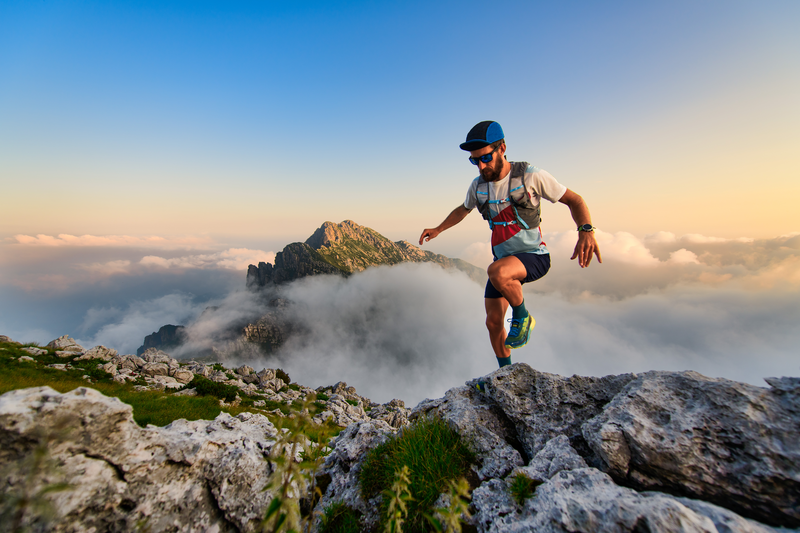
94% of researchers rate our articles as excellent or good
Learn more about the work of our research integrity team to safeguard the quality of each article we publish.
Find out more
REVIEW article
Front. Pharmacol. , 23 July 2024
Sec. Respiratory Pharmacology
Volume 15 - 2024 | https://doi.org/10.3389/fphar.2024.1360607
Asthma is a common chronic inflammatory disease of the lungs and airway, yet its inflammatory subtypes and potential pathogenesis have not been completely elucidated and require further study. With advances in epigenetic development, methylation has emerged as a new direction for identifying and decoding the occurrence and subtype manifestations of asthma. N6-methyladenosine (m6A), an RNA methylation modification occurring in the N6-position of adenosine, is a prevalent epigenetic modification observed in eukaryotes. It exerts significant control over mRNA metabolism by regulating alternative splicing, stability, export, and translation. The dynamic process of m6A methylation plays a crucial role in the pathogenesis of asthma and is tightly regulated by three types of regulators: writers, readers, and erasers. This article provides a comprehensive review of the association between m6A regulators and the pathogenesis of inflammatory subtypes of asthma, such as involvement of inflammatory cells and related inflammatory response. Furthermore, the findings presented herein provide new insights and a solid foundation for further research on m6A mRNA methylation as biomarkers for the diagnosis and development of personalized treatment for different subtypes of asthma, particularly neutrophilic asthma and eosinophilic asthma.
Asthma is a common heterogeneous disease of the respiratory system, and chronic inflammation, allergic reactions, and airway hyperresponsiveness are the main causes of asthma-related morbidity. Despite its prevalence, the inflammatory subtypes and their potential pathogenesis of asthma have not yet been fully elucidated. Hence, we aimed to review previous studies in order to highlight key findings and discover new insights that will guide future research in these areas.
Currently, the internationally applied Global Initiative for Asthma guidelines categorize patients with asthma into four inflammatory subtypes according to a cell-based classification of the inflammatory infiltrate in their sputum: eosinophilic asthma (EA), neutrophilic asthma (NA), mixed granulocyte asthma (MA), and oligo-granulocyte asthma (PA) (Simpson et al., 2006). The EA subtype manifests as the secretion of Th2-type cytokines (IL-4, IL-5, IL-13) and the expression of type 2 innate lymphocytes (ILC2), downstream of which a Th2-type immune response occurs, and then IL-4 stimulates the expression of polyfilament proteins in bronchial epithelial cells, which are important genes for the development and progression of EA (Carr et al., 2018; Gao et al., 2021). Treatment with steroids can be effective for asthmatics with a clear diagnosis of EA, but early diagnosis of EA and precise targeting of EA targets is more effective for patients with severe hormonal complications (Carr et al., 2018). The NA subtype is associated with non-Th2 asthma and is primarily driven by neutrophil extracellular traps (NETs) with large numbers of neutrophils infiltrating the blood and sputum (Hudey et al., 2020; Xie et al., 2022). Inflammatory factors such as IL-1β, IL-6, IL-12, and IL-17 play important roles in the development of NA and steroid resistance, and are also associated with the activation of NLRP3 vesicles and regulating the NF-kB inflammatory signaling pathway during inflammation in asthma (Sánchez-Ovando et al., 2021; Williams et al., 2021; Chen et al., 2022; Chang et al., 2023). Relevant markers for a definitive diagnosis of NA are lacking, and NA is resistant to steroid hormone therapy, making conventional asthma medications less effective (Chung, 2016; Mogensen et al., 2020). The MA subtype presents with 80% neutrophils, 10% eosinophils, and worse lung function in the airways. The mechanism underlying the pathogenesis of MA has not been clarified, but it may be associated with neutrophil inflammation and inflammatory factors such as IL-35 and IL-17A (Li et al., 2020; Menson et al., 2020; Hastie et al., 2021). The PA subtype is induced by sputum showing no significant increase in eosinophilic and neutrophilic inflammation in the airways, with an elevated sputum macrophage count; however, a stable consensus on the pathogenesis of PA has not yet been reached (Olgac et al., 2021). Tliba et al. suggested that PA airway obstruction may result from a pulmonary inflammatory mechanism of hypertrophy and dysfunction of airway smooth muscle tissue; however, PA is insensitive to hormonal treatment (Ntontsi et al., 2017; Tliba and Panettieri, 2019). Further research on targets for asthma subtypes is needed to further refine the diagnosis and treatment of each subtype classification.
The most common epigenetic of eukaryotic RNA modifications, m6A modifications were first identified in 1974 for their unique distribution in mRNAs (Tang et al., 2021; Desrosiers et al., 1974; Lence et al., 2019), in which m6A methylation sites bind reversibly to various proteins, act at the post-transcriptional stage, affect mRNA stability, are involved in almost all biological activities in the human body, and have been strongly implicated in diseases of the lungs, heart, intestines, brain, muscles, and other organs (Tang et al., 2021; Xiong et al., 2021; Wang et al., 2022; Fei et al., 2022). Relying on a series of regulatory factors, m6A regulatory factors carry out various functions and roles. The three main types of functional regulators involved in m6A methylation are methyltransferase, methylated reading proteins, and demethylation enzymes, which are known as writes, readers, and erases, respectively, and provide a synergistic effect on the progression of inflammation, tumors, and other related diseases (Yang et al., 2018; Zaccara et al., 2019). m6A is assembled by a multicomponent methyltransferase complex, which next recognizes the target mRNA in the presence of binding proteins. Upon binding to the target mRNA, the m6A modification regulates the transcription process and metabolism of the RNA, including selective splicing, exportation, stability, and further translation (Yang et al., 2018). The presence of demethylase allows the mRNA-bound m6A to detach at the right time (Jia et al., 2011; Zheng et al., 2013). There are 27 widely recognized regulatory factors, including writes: cbl proto-oncogene like 1 (CBLL1), methyltransferase-like 14 (METTL14), methyltransferase-like 3 (METTL3), zinc finger CCCH-type-containing 13 (ZC3H13), the aberrant expression of the zinc finger protein 217 (ZNF217), RNA-binding motif protein 15B (RBM15B), Wilms’ tumor 1-associating protein (WTAP), RNA-binding motif protein 15 (RBM15), and virus-like m6A methyltransferase-associated protein (KIAA1429); readers: YT521-B homology (YTH) domain (YTHDF1, YTHDF2, YTHDF3, YTHDC1, YTHDC2), eukaryotic translation initiation factor 3 subunit A (EIF3A), eukaryotic translation initiation factor 3 subunit B (EIF3B), heterogeneous nuclear ribonucleoprotein A2B1 (HNRNPA2B1), heterogeneous nuclear ribonucleoprotein C (HNRNPC), IGF2 mRNA binding proteins (IGF2BP1/2/3) families, leucine rich pentatricopeptide repeat containing (LRPPRC), fragile X messenger ribonucleoprotein 1 (FMR1), and ELAV-like RNA binding protein 1 (ELAVL1); and erasers: alkB homolog 5 (ALKBH5) and fat mass and obesity-associated protein (FTO) (Yang et al., 2018; Sun et al., 2021; Deng et al., 2022; Mo et al., 2022). According to the expression of 27 m6A regulators, Non-negative matrix factorization (NMF) was performed to identify three different m6A modification patterns, with FMR1, IGF2BP2, and YTHDC2 accounting for the largest proportion of patterns 1, 2, and 3, respectively. Different patterns play different roles in the infiltration of asthma inflammatory cells, which can be referred to as Modes 1, 2, and 3. Mode one showed higher expression levels of γ-T cells, TH17, macrophages, eosinophils, mast cells, and neutrophils; Mode two affected the infiltration of NK, DC, and monocytes; and Mode three regulated the infiltration of CD8+ T cells and TH1 cells in asthma (Sun et al., 2021).
In the presence of regulatory factors, m6A is involved in inflammatory and lung diseases such as asthma, respiratory distress syndrome, pneumonia, and lung cancer (Chao et al., 2020; Kim et al., 2021; Li et al., 2021; Fei et al., 2022). In asthma inflammatory subtypes, m6A methylation regulates macrophage and T-cell differentiation as well as eosinophil, neutrophil, natural killer (NK), and dendritic cell (DC) infiltration in inflammatory responses to different regulatory factors (Table 1), and regulates inflammatory pathways such as nuclear factor-κB (NF-ΚB) and JAK/STATA (Table 2), which are features used for the classification of asthma inflammatory subtypes (Wang et al., 2019a; Ma et al., 2021; Sun et al., 2021; Cai et al., 2022; Liu et al., 2022).
Table 1. Regulatory factors and their roles in cellular functions and inflammatory subtypes of asthma.
This article provides a review of the specific mechanisms by which m6A methylation and its associated regulators are involved in the inflammatory response, formation, pathogenesis, and prognosis of asthma and its inflammatory subtypes (Figures 1–3).
m6A mRNA methylation is widely involved in various biological functions of the human body, especially in inflammatory and immune responses, and plays an important role in the onset and progression of asthma. By analyzing the dataset of childhood asthma patients obtained from the Gene Expression Omnibus (GEO) database, 11 regulators closely related to asthma were obtained, including: YTHDC1, HNRNPC, YTHDC2, FMR1, YTHDF3, HNRNPA2B1, KIAA1429, METTL3, WTAP, RBM15B, and ZC3H13, of which RBM15B expression was significantly upregulated in asthma, while all other regulators were downregulated (Dai et al., 2021). Further analysis of the above 11 m6A regulators using consistent clustering revealed that the expressions of YTHDC1, HNRNPC, FMR1, HNRNPA2B1, KIAA1429, METTL3, RBM15B, and ZC3H13 were associated with Th1 immunity, and the expression of YTHDF3 was associated with Th2 immunity (Xiong et al., 2024). Th1 immunity and Th2 immunity are the immune mechanisms of NA and EA, respectively (Hudey et al., 2020; Gao et al., 2021). A combined analysis of m6A methylation and gene expression in asthma lung tissues demonstrated that m6A methylation is involved in Th1 cell differentiation and other pathological mechanisms that have been correlated to asthma airway hyperresponsiveness, inflammatory and immune responses in asthma, and asthma inflammatory subtypes, although the specific mechanisms involved need to be further investigated (Teng et al., 2021).
