- 1School of Pharmacy/School of Modern Chinese Medicine Industry, Chengdu University of Traditional Chinese Medicine, Chengdu, China
- 2State Key Laboratory of Southwestern Chinese Medicine Resources, Chengdu University of Traditional Chinese Medicine, Chengdu, China
Backgrounds: Ulcerative colitis (UC) is a form of chronic inflammatory bowel disease, and UC diagnosis rates continue to rise throughout the globe. The research and development of new drugs for the treatment of UC are urgent, and natural compounds are an important source. However, there is a lack of systematic summarization of natural compounds and their mechanisms for the treatment of UC.
Methods: We reviewed the literature in the databases below from their inception until July 2023: Web of Science, PubMed, China National Knowledge Infrastructure, and Wanfang Data, to obtain information on the relationship between natural compounds and UC.
Results: The results showed that 279 natural compounds treat UC through four main mechanisms, including regulating gut microbiota and metabolites (Mechanism I), protecting the intestinal mucosal barrier (Mechanism II), regulating intestinal mucosal immune response (Mechanism III), as well as regulating other mechanisms (Mechanism Ⅳ) such as cellular autophagy modulation and ferroptosis inhibition. Of these, Mechanism III is regulated by all natural compounds. The 279 natural compounds, including 62 terpenoids, 57 alkaloids, 52 flavonoids, 26 phenols, 19 phenylpropanoids, 9 steroids, 9 saponins, 8 quinonoids, 6 vitamins, and 31 others, can effectively ameliorate UC. Of these, terpenoids, alkaloids, and flavonoids have the greatest potential for treating UC. It is noteworthy to highlight that a total of 54 natural compounds exhibit their therapeutic effects by modulating Mechanisms I, II, and III.
Conclusion: This review serves as a comprehensive resource for the pharmaceutical industry, researchers, and clinicians seeking novel therapeutic approaches to combat UC. Harnessing the therapeutic potential of these natural compounds may significantly contribute to the improvement of the quality of life of patients with UC and promotion of disease-modifying therapies in the future.
1 Introduction
Ulcerative colitis (UC) is an idiopathic, chronic, inflammatory bowel disease (IBD) characterized by continuous inflammation starting from the rectum (Hoivik et al., 2013; Conrad et al., 2014; Nanki et al., 2019). World Health Organization has classified UC as a clinically intractable disease. Its global prevalence and incidence have been increasing with time; currently, its incidence and prevalence are 8–10 cases/100,000 subjects and 150–200 cases/100,000 subjects, respectively (da Silva et al., 2014; Ungaro et al., 2017). The annual UC treatment costs (direct and indirect) are estimated to be approximately US$8.1–14.9 billion and €12.5–29.1 billion in the United States and Europe, respectively (Cohen et al., 2010).
UC is primarily treated with medicines, including aminosalicylates, immunomodulators, steroids, and biologics. However, due to potential adverse reactions and reduced efficiency of standard therapies, a comprehensive search for the identification of novel and natural medicines has been initiated to replace or complement present treatment options (Pastorelli et al., 2009; Wan et al., 2014). Many researchers are now turning to natural resources to seek effective compounds that can be used against UC (Cao et al., 2019).
Currently, there are some reviews on natural compounds and UC, such as summarizing some natural compounds or a class of compounds. These studies are significant for finding drugs for UC, but there is still a lack of systematic summaries. Therefore, this study reviews the current progress made in the intervention of natural compounds in UC, and provides a complete overview of natural compounds and their mechanisms of action. More importantly, we hope that such a systematic summary will lead to important natural compounds and mechanisms of action for the treatment of UC. This review serves as a comprehensive resource for the pharmaceutical industry, researchers, and clinicians seeking novel therapeutic approaches to combat UC. Harnessing the therapeutic potential of these natural compounds may significantly contribute to the improvement of the quality of life of patients with UC and promotion of disease-modifying therapies in the future.
2 The etiology of UC
The most accepted hypothesis states that UC pathogenesis comprises complex communications between, external, immunological, and gut microbial factors in a genetically susceptible host (Figure 1) (Abraham et al., 2017; Glassner et al., 2020).
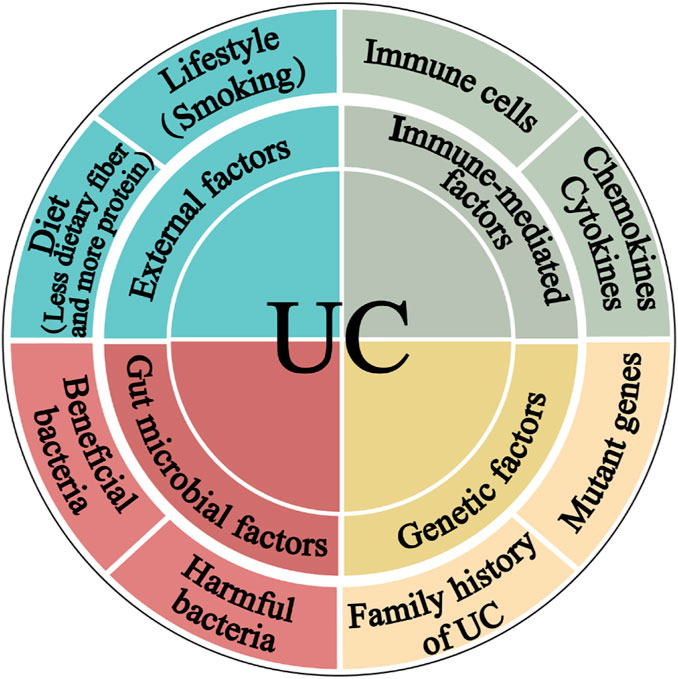
Figure 1. The pathogenesis of ulcerative colitis. The four main components linked to epithelial barrier abnormalities that drive the ulcerative colitis pathogenic mechanism are external factors, immune dysregulation, gut microbiota, and genetic inheritance.
2.1 External factors
The diet structure of modern people is constantly changing. People are gradually consuming less dietary fiber, whereas increasing meat, egg, and milk product intake, is the main reason for the increase in the prevalence of UC. Furthermore, although smoking cigarettes is a critical Crohn’s disease (CD) risk factor, quitting it has been linked to UC. According to a meta-analysis, smoking is more protective against UC than not smoking (Mahid et al., 2006). UC individuals who smoked had a milder disease course than non-smokers. UC is harsher for those who stop smoking. It may be mediated by carbon monoxide that can suppress interleukin-10 (IL-10) through a heme oxygenase (HO)-1-dependent pathway in UC mice (Sheikh et al., 2011).
2.2 Immune-mediated factors
The immune response is intricately associated with the pathophysiology of UC. The buildup of innate lymphoid cells (ILC), natural killer (NK) cells, macrophages, dendritic cells, neutrophils, and abnormal T and B cells inside the intestinal mucosa, along with the production of chemokines and cytokines that may trigger an inflammatory response. This inflammatory process can lead to the disruption of the intestinal mucosa and ultimately result in the development of UC (Liu Y. et al., 2022).
2.3 Gut microbial factors
The gut microbiota directly impacts the maintenance of homeostasis in the intestinal pro-inflammatory and anti-inflammatory responses. Germ-free conditions prevent the development of colitis in genetically susceptible mice (Veltkamp et al., 2001). Moreover, the introduction of proinflammatory bacteria or microbiota from patients with UC into healthy mice can induce inflammation (Ohkusa et al., 2003), while colonization of mice with intestinal microbiota from donors with IBD exacerbates colitis by modulating immune responses (Britton et al., 2019).
2.4 Genetic factors
Genetic factors have also been linked with UC. 12% of UC patients have a family history of IBD (Childers et al., 2014). Genome-wide association studies have identified 200 risk loci for IBD to date, with most genes contributing to both UC and CD phenotypes (Jostins et al., 2012; Liu et al., 2015). Examples of loci associated with increased UC susceptibility include human leukocyte antigen and genes associated with barrier function, such as HNF4A and CDH1 (Consortium et al., 2009). In addition, with increasing knowledge about UC pathogenesis, natural compounds have become a research hotspot because of their more efficient application prospects for preventing and mitigating UC occurrence and development.
3 The mechanism of natural compounds in intervention UC
We reviewed the scientific papers in the databases below from their inception to July 2023 to identify the studies relevant to the mechanism and activity of natural compounds against UC: PubMed, Web of Science, Wanfang Data, and the China National Knowledge Infrastructure. The present study provides a comprehensive summary of 279 natural compounds demonstrated to treat UC through various mechanisms primarily. These mechanisms include regulating gut microbiota and metabolites (Mechanism I), protecting the intestinal mucosal barrier (Mechanism II), regulating intestinal mucosal immune response (Mechanism III), as well as the other mechanisms (Mechanism Ⅳ) such as cellular autophagy modulation and ferroptosis inhibition (as depicted in Figure 2; Supplementary Table S1). It is noteworthy to highlight that Mechanism III is regulated by all natural compounds; Mechanisms II and III can be modulated by at least half of the compounds. Research on these mechanisms may give information on the etiology of UC.
3.1 Regulating gut microbiota and metabolites
The available evidence indicates that UC is an increased immune response in the mucosal lining, which is triggered by an imbalance in particular gut bacteria. This condition is defined by an abnormal composition of the microbiota and the presence of bacterial products. According to the data shown in Supplementary Table S1, there has been extensive research conducted on natural compounds to investigate their prebiotic qualities. These compounds have been found to have an impact on the makeup of the microbiota and its metabolites, as well as the prevention of colonization by intestinal pathogens and the reduction of the risk of recurrence of ulcerative colitis, as illustrated in Figure 3.
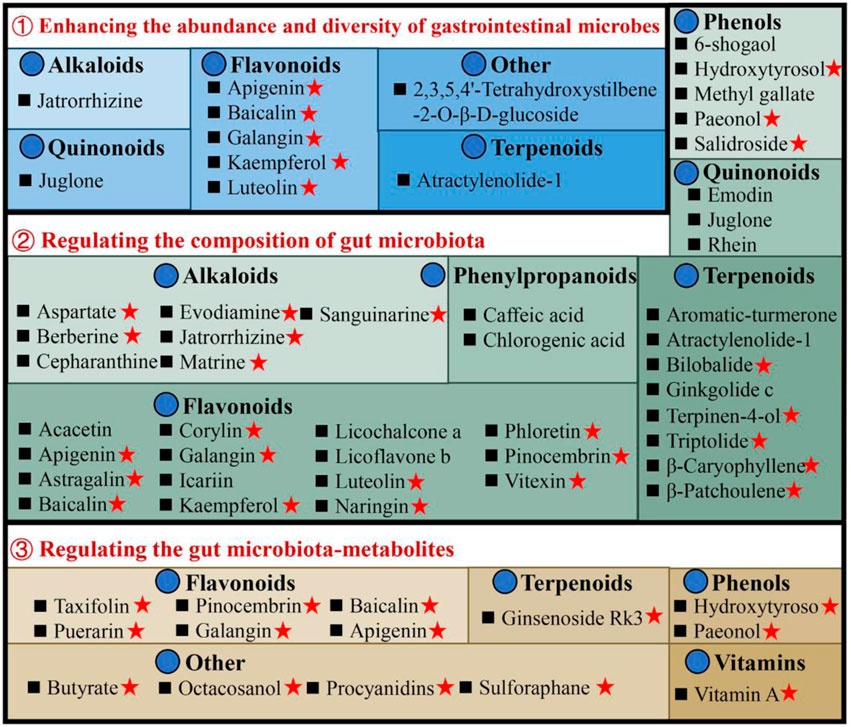
Figure 3. Illustrates the utilization of natural compounds in the management of ulcerative colitis through the modulation of gut microbiota and metabolites. Red pentagrams indicate compounds involved in Mechanism Ⅰ, Ⅱ, and Ⅲ. The natural compounds involved in this paper are shown in Supplementary Table S1.
3.1.1 Enhancing the abundance and diversity of gastrointestinal microbes
Patients with UC exhibit a diminished diversity of gut microbes, and an imbalance in the composition of the microbiome continues throughout the progression of the disease (Fang et al., 2021). Microbial diversity and community richness of species are reflected by Shannon and Simpson’s indexes and abundance-based coverage estimator (ACE) and Chao1 indexes, respectively (Wang et al., 2022). Research shows that atractylenolide Ⅰ (Qu et al., 2022), kaempferol (Qu et al., 2021), and 6-shogaol (Wei et al., 2022) indicated a substantial elevation of the Shannon, Simpson, and Chao1 indexes in the UC mice intestinal flora. Ginkgolide C (Xu et al., 2022), hydroxytyrosol (Miao, 2022), jatrorrhizine (Zhang et al., 2022), luteolin (Li et al., 2021), and sauchinone (Wu et al., 2023) enhanced the diversity and abundance of UC intestinal flora by increasing Chao1 and Shannon indexes. Whereas apigenin (Fu et al., 2022), berberine (Wei et al., 2022), docosapentaenoic acid (Dong et al., 2022a), ginsenoside Rg1 (Cheng et al., 2022; Long et al., 2022), and 2,3,5,4′-tetrahydroxystilbene-2-O-β-D-glucoside (He et al., 2021) elevated the abundance and diversity by Chao1 and ACE indexes upregulation.
3.1.2 Regulating the composition of gut microbiota
The composition of gut microbiota in individuals with UC or animal models has exhibited considerable heterogeneity across different studies. In general, when comparing the microbiota of individuals or animals in good health, it is observed that there is a reduction in the abundance of beneficial bacteria and an increase in the prevalence of harmful bacteria. Seven alkaloids [aspartate (Hu et al., 2022), berberine (Li et al., 2022a), cepharanthine (Wang et al., 2022), evodiamine (Wang et al., 2020), jatrorrhizine (Zhang et al., 2022), matrine (Yao et al., 2021), and sanguinarine (Li et al., 2022b)], 15 flavonoids [acacetin (Ren et al., 2021), apigenin (Fu et al., 2022), astragalin (Peng et al., 2020), corylin (Wang et al., 2023), galangin (Xuan et al., 2020), icariin (Zhang et al., 2021a), kaempferol (Qu et al., 2021), luteolin (Li et al., 2021), licoflavone B (Zhang et al., 2022b), licochalcone A (Zhang et al., 2021), α-mangostin (Gutierrez-Orozco et al., 2014), naringin (Cao et al., 2021), pinocembrin (Yue et al., 2020), phloretin (Wu et al., 2019), and vitexin (Zhang et al., 2022c)], 6 phenols [hydroxytyrosol (Miao, 2022), methyl gallate (Zhou et al., 2022), paeonol (Zheng et al., 2022), prim-O-Glucosylcimifugin (Yin et al., 2022), salidroside (Liu et al., 2023), and 6-shogaol (Wei et al., 2022)], 2 quinonoids [juglone (Hua et al., 2021), rhein (Dong et al., 2022)], 8 terpenoids [atractylenolide-1 (Qu et al., 2022), aromatic-turmerone (Li et al., 2022), bilobalide (Zhang et al., 2021c), β-caryophyllene (Yeom et al., 2022), ginkgolide C (Xu et al., 2022), β-patchoulene (Liu et al., 2020), triptolide (Wu et al., 2020) and terpinen-4-ol (Zhang et al., 2017a)], 2 phenylpropanoids [caffeic acid (Zhang et al., 2016), chlorogenic acid (Niu et al., 2022)] could stimulates the propagation of beneficial bacteria and reduces some pathogenic bacteria. For instance, the administration of berberine in mice with DSS-induced UC has been found to induce a range of protective effects (Zhang et al., 2017; Jiang et al., 2021; Zheng et al., 2021; Li et al., 2022a). These effects include the mitigation of colon inflammation and oxidative stress, restoration of the epithelial barrier’s functionality, and improvement of the gut microenvironment. Specifically, berberine supplementation has been observed to increase the abundance of Bacillibacteria, Bacteroides fragilis, Eubacterium, Lactobacillales, and Lactobacillus/Lactococcus. Conversely, it has been found to decrease the levels of Akkermansia muciniphila, Bacteroides, Desulfovibrio, Enterobacteriaceae, Segmented flamentous bacteria, Verrucomicrobiae, and Verrucomicrobiales.
3.1.3 Regulating the gut microbiota-metabolites
The metabolites of gut microbiota, including tryptophan, bile acids, and short-chain fatty acids (SCFAs), affect UC development. Most of the current research has focused primarily on the effects of SCFAs. The research indicates reduced SCFA-producing bacteria, including Clostridium clusters IV and XIVb, Faecalibacterium, Leuconostocaceae, Odoribacter, and Roseburia in UC patients (Kostic et al., 2014). Moreover, recently many natural compounds, for instance, apigenin (Fu et al., 2022), baicalin (Zhu et al., 2020), evodiamine (Shen et al., 2019), galangin (Xuan et al., 2020), ginsenoside Rg1 (Long et al., 2022), hydroxytyrosol (Miao, 2022), octacosanol (Miao et al., 2022), pinocembrin (Hu et al., 2019), paeonol (Zheng et al., 2022), procyanidins (Huang et al., 2022), sulforaphane (Zhang et al., 2020), and vitamin A (Pang et al., 2021), could increase SCFA-producing bacteria in UC models. For example, taxifolin can ameliorate DSS-induced colitis by altering gut microbiota to increase the production of SCFAs (Li et al., 2022). Furthermore, SCFAs function by stimulating G-protein-coupled receptors (GPCR) and suppressing histone deacetylases (Kostic et al., 2014). It is reported that taxifolin can increase the level of GPR41 and GPR43 in the colon, and increase the level of the content of SCFAs, thereby reducing DSS-induced intestinal inflammatory reaction and protecting the intestinal mucosa (Li et al., 2022).
The present study has specifically examined the impact of pharmaceutical substances on the composition and diversity of the gastrointestinal microbiota. However, the gut microbiota exerts a significant influence on the chemical alteration, pharmacological action, and metabolic mechanisms of natural compounds (Zhao et al., 2022a). Certain gut microorganisms possess the ability to break down and convert organic substances, resulting in the production of metabolites and functional chemicals that exhibit physiological actions that are not naturally generated by the host organism (Koppel et al., 2017). There is currently a significant amount of research being dedicated to comprehending the distinct ways in which microorganisms alter natural products and the consequent effects of these metabolites on the health of the host organism (Luca et al., 2020). This is a matter that warrants further investigation in our research.
3.2 Protecting intestinal mucosal barrier
The intestinal mucosal barrier damage is a crucial UC characteristic (Ungaro et al., 2017). Complete healing of intestinal mucosa is the most desired goal in UC treatment (Du et al., 2020). As shown in Supplementary Table S1, natural compounds can improve the barrier function of the UC mucosa through multiple perspectives, these include upregulation of the expression of tight junction protein, reduction in the intestinal mucosal, permeability, regulation of the intestinal mucus function, reduction of oxidative stress, and protection of the intestinal epithelial cells (Figure 4).
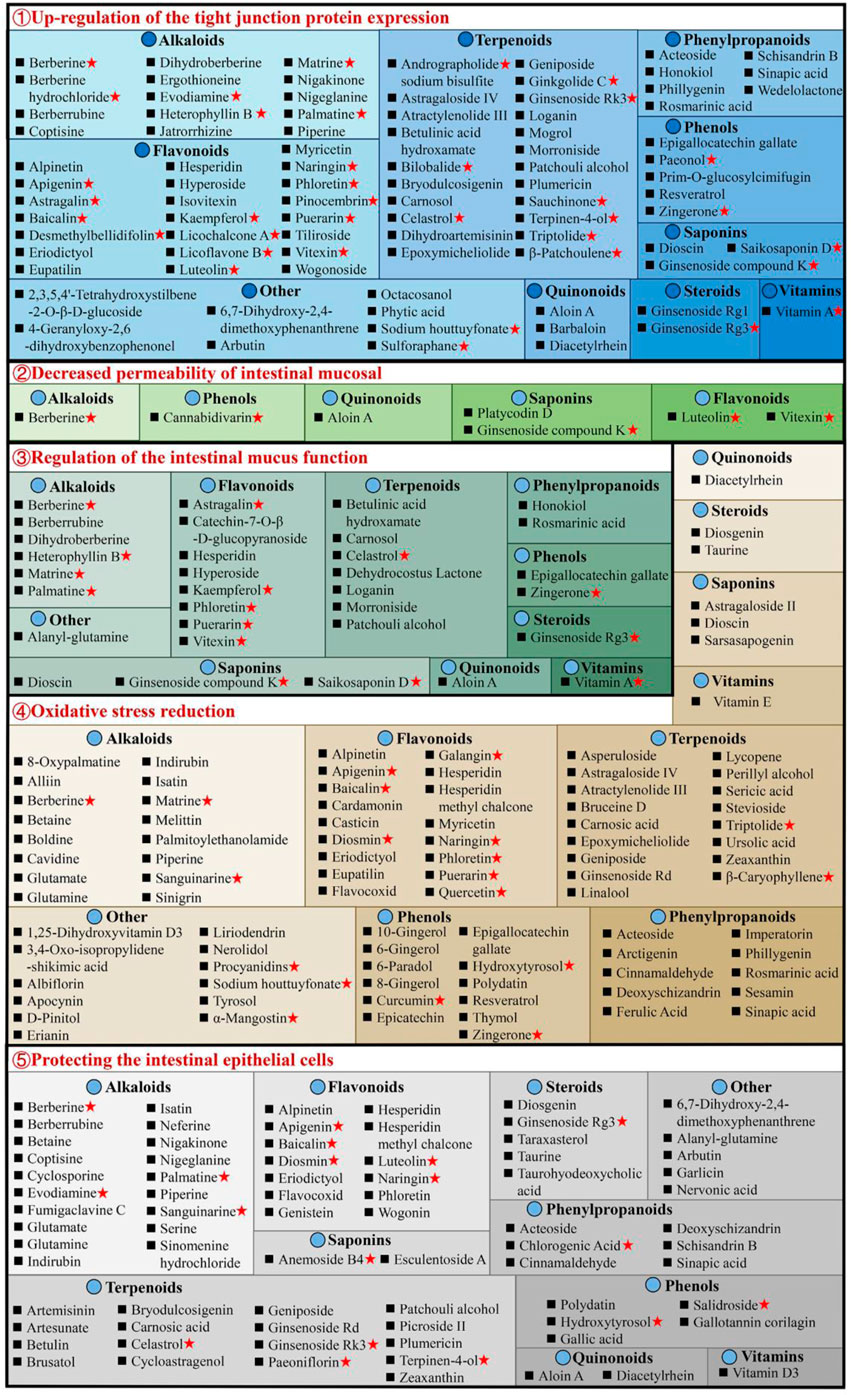
Figure 4. Natural compounds against ulcerative colitis via intestinal mucosal barrier protection. Red pentagrams indicate compounds involved in Mechanism Ⅰ, Ⅱ, and Ⅲ. The natural compounds involved in this paper are shown in Supplementary Table S1.
