- 1College of Civil and Transportation Engineering, Shenzhen University, Shenzhen, China
- 2Institute for Advanced Study, Shenzhen University, Shenzhen, China
- 3Institute of Microscale Optoelectronics, Shenzhen University, Shenzhen, China
- 4Southern Marine Science and Engineering Guangdong Laboratory, Zhuhai, China
Antibiotic-resistant bacteria are rapidly emerging, and the increasing prevalence of multidrug-resistant (MDR) Acinetobacter baumannii poses a severe threat to humans and healthcare organizations, due to the lack of innovative antibacterial drugs. Endolysins, which are peptidoglycan hydrolases encoded by a bacteriophage, are a promising new family of antimicrobials. Endolysins have been demonstrated as an effective therapeutic agent against bacterial infections of A. baumannii and many other Gram-positive and Gram-negative bacteria. Endolysin research has progressed from basic in vitro characterization to sophisticated protein engineering methodologies, including advanced preclinical and clinical testing. Endolysin are therapeutic agent that shows antimicrobial properties against bacterial infections caused by drug-resistant Gram-negative bacteria, there are still barriers to their implementation in clinical settings, such as safety concerns with outer membrane permeabilizers (OMP) use, low efficiency against stationary phase bacteria, and stability issues. The application of protein engineering and formulation techniques to improve enzyme stability, as well as combination therapy with other types of antibacterial drugs to optimize their medicinal value, have been reviewed as well. In this review, we summarize the clinical development of endolysin and its challenges and approaches for bringing endolysin therapies to the clinic. This review also discusses the different applications of endolysins.
1 Antimicrobial resistance
The discovery of antibiotics transformed medicine, greatly increasing the quality of life, extending human lifespans, and enabling numerous complicated medical procedures and medicines, all while maintaining healthy lives and boosting public health. Unfortunately, genetically encoded antimicrobial resistance (AMR) has increased as discoveries have reduced, particularly in the early part of this century. Nonetheless, there have always been infectious factors when antibiotics have failed, and these keep outnumbering antibiotic resistance as a cause of morbidity and mortality (de la Fuente-Nunez et al., 2023). The resistance to antibiotics is a result of bacterial evolution, which protects bacteria from drugs that are harmful to their survival. It is a subset of AMR, a broader term for the evolution of resistance to naturally occurring agents or targeted medications in bacteria. AMR is linked to antibiotic use and becomes worse by antibiotic misuse and overuse in the medical sector. Increased resistance in pathogenic bacteria poses several critical public health problems, including severe and protracted illness, more hospitalizations and complications, and higher rates, all of which result in a significant cost burden (Behling et al., 2023). In recent decades, worldwide use of veterinary antibiotics has decreased, although worldwide clinical use of antibiotics remains high, and overuse exists in low- and middle-income nations. Although the majority of countries have implemented national action plans (NAPs) to actively address antibiotic resistance, actual AMR measures range significantly (Ding et al., 2023). In 2015, the 68th World Health Organization (WHO) assembly passed the global action plan on AMR to support action against resistance to antibiotics, and as a result, several nations and regions have implemented AMR mitigation strategies. The library of AMR NAPs was evaluated on 31 January 2023, and 134 countries (of which 40 were written in a language other than English) had officially approved NAPs, which includes 69% of the world’s 194 countries (Ding et al., 2023). As a result of antibiotic overuse and misuse, an increasing number of resistant bacteria have been isolated from the healthcare system and the environment. The rapid genetic material and resistance genes exchange among bacterial strains encourages the spread of AMR (Loh et al., 2021). Antibiotic resistance has been a worldwide challenge that could make a massive impact on both healthcare systems and the economic system. To fill the significant gap left by a high rate of resistance development and an inadequately filled antibiotic development pipeline, the WHO has called for the urgent development of novel antibacterial agents (Abdelkader et al., 2022). A novel alternative antimicrobial agent must be developed immediately to combat the severe threat of MDR bacterial infections due to the decline in antibiotic efficacy and the lack of novel antibiotics, pushing us closer to a post-antibiotic era (Chu et al., 2022). The bacteria that are part of ESKAPE (Enterococcus faecium, Staphylococcus aureus, Kleibsiella pneumoniae, A. baumannii, P. aeruginosa and Enterobacter species) are important drug-resistant pathogens. Among these, Acinetobacter baumannii is regarded as a global problem because of its potential to gain antibiotic resistance at a staggering rate (Rai and Kumar, 2022). A. baumannii is a significant nosocomial and opportunistic Gram-negative bacterium, causing infections worldwide, especially in intensive care units (ICU). It can cause numerous hospital- and community-acquired infections, such as ventilator-associated pneumonia, bacteremia, wound infections, and meningitis. It is clinically significant because of its capacity to survive for long periods on the substrate surface and its resistance to multiple drugs, making it the most prevalent and high alert pathogen on the WHO list of critical pathogens. This bacterium has been recognized to flout available therapeutic options. Alternative approaches to medicating A. baumannii infections are vitally important (Oyejobi et al., 2022a). The WHO has classified carbapenem-resistant A. baumannii (CRAB) as an urgently needed pathogen with limited therapy choices, thus there is an urgent need for research and development of new antibiotics as well as various alternative treatment strategies (Jansen et al., 2018). AMR highlighted that the present therapeutic pipeline is insufficient to address the AMR issue, particularly for three Gram-negative carbapenem-resistant, important priority pathogens: Pseudomonas aeruginosa, A. baumannii, and Enterobacteriaceae. Despite an increase in the number of antibiotics targeting Gram-negative infections in the clinical pipeline, most of them are variants of existing ones, serving as a temporary remedy because resistance to these evolved medications is likely to emerge quickly. Out of forty-two novel drugs for treatment that target priority pathogens, just two drugs from the same class (siderophore-cephalosporins) target the three Gram-negative critical priority pathogens (Gutiérrez and Briers, 2021). With a novel mode of action, bacteriophage-encoded endolysin has developed into the most effective class of bactericidal biological agents currently undergoing clinical testing (WHO (Organization, 2019). Endolysin were initially denied from use against Gram-negative pathogens due to their impermeable outer membrane, but they are now being developed as effective therapeutic agent against these critical priority pathogens. In principle, the three routes of investigation have been recently explored and advanced to varying degrees, including the use of endolysin that possess intrinsic activity due to a positively charged C-terminus that destabilizes the outer membrane, the use of physical or chemical methods to disrupt the outer membrane integrity, and protein engineering to provide the endolysin with the tools it needs to overcome the outer membrane (Gutiérrez and Briers, 2021). In this review article, we have specifically addressed the use of endolysin against A. baumannii and we have addressed and presented A. baumannii as a case study model of the use of endolysin as a new therapeutic agent. We have also highlighted the issues and barriers of transforming endolysin from the lab to the clinic and the strategies to overcome these issues of endolysin to be used as a novel therapeutic drug.
1.1 Endolysins’ structure and enzymatic activity
Endolysins are proteins that are expressed late in the bacteriophage virus’s lytic cycle. After the bacteriophage completes its lytic cycle within the host, endolysins break down the host’s cell wall by splitting the host’s peptidoglycan to release offspring virions (Abdelrahman et al., 2021b). Gram-positive bacteriophage endolysins have a modular structure, with EADs at the N-terminus and CBDs attached at the C-terminus via a linker. Endolysins have enzymatic hydrolysis and substrate identification capabilities due to the presence of EADs and CBDs. Typically, modular endolysins have one or two EADs at the N-terminal and CBD at the C-terminal connected by a flexible region known as the linker (Gondil et al., 2021b). The N-terminal EAD of modular endolysins cleaves certain peptidoglycan bonds in the host bacterium’s murein layer, while the C-terminal CBD identifies and connects to numerous epitopes in the cell wall for optimal binding of the EAD’s catalytic actions (Wang et al., 2023). The bacteriophage-derived endolysins of Gram-negative hosts can be structured in different kinds of ways, although most of them have a basic globular EAD domain without a CBD. The latest study also discovered Gram-negative endolysins with globular structures, one or two CBDs at the N-terminus, and the EAD module at the end of the C-terminal region (Briers et al., 2007). Endolysins are categorized based on their cleavage site. These enzymes include lysozymes (N-acetylmuramidases), glycosidases (N-acetyl--d-glucosamidases), N-acetylmuramoyl-l-alanine amidases, and L-alanoyl-d-glutamate endopeptidases (Khan et al., 2023b). Endolysins, which are composed of one of these four N-terminals connected to a distinct cell wall-binding domain, also be known as bacteriophage-encoded cell wall hydrolases (Murray et al., 2021).
Glycosidases: The polymeric structures of N-acetylmuramic acids (MurNAc) and N-acetylglucosamines (GlcNAc) are linked by −1,4 glycosidic linkages that are split by glycosidases. N-acetyl--D-muramidase’s split bonds within GlcNAc and MurNAc residues. N-acetyl--D-glucosidases split bonds within GlcNAc and MurNAc residues (Matamp and Bhat, 2019). Like the other two glycosidases, transglycosylases cleave −1,4 bonds within GlcNAc and MurNAc, but they additionally play a role in an intramolecular mechanism that results in the production of a 1,6-anhydro ring at the MurNAc residue (Rodríguez-Rubio et al., 2016a). Lysozymes, also known as N-acetylmuramidases, eradicate bacteria by specialized hydrolysis. Glycosidases, or N-acetyl-β-d-glucosamidases, control the hydrolysis of the glycosidic link. Glycosidic linkages β-1,4 bind the NAG (N-acetylglucosamine) and NAM (N-acetylmuramic acid) monomers of peptidoglycan polymers together. Lysozyme acts to break the structural integrity of the peptidoglycan cell wall by hydrolyzing these connections. This results in an imbalance in the turgor pressure, which kills the bacterial cell (Ragland and Criss, 2017).
Amidases: The mechanism by which N-acetylmuramoyl-L-alanine amidases, also referred to as peptidoglycan amidases act is to break the amide bond that separates the glycan strand from the stem peptide that is located between the L-alanine and N-acetylmuramic acid residues (Abdelrahman et al., 2021b).
Endopeptidases: Enzymes called endopeptidases dissolve the bonds that hold two amino acids together in the stem peptide. Both L-alanoyl-d-glutamate endopeptidases and interpeptide bridge-specific endopeptidases target the peptide that makes up the L-alanoyl-d-glutamate link. Between stem peptides or inside an interpeptide bridge, bonds can break (Rodríguez-Rubio et al., 2016a).
