- 1New York Medical College, Valhalla, NY, United States
- 2Department of Biomedical Engineering, Stony Brook University, Stony Brook, NY, United States
- 3Department of Psychology, Stony Brook University, Stony Brook, NY, United States
- 4National Institute on Alcohol Abuse and Alcoholism, Bethesda, MD, United States
Cocaine is a highly addictive drug, and its use is associated with adverse medical consequences such as cerebrovascular accidents that result in debilitating neurological complications. Indeed, brain imaging studies have reported severe reductions in cerebral blood flow (CBF) in cocaine misusers when compared to the brains of healthy non-drug using controls. Such CBF deficits are likely to disrupt neuro-vascular interaction and contribute to changes in brain function. This review aims to provide an overview of cocaine-induced CBF changes and its implication to brain function and to cocaine addiction, including its effects on tissue metabolism and neuronal activity. Finally, we discuss implications for future research, including targeted pharmacological interventions and neuromodulation to limit cocaine use and mitigate the negative impacts.
1 Introduction
Cocaine disrupts brain function, including resting state activity, response to stimulation, and functional connectivity between brain regions. These functional impairments might result from (or be associated with) decreases in cerebral blood flow (CBF) observed in cocaine misusers and in laboratory animals with chronic cocaine exposures. Though the mechanisms underlying cocaine-induced CBF reductions are not fully understood they are likely to reflect: 1) direct vasoconstrictive effects of cocaine and 2) indirect effects from reduced neural activity and metabolic demand (Volkow et al., 1988). The severity, brain regional locations, and the mechanism underlying cocaine’s CBF reductions in turn will affect the characteristics of the brain dysfunction. Here we summarize our preclinical findings using in-vivo optical neuroimaging along with a literature review of preclinical and clinical studies. This review aims to provide an overview of cocaine-induced changes in the brain’s CBF and metabolism, which may result in brain dysfunction and contribute to neurotoxicity and cognitive deficits that perpetuate addictive behaviors.
2 Literature search strategy
The goal of this literature review was to explore cocaine’s influence on CBF and whether it was associated with brain metabolic and/or functional changes, including long-term neurological complications. To conduct this review, we searched through publications in both preclinical and clinical research through the databases (PubMed, Embase, and Web of Science) focusing mostly on the past 10 years (2013–2023). The details of search keywords and the numbers of relevant articles identified are listed in Table 1, including our publications. Additionally, to ensure identification of all relevant literature, we utilized Chat GPT 3.5 to identify studies we might have missed. The major findings from these articles are summarized in Figure 1.
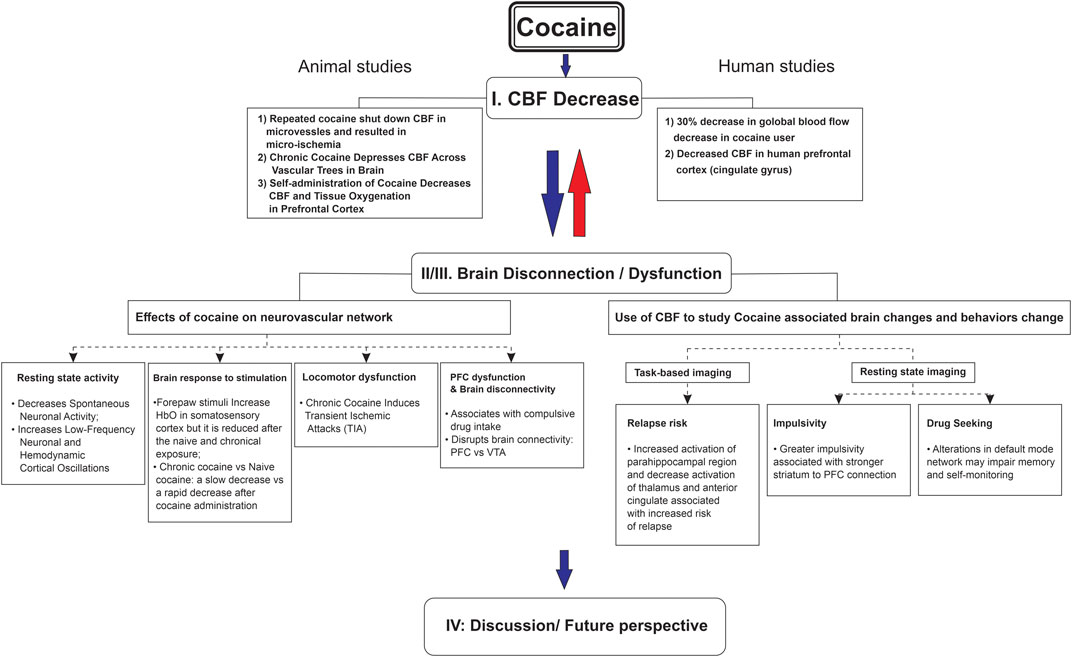
FIGURE 1. Cocaine-induced cerebral blood flow decrease associated with brain dysfunction from neurovascular imaging to human addictive behavior.
3 Cocaine decreases cerebral blood flow
Cocaine’s toxicity and vascular effects have been investigated for more than 3 decades. A recent review (Bachi et al., 2017), proposed that acute cocaine-induced vasoconstriction is due to endothelin-1 release and a decrease of nitric oxide (a blood vessel dilator) (Saez et al., 2011; Saez et al., 2014). Cocaine-induced vasoconstriction caused deformation and damage of blood vessels, reducing cerebral blood flow (CBF). For example, cocaine exposure reduced cerebrovascular pulsatility and CBF in mice assessed with two-photon microscopy (Chen et al., 2020), and cocaine-induced CBF decrease was also detected in non-human primate subjects with positron emission tomography (PET) imaging (Howell et al., 2002). Additionally, a study using a two-sensor electrochemical recording method (Thomas et al., 2021) reported cocaine decreased oxygen levels in the nucleus accumbens (NAc) of rodents.
We have used advanced multimodality optical imaging techniques (including multi-wavelength optical imaging and optical coherence tomography) to study the effects of acute and chronic cocaine on vascular networks in the rodent brain using noncontingent and self-administration models. This multimodality optical approach allowed us to perform high-resolution in-vivo imaging of cerebral vessels; quantification of CBF and metabolism by assessing oxygenated and deoxygenated hemoglobin; and to record neuronal activity by measuring changes in intracellular calcium. Our in-vivo preclinical studies have led to the following findings.
3.1 Cocaine curtailed CBF velocity in microvessels and led to microischemia with repeated exposure
Ultrahigh-resolution Optical Doppler Tomography (μODT) (Ren et al., 2012a; Ren et al., 2012b; You et al., 2014) enabled tracker-free three-dimensional (3D) angiography of cerebral microvasculature. To quantify simultaneous changes in the vascular network and CBF in mice expose to cocaine, we implemented a phase-intensity-mapping algorithm (Yuan et al., 2011). When analyzed using the enhanced 3D μODT, microvessels in the mouse cerebral cortex demonstrated a 70% decrease in CBFv 2–3 min after acute cocaine administration. Following this reduction, the recovery varied by vessel type (venules: 12 min, arterioles: 5 min) with enhancement of these changes following chronic cocaine treatment. Interestingly, capillaries were unique in that their time to recovery ranged from 4–20 min. While no hemorrhage was observed in association to these vascular changes, repeated cocaine administration worsened local ischemia and vasoconstriction (Ren et al., 2012a). The response of cerebral vessels to cocaine supports its role in the development of cerebral micro-ischemia. Coupled with repeated injury due to chronic cocaine consumption, the ischemic environment may contribute to cocaine’s neurotoxic effects.
3.2 Chronic cocaine reduced CBFv across cerebral vascular trees
The cerebral microvascular networks (including capillary beds) along with larger-size branch vessels can be imaged with μODT, enabling quantification of CBFv changes (Ren et al., 2012a; Pan et al., 2014; You et al., 2014). Comparison of μODT images between control mice (not exposed to cocaine) and mice exposed to chronic cocaine showed dramatic flow decreases with chronic cocaine exposure (You et al., 2014). Specifically, CBFv decreased 22.5% ± 8.7% (p < 0.05) in arterial vessels, 68.4% ± 6.0% (p < 0.05) in venules and 49.1% ± 17.9% in the capillary networks. Similar experiments in rats showed that chronic cocaine exposure over 2–4 weeks significantly decreased CBFv across the cerebrovascular network in the somatosensory cortex (Zhang et al., 2016). A comparison between the two cocaine groups showed that CBFv in small vessels was significantly lower after 4 weeks than after 2 weeks of cocaine exposure. Therefore, these results show that chronic cocaine decreased CBFv across the vascular tree of various vessel sizes in the cerebral cortex of mice and rats.
3.3 Self-administration of cocaine decreased CBFv and tissue oxygenation in the prefrontal cortex
Rodent models of cocaine self-administration have been used to study addiction-like behaviors. In fact, methods that lead to high levels of cocaine intake in rats have been shown to be relevant to cocaine addiction in humans (Roberts et al., 2007). Behaviors associated with cocaine addiction, including tolerance, increase intake, and motivation are emulated in a rodent model that gives unrestricted extended access for cocaine self-administration. (Koob and Bloom, 1988; Koob and Volkow, 2010). Further emphasizing the transition from drug use to addiction, Vanderschuren and Everitt highlighted that drug-seeking behaviors in rodents become particularly compulsive after extended access to cocaine (Vanderschuren and Everitt, 2004).
Substance use disorder (SUD), as defined by DSM 5, includes compulsive behavior where the subject continues to invest significant amount of time seeking and consuming a substance despite awareness of the adverse consequences (Association, 2000; Association, 2013). Compulsive behavior is also being considered for inclusion into the International Diagnosis Codes (ICD-11) (Matone et al., 2022). Compulsive drug consumption is recapitulated in the Long-access (LgA; a daily 6-h of access session) self-administration model. Specifically, compared to a more time restricted access to the drug model (Short-Access or ShA, e.g., a daily 1-h session), extended access leads to increased cocaine intake and cocaine seeking (Wee and Koob, 2010). We conducted quantitative CBFv and angiographic imaging of neurovascular networks in the prefrontal cortex (PFC) of LgA animals and compared to yoked control (i.e., saline infusion instead of cocaine). We found that CBFv in the PFC of the LgA animals were much lower than in controls throughout the cerebrovascular tree. Moreover, CBFv in the PFC was negatively associated with cocaine doses self-administered by the animals, such that rats who consumed the largest doses had the greatest CBFv decreases (Du et al., 2020). These findings of CBFv reductions in PFC of animals undergoing self-administration of large cocaine doses are consistent with those observed in cocaine-addicted individuals.
The optical imaging findings revealed sensitization to cocaine-induced CBFv reductions, such that an acute cocaine challenge triggered significantly larger and longer-lasting CBFv reductions and Δ[HbO2] decreases in LgA than in drug naïve animals. Indeed, cocaine-induced decreases in Δ[HbO2] in LgA animals were double to those in controls (i.e., 20.7% versus 10.8%). Human studies with near infrared optical imaging have revealed that decreases in brain oxygen saturation of 13% were associated with EEG changes characteristic of cerebral ischemia in 97% of cases (Al-Rawi and Kirkpatrick, 2006). Considering that chronically exposed animals had a 20.7% reduction in Δ[HbO2] after acute cocaine dose, this indicates that these reductions are well within clinical values associated with ischemia.
Clinical studies have also reported that chronic cocaine exposures can cause neurological complications such as stroke. Chronic cocaine misuse has been consistently shown to lead to deficits in CBF including cortical perfusion (Volkow et al., 1988; Kosten, 1998; Lukas, 2014). Both positron emission tomography (PET) and single photon emission computed tomography (SPECT) studies have shown that chronic cocaine misusers had perfusion deficits that appeared similar to those seen after a stroke or head injury (Levin et al., 1994). The abnormalities in CBF have been associated with alterations in neuropsychological function and neurologic symptoms in cocaine misusers (Pascual-Leone et al., 1991a; 1991b; Strickland et al., 1993; Strickland et al., 1998; Bartzokis et al., 1999) including cocaine-induced strokes even among young misusers (Desai et al., 2017). The mechanism underlying cocaine-induced reductions in cerebral blood flow is not fully understood, yet studies have documented negative effects of cocaine on blood vessels, including endothelial damage and vasculature alterations, which are likely to contribute to neurotoxicity (Dressler et al., 1990; Diercks et al., 2008; Finkel and Marhefka, 2011).
4 Effects of cocaine on neurovascular networks
Cocaine-induced vasoconstriction reduces cerebral blood flow, but further reductions could occur through cocaine’s neuronal effects, which may affect neurovascular coupling (Ren et al., 2012a; You et al., 2017; Du et al., 2020). Optical imaging has made it possible to investigate in greater detail the effects of cocaine on neurovascular coupling (Ren et al., 2012a; You et al., 2017; Du et al., 2020).
