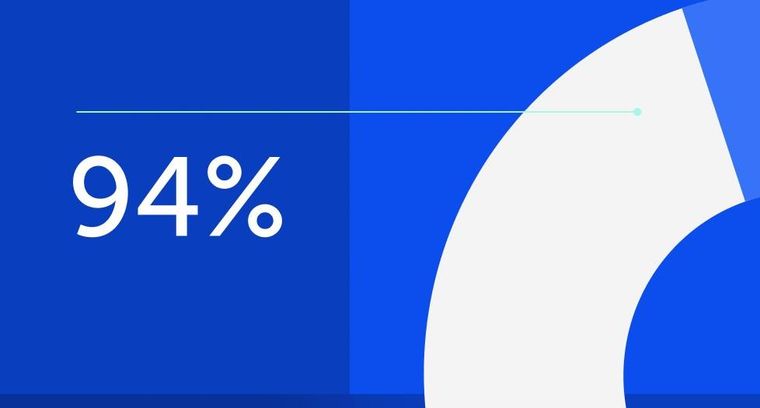
94% of researchers rate our articles as excellent or good
Learn more about the work of our research integrity team to safeguard the quality of each article we publish.
Find out more
REVIEW article
Front. Pharmacol., 04 June 2024
Sec. Ethnopharmacology
Volume 15 - 2024 | https://doi.org/10.3389/fphar.2024.1393209
This article is part of the Research TopicTraditional Processing Methods in Ethnopharmacology: Enhancing Therapeutic Effects and Unveiling Mechanisms of ActionView all 11 articles
Background and objectives: Acute mountain sickness (AMS) is a pathology with different symptoms in which the organism is not adapted to the environment that occurs under the special environment of high altitude. Its main mechanism is the organism’s tissue damage caused by acute hypobaric hypoxia. Traditional Chinese medicine (TCM) theory focuses on the holistic concept. TCM has made remarkable achievements in the treatment of many mountain sicknesses. This review outlines the pathogenesis of AMS in modern and traditional medicine, the progress of animal models of AMS, and summarizes the therapeutic effects of TCM on AMS.
Methods: Using the keywords “traditional Chinese medicine,” “herbal medicine,” “acute mountain sickness,” “high-altitude pulmonary edema,” “high-altitude cerebral edema,” “acute hypobaric hypoxia,” and “high-altitude,” all relevant TCM literature published up to November 2023 were collected from Scopus, Web of Science, PubMed, and China National Knowledge Infrastructure databases, and the key information was analyzed.
Results: We systematically summarised the effects of acute hypobaric hypoxia on the tissues of the organism, the study of the methodology for the establishment of an animal model of AMS, and retrieved 18 proprietary Chinese medicines for the clinical treatment of AMS. The therapeutic principle of medicines is mainly invigorating qi, activating blood and removing stasis. The components of botanical drugs mainly include salidroside, ginsenoside Rg1, and tetrahydrocurcumin. The mechanism of action of TCM in the treatment of AMS is mainly through the regulation of HIF-1α/NF-κB signaling pathway, inhibition of inflammatory response and oxidative stress, and enhancement of energy metabolism.
Conclusion: The main pathogenesis of AMS is unclear. Still, TCM formulas and components have been used to treat AMS through multifaceted interventions, such as compound danshen drip pills, Huangqi Baihe granules, salidroside, and ginsenoside Rg1. These components generally exert anti-AMS pharmacological effects by inhibiting the expression of VEGF, concentration of MDA and pro-inflammatory factors, down-regulating NF-κB/NLRP3 pathway, and promoting SOD and Na + -K + -ATPase activities, which attenuates acute hypobaric hypoxia-induced tissue injury. This review comprehensively analyses the application of TCM in AMS and makes suggestions for more in-depth studies in the future, aiming to provide some ideas and insights for subsequent studies.
China is the country with the largest plateau area in the world, and its area with an altitude greater than 2500 m accounts for more than a quarter of its land area. Compared with the plains, the high altitude condition has the characteristics of low air pressure, low oxygen partial pressure, coldness, strong ultraviolet rays, and dry climate. Among them, hypoxia has non-specific effects on the body’s functions and metabolism and is the most important factor affecting the human body in the high altitude conditions (Richalet et al., 2023). Acute mountain sickness (AMS) refers to the condition that occurs due to low oxygen when people rush from plain areas or low-altitude areas to high-altitude areas. It is mainly headache, and may be accompanied by dizziness, loss of appetite, and nausea. A clinical syndrome with symptoms such as vomiting, palpitation, insomnia, fatigue, and fatigue (Burtscher et al., 2023). From the perspective of medical altitude (high altitude), 3,000 m above sea level was once used as the plateau limit. However, at the sixth International Conference on High Altitude Medicine held in Xining, Qinghai in 2004, 2,500 m above sea level was redefined as the medical altitude limit. Once one rushes from the mainland to an area above 2,500 m above sea level, AMS may occur (Wu, 2005; Luks et al., 2010). Although AMS is not fatal in most cases, in severe cases, it can progress to high altitude pulmonary edema (HAPE) and high altitude cerebral edema (HACE), both of which are also called acute severe mountain sickness. Generally not self-healing and can be life-threatening (West, 2012; Luks et al., 2017).
Currently, a large number of people travel from plain areas to high altitude areas for tourism, work, sports competitions and performing tasks. The incidence of AMS is closely related to altitude. According to statistics, the incidence rate of AMS in people who have not acclimated to hypobaric hypoxia is about 10%–25% after they quickly arrive at a high-altitude of 2500 m from the plains, and the symptoms are mild. The incidence rate of AMS after quickly reaching high altitude areas of 4500–5500 m is about 50%–85% and may affect mobility. In severe cases, it can cause severe altitude sickness such as HAPE and HACE (Norris et al., 2012; Garrido et al., 2021). Therefore, it is necessary to conduct in-depth research on the pathogenesis and drug treatment for AMS. The overall occurrence of AMS among participants was 73.5% (23.2% mild, 50.3% moderate–severe) (Cobb et al., 2021). Eleven of 21 volunteers had AMS and lower resting Blood oxygen saturation (SaO2) levels while ascending to high altitude (Fayazi et al., 2023).
Under acute hypobaric hypoxia, the body adapts to the external environment by changing a series of gene expressions, and hypoxia inducible factor-1 (HIF-1) is the most important transcription factor that regulates cellular adaptation to hypoxia, which plays a key role in cellular perception and acclimatation to the change of oxygen concentration (Li et al., 2020a). HIF-1 is a heterodimer consisting of two subunits: HIF-1α and HIF-1β. Under normoxia, HIF-1 was hydroxylated by oxygen and proline hydroxylase (PHD), and the hydroxylated modified HIF-1α was rapidly degraded after binding to oncoproteins, resulting in the inability of HIF-1α to be induced to be expressed (Bw et al., 2013). In hypobaric hypoxia, respiratory metabolism is enhanced, increasing reactive oxygen species (ROS) radicals in the electron transport chain complex III, which inactivates PHD, and the inactivated PHD can affect the stability of HIF-1α protein through the phosphorylation pathway (Bw et al., 2013). Additionally, under acute hypobaric hypoxia, degradation of HIF-1α is reduced, allowing it to stabilize and accumulate in the cell, where it then binds to HIF-1β and translocates to the nucleus, activating multiple downstream genes (Dzhalilova and Makarova, 2020). These genes are involved in promoting erythropoiesis (increased production of the erythropoietin EPO), increasing angiogenesis (upregulation of the vascular endothelial growth factor VEGF), and reprogramming cellular energy metabolism (increased expression of glycolytic enzymes) (Lappin and Lee, 2019). The mechanisms of HIF-1a involved in acute hypobaric hypoxia include the ROS pathway, the Toll-like receptor 4 (TLR4) signaling pathway, and the autophagy pathway (El Alam et al., 2022). Targeted inhibition on different pathways may be effective in attenuating acute hypobaric hypoxia-induced multiple tissue injuries.
With its multi-compounds and multi-target properties, traditional Chinese medicine (TCM) has been widely used as an alternative medical treatment for various diseases (Gan et al., 2023). Studies have shown that TCM has good clinical efficacy in treating AMS, and can significantly increase the levels of hemoglobin, hematocrit and antioxidant factors, and improve the ability to adapt to high altitude exposure (Li et al., 2020b). Therefore, this paper reviews the effects of acute hypobaric hypoxia on the organism, the pathogenesis of AMS, animal models of AMS, and the efficacy and mechanism of action of Chinese medicine in the treatment of AMS.
Literature searches were conducted through Scopus, Web of Science, PubMed, and China National Knowledge Infrastructure databases using the terms “traditional Chinese medicine,” “herbal medicine,” “acute mountain sickness,” “high-altitude pulmonary edema,” “high-altitude cerebral edema,” “acute hypobaric hypoxia,” and “high-altitude,” as well as various combinations of these keywords. We reviewed the retrieved literature for the pathogenesis of AMS and the efficacy and mechanism of action of TCM on AMS. Relevant studies on the mechanisms of AMS since the establishment of the repository up to November 2023 were selected to ensure completeness of the review and to provide support.
1) Clinical and animal studies of TCM in relation to AMS, HAPE, HACE; 2) Studies on acute high altitude hypobaric hypoxia associated with TCM; 3) Clinical study of TCM for AMS.
1) Studies related to simple hypoxia; 2) AMS-related questionnaire survey and Meta-analysis studies; 3) Clinical studies on non-Chinese medicine related drugs for AMS treatment.
