- 1Department of Experimental Physiology and Pathophysiology, Medical University of Bialystok, Bialystok, Poland
- 2Department of Pharmacology and Toxicology, University of Bonn, Bonn, Germany
Ischemic heart disease, associated with high morbidity and mortality, represents a major challenge for the development of drug-based strategies to improve its prognosis. Results of pre-clinical studies suggest that agonists of cannabinoid CB2 receptors and multitarget cannabidiol might be potential cardioprotective strategies against ischemia-reperfusion injury. The aim of our study was to re-evaluate the cardioprotective effects of cannabinoids against ischemia-reperfusion injury according to the IMproving Preclinical Assessment of Cardioprotective Therapies (IMPACT) criteria published recently by the European Union (EU) CARDIOPROTECTION COST ACTION. To meet the minimum criteria of those guidelines, experiments should be performed (i) on healthy small animals subjected to ischemia with reperfusion lasting for at least 2 hours and (ii) confirmed in small animals with comorbidities and co-medications and (iii) in large animals. Our analysis revealed that the publications regarding cardioprotective effects of CB2 receptor agonists and cannabidiol did not meet all three strict steps of IMPACT. Thus, additional experiments are needed to confirm the cardioprotective activities of (endo)cannabinoids mainly on small animals with comorbidities and on large animals. Moreover, our publication underlines the significance of the IMPACT criteria for a proper planning of preclinical experiments regarding cardiac ischemia-reperfusion injury.
1 Introduction
The energy supply of the heart is very high and mainly depends on aerobic metabolism. The high oxygen consumption is further increased by rises in heart rate, contractility and ventricular wall. For this reason, a proper maintenance of the balance between oxygen supply and consumption is extremely important; changes in this balance may result in ischemia (Boyette and Manna, 2023). Sudden myocardial ischemia (I) is usually caused by the acute rupture of an atherosclerotic plaque and the obstruction of a coronary artery leading to an acute coronary syndrome and acute myocardial infarction (AMI) (Basalay et al., 2020). When blood flow and oxygen delivery is limited, anaerobic metabolic processes will be activated finally resulting in damage to the cardiac tissue. Reperfusion (R), which restores the blood supply to the cardiac muscle, halts the progression of myocardial ischemic injury but simultaneously produces sublethal to lethal reperfusion injury in marginally viable myocardium aggravating its damage. In consequence, both events lead to the myocardial ischemia/reperfusion (I/R) injury (Buja, 2023; Wang et al., 2023). Multiple mechanisms are involved in the pathogenesis of the ischemic and reperfusion period including changes in cell metabolism, impairment of mitochondrial function, enhanced inflammatory response, disability of autophagy, platelet-dependent activation of leukocytes, escalating overproduction of reactive oxygen species (ROS) and intracellular calcium overload (Kalogeris et al., 2016; Buja, 2023; Ferdinandy et al., 2023; Liu et al., 2023).
2 Cannabinoids and the heart
Numerous compounds including cannabinoids have been suggested for protection of the heart against I/R injury. Cannabinoids can be divided into three groups, i.e., (i) phytocannabinoids found in the cannabis plants Cannabis sativa or indica, including psychoactive Δ9-tetrahydrocannabinol (∆9-THC) and non-psychoactive cannabidiol (CBD), (ii) synthetic cannabinoids, e.g., WIN55212-2 and HU-210 and (iii) endocannabinoids (ECBs), such as anandamide (AEA) and 2-arachidonoylglycerol (2-AG). Removal of the endocannabinoids from the biophase occurs by degradation (e.g., by fatty acid amide hydrolase, FAAH, and monoacylglycerol lipase, MAGL) and cannabinoid reuptake [Figure 1, (Toczek and Malinowska, 2018; Maccarrone et al., 2023),]. Cannabinoids act, although with strongly differing affinity, via the G protein-coupled classical cannabinoid receptors (CBRs) CB1 and CB2 (Figure 1), some G protein-coupled orphan receptors (GPRs, e.g., GPR55), transient receptor potential vanilloid 1 (TRPV1) receptors and nuclear peroxisome proliferator-activated receptors (PPARs).
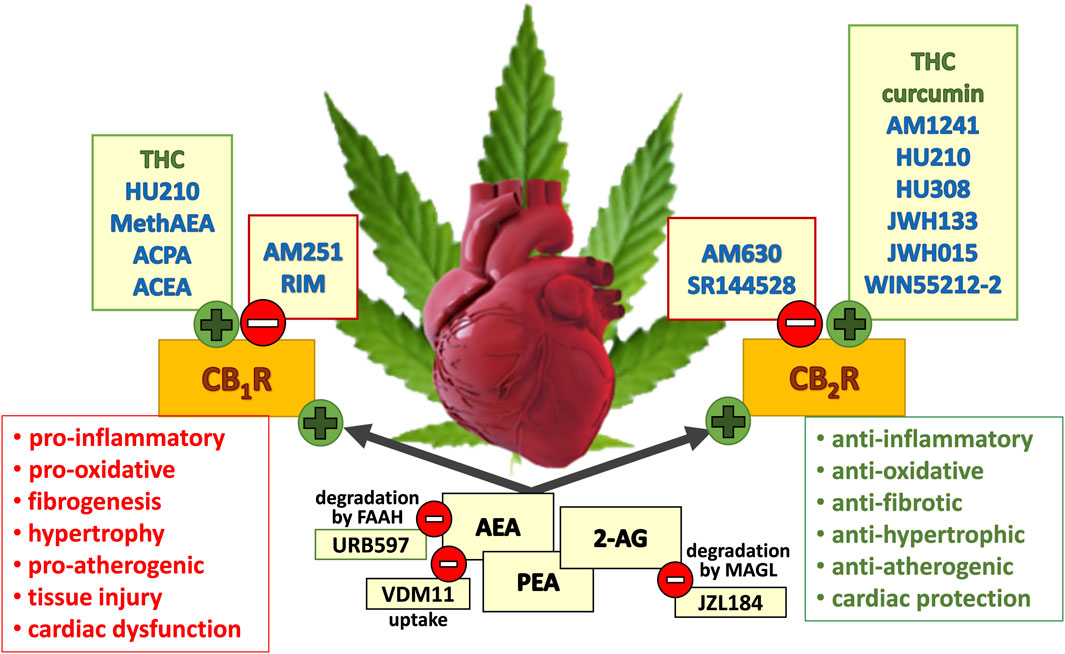
Figure 1. Potential beneficial and detrimental effects of the (endo)cannabinoids considered in this review and mediated via CB1R (cannabinoid receptor type 1) and CB2R activation (cannabinoid receptor type 2). Green circles with a plus sign describe (partial) agonism at the respective receptor; red circles with a minus sign describe antagonism, inverse agonism or inhibition at the respective mechanism (degradation or uptake). Synthetic, plant-derived compounds and endocannabinoids are written in blue, green, and black font, respectively. 2-AG, 2-arachidonoylglycerol; ACEA, arachidonyl-2′-chloroethylamide; ACPA, arachidonylcyclopropylamide; AEA, anandamide; FAAH, fatty acid amide hydrolase; MAGL, monoacylglycerol lipase; MethAEA, methanandamide; PEA, N-palmitoylethanolamide; RIM, rimonabant; THC, Δ9-tetrahydrocannabinol. The scheme is based on figures shown by Pacher and Haskó 2008; Steffens and Pacher 2012; Tang et al., 2021; Weresa et al., 2022; Rorabaugh et al., 2023; Maccarrone et al., 2023.
Gene and/or protein expression of CB1Rs and CB2Rs has been detected in the left human ventricle, right atrium, coronary artery endothelial and smooth muscle cells and epicardial adipose tissue. Cannabinoid receptors have also been identified in hearts of the guinea pig and in the left ventricle and left atrium of rat and mouse (Rajesh et al., 2010; 2022; Szekeres et al., 2018; Weresa et al., 2022). CB1Rs are localized on arterial and capillary endothelial cells, whereas CB2Rs are found on cardiomyocytes and endothelial cells of larger arteries (Lépicier et al., 2007). Moreover, presynaptic CB1Rs are also present on sympathetic nerve endings innervating human (Molderings et al., 1999) and rat (Ishac et al., 1996) heart. CB2Rs are also localized in rodent myocardial fibroblasts, B cells, and T cells (Defer et al., 2009; Puhl, 2020). CB1Rs were also identified in mice cardiac fibroblasts (Valenta et al., 2018) although their function has not been evaluated.
Depending on the type of cannabinoid receptor, its stimulation causes opposite effects (Pacher et al., 2018; Rorabaugh et al., 2023). Thus, CB1R activation induces a negative inotropic effect (also in humans: Bonz et al., 2003; Sterin-Borda et al., 2005), decreases noradrenaline release from the cardiac sympathetic nerve endings (Ishac et al., 1996; Molderings et al., 1999), leads to vasodilatation of rat coronary artery (e.g., Szekeres et al., 2018), stimulates oxidative stress and cell death in human endothelial cells and cardiomyocytes (Rajesh et al., 2010), increases smooth muscle proliferation and promotes vascular inflammation, atherosclerosis and cardiac injury (Pacher et al., 2018). On the other hand, CB2R stimulation produces a positive inotropic effect (e.g., Sterin-Borda et al., 2005), decreases the acute inflammatory response and consequent oxidative stress in immune and activated endothelial cells (Rajesh et al., 2022) and reduces cardiac fibrosis by diminishing fibroblast-myoblast transformation and collagen production (Defer et al., 2009).
Proper cannabinoid signalling might play a protective role against cardiac injury caused by an imbalance in oxygen supply and demand. As shown in Figure 1, activation of CB1Rs leads to deleterious consequences resulting from enhancement in oxidative stress, immune cell migration, inflammation, proliferation and fibrosis. On the other hand, CB2 receptor activation mainly has a beneficial effect by an influence opposite in direction to that of the CB1Rs (Pacher et al., 2018; Smoum et al., 2022). However, despite previously observed cardioprotective effects mediated mainly via CB2 receptor activation [for review, see (Lamontagne et al., 2006; Pacher and Haskó, 2008; Steffens and Pacher, 2012; Maslov et al., 2016; Tang et al., 2021; Rorabaugh et al., 2023; More et al., 2024)] the number of studies reporting cardioprotective effects of cannabinoids in different cardiac disorders seems to decline. The previous studies are not even included in the most recent analysis of multitarget strategies proposed to reduce myocardial I/R injury (Davidson et al., 2019; Heusch, 2023). For better understanding the obstacles for the translation from successful preclinical studies on cardioprotection to clinical practice, the COST ACTION cardioprotection consortium proposed an in vivo set of step-by-step criteria. This approach is termed IMproving Preclinical Assessment of Cardioprotective Therapies (IMPACT) and its aim is to improve the likelihood of translating novel cardioprotective interventions into the clinical setting for patient benefit [(Lecour et al., 2021); for details, see below]. Although the IMPACT criteria primarily consider infarct size in experiments in vivo, we decided to extend our analysis to other effects, such as inflammation or cardiac contractility to assay the possible broad spectrum of cardiac effects of cannabinoids.
3 Aims of the study and methodological approach
The first aim of this review is to summarize the current knowledge about the potential cardioprotective role of the (endo)cannabinoid system under hypoxia, ischemia and reperfusion. Data from humans with coronary heart disease (mainly determinations of endocannabinoid levels) are described first (Table 1), followed by animal studies in which the putative cardioprotective role of cannabinoids in ischemic preconditioning is considered (Table 2). Then, we describe in vivo experiments on animals exposed to temporary (I/R studies) or permanent occlusion (acute myocardial infarction studies) of the left descending artery (LAD) (Tables 3, 4) and in vitro experiments on isolated cardiomyocytes undergoing hypoxia and on isolated hearts subjected to LAD occlusion, low-flow or global no-flow (Table 5). In the second part of the review, we evaluate whether the in vivo models and experimental protocols considered here (Tables 3, 4) fulfil the IMPACT criteria and allow translation of the cardioprotective effects of cannabinoids in I/R injury to clinical settings.