1.2 A. baumannii resistance and a lack of therapeutic alternatives
Treatment of A. baumannii infections is complex due to its high capacity for acquiring resistance genes and its natural resistance to a wide range of antibiotics (Poirel et al., 2011). Acinetobacter baumannii infections are frequently treated with colistin as a last-line antibiotic. Still, an increasing number of reports on colistin-resistant strains indicate that the era of antibiotics is challenging (Lim et al., 2019). Carbapenems were once the primary therapy for MDR A. baumannii infections, but their use has resulted in an increase in carbapenem resistance in recent years (Nordmann and Poirel, 2019). Polymyxins are currently routinely used as the antibiotics of choice for treating MDR A. baumannii infections, despite initially being prohibited due to systemic toxicities (nephrotoxicity and neurotoxicity) (Piperaki et al., 2019). Extensive drug-resistant (XDR) A. baumannii is classified as an isolate resistant to three or more of the antibiotic classes (penicillin’s and cephalosporins, which include inhibitor combinations, fluoroquinolones, and aminoglycosides, with carbapenem resistance in the majority of cases). In contrast, pan-drug-resistant (PDR) A. baumannii is an XDR isolate resistant to polymyxins and tigecycline (Mulani et al., 2019). Antibiotic resistance mechanisms fall into three categories. First, resistance can be developed by decreasing membrane permeability or increasing antibiotic efflux, thereby blocking access to the target. Secondly, bacteria can safeguard the antibiotic receptor through genetic mutation or post-translational modification. Finally, antibiotics can be immediately inactivated through hydrolysis or alteration (Blair et al., 2015). One of Acinetobacter’s most powerful weapons is its remarkable genetic adaptation, which allows for rapid genetic changes and rearrangements, as well as the incorporation of foreign determinants carried by mobile genetic components. Insertion sequences are regarded as one of the primary mechanisms shaping bacterial genomes and, ultimately, evolution (Vrancianu et al., 2020). Furthermore, A. baumannii can form biofilms, extending its ability to survive on healthcare equipment, such as ventilators in intensive care units (ICUs) (Pakharukova et al., 2018). However, the link between biofilm development and antibiotic resistance is currently unclear (Yang et al., 2019). Many therapeutic options have been failed by A. baumannii, making treatment of these infections difficult and, in some circumstances, impossible. Given the scarcity of treatment alternatives, there is a need for an innovative strategy and rethinking of remedies for combating this bacterium (Oyejobi et al., 2022b).
1.3 Endolysins are a new class of therapeutic products against A. baumannii infections
The emergence of antibiotic-resistant A. baumannii necessitates the development of alternative treatment options, with many global pharmaceutical industry players strategically choosing to discontinue or outsource novel antibiotic discovery programs. One of the most promising methods is endolysin therapy, which uses endolysin to combat bacterial pathogens (Khan et al., 2021). Endolysins are peptidoglycan hydrolytic enzymes encoded by bacteriophages that have great potential as a new class of antimicrobial therapeutic agents for multidrug-resistant bacteria (Gondil et al., 2021a). Due to the widespread emergence of antibiotic resistance, endolysins have drawn more attention as an alternative therapy. There is strong evidence that endolysins can externally degrade peptidoglycan without the aid of a bacteriophage. Their incorporation into therapeutic approaches has consequently created new opportunities for treating bacterial infections in the human, veterinary, agricultural, and biotechnology fields (Abdelrahman et al., 2021a). Endolysins are a desirable alternative due to their lytic potential against various bacterial species that cause human infections. Endolysins are the most successful alternative therapy for treating and curing MDR bacteria. According to reports numerous endolysins have been used successfully to treat bacteria resistant to antibiotics. The antibacterial treatment through bacteriophage-derived endolysin is tested in animal experiment models. Due to their extraordinary therapeutic potential against multidrug-resistant bacteria, endolysin has attracted much attention in recent years (Mehmood Khan et al., 2023). Endolysin are bacteriophage-derived proteins which have high specificity for bacteria, they have no detrimental effects on human or animal cells. Endolysin lacks many features that make antibiotics superior to them, such as no resistance and high specificity (Abdelrahman et al., 2021a; Gondil et al., 2021b). Most bacteriophage endolysins indicate near-species specificity, which is thought to be one of their most beneficial features in this age of broad-range resistance to antibiotics as it avoids selective pressure on naturally occurring beneficial microbiota. Furthermore, resistance to endolysins is improbable for a variety of reasons, Endolysins have evolved to attach to and break highly conserved components in the cell wall of the pathogenic bacteria, secondly, as the bacteriophage and host bacteria coevolution occurs so there are fewer chances of resistance development to endolysin. Furthermore, because endolysins are applied externally and act on the cell wall rather than entering the bacterial cell, they avoid the majority of possible resistance mechanisms (e.g., active efflux from the cell or decreased membrane permeability) that contribute to resistance to most conventional antibiotics. Several endolysins include two catalytic domains that hydrolyze distinct bonds in the peptidoglycan, which is thought to limit the likelihood of resistance formation (Haddad Kashani et al., 2018).
Endolysin LysAB1245 showed broad lytic range efficacy against A. baumannii isolates from various capsular variants. Furthermore, LysAB1245 exhibited quick antibacterial activity and stability under different ranges of pH and temperature conditions. This study tested the possibility of LysAB1245 as a novel therapeutic agent for the treatment of MDR A. baumannii bacterial infections (Soontarach et al., 2024). Table 1 shows the summary of the therapeutic potentials of bacteriophage derived endolysins against A. baumannii.
1.4 Evaluation of endolysin against A. baumannii
Establishing the in vivo safety and efficacy of phage-encoded endolysin as medicinal products is an essential step in their development (Lai et al., 2020a). Endolysin can be administered by various methods, such as intravenously and intraperitoneal injections, topical treatments (creams, ointments, and gels), and trans nasal, vaginal, and oral delivery systems (Abdelrahman et al., 2021b). Endolysins, which target pathogens in various tissues and organs, are currently in clinical trials. Schmelcher et al. have briefly addressed the systemic use of endolysins to treat bacteria-related infections of the bloodstream, organs, and tissues (Schmelcher M. and Loessner M. J. J. C. O. I. B., 2021). In an A. baumannii mouse model of burn wound infection, a single dosage of 14 µg/mouse of endolysin LysP53 resulted in a 3-log reduction in bacterial load. The findings suggested that LysP53 can be a promising choice for the treatment of topical infections caused by A. baumannii and other Gram-negative bacteria (Li et al., 2021). ElyA1 was used to treat skin infections of A. baumannii in Galleria mellonella. ElyA1 showed a confirmed significant reduction in the number of bacteria (Blasco et al., 2020a). The P307SQ-8C reduced the bacterial burden by two logs in a mice model of A. baumannii cutaneous infection in 2 h (Thandar et al., 2016). LysAB2 P3 reduced bacterial burden in ascites and blood by 13-fold and 27-fold, respectively, in a mouse intraperitoneal infection model 4 h after bacterial injection. Furthermore, LysAB2 P3 saved 60% of mice infected with A. baumannii from deadly bacteremia (Peng et al., 2017). Repeated exposure to endolysins LysAm24, LysAp22, LysECD7, and LysSi3 that target Gram-negative bacteria are tested to determine the development of resistance. These endolysins function well in animal models of wound and burn skin infections, have a broad spectrum of activity, and are active against both planktonic bacteria and bacterial biofilms. Regarding safety, these enzymes do not cause cytotoxicity, do not contribute to the development of resistance, and do not disrupt the natural intestinal microbiota in vivo (Vasina et al., 2021). Endolysins specifically prevent specific species or subspecies of pathogenic bacteria, without disrupting the surrounding normal microbiota (Yoong et al., 2004). The endolysin PlyV12 causes less disruption of the normal microbiota when used to treat antibiotic-resistant bacterial strains and they do not transfer resistance genes or bacterial toxins and do not eliminate the colonized beneficial normal flora of mucous membranes (Rahimzadeh et al., 2018).
1.5 Endolysin against A. baumannii biofilm
Due to several resistance mechanisms, including biofilm formation, A. baumannii can survive and spread in the hospital environment (Salmani et al., 2020). Endolysin chemotherapy has a significant antibacterial effect on biofilm-associated bacteria, which are difficult to eliminate due to their minimal metabolic activity (Chang et al., 2022). Endolysin Abtn-4 treatment inhibited A. baumannii biofilm growth by more than 30%, suggesting that endolysin is a possibly effective antibacterial agent in regulating biofilm development (Yuan et al., 2021). The pre-treatment of catheters with endolysin PlyF307 decreased A. baumannii biofilm on the surface by 1.6 log10, resulting in a substantial decrease in total biofilm growth (Lood et al., 2015a). In an additional in vivo study, Lood et al. investigated the efficiency of PlyF307 in eliminating biofilm from subcutaneously implanted catheters colonized with 2-day-old A. baumannii biofilms in the intestines of mice. Their findings revealed that mice with fatal A. baumannii bacteremia were saved by PlyF307 (Lood et al., 2015a). Endolysin anti-biofilm activity should be evaluated in future studies implementing more clinically relevant models such as multispecies biofilm matrices and flow cell-based biofilm models, as well as pre-treatment processes on diverse substrates and surfaces in hospitals (Chang et al., 2022). The endolysin LysAB1245 showed broad lytic spectrum efficacy against MDR A. baumannii isolates from various main capsular types. Furthermore, LysAB1245 has the potential for use with nosocomial MDR A. baumannii infections and can control the biofilms in the clinical healthcare environment (Soontarach et al., 2024).
1.6 Synergistic effects of endolysin and antibiotic against A. baumannii
The outer membrane of Gram-negative bacteria acts as a barrier for many endolysins, and very few endolysins with exogenous activity against Gram-negative bacteria have been observed. Endolysins can target Gram-negative bacteria if the outer membrane is first permeated with chemicals such as Ethylenediaminetetraacetic acid (EDTA), which destabilizes the lipopolysaccharides of the outer membrane; but the combined use of endolysin and EDTA is restricted to superficial treatment of localized infection (Briers et al., 2011; Thummeepak et al., 2016b). Several studies have tried enhancing the muralytic effect of endolysins by using them with different antibiotics to take on their combined synergistic effects (Thummeepak et al., 2016b; Letrado et al., 2018). Using endolysin and antibiotics together to target Gram-negative bacteria has shown some promising outcomes (Lai et al., 2020a). The synergistic effect of LysABP-01 endolysin with seven commonly prescribed antibiotics against the MDR strain of A. baumannii was assessed. LysABP-01 and colistin together had a synergistic effect in vitro, with a fractional inhibitory concentration index ranging from 0.156 to 0.188. The encouraging findings demonstrated that colistin and LysABP-01’s minimum inhibitory concentrations (MICs) were lowered by up to 8 and 32 fold, respectively (Thummeepak et al., 2016a). Colistin, a ‘last-resort’ antibiotic, is commonly used to treat infections caused by MDR Gram-negative bacteria. However, due to its nephrotoxicity and neurotoxicity, the dose of colistin is extremely restrictive (Nation and Li, 2009). As a result, combining colistin with lysins may help optimize the clinical use of colistin at a lower dose. Blasco et al. recently demonstrated the synergistic effects of the endolysin ElyA1 and colistin in targeting various MDR Gram-negative bacteria including A. baumannii, in both in vitro and in vivo conditions. When colistin was combined with ELyA1, the MIC of colistin was lowered by at least 4-fold for all tested A. baumannii strains (Blasco et al., 2020a). In both skin and lung infection models, the in vivo survival rate for A. baumannii infected G. mellonella and bacterial reduction of infected mice treated with ELyA1 and colistin combination was considerably greater than that of the colistin-alone. Colistin can operate as an OMPto break the integrity of the OM, allowing lysin access to the PG substrates. Simultaneously, peptidoglycan cleavage by endolysin may increase antibiotic absorption and hence promote antibiotic action (Gerstmans et al., 2018a). These positive results show that combining endolysin and antibiotics has the potential to resensitize bacterial pathogens with drug resistance to antibiotics. And to slow the spread of antibiotic resistance by using fewer antibiotic treatments of low dosages (Lai et al., 2020a). Combined use of endolysin LysAB-vT2-fusion with colistin, polymyxin B, or copper showed synergistic against A. baumannii, which may be used for the control of A. baumannii infection (Sitthisak et al., 2023b).
