- 1Guang’anmen Hospital, China Academy of Chinese Medical Sciences, Beijing, China
- 2First Teaching Hospital of Tianjin University of Traditional Chinese Medicine (National Clinical Research Center for Chinese Medicine Acupuncture and Moxibustion), Tianjin, China
- 3Henan Province Hospital of Traditional Chinese Medicine (The Second Affiliated Hospital of Henan University of Traditional Chinese Medicine), Zhengzhou, Henan, China
Significant advances in chemotherapy drugs have reduced mortality in patients with malignant tumors. However, chemotherapy-related cardiotoxicity increases the morbidity and mortality of patients, and has become the second leading cause of death after tumor recurrence, which has received more and more attention in recent years. Arrhythmia is one of the common types of chemotherapy-induced cardiotoxicity, and has become a new risk related to chemotherapy treatment, which seriously affects the therapeutic outcome in patients. Traditional Chinese medicine has experienced thousands of years of clinical practice in China, and has accumulated a wealth of medical theories and treatment formulas, which has unique advantages in the prevention and treatment of malignant diseases. Traditional Chinese medicine may reduce the arrhythmic toxicity caused by chemotherapy without affecting the anti-cancer effect. This paper mainly discussed the types and pathogenesis of secondary chemotherapeutic drug-induced arrhythmia (CDIA), and summarized the studies on Chinese medicine compounds, Chinese medicine Combination Formula and Chinese medicine injection that may be beneficial in intervention with secondary CDIA including atrial fibrillation, ventricular arrhythmia and sinus bradycardia, in order to provide reference for clinical prevention and treatment of chemotherapy-induced arrhythmias.
1 Introduction
Cancer is currently the leading cause of human disease-related death worldwide, and the incidence and mortality of cancer are increasing year by year (Sung et al., 2021). Major advances in chemotherapy drugs have reduced the mortality of malignant tumors, however, chemotherapy-related cardiac toxicity has increased the morbidity and mortality of patients, and has become the second leading cause of death after tumor recurrence, which has received more and more attention in recent years (Curigliano et al., 2016; Zamorano et al., 2016). Arrhythmia is one of the common types of chemotherapy-induced cardiotoxicity and has become a new risk associated with chemotherapy (Alexandre et al., 2021; Garg and Fradley, 2021), seriously affecting patient outcomes (Tamargo et al., 2015). In addition, the incidence of cancer is closely related to increasing age, so the prevention and treatment of chemotherapeutic drug-induced arrhythmia (CDIA) appear to be very important, especially in improving the quality of life of elderly patients. CDIA can be classified into the primary (Drugs directly disrupt specific molecular targets or signaling pathways that regulate the electrophysiological properties of the heart muscle) and the secondary (caused by ischemia, inflammation, oxidative stress, ER stress, apoptosis damage to the endocardium/myocardium/pericardium, which is a secondary phenomenon) (Buza et al., 2017). Common drugs that cause primary CDIA are Anthracyclines (Buza et al., 2017), Immunomodulatoryb Drugs (Rajkumar et al., 2003), Multitargeted Tyrosine Kinase Inhibitors (Buza et al., 2017). Common drugs that cause secondary CDIA are Anthracyclines (Buza et al., 2017), Antimetabolites (Khan et al., 2012), Platinum Compounds (Thix et al., 2009), Proteasome Inhibitors (Buza et al., 2017), Multitargeted Tyrosine Kinase Inhibitors (Buza et al., 2017), Vascular Endothelial Growth Factor Signaling Pathway Inhibitors (Buza et al., 2017), Human Epidermal Growth Factor Receptor 2/neu Inhibitors (Piotrowski et al., 2012), Immune Check Point Inhibitors (Heinzerling et al., 2016; Behling et al., 2017). The secondary CDIA is much more common in the clinic (see Table 1 for CDIA classification and underlying mechanisms).
Traditional Chinese medicine has experienced thousands of years of clinical practice in China, and has accumulated a wealth of medical theories and treatment formulas, which has unique advantages in the prevention and treatment of malignant diseases, such as arsenic trioxide in the treatment of acute promyelocytic leukemia (Chen et al., 2021; Kayser et al., 2021). Traditional Chinese medicine may reduce the arrhythmic toxicity caused by chemotherapy without affecting the anti-cancer effect (Yang et al., 2018). This paper mainly discussed the types and pathogenesis of secondary CDIA, and summarized the preclinical studies of Chinese medicine compounds, Chinese medicine Combination Formula and Chinese medicine injection on the cause of chemotherapeutic drugs-induced cardiotoxicity, in order to provide reference for clinical prevention and treatment of CDIA.
2 Types of arrhythmias caused by chemotherapy drugs
The potential cardiotoxicity of chemotherapy drugs can lead to a variety of arrhythmias (tachyarrhythmic or bradyarrhythmic). The actual incidence of CDIA may be far underestimated because routine cardiac monitoring are not performed or only 12-lead electrocardiograms during clinical therapy (Herrmann, 2020). It is crucial for cardiologists and oncologists to recognize the causal association between chemotherapeutic agents and arrhythmias and to target prevention and treatment for cancer patients.
