- 1Innovative Institute of Chinese Medicine and Pharmacy, Chengdu University of Traditional Chinese Medicine, Chengdu, China
- 2School of Pharmacy, Zhejiang Chinese Medical University, Hangzhou, China
Osteoporosis characterized by decreased bone density and mass, is a systemic bone disease with the destruction of microstructure and increase in fragility. Osteoporosis is attributed to multiple causes, including aging, inflammation, diabetes mellitus, and other factors induced by the adverse effects of medications. Without treatment, osteoporosis will further progress and bring great trouble to human life. Due to the various causes, the treatment of osteoporosis is mainly aimed at improving bone metabolism, inhibiting bone resorption, and promoting bone formation. Although the currently approved drugs can reduce the risk of fragility fractures in individuals, a single drug has limitations in terms of safety and effectiveness. By contrast, traditional Chinese medicine (TCM), a characteristic discipline in China, including syndrome differentiation, Chinese medicine prescription, and active ingredients, shows unique advantages in the treatment of osteoporosis and has received attention all over the world. Therefore, this review summarized the pathogenic factors, pathogenesis, therapy limitations, and advantages of TCM, aiming at providing new ideas for the prevention and treatment of OP.
1 Introduction
Osteoporosis (OP), characterized by the decrease in bone mineral density (BMD) and disorders of the bone microstructure, is a chronic metabolic bone disease (Noh et al., 2020). OP is a result of the imbalance of bone homeostasis that is maintained by coordinated cycles of bone resorption and formation and can contribute to the increase in bone fragility as well as the risk of fractures (El-Gazzar and Högler, 2021; Zhang et al., 2023). These fragility fractures lead to a disproportionately high mortality rate and drastically reduce the quality of life, all of which make osteoporosis a growing and enormous threat to public health that affects 200 million people worldwide (Muñoz et al., 2020). From the perspective of etiology, osteoporosis can be divided into two types: primary and secondary (Eastell et al., 2016; Compston et al., 2019). Among them, primary osteoporosis is a result of aging, and its most common form is postmenopausal osteoporosis (PMOP), which is caused by the decrease in estrogen secretion after menopause (Compston et al., 2019), while secondary osteoporosis occurs when BMD is reduced by other factors such as drugs (Compston et al., 2019; S., 2011). Based on various induction factors of osteoporosis, a series of therapeutic drugs for osteoporosis have been developed for clinical practice, including estrogen, calcitonin, bisphosphonates, and teriparatide (Khosla and Hofbauer, 2017). Among these, estrogens, used in the prevention and therapy of osteoporosis, have certain side effects, including increased risk of cardiovascular events and breast cancer (Rossouw et al., 2002). Calcitonin was developed based on animal and human studies and is now rarely used for the treatment of osteoporosis due to its limited efficacy on osteoporosis and concerns that its long-term use may increase the risk of cancer (Chesnut et al., 2000). In addition, bisphosphonates are the most widely used drugs, but poor adherence is a major limiting factor for their treatment of osteoporosis, which is largely associated with gastrointestinal adverse events (Cramer et al., 2007). Moreover, treatment with high doses of teriparatide may increase the risk of developing osteosarcoma in growing rodents (Vahle et al., 2016). Therefore, it is urgent to seek some effective drugs with few side effects for the treatment of osteoporosis. Despite some progress in the treatment of osteoporosis, the side effects of these drugs are of concern (Khosla and Hofbauer, 2017). Therefore, exploring the pathogenesis of OP and finding its therapeutic drugs are crucial.
OP is closely associated with aging, endocrine diseases, chronic kidney diseases, gastrointestinal diseases, and so on (Lane, 2006). It is worth mentioning that osteoporosis is often accompanied by pain, spinal deformity, and fracture, even limitation of movement and disability, all of which seriously affect the quality of daily life (Tsai et al., 2019). Under the inducement of these factors, the formation of OP is associated with osteoblasts that maintain functional cells of bone formation and osteoclasts that participate in bone resorption. Osteoblasts are the main functional cells in bone for bone resorption. Osteoblasts play an important role in the synthesis, secretion, and mineralization of the bone matrix, while osteoclasts, known as bone-resorbing cells, are a component of bone tissue and mainly regulate the function of bone resorption (Chotiyarnwong and McCloskey, 2020). In OP, osteoclasts accomplish the transformation from bone resorption to bone formation by transmitting coupling signals to osteoblasts (Ikebuchi et al., 2018). During this process, the receptor activator of NF-κB (RANK)/receptor activator of the NF-κB ligand (RANKL)/osteoprotegerin (OPG) axis plays a key role (Zhao et al., 2020). Studies showed that the inhibition of RANKL can be a therapeutic strategy for excessive bone resorption, such as recombinant, which is still in the research stage due to its uncertain side effects. Therefore, it is urgent to seek some effective drugs with few side effects for the treatment of OP (He et al., 2019).
Traditional Chinese medicine (TCM), with few side effects, has unique advantages in the treatment of chronic disease. Studies showed that TCM has a long history in the prevention and treatment of OP (Zhang et al., 2016), such as Eucommiae Folium, Cornus officinalis, and Radix Angelicae sinensis. Moreover, TCM treatment based on syndrome differentiation is the accumulation of clinical practice gathered over centuries (Fu et al., 2021) and has specific advantages in the treatment of OP. In this review, we summarized the pathogenic factors, pathogenesis, therapy limitations, and advantages of TCM, aiming at providing new ideas for the prevention and treatment of OP.
2 Pathogenic factors of osteoporosis
2.1 Aging is a major cause of osteoporosis
The skeletal system grows rapidly, mainly from the postnatal period to puberty after birth and adolescence, reaches its peak at about 35 years of age (Wayne Sampson, 2002), and then, gradually decreases with age in both men and women (Yamakawa et al., 2020). Therefore, aging is a baseline risk factor in the development of OP and bone fracture, as well as a predictor of poor outcomes after fracture (VanderWalde and Hurria, 2011). In women, primary OP, also called postmenopausal OP, is mainly induced by menopause plus the cessation of ovarian function, specifically, a decline in postmenopausal ovarian endocrine function, resulting in the decrease in Es level and leading to greater bone resorption than bone formation (Wu et al., 2021). Of note, up to one-third of fragility fractures occur in older men and are usually accompanied by severe osteoporotic fractures, especially hip fractures. Due to these problems, OP in the elderly is an important threat to the life quality of individual patients and a huge burden to society (Kaufman, 2021). Therefore, exploring the pathogenesis of aging-related OP is crucial for the treatment of OP. Qadir et al. stated that due to aging, bone marrow stromal cells were more likely to differentiate into adipocytes rather than osteoblasts, which contributes to the decrease in bone formation, leading to the development of senile OP (Qadir et al., 2020). Moreover, with aging, the excessive accumulation of reactive oxygen species (ROS), interleukin-6 (IL-6), tumor necrosis factor-α (TNF-α), and other cytokines in cells will affect the differentiation of osteoclasts and the formation of osteoblasts, leading to osteoporotic bone loss (Yu and Wang, 2016). Therefore, inflammation is another cause of osteoporosis.
2.2 Inflammation is involved in the development of osteoporosis
Innate immune cells are the major source of proinflammatory factors, such as IL-6 and TNF-α, and can immediately respond to various challenges in the body, which has been considered one of the main inducements of skeletal diseases (Hato and Dagher, 2015). In OP, osteoblasts undergo programmed necrosis and release NOD-like receptor protein 3 (NLRP3), thereby resulting in inflammatory responses. During these processes, IL-1β and IL-18 were cleaved by caspase-1 and turned into mature forms that could be released into the extracellular environment, which promoted excessive bone resorption (Vijayaraj et al., 2021). Moreover, other immune cells, such as DCs, macrophages, and monocytes, can also take part in osteoclast formation because they share a common developmental niche (Ponzetti and Rucci, 2019). Analogous eosinophils, mast cells, and neutrophils could also contribute to the development of OP (Yu et al., 2015; Ragipoglu et al., 2020). It can be seen that inflammation plays a critical role in OP due to its role in bone loss and osteoblast function (Amarasekara et al., 2015), which is characterized by BMD reduction and the production of cytokines in diseases such as periodontitis (Yu and Wang, 2022) and rheumatoid arthritis (RA) (Forsblad D'Elia et al., 2003).
2.3 OP is often a complication of diabetes mellitus
OP is also closely associated with diabetes mellitus (DM), which is characterized by polyphagia, polyuria, and hyperglycemia (Fang et al., 2021). DM is usually divided into two types: type 1 diabetes mellitus (T1DM) and type 2 diabetes. Among them, T1DM is known as insulin-dependent diabetes and can induce bone loss due to calcium and phosphorus imbalance (Lecka-Czernik, 2017), while type 2 diabetes is non-insulin-dependent diabetes mellitus that is considered a risk factor for OP (Barrett-Connor E, 1992), mainly due to that increased obesity in diabetic patients will affect the function of osteoblasts and osteoclasts (Rathinavelu et al., 2018). In addition, a variety of chronic complications related to diabetes mellitus, such as diabetic liver disease (fatty liver), diabetic nephropathy (Paschou et al., 2017), microvascular disease (Samakkarnthai et al., 2020), and diabetic neuropathy (Paschou et al., 2017), can also contribute to the development of OP.
2.4 Other factors contribute to osteoporosis
In addition, other factors, such as cerebral apoplexy, breast cancer (Sozel and Yilmaz, 2021), decompensated cirrhosis (Yang and Kim, 2021), gastrointestinal disease (Klaus et al., 2002), bowel disease, celiac disease, and hyperuricemia (Lee et al., 2021) and its induction divisors (smoking, alcohol, virus, etc.) (Lo et al., 2020), can also contribute to various degrees of osteoporosis. It is worth mentioning that the current treatment drugs for the above diseases include rosiglitazone, bisphosphonate (Sheu et al., 2022), unfractionated heparin, and low-molecular-weight heparin (Zhang B. et al., 2021). Proton pump inhibitors also play critical roles in the development of OP. In addition, a study showed that weight was also closely associated with the incidence of OP (Andreoli et al., 2011), which is due to that a high body mass index is related to high BMD and reduction of fracture risk in postmenopausal females (Tariq et al., 2017). Furthermore, other factors that affect body weight are also involved in the development of OP, such as nutrition and exercise. To be specific, malnutrition caused by low-protein diets can reduce the expression of insulin-like growth factor 1 (IGF-1), suppress the absorption of intestinal calcium and phosphorus, and inhibit bone calcification (Muñoz and Argente, 2002); exercise can regulate the biological activity of osteoblasts, increase mass accumulation of bone, and prolong bone turnover rate; furthermore, long-term plus regular weight-bearing exercise can increase BMD and reduce bone loss (Stein and Shane, 2003) (Figure 1).
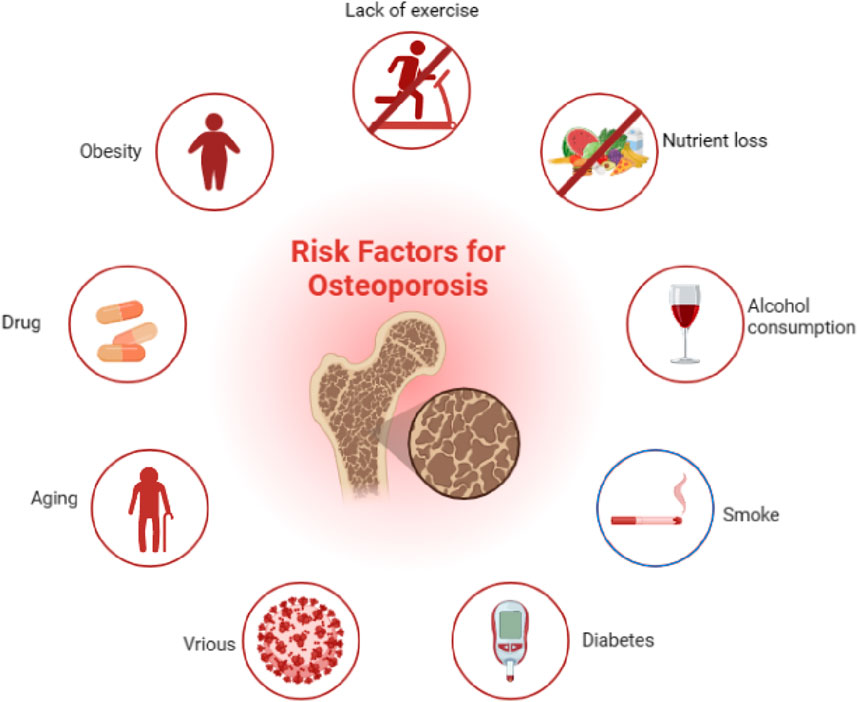
Figure 1. Pathogenic factors of osteoporosis, such as aging, inflammation, and diabetes mellitus, could contribute to the development of osteoporosis.
