- 1Department of Anesthesiology, West China Hospital, Sichuan University, Chengdu, Sichuan, China
- 2Laboratory of Anesthesia and Critical Care Medicine, National-Local Joint Engineering Research Centre of Translational Medicine of Anesthesiology, West China Hospital, Sichuan University, Chengdu, Sichuan, China
Sophocarpine is a natural compound that belongs to the quinolizidine alkaloid family, and has a long history of use and widespread distribution in traditional Chinese herbal medicines such as Sophora alopecuroides L., Sophora flavescens Ait., and Sophora subprostrata. This article aims to summarize the pharmacology, pharmacokinetics, and toxicity of sophocarpine, evaluate its potential pharmacological effects in various diseases, and propose the necessity for further research and evaluation to promote its clinical application. A large number of studies have shown that it has anti-inflammatory, analgesic, antiviral, antiparasitic, anticancer, endocrine regulatory, and organ-protective effects as it modulates various signaling pathways, such as the NF-κB, MAPK, PI3K/AKT, and AMPK pathways. The distribution of sophocarpine in the body conforms to a two-compartment model, and sophocarpine can be detected in various tissues with a relatively short half-life. Although the pharmacological effects of sophocarpine have been confirmed, toxicity and safety assessments and reports on molecular mechanisms of its pharmacological actions have been limited. Given its significant pharmacological effects and potential clinical value, further research and evaluation are needed to promote the clinical application of sophocarpine.
Introduction
Natural products have played vital roles in pharmacotherapy. They can be obtained from various species ranging from mosses to flowering plants, animals, or microorganisms and have offered unique chemical templates or novel scaffolds for drug discovery (Atanasov et al., 2021). Many natural products have active pharmacological properties and possess therapeutic value (Guo et al., 2022). Historically, these medicinal plants have long been used for treating various human diseases in traditional Chinese medicine. Among such products, the alkaloids found in the bark, root, leaf, seed, flower, or other parts of the plant are among the largest and most important groups of naturally occurring organic molecules, and constitute the active ingredients of the crude medical source (Omar et al., 2021). Over 27,000 different alkaloids are listed in the Dictionary of Natural Products (DNP), and more than 70% of these are from plants. The genus Sophora exhibits a wide distribution across North America and Eurasia, encompassing several plant species that have long been employed in traditional herbal medicine, such as Sophora alopecuroides L. and Sophora flavescens Ait. The primary alkaloidal constituents of Sophora are presented in Table 1. Quinolizidine alkaloids, as the major active ingredients in the genus Sophora, are divided into different classes of alkaloids, including matrine-, lupinine-, lupanine-, macrocyclic bisquinolizidine-, biphenylquinolizidine lactone-, cytosine-, sparteine-, tetrahydrocytisine-, and anagyrine-type alkaloids. Among these compounds, matrine is the most common type, accounting for 13.6% of the total quinolizidine alkaloids (Cely-Veloza et al., 2023). Sophocarpine (C15H22N2O, CAS No. 145572-44-7) (Figure 1), also known as 13,14-didehydromatridin-15-one, is a tetracyclic matrine-type quinolizidine alkaloid found in Sophora flavescens Ait., Sophora alopecuroides L., Sophora viciifolia., Sophora tonkinensis Gagnep., and Daphniphyllum oldhamii (Hemsl.) K. Rosenthal. These medicinal plants have been used in healthcare for centuries, especially in East Asia.
The beneficial effect of sophocarpine has been widely explored. Many modern pharmacological studies have revealed its multiple bioactive effects, such as anticancer (Luo et al., 2019), anti-inflammatory (Gao et al., 2012), antiviral (Gao et al., 2013; Sang et al., 2017), analgesic (Wang et al., 2021b), and organ protection (Li et al., 2014; Hou et al., 2020) or addressing vessel dysfunction (Fang et al., 2020) effects. Moreover, it also has an antidiabetic effect on type 1 diabetes (Su et al., 2021). Furthermore, sophocarpine exhibits great benefits in agriculture. Sophocarpine may be used as a natural control agent for the invasive and dangerous red imported fire ants (Solenopsis invicta) (Tian and Zhang, 2023). Meanwhile, sophocarpine also has aphicidal effects on pea aphids (Acyrthosiphon pisum) (Ma et al., 2020). In addition, sophocarpine is an ideal starting material for drug synthesis and structural modification due to its unique chemical structure with an α,β-unsaturated carbonyl group, which may provide a flexible reactive site for nucleophiles (Hu et al., 2010).
As a main bioactive component of quinolizidine alkaloids, sophocarpine has been widely studied in recent years. However, the pharmacological activities and underlying mechanism of sophocarpine have not been reviewed systematically. To further understand its progress, this review aims to provide up-to-date knowledge on the pharmacology, pharmacokinetics and toxicity of sophocarpine, hopefully providing valuable insights into its therapeutic potential in clinical settings. We believe this review will be helpful in understanding the key characteristics of sophocarpine and may offer constructive suggestions for further studies.
Pharmacological activities
Sophocarpine and inflammation
Sophora alopecuroides L. and its active components have a long history as a traditional folk medicine in China for the treatment of inflammation. Rheumatoid arthritis is a chronic systemic inflammatory autoimmune disease that can affect the joints (Sparks, 2019). Zhu’s group further confirmed that sophocarpine may be a potential drug for treating rheumatoid arthritis, demonstrating its ability to suppress the expression of proinflammatory cytokines and downregulate the MAPK and NF-κB signaling pathways (Zhu and Zhu, 2017). Similarly, Wu et al. have reported that sophocarpine can also attenuate inflammatory factors such as IL-6 and TNF-α in both in vivo and in vitro models of osteoarthritis-related inflammatory cartilage degeneration, with mechanisms involving the PI3K/AKT and NF-κB signaling pathways (Wu et al., 2019). Implant loosening can occur after long-term total joint arthroplasty. Using both in vivo and in vitro models, Zhou et al. reported that sophocarpine could prevent implant loosening by suppressing osteoclastogenesis and bone resorption. The molecular mechanism may involve the NF-κB signaling pathway. Therefore, sophocarpine may be used as a potential reagent for treating prosthesis loosening (Zhou et al., 2018). It is evident that targeting the NF-κB signaling pathway, along with upstream pathways such as PI3K/AKT and MAPK signaling pathways represents crucial therapeutic strategies for managing osteoarthritis using sophocarpine. However, it should be noted that other pathways such as the HIF2α (Saito et al., 2010) and Wnt/β-catenin (Monteagudo et al., 2022) pathways, have been demonstrated to significantly influence the pathogenesis of osteoarthritis. It remains uncertain whether these pathways directly contribute to the therapeutic effects of sophocarpine.
Lipopolysaccharide (LPS) is a component of the gram-negative bacterial outer membrane and may cause inflammation. The effect of sophocarpine on LPS-induced inflammatory responses in RAW 264.7 cells was investigated. The results indicated that sophocarpine has anti-inflammatory effects in vitro, which may be associated with the MAPK and NF-kB signaling pathways (Gao et al., 2012). Similarly, He et al. showed that sophocarpine reduced the levels of IL-6 and TNF-α in RAW 264.7 cells exposed to LPS and decreased the inflammatory response in zebrafish exposed to CuSO4, indicating that sophocarpine was effective in ameliorating inflammation both in vitro and in vivo (He et al., 2019). Ulcerative colitis is a chronic inflammatory bowel disease (Gajendran et al., 2019). Sophocarpine significantly ameliorated dextran sulfate sodium salt (DSS)- induced colitis by regulating pro- and anti-inflammatory cytokine production (Wang et al., 2012) and TLR4/MAPK and K2/STAT3 signaling pathway activation (Zhang et al., 2015). Similarly, Jiang et al. conducted a screening of five compounds (sophocarpine, sophoridine, cytisine, aloperine, and matrine) derived from Sophora flavescens Ait. and observed that sophocarpine demonstrated the most significant anti-inflammatory effect. Furthermore, they identified that sophocarpine mitigated DSS-induced colitis inflammation and intestinal fibrosis by modulating the SIRT 1/NF-κB p65 signaling pathway, reversing the senescence-associated secretory phenotype (SASP) and fibroblast-into-myofibroblast transition (FMT) of fibroblasts, as well as maintaining intestinal mucosal homeostasis (Jiang et al., 2024). In a rat model of 2,4,6-trinitrobenzene sulfonic acid-induced colitis, the total alkaloid Sophora alopecuroides (TASA), whose main ingredient is sophocarpine, was found to exert a strong anti-inflammatory effect by upregulating the levels of CD4+CD25+ Tregs and IL-10 in the colon and peripheral blood (Zhou et al., 2010a). Notably, sophocarpine has anti-asthmatic activity in an ovalbumin-induced mouse asthma model, as evidenced by the regulation of Th1/Th2 cytokine production, reduced pulmonary damage, inflammatory cell infiltration and decreased serum levels of IgE levels (Zhi et al., 2021). The aforementioned study suggested that sophocarpine exhibits remarkable efficacy in combating a wide range of inflammatory diseases, and is therefore a highly promising candidate for the development of novel anti-inflammatory strategies. However, it is worth noting that there is a dearth of pertinent research on the role of sophocarpine in other inflammatory conditions such as pneumonia, esophagitis, and gastritis. However, further investigations are needed to elucidate the pharmacological effects and intricate mechanisms of action underlying the effects of sophocarpine in these pathological conditions.
Sophocarpine and virus
Sophocarpine reportedly has anti-hepatitis B virus (HBV) activity. A study comparing the anti-HBV effects of different alkaloids showed that HepG2.2.15 cells exposured to 0.4 or 1.6 mM sophocarpine had more effective decreases in hepatitis B surface antigen (HBsAg) levels in the medium than did those exposed to sophoridine or lamivudine, and sophocarpine produced the greatest reduction in HBsAg levels after 24 h of exposure. Moreover, sophocarpine concentration-dependently reduced the level of HBV DNA in culture media (Chen et al., 2016). Ding et al. isolated the ingredients of the roots of Sophora flavescens Ait. Using chromatography and found that sophocarpine was one of the major components. These authors further confirmed its anti-HBV activity, as evidenced by reduced HBsAg and hepatitis B “e” antigen (HBeAg) secretion in the HepG2 2.2.15 cell line (Ding et al., 2006). Liu et al. showed similar results that the co-administration of all four kinds of matrine type alkaloids (sophocarpine, oxymatrine, matrine, sophoridine) with thymopolypeptides could inhibit HBsAg and HBeAg secretion and HBV DNA replication in HepG2.2.15 cells (Liu et al., 2016). The studies mentioned above illustrate the significant anti-HBV activity of sophocarpine. However, these findings are currently limited to cell-based experimental models, thus, confirmation of the independent anti-HBV activity of sophocarpine in in vivo is still lacking. Furthermore, the specific molecular mechanisms through which sophocarpine counteracts HBV infection, including its potential involvement in established anti-HBV pathways such as liver X receptor pathways (Zeng et al., 2020), AMPK-ULK1 pathway (Wang et al., 2021d), and the cGAS-STING pathway (Zhao et al., 2023), have not been identified. Therefore, further investigations are warranted to address these pertinent issues.
Additionally, sophocarpine was found to attenuate liver injury in patients with concanavalin A-induced hepatitis. The protective effect of these agents was related to the inhibition of proinflammatory cytokines, chemokines, and the IFN-γ/STAT1 signaling pathway (Sang et al., 2017). Furthermore, N-substituted sophocarpinic acid derivatives, such as (E)-β, γ-N-(benzenesulfonyl) sophocarpinic acids, were shown to have anti-enteric activities against coxsackievirus. Notably, the inhibitory effect of (E)-12-N-(m-cyanobenzenesulfonyl)-β,γ-sophocarpinic acid against coxsackievirus B3 (CVB3) and coxsackievirus B6 (CVB6) in Vero cells is particularly noteworthy, and it can be safely administered orally, exhibiting an AUC value of 7.29 μM h. The maximum concentration (Cmax) in plasma was 4.54 μM, indicating rapid absorption with a maximum time (Tmax) of 0.5 h and a conducive half-time (t1/2) of 1.17 h. Additionally, the mean residence time (MRT) in rats was 1.5 h, and the LD50 in mice was found to exceed >1,000 mg kg−1 (Gao et al., 2013). Additionally, sophocarpine could inhibit human herpesvirus 6 (HHV-6) replication with selective indices of 184 and 183 (Qavi et al., 2002). Enterovirus 71 (EV71) is a major cause of hand, foot and mouth disease (HFMD) in children (Nayak et al., 2022). Jin’s group demonstrated that sophocarpine effectively inhibited the attachment and penetration of EV71, therefore preventing the entry of the virus into the cells. It also suppressed the replication of viral genomic RNA, suggesting that sophocarpine has anti-EV71 infection activity (Jin et al., 2017). Zhang et al. used a network pharmacology analysis technique followed by experimental validation and reported that sophocarpine may have potential therapeutic effects on coronavirus disease 2019 (COVID-19) by mediating cytokine release and the nuclear factor NF-κB signaling pathway (Zhang and Zhang, 2022). In brief, these reports indicate that sophocarpine may be a promising agent for the management of a wide range of viral infections.
Sophocarpine and parasites
Parasitic diseases have long posed a substantial threat to public health, particularly in tropical regions where their prevalence remains alarmingly high. Consequently, there is an urgent need for novel antiparasitic strategies to effectively address the current situation (Pink et al., 2005). Cystic echinococcosis (CE) is a persistent parasitic affliction caused by the larval stage of E. granulosus sensu lato, which impacts both animals and humans alike. The ability of this disease to infect both human and livestock populations, coupled with the inadequate efficacy of existing therapeutic interventions, exacerbates its transmission dynamics while inflicting significant economic losses and compromising patient wellbeing. Luo et al. found that the water-soluble alkaloids E2-a from Sophora moorcroftiana (Benth.) Benth. ex Baker seeds could reduce cyst weight and stimulate a specific immune response targeting T cells in protoscolex-infected mice, suggesting that the E2-a fraction may be used as a potential therapeutic agent against E. granulosus infection (Luo et al., 2018). E2-a primarily comprises two crucial constituents, matrine and sophocarpine. However, there is currently no research validating the efficacy of either sophocarpine or matrine alone in combating E. granulosus infection. Furthermore, it remains unclear whether sophocarpine has therapeutic potential against other parasitic diseases such as leishmaniasis, filariasis, and malaria. In conclusion, sophocarpine represents a promising drug candidate that can serve as a key monomer or fundamental framework for developing novel antiparasitic drugs; hence, further investigations are necessary to elucidate its relevant pharmacological effects and mechanisms of action.
