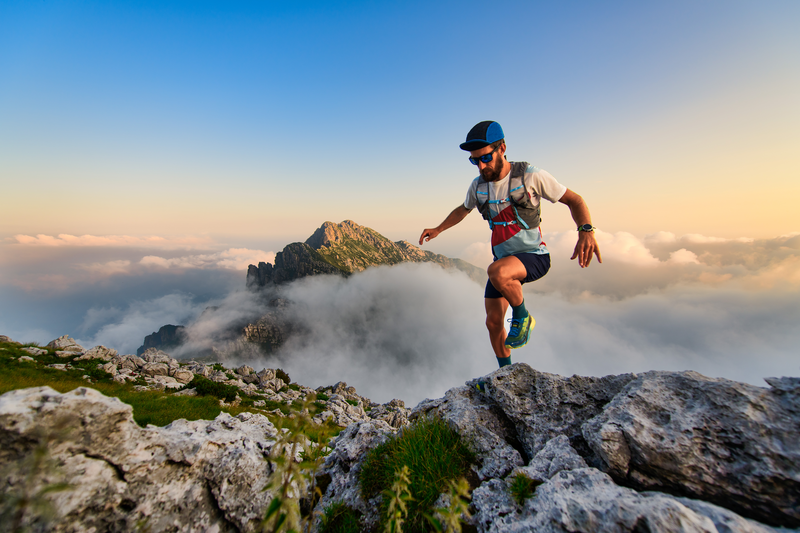
95% of researchers rate our articles as excellent or good
Learn more about the work of our research integrity team to safeguard the quality of each article we publish.
Find out more
ORIGINAL RESEARCH article
Front. Pharmacol. , 23 February 2024
Sec. Gastrointestinal and Hepatic Pharmacology
Volume 15 - 2024 | https://doi.org/10.3389/fphar.2024.1358262
This article is part of the Research Topic Novel Insights into Liver Injury: Mechanisms, Pathophysiology, and Therapeutic Strategies View all 7 articles
Valproic acid (VPA) has been widely used as an antiepileptic drug for decades. Although VPA is effective and well-tolerated, long-term VPA treatment is usually associated with hepatotoxicity. However, the underlying mechanisms of VPA-caused hepatotoxicity remain unclear. In this study, a total of 157 pediatric patients with epilepsy were recruited and divided into normal liver function (NLF, 112 subjects) group and abnormal liver function (ABLF, 45 subjects) group. We observed that MTHFR A1298C and MTHFR C677T variants may be linked to VPA-induced liver dysfunction (p = 0.001; p = 0.023, respectively). We also found that the MTHFR A1298C polymorphism was associated with a higher serum Hcy level (p = 0.001) and a lower FA level (p = 0.001). Moreover, the serum Hcy levels was strongly correlated with the GSH and TBARS concentrations (r = −0.6065, P < 0.001; r = 0.6564, P < 0.001, respectively). Furthermore, logistic analysis indicated that MTHFR A1298C/C677T polymorphisms and increased Hcy concentrations may be risk factors for VPA-induced liver dysfunction. These results suggested that individual susceptibility to VPA-induced liver dysfunction may result from MTHFR A1298C/C677T polymorphisms and increased Hcy levels. This study may be helpful for the prevention and guidance of VPA-induced liver dysfunction.
Epilepsy, one of the most common central nervous system disorders, affects more than 70 million people worldwide (Thijs et al., 2019). Epilepsy is usually characterized by the recurrence of unprovoked seizures, leading to neurological injury as well as psychosocial and cognitive consequences. For most epileptic patients, anti-epileptic drugs (AEDs) treatment is a priority modality. Valproic acid (VPA), a broad-spectrum AED, has been prescribed predominantly for the treatment of epilepsy and bipolar disorder for decades (Mishra et al., 2021). Although VPA was confirmed to be effective and well-tolerated, long-term VPA treatment is usually accompanied by hepatotoxicity (Guo et al., 2019; Ezhilarasan and Mani, 2022) However, the underlying mechanism for VPA-induced hepatotoxicity is not fully understood.
Accumulating evidence indicates that one-carbon metabolism (OCM) is involved in the progression of alcoholic liver disease (ALD) and nonalcoholic fatty liver disease (NAFLD) (Tsuchiya et al., 2012; Radziejewska et al., 2020). One-carbon (1C) metabolism, mediated by folate cofactors and co-substrates [Vitamin B6 (VB6), Vitamin B12 (VB12)], serves multiple biological processes (Ducker and Rabinowitz, 2017). In detail, folate and methionine cycles are included in the progression of OCM (Raghubeer and Matsha, 2021). Specifically, dietary folate acid (FA) is converted and reduced to tetrahydrofolate (THF). THF is converted to 5,10-methyleneTHF and then to 5-methylTHF via methylene tetrahydrofolate reductase (MTHFR) in the folate cycle (Petrone et al., 2021). 5-methylTHF is used as a methyl donor to generate methionine and its subsequent products [such as S-adenosyl Methionine, S-adenosyl homocysteine (Hcy) and Hcy] in the methionine cycle. This reaction is catalyzed by methyltransferase (MTR) and methionine synthase reductase (MTRR) with VB12 serving as a cofactor. Meanwhile, Hcy can also entre the transsulfuration pathway to synthesize glutathione (GSH) to defend against redox reactions (Figure 1) (McBean, 2012). However, there are limited data about its relationship with VPA-induced liver disease.
