- 1Department of Pharmacy, People’s Hospital of Zhongjiang County, Deyang, China
- 2Department of Pharmacy and Evidence-Based Pharmacy Center, West China Second University Hospital, Chengdu, China
- 3Key Laboratory of Birth Defects and Related Diseases of Women and Children (Sichuan University), Ministry of Education, Chengdu, China
Background: Oseltamivir and baloxavir marboxil are the two primary oral drugs approved by the Food and Drug Administration (FDA) for treating influenza. Limited real-world evidence exists on their adverse events in children. The purpose of this study was to explore the adverse event (AE) profiles of oseltamivir and baloxavir marboxil in children based on the U.S. Food and Drug Administration Adverse Event Reporting System (FAERS) database.
Methods: FAERS reports were collected and analyzed from the first quarter of 2019 to the third quarter of 2023. Disproportionality analyses, including the reporting odds ratio (ROR), the proportional reporting ratio (PRR), the Bayesian confidence propagation neural network (BCPNN), and the multi-item gamma Poisson shrinker (MGPS) algorithms, were employed in data mining to quantify the signals of oseltamivir and baloxavir marboxil-related AEs.
Results: A total of 464 reports of AEs to oseltamivir as the “primary suspect (PS)” and 429 reports of AEs to baloxavir marboxil as the “PS” were retrieved in pediatric patients. A total of 100 oseltamivir-induced AE signals were detected in 17 system organ classes (SOCs), and 11 baloxavir marboxil-induced AE signals were detected in 6 SOCs after complying with the four algorithms simultaneously. Categorized and summarized by the number of reports of involvement in each SOC, the top 3 for oseltamivir were psychiatric disorders, gastrointestinal disorders, general disorders and site-of-administration conditions, respectively. The top 3 for baloxavir marboxil were injury, poisoning and surgical complications, general disorders and site of administration conditions, and psychiatric disorders, respectively.
Conclusion: Our study identifies potential new AE signals for oseltamivir and provides a broader understanding of the safety of oseltamivir and baloxavir marboxil in children.
1 Introduction
Acute respiratory diseases are common in children, and one of the common pathogens of influenza virus (Heikkinen, 2006). Influenza virus infections can occur in children of all ages from birth to 18 years of age (Fowlkes et al., 2014; Caini et al., 2018). While this condition is typically self-limiting in healthy adults, it may lead to severe complications in children (Paules and Subbarao, 2017). The younger the child, the sicker the flu and the higher the rate of complications (Principi and Esposito, 2016; Uyeki, 2020). In addition, the high incidence of seasonal influenza in school-age children has led to a significant spread of influenza viruses (Rotrosen and Neuzil, 2017; Nayak, Hoy, and Gordon, 2021), and has imposed a socioeconomic burden on affected children and families (Principi et al., 2003; Ambrose and Antonova, 2014; McLean et al., 2017). And anti-influenza viral drugs have been shown to be effective in preventing and treating influenza by reducing the duration and severity of illness (Fiore et al., 2011).
Oseltamivir and baloxavir marboxil are the two most commonly used oral agents approved by the FDA for the treatment of influenza. Oseltamivir is a neuraminidase inhibitor (NAI) that prevents the influenza virus from replicating and reduces infectiousness. It effectively shortens the time to symptom relief (Jefferson et al., 2014), hospitalization rates, and the incidence of complications in pediatric influenza patients (Peters et al., 2008). Oseltamivir is a first-line drug for the prevention and treatment of influenza (Committee on Infectious Diseases, 2023). Baloxavir marboxil is the first FDA approval of the world’s first anti-influenza virus drug based on a completely new mechanism in nearly 20 years. It was approved for marketing in Japan and the United States in 2018. Baloxavir marboxil is a novel cap-dependent endonuclease inhibitor. Unlike neuraminidase inhibitors that block viral release from infected host cells, baloxavir marboxil blocks influenza virus proliferation by inhibiting viral messenger Ribonucleic Acid (mRNA) transcription (F.G. Hayden et al., 2018).
However, there needs to be more data evaluating the safety of oseltamivir and baloxavir marboxil in children, and findings on the safety of oseltamivir and baloxavir are inconsistent. A meta-analysis that included three randomized controlled trials (RCTs) noted that baloxavir marboxil appears to be a relatively safe anti-influenza drug compared to oseltamivir (Kuo et al., 2021). In contrast, a recent study, which included 200 patients aged 14–85 years with a diagnosis of influenza A, showed that the difference in the incidence of adverse events between the two groups of patients treated with baloxavir marboxil and oseltamivir was not statistically significant (Qiu et al., 2024). These discrepancies highlight the need for more comprehensive safety evaluations, particularly in pediatric populations. Clinical trial results may not always reflect real-world outcomes (Ma et al., 2021), and women are increasingly acknowledged as a risk factor for significant side effects with clinical relevance (Franconi and Campesi, 2014). Therefore, there is an urgent need for pharmacovigilance studies to examine the adverse reaction profiles of oseltamivir and baloxavir marboxil in children.
The FAERS database is a valuable resource for post-marketing surveillance and early detection of drug safety problems (Feng et al., 2022). Although it is impossible to explain the causal relationship between drugs and AEs, disproportionality analysis in the spontaneous reporting of adverse drug reactions database remains a validated quantitative methodology for pharmacovigilance signal detection (Cutroneo et al., 2024). In-depth analysis of the FAERS data in this study allowed for a comprehensive assessment of the safety of oseltamivir and baloxavir marboxil in a real-world pediatric population, thereby increasing clinical awareness, enhancing proactive surveillance, and promoting safer medication use.
2 Materials and methods
2.1 Data sources
The FAERS database is a free U.S. database where health professionals, consumers, manufacturers, and others may voluntarily submit AE reports to help the FDA monitor the safety of medicines and biologics after they have been marketed (Cirmi et al., 2020; Hu et al., 2020). FAERS has been noted for its capability to identify early safety concerns, especially for recently authorized medications and uncommon adverse reactions (Harpaz et al., 2013; Fukazawa et al., 2018). The FAERS database is updated every 3 months, and anybody may freely access and download the data from the FDA website. The FAERS database contains seven modules corresponding to seven aspects of the main content, including patient demographic and administrative information (DEMO), report source (RPSR), drug information (DRUG), adverse events (REAC), patient outcomes (OUTC), indications for drug administration (INDI), and therapy start states and end dates for the reported drugs (THER). In this study, data on baloxavir marboxil and oseltamivir were extracted from the FAERS database for the period January 2019 to September 2023 (the most recent data available in the FAERS database) and all data were imported into MySQL 8.0 for analysis.
A total of 8,430,706 AE reports were retrieved from the FAERS database. We used a two-step deduplication process to ensure the uniqueness of the reports. First, we downloaded the deleted data from the deleted files from the FAERS database, and then performed the deduplication process according to the FDA recommendations by selecting the higher primaryid when the caseid and FDA_DT are the same, and selecting the most recent FDA_DT when the caseid are the same (Sakaeda et al., 2013), which ultimately reduced the number of reports to 7,186,915 (Figure 1). We further filtered the dataset to include only those reports in which oseltamivir or baloxavir marboxil was the PS drug. This means that in all of our study’s AE reports, oseltamivir or baloxavir marboxil were the only drugs coded as “PS” in the role_cod field. This improves the reliability of the study results.
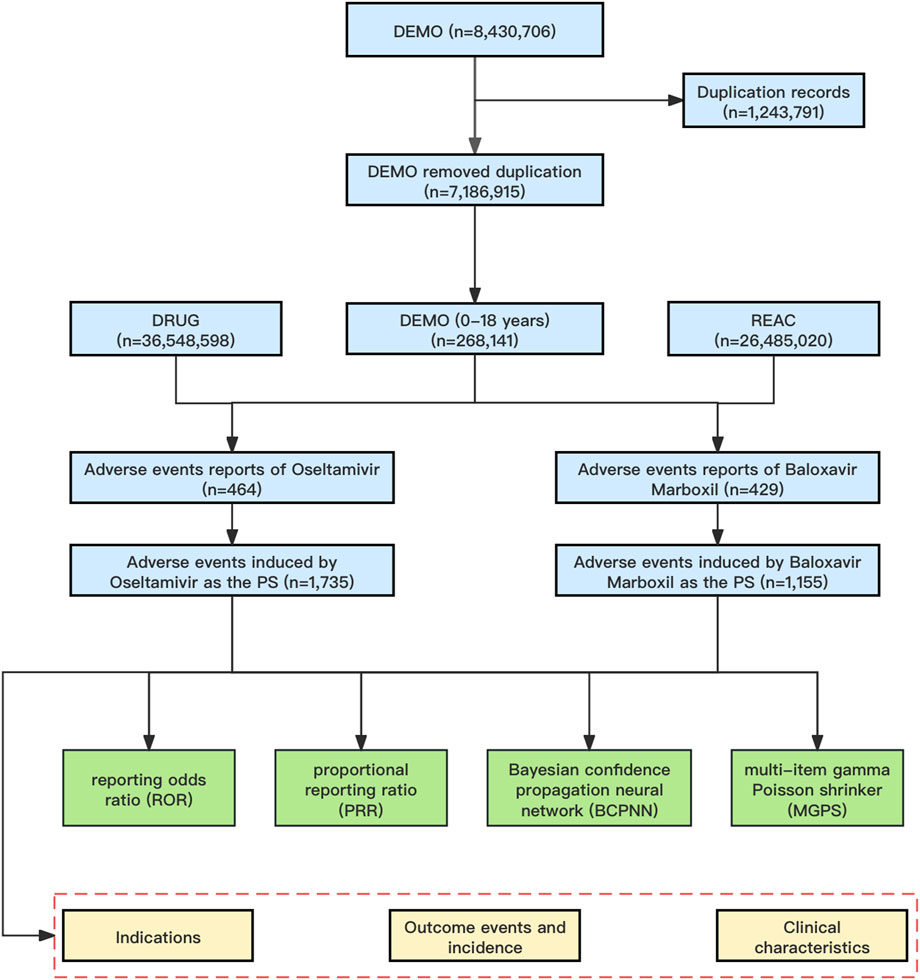
Figure 1. The flowchart of identifying oseltamivir and baloxavir marboxil AEs in FAERS database. Abbreviations: FAERS, United States Food and Drug Administration Adverse Event Reporting System; DEMO, demographic and administrative information file; DRUG, drug information file; REAC, adverse events file; PS, Primary Suspect.
