- Key Laboratory of Joint Diagnosis and Treatment of Chronic Liver Disease and Liver Cancer of Lishui, Wenzhou Medical University Lishui Hospital, Lishui People’s Hospital, Lishui, Zhejiang, China
This work aimed to explore the mechanisms underlying the interaction of the active furanocoumarins in P. corylifolia on tofacitinib both in vivo and in vitro. The concentration of tofacitinib and its metabolite M8 was determined using UPLC-MS/MS. The peak area ratio of M8 to tofacitinib was calculated to compare the inhibitory ability of furanocoumarin contained in the traditional Chinese medicine P. corylifolia in rat liver microsomes (RLMs), human liver microsomes (HLMs) and recombinant human CYP3A4 (rCYP3A4). We found that bergapten and isopsoralen exhibited more significant inhibitory activity in RLMs than other furanocoumarins. Bergapten and isopsoralen were selected to investigate tofacitinib drug interactions in vitro and in vivo. Thirty rats were randomly allocated into 5 groups (n = 6): control (0.5% CMC-Na), low-dose bergapten (20 mg/kg), high-dose bergapten (50 mg/kg), low-dose isopsoralen (20 mg/kg) and ketoconazole. 10 mg/kg of tofacitinib was orally intervented to each rat and the concentration level of tofacitinib in the rats were determined by UPLC-MS/MS. More imporrantly, the results showed that bergapten and isopsoralen significantly inhibited the metabolism of tofacitinib metabolism. The AUC(0-t), AUC(0-∞), MRT(0-t), MRT(0-∞) and Cmax of tofacitinib increased in varying degrees compared with the control group (all p < 0.05), but CLz/F decreased in varying degrees (p < 0.05) in the different dose bergapten group and isopsoralen group. Bergapten, isopsoralen and tofacitinib exhibit similar binding capacities with CYP3A4 by AutoDock 4.2 software, confirming that they compete for tofacitinib metabolism. P. corylifolia may considerably impact the metabolism of tofacitinib, which can provide essential information for the accurate therapeutic application of tofacitinib.
1 Introduction
Multiple drugs taken together cause DDIs, which further produce numerous side effects. In addition, DDIs may further induce adverse drug reactions (ADRs) (Zhang et al., 2020). Several drugs are frequently administered to patients with multiple disorders to boost their therapeutic effects. However, drug interactions may not always work and raise the risk of severe consequences or death. The primary factor contributing to DDIs is alterations in pharmacokinetics and pharmacodynamics (Aronson, 2004). Growing evidence indicates that the modulation of cytochrome P450 enzymes (CYP450s) through inhibition and induction can alter the pharmacokinetic characteristics of drugs, serving as a fundamental mechanism for drug interactions (Lin and Lu, 1998; Lin, 2006). Most drugs pass through metabolism by CYP oxidation (Hardman, 2001). The CYP3A4 enzyme found in the liver and intestines is highly prevalent and plays a crucial role in metabolizing approximately half of all drugs available on the market (Zhou, 2008). There is increasing evidence that drugs and/or exogenous substances stimulate or obstruct the activity and generation of CYP3A4, resulting in toxicity (Sabers, 2008). The combination of tofacitinib and ketoconazole, a CYP3A4 inhibitor, may prolong the QT interval and result in arrhythmia and mortality (Mathews et al., 1991). Accumulating evidence indicates that DDIs significantly contribute to preventable disease and death in modern healthcare (Day et al., 2017).
Tofacitinib (CP-690550) is a small-molecule oral JAK kinase inhibitor that can be used for long-term treatment (Palasik and Wang, 2021). Multiple studies have revealed that tofacitinib obstructs intracellular JAK kinase, cytokine receptor recruitment and STAT phosphorylation to hinder STAT from being activated into the nucleus, impede intracellular JAK pathway signaling, alleviate immune cell activation and pro-inflammatory factor release and inflammatory cascade (Meyer et al., 2010; Dhillon, 2017). Rheumatoid arthritis (RA) is reagred as a systemic chronic immune-mediated inflammatory disorder that affects multiple joints throughout the body. The JAK signal transduction factor and the transcription activation pathway play a crucial role in the pathogenesis of RA. Prior studies have revealed that tofacitinib can remarkably reduce bone marrow edema in patients with RA and ameliorate the progression of structural impairment (Conaghan et al., 2016). Tofacitinib has been approved for the treatment of RA in both the United States and Europe (Karaman et al., 2008).
The origins of traditional medicine for the treatment of diseases may be tracked over several thousands of years. Traditional Chinese Medicine is frequently combined with synthetic drugs to treat diseases in modern medicine. P. corylifolia, a traditional Chinese medicine, exhibits remarkable anti-inflammatory actions by modulating inflammatory signaling pathways, mitigating the generation and secretion of inflammatory mediators and suppressing the infiltration of inflammatory cells (Chen et al., 2023). Current evidence indicates that P. corylifolia potentially delivers therapeutic applications in treating inflammatory diseases including RA (Pai et al., 2021; Wang et al., 2022). A pioneering study has revealed that psoralen possesses remarkable anti-inflammatory properties and enhances the proliferation of MDSCs (Pai et al., 2021). Due to the significance of tofacitinib in treating RA and the anti-arthritis potential of psoralen, drug interaction is essential. P. corylifolia contains coumarins (Alam et al., 2018), which exert multiple pharmacological actions including anti-inflammatory, anti-tumor, anti-bacterial and antioxidant properties (Sharifi-Rad et al., 2021). The compounds found in P. corylifolia such as psoralen, isopsoralen, bergapten, psoralidin, xanthotoxol, and 8-methoxypsoralen, have been proven to possess diverse pharmacological actions including anti-inflammatory and anti-oxidation properties (Chen et al., 2023). Resarch suggests that psoralen may efficaciously alleviate ulcerative colitis inflammation (Zx, 2020). In addition, psoralen obstructs MMP-13 protein synthesis, promoting chondrocyte growth and protecting against TNF-α-induced gene dysregulation (Wang et al., 2019). More importantly, psoralen can decrease MMP-1/2/3/9/12/13 gene expressions and attenuate MMP-13 protein synthesis, which may enhance chondrocyte proliferation and cartilage-associated gene expression. In addition, psoralen protects chondrocytes against TNF-α-induced gene expression abnormalities. Studies have indicated that isopsoralen can effectively mitigate inflammation by suppressing the release of inflammatory factors in vivo and in vitro (Kong et al., 2017; Han et al., 2021; Liang et al., 2021). Bergapten (5-methoxy-psoralen) has been proven to have promising anti-inflammatory and analgesic actions (Yeo et al., 2000).
Furanocoumarins are commonly found in a variety of plants in nature. Certain furancoumarins can interact with the intestinal cytochrome P450 isomer CYP3A4, which has been determined in extensive research (Yeo et al., 2000; Nijsten and Stern, 2003; Stern, 2012). Furanocoumarins in grapefruit may strongly inhibit CYP3A4, which might affect the pharmacokinetic characteristics of multiple drugs and induce drug interactions (Ohnishi et al., 2000). Furanocoumarins in P. corylifolia interact with CYP3A4, which can alter pharmacokinetics and pharmacodynamics. Pioneering research revealed that resveratrol could increase plasma exposure to tofacitinib by impeding CYP3A4 enzyme (Ye et al., 2023). Tofacitinib used with moderate or severe renal and hepatic impairment must now be adjusted to 5 mg per day orally (Vyas et al., 2013). The primary adverse effects of tofacitinib included infection, gastrointestinal disturbances including perforation, increased liver enzyme levels and dyslipidemia (Moriana et al., 2022). Prolonged or high-dose administration of tofacitinib for treating RA elevates the likelihood of opportunistic infections related to immunosuppression (Beaugerie et al., 2020) and susceptibility to cardiovascular disease and malignancies (Charles-Schoeman et al., 2023; Curtis et al., 2023). Tofacitinib has been found to promote herpes zoster infection in a dose—and age-dependent manner (Beaugerie et al., 2020). A study conducted by Cox revealed that the augmented risk of severe infection in patients with RA treated with tofacitinib was attributed to the dosage of the drugs (Cohen et al., 2020). Tofacitinib dose limits may lower expectations when combined with other medicines due to tolerability and safety (Serhal and Edwards, 2019). P. corylifolia, a new RA medication, requires clinical and product development. In order to understand the underlying mechanism of DDIs, furocoumarins in P. corylifolia need to be investigated in combination with other drugs.
