- 1Department of Anesthesiology, Pharmacology and Therapeutics, Faculty of Medicine, University of British Columbia (UBC), Vancouver, BC, Canada
- 2British Columbia Mental Health and Substance Use Services Research Institute, Vancouver, BC, Canada
- 3Department of Psychiatry, Faculty of Medicine, Canada Faculty of Pharmaceutical Sciences, UBC, Vancouver, BC, Canada
One of the most important developments in psychopharmacology in the past decade has been the emergence of novel treatments for mood disorders, such as psilocybin for treatment-resistant depression. Psilocybin is most commonly found in different species of mushroom; however, the literature on mushroom and fungus extracts with potential antidepressant activity extends well beyond just psilocybin-containing mushrooms, and includes both psychedelic and non-psychedelic species. In the current review, we systematically review the preclinical literature on mushroom and fungus extracts, and their effects of animal models of depression and tests of antidepressant activity. The PICO structure, PRISMA checklist and the Cochrane Handbook for systematic reviews of intervention were used to guide the search strategy. A scoping search was conducted in electronic databases PubMed, CINAHL, Embase and Web of Science. The literature search identified 50 relevant and suitable published studies. These included 19 different species of mushrooms, as well as seven different species of other fungi. Nearly all studies reported antidepressant-like effects of treatment with extracts. Treatments were most commonly delivered orally, in both acute and chronically administered studies to predominantly male rodents. Multiple animal models of depression were used, the most common being unpredictable chronic mild stress, while the tail suspension test and forced swim test were most frequently used as standalone antidepressant screens. Details on each experiment with mushroom and fungus species are discussed in detail, while an evaluation is provided of the strengths and weaknesses of these studies.
Introduction
Mood disorders remain among the most prevalent and disabling of all psychiatric conditions. They also represent one of the leading causes of worldwide disease burden (Friedrich, 2017; Collaborators, 2022). While many individuals affected by mood disorders respond well to treatment, a significant proportion of people show either partial or no response to antidepressant therapies (McLachlan, 2018). If an individual fails to respond to two or more trials of standard antidepressant pharmacotherapy, they may be considered “treatment-resistant” (Voineskos et al., 2020). Furthermore, many individuals may show a therapeutic response to antidepressant treatment but suffer side-effects that significantly reduce their quality of life (Teng et al., 2022), resulting in reduced treatment adherence (Hung, 2014; Rossom et al., 2016).
Clinical treatment options for those who do not respond well to standard antidepressant therapies have historically remained limited. However, in recent years, several landmark studies have reported that administration of psychedelic drugs under controlled conditions, typically in combination with psychotherapy, can significantly reduce depressive symptoms (Griffiths et al., 2016; Ross et al., 2016; Palhano-Fontes et al., 2019; Davis et al., 2021; Eisenstein, 2022; Goodwin et al., 2022). Importantly, this includes individuals with treatment-resistant depression (Carhart-Harris et al., 2016). Additionally, in clinical trials reported to-date, the side-effect profile of these compounds has appeared relatively benign (Eisenstein, 2022) with no evidence of some of the side-effects associated with other psychotropic medications, such as weight gain and metabolic dysregulation (Boyda et al., 2013; Boyda et al., 2021; Sepúlveda-Lizcano et al., 2023).
While the use of the term “psychedelic” has no official definition, it typically refers to a drug that is able to alter perception, thoughts, feelings and consciousness in humans (Hosanagar et al., 2021). Psychedelic drugs are commonly categorized as either “classical” or “atypical” (Kamal et al., 2023). The former category represents drugs with agonism or partial agonism at the serotonergic 5-HT2A receptor, and includes tryptamines (such as psilocybin and DMT), ergolines (such as LSD) and phenethylamines (such as mescaline) (Kelmendi et al., 2022). The atypical psychedelics have diverse mechanisms of action (Aleksandrova and Phillips, 2021), which are not primarily at the 5-HT2A receptor, and include drugs such as ketamine, ibogaine, muscimol and salvinorin A (Kelmendi et al., 2022). At this point, it is important to note that many compounds from both classes of psychedelic drugs have their origins in commonly available mushrooms and other fungi.
Mushrooms and other fungi
Mushrooms are generally defined as the spore-producing fruiting body of a fungus. Traditional medicine has used mushrooms, and fungi in general, in medical treatment for centuries (Yadav and Negi, 2021; Gravina et al., 2023), taking advantage of their numerous perceived therapeutic benefits. Such properties have been reported to include antimicrobial (Moussa et al., 2022), antibacterial, antioxidant, hepatoprotective (Venturella et al., 2021), and antitumor (Pandya et al., 2019) effects. More recently, researchers have investigated “medicinal” mushrooms as potential alternatives or complements to mainstream antidepressant treatments. For example, non-psychedelic species such as Hericium erinaceus and Ganoderma lucidum have been noted as having mood-improving qualities in humans (Nagano et al., 2010; Fijałkowska et al., 2022), although head-to-head trials comparing effects against standard antidepressant pharmacotherapies are lacking. Nevertheless, the increasing body of evidence which indicates that psilocybin (a psychedelic compound found in many species of mushrooms (Strauss et al., 2022)) has potent antidepressant effects, including in those with treatment-resistant depression (Haikazian et al., 2023; Simonsson et al., 2023), supports the notion that mushrooms and other fungi may hold significant therapeutic potential in this area. However, given the enormous number of potential species of mushroom and other fungi that could have antidepressant effects, measured against the tremendous costs associated with conducting clinical trials in humans, it is critical to determine which mushroom and fungus species and their derivatives represent the best preclinical leads for further development. In this context, it is vitally important to understand which species have already demonstrated efficacy in preclinical animal models of depression and specific screens for antidepressant activity. The purpose of the present scoping review is therefore to systematically identify which mushroom and fungus species have been tested for antidepressant effects in specific preclinical models, and to summarize and evaluate the results of these studies.
Materials and methods
The PICO structure, PRISMA checklist and the Cochrane Handbook (Higgins and Green, 2011) for systematic reviews of intervention were used to guide the search strategy. A scoping search was conducted in electronic databases PubMed, CINAHL (via EBSCO), Embase (via Ovid), and Web of Science, as previously (Tse et al., 2014; Yuen et al., 2021; Lian et al., 2022). One preprint source was found as a suggestion under another article and later located on Google Scholar. The latest literature search was conducted on 19 December 2023.
A combination of 26 individual search terms were used with the following keywords: “mushroom” or “mushrooms” or “fungus” and “depress*” or “antidepress*” and “animal” or “animal model”. Filters excluding human studies or non-article sources were applied as needed. Searches were also conducted using specific behavioural models/tests or mushroom species as keywords. Studies were limited to those using rodent species as those reflect the expertise of the authors (Lu et al., 2005; Barr et al., 2006; Hill et al., 2007; Boyda et al., 2014); however, it is important to note that other species, such as zebrafish, represent additional valid animal models of antidepressant efficacy (Braun et al., 2024).
Studies were included if they met the following criteria: 1) studies tested a mushroom, fungus, or relevant mushroom derivative, and; 2) used a rodent model or behavioural test of depression or screen of antidepressant activity. Studies were excluded if they 1) were not published in English, or; 2) were not full text original research studies (i.e., conference abstracts, review papers).
A total of 546 articles were identified using Covidence (www.covidence.org), with 241 duplicates removed, leaving 305 articles to be screened. After title and abstract screening, 237 were deemed irrelevant, leaving 68 studies for eligibility assessment. After full text review, 18 studies were excluded, leaving 50 studies in the final database. Figure 1 outlines a PRISMA flowchart of the study selection process.
Abstracts and full texts were screened by GK and CKW. Data was extracted independently by GK and CKW with key variables extrapolated and outlined in Supplementary Table S1. Any discrepancies throughout the process were brought to consensus by GK and CKW with the assistance of AMB if required.
Results
The literature search identified 50 relevant and suitable published studies. These included 19 different species of mushrooms see Table 1, as well as seven different species of other fungi see Table 2; there were also three studies that used compounds which are common to multiple mushroom species.

Table 1. Summary of rodent depression models and behavioural tests used to screen for antidepressant effects in different mushroom species. Subchronic and chronic treatment schedules include daily administration of drug unless otherwise stated.
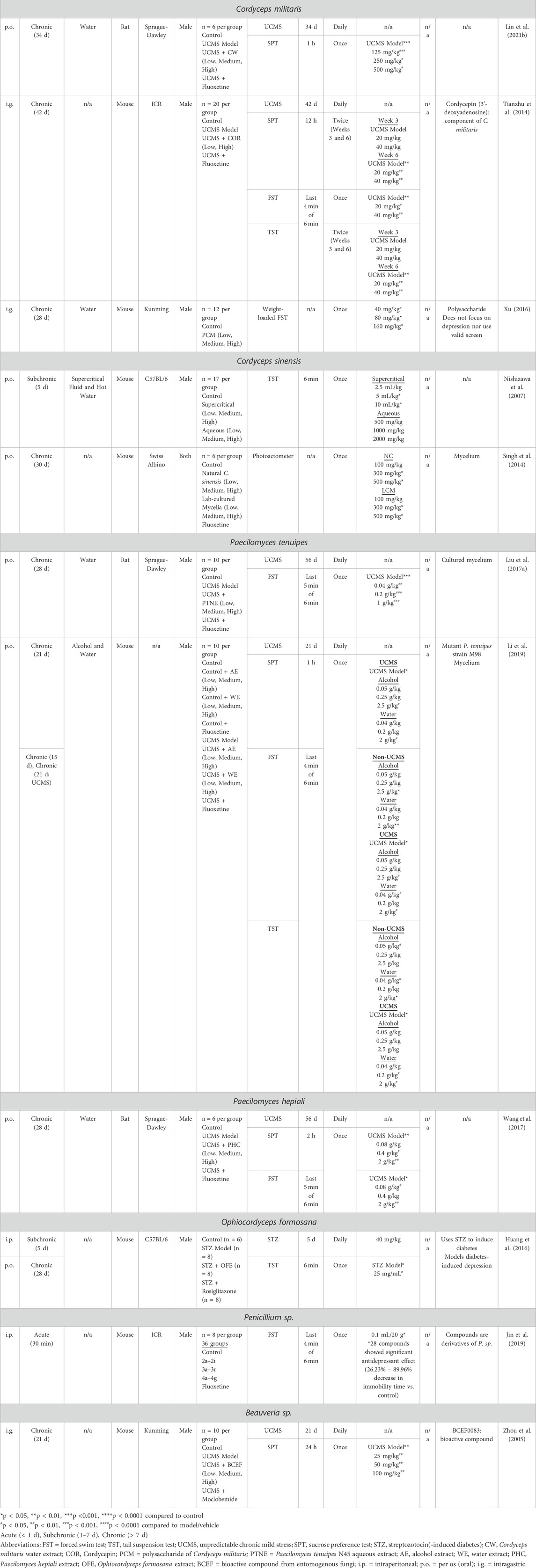
Table 2. Summary of rodent depression models and behavioural tests used to screen for antidepressant effects in different non-mushroom species of fungi. Subchronic and chronic treatment schedules include daily administration of drug unless otherwise stated.