2.2 Procedures
We used FDA-approved generic and trade names to identify AEs in Drug files by fuzzy matching, including oseltamivir (TAMIFLU) AND baloxavir marboxil (XOFLUZA). To improve the accuracy of the analysis, we restricted the analysis to reports with a drug role_cod of “PS (primary suspect)” in the Drug file. AEs in FAERS are coded by the preferred term (PT) in the Standardized Medical Dictionary (MedDRA) terminology (Brown et al., 1999), which is structured at five levels: system organ class (SOC), high level group term (HLGT), high level term (HLT), PT, and lowest level term (LLT). In addition, different PTs can be combined to define specific clinical syndromes using an algorithm known as a standardized MedDRA query.
2.3 Data mining
Disproportionality analysis is a key tool in pharmacovigilance research used to detect medication-related AEs by comparing the occurrence of AEs between a certain medicine and all other drugs (Hu et al., 2020). The general principle is that a meaningful signal is considered to be generated when the incidence of a specific AE for a particular drug is significantly higher than the background frequency in the database and reaches a certain threshold or criterion. The study utilized four algorithms: proportional reporting ratio (PRR), reporting odds ratio (ROR), Bayesian confidence propagation neural network (BCPNN), and multi-item gamma Poisson shrinker (MGPS) (van Puijenbroek et al., 2002; Song et al., 2020). PRR and ROR are examples of frequencyist approaches, which do not use Bayesian principles. On the other hand, BCPNN and MGPS are Bayesian methods (Sakaeda et al., 2013). Information Components (IC) are utilized within the BCPNN tool to quantify disproportionality (Bate, 2007). The utilization of MGPS analysis is a well-acknowledged approach for mitigating the occurrence of inaccurate positive findings. It accomplishes this by employing a Bayesian shrinkage estimator to modify the observed/expected ratio. This adjustment results in smaller risk estimates with narrower confidence intervals, even when the event counts are low (Napoli et al., 2014). The two Bayesian approaches, BCPNN and MGPS, were deemed valuable because of their ability to identify distinct signals, even in cases where there were few reports of AEs for a specific medication (Nomura et al., 2015). In general, an increase in the value of the four parameters leads to a corresponding increase in the strength of the signal value. The precise formulas and criteria used by the four algorithms for detecting positive safety signals were deemed valuable. The presence of the four algorithms is demonstrated in Table 1. To ensure the findings’ dependability, we selected the AE signals that meet the thresholds of all four methods simultaneously for the investigation.
2.4 Subgroup analysis
We performed subgroup analyses according to the gender of the patients (female and male) to study the differences in AEs due to oseltamivir versus baloxavir marboxil across different genders.
3 Results
3.1 Descriptive analysis
From January 2019 through September 2023, a total of 8,430,706 AE reports were recorded in the FAERS database, which was reduced to 7,186,915 AE reports by removing duplicates, and then further screened to identify 268,141 AE reports aged 0–18 years. In the present study, 464 reports identified oseltamivir as the PS, corresponding to a total of 1,735 AEs attributed to oseltamivir. Similarly, for baloxavir marboxil, 429 reports identified it as the PS, with a total of 1,155 AEs linked to baloxavir marboxil as the suspected drug. The mean age of pediatric patients treated with oseltamivir was 8.28 years while the mean age of patients treated with baloxavir marboxil was 10.52 years. The highest number of AEs were reported in the age group of 6–12 years with 187 (40.30%) for oseltamivir and 322 (75.06%) for baloxavir marboxil. In addition, the highest number of reports from consumers were 267 cases (57.54%) for oseltamivir and 279 cases (65.03%) for baloxavir marboxil. As for the countries where the adverse events were reported, the countries with the highest number of reports were all from the United States, which were oseltamivir 295 cases (63.58%) and baloxavir marboxil 320 cases (74.59%), respectively. See Table 2 for details. 194 patients treated with oseltamivir reported 579 concomitant medications, while 69 patients treated with baloxavir marboxil reported 156 concomitant medications. Table 3 lists concomitant medications with oseltamivir and baloxavir marboxil (the top five). The most used concomitant drugs were all acetaminophen.
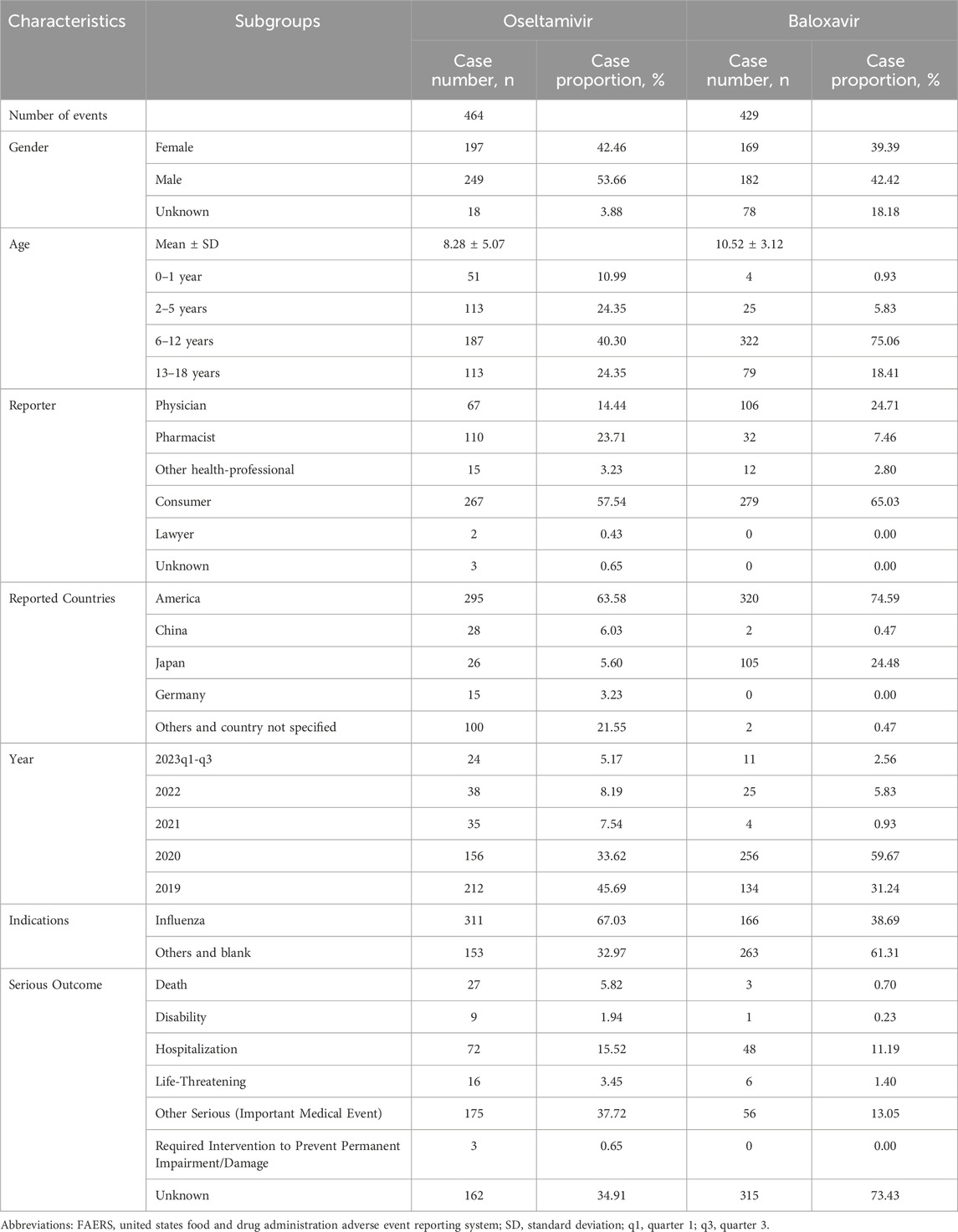
Table 2. Clinical characteristics of reports with oseltamivir and baloxavir marboxil from the FAERS database (January 2019 to September 2023).