2 Clinical development of endolysin
Protein prescription drugs are a rapidly growing sector of the pharmaceutical industry. According to THPdb, a curated database of FDA-approved therapeutic proteins and peptides, there are 239 approved proteins and peptides, as well as 380 recognized variants of these proteins/peptides (Usmani et al., 2017; Wishart et al., 2018). Protein enzymes have significant advantages as medications because of their high selectivity, proteinaceous composition, which eliminates chemical toxicity, and tremendous potential for modification and consequent development (Labrou, 2019). Endolysins have been recognized by the WHO as a novel, non-traditional antimicrobial (WHO (Organization, 2022). Several biotechnology and pharmaceutical companies have been performing human clinical trials utilizing endolysins. ContraFect, a biotech company located at Rockefeller University, obtained ownership rights to nine phage-derived endolysin. This company particularly focuses on endolysin therapy for bacterial infectious diseases. ContraFect conducts a phase III clinical medicine trial on endolysin (identifier#: NCT04160468). At the same time, iNtRON Biopharma completed a phase III clinical trial of N-Rephasin Sal200 in 2021. (clinicalTrials.gov, accessed on 21 June 2022, identifier#: NCT03089697). In February 2020, the FDA recognized endolysin innovations as antibacterial biological drugs by recognizing the ContraFect endolysin in the phase III study as “Breakthrough Therapy.” Pre-clinical development of additional endolysins is now underway in both industries and universities (Harhala et al., 2022). Exebacase “Endolysin CF-301” is a recombinant endolysin developed by ContraFects for use against a variety of Streptococcus and Staphylococcus species in the treatment of infective endocarditis in humans. This endolysin was the first of its class to enter human clinical trials in the United States. In phase II clinical studies, endolysin enhanced the recovery rate of methicilin-resistant S. aureus (MRSA) induced infectious endocarditis by 42.8% when used in combination with regular antibiotic treatment (Watson et al., 2019). Another phage-derived endolysin that has shown clinical efficacy is SAL200. SAL200, also known as N-Rephasin® SAL200, was found to be an acceptable medication for intravenous administration in this trial. Thirty-four healthy male volunteers received SAL200 at varying doses, There were no significant adverse effects noted during the endolysin pharmacokinetics study in the human body and its tolerance to intravenous administration (Jun et al., 2017).
3 Challenges of endolysin therapy transferring from the lab to the clinic
Totté et al. reported three clinical cases in which patients with persistent and recurring S. aureus-related skin infections were successfully treated with Staphefekt SA.100. Staphefekt SA.100 is a synthetic phage endolysin designed for topical skin application. Staphefekt is currently registered as a (class 1) medical device in Europe and is readily accessible over the counter as a cetomacrogol-based cream and gel (Totté et al., 2017). Although increased interest has been directed to investigating the potential of endolysin to target MDR Gram-negative bacteria, there are several challenges to its clinical application. These include questions about the safety of OMPs with endolysin, the application of outcomes from animal models of acute infection to clinical practice, and the effectiveness and stability of endolysin. Protein engineering and formulation design for effective distribution and stabilization of Gram-negative endolysin could be some options for advancing Gram-negative endolysin development for clinical testing before regulatory authorization (Lai et al., 2020a).
Figure 1 describes the current challenges of endolysin use in the clinic and healthcare market.
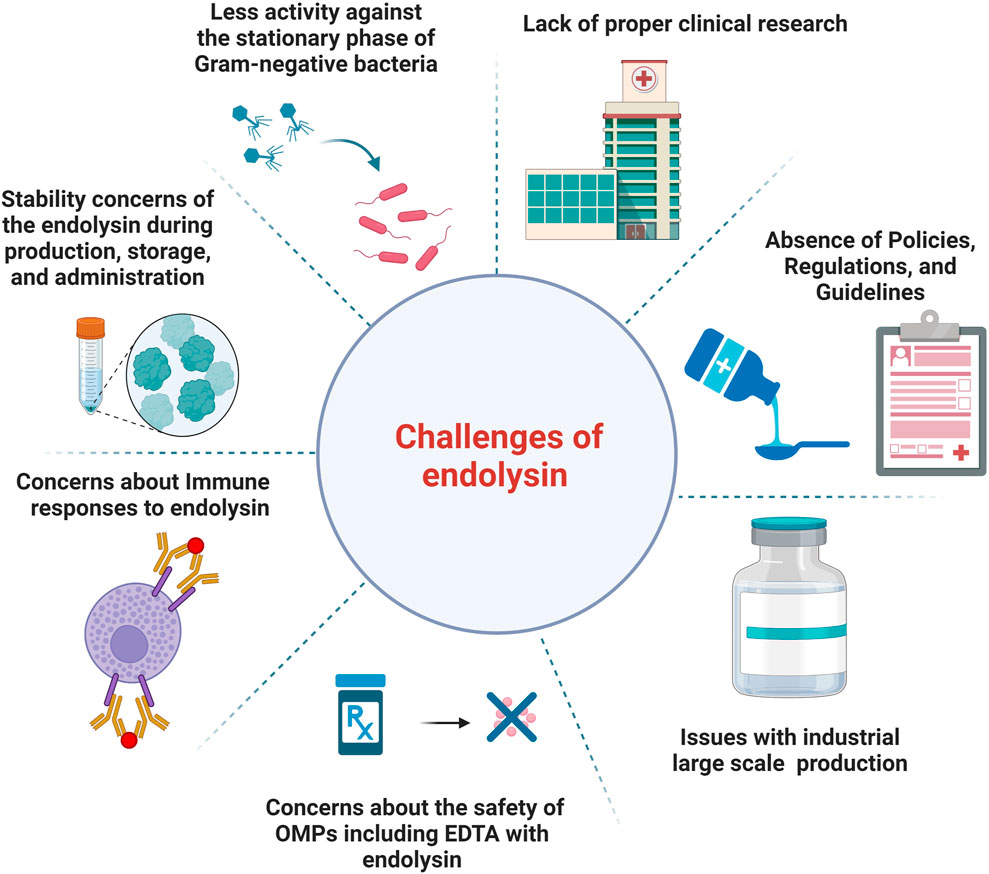
Figure 1. An illustration of the current challenges of endolysins use in the clinic and healthcare market.
3.1 Concerns about the safety of OMPs with endolysin
In Gram-negative bacteria, the outer membrane functions as a barrier to several endolysins.
Few endolysins with exogenous activity against Gram-negative bacteria have been reported and the majority are synthetically engineered endolysins (Lai et al., 2011b; Briers et al., 2014a; Briers et al., 2014b; Lim et al., 2014; Lood et al., 2015; Soontarach et al., 2024).
OMPs have the potential to significantly increase the antibacterial activity of endolysin while also broadening their host spectrum. However, the in-vivo study of the efficacy of endolysin and OMPs in combination is rarely described in the literature. The harmful effects of the most often used OMPs such as EDTA through various delivery routes, such as oral, intravenous, intraperitoneal, topical, and inhalation, have been investigated (Lanigan and Yamarik, 2002). EDTA is cytotoxic, slightly genotoxic, and has an anticoagulant effect. It has been observed that the low EDTA dose required to cause toxicity in rats was 750 mg/kg/day. As a result, the use of EDTA with endolysin should be restricted to topical applications because it cannot affect the skin and does not produce skin hypersensitivity (Lanigan and Yamarik, 2002). Citric acid is also used as an OMP, but despite having a much better safety profile than EDTA, more testing is required because citric acid also has hazardous consequences, When used in combination with endolysin, particularly for the inhalation route, as it has been linked to animal obstruction of the airway and cause reactions in humans coughing (Allott et al., 1980). With further work being done on combining endolysin with polycationic peptides to enable them to target Gram-negative bacteria, their therapeutic use should be carefully evaluated. This is because endolysin with OM-disrupting characteristics or those coupled with peptide OMPs may also interact with eukaryotic cell membranes, raising safety issues (Schmelcher et al., 2012).
3.2 Weak effectiveness against the stationary phase of Gram-negative bacteria
The log-growth phase bacteria are more susceptible to endolysin treatments than the stationary phase bacteria. Oliveira et al. presented that the lower bactericidal efficiency against the bacterial stationary cells is due to modifications to the structure in the outer membrane, such as LPS biosynthesis, which affects endolysin permeability that even in the presence of OMPs, or chemical modifications of the peptidoglycan compositions (Oliveira et al., 2014). These alterations could aid bacterial cells in coping with endolysins via acetylation, N-deacetylation, and amidation (Typas et al., 2012). A significant number of findings show anti-bacterial efficacy against the log-phase bacteria. To overcome this research gap, and to completely investigate the potency of endolysin against Gram-negative bacteria, further research is required to evaluate the antibacterial efficiency of endolysins against the stationary phase bacteria. Regarding the concern of the resistance of bacteria to endolysin; The resistance development of endolysin was evaluated in which bacterial strains were repeatedly challenged with subinhibitory doses of endolysins or artilysins failed to give rise to resistant mutants, although bacterial strains exposed to control antibiotics got resistance. The probability of developing resistance against endolysins and artilysins is thus regarded as minimal, especially when compared to standard antibiotics (Fischetti, 2005; Briers et al., 2014a).
3.3 Concerns about stability
The stability of the endolysin during production, storage, and administration is essential for its prospective use as an antibacterial agent. Several stability concerns of Gram-negative endolysins have been observed in preclinical studies and must be solved for further research and development (Lai et al., 2020a). The oral delivery route is the most widely used for gut-targeted medicines and is also the most well-liked by patients. Most medications available on the market are used orally and come in tablet or liquid form. This is not without its challenges, though. Because medications of this kind have to pass through the stomach and digestive tract, they are instantly exposed to a variety of enzymes, varying pH levels, and mechanical digestion. All of these may have an impact on the drug’s molecular integrity and systemic bioavailability (Murray et al., 2021). Endolysins are proteinaceous, therefore they can be swiftly damaged by these processes, rendering them ineffective. Stomach acid has the potential to damage the structure of several endolysins. Proteases such as trypsin, chymotrypsin, pepsin, and peptidase degrade proteins. Many endolysins contain cleavage sites that these enzymes can target (Bruno et al., 2013).
3.3.1 Inactivation of endolysin in physiological conditions
Environmental conditions such as salts and proteins are well recognized to have a major impact on the activity of Gram-negative endolysins. Khan et al. reported that the A. baumannii endolysins LysAB54 entirely loses its antibacterial efficacy in the serum, limiting its use to topical infections such as burn wounds or joint infections. One possible explanation for the reduction of LysAB54 activity in the serum is endolysin ion exchange, which might potentially neutralize endolysin antibacterial activity (Khan et al., 2021). Endolysin inactivation in human serum could be associated with the conjugation and passivation of positively charged peptides on endolysin by negatively charged serum molecules. The neutralized cationic domain may result in the lack of intrinsic OM permeabilizing activity (Yang et al., 2015).
3.3.2 Thermostability
Because of their proteinaceous composition, endolysins are temperature-sensitive. Temperature effects on endolysin activity have been extensively studied since protein thermostability can affect functionality and storage stability. Most endolysin perform best activities between 30°C and 40 °C, making them useful for clinical use (Lai et al., 2020b).
3.3.3 Storage stability
Drugs with a moderately extended shelf life (12 months) under refrigeration (2°C–8°C) or at room temperature (20°C–25°C) are preferable for commercial viability. Products for freezer storage (−20°C ± 5°C) should have at least a 12-month shelf-life (Guideline, 2003). However, there is a lack of information regarding the Gram-negative endolysin storage stability, and what little that is available only covers short-term storage—from 1 week to six months—under various storage environments (Lai et al., 2020b).