Sophocarpine and cancer
The strong chemotherapeutic capacities of medicinal herbs and their derivative phytocompounds have been repeatedly confirmed by experimental and clinical studies on various cancer types (Huang et al., 2018). It can effectively improve the quality of life, survival and outcome of cancer patients (Luo et al., 2019). A large number of studies have confirmed the antitumor effect of sophocarpine. It may enhance antitumor immunity when used alone or in combination with other therapeutics. Based on the literature, sophocarpine has been shown to have anticancer effects on lung cancer, colorectal cancer, cervical cancer, head and neck cancer, prostate cancer, myeloma and liver cancer. In a study exploring the efficiency of sophocarpine in treating non-small cell lung cancer (NSCLC), the authors used a systems pharmacology and bioinformatics approach, in combination with C57/BL6 mice and different cell lines, including human NSCLC cell lines (NCI-H1975 and A549) and mouse Lewis lung carcinoma cell lines, and found that the combination of sophocarpine and an anti-PD-L1 antibody significantly inhibited tumor growth via a mechanism involving the ADORA1-ATF3-PD-L1 axis (Chen et al., 2022b). To evaluate the antitumor effects of different alkaloids, Lin et al. used human cancer cell lines of differing tissue origins. They found that sophocarpine (IC50: 3.68 mM) had a selective effect on different types of cancer, and had a significant inhibitory effect on lung cancer A549 cells (Lin et al., 2011). Moreover, sophocarpine was shown to inhibit colorectal cancer cell proliferation, invasion, and migration via a mechanism involving downregulation of the MEK/ERK/VEGF pathway, while overexpression of MEK reversed the beneficial effect of sophocarpine (Wang et al., 2019a). Meanwhile, the inhibitory effect of oxaliplatin on colorectal cancer liver metastasis could be further potentiated by the administration of sophocarpine. Yang et al. demonstrated that sophocarpine enhances the anti-proliferative, anti-invasion, and anti-migration effects of oxaliplatin on LoVo human colon cancer cells in vitro, and augments the inhibitory effect of oxaliplatin on nude mice with colon cancer liver metastasis (CCLM) in vivo (Yang et al., 2021c). Furthermore, sophocarpine dose-dependently inhibited the growth of the gastric cancer cell line MKN45 and BGC-823 by interrupting cell cycle progression, inhibiting proliferation and apoptosis. The mechanism underlying this effect was associated with autophagy induction and the regulation of the PTEN/PI3K/AKT pathway. However, unlike in previous studies of gastrointestinal tract cancer, no effect on inhibiting invasion or migration of cancer cells was mentioned, and whether the regulatory effect of p53, Bax, and Bcl-2 was related to the PTEN/PI3K/AKT pathway deserves further research (Huang et al., 2019).
Cancer cachexia is a multifactorial syndrome that leads to high morbidity and mortality in patients with advanced cancer. Zhang et al. tested the therapeutic effect of different kinds of alkaloids, including matrine, oxymatrine, sophocarpine, sophoramine, and sophoridine, on cachexia-related symptoms induced by colon-26 adenocarcinoma (C26). They found that sophocarpine exerted the most potent inhibitory effect on TNF-α and IL-6 production in both RAW264.7 cells and murine primary macrophages and had a better therapeutic effect on attenuating cachexia symptoms (Zhang et al., 2008). In addition, Li et al. extracted and purified total alkaloids from Sophora alopecuroides L. using macroporous resin and found that their active components, including sophocarpine, could effectively reduce the proliferation and apoptosis of human cervical tumor HeLa cells; However, it was not clear whether this effect occurred through the inhibition of the release of inflammatory factors by cancer cells or host cells (Li et al., 2016). In a study exploring the chemopreventive effect of sophocarpine, treatment of the head and neck squamous carcinoma cell lines UM-SCC-22B and UM-SCC-47 with sophocarpine resulted in a dose-dependent inhibition of proliferation, migration, and invasion. In addition, sophocarpine treatment activated p38 MAPK and repressed miR-21 expression by specifically blocking Dicer processing of premiR-21 to mature miR-21. Sophocarpine upregulated phosphatase and tensin homolog (PTEN), a target gene of miR-21, causing the inhibition of epithelial-mesenchymal transition in cancer cells. One study suggested that sophocarpine may be a potent miR-21 inhibitor for cancer treatment (Liu et al., 2017a). The antitumor effect of sophocarpine on prostate cancer was explored. Wang et al. used a comprehensive 2D PC-3 cell membrane chromatography (CMC) system to identify anti-prostate cancer components, including sophocarpine, and found that sophocarpine effectively inhibited epidermal growth factor-induced prostate cancer (PC-3) cell proliferation and induced apoptosis in a dose-dependent manner (Wang et al., 2017). Researchers further demonstrated that sophocarpine treatment suppressed the proliferation, migration and invasion of two castration-resistant prostate cancer cell lines, DU145 and PC-3 by deactivating the PI3K/AKT/mTOR signaling pathway (Weng et al., 2022a). In a study of myeloma, sophocarpine triflorohydrazone was found to inhibit KRASA12 and AMO-1 myeloma cell proliferation by promoting the expression of proapoptotic proteins and activation of Notch3-p53 signaling (Wang et al., 2021e). Consistently, research conducted by Zhang’s group tested the efficacy of sophocarpine against hepatoma cells and cancer stem cells. They found that sophocarpine exerts its antitumor effects by mediating the AKT/GSK3β/β-catenin axis and inhibiting epithelial-to-mesenchymal transition (EMT) induced by TGF-β (Zhang et al., 2016).
In summary, these basic studies have shown that sophocarpine has strong anticancer effects and thus may serve as a potential anticancer agent in clinical settings (Figure 2). Additionally, during development, sophocarpine can act against tumor cells by regulating proliferation, apoptosis, invasion, metastasis, and the tumor microenvironment, among other processes. However, most of those studies focused only on one aspect, and it is necessary to evaluate whether sophocarpine can exert multifaceted effects on various types of tumors.
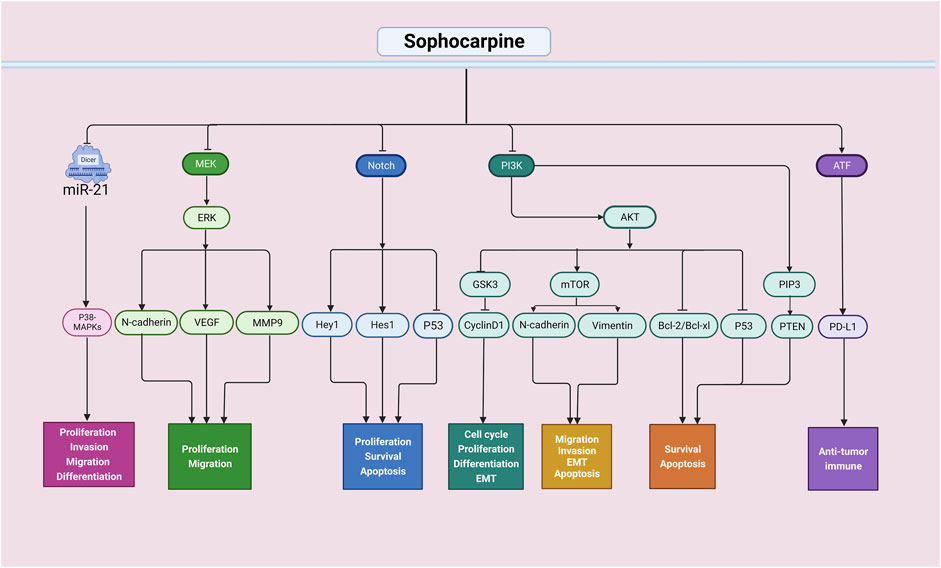
Figure 2. Antitumor effects of sophocarpine through multiple signaling pathways. The antitumor activity of sophocarpine is mainly achieved by interfering with multiple signaling pathways in tumor cells. It can inhibit the proliferation and invasion of tumor cells, as well as their migration and metastasis. Additionally, sophocarpine can regulate the apoptotic signaling pathway in tumor cells, promoting their apoptosis and thus inhibiting tumor growth and spread. Furthermore, sophocarpine also exhibited immunomodulatory effects on tumors. Overall, as a natural compound, sophocarpine has promising potential in the field of cancer treatment.
Sophocarpine and heart disease
Heart disease has emerged as a paramount global health concern, with its burden steadily escalating over the past decades. Based on pertinent research estimates, heart disease is projected to persist as the predominant contributor to the world’s disease burden in forthcoming decades (Roth et al., 2020). In recent years, natural agents have received increased amounts of attention as cardioprotective agents.
Cardiac electrical activity is determined by different ion channels in cardiac myocytes. Cardiac arrhythmia occurs due to alterations in ion channel function (Katz, 1993). Sophocarpine was shown to have antiarrhythmic activity (Figure 3). It could prolong the action potential duration of guinea pig papillary muscle (Zhu et al., 1989). However, this study did not explore the potential mechanism by which sophocarpine exerts its antiarrhythmic effect through modulation of ion channel function. Subsequently, sophocarpine was found to inhibit late sodium current, the Na+/Ca2+ exchanger current, diastolic calcium concentration, and ventricular muscle contractility in rabbit ventricular myocytes (Zhang et al., 2012). Yang et al. further examined the electrophysiological effects of sophocarpine on cardiac channel currents and reported that sophocarpine was effective at modulating sodium, calcium and potassium channel currents (Yang et al., 2011). The human ether-a-go-go-related gene (hERG), also known as the KCNH2 gene, encodes the Kv11.1 protein, which serves as the α subunit of a voltage-sensitive potassium channel. The hERG channel plays a pivotal role in modulating the repolarization phase of cardiac action potentials by precisely regulating the rapid delayed rectifier K+ current (Ikr). This regulation effectively influences both the action potential duration (APD) and QT interval observed via electrocardiograms, thereby impacting the occurrence of cardiac arrhythmias (Vandenberg et al., 2012; Butler et al., 2019). Using the whole-cell patch-clamp technique, Qi et al. found that sophocarpine inhibited transfected human ether-a-go-go-related gene (hERG) channels in a concentration-dependent manner by influencing the inactivation state (Qi et al., 2008). In addition, sophocarpine had no effect on the generation and trafficking of the hERG protein (Zhao et al., 2008). These authors further compared the effects of sophocarpine and sophoridine on hERG channels and showed that sophocarpine acted as a more potent hERG K+ channel blocker than sophoridine (Zhao et al., 2009). These studies consistently demonstrated that sophocarpine exerts antiarrhythmic effects through the inhibition of the hERG channel. However, further investigates of alternative cell types, such as cardiac myocytes or in vitro animal tissues, and comparisons of the findings with those obtained from HEK293 cells are needed to enhance the scientific rigor and broaden the scope of this research. In addition, aloperine, another alkaloid derived from Sophora flavescens Ait., has been scientifically proven to possess potent antiarrhythmic effects (Hu et al., 2023) and to act as a natural KCNQ5 agonist (Manville et al., 2019). Hence, it is intriguing to explore whether sophocarpine also modulates other currents such as the slow delayed rectifier K+ current (Iks), transient outward K+ current (Ito), or other α subunits, such as the KCNQ1 gene and its upstream regulatory genes of the KCNE family, in addition to regulating IKr currents associated with the hERG. Moreover, conducting further in vivo experiments utilizing appropriate animal models such as mice or rats would be imperative for validating the antiarrhythmic resistance effect of sophocarpine.
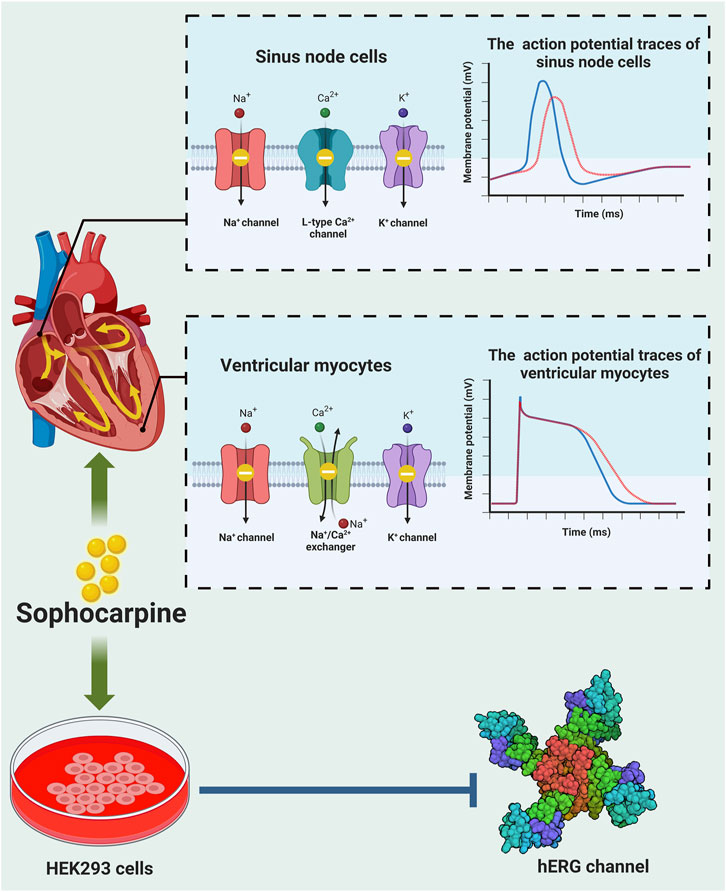
Figure 3. Anti-arrhythmic effects of sophocarpine. Sophocarpine has the property of blocks multiple ion channels, and its antiarrhythmic function is the result of its combined effect on these ion channels. Top: When sophocarpine was used to conduct action potential experiments on the sinoatrial node and ventricular muscles, it is found to significantly inhibit potassium, sodium, calcium ion channels and sodium-calcium exchangers (left), leading to a decrease in action potential (AP), prolongation of action potential duration (APD) and decrease in the action potential peak (right). blue strand: normal AP trace; red strand: AP trace after treatment with sophocarpine. Bottom: Sophocarpine can inhibit the hERG channel (PDB:7CN0) -mediated potassium ion tail current in HEK293 cells. These effects reverse the arrhythmias induced by isoproterenol.
