- Department of Pharmacy, West China Hospital, Sichuan University, Chengdu, Sichuan, China
Background: Voriconazole plasma concentration exhibits significant variability and maintaining it within the therapeutic range is the key to enhancing its efficacy. We conducted a systematic review and meta-analysis to estimate the prevalence of patients achieving the therapeutic range of plasma voriconazole concentration and identify associated factors.
Methods: Eligible studies were identified through the PubMed, Embase, Cochrane Library, and Web of Science databases from their inception until 18 November 2023. We conducted a meta-analysis using a random-effects model to determine the prevalence of patients who reached the therapeutic plasma voriconazole concentration range. Factors associated with plasma voriconazole concentration were summarized from the included studies.
Results: Of the 60 eligible studies, 52 reported the prevalence of patients reaching the therapeutic range, while 20 performed multiple linear regression analyses. The pooled prevalence who achieved the therapeutic range was 56% (95% CI: 50%–63%) in studies without dose adjustment patients. The pooled prevalence of adult patients was 61% (95% CI: 56%–65%), and the pooled prevalence of children patients was 55% (95% CI: 50%–60%) The study identified, in the children population, several factors associated with plasma voriconazole concentration, including age (coefficient 0.08, 95% CI: 0.01 to 0.14), albumin (−0.05 95% CI: −0.09 to −0.01), in the adult population, some factors related to voriconazole plasma concentration, including omeprazole (1.37, 95% CI 0.82 to 1.92), pantoprazole (1.11, 95% CI: 0.17–2.04), methylprednisolone (−1.75, 95% CI: −2.21 to −1.30), and dexamethasone (−1.45, 95% CI: −2.07 to −0.83).
Conclusion: The analysis revealed that only approximately half of the patients reached the plasma voriconazole concentration therapeutic range without dose adjustments and the pooled prevalence of adult patients reaching the therapeutic range is higher than that of children. Therapeutic drug monitoring is crucial in the administration of voriconazole, especially in the children population. Particular attention may be paid to age, albumin levels in children, and the use of omeprazole, pantoprazole, dexamethasone and methylprednisolone in adults.
Systematic Review Registration: https://www.crd.york.ac.uk/prospero/display_record.php?ID=CRD42023483728.
1 Introduction
The issue of invasive fungal infections is significant in contemporary medicine, notably impacting human health, especially among patients with compromised immune systems, resulting in increased morbidity and mortality (Hope et al., 2013). Voriconazole, a second-generation triazole, demonstrates broad-spectrum antifungal activity against Candida, C. neoformans, Aspergillus, various dimorphic fungi, and other medically significant fungi (Sabatelli et al., 2006). As an azole antifungal agent, it is prescribed for treating and preventing invasive fungal infections, particularly invasive aspergillosis (Tissot et al., 2017; Walsh et al., 2008; Patterson et al., 2016).
Voriconazole demonstrates linear pharmacokinetics in children, whereas its pharmacokinetics are nonlinear in adults (Chen X. et al., 2022; Theuretzbacher et al., 2006). As the dosage increases, the clearance of the drug does not necessarily increase linearly, potentially being affected by saturation effects or other enzymatic activities (Ludden, 1991). This non-linearity renders the drug more complex and increases the challenge of adjusting dosages and predicting drug concentrations in clinical settings. In addition to its non-linear pharmacokinetics, drug interactions, CYP2C19 genotype, and liver function are potential influences on plasma levels of voriconazole. A correlation between voriconazole exposure and response has been confirmed (Dolton and Mclachlan, 2014). Low voriconazole concentration may elevate the risk of treatment failure, while higher concentrations correlate with heightened toxicity (Elewa et al., 2015). Consequently, maintaining an ideal voriconazole plasma concentration is important but difficult in both children and adults.
A randomized controlled trial indicated significantly better outcomes (complete or partial response) in patients receiving therapeutic drug monitoring (TDM) compared to those not receiving TDM (Park et al., 2012). Therefore, performing TDM on voriconazole is helpful in maintaining the ideal plasma concentration of voriconazole. For TDM of voriconazole, trough concentration is commonly measured (Ashbee et al., 2014). The recommended therapeutic range of voriconazole plasma concentration varies in different regions. A meta-analysis suggested that maintaining the trough concentration of voriconazole between 0.5 and 3.0 mg/L optimizes clinical efficacy while minimizing hepatotoxicity (Jin et al., 2016). Substantial studies indicated that voriconazole trough concentrations maintained above 1.0 mg/L and below 4.0 mg/L are effective and safe (Hamada et al., 2012; Troke et al., 2011; Jeans et al., 2012; Ashbee et al., 2014). These studies (Hamada et al., 2012; Troke et al., 2011; Jeans et al., 2012; Ashbee et al., 2014; Park et al., 2012; Jin et al., 2016), highlight that maintaining specific therapeutic ranges of plasma voriconazole concentration increases the likelihood of therapeutic success. Early TDM to achieve specific range contributes significantly to treatment success (Resztak et al., 2021).
Multiple factors have been confirmed to be associated with interindividual variability in voriconazole concentrations (e.g., CYP2C19 genotype), and several cross-sectional studies have investigated the prevalence of patients achieving therapeutic range and the factors associated with voriconazole plasma concentration. However, there are no evidence-based medical studies summarizing the prevalence of patients reaching the specific therapeutic range of plasma concentration the factors affecting the plasma concentration of voriconazole. Therefore, this systematic review and meta-analysis aggregated data to estimate the prevalence of patients achieving the therapeutic range of plasma voriconazole concentration. Additionally, it analyzed various factors influencing plasma voriconazole levels. The findings could assist clinicians in making more informed treatment decisions. With further research and the accumulation of medical evidence, clinicians can optimize voriconazole use to improve patient outcomes and survival rates.
2 Methods
This study was conducted according to the Meta-analysis of Observational Studies in Epidemiology (MOOSE) guidelines (Stroup et al., 2000) and followed the Preferred Reporting Items for Systematics Reviews and Meta-Analyses guidelines (Moher et al., 2015). The protocol of this review was registered in PROSPERO (CRD42023483728).
2.1 Search strategy
A preliminary scoping search was conducted in PubMed, the Cochrane Library, Web of Science, and Embase from their inception until 18 November 2023. The search strategy employed a combination of Medical Subject Headings (MeSH) terms and free-text terms. Detailed search strategies are shown in Supplementary Table S1.
2.2 Selection criteria
Studies were eligible for inclusion if they: 1) involved patients undergoing voriconazole treatment; 2) reported the number of patients achieving therapeutic plasma concentrations of voriconazole or provided coefficients and standard errors from multiple linear regression analyses; 3) were published in English; and 4) utilized cross-sectional or cohort study designs. Exclusion criteria were 1) conference abstracts, reviews, letters, or commentaries; and 2) studies where data extraction was infeasible or if the independent variable in the multiple linear regression analysis was not clearly defined.
2.3 Study selection
Two reviewers (XQ Li and QZ Hu) independently screened the titles and abstracts of the preliminarily included studies, adhering to the predefined inclusion and exclusion criteria. In instances where a study’s eligibility was unclear from the abstract alone, a comprehensive assessment of the full text was conducted. Any discrepancies during the screening process were resolved by consulting a third senior investigator (T Xu).
2.4 Data extraction
Data extraction from the included literature was independently conducted by two reviewers (XQ Li and QZ Hu). The following information was collected: author(s), publication year, country of study, study type, patient age and sex demographics, type of treatment settings, method of voriconazole measurement, duration of treatment, number of patients enrolled, number of blood samples analyzed, number of patients achieving the therapeutic range of plasma voriconazole concentration, the execution of multiple linear regression analyses, and, where applicable, coefficients and standard errors derived from these regressions.
2.5 Quality assessment
Two reviewers (XQ Li and QZ Hu) assessed the quality of the included studies using the Agency for Healthcare Research and Quality (AHRQ) criteria, which comprise 11 items. Each item was rated as “yes,” “no,” or “unclear.” A “yes” response earned 1 point, while a “no” or “unclear” received no points. Based on their total scores, the studies were categorized into three quality levels: 0–3 indicated low quality, 4–7 moderate quality, and 8–11 high quality.
2.6 Statistical analysis
A meta-analysis was conducted to evaluate the prevalence of patients who achieved the therapeutic range of plasma voriconazole concentration, using STATA version 15. The pooled prevalence was reported proportionally, with 95% confidence intervals (CIs). Heterogeneity between studies was assessed using the χ2 test and the I2 statistic, with an I2 value >50% suggesting significant heterogeneity. Accordingly, the Der Simonian-Laird random-effects model was applied to analyze such cases. A sensitivity analysis was performed to ensure the robustness of the meta-analysis findings. The subgroup analysis was performed based on the study cohort (adults vs. children) and the method of dose adjustments. The potential for publication bias was evaluated using Egger’s test, Begg’s test, and the trim-and-fill method. Additionally, for instances where two or more studies with children or adults population reported coefficients and standard errors (SEs) from multiple linear regression analyses, we summarized these coefficients and SEs using a random-effects model, following the approach outlined by Rolden et al. (2014).
