- 1Guangzhou Municipal and Guangdong Provincial Key Laboratory of Molecular Target & Clinical Pharmacology, The NMPA and State Key Laboratory of Respiratory Disease, School of Pharmaceutical Sciences and the Fifth Affiliated Hospital, Guangzhou Medical University, Guangzhou, China
- 2School of Chinese Medicine, Hong Kong Baptist University, Kowloon, Hong Kong SAR, China
- 3School of Pharmacy, Mongolian National University of Medical Sciences, Ulaanbaatar, Mongolia
- 4Deraya Center for Scientific Research, Deraya University, New Minia, Egypt
- 5Department of Pharmacognosy, Faculty of Pharmacy, Minia University, Minia, Egypt
- 6Ministry of Education Engineering Research Center of Tibetan Medicine Detection Technology, Xizang Minzu University, Xianyang, China
- 7The Affiliated TCM Hospital, Guangzhou Medical University, Guangzhou, China
- 8The Affiliated Qingyuan Hospital, Guangzhou Medical University, Qingyuan, China
Cancer stands as a prominent global cause of death. One of the key reasons why clinical tumor chemotherapy fails is multidrug resistance (MDR). In recent decades, accumulated studies have shown how Natural Product-Derived Compounds can reverse tumor MDR. Discovering novel potential modulators to reduce tumor MDR by Natural Product-Derived Compounds has become a popular research area across the globe. Numerous studies mainly focus on natural products including flavonoids, alkaloids, terpenoids, polyphenols and coumarins for their MDR modulatory activity. Natural products reverse MDR by regulating signaling pathways or the relevant expressed protein or gene. Here we perform a deep review of the previous achievements, recent advances in the development of natural products as a treatment for MDR. This review aims to provide some insights for the study of multidrug resistance of natural products.
1 Introduction
According to the World Health Organization (WHO) and International Agency for Cancer Research, there were approximately 19.3 million new cases of cancer in the past (Rudnicka et al., 2020), along with around 10 million cancer-related deaths (Sung et al., 2021). Cancer was one of the leading causes of mortality worldwide, and there were likely 28.4 million new cases by the year 2040 (Siegel et al., 2023). Chemotherapy could be confidently recommended for first-line treatment. MDR was a phenomenon in which cancer cells acquired cross-resistance to structurally and functionally related different types of anticancer drugs. However, the emergence of drug resistance made chemotherapy much less effective (Lu et al., 2022). Scientists have shown an interest in natural products (Zhang et al., 2020) because of their superiority in terms of rich resources, lack of negative side effects, and variety of components. Various biological actions are demonstrated by natural compounds, such as anti-tumor (Mans et al., 2000), anti-microbial (Chakrawarti et al., 2016; Martinelli et al., 2021; Papadochristopoulos et al., 2021), antioxidant (Arulselvan et al., 2016; Huang et al., 2017), anti-inflammatory (Arulselvan et al., 2016; Huang et al., 2017), anti-diabetic (Chen W. et al., 2019; Luo et al., 2019), anti-hypertensive (Fang et al., 2021; Gupta et al., 2022), anti-atherogenic (Penson and Banach, 2021), gastro-protective (Tamaddonfard et al., 2019), anti-platelets (Iqbal et al., 2022), anti-thrombotic (Csikós et al., 2021; Junren et al., 2021; Yen et al., 2022) vital effects on reversing MDR (Guo et al., 2017; Wang et al., 2018; Zhao et al., 2020).
The application of natural products in MDR has received much attention recently. This review examines the scientific advances on using natural products to treat tumors with MDR. We discuss the mechanisms of action of various kinds of natural products, including as flavonoids (Vissenaekens et al., 2022), alkaloids (Liu C. et al., 2019), terpenoids (Kuang et al., 2021), polyphenols (Luca et al., 2020), and coumarins (Xiaokaiti and Li, 2020; Giovannuzzi et al., 2022).
2 The mechanism of MDR
MDR refers to a cancer cell’s sensitivity to various anti-cancer medication therapies (Assaraf et al., 2019). Nevertheless, setting out the factors related to MDR could benefit the administration of antitumor drugs and curation (Lu et al., 2022). This paper describes the mechanisms of MDR, such as increasing drug efflux, altering drug targets, increasing DNA damage repair, MDR-related factors or signaling pathways, non-coding RNA (ncRNA)-mediated multidrug resistance, and autophagy and tumor microenvironment effects.
2.1 Increasing of drug efflux
Cancer cells exhibiting MDR typically increase the efflux of drug molecules, which reduces the chemosensitivity to anticancer medicines (Mohammad et al., 2018; Abdelfatah et al., 2021). Some genes associated with multidrug resistance in cancer are very unstable. For example, the CDK6 and CDK6-PI3K axes act synergistically in regulating ABCB1/P-gp-mediated expression of MDR (Zhang L. et al., 2022). It has been noted that long-term use of chemotherapy medicines increases the expression of the ABC transporter, thereby raising the risk of MDR. The ABC transporter by ATP hydrolysis move substrates outward against a concentration gradient (Jacobo-Albavera et al., 2021). For this reason, ATP-binding cassette sub-family B member 1, also known as P-glycoprotein (ABCB1/P-gp), ATP-binding cassette sub-family C member 1/Multidrug resistance-associated proteins (ABCC1/MRPs), and ATP-binding cassette sub-family G member 2/Breast cancer resistance protein (ABCG2/BCRP) are the most important drug transporters.
P-gp is present in both healthy and malignant cells (Sarkadi et al., 2006). The transmembrane structural domain and the nucleotide-binding domain are the two primary structural domains that make up the P-gp transporter. The ABC protein’s structure may change upon binding to ATP, leading to concurrent change in the substrate binding site, which causes the substrate drug to be expelled from the cell. Different chemotherapeutic medicines bind to P-gp, which modifies the structure of P-gp and releases the drug into the extracellular space as ATP is digested (Assaraf et al., 2019) (Figure 1).
In various cancer types, such as lung, breast and prostate cancer, the expression of MRPs and BCRPs leads to resistance drugs (Ambudkar et al., 2003; Goel and Aggarwal, 2010). MRPs have the same structure and function as P-gp, but MRPs only transport medicines that have undergone glutathione (GSH) modification, and they have an impact on when pharmaceuticals are metabolically activated (Liu, 2009). Drugs are effluxed after MRPs create a complex with glutathione, glucuronate, or sulfate. Etoposide, doxorubicin (Dox), vincristine (VCR), and epirubicin (EPI) are among the drugs that are susceptible to MRPs (Liu, 2009). BCRP structure is homodimeric formed two-half transporters joined by a disulfide bond (Figure 1). Such drugs as mitoxantrone, topotecan, Dox, irinotecan, EPI, and flavopiridol are sensitive to BCRP (Ambudkar et al., 2003; Robey et al., 2007).
2.2 Altering the drug targets
Creizotinib resistance in lung adenocarcinoma, caused by an acquired mutation in the glycine-to-arginine substitution at codon 2032 in the structural domain of reactive oxygen species (ROS) proto-oncogene 1 (ROS1) kinase (Awad et al., 2013). Similarly, secondary EGFR mutations in the outer structural domain S492R lead to cetuximab resistance by preventing EGFR antibodies from binding to their target sites in colon cancer (Montagut et al., 2012) (Figure 1).
2.3 Increase in DNA damage repair
A major cause of tumor progression, is persistent genetic mutations have been occurring in the genome of cancer cells. The DNA damage response (DDR) is essential to protect cells from the large amount of oxidative damage to which they are periodically subjected by cellular damage (Groelly et al., 2023). DDR and cell cycle checkpoints are interlock signaling networks that can impede the cell cycle and transmission of genetic information to daughter cells to ensure genomic integrity (Maleki Dana et al., 2022). Cell cycle checkpoints enable the orderly progression of cell cycle events and avoid the development of genomic instability-related diseases such as cancer.
The G1 checkpoint is dysregulated in the majority of cancer cells, making them reliant on the S and G2 checkpoints, specifically ATR-CHK1. Cancer enhance their DNA repair system and promote survival by activating the ATR-CHK1 pathway. Chemotherapeutic drugs that target replicating DNA cells activate the ATR-CHK1 pathway (da Costa et al., 2023). Additionally, inhibitors of ATR-CHK1 have been reported to decrease the levels of P-gp (Ahmed et al., 2022).
Natural products, including curcumin, mangostin, resveratrol, and carnosine, block the G1-S phase but not affect the cell division cycle of proliferating healthy cells. Studies have shown that resveratrol, mangostin, and carnosine are agonists of ATR-CHK1, and kaempferol, curcumin, raffinose, and caffeine are inhibitors of ATR-CHK1, and these phytochemicals may help overcome tumor resistance (Ahmed et al., 2022). PU-1 is a sesquiterpenoid derived from Asteraceae that inhibits the growth of drug-resistant tumor cells through DNA damage, G2/M cell cycle blockade and apoptosis (Hegazy et al., 2021) (Figure 1).
2.4 MDR-related factors or signaling pathways
Some transcription factors aid in the growth and resistance to chemotherapy of drug-resistant cancer cells. The transcription factor Nrf2 aids to the resistance to chemotherapy and to proliferation of cancer cells by control the expression of cytoprotective and antioxidant enzymes (Ghareghomi et al., 2022; Panieri et al., 2022). Another important Five transcription factors that make up NF-κB can attach to responsive gene promoter regions and control biological activities such DNA transcription, cytokine generation, and cell survival. Apoptosis suppression, tumor cell proliferation, and treatment resistance are all caused by activated NF-κB. Numerous cancer forms exhibit abnormal factor regulation, which is exacerbated by the presence of the majority of anticancer medications in the cancer cells (Vlahopoulos, 2017; Xia et al., 2018) (Figure 1).
2.5 Non-coding RNA (ncRNA)-mediated multidrug resistance
Almost all cell processes, including transcription, proliferation, apoptosis, and differentiation, have been shown to be significantly regulated by non-coding RNAs (ncRNAs), particularly microRNA (Zou et al., 2017; Yang et al., 2021), long non-coding RNA (lncRNA) (Singh et al., 2022), and circular RNA. They also play a significant role in the regulation of cancer drug resistance (Leng et al., 2022). The expression of specific target genes, including Bcl-2, MDR1/ABCB1, and MRP1/ABCC1, which regulate apoptosis, autophagy, drug efflux, epithelial to mesenchymal transition (EMT), and cancer stem cells (CSCs), leads to the development of MDR. The interaction of ncRNAs with DNA, RNA, and proteins is the foundation for all of these mechanisms (Zhou et al., 2022a; Li et al., 2022) (Figure 1).
2.6 Autophagy and tumor microenvironment effects
2.6.1 Autophagy
Autophagy breaks down damaged organelles and stops cells from accumulating too many anticancer drugs, which encourages the growth of multidrug resistance in cancer cells (Chang and Zou, 2020). However, autophagy in the cellular surroundings can also eliminate MDR tumor cells, thereby enhancing the efficacy of chemotherapy. This process is regulated by various autophagy-related pathways and components, including ATG16L1, ATG5, the PI3K/AKT/mTOR pathway, AMP-activated protein kinase (AMPK), the miR199a-5p/p62 axis, p53, TFEB, and NSAIDs (Debnath et al., 2023). The activation of autophagy facilitates the removal of drug-resistant tumor cells, thus improving the overall response to chemotherapy (Li et al., 2017; Rakesh et al., 2022; Xing et al., 2022) (Figure 1).
2.6.2 Tumor microenvironment (TME)
Low extracellular pH, increased ROS concentrations, hypoxia, and the overexpression of certain proteases and factors are TME characteristics (Barkley et al., 2022). TME has its own blood supply, lymphatic and neurological systems, stroma, immune cells, and Extracellular Matrix (ECM) for each person with a specific tumor (Tiwari et al., 2022) (Figure 1).
Under hypoxic conditions, TME demonstrates chemoresistance and reduces drug-induced cytotoxicity, which encourages cancer growth and spread. For optimum activity, several anticancer medications like Cisplatin (DDP), etoposide, and gemcitabine require oxygen (Zhang W. et al., 2022). A key factor in hypoxia-induced chemoresistance is the HIF protein. Under hypoxic conditions, Hif-1α encodes P-gp and increases the expression of MRP1, BCRP, and LRP. Additionally, Hif-1α supports DNA repair processes and inhibits the effects of chemotherapeutic drugs (Emran et al., 2022).
Another important component of MDR is the control of pH. According to research, MCF-7 cells are more resistant to the effects of chemotherapeutic medicines when the extracellular pH is lower (Tavares-Valente et al., 2013). The ATP-rich tumor microenvironment is associated with cancer drug resistance, and cancer cells are able to take up extracellular ATP (eATP) through macrocytosis to increase intracellular ATP (iATP) levels and enhance drug resistance. Elevated iATP upregulated ABC transporter efflux activity in A549 and SK-Hep-1 cells, as well as PDGFRα and protein phosphorylation in the PDGFR-mediated Akt-mTOR and Raf-MEK signaling pathways in A549 cells (Wang et al., 2017).
3 Natural product—derived compounds overcome cancer drug resistance mechanisms
Based on various studies, natural compounds do work as multifunctional agents that can control the main causes of MDR. Below, we outline how certain natural compounds, such as flavonoids, alkaloids, terpenoids, polyphenols, and coumarins, can contribute to reducing MDR.
3.1 Flavonoids
In many different sections of plants, the most prevalent and significant secondary metabolites are flavonoids. Flavonoids, iso-flavonoids, and neo-flavonoids are the three types of flavonoids that can be distinguished by the presence of 2-phenylchromone ketone (Eichhorn and Efferth, 2012). These natural metabolites are frequently employed in clinical settings due to their anti-mutagenic, anti-oxidative, anti-inflammatory, and anti-carcinogenic effects as well as their ability to control important cellular enzymes (Kumar and Jaitak, 2019) (Figure 2; Table 1).
Dihydromyricetin (DMY), a naturally occurring flavonoid derived from Vitis heyneana, a traditional Chinese medicine plant. By reducing MDR1 mRNA and protein expression to 5-FU cytotoxicity, DMY decreases MRP2 expression and its promoter activity in HCT116/Oxaliplatin (OXA)and HCT8/VCR cells as well as sensitized SGC7901/5-FU cells (Wang et al., 2021). In order to reestablish chemosensitivity in CRC cells, DMY suppresses the Nrf2/MRP2 signaling pathway (Wang et al., 2021). DMY also activates p53 and induces apoptosis in paclitaxel (PTX)- and Dox-resistant OvCa cells (Xu et al., 2017).