4.1 Cocaine’s effects on resting-state brain activity
Multiple imaging modalities have been developed to investigate brain function using different methods to quantify neuronal firing and hemodynamics. Functional MRI (fMRI) utilizes the magnetic properties of hemoglobin to assess neuronal activity through the BOLD signal whereas tools such as electrophysiology directly measure local field potentials (FP) within a neuron. Additionally, optical technologies such as optical intrinsic signal imaging (OISI) and optical intracellular calcium fluorescence imaging (OFI) quantify neuronal and hemodynamic activity using light. The dynamic activity recorded by these different methodologies can be decomposed into unique oscillations at specific frequencies. All of these technologies demonstrate underlying Low-frequency oscillations (LFOs) in spontaneous neuronal activity and cerebral hemodynamic fluctuations (fMRI: (Fransson, 2005), OISI: (Du et al., 2014; Rayshubskiy et al., 2014), OFI: (Du et al., 2014; Vincze et al., 2018), FP: (Obrig et al., 2000; Buzsaki and Draguhn, 2004; Rayshubskiy et al., 2014)).
The generation of functional connectivity brain maps obtained during resting state conditions is based on the assumption that LFOs in neuronal activity are reflected in the hemodynamic LFOs as the neuronal and cerebral vessels are thought to be tightly linked through neurovascular coupling (Biswal et al., 1995; Fair et al., 2007; Raichle and Snyder, 2007). A study that used single electrode recording and optical intrinsic signal imaging showed that resting-state hemodynamic oscillations were regionally coupled to the multiunit activity (MUA) of a neuronal population (Ma et al., 2016). To assess if cocaine disrupted neurovascular coupling between neuronal and vascular LFOs, we simultaneously measured LFOs of neuronal activity and hemodynamics in naïve and chronic cocaine rats. Animals were tested at baseline and after acute cocaine. Specifically, we measured local field potentials and cerebral blood flow LFOs (0–1 Hz) in the somatosensory cortex of naïve and chronic cocaine (2 weeks) rats at baseline and during cocaine intoxication.
Our study showed that cocaine reduced spontaneous neuronal firing rates while synchronizing neuronal activity and enhancing LFOs in the somatosensory cortex. Reduced spontaneous neuronal firing with cocaine was consistent with prior findings from our group (Chen et al., 2016) and that of others (Bekavac and Waterhouse, 1995). The reduction in spontaneous neuronal activity has been interpreted to reflect cocaine’s dopaminergic enhancing effects (perhaps also its noradrenergic actions) (Bunney et al., 1973; Ritz et al., 1987; Einhorn et al., 1988). In the PFC, dopamine (DA) has been shown to suppress spontaneous neuronal firing of pyramidal neurons, an effect that was enhanced by cocaine (Kroener et al., 2009). By decreasing neuronal background firing, stimulants, such as cocaine, may improve detection of salient stimuli leading to enhanced attention. (Volkow et al., 2008; Arnsten, 2009). However, with chronic cocaine exposure, the suppression of spontaneous neuronal activity in the ventromedial PFC or anterior cingulate cortex could also contribute to compulsive cocaine intake in addiction by promoting inflexible behaviors (Volkow et al., 2011). An enhanced signal-to-noise ratio to a conditioned stimulus (cocaine or its cues) could produce an accentuated response to it while reducing the influence of weaker competitive stimuli. This would further strengthen conditioning while reducing the salience of nonrelated drug stimuli accounting in part for the behavioral inflexibility observed in individuals suffering from addiction (Wise and Kiyatkin, 2011; Volkow et al., 2013).
In addition to depressing spontaneous neuronal spiking activity, acute cocaine synchronized the fluctuation of the FP events in the LFO band (0.03–0.15 Hz), narrowing the power spectral distribution of the FP event rate. In naïve animals, cocaine depressed neuronal oscillation frequencies >0.15 Hz that recovered 20 min following cocaine administration. Chronically treated animals had a similar suppression of frequencies > 0.15 Hz following a cocaine challenge but the suppression was more prominent and persisted for up to 30 min. At baseline, chronically treated animals had accentuated LFOs suggesting that the effects of cocaine persisted up to 24 h after last injection. These findings are consistent with previous reports in the globus pallidus of increases in LFOs (0.017–0.1 Hz) in neuronal activity in the presence of cocaine and other dopamine agonists (Ruskin et al., 1999).
We observed that while in naïve animals the effects of acute cocaine on CBF fluctuation spectra were similar to those on neuronal activity, in the chronic animals, acute cocaine further narrowed the spectral distribution of neuronal LFOs but it did not further change hemodynamic LFOs. We interpreted this to reflect decreased hemodynamic reactivity to further changes in neuronal activity in chronic cocaine animals. This neurovascular uncoupling might reflect the marked and persistent vasoconstriction associated with chronic cocaine exposure (Yin et al., 2017), which might limit the hemodynamic responses associated with neuronal oscillations.
In summary, acute and chronic cocaine administration depressed neuronal activity in the somatosensory cortex but increased LFP oscillations in neuronal activity and CBF fluctuations. The changes of neuronal LFOs were spectral-temporally correlated with the changes of CBF LFOs in the resting state in naïve animals, demonstrating neurovascular coupling in response to an acute cocaine challenge. In contrast, the LFO changes in neuronal activity and CBF fluctuations deviated from a linear relationship in chronic cocaine-exposed animals, suggestive of hemodynamic uncoupling from neuronal activity under resting state conditions.
4.2 Cocaine’s effects on brain response to stimulation
Many researchers have studied cocaine effects at the molecular level including in proteins (Martinez et al., 2014; Jin et al., 2015; Wiskerke et al., 2016; Hurley et al., 2017; Coyle and Balu, 2018), genetics/epigenetics (Hachem-Delaunay et al., 2015), and their relationship to behavior (Antoniazzi et al., 2014; Lespine et al., 2019). However, cocaine’s effects on neurovascular networks and their functional consequences have not been fully investigated.
Functional MRI allows for the study of regional patterns of brain activation triggered by tasks, stimuli, and drugs such as cocaine. Activation and deactivation as assess by BOLD in fMRI assumes that there is a strong correlation between neuronal and hemodynamic activity through neurovascular coupling (Logothetis et al., 2001; Li and Freeman, 2007). Cocaine, a highly vasoactive substance, interferes with the tight coupling between neurons and cerebral vessels and may confound data collected on neuronal activation through BOLD (Iannetti and Wise, 2007). One study by Gollub et al. found that while, the fMRI measurements of the visual cortex before and after cocaine administration showed no change in BOLD, the use of the FAIR (flow-sensitive alternating inversion recovery) method demonstrated decreased CBF (Gollub et al., 1998). Consistent with the reduction in CBF seen with FAIR, studies have also found decreased functional connectivity, which was interpreted as a reduction in neuronal activity (Li et al., 2000). Therefore, cocaine effects on cerebrovascular reactivity may complicate the interpretation of BOLD contrast fMRI as well as results from other imaging studies that rely on CBF measurements.
To distinguish cocaine-induced vascular effects from its neuronal actions, we combined Laser Doppler Flowmetry (LDF) with electroencephalography (EEG) to simultaneously measure CBF (reflecting neurovascular hemodynamics) and local field potential (LFP, reflecting neuronal activities) in the rat somatosensory cortex (Chen et al., 2016) during resting state (i.e., non-stimulation state) and during electrical forepaw stimulation before and after acute cocaine. Neuronal activity was random during the resting state but synchronized during forepaw stimulation. Cocaine depressed both resting-state LFP and CBF and these changes were strongly correlated with each other (r = 0.81, p < 0.001) supporting that neurovascular coupling was maintained. In contrast, acute cocaine disrupted the neurovascular coupling following forepaw stimulation, as the CBF increase was attenuated by 50% for ∼20 min. The disruption of neurovascular coupling following forepaw stimulation but not in the resting state, suggests that distinct mechanisms may control neurovascular regulation in response to stimulation as compared to at rest. Alternatively, it might reflect that the magnitude of the spontaneous flow increase while at rest is much smaller than during activation. Therefore, despite the vasoconstriction, the vessels can still accommodate the small spontaneous flow change but not the larger increases with stimulation. Our studies demonstrate that when interpreting fMRI studies in chronic cocaine users and controls, it may be necessary to consider the hemodynamic and vascular state as CBF changes in response to stimuli may be dependent on background CBF conditions.
To further characterize chronic cocaine’s effects, we measured the CBF responses along with oxygenated-hemoglobin (HbO2) and deoxygenated-hemoglobin (HbR) dynamics to forepaw electrical stimulation in naive and chronic cocaine treated animals. Our experiments showed that stimulation-evoked tissue HbO2 response decreased after cocaine in both control (naïve) and chronic groups compared to their baselines (prior to acute cocaine administration) and did not recover until 28 min post cocaine. The HbO2 decrease in the chronic cocaine group was slower (minimum value ∼16 min after cocaine) than in the control group (minimum value at 4–8 min). A CBF decrease was also observed after acute cocaine that partially recovered in the control group at 28 min and fully recovered in the chronic group at around 20 min. However, the chronically treated group had lower basal CBF (presumably due to persistent vasoconstriction), thus, even after recovery from acute cocaine the CBF was still lower than the basal levels in control rats. Similarly, hemoglobin responses were weaker in the chronic cocaine group compared to the control group during the baseline period, which indicated that chronic cocaine accentuated neurovascular decoupling and dysfunction. (Du et al., 2020).
Taken together, these findings revealed that cocaine attenuated blood flow and depressed spontaneous neuronal activity at rest. Following stimulation, it did not change the neuronal response but reduced CBF responses, indicating that neurovascular coupling during stimulation was temporarily disrupted by cocaine. Neurovascular uncoupling could contribute to cocaine’s neurotoxicity, particularly for stimulation conditions when CBF might be insufficient to sustain the energetic demands of neuronal tissue (Chen et al., 2016).
4.3 Chronic cocaine interferes with cerebrovascular physiology
The risk of cocaine use leading to cerebrovascular complications has been extensively reported (Treadwell and Robinson, 2007; Cheng et al., 2016). Cocaine intake activates the sympathetic system, leading to hypertension, tachycardia, and increased metabolic demand, all of which can contribute to vascular events (Daras et al., 1994). Such activation is particularly concerning given that hypertension is a known risk factor for cerebrovascular diseases.
Cocaine can also disrupt the integrity of the blood-brain barrier, making the central nervous system more susceptible to toxins and potentially leading to inflammatory responses (Fiala et al., 1998). The blood-brain barrier acts as a protective shield, preventing harmful substances from entering the brain. Disruption of this barrier could lead to increased susceptibility to infections and other pathologies.
Chronic use of cocaine has been associated with arterial stiffness, which would further enhance the risk of cerebrovascular accidents. Arterial stiffness makes blood vessels less capable of expanding and contracting, decreasing flow (Kozor et al., 2014). This in turn increases the risk of ischemic events as reduced flow can starve brain tissues of essential oxygen and nutrients.
In the context of treatment, understanding these cocaine-induced cerebrovascular changes is crucial. Use of neuroimaging, including fMRI, has allowed investigators to monitor real-time brain activity and vascular responses relevant to cocaine’s cerebrovascular effects (Kaufman et al., 1998).
In conclusion, it is clear that cocaine use poses a significant risk to cerebrovascular health, not just because of its direct effects but also due to the cascade of physiological responses it triggers. Comprehensive studies, innovative imaging techniques, and a deeper understanding of the physiological changes induced by cocaine are paramount in designing effective treatments and interventions.
4.4 Cocaine disrupted brain connectivity
The PFC is modulated by dopaminergic and glutamatergic neurons that project from the ventral tegmental area (VTA) and its disruption might facilitate impulsive behaviors as occurs during cocaine intoxication. The involvement of the dorsal lateral PFC and anterior cingulate cortex (ACC) in self-regulation and decision making and its relevance to impulsive behaviors (Goldstein and Volkow, 2011) and addiction has been extensively investigated (Baler and Volkow, 2006). To study this, we assessed the effects of acute cocaine (30 mg/kg, i.p.) on the reactivity of the PFC to VTA stimulation (Park et al., 2022) using a genetically encoded calcium indicator (GCaMP6f), which allowed us to track neural activity (Chen et al., 2013), in medial PFC (mPFC) in response to “tonic-like” (5 Hz) and “phasic-like” (50 Hz) electrical VTA stimulation. The 5 Hz and 50 Hz stimuli were used to mimic DA neuronal tonic and phasic firing in the VTA, respectively (Schultz, 2007). We wanted to distinguish the effects of tonic dopamine firing (1–5 Hz), which contributes to low stable levels of extracellular DA necessary for sustained processing and motivation to those from phasic firing (40–50 Hz), which occurs in bursts and induces DA concentration spikes that are relevant to reward and conditioning (Grace and Bunney, 1984; Robinson et al., 2004; Schultz, 2016) including to drugs (Berridge and Robinson, 1998). In contrast, tonic stimulation of VTA DA neurons attenuates drug consumption (Bass et al., 2013). The high temporal and spatial resolutions of optical imaging techniques have greatly advanced our understanding of neural circuits (Knopfel, 2012). This includes giving researchers the ability to capture single neuronal Ca2+ transients from individual stimuli with “tonic-like” stimulation and neuronal activation evoked by “phasic-like” VTA stimulation.