3 Result
3.1 Study selection
A comprehensive search across four databases yielded 3,292 literature references. Following the exclusion of 1,154 duplicates, 2,138 studies underwent title and abstract screening. Ultimately, 60 studies were selected for qualitative synthesis based on a full-text review (Aiuchi et al., 2022; Allegra et al., 2018; Bartelink et al., 2013; Benedict et al., 2023; Blanco-Dorado et al., 2019; Boast et al., 2016; Cabral-Galeano et al., 2015; Chaudhri et al., 2020; Chen C. Y. et al., 2022; Chen J. et al., 2022; Chen T. T. et al., 2022; Chen X. et al., 2022; Cheng et al., 2020; Choi et al., 2013; Chuwongwattana et al., 2016; Cojutti et al., 2016; Dolton et al., 2012; Dorado et al., 2020; Dote et al., 2016; Duehlmeyer et al., 2021; Ebrahimpour et al., 2017; Fan et al., 2022; Hashemizadeh et al., 2017; Hoenigl et al., 2013; Hu et al., 2018; Hu et al., 2023; Huang et al., 2023; Huang et al., 2020; Jia et al., 2021; Kang et al., 2015; Kim et al., 2014; Lempers et al., 2019; Li et al., 2020; Li et al., 2023; Liu et al., 2017; Mafuru et al., 2021; Miao et al., 2019; Myrianthefs et al., 2010; Pieper et al., 2012; Ronda et al., 2023; Ruiz et al., 2019; Saini et al., 2014; Shao et al., 2017; Shen et al., 2022; Soler-Palacin et al., 2012; Takahashi et al., 2020; Tian et al., 2021; Troke et al., 2011; Valle-T-Figueras et al., 2021; Wei et al., 2019; Yan et al., 2018; Yang et al., 2023; Ye et al., 2022; Yi et al., 2017; Zeng et al., 2020; Zhang et al., 2023; Zhao et al., 2021a; Zhao et al., 2021b; Zhao Y. C. et al., 2021; Zhou et al., 2020). Of the 60 articles, 58 studies were included in the quantitative synthesis. Figure 1 shows the specific screening process of the literature.
3.2 Study characteristics
All included studies were cross-sectional studies. The characteristics of the included studies are detailed in Table 1. Of these, 20 studies performed multiple linear regression and reported coefficients (Allegra et al., 2018; Chen T. T. et al., 2022; Chen W. Q. et al., 2022; Cojutti et al., 2016; Dolton et al., 2012; Dorado et al., 2020; Dote et al., 2016; Fan et al., 2022; Hashemizadeh et al., 2017; Hu et al., 2018; Hu et al., 2023; Mafuru et al., 2021; Ronda et al., 2023; Takahashi et al., 2020; Tian et al., 2021; Ye et al., 2022; Zeng et al., 2020; Zhao et al., 2021a; Zhao et al., 2021b; Zhao Y. C. et al., 2021). In contrast, 53 studies provided data on the number of patients achieving the therapeutic range of plasma voriconazole concentration (Aiuchi et al., 2022; Bartelink et al., 2013; Benedict et al., 2023; Boast et al., 2016; Cabral-Galeano et al., 2015; Chaudhri et al., 2020; Chen C. Y. et al., 2022; Chen J. et al., 2022; Chen T. T. et al., 2022; Chen X. et al., 2022; Cheng et al., 2020; Choi et al., 2013; Chuwongwattana et al., 2016; Dorado et al., 2020; Duehlmeyer et al., 2021; Ebrahimpour et al., 2017; Hoenigl et al., 2013; Hu et al., 2018; Hu et al., 2023; Huang et al., 2023; Huang et al., 2020; Jia et al., 2021; Kang et al., 2015; Kim et al., 2014; Lempers et al., 2019; Li et al., 2020; Li et al., 2023; Miao et al., 2019; Myrianthefs et al., 2010; Pieper et al., 2012; Ronda et al., 2023; Ruiz et al., 2019; Saini et al., 2014; Shao et al., 2017; Shen et al., 2022; Soler-Palacin et al., 2012; Takahashi et al., 2020; Tian et al., 2021; Troke et al., 2011; Valle-T-Figueras et al., 2021; Wei et al., 2019; Yan et al., 2018; Yang et al., 2023; Ye et al., 2022; Yi et al., 2017; Zeng et al., 2020; Zhang et al., 2023; Zhao et al., 2021b; Zhao Y. C. et al., 2021; Zhou et al., 2020; Blanco-Dorado et al., 2020).
The total count of patients and blood samples in these studies was 7,319 and 14,646, respectively. The recommended concentration ranges for voriconazole varied between studies and included 1.0–5.5 mg/L (n = 24), 1.0–5.0 mg/L (n = 10), 0.5–5.0 mg/L (n = 3), 1.5–5.5 mg/L (n = 3), 2.0–5.5 mg/L (n = 3), 1.0–4.0 mg/L (n = 2), and 1.0–6.0 mg/L (n = 2). Additionally, there was one study each for the ranges 1.5–4.0 mg/L, 0.5–5.5 mg/L, and 1.5–5.0 mg/L. Regarding dose adjustments, 33 studies implemented them, 16 did not, and 3 did not specify. Among the 33 studies that adjusted doses, one followed Australian guideline, three adhered to Chinese guidelines, 16 based adjustments on TDM, clinical efficacy, adverse events, and clinician experience, 13 did not specify the adjustment methodology, and only five studies explicitly recorded their dose adjustment plan. Details of dose adjustments are shown in Supplementary Table S2.
3.3 Study quality assessment
The 60 studies included in this analysis were all cross-sectional studies. Regarding the quality assessment based on the AHRQ scoring system, each study scored 5 or higher. Specifically, 4 studies were categorized as high quality, while the remaining 56 were considered moderate quality. Of these, 4 studies scored 8, 30 scored 7, 24 scored 6, and only 2 scored 5. Detailed results of the quality assessment for each study are shown in Supplementary Table S3.
3.4 Prevalence of patients reaching the therapeutic range of plasma concentration
Among the 60 included studies, 52 reported the prevalence of patients who achieved the plasma voriconazole concentration therapeutic range. The highest and lowest recorded prevalences were 0.84 and 0.33, respectively. A sensitivity analysis led to the exclusion of one study that significantly skewed the results, as shown in Figure 2. After removing this outlier, the pooled prevalence of patients reaching therapeutic voriconazole concentration was 59% (95% CI: 56%–62%). In the subgroup analysis according to the method of dose adjustment, the pooled prevalence was 56% (95% CI: 50%–63%) in studies without dose adjustment and 61% (95% CI: 56%–66%) in studies with dose adjustment according to TDM, and the pooled prevalence of guideline-adjusted dose studies was 62% (95% CI: 58%–66%), as shown in Figure 3. In the subgroup analysis according to study cohort, the pooled prevalence of adult patients was 61% (95% CI: 56%–65%), and the pooled prevalence of children patients was 55% (95% CI: 50%–60%), as shown in Figure 4.
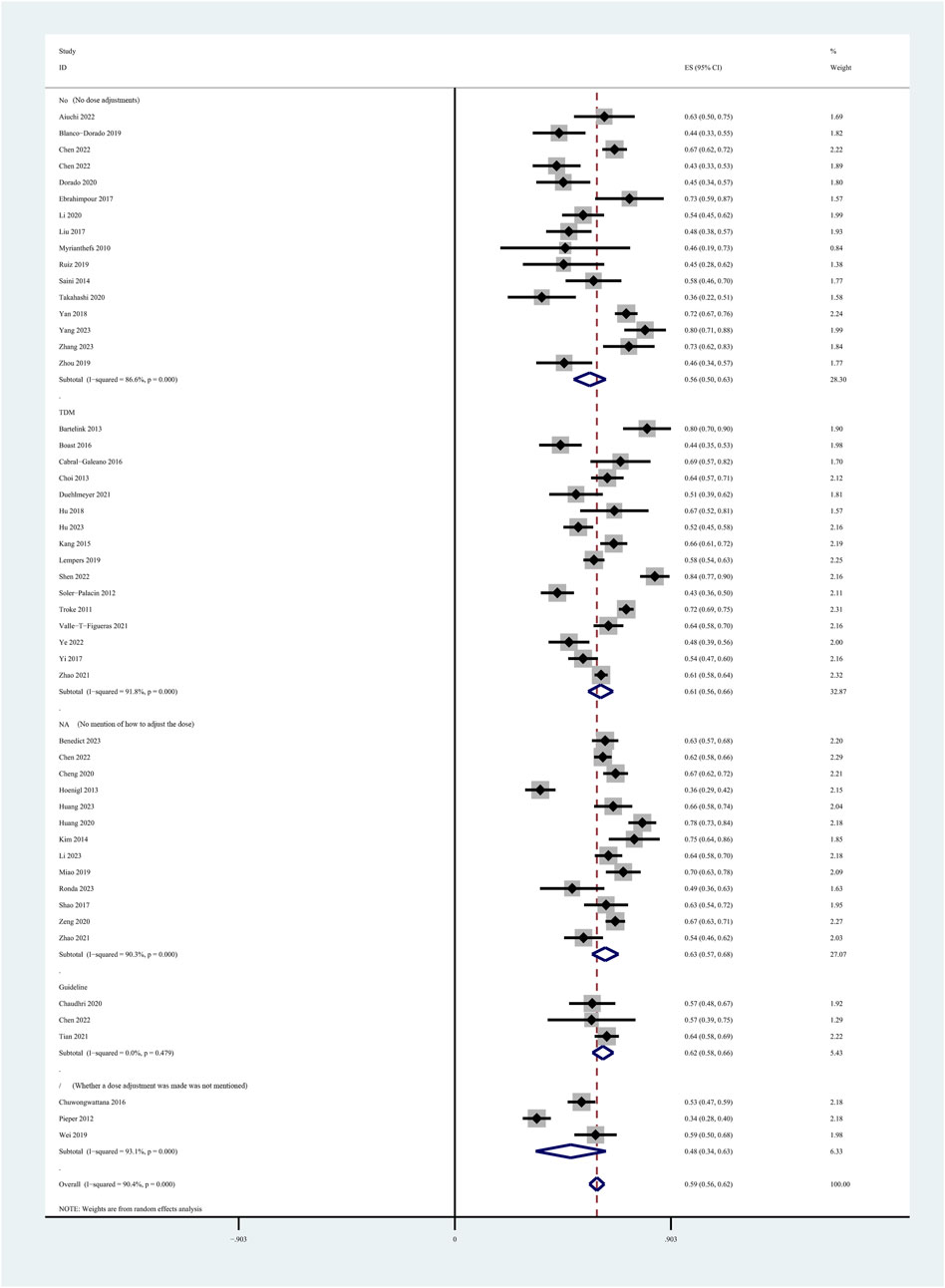
Figure 3. Prevalence of patients reaching the therapeutic range of voriconazole plasma concentration across the method of dose adjustment.
