6 min with tape placed approximately 1 cm from the tip of their tails, and were considered immobile when they hung passively and were completely motionless (any mouse that climbed on its tail was excluded from further analysis). For FST, mice were placed in a clear glass cylinder (height 25 cm and diameter 10 cm) filled to 10 cm with water at 25°C ± 1°C for 6 min. They were considered immobile when they floated in the water without struggling, making only the movement necessary to keep their heads above the water. The duration of immobility during the last 4 min of floating in the TST or forced swimming in the FST was recorded with a video (Anhui Zhenghua Biological Instrument Equipment Co. Ltd, Huaibei, China).
The SPT was performed following one of our previous studies (Lu et al., 2022). Mice were given the choice to drink from two bottles in individual cages, one containing 1% sucrose solution and the other containing water, and were acclimated to the two-bottle conditions for 2 days. The position of the two bottles was changed every 6 h to prevent side preference. On the test day, after 24 h of food and water deprivation, the mice were first acclimated to the test room for 20 min, and then exposed to the weighed bottles for 6 h, during which time the position of the bottles was exchanged. Sucrose preference was calculated as the percentage of sucrose solution consumed relative to total fluid intake.
The OFT was performed following one of our previous studies (Li et al., 2020). Mice were acclimated to the test room for 20 min before the session began in a dimly lit environment with a red light bulb (50 W) on the ceiling. During the test, each mouse, initially placed in the center area, was allowed to move freely in a cubic chamber (360 (W)×360 (H)×360 (D) mm) for 5 min. The time spent in the central area and the total distance of the mice in the open field were recorded by the automatic analysis system (Anhui Zhenghua Biological Instrument Equipment Co. Ltd, Huaibei, China). The apparatus in each instrument was thoroughly cleaned with 70% ethanol after each trial to remove olfactory cues.
Quantitative real-time PCR
24 h after the last probiotic treatment, the mice were killed by rapid decapitation. The hippocampus and medial prefrontal cortex (from the same animals), two brain regions with severe damage associated with depression (Price and Duman, 2020), were immediately harvested. Total RNA in these tissues was extracted using an RNeasy Mini Kit according to the manufacturer’s instructions (Qiagen, GmbH, Hilden, Germany). The first strand of cDNA was generated using a reverse transcription system (Promega, Madison, WI, USA). Real-time PCR was performed using a reaction system containing 1 × Faststart SYBR Green Master Mix (Roche Molecular Biochemicals), 2 μL diluted cDNA, 2 mM MgCl2, and 0.5 μM primers. Primers for TNF-α, IL-1β, IL-6, IL-4, IL-10, chitinase-3-like protein 3 (CHI3L1, also named Ym-1), and GAPDH are as follows: TNF-α, 5′-CTGTGAAGGGAATGGGTGTT-3′ F), 5′-GGTCAC TGTCCCAGCATCTT-3′ (R); IL-β, 5′-TGGAAAAGC GGTTTGTC TTC-3′ F), 5′-TACCAGTTGGGGAACTCTGC-3′ (R); IL-6: 5′-AGAGATACAAAGAAATGATGGA-3′ (F), 5′-AGCTATGGTACTCCACAAGACCA-3′ (R); IL-4, 5′-CAGCTAGTTGTCATCCTGCTCTTC-3′ (F), 5′- GCCGATGATCTCTCTCAAGTGA-3′ (R); IL-10, 5′-GGCAGAGAACCATGGCCCAGAA-3′ (F), 5′-AATCGATGACAGCGCCTCAGCC-3′ (R); Ym-1, 5′-TCACTTACACACATGAGCAAGAC-3′ (F), 5′- CGGTTCTGAGGAGTAGAGACCA-3′ (R); GAPDH, 5′-GGCCT TCCGTGTTCCTAC-3′ (F), 5′-TGTCATCATATCTGGCAGGTT-3′ (R). PCR products were detected by observing the increase in the fluorescence intensity of the double-stranded DNA-binding dye SYBR Green. Gene expression analysis was performed using the 2−ΔΔCt method (Livak and Schmittgen, 2001). Samples were assayed in triplicate, and values were normalized to the housekeeping gene GAPDH.
Detection of BDNF concentrations
BDNF concentrations in the hippocampus and medial prefrontal cortex were measured using the BDNF DuoSet Kit (R&D System; DY248) according to the manufacturer’s protocol. In brief, a 96-well microplate was coated (overnight at room temperature) with 100 μL per well of diluted capture antibody, followed by careful washing on the second day. The plates were then blocked with reagent diluent (300 μL, 1 h at room temperature). Then, 100 μL of the sample or standards in reagent dilution were added to the prepared wells and incubated for 2 h at room temperature. Then, the detection antibody (100 μL), the working dilution of streptavidin-HRP (100 μL), the substrate solution (100 μL), and the stop solution (50 μL) were added to each well in turn. Finally, the optical density of each well was determined using a microplate reader set at 450 nm (Molecular Devices, Sunnyvale, CA, USA).
Detection of pro-inflammatory and anti-inflammatory cytokine concentrations
The concentrations of IL-1β, IL-6, TNF-α, IL-4, and IL-10 in the hippocampus and medial prefrontal cortex were determined according to the manufacturer’s protocol using the corresponding commercial kits (Proteintech, Wuhan, China). The concentrations of IL-1β, IL-6, TNF-α, IL-4, and IL-10 were expressed as picograms per Gram of tissue (pg/g tissue).
Detection of nitrite contents
Nitrite levels in the hippocampus and medial prefrontal cortex were measured according to the manufacturer’s protocol (Bi Yuntian Biological Technology Institution, Shanghai, China). In brief, we first diluted the standard sample with tissue lysis buffer containing 50 mM Tris-HCl (pH 7.4), 1 mM EDTA, 100 mM NaCl, 20 mM NaF, and 3 mM Na3VO4 with 1% NP-40, and then added the samples and reaction solutions to a 96-well microplate in the following order: 150 μL of the samples (tissue supernatant in the lysis buffer), 20 μL of the Griess Reagent, and 130 μL of de-ionized water. Nitrite concentration was determined using a spectrophotometric M2 microplate reader (548 nm, Molecular Devices, Sunnyvale, CA, USA) based on a standard curve (0–100 μM/L) derived from NaNO2.
Detection of malondialdehyde (MDA) and reduced glutathione (GSH) content
The levels of MDA and GSH were determined by the method used in one of our previous studies (Yang et al., 2017) by homogenizing brain tissue in 0.1 M phosphate buffer (pH 7.4) (10% w/v). MDA and GSH levels were expressed as μM/g wet tissue. Brain protein was measured according to the Lowry method (Lowry et al., 1951) and was expressed as μM/mg of protein concentration.
Statistical analysis
Statistical analyses were performed using Graphpad Prism eight from Graphpad Software, Inc. in CA, USA. Additionally, the Kolmogorov-Smirnov test was used to examine the pattern and distribution of the data; Grubbs’ outlier test was used to further identify and exclude potential outliers in the data. The normality of distribution and sphericity were checked using the Mauchly and Shapiro-Wilk tests. The differences between the means of these data were evaluated using a two-way analysis of variance (ANOVA), and wherever necessary, the Bonferroni post hoc test was used to evaluate isolated comparisons. In an experiment, if two factors do not interact in the ANOVA and there are main effects on both factors, the main effects should be determined by averaging the effect of one factor over the levels of the other. This problem can be solved using a post hoc t-test, described by Wei et al., to further compare the factors that do not interact (Wei et al., 2012). p values < 0.05 were considered statistically significant. Data are presented as mean ± standard error of the mean (SEM).
Results
BLLL formula reverses CUS-induced depression-like behaviors in mice
First, we evaluated the dose-dependent effect of the BLLL formula on CUS-induced behavioral changes in TST, FST, SPT, and OFT in mice. The experimental procedure is shown in Figure 1A. In the TST, the two-way ANOVA showed significant effects for CUS exposure (F1,72 = 20.92, p < 0.001), BLLL formula treatment (F3,72 = 7.27, p < 0.001), and CUS × BLLL formula interaction (F3,72 = 3.98, p < 0.05) (Figure 1B). For FST, the two-way ANOVA showed significant effects for CUS exposure (F1,72 = 13.93, p < 0.001), BLLL formula treatment (F3,72 = 4.12, p < 0.01), and CUS × BLLL formula interaction (F3,72 = 2.94, p < 0.01) (Figure 1C). Post-hoc analysis revealed that 10-day BLLL formula treatment at a dose of 8 × 1010 CFU/kg, but not 2 or 4 × 1010 CFU/kg, reversed CUS-induced increase in immobility time in TST (Figure 1B) and FST (Figure 1C) in mice.
In the SPT, the two-way ANOVA showed significant effects for CUS exposure (F1,72 = 36.18, p < 0.001), BLLL formula treatment (F3,72 = 2.83, p < 0.05), and the CUS × BLLL formula interaction (F3,72 = 4.34, p < 0.01) (Figure 1D). Post-hoc analysis showed that 10-day BLLL formula treatment at a dose of 8 × 1010 CFU/kg, but not 2 or 4 × 1010 CFU/kg, reversed CUS-induced decrease in sucrose intake in mice in SPT (Figure 1D).
To rule out the influence of locomotor activity on mouse behaviors, the total distance of mice in the OFT was evaluated. The two-way ANOVA showed no significant effects for CUS exposure (F1,72 = 0.02, p = 0.89), BLLL formula treatment (F3,72 = 0.05, p = 0.99), and the CUS × BLLL formula interaction (F3,72 = 0.14, p = 0.94) (Figure 1E). Post-hoc analysis revealed that 10-day BLLL formula treatment at a dose of either 2, four or 8 × 1010 CFU/kg had not affect the total distance of mice in OFT (Figure 1E).
BLLL formula reverses CUS-induced enhancement of pro-inflammatory response in the hippocampal and medial prefrontal cortex in mice
Next, we evaluated the effect of 10 days of BLLL formula treatment on CUS-induced enhancement of pro-inflammatory responses in the hippocampus and medial prefrontal cortex in mice. To this end, we first evaluated the effect of the BLLL formula on pro-inflammatory cytokine mRNA expression in the hippocampus and medial prefrontal cortex in mice treated with or without the BLLL formula. A two-way ANOVA for levels of IL-1β, IL-6, and TNF-α mRNA in the hippocampus showed significant effects for CUS exposure (IL-1β mRNA: F1,28 = 21.33, p < 0.001, IL-6 mRNA: F1,28 = 22.69, p < 0.001, TNF-α mRNA: F1,28 = 24.96, p < 0.001), BLLL formula treatment (IL-1β mRNA: F1,28 = 12.43, p < 0.01; IL-6 mRNA: F1,28 = 5.82, p < 0.05; TNF-α mRNA: F1,28 = 11.47, p < 0.01), and the CUS × BLLL formula interaction (IL-1β mRNA: F1,28 = 8.74, p < 0.01; IL-6 mRNA: F1,28 = 6.76, p < 0.05; TNF-α mRNA: F1,28 = 13.03, p < 0.01) (Figures 2A–C). Post-hoc analysis revealed that 10-day BLLL formula treatment at a dose of 8 × 1010 CFU/kg reversed CUS-induced increases in the expression levels of IL-1β (Figure 2A), IL-6 (Figure 2B), and TNF-α (Figure 2C) mRNA in the hippocampus.