Under acute hypobaric hypoxia, the body will naturally increase cardiac output to ensure the supply of oxygen to vital organs, resulting in a faster heartbeat and higher blood pressure. At the same time, to increase the efficiency of oxygen transport, the red blood cells in the blood will increase, making the blood viscosity increase, leading to a decrease in the speed of blood flow, which in turn affects the delivery of oxygen (Richalet et al., 2023). The increased blood viscosity will also lead to a decrease in the deformation ability of red blood cells and impeded blood circulation. Particularly in the microvasculature, this may lead to microcirculatory disturbances that affect the supply of oxygen and nutrients to the tissues. In addition, cerebral vasodilatation occurs in hypobaric hypoxia environments to increase cerebral blood flow to compensate for the lack of oxygen, but this may lead to damage to the blood-brain barrier, resulting in elevated intracranial pressure, as shown in Figure 1. Elevated intracranial pressure, if not properly controlled, can further progress to HACE (Wilson et al., 2009; Turner et al., 2021). This increase in intracranial pressure is a key cause of major AMS symptoms such as headache, nausea, fatigue, and insomnia. In addition to this, due to the hypoxic environment, the pulmonary blood vessels also constrict, which can lead to an increase in pulmonary blood pressure, and in the long term may result in the formation of HAPE (El Alam et al., 2022).
Hypobaric hypoxia is known to regulate inflammation (Bhattacharya et al., 2021). Inflammation is considered an important risk factor for the occurrence of AMS, and it plays a crucial role in the physiological response to hypoxia (El Alam et al., 2022; Pham et al., 2022). Hypobaric hypoxia exposure can lead to changes in the inflammatory profile (Pham et al., 2022). Studies have shown that the concentrations of pro-inflammatory cytokines and inflammatory markers such as C-reactive protein, interleukin-1β (IL-1β), IL-6, IL-17F, and CCL8 in acutely exposed individuals at high altitudes are significantly higher than at sea level, while the concentrations of the anti-inflammatory cytokine IL-10 are significantly lower than at sea level (Song et al., 2016; Liu et al., 2017). Meanwhile, in the HACE model induced by low-dose lipopolysaccharide (LPS, 0.5 mg/kg) combined with acute hypobaric hypoxia exposure (6000 m, exposure for 24 h), the level of TNF-α, IL-1β, and IL-6 in plasma and hippocampal tissue increased significantly, and the brain water content increased. These results demonstrate that hypobaric hypoxia augments LPS-induced inflammation and induces the occurrence and development of HACE in mice (Zhou et al., 2017).
In addition, Pham et al. showed that several inflammation-related genes were significantly upregulated in participants' peripheral blood after 1 day of hypobaric hypoxia exposure (3800 m), including HMGB1 (high mobility group box 1), calprotectin (S100A8), TLR4 and LY96 (Immunogenic cell death-related hub genes) (Pham et al., 2022). HMGB1 is a damage-associated molecular pattern (DAMP) molecule that amplifies immune responses during tissue damage (Pham et al., 2022). Additionally, hypobaric hypoxia can stimulate immune cells, such as macrophages and lymphocytes, to release a variety of inflammatory mediators, including tumor necrosis factor-alpha (TNF-α), IL-1, IL-6 and nitric oxide (NO), as shown in Figure 1. These inflammatory factors not only increase vascular permeability, leading to edema; they may also trigger systemic or local inflammatory responses (El Alam et al., 2022). The prolonged inflammatory response may also lead to tissue damage, further exacerbating the severity of acute mountain sickness. It has been shown that acute hypobaric hypoxia responders have higher levels of IL-1 receptor agonist (IL-1RA), heat-shock protein 70 (HSP-70), and adrenomedullin (Julian et al., 2011). In addition, macrophage inflammatory protein 1β (IL-1β) was higher in patients with acute hypobaric hypoxia response compared to the non-plateau-responsive population (Wang et al., 2018), suggesting that prevention of acute high altitude condition response may be aided by anti-inflammation. Bhattacharya et al. explored potential targets for regulating inflammation by genotyping the whole blood of Tibetan population in hypobaric hypoxia exposure areas, and proposed that enhancing PHD2 activity may be an important method to reduce inflammation caused by hypoxia (Bhattacharya et al., 2021). Therefore, inhibiting the inflammation caused by hypoxia may be used as a therapeutic strategy to reduce the occurrence of AMS disease.
Oxidative stress can cause an inflammatory response, and in turn, the inflammatory response itself can also trigger oxidative stress, and both promote and influence each other (Liao et al., 2023). Hypobaric hypoxia exposure-induced oxidative stress is a key pathological mechanism for AMS (Li et al., 2024). Oxidative stress injury is ubiquitous in the pathogenesis of AMS, HACE, and HAPE, and has become a hot topic of research in hypobaric hypoxic in recent years (Pena et al., 2022). Studies have shown that when exposed to acute hypobaric hypoxic (above 4500 m), the pO2 gradient decreases dramatically, and oxygen can activate the oxidative stress response by regulating the STAT3-RXR-Nrf2 signaling pathway, promoting reactive oxygen species (ROS) generation (Paul et al., 2018). Interestingly, high altitude hypoxia increases endoplasmic reticulum stress and oxidative stress (Hu et al., 2021). Whereas hypoxia-induced oxidative stress mediates endoplasmic reticulum dysfunction, which leads to the accumulation of unfolded proteins (Pena et al., 2022); this dysregulation contributes to the acceleration of multiple processes associated with hypoxic inflammatory pathways. Additionally, Himadri et al. found that rats exposed to a simulated altitude of 25,000 ft for 24 h developed HACE, with increased levels of ROS and MDA, decreased levels of SOD and GSH-Px, increased oxidative stress, and upregulation of NF-κB in brain tissues (Himadri et al., 2010). Moreover, oxidative stress and immune responses are rapidly initiated in rats under hypobaric hypoxic stress; however, with prolonged hypoxic stress, excessive oxidative stress further stimulates the immune system in the body and releases large amounts of inflammatory factors that accumulate in the body (Pu et al., 2022). This, in turn, may lead to an inflammatory storm and further damage to lung tissue resulting in AMS (Pu et al., 2022). Therefore, eliminating oxidative stress with an anti-oxidative and anti-inflammatory agent could be an important strategy to use as prophylaxis and in the treatment of AMS (Pena et al., 2020; Pena et al., 2022).
Hypoxia induces autophagy, which plays an important role in the protection of the body against tissue damage. Hu Y et al. found that prolonged high altitude hypoxia can lead to enhanced basal autophagy (Hu et al., 2021). Autophagy flux was increased in high altitude hypoxic environment, as evidenced by an increase in the ratio of microtubule-associated protein 1 light chain type 3 II/I (LC3-II/I) and an increase in the expression of LC3-II (Masschelein et al., 2014). In addition, Zhao Z et al. also found that prolonged exposure to high altitude hypoxia resulted in increased lung injury, formation of autophagosomes with a double-membrane structure, and increased levels of Beclin-1 and LC3-II in alveolar tissues compared with controls (Zhong et al., 2022). However, autophagy is a double activity. Acute hypobaric hypoxia may also inhibit levels of autophagy. Interestingly, using a hypoxic chamber (4000 m, 56.0 kPa, 6000 m, 47.0 kPa, and 8000 m, 36.0 kPa), Dai S et al. demonstrated that acute hypoxic exposure impaired autophagic activity in early and late stages of the mouse brain and partially contributed to hypoxia-induced oxidative stress, neuronal loss, and brain damage (Dai et al., 2024). This result confirms that enhanced autophagy activity attenuates neurological deficits after oxidative stress and exposure to hypobaric hypoxia (Dai et al., 2024). Therefore, whether to inhibit autophagy or to promote autophagy levels for the treatment of tissue damage induced by high altitude hypoxia requires an individualised comprehensive analysis.
The development of AMS is considered to be a multifactorial process, and blood-brain barrier (BBB) dysfunction caused by acute hypobaric hypoxia and the resulting vasogenic edema are considered to be one of the potential mechanisms. The BBB is a highly selective semipermeable membrane barrier that exists between the brain and blood and maintains homeostasis in the brain’s internal environment (Neuwelt et al., 2011). The function of the BBB depends on the integrity of the neurovascular unit (NVU), which consists of endothelial cells, perivascular astrocytes, neurons, and microglia (Hourfar et al., 2023). These tight junctions between cells are essential for maintaining the integrity of the BBB and these tight junctions are regulated by several types of proteins such as intracellular occluder band-1 (ZO-1), occludin, claudins, and junctional adhesion molecules (Luo et al., 2024). Disruption of the tight junction induced by hypoxia is an important cause of BBB impairment and cerebral edema (Chen et al., 2020). Hypoxia-induced disruption of endothelial tight junctions triggers BBB injury and induces vasogenic edema (Xue et al., 2022). Studies have shown that hypoxia (1% O2 for 24 h) upregulates CAV-1 transcription through activation of NRF1 in endothelial cells, which induces internalization of claudin-5 and autophagic degradation, and accordingly, these effects lead to BBB disruption and trigger HACE (Xue et al., 2022). Moreover, hypoxia enhances lipopolysaccharide (LPS)-induced inflammatory responses and triggers HACE in mice (Zhou et al., 2017). LPS-induced systemic inflammatory responses rapidly exacerbated cerebral edema after acute hypobaric hypoxia exposure by disrupting the integrity of the blood-brain barrier and activating microglia, and by increasing water permeability through the accumulation of aquaporin-4 (AQP4) (Zhou et al., 2017). Furthermore, NB-3 (contactin-6) is a neural recognition molecule that also plays a key role in the development of HACE. Studies have shown that NB-3 is expressed in neurons and endothelial cells, and that deletion of NB-3 decreases levels of tight junction proteins, which disrupts BBB integrity and exacerbates HACE and BBB leakage (Zhou et al., 2017).