Characteristics of animals used and drug administration
The species used in all of the animal models were limited to rats and mice: we did not find instances of other rodent species that have been utilized as antidepressant screens (Kramer et al., 1998; Alo et al., 2019). Fourteen of the studies used rats (Matsuzaki et al., 2013; Minami et al., 2013; Tan et al., 2016; Liu C. et al., 2017; Song et al., 2017; Wang et al., 2017; Huang et al., 2020; Nascimento et al., 2020; Lin et al., 2021a; Zhang L. et al., 2021; Lin et al., 2021b; Anuar et al., 2022; Cheng, 2023; Rakoczy et al., 2023), while the remaining 36 studies used mice to test for antidepressant-like effects (Zhou et al., 2005; Nishizawa et al., 2007; Koo et al., 2008; Singh et al., 2014; Tianzhu et al., 2014; Socala et al., 2015; Yao et al., 2015; Gupta et al., 2016; Huang et al., 2016; Nakamichi et al., 2016; Song et al., 2016; Xu, 2016; Bao et al., 2017; Liu Y. et al., 2017; Lin et al., 2017; Chiu et al., 2018; Mahmoudi et al., 2018; Ryu et al., 2018; Jin et al., 2019; Li et al., 2019; Song et al., 2020; Li H. et al., 2021; Li TJ. et al., 2021; Zhang T. et al., 2021; Chong et al., 2021; Hossen et al., 2021; Park et al., 2021; Singh et al., 2021; Zhao et al., 2021; Chou et al., 2022; Mi et al., 2022; Xin et al., 2022; Yu et al., 2022; Ezurike et al., 2023; Zhao et al., 2023; Hernandez-Leon et al., 2024). In terms of strains, 10 of the rat studies used Sprague-Dawley, three included Wistar and one used Long-Evans rats. For the mice studies, the most commonly used strain was the C57BL/6 strain and sub-strains (12 studies), followed by Institute for Cancer Research mice (seven studies), Swiss Albino and Kunming mice (six studies each) and one study each with BALB/c, CD1, Swiss Webster and NMRI strains. Two studies did not mention the specific strains used (Li et al., 2019; Park et al., 2021). The overwhelming majority of rat studies used male rats (12 studies) compared to female rats (two studies (Minami et al., 2013; Anuar et al., 2022)). All mice studies utilized males, most of which included males only, while five studies used both male and female mice (Singh et al., 2014; Song et al., 2016; Liu Y. et al., 2017; Hossen et al., 2021; Ezurike et al., 2023), and one study did not specify sex (Park et al., 2021). Thus, only 14% of studies used female animals in their investigation.
Administration of mushroom or fungus derivatives to animals was mostly through a single route of administration, although a handful of studies used two different routes of administration (Yao et al., 2015; Huang et al., 2016; Chou et al., 2022; Yu et al., 2022; Hernandez-Leon et al., 2024). The most common route of administration was oral (per os, p.o.), which accounted for more than 50% of studies (29 of 55 instances of administration). Second most common was treatment by intraperitoneal (i.p.) injection (13 instances), followed by intragastric (i.g.) administration (7 instances). Extracts were administered to animals in their food in three separate studies (Nakamichi et al., 2016; Bao et al., 2017; Anuar et al., 2022), by subcutaneous (s.c.) injection in two studies (Chou et al., 2022; Yu et al., 2022), and by intravenous (i.v.) administration in one study (Zhang L. et al., 2021).
The methods of extraction of mushroom and fungus derivatives was reported in 28 studies. Methods included use of both polar and non-polar solvents, with the most common ones including water and various alcohols. For many of the studies where complex extraction procedures were involved, including with non-polar solvents, it was not possible to determine if the extracts that were administered to animals also contained traces of these solvents (e.g., (Singh et al., 2021)), which could feasibly have an effect on behaviour.
The duration of drug treatment varied significantly across the studies, from acute doses with behavioural testing 30 min later (Socala et al., 2015; Mahmoudi et al., 2018; Jin et al., 2019; Zhang T. et al., 2021; Hossen et al., 2021; Park et al., 2021; Hernandez-Leon et al., 2024), up to 92 days of continuous administration (Anuar et al., 2022). Of the 50 studies, 13 were acute (treatment over a span of <24 h) (Matsuzaki et al., 2013; Socala et al., 2015; Yao et al., 2015; Gupta et al., 2016; Lin et al., 2017; Mahmoudi et al., 2018; Jin et al., 2019; Zhang T. et al., 2021; Hossen et al., 2021; Park et al., 2021; Singh et al., 2021; Rakoczy et al., 2023; Hernandez-Leon et al., 2024), six were sub-acute (1–7 days) (Nishizawa et al., 2007; Song et al., 2016; Bao et al., 2017; Nascimento et al., 2020; Song et al., 2020; Yu et al., 2022), and the remaining 31 studies involved chronic treatment (>7 days). The mean duration of treatment for the chronic studies was 30.4 (±16.7) days for the longest treated group in each study (some studies had varying durations of treatment depending on the group). The modal and median periods of treatment for chronic studies were both 28 days. Rats were more likely to be treated chronically, with only two of the 14 rat studies involving acute treatment (Matsuzaki et al., 2013; Rakoczy et al., 2023).
Animal models of depression and tests of antidepressant activity
A variety of animal models of depression and antidepressant screens were used to examine mushroom and fungus antidepressant efficacy. By far the most common animal model used to induce a depressive-like phenotype in rodents was the unpredictable chronic mild stress paradigm (UCMS), with 14 studies implementing this model (Zhou et al., 2005; Tianzhu et al., 2014; Tan et al., 2016; Liu C. et al., 2017; Song et al., 2017; Wang et al., 2017; Li et al., 2019; Huang et al., 2020; Lin et al., 2021a; Zhang L. et al., 2021; Lin et al., 2021b; Zhao et al., 2021; Xin et al., 2022; Cheng, 2023); rats were used in the majority (9) of these studies. The second most frequent model involved the use of chronic restraint stress, in four mouse studies (Nakamichi et al., 2016; Chiu et al., 2018; Chong et al., 2021; Zhao et al., 2023). Two rat studies used ovariectomy procedures to model menopausal depression (Minami et al., 2013; Anuar et al., 2022), while high-dose corticosterone was administered to mice in two studies (Chou et al., 2022; Yu et al., 2022). Other models included the use of lipopolysaccharide (Yao et al., 2015), chronic social defeat stress (Li H. et al., 2021), maternal separation (Mi et al., 2022), ethanol binge drinking (Nascimento et al., 2020) and streptozotocin to model diabetes-induced depression (Huang et al., 2016). To determine that a depressive-like state had been induced by the animal models, which could then be reversed by compounds with antidepressant activity, behavior was predominantly assessed with three main tests, which included the forced swim test (FST) (19 studies), tail suspension test (TST) (13 studies) and sucrose preference test (SPT) (16 studies)—multiple studies used two or more of these tasks. One study assessed behavior in the splash test as well as nest building (Mi et al., 2022), while one study measured locomotor activity and neuromuscular endurance (Singh et al., 2014). Twenty one of the 50 studies did not use an animal model of depression per se, and tested antidepressant activity solely with standalone antidepressant screens. This included 18 studies which used the FST and 10 that used the TST (seven studies used both); only two of these 21 studies used rats (Matsuzaki et al., 2013; Rakoczy et al., 2023).
Antidepressant effects of mushroom extracts
The Kingdom Fungi encompasses many known species which can be further classified into subgroups by the mechanism with which they reproduce and disseminate their spores (Boundless, 2024). Fungi subcategories include mushrooms, as well as other fungi such as moulds and yeasts. Mushrooms from the genus Psilocybe are of particular interest as many from the genus are known to contain the psychoactive compounds psilocybin and psilocin. This includes the species Psilocybe cubensis, which has been demonstrated to be able to alleviate depression and anxiety symptoms in clinical trials (Ross et al., 2016; Goodwin et al., 2022). Other mushrooms species such as H. erinaceus and G. lucidum do not necessarily contain psychoactive compounds, but are still of interest in models and studies of depression. Most research investigating the use of medicinal mushrooms and their extracts to treat depression has been in preclinical settings, rather than in clinical trials.