Moreover, in a rat model of myocardial ischemia and reperfusion injury, sophocarpine was found to limit infarct size post-I/R, accompanied by decreased serum levels of TNF-α, IL-6, IL-10, and myeloperoxidase (MPO) activity, indicating reduced inflammation. The authors further proved that NF-κB inactivation may play a role in sophocarpine-induced cardioprotection (Li et al., 2011). Additionally, Zhang et al. conducted a study on the therapeutic potential of sophocarpine in mitigating doxorubicin-induced heart injury in mice and H9C2 cells. Their findings demonstrated that sophocarpine effectively ameliorated doxorubicin-induced cardiac dysfunction, while concurrently reducing levels of creatine kinase (CK), creatine kinase-MB (CK-MB), lactate dehydrogenase (LDH), as well as oxidative stress markers such as malondialdehyde (MDA), superoxide dismutase (SOD), and glutathione (GSH) in mouse serum. Furthermore, sophocarpine was shown to decrease apoptosis and oxidative stress levels in both mice and H9C2 cells through activation of the Nrf 2/HO-1 signaling pathway at a molecular level (Zhang et al., 2024). Similarly, in an overload-induced cardiac fibrosis rat model, sophocarpine was effective at attenuating cardiac fibrosis, as indicated by reduced heart weight to body weight ratio and improved hemodynamics. It decreases the levels of proinflammatory cytokines, collagen content, and matrix metalloproteinases (MMPs) by modulating the NF-κB signaling pathway (Li et al., 2014). It has been shown that sophocarpine can alleviate sepsis-induced cardiomyopathy. It effectively inhibited LPS-induced cardiac inflammation, oxidative stress, apoptosis, and autophagy. A follow-up mechanistic study suggested that sophocarpine protects cells by inhibiting TLR-4/NF-κB while activating the Nrf2/HO-1 signaling pathway (Fu et al., 2023). These studies provide evidence that sophocarpine has cardioprotective effects on diverse forms of damage by mitigating inflammation, attenuating oxidative stress, and engaging multiple signaling pathways and mechanisms. Further investigation is warranted to elucidate the therapeutic and protective effects of sophocarpine on other types of heart diseases such as myocardial hypertrophy and ventricular remodeling, as well as to uncover the underlying mechanisms of action.
Taken together, these studies not only identified sophocarpine as a potential antiarrhythmic agent but also provided evidence for its potential therapeutic value in treating cardiac fibrosis as well as different types of cardiac injury.
Sophocarpine and vascular disease
The accumulation of oxidized low-density lipoprotein (ox-LDL) leads to endothelial cell injury and has been recognized as the major factor in the development of arterial atherosclerosis (Poznyak et al., 2020). Sophocarpine was found to attenuate ox-LDL-induced endothelial cell dysfunction and apoptosis through suppression of the NF-κB pathway in human aortic endothelial cells (Fang et al., 2020). Furthermore, sophocarpine could produce endothelial protective effects against advanced glycation end product-induced reactive oxygen species-mediated apoptosis by targeting MKK3 and p38MAPK signaling (Liu et al., 2017b). Balloon angioplasty is a general interventional technique applied for treating coronary arteries and endovascular vessel-related disease. However, in-stent restenosis often occurs and may lead to prolonged hospitalization and increased mortality (Katsanos et al., 2018). In a rat model, Yang et al. reported that sophocarpine effectively alleviated intimal hyperplasia following balloon injury via the modulation of inflammation-related signals (Yang et al., 2021a). In addition, sophocarpine alleviated the restenosis induced by cigarette smoke in rats postangioplasty (Yang et al., 2020). These studies support the idea that sophocarpine is capable of reducing endothelial cell injury and maintaining vessel integrity and therefore may be used to treat atherosclerosis or vessel dysfunction after balloon angioplasty. However, the role of sophocarpine in hypertension, a prevalent chronic disease, has not been fully elucidated. Conversely, extensive research has demonstrated the vasodilatory effects and reduction in vascular pressure associated with matrine and aloperine extracted from Sophora flavescens Ait. (Zheng et al., 2009; Shan et al., 2023). Given that sophocarpine can regulate multiple ion channels and safeguard vascular endothelium, it unequivocally holds potential for antihypertensive effects. Nevertheless, further investigation is imperative to substantiate this assertion.
Hepatic protective activity
Sophocarpine was shown to have a hepatoprotective effect on different types of liver injury. It was shown to attenuate hepatic oxidative reactions, inflammation, and apoptosis induced by lipopolysaccharide via a mechanism related to suppressing the CYP2E/Nrf2/ROS and PI3K/AKT pathways (Zhengyu et al., 2018). In a mouse cecal ligation and puncture model, sophocarpine alleviated septic liver injury, as evidenced by reduced serum levels of aspartate aminotransferase (AST) and alanine aminotransferase (ALT) and inflammatory responses. Sophocarpine-induced the nucleotide-binding oligomerization domain, leucine rich repeat and pyrin domain containing 3 (NLRP3) degradation by autophagy may be responsible for its protective effects (Hou et al., 2020). The above two studies employed distinct murine models of septic liver injury, providing ample evidence supporting the protective effect of sophocarpine against this injury through multiple mechanisms. Another study conducted by Huang et al. showed that sophocarpine could inhibit NK cell activity and thus attenuate the immunological liver injury induced by Poly I:C/D-GalN in C57BL/6 mice (Huang et al., 2016). Further investigations into the regulatory effects of sophocarpine on other immune cells, including T cells and macrophages, are important for elucidating the role of sophocarpine.
Using two types of injury models (dimethylnitrosamine injection and bile duct ligation), sophocarpine was found to suppress hepatic fibrosis and inhibit the activation and proliferation of hepatic stellate cells (Qian et al., 2014). Additionally, sophocarpine also has an anti-nonalcoholic steatohepatitis effect (Song et al., 2011), and the mechanism may involve the activation of the AMPK signaling pathway (Song et al., 2013). Later, the same group further demonstrated that sophocarpine treatment reduced Toll-like receptor 4 expression levels and suppressed proinflammatory cytokine synthesis in oleic acid-induced steatotic hepatocytes (Song et al., 2015).
Neuroprotective activity
Using a rat model of transient focal cerebral ischemia, sophocarpine was shown to ameliorate brain damage, as indicated by reduced infarction and apoptosis, accompanied by improved neurological scores. The observed positive outcomes could potentially be attributed to the inhibitory action on acid-sensing ion channel 1 (ASIC1) and potent anti-apoptotic effects exerted by sophocarpine, thereby suggesting a plausible mechanism underlying its beneficial effects (Yifeng et al., 2011). Zhu et al. used β-amyloid to induce PC12 neuronal cell damage and evaluated the protective effect of sophocarpine. The authors found that the favorable effect of sophocarpine was associated with inhibition of NF-κB nuclear translocation (Zhu et al., 2021). The role of sophocarpine in improving neurological functions and cognitive performance was studied by Ye et al. They found that sophocarpine alleviated cognitive impairment and reduced neural loss via modulation of the inflammatory pathway in a mouse model of Alzheimer’s disease (Ye et al., 2021). The next step in research may involve the assessment of sophocarpine across various dosages and administration routes to investigate its potential role in the treatment of Alzheimer’s disease. In conclusion, despite the limitations of the current research, it is indisputable that sophocarpine will exhibit neuroprotective effects and hold promise as a novel therapeutic agent for major neurological disorders such as cerebral ischemia and Alzheimer’s disease.
Pulmonary protective activity
The pulmonary protective effects of sophocarpine on acute lung injury (ALI) was evaluated in two studies. Han et al. used a mouse model of LPS-induced ALI and reported that sophocarpine treatment reduced the lung wet/dry ratio, pathological changes, and inflammatory responses (Han et al., 2006). The same results were obtained in another study carried out by Lu et al., who similarly evaluated the protective effect of sophocarpine in a mouse model change and additionally discovered sophocarpine decreased myeloperoxidase (MPO) activity and malondialdehyde (MDA) content. The underlying mechanism may be attributed to the modulation of TLR4 expression and NF-κB and MAPK activation (Lu et al., 2019). Patients suffering acute lung injury have high morbidity and mortality rates. Its pathogenesis is unclear, and treatment options are limited. Although the pulmonary protective effect of sophocarpine was confirmed, whether sophocarpine is still protective against other types of ALI, such as transfusion-, pulmonary ischemia/reperfusion injury-, or ventilator-induced ALI, would be interesting topics in the future.
Renal protective activity
It has been shown that in a mouse model of isoproterenol (ISO)-induced kidney injury, sophocarpine could alleviate kidney injury by reducing the serum levels of serum creatinine (SCr) and blood urea nitrogen (BUN), inhibiting inflammatory cytokine release, preventing fibrosis, and reducing apoptosis and oxidative stress. The TLR-4/NF-κB, TGF-β1/Smad3, and Nrf2/HO-1 signaling pathways may participate in sophocarpine-mediated renal protection (Zhou et al., 2022). Lupus nephritis is a complication of the autoimmune disease systemic lupus erythematosus (Anders et al., 2020). Sophocarpine effectively protected kidneys against lupus nephritis in MRL/lpr mice. It reduced the renal inflammatory response and inhibited NLRP3 inflammasome activation. The underlying mechanism may be associated with the IKKS-NF-κB pathway (Li et al., 2018). Although information regarding sophocarpine-induced renal protection is limited, these studies may shed light on novel therapeutic options for treating kidney disease with sophocarpine.
Sophocarpine and morphine withdrawal syndrome
The effect of Sophora alopecuroides var. alopecuroides seeds on morphine withdrawal syndrome was evaluated. The authors compared the pharmacological effects of the seed total extract, alkaloid fraction and major compound matrine to those of saline and methadone on mice with morphine withdrawal syndrome and found that all the abovementioned reagents decreased jumping and diarrhea. They further used gas chromatography‒mass spectrometry to analyze the main ingredients involved in the extraction of alkaloids and found that sophocarpine is one of the major constituents (Kianbakht and Hashem Dabaghian, 2016). The same group subsequently conducted a randomized, double-blind, and placebo-controlled clinical trial on abstinent opium addicts who took alkaloid components of S. alopecuroides extract capsules once daily for 8 consecutive days. The clinical opiate withdrawal scale (COWS) was used to assess withdrawal symptoms. Patients treated with extract capsules had lower COWS scores than did those in the placebo group. Moreover, it did not affect blood parameters. The extract may be a potential therapeutic agent for acute opioid withdrawal symptoms (Kianbakht et al., 2020). The utilization of traditional Chinese herbal extracts in the management of opiate withdrawal syndrome represents a pioneering and noteworthy area of research. Future investigations should further elucidate the potential underlying mechanisms of action of these compounds, as well as explore the therapeutic effects of specific compounds such as matrine and sophocarpine on opiate withdrawal syndrome. It is crucial to investigate whether these effects are associated with μ, κ, and δ opioid receptors or nicotinic receptors (Rahman et al., 2014). Furthermore, considering the global prevalence of methamphetamine addiction and alcohol dependence, it is worthwhile to further explore whether S. alopecuroides extract or sophocarpine could serve as treatment options for these conditions.
Sophocarpine and diabetes
Su et al. tested the antidiabetic effect of sophocarpine in a mouse model of streptozotocin (STZ)-induced type 2 diabetes. They found that sophocarpine regulated blood glucose, insulin, LDL-c content and HDL-c levels while concurrently mitigating pancreatic injury and effectively impeding the advancement of type 2 diabetes by attenuating peroxisome proliferator-activated receptor gamma (PPARγ) activity in mice with type 2 diabetes (Su et al., 2021). Although sophocarpine was shown to have beneficial effects in this study, further animal and human studies are needed to confirm and evaluate the role of sophocarpine in both type 1 and type 2 diabetes.
Sophocarpine and analgesia
Using different mouse models, such as carrageenan-induced rat hind paw edema, xylene-induced mouse ear edema and acetic acid-induced mouse vascular permeation, sophocarpine was found to possess analgesic and anti-inflammatory properties (Gao et al., 2009) and anti-inflammatory activities via the possible mechanism of affecting Ca2+ influx and inhibiting the secretion of inflammatory factors (Wang et al., 2021b). The effect of sophocarpine on neuropathic pain induced by chronic constriction injury was further evaluated by Jin et al. These authors showed that sophocarpine was effective at increasing the mechanical withdrawal threshold (MWT), thermal withdrawal latency (TWL), and tail-curling latency while reducing the cold withdrawal threshold (CWT) (Jin et al., 2019). The pharmacological effects and mechanism of action of sophocarpine are listed in Table 2.
Formulas containing of sophocarpine
In Chinese medicine, traditional herbal formulas consist of combinations of various herbs that contain multiple active ingredients that work synergistically to exert therapeutic effects. Among these complex formulas, such as the Kaihoujian recipe, Kangfuxiaoyan suppository, and Qingluoyin formula, sophocarpine has been identified as the principal active ingredient.
The Kaihoujian recipe originates from Miao traditional medicine in China and has a long history. It is composed of Shan Dou Gen (Sophorae Tonkinensis Radix et Rhizoma—Sophora tonkinensis Gagnep. [Fabaceae]), Ba Zhao Jin Long (Ardisia crispa [Thunb.] A.DC. [Primulaceae]), Chan Tui (Cicadae Periostracum—Cryptotympana pustulata Fabricius [Homoptera]), and Bo He Nao (Menthol—Mentha haplocalyx Briq. [Lamiaceae]). Kaihoujian spray, derived from the original formula of Kaihoujian, is primarily employed for the treatment of acute pharyngitis and acute tonsillitis; this spray results in direct mucous membrane absorption, yielding effective outcomes with minimal adverse effects and has gained extensive use. By employing a combination of gray correlation analysis and network pharmacology analysis, Chen et al. further substantiated sophocarpine as the principal active anti-inflammatory constituent in Kaihoujian spray. Kaihoujian spray effectively mitigated NO production in LPS-induced RAW264.7 cells (Chen et al., 2022a). Additionally, Pang’s research group demonstrated that the application of Kaihoujian spray can effectively reduce the count of white blood cells and the levels of inflammatory factors such as IL-1β and MCP-1 in the bloodstream. The therapeutic efficacy of this treatment for acute pharyngitis may be mediated through the PI3K-AKT, NF-κB, and Toll-like receptor signaling pathways (Pang et al., 2023).