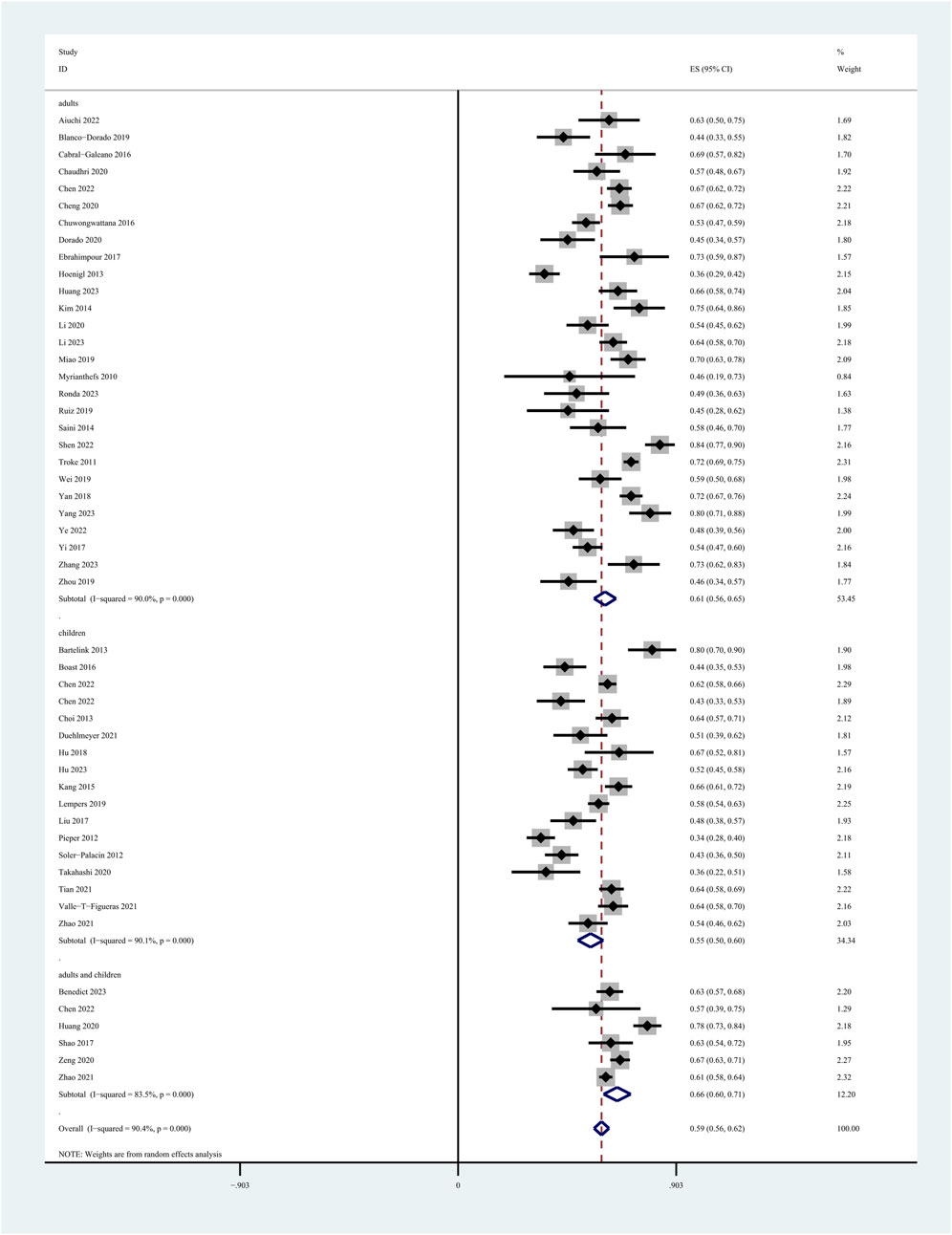
Figure 4. Prevalence of patients reaching the therapeutic range of voriconazole plasma concentration across study cohort.
The meta-analysis revealed high heterogeneity in all included studies. To investigate the sources of this heterogeneity, a meta-regression was performed, considering factors such as sex, country, dose adjustments, therapeutic range, type of treatment settings, and the analytical method used for measuring voriconazole. The results identified the therapeutic range, sex, and analytical method as significant contributors to the heterogeneity. Detailed findings from the meta-regression are presented in Supplementary Figure S1.
3.5 Associated factors with voriconazole concentration
Of the 60 studies, 20 conducted multiple linear regression and reported coefficients. However, only 17 studies provided coefficients and standard errors derived from regression analyses. Table 2 details the specific factors reported in each study. Due to consistency in units and references between studies, we pooled and analysed respectively the coefficients and standard errors of associated factors about plasma voriconazole concentration in children and adult cohorts. These factors included age, sex, dosage, administration modes (oral and intravenous), albumin (ALB), blood urea nitrogen (BUN), total bilirubin (TBil), alanine aminotransferase (ALT), aspartate aminotransferase (AST), use of proton pump inhibitors (PPIs), omeprazole, pantoprazole, glucocorticoids, methylprednisolone, and dexamethasone. Figure 5 presents details of all the potential confounders identified in these studies.
3.5.1 Age
For the children cohort, among the 21 studies, 6 examined the association between age and voriconazole plasma concentration. However, due to one study did not report the standard error of its coefficient, we pooled the coefficients of only 5 studies. The resulting pooled coefficient was 0.08 (95% CI: 0.01–0.14), indicating a statistically significant association. For the adult cohort, 5 of the 20 studies explored the correlation between age and plasma concentration of voriconazole. However, as one study lacked the report of its coefficient’s standard error, we combined only the coefficients from 4 studies. The pooled coefficient for this analysis was 0.02 (95% CI: 0.00–0.04), but this result was not statistically significant.
3.5.2 Sex
In terms of sex (females), four studies investigated its relationship with voriconazole plasma concentration in children. The relationship between sex (females) and voriconazole plasma concentration in adults was examined in two studies. We pooled the coefficients and standard errors from the children and adults, respectively. The pooled coefficients for this analysis were −0.16 (95% CI: −0.46 to 0.14) for children and −0.08 (95% CI: −0.44 to 0.28) for adults, but these results were not statistically significant.
3.5.3 Weight
In the children cohort and the adult cohort, respectively, three studies reported both coefficients and standard errors from multiple linear regression analyses. When these coefficients and standard errors were pooled separately, the resulting coefficients were −0.01 (95% CI: −0.05 to 0.02) for children and −0.01 (95% CI: −0.03 to 0.01) for adult, both of which lacked statistical significance.
3.5.4 Voriconazole dose
The voriconazole doses administered to adults and children varied, requiring separate pooling of the adult dose, the dose for children, and their respective plasma concentrations. The pooled coefficients for dose (mg) and dose (mg/kg) were 0.01 (95% CI: 0.00–0.02) and 0.23 (95% CI: −0.15 to 0.62), neither of which was statistically significant.
3.5.5 Route of administration
This study investigated the correlation between plasma voriconazole concentration and intravenous administration in children, as well as the correlation with oral administration in adults. The pooled coefficients for these were −0.02 (95% CI: −0.58 to 0.80) and −0.72 (95% CI: −1.63 to 0.19), respectively, with no statistical significance observed in either case.
3.5.6 Albumin
Only two studies confirmed the relationship between albumin and voriconazole plasma concentration in children. The pooled coefficient was −0.05 (95% CI: −0.09 to −0.01), indicating a statistically significant association.
3.5.7 Liver function
Several indicators of liver function were considered, but the study focused on the correlations between TBil, ALT, AST, and voriconazole concentration in children. The pooled coefficients for these indicators were 0.17 (95% CI: −0.30 to 0.65), 0.00 (95% CI: −0.01 to 0.00), and 0.01 (95% CI: 0.00–0.01), respectively. However, none showed statistically significant correlations.
3.5.8 Drug combinations
Three studies explored the correlation between PPIs and voriconazole plasma concentration in adults, but the pooled estimate did not show statistical significance. For specific PPIs, two studies focused on omeprazole in relation to voriconazole plasma concentration in adults, and another two on pantoprazole in relation to voriconazole plasma concentration in adults. The pooled coefficients were 1.37 (95% CI 0.82–1.92) for omeprazole and 1.11 (95% CI: 0.17–2.04) for pantoprazole. These results suggest that omeprazole and pantoprazole increase voriconazole plasma concentration by 1.37-fold and 1.11-fold, respectively.
Two studies on glucocorticoids were pooled to assess their association with plasma voriconazole concentration in children. The pooled coefficient was −0.51 (95% CI: −1.66 to 2.67). Three studies on glucocorticoids were pooled to assess their association with plasma voriconazole concentration in adults. The pooled coefficient was −1.33 (95% CI: −3.25 to 0.58). But the results all did not show statistical significance. Two studies focused on methylprednisolone in correlation to voriconazole plasma concentration in adults, and another two on dexamethasone in correlation to voriconazole plasma concentration in adults. The pooled coefficients were −1.75 (95% CI: −2.21 to −1.30) for methylprednisolone and −1.45 (95% CI: −2.07 to −0.83) for dexamethasone, indicating that the voriconazole plasma concentration decreased by 1.75-fold and 1.45-fold, respectively, with the concurrent use of these glucocorticoids in adults.
3.5.9 Inflammation and genetic factor
Only one study established a correlation between C-reactive protein (CRP) and plasma voriconazole concentration; therefore, we did not pool the coefficient and standard error for this parameter. Among the 20 studies, 11 reported a correlation between the CYP2C19 genotype or phenotype and plasma voriconazole concentration. However, the coefficients and standard errors of these studies were not pooled.