Recent studies have confirmed that FTO, acting as an eraser, downregulated the m6A modification on IKBKB mRNA in a mouse asthma model, thereby enhancing its stability and leading to the upregulation of IKBKB expression, which effectively attenuated epithelial barrier damage in PM2.5-exposed cells by regulating NF-κB signaling (Xiong et al., 2024). In a human asthma lung tissue model, the YTHDF1 gene binds to the cyclin 1 (CCND1) mRNA modification site, thereby stimulating the proliferation and migration of airway smooth muscle cells, which are involved in airway spasm and contraction during asthma pathogenesis (Wang et al., 2023a). Meanwhile, in human and mouse airway epithelial cells, FTO knockout caused deletion of airway epithelial ciliated cells and exhibited asthmatic airway inflammatory manifestations with upregulation of IL-13 expression and downregulation of IL-12b expression, which may have been related to a modification in the expression of Foxj1 mRNA, a major regulator of motor ciliogenesis, by FTO-m6A (Kim et al., 2021). Leoni et al. identified enhanced mast cell responses to acute stimuli in mice knocked out of METTL3 and METTL14 genes, which may have been directly related to the fact that deletion of METTL3 and METTL14 increases mast cell effector center factors (TNF and IL-13) (Leoni et al., 2023). It is well known that the immune response occurring in mast cells mediated by immunoglobulin E is an effective stimulus for pathological changes in asthma (Kaliner, 1989). Deletion of YTHDF2 promotes demethylation of histone H3 lysine-27 trimethylation (H3K27me3) in response to bacterial-induced inflammation, which leads to enhanced production of pro-inflammatory cytokines and facilitates co-transcriptional deposition of m6A (Wu et al., 2020). In studies of lung tissue from asthmatic mice, H3K27me3 demethylation was found to regulate the contractile function and migratory reproduction function of airway smooth muscle in human asthma disease, as well as inhibit the expression of inflammatory factors IL-6 and IL-12B, which are involved in the regulation of the cellular inflammatory response as well as the development of asthma (Yu et al., 2018). METTL3 synergizes with YTHDF2 to promote PGC-1α mRNA degradation and affects monocyte inflammation and mitochondrial metabolism in a monocyte inflammation model (Zhang et al., 2021). In human and mouse asthma disease, PGC-1α expression was shown to be significantly upregulated in bronchial smooth muscle and was an important factor in the proliferation of asthmatic airway smooth muscle and impaired airway epithelial barrier dysfunction (Trian et al., 2007; Saito et al., 2021; Huang et al., 2023). In alveolar epithelial cells from mice with bronchopulmonary dysplasia, inhibition of YTHDC1 led to a decrease in IL-33 mRNA and protein expression, which in turn inhibited alveolar epithelial cell apoptosis and alleviated lung injury induced by hyperoxia treatment for bronchopulmonary dysplasia (Bao et al., 2023). Enrichment analysis and scoring against gene set library in the Kyoto Encyclopedia of Genes and Genomes database clarified that the genetic signature of IL-33 activation is elevated in neutrophilic and mixed granulocytic asthma (Badi et al., 2023).
In summary, m6A methylation regulates the release of certain asthma inflammatory factors, the contraction of airway smooth muscle cells, and the development of asthma subtypes, which are further mediated by regulatory factors such as YTHDF1, YTHDF2, YTHDC1, FTO, and METTL3, which have emerged as new targets for the treatment and prognosis of asthma.
m6A is involved in macrophage inflammatory response and polarization mediated by regulatory factors, and macrophages can be polarized into M1 and M2 macrophages. M1 macrophages promote the expression of Th1 and Th17 inflammatory cells and chemokines, and are involved in the development of NA and its steroid resistance, whereas M2 macrophages activate Th2 cells, promote the infiltration of lung eosinophils, and are involved in the development of EA (Saradna et al., 2018). Among them, the regulatory factors YTHDF1, YTHDF2, METTL3, METTL14, and FTO may play crucial roles in mediating m6A regulation of macrophage polarization and participate in the mechanisms underlying the formation of NA and EA subtypes.
YTHDF1 gene expression was upregulated in human macrophages infected by syphilis, whereas YTHDF1 knockdown upregulated the expression of M1 markers (CD86 and iNOS) and downregulated M2 markers (CD206 and ARG1); during the course of infection, m6A-YTHDF1 promoted the inhibitory factor of the important macrophage inflammatory pathway JAK2/STAT3-mRNA translation, thus negatively regulating macrophage-associated inflammatory responses and the secretion of the inflammatory factor IL-1β (Li et al., 2022). Blockade of IL-1β modulated Th17/Treg immune imbalance and attenuated neutrophil airway inflammation in an ovalbumin-induced mouse model of asthma (Chen et al., 2022). Studies in septicemic rats indicated that YTHDF1-m6A directly acts on the JAK2/STAT3 signaling pathway in macrophages, thereby inducing the upregulation of IL-17 expression, affecting Th1/Th17 balance and regulating inflammatory responses in macrophages (Xing et al., 2021). The JAK2/STAT3 signaling pathway in macrophages is an important target for allergic asthma development and is involved in important asthma pathological processes such as the activation of mast cells and the promotion of bronchial smooth muscle thickening and airway remodeling (Qu et al., 2022; Wang et al., 2022; Bai et al., 2023). Inhibition of the JAK/STAT pathway reduces neutrophil activation and improves the anti-inflammatory effects of corticosteroids in asthma patients (Milara et al., 2022). Considered together, m6A-YTHDF1 plays a complex role in the pathological process of inflammatory response in the NA asthma subtype through the regulation of the macrophage JAK2/STAT3 signaling pathway.
Yu et al. found for the first time that YTHDF2 expression was upregulated in lipopolysaccharide (LPS)-induced macrophage inflammatory response and regulated the inflammatory response through the YTHDF2-MAP2K4/MAP4K4 mRNA-p38 MAPK/p65 NF-κB/ERK-IL-6/IL-12/IL-1β pathway to regulate inflammatory response in macrophages (Yu et al., 2019). p38 MAPK, IL-6, and IL-1β are all important targets of the inflammatory response in NA asthma and are involved in the mechanism of steroid resistance in NA therapy (Sánchez-Ovando et al., 2021; Chen et al., 2022; Xie et al., 2022). In a mouse model of neutrophilic allergic airway inflammation, exogenous IL-25 ameliorated neutrophilia in the airway by inhibiting macrophage M1 polarization as well as IL-12 expression (Chang et al., 2023). Subsequently, the relationship between YTHDF2 and macrophage M1 and M2 polarization was first identified in a study of the macrophage polarization model. YTHDF2 induced M2 macrophage polarization by promoting the degradation of p53 mRNA, and inhibited M1 macrophage polarization by inhibiting the NF-κB, p38 and JNK signaling pathways (Cai et al., 2022). On the other hand, in mouse macrophages, YTHDF2 interacted with the RNA-binding motif 4 (RBM4) to promote YTHDF2-m6A degradation of STAT1 mRNA, which in turn inhibited IFN-r-, IL-6-, and IL-1β-induced macrophage M1 polarization but did not affect IL-4-induced M2 macrophage polarization (Huangfu et al., 2020). In summary, m6A-YTHDF2 inhibits M1 polarization and promotes M2 polarization by acting on signaling pathways such as NF-κB, MAPK, and STAT in macrophages, thereby playing an important role in the mechanism of asthma subtypes and targeted therapy.
METTL3-m6A suppresses the macrophage inflammatory response induced by LPS by inhibiting the NF-κB signaling factor to downregulate IL-6 and TNF-α expression (Wang et al., 2019). Another study of LPS-induced macrophages found that METTL3-m6A targeting of Irakm mRNA/TLR4 signaling in macrophages promoted macrophage activation (Tong et al., 2021) Recent m6A-seq sequencing of peripheral blood cells from children with allergic asthma revealed that METTL3 is lowly expressed in monocyte-derived macrophages from children with allergic asthma (Han et al., 2023). Further animal experiments confirmed that the above may be associated with the inhibition of M2 macrophage activation by METTL3-m6A through PI3K/AKT and JAK/STAT6 signaling, a process dependent on the degradation of PTX3 mRNA by METTL3-YTHDF3-m6A (Han et al., 2023). In pediatric pneumonia peripheral blood mononuclear cell and human embryonic lung cell models, downregulation of METTL3 modified the EZH2 gene, which in turn regulated the JAK2/STAT3 signaling pathway, inhibited the expression of IL-6 and TNF-α, and participated in the development of LPS-induced inflammatory response (Yang et al., 2021). CircN4bp1 mRNA may be upregulated in sepsis-induced acute respiratory distress syndrome macrophages with modification of METTL3-m6A, which further promotes M1 macrophage activation but inhibits M2 macrophage polarization through the CircN4bp1-miR-138–5p/EZH2 axis (Zhao et al., 2021). In a mouse model of asthma, EZH2 promoted asthma development through the FOXO3-miR-34b-BTG2 axis (Liu et al., 2021). In summary, the results of different studies on the role of METTL3-m6A in macrophage inflammation and activation are varied, which may be related to the multi-targets of METTL3-m6A and the complexity of the biological microenvironment, but it may be involved in macrophage differentiation and the development of asthma subtypes.
In macrophages from acutely bacterially infected mice, METTL14 was involved in macrophage activation through the METTL14/YTHDF1-SOCS1-TLR4-p65 NF-κB axis, which promoted the production of inflammatory factors TNF-α, IL-6, and inflammatory factor storm, and downregulated macrophage responses to acute bacterial infection in mice (Du et al., 2020). In a stroke model, depletion of METTL14 inhibited M1 macrophage polarization and promoted M1 to M2 conversion, which in turn attenuated brain injury (Li et al., 2023). In the inflammatory response of macrophages in atherosclerosis, m6A-METTL14 directly acted on the p65 NF-κB pathway to regulate IL-6 transcription, which in turn induced macrophage M2 polarization (Zheng et al., 2022), which is similar to the mechanism by which YTHDF2 mediates macrophage effects on the inflammatory subtype of asthma; therefore, it would be worthwhile to further investigate whether this mechanism is synergistically accomplished by both factors.
Unlike other regulators, FTO promoted both M1 and M2 polarization in a mouse macrophage model, which may have been related to the fact that FTO promotes the phosphorylation levels of IKKα/β, IκBα, and p65 in the NF-κB signaling pathway while maintaining the mRNA stability of STAT1, STAT6, and PPAR-γ (Gu et al., 2020). During the macrophage inflammatory response in mice, m6A levels were expressed at higher levels in the harder microenvironment than in the rest of the microenvironment, and inhibition of the FTO gene promoted the expression of SOCS1 mRNA, a process that required YTHDF1-m6A mediation, while high expression of SOCS1 further inhibited the phosphorylation of STAT1 and p65 as well as the expression of inflammatory factors (IL-1β, IL-6), thereby regulating the inflammatory response and functional activation of macrophages in different tissue microenvironments (Hu et al., 2022). FTO targets macrophage polarization and inflammatory responses and may influence the mechanism of asthma subtype formation in different microenvironments.
m6A in inflammatory response may be involved in macrophage polarization through modification of NLRP3 mRNA in regulating inflammatory response and inflammatory subtypes. Liu et al. found that NLPR3 promotes M2 macrophage polarization and inhibits inflammatory response under the regulation of ubiquitin-specific protease 19 and autophagy in a series of animal and cellular experiments (Liu et al., 2021). On the one hand, M2 macrophages promote Th2 cell activation in asthma, and on the other hand, NLRP3 is an important transcription factor for Th2 cell differentiation and induces Th2 inflammatory responses in asthmatic mice (Bruchard et al., 2015). However, inhibition of NLRP3 inflammatory vesicles attenuated neutrophil airway inflammation in asthmatic neutrophil airway inflammation in a mouse model of neutrophil airway inflammation and in human asthmatics (Liu et al., 2023). The roles of METTL14, METTL3, and YTHDF1 in NLRP3 and macrophage polarization and macrophage inflammation are described below (Figure 4).
Animal studies revealed that METTL14 levels were upregulated in macrophages from ischemic stroke rats, and KAT3B expression was enhanced by m6A modification of KAT3B mRNA, whereas knockdown of the METTL14 gene promoted macrophage switching from M1 to M2 via KAT3B-STING signaling and further inhibited NLRP3 inflammatory vesicles in macrophages to decrease IL-1β levels and alleviate brain injury (Li et al., 2023). Recent studies have shown that METTL14 was significantly upregulated in macrophages in a mouse model of lung injury (ALI), and that METTL14-m6A modified the NLRP3 transcript, which was subsequently recognized by IGF2BP2 to stabilize the expression of NLRP3 mRNA, thereby promoting the secretion of IL-1β and IL-18, leading to lung injury (Cao et al., 2024).