3.2.1 Upregulation of the tight junction protein expression
The tight junctions (TJs) are present between epithelial cells in the junctions’ apical region and comprise multiple proteins, such as claudins, junctional adhesion molecules, occludin, and tricellulin (Pan et al., 2023). TJs are an intestinal mucosal mechanical barrier required for the maintenance of intestinal epithelium integrity and intestinal mucosal permeability by modulating ions and molecules’ entrance into the paracellular channels (Suzuki, 2013). The destruction or reduction of TJ proteins can disrupt the gastrointestinal mucosal barrier, causing UC and other intestinal disorders. However, some natural compounds, such as 8 alkaloids [berberine hydrochloride (Zhu et al., 2019a), berberrubine (Yu et al., 2018), coptisine (Wang et al., 2021a), dihydroberberine (Li et al., 2021), evodiamine (Shen et al., 2019), matrine (Yan et al., 2020), nigakinone (Liu et al., 2023), and piperine (Guo et al., 2020)], 8 flavonoids [apigenin (Fu et al., 2022), kaempferol (Qu et al., 2021), licochalcone A (Zhang et al., 2021), licoflavone B (Zhang et al., 2022b), phloretin (Zhang Z. et al., 2019), pinocembrin (Hu et al., 2019), puerarin (Wu et al., 2020), and wogonoside (Huang et al., 2020)], 2 phenylpropanoids [honokiol (Wang and Wang, 2022), sinapic acid (Qian et al., 2020)], 5 terpenoids [carnosol (Xu et al., 2022b), ginsenoside Rk3 (Tian et al., 2020), patchouli alcohol (Wu et al., 2020), plumericin (Rapa et al., 2021), and sauchinone (Wu et al., 2023)], arbutin (Zhang et al., 2021), sodium houttuyfonate (Cheng et al., 2023), can promote TJ proteins expression in UC animals, such as claudin-1, occludin, and zona occludens 1 (ZO-1), thus efficiently prevent the paracellular permeability disruption. Additionally, berberrubine (Yu et al., 2018), coptisine (Wang et al., 2021a), dihydroberberine (Li et al., 2021), patchouli alcohol (Wu et al., 2020), and palmatine (Zhang et al., 2018) promote ZO-2 protein levels in UC animals. Multiple researches are investigating UC alleviation by alkaloids and flavonoids, which upregulate TJ proteins.
3.2.2 Decreased permeability of intestinal mucosal
The permeability of the intestinal mucosa controls the transport of molecular substances across the epithelium of the intestinal mucosa by the process of simple diffusion. Increased mucosal permeability (Nakarai et al., 2012) has been reported in UC patients (Wang et al., 2022), allowing the entrance of intestinal pathogens as well as their toxic metabolites in the liver, lymph, peripheral tissues, and blood, causing enhanced oxidative stress and inflammation. Intestinal permeability allows accurate, direct, and quantitative evaluation of the colonic epithelial barrier (Huang et al., 2016). Generally, FITC-dextran (fluorescein isothiocyanate dextran) permeability is utilized for the elucidation of epithelium integrity. It has recently been revealed that after taking FITC-dextran orally, the serum of DSS mice had markedly increased FITC-dextran levels (Zhang et al., 2022c). Interestingly, berberine (Zheng et al., 2021), cannabidivarin (Pagano et al., 2019), dioscin (Cai et al., 2021), ginsenoside compound K (Wang et al., 2022), luteolin (Xie et al., 2022), platycodin D (Guo et al., 2021), vitexin (Zhang et al., 2022c), and wogonoside (Huang et al., 2020), can decrease serum FITC-dextran level in UC animals.
3.2.3 Regulation of the intestinal mucus function
The structure of the intestinal mucus is composed of the glycoprotein network containing host-specific glycan that prevents the interaction of bacteria and epithelium, inhibits infection, and modulates the balance between exogenous stimulation and immune function (Johansson et al., 2014). Intestinal mucosal layer dysfunction compromises intestinal epithelium integrity and enhances pathogenic susceptibility. The main intestinal mucosal component is mucin (Johansson et al., 2011). During active UC, there are decreased goblet cells in the colon epithelium, the protective mucus layer thickness reduces, and the mucus levels in mucin, glycosylation, and phosphatidylcholine alters. Alterations in the levels of colon proteins, such as trefoil factor 3 (Tff3), mucin 1 (Muc1), and Muc2, increase susceptibility to chronic inflammation, indicating mucins’ importance in intestinal barrier repair. The research suggests that 7 flavonoids [astragalin (Peng et al., 2020), catechin-7-O-β-D-glucopyranoside (Kook et al., 2015), hyperoside (Cheng et al., 2021), kaempferol (Park et al., 2012), puerarin (Wu et al., 2020), phloretin (Wu et al., 2019), and vitexin (Zhang et al., 2022c)], 8 alkaloids [berberrubine (Yu et al., 2018), berberine (Dong et al., 2022c), dihydroberberine (Li et al., 2021), evodiamine (Wang et al., 2020), heterophyllin B (Chen et al., 2022), matrine (Yan et al., 2020), palmatine (Zhang et al., 2018), and tryptophan (Islam et al., 2017)], 8 terpenoids [betulinic acid hydroxamate (Prados et al., 2021), carnosol (Xu et al., 2022b), celastrol (Li et al., 2022), dehydrocostus lactone (Zhou et al., 2020), ginsenoside Rg3 (Liu et al., 2023), loganin (Yuan et al., 2020), morroniside (Yuan et al., 2020), and patchouli alcohol (Wu et al., 2020)], 2 phenols [epigallocatechin gallate (Diwan and Sharma, 2022), zingerone (Zhang et al., 2022)], aloin A (Jiang et al., 2022), alanyl-glutamine (Hou et al., 2013), rosmarinic acid (Formiga et al., 2020), saikosaponin D (Li et al., 2020) and vitamin A (Pang et al., 2021) can effectively enhance the colon tissue expression of mucus-linked mucins and Tff3 in the UC mice to improve the function of colonic barrier.
3.2.4 Oxidative stress reduction
Increased oxidative stress causes colonic mucosal barrier activity loss and a marked reduction in TJ proteins, thus enhancing the risk for the development of UC. In the intestine, inflammation and oxidative stress together disrupt the mucosal redox balance and promotes apoptosis of intestinal epithelial cell (IEC) (Seo et al., 2014). It has been indicated that bruceine D (Dou et al., 2018) and casticin (Ma et al., 2018) reduce malondialdehyde (MDA) and reactive oxygen species (ROS) and enhances glutathione (GSH) and superoxide dismutase to alleviate the damage caused by oxidative stress damage in colon tissues and UC symptoms in animals. Stevioside can alleviate colonic epithelium oxidative damage by UC, including ROS reduction and intestinal mucosal GSH consumption and elevating the enzyme activity of catalase (CAT), GSH (Mostafa et al., 2020), and heme oxygenase-1 (HO-1). Furthermore, there are many compounds, including atractylenolide III (Han et al., 2022), astragaloside IV (Zhong et al., 2022), asperuloside (Chen et al., 2021), alpinetin (Tan and Zheng, 2018), acteoside (Guo et al., 2022), brusatol (Zhou et al., 2018), bruceine D (Dou et al., 2018), betaine (Chen et al., 2022), berberine (Zhang et al., 2017), baicalin (Yao et al., 2016), carnosic acid (Yang et al., 2017), D-pinitol (Lin et al., 2021), epoxymicheliolide (He et al., 2022), geniposide (Yang et al., 2020), galangin (Sangaraju et al., 2019), 6-gingerol (Ajayi et al., 2018), hydroxytyrosol (Elmaksoud et al., 2021), isatin (Socca et al., 2014), imperatorin (Luo and Luo, 2021), lycopene (Tekeli et al., 2018; Li et al., 2021; Yin et al., 2023), naringenin (Al-Rejaie et al., 2013), 8-oxypalmatine (Cheng et al., 2022), and 3,4-Oxo-isopropylidene-shikimic acid (Xing et al., 2012), puerarin (Jeon et al., 2020), sesamin (Bai et al., 2019), syringic acid (Fang et al., 2019), stevioside (Alavala et al., 2019; Mostafa et al., 2020), sinigrin (Kotipalli et al., 2023), sinapic acid (Qian et al., 2020), tyrosol (Guvenc et al., 2019), vitamin C (Yan et al., 2015), wogonin (Zhou et al., 2022), et al. that decrease colonic epithelium oxidative damage by UC.
3.2.5 Protecting the intestinal epithelial cells
The IECs have rapid renewal capability (Krndija et al., 2019), ensuring normal digestion and barrier activity, and are based on non-inflammatory apoptosis. At UC onset, IEC travels to the damaged area to maintain the intestinal barrier’s integrity (Maria-Ferreira et al., 2018). However, the excessive apoptosis and uncontrolled IEC inflammation are primarily responsible for impaired intestinal mucosal barrier activity in UC. Caspase is the most critical protease associated with apoptosis; Bax and Bcl-2 are essential apoptosis modulatory genes. Some natural compounds, such as 5 alkaloids [berberine (Jia et al., 2020), coptisine (Wang et al., 2021a), indirubin (Gao et al., 2018), isatin (Gao et al., 2018), and palmatine (Zhang et al., 2018)], 3 flavonoids [baicalin (Shen et al., 2019b), hesperidin (Shafik et al., 2019), and wogonin (Zhou et al., 2022)], 2 phenols [hydroxytyrosoland (Elmaksoud et al., 2021), polydatin (Lv et al., 2018)], 4 phenylpropanoids [acteoside (Guo et al., 2022), chlorogenic acid (Gao et al., 2019), deoxyschizandrin (Zhang et al., 2016; Yu and Qian, 2021), and sinapic acid (Shahid et al., 2022)], 3 steroids [taraxasterol (Che et al., 2019), taurine (Giris et al., 2008), and taurohyodeoxycholic acid (Laukens et al., 2014)], 4 terpenoids [cycloastragenol (Bagalagel et al., 2022), plumericin (Rapa et al., 2021), paeoniflorin (Gu et al., 2017), and patchouli alcohol (Qu et al., 2017)] and arbutin (Zhang et al., 2021), diacetylrhein (Zohny et al., 2022), glutamate (Li et al., 2014), and nervonic acid (Yuan et al., 2023), have indicated UC improvement, decreasing the expression of Bax, caspase-3, and caspase-9, whereas increasing Bcl-2 in epithelial cells. Meanwhile, anemoside B4 (Zhang et al., 2021), bryodulcosigenin (Li et al., 2022), and berberrubine (Yu et al., 2018) could decrease the ratio of Bax/Bcl-2 and caspase-3, while artesunate (Yin et al., 2020) increasing the ratio of Bcl-2/Bax and decreasing caspase-3. Diosgenin (Tang et al., 2020) can protect against colonic apoptosis by downregulating the Bax/Caspase-1 pathway. In addition, cyclosporine protects against epithelial apoptosis linked with increased tumor growth factor-β-related signaling (Satoh et al., 2009).
3.3 Regulating intestinal mucosal immune response
The intestinal mucosal immunological disorder is the essential factor for UC pathogenesis, characterized by innate immune system alterations, adaptive immune system activation, increased pro-inflammatory mediators, and anti-inflammatory signals inhibition, causing chronic intestinal inflammation. Currently, 283 natural compounds have been indicated to improve mucosal immune response in UC, primarily by regulating cytokine, inflammatory signaling pathways, and immune cells as shown in Figure 5.
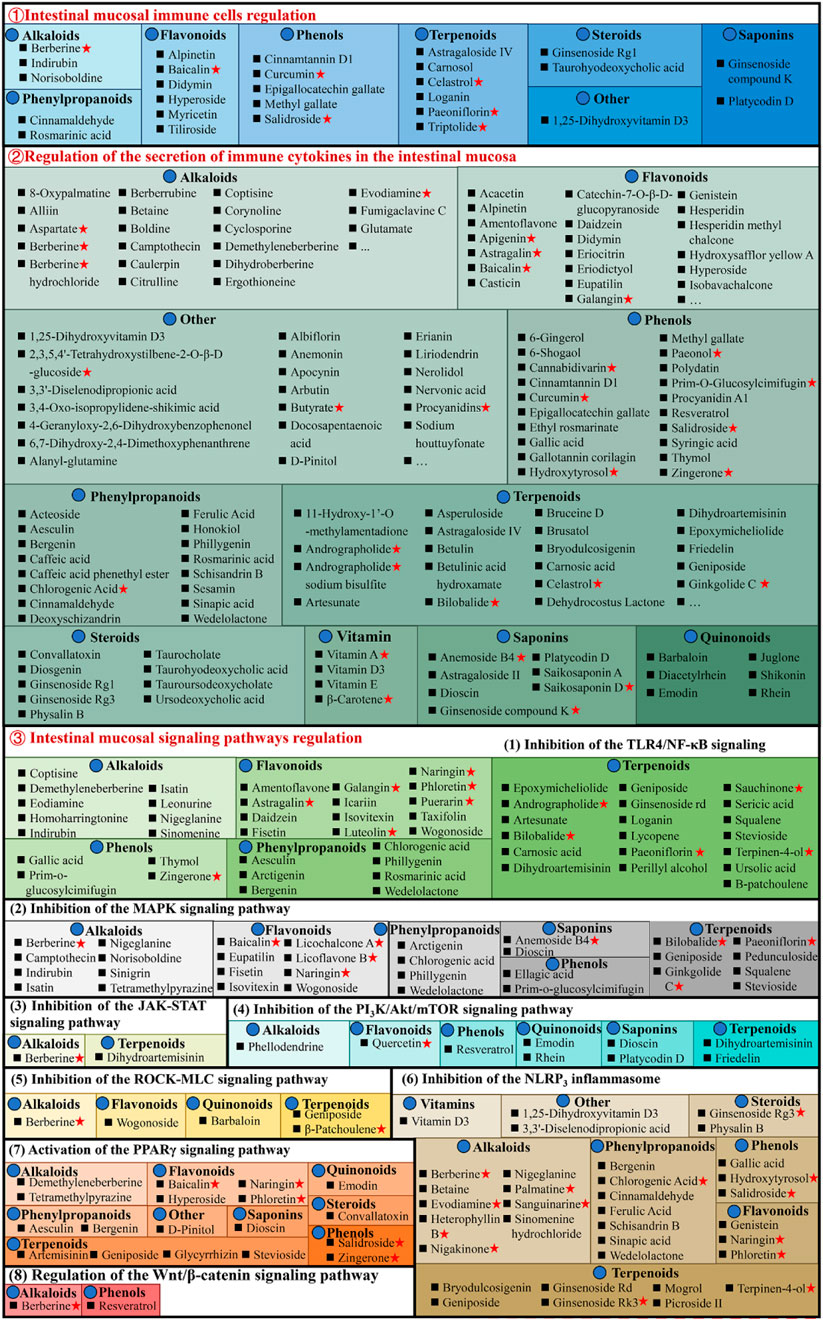
Figure 5. Natural compounds against ulcerative colitis via regulation of intestinal mucosal immune response. Red pentagrams indicate compounds involved in Mechanism Ⅰ, Ⅱ, and Ⅲ. The natural compounds involved in this paper are shown in Supplementary Table S1.
3.3.1 Regulating intestinal mucosal immune cells
Various cell types, including antigen-presenting (dendritic cells and macrophages) and effector and regulatory T cells, are critically linked with UC pathogenesis, as they promote or inhibit inflammation. Macrophages are essential for intestinal homeostasis and the pathology of IBD. Generally, persistent M1 macrophage activation causes excessive stimulation of pro-inflammatory cytokines to release, causing an imbalance of colonic homeostasis and barrier disruption (Bain et al., 2013). Whereas M2 macrophages stimulate anti-inflammatory cytokines to alleviate UC progression (Formentini et al., 2017). It has been reported that didymin (Lv et al., 2021), 1,25-dihydroxyvitamin D3 (Cao et al., 2020), ginsenoside Rg1 (Long et al., 2022), loganin (Liu et al., 2020), methyl gallate (Zhou et al., 2022), platycodin D (Guo et al., 2021), triptolide (Tang et al., 2020), and tiliroside (Zhuang et al., 2021) suppresses M1 macrophages activation and promote M2 macrophages, thereby alleviating UC. In addition, baicalin (Zou et al., 2015), cinnamtannin D1 (Yang et al., 2022), curcumin (Wang et al., 2022), cinnamaldehyde (Qu et al., 2021), epigallocatechin gallate (Xu et al., 2015), ginsenoside compound K (Wang et al., 2022), hyperoside (Cheng et al., 2021), paeoniflorin (Zheng et al., 2020), and salidroside (Liu et al., 2023) can ameliorate chronic intestinal inflammation in UC, and its mechanism that promote intestinal mucosal immune imbalance, thereby regulating Th17/Treg balance.
Interestingly, these compounds are mainly phenols. The endoscopic findings consistently indicate that supplementation with phenols has demonstrated benefits in individuals with IBD. However, to acquire a more comprehensive understanding of the influence of phenols, it is necessary to conduct long-term trials that incorporate both clinical and mechanistic investigations (Hagan et al., 2021).
3.3.2 Regulating the secretion of immune cytokines in the intestinal mucosa
In UC patients’ intestines, increased pro-inflammatory cytokines cause persistent mucosal inflammation and are directly linked with UC pathogenesis. The mucosal immune system is the primary factor affecting intestinal injury and inflammation, and with cytokines, it modulates inflammation (Ardizzone and Bianchi Porro, 2005). Therefore, cytokines are a logical UC target that can be modulated by specific inhibitors. The literature has indicated that chemokine ligand 5 (CCL5), cyclooxygenase (COX-2), IL-1β, IL-6, IL-8, IL-17, interferon-γ (IFN-γ), inducible nitric oxide synthase (iNOS), myeloperoxidase (MPO), nitric oxide (NO), and tumor necrosis factor alpha (TNF-α) expression enhanced in UC animal models, while anti-inflammatory cytokines secretion such as Arg-1, IL-10, and IL-4 decreased. Most natural compounds compiled here can regulate these cytokines to treat UC. Taurohyodeoxycholic acid (TA), a natural 6α-hydroxylated bile acid with hydrophilic properties, is the main component of traditional Chinese medicine (TCM) Pulvis Fellis Suis. TA can modulate multiple cytokines in UC, including CXC motif chemokine ligand 2 (Cxcl2), IL-1β, IL-4, IL-6, IL-10, IL-17A, IL-21, IL-22, IFN-γ, MPO, and TNF-α for maintaining the immune balance of the body (He et al., 2011; Laukens et al., 2014; Lv et al., 2023).
3.3.3 Regulating intestinal mucosal signaling pathways
The pathogenesis of UC is associated with multiple complex inflammatory signaling pathways. Natural compounds directly or indirectly interact with the immune system, stimulating different molecular and cellular pathways and producing anti-inflammatory effects. Therefore, UC prevention and therapy by natural molecules regulate one or more complicated signaling pathways.
3.3.3.1 Inhibition of the TLR4/NF-κB signaling pathway
NF-κB is a transcription factor that stimulates inflammatory cytokines’s genetic transcription and is linked with multiple inflammatory diseases. Physiologically, inactive NF-κB interacts with cytoplasmic inhibitor protein IkappaB (IκB). During inflammation, IκB undergoes phosphorylation and degradation, dissociating NF-κB and translocating it from the cytoplasm to the nucleus, thereby activating downstream gene transcription, such as pro-inflammatory cytokines and iNOS. Therefore, NF-κB inhibition is an efficient strategy to prevent UC and inflammatory cytokines release in UC patients. It is reported that 14 alkaloids [corynoline (Zhang et al., 2023), cavidine (Niu et al., 2015), caulerpin (Lucena et al., 2018), coptisine (Wang et al., 2021a), demethyleneberberine (Zhao et al., 2022b), evodiamine (Shen et al., 2019), homoharringtonine (Liu et al., 2022), isatin (Gao et al., 2018), indirubin (Gao et al., 2018), leonurine (Zheng et al., 2021), melittin (Ahmedy et al., 2020), nigeglanine (Gao et al., 2019), platycodin D (Guo et al., 2021), and sinomenine (Zhou et al., 2023)], 16 flavonoids [astragalin (Peng et al., 2020), and amentoflavone (Sakthivel and Guruvayoorappan, 2013), baicalin (Feng et al., 2014), daidzein (Shen et al., 2019c), eupatilin (Zhou et al., 2018), euptailin (Zhou et al., 2018), fisetin (Sahu et al., 2016), galangin (Sangaraju et al., 2019), icariin (Zhang et al., 2021a), licochalcone A (Liu et al., 2018), luteolin (Li et al., 2021), α-mangostin (You et al., 2017), naringin (Cao et al., 2018), puerarin (Jeon et al., 2020), phloretin (Zhang et al., 2019), and taxifolin (Li W. et al., 2022)], 6 phenols [epicatechin (Zhang et al., 2016), gallic acid (Zhu et al., 2019b), polydatin (Yao et al., 2011), prim-o-glucosylcimifugin (Yin et al., 2022), thymol (Chamanara et al., 2019), and zingerone (Zhang et al., 2022)], 7 phenylpropanoids [arctigenin (Wu et al., 2014), aesculin (Tiana et al., 2019), bergenin (Wang et al., 2018), caffeic acid (Zhang et al., 2016), chlorogenic acid (Zeng et al., 2020), phillygenin (Xue et al., 2023), and wedelolactone (Wei et al., 2017)], 25 terpenoids [astragaloside IV (Wu and Chen, 2019), astragalin (Peng et al., 2020), asperuloside (Chen et al., 2021), artesunate (Chen et al., 2019), bilobalide (Zhang et al., 2021c), brusatol (Zhou et al., 2018), carnosic acid (Yang et al., 2017), β-carotene (Zhu et al., 2021), diosgenin (Tang et al., 2020), dihydroartemisinin (Li N. et al., 2019), epoxymicheliolide (He et al., 2022), geniposide (Yang et al., 2020), ginkgolide C (Xu D. et al., 2022), ginsenoside Rd (Qu et al., 2022), patchouli alcohol (Wu et al., 2020), parthenolide (Zhao et al., 2012), picroside II (Yao et al., 2022), paeoniflorin (Gu et al., 2017), rographolide (Zhang et al., 2020), stevioside (Alavala et al., 2019), squalene (Sanchez-Fidalgo et al., 2015), sericic acid (Lifei et al., 2023), sauchinone (Wu et al., 2023), terpinen-4-ol (Zhang et al., 2017a), and ursolic acid (Liu et al., 2016)], and 2 steroids [convallatoxin (Li et al., 2019), physalin B (Zhang et al., 2020)], 2 aponins [dioscin (Cai et al., 2021), saikosaponin A (Zhou et al., 2019)] and albiflorin (Wang et al., 2023), erianin (Dou et al., 2020), 4-geranyloxy-2,6-dihydroxybenzophenonel (Wang et al., 2023), liriodendrin (Zhang et al., 2017c), nervonic acid (Yuan et al., 2023), and vitamin C (Kondo et al., 2019) have been indicated to improve UC related systemic symptoms by suppressing NF-κB inflammatory signaling pathway.