2.1 Atrial fibrillation
Atrial fibrillation (AF) is a common cardiotoxicity of anticancer therapy in cancer patients (Tamargo et al., 2015), especially in the elderly on combination drugs, in patients with advanced cancer, and in patients undergoing active treatment (O'Neal et al., 2015), where the development of AF is a poor prognostic factor (Tamargo et al., 2015). The tyrosine kinase inhibitor (TKI) ibrutinib has the strongest correlation with atrial fibrillation (paroxysmal and persistent), which can occur in 3%–16% (mean 8%) of patients with cancer (Leong et al., 2016; Thompson et al., 2016; Yun et al., 2017; Ye et al., 2021), with incidence rates ranging from 10% to 16% with longer follow-up (Burger et al., 2015; Byrd et al., 2015; Wang et al., 2015; Leong et al., 2016; Brown et al., 2017), and leads to early discontinuation of therapy in 46% of patients with cancer (Byrd et al., 2015). Anthracyclines at standard doses can induce both acute and delayed AF, with the type of AF being predominantly persistent (Ando et al., 2000; Kilickap et al., 2007; Guglin et al., 2009; Amioka et al., 2016). Cisplatin, when administered intravenously, intrapericardial, or intrathoracically, can cause acute AF in 10%–32% of patients (Tomkowski and Filipecki, 1997; Tomkowski et al., 2004; Bischiniotis et al., 2005; Lara et al., 2005; Richards et al., 2006; Tilleman et al., 2009; Zellos et al., 2009). A systematic review showed that standard doses of gemcitabine, immune checkpoint inhibitors, Marfan, abiraterone acetate, and rituximab can increase the incidence of atrial fibrillation in elderly patients, those with reduced renal function, or those with hypertension (Olivieri et al., 1998; Phillips et al., 2004; Mileshkin et al., 2005; Feliz et al., 2011; Passalia et al., 2013; Sharma et al., 2017; Hilmi et al., 2020; Mascolo et al., 2023). In addition, high doses of cyclophosphamide and isocyclophosphamide increase the risk of paroxysmal AF (Kupari et al., 1990; Quezado et al., 1993).
2.2 Ventricular arrhythmias
Arsenic trioxide is considered to be the most dangerous drug causing QTc interval prolongation, as tip-twisting ventricular tachycardia is a very problematic malignant arrhythmic event (Roboz et al., 2014; Zamorano et al., 2016). Immune checkpoint inhibitors can cause ventricular arrhythmias in 5%–10% of patients and lead to a 40% mortality rate (Drugs FDA: 2023 FDA Approved Drug Products. Retrieved September 25). Paclitaxel and ibrutinib can lead to polymorphic ventricular tachycardia, ventricular fibrillation, and even sudden death (Arbuck et al., 1993; Wallace et al., 2016), in a significant dose-dependent manner (Brouty-Boye et al., 1995). 5-Fluorouracil can lead to a significant increase in premature ventricular contraction and ventricular tachycardia events in patients (Yilmaz et al., 2007; Khan et al., 2012; Polk et al., 2013). Adriamycin in combination with 5-fluorouracil can lead to an increase in the incidence of premature ventricular contractions from 3% to 24% (Steinberg et al., 1987). Anthracyclines can lead to acute ventricular arrhythmic events in patients (Tamargo et al., 2015).
2.3 Sinus bradycardia
Sinus bradycardia is an important risk factor for prolonged QT interval and the development of lethal polymorphic ventricular tachycardia (Roden, 2016; Zamorano et al., 2016). It is often underestimated clinically, because of its low incidence and the fact that most patients are asymptomatic; therefore, the focus should be on the potentially harmful effects of sinus bradycardia in cancer patients (Font et al., 2023). Thalidomide causes sinus bradycardia (heart rate of 30–50 beats/min) in 53% of patients with multiple myeloma, heart rate as low as 30 beats/min in eight patients with symptoms of severe weakness and hypotension, and syncope in 12% of patients, one-third of whom were fitted with pacemakers (Fahdi et al., 2004; Barlogie et al., 2006; Herrmann, 2020). Sinus cardiac arrest has been reported with ibrutinib (Mathur et al., 2017). A systematic review demonstrated that immune checkpoint inhibitors can cause atrioventricular block or conduction disease in 10% of patients, leading to a 50% mortality rate (Mir et al., 2018). The mean maximum heart rate in patients with non-small cell lung cancer with crizotinib decreased to 25 beats/min, with 18.8% of patients having a heart rate of 45–49 beats/min (Ou et al., 2016).
3 Molecular mechanisms of chemotherapy-related cardiotoxicity
The mechanism of arrhythmia induced by chemotherapeutic agents is complex. This article focuses on CDIA secondary to myocardial injury or cardiac cytotoxicity. Indeed, the secondary CDIA is the result of multifactorial interactions, mainly targeting cell survival and death pathways, and is associated with multiple molecular and signaling pathways, with oxidative stress and inflammation being the most common mechanisms, followed by apoptosis, autophagy, endoplasmic reticulum stress and abnormalities in myocardial energy metabolism, which together contribute to the onset and development of arrhythmias (Figures 1, 2).