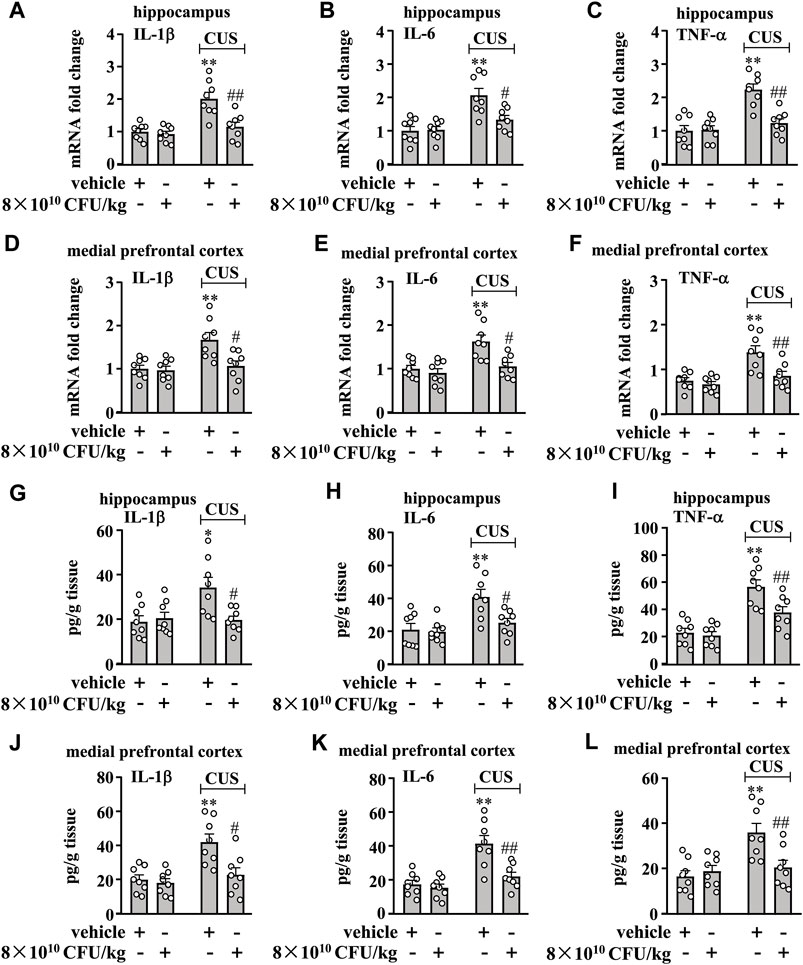
Figure 2. Effect of BLLL formula on pro-inflammatory responses in the brain in chronically stressed mice. (A–C) Quantitative analysis shows that 10 days of BLLL formula treatment (8 × 1010 CFU/kg) reversed CUS-induced increases in the mRNA expression levels of IL-1β (A), IL-6 (B), and TNF-α (C) in the hippocampus in mice (n = 8, **p < 0.01 vs. vehicle; #p < 0.05 or ##p < 0.01 vs vehicle + CUS). (D–F) Quantitative analysis shows that 10 days of BLLL formula treatment (8 × 1010 CFU/kg) reversed CUS-induced increases in the mRNA expression levels of IL-1β (D), IL-6 (E), and TNF-α (F) in the medial prefrontal cortex in mice (n = 8, **p < 0.01 vs vehicle; #p < 0.05 or ##p < 0.01 vs. vehicle + CUS). (g–i) Quantitative analysis shows that 10 days of BLLL formula treatment (8 × 1010 CFU/kg) reversed CUS-induced increases in levels of IL-1β (G), IL-6 (H), and TNF-α (I) in the hippocampus in mice (n = 8, *p < 0.05 or **p < 0.01 vs vehicle; #p < 0.05 or ##p < 0.01 vs vehicle + CUS). (J–L) Quantitative analysis shows that 10 days of BLLL formula treatment (8 × 1010 CFU/kg) reversed CUS-induced increases in levels of IL-1β (J), IL-6 (K), and TNF-α (L) in the medial prefrontal cortex in mice (n = 8, **p < 0.01 vs. vehicle; #p < 0.05 or ##p < 0.01 vs. vehicle + CUS). Data are shown as mean ± SEM.
For the levels of IL-1β, IL-6, and TNF-α mRNA in the medial prefrontal cortex, the ANOVA showed significant effects for CUS exposure (IL-1β mRNA: F1,28 = 10.38, p < 0.01, IL-6 mRNA: F1,28 = 13.30, p < 0.01, TNF-α mRNA: F1,28 = 17.37, p < 0.001), BLLL formula treatment (IL-1β mRNA: F1,28 = 7.22, p < 0.05; IL-6 mRNA: F1,28 = 10.01, p < 0.01; TNF-α mRNA: F1,28 = 9.46, p < 0.01), and the CUS × BLLL formula interaction (IL-1β mRNA: F1,28 = 5.91, p < 0.05; IL-6 mRNA: F1,28 = 4.89, p < 0.05; TNF-α mRNA: F1,28 = 5.13, p < 0.05) (Figures 2D–F). Post-hoc analysis revealed that 10-day BLLL formula treatment at a dose of 8 × 1010 CFU/kg could reverse CUS-induced increase in the expression levels of IL-1β (Figure 2D), IL-6 (Figure 2E), and TNF-α (Figure 2F) mRNA in the medial prefrontal cortex.
Next, we evaluated the effect of the BLLL formula on pro-inflammatory cytokine protein levels in the hippocampus and medial prefrontal cortex in mice treated with or without the BLLL formula. A two-way ANOVA for levels of IL-1β, IL-6, and TNF-α in the hippocampus showed significant effects for CUS exposure (IL-1β: F1,28 = 5.50, p < 0.05, IL-6: F1,28 = 13.31, p < 0.01, TNF-α: F1,28 = 40.99, p < 0.001), BLLL formula treatment (IL-1β: F1,28 = 4.47, p < 0.05; IL-6: F1,28 = 5.99, p < 0.05; TNF-α: F1,28 = 6.97, p < 0.05), and the CUS × BLLL formula interaction (IL-1β: F1,28 = 7.04, p < 0.05; IL-6: F1,28 = 4.25, p < 0.05; TNF-α: F1,28 = 4.62, p < 0.05) (Figures 2G–I). Post-hoc analysis revealed that 10-day BLLL formula treatment at a dose of 8 × 1010 CFU/kg reversed CUS-induced increases in the expression levels of IL-1β (Figure 2G), IL-6 (Figure 2H), and TNF-α (Figure 2I) in the hippocampus.
For the levels of IL-1β, IL-6, and TNF-α in the medial prefrontal cortex, the ANOVA showed significant effects for CUS exposure (IL-1β: F1,28 = 13.43, p < 0.01, IL-6: F1,28 = 24.14, p < 0.001, TNF-α: F1,28 = 11.43, p < 0.01), BLLL formula treatment (IL-1β: F1,28 = 8.39, p < 0.01; IL-6: F1,28 = 11.72, p < 0.01; TNF-α: F1,28 = 4.33, p < 0.05), and the CUS × BLLL formula interaction (IL-1β: F1,28 = 5.60, p < 0.05; IL-6: F1,28 = 7.79, p < 0.01; TNF-α: F1,28 = 8.28, p < 0.01) (Figures 2J,K). Post-hoc analysis revealed that 10-day BLLL formula treatment at a dose of 8 × 1010 CFU/kg could reverse CUS-induced increase in the expression levels of IL-1β (Figure 2J), IL-6 (Figure 2K), and TNF-α (Figure 2L) in the medial prefrontal cortex.
BLLL formula reverses CUS-induced impairment of the anti-inflammatory responses in the hippocampal and medial prefrontal cortex in mice
We also evaluated the effect of 10-day BLLL formula treatment on anti-inflammatory responses in the hippocampus and prefrontal cortex in chronically-stressed mice. A two-way ANOVA for levels of IL-4, IL-10, and Ym-1 mRNA in the hippocampus revealed significant effects for CUS exposure (IL-4 mRNA: F1,28 = 16.74, p < 0.001, IL-10 mRNA: F1,28 = 24.78, p < 0.001, Ym-1 mRNA: F1,28 = 4.46, p < 0.05) and BLLL formula treatment (IL-4 mRNA: F1,28 = 4.46, p < 0.05; IL-10 mRNA: F1,28 = 5.51, p < 0.05; Ym-1 mRNA: F1,28 = 11.33, p < 0.01), but not for CUS × BLLL formula interaction (IL-4 mRNA: F1,28 = 0.59, p = 0.45; IL-10 mRNA: F1,28 = 0.90, p = 0.35; Ym-1 mRNA: F1,28 = 2.87, p = 0.10) (Figures 3A–C). Post-hoc analysis revealed that 10-day BLLL formula treatment at the dose of 8 × 1010 CFU/kg reversed CUS-induced decrease in the expression levels of IL-4 (Figure 3A), IL-10 (Figure 3B), and Ym-1 (Figure 3C) mRNA in the hippocampus.