Our results showed that “tonic-like” VTA stimulation induced a rapid increase in neuronal Ca2+ in mPFC followed by a plateau and recovery upon termination of stimulation. However, after cocaine, the mPFC sensitivity to “tonic-like” VTA stimulation was attenuated. Such perturbations in neural responsiveness might underlie the alterations in decision-making and heightened impulsivity during cocaine intoxication (Jentsch and Taylor, 1999). Impulsivity, is the tendency to act on impulse with no regard to consequence and is described as a lack of behavioral inhibition by DSM 5 (Association, 2013). As a multidimensional construct, impulsivity includes a range of “actions that are poorly conceived, prematurely expressed, unduly risky, or inappropriate to the situation and that often result in undesirable outcomes” (Daruna and Barnes, 1993). One particularly relevant dimension of impulsivity for understanding the neural impact of substance use involves the concept of disrupted inhibitory control and is strongly associated with substance use disorders (Jentsch and Pennington, 2014; Kozak et al., 2019). “Phasic-like” stimulation evoked a rapid Ca2+ fluorescence increases in the mPFC with an immediate decay process and cocaine further shortened the recovery time. Cocaine’s modulation of this response hints at its profound impact on reward-related neuronal processes (Hyman et al., 2006). These changes in mPFC might contribute to cocaine binging during intoxication and is consistent with findings of decreased tonic DA function in cocaine misusers (Volkow et al., 1997; Martinez et al., 2007; Volkow et al., 2014). This impaired PFC function could also contribute to drug-seeking and relapse (Everitt and Robbins, 2005).
4.5 Cocaine-induced ischemia in PFC is associated with escalation of cocaine intake
Chronic cocaine exposure in rats has been associated with loss of neurons in the PFC (George et al., 2008). Within the PFC, the prelimbic cortex (PrL) is involved in controlling drug-seeking behavior (Martin-Garcia et al., 2014), as is the orbitofrontal cortex (OFC) (Limpens et al., 2015). Reduced neural activity in PrL and OFC have been linked with persistent seeking behaviors and are likely to contribute to compulsive aspects of addiction (Limpens et al., 2015).
Deficits in PFC including OFC and dorsal ACC (analogue of rodent PrL) are associated with the development of compulsive cocaine use in humans (Rapinesi et al., 2016). Chronic cocaine consumption alters the normal striato-cortical circuitry leading to prefrontal dysfunction (Volkow and Morales, 2015). In additional to the neural circuitry adaptions, cocaine-induced alterations of CBF and the cerebrovasculature are also likely to play a role in hypofrontality.
Indeed, cocaine misusers are at higher risk of ischemic and hemorrhagic strokes in the brain than non-users (Levine et al., 1987; Tuchman et al., 1987; Levine et al., 1991) and imaging studies in cocaine misusers have documented marked decreases in CBF, which are most prominent in PFC (Volkow et al., 1988).
As discussed in prior sections, rodent studies have shown that chronic cocaine triggers vasoconstriction, reduces CBF, and results in cerebral ischemia (Zhang et al., 2016). By applying integrated optical imaging to investigate these phenomena, we documented that despite significant proliferation of blood vessels in areas of vasoconstriction (Zhang et al., 2016; Allen et al., 2019; Du et al., 2020), CBF remained reduced even after 1 month of cocaine detoxification (Du et al., 2020). This could explain why deficits in executive control can persist in cocaine users even months after drug discontinuation (Rezapour et al., 2016).
5 Clinical studies of cocaine’s impact on CBF
It has been well established that chronic use of cocaine leads to global and regional CBF decreases in the brain. Volkow et al. using PET first reported on this finding, revealing that chronic cocaine users had significant decreases in CBF relative to non-users (Volkow et al., 1988). Following this work, Wallace et al. quantified the change in relative CBF following 40 mg of intravenous (IV) cocaine in patients with cocaine use disorder (CUD). These patients demonstrated CBF reductions of 30% within the anterior cingulate cortex (Wallace et al., 1996). As summarized in Table 2, recent literature has replicated these findings. For example, Luo et al. used a stop signal task to study the impact of cocaine on brain regions involved in error processing and how these changes may predict relapse. They found that decreased flow to higher level processing centers of the brain such as the dorsal ACC, predicted early relapse following discharge (Luo et al., 2013). This is consistent with the findings reported above in preclinical models of attenuated blood flow in PFC of chronically treated animals.
5.1 Relapse and remission
A recent meta-analysis looking at functional studies from 2000–2017 using CBF to identify brain structures involved in relapse and remission identified only 3 papers that specifically focused on cocaine use disorders (CUD) (Forster et al., 2018). These papers identified different regions whose activation or inhibition predispose patients to increased risk of relapse or remission. Activation of the left posterior hippocampus as determined by relative CBF increases was the only difference observed in voxel-wise whole brain analysis in CUD patients that predicted relapse within 30 days of discharge (Adinoff et al., 2015).
In contrast, Luo et al. reported sex-specific decreases associated with relapse. In females, attenuated dorsal ACC and thalamus activity predicted earlier relapse, while in males, it was the decreased activity in left insula and ACC (Luo et al., 2013). The likelihood of relapse was predicted with an area under the curve of 0.85 for a receiver operating analysis. Another review by Jasinska et al. undertook a comprehensive survey of human neuroimaging results, pointing out factors modulating neural reactivity to drug cues in addiction, including cocaine (Jasinska et al., 2014).
5.2 Alterations in resting state of cocaine users and PFC dysfunction
While rodent studies have help determine how different subregions, of the PFC contribute to the development of CUD, translating these findings from rodents to humans has been challenging as the rodent networks need to be identified and mapped to the human brain (Box 1). Therefore, to determine how chronic cocaine use, abstinence, and relapse alters normal human brain function, clinical functional imaging studies have investigated resting functional connectivity. Hu et al., used fMRI to measure resting functional connectivity in 56 patients with CUD and reported that patients with higher impulsivity scores (BIS-11) or who used cocaine recently had increased connectivity between the striatum and dorsal lateral PFC (Hu et al., 2015).
There is increased recognition that bi-directional signaling between the PFC, nucleus accumbens (NAc), and VTA are crucial to drug reward and addiction. Significant alterations in this network have been reported in CUD. For example, whereas former patients with CUD showed decreased connectivity between the right NAc and the dorsal PFC (Berlingeri et al., 2017), increased connectivity between PFC and VTA and between NAc and mPFC was found in non-treatment seeking individuals with CUD (Ray et al., 2016). Insights by Volkow et al. further supported the notion of unbalanced neuronal circuits in addiction, with enhanced reactivity of circuits associated with saliency, emotion, and reduced function of executive networks in cocaine misusers (Volkow et al., 2013).
BOX 1 Prefrontal cortical correlates between rodents and humans
In order to study the neurobiological changes associated with the transition from voluntary to compulsive use, rodent models have been heavily utilized as a model of addiction. These pre-clinical studies have provided insights into the functional role of specific brain regions and their contribution to CUD and have implicated the PFC in the neurobiology of addiction including a role in risk for drug use and substance use disorders.
Different strategies have been employed to try to determine homology between rodent and human PFC based on functional connectivity or on cellular structure (Heilbronner et al., 2016; Preuss, 1995). Within the rodent PFC, there are four main structures of interest for drug addiction: the anterior cingulate gyrus, the prelimbic cortex, the infralimbic cortex, and the orbitofrontal cortex.
The anterior cingulate gyrus in rodents is thought to correspond to Brodmann areas 24, 25, and 32 in humans and to contribute to attention allocation, and salience (Paus, 2001; Ceceli et al., 2022). In BOLD imaging, ACC activation was found to be positively associated with strength of cocaine craving with exposure to cocaine-associated cues (Goldstein et al., 2009).
The medial prefrontal cortex in the rodent brain can be further subdivided into the Prelimbic (Prl) and infralimbic (IL) cortex. Studies suggest that these two regions play antagonistic roles with the Prl contributing to cocaine seeking and relapse whereas, the IL works to inhibit actions and promote drug extinction (Nett and LaLumiere, 2021; Moorman and Aston-Jones, 2023). Moreover, while the homolog of the IL in humans has been identified as the ventromedial prefrontal cortex (Brodmann area 25), there appears to be no clear correlation for the Prl (Nett and LaLumiere, 2021). Depending on the study, the Prl is either correlated with the dorsal lateral prefrontal cortex (Brodmann are 9 and 46) or the AAC in Brodmann area 32 (Heilbronner et al., 2016).
Lastly, the orbitofrontal cortex, whose homolog is the orbital frontal cortex (Brodmann areas 10, 11, 47) is involved with prediction and prediction error (Stalnaker et al., 2015). This brain region is thought to integrate different possible outcomes given a situation an individual has never experienced before (Levy and Glimcher, 2012). Following the event, the OFC might process the outcome and determine where errors were made in its prediction calculation in a process named “Credit error” (Lak et al., 2014). In addition, impaired OFC processing may lead to incorrect value attribution and the decision to pursue cocaine triggering relapse.
5.3 Limitations of clinical Studies
While current clinical studies have provided important insight into CUD pathology and the underlying changes in brain functional activity and CBF, there are limitations shared by these investigations. One limitation is the relatively small samples size of these clinical neuroimaging studies such that the largest size of the reviewed studies included 97 patients (Table 2). Thus, pooling of data through meta-analysis, is necessary to draw more robust conclusions. These studies are also confounded by variability in length of cocaine use prior to the investigation. Patients within the same study may therefore have different degrees of functional changes associated with length of drug misuse. Lastly, clinical studies are limited in that they can only study misusers after a varying period of use. While pre-clinical studies can investigate the pathophysiology and neuropathology of addiction as it evolves from first drug exposure to compulsive use, clinical studies are unable to examine the early stages of addiction due to difficulties in identifying patients early in the transition from voluntary to compulsive use. Ongoing large prospective brain imaging studies of adolescents as they transition into adulthood will allow us to investigate brain patterns that might help predict future drug use and addiction (Volkow et al., 2018).
6 Involvement of astrocytes in neurovascular regulation and Cocaine’s actions
Changes in brain function and CBF associated with cocaine may reflect adaptations in neurovascular coupling, a dynamic and complex system which allows for communication between neurons and blood vessels and regulates what crosses into the brain via the blood brain barrier. The exact mechanism of communication between neurons and cerebral vasculature remains unclear. Some literature suggests that the demand for increased blood flow is driven by a deficit of energy resources such as ATP and oxygen while others suggest that it is triggered by intracellular signaling resulting from synaptic activity (e.g., Ca2+) (Attwell et al., 2010; Mishra, 2017).
Astrocytes are a critical component of neurovascular coupling uniquely positioned between neurons and the vasculature. Their end-feet wrap around cerebral vessels contributing to the blood brain barrier while their processes interact with neurons and other astrocytes to create a functional syncytium (Mishra et al., 2016). Previous investigations into how astrocytes influence vessel diameter have established that increases in astrocytic Ca2+ in response to neurotransmitters such as glutamate leads to the release of vasoactive compounds that influence cerebral vessel tone (Simard et al., 2003; Gordon et al., 2008; Mishra et al., 2016). One of these compounds, 20-hydroxytetraenoic acid (20-HETE), is hypothesized to stimulate vasodilation by allowing Ca2+ entry into vascular smooth muscle cells via L-type Ca2+ channels (Rousseau et al., 2005). These same channels have been previously shown to attenuate the reduction of oxygenated hemoglobin in rats exposed to chronic cocaine (Du et al., 2021). However, the relationship between vascular dynamics and astrocytic activity with cocaine exposure have not been clarified. We have performed a series of studies to test the role of astrocytic Ca2+ accumulation in cocaine effects in the vasculature including neurovascular coupling dynamics and shown that cocaine effects on astrocytic activity modulate vascular reactivity (Liu et al., 2022).
Taken together, the current literature on CUD supports tripartite pathophysiology with neuronal, vascular and astrocytic contributions. Because of astrocytes proximity and interaction with neurons and cerebral vessels, they present a possible target for therapeutic intervention. However, there is still a substantial gap in the literature regarding the role of astrocytes in regulative vasculature in CUD. At the time of writing, a PubMed search using the MeSH terms “Cocaine-Related Disorders,” “Astrocytes,” and “Cerebrovascular Circulation” yielded only one publication by Liu et al. In this investigation, the team utilized genetically encoded calcium indicators and chemogenetics to manipulate astrocytes and observed its impact on cocaine-induced changes. They found that while neurons had a greater increase in intracellular calcium following cocaine, astrocytic calcium stayed elevated longer and was correlated with the flow of oxygenated hemoglobin in cerebral arteries. Importantly, inhibition of astrocytes using DREADD (Gi) minimized cocaine-induced vasoconstriction (Liu et al., 2022). This data demonstrated that astrocyte activation is critical for modulating vascular reactivity to cocaine in the brain parenchyma.