In related studies, it was found that in the ALI mouse model, METTL3-m6A mediated the expression of apolipoprotein C3 (ApoC3) mRNA in macrophages and promoted the activation of NLRP3 inflammatory vesicles, which led to macrophage pyroptosis and the release of inflammatory factors such as IL-1β, thus exacerbating the lung injury (Pu et al., 2024). On the other hand, METTL3-m6A inhibited the stability of the TTC4 gene, further inhibiting the mitochondrial damage occurring in the HSP70/ROS/NLRP3/Caspase-1 signaling pathway, and reduced the levels of inflammatory factors such as IL-1β, IL-6, thus reducing the inflammation and scorched death of macrophages in the ALI mouse model (Chen et al., 2023). Animal studies confirmed that IL-18 may initiate asthma-induced eosinophil production and airway obstruction (Mishra et al., 2022). In a mouse model of knee osteoarthritis, extracellular vesicles inhibited the downregulation of NLRP3 expression activity via modification of NLRP3 mRNA by METTL3-m6A in macrophages and inhibited the secretion of IL-1β and IL-18 inflammatory factors and inflammatory responses (Zhou et al., 2022). Recent studies have confirmed that METTL3-MA modification promotes Mycobacterium tuberculosis-induced NLRP3 activation and inflammatory responses in mouse macrophages and promotes the secretion of inflammatory factors such as IL-1β, IL-18, IL-6, and TNF-α (Han et al., 2024).
Additional in vivo and in vitro experimental studies have shown that TYHDF1 is upregulated under sepsis environmental conditions and mediates m6A modification of WWP1 mRNA in macrophage cell line RAW264.7 cells, which promotes the translation of WWP1 and consequently inhibits apoptosis and the release of the IL-1β inflammatory factor via the WWP1/NLRP3/caspase-1 axis (Zhang S. et al., 2022). In another study in septic mice, YTHDF1-m6A methylation directly promoted the translation of NLRP3 mRNA and induced IL-1β production in macrophages (Hao et al., 2022). In bacterially infected mouse macrophages, YTHDF1 promoted NLRP3 expression in macrophages and IL-1β production during bacterial infection (Hao et al., 2022).
m6A methylation acts as an upstream signal to promote or inhibit the activity of NLRP3 inflammatory vesicles, which regulates M2 polarization and the secretion of inflammatory factors in macrophages; therefore, further study of m6A-NLRP3-macrophage-asthma is of great significance in providing precisely targeted therapy to EA and NA.
In summary, m6A exerts its methylation function with the assistance of the above regulatory factors to regulate M1 and M2 polarization and inflammatory responses in macrophages, thereby affecting the development of asthma subtypes. Although the conclusions we obtained seem to be contradictory, which may be related to the cellular microenvironment and hardness, further study is needed to clarify the specific mechanism.
m6A is closely related to the differentiation and activity function of T cell subsets, and the type of inflammatory response induced can be categorized into Th1, Th17, and Th2 inflammatory responses based on T cell subsets (Luo et al., 2022), such as Th2 regulates EA (Borish, 2016). Th17 and Th1 coordinate neutrophil recruitment to the lungs, induce steroid resistance in NA asthma, and are the main inflammatory cells involved in the development of the NA subtype (Sánchez-Ovando et al., 2021; Luo et al., 2022).
METTL3 was shown to be highly expressed in CD4+ T cells obtained from the peripheral blood of asthma patients, which may have been due to the fact that METTL3 synergizes with YTHDF2 to mediate a reduction of Foxp3 mRNA stability by m6A, leading to dysregulation of Treg/Th17 homeostasis and regulating inflammatory responses in asthma (Fan et al., 2022). Animal studies have shown that deletion of the METTL3 gene in T cells affects the differentiation and maturation of naïve T cells, thus leading to a decrease in the production of Th1 and Th17 cells and an increase in Th2 cells, mainly due to the fact that METTL3-m6A has the ability to regulate the balance of the two basic signaling pathways of T cell homeostasis (IL-17/JAK-STAT and TCR/ERK/AKT) (Li et al., 2017). Recent studies have shown that low METTL3 expression in the peripheral blood of Th2 asthma patients is correlated with disease severity, and further animal experiments confirmed that decreasing METTL3-m6A methylation activity regulated CD4+ T cell activity by increasing the stability of SOX5 mRNA in bronchial epithelial cells, which in turn upregulated GATA3/Th2 activity, inhibited T-bet/Th1 activity, and promoted the development of Th2 asthma (Chen et al., 2024). In an autoimmune encephalomyelitis model, METTL3 maintained Th17 differentiation and activity by targeting and modifying the stability of SOCS1 and SOCS3 mRNAs in Th17 cells (Wang et al., 2023). In T cells sampled from patients with autoimmune uveitis (EAU), METTL3, assisted by YTHDC2 was targeted to inhibit Th17 function and inflammatory responses via the m6A-ASH1L mRNA- IL-17A/17F/23R-Th17 pathway (Zhao et al., 2023). In acute infection models, deletion of METTL3 in T cells has been shown to impair T-bet expression and inhibit Th1 cell differentiation (Yao et al., 2021). In contrast, studies of immune rejection revealed that METTL3 deficiency inhibited T-bet expression and impaired Th1 cell differentiation in allogeneic T cell responses (Li et al., 2022). T-bet is a key transcription factor required for Th1 cell differentiation and is inextricably linked to NA development (Kallies and Good-Jacobson, 2017). METTL3-m6A regulates Th1 and Th17 differentiation and activity, and its role in NA asthma inflammatory subtypes should be explored in depth.
Knockout of the FTO gene in mouse lung tissue cells resulted in significant asthma-like inflammation in mice, as evidenced by upregulation of Th2 cell marker IL13 mRNA levels and downregulation of Th1 cell marker IL12b and the T cell antibody CD8b1 (Kim et al., 2021). In contrast, in a mouse model of spontaneous colitis, mouse METTL14 gene deficiency caused upregulation of Th1 and Th17 cells (Lu et al., 2020). In the EAU model, deletion of ALKBH5 in T cells inhibited the IL-17 signaling pathway and IFN-γ secretion in CD4+ T cells, while specifically suppressing the ability of T cells to recruit neutrophils to the central nervous system during neuroinflammation (Zhou et al., 2021). FTO, ALKBH5, and METTL14 are thought to regulate T-cell differentiation and inflammatory response, thus having a potential role in the mechanism of different subtypes of asthma disease.
Under the multiple modes of action of regulatory factors, m6A plays a complex regulatory role in the differentiation and function of Th cell subpopulations, and in-depth studies are needed regarding its role in regulating the balance of Th2/Th17 and thus its involvement in the diagnosis and management of asthma subtypes.
Th17- and Th1-induced non-Th2 inflammatory responses drive the development of NA, which is mainly characterized by sputum-induced neutrophilia and infiltration of inflammatory factors such as IL-1β, IL-6, IL-12, and IL-17. In LPS-induced inflammation in mice, METTL3-m6A modified TLR4 mRNA to induce TLR4 protein expression in neutrophils, which further enhanced the migration of bone marrow neutrophils into the bloodstream via TLR4/CXCL2 and promoted TNF-α, IL-6, and IL-1β inflammatory factors in neutrophils via TLR4/NF-κB signaling pathway production. (Luo et al., 2023). ALKBH5 expression was downregulated in neutrophils sampled from septicemia mice, and ALKBH5-m6A methylation maintained the intrinsic ability of neutrophils to undergo chemotaxis toward the site of infection, ensuring their antimicrobial and immune functions, due to the function of ALKBH5-m6A in maintaining CXCR2 mRNA stability (Liu et al., 2022). Inhibition of the chemokine CXCR2 is involved in peripheral neutrophil migration and ameliorates neutrophil-dependent airway inflammation in asthmatics and asthmatic mice (Mattos et al., 2020; Zhu et al., 2020).
The process by which neutrophils release elastase, histone G, myeloperoxidase, and DNA in response to infection is known as NETs, which creates anti-infective effects in the organism and can cause tissue damage (Lee and Grinstein, 2004; Silva et al., 2021). The release of NETs is a key upstream signal that induces the onset of NA and significantly correlates with the severity of asthma development (Wan et al., 2020; Janssen et al., 2022). NETs may target m6A to regulate iron death and autophagy, thus participating in NA.
PPARγ inhibits Th17 responses by modulating reactive oxygen species (ROS) signaling in an LPS-induced asthma mouse model (Miao et al., 2021). GPX4-induced iron death occurs to exacerbate the progression of asthma disease in humans, and inhibition of GPX4 gene expression attenuates bronchial epithelial cell damage and NA progression in mice (Bao et al., 2022; Nagasaki et al., 2022). In a mouse model of sepsis, NETs induced iron death in alveolar epithelial cells through activation of METTL3-m6A modification, a pathway that contributes to lung injury and systemic inflammation by promoting iron death via the NETs/p300/H3K27ac/METTL3-m6A/IGF2BP2-m6A/HIF-1α/GPX4/ROS pathway (Zhang et al., 2023). In a mouse model of sepsis-associated acute lung injury (SI-ALI), NETs induced METTL3-m6A modification of GPX4 mRNA, which inhibited GPX4 expression by reducing GPX4 mRNA stability and promoted iron death in alveolar epithelial cells, while NETs specifically exacerbated iron death through the TLR9/MyD88/NF-kβ/METTL3-m6A/GPX4/ROS pathway to exacerbate iron death and contribute to the development of lung injury (Zhang et al., 2022). In a mouse model of acute kidney injury, knockdown of METTL3 reduced the downregulation of GPX4 expression and upregulation of ROS expression in LPS-treated HK-2 cells, promoting iron death (Hu et al., 2023). Considered together, m6A methylation may be involved in the mechanism of NA asthma by inducing the onset of iron death via GPX4/ROS under the NETs activation.
In asthmatics, increased autophagy plays a role in promoting Th2-type immune response and eosinophilic inflammation, whereas decreased autophagy plays an important role in NA (Barnes et al., 2022). In a mouse model of SI-ALI, NETs contribute to alveolar epithelial cell injury by causing impaired autophagic flux through the METTL3-m6A/Sirt1 mRNA pathway (Qu et al., 2022). In a mouse model of osteoarthritis, METTL3 mediates m6A regulation of autophagy-associated 7 (ATG7) mRNA stability to impair autophagic flux in a YTHDF2-dependent manner (Chen et al., 2022). In animal experiments, METTL3 silencing enhanced autophagic flux and inhibited apoptosis in ischemia-reperfusion-treated cardiomyocytes (Song et al., 2019). Considered together, the process of m6A methylation targeting NETs to promote NA may be related to autophagy.
Accordingly, we hypothesized that m6A could serve as an intermediate target for NETs-induced asthma development, which is involved in neutrophil aggregation in asthma disease and is an important target for the mechanism underlying NA subtype development.
Infiltration of eosinophils in sputum induction is an important criterion for the diagnosis of EA (Simpson et al., 2006; Komlósi et al., 2022). According to the data analysis conducted by Sun et al., there was a significant correlation between YTHDF3 and EIF3B and the infiltration of eosinophils in severe asthma, with the expression of YTHDF3 being positively correlated and the expression of EIF3B being negatively correlated (Sun et al., 2021). However, there have been no laboratory studies conducted on the specific mechanisms of YTHDF3 and EIF3B with eosinophils and EA. However, recent studies in mice with allergic rhinitis found that METTL3-m6A methylation enhances circMIRLET7BHG expression, and circMIRLET7BHG in mouse epithelial cells contributes to OVA-induced allergic symptoms by modulating inflammatory responses such as eosinophil infiltration (Zhan et al., 2023).
m6A methylation is unequivocally associated with eosinophil infiltration and the development of EA in asthma; however, more detailed studies on the specific mechanisms and pathological processes of m6A-induced EA development are needed by researchers.