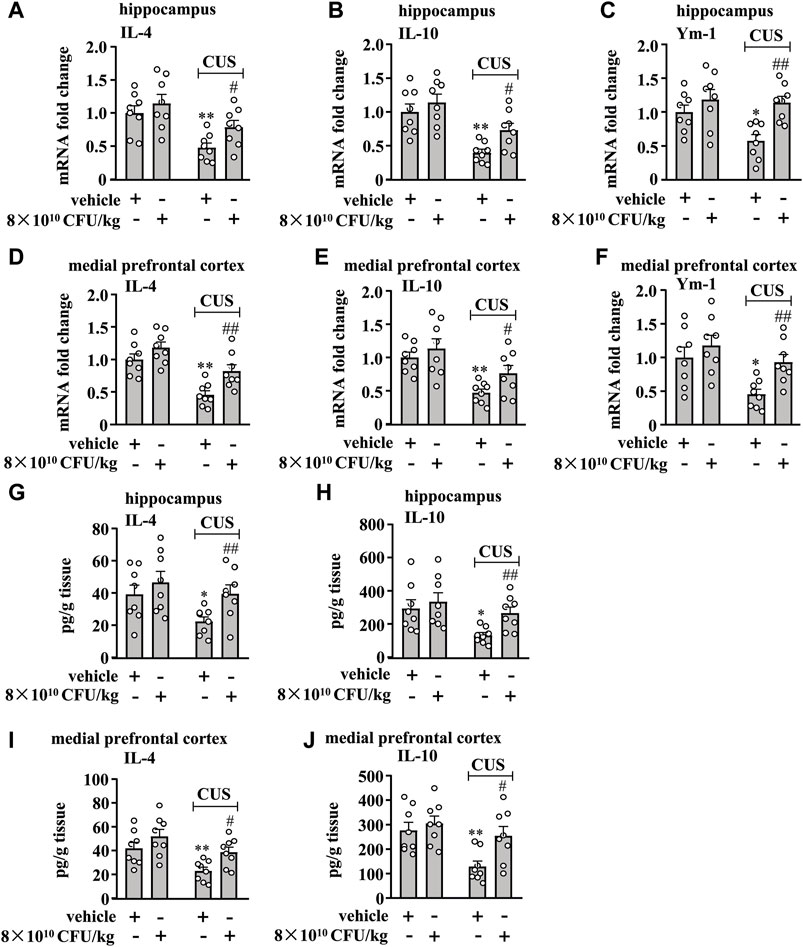
Figure 3. Effect of BLLL formula on anti-inflammatory responses in the brain in chronically stressed mice. (A–C) Quantitative analysis shows that 10 days of BLLL formula treatment (8 × 1010 CFU/kg) reversed CUS-induced decreases in the mRNA expression levels of IL-4 (A), IL-10 (B), and Ym-1 (C) in the hippocampus in mice (n = 8, *p < 0.05 or **p < 0.01 vs vehicle; #p < 0.05 or ##p < 0.01 vs. vehicle + CUS). (D–F) Quantitative analysis shows that 10 days of BLLL formula treatment (8 × 1010 CFU/kg) reversed CUS-induced decreases in the mRNA expression levels of IL-4 (D), IL-10 (E), and Ym-1 (F) in the medial prefrontal cortex in mice (n = 8, *p < 0.05 or *p < 0.01 vs vehicle; #p < 0.05 or ##p < 0.01 vs vehicle + CUS). (G, H) Quantitative analysis shows that 10 days of BLLL formula treatment (8 × 1010 CFU/kg) reversed CUS-induced decreases in levels of IL-4 (G) and IL-10 (H) in the hippocampus in mice (n = 8, *p < 0.05 vs vehicle; ##p < 0.01 vs vehicle + CUS). (I, J) Quantitative analysis shows that 10 days of BLLL formula treatment (8 × 1010 CFU/kg) reversed CUS-induced decreases in levels of IL-4 (I) and IL-10 (J) in the medial prefrontal cortex in mice (n = 8, **p < 0.01 vs vehicle; #p < 0.05 vs vehicle + CUS). Data are shown as mean ± SEM.
For the levels of IL-4, IL-10, and Ym-1 mRNA in the medial prefrontal cortex, the ANOVA showed significant effects for CUS exposure (IL-4 mRNA: F1,28 = 29.32, p < 0.001, IL-10 mRNA: F1,28 = 18.64, p < 0.001, Ym-1 mRNA: F1,28 = 9.98, p < 0.01) and BLLL formula treatment (IL-4 mRNA: F1,28 = 10.87, p < 0.01; IL-10 mRNA: F1,28 = 4.26, p < 0.05; Ym-1 mRNA: F1,28 = 6.78, p < 0.05), but not for the CUS × BLLL formula interaction (IL-4 mRNA: F1,28 = 1.18, p = 0.29; IL-10 mRNA: F1,28 = 0.59, p = 0.45; Ym-1 mRNA: F1,28 = 1.36, p = 0.25) (Figures 3D–F). Post-hoc analysis revealed that 10-day BLLL formula treatment at the dose of 8 × 1010 CFU/kg also reversed CUS-induced decrease in the expression levels of IL-4 (Figure 3D), IL-10 (Figure 3E), and Ym-1 (Figure 3F) mRNA in the medial prefrontal cortex.
We also evaluated the effect of 10-day BLLL formula treatment on CUS-induced decrease in IL-4 and IL-10 protein levels in the hippocampus and medial prefrontal cortex in mice. The two-way ANOVA for the levels of IL-4 and IL-10 in the hippocampus showed significant effects for CUS exposure (IL-4: F1,28 = 4.81, p < 0.05, IL-10: F1,28 = 7.55, p < 0.05) and BLLL formula treatment (IL-4: F1,28 = 5.26, p < 0.05; IL-10: F1,28 = 4.29, p < 0.05), but not for the CUS × BLLL formula interaction (IL-4: F1,28 = 0.79, p = 0.38; IL-10: F1,28 = 1.27, p = 0.27) (Figures 3G, H). The two-way ANOVA for the levels of IL-4 and IL-10 in the medial prefrontal cortex showed significant effects for CUS exposure (IL-4: F1,28 = 11.51, p < 0.01, IL-10: F1,28 = 9.96, p < 0.01) and BLLL formula treatment (IL-4: F1,28 = 7.54, p < 0.05; IL-10: F1,28 = 5.95, p < 0.05), but not for the CUS × BLLL formula interaction (IL-4: F1,28 = 0.36, p = 0.56; IL-10: F1,28 = 2.43, p = 0.13) (Figures 3I, J). Post-hoc analysis revealed that 10-day BLLL formula treatment at the dose of 8 × 1010 CFU/kg reversed CUS-induced decrease in levels of IL-4 (Figures 3G, I) and IL-10 (Figures 3H, J) in the hippocampus and medial prefrontal cortex.
BLLL formula reverses CUS-induced changes in markers indicative of oxido-nitrosative stress in the hippocampus and medial prefrontal cortex
We then examined the effect of the 10-day BLLL formula treatment on markers indicative of oxido-nitrosative stress in the hippocampus and medial prefrontal cortex in chronically stressed mice. A two-way ANOVA for nitrite levels in the hippocampus and medial prefrontal cortex showed significant effects for CUS exposure (hippocampus: F1,28 = 38.99, p < 0.001, cortex: F1,28 = 39.14, p < 0.001), BLLL formula treatment (hippocampus: F1,28 = 6.48, p < 0.05, cortex: F1,28 = 20.76, p < 0.001), and CUS × BLLL formula interaction (hippocampus: F1,28 = 4.91, p < 0.05, cortex: F1,28 = 18.77, p < 0.001) (Figures 4A, B). Post-hoc analysis revealed that 10-day BLLL formula treatment at a dose of 8 × 1010 CFU/kg reversed CUS-induced increase in nitrite levels in the hippocampus (Figure 4A) and medial prefrontal cortex (Figure 4B).
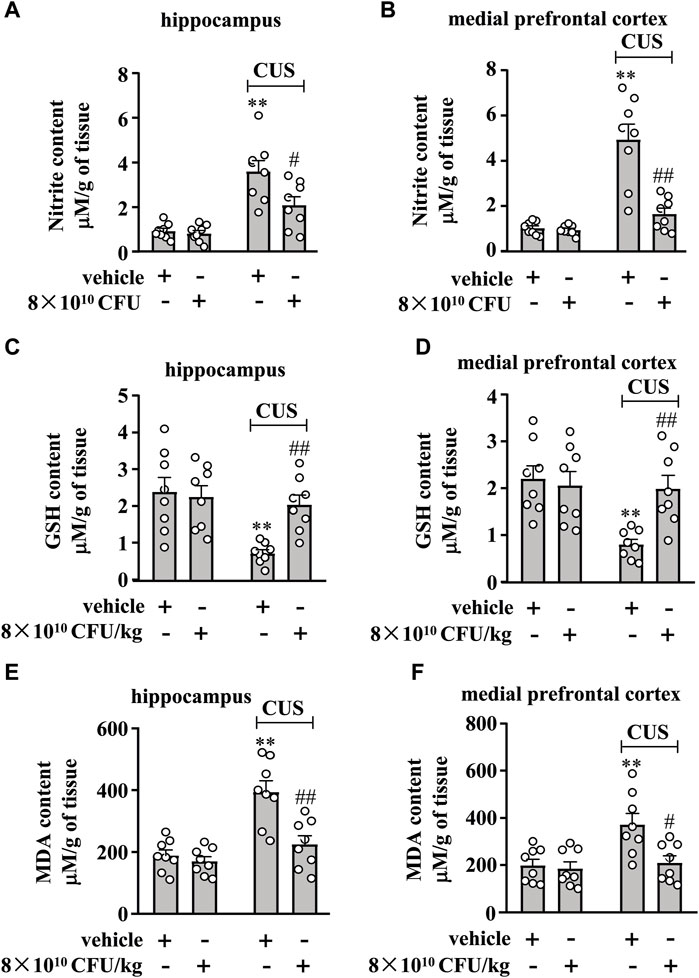
Figure 4. Effect of BLLL formula on CUS-induced changes in nitrite, GSH, and MDA content in the hippocampus and medial prefrontal cortex. (A, B) Quantitative analysis shows that BLLL formula treatment (8 × 1010 CFU/kg) reversed CUS-induced increases in levels of nitrite in the hippocampus (A) and medial prefrontal cortex (B) in mice (n = 8, **p < 0.01 vs vehicle; ##p < 0.01 vs vehicle + CUS). (C, D) Quantitative analysis shows that BLLL formula treatment (8 × 1010 CFU/kg) reversed CUS-induced decreases in contents of GSH in the hippocampus and medial prefrontal cortex in mice (n = 8, **p < 0.01 vs. vehicle; ##p < 0.01 vs. vehicle + CUS). (E, F) Quantitative analysis shows that BLLL formula treatment (8 × 1010 CFU/kg) reversed CUS-induced increases in contents of MDA in the hippocampus and medial prefrontal cortex in mice (n = 8, **p < 0.01 vs vehicle; #p < 0.05 or ##p < 0.01 vs vehicle + CUS). Data are shown as mean ± SEM.