Of the 19 species of mushroom tested for antidepressant-like activity in the current review, the most common one was G. lucidum, in nine studies Table 1. Two studies used UCMS and reported 28-day treatment with doses of 100–500 mg/kg, p.o. exerted antidepressant-like effects in the SPT (Cheng, 2023) and both the SPT and FST (Zhao et al., 2021). A 5 mg/kg, i.p. dose in mice exerted antidepressant-like effects in the TST and FST after chronic social defeat stress (Li H. et al., 2021), while effects in mice subjected to the maternal separation model were reversed with a 21-day treatment with 40 mg/kg, i.p. of extract (Mi et al., 2022); 100 mg/kg, p.o. also reversed immobility in the FST in a binge-alcohol model (Nascimento et al., 2020). Antidepressant screens found positive effects with chronic doses of 100–1,000 mg/kg, p.o. in the FST and TST (Matsuzaki et al., 2013; Socala et al., 2015; Singh et al., 2021; Ezurike et al., 2023). Significant antidepressant-like effects were observed with the UCMS model with Ganoderma sp. extracts (21-day, 20–30 mg/kg, i.v.) (Zhang L. et al., 2021); in this study, the authors did not specify with species of Ganoderma the active compound ganoderic acid-a was extracted from.
Hericium erinaceus was examined in seven studies. Extracts (25 mg/kg, i.p. and 200–400 mg/kg, p.o.) for 28 days reversed the effects of chronic restraint stress in the SPT, TST (Chong et al., 2021) and FST (Chiu et al., 2018). Doses of 12–24 mg (combined with Chlorella Vulgaris), p.o. for 21 days significantly reversed immobility in the FST caused by treatment with high dose corticosterone (Chou et al., 2022). A single oral dose of 200 mg/kg reversed increased immobility in the FST and TST caused by lipopolysaccharide (Yao et al., 2015), while 28-day administration at 20–60 mg/kg, p.o. decreased immobility in the TST and FST (Ryu et al., 2018).
For other mushroom species examined, effects were observed with the UCMS model with Marasmius androsaceus (28-day, 30–150 mg/kg, p.o.), Poria cocos (35-day, 100–300 mg/kg, p.o.) (Huang et al., 2020), Armillaria mellea (35-day, 250–1,000 mg/kg, p.o.) (Lin et al., 2021a), Agaricus brasiliensis (30-day, 3,000 mg/kg, p.o.) (Xin et al., 2022) and Xylaria sp. (28-day, 500–2000 mg/kg, i.g.) (Tan et al., 2016). Other animal models included antidepressant-like effects in a model of menopausal depression (Pleurotus eryngii, 79-day, 500 mg/kg, p.o.) (Minami et al., 2013), chronic restraint stress (Pleurotus citrinopileatus, 14-day, 1,200 mg/kg, in food) (Nakamichi et al., 2016) (M. androsaceus, 14-day, i.g.) (Zhao et al., 2023) and high-dose corticosterone (P-coumaric acid–compound found in some mushrooms, 3-day, 75 mg/kg, i.p.) (Yu et al., 2022).
As an antidepressant screen, studies using the standalone FST and TST reported significant antidepressant-like effects with Ganoderma applanatum, Ganoderma philippii, and Ganoderma brownii (single dose, 100–400 mg/kg, p.o.) (Singh et al., 2021), Grifola frondosa (1/5-days, in a 1:1-1:4 ratio of Griflola frondosa powder to rat chow ratio) while Pleurotus ostreatus had no effect in the same study (Bao et al., 2017), P. cubensis (single dose. 1,000 mg/kg, p.o. (Hernandez-Leon et al., 2024), and single dose 10–40 mg/kg, i.p., combined with ketamine) (Mahmoudi et al., 2018), P. eryngii (single dose, 20 mg/kg, i.p.) (Park et al., 2021), M. androsaceus (7-day, 50–250 mg/kg, p.o.) (Song et al., 2016; Song et al., 2020), Lentinula edodes (single dose 10 ml/kg p.o., [30% water soluble chitosan, 30% Allium sativum extract, 30% L. edodes extract, 0.5% Dioscorea Batatas extract, 0.5% bamboo salt extract]) (Koo et al., 2008), A. mellea (single dose, 5–20 mg/kg, i.p.) (Zhang T. et al., 2021), as well as ergosterol and derivatives (single dose, 0.1–20 mg/kg, i.p.) (Lin et al., 2017), and the mushroom extracts psilocybin and norbaeocystin (three doses over 24 h, 1 mg/kg, i.g.) (Rakoczy et al., 2023). No antidepressant effect was observed for Collybolide (a fungal metabolite; 2 mg/kg, i.p.) extract (Gupta et al., 2016).
Antidepressant effects of fungus extracts
For the seven species of fungus that do not produce mushrooms, antidepressant activity was examined using the UCMS model in six studies Table 2. Antidepressant-like effects on the SPT and/or FST were observed with Cordyceps militaris (34-day, 125–500 mg/kg, p.o.) (Lin et al., 2021b) and (42-day, 20–40 mg/kg, i.g.) (Tianzhu et al., 2014), Paecilomyces tenuipes (28-day, 40–1,000 mg/kg, p.o.) (Liu C. et al., 2017) and (21-day, 40–2,500 mg/kg, p.o.) (Li et al., 2019), Paecilomyces hepiali (28-day, 80–2000 mg/kg, p.o.) (Wang et al., 2017) and Beauveria sp. (21-day, 25–100 mg/kg, i.g.) (Zhou et al., 2005). Treatment with Ophiocordyceps formosana (28-day, 2.5 mg, p.o.) reversed TST deficits in a streptozotocin-induced model of diabetic depression (Huang et al., 2016). Three studies used standalone animal antidepressant screens, in which Cordyceps sinensis decreased immobility in the TST (5-day, 5–10 ml/kg, p.o.) (Nishizawa et al., 2007) and locomotor activity (30-day, 300–500 mg/kg, p.o.) (Singh et al., 2014), while a wide range of Penicillium sp. derivatives (single dose, 30 mg/kg, i.p.) were active in the FST (Jin et al., 2019).
Discussion
In the current analysis, we have summarized the main findings from a scoping review of the effects of mushroom and fungus extracts in preclinical tests of antidepressant efficacy. While this topic covers a broad range of compounds and techniques, several important themes are evident. Firstly, a large number of different species exhibit antidepressant-like activity, including 19 species of mushrooms and seven species of other fungi. For each of these, there can be multiple derivatives with their own antidepressant-like effects; for example, one study with Penicillium sp. identified 28 individual compounds with antidepressant-like effects in the FST (Jin et al., 2019), including some with more potent effects than the positive control fluoxetine. Thus, it appears that there is significant potential for novel compounds with antidepressant activity within these organisms. While this includes mushrooms with extracts that have traditionally been associated with psychoactive properties, such as P. cubensis, other novel compounds were identified with antidepressant-like effects. For example, P-coumaric acid was found to exhibit antidepressant-like effects after high dose corticosterone treatment (Yu et al., 2022); and was previously reported to exert pro-cognitive and anxiolytic effects in rodents (Scheepens et al., 2014; Kim et al., 2017; Ghaderi et al., 2022). Several of the species evaluated in this review have been tested in humans, confirming benefits for clinical depression. The antidepressant effects of psilocybin and psilocin, which are present in multiple of the current mushroom species are now well established (Griffiths et al., 2016; Ross et al., 2016; Davis et al., 2021; Eisenstein, 2022; Goodwin et al., 2022). In addition, one study showed that menopausal women experienced a reduction in depression and anxiety after 4 weeks of Hericium erinaceus intake (Nagano et al., 2010) while another showed a non-significant trend of reduced depression in women with fibromyalgia who received micromilled G. lucidum carpophores for 6 weeks (Pazzi et al., 2020).
Secondly, viewed as a whole, there are a number of both strengths and limitations within this literature. A positive is that the majority of studies administered compounds orally. While for many, use of oral gavage on a daily basis is technically more challenging than i.p. or s.c. drug administration in rodents (Turner et al., 2011), it strongly increases the translational validity of the studies, as human trials will be likely to use the same route of administration and be affected by similar pharmacokinetic processes, such as first-pass metabolism and low bioavailability (Bicker et al., 2020). It is also promising that antidepressant-like effects were observed across a wide duration of treatments with psychedelic and non-psychedelic-containing mushrooms and other fungi. Psychedelic compounds generally induce rapid drug tolerance upon repeated administration (Baumeister et al., 2014; Huang et al., 2022), where 5-HT2A receptor desensitization and/or downregulation leads to functional tolerance that can last several days (Buchborn et al., 2015; de la Fuente Revenga et al., 2022). However, observations of antidepressant-like effects weeks after treatment indicate that therapeutic effects may be sustained with these compounds (Aleksandrova and Phillips, 2021; Kelmendi et al., 2022). Various psychedelics have been reported to enhance neuroplasticity (synapto- and dendritogenesis) in frontocorticolimbic circuitry and increase functional connectivity in the brain, presumably reversing structural and functional deficits in depression (Aleksandrova and Phillips, 2021; Kelmendi et al., 2022). These psychedelic-induced structural and functional changes have been shown to last for weeks to months in animal models and/or humans and are thought to underlie the sustained therapeutic efficacy of these compounds (Aleksandrova and Phillips, 2021; Kelmendi et al., 2022).