FIGURE 1. Schematic of One-Carbon metabolism related folate cycle, methionine cycle and transsulfration pathway. MTHFR, methylene tetrahydrofolate reductase; MTR, methionine synthase; MTRR, methionine synthase reductase CBS, cystathionine beta-synthase; ROS, reactive oxygen species.
Previous study indicated that VPA treatment disrupted the homeostasis of OCM in epileptic patients (Ni et al., 2018a). Additionally, another study indicated that patients underwent long-term VPA treatment were more susceptible to OCM dysmetabolism than general population (Ni et al., 2017). However, due to the complicated compositions of OCM, the mechanism in susceptibility to VPA-induced liver dysfunction remains elusive. On the one hand, OCM-related genetic polymorphisms, including MTHFR (C677T, rs1801133) and MTR (A2756G, rs1805087) were associated with DNA methylation in VPA-treated patients (Vurucu et al., 2008; Ni et al., 2018b). Moreover, MTHFR A1298C and MTRR A66G were also reported to be involved in the progression of liver diseases (Kasapoglu et al., 2015; Peres et al., 2016). On the other hand, VPA therapy (once or long-term) altered the levels of OCM-associated nutrients (FA, Hcy and VB12) (Gidal et al., 2005; Zhu et al., 2022). Taken together, OCM-related genetic variants and nutrient levels are highly correlated with VPA treatment in epileptic patients. However, to the best of our knowledge, there is no direct evidence supporting the association between OCM and VPA-induced liver dysfunction. Alternatively, numerous studies have demonstrated that Hcy can induce oxidative stress in molecular and cellular aspects (Perna and De Santo, 2003; Scherer et al., 2011). Moreover, oxidative stress is recognized as a key inducer of the progression of VPA-induced liver dysfunction (Chang and Abbott, 2006). Based on these findings, we hypothesize that OCM-related nutrients, genetic polymorphism and oxidative stress may contribute to VPA-induced liver dysfunction, but the mechanism underlying VPA-induced liver dysfunction remain unclear.
In this study, we systematically explored the associations between OCM-related genetic polymorphisms and nutrient levels as well as oxidative stress with VPA-induced liver dysfunction in epileptic patients.
This study included 157 patients with a diagnosis of symptomatic epilepsy based on the etiologic classification of epilepsy (Fisher et al., 2014). All patients were recruited at Yuhuangding Hospital between December 2021 to February 2023. For each patient, demographic characteristics (age, BMI, VPA dose, concomitant drug therapy and liver function) were recorded. In addition, patients were divided into a normal liver function [NLF, the levels of all liver function indicators did not exceed the upper limit of normal (ULN), 112 subjects] group and an abnormal liver function (ABLF, at least one indicators exceed two-times of the ULN, 45 subjects) group according to liver function indicators as described previously (Chen et al., 2019). Meanwhile, patients with the following conditions were excluded from this study: 1) pre-existing of ABLF; 2) hyperhomocysteinemia; 3) other potential causes of liver disease including hepatitis A/B/C, HIV- positive or metabolic disease 4) smoking, alcohol or drug abuse; 5) less than 3-month of VPA-based treatment.
This study was approved by the Ethics Committee of the Affiliated Yuhuangding Hospital of Qingdao University (No. QDU-HEC-2023108). Informed consents were signed by patients or their legal guardians. Sample handling and data analysis protocols followed the clinical principles of Yuhuangding Hospital.
After fasting overnight for approximately 10 h, venous blood was collected from each patient using sterile clot activator tubes. Blood samples were centrifuged at 3500 rpm for 5 min and then analyzed within 30 min or stored at −80 °C for later analysis.
Liver function indicators, such as alanine aminotransferase (ALT), aspartate aminotransferase (AST), alkaline phosphatase (ALP), gamma-glutamyltransferase (γ-GT), total bilirubin (TBiL), total protein (TP), albumin (ALB) and Hcy were determined by an automatic biochemistry analyzer (Au5800, Beckman Coulter, United States). The FA and VB12 concentrations were determined by chemiluminescent immunoassay using automated techniques (e601, COBAS, Roche, Germany) based on the protocols given by the manufacturer.
In this study, patients underwent more than 3 months of VPA-based therapy to ensure a steady-state VPA concentration. Ethylenediaminetetraacetic acid (EDTA)-2Na tubes were used to obtain venous blood samples from each patient. To measure the steady-state VPA concentration, blood samples were collected just before the last VPA administration. Subsequently, the VPA concentration was quantitatively measured using an automatic fluorescence immunoassay system (Abbott, Chicago, United States) as described previously (Ma et al., 2019).
A total of 5 mL of peripheral blood was obtained from each participant using an EDTA tube and stored at −20 °C. Genomic DNA was extracted by DNA Extraction Kit (OMEGA, Norcross, United States) according to the manufacturer’s protocols. The MTHFR A1298C, MTHFR C677T, MTR A2756G and MTRR A66G polymorphisms were detected directly by DNA sequencing after PCR amplification using an automatic genetic analyzer (Applied Biosystems, United States). Details of genetic polymorphisms and sequences of primers used for genotyping procedures are presented in Supplementary Table S1.