Apigenin (API), a common dietary flavonoid, inhibits P-gp and BCRP, increasing cellular uptake of anticancer drugs such as Dox or TXT in MDR (Noorian et al., 2022). API is reported to suppress cell growth, clonogenicity, and invasiveness in CSCs. In human CD44+ prostate CSCs, API can upregulate caspase-8, apaf-1, and p53 mRNA expression, downregulate Bcl-2, sharpin, and survival, and increase the effectiveness of DDP (Erdogan et al., 2016; Erdogan et al., 2017). API suppresses STAT3, Akt, and MAPK in glioblastoma multiforme U87MG and U373MG cells in other CSCs(Kim B. et al., 2016). A549/OXA/bleomycin (BLM) and H1299/OXA/BLM cells may undergo a large rise in apoptosis as a result of API’s ability to activate p53 and pro-apoptotic proteins (Chen et al., 2016). Acacetin, an O-methylated API, inhibits the MDR1 gene at the mRNA level in NSCLC model cell lines A549/OXA/BLM and H1299/OXA/BLM (Punia et al., 2017; Singh et al., 2020).
Wogonin (WOG), a compound known as 5,7-dihydroxy-8-methoxyflavone, is found in fruits, vegetables, and certain medicinal plants. It possesses a wide range of biological activities, including anti-cancer, anti-inflammatory, and the treatment of bacterial and viral infections (Huynh et al., 2020). By preventing P-gp’s expression and functional activity, WOG sensitizes Dox-resistant K562/A02 cells (Xu et al., 2014). By lowering the expression of HIF-1α in HCT116/DDP cells, WOG suppresses the PI3K/Akt signaling pathway and increases cytotoxicity when combined with medicines like DDP, Dox, and PTX (He et al., 2013). WOG potentiates apoptosis and inhibits autophagy by regulating AKR1C1/1C2 and TNF-α (Huynh et al., 2017b). WOG blocks the IGF-1R/AKT signaling pathway in human breast cancer, increasing the cytotoxicity of sorafenib and Dox (Rong et al., 2017). In human osteosarcoma CSCs with anti-CD133 (Yang et al., 2022), WOG behavior demonstrate apoptosis through downregulating MMP-9 expression, which inhibits mobility and stops cell renewal (Huynh et al., 2017a). In response to internal and external stressors brought on by ROS, Nrf2 acts as a transcription factor by up-regulating antioxidant proteins (Leinonen et al., 2014; Sajadimajd and Khazaei, 2018). WOG induces ROS accumulation and further sensitizes TRAIL-induced apoptosis in A549 cells (Yang et al., 2013). Additionally, WOG increases ROS buildup, which increases intracellular ROS, and inhibits Nrf2 nuclear translocation via inactivating NF-κB (Ye et al., 2019). In DDP-resistant HNC cells, WOG inhibited Nrf2 and glutathione S-transferase P, increasing intracellular ROS (Kim E. H. et al., 2016).
Kaempferol (KAE), 3,4′,5,7-tetrahydroxyflavone, is a secondary metabolite found in many plants, and traditional medicines. KAE can inhibit ABCB1/P-gp through enhancing the capacity of ATPase (Felice et al., 2022), and also as an ABCG2/BCRP substrate, it can inhibit ABCG2/BCRP upregulation. KAE appears to have potential synergies with DDP, inhibits the mRNA levels of MRPs and cMyc in OVCAR-3 cells (Amjad et al., 2022). KAE promotes the development of miR-326, suppresses the process of glycolysis, and the resistence of HCT8-R cells to 5-Fluorouracil (5-FU) (Wu et al., 2022). KAE may increase the capacity of chronic myeloid leukemia (CML) cells to withstand the pro-apoptotic effects of anti-TRAIL antibodies (Saraei et al., 2022). Additionally, in human LS174-R colon cancer cells that are resistant to 5-FU, KAE suppresses reactive oxygen species and modifies the expression of JAK/STAT3, MAPK, PI3K/AKT, and NF-κB (Riahi-Chebbi et al., 2019).
Quercetin can be found in numerous fruits such as apples, berries (such as blueberries and cranberries), citrus fruits (such as oranges and lemons), and grapes. It is also present in vegetables such as onions, broccoli, kale, and tomatoes. Additionally, quercetin can be found in leaves of plants such as tea leaves and grains like buckwheat. In 5-FU-resistant BEL/5-FU cells, QUE would prevent ABCB1/P-gp and MRPs from functioning and expressing (Chen et al., 2018). Dox, PTX, VCR, and QUE combined treatment substantially reduces ABCB1/P-gp expression and eliminates breast CSCs (Li S. et al., 2018). Additionally, quercetin suppresses colorectal and breast CSCs (Azizi et al., 2022). QUE can control HIF-1α, which re-sensitizes 4T1 cells, MCF-7/Dox cells, and HCT116 cancer cells to DDP and etoposide (Kim et al., 2012). Through the miR-217-KRAS axis, QUE boosts osteosarcoma 143B cells’ sensitivity to the chemotherapy drug DDP (Zhang X. et al., 2015).
Collateral sensitivity (CS) involves the exploration of medications that specifically induce a higher level of cytotoxicity in MDR cells compared to the original non-resistant cells (Efferth et al., 2020). Galangin (GA), which has been extracted from the root of Alpinia galanga, has greater inhibitory effects on MDR cells (Lorendeau et al., 2014), and demonstrates collateral sensitivity (Lorendeau et al., 2017). A study found that GA increased the p53-dependent apoptotic pathway in ovarian cancer cells A2780/CP70/DDP and OVCAR-3/DDP, favorably inducing apoptosis compared to normal ovarian cells (Huang et al., 2020). GA through inactivating p-STAT3/p65 and Bcl-2 pathways attenuates DDP-induced resistance in A549 cells (Yu et al., 2018).
An isoflavone called puerarin (PU) was isolated from the plant Pueraria lobata (Willd.) Ohwi. There is a study by Li et al. which shows that PU suppresses Akt and JNK phosphorylation and promotes death in K562/Dox cells, as well as that autophagy makes tumor cells more resistant to anticancer drugs (Liu Q. et al., 2021). By inhibiting the NF-κB pathway and reducing MDR1 expression, PU sensitized K562/Dox cells (Liu Q. et al., 2021).
The primary daidzein metabolites genistein (IFG) and 7,3′,4′-trihydroxyisoflavone (TDI), which are present in fruits, nuts, and soy, also exhibit anti-Nrf2 and anti-ROS properties. The degree of methylation in the Keep1 promoter region is controlled by IFG, which decreases nucleus transcription and raises ROS in A549 cells (Cort et al., 2016; Liu et al., 2016). IFG and radiation are both effective in increasing cell apoptosis in A549 cells. TDI downregulation of ABCB1/P-gp and MRPs leads to a significant increase in EPI accumulation and attenuation of EPI resistance in HeLa cells (Hummelova et al., 2015). The methylation of some cancer-related genes may be hijacked by cancer cells to promote tumorigenesis. Research shows that IFG (0.5–10 μM) may lower the level of methyl DNA transference (MNDF). IFG (0.5–10 μM) significantly decreased the methylation of the Estrogen receptor β (ER-β) promoter in prostate cancer (PCa), ER-β which has an inducing effect on PCa cellular metabolism (Ji et al., 2022). IFG regulates caspase-3 and p38MAPK pathways and induces apoptosis in PC3 prostate cancer cells (Song et al., 2020).
Luteolin (LU), 3′,4′,5,7-Tetrahydroxyflavone, is a compound that is abundantly found in leaves and aromatic flowering plants. It possesses anti-inflammation, anti-allergy, and anti-cancer properties, and can function as either an antioxidant or a pro-oxidant biochemically (Lo et al., 2012). Additionally, LU has the power to dramatically decrease Nrf2 and enhance the cytotoxicity of DDP in KKU-100 cholangiocarcinoma cells; HCT116/OXA and SW620/OXA cells; A549/BLM/Dox cells and in MDA-MB 231/Dox cells. By increasing the expression of the epithelial biomarker E-cadherin, LU can reverse EMT. It also suppresses Hif-1α signaling in cervical cancer cell lines to prevent the invasivity from being activated (Imran et al., 2019). In pancreatic cancer cells, LU induces apoptosis by blocking the K-RAS/GSK-3β/NF-κB signaling pathway (Imran et al., 2019).
Honey, propolis, and the passion flower Passiflora caerulea all contain chrysin, which is a 5,7-dihydroxyflavone. Chrysin has the power to stop the efflux of ABCB1/P-gp from MDA-MB-231/mitoxantrone cells and regulate the transport of nitrofurantoin through ABCG2/BCRP from BCRP-overexpressing MCF-7 cells. Moreover, chromatin sensitizes BCRP-transfected cells via stimulating ATPase (Ahmed-Belkacem et al., 2005).
Due to the pseudo-dimeric shape of ABC transporters, Synthetic compounds were synthesized from natural flavonoids using a “click chemistry” method to efficiently produce a variety of triazole-bridged homo- and heteroflavonoid dimers. Ac15(Az8)2, a flavonoid dimer, inhibits BCRP potently, safely, and specifically. Through the inhibition of BCRP-ATPase activity and drug efflux in S1M180/topotecan cells, Ac15(Az8)2 restored intracellular drug accumulation, according to mechanistic investigations (Chong et al., 2022).
3.2 Alkaloids
Alkaloids are also secondary metabolites that are found in a variety of plants, fungi, and bacteria worldwide. The presence of one or more basic nitrogens, often in a heterocyclic ring, and significant pharmacological activity characterize an alkaloid. Their P-gp inhibitory function is likewise influenced by the basic nitrogen atoms (Gonçalves et al., 2020) (Figure 3; Table 1).
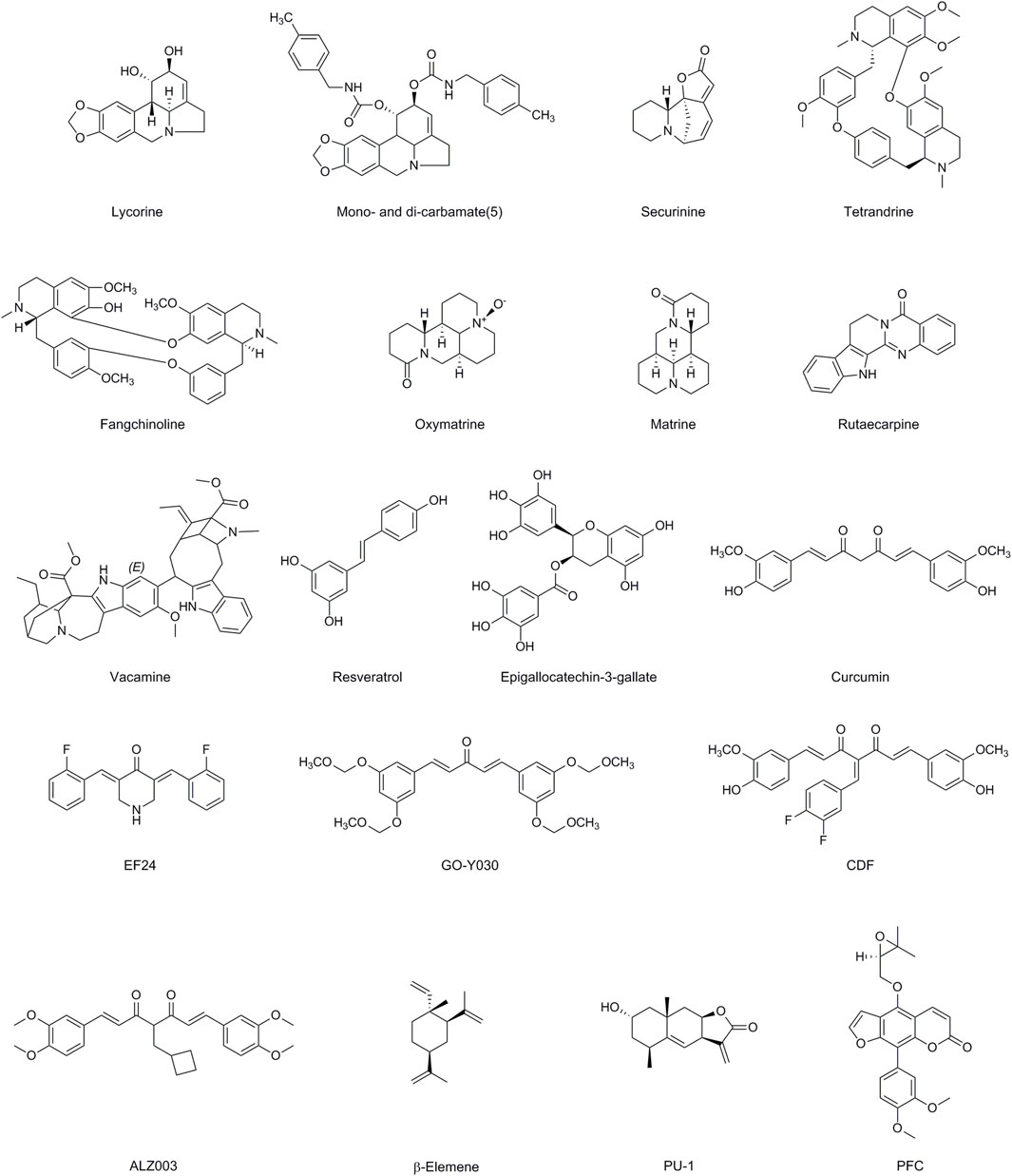
Figure 3. Chemical structures of Alkaloids, Polyphenols, Terpenoids and Coumarins having MDR modulatory activity.
Lycorine (LYC) is a commonly used alkaloids extracted from the bulb of Lycoris radiate, recognized for its various biological effects, which include anticancer, antiviral, antibacterial, and anti-inflammatory activities (Roy et al., 2018). TCRP1 is a new candidate for a human gene that is associated with chemotherapeutic resistance. It is expressed extensively in various types of cancer cells and is associated with chemotherapeutic resistance (Liu X. et al., 2019). LYC lowers the levels of tongue cancer and Hepatocellular Carcinoma (HCC) cells TCRP1 protein by promoting the degradation pathway of TCRP1 protein, which translates into repression of Akt/mTOR signaling, and therefore activates apoptotic and autophagic abilities (Yu H. et al., 2017). Bioinformatics analysis revealed that upregulation of FABP5 expression facilitates acute myeloid leukemia (AML) cell viability, protects AML cells from apoptosis. LYC downregulated the expression levels of FABP5 and its target PPARγ, impaired AML cell viability, and triggered apoptosis (Liang et al., 2023). By performing extracellular, cytoplasmic, and nuclear roles, HMGB1 is crucial for stress signaling as well as for the activation of autophagy. Because autophagy is inhibited in human bone marrow CD138 primary myeloma cells and multiple myeloma (MM) cell lines, LYC-induced proteasomal degradation of HMGB1 inhibits the activation of the MEK-ERK signaling pathway and Bcl-2 phosphorylation declines, leading to the constitutive association of Bcl-2 with Beclin-1 (Roy et al., 2016). LYC inhibits EGF-induced JAK/STAT signaling as well as various downstream STAT3 targets, such as cyclin D1, Bcl-2, Bcl-xL, matrix metalloproteinase 2 (MMP2), and the EMT promoter Twist, which lowers prostate cancer cell line proliferation, migration, invasion (Hu et al., 2015). Amaryllidaceae alkaloids were investigated as MDR reversibles in human colon cancer cells by derivatizing alkaloid hydroxyl groups into mono- and di-carbamates. Di-carbamates that contain phenethyl or benzyl moieties were found to be more potent inhibitors than verapamil. The collateral sensitivity of a number of derivatives also suggested a dual role in reversing P-gp-mediated MDR (Sancha et al., 2023).
