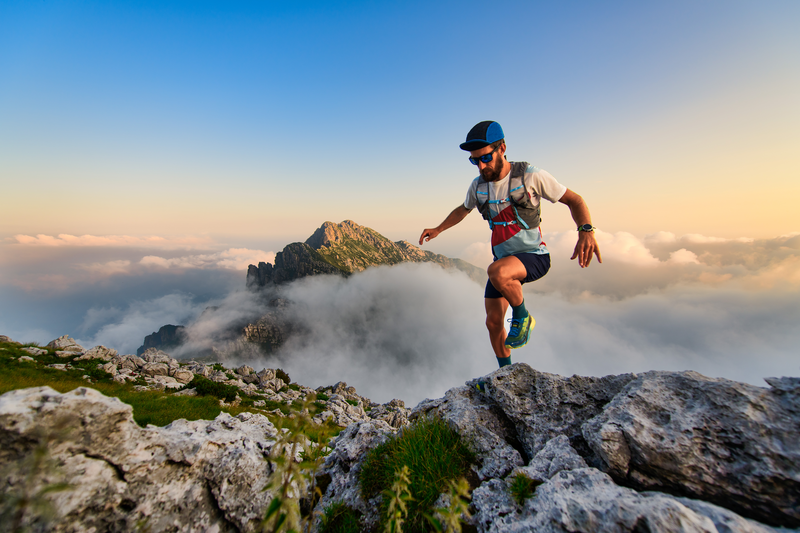
94% of researchers rate our articles as excellent or good
Learn more about the work of our research integrity team to safeguard the quality of each article we publish.
Find out more
ORIGINAL RESEARCH article
Front. Pharmacol. , 09 April 2024
Sec. Experimental Pharmacology and Drug Discovery
Volume 15 - 2024 | https://doi.org/10.3389/fphar.2024.1343306
This article is part of the Research Topic Cellular and Molecular Mechanisms in Metabolic Disorders: Role of Inflammation and Oxidative Stress View all 9 articles
Introduction: Vernonia anthelmintica (L.) Willd. is a traditional treatment for vitiligo in Xinjiang. However, its therapeutic mechanism remains unclear owing to its complex composition and limited research on its chemical profile.
Methods: We employed a targeted metabolome approach, combining selective reaction monitoring/multiple response monitoring (SRM/MRM) with high-performance liquid chromatography and MRM mass spectrometry to quantitatively analyze the flavonoid constituents of Vernonia anthelmintica. We also used network pharmacology and molecular docking to identify potential vitiligo-linked compounds and targets of V. anthelmintica seeds. Additionally, we assessed HaCaT cell proliferation by AAPH-induced, alongside changes in SOD activity and MDA content, following treatment with V. anthelmintica components. Finally, flow cytometry was used to detect apoptosis and ROS levels.
Results and Discussion: We identified 36 flavonoid compounds in V. anthelmintica seeds, with 14 compounds exhibiting druggability. AKT1, VEGFA, ESR1, PTGS2, and IL2 have been identified as key therapeutic target genes, with PI3K/AKT signaling being an important pathway. Notably, kaempferol, one of the identified compounds, exhibited high expression in network pharmacology analysis. Kaempferol exhibited a strong binding affinity to important targets. Further, kaempferol enhanced HaCaT cell viability, inhibited apoptosis, reduced MDA levels, suppressed ROS activity, and upregulated SOD activity, increase the expression of cellular antioxidant genes, including HO-1, GCLC, GCLM, Nrf2, NQO1 and Keap1, providing significant protection against oxidative stress damage in vitro. Here, we present the first comprehensive study integrating SRM/MRM approaches and network analysis to identify active flavonoid compounds within V. anthelmintica (L.) Willd. Moreover, we revealed that its active ingredient, kaempferol, offers protection against AAPH-induced damage in keratinocytes, highlighting its potential as a clinical resource.
Vitiligo stands as the most common skin depigmentation disease, characterized by chronic depigmentation and milk-white lesions on the skin, with a global prevalence of 0.5%–2% (Krüger and Schallreuter, 2012). Vitiligo can have a detrimental impact on appearance, affect social interaction, work experiences, marriage prospects, thereby resulting in social discrimination, which can promote the development of anxiety, depression, and other mental health disorders (Dabas et al., 2020; Wang et al., 2022). Clinically, vitiligo often co-occurs with hyperthyroidism, diabetes, alopecia areata, and other autoimmune disorders and has the capacity to induce the onset of psoriasis, malignant tumors, bronchial asthma, and other diseases (Amer and Gao, 2016; Zhang et al., 2022).
Oxidative stress damage is pivotal in the etiology of vitiligo, being considered as one of the most crucial initiators of this condition (Di Dalmazi et al., 2016; Chen et al., 2021). The secretion of growth factors, release of pro-inflammatory cytokines, and activation of inflammasomes within keratinocytes can exacerbate oxidative stress and promote immune responses, thus inducing or exacerbating the development of vitiligo (Chen et al., 2019). Therefore, antioxidant therapy has emerged as a promising strategy for the treatment of vitiligo.
Vernonia anthelmintica (L.) Willd. is an annual herb of the Compositae family. Notably, its habitat is exclusive to Kashgar, Aksu, and other areas in Xinjiang and India (Zhou et al., 2012; Hu et al., 2023). However, the chemical composition of Vernonia anthelmintica (L.) Willd. is complex and remains relatively unexplored worldwide. Previous studies have shown that the most effective ingredients for treating of vitiligo were flavonoids (Orhan and Khan, 2014; Liu-Smith and Meyskens, 2016). However, current investigations into V. anthelmintica (L.) Willd., have predominantly centered on total flavonoid extraction, with few studies focusing on individual flavonoid monomers and their respective therapeutic targets. Recent studies have suggested that kaempferol may be the primary active component of V. anthelmintica (L.) Willd. (Liu-Smith and Meyskens, 2016). Kaempferol is a flavonoid, commonly found in various Chinese plants, including tea, legumes, and plant-derived foods. Kaempferol has been demonstrated to play an important role in inflammatory diseases such as rheumatoid arthritis, systemic lupus erythematosus, and ankylosing spondylitis (Du et al., 2018; Pan et al., 2018; Wang et al., 2019a). However, the precise molecular mechanisms underlying the role of kaempferol in treating vitiligo remain unclear.
The targeted metabolome selective reaction/multiple response monitoring (SRM/MRM) approach enabling the detection and analysis of specific metabolite groups, yields absolute quantitative results for target metabolites. This method holds significant value, particularly in elucidating the complex compositions of traditional Chinese medicine (Awasthi et al., 2018). Network pharmacology is a technique that establishes associations between drugs and diseases by constructing intricate networks, offering insights into the potential mechanism of action of these medications (Wang et al., 2021; Ko et al., 2022; Noor et al., 2022; Liu et al., 2023). The integration of network pharmacology and molecular docking analyses emerges as an important approach for exploring key compounds and targets intricate relationships.