The concentration of GSH was quantitatively measured according to the protocol given by Chien’s study (Chien et al., 2016). Briefly, 10 μL of serum was mixed in 990 μL cold phosphate (10 μM)/EDTA (5 μM) buffer. Then, add 1 volume ice trichloroacetic acid (TCA) into 5 volumes of sample, and centrifuge samples at 12,000 g for 5 min. Next, the supernatant was neutralized by NaHCO3 and measured spectrophotometrically at 405 nm. Serum SOD activity and TBARS concentrations were determined by Beckman Coulter ACCESS ® (Brea, CA, United States) and suitable kits according to the manufacturer’s instructions.
The statistical analysis in this study was performed using SPSS (version 20.0; IBM, United States). For demographic characteristic analysis, Student’s t-test was used to evaluate significant differences between the NLF and ABLF groups. The statistical significance of the differences in VPA concentration and concomitant drug were determined by Student’s t-test or Fisher’s exact test. Comparisons of genotype frequencies were performed by χ2 (chi-square) tests. Logistic regression was used to evaluate the risk factors for VPA-induced liver dysfunction. Data are presented as the mean ± standard deviation. p-value less than 0.05 indicated a statistically significant difference.
This study included 157 pediatric patients (aged: 6.93 ± 6.02 years in the ABLF group and 6.16 ± 4.80 years in the NLF group) with VPA-based therapy. Patients were divided into ABLF and NLF groups based on the results of liver function tests. The demographic characteristics of ABLF and NLF patients are summarized and listed in Table 1. Patients in ABLF group exhibited significantly higher ALT, AST, γ-GT and TBiL levels than those in NLF group (P < 0.05). Moreover, no differences in age, BMI, TP, ALB or globulin (GLO) levels were observed between ABLF and NLF groups (Table 1).
In this study, all patients underwent VPA-based therapy (monotherapy or polytherapy). We recorded the VPA dose and concomitant drug used for each patient in ABLF and NLF groups. Moreover, we analyzed the VPA concentration by an automatic fluorescence immunoassay system. As shown in Table 2, there were no significant differences in VPA dose, VPA concentration or adjusted VPA concentration between two groups, suggesting that neither the VPA dose nor the concentration may be directly associated with VPA-induced liver dysfunction.
TABLE 2. Comparisons of VPA concentrations, concomitant drugs and OCM-related nutrients in ABLF and NLF groups.
In addition, carbamazepine (ABLF: 2, NLF: 4), clonazepam (NLF: 2), lamotrigine (ABLF: 2, NLF: 5), levetiracetam (ABLF: 1, NLF: 3), topiramate (ABLF: 2, NLF: 4) and oxcarbazepine (NLF: 1) were combined with VPA therapy. However, no significant association was found between concomitant drugs and liver dysfunction in the ABLF or NLF groups (Table 2).
To evaluate the association between OCM and VPA-induced liver dysfunction, we first analyzed the levels of OCM-related nutrients in the ABLF and NLF groups. As shown in Table 2, there was a clear separation between the two groups. Patients in ABLF group had significantly higher Hcy level than that in NLF group (P < 0.05). In contrast, the FA and VB12 concentrations were significantly lower in ABLF group than those in NLF group (P < 0.05).
Then, we explored the effect of VPA treatment on OCM, correlation analysis was performed and exhibited in Figure 2. As shown in Figure 2, there was a significant negative correlation between the plasma VPA concentration and FA concentration (r = −0.2962, P < 0.05), and a significant positive correlation between the plasma VPA concentration and Hcy concentration (r = 0.4387, P < 0.05). Interestingly, although the VB12 concentration displayed a separation between ABLF and NLF groups, there was no significant correlation between the plasma VPA concentration and VB12 concentration (p > 0.05). These results suggest that OCM pathway contributes to VPA-induced liver dysfunction.
FIGURE 2. Associations between plasma VPA concentrations and OCM-related nutrition levels of all subjects (ABLF and NLF groups). Relationships between (A) plasma VPA concentrations and folate levels. (B) plasma VPA concentrations and homocysteine levels. (C) plasma VPA concentrations and Vitamin B12 levels.
To further elucidate the association between OCM and VPA-induced liver dysfunction, we analyzed the OCM pathway-related genetic variants (MTHFR A1298C, MTHFR C677T, MTR A2756G and MTRR A66G) in the ABLF and NLF groups. The Hardy-Weinberg equilibrium test and allele frequencies of the four genes are listed in Supplementary Table S2. The associations between the four gene polymorphisms and VPA-induced liver dysfunction are listed in Table 3. In detail, the frequencies of AC and CC genotypes in MTHFR A1298C were significantly higher in ABLF group than those in NLF group (48.9% versus 31.2%, p = 0.004; 15.5% versus 1.8%, p = 0.001, respectively). Meanwhile, there was a significantly increased frequency of TT genotype of MTHFR C677T in ABLF group when compared to NLF group (15.6% versus 6.3%, p = 0.023). However, there were no statistically significant correlations between the MTR A2756G or MTRR A66G genotype and VPA-associated liver dysfunction (p > 0.05, Table 3). These results suggested that MTHFR A1298C and MTHFR C677T may be risk factors for VPA-induced liver dysfunction.