Securinega alkaloids (SA) are indolizidine alkaloids derived from the Asian plant Securinega suffruticosa’s leaf and root. SA Several activities have been documented, including antiproliferative activity, leukemia differentiation induction activity, MDR reversal activity, antimalarial activity, and antibacterial activity (Hou et al., 2023). Recent studies have found altering securinine at the C15 sites increases the ability to reverse drug resistance caused by several drugs, whereas derivatives with a bivalent mimic attached to the C15 site increase the ability to induce differentiation and reverse drug resistance caused by P-gp. Mechanism investigations indicated MDR reversal action in HepG2/Doxvia reduction of P-gp function against Dox (Hou et al., 2023).
The bisbenzyl isoquinoline alkaloid tetrandrine (TET), which was isolated from the Chinese plant Stephania tetrandra (Han-Fang-Chi), has anticancer effects because it inhibits cell proliferation and induces apoptosis (Zhou et al., 2022b). Previous research has shown that TET and its derivatives can reverse MDR caused by osteosarcoma/Dox. TET may significantly increase intracellular chemotherapeutic drug concentration by changing the expression of the MDR1 gene and P-gp, and can be used in combination with chemotherapy drugs to significantly inhibit P-gp expression (Zhou et al., 2022b). TET inhibits drug efflux caused by MDR1 overexpression and has anti-MDR action in Hep-2/VCR cells (Li Y. et al., 2020). TET decreased transporter protein mRNA and protein levels suppress MRP overexpression in MDR human esophageal squamous carcinoma YES-2/DDP cells and epidermis-like k2–mrp1 cancer cells (Wang et al., 2018). Molecular dynamics simulations were employed to design OY-101, a novel chemical compound derived from the modification of natural TET. OY-101 demonstrated selective and potent inhibition of P-gp (Zeng et al., 2023). Fangchinoline (FAN) is a prominent bisbenzylisoquinoline (BBIQ) alkaloid derived from the roots of S. tetrandra in the Menispermaceae family that consists of two benzylisoquinoline units joined by oxygen bridges. FAN has been shown to reverse MDR in Caco-2 and CEM/Dox5000 cancer cells when combined with Dox (Chan et al., 2021).
Oxymatrine (OMA) and matrine (MA), as natural compounds derived from Sophora flavescens, have been reported to possess a wide range of pharmacological properties, including anti-inflammatory, antiviral, anti-tumor, and immunomodulatory effects. In addition to considerably reversing cellular MDR, enhancing apoptotic induction, suppressing MRP1 expression, and inactivating NF-κB signaling by lowering phosphorylated p65, OMA alone or in conjunction with 5-FU significantly reversed cellular MDR (Du and Shim, 2016). One of the signaling pathways responsible for 5-FU resistance in CRC is NF κB. Combining OMA and OXA improved both the in vitro and in vivo anticancer effects of OXA in CRC cancer cells. These additive effects were attained by suppressing the NF κB and PI3K/AKT/mTOR signaling pathways, which decreased the treatment resistance of CRC cancer cells. OMA is known to inhibit the NF κB pathway, so its combination with OXA could provide an enhanced therapeutic benefit (Chen M. H. et al., 2021). LRP, or lung resistance protein, is a cytoplasmic vault protein that plays a role in both the vesicular sequestration of drugs in the cytoplasm and their translocation from the nucleus to the cytoplasm. MA reversed drug resistance in OXA-resistant HT-29/OXA cells, increased HT-29/OXA cells’ sensitivity to OXA in a dose-dependent manner, and significantly decreased LRP and P-gp expression in HT-29/OXA cells at the mRNA and protein levels. In K562/Dox cells, MA causes a dose-dependent stoppage of the cell cycle at the G0/G1 phase, which promotes autophagy (Li Z. et al., 2020).
Rutaecarpine (Rut), a bioactive alkaloid found in Evodia rutaecarpa, has been associated with various pharmacological effects such as analgesic, anticancer, and anti-inflammatory properties. On P-gp-overexpressing MCF-7/Dox and A549/PTX cells, Rut dose-dependently improved the effectiveness of Dox, PTX, and colchicine. Since the ubiquitination pathway plays a major role in protein degradation, the E3 ubiquitin ligase MARCH8 is an ABCB1/P-gp substrate. MARCH8 interacts with ABCB1/P-gp to promote ubiquitination and degradation (Zhang et al., 2023). Rut can boost MARCH8 expression, which encourages the degradation of ABCB1/P-gp (Zou et al., 2021). Castration-resistant prostate cancer (CRPC) is largely brought on by androgen receptor splice variation 7 (AR-V7). Rut restores the susceptibility of castration-resistant prostate cancer to anti-androgen therapy in vitro and in vivo by specifically inducing AR-V7 protein degradation via K48-linked ubiquitination (Liao et al., 2020).
A bisindole alkaloid known as voacamine (VOA) was discovered in the Voacanga and Peschiera species of the Apocynaceae family. VOA possesses a variety of biological qualities, including antibacterial action, resistance to Plasmodium falciparum, strong neuroprotective activity against Alzheimer’s disease, and the ability to inhibit the mutagenicity brought on by several genotoxic substances (Theissinger et al., 2023). VOA was an effective substrate for P-gp and acted as a competitive antagonist to obstruct P-gp-mediated drug export. When VOA was administered to U-2 OS-DX/Dox cells, laser scanning confocal microscopy (LSCM) studies showed a disorganizing effect on microtubules (Condello et al., 2020).
3.3 Polyphenols
A massive family of 10,000 plant compounds known as polyphenols is mostly present in fruits, green and black tea, coffee, red wine, chocolate, and seeds (Zhang Y. et al., 2022). Polyphenols frequently have three-membered flavan ring structures. In the body, free radicals primarily fall into two categories: ROS and reactive nitrogen species (RNS). The involvement of the B rings in scavenging ROS/RNS and the capacity of hydroxyl groups connected to benzene rings to donate a hydrogen atom or an electron to free radicals are essential elements of the mechanism underpinning polyphenol action. Cell death, EMT, ROS, DNA repair procedures, CSCs, and epigenetics [such as MicroRNAs (miRNAs)] are some other targets that polyphenols affect in order to combat chemoresistance in cancer cells (Wang et al., 2022) (Figure 3; Table 1).
Numerous cancers, including bladder, prostate, breast, lung, glioblastoma, colon, and ovarian, are affected by resveratrol (RES) in terms of apoptosis (Ren et al., 2021). Under the regulation of MRP1, various endogenous and xenobiotic substrates are absorbed and eliminated. Due to the elevated levels of MRP1 gene expression in Dox-resistant acute myeloid leukemia (AML)-2/DX300 cells, its expression may lead to a decrease in drug cellular absorption (Chen J. et al., 2019). RES with Dox decreases Dox IC50 from 0.96 ± 0.02 M to 0.52 ± 0.05 M in HCT 116 colorectal cancer cells, increases Dox intracellularly, and inhibits the efflux action of P-gp (Wang et al., 2020). Inhibitory the PI3K/Akt/mTOR pathway causes the inhibitory impact of RES over P-gp in K562/Dox cells (Khan et al., 2020). RES recognize miR-122-5p and regulates Bcl-2 and CDKs, resulting in the chemosensitization of Dox-resistant breast cancer MCF-7 cells (ALkharashi, 2023). In DDP-resistant human oral cancer CAR cells, RES therapy promotes the expression of autophagy-related genes, such as Beclin-1 Atg12 and LC3-II, at the mRNA level and increases AMPK phosphorylation. This results in regulated autophagy and pro-apoptosis-related signals to be send (Chang et al., 2017). RES dramatically reduced the activation of tumor-promoting factors (NF-κB, MMP-9, CXCR4) and epithelial-to-mesenchymal transition-factors (increased vimentin and slug, decreased E-cadherin) in TNF-induced activation of CRC cells by preventing EMT and CSC formation (Buhrmann et al., 2018). RES treatment results in elevated levels of DNA topoisomerase-II (TOPO), an enzyme that is commonly found in malignancies and plays a crucial role in maintaining DNA structure during transcription and DNA replication. Furthermore, the RES-treated group exhibited significantly higher levels of Topo-II compared to other groups. It appears that RES promotes drug-induced DNA damage in Dox-resistant PUMC-91/Dox cells (Skok et al., 2020; Choi et al., 2022). 5-FU resistant (5-FU-R) cells may exhibit resistance to the DNA-damaging chemical 1,3-bis(2-chloroethyl)-1-nitrosourea (BCNU). However, when RES and BCNU are combined, it is enhance the sensitivity and induce DNA damage in 5-FU-R cells (Choi et al., 2022).
A significant polyphenolic component of green tea called epigallocatechin-3-gallate (EGCG) has several beneficial properties, including the capacity to lower stress, regulate metabolism, prevent cancer, and offer protection from various diseases. In drug-resistant KB-A1 cells, EGCG has been found to alter P-gp activity and increase intracellular Dox concentration (Tang et al., 2017; Li H. et al., 2018). The primary copper influx transporter CTR1 is in charge of copper’s resistance to platinum. Because EGCG raises CTR1 expression at the mRNA and protein levels in ovarian cancer cells and upregulates the rapid DDP-induced degradation of CTR1, OVCAR3 and SKOV3 ovarian cancer cells are more vulnerable to DDP when it is present (Wang et al., 2015). Ovarian cancer and non-small-cell lung cancer (NSCLC) cells were made more susceptible to DDP when supplemented with EGCG by increasing ROS generation and CTR1 expression through activating the ERK1/2/NEAT1 pathway (Chen A. et al., 2020). Through suppression of the Bcr/Abl oncoprotein and control of its downstream p38-MAPK/JNK and JAK2/STAT3/AKT pathways, EGCG was able to decrease cell proliferation and cause apoptosis in CML (Xiao et al., 2019). The DDP-induced DNA damage is substantially repaired by the 5′-3′ structure-specific endonuclease ERCC1/XPF. A DNA-endonuclease incision test based on fluorescence was used to identify (Heyza et al., 2018). EGCG exhibits inhibitory effects on colorectal CSCs and lung CSCs. It downregulates the activation of the Wnt/β-catenin pathway (Chen et al., 2017; Fujiki et al., 2018).
Curcumin (Cur) is an active polyphenolic pigment obtained from the rhizomes of Curcuma longa. Cur is typically used for its antioxidant, anti-inflammatory, wound-healing, and anti-carcinogenesis qualities that halt the onset or progression of cancer (Tomeh et al., 2019). The inhibition of ABC family transporters by Cur in various cancer cells causes drug accumulation within cancer cells (Dong et al., 2023). Cur is a helpful medicine when used in conjunction with significant chemotherapeutic medications to combat MDR. Cur causes DNA damage in several cell lines and inhibits particular DNA repair enzymes. Rad51-dependent homologous recombination is a crucial DNA repair pathway that enables cancer cells to develop resistance to medications that target tumor DNA damage. However, Cur has been found to lower the expression of Rad51, leading to DNA damage in cancer cells (Zhao et al., 2018; Wong, 2021). According to reports, Cur is a powerful DNA hypomethylation agent that inhibits DNMT1 activity by covalently attaching to and inhibiting the catalytic thiol group of cysteine (C1226) (Wu et al., 2016; Tong et al., 2020). By preventing the expression of DNMT1, Cur slows down cell growth and triggers apoptosis in hepatocellular carcinoma (Liu et al., 2017). Numerous varieties of multidrug resistant cancer cells were revealed to have improper NF-κB regulation. The expression of genes regulated by NF-κB is decreased as a result of Cur’s suppression of NF-κB activity and prevention of NF-κB binding to DNA (Zhou et al., 2017). Cur reduces IκBα kinase activity in human head and neck squamous cell carcinoma cell lines, which blocks NF-κB activity. By blocking the PI3K/AKT pathway, which reduces NF-κB expression, Cur increases the sensitivity of cancer cells to treatment (Mortezaee et al., 2019a; Villegas et al., 2021; Abadi et al., 2022). Cur may demethylate in the MEG3 promoter area in the A2780cp ovarian cancer cell line, which downregulates miR-214 and, indirectly, lowers DDP resistance (Zhou et al., 2017). Liu et al. proposed a potential regulatory network involving HOX transcript antisense RNA (HOTAIR), miR-20a-5p, and Wilms’ tumor 1 (WT1). Their findings demonstrated that Cur inhibits the levels of WT1 in human acute myeloid leukemia cells (HL-60) or HL-60/Dox cells. They also observed that the suppression of miR-20a-5p, resulting in increased WT1 expression, attenuated the effect of Cur on the resistance of leukemia cells to Dox. These results indicate that Cur inhibits the resistance of tumor cells to Dox by targeting the HOTAIR/miR-20a-5p/WT1 axis. Cur slows the proliferation of cancer cells, as evidenced by the downregulation of NF-κB in mantle cell lymphoma, oral MCF-7, and non-small-cell lung carcinoma (Meiyanto et al., 2014). Additionally, the activation of redox processes within cells leads to the generation of ROS, which upregulates the apoptotic receptors on the membrane of tumor cells (Mortezaee et al., 2019b). Cur decreases tumor cell growth and increases apoptosis by upregulating the expression and activity of p53, attenuating the regulation of antiapoptosis PI3K signaling and MAPKs to boost endogenous ROS generation, and overexpressing antiapoptosis genes such Bcl-2 (Mortezaee et al., 2019b). By controlling oxidative stress, modulating fibrosis, activating SIRT1, and encouraging cellular apoptosis, Cur may kill MCF7/TH, HCT116R, and A549/Dox cancer cells (Gabr et al., 2022). A therapeutic target, nicotinamide N-methyltransferase (NNMT) has a variety of effects on CRC aggressiveness and 5-FU resistance (Abadi et al., 2022). Cur has the ability to reduce NNMT and p-STAT3 expression. Particularly in CRC cell lines with significant NNMT expression, Cur may also lessen ROS generation, G2/M phase cell cycle arrest, and cell growth (Keyvani-Ghamsari et al., 2020; Gabr et al., 2022).