Hypobaric hypoxia also activates multiple inflammation-related signaling pathways. These include the nuclear factor-κB (NF-κB) pathway and the NOD-like receptor thermal protein domain associated protein 3 (NLRP3) pathway, which are commonly associated with stress and inflammatory responses (Jiang et al., 2022; Pu et al., 2022). NF-κB is an important intracellular transcription factor that is essential for regulating inflammatory responses. Under hypobaric hypoxia conditions, NF-κB can be activated and translocated to the nucleus, activating a variety of inflammatory response genes, such as cytokines, chemokines, and adhesion molecules (Pena et al., 2020). An increase in these factors can lead to an inflammatory response in cells and tissues, promoting leukocyte infiltration and producing edema. Zeng et al. found that acute hypobaric hypoxia (6000 m, exposure for 3 days) induced a significant increase of TLR4, NLRP3, p-NF-κB p65, caspase-1 protein and gene expressions in lung tissue samples and induced acute lung injury (Zeng et al., 2024). Additionally, Jia et al. found that NLRP3, gasdermin D (GSDMD), cleaved caspase-1, and cleaved IL-1β levels were upregulated in acute hypobaric hypoxia rats (6000 m, exposure for 3 days), suggesting that acute hypobaric hypoxia could activate the NLRP3/caspase-1 signaling pathway in heart tissue of acute hypobaric hypoxia-induced heart injury rats (Jia et al., 2023). Mechanistically, acute hypobaric hypoxia (6000 m, exposure for 3 days) aggravates oxidative stress and upregulates (pro)-inflammatory cytokines, activating NLRP3 inflammasome-mediated pyroptosis (Shen et al., 2023). Similaryly, hypobaric hypoxia (5000 m, exposure for 7 days) can induce retinal pyroptosis in rats by inducing activation of NLRP3 inflammasome and release of proinflammatory cytokines (Xin et al., 2022).
Cellular sensing and adaptation to changes in external oxygen concentration are important active processes in living organisms. Oxygen homeostasis is essential for the maintenance of body function. The disruption of oxygen homeostasis can lead to abnormal biological functions such as gene expression, energy metabolism, vascular regeneration, and stem cell proliferation and differentiation (Ghoneum et al., 2020). Carotid body is a sensory organ that monitors the concentration of oxygen in arterial blood. Decreased oxygen concentration in arterial blood stimulates neural activity in the carotid body, triggering reflex stimulation of respiration and blood pressure, which are essential for the maintenance of homeostasis in a hypoxia environment (Peng et al., 2023). Hydrogen sulfide (H2S) signaling is associated with hypoxic activation of carotid bodies (Peng et al., 2023). It is suggested that H2S is involved in sensing and response to hypoxia in multi-tissues that possess the ability to sense hypoxia (Wu et al., 2015). Using a hypobaric hypoxia animal model, it was demonstrated that exposure of animals to hypobaric hypoxia (7620 m, exposure for 1, 3, and 7 days) resulted in a significant decrease in H2S levels in the brain (Mishra et al., 2020). After exposure to acute hypobaric hypoxia (5260 m, for 1 day), H2S levels decreased significantly, while hypobaric hypoxia promoted glycolysis and dysregulated the pentose phosphate pathway, as well as purine catabolism, glutathione homeostasis, arginine/nitric oxide and sulfur/H2S metabolisms (D’Alessandro et al., 2016). Under hypobaric hypoxia exposure conditions (7620 m, 8% O2, for 7 days), increasing H2S levels inhibited hypobaric hypoxia-induced apoptosis in hippocampal neurons and also significantly protected glial vascular homeostasis and key neurophysiological functions (such as cerebral blood flow, functional congestion, and spatial memory) (Kumar et al., 2016a).
Mechanistically, H2S regulates intracellular O2 homeostasis and inhibits hypoxia-induced HIF-1 activation in a VHL- and mitochondria-dependent manner (Kai et al., 2012). Reduction of endogenous H2S increased pulmonary respiratory membrane permeability in HAPE rats and leads to increased protein exudation (Wang et al., 2017). This suggests that the reduction of H2S in vivo exacerbates the damage to lung respiratory membranes caused by acute hypobaric hypoxia. Moreover, Yang et al. found that in the early stage of exposure to acute hypobaric hypoxia, the stress of plasma H2S production was increased and pulmonary arterial pressure was elevated, suggesting that exogenous supplementation of H2S could delay acute hypobaric hypoxia-induced pulmonary arterial pressure elevation (Yang et al., 2011). Therefore, H2S can be used as a promising molecule to provide a reference for the development of AMS drugs.
AMS is mainly caused by acute hypobaric hypoxia stimulation (Irarrázaval et al., 2017). Therefore, the animal model of AMS can be established in high altitude condition simulation chamber or plateau field. The model can be established by feeding in the hypobaric hypoxic chamber or animal treadmill-assisted sports training (Huo et al., 2021). Blood oxygen saturation (SaO2), blood gas, inflammatory factors, tissue water content, and pathological sections are commonly used to evaluate the success of the animal model (Wang et al., 2018; Huo et al., 2021; Peng et al., 2022).
Interestingly, some of the animal experimental conditions for high-altitude hypobaric hypoxia injury are consistent with the AMS animal model. The difference is the final detection index and evaluation method. Zeng Y et al. established an animal model of lung injury from hypobaric hypoxia in a high altitude condition, which involved exposing SD rats to hypoxia at an altitude of 6000 m, with an ascent rate of 10 m/s, and continuous exposure to hypoxia for 3 days (Zeng et al., 2024). Additionally, Zhang P et al. chose cynomolgus monkeys to establish an acute hypobaric hypoxia brain injury model, and the specific steps: altitude simulation was started at 320 m, then suspended at 3000 m, 4500 m, and 6000 m for 50 min each, and finally maintained at 7500 m for an initial 48 h, with a rate of ascent of 3 m/s (Zhang et al., 2021). Detailed AMS animal model construction methods and animal type information are shown in Table 1.
The body inhales insufficient pure air from the external high altitude conditions, which can easily lead to shortness of qi or qi deficiency in the body. In traditional Chinese medicine (TCM) theory, “qi” is an important concept, which refers to the manifestation of life activity and vitality and is one of the basic substances that make up everything in the universe. When stepping into the high altitude condition from the sea level, the body is suddenly stimulated by the cold and lack of oxygen, the body’s zongqi is disordered, the spleen and stomach cannot be adequately moistened, nausea and vomiting easily. Insufficient qi in the high altitude condition results in insufficient zong qi, creating qi deficiency. Qi deficiency leads to blood stasis and insufficient blood supply to the brain, resulting in weakness and tiredness, and attacks of vertigo (Gao et al., 2023). TCM theory suggests that the etiology of this disease is a combination of qi deficiency, blood stasis, dampness stagnation, and phlegm and blood stasis obstruction (Feng et al., 2013). Most doctors believe that the cause of AMS is mainly divided into external and internal causes. The external causes are caused by the terrestrial qi and the celestial qi, which include lack of pure air, severe cold, and dry climate in the altitude. The internal causes are caused by the deficiency of qi in the human body (Zhang and Zhang, 1993; Li et al., 2012; Feng et al., 2013).
Although the concept of altitude diseases is not explicitly mentioned in the ancient books of Tibetan medicine, Tibetan medicine itself comes from the actual life of altitude people, so its essence is altitude medicine. Tibetan medicine is a great medical system formed by the people in the high-altitude hypoxia region in the struggle against various diseases and harsh natural environments (Liu et al., 2018a).
According to Tibetan medical theory and “Four Tantras”《四部医典》, there are three major factors in the human body ("Dragon”, “Chiba”, “Pegan”), seven major material bases (dietary essence, blood, meat, fat, bone, bone marrow, sperm), and three excretions (stool, urine, sweat), and the three major factors govern the movement of the seven major material bases and the three excretions (Zhong et al., 2022). The most essential cause of AMS is three major factors and seven major material dysfunctions (Zhang and Zhang, 1993; Feng et al., 2013).
According to the theory of TCM and the principle of pattern differentiation and treatment, the main TCM pattern types of AMS are a pattern of Qi deficiency with blood stasis, and the main principle of treatment is activating Qi, activating blood circulation, and resolving phlegm (Feng et al., 2013; Wu et al., 2023). The clinical applications of TCM are based on compound danshen dripping pill (复方丹参滴丸), huangqi injection (黄芪注射液), shulikang capsule (舒理康胶囊), duoxuekang capsule (多血康胶囊), and xuefu zhuyu decoction (血府逐瘀汤). Commonly used botanical drugs are mainly Astragalus mongholicus Bunge (Huangqi), Salvia miltiorrhiza Bunge (Danshen), Panax ginseng C.A. Mey. (Renshen), and Rhodiola rosea L. (Hongjingtian). The TCM pattern types of AMS and the application of TCM are shown in Table 2.
In addition, the clinical evidence of TCM for several typical AMS is summarized in Table 2. A total of 18 representative TCMs, including 6 capsules, 3 injections, 3 pills, 2 tablets, 2 granules, 1 oral solution, and 1 acupuncture were compiled and summarized. TCM formulas can improve exercise tolerance and quality of life and relieve clinical symptoms. This provides a reference and basis for clinical use and further pharmacological studies based on the results of the analysis of TCM pattern types and TCM application in AMS.
According to the data in Table 2, among a total of 33 botanical drugs used clinically, and the top 10 botanical drugs ranked by their relatively high frequency of use are shown in Table 3. Table 3 shows that the most frequently used botanical drugs were Salvia miltiorrhiza Bunge (Danshen). The type of botanical drugs is mainly invigorating qi (huangqi, dangshen, hongjingtian, renshen), activating blood and removing stasis (danshen, chuanxiong) and nourishing Yin (Beishashen, Gouqizi), as shown in Supplementary Table S1. The properties of botanical drugs are mainly warm, calm, and cold. The flavor of botanical drugs is mainly sweet, bitter, and pungent. Specific botanical drugs information is shown in Table 3.