Astrocytes are also uniquely integrated into the dopamine reward circuitry with subgroups of astrocytes only responding to dopamine-1 receptor (D1R) medium spiny neurons (MSNs) or to dopamine-2 receptor (D2R) MSNs (Martin et al., 2015; Graziane et al., 2016). Astrocytes are also directly sensitive to changes in dopamine as optogenetic stimulation of the VTA triggering the release of DA within the reward circuity increased astrocytic calcium via astrocyte D1R or inhibition of astrocytic calcium via astrocyte D2Rs (Xin et al., 2019; Corkrum et al., 2020). Changes in the structure of astrocytes have also been associated with specific drug behaviors. In rodent models of cocaine self-administration, astrocytes in the NAc had smaller volumes and surface area following extinction of self-administration; whereas astrocytes in animals that did not undergo extinction did not show changes (Testen et al., 2018).
7 Discussion and future perspectives
Both pre-clinical and clinical investigations of chronic cocaine have documented decreased CBF in PFC, which may contribute to impulsive and compulsive behaviors (Tables 1, 2). Studies provide evidence that both reduced DA signaling and adaptations in the cerebral vasculature contributes to hypofrontality (Volkow and Morales, 2015; Zhang et al., 2016). Indeed, in our preclinical studies increases in neuronal calcium and decreases in oxygenated hemoglobin during acute cocaine intoxication in PFC correlated with cocaine intake. Moreover, pretreatment with an L-type calcium channel blocker attenuated these effects and was associated with reduced cocaine self-administration. These results provide evidence that prefrontal dysfunction mediated by cocaine induced changes in vascular physiology and in neuron/astrocytic activity contribute to addictive behaviors, such as compulsive drug intake (Du et al., 2020).
7.1 Recovery of neurovascular coupling following abstinence in humans
While the deficits in neurovascular coupling and its contribution to addiction associated behaviors have been examined, there is limited clinical literature on the recovery of this system following abstinence. A systemic review in 2022 that focused on structural and functional recovery following abstinence identified only 5 longitudinal imaging studies on cocaine abstinence (Alper et al., 1998; Moeller et al., 2012; Balodis et al., 2016; Parvaz et al., 2017a; Parvaz et al., 2017b; Parvaz et al., 2022). These studies investigated functional changes following cocaine withdrawal using BOLD or EEG as well as grey matter volume.
An investigation of the effects of chronic cocaine in the main cerebral arteries (Middle Cerebral Artery (MCA), Anterior Cerebral Artery (ACA), and Posterior Cerebral Artery (PCA)) using transcranial doppler (TCD) reported that cocaine users had decreased flow velocity with increased pulsatility index (PI). This pattern is suggestive of cerebrovascular disease caused by increased resistance in the small cortical vessels consistent with pre-clinical observations. As described above, animals models of chronic cocaine show constriction, and ultimately loss, of small vasculature in the brain parenchyma. Interestingly, following 30 days of monitored withdrawal in a closed psychiatric facility, repeat TCD showed only minor improvement in MCA velocity and no change in PI indicative of lack of recovery in the cerebrovasculature following this short abstinence window (Herning et al., 1999). This is consistent with reported investigations in mice, which found no change in cerebral hemodynamics or neovascular density following 30 days of withdrawal after 1 month of cocaine treatment.
However, longer periods of abstinence have shown more promising results regarding functional recovery. In Connolly et al. short-term abstainers (2.4 weeks) and long-term abstainers (69 weeks) in a semi-closed recovery unit underwent functional MRI to investigate cognitive impact of long-term abstinence. Using a GO/NOGO task, patients cognitive performance was assessed including their ability to successfully inhibit and errors of commission. While both groups showed increased inhibitory activity, short-term abstinent patients had increased activity in the dorsal regions of the frontal gyri while the longer-term abstainers showed increased activity in the inferior gyri, an area strongly associated with response inhibition (Connolly et al., 2012). Although limited, current investigations suggest that there is improvement in prefrontal function following abstinence with decreasing levels of impulsivity. However, it remains unclear whether this is a recovery in neuronal or astrocytic function or more closely associated with a recovery in cerebral vasculature.
7.2 Effects of cocaine on the blood brain barrier
The Blood-Brain Barrier (BBB), composed of astrocytes and endothelial cells regulates the passage of substances between the circulation and CNS (Obermeier et al., 2013; Banks, 2016). This barrier protects the brain from pathogens and regulates homeostasis. Substances of abuse, such as cocaine, have been found to increase the permeability of the BBB, allowing for the entry of toxins and contributing to neurological diseases such as HIV-associated Neurocognitive Disorder (HAND) (Fiala et al., 2005; Kousik et al., 2012).
Current literature supports that cocaine’s effects on the BBB involve various pathways. For example, chronic cocaine alters the surrounding extracellular matrix and damages the basement membrane of endothelial cells through iNOS-dependent upregulation of metalloproteinases (Sharma et al., 2009; Smith et al., 2014; Ysrayl et al., 2019). Other studies have suggested that the degeneration of the BBB is associated with cocaine-induced activation of the platelet-derived endothelial growth factor pathway. Activation of this cascade triggers a proteolytic signaling ultimately increasing cellular membrane permeability (Yao et al., 2011; Pimentel et al., 2020). Lastly, glial cells have also been implicated in damaging the BBB through cocaine-induced increase in pro-inflammatory cytokines, which increase astroglial activation, thus decreasing BBB integrity (Yao et al., 2011; Yang et al., 2016). These same cytokines, which contribute to BBB damage, are also responsible for accelerating HAND, as these molecules increase recruitment of monocytic cells that carry the HIV virus into the brain parenchyma (Dhillon et al., 2008).
7.3 Neuronal-astrocytic interactions and their role in cocaine-induced toxicity and addiction
Though for a long time it was thought that astrocytes only provided structure and nutrients to surrounding neurons, there is a much better understanding of the diverse roles of astrocytes in brain function including regulation of the synaptic microenvironment and modulation of CBF in response to neural demand (neurovascular coupling). Astrocytes display unique signaling dynamics in which calcium oscillations can propagate between astrocytes that are thought to represent gliotransmission (Xu et al., 2010; Srinivasan et al., 2015; Hanani and Verkhratsky, 2021). The regulation of glutamate, a bidirectional neuronal and glial transmitter, has been specifically implicated in addictive behaviors. The development of drug seeking and reinstatement have been linked to disruptions in astrocytic glutamate within the NAc. Both chemogenetic and pharmacological manipulation of glutamate transporters ameliorated these behaviors (Kenny et al., 2005; Miguens et al., 2008; Li et al., 2018). Specifically, activation of excitatory DREADD (Gq) selectively expressed in astrocytes in NAC prevented cue-induced reinstatement following extinction (Scofield et al., 2015). It is hypothesized that astrocytes enable increased intrasynaptic glutamate to feed back onto presynaptic inhibitory glutamate receptors preventing overexcitation by limiting the release of this excitatory transmitter (Knackstedt et al., 2009). In the absence of astrocytic stimulation, such as with chronic cocaine exposure, the excess of synaptic glutamate from the loss of presynaptic mediated inhibition of release may contribute to cocaine’s neurotoxicity through glutamate mediated excitotoxicity (Olney et al., 1991).
Acute cocaine triggers global cerebral vasoconstriction and decreased CBF and chronic use leads to vascular remodeling including angiogenesis to compensate for hypoxic injury. Astroglia appear to regulate vascular tone as their chemogenetic inhibition vasodilates cerebral blood vessels and increases CBF. Following a cocaine challenge, their inhibition abolishes cocaine-induced vasoconstriction and attenuates the reductions in CBF while also limiting neuronal calcium influx (Pan et al., 2023). Given astrocytes control of the local synaptic environment and vascular tone, they offer a promising new target for treatment of cocaine-induced neuronal toxicity and hypoxia.
7.4 Translation of discoveries into treatments
Over the past 10 years, there have been significant investments into the development of treatments for CUD (Supplementary Table S1). Advancements in the treatment of CUD have focused on either stimulating or inhibiting various types of dopamine (DA) receptors (i.e., D1R, D2R, and D3R) through agonists or antagonist but findings are inconclusive. Following a similar strategy to that of methadone as a replacement for opioid use, the utilization of amphetamine to treat CUD patients has been explored. The increase monoamines triggered by amphetamine might decrease cocaine seeking by increasing tonic DA levels and preventing burst firing (Grace, 1991; Grabowski et al., 2004; Brandt et al., 2021). Indeed, clinical trials have shown that dextro-amphetamine decreased cocaine intake in CUD patients (Shearer et al., 2003). Other medications such as modafinil, which enhances DA by inhibiting DA transporter (Volkow et al., 2009), have demonstrated mixed results in a meta-analysis of 11 randomized control trials with seven studies showing no benefit over placebo (Sangroula et al., 2017).
Transcranial magnetic stimulation (TMS) of the prefrontal cortex has also been studied for its potential to treat CUD. TMS can impact cortical functional activity and long-term potentiation or depression (Sanna et al., 2019). Targeted high frequency stimulation of the prefrontal cortex of rodents’ triggers release of DA in striatal regions with this effect paralleled in clinical studies showing that prefrontal stimulation released DA in the ipsilateral caudate nucleus. Behaviorally, repeated high frequency stimulation of the dorsal lateral prefrontal cortex of patients with CUD has led to decreased rates of relapse and reduced cocaine craving (Terraneo et al., 2016).
In addition to therapeutics that directly target the DA system, other pharmacological non-dopamine targets have been investigated to treat CUD. For example, Topiramate, an FDA approved medication for epilepsy and migraines that acts as a glutamate antagonist and γ-aminobutyric acid (GABA) agonist, increased abstinence in patients with CUD (Johnson et al., 2013). However, the effects were small and concerns regarding topiramate’s cognitive side effects mitigated interest in this medication (Rass et al., 2015). Disulfiram, an approved medication for alcohol use disorder has also been investigated for CUD and shown a small effect in improving abstinence (Traccis et al., 2024), but its high toxicity has limited further developments. Finally, bupropion, an FDA approved treatment for depression was also found to increase rates of abstinence among users, but its effects are small and findings inconsistent (Chen et al., 2020; Traccis et al., 2024).
More recently, pre-clinical studies on the impact of cocaine on the cerebrovasculature and neuronal calcium have revealed potential new treatments for CUD. These new potential therapies target channels associated with the pathophysiology of cocaine’s neurotoxicity and cocaine-induced cerebral ischemia. In a rat self-administration model, the L-type calcium channel blocker nifedipine blunted neuronal calcium accumulation and attenuated the reduction in tissue oxygenation following a cocaine challenge. Animals treated with nifedipine also displayed decreased cocaine intake and loss of cocaine reinstatement following extinction (Du et al., 2020; Du et al., 2021).
The efficacy of NMDA antagonist for treatment of CUD has also been investigated. Cocaine naïve rodents pretreated with memantine (NDMA antagonist) prior to a cocaine challenge showed a shortened duration of calcium influx with improved cerebral hemodynamics as compared to controls (Du et al., 2022). Taken together, these pre-clinical findings suggest that L-type calcium channels blockers and NMDA antagonists have potential as treatment to alleviate neuronal damage caused by increased calcium influx and chronic ischemia.
8 Conclusion
In this review, we sought to evaluate reductions in CBF as a potential mechanism in the relationship between cocaine and long-term neurological complications. In doing so, we explored the current work and understanding of how cocaine perturbations of the vascular system disrupt hemodynamic interactions with neuronal cells relevant to brain functional changes including changes in functional connectivity. Pre-clinical work has characterized the cerebral vascular response following acute cocaine and the adaptations to chronic cocaine exposures that are relevant for understanding the clinical findings of CBF and functional connectivity disruptions in CUD. Though significant advances have been made in delineating the effects of cocaine in the brain, further investigations are needed to better understand the pathophysiology of CUD including 1) characterization of the complex changes in neuronal and neurotransmitter activity that occurs with chronic use; 2) expanding the investigation on the roles of astrocytes in regulating the cerebral vasculature and neuronal activity; and 3) exploring the local microenvironment in the reactivity of neurons and astrocytes.
Author contributions
KC: Data curation, Writing–original draft, Writing–review and editing. KP: Data curation, Writing–original draft. YP: Conceptualization, Funding acquisition, Methodology, Resources, Supervision, Writing–review and editing. CL: Conceptualization, Methodology, Writing–review and editing. NDV: Conceptualization, Funding acquisition, Methodology, Resources, Writing–review and editing. CD: Conceptualization, Funding acquisition, Methodology, Resources, Supervision, Writing–original draft, Writing–review and editing.
Funding
The author(s) declare financial support was received for the research, authorship, and/or publication of this article. This work was supported in part by National Institutes of Health (NIH) grants RF1DA048808 (YP and CD), 2R01 DA029718 (CD and YP) and R21 DA057699 (YP and CD) and NIH’s Intramural Program of NIAAA (NDV). The authors would like to thank NIDA’s Drug Supply Program for providing cocaine used in the preclinical images.
Conflict of interest
The authors declare that the research was conducted in the absence of any commercial or financial relationships that could be construed as a potential conflict of interest.
The author(s) declared that they were an editorial board member of Frontiers, at the time of submission. This had no impact on the peer review process and the final decision.