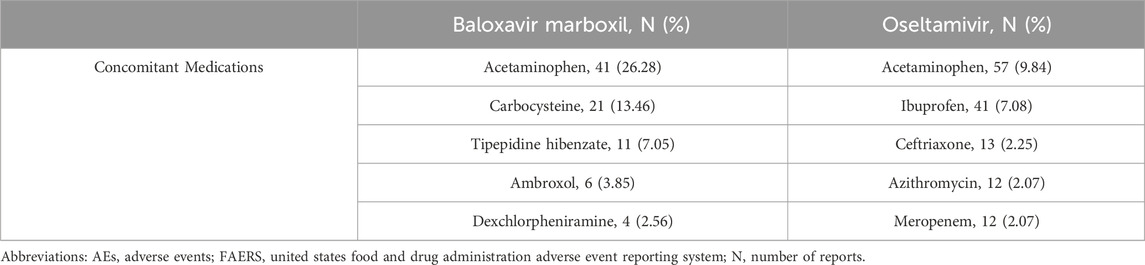
Table 3. Top five concomitant medications for oseltamivir and baloxavir marboxil AEs from the FAERS database.
3.2 Signal distribution at the SOC level
In this study, we categorized the signaling PTs by SOC and mined out oseltamivir 100 AE signals involving 17 SOCs, baloxavir marboxil 11 AE signals involving 6 SOCs. We categorized and summarized by the cumulative number of reports for each SOC (Figure 2), which showed that the top 3 for oseltamivir were psychiatric disorders (43.41%), gastrointestinal disorders (13.90%), general disorders and administration site conditions (10.12%). The top 3 for baloxavir marboxil were injury, poisoning and procedural complications (60.44%), general disorders and administration site conditions (33.62%), psychiatric disorders (3.21%).
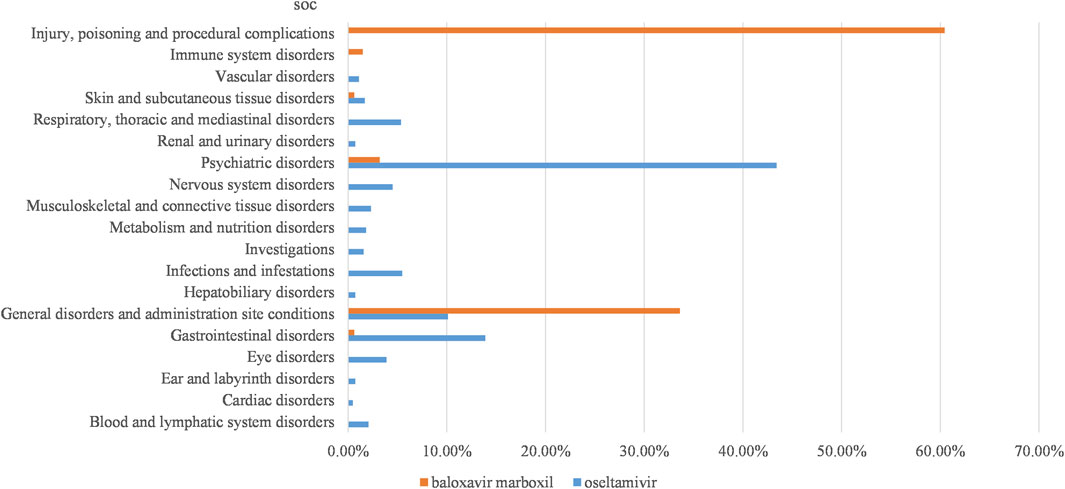
Figure 2. Proportion of reported cases of oseltamivir and baloxavir marboxil involving systemic AEs. Abbreviations: SOC, organ system classification.
3.3 Signal at the PT level
Through data mining, we found large differences in the number and intensity of AEs and signals between oseltamivir and baloxavir marboxil at the PT level.
SOCs commonly implicated in adverse event signaling for oseltamivir and baloxavir marboxil include gastrointestinal disorders, general disorders and administration site conditions, skin and subcutaneous tissue disorders, and psychiatric disorders (Figure 3 illustrates the signal strength of the ROR). Our signal mining results showed that baloxavir marboxil had a favorable safety profile, with the most reported no adverse event, with 268 cases reported, with signal intensities of ROR 54.84 (46.89–64.15), PRR 34.14 (8015.30), IC 4.97 (4.62), EBGM 31.43 (26.87). In addition, it is of interest to note that in SOC: psychiatric disorders, 21 of the children treated with baloxavir marboxil reported abnormal behavior with signal intensities of ROR 12.28 (7.89–19.11), PRR 11.75 (203.40), IC 3.53 (2.37), EBGM 11.54 (7.42). 5 cases reported delirium with signal intensities of ROR 9.01 (3.71–21.89), PRR 8.91 (34.67), IC 3.14 (0.69), EBGM 8.80 (3.62). In contrast, 34 of the children treated with oseltamivir reported abnormal behavior with signal intensities of ROR 20.08 (14.09–28.62), PRR 18.68 (553.34), IC 4.18 (3.14), EBGM 18.13 (12.72). Delirium was reported in 17 cases with signal intensity of ROR 30.44 (18.54–49.99), PRR 29.36 (443.77), IC 4.81 (2.83), and EBGM 27.99 (17.04).
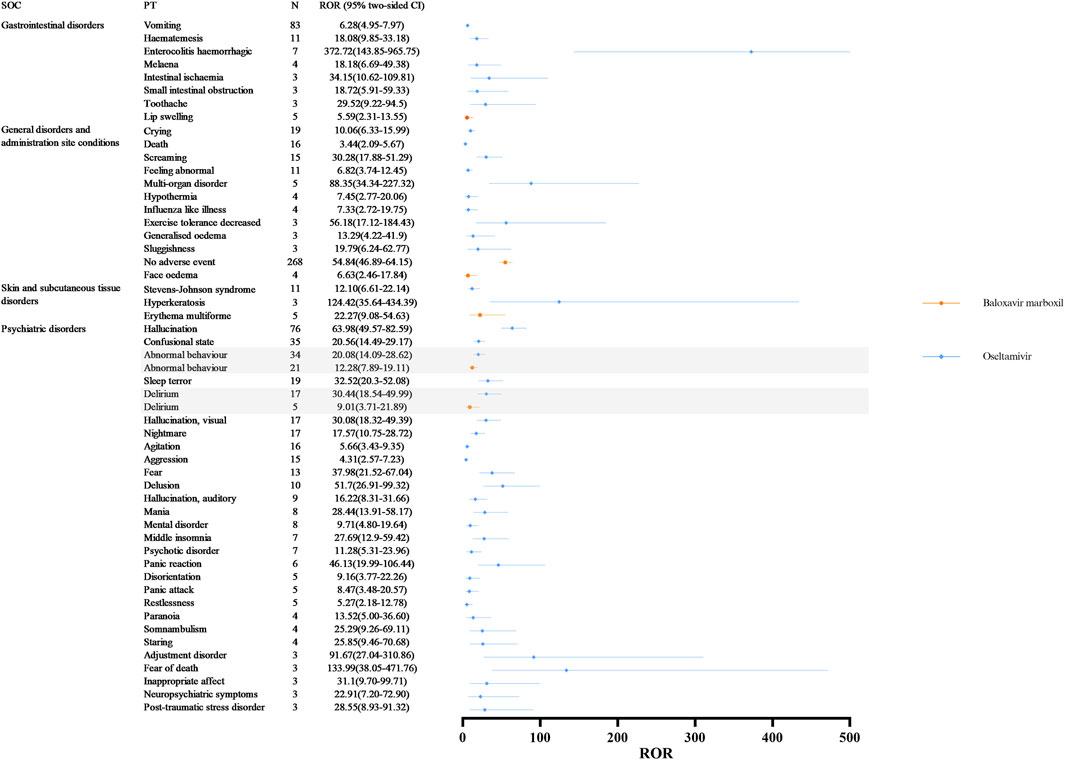
Figure 3. Differences in adverse event signaling in SOC Co-Involving oseltamivir and baloxavir marboxil at the PT Level. Abbreviations: SOC, organ system classification; PT, preferred term; N, number of reports; CI, Confidence Internal; ROR, reporting odds ratio.
Table 4 lists 5 AE signals at the PT level that were present in the two SOCs that were individually accumulated by baloxavir marboxil. Off-label use and medication errors associated with baloxavir marboxil were found to be cause for alarm in this study. Among them, 263 cases reported off label use, with signal intensities of ROR 5.60 (4.80–6.54), PRR 3.85 (610.84), IC 1.94 (1.72), and EBGM 3.82 (3.28). Intentional product use issue was reported in 203 cases with signal intensities of ROR 97.43 (81.73–116.14), PRR 66.45 (11374.74), IC 5.85 (5.28), EBGM 57.57 (48.30). Product administered to patient of inappropriate age was reported in 15 cases with signal intensities of ROR 6.16 (3.67–10.33), PRR 5.99 (62.03), IC 2.57 (1.47), EBGM 5.94 (3.54). In addition, medication error was reported in 8 cases with signal intensities of ROR 6.36 (3.15–12.84), PRR 6.26 (35.10), IC 2.63 (1.00), and EBGM 6.21 (3.07).