NK cells are one of the inflammatory bases of asthma disease development (Komlósi et al., 2022). An animal study showed that YTHDF2, which is upregulated in NK cells that are activated by cytokines or viral infection, maintained NK cell homeostasis and terminal maturation, regulated NK cell trafficking, and promoted the intrinsic function of NK cells through the formation of a STAT5-YTHDF2–positive feedback loop (Ma et al., 2021). In mouse spleen FTO-deficient NK cells, the expressions of IL-2, IL-15, and the JAK/STAT signaling pathway were significantly elevated, mainly due to the fact that FTO regulates the stability of SOCS3 mRNA through demethylation to modulate NK cell homeostasis and antitumor immunity (Kim et al., 2023). IL-2 inhibited the expression of IL-17A in TLR9-induced asthmatic mice (Murakami et al., 2020). Further studies are needed to demonstrate the mechanisms linking m6A and NK cells in asthma, which would lead to the discovery of new strategies for asthma treatment.
m6A plays a complex role in the activation and function of DC cells, which are involved in asthma inflammation. DC cell apoptosis and activation are essential for maintaining the Th2/Th17 balance in asthma. Depending on the degree of their maturation and route of action, DCs can play different roles in inflammatory progression in NA and EA (Park et al., 2020; Izumi et al., 2021; Deng et al., 2022).
Animal studies revealed that the reduced levels of asthma-associated inflammatory factors IL-6 and IL-12 mRNA in DC cells knocked down for METTL3 may have been related to a defective TLR4/NF-κB signaling pathway caused by the deletion of METTL3. In addition, METTL3-m6A upregulated the translation of key DC transcripts (CD40, CD80, and TLR signaling junctions) to promote DC maturation and activation (Wang et al., 2019a). Animal studies revealed that m6A modified lncRNA lnc-Dpf3 demethylation and upregulated lnc-Dpf3 interacting with the transcription factor hypoxia-inducible factor 1-alpha (HIF-1alpha), which suppressed the migratory capacity of DCs and inhibited the expression of inflammatory factors associated with IFN-γ and IL-17 in Th1 and Th17 cells (Liu et al., 2019).
The role of m6A methylation in the function of DC cells is contradictory, but the role of m6A-targeted DCs in regulating Th2/Th17 homeostasis is important for the progression of inflammatory subtypes in asthma and remains to be investigated.
Screening of the GEO database identified six m6A-related genes (FTO, IGF2BP2, RBM15, RBMX, WTAP, and YTHDC1) that are significantly dysregulated in asthma disease, and further using the CMap database, we found that resveratrol may target these dysregulated m6A genes and could serve as a potential therapeutic agent for asthma (Mo et al., 2022).
Glucocorticoids are the most commonly used drugs for the treatment of asthma, although steroid resistance is a challenge in the NA subtype, which reduces the therapeutic effect. After nasal mucosal drug sensitivity analysis, methylprednisolone was found to be closely related to METTL14 expression, and topical nasal methylprednisolone can potentially improve asthma respiratory allergy symptoms by regulating METTL14 expression (Wang et al., 2023). Inhibition of ALKBH5-m6A modification ameliorates glucocorticoid resistance in T-cell acute lymphoblastic leukemia, which may be related to modification of the resistance gene USP1 by ALKBH5-m6A (Gong et al., 2021). In a mouse model of nonalcoholic fatty liver disease, FTO knockdown significantly attenuated dexamethasone-induced fatty liver in mice, which may be related to glucocorticoid receptor–mediated FTO trans-activation and m6A demethylation (Hu et al., 2020). These results indicate the potential mechanism of action of m6A on glucocorticoids, which establishes an important direction for addressing steroid resistance in asthma.
In animal experiments, inhibition of METTL3 in CD4+ T cells of STM2457-treated mice mediated downregulation of m6A levels, which in turn suppressed T-bet expression and inhibited Th1 differentiation of CD4+ T cells (Li et al., 2022). In a mouse model of viral infection, METTL3-m6A regulated T-bet expression to regulate the CD8+ T cell effector differentiation function (Guo et al., 2023). In a mouse model of asthma, dexamethasone and hemp mustard calming barb (Majie cataplasm) modulated the Th1/Th2 balance of asthmatic mice by regulating T-bet, which was significantly reduced in dexamethasone-treated asthmatic mice and significantly increased in M. cataplasm-treated asthmatic mice (Ji et al., 2020). In an OVA-induced asthma mouse model, locust yellow regulated Th1/Th2 and Treg/Th17 homeostasis by acting on the Th1 transcription factor T-bet (Liang et al., 2017). Thus, m6A may precisely treat asthma inflammatory subtypes by targeting T-bet transcription factors.
m6A can participate in the inflammatory response of asthma-associated inflammatory cells through the TLR4/NF-ΚB pathway. For example, METTL14/YTHDF1-m6A is involved in macrophage activation and the release of inflammatory factor IL-6 via TLR4/NF-ΚB in bacteria-infected mice (Du et al., 2020). In animal experiments, METTL3-m6A promoted IL-6, IL-1β production in neutrophils as well as reduced IL-6 and IL-12 levels in DC cells through the TLR4/NF-κB signaling pathway (Wang et al., 2019a; Luo et al., 2023). Inhibition of the TLR4/NF-ΚB pathway by FTO-m6A in turn inhibited TNFα and IL6 expression in the microglia in a mouse model of EAU (He et al., 2023). Meanwhile, Dubey et al. discovered that in cardiomyocytes from LPS-induced endotoxemia mice, FTO expression downregulated while increasing the overall m6A-RNA methylation level, which promoted the expression of IL-6, IL-1β, and TNF-α proinflammatory factors that aggravated myocardial injury (Dubey et al., 2022).
In a rat asthma model, Yanghe Pingchuan granules, traditional Chinese medicine, alleviated bronchial asthma airway inflammation by blocking the TLR4/NF-κB/NRLP3 signaling pathway to reduce the levels of inflammatory factors IL-1β and IL-18 in rat bronchial tissues (Pan et al., 2022). In addition, in a mouse model of OVA/LPS-induced neutrophilic airway inflammation in asthma, MUC1-CT attenuated neutrophilic airway inflammation in asthma and resisted steroid resistance through inhibition of the TLR4/NF-κB pathway (Liu et al., 2023). Dexmedetomidine (DEX) is a highly selective α2 adrenergic receptor agonist, which may ameliorate the Th2 inflammatory response in asthmatic mice by inhibiting TLR4/NF-κB (Zhou et al., 2023). Another related study confirmed that DEX attenuates asthma airway hyperresponsiveness and airway inflammation by reducing Th2-type cytokine production through inhibition of TLR4/NF-κB signaling in an OVA-induced asthma model in mice.
Considered together, m6A may pave the way for the treatment of inflammatory subtypes of asthma disease by modulating TLR4/NF-ΚB-related pathways, which may become a new therapeutic approach.
In a human cytomegalovirus infection model, vitamin D3 inhibited METTL3-m6A-mediated apoptosis by downregulating METTL3 through inhibiting AMPK activation (Zhu et al., 2022). Vitamin D3 (VitD3) is important in the adjunctive treatment of asthma, and supplementation with VitD3 induced an enhanced blockade of the antibody response and suppressed the eosinophilic inflammatory response as well as the expression of IL-10 in lung tissues (Hesse et al., 2020). In vitro experiments demonstrated that in lung fibroblasts, VitD3 may increase the efficacy of beclomethasone 17-propionate and montelukast sodium in resisting asthmatic airway remodeling at the mRNA level (Sobczak and Pawliczak, 2022).
In oxLDL-induced THP1 monocyte inflammation, METTL3/YTHDF2-m6A promotes the degradation of PGC-1α mRNA and facilitates monocyte inflammation and mitochondrial metabolism (Zhang et al., 2021). In a rat model of insulin resistance, METTL14 and YTHDF2 may coordinately mediate m6A methylation to regulate PGC-1α mRNA translation and stability, which induces iron death and insulin resistance (Zhang et al., 2023). PGC-1α mRNA is a key target in the mechanism of asthma, and in a mouse model of asthma airway smooth muscle cell proliferation, icariin increased activation of the SIRT1/AMPK/PGC-1α axis by inhibiting the expression of miR-138-5, ameliorating asthma oxidative stress and airway remodeling (Huang et al., 2023). Additional in vitro experiments have demonstrated that montelukast and zafirlukast enhance mitochondrial biogenesis and strengthen mitochondrial function by promoting the expression of PGC-1α mRNA in human bronchial epithelial cells, which is a novel pharmacological route for the treatment of asthma (Wang et al., 2019c; Ren et al., 2021).
In conclusion, m6A is a new target for the treatment of asthma, which is expected to address the role of NA steroid resistance and precisely target NA and EA for the treatment of asthma disease.
Su et al. (Sun et al., 2021) used 87 healthy controls and 344 severe asthma cases from the Unbiased Biomarkers for Predicting Outcomes of Respiratory Diseases (U-BIOPRED) study to systematically assess the pattern of m6A modifications mediated by 27 m6A modifiers, as well as the impact of their immune microenvironmental characteristics, by using the Wilcox test, R language for the 27 modifiers, logistic regression, and bioinformatics analysis such as COX regression analysis, which led to the discovery that YTHDF3 and YTHDC1 regulators play a crucial roles in severe asthma and can influence the prognosis of severe asthma. Another similar study extensively included RNA-modified regulatory factors and found that m6A RNA methylation of writer WTAP is closely associated with the development of severe asthma (Lin et al., 2022). Nutritional status largely influences the severity and prognosis of asthma. A recent META regression analysis study suggested that leptin levels were significantly higher in severe asthma cases than in mild asthma cases in the overall population and in Asians, independent of age and the sex ratio of the overall population, and may be a risk predictor and prognostic marker of asthma, which may be related to the association of leptin with obesity and body mass index in asthmatics (Wang et al., 2023d). Subsequent animal studies indicated that in an obese mouse model, FTO acted on CX3Cl1 mRNA to promote its de-m6A methylation, enhance its stability and half-life, and further participate in the hypothalamic leptin resistance function through the upregulation of CX3CL1/CX3CR1/SOCS3 (Liu et al., 2024). A multicenter cohort study confirmed the role of TGF-β in severe asthma and that its plasma levels were not affected by oral steroid medications (Sparreman et al., 2022). Another cellular assay confirmed that TGF-β can be an important factor for inducing epithelial-mesenchymal transition in human lung epithelial cells, which promotes the development of severe asthma and airway remodeling, greatly affecting the prognosis of asthma disease (Cheng et al., 2022). Another animal study indicated that in LPS-induced mouse Kupffer cells, METTL3/METTL14-m6A modification of TGF-β1 mRNA promoted the expressions of TGF-β1, IL-1β, and IL-6 as well as the development of hepatic fibrosis (Feng et al., 2021). Considered together, the m6A regulators YTHDF3, YTHDC1, WTAP, FTO, METTL3, and ETTL14 may have a significant effect on the prognosis of asthma, but studies on m6A methylation, severe asthma, and the prognosis of asthma are still in the initial stage and require further exploration by researchers.
m6A methylation plays an important role in the transcription and translation of many genes in the body in the presence of its different regulators, through which it becomes involved in the pathogenesis of asthma through a wide range of pathways, thereby affecting the inflammatory subtypes of asthma and steroid resistance during asthma treatment (Yang et al., 2018; Sun et al., 2021). Under the action of different cellular microenvironments and regulatory factors, m6A may produce multiple different or even contradictory effects and participate in the mechanisms of the development of inflammatory subtypes of asthma. Here, we discuss how m6A regulatory factors regulate the pathogenesis and treatment of different subtypes (EA, NA) of asthma disease, which may be of great value as important biomarkers for the prognosis and treatment of asthma subtypes.
Although we have gained a deeper understanding of asthma subtypes based on m6A-related mechanisms, the current study still has some limitations. First, the complex roles that m6A regulators play in different inflammatory cells make it difficult to clearly elucidate how they interact with each other to regulate the pathologic process of asthma subtypes, which requires us to understand their indispensable roles from a higher perspective. In addition, although there have been some studies on m6A-related combination drugs to improve asthma symptoms and steroid resistance, most of them remain at the mechanistic level, and clinical applications need to be further investigated.
m6A is a new way to diagnose asthma subtype classification and personalize treatment based on the subtypes of asthma. Different subtypes of asthma are regulated by different m6A-related genes, so exploration of these genes will guide the development of precise targeted therapies. The complex relationship between m6A regulators, asthma subtypes, and the specific mechanisms involved is an important direction for the researchers in the future.
GG: Writing–original draft. YH: Writing–original draft, Writing–review and editing. PG: Formal Analysis, Writing–review and editing. CW: Writing–review and editing.
The author(s) declare that financial support was received for the research, authorship, and/or publication of this article. This review was supported by R&D project on key technologies for social development (20240304055SF and 20240304201SF).