Publisher’s note
All claims expressed in this article are solely those of the authors and do not necessarily represent those of their affiliated organizations, or those of the publisher, the editors and the reviewers. Any product that may be evaluated in this article, or claim that may be made by its manufacturer, is not guaranteed or endorsed by the publisher.
Supplementary material
The Supplementary Material for this article can be found online at: https://www.frontiersin.org/articles/10.3389/fphar.2024.1357422/full#supplementary-material
References
Adinoff, B., Gu, H., Merrick, C., McHugh, M., Jeon-Slaughter, H., Lu, H., et al. (2015). Basal hippocampal activity and its functional connectivity predicts cocaine relapse. Biol. Psychiatry 78 (7), 496–504. doi:10.1016/j.biopsych.2014.12.027
Allen, C. P., Park, K., Li, A., Volkow, N. D., Koob, G. F., Pan, Y., et al. (2019). Enhanced neuronal and blunted hemodynamic reactivity to cocaine in the prefrontal cortex following extended cocaine access: optical imaging study in anesthetized rats. Addict. Biol. 24 (3), 485–497. doi:10.1111/adb.12615
Alper, K. R., Prichep, L. S., Kowalik, S., Rosenthal, M. S., and John, E. R. (1998). Persistent QEEG abnormality in crack cocaine users at 6 months of drug abstinence. Neuropsychopharmacology 19 (1), 1–9. doi:10.1016/S0893-133X(97)00211-X
Al-Rawi, P. G., and Kirkpatrick, P. J. (2006). Tissue oxygen index: thresholds for cerebral ischemia using near-infrared spectroscopy. Stroke 37 (11), 2720–2725. doi:10.1161/01.STR.0000244807.99073.ae
Antoniazzi, C. T., Boufleur, N., Pase, C. S., Kuhn, F. T., Dias, V. T., Segat, H. J., et al. (2014). Tactile stimulation and neonatal isolation affect behavior and oxidative status linked to cocaine administration in young rats. Behav. Process. 103, 297–305. doi:10.1016/j.beproc.2014.01.011
Arnsten, A. F. (2009). Toward a new understanding of attention-deficit hyperactivity disorder pathophysiology: an important role for prefrontal cortex dysfunction. CNS Drugs 23, 33–41. Suppl 1. doi:10.2165/00023210-200923000-00005
Attwell, D., Buchan, A. M., Charpak, S., Lauritzen, M., Macvicar, B. A., and Newman, E. A. (2010). Glial and neuronal control of brain blood flow. Nature 468 (7321), 232–243. doi:10.1038/nature09613
Bachi, K., Mani, V., Jeyachandran, D., Fayad, Z. A., Goldstein, R. Z., and Alia-Klein, N. (2017). Vascular disease in cocaine addiction. Atherosclerosis 262, 154–162. doi:10.1016/j.atherosclerosis.2017.03.019
Baler, R. D., and Volkow, N. D. (2006). Drug addiction: the neurobiology of disrupted self-control. Trends Mol. Med. 12 (12), 559–566. doi:10.1016/j.molmed.2006.10.005
Balodis, I. M., Kober, H., Worhunsky, P. D., Stevens, M. C., Pearlson, G. D., Carroll, K. M., et al. (2016). Neurofunctional reward processing changes in cocaine dependence during recovery. Neuropsychopharmacology 41 (8), 2112–2121. doi:10.1038/npp.2016.11
Banks, W. A. (2016). From blood-brain barrier to blood-brain interface: new opportunities for CNS drug delivery. Nat. Rev. Drug Discov. 15 (4), 275–292. doi:10.1038/nrd.2015.21
Bartzokis, G., Beckson, M., Hance, D. B., Lu, P. H., Foster, J. A., Mintz, J., et al. (1999). Magnetic resonance imaging evidence of "silent" cerebrovascular toxicity in cocaine dependence. Biol. Psychiatry 45 (9), 1203–1211. doi:10.1016/s0006-3223(98)00228-5
Bass, C. E., Grinevich, V. P., Gioia, D., Day-Brown, J. D., Bonin, K. D., Stuber, G. D., et al. (2013). Optogenetic stimulation of VTA dopamine neurons reveals that tonic but not phasic patterns of dopamine transmission reduce ethanol self-administration. Front. Behav. Neurosci. 7, 173. doi:10.3389/fnbeh.2013.00173
Bekavac, I., and Waterhouse, B. D. (1995). Systemically administered cocaine selectively enhances long-latency responses of rat barrel field cortical neurons to vibrissae stimulation. J. Pharmacol. Exp. Ther. 272 (1), 333–342.
Berlingeri, M., Losasso, D., Girolo, A., Cozzolino, E., Masullo, T., Scotto, M., et al. (2017). Resting state brain connectivity patterns before eventual relapse into cocaine abuse. Behav. Brain Res. 327, 121–132. doi:10.1016/j.bbr.2017.01.002
Berridge, K. C., and Robinson, T. E. (1998). What is the role of dopamine in reward: hedonic impact, reward learning, or incentive salience? Brain Res. Brain Res. Rev. 28 (3), 309–369. doi:10.1016/s0165-0173(98)00019-8
Biswal, B., Yetkin, F. Z., Haughton, V. M., and Hyde, J. S. (1995). Functional connectivity in the motor cortex of resting human brain using echo-planar MRI. Magn. Reson Med. 34 (4), 537–541. doi:10.1002/mrm.1910340409
Brandt, L., Chao, T., Comer, S. D., and Levin, F. R. (2021). Pharmacotherapeutic strategies for treating cocaine use disorder-what do we have to offer? Addiction 116 (4), 694–710. doi:10.1111/add.15242
Bunney, B. S., Aghajanian, G. K., and Roth, R. H. (1973). Comparison of effects of L-dopa, amphetamine and apomorphine on firing rate of rat dopaminergic neurones. Nat. New Biol. 245 (143), 123–125. doi:10.1038/newbio245123a0
Buzsaki, G., and Draguhn, A. (2004). Neuronal oscillations in cortical networks. Science 304 (5679), 1926–1929. doi:10.1126/science.1099745
Ceceli, A. O., Bradberry, C. W., and Goldstein, R. Z. (2022). The neurobiology of drug addiction: cross-species insights into the dysfunction and recovery of the prefrontal cortex. Neuropsychopharmacology 47 (1), 276–291. doi:10.1038/s41386-021-01153-9
Chen, T. W., Wardill, T. J., Sun, Y., Pulver, S. R., Renninger, S. L., Baohan, A., et al. (2013). Ultrasensitive fluorescent proteins for imaging neuronal activity. Nature 499 (7458), 295–300. doi:10.1038/nature12354
Chen, W., Huang, P., Zeng, H., Lin, J., Shi, Z., and Yao, X. (2020). Cocaine-induced structural and functional impairments of the glymphatic pathway in mice. Brain Behav. Immun. 88, 97–104. doi:10.1016/j.bbi.2020.04.057
Chen, W., Liu, P., Volkow, N. D., Pan, Y., and Du, C. (2016). Cocaine attenuates blood flow but not neuronal responses to stimulation while preserving neurovascular coupling for resting brain activity. Mol. Psychiatry 21 (10), 1408–1416. doi:10.1038/mp.2015.185
Cheng, Y.-C., Ryan, K. A., Qadwai, S. A., Shah, J., Sparks, M. J., Wozniak, M. A., et al. (2016). Cocaine use and risk of ischemic stroke in young adults. Stroke 47 (4), 918–922. doi:10.1161/STROKEAHA.115.011417
Connolly, C. G., Foxe, J. J., Nierenberg, J., Shpaner, M., and Garavan, H. (2012). The neurobiology of cognitive control in successful cocaine abstinence. Drug Alcohol Depend. 121 (1-2), 45–53. doi:10.1016/j.drugalcdep.2011.08.007
Corkrum, M., Covelo, A., Lines, J., Bellocchio, L., Pisansky, M., Loke, K., et al. (2020). Dopamine-evoked synaptic regulation in the nucleus accumbens requires astrocyte activity. Neuron 105 (6), 1036–1047. e1035. doi:10.1016/j.neuron.2019.12.026
Coyle, J. T., and Balu, D. T. (2018). The role of serine racemase in the pathophysiology of brain disorders. Adv. Pharmacol. 82, 35–56. doi:10.1016/bs.apha.2017.10.002
Daras, M., Tuchman, A. J., Koppel, B. S., Samkoff, L. M., Weitzner, I., and Marc, J. (1994). Neurovascular complications of cocaine. Acta Neurol. Scand. 90 (2), 124–129. doi:10.1111/j.1600-0404.1994.tb02691.x
Daruna, J. H., and Barnes, P. A. (1993). “A neurodevelopmental view of impulsivity,” in The impulsive client: theory, research, and treatment (Massachusetts, United States: American Psychological Association), 23–37. doi:10.1037/10500-002
Desai, R., Patel, U., Rupareliya, C., Singh, S., Shah, M., Patel, R. S., et al. (2017). Impact of cocaine use on acute ischemic stroke patients: insights from nationwide inpatient sample in the United States. Cureus 9 (8), e1536. doi:10.7759/cureus.1536
Dhillon, N. K., Peng, F., Bokhari, S., Callen, S., Shin, S. H., Zhu, X., et al. (2008). Cocaine-mediated alteration in tight junction protein expression and modulation of CCL2/CCR2 axis across the blood-brain barrier: implications for HIV-dementia. J. Neuroimmune Pharmacol. 3 (1), 52–56. doi:10.1007/s11481-007-9091-1
Diercks, D. B., Fonarow, G. C., Kirk, J. D., Jois-Bilowich, P., Hollander, J. E., Weber, J. E., et al. (2008). Illicit stimulant use in a United States heart failure population presenting to the emergency department (from the acute decompensated heart failure national registry emergency module). Am. J. Cardiol. 102 (9), 1216–1219. doi:10.1016/j.amjcard.2008.06.045
Dressler, F. A., Malekzadeh, S., and Roberts, W. C. (1990). Quantitative analysis of amounts of coronary arterial narrowing in cocaine addicts. Am. J. Cardiol. 65 (5), 303–308. doi:10.1016/0002-9149(90)90292-9
Du, C., Hua, Y., Clare, K., Park, K., Allen, C. P., Volkow, N. D., et al. (2022). Memantine attenuates cocaine and neuroHIV neurotoxicity in the medial prefrontal cortex. Front. Pharmacol. 13, 895006. doi:10.3389/fphar.2022.895006
Du, C., Park, K., Allen, C. P., Hu, X. T., Volkow, N. D., and Pan, Y. (2021). Ca(2+) channel blockade reduces cocaine's vasoconstriction and neurotoxicity in the prefrontal cortex. Transl. Psychiatry 11 (1), 459. doi:10.1038/s41398-021-01573-7
Du, C., Volkow, N. D., Koretsky, A. P., and Pan, Y. (2014). Low-frequency calcium oscillations accompany deoxyhemoglobin oscillations in rat somatosensory cortex. Proc. Natl. Acad. Sci. U. S. A. 111 (43), E4677–E4686. doi:10.1073/pnas.1410800111
Du, C., Volkow, N. D., You, J., Park, K., Allen, C. P., Koob, G. F., et al. (2020). Cocaine-induced ischemia in prefrontal cortex is associated with escalation of cocaine intake in rodents. Mol. Psychiatry 25 (8), 1759–1776. doi:10.1038/s41380-018-0261-8
Einhorn, L. C., Johansen, P. A., and White, F. J. (1988). Electrophysiological effects of cocaine in the mesoaccumbens dopamine system: studies in the ventral tegmental area. J. Neurosci. 8 (1), 100–112. doi:10.1523/JNEUROSCI.08-01-00100.1988
Everitt, B. J., and Robbins, T. W. (2005). Neural systems of reinforcement for drug addiction: from actions to habits to compulsion. Nat. Neurosci. 8 (11), 1481–1489. doi:10.1038/nn1579