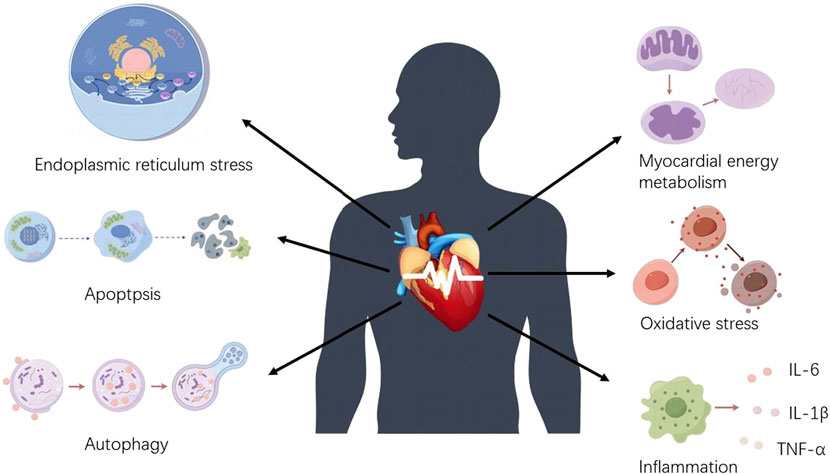
Figure 1. Mechanisms of chemotherapeutic drug-induced arrhythmias. Chemotherapeutic drug-induced arrhythmias are mainly caused by increased oxidative stress, abnormal myocardial energy metabolism, endoplasmic reticulum stress disorder, apoptosis, inflammatory stimuli, and autophagy dysfunction.
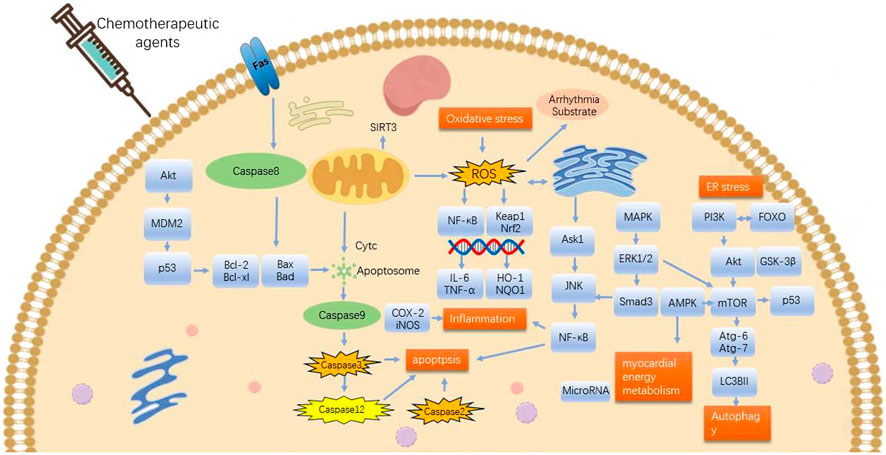
Figure 2. Mechanisms involved in potential effects of TCMs on arrhythmia originating from chemotherapeutic drugs-induced cardiac cytotoxicity. ROS, reactive oxygen species; MAPK, mitogen-activated protein kinase; Nrf2, nuclear factor E2-related factor-2; HO-1, heme oxygenase-1; Keap1, E3 ligase junction Kelch-like ECH-associated protein 1; ER stress, endoplasmic reticulum stress; JNK,c-Jun N-terminal kinase. Pro-survival: Bcl-2, HO-1, AMPK, PI3K, Akt, mTOR, p-AMPK, SIRT3, microRNA, GSK-3β, Nrf2, NQO1, ERK1/2. Pro-death: Keap1, Bid, GSK-3β, caspase-8, caspase-9, ROS, COX-2, iNOS, NF-κB, TNF-α, IL-6, Bax, Caspase-3, ERK1/2, p53, MAPK, FOXO, p38-MAPK, TGFβ1, αSMA, FFA, caspase-12, caspase-2, ERS, JNK-mTOR.