We also examined the effect of 10 days of BLLL treatment on GSH and MDA contents in the hippocampus and medial prefrontal cortex of chronically stressed mice. A two-way ANOVA for GSH content in the hippocampus and medial prefrontal cortex showed significant effects for CUS exposure (hippocampus: F1,28 = 10.94, p < 0.01, cortex: F1,28 = 8.69, p < 0.01), BLLL formula treatment (hippocampus: F1,28 = 4.33, p < 0.05, cortex: F1,28 = 4.40, p < 0.05), and the CUS × BLLL formula interaction (hippocampus: F1,28 = 6.68, p < 0.05, cortex: F1,28 = 7.19, p < 0.05) (Figures 4C, D). Post-hoc analysis revealed that 10-day BLLL formula treatment at a dose of 8 × 1010 CFU/kg reversed CUS-induced decrease in GSH content in the hippocampus (Figure 4C) and medial prefrontal cortex (Figure 4D).
For MDA content in the hippocampus and medial prefrontal cortex, the ANOVA showed significant effects for CUS exposure (hippocampus: F1,28 = 25.31, p < 0.001, cortex: F1,28 = 8.96, p < 0.01), BLLL formula treatment (hippocampus: F1,28 = 13.10, p < 0.01, cortex: F1,28 = 7.07, p < 0.05), and CUS × BLLL formula interaction (hippocampus: F1,28 = 8.37, p < 0.01, cortex: F1,28 = 5.05, p < 0.05) (Figures 4E, F). Post-hoc analysis revealed that 10-day BLLL formula treatment at a dose of 8 × 1010 CFU/kg reversed the CUS-induced increase in MDA content in the hippocampus (Figure 4E) and medial prefrontal cortex (Figure 4F).
BLLL formula reverses CUS-induced decrease in BDNF levels in the hippocampal and medial prefrontal cortex
Finally, we evaluated the effect of 10-day BLLL formula treatment on BDNF content in the hippocampus and medial prefrontal cortex in chronically-stressed mice. A two-way ANOVA for the change of BDNF content in the hippocampus and medial prefrontal cortex showed significant effects for CUS exposure (hippocampus: F1,28 = 19.66, p < 0.001, cortex: F1,28 = 10.75, p < 0.01), BLLL formula treatment (hippocampus: F1,28 = 5.99, p < 0.01, cortex: F1,28 = 5.11, p < 0.05), and CUS × BLLL formula interaction (hippocampus: F1,28 = 7.11, p < 0.05, cortex: F1,28 = 10.04, p < 0.01) (Figures 5A, B). Post-hoc analysis revealed that 10-day BLLL formula treatment at a dose of 8 × 1010 CFU/kg reversed CUS-induced decrease in BDNF content in the hippocampus (Figure 5A) and medial prefrontal cortex (Figure 5B).
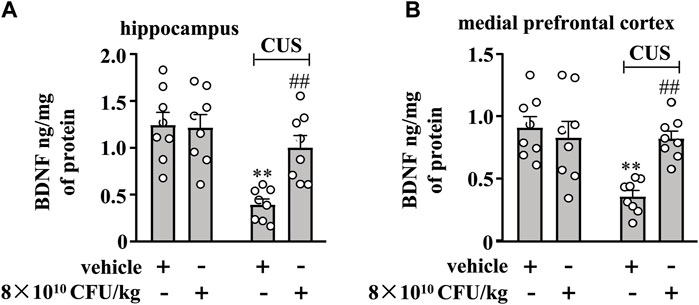
Figure 5. Effect of BLLL formula on CUS-induced decreases in levels of BDNF in the hippocampus and medial prefrontal cortex. (A, B) Quantitative analysis shows that BLLL formula treatment (8 × 1010 CFU/kg) reversed CUS-induced decreases in levels of BDNF protein in the hippocampus (A) and medial prefrontal cortex (B) in mice (n = 8, **p < 0.01 vs. vehicle; ##p < 0.01 vs. vehicle + CUS). Data are shown as mean ± SEM.
Discussion
One of the main contributions of the present study was the identification of an apparent antidepressant-like effect of a new probiotic formula consisting of four types of probiotics B. breve, L. plantarum, L. paracasei, and L. helveticus (called BLLL formula) in mice subjected to chronic stress in behavioral tests such as TSF, FST, and SPT. The antidepressant-like effect of BLLL was significant when administered at 8 × 1010 CFU/kg for 10 days. The reduction in immobility in TST and FST in the BLLL-treated mice was not attributed to changes in their endogenous spontaneous locomotor activity, indicating that the formula indeed has an antidepressant-like effect. This hypothesis was further supported by the finding that continuous administration of the BLLL formula for 10 days reversed the CUS-induced reduction in sucrose intake in mice. As in-depth studies on the regulatory effects of probiotics on the nervous system are ongoing worldwide, supplementing probiotics with neuroprotective effects is now considered a potential strategy for the treatment of nervous system disorders, including depression (Aghamohammad et al., 2023; Varesi et al., 2023). Our results represent a potential option for this purpose and may be of interest to those aiming to develop new antidepressants based on probiotics. It is worth noting that the antidepressant-like effects of the individual components of BLLL have already been described in multiple prior studies (Maehata et al., 2019; Tian et al., 2019; Tian et al., 2020; Partrick et al., 2021; Xu et al., 2022; Zhu R. et al., 2023; Kwon et al., 2023; Ma et al., 2024). As the efficacy of probiotics is both strain-specific and outcome-specific (McFarland et al., 2021), the supplementation of a multi-strain probiotic formula may have additive or synergistic effects that require thorough investigation in future studies.
In recent decades, there have been numerous reports on the therapeutic effects of probiotics on depressive behavior in both animals and humans (Huang et al., 2016; Park et al., 2018; Kosuge et al., 2021; Tian et al., 2022). For example, B. breve has been shown to have significant antidepressant-like effects in chronically stressed C57BL/6J mice (Tian et al., 2020; Sushma et al., 2023). The administration of L. paracasei and L. plantarum has been found to prevent stress-induced anxiety- and depression-like behaviors in Swiss mice (Stenman et al., 2020). Lactobacillus rhamnosus, combined with L. helveticus, has been reported to alleviate anxiety- and depression-like behaviors in B- and T-cell-deficient Rag1−/− mice (Smith et al., 2014). All of these studies begin with the administration of probiotics before or at the onset of stress and continue for four or 5 weeks until the end of chronic stress [63–65]. However, it is worth noting that it is almost impossible to administer medication or probiotics before or at the onset of stress. Our study holds greater clinical significance because the probiotic formula was administered after stress stimulation was discontinued. In addition, while numerous prior studies focus on a single strain, our investigation explored the impact of a mixture of four stains of probiotics on depression-like behaviors in animals. Although the mechanisms underlying the antidepressant-like effect of probiotics on depression are still largely unclear, many studies have reported that metabolites produced by probiotics in the gut may mediate these effects. According to the dose-dependent theory, it is reasonable to assume that the efficacy of the antidepressant-like effects of probiotics could be influenced by the dosage used. The mixture of different strains of probiotics may enhance the antidepressant-like effects of probiotics by producing more anti-inflammatory and anti-oxidative agents. These hypotheses should be investigated in future studies.
The development of new antidepressants remains a challenge due to the unclear biological mechanisms underlying depression. One possible reason for this could be that too much attention is paid to neuronal dysfunction. In recent years, numerous studies have investigated the connection between depression and neuroinflammation as well as oxido-nitrosative stress (Sulakhiya et al., 2014; Yang et al., 2017; Dantzer et al., 2018; Himmerich et al., 2019; Nowak et al., 2019; Osimo et al., 2020; Cosma et al., 2021; Perry et al., 2021). Chronic stress in animals, such as CUS, chronic social defeat stress, and chronic restraint stress, can lead to an increase in pro-inflammatory cytokines in the brain, like IL-6, IL-1β, and TNF-α. These cytokines could trigger microglial hyper-activation, neuroinflammation, and impairment of the BDNF pathway, ultimately leading to neuronal damage and the development of depressive symptoms that can be assessed by behavioral experiments such as TST, FST, and SPT. Furthermore, the direct infusion of pro-inflammatory cytokines such as IL-1β and TNF-α into the brain has been shown to induce depression-like behaviors in rodents (Anisman et al., 2008; Hayley et al., 2013). In humans, administrating pro-inflammatory substance LPS can promote an increase of pro-inflammatory cytokines in serum, thereby triggering illness and depression-like behaviors (Maes et al., 2008; Benson et al., 2017). Here, we found that 35-day CUS exposure elicited a significant increase in pro-inflammatory cytokine levels (such as IL-6, IL-1β, and TNF-α) in the hippocampus and medial prefrontal cortex of mice while also reducing the levels of anti-inflammatory factors (such as IL-4, IL-10, and Ym-1) in these brain regions. BLLL administration suppressed the CUS-induced increase in IL-6, IL-1β, and TNF-α and the CUS-induced decrease in IL-4, IL-10, and Ym-1 in the hippocampus and medial prefrontal cortex. IL-4 and IL-10 are typical factors with anti-inflammatory effects. They can transform inflammatory cells such as macrophages and microglia from a pro-inflammatory state to an anti-inflammatory state, which could be beneficial for tissue remodeling, repair, and neuroprotection. Ym1, a secreted and self-crystallizing protein, is encoded by a gene that was cloned from mouse peritoneal-activated macrophages (Chang et al., 2001) and is also expressed in microglia (Li et al., 2024), glandular gastric epithelium (Ward et al., 2001) or neutrophils (Harbord et al., 2002). As an inflammation-responsive protein (Raes et al., 2002), increased Ym-1 expression can help limit the progression of inflammation and can be further enhanced by interleukin four-induced gene-1 (Yue et al., 2015). Under BLLL treatment, the rise in Ym-1 in CUS-exposed mice could shift the neuroinflammatory responses in the brain towards an anti-inflammatory phenotype, thereby inducing an antidepressant-like effect. The relationship between Ym-1 and IL-4 or IL-10 should be carefully investigated in future studies.
Our results also showed that CUS caused a significant reduction in BDNF levels in the hippocampus and medial prefrontal cortex and that these effects of CUS were reversed by administration of the BLLL formula. This finding is in strong agreement with previous reports confirming the link between neuroinflammation and the BDNF signaling pathway (Calabrese et al., 2014; Yang et al., 2017). Considering that pro-inflammatory cytokines have been widely described as mediators of immobile and anhedonic behavior in TST, FST, and SPT, we concluded that administration of BLLL could attenuate CUS-induced depression-like behavior in mice in part by suppressing neuroinflammation that accompanied the restoration of BDNF expression. However, further investigation is required to understand how the BLLL formula affects the production of pro-inflammatory cytokines and BDNF in the brain. We also can not overlook the possibility that the BLLL formula could influence the expression of pro-inflammatory cytokines and BDNF in the brain in an independent manner. Additionally, since microglia can also produce BDNF, it needs to clarify whether the increased BDNF levels in the brain of CUS mice after administration of BLLL originate from microglia. These questions should be addressed in future studies.