Furthermore, the Toll-like receptor 4 (TLR4) is an essential signaling pathway associated with colon inflammation (Rashidian et al., 2020). As an innate immune receptor, TLR4 is activated during inflammation after gut pathogen-associated molecular patterns (PAMPs) recognition, conformation alterations, and dimerization. Activated TLR4 is then recruited at aptamer, activating NF-κB. Much research suggests that colitis is linked with excessive activation of the TLR4/NF-κB signaling pathway (Rashidian et al., 2016; Liu et al., 2017). It is reported that the baicalin (Cui et al., 2014; Feng et al., 2014), berberine (Zhang et al., 2011), cinnamaldehyde (Tan et al., 2023), deoxyschizandrin (Zhang et al., 2016), eriodictyol (Hu et al., 2021), emodin (Xu et al., 2021), honokiol (Wang et al., 2022), hypaconitine (Zhang et al., 2011), hydroxysafflor yellow A (Feng et al., 2022), honokiol (Wang et al., 2022), methyl gallate (Zhou et al., 2022), naringenin (Dou et al., 2013), perillyl alcohol (Puppala et al., 2022), vitexin (Duan et al., 2020), and vitexin (Duan et al., 2020) have been linked with the inhibition of the TLR4/NF-κB signaling pathway and inflammatory cytokines, thereby exerting anti-UC effect.
3.3.3.2 Inhibition of the MAPK signaling pathway
MAPKs family comprises evolutionarily conserved serine/threonine protein kinases, which regulate cellular pathways, such as inflammation-related genes. Among these, stimulation of extracellular-signal-regulated kinases 1/2(ERK-1/2), c-Jun N-terminal kinase (JNK), and p38 kinase (p38) promotes cell apoptosis and aggravates intestinal inflammation. Much research has been published indicating that natural compounds, 7 alkaloids [camptothecin (Wang et al., 2021b), isatin (Gao et al., 2018), indirubin (Gao et al., 2018), melittin (Ahmedy et al., 2020), nigeglanine (Gao et al., 2019), sinigrin (Kotipalli et al., 2023), and tetramethylpyrazine (He et al., 2012)], 5 flavonoids [baicalin (Liang et al., 2019), eupatilin (Zhou et al., 2018), licoflavone B (Zhang et al., 2022b), licochalcone A (Zhang et al., 2021), and naringin (Cao et al., 2018)], 8 terpenoids [bilobalide (Zhang et al., 2021c), ginkgolide C (Xu et al., 2022), geniposide (Lu et al., 2021), pedunculoside (Liu et al., 2020), squalene (Sanchez-Fidalgo et al., 2015), stevioside (Alavala et al., 2019), squalene (Sanchez-Fidalgo et al., 2015), and ursolic acid (Sheng et al., 2021)], 4 phenylpropanoids [arctigenin (Wu et al., 2014), chlorogenic acid (Gao et al., 2019), phillygenin (Xue et al., 2023), and wedelolactone (Wei et al., 2017)], albiflorin (Wang et al., 2023), atractylodin (Qu et al., 2021), β-carotene (Zhu et al., 2021), dioscin (Cai et al., 2021), α-mangostin (You et al., 2017), nervonic acid (Yuan et al., 2023), and prim-O-Glucosylcimifugin (Yin et al., 2022) have suppressive effect on MAPK pathway, reducing the expression and inflammatory mediators release.
3.3.3.3 Inhibition of the JAK-STAT signaling pathway
The janus kinase/signal transducer and activator of tranions (JAK/STAT) is a common signaling pathway for transducing signals from various cytokines, which widely regulate cell growth, differentiation, inflammation, apoptosis, and other mechanisms. berberine (Zhang et al., 2017), dihydroartemisinin (Jiang et al., 2021), and erianin (Dou et al., 2020) can ameliorate UC’s intestinal mucosal inflammation by downregulating phosphorylated Janus kinase 2 (p-JAK2), JAK2, phosphorylated signal transducer and activator of transcription 3 (p-STAT3), and signal transducer and activator of transcription 3 (STAT3) expression.
3.3.3.4 Inhibition of the PI3K/Akt/mTOR signaling pathway
Studies have shown that the PI3K/AKT signaling pathway plays an important role in the occurrence of UC (Dong et al., 2022). The inflammatory response can be alleviated by blocking this signal transduction pathway, thus presenting a promising target for treating UC. Interestingly, dihydroartemisinin (Jiang et al., 2021), glutamine (Yan et al., 2020), ihydroartemisinin (Li et al., 2019), luteolin (Vukelic et al., 2020), platycodin D (Guo et al., 2021), quercetin (Zhang et al., 2023), and rhein (Dong et al., 2022) attenuate DSS-induced colitis via PI3K/AKT signaling pathway inhibition. Additionally, mTOR, a downstream target of PI3K/AKT, primarily modulates cell growth and metabolism, promoting anabolism, including ribosome biogenesis and synthesizing nucleotides, proteins, fatty acids, and lids, and inhibiting catabolism. P-mTOR upregulation in the colon tissues of DSS-induced UC rats causes autophagy dysfunction. However, alpinetin (Miao et al., 2019), dioscin (Li et al., 2022), friedelin (Shi et al., 2021), phellodendrine (Su et al., 2021), and rhein (Dong et al., 2022) reverse this effect, return mTOR to normal levels, and inhibit inflammatory cascade, thereby improving intestinal inflammation.
3.3.3.5 Inhibition of the ROCK-MLC signaling pathway
The Ras homologous protein A-Rho kinase (RhoA-ROCK) signaling pathway modulates TJ synthesis, polymerization, and epithelial cell gap permeability, typically linked with the ROCK-MLC pathway (Liu et al., 2020). ROCKs is a serine-threonine kinase family member, including Rho-associated kinase 1 (ROCK1) and ROCK2. ROCK1 directly modulates myosin light chain 2 (MLC2) activation and myosin contraction for TJ depolymerization, accompanied by increased intercellular permeability (Fu et al., 2019). According to a study, UC animals have increased ROCK1 and MLC2 phosphorylation in the colon; however, barbaloin (Gai et al., 2019), geniposide (Xu et al., 2017), and β-patchoulene (Liu et al., 2020) substantially downregulate them to improve the colonic barrier.
3.3.3.6 Inhibition of the NLRP3 inflammasome
Recently, it was observed that single nucleotide polymorphisms (SNPs) in genes encoding the NOD-like receptor protein 3 (NLRP3) are associated with IBD susceptibility. NLRP3 belongs to the NOD-like receptor (NLR) family (Kanneganti et al., 2007; Abraham et al., 2017). It is a cytosolic platform protein that assembles inflammasome, a protein complex involved in proteolytic maturation and release of IL-1 and IL-18 pro-inflammatory cytokines (Martinon et al., 2002; Villani et al., 2009). NLRP3 inflammasome regulates various inflammatory and autoimmune disorders (Cao et al., 2020). There were 11 alkaloids [betaine (Chen et al., 2022), berberine (Li et al., 2020), demethyleneberberine (Zhao et al., 2022b), evodiamine (Shen et al., 2019), heterophyllin B (Chen et al., 2022), nigeglanine (Gao et al., 2019), nigakinone (Liu et al., 2023), 8-Oxypalmatine (Cheng et al., 2022), palmatine (Mai et al., 2019), sinomenine hydrochloride (Zhou et al., 2021), and sanguinarine (Li et al., 2022b)], 7 phenylpropanoids [bergenin (Lopes de Oliveira et al., 2019), cinnamaldehyde (Tan et al., 2023), chlorogenic acid (Zeng et al., 2020), ferulic acid (Yu S. et al., 2023), sinapic acid (Qian et al., 2020), schisandrin B (Zhang et al., 2021), and wedelolactone (Wei et al., 2017)], 9 terpenoids [brusatol (Zhou et al., 2018), bryodulcosigenin (Li et al., 2022), ginsenoside Rk3 (Tian et al., 2020), ginsenoside Rg3 (Liu et al., 2023), ginsenoside Rd (Liu C. et al., 2018), geniposide (Pu et al., 2020), mogrol (Liang et al., 2021), picroside II (Yao et al., 2022), and terpinen-4-ol (Zhang et al., 2017a)], 2 flavonoids [naringin (Cao et al., 2018), phloretin (Zhang et al., 2019)], 3 phenols [gallic acid (Yu et al., 2023), hydroxytyrosol (Miao, 2022), and salidroside (Liu et al., 2019)], diacetylrhein (Zohny et al., 2022), dioscin (Cai et al., 2021), 1,25-dihydroxyvitamin D3 (Cao et al., 2020), 3,3′-diselenodipropionic acid (Zheng et al., 2023), physalin B (Zhang et al., 2020), and vitamin D3 (Gao et al., 2023) have been linked with the alleviation of UC via NLRP3 inhibition.
3.3.3.7 Activation of the PPARγ signaling pathway
PPARγ is a transcriptional factor expressed mainly in colonic epithelial cells, and UC, its expressions are reduced (Su et al., 1999; Aoyagi et al., 2010). PPARγ activation decreases UC-mediated NF-κB pathway stimulation and inflammatory cytokines (IL-6, IL-1β, and TNF-α) expression (Aoyagi et al., 2010). Furthermore, targeted PPARγ expression alteration enhances mice’s susceptibility towards DSS-induced colitis (Shah et al., 2007; Aoyagi et al., 2010). However, natural compounds, artemisinin (Jia et al., 2022), baicalin (Xu et al., 2021), bergenin (Wang et al., 2018), aesculin (Tiana et al., 2019), convallatoxin (Li et al., 2019), dioscin (Wu et al., 2021), d-pinitol (Lin et al., 2021), demethyleneberberine (Zhao et al., 2022b), emodin (Luo et al., 2022), glycyrrhizin (Sethuraman et al., 2015), geniposide (Zhang et al., 2017d), honokiol (Wang et al., 2022), hyperoside (Cheng et al., 2021), luteolin (Li et al., 2021), naringin (Cao et al., 2018), phloretin (Zhang et al., 2019), salidroside (Liu et al., 2019), stevioside (Mostafa et al., 2020), tetramethylpyrazine (He et al., 2012), and zingerone (Zhang et al., 2022), can upregulate PPARγ expression to alleviate UC.
3.3.3.8 Regulation of the Wnt/β-catenin signaling pathway
It has been observed that the Wnt signaling pathway substantially affects epithelial cell proliferation to repair mechanical barriers (Kuhnert et al., 2004). Wnt modulates β-catenin expression and is involved in the pathological and physiological mechanisms of injury (Whyte et al., 2012). Multiple research indicates that berberine (Dong et al., 2022c) and 6-gingerol (Ajayi et al., 2018) alleviate UC by maintaining intestinal mucosal barrier function and structure and function, regulating the homeostasis of intestinal mucosal immunity via the Wnt/β-catenin pathway.
3.4 Regulating other mechanisms
3.4.1 Regulating cellular autophagy
One of the cellular self-protection mechanisms is autophagy, which is a self-protective mechanism that maintains homeostasis. It is an evolutionarily conserved mechanism that starts with the generation of a double-membrane autophagosome with cytoplasmic contents (Xie et al., 2020). It is essential for maintaining intestinal homeostasis, modulation of gut ecology, appropriate intestinal immune responses, and microbial protection. It has been suggested that autophagy can substantially suppress cells’ inflammatory reactions (Lin et al., 2019; Larabi et al., 2020). Notably, natural compounds [i.e., alpinetin (Miao et al., 2019), berberine (Xu et al., 2022c), curcumin (Zhang et al., 2019), dioscin (Li et al., 2022), friedelin (Shi et al., 2021), galangin (Xuan et al., 2020), luteolin (Vukelic et al., 2020), palmatine (Mai et al., 2019), procyanidin A1 (Zhang et al., 2022), resveratrol (Pan et al., 2020)], and salidroside (Liu et al., 2023) can improve autophagy and reduce inflammation in the intestinal disorders. Berberine alleviates DSS-induced UC and suppresses the expression and release of lysozyme by stimulating autophagy via adenosine 5‘-monophosphate (AMP)-triggered protein kinase (AMP-activated protein kinase) (AMPK)/mammalian target of rapamycin (mechanistic target of rapamycin kinase) (MTOR)/unlike autophagy activating kinase 1 ULK1 (unc-51 like autophagy activating kinase 1) pathway (Foerster et al., 2022).
3.4.2 Inhibiting ferroptosis
In 2012, ferroptosis was formally stated as an iron-dependent, non-apoptotic cell death manifested by the accumulation of lipid peroxidation products and the depletion of membrane polyunsaturated fatty acid (Dixon et al., 2012). It is characterized by lipid peroxidation, iron accumulation, and increased ROS generation. Iron sagging includes iron deposition, increased lipid peroxidation, reduced GSH, inactivation of glutathione peroxidase 4 (GPX4), and enhanced lipoxygenase (LOX), all of which are linked with UC pathogenesis (Huang et al., 2022). These findings validate that ferroptosis inhibition might be a novel target for treating UC (Wang et al., 2020; Chen et al., 2021; Dong et al., 2021; Tang et al., 2021). β-Caryophyllene is widely found in various plant essential oils, and its flavor and fragrance resembles bicyclic sesquiterpene (Jha et al., 2021). A study revealed that β-caryophyllene acts as an inhibitor of ferroptosis that represses lipid peroxidation and inflammation, thereby alleviating UC (Wu et al., 2022).
3.4.3 Regulating metabolism pathway
The literature suggests that metabolic reprogramming can regulate the activation of macrophages. The metabolic signals furnish energy and polarize macrophages. M1 macrophages substantially depend on glycolytic metabolism, whereas M2 primarily depends on oxidative phosphorylation (Saha et al., 2017). Glucose is converted to pyruvate and lactic acid glycolysis via a series of cytoplasmic enzymes. Pyruvate dehydrogenase kinase 1 (PDK1) knockdown is a key modulator enzyme of glucose metabolism, reducing M1 but enhancing M2 macrophage activation (Tan et al., 2015). Glycolysis inhibitor 2-deoxy-D-glucose (2-DG) reduces M1 macrophage activation and pro-inflammatory cytokines secretion (Wang et al., 2018). It has been revealed that tiliroside alleviates UC by restoring the M1/M2 macrophage balance via the HIF-1α/glycolysis pathway (Zhuang et al., 2021).
3.4.4 Inhibiting endoplasmic reticulum stress responses
The endoplasmic reticulum (ER) is an essential cellular organelle with multiple functions to store free calcium and synthesize, mature, and transport various lipids, proteins, sterols, etc. Because of multiple cellular factors, proteins are unable to fold correctly, resulting in the accumulation of newly synthesized unfolded proteins in cells, thereby promoting ER stress (Song et al., 2021). Much research indicates that ER stress is associated with UC progression. Highly secretory cells, such as intestinal paneth and goblet cells, are specifically impressionable to ER stress (Kaser et al., 2010). Inhibition of ER stress responses is thus an important therapeutic rationale for UC. Limonin might be utilized for this purpose as it blocks the PERK-ATF4-CHOP pathway of ER stress (Song et al., 2021). Furthermore, berberine (Shen et al., 2020) and artesunate (Yin et al., 2021) reduce ER stress-related marker proteins (glucose-regulated protein, GRP78, C/EBP-homologous protein, CHOP) to treat UC.
4 Analysis of important natural compounds
Since pharmacotherapy based on a single target has been insufficient for drug development in complex diseases, the emerging multi-target approach is a promising strategy for the search of new drug candidates. Therefore, we analyzed the relationship between the 279 natural compounds and mechanisms covered in this review. The 279 natural compounds, including 62 terpenoids, 57 alkaloids, 52 flavonoids, 26 phenols, 19 phenylpropanoids, 9 steroids, 9 saponins, 8 quinonoids, 6 vitamins, and 31 others, can effectively ameliorate UC. Of these, terpenoids, alkaloids, and flavonoids have the greatest potential for treating UC. It is noteworthy to highlight that a total of 54 compounds are linked to Mechanism Ⅰ, Ⅱ, and Ⅲ; 151 compounds are associated with Mechanism Ⅰ and Ⅱ; 18 compounds are associated with Mechanism Ⅱ and Ⅲ; 4 compounds are associated with Mechanism Ⅰ; 50 compounds are associated with Mechanism Ⅱ; 2 compounds are related to Mechanism Ⅲ (Figure 6).
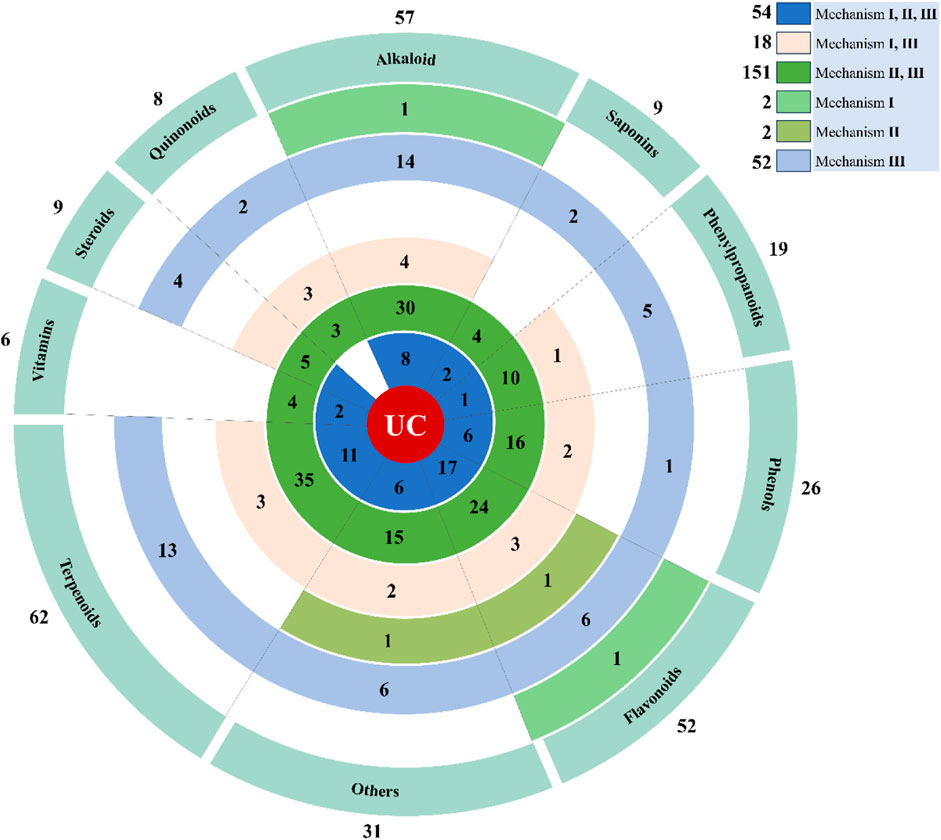
Figure 6. Displays the total amount of natural compounds associated with various mechanisms. The natural compounds involved in this paper are shown in Supplementary Table S1.
Furthermore, we conducted a comprehensive search of the Pubchem and Drugbank databases to obtain pertinent data regarding the clinical studies associated with the aforementioned natural compounds. Consequently, a total of 6 compounds (andrographolide, berberine, berberine hydrochloride, butyrate, curcumin, and diosmin) for the therapeutic management of UC were identified to be either in the clinical stage of development or already available on the market (Table 1). Interestingly, the vast majority of these compounds can alleviate UC by Mechanism Ⅰ, Ⅱ, and Ⅲ. This indicates that we should pay more attention to the compounds with multiple mechanisms in the follow-up UC drug research (Figure 7).
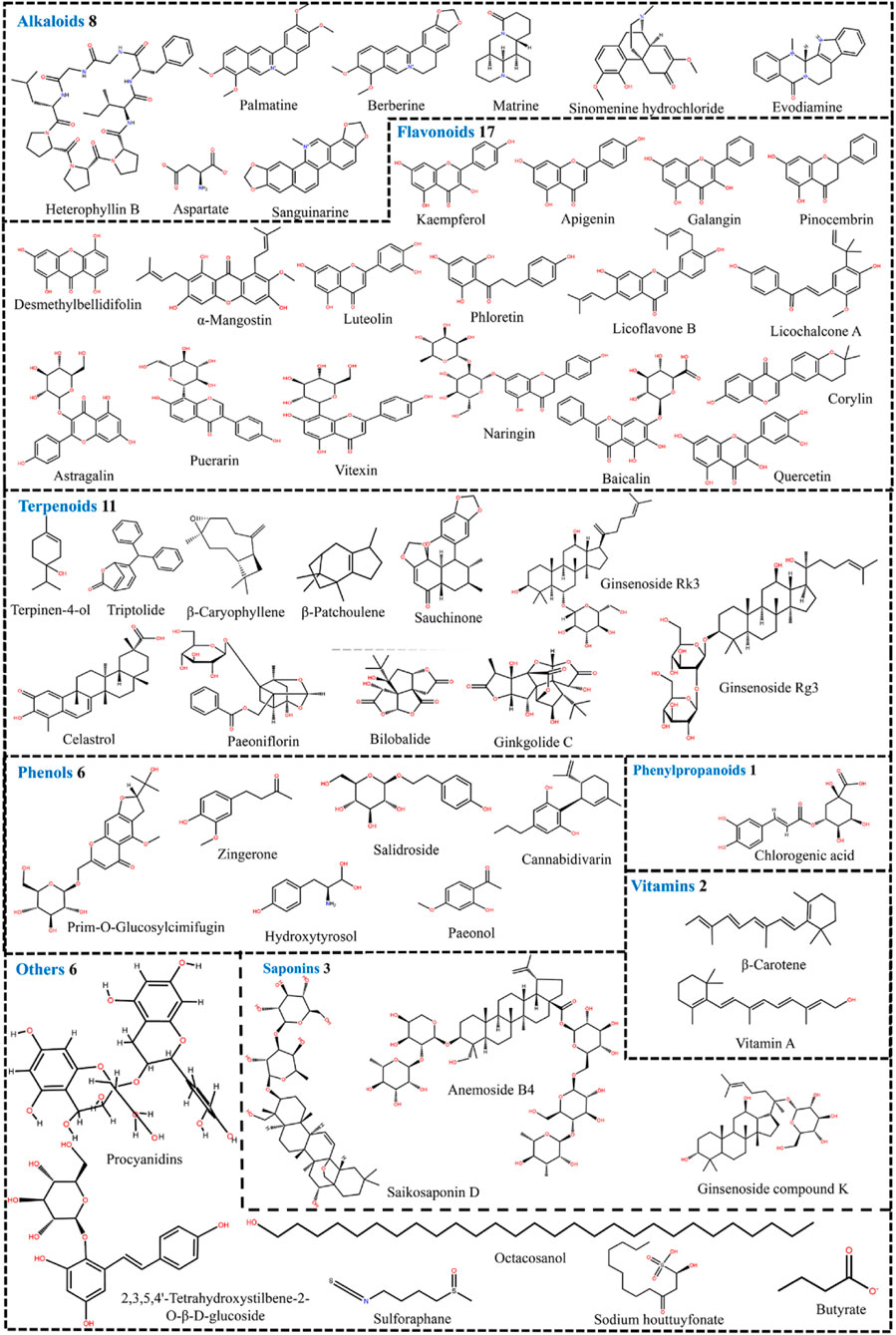
Figure 7. 54 natural compounds that can treat ulcerative colitis by regulating multiple mechanisms (Mechanism Ⅰ, Ⅱ, and Ⅲ).