The authors declare that the research was conducted in the absence of any commercial or financial relationships that could be construed as a potential conflict of interest.
All claims expressed in this article are solely those of the authors and do not necessarily represent those of their affiliated organizations, or those of the publisher, the editors and the reviewers. Any product that may be evaluated in this article, or claim that may be made by its manufacturer, is not guaranteed or endorsed by the publisher.
Badi, Y. E., Salcman, B., Taylor, A., Rana, B., Kermani, N. Z., Riley, J. H., et al. (2023). IL1RAP expression and the enrichment of IL-33 activation signatures in severe neutrophilic asthma. Allergy 78 (1), 156–167. doi:10.1111/all.15487
Bai, H., Xue, Z., Zhang, W., Feng, C., Zhou, Z., Hu, S., et al. (2023). α-Asarone alleviates allergic asthma by stabilizing mast cells through inhibition of ERK/JAK2-STAT3 pathway. Biofactors 49 (1), 140–152. doi:10.1002/biof.1879
Bao, C., Liu, C., Liu, Q., Hua, L., Hu, J., Li, Z., et al. (2022). Liproxstatin-1 alleviates LPS/IL-13-induced bronchial epithelial cell injury and neutrophilic asthma in mice by inhibiting ferroptosis. Int. Immunopharmacol. 109, 108770. doi:10.1016/j.intimp.2022.108770
Bao, T., Liu, X., Hu, J., Ma, M., Li, J., Cao, L., et al. (2023). Recruitment of PVT1 enhances YTHDC1-mediated m6A modification of IL-33 in hyperoxia-induced lung injury during bronchopulmonary dysplasia. Inflammation 47, 469–482. doi:10.1007/s10753-023-01923-1
Barnes, P. J., Baker, J., and Donnelly, L. E. (2022). Autophagy in asthma and chronic obstructive pulmonary disease. Clin. Sci. Lond. Engl. 1979 136 (10), 733–746. doi:10.1042/CS20210900
Borish, L. (2016). The immunology of asthma: asthma phenotypes and their implications for personalized treatment. Ann. Allergy Asthma Immunol. 117 (2), 108–114. doi:10.1016/j.anai.2016.04.022
Bruchard, M., Rebé, C., Derangère, V., Togbé, D., Ryffel, B., Boidot, R., et al. (2015). The receptor NLRP3 is a transcriptional regulator of TH2 differentiation. Nat. Immunol. 16 (8), 859–870. doi:10.1038/ni.3202
Cai, L., Li, D., Feng, Z., Gu, X., Xu, Q., and Li, Q. (2022). YTHDF2 regulates macrophage polarization through NF-κB and MAPK signaling pathway inhibition or p53 degradation. Dis. markers 2022, 3153362. doi:10.1155/2022/3153362
Cao, F., Chen, G., Xu, Y., Wang, X., Tang, X., Zhang, W., et al. (2024). METTL14 contributes to acute lung injury by stabilizing NLRP3 expression in an IGF2BP2-dependent manner. Cell Death Dis. 15 (1), 43. doi:10.1038/s41419-023-06407-6
Cao, X., Shu, Y., Chen, Y., Xu, Q., Guo, G., Wu, Z., et al. (2021). Mettl14-Mediated m6A modification facilitates liver regeneration by maintaining endoplasmic reticulum homeostasis. Cell Mol. Gastroenterol. Hepatol. 12 (2), 633–651. doi:10.1016/j.jcmgh.2021.04.001
Carr, T. F., Zeki, A. A., and Kraft, M. (2018). Eosinophilic and noneosinophilic asthma. Am. J. Respir. Crit. care Med. 197 (1), 22–37. doi:10.1164/rccm.201611-2232PP
Chang, C., Chen, G., Wu, W., Chen, D., Chen, S., Gao, J., et al. (2023). Exogenous IL-25 ameliorates airway neutrophilia via suppressing macrophage M1 polarization and the expression of IL-12 and IL-23 in asthma. Respir. Res. 24 (1), 260. doi:10.1186/s12931-023-02557-5
Chao, Y., Shang, J., and Ji, W. (2020). ALKBH5-m6A-FOXM1 signaling axis promotes proliferation and invasion of lung adenocarcinoma cells under intermittent hypoxia. Biochem. Biophys. Res. Commun. 521 (2), 499–506. doi:10.1016/j.bbrc.2019.10.145
Chen, L., Hou, W., Liu, F., Zhu, R., Lv, A., Quan, W., et al. (2022a). Blockade of NLRP3/caspase-1/IL-1β regulated Th17/treg immune imbalance and attenuated the neutrophilic airway inflammation in an ovalbumin-induced murine model of asthma. J. Immunol. Res. 2022, 9444227. doi:10.1155/2022/9444227
Chen, P., Liu, J., Sun, K., Wang, L., Li, X., Li, X., et al. (2023). Methylation of TTC4 interaction with HSP70 inhibits pyroptosis in macrophages of sepsis-induced lung injury by NLRP3 inflammation. Am. J. Cancer Res. 13 (11), 5122–5137.
Chen, S., Chen, Z., Deng, Y., Zha, S., Yu, L., Li, D., et al. (2022b). Prevention of IL-6 signaling ameliorates toluene diisocyanate-induced steroid-resistant asthma. Allergol. Int. 71 (1), 73–82. doi:10.1016/j.alit.2021.07.004
Chen, X., Gong, W., Shao, X., Shi, T., Zhang, L., Dong, J., et al. (2022c). METTL3-mediated m6A modification of ATG7 regulates autophagy-GATA4 axis to promote cellular senescence and osteoarthritis progression. Ann. rheumatic Dis. 81 (1), 87–99. doi:10.1136/annrheumdis-2021-221091
Chen, Z., Shang, Y., Zhang, X., Duan, W., Li, J., Zhu, L., et al. (2024). METTL3 mediates SOX5 m6A methylation in bronchial epithelial cells to attenuate Th2 cell differentiation in T2 asthma. Heliyon 10 (7), e28884. doi:10.1016/j.heliyon.2024.e28884
Cheng, W.-H., Kao, S.-Y., Chen, C.-L., Yuliani, F. S., Lin, L.-Y., Lin, C.-H., et al. (2022). Amphiregulin induces CCN2 and fibronectin expression by TGF-β through EGFR-dependent pathway in lung epithelial cells. Respir. Res. 23 (1), 381. doi:10.1186/s12931-022-02285-2
Chung, K. F. (2016). Asthma phenotyping: a necessity for improved therapeutic precision and new targeted therapies. J. Intern Med. 279 (2), 192–204. doi:10.1111/joim.12382
Dai, B., Sun, F., Cai, X., Li, C., Liu, H., and Shang, Y. (2021). Significance of RNA N6-methyladenosine regulators in the diagnosis and subtype classification of childhood asthma using the gene expression Omnibus database. Front. Genet. 12, 634162. doi:10.3389/fgene.2021.634162
Deng, N., Zuo, X., Lin, Q., Wang, T., Li, Y., Zhong, J., et al. (2022). Low-dose 5-fluorouracil ameliorates Th2 responses through the induction of apoptotic cell death of lung monocyte-derived dendritic cells in asthma. Biomed. Pharmacother. 156, 113875. doi:10.1016/j.biopha.2022.113875
Desrosiers, R., Friderici, K., and Rottman, F. (1974). Identification of methylated nucleosides in messenger RNA from Novikoff hepatoma cells. Proc. Natl. Acad. Sci. U. S. A. 71 (10), 3971–3975. doi:10.1073/pnas.71.10.3971
Du, J., Liao, W., Liu, W., Deb, D. K., He, L., Hsu, P. J., et al. (2020). N6-Adenosine methylation of Socs1 mRNA is required to sustain the negative feedback control of macrophage activation. Dev. Cell 55 (6), 737–753.e7. doi:10.1016/j.devcel.2020.10.023
Dubey, P. K., Patil, M., Singh, S., Dubey, S., Ahuja, P., Verma, S. K., et al. (2022). Increased m6A-RNA methylation and FTO suppression is associated with myocardial inflammation and dysfunction during endotoxemia in mice. Mol. Cell Biochem. 477 (1), 129–141. doi:10.1007/s11010-021-04267-2
Fan, L., Wu, J., Wang, H., Chen, Q., He, X., Wang, Q., et al. (2022). METTL3-Mediated N6-methyladenosine methylation modifies Foxp3 mRNA levels and affects the Treg cells proportion in peripheral blood of patients with asthma. Ann. Clin. Lab. Sci. 52 (6), 884–894.
Fei, L., Sun, G., Sun, J., and Wu, D. (2022). The effect of N6-methyladenosine (m6A) factors on the development of acute respiratory distress syndrome in the mouse model. Bioengineered 13 (3), 7622–7634. doi:10.1080/21655979.2022.2049473
Feng, Y., Dong, H., Sun, B., Hu, Y., Yang, Y., Jia, Y., et al. (2021). METTL3/METTL14 transactivation and m6A-dependent TGF-β1 translation in activated kupffer cells. Cell Mol. Gastroenterol. Hepatol. 12 (3), 839–856. doi:10.1016/j.jcmgh.2021.05.007
Gao, W., Gong, J., Mu, M., Zhu, Y., Wang, W., Chen, W., et al. (2021). The pathogenesis of eosinophilic asthma: a positive feedback mechanism that promotes Th2 immune response via filaggrin deficiency. Front. Immunol. 12, 672312. doi:10.3389/fimmu.2021.672312
Gong, H., Liu, L., Cui, L., Ma, H., and Shen, L. (2021). ALKBH5-mediated m6A-demethylation of USP1 regulated T-cell acute lymphoblastic leukemia cell glucocorticoid resistance by Aurora B. Mol. Carcinog. 60 (9), 644–657. doi:10.1002/mc.23330
Gu, X., Zhang, Y., Li, D., Cai, H., Cai, L., and Xu, Q. (2020). N6-methyladenosine demethylase FTO promotes M1 and M2 macrophage activation. Cell Signal 69, 109553. doi:10.1016/j.cellsig.2020.109553
Guo, W., Wang, Z., Zhang, Y., Li, Y., Du, Q., Zhang, T., et al. (2023). Mettl3-dependent m6A modification is essential for effector differentiation and memory formation of CD8+ T cells. Sci. Bull. (Beijing) 69, 82–96. doi:10.1016/j.scib.2023.11.029
Han, L., Tieliwaerdi, N., and Li, X. (2024). METTL3-deficiency m6A-dependently degrades MALAT1 to suppress NLRP3-mediated pyroptotic cell death and inflammation in Mycobacterium tuberculosis (H37Ra strain)-infected mouse macrophages. Tuberc. (Edinb). 146, 102502. doi:10.1016/j.tube.2024.102502
Han, X., Liu, L., Huang, S., Xiao, W., Gao, Y., Zhou, W., et al. (2023). RNA m6A methylation modulates airway inflammation in allergic asthma via PTX3-dependent macrophage homeostasis. Nat. Commun. 14 (1), 7328. doi:10.1038/s41467-023-43219-w
Hao, W. Y., Lou, Y., Hu, G. Y., Qian, C. Y., Liang, W. R., Zhao, J., et al. (2022). RNA m6A reader YTHDF1 facilitates inflammation via enhancing NLRP3 translation. Biochem. Biophys. Res. Commun. 616, 76–81. doi:10.1016/j.bbrc.2022.05.076
Hastie, A. T., Mauger, D. T., Denlinger, L. C., Coverstone, A., Castro, M., Erzurum, S., et al. (2021). Mixed sputum granulocyte longitudinal impact on lung function in the severe asthma research program. Am. J. Respir. Crit. care Med. 203 (7), 882–892. doi:10.1164/rccm.202009-3713OC
He, M., Li, D., Fang, C., and Xu, Q. (2022). YTHDF1 regulates endoplasmic reticulum stress, NF-κB, MAPK and PI3K-AKT signaling pathways in inflammatory osteoclastogenesis. Arch. Biochem. Biophys. 732, 109464. doi:10.1016/j.abb.2022.109464
