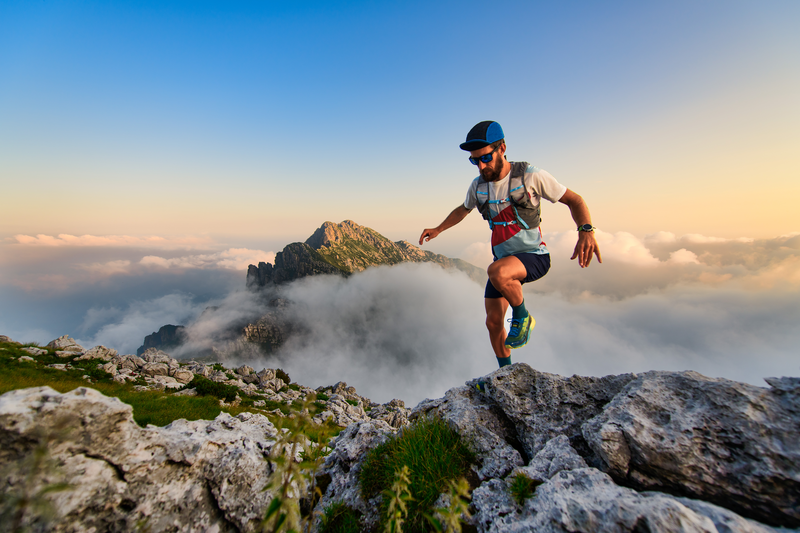
94% of researchers rate our articles as excellent or good
Learn more about the work of our research integrity team to safeguard the quality of each article we publish.
Find out more
ORIGINAL RESEARCH article
Front. Pharmacol. , 19 February 2024
Sec. Renal Pharmacology
Volume 15 - 2024 | https://doi.org/10.3389/fphar.2024.1353029
Introduction: Nephrotoxicity represents a major complication of using doxorubicin (DOX) in the management of several types of cancers. Increased oxidative stress and the activation of inflammatory mediators play outstanding roles in the development of DOX-induced kidney damage. This study aimed to investigate whether the two pathways of incretin-based therapy, glucagon-like peptide-1 receptor agonist (presented as semaglutide, SEM) and dipeptidyl peptidase-4 inhibitor (presented as alogliptin, ALO), differentially protect against DOX-induced nephrotoxicity in rats and to clarify the underlying molecular mechanisms.
Methods: Adult male rats were divided into six groups: control (received the vehicle), DOX (20 mg/kg, single I.P. on day 8), DOX + ALO (20 mg/kg/day, P.O. for 10 days), DOX + SEM (12 μg/kg/day, S.C. for 10 days), ALO-alone, and SEM-alone groups. At the end of the study, the animals were sacrificed and their kidney functions, oxidative stress, and inflammatory markers were assessed. Kidney sections were also subjected to histopathological examinations.
Results: The co-treatment with either ALO or SEM manifested an improvement in the kidney functions, as evidenced by lower serum concentrations of creatinine, urea, and cystatin C compared to the DOX group. Lower levels of MDA, higher levels of GSH, and increased SOD activity were observed in either ALO- or SEM-treated groups than those observed in the DOX group. DOX administration resulted in decreased renal expressions of sirtuin 1 (SIRT1) and Nrf2 with increased NF-κB and TNF-α expressions, and these effects were ameliorated by treatment with either ALO or SEM.
Discussion: Co-treatment with either ALO or SEM showed a renoprotective effect that was mediated by their antioxidant and anti-inflammatory effects via the SIRT1/Nrf2/NF-κB/TNF-α pathway. The fact that both pathways of the incretin-based therapy demonstrate an equally positive effect in alleviating DOX-induced renal damage is equally noteworthy.
The number of cancer patients worldwide is rapidly increasing as it was reported that more than 19 million people have been diagnosed with cancer in 2020 (Ferlay et al., 2021; Global cancer Observatory, 2023). Doxorubicin (DOX) is an anthracycline anticancer agent which is widely used in clinical practice. It treats a variety of tumors, including breast cancer, lung cancer, prostate cancer, liver cancer, Kaposi’s sarcoma, osteosarcomas, and hematological malignancies, such as leukemia and lymphomas (Carvalho et al., 2009; Alghorabi et al., 2019). Nevertheless, DOX’s clinical application is linked to dose-dependent adverse effects on the kidney, heart, and blood, posing a significant clinical challenge that could curtail its effectiveness. It was reported that DOX can cause nephrotic syndrome and acute renal damage (with an incidence ratio of 4.5%) that may persist after drug discontinuation. It can damage the glomeruli and the tubules in addition to causing interstitial inflammation and fibrosis (Carvalho, et al., 2009; Carron et al., 2014; Yemm et al., 2019; Chang et al., 2023). The exact mechanism underlying DOX-induced nephropathy is not fully established. However, many studies reported that oxidative stress, mitochondrial dysfunction, and inflammation are involved in DOX-induced renal damage (Rashid et al., 2013).
Incretin-based therapies, such as glucagon-like peptide-1 receptor agonists (GLP-1RAs) and dipeptidyl peptidase-4 (DPP-4) inhibitors, have become crucial in the treatment of type 2 diabetes mellitus. However, recent studies have revealed the pleiotropic effects of incretin-based therapies beyond the glycemic control, which includes antioxidant, anti-inflammatory, and immunomodulatory effects (Radbakhsh et al., 2021; Yaribeygi et al., 2021). Furthermore, these treatments have displayed nephroprotective properties in diabetic patients (Vaghasiya et al., 2011; Muskiet et al., 2014; Esaki et al., 2017; Yaribeygi et al., 2021; Youssef et al., 2021). Additionally, their beneficial effects on kidney protection extend beyond diabetic models, as demonstrated by their ability to mitigate cisplatin-induced nephropathy (Katagiri et al., 2013; Iwakura et al., 2020) in various experimental contexts. This study aimed to explore the potential protective effects of semaglutide, a GLP-1RA, and alogliptin, a DPP-4 inhibitor, against DOX-induced nephrotoxicity. Additionally, it sought to elucidate the signaling pathways that might be responsible for these observed effects.
