- 1Guangdong Provincial Key Laboratory of Veterinary Pharmaceutics Development and Safety Evaluation, College of Veterinary Medicine, South China Agricultural University, Guangzhou, China
- 2National Laboratory of Safety Evaluation (Environmental Assessment) of Veterinary Drugs, South China Agricultural University, Guangzhou, China
- 3National Risk Assessment Laboratory for Antimicrobial Resistance of Animal Original Bacteria, South China Agricultural University, Guangzhou, China
Background: The rapid spread of bacteria with plasmid-mediated resistance to antibiotics poses a serious threat to public health. The search for potential compounds that can increase the antibacterial activity of existing antibiotics is a promising strategy for addressing this issue.
Methods: Synergistic activity of the FDA-approved agent oxethazine combined with colistin was investigated in vitro using checkerboard assays and time-kill curves. The synergistic mechanisms of their combination of oxethazine and colistin was explored by fluorescent dye, scanning electron microscopy (SEM) and LC-MS/MS. The synergistic efficacy was evaluated in vivo by the Galleria mellonella and mouse sepsis models.
Results: In this study, we found that oxethazine could effectively enhance the antibacterial activity of colistin against both mcr-positive and -negative pathogens, and mechanistic assays revealed that oxethazine could improve the ability of colistin to destruct bacterial outer membrane and cytoplasmic membrane permeability. In addition, their combination triggered the accumulation of reactive oxygen species causing additional damage to the membrane structure resulting in cell death. Furthermore, oxethazine significantly enhanced the therapeutic efficacy of colistin in two animal models.
Conclusion: These results suggested that oxethazine, as a promising antibiotic adjuvant, can effectively enhance colistin activity, providing a potential strategy for treating multidrug-resistant bacteria.
1 Introduction
The discovery and clinical use of antibiotics was one of the highlights of the 20th century, which resulted in an average increase in human life expectancy of 23 years (Katz and Baltz, 2016; Hutchings et al., 2019). However, as a double-edged sword, antimicrobial resistance caused by overuse of antibiotics is growing more serious and the therapeutic alternatives are becoming more difficult due to multidrug-resistant (MDR) bacteria (McEwen and Collignon, 2018; Pariente and PLOS Biology Staff Editors, 2022). Furthermore, the World Health Organization (WHO) considers it to be one of the top 10 public health threats (Pariente and PLOS Biology Staff Editors, 2022), with MDR Gram-negative bacteria being of particular concern (Silvestri and van Saene, 2010). The permeability barrier of outer membrane (OM) of Gram-negative bacteria makes it difficult for the drugs to enter the cell and reach the site of action (Pagès et al., 2008; MacNair and Brown, 2020). Despite this, the majority of antibiotics currently in use to treat Gram-negative bacteria are from classes discovered before the 1970s (Lewis, 2013; Cook and Wright, 2022). Only two new antibiotics have been approved in the last 20 years, both against Gram-positive bacteria (MacNair and Brown, 2020). Despite the urgent need for new antibiotics to treat MDR Gram-negative infections, no new Gram-negative antibiotics have been identified since the quinolones (Lewis, 2020; MacNair and Brown, 2020). Therefore, developing new therapeutic strategies to tackle antibiotic resistance in Gram-negative bacteria is critical.
Combination therapies offer a promising strategy to overcome the antibiotic resistance crisis (Bailey et al., 2022). Combination therapy was discovered in the 1950s to combat single-drug resistance (Jayaraman et al., 2010; Kerantzas and Jacobs, 2017), and it has proven to be more effective than monotherapy (Bailey et al., 2022). Moreover, combination therapy is an effective strategy to reduce the time and cost of developing traditional antibiotics (Liu et al., 2021). Colistin has been used as a last resort against MDR Gram-negative pathogens (Paterson and Harris, 2016; Yin et al., 2021). The preventive use of colistin as a food additive led to an increase in colistin resistance rates in the livestock and poultry industry (Sun et al., 2020). The mcr-1 gene encodes phosphoethanolamine (pEtN) transferase that transfers pEtN to lipid A, resulting in colistin resistance (Liu et al., 2023). Notably, the antibacterial efficacy of colistin has been threatened by the emergence of the mobile drug resistance gene mcr-1 since 2015 (Liu et al., 2016; Yin et al., 2021). Therefore, it is vital to find colistin adjuvants for treating infections caused by colistin-resistant bacteria.
Oxethazaine is a stronger local anaesthetic than cocaine and procaine (Seifter et al., 1962). Due to the unique chemical structure (‘double-anesthetic’) (Figure 1A), oxethazine can maintain function in the low acid environment of pH 1 (Seifter et al., 1962; Bao et al., 2022). Therefore, oxethazaine is frequently used to treat the pain produced by chronic gastritis and duodenal ulcers (Bao et al., 2022). Besides, oxethazaine has also been shown to inhibit proliferation and migration of esophageal cancer cells (Bao et al., 2022). Moreover, oxethazaine has been confirmed to suppress chronic hepatitis B virus replication and capsid assembly (Zhang et al., 2016). However, oxethazaine has rarely been reported in treatment of pathogenic bacteria. Herein, we found that oxethazaine enhanced the activity of colistin against MDR Gram-negative pathogens through various modes of action. This study provides a therapeutic strategy for combating MDR bacteria.
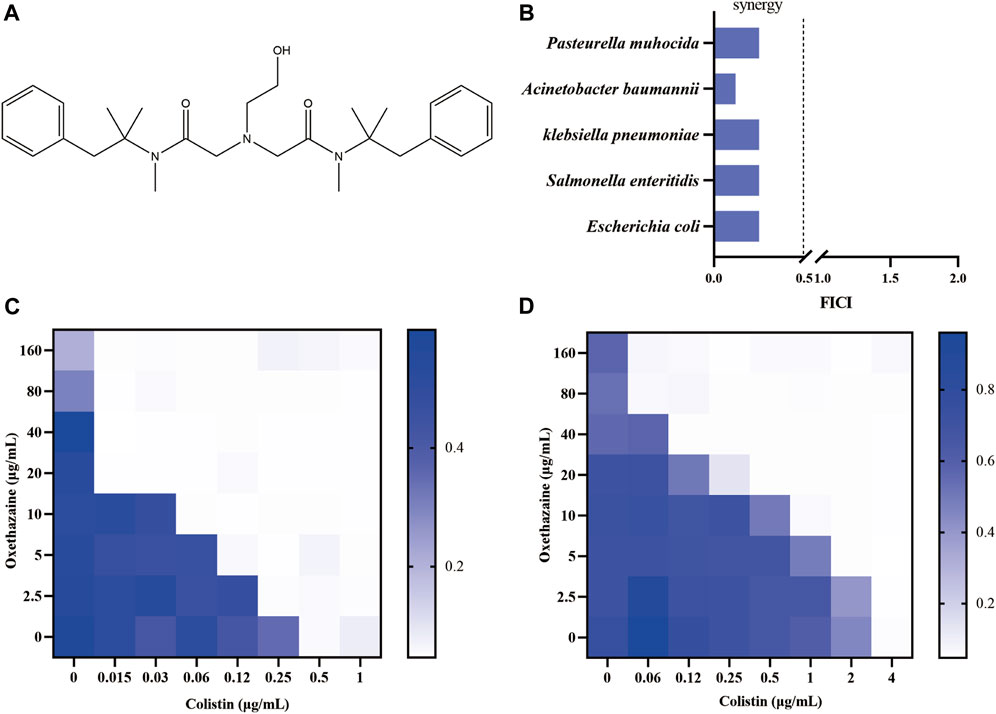
Figure 1. Oxethazaine increased colistin activity against both colistin-resistant and -sensitive E. coli. (A) Chemical structure of oxethazaine. (B) FIC index of oxethazaine combined with colistin against diverse Gram-negative bacteria. Synergy is defined as FICI ≤0.5. (C) and (D) Chequerboard broth microdilution assays between oxethazaine and colistin against E. coli ATCC 25922 and SHP45, respectively. The results of the checkerboard broth microdilution assay were measured by absorbance at 600 nm. Data represent the average of three biological replicates.
