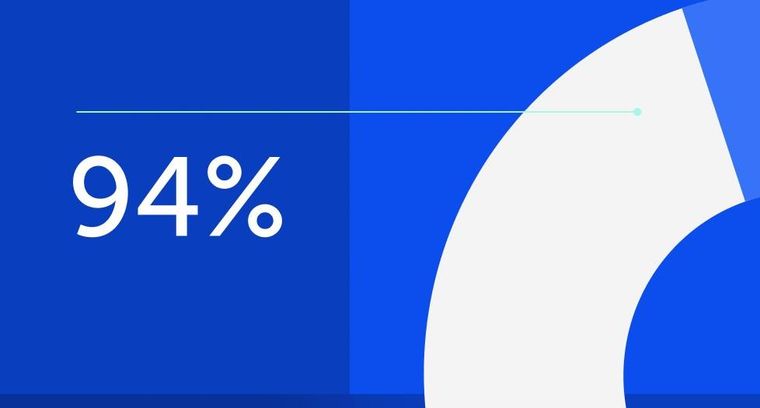
94% of researchers rate our articles as excellent or good
Learn more about the work of our research integrity team to safeguard the quality of each article we publish.
Find out more
MINI REVIEW article
Front. Pharmacol., 07 March 2024
Sec. Pharmacology of Anti-Cancer Drugs
Volume 15 - 2024 | https://doi.org/10.3389/fphar.2024.1355533
This article is part of the Research TopicDrug Discovery in Cancer Research: Success Stories and Open ChallengesView all 26 articles
Brazilin is the main compound in Caesalpinia sappan and Haematoxylum braziletto, which is identified as a homoisoflavonoid based on its molecular structure. These plants are traditionally used as an anti-inflammatory to treat fever, hemorrhage, rheumatism, skin problems, diabetes, and cardiovascular diseases. Recently, brazilin has increased its interest in cancer studies. Several findings have shown that brazilin has cytotoxic effects on colorectal cancer, breast cancer, lung cancer, multiple myeloma, osteosarcoma, cervical cancer, bladder carcinoma, also other cancers, along with numerous facts about its possible mechanisms that will be discussed. Besides its flavonoid content, brazilin is able to chelate metal ions. A study has proved that brazilin could be used as an antituberculosis agent based on its ability to chelate iron. This possible iron-chelating of brazilin and all the studies discussed in this review will lead us to the statement that, in the future, brazilin has the potency to be a chemo-preventive and anticancer agent. The article review aimed to determine the brazilin mechanism and pathogenesis of cancer.
Cancer is the second-leading cause of death among noncommunicable diseases (NCDs), after cardiovascular disease (Cadoná et al., 2022). As of 2022, there were 19.9 million incidents of cancer overall, with 9.7 million of those cases resulting in death (IARC, 2024). According to the Global Burden of Cancer Study (GLOBOCAN), the global incidence of cancer will rapidly reach 30.2 million cases by 2040, with a mortality rate of 16.3 million cases (Sung et al., 2021). Cancer is the largest cause of death in Asia, which accounts for 49.3% of all deaths (Arnold et al., 2022).
Cancer research has always been complex due to its complexity. Despite the fact that numerous treatment options are available, their effectiveness is dependent on the stage and form of the disease. Considering the different therapeutic methods, meticulous surgical excision of aggressive tissues or tumors, chemotherapy, radiation therapy, and immunotherapy are commonly used. Surgery and radiotherapy have local effects, whereas chemotherapy and targeted therapy have systemic effects. Whether these medicines are employed individually or in combination with other treatments depends on the type and stage of cancer (Muhammad et al., 2022). They could trigger processes that promote medication resistance (Naeem et al., 2022). Combinations with additional treatments (for example, radiation therapy and conventional chemotherapy) will probably kill both normal and malignant cells, resulting in substantial hematological toxicities and tissue damage (Sharifi-Rad et al., 2021).
Historically, natural products (NPs) have played an essential role in drug discovery. Compared to conventional synthetic compounds, NPs have unique properties that provide advantages and difficulties in drug discovery. NPs are the most abundant source of high chemical diversity and structural complexity. Attempts to identify interesting therapeutic chemicals from natural sources may be one explanation for this (Feher and Schmidt, 2003; Mangal et al., 2013; Atanasov et al., 2015). Approximately 80% of NPs have previously been developed for cancer treatment (Newman and Cragg, 2016). Phenolic compounds, one of the NPS, which are molecular functional compounds that target multiple signaling pathways involved in activation or transformation of cells (Bakrim et al., 2022). Flavonoids exhibit a variety of anticancer properties, including the modification of ROS-scavenging enzyme activities, cell cycle arrest, the induction of apoptosis and autophagy, and the inhibition of cancer cell growth and invasion (Kopustinskiene et al., 2020).
Caesalpinia sappan is a medicinal plant with many flavonoids (Nguyen et al., 2020). Caesalpinia sappanwood’s high flavonoid concentration supports anti-cancer research. The primary flavonoid ingredient isolated from C. sappanwood is brazilin (Mottaghipisheh and Stuppner, 2021). Brazilin can also be found in heartwood trees, including brazilwood (Caesalpinia echinata) and bralette (Caesalpinia Violaceae) (Dapson and Bain, 2015). In several studies, we found that brazilin has the ability to treat several types of cancer, such as breast cancer (Jenie et al., 2018; Hermawan and Putri, 2020; Jang et al., 2020; Chatterjee et al., 2022; Haryanti et al., 2022; Yang et al., 2023), colorectal cancer (Handayani et al., 2017), multiple myeloma (Kim et al., 2012), osteosarcoma (Kang et al., 2018), lung cancer (Suyatmi et al., 2022), cervical cancer (Kitdamrongtham et al., 2013; Jeon et al., 2014), bladder carcinoma (Zhang et al., 2015; Zhang et al., 2018), and others (Lee et al., 2013; Mitani et al., 2013; Zhang et al., 2014; Bello-Martínez et al., 2017; He et al., 2017; Correia Soeiro et al., 2022; Yan et al., 2022).
A study stated that Brazilin substances can be used to chelate iron because of their structure, which reacts with metal ions such as iron (Fe) to form a stable complex (Safitri et al., 2022). Iron chelators have long been considered potential anticancer agents (Kulp et al., 1996; Kovář et al., 2001). Iron is a major substance essential in cell growth, metabolism, and replication. Metabolism of iron is regulated in cells with cancer to cope with greater replicative demands (Theil and Goss, 2009). Iron chelating substance were initially created to treat iron overload disorders, but their potential for anticancer use is becoming increasingly apparent (Ibrahim and OSullivan, 2020).
Based on the mentioned evidence, this article review aims to determine the future potential of brazilin as an anticancer agent and its mechanism to conclude whether there is a relationship between the activity of iron chelation from the brazilin compound and cancer pathogenesis.
Relevant articles were obtained from the PubMed database with the terms “brazilin” and “cancer.” In total, 32 articles were identified. We exclude 11 articles that are not in English, not review articles, not Brazilin, and not cancer studies. Therefore, the rest 21 articles were included. The relevant research articles published without any time limit were included according to the criteria.
