- 1Xiyuan Hospital, Cohina Academy of Chinese Medical Sciences, Beijing, China
- 2Cardiovascular Diseases Center, Xiyuan Hospital, China Academy of Chinese Medical Sciences, Beijing, China
Purpose: This study aimed to assess the efficacy and safety of Panax notoginseng saponin (PNS) injection, when combined with conventional treatment (CT), for acute myocardial infarction (AMI).
Methods: Comprehensive searches were conducted in seven databases from inception until 28 September 2023. The search aimed to identify relevant randomized controlled trials (RCTs) focusing on PNS injection in the context of AMI. This meta-analysis adhered to the PRISMA 2020 guidelines, and its protocol was registered with PROSPERO (number: CRD42023480131).
Result: Twenty RCTs involving 1,881 patients were included. The meta-analysis revealed that PNS injection, used adjunctively with CT, significantly improved treatment outcomes compared to CT alone, as evidenced by the following points: (1) enhanced total effective rate [OR = 3.09, p < 0.05]; (2) decreased incidence of major adverse cardiac events [OR = 0.32, p < 0.05]; (3) reduction in myocardial infarct size [MD = −6.53, p < 0.05]; (4) lower ST segment elevation amplitude [MD = −0.48, p < 0.05]; (5) mitigated myocardial injury as indicated by decreased levels of creatine kinase isoenzymes [MD = −11.19, p < 0.05], cardiac troponin T [MD = −3.01, p < 0.05], and cardiac troponin I [MD = −10.72, p < 0.05]; (6) enhanced cardiac function, reflected in improved brain natriuretic peptide [MD = −91.57, p < 0.05], left ventricular ejection fraction [MD = 5.91, p < 0.05], left ventricular end-diastolic dimension [MD = −3.08, p < 0.05], and cardiac output [MD = 0.53, p < 0.05]; (7) reduced inflammatory response, as shown by lower levels of C-reactive protein [MD = −2.99, p < 0.05], tumor necrosis factor-α [MD = −6.47, p < 0.05], interleukin-6 [MD = −24.46, p < 0.05], and pentraxin-3 [MD = −2.26, p < 0.05]; (8) improved vascular endothelial function, demonstrated by decreased endothelin-1 [MD = −20.56, p < 0.05] and increased nitric oxide [MD = 1.33, p < 0.05]; (9) alleviated oxidative stress, evidenced by increased superoxide dismutase levels [MD = 25.84, p < 0.05]; (10) no significant difference in adverse events [OR = 1.00, p = 1.00].
Conclusion: This study highlighted the efficacy and safety of adjunctive PNS injections in enhancing AMI patient outcomes beyond CT alone. Future RCTs need to solidify these findings through rigorous methods.
Systematic Review Registration: (https://www.crd.york.ac.uk/PROSPERO/), identifier (CRD42023480131)
1 Introduction
Acute myocardial infarction (AMI) represents irreversible myocardial damage, typically precipitated by the rupture of coronary artery plaque (Byrne et al., 2023). Annually, it is responsible for over 2.4 million fatalities in the United States, 4 million in Europe and northern Asia, and affects more than 7 million individuals worldwide (Yeh et al., 2010; Nichols et al., 2014). As a significant contributor to global disability and mortality, AMI poses a critical challenge in contemporary medicine (Reed et al., 2017). Treatment strategies for AMI prioritize the restoration of coronary flow to enable myocardial tissue reperfusion, primarily through percutaneous coronary intervention (PCI) and pharmacological thrombolysis. This approach is complemented by the administration of several medications. These include antiplatelet agents, antianginal agents, anticoagulants, and vasodilators. Vasodilators are also used to enhance cardiomyocyte nutrition. Additionally, beta-blockers and calcium antagonists are administered to reduce myocardial oxygen consumption (Montecucco et al., 2016; Reed et al., 2017; Sanchis et al., 2023). Although reperfusion alleviates ischemic myocardial injury, it can also cause specific, irreversible damage to cardiomyocytes (Gong et al., 2021; Murphy and Goldberg, 2022). Furthermore, existing treatments fall short in restoring the cardiac structure and function during prolonged AMI therapy, resulting in persistently high morbidity and mortality rates despite conventional drug therapy (Heusch and Gersh, 2017).
Panax notoginseng (PN), known as the root of Panax notoginseng (Burk.) F. H. Chen, is a botanical drug with an extensive history of medicinal use in traditional Chinese medicine (TCM) in China (Wang et al., 2016). Over centuries, PN has demonstrated significant efficacy in managing internal and external bleeding and ameliorating blood stasis, leading to its widespread use in clinical practice for the treatment of cardiovascular diseases (CVDs) (Liu et al., 2020). Panax notoginseng saponins (PNS), the freeze-dried extract of PN, comprises metabolites such as notoginsenoside R1 and ginsenosides Rb1, Rg1, Rd, and Re, as identified by high-performance liquid chromatography fingerprint analysis (Peng et al., 2018) (Figure 1) (Sun et al., 2020a). PNS formulations, including Xuesaitong, Xueshuantong , and Lulutong injections, have been clinically validated through trials and fundamental studies. These investigations substantiate PNS’s efficacy in reducing cardiac damage, mitigating inflammation, and inhibiting platelet aggregation (Zhou et al., 2018a; Wang et al., 2021a; Li et al., 2022; Wang et al., 2022; Zeng et al., 2023).
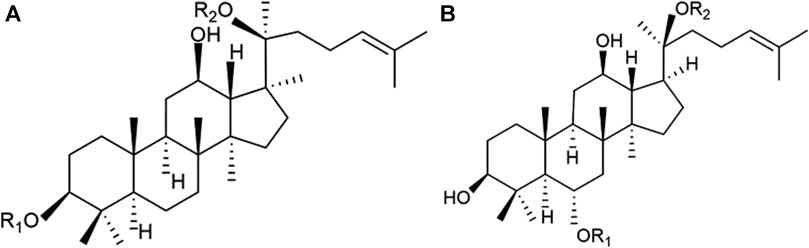
Figure 1. Chemical structures of the main saponins in PNS. (A) 20(S)-protopanaxatriol saponins, including notoginsenoside R1, ginsenoside Rg1, and Re, and (B) 20(S)- protopanaxadiol saponins, including ginsenoside Rb1 and Rd.
In recent years, there have been an increasing amount of randomized controlled trials (RCTs) using PNS injection to treat AMI. However, no comprehensive literature review or meta-analysis has assessed the efficacy and safety of these interventions. Consequently, this study aims to elucidate the cardioprotective effects of PNS injection in AMI patients.
2 Methods
2.1 Search strategy
Comprehensive searches were conducted in PubMed, EMBASE, Web of Science, Cochrane Library, Chinese National Knowledge Infrastructure, Wanfang, and VIP databases from inception until 28 September 2023. The search expression used in MEDLINE was (“acute myocardial infarction” OR “AMI” OR “st elevated myocardial infarction” OR “STEMI”) AND (“panax notoginseng saponins” OR “PNS” OR “sanqi” OR “sanchi” OR “xuesaitong” OR “xueshuantong”) AND (“randomized controlled trial” OR “RCT”). The detailed search strategies for all databases are presented in Supplementary Table S1. Titles, abstracts, and full texts were independently reviewed and cross-checked by two investigators (Pengfei Chen and Deng Pan), and any disagreement was decided by the senior reviewer (Dazhuo Shi).
2.2 Inclusion criteria
2.2.1 Type of study
The study type was RCT, with no restrictions on languages, publication date, or publication status.