2 Methods
2.1 Cell culture and drugs
African green monkey kidney cells (Vero) were purchased from the American Type Culture Collection (ATCC) and cultured in Dulbecco’s modified Eagle’s medium (Gibco) supplemented with 10% heat-inactivated FBS (Invitrogen), 1% penicillin–streptomycin and 1% sodium pyruvate (Sigma-Aldrich) at 37°C in a 5% CO2 atmosphere. Oxethazaine (CAS no. 126-27-2) and colistin sulfate salt (CAS no. 1264-72-8) were purchased from GlpBio and Sigma-Aldrich, respectively.
2.2 Strains
The standard strains for this study were purchased from the China General Microbiological Culture Collection Center (CGMCC), Escherichia coli SHP45 and other clinical strains were isolated and preserved by our laboratory. MG1655-ΔwaaC was previously constructed in our laboratory (Li et al., 2023).
2.3 Antimicrobial susceptibility test
The clinical and laboratory standards institute (CLSI 2021) guideline was used to determine the minimum inhibitory concentration (MIC) of oxethazaine and other antibiotics (CLSI, 2021). The combined effect of oxethazaine with other antibiotics was determined using the checkerboard method. Briefly, the single colonies were cultured in Mueller–Hinton broth (Qingdao Haibo Biotechnology Co., Ltd.) and then diluted to 106 CFU/mL. Antibiotics were serial diluted 2-fold in Mueller–Hinton broth, and an equal volume of bacterial suspension (106 CFU/mL) was added in a 96-well microtiter. The MIC values were defined as the lowest antibiotic concentrations with no visible bacterial growth after incubation at 37°C for 18 h. The FICI value is calculated from the MIC value of the drug alone. The specific calculation method of the FICI is as follows:
MICa is the MIC of A alone, MICab is the MIC of compound A in combination with compound B, MICb is the MIC of compound B alone, and MICba is the MIC of compound B in combination with compound A. FICI ≤0.5 was deemed as synergistic (Song et al., 2020).
2.4 Safety assay
Sterile sheep red blood cells (RBC) were used to evaluate hemolytic activity as previously described with slight modifications (Liu et al., 2020). In brief, RBC were washed with PBS for three times, then 8% suspension of RBC was treated with colistin (0.12, 0.25, 0.5, 1, 4, 8 and 16 μg/mL) with or without oxethazaine (20 μg/mL). At the same time, PBS and Triton X-100 were used as the negative and positive control, respectively. After 1 h incubation at 37°C, the cells were centrifuged at 3,500 g for 8 min, and the absorbance of the supernatant was determined at 576 nm. The haemolysis rate was calculated according to the absorbance of positive control and the blank control. All experiments were carried out at least twice.
The cytotoxicity of Vero cells was detected by cell counting kit-8 (CCK-8) assay (Solarbio life sciences) based on previous report (Zhang et al., 2023). The cells were plated in a 96-well plate at a density of 3 × 104 cells per well and treated with colistin (0.5, 1, 2, 4, 16, 32, 64 and 16 μg/mL) or the combination of colistin (0.5, 1, 2, 4, 16, 32, 64 and 16 μg/mL) and oxethazaine (20 μg/mL). After cultured 4 h at 37°C and 5% CO2, 10% CCK-8 solution was added to 100 μL of medium in each well and the culture maintained for an additional 2 h, cells were analyzed with absorbance at 450 nm.
2.5 Time-dependent killing assay
An overnight culture of E. coli ATCC 25922 and E coli SHP45 was diluted 1:1000 in fresh LB broth (Qingdao Haibo Biotechnology Co., Ltd.), and 2 mL bacterial suspension was placed in the 10 mL centrifuge tube. Then the bacteria were treated with 0.5×MIC of colistin, oxethazaine (20 μg/mL) or the combination of colistin and oxethazaine. After incubated at 37°C, 180 rpm for 0, 2, 4, 8, 12, 16, 20 and 24 h, 100 μL of bacterial suspension were collected and ten-fold serially diluted in PBS, and the suspensions were plated on LBA plates. After overnight cultivation at 37°C, bacterial colonies were calculated. Experiments were performed in biological triplicate.
2.6 Microscopic observation of LIVE/DEAD staining
A LIVE/DEAD BacLight bacterial viability kit was used to evaluate the synergistic antimicrobial effect of colistin and oxethazaine. An overnight culture of E. coli ATCC 25922 was adjusted to OD600 of 0.5 and incubated with colistin, oxethazaine or their combination. After 30 min, the bacterial cells were washed with PBS and LIVE/DEAD dye was added. The images of E. coli were observed using a confocal fluorescence microscope (Leica, Germany). In addition, the dead cells were observed at excitation wavelength 535 nm and emission wavelength 615 nm with an EnSight multimode plate reader.
2.7 Outer membrane integrity assay
The integrity of the OM was evaluated using NPN fluorescent dye (Aladdin, China) (Liu et al., 2020; Song et al., 2020). After incubation overnight at 37°C, the E. coli ATCC 25922 were washed three times with 5 mM HEPES (5 mM glucose). After 20 min incubation with the NPN dye, the colistin alone, oxethazaine alone, or their combination was added to the bacterial suspensions. After treatment in the dark at 37°C for 30 min, the fluorescence intensity was determined with the excitation wavelength of 350 nm and the emission wavelength of 420 nm with an EnSight multimode plate reader.
2.8 Cytoplasmic membrane permeability assay
Propidium iodide PI (Beyotime, China) was used to evaluated the cytoplasmic membrane permeability (Liu et al., 2020). Briefly, overnight cultures of E. coli ATCC 25922 were washed three times with PBS and resuspended with PBS to approximately an OD600 nm of 0.5. The bacterial suspensions were cultured with propidium iodide PI at 37°C for 20 min, followed by the addition of colistin (0, 1, 2 and 4 μg/mL) alone or combined with 20 μg/mL oxethazaine. After incubation for 30 min at 37°C, fluorescence intensity was monitored using the EnSight® Multimode Plate reader at an excitation wavelength of 535 nm and an emission wavelength of 615 nm. Experiments were performed in biological triplicate.
2.9 Cytoplasmic membrane potential
The fluorescent probe DiSC3 (Silvestri and van Saene, 2010) (Aladdin, China) was used to analyze the membrane potential of E. coli ATCC 25922 (Song et al., 2021). In brief, bacteria were grown on LB broth to reach the exponential phase, then washed twice with PBS and adjusted to OD600 of 0.5. After incubation of the bacterial suspension with DiSC3 (Silvestri and van Saene, 2010) (0.5 µM) for 20 min, varying concentrations of colistin (0, 1, 2 and 4 μg/mL) with or without oxethazaine were added, and the fluorescence intensity was determined with the excitation wavelength at 622 nm and emission wavelength at 670 nm with an EnSight multimode plate reader. All tests included biological replicates.
2.10 ROS measurement
The levels of ROS in E. coli ATCC 25922 were measured using the fluorescence probe 2′,7′-dichlorodihydro-fluorescein diacetate (DCFH-DA) (Beyotime, China) with the excitation wavelength at 488 nm and the emission wavelength at 525 nm (Song et al., 2020). In brief, the bacteria were resuspended in PBS to an OD600 of 0.5, then the DCFH-DA probe was added to a final concentration of 10 µM. After the bacteria were incubated at 37°C for 20 min, different concentrations of colistin (0, 1, 2 and 4 μg/mL) in the presence or absence of 20 μg/mL oxethazaine was added and the fluorescence intensity was detected.
2.11 Scanning electron microscopy (SEM)
The bacterial morphology was observed by the SEM. Briefly, E. coli ATCC 25922 in mid-log phase was washed three times with PBS, and resuspended to 107 CFU. Colistin (0.5×MIC), oxethazaine (20 μg/mL) or their combination (0.5×MIC colistin+20 μg/mL oxethazaine) were added and incubated at 37°C for 4 h. Then samples were washed with PBS and fixed overnight in 2.5% glutaraldehyde solution at 4°C. The bacteria were dehydrated with different gradients of ethanol, and the bacterial morphology was observed by the SEM (Hitachi, Japan) after vacuum drying.