Cur plays a wide range of roles in cancer cells’ multidrug resistance, but its clinical application is challenging. Cur’s weak solubility, minimal absorption, restricted tissue distribution, and quick metabolism are its significant drawbacks. Generating novel Cur analogues is one method to solve these issues. EF24 is a developed Cur analog. By suppressing NF-κB, HIF-1α, controlling the creation of ROS, and controlling important genes by miRNA, EF24 prevented cancer cells from going through the cell cycle and caused apoptosis (Bisht et al., 2016; Ibáñez Gaspar and McMorrow, 2023). EF24 makes ovarian cancer resistant cells more susceptible to DDP by causing an overexpression of the p53 and p21 proteins in the G2/M checkpoint. In DDP-resistant cells, it also induces apoptosis by promoting PTEN phosphorylation and inhibiting Akt, a resistance cell (Su et al., 2023). Go-Y030, EF24 analog of Cur, can stop K562/mitoxantrone cells from producing mitoxantrone and pheophorbide A from ABCG2/BCRP (Murakami et al., 2017). Another Cur homologue with better bioavailability is CDF. Pancreatic cancer cells underwent apoptosis and had their NF-κB activity inhibited when CDF and gemcitabine were combined (Lee et al., 2018). Additionally, PTEN expression was increased while overexpressed miR-21 expression was decreased by CDF, which prevented cells resistant to gemcitabine from undergoing cellular arrest (Lee et al., 2018). The androgen receptor (AR) in prostate cancer has been reported to promote treatment resistance. In vitro and in vivo, a Cur analog named ALZ003 significantly lowers the survival of TMZ-sensitive and -resistant glioblastoma by triggering FBXL2-mediated AR ubiquitination, which leads to its degredation (Bott et al., 2016; Chen T. C. et al., 2020).
3.4 Terpenoids and Coumarins
3.4.1 Terpenoids
Terpenoid is a class of natural compounds that is both extensively studied and structurally diverse. Terpenoids are classified into monoterpenoids (C10), sesquiterpenoids (C15), diterpenoids (C20), sesterterpenoids (C25), triterpenoids (C30), tetraterpenes (C40), and polyterpenes based on the number of isoprene units present in the parent structure. Terpenoids display numerous medicinal benefits, such as hypoglycemia, liver protection, antibacterial, anti-inflammatory, and anti-tumor characteristics (Kumar and Jaitak, 2019) (Figure 3; Table 1).
The sesquiterpene chemical β-Elemene (β-ELE), derived from Curcuma Rhizoma, exhibits properties such as inhibiting cell proliferation, arresting the cell cycle, inducing cell death, and reversing MDR in chemotherapy. β-ELE may be able to overcome drug resistance in human NSCLC A549/ER cells that are resistant to erlotinib (ER) in vitro by lowering P-gp expression, suppressing P-gp dependent drug efflux, and increasing intracellular concentrations of anticancer drugs (Lin et al., 2018). MiRNAs found in exosomes, function in the mechanism of intercellular communication and modify chemosensitivity. The intercellular transfer of certain miRNAs is partly responsible for the MDR of tumor cells. The ability of MDR Breast Cancer Anti-Estrogen (BCA) cells to propagate drug resistance is determined by the exosomes they produce. Recent research has demonstrated that β-ELE influences exosome content, affects the expression of certain MDR-related miRNAs, and reduces the exosome-mediated transmission of drug resistance, thereby enhancing the cells’ capacity to overcome MDR (Zhang J. et al., 2015). In the exosomes of multidrug-resistant gastric cancer cells, miR-1323 is significantly expressed, which encourages EMT of gastric cancer sensitive cells and enhances their capacity for invasion and migration (Tan et al., 2021). The expression of Cbl-b is likewise suppressed by miR-1323, resulting in the attenuation of drug resistance in cancer cells with MDR. By reversing the drug resistance and metastasis generated by exosomes, β-ELE reduces the ability of SGC7901 cells to resist drugs and migration (Tan et al., 2021). In terms of internal miRNA-29a and miRNA222, human MCF-7 cells that were resistant to Docetaxel (MCF-7/Doc) and Dox (MCF-7/Dox), respectively, were significantly downregulated by β-ELE. The tumor cells’ medication resistance was overcome by suppression of the PI3K-AKT signaling pathway. PTEN, a common tumor suppressor gene that blocks the PI3K-AKT signaling pathway, is the two miRNAs’ target gene and is significantly increased after miRNA-29a and miRNA222 are downregulated (Hu et al., 2019). A549/DDP cells underwent apoptosis when exposed to β-ELE because it decreased the mitochondrial membrane potential and increased intracellular ROS levels which may cause apoptosis and mitochondrial damage (Liskova et al., 2021) By increasing Caspase-3 protein expression, β-ELE may be used to overcome gastric cancer resistance (Low et al., 2021). β-ELE can additionally reduce chemoresistance in lung cancer by inhibiting the paracrine activities mediated by cyclin-dependent kinase inhibitor P21, which are regulated by CDK8. SPC-A1/DDP, a DDP-resistant lung cancer cell line, is more susceptible to apoptosis and medication treatment when β-ELE which is accomplished via fostering Beclin-1-induced autophagy (Li et al., 2021).
PU-1 is a sesquiterpene with the α-methylene-γ-lactone moiety that has been isolated from numerous plant species of the genera Inula and the genus Pulicaria. It might have anti-inflammatory and anti-cancer effects. In CCRF-CEM leukemic cells, the PI3K/AKT pathway prevents the development of drug-resistant tumor cells by causing DNA damage, obstructing the G2/M cell cycle, and triggering apoptosis. Resazurin reduction tests showed that PU-1 suppressed this pathway (Hegazy et al., 2021).
3.4.2 Coumarins
Coumarins are a class of organic compounds that are widely distributed in nature, including in plants such as fruits, vegetables, and herbs. They are characterized by a benzene ring fused to an alpha-pyrone ring. The phenylfurocoumarin derivative (R)-9-(3,4-dimethoxyphenyl)-4-((3,3-dimethyloxiran-2-yl)methoxy)-7H-furo [3,2-g]chromen-7-one (PFC) in HCT-116/BCRP colon cancer cells, drastically lowers the IC50 of SN-38 while inhibiting ABCG2/BCRP-mediated drug transport function. Additionally, in the ABCG2/BCRP-overexpressing HCT-116/BCRP cell xenograft mice model, PD-stimulated ABCG2/BCRP-mediated ATP hydrolysis reduced irinotecan resistance without resulting in toxicity (Kokubo et al., 2021). In a recent study, fifteen sesquiterpene coumarins were extracted and purified from various Ferula species and tested for their ability to reverse MDR. The study found that the sesquiterpene coumarins enhanced Dox cytotoxicity in MCF-7/Dox cells, which are Dox-resistant derivatives of MCF-7 cells that overexpress the P-gp protein. Further analysis of the structure-activity relationship of these sesquiterpene coumarins indicated that ring-opened drimane-type compounds, specifically farnesiferol B, farnesiferol C, and lehmferin, exhibited the most potent inhibitory effects on P-gp pump efflux. These compounds could be considered as lead scaffolds for future modifications to improve their efficacy in reversing MDR (Kasaian et al., 2015) (Figure 3; Table 1).
4 Conclusion
Drug resistance is usually the reason why cancer treatments fail, despite major advancements in the manufacturing of new chemotherapeutic medications. Researchers have started taking conventional treatments like natural components more into consideration recently because of their reduced cost and adverse effects. Numerous studies have shown that the efficacy of natural products can influence various elements of cancer medication resistance. As described in this review, natural products have significant effects in overcoming drug resistance and enhancing the efficacy of chemotherapy. Natural products modulate inhibition of ABC transporters, increase in DNA damage, regulate ncRNA-mediated multidrug resistance, activate apoptotic cells, regulate the expression of metabolic enzymes, and chemosensitivity in various types of cancers both in vitro and in vivo.
Natural products are easily obtainable, but improving their qualities requires considering factors such as biological availability, biocompatibility, and half-life to enhance clinical efficacy and reduce risks to patients. To create more efficient antitumor drug delivery systems, the structure of tumor drugs is utilized to optimize drug properties. Additionally, computer-aided drug design can help in the development of natural products by predicting drug targets, modifying drug structure, and predicting toxicity. The focus of cancer therapeutic medication research is now on creating safe and effective natural products with fewer harmful side effects.
Patients who have developed medication resistance are being treated in clinical settings using a multi-targeted approach. For example, MET amplification causes cells to bypass EGFR and activate the PI3K/AKT pathway, allowing them to resist the effects of the EGFR inhibitors and develop resistance. For patients who have both MET amplification and EGFR mutations, the best treatment strategy is to use a dual-targeted EGFR/MET regimen to inhibit both EGFR and MET. To delay the development of resistance in KRAS-G12C patients, combination therapy is likely to become the preferred approach. When compared to mono-therapeutic regimens, combination techniques can significantly increase a therapy’s safety and effectiveness, particularly if the combined medications have different modes of action. The creation of biomarker-driven therapeutics is the result of the identification of certain resistance mechanisms. Both targeting tactics that anticipate the selection of resistant and combinatorial strategies that target multiple resistance nodes are being considered. Clinical research on natural products currently available indicate that they are primarily adjuvant medications. For instance gemcitabine coupled with celecoxib and curcumin in the treatment of patients with pancreatic cancer, in a prospective phase II trial, the safety and efficacy of curcumin (2000 mg/day in four capsules of 500 mg each) and gemcitabine (10 mg/m2) were evaluated in 44 patients with advanced and metastatic pancreatic cancer. The data showed a median progression-free survival and overall survival of 8.4 and 10.2 months, respectively. These findings suggest that the combination of gemcitabine and curcumin phytosome can safely and effectively increase the rate of response to first-line treatment for advanced pancreatic cancer. Natural substances may act as sensitizing agents in the therapy of cancer when used in combination, according to a growing body of research. In order to bring more effective treatments for reversing MDR to the clinic, it is crucial to uncover more potent and less dangerous chemicals and research their pharmacological mechanisms using cutting-edge technology, such as high-throughput screening methods, next-generation sequencing, advanced imaging techniques, and computational modeling. These technologies enable researchers to analyze complex biological systems at a molecular level, identify novel drug targets, and design innovative therapeutic strategies. By harnessing cutting-edge technology, scientists can accelerate the discovery and development of new drugs that can overcome multidrug resistance and improve patient outcomes. In order to encourage their clinical application and offer fresh ideas for the treatment of tumor drug resistance, this review covers the research progress of natural products in tumor drug resistance during the past few years.
Author contributions
J-YZ: Conceptualization, Data curation, Formal Analysis, Writing–original draft. Q-LC: Writing–original draft, and Writing–review and editing. X-CL: Data curation, Writing–original draft, and Writing–review and editing. DD: Writing–original draft, and Writing–review and editing. UA: Writing–original draft, and Writing–review and editing. H-YL: Writing–original draft, and Writing–review and editing. TB: Writing–original draft, and Writing–review and editing. H-BC: Writing–original draft, and Writing–review and editing. Y-QW: Supervision, Formal Analysis, Writing–original draft, and Writing–review and editing. J-YZ: Funding acquisition, Resources, Supervision, Writing–original draft, and Writing–review and editing.
Funding
The author(s) declare financial support was received for the research, authorship, and/or publication of this article. This work was supported by Fund of Guangdong Science and Technology Department (2022A0505050080), the National Key R&D Program of China (2021YFE0202000), the Fund of Guangzhou Science and Technology Program (202002030410), Innovation and Technology Fund in Hong Kong (MHP/023/20), the Affiliated Qingyuan Hospital of Guangzhou Medical University (202301-301), Ministry of Education Engineering Research Center of Tibetan Medicine Detection Technology (2022-ZYZXK07), Guangzhou Medical University Undergraduates project (pdjh 2022b0425, 02-410-2206301).
Conflict of interest
The authors declare that the research was conducted in the absence of any commercial or financial relationships that could be construed as a potential conflict of interest.
Publisher’s note
All claims expressed in this article are solely those of the authors and do not necessarily represent those of their affiliated organizations, or those of the publisher, the editors and the reviewers. Any product that may be evaluated in this article, or claim that may be made by its manufacturer, is not guaranteed or endorsed by the publisher.