He, S., Li, W., Wang, G., Wang, X., Fan, W., Zhang, Z., et al. (2023). FTO-mediated m6A modification alleviates autoimmune uveitis by regulating microglia phenotypes via the GPC4/TLR4/NF-κB signaling axis. Genes and Dis. 10 (5), 2179–2193. doi:10.1016/j.gendis.2022.09.008
Hesse, L., Petersen, A. H., Oude Elberink, J. N. G., van Oosterhout, A. J. M., and Nawijn, M. C. (2020). 1,25(OH)2VitD3 supplementation enhances suppression of grass pollen-induced allergic asthma by subcutaneous and sublingual immunotherapy in a mouse model. Sci. Rep. 10 (1), 8960. doi:10.1038/s41598-020-65946-6
Hu, C., Zhang, B., and Zhao, S. (2023). METTL3-mediated N6-methyladenosine modification stimulates mitochondrial damage and ferroptosis of kidney tubular epithelial cells following acute kidney injury by modulating the stabilization of MDM2-p53-LMNB1 axis. Eur. J. Med. Chem. 259, 115677. doi:10.1016/j.ejmech.2023.115677
Hu, Y., Feng, Y., Zhang, L., Jia, Y., Cai, D., Qian, S.-B., et al. (2020). GR-mediated FTO transactivation induces lipid accumulation in hepatocytes via demethylation of m6A on lipogenic mRNAs. RNA Biol. 17 (7), 930–942. doi:10.1080/15476286.2020.1736868
Hu, Z., Li, Y., Yuan, W., Jin, L., Leung, W. K., Zhang, C., et al. (2022). N6-methyladenosine of Socs1 modulates macrophage inflammatory response in different stiffness environments. Int. J. Biol. Sci. 18 (15), 5753–5769. doi:10.7150/ijbs.74196
Huang, Y.-F., Ou, G.-C., Ma, S.-H., Liu, M.-W., and Deng, W. (2023). Effect of icariin on the H2O2-induced proliferation of mouse airway smooth muscle cells through miR-138-5p regulating SIRT1/AMPK/PGC-1α axis. Int. J. Immunopathol. Pharmacol. 37, 3946320231151515. doi:10.1177/03946320231151515
Huangfu, N., Zheng, W., Xu, Z., Wang, S., Wang, Y., Cheng, J., et al. (2020). RBM4 regulates M1 macrophages polarization through targeting STAT1-mediated glycolysis. Int. Immunopharmacol. 83, 106432. doi:10.1016/j.intimp.2020.106432
Hudey, S. N., Ledford, D. K., and Cardet, J. C. (2020). Mechanisms of non-type 2 asthma. Curr. Opin. Immunol. 66, 123–128. doi:10.1016/j.coi.2020.10.002
Izumi, G., Nakano, H., Nakano, K., Whitehead, G. S., Grimm, S. A., Fessler, M. B., et al. (2021). CD11b+ lung dendritic cells at different stages of maturation induce Th17 or Th2 differentiation. Nat. Commun. 12 (1), 5029. doi:10.1038/s41467-021-25307-x
Janssen, P., Tosi, I., Hego, A., Maréchal, P., Marichal, T., and Radermecker, C. (2022). Neutrophil extracellular traps are found in bronchoalveolar lavage fluids of horses with severe asthma and correlate with asthma severity. Front. Immunol. 13, 921077. doi:10.3389/fimmu.2022.921077
Ji, W., Zhang, Q., Shi, H., Dong, R., Ge, D., Du, X., et al. (2020). The mediatory role of Majie cataplasm on inflammation of allergic asthma through transcription factors related to Th1 and Th2. Chin. Med. 15, 53. doi:10.1186/s13020-020-00334-w
Jia, G., Fu, Y., Zhao, X., Dai, Q., Zheng, G., Yang, Y., et al. (2011). N6-methyladenosine in nuclear RNA is a major substrate of the obesity-associated FTO. Nat. Chem. Biol. 7 (12), 885–887. doi:10.1038/nchembio.687
Kaliner, M. (1989). Asthma and mast cell activation. J. Allergy Clin. Immunol. 83 (2 Pt 2), 510–520. doi:10.1016/0091-6749(89)90031-6
Kallies, A., and Good-Jacobson, K. L. (2017). Transcription factor T-bet orchestrates lineage development and function in the immune system. Trends Immunol. 38 (4), 287–297. doi:10.1016/j.it.2017.02.003
Kim, H., Lee, Y. S., Kim, S. M., Jang, S., Choi, H., Lee, J. W., et al. (2021). RNA demethylation by FTO stabilizes the FOXJ1 mRNA for proper motile ciliogenesis. Dev. Cell 56 (8), 1118–1130.e6. doi:10.1016/j.devcel.2021.03.006
Kim, S.-M., Oh, S.-C., Lee, S.-Y., Kong, L.-Z., Lee, J.-H., and Kim, T.-D. (2023). FTO negatively regulates the cytotoxic activity of natural killer cells. EMBO Rep. 24 (4), e55681. doi:10.15252/embr.202255681
Komlósi, Z. I., van de Veen, W., Kovács, N., Szűcs, G., Sokolowska, M., O'Mahony, L., et al. (2022). Cellular and molecular mechanisms of allergic asthma. Mol. Asp. Med. 85, 100995. doi:10.1016/j.mam.2021.100995
Kong, Y., Zhang, Y., Cai, Y., Li, D., Yi, B., and Xu, Q. (2022). METTL3 mediates osteoblast apoptosis by regulating endoplasmic reticulum stress during LPS-induced inflammation. Cell Signal 95, 110335. doi:10.1016/j.cellsig.2022.110335
Lan, J., Xu, B., Shi, X., Pan, Q., and Tao, Q. (2022). WTAP-mediated N(6)-methyladenosine modification of NLRP3 mRNA in kidney injury of diabetic nephropathy. Cell. Mol. Biol. Lett. 27 (1), 51. doi:10.1186/s11658-022-00350-8
Lee, W. L., and Grinstein, S. (2004). Immunology. The tangled webs that neutrophils weave. Science 303 (5663), 1477–1478. doi:10.1126/science.1095484
Lence, T., Paolantoni, C., Worpenberg, L., and Roignant, J.-Y. (2019). Mechanistic insights into m6A RNA enzymes. Biochim. Biophys. Acta Gene Regul. Mech. 1862 (3), 222–229. doi:10.1016/j.bbagrm.2018.10.014
Leoni, C., Bataclan, M., Ito-Kureha, T., Heissmeyer, V., and Monticelli, S. (2023). The mRNA methyltransferase Mettl3 modulates cytokine mRNA stability and limits functional responses in mast cells. Nat. Commun. 14 (1), 3862. doi:10.1038/s41467-023-39614-y
Li, H.-B., Tong, J., Zhu, S., Batista, P. J., Duffy, E. E., Zhao, J., et al. (2017). m6A mRNA methylation controls T cell homeostasis by targeting the IL-7/STAT5/SOCS pathways. Nature 548 (7667), 338–342. doi:10.1038/nature23450
Li, S., Zou, D., Chen, W., Britz, G. W., Liu, Z., and Weng, Y. L. (2022b). METTL3 inhibition reduces N(6) -methyladenosine levels and prevents allogeneic CD4(+) T-cell responses. Immunol. Cell Biol. 100 (9), 718–730. doi:10.1111/imcb.12581
Li, S.-X., Yan, W., Liu, J.-P., Zhao, Y.-J., and Chen, L. (2021). Long noncoding RNA SNHG4 remits lipopolysaccharide-engendered inflammatory lung damage by inhibiting METTL3 - mediated m6A level of STAT2 mRNA. Mol. Immunol. 139, 10–22. doi:10.1016/j.molimm.2021.08.008
Li, W., Gao, R., Xin, T., and Gao, P. (2020). Different expression levels of interleukin-35 in asthma phenotypes. Respir. Res. 21 (1), 89. doi:10.1186/s12931-020-01356-6
Li, Y., Li, J., Yu, Q., Ji, L., and Peng, B. (2023). METTL14 regulates microglia/macrophage polarization and NLRP3 inflammasome activation after ischemic stroke by the KAT3B-STING axis. Neurobiol. Dis. 185, 106253. doi:10.1016/j.nbd.2023.106253
Li, Z., Teng, M., Jiang, Y., Zhang, L., Luo, X., Liao, Y., et al. (2022a). YTHDF1 negatively regulates Treponema pallidum-induced inflammation in THP-1 macrophages by promoting SOCS3 translation in an m6A-dependent manner. Front. Immunol. 13, 857727. doi:10.3389/fimmu.2022.857727
Liang, P., Peng, S., Zhang, M., Ma, Y., Zhen, X., and Li, H. (2017). Huai Qi Huang corrects the balance of Th1/Th2 and Treg/Th17 in an ovalbumin-induced asthma mouse model. Biosci. Rep. 37 (6). doi:10.1042/BSR20171071
Lin, Q., Ni, H., Zheng, Z., Zhong, J., and Nie, H. (2022). Cross-talk of four types of RNA modification writers defines the immune microenvironment in severe asthma. Ann. N. Y. Acad. Sci. 1514 (1), 93–103. doi:10.1111/nyas.14782
Liu, B., Sun, H., Wang, J., Liu, H., and Zhao, C. (2021a). Potential role for EZH2 in promotion of asthma through suppression of miR-34b transcription by inhibition of FOXO3. Lab. Invest. 101 (8), 998–1010. doi:10.1038/s41374-021-00585-7
Liu, C., Chen, H., Tao, X., Li, C., Li, A., and Wu, W. (2023b). ALKBH5 protects against stroke by reducing endoplasmic reticulum stress-dependent inflammation injury via the STAT5/PERK/EIF2α/CHOP signaling pathway in an m6A-YTHDF1-dependent manner. Exp. Neurol. 372, 114629. doi:10.1016/j.expneurol.2023.114629
Liu, J., Zhang, X., Chen, K., Cheng, Y., Liu, S., Xia, M., et al. (2019). CCR7 chemokine receptor-inducible lnc-dpf3 restrains dendritic cell migration by inhibiting HIF-1α-Mediated glycolysis. Immunity 50 (3), 600–615. doi:10.1016/j.immuni.2019.01.021
Liu, L., Zhou, L., Wang, L., Mao, Z., Zheng, P., Zhang, F., et al. (2023a). MUC1 attenuates neutrophilic airway inflammation in asthma by reducing NLRP3 inflammasome-mediated pyroptosis through the inhibition of the TLR4/MyD88/NF-κB pathway. Respir. Res. 24 (1), 255. doi:10.1186/s12931-023-02550-y
Liu, S., Song, S., Wang, S., Cai, T., Qin, L., Wang, X., et al. (2024). Hypothalamic FTO promotes high-fat diet-induced leptin resistance in mice through increasing CX3CL1 expression. J. Nutr. Biochem. 123, 109512. doi:10.1016/j.jnutbio.2023.109512
Liu, T., Wang, L., Liang, P., Wang, X., Liu, Y., Cai, J., et al. (2021b). USP19 suppresses inflammation and promotes M2-like macrophage polarization by manipulating NLRP3 function via autophagy. Cell Mol. Immunol. 18 (10), 2431–2442. doi:10.1038/s41423-020-00567-7
Liu, Y., Song, R., Zhao, L., Lu, Z., Li, Y., Zhan, X., et al. (2022). m6A demethylase ALKBH5 is required for antibacterial innate defense by intrinsic motivation of neutrophil migration. Signal Transduct. Target Ther. 7 (1), 194. doi:10.1038/s41392-022-01020-z
Lu, T. X., Zheng, Z., Zhang, L., Sun, H.-L., Bissonnette, M., Huang, H., et al. (2020). A new model of spontaneous colitis in mice induced by deletion of an RNA m6A methyltransferase component METTL14 in T cells. Cell Mol. Gastroenterol. Hepatol. 10 (4), 747–761. doi:10.1016/j.jcmgh.2020.07.001
Luo, S., Liao, C., Zhang, L., Ling, C., Zhang, X., Xie, P., et al. (2023). METTL3-mediated m6A mRNA methylation regulates neutrophil activation through targeting TLR4 signaling. Cell Rep. 42 (3), 112259. doi:10.1016/j.celrep.2023.112259
Luo, W., Hu, J., Xu, W., and Dong, J. (2022). Distinct spatial and temporal roles for Th1, Th2, and Th17 cells in asthma. Front. Immunol. 13, 974066. doi:10.3389/fimmu.2022.974066
Ma, S., Yan, J., Barr, T., Zhang, J., Chen, Z., Wang, L.-S., et al. (2021). The RNA m6A reader YTHDF2 controls NK cell antitumor and antiviral immunity. J. Exp. Med. 218 (8), e20210279. doi:10.1084/jem.20210279