3 Treatment of OP
Due to the variety of pathogenic factors of OPG, the application of TCM and Western medicine in the treatment of OP is also very different. For Western medicine, the treatment strategy for OP is mainly to prevent fractures, and reducing bone resorption or stimulating bone formation in this process are common means (Gennari et al., 2020). Based on this, the main therapeutic drugs for OP are anti-absorption drugs and anabolic agents (Li et al., 2021). Among them, bisphosphonates are the most widely used anti-bone resorption drugs, which can reduce bone turnover markers to a lower concentration before menopause and have achieved considerable results in reducing the fracture rate and the treatment of OP (Eastell and Szulc, 2017). However, the study revealed that the patients who were treated with bisphosphonates for 3 years or more were at an increased risk for osteonecrosis of the jaw (ONJ) and atypical femoral fractures (AFFs), but the absolute risks were low (Ayers et al., 2023). Moreover, poor adherence to bisphosphonate therapy is a major limiting factor in OP treatment, which is largely associated with gastrointestinal adverse events (Pagnotti et al., 2019). In addition, treatment using bisphosphonates can also cause other side effects, such as fever and myalgia, especially after the treatment is started (Ayers et al., 2023). It is worth noting that these side reactions caused by bisphosphonates can be alleviated by TCM, which is due to the characteristics of TCM with multiple approaches, multiple targets, and the four diagnostic methods of TCM, namely, observation, listening, asking, and cutting (Zhang et al., 2016).
Compared with the single treatment using bisphosphonates, TCM can develop personalized treatment plans according to the different physiques of patients. Specifically, for patients with poor spleen and stomach function, accompanied by general weakness, drugs with properties of strengthening the spleen and replenishing qi can be used; for patients with spleen and stomach disorders, accompanied by diarrhea and abdominal pain, drugs with an effect of strengthening the spleen and stopping diarrhea can be used; TCM can also improve blood circulation, promote the delivery of bone nutrients, and alleviate pain in patients with OP through methods such as acupuncture and moxibustion (Zhuo et al., 2022). Therefore, TCM has unique advantages in the treatment of OP, including alleviating symptoms, improving prognosis, and reducing fracture incidence.
However, the mechanism and pharmacodynamic components of TCM on OP treatment are still unclear, and the main limiting factors of its development are also problems that need to be solved urgently.
4 TCM in the treatment of OP based on syndrome differentiation
TCM has a long history of being used to prevent and treat osteoporosis. According to the pathology and clinical manifestations of OP in modern medicine, OP in TCM can be classified into the scope of “ostealgia (Gu bi)” and “atrophic debility of bones (Gu wei)” according to “Nei jing.” Among them, kidney deficiency, blood stasis, and qi and yin deficiency are the main pathogenesis, and the disease location is bone. The nature is “deficiency of kidney essence, spleen deficiency, and nourishment loss,” whose features are blood stasis block (Mohammad et al., 2018). For the treatment of this disease, some TCM preparations, acupoint application, acupuncture, massage, and other TCM therapies are also applied to improve BMD. According to the TCM theory, bone diseases are closely related to the health status of the kidney. Therefore, people with kidney qi deficiency and kidney yin deficiency are more prone to suffer from OP. Traditional treatments mainly use the method of tonifying the kidney and strengthening the bone based on syndrome differentiation. In addition to drug treatment, acupuncture and acupoint application can effectively prevent and treat the disease. The research of single Chinese medicine and effective components mainly involves Epimedii Folium, Eucommiae Cortex, and Salviae Miltiorrhizae Radix et Rhizoma (Figure 2).
4.1 Herbal extracts
Chinese herbal medicine formulations with the advantages of multiple components and targets have been widely considered by researchers in the treatment of chronic disease (Cui et al., 2010). Bellavia et al. revealed the potential effects of flavonoids in bone resorption and promoting bone formation via a review of literature records in the last 5 years (Bellavia et al., 2021). In addition, saponins, iridoid glycosides, and lignans in Chinese herbal medicine also showed certain therapeutic effects on OP, whose mechanism was associated with Wnt/β-catenin, BMP/Smad, and mitogen-activated protein kinase (MAPK) pathways, RANKL/OPG signaling, and others (Zhang et al., 2016). For example, the dry leaf of Epimedium brevicornu Maxim. has a long history in the treatment of bone diseases in China due to its effect of tonifying kidney yang and strengthening muscles and bones. According to modern research, total flavonoids of Epimedium brevicornu Maxim. can treat osteoporotic distal radius fractures (Xue et al., 2016). Icariin (ICA), as the main active flavonoid glycoside in Epimedium brevicornu Maxim., has the effect of enhancing osteogenic activity through the regulation of the JNK/c-Jun signaling pathway, Wnt/β-catenin pathway, and Notch signaling pathway (Huang et al., 2020; Xu et al., 2019; Yu et al., 2020). In addition, Eucommiae Folium extract can promote the osteogenic differentiation of bone marrow mesenchymal stem cells (BMSCs) by regulating the Wnt/β-catenin signaling pathway and decreasing RANKL-induced bone resorption-related genes, such as TRAP (Cheng et al., 2019). The aqueous extract of Eucommiae Folium after salt roasting can promote the proliferation and differentiation of MC3T3-E1 cells, elevate the OPG level, and inhibit the secretion and expression of RANKL protein by regulating ERK, NF-κB, AKT pathways (Guan et al., 2021). Furthermore, Salviae Miltiorrhizae Radix et Rhizoma, also known as Danshen in Chinese, has a long history of being used to treat bone disorders (Guo et al., 2014). With the development of modern analytical techniques, many compounds have been isolated and identified from Danshen. Tanshinol is one of these compounds, and importantly, it can also play a role in reducing bone formation disorders through KLF15/PPARγ2 signaling (Yang Y.-j. et al., 2018). In addition, tanshinones are thought to inhibit osteoclast differentiation and may be a candidate for the treatment of OP (Lee et al., 2005). Moreover, Danshen has been reported to combine with Puerariae Lobatae Radix to alleviate OP through autophagy and oxidative stress-mediated osteoclast differentiation (Qin et al., 2021). Drynariae Rhizoma is introduced to improve glucocorticoid-induced OP by regulating the activity of osteoblasts and osteoclasts. Naringin has been identified as an effective anti-OP component of Drynariae Rhizoma (Peng C.-H. et al., 2022). Like naringin, the other five flavonoids, namely, aglycones, kurarinone, kushennol F, xanthogalenol, and sophoraflavanone G, have a potential protective effect against ovariectomized-induced osteoporosis, which may be related to the activation of endoplasmic reticulum signaling pathways (Wang et al., 2011). Achyranthis Bidentatae Radix is a TCM used to treat OP (Zhang M. et al., 2018). Polysaccharides have been the most studied in all components of Achyranthis Bidentatae Radix, and it has been reported that these polysaccharides can promote bone formation and, thus, play a potential role in anti-OP therapy (Zhang S. et al., 2018). In addition, Dipsaci Radix is a typical Chinese medicine used to treat OP by regulating immune-related pathways. In this study, ursolic acid and beta-sitosterol were shown to be the effective compounds (Zhang W. et al., 2019). Sweroside is the major active iridoid glycoside isolated from Dipsaci Radix. It has been reported that sweroside can exert beneficial effects on anti-OP by interacting with the membrane estrogen receptor-α and GPR30 to activate the p38 signaling pathway (Wu et al., 2020). As a triterpenoid saponin, asperosaponin VI exhibits anti-osteoclastogenic activity by inhibiting RANKL-induced osteoclast differentiation and function (Liu et al., 2019). Some compounds in Psoraleae Fructus display anti-OP activity by activating the ER-Wnt-β-catenin signaling pathway, among which isoflavones have the strongest activity (Cai et al., 2021). As a representative flavonoid, corylifol A can reduce ROS production through the activation of Nrf2, leading to the inhibition of osteoclast production and activation (Li et al., 2024). Similarly, corylin is also a flavonoid in Psoraleae Fructus, which increases the expression of osteogenic markers such as Runt-related transcription factor 2 (Runx2), osterix, type I collagen (Col1), and alkaline phosphatase (ALP), thus targeting the treatment of osteoblast-mediated OP (Yu TX. Y. et al., 2020). Cistanches Herba is an edible Chinese medicine that inhibits the RANKL/Rank-induced activation of downstream NF-κB and PI3K/AKT pathways and blocks the activity of the key osteoclastogenic proteins NFAT2 and c-Fos (Zhang B. et al., 2019). Cistanches Herba polysaccharide reduces RANKL-mediated ROS production in osteoclasts, which impairs osteoclastogenesis and bone resorption (Song et al., 2018). Cistanoside A is a phenylethanol glycoside compound isolated from Cistanches Herba, which has the potential to treat OP by downregulating TRAF6 (Xu et al., 2017). In summary, numerous TCM formulations and compounds derived from them have showed potential therapeutic effects in the prevention and treatment of OP (Table 1).
4.2 Traditional Chinese medicine formula
“Shen nong ben cao jing” recorded that medicine should be in harmony with the king and minister (Jun–chen–zuo–shi). Due to this theory, TCM preparations consisting of multiple herbs have attracted increasing international attention because of their characteristics and curative effects. Among these, OP Liuwei Dihuang pill (LWD), which mainly consists of Rehmannia glutinosa Libosch, Paeonia suffruticosa Andr, Dioscorea opposita Thunb., Poria cocos (Schw.) Wolf, Alisma orientale (Sam.) Juz., and Cornus officinalis Sieb. et Zucc., was used in the treatment of OP, showing that miR-574 plays critical roles in osteoporosis, and kaempferol and quercetin actives may be the ingredients of LWD targeting MAPK1 to mediate MiR-574, thereby regulating the bone microenvironment and improving OP (Liu et al., 2022). Additionally, Shuai Bo et al. found that Qing’e Pill (QEP), consisting of Eucommia ulmoides Oliv., Psoralea corylifolia L., Juglans regia L., and Allium sativum L, could improve the microstructure of cancellous bone in ovariectomized mice by increasing the expression of β-catenin. Further study revealed that QEP could prevent osteoblast ferroptosis and increase osteogenesis (Shuai et al., 2019; Hao et al., 2022). Yangyang et al. confirmed that Yishen Bugu Ye (YSBGY) showed potential anti-osteoporotic effects through the modulation of the osteoblast/osteoclast balance and serum concentrations of inflammatory factors (Li et al., 2020). As a famous Chinese medicine preparation, Erzhi Wan (EZW) has a favorable anti-OP potential, mainly through inhibiting osteoclast bone absorption (Zhang et al., 2008). Zuogui Pill (ZGP) is a classic kidney-tonifying drug that can promote the osteogenic differentiation of bone marrow mesenchymal stem cells, which provides a scientific basis for its effective treatment of OP (Yang et al., 2018a). In addition, details of other traditional Chinese medicine formulas used in OP therapy are shown in Table 2.
5 Therapeutic mechanism of TCM on anti-osteoporosis
Although TCM has certain advantages in the treatment of osteoporosis, its therapeutic mechanism has not been fully elucidated, which is undoubtedly a huge challenge for researchers. In this study, we summarize and generalize the signaling pathways involved in OP, which may provide a certain theoretical basis for further elucidation of OP treatment with TCM. According to previous studies, Wnt/β-catenin, BMP-SMAD, MAPK, and RANK/NF-κB/OPG play a key role in OP (Wang et al., 2022; Wei et al., 2022) (Table3).
5.1 Wnt/β-catenin signaling pathway
Wnt signaling is a critical signal transduction pathway and mainly regulates embryonic development and tissue regeneration (Nusse and Clevers, 2017). As a kind of secretory glycoprotein, Wnt-regulating signaling was associated with multiple genes and various receptors, all of which could regulate canonical β-catenin-dependent and non-canonical β-catenin-independent pathways (Wang et al., 2019). Among them, the canonical Wnt signaling pathway could be regulated at many levels, including negative regulation. During this process, DKK1, a negative regulator, can bind to the LRP receptor, thereby suppressing the Wnt signaling pathway. When cells were not exposed to Wnt signaling, major signaling components, such as β-catenin and receptors, were in a closed state (Nusse, 2012). When it was at an active state, Wnt signaling could activate the intracellular protein DVL and inhibit the degradation activity of the β-catenin degradation complex formed by GSK-3β. In non-canonical β-catenin-independent signaling pathways, Wnt could induce cytoskeletal re-arrangement through the activation of GTPase, including Rho and Rac (van Amerongen et al., 2008).