Kangfuxiaoyan suppository, a traditional Chinese medicine, is primarily composed of Ku Shen (Radix Sophorae Flavescentis—Sophora flavescens Aiton [Fabaceae]), Chuan Xin Lian (Andrographis Herba—Andrographis paniculata [Burm.f.] Wall. ex Nees [Acanthaceae]), Zi Cao (Arnebiae Radix—Arnebia euchroma [Royle ex Benth.] I. M. Johnst. and Arnebia guttata Bunge [Boraginaceae]), Bai Jiang Cao (Herba Patriniae—Patrinia scabiosaefolia Fisch. and Patrinia villosa Juss. [Caprifoliaceae]), Pu Gong Ying (Taraxaci Herba—Taraxacum mongolicum Hand. -Mazz. and Taraxacum borealisinense Kitam. [Asteraceae]), Zi Hua Di Ding (Violae Herba—Viola yedoensis Makino [Violaceae]), Lu Hui (Aloe Vera—Aloe barbadensis Miller and Aloe ferox Miller [Asphodelaceae]), Zhu Dan Fen (Suis Fellis Pulvis—Sus scrofa domestica Brisson. [Suidae]). This formulation has been developed for for rectal administration and is marketed in China as a therapeutic option for chronic pelvic inflammatory disease. Zhang et al. conducted metabolomic and network pharmacology analysis to reveal the efficacy of Kangfuxiaoyan suppository in reversing the expression of uterine inflammation markers such as IL-1 and IL-6. Furthermore, it exhibited regulatory effects on pivotal targets such as ARG1, NOS2, NOS3, and its principal constituent sophocarpine, suggesting its potential role in mitigating the inflammatory response (Zhang et al., 2022). Although metabolomics and network pharmacology analysis have shown great potential, this formula needs further exploration in experimental models.
The herbal formula Qingluoyin, composed of Ku Shen (Radix Sophorae Flavescentis—Sophora flavescens Aiton [Fabaceae]), Qing Feng Teng (Sinomenii Caulis—Sinomenium acutum [Thunb.] Rehd et Wils. and S. acutum [Thunb.] Rehd et Wils. var. cinereum Rehd. et Wils [Menispermaceae]), Huang Bo (Phellodendri Chinensis Cortex—Phellodendron chinense C.K. Schneid. [Rutaceae]), Fen Bi Xie (Dioscoreae Hypoglaucae Rhizome—Dioscorea hypoglauca Palib. [Dioscoreaceae]), is a classic Chinese herbal formula with a clinical application history of more than 40 years, exhibits significant efficacy in treating hot syndrome-related rheumatoid arthritis. The major bioactive compound in Qingluoyin has been identified as sophocarpine, which primarily acts on rheumatoid arthritis by modulating T cells and monocytes through disruption of their interaction. This modulation is achieved by inhibiting the phosphorylation of JNK and p65, resulting in decreased expression of iNOS and IL-1β, thereby suppressing the production of inflammatory factors such as IL-6 and IL-1β (Wang et al., 2021a). Furthermore, Wang discovered that Qingluoyin exerts anti-inflammatory effects in rats with rheumatoid arthritis by upregulating PPAR-γ expression, thereby modulating monocyte/macrophage polarization and adipocyte differentiation (Wang et al., 2021c).
Similarly, the Suduxing formula is also a combination of traditional Chinese herbal medicines, that have been adapted from the potent anti-HIV drug Su-du injection. Liu’s research team discovered that sophocarpine, a major constituent of Suduxing, significantly reduces the levels of HBsAg, HBeAg, and HBV DNA. These findings demonstrated potent anti-HBV activity against both wild-type and entecavir-resistant strains of HBV. Additionally, the observed anti-HBV activity is likely attributed to pivotal molecules including CCNA2, ATF4, FAS, and CDKN1A (Liu et al., 2018).
Compound kushen injection, a traditional Chinese herbal formula, has received approval from the National Medical Products Administration (NMPA) for its efficacy in managing malignant tumor-related pain and bleeding, as well as alleviating chemotherapy-induced adverse reactions. Its mechanism of action involves targeting the transient receptor potential ion channel (TRPV1) pathway. It consists of two medicinal herbs—Ku Shen (Radix Sophorae Flavescentis—Sophora flavescens Aiton [Fabaceae]), Tu Fu Ling (Rhizoma Smilacis Glabrae—Smilax glabra Roxb. [Smilacaceae]). The main active ingredients include matrine, oxymatrine, sophocarpine, and oxysophocarpine. Current research increasingly demonstrates the remarkable antitumor effects of compound Kushen injection and its principal constituents, such as matrine, oxymatrine, sophocarpine, and oxysophocarpine (Gao et al., 2021; Yang et al., 2022). Yang et al. performed a clinical meta-analysis and reported that compound Kushen injection effectively relieved liver fibrosis and cirrhosis in hepatitis patients. Then, they used two preclinical animal models and found that Kushen injection (1, 2.5, 5.0, and 7.5 mL/kg) suppressed HSC activation, protecting the liver against hepatic fibrosis and hepatocarcinogenesis by targeting TGF-β/Smad signaling. The authors identified sophocarpine as one of the most potent antifibrotic ingredients in Ku-Shen (Yang et al., 2021b). However, it remains unclear whether other active ingredients, such as oxymatrine, matrine, and oxysophocarpine, synergistically interact with sophocarpine to exert their biological effects. Therefore, further investigations are warranted to elucidate these aspects.
The aforementioned traditional Chinese medicine formulas, which contain sophocarpine as the primary active ingredient, have exhibited diverse therapeutic effects (Table 3). However, further investigations are warranted to ascertain whether sophocarpine has the highest potency among these alkaloids. Moreover, in comparison to individual components, combinations of multiple ingredients may either augment or diminish pharmacological effects. Henceforth, it is imperative to explore the potential interactions between sophocarpine and other compounds along with their specific mechanisms of action in order to elucidate the underlying principles governing traditional Chinese herbal formulae and provide a theoretical foundation for subsequent drug development endeavors.
Association of sophocarpine with inflammatory cytokines, NF-κB signaling, and p38MAPK signaling
Sophocarpine regulates inflammatory cytokines
Inflammation is an immune response triggered by various harmful stimuli. A properly regulated inflammatory response can effectively shield the body against external stimuli, whereas an excessive inflammatory response can exacerbate the initial injury and consequently mediate a range of pathophysiological processes (Dinarello, 2010). Cytokines serve as crucial immune mediators that regulate inflammatory responses in diverse diseases and are primarily categorized into proinflammatory cytokines and anti-inflammatory cytokines. Among these cytokines, common proinflammatory cytokines, such as TNF-α, IL-6, and IL-1β, predominantly contribute to the progression of inflammation in diseases; conversely, anti-inflammatory cytokines, such as IL-10, IL-4, and transforming growth factor beta (TGF-β), exert opposing effects (Yahfoufi et al., 2018). The dynamic equilibrium between proinflammatory cytokines and anti-inflammatory cytokines constitutes a vital component for maintaining immune homeostasis within the body (Khatami et al., 2016). Sophocarpine has been demonstrated to modulate various proinflammatory and anti-inflammatory cytokines through multiple pathways, exerting significant biological effects. In the context of inflammatory diseases affecting the osteoarticular system (Zhu and Zhu, 2017; Wu et al., 2019) and colitis models (Wang et al., 2012; Zhang et al., 2015), sophocarpine primarily exerts its anti-inflammatory effects by downregulating the levels of key proinflammatory factors such as IL-6, TNF-α, IL-1β, and IL-12. However, in a mouse model of asthma investigated by Zhi et al., sophocarpine was found to mitigate lung injury by modulating the expression of IL-4, IL-5, and INF-γ (Zhi et al., 2021). Furthermore, in LPS-induced lung injury models, sophocarpine predominantly targets the regulation of IL-6, TNF-α, and IL-8 as major therapeutic targets (Han et al., 2006; Lu et al., 2019), demonstrating its multifaceted therapeutic potential. In addition, Sang et al. (Sang et al., 2017) demonstrated that sophocarpine exhibited antiviral and hepatoprotective effects in a Concanavalin A-induced hepatitis mouse model by reducing the levels of the proinflammatory cytokines IFN-γ and TNF-α. In nonviral liver diseases such as septic liver injury (Zhengyu et al., 2018; Hou et al., 2020) and nonalcoholic steatohepatitis (Song et al., 2011; Song et al., 2013), sophocarpine exerts its liver protective effects through the modulation of key inflammatory cytokines, including IL-6, TNF-α, TGF-β1, and IL-1β. In the field of cardiovascular disease, sophocarpine effectively modulates proinflammatory mediators, including TGF-β, IL-6, IL-1β, and TNF-α, and proinflammatory vascular adhesion molecules, such as VCAM-1 and ICAM-1. This regulation contributes to the therapeutic potential of sophocarpine in treating atherosclerosis (Fang et al., 2020) and restenosis after angioplasty (Yang et al., 2020; Yang et al., 2021a). Few studies have explored the impact of sophocarpine on tumors through its modulation of inflammatory cytokines. Nevertheless, evidence suggests that sophocarpine can mitigate cancer-induced cachexia by concurrently reducing the levels of TNF-α and IL-6 in RAW264.7 cells and macrophages (Zhang et al., 2008), indicating the possible involvement of inflammatory cytokines in the antitumor effects exerted by sophocarpine. Further investigations are warranted to elucidate the precise underlying mechanisms involved.
Sophocarpine and NF-κB signaling
Nuclear factor kappa B (NF-κB) is a transcription factor family that orchestrates inflammatory responses and governs diverse functions, including immunity, cell proliferation, and cell differentiation (Mitchell et al., 2016). The NF-κB pathway is involved in numerous diseases characterized by inflammation, including cancer and ischemia‒reperfusion injury in various organs (Guijarro and Egido, 2001; Hoesel and Schmid, 2013). Concurrently, an increasing body of research has demonstrated that herbal ingredients can elicit multiple biological effects, such as anti-inflammatory effects, antitumor activity, and organ protection, through modulation of the NF-κB signaling cascade (Ye et al., 2020; Dong et al., 2022; Lin et al., 2022). Currently, sophocarpine has emerged as a potent modulator that targets this pivotal NF-κB pathway to exert its therapeutic effects across diverse disease contexts. In the context of the nervous system, sophocarpine has been shown to enhance pain tolerance toward various stimuli in a mouse model by downregulating NF-κB phosphorylation levels, thereby exhibiting therapeutic potential for neuropathic pain (Jin et al., 2019). In terms of specific nerve cells, Zhu et al. demonstrated that sophocarpine intervention in a β-amyloid injury model established in PC12 cells can mitigate damage caused by β-amyloid through NF-κB nuclear translocation, further substantiating the association between sophocarpine and neuroprotection via the NF-κB pathway (Zhu et al., 2021). In addition to its neuroprotective effects, sophocarpine has been shown to have protective effects against septic liver injury (Zhengyu et al., 2018), nonalcoholic steatohepatitis (Song et al., 2015), and LPS-induced lung injury (Lu et al., 2019) through the inhibition of NF-κB and JNK-related pathways. This mechanism leads to a reduction in inflammatory factors within the body, alleviation of oxidative stress, and ultimately safeguards the liver and lungs from damage caused by relevant detrimental factors. In the context of kidney function, NF-κB serves as a pivotal pathway for sophocarpine in addressing isoproterenol (iso)-induced kidney injury (Zhou et al., 2022) while also exhibiting potential in managing autoimmune disorders such as lupus nephritis. By diminishing the production of anti-dsDNA antibodies and reducing immune complex deposition within renal tissues, NF-κB significantly contributes to the therapeutic efficacy of sophocarpine treatment (Li et al., 2018). Moreover, in the context of heart disease, sophocarpine not only ameliorates ischemia‒reperfusion injury resulting from postinterventional treatment for myocardial infarction (Li et al., 2011) but also exhibits a favorable mitigating effect on restenosis following revascularization. The therapeutic application of sophocarpine in these two conditions involves modulation of the NF-κB pathway and ultimately aims to reduce inflammatory mediators such as IL-6 and IL-1β (Yang et al., 2021a). In conclusion, NF-κB serves as a pivotal signaling molecule through which sophocarpine exerts multiorgan-protective and anti-inflammatory effects and combats autoimmune diseases. Additional investigations into the interplay between sophocarpine and NF-κB in alternative pathological states are warranted.
Sophocarpine and p38MAPK signaling
The mitogen-activated protein kinase (MAPK) signaling pathway plays a crucial role in regulating diverse biological processes within the human body, including cell survival, differentiation, proliferation, inflammation, and apoptosis (Pearson et al., 2001). Among the MAPKs identified in organisms, three prominent ones include signal-regulated kinase (ERK), c-Jun N-terminal kinase (JNK), and p38 kinase (Wei et al., 2021). Specifically, the p38MAPK signaling pathway, which is an integral member of the MAPK family consisting of four subtypes (P38α, P38β, P38γ, and P38δ), actively participates in essential mechanisms such as the regulation of inflammation, the induction of cellular apoptosis, and the modulation of autophagy (Han et al., 1994). Moreover, the p38MAPK signaling pathway is highly important for exploring the potential of traditional Chinese medicine extracts, and an increasing body of research has revealed promising associations between traditional Chinese medicine and this pathway (Chang and Xiong, 2020; Li et al., 2023), including sophocarpine. In a murine model of septic liver injury, sophocarpine effectively suppressed the p38/JNK signaling pathway, mitigated oxidative stress, and upregulated the expression of anti-inflammatory factors such as superoxide dismutase (SOD), catalase (CAT), and glutathione (GSH) to exert hepatoprotective effects (Zhengyu et al., 2018). In addition, sophocarpine is closely associated with the p38MAPK signaling pathway in cardiovascular diseases. Li et al. conducted a study on rats to investigate the effects of sophocarpine on myocardial ischemia‒reperfusion and reported a reduction in the phosphorylation of P38/JNK and decreased infiltration of central granulocytes, myeloperoxidase (MPO) activity, and infarct area (Li et al., 2011). Similarly, Liu et al. evaluated the impact of sophocarpine on endothelial apoptosis by isolating rat aortas and culturing rat aortic endothelial cells. They discovered that sophocarpine exerts antiapoptotic effects through p38MAPK/Nrf2 signaling by reducing ROS production, which is potentially regulated upstream by MKK3/6 (Liu et al., 2017b). Yang et al. obtained similar results in their intervention with sophocarpine in a rat restenosis after angioplasty model and observed a decrease in IL-1β and TNF-a levels involved in its vascular protective effect (Yang et al., 2020). Additionally, in their investigation of the therapeutic potential of sophocarpine against tumors, Liu et al. discovered that sophocarpine effectively hinders the maturation of miRNA-21 through the p38MAPK signaling pathway, thereby suppressing the proliferation, invasion, and migration of head and neck squamous cell carcinoma (HNSCC) (Liu et al., 2017a). Based on these findings, it can be inferred that while the precise relationship between sophocarpine and the p38MAPK signaling pathway remains unclear in other disease contexts, it is evident that this pathway plays a crucial role in mediating the biological effects of sophocarpine.