3.4 Concerns about immune responses to endolysins
One of the limitations of endolysin is its short in vivo half-life, caused by the inflammatory response of cytokines and neutralizing antibodies. Endolysin elicits an immune system response when used repeatedly, and as a result of the immune response, it loses its enzyme-mediated lytic activity in vivo (Nau and Eiffert, 2002; Jado et al., 2003; Abdelrahman et al., 2021a). Raz et al. described a novel approach to discovering immune-derived engineered endolysins. The engineering of CBDs of different endolysins fusions to the Fc receptor of human IgG antibodies. The engineered lysin called Lysibodies binds to S. aureus cells and opsonizes them, causing immune complement system activation, which can cause phagocytosis and clearance of the bacteria. Through this approach, endolysin can be used to target an immune response toward pathogenic bacteria (Raz et al., 2018). A similar approach without using antibody fragments was described for a protein derived from the CBD of the endolysin PlyV12 (Yang et al., 2018). As some of the Gram-negative endolysin enter preclinical development, concerns about immunogenicity, toxicity from lipopolysaccharide release during the bactericidal process, and pharmacokinetic issues due to the complexity of the Gram-negative cell wall must be addressed (Ghose and Euler, 2020). We suggested a strategy to minimize the body’s immune responses to endolysin. Engineered endolysins should be designed with human immune cells. When such proteins are expressed, the immune cells will recognize them as their body proteins, so there will be fewer immune reactions to these foreign proteins and less chance of the clearance of such foreign proteins in the body.
3.5 Lack of proper clinical research
In February 2020, the FDA recognized endolysin innovations as antibacterial biological drugs in the phase iii study as “Breakthrough Therapy” (Harhala et al., 2022). However, the fundamental obstacle to the use of endolysins as a therapeutic agent is the lack of proper clinical research to provide an excellent scientific fundamental basis for approval. We suggested that additional research on endolysins affecting their safety in humans will be beneficial to get past the reservations point of view on endolysins’ usage and create the legal framework for the approval of endolysins. Preclinical trials of more endolysins are needed to bring endolysin into the medical market for treatment purposes.
3.6 Absence of policies, regulations, and guidelines
Numerous potential endolysin applications are currently being reported in medicine, but there are no published guidelines and regulations for endolysins. Early and effective communication with the relevant authorities is essential to establish endolysin regulatory pathways in their use as a medicine in the health sector and medicine industry. However, there are currently no clear legal principles or laws governing the use of endolysins.
3.7 Issues with large-scale industrial production
Endolysins have made great progress towards clinical application as novel antibacterial treatments. Another major problem that must be addressed is the large-scale industrial manufacture of endolysin. The present cost of endolysin production is projected to be expensive, which may be a major barrier to the use of endolysins as antibacterial replacements (Oliveira et al., 2012). The high cost arises mostly from the necessity for the development of recombination engineering, effective, and safe expression platforms for endolysin expression and purification. As a result, technical techniques to increase the solubility and expression levels of target endolysins, as well as cost-effective expression systems, are required to make endolysins commercially acceptable antibacterial agents worth investing in (Lee et al., 2023). When transferring the therapeutic endolysin expression and purification from the lab to the industrial scale, manufacturing costs and safety concerns must be addressed. This applies to the entire manufacturing process, from the selection of the expression host. From the beginning of protein expression until the last stages of processing, there are numerous chances to lower production costs and improve safety. Cell harvesting, cell lysis, and purification are examples of downstream processing procedures that are carried out once endolysins have been engineered and reassembled in a particular expression system. Toxic effects must be avoided, especially when it comes to protein expression in bacteria where endotoxin must be eliminated. Furthermore, early in the production process, choosing the best expression hosts—such as bacteria, yeast, or plants—is a crucial step toward achieving overexpression and cheap expression costs. Because they are inexpensive to produce, plants have been suggested as potential hosts for recombinant protein production. Furthermore, plant-produced proteins are likely safer than those made from bacteria or animal cells (Magnusdottir et al., 2013).
3.7.1 High dose of endolysin needed for efficient killing of Gram-negative bacteria
The majority of Gram-negative bacteria require dosages of 100–500 μg/mL of endolysin to be eradicated, which is significantly higher than that of their Gram-positive counterparts, which commonly need doses of less than 10 μg/mL (Abouhmad et al., 2016; Gerstmans et al., 2018b). The development of commercially viable products might encounter production and formulation issues due to the high concentration of endolysin needed. Reducing the amount of endolysin dose by boosting the potency of Gram-negative endolysin is an efficient way to deal with this problem. Protein engineering could be able to perform this higher lytic activity, meaning that, in comparison to natural lysins, a lower concentration (<100 μg/mL) of modified endolysin is needed to exert effective bacterial killing. The logical design of engineered endolysin is crucial for successful modification to improve activity and stability. To attain this goal, knowledge of the endolysin tertiary or crystal structures will be required (Lai et al., 2020b).
3.8 Limited availability of in vivo data
So far, the effectiveness of Gram-negative endolysin in vivo has only been assessed using acute infection models. There are fewer documented chronic in vivo models for endolysin assessment, except for topical infection research investigations (Lai et al., 2020b). The current in vivo research on Gram-negative endolysin has been focused on assessing the rates of survival and/or reduction of bacterial burden to validate their effectiveness. Before continued research, issues with immunogenicity, pharmacokinetics, and the production of proinflammatory components during bacteriolysis must be resolved for the systemic use of Gram-negative endolysin. Many Gram-positive endolysins had problems being cleared by neutralizing antibodies because they were proteinaceous, which resulted in a relatively short half-life in vivo—between 4 and 40 min, depending on the type of endolysin (Gerstmans et al., 2018b).
4 Overcoming challenges of Gram-negative endolysins through genetic engineering
The majority of naturally occurring endolysins are lytic against Gram-positive bacteria. In Gram-negative bacteria, the outer membrane inhibits endolysin from accessing the target peptidoglycan. Various scientific and formulation solutions have been developed throughout the years for tackling the outer membrane barrier (De Maesschalck et al., 2020). Modular endolysins offer a unique opportunity for scientists to engineer proteins to change bacteriolytic activity, solubility, specificity, and various other physicochemical properties (Haddad Kashani et al., 2018). The shortening of a full-length enzyme, site-directed mutagenesis, or different fusions such as EADs with cell wall binding domain CBDs of distinct endolysin, virion-associated endolysin with CBD of a different endolysin, endolysin fusion with antimicrobial peptides are examples of engineering techniques (Love et al., 2018).
4.1 Formulation strategies
Formulation techniques include the use of different carrier systems. The use of OMP (chelators such as citric acid, lactic acid, malic acid, acetic acid, benzoic acid and EDTA in the formulation is useful in permeabilizing the outer membrane of Gram-negative bacteria to endolysins (Oliveira et al., 2016a). Using OMP is the simplest way to get over the outer membrane barrier. The most often utilized permeabilizer in this scenario is EDTA, which works by chelating the outer membrane stabilizing divalent cations (Kocot et al., 2023).
4.2 Endolysin encapsulation system
Despite their therapeutic effects, bacteriophages and endolysins face some practical challenges posed by the host system, such as low bioavailability, losing activity, non-targeted delivery, rapid elimination by the retinal endothelial system, and antibody-mediated deactivation. Against this context, there has been an increase of interest among scientists to investigate the possibilities of delivery systems for encapsulating bacteriophages and endolysins. In recent years, a multitude of bacteriophage and endolysin encapsulation strategies have been reported (Loh et al., 2021). Similarly, nanoencapsulation can help improve the therapeutic potential by not only shielding endolysin from degradation but also allowing for continuous release, potentially boosting stability, shelf life, and therapeutic efficacy (Gondil and Chhibber, 2021). Liposomes’ ability to penetrate bacterial outer membranes through membrane fusion is also a viable way for delivering endolysin to Gram-negative bacteria. The authors achieved successful lysis of both Salmonella typhimurium and E. coli with endolysin BSP16Lys-containing liposomes without the need for a membrane permeabilizer (Bai et al., 2019b). These examples highlight the need to utilize these existing strategies to improve the potential of endolysin as an effective and sustainable therapy.
4.3 Endolysin delivery using liposomes
It has been observed that endolysin encapsulated in liposomes enhances the efficacy of endolysins against Gram-negative bacteria (Bai et al., 2019a). The method of packing lytic proteins derived from bacteriophage in the structure of liposomes appears to be a promising weapon against Gram-negative bacteria. Like dendrimers, liposomes can also have a protective role that maintains the endolysin activity (Kocot et al., 2023).
4.4 Endolysin delivery using chitosan nanoparticles
Encapsulation in alginate-chitosan nanoparticles is another strategy for increasing the efficacy of endolysin, particularly for use in therapy (Gondil and Chhibber, 2021). Chitosan-based formulations provide benefits over alternative delivery systems in that they boost therapeutic agent bioavailability while also properly removing from the host after the release of the agent (Gondil and Chhibber, 2021).
4.5 Breaching the barrier of the outer membrane of Gram-negative bacteria
Because the outer membrane (OM) of Gram-negative bacteria prevents externally applied endolysins from accessing peptidoglycan, controlling the growth of these Gram-negative pathogenic bacteria is difficult (Sisson et al., 2024b).
4.5.1 Synergy through permeabilizing molecules
Chemical permeabilizers are a useful tool for facilitating endolysins’ entry into the periplasm. Divalent metal ion (Mg2+ and Ca2+) chemical chelate stabilizes the lipopolysaccharide (LPS) layer, degrades and permeabilizes the OM, and makes the peptidoglycan accessible to endolysins (Oliveira et al., 2016b). Citric and malic acids are examples of organic acids that permeabilize the OM and improve endolysins’ ability to kill Gram-negative bacteria (Sisson et al., 2024a). Endolysins work in combination with additional compounds that disrupt the OM. Oregano and thyme essential oils, carvacrol, have been shown to enhance endolysin activity against Gram-negative bacteria and could cause the breakdown of lipopolysaccharides. Moreover, colistin, polymyxin B, and ε-poly-L-lysine, which are cationic chemicals, work in combination with endolysins by either dissolving or competitively displacing OM cations. In summary, many chemicals allow endolysins to access peptidoglycan and promote cell lysis by damaging or permeabilizing the OM (Blasco et al., 2020b; Ning et al., 2021; Hong and Myung, 2022).
4.5.2 Synergy through bacterial receptors
An alternate method for increasing endolysin activity is to enhance its attachment to and permeation of the OM. Adding endolysins to proteins allows them to attach to bacterial cell surface receptors. For example, ‘Lysocins’ are endolysin-bacteriocin fusions (Martínez et al., 2016; Heselpoth et al., 2019). Some bacteriocins produce pores in a membrane by binding to OM phospholipids and specific surface components, such as receptors and transporters, allowing the endolysin to pass through to the peptidoglycan (Martínez et al., 2016). Innolysins include the attachment of endolysin EADs to phage receptor-binding proteins (RBPs) and provide a second way of breaking through the OM (Zampara et al., 2020).
4.6 Engineering of endolysin with antimicrobial peptide
Some endolysins contain peptides, typically at their C-terminus, that have a strong antibacterial activity that is not dependent on peptidoglycan breakdown. These antimicrobial peptides (AMPs) are responsible for some endolysins natural capacity to penetrate the outer membrane of Gram-negative bacteria (Szadkowska et al., 2022; Kocot et al., 2023). Predicting AMP-like regions is a good technique for designing endolysin with an intrinsic antibacterial activity that can serve as a framework for further engineering (Kocot et al., 2023).