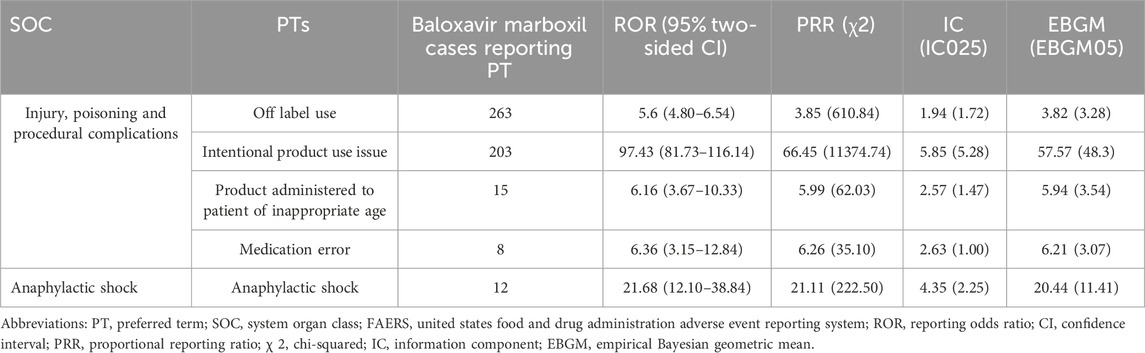
Table 4. The signal strength at the PT level for baloxavir marboxil-accumulated only SOC in FAERS database.
The 12 SOCs that were individually accrued by oseltamivir contained 53 AE signals at the PT level (Table 5). This study first detected some signals of AEs for which the oseltamivir insert gives a warning. 11 of them reported Stevens-Johnson syndrome (SJS) with signal intensities of ROR 12.10 (6.61–22.14), PRR 11.84 (107.18), IC 3.54 (1.80), and EBGM 11.62 (6.35). In the SOC “Infections and infestations,” this study detected AE signals at the PT level, including cytomegalovirus infection reactivation, central nervous system infection, pneumonia necrotising, and pneumonia streptococcal. It is noteworthy that, in the SOC “eye disorders,” we identified 8 unexpected AE signals beyond the drug insert. Among them, 3 cases reported acute macular outer retinopathy, with a remarkably high signal strength, having values of ROR 1741.93 (180.85–16777.66), PRR 1730.67 (1296.52), IC 8.76 (0.70), and EBGM 433.42 (45.00).
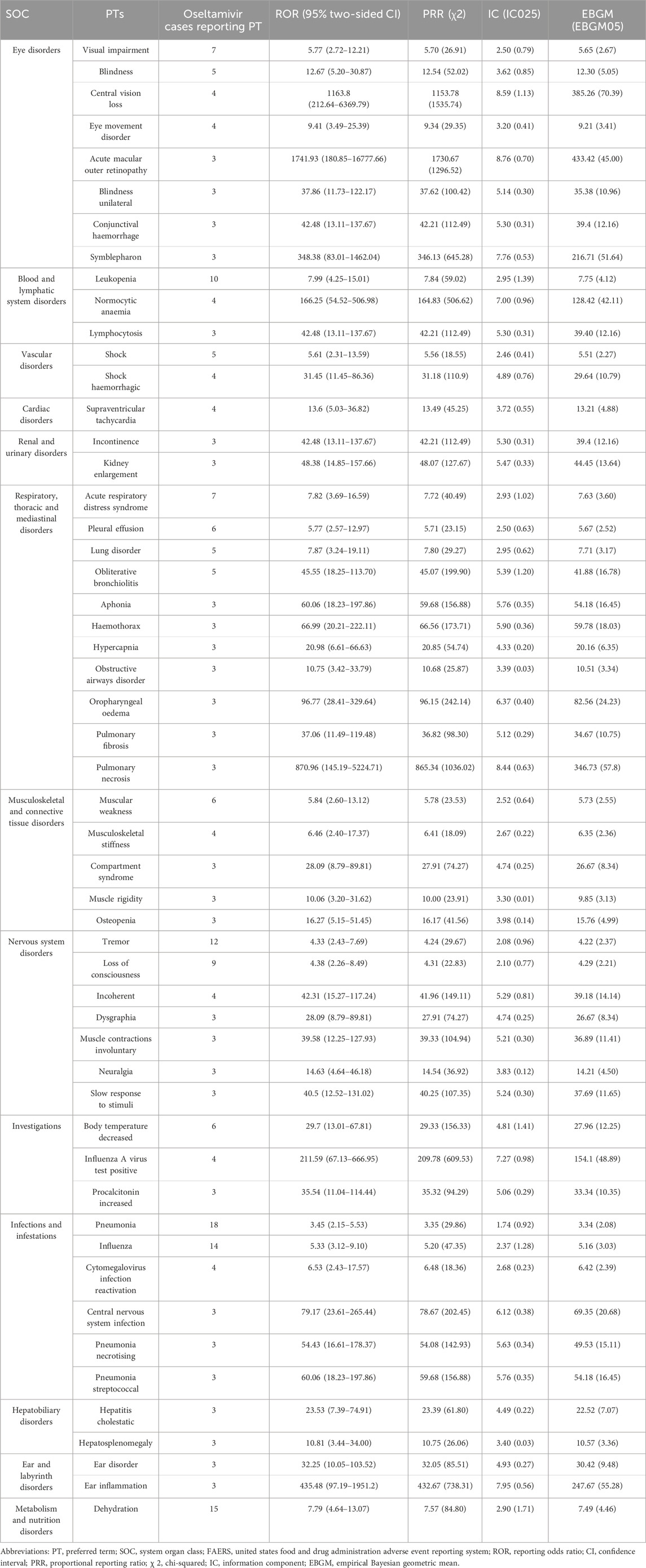
Table 5. The signal strength at the PT level for oseltamivir-accumulated only SOC in FAERS database.
3.4 Subgroup analysis by gender
To further investigate the differences in adverse event signaling between male and female children, we stratified the analysis by gender for children receiving oseltamivir and baloxavir, respectively. Figure 4 demonstrates the distribution of AE signal intensities based on the ROR calculation method for baloxavir marboxil. Overall, the signal of AEs due to baloxavir marboxil was approximately the same in male and female pediatric patients. However, it is of concern that anaphylactic shock was reported in 10 male children with signal intensities of ROR 55.33 (29.22–104.80), PRR 52.35 (502.33), IC 5.70 (2.34), and EBGM 52.16 (27.54). In contrast, in female children no such AE signal was found. For oseltamivir, Figures 5A,B show the distribution of AE signals between genders. Overall, there were more AE signals in girls than boys. Alarmingly, in skin and subcutaneous tissue disorders, SJS was reported in 11 girls with signal intensities of ROR 87.07 (47.33–160.16), PRR 82.26 (879.74), IC 6.36 (2.59), and EBGM 81.91 (44.53). In addition, four girls reported toxic epidermal necrolysis (TEN) with the signal intensity of ROR 36.84 (13.67–99.24), PRR 36.11 (136.36), IC 5.17 (0.78), and EBGM 36.04 (13.38). Supplementary Table 1 through 4 display the results of the four signal calculation methods.
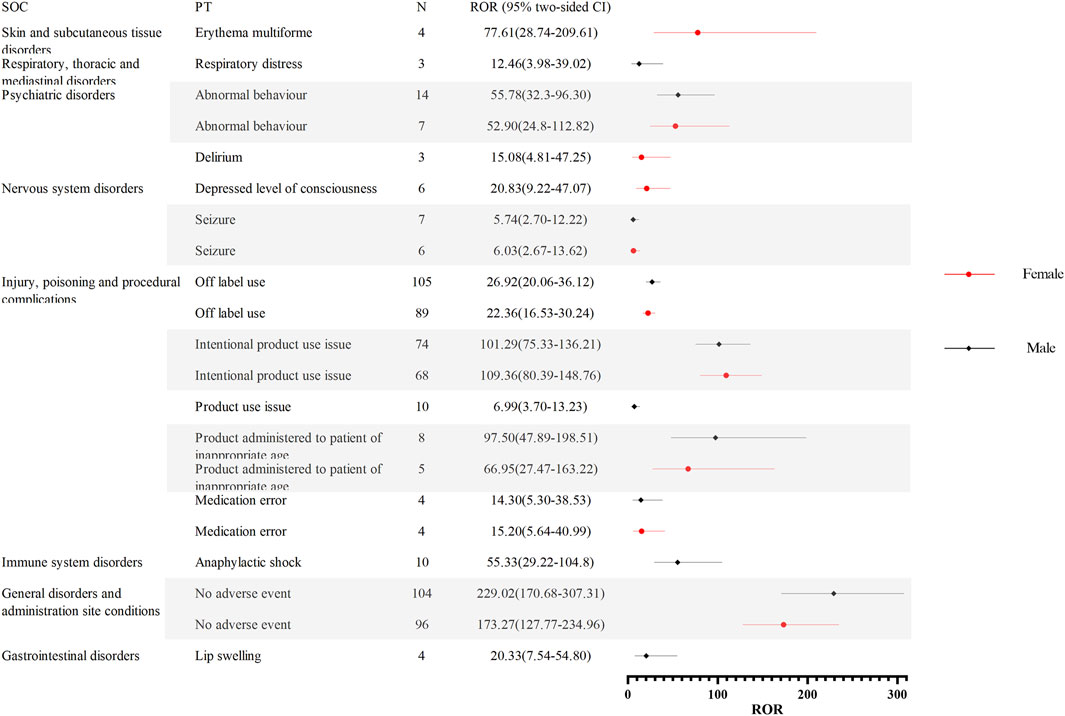
Figure 4. Distribution of AE signal intensities for baloxavir marboxil in male and female children based on ROR calculation method. Abbreviations: AE, adverse event; ROR, reporting odds ratio; SOC, organ system classification; PT, preferred term; N, number of reports; CI, Confidence Internal.