Wnt/β-catenin could regulate bone metabolism by controlling the differentiation and function of mesenchymal stem cells (Zhu et al., 2019), adipose-derived stem cells (Shao et al., 2017), osteoblasts, and osteoclasts. The specific mechanism of action was as follows: Runx2, a specific transcription factor, plays a vital role in osteoblast differentiation and chondrocyte maturation (Komori, 2018). Cai et al. found that Wnt/β-catenin could directly regulate the expression of Runx2, thereby promoting vascular smooth muscle cells to transdifferentiation (Cai et al., 2016). In addition, Runx2 could cooperate with the forkhead box protein O3 transcription factor (FoxO3) that belongs to a subclass of forkhead transcription factors (Yuan et al., 2022). Moreover, Runx2 could cross-conduce with the Wnt signal, all of which were involved in the elimination of superoxide, thereby remodeling normal bone. In addition, miR-29a, a downstream factor of Wnt/β-catenin, could ameliorate age-induced osteoblast loss and OP by targeting Dnmt3b-mediated FoxO3 methylation, upregulating the expressions of antioxidant proteins and DNA methylation (Lian et al., 2021). Similarly, FoxO1 was also involved in the degradation of spontaneous cartilage and the formation of osteoarthritis. Matsuzaki et al. found that the ectopic expression of FoxO1 could synergize with the stimulation of transforming growth factor-β (TGF-β), leading to the differentiation of macrophages along with the release of IL-1β (Matsuzaki et al., 2018). In addition, TGF-βin osteoclast can induce the secretion of Wnt1, thereby combining bone resorption with bone formation (Weivoda et al., 2016). Moreover, the paracrine secretion of DKK1 (a Wnt inhibitor) induced by TGF-β is essential for osteoclastogenesis. Subsequently, Esposito found that TGF-β could induce the biomolecular aggregation of DACT1, which suppresses the Wnt signaling pathway and promotes bone metastasis (Esposito et al., 2021). Furthermore, Zhang et al. found that insulin inhibited autophagy and promoted premature aging through the TGF-β pathway, thus inhibiting BMSC osteogenesis. (Zhang et al., 2020). IGF could directly affect the differentiation of osteoblasts and enhance the function of mature osteoblasts, thereby promoting the formation of bone collagen and bone matrix (Xian et al., 2012). During this process, IGF-1 antagonized the Wnt/β-catenin signaling pathway by catalyzing the transcription of Axin2 and stabilizing the Axin1 protein. Meanwhile, the IGF-1 receptor can phosphorylate and degrade β-catenin, activate GSK-3β, and degrade insulin receptor substrate 1, all of which contribute to glucose and bone metabolism (E., 2018; Lindsey and Mohan, 2016; Zhang B. et al., 2019) (Figure 3). Additionally, the physiological dose of glucocorticoid can stimulate the differentiation of osteoblasts, thereby inhibiting bone formation through BMPs and other classical pathways (Compston, 2018).
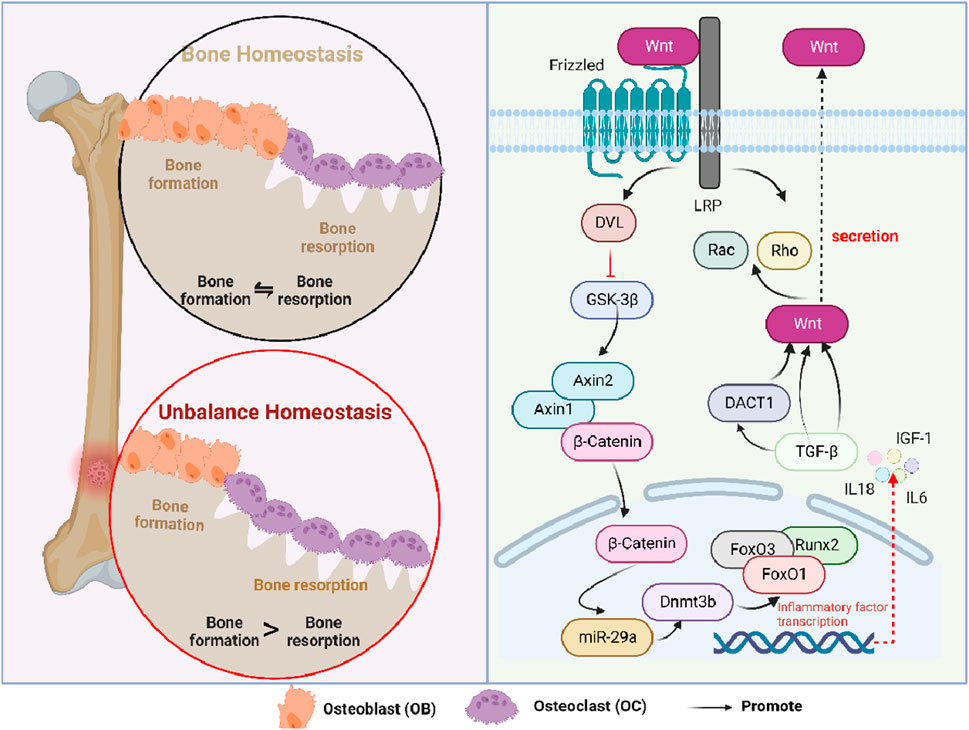
Figure 3. Role of the Wnt/β-catenin signaling pathway in osteoporosis. Interleukin, IL; insulin-like growth factor-1, IGF-1; lipoprotein receptor-related protein, LRP; disheveled, DVL; glycogen synthase kinase-3β, GSK-3β; DNA methyltransferase 3B, Dnmt3b; runt-related transcription factor 2, Runx2; forkhead box O, FoxO; dickkopf-1, DKK1; disheveled binding antagonist of beta-catenin 1, DACT1; and transforming growth factor-beta, TGF-β.
5.2 TGF-β/BMP signaling pathway
Similar to the role of the Wnt/β-catenin signaling pathway, the TGF-β/BMP signaling pathway also plays a crucial regulatory role in the body, especially in the homeostasis of postnatal bone and differentiation of mesenchymal cells into osteoblasts (Chen G. et al., 2012; Wu et al., 2016), which may be due to DNA synthesis and cell replication. Specifically, there are three main forms of TGF-β in mammals: TGF-β1, TGF-β2, and TGF-β3 (Derynck and Erine, 2019), all of which could be activated and bind to TGF-β I receptors (TβRI) and two type II receptors (TβRII). Subsequently, TGF-β could transmit its signals to the Smad signaling pathway both in canonical-dependent and non-canonical-independent forms (Wu et al., 2016). For example, Smad2/3 could be activated by TGF-β, regulating the TGF-β-induced differentiation of chondrocyte and osteoblast through the Smad pathway. Moreover, Smad2/3 also recruited HDACs 4/5 and inhibited Runx2 function, all of which could participate in osteoblast differentiation (Kang et al., 2005), while in the Smad-independent pathway, TGF-β accelerates the proliferation and differentiation of osteoblast by regulating MAPK and Smad2/3 signaling pathways (Matsunobu et al., 2009). In addition, MAPK could positively regulate the function of Runx2, contributing to the differentiation of MSCs (Li et al., 2009).
In bone, BMP signals were also mediated by their receptors and formed complex bodies with them, further regulating the transcription of related genes involved in the differentiation of osteoblast and formation of bone (Wu et al., 2016). Among BMPs, BMP-2 could promote osteogenesis by regulating the expression of Runx2, ALP, and integrin-binding sialoprotein and activate osteoclast through the upregulation of TNF-α and NF-κB ligands (Ingwersen et al., 2022). Moreover, BMP/Smad1 could regulate the activity of atonal homolog 8 and inhibit the expression ratio of RANKL/OPG, thereby regulating the osteoclast number negatively and promoting bone resorption and loss (Yahiro et al., 2020) (Figure 4).
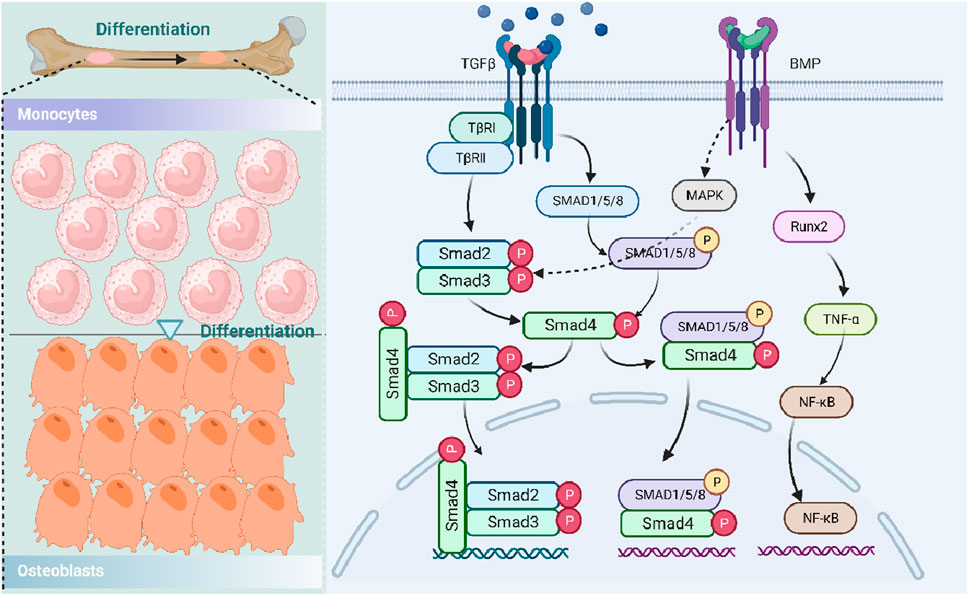
Figure 4. Role of the TGF-β/BMP signaling pathway in osteoporosis. Transforming growth factor-beta, TGF-β; TGF-β type I receptor, TβR; runt-related transcription factor 2, Runx2; histone deacetylases, HDACs; mitogen-activated protein kinase, MAPK; bone morphogenetic protein, BMP; tumor necrosis factor-α, TNF-α; and nuclear factor-κB, NF-κB.
5.3 MAPK signaling pathway
MAPK is a class of conserved serine/threonine protein kinases and plays a role in cell proliferation, differentiation, and apoptosis (Zhang W, 2003). In mammals, three families of MAPK have been identified: JNK kinase, extracellular signal-regulated protein kinase (ERK), and p38 MAPK. Among these, the MAPK-mediated pathway is within an enzymatic cascade, which comprises at least three continuously activated enzymes (Widmann et al., 1999).
In OP, the MAPK pathway was related to the osteoclastogenesis as well as bone resorption, which was due to its role in the phosphorylation of ERK1/2 that could, in sequence, regulate the transcription and expression of the main osteoclast transcription factor, such as a recombinant nuclear factor of activated T-cells, cytoplasmic 1 (NFATc1) (Long et al., 2022). In addition, Ren et al. found that the expression of MAPK/p38MAPK could be increased by RUNX2, along with the increases of ALP, OCN, and OSX, all of which were associated with the osteoblast differentiation and downregulation of autophagy genes, including Beclin-1, ATG1, and p62 (Ren et al., 2022). In addition, the MAPK signaling pathway also takes part in high-glucose-caused osteoclast differentiation, which was associated with Dickkopf-1 and tartrate-resistant acid phosphatase 5B (TRAP5b), c-terminal telopeptides of type 1 (CTX1), cathepsin K, and Nqo1 (Ren et al., 2022). Moreover, downstream of MAPK, pathways such as P38, ERK, and JNK can be activated by AGEs and contribute to the release of TNF-α, IL-1β, and IL-6 (Wang et al., 2020) (Figure 5).