DOX was obtained from Novartis, Switzerland. Alogliptin was obtained from Hikma Pharmaceuticals, London, United Kingdom. Semaglutide was obtained from Novo Nordisk, Denmark. Carboxymethyl cellulose sodium salt (CMC) was purchased from Oxford Lab Chem, India.
Adult male Wistar rats (160–220 g) were obtained from the Helwan Farm belonging to VACSERA Co., Cairo, Egypt. The animals were exposed to dark/light cycles of 12 h with free access to food and water and were kept for 2 weeks for acclimatization. All the experiments have been approved by the Research Ethics Committee for the laboratory animal’s care and use, Faculty of Pharmacy, Minia University, under the approval number: MPEC (230105).
The rats were randomly divided into six groups (n = 6, each), as summarized in Figure 1.
1-Control group: The rats were subcutaneously injected with normal saline and received 0.5% w/v carboxymethyl cellulose, the vehicle for alogliptin orally for 10 days. They were injected with saline I.P. on day 8.
2-Alogliptin (ALO) group: The rats received alogliptin (20 mg/kg/day, P.O.) suspended in 0.5% CMC for 10 days and normal saline I.P. on day 8.
3-Semaglutide (SEM) group: The rats received semaglutide (12 μg/kg/day, S.C.) for 10 days and normal saline I.P. on day 8.
4-Doxorubicin (DOX) group: The rats were given a single dose of DOX (20 mg/kg/day, I.P.) on day 8 and received the vehicles of semaglutide and alogliptin for 10 days.
5-Doxorubicin and alogliptin group (DOX + ALO): The rats received alogliptin (20 mg/kg/day, P.O.) for 10 days and DOX (20 mg/kg/day, I.P.) on day 8.
6-Doxorubicin and semaglutide group (DOX + SEM): The rats received semaglutide (12 μg/kg/day, S.C.) for 10 days and DOX (20 mg/kg/day, I.P.) on day 8.
The DOX, ALO, and SEM dosing regimen utilized in this study has been employed by other investigators (Kabel 2018; Qian et al., 2020; Al-Kuraishy et al., 2022) and also in the pilot experimental trials of the present study.
Twenty-four hours after the last dose, the rats were anesthetized using (1 g/kg) urethane by intraperitoneal injection after measuring the body weight, and then, blood samples were collected by decapitation and centrifuged at 3,500 rpm for 10 min for the separation of the serum. Fresh serum samples were used for the assessment of kidney function tests (creatinine and urea concentration). Other serum samples were stored at −80°C for the assessment of the cystatin C concentration. The kidneys were rapidly excised and washed in cold saline. The separated kidneys were dried on filter paper and weighed, and the kidney index was calculated according to the following equation:
Sections of the left kidney of each group were preserved in 10% formalin, embedded in paraffin blocks, and then sectioned into 4–6-μm-thick sections and stained with hematoxylin and eosin (H&E) for histopathological evaluations, according to Bancroft and Gamble (2008).
The rest of the kidneys were directly frozen in liquid nitrogen and kept at −80°C until the time of the analysis. Kidney tissues were homogenized in cold PBS and centrifuged at 10,000 rpm for 10 min, and the supernatant was used for biochemical analyses.
Serum levels of creatinine, urea, and cystatin C were used to evaluate the nephrotoxicity induced by DOX. Creatinine and urea concentrations were assessed spectrophotometrically using commercially available kits (Biodiagnostic, Cairo, Egypt), while the cystatin C concentration was measured using the commercially available ELISA Kit from Elabscience Biotechnology Co., Ltd., Wuhan, China; catalog no. E-EL-R0304.
The concentration of malondialdehyde, which is a product of lipid peroxidation, was used as a marker of oxidative stress. It was measured using the TBARS method described by Buege and Aust (1978). In addition, reduced glutathione and superoxide dismutase were also measured as antioxidant markers. Reduced glutathione was measured using the method described by Ellman (1959), while SOD activity was measured according to the method described by Marklund (1985).
Kidney tissues were homogenized in ice-cold Tris buffer and the protease inhibitor cocktail (Biospes, China) at 4°C for 30 min using the method described by Ali et al. (2018) and then centrifuged at 10,000 × g for 10 min. The total protein concentrations were measured according to the Biuret method (WANG et al., 1996). A measure of 30 μg of protein was loaded in each lane and resolved by 10% SDS-polyacrylamide gel electrophoresis and then transferred to the PVDF membrane (Millipore, Merck, United States) using semi-dry transfer methods (Towbin et al., 1979). Five percent non-fat milk in the TBST buffer was used as a blocking solution for 1 h at room temperature, and then, the membrane was incubated overnight with primary antibodies at 4°C. The antibodies used were anti- NF-kB p65 (1:500), anti-Nrf2 (1:500), anti-SIRT1 (1:500) (Chongqing Biospes Co., Ltd., China), and anti-TNF-α (1:500) (Santa Cruz Biotechnology, Inc., sc-52746). Then, the membranes were incubated with the alkaline phosphatase-conjugated secondary antibody (Biospes, China, dilution 1:5,000) for 1 h. The BCIP/NBT substrate detection kit (Genemed Biotechnologies, United States) was used to visualize the bands. The obtained bands were analyzed using ImageJ® software (National Institutes of Health, Bethesda, United States) in relation to the loading control β-actin bands.
H&E stained sections of kidneys from each group were examined by a histopathologist, in a blinded manner, under a light microscope, and the damage was assessed according to Jablonski et al. (1983)’s scoring system.
Data were expressed as mean ± S.E.M and evaluated using one-way ANOVA, followed by Tukey’s multiple comparison post hoc test. A p-value less than 0.05 was considered statistically significant. Prism 9 software (GraphPad Software Inc., San Diego, CA) was used for data analysis.