2.2.2 Research object
All patients meeting any one of the current or previous diagnostic criteria for AMI were included. According to the Fourth Universal Definition of Myocardial Infarction (2018) standard (Thygesen et al., 2019), AMI is defined as acute myocardial injury [increased and/or decreased serum cardiac troponin (cTn), with at least once higher than the upper limit of the normal value (99 percentile of the upper limit of the reference value)], and there is at least one evidence of myocardial ischemia: (1) myocardial ischemic symptoms; (2) new ST-T changes or a new left bundle branch block (LBBB) observed in electrocardiogram (ECG); (3) a pathological Q-wave appeared in ECG; (4) imaging showed new loss of viable myocardium or new regional wall motion abnormalities; and (5) coronary angiography or autopsy confirmed thrombus in the coronary artery.
2.2.3 Intervention
The control group (CG) received conventional treatment (CT), which was the first-line therapy regimens recommended in the current guideline. The experimental group (EG) received PNS injection plus CT, and the courses and dosages of PNS injection were not limited.
2.2.4 Outcome indicators
The primary efficacy outcomes were total effective rates and major adverse cardiac events (MACEs). The secondary efficacy outcomes were (1) myocardial infarct size (MIS); (2) ST segment elevation amplitude; (3) myocardial injury markers: creatine kinase isoenzymes (CK-MB), cardiac troponin I (cTnI), and cardiac troponin T (cTnT); (4) cardiac function index: brain natriuretic peptide (BNP), left ventricular ejection fraction (LVEF), left ventricular end-diastolic dimension (LVEDD), and cardiac output (CO); (5) inflammatory response markers: C-reactive protein (CRP), tumor necrosis factor-α (TNF-α), interleukin-6 (IL-6), and pentraxin-3 (PTX-3); (6) vascular endothelial function: endothelin-1 (ET-1) and nitric oxide (NO); and (7) oxidative stress: superoxide dismutase (SOD). The safety outcome was adverse events.
2.3 Exclusion criteria
The exclusion criteria are as follows: (1) the intervention included other TCM (oral PNS, other Chinese medicine injections, and oral Chinese patent medicines) other than PNS injection; (2) research data had obvious deficiencies or serious errors; (3) patients with severe comorbidities such as severe heart failure, severe arrhythmia, and severe liver and renal dysfunction; and (4) duplicate publication.
2.4 Data extraction
The document information extraction table was established by Excel software, including basic characteristics of trials (first author, publication year, journal, and country), characteristics of patients (sample size, gender, age, and medical history), PNS injection and CT treatments (initial time, dosage, frequency, duration, and mean follow-up time), and outcome indicators.
2.5 Bias assessment
Two investigators (Pengfei Chen and Deng Pan) independently evaluated the quality assessment and risk of bias using the Cochrane Risk of Bias Tool 2.0, according to the following six aspects: (1) random sequence generation; (2) allocation concealment; (3) blinding (patients, medical staff, outcome evaluations, and data analysis); (4) incompletion of the outcome report (important indicators and follow-up rate); (5) selective reporting; and (6) other biases (such as baseline imbalance and suspected fraud. The two investigators’ answer of “yes” indicated a lower risk of bias, “no” indicated a higher risk of bias, and “unclear” indicated an uncertain risk of bias.
2.6 Statistical analysis
Statistical analysis was conducted using RevMan 5.4 software. Odds ratios (ORs) were used to represent categorical variables, and mean differences (MDs) were used for continuous variables while calculating 95% confidence intervals (CIs). The Cochrane’s Q test and the I2-value were used to assess the statistical heterogeneity, where p > 0.1 or I2 ≤ 50% suggested a favorable homogeneity among studies, and a fixed-effects model was used; p ≤ 0.1 or I2>50% suggested significant heterogeneity, so a random-effects model was used. When only two trials were included, although the heterogeneity was not significant, a random-effects model was selected to ensure the accuracy of the results. For sensitivity analysis, one study was omitted at a time to find the source of publication for heterogeneity, such as interventions, gender, age, and outcome indicators. When the number of trials was ≥10, we used the funnel chart to test whether there was publication bias. Although planned, we did not check the publication bias using Egger’s or Begg’s test as these tests were unreliable when fewer than 10 trials were included.
3 Results
3.1 Study search
The process of the database search and study identification is presented in Figure 2. The initial database search identified 406 records. We excluded 245 duplicate records and 124 ineligible records by screening titles and abstracts. Of the remaining 37 articles that underwent full-text review, 17 were further excluded because of inaccurate data, ineligible interventions, and non-RCT study design. The exclusion studies and reasons are presented in Supplementary Table S2. Finally, 20 RCTs (Gan et al., 2010; Fu et al., 2014a; Fu et al., 2014b; Fan et al., 2015; Qiao et al., 2016; Feng et al., 2017; Xie, 2017; Liu et al., 2018; Wang, 2018; Xin and Qian, 2018; Zhou, 2018; Chen, 2019; Guo, 2019; Zhang, 2019; Zhang and Du, 2019; Zhong and Zheng, 2019; Sun et al., 2020b; Sun, 2021; Ji and Liu, 2022; Yang et al., 2022) were included.
3.2 Basic information
All 20 RCTs were published in China from 2010 to 2023. The total sample size was 1,881 cases, with a maximum of 138 cases and a minimum of 39 cases. In total, there were 943 cases in the EG and 938 cases in the CG. According to the ConPhyMP guidelines (Heinrich et al., 2022), all included RCTs focused on “type A” extracts (documented in the national pharmacopeia with licensed applications). The composition, source, and chemical characteristics of PNS injection used in the included trials are presented in Supplementary Table S3. The other details are shown in Table 1.
3.3 Literature quality evaluation
Regarding randomization, 13 RCTs (Fu et al., 2014a; Fu et al., 2014b; Fan et al., 2015; Xie, 2017; Liu et al., 2018; Wang, 2018; Xin and Qian, 2018; Zhou, 2018; Chen, 2019; Zhang, 2019; Sun et al., 2020b; Sun, 2021; Yang et al., 2022) generated random sequences using a random number table, which were rated as “low risk.” The remaining trials did not report the specific randomized method, resulting in an “unknown risk.” None of the RCTs reported the assignment hiding scheme, and the predictable future assignments could have led to the failure of randomization. Therefore, we rate the allocation concealment of all RCTs as “unknown risk”. Whether or not outcomes were measured by a blinded assessor could not be established because of the lack of information. Therefore, we rate the blinding of all RCTs as “unknown risk”. Two RCTs (Fu et al., 2014a; Feng et al., 2017) were rated as “high risk” due to incomplete data, which did not indicate the sample size and average age, while the others were rated as “low risk.” The results of all study reports were rated as “low risk” without selective reporting. No other bias was reported in any RCTs and was rated as “unknown risk” (Figure 3).
3.4 Main efficacy outcomes
3.4.1 Total effective rate
Four RCTs (Xie, 2017; Zhou, 2018; Sun et al., 2020b; Ji and Liu, 2022) were reported on the total effective rate of PNS injection plus CT versus CT. The heterogeneity was not significant (p = 0.93, I2 = 0%), so a fixed-effects model was used. The results presented in Figure 4 show that the total effective rate in the EG is significantly higher than that in the CG, with statistically significant differences [OR = 3.09, 95% CI: 1.67 to 5.72, p < 0.05].
3.4.2 MACEs
Eight RCTs (Gan et al., 2010; Fu et al., 2014a; Fu et al., 2014b; Fan et al., 2015; Xie, 2017; Xin and Qian, 2018; Zhang, 2019; Ji and Liu, 2022; Yang et al., 2022) investigated the incidence of MACEs. As the heterogeneity was significant (p = 0.01, I2 = 60%), a random-effects model was used. The results presented in Figure 5 show that the incidence of MACEs in the EG is significantly lower than that in the CG, and the differences were statistically significant [OR = 0.32, 95% CI: 0.16 to 0.64, p < 0.05].