While many other TCM therapies, such as acupuncture and bloodletting, are effective in clinical applications for tissue damage caused by hypobaric hypoxia (Wang et al., 2022; Li et al., 2023a), this review focuses only on herbal treatments. Classical prescriptions or TCM formulas, as the main means of clinical treatment in modern TCM, are an important breakthrough point for the inheritance and innovative development of TCM (Xu et al., 2023). Currently, research on TCM formulas for the treatment of AMS is mainly based on the compound danshen drip pills (CDDP) (Yan et al., 2022). Several studies have confirmed that CDDP prevents hypobaric hypoxia induced tissue damage by reducing erythrocyte aggregation and hemorheology and promoting the expression of AQP1 and Nrf2 (Hu et al., 2021). Moreover, Fu et al. found that qi-long-tian formula extract attenuated the inflammatory response in AMS model rats (6000 m, exposed to a Fenglei hypobaric hypoxic chamber for 72 h) by inhibiting HIF-1a, VEGF, suppressing the expression of inflammation-associated effectors (MMP9 and TIMP1), inhibiting the expression of target genes downstream of IL-17 (Ccl2, MMP9, S100a9, and S100a8), and attenuating lung injury and immune cell infiltration (Fu et al., 2021). Our team also demonstrated that modified siwu decoction has a favorable protective effect against hypobaric hypoxia by down-regulating proteins associated with oxidative stress and the ubiquitin-proteasome system ((Superoxide dismutase 1, SOD1), (Catalase, CAT), GSTP1 and PRDX1), as well as by ameliorating hypoxia-induced upregulation of MDA and disturbances in energy and lipid metabolism (Tu et al., 2023). Meanwhile, Zeng Y et al. found that Huangqi Baihe Granules could significantly enhance lung function, reduce lung wet/dry ratio, alleviate lung tissue injury, inhibit the formation of ROS and MDA, and increase SOD activity and GSH expression, and its mechanism of action may be to inhibit the activation of TLR4/NF-κB p65/NLPR3 signaling pathway and reduce the release of downstream pro-inflammatory cytokines in lung tissue (Zeng et al., 2024).
In addition, studies on single TCMs for the treatment of AMS are mainly based on Rhodiola rosea L. (Hongjingtian), and it has been reported that chemical components salidroside can coordinate metabolic reprogramming by modulating the HIF-1α signaling pathway in AMS (Yan et al., 2021). Xie et al. found that Rhodiola rosea L. extract significantly increased SOD, GSH-Px, T-AO, and Na + -K + -ATPcase levels, decreased NF-α, IL-1β, IL-6, MDA, and LDH levels, and inhibited the NF-κB/NLRP3 signaling pathway, which further corrected the imbalance in energy metabolism and maintained the integrity of the BBB, thereby alleviates hypobaric hypoxia-induced brain injury (8000 m, exposed to a FLYDWC50-II C Fenglei hypobaric hypoxic chamber for 48 h) (Xie et al., 2022). Salidroside (Sal), the active chemical components of Rhodiola rosea L., has been reported to have potential protective effects against tissue damage caused by acute hypobaric hypoxia. Studies have shown that Sal attenuates acute hypobaric hypoxia-induced brain oxidative stress injury, inflammatory response, and BBB damage (Hou et al., 2024). Mechanically, Sal reduced the levels of ROS and MDA and the levels of TNF-α, IL-1β, and IL-6, and increased the activities of SOD and GSH-Px, thereby attenuating hypobaric hypoxia-induced pathological injury and oxidative stress (Jiang et al., 2022). In addition, Sal partially promoted energy metabolism and increased the activities of Na + -K + -ATPase and Ca2+-Mg2+-ATPase. The mechanism of action is related to inhibition of the NF-κB/NLRP3 pathway (Jiang et al., 2022).
Our team’s previous study found that polysaccharide extracted from Potentilla anserina L could inhibit oxidative stress and inflammatory responses to treat and prevent HACE and HAPE, and its mechanism of action may be to block the activation of the NF-κB and HIF-1α signaling pathways, which inhibits the production of downstream pro-inflammatory cytokines (IL-1β, IL-6, TNF-α, and VEGF) (Shi et al., 2019; Shi et al., 2019). Meanwhile, Pan Y et al. showed that tetrahydrocurcumin significantly reduced the expression of VEGF (AMS biomarker), MMP-9 and NF-κB, and effectively attenuated cerebral edema and inflammation induced by acute high-altitude hypobaric hypoxia (Pan et al., 2020; Nourkami-Tutdibi et al., 2023). Moreover, Zha et al. established an animal model of AMS (6000 m, 10 m/s rate of ascent, continuous exposure to hypoxia for 24 h) and found that ginsenoside Rg1 reduced the wet-to-dry ratio of lung tissues, improved the pathological changes of lung tissues, decreased the MDA content and NOS viability, elevated the CAT and Na + -K + -ATPase viability, and decreased the IL-6 and TNF-α levels (Arriaza et al., 2022). As the main active chemical component of Panax notoginseng saponins, notoginsenoside R1 attenuated inflammation and oxidative stress, reduced lung dry-to-wet ratio, alleviated the lung tissue damage, and ameliorated arterial blood gas changes in acute hypobaric hypoxia-induced HAPE rats (6000 m, exposed to a ProOx-830 hypobaric hypoxic chamber for 48 h), and its mechanism of action may be that notoginsenoside R1 promoted the activation of the ERK1/2-P90rsk-BAD signaling pathway (Pei et al., 2023). Details of TCM formulas, the specific simple botanical drugs, active components, modes of administration, experimental models, mimic altitudes, dosages, and pharmaceutical effects are shown in Table 4. The mechanism of action of TCM intervention for AMS is shown in Figure 2.
AMS is a typical high altitude condition-specific hypoxic illness with a quick onset and progression. Even though fundamental and clinical research on AMS has advanced significantly, numerous scientific issues still need to be resolved. 1) Initial diagnosis of AMS is difficult and there is a lack of targeted treatment. Acetazolamide is currently the main drug used to treat AMS, but blind use of acetazolamide without a clear diagnosis of the disease can often lead to side effects. 2) The effects of different altitudes on the function of multiple tissues and organs of the body remain elusive. Studies have shown that different altitudes directly affect hepatic perfusion, with hepatic arterial perfusion increasing with altitude (Shi, 2011). 3) The basic experimental models of AMS are mainly animal-based, and the index parameters of model construction success are still not unified, so it is not possible to judge the success rate of the model effectively and quickly. In addition, animal experiments are time-consuming, so there is a need for cellular models for drug screening to facilitate rapid and effective drug screening.
With the rapid development of tourism and mountaineering in the plateau and the construction of the plateau economy, more and more people from the plains will enter the high altitude conditions, which can cause AMS if no precautions are taken. This raises important questions about how to effectively prevent and treat AMS. Due to its multi-component, multi-target and multi-pathway action characteristics, TCM can better respond to the multi-system dysfunction caused by the complex hypobaric hypoxia environment. At the same time, TCM has long-term experience in human use, has better clinical safety, lower toxicity and side effects, and is often used in combination with chemical medicine to achieve synergistic effect. In addition, the research of TCM with both medicinal and edible values in the prevention and treatment of AMS diseases has also attracted much attention, such as Rhodiola rosea L., Tibetan Brassica rapa L., Tibetan Hippophae rhamnoides L. (HIPPOPHAE FRUCTUS) and so on. In the future, this will remain a hot spot in the research of TCM against AMS disease. According to TCM theory and practice, the etiology and pathogenesis of AMS are mainly “qi deficiency and blood stasis” and “qi stagnation and blood stasis”. TCM for treating AMS is based on “invigorating qi botanical drugs” and “botanical drugs for activating blood circulation and removing blood stasis”. At present, there is some efficacy in TCM formulas, active chemical components, and botanical drugs extracts in the treatment of AMS, and there is some research on the mechanism of action of related chemical components. Compound danshen dripping pill, Huangqi Baihe granules, salidroside, ginsenoside Rg1 and tetrahydrocurcumin have better ability in anti-AMS. These chemical components generally exert anti-AMS pharmacological effects by inhibiting the expression of VEGF (AMS marker), MDA, and pro-inflammatory factors (TNF-α, IL-1β, and IL-6), down-regulating NF-κB/NLRP3 pathway, and promoting SOD and Na + -K + -ATPase activities, which attenuates acute hypobaric hypoxia-induced tissue injury. This review focuses on the relationship between AMS and hemodynamic and hemorheological stress, changes in cytokines and inflammatory cytokines, regulation of related signaling pathways, blood-brain barrier damage, oxidative stress injury, autophagy, and summarises the establishment of AMS animal model methods. Although TCM has the potential to regulate AMS caused by hypobaric hypoxia, many studies still have some limitations. 1) The effective dose, maximum tolerated intake, and taking time for clinical treatment of TCM formulas are still uncertain. 2) In single-dose studies of TCM formula extracts or crude drugs, the pharmacological effects are still controversial. 3) As for the chemical components of TCM, except for in-depth research on components such as salidroside and ginsenoside, there are few studies on other effective chemical components of TCM. Subsequent screening and comparison of the anti-hypoxic activity of TCM chemical components is needed. 4) Due to the multi-component and multi-target characteristics of TCM, the research on TCM against acute hypobaric hypoxia in recent years has remained at the level of animal experiments, with few clinical studies. 5) There has been limited progress in translating research results into clinical applications. Future efforts should focus on exploring clinical treatment methods using TCM chemical components (salidroside, ginsenoside, etc.), such as exploring new drug delivery methods, which can improve drug targeting, stability, bioavailability, and exert its biological activity. In conclusion, this review summarizes the potential of TCM to treat AMS, but its specific mechanisms deserve further study. The solution to this problem will be beneficial to the long-term development of TCM in the treatment of body damage diseases induced by hypobaric hypoxia.