Brazilin, one of the main compounds originating from fractionation of the heartwood extract of C. sappan and Haematoxylum brasiletto, is most widely distributed in Southeast Asia and America (Toegel et al., 2012). Traditionally, brazilin has been taken as a red dye for cosmetics, beverages, fabrics, and food in Malaysia, China, Thailand, Mexico and Vietnam because it produces a red color (Nava-Tapia et al., 2022).
Brazilin is an organic compound of the homoisoflavonoid type, named by IUPAC as (6aS,11bR)-7,11b-dihydro-6H-indeno [2,1-c]chromene-3,6a,9,10-tetrol, with molecular formula C16H14O5 and the molecular weight 286.28 g/mol (National Center for Biotechnology Information, 2023). It may also be named as natural red, braziletto, or brasilin (Edwards et al., 2003). Brazilin is a colorless phenolic compound consisting of one pyrone, one five-membered ring, and two aromatic rings (Rondão et al., 2013). However, the hydroxyl group of the brazilin structure is readily oxidized. It can be converted into carbonyl groups, leading to transformation of the structure and forming a colored substance called Brazilein (Harborne et al., 2013).
Brazilin is the main component of the crude dye, and brazilein is a polyphenolic compound that may be separated in large amounts by exposing the organic extract to air and light, which oxidizes the hydroxyl of brazilin to a carbonyl group. Brazilin and brazilein are tetracyclic. The aromatic ring attached to the pyrone ring should originate in the acetate pathway, while the aromatic ring bonded to the five-membered ring in the shikimic acid pathway (de Oliveira et al., 2002).
Traditionally, extracts of C. sappan and H. brasiletto have been applied to fever, hemorrhage, diabetes, skin problems, cardiovascular diseases, and diabetes as an anti-inflammatory because of their potential for medicinal use (Pawar et al., 2008; Nirmal and Panichayupakaranant, 2015; Mueller et al., 2016; Hwang and Shim, 2018). Specifically, as a major compound of both plants, brazilin possesses various pharmacological activities. Brazilin is a significant inhibitor of nitrite oxide (NO) production. It is a valuable therapy for antioxidants, anti-inflammation, and vascular relaxation (Hu et al., 2003; Sasaki et al., 2007). Brazilin significantly inhibited J444.1 cell line nitrite oxide (NO) generation produced by lipopolysaccharide (LPS). It has been discovered that brazilin significantly lowers iNOS gene expression at 100 μM, while its derivative, brazilein, does so even at 10 µM54. As an essential relaxing factor in the circulatory system, brazilin increases NO production, NOS activity, and extracellular Ca2+ influx in human umbilical vein endothelial cells (Hu et al., 2003). Compared to its derivate, Brazilein, which has activity to reducing liver damage that caused by excess iron, increasing cytotoxicity and apoptosis in T47D cells, and inhibiting NFκB1/p50 in human osteoarthritic (Safitri et al., 2016; Utomo et al., 2018; Weinmann et al., 2018). Brazilein also can be invented as potential antibacterial agent against Escherichia coli MDR, a sensitive antibiotic using in silico method (Krihariyani et al., 2020).
Brazilin has antimicrobial effects through decreasing DNA and protein production. Brazilin inhibited the growth of bacteria that cause methicillin-resistant Staphylococcus aureus (MRSA), dental caries (Streptococcus mutans), periodontal disease (Prevotella intermedia), acne (Propionibacterium acnes), and strep throat (Group A strep) (Xu and Lee, 2004). Brazilin has an increased pyruvate kinase activity mechanism. In addition, it may play a role in the anti-gluconeogenic action of brazilin. Brazilin enhanced the levels of 6-phosphofructo-2-kinase (PFK-2), fructose-6-phosphate (F-6-P), and hexose-6-phosphate (H-6-P) extensively (You et al., 2005).
Brazilin has been studied in several types of cancer, such as cervical cancer, cervical squamous cell carcinoma, breast cancer, colorectal adenocarcinoma, colorectal cancer, colon cancer, hepatocellular carcinoma, lung adenocarcinoma, and sarcoma. A compound’s ability to impede biological or biochemical function may be measured through its half-maximal inhibitory concentration (IC50) (Hendriks, 2010). Drug potency, or the quantity of a drug required to have a therapeutic effect, is correlated with the IC50 value. Like a drug, the IC50 value of a natural compound must be determined to know the cytotoxicity. The lower the IC50 value, the more cytotoxic the substance is (Meyer et al., 2019). Based on its IC50 value from different types of cancer cell lines (Bello-Martínez et al., 2017; Handayani et al., 2017; Haryanti et al., 2022; Jenie et al., 2018; Suyatmi et al., 2022; Yang et al., 2023; Zhang et al., 2014), brazilin has a strong ability to treat breast cancer on 4T1 cell lines with the measured IC50 value of 3.7 µM. The methods used in several studies to know the mechanism of brazilin to treat several types of cancer are shown in Table 1.
In treating cancer, brazilin has different types of mechanisms, but the general mechanism is to induce apoptosis and inhibit cell proliferation. Most of the studies were carried out using breast cancer cell lines. Brazilin inhibits cell proliferation, migration, and invasion as the primary therapy and co-therapy with doxorubicin (Jenie et al., 2018; Haryanti et al., 2022). Hemin-induced heme oxygenase-1 (HO-1) in breast cancer cells is slightly inhibited by brazilin (Jang et al., 2020). Besides, HO-1 has cytoprotective properties to promote cancer progression in cancer cells, yet HO-1 overactivation also promotes unconventional ferroptosis due to an accumulation of prooxidant-free iron (Nitti et al., 2021). Other findings were obtained to help arrange possible molecular mechanisms of brazilin as a novel anticancer agent. Brazilin suppressed the activity of transcription factors called histone deacetylases (HDACs), which are involved in controlling cell cycle arrest and apoptosis in multiple myeloma (Kim et al., 2012).
An in silico study predicted several targets that brazilin inhibited. In triple breast cancer, brazilin was found to attach more firmly and effectively to MMP-2, MMP-9, and PTGS2 compared to its native ligand (Haryanti et al., 2022). These enzymes are involved in cell migration and metastasis (Webb et al., 2017; Ercolano et al., 2019). According to these findings, brazilin could potentially disrupt the activities of the enzymes by binding to their active sites (Haryanti et al., 2022).
Brazilin has also shown its activity in several other types of cancer, but its mechanism has not yet been studied further. Brazilin mechanisms are limited to in silico, in vitro, and in vivo. No clinical research has ever been done. Apoptotic-related pathways are still the most interesting to explore for a new potential anticancer agent. Every discovery is valuable to guide us for new pathways regarding anticancer therapy mechanisms by brazilin. Of all the findings about brazilin mechanisms towards cancer, the iron chelation mechanism has not been mentioned yet. In the meantime, using iron chelators as an adjuvant cancer treatment is becoming more popular, even though they have been developed initially to treat iron overload disorders (Wang et al., 2019; Safitri et al., 2022).