While not necessarily a weakness, an extremely wide range of doses of extracts were tested in the current studies. From Tables 1, 2, these range from 1 mg/kg (Li H. et al., 2021; Rakoczy et al., 2023) to 3,000 mg/kg (Xin et al., 2022). Part of this reflects the effects of different routes of administration. Most of the extracts were administered orally, which is associated with a need for higher dosing, and therefore many of these studies included doses in the hundreds of milligrams per kilogram. But this wide range of dosing also represents the likelihood that many of the extracts were in early stage development, where the active compounds are unknown, and so whole product, heterogeneous extracts are used where the efficacy of active compounds may be modified through both pharmacodynamic (e.g., receptor antagonism) and pharmacokinetic (e.g., absorption) processes by many inactive compounds. Thus, such studies are early-stage screens as part of an iterative process (Reis et al., 2017), and in the case of positive effects in the antidepressant screen, this will lead to refinement of extracts by further chemical analysis and result in greater potency, with a lower dose needed.
Multiple different animal models of depression and antidepressant screens were used to test for antidepressant-like effects. Although there is no universally accepted definition, animal models of depression are typically more complex and chronic than antidepressant screens, and are used to emulate some feature(s) of depression, such as its symptoms (face validity) or underlying biology (construct validity) (Geyer et al., 1995; Willner, 1984; Belzung and Lemoine, 2011; van den Berg, 2022). By contrast, antidepressant screens such as the TST and FST are acute and were originally designed to identify novel antidepressant compounds (predictive validity) without regard for similarity to the human condition (Commons et al., 2017). The most commonly used animal model of depression in the present studies was the UCMS paradigm, which is based on the development of anhedonia following exposure to chronic, variable stressors (Willner, 2017; Nollet, 2021). The model has strong theoretical appeal, based on the chronic onset of the antidepressant response, and performs well on key measures of validity (Willner, 1997). Nevertheless, the model has been criticized on both theoretical and practical grounds (Forbes et al., 1996; Barr and Phillips, 1998; Planchez et al., 2019; Markov and Novosadova, 2022), although a recent meta-analysis supported the utility of the model when specifically measuring anhedonia (Antoniuk et al., 2019). Thus, greater confidence should be placed in those studies with mushroom and fungus extracts that measured anhedonia (such as with the SPT) than those that did not. Alternate models of depression were also conducted, such as chronic social defeat stress (Li H. et al., 2021) and maternal separation (Mi et al., 2022), but typically only in a single study; given the importance of reproducibility within this field (Petković and Chaudhury, 2022), the literature will benefit from similar findings from alternate groups, or reproduction by the same groups themselves. Additionally, there are a number of other well-established and commonly used animal models of depression that should be used to assess antidepressant activity with these extracts, including surgical, pharmacological and genetic models (Barr and Phillips, 2002; Song and Leonard, 2005; Barr et al., 2011; Overstreet, 2012; Overstreet and Wegener, 2013; Vollmayr and Gass, 2013; Hendriksen et al., 2015; Czéh et al., 2016; Aleksandrova et al., 2019).
Slightly under half of the studies (22) utilized antidepressant screens such as the FST and TST, rather than models of depression. In most cases, these studies were methodologically sound, and used the appropriate controls, such as concurrent testing for locomotor activity and positive drug controls (Bogdanova et al., 2013; Yankelevitch-Yahav et al., 2015). However, several studies utilized variants of the FST, such as the “weight-loaded” FST (Xu, 2016; Liu Y. et al., 2017), whose validity is less well determined, while one study ascribed antidepressant-like effects based on changes in locomotor activity (Singh et al., 2014), which is a behavior with low specificity for depression. An additional concern was the small proportion of female animals tested, given that major depression is twice as common in women as in men: this issue is prevalent in the field of animal models of neuropsychiatric disorders as a whole (Kokras and Dalla, 2014), but future studies in this area should consider including female animals (Gobinath et al., 2018). Overall, however, the present review suggests that there is significant potential for novel antidepressant drug development with mushroom and fungus extracts provided that models and screens are conducted with high integrity.
Author contributions
CW: Conceptualization, Data curation, Formal Analysis, Investigation, Methodology, Validation, Visualization, Writing–original draft, Writing–review and editing. GK: Conceptualization, Data curation, Formal Analysis, Investigation, Methodology, Validation, Visualization, Writing–original draft, Writing–review and editing. LA: Formal Analysis, Writing–original draft, Writing–review and editing. WP: Conceptualization, Funding acquisition, Investigation, Project administration, Resources, Supervision, Writing–original draft, Writing–review and editing. AB: Conceptualization, Formal Analysis, Funding acquisition, Investigation, Methodology, Project administration, Resources, Supervision, Validation, Writing–original draft, Writing–review and editing.
Funding
The author(s) declare that financial support was received for the research, authorship, and/or publication of this article. AB was supported by a grant from NSERC. CW and GK were supported by BioTalent Canada with financial support from Translational Life Sciences.
Conflict of interest
The authors declare that the research was conducted in the absence of any commercial or financial relationships that could be construed as a potential conflict of interest.
Publisher’s note
All claims expressed in this article are solely those of the authors and do not necessarily represent those of their affiliated organizations, or those of the publisher, the editors and the reviewers. Any product that may be evaluated in this article, or claim that may be made by its manufacturer, is not guaranteed or endorsed by the publisher.
Supplementary material
The Supplementary Material for this article can be found online at: https://www.frontiersin.org/articles/10.3389/fphar.2024.1387158/full#supplementary-material
References
Aleksandrova, L. R., and Phillips, A. G. (2021). Neuroplasticity as a convergent mechanism of ketamine and classical psychedelics. Trends Pharmacol. Sci. 42 (11), 929–942. Epub 2021/09/28. doi:10.1016/j.tips.2021.08.003
Aleksandrova, L. R., Wang, Y. T., and Phillips, A. G. (2019). Evaluation of the Wistar-Kyoto rat model of depression and the role of synaptic plasticity in depression and antidepressant response. Neurosci. Biobehav Rev. 105, 1–23. Epub 2019/07/25. doi:10.1016/j.neubiorev.2019.07.007
Alo, R., Zizza, M., Fazzari, G., Facciolo, R. M., and Canonaco, M. (2019). Genistein modifies hamster behavior and expression of inflammatory factors following subchronic unpredictable mild stress. Neuroendocrinology 108 (2), 98–108. doi:10.1159/000495209
Antoniuk, S., Bijata, M., Ponimaskin, E., and Wlodarczyk, J. (2019). Chronic unpredictable mild stress for modeling depression in rodents: meta-analysis of model reliability. Neurosci. Biobehav Rev. 99, 101–116. Epub 2018/12/12. doi:10.1016/j.neubiorev.2018.12.002
Anuar, A. M., Minami, A., Matsushita, H., Ogino, K., Fujita, K., Nakao, H., et al. (2022). Ameliorating effect of the edible mushroom Hericium erinaceus on depressive-like behavior in ovariectomized rats. Biol. Pharm. Bull. 45 (10), 1438–1443. Epub 2022/10/03. doi:10.1248/bpb.b22-00151
Bao, H., Ran, P., Sun, L., Hu, W., Li, H., Xiao, C., et al. (2017). Griflola frondosa (GF) produces significant antidepressant effects involving AMPA receptor activation in mice. Pharm. Biol. 55 (1), 299–305. Epub 2016/12/13. doi:10.1080/13880209.2016.1235590
Barr, A. M., Boyda, H. N., and Procyshyn, R. M. (2011). “Withdrawal,” in Animal models of drug addiction. Editor M. C. Olmstead (Totowa, NJ: Humana Press), 431–459.
Barr, A. M., and Phillips, A. G. (1998). Chronic mild stress has no effect on responding by rats for sucrose under a progressive ratio schedule. Physiol. Behav. 64 (5), 591–597. PubMed PMID: 9817568. doi:10.1016/s0031-9384(98)00060-2
Barr, A. M., and Phillips, A. G. (2002). Increased successive negative contrast in rats withdrawn from an escalating-dose schedule of D-amphetamine. Pharmacol. Biochem. Behav. 71 (1-2), 293–299. PubMed PMID: 11812535. doi:10.1016/s0091-3057(01)00664-5
Barr, A. M., Powell, S. B., Markou, A., and Geyer, M. A. (2006). Iloperidone reduces sensorimotor gating deficits in pharmacological models, but not a developmental model, of disrupted prepulse inhibition in rats. Neuropharmacology 51 (3), 457–465. PubMed PMID: 16762376. doi:10.1016/j.neuropharm.2006.04.004
Baumeister, D., Barnes, G., Giaroli, G., and Tracy, D. (2014). Classical hallucinogens as antidepressants? A review of pharmacodynamics and putative clinical roles. Ther. Adv. Psychopharmacol. 4 (4), 156–169. Epub 2014/08/02. doi:10.1177/2045125314527985
Belzung, C., and Lemoine, M. (2011). Criteria of validity for animal models of psychiatric disorders: focus on anxiety disorders and depression. Biol. mood anxiety Disord. 1 (1), 9. Epub 2011/01/01. doi:10.1186/2045-5380-1-9
Bicker, J., Fortuna, A., Alves, G., and Falcão, A. (2020). Nose-to-brain delivery of natural compounds for the treatment of central nervous system disorders. Curr. Pharm. Des. 26 (5), 594–619. Epub 2020/01/16. doi:10.2174/1381612826666200115101544
Bogdanova, O. V., Kanekar, S., D'Anci, K. E., and Renshaw, P. F. (2013). Factors influencing behavior in the forced swim test. Physiol. Behav. 118, 227–239. Epub 2013/05/21. doi:10.1016/j.physbeh.2013.05.012
Boundless (2024) Fungi reproduction: UC Davis office of the provost. Available at: https://bio.libretexts.org/Bookshelves/Introductory_and_General_Biology/Book%3A_General_Biology_(Boundless)/24%3A_Fungi/24.01%3A_Characteristics_of_Fungi/24.1C%3A_Fungi_Reproduction (updated 4/18/2024).