Figure 2 shows that the administration of DOX, 20 mg/kg, significantly increased the kidney index compared to the control group (p <0.05). Co-treatment with ALO or SEM with DOX ameliorated the DOX-induced increase in the kidney index. On the other hand, there was no notable change in the kidney index among the control group, ALO alone-treated group, or SEM alone-treated group.
FIGURE 2. Effect of incretin-based therapies (alogliptin and semaglutide) and doxorubicin (DOX) on the kidney index. Data are represented as mean ± SEM. * is significant compared to the control group at p <0.05. # is significant compared to the DOX group at p <0.05.
Renal damage induced by DOX was evident by the significant (p <0.05) elevation of the serum levels of creatinine, cystatin C, and urea, compared to the control group. Co-treatment with either alogliptin or semaglutide showed significant (p <0.05) improvement in DOX-induced renal dysfunction, as shown in Figure 3.
FIGURE 3. Aligned dot plots showing the effect of incretin-based therapies (alogliptin and semaglutide) on the DOX-induced renal dysfunction: (A) serum creatinine, (B) urea, and (C) cystatin C concentrations. Data are represented as mean ± SEM. * is significant compared to the control group at p <0.05, and # is significant compared to the DOX group at p <0.05.
The administration of DOX (20 mg/kg) resulted in a significant (p <0.05) increase in the MDA level and a significant decline in the reduced glutathione level and SOD activity in renal tissues compared to that in the control group. On the other hand, treatment with either alogliptin or semaglutide significantly (p <0.05) ameliorated these changes compared to the DOX alone-treated group, as illustrated in Figure 4.
FIGURE 4. Aligned dot plots showing the effect of DOX and incretin-based therapies (alogliptin and semaglutide) on the renal levels of MDA (A), GSH (B), and SOD (C). Data are represented as mean ± SEM. * is significant compared to the control group at p <0.05. # is significant compared to the DOX group at p <0.05.
Figure 5 shows that the administration of DOX, 20 mg/kg, caused a significant (p <0.05) decline in the renal expression of Nrf2 compared to the control group, while the concomitant administration of either alogliptin or semaglutide with DOX significantly (p <0.05) prevented this decline.
FIGURE 5. Western blot analysis of the effect of DOX and incretin-based therapies (alogliptin and semaglutide) on the renal expression of Nrf2. Nrf2 and β-actin bands of each group are shown (A), along with the bar chart of their densitometric analysis (B). Data are represented as mean ± SEM. * is significant compared to the control group at p <0.05. # is significant compared to the DOX group at p <0.05.
Figure 6 illustrates the effect of DOX administration and concomitant treatment with either alogliptin or semaglutide on the renal expression of sirtuin 1. Treatment with DOX resulted in the significant (p <0.05) downregulation of the sirtuin 1 expression compared to that in the control group. On the other hand, treatment with either alogliptin or semaglutide, concomitantly with DOX, significantly (p <0.05) prevented the DOX-induced decline in the SIRT1 renal level.
FIGURE 6. Western blot analysis of the effect of DOX and incretin-based therapies (alogliptin and semaglutide) on the renal expression of SIRT1. SIRT1 and β-actin bands of each group are shown (A), along with their bar chart of their densitometric analysis (B). Data are represented as mean ± SEM. * is significant compared to the control group at p <0.05. # is significant compared to the DOX group at p <0.05.
DOX administration resulted in the significant (p <0.05) upregulation of the renal level of NF-κB compared to that in the control group. Co-treatment with alogliptin or semaglutide significantly (p <0.05) abrogated the increase in the NF-κB renal expression induced by DOX, as illustrated in Figure 7.
FIGURE 7. Western blot analysis of the effect of DOX and incretin-based therapies (alogliptin and semaglutide) on the renal expression of NF-κB, showing NF-κB bands and the corresponding β-actin bands of each group (A) and the bar chart of their densitometric analysis (B). Data are represented as mean ± SEM. * is significant compared to the control group at p <0.05. # is significant compared to the DOX group at p <0.05.
Compared to the control group, the renal expression of TNF-α was significantly (p <0.05) increased in the DOX-treated group. This effect was significantly (p <0.05) abrogated by concomitant treatment with either alogliptin or semaglutide, as shown in Figure 8.
FIGURE 8. Western blot analysis of the effect of DOX and incretin-based therapies (alogliptin and semaglutide) on the renal expression of TNF-α, showing TNF-α bands and the corresponding β-actin bands of each group (A) and bar chart of their densitometric analysis (B). Data are represented as mean ± SEM. * is significant compared to the control group at p <0.05. # is significant compared to the DOX group at p <0.05.
The kidney tissue section of the control and ALO- and SEM-treated groups showed a normal histological structure of the renal tubular epithelial lining. No inflammation or necrosis was observed. There is no visible interstitial damage/abnormality. The blood vessels showed a uniform endothelium with no swelling or disruption of the endothelial cells. The glomerulus appeared intact with thin-walled Bowman’s capsules and no tuft retraction (Figures 9Aa,b, Ba,b, Ca,b). On the other side, the kidney sections of the DOX-treated group revealed varying degrees of tubular epithelial cell damage. Some renal tubules showed the necrosis of their epithelial lining with intratubular albuminous casts. The renal blood vessel showed the loss of the endothelial lining and perivascular edema. Glomeruli showed a marked retraction of capillary tufts and the widening of Bowman’s space. An inflammatory reaction appeared in the form of the congestion of blood capillaries and mononuclear cell infiltration of the interstitial compartment, with necrosis in up to 60% of the cells (Figures 9Da, b). Kidney sections of the DOX + ALO group showed a loss of the tubular epithelial brush border in less than 25% of tubular cells with the integrity of a basal membrane. The renal blood vessel showed endothelial swelling. Glomeruli showed the mild retraction of capillary tufts and the widening of Bowman’s space, causing inflammation and hemorrhage in less than 25% of the tissue (Figures 9Ea, b). Kidney sections of DOX + SEM showed an intact tubular epithelial lining without notable pathological alterations. The renal blood vessel showed an intact endothelial lining. Glomeruli showed the mild thickening of Bowman’s capsule without the retraction of capillary tufts and the absence of inflammation, congestion of blood capillaries, hemorrhage, and tubular necrosis (Figures 9Fa, b). Figure 9G represents a bar chart of histopathological damage scoring of each group, revealing that the administration of DOX induced significant histopathological damage, while treatment with either alogliptin or semaglutide showed improvements in DOX-induced damage.