Therefore, here, we employed the SRM/MRM method to isolate 36 flavonoid compounds from V. anthelmintica (L.) Willd. Subsequently, we conducted a systematic network analysis to investigate the relationships between herbs, bioactive compounds, and putative target genes associated with V. anthelmintica (L.) Willd. Compounds that exhibited druggability were then screened for oral bioavailability (OB) and drug-likeness (DL). Putative target genes of these compounds and vitiligo-related genes were subsequently retrieved from public databases. By constructing a network that links the compounds with druggability derived from V. anthelmintica (L.) Willd. with their respective target genes, we aimed to identify key compounds and potential targets implicated in the treatment of vitiligo. Overall, this study demonstrated that kaempferol holds significant potential as a novel treatment strategy for vitiligo.
Kaempferol, AAPH, superoxide dismutase (SOD), malondialdehyde (MDA), and cytotoxicity assay (CCK-8) kits were obtained from Solarbio (Beijing, China). 3,5-dimethylphenol was obtained from Life Technologies (Carlsbad, CA, United States). Phosphate-buffered saline (PBS) was procured from Biological Industries (Ridgefield, CT, United States). Penicillin–streptomycin solution was obtained from ScienCell (San Diego, CA, United States). Dulbecco’s modified Eagle’s medium (DMEM) and trypticase were purchased from HyClone (Logan, UT, United States). Fetal bovine serum (FBS) was procured from Gibco (Waltham, MA, United States). A BCA Protein Quantitation Kit was obtained from Thermo Fisher Scientific (Waltham, MA, United States).
Vernonia anthelmintica (L.) Willd. was procured from the Eric Uygur medical room in Urumqi (Xinjiang Province, China). Subsequently, the chief pharmacist of the Pharmaceutical Research Institute of the Xinjiang Uygur Autonomous Region identified this sample as dried mature seeds of V. anthelmintica (L.) Willd. The extraction process involved placing the seed powder in a sealed contained and adding 50 mL of 60% ethanol solution. This mixture was then subjected to ultrasonic extraction at 40°C for 50 min, followed by filtration. Subsequently, 40 mL of 60% ethanol solution was added to the residue before further ultrasonic extraction at 40°C for 20 min, followed by filtration. The filtrates from both steps were then combined, before a final filtration with 10 mL of hot ethanol solution (60% volume fraction, 56°C preheat). The resulting filtrate was then cooled down to 25°C and a 60% ethanol solution was adjusted to 100 mL. Finally, the sample was shaken and left to stand for future use.
First, we analyzed the V. anthelmintica (L.) Willd. extract using SRM/MRM. Initially, 500 µL of the sample was placed in a 2-mL centrifuge tube, for centrifugation at 4000 rpm for 5 min. The supernatant was then removed, with 5 µL aliquots being placed into a 96 well plate for mass analysis. The sample was then placed in an ACQUITY UPLC I-Class system (Waters, American), for SRM/MRM under the following conditions: An autosampler temperature of 4°C; Mobile phase A and B of 0.1% formic acid in water and acetonitrile, respectively. The gradient elution procedure used was as follows: B increased from 5% to 20% between 0 and 3 min; B was then maintained at 20% between 3 and 4.3 min; at 4.3–9 min B increased from 20% to 45%; then, B increased from 45% to 98% between 9 and 11 min; at 11–13 min B was maintained at 98%; B then decreased from 98% to 5% between 13 and 13.1 min; and, finally, B was maintained at 5% at 13.1–15 min. The flow rate of this system was set at 400 μL/min, with an injection volume of 5 µL.
For mass spectrometry (MS), a Sciex 5500 QTRAP, mass spectrometer (AB SCIEX, American) was used, which was capable of operating in both positive and negative ion switching modes. The positive ion source parameters were as follows: source temperature, 550°C; Gas 1, 55 psi; Gas 2, 50 psi; curtain gas (CRU), 30 psi; ion spray voltage floating (ISVF), 5–500 V. The negative ion source parameters were as follows: source temperature, 550°C; Gas 1, 55 psi; Gas 2, 50 psi; CRU, 30 psi; ISVF, 4500 V.
In this study, active ingredients of V. anthelmintica (L.) Willd. were screened using the Traditional Chinese Medicine Systems Pharmacology database (https://tcmsp-e.com/tcmsp.php, accessed 20 March 2023). Oral bioavailability (OB) and drug-likeness (DL) were considered the most important pharmacokinetic parameters for absorption, distribution, metabolism, and excretion (ADME). Therefore, these active ingredients were screened using the following filter criteria: OB ≥ 30% and DL ≥ 0.18. Complete compound information was then collected, including the Mol ID, structure, and relevant target names. Meanwhile, disease-related targets were retrieved using the DisGeNET platform (https://www.disgenet.org/, accessed on 21 March 2023), which identified gene–disease associations liked to the term “vitiligo.” The targets were normalized using the UniProt database (https://www.uniprot.org/, accessed on 21 March 2023), with “human species” and “reviewed” filter criteria. To identify common targets of active ingredients and vitiligo, the screened active ingredients targets and vitiligo targets were visualized in a Venn diagram (http://bioinformatics.psb.ugent.be/webtools/Venn/, accessed on 29 March 2023).
To explore the interactions between the targets of active ingredients that treat vitiligo, we imported the common targets of V. anthelmintica (L.) Willd. active ingredients and vitiligo into an online platform, STRING (version 11.0.6, https://cn.string-db.org/, accessed on 29 March 2023), which was used to construct a PPI network. The PPI network results were then analyzed and visualized using Cytoscape v.3.9.1. Further, a Cytoscape plug-in, Centiscape 2.2, was used to identify high-interaction targets based on the closeness unDir, betweenness unDir, and degree unDir thresholds.
GO and KEGG pathway enrichment analyses were conducted using the Metascape platform (http://metascape.org/gp/index.html#/main/step1, accessed 29 March 2023). The primary GO terms associated with potential targets were analyzed, identifying biological process (BP), cellular component (CC), and molecular function (MF) enrichment. KEGG pathways analysis was then performed to identify enriched pathways. The significant enrichment terms (p ≤ 0.01) were then visualized using an online platform (http://www.bioinformatics.com.cn/, accessed on 29 March 2023).
The 2D structures of the identified active ingredients were downloaded from PubChem (https://pubchem.ncbi.nlm.nih.gov/, accessed on 30 March 2023), converted into 3D structures using Chem3D software, and saved in a docking ligand mol2 format. Alternatively, the 3D structures of the previously identified key proteins were obtained from the PSCB PDB platform (https://www.rcsb.org/, accessed 30 March 2023). The key proteins were removed from the organic solvents associated with them before and adding hydrogen using PyMOL software. AutoDock software (v. 4.2.6) was then used for molecular docking simulation. Finally, the molecular docking results were visualized using PyMOL software.