In this study, UPLC-MS/MS was utilized to determine tofacitinib and its metabolite M8 and to investigate the interaction of furocoumarin in psoralen in rat liver microsomes (RLMs), human liver microsomes (HLMs) and recombinant human CYP3A4 (rCYP3A4). First, eight furocoumarins in psoralen were evaluated for inhibitory activity and bergapten and isopsoralen were shown to be significant inhibitors. Berkapten and isopsoralen inhibited tofacitinib in RLMs, HLMs, and rCYP3A4, with a higher pre-inhibitory impact. Therefore, we investigated the in vivo interactions of bergapten, isopsoralen and tofacitinib. Our study focused on the pharmacokinetic properties of tofacitinib and the suppressory effects of bergapten and isopsoralen on tofacitinib. The binding interaction of bergapten and furanocoumarin with rCYP3A4 was finally confirmed employing molecular docking analysis to understand and anticipate the probability of interaction.
2 Materials and methods
2.1 Chemicals, reagents and biological samples
Tofacitinib (purity >98%) was purchased from Beijing InnoChem Science & Technology Co., Ltd. Psoralen, isopsoralen, psoralidin, bergapten, 8-methoxypsoralen and xanthotoxol were purchased from Chengdu Must Bio-technology Co., Ltd.Midazolam (internal standard (IS); purity >98%) was purchased from the Tianjin King York Pharmaceutical Co. Ltd. (Tianjin, China). HLMs was purchased from Wuhan PrimeTox biomedicine technology Co., Ltd. (Wuhan, China). rCYP3A4 was gifted from Beijing Hospital. Acetonitrile and methanol were bought from Merck Co., Ltd. (Darmstadt, Germany). Carboxymethylcellulose sodium salt (CMC-Na) was purchased from Sinopharm Chemical Reagent Co., Ltd. (Shanghai China). Formic acid was bought from Sigma-Aldrich (St. Louis, MO, United States). Ultrapure water was obtained using a Milli-Q water purification system (Millipore, Billerica, MA, United States). RLMs was prepared in our laboratory. All other chemicals and biological substances were of analytical grade or higher.
2.2 Instruments and operation conditions
The determination of tofacitinib parameters was executed by employing a UPLC-MS/MS system, which possessed an ACQUITY I Class UPLC and a XEVO TQD triple quadrupole mass spectrometer (Waters Corp., Milford, MA, United States). Chromatographic analysis of tofacitinib was conducted with a CORTECS C18 column (2.1 × 50 mm, 1.6 µm) maintained at 40°C. The mobile phase included 0.1% formic acid, 5 mM ammonium formate and acetonitrile. Acetonitrile was eluted from 10% to 30% over 0.5–1.0 min; maintained at 95% for 1.0–2.0 min and then decreased to 10% over 2.0–2.3 min. The flow rate was 0.4 mL/min and the total run time was 3 min. Multiple positive reactions were monitored in mass scan mode. The parent ion and daughter ion were m/z 313.18→149.03 for tofacitinib, m/z 299.16→98.10 for tofacitinib M8 and m/z 325.98→291.07 for IS. Tofacitinib, M8 and IS were defined as 40 V, 40 V, and 50 V cone voltages and 30 V, 30 V, and 26 V collision energies, respectively.
2.3 The inhibitory effect of bergapten and isopsoralen on tofacitinib in vitro and the mechanism of action
Tofacitinib, 30 mg/mL RLMs, 20 mg/mL HLMs, or 2.68 mg/mL rCYP3A4, 0.1 mM PBS (pH7.4), 20 mM (Reduced) Nicotinamide Adenine Dinucleotide Phosphate (NADPH), bergapten or isopsoralen, or not included were added to 200 μL incubation. 100 μM psoralen, isopsoralen, psoralidin, bergapten, 8-methoxypsoralen and xanthotoxol were incubated with 0.3 mg/mL RLMs. Bergapten and Isopsoralen were found to have high RLMs inhibitory activity after determining the content of M8, a metabolite of tofacitinib. To determine IC50, the concentrations of bergapten and isopsoralen used were 0.01, 0.1, 1, 5, 10, 50, and 100 μM and tofacitinib was used at concentrations approximating Km values. To determine the inhibitory characteristic of Ki, tofacitinib was incubated at 1/4, 1/2, 1, 2 times the Km value and bergapten and isopsoralen at 1/4, 1/2, 1, 2 times the IC50 value. All except NADPH were mixed on ice. Afterward, the mixture was incubated at 37°C for 5 min. Next, the incubation was extended for an additional 30 min following the addition of NADPH to initiate the reaction. Acetonitrile containing midazolam was added for quenching and 200 ng/mL midazolam was used as the normal solution. After vortexing, the mixture content was adequeatly centrifuged at 13,000 rpm for 5 min and 150 μL of the supernatant was stored in a sample injection bottle for UPLC-MS/MS analysis.
2.4 Molecular docking simulations
The X-ray crystal structure of CYP3A4 (PDB ID: 2J0D) was found using PDB (Protein Data Bank, http://www.rcsb.org/pdb). In Chem3D Ultra 14.0, the three-dimensional structure of tofacitinib, bergapten and isopsoralen were optimized for energy minimization. The smile file for each ligand was then converted to PDBQT format utilizing AutoDockTools-1.5.6 software (AutoDock 4.2 software, The Scripps Research Institute, United States). Before the docking analysis, the CYP3A4 protein structure underwent modifications including removing water, the primary inhibitor and ligand molecules. After adding hydrogen atoms and Kollman charge, AutoDockTools-1.5.6 converted the structure to PDBQT. This ligand docking selected the standard precision mode, which pairs the ligand to the active site of the target protein. For the 10-GA docking simulation, Lamarque GA with default settings was employed. Protein-ligand interactions were studied by the protein-ligand Interaction Profile and PyMOL.
2.5 The pharmacokinetic interactions of tofacitinib with bergapten and isopsoralen in vivo
Male Sprague Dawley (SD) rats (average weighing 220–250) g were purchased from the laboratory animal center of Wenzhou Medical University, Zhejiang Province, China. Animals were maintained in a controlled environment with a 12 h light-dark cycle at 20°C–25°C and 55% ± 15% relative humidity. Diet intake was not allowed for a period of 12 h prior to the experiment. Then, water was allowed to be free and food was provided after the experiment. All the experimental procedures and protocols were reviewed and approved by the animal ethics committee of Wenzhou Medical University according to the guide for the care and use of laboratory animals (xmsq2021-0409). 36 male rats aged 8–10 weeks were randomly selected and divided into six groups for gavage. The group which was orally administered 40 mg/kg ketoconazole and 10 mg/kg tofacitinib acted as the positive control group and the group which was only orally administered 10 mg/kg tofacitinib acted as the negative group. Another four groups were orally administered 20 mg/kg bergapten, 50 mg/kg of bergapten, 20 mg/kg of isopsoralen and 50 mg/kg of isopsoralen, respectively, except 10 mg/kg of tofacitinib. Blood (300 μL) was collected from the tail veins into 1.5 mL tubes at 5, 15, and 30 min and 1, 2, 3, 4, 6, 8, 12, and 24 h after administering the drugs. The supernatant was collected and stored at −20°C after centrifugation at 4000 rpm for 10 min. The samples were restored to RT before analysis. 50 μL sample was accurately drawn to 1.5 mL of EP tube, 150 μL of midazolam internal standard solution (200 ng/mL) was added to the tube, then placed and vortexed for 15 s. After centrifugation at 13, 000 rpm for 15 min, 150 µL of supernatant was prepared for the UPLC-MS/MS system to analyze.