3.6 Publication bias
We used the Egger test, the Begg test, and the trim-and-fill method to assess publication bias in the pooled estimate of the prevalence of patients reaching the therapeutic range of plasma voriconazole concentration. Begg’s test yielded a result of 0.127, and Egger’s test showed 0.045. Although the Egger test result did not exceed the threshold of 0.05, it was close to this limit. Consequently, the trim-and-fill method was applied to further the analysis. Using the linear method with two iterations, the software estimated that no studies were missing, suggesting an absence of publication bias. The detailed results of the trim-and-fill method are presented in in Supplementary Figure S2.
4 Discussion
The high pharmacokinetic variability of voriconazole and the benefits of maintaining its’ plasma concentration within the therapeutic range to improve efficacy and safety are well recognized. This study represents the first effort to pool data on the prevalence of patients who achieve this therapeutic range, underscoring the vital importance of TDM in voriconazole. Our analysis of the factors that influence plasma voriconazole concentration offers valuable evidence to healthcare decision-makers, helping to develop more effective voriconazole treatment strategies.
In the studies included in our analysis, the prevalence of patients reaching the therapeutic range of voriconazole plasma concentration varied widely, ranging from 33% to 84%. This variance can be partly attributed to the recommended therapeutic range of voriconazole plasma concentration varies in different regions. For example, guidelines in Japan, the United Kingdom, Australia, and the United States typically recommend a range of 1.0–5.5 mg/L for voriconazole (Ashbee et al., 2014; Hamada et al., 2013; Pappas et al., 2016; Chau et al., 2021), while the Canadian guideline suggests 1.5–5.0 mg/L (Laverdiere et al., 2014), and in China, it is defined as 0.5–5.5 mg/L (Chen et al., 2018). Our study included studies that adopted nine different therapeutic ranges for plasma voriconazole concentration. Although the therapeutic range, sex, and the analytical method for voriconazole measurement were identified as significant factors affecting heterogeneity through meta-regression, other contributors could not be completely excluded. These may include variations in treatment duration, types of treatment settings, and the purpose of voriconazole use (prophylaxis or treatment), all of which could influence achieving the effective and safe therapeutic concentration. Additionally, disparities in study design and patient populations might also affect the prevalence of patients reaching the effective and safe therapeutic range of voriconazole concentrations.
The pooled prevalence was higher in studies with dose adjustments in accordance with TDM and guidelines than in studies without dose adjustments. The results suggested that dose adjustment according to TDM and guidelines is helpful to improve the prevalence of patients reaching concentrations within the effective and safe range. Since most of the studies were retrospective, many of them only mentioned the CYP2C19 genotype testing, but did not mention whether the dose was adjusted according to the genotype. This prevented us from determining the effect of dose adjustment based on CYP2C19 genotype on patients achieving therapeutic range. After subgroup analysis according to the study population, the pooled prevalence of adult patients reaching the therapeutic range is higher than that of children. In adults, the physiological maturity and stability facilitate achieving the effective and safe therapeutic range of voriconazole plasma concentration. In contrast, children exhibit a lower prevalence of reaching the target concentration due to the incomplete development of their metabolic enzyme systems, variations in drug distribution and absorption, and greater individual physiological variability.
Numerous factors influence plasma voriconazole concentration, as highlighted in our study, which included 21 studies that performed multiple linear regression analyses to explore these relationships. Factors associated with plasma voriconazole concentration identified in the literature included primarily demographic characteristics (age, sex, weight, ethnicity, etc.), genetic factors (such as CYP2C19 gene polymorphism), drug interactions (with PPIs, glucocorticoids, etc.), administration methods (oral, intravenous), and dosage. However, not all of these factors were found to have a significant correlation with plasma voriconazole concentration. Although our study did not pool coefficients and standard errors between the CYP2C19 genotype or phenotype and voriconazole plasma concentration, the impact of CYP2C19 on voriconazole plasma concentration is well established. A clinical study has shown that the plasma concentration of voriconazole is influenced by CYP2C19 genotype in both children and adults (Hu et al., 2023). CYP2C19 is crucial in voriconazole metabolism and exhibits a significant genetic polymorphism (Dean, 2012). Poor metabolizers show an approximately four-fold increase in voriconazole exposure compared to homozygous normal metabolizers (Dean, 2012). Heterozygous normal metabolizers experience, on average, a two-fold increase in exposure compared to their homozygous normal counterparts (Dean, 2012). Single nucleotide polymorphisms (SNPs) in the CYP2C19 gene account for approximately 50%–55% of variability in voriconazole metabolism (Murayama et al., 2007). Both the Clinical Pharmacogenetics Implementation Consortium (CPIC) and the Dutch Pharmacogenetics Working Group (DWPG) recommend adjusting the voriconazole dosing regimen based on the CYP2C19 genotype (Moriyama et al., 2017; Swen et al., 2011). Therefore, it is recommended that both adults and children should adjust the voriconazole dosing regimen based on CYP2C19 genotype.
We observed a weak correlation between age and voriconazole plasma concentration in children, but not in adults. This difference may be attributed to differences in drug metabolism and physiological characteristics between the two groups. Moreover, since children’s physiological and metabolic systems are not fully developed, their response to medication may change as they age. A systematic review showed that the dosing of voriconazole in pediatric patients was age-dependent, with younger children requiring higher doses to reach target concentration compared to older children (Hu et al., 2024).
Several studies have highlighted the impact of inflammation on voriconazole metabolism (Liang et al., 2022; Bolcato et al., 2021). Inflammatory cytokines, such as IL-6 and IL-8, can modulate drug-metabolizing enzymes and transporters (DMETs) (Stanke-Labesque et al., 2020), affecting the expression of CYP2C19 and CYP34A, influencing voriconazole plasma concentration (Vreugdenhil et al., 2018; Gautier-Veyret et al., 2017; Klein et al., 2015; Li et al., 2014). CRP, an inflammation indicator, was shown to influence the plasma concentration of voriconazole by impacting CYP2C19 activity (Van Wanrooy et al., 2014; Ruiz et al., 2019; Simon et al., 2021). However, our analysis was limited by the small number of studies addressing CPR, IL-6, and IL-8. Consequently, we could not pool the coefficients from multiple linear regressions for a random-effects model. A systematic review indicated that the level of inflammation (CRP levels) can significantly affect voriconazole plasma concentration in adults (Li et al., 2022). However, a clinical study identified variations in the correlation between CRP levels and voriconazole plasma concentration among pediatric patients across different age groups (Luo et al., 2021). The impact of inflammation (CRP levels) on voriconazole metabolism in children should need further analysis in relation to age groups, which requires a further exploration with a larger sample size.
In our analysis, we found a weak correlation between albumin and voriconazole plasma concentrations in children. Regarding the correlation between albumin and plasma concentration of voriconazole in adults, this article could not conduct research, but some studies showed that hypoproteinemia affected the plasma concentration of voriconazole in adults (Li et al., 2023; Chantharit et al., 2020; Khan-Asa et al., 2020). The inflammatory cytokine IL-6 increased CRP synthesis and decreased albumin synthesis in the liver (Bologa et al., 1998), likely leading to higher voriconazole plasma concentrations due to elevated CRP levels rather than reduced albumin.
Voriconazole is predominantly metabolized in the liver through oxidative processes mediated by CYP450 enzymes, including CYP2C19, CYP3A4, and CYP2C9 (Ashbee et al., 2014). Liver injury may cause metabolic abnormalities and increased exposure, affecting plasma voriconazole concentration. Hepatocellular injury is often indicated by elevated levels of ALT and AST (Kwo et al., 2017). Due to the exclusion of patients with abnormal liver function in some included studies and the limited number of studies that conducted multiple linear regression to assess correlations between AST, ALT, Child-Pugh class, and plasma voriconazole concentration, our study did not observe a definitive correlation between these liver function indicators and plasma voriconazole concentration in the children and adult population. However, we recommend dose reduction or avoidance of voriconazole in patients with impaired liver function (Hamada et al., 2013; Limper et al., 2011). The Japanese guideline (Hamada et al., 2013) recommends maintaining the loading dose but halving the maintenance dose for patients with severe liver disease classified as Child-Pugh classes A and B, while voriconazole is not recommended for those in class C. Similarly, United Kingdom, Canada, the United States, and Australia guidelines generally do not recommend antifungal therapy for patients with severe liver insufficiency (Ashbee et al., 2014; Laverdiere et al., 2014; Chau et al., 2021; Pappas et al., 2016). The Chinese guideline (Chen et al., 2018) advises against using voriconazole as an initial therapy for patients with severe liver disease, recommending close monitoring of plasma concentration and liver function if its use is deemed necessary.
The correlation between plasma voriconazole concentration and PPIs in adults were not found in our study, however, we found that voriconazole plasma concentration was positively correlated with omeprazole and pantoprazole in adults. Additionally, we also did not observe the relationship between plasma voriconazole concentration and glucocorticoids in children and adults, however, a negatively correlated between methylprednisolone, dexamethasone and voriconazole plasma concentration in adults was found in our study. Voriconazole inhibits the activity of CYP2C19, CYP3A4, and CYP2C9, resulting in several clinically significant drug interactions (Ashbee et al., 2014). PPIs(Klotz et al., 2004; Li et al., 2004) and glucocorticoids (Czock et al., 2005; Chen et al., 2003) are known to affect CYP2C19 activity. However, neither PPIs nor glucocorticoids seem to affect the pharmacokinetics of voriconazole (Shi et al., 2019). Some studies indicated that glucocorticoids and PPIs can impact plasma voriconazole concentration (Jia et al., 2021; Li et al., 2004). Rabeprazole and lansoprazole are less potent CYP450 inhibitors compared to omeprazole and pantoprazole (Li et al., 2004), and another study found that voriconazole plasma concentration decreased significantly with dexamethasone or methylprednisolone, but less so with prednisone or prednisolone (Jia et al., 2021). Our analysis did not find a correlation between the PPIs as a group, glucocorticoids as a group and plasma voriconazole concentration, possibly due to the inclusion of different types of PPIs and glucocorticoids in the reviewed studies. In summary, the effects of PPIs and glucocorticoids on voriconazole pharmacokinetics are disputed. Indeed, substantial studies showed that the type of PPI and dose (or glucocorticoid) significantly impact on voriconazole exposure (Qi et al., 2017; Jia et al., 2021). Drug interactions may occur in both adults and children; thus, attention should be paid to the combined use of glucocorticoids or PPIs, including consideration of the type and dose of these medications.