3.5 Secondary efficacy outcomes
3.5.1 MIS
Three RCTs (Fu et al., 2014b; Zhong and Zheng, 2019; Sun et al., 2020b) examined the MIS. The statistical heterogeneity among them was substantial (p < 0.01, I2 = 89%), so a random-effects model was used. As shown in the results, a statistical difference was found between the two groups, which meant that the MIS in the EG was significantly lower than that in the CG [MD = −6.53, 95% CI: −8.81 to −4.24, p < 0.05] (Figure 6).
3.5.2 ST segment elevation amplitude
Two RCTs (Gan et al., 2010; Feng et al., 2017) examined the ST segment elevation amplitude. Although the heterogeneity was not significant between the two studies (p = 0.92, I2 = 0%), the random-effects model was used. The results indicated that PNS injection plus CT significantly decreases the ST segment elevation amplitude compared with CT alone [MD = −0.48, 95% CI: −0.94 to −0.02, p < 0.05] (Figure 7).
3.5.3 Myocardial injury
Six RCTs (Xie, 2017; Liu et al., 2018; Zhou, 2018; Chen, 2019; Guo, 2019; Ji and Liu, 2022) reported on CK-MB. The meta-analysis revealed that the CK-MB levels in the EG were significantly lower than those in the CG [MD = −11.19, 95% CI: −20.88 to −1.50, p < 0.05; I2 = 61%]. Five RCTs (Fan et al., 2015; Liu et al., 2018; Zhou, 2018; Chen, 2019; Guo, 2019) addressed the cTnT, with the meta-analysis indicating that cTnT levels in the EG were significantly reduced compared to those in the CG [MD = −10.72, 95% CI: −19.37 to −2.06, p < 0.05; I2 = 87%]. Additionally, two RCTs (Xie, 2017; Ji and Liu, 2022) focused on cTnI, and the meta-analysis showed that cTnI levels in the EG were notably lower than those in the CG [MD = −3.01, 95% CI: −5.68 to −0.33, p < 0.05; I2 = 0%] (Figure 8).
3.5.4 Cardiac function
Five RCTs (Fu et al., 2014a; Liu et al., 2018; Zhou, 2018; Chen, 2019; Guo, 2019) reported on BNP. The meta-analysis showed that the BNP in the EG is significantly lower than that in the CG [MD = −91.57, 95% CI: −130.27 to −52.86, p < 0.05; I2 = 68%]. Thirteen RCTs (Fu et al., 2014b; Xie, 2017; Liu et al., 2018; Wang, 2018; Zhou, 2018; Chen, 2019; Guo, 2019; Zhang and Du, 2019; Zhong and Zheng, 2019; Sun et al., 2020b; Sun, 2021; Ji and Liu, 2022; Yang et al., 2022) focused on the LVEF, revealing that LVEF in the EG was significantly increased compared to that in the CG [MD = 5.91, 95% CI: 4.23 to 7.59, p < 0.05; I2 = 90%]. Nine RCTs (Xie, 2017; Liu et al., 2018; Wang, 2018; Zhou, 2018; Chen, 2019; Guo, 2019; Zhang and Du, 2019; Sun, 2021; Ji and Liu, 2022) focused on the LVEDD, revealing that LVEDD in the EG was significantly reduced compared to that in the CG [MD = −3.08, 95% CI: −4.34 to −1.82, p < 0.05; I2 = 84%]. Additionally, two RCTs (Sun et al., 2020b; Yang et al., 2022) reported on CO. Meta-analysis showed that the CO in EG is significantly higher than that in the CG [MD = 0.53, 95% CI: 0.27 to 0.79, p < 0.05; I2 = 0%] (Figure 9).
3.5.5 Inflammatory response
Seven RCTs (Qiao et al., 2016; Liu et al., 2018; Xin and Qian, 2018; Zhou, 2018; Zhang, 2019; Zhang and Du, 2019; Sun, 2021) examined the CRP levels. The meta-analysis demonstrated that the CRP levels in the EG were significantly lower than those in the CG [MD = −2.99, 95% CI: −5.36 to −0.61, p < 0.05; I2 = 98%]. Four RCTs (Xin and Qian, 2018; Zhou, 2018; Zhang, 2019; Sun, 2021) investigated TNF-α, revealing that TNF-α levels in the EG were significantly reduced compared to those in the CG [MD = −6.47, 95% CI: −8.76 to −4.18, p < 0.05; I2 = 62%]. Two RCTs (Qiao et al., 2016; Zhou, 2018) reported on IL-6, and the meta-analysis indicated that IL-6 levels in the EG were significantly lower than in the CG [MD = −24.46, 95% CI: −35.99 to −12.94, p < 0.05; I2 = 91%]. Similarly, two RCTs (Liu et al., 2018; Zhou, 2018) focused on PTX-3, and the meta-analysis showed that PTX-3 levels in the EG were significantly reduced compared to those in the CG [MD = −2.26, 95% CI: −3.21 to −1.31, p < 0.05; I2 = 0%] (Figure 10).
3.5.6 Vascular endothelial function
Three RCTs (Fan et al., 2015; Xie, 2017; Zhong and Zheng, 2019) investigated the ET-1, revealing that ET-1 levels in the EG were significantly reduced compared to those in the CG [MD = −20.56, 95% CI: −36.19 to −4.93, p < 0.05; I2 = 98%]. Similarly, Zhong and Zheng (2019) reported on NO. The result showed that the NO level in the EG is higher than that in the CG [MD = 1.33, 95% CI: 0.92 to 1.74, p < 0.05] (Figure 11).
3.5.7 Oxidative stress
Two RCTs (Fan et al., 2015; Xie, 2017) reported on SOD. The results indicated that PNS injection plus CT significantly increases the SOD levels compared with CT alone. [MD = 25.84, 95% CI: 16.44 to 35.52, p < 0.05; I2 = 0%, random-effects model] (Figure 12).
3.6 Safety outcomes
Four RCTs (Liu et al., 2018; Wang, 2018; Chen, 2019; Sun, 2021) assess the adverse effects. Given the non-significant heterogeneity (p = 0.73, I2 = 0%), a fixed-effects model was employed for the analysis. Figure 13 illustrates that the incidence of adverse events did not significantly differ between the EG and CG [OR = 1.00, 95% CI: 0.49 to 2.06, p = 1.00]. Regarding bleeding events, Fan et al. (2015) observed three instances in the CG and one in the EG. Xie (2017) reported two severe bleeding events in the CG, while the EG recorded none. There was no significant difference in the incidence of bleeding events between the two groups (p = 0.16).
3.7 Subgroup analysis
Subgroup analysis stratified by the reperfusion strategies (PCI or thrombolysis) indicated that PNS injection could enhance the total effective rate [OR = 2.57, 95% CI: 1.14 to 5.78, p < 0.05] and decrease MACEs [OR = 0.38, 95% CI: 0.21 to 0.67, p < 0.05] in patients undergoing PCI. Likewise, PNS injection was associated with an increased total effective rate [OR = 3.89, 95% CI: 1.49 to 10.14, p < 0.05] and a reduction in MACEs [OR = 0.24, 95% CI: 0.04 to 1.39, p < 0.05] in patients who underwent thrombolysis (Figure 14).