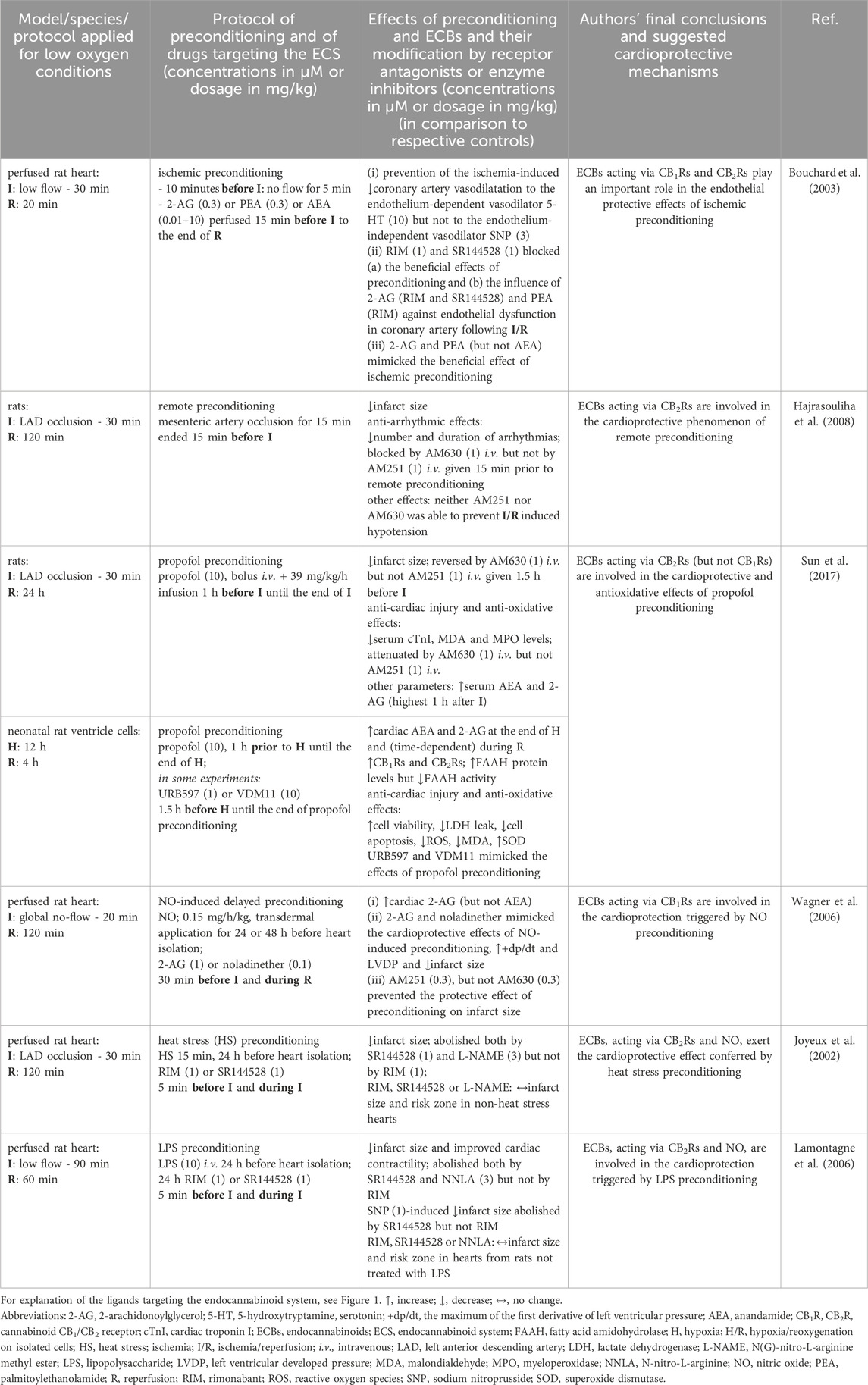
Table 2. Cardioprotective role of endocannabinoids in various preconditioning strategies in vivo and in vitro.
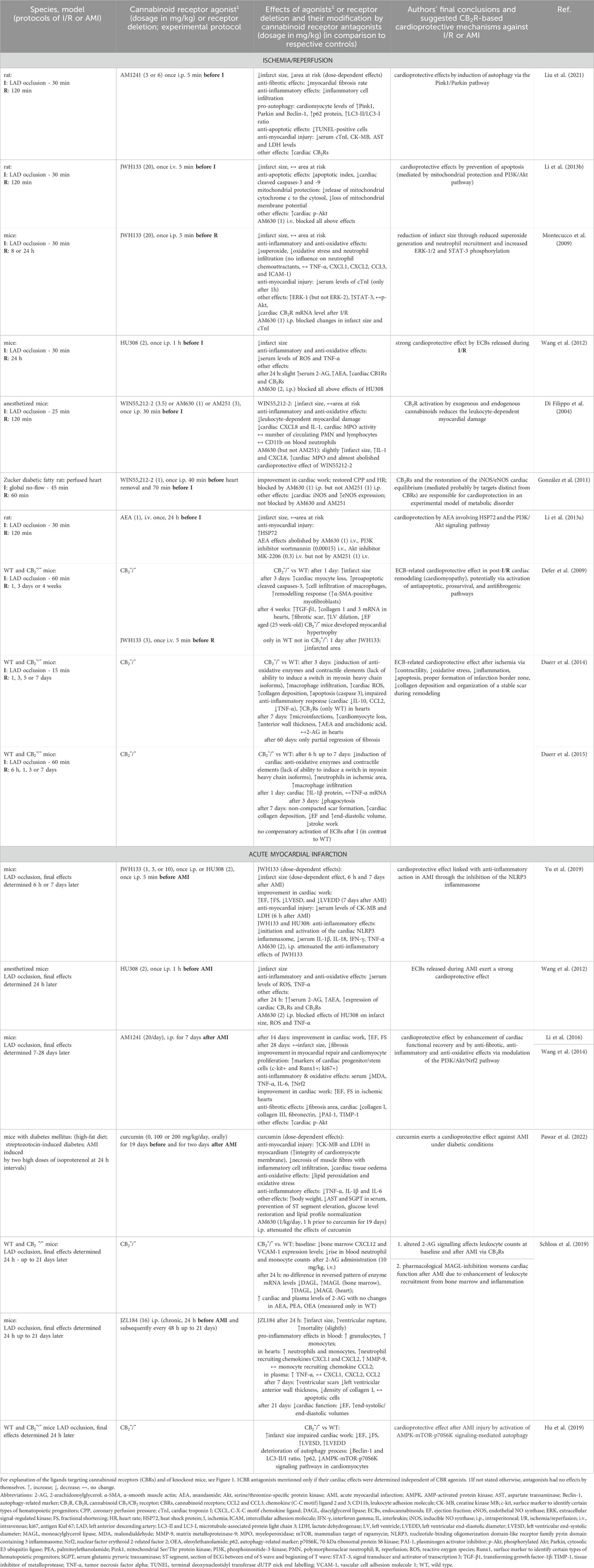
Table 3. Cannabinoid CB2R-based cardioprotective actions of cannabinoids in in vivo models of ischemia/reperfusion and acute myocardial infarction.
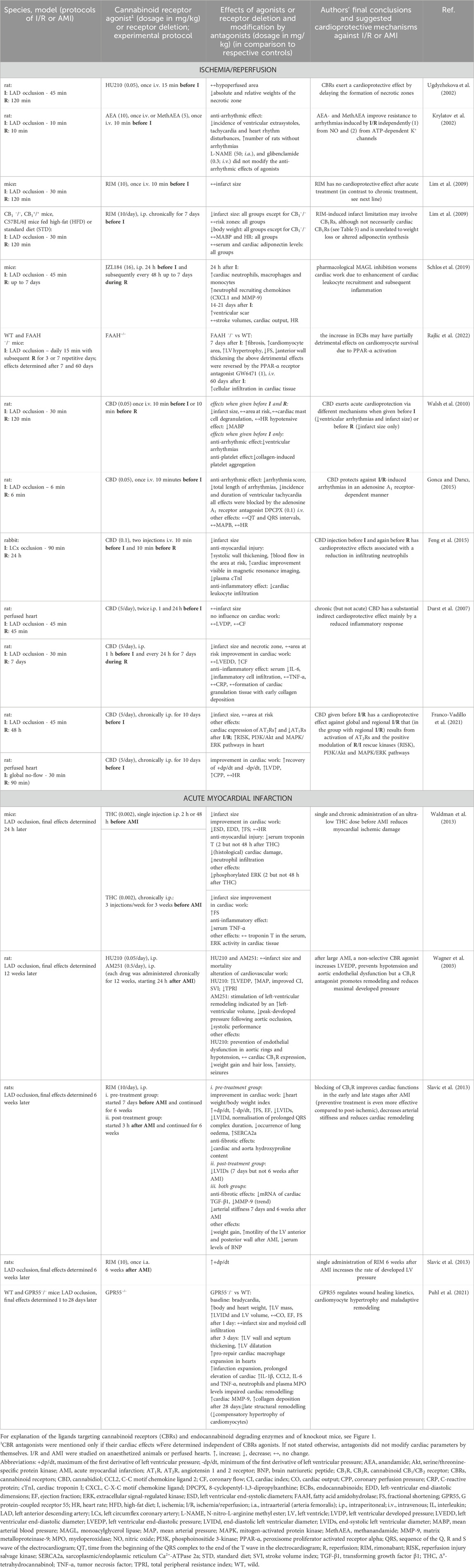
Table 4. Cardioprotective actions of cannabinoids in in vivo models of ischemia/reperfusion and acute myocardial infarction by CB2R-independent mechanisms.
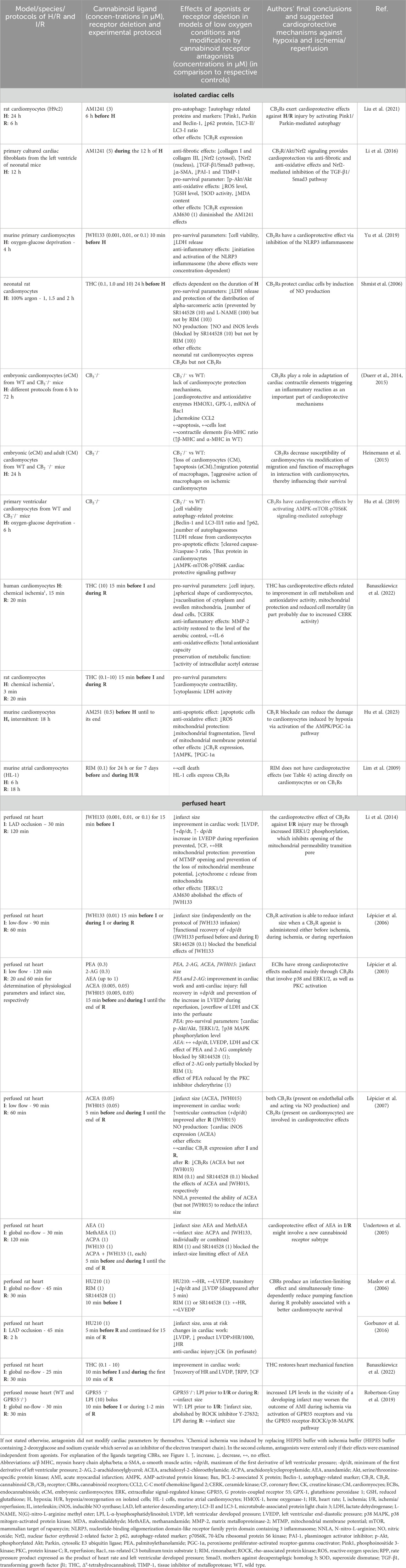
Table 5. Cardioprotective effects of cannabinoids in different models of low oxygen conditions in isolated cardiac cells and heart preparations.
To find the relevant articles dealing with the cardioprotective effects of cannabinoids against ischemia-reperfusion injury, we performed a comprehensive search in the PubMed, Medline and EMBASE databases (closed in February 2024). Because there is a substantial amount of research in the databases that deal with ischemia in the nervous system or perinatal hypoxia-ischemia, we added the word “cardiac” to each search. The following key phrases were used: “cardiac ischemia cannabinoid” (which yielded 205 results), “cardiac hypoxia cannabinoid” (21 results), “cardiac preconditioning cannabinoid” (16 results), “human cardiac ischemia cannabinoid” (130 results) and “myocardial infarction cannabinoid” (180 results). Based on the approved therapeutic uses of cannabidiol, search phrases also included “cannabidiol” coupled to “cardiac ischemia”, “cardiac hypoxia” and “myocardial infarction” (a total of 35 results). Titles, abstracts, and full texts of the identified papers were analyzed, and duplicate articles or those with non-relevant content were excluded; only articles in English were considered. In total, 52 publications were included in this review, which are summarized in Table 1-5. A schematic representation of the typical protocols for ischemia, hypoxia or acute myocardial infarction used in the above studies is given in Figure 2.
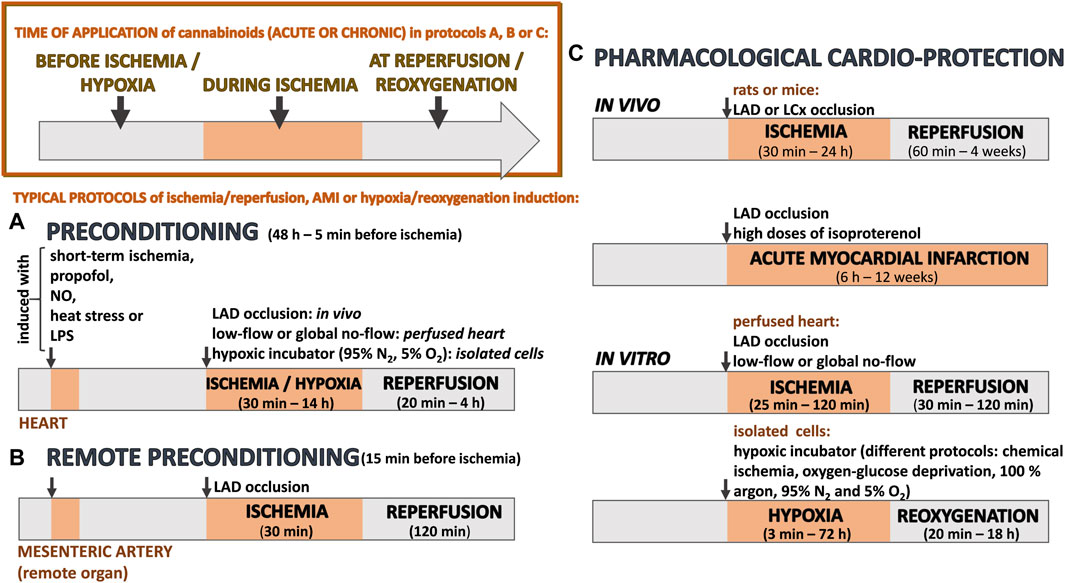
Figure 2. A schematic representation of typical protocols for ischemia, hypoxia or acute myocardial infarction (AMI) that have been used to examine whether (endo)cannabinoids have cardioprotective effects during various preconditioning strategies (A, B) or when administered to assess their pharmacological effects (C). At the top of the image (left part), the time of application displays different approaches to administer (endo)cannabinoids. For results, see Tables 1-5. LAD, left anterior descending artery; LCx, left circumflex coronary artery; NO, nitric oxide; LPS, lipopolysaccharide.