3.1 Oxidative stress
Oxidative stress (OS) describes a state of imbalance between antioxidants and oxidants in the body, which is triggered when large amounts of Reactive oxygen species (ROS) and their metabolites accumulate intracellularly. Cardiomyocytes are sensitive to chemotherapeutic drug-induced oxidative stress injury because of the abundance of mitochondria, which not only generate ROS but are also the target of ROS-activated injury. OS can cause arrhythmias by altering ion channels, leading to mitochondrial dysfunction and abnormal energy metabolism in cardiomyocytes, inducing cardiac electrical remodeling. Therefore, OS is considered to be the main mechanism causing cytotoxicity. ROS along with OS is a key contributor to arrhythmias and it plays a crucial role in the progression of AF (Barangi et al., 2018; Mason et al., 2020; Yang et al., 2020). It also causes life-threatening arrhythmias such as ventricular tachycardia and ventricular fibrillation (Szyller et al., 2022) and is considered to be the primary mechanism causing cytotoxicity (Sies, 2015; Sinha and Dabla, 2015; Tocchetti et al., 2019; Zhao et al., 2023). Chemotherapy leads to ROS production through multiple pathways, and overproduction of ROS is considered the most prevalent theoretical pathway by which chemotherapy triggers cardiotoxicity (Rochette et al., 2015; Curigliano et al., 2016; Yang et al., 2018; Yang et al., 2020). Available studies suggest a direct or indirect relationship between OS and anticancer drug-induced cardiotoxicity (Zhang et al., 2019; Attanasio et al., 2021). Literature has reported that 5-fluorouracil (5-FU) (Focaccetti et al., 2015) can lead to elevated intracellular OS in cardiomyocytes, thereby causing damage to cardiomyocytes (Durak et al., 2000; Lamberti et al., 2014; Polk et al., 2014; Sara et al., 2018; Rapa et al., 2021). Elevated OS is also an important factor in doxorubicin (Dox)-induced cardiotoxicity (Harstad and Klaassen, 2002; Ghibu et al., 2012; Hussein, 2012; Vejpongsa and Yeh, 2014; Akolkar et al., 2017). Dox promotes the production of ROS in cells and induces cell poisoning (Carresi et al., 2018; Wei et al., 2018), while high levels of ROS promote autophagy and apoptosis by activating p53 (Saha et al., 2014; Shi et al., 2014) and MAPK (Beccafico et al., 2015; Liu et al., 2015) signaling pathways. Nuclear factor E2-related factor-2 (Nrf2), an important transcription factor regulating OS (Zhao et al., 2023), coordinates antioxidant and cytoprotective effects (Wang et al., 2015; Zhao et al., 2023), and is protective against Dox-induced myocardial OS (Yarmohammadi et al., 2021). Nrf2 can enhance endogenous antioxidant protection mechanisms during OS development and through the activation of heme oxygenase-1 (HO-1) for OS protection (Edwardson et al., 2015). Deletion or inhibited expression of Nrf2 leads to abnormal cardiomyocyte remodeling and increased Oxidative Stress (Singh et al., 2015). The Keap1-Nrf2 pathway formed by Nrf2 and its major negative regulator, the E3 ligase junction Kelch-like ECH-associated protein 1 (Keap1), is an important endogenous antioxidant signaling pathway important in attenuating myocardial injury (Li et al., 2014; Wang et al., 2015; Lu et al., 2016; Cuadrado et al., 2018), which may be a therapeutic target for attenuating Dox-induced cardiotoxicity through activation of cellular-level antioxidant defense mechanisms in cardiomyocytes (Arunachalam et al., 2021).
3.2 Myocardial energy metabolism
Myocardial energy metabolism is a key factor affecting cardiac function (Doenst et al., 2013; Lopaschuk et al., 2021). Highly active cardiomyocytes must have sufficient energy metabolism to maintain normal function (Yu et al., 2023). Mitochondria are the center of energy metabolism in the organism, and in recent years, it has been found that decreased mitochondrial function caused by abnormal regulation of mitochondrial mass may play an important role in cardiac damage caused by disorders of energy metabolism (Li et al., 2023). AMPK, as the main energy receptor of the cell, plays a central role in this process, just like a “fuel gauge" (Hardie and Carling, 1997), is recognized as an important kinase in the regulation of myocardial energy metabolism, which shows metabolic changes in heart failure (Kitani et al., 2019). Activation of AMPK promotes energy metabolism, increases antioxidant enzyme activity, decreases OS, protects cardiomyocytes from apoptosis, promotes autophagy, and attenuates inflammatory responses (Cai et al., 2022; Zhang et al., 2022; Pasini et al., 2023). Cardiotoxic chemotherapeutic agents have been shown to impair the intracellular mechanisms controlling cardiac metabolism (Fazzini et al., 2022). AMPK has now been found to lie at the control point for many of the mechanisms that have been shown to be associated with Dox cardiotoxicity (Timm and Tyler, 2020). Dox markedly inhibits AMPK activity in the myocardium and can exacerbate myocardial toxicity (Timm and Tyler, 2020). Previous studies have found that trastuzumab causes mitochondrial dysfunction in the human induced pluripotent stem cell model (iPSC-CM) and affects the cardiac energy metabolism pathway (Necela et al., 2017; Kitani et al., 2019), which is consistent with the role of the AMPK pathway in Dox-induced myocardial toxicity (Sharma et al., 2018).
3.3 Endoplasmic reticulum stress
The endoplasmic reticulum (ER) is a structurally and functionally diverse membrane-bound organelle in the cytoplasm of eukaryotic cells, which is mainly involved in protein synthesis, folding, maturation, modification, transport and calcium homeostasis (Omidkhoda et al., 2019; Shakeri et al., 2019; Yang et al., 2020; Yarmohammadi et al., 2021). Endoplasmic reticulum stress (ERS) is a “double-edged sword,” which is an adaptive response to maintain cell survival by regulating endoplasmic reticulum function. Prolonged severe or persistent endoplasmic reticulum stress and protein misfolding can induce inflammatory responses and apoptosis, and promote myocardial remodeling (Oakes and Papa, 2015; Narezkina et al., 2021; Ren et al., 2021; Ren et al., 2022). Endoplasmic reticulum stress (ERS) plays a key role in a variety of cardiovascular diseases, including fatal arrhythmias (Sirish et al., 2021; Hamilton and Terentyev, 2022). Recent studies have documented that ERS plays a crucial role in the pathophysiologic basis of AF (Sirish et al., 2021). It has been shown that the ERS pathway regulates a variety of myocardial functions such as inflammatory response, apoptosis, and autophagy. Chronic ERS interferes with the redox state in the endoplasmic reticulum and reduces the expression of myocardial ion channel proteins, which leads to arrhythmogenesis (Hamilton and Terentyev, 2022). The importance of ER and its signaling pathway in Dox-induced cardiotoxicity concerning inflammation, apoptosis, and autophagy suggests that it may be a key factor in the cardiotoxic effects of Dox-induced cardiovascular toxicity (Minamino et al., 2010; Abdel-Daim et al., 2017; Akolkar et al., 2017; Wang et al., 2018; Yarmohammadi et al., 2021). Thus, control of ER and its signaling pathways can significantly slow the development of Dox-induced cardiotoxicity (Yarmohammadi et al., 2021).