Mattos, M. S., Ferrero, M. R., Kraemer, L., Lopes, G. A. O., Reis, D. C., Cassali, G. D., et al. (2020). CXCR1 and CXCR2 inhibition by ladarixin improves neutrophil-dependent airway inflammation in mice. Front. Immunol. 11, 566953. doi:10.3389/fimmu.2020.566953
Meng, L., Lin, H., Huang, X., Weng, J., Peng, F., and Wu, S. (2022). METTL14 suppresses pyroptosis and diabetic cardiomyopathy by downregulating TINCR lncRNA. Cell Death Dis. 13 (1), 38. doi:10.1038/s41419-021-04484-z
Menson, K. E., Mank, M. M., Reed, L. F., Walton, C. J., Van Der Vliet, K. E., Ather, J. L., et al. (2020). Therapeutic efficacy of IL-17A neutralization with corticosteroid treatment in a model of antigen-driven mixed-granulocytic asthma. Am. J. Physiol. Lung Cell Mol. Physiol. 319 (4), L693–L709. doi:10.1152/ajplung.00204.2020
Miao, Y., Zheng, Y., Geng, Y., Yang, L., Cao, N., Dai, Y., et al. (2021). The role of GLS1-mediated glutaminolysis/2-HG/H3K4me3 and GSH/ROS signals in Th17 responses counteracted by PPARγ agonists. Theranostics 11 (9), 4531–4548. doi:10.7150/thno.54803
Milara, J., Ballester, B., de Diego, A., Calbet, M., Ramis, I., Miralpeix, M., et al. (2022). The pan-JAK inhibitor LAS194046 reduces neutrophil activation from severe asthma and COPD patients in vitro. Sci. Rep. 12 (1), 5132. doi:10.1038/s41598-022-09241-6
Mishra, A., Majid, D., Kandikattu, H. K., Yadavalli, C. S., and Upparahalli Venkateshaiah, S. (2022). Role of IL-18-transformed CD274-expressing eosinophils in promoting airway obstruction in experimental asthma. Allergy 77 (4), 1165–1179. doi:10.1111/all.15180
Mo, B.-W., Li, X.-M., Li, S.-M., Xiao, B., Yang, J., and Li, H.-M. (2022). m6A echoes with DNA methylation: coordinated DNA methylation and gene expression data analysis identified critical m6A genes associated with asthma. Gene 828, 146457. doi:10.1016/j.gene.2022.146457
Mogensen, I., James, A., and Malinovschi, A. (2020). Systemic and breath biomarkers for asthma: an update. Curr. Opin. Allergy Clin. Immunol. 20 (1), 71–79. doi:10.1097/ACI.0000000000000599
Murakami, Y., Ishii, T., Nunokawa, H., Kurata, K., Narita, T., and Yamashita, N. (2020). TLR9-IL-2 axis exacerbates allergic asthma by preventing IL-17A hyperproduction. Sci. Rep. 10 (1), 18110. doi:10.1038/s41598-020-75153-y
Nagasaki, T., Schuyler, A. J., Zhao, J., Samovich, S. N., Yamada, K., Deng, Y., et al. (2022). 15LO1 dictates glutathione redox changes in asthmatic airway epithelium to worsen type 2 inflammation. J. Clin. Invest. 132 (1), e151685. doi:10.1172/JCI151685
Ntontsi, P., Loukides, S., Bakakos, P., Kostikas, K., Papatheodorou, G., Papathanassiou, E., et al. (2017). Clinical, functional and inflammatory characteristics in patients with paucigranulocytic stable asthma: comparison with different sputum phenotypes. Allergy 72 (11), 1761–1767. doi:10.1111/all.13184
Olgac, M., Guler, S. D., Demir, S., Unal, D., Ertek, B., Ozseker, Z. F., et al. (2021). Paucigranulocytic asthma: do sputum macrophages matter? Allergy Asthma Proc. 42 (6), 530–536. doi:10.2500/aap.2021.42.210060
Pan, L., Chen, Y., Jiang, Y., Sun, Y., Han, Y., and Wang, Y. (2022). Yanghe pingchuan granules alleviate airway inflammation in bronchial asthma and inhibit pyroptosis by blocking the TLR4/NF-κB/NRLP3 signaling pathway. Mediat. Inflamm. 2022, 6561048. doi:10.1155/2022/6561048
Park, S. C., Kim, H., Bak, Y., Shim, D., Kwon, K. W., Kim, C. H., et al. (2020). An alternative dendritic cell-induced murine model of asthma exhibiting a robust Th2/Th17-skewed response. Allergy Asthma Immunol. Res. 12 (3), 537–555. doi:10.4168/aair.2020.12.3.537
Pu, Z., Wang, W., Xie, H., and Wang, W. (2024). Apolipoprotein C3 (ApoC3) facilitates NLRP3 mediated pyroptosis of macrophages through mitochondrial damage by accelerating of the interaction between SCIMP and SYK pathway in acute lung injury. Int. Immunopharmacol. 128, 111537. doi:10.1016/j.intimp.2024.111537
Qu, M., Chen, Z., Qiu, Z., Nan, K., Wang, Y., Shi, Y., et al. (2022b). Neutrophil extracellular traps-triggered impaired autophagic flux via METTL3 underlies sepsis-associated acute lung injury. Cell death Discov. 8 (1), 375. doi:10.1038/s41420-022-01166-3
Qu, T., Huang, B., Lei, L., and Luo, S. (2022a). Cetirizine inhibits activation of JAK2-STAT3 pathway and mast cell activation in lung tissue of asthmatic mice. Xi Bao Yu Fen Zi Mian Yi Xue Za Zhi 38 (3), 193–198.
Ren, P., Gong, F., Chang, L., Hong, X., Xing, L., and Zhang, H. (2021). Zafirlukast promotes mitochondrial respiration by stimulating mitochondrial biogenesis in human bronchial epithelial cells. J. Mol. Histol. 52 (4), 643–650. doi:10.1007/s10735-021-09974-0
Saito, T., Ichikawa, T., Numakura, T., Yamada, M., Koarai, A., Fujino, N., et al. (2021). PGC-1α regulates airway epithelial barrier dysfunction induced by house dust mite. Respir. Res. 22 (1), 63. doi:10.1186/s12931-021-01663-6
Sánchez-Ovando, S., Simpson, J. L., Barker, D., Baines, K. J., and Wark, P. A. B. (2021). Transcriptomics of biopsies identifies novel genes and pathways linked to neutrophilic inflammation in severe asthma. Clin. Exp. Allergy J. Br. Soc. For Allergy Clin. Immunol. 51 (10), 1279–1294. doi:10.1111/cea.13986
Saradna, A., Do, D. C., Kumar, S., Fu, Q.-L., and Gao, P. (2018). Macrophage polarization and allergic asthma. Transl. Res. 191, 1–14. doi:10.1016/j.trsl.2017.09.002
Silva, C. M. S., Wanderley, C. W. S., Veras, F. P., Sonego, F., Nascimento, D. C., Gonçalves, A. V., et al. (2021). Gasdermin D inhibition prevents multiple organ dysfunction during sepsis by blocking NET formation. Blood 138 (25), 2702–2713. doi:10.1182/blood.2021011525
Simpson, J. L., Scott, R., Boyle, M. J., and Gibson, P. G. (2006). Inflammatory subtypes in asthma: assessment and identification using induced sputum. Respirology 11 (1), 54–61. doi:10.1111/j.1440-1843.2006.00784.x
Sobczak, M., and Pawliczak, R. (2022). Does vitamin D work synergistically with anti-asthmatic drugs in airway remodeling? Int. J. Mol. Sci. 23 (21), 12798. doi:10.3390/ijms232112798
Song, H., Feng, X., Zhang, H., Luo, Y., Huang, J., Lin, M., et al. (2019). METTL3 and ALKBH5 oppositely regulate m6A modification of TFEB mRNA, which dictates the fate of hypoxia/reoxygenation-treated cardiomyocytes. Autophagy 15 (8), 1419–1437. doi:10.1080/15548627.2019.1586246
Sparreman, M. M., Kolmert, J., Andersson, L. I., Östling, J., Knowles, R. G., Gómez, C., et al. (2022). Plasma proteins elevated in severe asthma despite oral steroid use and unrelated to Type-2 inflammation. Eur. Respir. J. 59 (2), 2100142. doi:10.1183/13993003.00142-2021
Subbarayalu, P., Yadav, P., Timilsina, S., Medina, D., Baxi, K., Hromas, R., et al. (2023). The RNA demethylase ALKBH5 maintains endoplasmic reticulum homeostasis by regulating UPR, autophagy, and mitochondrial function. Cells 12 (9), 1283. doi:10.3390/cells12091283
Sun, D., Yang, H., Fan, L., Shen, F., and Wang, Z. (2021). m6A regulator-mediated RNA methylation modification patterns and immune microenvironment infiltration characterization in severe asthma. J. Cell. Mol. Med. 25 (21), 10236–10247. doi:10.1111/jcmm.16961
Tang, Y., Chen, K., Song, B., Ma, J., Wu, X., Xu, Q., et al. (2021). m6A-Atlas: a comprehensive knowledgebase for unraveling the N6-methyladenosine (m6A) epitranscriptome. Nucleic Acids Res. 49 (D1), D134–D143. doi:10.1093/nar/gkaa692
Teng, F., Tang, W., Wuniqiemu, T., Qin, J., Zhou, Y., Huang, X., et al. (2021). N (6)-methyladenosine methylomic landscape of lung tissues in murine acute allergic asthma. Front. Immunol. 12, 740571. doi:10.3389/fimmu.2021.740571
Tliba, O., and Panettieri, R. A. (2019). Paucigranulocytic asthma: uncoupling of airway obstruction from inflammation. J. Allergy Clin. Immunol. 143 (4), 1287–1294. doi:10.1016/j.jaci.2018.06.008
Tong, J., Wang, X., Liu, Y., Ren, X., Wang, A., Chen, Z., et al. (2021). Pooled CRISPR screening identifies m6A as a positive regulator of macrophage activation. Sci. Adv. 7 (18), eabd4742. doi:10.1126/sciadv.abd4742
Trian, T., Benard, G., Begueret, H., Rossignol, R., Girodet, P.-O., Ghosh, D., et al. (2007). Bronchial smooth muscle remodeling involves calcium-dependent enhanced mitochondrial biogenesis in asthma. J. Exp. Med. 204 (13), 3173–3181. doi:10.1084/jem.20070956
Wan, R., Jiang, J., Hu, C., Chen, X., Chen, C., Zhao, B., et al. (2020). Neutrophil extracellular traps amplify neutrophil recruitment and inflammation in neutrophilic asthma by stimulating the airway epithelial cells to activate the TLR4/NF-κB pathway and secrete chemokines. Aging (Albany NY) 12 (17), 16820–16836. doi:10.18632/aging.103479
Wang, H., Cheng, Y., Liu, Y., Shi, J., and Cheng, Z. (2019c). Montelukast promotes mitochondrial biogenesis via CREB/PGC-1α in human bronchial epithelial cells. Artif. Cells Nanomed Biotechnol. 47 (1), 4234–4239. doi:10.1080/21691401.2019.1687502
Wang, H., Hu, X., Huang, M., Liu, J., Gu, Y., Ma, L., et al. (2019a). Mettl3-mediated mRNA m6A methylation promotes dendritic cell activation. Nat. Commun. 10 (1), 1898. doi:10.1038/s41467-019-09903-6
Wang, J., Huang, J., and Fang, L. (2022a). Inhibition of TLR4 suppresses the inflammatory response in inflammatory bowel disease (IBD) by modulating the PDK1-induced metabolism reprogramming via a m6A-denpendent manner. Comput. Math. methods Med. 2022, 1335562. doi:10.1155/2022/1335562
Wang, J., Wang, L., Tian, X., and Luo, L. (2023a). N6-methyladenosine reader YTHDF1 regulates the proliferation and migration of airway smooth muscle cells through m6A/cyclin D1 in asthma. PeerJ 11, e14951. doi:10.7717/peerj.14951