Many synthetic drugs are currently in use to treat UC such as 5-aminosalicylic acid (5-ASA) (Hossen et al., 2020). Therapeutic mechanisms of 5-ASA for UC include inhibition of cyclooxygenases and lipoxygenase, activation of peroxisome proliferator activated receptor γ, inhibition of T-cell proliferation and activation, reduction of chemotaxis, adhesion and phagocytosis, inhibition of nuclear factor-κβ (Hauso et al., 2015). Overall, 5-ASA appears to exert its therapeutic effect by topical action on the affected areas of inflammation. This is the same as one of the mechanisms (Mechanism III) by which natural compounds treat UC. However, the mechanism of natural compounds against UC is more complex compared to synthetic drugs. Furthermore, 5-ASA have some drawbacks as long-term use results in side effects including nausea, vomiting, fatigue, diarrhea, abdominal pain, pulmonary fibrosis, etc (Rogler, 2010). For centuries, herbal treatments have shown their potential to ameliorate countless diseases and disorders with no or fewer side effects. In conclusion, natural compounds have a richer mechanism for treating UC than synthetic drugs, and natural compounds are more abundantly available and have fewer side effects.
5 Concluding remarks and future directions
This review provides a comprehensive overview of the protective effects exhibited by natural substances against UC, while also delving into their probable mechanisms of action in mitigating colitis. Results indicated that 279 natural compounds (62 terpenoids, 57 alkaloids, 52 flavonoids, 26 phenols, 19 phenylpropanoids, 9 steroids, 9 saponins, 8 quinonoids, 6 vitamins, and 31 others) can act on various mechanisms to improve UC, such as regulating gut microbiota and metabolites (Mechanisms I), protecting the intestinal mucosal barrier (Mechanisms II), regulating intestinal mucosal immune response (Mechanisms III), as well as the other mechanisms (cellular autophagy modulation and ferroptosis inhibition). More importantly, (1) 54 natural compounds exhibit their therapeutic effects by modulating Mechanisms I, II, and III, which can be used to develop multitargeted drugs for UC; Terpenoids, alkaloids, and flavonoids have the greatest potential for treating UC. (2) Mechanism III is regulated by all natural compounds; Mechanisms II and III can be modulated by at least half of the compounds, which may give information on the etiology of UC. In conclusion, this review serves as a comprehensive resource for the pharmaceutical industry, researchers, and clinicians seeking novel therapeutic approaches to combat UC. Harnessing the therapeutic potential of these natural compounds may significantly contribute to the improvement of the quality of life of patients with UC and promotion of disease-modifying therapies in the future.
This review fails to resolve some issues and requires further research and refined methodology to provide evidence for the natural compound’s therapeutic efficacy. The limitations include: (1) Disadvantages including reduced water insolubility, oral bioavailability, rapid metabolism, and increased degradation limit the clinical use of various natural compounds. However, different drug delivery strategies can resolve these issues. (2) Clinical trials are required to assess natural compounds' safety and efficacy profiles, the elucidation criteria of which are not uniform for UC, and the treatment mechanism is not thoroughly studied. Research requires standardization and rationalization to improve UC’s therapeutic effect and promote new drug development. (3) Co-treatment of natural compounds and other drugs should be studied for improved treatment. Furthermore, applying targeted preparations would benefit the targeted delivery of natural compounds with an increased curative effect and potential.
Author contributions
YH: Methodology, Software, Writing–original draft, Writing–review and editing. QW: Software, Writing–review and editing. SL: Investigation, Resources, Software, Writing–review and editing. XL: Data curation, Software, Writing–review and editing. SY: Data curation, Software, Writing–review and editing. RZ: Data curation, Software, Writing–review and editing. CF: Conceptualization, Supervision, Writing–original draft, Writing–review and editing. ZZ: Conceptualization, Software, Supervision, Writing–original draft, Writing–review and editing.
Funding
The author(s) declare that financial support was received for the research, authorship, and/or publication of this article. This work was supported by the National Natural Science Foundation of China (U21A20409, No. 81803742), the Science and Technology Plan Project of Sichuan Province (2021YJ0251), Special Foundation for Scientific Research on Traditional Chinese Medicine of Sichuan Provincial Administration of Traditional Chinese Medicine (2023MS600), Youth Foundation Talent Special Project of Xinglin Scholars’ of Chengdu University of Traditional Chinese Medicine (QJRC2022027), Young Teachers Special Foundation of School of Pharmacy/School of Modern Chinese Medicine Industry of Chengdu University of Traditional Chinese Medicine (2022JJRC04), the fellowship from China Scholarship Council (No. 202308510149), the Natural Science Foudation of Sichuan Province (2024NSFSC0712), Young Teachers Special Foundation of School of Pharmacy/School of Modern Chinese Medicine Industry of Chengdu University of Traditional Chinese Medicine (2024JCRC04), and the authors also thank the support of the experimental equipments from the State Key Laboratory of CDUTCM.
Conflict of interest
The authors declare that the research was conducted in the absence of any commercial or financial relationships that could be construed as a potential conflict of interest.
Publisher’s note
All claims expressed in this article are solely those of the authors and do not necessarily represent those of their affiliated organizations, or those of the publisher, the editors and the reviewers. Any product that may be evaluated in this article, or claim that may be made by its manufacturer, is not guaranteed or endorsed by the publisher.
Supplementary material
The Supplementary Material for this article can be found online at: https://www.frontiersin.org/articles/10.3389/fphar.2024.1394124/full#supplementary-material
References
Abraham, B. P., Ahmed, T., and Ali, T. (2017). Inflammatory bowel disease: pathophysiology and current therapeutic approaches. Handb. Exp. Pharmacol. 239, 115–146. doi:10.1007/164_2016_122
Ahmedy, O. A., Ibrahim, S. M., Salem, H. H., and Kandil, E. A. (2020). Antiulcerogenic effect of melittin via mitigating TLR4/TRAF6 mediated NF-κB and p38MAPK pathways in acetic acid-induced ulcerative colitis in mice. Chem. Biol. Interact. 331, 109276. doi:10.1016/j.cbi.2020.109276
Ajayi, B. O., Adedara, I. A., and Farombi, E. O. (2018). Protective mechanisms of 6-gingerol in dextran sulfate sodium-induced chronic ulcerative colitis in mice. Hum. Exp. Toxicol. 37, 1054–1068. doi:10.1177/0960327117751235
Alavala, S., Sangaraju, R., Nalban, N., Sahu, B. D., Jerald, M. K., Kilari, E. K., et al. (2019). Stevioside, a diterpenoid glycoside, shows anti-inflammatory property against Dextran Sulphate Sodium-induced ulcerative colitis in mice. Eur. J. Pharmacol. 855, 192–201. doi:10.1016/j.ejphar.2019.05.015
Al-Rejaie, S. S., Abuohashish, H. M., Al-Enazi, M. M., Al-Assaf, A. H., Parmar, M. Y., and Ahmed, M. M. (2013). Protective effect of naringenin on acetic acid-induced ulcerative colitis in rats. World J. Gastroenterol. 19, 5633–5644. doi:10.3748/wjg.v19.i34.5633
Aoyagi, Y., Nagata, S., Kudo, T., Fujii, T., Wada, M., Chiba, Y., et al. (2010). Peroxisome proliferator-activated receptor gamma 2 mutation may cause a subset of ulcerative colitis. Pediatr. Int. 52, 729–734. doi:10.1111/j.1442-200X.2010.03195.x
Ardizzone, S., and Bianchi Porro, G. (2005). Biologic therapy for inflammatory bowel disease. Drugs 65, 2253–2286. doi:10.2165/00003495-200565160-00002
Bagalagel, A., Diri, R., Noor, A., Almasri, D., Bakhsh, H. T., Kutbi, H. I., et al. (2022). The therapeutic effects of cycloastragenol in ulcerative colitis by modulating SphK/MIP-1α/miR-143 signalling. Basic Clin. Pharmacol. Toxicol. 131, 406–419. doi:10.1111/bcpt.13788
Bai, X., Gou, X., Cai, P., Xu, C., Cao, L., Zhao, Z., et al. (2019). Sesamin enhances nrf2-mediated protective defense against oxidative stress and inflammation in colitis via AKT and ERK activation. Oxid. Med. Cell. Longev. 2019, 2432416. doi:10.1155/2019/2432416
Bain, C. C., Scott, C. L., Uronen-Hansson, H., Gudjonsson, S., Jansson, O., Grip, O., et al. (2013). Resident and pro-inflammatory macrophages in the colon represent alternative context-dependent fates of the same Ly6Chi monocyte precursors. Mucosal Immunol. 6, 498–510. doi:10.1038/mi.2012.89
Britton, G. J., Contijoch, E. J., Mogno, I., Vennaro, O. H., Llewellyn, S. R., Ng, R., et al. (2019). Microbiotas from humans with inflammatory bowel disease alter the balance of gut Th17 and RORγt+ regulatory T cells and exacerbate colitis in mice. Immunity 50, 212–224. doi:10.1016/j.immuni.2018.12.015
Cai, J., Liu, J., Fan, P., Dong, X., Zhu, K., Liu, X., et al. (2021). Dioscin prevents DSS-induced colitis in mice with enhancing intestinal barrier function and reducing colon inflammation. Int. Immunopharmacol. 99, 108015. doi:10.1016/j.intimp.2021.108015
Cao, H., Liu, J., Shen, P., Cai, J., Han, Y., Zhu, K., et al. (2018). Protective effect of naringin on DSS-induced ulcerative colitis in mice. J. Agric. Food Chem. 66, 13133–13140. doi:10.1021/acs.jafc.8b03942
Cao, R., Ma, Y., Li, S., Shen, D., Yang, S., Wang, X., et al. (2020). 1,25(OH)(2)D-3 alleviates DSS-induced ulcerative colitis via inhibiting NLRP3 inflammasome activation. J. Leukoc. Biol. 108, 283–295. doi:10.1002/jlb.3ma0320-406rr
Cao, R., Wu, X., Guo, H., Pan, X., Huang, R., Wang, G., et al. (2021). Naringin exhibited therapeutic effects against DSS-induced mice ulcerative colitis in intestinal barrier-dependent manner. Molecules 26, 6604. doi:10.3390/molecules26216604
Cao, S., Ye, S., Wang, W., Wang, B., Zhang, T., and Pu, Y. (2019). Progress in active compounds effective on ulcerative colitis from Chinese medicines. Chin. J. Nat. Med. 17, 81–102. doi:10.1016/s1875-5364(19)30012-3
Chamanara, M., Abdollahi, A., Rezayat, S. M., Ghazi-Khansari, M., Dehpour, A., Nassireslami, E., et al. (2019). Thymol reduces acetic acid-induced inflammatory response through inhibition of NF-kB signaling pathway in rat colon tissue. Inflammopharmacology 27, 1275–1283. doi:10.1007/s10787-019-00583-8
Che, L., Li, Y., Song, R., Qin, C., Hao, W., Wang, B., et al. (2019). Anti-inflammatory and anti-apoptosis activity of taraxasterol in ulcerative colitis in vitro and in vivo. Exp. Ther. Med. 18, 1745–1751. doi:10.3892/etm.2019.7736
Chen, C., Liang, H., Wang, J., Ren, G., Li, R., Cui, Z.-G., et al. (2022a). Heterophyllin B an active cyclopeptide alleviates dextran sulfate sodium-induced colitis by modulating gut microbiota and repairing intestinal mucosal barrier via AMPK activation. Mol. Nutr. Food Res. 66, e2101169. doi:10.1002/mnfr.202101169
Chen, L., Liu, D., Mao, M., Liu, W., Wang, Y., Liang, Y., et al. (2022b). Betaine ameliorates acute sever ulcerative colitis by inhibiting oxidative stress induced inflammatory pyroptosis. Mol. Nutr. Food Res. 66, e2200341. doi:10.1002/mnfr.202200341
Chen, Y., Wang, J., Li, J., Zhu, J., Wang, R., Xi, Q., et al. (2021b). Astragalus polysaccharide prevents ferroptosis in a murine model of experimental colitis and human Caco-2 cells via inhibiting NRF2/HO-1 pathway. Eur. J. Pharmacol. 911, 174518. doi:10.1016/j.ejphar.2021.174518
Chen, Y. E., Xu, S. J., Lu, Y. Y., Chen, S. X., Du, X. H., Hou, S. Z., et al. (2021a). Asperuloside suppressing oxidative stress and inflammation in DSS-induced chronic colitis and RAW 264.7 macrophages via Nrf2/HO-1 and NF-κB pathways. Chem. Biol. Interact. 344, 109512. doi:10.1016/j.cbi.2021.109512
Chen, Y.-X., Zhang, X.-Q., Yu, C.-G., Huang, S.-L., Xie, Y., Dou, X.-T., et al. (2019). Artesunate exerts protective effects against ulcerative colitis via suppressing Toll-like receptor 4 and its downstream nuclear factor-kappa B signaling pathways. Mol. Med. Rep. 20, 1321–1332. doi:10.3892/mmr.2019.10345
Cheng, C., Zhang, W., Zhang, C., Ji, P., Wu, X., Sha, Z., et al. (2021). Hyperoside ameliorates DSS-induced colitis through MKRN1-mediated regulation of PPARγ signaling and Th17/treg balance. J. Agric. Food Chem. 69, 15240–15251. doi:10.1021/acs.jafc.1c06292
Cheng, H., Liu, J., Zhang, D., Wang, J., Tan, Y., Feng, W., et al. (2022a). Ginsenoside Rg1 alleviates acute ulcerative colitis by modulating gut microbiota and microbial tryptophan metabolism. Front. Immunol. 13, 817600. doi:10.3389/fimmu.2022.817600
Cheng, J., Ma, X., Zhang, H., Wu, X., Li, M., Ai, G., et al. (2022b). 8-Oxypalmatine, a novel oxidative metabolite of palmatine, exhibits superior anti-colitis effect via regulating Nrf2 and NLRP3 inflammasome. Biomed. Pharmacother. 153, 113335. doi:10.1016/j.biopha.2022.113335
Cheng, T., Xu, C., Wu, D., Yan, G., Wang, C., Wang, T., et al. (2023). Sodium houttuyfonate derived from Houttuynia cordata Thunb improves intestinal malfunction via maintaining gut microflora stability in Candida albicans overgrowth aggravated ulcerative colitis. Food. Funct. 14, 1072–1086. doi:10.1039/d2fo02369e
Childers, R. E., Eluri, S., Vazquez, C., Weise, R. M., Bayless, T. M., and Hutfless, S. (2014). Family history of inflammatory bowel disease among patients with ulcerative colitis: a systematic review and meta-analysis. J. Crohns Colitis 8, 1480–1497. doi:10.1016/j.crohns.2014.05.008
Cohen, R. D., Yu, A. P., Wu, E. Q., Xie, J., Mulani, P. M., and Chao, J. (2010). Systematic review: the costs of ulcerative colitis in Western countries. Pharmacol. Ther. 31, 693–707. doi:10.1111/j.1365-2036.2010.04234.x
Conrad, K., Roggenbuck, D., and Laass, M. W. (2014). Diagnosis and classification of ulcerative colitis. Autoimmun. Rev. 13, 463–466. doi:10.1016/j.autrev.2014.01.028
Consortium, U. I. G., Barrett, J. C., Lee, J. C., Lees, C. W., Prescott, N. J., Anderson, C. A., et al. (2009). Genome-wide association study of ulcerative colitis identifies three new susceptibility loci, including the HNF4A region. Nat. Genet. 41, 1330–1334. doi:10.1038/ng.483
Cui, L., Feng, L., Zhang, Z. H., and Jia, X. B. (2014). The anti-inflammation effect of baicalin on experimental colitis through inhibiting TLR4/NF-kappa B pathway activation. Int. Immunopharmacol. 23, 294–303. doi:10.1016/j.intimp.2014.09.005
da Silva, B. C., Lyra, A. C., Rocha, R., and Santana, G. O. (2014). Epidemiology, demographic characteristics and prognostic predictors of ulcerative colitis. World J. Gastroenterol. 20, 9458–9467. doi:10.3748/wjg.v20.i28.9458
Diwan, B., and Sharma, R. (2022). Green tea EGCG effectively alleviates experimental colitis in middle-aged male mice by attenuating multiple aspects of oxi-inflammatory stress and cell cycle deregulation. Biogerontology 23, 789–807. doi:10.1007/s10522-022-09976-9
Dixon, S. J., Lemberg, K. M., Lamprecht, M. R., Skouta, R., Zaitsev, E. M., Gleason, C. E., et al. (2012). Ferroptosis: an iron-dependent form of nonapoptotic cell death. Cell 149, 1060–1072. doi:10.1016/j.cell.2012.03.042
Dong, L., Du, H., Zhang, M., Xu, H., Pu, X., Chen, Q., et al. (2022b). Anti-inflammatory effect of Rhein on ulcerative colitis via inhibiting PI3K/Akt/mTOR signaling pathway and regulating gut microbiota. Phytother. Res. 36, 2081–2094. doi:10.1002/ptr.7429
Dong, S., Lu, Y., Peng, G., Li, J., Li, W., Li, M., et al. (2021). Furin inhibits epithelial cell injury and alleviates experimental colitis by activating the Nrf2-Gpx4 signaling pathway. Dig. Liver Dis. 53, 1276–1285. doi:10.1016/j.dld.2021.02.011
Dong, Y., Fan, H., Zhang, Z., Jiang, F., Li, M., Zhou, H., et al. (2022c). Berberine ameliorates DSS-induced intestinal mucosal barrier dysfunction through microbiota-dependence and Wnt/β-catenin pathway. Int. J. Biol. Sci. 18, 1381–1397. doi:10.7150/ijbs.65476
Dong, Y., Huang, C., Yang, J., Zheng, Z., and Dai, Z. (2022a). Docosapentaenoic acid (DPA, 22:5n-3) alleviates ulcerative colitis via modification of gut microbiota and their metabolism. Nutrients 14, 4204. doi:10.3390/nu14194204
Dou, B., Hu, W., Song, M., Lee, R. J., Zhang, X., and Wang, D. (2020). Anti -inflammation of Erianin in dextran sulphate sodium-induced ulcerative colitis mice model via collaborative regulation of TLR4 and STAT3. Chemico-Biological Interact. 324, 109089. doi:10.1016/j.cbi.2020.109089
Dou, W., Zhang, J., Sun, A., Zhang, E., Ding, L., Mukherjee, S., et al. (2013). Protective effect of naringenin against experimental colitis via suppression of Toll-like receptor 4/NF-kappa B signalling. Br. J. Nutr. 110, 599–608. doi:10.1017/s0007114512005594
Dou, Y. X., Zhou, J. T., Wang, T. T., Huang, Y. F., Chen, V. P., Xie, Y. L., et al. (2018). Self-nanoemulsifying drug delivery system of bruceine D: a new approach for anti-ulcerative colitis. Int. J. Nanomedicine 13, 5887–5907. doi:10.2147/IJN.S174146
Du, G., Xiong, L., Li, X., Zhuo, Z., Zhuang, X., Yu, Z., et al. (2020). Peroxisome elevation induces stem cell differentiation and intestinal epithelial repair. Dev. Cell 53, 169–184. doi:10.1016/j.devcel.2020.03.002
Duan, S., Du, X., Chen, S., Liang, J., Huang, S., Hou, S., et al. (2020). Effect of vitexin on alleviating liver inflammation in a dextran sulfate sodium (DSS)-induced colitis model. Biomed. Pharmacother. 121, 109683. doi:10.1016/j.biopha.2019.109683
Elmaksoud, H. A. A., Motawea, M. H., Desoky, A. A., Elharrif, M. G., and Ibrahimi, A. (2021). Hydroxytyrosol alleviate intestinal inflammation, oxidative stress and apoptosis resulted in ulcerative colitis. Biomed. Pharmacother. 142, 112073. doi:10.1016/j.biopha.2021.112073
Fang, J., Wang, H., Zhou, Y., Zhang, H., Zhou, H., and Zhang, X. (2021). Slimy partners: the mucus barrier and gut microbiome in ulcerative colitis. Exp. Mol. Med. 53, 772–787. doi:10.1038/s12276-021-00617-8
Fang, W., Zhu, S., Niu, Z., and Yin, Y. (2019). The protective effect of syringic acid on dextran sulfate sodium-induced experimental colitis in BALB/c mice. Drug Dev. Res. 80, 731–740. doi:10.1002/ddr.21524
Feng, J. S., Guo, C. C., Zhu, Y. Z., Pang, L. P., Yang, Z., Zou, Y., et al. (2014). Baicalin down regulates the expression of TLR4 and NFkB-p65 in colon tissue in mice with colitis induced by dextran sulfate sodium. Int. J. Clin. Exp. Med. 7, 4063–4072.