The associations between OCM-related genetic polymorphisms and nutrient levels (FA, Hcy and vitamin B12) in ABLF and NLF patients were analyzed. As shown in Table 4, we observed that the MTHFR A1298C polymorphism was associated with serum Hcy (AA: 7.25 ± 1.70; AC: 8.16 ± 1.93; CC: 9.63 ± 3.81, p = 0.001) and FA (AA: 19.17 ± 2.89; AC: 17.92 ± 3.70; CC: 17.97 ± 3.56, p = 0.001) levels. In addition, the MTHFR CC genotype resulted in higher Hcy levels (P < 0.01) and lower FA levels (P < 0.01) than the AA genotype (Figure 3). Interestingly, although MTHFR C677T showed a different genotype frequency between the ABLF and NLF groups, the differences in the MTHFR C677T polymorphism among OCM-related nutrient levels were not statistically significant (p > 0.05, Table 4; Figure 3).
TABLE 4. Effects of OCM-related SNPs on Hcy, Folate and Vitamin B12 levels in patients with epilepsy.
FIGURE 3. Effect of genetic polymorphisms on OCM-related nutrition levels. (A) MTHFR A1298C variant and serum Hcy levels. (B) MTHFR A1298C variant and serum FA levels. (C) MTHFR A1298C variant and serum Vitamin B12 levels. (D) MTHFR C677T variant and serum Hcy levels. (E) MTHFR C677T variant and serum FA levels. (F) MTHFR C677T variant and serum Vitamin B12 levels. *p < 0.05, **p < 0.01.
We further analyzed the associations between MTR A2756G and MTRR A66G polymorphisms and OCM-related nutrition levels. However, there were no statistically significant differences between MTR A2756G and MTRR A66G polymorphisms in OCM-related nutrition levels (p > 0.05, Supplementary Table S3).
Oxidative stress is well known to be involved in the progression of VPA-associated liver dysfunction (Chang and Abbott, 2006; Gai et al., 2020). Hence, we examined oxidative stress-related parameters. As shown in Table 5, a significantly higher TBARS concentration and a lower GSH concentration were observed in ABLF patients than in NLF patients (P < 0.05). These results demonstrated that oxidative stress is associated with VPA-induced liver dysfunction.
Considering the potential link between VPA administration and oxidative stress, we next performed correlation analysis to explore the influences of VPA concentration and oxidative stress. In this study, the VPA concentrations was significantly positively correlated with TBARS concentration (r = 0.2694, p = 0.006) and a significantly negative correlation with GSH concentration (r = −0.2233, p = 0.0049, Figures 4A,B), suggesting that the VPA concentration is associated with oxidative stress.
FIGURE 4. Association among plasma VPA concentration, serum Hcy levels and oxidative stress parameters of all subjects (ABLF and NLF groups). Relationships between (A) plasma VPA concentrations and GSH levels. (B) plasma VPA concentrations and TBARS levels. (C) serum Hcy concentration and GSH levels. (D) serum Hcy concentration and TBARS levels.
Importantly, Hcy is involved in redox signaling via regulating ROS generation and GSH levels (Gomez et al., 2011). For this reason, we analyzed the correlation between Hcy levels and oxidative stress. As shown in Figures 4C,D, we found that Hcy concentration exhibited highly correlated with GSH and TBARS concentrations (r = −0.6065, P < 0.0001; r = 0.6564, P < 0.0001, respectively). Based on these findings, Hcy-related oxidative stress might be involved in the pathogenesis of VPA-induced liver dysfunction.
To further clarify the associations of OCM-related nutrients and genetic factors with VPA-induced liver dysfunction, logistic regression analysis was performed. As shown in Table 6, the MTHFR A1298C polymorphism (r = 1.646, p = 0.001), MTHFR C677T polymorphism (r = 1.162, p = 0.007) and Hcy level (r = 1.334, p = 0.030) exhibited a significant positive correlation with VPA-associated liver dysfunction. Moreover, there were significant negative correlations between FA, GSH concentrations and VPA-induced liver dysfunction (r = −0.602, p = 0.025; r = −1.258, p = 0.006, respectively). Interestingly, although TBARS concentrations displayed only a marginally significant association with VPA-caused liver dysfunction (p = 0.067), there was a 2.6-fold (95% CI: 0.693–6.436) increased risk for liver dysfunction progression. These results indicated that MTHFR A1298C/C677T polymorphisms and GSH/Hcy/FA concentrations may be risk factors for VPA-induced liver dysfunction.
Accumulating evidence indicate that dysregulation of OCM is associated with NAFLD, but rarely reported in VPA-induced liver disease (de Carvalho et al., 2013; Fernández-Ramos et al., 2022). In this study, patients with long-term VPA treatment exhibited dysmetabolism of OCM, leading to alternations in OCM-associated nutrients and increased risk of liver dysfunction. Moreover, we demonstrated that MTHFR A1298C and MTHFR C677T polymorphisms contributed to VPA-induced liver dysfunction in patients with epilepsy. To the best of our knowledge, this study first revealed the association between OCM and VPA-induced liver dysfunction.