2.12 LC–MS/MS analysis
The LC–MS/MS assay was prepared according to described earlier, with minor modifications (Lee et al., 2013). A C18 column (5 μm, 150 mm × 2.0 mm I.D.) was used for chromatography and maintained at 40°C. After the bacteria were treated with 20 μg/mL oxethazaine alone or combined with 1 μg/mL colistin, the accumulation of oxethazaine in E. coli was performed on the LCMS-8050 system (Shimadzu, Kyoto, Japan).
2.13 Galleria mellonella infection model
The synergistic antibacterial effect of oxethazaine and colistin was evaluated in the Galleria mellonella larvae infection model. The larvae of G. mellonella were randomly divided into four groups (n = 10 per group), and injected with 10 μL of 9.8 × 104 CFUs E coli SHP45 suspension. After 1 h, G. mellonella were subjected to antibiotic treatment, including 20 mg/kg oxethazaine, 2 mg/kg colistin or the combination of 20 mg/kg oxethazaine and 2 mg/kg colistin. At the same time, PBS was used as the control group. Survival of G. mellonella in each group was observed.
2.14 Mouse sepsis infection model
6–8 weeks old female BALB/C mice (20 ± 2 g) were used for mouse peritonitis-sepsis model. Mice randomly assigned into five groups (n = 8 per group), mice were acclimated for 7 days before infection in the Laboratory Animal Center of South China Agricultural University. Then mice were infected with 200 μL of 1.8 × 108 CFU E coli SHP45 suspension via intraperitoneal injection. After 1 h post infection, mice were treated with PBS, colistin (2 mg/kg), oxethazaine (20 mg/kg), or the combination of 2 mg/kg colistin with 20 mg/kg oxethazaine. The number of survival mice in each group was observed. Once the infected mice died, the liver, spleen, and kidney were removed and analyzed bacterial loading and subjected to histological analysis. After 5 days of experimentation, the remaining animals were euthanized by cervical dislocation according to animal welfare related guidelines. The organs were collected under aseptic conditions, one part of the organs was used for homogenization and other for histological analysis (Valiante et al., 2015; Xie et al., 2020).
2.15 Statistical analysis
GraphPad Prism 9.0 software was used for statistical analyses. All data are expressed as a mean ± standard deviation (SD). p-values were calculated by one-way ANOVA among multiple groups or t-test between two groups. *p < 0.05, **p < 0.01.
3 Results
3.1 Synergy of oxethazaine with colistin against gram-negative pathogens
Firstly, the potential efficacy of oxethazaine and existing antibiotics was evaluated using checkerboard dilution assays. Interestingly, we observed synergistic activity between oxethazaine and colistin against both colistin-resistant E. coli SHP45 and colistin-sensitive ATCC 25922, whereas oxethazaine alone had no antibacterial activity (Figures 1C,D). To evaluate whether the synergistic antibacterial activity was specific, six species of Gram-negative bacteria were used to determine the synergistic effect of oxethazine and colistin. As expected, oxethazaine combined with colistin showed a strong synergistic effect against Gram-negative bacteria (Figure 1B, Supplementary Table S1), suggesting that oxethazaine was a potent antibiotic adjuvant to restore the antibacterial activity of colistin.
3.2 Oxethazaine potentiates the activity of colistin in vitro without obvious toxicity
To further confirm the synergistic antibacterial activity of oxethazaine and colistin, the time-killing experiments were performed on mcr-positive or -negative E. coli. We found that either 20 μg/mL oxethazaine alone or 0.5×MIC colistin alone showed little bactericidal activity, whereas the combination of oxethazaine and colistin rapidly reduced the number of bacteria with more than 2log10 CFU (Figures 2A,B). Furthermore, the synergistic effect was also confirmed by LIVE/DEAD staining, which stains dead bacteria red and viable bacteria green. Compared with colistin or oxethazaine alone, the proportion of dead bacteria increased in the combination group (0.5×MIC colistin+20 μg/mL oxethazaine) (Figures 2C,D).
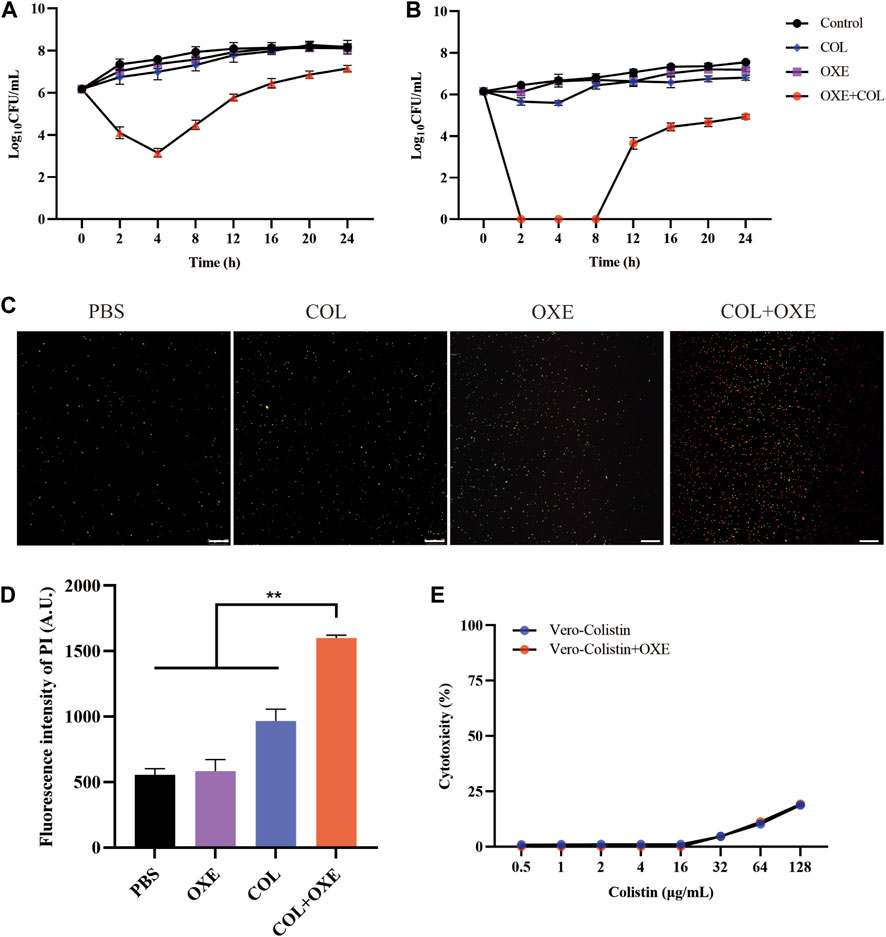
Figure 2. Synergistic antibacterial activity of colistin combined with oxethazaine in vitro. Time–kill assays of mcr-positive E. coli SHP45 (A) and mcr-negative E. coli ATCC 25922 (B) was conducted with 0.5×MIC colistin alone, 20 μg/mL oxethazaine alone or their combination (0.5×MIC colistin+20 μg/mL oxethazaine). The number of bacteria per milliliter was measured at different time points within 24 h. (C) Fluorescent images of stained bacteria were observed with a confocal fluorescence microscope. E. coli ATCC 25922 was treated with 0.5×MIC colistin, 20 μg/mL oxethazaine or the combination of colistin and oxethazaine (0.5×MIC colistin+20 μg/mL oxethazaine). Scar bar, 25 µm. (D) The proportion of dead cells was represented by the fluorescence value. The fluorescence intensity was determined with the excitation wavelength of 535 nm and the emission wavelength of 615 nm. (E) Cytotoxicity of Vero cells was evaluated by CCK-8. Data are expressed as the mean ± SD of three independent experiments. COL, colistin alone; OXE, oxethazaine alone; COL + OXE, the combination of colistin and oxethazaine.