Although our study provides a comprehensive quantitative summary of the prevalence of patients who achieve the therapeutic range of plasma voriconazole concentration and associated factors, it is important to recognize several limitations. First, the included studies covered a global scale, including various medical practices, economic conditions, geographic locations, and cultural backgrounds. These factors could influence the reported prevalence of patients who reached the therapeutic voriconazole concentration. Second, the studies exhibited high heterogeneity, possibly due to variations in sample size, study populations, sex distribution, indications for voriconazole use, dose adjustments, and concentration measurement methodologies. Third, the restriction to studies published in English might have led to an underestimation or overestimation of prevalence and limited the pooling of several factors. Some factors in our study were only analyzed in two studies, raising concerns about possible bias in these results. Therefore, further research is warranted to better understand the factors associated with voriconazole plasma concentration.
5 Conclusion
The analysis revealed that only approximately half of the patients reached the plasma voriconazole concentration therapeutic range without dose adjustments and the pooled prevalence of adult patients reaching the therapeutic range is higher than that of children. The meta-analysis identified factors associated with voriconazole plasma concentration in children, including age, albumin levels. Meanwhile, it also identified the factors related to plasma voriconazole concentration in adults, including the use of omeprazole, pantoprazole, dexamethasone and methylprednisolone.
Data availability statement
The original contributions presented in the study are included in the article/Supplementary Material, further inquiries can be directed to the corresponding author.
Author contributions
XL: Writing–original draft. QH: Writing–original draft. TX: Writing–review and editing.
Funding
The author(s) declare that financial support was received for the research, authorship, and/or publication of this article. This research was supported by National Key Clinical Specialties Construction Program.
Conflict of interest
The authors declare that the research was conducted in the absence of any commercial or financial relationships that could be construed as a potential conflict of interest.
Publisher’s note
All claims expressed in this article are solely those of the authors and do not necessarily represent those of their affiliated organizations, or those of the publisher, the editors and the reviewers. Any product that may be evaluated in this article, or claim that may be made by its manufacturer, is not guaranteed or endorsed by the publisher.
Supplementary material
The Supplementary Material for this article can be found online at: https://www.frontiersin.org/articles/10.3389/fphar.2024.1368274/full#supplementary-material
References
Aiuchi, N., Nakagawa, J., Sakuraba, H., Takahata, T., Kamata, K., Saito, N., et al. (2022). Impact of polymorphisms of pharmacokinetics-related genes and the inflammatory response on the metabolism of voriconazole. Pharmacol. Res. and Perspect. 10, 11. doi:10.1002/prp2.935
Allegra, S., Fatiguso, G., Francia, S., Pirro, E., Carcieri, C., Cusato, J., et al. (2018). Pharmacogenetic of voriconazole antifungal agent in pediatric patients. Pharmacogenomics 19, 913–925. doi:10.2217/pgs-2017-0173
Ashbee, H. R., Barnes, R. A., Johnson, E. M., Richardson, M. D., Gorton, R., and Hope, W. W. (2014). Therapeutic drug monitoring (TDM) of antifungal agents: guidelines from the British Society for Medical Mycology. J. Antimicrob. Chemother. 69, 1162–1176. doi:10.1093/jac/dkt508
Bartelink, I. H., Wolfs, T., Jonker, M., De Waal, M., Egberts, T. C., Ververs, T. T., et al. (2013). Highly variable plasma concentrations of voriconazole in pediatric hematopoietic stem cell transplantation patients. Antimicrob. Agents Chemother. 57, 235–240. doi:10.1128/AAC.01540-12
Benedict, K., Gold, J. A. W., Toda, M., Thompson, G. R., Wiederhold, N. P., and Smith, D. J. (2023). Low rates of antifungal therapeutic drug monitoring among inpatients who received itraconazole, posaconazole, or voriconazole, United States, 2019-2021. Open Forum Infect. Dis. 10, ofad389. doi:10.1093/ofid/ofad389
Blanco-Dorado, S., Cea-Arestin, C., Gonzalez Carballo, A., Latorre-Pellicer, A., Maronas Amigo, O., Barbeito Castineiras, G., et al. (2019). An observational study of the efficacy and safety of voriconazole in a real-life clinical setting. J. Chemother. 31 (1), 49–57. doi:10.1080/1120009X.2018.1524085
Blanco-Dorado, S., Maroñas, O., Latorre-Pellicer, A., Rodríguez Jato, M. T., López-Vizcaíno, A., Gómez Márquez, A., et al. (2020). Impact of CYP2C19 genotype and drug interactions on voriconazole plasma concentrations: a Spain pharmacogenetic-pharmacokinetic prospective multicenter study. Pharmacotherapy 40, 17–25. doi:10.1002/phar.2351
Boast, A., Curtis, N., Cranswick, N., and Gwee, A. (2016). Voriconazole dosing and therapeutic drug monitoring in children: experience from a paediatric tertiary care centre. J. Antimicrob. Chemother. 71, 2031–2036. doi:10.1093/jac/dkw056
Bolcato, L., Khouri, C., Veringa, A., Alffenaar, J. W. C., Yamada, T., Naito, T., et al. (2021). Combined impact of inflammation and pharmacogenomic variants on voriconazole trough concentrations: a meta-analysis of individual data. J. Clin. Med. 10, 2089. doi:10.3390/jcm10102089
Bologa, R. M., Levine, D. M., Parker, T. S., Cheigh, J. S., Serur, D., Stenzel, K. H., et al. (1998). Interleukin-6 predicts hypoalbuminemia, hypocholesterolemia, and mortality in hemodialysis patients. Am. J. Kidney Dis. 32, 107–114. doi:10.1053/ajkd.1998.v32.pm9669431
Cabral-Galeano, E., Ruiz-Camps, I., Len-Abad, O., Pou-Clavé, L., Sordé-Masip, R., Meije-Castillo, Y., et al. (2015). Clinical usefulness of therapeutic drug monitoring of voriconazole in a university hospital. Enferm. Infecc. Microbiol. Clin. 33, 298–302. doi:10.1016/j.eimc.2014.09.005
Chantharit, P., Tantasawat, M., Kasai, H., and Tanigawara, Y. (2020). Population pharmacokinetics of voriconazole in patients with invasive aspergillosis: serum albumin level as a novel marker for clearance and dosage optimization. Ther. Drug Monit. 42, 872–879. doi:10.1097/FTD.0000000000000799
Chau, M. M., Daveson, K., Alffenaar, J. C., Gwee, A., Ho, S. A., Marriott, D. J. E., et al. (2021). Consensus guidelines for optimising antifungal drug delivery and monitoring to avoid toxicity and improve outcomes in patients with haematological malignancy and haemopoietic stem cell transplant recipients, 2021. Intern Med. J. 51 (Suppl. 7), 37–66. doi:10.1111/imj.15587
Chaudhri, K., Stocker, S. L., Williams, K. M., Mcleay, R. C., Marriott, D. J. E., Di Tanna, G. L., et al. (2020). Voriconazole: an audit of hospital-based dosing and monitoring and evaluation of the predictive performance of a dose-prediction software package. J. Antimicrob. Chemother. 75, 1981–1984. doi:10.1093/jac/dkaa098
Chen, C. Y., Xu, T., Zhou, K. T., and Zhu, S. Y. (2022). Factors affecting voriconazole concentration to dose ratio changes according to route of administration. Eur. J. Hosp. Pharm. 5. doi:10.1136/ejhpharm-2021-003173
Chen, J., Wu, Y., He, Y., Feng, X., Ren, Y., and Liu, S. (2022). Combined effect of CYP2C19 genetic polymorphisms and C-reactive protein on voriconazole exposure and dosing in immunocompromised children. Front. Pediatr. 10. doi:10.3389/fped.2022.846411
Chen, K., Zhang, X., Ke, X., Du, G., Yang, K., and Zhai, S. (2018). Individualized medication of voriconazole: a practice guideline of the division of therapeutic drug monitoring, Chinese pharmacological society. Ther. Drug Monit. 40, 663–674. doi:10.1097/FTD.0000000000000561
Chen, T. T., Wu, S. F., Zhang, H. T., Zhang, Q. Q., and Lin, Z. Q. (2022). Therapeutic drug monitoring of voriconazole in AIDS patients. Lat. Am. J. Pharm. 41, 2020–2026. doi:10.21203/rs.3.rs-1010995/v1
Chen, W. Q., Wang, X. X., Li, B., Qin, W., Li, S., Wang, X. X., et al. (2022). Effects of voriconazole exposure on the pharmacokinetics of tacrolimus in lung transplantation patients, based on therapeutic drug monitoring data. J. Clin. Pharmacol. 62, 1310–1320. doi:10.1002/jcph.2066
Chen, X., Xiao, Y., Li, H., Huang, Z., Gao, J., Zhang, X., et al. (2022). Therapeutic drug monitoring and CYP2C19 genotyping guide the application of voriconazole in children. Transl. Pediatr. 11 (8), 1311–1322. doi:10.21037/tp-22-156
Chen, Y., Ferguson, S. S., Negishi, M., and Goldstein, J. A. (2003). Identification of constitutive androstane receptor and glucocorticoid receptor binding sites in the CYP2C19 promoter. Mol. Pharmacol. 64, 316–324. doi:10.1124/mol.64.2.316
Cheng, L., Xiang, R. F., Liu, F., Li, Y. L., Chen, H. L., Yao, P., et al. (2020). Therapeutic drug monitoring and safety of voriconazole in elderly patients. Int. Immunopharmacol. 78, 106078. doi:10.1016/j.intimp.2019.106078
Choi, S. H., Lee, S. Y., Hwang, J. Y., Lee, S. H., Yoo, K. H., Sung, K. W., et al. (2013). Importance of voriconazole therapeutic drug monitoring in pediatric cancer patients with invasive aspergillosis. Pediatr. Blood Cancer 60, 82–87. doi:10.1002/pbc.24262
Chuwongwattana, S., Jantararoungtong, T., Chitasombat, M. N., Puangpetch, A., Prommas, S., Dilokpattanamongkol, P., et al. (2016). A prospective observational study of CYP2C19 polymorphisms and voriconazole plasma level in adult Thai patients with invasive aspergillosis. Drug Metab. Pharmacokinet. 31, 117–122. doi:10.1016/j.dmpk.2015.12.005
Cojutti, P., Candoni, A., Forghieri, F., Isola, M., Zannier, M. E., Bigliardi, S., et al. (2016). Variability of voriconazole trough levels in haematological patients: influence of comedications with cytochrome P450(CYP) inhibitors and/or with CYP inhibitors plus CYP inducers. Basic Clin. Pharmacol. Toxicol. 118, 474–479. doi:10.1111/bcpt.12530
Czock, D., Keller, F., Rasche, F. M., and Häussler, U. (2005). Pharmacokinetics and pharmacodynamics of systemically administered glucocorticoids. Clin. Pharmacokinet. 44, 61–98. doi:10.2165/00003088-200544010-00003
Dean, L. (2012). “Voriconazole therapy and CYP2C19 genotype,” in Medical genetics summaries. Editors V. M. Pratt, S. A. Scott, M. Pirmohamed, B. Esquivel, B. L. Kattman, and A. J. Malheiro (Bethesda (MD): National Center for Biotechnology Information).