In this study, human keratinocytes (HaCaT; Kunming Cell Bank, Chinese Academy of Sciences, China) were cultured in DMEM supplemented with 10% FBS and a 1% penicillin–streptomycin solution. These cells cultured grown at 37°C in a humidified atmosphere with 5% CO2 and 10% a copper sulfate solution. Subsequent experiments were performed 24 h after the cells were seeded. Specifically, HaCaT cells were seeded at a density of 8×104 cells/well into 96-well plates for 12 h and treated with various concentrations (10, 15, 20, 25, 30, 40, 50, or 60 mM) of AAPH. Alternatively, a subset of HaCaT cells was pre-treated with various concentrations (10, 20, 30, 40, 60, 80, or 100 µM) of kaempferol within the medium for 12 h to determine the optimal treatment concentration. Subsequently, the pre-treated cells were cultured for an additional 12 h with 25 mM AAPH to induce oxidative damage. Three replicates were conducted per group for all the experiments; untreated cells and cells treated solely with AAPH were used as control groups.
To evaluate the cellular toxicity of AAPH and the protective effects of kaempferol, we employed the CCK-8 assay on HaCaT cells. After treatment with the aforementioned concentrations of AAPH and kaempferol, 10 µL of the CCK-8 kit solution was added to each well. Subsequently, the cells were incubated for 2 h at 37°C. The absorbance of each sample was measured using a microplate reader (Thermo, Multiskanm FC, American).
Cold PBS was used to collect kaempferol-treated and untreated HaCaT cell samples following centrifugation at 157 g for 3 min, according to the instructions provided in the respective enzyme kits. These cells were then homogenized with cold PBS, and corresponding commercial kits were used to extract the supernatant; this supernatant was then analyzed for MDA and SOD activity levels.
To assess the protective effect of kaempferol against oxidative stress–induced apoptosis in HaCaT cells, we employed a Hoechst assay. Cells pre-treated with 10, 20, and 30 µM kaempferol were used as the experimental groups for this assay. After cell treatment, 5 μg/mL Hoechst staining solution was added to the samples, before incubation for 10–15 min at 25°C in the dark. Subsequently, 400 µL of 1× binding buffer was added to the samples, ensuring thorough mixing before being placed on ice. Finally, cellular images were taken within 1 h under an Olympus fluorescence microscope. This experiment was repeated thrice.
In this study, SOD activity in HaCaT cells was evaluated using the tetrazolium nitro blue method. First, HaCaT cells in the logarithmic growth stage were prepared by digestion and PBS washing. The cell suspensions were then centrifuged at 157 g for 5 min. HaCaT cells were seeded in 6-well plates at a density of 1×106 cells/well, and cultured for 12 h at 37°C in a humidified atmosphere with 5% CO2. When the cells had adhered to the wells and reached a density of approximately 80%, they were treated with various concentrations (10, 20, or 30 µM) of kaempferol for 12 h. Subsequently, the pre-treated cells were cultured for an additional 12 h with 25 mM AAPH. The cell suspensions were then mixed thoroughly using a vortex mixer (ice bath; power, 20%/200 W; ultrasound, 3-s, 10-s intervals, 30 repeats) before centrifugation at 8000 g at 4°C for 10 min. Subsequently, the corresponding reagents were added to the cell suspension according to the manufacturer’s instructions. Finally, the mixture was thoroughly mixed before culturing at 37°C for 30 min. Cell suspension samples were then placed in a 1-mL glass cuvette, and maximal absorbance was measured at 560 nm using a spectrophotometer (Thermo, Multiskanm FC, American). One unit of SOD activity was defined as the amount of enzyme required for 50% activity inhibition.
In this study, MDA concentration was used to assess lipid peroxidation in the HaCaT cells. First, the cell suspensions were mixed thoroughly using a vortex mixer (ice bath; power, 20%/200 W; ultrasound 3-s, 10-s intervals, 30 repeats) before centrifugation at 8000 g at 4°C for 10 min. The corresponding reagents were then added to the cell suspensions according to the manufacturer’s instructions. Subsequently, the mixture was thoroughly mixed and incubated at 100°C for 60 min, cooled in an ice bath, and centrifuged at 10,000 ×g for 10 min. Finally, 200 µL of each supernatant was transferred into a microglass cuvette or 96-well plate; maximal absorbance was then determined at 450 nm, 532 nm, and 600 nm using a spectrophotometer. MDA content was calculated according to the identified protein concentration.
All statistical analyses were conducted using SPSS (version 22.0; IBM Corp., Armonk, NY, United States). Statistical significance was set at p < 0.05.
Ethanolic extracts obtained from V. anthelmintica (L.) Willd. Seeds were subjected to quantitative flavonoid testing, which revealed a total flavonoid content of 0.97 g per 100 g. Then, we conducted SRM MS analysis following high-performance liquid chromatography and SRM/MRM; this analysis identified 36 flavonoid compounds. These compounds were subsequently categorized according to their structure: flavonoid glycosides (9/36), isoflavonoids (9/36), flavans (7/36), flavones (5/36), and others (6/36). Notably, all of the resultant curves exhibited excellent linear regressions with coefficients of determination (R2) ranging from 0.9963 to 0.9999 (Figure 1). The top five compounds with the highest contents were saccarol (12650.98 ng/100 g), isquercitrin (7293.86 ng/100 g), meltein (4503.36 ng/100 g), violet (4320.83 ng/100 g), and naringenin (3390.59 ng/100 g).
Figure 1. XIC profile of the standard mixture. The abscissa represents the time (min), the vertical coordinates represents ionic intensity.
Overall, 36 flavonoid compounds were obtained from the ethanolic extract of V. anthelmintica (L.) Willd. Seeds. To identify the active ingredient of this herb, we utilized databases to screen bioactive compounds and ADME properties in V. anthelmintica (L.) Willd. When the screening criteria (OB ≥ 30%; DL ≥ 0.18) were applied, we identified 14 compounds with druggability in V. anthelmintica (L.) Willd. (Table 1).
Table 1. List of 14 compounds with druggability, identified using network pharmacology analysis of Vernonia anthelmintica (L.) Willd. seeds.
In this study, we obtained a total of 1286 vitiligo-related genes from various databases, including GeneCards, OMIM, and TTD. Subsequently, we identified a total of 211 target genes associated with the 14 compounds selected for further analysis, eliminating any overlapping targets obtained from the GeneCards, PubChem, and Swiss target prediction databases. After identifying the compound-target vitiligo-related genes, we visualized the corresponding compound-target interaction network, constructing a network that consisted of 227 nodes and 963 edges (Figure 2A). The five compounds with the highest degree of interaction in this network were kaempferol, isorhamnetin, kaempferide, quercetin, and liquiritigenin (degree = 101). Further, by taking the intersection between compound–target genes and vitiligo-related genes from the Wayne database, we identified the compounds target and vitiligo-related gene set (Figure 2B); this consisted of 43 common targets, which were used for further analysis.