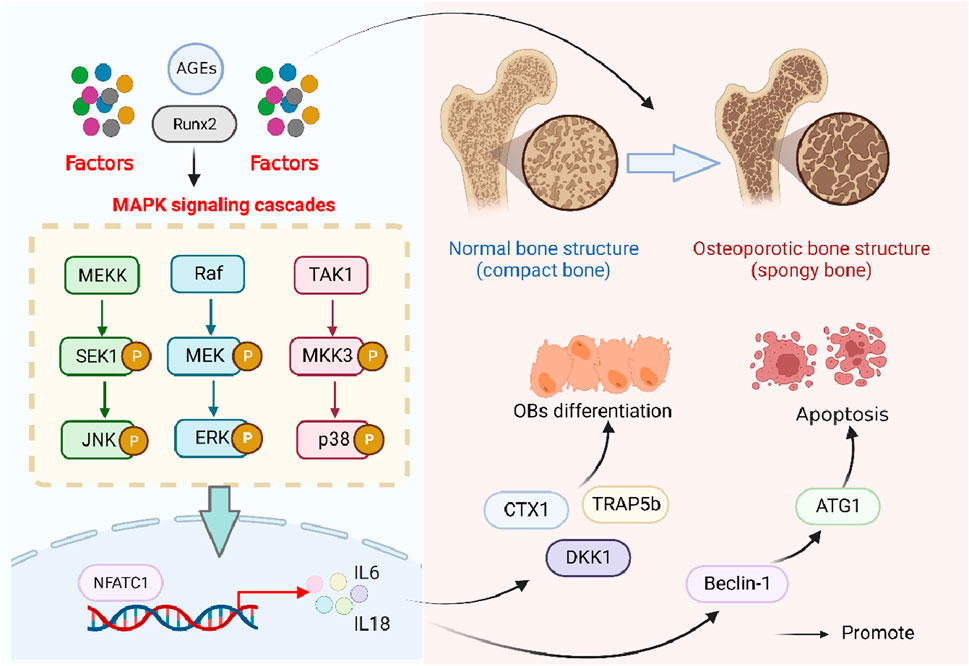
Figure 5. Role of the MAPK signaling pathway in osteoporosis. Advanced glycation end products, AGEs; runt-related transcription factor 2, Runx2; SAPK/Erk kinase 1, SEK1; Jun N-terminal kinase, JNK; mitogen-activated protein kinase kinase, MEK; mitogen-activated protein kinase kinase kinase, MEKK1; extracellular signal-regulated kinases, ERK; transforming growth factor-β-activated kinase 1, TAK1; MAP kinase kinase-3, MKK3; C-terminal telopeptide of type I collagen, CTX1; tartrate-resistant acid phosphatase isoform 5b, TRAP5b; dickkopf-1, Dkk1; anti-human t-lymphocyte globulin 1, ATG1; nuclear factor of activated T-cells 1, NFATC1; and interleukin, IL.
5.4 RANKL/NF-κB/OPG signaling pathway
The RANKL/NF-kB/OPG pathway, mainly involved in osteoclast formation (Whyte, 2006), is a process initiated by the binding of RANKL to RANK (Yang W. et al., 2021). RNAKL, known as TNF superfamily member 11 (TNFSF11), mainly regulates the differentiation of osteoclasts and the formation of OP (Luo et al., 2016). During osteoclast differentiation, leucine-rich repeat-containing G-protein-coupled receptor 4 (LGR4) (a receptor of RANKL) could competitively bind RANKL to RANK and block classical RANK way, along with activations of the glycogen synthase kinase-3 (GSK3)-β, α subunit of inhibitory G protein (Gαq), and NFATC1 (Luo et al., 2016). In addition, TNF could regulate the activation of the NF-κB pathway through the expressions of FoxO1, Sod2, and catalase and accumulation of ROS (Liao et al., 2016); under normal conditions, NF-κB can form a dimer with IκBα in the cytoplasm; upon stimulation, the dimer was dissociated, along with NF-κB entering the nucleus and IκBα phosphorylation, resulting in the synthesis and release of inflammatory factors, all of which promoted osteoclast formation, osteoclast differentiation, and osteolysis (Zou et al., 2020). Additionally, long-term use or overdose of glucocorticoids contribute to the formation of osteoclast and expressions of RANKL and macrophage colony-stimulating factor (MCSF), inhibiting the level of serum OPG and activating osteoclasts (Chotiyarnwong and McCloskey, 2020). Moreover, estrogen could promote the accumulation of superoxide involved in bone remodeling and promote osteogenic differentiation. In postmenopausal osteoporosis, deficiency of estrogen led to unbalanced bone homeostasis, a decrease in OPG expression, and increases in RANKL and M-CSF (Karim et al., 2021) (Figure 6).
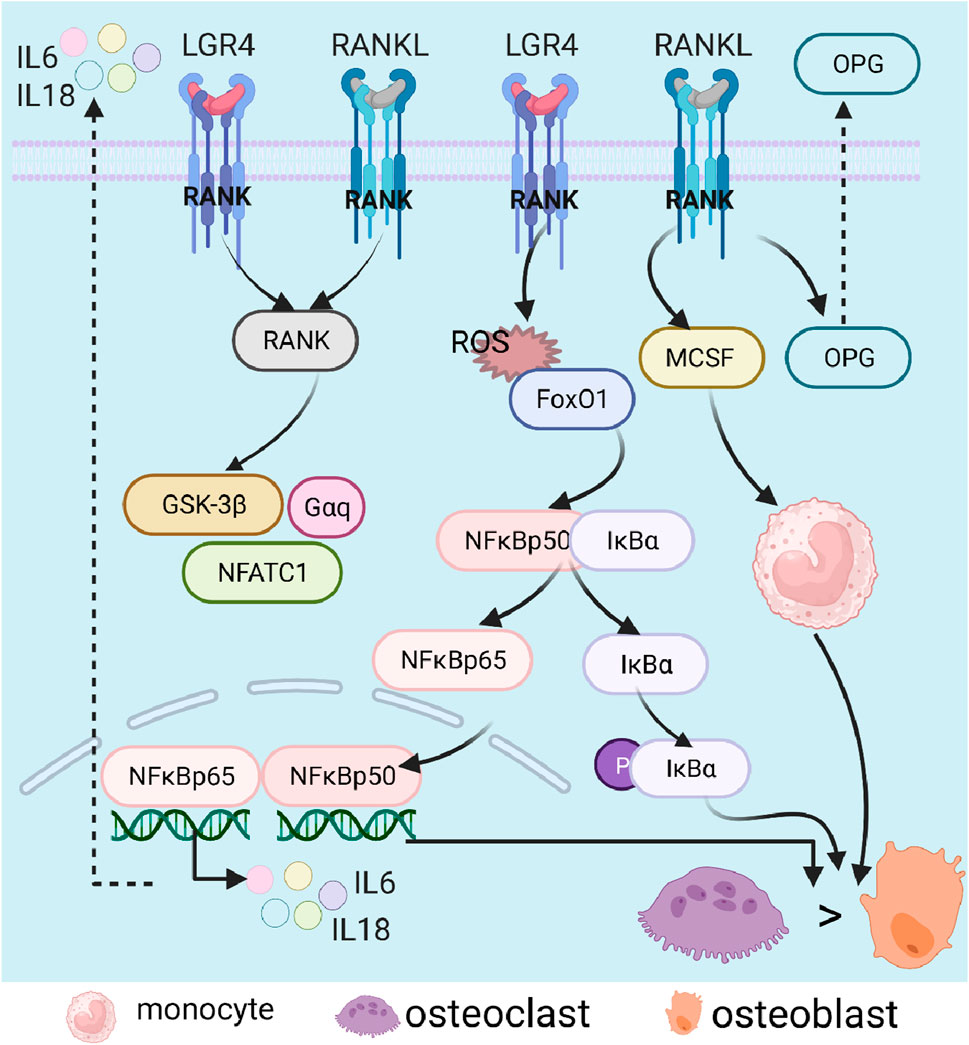
Figure 6. Role of the RANK/NF-κB (RANKL)/OPG signaling pathway in osteoporosis. Nuclear factor-κB, NF-κB; nuclear factor kappa B, RANK; nuclear factor-κB ligand, RANKL; inhibitor of NF-κB α, IκBα; osteoprotegerin, OPG; interleukin, IL; glycogen synthase kinase-3β, GSK-3β; nuclear factor of activated T-cells 1, NFATC1; forkhead box O, FoxO; reactive oxygen species, ROS; and macrophage colony-stimulating factor, MCSF.
5.5 Other pathways related to the pathogenesis of osteoporosis
In addition to the above signal paths, relevant research showed that PI3K/AKT/mTOR, ERα-AMPK, GH/IGF (Lindsey and Mohan, 2016), and calcium signaling pathways also participate in OP pathogenesis. The osteoblast-related gene expressions, including Alp, cbfa1, Col1a1, and osteocalcin (OCN), can be upregulated by the phosphorylation of PI3K and Akt (Xie et al., 2022). Low levels of glucocorticoids can promote the apoptosis and autophagy of OB through inhibiting the mammalian target of rapamycin (mTOR) pathway (Chotiyarnwong and McCloskey, 2020). ER, including ER-α and ER-β, can directly increase the activity of LKBl, which is the most important upstream protein kinase of AMPK. In addition, the mutual promotion of AMPK and Sirt1 can also modulate the autophagy or apoptosis of osteoblasts, suggesting that the ERα-AMPK-Sirt1 signaling pathway may play an important role in OP (Xiao et al., 2022). Furthermore, growth hormone facilitated longitudinal bone growth primarily via the production of hepatic IGF-I, and the growth hormone receptor was activated and then induced the phosphorylation of the Janus kinase (JAK)-signal transducers and activators of transcription (STAT) pathway (Lindsey and Mohan, 2016). Of note, the JAK-STAT pathway can disrupt normal bone remodeling by targeting osteoclasts and osteoblasts in the joint and in the joint exoskeleton (Damerau et al., 2020).
6 Clinical or preclinical studies of TCM in OP treatment
TCM has unique advantages in the treatment of osteoporosis, which mainly lies in the overall regulation of the balance of yin and yang of the human body (Hu et al., 2019), fundamentally improving bone metabolism and repair, and preventing and treating the occurrence and development of OP from various aspects (Qian et al., 2021). As we mentioned, TCM has made certain progress in the treatment of OP, which is not only reflected in preclinical research but has also achieved good results in clinical research. At present, the National Medical Products Administration has approved many Chinese patent drugs for the treatment of OP in China. Among them, the Xianling Gubao capsule (tablet) (Xiao et al., 2022), Gushukang capsule (granule) (Li et al., 2022), Jintiange capsule (Liang et al., 2022), and Qianggu capsule (Huang et al., 2022) are more widely used. In addition, the characteristic therapy of OP in Chinese medicine includes acupuncture, moxibustion, treatment by way of pasting on acupuncture points, massage, and so on (Peng Z. et al., 2022). To sum up, there are various methods for the treatment of OP by TCM, but it is worth noting that appropriate therapies should be selected according to different syndrome types under the guidance of the TCM theory.
7 Conclusion and future prospects
The pathogenic factors and related pathways in OP reported in this review provide a basis for better elucidating the pathogenesis of osteoporosis. TCM, including syndrome differentiation, single herbs, and prescription, shows a unique advantage in the treatment of osteoporosis, which undoubtedly points out the direction for researchers. However, besides the abovementioned diseases related to osteoporosis, whether there are other diseases that can affect OP or not needs to be studied.
Although TCM has certain advantages in the treatment of osteoporosis, the material basis is unclear, which brings challenges to clinical application. Compared with the single target of chemical drugs, the multi-pathway and multi-target characteristics of TCM are not only advantages but also disadvantages, which is also a major challenge for the internationalization of TCM. This review summarizes the potential of TCM in the treatment of osteoporosis, but its specific mechanism is worth further research. The solution to this problem will be beneficial to the long-term development of TCM in the treatment of osteoporosis.
Author contributions
GC: writing–original draft. SH: writing–original draft. YN: writing–original draft. XD: writing–review and editing. CD: writing–review and editing. LW: writing–review and editing. ZW: writing–review and editing. XS: writing–review and editing. QY: writing–review and editing. JS: writing–review and editing. MH: writing–review and editing. XH: writing–review and editing.
Funding
The authors declare that no financial support was received for the research, authorship, and/or publication of this article.
Conflict of interest
The authors declare that the research was conducted in the absence of any commercial or financial relationships that could be construed as a potential conflict of interest.
Publisher’s note
All claims expressed in this article are solely those of the authors and do not necessarily represent those of their affiliated organizations, or those of the publisher, the editors, and the reviewers. Any product that may be evaluated in this article, or claim that may be made by its manufacturer, is not guaranteed or endorsed by the publisher.