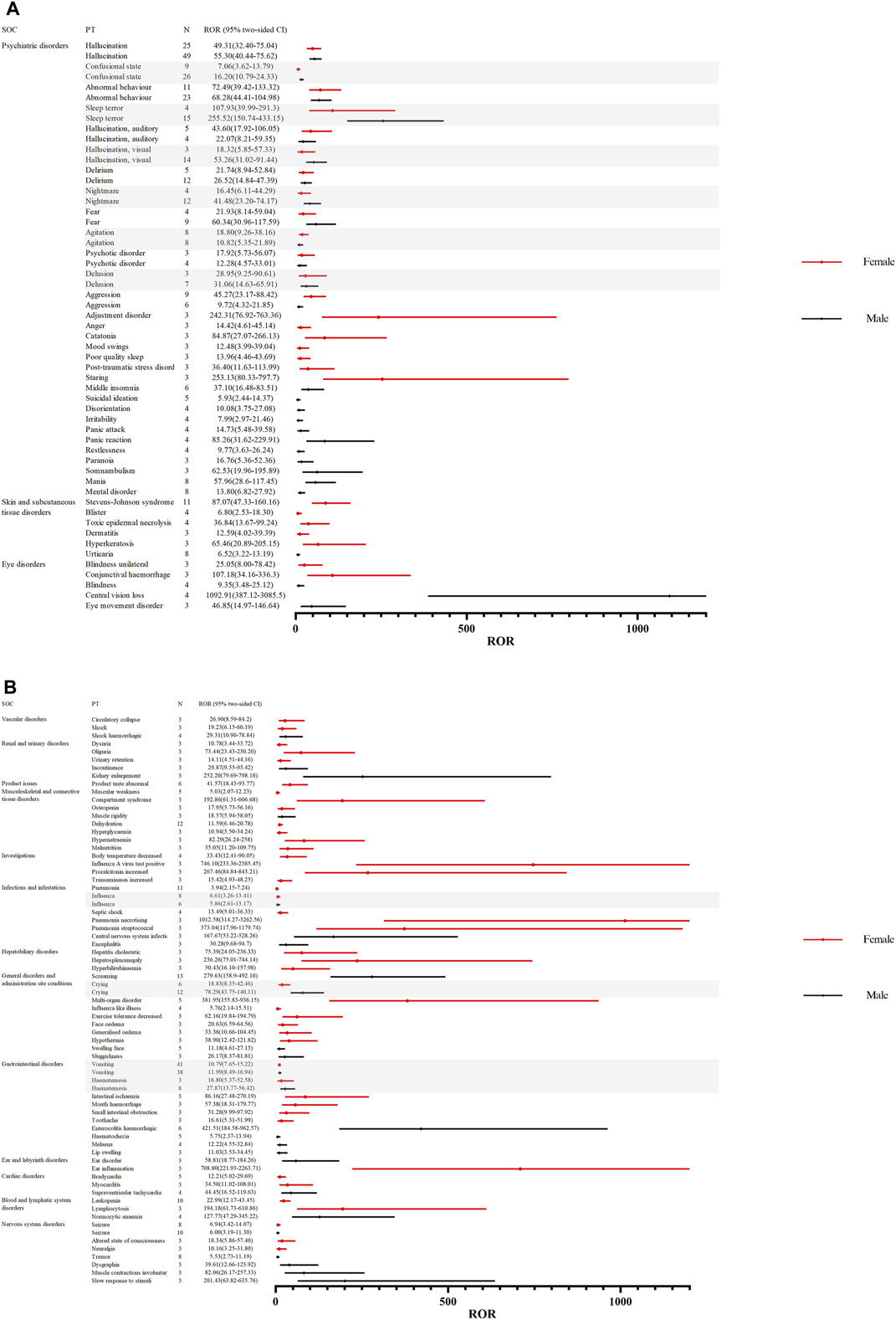
Figure 5. (A). Distribution of AE signal intensities for oseltamivir in male and female children based on ROR calculation method. Abbreviations: AE, adverse event; ROR, reporting odds ratio; SOC, organ system classification; PT, preferred term; N, number of reports; CI, Confidence Internal. (B). Distribution of AE signal intensities for oseltamivir in male and female children based on ROR calculation method .
4 Discussion
This is the first and most thorough post-marketing pharmacovigilance study of oseltamivir and baloxavir marboxil in children using the FAERS database. Our study reveals real-world differences in AEs that occur in children treated with oseltamivir and baloxavir marboxil.
Baloxavir inhibits the endonuclease activity of the polymerase acidic (PA) protein, an influenza virus-specific enzyme in the viral RNA polymerase complex required for viral gene transcription, resulting in inhibition of influenza virus replication (Heo, 2018). Baloxavir marboxil is effective against oseltamivir-resistant influenza viruses and may act synergistically with neuraminidase inhibitors. Oseltamivir has a half-life of 1–3 h. The half-life of baloxavir can reach 79 h (Table 6 summarizes the pharmacokinetic (PK) properties of baloxavir and oseltamivir) (Abraham et al., 2020). This implies that baloxavir marboxil only requires a single dose. Among children treated with baloxavir marboxil, we detected an AE signal for medication error. Medication errors are a major cause of harm in the healthcare system and can be prevented (Mirosevic Skvrce et al., 2020). Medication errors are one of the most common causes of pediatric AEs. They are more likely to occur in pediatric patients than adults, and dosing errors are the most common cause (Mirosevic Skvrce et al., 2024). Previous studies have pointed out that the PT “no adverse event” was frequently recorded in children and adolescent age groups along with medication error PTs with signals of disproportionate reporting, and pediatric patients are more vulnerable to the harm caused by medication errors (Carnovale et al., 2018), which is consistent with our findings. For children with influenza treated with baloxavir marboxil, the drug needs to be administered only once during the entire course of the illness. This is compared to traditional dosing methods (e.g., oseltamivir needs to be given twice daily for 5 days). Baloxavir marboxil‘s unique dosing frequency undoubtedly increases the risk of medication errors. Therefore, medication education for children and their families is essential.
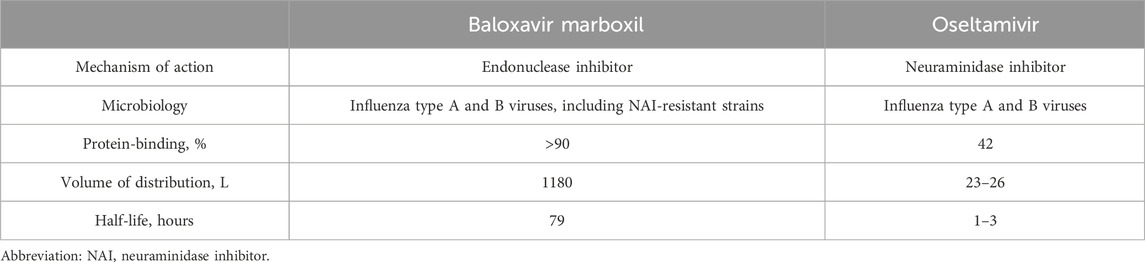
Table 6. Comparison of baloxavir marboxil and oseltamivir Mechanism of Action, Microbiology, and Pharmacokinetics.
In addition, there was an AE signal for off-label use in children treated with baloxavir marboxil. Off-label use is common and usually legal, unless it violates ethical guidelines or safety regulations. The reason for this is usually to fulfill a patient’s medical need or to make an innovative drug available to the patient, especially if there are no other better options available (Wu and Wu, 2014; Rusz et al., 2021). Far fewer drugs are approved for use in children than are approved for use in adults. Therefore, off-label drug use in children is more common. Moreover, off-label drug use in children typically lacks the same level of evidence as off-label use authorized for adults (Kaley et al., 2019). The minimum age for approved use of baloxavir varies in different countries, with the European Union recommending it for patients aged 1 year and older with influenza, and Japan allowing it to be used in children aged <12 years and weighing ≥10 kg with influenza. However, currently, the FDA and countries like China only approve the use of baloxavir marboxil in children aged 5 and above. For oseltamivir, the age range for its use is wider, as the FDA approved it for use in children ≥3 months of age. For children under 5 years old with influenza virus infections resistant to neuraminidase inhibitors, baloxavir marboxil may be a reliable alternative. Consequently, the issue of off-label use is inevitably raised. If the benefits outweigh the risks upon evaluation, reasonable and clinically appropriate off-label prescriptions for children should be considered (Schrier et al., 2020; van der Zanden et al., 2021).
In our study of oseltamivir, a number of AE signals consistent with warnings and precautions in the drug’s labeling were identified, including severe skin/allergic reactions, neuropsychiatric events, and bacterial infections, which confirms the reliability of our findings. However, of the 8 AE signals in SOC: eye disorders not mentioned in the oseltamivir instructions, acute macular outer retinopathy is particularly alarming. Acute macular neuroretinopathy (AMN) is a rare retinal disorder characterized by acute, symptomatic photopsias and paracentral scotomas associated with mild vision loss (Bos and Deutman, 1975). It has now been shown that acute influenza virus circulating cytokine levels are elevated during acute influenza virus infection (Bian et al., 2014), and elevated levels of tumor necrosis factor alpha (TNFα) may damage retinal photoreceptors in vitro and lead to mitochondrial dysfunction and visual datasource cell death in models of ischemic brain injury (Nakazawa et al., 2011; Doll et al., 2015). Another study also noted that approximately 50% of the 101 cases of AMN were associated with prior influenza illness (Bhavsar et al., 2016). However, to date, there has been no robust evidence linking AMN to influenza. Given the exploratory nature of our study, the detection of a signal for acute macular outer retinopathy warrants further investigation. While we identified this potential association, it is important to interpret these findings with caution. Additional research is needed to confirm any causal relationship and to understand the underlying mechanisms.