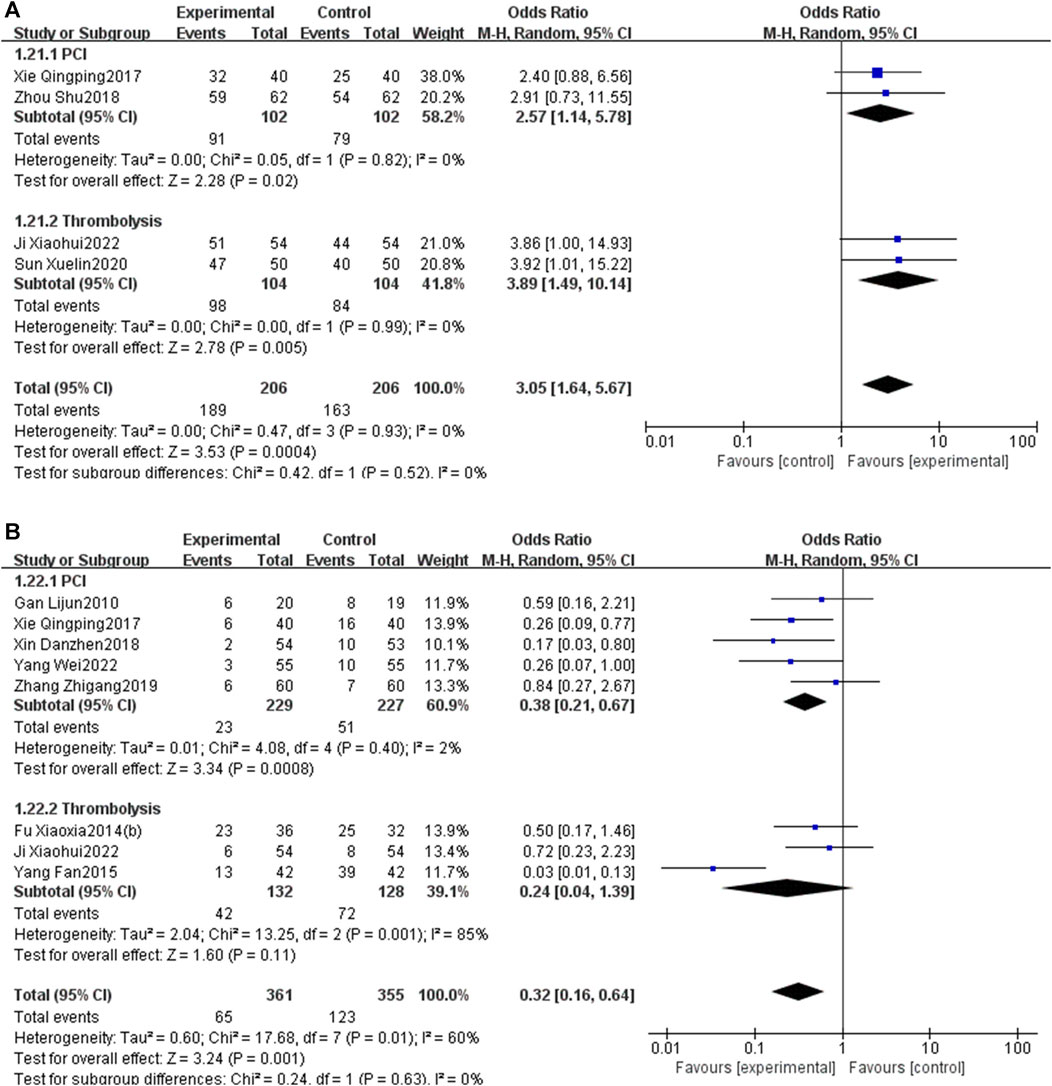
Figure 14. Subgroup analysis stratified by the reperfusion strategies [(A) total effective rate; (B) MACEs].
The subgroup analysis, stratified by the duration of PNS injection (7 days or 14 days), indicated that a 7-day PNS injection was associated with an increased total effective rate [OR = 2.84, 95% CI: 1.27 to 6.37, p < 0.05] and a reduction in MACEs [OR = 0.41, 95% CI: 0.23 to 0.72, p < 0.05]. Similarly, a 14-day PNS injection enhanced the total effective rate [OR = 3.39, 95% CI: 1.29 to 8.90, p < 0.05] and decreased MACEs [OR = 0.17, 95% CI: 0.02 to 1.22, p < 0.05] (Figure 15).
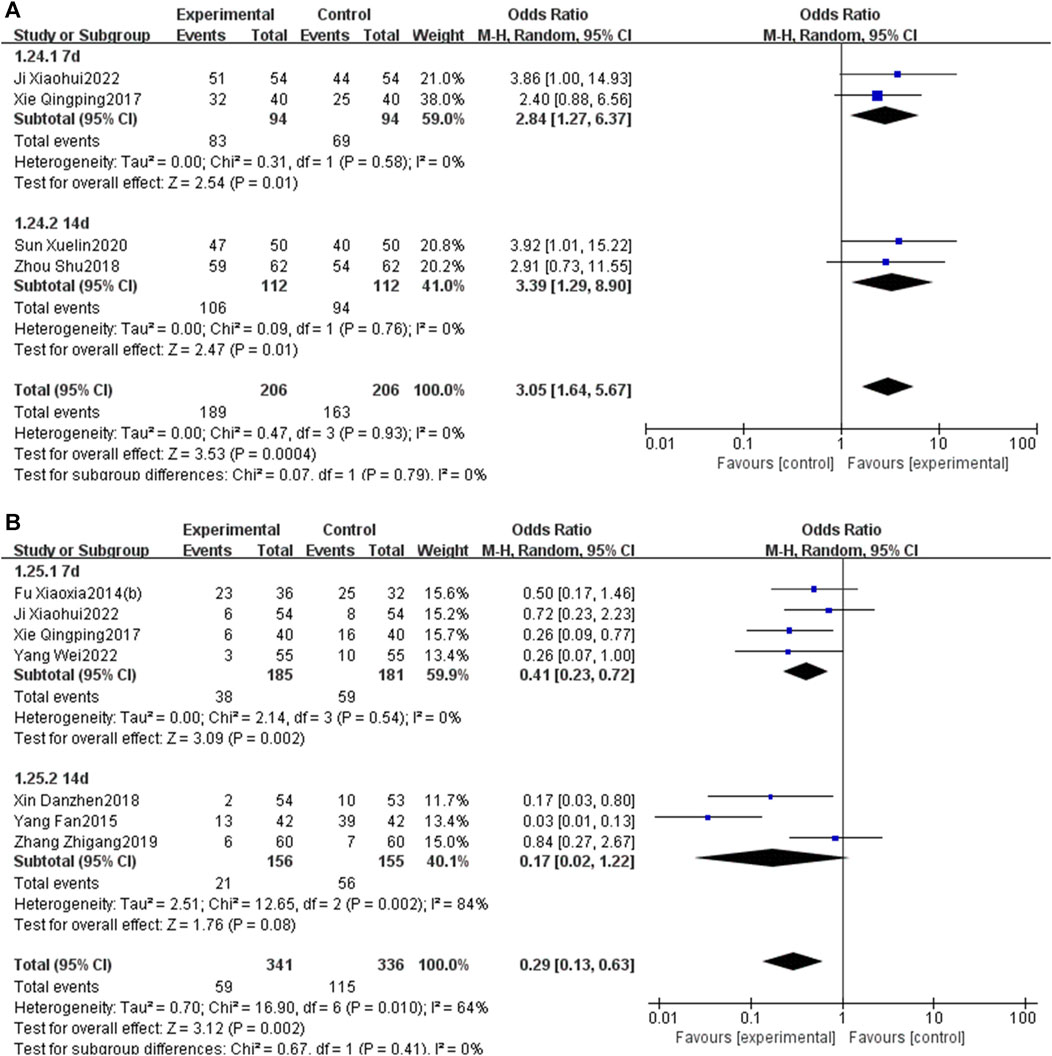
Figure 15. Subgroup analysis stratified by the courses of PNS injection [(A) total effective rate; (B) MACEs].