References
Amarasekara, D. S., Yu, J., and Rho, J. (2015). Bone loss triggered by the cytokine network in inflammatory autoimmune diseases. J. Immunol. Res. 2015, 832127. doi:10.1155/2015/832127
An, J., Yang, H., Zhang, Q., Liu, C., Zhao, J., Zhang, L., et al. (2016). Natural products for treatment of osteoporosis: the effects and mechanisms on promoting osteoblast-mediated bone formation. Life Sci. 147, 46–58. doi:10.1016/j.lfs.2016.01.024
Andreoli, A., Bazzocchi, A., Celi, M., Lauro, D., Sorge, R., Tarantino, U., et al. (2011). Relationship between body composition, body mass index and bone mineral density in a large population of normal, osteopenic and osteoporotic women. Radiol. Med. 116, 1115–1123. doi:10.1007/s11547-011-0689-2
Ayers, C., Kansagara, D., Lazur, B., Fu, R., Kwon, A., and Harrod, C. (2023). Effectiveness and safety of treatments to prevent fractures in people with low bone mass or primary osteoporosis: a living systematic review and network meta-analysis for the American college of physicians. Ann. Intern Med. 176, 182–195. doi:10.7326/M22-0684
Barrett-Connor, E. H. T., and Holbrook, T. L. (1992). Sex differences in osteoporosis in older adults with non-insulin-dependent diabetes mellitus. JAMA 268, 3333–3337. doi:10.1001/jama.1992.03490230063029
Bellavia, D., Dimarco, E., Costa, V., Carina, V., De Luca, A., Raimondi, L., et al. (2021). Flavonoids in bone erosive diseases: perspectives in osteoporosis treatment. Trends Endocrinol. Metab. 32, 76–94. doi:10.1016/j.tem.2020.11.007
Cai, T., Sun, D., Duan, Y., Wen, P., Dai, C., Yang, J., et al. (2016). WNT/β-catenin signaling promotes VSMCs to osteogenic transdifferentiation and calcification through directly modulating Runx2 gene expression. Exp. Cell Res. 345, 206–217. doi:10.1016/j.yexcr.2016.06.007
Cai, X.-y., Zhang, Z.-j., Xiong, J.-l., Yang, M., and Wang, Z.-t. (2021). Experimental and molecular docking studies of estrogen-like and anti-osteoporosis activity of compounds in Fructus Psoraleae. J. Ethnopharmacol. 276, 114044. doi:10.1016/j.jep.2021.114044
Cao, H. J., Li, C. R., Wang, L. Y., Ziadlou, R., Grad, S., Zhang, Y., et al. (2019). Effect and mechanism of psoralidin on promoting osteogenesis and inhibiting adipogenesis. Phytomedicine 61, 152860. doi:10.1016/j.phymed.2019.152860
Chen, G., Deng, C., and Li, Y. P. (2012a). TGF-β and BMP signaling in osteoblast differentiation and bone formation. Int. J. Biol. Sci. 8, 272–288. doi:10.7150/ijbs.2929
Chen, G., Wang, C., Wang, J., Yin, S., Gao, H., Xiang, L. U., et al. (2016). Antiosteoporotic effect of icariin in ovariectomized rats is mediated via the Wnt/β-catenin pathway. Exp. Ther. Med. 12, 279–287. doi:10.3892/etm.2016.3333
Chen, H., Wu, M., and Kubo, K. (2012b). Combined treatment with a traditional Chinese medicine, Hachimi-jio-gan (Ba-Wei-Di-Huang-Wan) and alendronate improves bone microstructure in ovariectomized rats. J. Ethnopharmacol. 142, 80–85. doi:10.1016/j.jep.2012.04.017
Cheng, C. F., Chien-Fu Lin, J., Tsai, F. J., Chen, C. J., Chiou, J. S., Chou, C. H., et al. (2019). Protective effects and network analysis of natural compounds obtained from Radix dipsaci, Eucommiae cortex, and Rhizoma drynariae against RANKL-induced osteoclastogenesis in vitro. J. Ethnopharmacol. 244, 112074. doi:10.1016/j.jep.2019.112074
Chesnut, C. H., Silverman, S., Andriano, K., Genant, H., Gimona, A., Harris, S., et al. (2000). A randomized trial of nasal spray salmon calcitonin in postmenopausal women with established osteoporosis: the prevent recurrence of osteoporotic fractures study. PROOF Study Group. Am. J. Med. 109, 267–276. doi:10.1016/s0002-9343(00)00490-3
Chotiyarnwong, P., and McCloskey, E. V. (2020). Pathogenesis of glucocorticoid-induced osteoporosis and options for treatment. Nat. Rev. Endocrinol. 16, 437–447. doi:10.1038/s41574-020-0341-0
Compston, J. (2018). Glucocorticoid-induced osteoporosis: an update. Endocrine 61, 7–16. doi:10.1007/s12020-018-1588-2
Compston, J. E., McClung, M. R., and Leslie, W. D. (2019). Osteoporosis. Lancet 393, 364–376. doi:10.1016/S0140-6736(18)32112-3
Cramer, J. A., Gold, D. T., Silverman, S. L., and Lewiecki, E. M. (2007). A systematic review of persistence and compliance with bisphosphonates for osteoporosis. Osteoporos. Int. 18, 1023–1031. doi:10.1007/s00198-006-0322-8
Cui, X., Trinh, K., and Wang, Y. J. (2010). Chinese herbal medicine for chronic neck pain due to cervical degenerative disc disease. Spine (Phila Pa 1976) 35, 2121–2127. doi:10.1097/BRS.0b013e3181edfd17
Damerau, A., Gaber, T., Ohrndorf, S., and Hoff, P. (2020). JAK/STAT activation: a general mechanism for bone development, homeostasis, and regeneration. Int. J. Mol. Sci. 21, 9004. doi:10.3390/ijms21239004
Derynck, R., and Erine, H. B. (2019). Specificity, versatility, and control of TGF-β family signaling. Sci. Signal 12, eaav5183. doi:10.1126/scisignal.aav5183
Eastell, R., O'Neill, T. W., Hofbauer, L. C., Langdahl, B., Reid, I. R., Gold, D. T., et al. (2016). Postmenopausal osteoporosis. Nat. Rev. Dis. Prim. 2, 16069. doi:10.1038/nrdp.2016.69
Eastell, R., and Szulc, P. (2017). Use of bone turnover markers in postmenopausal osteoporosis. Lancet Diabetes Endocrinol. 5, 908–923. doi:10.1016/S2213-8587(17)30184-5
El-Gazzar, A., and Högler, W. (2021). Mechanisms of bone fragility: from osteogenesis imperfecta to secondary osteoporosis. Int. J. Mol. Sci. 22, 625. doi:10.3390/ijms22020625
Ernesto, C. (2018). Management of endocrine disease: novel anabolic treatments for osteoporosis. Eur. J. Endocrinol. 178, R33–R44. doi:10.1530/eje-17-0920
Esposito, M., Fang, C., Cook, K. C., Park, N., Wei, Y., Spadazzi, C., et al. (2021). TGF-β-induced DACT1 biomolecular condensates repress Wnt signalling to promote bone metastasis. Nat. Cell Biol. 23, 257–267. doi:10.1038/s41556-021-00641-w
Fang, P., She, Y., Han, L., Wan, S., Shang, W., Zhang, Z., et al. (2021). A promising biomarker of elevated galanin level in hypothalamus for osteoporosis risk in type 2 diabetes mellitus. Mech. Ageing Dev. 194, 111427. doi:10.1016/j.mad.2020.111427
Forsblad D'Elia, H. L. A., Waltbrand, E., Kvist, G., Mellström, D., Saxne, T., Ohlsson, C., et al. (2003). Radiographic joint destruction in postmenopausal rheumatoid arthritis is strongly associated with generalised osteoporosis. Ann. Rheum. Dis. 62, 617–623. doi:10.1136/ard.62.7.617
Fu, Q., Hu, S., Yang, J., Hao, X., Zhu, B., Wang, Q., et al. (2010). Comparison of effects of Wujia Bugu decoction and alendronate sodium on protection the bone loss of hindlimb unloaded rats. Zhongguo gu shang = China J. Orthop. traumatology 23, 524–528.
Fu, R., Li, J., Yu, H., Zhang, Y., Xu, Z., and Martin, C. (2021). The Yin and Yang of traditional Chinese and western medicine. Med. Res. Rev. 41, 3182–3200. doi:10.1002/med.21793
Gan, D., Xu, X., Chen, D., Feng, P., and Xu, Z. (2019). Network pharmacology-based pharmacological mechanism of the Chinese medicine rhizoma drynariae against osteoporosis. Med. Sci. Monit. 25, 5700–5716. doi:10.12659/MSM.915170
Ge, X., and Zhou, G. (2021). Protective effects of naringin on glucocorticoid-induced osteoporosis through regulating the PI3K/Akt/mTOR signaling pathway. Am. J. Transl. Res. 13, 6330–6341.
Gennari, L., Merlotti, D., Falchetti, A., Eller Vainicher, C., Cosso, R., and Chiodini, I. (2020). Emerging therapeutic targets for osteoporosis. Expert Opin. Ther. Targets 24, 115–130. doi:10.1080/14728222.2020.1726889
Gong, W., Zhang, N., Cheng, G., Zhang, Q., He, Y., Shen, Y., et al. (2019). Rehmannia glutinosa Libosch extracts prevent bone loss and architectural deterioration and enhance osteoblastic bone formation by regulating the IGF-1/PI3K/mTOR pathway in streptozotocin-induced diabetic rats. Int. J. Mol. Sci. 20, 3964. doi:10.3390/ijms20163964
Guan, M., Pan, D., Zhang, M., Leng, X., Yao, B., and Elvy Suhana, M. R. (2021). The aqueous extract of Eucommia leaves promotes proliferation, differentiation, and mineralization of osteoblast-like mc3t3-E1 cells. Evidence-Based Complementary Altern. Med. 2021, 3641317. doi:10.1155/2021/3641317
Guo, Y., Li, Y., Xue, L., Severino, R. P., Gao, S., Niu, J., et al. (2014). Salvia miltiorrhiza: an ancient Chinese herbal medicine as a source for anti-osteoporotic drugs. J. Ethnopharmacol. 155, 1401–1416. doi:10.1016/j.jep.2014.07.058
Hao, J., Bei, J., Li, Z., Han, M., Ma, B., Ma, P., et al. (2022). Qing`e Pill Inhibits Osteoblast Ferroptosis via ATM Serine/Threonine Kinase (ATM) and the PI3K/AKT Pathway in Primary Osteoporosis. Front. Pharmacol. 13, 902102. doi:10.3389/fphar.2022.902102
Hato, T., and Dagher, P. C. (2015). How the innate immune system senses trouble and causes trouble. Clin. J. Am. Soc. Nephrol. 10, 1459–1469. doi:10.2215/CJN.04680514
He, J., Li, X., Wang, Z., Bennett, S., Chen, K., Xiao, Z., et al. (2019). Therapeutic anabolic and anticatabolic benefits of natural Chinese medicines for the treatment of osteoporosis. Front. Pharmacol. 10, 1344. doi:10.3389/fphar.2019.01344
Hu, Q., Yu, T., Li, J., Yu, Q., Zhu, L., and Gu, Y. (2019). End-to-End syndrome differentiation of Yin deficiency and Yang deficiency in traditional Chinese medicine. Comput. Methods Programs Biomed. 174, 9–15. doi:10.1016/j.cmpb.2018.10.011
Hua, S., and Zhang, X. (2019). Effects of Achyranthes bidentata alcohol on proliferation capacity of osteoblasts and miRNA in Runx2. Exp. Ther. Med. 18, 1545–1550. doi:10.3892/etm.2019.7723
Huang, A. Y., Xiong, Z., Liu, K., Chang, Y., Shu, L., Gao, G., et al. (2022). Identification of kaempferol as an OSX upregulator by network pharmacology-based analysis of qianggu Capsule for osteoporosis. Front. Pharmacol. 13, 1011561. doi:10.3389/fphar.2022.1011561
Huang, M., Wang, Y., and Peng, R. (2020). Icariin alleviates glucocorticoid-induced osteoporosis through EphB4/ephrin-B2 Axis. Evid. Based Complement. Altern. Med. 2020, 2982480. doi:10.1155/2020/2982480
Ikebuchi, Y., Aoki, S., Honma, M., Hayashi, M., Sugamori, Y., Khan, M., et al. (2018). Coupling of bone resorption and formation by RANKL reverse signalling. Nature 561, 195–200. doi:10.1038/s41586-018-0482-7
Ingwersen, L. C., Frank, M., Naujokat, H., Loger, K., Bader, R., and Jonitz-Heincke, A. (2022). BMP-2 long-term stimulation of human pre-osteoblasts induces osteogenic differentiation and promotes transdifferentiation and bone remodeling processes. Int. J. Mol. Sci. 23, 3077. doi:10.3390/ijms23063077