Figure 2. Primary active components of Vernonia anthelmintica (L.) Willd. and the prediction of biological targets involved in the treatment of vitiligo. (A) Network of interactions between active ingredients, action targets, and vitiligo. The circle represents the potential action targets of active ingredients; the rhombus represents the active ingredients of V. anthelmintica (L.) Willd.; and the triangle represents vitiligo. (B) Identification of 43 overlapping differentially expressed genes associated with the targets of vitiligo (blue) and the pharmaceutical components of V. anthelmintica (L.) Willd. (yellow). (C) Protein–protein interaction network diagram of V. anthelmintica (L.) Willd. intervention disease targets; the thicker the connected edges, the greater the interaction between the two nodes. (D) Top 10 genes associated with V. anthelmintica (L.). Willd. in the treatment of vitiligo; deeper shades of red indicate higher degree values. (E) GO enrichment analysis of the vitiligo-related disease targets associated with the primary active components of Vernonia anthelmintica (L.) Willd. (F) KEGG pathway enrichment analysis of the vitiligo-related disease targets associate with the primary active components of V. anthelmintica (L.) Willd.
As shown in Figure 2C, the PPI network contained candidate targets of pharmaceutical ingredients for treating vitiligo and its interacting proteins. This network comprehensively summarizes the internal network of the pharmaceutical ingredients of V. anthelmintica (L.) Willd. involved in treating vitiligo. Finally, the NetworkAnalyzer and CytoNCA functions in Cytoscape were used to identify the core targets by analyzing various key properties, including the closeness, degree, and betweenness centrality (BC). In this analysis, a greater degree value indicates a more significant role in the network. r. The top five nodes with the highest degree values were determined to be AKT1 (d = 60), VEGF-A (d = 50), ESR1 (d = 44), IL2 (d = 30), and MPO (d = 24). The degree values of the top 10 nodes are shown in Figure 2D. Therefore, these core targets are postulated to play a major role in the treatment of vitiligo using V. anthelmintica (L.) Willd.
GO enrichment analysis revealed that the biological processes associated with the targets of the active components in V. anthelmintica (L.) Willd. (Figure 2E), and vitiligo primarily involve responses to inorganic substances, regulation of mitochondrial membrane potential, and mitochondrial organization. The cell components identified were predominantly enriched in the phosphatidylinositol 3-kinase complex, the extrinsic component of the membrane, and the protein–DNA complex. The associated molecular functions primarily involved protein homodimerization, steroid binding, and transcription coactivator binding.
Biological pathway enrichment analysis of the target genes was also performed using the KEGG database. The enriched pathways identified by KEGG analysis included the PI3K−Akt signaling pathway, chemical carcinogenesis receptor activation, the estrogen signaling pathway, endocrine resistance, and C-type lectin receptor signaling pathways. Among them, the PI3K-AKT signaling pathway was determined to be particularly important. As shown in Figure 2F, the PI3K-AKT signaling pathway was linked to several key functions, including apoptosis, cell proliferation, cell cycle regulation, protein synthesis, and metabolism. Furthermore, it exhibits synergistic crosstalk with various signaling pathways, including the VEGF, MAPK, JAK/STAT, NFκB signaling pathways.
Kaempferol (d = 101) was identified as one of the compounds with the highest degree of association with the constructed networks, according to the network pharmacology analysis, with OB and DL values of 41.88% and 0.24, respectively. Furthermore, in this study, we used detected a kaempferol content of 62.96 ng per 100 g of V. anthelmintica (L.) Willd.seed ethanolic extract by MRM approach. However, the specific mechanism of action remains unclear. Next, we conducted molecular docking simulations to explore the binding interactions between kaempferol and five target proteins (AKT1, VEGFA, ESR1, PTGS2, and IL2) that exhibited the highest degree of association with the established networks (Figure 3).
Figure 3. Pharmacological targets of kaempferol in vitiligo. Molecular docking revealed the binding of kaempferol to AKT1 (A), MMP2 (B), ESR1 (C), IL2 (D), PTGS2 (E) and MPO (F).
The molecular docking analysis used in this study simulated the binding of kaempferol to the potential key targets, AKT1, MMP2, ESR1, IL2, PTGS2, and MPO. Diagrams of the ligand and potential target docking results are shown in Figure 3. Overall, kaempferol was able to bind to the ARG-86, GLU-85, GLY-16, THR-87, and ARG-67 residues of AKT1 with a binding energy of −6.42 kcal·mol-1. The binding energy of Kaempferol to MMP2 residues, including CYS-29, SER-25, ARG-39, and THR-31, was −5.14 kcal·mol-1.
To investigate the role of kaempferol in the treatment of vitiligo, we constructed a vitiligo oxidative stress model. We first treated HaCaT cells with different concentrations of AAPH for 24 h in vitro. In terms of cell morphology, cells in the oxidative stress model group compare with control group displayed slightly elliptical, oval, or round morphology, with blurred edges and reduced adherence (Figure 4B). Nonetheless, after intervention with kaempferol at different concentrations (10, 20, and 30 mM), there was partial restoration of cell morphology (Figures 4C–E). The cell viability results revealed a concentration-dependent reduction in cell viability as the concentration of AAPH increased (Figure 5A). Moreover, cell viability in the AAPH-alone group (>25 mM) was significantly lower than that of the control group (no AAPH treatment), indicating that AAPH induces cytotoxic damage in HaCaT cells (p < 0.001). Additionally, the half-maximal inhibitory concentration (IC50) value of AAPH was determined to be approximately 25 mM. Therefore, 25 mM AAPH was used in future experiments to establish a HaCaT cell oxidative stress model. Subsequently, we confirmed that the concentration of kaempferol treatment can effect HaCaT cell viability (Figure 5B).
Figure 4. Effect of different kaempferol concentrations on the morphology of HaCaT cells (magnification, ×100). (A) Control, untreated HaCaT cells. (B) HaCaT cells treated with 25 mM AAPH for 24 h (C–E) HaCaT cells pretreated with (C) 10 μM, (D) 20 µM or (E) 30 µM kaempferol for 24 h, followed by incubation with 25 mM AAPH for 24 h.
Figure 5. Effect of kaempferol treatment on the viability and apoptotic rate of HaCaT cells under AAPH-induced cytotoxicity. (A) HaCaT cells treated with AAPH (0–60 mM) for 24 h (B) HaCaT cells treated with kaempferol (0–100 µM) for 24 h (C) HaCaT cells pretreated with kaempferol (10, 20, 30, or 40 µM) for 24 h, followed by incubation with 25 mM AAPH for 24 h. (D) Apoptotic rate of HaCaT cells treated with AAPH assessed by PI staining and flow cytometry. (E) ROS activity, (F) SOD activity, and (G) MDA content in HaCaT cells. Control, untreated HaCaT cells; AAPH, cells treated with 25 mM AAPH; in the experimental groups, cells were treated with 10, 20, or 30 µM kaempferol for 24 h. The values presented indicate the mean ± SEM of three independent experiments. Effects of kaempferol on AAPH-induced **p < 0.01,***p < 0.001, ****p < 0.0001 versus control; #p < 0.05, ##p < 0.01, ####p < 0.0001 versus AAPH-treated cells. AAPH, 2,2-azobis (2-methylpropionamidine) dihydrochloride; KAE, kaempferol; SEM, standard error of the mean.