Feng, Z., Zhou, P., Wu, X., Zhang, J., and Zhang, M. (2022). Hydroxysafflor yellow A protects against ulcerative colitis via suppressing TLR4/NF-kappa B signaling pathway. Chem. Biol. Drug Des. 99, 897–907. doi:10.1111/cbdd.14045
Foerster, E. G., Mukherjee, T., Cabral-Fernandes, L., Rocha, J. D. B., Girardin, S. E., and Philpott, D. J. (2022). How autophagy controls the intestinal epithelial barrier. Autophagy 18, 86–103. doi:10.1080/15548627.2021.1909406
Formentini, L., Santacatterina, F., Nunez de Arenas, C., Stamatakis, K., Lopez-Martinez, D., Logan, A., et al. (2017). Mitochondrial ROS production protects the intestine from inflammation through functional M2 macrophage polarization. Cell Rep. 19, 1202–1213. doi:10.1016/j.celrep.2017.04.036
Formiga, R. O., Alves Junior, E. B., Vasconcelos, R. C., Bernardo Guerra, G. C., de Araujo, A. A., de Carvalho, T. G., et al. (2020). p-Cymene and rosmarinic acid ameliorate TNBS-induced intestinal inflammation upkeeping ZO-1 and MUC-2: role of antioxidant system and immunomodulation. Int. J. Mol. Sci. 21, 5870. doi:10.3390/ijms21165870
Fu, C., Hao, S., Xu, X., Zhou, J., Liu, Z., Lu, H., et al. (2019). Activation of SIRT1 ameliorates LPS-induced lung injury in mice via decreasing endothelial tight junction permeability. Acta Pharmacol. Sin. 40, 630–641. doi:10.1038/s41401-018-0045-3
Fu, R., Wang, L., Meng, Y., Xue, W., Liang, J., Peng, Z., et al. (2022). Apigenin remodels the gut microbiota to ameliorate ulcerative colitis. Front. Nutr. 9, 1062961. doi:10.3389/fnut.2022.1062961
Gai, L., Chu, L., Xia, R., Chen, Q., and Sun, X. (2019). Barbaloin attenuates mucosal damage in experimental models of rat colitis by regulating inflammation and the AMPK signaling pathway. Med. Sci. Monit. 25, 10045–10056. doi:10.12659/msm.918935
Gao, H., Zhou, H., Zhang, Z., Gao, J., Li, J., and Li, X. (2023). Vitamin D3 alleviates inflammation in ulcerative colitis by activating the VDR-NLRP6 signaling pathway. Front. Immunol. 14, 1135930. doi:10.3389/fimmu.2023.1135930
Gao, W., Wang, C., Yu, L., Sheng, T., Wu, Z., Wang, X., et al. (2019a). Chlorogenic acid attenuates dextran sodium sulfate-induced ulcerative colitis in mice through MAPK/ERK/JNK pathway. Biomed. Res. Int. 2019, 6769789. doi:10.1155/2019/6769789
Gao, W. Y., Zhang, L. D., Wang, X. Q., Yu, L., Wang, C. H., and Gong, Y. (2018). The combination of indirubin and isatin attenuates dextran sodium sulfate induced ulcerative colitis in mice. Biochem. Cell Biol. 96, 636–645. doi:10.1139/bcb-2018-0041
Gao, X.-J., Tang, B., Liang, H.-H., Yi, L., and Wei, Z.-G. (2019b). The protective effect of nigeglanine on dextran sulfate sodium-induced experimental colitis in mice and Caco-2 cells. J. Cell. Physiol. 234, 23398–23408. doi:10.1002/jcp.28909
Giris, M., Depboylu, B., Dogru-Abbasoglu, S., Erbil, Y., Olgac, V., Alis, H., et al. (2008). Effect of taurine on oxidative stress and apoptosis-related protein expression in trinitrobenzene sulphonic acid-induced colitis. Clin. Exp. Immunol. 152, 102–110. doi:10.1111/j.1365-2249.2008.03599.x
Glassner, K. L., Abraham, B. P., and Quigley, E. M. M. (2020). The microbiome and inflammatory bowel disease. J. Allergy Clin. Immunol. 145, 16–27. doi:10.1016/j.jaci.2019.11.003
Gu, P., Zhu, L., Liu, Y., Zhang, L., Liu, J., and Shen, H. (2017). Protective, effects of paeoniflorin on TNBS-induced ulcerative colitis through inhibiting NF-kappaB pathway and apoptosis in mice. Int. Immunopharmacol. 50, 152–160. doi:10.1016/j.intimp.2017.06.022
Guo, G., Shi, F., Zhu, J., Shao, Y., Gong, W., Zhou, G., et al. (2020). Piperine, a functional food alkaloid, exhibits inhibitory potential against TNBS-induced colitis via the inhibition of IκB-α/NF-κB and induces tight junction protein (claudin-1, occludin, and ZO-1) signaling pathway in experimental mice. Hum. Exp. Toxicol. 39, 477–491. doi:10.1177/0960327119892042
Guo, R., Meng, Q., Wang, B., and Li, F. (2021). Anti-inflammatory effects of Platycodin D on dextran sulfate sodium (DSS) induced colitis and E. coli Lipopolysaccharide (LPS) induced inflammation. Int. Immunopharmacol. 94, 107474. doi:10.1016/j.intimp.2021.107474
Guo, W., Wang, X., Liu, F., Chen, S., Wang, S., Zhang, Q., et al. (2022). Acteoside alleviates dextran sulphate sodium-induced ulcerative colitis via regulation of the HO-1/HMGB1 signaling pathway. Mol. Med. Rep. 26, 360. doi:10.3892/mmr.2022.12877
Gutierrez-Orozco, F., Thomas-Ahner, J. M., Berman-Booty, L. D., Galley, J. D., Chitchumroonchokchai, C., Mace, T., et al. (2014). Dietary α-mangostin, a xanthone from mangosteen fruit, exacerbates experimental colitis and promotes dysbiosis in mice. Mol. Nutr. Food Res. 58, 1226–1238. doi:10.1002/mnfr.201300771
Guvenc, M., Cellat, M., Ozkan, H., Tekeli, I. O., Uyar, A., Gokcek, I., et al. (2019). Protective effects of tyrosol against DSS-induced ulcerative colitis in rats. Inflammation 42, 1680–1691. doi:10.1007/s10753-019-01028-8
Hagan, M., Hayee, B. H., and Rodriguez-Mateos, A. (2021). (Poly)phenols in inflammatory bowel disease and irritable bowel syndrome: a review. Molecules 26, 1843. doi:10.3390/molecules26071843
Han, J., Li, W., Shi, G., Huang, Y., Sun, X., Sun, N., et al. (2022). Atractylenolide III improves mitochondrial function and protects against ulcerative colitis by activating AMPK/SIRT1/PGC-1α. Mediat. Inflamm. 2022, 9129984. doi:10.1155/2022/9129984
Hauso, Ø., Martinsen, T. C., and Waldum, H. (2015). 5-Aminosalicylic acid, a specific drug for ulcerative colitis. Scand. J. Gastroenterol. 50, 933–941. doi:10.3109/00365521.2015.1018937
He, J., Liang, J., Zhu, S., Zhao, W., Zhang, Y., and Sun, W. (2011). Protective effect of taurohyodeoxycholic acid from Pulvis Fellis Suis on trinitrobenzene sulfonic acid induced ulcerative colitis in mice. Eur. J. Pharmacol. 670, 229–235. doi:10.1016/j.ejphar.2011.08.036
He, J., Liu, L., Liu, X., Chen, H., Liu, K., Huang, N., et al. (2022). Epoxymicheliolide prevents dextran sulfate sodium-induced colitis in mice by inhibiting TAK1-NF-κB pathway and activating Keap1-NRF2 signaling in macrophages. Int. Immunopharmacol. 113, 109404. doi:10.1016/j.intimp.2022.109404
He, X., Liu, J., Long, G., Xia, X.-H., and Liu, M. (2021). 2,3,5,4'-Tetrahydroxystilbene-2-O-β-D-glucoside, a major bioactive component from Polygoni multiflori Radix (Heshouwu) suppresses DSS induced acute colitis in BALb/c mice by modulating gut microbiota. Biomed. Pharmacother. 137, 111420. doi:10.1016/j.biopha.2021.111420
He, X., Zheng, Z., Yang, X., Lu, Y., Chen, N., and Chen, W. (2012). Tetramethylpyrazine attenuates PPAR-gamma antagonist-deteriorated oxazolone-induced colitis in mice. Mol. Med. Rep. 5, 645–650. doi:10.3892/mmr.2011.721
Hoivik, M. L., Moum, B., Solberg, I. C., Henriksen, M., Cvancarova, M., Bernklev, T., et al. (2013). Work disability in inflammatory bowel disease patients 10 years after disease onset: results from the IBSEN Study. Gut 62, 368–375. doi:10.1136/gutjnl-2012-302311
Hossen, I., Hua, W., Ting, L., Mehmood, A., Jingyi, S., Duoxia, X., et al. (2020). Phytochemicals and inflammatory bowel disease: a review. Crit. Rev. Food Sci. Nutr. 60, 1321–1345. doi:10.1080/10408398.2019.1570913
Hou, Y.-C., Chu, C.-C., Ko, T.-L., Yeh, C.-L., and Yeh, S.-L. (2013). Effects of alanyl-glutamine dipeptide on the expression of colon-inflammatory mediators during the recovery phase of colitis induced by dextran sulfate sodium. Eur. J. Nutr. 52, 1089–1098. doi:10.1007/s00394-012-0416-3
Hu, L., Wu, C., Zhang, Z., Liu, M., Prasad, E. M., Chen, Y., et al. (2019). Pinocembrin protects against dextran sulfate sodium-induced rats colitis by ameliorating inflammation, improving barrier function and modulating gut microbiota. Front. Physiol. 10, 908. doi:10.3389/fphys.2019.00908
Hu, L.-H., Liu, J.-Y., and Yin, J.-B. (2021). Eriodictyol attenuates TNBS-induced ulcerative colitis through repressing TLR4/NF-kB signaling pathway in rats. Kaohsiung J. Med. Sci. 37, 812–818. doi:10.1002/kjm2.12400
Hu, X., He, X., Peng, C., He, Y., Wang, C., Tang, W., et al. (2022). Improvement of ulcerative colitis by aspartate via RIPK pathway modulation and gut microbiota composition in mice. Nutrients 14, 3707. doi:10.3390/nu14183707
Hua, Y., Liu, R., Lu, M., Guan, X., Zhuang, S., Tian, Y., et al. (2021). Juglone regulates gut microbiota and Th17/Treg balance in DSS-induced ulcerative colitis. Int. Immunopharmacol. 97, 107683. doi:10.1016/j.intimp.2021.107683
Huang, B., Wang, L., Liu, M., Wu, X., Lu, Q., and Liu, R. (2022a). The underlying mechanism of A-type procyanidins from peanut skin on DSS-induced ulcerative colitis mice by regulating gut microbiota and metabolism. J. Food Biochem. 46, e14103. doi:10.1111/jfbc.14103
Huang, J., Zhang, J., Ma, J., Ma, J., Liu, J., Wang, F., et al. (2022b). Inhibiting ferroptosis: a novel approach for ulcerative colitis therapeutics. Oxid. Med. Cell. Longev. 2022, 9678625. doi:10.1155/2022/9678625
Huang, S., Fu, Y., Xu, B., Liu, C., Wang, Q., Luo, S., et al. (2020). Wogonoside alleviates colitis by improving intestinal epithelial barrier function via the MLCK/pMLC2 pathway. Phytomedicine 68, 153179. doi:10.1016/j.phymed.2020.153179
Huang, X. R., Wang, C. D., Wang, R. X., and Jiao, H. X. (2016). Changes of colonic permeability and its correlation with TNF-α, NF-κB p65 in ulceration colitis mice. Chin. J. Appl. Physiol. 32, 112–115. doi:10.13459/j.cnki.cjap.2016.02.005
Islam, J., Sato, S., Watanabe, K., Watanabe, T., Ardiansyah, H. K., Aoyama, Y., et al. (2017). Dietary tryptophan alleviates dextran sodium sulfate-induced colitis through aryl hydrocarbon receptor in mice. J. Nutr. Biochem. 42, 43–50. doi:10.1016/j.jnutbio.2016.12.019
Jeon, Y.-D., Lee, J.-H., Lee, Y.-M., and Kim, D.-K. (2020). Puerarin inhibits inflammation and oxidative stress in dextran sulfate sodium-induced colitis mice model. Biomed. Pharmacother. 124, 109847. doi:10.1016/j.biopha.2020.109847
Jha, N. K., Sharma, C., Hashiesh, H. M., Arunachalam, S., Meeran, M. N., Javed, H., et al. (2021). β-Caryophyllene, A natural dietary CB2 receptor selective cannabinoid can be a candidate to target the trinity of infection, immunity, and inflammation in COVID-19. Front. Pharmacol. 12, 590201. doi:10.3389/fphar.2021.590201
Jia, L., Xue, K., Liu, J., Habotta, O. A., Hu, L., and Abdel Moneim, A. E. (2020). Anticolitic effect of berberine in rat experimental model: impact of PGE2/p38 MAPK pathways. Mediat. Inflamm. 2020, 9419085. doi:10.1155/2020/9419085
Jia, X., Gao, Y., Liu, L., Guo, Y., Wang, J., Ma, H., et al. (2022). Artemisinin alleviates intestinal inflammation and metabolic disturbance in ulcerative colitis rats induced by DSS. Evid. Based Complement. Altern. Med. 2022, 6211215. doi:10.1155/2022/6211215
Jiang, H., Shi, G.-F., Fang, Y.-X., Liu, Y.-Q., Wang, Q., Zheng, X., et al. (2022). Aloin A prevents ulcerative colitis in mice by enhancing the intestinal barrier function via suppressing the Notch signaling pathway. Phytomedicine 106, 154403. doi:10.1016/j.phymed.2022.154403
Jiang, M., Zhong, G., Zhu, Y., Wang, L., He, Y., Sun, Q., et al. (2021b). Retardant effect of dihydroartemisinin on ulcerative colitis in a JAK2/STAT3-dependent manner. Acta Biochim. Biophys. Sin. 53, 1113–1123. doi:10.1093/abbs/gmab097
Jiang, Y., Zhao, L., Chen, Q., and Zhou, L. (2021a). Exploring the mechanism of berberine intervention in ulcerative colitis from the perspective of inflammation and immunity based on systemic pharmacology. Evid. Based Complement. Altern. Med. 2021, 9970240. doi:10.1155/2021/9970240
Johansson, M. E. V., Ambort, D., Pelaseyed, T., Schütte, A., Gustafsson, J. K., Ermund, A., et al. (2011). Composition and functional role of the mucus layers in the intestine. Cell. Mol. Life Sci. 68, 3635–3641. doi:10.1007/s00018-011-0822-3
Johansson, M. E. V., Gustafsson, J. K., Holmen-Larsson, J., Jabbar, K. S., Xia, L. J., Xu, H., et al. (2014). Bacteria penetrate the normally impenetrable inner colon mucus layer in both murine colitis models and patients with ulcerative colitis. Gut 63, 281–291. doi:10.1136/gutjnl-2012-303207
Jostins, L., Ripke, S., Weersma, R. K., Duerr, R. H., McGovern, D. P., Hui, K. Y., et al. (2012). Host–microbe interactions have shaped the genetic architecture of inflammatory bowel disease. Nature 491, 119–124. doi:10.1038/nature11582
Kanneganti, T. D., Lamkanfi, M., and Nunez, G. (2007). Intracellular NOD-like receptors in host defense and disease. Immunity 27, 549–559. doi:10.1016/j.immuni.2007.10.002
Kaser, A., Martinez-Naves, E., and Blumberg, R. S. (2010). Endoplasmic reticulum stress: implications for inflammatory bowel disease pathogenesis. Curr. Opin. Gastroenterol. 26, 318–326. doi:10.1097/MOG.0b013e32833a9ff1
Kondo, K., Hiramoto, K., Yamate, Y., Goto, K., Sekijima, H., and Ooi, K. (2019). Ameliorative effect of high-dose vitamin C administration on dextran sulfate sodium-induced colitis mouse model. Biol. Pharm. Bull. 42, 954–959. doi:10.1248/bpb.b18-00967
Kook, S.-H., Choi, K. C., Cho, S.-W., Chod, H.-K., Lee, K. D., and Lee, J.-C. (2015). Catechin-7-O-β-D-glucopyranoside isolated from the seed of Phaseolus calcaratus Roxburgh ameliorates experimental colitis in rats. Int. Immunopharmacol. 29, 521–527. doi:10.1016/j.intimp.2015.10.003
Koppel, N., Rekdal, V. M., and Balskus, E. P. (2017). Chemical transformation of xenobiotics by the human gut microbiota. Science 356, eaag2770. doi:10.1126/science.aag2770
Kostic, A. D., Xavier, R. J., and Gevers, D. (2014). The microbiome in inflammatory bowel disease: current status and the future ahead. Gastroenterology 146, 1489–1499. doi:10.1053/j.gastro.2014.02.009
Kotipalli, R. S. S., Tirunavalli, S. K., Pote, A. B., Sahu, B. D., Kuncha, M., Jerald, M. K., et al. (2023). Sinigrin attenuates the dextran sulfate sodium-induced colitis in mice by modulating the MAPK pathway. Inflammation 46, 787–807. doi:10.1007/s10753-022-01780-4
Krndija, D., El Marjou, F., Guirao, B., Richon, S., Leroy, O., Bellaiche, Y., et al. (2019). Active cell migration is critical for steady-state epithelial turnover in the gut. Science 365, 705–710. doi:10.1126/science.aau3429
Kuhnert, F., Davis, C. R., Wang, H. T., Chu, P., Lee, M., Yuan, J., et al. (2004). Essential requirement for Wnt signaling in proliferation of adult small intestine and colon revealed by adenoviral expression of Dickkopf-1. Proc. Natl. Acad. Sci. U. S. A. 101, 266–271. doi:10.1073/pnas.2536800100
Larabi, A., Barnich, N., and Nguyen, H. T. T. (2020). New insights into the interplay between autophagy, gut microbiota and inflammatory responses in IBD. Autophagy 16, 38–51. doi:10.1080/15548627.2019.1635384
Laukens, D., Devisscher, L., Van den Bossche, L., Hindryckx, P., Vandenbroucke, R. E., Vandewynckel, Y. P., et al. (2014). Tauroursodeoxycholic acid inhibits experimental colitis by preventing early intestinal epithelial cell death. Lab. Invest. 94, 1419–1430. doi:10.1038/labinvest.2014.117
Li, B., Du, P., Du, Y., Zhao, D., Cai, Y., Yang, Q., et al. (2021a). Luteolin alleviates inflammation and modulates gut microbiota in ulcerative colitis rats. Life Sci. 269, 119008. doi:10.1016/j.lfs.2020.119008
Li, C., Dong, N., Wu, B., Mo, Z., Xie, J., and Lu, Q. (2021b). Dihydroberberine, an isoquinoline alkaloid, exhibits protective effect against dextran sulfate sodium-induced ulcerative colitis in mice. Phytomedicine 90, 153631. doi:10.1016/j.phymed.2021.153631
Li, C., Zhang, W., Wu, X., Cai, Q., Tan, Z., Hong, Z., et al. (2022c). Aromatic-turmerone ameliorates DSS-induced ulcerative colitis via modulating gut microbiota in mice. Inflammopharmacology 30, 1283–1294. doi:10.1007/s10787-022-01007-w
Li, H., Fan, C., Lu, H., Feng, C., He, P., Yang, X., et al. (2020b). Protective role of berberine on ulcerative colitis through modulating enteric glial cells-intestinal epithelial cells-immune cells interactions. Acta Pharm. Sin. B 10, 447–461. doi:10.1016/j.apsb.2019.08.006
Li, H., Pang, B., Nie, B., Qu, S., Zhang, K., Xu, J., et al. (2022g). Dioscin promotes autophagy by regulating the AMPK-mTOR pathway in ulcerative colitis. Immunopharmacol. Immunotoxicol. 44, 238–246. doi:10.1080/08923973.2022.2037632
Li, M., Guo, W., Dong, Y., Wang, W., Tian, C., Zhang, Z., et al. (2022e). Beneficial effects of celastrol on immune balance by modulating gut microbiota in experimental ulcerative colitis mice. Genomics Proteomics Bioinforma. 20, 288–303. doi:10.1016/j.gpb.2022.05.002
Li, M. Y., Zhang, Z. H., Wang, Z., Zuo, H. X., Wang, J. Y., Xing, Y., et al. (2019b). Convallatoxin protects against dextran sulfate sodium-induced experimental colitis in mice by inhibiting NF-κB signaling through activation of PPARγ. Pharmacol. Res. 147, 104355. doi:10.1016/j.phrs.2019.104355
Li, N., Sun, W., Zhou, X., Gong, H., Chen, Y., Chen, D., et al. (2019a). Dihydroartemisinin protects against dextran sulfate sodium-induced colitis in mice through inhibiting the PI3K/AKT and NF-κB signaling pathways. Biomed. Res. Int. 2019, 1415809–1415812. doi:10.1155/2019/1415809
Li, P., Wu, M., Xiong, W., Li, J., An, Y., Ren, J., et al. (2020a). Saikosaponin-d ameliorates dextran sulfate sodium-induced colitis by suppressing NF-κB activation and modulating the gut microbiota in mice. Int. Immunopharmacol. 81, 106288. doi:10.1016/j.intimp.2020.106288
Li, R., Chen, C., Liu, B., Shi, W., Shimizu, K., and Zhang, C. (2022f). Bryodulcosigenin a natural cucurbitane-type triterpenoid attenuates dextran sulfate sodium (DSS)-induced colitis in mice. Phytomedicine 94, 153814. doi:10.1016/j.phymed.2021.153814
Li, T. T., Zhang, J. F., Fei, S. J., Zhu, S. P., Zhu, J. Z., Qiao, X., et al. (2014). Glutamate microinjection into the hypothalamic paraventricular nucleus attenuates ulcerative colitis in rats. Acta Pharmacol. Sin. 35, 185–194. doi:10.1038/aps.2013.140
Li, W., Zhang, L., Xu, Q., Yang, W., Zhao, J., Ren, Y., et al. (2022d). Taxifolin alleviates DSS-induced ulcerative colitis by acting on gut microbiome to produce butyric acid. Nutrients 14, 1069. doi:10.3390/nu14051069
Li, X., Wu, X., Wang, Q., Xu, W., Zhao, Q., Xu, N., et al. (2022b). Sanguinarine ameliorates DSS induced ulcerative colitis by inhibiting NLRP3 inflammasome activation and modulating intestinal microbiota in C57BL/6 mice. Phytomedicine 104, 154321. doi:10.1016/j.phymed.2022.154321
Li, X., Xu, S., Zhang, Y., Li, K., Gao, X.-J., and Guo, M.-Y. (2022a). Berberine depresses inflammation and adjusts smooth muscle to ameliorate ulcerative colitis of cats by regulating gut microbiota. Microbiol. Spectr. 10, e0320722. doi:10.1128/spectrum.03207-22