2.6 Data analysis
Pharmacokinetic data were analyzed by a non-compartmental method using Drug and Statistics (DAS) software (version 3.2.8). The plasma concentration at different times was expressed as mean ± SD and the drug concentration-time curve was obtained based on the mean concentration of 6 mice assessed at each time-point. Km, Vmax, and IC50 values were performed using Prism software v.8 (GraphPad). Statistical analysis was conducted using Student's t-test. A statistically significant difference was considered when p < 0.05. All in vitro experiments were repeated three times, and six rats in each group for in vivo experiments.
3 Results
3.1 Characterization of the activity of RLMs, HLMs, and rCYP3A4 in tofacitinib treatment and screening of inhibitors
The Michaelis-Menten curves and Michaelis kinetic parameters of tofacitinib in RLMs, HLMs and rCYP3A4 were shown in Figure 1A; Table 1. There is no significant difference between RLMs, HLMs, and rCYP3A4 based on changes in maximum velocity of reaction (Vmax). Our data indicated that the Km of tofacitinib in RLMs, HLMs and rCYP3A4 were 76.25 ± 4.514 μM, 65.85 ± 4.749 μM and 1 0.61 ± 1.667 μM. Figure 1B demonstrated the inhibitory effectiveness of six furanocoumarins on RLMs. Among them, bergapten and isopsoralen were selected for their potent inhibitory activity. And the results were statistically significant compared to the control group (p < 0.05).
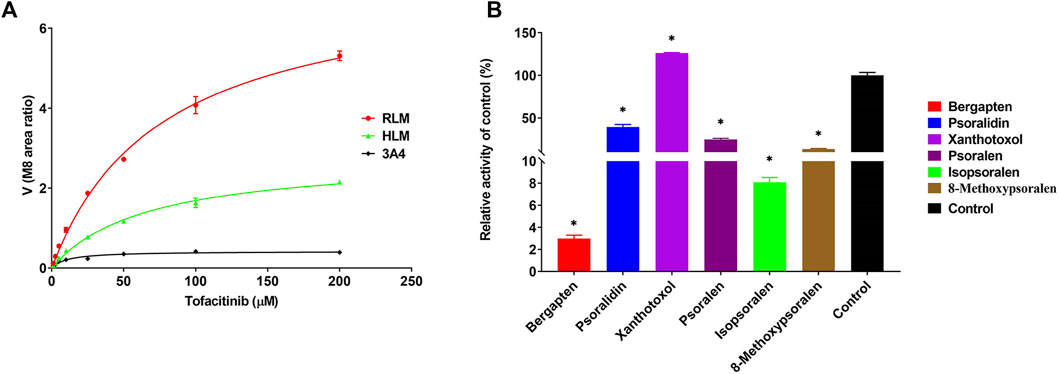
Figure 1. (A) Michaelis-Menten plots of enzymatic activity of RLMs, HLMs and rCYP3A4 on tofacitinib metabolism. Data are presented as the mean ± standard deviation of three experiments. (B) Screening of bergapten, psoralidin, xanthotoxol, psoralen, isopsoralen, and 8-methoxypsoralen in RLMs. Values are mean ± SD, n = 3. *p < 0.05, significant difference from control group.
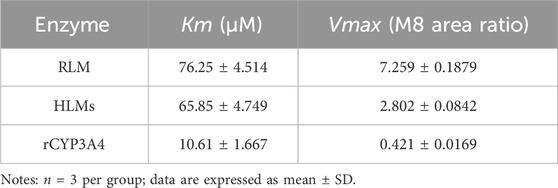
Table 1. Kinetic parameters for the activity of RLMs, HLMs, and rCYP3A4 variants on tofacitinib metabolism.
3.2 Bergapten potently inhibits the metabolism of tofacitinib in RLMs, HLMs, and rCYP3A4 with the competitive mechanism
Figure 2 shows IC50 curves and a Lineweaver-Burk diagram of tofacitinib inhibited by bergapten in RLMs, HLMs and rCYP3A4. As given in Table 2, the results displayed that tofacitinib was restrained by bergapten in RLMs, HLMs and rCYP3A4 with IC50 values of 2.619 μM and 2.647 μM, 1.424 μM, respectively. All Km and Vmax values of bergapten on tofacitinib metabolism in RLMs, HLMs and rCYP3A4 are shown in Table 3. The Ki values of bergapten in RLMs, HLMs and rCYP3A4 were 0.4429 μM, 0.1893 μM, and 1.223 μM, respectively. The dynamic parameters of competitive inhibition are characterized by the increase of Km value and no change of Vmax. The findings revealed that bergapten competitively inhibited the metabolism of tofacitinib in RLMs, HLMs, and rCYP3A4 (Figure 3).
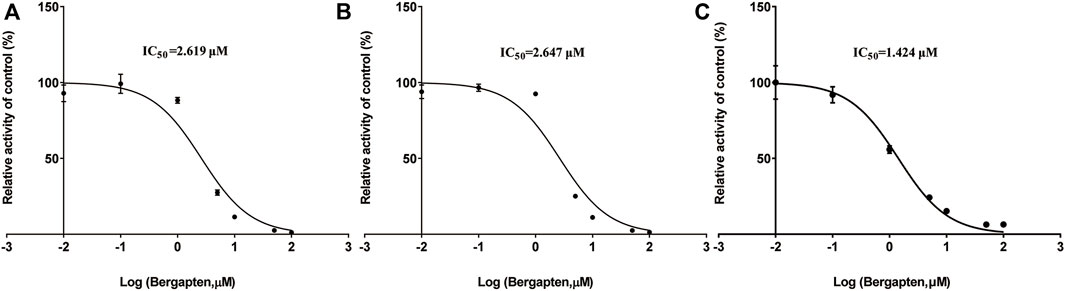
Figure 2. (A) IC50 of the inhibition of tofacitinib in RLMs by bergapten; (B) IC50 of the inhibition of tofacitinib in HLMs by bergapten; (C) IC50 of the inhibition of tofacitinib in rCYP3A4 by bergapten. Values are mean ± SD, n = 3.
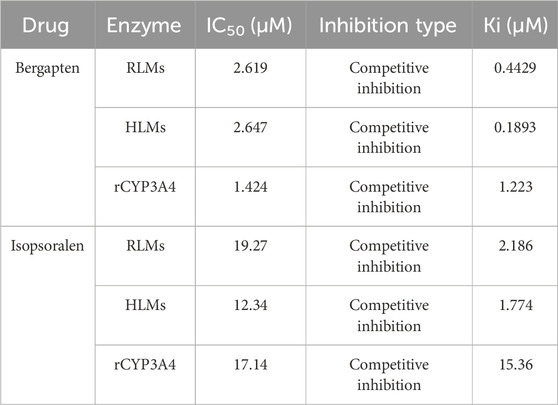
Table 2. The IC50 values and inhibitory effects of bergapten and isopsoralen on tofacitinib metabolism in RLMs, HLMs, and rCYP3A4.