4 Human cardiac disorders modify endocannabinoid levels
The endocannabinoid system is activated during various cardiac disorders in humans (Table 1). Thus, higher endocannabinoid levels (2-AG, AEA or both) were found in patients with coronary artery disease (CAD) and at the ruptured plaque site of the infarct-related coronary artery in patients with AMI (Maeda et al., 2009; Wang et al., 2012) and CAD (Sugamura et al., 2009; Jehle et al., 2019) in comparison to peripheral arteries. Interestingly, the endocannabinoid level was higher in the coronary artery of patients with non-ST-elevation myocardial infarction (NSTEMI) compared to CAD (Jehle et al., 2019) and in the blood of patients with severe chronic heart failure (CHF) in comparison to controls (Weis et al., 2010). Note that, in the latter study, CHF might have been due to ischemia or to other reasons.
As shown in Table 1, there are differences as to which endocannabinoid is enhanced under pathological conditions even within the same disease. Thus, in AMI, the 2-AG level in the infarct-related coronary artery was higher in the paper by Wang et al. (2012) but undetectable in the publication by Maeda et al. (2009). The above discrepancy probably results from methodological differences. For example, Jehle et al. (2019) underlined the influence of the site of blood sampling, i.e., venous (Sugamura et al., 2009) versus arterial (Jehle et al., 2019). Of course, one cannot exclude a higher heterogeneity of the experimental groups in humans than in experimental animals. Importantly, as mentioned above, the endocannabinoid levels (AEA and 2-AG) in infarct-related coronary arteries were higher than those in blood samples drawn from distinct locations.
Additionally, in coronary atherectomy samples, CB1R mRNA expression was more markedly increased in patients with unstable angina than in those with stable angina (Sugamura et al., 2009). The expression of CB1Rs and CB2Rs was also studied in human left ventricular myocardium of patients with chronic heart failure (CHF) and controls (Weis et al., 2010). In healthy myocardium, mRNA transcripts of CB1Rs and CB2Rs were expressed similarly whereas in the myocardium of CHF patients a shift of the CB1/CB2R ratio towards the expression of CB2Rs was observed (Table 1).
An abnormal lipid profile belongs to the risk factors important for cardiovascular disease. Targeted lipidomics analysis showed the existence of two subgroups within the ischemic end-stage failing human left ventricle, the first one resembling controls and the second one presenting with an altered lipid profile. Interestingly, only in the second subgroup decreased 2-AG and increased AEA, N-acylethanolamine and free fatty acids levels as well as a robust reduction in cardiac MAGL activity occurred (van Esbroeck et al., 2020).
The question arises, whether activation of the endocannabinoid system in humans is beneficial or has pathological relevance in myocardial infarction. Unfortunately, we are not able to answer this question based on only few publications related to rather small patient groups (Table 1). Data obtained on cardiac experimental models suggest mainly protective effects of endocannabinoids (see below). On the other hand, an increase in endocannabinoid levels in humans (Table 1) was accompanied by enhanced serum levels of reactive oxygen species (ROS) and tumor necrosis factor-α (TNF-α) in infarct-related coronary artery (Wang et al., 2012) or correlated with peak troponin and IL-6 levels (Jehle et al., 2019) but correlations, of course, do not prove a causal relationship.
5 Cardioprotective role of endocannabinoids during preconditioning
Almost four decades ago, Murry and colleagues discovered that brief episodes of ischemia followed by short-lasting reperfusion before a sustained period of coronary artery occlusion reduced infarct size in dogs (Murry et al., 1986). This was the beginning of the development of research on “conditioning” phenomena used to reduce infarct size by brief periods of I/R on the heart. Ischemic preconditioning is considered the gold standard of cardioprotection (Basalay et al., 2020). In remote preconditioning, brief episodes of I/R are administered to organs or tissues other than the heart and can be easily applied in a non-invasive way, e.g., using a blood pressure cuff on an arm or a leg (Lang and Kim, 2022; Penna et al., 2022; Comità et al., 2023; Ferdinandy et al., 2023; Zhao et al., 2023). Another way to protect the heart against the consequences of I/R injury is pharmacological conditioning (i.e., volatile anesthetics, opioids or α2-adrenoceptor agonists), a concept that is based on the administration of specific drugs mimicking the effect of ischemic preconditioning (Roth et al., 2021; Penna et al., 2022; Ferdinandy et al., 2023). Controlled occlusions or pharmacological interventions activate a molecular self-defense program which delays the progression of infarction. The subsequent activation of multiple endogenous cardioprotective mechanisms including adenosine, protein kinase, calcium signalling, nitric oxide release, reduction in ROS but also the involvement of autacoids, hormones, neurotransmitters and cytokines lead to cardioprotection and reduce infarct size (Penna et al., 2022; Heusch, 2023).
The endocannabinoid system is suggested to play a role in preconditioning-induced cardioprotection as well. Importantly, its involvement was confirmed in different models of preconditioning (Table 2): ischemic preconditioning (Bouchard et al., 2003), remote preconditioning (Hajrasouliha et al., 2008), propofol- (Sun et al., 2017), NO- (Wagner et al., 2006), heat stress- (Joyeux et al., 2002) and LPS-induced preconditioning (Lamontagne et al., 2006) that led to the reduction in the infarct size (Lagneux and Lamontagne, 2001; Joyeux et al., 2002; Wagner et al., 2006; Sun et al., 2007; Hajrasouliha et al., 2008). Moreover, they were associated with prevention of ischemia-induced endothelial dysfunction (Bouchard et al., 2003), anti-oxidative (Sun et al., 2017), anti-arrhythmic effects (Hajrasouliha et al., 2008) and an improvement in cardiac contractility (Wagner et al., 2006).
The involvement of ECBs in the above studies was confirmed by three approaches (Table 2). Firstly, propofol- (Sun et al., 2017) and NO-preconditioning (Wagner et al., 2006) enhanced the release of AEA and 2-AG in heart and serum in in vivo studies in mice after LAD occlusion and in isolated neonatal rat ventricle cells exposed to hypoxia (Sun et al., 2017) and of 2-AG in perfused rat heart (Wagner et al., 2006). Secondly, the beneficial effect of propofol was mimicked by the FAAH inhibitor URB597 and the selective endocannabinoid reuptake inhibitor VDM11 (Sun et al., 2017), drugs that increase endocannabinoid levels, and the effect of ischemic preconditioning (Bouchard et al., 2003) was also obtained with 2-AG and palmitoylethanolamide (PEA). Moreover, 2-AG and its metabolically stable derivative noladinether mimicked the cardioprotective effects of NO-induced preconditioning (Wagner et al., 2006). Thirdly, the beneficial effect of preconditioning and ECBs was reversible and blocked mainly by the CB2R antagonists SR144528 and AM630 (Lagneux and Lamontagne, 2001; Joyeux et al., 2002; Bouchard et al., 2003; Sun et al., 2017). However, a contribution of CB1Rs is also suggested due to the blockade of beneficial effects by AM251 (Wagner et al., 2006) or rimonabant (Bouchard et al., 2003).
6 Cardioprotection in experimental myocardial ischemia/reperusion injury and acute myocardial infarction
6.1 Endocannabinoids
As described in the two previous chapters, alterations (mostly increases) in the levels of the endocannabinoids AEA and 2-AG occurred in humans suffering from coronary heart disease (Table 1) and in animals subjected to preconditioning prior to experimental cardiac ischemia (Table 2). Although the endocannabinoids may just accompany ischemia, some of the above studies suggest that they contribute to the pathophysiological events or serve as a counteracting system. There is a big body of studies on experimental animals in vivo (Tables 3, 4) and in vitro (Table 5) which provides additional evidence that alterations of endocannabinoid levels and the cellular mechanisms involved in ischemia are associated. In order to get an idea which role the endogenously formed endocannabinoids play, experiments with selective cannabinoid receptor antagonists, inhibitors of endocannabinoid degradation and knockout animals are interesting. In the second subsection, the question will be addressed how exogenously added endocannabinoids like AEA and 2-AG influence ischemia in vivo and in vitro.
6.1.1 Effects of endogenously formed endocannabinoids?
In order to detect the possible functional role of either type of receptor, the use of selective CB1R and CB2R antagonists is a splendid technique. One can expect that the available antagonists (Figure 1) will cause an effect opposite in direction to that of the endocannabinoid(s). Even if the receptors are not exposed to an increased endocannabinoid level but are constitutively active, drugs like rimonabant or AM630 will reveal an endogenous tone since they are also inverse agonists (Maccarrone et al., 2023).
Some studies with CB1R antagonists suggest that CB1R activation has a detrimental influence. Thus, when the CB1R antagonist rimonabant was given once a day for 7 days before I/R, infarct size was reduced in wild-type CB1+/+ but not in CB1−/– mice (Lim et al., 2009). Moreover, its administration for 7 days before or 6 h after AMI and its continuous use for 6 weeks improved cardiac contractility (by increasing the rate of developed left ventricle (LV) pressure) and reduced arterial stiffness and cardiac remodeling; preventive chronic treatment with rimonabant was more effective in comparison to post-ischemic administration (Slavic et al., 2013). On the other hand, AM251, another CB1R antagonist, which was started 24 h after AMI and continued for 12 weeks, failed to affect infarct size but promoted cardiac remodeling (Wagner et al., 2003). The same drug, however, reduced the damage of murine cardiomyocytes undergoing hypoxia by decreasing apoptosis, oxidative stress and mitochondrial injury (Hu et al., 2023). Incubation of murine atrial cardiomyocytes with rimonabant failed to inhibit cell death during H/R (Lim et al., 2009). Note that in most of the studies of Tables 4, 5 the effect of CB1R antagonists, given alone, was not examined.
Only one CB2R antagonist-based study suggests that endogenous CB2R activation has a beneficial effect. Thus, single administration of the CB2R antagonist AM630 before ischemia increased the infarct size and oxidative and inflammation parameters in anaesthetized mice (Di Filippo et al., 2004). Note that in most of the publications, CB1R and CB2R antagonists did not modify cardiac parameters by themselves (Tables 3–5).
The use of inhibitors of the degradation of endocannabinoids, e.g., of URB597, an inhibitor of the AEA-degrading enzyme FAAH, or of JZL184, an inhibitor of the 2-AG-degrading enzyme MAGL (Figure 1), represents another means to identify an interplay between endocannabinoids and mechanisms involved in ischemia. One has, however, to consider that the endocannabinoid level reached under blockade of the degrading enzyme may not reflect the true level occurring under ischemia. The experiments by Schloss et al. (2019) show that higher plasma and cardiac levels of 2-AG occur in mice after AMI and that 2-AG has an unfavorable CB2R-mediated effect in I/R and AMI in mice. Administration of JZL184 further enhanced cardiac neutrophil and monocyte recruitment and inflammation, enhanced infarct size, impaired ventricular remodeling, increased ventricular fibrosis and finally increased mortality (Table 3).
Knockout mice represent a third approach to identify a functional role of endocannabinoids in ischemia and AMI. Studies were performed with CB2−/–, FAAH−/− and GPR55−/− mice (GPR55 is an orphan G protein-coupled receptor activated by endocannabinoids (Guerrero-Alba et al., 2019; Puhl et al., 2021). One has to admit that knockout mice have the disadvantage that compensatory alterations may occur due to the life-long absence of a receptor or an enzyme.
CB2R deficiency in mice subjected to ischemia/reperfusion injury or AMI led to detrimental cardiac effects such as worse prognosis of cardiac infarction and profound I/R injury as heralded by an increase in infarct size, apoptosis and remodeling, fibrosis, collagen deposition, altered systolic and diastolic function and decreases in ejection fraction (EF), fractional shortening (FS) and stroke work (Defer et al., 2009; Duerr et al., 2014; 2015; Hu et al., 2019). These changes were accompanied by an increase in macrophage and neutrophil infiltrations, a decrease in anti-oxidative enzymes (Duerr et al., 2015), impaired cardiac work and a deterioration of the autophagy process (Hu et al., 2019) (Table 3). A similar picture emerged on cardiac cells isolated from CB2−/– mice undergoing hypoxia (Table 5). Like in the in vivo experiments, one can observe detrimental cardiac effects such as the lack of decrease in levels of anti-oxidative enzymes and pro-autophagy proteins with a concomitant increase of LDH release, production of apoptotic factors and loss of cardiomyocytes (Duerr et al., 2014; 2015; Heinemann et al., 2015; Hu et al., 2019). Summarizing, all above experiments on knockout CB2−/– mice clearly suggest the cardioprotective role of CB2R against I/R injury.
In FAAH-deficient mice, cardiac work after I/R deteriorated and manifested with an increase in fibrosis, left ventricle and cardiomyocyte hypertrophy, wall thickening and a decrease in fractional shortening (Rajlic et al., 2022), Table 4]. These detrimental cardiac effects evoked by enhanced endocannabinoid tone were reversed by the peroxisome proliferator-activated receptor α (PPARα) antagonist GW6471 suggesting the involvement of PPARα receptors [(Rajlic et al., 2022) Table 4].