References
Abadi, A. J., Mirzaei, S., Mahabady, M. K., Hashemi, F., Zabolian, A., Hashemi, F., et al. (2022). Curcumin and its derivatives in cancer therapy: potentiating antitumor activity of cisplatin and reducing side effects. Phytother. Res. 36 (1), 189–213. doi:10.1002/ptr.7305
Abdelfatah, S., Bockers, M., Asensio, M., Kadioglu, O., Klinger, A., Fleischer, E., et al. (2021). Isopetasin and S-isopetasin as novel P-glycoprotein inhibitors against multidrug-resistant cancer cells. Phytomedicine 86, 153196. doi:10.1016/j.phymed.2020.153196
Ahmed, S., Alam, W., Aschner, M., Alsharif, K. F., Albrakati, A., Saso, L., et al. (2022). Natural products targeting the ATR-CHK1 signaling pathway in cancer therapy. Biomed. Pharmacother. 155, 113797. doi:10.1016/j.biopha.2022.113797
Ahmed-Belkacem, A., Pozza, A., Muñoz-Martínez, F., Bates, S. E., Castanys, S., Gamarro, F., et al. (2005). Flavonoid structure-activity studies identify 6-prenylchrysin and tectochrysin as potent and specific inhibitors of breast cancer resistance protein ABCG2. Cancer Res. 65 (11), 4852–4860. doi:10.1158/0008-5472.Can-04-1817
Alkharashi, N. A. (2023). Efficacy of resveratrol against breast cancer and hepatocellular carcinoma cell lines. Saudi Med. J. 44 (3), 246–252. doi:10.15537/smj.2023.44.3.20220768
Ambudkar, S., Kimchi-Sarfaty, C., Sauna, Z., and Gottesman, M. (2003). P-glycoprotein: from genomics to mechanism. Oncogene 22 (47), 7468–7485. doi:10.1038/sj.onc.1206948
Amjad, E., Sokouti, B., and Asnaashari, S. (2022). A systematic review of anti-cancer roles and mechanisms of kaempferol as a natural compound. Cancer Cell Int. 22 (1), 260. doi:10.1186/s12935-022-02673-0
Arulselvan, P., Fard, M. T., Tan, W. S., Gothai, S., Fakurazi, S., Norhaizan, M. E., et al. (2016). Role of antioxidants and natural products in inflammation. Oxid. Med. Cell Longev. 2016, 5276130. doi:10.1155/2016/5276130
Assaraf, Y. G., Brozovic, A., Gonçalves, A. C., Jurkovicova, D., Linē, A., Machuqueiro, M., et al. (2019). The multi-factorial nature of clinical multidrug resistance in cancer. Drug Resist Updat 46, 100645. doi:10.1016/j.drup.2019.100645
Awad, M. M., Katayama, R., McTigue, M., Liu, W., Deng, Y. L., Brooun, A., et al. (2013). Acquired resistance to crizotinib from a mutation in CD74-ROS1. N. Engl. J. Med. 368 (25), 2395–2401. doi:10.1056/NEJMoa1215530
Azizi, E., Fouladdel, S., Komeili Movahhed, T., Modaresi, F., Barzegar, E., Ghahremani, M. H., et al. (2022). Quercetin effects on cell cycle arrest and apoptosis and doxorubicin activity in T47D cancer stem cells. Asian Pac J. Cancer Prev. 23 (12), 4145–4154. doi:10.31557/apjcp.2022.23.12.4145
Barkley, D., Moncada, R., Pour, M., Liberman, D. A., Dryg, I., Werba, G., et al. (2022). Cancer cell states recur across tumor types and form specific interactions with the tumor microenvironment. Nat. Genet. 54 (8), 1192–1201. doi:10.1038/s41588-022-01141-9
Bisht, S., Schlesinger, M., Rupp, A., Schubert, R., Nolting, J., Wenzel, J., et al. (2016). A liposomal formulation of the synthetic curcumin analog EF24 (Lipo-EF24) inhibits pancreatic cancer progression: towards future combination therapies. J. Nanobiotechnology 14 (1), 57. doi:10.1186/s12951-016-0209-6
Bott, L. C., Badders, N. M., Chen, K. L., Harmison, G. G., Bautista, E., Shih, C. C., et al. (2016). A small-molecule Nrf1 and Nrf2 activator mitigates polyglutamine toxicity in spinal and bulbar muscular atrophy. Hum. Mol. Genet. 25 (10), 1979–1989. doi:10.1093/hmg/ddw073
Buhrmann, C., Yazdi, M., Popper, B., Shayan, P., Goel, A., Aggarwal, B. B., et al. (2018). Resveratrol chemosensitizes TNF-β-induced survival of 5-FU-treated colorectal cancer cells. Nutrients 10 (7), 888. doi:10.3390/nu10070888
Chakrawarti, L., Agrawal, R., Dang, S., Gupta, S., and Gabrani, R. (2016). Therapeutic effects of EGCG: a patent review. Expert Opin. Ther. Pat. 26 (8), 907–916. doi:10.1080/13543776.2016.1203419
Chan, E. W. C., Wong, S. K., and Chan, H. T. (2021). An overview on the chemistry, pharmacology and anticancer properties of tetrandrine and fangchinoline (alkaloids) from Stephania tetrandra roots. J. Integr. Med. 19 (4), 311–316. doi:10.1016/j.joim.2021.01.001
Chang, C. H., Lee, C. Y., Lu, C. C., Tsai, F. J., Hsu, Y. M., Tsao, J. W., et al. (2017). Resveratrol-induced autophagy and apoptosis in cisplatin-resistant human oral cancer CAR cells: a key role of AMPK and Akt/mTOR signaling. Int. J. Oncol. 50 (3), 873–882. doi:10.3892/ijo.2017.3866
Chang, H., and Zou, Z. (2020). Targeting autophagy to overcome drug resistance: further developments. J. Hematol. Oncol. 13 (1), 159. doi:10.1186/s13045-020-01000-2
Chen, A., Jiang, P., Zeb, F., Wu, X., Xu, C., Chen, L., et al. (2020a). EGCG regulates CTR1 expression through its pro-oxidative property in non-small-cell lung cancer cells. J. Cell. physiology 235 (11), 7970–7981. doi:10.1002/jcp.29451
Chen, F., Zhuang, M., Zhong, C., Peng, J., Wang, X., Li, J., et al. (2015). Baicalein reverses hypoxia-induced 5-FU resistance in gastric cancer AGS cells through suppression of glycolysis and the PTEN/Akt/HIF-1α signaling pathway. Oncol. Rep. 33 (1), 457–463. doi:10.3892/or.2014.3550
Chen, J., Tian, B., Zhou, C., Sun, J., Lin, L., Jin, S., et al. (2019a). A novel resveratrol-arsenic trioxide combination treatment synergistically induces apoptosis of adriamycin-selected drug-resistant leukemia K562 cells. J. Cancer 10 (22), 5483–5493. doi:10.7150/jca.34506
Chen, M., Wang, X., Zha, D., Cai, F., Zhang, W., He, Y., et al. (2016). Apigenin potentiates TRAIL therapy of non-small cell lung cancer via upregulating DR4/DR5 expression in a p53-dependent manner. Sci. Rep. 6, 35468. doi:10.1038/srep35468
Chen, M. H., Gu, Y. Y., Zhang, A. L., Sze, D. M., Mo, S. L., and May, B. H. (2021a). Biological effects and mechanisms of matrine and other constituents of Sophora flavescens in colorectal cancer. Pharmacol. Res. 171, 105778. doi:10.1016/j.phrs.2021.105778
Chen, T. C., Chuang, J. Y., Ko, C. Y., Kao, T. J., Yang, P. Y., Yu, C. H., et al. (2020b). AR ubiquitination induced by the curcumin analog suppresses growth of temozolomide-resistant glioblastoma through disrupting GPX4-Mediated redox homeostasis. Redox Biol. 30, 101413. doi:10.1016/j.redox.2019.101413
Chen, W., Balan, P., and Popovich, D. G. (2019b). Review of ginseng anti-diabetic studies. Molecules 24 (24), 4501. doi:10.3390/molecules24244501
Chen, Y., Wang, X. Q., Zhang, Q., Zhu, J. Y., Li, Y., Xie, C. F., et al. (2017). (-)-Epigallocatechin-3-Gallate inhibits colorectal cancer stem cells by suppressing wnt/β-catenin pathway. Nutrients 9 (6), 572. doi:10.3390/nu9060572
Chen, Y., Zhang, J., Zhang, M., Song, Y., Zhang, Y., Fan, S., et al. (2021b). Baicalein resensitizes tamoxifen-resistant breast cancer cells by reducing aerobic glycolysis and reversing mitochondrial dysfunction via inhibition of hypoxia-inducible factor-1α. Clin. Transl. Med. 11 (11), e577. doi:10.1002/ctm2.577
Chen, Z., Huang, C., Ma, T., Jiang, L., Tang, L., Shi, T., et al. (2018). Reversal effect of quercetin on multidrug resistance via FZD7/β-catenin pathway in hepatocellular carcinoma cells. Phytomedicine 43, 37–45. doi:10.1016/j.phymed.2018.03.040
Choi, C. Y., Lim, S. C., Lee, T. B., and Han, S. I. (2022). Molecular basis of resveratrol-induced resensitization of acquired drug-resistant cancer cells. Nutrients 14 (3), 699. doi:10.3390/nu14030699
Chong, T. C., Wong, I. L. K., Cui, J., Law, M. C., Zhu, X., Hu, X., et al. (2022). Characterization of a potent, selective, and safe inhibitor, Ac15(az8)(2), in reversing multidrug resistance mediated by breast cancer resistance protein (BCRP/ABCG2). Int. J. Mol. Sci. 23 (21), 13261. doi:10.3390/ijms232113261
Condello, M., Pellegrini, E., Multari, G., Gallo, F. R., and Meschini, S. (2020). Voacamine: alkaloid with its essential dimeric units to reverse tumor multidrug resistance. Toxicol Vitro 65, 104819. doi:10.1016/j.tiv.2020.104819
Cort, A., Ozben, T., Saso, L., De Luca, C., and Korkina, L. (2016). Redox control of multidrug resistance and its possible modulation by antioxidants. Oxid. Med. Cell Longev. 2016, 4251912. doi:10.1155/2016/4251912
Csikós, E., Horváth, A., Ács, K., Papp, N., Balázs, V. L., Dolenc, M. S., et al. (2021). Treatment of benign prostatic hyperplasia by natural drugs. Molecules 26 (23), 7141. doi:10.3390/molecules26237141
da Costa, A., Chowdhury, D., Shapiro, G. I., D'Andrea, A. D., and Konstantinopoulos, P. A. (2023). Targeting replication stress in cancer therapy. Nat. Rev. Drug Discov. 22 (1), 38–58. doi:10.1038/s41573-022-00558-5
Debnath, J., Gammoh, N., and Ryan, K. M. (2023). Autophagy and autophagy-related pathways in cancer. Nat. Rev. Mol. Cell Biol. 24 (8), 560–575. doi:10.1038/s41580-023-00585-z
Dong, J., Yuan, L., Hu, C., Cheng, X., and Qin, J. J. (2023). Strategies to overcome cancer multidrug resistance (MDR) through targeting P-glycoprotein (ABCB1): an updated review. Pharmacol. Ther. 249, 108488. doi:10.1016/j.pharmthera.2023.108488
Du, B., and Shim, J. S. (2016). Targeting epithelial-mesenchymal transition (EMT) to overcome drug resistance in cancer. Molecules 21 (7), 965. doi:10.3390/molecules21070965
Efferth, T., Saeed, M. E. M., Kadioglu, O., Seo, E. J., Shirooie, S., Mbaveng, A. T., et al. (2020). Collateral sensitivity of natural products in drug-resistant cancer cells. Biotechnol. Adv. 38, 107342. doi:10.1016/j.biotechadv.2019.01.009
Eichhorn, T., and Efferth, T. (2012). P-glycoprotein and its inhibition in tumors by phytochemicals derived from Chinese herbs. J. Ethnopharmacol. 141 (2), 557–570. doi:10.1016/j.jep.2011.08.053
Emran, T. B., Shahriar, A., Mahmud, A. R., Rahman, T., Abir, M. H., Siddiquee, M. F., et al. (2022). Multidrug resistance in cancer: understanding molecular mechanisms, immunoprevention and therapeutic approaches. Front. Oncol. 12, 891652. doi:10.3389/fonc.2022.891652
Erdogan, S., Doganlar, O., Doganlar, Z. B., Serttas, R., Turkekul, K., Dibirdik, I., et al. (2016). The flavonoid apigenin reduces prostate cancer CD44(+) stem cell survival and migration through PI3K/Akt/NF-κB signaling. Life Sci. 162, 77–86. doi:10.1016/j.lfs.2016.08.019
Erdogan, S., Turkekul, K., Serttas, R., and Erdogan, Z. (2017). The natural flavonoid apigenin sensitizes human CD44(+) prostate cancer stem cells to cisplatin therapy. Biomed. Pharmacother. 88, 210–217. doi:10.1016/j.biopha.2017.01.056
Fang, C. Y., Lou, D. Y., Zhou, L. Q., Wang, J. C., Yang, B., He, Q. J., et al. (2021). Natural products: potential treatments for cisplatin-induced nephrotoxicity. Acta Pharmacol. Sin. 42 (12), 1951–1969. doi:10.1038/s41401-021-00620-9
Felice, M. R., Maugeri, A., De Sarro, G., Navarra, M., and Barreca, D. (2022). Molecular pathways involved in the anti-cancer activity of flavonols: a focus on myricetin and kaempferol. Int. J. Mol. Sci. 23 (8), 4411. doi:10.3390/ijms23084411
Ferreira, A., Rodrigues, M., Fortuna, A., Falcão, A., and Alves, G. (2018a). Flavonoid compounds as reversing agents of the P-glycoprotein-mediated multidrug resistance: an in vitro evaluation with focus on antiepileptic drugs. Food Res. Int. 103, 110–120. doi:10.1016/j.foodres.2017.10.010
Ferreira, A., Santos, A. O., Falcão, A., and Alves, G. (2018b). In vitro screening of dual flavonoid combinations for reversing P-glycoprotein-mediated multidrug resistance: focus on antiepileptic drugs. Food Chem. Toxicol. 111, 84–93. doi:10.1016/j.fct.2017.11.004
Fujiki, H., Watanabe, T., Sueoka, E., Rawangkan, A., and Suganuma, M. (2018). Cancer prevention with green tea and its principal constituent, EGCG: from early investigations to current focus on human cancer stem cells. Mol. Cells 41 (2), 73–82. doi:10.14348/molcells.2018.2227
Gabr, S. A., Elsaed, W. M., Eladl, M. A., El-Sherbiny, M., Ebrahim, H. A., Asseri, S. M., et al. (2022). Curcumin modulates oxidative stress, fibrosis, and apoptosis in drug-resistant cancer cell lines. Life (Basel) 12 (9), 1427. doi:10.3390/life12091427
Ghareghomi, S., Habibi-Rezaei, M., Arese, M., Saso, L., and Moosavi-Movahedi, A. A. (2022). Nrf2 modulation in breast cancer. Biomedicines 10 (10), 2668. doi:10.3390/biomedicines10102668