Brazilin belongs to the class of flavonoids that are likely to interact with metals, mainly Fe (Kejík et al., 2021). A study has found that brazilin could be used as an antituberculosis because of its mechanism of inhibiting Mycobacterium tuberculosis (Mtb) extracellularly by iron chelation (Safitri et al., 2022). Iron overload removal and prevention are the main goals of chelation therapy. By chelating extra iron, iron levels can be maintained at normal levels. Iron chelation therapy was first developed for transfusion-dependent anemias, including myelodysplasia, sickle cell disease, and thalassemia. However, this is only a small portion of the potential spectrum of activity for iron chelators (Porter, 2001). Transferrin (Tf), a protein with a strong affinity for iron, carries iron in plasma. After the iron transferrin complex attaches to the cell surface’s transferrin receptor 1 (TfR1), the complex is internalized through receptor-mediated endocytosis, and endosomal acidification releases the iron from Tf (Hentze et al., 2004). On the other hand, cancer cells have a few different mechanisms to keep the balance of iron within their cells. In neoplastic cells, iron metabolism is altered in order to fulfill higher replicative needs. Numerous processes contribute to the increased iron uptake in neoplastic cells, but the most prominent one is the increased protein expression of the TfR1 receptor, which has been found in several cases, including renal, colorectal, liver, breast, and lung cancer (Kindrat et al., 2016; Greene et al., 2017; Horniblow et al., 2017; Rychtarcikova et al., 2017). In these neoplasms, its level has been connected to the growth of the tumor (Brookes et al., 2006). Numerous neoplasms have also been reported to have elevated levels of the homologous TfR2 (Calzolari et al., 2007). It has been shown in melanoma and hepatoma cell lines that intake occurs via non-receptor-mediated pinocytosis once TfR1 is saturated (Richardson and Baker, 1994; Trinder et al., 1996).
Iron chelators that are clinically approved are as follows: Deferoxamine (DFO) (Kontoghiorghes et al., 1987; Hernlem et al., 1996), Deferiprone (L1) (Rombos et al., 2000; Cohen et al., 2003; Hoffbrand et al., 2003), and Deferasirox (DFX) (Gaboriau et al., 2010). Iron chelators were initially developed to treat iron overload disorders like thalassemia, but there is increasing interest in their potential as adjunctive therapy for cancer. The combination of iron-chelating agents like DFO or DFX with cisplatin, doxorubicin, and carboplatin has been shown to increase the cytotoxic effects of these chemotherapeutics in some studies (Wang et al., 2019; Safitri et al., 2022). Another natural compound that has iron chelator activity is curcumin. Curcumin shows great potential as a therapeutic substance and is being studied in humans for a number of diseases, such as psoriasis, pancreatic cancer, multiple myeloma, colon cancer, and myelodysplastic syndromes. Curcumin inhibits the development and progression of cancer by targeting different stages in the malignant process (Hatcher et al., 2009).
Due to the bidentate ligand, a strong metal cation scavenger that can tightly bind iron (III) at pH 7, brazilin also happens to have a catechol group that may chelate iron, according to various research (Hruby et al., 2021). Brazilin’s structure shares a few similarities to that of DFO, a hexadentate compound that can bind iron in a 1:1 ratio to form a stable complex that prevents the free radicals that iron produces (Zhou et al., 2012). An iron chelator’s potency is determined by how well it can bind transferrin-bound iron that is not circulating in the plasma. Among other iron chelators, L1 is considered very effective in iron chelating (Maskoen et al., 2016). The substance has the benefit of quickly penetrating membranes to remove potentially harmful iron from tissues since the Fe(III) chelate of L1 has no net charge (Kattamis et al., 2006). To chelate one iron atom, L1 molecules are required (Kontoghiorghes et al., 1987; Merson and Olivier, 2002). Structurally, brazilin has properties similar to L1 due to its bidentate structure, indicating that brazilin can bind iron with the same ratio as L1. Mechanism prediction of brazilin as iron chelators is shown in Figure 1.
FIGURE 1. Brazilin (National Center for Biotechnology Information, 2024b) and Brazilein ((National Center for Biotechnology Information, 2024a) structure (A), Brazilin predicted mechanism as iron chelators in cancer cells (B). Illustration refers to the common mechanism of clinically approved iron chelators (i.e., Deferoxamine (DFO)). DFO and other compounds with similar structures have poor pharmacokinetics profiles. Conjugation with drug carriers, such as polymers, could help increase therapeutical efficacy (Komoto et al., 2021). Brazilin with carriers (polymer, plasmid, etc.) entering cell cytoplasm via endocytosis. Iron uptake involves Transferrin that binds two irons and enters cells by interaction with the Transferrin Receptor (TfR1). Iron is typically transported by DMT1 toward the labile iron pool (LIP) and then scavenged and stored by Ferritin (Pfeifhofer-Obermair et al., 2018). In cancer cells, the requirements of iron are relatively high, and there is also an accumulation of free iron inside cells caused by Hepcidin (HAMP) binding to Ferroportin (FPN), which is beneficial for iron export to maintain iron homeostasis (Bystrom et al., 2014). Iron chelation occurs inside cells toward free labile iron (Fe2+). Thereby, storage and utilization of iron are inhibited by this chelation mechanism. Further research is recommended to clarify whether brazilin promotes pro-oxidant and iron depletion effects. Abbreviations: DMT1, divalent metal transporter 1; Fe-S, iron sulfide; HAMP, hepcidin antimicrobial peptide; ROS, reactive oxygen species; STEAP3, six-transmembrane epithelial antigen of prostate 3; Tf, transferrin.
The development of iron chelators as therapeutic agents can also be useful anticancer agents (Buss et al., 2005) either by depleting iron in the tumor or by causing selective oxidative stress in the tumor due to redox perturbations in its environment (Hatcher et al., 2009; Fibach and Rachmilewitz, 2017). One of the metabolic characteristics of malignant cancer cells is dysregulated iron homeostasis, where iron is crucial for the growth, survival, proliferation, and metastasis of tumors at every stage of the process (Ludwig et al., 2015). Tumor cells are more susceptible to iron deficiency than normal cells because they rely heavily on iron for development and proliferation (Bystrom and Rivella, 2015). Iron reductase, primarily found in some members of the metal reductases six-transmembrane epithelial antigen of the prostate (STEAP1-4) family, reduces Fe3+ to Fe2+ in the endosome. Many human cancer types, including breast, colon, prostate, cervix, bladder, pancreatic, testis, ovary and Ewing sarcoma, have high expression levels of STEAP1 and STEAP2. In malignant gliomas, STEAP3 is overexpressed, and STEAP3 knockdown suppresses glioma cell metastasis, proliferation, and clonality, in vitro and tumor growth in vivo. Under hypoxic conditions, STEAP4 is activated, which increases the incidence of colitis-associated colon cancer in mice models, enhances the formation of reactive oxygen species (ROS), and causes an iron imbalance in the mitochondria (Wang et al., 2019).