Toxicity is one of the key factors limiting the clinical application of combination therapy. Therefore, we subsequently evaluated whether the addition of oxethazaine would increase toxicity. Surprisingly, we found no significant increase in hemolytic activity of Sterile sheep red blood cells (RBC) after treatment with the combination of colistin and oxethazaine (Supplementary Figure S1). Moreover, Vero cells were also used to evaluate the cytotoxicity of colistin with or without oxethazaine. Compared to colistin alone, no increased cytotoxicity was observed in the Vero cells after exogenous addition of oxethazaine (Figure 2E). These results indicated that oxethazaine exhibited a robust potentiating effect on the colistin activity without increased toxicity.
3.3 Oxethazaine plays a key role in the synergetic effect with colistin
Bacterial structural morphology is critical for bacterial viability (Srivastava et al., 2020), thus the morphological changes was evaluated by scanning electron microscopy (SEM) analysis. After treatment with either oxethazaine or colistin alone, a slight morphological change was observed. In comparison, the cell surface appeared strongly sunken and shrinkage after treated with the oxethazaine combined with colistin (Figure 3A). Therefore, we hypothesized that the combination might have an effect on the bacterial membranes. To confirm this hypothesis, we first evaluated the effect on the OM using the fluorescent dye N-phenyl-1-naphthylamine (NPN) (Song et al., 2020). As expected, the fluorescence value of colistin was significantly increased in the presence of oxethazaine (p < 0.05), whereas only subtle changes in fluorescence intensity was detected after treated with oxethazaine alone (Figure 3B) (p > 0.05). In addition, oxethazaine restored antibacterial activity against LPS-deleted strain MG1655-ΔwaaC (Table 1), indicating that the OM acts as a permeability barrier to prevent oxethazaine from entering the cell. Thus, the intracellular accumulation of agents was detected by LC-MS/MS (Figure 4C, Supplementary Table S2), and we found that colistin significantly promoted the accumulation of oxethazaine (Figure 4A) (p < 0.05). Moreover, cytoplasmic membrane (CM) integrity and membrane potential was significantly dissipated in the combination group (Figures 3C,D) (p < 0.05).
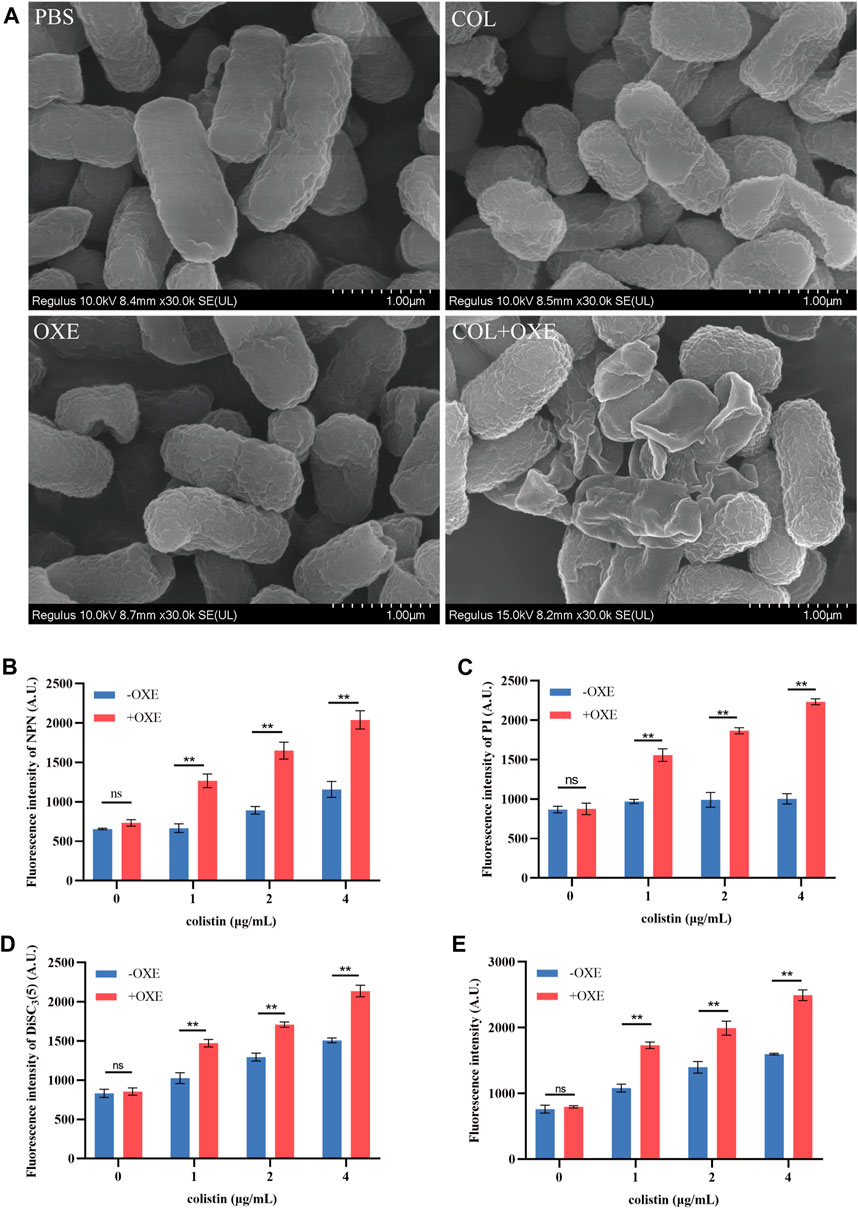
Figure 3. Oxethazaine potentiated the activity of colistin against E. coli ATCC 25922. (A) Bacterial morphology of E. coli ATCC 25922 after exposure to colistin alone, oxethazaine alone or their combination by SEM analysis. Scar bar, 1 µm. (B) The outer membrane permeability was measured by the fluorescence intensity of 1-N-phenylnaphthylamine (NPN) after treated with the different concentrations of colistin alone or combined with 20 μg/mL oxethazaine. Fluorescence was measured with the excitation/emission wavelength at 350 nm/420 nm. (C) The cytoplasmic membrane permeability was evaluated under the different concentrations of colistin with or without 20 μg/mL oxethazaine. Fluorescence dye propidium iodide (PI) was used to determined the permeability of cytoplasmic membrane. (D) The membrane potential of colistin with or without 20 μg/mL oxethazaine was evaluated by the fluorescence dye DiSC3(5). (E) The effect of colistin on the ROS accumulation in E. coli in the absence or presence of 20 μg/mL oxethazaine. All data are presented as mean ± SD and the significances were determined by nonparametric one-way ANOVA (*p < 0.05, **p < 0.01). OXE, oxethazaine.
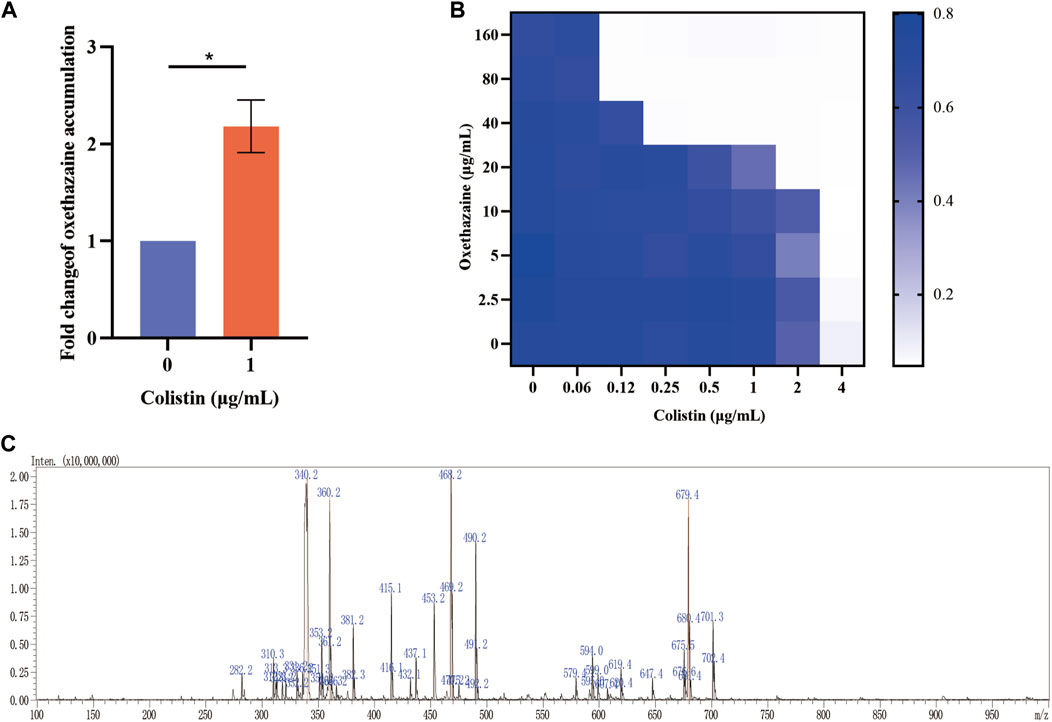
Figure 4. Oxethazaine plays a key role in the synergetic effect with colistin. (A) The accumulation of oxethazaine was conducted in the presence or absence of colistin. (B) Effects of NAC (6 mmol/L) on the synergistic effect of oxethazaine against Gram-negetive bacteria. (C) The MS spectrum of oxethazaine in positive ion mode. All date were presented as mean ± SD. p values were calculated with an unpaired, two-tailed Student’s t-test. *p < 0.05.