3.4 Apoptosis
Apoptosis is a highly regulated mode of programmed cell death (Shabalala et al., 2017), in which nucleic acid endonucleases and cysteine-aspartate proteases (caspases) are the most critical enzymes mediating apoptosis, and either insufficient or excessive apoptosis can lead to a variety of diseases. Apoptosis plays an important role in cardiovascular diseases (Michalson et al., 2018; Yang et al., 2020). It has been shown that in cardiomyocytes and endothelial cells, elevated reactive oxygen species triggers caspase-3 and activates apoptosis (Eskandari et al., 2015; Focaccetti et al., 2015; Rawat et al., 2021). Cisplatin opens mitochondrial permeability channels, allowing intra-mitochondrial Cyt-c to enter the cytosol, initiating a mitochondria-dependent pathway and inducing apoptosis (Kim et al., 2003). p53 is an important regulator of cell death and has long been known to be associated with Dox-induced myocardial toxicity (Christidi and Brunham, 2021). Dox can activate the p53 pathway to initiate endogenous, exogenous, and endoplasmic reticulum-associated apoptotic pathways, thereby inhibiting cardiomyocyte apoptosis (Yarmohammadi et al., 2021). In transcriptomic studies, p53 has been shown to be a key transcriptomic regulator of cardiotoxicity (McSweeney et al., 2019). Increased expression of p53-mediated cell death receptors regulates cardiomyocyte apoptosis, autophagy, and leads to cardiac atrophy. It has been reported in the literature that Dox activates p53 in mitochondria, which in turn induces cardiomyocyte apoptosis. It has been found that p53 is overexpressed in cardiomyocytes and can promote apoptosis through transcriptional activation into the nucleus (Wang et al., 2013). Conversely, inhibition of p53 activity promotes apoptosis in cardiomyocytes at the later stages of Dox treatment (Guo et al., 2023). The expression and regulation of Bcl-2 family genes have a decisive influence on the occurrence of apoptosis, among which Bax and Bcl-2 have an important role in the regulation of mitochondrial apoptosis (Qi et al., 2022). These proteins may regulate apoptosis through the PI3K/Akt pathway and the P53 pathway (Wang et al., 2015; Wenningmann et al., 2019). Dox upregulates the expression of Bax and Caspase-9 and downregulates the expression of the Bcl-2 gene, which induces apoptosis in rat myocardial H9c2 cells (Han et al., 2008; Mei et al., 2015; Qi et al., 2022). It has been shown that the mitogen-activated protein kinase (MAPK) signaling pathway plays an important role in cell proliferation, differentiation, and apoptosis (Kim and Choi, 2015; Zhao et al., 2022), c-Jun N-terminal kinase (JNK) belongs to the MAPK family, and Dox induces apoptosis through the JNK and MAPK signaling pathways (Das et al., 2011). It is known that nuclear factor-κB (NF-κB) activation and MAPK signaling pathway can activate pro-apoptotic events leading to apoptosis (Sahu et al., 2019). However, Dox-induced cardiomyocyte apoptosis is associated with NF-κB activation (Wang et al., 2002), and myocardial apoptosis is the predominant mode of Dox-induced cardiomyocyte death in in vitro and in vivo models (Christidi and Brunham, 2021).
3.5 Autophagy
Autophagy is an important mode of energy catabolism in the organism (Gatica et al., 2015), whose main function is to recycle damaged or unwanted cellular components, thereby maintaining cell viability, and is often considered a protective mechanism (Koleini and Kardami, 2017). Autophagy is involved in maintaining intracellular homeostasis in most types of cardiovascular cells, especially cardiomyocytes (Taneike et al., 2010; Gottlieb and Mentzer, 2013; Xiao et al., 2019; Luan et al., 2021; Elshazly et al., 2022). Several studies have shown that under normal conditions, decreased levels of autophagy are an adaptive response of the body to the heart to prevent cardiomyocyte death (Mei et al., 2015). However, cardiomyocyte autophagy dysfunction is closely associated with the development of arrhythmias (Luan et al., 2021). Autophagy, as a regulatory mechanism, is highly conserved throughout the life cycle of yeast and humans (Bestion et al., 2023), and plays a large role in tumorigenesis (Amaravadi et al., 2016; Udristioiu and Nica-Badea, 2019; Zhang et al., 2023). Anthracycline-induced myocardial damage is closely related to autophagy (Koleini and Kardami, 2017). Indeed, Dox promotes cardiac autophagy and contributes to the pathogenesis of the resulting cardiotoxicity (Wang et al., 2014), and autophagy dysfunction is an important cause of excessive cardiomyocyte death (Bartlett et al., 2017). It has been shown that dysregulated (or excessive) autophagy can be inhibited to achieve a reduction in DOX toxicity (Yin et al., 2018; Lv et al., 2020). The phosphatidylinositol 3-kinase/Akt/mammalian target of the rapamycin (PI3K/Akt/mTOR) pathway is thought to be a central regulatory element in the autophagy process (Sun et al., 2013). Dox regulate upstream regulatory aspects of autophagy, such as mTOR, AMPK, etc., whereas PI3K is also activated in Dox cardiomyotoxic rats (Bartlett et al., 2017).