Deferoxamine (DFO) and Deferasirox (DFX) are widely used for iron overload disease in cancer therapy (Ibrahim and OSullivan, 2020). Among all iron chelators available on market, DFX is the first-choice iron chelator used globally to treat non-transfusion-dependent thalassemia syndromes in patients from age of 10 and above, along with chronic iron overload on by blood transfusions in patients from age of 2 (Piga et al., 2006). DFO chelates non-transferrin bound iron (free iron), hemosiderin, and iron in transit between transferrin and ferritin (labile chelating iron pool). DFO is able to directly attach to iron and remove it away from heart cells, but it is unable to bind iron that has already been integrated into other molecules, such as hemoglobin, transferrin, or cytochromes (Hershko et al., 2001; Komoto et al., 2021). However, DFX preferentially binds to iron in its oxidized ferric (Fe3+) state than to the reduced or ferrous (Fe2+) state. Every DFX molecule binds two ferric irons (Valentovic and Enna, 2008). Additionally, it was discovered that DFX caused apoptosis by reducing ER stress responses (Kim et al., 2016). Based on previous discussion, we conclude that brazilin has the potential ability to chelate iron. Concerning its activity, it has previously been developed as a targeted anticancer therapy. As a result, brazilin can potentially be an anticancer agent through the iron chelation mechanism.
Brazilin has different mechanisms in treating cancer, but mainly induces apoptosis and inhibits cell proliferation. Most of the studies were carried out using breast cancer cell lines. Brazilin inhibits cell proliferation, migration, and invasion as the primary therapy and co-therapy with doxorubicin (Jenie et al., 2018; Haryanti et al., 2022). Brazilian mechanism studies are limited to in silico and in vivo. Every discovery is valuable to guide us for new pathways regarding anticancer therapy mechanisms by brazilin. The iron chelation mechanism in brazilin to treat cancer is not mentioned yet. Iron chelators were originally created to treat iron overload problems, however there has been rising interest in using them as adjuvant therapy for cancer (Wang et al., 2019; Safitri et al., 2022).
Other pathways are present in cancer cells for keeping the equilibrium of iron within the cell. In neoplastic cells, iron metabolism is altered to satisfy higher replicative needs. Numerous processes contribute to the increased iron uptake in neoplastic cells, but the most prominent one is the increased protein expression of the TfR1 receptor, which has been found in several cases, including renal, colorectal, liver, breast, and lung cancer (Kindrat et al., 2016; Greene et al., 2017; Horniblow et al., 2017; Rychtarcikova et al., 2017). Brazilin’s bidentate ligand, a strong metal cation scavenger that can tightly bind iron (III) at pH 7, is responsible for the catechol group’s ability to chelate iron (Hruby et al., 2021). Brazilin’s structure shares a few similarities to that of deferoxamine (DFO), a hexadentate compound that can bind iron in a 1:1 ratio to form a stable complex that prevents the free radicals that iron produces (Zhou et al., 2012).
In conclusion, we have found that brazilin has activities toward cancer pathogenesis via apoptosis mechanism and cell cycle arrest. There is a study stating that brazilin has the ability to chelate iron as an antituberculosis agent. The development of iron chelators also can be useful as anticancer agents. Throughout the whole process of tumor growth, survival, proliferation, and metastasis, iron is essential. Therefore, brazilin has the potential as an anticancer agent through the iron chelation mechanism. However, further research and investigations must be conducted on brazilin to confirm this finding.
CR: Data curation, Methodology, Writing–original draft. SZ: Data curation, Methodology, Writing–original draft. AF: Data curation, Formal Analysis, Methodology, Visualization, Writing–original draft, Writing–review and editing. RA: Conceptualization, Formal Analysis, Funding acquisition, Methodology, Project administration, Supervision, Visualization, Writing–original draft, Writing–review and editing.
The author(s) declare that financial support was received for the research, authorship, and/or publication of this article. Universitas Padjadjaran funded this research study under the Universitas Padjadjaran Research Grant Scheme.
Universitas Padjadjaran funded this research study under the Universitas Padjadjaran Research Grant Scheme.
The authors declare that the research was conducted in the absence of any commercial or financial relationships that could be construed as a potential conflict of interest.
All claims expressed in this article are solely those of the authors and do not necessarily represent those of their affiliated organizations, or those of the publisher, the editors and the reviewers. Any product that may be evaluated in this article, or claim that may be made by its manufacturer, is not guaranteed or endorsed by the publisher.
Arnold, M., Morgan, E., Rumgay, H., Mafra, A., Singh, D., Laversanne, M., et al. (2022). Current and future burden of breast cancer: global statistics for 2020 and 2040. Breast 66, 15–23. doi:10.1016/j.breast.2022.08.010
Atanasov, A. G., Waltenberger, B., Pferschy-Wenzig, E. M., Linder, T., Wawrosch, C., Uhrin, P., et al. (2015). Discovery and resupply of pharmacologically active plant-derived natural products: a review. Biotechnol. Adv. 33 (8), 1582–1614. doi:10.1016/j.biotechadv.2015.08.001
Bakrim, S., El Omari, N., El Hachlafi, N., Bakri, Y., Lee, L. H., and Bouyahya, A. (2022). Dietary phenolic compounds as anticancer natural drugs: recent update on molecular mechanisms and clinical trials. Foods 11 (21), 3323. doi:10.3390/foods11213323
Bello-Martínez, J., Jiménez-Estrada, M., Rosas-Acevedo, J. L., Avila-Caballero, L. P., Vidal-Gutierrez, M., Patiño-Morales, C., et al. (2017). Antiproliferative activity of Haematoxylum brasiletto H. Karst. Pharmacogn. Mag. 13 (50), S289-S293–S293. doi:10.4103/pm.pm_466_16
Brookes, M. J., Hughes, S., Turner, F. E., Reynolds, G., Sharma, N., Ismail, T., et al. (2006). Modulation of iron transport proteins in human colorectal carcinogenesis. Gut 55 (10), 1449–1460. doi:10.1136/gut.2006.094060
Buss, J., Torti, F., and Torti, S. (2005). The role of iron chelation in cancer therapy. Curr. Med. Chem. 10 (12), 1021–1034. doi:10.2174/0929867033457638
Bystrom, L. M., Guzman, M. L., and Rivella, S. (2014). Iron and reactive oxygen species: friends or foes of cancer cells? Antioxidants Redox Signal 20 (12), 1917–1924. doi:10.1089/ars.2012.5014
Bystrom, L. M., and Rivella, S. (2015). Cancer cells with irons in the fire. Free Radic. Biol. Med. 79, 337–342. doi:10.1016/j.freeradbiomed.2014.04.035