ZW: Writing–original draft, Writing–review and editing. YW: Funding acquisition, Writing–original draft, Writing–review and editing. RG: Investigation, Writing–original draft. JC: Conceptualization, Formal Analysis, Writing–original draft. YC: Data curation, Software, Writing–original draft. ML: Formal Analysis, Supervision, Writing–original draft. YG: Supervision, Writing–review and editing.
The author(s) declare that financial support was received for the research, authorship, and/or publication of this article. This work was supported by the Innovation Team and Talents Cultivation Program of National Administration of Traditional Chinese Medicine (ZYYCXTD-D-202207), the National Natural Science Foundation of China (82204774).
The authors declare that the research was conducted in the absence of any commercial or financial relationships that could be construed as a potential conflict of interest.
All claims expressed in this article are solely those of the authors and do not necessarily represent those of their affiliated organizations, or those of the publisher, the editors and the reviewers. Any product that may be evaluated in this article, or claim that may be made by its manufacturer, is not guaranteed or endorsed by the publisher.
The Supplementary Material for this article can be found online at: https://www.frontiersin.org/articles/10.3389/fphar.2024.1393209/full#supplementary-material
AMS, acute mountain sickness; TCM, traditional Chinese medicine; HACE, high altitude cerebral edema; HAPE, high altitude pulmonary edema; NF-κB, nuclear factor-κB; AMPK, AMP-activated protein kinase; ROS, Reactive oxygen species; SaO2, Blood oxygen saturation; Hb, Hemoglobin; RBC, Red blood cell; CDDP, Compound danshen dripping pill; TNF-α, Tumour necrosis factor-α; VEGF, Vascular endothelial-derived growth factor; MDA, malondialdehyde; SOD, Superoxide dismutase; GSH-Px, Glutathione peroxidase; mTOR, mammalian target of rapamycin; NLRP3, nod-like receptor protein 3; BBB, blood-brain barrier; LPS, lipopolysaccharide; Glu, Glucose; ATP, adenosine triphosphate; IL-6, interleukin 6.
Arriaza, K., Cuevas, C., Pena, E., Siques, P., and Brito, J. (2022). Impact of zinc on oxidative signaling pathways in the development of pulmonary vasoconstriction induced by hypobaric hypoxia. Int. J. Mol. Sci. 23, 6974. doi:10.3390/ijms23136974
Bai, C., She, J., Goolaerts, A., Song, Y., Shen, C., Shen, J., et al. (2010). Stress failure plays a major role in the development of high-altitude pulmonary oedema in rats. Eur. Respir. J. 35, 584–591. doi:10.1183/09031936.00001709
Bailly, C. (2021). Anticancer properties of lobetyolin, an essential component of radix codonopsis (dangshen). Nat. Prod. Bioprospect 11, 143–153. doi:10.1007/s13659-020-00283-9
Bhattacharya, S., Shrimali, N. M., Mohammad, G., Koul, P. A., Prchal, J. T., and Guchhait, P. (2021). Gain-of-function Tibetan PHD2D4E;C127S variant suppresses monocyte function: a lesson in inflammatory response to inspired hypoxia. EBioMedicine 68, 103418. doi:10.1016/j.ebiom.2021.103418
Burtscher, J., Swenson, E. R., Hackett, P. H., Millet, G. P., and Burtscher, M. (2023). Flying to high-altitude destinations: is the risk of acute mountain sickness greater? J. Travel Med. 30, taad011. doi:10.1093/jtm/taad011
Bw, W., A, K., U, B., and P, C. (2013). Emerging novel functions of the oxygen-sensing prolyl hydroxylase domain enzymes. Trends Biochem. Sci. 38, 3–11. doi:10.1016/j.tibs.2012.10.004
Chen, Y. Y., Liu, Q. P., An, P., Jia, M., Luan, X., Tang, J. Y., et al. (2022). Ginsenoside Rd: a promising natural neuroprotective agent. Phytomedicine 95, 153883. doi:10.1016/j.phymed.2021.153883
Chen, Z., Liu, L., Gao, C., Chen, W., Vong, C. T., Yao, P., et al. (2020). Astragali Radix (Huangqi): a promising edible immunomodulatory herbal medicine. J. Ethnopharmacol. 258, 112895. doi:10.1016/j.jep.2020.112895
Cheng, T. O. (2007). Cardiovascular effects of danshen. Int. J. Cardiol. 121, 9–22. doi:10.1016/j.ijcard.2007.01.004
Chinese Pharmacopoeia Commission Pharmacopoeia of the People’s Republic of China (2020) Chinese Pharmacopoeia commission Pharmacopoeia of the People’s Republic of China, 1. Beijing: People’s Medical Publishing House.
Cobb, A. B., Levett, D. Z. H., Mitchell, K., Aveling, W., Hurlbut, D., Gilbert-Kawai, E., et al. (2021). Physiological responses during ascent to high altitude and the incidence of acute mountain sickness. Physiol. Rep. 9, e14809. doi:10.14814/phy2.14809
Cui, J., Wu, P., Wang, F., Zhang, Y., Li, Q., Liu, Y., et al. (2014). Clinical observation of Xifengzhitong granule on Acute Mountain sickness. J. High Alt. Med. 24, 8–11.
Cui, J., Zhang, Y., Li, Q., Wu, P., Wang, F., and Li, B. (2015). Effect of thrombolytic capsule on prevention and treatment of Acute Mountain sickness. Med. J. Natl. Defending Forces Southwest China 25, 662–665. doi:10.3969/j.issn.1004-0188.2015.06.033
Dai, S., Feng, Y., Lu, C., Zhang, H., Ma, W., Xie, W., et al. (2024). Impairment of autophagic flux after hypobaric hypoxia potentiates oxidative stress and cognitive function disturbances in mice. Neurosci. Bull. 40, 35–49. doi:10.1007/s12264-023-01099-6
D’Alessandro, A., Nemkov, T., Sun, K., Liu, H., Song, A., Monte, A. A., et al. (2016). AltitudeOmics: red blood cell metabolic adaptation to high altitude hypoxia. J. Proteome Res. 15, 3883–3895. doi:10.1021/acs.jproteome.6b00733
Du, S., Miao, S., and Wang, K. (2004). Clinical efficacy analysis of Rhodiola rosea in the treatment of Acute Mountain sickness. Med. J. Natl. Defending Forces Southwest China 14, 615–616.
Dzhalilova, D., and Makarova, O. (2020). Differences in tolerance to hypoxia: physiological, biochemical, and molecular-biological characteristics. Biomedicines 8, 428. doi:10.3390/biomedicines8100428
El Alam, S., Pena, E., Aguilera, D., Siques, P., and Brito, J. (2022). Inflammation in pulmonary hypertension and edema induced by hypobaric hypoxia exposure. Int. J. Mol. Sci. 23, 12656. doi:10.3390/ijms232012656
Fang, Y. (2008). Study on the curative effect of xingnaojing injection for Acute Mountain sickness. Sichuan Med. J. 24, 853–854. doi:10.3969/j.issn.1004-0501.2008.07.023
Fang, Y. (2011). Clinical effictive observation of Danhong injection in treating Acute Mountain sickness. J. Mod. Med. Health 27, 2909–2910.
Fayazi, B., Tadibi, V., and Ranjbar, K. (2023). The role of hypoxia related hormones responses in acute mountain sickness susceptibility individuals unaccustomed to high altitude. PLoS One 18, e0292173. doi:10.1371/journal.pone.0292173
Feng, B., Liu, Z., Xing, Y., Gao, A., Huang, J., Zhu, H., et al. (2013). Traditional Chinese medical recognition on mountain sickness. China J. Traditional Chin. Med. Pharm. 28, 3475–3479.
Fu, X., Yang, C., Chen, B., Zeng, K., Chen, S., and Fu, Y. (2021). Qi-Long-Tian formula extract alleviates symptoms of acute high-altitude diseases via suppressing the inflammation responses in rat. Respir. Res. 22, 52. doi:10.1186/s12931-021-01645-8
Gan, X., Shu, Z., Wang, X., Yan, D., Li, J., Ofaim, S., et al. (2023). Network medicine framework reveals generic herb-symptom effectiveness of traditional Chinese medicine. Sci. Adv. 9, eadh0215. doi:10.1126/sciadv.adh0215
Gao, J., Zhang, Z., Yan, J., Ge, Y., Ma, Z., and Gao, Y. (2023). Evaluation of animal models of high altitude qi deficiency and blood stasis syndrome and research progress in therapeutic drugs. Chin. J. Pharmacovigil. 20, 1189–1194. doi:10.19803/j.1672-8629.20230120
Garrido, E., Botella de Maglia, J., and Castillo, O. (2021). Acute, subacute and chronic mountain sickness. Rev. Clin. Esp. 221, 481–490. doi:10.1016/j.rce.2019.12.013
Geng, Y., Yang, J., Cheng, X., Han, Y., Yan, F., Wang, C., et al. (2022). A bioactive gypenoside (GP-14) alleviates neuroinflammation and blood brain barrier (BBB) disruption by inhibiting the NF-κB signaling pathway in a mouse high-altitude cerebral edema (HACE) model. Int. Immunopharmacol. 107, 108675. doi:10.1016/j.intimp.2022.108675
Ghoneum, A., Abdulfattah, A. Y., Warren, B. O., Shu, J., and Said, N. (2020). Redox homeostasis and metabolism in cancer: a complex mechanism and potential targeted therapeutics. Int. J. Mol. Sci. 21, 3100. doi:10.3390/ijms21093100
Gongga, L., Ciren, W., Suolang, Y., Baima, D., and Duoji, Z. (2015). Clinical observation of Rhodiola rosea in the treatment of Acute Mountain sickness. China Pharm. 26, 2818–2820.