Fair, D. A., Schlaggar, B. L., Cohen, A. L., Miezin, F. M., Dosenbach, N. U., Wenger, K. K., et al. (2007). A method for using blocked and event-related fMRI data to study "resting state" functional connectivity. Neuroimage 35 (1), 396–405. doi:10.1016/j.neuroimage.2006.11.051
Fiala, M., Eshleman, A. J., Cashman, J., Lin, J., Lossinsky, A. S., Suarez, V., et al. (2005). Cocaine increases human immunodeficiency virus type 1 neuroinvasion through remodeling brain microvascular endothelial cells. J. Neurovirol 11 (3), 281–291. doi:10.1080/13550280590952835
Fiala, M., Gan, X. H., Zhang, L., House, S. D., Newton, T., Graves, M. C., et al. (1998). Cocaine enhances monocyte migration across the blood-brain barrier. Cocaine's connection to AIDS dementia and vasculitis? Adv. Exp. Med. Biol. 437, 199–205. doi:10.1007/978-1-4615-5347-2_22
Finkel, J. B., and Marhefka, G. D. (2011). Rethinking cocaine-associated chest pain and acute coronary syndromes. Mayo Clin. Proc. 86 (12), 1198–1207. doi:10.4065/mcp.2011.0338
Forster, S. E., Dickey, M. W., and Forman, S. D. (2018). Regional cerebral blood flow predictors of relapse and resilience in substance use recovery: a coordinate-based meta-analysis of human neuroimaging studies. Drug Alcohol Depend. 185, 93–105. doi:10.1016/j.drugalcdep.2017.12.009
Fransson, P. (2005). Spontaneous low-frequency BOLD signal fluctuations: an fMRI investigation of the resting-state default mode of brain function hypothesis. Hum. Brain Mapp. 26 (1), 15–29. doi:10.1002/hbm.20113
George, O., Mandyam, C. D., Wee, S., and Koob, G. F. (2008). Extended access to cocaine self-administration produces long-lasting prefrontal cortex-dependent working memory impairments. Neuropsychopharmacology 33 (10), 2474–2482. doi:10.1038/sj.npp.1301626
Goldstein, R. Z., Alia-Klein, N., Tomasi, D., Carrillo, J. H., Maloney, T., Woicik, P. A., et al. (2009). Anterior cingulate cortex hypoactivations to an emotionally salient task in cocaine addiction. Proc. Natl. Acad. Sci. U. S. A. 106 (23), 9453–9458. doi:10.1073/pnas.0900491106
Goldstein, R. Z., and Volkow, N. D. (2011). Dysfunction of the prefrontal cortex in addiction: neuroimaging findings and clinical implications. Nat. Rev. Neurosci. 12 (11), 652–669. doi:10.1038/nrn3119
Gollub, R. L., Breiter, H. C., Kantor, H., Kennedy, D., Gastfriend, D., Mathew, R. T., et al. (1998). Cocaine decreases cortical cerebral blood flow but does not obscure regional activation in functional magnetic resonance imaging in human subjects. J. Cereb. Blood Flow. Metab. 18 (7), 724–734. doi:10.1097/00004647-199807000-00003
Gordon, G. R., Choi, H. B., Rungta, R. L., Ellis-Davies, G. C., and MacVicar, B. A. (2008). Brain metabolism dictates the polarity of astrocyte control over arterioles. Nature 456 (7223), 745–749. doi:10.1038/nature07525
Grabowski, J., Rhoades, H., Stotts, A., Cowan, K., Kopecky, C., Dougherty, A., et al. (2004). Agonist-like or antagonist-like treatment for cocaine dependence with methadone for heroin dependence: two double-blind randomized clinical trials. Neuropsychopharmacology 29 (5), 969–981. doi:10.1038/sj.npp.1300392
Grace, A. A. (1991). Phasic versus tonic dopamine release and the modulation of dopamine system responsivity: a hypothesis for the etiology of schizophrenia. Neuroscience 41 (1), 1–24. doi:10.1016/0306-4522(91)90196-u
Grace, A. A., and Bunney, B. S. (1984). The control of firing pattern in nigral dopamine neurons: burst firing. J. Neurosci. 4 (11), 2877–2890. doi:10.1523/JNEUROSCI.04-11-02877.1984
Graziane, N. M., Sun, S., Wright, W. J., Jang, D., Liu, Z., Huang, Y. H., et al. (2016). Opposing mechanisms mediate morphine- and cocaine-induced generation of silent synapses. Nat. Neurosci. 19 (7), 915–925. doi:10.1038/nn.4313
Hachem-Delaunay, S., Fournier, M. L., Cohen, C., Bonneau, N., Cador, M., Baunez, C., et al. (2015). Subthalamic nucleus high-frequency stimulation modulates neuronal reactivity to cocaine within the reward circuit. Neurobiol. Dis. 80, 54–62. doi:10.1016/j.nbd.2015.05.007
Hanani, M., and Verkhratsky, A. (2021). Satellite glial cells and astrocytes, a comparative review. Neurochem. Res. 46 (10), 2525–2537. doi:10.1007/s11064-021-03255-8
Heilbronner, S. R., Rodriguez-Romaguera, J., Quirk, G. J., Groenewegen, H. J., and Haber, S. N. (2016). Circuit-based corticostriatal homologies between rat and primate. Biol. Psychiatry 80 (7), 509–521. doi:10.1016/j.biopsych.2016.05.012
Herning, R. I., King, D. E., Better, W. E., and Cadet, J. L. (1999). Neurovascular deficits in cocaine abusers. Neuropsychopharmacology 21 (1), 110–118. doi:10.1016/S0893-133X(98)00141-9
Howell, L. L., Hoffman, J. M., Votaw, J. R., Landrum, A. M., Wilcox, K. M., and Lindsey, K. P. (2002). Cocaine-induced brain activation determined by positron emission tomography neuroimaging in conscious rhesus monkeys. Psychopharmacol. Berl. 159 (2), 154–160. doi:10.1007/s002130100911
Hu, Y., Salmeron, B. J., Gu, H., Stein, E. A., and Yang, Y. (2015). Impaired functional connectivity within and between frontostriatal circuits and its association with compulsive drug use and trait impulsivity in cocaine addiction. JAMA Psychiatry 72 (6), 584–592. doi:10.1001/jamapsychiatry.2015.1
Hurley, S. W., West, E. A., and Carelli, R. M. (2017). Opposing roles of rapid dopamine signaling across the rostral-caudal Axis of the nucleus accumbens shell in drug-induced negative affect. Biol. Psychiatry 82 (11), 839–846. doi:10.1016/j.biopsych.2017.05.009
Hyman, S. E., Malenka, R. C., and Nestler, E. J. (2006). Neural mechanisms of addiction: the role of reward-related learning and memory. Annu. Rev. Neurosci. 29, 565–598. doi:10.1146/annurev.neuro.29.051605.113009
Iannetti, G. D., and Wise, R. G. (2007). BOLD functional MRI in disease and pharmacological studies: room for improvement? Magn. Reson Imaging 25 (6), 978–988. doi:10.1016/j.mri.2007.03.018
Jasinska, A. J., Stein, E. A., Kaiser, J., Naumer, M. J., and Yalachkov, Y. (2014). Factors modulating neural reactivity to drug cues in addiction: a survey of human neuroimaging studies. Neurosci. Biobehav Rev. 38, 1–16. doi:10.1016/j.neubiorev.2013.10.013
Jentsch, J. D., and Pennington, Z. T. (2014). Reward, interrupted: inhibitory control and its relevance to addictions. Neuropharmacology 76 (0 0), 479–486. Pt B. doi:10.1016/j.neuropharm.2013.05.022
Jentsch, J. D., and Taylor, J. R. (1999). Impulsivity resulting from frontostriatal dysfunction in drug abuse: implications for the control of behavior by reward-related stimuli. Psychopharmacol. Berl. 146 (4), 373–390. doi:10.1007/pl00005483
Jin, J. L., Liou, A. K., Shi, Y., Yin, K. L., Chen, L., Li, L. L., et al. (2015). CART treatment improves memory and synaptic structure in APP/PS1 mice. Sci. Rep. 5, 10224. doi:10.1038/srep10224
Kaufman, M. J., Levin, J. M., Ross, M. H., Lange, N., Rose, S. L., Kukes, T. J., et al. (1998). Cocaine-induced cerebral vasoconstriction detected in humans with magnetic resonance angiography. JAMA 279 (5), 376–380. doi:10.1001/jama.279.5.376
Kenny, P. J., Boutrel, B., Gasparini, F., Koob, G. F., and Markou, A. (2005). Metabotropic glutamate 5 receptor blockade may attenuate cocaine self-administration by decreasing brain reward function in rats. Psychopharmacol. Berl. 179 (1), 247–254. doi:10.1007/s00213-004-2069-2
Knackstedt, L. A., LaRowe, S., Mardikian, P., Malcolm, R., Upadhyaya, H., Hedden, S., et al. (2009). The role of cystine-glutamate exchange in nicotine dependence in rats and humans. Biol. Psychiatry 65 (10), 841–845. doi:10.1016/j.biopsych.2008.10.040
Knopfel, T. (2012). Genetically encoded optical indicators for the analysis of neuronal circuits. Nat. Rev. Neurosci. 13 (10), 687–700. doi:10.1038/nrn3293
Koob, G. F., and Bloom, F. E. (1988). Cellular and molecular mechanisms of drug dependence. Science 242 (4879), 715–723. doi:10.1126/science.2903550
Koob, G. F., and Volkow, N. D. (2010). Neurocircuitry of addiction. Neuropsychopharmacology 35 (1), 217–238. doi:10.1038/npp.2009.110
Kosten, T. R. (1998). Addiction as a brain disease. Am. J. Psychiatry 155 (6), 711–713. doi:10.1176/ajp.155.6.711
Kousik, S. M., Napier, T. C., and Carvey, P. M. (2012). The effects of psychostimulant drugs on blood brain barrier function and neuroinflammation. Front. Pharmacol. 3, 121. doi:10.3389/fphar.2012.00121
Kozak, K., Lucatch, A. M., Lowe, D. J. E., Balodis, I. M., MacKillop, J., and George, T. P. (2019). The neurobiology of impulsivity and substance use disorders: implications for treatment. Ann. N. Y. Acad. Sci. 1451 (1), 71–91. doi:10.1111/nyas.13977
Kozor, R., Grieve, S. M., Buchholz, S., Kaye, S., Darke, S., Bhindi, R., et al. (2014). Regular cocaine use is associated with increased systolic blood pressure, aortic stiffness and left ventricular mass in young otherwise healthy individuals. PLOS ONE 9 (4), e89710. doi:10.1371/journal.pone.0089710
Kroener, S., Chandler, L. J., Phillips, P. E., and Seamans, J. K. (2009). Dopamine modulates persistent synaptic activity and enhances the signal-to-noise ratio in the prefrontal cortex. PLoS One 4 (8), e6507. doi:10.1371/journal.pone.0006507
Lak, A., Costa, G. M., Romberg, E., Koulakov, A. A., Mainen, Z. F., and Kepecs, A. (2014). Orbitofrontal cortex is required for optimal waiting based on decision confidence. Neuron 84 (1), 190–201. doi:10.1016/j.neuron.2014.08.039
Lespine, L. F., Plenevaux, A., and Tirelli, E. (2019). Wheel-running exercise before and during gestation against acute and sensitized cocaine psychomotor-activation in offspring. Behav. Brain Res. 363, 53–60. doi:10.1016/j.bbr.2019.01.049
Levin, J. M., Holman, B. L., Mendelson, J. H., Teoh, S. K., Garada, B., Johnson, K. A., et al. (1994). Gender differences in cerebral perfusion in cocaine abuse: technetium-99m-HMPAO SPECT study of drug-abusing women. J. Nucl. Med. 35 (12), 1902–1909.