Pharmacokinetics
Pharmacokinetic research is crucial for the application and prospects of sophocarpine. After a single oral administration of 200 mg/kg of sophocarpine to fasting rabbits, the Cmax was 11.64 ± 1.28 mg L−1, the Tmax was 40.95 ± 8.35 min, and the AUC was 1,475.72 ± 326.5 mg·min/L, respectively (Bao-xin, 2009). While in another study, rapid intravenous injection of 10 mg/kg sophocarpine in rabbits resulted in rapid distribution in the body, with a half-life of 6.0 min, an elimination half-life of 82.6 min, and a pseudoequilibrium time of approximately 30 min. The mean residence time (MRT) was 106 min, and the mean volume of distribution was 2.992 L/kg. The drug was found to be distributed specifically, primarily in the peripheral compartment (Liu et al., 1987). When administered via tail vein injection to rats, sophocarpine exhibited widespread distribution in the body, with peak tissue concentrations observed within 5–20 min, and the highest levels found in the kidneys, followed by the liver, gastrointestinal tract, and lungs. Detectable levels in the brain and adipose tissues suggested penetration of the blood‒brain barrier (Liu and Huang, 1988). The plasma clearance rate was 9.15 ± 2.58 mL/min, with 32.0% ± 7.0% of the excreted sophocarpine remaining unchanged in the urine within 24 h, indicating a renal clearance fraction of 0.32 ± 0.07. The hepatic and renal clearance rates were 6.21 and 2.94 mL/min, respectively (Liu and Huang, 1988). Sophocarpine was found to inhibit the activity of CYP3A4 and 2C9 (Lin et al., 2015; Zhang et al., 2019), leading to significant alterations in the pharmacokinetic parameters of coadministered drugs such as umbralisib. However, Weng et al. reported that co-administration of umbralisib with sophocarpine led to a significant increase in the metabolism of umbralisib in rats, resulting in alterations to the pharmacokinetic parameters of umbralisib. Specifically, there were significant reductions in the area under the curve for plasma concentration from zero to last measurable plasma sample time (AUC0-t) and the area under the curve for plasma concentration from zero to infinity (AUC0-∞), as well as significant decreases in Cmax and Tmax (Weng et al., 2022b). These alterations cannot be attributed to inhibition of CYP3A4 and 2C9 activity, indicating a potential interaction between sophocarpine and umbralisib that warrants further investigation in future studies.
To improve the absorption and bioavailability of sophocarpine, one study utilized an exosome delivery system containing sophocarpine from Sophora extract, which enables enhanced penetration through the stratum corneum into deeper layers of the skin, resulting in significantly greater drug flux and absorption rate (Zhou et al., 2010b). Peng et al. discovered that the traditional Chinese medicinal formula, Kushen recipe, containing sophocarpine, exhibited limited permeability in aqueous solution but showed potential for treating skin-related conditions such as pruritus and eczema. They conducted a study to assess the transdermal parameters of the four main alkaloids (matrine, oxymatrine, sophocarpine and oxysophocarpine) in Kushen recipe. They observed that oxymatrine and oxysophocarpine exhibited strong polarity and poor lipid solubility, leading to low skin permeability. To address this issue, the authors employed techniques involving ethanol extraction and purification with absorbent resin to obtain purer alkaloids from Sophora flavescens Ait. These purified alkaloids were then combined with carbomer and essential oil to formulate a gel agent. The essential oil used was extracted from Schizonepeta tenuifolia Briq, containing main components such as menthone, menthol, and pulegone which enhanced the percutaneous efficacy of Sophora alkaloids. Ultimately, these methods resulted in improved permeability of Kushen recipe and significantly enhanced transdermal parameters of the four alkaloids in rat experiments after 48 h (Peng et al., 2007). Furthermore, a patented cocrystal compound has jointly improved the water solubility of rhein and sophocarpine, thereby enabling better absorption and more rapid attainment of effective drug blood concentration for enhanced therapeutic effects of the medication (Lv et al., 2024). While specific pharmacokinetic parameters were not explicitly mentioned in some articles, considering the various forms of entry of sophocarpine into the body, we have summarized the information about related parameters in the table below (Table 4).
Although sophocarpine can be absorbed in various forms to exert its pharmacological effects, current pharmacokinetic studies are predominantly conducted via intravenous administration, and the pharmacokinetic parameters for oral administration are still lacking and warrant further exploration. Additionally, like other drugs, sophocarpine requires further investigation to improve its in vivo efficacy; for instance, sophocarpine can be absorbed through the skin, thus exerting antipruritic and anti-inflammatory effects, and future efforts should aim to enhance its subcutaneous absorption, which is currently low. Furthermore, the insufficiency of clinical trials on sophocarpine in mammals and humans needs to be addressed to facilitate its clinical application.
Toxicity
Sophocarpine is a major ingredient in many traditional Chinese medicines. It has been widely used in China. However, the toxicity and safety profile of sophocarpine have not been well characterized to date. In general, it has been found that sophocarpine potentially induces neurotoxicity and cardiotoxicity (Figure 4). Lu et al. studied the developmental toxicity and neurotoxicity of sophocarpine in zebrafish embryos/larvae and reported that sophocarpine had neurotoxic effects with ED50 and LD50 values of 87.1 and 166 mg/L, respectively. It induces teratogenic and lethal effects on zebrafish embryos, alters spontaneous movement and inhibites swimming behavior (Lu et al., 2014). Moreover, using a cardio nonlabeled cell function analysis and culture system (Cardio-NLCS), Wang et al. showed that sophocarpine dose-dependently affects the impedance and extracellular field potential (EFP) of human-induced pluripotent stem cell-derived cardiomyocytes (hiPSC-CMs), suggesting that sophocarpine may have cardiotoxic effects. Furthermore, the mechanism was associated with the disturbance of calcium homeostasis and oxidative stress (Wang et al., 2019b).
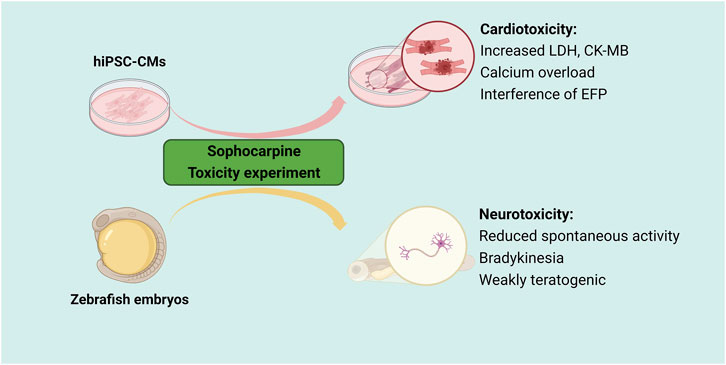
Figure 4. Neurotoxicity and cardiotoxicity of sophocarpine. When zebrafish embryos are exposed to sophocarpine, their behavioral assessments show a decrease in activity and coordination, along with abnormal movement and swimming performance. In cardiac muscle cells, the cardiotoxicity of sophocarpine is associated with disruptions in calcium homeostasis and oxidative stress, leading to pathological apoptosis. These findings collectively indicate that sophocarpine may have adverse effects on the nervous system and the heart.
In the future, toxicological research on sophocarpine must continue to occur to ensure its safety and efficacy in clinical applications. Although initial experiments have demonstrated its therapeutic potential, the chronic toxicity associated with long-term use, particularly its potential impact on the liver and kidneys, remains unclear. It will be necessary to further explore the dose‒response relationship of sophocarpine, and metabolic changes following prolonged exposure, and how this relationship affects specific biomarkers and molecular pathways.
Discussion and future prospects
Sophocarpine can be found in various types of traditional herbal medicines. It is a well-recognized matrine-type quinolizidine alkaloid with an established chemical structure. A large number of studies have confirmed its potential pharmacological benefits. This review systematically summarized the pharmacological activities of sophocarpine. Sophocarpine has beneficial effects on various cancer types, such as lung cancer, gastric cancer, colon cancer, cervical cancer, prostate cancer, liver cancer, myeloma, and head and neck cancer. The mechanism may involve signaling pathway inactivation or apoptosis inhibition. In terms of inflammation, sophocarpine has strong anti-inflammatory effects on pharyngitis, rheumatoid arthritis, osteoarthritis, colitis and toxicity-induced inflammatory responses. Sophocarpine has excellent antiviral effects on viral hepatitis, coxsackievirus, and enterovirus 71. Although sophocarpine has significant pharmacological effects, its clinical value has not been fully elucidated. Most of the related reports were observational rodent or cell culture studies, and clinical explorations of the pharmacological activities of these materials are not sufficient. Moreover, pharmacological parameters, such as dose range, minimum active concentration and duration still merit further exploration. Therefore, to promote the clinical application of sophocarpine, different human disease models or various clinical occasions may be needed to improve the clinical efficacy of sophocarpine therapy. Additionally, although sophocarpine has been proven to exert protective effects on multiple organs, it is still unclear whether it can be effective for treating other specific organ diseases. In the future, this protective effect will need to be further validated in various disease models. In addition to in vitro and ex vivo studies, positive or negative control experiments and experiments on large animals are needed due to differences between humans and rodents.
In terms of pharmacokinetics, it has been found in an article that the distribution of sophocarpine within the body of rabbits conforms to a two-compartment model (Liu et al., 1987). It has a short half-life and is primarily excreted through urine. After intravenous injection, it is widely distributed in various tissues within the body, and thereby has protective effects on multiple organs. However, the high accumulation of NGAL in renal tissues suggests the need for cautious consideration when administering this compound to patients with renal impairment (Liu and Huang, 1988). Currently, there is limited research on the metabolism and transformation of sophocarpine within the body, and further study is necessary to determine its pharmacokinetic characteristics and design different forms of biological preparations to enhance the activity and protective properties of sophocarpine. Additionally, studies have revealed that sophocarpine can alter the pharmacokinetic properties of the antitumor drug umbralisib in mice (Weng et al., 2022b), indicating the need for future research on herb-drug interactions involving sophocarpine to improve the concentration of corresponding drugs in the body or to prevent excessive accumulation.
Furthermore, the reported pharmacological studies regarding organ protection by sophocarpine have focused mainly on cardiovascular diseases. Scattered studies have indicated that sophocarpine may protect other vital organs, such as the liver, kidney, brain, and lung. There are different types of organ injury, such as ischemia/reperfusion injury, toxicity-induced acute organ injury, and transfusion-induced organ injury. Current studies have been conducted only on several specific types of injuries, and there are still large knowledge gaps that need to be filled in future studies.
A clinically feasible agent must have been tested in both clinical and toxicological studies. The promising safety and toxicity of the candidate agent have yet to be assessed. However, there are a limited number of studies on sophocarpine-induced toxicity in the current review. One study focused on developmental toxicity and neurotoxicity in zebrafish (Danio rerio) embryos/larvae (Lu et al., 2014), while another researcher studied cardiotoxic effects in human-induced pluripotent stem cell-derived cardiomyocytes (Wang et al., 2019b). Notably, other alkaloids, such as matrine and sophoridine, were reported to be hepatotoxic, to cause developmental toxicity, neurotoxicity and reproductive toxicity (Li et al., 2021; Wang et al., 2022b). Therefore, because of their toxicity, the therapeutic application of these alkaloids must be taken seriously. Unfortunately, it is currently unknown whether sophocarpine has toxic effects in vivo. In addition, there is no information regarding target organ toxicity across the species. Moreover, it would be interesting to determine whether combination medication would offer a more potent therapeutic effect while reducing toxicity and complications.
Although the pharmacological effect of sophocarpine was described in this review, current preclinical works have focused mainly on verifying the therapeutic effect of sophocarpine on various disease models or cell lines. Most of those studies were performed without sufficient mechanistic studies. Identification of the target molecular mechanism responsible for the pharmacological action will aid in the development of new therapeutic strategies. However, the target or mechanism of action is not clearly defined in most related studies. The lack of mechanistic insight, however, seems to be a common issue for sophocarpine-based studies. We believe that a better understanding of the mechanism underlying the pharmacological response is vital for promoting the clinical application of sophocarpine.
Author contributions
SW: Data curation, Formal Analysis, Investigation, Validation, Visualization, Writing–original draft, Writing–review and editing. JX: Data curation, Formal Analysis, Investigation, Validation, Visualization, Writing–original draft, Writing–review and editing. FJ: Data curation, Formal Analysis, Validation, Writing–review and editing. JL: Conceptualization, Methodology, Supervision, Writing–review and editing. ZH: Conceptualization, Data curation, Formal Analysis, Funding acquisition, Investigation, Methodology, Project administration, Supervision, Validation, Visualization, Writing–original draft, Writing–review and editing.
Funding
The author(s) declare that financial support was received for the research, authorship, and/or publication of this article. This work was supported by the Sichuan University West China Hospital setup funding to ZH.
Acknowledgments
Figures were created with BioRender.com.
Conflict of interest
The authors declare that the research was conducted in the absence of any commercial or financial relationships that could be construed as a potential conflict of interest.
Publisher’s note
All claims expressed in this article are solely those of the authors and do not necessarily represent those of their affiliated organizations, or those of the publisher, the editors and the reviewers. Any product that may be evaluated in this article, or claim that may be made by its manufacturer, is not guaranteed or endorsed by the publisher.