Boyda, H. N., Procyshyn, R. M., Pang, C. C., Hawkes, E., Wong, D., Jin, C. H., et al. (2013). Metabolic side-effects of the novel second-generation antipsychotic drugs asenapine and iloperidone: a comparison with olanzapine. PLoS One 8 (1), e53459. PubMed PMID: 23326434. doi:10.1371/journal.pone.0053459
Boyda, H. N., Procyshyn, R. M., Tse, L., Yuen, J. W. Y., Honer, W. G., and Barr, A. M. (2021). A comparison of the metabolic side-effects of the second-generation antipsychotic drugs risperidone and paliperidone in animal models. PLoS One 16 (1), e0246211. Epub 2021/01/29. doi:10.1371/journal.pone.0246211
Boyda, H. N., Ramos-Miguel, A., Procyshyn, R. M., Topfer, E., Lant, N., Choy, H. H., et al. (2014). Routine exercise ameliorates the metabolic side-effects of treatment with the atypical antipsychotic drug olanzapine in rats. Int. J. Neuropsychopharmacol. 17 (1), 77–90. PubMed PMID: 23953063. doi:10.1017/S1461145713000795
Braun, D., Rosenberg, A. M., Rabaniam, E., Haruvi, R., Malamud, D., Barbara, R., et al. (2024). High-resolution tracking of unconfined zebrafish behavior reveals stimulatory and anxiolytic effects of psilocybin. Mol. Psychiatry. Epub 2024/01/18. doi:10.1038/s41380-023-02391-7
Buchborn, T., Schröder, H., Dieterich, D. C., Grecksch, G., and Höllt, V. (2015). Tolerance to LSD and DOB induced shaking behaviour: differential adaptations of frontocortical 5-HT(2A) and glutamate receptor binding sites. Behav. Brain Res. 281, 62–68. Epub 2014/12/17. doi:10.1016/j.bbr.2014.12.014
Carhart-Harris, R. L., Bolstridge, M., Rucker, J., Day, C. M., Erritzoe, D., Kaelen, M., et al. (2016). Psilocybin with psychological support for treatment-resistant depression: an open-label feasibility study. Lancet Psychiatry 3 (7), 619–627. Epub 2016/05/24. doi:10.1016/s2215-0366(16)30065-7
Cheng, H. Y. (2023). Ganoderma lucidum ameliorated depression-like behavior in an unpredictable chronic mild stress rat model via regulation of monoamines. Res. Square. 10.21203/rs.3.rs-2389771/v1 Available at: https://www.researchsquare.com/article/rs-2389771/v1.
Chiu, C. H., Chyau, C. C., Chen, C. C., Lee, L. Y., Chen, W. P., Liu, J. L., et al. (2018). Erinacine A-enriched Hericium erinaceus mycelium produces antidepressant-like effects through modulating BDNF/PI3K/Akt/GSK-3β signaling in mice. Int. J. Mol. Sci. 19 (2), 341. Epub 2018/01/25. doi:10.3390/ijms19020341
Chong, P. S., Poon, C. H., Roy, J., Tsui, K. C., Lew, S. Y., Phang, M. W. L., et al. (2021). Neurogenesis-dependent antidepressant-like activity of Hericium erinaceus in an animal model of depression. Chin. Med. 16 (1), 132. Epub 2021/12/09. doi:10.1186/s13020-021-00546-8
Chou, M. Y., Ho, J. H., Huang, M. J., Chen, Y. J., Yang, M. D., Lin, L. H., et al. (2022). Potential antidepressant effects of a dietary supplement from the chlorella and lion's mane mushroom complex in aged SAMP8 mice. Front. Nutr. 9, 977287. Epub 2022/09/20. doi:10.3389/fnut.2022.977287
Collaborators, G. M. D. (2022). Global, regional, and national burden of 12 mental disorders in 204 countries and territories, 1990-2019: a systematic analysis for the Global Burden of Disease Study 2019. Lancet Psychiatry 9 (2), 137–150. Epub 2022/01/14. doi:10.1016/s2215-0366(21)00395-3
Commons, K. G., Cholanians, A. B., Babb, J. A., and Ehlinger, D. G. (2017). The rodent forced swim test measures stress-coping strategy, not depression-like behavior. ACS Chem. Neurosci. 8 (5), 955–960. Epub 2017/03/14. doi:10.1021/acschemneuro.7b00042
Czéh, B., Fuchs, E., Wiborg, O., and Simon, M. (2016). Animal models of major depression and their clinical implications. Prog. Neuropsychopharmacol. Biol. Psychiatry 64, 293–310. Epub 2015/04/22. doi:10.1016/j.pnpbp.2015.04.004
Davis, A. K., Barrett, F. S., May, D. G., Cosimano, M. P., Sepeda, N. D., Johnson, M. W., et al. (2021). Effects of psilocybin-assisted therapy on major depressive disorder: a randomized clinical trial. JAMA psychiatry 78 (5), 481–489. Epub 2020/11/05. doi:10.1001/jamapsychiatry.2020.3285
de la Fuente Revenga, M., Jaster, A. M., McGinn, J., Silva, G., Saha, S., and González-Maeso, J. (2022). Tolerance and cross-tolerance among psychedelic and nonpsychedelic 5-HT(2A) receptor agonists in mice. ACS Chem. Neurosci. 13 (16), 2436–2448. Epub 2022/07/29. doi:10.1021/acschemneuro.2c00170
Eisenstein, M. (2022). The psychedelic escape from depression. Nature 609 (7929), S87–S89. Epub 2022/09/29. doi:10.1038/d41586-022-02872-9
Ezurike, P. U., Odunola, E., Oke, T. A., Bakre, A. G., Olumide, O., Odetoye, O., et al. (2023). Ganoderma lucidum ethanol extract promotes weight loss and improves depressive-like behaviors in male and female Swiss mice. Physiol. Behav. 265, 114155. Epub 2023/03/13. doi:10.1016/j.physbeh.2023.114155
Fijałkowska, A., Jędrejko, K., Sułkowska-Ziaja, K., Ziaja, M., Kała, K., and Muszyńska, B. (2022). Edible mushrooms as a potential component of dietary interventions for major depressive disorder. Foods (Basel, Switz.) 11 (10), 1489. Epub 2022/05/29. doi:10.3390/foods11101489
Forbes, N. F., Stewart, C. A., Matthews, K., and Reid, I. C. (1996). Chronic mild stress and sucrose consumption: validity as a model of depression. Physiol. Behav. 60 (6), 1481–1484. Epub 1996/12/01. doi:10.1016/s0031-9384(96)00305-8
Friedrich, M. J. (2017). Depression is the leading cause of disability around the world. Jama 317 (15), 1517. Epub 2017/04/19. doi:10.1001/jama.2017.3826
Geyer, M. A., and Markou, A. (1995). “Animal models of psychiatric disorders,” in Psychopharmacology: the fourth generation of progress. Psychopharmacology: the fourth generation of progress. Editors F. E. Bloom, and D. J. Kupfer (New York: Raven Press), 787–798.
Ghaderi, S., Gholipour, P., Komaki, A., Salehi, I., Rashidi, K., Esmaeil Khoshnam, S., et al. (2022). p-Coumaric acid ameliorates cognitive and non-cognitive disturbances in a rat model of Alzheimer's disease: the role of oxidative stress and inflammation. Int. Immunopharmacol. 112, 109295. Epub 2022/10/05. doi:10.1016/j.intimp.2022.109295
Gobinath, A. R., Wong, S., Chow, C., Lieblich, S. E., Barr, A. M., and Galea, L. A. M. (2018). Maternal exercise increases but concurrent maternal fluoxetine prevents the increase in hippocampal neurogenesis of adult offspring. Psychoneuroendocrinology 91, 186–197. Epub 2018/03/27. doi:10.1016/j.psyneuen.2018.02.027
Goodwin, G. M., Aaronson, S. T., Alvarez, O., Arden, P. C., Baker, A., Bennett, J. C., et al. (2022). Single-dose psilocybin for a treatment-resistant episode of major depression. N. Engl. J. Med. 387 (18), 1637–1648. Epub 2022/11/03. doi:10.1056/NEJMoa2206443
Gravina, A. G., Pellegrino, R., Auletta, S., Palladino, G., Brandimarte, G., D'Onofrio, R., et al. (2023). Hericium erinaceus, a medicinal fungus with a centuries-old history: evidence in gastrointestinal diseases. World J. gastroenterology 29 (20), 3048–3065. Epub 2023/06/22. doi:10.3748/wjg.v29.i20.3048
Griffiths, R. R., Johnson, M. W., Carducci, M. A., Umbricht, A., Richards, W. A., Richards, B. D., et al. (2016). Psilocybin produces substantial and sustained decreases in depression and anxiety in patients with life-threatening cancer: a randomized double-blind trial. J. Psychopharmacol. 30 (12), 1181–1197. Epub 2016/12/03. doi:10.1177/0269881116675513
Gupta, A., Gomes, I., Bobeck, E. N., Fakira, A. K., Massaro, N. P., Sharma, I., et al. (2016). Collybolide is a novel biased agonist of κ-opioid receptors with potent antipruritic activity. Proc. Natl. Acad. Sci. U. S. A. 113 (21), 6041–6046. Epub 2016/05/11. doi:10.1073/pnas.1521825113
Haikazian, S., Chen-Li, D. C. J., Johnson, D. E., Fancy, F., Levinta, A., Husain, M. I., et al. (2023). Psilocybin-assisted therapy for depression: a systematic review and meta-analysis. Psychiatry Res. 329, 115531. Epub 2023/10/16. doi:10.1016/j.psychres.2023.115531
Hendriksen, H., Korte, S. M., Olivier, B., and Oosting, R. S. (2015). The olfactory bulbectomy model in mice and rat: one story or two tails? Eur. J. Pharmacol. 753, 105–113. Epub 2014/12/03. doi:10.1016/j.ejphar.2014.10.033
Hernandez-Leon, A., Escamilla-Orozco, R. I., Tabal-Robles, A. R., Martínez-Vargas, D., Romero-Bautista, L., Escamilla-Soto, G., et al. (2024). Antidepressant- and anxiolytic-like activities and acute toxicity evaluation of the Psilocybe cubensis mushroom in experimental models in mice. J. Ethnopharmacol. 320, 117415. Epub 2023/11/18. doi:10.1016/j.jep.2023.117415
Higgins, J. P. T., and Green, S. (2011) Cochrane Handbook for systematic reviews of interventions. Chichester (UK): John Wiley & Sons.