Finally, GPR55 deficiency had no influence on infarct size in mice in vivo (Puhl et al., 2021). Nonetheless, GPR55 receptors had favourable effects on left ventricular load, compensatory hypertrophy, wound healing and maladaptation after AMI in that study (Table 4).
6.1.2 Effects of exogenously administered endocannabinoids
Unfortunately, there are only few publications in which the effects of exogenously added AEA and 2-AG were examined in experimental models of I/R, AMI and hypoxia (Table 3-5). Thus, in rats with LAD occlusion AEA and/or its stable analogue methanandamide (MetAEA) reduced infarct size (Li et al., 2013a) and had an antiarrhythmic effect by improving myocardial resistance to arrhythmias induced by I/R (Krylatov et al., 2002). Similarly, in experiments on the isolated rat heart, 2-AG, PEA (Lépicier et al., 2003), AEA and MethAEA (Undertown et al., 2005) diminished infarct size and 2-AG and PEA improved cardiac work and had a beneficial effect against cardiac injury, as reflected by a decrease in lactate dehydrogenase (LDH) and creatine kinase (CK) levels in the perfusate (Lépicier et al., 2003).
The interaction of the endogenous cannabinoids with selective cannabinoid receptor antagonists was studied in three of the latter four studies (Tables 3–5). Thus, the infarct-limiting effect of AEA was antagonized by a CB2R antagonist (AM630 or SR144528) in the studies of Li et al. (2013a) and Undertown et al. (2005) whereas a CB1R antagonist (AM251 or RIM) had an antagonistic effect in the study of Undertown et al. (2005) only. In the study of Lépicier et al. (2003), the infarct-limiting effect of 2-AG and PEA was fully blocked by the CB2R antagonist SR144528 and the effect of 2-AG was also partially blocked by the CB1R antagonist RIM.
6.2 Synthetic cannabinoids
6.2.1 Cardioprotection via cannabinoid CB2 receptors
As mentioned above, some of the studies described in Table 2 and experiments with rimonabant and CB2R-deficient mice (Tables 3, 5) suggest a cardioprotective role of CB2R activation. This view is further corroborated by in vivo experiments with animals exposed to I/R injury and treated with selective and unselective agonists of CB2Rs (Table 3). The latter agonists reduced infarct size, which is the most robust endpoint of cardioprotection studies in experimental models of myocardial ischemia. Thus, as shown in Table 3, AM1241 (Liu et al., 2021), JWH133 (Defer et al., 2009; Montecucco et al., 2009; Li et al., 2013b), HU308 (Wang et al., 2012) and WIN55,515-2 (Di Filippo et al., 2004) diminished infarct size in I/R models. Similarly, a limitation of infarct size was observed after administration of JWH133 (Yu et al., 2019) and HU308 (Wang et al., 2012) after AMI. One should keep in mind, that myocardial infarction is properly expressed as percentage of the area at risk which represents the myocardial perfusion bed distal to an occluded artery (Gimelli and Rovai, 2013; Heusch, 2019). Unfortunately, the reduction in infarct size in cannabinoid studies (Tables 3-5) was not correlated with the reduction of the area at risk (with one exception in the study of Liu et al. (2021). Additionally, Wang et al. (2012) expressed myocardial infarct size as a percentage of the infarct area over total LV area.
In addition to the reduction in infarct size, CB2R agonists showed other cardioprotective effects. Thus, an anti-fibrotic effect associated with a decrease in cardiac collagen content, fibronectin and other factors implicated in the fibrotic response to injury was demonstrated in the studies of Liu et al. (2021) and Wang et al. (2014). Anti-inflammatory effects were noticed as well and correlated with a reduction in inflammatory cell infiltration (Montecucco et al., 2009; Liu et al., 2021), serum and cardiac inflammatory cytokines (Wang et al., 2012; Wang et al., 2014; Li et al., 2016; Yu et al., 2019) and the level of the NLRP3 inflammasome [which controls proinflammatory processes; (Yu et al., 2019);]. Another beneficial consequence of CB2R activation was the decrease in oxidative stress (Montecucco et al., 2009; Wang et al., 2012; Wang et al., 2014; Li et al., 2016), the reduction in apoptosis (Li et al., 2013b; Liu et al., 2021) and the augmentation of autophagy (Liu et al., 2021). The above effects were closely correlated with the decrease in enzymes reflecting cardiac injury like serum troponin and creatine kinase (Montecucco et al., 2009; Yu et al., 2019; Liu et al., 2021) and the improvement in cardiac work (Wang et al., 2014; Li et al., 2016).
The involvement of CB2Rs in the cardioprotective effects of synthetic cannabinoids against I/R and AMI was confirmed in experiments with CB2R antagonists (Table 3). Thus, the beneficial cardiac effects of JWH133 (Montecucco et al., 2009; Li et al., 2013b; Yu et al., 2019) and HU308 (Wang et al., 2012) were diminished by the CB2R antagonist AM630. The use of CB2R antagonists also allowed to decide that compounds possessing affinity for both CB1Rs and CB2Rs [WIN55,212-2; (Di Filippo et al., 2004; González et al., 2011); and AEA; (Li et al., 2013b);] act through CB2Rs since the improvement in cardiac work and coronary pressure and the decrease in infarct size and inflammatory responses induced by unselective agonists were attenuated or abolished by AM630 but not by the CB1R antagonist AM251. In this context, also the beneficial effect of curcumin, which reduced biochemical markers of cardiac injury, oxidative stress and inflammation against AMI in mice with streptozotocin-induced diabetes mellitus should be mentioned. Its effects were antagonized by AM630 (Pawar et al., 2022); CB1R antagonist not studied]. Curcumin is a polyphenol derived from the perennial plant Curcuma longa, which interacts with CB2Rs as shown in molecular docking studies (Pawar et al., 2022). A meta-analysis of 37 preclinical studies involving 771 rats or mice confirmed recently that curcumin exerts an excellent potential for the treatment of myocardial I/R injury in animal models. Surprisingly, the authors did not mention cannabinoid receptors in their publication at all (Zeng et al., 2023).
Cardioprotective effects of CB2R agonists against I/R injury have also been shown in vitro in cardiac cells and in isolated heart preparations subjected to hypoxia and ischemia, respectively (Table 5). In rat cardiomyocytes undergoing hypoxia with subsequent reoxygenation, incubation of cells with AM1241 (Liu et al., 2021) had a beneficial cardiac influence against H/R injury; so, it increased the production of pro-autophagy-related proteins and decreased the production of collagen, other pro-fibrotic factors and ROS (Li et al., 2016). In murine cardiomyocytes JWH133 increased cell survival and diminished hypoxia-dependent increase in the NLRP3 inflammasome (Yu et al., 2019). Moreover, in studies on isolated rat heart, JWH133, JWH015 and AM1241 decreased infarct size and improved cardiac recovery and work in hearts subjected to LAD occlusion (Li et al., 2014) or to a low-flow protocol (Lépicier et al., 2003; 2006; 2007). Only in the study by Undertown et al. (2005), JWH133 did not diminish infarct size although a 10-fold higher concentration was used when compared to the above studies. The authors started heart perfusion with JWH133 only 5 min before ischemia (Undertown et al., 2005); this time interval had proven to be sufficient for demonstration of the beneficial influence of JWH015 (Lépicier et al., 2007), AEA and MethAEA (Undertown et al., 2005) (Table 5). The results with JWH133 are surprising since, as mentioned above, AEA showed an infarct-limiting effect in the study of Undertown et al. (2005).
Final proof for the involvement of CB2Rs in the cardioprotection against I/R injury in vitro was obtained by the use of CB2R antagonists (Table 5). Thus, AM630 and/or SR144528 diminished the beneficial effects of JWH133 and/or JWH015 in the isolated rat heart, respectively (Lépicier et al., 2007; Li et al., 2014).
The results obtained with the nonselective agonist HU210 shall be discussed separately. HU210 decreased the weight of the necrotic zone without affecting hypoperfused area flow in rats after I/R (Ugdyzhekova et al., 2002), improved cardiac work recovery and decreased infarct size, area at risk and cardiac injury (visible as a decrease in the level of creatinine kinase in the perfusate) after I/R (Maslov et al., 2006; Gorbunov et al., 2016). Unfortunately, in none of the latter studies, the exact type(s) of cannabinoid receptor(s) has been determined. In the study of Wagner et al. (2003), twelve week-administration of this agonist started 24 h after AMI failed to affect infarct size and mortality in rats but improved cardiac contractility and prevented endothelial dysfunction in aortic rings and hypotension whereas the CB1R antagonist AM251 promoted cardiac remodeling. One has to consider that the fact that HU210 had a cardioprotective and AM251 a detrimental effect (Wagner et al., 2003) does not prove that HU210 acted via CB1Rs; again, interaction experiments with a CB2R antagonist have not been performed.
The possibility that CB2Rs, e.g., activated by HU-210, interact with β1-adrenoceptors (β-ARs) that play an important role in the regulation of cardiac tolerance to ischemia and reperfusion (e.g., Maslov et al., 2024) had to be considered. Interestingly, HU-210 and WIN55212-2 diminished the positive inotropic and chronotropic effects of the non-selective β-AR agonist, isoprenaline, and reduced the isoprenaline-stimulated increase in cAMP formation in isolated rat hearts (Maslov et al., 2004) and neonatal cardiomyocytes (Liao et al., 2013). Regarding cardiac ischemia-mediated injury, only one study on rats has been carried out, which reveals that the chronic administration of β-caryophyllene (BCP), a naturally occurring dietary cannabinoid (50 mg/kg, orally; twice daily for 10 days), diminished the isoprenaline-induced myocardial injury (including impaired cardiac function, increased levels of serum cardiac marker enzymes, and enhanced oxidative stress). In that study, isoprenaline (85 mg/kg) was given at an interval of 24 h for 2 days (ninth and 10th day) and its effect was partially sensitive to the CB2R antagonist AM630 (1 mg/kg given i. p. chronically prior to BCP treatment for 10 days) (Meeran et al., 2021).
Unfortunately, the combination of a CB2R agonist and a β-AR antagonist has so far not been studied in an animal model of cardiac ischemia-mediated injury. One should keep in mind that acute and chronic treatment with β-AR antagonists is frequently used to improve the outcome of the acute (Giannakopoulos and Noble, 2021) and chronic (Kim et al., 2020) phase of acute myocardial infarction in humans. It would be interesting to know whether the beneficial effect of β-AR antagonists (which block a Gs protein-coupled receptor) can be further increased by a CB2R agonist (which activates a Gi protein-coupled receptor).
6.2.2 Cardioprotection via other receptors/mechanisms
The putative involvement of other types of receptors, and in particular of CB1Rs, in the cardioprotective effects of synthetic cannabinoids during ischemia has been demonstrated in a few publications in vivo (Table 4) and in vitro (Table 5). The results regarding the role of CB1Rs in I/R injury are inconsistent. It has already been mentioned above that studies with CB1R antagonists suggest that CB1Rs have a beneficial (Lim et al., 2009)–in vivo experiments only; (Wagner et al., 2003; Slavic et al., 2013);] or detrimental effect (Hu et al., 2023) or no effect at all [(Lim et al., 2009)–in vitro experiments only)].
In one study, a synthetic selective CB1R agonist has been administered. Arachidonyl-2-chloroethylamide (ACEA) diminished infarct size in isolated rat heart in a manner sensitive to the CB1R antagonist rimonabant (Lépicier et al., 2007).
Apart from CB2Rs and CB1Rs, other receptors may come into play during I/R. As mentioned above, experiments with GPR55-deficient mice suggest that this receptor [activated by endocannabinoids but mainly by the endogenous agonist L-α-lysophosphatidylinositol, LPI; (Puhl, 2020);] has cardioprotective properties (Puhl et al., 2021) (Table 4). In another study on GPR55-deficient mice, Robertson-Gray et al. (2019) showed that LPI administration before ischemia (but not reperfusion) increased infarct size in the perfused heart of wild-type mice but not in mice with deletion of GPR55 receptors (Table 5). A satisfactory explanation for the discrepancy between the studies of Puhl et al. (2021) and Robertson-Gray et al. (2019) cannot be given.
6.3 Phytocannabinoids
Although Cannabis sativa contains numerous cannabinoids, Δ9-tetrahydrocannabinol and cannabidiol prevail. Their potential cardioprotective effects were examined in a series of studies.
The use of Δ9-tetrahydrocannabinol (THC) in cardiac I/R injury has been controversial and is strongly limited by its psychoactive properties (Leinen et al., 2023) (Table 4, 5). An ultra-low dose of THC (0.002 mg/kg, intraperitoneally) decreased both infarct size (given as a single bolus and chronically for 3 weeks before AMI) and cardiac damage in mice treated with this agent before AMI (Waldman et al., 2013). In line with the above report are the results of Shmist et al. (Shmist et al., 2006) who found that a 24 h incubation of rat cardiomyocytes undergoing hypoxia with THC protected cells from injury in an NO-dependent manner. Additionally (Banaszkiewicz et al., 2022), incubation of murine cardiomyocytes with THC under chemical hypoxia increased cardiomyocyte contractility and cytoplasmic LDH activity. Importantly, in human cardiomyocytes subjected to chemical hypoxia THC also exerted cardioprotective effects related to an improvement in cell metabolism and antioxidative activity, mitochondrial protection and a decrease in cell mortality. Moreover, THC improved recovery of the isolated rat heart after I/R.