The CCK-8 results revealed the application of kaempferol at concentrations ranging from 10 to 30 µM led to a significant increase in cell viability within AAPH-treated cells, without any apparent cytotoxic effects; in particular, treatment with 20 µM kaempferol exhibit the highest increase in cell viability and provide the highest cytoprotective effect (Figure 5C).
To investigate the effects of kaempferol pretreatment on AAPH-induced apoptosis in HaCaT cells, we conducted propidium iodide (PI) staining (Figure 5D). The corresponding results indicated that the apoptosis rates for the control group, AAPH-alone group, AAPH with 10 μM, 20 µM kaempferol and 30 µM Kaempferol were 14.87% ± 0.35%, 28.03% ± 0.24%, 32.53% ± 0.42%, 14.47% ± 0.42% and 20.37% ± 0.32%, respectively. Thus, when compared to the AAPH-alone group, pre-treatment with 20 µM kaempferol yielded the most significant reduction in apoptosis.
Next, we evaluated the effects of different concentrations of AAPH on MDA content, SOD enzyme activity, and ROS activity in HaCaT cells. In comparison to the control group, ROS activity (Figure 5E) and SOD level (Figure 5F) were significantly reduced, while MDA content (Figure 5G) was significant increased in HaCaT Cells that were exposed to 25 mM AAPH for 24 h. Nonetheless, pretreatment with 10, 20, or 30 µM kaempferol led to a significant reduction in the elevated MDA content and ROS activity, while increasing the decreased SOD level induced by AAPH. These findings suggest that kaempferol may ameliorate the oxidative and antioxidant imbalances induced by AAPH through its effects on MDA, SOD, and ROS in HaCaT cells.
Finally, we evaluated the effects of kaempferol on the expression of HO-1, GCLC, GCLM, Nrf2, AKT, NQO1, Keap1 and PI3K. Compared with the control group, 25 mM AAPH can increase the cellular expression levels of HO-1, GCLC, GCLM, Nrf2, NQO1 and Keap1 mRNA, and decrease AKT and PI3K mRNA (Figure 6). Low doses of kaempferol increased Nrf 2, HO-1 and GCLC mRNA expression levels in HaCaT cells, and high doses of KP only increased the intracellular Nrf2 mRNA expression levels. Nrf two protein expression in HaCaT compared with the control group. Different doses of KP can increase the intracellular Nrf two protein expression in a concentration-dependent manner as compared to the model group (Figure 6I).
Figure 6. Effects of kaempferol on the expression of (A) HO-1, (B) GCLC, (C) GCLM, (D) Nrf2, (E) AKT, (F) NQO1, (G) Keap1 and (H) PI3K. (I) Effect of kaempferol on the expression of Nrf2 protein in HaCaT cells. AAPH, cells treated with 25 mM AAPH; in the experimental groups, cells were treated with 10 or 30 µM kaempferol for 24 h**p < 0.01,***p < 0.001, ****p < 0.0001 versus control. AAPH, 2,2-azobis (2-methylpropionamidine) dihydrochloride; KAE, kaempferol; SEM, standard error of the mean.
Vitiligo is a common, acquired, and progressive disorder, characterized by depigmentation of the skin. The complex pathogenesis of vitiligo, oxidative stress injury is a key factor involved in the autoimmune response of vitiligo (Li et al., 2017). Therefore, reducing the level of oxidative stress is an important topic in vitiligo treatment. In Xinjiang, V. anthelmintica (L.) Willd has been commonly used to increase melanin production (Zhou et al., 2012), flavonoids and caffeic acid compounds have been identified as some of the most effective components for vitiligo treatment (Tuerxuntayi et al., 2014). Flavonoids, in particular, have received increasing attention due to their antioxidant and anti-inflammatory properties (Panche et al., 2016). Evidence suggests that the flavonoid active compounds have been isolated and identified, such as isorhamnetin, Butin and luteolin. These flavonoids can reduce ROS accumulation and promote melanogenesis (Lai et al., 2021; Hu et al., 2023). However, the current anti-vitiligo treatment for Vernonia anthelmintica (L.) Willd. is in preliminary stages, exact therapeutic effect and the treatment targets of the active ingredient have not yet been established.
To the best of our knowledge, this is the first comprehensive study that combines SRM/MRM approaches with network analysis to explore the active flavonoid compounds within V. anthelmintica (L.) Willd. Overall, our study highlights the protective effects of the active ingredient of V. anthelmintica (L.) Willd., kaempferol, on keratinocytes in mitigating AAPH-induced damage. In the present study, we identified 36 flavonoids within V. anthelmintica (L.)Willd seeds. Furthermore, by employing network pharmacology, we identified bioactive compounds and conducted ADME screening of these 36 isolated flavonoids, which ultimately revealed the presence of 14 compounds with druggability, including kaempferol, kaempferide, and eriodictyol. In total, we identified 1286 vitiligo-related genes and 211 target genes associated with the 14 identified compounds, which were used to, ultimately, identify a total of 43 component–vitiligo common targets.
Here, we employed GO analysis to investigate the 43 previously identified common targets of V. anthelmintica (L.) Willd. that are associated with to vitiligo. These critical targets were mainly involved in processes related to oxidative stress, inflammation, cell growth, metabolism and immunity. Notably, biological processes such as the regulation of inorganic substances, mitochondrial membrane potential, and mitochondrial organization were significantly enriched. Mitochondria are not only recognized as the source of ROS; they are also the primary organelles susceptible to the harmful effects of oxidative stress. Any impairment of mitochondrial function can subsequently affect melanocyte survival (Zhou et al., 2018). Increasing evidence has demonstrated that mitochondria are crucial in oxidative stress–mediated melanocyte apoptosis, highlighting mitochondria as potential targets for clinical vitiligo therapy (Wang et al., 2019b; Jiang et al., 2019).