Hill, M. N., Barr, A. M., Ho, W. S., Carrier, E. J., Gorzalka, B. B., and Hillard, C. J. (2007). Electroconvulsive shock treatment differentially modulates cortical and subcortical endocannabinoid activity. J. Neurochem. 103 (1), 47–56. Epub 2007/06/15. doi:10.1111/j.1471-4159.2007.04688.x
Hosanagar, A., Cusimano, J., and Radhakrishnan, R. (2021). Therapeutic potential of psychedelics in the treatment of psychiatric disorders, Part 1: psychopharmacology and neurobiological effects. J. Clin. Psychiatry 82 (2), 20ac13786. Epub 2021/05/15. doi:10.4088/JCP.20ac13786
Hossen, S. M. M., Islam, M. J., Hossain, M. R., Barua, A., Uddin, M. G., and Emon, N. U. (2021). CNS anti-depressant, anxiolytic and analgesic effects of Ganoderma applanatum (mushroom) along with ligand-receptor binding screening provide new insights: multi-disciplinary approaches. Biochem. biophysics Rep. 27, 101062. Epub 2021/07/22. doi:10.1016/j.bbrep.2021.101062
Huang, C. W., Hong, T. W., Wang, Y. J., Chen, K. C., Pei, J. C., Chuang, T. Y., et al. (2016). Ophiocordyceps formosana improves hyperglycemia and depression-like behavior in an STZ-induced diabetic mouse model. BMC Complement. Altern. Med. 16 (1), 310. Epub 2016/08/25. doi:10.1186/s12906-016-1278-7
Huang, J., Pham, M., Panenka, W. J., Honer, W. G., and Barr, A. M. (2022). Chronic treatment with psilocybin decreases changes in body weight in a rodent model of obesity. Front. Psychiatry 13, 891512. Epub 2022/06/07. doi:10.3389/fpsyt.2022.891512
Huang, Y. J., Hsu, N. Y., Lu, K. H., Lin, Y. E., Lin, S. H., Lu, Y. S., et al. (2020). Poria cocos water extract ameliorates the behavioral deficits induced by unpredictable chronic mild stress in rats by down-regulating inflammation. J. Ethnopharmacol. 258, 112566. Epub 2020/01/14. doi:10.1016/j.jep.2020.112566
Hung, C. I. (2014). Factors predicting adherence to antidepressant treatment. Curr. Opin. Psychiatry 27 (5), 344–349. Epub 2014/07/18. doi:10.1097/yco.0000000000000086
Jin, Q., Fu, Z., Guan, L., and Jiang, H. (2019). Syntheses of benzo[d]Thiazol-2(3H)-One derivatives and their antidepressant and anticonvulsant effects. Mar. drugs 17 (7), 430. Epub 2019/07/26. doi:10.3390/md17070430
Kamal, S., Jha, M. K., and Radhakrishnan, R. (2023). Role of psychedelics in treatment-resistant depression. Psychiatr. Clin. North Am. 46 (2), 291–305. Epub 2023/05/07. doi:10.1016/j.psc.2023.02.004
Kelmendi, B., Kaye, A. P., Pittenger, C., and Kwan, A. C. (2022). Psychedelics. Curr. Biol. 32 (2), R63–R67. Epub 2022/01/26. doi:10.1016/j.cub.2021.12.009
Kim, H. B., Lee, S., Hwang, E. S., Maeng, S., and Park, J. H. (2017). p-Coumaric acid enhances long-term potentiation and recovers scopolamine-induced learning and memory impairments. Biochem. Biophys. Res. Commun. 492 (3), 493–499. Epub 2017/08/24. doi:10.1016/j.bbrc.2017.08.068
Kokras, N., and Dalla, C. (2014). Sex differences in animal models of psychiatric disorders. Br. J. Pharmacol. 171 (20), 4595–4619. Epub 2014/04/05. doi:10.1111/bph.12710
Koo, H. N., Um, J. Y., Kim, H. M., Lee, E. H., Sung, H. J., Kim, I. K., et al. (2008). Effect of pilopool on forced swimming test in mice. Int. J. Neurosci. 118 (3), 365–374. Epub 2008/02/27. doi:10.1080/00207450701593145
Kramer, M. S., Cutler, N., Feighner, J., Shrivastava, R., Carman, J., Sramek, J. J., et al. (1998). Distinct mechanism for antidepressant activity by blockade of central substance P receptors. Science. 281 (5383), 1640–1645. PubMed PMID: 9733503. doi:10.1126/science.281.5383.1640
Li, H., Xiao, Y., Han, L., Jia, Y., Luo, S., Zhang, D., et al. (2021a). Ganoderma lucidum polysaccharides ameliorated depression-like behaviors in the chronic social defeat stress depression model via modulation of Dectin-1 and the innate immune system. Brain Res. Bull. 171, 16–24. Epub 2021/03/12. doi:10.1016/j.brainresbull.2021.03.002
Li, T. J., Lee, T. Y., Lo, Y., Lee, L. Y., Li, I. C., Chen, C. C., et al. (2021b). Hericium erinaceus mycelium ameliorate anxiety induced by continuous sleep disturbance in vivo. BMC complementary Med. Ther. 21 (1), 295. Epub 2021/12/07. doi:10.1186/s12906-021-03463-3
Li, Y., Han, L., Lu, T., Noman, M., Qiang, W., Lan, X., et al. (2019). Antidepressant-like activities of extracts of the fungus Paecilomyces tenuipes M98. Psychiatry Clin. Psychopharmacol. 29 (4), 872–879. doi:10.1080/24750573.2019.1691352
Lian, L., Kim, D. D., Procyshyn, R. M., Cázares, D., Honer, W. G., and Barr, A. M. (2022). Long-acting injectable antipsychotics for early psychosis: a comprehensive systematic review. PLoS One 17 (4), e0267808. Epub 2022/04/30. doi:10.1371/journal.pone.0267808
Lin, M., Li, H., Zhao, Y., Cai, E., Zhu, H., Gao, Y., et al. (2017). Ergosteryl 2-naphthoate, an ergosterol derivative, exhibits antidepressant effects mediated by the modification of GABAergic and glutamatergic systems. Mol. (Basel, Switz.) 22 (4), 565. Epub 2017/04/01. doi:10.3390/molecules22040565
Lin, Y. E., Chen, Y. C., Lu, K. H., Huang, Y. J., Panyod, S., Liu, W. T., et al. (2021b). Antidepressant-like effects of water extract of Cordyceps militaris (Linn.) Link by modulation of ROCK2/PTEN/Akt signaling in an unpredictable chronic mild stress-induced animal model. J. Ethnopharmacol. 276, 114194. Epub 2021/05/12. doi:10.1016/j.jep.2021.114194
Lin, Y. E., Wang, H. L., Lu, K. H., Huang, Y. J., Panyod, S., Liu, W. T., et al. (2021a). Water extract of Armillaria mellea (Vahl) P. Kumm. Alleviates the depression-like behaviors in acute- and chronic mild stress-induced rodent models via anti-inflammatory action. J. Ethnopharmacol. 265, 113395. Epub 2020/09/22. doi:10.1016/j.jep.2020.113395
Liu, C., Wang, J., Xu, S., An, S., Tang, S., He, J., et al. (2017a). Paecilomyces tenuipes extract prevents depression-like behaviors in chronic unpredictable mild stress-induced rat model via modulation of neurotransmitters. Mol. Med. Rep. 16 (2), 2172–2178. Epub 2017/06/29. doi:10.3892/mmr.2017.6807
Liu, Y., Li, L., An, S., Zhang, Y., Feng, S., Zhao, L., et al. (2017b). Antifatigue effects of antrodia cinnamomea cultured mycelium via modulation of oxidative stress signaling in a mouse model. Biomed. Res. Int. 2017, 9374026. Epub 2017/04/21. doi:10.1155/2017/9374026
Lu, X., Barr, A. M., and Bartfai, T. (2005). Galanin receptors as novel drug targets for the treatment of depression and anxiety. Drug Dev. Res. 65 (4), 227–236. doi:10.1002/ddr.20026
Mahmoudi, E., Faizi, M., Hajiaghaee, R., and Razmi, A. (2018). Alteration of depressive-like behaviors by Psilocybe cubensis alkaloid extract in mice: the role of glutamate pathway. Res. J. Pharmacogn. 5 (2), 17–24. doi:10.22127/rjp.2018.58486
Markov, D. D., and Novosadova, E. V. (2022). Chronic unpredictable mild stress model of depression: possible sources of poor reproducibility and latent variables. Biology 11 (11), 1621. Epub 2022/11/12. doi:10.3390/biology11111621
Matsuzaki, H., Shimizu, Y., Iwata, N., Kamiuchi, S., Suzuki, F., Iizuka, H., et al. (2013). Antidepressant-like effects of a water-soluble extract from the culture medium of Ganoderma lucidum mycelia in rats. BMC Complement. Altern. Med. 13, 370. Epub 2013/12/29. doi:10.1186/1472-6882-13-370
McLachlan, G. (2018). Treatment resistant depression: what are the options? Bmj 363, k5354. Epub 2018/12/20. doi:10.1136/bmj.k5354
Mi, X., Zeng, G. R., Liu, J. Q., Luo, Z. S., Zhang, L., Dai, X. M., et al. (2022). Ganoderma lucidum triterpenoids improve maternal separation-induced anxiety- and depression-like behaviors in mice by mitigating inflammation in the periphery and brain. Nutrients 14 (11), 2268. Epub 2022/06/11. doi:10.3390/nu14112268
Minami, A., Matsushita, H., Horii, Y., Ieno, D., Matsuda, Y., Saito, M., et al. (2013). Improvement of depression-like behavior and memory impairment with the ethanol extract of Pleurotus eryngii in ovariectomized rats. Biol. Pharm. Bull. 36 (12), 1990–1995. Epub 2013/12/03. doi:10.1248/bpb.b13-00657
Moussa, A. Y., Fayez, S., Xiao, H., and Xu, B. (2022). New insights into antimicrobial and antibiofilm effects of edible mushrooms. Food Res. Int. Ott.( Ont) 162 (Pt A), 111982. Epub 2022/12/04. doi:10.1016/j.foodres.2022.111982
Nagano, M., Shimizu, K., Kondo, R., Hayashi, C., Sato, D., Kitagawa, K., et al. (2010). Reduction of depression and anxiety by 4 weeks Hericium erinaceus intake. Biomed. Res. (Tokyo, Jpn.) 31 (4), 231–237. Epub 2010/09/14. doi:10.2220/biomedres.31.231