3.6 Inflammation
Continued and uncontrolled inflammatory response can result in cardiac failure, arrhythmias, and even sudden death (Liu et al., 2016; Hiram et al., 2021). 2019 Novel coronavirus (COVID-19) is an important systemic systemic inflammatory disease that is closely associated with the onset and development of several cardiac arrhythmias (Gawałko et al., 2020; Coromilas et al., 2021). Several studies have confirmed that inflammation is strongly linked to AF, and inflammatory signaling in atrial cardiomyocytes is activated in both animal experimental models and in patients with AF (Elahi et al., 2008; Patel et al., 2010; Pastori et al., 2018; Dobrev et al., 2023). NF-κB has been suggested to be a key transcriptional regulator of pro-inflammatory factors (Tam et al., 2012). The accumulation of large amounts of ROS further triggers the NF-κB pathway to release inflammatory factors such as TNF-α and IL-6 (Rahman, 2002), triggering a series of inflammatory responses (Abd El-Aziz et al., 2012). Inflammatory changes are common in AF triggered by tumor therapy, and it has been shown that inflammation may be a hidden mechanism of Dox-induced cardiotoxicity (Thandavarayan et al., 2015; Monkkonen and Debnath, 2018). Dox significantly activates the NF-κB signaling pathway, which induces myocardial inflammation in mice [9]. During the course of chemotherapy with gemcitabine versus vincristine, the presence of atrial flutter and AF is more common, which is associated with the activation of the NF-κB pathway (Qanungo et al., 2014). Growing evidence suggests that melphalan has the potential to trigger the NF-κB pathway and increase pro-inflammatory cytokines in myocardial tissue, further triggering the development of arrhythmias (Qing et al., 2006; Baumann et al., 2008; Salminen et al., 2012; Amin et al., 2020; Lan et al., 2020; Rawat et al., 2021). Inflammation is a key factor in cisplatin-induced cardiotoxicity, and increasing evidence supports that cisplatin enhances the release of inflammatory cytokines and chemokines, and that inflammation-associated genes are mainly regulated by the transcription factor NF-κB. NF-κB activation in cisplatin-induced cardiac injury leads to the expression of tumor necrosis factor α in cardiomyocytes, causing cardiac remodeling (Chowdhury et al., 2016; Manohar and Leung, 2018). The signaling pathway of Toll-like Receptor 4 (TLR4) plays a central role in Dox-induced cardiac inflammation (Sumneang et al., 2023), which may be consistent with the mechanism of arrhythmia induced by ibrutinib.
4 Effects of Chinese herbal medicine and its Constituent compounds against arrhythmogenic injury induced by chemotherapeutic agents
Malignant tumors and cardiovascular diseases are two of the most common major diseases in the world today, and cardiovascular toxicity brought about by chemotherapeutic drug treatment has become an important threat to the life and safety of cancer patients. In particular, secondary CDIA caused by chemotherapeutic agents are very common. In recent years, it has been found that traditional Chinese medicine has the ability to antagonize the myocardial injury caused by chemotherapeutic drugs without affecting the efficacy of antitumor drugs. However, the mechanism of Chinese herbal medicines against chemotherapy drug-induced myocardial injury and potential intervention with the secondary arrhythmia is still not fully elucidated. In this regard, we have reviewed the research on chemotherapy-induced arrhythmogenic cardiotoxicity in Chinese herbal medicines and their components at home and abroad to provide a theoretical basis for the clinical application of Chinese herbal medicines to prevent and treat chemotherapy-induced myocardial toxicity (Figure 2; Table 2).