Guo, P., Luo, H., Fan, Y., Luo, Y., and Zhou, Q. (2013). Establishment and evaluation of an experimental animal model of high altitude cerebral edema. Neurosci. Lett. 547, 82–86. doi:10.1016/j.neulet.2013.05.008
Himadri, P., Kumari, S. S., Chitharanjan, M., and Dhananjay, S. (2010). Role of oxidative stress and inflammation in hypoxia-induced cerebral edema: a molecular approach. High Alt. Med. Biol. 11, 231–244. doi:10.1089/ham.2009.1057
Hou, Y., Fan, F., Xie, N., Zhang, Y., Wang, X., and Meng, X. (2024). Rhodiola crenulata alleviates hypobaric hypoxia-induced brain injury by maintaining BBB integrity and balancing energy metabolism dysfunction. Phytomedicine 128, 155529. doi:10.1016/j.phymed.2024.155529
Hourfar, H., Aliakbari, F., Aqdam, S. R., Nayeri, Z., Bardania, H., Otzen, D. E., et al. (2023). The impact of α-synuclein aggregates on blood-brain barrier integrity in the presence of neurovascular unit cells. Int. J. Biol. Macromol. 229, 305–320. doi:10.1016/j.ijbiomac.2022.12.134
Hu, Y., Sun, J., Wang, T., Wang, H., Zhao, C., Wang, W., et al. (2021). Compound Danshen Dripping Pill inhibits high altitude-induced hypoxic damage by suppressing oxidative stress and inflammatory responses. Pharm. Bio 59, 1585–1593. doi:10.1080/13880209.2021.1998139
Huang, X., Zhou, Y., Zhao, T., Han, X., Qiao, M., Ding, X., et al. (2015). A method for establishing the high-altitude cerebral edema (HACE) model by acute hypobaric hypoxia in adult mice. J. Neurosci. Methods 245, 178–181. doi:10.1016/j.jneumeth.2015.02.004
Huo, Y., Zhao, A., Li, X., Li, J., and Wang, R. (2021). Animal models of acute plateau disease. Chin. Pharmacol. Bull. 37, 26–30. doi:10.3969/j.issn.1001-1978.2021.01.006
Irarrázaval, S., Allard, C., Campodónico, J., Pérez, D., Strobel, P., Vásquez, L., et al. (2017). Oxidative stress in acute hypobaric hypoxia. High Alt. Med. Biol. 18, 128–134. doi:10.1089/ham.2016.0119
Jia, N., Shen, Z., Zhao, S., Wang, Y., Pei, C., Huang, D., et al. (2023). Eleutheroside E from pre-treatment of Acanthopanax senticosus (Rupr.etMaxim.) Harms ameliorates high-altitude-induced heart injury by regulating NLRP3 inflammasome-mediated pyroptosis via NLRP3/caspase-1 pathway. Int. Immunopharmacol. 121, 110423. doi:10.1016/j.intimp.2023.110423
Jiang, S., Fan, F., Yang, L., Chen, K., Sun, Z., Zhang, Y., et al. (2022). Salidroside attenuates high altitude hypobaric hypoxia-induced brain injury in mice via inhibiting NF-κB/NLRP3 pathway. Eur. J. Pharmacol. 925, 175015. doi:10.1016/j.ejphar.2022.175015
Jiao, L., Liu, Y., Xu, C., Wang, J., Liu, J., Tian, Y., et al. (2023). Protective effect of Taikong Yangxin pills against hypoxia when rush into plateau. Manned Spacefl. 29, 336–344. doi:10.16329/j.cnki.zrht.2023.03.006
Julian, C. G., Subudhi, A. W., Wilson, M. J., Dimmen, A. C., Pecha, T., and Roach, R. C. (2011). Acute mountain sickness, inflammation, and permeability: new insights from a blood biomarker study. J. Appl. Physiology 111, 392–399. doi:10.1152/japplphysiol.00391.2011
Kai, S., Tanaka, T., Daijo, H., Harada, H., Kishimoto, S., Suzuki, K., et al. (2012). Hydrogen sulfide inhibits hypoxia- but not anoxia-induced hypoxia-inducible factor 1 activation in a von hippel-lindau- and mitochondria-dependent manner. Antioxid. Redox Signal 16, 203–216. doi:10.1089/ars.2011.3882
Kumar, G., Chhabra, A., Mishra, S., Kalam, H., Kumar, D., Meena, R., et al. (2016a). H2S regulates hypobaric hypoxia-induced early glio-vascular dysfunction and neuro-pathophysiological effects. EBioMedicine 6, 171–189. doi:10.1016/j.ebiom.2016.03.002
Kumar, K., Sharma, S., Vashishtha, V., Bhardwaj, P., Kumar, A., Barhwal, K., et al. (2016b). Terminalia arjuna bark extract improves diuresis and attenuates acute hypobaric hypoxia induced cerebral vascular leakage. J. Ethnopharmacol. 180, 43–53. doi:10.1016/j.jep.2016.01.002
Lappin, T. R., and Lee, F. S. (2019). Update on mutations in the HIF: EPO pathway and their role in erythrocytosis. Blood Rev. 37, 100590. doi:10.1016/j.blre.2019.100590
Li, H., and Qian, D. (2011). Clinical observation of Xingnaojing injection in the treatment of high altitude cerebropathy. Shaanxi J. Traditional Chin. Med. 32, 162–163.
Li, M., Lu, B., Qi, X., Wang, P., and Qin, L. (2023a). Protective effect of Danqijing granules on acute high altitude exposure: a field cohort study on plateau. Acad. J. Chin. PLA Med. Sch., 1–6.
Li, S., Xu, N., Fang, Q., Cheng, X., Chen, J., Liu, P., et al. (2023b). Glehnia littoralis Fr. Schmidtex Miq.: a systematic review on ethnopharmacology, chemical composition, pharmacology and quality control. J. Ethnopharmacol. 317, 116831. doi:10.1016/j.jep.2023.116831
Li, X., Zhang, J., Liu, G., Wu, G., Wang, R., and Zhang, J. (2024). High altitude hypoxia and oxidative stress: the new hope brought by free radical scavengers. Life Sci. 336, 122319. doi:10.1016/j.lfs.2023.122319
Li, X., Zhang, Q., Nasser, M. I., Xu, L., Zhang, X., Zhu, P., et al. (2020a). Oxygen homeostasis and cardiovascular disease: a role for HIF? Biomed. Pharmacother. 128, 110338. doi:10.1016/j.biopha.2020.110338
Li, Y. (2016). Observation on the therapeutic effect of compound dangshen tablet on Acute Mountain sickness. Qinghai Med. J. 46, 14–15.
Li, Y., Wang, D., Ma, H., and Bao, Q. (2012). Discussion on traditional Chinese medicine syndrome types of high altitude disease. Liaoning J. Traditional Chin. Med. 39, 653–656. doi:10.13192/j.ljtcm.2012.04.82.liyq.029
Li, Z., Guo, J., Liu, C., Shi, Y., Li, Y., Wang, J., et al. (2020b). Compound danshen dripping pill promotes adaptation to acute high-altitude exposure. High. Alt. Med. Biol. 21, 258–264. doi:10.1089/ham.2019.0126
Liao, Y., Chen, Z., Yang, Y., Shen, D., Chai, S., Ma, Y., et al. (2023). Antibiotic intervention exacerbated oxidative stress and inflammatory responses in SD rats under hypobaric hypoxia exposure. Free Radic. Biol. Med. 209, 70–83. doi:10.1016/j.freeradbiomed.2023.10.002
Lin, Y., and Xiong, J. (2008). Clinical observation of Sankang capsule on Acute Mountain reaction. Clin. J. Med. Officers 36, 831–832.
Lin, Y., Xiong, J., Zhang, H., Yin, T., Peng, Y., Zhang, Y., et al. (2008). Observation of curative effect of Shulikang capsule on acute mountain sickness. J. Mil. Surg. Southwest China 10, 45–46.
Liu, B., Chen, J., Zhang, L., Gao, Y., Cui, J., Zhang, E., et al. (2017). IL-10 dysregulation in Acute Mountain sickness revealed by transcriptome analysis. Front. Immunol. 8, 628. doi:10.3389/fimmu.2017.00628
Liu, H., Zhao, C., Zhang, W., Zhang, Y., and Nie, J. (2018a). Study on medication laws of Tibetan medicine in treatment of plateau diseases based on data mining technology. China J. Chin. Materia Medica 43, 1726–1731. doi:10.19540/j.cnki.cjcmm.20180112.001
Liu, Y., Liu, T., Ding, K., Liu, Z., Li, Y., He, T., et al. (2018b). Phospholipase Cγ2 signaling cascade contribute to the antiplatelet effect of notoginsenoside fc. Front. Pharmacol. 9, 1293. doi:10.3389/fphar.2018.01293
Luks, A. M., McIntosh, S. E., Grissom, C. K., Auerbach, P. S., Rodway, G. W., Schoene, R. B., et al. (2010). Wilderness medical society consensus guidelines for the prevention and treatment of acute altitude illness. Wilderness & Environ. Med. 21, 146–155. doi:10.1016/j.wem.2010.03.002
Luks, A. M., Swenson, E. R., and Bärtsch, P. (2017). Acute high-altitude sickness. Eur. Respir. Rev. 26, 160096. doi:10.1183/16000617.0096-2016
Luo, M., He, J., Yin, L., Zhan, P., Zhao, Z., Xiong, H., et al. (2024). Borneol exerts its antipruritic effects by inhibiting TRPA1 and activating TRPM8. J. Ethnopharmacol. 322, 117581. doi:10.1016/j.jep.2023.117581
Ma, D., Wang, L., Jin, Y., Gu, L., Yin, G., Wang, J., et al. (2022). Chemical characteristics of Rhodiola Crenulata and its mechanism in acute mountain sickness using UHPLC-Q-TOF-MS/MS combined with network pharmacology analysis. J. Ethnopharmacol. 294, 115345. doi:10.1016/j.jep.2022.115345
Ma, H., Fan, P., Jing, L., Yao, J., He, X., Yang, Y., et al. (2011). Anti-hypoxic activity at simulated high altitude was isolated in petroleum ether extract of Saussurea involucrata. J. Ethnopharmacol. 137, 1510–1515. doi:10.1016/j.jep.2011.08.037
Ma, H., Wu, J., Gao, R., Li, L., Fan, P., Jing, L., et al. (2013). Establishment of an animal model for Acute Mountain sickness with A decompression chamber. Pharm. J. Chin. PLA 29, 301–304.