FIGURE 9. Photomicrographs of the kidney tissue sections of the control group ((Aa), X200 and (Ab), X400), alogliptin group ((Ba), X200 and (Bb), X400), and semaglutide group ((Ca), X200 and (Cb), X400), showing their normal histological structure; the DOX group ((Da), X200 and (Db), X400) shows a tubular form with necrosis with the intratubular albuminous casts (arrow) and the marked retraction of capillary tufts and the widening of Bowman’s space (red arrow). The DOX + ALO group ((Ea), X200 and (Eb), X400) shows the loss of the tubular epithelial brush border with the integrity of the basal membrane (arrow), along with the mild retraction of capillary tufts and the widening of Bowman’s space (red arrow). The DOX + SEM group ((Fa), X200, (Fb), X400) shows the intact tubular epithelial lining (arrow) and the mild thickening of Bowman’s capsule without the retraction of capillary tufts (red arrow), and the bar chart (G) represents the total damage score of each group. Data are represented as mean ± SEM. * is significant compared to the control group at p <0.05. # is significant compared to the DOX group at p <0.05.
Doxorubicin is a widely used anthracycline antineoplastic agent; however, its renal adverse effects may limit its use (Ayla et al., 2011). The exact mechanism responsible for DOX-induced renal damage is not fully understood; however, many studies have emphasized the role of oxidative imbalance in DOX-induced nephrotoxicity (Deman et al., 2001; Lebrecht et al., 2004; Carvalho, et al., 2009; Afsar et al., 2020). DOX damages the mitochondrial DNA and decreases the activities of mitochondrial complexes I and IV, leading to mitochondrial dysfunction, accumulation of the ROS, and a rapid decline in renal functions (Okuda et al., 1986; Lebrecht, et al., 2004). In addition, studies showed that DOX administration leads to increased lipid peroxidation and a decline in renal enzymatic and non-enzymatic antioxidants (Mansour et al., 1999; Afsar, et al., 2020). These studies agreed with the observed results in this study as DOX administration resulted in increased lipid peroxidation, a lower concentration of reduced glutathione, and decreased SOD activity in renal tissues. In addition, treatment with DOX decreased the renal expression of Nrf2, a master regulator of oxidative status, which empowers the cellular defenses against oxidative stress by increasing the transcription of antioxidant enzymes, such as superoxide dismutase, glutathione peroxidase, and glutathione S-transferase (Narasimhan and Rajasekaran, 2017). Interestingly, decreased Nrf2 paralleled the decrease in the antioxidant activities in the kidney tissues. Importantly, the activity of the Nrf2/antioxidant responsive element (ARE) pathway can be enhanced by SIRT1, a mammalian sirtuin capable of deacetylating various histone and non-histone proteins with resultant antioxidant, anti-inflammatory, and antiapoptotic effects (Haigis and Sinclair, 2010; Huang et al., 2013). Thus, we hypothesized that the DOX-mediated increase in oxidative stress is related to decreased Nrf2 and SIRT1 expressions.
The observed increase in oxidative stress parameters coupled with the decline in the renal expression of Nrf2 and SIRT1 was diminished by treatment with either alogliptin or semaglutide. This agrees with the reported data by Xiang et al. (2023), who showed the activating effect of semaglutide on the SIRT1 pathway in C2C12 myotube cells. The same effect was observed using alogliptin against cyclophosphamide-induced liver injury (Salama et al., 2019). In addition, a previous study by Abdel-Latif et al. (2020) showed that low-dose lixisenatide treatment was able to protect against early diabetic nephropathy by causing a significant decrease in renal malondialdehyde and the total nitrite levels along with a marked rise in the total antioxidant capacity. On account of these findings, we supposed that the use of the incretin-based therapy concurrently with DOX could mitigate the increase in renal oxidative stress via the upregulation of renal Nrf2 and SIRT1 expressions. As a result of this oxidative imbalance, renal functions declined, and this was observed by the increase in serum creatinine and urea, as well as cystatin C levels (which are the biomarkers of kidney function that are usually monitored to assess the glomerular function). In addition, marked alterations were observed in the histological examination, including tubular necrosis, cast formation, perivascular edema, glomerular tuft retraction with the widening of Bowman’s space, congestion, and inflammatory cell infiltration, with a marked increase in the renal index of the DOX-treated group. These results agree with the previous studies (Heeba and Mahmoud, 2016; Hazem et al., 2022). Previous reports have supported the role of GLP-1RAs on diabetic kidney diseases studied until now; the mechanisms that underlie such effects are to be clarified. It has been found that GLP-1 receptors are abundantly expressed in the glomerulus and the proximal convoluted tubule in kidney tissues, which explains their role in modulating the renal physiological and pathophysiological functions (Crajoinas et al., 2011; Dieter et al., 2018). However, treatment with either alogliptin or semaglutide ameliorated DOX-induced renal damage, as manifested by the decrease in serum creatinine, urea, and cystatin C concentrations, in addition to the observed improvement in the histopathological changes in renal tubules, glomeruli, and interstitial tissues compared to the DOX-treated group.