The integrity of the membrane structure is critical for several bacterial biosynthetic processes (Wang et al., 2022). Notably, we observed that the accumulation of bacterial reactive oxygen species (ROS) was enhanced in a dose-dependent manner after the addition of oxethazaine (Figure 3E). Moreover, exogenous addition of N-acetylcysteine (NAC, 6 mmol/L) can reduce the synergistic effect of oxethazaine combined with colistin (Figure 4B), revealing that ROS play a critical role in their synergistic process. These results indicated that oxethazaine enhanced the antibacterial activity of colistin by multiple modes of action (Figure 5).
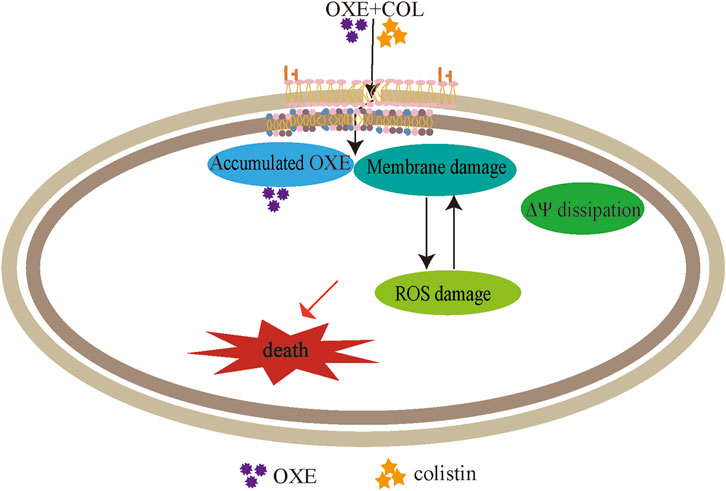
Figure 5. The synergistic mechanism of oxethazaine combined with colistin against MDR pathogens. Oxethazine improves the ability of colistin to destruct bacterial outer membrane and cytoplasmic membrane permeability. At the moment, the combination of colistin and oxethazaine dissipates the membrane potential of cytoplasmic membrane and promotes the accumulation of oxethazaine. In addition, their combination triggers the accumulation of ROS, causing additional damage to the membrane structure resulting in cell death. OXE, oxethazaine.
3.4 The efficacy of the combination in vivo
Given the strong potentiation of oxethazaine on colistin in vitro, we further assessed whether their combination had potential efficacy in vivo infected with E. coli SHP45 using the G. mellonella model and the mouse peritonitis/sepsis model. As expected, the survival rate of infected larvae was increased after treatment with the combination of oxethazaine and colistin (20 mg/kg+2 mg/kg), whereas the monotreatment only displayed a slight therapeutic effect (Figure 6A). Accordingly, the significant therapeutic efficacy was also confirmed in the mouse model. 75.0% of the mice were protected in the therapy of 20 mg/kg oxethazaine combined with 2 mg/kg colistin. In contrast, all mice in the PBS and oxethazaine monotherapy groups died within 3 days (Figure 6B). Besides, the pathological changes in each organ were alleviated accordingly (Figure 6C). Moreover, compared with the monotherapy groups, combinational therapy significantly reduced the bacterial burden in various organs of mice (Supplementary Figure S2). These findings revealed that oxethazaine effectively rescued colistin activity to combat the MDR E. coli in vivo.
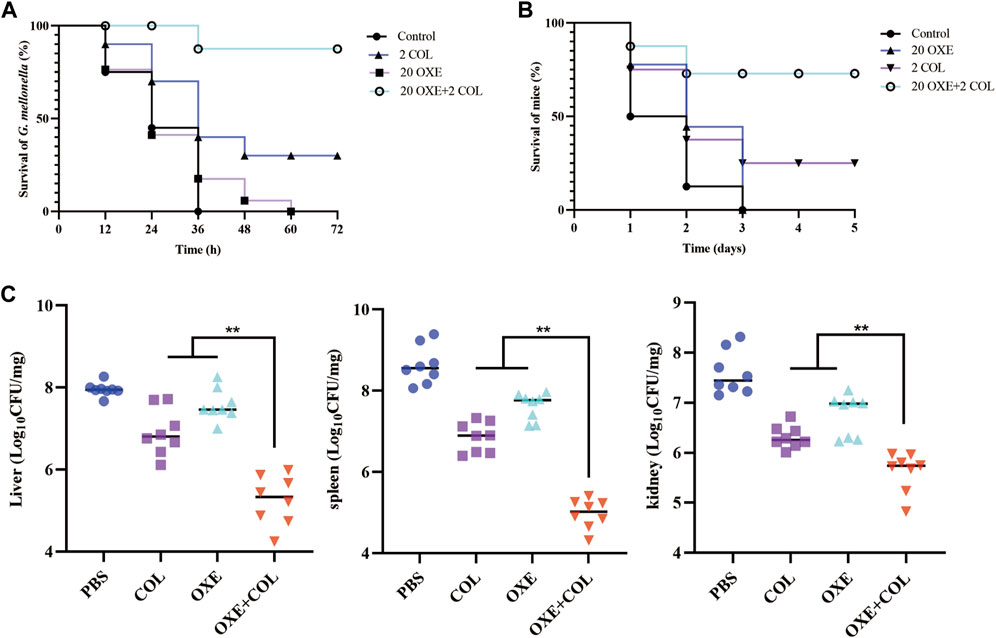
Figure 6. Oxethazaine rescued the therapeutic effect of colistin in animal infection models. (A) Survival rates of G. mellonella larva (n = 10 per group) treated with 20 mg/kg oxethazaine alone, 2 mg/kg colistin alone or their combination (20 mg/kg oxethazaine +2 mg/kg colistin). (B) Survival rates of peritonitis-sepsis mice (n = 8 per group) infected with 1.8 × 108 CFU E. coli SHP45. The combination therapy of oxethazaine and colistin (20 mg/kg+2 mg/kg) improved the Survival rates of mice. (C) Bacterial loads in different organs (liver, spleen and kidney) were counted in mouse infection model. p values were calculated by nonparametric one-way ANOVA (*p < 0.05, **p < 0.01). COL, 2 mg/kg colistin alone; OXE, 20 mg/kg oxethazaine alone; COL + OXE, the combination of 2 mg/kg colistin and 20 mg/kg oxethazaine.
4 Discussion
The dissemination of resistant pathogens far outpaces development rate of new antibiotics. Furthermore, there are few antibiotics against Gram-negative bacteria in the drug discovery pipeline (Li et al., 2014). Therefore, there is an urgent need to develop antibiotic adjuvant strategies to substantially fill the vacancy of new antibiotics. Antimicrobial adjuvants not only extend the life of existing antibiotics but also broaden the antimicrobial spectrum (Allen and Brown, 2019; Liu et al., 2021). The classic example is the antibiotic adjuvant β–lactamase inhibitor clavulanic acid, which has a potentiating effect on β-lactam antibiotic therapy (Worthington and Melander, 2013). Given the global prevalence of plasmid-mediated acquired colistin resistance (Wang et al., 2018), the discovery of effective colistin adjuvants is critical.