The field of medicine is gradually recognizing the impact of gender on treatment outcomes, and women are increasingly recognized as risk factors for developing side effects with significant consequences (Franconi and Campesi, 2014). Furthermore, research indicates that age and gender influence the frequency of unprompted complaints about adverse reactions (Holm et al., 2017). As a result, in this study, we investigated the use of oseltamivir and baloxavir marboxil with real-world AE signals. Based on the study’s findings, we observed signals of AEs caused by oseltamivir only in female patients: SJS and TEN. SJS/TEN is a severe skin-mucosal reaction, caused mainly by drugs, characterized by blistering and generalized epidermolysis bullosa (White et al., 2018). In the United States, the estimated incidence of SJS, overlapping SJS/TEN, and TEN in children was 5.3 cases per 1 million, 0.8 cases per 1 million, and 0.4 cases per 1 million, respectively (Hsu et al., 2017). In addition, the results of one study indicate that SJS/TEN is more prevalent in women, with a male-to-female ratio of approximately 1:2 (Sekula et al., 2013). Therefore, healthcare professionals and patients’ families should be more alert to the occurrence of such cutaneous severe AEs in female children treated with oseltamivir. As for children treated with baloxavir, it is of concern that the AE signal for anaphylactic shock was only seen in male patients. Anaphylactic shock is a fatal allergic reaction (Li et al., 2022). Compared to adult data, reports of drug-induced anaphylactic reactions (DIA) in children are rare (Bianchi et al., 2024). DIA is primarily an IgE-mediated tachyphylactic reaction leading to the degranulation of mast cells and basophils and the release of pro-inflammatory mediators (Pichler, 2003). The pathogens that cause drug-induced systemic anaphylactic reactions may be different depending on the country and the way the data is collected. Antibiotics (mainly penicillins and cephalosporins) are usually the drugs most often linked to fatal drug-induced anaphylactic reactions. Still, other drugs like neuromuscular blocking agents and radiographic contrast agents have also been linked (Turner et al., 2017). In a French study of severe allergies associated with neuromuscular blockers, men were a risk factor associated with fatal outcomes (Reitter et al., 2014). Although the mechanisms involved have not been elucidated, our study still reveals a high association with anaphylactic shock in male children treated with baloxavir marboxil, which emphasizes the importance of close monitoring and follow-up of this severe allergic reaction, especially in male children treated with baloxavir marboxil.
Combination therapy is often considered a promising strategy to circumvent treatment resistance. When used together, drugs that work against different viral proteins or host factors may help stop the development of resistant strains even more than when they are used alone (F. Hayden, 2009; Govorkova and Webster, 2010). Preclinical studies have shown that nizoralnit or itraconazole combined with oseltamivir can be more effective at killing viruses than oseltamivir alone (Belardo et al., 2015; Schloer et al., 2020). As for baloxavir, the results of the current preclinical study showed that combination therapy with oseltamivir or famciclovir increased in vitro antiviral activity (Checkmahomed et al., 2020), combination therapy with influenza drugs with different mechanisms of action decreased the selection pressure for viruses with reduced drug susceptibility (Hamza et al., 2021; Park et al., 2021; Koszalka et al., 2022). However, many challenges remain in managing the risk of drug interactions in clinical practice. Combination drug interactions may occur at the PK level, where a drug can affect the absorption, metabolism, elimination, induction, or inhibition of other drug metabolizing enzymes. This may result in changes in drug concentration, leading to toxicity or reduced efficacy (van Hasselt and Iyengar, 2019). An RCT involving 366 patients demonstrated a well-tolerated combination of baloxavir marboxil and NAIs compared to NAIs alone, with no new safety signals observed (Kumar et al., 2022). In the present study, only three cases of AE with baloxavir marboxil as the PS drug and oseltamivir as a co-administration and two cases of AE with oseltamivir as the PS drug and baloxavir marboxil as a co-administration were reported (Supplementary Tables S5, S6). Therefore, we were unable to assess whether the combination of the two would increase the incidence of adverse events or lead to new AE signals. More high-quality studies are required in the future to examine the safety of oseltamivir and baloxavir marboxil.
We analyzed AE signals linked to oseltamivir and baloxavir marboxil using the FAERS database for disproportionate analysis. This approach displays strong extrapolation skills, successfully overcoming the constraints of small sample numbers and short observation periods in clinical studies. However, it is crucial to recognize and address some limitations. First, FAERS is a spontaneous reporting system that gathers data from many nations and professions. It may include missing or inaccurate information, which might introduce bias in the study. Second, the lack of the total number of pediatric patients treated with oseltamivir or baloxavir marboxil made it impossible to calculate the incidence of each AE. Third, this study did not establish a direct cause-and-effect relationship between drugs and AEs. Disproportionate analyses only indicate the strength of a signal statistically, without quantifying risk or causality. Further research is required to confirm the experimental findings. Finally, although we performed data cleaning and de-duplication operations as recommended by the FDA, we may still retain potential duplicate entries that may exaggerate the strength of certain AE signals (Schilder et al., 2023; Cutroneo et al., 2024). Despite these limitations, our findings offer valuable insights for healthcare providers and patients regarding the monitoring of AEs associated with oseltamivir and baloxavir marboxil in children.
5 Conclusion
This pharmacovigilance study explored reports of AEs associated with oseltamivir and baloxavir marboxil use in children in the FAERS database. This long-term post-marketing drug safety evaluation provides an overview of the safety profiles of oseltamivir and baloxavir marboxil in children. Our exploratory findings suggest an AE signal of acute macular outer retinopathy in children treated with oseltamivir, indicating a need for further investigation to confirm or reject this association. For children treated with baloxavir, the frequency of administration poses a risk of medication errors, highlighting the importance of proper medication education.
Data availability statement
The raw data supporting the conclusions of this article will be made available by the authors, without undue reservation.
Author contributions
WW: Conceptualization, Data curation, Formal Analysis, Funding acquisition, Investigation, Methodology, Project administration, Resources, Software, Supervision, Validation, Visualization, Writing–original draft, Writing–review and editing. LH: Data curation, Formal Analysis, Investigation, Writing–original draft, Writing–review and editing. YB: Writing–review and editing, Visualization, Supervision. EC: Writing–review and editing, Conceptualization, Data curation, Formal Analysis. JL: Conceptualization, Data curation, Formal Analysis, Funding acquisition, Investigation, Methodology, Project administration, Resources, Software, Supervision, Validation, Visualization, Writing–original draft, Writing–review and editing.
Funding
The author(s) declare that no financial support was received for the research, authorship, and/or publication of this article.
Conflict of interest
The authors declare that the research was conducted in the absence of any commercial or financial relationships that could be construed as a potential conflict of interest.
Publisher’s note
All claims expressed in this article are solely those of the authors and do not necessarily represent those of their affiliated organizations, or those of the publisher, the editors and the reviewers. Any product that may be evaluated in this article, or claim that may be made by its manufacturer, is not guaranteed or endorsed by the publisher.
Supplementary material
The Supplementary Material for this article can be found online at: https://www.frontiersin.org/articles/10.3389/fphar.2024.1391003/full#supplementary-material
References
Abraham, G. M., Morton, J. B., and Saravolatz, L. D. (2020). Baloxavir: a novel antiviral agent in the treatment of influenza. Clin. Infect. Dis. 71 (7), 1790–1794. doi:10.1093/cid/ciaa107
Ambrose, C. S., and Antonova, E. N. (2014). The healthcare and societal burden associated with influenza in vaccinated and unvaccinated European and Israeli children. Eur. J. Clin. Microbiol. Infect. Dis. 33 (4), 569–575. doi:10.1007/s10096-013-1986-6
Bate, A. (2007). Bayesian confidence propagation neural network. Drug Saf. 30 (7), 623–625. doi:10.2165/00002018-200730070-00011
Belardo, G., Cenciarelli, O., La Frazia, S., Rossignol, J. F., and Santoro, M. G. (2015). Synergistic effect of nitazoxanide with neuraminidase inhibitors against influenza A viruses in vitro. Antimicrob. Agents Chemother. 59 (2), 1061–1069. doi:10.1128/aac.03947-14
Bhavsar, K. V., Lin, S., Rahimy, E., Joseph, A., Freund, K. B., Sarraf, D., et al. (2016). Acute macular neuroretinopathy: a comprehensive review of the literature. Surv. Ophthalmol. 61 (5), 538–565. doi:10.1016/j.survophthal.2016.03.003
Bian, J. R., Nie, W., Zang, Y. S., Fang, Z., Xiu, Q. Y., and Xu, X. X. (2014). Clinical aspects and cytokine response in adults with seasonal influenza infection. Int. J. Clin. Exp. Med. 7 (12), 5593–5602.