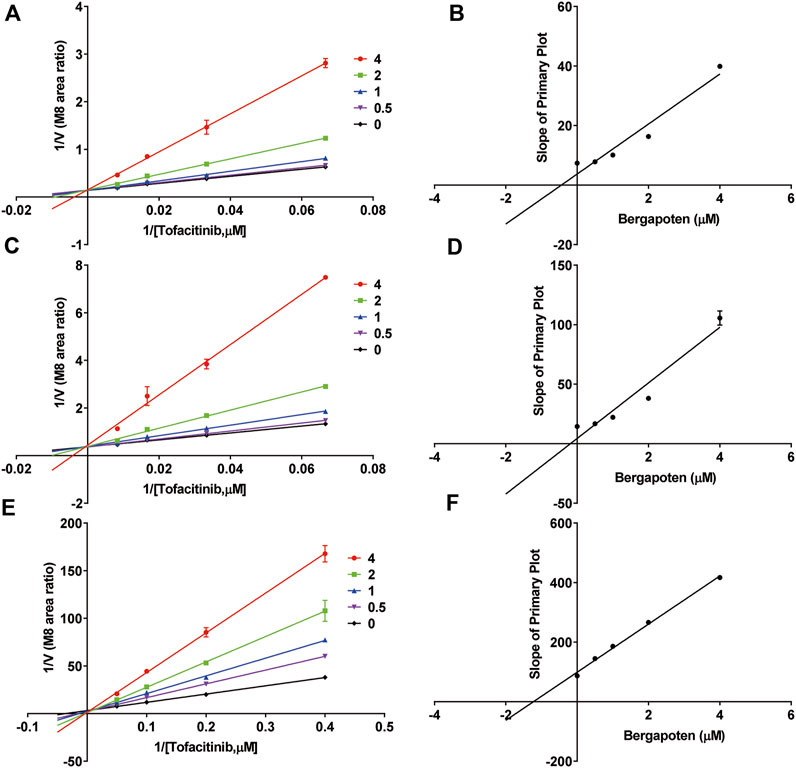
Figure 3. Inhibitory mechanism of bergapten against tofacitinib in (A,B) RLMs, (C,D) HLMs and (E,F) rCYP3A4. Values are mean ± SD, n = 3.
3.3 Ispsoralen potently blocks tofacitinib metabolism in RLMs, HLMs, and rCYP3A4 with the competitive mechanism
Figure 4; Table 2 exhibited the IC50 curves, Lineweaver-Burk plot and the IC50 and Ki values of tofacitinib metabolism by isopsoralen in RLMs, HLMs and rCYP3A4. The IC50 values of Isopsoralen were 12.34 μM and 17.17 μM, 19.27 μM. Table 4 shows Km and Vmax values of ispsoralen on tofacitinib metabolism in RLMs, HLMs and rCYP3A4. Isopsoralen exhibited competitive suppression of tofacitinib metabolism in RLMs, HLMs and rCYP3A4, with Ki values of 15.36 μM, 1.744 μM, and 2.186 μM (Figure 5).
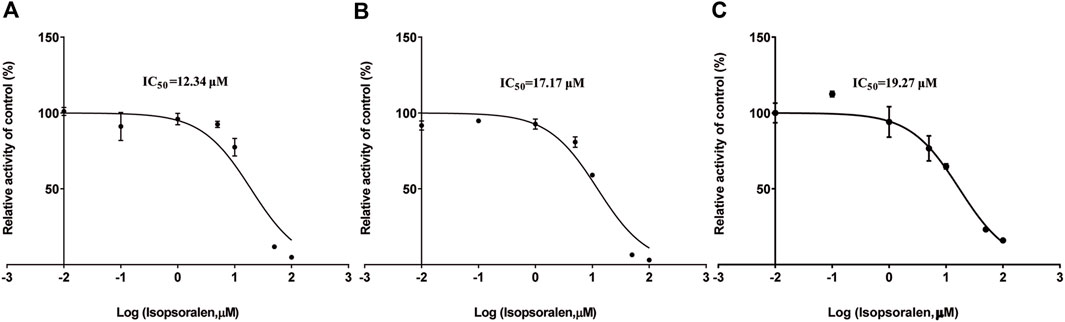
Figure 4. (A) IC50 of tofacitinib inhibition in RLMs by isopsoralen; (B) IC50 of tofacitinib inhibition in HLMs by isopsoralen; (C) IC50 of tofacitinib inhibition in rCYP3A4 by isopsoralen.
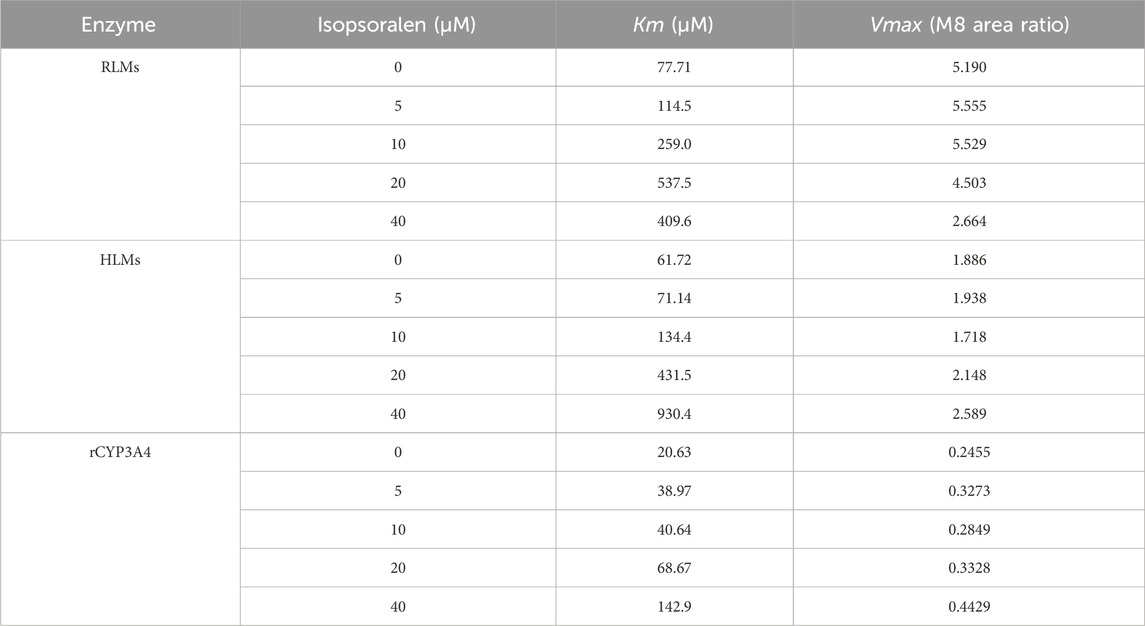
Table 4. The Km and Vmax values of isopsoralen on tofacitinib metabolism in RLMs, HLMs, and rCYP3A4.
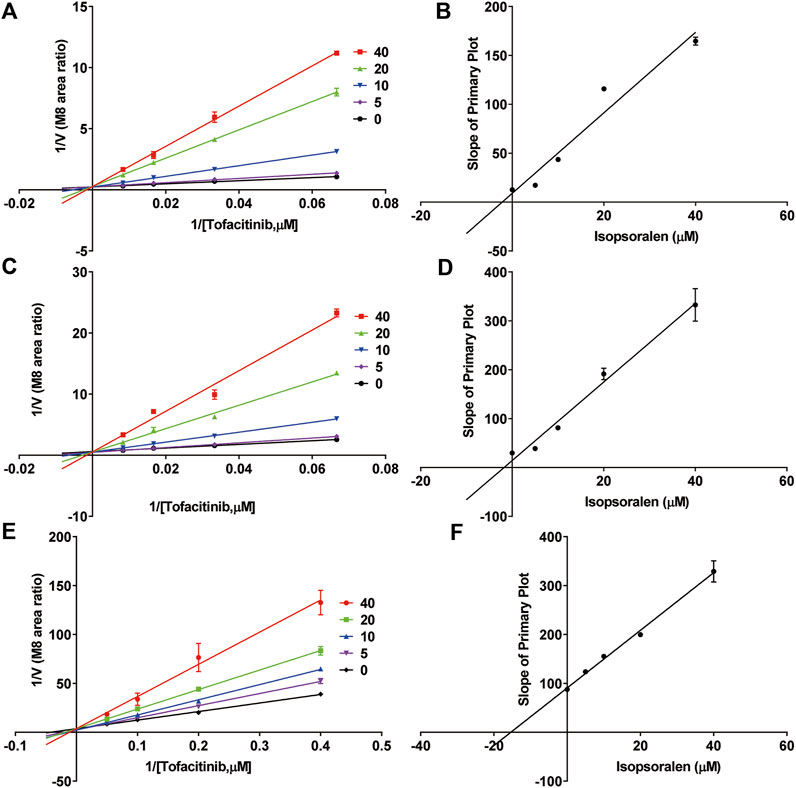
Figure 5. Inhibitory mechanism of isopsoralen against tofacitinib in (A,B) RLMs, (C,D) HLMs and (E,F) rCYP3A4. Values are mean ± SD, n = 3.