Kang, J. S., Alliston, T., Delston, R., and Derynck, R. (2005). Repression of Runx2 function by TGF-beta through recruitment of class II histone deacetylases by Smad3. EMBO J. 24, 2543–2555. doi:10.1038/sj.emboj.7600729
Karim, K., Giribabu, N., and Salleh, N. (2021). Marantodes pumilum Var Alata (Kacip Fatimah) ameliorates derangement in RANK/RANKL/OPG pathway and reduces inflammation and oxidative stress in the bone of estrogen-deficient female rats with type-2 diabetes. Phytomedicine 91, 153677. doi:10.1016/j.phymed.2021.153677
Kaufman, J. M. (2021). Management of osteoporosis in older men. Aging Clin. Exp. Res. 33, 1439–1452. doi:10.1007/s40520-021-01845-8
Khosla, S., and Hofbauer, L. C. (2017). Osteoporosis treatment: recent developments and ongoing challenges. Lancet Diabetes and Endocrinol. 5, 898–907. doi:10.1016/S2213-8587(17)30188-2
Klaus, J. A. G., Steinkamp, M., Brückel, J., Rieber, A., Adler, G., Reinshagen, M., et al. (2002). High prevalence of osteoporotic vertebral fractures in patients with Crohn's disease. Gut 51, 654–658. doi:10.1136/gut.51.5.654
Komori, T. (2018). Runx2, an inducer of osteoblast and chondrocyte differentiation. Histochem Cell Biol. 149, 313–323. doi:10.1007/s00418-018-1640-6
Lane, N. E. (2006). Epidemiology, etiology, and diagnosis of osteoporosis. Am. J. Obstet. Gynecol. 194, S3–S11. doi:10.1016/j.ajog.2005.08.047
Lecka-Czernik, B. (2017). Diabetes, bone and glucose-lowering agents: basic biology. Diabetologia 60, 1163–1169. doi:10.1007/s00125-017-4269-4
Lee, H. W., Suh, J. H., Kim, H.-N., Kim, A. Y., Park, S. Y., Shin, C. S., et al. (2008). Berberine promotes osteoblast differentiation by Runx2 activation with p38 MAPK. J. Bone Mineral Res. 23, 1227–1237. doi:10.1359/jbmr.080325
Lee, J. W., Kwon, B. C., and Choi, H. G. (2021). Analyses of the relationship between hyperuricemia and osteoporosis. Sci. Rep. 11, 12080. doi:10.1038/s41598-021-91570-z
Lee, S. R., Jeon, H., Kwon, J. E., Suh, H., Kim, B. H., Yun, M. K., et al. (2020). Anti-osteoporotic effects of Salvia miltiorrhiza Bunge EtOH extract both in ovariectomized and naturally menopausal mouse models. J. Ethnopharmacol. 258, 112874. doi:10.1016/j.jep.2020.112874
Lee, S. Y., Choi, D. Y., and Woo, E. R. (2005). Inhibition of osteoclast differentiation by tanshinones from the root of Salvia miltiorrhiza bunge. Archives pharmacal Res. 28, 909–913. doi:10.1007/BF02973876
Li, H., Deng, W., Yang, J., Lin, Y., Zhang, S., Liang, Z., et al. (2024). Corylifol A suppresses osteoclastogenesis and alleviates ovariectomy-induced bone loss via attenuating ROS production and impairing mitochondrial function. Biomed. Pharmacother. 171, 116166. doi:10.1016/j.biopha.2024.116166
Li, J., Zhao, Z., Yang, J., Liu, J., Wang, J., Li, X., et al. (2009). p38 MAPK mediated in compressive stress-induced chondrogenesis of rat bone marrow MSCs in 3D alginate scaffolds. J. Cell Physiol. 221, 609–617. doi:10.1002/jcp.21890
Li, S.-S., He, S.-H., Xie, P.-Y., Li, W., Zhang, X.-X., Li, T.-F., et al. (2021). Targeting the wnt/β-catenin signaling pathway as a potential therapeutic strategy in renal tubulointerstitial fibrosis. Front. Pharmacol. 12, 719880. doi:10.3389/fphar.2021.719880
Li, X.-L., Wang, L., He, M.-C., Li, W.-X., Zhang, J.-L., Fu, Y.-F., et al. (2022). A clinical herbal prescription Gu-Shu-Kang capsule exerted beneficial effects on the musculoskeletal system of dexamethasone-treated mice by acting on tissue IGF-1 signalling pathway. Pharm. Biol. 60, 2098–2109. doi:10.1080/13880209.2022.2132029
Li, Y., Zhang, Y., Meng, W., Li, Y., Huang, T., Wang, D., et al. (2020). The antiosteoporosis effects of yishen Bugu Ye based on its regulation on the differentiation of osteoblast and osteoclast. Biomed. Res. Int. 2020, 9467683. doi:10.1155/2020/9467683
Lian, W. S., Wu, R. W., Chen, Y. S., Ko, J. Y., Wang, S. Y., Jahr, H., et al. (2021). MicroRNA-29a mitigates osteoblast senescence and counteracts bone loss through oxidation resistance-1 control of FoxO3 methylation. Antioxidants (Basel) 10, 1248. doi:10.3390/antiox10081248
Liang, H., Wang, O., Cheng, Z., Xia, P., Wang, L., Shen, J., et al. (2022). Jintiange combined with alfacalcidol improves muscle strength and balance in primary osteoporosis: a randomized, double-blind, double-dummy, positive-controlled, multicenter clinical trial. J. Orthop. Transl. 35, 53–61. doi:10.1016/j.jot.2022.05.002
Liao, L., Su, X., Yang, X., Hu, C., Li, B., Lv, Y., et al. (2016). TNF-Α inhibits FoxO1 by upregulating miR-705 to aggravate oxidative damage in bone marrow-derived mesenchymal stem cells during osteoporosis. Stem Cells 34, 1054–1067. doi:10.1002/stem.2274
Lin, Z., Xiong, Y., Hu, Y., Chen, L., Panayi, A. C., Xue, H., et al. (2021). Polydatin ameliorates osteoporosis via suppression of the mitogen-activated protein kinase signaling pathway. Front. Cell Dev. Biol. 9, 730362. doi:10.3389/fcell.2021.730362
Lindsey, R. C., and Mohan, S. (2016). Skeletal effects of growth hormone and insulin-like growth factor-I therapy. Mol. Cell. Endocrinol. 432, 44–55. doi:10.1016/j.mce.2015.09.017
Liu, K., Liu, Y., Xu, Y., Nandakumar, K. S., Tan, H., He, C., et al. (2019). Asperosaponin VI protects against bone destructions in collagen induced arthritis by inhibiting osteoclastogenesis. Phytomedicine 63, 153006. doi:10.1016/j.phymed.2019.153006
Liu, M. M., Lv, N. N., Geng, R., Hua, Z., Ma, Y., Huang, G. C., et al. (2022). Uncovering the key miRNAs and targets of the liuwei Dihuang pill in diabetic nephropathy-related osteoporosis based on weighted gene Co-expression network and network pharmacology analysis. Endocr. Metab. Immune Disord. Drug Targets 22, 274–289. doi:10.2174/1871530321666210215161921
Lo, B., Holm, J. P., Vester-Andersen, M. K., Bendtsen, F., Vind, I., and Burisch, J. (2020). Incidence, risk factors and evaluation of osteoporosis in patients with inflammatory bowel disease: a Danish population-based inception cohort with 10 Years of follow-up. J. Crohns Colitis 14, 904–914. doi:10.1093/ecco-jcc/jjaa019
Long, F., Chen, R., Su, Y., Liang, J., Xian, Y., Yang, F., et al. (2022). Epoxymicheliolide inhibits osteoclastogenesis and resists OVX-induced osteoporosis by suppressing ERK1/2 and NFATc1 signaling. Int. Immunopharmacol. 107, 108632. doi:10.1016/j.intimp.2022.108632
Luo, J., Yang, Z., Ma, Y., Yue, Z., Lin, H., Qu, G., et al. (2016). LGR4 is a receptor for RANKL and negatively regulates osteoclast differentiation and bone resorption. Nat. Med. 22, 539–546. doi:10.1038/nm.4076
Matsunobu, T., Torigoe, K., Ishikawa, M., de Vega, S., Kulkarni, A. B., Iwamoto, Y., et al. (2009). Critical roles of the TGF-beta type I receptor ALK5 in perichondrial formation and function, cartilage integrity, and osteoblast differentiation during growth plate development. Dev. Biol. 332, 325–338. doi:10.1016/j.ydbio.2009.06.002
Matsuzaki, A.-G. O., Mokuda, S., Nagira, K., Olmer, M., Gamini, R., Miyata, K., et al. (2018). FoxO transcription factors modulate autophagy and proteoglycan 4 in cartilage homeostasis and osteoarthritis. Sci. Transl. Med. 10, eaan0746. doi:10.1126/scitranslmed.aan0746
Mo, H., Zhang, N., Li, H., Li, F., and Pu, R. (2019). Beneficial effects of Cuscuta chinensis extract on glucocorticoid-induced osteoporosis through modulation of RANKL/OPG signals. Braz. J. Med. Biol. Res. 52, e8754. doi:10.1590/1414-431X20198754
Mohammad, N. A., Razaly, N. I., Mohd Rani, M. D., Mohd Aris, M. S., and Mohd Effendy, N. (2018). An evidence-based review: the effects of Malaysian traditional herbs on osteoporotic rat models. Malays. J. Med. Sci. 25, 6–30. doi:10.21315/mjms2018.25.4.2
Muñoz, J., Akhavan, N. S., Mullins, A. P., and Arjmandi, B. H. (2020). Macrophage polarization and osteoporosis: a review. Nutrients 12, 2999. doi:10.3390/nu12102999
Muñoz, M. T., and Argente, J. (2002). Anorexia nervosa in female adolescents: endocrine and bone mineral density disturbances. Eur. J. Endocrinol. 147, 275–286. doi:10.1530/eje.0.1470275
Noh, J. Y., Yang, Y., and Jung, H. (2020). Molecular mechanisms and emerging therapeutics for osteoporosis. Int. J. Mol. Sci. 21, 7623. doi:10.3390/ijms21207623
Nusse, R. (2012). Wnt signaling. Cold Spring Harb. Perspect. Biol. 4, a011163. doi:10.1101/cshperspect.a011163
Nusse, R., and Clevers, H. (2017). Wnt/β-Catenin signaling, disease, and emerging therapeutic modalities. Cell 169, 985–999. doi:10.1016/j.cell.2017.05.016
Pagnotti, G. M., Styner, M., Uzer, G., Patel, V. S., Wright, L. E., Ness, K. K., et al. (2019). Combating osteoporosis and obesity with exercise: leveraging cell mechanosensitivity. Nat. Rev. Endocrinol. 15, 339–355. doi:10.1038/s41574-019-0170-1
Park, B., Song, H. S., Kwon, J. E., Cho, S. M., Jang, S. A., Kim, M. Y., et al. (2017). Effects of Salvia miltiorrhiza extract with supplemental liquefied calcium on osteoporosis in calcium-deficient ovariectomized mice. BMC Complement. Altern. Med. 17, 545. doi:10.1186/s12906-017-2047-y
Paschou, S. A., Dede, A. D., Anagnostis, P. G., Vryonidou, A., Morganstein, D., and Goulis, D. G. (2017). Type 2 diabetes and osteoporosis: a guide to optimal management. J. Clin. Endocrinol. Metab. 102, 3621–3634. doi:10.1210/jc.2017-00042
Peng, C.-H., Lin, W.-Y., Li, C.-Y., Dharini, K. K., Chang, C.-Y., Hong, J.-T., et al. (2022a). Gu Sui Bu (Drynaria fortunei J. Sm.) antagonizes glucocorticoid-induced mineralization reduction in zebrafish larvae by modulating the activity of osteoblasts and osteoclasts. J. Ethnopharmacol. 297, 115565. doi:10.1016/j.jep.2022.115565
Peng, Z., Xu, R., and You, Q. (2022b). Role of traditional Chinese medicine in bone regeneration and osteoporosis. Front. Bioeng. Biotechnol. 10, 911326. doi:10.3389/fbioe.2022.911326
Ponzetti, M., and Rucci, N. (2019). Updates on osteoimmunology: what's new on the cross-talk between bone and immune system. Front. Endocrinol. (Lausanne) 10, 236. doi:10.3389/fendo.2019.00236
Qadir, A., Liang, S., Wu, Z., Chen, Z., Hu, L., and Qian, A. (2020). Senile osteoporosis: the involvement of differentiation and senescence of bone marrow stromal cells. Int. J. Mol. Sci. 21, 349. doi:10.3390/ijms21010349