Bianchi, A., Valluzzi, R., Crisafulli, G., Bottau, P., Caimmi, S., Franceschini, F., et al. (2024). Drug-induced anaphylaxis in children. Biomedicines 12 (3), 527. doi:10.3390/biomedicines12030527
Bos, P. J., and Deutman, A. F. (1975). Acute macular neuroretinopathy. Am. J. Ophthalmol. 80 (4), 573–584. doi:10.1016/0002-9394(75)90387-6
Brown, E. G., Wood, L., and Wood, S. (1999). The medical dictionary for regulatory activities (MedDRA). Drug Saf. 20 (2), 109–117. doi:10.2165/00002018-199920020-00002
Caini, S., Spreeuwenberg, P., Kusznierz, G. F., Rudi, J. M., Owen, R., Pennington, K., et al. (2018). Distribution of influenza virus types by age using case-based global surveillance data from twenty-nine countries, 1999-2014. BMC Infect. Dis. 18 (1), 269. doi:10.1186/s12879-018-3181-y
Carnovale, C., Mazhar, F., Pozzi, M., Gentili, M., Clementi, E., and Radice, S. (2018). A characterization and disproportionality analysis of medication error related adverse events reported to the FAERS database. Expert Opin. Drug Saf. 17 (12), 1161–1169. doi:10.1080/14740338.2018.1550069
Checkmahomed, L., Padey, B., Pizzorno, A., Terrier, O., Rosa-Calatrava, M., Abed, Y., et al. (2020). In vitro combinations of baloxavir Acid and other inhibitors against seasonal influenza A viruses. Viruses 12 (10), 1139. doi:10.3390/v12101139
Cirmi, S., El Abd, A., Letinier, L., Navarra, M., and Salvo, F. (2020). Cardiovascular toxicity of tyrosine kinase inhibitors used in chronic myeloid leukemia: an analysis of the FDA adverse event reporting system database (FAERS). Cancers (Basel) 12 (4), 826. doi:10.3390/cancers12040826
Committee on Infectious Diseases (2023). Recommendations for prevention and control of influenza in children, 2023–2024. Pediatrics 152 (4), e2023063773. doi:10.1542/peds.2023-063773
Cutroneo, P. M., Sartori, D., Tuccori, M., Crisafulli, S., Battini, V., Carnovale, C., et al. (2024). Conducting and interpreting disproportionality analyses derived from spontaneous reporting systems. Front. Drug Saf. Regul. 3, 1323057. doi:10.3389/fdsfr.2023.1323057
Doll, D. N., Rellick, S. L., Barr, T. L., Ren, X., and Simpkins, J. W. (2015). Rapid mitochondrial dysfunction mediates TNF-alpha-induced neurotoxicity. J. Neurochem. 132 (4), 443–451. doi:10.1111/jnc.13008
Feng, Z., Li, X., Tong, W. K., He, Q., Zhu, X., Xiang, X., et al. (2022). Real-world safety of PCSK9 inhibitors: a pharmacovigilance study based on spontaneous reports in FAERS. Front. Pharmacol. 13, 894685. doi:10.3389/fphar.2022.894685
Fiore, A. E., Fry, A., Shay, D., Gubareva, L., Bresee, J. S., Uyeki, T. M., et al. (2011). Antiviral agents for the treatment and chemoprophylaxis of influenza --- recommendations of the Advisory Committee on Immunization Practices (ACIP). MMWR Recomm. Rep. 60 (1), 1–24.
Fowlkes, A., Giorgi, A., Erdman, D., Temte, J., Goodin, K., Di Lonardo, S., et al. (2014). Viruses associated with acute respiratory infections and influenza-like illness among outpatients from the Influenza Incidence Surveillance Project, 2010-2011. J. Infect. Dis. 209 (11), 1715–1725. doi:10.1093/infdis/jit806
Franconi, F., and Campesi, I. (2014). Sex and gender influences on pharmacological response: an overview. Expert Rev. Clin. Pharmacol. 7 (4), 469–485. doi:10.1586/17512433.2014.922866
Fukazawa, C., Hinomura, Y., Kaneko, M., and Narukawa, M. (2018). Significance of data mining in routine signal detection: analysis based on the safety signals identified by the FDA. Pharmacoepidemiol Drug Saf. 27 (12), 1402–1408. doi:10.1002/pds.4672
Govorkova, E. A., and Webster, R. G. (2010). Combination chemotherapy for influenza. Viruses 2 (8), 1510–1529. doi:10.3390/v2081510
Hamza, H., Shehata, M. M., Mostafa, A., Pleschka, S., and Planz, O. (2021). Improved in vitro efficacy of baloxavir marboxil against influenza A virus infection by combination treatment with the mek inhibitor ATR-002. Front. Microbiol. 12, 611958. doi:10.3389/fmicb.2021.611958
Harpaz, R., DuMouchel, W., LePendu, P., Bauer-Mehren, A., Ryan, P., and Shah, N. H. (2013). Performance of pharmacovigilance signal-detection algorithms for the FDA adverse event reporting system. Clin. Pharmacol. Ther. 93 (6), 539–546. doi:10.1038/clpt.2013.24
Hayden, F. (2009). Developing new antiviral agents for influenza treatment: what does the future hold? Clin. Infect. Dis. 48 (Suppl. 1), S3–S13. doi:10.1086/591851
Hayden, F. G., Sugaya, N., Hirotsu, N., Lee, N., de Jong, M. D., Hurt, A. C., et al. (2018). Baloxavir marboxil for uncomplicated influenza in adults and adolescents. N. Engl. J. Med. 379 (10), 913–923. doi:10.1056/NEJMoa1716197
Heikkinen, T. (2006). Influenza in children. Acta Paediatr. 95 (7), 778–784. doi:10.1080/08035250600612272
Heo, Y. A. (2018). Baloxavir: first global approval. Drugs 78 (6), 693–697. doi:10.1007/s40265-018-0899-1
Holm, L., Ekman, E., and Jorsäter Blomgren, K. (2017). Influence of age, sex and seriousness on reporting of adverse drug reactions in Sweden. Pharmacoepidemiol. Drug Saf. 26 (3), 335–343. doi:10.1002/pds.4155
Hsu, D. Y., Brieva, J., Silverberg, N. B., Paller, A. S., and Silverberg, J. I. (2017). Pediatric Stevens-Johnson syndrome and toxic epidermal necrolysis in the United States. J. Am. Acad. Dermatol. 76 (5), 811–817. doi:10.1016/j.jaad.2016.12.024
Hu, Y., Gong, J., Zhang, L., Li, X., Li, X., Zhao, B., et al. (2020). Colitis following the use of immune checkpoint inhibitors: a real-world analysis of spontaneous reports submitted to the FDA adverse event reporting system. Int. Immunopharmacol. 84, 106601. doi:10.1016/j.intimp.2020.106601
Jefferson, T., Jones, M. A., Doshi, P., Del Mar, C. B., Hama, R., Thompson, M. J., et al. (2014). Neuraminidase inhibitors for preventing and treating influenza in adults and children. Cochrane Database Syst. Rev. 2014 (4), Cd008965. doi:10.1002/14651858.CD008965.pub4
Kaley, V. R., Aregullin, E. O., Samuel, B. P., and Vettukattil, J. J. (2019). Trends in the off-label use of β-blockers in pediatric patients. Pediatr. Int. 61 (11), 1071–1080. doi:10.1111/ped.14015
Koszalka, P., George, A., Dhanasekaran, V., Hurt, A. C., and Subbarao, K. (2022). Effect of baloxavir and oseltamivir in combination on infection with influenza viruses with PA/I38T or PA/E23K substitutions in the ferret model. mBio 13 (4), e0105622. doi:10.1128/mbio.01056-22
Kumar, D., Ison, M. G., Mira, J. P., Welte, T., Hwan Ha, J., Hui, D. S., et al. (2022). Combining baloxavir marboxil with standard-of-care neuraminidase inhibitor in patients hospitalised with severe influenza (FLAGSTONE): a randomised, parallel-group, double-blind, placebo-controlled, superiority trial. Lancet Infect. Dis. 22 (5), 718–730. doi:10.1016/s1473-3099(21)00469-2
Kuo, Y. C., Lai, C. C., Wang, Y. H., Chen, C. H., and Wang, C. Y. (2021). Clinical efficacy and safety of baloxavir marboxil in the treatment of influenza: a systematic review and meta-analysis of randomized controlled trials. J. Microbiol. Immunol. Infect. 54 (5), 865–875. doi:10.1016/j.jmii.2021.04.002
Li, X., Ma, Q., Yin, J., Zheng, Y., Chen, R., Chen, Y., et al. (2022). A clinical practice guideline for the emergency management of anaphylaxis (2020). Front. Pharmacol. 13, 845689. doi:10.3389/fphar.2022.845689
Ma, Z., Sun, X., Zhao, Z., Lu, W., Guo, Q., Wang, S., et al. (2021). Risk of pneumonitis in cancer patients treated with PARP inhibitors: a meta-analysis of randomized controlled trials and a pharmacovigilance study of the FAERS database. Gynecol. Oncol. 162 (2), 496–505. doi:10.1016/j.ygyno.2021.05.012
McLean, H. Q., Peterson, S. H., King, J. P., Meece, J. K., and Belongia, E. A. (2017). School absenteeism among school-aged children with medically attended acute viral respiratory illness during three influenza seasons, 2012-2013 through 2014-2015. Influenza Other Respir. Viruses 11 (3), 220–229. doi:10.1111/irv.12440
Mirosevic Skvrce, N., Galic, I., Pacadi, C., Kandzija, N., and Mucalo, I. (2020). Adverse drug reactions that arise from the use of medicinal products outside the terms of the marketing authorisation. Res. Soc. Adm. Pharm. 16 (7), 928–934. doi:10.1016/j.sapharm.2019.10.003
Mirosevic Skvrce, N., Omrcen, L., Pavicic, M., and Mucalo, I. (2024). Root cause analysis of medication errors of the most frequently involved active substances in paediatric patients. Res. Soc. Adm. Pharm. 20 (2), 99–104. doi:10.1016/j.sapharm.2023.10.005
Nakazawa, T., Kayama, M., Ryu, M., Kunikata, H., Watanabe, R., Yasuda, M., et al. (2011). Tumor necrosis factor-alpha mediates photoreceptor death in a rodent model of retinal detachment. Invest. Ophthalmol. Vis. Sci. 52 (3), 1384–1391. doi:10.1167/iovs.10-6509
Napoli, A. A., Wood, J. J., Coumbis, J. J., Soitkar, A. M., Seekins, D. W., and Tilson, H. H. (2014). No evident association between efavirenz use and suicidality was identified from a disproportionality analysis using the FAERS database. J. Int. AIDS Soc. 17 (1), 19214. doi:10.7448/ias.17.1.19214
Nayak, J., Hoy, G., and Gordon, A. (2021). Influenza in children. Cold Spring Harb. Perspect. Med. 11 (1), a038430. doi:10.1101/cshperspect.a038430
Nomura, K., Takahashi, K., Hinomura, Y., Kawaguchi, G., Matsushita, Y., Marui, H., et al. (2015). Effect of database profile variation on drug safety assessment: an analysis of spontaneous adverse event reports of Japanese cases. Drug Des. Devel. Ther. 9, 3031–3041. doi:10.2147/dddt.S81998
Park, J. H., Kim, B., Antigua, K. J. C., Jeong, J. H., Kim, C. I., Choi, W. S., et al. (2021). Baloxavir-oseltamivir combination therapy inhibits the emergence of resistant substitutions in influenza A virus PA gene in a mouse model. Antivir. Res. 193, 105126. doi:10.1016/j.antiviral.2021.105126
Paules, C., and Subbarao, K. (2017). Influenza. Lancet 390 (10095), 697–708. doi:10.1016/s0140-6736(17)30129-0
Peters, P. H., Moscona, A., Schulman, K. L., and Barr, C. E. (2008). Study of the impact of oseltamivir on the risk for pneumonia and other outcomes of influenza, 2000-2005. Medscape J. Med. 10 (6), 131.