3.4 Molecular docking analysis of bergapten and isopsoralen on CYP3A4
The results of molecular docking and binding energy calculations are presented in Figure 6. Bergapten, isopsoralen and tofacitinib were well-docked at the active sites of CYP3A4. Molecular docking analysis has shown that tofacitinib, bergapten and isopsoralen establish hydrogen bond interactions with amino acid residues on CYP3A4 through hydrogen bonding. The CYP3A4 binding energies of tofacitinib, bergapten and isopsoralen were −6.8, −6.2, and −6.7 kcal/mol.
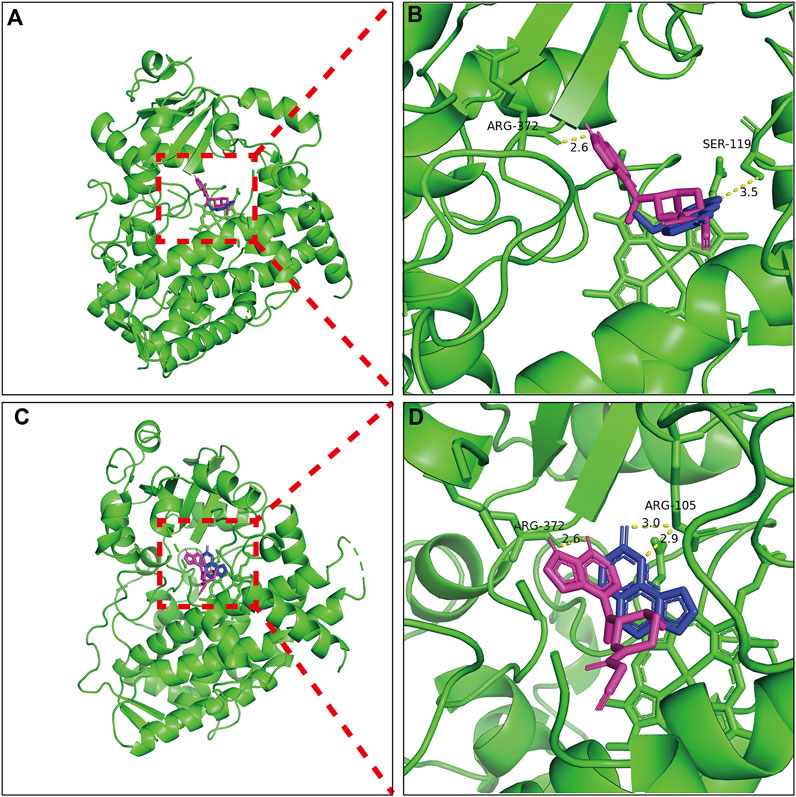
Figure 6. Molecular docking scheme of tofacitub and bergapten (A) and isopsoralen (C); Action site between bergapten (B), isopsoralen (D) and tofacitinib and CYP3A4 via hydrogen bonding.
3.5 Effects of BGZs on the pharmacokinetics of tofacitinib in vivo
Figures 7A, B showed the mean plasma concentration-time curve of the bergapten or isopsoralen treatment group or control group after oral supplementation of tofacitinib. The pharmacokinetic properties of tofacitinib were evaluated using a non-compartmental model, as presented in Tables 5, 6. We observed that tofacitinib was rapidly absorbed by the plasma and reached its maximum concentration of 509.99 mg/L at 0.5 h following oral administration. Compared with the control group, the AUC (0-t), AUC (0-∞), MRT (0-t), MRT (0-∞) and Cmax of tofatinib increased in varying degrees (all p < 0.05), but CLz/F decreased in varying degrees (p < 0.05) in the bergapten group and isopsoralen group in a dose-dependent manner. Intriguingly, there was no statistically significant difference in changes in t1/2z and Tmax (p > 0.05). Collectively, these findings indicated that bergapten and isopsoralen inhibited the metabolism of tofacitinib in a dose-dependent manner.
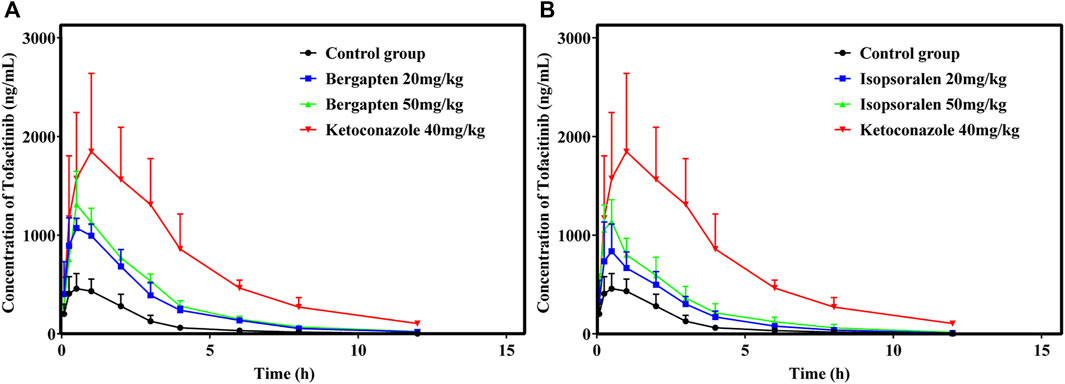
Figure 7. Mean plasma concentration–time curves of tofacitinib in different treatment groups. Group (A), bergapten; group (B), isopsoralen (n = 6).
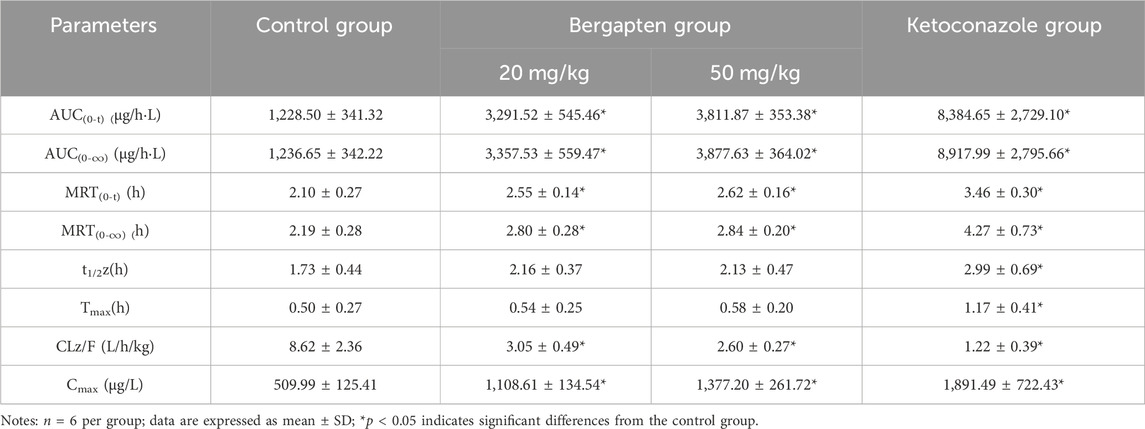
Table 5. Main pharmacokinetic parameters of tofacitinib in bergapten group and control group in rats.