Ma, R., Lu, D., Xie, Q., Yuan, J., Ren, M., Li, Y., et al. (2023). l-Borneol and d-Borneol promote transdifferentiation of astrocytes into neurons in rats by regulating Wnt/Notch pathway to exert neuroprotective effect during recovery from cerebral ischaemia. Phytomedicine 109, 154583. doi:10.1016/j.phymed.2022.154583
Masschelein, E., Van Thienen, R., D’Hulst, G., Hespel, P., Thomis, M., and Deldicque, L. (2014). Acute environmental hypoxia induces LC3 lipidation in a genotype-dependent manner. FASEB J. 28, 1022–1034. doi:10.1096/fj.13-239863
Mishra, S., Kumar, G., Chhabra, A., Sethy, N. K., Jain, N., Meena, R. N., et al. (2020). Cysteine becomes conditionally essential during hypobaric hypoxia and regulates adaptive neuro-physiological responses through CBS/H2S pathway. Biochim. Biophys. Acta Mol. Basis Dis. 1866, 165769. doi:10.1016/j.bbadis.2020.165769
Moraga, F. A., Flores, A., Serra, J., Esnaola, C., and Barriento, C. (2007). Ginkgo biloba decreases Acute Mountain sickness in people ascending to high altitude at ollagüe (3696m) in northern Chile. Wilderness Environ. Med. 18, 251–257. doi:10.1580/06-WEME-OR-062R2.1
Neuwelt, E. A., Bauer, B., Fahlke, C., Fricker, G., Iadecola, C., Janigro, D., et al. (2011). Engaging neuroscience to advance translational research in brain barrier biology. Nat. Rev. Neurosci. 12, 169–82. doi:10.1038/nrn2995
Niu, W., Wang, Y., Cao, Z., Yu, S., and Zhang, L. (2006a). Effects of Shulikang Capsule on heart rate of young men exposed rapidly to high altitude. Med. J. Natl. Defending Forces Southwest China 16, 493–495. doi:10.3969/j.issn.1004-0188.2006.05.011
Niu, W., Wang, Y., Cao, Z., Yu, S., and Zhang, L. (2006b). Experimental Effects of Shulikang capsule on prevention of acute high altitude reaction. J. High Alt. Med. 16, 2–4. doi:10.3969/j.issn.1007-3809.2006.03.002
Norris, J. N., Viirre, E., Aralis, H., Sracic, M. K., Thomas, D., and Gertsch, J. H. (2012). High altitude headache and Acute Mountain sickness at moderate elevations in a military population during battalion-level training exercises. Mil. Med. 177, 917–923. doi:10.7205/MILMED-D-12-00007
Nourkami-Tutdibi, N., Küllmer, J., Dietrich, S., Monz, D., Zemlin, M., and Tutdibi, E. (2023). Serum vascular endothelial growth factor is a potential biomarker for acute mountain sickness. Front Physiol. 14, 1083808. doi:10.3389/fphys.2023.1083808
Ou, C., Geng, T., Wang, J., Gao, X., Chen, X., Luo, X., et al. (2020). Systematically investigating the pharmacological mechanism of Dazhu Hongjingtian in the prevention and treatment of acute mountain sickness by integrating UPLC/Q-TOF-MS/MS analysis and network pharmacology. J. Pharm. Biomed. Anal. 179, 113028. doi:10.1016/j.jpba.2019.113028
Pan, Y., Zhang, Y., Yuan, J., Ma, X., Zhao, Y., Li, Y., et al. (2020). Tetrahydrocurcumin mitigates acute hypobaric hypoxia-induced cerebral oedema and inflammation through the NF-κB/VEGF/MMP-9 pathway. Phytother. Res. 34, 2963–2977. doi:10.1002/ptr.6724
Park, S. H., Chung, S., Chung, M.-Y., Choi, H.-K., Hwang, J.-T., and Park, J. H. (2022). Effects of Panax ginseng on hyperglycemia, hypertension, and hyperlipidemia: a systematic review and meta-analysis. J. Ginseng Res. 46, 188–205. doi:10.1016/j.jgr.2021.10.002
Paul, S., Gangwar, A., Bhargava, K., and Ahmad, Y. (2018). STAT3-RXR-Nrf2 activates systemic redox and energy homeostasis upon steep decline in pO2 gradient. Redox Biol. 14, 423–438. doi:10.1016/j.redox.2017.10.013
Pei, C., Jia, N., Wang, Y., Zhao, S., Shen, Z., Shi, S., et al. (2023). Notoginsenoside R1 protects against hypobaric hypoxia-induced high-altitude pulmonary edema by inhibiting apoptosis via ERK1/2-P90rsk-BAD ignaling pathway. Eur. J. Pharmacol. 959, 176065. doi:10.1016/j.ejphar.2023.176065
Pena, E., Brito, J., El Alam, S., and Siques, P. (2020). Oxidative stress, kinase activity and inflammatory implications in right ventricular hypertrophy and heart failure under hypobaric hypoxia. Int. J. Mol. Sci. 21, 6421. doi:10.3390/ijms21176421
Pena, E., El Alam, S., Siques, P., and Brito, J. (2022). Oxidative stress and diseases associated with high-altitude exposure. Antioxidants 11, 267. doi:10.3390/antiox11020267
Peng, Y., Yin, H., Li, S., and Yang, H. (2022). Transcriptome of pituitary function changes in rat model of high altitude cerebral edema. Genomics 114, 110519. doi:10.1016/j.ygeno.2022.110519
Peng, Y. J., Nanduri, J., Wang, N., Kumar, G. K., Bindokas, V., Paul, B. D., et al. (2023). Hypoxia sensing requires H2S-dependent persulfidation of olfactory receptor 78. Sci. Adv. 9, eadf3026. doi:10.1126/sciadv.adf3026
Pham, K., Frost, S., Parikh, K., Puvvula, N., Oeung, B., and Heinrich, E. C. (2022). Inflammatory gene expression during acute high-altitude exposure. J. Physiol. 600, 4169–4186. doi:10.1113/JP282772
Pu, X., Li, F., Lin, X., Wang, R., and Chen, Z. (2022). Oxidative stress and expression of inflammatory factors in lung tissue of acute mountain sickness rats. Mol. Med. Rep. 25, 49. doi:10.3892/mmr.2021.12565
Qian, W., Cai, X., Qian, Q., Wang, D., and Zhang, L. (2020). Angelica sinensis polysaccharide suppresses epithelial-mesenchymal transition and pulmonary fibrosis via a DANCR/AUF-1/FOXO3 regulatory Axis. Aging Dis. 11, 17–30. doi:10.14336/AD.2019.0512
Richalet, J.-P., Hermand, E., and Lhuissier, F. J. (2023). Cardiovascular physiology and pathophysiology at high altitude. Nat. Rev. Cardiol. 21, 75–88. doi:10.1038/s41569-023-00924-9
Roncin, J. P., Schwartz, F., and D’Arbigny, P. (1996). EGb 761 in control of acute mountain sickness and vascular reactivity to cold exposure. Aviat. Space Environ. Med. 67, 445–452.
Ruan, H. (2009). Compound Danshen Dripping Pill combined with aminophylline for prevention of acute mild altitude sickness. Mod. J. Integr. Traditional Chin. West. Med. 18, 4090–4091.
Shen, J. (2019). Hongjingtian combined with dexamethasone and albuterol aerosol in the treating 60 cases of Acute Mountain sickness. West. J. Traditional Chin. Med. 32, 80–82.
Shen, Z., Huang, D., Jia, N., Zhao, S., Pei, C., Wang, Y., et al. (2023). Protective effects of Eleutheroside E against high-altitude pulmonary edema by inhibiting NLRP3 inflammasome-mediated pyroptosis. Biomed. Pharmacother. 167, 115607. doi:10.1016/j.biopha.2023.115607
Shi, B., Cui, Q., Feng, Z., and Li, J. (2019). Mining potential drugs for prevention and treatment of acute mountain sickness based on bioinformatics approach. Chin. J. Clin. Healthc. 22, 503–507.
Shi, X. (2011) MSCT study of liver hemodynamics and volume in normal adults at different altitudes. China: Qinghai University.
Song, T.-T., Bi, Y.-H., Gao, Y.-Q., Huang, R., Hao, K., Xu, G., et al. (2016). Systemic pro-inflammatory response facilitates the development of cerebral edema during short hypoxia. J. Neuroinflammation 13, 63. doi:10.1186/s12974-016-0528-4
Su, H.-F., Shaker, S., Kuang, Y., Zhang, M., Ye, M., and Qiao, X. (2021). Phytochemistry and cardiovascular protective effects of huang-qi (astragali radix). Med. Res. Rev. 41, 1999–2038. doi:10.1002/med.21785
Tang, Y., Song, H., and Fei, J. (2013). Effect of Shengnaokang pills on prevention and treatment of Acute Mountain sickness. People’s Mil. Surg. 56, 46–47.