Li, Y., Pan, X., Yin, M., Li, C., and Han, L. (2021c). Preventive effect of lycopene in dextran sulfate sodium-induced ulcerative colitis mice through the regulation of TLR4/TRIF/NF-κB signaling pathway and tight junctions. J. Agric. Food Chem. 69, 13500–13509. doi:10.1021/acs.jafc.1c05128
Liang, H., Cheng, R., Wang, J., Xie, H., Li, R., Shimizu, K., et al. (2021). Mogrol, an aglycone of mogrosides, attenuates ulcerative colitis by promoting AMPK activation. Phytomedicine 81, 153427. doi:10.1016/j.phymed.2020.153427
Liang, S., Deng, X., Lei, L., Zheng, Y., Ai, J., Chen, L., et al. (2019). The comparative study of the therapeutic effects and mechanism of baicalin, baicalein, and their combination on ulcerative colitis rat. Front. Pharmacol. 10, 1466. doi:10.3389/fphar.2019.01466
Lifei, L., Zhang, J., Li, X., Zhu, Y., Wang, Y., and Liu, D. (2023). Sericic acid ameliorates DSS-induced ulcerative colitis in mice by modulating the NF-kappa B and Nrf2 pathways. Curr. Mol. Pharmacol. 16, 759–770. doi:10.2174/1874467215666220928100319
Lin, Y., Jiang, M., Chen, W., Zhao, T., and Wei, Y. (2019). Cancer and ER stress: mutual crosstalk between autophagy, oxidative stress and inflammatory response. Biomed. Pharmacother. 118, 109249. doi:10.1016/j.biopha.2019.109249
Lin, Y., Wu, Y., Su, J., Wang, M., Wu, X., Su, Z., et al. (2021). Therapeutic role of d-pinitol on experimental colitis via activating Nrf2/ARE and PPAR-γ/NF-κB signaling pathways. Food. Funct. 12, 2554–2568. doi:10.1039/d0fo03139a
Liu, B., Piao, X., Guo, L., Liu, S., Chai, F., and Gao, L. (2016). Ursolic acid protects against ulcerative colitis via anti-inflammatory and antioxidant effects in mice. Mol. Med. Rep. 13, 4779–4785. doi:10.3892/mmr.2016.5094
Liu, C., Wang, J., Yang, Y., Liu, X., Zhu, Y., Zou, J., et al. (2018b). Ginsenoside Rd ameliorates colitis by inducing p62-driven mitophagy-mediated NLRP3 inflammasome inactivation in mice. Biochem. Pharmacol. 155, 366–379. doi:10.1016/j.bcp.2018.07.010
Liu, D., Huo, X., Gao, L., Zhang, J., Ni, H., and Cao, L. (2018a). NF-κB and Nrf2 pathways contribute to the protective effect of Licochalcone A on dextran sulphate sodium-induced ulcerative colitis in mice. Biomed. Pharmacother. 102, 922–929. doi:10.1016/j.biopha.2018.03.130
Liu, D., Tian, Q., Liu, K., Ren, F., Liu, G., Zhou, J., et al. (2023c). Ginsenoside Rg3 ameliorates DSS-induced colitis by inhibiting NLRP3 inflammasome activation and regulating microbial homeostasis. J. Agric. Food Chem. 71, 3472–3483. doi:10.1021/acs.jafc.2c07766
Liu, F., Yao, Y., Wang, Q., Zhang, F., Wang, M., Zhu, C., et al. (2023b). Nigakinone alleviates DSS-induced experimental colitis via regulating bile acid profile and FXR/NLRP3 signaling pathways. Phytother. Res. 37, 15–34. doi:10.1002/ptr.7588
Liu, H., Xiong, J., He, T., Xiao, T., Li, Y., Yu, Y., et al. (2017). High uric acid-induced epithelial-mesenchymal transition of renal tubular epithelial cells via the TLR4/NF-kB signaling pathway. Am. J. Nephrol. 46, 333–342. doi:10.1159/000481668
Liu, J., Cai, J., Fan, P., Zhang, N., and Cao, Y. (2019). The abilities of salidroside on ameliorating inflammation, skewing the imbalanced nucleotide oligomerization domain-like receptor family pyrin domain containing 3/autophagy, and maintaining intestinal barrier are profitable in colitis. Front. Pharmacol. 10, 1385. doi:10.3389/fphar.2019.01385
Liu, J., Shi, L., Huang, W., Zheng, Z., Huang, X., and Su, Y. (2022b). Homoharringtonine attenuates dextran sulfate sodium-induced colitis by inhibiting NF-kappa B signaling. Mediat. Inflamm. 2022, 3441357. doi:10.1155/2022/3441357
Liu, J. Z., van Sommeren, S., Huang, H., Ng, S. C., Alberts, R., Takahashi, A., et al. (2015). Association analyses identify 38 susceptibility loci for inflammatory bowel disease and highlight shared genetic risk across populations. Nat. Genet. 47, 979–986. doi:10.1038/ng.3359
Liu, K., Li, G., Guo, W., and Zhang, J. (2020c). The protective effect and mechanism of pedunculoside on DSS (dextran sulfate sodium) induced ulcerative colitis in mice. Int. Immunopharmacol. 88, 107017. doi:10.1016/j.intimp.2020.107017
Liu, S., Shen, H., Li, J., Gong, Y., Bao, H., Zhang, J., et al. (2020b). Loganin inhibits macrophage M1 polarization and modulates sirt1/NF-kappa B signaling pathway to attenuate ulcerative colitis. Bioengineered 11, 628–639. doi:10.1080/21655979.2020.1774992
Liu, X., Zhou, M., Dai, Z., Luo, S., Shi, Y., He, Z., et al. (2023a). Salidroside alleviates ulcerative colitis via inhibiting macrophage pyroptosis and repairing the dysbacteriosis-associated Th17/Treg imbalance. Phytother. Res. 37, 367–382. doi:10.1002/ptr.7636
Liu, Y., Li, B. G., Su, Y. H., Zhao, R. X., Song, P., Li, H., et al. (2022a). Potential activity of traditional Chinese medicine against ulcerative colitis: a review. J. Ethnopharmacol. 289, 115084. doi:10.1016/j.jep.2022.115084
Liu, Y., Wu, J., Chen, L., Wu, X., Gan, Y., Xu, N., et al. (2020a). β-patchoulene simultaneously ameliorated dextran sulfate sodium-induced colitis and secondary liver injury in mice via suppressing colonic leakage and flora imbalance. Biochem. Pharmacol. 182, 114260. doi:10.1016/j.bcp.2020.114260
Long, J., Liu, X. K., Kang, Z. P., Wang, M. X., Zhao, H. M., Huang, J. Q., et al. (2022). Ginsenoside Rg1 ameliorated experimental colitis by regulating the balance of M1/M2 macrophage polarization and the homeostasis of intestinal flora. Eur. J. Pharmacol. 917, 174742. doi:10.1016/j.ejphar.2022.174742
Lopes de Oliveira, G. A., Alarcon-de-la-Lastra, C., Angeles Rosillo, M., Castejon Martinez, M. L., Sanchez-Hidalgo, M., Rolim Medeiros, J. V., et al. (2019). Preventive effect of bergenin against the development of TNBS-induced acute colitis in rats is associated with inflammatory mediators inhibition and NLRP3/ASC inflammasome signaling pathways. Chemico-Biological Interact. 297, 25–33. doi:10.1016/j.cbi.2018.10.020
Lu, Y., Chen, J., He, X., Xu, S., Chen, Y.-E., Gao, J., et al. (2021). Combined administration of vitamin D-3 and geniposide is less effective than single use of vitamin D-3 or geniposide in the treatment of ulcerative colitis. Front. Pharmacol. 12, 714065. doi:10.3389/fphar.2021.714065
Luca, S. V., Macovei, I., Bujor, A., Miron, A., Skalicka-Wozniak, K., Aprotosoaie, A. C., et al. (2020). Bioactivity of dietary polyphenols: the role of metabolites. Crit. Rev. Food Sci. Nutr. 60, 626–659. doi:10.1080/10408398.2018.1546669
Lucena, A. M. M., Souza, C. R. M., Jales, J. T., Guedes, P. M. M., de Miranda, G. E. C., de Moura, A. M. A., et al. (2018). The bisindole alkaloid caulerpin, from seaweeds of the genus caulerpa, attenuated colon damage in murine colitis model. Mar. Drugs. 16, 318. doi:10.3390/md16090318
Luo, M., and Luo, Y. (2021). Imperatorin relieved ulcerative colitis by regulating the nrf-2/ARE/HO-1 pathway in rats. Inflammation 44, 558–569. doi:10.1007/s10753-020-01353-3
Luo, S., He, J., Huang, S., Wang, X., Su, Y., Li, Y., et al. (2022). Emodin targeting the colonic metabolism via PPAR gamma alleviates UC by inhibiting facultative anaerobe. Phytomedicine 104, 154106. doi:10.1016/j.phymed.2022.154106
Lv, L., Chen, Z., Bai, W., Hao, J., Heng, Z., Meng, C., et al. (2023). Taurohyodeoxycholic acid alleviates trinitrobenzene sulfonic acid induced ulcerative colitis via regulating Th1/Th2 and Th17/Treg cells balance. Life Sci. 318, 121501. doi:10.1016/j.lfs.2023.121501
Lv, Q., Xing, Y., Liu, Y., Chen, Q., Xu, J., Hu, L., et al. (2021). Didymin switches M1-like toward M2-like macrophage to ameliorate ulcerative colitis via fatty acid oxidation. Pharmacol. Res. 169, 105613. doi:10.1016/j.phrs.2021.105613
Lv, T., Shen, L., Yang, L., Diao, W., Yang, Z., Zhang, Y., et al. (2018). Polydatin ameliorates dextran sulfate sodium-induced colitis by decreasing oxidative stress and apoptosis partially via Sonic hedgehog signaling pathway. Int. Immunopharmacol. 64, 256–263. doi:10.1016/j.intimp.2018.09.009
Ma, J., Yin, G., Lu, Z., Xie, P., Zhou, H., Liu, J., et al. (2018). Casticin prevents DSS induced ulcerative colitis in mice through inhibitions of NF-κB pathway and ROS signaling. Phytother. Res. 32, 1770–1783. doi:10.1002/ptr.6108
Mahid, S. S., Minor, K. S., Soto, R. E., Hornung, C. A., and Galandiuk, S. (2006). Smoking and inflammatory bowel disease: a meta-analysis. Mayo Clin. Proc. 81, 1462–1471. doi:10.4065/81.11.1462
Mai, C.-T., Wu, M.-M., Wang, C.-L., Su, Z.-R., Cheng, Y.-Y., and Zhang, X.-J. (2019). Palmatine attenuated dextran sulfate sodium (DSS)-induced colitis via promoting mitophagy-mediated NLRP3 inflammasome inactivation. Mol. Immunol. 105, 76–85. doi:10.1016/j.molimm.2018.10.015
Maria-Ferreira, D., Nascimento, A. M., Cipriani, T. R., Santana, A. P., Watanabe, P. D., Ana, D., et al. (2018). Rhamnogalacturonan, a chemically-defined polysaccharide, improves intestinal barrier function in DSS-induced colitis in mice and human Caco-2 cells. Sci. Rep. 8, 12261. doi:10.1038/s41598-018-30526-2
Martinon, F., Burns, K., and Tschopp, J. (2002). The inflammasome: a molecular platform triggering activation of inflammatory caspases and processing of proIL-beta. Mol. Cell 10, 417–426. doi:10.1016/s1097-2765(02)00599-3
Miao, F. (2022). Hydroxytyrosol alleviates dextran sodium sulfate-induced colitis by inhibiting NLRP3 inflammasome activation and modulating gut microbiota in vivo. Nutrition 97, 111579. doi:10.1016/j.nut.2021.111579
Miao, S., Lu, Q., Zhou, Y., Chang, Y., Xu, T., and Zhu, M. (2022). Oral administration of octacosanol modulates the gut bacteria and protects the intestinal barrier in ulcerative colitis mice. J. Food Biochem. 46, e14284. doi:10.1111/jfbc.14284
Miao, Y., Lv, Q., Qiao, S., Yang, L., Tao, Y., Yan, W., et al. (2019). Alpinetin improves intestinal barrier homeostasis via regulating AhR/suv39h1/TSC2/mTORC1/autophagy pathway. Toxicol. Appl. Pharmacol. 384, 114772. doi:10.1016/j.taap.2019.114772
Mostafa, A. F., Elalfy, M. M., Shata, A., and Elhadidy, M. G. (2020). Prophylactic effect of aquatic extract of stevia on acetic acid induced-ulcerative colitis in male rats: a possible role of Nrf2 and PPARγ. J. Basic Clin. Physiol. Pharmacol. 32, 1093–1104. doi:10.1515/jbcpp-2020-0039
Nakarai, H., Yamashita, A., Nagayasu, S., Iwashita, M., Kumamoto, S., Ohyama, H., et al. (2012). Adipocyte-macrophage interaction may mediate LPS-induced low-grade inflammation: potential link with metabolic complications. Innate Immun. 18, 164–170. doi:10.1177/1753425910393370
Nanki, K., Fujii, M., Shimokawa, M., Matano, M., Nishikori, S., Date, S., et al. (2019). Somatic inflammatory gene mutations in human ulcerative colitis epithelium. Nature 577, 254–259. doi:10.1038/s41586-019-1844-5
Niu, W., Chen, Y., Wang, L., Li, J., Cui, Z., Lv, J., et al. (2022). The combination of sodium alginate and chlorogenic acid enhances the therapeutic effect on ulcerative colitis by the regulation of inflammation and the intestinal flora. Food. Funct. 13, 10710–10723. doi:10.1039/d2fo01619b
Niu, X., Zhang, H., Li, W., Wang, Y., Mu, Q., Wang, X., et al. (2015). Protective effect of cavidine on acetic acid-induced murine colitis via regulating antioxidant, cytokine profile and NF-κB signal transduction pathways. Chem. Biol. Interact. 239, 34–45. doi:10.1016/j.cbi.2015.06.026
Ohkusa, T., Okayasu, I., Ogihara, T., Morita, K., Ogawa, M., and Sato, N. (2003). Induction of experimental ulcerative colitis by Fusobacterium varium isolated from colonic mucosa of patients with ulcerative colitis. Gut 52, 79–83. doi:10.1136/gut.52.1.79
Pagano, E., Romano, B., Iannotti, F. A., Parisi, O. A., D'Armiento, M., Pignatiello, S., et al. (2019). The non-euphoric phytocannabinoid cannabidivarin counteracts intestinal inflammation in mice and cytokine expression in biopsies from UC pediatric patients. Pharmacol. Res. 149, 104464. doi:10.1016/j.phrs.2019.104464
Pan, H.-H., Zhou, X.-X., Ma, Y.-Y., Pan, W.-S., Zhao, F., Yu, M.-S., et al. (2020). Resveratrol alleviates intestinal mucosal barrier dysfunction in dextran sulfate sodium-induced colitis mice by enhancing autophagy. World J. Gastroenterol. 26, 4945–4959. doi:10.3748/wjg.v26.i33.4945
Pan, Y. Y., Deng, Y., Su, S., Yin, J. H., Chen, Y. H., Wang, L. C., et al. (2023). Structure composition and intracellular transport of clathrin-mediated intestinal transmembrane tight junction protein. Inflammation 46, 18–34. doi:10.1007/s10753-022-01724-y
Pang, B., Jin, H., Liao, N., Li, J., Jiang, C., and Shi, J. (2021). Vitamin A supplementation ameliorates ulcerative colitis in gut microbiota-dependent manner. Food Res. Int. 148, 110568. doi:10.1016/j.foodres.2021.110568
Park, M.-Y., Ji, G. E., and Sung, M.-K. (2012). Dietary kaempferol suppresses inflammation of dextran sulfate sodium-induced colitis in mice. Dig. Dis. Sci. 57, 355–363. doi:10.1007/s10620-011-1883-8
Pastorelli, L., Pizarro, T. T., Cominelli, F., and Vecchi, M. (2009). Emerging drugs for the treatment of ulcerative colitis. Drugs 14, 505–521. doi:10.1517/14728210903146882
Peng, L., Gao, X., Nie, L., Xie, J., Dai, T., Shi, C., et al. (2020). Astragalin attenuates dextran sulfate sodium (DSS)-Induced acute experimental colitis by alleviating gut microbiota dysbiosis and inhibiting NF-kappa B activation in mice. Front. Immunol. 11, 2058. doi:10.3389/fimmu.2020.02058
Prados, M. E., Garcia-Martin, A., Unciti-Broceta, J. D., Palomares, B., Collado, J. A., Minassi, A., et al. (2021). Betulinic acid hydroxamate prevents colonic inflammation and fibrosis in murine models of inflammatory bowel disease. Acta Pharmacol. Sin. 42, 1124–1138. doi:10.1038/s41401-020-0497-0
Pu, Z., Liu, Y., Li, C., Xu, M., Xie, H., and Zhao, J. (2020). Using network pharmacology for systematic understanding of geniposide in ameliorating inflammatory responses in colitis through suppression of NLRP3 inflammasome in macrophage by AMPK/Sirt1 dependent signaling. Am. J. Chin. Med. 48, 1693–1713. doi:10.1142/s0192415x20500846
Puppala, E. R., Aochenlar, S. L., Shantanu, P. A., Ahmed, S., Jannu, A. K., Jala, A., et al. (2022). Perillyl alcohol attenuates chronic restraint stress aggravated dextran sulfate sodium-induced ulcerative colitis by modulating TLR4/NF-kappa B and JAK2/STAT3 signaling pathways. Phytomedicine 106, 154415. doi:10.1016/j.phymed.2022.154415
Qian, B., Wang, C., Zeng, Z., Ren, Y., Li, D., and Song, J.-L. (2020). Ameliorative effect of sinapic acid on dextran sodium sulfate- (DSS-) induced ulcerative colitis in kunming (KM) mice. Oxid. Med. Cell. Longev. 2020, 8393504. doi:10.1155/2020/8393504
Qu, B., Cao, T., Wang, M., Wang, S., Li, W., Li, H., et al. (2022b). Ginsenosides Rd monomer inhibits proinflammatory cytokines production and alleviates DSS-colitis by NF-κB and P38MAPK pathways in mice. Immunopharmacol. Immunotoxicol. 44, 110–118. doi:10.1080/08923973.2021.2012482
Qu, C., Yuan, Z. W., Yu, X. T., Huang, Y. F., Yang, G. H., Chen, J. N., et al. (2017). Patchouli alcohol ameliorates dextran sodium sulfate-induced experimental colitis and suppresses tryptophan catabolism. Pharmacol. Res. 121, 70–82. doi:10.1016/j.phrs.2017.04.017
Qu, L., Lin, X., Liu, C., Ke, C., Zhou, Z., Xu, K., et al. (2021c). Atractylodin attenuates dextran sulfate sodium-induced colitis by alleviating gut microbiota dysbiosis and inhibiting inflammatory response through the MAPK pathway. Front. Pharmacol. 12, 665376. doi:10.3389/fphar.2021.665376
Qu, L., Shi, K., Xu, J., Liu, C., Ke, C., Zhan, X., et al. (2022a). Atractylenolide-1 targets SPHK1 and B4GALT2 to regulate intestinal metabolism and flora composition to improve inflammation in mice with colitis. Phytomedicine 98, 153945. doi:10.1016/j.phymed.2022.153945
Qu, S., Chen, L., Wen, X., Zuo, J., Wang, X., Lu, Z., et al. (2021b). Suppression of Th17 cell differentiation via sphingosine-1-phosphate receptor 2 by cinnamaldehyde can ameliorate ulcerative colitis. Biomed. Pharmacother. 134, 111116. doi:10.1016/j.biopha.2020.111116
Qu, Y., Li, X., Xu, F., Zhao, S., Wu, X., Wang, Y., et al. (2021a). Kaempferol alleviates murine experimental colitis by restoring gut microbiota and inhibiting the LPS-TLR4-NF-κb Axis. Front. Immunol. 12, 679897. doi:10.3389/fimmu.2021.679897
Rapa, S. F., Di Paola, R., Cordaro, M., Siracusa, R., D'Amico, R., Fusco, R., et al. (2021). Plumericin protects against experimental inflammatory bowel disease by restoring intestinal barrier function and reducing apoptosis. Biomedicines 9, 67. doi:10.3390/biomedicines9010067
Rashidian, A., Dejban, P., Karami Fard, K., Abdollahi, A., Chamanara, M., Dehpour, A., et al. (2020). Bupropion ameliorates acetic acid-induced colitis in rat: the involvement of the TLR4/NF-kB signaling pathway. Inflammation 43, 1999–2009. doi:10.1007/s10753-020-01273-2
Rashidian, A., Muhammadnejad, A., Dehpour, A. R., Mehr, S. E., Akhavan, M. M., Shirkoohi, R., et al. (2016). Atorvastatin attenuates TNBS-induced rat colitis: the involvement of the TLR4/NF-kB signaling pathway. Inflammopharmacology 24, 109–118. doi:10.1007/s10787-016-0263-6
Ren, J., Yue, B., Wang, H., Zhang, B., Luo, X., Yu, Z., et al. (2021). Acacetin ameliorates experimental colitis in mice via inhibiting macrophage inflammatory response and regulating the composition of gut microbiota. Front. Physiol. 11, 577237. doi:10.3389/fphys.2020.577237
Rogler, G. (2010). Gastrointestinal and liver adverse effects of drugs used for treating IBD. Best. Pract. Res. Clin. Gastroenterol. 24, 157–165. doi:10.1016/j.bpg.2009.10.011
Saha, S., Shalova, I. N., and Biswas, S. K. (2017). Metabolic regulation of macrophage phenotype and function. Immunol. Rev. 280, 102–111. doi:10.1111/imr.12603
Sahu, B. D., Kumar, J. M., and Sistla, R. (2016). Fisetin, a dietary flavonoid, ameliorates experimental colitis in mice: relevance of NF-kappa B signaling. J. Nutr. Biochem. 28, 171–182. doi:10.1016/j.jnutbio.2015.10.004
Sakthivel, K. M., and Guruvayoorappan, C. (2013). Amentoflavone inhibits iNOS, COX-2 expression and modulates cytokine profile, NF-kappa B signal transduction pathways in rats with ulcerative colitis. Int. Immunopharmacol. 17, 907–916. doi:10.1016/j.intimp.2013.09.022
Sanchez-Fidalgo, S., Villegas, I., Angeles Rosillo, M., Aparicio-Soto, M., and Alarcon-de-la-Lastra, C. (2015). Dietary squalene supplementation improves DSS-induced acute colitis by downregulating p38 MAPK and NFkB signaling pathways. Mol. Nutr. Food Res. 59, 284–292. doi:10.1002/mnfr.201400518