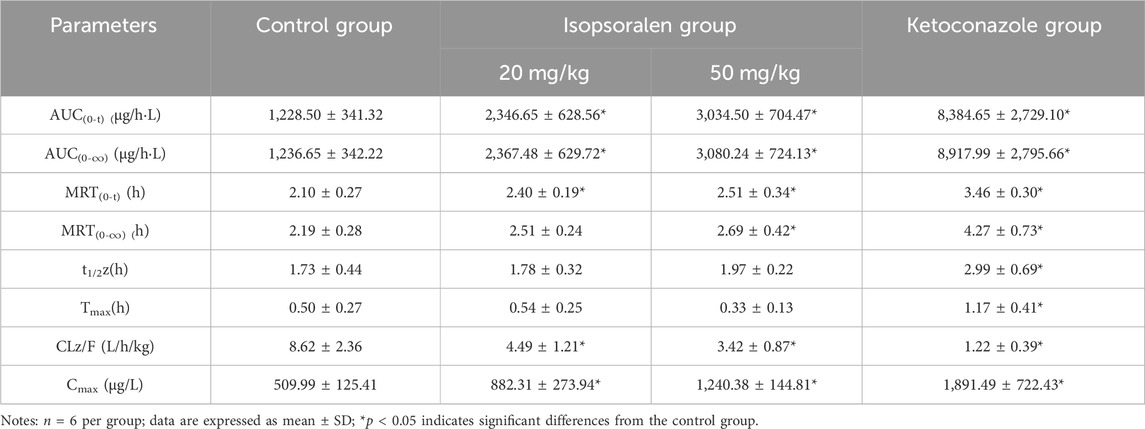
Table 6. Main pharmacokinetic parameters of tofacitinib in isopsoralen group and control group in rats.
4 Discussion
Tofacitinib is mainly metabolized by cytochrome P450 (CYP) 3A4 in the human body, followed by CYP2C19 metabolism (Veeravalli et al., 2020). Approximately 70% of tofacitinib is eliminated by a non-renal mechanism, whereas the remaining 30% is eliminated through the renal system (Bannwarth et al., 2013; Abdulrahim et al., 2019). Researchers found that the drug bergapten in P. corylifolia strongly blocks the activity of the CYP3A4 enzyme (Ho et al., 2001). Previous studies have found that isopsoralen can disrupt the activity of human recombinant CYP3A4 enzyme in vitro (Liu and Flynn, 2015; Hai et al., 2017). In this study, we measured the content of tofacitinib and its metabolite M8 to investigate the interaction of bergapten and isopsoralen with tofacitinib in rat liver microsomes, human liver microsomes and rCYP3A4. The results exhibited that bergapten and isopsoralen inhibited the metabolism of tofacitinib in rCYP 3A4, RLMs and HLMs. The relationship between substrates and inhibitors can be divided into three categories: competitive inhibition, non competitive inhibition, and uncompetitive inhibition. The dynamic parameters of competitive inhibition are characterized by the increase of Km value and no change of Vmax. Tofacitinib was shown to inhibit rCYP3A4, RLMs and HLMs competitively by bergapten and isopsoralen based on Km, Vmax and the Lineweaver-Burk plotting of double reciprocal plotting of the Mi equation. According to the literature, the risk of DDI is highest at ki value <1 µM, low at >50 µM and medium at 1–50 µM (Bjornsson et al., 2003a).Therefor, the risk of DDI between bergapten and tofacitinib was medium in 3A4 and high risk in RLMs and HLMs. In CYP3A4, RLMs and HLMs, the risk of DDI of isopsoralen and tofacitinib was medium.
The oral intervention of tofacitinib (10–100 mg/kg) to male Sprague-Dawley rats showed dose-dependent AUC above 50 mg/kg. Therefore, we selected 10 mg as the oral dose of tofacitinib (Lee and Kim, 2019). The ratio of AUCI/AUC greater than 2 indicates a high risk of DDI caused by drug interactions (Tucker et al., 2001; Bjornsson et al., 2003b). The AUCI/AUC ratios for bergapten at 20 mg/kg and 50 mg/kg were 2.7 and 3.1, respectively. Similarly, the AUCI/AUC ratios for isopsoralen were 1.91 and 2.49. Isopsoralen and bergapten increase tofacitinib-induced DDI risk. The AUC(0-∞) (p < 0.05), Cmax (p < 0.05) and MRT(0-∞) of tofacitinib were augmented and CLz/F (p < 0.05) was decreased in a dose-dependent manner after the oral intervention of bergapten and isopsoralen in vivo. Taken together, the results suggested that bergapten and isopsoralen might inhibit the metabolism of tofacitinib through CYP3A4 in vivo. And the risk of adverse reactions when bergapten or isopsoralen are combined with tofacitinib may increase due to an increase in the dosage of bergapten and isopsoralen.
Hydrogen bonding stabilizes energetically favorable ligands in protein structures. The interaction energy between target 3D structures and ligand molecules must be quantified to analyze the affinity of the binding (Patil et al., 2010). At present, it is generally believed that when the binding energy value is less than −5 kJ/mol, the binding between ligand and receptor is relatively stable, and the lower the binding energy values, the lower the conformational stability energy, and the greater the possibility of interaction between receptor and ligand. According to the formula 1 kJ = 0.24 kcal, the unit conversion between kcal/mol and kJ/mol shows that the binding energy of bergapten, isopsoralen, tofacitinib and CYP3A4 is less than −5 kJ/mol. The binding energy of bergapten, isopsoralen and tofacitinib is almost the same, which indicates that in the interaction with CYP3A4, bergapten and isopsoralen have similar stable binding ability to CYP3A4 as tofacitinib. In addition, according to the molecular docking results, it can be seen that the binding sites of bergapten and isopsoralen with CYP3A4 are similar to those of tofacitinib. This confirms the results of in vitro and in vivo studies that bergapten and isopsoralen may competitively inhibit tofacitinib metabolism in CYP3A4.
The dosage and metabolism of DDIs might produce adverse drug reactions. It is widely believed that drug metabolism plays an important role in drug safety. Therefore, it is necessary to evaluate the risk of adverse reactions between drugs due to dosage and metabolism. This study confirmed the inhibitory effect of bergapten and isopsoralen on the metabolism of tofacitinib in vitro and in vivo. The CYP450 enzyme inhibitors burgapten and isopsoralen increase blood tofacitinib levels in vivo. P. corylifolia possesses significant application potential owing to its abundant pharmacological action. This indicates that when we are using tofacitinib and P. corylifolia for combined treatment of rheumatoid arthritis, attention should be paid to the dosage of P. corylifolia intake.
Tofacitinib and bergapten/isopsoralen have significant interactions, which can be used to guide clinical medication and treatment in patients with RA. While further studies are required due to rat-human species differences.
5 Conclusion
DDIs may result in adverse drug reactions depending on dosage and metabolism. Drug metabolism is a crucial factor in maintaining drug toxicity. This study confirmed the inhibitory effect of bergapten and isopsoralen on CYP3A4 in vitro and vivo. We found that bergapten and isopsoralen exhibited distinct regulatory impacts on the metabolism of tofacitinib in RLM, HLM and CYP3A4. Our results also revealed that berkatten and isopsoralen inhibited CYP450 enzymes to enhance blood tofacitinib levels in vivo. Tofacitinib exposure may lead to more severe and frequent adverse events. Therefore, when using tofacitinib to treat rheumatoid arthritis, it is necessary to pay attention to or adjust the dose of Chinese herbs containing bergapten and isopsoralen, so as to reduce the occurrence of adverse reactions caused by the increase of tofacitinib blood concentration. This study provides a theoretic basis for the combination of P. corylifolia and tofacitinib. In addition to P. corylifolia, there are also many other traditional Chinese medicines that contain bergapten and isopsoralen, such as Coastal Glehnia Root and Saposhnikoviae Radiox.This study also provides significance for combining use of tofacitinib and traditional Chinese medicine containing this type of coumarin in the treatment of diseases.
Data availability statement
The original contributions presented in the study are included in the article/Supplementary material, further inquiries can be directed to the corresponding authors.
Ethics statement
The animal study was approved by the animal ethics committee of Wenzhou Medical University. The study was conducted in accordance with the local legislation and institutional requirements.
Author contributions
YW: Conceptualization, Writing–original draft. QZ: Methodology, Supervision, Visualization, Writing–review and editing. HW: Data curation, Formal Analysis, Methodology, Writing–original draft. WS: Methodology, Writing–original draft. JW: Formal Analysis, Visualization, Writing–original draft. AM: Writing–review and editing. PG: Investigation, Software, Validation, Writing–original draft. YZ: Funding acquisition, Writing–review and editing. SW: Conceptualization, Investigation, Resources, Validation, Writing–review and editing.