However, Gram-negative bacteria are difficult to regulate due to the presence of an outer membrane that protects the peptidoglycan layer from enzymatic breakdown. To surpass this threshold, the fusion of endolysin with a sensitizer peptide is known to extend efficacy by disrupting the outer membrane of Gram-negative bacteria (Son et al., 2023). The sensitizer peptide-fused endolysin Lys1S-L9P has highly effective bactericidal activity against various MDR Gram-negative bacteria. Lys1S-L9P displayed strong antibacterial action against MDR Gram-negative bacteria in the G. mellonella in vivo model, with no harmful effect. Sensitizer peptide-fused endolysin could be a useful biocontrol agent against MDR Gram-negative bacteria (Son et al., 2023).
5 Applications of endolysins
5.1 Endolysin is a disinfectant agent in the healthcare environment
A. baumannii is a leading contributor to nosocomial infections; it is becoming more and more linked to different epidemics and is a serious worry in intensive care units of hospitals across the globe (Almasaudi, 2018). Nosocomial infections resulting from multidrug-resistant bacteria are life-threatening (Choi et al., 2022). Secondly, the formation of bacterial biofilms on medical equipment is one of the biggest problems in the healthcare system (Gutiérrez et al., 2012). Bacteria trapped in biofilms are less sensitive because they grow more slowly and have restricted access to antibiotics and disinfectants (Davies, 2003). The bacteria-reduced susceptibility to antimicrobial agents within the biofilm that exhibited a more resistant phenotypic condition has also been linked to recurring infections (Lewis, 2007). Endolysins break down the bacterial cell wall peptidoglycan and could potentially help in controlling the spread of infections of MDR bacteria seen in hospitals (Choi et al., 2022). This revealed the endolysin potential as an environmentally friendly replacement for hospital-use harmful chemical disinfectants (Choi et al., 2022).
5.2 Role of endolysin in therapeutic protein delivery
Vaccination, which stimulates the immune system to enhance adaptive immunity through the injection of antigenic material, is one of the greatest public health achievements. Viral nanoparticles (VNPs) have been a focus of recent research as delivery vehicles for protein and peptide-based vaccinations (Rodríguez-Rubio et al., 2016b). Bacteriophage-derived nanoparticles have drawn the attention of researchers since foreign peptides or proteins can be expressed on the surface of phages as fusion proteins. As a result, phage-displayed peptides or proteins have been proven to be functionally and immunologically active, making them appropriate for vaccine development (Hamzeh-Mivehroud et al., 2013). Endolysin can also be employed as a binding agent for displaying heterologous proteins on the surfaces of bacteria in the hunt for live vaccination delivery systems. The endolysin CBDs, can be fused with various other proteins such as antigens to show them at the bacterial surface while retaining their structure and activity (Loessner et al., 2002). Surface display technology based on bacteriophage-derived endolysins has been developed using lactic acid bacteria (Hu et al., 2010; Ribelles et al., 2013). It has been shown that the external addition of the E7 antigen from human papillomavirus type-16 (HPV-16) when fixed on the surface of lactic acid bacteria by the CBD of Lactobacillus casei A2 phage endolysin, secure mice from an HPV-16 attack and its associated tumors. After being immunized via intranasal vaccination with lactic acid bacteria expressing E7 antigen, more than 60% of the mice remained tumor-free. Thus, the surface display of endolysins CBDs is a fascinating method for effectively and safely delivering therapeutic proteins (Ribelles et al., 2013). Figure 2, illustrates the applications of bacteriophage-derived endolysin.
6 Conclusion
Due to the lack of antibiotic development for Gram-negative bacterial infections, in a scenario in which no curative therapies are available, there is an urgent need to find innovative antibacterial drugs. While bacteriophage-encoded endolysin has been regarded as an alternative therapeutic agent against bacterial infections. For Gram-positive bacteria, there are various ongoing clinical trials in Phase I and Phase III, while endolysin development against Gram-negative bacterial infections is lagging. The presence of an outer membrane on the Gram-negative bacteria is a key barrier to its development because it prevents endolysin from accessing the underlying peptidoglycan substrates, limiting their potency. For this reason, increasing interest has been focused on permeabilizing endolysin that exerts lytic activity against Gram-negative bacteria throughout the outer membrane. Endolysin with synergistic effects with other antibacterial drugs against A. baumannii are summarized and described. To move clinical development forward, several challenges must be overcome, including safety concerns about the Gram-negative bacteria membrane-disrupting permeabilizers including EDTA, a lack of knowledge about immunogenicity and pharmacokinetics, lower potency against the stationary phase of Gram-negative bacteria and stability issues in serum and blood. While further in vivo research is needed to understand the safety and distribution profiles of endolysin after administration, protein engineering and formulation sciences are promising ways to improve the efficacy and stability of Gram-negative endolysin.
Author contributions
FK: Writing–review and editing, Writing–original draft, Investigation, Funding acquisition, Conceptualization. FR: Writing–review and editing, Writing–original draft, Software, Investigation, Conceptualization. YY: Writing–review and editing, Software, Investigation, Formal Analysis. BL: Writing–review and editing, Supervision, Investigation, Funding acquisition, Conceptualization. RZ: Writing–review and editing, Supervision, Resources, Project administration, Funding acquisition, Conceptualization.
Funding
The author(s) declare that financial support was received for the research, authorship, and/or publication of this article. This work is financially supported by the Research Fund for International Young Scientists, Grant number 32250410292, from the National Natural Science Foundation of China (NSFC) to FK. This study was also supported by the Southern Marine Science and Engineering Guangdong Laboratory (Zhuhai) (SML2023SP218), the Guangdong Major Project of Basic and Applied Basic Research (2023B0303000017) and the Innovation Team Project of Universities in Guangdong Province (No. 2023KCXTD028).
Acknowledgments
BioRender.com was used to generate figures.
Conflict of interest
The authors declare that the research was conducted in the absence of any commercial or financial relationships that could be construed as a potential conflict of interest.
Publisher’s note
All claims expressed in this article are solely those of the authors and do not necessarily represent those of their affiliated organizations, or those of the publisher, the editors and the reviewers. Any product that may be evaluated in this article, or claim that may be made by its manufacturer, is not guaranteed or endorsed by the publisher.
References
Abdelkader, K., Gutiérrez, D., Grimon, D., Ruas-Madiedo, P., Lood, C., Lavigne, R., et al. (2020). Lysin LysMK34 of Acinetobacter baumannii bacteriophage PMK34 has a turgor pressure-dependent intrinsic antibacterial activity and reverts colistin resistance. Appl. Environ. Microbiol. 86, e01311-20–e01320. doi:10.1128/AEM.01311-20
Abdelkader, K., Gutiérrez, D., Tamés-Caunedo, H., Ruas-Madiedo, P., Safaan, A., Khairalla, A. S., et al. (2022). Engineering a lysin with intrinsic antibacterial activity (LysMK34) by cecropin a fusion enhances its antibacterial properties against Acinetobacter baumannii. Appl. Environ. Microbiol. 88, 01515211. doi:10.1128/AEM.01515-21
Abdelrahman, F., Easwaran, M., Daramola, O. I., Ragab, S., Lynch, S., Oduselu, T. J., et al. (2021a). Phage-encoded endolysins. Antibiotics 10, 124. doi:10.3390/antibiotics10020124
Abdelrahman, F., Easwaran, M., Daramola, O. I., Ragab, S., Lynch, S., Oduselu, T. J., et al. (2021b). Phage-encoded endolysins. Antibiot. (Basel). 10, 124. doi:10.3390/antibiotics10020124
Abouhmad, A., Mamo, G., Dishisha, T., Amin, M., and Hatti-Kaul, R. J. J. O. a.M. (2016). T4 lysozyme fused with cellulose-binding module for antimicrobial cellulosic wound dressing materials. J. Appl. Microbiol. 121, 115–125. doi:10.1111/jam.13146
Allott, C., Evans, D., and Marshall, P. (1980). A model of irritant-induced bronchoconstriction in the spontaneously breathing Guinea-pig. Br. J. Pharmacol. 71, 165–168. doi:10.1111/j.1476-5381.1980.tb10921.x
Almasaudi, S. B. (2018). Acinetobacter spp. as nosocomial pathogens: epidemiology and resistance features. Saudi J. Biol. Sci. 25, 586–596. doi:10.1016/j.sjbs.2016.02.009
Bai, J., Jeon, B., and Ryu, S. (2019a). Effective inhibition of Salmonella Typhimurium in fresh produce by a phage cocktail targeting multiple host receptors. Food Microbiol. 77, 52–60. doi:10.1016/j.fm.2018.08.011
Bai, J., Yang, E., Chang, P.-S., and Ryu, S. (2019b). Preparation and characterization of endolysin-containing liposomes and evaluation of their antimicrobial activities against gram-negative bacteria. Enzyme Microb. Technol. 128, 40–48. doi:10.1016/j.enzmictec.2019.05.006
Behling, A. H., Wilson, B. C., Ho, D., Virta, M., O’sullivan, J. M., and Vatanen, T. J. C. O. I. M. (2023). Addressing antibiotic resistance: computational answers to a biological problem? Curr. Opin. Microbiol. 74, 102305. doi:10.1016/j.mib.2023.102305
Blair, J. M., Webber, M. A., Baylay, A. J., Ogbolu, D. O., and Piddock, L. J. J. N. R. M. (2015). Molecular mechanisms of antibiotic resistance. Nat. Rev. Microbiol. 13, 42–51. doi:10.1038/nrmicro3380
Blasco, L., Ambroa, A., Trastoy, R., Bleriot, I., Moscoso, M., Fernández-Garcia, L., et al. (2020a). In vitro and in vivo efficacy of combinations of colistin and different endolysins against clinical strains of multi-drug resistant pathogens. Sci. Rep. 10, 7163. doi:10.1038/s41598-020-64145-7
Blasco, L., Ambroa, A., Trastoy, R., Bleriot, I., Moscoso, M., Fernández-Garcia, L., et al. (2020b). In vitro and in vivo efficacy of combinations of colistin and different endolysins against clinical strains of multi-drug resistant pathogens. Sci. Rep. 10, 7163. doi:10.1038/s41598-020-64145-7
Briers, Y., Volckaert, G., Cornelissen, A., Lagaert, S., Michiels, C. W., Hertveldt, K., et al. (2007). Muralytic activity and modular structure of the endolysins of Pseudomonas aeruginosa bacteriophages phiKZ and EL. Mol. Microbiol. 65, 1334–1344. doi:10.1111/j.1365-2958.2007.05870.x
Briers, Y., Walmagh, M., Grymonprez, B., Biebl, M., Pirnay, J.-P., Defraine, V., et al. (2014a). Art-175 is a highly efficient antibacterial against multidrug-resistant strains and persisters of Pseudomonas aeruginosa. Antimicrob. Agents Chemother. 58, 3774–3784. doi:10.1128/AAC.02668-14
Briers, Y., Walmagh, M., and Lavigne, R. J. J. O. a.M. (2011). Use of bacteriophage endolysin EL188 and outer membrane permeabilizers against Pseudomonas aeruginosa. J. Appl. Microbiol. 110, 778–785. doi:10.1111/j.1365-2672.2010.04931.x
Briers, Y., Walmagh, M., Van Puyenbroeck, V., Cornelissen, A., Cenens, W., Aertsen, A., et al. (2014b). Engineered endolysin-based “Artilysins” to combat multidrug-resistant gram-negative pathogens. mBio 5, e01379–e01314. doi:10.1128/mBio.01379-14
Bruno, B. J., Miller, G. D., and Lim, C. S. J. T. D. (2013). Basics and recent advances in peptide and protein drug delivery. Ther. Deliv. 4, 1443–1467. doi:10.4155/tde.13.104
Chang, R. Y. K., Nang, S. C., Chan, H.-K., and Li, J. (2022). Novel antimicrobial agents for combating antibiotic-resistant bacteria. Adv. Drug Deliv. Rev. 187, 114378. doi:10.1016/j.addr.2022.114378
Chen, X., Liu, M., Zhang, P., Leung, S. S. Y., and Xia, J. (2021). Membrane-permeable antibacterial enzyme against multidrug-resistant Acinetobacter baumannii. ACS Infect. Dis. 7, 2192–2204. doi:10.1021/acsinfecdis.1c00222
Choi, Y.-J., Kim, S., Bae, S., Kim, Y., Chang, H.-H., and Kim, J. (2022). Antibacterial effects of recombinant endolysins in disinfecting medical equipment: a pilot study. Front. Microbiol. 12, 773640. doi:10.3389/fmicb.2021.773640
Chu, J. J. K., Poh, W. H., Hasnuddin, N. T. B., Hew, E. Y., Dam, L. C., Sahili, A. E., et al. (2022). Novel phage lysin Abp013 against Acinetobacter baumannii. Antibiotics 11, 169. doi:10.3390/antibiotics11020169
Davies, D. (2003). Understanding biofilm resistance to antibacterial agents. Nat. Rev. Drug Discov. 2, 114–122. doi:10.1038/nrd1008
Defraine, V., Schuermans, J., Grymonprez, B., Govers, S. K., Aertsen, A., Fauvart, M., et al. (2016). Efficacy of artilysin Art-175 against resistant and persistent Acinetobacter baumannii. Antimicrob. agents Chemother. 60, 3480–3488. doi:10.1128/AAC.00285-16
De La Fuente-Nunez, C., Cesaro, A., and Hancock, R. E. J. D. R. U. (2023). Antibiotic failure: beyond antimicrobial resistance, 101012.