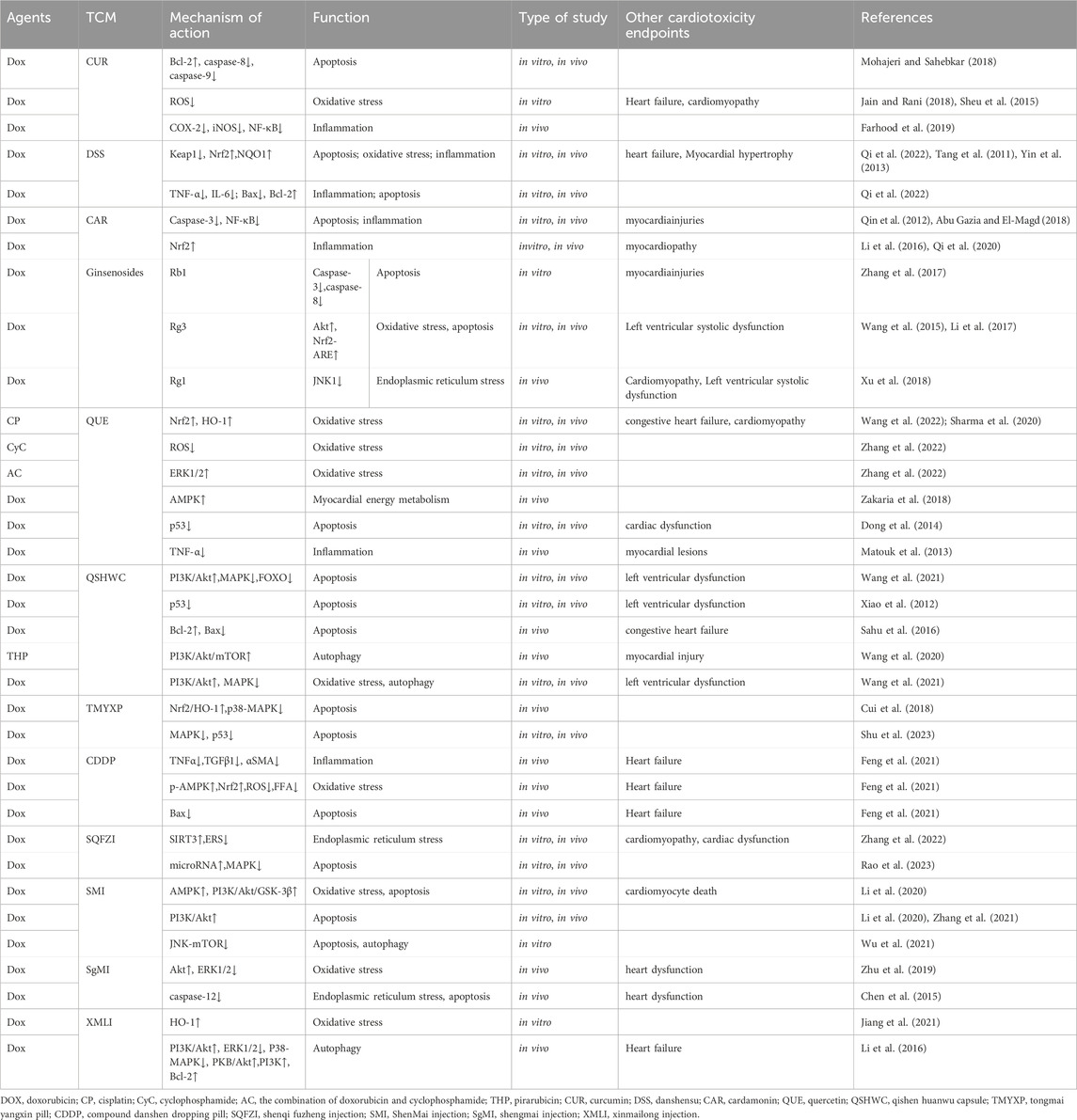
Table 2. Effects and mechanisms of TCM on chemotherapy-related myocardial injury and resultant arrhythmia.
4.1 Chinese medicinal compounds
4.1.1 Curcumin
Curcumin (CUR) is a naturally occurring polyphenol derived from the turmeric plant (Yu et al., 2019), that is insoluble in water (Farhood et al., 2019; Najafi et al., 2020). This natural polyphenol is the key active substance in turmeric (Wang et al., 2015), which is a natural antioxidant (Bahadır et al., 2018). CUR exhibits a variety of biological and pharmacological properties, and the protection of cardiomyocytes by non-toxic doses of CUR is mainly achieved by enhancing its antioxidant function, modulating cell death, and exerting anti-inflammatory effects (Jain and Rani, 2018; Mohajeri and Sahebkar, 2018; Armandeh et al., 2022; Zhang and Wu, 2022). CUR is a well-known anticancer drug that regulates cell proliferation and apoptosis at multiple levels (Mohajeri and Sahebkar, 2018). CUR reduces the side effects of chemotherapy and improves the sensitivity of chemotherapeutic agents (Zhang and Wu, 2022). Pretreatment with CUR can significantly alleviate Dox-induced cardiomyocyte death by increasing Bcl-2 and decreasing caspase-8 and caspase-9 levels (Mohajeri and Sahebkar, 2018). The Bax/Bcl-2 ratio was significantly decreased after 2 h of CUR pretreatment; furthermore, CUR inhibited Dox-mediated ROS generation (Sheu et al., 2015; Jain and Rani, 2018). CUR also acts on a variety of inflammatory mediators, such as cyclo-oxygenase-2 (COX-2), inducible nitric oxide synthase (iNOS), and NF-κB, to reduce the secretion of inflammatory factors, decrease chronic free radical production and attenuates tissue toxicity (Farhood et al., 2019). However, contradictory findings were reported by Hosseinzadeh et al. (2011), with the in vitro study results showing that pretreatment with CUR at nontoxic concentrations (5–15 µM) for 1 h significantly potentiated DOX-induced apoptosis in rat H9c2 cardiac muscle cells through downregulation of Bcl-2 (an increase in Bax/Ccl-2 ratio), upregulation of caspase-8 and caspase-9, and an increase in ROS generation by Dox. Potentiation of Dox-induced death of H9c2 cells was also confirmed by Jain and Rani (2018) when treating CUR with DOX concurrently, but not after pretreatment of CUR. Therefore, the clinic use of CUR, especially the dosing regimen, to mitigate Dox-induced cardiotoxicity in cancer patients needs to be re-evaluated.