Aside from neuroinflammation, oxidative and nitrosative stress may also play an important role in depression (Sulakhiya et al., 2014; Yang et al., 2017). It has been reported that the over-activated microglia and innate immune system increase oxidative and nitrosative stress (Morris et al., 2018; Bittencourt et al., 2022). Direct administration of pro-inflammatory cytokines such as TNF-α and IL-6 to mice also increases reactive oxygen species (ROS) by increasing the production of NO (Madrigal et al., 2002). The increased NO, along with ROS, causes oxido-nitrosative stress, which leads to neurotoxic effects through various mechanisms, such as the reduction of BDNF expressions and monoamine levels (Kasala et al., 2014; Amiry et al., 2023). This opinion was supported by our data, as some markers that reflect the status of oxidative and nitrosative stress, such as MDA, GSH, and nitrite, were significantly altered by CUS exposure. Increased MDA and nitrite levels reflect higher oxidative and nitrosative status, while decreased GSH levels indicate lower antioxidant status (Rongzhu et al., 2009; Borges et al., 2015). Reversing the deleterious changes in these markers would be beneficial for the treatment of major depression. Our studies showed that administration of the BLLL formula significantly reversed the CUS-induced increase in MDA and nitrite levels and the CUS-induced decrease in GSH levels in the hippocampus and prefrontal cortex. These findings suggest that the suppression of oxido-nitrosative stress may be a key mechanism for the reversal of depression-like behavior using the BLLL formula. Whether the antioxidant effect of the BLLL formula is related to its anti-neuroinflammatory effect should be investigated in future studies.
It is worth noting that the same dose and duration of BLLL treatment has no effect on the behavior of non-stressed control mice in the TST, FST, and SPT, as well as on the levels of IL-1β, IL-6, TNF-α, IL-4, IL-10, Ym-1, nitrite, MDA, GSH, BDNF in the hippocampus and medial prefrontal cortex of the non-stressed control mice. It is interesting to consider why BLLL only affects the above parameters in chronically stressed mice. Since chronic stress exposure is a pathological stimulation, we hypothesize that 35-day CUS stimulation may disrupt the homeostasis of some targets that mediate the production of pro-inflammatory cytokines and the progression of oxidative and nitrosative stress, such as nuclear factor-kappa B (NF-κB) and nuclear factor erythroid-derived 2-like 2 (Nrf2), thereby promoting the development of depression-like behaviors. Numerous studies have reported that targets such as NF-κB and Nrf2 could be affected by different types of probiotics in animal models of depression (Gao et al., 2021; Zhu L. et al., 2023; Fatima et al., 2023; Rezaie et al., 2024). In future studies, we will further investigate how exactly the progression of neuroinflammation and oxidative stress in the brain is affected under chronic stress conditions.
There are some limitations to this study that need to be acknowledged. First, we only investigated the antidepressant-like effect of BLLL in male mice but not in female mice. This is very important because female and male mice usually display different behavioral and biological responses to stress. For instance, in the helplessness model, female animals can learn to escape from stressful tasks and, therefore, do not exhibit helplessness behavior (Heinsbroek et al., 1991). In some other studies, female animals have shown a delayed return to baseline corticosterone levels after abnormal activation of the hypothalamic-pituitary-adrenal axis (Viau et al., 2005; Dudek et al., 2021). In addition, female animals can demonstrate a more efficient anti-inflammatory adaptation to chronic stress (Bollinger et al., 2020). In future studies, we will investigate whether the antidepressant-like effect of BLLL also applies to female animals. Second, in the present study, we only examined the parameters reflecting neuroinflammation and oxidative stress in the hippocampus and medial prefrontal cortex to illustrate the mechanisms underlying the antidepressant-like effect of BLLL. However, there are many other brain regions, such as the amygdala, nucleus accumbens, and hypothalamus, that respond to neuroinflammation and oxidative stress and may be associated with depression (Wang J. et al., 2022; Xu et al., 2023; Zhang et al., 2023). Therefore, future studies should investigate the cellular and regional basis for the antidepressant-like effects of BLLL. Furthermore, the BLLL probiotics supplement used here can directly influence the gut microbiota. To this end, future studies should also explore other mechanisms, besides anti-neuroinflammation and anti-oxidative stress, that are related to gut dysbiosis and the brain-gut axis, as well as the absorption of the metabolites associated with BLLL administration.
Conclusion
Our results show that repeated administration of the BLLL formula, a newly developed probiotic consisting of B. breve, L. plantarum, L. paracasei, and L. helveticus, can reverse chronic stress-induced depression-like behavior in mice, which is achieved by reducing neuroinflammation and oxido-nitrosative stress, and by increasing BDNF levels in the brain (Figure 6). Given the close association between gut microbiota dysfunction and the development of depression (Huang et al., 2016; Park et al., 2018; Kosuge et al., 2021; Tian et al., 2022), as well as the individual components of the BLLL formula having demonstrated apparent antidepressant-like effects in patients with depression (Rode et al., 2022; Duarte et al., 2023; Önning et al., 2023; Sarkawi et al., 2024), our findings suggest that the BLLL formula used in the present study has the potential to be developed as a new drug for the treatment of depression in the clinic. However, before this goal can be achieved, further studies should be conducted to clarify the effects, metabolism, conversion, stability, and safety of the BLLL formula in humans.
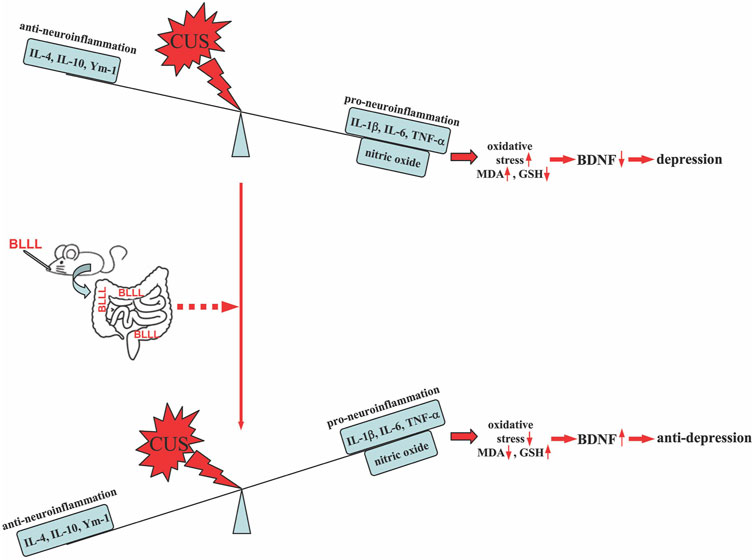
Figure 6. A schematic illustration showing that oral administration of BLLL reverses chronic stress-induced depression-like behavior in mice by converting the neuroinflammatory responses to an anti-inflammatory state, as indicated by the decrease in the levels of IL-1β, IL-6, and TNF-α and the increase in the levels of IL-4, IL-10, and Ym-1 in the brain. This would subsequently rebalance the levels of MDA, GSH, and BDNF in the brains of chronically stressed mice.
Data availability statement
The raw data supporting the conclusions of this article will be made available by the authors, without undue reservation.
Ethics statement
The animal study was approved by the Animal Ethics Committee of Nantong University. The study was conducted in accordance with the local legislation and institutional requirements.
Author contributions
MY: Writing–original draft, Writing–review and editing. FJ: Writing–original draft, Writing–review and editing. CH: Writing–original draft, Writing–review and editing. FL: Writing–original draft, Writing–review and editing. CZ: Writing–original draft, Writing–review and editing. YZ: Writing–review and editing. RW: Writing–original draft, Writing–review and editing. KM: Writing–original draft, Writing–review and editing. XL: Writing–original draft, Writing–review and editing. HW: Writing–original draft, Writing–review and editing.
Funding
The author(s) declare that financial support was received for the research, authorship, and/or publication of this article. This work was supported by the National Natural Science Foundation of China (82271376), the Science and Education Project of Suzhou (KJXW2023076), the Science and Technology Project of Changzhou City (CJ20230085), the Natural Science Foundation of Jiangsu Province (BK20221375), and the Natural Science Foundation of the Higher Education Institutions of Jiangsu Province (23KJB310020).
Acknowledgments
We thank the foundation from the National Natural Science Foundation of China (82271376), the Science and Education Project of Suzhou (KJXW2023076), the Science and Technology Project of Changzhou City (CJ20230085), the Natural Science Foundation of Jiangsu Province (BK20221375), and the Natural Science Foundation of the Higher Education Institutions of Jiangsu Province (23KJB310020).
Conflict of interest
The authors declare that the research was conducted in the absence of any commercial or financial relationships that could be construed as a potential conflict of interest.
Publisher’s note
All claims expressed in this article are solely those of the authors and do not necessarily represent those of their affiliated organizations, or those of the publisher, the editors and the reviewers. Any product that may be evaluated in this article, or claim that may be made by its manufacturer, is not guaranteed or endorsed by the publisher.