In addition to the reported and abovementioned role for oxidative stress, some studies have suggested the role of inflammation in the pathogenesis of DOX-induced nephropathy (Zhang et al., 2017; Ibrahim et al., 2020). Their suggestion agreed with the observed upregulation of NF-κB and TNF-α in the renal tissues of the DOX-treated group in this study. It has been found that the activity of NF-κB is enhanced by various pro-inflammatory cytokines, such as TNF-α (Beg et al., 1993). Furthermore, the activation of NF-κB leads to the transcription of many genes encoding the pro-inflammatory cytokines, such as TNF-α and different interleukins (Liu et al., 2017). This crosstalk between NF-κB and TNF-α was also observed by Kagoya et al. (2014). Notably, a correlation between NF-κB and Nrf2 exists; it has also been reported that Nrf2 mitigates inflammation either by blocking the translocation and activation of NF-κB or by directly inhibiting the pro-inflammatory cytokine gene transcription (He et al., 2020). In the present study, co-treatment with either alogliptin or semaglutide ameliorated the coupled upregulation of NF-κB/TNF-α induced by DOX. These results are in accordance with other studies reporting the anti-inflammatory effects of GLP-1RA, semaglutide, in different models, such as obesity, multiple sclerosis, and diabetes (Tan and Tan, 2019; Pan et al., 2023; Sadek et al., 2023), and the DPP-4 inhibitor, alogliptin, in lipopolysaccharide-induced neuroinflammation (El-Sahar et al., 2021). It is also worth mentioning that alogliptin showed the protective effect against DOX-induced testicular dysfunction through decreasing oxidative stress, inflammation, and apoptosis (Kabel, 2018). Accordingly, it appeared that treatment with alogliptin or semaglutide succeeded in preventing the DOX-induced renal damage. The observed nephroprotective effect of alogliptin and semaglutide in this study may be mediated by the antioxidant and anti-inflammatory effects of both drugs by modulating the SIRT1/Nrf2/NF-κB/TNF-α signaling pathway, with no superiority of either drug above the other with respect to the doses used in this study. This nephroprotective effect was also observed using other DPP4is, such as sitagliptin, saxagliptin, vildagliptin and linagliptin (Jo et al., 2018; Mostafa et al., 2021), and the GLP1RA, lixisenatide (Guo et al., 2019).
In conclusion, augmenting the GLP-1 pathway either by DPP-4 inhibitors or by directly using GLP-1RAs with the resultant antioxidant and anti-inflammatory effect represents a promising strategy for preventing DOX-induced nephrotoxicity. However, further clinical studies are needed to validate the potential significance of this study and to find the proper therapeutic implementation in ameliorating the adverse effects of anti-cancer drugs.
The raw data supporting the conclusion of this article will be made available by the authors, without undue reservation.
The animal study was approved by the Research Ethics Committee for the laboratory animal’s care and use, Faculty of Pharmacy, Minia University. The study was conducted in accordance with the local legislation and institutional requirements.
SB: writing–original draft, writing–review and editing, data curation, formal analysis, investigation, methodology, and validation. AM: data curation, formal analysis, investigation, methodology, validation, writing–original draft, conceptualization, project administration, supervision, visualization, and writing–review and editing. AA: data curation, formal analysis, investigation, methodology, validation, writing–original draft, conceptualization, project administration, supervision, visualization, and writing–review and editing. GH: conceptualization, data curation, formal analysis, investigation, methodology, project administration, software, supervision, validation, writing–original draft, and writing–review and editing.
The author(s) declare that financial support was received for the research, authorship, and/or publication of this article. The study received partial funding from College of Medicine, University of Sharjah for AA.
The authors declare that the research was conducted in the absence of any commercial or financial relationships that could be construed as a potential conflict of interest.
All claims expressed in this article are solely those of the authors and do not necessarily represent those of their affiliated organizations, or those of the publisher, the editors, and the reviewers. Any product that may be evaluated in this article, or claim that may be made by its manufacturer, is not guaranteed or endorsed by the publisher.
Abdel-Latif, R. G., Ahmed, A. F., and Heeba, G. H. (2020). Low-dose lixisenatide protects against early-onset nephropathy induced in diabetic rats. Life Sci. 263, 118592. Epub 2020/10/17. doi:10.1016/j.lfs.2020.118592
Afsar, T., Razak, S., Almajwal, A., and Al-Disi, D. (2020). Doxorubicin-induced alterations in kidney functioning, oxidative stress, DNA damage, and renal tissue morphology; Improvement by Acacia hydaspica tannin-rich ethyl acetate fraction. Saudi J. Biol. Sci. Sep. 27, 2251–2260. Epub 2020/09/05. doi:10.1016/j.sjbs.2020.07.011
Alghorabi, A. A., Kabel, A. M., and Elmaaboud, M. (2019). Doxorubicin: insights into dynamics, clinical uses and adverse effects. J. Cancer Res. Treat. 7, 17–20. doi:10.12691/jcrt-7-1-3
Ali, F. E. M., Bakr, A. G., Abo-youssef, A. M., Azouz, A. A., and Hemeida, R. A. M. (2018). Targeting Keap-1/Nrf-2 pathway and cytoglobin as a potential protective mechanism of diosmin and pentoxifylline against cholestatic liver cirrhosis. Life Sci. 207, 50–60. doi:10.1016/j.lfs.2018.05.048
Al-Kuraishy, H. M., Issa, H. K., Al-Gareeb, A. I., El-Bouseary, M. M., Youssef, A., Abdelaziz, A. S., et al. (2022). The role of ivabradine in doxorubicin-induced cardiotoxicity: exploring of underlying argument. Inflammopharmacology. Dec 30, 2441–2446. Epub 2022/10/12. doi:10.1007/s10787-022-01082-z
Ayla, S., Seckin, I., Tanriverdi, G., Cengiz, M., Eser, M., Soner, B. C., et al. (2011). Doxorubicin induced nephrotoxicity: protective effect of nicotinamide. Int. J. Cell Biol. 2011, 390238. Epub 2011/07/27. doi:10.1155/2011/390238
Bancroft, J. D., and Gamble, M. (2008). Theory and practice of histological techniques. Amsterdam, Netherlands: Elsevier health sciences.