Nakamichi, N., Nakayama, K., Ishimoto, T., Masuo, Y., Wakayama, T., Sekiguchi, H., et al. (2016). Food-derived hydrophilic antioxidant ergothioneine is distributed to the brain and exerts antidepressant effect in mice. Brain Behav. 6 (6), e00477. Epub 2016/05/03. doi:10.1002/brb3.477
Nascimento, C. P., Luz, D. A., da Silva, C. C. S., Malcher, C. M. R., Fernandes, L. M. P., Dalla Santa, H. S., et al. (2020). Ganoderma lucidum ameliorates neurobehavioral changes and oxidative stress induced by ethanol binge drinking. Oxidative Med. Cell. Longev. 2020, 2497845. Epub 2020/08/18. doi:10.1155/2020/2497845
Nishizawa, K., Torii, K., Kawasaki, A., Katada, M., Ito, M., Terashita, K., et al. (2007). Antidepressant-like effect of Cordyceps sinensis in the mouse tail suspension test. Biol. Pharm. Bull. 30 (9), 1758–1762. Epub 2007/09/11. doi:10.1248/bpb.30.1758
Nollet, M. (2021). Models of depression: unpredictable chronic mild stress in mice. Curr. Protoc. 1 (8), e208. doi:10.1002/cpz1.208
Overstreet, D. H. (2012). Modeling depression in animal models. Methods Mol. Biol. 829, 125–144. Epub 2012/01/11. doi:10.1007/978-1-61779-458-2_7
Overstreet, D. H., and Wegener, G. (2013). The flinders sensitive line rat model of depression--25 years and still producing. Pharmacol. Rev. 65 (1), 143–155. Epub 2013/01/16. doi:10.1124/pr.111.005397
Palhano-Fontes, F., Barreto, D., Onias, H., Andrade, K. C., Novaes, M. M., Pessoa, J. A., et al. (2019). Rapid antidepressant effects of the psychedelic ayahuasca in treatment-resistant depression: a randomized placebo-controlled trial. Psychol. Med. 49 (4), 655–663. Epub 2018/06/16. doi:10.1017/s0033291718001356
Pandya, U., Dhuldhaj, U., and Sahay, N. S. (2019). Bioactive mushroom polysaccharides as antitumor: an overview. Nat. Prod. Res. 33 (18), 2668–2680. Epub 2018/05/05. doi:10.1080/14786419.2018.1466129
Park, Y. S., Jang, S., Lee, H., Kang, S., Seo, H., Yeon, S., et al. (2021). Identification of the antidepressant function of the edible mushroom Pleurotus eryngii. J. fungi (Basel, Switz.) 7 (3), 190. Epub 2021/04/04. doi:10.3390/jof7030190
Pazzi, F., Adsuar, J. C., Domínguez-Muñoz, F. J., García-Gordillo, M. A., Gusi, N., and Collado-Mateo, D. (2020). Ganoderma lucidum effects on mood and health-related quality of life in women with fibromyalgia. Healthc. (Basel, Switz.) 8 (4), 520. Epub 2020/12/04. doi:10.3390/healthcare8040520
Petković, A., and Chaudhury, D. (2022). Encore: behavioural animal models of stress, depression and mood disorders. Front. Behav. Neurosci. 16, 931964. Epub 2022/08/26. doi:10.3389/fnbeh.2022.931964
Planchez, B., Surget, A., and Belzung, C. (2019). Animal models of major depression: drawbacks and challenges. J. neural Transm. (Vienna) 126 (11), 1383–1408. Epub 2019/10/05. doi:10.1007/s00702-019-02084-y
Rakoczy, R. J., Runge, G. N., Sen, A. K., Sandoval, O., Nguyen, Q., Roberts, B. R., et al. (2023). Pharmacological and behavioral effects of tryptamines present in psilocybin-containing mushrooms. bioRxiv 2023. doi:10.1101/2023.10.19.563138
Reis, F. S., Martins, A., Vasconcelos, M. H., Morales, P., and Ferreira, ICFR (2017). Functional foods based on extracts or compounds derived from mushrooms. Trends Food Sci. Technol. 66, 48–62. doi:10.1016/j.tifs.2017.05.010
Ross, S., Bossis, A., Guss, J., Agin-Liebes, G., Malone, T., Cohen, B., et al. (2016). Rapid and sustained symptom reduction following psilocybin treatment for anxiety and depression in patients with life-threatening cancer: a randomized controlled trial. J. Psychopharmacol. 30 (12), 1165–1180. Epub 2016/12/03. doi:10.1177/0269881116675512
Rossom, R. C., Shortreed, S., Coleman, K. J., Beck, A., Waitzfelder, B. E., Stewart, C., et al. (2016). Antidepressant adherence across diverse populations and healthcare settings. Depress Anxiety 33 (8), 765–774. Epub 2016/06/21. doi:10.1002/da.22532
Ryu, S., Kim, H. G., Kim, J. Y., Kim, S. Y., and Cho, K. O. (2018). Hericium erinaceus extract reduces anxiety and depressive behaviors by promoting hippocampal neurogenesis in the adult mouse brain. J. Med. food 21 (2), 174–180. Epub 2017/11/02. doi:10.1089/jmf.2017.4006
Scheepens, A., Bisson, J. F., and Skinner, M. (2014). p-Coumaric acid activates the GABA-A receptor in vitro and is orally anxiolytic in vivo. Phytotherapy Res. PTR 28 (2), 207–211. Epub 2013/03/28. doi:10.1002/ptr.4968
Sepúlveda-Lizcano, L., Arenas-Villamizar, V. V., Jaimes-Duarte, E. B., García-Pacheco, H., Paredes, C. S., Bermúdez, V., et al. (2023). Metabolic adverse effects of psychotropic drug therapy: a systematic review. Eur. J. investigation health, Psychol. Educ. 13 (8), 1505–1520. Epub 2023/08/25. doi:10.3390/ejihpe13080110
Simonsson, O., Carlbring, P., Carhart-Harris, R., Davis, A. K., Nutt, D. J., Griffiths, R. R., et al. (2023). Assessing the risk of symptom worsening in psilocybin-assisted therapy for depression: a systematic review and individual participant data meta-analysis. Psychiatry Res. 327, 115349. Epub 2023/08/01. doi:10.1016/j.psychres.2023.115349
Singh, K. P., Meena, H. S., and Negi, P. S. (2014). Enhancement of neuromuscular activity by natural specimens and cultured mycelia of Cordyceps sinensis in mice. Indian J. Pharm. Sci. 76 (5), 458–461. doi:10.4103/0250-474X.143105
Singh, R., Shri, R., Singh, A. P., and Dhingra, G. S. (2021). Valorization of Ganoderma species: chemical characterization and antidepressant-like activity. Waste Biomass Valorization 12 (4), 2025–2036. doi:10.1007/s12649-020-01157-4
Socala, K., Nieoczym, D., Grzywnowicz, K., Stefaniuk, D., and Wlaz, P. (2015). Evaluation of anticonvulsant, antidepressant-and anxiolytic-like effects of an aqueous extract from cultured mycelia of the lingzhi or reishi medicinal mushroom Ganoderma lucidum (higher basidiomycetes) in mice. Int. J. Med. mushrooms 17 (3), 209–218. Epub 2015/05/09. doi:10.1615/intjmedmushrooms.v17.i3.10
Song, C., and Leonard, B. E. (2005). The olfactory bulbectomised rat as a model of depression. Neurosci. Biobehav Rev. 29 (4-5), 627–647. Epub 2005/06/01. doi:10.1016/j.neubiorev.2005.03.010
Song, J., Geng, X., Su, Y., Zhang, X., Tu, L., Zheng, Y., et al. (2020). Structure feature and antidepressant-like activity of a novel exopolysaccharide isolated from Marasmius androsaceus fermentation broth. Int. J. Biol. Macromol. 165 (Pt B), 1646–1655. Epub 2020/10/12. doi:10.1016/j.ijbiomac.2020.10.015
Song, J., Wang, X., Huang, Y., Qu, Y., Teng, L., Wang, D., et al. (2017). Antidepressant-like effects of Marasmius androsaceus metabolic exopolysaccharides on chronic unpredictable mild stress-induced rat model. Mol. Med. Rep. 16 (4), 5043–5049. Epub 2017/08/03. doi:10.3892/mmr.2017.7145
Song, J., Xing, G., Cao, J., Teng, L., Li, C., Meng, Q., et al. (2016). Investigation of the antidepressant effects of exopolysaccharides obtained from Marasmius androsaceus fermentation in a mouse model. Mol. Med. Rep. 13 (1), 939–946. Epub 2015/12/10. doi:10.3892/mmr.2015.4584
Strauss, D., Ghosh, S., Murray, Z., and Gryzenhout, M. (2022). An overview on the taxonomy, phylogenetics and ecology of the psychedelic genera Psilocybe, panaeolus, pluteus and gymnopilus. Front. For. Glob. Change 5. doi:10.3389/ffgc.2022.813998
Tan, Y. F., Liao, Z. L., Qiu, Y. J., Zhu, J. P., and Yu, E. Y. (2016). Possible involvement of L-arginine-nitric oxide (NO)-cyclic guanosine monophosphate (cGMP) signaling pathway in the antidepressant-like effect of Wuling mycelia powder in rat. Biomed. Pharmacother. 78, 60–65. Epub 2016/02/24. doi:10.1016/j.biopha.2015.12.016
Teng, T., Zhang, Z., Yin, B., Guo, T., Wang, X., Hu, J., et al. (2022). Effect of antidepressants on functioning and quality of life outcomes in children and adolescents with major depressive disorder: a systematic review and meta-analysis. Transl. psychiatry 12 (1), 183. Epub 2022/05/05. doi:10.1038/s41398-022-01951-9
Tianzhu, Z., Shihai, Y., and Juan, D. (2014). Antidepressant-like effects of cordycepin in a mice model of chronic unpredictable mild stress. Evidence-based complementary Altern. Med. eCAM. 2014, 438506. Epub 2015/01/15. doi:10.1155/2014/438506
Tse, L., Procyshyn, R. M., Fredrikson, D. H., Boyda, H. N., Honer, W. G., and Barr, A. M. (2014). Pharmacological treatment of antipsychotic-induced dyslipidemia and hypertension. Int. Clin. Psychopharmacol. 29 (3), 125–137. PubMed PMID: 24169026. doi:10.1097/YIC.0000000000000014
Turner, P. V., Brabb, T., Pekow, C., and Vasbinder, M. A. (2011). Administration of substances to laboratory animals: routes of administration and factors to consider. J. Am. Assoc. Laboratory Animal Sci. JAALAS. 50 (5), 600–613.