Further subgroup analysis, stratified by the initial time of the injection (before reperfusion or after reperfusion), revealed that administering PNS prior to reperfusion was linked to a decrease in MACEs [OR = 0.51, 95% CI: 0.27 to 0.94, p < 0.05] and an increase in LVEF [MD = 6.65, 95% CI: 4.06 to 9.25, p < 0.05]. Conversely, administering PNS post-reperfusion was also correlated with a reduction in MACEs [OR = 0.21, 95% CI: 0.06 to 0.70, p < 0.05] and an elevation in LVEF [MD = 4.97, 95% CI: 3.36 to 6.58, p < 0.05] (Figure 16).
An additional subgroup analysis, stratified by the duration of the follow-up, established that the incidence of MACEs in the EG was significantly lower than that in the CG at a 6-month checkpoint [OR = 0.44, 95% CI: 0.21 to 0.89, p < 0.05]. However, no significant differences were observed at the 14-day [OR = 0.41, 95% CI: 0.08 to 2.01, p = 0.27] and 1-month intervals [OR = 0.43, 95% CI: 0.16 to 1.14, p = 0.09] (Figure 17).
3.8 Sensitivity analysis
When the heterogeneity was significant, we performed a sensitivity analysis, including MACEs, MIS, CK-MB, cTnT, BNP, LVEF, LVEDD, CRP, TNF-α, and ET-1. Sensitivity analysis found that the reasons may lie in the heterogeneity of trials with respect to the sample size, the age range of participants, PNS dosing protocols, duration of the intervention, and the outcome measures used. After excluding these studies, the heterogeneity significantly reduced, and the results showed a limited impact (Supplementary Table S4). Therefore, these results of meta-analysis were stable and reliable.
3.9 Publication bias
We performed the publication bias for LVEF. As the number of trials was ≥10, we used the funnel chart to determine whether there was publication bias. The results showed that the points of the effects of each trial show a symmetrical inverted funnel distribution, suggesting that the possibility of publication bias was small (Figure 18).
4 Discussion
4.1 Summary of findings
To the best of our knowledge, this is the first meta-analysis that systematically assesses the efficacy and safety of PNS injection in the treatment of AMI. The findings indicate a significant enhancement in the total effective rate and a reduction in MACEs at 6 months, ST segment elevation, and MIS in AMI patients treated with PNS injection. Additionally, PNS injections were found to improve cardiac and vascular endothelial functions and mitigate myocardial injury, inflammatory responses, and oxidative stress, likely attributing to the protective mechanism of PNS in AMI. Safety assessments suggest that PNS does not increase the incidence of adverse events, particularly bleeding. These results demonstrate the efficacy and safety of PNS injection, offering improved functional outcomes in AMI patients compared to CT alone and potentially presenting a promising treatment modality for AMI. Subgroup analysis further revealed that regardless of the variation in reperfusion strategies (PCI or thrombolysis), initiation timing (pre or post-reperfusion), and duration of treatment (7 or 14 days), PNS exhibited significant therapeutic benefits and favorable long-term prognoses in AMI management. Consequently, PNS is advocated as a secondary treatment in reperfusion AMI patients. The decision to initiate PNS immediately post-reperfusion should consider the risk of bleeding, with a recommended treatment duration of 1–2 weeks.
4.2 Mechanism of PNS in AMI
Apoptosis, occurring within hours after the occurrence of AMI, is a primary factor contributing to myocardial injury (Zhou et al., 2018b). This process is intricately linked to the Bcl-2 gene (Bcl-2) and Bcl-2-associated X protein (Bax). PNS is shown to mitigate ultrastructural damage in cardiomyocytes, suppress Bax expression, and enhance the Bcl-2/Bax ratio, thereby reducing cardiomyocyte apoptosis induced by myocardial injury (Zhang et al., 2022). Liu et al. (2019) reported that PNS promotes cardiomyocyte autophagy via the phosphorylation of AMPK and CaMKII, conferring cardioprotection in AMI. Furthermore, PNS has been observed to prevent the decline in mitochondrial membrane potential triggered by hypoxia and serum glucose starvation (SGOD) in vitro, inhibiting apoptosis in H9c2 cells. The anti-apoptotic property of PNS may be associated with the upregulation of miR-21 and the inhibition of the PI3K/Akt signaling pathway (Chen et al., 2011; Chen et al., 2019).
Frequent inflammatory reactions aggravate ischemia-reperfusion injury post-AMI, leading to an elevation in MIS (Toldo and Abbate, 2018). TNF-α and IL-6 are the primary inflammatory mediators (Neumann et al., 1995; Frangogiannis, 2014). Fundamental research has demonstrated that PNS can decrease the levels of inflammatory mediators such as nuclear factor kappa B (NF-κB), TNF-α, and IL-6 in rat models of AMI. This reduction mitigates the extent of inflammatory infiltration and myocardial cell edema (Pan et al., 2015; Ning et al., 2020). Sun et al. (2013) and Zhong et al. (2015) corroborated that notoginsenoside R1, a significant phytoestrogen, mitigates H9c2 cell injury by diminishing inflammatory responses and apoptosis in both in vivo and in vitro experiments.
Oxidative stress injury critically contributes to irreversible and severe damage to cardiomyocytes in AMI (Neri et al., 2015). Experimental findings (Yamada et al., 2019; Wang et al., 2020) confirm that PNS curtails the generation of oxygen-free radicals in cardiomyocytes post-AMI. This modulation alleviates the imbalance between oxidative and antioxidative mechanisms, ameliorating oxidative stress injury and safeguarding the damaged myocardium. Yu et al. (2016) verified through in vivo and in vitro studies that PNS scavenges oxygen-free radicals, augments the activity of antioxidant enzymes, decreases the expression of endoplasmic reticulum stress-associated proteins, and suppresses the expression of proteins linked to pro-apoptosis.
Alterations in the structure and function of vascular endothelial cells are intimately associated with AMI onset. PNS inhibits the apoptosis of rat vascular endothelial cells by downregulating caspase-3 expression and upregulating the Bcl-2 gene. It enhances endothelium-dependent vasodilation function by elevating NO levels and reducing ET-1 levels (Kwan and Kwan, 2000; Qiao et al., 2015; Zhang et al., 2021). Certain studies (Yang et al., 2016; Zhou et al., 2019) have suggested that angiogenesis induced by PNS post-AMI might correlate with the upregulation of vascular endothelial growth factor A (VEGFA) gene expression. PNS notably promotes the VEGF-stimulated migration of human umbilical vein endothelial cells (HUVECs) and induces approximately a three-fold increase in VEGF mRNA expression.
Platelet activation is a direct precursor to platelet aggregation, potentially leading to AMI. PNS exerts a notable inhibitory effect on platelet-activating factor CD62P and platelet membrane glycoprotein IIb/IIIa. This effect significantly counteracts platelet aggregation and adhesion, enhances fibrinolytic activity, and decelerates thrombosis (Lau et al., 2009). Studies indicate that PNS not only reduces platelet aggregation but also, when used with aspirin, enhances its antiplatelet effect and protects the gastric mucosa. This effect is likely due to PNS’s impact on the metabolic pathways of cyclooxygenase-1 and cytochrome enzymes in the platelet arachidonic acid (AA) pathway (Wang et al., 2021b; Wang et al., 2021c; Dai et al., 2022). Zhong and Zheng (2019) observed that the platelet aggregation rate in the PNS plus CT group was significantly lower than that in the CT group 2 h post-thrombolysis, although no significant difference was noted at 10 h. Although our study did not include indicators related to platelet function and coagulation, future research may address this gap.
4.3 Advantages and limitations
The advantages of this meta-analysis included the following. First, a comprehensive search was conducted in a variety of databases without language and time restrictions. Second, independent study selection, data extraction, and bias assessment by two investigators minimized errors. Third, sensitivity analyses did not significantly affect the results, indicating the results were credible. Fourth, multiple subgroup analyses were conducted to assess potential influencing factors and reduce heterogeneity.