References
Anders, H. J., Saxena, R., Zhao, M. H., Parodis, I., Salmon, J. E., and Mohan, C. (2020). Lupus nephritis. Nat. Rev. Dis. Prim. 6, 7. doi:10.1038/s41572-019-0141-9
Atanasov, A. G., Zotchev, S. B., Dirsch, V. M., International Natural Product Sciences, T., and Supuran, C. T. (2021). Natural products in drug discovery: advances and opportunities. Nat. Rev. Drug Discov. 20, 200–216. doi:10.1038/s41573-020-00114-z
Bian, K., and Toda, N. (1988). Effects of sophoramine, an alkaloid from Sophora alopecuroides on isolated dog blood vessels. J. Ethnopharmacol. 24, 167–178. doi:10.1016/0378-8741(88)90148-1
Butler, A., Helliwell, M. V., Zhang, Y., Hancox, J. C., and Dempsey, C. E. (2019). An update on the structure of hERG. Front. Pharmacol. 10, 1572. doi:10.3389/fphar.2019.01572
Cely-Veloza, W., Kato, M. J., and Coy-Barrera, E. (2023). Quinolizidine-type alkaloids: chemodiversity, occurrence, and bioactivity. ACS Omega 8, 27862–27893. doi:10.1021/acsomega.3c02179
Chang, M. X., and Xiong, F. (2020). Astaxanthin and its effects in inflammatory responses and inflammation-associated diseases: recent advances and future directions. Mol. Basel, Switz. 25, 5342. doi:10.3390/molecules25225342
Chen, J. X., Shen, H. H., Niu, M., Guo, Y. M., Liu, X. Q., Han, Y. Z., et al. (2016). Anti-hepatitis B virus effect of matrine-type alkaloid and involvement of p38 mitogen-activated protein kinase and tumor necrosis factor receptor-associated factor 6. Virus Res. 215, 104–113. doi:10.1016/j.virusres.2015.12.005
Chen, J., Liu, Y., Gai, X., Ye, Q., Zhou, S., Tian, C., et al. (2022a). Studies on quality markers of kaihoujian spray for anti-inflammation based on gray correlation analysis strategy. Evid. Based Complement. Altern. Med. 2022, 1273066. doi:10.1155/2022/1273066
Chen, S., Ma, S., Wang, H., Shao, X., Ding, B., Guo, Z., et al. (2022b). Unraveling the mechanism of alkaloids from Sophora alopecuroides Linn combined with immune checkpoint blockade in the treatment of non-small cell lung cancer based on systems pharmacology. Bioorg Med. Chem. 64, 116724. doi:10.1016/j.bmc.2022.116724
Dinarello, C. A. (2010). Anti-inflammatory agents: present and future. Cell. 140, 935–950. doi:10.1016/j.cell.2010.02.043
Ding, P. L., Liao, Z. X., Huang, H., Zhou, P., and Chen, D. F. (2006). (+)-12alpha-Hydroxysophocarpine, a new quinolizidine alkaloid and related anti-HBV alkaloids from Sophora flavescens. Bioorg Med. Chem. Lett. 16, 1231–1235. doi:10.1016/j.bmcl.2005.11.073
Dong, X., Jiang, J., Lin, Z., Wen, R., Zou, L., Luo, T., et al. (2022). Nuanxinkang protects against ischemia/reperfusion-induced heart failure through regulating IKKβ/IκBα/NF-κB-mediated macrophage polarization. Phytomedicine Int. J. Phytotherapy Phytopharm. 101, 154093. doi:10.1016/j.phymed.2022.154093
Fang, H., Bo, T., Zi, X., Tan, D., Liu, X., Jiang, C., et al. (2020). Sophocarpine exert protective effect against ox-LDL-induced endothelial damage via regulating NF-κB signaling pathway. Biosci. Biotechnol. Biochem. 84, 2104–2112. doi:10.1080/09168451.2020.1787813
Fu, Y., Zhang, H. J., Zhou, W., Lai, Z. Q., and Dong, Y. F. (2023). The protective effects of sophocarpine on sepsis-induced cardiomyopathy. Eur. J. Pharmacol. 950, 175745. doi:10.1016/j.ejphar.2023.175745
Gajendran, M., Loganathan, P., Jimenez, G., Catinella, A. P., Ng, N., Umapathy, C., et al. (2019). A comprehensive review and update on ulcerative colitis. Dis. Mon. 65, 100851. doi:10.1016/j.disamonth.2019.02.004
Gao, L. M., Tang, S., Wang, Y. X., Gao, R. M., Zhang, X., Peng, Z. G., et al. (2013). Synthesis and biological evaluation of N-substituted sophocarpinic acid derivatives as coxsackievirus B3 inhibitors. ChemMedChem 8, 1545–1553. doi:10.1002/cmdc.201300224
Gao, Y., Hai, L., Kang, Y., Qin, W., Liu, F., Cai, R., et al. (2021). Compound kushen injection induces immediate hypersensitivity reaction through promoting the production of platelet-activating factor via de novo pathway. Front. Pharmacol. 12, 768643. doi:10.3389/fphar.2021.768643
Gao, Y., Jiang, W., Dong, C., Li, C., Fu, X., Min, L., et al. (2012). Anti-inflammatory effects of sophocarpine in LPS-induced RAW 264.7 cells via NF-κB and MAPKs signaling pathways. Toxicol Vitro 26, 1–6. doi:10.1016/j.tiv.2011.09.019
Gao, Y., Li, G., Li, C., Zhu, X., Li, M., Fu, C., et al. (2009). Anti-nociceptive and anti-inflammatory activity of sophocarpine. J. Ethnopharmacol. 125, 324–329. doi:10.1016/j.jep.2009.06.036
Guijarro, C., and Egido, J. (2001). Transcription factor-kappa B (NF-kappa B) and renal disease. Kidney Int. 59, 415–424. doi:10.1046/j.1523-1755.2001.059002415.x
Guo, L., Yao, H., Chen, W., Wang, X., Ye, P., Xu, Z., et al. (2022). Natural products of medicinal plants: biosynthesis and bioengineering in post-genomic era. Hortic. Res. 9, uhac223. doi:10.1093/hr/uhac223
Han, J., Lee, J. D., Bibbs, L., and Ulevitch, R. J. (1994). A MAP kinase targeted by endotoxin and hyperosmolarity in mammalian cells. Sci. (New York, N.Y.) 265, 808–811. doi:10.1126/science.7914033
Han, Y., Zhou, Y., and Liu, Q. (2006). Antiendotoxic effects of Sophora alopecuroides L. Zhong Yao Cai 29, 1066–1069.
He, L. J., Liu, J. S., Luo, D., Zheng, Y. R., Zhang, Y. B., Wang, G. C., et al. (2019). Quinolizidine alkaloids from Sophora tonkinensis and their anti-inflammatory activities. Fitoterapia 139, 104391. doi:10.1016/j.fitote.2019.104391
Hoesel, B., and Schmid, J. A. (2013). The complexity of NF-κB signaling in inflammation and cancer. Mol. Cancer 12, 86. doi:10.1186/1476-4598-12-86
Hou, N., Dai, X., Lu, W., Yang, H., Yu, H., Liu, J., et al. (2020). Sophocarpine attenuates septic liver injury through suppression of the NLRP3 inflammasome via autophagy-mediated degradation. Exp. Ther. Med. 20, 249. doi:10.3892/etm.2020.9379
Huang, M. Y., Zhang, L. L., Ding, J., and Lu, J. J. (2018). Anticancer drug discovery from Chinese medicinal herbs. Chin. Med. 13, 35. doi:10.1186/s13020-018-0192-y
Huang, Y. Q., Li, P. Y., Wang, J. B., Zhou, H. Q., Yang, Z. R., Yang, R. C., et al. (2016). Inhibition of sophocarpine on poly I: C/D-GalN-Induced immunological liver injury in mice. Front. Pharmacol. 7, 256. doi:10.3389/fphar.2016.00256
Huang, Y., Chen, X., Guo, G., Guo, W., Ma, Q., and Yuan, J. (2019). Sophocarpine inhibits the growth of gastric cancer cells via autophagy and apoptosis. Front. Biosci. Landmark Ed. 24, 616–627. doi:10.2741/4740
Hu, H., Wang, S., Zhang, C., Wang, L., Ding, L., Zhang, J., et al. (2010). Synthesis and in vitro inhibitory activity of matrine derivatives towards pro-inflammatory cytokines. Bioorg Med. Chem. Lett. 20, 7537–7539. doi:10.1016/j.bmcl.2010.09.075
Hu, Z., Li, J., Liu, Q., Manville, R. W., and Abbott, G. W. (2023). The plant-derived alkaloid aloperine prevents ischemia/reperfusion injury-induced sudden cardiac death. Faseb J. 37, e22999. doi:10.1096/fj.202300253R
Jiang, R., Fang, Z., Lai, Y., Li, L., Tan, J., Yu, C., et al. (2024). Sophocarpine alleviates intestinal fibrosis via inhibition of inflammation and fibroblast into myofibroblast transition by targeting the Sirt1/p65 signaling axis. Eur. J. Pharmacol. 967, 176318. doi:10.1016/j.ejphar.2024.176318
Jiang, W., Tang, M., Yang, L., Zhao, X., Gao, J., Jiao, Y., et al. (2022). Analgesic alkaloids derived from traditional Chinese medicine in pain management. Front. Pharmacol. 13, 851508. doi:10.3389/fphar.2022.851508
Jiao, Y.-F., Lu, M., Zhao, Y.-P., Liu, N., Niu, Y.-T., Niu, Y., et al. (2018). N-methylcytisine ameliorates dextran-sulfate-sodium-induced colitis in mice by inhibiting the inflammatory response. Mol. Basel, Switz. 23, 510. doi:10.3390/molecules23030510
Jin, S., Xu, S., Wang, R., Wang, T., Guo, K., Guo, L., et al. (2019). Sophocarpine attenuates chronic constriction sciatic nerve injury-induced neuropathic pain in mice by inhibiting the HMGB1/TLR4/NF-κB signaling pathway. Iran. Red Crescent Med. J. 21. doi:10.5812/ircmj.94716
Jin, Z., Yang, L., Ding, G., Yang, G., Han, Y., Zhang, X., et al. (2017). Sophocarpine against enterovirus 71 in vitro. Exp. Ther. Med. 14, 3792–3797. doi:10.3892/etm.2017.4958
Katsanos, K., Spiliopoulos, S., Kitrou, P., Krokidis, M., and Karnabatidis, D. (2018). Risk of death following application of paclitaxel-coated balloons and stents in the femoropopliteal artery of the leg: a systematic review and meta-analysis of randomized controlled trials. J. Am. Heart Assoc. 7, e011245. doi:10.1161/JAHA.118.011245
Katz, A. M. (1993). Cardiac ion channels. N. Engl. J. Med. 328, 1244–1251. doi:10.1056/NEJM199304293281707
Khatami, M., Wolff, K., Pohl, O., Ejtehadi, M. R., and Stark, H. (2016). Active Brownian particles and run-and-tumble particles separate inside a maze. Sci. Rep. 6, 37670. doi:10.1038/srep37670
Kianbakht, S., Hajiaghaee, R., and Akhondzadeh, S. (2020). Efficacy and safety of Sophora alopecuroides var. alopecuroides seed extract for opioid detoxification: a randomized, double-blind, and placebo-controlled clinical trial. Phytother. Res. 34, 1108–1113. doi:10.1002/ptr.6578
Kianbakht, S., and Hashem Dabaghian, F. (2016). Sophora alopecuroides L. var. alopecuroides alleviates morphine withdrawal syndrome in mice: involvement of alkaloid fraction and matrine. Iran. J. Basic Med. Sci. 19, 1090–1095.
Lan, X., Zhao, J., Zhang, Y., Chen, Y., Liu, Y., and Xu, F. (2020). Oxymatrine exerts organ- and tissue-protective effects by regulating inflammation, oxidative stress, apoptosis, and fibrosis: from bench to bedside. Pharmacol. Res. 151, 104541. doi:10.1016/j.phrs.2019.104541
Li, J. G., Yang, X. Y., and Huang, W. (2016). Total alkaloids of Sophora alopecuroides inhibit growth and induce apoptosis in human cervical tumor HeLa cells in vitro. Pharmacogn. Mag. 12, S253–S256. doi:10.4103/0973-1296.182157
Li, C., Gao, Y., Tian, J., Shen, J., Xing, Y., and Liu, Z. (2011). Sophocarpine administration preserves myocardial function from ischemia-reperfusion in rats via NF-κB inactivation. J. Ethnopharmacol. 135, 620–625. doi:10.1016/j.jep.2011.03.052
Li, G., Zhang, H., Lai, H., Liang, G., Huang, J., Zhao, F., et al. (2023). Erianin: a phytoestrogen with therapeutic potential. Front. Pharmacol. 14, 1197056. doi:10.3389/fphar.2023.1197056
Li, J., Li, L., Chu, H., Sun, X., and Ge, Z. (2014). Oral sophocarpine protects rat heart against pressure overload-induced cardiac fibrosis. Pharm. Biol. 52, 1045–1051. doi:10.3109/13880209.2013.877038
Lin, Z. X., Che, C. T., Lee, S. S., Chan, R. C., Ip, P. S., and Yang, J. M. (2015). Sophora flavescens (Ku-Shen) as a booster for antiretroviral therapy through cytochrome P450 3A4 inhibition. Hong Kong Med. J. 21 (Suppl. 7), S18–S21.
Lin, Y., He, F., Wu, L., Xu, Y., and du, Q. (2022). Matrine exerts pharmacological effects through multiple signaling pathways: a comprehensive review. Drug Des. Dev. Ther. 16, 533–569. doi:10.2147/DDDT.S349678
Lin, Z., Huang, C. F., Liu, X. S., and Jiang, J. (2011). In vitro anti-tumour activities of quinolizidine alkaloids derived from Sophora flavescens Ait. Basic Clin. Pharmacol. Toxicol. 108, 304–309. doi:10.1111/j.1742-7843.2010.00653.x
Liu, X. D., and Huang, S. K. (1988). Linear system analysis and physiological model in estimating disposition kinetics of sophocarpine in rats. Yao Xue Xue Bao 23, 481–489.