References
Aghamohammad, S., Hafezi, A., and Rohani, M. (2023). Probiotics as functional foods: how probiotics can alleviate the symptoms of neurological disabilities. Biomed. Pharmacother. 163, 114816. doi:10.1016/j.biopha.2023.114816
Amiry, G. Y., Haidary, M., Azhdari-Zarmehri, H., Beheshti, F., and Ahmadi-Soleimani, S. M. (2023). Omega-3 fatty acids prevent nicotine withdrawal-induced exacerbation of anxiety and depression by affecting oxidative stress balance, inflammatory response, BDNF and serotonin metabolism in rats. Eur. J. Pharmacol. 947, 175634. doi:10.1016/j.ejphar.2023.175634
Anisman, H., Gibb, J., and Hayley, S. (2008). Influence of continuous infusion of interleukin-1beta on depression-related processes in mice: corticosterone, circulating cytokines, brain monoamines, and cytokine mRNA expression. Psychopharmacol. Berl. 199 (2), 231–244. doi:10.1007/s00213-008-1166-z
Barberio, B., Zamani, M., Black, C. J., Savarino, E. V., and Ford, A. C. (2021). Prevalence of symptoms of anxiety and depression in patients with inflammatory bowel disease: a systematic review and meta-analysis. Lancet Gastroenterol. Hepatol. 6 (5), 359–370. doi:10.1016/S2468-1253(21)00014-5
Benson, S., Engler, H., Wegner, A., Rebernik, L., Spreitzer, I., Schedlowski, M., et al. (2017). What makes you feel sick after inflammation? Predictors of acute and persisting physical sickness symptoms induced by experimental endotoxemia. Clin. Pharmacol. Ther. 102 (1), 141–151. doi:10.1002/cpt.618
Bittencourt, A., Brum, P. O., Ribeiro, C. T., Gasparotto, J., Bortolin, R. C., de Vargas, A. R., et al. (2022). High fat diet-induced obesity causes a reduction in brain tyrosine hydroxylase levels and non-motor features in rats through metabolic dysfunction, neuroinflammation and oxidative stress. Nutr. Neurosci. 25 (5), 1026–1040. doi:10.1080/1028415X.2020.1831261
Bollinger, J. L., Horchar, M. J., and Wohleb, E. S. (2020). Diazepam limits microglia-mediated neuronal remodeling in the prefrontal cortex and associated behavioral consequences following chronic unpredictable stress. Neuropsychopharmacology 45 (10), 1766–1776. doi:10.1038/s41386-020-0720-1
Bollini, P., Pampallona, S., Tibaldi, G., Kupelnick, B., and Munizza, C. (1999). Effectiveness of antidepressants. Meta-analysis of dose-effect relationships in randomised clinical trials. Br. J. Psychiatry 174, 297–303. doi:10.1192/bjp.174.4.297
Borges, C. G., Canani, C. R., Fernandes, C. G., Zanatta, A., Seminotti, B., Ribeiro, C. A., et al. (2015). Reactive nitrogen species mediate oxidative stress and astrogliosis provoked by in vivo administration of phytanic acid in cerebellum of adolescent rats: a potential contributing pathomechanism of cerebellar injury in peroxisomal disorders. Neuroscience 304, 122–132. doi:10.1016/j.neuroscience.2015.07.028
Calabrese, F., Rossetti, A. C., Racagni, G., Gass, P., Riva, M. A., and Molteni, R. (2014). Brain-derived neurotrophic factor: a bridge between inflammation and neuroplasticity. Front. Cell. Neurosci. 8, 430. doi:10.3389/fncel.2014.00430
Chang, N. C., Hung, S. I., Hwa, K. Y., Kato, I., Chen, J. E., Liu, C. H., et al. (2001). A macrophage protein, Ym1, transiently expressed during inflammation is a novel mammalian lectin. J. Biol. Chem. 276 (20), 17497–17506. doi:10.1074/jbc.M010417200
Cosma, N. C., Usekes, B., Otto, L. R., Gerike, S., Heuser, I., Regen, F., et al. (2021). M1/M2 polarization in major depressive disorder: disentangling state from trait effects in an individualized cell-culture-based approach. Brain Behav. Immun. 94, 185–195. doi:10.1016/j.bbi.2021.02.009
Crawford, A. A., Lewis, S., Nutt, D., Peters, T. J., Cowen, P., O'Donovan, M. C., et al. (2014). Adverse effects from antidepressant treatment: randomised controlled trial of 601 depressed individuals. Psychopharmacol. Berl. 231 (15), 2921–2931. doi:10.1007/s00213-014-3467-8
Cussotto, S., Aouizerate, B., and Capuron, L. (2021). From gut to brain: microbiota depletion in mice as a tool to explore causality. Brain Behav. Immun. 94, 4–5. doi:10.1016/j.bbi.2021.02.029
Dantzer, R., Cohen, S., Russo, S. J., and Dinan, T. G. (2018). Resilience and immunity. Brain Behav. Immun. 74, 28–42. doi:10.1016/j.bbi.2018.08.010
Dinan, T. G., and Cryan, J. F. (2019). Gut microbes and depression: still waiting for Godot. Brain Behav. Immun. 79, 1–2. doi:10.1016/j.bbi.2019.02.007
Du, R. H., Tan, J., Sun, X. Y., Lu, M., Ding, J. H., and Hu, G. (2016). Fluoxetine inhibits NLRP3 inflammasome activation: implication in depression. Int. J. Neuropsychopharmacol. 19 (9), pyw037. doi:10.1093/ijnp/pyw037
Duarte, L. J., Manassi, C., Magnani, M., Cruz, A. G. D., Pimentel, T. C., and Verruck, S. (2023). Lactiplantibacillus plantarum as a promising adjuvant for neurological disorders therapy through the brain-gut axis and related action pathways. Crit. Rev. Food Sci. Nutr. 11, 1–13. doi:10.1080/10408398.2023.2280247
Ducray, H. A. G., Globa, L., Pustovyy, O., Roberts, M. D., Rudisill, M., Vodyanoy, V., et al. (2020). Prevention of excessive exercise-induced adverse effects in rats with Bacillus subtilis BSB3. J. Appl. Microbiol. 128 (4), 1163–1178. doi:10.1111/jam.14544
Dudek, K. A., Dion-Albert, L., Kaufmann, F. N., Tuck, E., Lebel, M., and Menard, C. (2021). Neurobiology of resilience in depression: immune and vascular insights from human and animal studies. Eur. J. Neurosci. 53 (1), 183–221. doi:10.1111/ejn.14547
Fatima, S., Altwaijry, H., Abulmeaty, M. M. A., Abudawood, M., Siddiqi, N. J., Alrashoudi, R. H., et al. (2023). Combined supplementation of Clostridium butyricum and Bifidobacterium infantis diminishes chronic unpredictable mild stress-induced intestinal alterations via activation of nrf-2 signaling pathway in rats. Int. J. Mol. Sci. 24 (9), 8264. doi:10.3390/ijms24098264
Fitri, L. E., Sardjono, T. W., Winaris, N., Pawestri, A. R., Endharti, A. T., Norahmawati, E., et al. (2023). Bifidobacterium longum administration diminishes parasitemia and inflammation during Plasmodium berghei infection in mice. J. Inflamm. Res. 16, 1393–1404. doi:10.2147/JIR.S400782
Furukawa, Y., Hamza, T., Cipriani, A., Furukawa, T. A., Salanti, G., and Ostinelli, E. G. (2022). Optimal dose of aripiprazole for augmentation therapy of antidepressant-refractory depression: preliminary findings based on a systematic review and dose-effect meta-analysis. Br. J. Psychiatry 221 (2), 440–447. doi:10.1192/bjp.2021.165
Gao, H., Li, Y., Sun, J., Xu, H., Wang, M., Zuo, X., et al. (2021). Saccharomyces boulardii ameliorates dextran sulfate sodium-induced ulcerative colitis in mice by regulating NF-κB and Nrf2 signaling pathways. Oxid. Med. Cell. Longev. 28 (2021), 1622375. doi:10.1155/2021/1622375
Gao, K., Chen, C. L., Ke, X. Q., Yu, Y. X., Chen, S., Liu, G. C., et al. (2022). Ingestion of Lactobacillus helveticus WHH1889 improves depressive and anxiety symptoms induced by chronic unpredictable mild stress in mice. Benef. Microbes 13 (6), 473–488. doi:10.3920/BM2022.0052
Harbord, M., Novelli, M., Canas, B., Power, D., Davis, C., Godovac-Zimmermann, J., et al. (2002). Ym1 is a neutrophil granule protein that crystallizes in p47phox-deficient mice. J. Biol. Chem. 277 (7), 5468–5475. doi:10.1074/jbc.M110635200
Hayley, S., Scharf, J., and Anisman, H. (2013). Central administration of murine interferon-α induces depressive-like behavioral, brain cytokine and neurochemical alterations in mice: a mini-review and original experiments. Brain Behav. Immun. 31, 115–127. doi:10.1016/j.bbi.2012.07.023
Heinsbroek, R. P., Van Haaren, F., Van de Poll, N. E., and Steenbergen, H. L. (1991). Sex differences in the behavioral consequences of inescapable footshocks depend on time since shock. Physiol. Behav. 49 (6), 1257–1263. doi:10.1016/0031-9384(91)90360-z
Himmerich, H., Patsalos, O., Lichtblau, N., Ibrahim, M. A. A., and Dalton, B. (2019). Cytokine research in depression: principles, challenges, and open questions. Front. Psychiatry 10, 30. doi:10.3389/fpsyt.2019.00030
Huang, R., Wang, K., and Hu, J. (2016). Effect of probiotics on depression: a systematic review and meta-analysis of randomized controlled trials. Nutrients 8 (8), 483. doi:10.3390/nu8080483
Ji, C., Tang, Y., Zhang, Y., Li, C., Liang, H., Ding, L., et al. (2022). Microglial glutaminase 1 deficiency mitigates neuroinflammation associated depression. Brain Behav. Immun. 99, 231–245. doi:10.1016/j.bbi.2021.10.009
Kasala, E. R., Bodduluru, L. N., Maneti, Y., and Thipparaboina, R. (2014). Effect of meditation on neurophysiological changes in stress mediated depression. Complement. Ther. Clin. Pract. 20 (1), 74–80. doi:10.1016/j.ctcp.2013.10.001
Kosuge, A., Kunisawa, K., Arai, S., Sugawara, Y., Shinohara, K., Iida, T., et al. (2021). Heat-sterilized Bifidobacterium breve prevents depression-like behavior and interleukin-1β expression in mice exposed to chronic social defeat stress. Brain Behav. Immun. 96, 200–211. doi:10.1016/j.bbi.2021.05.028
Kraus, C., Castren, E., Kasper, S., and Lanzenberger, R. (2017). Serotonin and neuroplasticity - links between molecular, functional and structural pathophysiology in depression. Neurosci. Biobehav Rev. 77, 317–326. doi:10.1016/j.neubiorev.2017.03.007
Kwon, H., Lee, E. H., Choi, J., Park, J. Y., Kim, Y. K., and Han, P. L. (2023). Extracellular vesicles released by Lactobacillus paracasei mitigate stress-induced transcriptional changes and depression-like behavior in mice. Exp. Neurobiol. 32 (5), 328–342. doi:10.5607/en23024
Li, F., Xiang, H., Lu, J., Chen, Z., Huang, C., and Yuan, X. (2020). Lycopene ameliorates PTSD-like behaviors in mice and rebalances the neuroinflammatory response and oxidative stress in the brain. Physiol. Behav. 224, 113026. doi:10.1016/j.physbeh.2020.113026
Li, R., Song, M., Zheng, Y., Zhang, J., Zhang, S., and Fan, X. (2024). Naoxueshu oral liquid promotes hematoma absorption by targeting CD36 in M2 microglia via TLR4/MyD88/NF-κB signaling pathway in rats with intracerebral hemorrhage. J. Ethnopharmacol. 319 (Pt 1), 117116. doi:10.1016/j.jep.2023.117116
Liu, L., Wang, H., Zhang, H., Chen, X., Zhang, Y., Wu, J., et al. (2022). Toward a deeper understanding of gut microbiome in depression: the promise of clinical applicability. Adv. Sci. (Weinh) 9 (35), e2203707. doi:10.1002/advs.202203707
Liu, Y., Tang, W., Ji, C., Gu, J., Chen, Y., Huang, J., et al. (2021). The selective SIK2 inhibitor ARN-3236 produces strong antidepressant-like efficacy in mice via the hippocampal CRTC1-CREB-BDNF pathway. Front. Pharmacol. 11, 624429. doi:10.3389/fphar.2020.624429
Livak, K. J., and Schmittgen, T. D. (2001). Analysis of relative gene expression data using real-time quantitative PCR and the 2(-Delta Delta C(T)) Method. Methods 25 (4), 402–408. doi:10.1006/meth.2001.1262
Lowry, O. H., Rosebrough, N. J., Farr, A. L., and Randall, R. J. (1951). Protein measurement with the Folin phenol reagent. J. Biol. Chem. 193 (1), 265–275. doi:10.1016/s0021-9258(19)52451-6
Lu, X., Liu, H., Cai, Z., Hu, Z., Ye, M., Gu, Y., et al. (2022). ERK1/2-dependent BDNF synthesis and signaling is required for the antidepressant effect of microglia stimulation. Brain Behav. Immun. 106, 147–160. doi:10.1016/j.bbi.2022.08.005
Ma, J., Wang, J., Wang, G., Wan, Y., Li, N., Luo, L., et al. (2024). The potential beneficial effects of Lactobacillus plantarum GM11 on rats with chronic unpredictable mild stress-induced depression. Nutr. Neurosci. 27 (5), 413–424. doi:10.1080/1028415X.2023.2205742
Madrigal, J. L., Hurtado, O., Moro, M. A., Lizasoain, I., Lorenzo, P., Castrillo, A., et al. (2002). The increase in TNF-alpha levels is implicated in NF-kappaB activation and inducible nitric oxide synthase expression in brain cortex after immobilization stress. Neuropsychopharmacology 26 (2), 155–163. doi:10.1016/S0893-133X(01)00292-5
Maehata, H., Kobayashi, Y., Mitsuyama, E., Kawase, T., Kuhara, T., Xiao, J. Z., et al. (2019). Heat-killed Lactobacillus helveticus strain MCC1848 confers resilience to anxiety or depression-like symptoms caused by subchronic social defeat stress in mice. Biosci. Biotechnol. Biochem. 83 (7), 1239–1247. doi:10.1080/09168451.2019.1591263
Maes, M., Kubera, M., and Leunis, J. C. (2008). The gut-brain barrier in major depression: intestinal mucosal dysfunction with an increased translocation of LPS from gram negative enterobacteria (leaky gut) plays a role in the inflammatory pathophysiology of depression. Neuro Endocrinol. Lett. 29 (1), 117–124.