Giovannuzzi, S., De Luca, V., Nocentini, A., Capasso, C., and Supuran, C. T. (2022). Coumarins inhibit eta-class carbonic anhydrase from Plasmodium falciparum. J. Enzyme Inhib. Med. Chem. 37 (1), 680–685. doi:10.1080/14756366.2022.2036986
Goel, A., and Aggarwal, B. (2010). Curcumin, the golden spice from Indian saffron, is a chemosensitizer and radiosensitizer for tumors and chemoprotector and radioprotector for normal organs. Nutr. cancer 62 (7), 919–930. doi:10.1080/01635581.2010.509835
Gonçalves, B. M. F., Cardoso, D. S. P., and Ferreira, M. J. U. (2020). Overcoming multidrug resistance: flavonoid and terpenoid nitrogen-containing derivatives as ABC transporter modulators. Molecules 25 (15), 3364. doi:10.3390/molecules25153364
Groelly, F. J., Fawkes, M., Dagg, R. A., Blackford, A. N., and Tarsounas, M. (2023). Targeting DNA damage response pathways in cancer. Nat. Rev. Cancer 23 (2), 78–94. doi:10.1038/s41568-022-00535-5
Guo, B. J., Bian, Z. X., Qiu, H. C., Wang, Y. T., and Wang, Y. (2017). Biological and clinical implications of herbal medicine and natural products for the treatment of inflammatory bowel disease. Ann. N. Y. Acad. Sci. 1401 (1), 37–48. doi:10.1111/nyas.13414
Gupta, A. K., Talukder, M., and Bamimore, M. A. (2022). Natural products for male androgenetic alopecia. Dermatol Ther. 35 (4), e15323. doi:10.1111/dth.15323
He, L., Lu, N., Dai, Q., Zhao, Y., Zhao, L., Wang, H., et al. (2013). Wogonin induced G1 cell cycle arrest by regulating Wnt/β-catenin signaling pathway and inactivating CDK8 in human colorectal cancer carcinoma cells. Toxicology 312, 36–47. doi:10.1016/j.tox.2013.07.013
Hegazy, M. F., Dawood, M., Mahmoud, N., Elbadawi, M., Sugimoto, Y., Klauck, S. M., et al. (2021). 2α-Hydroxyalantolactone from Pulicaria undulata: activity against multidrug-resistant tumor cells and modes of action. Phytomedicine 81, 153409. doi:10.1016/j.phymed.2020.153409
Heyza, J. R., Arora, S., Zhang, H., Conner, K. L., Lei, W., Floyd, A. M., et al. (2018). Targeting the DNA repair endonuclease ERCC1-XPF with green tea polyphenol epigallocatechin-3-gallate (EGCG) and its prodrug to enhance cisplatin efficacy in human cancer cells. Nutrients 10 (11), 1644. doi:10.3390/nu10111644
Hou, W., Huang, H., Wu, X. Q., and Lan, J. X. (2023). Bioactivities and mechanism of action of securinega alkaloids derivatives reported prior to 2022. Biomed. Pharmacother. 158, 114190. doi:10.1016/j.biopha.2022.114190
Hu, M., Peng, S., He, Y., Qin, M., Cong, X., Xing, Y., et al. (2015). Lycorine is a novel inhibitor of the growth and metastasis of hormone-refractory prostate cancer. Oncotarget 6 (17), 15348–15361. doi:10.18632/oncotarget.3610
Hu, M., Zhu, S., Xiong, S., Xue, X., and Zhou, X. (2019). MicroRNAs and the PTEN/PI3K/Akt pathway in gastric cancer (Review). Oncol. Rep. 41 (3), 1439–1454. doi:10.3892/or.2019.6962
Huang, G., Mei, X., and Hu, J. (2017). The antioxidant activities of natural polysaccharides. Curr. Drug Targets 18 (11), 1296–1300. doi:10.2174/1389450118666170123145357
Huang, H., Chen, A. Y., Ye, X., Guan, R., Rankin, G. O., and Chen, Y. C. (2020). Galangin, a flavonoid from lesser galangal, induced apoptosis via p53-dependent pathway in ovarian cancer cells. Molecules 25 (7), 1579. doi:10.3390/molecules25071579
Hummelova, J., Rondevaldova, J., Balastikova, A., Lapcik, O., and Kokoska, L. (2015). The relationship between structure and in vitro antibacterial activity of selected isoflavones and their metabolites with special focus on antistaphylococcal effect of demethyltexasin. Lett. Appl. Microbiol. 60 (3), 242–247. doi:10.1111/lam.12361
Huynh, D. L., Kwon, T., Zhang, J. J., Sharma, N., Gera, M., Ghosh, M., et al. (2017a). Wogonin suppresses stem cell-like traits of CD133 positive osteosarcoma cell via inhibiting matrix metallopeptidase-9 expression. BMC Complement. Altern. Med. 17 (1), 304. doi:10.1186/s12906-017-1788-y
Huynh, D. L., Ngau, T. H., Nguyen, N. H., Tran, G. B., and Nguyen, C. T. (2020). Potential therapeutic and pharmacological effects of Wogonin: an updated review. Mol. Biol. Rep. 47 (12), 9779–9789. doi:10.1007/s11033-020-05972-9
Huynh, D. L., Sharma, N., Kumar Singh, A., Singh Sodhi, S., Zhang, J. J., Mongre, R. K., et al. (2017b). Anti-tumor activity of wogonin, an extract from Scutellaria baicalensis, through regulating different signaling pathways. Chin. J. Nat. Med. 15 (1), 15–40. doi:10.1016/s1875-5364(17)30005-5
Ibáñez Gaspar, V., and McMorrow, T. (2023). The curcuminoid EF24 in combination with TRAIL reduces human renal cancer cell migration by decreasing MMP-2/MMP-9 activity through a reduction in H(2)O(2). Int. J. Mol. Sci. 24 (2), 1043. doi:10.3390/ijms24021043
Imran, M., Rauf, A., Abu-Izneid, T., Nadeem, M., Shariati, M. A., Khan, I. A., et al. (2019). Luteolin, a flavonoid, as an anticancer agent: a review. Biomed. Pharmacother. 112, 108612. doi:10.1016/j.biopha.2019.108612
Iqbal, H., Menaa, F., Khan, N. U., Razzaq, A., Khan, Z. U., Ullah, K., et al. (2022). Two promising anti-cancer compounds, 2-hydroxycinnaldehyde and 2- benzoyloxycinnamaldehyde: where do we stand? Comb. Chem. High. Throughput Screen 25 (5), 808–818. doi:10.2174/1386207324666210216094428
Jacobo-Albavera, L., Domínguez-Pérez, M., Medina-Leyte, D. J., González-Garrido, A., and Villarreal-Molina, T. (2021). The role of the ATP-binding cassette A1 (ABCA1) in human disease. Int. J. Mol. Sci. 22 (4), 1593. doi:10.3390/ijms22041593
Ji, X., Liu, K., Li, Q., Shen, Q., Han, F., Ye, Q., et al. (2022). A mini-review of flavone isomers apigenin and genistein in prostate cancer treatment. Front. Pharmacol. 13, 851589. doi:10.3389/fphar.2022.851589
Junren, C., Xiaofang, X., Huiqiong, Z., Gangmin, L., Yanpeng, Y., Xiaoyu, C., et al. (2021). Pharmacological activities and mechanisms of hirudin and its derivatives - a review. Front. Pharmacol. 12, 660757. doi:10.3389/fphar.2021.660757
Kasaian, J., Mosaffa, F., Behravan, J., Masullo, M., Piacente, S., Ghandadi, M., et al. (2015). Reversal of P-glycoprotein-mediated multidrug resistance in MCF-7/Adr cancer cells by sesquiterpene coumarins. Fitoterapia 103, 149–154. doi:10.1016/j.fitote.2015.03.025
Keyvani-Ghamsari, S., Khorsandi, K., and Gul, A. (2020). Curcumin effect on cancer cells' multidrug resistance: an update. Phytother. Res. 34 (10), 2534–2556. doi:10.1002/ptr.6703
Khan, K., Quispe, C., Javed, Z., Iqbal, M. J., Sadia, H., Raza, S., et al. (2020). Resveratrol, curcumin, paclitaxel and miRNAs mediated regulation of PI3K/Akt/mTOR pathway: go four better to treat bladder cancer. Cancer Cell Int. 20 (1), 560. doi:10.1186/s12935-020-01660-7
Kim, B., Jung, N., Lee, S., Sohng, J. K., and Jung, H. J. (2016a). Apigenin inhibits cancer stem cell-like phenotypes in human glioblastoma cells via suppression of c-met signaling. Phytother. Res. 30 (11), 1833–1840. doi:10.1002/ptr.5689
Kim, E. H., Jang, H., Shin, D., Baek, S. H., and Roh, J. L. (2016b). Targeting Nrf2 with wogonin overcomes cisplatin resistance in head and neck cancer. Apoptosis 21 (11), 1265–1278. doi:10.1007/s10495-016-1284-8
Kim, H. S., Wannatung, T., Lee, S., Yang, W. K., Chung, S. H., Lim, J. S., et al. (2012). Quercetin enhances hypoxia-mediated apoptosis via direct inhibition of AMPK activity in HCT116 colon cancer. Apoptosis 17 (9), 938–949. doi:10.1007/s10495-012-0719-0
Kokubo, S., Ohnuma, S., Murakami, M., Kikuchi, H., Funayama, S., Suzuki, H., et al. (2021). A phenylfurocoumarin derivative reverses ABCG2-mediated multidrug resistance in vitro and in vivo. Int. J. Mol. Sci. 22 (22), 12502. doi:10.3390/ijms222212502
Kuang, Y., Li, B., Wang, Z., Qiao, X., and Ye, M. (2021). Terpenoids from the medicinal mushroom Antrodia camphorata: chemistry and medicinal potential. Nat. Prod. Rep. 38 (1), 83–102. doi:10.1039/d0np00023j
Kumar, A., and Jaitak, V. (2019). Natural products as multidrug resistance modulators in cancer. Eur. J. Med. Chem. 176, 268–291. doi:10.1016/j.ejmech.2019.05.027
Lee, G., Joung, J. Y., Cho, J. H., Son, C. G., and Lee, N. (2018). Overcoming P-Glycoprotein-Mediated multidrug resistance in colorectal cancer: potential reversal agents among herbal medicines. Evid. Based Complement. Altern. Med. 2018, 3412074. doi:10.1155/2018/3412074
Leinonen, H. M., Kansanen, E., Pölönen, P., Heinäniemi, M., and Levonen, A. L. (2014). Role of the Keap1-Nrf2 pathway in cancer. Adv. Cancer Res. 122, 281–320. doi:10.1016/b978-0-12-420117-0.00008-6
Leng, S., Qu, H., Lv, X., and Liu, X. (2022). Role of ncRNA in multiple myeloma. Biomark. Med. 16 (16), 1181–1191. doi:10.2217/bmm-2022-0349
Li, H., Krstin, S., and Wink, M. (2018a). Modulation of multidrug resistant in cancer cells by EGCG, tannic acid and curcumin. Phytomedicine 50, 213–222. doi:10.1016/j.phymed.2018.09.169
Li, H., Lei, Y., Li, S., Li, F., and Lei, J. (2022). MicroRNA-20a-5p inhibits the autophagy and cisplatin resistance in ovarian cancer via regulating DNMT3B-mediated DNA methylation of RBP1. Reprod. Toxicol. 109, 93–100. doi:10.1016/j.reprotox.2021.12.011
Li, J., Duan, B., Guo, Y., Zhou, R., Sun, J., Bie, B., et al. (2018b). Baicalein sensitizes hepatocellular carcinoma cells to 5-FU and Epirubicin by activating apoptosis and ameliorating P-glycoprotein activity. Biomed. Pharmacother. 98, 806–812. doi:10.1016/j.biopha.2018.01.002
Li, S., Zhao, Q., Wang, B., Yuan, S., Wang, X., and Li, K. (2018c). Quercetin reversed MDR in breast cancer cells through down-regulating P-gp expression and eliminating cancer stem cells mediated by YB-1 nuclear translocation. Phytother. Res. 32 (8), 1530–1536. doi:10.1002/ptr.6081
Li, X., Yang, K. B., Chen, W., Mai, J., Wu, X. Q., Sun, T., et al. (2021). CUL3 (cullin 3)-mediated ubiquitination and degradation of BECN1 (beclin 1) inhibit autophagy and promote tumor progression. Autophagy 17 (12), 4323–4340. doi:10.1080/15548627.2021.1912270
Li, Y., Li, D., Wang, P., Zhu, W., and Yin, W. (2020a). Tetrandrine partially reverses multidrug resistance of human laryngeal cancer cells. J. Int. Med. Res. 48 (8), 300060520944706. doi:10.1177/0300060520944706
Li, Y. J., Lei, Y. H., Yao, N., Wang, C. R., Hu, N., Ye, W. C., et al. (2017). Autophagy and multidrug resistance in cancer. Chin. J. Cancer 36 (1), 52. doi:10.1186/s40880-017-0219-2
Li, Z., Wang, N., Yue, T., and Liu, L. (2020b). Matrine reverses the drug resistance of K562/ADM cells to ADM and VCR via promoting autophagy. Transl. Cancer Res. 9 (2), 786–794. doi:10.21037/tcr.2019.12.11
Liang, X., Fu, W., Peng, Y., Duan, J., Zhang, T., Fan, D., et al. (2023). Lycorine induces apoptosis of acute myeloid leukemia cells and inhibits triglyceride production via binding and targeting FABP5. Ann. Hematol. 102 (5), 1073–1086. doi:10.1007/s00277-023-05169-7
Liao, Y., Liu, Y., Xia, X., Shao, Z., Huang, C., He, J., et al. (2020). Targeting GRP78-dependent AR-V7 protein degradation overcomes castration-resistance in prostate cancer therapy. Theranostics 10 (8), 3366–3381. doi:10.7150/thno.41849
Lin, L., Li, L., Chen, X., Zeng, B., and Lin, T. (2018). Preliminary evaluation of the potential role of β-elemene in reversing erlotinib-resistant human NSCLC A549/ER cells. Oncol. Lett. 16 (3), 3380–3388. doi:10.3892/ol.2018.8980
Liskova, A., Samec, M., Koklesova, L., Kudela, E., Kubatka, P., and Golubnitschaja, O. (2021). Mitochondriopathies as a clue to systemic disorders-analytical tools and mitigating measures in context of predictive, preventive, and personalized (3P) medicine. Int. J. Mol. Sci. 22 (4), 2007. doi:10.3390/ijms22042007
Liu, C., Yang, S., Wang, K., Bao, X., Liu, Y., Zhou, S., et al. (2019a). Alkaloids from traditional Chinese medicine against hepatocellular carcinoma. Biomed. Pharmacother. 120, 109543. doi:10.1016/j.biopha.2019.109543
Liu, F. (2009). Mechanisms of chemotherapeutic drug resistance in cancer therapy--a quick review. Taiwan. J. obstetrics Gynecol. 48 (3), 239–244. doi:10.1016/s1028-4559(09)60296-5
Liu, J., Li, M., Wang, Y., and Luo, J. (2017). Curcumin sensitizes prostate cancer cells to radiation partly via epigenetic activation of miR-143 and miR-143 mediated autophagy inhibition. J. Drug Target 25 (7), 645–652. doi:10.1080/1061186x.2017.1315686