Funding
The author(s) declare that financial support was received for the research, authorship, and/or publication of this article. This work was supported by the Public Welfare Technology Research Funding Project of Zhejiang (LTGY24H100002 and LTGY23H160034), Public Welfare Technology Research Funding Project of Lishui (2021SJZC022, 2020GYX18, 2022SJZC074, 2022SJZC079 and 2020GYX23) and Key Research and Development Project of Lishui (2021ZDYF13, 2021ZDYF15, and 2022ZDYF23).
Conflict of interest
The authors declare that the research was conducted in the absence of any commercial or financial relationships that could be construed as a potential conflict of interest.
Publisher’s note
All claims expressed in this article are solely those of the authors and do not necessarily represent those of their affiliated organizations, or those of the publisher, the editors and the reviewers. Any product that may be evaluated in this article, or claim that may be made by its manufacturer, is not guaranteed or endorsed by the publisher.
References
Abdulrahim, H., Sharlala, H., and Adebajo, A. O. (2019). An evaluation of tofacitinib for the treatment of psoriatic arthritis. Expert Opin. Pharmacother. 20 (16), 1953–1960. doi:10.1080/14656566.2019.1657404
Alam, F., Khan, G. N., and Asad, M. (2018). Psoralea corylifolia L: ethnobotanical, biological, and chemical aspects: a review. Phytother. Res. 32 (4), 597–615. doi:10.1002/ptr.6006
Aronson, J. K. (2004). Classifying drug interactions. Br. J. Clin. Pharmacol. 58 (4), 343–344. doi:10.1111/j.1365-2125.2004.02244.x
Bannwarth, B., Kostine, M., and Poursac, N. (2013). A pharmacokinetic and clinical assessment of tofacitinib for the treatment of rheumatoid arthritis. Expert Opin. Drug Metab. Toxicol. 9 (6), 753–761. doi:10.1517/17425255.2013.789500
Beaugerie, L., Rahier, J. F., and Kirchgesner, J. (2020). Predicting, preventing, and managing treatment-related complications in patients with inflammatory bowel diseases. Clin. Gastroenterol. Hepatol. 18 (6), 1324–1335. doi:10.1016/j.cgh.2020.02.009
Bjornsson, T. D., Callaghan, J. T., Einolf, H. J., Fischer, V., Gan, L., Grimm, S., et al. (2003a). The conduct of in vitro and in vivo drug-drug interaction studies: a Pharmaceutical Research and Manufacturers of America (PhRMA) perspective. Drug Metab. Dispos. 31 (7), 815–832. doi:10.1124/dmd.31.7.815
Bjornsson, T. D., Callaghan, J. T., Einolf, H. J., Fischer, V., Gan, L., Grimm, S., et al. (2003b). The conduct of in vitro and in vivo drug-drug interaction studies: a PhRMA perspective. J. Clin. Pharmacol. 43 (5), 443–469. doi:10.1177/0091270003043005001
Charles-Schoeman, C., Buch, M. H., Dougados, M., Bhatt, D. L., Giles, J. T., Ytterberg, S. R., et al. (2023). Risk of major adverse cardiovascular events with tofacitinib versus tumour necrosis factor inhibitors in patients with rheumatoid arthritis with or without a history of atherosclerotic cardiovascular disease: a post hoc analysis from ORAL Surveillance. Ann. Rheum. Dis. 82 (1), 119–129. doi:10.1136/ard-2022-222259
Chen, L., Chen, S., Sun, P., Liu, X., Zhan, Z., and Wang, J. (2023). Psoralea corylifolia L.: a comprehensive review of its botany, traditional uses, phytochemistry, pharmacology, toxicology, quality control and pharmacokinetics. Chin. Med. 18 (1), 4. doi:10.1186/s13020-022-00704-6
Cohen, S. B., Tanaka, Y., Mariette, X., Curtis, J. R., Lee, E. B., Nash, P., et al. (2020). Long-term safety of tofacitinib up to 9.5 years: a comprehensive integrated analysis of the rheumatoid arthritis clinical development programme. RMD Open 6 (3), e001395. doi:10.1136/rmdopen-2020-001395
Conaghan, P. G., Østergaard, M., Bowes, M. A., Wu, C., Fuerst, T., van der Heijde, D., et al. (2016). Comparing the effects of tofacitinib, methotrexate and the combination, on bone marrow oedema, synovitis and bone erosion in methotrexate-naive, early active rheumatoid arthritis: results of an exploratory randomised MRI study incorporating semiquantitative and quantitative techniques. Ann. Rheum. Dis. 75 (6), 1024–1033. doi:10.1136/annrheumdis-2015-208267
Curtis, J. R., Yamaoka, K., Chen, Y. H., Bhatt, D. L., Gunay, L. M., Sugiyama, N., et al. (2023). Malignancy risk with tofacitinib versus TNF inhibitors in rheumatoid arthritis: results from the open-label, randomised controlled ORAL Surveillance trial. Ann. Rheum. Dis. 82 (3), 331–343. doi:10.1136/ard-2022-222543
Day, R. O., Snowden, L., and McLachlan, A. J. (2017). Life-threatening drug interactions: what the physician needs to know. Intern Med. J. 47 (5), 501–512. doi:10.1111/imj.13404
Dhillon, S. (2017). Tofacitinib: a review in rheumatoid arthritis. Drugs 77 (18), 1987–2001. doi:10.1007/s40265-017-0835-9
Hai, Y., Feng, S., Wang, L., Ma, Y., Zhai, Y., Wu, Z., et al. (2017). Coordination mechanism and bio-evidence: reactive γ-ketoenal intermediated hepatotoxicity of psoralen and isopsoralen based on computer approach and bioassay. Molecules 22 (9), 1451. doi:10.3390/molecules22091451
Han, Y., Wang, J., Li, S., Li, Y., Zhang, Y., Zhang, R., et al. (2021). Isopsoralen ameliorates rheumatoid arthritis by targeting MIF. Arthritis Res. Ther. 23 (1), 243. doi:10.1186/s13075-021-02619-3
Hardman, J. G., Limbird, L. E., and Goodman, A. G. (2001). Goodman and Gilman’s the pharmacological basis of therapeutics. [M].
Ho, P. C., Saville, D. J., and Wanwimolruk, S. (2001). Inhibition of human CYP3A4 activity by grapefruit flavonoids, furanocoumarins and related compounds. J. Pharm. Pharm. Sci. 4 (3), 217–227.