van den Berg, H. (2022). Evaluating the validity of animal models of mental disorder: from modeling syndromes to modeling endophenotypes. Hist. Philosophy Life Sci. 44 (4), 59. doi:10.1007/s40656-022-00537-4
Venturella, G., Ferraro, V., Cirlincione, F., and Gargano, M. L. (2021). Medicinal mushrooms: bioactive compounds, use, and clinical trials. Int. J. Mol. Sci. 22 (2), 634. Epub 2021/01/14. doi:10.3390/ijms22020634
Voineskos, D., Daskalakis, Z. J., and Blumberger, D. M. (2020). Management of treatment-resistant depression: challenges and strategies. Neuropsychiatr. Dis. Treat. 16, 221–234. Epub 2020/02/06. doi:10.2147/ndt.s198774
Vollmayr, B., and Gass, P. (2013). Learned helplessness: unique features and translational value of a cognitive depression model. Cell. tissue Res. 354 (1), 171–178. Epub 2013/06/14. doi:10.1007/s00441-013-1654-2
Wang, J., Liu, Y., Li, L., Qi, Y., Zhang, Y., Li, L., et al. (2017). Dopamine and serotonin contribute to Paecilomyces hepiali against chronic unpredictable mild stress induced depressive behavior in Sprague Dawley rats. Mol. Med. Rep. 16 (4), 5675–5682. Epub 2017/08/30. doi:10.3892/mmr.2017.7261
Willner, P. (1984). The validity of animal models of depression. Psychopharmacol. Berl. 83 (1), 1–16. Epub 1984/01/01. doi:10.1007/bf00427414
Willner, P. (1997). Validity, reliability and utility of the chronic mild stress model of depression: a 10-year review and evaluation. Psychopharmacol. Berl. 134 (4), 319–329. Epub 1998/02/06. doi:10.1007/s002130050456
Willner, P. (2017). The chronic mild stress (CMS) model of depression: history, evaluation and usage. Neurobiol. stress 6, 78–93. Epub 2017/02/24. doi:10.1016/j.ynstr.2016.08.002
Xin, Y., Zhang, Y., and Zhang, X. (2022). Antidepressant effect of water extract of royal sun medicinal mushroom, Agaricus brasiliensis (agaricomycetes), in mice exposed to chronic unpredictable mild stress. Int. J. Med. mushrooms 24 (4), 63–73. Epub 2022/06/14. doi:10.1615/IntJMedMushrooms.2022042726
Xu, Y. F. (2016). Effect of polysaccharide from Cordyceps militaris (ascomycetes) on physical fatigue induced by forced swimming. Int. J. Med. mushrooms 18 (12), 1083–1092. Epub 2017/01/18. doi:10.1615/IntJMedMushrooms.v18.i12.30
Yadav, D., and Negi, P. S. (2021). Bioactive components of mushrooms: processing effects and health benefits. Food Res. Int. Ott.( Ont) 148, 110599. Epub 2021/09/12. doi:10.1016/j.foodres.2021.110599
Yankelevitch-Yahav, R., Franko, M., Huly, A., and Doron, R. (2015). The forced swim test as a model of depressive-like behavior. J. Vis. Exp. (97), 52587. Epub 2015/04/14. doi:10.3791/52587
Yao, W., Zhang, J. C., Dong, C., Zhuang, C., Hirota, S., Inanaga, K., et al. (2015). Effects of amycenone on serum levels of tumor necrosis factor-α, interleukin-10, and depression-like behavior in mice after lipopolysaccharide administration. Pharmacol. Biochem. Behav. 136, 7–12. Epub 2015/07/08. doi:10.1016/j.pbb.2015.06.012
Yu, X. D., Zhang, D., Xiao, C. L., Zhou, Y., Li, X., Wang, L., et al. (2022). P-coumaric acid reverses depression-like behavior and memory deficit via inhibiting AGE-RAGE-mediated neuroinflammation. Cells 11 (10), 1594. Epub 2022/05/29. doi:10.3390/cells11101594
Yuen, J. W. Y., Kim, D. D., Procyshyn, R. M., Panenka, W. J., Honer, W. G., and Barr, A. M. (2021). A focused review of the metabolic side-effects of clozapine. Front. Endocrinol. 12, 609240. Epub 2021/03/16. doi:10.3389/fendo.2021.609240
Zhang, L., Zhang, L., and Sui, R. (2021a). Ganoderic acid A-mediated modulation of microglial polarization is involved in depressive-like behaviors and neuroinflammation in a rat model of post-stroke depression. Neuropsychiatr. Dis. Treat. 17, 2671–2681. Epub 2021/08/24. doi:10.2147/ndt.s317207
Zhang, T., Du, Y., Liu, X., Sun, X., Cai, E., Zhu, H., et al. (2021b). Study on antidepressant-like effect of protoilludane sesquiterpenoid aromatic esters from Armillaria Mellea. Nat. Prod. Res. 35 (6), 1042–1045. Epub 2019/05/29. doi:10.1080/14786419.2019.1614577
Zhao, J., Zeng, X., Liu, J., Liu, X., Liu, Z., Wang, B., et al. (2023). Marasmius androsaceus mitigates depression-exacerbated intestinal radiation injuries through reprogramming hippocampal miRNA expression. Biomed. Pharmacother. 165, 115157. Epub 2023/07/17. doi:10.1016/j.biopha.2023.115157
Zhao, S., Rong, C., Gao, Y., Wu, L., Luo, X., Song, S., et al. (2021). Antidepressant-like effect of Ganoderma lucidum spore polysaccharide-peptide mediated by upregulation of prefrontal cortex brain-derived neurotrophic factor. Appl. Microbiol. Biotechnol. 105 (23), 8675–8688. Epub 2021/10/31. doi:10.1007/s00253-021-11634-y
Zhou, L. L., Ming, L., Ma, C. G., Cheng, Y., and Jiang, Q. (2005). Antidepressant-like effects of BCEF0083 in the chronic unpredictable stress models in mice. Chin. Med. J. Engl. 118 (11), 903–908.
Glossary
Keywords: animal model, antidepressant, fungus, mushroom, preclinical
Citation: Wang CK, Kim G, Aleksandrova LR, Panenka WJ and Barr AM (2024) A scoping review of the effects of mushroom and fungus extracts in rodent models of depression and tests of antidepressant activity. Front. Pharmacol. 15:1387158. doi: 10.3389/fphar.2024.1387158
Received: 16 February 2024; Accepted: 02 May 2024;
Published: 03 June 2024.
Edited by:
Christina Dalla, National and Kapodistrian University of Athens, GreeceReviewed by:
Alexia Polissidis, Biomedical Research Foundation of the Academy of Athens (BRFAA), GreeceCopyright © 2024 Wang, Kim, Aleksandrova, Panenka and Barr. This is an open-access article distributed under the terms of the Creative Commons Attribution License (CC BY). The use, distribution or reproduction in other forums is permitted, provided the original author(s) and the copyright owner(s) are credited and that the original publication in this journal is cited, in accordance with accepted academic practice. No use, distribution or reproduction is permitted which does not comply with these terms.
*Correspondence: Alasdair M. Barr, al.barr@ubc.ca
†ORCID: Alasdair M. Barr, orcid.org/0000-0002-3407-1574
‡These authors have contributed equally to this work to this manuscript