Tu, B., Wang, Y., Wu, Z., Zhou, W., Tang, X., Zhang, C., et al. (2023). DIA-based serum proteomics revealed the protective effect of modified siwu decoction against hypobaric hypoxia. J. Ethnopharmacol. 319, 117303. doi:10.1016/j.jep.2023.117303
Turner, R. E. F., Gatterer, H., Falla, M., and Lawley, J. S. (2021). High-altitude cerebral edema: its own entity or end-stage acute mountain sickness? J. Appl. Physiol. 131, 313–325. doi:10.1152/japplphysiol.00861.2019
Wang, C., Jiang, H., Duan, J., Chen, J., Wang, Q., Liu, X., et al. (2018). Exploration of acute phase proteins and inflammatory cytokines in early stage diagnosis of Acute Mountain sickness. High Alt. Med. Biol. 19, 170–177. doi:10.1089/ham.2017.0126
Wang, C., Yan, M., Duan, J., Chen, J., and Huang, J. (2015). Reproduction of a rat model of acute high-altitude sickness and evaluation of its related indexes. Med. J. Chin. PLA 40, 716–721.
Wang, X., Liu, C., Zhang, H., Guo, X., and Zhang, W. (2017). Effect of hydrogen sulfide on pulmonary respiratory membrane permeability in rats with experimental high altitude pulmonary edema. Chin. High Alt. Med. Biol. 38, 86–90. doi:10.13452/j.cnki.jqmc.2017.02.003
Wang, X., Sun, H., Cui, L., Wang, X., Ren, C., Tong, Z., et al. (2022). Acute high-altitude hypoxia exposure causes neurological deficits via formaldehyde accumulation. CNS Neurosci. Ther. 28, 1183–1194. doi:10.1111/cns.13849
Wang, Z. (1994). Acupuncture for Acute Mountain sickness 56 cases. Chin. Acupunct. Moxibustion, 338–339.
West, J. (2012). High-altitude medicine. Am. J. Respir. Crit. Care Med. 186, 1229–1237. doi:10.1164/rccm.201207-1323CI
Wilson, M. H., Newman, S., and Imray, C. H. (2009). The cerebral effects of ascent to high altitudes. Lancet Neurol. 8, 175–191. doi:10.1016/S1474-4422(09)70014-6
Wu, B., Teng, H., Zhang, L., Li, H., Li, J., Wang, L., et al. (2015). Interaction of hydrogen sulfide with oxygen sensing under hypoxia. Oxid. Med. Cell. Longev. 2015, 758678. doi:10.1155/2015/758678
Wu, T. (2005). Chronic mountain sickness on the Qinghai-Tibetan plateau. Chin. Med. J. Engl. 118, 161–168.
Wu, Z., Xu, H., Wang, Y., Tu, B., Tang, X., Li, M., et al. (2023). Visual analysis of studies on traditional Chinese medicine for prevention and treatment of highaltitude disease. J. Environ. Occup. Med. 40, 1297–1306.
Xie, N., Fan, F., Jiang, S., Hou, Y., Zhang, Y., Cairang, N., et al. (2022). Rhodiola crenulate alleviates hypobaric hypoxia-induced brain injury via adjusting NF-κB/NLRP3-mediated inflammation. Phytomedicine 103, 154240. doi:10.1016/j.phymed.2022.154240
Xie, Y., and Guo, X. (2004). Treatment of Acute Mountain reaction with Astragalus injection in 200 cases. Chin. J. Integr. Traditional West. Med. 24, 1049.
Xin, X., Yang, K., Liu, H., and Li, Y. (2022). Hypobaric hypoxia triggers pyroptosis in the retina via NLRP3 inflammasome activation. Apoptosis 27, 222–232. doi:10.1007/s10495-022-01710-7
Xu, H., Li, S., Liu, J., Cheng, J., Kang, L., Li, W., et al. (2023). Bioactive compounds from Huashi Baidu decoction possess both antiviral and anti-inflammatory effects against COVID-19. Proc. Natl. Acad. Sci. U.S.A. 120, e2301775120. doi:10.1073/pnas.2301775120
Xue, Y., Wang, X., Wan, B., Wang, D., Li, M., Cheng, K., et al. (2022). Caveolin-1 accelerates hypoxia-induced endothelial dysfunction in high-altitude cerebral edema. Cell. Commun. Signal 20, 160. doi:10.1186/s12964-022-00976-3
Yan, J., Ruan, P., Zhang, Z., Ge, Y., Gao, J., Tan, H., et al. (2022). The effects and mechanism of compound Danshen driping pills on acute mountain sickness in rats. Chin. J. Clin. Pharmacol. 38, 2736–2740. doi:10.13699/j.cnki.1001-6821.2022.22.017
Yan, X., Liu, J., Zhu, M., Liu, L., Chen, Y., Zhang, Y., et al. (2021). Salidroside orchestrates metabolic reprogramming by regulating the Hif-1α signalling pathway in acute mountain sickness. Pharm. Biol. 59, 1540–1550. doi:10.1080/13880209.2021.1992449
Yang, H., Jin, G., Ma, Q., Liu, S., Li, S., and Ge, R. (2011). POTENTIAL ROLE OF EXOGENOUS HYDROGEN SULFIDE ON PULMONARY ARTERIAL PRESSURES IN RATS AT HIGH ALTITUDE. J. Qinghai Med. Coll. 32, 164–168+171. doi:10.13452/j.cnki.jqmc.2011.03.007
Yu, W., Yuan, J., Li, J., and Chen, Y. (2011). Observations on the efficacy of Shexiang Baoxin pill in the treatment of acute plateau reaction. Chin. J. Health Care Med. 13, 188+191.
Yu, Y., Ding, S., Xu, X., Yan, D., Fan, Y., Ruan, B., et al. (2023). Integrating network pharmacology and bioinformatics to explore the effects of dangshen (codonopsis pilosula) against hepatocellular carcinoma: validation based on the active compound luteolin. Drug Des. Devel Ther. 17, 659–673. doi:10.2147/DDDT.S386941
Zeng, Y., Cao, W., Huang, Y., Zhang, H., Li, C., He, J., et al. (2024). Huangqi Baihe Granules alleviate hypobaric hypoxia-induced acute lung injury in rats by suppressing oxidative stress and the TLR4/NF-κB/NLRP3 inflammatory pathway. J. Ethnopharmacol. 324, 117765. doi:10.1016/j.jep.2024.117765
Zhang, D., Zhang, Y., Nie, H., Zhang, R., Cui, J., Cheng, Y., et al. (2010). Protective effects of new compound codonopsis tablets against acute mountain sickness. Chin. J. Appl. Physiology 26, 148–152. doi:10.13459/j.cnki.cjap.2010.02.005
Zhang, D., Zhang, Y., Xie, Y., Ma, Y., Zhang, X., and Cui, J. (2005). Effect of Compound Dangshen capsule on prevention and treatment of acute mountain sickness. J. Prev. Med. Chin. People’s Liberation Army 23, 271–272.
Zhang, J., Wu, Y., Peng, X.-Y., Li, Q.-H., Xiang, X.-M., Zhu, Y., et al. (2021). The protective effect of a novel cross-linked hemoglobin-based oxygen carrier on hypoxia injury of Acute Mountain sickness in rabbits and goats. Front. Physiology 12, 690190–690213. doi:10.3389/fphys.2021.690190
Zhang, J., and Zhang, X. (1993). Extrinsic factors of mechanism and characteristics on altitude sickness. China J. Traditional Chin. Med. Pharm. 8, 336–337.
Zheng, H. L., Li, M. T., Zhou, T., Wang, Y. Y., Shang, E. X., Hua, Y. Q., et al. (2023). Protective effects of Lycium barbarum L. berry extracts against oxidative stress-induced damage of the retina of aging mouse and ARPE-19 cells. Food Funct. 14, 399–412. doi:10.1039/d2fo02788g
Zhong, G., Mi, M., Ge, Z., Dang, Z., and Zongba, B. (2022). Preliminary study on tongue diagnosis in Tibetan medicine four Tantras. China J. Traditional Chin. Med. Pharm. 37, 2303–2305.
Zhou, Y., Huang, X., Zhao, T., Qiao, M., Zhao, X., Zhao, M., et al. (2017). Hypoxia augments LPS-induced inflammation and triggers high altitude cerebral edema in mice. Brain Behav. Immun. 64, 266–275. doi:10.1016/j.bbi.2017.04.013
Keywords: traditional Chinese medicine, acute mountain sickness, hypobaric hypoxia, pathogenesis, mechanism
Citation: Wu Z, Wang Y, Gao R, Chen J, Chen Y, Li M and Gao Y (2024) Potential therapeutic effects of traditional Chinese medicine in acute mountain sickness: pathogenesis, mechanisms and future directions. Front. Pharmacol. 15:1393209. doi: 10.3389/fphar.2024.1393209
Received: 28 February 2024; Accepted: 06 May 2024;
Published: 04 June 2024.
Edited by:
Michael Heinrich, University College London, United KingdomReviewed by:
Bhuvnesh Kumar, Sharda University, IndiaCopyright © 2024 Wu, Wang, Gao, Chen, Chen, Li and Gao. This is an open-access article distributed under the terms of the Creative Commons Attribution License (CC BY). The use, distribution or reproduction in other forums is permitted, provided the original author(s) and the copyright owner(s) are credited and that the original publication in this journal is cited, in accordance with accepted academic practice. No use, distribution or reproduction is permitted which does not comply with these terms.
*Correspondence: Yue Gao, Z2FveXVlQGJtaS5hYy5jbg==; Maoxing Li, bGltYW94MjAyMEBhbGl5dW4uY29t
†These authors have contributed equally to this work and share first authorship
Disclaimer: All claims expressed in this article are solely those of the authors and do not necessarily represent those of their affiliated organizations, or those of the publisher, the editors and the reviewers. Any product that may be evaluated in this article or claim that may be made by its manufacturer is not guaranteed or endorsed by the publisher.
Research integrity at Frontiers
Learn more about the work of our research integrity team to safeguard the quality of each article we publish.