De Maesschalck, V., Gutiérrez, D., Paeshuyse, J., Lavigne, R., and Briers, Y. (2020). Advanced engineering of third-generation lysins and formulation strategies for clinical applications. Crit. Rev. Microbiol. 46, 548–564. doi:10.1080/1040841X.2020.1809346
Ding, D., Wang, B., Zhang, X., Zhang, J., Zhang, H., Liu, X., et al. (2023). The spread of antibiotic resistance to humans and potential protection strategies. Ecotoxicol. Environ. Saf. 254, 114734. doi:10.1016/j.ecoenv.2023.114734
Fischetti, V. a.J. T. I. M. (2005). Bacteriophage lytic enzymes: novel anti-infectives. Trends Microbiol. 13, 491–496. doi:10.1016/j.tim.2005.08.007
Gerstmans, H., Criel, B., and Briers, Y. (2018a). Synthetic biology of modular endolysins. Biotechnol. Adv. 36, 624–640. doi:10.1016/j.biotechadv.2017.12.009
Gerstmans, H., Criel, B., and Briers, Y. J. B. A. (2018b). Synthetic biology of modular endolysins. Biotechnol. Adv. 36, 624–640. doi:10.1016/j.biotechadv.2017.12.009
Ghose, C., and Euler, C. W. J. A. (2020). Gram-negative bacterial lysins. Antibiot. (Basel). 9, 74. doi:10.3390/antibiotics9020074
Gondil, V. S., and Chhibber, S. (2021). Bacteriophage and endolysin encapsulation systems: a promising strategy to improve therapeutic outcomes. Front. Pharmacol. 12, 675440. doi:10.3389/fphar.2021.675440
Gondil, V. S., Khan, F. M., Mehra, N., Kumar, D., Khullar, A., Sharma, T., et al. (2021a). “Clinical potential of bacteriophage and endolysin based therapeutics: a futuristic approach,” in Microbial products for health, environment and agriculture (Springer), 39–58.
Gondil, V. S., Khan, F. M., Mehra, N., Kumar, D., Khullar, A., Sharma, T., et al. (2021b). Clinical potential of bacteriophage and Endolysin based therapeutics: a futuristic approach, 39–58. Environment, and Agriculture.
Gutiérrez, D., and Briers, Y. J. C. O. I. B. (2021). Lysins breaking down the walls of Gram-negative bacteria, no longer a no-go. Curr. Opin. Biotechnol. 68, 15–22. doi:10.1016/j.copbio.2020.08.014
Gutiérrez, D., Delgado, S., Vázquez-Sánchez, D., Martínez, B., Cabo, M. L., Rodríguez, A., et al. (2012). Incidence of Staphylococcus aureus and analysis of associated bacterial communities on food industry surfaces. Appl. Environ. Microbiol. 78, 8547–8554. doi:10.1128/AEM.02045-12
Haddad Kashani, H., Schmelcher, M., Sabzalipoor, H., Seyed Hosseini, E., and Moniri, R. (2018). Recombinant endolysins as potential therapeutics against antibiotic-resistant Staphylococcus aureus: current status of research and novel delivery strategies. Clin. Microbiol. Rev. 31, e00071. doi:10.1128/CMR.00071-17
Hamzeh-Mivehroud, M., Alizadeh, A. A., Morris, M. B., Church, W. B., and Dastmalchi, S. (2013). Phage display as a technology delivering on the promise of peptide drug discovery. Drug Discov. today 18, 1144–1157. doi:10.1016/j.drudis.2013.09.001
Harhala, M. A., Gembara, K., Nelson, D. C., Miernikiewicz, P., and Dąbrowska, K. (2022). Immunogenicity of endolysin PlyC. Antibiotics 11, 966. doi:10.3390/antibiotics11070966
Heselpoth, R. D., Euler, C. W., Schuch, R., and Fischetti, V. a.J. a.A.Chemotherapy (2019). Lysocins: bioengineered antimicrobials that deliver lysins across the outer membrane of Gram-negative bacteria. Antimicrob. Agents Chemother. 63, e00342. doi:10.1128/AAC.00342-19
Hong, H.-W., Myung, H. J. F. I. M., Jang, J., Kim, M. S., and Song, M. (2022). Combination effect of engineered endolysin EC340 with antibiotics. Front. Microbiol. 13, 821936. doi:10.3389/fmicb.2022.821936
Hu, S., Kong, J., Kong, W., Guo, T., and Ji, M. (2010). Characterization of a novel LysM domain from Lactobacillus fermentum bacteriophage endolysin and its use as an anchor to display heterologous proteins on the surfaces of lactic acid bacteria. Appl. Environ. Microbiol. 76, 2410–2418. doi:10.1128/AEM.01752-09
Huang, G., Shen, X., Gong, Y., Dong, Z., Zhao, X., Shen, W., et al. (2014). Antibacterial properties of acinetobacter baumanniiphage Abp1 endolysin (PlyAB1). BMC Infect. Dis. 14, 681–688. doi:10.1186/s12879-014-0681-2
Jado, I., López, R., García, E., Fenoll, A., Casal, J., García, P., et al. (2003). Phage lytic enzymes as therapy for antibiotic-resistant Streptococcus pneumoniae infection in a murine sepsis model. J. Antimicrob. Chemother. 52, 967–973. doi:10.1093/jac/dkg485
Jansen, M., Wahida, A., Latz, S., Krüttgen, A., Häfner, H., Buhl, E. M., et al. (2018). Enhanced antibacterial effect of the novel T4-like bacteriophage KARL-1 in combination with antibiotics against multi-drug resistant Acinetobacter baumannii. Sci. Rep. 8, 14140. doi:10.1038/s41598-018-32344-y
Jun, S. Y., Jang, I. J., Yoon, S., Jang, K., Yu, K.-S., Cho, J. Y., et al. (2017). Pharmacokinetics and tolerance of the phage endolysin-based candidate drug SAL200 after a single intravenous administration among healthy volunteers. Antimicrob. agents Chemother. 61, e02629. doi:10.1128/AAC.02629-16
Khan, F. M., Chen, J.-H., Zhang, R., and Liu, B. J. F. I. M. (2023a). A comprehensive review of the applications of bacteriophage-derived endolysins for foodborne bacterial pathogens and food safety: recent advances, challenges, and future perspective. Front. Microbiol. 14, 1259210. doi:10.3389/fmicb.2023.1259210
Khan, F. M., Chen, J.-H., Zhang, R., and Liu, B. J. F. I. M. (2023b). A comprehensive review of the applications of bacteriophage-derived endolysins for foodborne bacterial pathogens and food safety: recent advances, challenges, and future perspective. Front. Microbiol. 14, 1259210. doi:10.3389/fmicb.2023.1259210
Khan, F. M., Gondil, V. S., Li, C., Jiang, M., Li, J., Yu, J., et al. (2021). A novel Acinetobacter baumannii bacteriophage endolysin LysAB54 with high antibacterial activity against multiple Gram-negative microbes. Front. Cell. Infect. Microbiol. 11, 637313. doi:10.3389/fcimb.2021.637313
Kim, S., Lee, D.-W., Jin, J.-S., and Kim, J. (2020). Antimicrobial activity of LysSS, a novel phage endolysin, against Acinetobacter baumannii and Pseudomonas aeruginosa. J. Glob. Antimicrob. Resist. 22, 32–39. doi:10.1016/j.jgar.2020.01.005
Kocot, A. M., Briers, Y., and Plotka, M. (2023). Phages and engineered lysins as an effective tool to combat Gram-negative foodborne pathogens. Compr. Rev. Food Sci. Food Saf. 22, 2235–2266. doi:10.1111/1541-4337.13145
Lai, M.-J., Lin, N.-T., Hu, A., Soo, P.-C., Chen, L.-K., Chen, L.-H., et al. (2011a). Antibacterial activity of Acinetobacter baumannii phage ϕAB2 endolysin (LysAB2) against both gram-positive and gram-negative bacteria. Appl. Microbiol. Biotechnol. 90, 529–539. doi:10.1007/s00253-011-3104-y
Lai, M.-J., Lin, N.-T., Hu, A., Soo, P.-C., Chen, L.-K., Chen, L.-H., et al. (2011b). Antibacterial activity of Acinetobacter baumannii phage ϕAB2 endolysin (LysAB2) against both gram-positive and gram-negative bacteria. Appl. Microbiol. Biotechnol. 90, 529–539. doi:10.1007/s00253-011-3104-y
Lai, M.-J., Soo, P.-C., Lin, N.-T., Hu, A., Chen, Y.-J., Chen, L.-K., et al. (2013). Identification and characterisation of the putative phage-related endolysins through full genome sequence analysis in Acinetobacter baumannii ATCC 17978. Int. J. Antimicrob. agents 42, 141–148. doi:10.1016/j.ijantimicag.2013.04.022