There were some limitations in this study. First, the quality of included RCTs was generally low, according to the collaboration tool. None of the 20 RCTs clearly described the method of allocation concealment and blinding, resulting in methodological deficiencies. Therefore, we assessed and reviewed the eligible RCTs by applying considerably high standards. Our meta-analysis attempted to secure methodological rigor and therefore draw unbiased conclusions. Second, all of 20 RCTs were written in Chinese and no English article was involved, and the publication biases could probably affect the results. Third, there were differences in CT of the 20 RCTs, which may increase heterogeneity between the included trials and influence the results. In addition, the heterogeneity in meta-analysis was significant. We used sensitivity and subgroup analyses to explore the source of heterogeneity.
5 Conclusion
This study highlighted the efficacy and safety of adjunctive PNS injection in enhancing AMI patient outcomes beyond CT alone. Future RCTs need to solidify these findings through rigorous methods, including double-blinding, enlarged sample sizes, the incorporation of multiple centers, and prolonged follow-up durations.
Data availability statement
The original contributions presented in the study are included in the article/Supplementary Material; further inquiries can be directed to the corresponding authors.
Author contributions
PC: data curation, software, and writing–original draft. ZG: writing–review. MG: formal analysis, software, and writing–original draft. DP: data curation and writing–original draft. HZ: software. JD: conceptualization and writing–review and editing. DS: conceptualization and writing–review and editing.
Funding
The author(s) declare that financial support was received for the research, authorship, and/or publication of this article. This work was supported by the project of Major New Drug Creation (No. 2018ZX09301-011-001).
Conflict of interest
The authors declare that the research was conducted in the absence of any commercial or financial relationships that could be construed as a potential conflict of interest.
Publisher’s note
All claims expressed in this article are solely those of the authors and do not necessarily represent those of their affiliated organizations, or those of the publisher, the editors, and the reviewers. Any product that may be evaluated in this article, or claim that may be made by its manufacturer, is not guaranteed or endorsed by the publisher.
Supplementary material
The Supplementary Material for this article can be found online at: https://www.frontiersin.org/articles/10.3389/fphar.2024.1353662/full#supplementary-material
References
Byrne, R. A., Rossello, X., Coughlan, J. J., Barbato, E., Berry, C., Chieffo, A., et al. (2023). 2023 ESC Guidelines for the management of acute coronary syndromes. Eur. Heart J. 44 (38), 3720–3826. doi:10.1093/eurheartj/ehad191
Chen, J., Xue, R., Li, L., Xiao, L. L., Shangguan, J., Zhang, W., et al. (2019). Panax notoginseng saponins protect cardiac myocytes against endoplasmic reticulum stress and associated apoptosis through mediation of intracellular calcium homeostasis. Front. Pharmacol. 10, 1013. doi:10.3389/fphar.2019.01013
Chen, S., Liu, J., Liu, X., Fu, Y., Zhang, M., Lin, Q., et al. (2011). Panax notoginseng saponins inhibit ischemia-induced apoptosis by activating PI3K/Akt pathway in cardiomyocytes. J. Ethnopharmacol. 137 (1), 263–270. doi:10.1016/j.jep.2011.05.011
Chen, Z. (2019). Effect of Xuesaitong injection on myocardial injury and cardiac function in patients with acute ST segment elevation myocardial infarction. Xizang Med. (01), 145–147.
Dai, L., Zhang, Y., Jiang, Y., and Chen, K. (2022). Panax notoginseng preparation plus aspirin versus aspirin alone on platelet aggregation and coagulation in patients with coronary heart disease or ischemic stroke: a meta-analysis of randomized controlled trials. Front. Pharmacol. 13, 1015048. doi:10.3389/fphar.2022.1015048
Fan, Y., Fu, X., and Li, Y. (2015). Application of Xueshuantong injection in the treatment of acute ST segment elevation myocardial infarction. China Med. Her. (04), 111–114.
Feng, G., Wei, Y., Bai, Y., Zhao, D., et al. (2017). Clinical effect of coronary injection of Panax notoginseng saponins during PCI in STEMI patients. J. Pract. Med. (02), 143–145. doi:10.14172/j.issn1671-4008.2017.02.016
Frangogiannis, N. G. (2014). The inflammatory response in myocardial injury, repair, and remodelling. Nat. Rev. Cardiol. 11 (5), 255–265. doi:10.1038/nrcardio.2014.28
Fu, X., Lu, J., Yang, F., and Xiao, W. (2014a). Preventive and therapeutic effect of Xueshuantong injection on reperfusion injury in acute myocardial infarction. Propr. Chin. Med. (05), 933–936.
Fu, X., Xiao, W., and Yang, F. (2014b). Effect of Xueshuantong injection on BNP level in patients with acute myocardial infarction. Today's Pharm. (4), 273–275.
Gan, L., Zhang, C., Zhang, M., Cheng, Y., Liao, Y., et al. (2010). Effect of intracoronary injection with xuesaitong in treating post-PCI slow-reflow phenomenon in patients with ST-segment elevation myocardial infarction. Chin. J. Integr. traditional Chin. West. Med. 30 (04), 348–351.
Gong, F. F., Vaitenas, I., Malaisrie, S. C., and Maganti, K. (2021). Mechanical complications of acute myocardial infarction: a review. JAMA Cardiol. 6 (3), 341–349. doi:10.1001/jamacardio.2020.3690
Guo, Q. (2019). To explore the clinical efficacy of Xuesaitong combined with PCI in the treatment of acute ST segment elevation myocardial infarction. Pract. Clin. integration traditional Chin. West. Med. (3), 62–63. doi:10.13638/j.issn.1671-4040.2019.03.030
Heinrich, M., Jalil, B., Abdel-Tawab, M., Echeverria, J., Kulić, Ž., McGaw, L. J., et al. (2022). Best Practice in the chemical characterisation of extracts used in pharmacological and toxicological research-The ConPhyMP-Guidelines. Front. Pharmacol. 13, 953205. doi:10.3389/fphar.2022.953205
Heusch, G., and Gersh, B. J. (2017). The pathophysiology of acute myocardial infarction and strategies of protection beyond reperfusion: a continual challenge. Eur. Heart J. 38 (11), 774–784. doi:10.1093/eurheartj/ehw224
Ji, X., and Liu, Z. (2022). Effect of alteplase combined with Xueshuantong on thrombolysis and myocardial injury in STEMI patients. Inn. Mong. Med. J. (06), 680–682. doi:10.16096/j.cnki.nmzyxzz.2022.54.06.011
Kwan, C. Y., and Kwan, T. K. (2000). Effects of Panax notoginseng saponins on vascular endothelial cells in vitro. Acta Pharmacol. Sin. 21 (12), 1101–1105.