Pichler, W. J. (2003). Delayed drug hypersensitivity reactions. Ann. Intern Med. 139 (8), 683–693. doi:10.7326/0003-4819-139-8-200310210-00012
Principi, N., and Esposito, S. (2016). Severe influenza in children: incidence and risk factors. Expert Rev. Anti Infect. Ther. 14 (10), 961–968. doi:10.1080/14787210.2016.1227701
Principi, N., Esposito, S., Marchisio, P., Gasparini, R., and Crovari, P. (2003). Socioeconomic impact of influenza on healthy children and their families. Pediatr. Infect. Dis. J. 22 (10 Suppl. l), S207–S210. doi:10.1097/01.inf.0000092188.48726.e4
Qiu, C., Cheng, F., Ye, X., Wu, Z., Ning, H., Liu, S., et al. (2024). Study on the clinical efficacy and safety of baloxavir marboxil tablets in the treatment of influenza A. Front. Med. (Lausanne) 11, 1339368. doi:10.3389/fmed.2024.1339368
Reitter, M., Petitpain, N., Latarche, C., Cottin, J., Massy, N., Demoly, P., et al. (2014). Fatal anaphylaxis with neuromuscular blocking agents: a risk factor and management analysis. Allergy 69 (7), 954–959. doi:10.1111/all.12426
Rotrosen, E. T., and Neuzil, K. M. (2017). Influenza: a global perspective. Pediatr. Clin. North Am. 64 (4), 911–936. doi:10.1016/j.pcl.2017.03.007
Rusz, C. M., Ősz, B. E., Jîtcă, G., Miklos, A., Bătrînu, M. G., and Imre, S. (2021). Off-label medication: from a simple concept to complex practical aspects. Int. J. Environ. Res. Public Health 18 (19), 10447. doi:10.3390/ijerph181910447
Sakaeda, T., Tamon, A., Kadoyama, K., and Okuno, Y. (2013). Data mining of the public version of the FDA adverse event reporting system. Int. J. Med. Sci. 10 (7), 796–803. doi:10.7150/ijms.6048
Schilder, J. M., Golembesky, A., Boyle, T. A. C., Ye, G. L., and Kuplast, J. (2023). Commentary: adverse event profiles of PARP inhibitors: analysis of spontaneous reports submitted to FAERS. Front. Pharmacol. 14, 1241524. doi:10.3389/fphar.2023.1241524
Schloer, S., Goretzko, J., Pleschka, S., Ludwig, S., and Rescher, U. (2020). Combinatory treatment with oseltamivir and itraconazole targeting both virus and host factors in influenza A virus infection. Viruses 12 (7), 703. doi:10.3390/v12070703
Schrier, L., Hadjipanayis, A., Stiris, T., Ross-Russell, R. I., Valiulis, A., Turner, M. A., et al. (2020). Off-label use of medicines in neonates, infants, children, and adolescents: a joint policy statement by the European Academy of Paediatrics and the European society for Developmental Perinatal and Pediatric Pharmacology. Eur. J. Pediatr. 179 (5), 839–847. doi:10.1007/s00431-019-03556-9
Sekula, P., Dunant, A., Mockenhaupt, M., Naldi, L., Bouwes Bavinck, J. N., Halevy, S., et al. (2013). Comprehensive survival analysis of a cohort of patients with Stevens-Johnson syndrome and toxic epidermal necrolysis. J. Invest. Dermatol. 133 (5), 1197–1204. doi:10.1038/jid.2012.510
Song, Y., Xu, Y. L., Lin, Y., Zhao, B., and Sun, Q. (2020). Fractures due to aromatase inhibitor therapy for breast cancer: a real-world analysis of FAERS data in the past 15 years. Oncol. Res. Treat. 43 (3), 96–102. doi:10.1159/000505376
Turner, P. J., Jerschow, E., Umasunthar, T., Lin, R., Campbell, D. E., and Boyle, R. J. (2017). Fatal anaphylaxis: mortality rate and risk factors. J. Allergy Clin. Immunol. Pract. 5 (5), 1169–1178. doi:10.1016/j.jaip.2017.06.031
Uyeki, T. M. (2020). High-risk groups for influenza complications. Jama 324 (22), 2334. doi:10.1001/jama.2020.21869
van der Zanden, T. M., Mooij, M. G., Vet, N. J., Neubert, A., Rascher, W., Lagler, F. B., et al. (2021). Benefit-risk assessment of off-label drug use in children: the bravo framework. Clin. Pharmacol. Ther. 110 (4), 952–965. doi:10.1002/cpt.2336
van Hasselt, J. G. C., and Iyengar, R. (2019). Systems pharmacology: defining the interactions of drug combinations. Annu. Rev. Pharmacol. Toxicol. 59, 21–40. doi:10.1146/annurev-pharmtox-010818-021511
van Puijenbroek, E. P., Bate, A., Leufkens, H. G., Lindquist, M., Orre, R., and Egberts, A. C. (2002). A comparison of measures of disproportionality for signal detection in spontaneous reporting systems for adverse drug reactions. Pharmacoepidemiol. Drug Saf. 11 (1), 3–10. doi:10.1002/pds.668
White, K. D., Abe, R., Ardern-Jones, M., Beachkofsky, T., Bouchard, C., Carleton, B., et al. (2018). SJS/TEN 2017: building multidisciplinary networks to drive science and translation. J. Allergy Clin. Immunol. Pract. 6 (1), 38–69. doi:10.1016/j.jaip.2017.11.023
Keywords: oseltamivir, baloxavir marboxil, FAERS, pharmacovigilance, signal detection
Citation: Wei W, Huang L, Bai Y, Chang E and Liu J (2024) The real-world safety of oseltamivir and baloxavir marboxil in children: a disproportionality analysis of the FDA adverse event reporting system. Front. Pharmacol. 15:1391003. doi: 10.3389/fphar.2024.1391003
Received: 26 February 2024; Accepted: 17 June 2024;
Published: 10 July 2024.
Edited by:
Svetlana Khaiboullina, University of Nevada, Reno, United StatesReviewed by:
Carla Carnovale, University of Milan, ItalySebastian Schloer, Leibniz-Institut für Experimentelle Virologie, Germany
Copyright © 2024 Wei, Huang, Bai, Chang and Liu. This is an open-access article distributed under the terms of the Creative Commons Attribution License (CC BY). The use, distribution or reproduction in other forums is permitted, provided the original author(s) and the copyright owner(s) are credited and that the original publication in this journal is cited, in accordance with accepted academic practice. No use, distribution or reproduction is permitted which does not comply with these terms.
*Correspondence: Jinfeng Liu, d2VpeXVleGkxMTIzNEAxNjMuY29t
†These authors have contributed equally to this work