Dolton, M. J., and Mclachlan, A. J. (2014). Voriconazole pharmacokinetics and exposure-response relationships: assessing the links between exposure, efficacy and toxicity. Int. J. Antimicrob. Agents 44, 183–193. doi:10.1016/j.ijantimicag.2014.05.019
Dolton, M. J., Ray, J. E., Chen, S. C. A., Ng, K., Pont, L. G., and Mclachlan, A. J. (2012). Multicenter study of voriconazole pharmacokinetics and therapeutic drug monitoring. Antimicrob. Agents Chemother. 56, 4793–4799. doi:10.1128/AAC.00626-12
Dorado, S. B., Amigo, O. M., Latorre-Pellicer, A., Jato, M. T. R., Lopez-Vizcaino, A., Marquez, A. G., et al. (2020). A multicentre prospective study evaluating the impact of proton-pump inhibitors omeprazole and pantoprazole on voriconazole plasma concentrations. Br. J. Clin. Pharmacol. 86, 1661–1666. doi:10.1111/bcp.14267
Dote, S., Sawai, M., Nozaki, A., Naruhashi, K., Kobayashi, Y., and Nakanishi, H. (2016). A retrospective analysis of patient-specific factors on voriconazole clearance. J. Pharm. Health Care Sci. 2 (1), 10. doi:10.1186/s40780-016-0044-9
Duehlmeyer, S., Klockau, C., Yu, D., and Rouch, J. (2021). Characterization of therapeutic drug monitoring practices of voriconazole and posaconazole at a pediatric hospital. J. Pediatr. Pharmacol. Ther. 26 (1), 26–32. doi:10.5863/1551-6776-26.1.26
Ebrahimpour, S., Namazi, S., Mohammadi, M., Nikbakht, M., Hadjibabaie, M., Masoumi, H., et al. (2017). Impact of CYP2C19 polymorphisms on serum concentration of voriconazole in iranian hematological patients. J. Res. Pharm. Pract. 6 (3), 151–157. doi:10.4103/jrpp.JRPP_17_31
Elewa, H., El-Mekaty, E., El-Bardissy, A., Ensom, M. H., and Wilby, K. J. (2015). Therapeutic drug monitoring of voriconazole in the management of invasive fungal infections: a critical review. Clin. Pharmacokinet. 54, 1223–1235. doi:10.1007/s40262-015-0297-8
Fan, X. H., Zhang, H., Wen, Z. P., Zheng, X. L., Yang, Y., and Yang, J. H. (2022). Effects of CYP2C19, CYP2C9 and CYP3A4 gene polymorphisms on plasma voriconazole levels in Chinese pediatric patients. Pharmacogenetics Genomics 32, 152–158. doi:10.1097/FPC.0000000000000464
Gautier-Veyret, E., Bailly, S., Fonrose, X., Tonini, J., Chevalier, S., Thiebaut-Bertrand, A., et al. (2017). Pharmacogenetics may influence the impact of inflammation on voriconazole trough concentrations. Pharmacogenomics 18, 1119–1123. doi:10.2217/pgs-2017-0054
Hamada, Y., Seto, Y., Yago, K., and Kuroyama, M. (2012). Investigation and threshold of optimum blood concentration of voriconazole: a descriptive statistical meta-analysis. J. Infect. Chemother. 18, 501–507. doi:10.1007/s10156-011-0363-6
Hamada, Y., Tokimatsu, I., Mikamo, H., Kimura, M., Seki, M., Takakura, S., et al. (2013). Practice guidelines for therapeutic drug monitoring of voriconazole: a consensus review of the Japanese Society of Chemotherapy and the Japanese Society of Therapeutic Drug Monitoring. J. Infect. Chemother. 19, 381–392. doi:10.1007/s10156-013-0607-8
Hashemizadeh, Z., Badiee, P., Malekhoseini, S. A., Shahraki, H. R., Geramizadeh, B., and Montaseri, H. (2017). Observational study of associations between voriconazole therapeutic drug monitoring, toxicity, and outcome in liver transplant patients. Antimicrob. Agents Chemother. 61, e01211–17. doi:10.1128/AAC.01211-17
Hoenigl, M., Duettmann, W., Raggam, R. B., Seeber, K., Troppan, K., Fruhwald, S., et al. (2013). Potential factors for inadequate voriconazole plasma concentrations in intensive care unit patients and patients with hematological malignancies. Antimicrob. Agents Chemother. 57, 3262–3267. doi:10.1128/AAC.00251-13
Hope, W., Natarajan, P., and Goodwin, L. (2013). Invasive fungal infections. Clin. Med. (Lond) 13, 507–510. doi:10.7861/clinmedicine.13-5-507
Hu, L., Dai, T. T., Zou, L., Li, T. M., Ding, X. S., and Yin, T. (2018). Therapeutic drug monitoring of voriconazole in children from a tertiary care center in China. Antimicrob. Agents Chemother. 62, e00955–18. doi:10.1128/AAC.00955-18
Hu, L., Huang, J., Li, Y., and He, G. (2024). Clinical application of voriconazole in pediatric patients: a systematic review. Ital. J. Pediatr. 50, 113. doi:10.1186/s13052-024-01684-z
Hu, L., Huang, Q., Huang, S., and Feng, Z. (2023). Therapeutic drug monitoring of voriconazole and CYP2C19 phenotype for dose optimization in paediatric patients. Eur. J. Clin. Pharmacol. 79, 1271–1278. doi:10.1007/s00228-023-03538-9
Huang, H. G., Wang, H. L., Lin, Y. K., Yi, Y. D., Liu, M., Dong, J. L., et al. (2023). Factors influencing and adverse reactions of voriconazole clearance in patients with hematological diseases. Zhongguo Shi Yan Xue Ye Xue Za Zhi 31, 562–567. doi:10.19746/j.cnki.issn.1009-2137.2023.02.036
Huang, Q., Liu, Q., Yin, T., Hu, L., Ding, H., Liu, S., et al. (2020). Effect of proton pump inhibitors on voriconazole concentrations in Chinese patients with malignant hematological diseases. Eur. J. Clin. Pharmacol. 76, 833–842. doi:10.1007/s00228-020-02841-z
Jeans, A. R., Howard, S. J., Al-Nakeeb, Z., Goodwin, J., Gregson, L., Warn, P. A., et al. (2012). Combination of voriconazole and anidulafungin for treatment of triazole-resistant aspergillus fumigatus in an in vitro model of invasive pulmonary aspergillosis. Antimicrob. Agents Chemother. 56, 5180–5185. doi:10.1128/AAC.01111-12
Jia, S. J., Gao, K. Q., Huang, P. H., Guo, R., Zuo, X. C., Xia, Q., et al. (2021). Interactive effects of glucocorticoids and cytochrome P450 polymorphisms on the plasma trough concentrations of voriconazole. Front. Pharmacol. 12, 666296. doi:10.3389/fphar.2021.666296
Jin, H., Wang, T., Falcione, B. A., Olsen, K. M., Chen, K., Tang, H., et al. (2016). Trough concentration of voriconazole and its relationship with efficacy and safety: a systematic review and meta-analysis. J. Antimicrob. Chemother. 71, 1772–1785. doi:10.1093/jac/dkw045
Kang, H. M., Lee, H. J., Cho, E. Y., Yu, K. S., Lee, H., Lee, J. W., et al. (2015). The clinical significance of voriconazole therapeutic drug monitoring in children with invasive fungal infections. Pediatr. Hematol. Oncol. 32, 557–567. doi:10.3109/08880018.2015.1088905
Khan-Asa, B., Punyawudho, B., Singkham, N., Chaivichacharn, P., Karoopongse, E., Montakantikul, P., et al. (2020). Impact of albumin and omeprazole on steady-state population pharmacokinetics of voriconazole and development of a voriconazole dosing optimization model in Thai patients with hematologic diseases. Antibiot. (Basel) 9, 574. doi:10.3390/antibiotics9090574
Kim, D. Y., Park, H. J., and Lee, Y. J. (2014). Factors affecting voriconazole plasma concentrations in patients with invasive fungal infections. Int. J. Clin. Pharmacol. Ther. 52, 209–216. doi:10.5414/CP202014
Klein, M., Thomas, M., Hofmann, U., Seehofer, D., Damm, G., and Zanger, U. M. (2015). A systematic comparison of the impact of inflammatory signaling on absorption, distribution, metabolism, and excretion gene expression and activity in primary human hepatocytes and HepaRG cells. Drug Metab. Dispos. 43, 273–283. doi:10.1124/dmd.114.060962
Klotz, U., Schwab, M., and Treiber, G. (2004). CYP2C19 polymorphism and proton pump inhibitors. Basic Clin. Pharmacol. Toxicol. 95, 2–8. doi:10.1111/j.1600-0773.2004.pto950102.x
Kwo, P. Y., Cohen, S. M., and Lim, J. K. (2017). ACG clinical guideline: evaluation of abnormal liver chemistries. Am. J. Gastroenterol. 112, 18–35. doi:10.1038/ajg.2016.517
Laverdiere, M., Bow, E. J., Rotstein, C., Autmizguine, J., Broady, R., Garber, G., et al. (2014). Therapeutic drug monitoring for triazoles: a needs assessment review and recommendations from a Canadian perspective. Can. J. Infect. Dis. Med. Microbiol. 25, 327–343. doi:10.1155/2014/340586
Lempers, V. J., Meuwese, E., Mavinkurve-Groothuis, A. M., Henriet, S., Van Der Sluis, I. M., Hanff, L. M., et al. (2019). Impact of dose adaptations following voriconazole therapeutic drug monitoring in pediatric patients. Med. Mycol. 57, 937–943. doi:10.1093/mmy/myz006