Beg, A. A., Finco, T. S., Nantermet, P. V., and Baldwin, A. S. (1993). Tumor necrosis factor and interleukin-1 lead to phosphorylation and loss of I kappa B alpha: a mechanism for NF-kappa B activation. Mol. Cell. Biol. 13, 3301–3310. doi:10.1128/mcb.13.6.3301
Buege, J. A., and Aust, S. D. (1978). “[30] Microsomal lipid peroxidation,” in Methods in enzymology (Amsterdam, Netherlands: Elsevier), 302–310.
Carron, P. L., Padilla, M., and Maurizi Balzan, J. (2014). Nephrotic syndrome and acute renal failure during pegylated liposomal doxorubicin treatment. Hemodial. Int. Oct. 18, 846–847. Epub 2014/07/22. doi:10.1111/hdi.12196
Carvalho, C., Santos, R. X., Cardoso, S., Correia, S., Oliveira, P. J., Santos, M. S., et al. (2009). Doxorubicin: the good, the bad and the ugly effect. Curr. Med. Chem. 16, 3267–3285. doi:10.2174/092986709788803312
Chang, W.-T., Wu, C.-C., Liao, I. C., Lin, Y.-W., Chen, Y.-C., Ho, C.-H., et al. (2023). Dapagliflozin protects against doxorubicin-induced nephrotoxicity associated with nitric oxide pathway—a translational study. Free Radic. Biol. Med. 208, 103–111. doi:10.1016/j.freeradbiomed.2023.08.013
Crajoinas, R. O., Oricchio, F. T., Pessoa, T. D., Pacheco, B. P., Lessa, L. M., Malnic, G., et al. (2011). Mechanisms mediating the diuretic and natriuretic actions of the incretin hormone glucagon-like peptide-1. Am. J. Physiol. Ren. Physiol. Aug 301, F355–F363. Epub 2011/05/20. doi:10.1152/ajprenal.00729.2010
Deman, A., Ceyssens, B., Pauwels, M., Zhang, J., Houte, K. V., Verbeelen, D. L., et al. (2001). Altered antioxidant defence in a mouse adriamycin model of glomerulosclerosis. Nephrol. Dial. Transplant. 16 1, 147–150. doi:10.1093/ndt/16.1.147
Dieter, B. P., Alicic, R. Z., and Tuttle, K. R. (2018). GLP-1 receptor agonists in diabetic kidney disease: from the patient-side to the bench-side. Am. J. Physiol. Ren. Physiol. 315, F1519–f1525. Epub 2018/08/16. doi:10.1152/ajprenal.00211.2018
Eleazu, C. O., Iroaganachi, M., Okafor, P. N., Ijeh, I. I., and Eleazu, K. C. (2013). Ameliorative potentials of ginger (Z. officinale Roscoe) on relative organ weights in streptozotocin induced diabetic rats. Int. J. Biomed. Sci. 9, 82–90. doi:10.59566/ijbs.2013.9082
Ellman, G. L. (1959). Tissue sulfhydryl groups. Archives Biochem. biophysics 82, 70–77. doi:10.1016/0003-9861(59)90090-6
El-Sahar, A. E., Shiha, N. A., El Sayed, N. S., and Ahmed, L. A. (2021). Alogliptin attenuates lipopolysaccharide-induced neuroinflammation in mice through modulation of TLR4/MYD88/NF-κB and miRNA-155/SOCS-1 signaling pathways. Int. J. Neuropsychopharmacol. 24, 158–169. Epub 2020/10/31. doi:10.1093/ijnp/pyaa078
Esaki, H., Tachi, T., Goto, C., Sugita, I., Kanematsu, Y., Yoshida, A., et al. (2017). Renoprotective effect of dipeptidyl peptidase-4 inhibitors in patients with type 2 diabetes mellitus. Front. Pharmacol. 8, 835. Epub 2017/12/01. doi:10.3389/fphar.2017.00835
Ferlay, J., Colombet, M., Soerjomataram, I., Parkin, D. M., Piñeros, M., Znaor, A., et al. (2021). Cancer statistics for the year 2020: an overview. Int. J. Cancer. 149, 778–789. doi:10.1002/ijc.33588
Global cancer Observatory (2023). Cancer today. Lyon, France: International agency for research on cancer: IARC.