Qian, D., Zhou, H., Fan, P., Yu, T., Patel, A., O'Brien, M., et al. (2021). A traditional Chinese medicine plant extract prevents alcohol-induced osteopenia. Front. Pharmacol. 12, 754088. doi:10.3389/fphar.2021.754088
Qin, H., Zhao, W., Jiao, Y., Zheng, H., Zhang, H., Jin, J., et al. (2021). Aqueous extract of salvia miltiorrhiza bunge-radix Puerariae herb pair attenuates osteoporosis in ovariectomized rats through suppressing osteoclast differentiation. Front. Pharmacol. 11, 581049. doi:10.3389/fphar.2020.581049
Qin, T., Wu, L., Hua, Q., Song, Z., Pan, Y., and Liu, T. (2020). Prediction of the mechanisms of action of Shenkang in chronic kidney disease: a network pharmacology study and experimental validation. J. Ethnopharmacol. 246, 112128. doi:10.1016/j.jep.2019.112128
Qu, N., Kuramasu, M., Nagahori, K., Ogawa, Y., Hayashi, S., Hirayanagi, Y., et al. (2020). Co-administration of the traditional medicines hachimi-jio-Gan and hochu-ekki-to can reverse busulfan-induced aspermatogenesis. Int. J. Mol. Sci. 21, 1716. doi:10.3390/ijms21051716
Ragipoglu, D., Dudeck, A., Haffner-Luntzer, M., Voss, M., Kroner, J., Ignatius, A., et al. (2020). The role of mast cells in bone metabolism and bone disorders. Front. Immunol. 11, 163. doi:10.3389/fimmu.2020.00163
Rathinavelu, S., Guidry-Elizondo, C., and Banu, J. (2018). Molecular modulation of osteoblasts and osteoclasts in type 2 diabetes. J. Diabetes Res. 2018, 6354787. doi:10.1155/2018/6354787
Ren, C., Xu, Y., Liu, H., Wang, Z., Ma, T., Li, Z., et al. (2022). Effects of runt-related transcription factor 2 (RUNX2) on the autophagy of rapamycin-treated osteoblasts. Bioengineered 13, 5262–5276. doi:10.1080/21655979.2022.2037881
Robert, S. W. (2011). Clinical practice. Glucocorticoid-induced bone disease. N. Engl. J. Med. 365, 62–70. doi:10.1056/NEJMcp1012926
Rossouw, J. E., Anderson, G. L., Prentice, R. L., LaCroix, A. Z., Kooperberg, C., Stefanick, M. L., et al. (2002). Risks and benefits of estrogen plus progestin in healthy postmenopausal women: principal results from the Women's Health Initiative randomized controlled trial. JAMA 288, 321–333. doi:10.1001/jama.288.3.321
Sakamoto, S., Sassa, S., Kudo, H., Suzuki, S., Mitamura, T., and Shinoda, H. (2000). Preventive effects of a herbal medicine on bone loss in rats treated with a GnRH agonist. Eur. J. Endocrinol. 143, 139–142. doi:10.1530/eje.0.1430139
Samakkarnthai, P., Sfeir, J. G., Atkinson, E. J., Achenbach, S. J., Wennberg, P. W., Dyck, P. J., et al. (2020). Determinants of bone material strength and cortical porosity in patients with type 2 diabetes mellitus. J. Clin. Endocrinol. Metab. 105, e3718–e3729. doi:10.1210/clinem/dgaa388
Shao, X. R., Lin, S. Y., Peng, Q., Shi, S. R., Li, X. L., Zhang, T., et al. (2017). Effect of tetrahedral DNA nanostructures on osteogenic differentiation of mesenchymal stem cells via activation of the Wnt/β-catenin signaling pathway. Nanomedicine 13, 1809–1819. doi:10.1016/j.nano.2017.02.011
Sheu, A., Bliuc, D., Tran, T., White, C. P., and Center, J. R. (2022). Fractures in type 2 diabetes confer excess mortality: the Dubbo osteoporosis epidemiology study. Bone 159, 116373. doi:10.1016/j.bone.2022.116373
Shuai, B., Zhu, R., Yang, Y. P., Shen, L., Xu, X. J., Ma, C., et al. (2019). Positive Effects of Qing'e Pill on Trabecular Microarchitecture and its Mechanical Properties in Osteopenic Ovariectomised Mice. Chin. J. Integr. Med. 25, 270–277. doi:10.1007/s11655-016-2604-0
Song, D., Cao, Z., Liu, Z., Tickner, J., Qiu, H., Wang, C., et al. (2018). Cistanche deserticola polysaccharide attenuates osteoclastogenesis and bone resorption via inhibiting RANKL signaling and reactive oxygen species production. J. Cell Physiol. 233, 9674–9684. doi:10.1002/jcp.26882
Song, N., Zhao, Z., Ma, X., Sun, X., Ma, J., Li, F., et al. (2017). Naringin promotes fracture healing through stimulation of angiogenesis by regulating the VEGF/VEGFR-2 signaling pathway in osteoporotic rats. Chem. Biol. Interact. 261, 11–17. doi:10.1016/j.cbi.2016.10.020
Sozel, H., and Yilmaz, F. (2021). Symptomatic hypocalcemia following a single dose of zoledronic acid in a patient with bone metastases secondary to breast cancer. J. Oncol. Pharm. Pract. 27, 494–497. doi:10.1177/1078155220940411
Stein, E., and Shane, E. (2003). Secondary osteoporosis. Endocrinol. Metabolism Clin. N. Am. 32, 115–134. doi:10.1016/s0889-8529(02)00062-2
Sun, C., Chen, X., Yang, S., Jin, C., Ding, K., and Chen, C. (2023). LBP1C-2 from Lycium barbarum alleviated age-related bone loss by targeting BMPRIA/BMPRII/Noggin. Carbohydrate Polymers 310.
Sun, X., Wei, B., Peng, Z., Fu, Q., Wang, C., Zhen, J., et al. (2019). Protective effects of Dipsacus asper polysaccharide on osteoporosis in vivo by regulating RANKL/RANK/OPG/VEGF and PI3K/Akt/eNOS pathway. Int. J. Biol. Macromol. 129, 579–587. doi:10.1016/j.ijbiomac.2019.02.022
Tang, X., Huang, Y., Fang, X., Tong, X., Yu, Q., Zheng, W., et al. (2023). Cornus officinalis: a potential herb for treatment of osteoporosis. Front. Med. 10, 1289144. doi:10.3389/fmed.2023.1289144
Tariq, S., Tariq, S., and Lone, K. P. (2017). Relationship of anthropometric measures with bone mineral density in postmenopausal non-osteoporotic, osteopenic and osteoporotic women. J. Pak Med. Assoc. 67, 590–594.
Tsai, J. N., Lee, H., David, N. L., Eastell, R., and Leder, B. Z. (2019). Combination denosumab and high dose teriparatide for postmenopausal osteoporosis (DATA-HD): a randomised, controlled phase 4 trial. Lancet Diabetes and Endocrinol. 7, 767–775. doi:10.1016/S2213-8587(19)30255-4
Vahle, J. L., Long, G. G., Sandusky, G., Westmore, M., Ma, Y. L., and Sato, M. (2016). Bone neoplasms in F344 rats given teriparatide [rhPTH(1-34)] are dependent on duration of treatment and dose. Toxicol. Pathol. 32, 426–438. doi:10.1080/01926230490462138
van Amerongen, R., Mikels, A., and Nusse, R. (2008). Alternative wnt signaling is initiated by distinct receptors. Sci. Signal 1, re9. doi:10.1126/scisignal.135re9
VanderWalde, A., and Hurria, A. (2011). Aging and osteoporosis in breast and prostate cancer. CA Cancer J. Clin. 61, 139–156. doi:10.3322/caac.20103
Vijayaraj, S. L., Feltham, R., Rashidi, M., Frank, D., Liu, Z., Simpson, D. S., et al. (2021). The ubiquitylation of IL-1β limits its cleavage by caspase-1 and targets it for proteasomal degradation. Nat. Commun. 12, 2713. doi:10.1038/s41467-021-22979-3
Wang, J., Yang, J., Tang, Z., Yu, Y., Chen, H., Yu, Q., et al. (2023). Curculigo orchioides polysaccharide COP70-1 stimulates osteogenic differentiation of MC3T3-E1 cells by activating the BMP and Wnt signaling pathways. Int. J. Biol. Macromol. 248, 125879. doi:10.1016/j.ijbiomac.2023.125879
Wang, M. Y., An, M. F., Fan, M. S., Zhang, S. S., Sun, Z. R., Zhao, Y. L., et al. (2022). FAEE exerts a protective effect against osteoporosis by regulating the MAPK signalling pathway. Pharm. Biol. 60, 467–478. doi:10.1080/13880209.2022.2039216
Wang, N., Xu, P., Wang, X., Yao, W., Wang, B., Wu, Y., et al. (2020). Timosaponin AIII attenuates inflammatory injury in AGEs-induced osteoblast and alloxan-induced diabetic osteoporosis zebrafish by modulating the RAGE/MAPK signaling pathways. Phytomedicine 75, 153247. doi:10.1016/j.phymed.2020.153247
Wang, W., Mao, J., Chen, Y., Zuo, J., Chen, L., Li, Y., et al. (2021). Naringin promotes osteogenesis and ameliorates osteoporosis development by targeting JAK2/STAT3 signalling. Clin. Exp. Pharmacol. Physiology 49, 113–121. doi:10.1111/1440-1681.13591
Wang, X., Zhen, L., Zhang, G., Wong, M.-S., Qin, L., and Yao, X. (2011). Osteogenic effects of flavonoid aglycones from an osteoprotective fraction of Drynaria fortunei—an in vitro efficacy study. Phytomedicine 18, 868–872. doi:10.1016/j.phymed.2011.01.022
Wang, Y., Cui, K., Zhao, H., Li, D., Wang, W., and Zhu, Y. (2009). Bushen Ningxin Decoction pharmacological serum promotes the proliferation and suppresses the apoptosis of murine osteoblasts through MAPK pathway. J. Ethnopharmacol. 122, 221–226. doi:10.1016/j.jep.2009.01.026
Wang, Y., Fan, X., Xing, L., and Tian, F. (2019). Wnt signaling: a promising target for osteoarthritis therapy. Cell Commun. Signal 17, 97. doi:10.1186/s12964-019-0411-x
Wayne Sampson, H. (2002). Alcohol and other factors affecting osteoporosis risk in women. Alcohol Res. Health 26, 292–298.
Wei, W., Peng, C., Gu, R., Yan, X., Ye, J., Xu, Z., et al. (2022). Urolithin A attenuates RANKL-induced osteoclastogenesis by co-regulating the p38 MAPK and Nrf2 signaling pathway. Eur. J. Pharmacol. 921, 174865. doi:10.1016/j.ejphar.2022.174865
Weivoda, M. M., Ruan, M., Pederson, L., Hachfeld, C., Davey, R. A., Zajac, J. D., et al. (2016). Osteoclast TGF-β receptor signaling induces Wnt1 secretion and couples bone resorption to bone formation. J. Bone Min. Res. 31, 76–85. doi:10.1002/jbmr.2586
Whyte, M. P. (2006). Paget's disease of bone and genetic disorders of RANKL/OPG/RANK/NF-kappaB signaling. Ann. N. Y. Acad. Sci. 1068, 143–164. doi:10.1196/annals.1346.016
Widmann, G. S., Jarpe, M. B., and Johnson, G. L. (1999). Mitogen-activated protein kinase: conservation of a three-kinase module from yeast to human. Physiol. Rev. 79, 143–180. doi:10.1152/physrev.1999.79.1.143
Wu, D., Cline-Smith, A., Shashkova, E., Perla, A., Katyal, A., and Aurora, R. (2021). T-cell mediated inflammation in postmenopausal osteoporosis. Front. Immunol. 12, 687551. doi:10.3389/fimmu.2021.687551
Wu, M., Chen, G., and Li, Y. P. (2016). TGF-β and BMP signaling in osteoblast, skeletal development, and bone formation, homeostasis and disease. Bone Res. 4, 16009. doi:10.1038/boneres.2016.9
Wu, Q.-c., Tang, X.-y., Dai, Z.-q., Dai, Y., Xiao, H.-h., and Yao, X.-s. (2020). Sweroside promotes osteoblastic differentiation and mineralization via interaction of membrane estrogen receptor-α and GPR30 mediated p38 signalling pathway on MC3T3-E1 cells. Phytomedicine 68, 153146. doi:10.1016/j.phymed.2019.153146
Xian, L., Wu, X., Pang, L., Lou, M., Rosen, C. J., Qiu, T., et al. (2012). Matrix IGF-1 maintains bone mass by activation of mTOR in mesenchymal stem cells. Nat. Med. 18, 1095–1101. doi:10.1038/nm.2793
Xiao, J., Li, W., Li, G., Tan, J., and Dong, N. (2022). STK11 overexpression prevents glucocorticoid-induced osteoporosis via activating the AMPK/SIRT1/PGC1α axis. Human Cell.