Li, A. P., Yang, Q., Vermet, H., Raoust, N., Klieber, S., and Fabre, G. (2014). Evaluation of human hepatocytes under prolonged culture in a novel medium for the maintenance of hepatic differentiation: results with the model pro-inflammatory cytokine interleukin 6. Drug Metab. Lett. 8, 12–18. doi:10.2174/187231280801140929155351
Li, H., Li, M., Yan, J., Gao, L., Zhou, L., Wang, Y., et al. (2020). Voriconazole therapeutic drug monitoring in critically ill patients improves efficacy and safety of antifungal therapy. Basic Clin. Pharmacol. Toxicol. 127, 495–504. doi:10.1111/bcpt.13465
Li, X., Lai, F., Jiang, Z., Li, M., Chen, Z., Cheng, J., et al. (2022). Effects of inflammation on voriconazole levels: a systematic review. Br. J. Clin. Pharmacol. 88, 5166–5182. doi:10.1111/bcp.15495
Li, X. Q., Andersson, T. B., Ahlström, M., and Weidolf, L. (2004). Comparison of inhibitory effects of the proton pump-inhibiting drugs omeprazole, esomeprazole, lansoprazole, pantoprazole, and rabeprazole on human cytochrome P450 activities. Drug Metab. Dispos. 32, 821–827. doi:10.1124/dmd.32.8.821
Li, Y. Y., Zhang, Y., Zhao, J. X., Bian, J. L., Zhao, Y. Y., Hao, X., et al. (2023). Combined impact of hypoalbuminemia and pharmacogenomic variants on voriconazole trough concentration: data from a real-life clinical setting in the Chinese population. J. Chemother. 11. doi:10.1080/1120009x.2023.2247208
Liang, Z., Yu, M., Liu, Z., Liu, F., Jia, C., Xiong, L., et al. (2022). Inflammation affects liver function and the metabolism of voriconazole to voriconazole-N-oxide in adult and elderly patients. Front. Pharmacol. 13, 835871. doi:10.3389/fphar.2022.835871
Limper, A. H., Knox, K. S., Sarosi, G. A., Ampel, N. M., Bennett, J. E., Catanzaro, A., et al. (2011). An official American Thoracic Society statement: treatment of fungal infections in adult pulmonary and critical care patients. Am. J. Respir. Crit. Care Med. 183, 96–128. doi:10.1164/rccm.2008-740ST
Liu, L., Zhou, X., Wu, T., Jiang, H., Yang, S., and Zhang, Y. (2017). Dose optimisation of voriconazole with therapeutic drug monitoring in children: a single-centre experience in China. Int. J. Antimicrob. Agents 49, 483–487. doi:10.1016/j.ijantimicag.2016.11.028
Ludden, T. M. (1991). Nonlinear pharmacokinetics: clinical Implications. Clin. Pharmacokinet. 20, 429–446. doi:10.2165/00003088-199120060-00001
Luo, X., Li, T., Hu, L., Liu, S., Zhao, H., Zhang, J., et al. (2021). Differential effects of C-reactive protein levels on voriconazole metabolism at three age groups in allogeneic hematopoietic cell transplant recipients. J. Chemother. 33, 95–105. doi:10.1080/1120009X.2020.1765604
Mafuru, M., Wu, S. L., Mayala, H., Msengwa, Z., Phillip, A., and Mgone, C. (2021). Analysis of combined effect of CYP2C19 genetic polymorphism and proton pump inhibitors coadministration on trough concentration of voriconazole. Pharmacogenomics and Personalized Med. 14, 1379–1389. doi:10.2147/PGPM.S329662
Miao, Q., Tang, J. T., Van Gelder, T., Li, Y. M., Bai, Y. J., Zou, Y. G., et al. (2019). Correlation of CYP2C19 genotype with plasma voriconazole exposure in South-western Chinese Han patients with invasive fungal infections. Med. Baltim. 98, e14137. doi:10.1097/MD.0000000000014137
Moher, D., Shamseer, L., Clarke, M., Ghersi, D., Liberati, A., Petticrew, M., et al. (2015). Preferred reporting items for systematic review and meta-analysis protocols (PRISMA-P) 2015 statement. Syst. Rev. 4, 1. doi:10.1186/2046-4053-4-1
Moriyama, B., Obeng, A. O., Barbarino, J., Penzak, S. R., Henning, S. A., Scott, S. A., et al. (2017). Clinical Pharmacogenetics implementation Consortium (CPIC) guidelines for CYP2C19 and voriconazole therapy. Clin. Pharmacol. Ther. 102, 45–51. doi:10.1002/cpt.583
Murayama, N., Imai, N., Nakane, T., Shimizu, M., and Yamazaki, H. (2007). Roles of CYP3A4 and CYP2C19 in methyl hydroxylated and N-oxidized metabolite formation from voriconazole, a new anti-fungal agent, in human liver microsomes. Biochem. Pharmacol. 73, 2020–2026. doi:10.1016/j.bcp.2007.03.012
Myrianthefs, P., Markantonis, S. L., Evaggelopoulou, P., Despotelis, S., Evodia, E., Panidis, D., et al. (2010). Monitoring plasma voriconazole levels following intravenous administration in critically ill patients: an observational study. Int. J. Antimicrob. Agents 35, 468–472. doi:10.1016/j.ijantimicag.2009.12.021
Pappas, P. G., Kauffman, C. A., Andes, D. R., Clancy, C. J., Marr, K. A., Ostrosky-Zeichner, L., et al. (2016). Clinical practice guideline for the management of candidiasis: 2016 update by the infectious diseases society of America. Clin. Infect. Dis. 62, e1–e50. doi:10.1093/cid/civ933
Park, W. B., Kim, N. H., Kim, K. H., Lee, S. H., Nam, W. S., Yoon, S. H., et al. (2012). The effect of therapeutic drug monitoring on safety and efficacy of voriconazole in invasive fungal infections: a randomized controlled trial. Clin. Infect. Dis. 55, 1080–1087. doi:10.1093/cid/cis599
Patterson, T. F., Thompson, G. R. 3rd, Denning, D. W., Fishman, J. A., Hadley, S., Herbrecht, R., et al. (2016). Practice guidelines for the diagnosis and management of aspergillosis: 2016 update by the infectious diseases society of America. Clin. Infect. Dis. 63, e1–e60. doi:10.1093/cid/ciw326
Pieper, S., Kolve, H., Gumbinger, H. G., Goletz, G., Wurthwein, G., and Groll, A. H. (2012). Monitoring of voriconazole plasma concentrations in immunocompromised paediatric patients. J. Antimicrob. Chemother. 67, 2717–2724. doi:10.1093/jac/dks258
Qi, F., Zhu, L., Li, N., Ge, T., Xu, G., and Liao, S. (2017). Influence of different proton pump inhibitors on the pharmacokinetics of voriconazole. Int. J. Antimicrob. Agents 49, 403–409. doi:10.1016/j.ijantimicag.2016.11.025
Resztak, M., Sobiak, J., and Czyrski, A. (2021). Recent advances in therapeutic drug monitoring of voriconazole, mycophenolic acid, and vancomycin: a literature review of pediatric studies. Pharmaceutics 13, 1991. doi:10.3390/pharmaceutics13121991
Rolden, H. J., Van Bodegom, D., Van Den Hout, W. B., and Westendorp, R. G. (2014). Old age mortality and macroeconomic cycles. J. Epidemiol. Community Health 68, 44–50. doi:10.1136/jech-2013-202544
Ronda, M., Llop-Talaveron, J. M., Fuset, M., Leiva, E., Shaw, E., Gumucio-Sanguino, V. D., et al. (2023). Voriconazole pharmacokinetics in critically ill patients and extracorporeal membrane oxygenation support: a retrospective comparative case-control study. Antibiotics-Basel 12, 1100. doi:10.3390/antibiotics12071100
Ruiz, J., Gordon, M., Villarreal, E., Peruccioni, M., Marques, M. R., Poveda-Andres, J. L., et al. (2019). Impact of voriconazole plasma concentrations on treatment response in critically ill patients. J. Clin. Pharm. Ther. 44, 572–578. doi:10.1111/jcpt.12817
Sabatelli, F., Patel, R., Mann, P. A., Mendrick, C. A., Norris, C. C., Hare, R., et al. (2006). In vitro activities of posaconazole, fluconazole, itraconazole, voriconazole, and amphotericin B against a large collection of clinically important molds and yeasts. Antimicrob. Agents Chemother. 50, 2009–2015. doi:10.1128/AAC.00163-06
Saini, L., Seki, J. T., Kumar, D., Atenafu, E. G., Cole, D. E. C., Wong, B. Y. L., et al. (2014). Serum voriconazole level variability in patients with hematological malignancies receiving voriconazole therapy. Can. J. Infect. Dis. and Med. Microbiol. 25, 271–276. doi:10.1155/2014/214813
Shao, B., Ma, Y., Li, Q., Wang, Y., Zhu, Z., Zhao, H., et al. (2017). Effects of cytochrome P450 3A4 and non-genetic factors on initial voriconazole serum trough concentrations in hematological patients with different cytochrome P450 2C19 genotypes. Xenobiotica 47 (12), 1121–1129. doi:10.1080/00498254.2016.1271960