Levine, S. R., Brust, J. C., Futrell, N., Brass, L. M., Blake, D., Fayad, P., et al. (1991). A comparative study of the cerebrovascular complications of cocaine: alkaloidal versus hydrochloride--a review. Neurology 41 (8), 1173–1177. doi:10.1212/wnl.41.8.1173
Levine, S. R., Washington, J. M., Jefferson, M. F., Kieran, S. N., Moen, M., Feit, H., et al. (1987). "Crack" cocaine-associated stroke. Neurology 37 (12), 1849–1853. doi:10.1212/wnl.37.12.1849
Levy, D. J., and Glimcher, P. W. (2012). The root of all value: a neural common currency for choice. Curr. Opin. Neurobiol. 22 (6), 1027–1038. doi:10.1016/j.conb.2012.06.001
Li, B., and Freeman, R. D. (2007). High-resolution neurometabolic coupling in the lateral geniculate nucleus. J. Neurosci. 27 (38), 10223–10229. doi:10.1523/JNEUROSCI.1505-07.2007
Li, S. J., Biswal, B., Li, Z., Risinger, R., Rainey, C., Cho, J. K., et al. (2000). Cocaine administration decreases functional connectivity in human primary visual and motor cortex as detected by functional MRI. Magn. Reson Med. 43 (1), 45–51. doi:10.1002/(sici)1522-2594(200001)43:1<45::aid-mrm6>3.0.co;2-0
Li, X., Peng, X. Q., Jordan, C. J., Li, J., Bi, G. H., He, Y., et al. (2018). mGluR5 antagonism inhibits cocaine reinforcement and relapse by elevation of extracellular glutamate in the nucleus accumbens via a CB1 receptor mechanism. Sci. Rep. 8 (1), 3686. doi:10.1038/s41598-018-22087-1
Limpens, J. H., Damsteegt, R., Broekhoven, M. H., Voorn, P., and Vanderschuren, L. J. (2015). Pharmacological inactivation of the prelimbic cortex emulates compulsive reward seeking in rats. Brain Res. 1628, 210–218. Pt A. doi:10.1016/j.brainres.2014.10.045
Liu, Y., Hua, Y., Park, K., Volkow, N. D., Pan, Y., and Du, C. (2022). Cocaine's cerebrovascular vasoconstriction is associated with astrocytic Ca(2+) increase in mice. Commun. Biol. 5 (1), 936. doi:10.1038/s42003-022-03877-w
Logothetis, N. K., Pauls, J., Augath, M., Trinath, T., and Oeltermann, A. (2001). Neurophysiological investigation of the basis of the fMRI signal. Nature 412 (6843), 150–157. doi:10.1038/35084005
Lukas, S. E. (2014). New perspectives on using brain imaging to study CNS stimulants. Neuropharmacology 87, 104–114. doi:10.1016/j.neuropharm.2014.07.011
Luo, X., Zhang, S., Hu, S., Bednarski, S. R., Erdman, E., Farr, O. M., et al. (2013). Error processing and gender-shared and -specific neural predictors of relapse in cocaine dependence. Brain 136, 1231–1244. Pt 4. doi:10.1093/brain/awt040
Ma, Y., Shaik, M. A., Kozberg, M. G., Kim, S. H., Portes, J. P., Timerman, D., et al. (2016). Resting-state hemodynamics are spatiotemporally coupled to synchronized and symmetric neural activity in excitatory neurons. Proc. Natl. Acad. Sci. U. S. A. 113 (52), E8463–E8471. doi:10.1073/pnas.1525369113
Martin, R., Bajo-Graneras, R., Moratalla, R., Perea, G., and Araque, A. (2015). Circuit-specific signaling in astrocyte-neuron networks in basal ganglia pathways. Science 349 (6249), 730–734. doi:10.1126/science.aaa7945
Martinez, D., Narendran, R., Foltin, R. W., Slifstein, M., Hwang, D. R., Broft, A., et al. (2007). Amphetamine-induced dopamine release: markedly blunted in cocaine dependence and predictive of the choice to self-administer cocaine. Am. J. Psychiatry 164 (4), 622–629. doi:10.1176/ajp.2007.164.4.622
Martinez, L. A., Peterson, B. M., Meisel, R. L., and Mermelstein, P. G. (2014). Estradiol facilitation of cocaine-induced locomotor sensitization in female rats requires activation of mGluR5. Behav. Brain Res. 271, 39–42. doi:10.1016/j.bbr.2014.05.052
Martin-Garcia, E., Courtin, J., Renault, P., Fiancette, J. F., Wurtz, H., Simonnet, A., et al. (2014). Frequency of cocaine self-administration influences drug seeking in the rat: optogenetic evidence for a role of the prelimbic cortex. Neuropsychopharmacology 39 (10), 2317–2330. doi:10.1038/npp.2014.66
Matone, A., Gandin, C., Ghirini, S., and Scafato, E. (2022). Alcohol and substance use disorders diagnostic criteria changes and innovations in ICD-11: an overview. Clin. Psychol. Eur. 4, e9539. Spec Issue. doi:10.32872/cpe.9539
Miguens, M., Del Olmo, N., Higuera-Matas, A., Torres, I., Garcia-Lecumberri, C., and Ambrosio, E. (2008). Glutamate and aspartate levels in the nucleus accumbens during cocaine self-administration and extinction: a time course microdialysis study. Psychopharmacol. Berl. 196 (2), 303–313. doi:10.1007/s00213-007-0958-x
Mishra, A. (2017). Binaural blood flow control by astrocytes: listening to synapses and the vasculature. J. Physiol. 595 (6), 1885–1902. doi:10.1113/JP270979
Mishra, A., Reynolds, J. P., Chen, Y., Gourine, A. V., Rusakov, D. A., and Attwell, D. (2016). Astrocytes mediate neurovascular signaling to capillary pericytes but not to arterioles. Nat. Neurosci. 19 (12), 1619–1627. doi:10.1038/nn.4428
Moeller, S. J., Tomasi, D., Woicik, P. A., Maloney, T., Alia-Klein, N., Honorio, J., et al. (2012). Enhanced midbrain response at 6-month follow-up in cocaine addiction, association with reduced drug-related choice. Addict. Biol. 17 (6), 1013–1025. doi:10.1111/j.1369-1600.2012.00440.x
Moorman, D. E., and Aston-Jones, G. (2023). Prelimbic and infralimbic medial prefrontal cortex neuron activity signals cocaine seeking variables across multiple timescales. Psychopharmacol. Berl. 240 (3), 575–594. doi:10.1007/s00213-022-06287-2
Nett, K. E., and LaLumiere, R. T. (2021). Infralimbic cortex functioning across motivated behaviors: can the differences be reconciled? Neurosci. Biobehav Rev. 131, 704–721. doi:10.1016/j.neubiorev.2021.10.002
Obermeier, B., Daneman, R., and Ransohoff, R. M. (2013). Development, maintenance and disruption of the blood-brain barrier. Nat. Med. 19 (12), 1584–1596. doi:10.1038/nm.3407
Obrig, H., Neufang, M., Wenzel, R., Kohl, M., Steinbrink, J., Einhaupl, K., et al. (2000). Spontaneous low frequency oscillations of cerebral hemodynamics and metabolism in human adults. Neuroimage 12 (6), 623–639. doi:10.1006/nimg.2000.0657
Olney, J. W., Labruyere, J., Wang, G., Wozniak, D. F., Price, M. T., and Sesma, M. A. (1991). NMDA antagonist neurotoxicity: mechanism and prevention. Science 254 (5037), 1515–1518. doi:10.1126/science.1835799
Pan, Y., Du, C., Park, K., Hua, Y., and Volkow, N. (2023). Astrocytes mediate cerebral blood flow and neuronal response to cocaine in prefrontal cortex. Res. Sq. doi:10.21203/rs.3.rs-2626090/v1
Pan, Y., You, J., Volkow, N. D., Park, K., and Du, C. (2014). Ultrasensitive detection of 3D cerebral microvascular network dynamics in vivo. Neuroimage 103, 492–501. doi:10.1016/j.neuroimage.2014.08.051
Park, K., Clare, K., Volkow, N. D., Pan, Y., and Du, C. (2022). Cocaine’s effects on the reactivity of the medial prefrontal cortex to ventral tegmental area stimulation: Optical imaging study in mice. Addiction 117 (8), 2242–2253. doi:10.1111/add.15869
Parvaz, M. A., Moeller, S. J., d'Oleire Uquillas, F., Pflumm, A., Maloney, T., Alia-Klein, N., et al. (2017a). Prefrontal gray matter volume recovery in treatment-seeking cocaine-addicted individuals: a longitudinal study. Addict. Biol. 22 (5), 1391–1401. doi:10.1111/adb.12403
Parvaz, M. A., Moeller, S. J., Malaker, P., Sinha, R., Alia-Klein, N., and Goldstein, R. Z. (2017b). Abstinence reverses EEG-indexed attention bias between drug-related and pleasant stimuli in cocaine-addicted individuals. J. Psychiatry Neurosci. 42 (2), 150358–150386. doi:10.1503/jpn.150358
Parvaz, M. A., Rabin, R. A., Adams, F., and Goldstein, R. Z. (2022). Structural and functional brain recovery in individuals with substance use disorders during abstinence: a review of longitudinal neuroimaging studies. Drug Alcohol Depend. 232, 109319. doi:10.1016/j.drugalcdep.2022.109319
Pascual-Leone, A., Dhuna, A., and Anderson, D. C. (1991a). Cerebral atrophy in habitual cocaine abusers: a planimetric CT study. Neurology 41 (1), 34–38. doi:10.1212/wnl.41.1.34
Pascual-Leone, A., Dhuna, A., and Anderson, D. C. (1991b). Longterm neurological complications of chronic, habitual cocaine abuse. Neurotoxicology 12 (3), 393–400.
Paus, T. (2001). Primate anterior cingulate cortex: where motor control, drive and cognition interface. Nat. Rev. Neurosci. 2 (6), 417–424. doi:10.1038/35077500
Pimentel, E., Sivalingam, K., Doke, M., and Samikkannu, T. (2020). Effects of drugs of abuse on the blood-brain barrier: a brief overview. Front. Neurosci. 14, 513. doi:10.3389/fnins.2020.00513
Preuss, T. M. (1995). Do rats have prefrontal cortex? The rose-woolsey-akert program reconsidered. J. Cogn. Neurosci. 7 (1), 1–24. doi:10.1162/jocn.1995.7.1.1
Raichle, M. E., and Snyder, A. Z. (2007). A default mode of brain function: a brief history of an evolving idea. Neuroimage 37 (4), 1083–1090. discussion 1097-1089. doi:10.1016/j.neuroimage.2007.02.041
Rapinesi, C., Del Casale, A., Di Pietro, S., Ferri, V. R., Piacentino, D., Sani, G., et al. (2016). Add-on high frequency deep transcranial magnetic stimulation (dTMS) to bilateral prefrontal cortex reduces cocaine craving in patients with cocaine use disorder. Neurosci. Lett. 629, 43–47. doi:10.1016/j.neulet.2016.06.049
Rass, O., Umbricht, A., Bigelow, G. E., Strain, E. C., Johnson, M. W., and Mintzer, M. Z. (2015). Topiramate impairs cognitive function in methadone-maintained individuals with concurrent cocaine dependence. Psychol. Addict. Behav. 29 (1), 237–246. doi:10.1037/adb0000027
Ray, S., Di, X., and Biswal, B. B. (2016). Effective connectivity within the mesocorticolimbic system during resting-state in cocaine users. Front. Hum. Neurosci. 10, 563. doi:10.3389/fnhum.2016.00563
Rayshubskiy, A., Wojtasiewicz, T. J., Mikell, C. B., Bouchard, M. B., Timerman, D., Youngerman, B. E., et al. (2014). Direct, intraoperative observation of ∼0.1 Hz hemodynamic oscillations in awake human cortex: implications for fMRI. Neuroimage 87, 323–331. doi:10.1016/j.neuroimage.2013.10.044
Ren, H., Du, C., and Pan, Y. (2012a). Cerebral blood flow imaged with ultrahigh-resolution optical coherence angiography and Doppler tomography. Opt. Lett. 37 (8), 1388–1390. doi:10.1364/OL.37.001388
Ren, H., Du, C., Yuan, Z., Park, K., Volkow, N. D., and Pan, Y. (2012b). Cocaine-induced cortical microischemia in the rodent brain: clinical implications. Mol. Psychiatry 17 (10), 1017–1025. doi:10.1038/mp.2011.160
Rezapour, T., DeVito, E. E., Sofuoglu, M., and Ekhtiari, H. (2016). Perspectives on neurocognitive rehabilitation as an adjunct treatment for addictive disorders: from cognitive improvement to relapse prevention. Prog. Brain Res. 224, 345–369. doi:10.1016/bs.pbr.2015.07.022
Ritz, M. C., Lamb, R. J., Goldberg, S. R., and Kuhar, M. J. (1987). Cocaine receptors on dopamine transporters are related to self-administration of cocaine. Science 237 (4819), 1219–1223. doi:10.1126/science.2820058
Roberts, D. C., Morgan, D., and Liu, Y. (2007). How to make a rat addicted to cocaine. Prog. Neuropsychopharmacol. Biol. Psychiatry 31 (8), 1614–1624. doi:10.1016/j.pnpbp.2007.08.028
Robinson, S., Smith, D. M., Mizumori, S. J., and Palmiter, R. D. (2004). Firing properties of dopamine neurons in freely moving dopamine-deficient mice: effects of dopamine receptor activation and anesthesia. Proc. Natl. Acad. Sci. U. S. A. 101 (36), 13329–13334. doi:10.1073/pnas.0405084101
Rousseau, E., Cloutier, M., Morin, C., and Proteau, S. (2005). Capsazepine, a vanilloid antagonist, abolishes tonic responses induced by 20-HETE on Guinea pig airway smooth muscle. Am. J. Physiol. Lung Cell Mol. Physiol. 288 (3), L460–L470. doi:10.1152/ajplung.00252.2004
Ruskin, D. N., Bergstrom, D. A., Kaneoke, Y., Patel, B. N., Twery, M. J., and Walters, J. R. (1999). Multisecond oscillations in firing rate in the basal ganglia: robust modulation by dopamine receptor activation and anesthesia. J. Neurophysiol. 81 (5), 2046–2055. doi:10.1152/jn.1999.81.5.2046