Patients with long-term VPA treatment are prone to OCM dysmetabolism, leading to hyperhomocysteinemia and DNA hypomethylation (Sener et al., 2006; Vurucu et al., 2008; Ni et al., 2018b). OCM is well known to be associated with chronic liver disease, but rarely reported in acute liver disease, and no report in VPA-induced liver disease (Woo et al., 2006; Tsuchiya et al., 2012; Pogribny et al., 2013). The relationship between OCM and VPA-induced liver dysfunction is unclear. In this study, ABLF patients showed lower levels of FA when compared to NLF patients (p = 0.001). Similarly, previous studies demonstrated that VPA treatment lowered FA levels by blocking the reuptake of folic acid, leading to a 25%–35% decrease in FA levels (Rubinchik-Stern et al., 2018; Reynolds and Green, 2020). Moreover, we also demonstrated that the levels of VB12 were also decreased in ABLF patients than NLF patients (p = 0.022). Interestingly, the concentration of VB12 level has a poor correlation with VPA concentration. In fact, not only VB12, but also VB6 was also confirmed to be involved in methionine cycle (Franco et al., 2022). Importantly, previous study reported that VB6 were positively associated with hepatic steatosis, as well as correlated with triglycerides, glucose, ALT and BMI (Ferro et al., 2017). For this reason, we hypothesize that VB6 may play as the major co-factor in OCM cycle (in patients with long-term VPA treatment). Further study and clinical validation are required to explore the implications of VPA treatment on OCM-related nutrients.
Hcy, an intermediate product of the methionine cycle in OCM, is an essential amino acid derived from dietary proteins (Kaplan et al., 2020). Previous study indicated that Hcy concentration was associated with the progression of liver disease (Chien et al., 2016). In this study, ABLF patients showed a higher level of Hcy than that in NLF patients. Meanwhile, Hcy level exhibited a significant positive correlation (r = 1.334, p = 0.030) with VPA-associated liver dysfunction. However, the underlying mechanism for increased Hcy concentration remain elusive. In fact, clinical trials confirmed that low levels of FA and VB12 may lead to elevated levels of Hcy (Apeland et al., 2002; Sener et al., 2006). Moreover, serum Hcy concentration is not only mediated by nutrient levels in OCM, but also regulated by OCM-related genetic polymorphisms. Specifically, the MTHFR C677T variant contributed to increased serum Hcy levels in VPA-treated patients (Vurucu et al., 2008). Meanwhile, numerous studies have indicated that the MTHFR A1298C and MTRR A66G variants are related to Hcy concentrations (Zidan et al., 2013; Li et al., 2020). In this study, we found that MTHFR A1298C (AC, OR: 1.342, 95% CI: 1.070–1.684; CC, OR: 3.709, 95% CI: 1.088–12.637) and MTHFR C677T (TT, OR: 1.587, 95% CI: 0.926–2.720) variants were linked to increased Hcy levels and contributed to the development of VPA-induced liver dysfunction.
Oxidative stress is a critical pathophysiological inducer of VPA-induced liver diseases (Ma et al., 2019; Ma et al., 2020). Hcy was reported to modulate oxidative stress via regulating the levels of GSH (Perna and De Santo, 2003). In this study, we found that the VPA concentration was significantly correlated with Hcy (r = 0.4387, p = 0.0001) concentration. Moreover, Hcy levels exhibited a strong correlation with GSH (r = −0.6065, P < 0.0001) and TBARS (r = 0.6564, P < 0.0001) levels in patients receiving VPA-based therapy, which is consistent with previous study (Chien et al., 2016). Based on these findings, we hypothesize that 1C dysmetabolism and increased Hcy levels may be involved in the progression of VPA-induced liver dysfunction via the oxidative stress pathway.
Finally, the limitations of this study should also be discussed. First, due to the low occurrence of liver toxicity during VPA therapy (1/600-1/800 in pediatric patients, 1/20,000 in the general population) (Perucca, 2002). The sample size in this study is not adequate (45 patients in ABLF group, 112 patients in NLF group). Second, other factors (such as environmental conditions, daily living habits and diet) that may also contribute to VPA-induced liver dysfunction, which were not excluded from this cross-sectional study.
In conclusion, this study demonstrated that the MTHFR A1298C and MTHFR C677T polymorphisms as well as increased serum Hcy levels contributed to the progression of VPA-induced liver dysfunction in epileptic patients via oxidative stress pathway.
The datasets presented in this study can be found in online repositories. The names of the repository/repositories and accession number(s) can be found below: China National Genebank (CNGB, https://db.cngb.org/cnsa/). The accession code is CNP0005334.
The studies involving humans were approved by Ethics Committee of the Affiliated Yuhuangding Hospital of Qingdao University. The studies were conducted in accordance with the local legislation and institutional requirements. Written informed consent for participation in this study was provided by the participants’ legal guardians/next of kin.