Karaman, M. W., Herrgard, S., Treiber, D. K., Gallant, P., Atteridge, C. E., Campbell, B. T., et al. (2008). A quantitative analysis of kinase inhibitor selectivity. Nat. Biotechnol. 26 (1), 127–132. doi:10.1038/nbt1358
Kong, L., Ma, R., Yang, X., Zhu, Z., Guo, H., He, B., et al. (2017). Psoralidin suppresses osteoclastogenesis in BMMs and attenuates LPS-mediated osteolysis by inhibiting inflammatory cytokines. Int. Immunopharmacol. 51, 31–39. doi:10.1016/j.intimp.2017.07.003
Lee, J. S., and Kim, S. H. (2019). Dose-dependent pharmacokinetics of tofacitinib in rats: influence of hepatic and intestinal first-pass metabolism. Pharmaceutics 11 (7), 318. doi:10.3390/pharmaceutics11070318
Liang, Y., Xie, L., Liu, K., Cao, Y., Dai, X., Wang, X., et al. (2021). Bergapten: a review of its pharmacology, pharmacokinetics, and toxicity. Phytother. Res. 35 (11), 6131–6147. doi:10.1002/ptr.7221
Lin, J. H. (2006). CYP induction-mediated drug interactions: in vitro assessment and clinical implications. Pharm. Res. 23 (6), 1089–1116. doi:10.1007/s11095-006-0277-7
Lin, J. H., and Lu, A. Y. (1998). Inhibition and induction of cytochrome P450 and the clinical implications. Clin. Pharmacokinet. 35 (5), 361–390. doi:10.2165/00003088-199835050-00003
Liu, Y., and Flynn, T. J. (2015). CYP3A4 inhibition by Psoralea corylifolia and its major components in human recombinant enzyme, differentiated human hepatoma HuH-7 and HepaRG cells. Toxicol. Rep. 2, 530–534. doi:10.1016/j.toxrep.2015.03.006
Mathews, D. R., McNutt, B., Okerholm, R., Flicker, M., and McBride, G. (1991). Torsades de pointes occurring in association with terfenadine use. Jama 266 (17), 2375–2376. doi:10.1001/jama.1991.03470170063023
Meyer, D. M., Jesson, M. I., Li, X., Elrick, M. M., Funckes-Shippy, C. L., Warner, J. D., et al. (2010). Anti-inflammatory activity and neutrophil reductions mediated by the JAK1/JAK3 inhibitor, CP-690,550, in rat adjuvant-induced arthritis. J. Inflamm. (Lond) 7, 41. doi:10.1186/1476-9255-7-41
Moriana, C., Moulinet, T., Jaussaud, R., and Decker, P. (2022). JAK inhibitors and systemic sclerosis: a systematic review of the literature. Autoimmun. Rev. 21 (10), 103168. doi:10.1016/j.autrev.2022.103168
Nijsten, T. E., and Stern, R. S. (2003). The increased risk of skin cancer is persistent after discontinuation of psoralen+ultraviolet A: a cohort study. J. Invest. Dermatol 121 (2), 252–258. doi:10.1046/j.1523-1747.2003.12350.x
Ohnishi, A., Matsuo, H., Yamada, S., Takanaga, H., Morimoto, S., Shoyama, Y., et al. (2000). Effect of furanocoumarin derivatives in grapefruit juice on the uptake of vinblastine by Caco-2 cells and on the activity of cytochrome P450 3A4. Br. J. Pharmacol. 130 (6), 1369–1377. doi:10.1038/sj.bjp.0703433
Pai, F. T., Lu, C. Y., Lin, C. H., Wang, J., Huang, M. C., Liu, C. T., et al. (2021). Psoralea corylifolia L. Ameliorates collagen-induced arthritis by reducing proinflammatory cytokines and upregulating myeloid-derived suppressor cells. Life (Basel) 11 (6), 587. doi:10.3390/life11060587
Palasik, B. N., and Wang, H. (2021). Tofacitinib, the First oral janus kinase inhibitor approved for adult ulcerative colitis. J. Pharm. Pract. 34 (6), 913–921. doi:10.1177/0897190020953019
Patil, R., Das, S., Stanley, A., Yadav, L., Sudhakar, A., and Varma, A. K. (2010). Optimized hydrophobic interactions and hydrogen bonding at the target-ligand interface leads the pathways of drug-designing. PLoS One 5 (8), e12029. doi:10.1371/journal.pone.0012029
Sabers, A. (2008). Pharmacokinetic interactions between contraceptives and antiepileptic drugs. Seizure 17 (2), 141–144. doi:10.1016/j.seizure.2007.11.012
Serhal, L., and Edwards, C. J. (2019). Upadacitinib for the treatment of rheumatoid arthritis. Expert Rev. Clin. Immunol. 15 (1), 13–25. doi:10.1080/1744666x.2019.1544892
Sharifi-Rad, J., Cruz-Martins, N., López-Jornet, P., Lopez, E. P., Harun, N., Yeskaliyeva, B., et al. (2021). Natural coumarins: exploring the pharmacological complexity and underlying molecular mechanisms. Oxid. Med. Cell Longev. 2021, 6492346. doi:10.1155/2021/6492346
Stern, R. S. (2012). The risk of squamous cell and basal cell cancer associated with psoralen and ultraviolet A therapy: a 30-year prospective study. J. Am. Acad. Dermatol 66 (4), 553–562. doi:10.1016/j.jaad.2011.04.004
Tucker, G. T., Houston, J. B., and Huang, S. M. (2001). Optimizing drug development: strategies to assess drug metabolism/transporter interaction potential-toward a consensus. Clin. Pharmacol. Ther. 70 (2), 103–114. doi:10.1067/mcp.2001.116891
Veeravalli, V., Dash, R. P., Thomas, J. A., Babu, R. J., Madgula, L. M. V., and Srinivas, N. R. (2020). Critical assessment of pharmacokinetic drug-drug interaction potential of tofacitinib, baricitinib and upadacitinib, the three approved janus kinase inhibitors for rheumatoid arthritis treatment. Drug Saf. 43 (8), 711–725. doi:10.1007/s40264-020-00938-z
Vyas, D., O'Dell, K. M., Bandy, J. L., and Boyce, E. G. (2013). Tofacitinib: the First Janus Kinase (JAK) inhibitor for the treatment of rheumatoid arthritis. Ann. Pharmacother. 47 (11), 1524–1531. doi:10.1177/1060028013512790
Wang, C., Al-Ani, M. K., Sha, Y., Chi, Q., Dong, N., Yang, L., et al. (2019). Psoralen protects chondrocytes, exhibits anti-inflammatory effects on synoviocytes, and attenuates monosodium iodoacetate-induced osteoarthritis. Int. J. Biol. Sci. 15 (1), 229–238. doi:10.7150/ijbs.28830
Wang, S., Du, Q., Sun, J., Geng, S., and Zhang, Y. (2022). Investigation of the mechanism of Isobavachalcone in treating rheumatoid arthritis through a combination strategy of network pharmacology and experimental verification. J. Ethnopharmacol. 294, 115342. doi:10.1016/j.jep.2022.115342
Ye, Z., Hu, J., Wang, J., Liu, Y. N., Hu, G. X., and Xu, R. A. (2023). The effect of Resveratrol on the pharmacokinetic profile of tofacitinib and the underlying mechanism. Chem. Biol. Interact. 374, 110398. doi:10.1016/j.cbi.2023.110398
Yeo, U. C., Shin, J. H., Yang, J. M., Park, K. B., Kim, M. M., Bok, H. S., et al. (2000). Psoralen-ultraviolet A-induced erythema: sensitivity correlates with the concentrations of psoralen in suction blister fluid. Br. J. Dermatol 142 (4), 733–739. doi:10.1046/j.1365-2133.2000.03419.x
Zhang, T., Leng, J., and Liu, Y. (2020). Deep learning for drug-drug interaction extraction from the literature: a review. Brief. Bioinform 21 (5), 1609–1627. doi:10.1093/bib/bbz087
Zhou, S. F. (2008). Drugs behave as substrates, inhibitors and inducers of human cytochrome P450 3A4. Curr. Drug Metab. 9 (4), 310–322. doi:10.2174/138920008784220664
Keywords: P. corylifolia, drug-drug interaction, cytochrome P450, pharmacokinetics, molecular docking
Citation: Wang Y, Zhou Q, Wang H, Song W, Wang J, Mamun AA, Geng P, Zhou Y and Wang S (2024) Effect of P. corylifolia on the pharmacokinetic profile of tofacitinib and the underlying mechanism. Front. Pharmacol. 15:1351882. doi: 10.3389/fphar.2024.1351882
Received: 07 December 2023; Accepted: 19 March 2024;
Published: 08 April 2024.
Edited by:
Rong Wang, People’s Liberation Army Joint Logistics Support Force 940th Hospital, ChinaCopyright © 2024 Wang, Zhou, Wang, Song, Wang, Mamun, Geng, Zhou and Wang. This is an open-access article distributed under the terms of the Creative Commons Attribution License (CC BY). The use, distribution or reproduction in other forums is permitted, provided the original author(s) and the copyright owner(s) are credited and that the original publication in this journal is cited, in accordance with accepted academic practice. No use, distribution or reproduction is permitted which does not comply with these terms.
*Correspondence: Shuanghu Wang, d2FuZ3NodWFuZ2h1QGxzdS5lZHUuY24=; Yunfang Zhou, enlmMjgwOEBsc3UuZWR1LmNu
†These authors have contributed equally to this work