Guo, N. F., Cao, Y. J., Chen, X., Zhang, Y., Fan, Y. P., Liu, J., et al. (2019). Lixisenatide protects doxorubicin-induced renal fibrosis by activating wNF-κB/TNF-α and TGF-β/Smad pathways. Eur. Rev. Med. Pharmacol. Sci. May 23, 4017–4026. Epub 2019/05/23. doi:10.26355/eurrev_201905_17832
Haigis, M. C., and Sinclair, D. A. (2010). Mammalian sirtuins: biological insights and disease relevance. Annu. Rev. Pathol. 5, 253–295. Epub 2010/01/19. doi:10.1146/annurev.pathol.4.110807.092250
Hazem, R. M., Antar, S. A., Nafea, Y. K., Al-Karmalawy, A. A., Saleh, M. A., and El-Azab, M. F. (2022). Pirfenidone and vitamin D mitigate renal fibrosis induced by doxorubicin in mice with Ehrlich solid tumor. Life Sci. 288, 120185. doi:10.1016/j.lfs.2021.120185
He, F., Antonucci, L., and Karin, M. (2020). NRF2 as a regulator of cell metabolism and inflammation in cancer. Carcinogenesis 41, 405–416. Epub 2020/04/30. doi:10.1093/carcin/bgaa039
Heeba, G. H., and Mahmoud, M. E. (2016). Dual effects of quercetin in doxorubicin-induced nephrotoxicity in rats and its modulation of the cytotoxic activity of doxorubicin on human carcinoma cells. Environ. Toxicol. May 31, 624–636. Epub 2014/11/21. doi:10.1002/tox.22075
Huang, K., Huang, J., Xie, X., Wang, S., Chen, C., Shen, X., et al. (2013). Sirt1 resists advanced glycation end products-induced expressions of fibronectin and TGF-β1 by activating the Nrf2/ARE pathway in glomerular mesangial cells. Free Radic. Biol. Med. Dec 65, 528–540. Epub 2013/07/31. doi:10.1016/j.freeradbiomed.2013.07.029
Ibrahim, K. M., Mantawy, E. M., Elanany, M. M., Abdelgawad, H. S., Khalifa, N. M., Hussien, R. H., et al. (2020). Protection from doxorubicin-induced nephrotoxicity by clindamycin: novel antioxidant, anti-inflammatory and anti-apoptotic roles. Naunyn-Schmiedeberg's Archives Pharmacol. 393, 739–748. doi:10.1007/s00210-019-01782-4
Iwakura, T., Fukasawa, H., Kitamura, A., Ishibuchi, K., Yasuda, H., and Furuya, R. (2020). Effect of dipeptidyl peptidase-4 inhibitors on cisplatin-induced acute nephrotoxicity in cancer patients with diabetes mellitus: a retrospective study. PLoS One 15, e0229377. Epub 2020/02/23. doi:10.1371/journal.pone.0229377
Jablonski, P., Howden, B. O., Rae, D. A., Birrell, C. S., Marshall, V. C., and Tange, J. (1983). An experimental model for assessment of renal recovery from warm ischemia. Transplant. Mar. 35, 198–204. Epub 1983/03/01. doi:10.1097/00007890-198303000-00002
Jo, C.-H., Kim, S., Park, J.-S., and Kim, G.-H. (2018). Anti-inflammatory action of sitagliptin and linagliptin in doxorubicin nephropathy. Kidney Blood Press. Res. 43, 987–999. doi:10.1159/000490688
Kabel, A. M. (2018). Zinc/alogliptin combination attenuates testicular toxicity induced by doxorubicin in rats: role of oxidative stress, apoptosis and TGF-β1/NF-κB signaling. Biomed. Pharmacother. Jan. 97, 439–449. Epub 2017/11/02. doi:10.1016/j.biopha.2017.10.144
Kagoya, Y., Yoshimi, A., Kataoka, K., Nakagawa, M., Kumano, K., Arai, S., et al. (2014). Positive feedback between NF-κB and TNF-α promotes leukemia-initiating cell capacity. J. Clin. Investigation 124, 528–542. doi:10.1172/JCI68101
Katagiri, D., Hamasaki, Y., Doi, K., Okamoto, K., Negishi, K., Nangaku, M., et al. (2013). Protection of glucagon-like peptide-1 in cisplatin-induced renal injury elucidates gut-kidney connection. J. Am. Soc. Nephrol. Dec 24, 2034–2043. Epub 2013/10/05. doi:10.1681/ASN.2013020134
Lebrecht, D., Setzer, B., Rohrbach, R., and Walker, U. A. (2004). Mitochondrial DNA and its respiratory chain products are defective in doxorubicin nephrosis. Nephrol. Dial. Transpl. Feb 19, 329–336. Epub 2004/01/23. doi:10.1093/ndt/gfg564
Liu, T., Zhang, L., Joo, D., and Sun, S.-C. (2017). NF-κB signaling in inflammation. Signal Transduct. Target. Ther. 2, 17023. doi:10.1038/sigtrans.2017.23
Mansour, M. A., El-Kashef, H. A., and Al-Shabanah, O. A. (1999). Effect of captopril on doxorubicin-induced nephrotoxicity in normal rats. Pharmacol. Res. Mar. 39, 233–237. Epub 1999/03/30. doi:10.1006/phrs.1998.0432
Marklund, S. L. (1985). Superoxide dismutase isoenzymes in tissues and plasma from New Zealand black mice, nude mice and normal BALB/c mice. Mutat. research/Fundamental Mol. Mech. Mutagen. 148, 129–134. doi:10.1016/0027-5107(85)90216-7
Mostafa, R. E., Morsi, A. H., and Asaad, G. F. (2021). Anti-inflammatory effects of saxagliptin and vildagliptin against doxorubicin-induced nephrotoxicity in rats: attenuation of NLRP3 inflammasome up-regulation and tubulo-interstitial injury. Res. Pharm. Sci. Oct. 16, 547–558. Epub 2021/09/16. doi:10.4103/1735-5362.323920
Muskiet, M. H., Smits, M. M., Morsink, L. M., and Diamant, M. (2014). The gut-renal axis: do incretin-based agents confer renoprotection in diabetes? Nat. Rev. Nephrol. Feb 10, 88–103. Epub 2014/01/01. doi:10.1038/nrneph.2013.272
Narasimhan, M., and Rajasekaran, N. S. (2017). Cardiac aging - benefits of exercise, Nrf2 activation and antioxidant signaling. Adv. Exp. Med. Biol. 999, 231–255. Epub 2017/10/13. doi:10.1007/978-981-10-4307-9_13
Okuda, S., Oh, Y., Tsuruda, H., Onoyama, K., Fujimi, S., and Fujishima, M. (1986). Adriamycin-induced nephropathy as a model of chronic progressive glomerular disease. Kidney Int. 29, 502–510. doi:10.1038/ki.1986.28
Pan, X., Yang, L., Wang, S., Liu, Y., Yue, L., and Chen, S. (2023). Semaglutide ameliorates obesity-induced cardiac inflammation and oxidative stress mediated via reduction of neutrophil Cxcl2, S100a8, and S100a9 expression. Mol. Cell. Biochem. doi:10.1007/s11010-023-04784-2