Liu, J. M., Li, M., Luo, W., and Sun, H. B. (2021a). Curcumin attenuates Adriamycin-resistance of acute myeloid leukemia by inhibiting the lncRNA HOTAIR/miR-20a-5p/WT1 axis. Lab. Invest. 101 (10), 1308–1317. doi:10.1038/s41374-021-00640-3
Liu, Q., Wang, C., Meng, Q., Wu, J., Sun, H., Sun, P., et al. (2021b). Puerarin sensitized K562/ADR cells by inhibiting NF-κB pathway and inducing autophagy. Phytother. Res. 35 (3), 1658–1668. doi:10.1002/ptr.6932
Liu, X., Mai, H., Jiang, H., Xing, Z., Peng, D., Kong, Y., et al. (2019b). FAM168A participates in the development of chronic myeloid leukemia via BCR-ABL1/AKT1/NFκB pathway. BMC Cancer 19 (1), 679. doi:10.1186/s12885-019-5898-4
Liu, X., Sun, C., Liu, B., Jin, X., Li, P., Zheng, X., et al. (2016). Genistein mediates the selective radiosensitizing effect in NSCLC A549 cells via inhibiting methylation of the keap1 gene promoter region. Oncotarget 7 (19), 27267–27279. doi:10.18632/oncotarget.8403
Lo, Y. L., Wang, W., and Ho, C. T. (2012). 7,3',4'-Trihydroxyisoflavone modulates multidrug resistance transporters and induces apoptosis via production of reactive oxygen species. Toxicology 302 (2-3), 221–232. doi:10.1016/j.tox.2012.08.003
Lorendeau, D., Dury, L., Genoux-Bastide, E., Lecerf-Schmidt, F., Simões-Pires, C., Carrupt, P. A., et al. (2014). Collateral sensitivity of resistant MRP1-overexpressing cells to flavonoids and derivatives through GSH efflux. Biochem. Pharmacol. 90 (3), 235–245. doi:10.1016/j.bcp.2014.05.017
Lorendeau, D., Dury, L., Nasr, R., Boumendjel, A., Teodori, E., Gutschow, M., et al. (2017). MRP1-dependent collateral sensitivity of multidrug-resistant cancer cells: identifying selective modulators inducing cellular glutathione depletion. Curr. Med. Chem. 24 (12), 1186–1213. doi:10.2174/0929867324666161118130238
Low, H. B., Wong, Z. L., Wu, B., Kong, L. R., Png, C. W., Cho, Y. L., et al. (2021). DUSP16 promotes cancer chemoresistance through regulation of mitochondria-mediated cell death. Nat. Commun. 12 (1), 2284. doi:10.1038/s41467-021-22638-7
Lu, S., Wu, L., Jian, H., Chen, Y., Wang, Q., Fang, J., et al. (2022). Sintilimab plus bevacizumab biosimilar IBI305 and chemotherapy for patients with EGFR-mutated non-squamous non-small-cell lung cancer who progressed on EGFR tyrosine-kinase inhibitor therapy (ORIENT-31): first interim results from a randomised, double-blind, multicentre, phase 3 trial. Lancet Oncol. 23 (9), 1167–1179. doi:10.1016/s1470-2045(22)00382-5
Luca, S. V., Macovei, I., Bujor, A., Miron, A., Skalicka-Wozniak, K., Aprotosoaie, A. C., et al. (2020). Bioactivity of dietary polyphenols: the role of metabolites. Crit. Rev. Food Sci. Nutr. 60 (4), 626–659. doi:10.1080/10408398.2018.1546669
Luo, Y., Peng, B., Wei, W., Tian, X., and Wu, Z. (2019). Antioxidant and anti-diabetic activities of polysaccharides from guava leaves. Molecules 24 (7), 1343. doi:10.3390/molecules24071343
Maleki Dana, P., Sadoughi, F., Mirzaei, H., Asemi, Z., and Yousefi, B. (2022). DNA damage response and repair in the development and treatment of brain tumors. Eur. J. Pharmacol. 924, 174957. doi:10.1016/j.ejphar.2022.174957
Mans, D. R., da Rocha, A. B., and Schwartsmann, G. (2000). Anti-cancer drug discovery and development in Brazil: targeted plant collection as a rational strategy to acquire candidate anti-cancer compounds. Oncologist 5 (3), 185–198. doi:10.1634/theoncologist.5-3-185
Martinelli, F., Perrone, A., Yousefi, S., Papini, A., Castiglione, S., Guarino, F., et al. (2021). Botanical, phytochemical, anti-microbial and pharmaceutical characteristics of hawthorn (crataegusmonogyna jacq.), rosaceae. Molecules 26 (23), 7266. doi:10.3390/molecules26237266
Meiyanto, E., Putri, D., Susidarti, R., Murwanti, R., and Fitriasari, A. (2014). Curcumin and its analogues (PGV-0 and PGV-1) enhance sensitivity of resistant MCF-7 cells to doxorubicin through inhibition of HER2 and NF-kB activation. Asian Pac. J. cancer Prev. 15 (1), 179–184. doi:10.7314/apjcp.2014.15.1.179
Meng, L., Xia, X., Yang, Y., Ye, J., Dong, W., Ma, P., et al. (2016). Co-encapsulation of paclitaxel and baicalein in nanoemulsions to overcome multidrug resistance via oxidative stress augmentation and P-glycoprotein inhibition. Int. J. Pharm. 513 (1-2), 8–16. doi:10.1016/j.ijpharm.2016.09.001
Mohammad, I. S., He, W., and Yin, L. (2018). Understanding of human ATP binding cassette superfamily and novel multidrug resistance modulators to overcome MDR. Biomed. Pharmacother. 100, 335–348. doi:10.1016/j.biopha.2018.02.038
Montagut, C., Dalmases, A., Bellosillo, B., Crespo, M., Pairet, S., Iglesias, M., et al. (2012). Identification of a mutation in the extracellular domain of the Epidermal Growth Factor Receptor conferring cetuximab resistance in colorectal cancer. Nat. Med. 18 (2), 221–223. doi:10.1038/nm.2609
Mortezaee, K., Najafi, M., Farhood, B., Ahmadi, A., Shabeeb, D., and Musa, A. (2019a). NF-κB targeting for overcoming tumor resistance and normal tissues toxicity. J. Cell. physiology 234 (10), 17187–17204. doi:10.1002/jcp.28504
Mortezaee, K., Salehi, E., Mirtavoos-Mahyari, H., Motevaseli, E., Najafi, M., Farhood, B., et al. (2019b). Mechanisms of apoptosis modulation by curcumin: implications for cancer therapy. J. Cell Physiol. 234 (8), 12537–12550. doi:10.1002/jcp.28122
Murakami, M., Ohnuma, S., Fukuda, M., Chufan, E. E., Kudoh, K., Kanehara, K., et al. (2017). Synthetic analogs of curcumin modulate the function of multidrug resistance-linked ATP-binding cassette transporter ABCG2. Drug Metab. Dispos. 45 (11), 1166–1177. doi:10.1124/dmd.117.076000
Noorian, M., Chamani, E., Salmani, F., Rezaei, Z., and Khorsandi, K. (2022). Effects of doxorubicin and apigenin on chronic myeloid leukemia cells (K562) in vitro: anti-proliferative and apoptosis induction assessments. Nat. Prod. Res. 37, 3335–3343. doi:10.1080/14786419.2022.2069765
Panieri, E., Pinho, S. A., Afonso, G. J. M., Oliveira, P. J., Cunha-Oliveira, T., and Saso, L. (2022). NRF2 and mitochondrial function in cancer and cancer stem cells. Cells 11 (15), 2401. doi:10.3390/cells11152401
Papadochristopoulos, A., Kerry, J. P., Fegan, N., Burgess, C. M., and Duffy, G. (2021). Natural anti-microbials for enhanced microbial safety and shelf-life of processed packaged meat. Foods 10 (7), 1598. doi:10.3390/foods10071598
Park, C. H., Han, S. E., Nam-Goong, I. S., Kim, Y. I., and Kim, E. S. (2018). Combined effects of baicalein and Docetaxel on apoptosis in 8505c anaplastic thyroid cancer cells via downregulation of the ERK and akt/mTOR pathways. Endocrinol. Metab. Seoul. 33 (1), 121–132. doi:10.3803/EnM.2018.33.1.121
Penson, P. E., and Banach, M. (2021). Natural compounds as anti-atherogenic agents: clinical evidence for improved cardiovascular outcomes. Atherosclerosis 316, 58–65. doi:10.1016/j.atherosclerosis.2020.11.015
Punia, R., Raina, K., Agarwal, R., and Singh, R. P. (2017). Acacetin enhances the therapeutic efficacy of doxorubicin in non-small-cell lung carcinoma cells. PLoS One 12 (8), e0182870. doi:10.1371/journal.pone.0182870
Rakesh, R., PriyaDharshini, L. C., Sakthivel, K. M., and Rasmi, R. R. (2022). Role and regulation of autophagy in cancer. Biochim. Biophys. Acta Mol. Basis Dis. 1868 (7), 166400. doi:10.1016/j.bbadis.2022.166400
Ren, B., Kwah, M. X., Liu, C., Ma, Z., Shanmugam, M. K., Ding, L., et al. (2021). Resveratrol for cancer therapy: challenges and future perspectives. Cancer Lett. 515, 63–72. doi:10.1016/j.canlet.2021.05.001
Riahi-Chebbi, I., Souid, S., Othman, H., Haoues, M., Karoui, H., Morel, A., et al. (2019). The Phenolic compound Kaempferol overcomes 5-fluorouracil resistance in human resistant LS174 colon cancer cells. Sci. Rep. 9 (1), 195. doi:10.1038/s41598-018-36808-z
Robey, R., Polgar, O., Deeken, J., To, K., and Bates, S. (2007). ABCG2: determining its relevance in clinical drug resistance. Cancer metastasis Rev. 26 (1), 39–57. doi:10.1007/s10555-007-9042-6
Rong, L. W., Wang, R. X., Zheng, X. L., Feng, X. Q., Zhang, L., Zhang, L., et al. (2017). Combination of wogonin and sorafenib effectively kills human hepatocellular carcinoma cells through apoptosis potentiation and autophagy inhibition. Oncol. Lett. 13 (6), 5028–5034. doi:10.3892/ol.2017.6059
Roy, M., Liang, L., Xiao, X., Feng, P., Ye, M., and Liu, J. (2018). Lycorine: a prospective natural lead for anticancer drug discovery. Biomed. Pharmacother. 107, 615–624. doi:10.1016/j.biopha.2018.07.147
Roy, M., Liang, L., Xiao, X., Peng, Y., Luo, Y., Zhou, W., et al. (2016). Lycorine downregulates HMGB1 to inhibit autophagy and enhances bortezomib activity in multiple myeloma. Theranostics 6 (12), 2209–2224. doi:10.7150/thno.15584
Rudnicka, E., Napierala, P., Podfigurna, A., Meczekalski, B., Smolarczyk, R., and Grymowicz, M. (2020). The World Health Organization (WHO) approach to healthy ageing. Maturitas 139, 6–11. doi:10.1016/j.maturitas.2020.05.018
Sajadimajd, S., and Khazaei, M. (2018). Oxidative stress and cancer: the role of Nrf2. Curr. Cancer Drug Targets 18 (6), 538–557. doi:10.2174/1568009617666171002144228
Sancha, S. A. R., Szemerédi, N., Spengler, G., and Ferreira, M. U. (2023). Lycorine carbamate derivatives for reversing P-glycoprotein-Mediated multidrug resistance in human colon adenocarcinoma cells. Int. J. Mol. Sci. 24 (3), 2061. doi:10.3390/ijms24032061
Saraei, R., Rahman, H. S., Soleimani, M., Asghari-Jafarabadi, M., Naimi, A., Hassanzadeh, A., et al. (2022). Kaempferol sensitizes tumor necrosis factor-related apoptosis-inducing ligand-resistance chronic myelogenous leukemia cells to apoptosis. Mol. Biol. Rep. 49 (1), 19–29. doi:10.1007/s11033-021-06778-z
Sarkadi, B., Homolya, L., Szakács, G., and Váradi, A. (2006). Human multidrug resistance ABCB and ABCG transporters: participation in a chemoimmunity defense system. Physiol. Rev. 86 (4), 1179–1236. doi:10.1152/physrev.00037.2005
Siegel, R. L., Miller, K. D., Wagle, N. S., and Jemal, A. (2023). Cancer statistics, 2023. CA Cancer J. Clin. 73 (1), 17–48. doi:10.3322/caac.21763
Singh, D., Assaraf, Y. G., and Gacche, R. N. (2022). Long non-coding RNA mediated drug resistance in breast cancer. Drug Resist Updat 63, 100851. doi:10.1016/j.drup.2022.100851
Singh, S., Gupta, P., Meena, A., and Luqman, S. (2020). Acacetin, a flavone with diverse therapeutic potential in cancer, inflammation, infections and other metabolic disorders. Food Chem. Toxicol. 145, 111708. doi:10.1016/j.fct.2020.111708
Skok, Ž., Zidar, N., Kikelj, D., and Ilaš, J. (2020). Dual inhibitors of human DNA topoisomerase II and other cancer-related targets. J. Med. Chem. 63 (3), 884–904. doi:10.1021/acs.jmedchem.9b00726
Song, Y. Y., Yuan, Y., Shi, X., and Che, Y. Y. (2020). Improved drug delivery and anti-tumor efficacy of combinatorial liposomal formulation of genistein and plumbagin by targeting Glut1 and Akt3 proteins in mice bearing prostate tumor. Colloids Surf. B Biointerfaces 190, 110966. doi:10.1016/j.colsurfb.2020.110966
Su, S. C., Hsin, C. H., Lu, Y. T., Chuang, C. Y., Ho, Y. T., Yeh, F. L., et al. (2023). EF-24, a curcumin analog, inhibits cancer cell invasion in human nasopharyngeal carcinoma through transcriptional suppression of matrix metalloproteinase-9 gene expression. Cancers (Basel) 15 (5), 1552. doi:10.3390/cancers15051552
Sung, H., Ferlay, J., Siegel, R. L., Laversanne, M., Soerjomataram, I., Jemal, A., et al. (2021). Global cancer statistics 2020: GLOBOCAN estimates of incidence and mortality worldwide for 36 cancers in 185 countries. CA Cancer J. Clin. 71 (3), 209–249. doi:10.3322/caac.21660
Tamaddonfard, E., Erfanparast, A., Farshid, A. A., Imani, M., Mirzakhani, N., Salighedar, R., et al. (2019). Safranal, a constituent of saffron, exerts gastro-protective effects against indomethacin-induced gastric ulcer. Life Sci. 224, 88–94. doi:10.1016/j.lfs.2019.03.054
Tan, T., Li, J., Luo, R., Wang, R., Yin, L., Liu, M., et al. (2021). Recent advances in understanding the mechanisms of elemene in reversing drug resistance in tumor cells: a review. Molecules 26 (19), 5792. doi:10.3390/molecules26195792