The CB2R antagonist SR144528 blocked the beneficial effects of THC in rat cardiomyocytes whereas the CB1R antagonist RIM failed to do so (Shmist et al., 2006). Unfortunately, the cannabinoid receptor(s) involved in the cardioprotective actions of THC in vivo (Waldman et al., 2013) and in vitro (Banaszkiewicz et al., 2022 has/have not been determined.
The non-intoxicating and well-tolerated multitarget cannabidiol (CBD) possesses a great therapeutic potential resulting from its strong anti-inflammatory, anti-oxidant and anticonvulsant properties (Atalay et al., 2019; Kicman and Toczek, 2020; Leinen et al., 2023). Few studies also suggest potential cardioprotective properties against I/R (Table 4; 5). Thus, chronic treatment with CBD diminished infarct size in experiments with cardiac I/R in rats and rabbits (Table 4) (Durst et al., 2007; Garberg et al., 2017). Interestingly, two single injections, given before I and R, were also effective in decreasing the infarct size (Walsh et al., 2010; Feng et al., 2015). By contrast, CBD given intraperitoneally in two single boluses to rats with subsequent I/R studied on the isolated perfused heart, failed to decrease the infarct size; this may suggest that CBD does not have an equivalent effect in vivo and in vitro and that complex systemic mechanisms are responsible for the positive CBD effect in vivo (Durst et al., 2007). Other beneficial effects of CBD in I/R studies include improvement in cardiac work in the isolated heart (Franco-Vadillo et al., 2021) and in experiments in vivo (Durst et al., 2007; Feng et al., 2015; Franco-Vadillo et al., 2021), anti-inflammatory (Durst et al., 2007; Feng et al., 2015) and antiarrhythmic effects (Walsh et al., 2010; Gonca and Darıcı, 2015) as well as inhibition of platelet aggregation (Walsh et al., 2010). In addition, CBD decreased the necrotic zone (but not the area at risk), and increased the blood flow in the area at risk (Durst et al., 2007; Feng et al., 2015) (Table 4).
The mechanisms responsible for the cardioprotective effects of CBD may include a reduction in inflammatory responses (Durst et al., 2007; Feng et al., 2015), modulation of the angiotensin-renin system (increase in the expression of AT2 receptors and prevention of an increase in AT1 receptors responsible for, e.g., vasoconstriction, inflammation and remodeling) and RISK pathway stimulation (Franco-Vadillo et al., 2021). The antiarrhythmic potency of CBD in I/R injury is due to adenosine A1 receptor activation (Gonca and Darıcı, 2015).
7 Potential cellular mechanisms of cardioprotective actions of cannabinoids in ischemia/reperfusion injury
It was not the purpose of this review to analyze the cellular mechanisms of cardioprotective cannabinoids (beyond the level of receptors) and for this reason they are summarized here only briefly. Tables 1-5 show that the potential cellular pathways of cardioprotective cannabinoids have been examined in few studies only.
Several recent reviews have highlighted cardioprotective mechanisms that can regulate mitochondrial function, autophagy processes, modulation of energy metabolism, inflammation and apoptosis, and protect from ischemia-reperfusion injury (Ferdinandy et al., 2023). They include activation of PI3K (phosphoinositide-3-kinase (PI3K) and Akt (protein kinase B) (Deng and Zhou, 2023), ERK1/2 (extracellular signal-regulated kinase) (Kong et al., 2019), Pink1/Parkin (PTEN-induced kinase 1 (PINK1) and the E3 ubiquitin ligase Parkin) (Yu and Miyamoto, 2021), AMPK (AMP-activated protein kinase) (Pakesh et al., 2022), p38 MAPK (p38 mitogen-activated protein kinase) (Ruiz et al., 2018) and TGF-β (transforming growth factor-β1)-dependent activation of Smad-dependent cascades (Humeres et al., 2022).
Regarding the cardioprotective effects of CB2R stimulation against I/R injury, the possible involvement of the pro-survival PI3K/Akt pathway (Li et al., 2013a; 2013b; Wang et al., 2014), the ERK1 pathway (Montecucco et al., 2009) and induction of autophagy via the Pink1/Parkin pathway (Liu et al., 2021) was shown. Moreover, in mice with deletion of CB2Rs deterioration of the autophagy process related to inhibition of cardiac protective AMPK signaling was described (Hu et al., 2019). In an in vitro study, the anti-fibrotic and anti-oxidative actions of CB2R activation leading to the inhibition of the TGF-β1/Smad3 pathway was shown (Li et al., 2016). Blockade of CB1Rs with AM251 reduced the damage of cardiomyocytes exposed to hypoxia by activation of the AMPK pathway (Hu et al., 2023). With respect to the cannabidiol-dependent cardioprotective actions against I/R injury, the PI3K/Akt and MAPK/ERK pathways were shown as the only cellular mechanisms (Franco-Vadillo et al., 2021). Conversely, the enhancement of cardiac injury during ischemia mediated via activation of GPR55 receptors was dependent on the activation of the ROCK/p38 MAPK (Rho-assisted protein kinase/p38 mitogen-activated protein kinase) pathway (Robertson-Gray et al., 2019). Consequently, further research is necessary to determine the exact cellular signaling pathways by which cannabinoids produce beneficial (or detrimental) effects on the heart.
Little information is so far available with respect to the cellular sources of ECBs during I/R or AMI. Endocannabinoids and enzymes involved in their synthesis were found in rat cardiac tissues, endothelial cells and macrophage-derived cell lines, which means that cardiac function and coronary perfusion might be modulated by endocannabinoids derived not only from cardiac tissues but also from circulating cells like macrophages and platelets (Pacher and Haskó, 2008; Puhl, 2020).
8 From preclinical studies to clinical settings–IMPACT criteria
Various drugs or surgical interventions have been shown to reduce infarct size and to improve cardiac function and healing in preclinical studies but despite the optimistic results none of the drugs/interventions could be implemented for preventing myocardial ischemia/reperfusion injury in patients with AMI (Bolli, 2021; Heusch, 2023). Promising cardioprotective effects have also been found in preclinical studies of cannabinoids including mainly CB2R agonists and the multitarget CBD (for review, see (Lamontagne et al., 2006; Pacher and Haskó, 2008; Steffens and Pacher, 2012; Maslov et al., 2016; Tang et al., 2021) but drugs for the use in humans have so far not been developed. The question arises, why successful preclinical results for the treatment of acute myocardial infarction do not translate well into clinical practice (Heusch, 2023)?
To solve the above problem, the European Union (EU) CARDIOPROTECTION COST ACTION published in 2021 gives step-by-step criteria for Improving Preclinical Assessment of Cardioprotective Therapies (IMPACT) that should be met to improve the likelihood of translating novel cardioprotective interventions into the clinical setting (Lecour et al., 2021). They consist of three steps and their minimum criteria are given here (Figure 3). STEP 1 means that experiments should be validated in one species of small animals (e.g., mouse, rat or rabbit) in a single centre in an acute I/R injury model (minimum of 2 h but preferably 24 h of reperfusion). The end-point of the study should be the measurement of infarct size relative to area at risk and possibly also coronary microvascular obstruction. STEP 2 requires validation in small animal models in the presence of at least one confounder since a variety of factors (age, sex, diabetes, hypertension, dyslipidemia) and co-medications (platelet inhibitors, anesthetics, anti-diabetic drugs, statins and nitrates) might interfere with the end-points of the treatment. STEP 3 includes criteria for validation in large animal models. The importance of experiments on small animals with comorbidities and co-medications as well as on large animals have recently been underlined also by other groups (Lindsey et al., 2021; Penna et al., 2022; Ferdinandy et al., 2023; Wang et al., 2023).
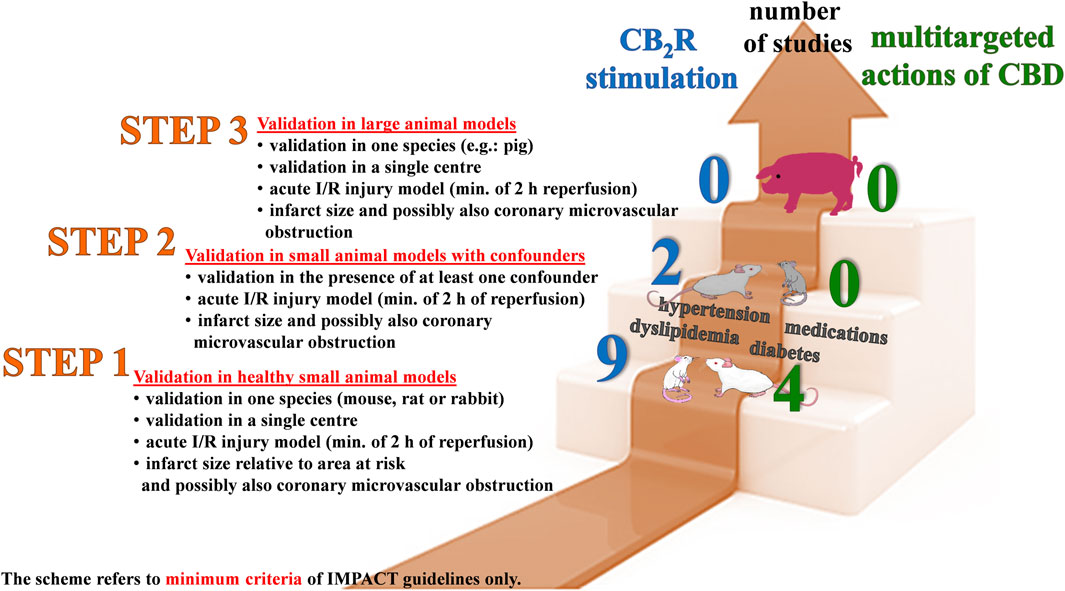
Figure 3. Are the studies regarding the cardioprotective effects of cannabinoid CB2 receptor (CB2R) agonistic drugs and cannabidiol (CBD) against cardiac ischemia/reperfusion (I/R) in accordance with the minimum (min.) criteria of the IMPACT (IMproving Preclinical Assessment of Cardioprotective Therapies) three-step schedule proposed by the European Union (EU) CARDIOPROTECTION COST ACTION (Lecour et al., 2021)? Our literature review, which is the basis for this scheme, shows that only 9 (blue numbers) and 4 (green numbers) of the studies examining the effects of CB2R activation and CBD, respectively, meet the minimum criteria of STEP 1. The minimum criteria of STEP 2 are partially met by two studies with CB2R activation only. Studies in large animals (STEP 3) are still lacking. For clarification: to meet STEP 2, experiments should be performed on small animals with con-founders such as co-morbidities (e.g., diabetes mellitus) or medications (used during surgery or for treatment of diseases) since these factors may influence the observed results.
Since IMPACT accepts in vivo preclinical studies only, we have checked the studies regarding cardioprotective effects of CB2R activation and collected in Table 3. Only nine studies met the criteria of STEP 1 (Di Filippo et al., 2004; Defer et al., 2009; Montecucco et al., 2009; Wang et al., 2012; Li et al., 2013a; 2013b; Duerr et al., 2014; 2015; Liu et al., 2023) The minimum criteria of STEP 2 were partially met by two studies only. Although the latter studies were performed on diabetic and/or fatty rats after I/R (González et al., 2011) or mice after AMI (Pawar et al., 2022) changes in infarct size were not determined. Finally, studies in large animals (STEP 3) are still lacking (Figure 3).
The EU CARDIOPROTECTION COST ACTION also stresses the importance of multitarget therapies as effective tools against myocardial ischemia/reperfusion injury (Davidson et al., 2019) With respect to the multitarget CBD we have found that only four among the seven papers in Table 4 met the minimum criteria of STEP 1 (Durst et al., 2007; Walsh et al., 2010; Feng et al., 2015). Studies on small animals with comorbidities or on large animals (STEP 2 and 3, respectively) have not been performed at all (Figure 3). The article by Garberg et al. (2016) on newborn piglets undergoing global hypoxia and treated with a high dose of CBD could not be considered in our analysis since cardiac ischemia was not quantified directly in that paper.
Apart from CBD and from drugs leading to CB2 receptor activation, only the study by Lim et al. (2009), dedicated to the cardioprotector effects of the CB1 receptor antagonist rimonabant, meets the criteria of STEP 1 and STEP 2 (Table 4).
Most of the studies in which potential cardioprotective properties of cannabinoids against I/R injury and AMI were examined took place before the IMPACT criteria were established. So, the experiments were conducted under experimental protocols in which reperfusion was not examined or, if so, was not long enough. Furthermore, compounds were administered prior to the onset of ischemia and the area of infarction was not always assessed in relation to the area at risk. Thus, the putative cardioprotective properties of cannabinoids against I/R and AMI should be re-evaluated in experiments on small animals under appropriate conditions and on large animals.
9 Limitations
Our literature review reveals that the protocols used to induce ischemia/hypoxia and the compounds under study extremely varied. Furthermore, most experiments were conducted on healthy rodents and cannabinoids were administered once only. Therefore, we were unable to give details to (1) the best timing of (endo)cannabinoid administration, i.e., before, during or after ischemia (from the clinical perspective, the best time for treatment is after ischemia or AMI, but this time of administration had been chosen by few of the studies only); (2) the effect of (endo)cannabinoids in animals with comorbidities and under chronic drug treatment; (3) the detrimental effects of the blockade of endocannabinoid degradation since only few studies of that type had been performed (Schloss et al., 2019; Rajlic et al., 2022). Moreover, the cardioprotective effects of (endo)cannabinoids in the human heart had been examined in few studies only (Table 1). Finally, in the light of the fact that the major heart receptors, the β-ARs, are essential for adjusting the heart to ischemia-reperfusion injury, it is unsatisfactory that the cross-talk between these receptors and the cannabinoid receptors had been examined in one study only (Meeran et al., 2021).