McFarland, L. V., Karakan, T., and Karatas, A. (2021). Strain-specific and outcome-specific efficacy of probiotics for the treatment of irritable bowel syndrome: a systematic review and meta-analysis. EClinicalMedicine 41, 101154. doi:10.1016/j.eclinm.2021.101154
Moller, H. J., Baldwin, D. S., Goodwin, G., Kasper, S., Okasha, A., Stein, D. J., et al. (2008). Do SSRIs or antidepressants in general increase suicidality? WPA Section on Pharmacopsychiatry: consensus statement. Eur. Arch. Psychiatry Clin. Neurosci. 258 (Suppl. 3), 3–23. doi:10.1007/s00406-008-3002-1
Morris, G., Reiche, E. M. V., Murru, A., Carvalho, A. F., Maes, M., Berk, M., et al. (2018). Multiple immune-inflammatory and oxidative and nitrosative stress pathways explain the frequent presence of depression in multiple sclerosis. Mol. Neurobiol. 55 (8), 6282–6306. doi:10.1007/s12035-017-0843-5
Mulaw, G., Muleta, D., Tesfaye, A., and Sisay, T. (2020). Protective effect of potential probiotic strains from fermented Ethiopian food against Salmonella typhimurium DT104 in mice. Int. J. Microbiol. 13 (2020), 7523629. doi:10.1155/2020/7523629
Nowak, W., Grendas, L. N., Sanmarco, L. M., Estecho, I. G., Arena, A. R., Eberhardt, N., et al. (2019). Pro-inflammatory monocyte profile in patients with major depressive disorder and suicide behaviour and how ketamine induces anti-inflammatory M2 macrophages by NMDAR and mTOR. EBioMedicine 50, 290–305. doi:10.1016/j.ebiom.2019.10.063
Önning, G., Montelius, C., Hillman, M., and Larsson, N. (2023). Intake of Lactiplantibacillus plantarum HEAL9 improves cognition in moderately stressed subjects: a randomized controlled study. Nutrients 15 (15), 3466. doi:10.3390/nu15153466
Osimo, E. F., Pillinger, T., Rodriguez, I. M., Khandaker, G. M., Pariante, C. M., and Howes, O. D. (2020). Inflammatory markers in depression: a meta-analysis of mean differences and variability in 5,166 patients and 5,083 controls. Brain Behav. Immun. 87, 901–909. doi:10.1016/j.bbi.2020.02.010
Park, C., Brietzke, E., Rosenblat, J. D., Musial, N., Zuckerman, H., Ragguett, R. M., et al. (2018). Probiotics for the treatment of depressive symptoms: an anti-inflammatory mechanism? Brain Behav. Immun. 73, 115–124. doi:10.1016/j.bbi.2018.07.006
Partrick, K. A., Rosenhauer, A. M., Auger, J., Arnold, A. R., Ronczkowski, N. M., Jackson, L. M., et al. (2021). Ingestion of probiotic (Lactobacillus helveticus and Bifidobacterium longum) alters intestinal microbial structure and behavioral expression following social defeat stress. Sci. Rep. 11 (1), 3763. doi:10.1038/s41598-021-83284-z
Perry, B. I., Upthegrove, R., Kappelmann, N., Jones, P. B., Burgess, S., and Khandaker, G. M. (2021). Associations of immunological proteins/traits with schizophrenia, major depression and bipolar disorder: a bi-directional two-sample mendelian randomization study. Brain Behav. Immun. 97, 176–185. doi:10.1016/j.bbi.2021.07.009
Price, R. B., and Duman, R. (2020). Neuroplasticity in cognitive and psychological mechanisms of depression: an integrative model. Mol. Psychiatry 25 (3), 530–543. doi:10.1038/s41380-019-0615-x
Raes, G., De Baetselier, P., Noël, W., Beschin, A., Brombacher, F., and Hassanzadeh, Gh G. (2002). Differential expression of FIZZ1 and Ym1 in alternatively versus classically activated macrophages. J. Leukoc. Biol. 71 (4), 597–602. doi:10.1189/jlb.71.4.597
Ramalho, J. B., Spiazzi, C. C., Bicca, D. F., Rodrigues, J. F., Sehn, C. P., da Silva, W. P., et al. (2022). Beneficial effects of Lactococcus lactis subsp. cremoris LL95 treatment in an LPS-induced depression-like model in mice. Behav. Brain Res. 426, 113847. doi:10.1016/j.bbr.2022.113847
Reyes-Martinez, S., Segura-Real, L., Gomez-Garcia, A. P., Tesoro-Cruz, E., Constantino-Jonapa, L. A., Amedei, A., et al. (2023). Neuroinflammation, microbiota-gut-brain Axis, and depression: the vicious circle. J. Integr. Neurosci. 22 (3), 65. doi:10.31083/j.jin2203065
Rezaie, N., Aghamohammad, S., Haj Agha Gholizadeh Khiavi, E., Khatami, S., Sohrabi, A., and Rohani, M. (2024). The comparative anti-oxidant and anti-inflammatory efficacy of postbiotics and probiotics through Nrf-2 and NF-kB pathways in DSS-induced colitis model. Sci. Rep. 14 (1), 11560. doi:10.1038/s41598-024-62441-0
Rode, J., Edebol Carlman, H. M. T., König, J., Repsilber, D., Hutchinson, A. N., Thunberg, P., et al. (2022). Probiotic mixture containing Lactobacillus helveticus, Bifidobacterium longum and Lactiplantibacillus plantarum affects brain responses toward an emotional task in healthy subjects: a randomized clinical trial. Front. Nutr. 9, 827182. doi:10.3389/fnut.2022.827182
Rongzhu, L., Suhua, W., Guangwei, X., Chunlan, R., Fangan, H., Suxian, C., et al. (2009). Effects of acrylonitrile on antioxidant status of different brain regions in rats. Neurochem. Int. 55 (7), 552–557. doi:10.1016/j.neuint.2009.05.009
Sampson, T. R., and Mazmanian, S. K. (2015). Control of brain development, function, and behavior by the microbiome. Cell. Host Microbe 17 (5), 565–576. doi:10.1016/j.chom.2015.04.011
Sarkawi, M., Raja Ali, R. A., Abdul Wahab, N., Abdul Rathi, N. D., and Mokhtar, N. M. (2024). A randomized, double-blinded, placebo-controlled clinical trial on Lactobacillus-containing cultured milk drink as adjuvant therapy for depression in irritable bowel syndrome. Sci. Rep. 14 (1), 9478. doi:10.1038/s41598-024-60029-2
Simpson, C. A., Diaz-Arteche, C., Eliby, D., Schwartz, O. S., Simmons, J. G., and Cowan, C. S. M. (2021). The gut microbiota in anxiety and depression - a systematic review. Clin. Psychol. Rev. 83, 101943. doi:10.1016/j.cpr.2020.101943
Smail, M. A., Smith, B. L., Nawreen, N., and Herman, J. P. (2020). Differential impact of stress and environmental enrichment on corticolimbic circuits. Pharmacol. Biochem. Behav. 197, 172993. doi:10.1016/j.pbb.2020.172993
Smith, C. J., Emge, J. R., Berzins, K., Lung, L., Khamishon, R., Shah, P., et al. (2014). Probiotics normalize the gut-brain-microbiota axis in immunodeficient mice. Am. J. Physiol. Gastrointest. Liver Physiol. 307 (8), G793–G802. doi:10.1152/ajpgi.00238.2014
Stenman, L. K., Patterson, E., Meunier, J., Roman, F. J., and Lehtinen, M. J. (2020). Strain specific stress-modulating effects of candidate probiotics: a systematic screening in a mouse model of chronic restraint stress. Behav. Brain Res. 379, 112376. doi:10.1016/j.bbr.2019.112376
Sulakhiya, K., Kumar, P., Jangra, A., Dwivedi, S., Hazarika, N. K., Baruah, C. C., et al. (2014). Honokiol abrogates lipopolysaccharide-induced depressive like behavior by impeding neuroinflammation and oxido-nitrosative stress in mice. Eur. J. Pharmacol. 744, 124–131. doi:10.1016/j.ejphar.2014.09.049
Sun, N., Ni, X., Wang, H., Xin, J., Zhao, Y., Pan, K., et al. (2020). Probiotic Lactobacillus johnsonii BS15 prevents memory dysfunction induced by chronic high-fluorine intake through modulating intestinal environment and improving gut development. Probiotics Antimicrob. Proteins 12 (4), 1420–1438. doi:10.1007/s12602-020-09644-9
Sushma, G., Vaidya, B., Sharma, S., Devabattula, G., Bishnoi, M., Kondepudi, K. K., et al. (2023). Bifidobacterium breve Bif11 supplementation improves depression-related neurobehavioural and neuroinflammatory changes in the mouse. Neuropharmacology 229, 109480. doi:10.1016/j.neuropharm.2023.109480
Tang, W. Q., Liu, Y., Ji, C. H., Gu, J. H., Chen, Y. M., Huang, J., et al. (2022). Virus-mediated decrease of LKB1 activity in the mPFC diminishes stress-induced depressive-like behaviors in mice. Biochem. Pharmacol. 197, 114885. doi:10.1016/j.bcp.2021.114885