Sangaraju, R., Nalban, N., Alavala, S., Rajendran, V., Jerald, M. K., and Sistla, R. (2019). Protective effect of galangin against dextran sulfate sodium (DSS)-induced ulcerative colitis in Balb/c mice. Inflamm. Res. 68, 691–704. doi:10.1007/s00011-019-01252-w
Satoh, Y., Ishiguro, Y., Sakuraba, H., Kawaguchi, S., Hiraga, H., Fukuda, S., et al. (2009). Cyclosporine regulates intestinal epithelial apoptosis via TGF-beta-related signaling. Am. J. Physiol. Gastrointest. Liver Physiol. 297, G514–G519. doi:10.1152/ajpgi.90608.2008
Seo, G. S., Jiang, W.-Y., Park, P.-H., Sohn, D. H., Cheon, J. H., and Lee, S. H. (2014). Hirsutenone reduces deterioration of tight junction proteins through EGFR/Akt and ERK1/2 pathway both converging to HO-1 induction. Biochem. Pharmacol. 90, 115–125. doi:10.1016/j.bcp.2014.05.006
Sethuraman, N., Swaminathan, S., Nelson, S. B., Palaninathan, P. S., Gopalan, T. K., and Velayudham, P. (2015). Modulation of PPARγ and TNFα by emu oil and glycyrrhizin in ulcerative colitis. Inflammopharmacology 23, 47–56. doi:10.1007/s10787-014-0226-8
Shafik, N. M., Gaber, R. A., Mohamed, D. A., and Ebeid, A. M. (2019). Hesperidin modulates dextran sulfate sodium-induced ulcerative colitis in rats: targeting sphingosine kinase-1- sphingosine 1 phosphate signaling pathway, mitochondrial biogenesis, inflammation, and apoptosis. J. Biochem. Mol. Toxicol. 33, e22312. doi:10.1002/jbt.22312
Shah, Y. M., Morimura, K., and Gonzalez, F. J. (2007). Expression of peroxisome proliferator-activated receptor-gamma in macrophage suppresses experimentally induced colitis. Am. J. Physiol. Gastrointest. Liver. Physiol. 292, G657–G666. doi:10.1152/ajpgi.00381.2006
Shahid, M., Raish, M., Ahmad, A., Bin Jardan, Y. A., Ansari, M. A., Ahad, A., et al. (2022). Sinapic acid ameliorates acetic acid-induced ulcerative colitis in rats by suppressing inflammation, oxidative stress, and apoptosis. Molecules 27, 4139. doi:10.3390/molecules27134139
Sheikh, S. Z., Hegazi, R. A., Kobayashi, T., Onyiah, J. C., Russo, S. M., Matsuoka, K., et al. (2011). An anti-inflammatory role for carbon monoxide and heme oxygenase-1 in chronic Th2-mediated murine colitis. J. Immunol. 186, 5506–5513. doi:10.4049/jimmunol.1002433
Shen, J., Cheng, J., Zhu, S., Zhao, J., Ye, Q., Xu, Y., et al. (2019b). Regulating effect of baicalin on IKK/IKB/NF-kB signaling pathway and apoptosis-related proteins in rats with ulcerative colitis. Int. Immunopharmacol. 73, 193–200. doi:10.1016/j.intimp.2019.04.052
Shen, J., Li, N., and Zhang, X. (2019c). Daidzein ameliorates dextran sulfate sodium-induced experimental colitis in mice by regulating NF-kappa B signaling. J. Environ. Pathol. Toxicol. Oncol. 38, 29–39. doi:10.1615/JEnvironPatholToxicolOncol.2018027531
Shen, P., Zhang, Z., Zhu, K., Cao, H., Liu, J., Lu, X., et al. (2019a). Evodiamine prevents dextran sulfate sodium-induced murine experimental colitis via the regulation of NF-kappa B and NLRP3 inflammasome. Biomed. Pharmacother. 110, 786–795. doi:10.1016/j.biopha.2018.12.033
Shen, Y., Liu, Y., Wang, Z., Ruan, X., Li, S., Ni, S., et al. (2020). Effect of berberine from coptis chinensis on apoptosis of intestinal epithelial cells in a mouse model of ulcerative colitis: role of endoplasmic reticulum stress. Evid. Based Complement. Altern. Med. 2020, 3784671. doi:10.1155/2020/3784671
Sheng, Q., Li, F., Chen, G., Li, J., Li, J., Wang, Y., et al. (2021). Ursolic acid regulates intestinal microbiota and inflammatory cell infiltration to prevent ulcerative colitis. J. Immunol. Res. 2021, 6679316. doi:10.1155/2021/6679316
Shi, B., Liu, S., Huang, A., Zhou, M., Sun, B., Cao, H., et al. (2021). Revealing the mechanism of friedelin in the treatment of ulcerative colitis based on network pharmacology and experimental verification. Evid. Based Complement. Altern. Med. 2021, 4451779. doi:10.1155/2021/4451779
Socca, E. A., Luiz-Ferreira, A., de Faria, F. M., de Almeida, A. C., Dunder, R. J., Manzo, L. P., et al. (2014). Inhibition of tumor necrosis factor-alpha and cyclooxigenase-2 by Isatin: a molecular mechanism of protection against TNBS-induced colitis in rats. Chem. Biol. Interact. 209, 48–55. doi:10.1016/j.cbi.2013.11.019
Song, C., Chen, J., Li, X., Yang, R., Cao, X., Zhou, L., et al. (2021). Limonin ameliorates dextran sulfate sodium-induced chronic colitis in mice by inhibiting PERK-ATF4-CHOP pathway of ER stress and NF-κB signaling. Int. Immunopharmacol. 90, 107161. doi:10.1016/j.intimp.2020.107161
Su, C. G., Wen, X., Bailey, S. T., Jiang, W., Rangwala, S. M., Keilbaugh, S. A., et al. (1999). A novel therapy for colitis utilizing PPAR-gamma ligands to inhibit the epithelial inflammatory response. J. Clin. Invest. 104, 383–389. doi:10.1172/JCI7145
Su, S., Wang, X., Xi, X., Zhu, L., Chen, Q., Zhang, H., et al. (2021). Phellodendrine promotes autophagy by regulating the AMPK/mTOR pathway and treats ulcerative colitis. J. Cell. Mol. Med. 25, 5707–5720. doi:10.1111/jcmm.16587
Suzuki, T. (2013). Regulation of intestinal epithelial permeability by tight junctions. Cell. Mol. Life Sci. 70, 631–659. doi:10.1007/s00018-012-1070-x
Tan, X., Wen, Y., Han, Z., Su, X., Peng, J., Chen, F., et al. (2023). Cinnamaldehyde ameliorates dextran sulfate sodium-induced colitis in mice by modulating TLR4/NF-kappa B signaling pathway and NLRP3 inflammasome activation. Chem. Biodivers. 20, e202200089. doi:10.1002/cbdv.202200089
Tan, Y., and Zheng, C. (2018). Effects of alpinetin on intestinal barrier function, inflammation and oxidative stress in dextran sulfate sodium-induced ulcerative colitis mice. Am. J. Med. Sci. 355, 377–386. doi:10.1016/j.amjms.2018.01.002
Tan, Z., Xie, N., Cui, H., Moellering, D. R., Abraham, E., Thannickal, V. J., et al. (2015). Pyruvate dehydrogenase kinase 1 participates in macrophage polarization via regulating glucose metabolism. J. Immunol. 194, 6082–6089. doi:10.4049/jimmunol.1402469
Tang, B., Zhu, J., Fang, S., Wang, Y., Vinothkumar, R., Li, M., et al. (2021). Pharmacological inhibition of MELK restricts ferroptosis and the inflammatory response in colitis and colitis-propelled carcinogenesis. Free Radic. Biol. Med. 172, 312–329. doi:10.1016/j.freeradbiomed.2021.06.012
Tang, B., Zhu, J., Zhang, B., Wu, F., Wang, Y., Weng, Q., et al. (2020b). Therapeutic potential of triptolide as an anti-inflammatory agent in dextran sulfate sodium-induced murine experimental colitis. Front. Immunol. 11, 592084. doi:10.3389/fimmu.2020.592084
Tang, X., Huang, G., Zhang, T., and Li, S. (2020a). Elucidation of colon-protective efficacy of diosgenin in experimental TNBS-induced colitis: inhibition of NF-κB/IkB-α and Bax/Caspase-1 signaling pathways. Biosci. Biotechnol. Biochem. 84, 1903–1912. doi:10.1080/09168451.2020.1776590
Tekeli, I. O., Atessahin, A., Sakin, F., Aslan, A., Ceribasi, S., and Yipel, M. (2018). Protective effects of conventional and colon-targeted lycopene and linalool on ulcerative colitis induced by acetic acid in rats. Inflammopharmacology 27, 313–322. doi:10.1007/s10787-018-0485-x
Tian, M., Ma, P., Zhang, Y., Mi, Y., and Fan, D. (2020). Ginsenoside Rk3 alleviated DSS-induced ulcerative colitis by protecting colon barrier and inhibiting NLRP3 inflammasome pathway. Int. Immunopharmacol. 85, 106645. doi:10.1016/j.intimp.2020.106645
Tiana, X., Peng, Z., Luo, S., Zhang, S., Li, B., Zhou, C., et al. (2019). Aesculin protects against DSS-Induced colitis though activating PPARγ and inhibiting NF-кB pathway. Eur. J. Pharmacol. 857, 172453. doi:10.1016/j.ejphar.2019.172453
Ungaro, R., Mehandru, S., Allen, P. B., Peyrin-Biroulet, L., and Colombel, J.-F. (2017). Ulcerative colitis. Lancet 389, 1756–1770. doi:10.1016/s0140-6736(16)32126-2
Veltkamp, C., Tonkonogy, S. L., de Jong, Y. P., Albright, C., Grenther, W. B., Balish, E., et al. (2001). Continuous stimulation by normal luminal bacteria is essential for the development and perpetuation of colitis in Tg(epsilon26) mice. Gastroenterology 120, 900–913. doi:10.1053/gast.2001.22547
Villani, A. C., Lemire, M., Fortin, G., Louis, E., Silverberg, M. S., Collette, C., et al. (2009). Common variants in the NLRP3 region contribute to Crohn's disease susceptibility. Nat. Genet. 41, 71–76. doi:10.1038/ng.285
Vukelic, I., Detel, D., Baticic, L., Potocnjak, I., and Domitrovic, R. (2020). Luteolin ameliorates experimental colitis in mice through ERK-mediated suppression of inflammation, apoptosis and autophagy. Food Chem. Toxicol. 145, 111680. doi:10.1016/j.fct.2020.111680
Wan, P., Chen, H., Guo, Y., and Bai, A. P. (2014). Advances in treatment of ulcerative colitis with herbs: from bench to bedside. World J. Gastroenterol. 20, 14099–14104. doi:10.3748/wjg.v20.i39.14099
Wang, F., Zhang, S., Jeon, R., Vuckovic, I., Jiang, X., Lerman, A., et al. (2018b). Interferon gamma induces reversible metabolic reprogramming of M1 macrophages to sustain cell viability and pro-inflammatory activity. EBioMedicine 30, 303–316. doi:10.1016/j.ebiom.2018.02.009
Wang, H., Zhang, M., Wen, X., He, L., Zhang, M., Zhang, J., et al. (2022b). Cepharanthine ameliorates dextran sulphate sodium-induced colitis through modulating gut microbiota. Microb. Biotechnol. 15, 2208–2222. doi:10.1111/1751-7915.14059
Wang, H.-Y., Ge, W., Liu, S.-Q., Long, J., Jiang, Q.-Q., Zhou, W., et al. (2022d). Curcumin inhibits T follicular helper cell differentiation in mice with dextran sulfate sodium (DSS)-Induced colitis. Am. J. Chin. Med. 50, 275–293. doi:10.1142/s0192415x22500100
Wang, K., Li, Y., Lv, Q., Li, X., Dai, Y., and Wei, Z. (2018a). Bergenin, acting as an agonist of PPAR gamma, ameliorates experimental colitis in mice through improving expression of SIRT1, and therefore inhibiting NF-kappa B-mediated macrophage activation. Front. Pharmacol. 8, 981. doi:10.3389/fphar.2017.00981
Wang, L., Shao, L., Chen, M.-Y., Wang, L., Zhang, W., Tan, F.-B., et al. (2022c). Effect of ginsenoside compound K on alleviating colitis via modulating gut microbiota. Chin. Med. 17, 146. doi:10.1186/s13020-022-00701-9
Wang, L., and Wang, J. (2022). Honokiol ameliorates DSS-induced mouse colitis by inhibiting inflammation and oxidative stress and improving the intestinal barrier. Oxid. Med. Cell. Longev. 2022, 1755608. doi:10.1155/2022/1755608
Wang, M., Li, Y., Su, J., Bai, J., Zhao, Z., and Sun, Z. (2023c). Protective effects of 4-geranyloxy-2,6-dihydroxybenzophenonel on DSS-induced ulcerative colitis in mice via regulation of cAMP/PKA/CREB and NF-kappa B signaling pathways. Phytother. Res. 37, 1330–1345. doi:10.1002/ptr.7689
Wang, M., Lin, L., Chen, Y., Zhong, Y., Lin, Y., Li, P., et al. (2020a). Evodiamine has therapeutic efficacy in ulcerative colitis by increasing Lactobacillus acidophilus levels and acetate production. Pharmacol. Res. 159, 104978. doi:10.1016/j.phrs.2020.104978
Wang, N., Kong, R., Han, W., Bao, W., Shi, Y., Ye, L., et al. (2022e). Honokiol alleviates ulcerative colitis by targeting PPAR-gamma-TLR4-NF-kappa B signaling and suppressing gasdermin-D-mediated pyroptosis in vivo and in vitro. Int. Immunopharmacol. 111, 109058. doi:10.1016/j.intimp.2022.109058
Wang, S., Liu, W., Wang, J., and Bai, X. (2020b). Curculigoside inhibits ferroptosis in ulcerative colitis through the induction of GPX4. Life Sci. 259, 118356. doi:10.1016/j.lfs.2020.118356
Wang, X., Su, L., Tan, J., Ding, T., and Yue, Y. (2023b). Albiflorin alleviates DSS-induced ulcerative colitis in mice by reducing inflammation and oxidative stress. Iran. J. Basic Med. Sci. 26, 48–56. doi:10.22038/ijbms.2022.66678.14624
Wang, Y., Liu, J., Huang, Z., Li, Y., Liang, Y., Luo, C., et al. (2021a). Coptisine ameliorates DSS-induced ulcerative colitis via improving intestinal barrier dysfunction and suppressing inflammatory response. Eur. J. Pharmacol. 896, 173912. doi:10.1016/j.ejphar.2021.173912
Wang, Y., Liu, K., Qi, Z., Chen, T., Yu, W., Jiang, Y., et al. (2021b). Therapeutic mechanism and effect of camptothecin on dextran sodium sulfate-induced ulcerative colitis in mice. J. Immunol. Res. 2021, 5556659. doi:10.1155/2021/5556659
Wang, Y. J., Li, Q. M., Zha, X. Q., and Luo, J. P. (2022a). Intervention and potential mechanism of non-starch polysaccharides from natural resources on ulcerative colitis: a review. Int. J. Biol. Macromol. 210, 545–564. doi:10.1016/j.ijbiomac.2022.04.208
Wang, Z.-J., Chen, L.-H., Xu, J., Xu, Q.-X., Xu, W., and Yang, X.-W. (2023a). Corylin ameliorates chronic ulcerative colitis via regulating the gut-brain axis and promoting 5-hydroxytryptophan production in the colon. Phytomedicine 110, 154651. doi:10.1016/j.phymed.2023.154651
Wei, H. L., Li, J. T., Chen, Z. G., and Yan, S. G. (2022). Experimental study on effects of berberine combined with 6-shogaol onintestinal inflammation and flora in mice with ulcerative colitis. China J. Chin. Mat. Med. 47, 4418–4427. doi:10.19540/j.cnki.cjcmm.20220413.401
Wei, W., Ding, M., Zhou, K., Xie, H., Zhang, M., and Zhang, C. (2017). Protective effects of wedelolactone on dextran sodium sulfate induced murine colitis partly through inhibiting the NLRP3 inflammasome activation via AMPK signaling. Biomed. Pharmacother. 94, 27–36. doi:10.1016/j.biopha.2017.06.071
Whyte, J. L., Smith, A. A., and Helms, J. A. (2012). Wnt signaling and injury repair. Cold Spring Harb. Perspect. Biol. 4, a008078. doi:10.1101/cshperspect.a008078
Wu, H., Rao, Q., Ma, G. C., Yu, X. H., Zhang, C. E., and Ma, Z. J. (2020a). Effect of triptolide on dextran sodium sulfate-induced ulcerative colitis and gut microbiota in mice. Front. Pharmacol. 11, 1652. doi:10.3389/fphar.2019.01652
Wu, K., Liu, X., Meng, X., Cao, L., Li, H., Bi, Y., et al. (2023). Sauchinone alleviates dextran sulfate sodium-induced ulcerative colitis via NAD(P)H dehydrogenase quinone 1/NF-kB pathway and gut microbiota. Front. Microbiol. 13, 1084257. doi:10.3389/fmicb.2022.1084257
Wu, M., Li, P., An, Y., Ren, J., Yan, D., Cui, J., et al. (2019). Phloretin ameliorates dextran sulfate sodium-induced ulcerative colitis in mice by regulating the gut microbiota. Pharmacol. Res. 150, 104489. doi:10.1016/j.phrs.2019.104489
Wu, M.-M., Wang, Q.-M., Huang, B.-Y., Mai, C.-T., Wang, C.-L., Wang, T.-T., et al. (2021). Dioscin ameliorates murine ulcerative colitis by regulating macrophage polarization. Pharmacol. Res. 172, 105796. doi:10.1016/j.phrs.2021.105796
Wu, S., and Chen, Z. (2019). Astragaloside IV alleviates the symptoms of experimental ulcerative colitis in vitro and in vivo. Exp. Ther. Med. 18, 2877–2884. doi:10.3892/etm.2019.7907
Wu, X., Yang, Y., Dou, Y., Ye, J., Bian, D., Wei, Z., et al. (2014). Arctigenin but not arctiin acts as the major effective constituent of Arctium lappa L. fruit for attenuating colonic inflammatory response induced by dextran sulfate sodium in mice. Int. Immunopharmacol. 23, 505–515. doi:10.1016/j.intimp.2014.09.026
Wu, Y., Li, Y., Ruan, Z., Li, J., Zhang, L., Lu, H., et al. (2020b). Puerarin rebuilding the mucus layer and regulating mucin-utilizing bacteria to relieve ulcerative colitis. J. Agric. Food Chem. 68, 11402–11411. doi:10.1021/acs.jafc.0c04119
Wu, Y. T., Zhong, L. S., Huang, C., Guo, Y. Y., Jin, F. J., Hu, Y. Z., et al. (2022). β-Caryophyllene acts as a ferroptosis inhibitor to ameliorate experimental colitis. Int. J. Mol. Sci. 23, 16055. doi:10.3390/ijms232416055
Wu, Z., Zeng, H., Zhang, L., Pu, Y., Li, S., Yuan, Y., et al. (2020c). Patchouli alcohol: a natural sesquiterpene against both inflammation and intestinal barrier damage of ulcerative colitis. Inflammation 43, 1423–1435. doi:10.1007/s10753-020-01219-8
Xie, J., Li, L., Deng, S., Chen, J., Gu, Q., Su, H., et al. (2020). Slit2/Robo1 mitigates DSS-induced ulcerative colitis by activating autophagy in intestinal stem cell. Int. J. Biol. Sci. 16, 1876–1887. doi:10.7150/ijbs.42331
Xie, X., Zhao, M., Huang, S., Li, P., Chen, P., Luo, X., et al. (2022). Luteolin alleviates ulcerative colitis by restoring the balance of NCR(-)ILC3/NCR(+)ILC3 to repairing impaired intestinal barrier. Int. Immunopharmacol. 112, 109251. doi:10.1016/j.intimp.2022.109251
Xing, J. F., Sun, J. N., Sun, J. Y., You, C. Y., Dong, K., Lv, J., et al. (2012). Protective effects of 3,4-oxo-isopropylidene-shikimic acid on experimental colitis induced by trinitrobenzenesulfonic acid in rats. Dig. Dis. Sci. 57, 2045–2054. doi:10.1007/s10620-012-2155-y
Xu, B., Huang, S., Chen, Y., Wang, Q., Luo, S., Li, Y., et al. (2021). Synergistic effect of combined treatment with baicalin and emodin on DSS-induced colitis in mouse. Phytother. Res. 35, 5708–5719. doi:10.1002/ptr.7230
Xu, B., Li, Y.-L., Xu, M., Yu, C.-C., Lian, M.-Q., Tang, Z.-Y., et al. (2017). Geniposide ameliorates TNBS-induced experimental colitis in rats via reducing inflammatory cytokine release and restoring impaired intestinal barrier function. Acta Pharmacol. Sin. 38, 688–698. doi:10.1038/aps.2016.168
Xu, D., Zhuang, L., Gao, S., Ma, H., Cheng, J., Liu, J., et al. (2022a). Orally administered ginkgolide C attenuates DSS-induced colitis by maintaining gut barrier integrity, inhibiting inflammatory responses, and regulating intestinal flora. J. Agric. Food Chem. 70, 14718–14731. doi:10.1021/acs.jafc.2c06177
Xu, X., Li, W., Yu, Z., Zhang, L., Duo, T., Zhao, Y., et al. (2022c). Berberine ameliorates dextran sulfate sodium-induced ulcerative colitis and inhibits the secretion of gut lysozyme via promoting autophagy. Metabolites 12, 676. doi:10.3390/metabo12080676
Xu, X., Zhang, G., Peng, K., Gao, Y., Wang, J., Gao, C., et al. (2022b). Carnosol maintains intestinal barrier function and mucosal immune homeostasis in DSS-induced colitis. Front. Nutr. 9, 894307. doi:10.3389/fnut.2022.894307
Xu, Z., Wei, C., Zhang, R., Yao, J., Zhang, D., and Wang, L. (2015). Epigallocatechin-3-gallate-induced inhibition of interleukin-6 release and adjustment of the regulatory T/T helper 17 cell balance in the treatment of colitis in mice. Exp. Ther. Med. 10, 2231–2238. doi:10.3892/etm.2015.2824
Xuan, H., Ou, A., Hao, S., Shi, J., and Jin, X. (2020). Galangin protects against symptoms of dextran sodium sulfate-induced acute colitis by activating autophagy and modulating the gut microbiota. Nutrients 12, 347. doi:10.3390/nu12020347
Xue, H., Li, J., Li, S., Guo, J., Yan, R., Chen, T., et al. (2023). Phillygenin attenuated colon inflammation and improved intestinal mucosal barrier in DSS-induced colitis mice via TLR4/src mediated MAPK and NF-kappa B signaling pathways. Int. J. Mol. Sci. 24, 2238. doi:10.3390/ijms24032238
Yan, H. Y., Wang, H. G., Zhang, X. L., Li, X. Q., and Yu, J. (2015). Ascorbic acid ameliorates oxidative stress and inflammation in dextran sulfate sodium-induced ulcerative colitis in mice. Int. J. Clin. Exp. Med. 8, 20245–20253.