Qian, P., Tian, H., Wang, Y., Lu, W., Li, Y., Ma, T., et al. (2020). A novel oral glucagon-like peptide 1 receptor agonist protects against diabetic cardiomyopathy via alleviating cardiac lipotoxicity induced mitochondria dysfunction. Biochem. Pharmacol. Dec 182, 114209. Epub 2020/08/30. doi:10.1016/j.bcp.2020.114209
Radbakhsh, S., Atkin, S. L., Simental-Mendia, L. E., and Sahebkar, A. (2021). The role of incretins and incretin-based drugs in autoimmune diseases. Int. Immunopharmacol. 98, 107845. doi:10.1016/j.intimp.2021.107845
Rashid, S., Ali, N., Nafees, S., Ahmad, S. T., Arjumand, W., Hasan, S. K., et al. (2013). Alleviation of doxorubicin-induced nephrotoxicity and hepatotoxicity by chrysin in Wistar rats. Toxicol. Mech. Methods 23, 337–345. Epub 2012/12/22. doi:10.3109/15376516.2012.759306
Sadek, M. A., Kandil, E. A., El Sayed, N. S., Sayed, H. M., and Rabie, M. A. (2023). Semaglutide, a novel glucagon-like peptide-1 agonist, amends experimental autoimmune encephalomyelitis-induced multiple sclerosis in mice: involvement of the PI3K/Akt/GSK-3β pathway. Int. Immunopharmacol. 115, 109647. doi:10.1016/j.intimp.2022.109647
Salama, R., Mohamed, A., Hamed, N., Ata, R., Noureldeen, A., and Hassan, M. (2019). Therapeutic benefit of alogliptin against cyclophosphamide-induced hepatic injury via targeting SIRT1/FoxO1 mechanistic pathway. Pharmacology 2019. doi:10.13140/RG.2.2.23429.58085
Tan, S. A., and Tan, L. (2019). Liraglutide and semaglutide attenuate inflammatory cytokines interferon-gamma, tumor necrosis factor-alpha, and interleukin-6: possible mechanism of decreasing cardiovascular risk in diabetes mellitus. J. Am. Coll. Cardiol. 73, 1866. doi:10.1016/s0735-1097(19)32472-6
Towbin, H., Staehelin, T., and Gordon, J. (1979). Electrophoretic transfer of proteins from polyacrylamide gels to nitrocellulose sheets: procedure and some applications. Proc. Natl. Acad. Sci. 76, 4350–4354. doi:10.1073/pnas.76.9.4350
Vaghasiya, J., Sheth, N., Bhalodia, Y., and Manek, R. (2011). Sitagliptin protects renal ischemia reperfusion induced renal damage in diabetes. Regul. Pept. 166, 48–54. Epub 2010/08/24. doi:10.1016/j.regpep.2010.08.007
Wang, S. F., Abouzied, M. M., and Smith, D. M. (1996). Proteins as potential endpoint temperature indicators for ground beef patties. J. Food Sci. 61, 5–7. doi:10.1111/j.1365-2621.1996.tb14713.x
Xiang, J., Qin, L., Zhong, J., Xia, N., and Liang, Y. (2023). GLP-1RA liraglutide and semaglutide improves obesity-induced muscle atrophy via SIRT1 pathway. Diabetes Metab. Syndr. Obes. 16, 2433–2446. Epub 2023/08/21. doi:10.2147/DMSO.S425642
Yaribeygi, H., Atkin, S. L., Montecucco, F., Jamialahmadi, T., and Sahebkar, A. (2021). Renoprotective effects of incretin-based therapy in diabetes mellitus. Biomed. Res. Int. 2021, 8163153. Epub 2021/09/03. doi:10.1155/2021/8163153
Yaribeygi, H., Maleki, M., Sathyapalan, T., Jamialahmadi, T., and Sahebkar, A. (2021). Antioxidative potentials of incretin-based medications: a review of molecular mechanisms. Oxid. Med. Cell Longev. 2021, 9959320. Epub 2021/05/20. doi:10.1155/2021/9959320
Yemm, K. E., Alwan, L. M., Malik, A. B., and Salazar, L. G. (2019). Renal toxicity with liposomal doxorubicin in metastatic breast cancer. J. Oncol. Pharm. Pract. 25, 1738–1742. doi:10.1177/1078155218798157
Youssef, N., Noureldein, M., Njeim, R., Ghadieh, H. E., Harb, F., Azar, S. T., et al. (2021). Reno-protective effect of GLP-1 receptor agonists in Type1 diabetes: dual action on TRPC6 and NADPH oxidases. Biomedicines 9, 1360. doi:10.3390/biomedicines9101360
Keywords: doxorubicin, nephrotoxicity, incretins, glucagon-like peptide-1, dipeptidyl peptidase-4 inhibitor
Citation: Botros SR, Matouk AI, Amin A and Heeba GH (2024) Comparative effects of incretin-based therapy on doxorubicin-induced nephrotoxicity in rats: the role of SIRT1/Nrf2/NF-κB/TNF-α signaling pathways. Front. Pharmacol. 15:1353029. doi: 10.3389/fphar.2024.1353029
Received: 09 December 2023; Accepted: 30 January 2024;
Published: 19 February 2024.
Edited by:
Luis A. Juncos, United States Department of Veterans Affairs, United StatesReviewed by:
Rodrigo O. Maranon, CCT CONICET Tucuman, ArgentinaCopyright © 2024 Botros, Matouk, Amin and Heeba. This is an open-access article distributed under the terms of the Creative Commons Attribution License (CC BY). The use, distribution or reproduction in other forums is permitted, provided the original author(s) and the copyright owner(s) are credited and that the original publication in this journal is cited, in accordance with accepted academic practice. No use, distribution or reproduction is permitted which does not comply with these terms.
*Correspondence: Gehan H. Heeba, Z2VoYW5faGVlYmFAbXUuZWR1LmVn; Amr Amin, YS5hbWluQHNoYXJqYWguYWMuYWU=
Disclaimer: All claims expressed in this article are solely those of the authors and do not necessarily represent those of their affiliated organizations, or those of the publisher, the editors and the reviewers. Any product that may be evaluated in this article or claim that may be made by its manufacturer is not guaranteed or endorsed by the publisher.
Research integrity at Frontiers
Learn more about the work of our research integrity team to safeguard the quality of each article we publish.