Tian, P., Chen, Y., Zhu, H., Wang, L., Qian, X., Zou, R., et al. (2022). Bifidobacterium breve CCFM1025 attenuates major depression disorder via regulating gut microbiome and tryptophan metabolism: a randomized clinical trial. Brain Behav. Immun. 100, 233–241. doi:10.1016/j.bbi.2021.11.023
Tian, P., O'Riordan, K. J., Lee, Y. K., Wang, G., Zhao, J., Zhang, H., et al. (2020). Towards a psychobiotic therapy for depression: Bifidobacterium breve CCFM1025 reverses chronic stress-induced depressive symptoms and gut microbial abnormalities in mice. Neurobiol. Stress 12, 100216. doi:10.1016/j.ynstr.2020.100216
Tian, P., Wang, G., Zhao, J., Zhang, H., and Chen, W. (2019). Bifidobacterium with the role of 5-hydroxytryptophan synthesis regulation alleviates the symptom of depression and related microbiota dysbiosis. J. Nutr. Biochem. 66, 43–51. doi:10.1016/j.jnutbio.2019.01.007
Tong, L., Gong, Y., Wang, P., Hu, W., Wang, J., Chen, Z., et al. (2017). Microglia loss contributes to the development of major depression induced by different types of chronic stresses. Neurochem. Res. 42 (10), 2698–2711. doi:10.1007/s11064-017-2270-4
Varesi, A., Campagnoli, L. I. M., Chirumbolo, S., Candiano, B., Carrara, A., Ricevuti, G., et al. (2023). The brain-gut-microbiota interplay in depression: a key to design innovative therapeutic approaches. Pharmacol. Res. 192, 106799. doi:10.1016/j.phrs.2023.106799
Viau, V., Bingham, B., Davis, J., Lee, P., and Wong, M. (2005). Gender and puberty interact on the stress-induced activation of parvocellular neurosecretory neurons and corticotropin-releasing hormone messenger ribonucleic acid expression in the rat. Endocrinology 146 (1), 137–146. doi:10.1210/en.2004-0846
Wang, J., Lai, S., Zhou, T., Xia, Z., Li, W., Sha, W., et al. (2022b). Progranulin from different gliocytes in the nucleus accumbens exerts distinct roles in FTD- and neuroinflammation-induced depression-like behaviors. J. Neuroinflammation 19 (1), 318. doi:10.1186/s12974-022-02684-8
Wang, L., Wang, R., Liu, L., Qiao, D., Baldwin, D. S., and Hou, R. (2019). Effects of SSRIs on peripheral inflammatory markers in patients with major depressive disorder: a systematic review and meta-analysis. Brain Behav. Immun. 79, 24–38. doi:10.1016/j.bbi.2019.02.021
Wang, Y., Gu, J. H., Liu, L., Liu, Y., Tang, W. Q., Ji, C. H., et al. (2021). Hippocampal PPARα plays a role in the pharmacological mechanism of vortioxetine, a multimodal-acting antidepressant. Front. Pharmacol. 12, 673221. doi:10.3389/fphar.2021.673221
Wang, Y., Hu, Z., Liu, H., Gu, Y., Ye, M., Lu, Q., et al. (2022a). Adolescent microglia stimulation produces long-lasting protection against chronic stress-induced behavioral abnormalities in adult male mice. Brain Behav. Immun. 105, 44–66. doi:10.1016/j.bbi.2022.06.015
Ward, J. M., Yoon, M., Anver, M. R., Haines, D. C., Kudo, G., Gonzalez, F. J., et al. (2001). Hyalinosis and Ym1/Ym2 gene expression in the stomach and respiratory tract of 129S4/SvJae and wild-type and CYP1A2-null B6, 129 mice. Am. J. Pathol. 158 (1), 323–332. doi:10.1016/S0002-9440(10)63972-7
Wei, J., Carroll, R. J., Harden, K. K., and Wu, G. (2012). Comparisons of treatment means when factors do not interact in two-factorial studies. Amino Acids 42 (5), 2031–2035. doi:10.1007/s00726-011-0924-0
Williams, N. T. (2010). Probiotics. Am. J. Health Syst. Pharm. 67 (6), 449–458. doi:10.2146/ajhp090168
Wu, Z. H., Fan, H., Gao, S. Y., Jin, Y. F., Chen, C., Jiang, B., et al. (2022). Antidepressant-like activity of oroxylin A in mice models of depression: a behavioral and neurobiological characterization. Front. Pharmacol. 13, 921553. doi:10.3389/fphar.2022.921553
Xiao, F., Dong, F., Li, X., Li, Y., Yu, G., Liu, Z., et al. (2022). Bifidobacterium longum CECT 7894 improves the efficacy of infliximab for DSS-induced colitis via regulating the gut microbiota and bile acid metabolism. Front. Pharmacol. 13, 902337. doi:10.3389/fphar.2022.902337
Xu, F. R., Wei, Z. H., Xu, X. X., Zhang, X. G., Wei, C. J., Qi, X. M., et al. (2023). The hypothalamic steroidogenic pathway mediates susceptibility to inflammation-evoked depression in female mice. J. Neuroinflammation 20 (1), 293. doi:10.1186/s12974-023-02976-7
Xu, M., Tian, P., Zhu, H., Zou, R., Zhao, J., Zhang, H., et al. (2022). Lactobacillus paracasei CCFM1229 and Lactobacillus rhamnosus CCFM1228 alleviated depression- and anxiety-related symptoms of chronic stress-induced depression in mice by regulating xanthine oxidase activity in the brain. Nutrients 14 (6), 1294. doi:10.3390/nu14061294
Yang, R., Wang, P., Chen, Z., Hu, W., Gong, Y., Zhang, W., et al. (2017). WY-14643, a selective agonist of peroxisome proliferator-activated receptor-α, ameliorates lipopolysaccharide-induced depressive-like behaviors by preventing neuroinflammation and oxido-nitrosative stress in mice. Pharmacol. Biochem. Behav. 153, 97–104. doi:10.1016/j.pbb.2016.12.010
Yue, Y., Huang, W., Liang, J., Guo, J., Ji, J., Yao, Y., et al. (2015). IL4I1 is a novel regulator of M2 macrophage polarization that can inhibit T cell activation via L-tryptophan and arginine depletion and IL-10 production. PLoS One 10 (11), e0142979. doi:10.1371/journal.pone.0142979
Zhang, W., Rutlin, J., Eisenstein, S. A., Wang, Y., Barch, D. M., Hershey, T., et al. (2023). Neuroinflammation in the amygdala is associated with recent depressive symptoms. Biol. Psychiatry Cogn. Neurosci. Neuroimaging 8 (9), 967–975. doi:10.1016/j.bpsc.2023.04.011
Zhu, L., Qiao, L., Dou, X., Song, X., Chang, J., Zeng, X., et al. (2023b). Lactobacillus casei ATCC 393 combined with vasoactive intestinal peptide alleviates dextran sodium sulfate-induced ulcerative colitis in C57BL/6 mice via NF-κB and Nrf2 signaling pathways. Biomed. Pharmacother. 165, 115033. doi:10.1016/j.biopha.2023.115033
Zhu, R., Fang, Y., Li, H., Liu, Y., Wei, J., Zhang, S., et al. (2023a). Psychobiotic Lactobacillus plantarum JYLP-326 relieves anxiety, depression, and insomnia symptoms in test anxious college via modulating the gut microbiota and its metabolism. Front. Immunol. 14, 1158137. doi:10.3389/fimmu.2023.1158137
Keywords: probiotic, depression, neuroinflammation, oxido-nitrosative stress, chronic stress
Citation: Ye M, Ji F, Huang C, Li F, Zhang C, Zhang Y, Wang R, Ma K, Lu X and Wang H (2024) A novel probiotic formula, BLLL, ameliorates chronic stress-induced depression-like behaviors in mice by reducing neuroinflammation and increasing neurotrophic factors. Front. Pharmacol. 15:1398292. doi: 10.3389/fphar.2024.1398292
Received: 09 March 2024; Accepted: 08 July 2024;
Published: 26 July 2024.
Edited by:
Karolina Pytka, Jagiellonian University Medical College, PolandReviewed by:
Eduardo Duarte-Silva, Northwestern University, United StatesSylvie Vancassel, INRA Centre Bordeaux-Aquitaine, France
Copyright © 2024 Ye, Ji, Huang, Li, Zhang, Zhang, Wang, Ma, Lu and Wang. This is an open-access article distributed under the terms of the Creative Commons Attribution License (CC BY). The use, distribution or reproduction in other forums is permitted, provided the original author(s) and the copyright owner(s) are credited and that the original publication in this journal is cited, in accordance with accepted academic practice. No use, distribution or reproduction is permitted which does not comply with these terms.
*Correspondence: Xu Lu, bHV4dTYwN0BudHUuZWR1LmNu; Hui Wang, d2FuZ2h1aXBoQG91dGxvb2suY29t
†These authors have contributed equally to this work and share first authorship