Lai, W. C. B., Chen, X., Ho, M. K. Y., Xia, J., and Leung, S. S. Y. (2020a). Bacteriophage-derived endolysins to target gram-negative bacteria. Int. J. Pharm. 589, 119833. doi:10.1016/j.ijpharm.2020.119833
Lai, W. C. B., Chen, X., Ho, M. K. Y., Xia, J., and Leung, S. S. Y. J. I. J. O. P. (2020b). Bacteriophage-derived endolysins to target gram-negative bacteria. Int. J. Pharm. 589, 119833. doi:10.1016/j.ijpharm.2020.119833
Lanigan, R. S., and Yamarik, T. A. (2002). Final report on the safety assessment of EDTA, calcium disodium EDTA, diammonium EDTA, dipotassium EDTA, disodium EDTA, TEA-EDTA, tetrasodium EDTA, tripotassium EDTA, trisodium EDTA, HEDTA, and trisodium HEDTA. Int. J. Toxicol. 21, 95–142. doi:10.1080/10915810290096522
Lee, C., Kim, H., and Ryu, S. J. C. R. I. F. S.Nutrition (2023). Bacteriophage and endolysin engineering for biocontrol of food pathogens/pathogens in the food: recent advances and future trends. Crit. Rev. Food Sci. Nutr. 63, 8919–8938. doi:10.1080/10408398.2022.2059442
Letrado, P., Corsini, B., Díez-Martínez, R., Bustamante, N., Yuste, J. E., and García, P. J. F. M. (2018). Bactericidal synergism between antibiotics and phage endolysin Cpl-711 to kill multidrug-resistant pneumococcus. Future Microbiol. 13, 1215–1223. doi:10.2217/fmb-2018-0077
Lewis, K. (2007). Persister cells, dormancy and infectious disease. Nat. Rev. Microbiol. 5, 48–56. doi:10.1038/nrmicro1557
Li, C., Jiang, M., Khan, F. M., Zhao, X., Wang, G., Zhou, W., et al. (2021). Intrinsic antimicrobial peptide facilitates a new broad-spectrum lysin LysP53 to kill Acinetobacter baumannii in vitro and in a mouse burn infection model. ACS Infect. Dis. 7, 3336–3344. doi:10.1021/acsinfecdis.1c00497
Lim, J., Jang, J., Myung, H., and Song, M. (2022). Eradication of drug-resistant Acinetobacter baumannii by cell-penetrating peptide fused endolysin. J. Microbiol. 60, 859–866. doi:10.1007/s12275-022-2107-y
Lim, J.-A., Shin, H., Heu, S., and Ryu, S. J. J. O. M.Biotechnology (2014). Exogenous lytic activity of SPN9CC endolysin against gram-negative bacteria. J. Microbiol. Biotechnol. 24, 803–811. doi:10.4014/jmb.1403.03035
Lim, S. M. S., Abidin, A. Z., Liew, S., Roberts, J., and Sime, F. (2019). The global prevalence of multidrug-resistance among Acinetobacter baumannii causing hospital-acquired and ventilator-associated pneumonia and its associated mortality: a systematic review and meta-analysis. J. Infect. 79, 593–600. doi:10.1016/j.jinf.2019.09.012
Loessner, M. J., Kramer, K., Ebel, F., and Scherer, S. (2002). C-terminal domains of Listeria monocytogenes bacteriophage murein hydrolases determine specific recognition and high-affinity binding to bacterial cell wall carbohydrates. Mol. Microbiol. 44, 335–349. doi:10.1046/j.1365-2958.2002.02889.x
Loh, B., Gondil, V. S., Manohar, P., Khan, F. M., Yang, H., and Leptihn, S. (2021). Encapsulation and delivery of therapeutic phages. Appl. Environ. Microbiol. 87, e01979-20–e01920. doi:10.1128/AEM.01979-20
Lood, R., Winer, B. Y., Pelzek, A. J., Diez-Martinez, R., Thandar, M., Euler, C. W., et al. (2015a). Novel phage lysin capable of killing the multidrug-resistant gram-negative bacterium Acinetobacter baumannii in a mouse bacteremia model. Antimicrob. agents Chemother. 59, 1983–1991. doi:10.1128/AAC.04641-14
Love, M. J., Bhandari, D., Dobson, R. C., and Billington, C. (2018). Potential for bacteriophage endolysins to supplement or replace antibiotics in food production and clinical care. Antibiotics 7, 17. doi:10.3390/antibiotics7010017
Magnusdottir, A., Vidarsson, H., Björnsson, J. M., and Örvar, B. L. J. T. I. B. (2013). Barley grains for the production of endotoxin-free growth factors. Trends Biotechnol. 31, 572–580. doi:10.1016/j.tibtech.2013.06.002
Martínez, B., Rodríguez, A., and Suárez, E. J. N. W. T. C. B. G. (2016). Antimicrobial peptides produced by bacteria: the bacteriocins, 15–38.
Matamp, N., and Bhat, S. G. J. M. (2019). Phage endolysins as potential antimicrobials against multidrug resistant Vibrio alginolyticus and Vibrio parahaemolyticus: current status of research and challenges ahead. Microorganisms 7, 84. doi:10.3390/microorganisms7030084
Mehmood Khan, F., Manohar, P., Singh Gondil, V., Mehra, N., Kayode Oyejobi, G., Odiwuor, N., et al. (2023). The applications of animal models in phage therapy: an update. Hum. Vaccines Immunother. 19, 2175519. doi:10.1080/21645515.2023.2175519
Mulani, M. S., Kamble, E. E., Kumkar, S. N., Tawre, M. S., and Pardesi, K. R. J. F. I. M. (2019). Emerging strategies to combat ESKAPE pathogens in the era of antimicrobial resistance: a review. Front. Microbiol. 10, 539. doi:10.3389/fmicb.2019.00539
Murray, E., Draper, L. A., Ross, R. P., and Hill, C. J. V. (2021). The advantages and challenges of using endolysins in a clinical setting. Viruses 13, 680. doi:10.3390/v13040680
Nation, R. L., and Li, J. (2009). Colistin in the 21st century. Curr. Opin. Infect. Dis. 22, 535–543. doi:10.1097/QCO.0b013e328332e672
Nau, R., and Eiffert, H. (2002). Modulation of release of proinflammatory bacterial compounds by antibacterials: potential impact on course of inflammation and outcome in sepsis and meningitis. Clin. Microbiol. Rev. 15, 95–110. doi:10.1128/cmr.15.1.95-110.2002
Ning, H.-Q., Lin, H., and Wang, J.-X. J. I. J. O. F. M. (2021). Synergistic effects of endolysin Lysqdvp001 and ε-poly-lysine in controlling Vibrio parahaemolyticus and its biofilms. Int. J. Food Microbiol. 343, 109112. doi:10.1016/j.ijfoodmicro.2021.109112
Nordmann, P., and Poirel, L. J. C. I. D. (2019). Epidemiology and diagnostics of carbapenem resistance in gram-negative bacteria. Clin. Infect. Dis. 69, S521–S528. doi:10.1093/cid/ciz824
Oliveira, H., Azeredo, J., Lavigne, R., and Kluskens, L. D. J. T. I. F. S. (2012). Bacteriophage endolysins as a response to emerging foodborne pathogens. Bacteriophage endolysins as a response Emerg. foodborne pathogens 28, 103–115. doi:10.1016/j.tifs.2012.06.016
Oliveira, H., Thiagarajan, V., Walmagh, M., Sillankorva, S., Lavigne, R., Neves-Petersen, M. T., et al. (2014). A thermostable Salmonella phage endolysin, Lys68, with broad bactericidal properties against gram-negative pathogens in presence of weak acids. PLoS One 9, e108376. doi:10.1371/journal.pone.0108376
Oliveira, H., Vilas Boas, D., Mesnage, S., Kluskens, L. D., Lavigne, R., Sillankorva, S., et al. (2016a). Structural and enzymatic characterization of ABgp46, a novel phage endolysin with broad anti-gram-negative bacterial activity. Front. Microbiol. 7, 208. doi:10.3389/fmicb.2016.00208
Oliveira, H., Vilas Boas, D., Mesnage, S., Kluskens, L. D., Lavigne, R., Sillankorva, S., et al. (2016b). Structural and enzymatic characterization of ABgp46, a novel phage endolysin with broad anti-gram-negative bacterial activity. Front. Microbiol. 7, 208. doi:10.3389/fmicb.2016.00208
Organization, W. H. (2019). 2019 Antibacterial agents in clinical development: an analysis of the antibacterial clinical development pipeline.
Organization, W. H. (2022). 2021 antibacterial agents in clinical and preclinical development: an overview and analysis.