Cadoná, F. C., Dantas, R. F., de Mello, G. H., and Silva-, F. P. (2022). Natural products targeting into cancer hallmarks: an update on caffeine, theobromine, and (+)-catechin. Crit. Rev. Food Sci. Nutr. 62 (26), 7222–7241. doi:10.1080/10408398.2021.1913091
Calzolari, A., Oliviero, I., Deaglio, S., Mariani, G., Biffoni, M., Sposi, N. M., et al. (2007). Transferrin receptor 2 is frequently expressed in human cancer cell lines. Blood Cells, Mol. Dis. 39 (1), 82–91. doi:10.1016/j.bcmd.2007.02.003
Chatterjee, B., Ghosh, K., Swain, A., Nalla, K. K., Ravula, H., Pan, A., et al. (2022). The phytochemical brazilin suppress DNMT1 expression by recruiting p53 to its promoter resulting in the epigenetic restoration of p21 in MCF7cells. Phytomedicine 95, 153885. doi:10.1016/j.phymed.2021.153885
Cohen, A. R., Galanello, R., Piga, A., De Sanctis, V., and Tricta, F. (2003). Safety and effectiveness of long-term therapy with the oral iron chelator deferiprone. Blood 102 (5), 1583–1587. doi:10.1182/blood-2002-10-3280
Correia Soeiro, M. de N., Vergoten, G., and Bailly, C. (2022). Molecular docking of brazilin and its analogs to barrier-to-autointegration factor 1 (BAF1). Ann. N. Y. Acad. Sci. 1511 (1), 154–163. doi:10.1111/nyas.14742
Dapson, R. W., and Bain, C. L. (2015). Brazilwood, sappanwood, brazilin and the red dye brazilein: from textile dyeing and folk medicine to biological staining and musical instruments. Biotech. Histochem 90 (6), 401–423. doi:10.3109/10520295.2015.1021381
de Oliveira, L. F. C., Edwards, H. G. M., Velozo, E. S., and Nesbitt, M. (2002). Vibrational spectroscopic study of brazilin and brazilein, the main constituents of brazilwood from Brazil. Vib. Spectrosc. 28 (2), 243–249. doi:10.1016/S0924-2031(01)00138-2
Edwards, H. G. M., De Oliveira, L. F. C., and Nesbitt, M. (2003). Fourier-transform Raman characterization of brazilwood trees and substitutes. Analyst 128 (1), 82–87. doi:10.1039/b209052j
Ercolano, G., De Cicco, P., Rubino, V., Terrazzano, G., Ruggiero, G., Carriero, R., et al. (2019). Knockdown of PTGS2 by CRISPR/CAS9 system designates a new potential gene target for melanoma treatment. Front. Pharmacol. 10, 1456. doi:10.3389/fphar.2019.01456
Feher, M., and Schmidt, J. M. (2003). Property distributions: differences between drugs, natural products, and molecules from combinatorial chemistry. J. Chem. Inf. Comput. Sci. 43 (1), 218–227. doi:10.1021/ci0200467
Fibach, E., and Rachmilewitz, E. A. (2017). Iron overload in hematological disorders. Press Medicale 46 (12P2), e296–e305. doi:10.1016/j.lpm.2017.10.007
Gaboriau, F., Leray, A. M., Ropert, M., Gouffier, L., Cannie, I., Troadec, M. B., et al. (2010). Effects of deferasirox and deferiprone on cellular iron load in the human hepatoma cell line HepaRG. BioMetals 23 (2), 231–245. doi:10.1007/s10534-009-9281-9
Greene, C. J., Attwood, K., Sharma, N. J., Gross, K. W., Smith, G. J., Xu, B., et al. (2017). Transferrin receptor 1 upregulation in primary tumor and downregulation in benign kidney is associated with progression and mortality in renal cell carcinoma patients. Oncotarget 8 (63), 107052–107075. doi:10.18632/oncotarget.22323
Handayani, S., Susidarti, R. A., Jenie, R. I., and Meiyanto, E. (2017). Two active compounds from Caesalpinia sappan L. in combination with cisplatin synergistically induce apoptosis and cell cycle arrest on WiDr cells. Adv. Pharm. Bull. 7 (3), 375–380. doi:10.15171/apb.2017.045
Harborne, J. B., Marby, H., and Marby, T. J. (2013). The flavonoids. London, New York: Chapman and Hall Ltd.
Haryanti, S., Zulfin, U. M., Salsabila, I. A., Wulandari, F., and Meiyanto, E. (2022). The cytotoxic and anti-migratory properties of Caesalpinia sappan and Ficus septica, in combination with doxorubicin on 4T1 TNBC cells with nephroprotective potential. Asian Pac. J. Cancer Prev. 23 (2), 743–752. doi:10.31557/APJCP.2022.23.2.743
Hatcher, H. C., Singh, R. N., Torti, F. M., and Torti, S. V. (2009). Synthetic and natural iron chelators: therapeutic potential and clinical use. Future Med. Chem. 1 (9), 1643–1670. doi:10.4155/fmc.09.121
He, Z. J., Zhu, F. Y., Li, S. S., Zhong, L., Tan, H. Y., and Wang, K. (2017). Inhibiting ROS-NF-κB-dependent autophagy enhanced brazilin-induced apoptosis in head and neck squamous cell carcinoma. Food Chem. Toxicol. 101, 55–66. doi:10.1016/j.fct.2017.01.002
Hendriks, B. S. (2010). Functional pathway pharmacology: chemical tools, pathway knowledge and mechanistic model-based interpretation of experimental data. Curr. Opin. Chem. Biol. 14 (4), 489–497. doi:10.1016/j.cbpa.2010.06.167
Hentze, M. W., Muckenthaler, M. U., and Andrews, N. C. (2004). Balancing acts: molecular control of mammalian iron metabolism. Cell 117 (3), 285–297. doi:10.1016/S0092-8674(04)00343-5
Hermawan, A., and Putri, H. (2020). Integrative bioinformatics analysis reveals potential target genes and TNFα signaling inhibition by brazilin in metastatic breast cancer cells. Asian Pac. J. Cancer Prev. 21 (9), 2751–2762. doi:10.31557/APJCP.2020.21.9.2751
Hernlem, B. J., Vane, L. M., and Sayles, G. D. (1996). Stability constants for complexes of the siderophore desferrioxamine b with selected heavy metal cations. Inorganica Chim. Acta 244 (2), 179–184. doi:10.1016/0020-1693(95)04780-8
Hershko, C., Konijn, A. M., Nick, H. P., Breuer, W., Cabantchik, Z. I., and Link, G. (2001). ICL670A: a new synthetic oral chelator: evaluation in hypertransfused rats with selective radioiron probes of hepatocellular and reticuloendothelial iron stores and in iron-loaded rat heart cells in culture. Blood 97 (4), 1115–1122. doi:10.1182/blood.V97.4.1115
Hoffbrand, A. V., Cohen, A., and Hershko, C. (2003). Role of deferiprone in chelation therapy for transfusional iron overload. Blood 102 (1), 17–24. doi:10.1182/blood-2002-06-1867
Horniblow, R. D., Bedford, M., Hollingworth, R., Evans, S., Sutton, E., Lal, N., et al. (2017). BRAF mutations are associated with increased iron regulatory protein-2 expression in colorectal tumorigenesis. Cancer Sci. 108 (6), 1135–1143. doi:10.1111/cas.13234
Hruby, M., Martínez, I. I. S., Stephan, H., Pouckova, P., Benes, J., and Stepanek, P. (2021). Chelators for treatment of iron and copper overload: shift from low-molecular-weight compounds to polymers. Polym. (Basel) 13 (22), 3969. doi:10.3390/polym13223969
Hu, C. M., Kang, J. J., Lee, C. C., Li, C. H., Liao, J. W., and Cheng, Y. W. (2003). Induction of vasorelaxation through activation of nitric oxide synthase in endothelial cells by brazilin. Eur. J. Pharmacol. 468 (1), 37–45. doi:10.1016/S0014-2999(03)01639-X
Hwang, H. S., and Shim, J. H. (2018). Brazilin and Caesalpinia sappan L. extract protect epidermal keratinocytes from oxidative stress by inducing the expression of GPX7. Chin. J. Nat. Med. 16 (3), 203–209. doi:10.1016/S1875-5364(18)30048-7
IARC (2024). Cancer today. Available at: https://gco.iarc.who.int/(Accessed February 2, 2024).