Lau, A. J., Toh, D. F., Chua, T. K., Pang, Y. K., Woo, S. O., and Koh, H. L. (2009). Antiplatelet and anticoagulant effects of Panax notoginseng: comparison of raw and steamed Panax notoginseng with Panax ginseng and Panax quinquefolium. J. Ethnopharmacol. 125 (3), 380–386. doi:10.1016/j.jep.2009.07.038
Li, H., Zhu, J., Xu, Y. W., Mou, F. F., Shan, X. L., Wang, Q. L., et al. (2022). Notoginsenoside R1-loaded mesoporous silica nanoparticles targeting the site of injury through inflammatory cells improves heart repair after myocardial infarction. Redox Biol. 54, 102384. doi:10.1016/j.redox.2022.102384
Liu, H., Lu, X., Hu, Y., and Fan, X. (2020). Chemical constituents of Panax ginseng and Panax notoginseng explain why they differ in therapeutic efficacy. Pharmacol. Res. 161, 105263. doi:10.1016/j.phrs.2020.105263
Liu, H., Qiao, Z., Ma, J., Zhang, L., Cao, H., Wang, H., et al. (2018). Clinical study of Xuesaitong combined with percutaneous coronary intervention in the treatment of acute ST segment elevation myocardial infarction. Study Cardiovasc. Dis. China 16 (8). doi:10.3969/j.issn.1672-5301.2018.08.021
Liu, Z., Wang, H., Hou, G., Cao, H., Zhao, Y., and Yang, B. (2019). Notoginsenoside R1 protects oxygen and glucose deprivation-induced injury by upregulation of miR-21 in cardiomyocytes. J. Cell Biochem. 120 (6), 9181–9192. doi:10.1002/jcb.28194
Montecucco, F., Carbone, F., and Schindler, T. H. (2016). Pathophysiology of ST-segment elevation myocardial infarction: novel mechanisms and treatments. Eur. Heart J. 37 (16), 1268–1283. doi:10.1093/eurheartj/ehv592
Murphy, A., and Goldberg, S. (2022). Mechanical complications of myocardial infarction. Am. J. Med. 135 (12), 1401–1409. doi:10.1016/j.amjmed.2022.08.017
Neri, M., Fineschi, V., Di Paolo, M., Pomara, C., Riezzo, I., Turillazzi, E., et al. (2015). Cardiac oxidative stress and inflammatory cytokines response after myocardial infarction. Curr. Vasc. Pharmacol. 13 (1), 26–36. doi:10.2174/15701611113119990003
Neumann, F. J., Ott, I., Gawaz, M., Richardt, G., Holzapfel, H., Jochum, M., et al. (1995). Cardiac release of cytokines and inflammatory responses in acute myocardial infarction. Circulation 92 (4), 748–755. doi:10.1161/01.cir.92.4.748
Nichols, M., Townsend, N., Scarborough, P., and Rayner, M. (2014). Cardiovascular disease in Europe 2014: epidemiological update. Eur. Heart J. 35 (42), 2929–2959. doi:10.1093/eurheartj/ehu378
Ning, K., Jiang, L., Hu, T., Wang, X., Liu, A., and Bao, Y. (2020). ATP-sensitive potassium channels mediate the cardioprotective effect of panax notoginseng saponins against myocardial ischaemia-reperfusion injury and inflammatory reaction. . Biomed. Res. Int. 2020, 3039184. doi:10.1155/2020/3039184
Pan, L., Zhang, Y., Lu, J., Geng, Z., Jia, L., Rong, X., et al. (2015). Panax notoginseng saponins ameliorates coxsackievirus B3-induced myocarditis by activating the cystathionine-γ-lyase/hydrogen sulfide pathway. J. Cardiovasc Transl. Res. 8 (9), 536–544. doi:10.1007/s12265-015-9659-8
Peng, M., Yi, Y. X., Zhang, T., Ding, Y., and Le, J. (2018). Stereoisomers of saponins in panax notoginseng (sanqi): a review. Front. Pharmacol. 9, 188. doi:10.3389/fphar.2018.00188
Qiao, Y., Zhang, P. J., Lu, X. T., Sun, W. w., Liu, G. l., Ren, M., et al. (2015). Panax notoginseng saponins inhibits atherosclerotic plaque angiogenesis by down-regulating vascular endothelial growth factor and nicotinamide adenine dinucleotide phosphate oxidase subunit 4 expression. Chin. J. Integr. Med. 21 (4), 259–265. doi:10.1007/s11655-014-1832-4
Qiao, Z., Gao, F., Xu, B., and Yang, J. (2016). Effect of Xuesaitong injection on inflammatory factors and matrix metalloproteinases in patients with acute myocardial infarction after PCI. J. Cardiovasc. Cerebrovasc. Dis. Integr. traditional Chin. West. Med. (20), 2394–2396. doi:10.3969/j.issn.1672.1349.2016.20.017
Reed, G. W., Rossi, J. E., and Cannon, C. P. (2017). Acute myocardial infarction. Lancet 389 (10065), 197–210. doi:10.1016/S0140-6736(16)30677-8
Sanchis, J., Bueno, H., Miñana, G., Guerrero, C., Martí, D., Martínez-Sellés, M., et al. (2023). Effect of routine invasive vs conservative strategy in older adults with frailty and non-ST-segment elevation acute myocardial infarction: a randomized clinical trial. JAMA Intern Med. 183 (5), 407–415. doi:10.1001/jamainternmed.2023.0047
Sun, A. (2021). Effect of Xuesaitong on inflammatory factors and cardiac function after PCI in patients with acute ST segment elevation myocardial infarction. J. chronic Venereol. (09), 1425–1427. doi:10.16440/J.CNKI.1674-8166.2021.09.40
Sun, B., Xiao, J., Sun, X. B., and Wu, Y. (2013). Notoginsenoside R1 attenuates cardiac dysfunction in endotoxemic mice: an insight into oestrogen receptor activation and PI3K/Akt signalling. Br. J. Pharmacol. 168 (7), 1758–1770. doi:10.1111/bph.12063
Sun, T., Wang, P., Deng, T., Tao, X., and Xu, Y. (2020a). Effect of panax notoginseng saponins on focal cerebral ischemia-reperfusion in rat models: a meta-analysis. Front. Pharmacol. 11, 572304. doi:10.3389/fphar.2020.572304
Sun, X., Cao, H., Kou, L., and Di, P. (2020b). Effect of Xuesaitong injection combined with Ateplase on Hcy, TM, PIC and thrombus load in patients with acute myocardial infarction. Study drug Eval. (7), 1363–1366. doi:10.7501/j.issn.1674-6376.2020.07.030
Thygesen, K., Alpert, J. S., Jaffe, A. S., Chaitman, B. R., Bax, J. J., Morrow, D. A., et al. (2019). Fourth universal definition of myocardial infarction (2018). Eur. Heart J. 40 (3), 237–269. doi:10.1093/eurheartj/ehy462
Toldo, S., and Abbate, A. (2018). The NLRP3 inflammasome in acute myocardial infarction. Nat. Rev. Cardiol. 15 (4), 203–214. doi:10.1038/nrcardio.2017.161
Wang, A., Li, Y., Wang, Z., Xin, G., You, Y., Sun, M., et al. (2022). Proteomic analysis revealed the pharmacological mechanism of Xueshuantong injection in preventing early acute myocardial infarction injury. Front. Pharmacol. 13, 1010079. doi:10.3389/fphar.2022.1010079
Wang, D., Lv, L., Xu, Y., Jiang, K., Chen, F., Qian, J., et al. (2021a). Cardioprotection of Panax Notoginseng saponins against acute myocardial infarction and heart failure through inducing autophagy. Biomed. Pharmacother. 136, 111287. doi:10.1016/j.biopha.2021.111287
Wang, L. (2018). Application value of Xuesaitong injection in interventional therapy of acute ST segment elevation myocardial infarction. Int. Med. Health Bull. (11), 1679–1682. doi:10.3760/cma.j.issn.1007-1245.2018.11.025
Wang, L., Chen, X., Wang, Y., Zhao, L., and Zhao, X. (2020). MiR-30c-5p mediates the effects of panax notoginseng saponins in myocardial ischemia reperfusion injury by inhibiting oxidative stress-induced cell damage. Biomed. Pharmacother. 125, 109963. doi:10.1016/j.biopha.2020.109963