10 Conclusion
A detailed review of all publications regarding cardiac effects of cannabinoids clearly shows that cannabinoids exert cardioprotective effects in cardiac preconditioning, I/R and AMI. Beneficial cardiac actions result mainly from the activation of CB2Rs although positive effects of the endocannabinoid AEA, the phytocannabinoid THC and the multitarget CBD were also described. Importantly, endocannabinoid levels were enhanced in acute and chronic cardiac disorders in humans including AMI.
In 2021 the strict step-by-step criteria for Improving Preclinical Assessement of Cardioprotective Therapies (IMPACT) have been established by the EU CARDIOPROTECTION COST ACTION (Lecour et al., 2021). In our evaluation of the papers regarding the cardioprotective effects of cannabinoids in the context of various protocols and models none of the publications so far met all IMPACT criteria. Thus, additional experiments are needed to confirm the cardioprotective activities of cannabinoids on small animals with comorbidities and on large animals. One should also keep in mind that it is necessary that the infarct size be expressed in relation to the area at risk. Additionally, as a result of our review of the literature, we have been able to identify the main directions in which intensive research should be conducted in order to gain a closer understanding of the cardioprotective effects of cannabinoids. Thus, the proper moment of administration of drugs (so far generally administered before ischemia, i.e., before the moment most difficult to predict for the patient) should be identified, since early reperfusion constitutes the critical time to perform life-saving interventions (Gibson, 2001; Heusch, 2020). Moreover, one should consider the use of a new generation of CB2Rs agonists (characterized, e.g., by a better solubility) that have shown promising results in preclinical studies related to analgesic and anti-inflammatory effects (Bryk and Starowicz, 2021; Whiting et al., 2022). With respect to the putative therapeutic application of FAAH or MAGL inhibitors (Toczek and Malinowska, 2018; Maccarrone et al., 2023) one should be particularly careful because chronic enhancement of the endocannabinoid tone caused detrimental cardiac effects in animal models (Schloss et al., 2019; Rajlic et al., 2022). Importantly, more new data regarding the cardiac endocannabinoid system in humans under pathological conditions are also needed.
Taken together, we believe that it is too early to place great hopes on the future cardioprotective application of cannabinoids (including CB2R agonists) in myocardial ischemia. Thus, tools and methods used for further studies must be well elaborated in order to meet the current shortcomings in our knowledge regarding the cardioprotective actions of cannabinoids against I/R injury.
Author contributions
AP-B: Writing–review and editing, Writing–original draft, Conceptualization. ES: Writing–review and editing. JW: Writing–original draft. BM: Writing–review and editing, Writing–original draft.
Funding
The author(s) declare that financial support was received for the research, authorship, and/or publication of this article. The study was supported by the Medical University of Bialystok (Poland, grant No B.SUB.23.396).
Conflict of interest
The authors declare that the research was conducted in the absence of any commercial or financial relationships that could be construed as a potential conflict of interest.
Publisher’s note
All claims expressed in this article are solely those of the authors and do not necessarily represent those of their affiliated organizations, or those of the publisher, the editors and the reviewers. Any product that may be evaluated in this article, or claim that may be made by its manufacturer, is not guaranteed or endorsed by the publisher.
References
Atalay, S., Jarocka-Karpowicz, I., and Skrzydlewska, E. (2019). Antioxidative and anti-inflammatory properties of cannabidiol. Antioxidants (Basel) 9, 21. doi:10.3390/antiox9010021
Banaszkiewicz, M., Tarwacka, P., Krzywonos-Zawadzka, A., Olejnik, A., Laprairie, R., Noszczyk-Nowak, A., et al. (2022). Δ9-Tetrahydrocannabinol (Δ9-THC) improves ischemia/reperfusion heart dysfunction and might serve as a cardioprotective agent in the future treatment. Front. Biosci. Landmark Ed. 27, 114. doi:10.31083/j.fbl2704114
Basalay, M. V., Yellon, D. M., and Davidson, S. M. (2020). Targeting myocardial ischaemic injury in the absence of reperfusion. Basic Res. Cardiol. 115, 63. doi:10.1007/s00395-020-00825-9
Bolli, R. (2021). CAESAR's legacy: a new era of rigor in preclinical studies of cardioprotection. Basic Res. Cardiol. 116, 33. doi:10.1007/s00395-021-00874-8
Bonz, A., Laser, M., Küllmer, S., Kniesch, S., Babin-Ebell, J., Popp, V., et al. (2003). Cannabinoids acting on CB1 receptors decrease contractile performance in human atrial muscle. J. Cardiovasc. Pharmacol. 41, 657–664. doi:10.1097/00005344-200304000-00020
Bouchard, J. F., Lépicier, P., and Lamontagne, D. (2003). Contribution of endocannabinoids in the endothelial protection afforded by ischemic preconditioning in the isolated rat heart. Life Sci. 72, 1859–1870. doi:10.1016/s0024-3205(02)02474-8
Boyette, L. C., and Manna, B. (2023). “Physiology, myocardial oxygen demand,” in StatPearls (Treasure Island (FL): StatPearls Publishing). PMID: 29763072.
Bryk, M., and Starowicz, K. (2021). Cannabinoid-based therapy as a future for joint degeneration. Focus on the role of CB2 receptor in the arthritis progression and pain: an updated review. Pharmacol. Rep. 73, 681–699. doi:10.1007/s43440-021-00270-y
Buja, L. M. (2023). Pathobiology of myocardial ischemia and reperfusion injury: models, modes, molecular mechanisms, modulation, and clinical applications. Cardiol. Rev. 31, 252–264. doi:10.1097/CRD.0000000000000440
Comità, S., Rubeo, C., Giordano, M., Penna, C., and Pagliaro, P. (2023). Pathways for cardioprotection in perspective: focus on remote conditioning and extracellular vesicles. Biol. (Basel) 12, 308. doi:10.3390/biology12020308
Davidson, S. M., Ferdinandy, P., Andreadou, I., Bøtker, H. E., Heusch, G., Ibáñez, B., et al. (2019). Multitarget strategies to reduce myocardial ischemia/reperfusion injury: JACC review topic of the week. J. Am. Coll. Cardiol. 73, 89–99. doi:10.1016/j.jacc.2018.09.086
Defer, N., Wan, J., Souktani, R., Escoubet, B., Perier, M., Caramelle, P., et al. (2009). The cannabinoid receptor type 2 promotes cardiac myocyte and fibroblast survival and protects against ischemia/reperfusion-induced cardiomyopathy. FASEB J. 23, 2120–2130. doi:10.1096/fj.09-129478
Deng, R. M., and Zhou, J. (2023). The role of PI3K/AKT signaling pathway in myocardial ischemia-reperfusion injury. Int. Immunopharmacol. 123, 110714. doi:10.1016/j.intimp.2023.110714
Di Filippo, C., Rossi, F., Rossi, S., and D’Amico, M. (2004). Cannabinoid CB2 receptor activation reduces mouse myocardial ischemia-reperfusion injury: involvement of cytokine/chemokines and PMN. J. Leukoc. Biol. 75, 453–459. doi:10.1189/jlb.0703303
Duerr, G. D., Heinemann, J. C., Gestrich, C., Heuft, T., Klaas, T., Keppel, K., et al. (2015). Impaired border zone formation and adverse remodeling after reperfused myocardial infarction in cannabinoid CB2 receptor deficient mice. Life Sci. 138, 8–17. doi:10.1016/j.lfs.2014.11.005
Duerr, G. D., Heinemann, J. C., Suchan, G., Kolobara, E., Wenzel, D., Geisen, C., et al. (2014). The endocannabinoid-CB2 receptor axis protects the ischemic heart at the early stage of cardiomyopathy. Basic Res. Cardiol. 109, 425. doi:10.1007/s00395-014-0425-x
Durst, R., Danenberg, H., Gallily, R., Mechoulam, R., Meir, K., Grad, E., et al. (2007). Cannabidiol, a nonpsychoactive cannabis constituent, protects against myocardial ischemic reperfusion injury. Am. J. Physiol. Heart Circ. Physiol. 293, H3602–H3607. doi:10.1152/ajpheart.00098.2007
Feng, Y., Chen, F., Yin, T., Xia, Q., Liu, Y., Huang, G., et al. (2015). Pharmacologic effects of cannabidiol on acute reperfused myocardial infarction in rabbits: evaluated with 3.0T cardiac magnetic resonance imaging and histopathology. J. Cardiovasc. Pharmacol. 66, 354–363. doi:10.1097/FJC.0000000000000287
Ferdinandy, P., Andreadou, I., Baxter, G. F., Bøtker, H. E., Davidson, S. M., Dobrev, D., et al. (2023). Interaction of cardiovascular nonmodifiable risk factors, comorbidities and comedications with ischemia/reperfusion injury and cardioprotection by pharmacological treatments and ischemic conditioning. Pharmacol. Rev. 75, 159–216. doi:10.1124/pharmrev.121.000348
Franco-Vadillo, A., Toledo-Blass, M., Rivera-Herrera, Z., Guevara-Balcazar, G., Orihuela-Rodriguez, O., Morales-Carmona, J. A., et al. (2021). Cannabidiol-mediated RISK PI3K/AKT and MAPK/ERK pathways decreasing reperfusion myocardial damage. Pharmacol. Res. Perspect. 9, e00784. doi:10.1002/prp2.784
Garberg, H. T., Solberg, R., Barlinn, J., Martinez-Orgado, J., Løberg, E. M., and Saugstad, O. D. (2017). High-dose cannabidiol induced hypotension after global hypoxia-ischemia in piglets. Neonatology 112, 143–149. doi:10.1159/000471786
Giannakopoulos, G., and Noble, S. (2021). Should we be using upstream Beta-blocker therapy for acute myocardial infarction? Curr. Cardiol. Rep. 23, 66. doi:10.1007/s11886-021-01494-3
Gibson, C. M. (2001). Time is myocardium and time is outcomes. Circulation 104, 2632–2634. doi:10.1161/circ.104.22.2632
Gimelli, A., and Rovai, D. (2013). Myocardium at risk: reasons and methods for measuring the extent. J. Nucl. Cardiol. 20, 23–26. doi:10.1007/s12350-012-9659-x
Gonca, E., and Darıcı, F. (2015). The effect of cannabidiol on ischemia/reperfusion-induced ventricular arrhythmias: the role of adenosine A1 receptors. J. Cardiovasc. Pharmacol. Ther. 20, 76–83. doi:10.1177/1074248414532013
González, C., Herradón, E., Abalo, R., Vera, G., Pérez-Nievas, B. G., Leza, J. C., et al. (2011). Cannabinoid/agonist WIN 55,212-2 reduces cardiac ischaemia–reperfusion injury in Zucker diabetic fatty rats: role of CB2 receptors and iNOS/eNOS. Diabetes Metab. Res. Rev. 27, 331–340. doi:10.1002/dmrr.1176
Gorbunov, A. S., Maslov, L. N., Tsibulnikov, S. Y., Khaliulin, I. G., Tsepokina, A. V., Khutornaya, M. V., et al. (2016). CB-receptor agonist HU-210 mimics the postconditioning phenomenon of isolated heart. Bull. Exp. Biol. Med. 162, 27–29. doi:10.1007/s10517-016-3536-6
Guerrero-Alba, R., Barragán-Iglesias, P., González-Hernández, A., Valdez-Moráles, E. E., Granados-Soto, V., Condés-Lara, M., et al. (2019). Some prospective alternatives for treating pain: the endocannabinoid system and its putative receptors GPR18 and GPR55. Front. Pharmacol. 9, 1496. doi:10.3389/fphar.2018.01496
Hajrasouliha, A. R., Tavakoli, S., Ghasemi, M., Jabehdar-Maralani, P., Sadeghipour, H., Ebrahimi, F., et al. (2008). Endogenous cannabinoids contribute to remote ischemic preconditioning via cannabinoid CB2 receptors in the rat heart. Eur. J. Pharmacol. 579, 246–252. doi:10.1016/j.ejphar.2007.09.034
Heinemann, J. C., Duerr, G. D., Keppel, K., Breitbach, M., Fleischmann, B. K., Zimmer, A., et al. (2015). CB2 receptor-mediated effects of pro-inflammatory macrophages influence survival of cardiomyocytes. Life Sci. 138, 18–28. doi:10.1016/j.lfs.2014.11.027
Heusch, G. (2019). Coronary microvascular obstruction: the new frontier in cardioprotection. Basic Res. Cardiol. 114, 45. doi:10.1007/s00395-019-0756-8
Heusch, G. (2020). Myocardial ischaemia-reperfusion injury and cardioprotection in perspective. Nat. Rev. Cardiol. 17, 773–789. doi:10.1038/s41569-020-0403-y
Heusch, G. (2023). Cardioprotection and its translation: a need for new paradigms? Or for new pragmatism? An opinionated retro- and perspective. J. Cardiovasc. Pharmacol. Ther. 28, 10742484231179613. doi:10.1177/10742484231179613
Hu, Y., Tao, Y., and Hu, J. (2019). Cannabinoid receptor 2 deletion deteriorates myocardial infarction through the down-regulation of AMPK-mTOR-p70S6K signaling-mediated autophagy. Biosci. Rep. 39, BSR20180650. doi:10.1042/BSR20180650
Hu, Z., Mu, S., Zhao, L., Dou, Z., Wang, P., Zhang, J., et al. (2023). Regulation of the CB1R/AMPK/PGC-1α signaling pathway in mitochondria of mouse hearts and cardiomyocytes with chronic intermittent hypoxia. Sleep. Breath. 28, 133–149. doi:10.1007/s11325-023-02863-8
Humeres, C., Venugopal, H., and Frangogiannis, N. G. (2022). Smad-dependent pathways in the infarcted and failing heart. Curr. Opin. Pharmacol. 64, 102207. doi:10.1016/j.coph.2022.102207
Ishac, E. J., Jiang, L., Lake, K. D., Varga, K., Abood, M. E., and Kunos, G. (1996). Inhibition of exocytotic noradrenaline release by presynaptic cannabinoid CB1 receptors on peripheral sympathetic nerves. Br. J. Pharmacol. 118, 2023–2028. doi:10.1111/j.1476-5381.1996.tb15639.x
Jehle, J., Goerich, H., Bindila, L., Lutz, B., Nickenig, G., and Tiyerili, V. (2019). Endocannabinoid 2-arachidonoylglycerol is elevated in the coronary circulation during acute coronary syndrome. PLoS One 14, e0227142. doi:10.1371/journal.pone.0227142
Joyeux, M., Arnaud, C., Godin-Ribuot, D., Demenge, P., Lamontagne, D., and Ribuot, C. (2002). Endocannabinoids are implicated in the infarct size-reducing effect conferred by heat stress preconditioning in isolated rat hearts. Cardiovasc. Res. 55, 619–625. doi:10.1016/s0008-6363(02)00268-7
Kalogeris, T., Baines, C. P., Krenz, M., and Korthuis, R. J. (2016). Ischemia/reperfusion. Compr. Physiol. 7, 113–170. doi:10.1002/cphy.c160006
Kicman, A., and Toczek, M. (2020). The effects of cannabidiol, a non-intoxicating compound of cannabis, on the cardiovascular system in health and disease. Int. J. Mol. Sci. 21, 6740. doi:10.3390/ijms21186740
Kim, J., Kang, D., Park, H., Kang, M., Park, T. K., Lee, J. M., et al. (2020). Long-term β-blocker therapy and clinical outcomes after acute myocardial infarction in patients without heart failure: nationwide cohort study. Eur. Heart J. 41, 3521–3529. doi:10.1093/eurheartj/ehaa376
Kong, T., Liu, M., Ji, B., Bai, B., Cheng, B., and Wang, C. (2019). Role of the extracellular signal-regulated kinase 1/2 signaling pathway in ischemia-reperfusion injury. Front. Physiol. 10, 1038. doi:10.3389/fphys.2019.01038
Krylatov, A. V., Uzhachenko, R. V., Maslov, L. N., Bernatskaya, N. A., Makriyannis, A., Mechoulam, R., et al. (2002). Endogenous cannabinoids improve myocardial resistance to arrhythmogenic effects of coronary occlusion and reperfusion: a possible mechanism. Bull. Exp. Biol. Med. 133, 122–124. doi:10.1023/a:1015574100494
Lagneux, C., and Lamontagne, D. (2001). Involvement of cannabinoids in the cardioprotection induced by lipopolysaccharide. Br. J. Pharmacol. 132, 793–796. doi:10.1038/sj.bjp.0703902
Lamontagne, D., Lépicier, P., Lagneux, C., and Bouchard, J. F. (2006). The endogenous cardiac cannabinoid system: a new protective mechanism against myocardial ischemia. Arch. Mal. Coeur. Vaiss. 99, 242–246.