Currently, a variety of colistin adjuvants have been discovered, including plant-derived (Wang et al., 2018; Song et al., 2021), chemically synthesized (Kathayat et al., 2020; Li et al., 2023), and FDA-approved (Stokes et al., 2017; Torres et al., 2018). Among these, the discovery of new uses for FDA approved old drugs is cheaper and faster than the discovery of new drugs from scratch (Boucher et al., 2013; Farha and Brown, 2019). Herein, we screened drugs that had a synergistic effect when combined with colistin from FDA-approved drugs, providing multiple strategies for the antibiotic selection pipeline. Fortunately, we found that oxethazaine, as a potential antibiotic adjuvant, could effectively restore the antibacterial activity of colistin against MDR E. coli. The time-killing assay confirmed further that the colistin combined with oxethazaine inhibited bacterial growth within 8 h compared with colistin alone. However, the observed bacterial regrowth was observed at later stages may be due to the half-life of colistin is 4 h (Bergen et al., 2010). In addition, the combination of oxethazaine and colistin showed a synergistic effect against all strains tested, which is consistent with previous report (Hind et al., 2019). However, the specific synergistic antibacterial effect in the combination of colistin and oxethazaine may be related to difference in the strains.
In the clinic, toxicity is an important evaluation index of drugs. Clinical therapy of colistin has historically been limited due to severe side effects (Spapen et al., 2011; Nang et al., 2021). As we all know, colistin was first discovered in the 1940s (Lim et al., 2010), but it was replaced by other antibiotics in the 1970s due to its nephrotoxicity (Wolinsky and Hines, 1962; Osei Sekyere et al., 2020). The most important significance of the discovery of colistin adjuvants is that they can increase the antibacterial activity of colistin, reducing the clinical dosage of colistin to be used in clinical treatment, thereby greatly reducing its toxicity and side effects. Notably, oxethazaine, an over-the-counter, has wide safety margins when administered orally, subcutaneously, intramuscularly, and rectally (Prado et al., 2017; Bao et al., 2022). When combined with colistin, oxethazaine had a negligible effect on colistin toxicity in vitro.
Bacterial membranes are a valuable target for bacterial eradication, especially persistent bacteria (Cui et al., 2016). Interestingly, we found that oxethazaine could increase the membrane damage of colistin (Figure 3B), which is similar to that of equisetin to enhance the activity of colistin (Zhang et al., 2021). Endogenous ROS are crucial for bactericidal agents and increased ROS can make bacteria more susceptible to antibiotics (Brynildsen et al., 2013; Van Acker and Coenye, 2017). Furthermore, the accumulation of ROS can aggravate membrane damage and further promote bacterial death (Rowe-Magnus et al., 2019). High levels of ROS can induce oxidative stress, resulting in the damage to lipids, DNA and cytoplasmic proteins (Yuan et al., 2014; Rowe-Magnus et al., 2019). More importantly, the accumulation of ROS further exacerbated membrane damage leading to bacterial death. The permeability barrier of the OM is an important reason why many drugs are ineffective against Gram-negative bacteria (Nikaido, 2003; May and Grabowicz, 2018; Theuretzbacher et al., 2020). Oxethazaine alone has no antibacterial activity against E. coli, but it has synergistic antibacterial activity against LPS deletion strains MG1655-ΔwaaC, indicating that OM might be a potential barrier preventing oxethazaine from reaching intracellular targets. Notably, this combination therapy remains effective in strains that are resistant to colistin due to chromosomal mutations (Lima et al., 2017; MacNair et al., 2018). Nevertheless, more in-depth synergistic mechanisms need to be studied in vitro. More importantly, the efficacy of combination therapy in vivo is crucial. Considering the high safety of oxethazaine (Prado et al., 2017; Bao et al., 2022), we believe it is an attractive lead compound as an adjuvant to colistin. However, more animal experiments should be carried out for clinical application in the future.
5 Conclusion
In this study, we found that FDA-approved oxethazaine restored the antibacterial activity of colistin against Gram-negative bacteria through multiple strategies, including enhancing the ability of colistin to disrupt OM and CM, promoting intracellular ROS accumulation and intracellular oxethazaine accumulation to exert antibacterial activity. Further, synergistic therapy of oxethazaine combined with colistin protected mice from mcr-positive E. coli bacterial infection in vivo. Oxethazaine is a potential candidate antimicrobial adjuvant candidate for colistin, providing an alternative strategy for the treatment of infections caused by MDR Gram-negative pathogens.
Data availability statement
The original contributions presented in the study are included in the article/Supplementary Material, further inquiries can be directed to the corresponding author.
Ethics statement
The animal study was approved by All experimental procedures were conducted in accordance with the South China Agricultural University (SCAU) Institutional Animal Welfare and Ethics guidelines. The study was conducted in accordance with the local legislation and institutional requirements.
Author contributions
JL: Data curation, Methodology, Writing–original draft. NH: Data curation, Formal Analysis, Writing–original draft. YL: Formal Analysis, Writing–original draft. FZ: Investigation, Writing–original draft. WX: Validation, Writing–review and editing. ZZ: Supervision, Writing–review and editing.
Funding
The author(s) declare financial support was received for the research, authorship, and/or publication of this article. This study was supported by the National Natural Science Foundation of China (Grant No. 32273057).
Conflict of interest
The authors declare that the research was conducted in the absence of any commercial or financial relationships that could be construed as a potential conflict of interest.
Publisher’s note
All claims expressed in this article are solely those of the authors and do not necessarily represent those of their affiliated organizations, or those of the publisher, the editors and the reviewers. Any product that may be evaluated in this article, or claim that may be made by its manufacturer, is not guaranteed or endorsed by the publisher.
Supplementary material
The Supplementary Material for this article can be found online at: https://www.frontiersin.org/articles/10.3389/fphar.2024.1363441/full#supplementary-material
References
Allen, R. C., and Brown, S. P. (2019). Modified antibiotic adjuvant ratios can slow and steer the evolution of resistance: Co-amoxiclav as a case study. MBio 10, e01831-19. doi:10.1128/mBio.01831-19
Bailey, J., Gallagher, L., Barker, W. T., Hubble, V. B., Gasper, J., Melander, C., et al. (2022). Genetic dissection of antibiotic adjuvant activity. MBio 13, e0308421. doi:10.1128/mbio.03084-21
Bao, Z., Li, A., Lu, X., Wang, Z., Yu, Y., Wu, W., et al. (2022). Oxethazaine inhibits esophageal squamous cell carcinoma proliferation and metastasis by targeting aurora kinase A. Cell Death Dis. 13, 189. doi:10.1038/s41419-022-04642-x
Bergen, P. J., Bulitta, J. B., Forrest, A., Tsuji, B. T., Li, J., and Nation, R. L. (2010). Pharmacokinetic/pharmacodynamic investigation of colistin against Pseudomonas aeruginosa using an in vitro model. Antimicrob. Agents Chemother. 54, 3783–3789. doi:10.1128/AAC.00903-09
Boucher, H. W., Talbot, G. H., Benjamin, D. K., Bradley, J., Guidos, R. J., Jones, R. N., et al. (2013). 10 x '20 Progress--development of new drugs active against gram-negative bacilli: an update from the Infectious Diseases Society of America. Clin. Infect. Dis. 56, 1685–1694. doi:10.1093/cid/cit152
Brynildsen, M. P., Winkler, J. A., Spina, C. S., MacDonald, I. C., and Collins, J. J. (2013). Potentiating antibacterial activity by predictably enhancing endogenous microbial ROS production. Nat. Biotechnol. 31, 160–165. doi:10.1038/nbt.2458
CLSI (2021). Performance standards for antimicrobial susceptibility testing. 31th ed. United States: Clinical and Laboratory Standards Institute.