Xiao, J., Zhang, G., Mai, J., He, Q., Chen, W., Li, J., et al. (2022). Bioinformatics analysis combined with experimental validation to explore the mechanism of XianLing GuBao capsule against osteoarthritis. J. Ethnopharmacol. 294, 115292. doi:10.1016/j.jep.2022.115292
Xie, Q., Xie, J., Dong, T., Su, J., Cai, D., Chen, J., et al. (2012). Effect of a derived herbal recipe from an ancient Chinese formula, Danggui Buxue Tang, on ovariectomized rats. J. Ethnopharmacol. 144, 567–575. doi:10.1016/j.jep.2012.09.041
Xie, X., Hu, L., Mi, B., Panayi, A. C., Xue, H., Hu, Y., et al. (2022). SHIP1 activator AQX-1125 regulates osteogenesis and osteoclastogenesis through PI3K/Akt and NF-κb signaling. Front. Cell Dev. Biol. 10, 826023. doi:10.3389/fcell.2022.826023
Xu, X., Zhang, Z., Wang, W., Yao, H., and Ma, X. (2017). Therapeutic effect of cistanoside A on bone metabolism of ovariectomized mice. Molecules 22, 197. doi:10.3390/molecules22020197
Xu, Y., Li, L., Tang, Y., Yang, J., Jin, Y., and Ma, C. (2019). Icariin promotes osteogenic differentiation by suppressing Notch signaling. Eur. J. Pharmacol. 865, 172794. doi:10.1016/j.ejphar.2019.172794
Xue, L., Jiang, Y., Han, T., Zhang, N., Qin, L., Xin, H., et al. (2016). Comparative proteomic and metabolomic analysis reveal the antiosteoporotic molecular mechanism of icariin from Epimedium brevicornu maxim. J. Ethnopharmacol. 192, 370–381. doi:10.1016/j.jep.2016.07.037
Yahiro, Y., Maeda, S., Morikawa, M., Koinuma, D., Jokoji, G., Ijuin, T., et al. (2020). BMP-induced Atoh8 attenuates osteoclastogenesis by suppressing Runx2 transcriptional activity and reducing the Rankl/Opg expression ratio in osteoblasts. Bone Res. 8, 32. doi:10.1038/s41413-020-00106-0
Yamakawa, H., Kusumoto, D., Hashimoto, H., and Yuasa, S. (2020). Stem cell aging in skeletal muscle regeneration and disease. Int. J. Mol. Sci. 21, 1830. doi:10.3390/ijms21051830
Yang, A., Yu, C., You, F., He, C., and Li, Z. (2018a). Mechanisms of zuogui pill in treating osteoporosis: perspective from bone marrow mesenchymal stem cells. Evidence-Based Complementary Altern. Med. 2018, 3717391–3717398. doi:10.1155/2018/3717391
Yang, A., Yu, C., You, F., He, C., and Li, Z. (2018b). Mechanisms of zuogui pill in treating osteoporosis: perspective from bone marrow mesenchymal stem cells. Evid. Based Complement. Altern. Med. 2018, 3717391. doi:10.1155/2018/3717391
Yang, F., Lin, Z. W., Huang, T. Y., Chen, T. T., Cui, J., Li, M. Y., et al. (2019). Ligustilide, a major bioactive component of Angelica sinensis, promotes bone formation via the GPR30/EGFR pathway. Sci. Rep. 9, 6991. doi:10.1038/s41598-019-43518-7
Yang, L., Fan, L., Wang, K., Chen, Y., Liang, L., Qin, X., et al. (2021a). Analysis of molecular mechanism of erxian decoction in treating osteoporosis based on formula optimization model. Oxid. Med. Cell Longev. 2021, 6641838. doi:10.1155/2021/6641838
Yang, W., Lu, X., Zhang, T., Han, W., Li, J., He, W., et al. (2021b). TAZ inhibits osteoclastogenesis by attenuating TAK1/NF-κB signaling. Bone Res. 9, 33. doi:10.1038/s41413-021-00151-3
Yang, Y. J., and Kim, D. J. (2021). An overview of the molecular mechanisms contributing to musculoskeletal disorders in chronic liver disease: osteoporosis, sarcopenia, and osteoporotic sarcopenia. Int. J. Mol. Sci. 22, 2604. doi:10.3390/ijms22052604
Yang, Y.-j., Zhu, Z., Wang, D.-t., Zhang, X.-l., Liu, Y.-y., Lai, W.-x., et al. (2018c). Tanshinol alleviates impaired bone formation by inhibiting adipogenesis via KLF15/PPARγ2 signaling in GIO rats. Acta Pharmacol. Sin. 39, 633–641. doi:10.1038/aps.2017.134
Yu, A. X., Xu, M. L., Yao, P., Kwan, K. K., Liu, Y. X., Duan, R., et al. (2020a). Corylin, a flavonoid derived from Psoralea Fructus, induces osteoblastic differentiation via estrogen and Wnt/β-catenin signaling pathways. FASEB J. 34, 4311–4328. doi:10.1096/fj.201902319RRR
Yu, B., and Wang, C. Y. (2016). Osteoporosis: the result of an 'aged' bone microenvironment. Trends Mol. Med. 22, 641–644. doi:10.1016/j.molmed.2016.06.002
Yu, B., and Wang, C. Y. (2022). Osteoporosis and periodontal diseases - an update on their association and mechanistic links. Periodontol 89, 99–113. doi:10.1111/prd.12422
Yu, T. X. Y., Luu, S., You, X., Li, B., Xia, J., Zhu, H., et al. (2020b). The shared KEGG pathways between icariin-targeted genes and osteoporosis. Aging (Albany NY) 12, 8191–8201. doi:10.18632/aging.103133
Yu, X. Y., Li, X. S., Li, Y., Liu, T., and Wang, R. T. (2015). Neutrophil-lymphocyte ratio is associated with arterial stiffness in postmenopausal women with osteoporosis. Arch. Gerontol. Geriatr. 61, 76–80. doi:10.1016/j.archger.2015.03.011
Yuan, S., Zhang, L., Ji, L., Zhong, S., Jiang, L., Wan, Y., et al. (2022). FoxO3a cooperates with RUNX1 to promote chondrogenesis and terminal hypertrophic of the chondrogenic progenitor cells. Biochem. Biophys. Res. Commun. 589, 41–47. doi:10.1016/j.bbrc.2021.12.008
Zhang, B., Yang, L. L., Ding, S. Q., Liu, J. J., Dong, Y. H., Li, Y. T., et al. (2019a). Anti-osteoporotic activity of an edible traditional Chinese medicine cistanche deserticola on bone metabolism of ovariectomized rats through RANKL/RANK/TRAF6-Mediated signaling pathways. Front. Pharmacol. 10, 1412. doi:10.3389/fphar.2019.01412
Zhang, Z. D., Ren, H., Wang, W. X., Shen, G. Y., Huang, J. J., Zhan, M. Q., et al. (2019b). IGF-1R/β-catenin signaling axis is involved in type 2 diabetic osteoporosis. J. Zhejiang Univ. Sci. B 20, 838–848. doi:10.1631/jzus.B1800648
Zhang, W., Xue, K., Gao, Y., Huai, Y., Wang, W., Miao, Z., et al. (2019c). Systems pharmacology dissection of action mechanisms of Dipsaci Radix for osteoporosis. Life Sci. 235, 116820. doi:10.1016/j.lfs.2019.116820
Zhang, B., Yuan, P., Xu, G., Chen, Z., Li, Z., Ye, H., et al. (2021a). DUSP6 expression is associated with osteoporosis through the regulation of osteoclast differentiation via ERK2/Smad2 signaling. Cell Death Dis. 12, 825. doi:10.1038/s41419-021-04110-y
Zhang, H., Xing, W.-W., Li, Y.-S., Zhu, Z., Wu, J.-Z., Zhang, Q.-Y., et al. (2008). Effects of a traditional Chinese herbal preparation on osteoblasts and osteoclasts. Maturitas 61, 334–339. doi:10.1016/j.maturitas.2008.09.023
Zhang, M., Wang, Y., Zhang, Q., Wang, C., Zhang, D., Wan, J.-B., et al. (2018a). UPLC/Q-TOF-MS-based metabolomics study of the anti-osteoporosis effects of Achyranthes bidentata polysaccharides in ovariectomized rats. Int. J. Biol. Macromol. 112, 433–441. doi:10.1016/j.ijbiomac.2018.01.204
Zhang, N.-D., Han, T., Huang, B.-K., Rahman, K., Jiang, Y.-P., Xu, H.-T., et al. (2016). Traditional Chinese medicine formulas for the treatment of osteoporosis: implication for antiosteoporotic drug discovery. J. Ethnopharmacol. 189, 61–80. doi:10.1016/j.jep.2016.05.025
Zhang, P., Lin, J., Xiao, T., Xu, R., Fu, Y., Zhang, Y., et al. (2020). Insulin impedes osteogenesis of BMSCs by inhibiting autophagy and promoting premature senescence via the TGF-β1 pathway. Aging (Albany NY) 12, 2084–2100. doi:10.18632/aging.102723
Zhang, S., Zhang, Q., Zhang, D., Wang, C., and Yan, C. (2018b). Anti-osteoporosis activity of a novel Achyranthes bidentata polysaccharide via stimulating bone formation. Carbohydr. Polym. 184, 288–298. doi:10.1016/j.carbpol.2017.12.070
Zhang, W., Zhou, X., Hou, W., Chen, E., Ye, C., Chen, M., et al. (2023). Reversing the imbalance in bone homeostasis via sustained release of SIRT-1 agonist to promote bone healing under osteoporotic condition. Bioact. Mater. 19, 429–443. doi:10.1016/j.bioactmat.2022.04.017
Zhang, W. L. H., and Liu, H. T. (2003). MAPK signal pathways in the regulation of cell proliferation in mammalian cells. Cell Res. 12, 9–18. doi:10.1038/sj.cr.7290105
Zhang, Y., Ma, J., and Zhang, W. (2021b). Berberine for bone regeneration: therapeutic potential and molecular mechanisms. J. Ethnopharmacol. 277, 114249. doi:10.1016/j.jep.2021.114249
Zhao, Y., Li, J., Liu, Y., Yu, K., Zhang, J., and Chen, X. (2007). Gu Ling Pian, a traditional Chinese medicine, regulates function and OPG/RANKL synthesis of osteoblasts via the p38 MAPK pathway. J. Pharm. Pharmacol. 59, 1167–1173. doi:10.1211/jpp.59.8.0016
Zhao, Y., Wang, H. L., Li, T. T., Yang, F., and Tzeng, C. M. (2020). Baicalin ameliorates dexamethasone-induced osteoporosis by regulation of the RANK/RANKL/OPG signaling pathway. Drug Des. Devel Ther. 14, 195–206. doi:10.2147/DDDT.S225516
Zhu, N., and Hou, J. (2020). Exploring the mechanism of action Xianlingubao Prescription in the treatment of osteoporosis by network pharmacology. Comput. Biol. Chem. 85, 107240. doi:10.1016/j.compbiolchem.2020.107240
Zhu, Y., Wang, Y., Jia, Y., Xu, J., and Chai, Y. (2019). Catalpol promotes the osteogenic differentiation of bone marrow mesenchymal stem cells via the Wnt/β-catenin pathway. Stem Cell Res. Ther. 10, 37. doi:10.1186/s13287-019-1143-y
Zhuo, Y., Li, M., Jiang, Q., Ke, H., Liang, Q., Zeng, L.-F., et al. (2022). Evolving roles of natural terpenoids from traditional Chinese medicine in the treatment of osteoporosis. Front. Endocrinol. (Lausanne) 13, 901545. doi:10.3389/fendo.2022.901545
Keywords: osteoporosis, pathogenesis, pathogenic factors, traditional Chinese medicine, treatment
Citation: Cao G, Hu S, Ning Y, Dou X, Ding C, Wang L, Wang Z, Sang X, Yang Q, Shi J, Hao M and Han X (2024) Traditional Chinese medicine in osteoporosis: from pathogenesis to potential activity. Front. Pharmacol. 15:1370900. doi: 10.3389/fphar.2024.1370900
Received: 15 January 2024; Accepted: 11 March 2024;
Published: 02 April 2024.
Edited by:
Rolf Teschke, Hospital Hanau, GermanyReviewed by:
Peng Zhang, Guangzhou University of Chinese Medicine, ChinaKun Chen, University of Science and Technology of China, China
Copyright © 2024 Cao, Hu, Ning, Dou, Ding, Wang, Wang, Sang, Yang, Shi, Hao and Han. This is an open-access article distributed under the terms of the Creative Commons Attribution License (CC BY). The use, distribution or reproduction in other forums is permitted, provided the original author(s) and the copyright owner(s) are credited and that the original publication in this journal is cited, in accordance with accepted academic practice. No use, distribution or reproduction is permitted which does not comply with these terms.
*Correspondence: Xin Han, xinhan@zcmu.edu.cn; Min Hao, hao_min0509@163.com
†These authors have contributed equally to this work