JZ: Conceptualization, Data curation, Formal Analysis, Investigation, Resources, Validation, Writing–original draft. ZW: Data curation, Formal Analysis, Investigation, Methodology, Software, Visualization, Writing–original draft. XS: Data curation, Formal Analysis, Investigation, Methodology, Resources, Software, Visualization, Writing–original draft, Writing–review and editing. DW: Data curation, Formal Analysis, Investigation, Software, Writing–original draft. XX: Data curation, Investigation, Resources, Writing–review and editing. LY: Conceptualization, Data curation, Formal Analysis, Investigation, Writing–review and editing. JD: Project administration, Supervision, Writing–original draft. ZZ: Data curation, Formal Analysis, Investigation, Writing–original draft. YQ: Data curation, Investigation, Writing–original draft. LM: Conceptualization, Data curation, Formal Analysis, Funding acquisition, Investigation, Methodology, Project administration, Resources, Supervision, Validation, Writing–original draft, Writing–review and editing.
The author(s) declare financial support was received for the research, authorship, and/or publication of this article. This study is supported by the Natural Science Foundation of Shandong Province grant (No. ZR2023QH039).
The authors declare that the research was conducted in the absence of any commercial or financial relationships that could be construed as a potential conflict of interest.
All claims expressed in this article are solely those of the authors and do not necessarily represent those of their affiliated organizations, or those of the publisher, the editors and the reviewers. Any product that may be evaluated in this article, or claim that may be made by its manufacturer, is not guaranteed or endorsed by the publisher.
The Supplementary Material for this article can be found online at: https://www.frontiersin.org/articles/10.3389/fphar.2024.1358262/full#supplementary-material
Apeland, T., Mansoor, M. A., Pentieva, K., McNulty, H., Seljeflot, I., and Strandjord, R. E. (2002). The effect of B-vitamins on hyperhomocysteinemia in patients on antiepileptic drugs. Epilepsy Res. 51 (3), 237–247. doi:10.1016/s0920-1211(02)00153-5
Chang, T. K. H., and Abbott, F. S. (2006). Oxidative stress as a mechanism of valproic acid-associated hepatotoxicity. Drug Metab. Rev. 38 (4), 627–639. doi:10.1080/03602530600959433
Chen, Y., Zhou, J., Xu, S., Liu, M., Wang, M., Ma, Y., et al. (2019). Association between the perturbation of bile acid homeostasis and valproic acid-induced hepatotoxicity. Biochem. Pharmacol. 170, 113669. doi:10.1016/j.bcp.2019.113669
Chien, Y.-W., Chen, Y.-L., Peng, H.-C., Hu, J. T., Yang, S. S., and Yang, S. C. (2016). Impaired homocysteine metabolism in patients with alcoholic liver disease in Taiwan. Alcohol 54, 33–37. doi:10.1016/j.alcohol.2016.06.002
de Carvalho, S. C. R., Muniz, M. T. C., Siqueira, M. D. V., Siqueira, E. R. F., Gomes, A. V., Silva, K. A., et al. (2013). Plasmatic higher levels of homocysteine in Non-alcoholic fatty liver disease (NAFLD). Nutr. J. 12 (1), 37. doi:10.1186/1475-2891-12-37
Ducker, G. S., and Rabinowitz, J. D. (2017). One-carbon metabolism in health and disease. Cell Metab. 25 (1), 27–42. doi:10.1016/j.cmet.2016.08.009
Ezhilarasan, D., and Mani, U. (2022). Valproic acid induced liver injury: an insight into molecular toxicological mechanism. Environ. Toxicol. Pharmacol. 2022 (95), 103967. doi:10.1016/j.etap.2022.103967
Fernández-Ramos, D., Lopitz-Otsoa, F., Millet, O., Alonso, C., Lu, S. C., and Mato, J. M. (2022). One carbon metabolism and S-adenosylmethionine in non-alcoholic fatty liver disease pathogenesis and subtypes. Livers 2 (4), 243–257. doi:10.3390/livers2040020
Ferro, Y., Carè, I., Mazza, E., Provenzano, F., Colica, C., Torti, C., et al. (2017). Protein and vitamin B6 intake are associated with liver steatosis assessed by transient elastography, especially in obese individuals. Clin. Mol. Hepatol. 23 (3), 249–259. doi:10.3350/cmh.2017.0019
Fisher, R. S., Acevedo, C., Arzimanoglou, A., Bogacz, A., Cross, J. H., Elger, C. E., et al. (2014). ILAE Official Report: a practical clinical definition of epilepsy. Epilepsia 55 (4), 475–482. doi:10.1111/epi.12550
Franco, C. N., Seabrook, L. J., Nguyen, S. T., Leonard, J. T., and Albrecht, L. V. (2022). Simplifying the B Complex: how vitamins B6 and B9 modulate one carbon metabolism in cancer and beyond. Metablites 12 (10), 961. doi:10.3390/metabo12100961