Lang, J. A., and Kim, J. (2022). Remote ischaemic preconditioning - translating cardiovascular benefits to humans. J. Physiol. 600, 3053–3067. doi:10.1113/JP282568
Lecour, S., Andreadou, I., Bøtker, H. E., Davidson, S. M., Heusch, G., Ruiz-Meana, M., et al. (2021). Improving preclinical assessment of cardioprotective therapies (IMPACT) criteria: guidelines of the EU-CARDIOPROTECTION COST Action. Basic Res. Cardiol. 116, 52. doi:10.1007/s00395-021-00893-5
Leinen, Z. J., Mohan, R., Premadasa, L. S., Acharya, A., Mohan, M., and Byrareddy, S. N. (2023). Therapeutic potential of cannabis: a comprehensive review of current and future applications. Biomedicines 11, 2630. doi:10.3390/biomedicines11102630
Lépicier, P., Bibeau-Poirier, A., Lagneux, C., Servant, M. J., and Lamontagne, D. (2006). Signaling pathways involved in the cardioprotective effects of cannabinoids. J. Pharmacol. Sci. 102, 155–166. doi:10.1254/jphs.crj06011x
Lépicier, P., Bouchard, J. F., Lagneux, C., and Lamontagne, D. (2003). Endocannabinoids protect the rat isolated heart against ischaemia. Br. J. Pharmacol. 139, 805–815. doi:10.1038/sj.bjp.0705313
Lépicier, P., Lagneux, C., Sirois, M. G., and Lamontagne, D. (2007). Endothelial CB1-receptors limit infarct size through NO formation in rat isolated hearts. Life Sci. 81, 1373–1380. doi:10.1016/j.lfs.2007.08.042
Li, Q., Guo, H. C., Maslov, L. N., Qiao, X. W., Zhou, J. J., and Zhang, Y. (2014). Mitochondrial permeability transition pore plays a role in the cardioprotection of CB2 receptor against ischemia-reperfusion injury. Can. J. Physiol. Pharmacol. 92, 205–214. doi:10.1139/cjpp-2013-0293
Li, Q., Shi, M., and Li, B. (2013a). Anandamide enhances expression of heat shock protein 72 to protect against ischemia-reperfusion injury in rat heart. J. Physiol. Sci. 63, 47–53. doi:10.1007/s12576-012-0228-5
Li, Q., Wang, F., Zhang, Y. M., Zhou, J. J., and Zhang, Y. (2013b). Activation of cannabinoid type 2 receptor by JWH133 protects heart against ischemia/reperfusion-induced apoptosis. Cell. Physiol. biochem. 31, 693–702. doi:10.1159/000350088
Li, X., Han, D., Tian, Z., Gao, B., Fan, M., Li, C., et al. (2016). Activation of cannabinoid receptor type II by AM1241 ameliorates myocardial fibrosis via nrf2-mediated inhibition of TGF-β1/smad3 pathway in myocardial infarction mice. Cell. Physiol. biochem. 39, 1521–1536. doi:10.1159/000447855
Liao, Y., Bin, J., Luo, T., Zhao, H., Ledent, C., Asakura, M., et al. (2013). CB1 cannabinoid receptor deficiency promotes cardiac remodeling induced by pressure overload in mice. Int. J. Cardiol. 167, 1936–1944. doi:10.1016/j.ijcard.2012.05.033
Lim, S. Y., Davidson, S. M., Yellon, D. M., and Smith, C. C. (2009). The cannabinoid CB1 receptor antagonist, rimonabant, protects against acute myocardial infarction. Basic Res. Cardiol. 104, 781–792. doi:10.1007/s00395-009-0034-2
Lindsey, M. L., Brunt, K. R., Kirk, J. A., Kleinbongard, P., Calvert, J. W., de Castro Brás, L. E., et al. (2021). Guidelines for in vivo mouse models of myocardial infarction. Am. J. Physiol. Heart Circ. Physiol. 321, H1056–H1073. doi:10.1152/ajpheart.00459.2021
Liu, W., Chen, C., Gu, X., Zhang, L., Mao, X., Chen, Z., et al. (2021). AM1241 alleviates myocardial ischemia-reperfusion injury in rats by enhancing Pink1/Parkin-mediated autophagy. Life Sci. 272, 119228. doi:10.1016/j.lfs.2021.119228
Liu, Y., Li, L., Wang, Z., Zhang, J., and Zhou, Z. (2023). Myocardial ischemia-reperfusion injury; molecular mechanisms and prevention. Microvasc. Res. 149, 104565. doi:10.1016/j.mvr.2023.104565
Maccarrone, M., Di Marzo, V., Gertsch, J., Grether, U., Howlett, A. C., Hua, T., et al. (2023). Goods and bads of the endocannabinoid system as a therapeutic target: lessons learned after 30 years. Pharmacol. Rev. 75, 885–958. doi:10.1124/pharmrev.122.000600
Maeda, N., Osanai, T., Kushibiki, M., Fujiwara, T., Tamura, Y., Oowada, S., et al. (2009). Increased serum anandamide level at ruptured plaque site in patients with acute myocardial infarction. Fundam. Clin. Pharmacol. 23, 351–357. doi:10.1111/j.1472-8206.2009.00679.x
Maslov, L. N., Khaliulin, I., Zhang, Y., Krylatov, A. V., Naryzhnaya, N. V., Mechoulam, R., et al. (2016). Prospects for creation of cardioprotective drugs based on cannabinoid receptor agonists. J. Cardiovasc. Pharmacol. Ther. 21, 262–272. doi:10.1177/1074248415612593
Maslov, L. N., Lasukova, O. V., Krylatov, A. V., Hanus, L. O., Pertwee, R., Ivanchuk, I. I., et al. (2006). Role of cannabinoid receptors in the regulation of cardiac contractility during ischemia/reperfusion. Bull. Exp. Biol. Med. 142, 557–561. doi:10.1007/s10517-006-0417-4
Maslov, L. N., Lasukova, O. V., Krylatov, A. V., Uzhachenko, R. V., and Pertwee, R. (2004). Selective cannabinoid receptor agonist HU-210 decreases pump function of isolated perfused heart: role of cAMP and cGMP. Bull. Exp. Biol. Med. 138, 550–553. doi:10.1007/s10517-005-0123-7
Maslov, L. N., Naryzhnaya, N. V., Voronkov, N. S., Kurbatov, B. K., Derkachev, I. A., Ryabov, V. V., et al. (2024). The role of β-adrenergic receptors in the regulation of cardiac tolerance to ischemia/reperfusion. Why do β-adrenergic receptor agonists and antagonists protect the heart? Fundam. Clin. Pharmacol., doi:10.1111/fcp.12988
Meeran, M. F. N., Laham, F., Azimullah, S., Sharma, C., Al Kaabi, A. J., Tariq, S., et al. (2021). β-Caryophyllene, a natural bicyclic sesquiterpene attenuates β-adrenergic agonist-induced myocardial injury in a cannabinoid receptor-2 dependent and independent manner. Free Radic. Biol. Med. 167, 348–366. doi:10.1016/j.freeradbiomed.2021.01.046
Molderings, G. J., Likungu, J., and Göthert, M. (1999). Presynaptic cannabinoid and imidazoline receptors in the human heart and their potential relationship. Naunyn Schmiedeb. Arch. Pharmacol. 360, 157–164. doi:10.1007/s002109900043
Montecucco, F., Lenglet, S., Braunersreuther, V., Burger, F., Pelli, G., Bertolotto, M., et al. (2009). CB(2) cannabinoid receptor activation is cardioprotective in a mouse model of ischemia/reperfusion. J. Mol. Cell. Cardiol. 46, 612–620. doi:10.1016/j.yjmcc.2008.12.014
More, S. A., Deore, R. S., Pawar, H. D., Sharma, C., Nakhate, K. T., Rathod, S. S., et al. (2024). CB2 cannabinoid receptor as a potential target in myocardial infarction: exploration of molecular pathogenesis and therapeutic strategies. Int. J. Mol. Sci. 25, 1683. doi:10.3390/ijms25031683
Murry, C. E., Jennings, R. B., and Reimer, K. A. (1986). Preconditioning with ischemia: a delay of lethal cell injury in ischemic myocardium. Circulation 74, 1124–1136. doi:10.1161/01.cir.74.5.1124
Pacher, P., and Haskó, G. (2008). Endocannabinoids and cannabinoid receptors in ischaemia-reperfusion injury and preconditioning. Br. J. Pharmacol. 153, 252–262. doi:10.1038/sj.bjp.0707582
Pacher, P., Steffens, S., Haskó, G., Schindler, T. H., and Kunos, G. (2018). Cardiovascular effects of marijuana and synthetic cannabinoids: the good, the bad, and the ugly. Nat. Rev. Cardiol. 15, 151–166. doi:10.1038/nrcardio.2017.130
Paskeh, M. D. A., Asadi, A., Mirzaei, S., Hashemi, M., Entezari, M., Raesi, R., et al. (2022). Targeting AMPK signaling in ischemic/reperfusion injury: from molecular mechanism to pharmacological interventions. Cell. Signal. 94, 110323. doi:10.1016/j.cellsig.2022.110323
Pawar, H. D., Mahajan, U. B., Nakhate, K. T., Agrawal, Y. O., Patil, C. R., Meeran, M. F. N., et al. (2022). Curcumin protects diabetic mice against isoproterenol-induced myocardial infarction by modulating CB2 cannabinoid receptors. Life (Basel) 12, 624. doi:10.3390/life12050624
Penna, C., Comità, S., Tullio, F., Alloatti, G., and Pagliaro, P. (2022). Challenges facing the clinical translation of cardioprotection: 35 years after the discovery of ischemic preconditioning. Vasc. Pharmacol. 144, 106995. doi:10.1016/j.vph.2022.106995
Puhl, S. L. (2020). Cannabinoid-sensitive receptors in cardiac physiology and ischaemia. Biochim. Biophys. Acta. Mol. Cell. Res. 1867, 118462. doi:10.1016/j.bbamcr.2019.03.009
Puhl, S. L., Hilby, M., Kohlhaas, M., Keidel, L. M., Jansen, Y., Hristov, M., et al. (2021). Haematopoietic and cardiac GPR55 synchronize post-myocardial infarction remodelling. Sci. Rep. 11, 14385. doi:10.1038/s41598-021-93755-y
Rajesh, M., Mukhopadhyay, P., Bátkai, S., Arif, M., Varga, Z. V., Mátyás, C., et al. (2022). Cannabinoid receptor 2 activation alleviates diabetes-induced cardiac dysfunction, inflammation, oxidative stress, and fibrosis. Geroscience 44, 1727–1741. doi:10.1007/s11357-022-00565-9
Rajesh, M., Mukhopadhyay, P., Haskó, G., Liaudet, L., Mackie, K., and Pacher, P. (2010). Cannabinoid-1 receptor activation induces reactive oxygen species-dependent and -independent mitogen-activated protein kinase activation and cell death in human coronary artery endothelial cells. Br. J. Pharmacol. 160, 688–700. doi:10.1111/j.1476-5381.2010.00712.x
Rajlic, S., Surmann, L., Zimmermann, P., Weisheit, C. K., Bindila, L., Treede, H., et al. (2022). Fatty acid amide hydrolase deficiency is associated with deleterious cardiac effects after myocardial ischemia and reperfusion in mice. Int. J. Mol. Sci. 23, 12690. doi:10.3390/ijms232012690
Robertson-Gray, O. J., Walsh, S. K., Ryberg, E., Jönsson-Rylander, A. C., Lipina, C., and Wainwright, C. L. (2019). l-α-Lysophosphatidylinositol (LPI) aggravates myocardial ischemia/reperfusion injury via a GPR55/ROCK-dependent pathway. Pharmacol. Res. Perspect. 7, e00487. doi:10.1002/prp2.487