Cook, M. A., and Wright, G. D. (2022). The past, present, and future of antibiotics. Sci. Transl. Med. 14, eabo7793. doi:10.1126/scitranslmed.abo7793
Cui, P., Niu, H., Shi, W., Zhang, S., Zhang, H., Margolick, J., et al. (2016). Disruption of membrane by colistin kills uropathogenic Escherichia coli persisters and enhances killing of other antibiotics. Antimicrob. Agents Chemother. 60, 6867–6871. doi:10.1128/AAC.01481-16
Farha, M. A., and Brown, E. D. (2019). Drug repurposing for antimicrobial discovery. Nat. Microbiol. 4, 565–577. doi:10.1038/s41564-019-0357-1
Hind, C. K., Dowson, C. G., Sutton, J. M., Jackson, T., Clifford, M., Garner, R. C., et al. (2019). Evaluation of a library of FDA-approved drugs for their ability to potentiate antibiotics against multidrug-resistant gram-negative pathogens. Antimicrob. Agents Chemother. 63, e00769-19. doi:10.1128/AAC.00769-19
Hutchings, M. I., Truman, A. W., and Wilkinson, B. (2019). Antibiotics: past, present and future. Curr. Opin. Microbiol. 51, 72–80. doi:10.1016/j.mib.2019.10.008
Jayaraman, P., Sakharkar, M. K., Lim, C. S., Tang, T. H., and Sakharkar, K. R. (2010). Activity and interactions of antibiotic and phytochemical combinations against Pseudomonas aeruginosa in vitro. Int. J. Biol. Sci. 6, 556–568. doi:10.7150/ijbs.6.556
Kathayat, D., Antony, L., Deblais, L., Helmy, Y. A., Scaria, J., and Rajashekara, G. (2020). Small molecule adjuvants potentiate colistin activity and attenuate resistance development in Escherichia coli by affecting pmrAB system. Infect. Drug Resist. 13, 2205–2222. doi:10.2147/IDR.S260766
Katz, L., and Baltz, R. H. (2016). Natural product discovery: past, present, and future. J. Industrial Microbiol. Biotechnol. 43, 155–176. doi:10.1007/s10295-015-1723-5
Kerantzas, C. A., and Jacobs, W. R. (2017). Origins of combination therapy for tuberculosis: lessons for future antimicrobial development and application. MBio 8, e01586-16. doi:10.1128/mBio.01586-16
Lee, S.-Y., You, I.-J., Kim, M.-J., Kwon, S.-H., Hong, S.-I., Kim, J.-H., et al. (2013). The abuse potential of oxethazaine: effects of oxethazaine on drug-seeking behavior and analysis of its metabolites in plasma and hair in animal models. Pharmacol. Biochem. Behav. 105, 98–104. doi:10.1016/j.pbb.2013.01.022
Lewis, K. (2013). Platforms for antibiotic discovery. Nat. Rev. Drug Discov. 12, 371–387. doi:10.1038/nrd3975
Lewis, K. (2020). The science of antibiotic discovery. Cell 181, 29–45. doi:10.1016/j.cell.2020.02.056
Li, J., Zhang, X., Han, N., Wan, P., Zhao, F., Xu, T., et al. (2023). Mechanism of action of isopropoxy benzene guanidine against multidrug-resistant pathogens. Microbiol. Spectr. 11, e0346922. doi:10.1128/spectrum.03469-22
Li, Y., Li, P.-K., Roberts, M. J., Arend, R. C., Samant, R. S., and Buchsbaum, D. J. (2014). Multi-targeted therapy of cancer by niclosamide: a new application for an old drug. Cancer Lett. 349, 8–14. doi:10.1016/j.canlet.2014.04.003
Lim, L. M., Ly, N., Anderson, D., Yang, J. C., Macander, L., Jarkowski, A., et al. (2010). Resurgence of colistin: a review of resistance, toxicity, pharmacodynamics, and dosing. Pharmacotherapy 30, 1279–1291. doi:10.1592/phco.30.12.1279
Lima, T. B., Silva, O. N., de Almeida, K. C., Ribeiro, S. M., Motta, D. O., Maria-Neto, S., et al. (2017). Antibiotic combinations for controlling colistin-resistant Enterobacter cloacae. J. Antibiotics 70, 122–129. doi:10.1038/ja.2016.77
Liu, J.-H., Liu, Y.-Y., Shen, Y.-B., Yang, J., Walsh, T. R., Wang, Y., et al. (2023). Plasmid-mediated colistin-resistance genes: mcr. Trends Microbiol. doi:10.1016/j.tim.2023.10.006
Liu, Y., Jia, Y., Yang, K., Li, R., Xiao, X., Zhu, K., et al. (2020). Metformin restores tetracyclines susceptibility against multidrug resistant bacteria. Adv. Sci. (Weinheim, Baden-Wurttemberg, Ger.) 7, 1902227. doi:10.1002/advs.201902227
Liu, Y., Tong, Z., Shi, J., Li, R., Upton, M., and Wang, Z. (2021). Drug repurposing for next-generation combination therapies against multidrug-resistant bacteria. Theranostics 11, 4910–4928. doi:10.7150/thno.56205
Liu, Y.-Y., Wang, Y., Walsh, T. R., Yi, L.-X., Zhang, R., Spencer, J., et al. (2016). Emergence of plasmid-mediated colistin resistance mechanism MCR-1 in animals and human beings in China: a microbiological and molecular biological study. Lancet Infect. Dis. 16, 161–168. doi:10.1016/S1473-3099(15)00424-7
MacNair, C. R., and Brown, E. D. (2020). Outer membrane disruption overcomes intrinsic, acquired, and spontaneous antibiotic resistance. MBio 11, e01615-20. doi:10.1128/mBio.01615-20
MacNair, C. R., Stokes, J. M., Carfrae, L. A., Fiebig-Comyn, A. A., Coombes, B. K., Mulvey, M. R., et al. (2018). Overcoming mcr-1 mediated colistin resistance with colistin in combination with other antibiotics. Nat. Commun. 9, 458. doi:10.1038/s41467-018-02875-z
May, K. L., and Grabowicz, M. (2018). The bacterial outer membrane is an evolving antibiotic barrier. Proc. Natl. Acad. Sci. U. S. A. 115, 8852–8854. doi:10.1073/pnas.1812779115
McEwen, S. A., and Collignon, P. J. (2018). Antimicrobial resistance: a one health perspective. Microbiol. Spectr. 6. doi:10.1128/microbiolspec.ARBA-0009-2017
Nang, S. C., Azad, M. A. K., Velkov, T., Zhou, Q. T., and Li, J. (2021). Rescuing the last-line polymyxins: achievements and challenges. Pharmacol. Rev. 73, 679–728. doi:10.1124/pharmrev.120.000020
Nikaido, H. (2003). Molecular basis of bacterial outer membrane permeability revisited. Microbiol. Mol. Biol. Rev. MMBR 67, 593–656. doi:10.1128/mmbr.67.4.593-656.2003
Osei Sekyere, J., Sephofane, A. K., and Mbelle, N. M. (2020). Comparative evaluation of CHROMagar COL-APSE, MicroScan walkaway, ComASP colistin, and colistin MAC test in detecting colistin-resistant gram-negative bacteria. Sci. Rep. 10, 6221. doi:10.1038/s41598-020-63267-2
Pagès, J.-M., James, C. E., and Winterhalter, M. (2008). The porin and the permeating antibiotic: a selective diffusion barrier in Gram-negative bacteria. Nat. Rev. Microbiol. 6, 893–903. doi:10.1038/nrmicro1994
Pariente, N.PLOS Biology Staff Editors (2022). The antimicrobial resistance crisis needs action now. PLoS Biol. 20, e3001918. doi:10.1371/journal.pbio.3001918
Paterson, D. L., and Harris, P. N. A. (2016). Colistin resistance: a major breach in our last line of defence. Lancet Infect. Dis. 16, 132–133. doi:10.1016/S1473-3099(15)00463-6
Prado, A. R., Yokaichiya, F., Franco, MKKD, Silva, C. M. G, Oliveira-Nascimento, L., Franz-Montan, M., et al. (2017). Complexation of oxethazaine with 2-hydroxypropyl-β-cyclodextrin: increased drug solubility, decreased cytotoxicity and analgesia at inflamed tissues. J. Pharm. Pharmacol. 69, 652–662. doi:10.1111/jphp.12703