Tang, H., Zeng, L., Wang, J., Zhang, X., Ruan, Q., Wang, J., et al. (2017). Reversal of 5-fluorouracil resistance by EGCG is mediate by inactivation of TFAP2A/VEGF signaling pathway and down-regulation of MDR-1 and P-gp expression in gastric cancer. Oncotarget 8 (47), 82842–82853. doi:10.18632/oncotarget.20666
Tavares-Valente, D., Baltazar, F., Moreira, R., and Queirós, O. (2013). Cancer cell bioenergetics and pH regulation influence breast cancer cell resistance to paclitaxel and doxorubicin. J. Bioenerg. Biomembr. 45 (5), 467–475. doi:10.1007/s10863-013-9519-7
Theissinger, K., Fernandes, C., Formenti, G., Bista, I., Berg, P. R., Bleidorn, C., et al. (2023). How genomics can help biodiversity conservation. Trends Genet. 39 (7), 545–559. doi:10.1016/j.tig.2023.01.005
Tiwari, A., Trivedi, R., and Lin, S. Y. (2022). Tumor microenvironment: barrier or opportunity towards effective cancer therapy. J. Biomed. Sci. 29 (1), 83. doi:10.1186/s12929-022-00866-3
Tomeh, M. A., Hadianamrei, R., and Zhao, X. (2019). A review of curcumin and its derivatives as anticancer agents. Int. J. Mol. Sci. 20 (5), 1033. doi:10.3390/ijms20051033
Tong, R., Wu, X., Liu, Y., Liu, Y., Zhou, J., Jiang, X., et al. (2020). Curcumin-induced DNA demethylation in human gastric cancer cells is mediated by the DNA-damage response pathway. Oxid. Med. Cell Longev. 2020, 2543504. doi:10.1155/2020/2543504
Villegas, C., Perez, R., Sterner, O., González-Chavarría, I., and Paz, C. (2021). Curcuma as an adjuvant in colorectal cancer treatment. Life Sci. 286, 120043. doi:10.1016/j.lfs.2021.120043
Vissenaekens, H., Criel, H., Grootaert, C., Raes, K., Smagghe, G., and Van Camp, J. (2022). Flavonoids and cellular stress: a complex interplay affecting human health. Crit. Rev. Food Sci. Nutr. 62 (31), 8535–8566. doi:10.1080/10408398.2021.1929822
Vlahopoulos, S. (2017). Aberrant control of NF-κB in cancer permits transcriptional and phenotypic plasticity, to curtail dependence on host tissue: molecular mode. Cancer Biol. Med. 14 (3), 254–270. doi:10.20892/j.issn.2095-3941.2017.0029
Wang, M., Liu, Z. F., Tang, H., and Chen, B. A. (2018). Application of alkaloids in reversing multidrug resistance in human cancers. Chin. J. Nat. Med. 16 (8), 561–571. doi:10.1016/S1875-5364(18)30093-1
Wang, Q., Yang, B., Wang, N., and Gu, J. (2022). Tumor immunomodulatory effects of polyphenols. Front. Immunol. 13, 1041138. doi:10.3389/fimmu.2022.1041138
Wang, X., Jiang, P., Wang, P., Yang, C. S., Wang, X., and Feng, Q. (2015). EGCG enhances cisplatin sensitivity by regulating expression of the copper and cisplatin influx transporter CTR1 in ovary cancer. PLoS One 10 (4), e0125402. doi:10.1371/journal.pone.0125402
Wang, X., Li, Y., Qian, Y., Cao, Y., Shriwas, P., Zhang, H., et al. (2017). Extracellular ATP, as an energy and phosphorylating molecule, induces different types of drug resistances in cancer cells through ATP internalization and intracellular ATP level increase. Oncotarget 8 (50), 87860–87877. doi:10.18632/oncotarget.21231
Wang, Y., Wang, W., Wu, X., Li, C., Huang, Y., Zhou, H., et al. (2020). Resveratrol sensitizes colorectal cancer cells to cetuximab by connexin 43 upregulation-induced Akt inhibition. Front. Oncol. 10, 383. doi:10.3389/fonc.2020.00383
Wang, Z., Sun, X., Feng, Y., Wang, Y., Zhang, L., Wang, Y., et al. (2021). Dihydromyricetin reverses MRP2-induced multidrug resistance by preventing NF-κB-Nrf2 signaling in colorectal cancer cell. Phytomedicine 82, 153414. doi:10.1016/j.phymed.2020.153414
Wong, K. K. (2021). DNMT1: a key drug target in triple-negative breast cancer. Semin. Cancer Biol. 72, 198–213. doi:10.1016/j.semcancer.2020.05.010
Wu, H., Du, J., Li, C., Li, H., Guo, H., and Li, Z. (2022). Kaempferol can reverse the 5-fu resistance of colorectal cancer cells by inhibiting PKM2-mediated glycolysis. Int. J. Mol. Sci. 23 (7), 3544. doi:10.3390/ijms23073544
Wu, H., Zhou, J., Zeng, C., Wu, D., Mu, Z., Chen, B., et al. (2016). Curcumin increases exosomal TCF21 thus suppressing exosome-induced lung cancer. Oncotarget 7 (52), 87081–87090. doi:10.18632/oncotarget.13499
Xia, L., Tan, S., Zhou, Y., Lin, J., Wang, H., Oyang, L., et al. (2018). Role of the NFκB-signaling pathway in cancer. OncoTargets Ther. 11, 2063–2073. doi:10.2147/ott.S161109
Xiao, X., Jiang, K., Xu, Y., Peng, H., Wang, Z., Liu, S., et al. (2019). (-)-Epigallocatechin-3-gallate induces cell apoptosis in chronic myeloid leukaemia by regulating Bcr/Abl-mediated p38-MAPK/JNK and JAK2/STAT3/AKT signalling pathways. Clin. Exp. Pharmacol. Physiol. 46 (2), 126–136. doi:10.1111/1440-1681.13037
Xiaokaiti, Y., and Li, X. (2020). Natural product regulates autophagy in cancer. Adv. Exp. Med. Biol. 1207, 709–724. doi:10.1007/978-981-15-4272-5_53
Xing, Y., Wei, X., Liu, Y., Wang, M. M., Sui, Z., Wang, X., et al. (2022). Autophagy inhibition mediated by MCOLN1/TRPML1 suppresses cancer metastasis via regulating a ROS-driven TP53/p53 pathway. Autophagy 18 (8), 1932–1954. doi:10.1080/15548627.2021.2008752
Xu, X., Zhang, Y., Li, W., Miao, H., Zhang, H., Zhou, Y., et al. (2014). Wogonin reverses multi-drug resistance of human myelogenous leukemia K562/A02 cells via downregulation of MRP1 expression by inhibiting Nrf2/ARE signaling pathway. Biochem. Pharmacol. 92 (2), 220–234. doi:10.1016/j.bcp.2014.09.008
Xu, Y., Wang, S., Chan, H. F., Lu, H., Lin, Z., He, C., et al. (2017). Dihydromyricetin induces apoptosis and reverses drug resistance in ovarian cancer cells by p53-mediated downregulation of survivin. Sci. Rep. 7, 46060. doi:10.1038/srep46060
Yang, J., Aljitawi, O., and Van Veldhuizen, P. (2022). Prostate cancer stem cells: the role of CD133. Cancers (Basel) 14 (21), 5448. doi:10.3390/cancers14215448
Yang, J., Hai, J., Dong, X., Zhang, M., and Duan, S. (2021). MicroRNA-92a-3p enhances cisplatin resistance by regulating krüppel-like factor 4-mediated cell apoptosis and epithelial-to-mesenchymal transition in cervical cancer. Front. Pharmacol. 12, 783213. doi:10.3389/fphar.2021.783213
Yang, L., Wang, Q., Li, D., Zhou, Y., Zheng, X., Sun, H., et al. (2013). Wogonin enhances antitumor activity of tumor necrosis factor-related apoptosis-inducing ligand in vivo through ROS-mediated downregulation of cFLIPL and IAP proteins. Apoptosis 18 (5), 618–626. doi:10.1007/s10495-013-0808-8
Ye, Q., Liu, K., Shen, Q., Li, Q., Hao, J., Han, F., et al. (2019). Reversal of multidrug resistance in cancer by multi-functional flavonoids. Front. Oncol. 9, 487. doi:10.3389/fonc.2019.00487
Yen, C., Zhao, F., Yu, Z., Zhu, X., and Li, C. G. (2022). Interactions between natural products and tamoxifen in breast cancer: a comprehensive literature review. Front. Pharmacol. 13, 847113. doi:10.3389/fphar.2022.847113
Yu, H., Qiu, Y., Pang, X., Li, J., Wu, S., Yin, S., et al. (2017a). Lycorine promotes autophagy and apoptosis via TCRP1/akt/mTOR Axis inactivation in human hepatocellular carcinoma. Mol. Cancer Ther. 16 (12), 2711–2723. doi:10.1158/1535-7163.Mct-17-0498
Yu, M., Qi, B., Xiaoxiang, W., Xu, J., and Liu, X. (2017b). Baicalein increases cisplatin sensitivity of A549 lung adenocarcinoma cells via PI3K/Akt/NF-κB pathway. Biomed. Pharmacother. 90, 677–685. doi:10.1016/j.biopha.2017.04.001
Yu, S., Gong, L. S., Li, N. F., Pan, Y. F., and Zhang, L. (2018). Galangin (GG) combined with cisplatin (DDP) to suppress human lung cancer by inhibition of STAT3-regulated NF-κB and Bcl-2/Bax signaling pathways. Biomed. Pharmacother. 97, 213–224. doi:10.1016/j.biopha.2017.10.059
Zeng, R., Yang, X. M., Li, H. W., Li, X., Guan, Y., Yu, T., et al. (2023). Simplified derivatives of tetrandrine as potent and specific P-gp inhibitors to reverse multidrug resistance in cancer chemotherapy. J. Med. Chem. 66 (6), 4086–4105. doi:10.1021/acs.jmedchem.2c02061
Zhang, J., Zhang, H. D., Yao, Y. F., Zhong, S. L., Zhao, J. H., and Tang, J. H. (2015a). β-Elemene reverses chemoresistance of breast cancer cells by reducing resistance transmission via exosomes. Cell Physiol. Biochem. 36 (6), 2274–2286. doi:10.1159/000430191
Zhang, L., Li, Y., Hu, C., Chen, Y., Chen, Z., Chen, Z. S., et al. (2022a). CDK6-PI3K signaling axis is an efficient target for attenuating ABCB1/P-gp mediated multi-drug resistance (MDR) in cancer cells. Mol. Cancer 21 (1), 103. doi:10.1186/s12943-022-01524-w
Zhang, L., Song, J., Kong, L., Yuan, T., Li, W., Zhang, W., et al. (2020). The strategies and techniques of drug discovery from natural products. Pharmacol. Ther. 216, 107686. doi:10.1016/j.pharmthera.2020.107686
Zhang, L., Ye, B., Chen, Z., and Chen, Z. S. (2023). Progress in the studies on the molecular mechanisms associated with multidrug resistance in cancers. Acta Pharm. Sin. B 13 (3), 982–997. doi:10.1016/j.apsb.2022.10.002
Zhang, W., Li, S., Li, C., Li, T., and Huang, Y. (2022b). Remodeling tumor microenvironment with natural products to overcome drug resistance. Front. Immunol. 13, 1051998. doi:10.3389/fimmu.2022.1051998
Zhang, X., Guo, Q., Chen, J., and Chen, Z. (2015b). Quercetin enhances cisplatin sensitivity of human osteosarcoma cells by modulating microRNA-217-KRAS Axis. Mol. Cells 38 (7), 638–642. doi:10.14348/molcells.2015.0037
Zhang, Y., Liu, K., Yan, C., Yin, Y., He, S., Qiu, L., et al. (2022c). Natural polyphenols for treatment of colorectal cancer. Molecules 27 (24), 8810. doi:10.3390/molecules27248810
Zhao, Q., Guan, J., Qin, Y., Ren, P., Zhang, Z., Lv, J., et al. (2018). Curcumin sensitizes lymphoma cells to DNA damage agents through regulating Rad51-dependent homologous recombination. Biomed. Pharmacother. 97, 115–119. doi:10.1016/j.biopha.2017.09.078
Zhao, Y., Yan, B., Wang, Z., Li, M., and Zhao, W. (2020). Natural polysaccharides with immunomodulatory activities. Mini Rev. Med. Chem. 20 (2), 96–106. doi:10.2174/1389557519666190913151632
Zhou, S., Li, J., Xu, H., Zhang, S., Chen, X., Chen, W., et al. (2017). Liposomal curcumin alters chemosensitivity of breast cancer cells to Adriamycin via regulating microRNA expression. Gene 622, 1–12. doi:10.1016/j.gene.2017.04.026
Zhou, X., Ao, X., Jia, Z., Li, Y., Kuang, S., Du, C., et al. (2022a). Non-coding RNA in cancer drug resistance: underlying mechanisms and clinical applications. Front. Oncol. 12, 951864. doi:10.3389/fonc.2022.951864
Zhou, X., Jin, N., and Chen, B. (2022b). Tetrandrine overcomes drug resistance mediated by bone marrow microenvironment by regulating the expression of P-glycoprotein in acute leukemia. Hematology 27 (1), 274–279. doi:10.1080/16078454.2022.2034256
Zou, T., Zeng, C., Qu, J., Yan, X., and Lin, Z. (2021). Rutaecarpine increases anticancer drug sensitivity in drug-resistant cells through MARCH8-dependent ABCB1 degradation. Biomedicines 9 (9), 1143. doi:10.3390/biomedicines9091143
Keywords: mechanism, natural product-derived compounds, cancer, multidrug resistance, MDR
Citation: Zou J-Y, Chen Q-L, Luo X-C, Damdinjav D, Abdelmohsen UR, Li H-Y, Battulga T, Chen H-B, Wang Y-Q and Zhang J-Y (2024) Natural products reverse cancer multidrug resistance. Front. Pharmacol. 15:1348076. doi: 10.3389/fphar.2024.1348076
Received: 01 December 2023; Accepted: 19 February 2024;
Published: 08 March 2024.
Edited by:
Yan Zhang, Shenyang Pharmaceutical University, ChinaReviewed by:
Radhika Amaradhi, University of Texas at San Antonio, United StatesBerna Dogan, Istanbul Technical University, Türkiye
Andleeb Sardar, Government College University, Pakistan
Copyright © 2024 Zou, Chen, Luo, Damdinjav, Abdelmohsen, Li, Battulga, Chen, Wang and Zhang. This is an open-access article distributed under the terms of the Creative Commons Attribution License (CC BY). The use, distribution or reproduction in other forums is permitted, provided the original author(s) and the copyright owner(s) are credited and that the original publication in this journal is cited, in accordance with accepted academic practice. No use, distribution or reproduction is permitted which does not comply with these terms.
*Correspondence: Davaadagva Damdinjav, ZGFndmFhMTk3MkB5YWhvby5jb20=; Hu-Biao Chen, aGJjaGVuQGhrYnUuZWR1Lmhr; Yu-Qing Wang, d2FuZ3l1cUBnemhtdS5lZHUuY24=; Jian-Ye Zhang, amlhbnllekAxNjMuY29t
†These authors have contributed equally to this work