KEGG pathway analysis further emphasized the relationship between these 43 common targets and vitiligo. Corresponding significantly enriched pathways included the PI3K-AKT signaling pathway, chemical carcinogenesis receptor activation, the estrogen signaling pathway, endocrine resistance, and the C-type lectin receptor signaling pathway. Notably, dysregulation in the PI3K/AKT signaling pathway has been implicated in various diseases associated with melanocytes, including melanoma, chloasma, and vitiligo. In melanocytic hyperproliferative diseases such as melanoma, the PI3K/AKT signaling pathway is overactivated, which promotes cell proliferation and inhibits apoptosis, facilitating tumor cell metastasis (Zhu et al., 2018). However, under H2O2-induced oxidative stress, the PI3K/AKT signaling pathway in melanocytes is inhibited, leading to increased apoptosis (Yang et al., 2016; Zhou et al., 2016). Activation of the PI3K-Akt signaling pathway protects against oxidative stress-induced cell death, often associated with the pathogenesis of vitiligo (Yang et al., 2016). The PI3K/Akt pathway can be activated by the basic fibroblast growth factor (bFGF) to increase cell proliferation, migration, and differentiation of several types of cells including melanocytes (Shi et al., 2016). This suggests that the identified compounds might also exert beneficial effects on vitiligo by activated PI3K/AKT signaling pathway. In summary, these findings indicate that 43 common targets identified in V. anthelmintica (L.) Willd. may be involved in vitiligo-related pathways, highlighting the potential of V. anthelmintica (L.) Willd. in the treatment of vitiligo. Thus, further study into these genes and pathways is certainly warranted.
The five key target genes (AKT1, VEGFA, ESR1, IL2, and MPO) identified by the compound–target network were closely related to the pathogenesis and/or treatment of vitiligo. In the present study, molecular docking simulations revealed that kaempferol exhibits high binding affinities with the five key target genes. AKT1 induces mitochondrial ROS production and mitophagy in macrophages; subsequently, AKT1-mediated mitophagy can then increase resistance to alveolar macrophage apoptosis and pulmonary fibrosis (Larson-Casey et al., 2016). Kaempferol, a flavonoid compound derived from plants, is known for its various functions, including antioxidant, anti-cancer, anti-inflammatory, and anti-apoptotic properties (Fouzder et al., 2021). Moreover, it was found to be one of the compounds with the highest degree of association (d = 101) in our network pharmacology analysis. While current literature on the direct impact of Kaempferol on mitochondrial function is limited, the implication of interaction with AKT1 indicates a potential pathway where Kaempferol could improve vitiligo by modulating mitochondrial and cell apoptosis. Further investigation is required to elucidate this mechanism and its clinical relevance. ESR1 has been reported as one of the proteins contributing to vitiligo susceptibility. Notably, this protein may be an important target for the successful treatment of vitiligo using a steroid–thyroid hormone mixture containing estrogen (Malhotra et al., 2018). VEGFA, also known as vascular endothelial growth factor A, is considered to be a particularly interesting candidate gene, which has been attributed to its polymorphisms, its correlation with autoimmune disorders, and its induction of angiogenic factor expression in vitiligo; moreover, VEGFA may be a potential target for late-onset vitiligo therapy (Almasi-Nasrabadi et al., 2019). IL2 has been implicated in the regulatory pathways of autoimmune diseases, including signaling and promotion of the growth and proliferation of T cells; therefore, this protein plays an important role in generating immune responses (Martins et al., 2020). Finally, MPO-specific markers are strongly associated with microscopic polyangiitis and other autoimmune diseases (Prendecki et al., 2021). Given that VEGFA, IL2, and MPO all play roles in autoimmune diseases, in this study, kaempferol has been shown to bind well with these molecules, which suggests a potential avenue for therapeutic interventions targeted at these molecular interactions in the context of vitiligo. Overall, numerous studies have indicated the association between these key target proteins and vitiligo, supporting the understanding that V. anthelmintica (L.) Willd. possesses antioxidant properties. Similarly, we demonstrated that kaempferol has good binding activity with these critical target proteins, including AKT1, ESR1, and PTGS2 (Jiang et al., 2022). Ultimately, these findings support the potential role of kaempferol as an active ingredient in V. anthelmintica (L.) Willd. for the treatment of vitiligo.
To further demonstrate the potential therapeutic effects of kaempferol on vitiligo, we performed in vitro cellular experiments. In these experiments, HaCaT cells were pretreated with kaempferol or left untreated before stimulation with AAPH to induce oxidative damage. Overall, pretreatment with kaempferol increased HaCaT cell viability, reduced apoptosis, lowered MDA content and ROS activity, and enhanced SOD activity. And kaempferol was also found to increase the expression of cellular antioxidant genes, including HO-1, GCLC, GCLM, Nrf2, NQO1 and Keap1. These findings indicate that kaempferol has the capacity to mitigate oxidative damage, offering promising prospects for its use as an antioxidant therapy for the treatment of vitiligo.
Despite the insights gained from network pharmacology, molecular docking, and in vitro experiments in identifying potential compounds and targets of V. anthelmintica (L.) Willd. in the context of vitiligo, this study has few limitations. First, the lack of in vivo analysis and limited in vitro experiments restricts the directly validation of the antioxidative effects and associated pathways of the identified compounds–target pairs. Furthermore, while the protective effects of kaempferol against AAPH-induced oxidative damage in HaCaT cells was investigated, further experiments and analysis are warranted to ascertain the exact impact of kaempferol on AAPH-induced melanocytes. Such investigations would, ultimately, facilitate the development of kaempferol as an antioxidant source and offer a more robust theoretical basis for targeted vitiligo therapy.
In conclusion, our research represents the first to comprehensive analysis of active flavonoid compounds in V. anthelmintica (L.) Willd via the combination of SRM/MRM and network analysis approaches. These results revealed the protective effects of its active ingredient, kaempferol, against AAPH-induced damage in keratinocytes. Moreover, compound-target network analysis identified 14 key compounds of V. anthelmintica (L.) Willd., including kaempferol, kaempferide, and eriodictyol; notably, these compounds exhibit significant druggability, with the potential to be used for the treatment of vitiligo. Further, we identified AKT1, VEGFA, ESR1, PTGS2, and IL2 as key therapeutic target genes, which possessed anti-oxidative properties. Among the significant pathway identified, the PI3K/AKT signaling pathway was of particular note. Moreover, kaempferol was identified as one of the key compounds in V. anthelmintica (L.) Willd. that exhibited a high degree of binding with these critical targets. In vitro cell experiments also showed that kaempferol increased HaCaT cell viability, reduced apoptosis, lowered MDA content and ROS activity, and enhanced SOD activity, effectively providing resistance against oxidative stress damage. In summary, this study highlights the promising potential of kaempferol as an antioxidant treatment for vitiligo.
The original contributions presented in the study are included in the article/Supplementary material, further inquiries can be directed to the corresponding author.
WH: Data curation, Formal Analysis, Methodology, Writing–original draft, Writing–review and editing. Hongjuan Wang: Formal Analysis, Software, Validation, Writing–original draft. Kaixiao Li: Formal Analysis, Methodology, Project administration, Writing–original draft. Zixian Lei: Data curation, Methodology, Project administration, Writing–original draft. FX: Formal Analysis, Methodology, Writing–review and editing. Jun Li: Data curation, Formal Analysis, Writing–original draft. XK: Conceptualization, Funding acquisition, Investigation, Resources, Writing–review and editing.
The author(s) declare financial support was received for the research, authorship, and/or publication of this article. This study was funded by the National Natural Science Foundation of China (82173406) and the Natural Science Foundation of Xinjiang Uygur Autonomous Region (2023D01C72).