Ibrahim, O., and OSullivan, J. (2020). Iron chelators in cancer therapy. BioMetals 33 (4-5), 201–215. doi:10.1007/s10534-020-00243-3
Jang, H. Y., Hong, O. Y., Chung, E. Y., Park, K. H., and Kim, J. S. (2020). Roles of JNK/NRF2 pathway on hemin-induced heme oxygenase-1 activation in MCF-7 human breast cancer cells. Med. Lith. 56 (6), 268. doi:10.3390/medicina56060268
Jenie, R. I., Handayani, S., Susidarti, R. A., Udin, L. Z., and Meiyanto, E. (2018). The cytotoxic and antimigratory activity of Brazilin-doxorubicin on MCF-7/HER2 cells. Adv. Pharm. Bull. 8 (3), 507–516. doi:10.15171/apb.2018.059
Jeon, J., Lee, J. H., Park, K. A., Byun, H. S., Lee, H., Lee, Y., et al. (2014). Brazilin selectively disrupts proximal IL-1 receptor signaling complex formation by targeting an IKK-upstream signaling components. Biochem. Pharmacol. 89 (4), 515–525. doi:10.1016/j.bcp.2014.04.004
Kang, Y., He, P., Wang, H., Ye, Y., Li, X., Xie, P., et al. (2018). Brazilin induces FOXO3A-dependent autophagic cell death by disturbing calcium homeostasis in osteosarcoma cells. Cancer Chemother. Pharmacol. 82 (3), 479–491. doi:10.1007/s00280-018-3633-5
Kattamis, A., Ladis, V., Berdousi, H., Kelekis, N. L., Alexopoulou, E., Papasotiriou, I., et al. (2006). Iron chelation treatment with combined therapy with deferiprone and deferioxamine: a 12-month trial. Blood Cells, Mol. Dis. 36 (1), 21–25. doi:10.1016/j.bcmd.2005.11.002
Kejík, Z., Kaplánek, R., Masařík, M., Babula, P., Matkowski, A., Filipenský, P., et al. (2021). Iron complexes of flavonoids-antioxidant capacity and beyond. Int. J. Mol. Sci. 22 (2), 646. doi:10.3390/ijms22020646
Kim, J. L., Lee, D. H., Na, Y. J., Kim, B. R., Jeong, Y. A., Lee, S. I., et al. (2016). Iron chelator-induced apoptosis via the ER stress pathway in gastric cancer cells. Tumor Biol. 37 (7), 9709–9719. doi:10.1007/s13277-016-4878-4
Kim, S. H., Kim, B., Kim, S. H., Jeong, S. J., Sohn, E. J., Jung, J. H., et al. (2012). Brazilin induces apoptosis and G2/M arrest via inactivation of histone deacetylase in multiple myeloma U266 cells. J. Agric. Food Chem. 60 (39), 9882–9889. doi:10.1021/jf302527p
Kindrat, I., Tryndyak, V., Conti, A. de, Shpyleva, S., Mudalige, T. K., Kobets, T., et al. (2016). MicroRNA-152-mediated dysregulation of hepatic transferrin receptor 1 in liver carcinogenesis. Oncotarget 7 (2), 1276–1287. doi:10.18632/oncotarget.6004
Kitdamrongtham, W., Manosroi, A., Akazawa, H., Gidado, A., Stienrut, P., Manosroi, W., et al. (2013). Potent anti-cervical cancer activity: synergistic effects of Thai medicinal plants in recipe N040 selected from the MANOSROI III database. J. Ethnopharmacol. 149 (1), 288–296. doi:10.1016/j.jep.2013.06.037
Komoto, K., Nomoto, T., El Muttaqien, S., Takemoto, H., Matsui, M., Miura, Y., et al. (2021). Iron chelation cancer therapy using hydrophilic block copolymers conjugated with deferoxamine. Cancer Sci. 112 (1), 410–421. doi:10.1111/cas.14607
Kontoghiorghes, G. J., Aldouri, M. A., Hoffbrand, A. V., Barr, J., Wonke, B., Kourouclaris, T., et al. (1987). Effective chelation of iron in beta thalassaemia with the oral chelator 1,2-dimethyl-3-hydroxypyrid-4-one. Br. Med. J. Clin. Res. Ed. 295 (6612), 1509–1512. doi:10.1136/bmj.295.6612.1509
Kopustinskiene, D. M., Jakstas, V., Savickas, A., and Bernatoniene, J. (2020). Flavonoids as anticancer agents. Nutrients 12 (2), 457. doi:10.3390/nu12020457
Kovář, J., Valenta, T., and Štýbrová, H. (2001). Differing sensitivity of tumor cells to apoptosis induced by iron deprivation in vitro. Vitr. Cell Dev. Biol. - Anim. 37 (7), 450–458. doi:10.1290/1071-2690(2001)037<0450:DSOTCT>2.0.CO;2
Krihariyani, D., Wasito, E. B., Isnaeni, I., Siswodihardjo, S., Yuniarti, W. M., and Kurniawan, E. (2020). In silico study on antibacterial activity and brazilein ADME of sappan wood (Caesalpinia sappan L.) against Escherichia coli (strain K12). Syst. Rev. Pharm. 11 (10), 290–296. doi:10.31838/srp.2020.10.49
Kulp, K. S., Green, S. L., and Vulliet, P. R. (1996). Iron deprivation inhibits cyclin-dependent kinase activity and decreases cyclin D/CDK4 protein levels in asynchronous MDA-MB-453 human breast cancer cells. Exp. Cell Res. 229 (1), 60–68. doi:10.1006/excr.1996.0343
Lee, D. Y., Lee, M. K., Kim, G. S., Noh, H. J., and Lee, M. H. (2013). Brazilin inhibits growth and induces apoptosis in human glioblastoma cells. Molecules 18 (2), 2449–2457. doi:10.3390/molecules18022449
Ludwig, H., Evstatiev, R., Kornek, G., Aapro, M., Bauernhofer, T., Buxhofer-Ausch, V., et al. (2015). Iron metabolism and iron supplementation in cancer patients. Wien Klin. Wochenschr 127 (23-24), 907–919. doi:10.1007/s00508-015-0842-3
Mangal, M., Sagar, P., Singh, H., Raghava, G. P. S., and Agarwal, S. M. (2013). NPACT: naturally occurring plant-based anti-cancer compound-activity-target database. Nucleic Acids Res. 41 (D1), D1124–D1129. doi:10.1093/nar/gks1047
Maskoen, A. M., Safitri, R., Milanda, T., Reniarti, L., and Fauziah, P. N. (2016). Iron chelation ability of granule sappan wood (Caesalpinia sappan, L.) extract on Iron-overloaded. Int. J. PharmTech Res. 9 (5), 299–305.