Oyejobi, G. K., Olaniyan, S. O., Yusuf, N.-A., Ojewande, D. A., Awopetu, M. J., Oyeniran, G. O., et al. (2022a). Acinetobacter baumannii: more ways to die. Microbiol. Res. 261, 127069. doi:10.1016/j.micres.2022.127069
Oyejobi, G. K., Olaniyan, S. O., Yusuf, N.-A., Ojewande, D. A., Awopetu, M. J., Oyeniran, G. O., et al. (2022b). Acinetobacter baumannii: More ways to die. Microbiol. Res. 261, 127069. doi:10.1016/j.micres.2022.127069
Pakharukova, N., Tuittila, M., Paavilainen, S., Malmi, H., Parilova, O., Teneberg, S., et al. (2018). Structural basis for Acinetobacter baumannii biofilm formation. Proc. Natl. Acad. Sci. U. S. A. 115, 5558–5563. doi:10.1073/pnas.1800961115
Peng, S.-Y., You, R.-I., Lai, M.-J., Lin, N.-T., Chen, L.-K., and Chang, K.-C. (2017). Highly potent antimicrobial modified peptides derived from the Acinetobacter baumannii phage endolysin LysAB2. Sci. Rep. 7, 11477. doi:10.1038/s41598-017-11832-7
Piperaki, E.-T., Tzouvelekis, L., Miriagou, V., and Daikos, G. J. C. M.Infection (2019). Carbapenem-resistant Acinetobacter baumannii: in pursuit of an effective treatment. Clin. Microbiol. Infect. 25, 951–957. doi:10.1016/j.cmi.2019.03.014
Poirel, L., Bonnin, R. A., and Nordmann, P. (2011). Genetic basis of antibiotic resistance in pathogenic Acinetobacter species. IUBMB life 63, 1061–1067. doi:10.1002/iub.532
Premetis, G. E., Georgakis, N. D., Stathi, A., and Labrou, N. E. (2023a). Metaviromics analysis of marine biofilm reveals a glycoside hydrolase endolysin with high specificity towards Acinetobacter baumannii. Biochimica Biophysica Acta (BBA)-Proteins Proteomics 1871, 140918. doi:10.1016/j.bbapap.2023.140918
Premetis, G. E., Stathi, A., Papageorgiou, A. C., and Labrou, N. E. (2023b). Characterization of a glycoside hydrolase endolysin from Acinetobacter baumannii phage Ab TZA1 with high antibacterial potency and novel structural features. FEBS J. 290, 2146–2164. doi:10.1111/febs.16686
Ragland, S. A., and Criss, A. K. J. P. P. (2017). From bacterial killing to immune modulation: recent insights into the functions of lysozyme. PLoS Pathog. 13, e1006512. doi:10.1371/journal.ppat.1006512
Rahimzadeh, G., Gill, P., and Rezai, M. S. J. J. O. P. R. (2018). Endolysins of bacteriophages as an anti-methicillin resistant staphylococcus aureus infection in children: a narrative review. J. Pediatr. Rev. 6, 36–43. doi:10.5812/jpr.11562
Rai, S., and Kumar, A. J. E. M. R. (2022). Bacteriophage therapeutics to confront multidrug-resistant Acinetobacter baumannii-a global health menace. Environ. Microbiol. Rep. 14, 347–364. doi:10.1111/1758-2229.12988
Raz, A., Serrano, A., Thaker, M., Alston, T., and Fischetti, V. A. (2018). Lysostaphin lysibody leads to effective opsonization and killing of methicillin-resistant Staphylococcus aureus in a murine model. Antimicrob. Agents Chemother. 62, e01056. doi:10.1128/AAC.01056-18
Ribelles, P., Benbouziane, B., Langella, P., Suárez, J. E., Bermúdez-Humarán, L. G., and Riazi, A. (2013). Protection against human papillomavirus type 16-induced tumors in mice using non-genetically modified lactic acid bacteria displaying E7 antigen at its surface. Appl. Microbiol. Biotechnol. 97, 1231–1239. doi:10.1007/s00253-012-4575-1
Rodríguez-Rubio, L., Gerstmans, H., Thorpe, S., Mesnage, S., Lavigne, R., Briers, Y. J. A., et al. (2016a). DUF3380 domain from a Salmonella phage endolysin shows potent N-acetylmuramidase activity. Appl. Environ. Microbiol. 82, 4975–4981. doi:10.1128/AEM.00446-16
Rodríguez-Rubio, L., Gutiérrez, D., Donovan, D. M., Martínez, B., Rodríguez, A., and García, P. (2016b). Phage lytic proteins: biotechnological applications beyond clinical antimicrobials. Crit. Rev. Biotechnol. 36, 542–552. doi:10.3109/07388551.2014.993587
Salmani, A., Mohsenzadeh, M., Pirouzi, A., and Khaledi, A. (2020). A comprehensive meta-analysis of antibiotic resistance pattern among biofilm production strains of Acinetobacter baumannii recovered from clinical specimens of patients. Gene Rep. 19, 100664. doi:10.1016/j.genrep.2020.100664
Schmelcher, M., Donovan, D. M., and Loessner, M. J. (2012). Bacteriophage endolysins as novel antimicrobials. Future Microbiol. 7, 1147–1171. doi:10.2217/fmb.12.97
Schmelcher, M., and Loessner, M. J. (2021a). Bacteriophage endolysins—extending their application to tissues and the bloodstream. Curr. Opin. Biotechnol. 68, 51–59. doi:10.1016/j.copbio.2020.09.012
Schmelcher, M., and Loessner, M. J. J. C. O. I. B. (2021b). Bacteriophage endolysins—extending their application to tissues and the bloodstream. Curr. Opin. Biotechnol. 68, 51–59. doi:10.1016/j.copbio.2020.09.012
Sisson, H. M., Fagerlund, R. D., Jackson, S. A., Briers, Y., Warring, S. L., Fineran, P. C. J. A., et al. (2024a). Antibacterial synergy between a phage endolysin and citric acid against the Gram-negative kiwifruit pathogen Pseudomonas syringae pv. Actinidiae. e01846-01823. doi:10.1128/aem.01846-23
Sisson, H. M., Jackson, S. A., Fagerlund, R. D., Warring, S. L., and Fineran, P. C. J. C. O. I. M. (2024b). Gram-negative endolysins: overcoming the outer membrane obstacle. Curr. Opin. Microbiol. 78, 102433. doi:10.1016/j.mib.2024.102433
Sitthisak, S., Manrueang, S., Khongfak, S., Leungtongkam, U., Thummeepak, R., Thanwisai, A., et al. (2023a). Antibacterial activity of vB_AbaM_PhT2 phage hydrophobic amino acid fusion endolysin, combined with colistin against Acinetobacter baumannii. Sci. Rep. 13, 7470. doi:10.1038/s41598-023-33822-8
Sitthisak, S., Manrueang, S., Khongfak, S., Leungtongkam, U., Thummeepak, R., Thanwisai, A., et al. (2023b). Antibacterial activity of vB_AbaM_PhT2 phage hydrophobic amino acid fusion endolysin, combined with colistin against Acinetobacter baumannii. Sci. Rep. 13, 7470. doi:10.1038/s41598-023-33822-8
Son, S. M., Kim, J., and Ryu, S. J. F. I. M. (2023). Development of sensitizer peptide-fused endolysin Lys1S-L9P acting against multidrug-resistant gram-negative bacteria. Front. Microbiol. 14, 1296796. doi:10.3389/fmicb.2023.1296796
Soontarach, R., Srimanote, P., Arechanajan, B., Nakkaew, A., Voravuthikunchai, S. P., and Chusri, S. J. P. O. (2024). Characterization of a novel bacteriophage endolysin (LysAB1245) with extended lytic activity against distinct capsular types associated with Acinetobacter baumannii resistance. PLoS One 19, e0296453. doi:10.1371/journal.pone.0296453
Szadkowska, M., Olewniczak, M., Kloska, A., Jankowska, E., Kapusta, M., Rybak, B., et al. (2022). A novel cryptic clostridial peptide that kills bacteria by a cell membrane permeabilization mechanism. Microbiol. Spectr. 10, 01657222. doi:10.1128/spectrum.01657-22
Thandar, M., Lood, R., Winer, B. Y., Deutsch, D. R., Euler, C. W., and Fischetti, V. A. (2016). Novel engineered peptides of a phage lysin as effective antimicrobials against multidrug-resistant Acinetobacter baumannii. Antimicrob. agents Chemother. 60, 2671–2679. doi:10.1128/AAC.02972-15
Thummeepak, R., Kitti, T., Kunthalert, D., and Sitthisak, S. (2016a). Enhanced antibacterial activity of acinetobacter baumannii bacteriophage øabp-01 endolysin (LysABP-01) in combination with colistin. Front. Microbiol. 7, 1402. doi:10.3389/fmicb.2016.01402
Thummeepak, R., Kitti, T., Kunthalert, D., and Sitthisak, S. J. F. I. M. (2016b). Enhanced antibacterial activity of Acinetobacter baumannii bacteriophage ØABP-01 endolysin (LysABP-01) in combination with colistin. Front. Microbiol. 7, 1402. doi:10.3389/fmicb.2016.01402
Totté, J. E., Van Doorn, M. B., and Pasmans, S. G. J. C. R. I. D. (2017). Successful treatment of chronic Staphylococcus aureus-related dermatoses with the topical endolysin Staphefekt SA.100: a report of 3 cases. 100 a Rep. 3 cases 9, 19–25. doi:10.1159/000473872
Typas, A., Banzhaf, M., Gross, C. A., and Vollmer, W. (2012). From the regulation of peptidoglycan synthesis to bacterial growth and morphology. Nat. Rev. Microbiol. 10, 123–136. doi:10.1038/nrmicro2677
Usmani, S. S., Bedi, G., Samuel, J. S., Singh, S., Kalra, S., Kumar, P., et al. (2017). THPdb: database of FDA-approved peptide and protein therapeutics. PloS one 12, e0181748. doi:10.1371/journal.pone.0181748
Vasina, D. V., Antonova, N. P., Grigoriev, I. V., Yakimakha, V. S., Nikiforova, M. A., Pochtovyi, A. A., et al. (2021). Discovering the potentials of four phage endolysins to combat gram-negative infections. Front. Microbiol. 12, 748718. doi:10.3389/fmicb.2021.748718
Vrancianu, C. O., Gheorghe, I., Czobor, I. B., and Chifiriuc, M. C. J. M. (2020). Antibiotic resistance profiles, molecular mechanisms and innovative treatment strategies of Acinetobacter baumannii. Microorganisms 8, 935. doi:10.3390/microorganisms8060935
Wang, M., Zhang, J., Wei, J., Jiang, L., Jiang, L., Sun, Y., et al. (2023). Phage-inspired strategies to combat antibacterial resistance, 1–16.
Watson, A., Oh, J. T., Sauve, K., Bradford, P. A., Cassino, C., and Schuch, R. (2019). Antimicrobial activity of exebacase (lysin CF-301) against the most common causes of infective endocarditis. Antimicrob. Agents Chemother. 63, e01078. doi:10.1128/AAC.01078-19
Wishart, D. S., Feunang, Y. D., Guo, A. C., Lo, E. J., Marcu, A., Grant, J. R., et al. (2018). DrugBank 5.0: a major update to the DrugBank database for 2018. Nucleic acids Res. 46, D1074-D1082–D1082. doi:10.1093/nar/gkx1037
Wu, M., Hu, K., Xie, Y., Liu, Y., Mu, D., Guo, H., et al. (2019). A novel phage PD-6A3, and its endolysin Ply6A3, with extended lytic activity against Acinetobacter baumannii. Front. Microbiol. 9, 3302. doi:10.3389/fmicb.2018.03302
Yang, C.-H., Su, P.-W., Moi, S.-H., and Chuang, L.-Y. J. M. (2019). Biofilm formation in Acinetobacter Baumannii: genotype-phenotype correlation. Molecules 24, 1849. doi:10.3390/molecules24101849
Yang, H., Wang, M., Yu, J., and Wei, H. J. F. I. M. (2015). Antibacterial activity of a novel peptide-modified lysin against Acinetobacter baumannii and Pseudomonas aeruginosa. Front. Microbiol. 6, 1471. doi:10.3389/fmicb.2015.01471
Yang, H., Xu, J., Li, W., Wang, S., Li, J., Yu, J., et al. (2018). Staphylococcus aureus virulence attenuation and immune clearance mediated by a phage lysin-derived protein. EMBO J. 37, e98045. doi:10.15252/embj.201798045
Yoong, P., Schuch, R., Nelson, D., and Fischetti, V. a.J. J. O. B. (2004). Identification of a broadly active phage lytic enzyme with lethal activity against antibiotic-resistant Enterococcus faecalis and Enterococcus faecium. J. Bacteriol. 186, 4808–4812. doi:10.1128/JB.186.14.4808-4812.2004
Yuan, Y., Li, X., Wang, L., Li, G., Cong, C., Li, R., et al. (2021). The endolysin of the Acinetobacter baumannii phage vB_AbaP_D2 shows broad antibacterial activity. Microb. Biotechnol. 14, 403–418. doi:10.1111/1751-7915.13594
Keywords: antimicrobial resistance, antimicrobial agent, A. baumannii, Gram-negative bacteria, endolysin
Citation: Khan FM, Rasheed F, Yang Y, Liu B and Zhang R (2024) Endolysins: a new antimicrobial agent against antimicrobial resistance. Strategies and opportunities in overcoming the challenges of endolysins against Gram-negative bacteria. Front. Pharmacol. 15:1385261. doi: 10.3389/fphar.2024.1385261
Received: 12 February 2024; Accepted: 16 April 2024;
Published: 20 May 2024.
Edited by:
Nikolaos E. Labrou, Agricultural University of Athens, GreeceReviewed by:
Sarunyou Chusri, Prince of Songkla University, ThailandShanmugaraj Gowrishankar, Alagappa University, India
Md. Sharifull Islam, Chinese Academy of Sciences (CAS), China
Pir Tariq Shah, Dalian University of Technology, China
Copyright © 2024 Khan, Rasheed, Yang, Liu and Zhang. This is an open-access article distributed under the terms of the Creative Commons Attribution License (CC BY). The use, distribution or reproduction in other forums is permitted, provided the original author(s) and the copyright owner(s) are credited and that the original publication in this journal is cited, in accordance with accepted academic practice. No use, distribution or reproduction is permitted which does not comply with these terms.
*Correspondence: Rui Zhang, ruizhang@szu.edu.cn; Bin Liu, liubin@szu.edu.cn