Wang, M. M., Xue, M., Xin, Z. H., Wang, Y. H., Li, R. J., Jiang, H. Y., et al. (2021c). Panax notoginseng saponin attenuates gastric mucosal epithelial cell injury induced by dual antiplatelet drugs through COX and PI3K/akt/VEGF-GSK-3β-RhoA network pathway. Chin. J. Integr. Med. 27 (11), 819–824. doi:10.1007/s11655-021-2854-3
Wang, T., Guo, R., Zhou, G., Zhou, X., Kou, Z., Sui, F., et al. (2016). Traditional uses, botany, phytochemistry, pharmacology and toxicology of Panax notoginseng (Burk.) F.H. Chen: a review. J. Ethnopharmacol. 188, 234–258. doi:10.1016/j.jep.2016.05.005
Wang, W., Yang, L., Song, L., Guo, M., Li, C., Yang, B., et al. (2021b). Combination of Panax notoginseng saponins and aspirin potentiates platelet inhibition with alleviated gastric injury via modulating arachidonic acid metabolism. Biomed. Pharmacother. 134, 111165. doi:10.1016/j.biopha.2020.111165
Xie, Q. (2017). Clinical effect of Xueshuantong injection on acute myocardial infarction and its effect on cardiac function. Pract. J. Cardiac, Cerebropulmonary Vasc. Dis. (03), 73–76. doi:10.3969/j.issn.1008-5971.2017.03.018
Xin, D., and Qian, L. (2018). Effect of Atto vastatin combined with Xuesaitong injection on inflammatory factors after PCI in patients with myocardial infarction. Chin. Rural. Med. (12), 39–40. doi:10.19542/j.cnki.1006-5180.001756
Yamada, Y., Minatoguchi, S., Endo, N., Kanamori, H., Kawasaki, M., Nishigaki, K., et al. (2019). Post-MI treatment with G-CSF and EPO-liposome with SLX repairs infarcted myocardium through EPCs mobilization and activation of prosurvival signals in rabbits. Pharmacol. Res. Perspect. 7 (1), e00451. doi:10.1002/prp2.451
Yang, B. R., Cheung, K. K., Zhou, X., Xie, R. F., Cheng, P. P., Wu, S., et al. (2016). Amelioration of acute myocardial infarction by saponins from flower buds of Panax notoginseng via pro-angiogenesis and anti-apoptosis. J. Ethnopharmacol. 181, 50–58. doi:10.1016/j.jep.2016.01.022
Yang, W., Liu, H., Chen, J., and Huang, Y. (2022). Clinical observation of Xueshuantong injection combined with recombinant human brain natriuretic peptide after emergency PCI in patients with acute ST segment elevation myocardial infarction. Tianjin Tradit. Chin. Med. (11), 1373–1378. doi:10.11656/j.issn.1672-1519.2022.11.03
Yeh, R. W., Sidney, S., Chandra, M., Sorel, M., Selby, J. V., and Go, A. S. (2010). Population trends in the incidence and outcomes of acute myocardial infarction. N. Engl. J. Med. 362 (23), 2155–2165. doi:10.1056/NEJMoa0908610
Yu, Y., Sun, G., Luo, Y., Wang, M., Chen, R., Zhang, J., et al. (2016). Cardioprotective effects of Notoginsenoside R1 against ischemia/reperfusion injuries by regulating oxidative stress- and endoplasmic reticulum stress-related signaling pathways. Sci. Rep. 6, 21730. doi:10.1038/srep21730
Zeng, J. J., Shi, H. Q., Ren, F. F., Zhao, X. S., Chen, Q. Y., Wang, D. J., et al. (2023). Notoginsenoside R1 protects against myocardial ischemia/reperfusion injury in mice via suppressing TAK1-JNK/p38 signaling. Acta Pharmacol. Sin. 44 (7), 1366–1379. doi:10.1038/s41401-023-01057-y
Zhang, T., Liu, W., Yang, J., Xu, H., Cao, Y., Guo, L., et al. (2022). Components of salvia miltiorrhiza and panax notoginseng protect pericytes against OGD/R-Induced injury via regulating the PI3K/AKT/mTOR and JNK/ERK/P38 signaling pathways. J. Mol. Neurosci. 72 (12), 2377–2388. doi:10.1007/s12031-022-02082-y
Zhang, X., Zhou, C., Miao, L., Tan, Y., Zhou, Y., Cheong, M. S., et al. (2021). Panax notoginseng protects against diabetes-associated endothelial dysfunction: comparison between ethanolic extract and total saponin. Oxid. Med. Cell Longev. 2021, 4722797. doi:10.1155/2021/4722797
Zhang, Z. (2019). Effect of Xuesaitong combined with western medicine on cardiac function and inflammatory factors after emergency PCI in patients with acute myocardial infarction. New tradit. Chin. Med. (6), 121–124. doi:10.13457/j.cnki.jncm.2019.06.036
Zhang, Z., and Du, P. (2019). Effects of Edaravone combined with Xuesaitong on hemorheology and inflammation in elderly patients with acute myocardial infarction. Guizhou Med. (12), 1921–1922.
Zhong, H., and Zheng, W. (2019). Effects of Xueshuantong injection combined with intravenous thrombolysis on platelet aggregation, vascular endothelial function and cardiac function in patients with acute myocardial infarction. J. Cardiovasc. Cerebrovasc. Dis. Integr. traditional Chin. West. Med. (22), 3561–3562. doi:10.12102/jssn.1672-1349.2019.22.028
Zhong, L., Zhou, X. L., Liu, Y. S., Wang, Y. M., Ma, F., Guo, B. L., et al. (2015). Estrogen receptor α mediates the effects of notoginsenoside R1 on endotoxin-induced inflammatory and apoptotic responses in H9c2 cardiomyocytes. Mol. Med. Rep. 12 (1), 119–126. doi:10.3892/mmr.2015.3394
Zhou, M. N., Delaveris, C. S., Kramer, J. R., Kenkel, J. A., Engleman, E. G., and Bertozzi, C. R. (2018b). N-carboxyanhydride polymerization of glycopolypeptides that activate antigen-presenting cells through dectin-1 and dectin-2. Angew. Chem. Int. Ed. Engl. 57 (12), 3137–3142. doi:10.1002/anie.201713075
Zhou, S. (2018). Study on the efficacy of Xuesaitong combined with western medicine in the treatment of AMI emergency PCI and its effect on cardiac function and inflammatory factors. Shaanxi Tradit. Chin. Med. (12), 1687–1690. doi:10.3969/j.issn.1000-7369.2018.12.009
Zhou, X., Li, Z. C., Chen, P. P., and Xie, R. F. (2019). Primary mechanism study of panax notoginseng flower (herb) on myocardial infarction in rats. Cardiol. Res. Pract. 2019, 8723076. doi:10.1155/2019/8723076
Keywords: Panax notoginseng saponins, acute myocardial infarction, meta-analysis, systematic review, randomized controlled trials
Citation: Chen P, Gao Z, Guo M, Pan D, Zhang H, Du J and Shi D (2024) Efficacy and safety of Panax notoginseng saponin injection in the treatment of acute myocardial infarction: a systematic review and meta-analysis of randomized controlled trials. Front. Pharmacol. 15:1353662. doi: 10.3389/fphar.2024.1353662
Received: 11 December 2023; Accepted: 16 February 2024;
Published: 21 March 2024.
Edited by:
Xuezhong Zhou, Beijing Jiaotong University, ChinaReviewed by:
Zhi Yong Du, Capital Medical University, ChinaXiao Li, Shandong Provincial Qianfoshan Hospital, China
Copyright © 2024 Chen, Gao, Guo, Pan, Zhang, Du and Shi. This is an open-access article distributed under the terms of the Creative Commons Attribution License (CC BY). The use, distribution or reproduction in other forums is permitted, provided the original author(s) and the copyright owner(s) are credited and that the original publication in this journal is cited, in accordance with accepted academic practice. No use, distribution or reproduction is permitted which does not comply with these terms.
*Correspondence: Dazhuo Shi, shidazhuo@126.com; Jianpeng Du, 13811518062@163.com
†These authors have contributed equally to this work