Liu, X. D., Huang, S. K., and Yuan, H. N. (1987). Determination of sophoramine and sophocarpine in rabbit plasma by capillary column gas chromatography and their pharmacokinetics. Zhongguo Yao Li Xue Bao 8, 474–477.
Liu, X. Q., Shen, H. H., Chen, J. X., Bai, Z. F., Wang, J. B., and Xiao, X. H. (2016). Thymopolypeptides combined with matrine type alkaloids suppress HBV replication. Zhongguo Zhong Yao Za Zhi 41, 1275–1281. doi:10.4268/cjcmm20160719
Liu, J., Xue, M., Huang, X., Wang, S., Jiang, Z., and Zhang, L. (2012). Pharmacokinetic of four alkaloids of Yanshu injection in Beagel dogs. Zhongguo Zhong Yao Za Zhi 37, 1845–1849. doi:10.4268/cjcmm20121232
Liu, W., Zhang, B., Chen, G., Wu, W., Zhou, L., Shi, Y., et al. (2017a). Targeting miR-21 with sophocarpine inhibits tumor progression and reverses epithelial-mesenchymal transition in head and neck cancer. Mol. Ther. 25, 2129–2139. doi:10.1016/j.ymthe.2017.05.008
Liu, Y., Yao, W., Si, L., Hou, J., Wang, J., Xu, Z., et al. (2018). Chinese herbal extract Su-duxing had potent inhibitory effects on both wild-type and entecavir-resistant hepatitis B virus (HBV) in vitro and effectively suppressed HBV replication in mouse model. Antivir. Res. 155, 39–47. doi:10.1016/j.antiviral.2018.04.017
Liu, Z., Lv, Y., Zhang, Y., Liu, F., Zhu, L., Pan, S., et al. (2017b). Matrine-type alkaloids inhibit advanced glycation end products induced reactive oxygen species-mediated apoptosis of aortic endothelial cells in vivo and in vitro by targeting MKK3 and p38MAPK signaling. J. Am. Heart Assoc. 6, e007441. doi:10.1161/JAHA.117.007441
Li, X., Tang, Z., Wen, L., Jiang, C., and Feng, Q. (2021). Matrine: a review of its pharmacology, pharmacokinetics, toxicity, clinical application and preparation researches. J. Ethnopharmacol. 269, 113682. doi:10.1016/j.jep.2020.113682
Li, X., Wang, M., Hong, H., Luo, C., Liu, Z., and Yang, R. (2018). Sophocarpine attenuates murine lupus nephritis via inhibiting NLRP3 inflammasome and NF-κB activation. Immunol. Res. 66, 521–527. doi:10.1007/s12026-018-9012-9
Li, Y., Wang, G., Liu, J., and Ouyang, L. (2020). Quinolizidine alkaloids derivatives from Sophora alopecuroides Linn: bioactivities, structure-activity relationships and preliminary molecular mechanisms. Eur. J. Med. Chem. 188, 111972. doi:10.1016/j.ejmech.2019.111972
Lu, Z. G., Li, M. H., Wang, J. S., Wei, D. D., Liu, Q. W., and Kong, L. Y. (2014). Developmental toxicity and neurotoxicity of two matrine-type alkaloids, matrine and sophocarpine, in zebrafish (Danio rerio) embryos/larvae. Reprod. Toxicol. 47, 33–41. doi:10.1016/j.reprotox.2014.05.015
Luo, H., Vong, C. T., Chen, H., Gao, Y., Lyu, P., Qiu, L., et al. (2019). Naturally occurring anti-cancer compounds: shining from Chinese herbal medicine. Chin. Med. 14, 48. doi:10.1186/s13020-019-0270-9
Luo, Y., Zhang, G., Liu, X., Yuan, M., Gao, Q., Gao, H., et al. (2018). Therapeutic and immunoregulatory effects of water-soluble alkaloids E2-a from Sophora moorcroftiana seeds as a novel potential agent against echinococcosis in experimentally protoscolex-infected mice. Vet. Res. 49, 100. doi:10.1186/s13567-018-0596-9
Lu, Y., Xu, D., Liu, J., and Gu, L. (2019). Protective effect of sophocarpine on lipopolysaccharide-induced acute lung injury in mice. Int. Immunopharmacol. 70, 180–186. doi:10.1016/j.intimp.2019.02.020
Lv, D. U., Yang, L. I. U., Wang, YANG, Song, ZHANG, and Yang, (2024). Eutectic crystal of rhein and sophocarpine, preparation method, composition and application thereof.
Manville, R. W., van der Horst, J., Redford, K. E., Katz, B. B., Jepps, T. A., and Abbott, G. W. (2019). KCNQ5 activation is a unifying molecular mechanism shared by genetically and culturally diverse botanical hypotensive folk medicines. Proc. Natl. Acad. Sci. U. S. A. 116, 21236–21245. doi:10.1073/pnas.1907511116
Ma, T., Shi, X., Ma, S., Ma, Z., and Zhang, X. (2020). Evaluation of physiological and biochemical effects of two Sophora alopecuroides alkaloids on pea aphids Acyrthosiphon pisum. Pest Manag. Sci. 76, 4000–4008. doi:10.1002/ps.5950
Mitchell, S., Vargas, J., and Hoffmann, A. (2016). Signaling via the NFκB system. Wiley Interdiscip. Rev. Syst. Biol. Med. 8, 227–241. doi:10.1002/wsbm.1331
Monteagudo, S., Cornelis, F. M. F., Wang, X., de Roover, A., Peeters, T., Quintiens, J., et al. (2022). ANP32A represses Wnt signaling across tissues thereby protecting against osteoarthritis and heart disease. Osteoarthr. Cartil. 30, 724–734. doi:10.1016/j.joca.2022.02.615
Nayak, G., Bhuyan, S. K., Bhuyan, R., Sahu, A., Kar, D., and Kuanar, A. (2022). Global emergence of Enterovirus 71: a systematic review. Beni Suef Univ. J. Basic Appl. Sci. 11, 78. doi:10.1186/s43088-022-00258-4
Omar, F., Tareq, A. M., Alqahtani, A. M., Dhama, K., Sayeed, M. A., Emran, T. B., et al. (2021). Plant-based indole alkaloids: a comprehensive overview from a pharmacological perspective. Molecules 26, 2297. doi:10.3390/molecules26082297
Pang, B., Zhao, R., Peng, B., Bao, L., Geng, Z., Li, S., et al. (2023). Pharmacological effects and mechanism of Kaihoujian Throat Spray (children's type) in the treatment of pediatric acute pharyngitis and tonsillitis. Heliyon 9, e17802. doi:10.1016/j.heliyon.2023.e17802
Pearson, G., Robinson, F., Beers Gibson, T., Xu, B. E., Karandikar, M., Berman, K., et al. (2001). Mitogen-activated protein (MAP) kinase pathways: regulation and physiological functions. Endocr. Rev. 22, 153–183. doi:10.1210/edrv.22.2.0428
Peng, C., Hu, J. H., Zhu, Q. G., Liu, J. Y., Qin, Z., and Wang, J. (2007). Studies on cutaneous permeation in vitro of Kushen recipe gel. Zhongguo Zhong Yao Za Zhi 32, 1870–1874.
Pink, R., Hudson, A., MourièS, M. A., and Bendig, M. (2005). Opportunities and challenges in antiparasitic drug discovery. Nat. Rev. Drug Discov. 4, 727–740. doi:10.1038/nrd1824
Poznyak, A. V., Nikiforov, N. G., Markin, A. M., Kashirskikh, D. A., Myasoedova, V. A., Gerasimova, E. V., et al. (2020). Overview of OxLDL and its impact on cardiovascular health: focus on atherosclerosis. Front. Pharmacol. 11, 613780. doi:10.3389/fphar.2020.613780
Qavi, H. B., Wyde, P. R., and Khan, M. A. (2002). In vitro Inhibition of HHV-6 replication by sophocarpines. Phytother. Res. 16, 154–156. doi:10.1002/ptr.949
Qi, Z. P., Shi, S. S., Zhao, X. L., Zhao, W. X., Bai, Y. L., Lu, Y. J., et al. (2008). Effect of sophocarpine on HERG K+ channels. Yao Xue Xue Bao 43, 44–49.
Qian, H., Shi, J., Fan, T. T., Lv, J., Chen, S. W., Song, C. Y., et al. (2014). Sophocarpine attenuates liver fibrosis by inhibiting the TLR4 signaling pathway in rats. World J. Gastroenterol. 20, 1822–1832. doi:10.3748/wjg.v20.i7.1822
Rahman, S., Engleman, E. A., and Bell, R. L. (2014). Nicotinic receptor modulation to treat alcohol and drug dependence. Front. Neurosci. 8, 426. doi:10.3389/fnins.2014.00426
Roth, G. A., Mensah, G. A., Johnson, C. O., Addolorato, G., Ammirati, E., Baddour, L. M., et al. (2020). Global burden of cardiovascular diseases and risk factors, 1990-2019: update from the GBD 2019 study. J. Am. Coll. Cardiol. 76, 2982–3021. doi:10.1016/j.jacc.2020.11.010
Saito, T., Fukai, A., Mabuchi, A., Ikeda, T., Yano, F., Ohba, S., et al. (2010). Transcriptional regulation of endochondral ossification by HIF-2alpha during skeletal growth and osteoarthritis development. Nat. Med. 16, 678–686. doi:10.1038/nm.2146
Sang, X. X., Wang, R. L., Zhang, C. E., Liu, S. J., Shen, H. H., Guo, Y. M., et al. (2017). Sophocarpine protects mice from ConA-induced hepatitis via inhibition of the IFN-Gamma/STAT1 pathway. Front. Pharmacol. 8, 140. doi:10.3389/fphar.2017.00140
Shan, X., Wang, J., Feng, H., Zhang, Z., Zheng, Q., Zhang, Q., et al. (2023). Aloperine protects pulmonary hypertension via triggering PPARγ signaling and inhibiting calcium regulatory pathway in pulmonary arterial smooth muscle cells. Am. J. Physiol. Cell. Physiol. 325, C1058–c1072. doi:10.1152/ajpcell.00286.2023
Song, C. Y., Shi, J., Zeng, X., Zhang, Y., Xie, W. F., and Chen, Y. X. (2013). Sophocarpine alleviates hepatocyte steatosis through activating AMPK signaling pathway. Toxicol Vitro 27, 1065–1071. doi:10.1016/j.tiv.2013.01.020
Song, C. Y., Zeng, X., Chen, S. W., Hu, P. F., Zheng, Z. W., Ning, B. F., et al. (2011). Sophocarpine alleviates non-alcoholic steatohepatitis in rats. J. Gastroenterol. Hepatol. 26, 765–774. doi:10.1111/j.1440-1746.2010.06561.x
Song, C. Y., Zeng, X., Wang, Y., Shi, J., Qian, H., Zhang, Y., et al. (2015). Sophocarpine attenuates toll-like receptor 4 in steatotic hepatocytes to suppress pro-inflammatory cytokines synthesis. J. Gastroenterol. Hepatol. 30, 405–412. doi:10.1111/jgh.12691
Sparks, J. A. (2019). Rheumatoid arthritis. Ann. Intern Med. 170, ITC1–ITC16. doi:10.7326/AITC201901010
Su, X., Miao, W., Li, L., Zheng, H., Hao, G., and du, L. (2021). Inhibition of type-2 diabetes mellitus development by sophocarpine through targeting PPARy-regulated gene expression. Dokl. Biochem. Biophys. 497, 137–143. doi:10.1134/S1607672921020150
Tian, Y., and Zhang, Z. (2023). Insecticidal activities of Sophora flavescens alt. Towards red imported fire ants (Solenopsis invicta buren). Toxins (Basel) 15, 105. doi:10.3390/toxins15020105
Vandenberg, J. I., Perry, M. D., Perrin, M. J., Mann, S. A., Ke, Y., and Hill, A. P. (2012). hERG K(+) channels: structure, function, and clinical significance. Physiol. Rev. 92, 1393–1478. doi:10.1152/physrev.00036.2011
Wang, D. D., Wu, X. Y., Dong, J. Y., Cheng, X. P., Gu, S. F., Olatunji, O. J., et al. (2021a). Qing-Luo-Yin alleviated experimental arthritis in rats by disrupting immune feedback between inflammatory T cells and monocytes: key evidences from its effects on immune cell phenotypes. J. Inflamm. Res. 14, 7467–7486. doi:10.2147/JIR.S346365
Wang, F. L., Wang, H., Wang, J. H., Wang, D. X., Gao, Y., Yang, B., et al. (2021b). Analgesic and anti-inflammatory activities of sophocarpine from Sophora viciifolia hance. Biomed. Res. Int. 2021, 8893563. doi:10.1155/2021/8893563
Wang, X. J., Deng, H. Z., Jiang, B., and Yao, H. (2012). The natural plant product sophocarpine ameliorates dextran sodium sulfate-induced colitis in mice by regulating cytokine balance. Int. J. Colorectal Dis. 27, 575–581. doi:10.1007/s00384-011-1352-z
Wang, H., Li, Y., Jiang, N., Chen, X., Zhang, Y., Zhang, K., et al. (2013). Protective effect of oxysophoridine on cerebral ischemia/reperfusion injury in mice. Neural Regen. Res. 8, 1349–1359. doi:10.3969/j.issn.1673-5374.2013.15.001
Wang, Q., Li, Y., Li, K.-W., and Zhou, C.-Z. (2022a). Sophoridine: a review of its pharmacology, pharmacokinetics and toxicity. Phytomedicine Int. J. Phytotherapy Phytopharm. 95, 153756. doi:10.1016/j.phymed.2021.153756
Wang, Q., Li, Y., Li, K. W., and Zhou, C. Z. (2022b). Sophoridine: a review of its pharmacology, pharmacokinetics and toxicity. Phytomedicine 95, 153756. doi:10.1016/j.phymed.2021.153756
Wang, Q., Wang, T., Zhu, L., He, N., Duan, C., Deng, W., et al. (2019a). Sophocarpine inhibits tumorgenesis of colorectal cancer via downregulation of MEK/ERK/VEGF pathway. Biol. Pharm. Bull. 42, 1830–1838. doi:10.1248/bpb.b19-00353
Wang, Q., Xu, J., Li, X., Zhang, D., Han, Y., and Zhang, X. (2017). Comprehensive two-dimensional PC-3 prostate cancer cell membrane chromatography for screening anti-tumor components from Radix Sophorae flavescentis. J. Sep. Sci. 40, 2688–2693. doi:10.1002/jssc.201700208