Merson, L., and Olivier, N. (2002). Orally active iron chelators. Blood Rev. 16 (2), 127–134. doi:10.1054/blre.2002.0009
Meyer, C. T., Wooten, D. J., Paudel, B. B., Bauer, J., Hardeman, K. N., Westover, D., et al. (2019). Quantifying drug combination synergy along potency and efficacy axes. Cell Syst. 8 (2), 97–108. doi:10.1016/j.cels.2019.01.003
Mitani, K., Takano, F., Kawabata, T., Allam, A. E., Ota, M., Takahashi, T., et al. (2013). Suppression of melanin synthesis by the phenolic constituents of sappanwood (Caesalpinia sappan). Planta Medica 79 (1), 37–44. doi:10.1055/s-0032-1327897
Mottaghipisheh, J., and Stuppner, H. (2021). A comprehensive review on chemotaxonomic and phytochemical aspects of homoisoflavonoids, as rare flavonoid derivatives. Int. J. Mol. Sci. 22 (5), 2735. doi:10.3390/ijms22052735
Mueller, M., Weinmann, D., Toegel, S., Holzer, W., Unger, F. M., and Viernstein, H. (2016). Compounds from Caesalpinia sappan with anti-inflammatory properties in macrophages and chondrocytes. Food Funct. 7 (3), 1671–1679. doi:10.1039/c5fo01256b
Muhammad, N., Usmani, D., Tarique, M., Naz, H., Ashraf, M., Raliya, R., et al. (2022). The role of natural products and their multitargeted approach to treat solid cancer. Cells 11 (14), 2209. doi:10.3390/cells11142209
Naeem, A., Hu, P., Yang, M., Zhang, J., Liu, Y., Zhu, W., et al. (2022). Natural products as anticancer agents: current status and future perspectives. Molecules 27 (23), 8367. doi:10.3390/molecules27238367
National Center for Biotechnology Information (2023). PubChem compound summary for CID 73384, brazilin. Available at: https://pubchem.ncbi.nlm.nih.gov/compound/Brazilin (Accessed June 7, 2023).
National Center for Biotechnology Information (2024a). PubChem compound summary for CID 6453902, brazilein. Available at: https://pubchem.ncbi.nlm.nih.gov/compound/Brazilein.
National Center for Biotechnology Information (2024b). PubChem compound summary for CID 73384, brazilin. Available at: https://pubchem.ncbi.nlm.nih.gov/compound/Brazilin.
Nava-Tapia, D. A., Cayetano-Salazar, L., Herrera-Zúñiga, L. D., Bello-Martínez, J., Mendoza-Catalán, M. A., and Brazilin, N.-T. N. (2022). Brazilin: biological activities and therapeutic potential in chronic degenerative diseases and cancer. Pharmacol. Res. 175, 106023. doi:10.1016/j.phrs.2021.106023
Newman, D. J., and Cragg, G. M. (2016). Natural products as sources of new drugs from 1981 to 2014. J. Nat. Prod. 79 (3), 629–661. doi:10.1021/acs.jnatprod.5b01055
Nguyen, V. B., Vu, B. D., Pham, G. K., Quang Le, B., Men, C. V., et al. (2020). Phenolic compounds from Caesalpinia sappan. Pharmacogn. J. 12 (2), 410–414. doi:10.5530/pj.2020.12.63
Nirmal, N. P., and Panichayupakaranant, P. (2015). Antioxidant, antibacterial, and anti-inflammatory activities of standardized brazilin-rich Caesalpinia sappan extract. Pharm. Biol. 53 (9), 1339–1343. doi:10.3109/13880209.2014.982295
Nitti, M., Ivaldo, C., Traverso, N., and Furfaro, A. L. (2021). Clinical significance of heme oxygenase 1 in tumor progression. Antioxidants 10 (5), 789. doi:10.3390/antiox10050789
Pawar, C. R., Landge, A. D., and Surana, S. J. (2008). Phytochemical and pharmacological aspects of Caesalpinia sappan. Journal of Pharmacy Research 1(2), 131–138.
Pfeifhofer-Obermair, C., Tymoszuk, P., Petzer, V., Weiss, G., and Nairz, M. (2018). Iron in the tumor microenvironment-connecting the dots. Front. Oncol. 8 (NOV), 549. doi:10.3389/fonc.2018.00549
Piga, A., Galanello, R., Forni, G. L., Cappellini, M. D., Origa, R., Zappu, A., et al. (2006). Randomized phase II trial of deferasirox (Exjade•, ICL670), a once-daily, orally-administered iron chelator, in comparison to deferoxamine in thalassemia patients with transfusional iron overload. Haematologica 91 (7), 873–880.
Porter, J. B. (2001). Practical management of iron overload. Br. J. Haematol. 115 (2), 239–252. doi:10.1046/j.1365-2141.2001.03195.x
Richardson, D. R., and Baker, E. (1994). Two saturable mechanisms of iron uptake from transferrin in human melanoma cells: the effect of transferrin concentration, chelators, and metabolic probes on transferrin and iron uptake. J. Cell Physiol. 161 (1), 160–168. doi:10.1002/jcp.1041610119
Rombos, Y., Tzanetea, R., Konstantopoulos, K., Simitzis, S., Zervas, C., Kyriaki, P., et al. (2000). Chelation therapy in patients with thalassemia using the orally active iron chelator deferiprone (L1). Haematologica 85 (2), 115–117.