Saez, C. G., Olivares, P., Pallavicini, J., Panes, O., Moreno, N., Massardo, T., et al. (2011). Increased number of circulating endothelial cells and plasma markers of endothelial damage in chronic cocaine users. Thromb. Res. 128 (4), e18–e23. doi:10.1016/j.thromres.2011.04.019
Saez, C. G., Pereira-Flores, K., Ebensperger, R., Panes, O., Massardo, T., Hidalgo, P., et al. (2014). Atorvastatin reduces the proadhesive and prothrombotic endothelial cell phenotype induced by cocaine and plasma from cocaine consumers in vitro. Arterioscler. Thromb. Vasc. Biol. 34 (11), 2439–2448. doi:10.1161/ATVBAHA.114.304535
Sangroula, D., Motiwala, F., Wagle, B., Shah, V. C., Hagi, K., and Lippmann, S. (2017). Modafinil treatment of cocaine dependence: a systematic review and meta-analysis. Subst. Use Misuse 52 (10), 1292–1306. doi:10.1080/10826084.2016.1276597
Sanna, A., Fattore, L., Badas, P., Corona, G., Cocco, V., and Diana, M. (2019). Intermittent theta burst stimulation of the prefrontal cortex in cocaine use disorder: a pilot study. Front. Neurosci. 13, 765. doi:10.3389/fnins.2019.00765
Schultz, W. (2007). Behavioral dopamine signals. Trends Neurosci. 30 (5), 203–210. doi:10.1016/j.tins.2007.03.007
Schultz, W. (2016). Dopamine reward prediction-error signalling: a two-component response. Nat. Rev. Neurosci. 17 (3), 183–195. doi:10.1038/nrn.2015.26
Scofield, M. D., Boger, H. A., Smith, R. J., Li, H., Haydon, P. G., and Kalivas, P. W. (2015). Gq-DREADD selectively initiates glial glutamate release and inhibits cue-induced cocaine seeking. Biol. Psychiatry 78 (7), 441–451. doi:10.1016/j.biopsych.2015.02.016
Sharma, H. S., Muresanu, D., Sharma, A., and Patnaik, R. (2009). Cocaine-induced breakdown of the blood-brain barrier and neurotoxicity. Int. Rev. Neurobiol. 88, 297–334. doi:10.1016/S0074-7742(09)88011-2
Shearer, J., Wodak, A., van Beek, I., Mattick, R. P., and Lewis, J. (2003). Pilot randomized double blind placebo-controlled study of dexamphetamine for cocaine dependence. Addiction 98 (8), 1137–1141. doi:10.1046/j.1360-0443.2003.00447.x
Simard, M., Arcuino, G., Takano, T., Liu, Q. S., and Nedergaard, M. (2003). Signaling at the gliovascular interface. J. Neurosci. 23 (27), 9254–9262. doi:10.1523/JNEUROSCI.23-27-09254.2003
Smith, A. C., Kupchik, Y. M., Scofield, M. D., Gipson, C. D., Wiggins, A., Thomas, C. A., et al. (2014). Synaptic plasticity mediating cocaine relapse requires matrix metalloproteinases. Nat. Neurosci. 17 (12), 1655–1657. doi:10.1038/nn.3846
Srinivasan, R., Huang, B. S., Venugopal, S., Johnston, A. D., Chai, H., Zeng, H., et al. (2015). Ca(2+) signaling in astrocytes from Ip3r2(-/-) mice in brain slices and during startle responses in vivo. Nat. Neurosci. 18 (5), 708–717. doi:10.1038/nn.4001
Stalnaker, T. A., Cooch, N. K., and Schoenbaum, G. (2015). What the orbitofrontal cortex does not do. Nat. Neurosci. 18 (5), 620–627. doi:10.1038/nn.3982
Strickland, T. L., Mena, I., Villanueva-Meyer, J., Miller, B. L., Cummings, J., Mehringer, C. M., et al. (1993). Cerebral perfusion and neuropsychological consequences of chronic cocaine use. J. Neuropsychiatry Clin. Neurosci. 5 (4), 419–427. doi:10.1176/jnp.5.4.419
Strickland, T. L., Miller, B. L., Kowell, A., and Stein, R. (1998). Neurobiology of cocaine-induced organic brain impairment: contributions from functional neuroimaging. Neuropsychol. Rev. 8 (1), 1–9. doi:10.1023/a:1025613322003
Terraneo, A., Leggio, L., Saladini, M., Ermani, M., Bonci, A., and Gallimberti, L. (2016). Transcranial magnetic stimulation of dorsolateral prefrontal cortex reduces cocaine use: a pilot study. Eur. Neuropsychopharmacol. 26 (1), 37–44. doi:10.1016/j.euroneuro.2015.11.011
Testen, A., Sepulveda-Orengo, M. T., Gaines, C. H., and Reissner, K. J. (2018). Region-specific reductions in morphometric properties and synaptic colocalization of astrocytes following cocaine self-administration and extinction. Front. Cell Neurosci. 12, 246. doi:10.3389/fncel.2018.00246
Thomas, S. A., Curay, C. M., and Kiyatkin, E. A. (2021). Relationships between oxygen changes in the brain and periphery following physiological activation and the actions of heroin and cocaine. Sci. Rep. 11 (1), 6355. doi:10.1038/s41598-021-85798-y
Traccis, F., Minozzi, S., Trogu, E., Vacca, R., Vecchi, S., Pani, P. P., et al. (2024). Disulfiram for the treatment of cocaine dependence. Cochrane Database Syst. Rev. 1 (1), CD007024. doi:10.1002/14651858.CD007024.pub2
Treadwell, S. D., and Robinson, T. G. (2007). Cocaine use and stroke. Postgrad. Med. J. 83 (980), 389–394. doi:10.1136/pgmj.2006.055970
Tuchman, A. J., Daras, M., Zalzal, P., and Mangiardi, J. (1987). Intracranial hemorrhage after cocaine abuse. JAMA 257 (9), 1175. doi:10.1001/jama.1987.03390090047010
Vanderschuren, L. J., and Everitt, B. J. (2004). Drug seeking becomes compulsive after prolonged cocaine self-administration. Science 305 (5686), 1017–1019. doi:10.1126/science.1098975
Vincze, J., Geyer, N., Diszhazi, G., Csernoch, L., Biro, T., Jona, I., et al. (2018). Laser induced calcium oscillations in fluorescent calcium imaging. Gen. Physiol. Biophys. 37 (3), 253–261. doi:10.4149/gpb_2017054
Volkow, N. D., Fowler, J. S., Logan, J., Alexoff, D., Zhu, W., Telang, F., et al. (2009). Effects of modafinil on dopamine and dopamine transporters in the male human brain: clinical implications. JAMA 301 (11), 1148–1154. doi:10.1001/jama.2009.351
Volkow, N. D., Koob, G. F., Croyle, R. T., Bianchi, D. W., Gordon, J. A., Koroshetz, W. J., et al. (2018). The conception of the ABCD study: from substance use to a broad NIH collaboration. Dev. Cogn. Neurosci. 32, 4–7. doi:10.1016/j.dcn.2017.10.002
Volkow, N. D., and Morales, M. (2015). The brain on drugs: from reward to addiction. Cell 162 (4), 712–725. doi:10.1016/j.cell.2015.07.046
Volkow, N. D., Mullani, N., Gould, K. L., Adler, S., and Krajewski, K. (1988). Cerebral blood flow in chronic cocaine users: a study with positron emission tomography. Br. J. Psychiatry 152, 641–648. doi:10.1192/bjp.152.5.641
Volkow, N. D., Tomasi, D., Wang, G. J., Logan, J., Alexoff, D. L., Jayne, M., et al. (2014). Stimulant-induced dopamine increases are markedly blunted in active cocaine abusers. Mol. Psychiatry 19 (9), 1037–1043. doi:10.1038/mp.2014.58
Volkow, N. D., Wang, G. J., and Baler, R. D. (2011). Reward, dopamine and the control of food intake: implications for obesity. Trends Cogn. Sci. 15 (1), 37–46. doi:10.1016/j.tics.2010.11.001
Volkow, N. D., Wang, G. J., Fowler, J. S., Logan, J., Gatley, S. J., Hitzemann, R., et al. (1997). Decreased striatal dopaminergic responsiveness in detoxified cocaine-dependent subjects. Nature 386 (6627), 830–833. doi:10.1038/386830a0
Volkow, N. D., Wang, G. J., Telang, F., Fowler, J. S., Logan, J., Childress, A. R., et al. (2008). Dopamine increases in striatum do not elicit craving in cocaine abusers unless they are coupled with cocaine cues. Neuroimage 39 (3), 1266–1273. doi:10.1016/j.neuroimage.2007.09.059
Volkow, N. D., Wang, G. J., Tomasi, D., and Baler, R. D. (2013). Unbalanced neuronal circuits in addiction. Curr. Opin. Neurobiol. 23 (4), 639–648. doi:10.1016/j.conb.2013.01.002
Wallace, E. A., Wisniewski, G., Zubal, G., vanDyck, C. H., Pfau, S. E., Smith, E. O., et al. (1996). Acute cocaine effects on absolute cerebral blood flow. Psychopharmacol. Berl. 128 (1), 17–20. doi:10.1007/s002130050104
Wee, S., and Koob, G. F. (2010). The role of the dynorphin-kappa opioid system in the reinforcing effects of drugs of abuse. Psychopharmacol. Berl. 210 (2), 121–135. doi:10.1007/s00213-010-1825-8
Wise, R. A., and Kiyatkin, E. A. (2011). Differentiating the rapid actions of cocaine. Nat. Rev. Neurosci. 12 (8), 479–484. doi:10.1038/nrn3043
Wiskerke, J., Schoffelmeer, A. N., and De Vries, T. J. (2016). Response contingency directs long-term cocaine-induced neuroplasticity in prefrontal and striatal dopamine terminals. Eur. Neuropsychopharmacol. 26 (10), 1667–1672. doi:10.1016/j.euroneuro.2016.08.013
Xin, W., Schuebel, K. E., Jair, K. W., Cimbro, R., De Biase, L. M., Goldman, D., et al. (2019). Ventral midbrain astrocytes display unique physiological features and sensitivity to dopamine D2 receptor signaling. Neuropsychopharmacology 44 (2), 344–355. doi:10.1038/s41386-018-0151-4
Xu, G., Wang, W., Kimelberg, H. K., and Zhou, M. (2010). Electrical coupling of astrocytes in rat hippocampal slices under physiological and simulated ischemic conditions. Glia 58 (4), 481–493. doi:10.1002/glia.20939
Yang, L., Yao, H., Chen, X., Cai, Y., Callen, S., and Buch, S. (2016). Role of sigma receptor in cocaine-mediated induction of glial fibrillary acidic protein: implications for HAND. Mol. Neurobiol. 53 (2), 1329–1342. doi:10.1007/s12035-015-9094-5
Yao, H., Duan, M., and Buch, S. (2011). Cocaine-mediated induction of platelet-derived growth factor: implication for increased vascular permeability. Blood 117 (8), 2538–2547. doi:10.1182/blood-2010-10-313593
Yin, W., Clare, K., Zhang, Q., Volkow, N. D., and Du, C. (2017). Chronic cocaine induces HIF-VEGF pathway activation along with angiogenesis in the brain. PLoS One 12 (4), e0175499. doi:10.1371/journal.pone.0175499
You, J., Du, C., Volkow, N. D., and Pan, Y. (2014). Optical coherence Doppler tomography for quantitative cerebral blood flow imaging. Biomed. Opt. Express 5 (9), 3217–3230. doi:10.1364/BOE.5.003217
You, J., Volkow, N. D., Park, K., Zhang, Q., Clare, K., Du, C., et al. (2017). Cerebrovascular adaptations to cocaine-induced transient ischemic attacks in the rodent brain. JCI Insight 2 (5), e90809. doi:10.1172/jci.insight.90809
Ysrayl, B. B., Balasubramaniam, M., Albert, I., Villalta, F., Pandhare, J., and Dash, C. (2019). A novel role of prolidase in cocaine-mediated breach in the barrier of brain microvascular endothelial cells. Sci. Rep. 9 (1), 2567. doi:10.1038/s41598-018-37495-6
Yuan, Z., Luo, Z., Volkow, N. D., Pan, Y., and Du, C. (2011). Imaging separation of neuronal from vascular effects of cocaine on rat cortical brain in vivo. Neuroimage 54 (2), 1130–1139. doi:10.1016/j.neuroimage.2010.08.045
Zhang, Q., You, J., Volkow, N. D., Choi, J., Yin, W., Wang, W., et al. (2016). Chronic cocaine disrupts neurovascular networks and cerebral function: optical imaging studies in rodents. J. Biomed. Opt. 21 (2), 26006. doi:10.1117/1.JBO.21.2.026006
Glossary
Keywords: cocaine, neurovascular coupling (NVC), neuroimaging, cerebral blood flow (CBF), addiction
Citation: Clare K, Park K, Pan Y, Lejuez CW, Volkow ND and Du C (2024) Neurovascular effects of cocaine: relevance to addiction. Front. Pharmacol. 15:1357422. doi: 10.3389/fphar.2024.1357422
Received: 18 December 2023; Accepted: 22 January 2024;
Published: 22 February 2024.
Edited by:
Juan J Canales, Victoria University of Wellington, New ZealandReviewed by:
Maria Bogdan, University of Medicine and Pharmacy of Craiova, RomaniaElena Martín-García, Pompeu Fabra University, Spain
Copyright © 2024 Clare, Park, Pan, Lejuez, Volkow and Du. This is an open-access article distributed under the terms of the Creative Commons Attribution License (CC BY). The use, distribution or reproduction in other forums is permitted, provided the original author(s) and the copyright owner(s) are credited and that the original publication in this journal is cited, in accordance with accepted academic practice. No use, distribution or reproduction is permitted which does not comply with these terms.
*Correspondence: Nora D. Volkow, bnZvbGtvd0BuaWRhLm5paC5nb3Y=; Congwu Du, Q29uZ3d1LkR1QHN0b255YnJvb2suZWR1