Wang, J., Yan, S., Lu, H., Wang, S., and Xu, D. (2019b). METTL3 attenuates LPS-induced inflammatory response in macrophages via NF-B signaling pathway. Mediat. Inflamm. 2019, 3120391. doi:10.1155/2019/3120391
Wang, J., Zhu, R., Shi, W., and Mao, S. (2023d). Predictive and prognostic value of leptin status in asthma. NPJ Prim. Care Respir. Med. 33 (1), 10. doi:10.1038/s41533-023-00332-z
Wang, R., Wang, Y., Liao, G., Chen, B., Panettieri, R. A., Penn, R. B., et al. (2022b). Abi1 mediates airway smooth muscle cell proliferation and airway remodeling via Jak2/STAT3 signaling. iScience 25 (2), 103833. doi:10.1016/j.isci.2022.103833
Wang, X., Chen, C., Sun, H., Mao, K., Yao, J., Zhang, W., et al. (2023b). m6A mRNA modification potentiates Th17 functions to inflame autoimmunity. Sci. China Life Sci. 66 (11), 2543–2552. doi:10.1007/s11427-022-2323-4
Wang, Y., Wang, J., Yan, Z., Liu, S., and Xu, W. (2023c). Microenvironment modulation by key regulators of RNA N6-methyladenosine modification in respiratory allergic diseases. BMC Pulm. Med. 23 (1), 210. doi:10.1186/s12890-023-02499-0
Williams, E. J., Negewo, N. A., and Baines, K. J. (2021). Role of the NLRP3 inflammasome in asthma: relationship with neutrophilic inflammation, obesity, and therapeutic options. J. Allergy Clin. Immunol. 147 (6), 2060–2062. doi:10.1016/j.jaci.2021.04.022
Wu, C., Chen, W., He, J., Jin, S., Liu, Y., Yi, Y., et al. (2020). Interplay of m6A and H3K27 trimethylation restrains inflammation during bacterial infection. Sci. Adv. 6 (34), eaba0647. doi:10.1126/sciadv.aba0647
Xie, Y., Abel, P. W., Casale, T. B., and Tu, Y. (2022). TH17 cells and corticosteroid insensitivity in severe asthma. J. Allergy Clin. Immunol. 149 (2), 467–479. doi:10.1016/j.jaci.2021.12.769
Xing, Y., Cheng, D., Shi, C., and Shen, Z. (2021). The protective role of YTHDF1-knock down macrophages on the immune paralysis of severe sepsis rats with ECMO. Microvasc. Res. 137, 104178. doi:10.1016/j.mvr.2021.104178
Xiong, A., He, X., Liu, S., Ran, Q., Zhang, L., Wang, J., et al. (2024). Oxidative stress-mediated activation of FTO exacerbates impairment of the epithelial barrier by up-regulating IKBKB via N6-methyladenosine-dependent mRNA stability in asthmatic mice exposed to PM2.5. Ecotoxicol. Environ. Saf. 272, 116067. doi:10.1016/j.ecoenv.2024.116067
Xiong, X., Hou, L., Park, Y. P., Molinie, B., Gregory, R. I., Kellis, M., et al. (2021). Genetic drivers of m6A methylation in human brain, lung, heart and muscle. Nat. Genet. 53 (8), 1156–1165. doi:10.1038/s41588-021-00890-3
Yang, Y., Hsu, P. J., Chen, Y.-S., and Yang, Y.-G. (2018). Dynamic transcriptomic m6A decoration: writers, erasers, readers and functions in RNA metabolism. Cell Res. 28 (6), 616–624. doi:10.1038/s41422-018-0040-8
Yang, Y., Yang, X., Wu, Y., and Fu, M. (2021). METTL3 promotes inflammation and cell apoptosis in a pediatric pneumonia model by regulating EZH2. Allergol. Immunopathol. Madr. 49 (5), 49–56. doi:10.15586/aei.v49i5.445
Yao, Y., Yang, Y., Guo, W., Xu, L., You, M., Zhang, Y.-C., et al. (2021). METTL3-dependent m6A modification programs T follicular helper cell differentiation. Nat. Commun. 12 (1), 1333. doi:10.1038/s41467-021-21594-6
Yu, J. T., Hu, X. W., Chen, H. Y., Yang, Q., Li, H. D., Dong, Y. H., et al. (2021). DNA methylation of FTO promotes renal inflammation by enhancing m(6)A of PPAR-α in alcohol-induced kidney injury. Pharmacol. Res. 163, 105286. doi:10.1016/j.phrs.2020.105286
Yu, Q., Yu, X., Zhao, W., Zhu, M., Wang, Z., Zhang, J., et al. (2018). Inhibition of H3K27me3 demethylases attenuates asthma by reversing the shift in airway smooth muscle phenotype. Clin. Exp. Allergy J. Br. Soc. For Allergy Clin. Immunol. 48 (11), 1439–1452. doi:10.1111/cea.13244
Yu, R., Li, Q., Feng, Z., Cai, L., and Xu, Q. (2019). m6A reader YTHDF2 regulates LPS-induced inflammatory response. Int. J. Mol. Sci. 20 (6), 1323. doi:10.3390/ijms20061323
Zaccara, S., Ries, R. J., and Jaffrey, S. R. (2019). Reading, writing and erasing mRNA methylation. Nat. Rev. Mol. Cell Biol. 20 (10), 608–624. doi:10.1038/s41580-019-0168-5
Zhan, J., Yang, J., Zheng, J., Qi, Y., Ye, Y., Chen, X., et al. (2023). CircMIRLET7BHG, upregulated in an m6A-dependent manner, induces the nasal epithelial barrier dysfunction in allergic rhinitis pathogenesis. Int. Immunopharmacol. 125 (Pt B), 111162. doi:10.1016/j.intimp.2023.111162
Zhang, H., Liu, J., Zhou, Y., Qu, M., Wang, Y., Guo, K., et al. (2022b). Neutrophil extracellular traps mediate m6A modification and regulates sepsis-associated acute lung injury by activating ferroptosis in alveolar epithelial cells. Int. J. Biol. Sci. 18 (8), 3337–3357. doi:10.7150/ijbs.69141
Zhang, H., Wu, D., Wang, Y., Guo, K., Spencer, C. B., Ortoga, L., et al. (2023a). METTL3-mediated N6-methyladenosine exacerbates ferroptosis via m6A-IGF2BP2-dependent mitochondrial metabolic reprogramming in sepsis-induced acute lung injury. Clin. Transl. Med. 13 (9), e1389. doi:10.1002/ctm2.1389
Zhang, J., Song, J., Liu, S., Zhang, Y., Qiu, T., Jiang, L., et al. (2023b). m6A methylation-mediated PGC-1α contributes to ferroptosis via regulating GSTK1 in arsenic-induced hepatic insulin resistance. Sci. Total Environ. 905, 167202. doi:10.1016/j.scitotenv.2023.167202
Zhang, S., Guan, X., Liu, W., Zhu, Z., Jin, H., Zhu, Y., et al. (2022a). YTHDF1 alleviates sepsis by upregulating WWP1 to induce NLRP3 ubiquitination and inhibit caspase-1-dependent pyroptosis. Cell death Discov. 8 (1), 244. doi:10.1038/s41420-022-00872-2
Zhang, X., Li, X., Jia, H., An, G., and Ni, J. (2021). The m6A methyltransferase METTL3 modifies PGC-1α mRNA promoting mitochondrial dysfunction and oxLDL-induced inflammation in monocytes. J. Biol. Chem. 297 (3), 101058. doi:10.1016/j.jbc.2021.101058
Zhao, D., Wang, C., Liu, X., Liu, N., Zhuang, S., Zhang, Q., et al. (2021). CircN4bp1 facilitates sepsis-induced acute respiratory distress syndrome through mediating macrophage polarization via the miR-138-5p/EZH2 Axis. Mediat. Inflamm. 2021, 7858746. doi:10.1155/2021/7858746
Zhao, L., Liu, Y., Ma, B., Liu, X., Wei, R., and Nian, H. (2023). METTL3 inhibits autoreactive Th17 cell responses in experimental autoimmune uveitis via stabilizing ASH1L mRNA. FASEB J. 37 (3), e22803. doi:10.1096/fj.202201548R
Zheng, G., Dahl, J. A., Niu, Y., Fedorcsak, P., Huang, C.-M., Li, C. J., et al. (2013). ALKBH5 is a mammalian RNA demethylase that impacts RNA metabolism and mouse fertility. Mol. Cell 49 (1), 18–29. doi:10.1016/j.molcel.2012.10.015
Zheng, Y., Li, Y., Ran, X., Wang, D., Zheng, X., Zhang, M., et al. (2022). Mettl14 mediates the inflammatory response of macrophages in atherosclerosis through the NF-κB/IL-6 signaling pathway. Cell. Mol. life Sci. CMLS 79 (6), 311. doi:10.1007/s00018-022-04331-0
Zhou, H., Shen, X., Yan, C., Xiong, W., Ma, Z., Tan, Z., et al. (2022). Extracellular vesicles derived from human umbilical cord mesenchymal stem cells alleviate osteoarthritis of the knee in mice model by interacting with METTL3 to reduce m6A of NLRP3 in macrophage. Stem Cell Res. Ther. 13 (1), 322. doi:10.1186/s13287-022-03005-9
Zhou, J., Zhang, X., Hu, J., Qu, R., Yu, Z., Xu, H., et al. (2021). m 6 A demethylase ALKBH5 controls CD4 + T cell pathogenicity and promotes autoimmunity. Sci. Adv. 7 (25). doi:10.1126/sciadv.abg0470
Zhou, Y., Du, X., Wang, Q., Xiao, S., Zhi, J., Gao, H., et al. (2023). Dexmedetomidine protects against airway inflammation and airway remodeling in a murine model of chronic asthma through TLR4/NF-κB signaling pathway. Mediat. Inflamm. 2023, 3695469. doi:10.1155/2023/3695469
Zhu, L.-M., Zeng, D., Lei, X.-C., Huang, J., Deng, Y.-F., Ji, Y.-B., et al. (2020). KLF2 regulates neutrophil migration by modulating CXCR1 and CXCR2 in asthma. Biochim. Biophys. Acta Mol. Basis Dis. 1866 (12), 165920. doi:10.1016/j.bbadis.2020.165920
Zhu, W., Zhang, H., and Wang, S. (2022). Vitamin D3 suppresses human cytomegalovirus-induced vascular endothelial apoptosis via rectification of paradoxical m6A modification of mitochondrial calcium uniporter mRNA, which is regulated by METTL3 and YTHDF3. Front. Microbiol. 13, 861734. doi:10.3389/fmicb.2022.861734
Keywords: N 6 -methyladenosine, asthma, asthma phenotype, inflammation, inflammatory disease
Citation: Gao G, Hao YQ, Wang C and Gao P (2024) Role and regulators of N6-methyladenosine (m6A) RNA methylation in inflammatory subtypes of asthma: a comprehensive review. Front. Pharmacol. 15:1360607. doi: 10.3389/fphar.2024.1360607
Received: 23 December 2023; Accepted: 03 June 2024;
Published: 23 July 2024.
Edited by:
Ahmed Nadeem, King Saud University, Saudi ArabiaReviewed by:
Ang Luo, Northwest A&F University, ChinaCopyright © 2024 Gao, Hao, Wang and Gao. This is an open-access article distributed under the terms of the Creative Commons Attribution License (CC BY). The use, distribution or reproduction in other forums is permitted, provided the original author(s) and the copyright owner(s) are credited and that the original publication in this journal is cited, in accordance with accepted academic practice. No use, distribution or reproduction is permitted which does not comply with these terms.
*Correspondence: Peng Gao, Z2FvcGVuZzEyMzRAc2luYS5jb20=
†These authors have contributed equally to this work and share senior authorship
Disclaimer: All claims expressed in this article are solely those of the authors and do not necessarily represent those of their affiliated organizations, or those of the publisher, the editors and the reviewers. Any product that may be evaluated in this article or claim that may be made by its manufacturer is not guaranteed or endorsed by the publisher.
Research integrity at Frontiers
Learn more about the work of our research integrity team to safeguard the quality of each article we publish.