The authors gratefully acknowledge the support of all partners involved in the study, and the support from the Dermatology Institute of the People’s Hospital of Xinjiang Uygur Autonomous Region. We would like to thank Shanghai Applied Protein Technology for the measurements and basic analysis of RNA-seq, and Editage (http://www.editage.cn) for their assistance with English language editing.
The authors declare that the research was conducted in the absence of any commercial or financial relationships that could be construed as a potential conflict of interest.
All claims expressed in this article are solely those of the authors and do not necessarily represent those of their affiliated organizations, or those of the publisher, the editors and the reviewers. Any product that may be evaluated in this article, or claim that may be made by its manufacturer, is not guaranteed or endorsed by the publisher.
Almasi-Nasrabadi, M., Amoli, M. M., Robati, R. M., Rajabi, F., and Parichehreh Dizaji, S. (2019). Is the +405 G/C single nucleotide polymorphism of the vascular endothelial growth factor (VEGF) gene associated with late-onset vitiligo? Int. J. Immunogenet 46 (4), 241–246. doi:10.1111/iji.12432
Amer, A. A., and Gao, X. H. (2016). Quality of life in patients with vitiligo: an analysis of the dermatology life quality index outcome over the past two decades. Int. J. Dermatol. 55, 608–614. doi:10.1111/ijd.13198
Awasthi, S., Maity, T., Oyler, B. L., Zhang, X., Goodlett, D. R., and Guha, U. (2018). Dataset describing the development, optimization and application of SRM/MRM based targeted proteomics strategy for quantification of potential biomarkers of EGFR TKI sensitivity. Data Brief. 19, 424–436. doi:10.1016/j.dib.2018.04.086
Chen, J., Li, S., and Li, C. (2021). Mechanisms of melanocyte death in vitiligo. Med. Res. Rev. 41, 1138–1166. doi:10.1002/med.21754
Chen, X., Guo, W., Chang, Y., Chen, J., Kang, P., Yi, X., et al. (2019). Oxidative stress-induced IL-15 trans-presentation in keratinocytes contributes to CD8+ T cells activation via JAK-STAT pathway in vitiligo. Free Radic. Biol. Med. 139, 80–91. doi:10.1016/j.freeradbiomed.2019.05.011
Dabas, G., Vinay, K., Parsad, D., Kumar, A., and Kumaran, M. S. (2020). Psychological disturbances in patients with pigmentary disorders: a cross-sectional study. J. Eur. Acad. Dermatol. Venereol. 34, 392–399. doi:10.1111/jdv.15987
Di Dalmazi, G., Hirshberg, J., Lyle, D., Freij, J. B., and Caturegli, P. (2016). Reactive oxygen species in organ-specific autoimmunity. Auto. Immun. Highlights 7, 11. doi:10.1007/s13317-016-0083-0
Du, W., An, Y., He, X., Zhang, D., and He, W. (2018). Protection of kaempferol on oxidative stress-induced retinal pigment epithelial cell damage. Oxid. Med. Cell. Longev. 2018, 1610751. doi:10.1155/2018/1610751
Fouzder, C., Mukhuty, A., and Kundu, R. (2021). Kaempferol inhibits Nrf2 signalling pathway via downregulation of Nrf2 mRNA and induces apoptosis in NSCLC cells. Arch. Biochem. Biophys. 697, 108700. doi:10.1016/j.abb.2020.108700
Hu, W., Zhang, J., Wang, H., Guan, M., Dai, L., Li, J., et al. (2023). Protective effects of isorhamnetin against H2O2-induced oxidative damage in HaCaT cells and comprehensive analysis of key genes. Sci. Rep. 13, 2498. doi:10.1038/s41598-023-27575-7
Jiang, W., Li, S., Chen, X., Zhang, W., Chang, Y., He, Y., et al. (2019). Berberine protects immortalized line of human melanocytes from H2O2-induced oxidative stress via activation of Nrf2 and Mitf signaling pathway. J. Dermatol. 94, 236–243. doi:10.1016/j.jdermsci.2019.03.007
Jiang, Y., Xie, Y. Z., Peng, C. W., Yao, K. N., Lin, X. Y., Zhan, S. F., et al. (2022). Modeling kaempferol as a potential pharmacological agent for COVID-19/PF co-occurrence based on bioinformatics and system pharmacological tools. Front. Pharmacol. 13, 865097. doi:10.3389/fphar.2022.865097
Ko, M., Kim, Y., Kim, H. H., Jeong, S., Ahn, D., Chung, S. J., et al. (2022). Network pharmacology and molecular docking approaches to elucidate the potential compounds and targets of Saeng-Ji-Hwang-Ko for treatment of type 2 diabetes mellitus. Comput. Biol. Med. 149, 106041. doi:10.1016/j.compbiomed.2022.106041
Krüger, C., and Schallreuter, K. U. (2012). A review of the worldwide prevalence of vitiligo in children/adolescents and adults. Int. J. Dermatol. 51, 1206–1212. doi:10.1111/j.1365-4632.2011.05377.x
Lai, Y., Feng, Q., Zhang, R., Shang, J., and Zhong, H. (2021). The great capacity on promoting melanogenesis of three compatible components in Vernonia anthelmintica (L.) willd. Int. J. Mol. Sci. 22, 4073. doi:10.3390/ijms22084073
Larson-Casey, J. L., Deshane, J. S., Ryan, A. J., Thannickal, V. J., and Carter, A. B. (2016). Macrophage Akt1 kinase-mediated mitophagy modulates apoptosis resistance and pulmonary fibrosis. Immunity 44, 582–596. doi:10.1016/j.immuni.2016.01.001
Li, S., Zhu, G., Yang, Y., Jian, Z., Guo, S., Dai, W., et al. (2017). Oxidative stress drives CD8+ T-cell skin trafficking in patients with vitiligo through CXCL16 upregulation by activating the unfolded protein response in keratinocytes. J. Allergy Clin. Immunol. 140, 177–189. doi:10.1016/j.jaci.2016.10.013
Liu, Y., Sun, W., Shen, N., Hao, W., Xin, H., Che, F., et al. (2023). Network pharmacology and molecular docking combined with widely targeted metabolomics to elucidate the potential compounds and targets of Euphorbia helioscopia seeds for the treatment of pulmonary fibrosis. Comput. Biol. Med. 160, 107007. doi:10.1016/j.compbiomed.2023.107007
Liu-Smith, F., and Meyskens, F. L. (2016). Molecular mechanisms of flavonoids in melanin synthesis and the potential for the prevention and treatment of melanoma. Mol. Nutr. Food Res. 60, 1264–1274. doi:10.1002/mnfr.201500822