Yan, S., Hui, Y., Li, J., Xu, X., Li, Q., and Wei, H. (2020b). Glutamine relieves oxidative stress through PI3K/Akt signaling pathway in DSS-induced ulcerative colitis mice. Iran. J. Basic Med. Sci. 23, 1124–1129. doi:10.22038/ijbms.2020.39815.9436
Yan, X., Lu, Q. G., Zeng, L., Li, X. H., Liu, Y., Du, X. F., et al. (2020a). Synergistic protection of astragalus polysaccharides and matrine against ulcerative colitis and associated lung injury in rats. World J. Gastroenterol. 26, 55–69. doi:10.3748/wjg.v26.i1.55
Yang, H., Yue, Y., Li, Y., Su, L., and Yan, S. (2020). Geniposide attenuates dextran sulfate sodium-induced colitis in mice via Nrf-2/HO-1/NF-κB pathway. Ann. Palliat. Med. 9, 2826–2836. doi:10.21037/apm-20-279
Yang, N., Xia, Z., Shao, N., Li, B., Xue, L., Peng, Y., et al. (2017). Carnosic acid prevents dextran sulfate sodium-induced acute colitis associated with the regulation of the Keap1/Nrf2 pathway. Sci. Rep. 7, 11036. doi:10.1038/s41598-017-11408-5
Yang, Y. X., Yuan, Y., and Xia, B. (2022). Cinnamtannin D1 ameliorates DSS-induced colitis by preventing Th17/Treg imbalance through activation of the AMPK/mTOR pathway. Allergol. Immunopathol. (Madr.) 50, 153–161. doi:10.15586/aei.v50i5.654
Yao, H., Shi, Y., Yuan, J., Sa, R., Chen, W., and Wan, X. (2021). Matrine protects against DSS-induced murine colitis by improving gut barrier integrity, inhibiting the PPAR-α signaling pathway, and modulating gut microbiota. Int. Immunopharmacol. 100, 108091. doi:10.1016/j.intimp.2021.108091
Yao, H., Yan, J., Yin, L., and Chen, W. (2022). Picroside II alleviates DSS-induced ulcerative colitis by suppressing the production of NLRP3 inflammasomes through NF-κB signaling pathway. Immunopharmacol. Immunotoxicol. 44, 437–446. doi:10.1080/08923973.2022.2054425
Yao, J., Cao, X., Zhang, R., Li, Y.-X., Xu, Z.-L., Zhang, D.-G., et al. (2016). Protective effect of baicalin against experimental colitis via suppression of oxidant stress and apoptosis. Pharmacogn. Mag. 12, 225–234. doi:10.4103/0973-1296.186342
Yao, J., Wang, J. Y., Liu, L., Zeng, W. S., Li, Y. X., Xun, A. Y., et al. (2011). Polydatin ameliorates DSS-induced colitis in mice through inhibition of nuclear factor-kappaB activation. Planta Med. 77, 421–427. doi:10.1055/s-0030-1250462
Yeom, J., Kim, S., and Park, S. (2022). Regulation of the gut microbiota and inflammation by β-caryophyllene extracted from cloves in a dextran sulfate sodium-induced colitis mouse model. Molecules 27, 7782. doi:10.3390/molecules27227782
Yin, S., Li, L., Tao, Y., Yu, J., Wei, S., Liu, M., et al. (2021). The inhibitory effect of artesunate on excessive endoplasmic reticulum stress alleviates experimental colitis in mice. Front. Pharmacol. 12, 629798. doi:10.3389/fphar.2021.629798
Yin, S., Yang, H., Tao, Y., Wei, S., Li, L., Liu, M., et al. (2020). Artesunate ameliorates DSS-induced ulcerative colitis by protecting intestinal barrier and inhibiting inflammatory response. Inflammation 43, 765–776. doi:10.1007/s10753-019-01164-1
Yin, Y., Liu, K., and Li, G. (2022). Protective effect of prim-O-glucosylcimifugin on ulcerative colitis and its mechanism. Front. Pharmacol. 13, 882924. doi:10.3389/fphar.2022.882924
Yin, Z., Wang, Q., and Cheng, H. (2023). Synergistic protective effect of interactions of quercetin with lycopene against ochratoxin A-induced ulcerative colitis. Appl. Biochem. Biotechnol. 195, 5253–5266. doi:10.1007/s12010-022-04287-8
You, B. H., Chae, H. S., Song, J., Ko, H. W., Chin, Y. W., and Choi, Y. H. (2017). α-Mangostin ameliorates dextran sulfate sodium-induced colitis through inhibition of NF-κB and MAPK pathways. Int. Immunopharmacol. 49, 212–221. doi:10.1016/j.intimp.2017.05.040
Yu, S., Qian, H., Zhang, D., and Jiang, Z. (2023a). Ferulic acid relieved ulcerative colitis by inhibiting the TXNIP/NLRP3 pathway in rats. Cell Biol. Int. 47, 417–427. doi:10.1002/cbin.11935
Yu, S., and Qian, H. H. (2021). Deoxyschizandrin treats mice with ulcerative colitis possibly via the TLR4/NF-κB signaling pathway. Am. J. Transl. Res. 13, 3856–3863.
Yu, T.-Y., Feng, Y.-M., Kong, W.-S., Li, S.-N., Sun, X.-J., Zhou, G., et al. (2023b). Gallic acid ameliorates dextran sulfate sodium-induced ulcerative colitis in mice via inhibiting NLRP3 inflammasome. Front. Pharmacol. 14, 1095721. doi:10.3389/fphar.2023.1095721
Yu, X. T., Xu, Y. F., Huang, Y. F., Qu, C., Xu, L. Q., Su, Z. R., et al. (2018). Berberrubine attenuates mucosal lesions and inflammation in dextran sodium sulfate-induced colitis in mice. PLoS ONE 13, e0194069. doi:10.1371/journal.pone.0194069
Yuan, J., Cheng, W., Zhang, G., Ma, Q., Li, X., Zhang, B., et al. (2020). Protective effects of iridoid glycosides on acute colitis via inhibition of the inflammatory response mediated by the STAT3/NF-кB pathway. Int. Immunopharmacol. 81, 106240. doi:10.1016/j.intimp.2020.106240
Yuan, S.-N., Wang, M.-X., Han, J.-L., Feng, C.-Y., Wang, M., Wang, M., et al. (2023). Improved colonic inflammation by nervonic acid via inhibition of NF-κB signaling pathway of DSS-induced colitis mice. Phytomedicine 112, 154702. doi:10.1016/j.phymed.2023.154702
Yue, B., Ren, J. Y., Yu, Z. L., Luo, X. P., Ren, Y. J., Zhang, J., et al. (2020). Pinocembrin alleviates ulcerative colitis in mice via regulating gut microbiota, suppressing TLR4/MD2/NF-kappa B pathway and promoting intestinal barrier. Biosci. Rep. 40. doi:10.1042/bsr20200986
Zeng, J., Zhang, D., Wan, X., Bai, Y., Yuan, C., Wang, T., et al. (2020). Chlorogenic acid suppresses miR-155 and ameliorates ulcerative colitis through the NF-kappa B/NLRP3 inflammasome pathway. Mol. Nutr. Food Res. 64, e2000452. doi:10.1002/mnfr.202000452
Zhang, C., Zhu, H., Jie, H., Ding, H., and Sun, H. (2021d). Arbutin ameliorated ulcerative colitis of mice induced by dextran sodium sulfate (DSS). Bioengineered 12, 11707–11715. doi:10.1080/21655979.2021.2005746
Zhang, H., Deng, A., Zhang, Z., Yu, Z., Liu, Y., Peng, S., et al. (2016c). The protective effect of epicatechin on experimental ulcerative colitis in mice is mediated by increasing antioxidation and by the inhibition of NF-κB pathway. Pharmacol. Rep. 68, 514–520. doi:10.1016/j.pharep.2015.12.011
Zhang, H., Lang, W., Li, S., Xu, C., Wang, X., Li, Y., et al. (2023a). Corynoline ameliorates dextran sulfate sodium-induced colitis in mice by modulating Nrf2/NF-κB pathway. Immunopharmacol. Immunotoxicol. 45, 26–34. doi:10.1080/08923973.2022.2112218
Zhang, H., Lang, W., Liu, X., Bai, J., Jia, Q., and Shi, Q. (2022e). Procyanidin A1 alleviates DSS-induced ulcerative colitis via regulating AMPK/mTOR/p70S6K-mediated autophagy. J. Physiol. Biochem. 78, 213–227. doi:10.1007/s13105-021-00854-5
Zhang, H., Wang, Y., Su, Y., Fang, X., and Guo, W. (2021c). The alleviating effect and mechanism of Bilobalide on ulcerative colitis. Food. Funct. 12, 6226–6239. doi:10.1039/d1fo01266e
Zhang, H., Zhuo, S., Song, D., Wang, L., Gu, J., Ma, J., et al. (2021a). Icariin inhibits intestinal inflammation of DSS-induced colitis mice through modulating intestinal flora abundance and modulating p-p65/p65 molecule. J. Gastroenterol. 32, 382–392. doi:10.5152/tjg.2021.20282
Zhang, J., Cao, L., Sun, Y., Qing, D.-G., Xu, X.-Q., Wang, J.-C., et al. (2021b). The regulatory effects of licochalcone A on the intestinal epithelium and gut microbiota in murine colitis. Molecules 26, 4149. doi:10.3390/molecules26144149
Zhang, J., Liang, F., Chen, Z., Chen, Y., Yuan, J., Xiong, Q., et al. (2022c). Vitexin protects against dextran sodium sulfate-induced colitis in mice and its potential mechanisms. J. Agric. Food Chem. 70, 12041–12054. doi:10.1021/acs.jafc.2c05177
Zhang, J., Xu, X., Li, N., Cao, L., Sun, Y., Wang, J., et al. (2022b). Licoflavone B, an isoprene flavonoid derived from licorice residue, relieves dextran sodium sulfate-induced ulcerative colitis by rebuilding the gut barrier and regulating intestinal microflora. Eur. J. Pharmacol. 916, 174730. doi:10.1016/j.ejphar.2021.174730
Zhang, J. L., Zhang, M. N., Wang, H. G., Yang, X. Z., and Yu, C. G. (2022a). Jatrorrhizine alleviates ulcerative colitis via regulating gut microbiota and NOS2 expression. Gut Pathog. 14, 41. doi:10.1186/s13099-022-00514-z
Zhang, L., Cao, N., Wang, Y., Wang, Y., Wu, C., Cheng, X., et al. (2020b). Improvement of oxazolone-induced ulcerative colitis in rats using andrographolide. Molecules 25, 76. doi:10.3390/molecules25010076
Zhang, L., Wang, Y., Tong, L., Sun, S., Liu, W., Zang, S., et al. (2017b). Berberine alleviates dextran sodium sulfate-induced colitis by improving intestinal barrier function and reducing inflammation and oxidative stress. Exp. Ther. Med. 13, 3374–3382. doi:10.3892/etm.2017.4402
Zhang, L., Xue, H., Zhao, G., Qiao, C., Sun, X., Pang, C., et al. (2019b). Curcumin and resveratrol suppress dextran sulfate sodium-induced colitis in mice. Mol. Med. Rep. 19, 3053–3060. doi:10.3892/mmr.2019.9974
Zhang, M., Long, Y., Sun, Y., Wang, Y., Li, Q., Wu, H., et al. (2011). Evidence for the complementary and synergistic effects of the three-alkaloid combination regimen containing berberine, hypaconitine and skimmianine on the ulcerative colitis rats induced by trinitrobenzene-sulfonic acid. Eur. J. Pharmacol. 651, 187–196. doi:10.1016/j.ejphar.2010.10.030
Zhang, Q., Wen, F., Sun, F., Xu, Z., Liu, Y., Tao, C., et al. (2023b). Efficacy and mechanism of quercetin in the treatment of experimental colitis using network pharmacology analysis. Molecules 28, 146. doi:10.3390/molecules28010146
Zhang, Q., Xu, N., Hu, X., and Zheng, Y. (2020c). Anti-colitic effects of Physalin B on dextran sodium sulfate-induced BALB/c mice by suppressing multiple inflammatory signaling pathways. J. Ethnopharmacol. 259, 112956. doi:10.1016/j.jep.2020.112956
Zhang, W., Wang, W., Shen, C., Wang, X., Pu, Z., and Yin, Q. (2021f). Network pharmacology for systematic understanding of Schisandrin B reduces the epithelial cells injury of colitis through regulating pyroptosis by AMPK/Nrf2/NLRP3 inflammasome. Aging-Us 13, 23193–23209. doi:10.18632/aging.203611
Zhang, W. F., Yang, Y., Su, X., Xu, D. Y., Yan, Y. L., Gao, Q., et al. (2016b). Deoxyschizandrin suppresses dss-induced ulcerative colitis in mice. Saudi. J. Gastroenterol. 22, 448–455. doi:10.4103/1319-3767.195552
Zhang, X.-J., Yuan, Z.-W., Qu, C., Yu, X.-T., Huang, T., Chen, P. V., et al. (2018). Palmatine ameliorated murine colitis by suppressing tryptophan metabolism and regulating gut microbiota. Pharmacol. Res. 137, 34–46. doi:10.1016/j.phrs.2018.09.010
Zhang, Y., Tan, L., Li, C., Wu, H., Ran, D., and Zhang, Z. (2020a). Sulforaphane alter the microbiota and mitigate colitis severity on mice ulcerative colitis induced by DSS. Amb. Express 10, 119. doi:10.1186/s13568-020-01053-z
Zhang, Y., Zha, Z., Shen, W., Li, D., Kang, N., Chen, Z., et al. (2021e). Anemoside B4 ameliorates TNBS-induced colitis through S100A9/MAPK/NF-κB signaling pathway. Chin. Med. 16, 11. doi:10.1186/s13020-020-00410-1
Zhang, Z., Cui, Y., Liu, S., Huang, J., Liu, Y., Zhou, Y., et al. (2022d). Short-term treatment with zingerone ameliorates dextran sulfate sodium-induced mouse experimental colitis. J. Sci. Food Agric. 102, 4873–4882. doi:10.1002/jsfa.11850
Zhang, Z., Li, S., Cao, H., Shen, P., Liu, J., Fu, Y., et al. (2019a). The protective role of phloretin against dextran sulfate sodium-induced ulcerative colitis in mice. Food. Funct. 10, 422–431. doi:10.1039/c8fo01699b
Zhang, Z., Li, Y., Shen, P., Li, S., Lu, X., Liu, J., et al. (2017d). Administration of geniposide ameliorates dextran sulfate sodium-induced colitis in mice via inhibition of inflammation and mucosal damage. Int. Immunopharmacol. 49, 168–177. doi:10.1016/j.intimp.2017.05.033
Zhang, Z., Shen, P., Lu, X., Li, Y., Liu, J., Liu, B., et al. (2017a). In vivo and in vitro study on the efficacy of Terpinen-4-ol in Dextran sulfate sodium-induced Mice experimental colitis. Front. Immunol. 8, 558. doi:10.3389/fimmu.2017.00558
Zhang, Z., Wu, X., Cao, S., Wang, L., Wang, D., Yang, H., et al. (2016a). Caffeic acid ameliorates colitis in association with increased Akkermansia population in the gut microbiota of mice. Oncotarget 7, 31790–31799. doi:10.18632/oncotarget.9306
Zhang, Z., Yang, L., Wang, B., Zhang, L., Zhang, Q., Li, D., et al. (2017c). Protective role of liriodendrin in mice with dextran sulphate sodium-induced ulcerative colitis. Int. Immunopharmacol. 52, 203–210. doi:10.1016/j.intimp.2017.09.012
Zhao, Y., Liu, P., Zhang, Y., Jiang, H., Luan, H., Xu, Y., et al. (2022b). Demethyleneberberine blocked the maturation of IL-1β in inflammation by inhibiting TLR4-mitochondria signaling. Int. Immunopharmacol. 113, 109319. doi:10.1016/j.intimp.2022.109319
Zhao, Y., Zhong, X., Yan, J., Sun, C., Zhao, X., and Wang, X. (2022a). Potential roles of gut microbes in biotransformation of natural products: an overview. Front. Microbiol. 13, 956378. doi:10.3389/fmicb.2022.956378
Zhao, Z. J., Xiang, J. Y., Liu, L., Huang, X. L., and Gan, H. T. (2012). Parthenolide, an inhibitor of the nuclear factor-κB pathway, ameliorates dextran sulfate sodium-induced colitis in mice. Int. Immunopharmacol. 12, 169–174. doi:10.1016/j.intimp.2011.11.007
Zheng, C., Wang, Y., Xu, Y., Zhou, L., Hassan, S., Xu, G., et al. (2021a). Berberine inhibits dendritic cells differentiation in DSS-induced colitis by promoting Bacteroides fragilis. Int. Immunopharmacol. 101, 108329. doi:10.1016/j.intimp.2021.108329
Zheng, J., Li, H., Zhang, P., Yue, S., Zhai, B., Zou, J., et al. (2022). Paeonol ameliorates ulcerative colitis in mice by modulating the gut microbiota and metabolites. Metabolites 12, 956. doi:10.3390/metabo12100956
Zheng, J.-Y., Xu, J.-Y., Zhang, L., Wang, Z.-M., Yin, X.-B., and Qin, L.-Q. (2023). Effect of 3,3 '-diselenodipropionic acid on dextran sodium sulfate-induced ulcerative colitis in mice. Biol. Trace Elem. Res. 201, 3961–3970. doi:10.1007/s12011-022-03491-1
Zheng, K., Jia, J., Yan, S., Shen, H., Zhu, P., and Yu, J. (2020). Paeoniflorin ameliorates ulcerative colitis by modulating the dendritic cell-mediated T(H)17/T(reg)balance. Inflammopharmacology 28, 1705–1716. doi:10.1007/s10787-020-00722-6
Zheng, S., Zhuang, T., Tang, Y., Wu, R., Xu, T., Leng, T., et al. (2021b). Leonurine protects against ulcerative colitis by alleviating inflammation and modulating intestinal microflora in mouse models. Exp. Ther. Med. 22, 1199. doi:10.3892/etm.2021.10633
Zhong, Y., Liu, W., Xiong, Y., Li, Y., Wan, Q., Zhou, W., et al. (2022). Astragaloside Ⅳ alleviates ulcerative colitis by regulating the balance of Th17/Treg cells. Phytomedicine 104, 154287. doi:10.1016/j.phymed.2022.154287
Zhou, F., Wang, N., Yang, L., Zhang, L.-C., Meng, L.-J., and Xia, Y.-C. (2019). Saikosaponin A protects against dextran sulfate sodium-induced colitis in mice. Int. Immunopharmacol. 72, 454–458. doi:10.1016/j.intimp.2019.04.024
Zhou, J., Wang, T., Dou, Y., Huang, Y., Qu, C., Gao, J., et al. (2018a). Brusatol ameliorates 2, 4, 6-trinitrobenzenesulfonic acid-induced experimental colitis in rats: involvement of NF-κB pathway and NLRP3 inflammasome. Int. Immunopharmacol. 64, 264–274. doi:10.1016/j.intimp.2018.09.008
Zhou, K., Cheng, R., Liu, B., Wang, L., Xie, H., and Zhang, C. (2018b). Eupatilin ameliorates dextran sulphate sodium-induced colitis in mice partly through promoting AMPK activation. Phytomedicine 46, 46–56. doi:10.1016/j.phymed.2018.04.033
Zhou, P., Lai, J., Li, Y., Deng, J., Zhao, C., Huang, Q., et al. (2022a). Methyl gallate alleviates acute ulcerative colitis by modulating gut microbiota and inhibiting TLR4/NF-kappa B pathway. Int. J. Mol. Sci. 23, 14024. doi:10.3390/ijms232214024
Zhou, Q., Zhang, W.-X., He, Z.-Q., Wu, B.-S., Shen, Z.-F., Shang, H.-T., et al. (2020). The possible anti-inflammatory effect of dehydrocostus lactone on DSS-induced colitis in mice. Evid. Based Complement. Altern. Med. 2020, 5659738. doi:10.1155/2020/5659738
Zhou, Y., Chen, S., Dai, Y., Wu, L., Jin, M., Zhao, J., et al. (2023). Sinomenine attenuated dextran sulfate sodium-induced inflammatory responses by promoting 14-3-3 theta protein and inhibiting NF-kappa B signaling. J. Ethnopharmacol. 303, 116037. doi:10.1016/j.jep.2022.116037
Zhou, Y., Chen, S., Gu, W., Sun, X., Wang, L., and Tang, L. (2021). Sinomenine hydrochloride ameliorates dextran sulfate sodium-induced colitis in mice by modulating the gut microbiota composition whilst suppressing the activation of the NLRP3 inflammasome. Exp. Ther. Med. 22, 1287. doi:10.3892/etm.2021.10722
Zhou, Y., Dou, F., Song, H., and Liu, T. (2022b). Anti-ulcerative effects of wogonin on ulcerative colitis induced by dextran sulfate sodium via Nrf2/TLR4/NF-κB signaling pathway in BALB/c mice. Environ. Toxicol. 37, 954–963. doi:10.1002/tox.23457
Zhu, L., Gu, P., and Shen, H. (2019a). Protective effects of berberine hydrochloride on DSS-induced ulcerative colitis in rats. Int. Immunopharmacol. 68, 242–251. doi:10.1016/j.intimp.2018.12.036
Zhu, L., Gu, P., and Shen, H. (2019b). Gallic acid improved inflammation via NF-kappa B pathway in TNBS-induced ulcerative colitis. Int. Immunopharmacol. 67, 129–137. doi:10.1016/j.intimp.2018.11.049
Zhu, L., Song, Y., Liu, H., Wu, M., Gong, H., Lan, H., et al. (2021). Gut microbiota regulation and anti-inflammatory effect of β-carotene in dextran sulfate sodium-stimulated ulcerative colitis in rats. J. Food Sci. 86, 2118–2130. doi:10.1111/1750-3841.15684
Zhu, L., Xu, L.-Z., Zhao, S., Shen, Z.-F., Shen, H., and Zhan, L.-B. (2020). Protective effect of baicalin on the regulation of Treg/Th17 balance, gut microbiota and short-chain fatty acids in rats with ulcerative colitis. Appl. Microbiol. Biotechnol. 104, 5449–5460. doi:10.1007/s00253-020-10527-w
Zhuang, H., Lv, Q., Zhong, C., Cui, Y., He, L., Zhang, C., et al. (2021). Tiliroside ameliorates ulcerative colitis by restoring the M1/M2 macrophage balance via the HIF-1α/glycolysis pathway. Front. Immunol. 12, 649463. doi:10.3389/fimmu.2021.649463
Zohny, M. H., Alrouji, M., Alhajlah, S., AlOmeir, O., Ewees, M. G. E., Ghaffar, D. M. A., et al. (2022). Diacetylrhein, an anthraquinone antiarthritic agent, suppresses dextran sodium sulfate-induced inflammation in rats: a possible mechanism for a protective effect against ulcerative colitis. Biomed. Pharmacother. 154, 113651. doi:10.1016/j.biopha.2022.113651
Zou, Y., Dai, S.-X., Chi, H.-G., Li, T., He, Z.-W., Wang, J., et al. (2015). Baicalin attenuates TNBS-induced colitis in rats by modulating the Th17/Treg paradigm. Arch. Pharm. Res. 38, 1873–1887. doi:10.1007/s12272-014-0486-2
Glossary
Keywords: ulcerative colitis, natural compounds, gut microbiota, intestinal mucosal barrier, intestinal immune responses
Citation: Huang Y, Wu Q, Li S, Lin X, Yang S, Zhu R, Fu C and Zhang Z (2024) Harnessing nature’s pharmacy: investigating natural compounds as novel therapeutics for ulcerative colitis. Front. Pharmacol. 15:1394124. doi: 10.3389/fphar.2024.1394124
Received: 06 March 2024; Accepted: 01 July 2024;
Published: 14 August 2024.
Edited by:
Claudio Ferrante, University of Studies G. d’Annunzio Chieti and Pescara, ItalyReviewed by:
Annalisa Chiavaroli, University of Studies G. d’Annunzio Chieti and Pescara, ItalyShi Xue Dai, Guangdong Provincial People’s Hospital, China
Copyright © 2024 Huang, Wu, Li, Lin, Yang, Zhu, Fu and Zhang. This is an open-access article distributed under the terms of the Creative Commons Attribution License (CC BY). The use, distribution or reproduction in other forums is permitted, provided the original author(s) and the copyright owner(s) are credited and that the original publication in this journal is cited, in accordance with accepted academic practice. No use, distribution or reproduction is permitted which does not comply with these terms.
*Correspondence: Zhen Zhang, emhhbmd6aGVuZHJAMTI2LmNvbQ==; Chaomei Fu, Y2hhb21laWZ1QDEyNi5jb20=