Gai, Z., Krajnc, E., Samodelov, S. L., Visentin, M., and Kullak-Ublick, G. A. (2020). Obeticholic acid ameliorates valproic acid-induced hepatic steatosis and oxidative stress. Mol. Pharmacol. 119, 118646. doi:10.1124/mol.119.118646
Gidal, B. E., Tamura, T., Hammer, A., and Vuong, A. (2005). Blood homocysteine, folate and vitamin B-12 concentrations in patients with epilepsy receiving lamotrigine or sodium valproate for initial monotherapy. Epilepsy Res. 64 (3), 161–166. doi:10.1016/j.eplepsyres.2005.03.005
Gomez, J., Sanchez-Roman, I., Gomez, A., Sanchez, C., Suarez, H., Lopez-Torres, M., et al. (2011). Methionine and homocysteine modulate the rate of ROS generation of isolated mitochondria in vitro. J. Bioenergetics Biomembr. 43 (4), 377–386. doi:10.1007/s10863-011-9368-1
Guo, H.-L., Jing, X., Sun, J.-Y., Hu, Y. H., Xu, Z. J., Ni, M. M., et al. (2019). Valproic acid and the liver injury in patients with epilepsy: an update. Curr. Pharm. Des. 25 (3), 343–351. doi:10.2174/1381612825666190329145428
Kaplan, P., Tatarkova, Z., Sivonova, M. K., Racay, P., and Lehotsky, J. (2020). Homocysteine and mitochondria in cardiovascular and cerebrovascular systems. Int. J. Mol. Sci. 21 (20), 7698–98. doi:10.3390/ijms21207698
Kasapoglu, B., Turkay, C., Yalcin, K. S., Kosar, A., and Bozkurt, A. (2015). MTHFR 677C/T and 1298A/C mutations and non-alcoholic fatty liver disease. Clin. Med. (Lond). 15 (3), 248–251. doi:10.7861/clinmedicine.15-3-248
Li, D., Zhao, Q., Zhang, C., Huang, X., Godfrey, O., and Zhang, W. (2020). Associations of MTRR A66G polymorphism and promoter methylation with ischemic stroke in patients with hyperhomocysteinemia. J. Gene Med. 22 (5), e3170. doi:10.1002/jgm.3170
Ma, L., Pan, Y., Sun, M., Shen, H., Zhao, L., and Guo, Y. (2019). Catalase C-262T polymorphism is a risk factor for valproic acid–induced abnormal liver function in Chinese patients with epilepsy. Ther. Drug Monit. 41 (1), 91–96. doi:10.1097/FTD.0000000000000574
Ma, L., Wang, Y., Chen, X., Zhao, L., and Guo, Y. (2020). Involvement of CYP2E1-ROS-CD36/DGAT2 axis in the pathogenesis of VPA-induced hepatic steatosis in vivo and in vitro. Toxicology 445 (445), 152585. doi:10.1016/j.tox.2020.152585
McBean, G. J. (2012). The transsulfuration pathway: a source of cysteine for glutathione in astrocytes. Amino Acids 42 (1), 199–205. doi:10.1007/s00726-011-0864-8
Mishra, M. K., Kukal, S., Paul, P. R., Bora, S., Singh, A., Kukreti, S., et al. (2021). Insights into structural modifications of valproic acid and their pharmacological profile. Molecules 27 (1), 104. doi:10.3390/molecules27010104
Ni, G., Qin, J., Chen, Z., Li, H., Zhou, J., Huang, M., et al. (2018b). Associations between genetic variation in one-carbon metabolism and leukocyte DNA methylation in valproate-treated patients with epilepsy. Clin. Nutr. 37 (1), 308–312. doi:10.1016/j.clnu.2017.01.004
Ni, G., Qin, J., Fang, Z., Chen, Y., Chen, Z., Zhou, J., et al. (2017). Increased homocysteine levels in valproate-treated patients with epilepsy: a meta-analysis. BMJ Open 4 (7), e004936. doi:10.1136/bmjopen-2014-004936
Ni, G., Qin, J., Li, H., Chen, Z., Zhou, Y., Fang, Z., et al. (2018a). Effects of antiepileptic drug monotherapy on one-carbon metabolism and DNA methylation in patients with epilepsy. PLoS One 10 (4), e0125656. doi:10.1371/journal.pone.0125656
Peres, N. P., Galbiatti-Dias, A. L., Castanhole-Nunes, M. M., da Silva, R. F., Pavarino, É. C., Goloni-Bertollo, E. M., et al. (2016). Polymorphisms of folate metabolism genes in patients with cirrhosis and hepatocellular carcinoma. World J. Hepatol. 18 (8), 1234–1243. doi:10.4254/wjh.v8.i29.1234
Perna, A. F., and De Santo, N. G. (2003). Homocysteine and oxidative stress. Amino Acids 25 (3-4), 409–417. doi:10.1007/s00726-003-0026-8
Perucca, E. (2002). Pharmacological and therapeutic properties of valproate: a summary after 35 years of clinical experience. CNS Drugs 16 (10), 695–714. doi:10.2165/00023210-200216100-00004
Petrone, I., Bernardo, P. S., dos Santos, E. C., and Abdelhay, E. (2021). MTHFR C677T and A1298C polymorphisms in breast cancer, gliomas and gastric cancer: a review. Genes 12, 587. doi:10.3390/genes12040587
Pogribny, I. P., Kutanzi, K., Melnyk, S., de Conti, A., Tryndyak, V., Montgomery, B., et al. (2013). Strain-dependent dysregulation of one-carbon metabolism in male mice is associated with choline- and folate-deficient diet-induced liver injury. FASEB J. 27 (6), 2233–2243. doi:10.1096/fj.12-227116