Rondão, R., Seixas De Melo, J. S., Pina, J., Melo, M. J., Vitorino, T., and Parola, A. J. (2013). Brazilwood reds: the (photo)chemistry of brazilin and brazilein. J. Phys. Chem. A 117 (41), 10650–10660. doi:10.1021/jp404789f
Rychtarcikova, Z., Lettlova, S., Tomkova, V., Korenkova, V., Langerova, L., Simonova, E., et al. (2017). Tumor-initiating cells of breast and prostate origin show alterations in the expression of genes related to iron metabolism. Oncotarget 8 (4), 6376–6398. doi:10.18632/oncotarget.14093
Safitri, R., Chaidir, L., Kuntana, Y. P., Syamsunarno, M. R. A. A., Yuliana, T., Utama, G. L., et al. (2022). Antituberculosis activity and iron chelation ability of brazilin isolated from Caesalpinia sappan L. Int. J. Adv. Sci. Eng. Inf. Technol. 12 (4), 1707–1713. doi:10.18517/IJASEIT.12.4.16210
Safitri, R., Ratningsih, N., Maskoen, A. M., Fauziah, P. N., and Panigoro, R. (2016). The effects of Caesalpinia sappan L. Extract granule to antioxidant activity in blood serum of wistar rat (rattus norvegicus) with excessive iron condition. Int. J. PharmTech Res. 9 (11), 497–512. doi:10.18502/kls.v3i6.1159
Sasaki, Y., Hosokawa, T., Nagai, M., and Nagumo, S. (2007). In vitro study for inhibition of NO production about constituents of Sappan lignum. Biol. Pharm. Bull. 30 (1), 193–196. doi:10.1248/bpb.30.193
Sharifi-Rad, J., Quispe, C., Patra, J. K., Singh, Y. D., Panda, M. K., Das, G., et al. (2021). Paclitaxel: application in modern oncology and nanomedicine-based cancer therapy. Oxid. Med. Cell Longev. 2021, 3687700. doi:10.1155/2021/3687700
Sung, H., Ferlay, J., Siegel, R. L., Laversanne, M., Soerjomataram, I., Jemal, A., et al. (2021). Global cancer statistics 2020: GLOBOCAN estimates of incidence and mortality worldwide for 36 cancers in 185 countries. CA Cancer J. Clin. 71 (3), 209–249. doi:10.3322/caac.21660
Suyatmi, S., Mudigdo, A., Purwanto, B., Indarto, D., Hakim, F. A., and Krisnawati, D. I. (2022). Brazilin isolated from caesalpina sappan wood induces intrinsic apoptosis on A549 cancer cell line by increasing p53, caspase-9, and caspase-3. Asian Pac. J. Cancer Prev. 23 (4), 1337–1343. doi:10.31557/APJCP.2022.23.4.1337
Theil, E. C., and Goss, D. J. (2009). Living with iron (and oxygen): questions and answers about iron homeostasis. Chem. Rev. 109 (10), 4568–4579. doi:10.1021/cr900052g
Toegel, S., Wu, S. Q., Otero, M., Goldring, M. B., Leelapornpisid, P., Chiari, C., et al. (2012). Caesalpinia sappan extract inhibits IL1β-mediated overexpression of matrix metalloproteinases in human chondrocytes. Genes Nutr. 7 (2), 307–318. doi:10.1007/s12263-011-0244-8
Trinder, D., Zak, O., and Aisen, P. (1996). Transferrin receptor-independent uptake of differic transferrin by human hepatoma cells with antisense inhibition of receptor expression. Hepatology 23 (6), 1512–1520. doi:10.1053/jhep.1996.v23.pm0008675172
Utomo, R. Y., Novarina, A., Tirtanirmala, P., Kastian, R. F., and Jenie, R. I. (2018). Enhancement of cytotoxicity and apoptosis induction of doxorubicin by brazilein containing fraction of secang (Caesalpinia sappan L.) on T47D cells. Indones. J. Cancer Chemoprevention 9 (1), 32–383. doi:10.14499/indonesianjcanchemoprev9iss1pp32-40
Valentovic, M. (2008). “Deferasirox,” in Bylund DBBT xPharm: TCPR. Editor S. J. Enna (Amsterdam, Netherlands: Elsevier), 1–4. doi:10.1016/B978-008055232-3.64057-7
Wang, L., Li, X., Mu, Y., Lu, C., Tang, S., Lu, K., et al. (2019a). The iron chelator desferrioxamine synergizes with chemotherapy for cancer treatment. J. Trace Elem. Med. Biol. 56, 131–138. doi:10.1016/j.jtemb.2019.07.008
Wang, Y., Yu, L., Ding, J., and Chen, Y. (2019b). Iron metabolism in cancer. Int. J. Mol. Sci. 20 (1), 95. doi:10.3390/ijms20010095
Webb, A. H., Gao, B. T., Goldsmith, Z. K., Irvine, A. S., Saleh, N., Lee, R. P., et al. (2017). Inhibition of MMP-2 and MMP-9 decreases cellular migration, and angiogenesis in in vitro models of retinoblastoma. BMC Cancer 17 (1), 434. doi:10.1186/s12885-017-3418-y
Weinmann, D., Mueller, M., Walzer, S. M., Hobusch, G. M., Lass, R., Gahleitner, C., et al. (2018). Brazilin blocks catabolic processes in human osteoarthritic chondrocytes via inhibition of NFKB1/p50. J. Orthop. Res. 36 (9), 2431–2438. doi:10.1002/jor.24013
Xu, H. X., and Lee, S. F. (2004). The antibacterial principle of Caesalpina sappan. Phyther Res. 18 (8), 647–651. doi:10.1002/ptr.1524
Yan, S., Lin, S., Chen, K., Yin, S., Peng, H., Cai, N., et al. (2022). Natural product library screens identify sanguinarine chloride as a potent inhibitor of telomerase expression and activity. Cells 11 (9), 1485. doi:10.3390/cells11091485
Yang, X., Liang, Y., Zhao, L., Chen, L., Yang, Y., Wang, J., et al. (2023). Brazilin inhibits the invasion and metastasis of breast cancer. Biol. Pharm. Bull. 46 (6), 773–780. doi:10.1248/bpb.b22-00637
You, E. J., Khil, L. Y., Kwak, W. J., Won, H. S., Chae, S. H., Lee, B. H., et al. (2005). Effects of brazilin on the production of fructose-2,6-bisphosphate in rat hepatocytes. J. Ethnopharmacol. 102 (1), 53–57. doi:10.1016/j.jep.2005.05.020
Zhang, Q., Liu, J. L., Qi, X. M., Qi, C. T., and Yu, Q. (2014). Inhibitory activities of Lignum Sappan extractives on growth and growth-related signaling of tumor cells. Chin. J. Nat. Med. 12 (8), 607–612. doi:10.1016/S1875-5364(14)60092-3
Zhang, T., Fan, X., Song, L., Ren, L., Ma, E., Zhang, S., et al. (2015). c-Fos is involved in inhibition of human bladder carcinoma T24 cells by brazilin. IUBMB Life 67 (3), 175–181. doi:10.1002/iub.1357
Zhang, T., He, J., Zhang, S., Wang, G., Ma, E., Wang, J., et al. (2018). Brazilin induces T24 cell death through c-Fos and GADD45β independently regulated genes and pathways. IUBMB Life 70 (11), 1101–1110. doi:10.1002/iub.1921
Keywords: brazilin, anticancer agent, cytotoxic effect, iron chelation, molecular mechanism
Citation: Raptania CN, Zakia S, Fahira AI and Amalia R (2024) Article review: Brazilin as potential anticancer agent. Front. Pharmacol. 15:1355533. doi: 10.3389/fphar.2024.1355533
Received: 14 December 2023; Accepted: 14 February 2024;
Published: 07 March 2024.
Edited by:
Wagdy Mohamed Eldehna, Kafrelsheikh University, EgyptReviewed by:
Haytham O. Tawfik, Tanta University, EgyptCopyright © 2024 Raptania, Zakia, Fahira and Amalia. This is an open-access article distributed under the terms of the Creative Commons Attribution License (CC BY). The use, distribution or reproduction in other forums is permitted, provided the original author(s) and the copyright owner(s) are credited and that the original publication in this journal is cited, in accordance with accepted academic practice. No use, distribution or reproduction is permitted which does not comply with these terms.
*Correspondence: Riezki Amalia, cmllemtpLmFtYWxpYUB1bnBhZC5hYy5pZA==
†These authors have contributed equally to this work
Disclaimer: All claims expressed in this article are solely those of the authors and do not necessarily represent those of their affiliated organizations, or those of the publisher, the editors and the reviewers. Any product that may be evaluated in this article or claim that may be made by its manufacturer is not guaranteed or endorsed by the publisher.
Research integrity at Frontiers
Learn more about the work of our research integrity team to safeguard the quality of each article we publish.