Rorabaugh, B. R., Guindon, J., and Morgan, D. J. (2023). Role of cannabinoid signaling in cardiovascular function and ischemic injury. J. Pharmacol. Exp. Ther. 387, 265–276. doi:10.1124/jpet.123.001665
Roth, S., Torregroza, C., Feige, K., Preckel, B., Hollmann, M. W., Weber, N. C., et al. (2021). Pharmacological conditioning of the heart: an update on experimental developments and clinical implications. Int. J. Mol. Sci. 22, 2519. doi:10.3390/ijms22052519
Ruiz, M., Coderre, L., Allen, B. G., and Des Rosiers, C. (2018). Protecting the heart through MK2 modulation, toward a role in diabetic cardiomyopathy and lipid metabolism. Biochim. Biophys. Acta. Mol. Basis. Dis. 1864, 1914–1922. doi:10.1016/j.bbadis.2017.07.015
Schloss, M. J., Horckmans, M., Guillamat-Prats, R., Hering, D., Lauer, E., Lenglet, S., et al. (2019). 2-Arachidonoylglycerol mobilizes myeloid cells and worsens heart function after acute myocardial infarction. Cardiovasc. Res. 115, 602–613. doi:10.1093/cvr/cvy242
Shmist, Y. A., Goncharov, I., Eichler, M., Shneyvays, V., Isaac, A., Vogel, Z., et al. (2006). Delta-9-tetrahydrocannabinol protects cardiac cells from hypoxia via CB2 receptor activation and nitric oxide production. Mol. Cell. Biochem. 283, 75–83. doi:10.1007/s11010-006-2346-y
Slavic, S., Lauer, D., Sommerfeld, M., Kemnitz, U. R., Grzesiak, A., Trappiel, M., et al. (2013). Cannabinoid receptor 1 inhibition improves cardiac function and remodelling after myocardial infarction and in experimental metabolic syndrome. J. Mol. Med. Berl. 91, 811–823. doi:10.1007/s00109-013-1034-0
Smoum, R., Grether, U., Karsak, M., Vernall, A. J., Park, F., Hillard, C. J., et al. (2022). Editorial: therapeutic potential of the cannabinoid CB2 receptor. Front. Pharmacol. 13, 1039564. doi:10.3389/fphar.2022.1039564
Steffens, S., and Pacher, P. (2012). Targeting cannabinoid receptor CB(2) in cardiovascular disorders: promises and controversies. Br. J. Pharmacol. 167, 313–323. doi:10.1111/j.1476-5381.2012.02042.x
Sterin-Borda, L., Del Zar, C. F., and Borda, E. (2005). Differential CB1 and CB2 cannabinoid receptor-inotropic response of rat isolated atria: endogenous signal transduction pathways. Biochem. Pharmacol. 69, 1705–1713. doi:10.1016/j.bcp.2005.03.027
Sugamura, K., Sugiyama, S., Nozaki, T., Matsuzawa, Y., Izumiya, Y., Miyata, K., et al. (2009). Activated endocannabinoid system in coronary artery disease and antiinflammatory effects of cannabinoid 1 receptor blockade on macrophages. Circulation 119, 28–36. doi:10.1161/CIRCULATIONAHA.108.811992
Sun, H. J., Lu, Y., Wang, H. W., Zhang, H., Wang, S. R., Xu, W. Y., et al. (2017). Activation of endocannabinoid receptor 2 as a mechanism of propofol pretreatment-induced cardioprotection against ischemia-reperfusion injury in rats. Oxid. Med. Cell. Longev. 2017, 2186383. doi:10.1155/2017/2186383
Szekeres, M., Nádasy, G. L., Soltész-Katona, E., and Hunyady, L. (2018). Control of myogenic tone and agonist induced contraction of intramural coronary resistance arterioles by cannabinoid type 1 receptors and endocannabinoids. Prostagl. Other Lipid Mediat 134, 77–83. doi:10.1016/j.prostaglandins.2017.10.001
Tang, X., Liu, Z., Li, X., Wang, J., and Li, L. (2021). Cannabinoid receptors in myocardial injury: a brother born to rival. Int. J. Mol. Sci. 22, 6886. doi:10.3390/ijms22136886
Toczek, M., and Malinowska, B. (2018). Enhanced endocannabinoid tone as a potential target of pharmacotherapy. Life Sci. 204, 20–45. doi:10.1016/j.lfs.2018.04.054
Ugdyzhekova, D. S., Krylatov, A. V., Bernatskaya, N. A., Maslov, L. N., Mechoulam, R., and Pertwee, R. G. (2002). Activation of cannabinoid receptors decreases the area of ischemic myocardial necrosis. Bull. Exp. Biol. Med. 133, 125–126. doi:10.1023/a:1015526217332
Underdown, N. J., Hiley, C. R., and Ford, W. R. (2005). Anandamide reduces infarct size in rat isolated hearts subjected to ischaemia-reperfusion by a novel cannabinoid mechanism. Br. J. Pharmacol. 146, 809–816. doi:10.1038/sj.bjp.0706391
Valenta, I., Varga, Z. V., Valentine, H., Cinar, R., Horti, A., Mathews, W. B., et al. (2018). Feasibility evaluation of myocardial cannabinoid type 1 receptor imaging in obesity: a translational approach. JACC Cardiovasc. Imaging. 11, 320–332. doi:10.1016/j.jcmg.2017.11.019
van Esbroeck, A. C. M., Varga, Z. V., Di, X., van Rooden, E. J., Tóth, V. E., Onódi, Z., et al. (2020). Activity-based protein profiling of the human failing ischemic heart reveals alterations in hydrolase activities involving the endocannabinoid system. Pharmacol. Res. 151, 104578. doi:10.1016/j.phrs.2019.104578
Wagner, J. A., Abesser, M., Harvey-White, J., and Ertl, G. (2006). 2-Arachidonylglycerol acting on CB1 cannabinoid receptors mediates delayed cardioprotection induced by nitric oxide in rat isolated hearts. J. Cardiovasc. Pharmacol. 47, 650–655. doi:10.1097/01.fjc.0000211752.08949.eb
Wagner, J. A., Hu, K., Karcher, J., Bauersachs, J., Schäfer, A., Laser, M., et al. (2003). CB(1) cannabinoid receptor antagonism promotes remodeling and cannabinoid treatment prevents endothelial dysfunction and hypotension in rats with myocardial infarction. Br. J. Pharmacol. 138, 1251–1258. doi:10.1038/sj.bjp.0705156
Waldman, M., Hochhauser, E., Fishbein, M., Aravot, D., Shainberg, A., and Sarne, Y. (2013). An ultra-low dose of tetrahydrocannabinol provides cardioprotection. Biochem. Pharmacol. 85, 1626–1633. doi:10.1016/j.bcp.2013.03.014
Walsh, S. K., Hepburn, C. Y., Kane, K. A., and Wainwright, C. L. (2010). Acute administration of cannabidiol in vivo suppresses ischaemia-induced cardiac arrhythmias and reduces infarct size when given at reperfusion. Br. J. Pharmacol. 160, 1234–1242. doi:10.1111/j.1476-5381.2010.00755.x
Wang, P. F., Jiang, L. S., Bu, J., Huang, X. J., Song, W., Du, Y. P., et al. (2012). Cannabinoid-2 receptor activation protects against infarct and ischemia-reperfusion heart injury. J. Cardiovasc. Pharmacol. 59, 301–307. doi:10.1097/FJC.0b013e3182418997
Wang, Q., Zuurbier, C. J., Huhn, R., Torregroza, C., Hollmann, M. W., Preckel, B., et al. (2023). Pharmacological cardioprotection against ischemia reperfusion injury-the search for a clinical effective therapy. Cells 12, 1432. doi:10.3390/cells12101432
Wang, Y., Ma, S., Wang, Q., Hu, W., Wang, D., Li, X., et al. (2014). Effects of cannabinoid receptor type 2 on endogenous myocardial regeneration by activating cardiac progenitor cells in mouse infarcted heart. Sci. China Life Sci. 57, 201–208. doi:10.1007/s11427-013-4604-z
Weis, F., Beiras-Fernandez, A., Sodian, R., Kaczmarek, I., Reichart, B., Beiras, A., et al. (2010). Substantially altered expression pattern of cannabinoid receptor 2 and activated endocannabinoid system in patients with severe heart failure. J. Mol. Cell. Cardiol. 48, 1187–1193. doi:10.1016/j.yjmcc.2009.10.025
Weresa, J., Pędzińska-Betiuk, A., Mińczuk, K., Malinowska, B., and Schlicker, E. (2022). Why do marijuana and synthetic cannabimimetics induce acute myocardial infarction in healthy young people? Cells 11, 1142. doi:10.3390/cells11071142
Whiting, Z. M., Yin, J., de la Harpe, S. M., Vernall, A. J., and Grimsey, N. L. (2022). Developing the cannabinoid receptor 2 (CB2) pharmacopoeia: past, present, and future. Trends Pharmacol. Sci. 43, 754–771. doi:10.1016/j.tips.2022.06.010
Yu, J. D., and Miyamoto, S. (2021). Molecular signaling to preserve mitochondrial integrity against ischemic stress in the heart: rescue or remove mitochondria in danger. Cells 10, 3330. doi:10.3390/cells10123330
Yu, W., Jin, G., Zhang, J., and Wei, W. (2019). Selective activation of cannabinoid receptor 2 attenuates myocardial infarction via suppressing NLRP3 inflammasome. Inflammation 42, 904–914. doi:10.1007/s10753-018-0945-x
Zeng, Y. F., Guo, Q. H., Wei, X. Y., Chen, S. Y., Deng, S., Liu, J. J., et al. (2023). Cardioprotective effect of curcumin on myocardial ischemia/reperfusion injury: a meta-analysis of preclinical animal studies. Front. Pharmacol. 14, 1184292. doi:10.3389/fphar.2023.1184292
Keywords: Cannabinoids, Cannabinoid receptor, Cannabidiol, Myocardial ischemia-reperfusion injury, Myocardial infarction, IMproving Preclinical assessment of Cardioprotective therapies (IMPACT)
Citation: Pędzińska-Betiuk A, Schlicker E, Weresa J and Malinowska B (2024) Re-evaluation of the cardioprotective effects of cannabinoids against ischemia-reperfusion injury according to the IMproving Preclinical Assessment of Cardioprotective Therapies (IMPACT) criteria. Front. Pharmacol. 15:1382995. doi: 10.3389/fphar.2024.1382995
Received: 06 February 2024; Accepted: 05 April 2024;
Published: 30 May 2024.
Edited by:
Gioacchino Calapai, University of Messina, ItalyReviewed by:
Worku Abebe, Augusta University, United StatesAndrea Elia, Temple University, United States
Copyright © 2024 Pędzińska-Betiuk, Schlicker, Weresa and Malinowska. This is an open-access article distributed under the terms of the Creative Commons Attribution License (CC BY). The use, distribution or reproduction in other forums is permitted, provided the original author(s) and the copyright owner(s) are credited and that the original publication in this journal is cited, in accordance with accepted academic practice. No use, distribution or reproduction is permitted which does not comply with these terms.
*Correspondence: Anna Pędzińska-Betiuk, YW5uYS5wZWR6aW5za2EtYmV0aXVrQHVtYi5lZHUucGw=