Rowe-Magnus, D. A., Kao, A. Y., Prieto, A. C., Pu, M., and Kao, C. (2019). Cathelicidin peptides restrict bacterial growth via membrane perturbation and induction of reactive oxygen species. MBio 10, e02021-19. doi:10.1128/mBio.02021-19
Seifter, J., Glassman, J. M., and Hudyma, G. M. (1962). Oxethazaine and related congeners: a series of highly potent local anesthetics. Proc. Soc. Exp. Biol. Med. Soc. Exp. Biol. Med. 109, 664–668. doi:10.3181/00379727-109-27300
Silvestri, L., and van Saene, H. K. F. (2010). Hospital-acquired infections due to gram-negative bacteria. N. Engl. J. Med. 363, 1483–1484. author reply 1483-1482. doi:10.1056/NEJMc1006641
Song, M., Liu, Y., Huang, X., Ding, S., Wang, Y., Shen, J., et al. (2020). A broad-spectrum antibiotic adjuvant reverses multidrug-resistant Gram-negative pathogens. Nat. Microbiol. 5, 1040–1050. doi:10.1038/s41564-020-0723-z
Song, M., Liu, Y., Li, T., Liu, X., Hao, Z., Ding, S., et al. (2021). Plant natural flavonoids against multidrug resistant pathogens. Adv. Sci. (Weinheim, Baden-Wurttemberg, Ger.) 8, e2100749. doi:10.1002/advs.202100749
Spapen, H., Jacobs, R., Van Gorp, V., Troubleyn, J., and Honoré, P. M. (2011). Renal and neurological side effects of colistin in critically ill patients. Ann. Intensive Care 1, 14. doi:10.1186/2110-5820-1-14
Srivastava, A., Murugaiyan, J., Garcia, J. A. L., De Corte, D., Hoetzinger, M., Eravci, M., et al. (2020). Combined methylome, transcriptome and proteome analyses document rapid acclimatization of a bacterium to environmental changes. Front. Microbiol. 11, 544785. doi:10.3389/fmicb.2020.544785
Stokes, J. M., MacNair, C. R., Ilyas, B., French, S., Côté, J.-P., Bouwman, C., et al. (2017). Pentamidine sensitizes Gram-negative pathogens to antibiotics and overcomes acquired colistin resistance. Nat. Microbiol. 2, 17028. doi:10.1038/nmicrobiol.2017.28
Sun, S., Gao, H., Liu, Y., Jin, L., Wang, R., Wang, X., et al. (2020). Co-existence of a novel plasmid-mediated efflux pump with colistin resistance gene mcr in one plasmid confers transferable multidrug resistance in Klebsiella pneumoniae. Emerg. Microbes Infect. 9, 1102–1113. doi:10.1080/22221751.2020.1768805
Theuretzbacher, U., Outterson, K., Engel, A., and Karlén, A. (2020). The global preclinical antibacterial pipeline. Nat. Rev. Microbiol. 18, 275–285. doi:10.1038/s41579-019-0288-0
Torres, N. S., Montelongo-Jauregui, D., Abercrombie, J. J., Srinivasan, A., Lopez-Ribot, J. L., Ramasubramanian, A. K., et al. (2018). Antimicrobial and antibiofilm activity of synergistic combinations of a commercially available small compound library with colistin against Pseudomonas aeruginosa. Front. Microbiol. 9, 2541. doi:10.3389/fmicb.2018.02541
Valiante, S., Falanga, A., Cigliano, L., Iachetta, G., Busiello, R. A., La Marca, V., et al. (2015). Peptide gH625 enters into neuron and astrocyte cell lines and crosses the blood-brain barrier in rats. Int. J. Nanomedicine 10, 1885–1898. doi:10.2147/IJN.S77734
Van Acker, H., and Coenye, T. (2017). The role of reactive oxygen species in antibiotic-mediated killing of bacteria. Trends Microbiol. 25, 456–466. doi:10.1016/j.tim.2016.12.008
Wang, C., Wang, J., Xue, K., Xiao, M., Wu, K., Lv, S., et al. (2022). Polarity-sensitive fluorescent probe for reflecting the packing degree of bacterial membrane lipids. Anal. Chem. 94, 3303–3312. doi:10.1021/acs.analchem.1c05268
Wang, R., van Dorp, L., Shaw, L. P., Bradley, P., Wang, Q., Wang, X., et al. (2018a). The global distribution and spread of the mobilized colistin resistance gene mcr-1. Nat. Commun. 9, 1179. doi:10.1038/s41467-018-03205-z
Wang, Y.-M., Kong, L.-C., Liu, J., and Ma, H.-X. (2018b). Synergistic effect of eugenol with Colistin against clinical isolated Colistin-resistant Escherichia coli strains. Antimicrob. Resist. Infect. Control 7, 17. doi:10.1186/s13756-018-0303-7
Wolinsky, E., and Hines, J. D. (1962). Neurotoxic and nephrotoxic effects of colistin in patients with renal disease. N. Engl. J. Med. 266, 759–762. doi:10.1056/NEJM196204122661505
Worthington, R. J., and Melander, C. (2013). Combination approaches to combat multidrug-resistant bacteria. Trends Biotechnol. 31, 177–184. doi:10.1016/j.tibtech.2012.12.006
Xie, D., Jiang, L., Lin, Y., and Liu, Z. (2020). Antioxidant activity of selenium-enriched Chrysomyia megacephala (Fabricius) larvae powder and its impact on intestinal microflora in D-galactose induced aging mice. BMC Complementary Med. Ther. 20, 264. doi:10.1186/s12906-020-03058-4
Yin, W., Ling, Z., Dong, Y., Qiao, L., Shen, Y., Liu, Z., et al. (2021). Mobile colistin resistance enzyme MCR-3 facilitates bacterial evasion of host phagocytosis. Adv. Sci. (Weinheim, Baden-Wurttemberg, Ger.) 8, e2101336. doi:10.1002/advs.202101336
Yuan, L., Dietrich, A. K., and Nardulli, A. M. (2014). 17β-Estradiol alters oxidative stress response protein expression and oxidative damage in the uterus. Mol. Cell. Endocrinol. 382, 218–226. doi:10.1016/j.mce.2013.09.023
Zhang, L., Liu, C., Xiao, Y., and Chen, X. (2016). Oxethazaine inhibits hepatitis B virus capsid assembly by blocking the cytosolic calcium-signalling pathway. J. General Virology 97, 1198–1209. doi:10.1099/jgv.0.000417
Zhang, Q., Chen, S., Liu, X., Lin, W., and Zhu, K. (2021). Equisetin restores colistin sensitivity against multi-drug resistant gram-negative bacteria. Antibiotics 10, 1263. doi:10.3390/antibiotics10101263
Keywords: antibiotic resistance, drug combination, colistin, oxethazine, gram-negative bacteria
Citation: Li J, Han N, Li Y, Zhao F, Xiong W and Zeng Z (2024) The synergistic antibacterial activity and mechanism of colistin-oxethazaine combination against gram-negative pathogens. Front. Pharmacol. 15:1363441. doi: 10.3389/fphar.2024.1363441
Received: 30 December 2023; Accepted: 01 March 2024;
Published: 21 March 2024.
Edited by:
Karunakaran Kalesh, Teesside University, United KingdomReviewed by:
Arijit Bhattacharya, Adamas University, IndiaJaviera Norambuena, Universidad de Playa Ancha, Chile
Copyright © 2024 Li, Han, Li, Zhao, Xiong and Zeng. This is an open-access article distributed under the terms of the Creative Commons Attribution License (CC BY). The use, distribution or reproduction in other forums is permitted, provided the original author(s) and the copyright owner(s) are credited and that the original publication in this journal is cited, in accordance with accepted academic practice. No use, distribution or reproduction is permitted which does not comply with these terms.
*Correspondence: Zhenling Zeng, emx6ZW5nQHNjYXUuZWR1LmNu