Shen, K., Gu, Y., Wang, Y., Lu, Y., Ni, Y., Zhong, H., et al. (2022). Therapeutic drug monitoring and safety evaluation of voriconazole in the treatment of pulmonary fungal diseases. Ther. Adv. Drug Saf. 13, 20420986221127503. doi:10.1177/20420986221127503
Shi, C., Xiao, Y., Mao, Y., Wu, J., and Lin, N. (2019). Voriconazole: a review of population pharmacokinetic analyses. Clin. Pharmacokinet. 58, 687–703. doi:10.1007/s40262-019-00735-7
Simon, F., Gautier-Veyret, E., Truffot, A., Chenel, M., Payen, L., Stanke-Labesque, F., et al. (2021). Modeling approach to predict the impact of inflammation on the pharmacokinetics of CYP2C19 and CYP3A4 substrates. Pharm. Res. 38, 415–428. doi:10.1007/s11095-021-03019-7
Soler-Palacin, P., Frick, M. A., Martin-Nalda, A., Lanaspa, M., Pou, L., Rosello, E., et al. (2012). Voriconazole drug monitoring in the management of invasive fungal infection in immunocompromised children: a prospective study. J. Antimicrob. Chemother. 67, 700–706. doi:10.1093/jac/dkr517
Stanke-Labesque, F., Gautier-Veyret, E., Chhun, S., and Guilhaumou, R. (2020). Inflammation is a major regulator of drug metabolizing enzymes and transporters: consequences for the personalization of drug treatment. Pharmacol. Ther. 215, 107627. doi:10.1016/j.pharmthera.2020.107627
Stroup, D. F., Berlin, J. A., Morton, S. C., Olkin, I., Williamson, G. D., Rennie, D., et al. (2000). Meta-analysis of observational studies in epidemiology: a proposal for reporting. Meta-analysis of Observational Studies in Epidemiology (MOOSE) group. Jama 283, 2008–2012. doi:10.1001/jama.283.15.2008
Swen, J. J., Nijenhuis, M., De Boer, A., Grandia, L., Maitland-Van Der Zee, A. H., Mulder, H., et al. (2011). Pharmacogenetics: from bench to byte--an update of guidelines. Clin. Pharmacol. Ther. 89, 662–673. doi:10.1038/clpt.2011.34
Takahashi, T., Smith, A. R., Jacobson, P. A., Fisher, J., Rubin, N. T., and Kirstein, M. N. (2020). Impact of obesity on voriconazole pharmacokinetics among pediatric hematopoietic cell transplant recipients. Antimicrob. Agents Chemother. 64, e00819–20. doi:10.1128/AAC.00653-20
Theuretzbacher, U., Ihle, F., and Derendorf, H. (2006). Pharmacokinetic/pharmacodynamic profile of voriconazole. Clin. Pharmacokinet. 45, 649–663. doi:10.2165/00003088-200645070-00002
Tian, X., Zhang, C., Qin, Z., Wang, D., Yang, J., and Zhang, X. (2021). Impact of CYP2C19 phenotype and drug-drug interactions on voriconazole concentration in pediatric patients. Antimicrob. Agents Chemother. 65, e0020721. doi:10.1128/AAC.00207-21
Tissot, F., Agrawal, S., Pagano, L., Petrikkos, G., Groll, A. H., Skiada, A., et al. (2017). ECIL-6 guidelines for the treatment of invasive candidiasis, aspergillosis and mucormycosis in leukemia and hematopoietic stem cell transplant patients. Haematologica 102, 433–444. doi:10.3324/haematol.2016.152900
Troke, P. F., Hockey, H. P., and Hope, W. W. (2011). Observational study of the clinical efficacy of voriconazole and its relationship to plasma concentrations in patients. Antimicrob. Agents Chemother. 55, 4782–4788. doi:10.1128/AAC.01083-10
Valle-T-Figueras, J. M., Miro, B. R., Carabante, M. I. B., Diaz-De-Heredia, C., Bofarull, J. V., Mendoza-Palomar, N., et al. (2021). Voriconazole use in children: therapeutic drug monitoring and control of inflammation as key points for optimal treatment. J. Fungi 7, 17. doi:10.3390/jof7060456
Van Wanrooy, M. J. P., Span, L. F. R., Rodgers, M. G. G., Van Den Heuvel, E. R., Uges, D. R. A., Van Der Werf, T. S., et al. (2014). Inflammation is associated with voriconazole trough concentrations. Antimicrob. Agents Chemother. 58, 7098–7101. doi:10.1128/AAC.03820-14
Vreugdenhil, B., Van Der Velden, W., Feuth, T., Kox, M., Pickkers, P., Van De Veerdonk, F. L., et al. (2018). Moderate correlation between systemic IL-6 responses and CRP with trough concentrations of voriconazole. Br. J. Clin. Pharmacol. 84, 1980–1988. doi:10.1111/bcp.13627
Walsh, T. J., Anaissie, E. J., Denning, D. W., Herbrecht, R., Kontoyiannis, D. P., Marr, K. A., et al. (2008). Treatment of aspergillosis: clinical practice guidelines of the Infectious Diseases Society of America. Clin. Infect. Dis. 46, 327–360. doi:10.1086/525258
Wei, X., Zhao, M., Fu, P., and Xiao, X. (2019). Risk factors associated with insufficient and potentially toxic voriconazole plasma concentrations: an observational study. J. Chemother. 31, 401–407. doi:10.1080/1120009X.2019.1646974
Yan, M., Wu, Z. F., Tang, D., Wang, F., Xiao, Y. W., Xu, P., et al. (2018). The impact of proton pump inhibitors on the pharmacokinetics of voriconazole in vitro and in vivo. Biomed. Pharmacother. 108, 60–64. doi:10.1016/j.biopha.2018.08.121
Yang, H., Du, R., Xing, X., Li, Y., and Qiu, B. (2023). Efficacy and influencing factor analysis of Voriconazole in the treatment of invasive fungal infections. Diagnostic Microbiol. Infect. Dis. 107 (3), 116047. doi:10.1016/j.diagmicrobio.2023.116047
Ye, Q., Yu, X., Chen, W., Li, M., Gu, S., Huang, L., et al. (2022). Impact of extracorporeal membrane oxygenation on voriconazole plasma concentrations: a retrospective study. Front. Pharmacol. 13, 972585. doi:10.3389/fphar.2022.972585
Yi, W. M., Schoeppler, K. E., Jaeger, J., Mueller, S. W., Maclaren, R., Fish, D. N., et al. (2017). Voriconazole and posaconazole therapeutic drug monitoring: a retrospective study. Ann. Clin. Microbiol. Antimicrob. 16, 60. doi:10.1186/s12941-017-0235-8
Zeng, G., Wang, L., Shi, L., Li, H., Zhu, M., Luo, J., et al. (2020). Variability of voriconazole concentrations in patients with hematopoietic stem cell transplantation and hematological malignancies: influence of loading dose, procalcitonin, and pregnane X receptor polymorphisms. Eur. J. Clin. Pharmacol. 76, 515–523. doi:10.1007/s00228-020-02831-1
Zhang, Y., Wu, R., Liu, F., Wang, Y., Zhang, J., Ji, C., et al. (2023). Factors influencing blood concentration of voriconazole and therapeutic drug monitoring in patients with child-pugh class C cirrhosis. J. Clin. Pharm. Ther. 2023, 1–10. doi:10.1155/2023/4240869
Zhao, Y., Hou, J., Xiao, Y., Wang, F., Zhang, B., Zhang, M., et al. (2021a). Predictors of voriconazole trough concentrations in patients with child-pugh class c cirrhosis: a prospective study. Antibiotics 10 (9), 1542. doi:10.3390/antibiotics10091130
Zhao, Y., Xiao, C., Hou, J., Wu, J., Xiao, Y., Zhang, B., et al. (2021b). A large sample retrospective study on the distinction of voriconazole concentration in asian patients from different clinical departments. Pharmaceuticals 14 (12), 1239. doi:10.3390/ph14121239
Zhao, Y. C., Zou, Y., Hou, J. J., Xiao, C. L., Zhang, B. K., Li, J. K., et al. (2021). Factors affecting voriconazole trough concentration and optimal maintenance voriconazole dose in Chinese children. Antibiotics-Basel 10, 1542. doi:10.3390/antibiotics10121542
Keywords: voriconazole, drug monitoring, voriconazole plasma concentration, factors, meta-analysis
Citation: Li X, Hu Q and Xu T (2024) Associated factors with voriconazole plasma concentration: a systematic review and meta-analysis. Front. Pharmacol. 15:1368274. doi: 10.3389/fphar.2024.1368274
Received: 10 January 2024; Accepted: 12 August 2024;
Published: 23 August 2024.
Edited by:
Anick Bérard, Montreal University, CanadaReviewed by:
Irene García García, University Hospital La Paz, SpainKatarzyna Kosicka-Noworzyń, Poznan University of Medical Sciences, Poland
Copyright © 2024 Li, Hu and Xu. This is an open-access article distributed under the terms of the Creative Commons Attribution License (CC BY). The use, distribution or reproduction in other forums is permitted, provided the original author(s) and the copyright owner(s) are credited and that the original publication in this journal is cited, in accordance with accepted academic practice. No use, distribution or reproduction is permitted which does not comply with these terms.
*Correspondence: Ting Xu, dGluZ3gyMDA5QDE2My5jb20=
†These authors share first authorship