Wang, R., Li, D. F., Hu, Y. F., Liao, Q., Jiang, T. T., Olatunji, O. J., et al. (2021c). Qing-Luo-Yin alleviated monocytes/macrophages-mediated inflammation in rats with adjuvant-induced arthritis by disrupting their interaction with (Pre)-Adipocytes through PPAR-γ signaling. Drug Des. Devel Ther. 15, 3105–3118. doi:10.2147/DDDT.S320599
Wang, R., Wang, M., Wang, S., Yang, K., Zhou, P., Xie, X., et al. (2019b). An integrated characterization of contractile, electrophysiological, and structural cardiotoxicity of Sophora tonkinensis Gapnep. in human pluripotent stem cell-derived cardiomyocytes. Stem Cell. Res. Ther. 10, 20. doi:10.1186/s13287-018-1126-4
Wang, X., Yang, J., Huang, P., Wang, D., Zhang, Z., Zhou, Z., et al. (2024). Cytisine: state of the art in pharmacological activities and pharmacokinetics. Biomed. Pharmacother. = Biomedecine Pharmacother. 171, 116210. doi:10.1016/j.biopha.2024.116210
Wang, Y., Han, M., Liu, S., Yuan, X., Zhao, J., Lu, H., et al. (2021d). S6K1 inhibits HBV replication through inhibiting AMPK-ULK1 pathway and disrupting acetylation modification of H3K27. Life Sci. 265, 118848. doi:10.1016/j.lfs.2020.118848
Wang, Y., Li, W., Huang, F., Wu, X., Chen, W., Dong, M., et al. (2021e). Synthesis of sophocarpine triflorohydrazone and its proliferation inhibition and apoptosis induction activity in myeloma cells through Notch3-p53 signaling activation. Environ. Toxicol. 36, 484–490. doi:10.1002/tox.23053
Wei, J., Liu, R., Hu, X., Liang, T., Zhou, Z., and Huang, Z. (2021). MAPK signaling pathway-targeted marine compounds in cancer therapy. J. Cancer Res. Clin. Oncol. 147, 3–22. doi:10.1007/s00432-020-03460-y
Weng, M., Shi, C., Han, H., Zhu, H., Xiao, Y., Guo, H., et al. (2022a). Sophocarpine inhibits tumor progression by antagonizing the PI3K/AKT/mTOR signaling pathway in castration-resistant prostate cancer. PeerJ 10, e14042. doi:10.7717/peerj.14042
Weng, Q., Lan, X., Wang, Y., Fan, C., Xu, R. A., and Zhang, P. (2022b). Effect of sophocarpine on the pharmacokinetics of umbralisib in rat plasma using a novel UPLC-MS/MS method. Front. Pharmacol. 13, 749095. doi:10.3389/fphar.2022.749095
Wu, D., Zhu, X., Kang, X., Huang, H., Yu, J., Pan, J., et al. (2019). The protective effect of sophocarpine in osteoarthritis: an in vitro and in vivo study. Int. Immunopharmacol. 67, 145–151. doi:10.1016/j.intimp.2018.11.046
Yahfoufi, N., Alsadi, N., Jambi, M., and Matar, C. (2018). The immunomodulatory and anti-inflammatory role of polyphenols. Nutrients 10, 1618. doi:10.3390/nu10111618
Yang, Y. S., Wen, D., and Zhao, X. F. (2021c). Sophocarpine can enhance the inhibiting effect of oxaliplatin on colon cancer liver metastasis-in vitro and in vivo. Naunyn Schmiedeb. Arch. Pharmacol. 394, 1263–1274. doi:10.1007/s00210-020-02032-8
Yang, Z. F., Li, C. Z., Wang, W., Chen, Y. M., Zhang, Y., Liu, Y. M., et al. (2011). Electrophysiological mechanisms of sophocarpine as a potential antiarrhythmic agent. Acta Pharmacol. Sin. 32, 311–320. doi:10.1038/aps.2010.207
Yang, G., Zeng, R., Song, X., Li, T., Liu, C., and Ni, L. (2020). Sophocarpine prevents cigarette smoke-induced restenosis in rat carotid arteries after angioplasty. Ann. Palliat. Med. 9, 1622–1630. doi:10.21037/apm-19-568
Yang, G., Zeng, R., Song, X., Liu, C., and Ni, L. (2021a). Sophocarpine alleviates injury-induced intima hyperplasia of carotid arteries by suppressing inflammation in a rat model. J. Clin. Med. 10, 5449. doi:10.3390/jcm10225449
Yang, Y., Lu, Y., Pei, T., Guo, B., Li, J., Wang, H., et al. (2022). Compound kushen injection in cancer treatments: efficacy, active ingredients, and mechanisms. Pharmacol. Res. - Mod. Chin. Med. 3, 100108. doi:10.1016/j.prmcm.2022.100108
Yang, Y., Sun, M., Li, W., Liu, C., Jiang, Z., Gu, P., et al. (2021b). Rebalancing TGF-β/Smad7 signaling via Compound kushen injection in hepatic stellate cells protects against liver fibrosis and hepatocarcinogenesis. Clin. Transl. Med. 11, e410. doi:10.1002/ctm2.410
Ye, J. Y., Hao, Q., Zong, Y., Shen, Y., Zhang, Z., and Ma, C. (2021). Sophocarpine attenuates cognitive impairment and promotes neurogenesis in a mouse model of Alzheimer's disease. Neuroimmunomodulation 28, 166–177. doi:10.1159/000508655
Ye, Y., Wang, Y., Yang, Y., and Tao, L. (2020). Aloperine suppresses LPS-induced macrophage activation through inhibiting the TLR4/NF-κB pathway. Inflamm. Res. Official J. Eur. Histamine Res. Soc. 69, 375–383. doi:10.1007/s00011-019-01313-0
Yifeng, M., Bin, W., Weiqiao, Z., Yongming, Q., Bing, L., and Xiaojie, L. (2011). Neuroprotective effect of sophocarpine against transient focal cerebral ischemia via down-regulation of the acid-sensing ion channel 1 in rats. Brain Res. 1382, 245–251. doi:10.1016/j.brainres.2011.01.004
Zeng, J., Wu, D., Hu, H., Young, J. A. T., Yan, Z., and Gao, L. (2020). Activation of the liver X receptor pathway inhibits HBV replication in primary human hepatocytes. Hepatology 72, 1935–1948. doi:10.1002/hep.31217
Zhang, H. J., Fu, Y., Zhang, H., Lai, Z. Q., and Dong, Y. F. (2024). Sophocarpine alleviates doxorubicin-induced heart injury by suppressing oxidative stress and apoptosis. Sci. Rep. 14, 428. doi:10.1038/s41598-023-51083-3
Zhang, H.-X., and Zhang, X. (2022). Network pharmacology and experimental validation identify the potential mechanism of sophocarpine for COVID-19. J. Med. Microbiol. 71. doi:10.1099/jmm.0.001538
Zhang, J. M., Zhu, Y. B., Deng, X., Wang, C. X., Luan, S. M., and Chen, Y. X. (2015). Mechanism of sophocarpine in treating experimental colitis in mice. Zhongguo Zhong Yao Za Zhi 40, 3081–3087.
Zhang, P. P., Wang, P. Q., Qiao, C. P., Zhang, Q., Zhang, J. P., Chen, F., et al. (2016). Differentiation therapy of hepatocellular carcinoma by inhibiting the activity of AKT/GSK-3β/β-catenin axis and TGF-β induced EMT with sophocarpine. Cancer Lett. 376, 95–103. doi:10.1016/j.canlet.2016.01.011
Zhang, H., Chen, L., Sun, X., Yang, Q., Wan, L., and Guo, C. (2020). Matrine: a promising natural product with various pharmacological activities. Front. Pharmacol. 11, 588. doi:10.3389/fphar.2020.00588
Zhang, J., Li, C., Zhang, J., and Zhang, F. (2019). In vitro inhibitory effects of sophocarpine on human liver cytochrome P450 enzymes. Xenobiotica 49, 1127–1132. doi:10.1080/00498254.2018.1468047
Zhang, S., Ma, J. H., Zhang, P. H., Luo, A. T., Ren, Z. Q., and Kong, L. H. (2012). Sophocarpine attenuates the Na(+)-dependent Ca2(+) overload induced by Anemonia sulcata toxin-increased late sodium current in rabbit ventricular myocytes. J. Cardiovasc Pharmacol. 60, 357–366. doi:10.1097/FJC.0b013e318262c932
Zhang, Y., Wang, S., Li, Y., Xiao, Z., Hu, Z., and Zhang, J. (2008). Sophocarpine and matrine inhibit the production of TNF-alpha and IL-6 in murine macrophages and prevent cachexia-related symptoms induced by colon26 adenocarcinoma in mice. Int. Immunopharmacol. 8, 1767–1772. doi:10.1016/j.intimp.2008.08.008
Zhang, Z., Xie, Z., Lv, S., Shi, Y., Zhai, C., Li, X., et al. (2022). Integrated metabolomics and network pharmacology study on the mechanism of kangfuxiaoyan suppository for treating chronic pelvic inflammatory disease. Front. Pharmacol. 13, 812587. doi:10.3389/fphar.2022.812587
Zhao, X. L., Gu, D. F., Qi, Z. P., Chen, M. H., Wei, T., Li, B. X., et al. (2009). Comparative effects of sophocarpine and sophoridine on hERG K+ channel. Eur. J. Pharmacol. 607, 15–22. doi:10.1016/j.ejphar.2009.02.013
Zhao, X. L., Qi, Z. P., Fang, C., Chen, M. H., Lv, Y. J., Li, B. X., et al. (2008). HERG K+ channel blockade by the novel antiviral drug sophocarpine. Biol. Pharm. Bull. 31, 627–632. doi:10.1248/bpb.31.627
Zhao, J., Xu, G., Hou, X., Mu, W., Yang, H., Shi, W., et al. (2023). Schisandrin C enhances cGAS-STING pathway activation and inhibits HBV replication. J. Ethnopharmacol. 311, 116427. doi:10.1016/j.jep.2023.116427
Zheng, J., Zheng, P., Zhou, X., Yan, L., Zhou, R., Fu, X. Y., et al. (2009). Relaxant effects of matrine on aortic smooth muscles of Guinea pigs. Biomed. Environ. Sci. 22, 327–332. doi:10.1016/S0895-3988(09)60063-5
Zhengyu, J., Yan, M., Lulong, B., Changli, W., Jinjun, B., and Xiaoming, D. (2018). Sophocarpine attenuates LPS-induced liver injury and improves survival of mice through suppressing oxidative stress, inflammation, and apoptosis. Mediat. Inflamm. 2018, 5871431. doi:10.1155/2018/5871431
Zhi, W., du, X., Li, Y., Wang, C., Sun, T., Zong, S., et al. (2021). Proteome profiling reveals the efficacy and targets of sophocarpine against asthma. Int. Immunopharmacol. 96, 107348. doi:10.1016/j.intimp.2020.107348
Zhou, C. H., Shi, Z. L., Meng, J. H., Hu, B., Zhao, C. C., Yang, Y. T., et al. (2018). Sophocarpine attenuates wear particle-induced implant loosening by inhibiting osteoclastogenesis and bone resorption via suppression of the NF-κB signalling pathway in a rat model. Br. J. Pharmacol. 175, 859–876. doi:10.1111/bph.14092
Zhou, H., Li, J., Sun, F., Wang, F., Li, M., Dong, Y., et al. (2020). A review on recent advances in aloperine research: pharmacological activities and underlying biological mechanisms. Front. Pharmacol. 11, 538137. doi:10.3389/fphar.2020.538137
Zhou, W., Fu, Y., and Xu, J.-S. (2022). Sophocarpine alleviates isoproterenol-induced kidney injury by suppressing inflammation, apoptosis, oxidative stress and fibrosis. Molecules 27, 7868. doi:10.3390/molecules27227868
Zhou, Y., Wang, H., Liang, L., Zhao, W. C., Chen, Y., and Deng, H. Z. (2010a). Total alkaloids of Sophora alopecuroides increases the expression of CD4+ CD25+ Tregs and IL-10 in rats with experimental colitis. Am. J. Chin. Med. 38, 265–277. doi:10.1142/S0192415X1000783X
Zhou, Y., Wei, Y., Liu, H., Zhang, G., and Wu, X. (2010b). Preparation and in vitro evaluation of ethosomal total alkaloids of Sophora alopecuroides loaded by a transmembrane pH-gradient method. AAPS PharmSciTech 11, 1350–1358. doi:10.1208/s12249-010-9509-6
Zhu, P. J., Liu, Y. C., Li, Z. Y., and Yu, D. Z. (1989). Electrophysiologic effects of sophocarpine on papillary muscle in Guinea pig. Zhongguo Yao Li Xue Bao 10, 227–229.
Zhu, L., and Zhu, L. (2017). Sophocarpine suppress inflammatory response in human fibroblast-like synoviocytes and in mice with collagen-induced arthritis. Eur. Cytokine Netw. 28, 120–126. doi:10.1684/ecn.2017.0400
Keywords: sophocarpine, pharmacology, pharmacokinetics, toxicity, review
Citation: Wei S, Xiao J, Ju F, Liu J and Hu Z (2024) A review on the pharmacology, pharmacokinetics and toxicity of sophocarpine. Front. Pharmacol. 15:1353234. doi: 10.3389/fphar.2024.1353234
Received: 10 December 2023; Accepted: 10 April 2024;
Published: 29 April 2024.
Edited by:
Tsong-Long Hwang, Chang Gung University of Science and Technology, TaiwanReviewed by:
Zhenglong Liu, Mayo Clinic, United StatesSutapa Biswas Majee, NSHM Knowledge Campus, India
Copyright © 2024 Wei, Xiao, Ju, Liu and Hu. This is an open-access article distributed under the terms of the Creative Commons Attribution License (CC BY). The use, distribution or reproduction in other forums is permitted, provided the original author(s) and the copyright owner(s) are credited and that the original publication in this journal is cited, in accordance with accepted academic practice. No use, distribution or reproduction is permitted which does not comply with these terms.
*Correspondence: Zhaoyang Hu, enlodUBob3RtYWlsLmNvbQ==