Malhotra, A. G., Jha, M., Singh, S., and Pandey, K. M. (2018). Construction of a comprehensive protein–protein interaction map for vitiligo disease to identify key regulatory elements: a systemic approach. Interdiscip. Sci. 10, 500–514. doi:10.1007/s12539-017-0213-z
Martins, L. T., Frigeri, H. R., de Castro, C. C. S., and Mira, M. T. (2020). Association study between vitiligo and autoimmune-related genes CYP27B1, REL, TNFAIP3, IL2 and IL21. Exp. Dermatol. 29, 535–538. doi:10.1111/exd.14100
Noor, F., Rehman, A., Ashfaq, U. A., Saleem, M. H., Okla, M. K., Al-Hashimi, A., et al. (2022). Integrating network pharmacology and molecular docking approaches to decipher the multi-target pharmacological mechanism of Abrus precatorius L. acting on diabetes. Pharmaceuticals-base 15 (4), 414. doi:10.3390/ph15040414
Orhan, I. E., and Khan, M. T. H. (2014). Flavonoid derivatives as potent tyrosinase inhibitors – a survey of recent findings between 2008–2013. Curr. Top. Med. Chem. 14, 1486–1493. doi:10.2174/1568026614666140523120741
Pan, D., Li, N., Liu, Y., Xu, Q., Liu, Q., You, Y., et al. (2018). Kaempferol inhibits the migration and invasion of rheumatoid arthritis fibroblast-like synoviocytes by blocking activation of the MAPK pathway. Int. Immunopharmacol. 55, 174–182. doi:10.1016/j.intimp.2017.12.011
Panche, A. N., Diwan, A. D., and Chandra, S. R. (2016). Flavonoids: an overview. J. Nutr. Sci. 5, e47. doi:10.1017/jns.2016.41
Prendecki, M., Gulati, K., Turner-Stokes, T., Bhangal, G., Chiappo, D., Woollard, K., et al. (2021). Characterisation of an enhanced preclinical model of experimental MPO-ANCA autoimmune vasculitis. J. Pathol. 255, 107–119. doi:10.1002/path.5746
Shi, H., Lin, B., Huang, Y., Wu, J., Zhang, H., Lin, C., et al. (2016). Basic fibroblast growth factor promotes melanocyte migration via activating PI3K/Akt-Rac1-FAK-JNK and ERK signaling pathways. IUBMB Life 68, 735–747. doi:10.1002/iub.1531
Tuerxuntayi, A., Liu, Y. Q., Tulake, A., Kabas, M., Eblimit, A., and Aisa, H. A. (2014). Kaliziri extract upregulates tyrosinase, TRP-1, TRP-2 and MITF expression in murine B16 melanoma cells. BMC Complement. Altern. Med. 14, 166. doi:10.1186/1472-6882-14-166
Wang, H., Chen, L., Zhang, X., Xu, L., Xie, B., Shi, H., et al. (2019a). Kaempferol protects mice from d-GalN/LPS-induced acute liver failure by regulating the ER stress-Grp78-CHOP signaling pathway. Biomed. Pharmacother. 111, 468–475. doi:10.1016/j.biopha.2018.12.105
Wang, J., Pan, Y., Wei, G., Mao, H., Liu, R., and He, Y. (2022). Damage-associated molecular patterns in vitiligo: igniter fuse from oxidative stress to melanocyte loss. Redox Rep. 27, 193–199. doi:10.1080/13510002.2022.2123864
Wang, X., Wang, Z. Y., Zheng, J. H., and Li, S. (2021). TCM network pharmacology: a new trend towards combining computational, experimental and clinical approaches. Chin. J. Nat. Med. 19, 1–11. doi:10.1016/S1875-5364(21)60001-8
Wang, Y., Li, S., and Li, C. (2019b). Perspectives of new advances in the pathogenesis of vitiligo: from oxidative stress to autoimmunity. Med. Sci. Monit. 25, 1017–1023. doi:10.12659/MSM.914898
Yang, B., Yang, Q., Yang, X., Yan, H. B., and Lu, Q. P. (2016). Hyperoside protects human primary melanocytes against H2O2-induced oxidative damage. Mol. Med. Rep. 13, 4613–4619. doi:10.3892/mmr.2016.5107
Zhang, J., Hu, W., Wang, P., Ding, Y., Wang, H., and Kang, X. (2022). Research progress on targeted antioxidant therapy and vitiligo. Oxid. Med. Cell. Longev. 2022, 1821780. doi:10.1155/2022/1821780
Zhou, J., An, X., Dong, J., Wang, Y., Zhong, H., Duan, L., et al. (2018). IL-17 induces cellular stress microenvironment of melanocytes to promote autophagic cell apoptosis in vitiligo. FASEB J. 32, 4899–4916. doi:10.1096/fj.201701242RR
Zhou, J., Ling, J., Song, J., Wang, Y., Feng, B., and Ping, F. (2016). Interleukin 10 protects primary melanocyte by activation of Stat-3 and PI3K/Akt/NF-κB signaling pathways. Cytokine 83, 275–281. doi:10.1016/j.cyto.2016.05.013
Zhou, J., Shang, J., Ping, F., and Zhao, G. (2012). Alcohol extract from Vernonia anthelmintica (L.) willd seed enhances melanin synthesis through activation of the p38 MAPK signaling pathway in B16F10 cells and primary melanocytes. J. Ethnopharmacol. 143, 639–647. doi:10.1016/j.jep.2012.07.030
Keywords: vitiligo, Vernonia anthelmintica, kaempferol, PI3K/AKT signaling, HaCaT cell
Citation: Hu W, Wang H, Li K, Lei Z, Xiang F, Li J and Kang X (2024) Identification of active compounds in Vernonia anthelmintica (L.) willd by targeted metabolome MRM and kaempferol promotes HaCaT cell proliferation and reduces oxidative stress. Front. Pharmacol. 15:1343306. doi: 10.3389/fphar.2024.1343306
Received: 23 November 2023; Accepted: 27 March 2024;
Published: 9 April 2024.
Edited by:
Md Saquib Hasnain, Palamau Institute of Pharmacy, India, IndiaReviewed by:
Munkhtuya Tumurkhuu, East Carolina University, United StatesCopyright © 2024 Hu, Wang, Li, Lei, Xiang, Li and Kang. This is an open-access article distributed under the terms of the Creative Commons Attribution License (CC BY). The use, distribution or reproduction in other forums is permitted, provided the original author(s) and the copyright owner(s) are credited and that the original publication in this journal is cited, in accordance with accepted academic practice. No use, distribution or reproduction is permitted which does not comply with these terms.
*Correspondence: Xiaojing Kang, ZHJrYW5neGo2NjZAMTYzLmNvbQ==
Disclaimer: All claims expressed in this article are solely those of the authors and do not necessarily represent those of their affiliated organizations, or those of the publisher, the editors and the reviewers. Any product that may be evaluated in this article or claim that may be made by its manufacturer is not guaranteed or endorsed by the publisher.
Research integrity at Frontiers
Learn more about the work of our research integrity team to safeguard the quality of each article we publish.