Radziejewska, A., Muzsik, A., Milagro, F. I., Martínez, J. A., and Chmurzynska, A. (2020). One-carbon metabolism and nonalcoholic fatty liver disease: the crosstalk between nutrients, microbiota, and genetics. Lifestyle Genom 13 (2), 53–63. doi:10.1159/000504602
Raghubeer, S., and Matsha, T. (2021). Methylenetetrahydrofolate (MTHFR), the one-carbon cycle, and cardiovascular risks. Nutrients 13 (12), 4562–62. doi:10.3390/nu13124562
Reynolds, E. H., and Green, R. (2020). Valproate and folate: congenital and developmental risks. Epilepsy & Behav. 108, 107068. doi:10.1016/j.yebeh.2020.107068
Rubinchik-Stern, M., Shmuel, M., Bar, J., Kovo, M., and Eyal, S. (2018). Adverse placental effects of valproic acid: studies in perfused human placentas. Epilepsia 59 (5), 993–1003. doi:10.1111/epi.14078
Scherer, E., da Cunha, A. A., Kolling, J., da Cunha, M. J., Schmitz, F., Sitta, A., et al. (2011). Development of an animal model for chronic mild hyperhomocysteinemia and its response to oxidative damage. Int. J. Dev. Neurosci. 29 (7), 693–699. doi:10.1016/j.ijdevneu.2011.06.004
Sener, U., Zorlu, Y., Karaguzel, O., Ozdamar, O., Coker, I., and Topbas, M. (2006). Effects of common anti-epileptic drug monotherapy on serum levels of homocysteine, Vitamin B12, folic acid and Vitamin B6. Seizure 15 (2), 79–85. doi:10.1016/j.seizure.2005.11.002
Thijs, R. D., Surges, R., O’Brien, T. J., and Sander, J. W. (2019). Epilepsy in adults. Lancet 393 (10172), 689–701. doi:10.1016/S0140-6736(18)32596-0
Tsuchiya, M., Ji, C., Kosyk, O., Shymonyak, S., Melnyk, S., Kono, H., et al. (2012). Interstrain differences in liver injury and one-carbon metabolism in alcohol-fed mice. Hepatology 56 (1), 130–139. doi:10.1002/hep.25641
Vurucu, S., Demirkaya, E., Kul, M., Unay, B., Gul, D., Akin, R., et al. (2008). Evaluation of the relationship between C677T variants of methylenetetrahydrofolate reductase gene and hyperhomocysteinemia in children receiving antiepileptic drug therapy. Prog. Neuropsychopharmacol. Biol. Psychiatry 32 (3), 844–848. doi:10.1016/j.pnpbp.2007.12.018
Woo, C. W., Prathapasinghe, G. A., Siow, Y., and O, K. (2006). Hyperhomocysteinemia induces liver injury in rat: protective effect of folic acid supplementation. Biochim. Biophys. Acta 1762 (7), 656–665. doi:10.1016/j.bbadis.2006.05.012
Zhu, S., Ni, G., Sui, L., Zhao, Y., Zhang, X., Dai, Q., et al. (2022). Genetic polymorphisms in enzymes involved in one-carbon metabolism and anti-epileptic drug monotherapy on homocysteine metabolism in patients with epilepsy. Front. Neurol. 2022 (12), 683275. doi:10.3389/fneur.2021.683275
Keywords: Valproic acid, one-carbon metabolism, MTHFR A1298C, MTHFR C677T, homocysteine, oxidative stress, liver dysfunction
Citation: Zhu J, Wang Z, Sun X, Wang D, Xu X, Yang L, Du J, Zhou Z, Qi Y and Ma L (2024) Associations between one-carbon metabolism and valproic acid-induced liver dysfunction in epileptic patients. Front. Pharmacol. 15:1358262. doi: 10.3389/fphar.2024.1358262
Received: 19 December 2023; Accepted: 13 February 2024;
Published: 23 February 2024.
Edited by:
Yang Xie, Harvard Medical School, United StatesReviewed by:
Xin Tong, Merck Sharp & Dohme Corp, United StatesCopyright © 2024 Zhu, Wang, Sun, Wang, Xu, Yang, Du, Zhou, Qi and Ma. This is an open-access article distributed under the terms of the Creative Commons Attribution License (CC BY). The use, distribution or reproduction in other forums is permitted, provided the original author(s) and the copyright owner(s) are credited and that the original publication in this journal is cited, in accordance with accepted academic practice. No use, distribution or reproduction is permitted which does not comply with these terms.
*Correspondence: Linfeng Ma, bWxmNjM0MDA4Mjk4QDE2My5jb20=
†ORCID: Linfeng Ma, orcid.org/0000-0002-9858-3085
‡These authors have contributed equally to this work and share first authorship
Disclaimer: All claims expressed in this article are solely those of the authors and do not necessarily represent those of their affiliated organizations, or those of the publisher, the editors and the reviewers. Any product that may be evaluated in this article or claim that may be made by its manufacturer is not guaranteed or endorsed by the publisher.
Research integrity at Frontiers
Learn more about the work of our research integrity team to safeguard the quality of each article we publish.