4.1.2 Danshensu
Danshen (Salvia miltiorrhiza Bge) is the root and rhizome of the herb Danshen in the family Labiatae, which is commonly used clinically against heart disease (Wang et al., 2017). Danshensu (DSS) is a water-soluble substance in Salvia miltiorrhiza, which mainly consists of catechol and lactic acid (Zhang et al., 2019). DSS has various pharmacological activities, such as antioxidant, anti-apoptotic, anti-myocardial ischemia, and attenuation of myocardial hypertrophy (Tang et al., 2011; Yin et al., 2013; Bao et al., 2018). Nrf2 deficiency aggravates Dox-induced myocardial toxicity and cardiac dysfunction (Bellezza et al., 2018; Wallace et al., 2020). Network pharmacological studies suggest that Keap1-Nrf2/NQO1 is a key factor contributing to Dox-induced cardiotoxicity. In animal experiments, DSS was able to effectively counteract Dox-induced cardiotoxicity by regulating the expression of Keap1-Nrf2/NQO1, exerting antioxidant, anti-inflammatory, and anti-apoptotic therapeutic effects, and thus attenuating Dox-induced cardiac injury (Yin et al., 2013; Qi et al., 2022). Literature reports that TNF-α, IL-6, etc., can induce inflammatory responses, and DSS alleviates Dox-induced myocardial injury by decreasing the expression of TNF-α, IL-6, etc. In addition, DSS effectively alleviated Dox-induced cardiomyocyte apoptosis, mainly by preventing the upregulation of Bax and the downregulation of Bcl-2. (Qi et al., 2022).
4.1.3 Cardamonin
Cardamonin (CAR) is a flavonoid compound isolated from traditional Chinese medicine and is widely found in a variety of herbs such as Myristica fragrans, Ginkgo biloba, and hickory, etc. This flavonoid compound has various pharmacological activities such as anti-inflammatory (Lee et al., 2006; Lee et al., 2012; Li et al., 2015; Wang et al., 2018), anti-tumor (Qin et al., 2012), anti-OS(Bajgai et al., 2011; Peng et al., 2017; Tan et al., 2021), etc. In different cells, CAR has different regulatory effects. In cardiomyocytes, CAR has been found to have antioxidant effects, which can inhibit the generation of ROS, induce apoptosis, and prevent cells from oxidative stress and inflammatory damage by up-regulating signaling pathways such as Nrf2 and NF-κB, thus protecting cardiomyocytes (Qi et al., 2020; Tan et al., 2021). In tumor cells, CAR can promote oxidative stress. CAR can inhibit the proliferation and migration of tumor cells by inhibiting the expression of NF-κB, subsequently enhancing the oxidative phosphorylation level of mitochondria, increasing the accumulation of ROS in tumor cells, inducing cell cycle arrest and apoptosis (Jin et al., 2019). CAR attenuates Dox-induced cardiomyocyte cytotoxicity by inhibiting apoptosis through downregulation of the Caspase-3, NF-κB pathway, and suppressing the inflammatory response (Qin et al., 2012; Abu Gazia and El-Magd, 2018). In the study of the Dox-triggered cardiotoxicity animal model, we found that by activating the Nrf2 signaling mechanism, CAR could effectively inhibit the OS response within the mouse myocardium, slow down the death of apoptotic cells, and inhibit the inflammatory response, which ultimately contributed to the reduction of myocardial injury (Li et al., 2016; Qi et al., 2020).
4.1.4 Ginsenosides
Ginsenosides are the most crucial active substances in ginseng, which have antioxidant, apoptosis inhibiting, autophagy regulating, and other cardiotoxicity-reducing effects (Hou et al., 2022; Lv et al., 2022). It has been found that Dox can cause apoptosis and autophagy in H9c2 cardiomyocytes, and ginsenoside Rb1 can attenuate Dox-induced myocardial injury by a mechanism that may be realized by inhibiting Dox-induced autophagy (Li et al., 2017; Zhang et al., 2017; Zhu et al., 2017). Meanwhile, Rb1 not only reduces the activities of caspase-3 and caspase-8 but also interrupts the apoptotic process of H9C2 cells (Zhu et al., 2017). Ginsenoside Rg3 (Rg3) is an anticancer active component of ginseng. According to studies, it possesses antioxidant, anti-apoptotic, and cardioprotective effects, and its mechanism of action is related to Akt activation and exerts antioxidant effects and inhibits apoptosis through activation of the Nrf2-ARE pathway (Wang et al., 2015; Li et al., 2017). Rg1 attenuates DOX-induced endoplasmic reticulum stress and autophagy in the hearts of mice by inhibiting the JNK1 signaling pathway (Xu et al., 2018).
4.1.5 Quercetin
Quercetin (QUE) is a polyhydroxyflavonoid widely found in plants and animals, and an increasing number of studies have confirmed its ability to effectively inhibit mitochondrial OS, suppress myocardial fibrosis, inhibit cardiomyocyte apoptosis, and improve myocardial remodeling (Zhou et al., 2022). QUE attenuates cisplatin and Dox-induced myocardial injury by activating the expression of Nrf 2, HO-1 (Sharma et al., 2020; Wang et al., 2022). QUE exhibits excellent antioxidant properties, which can reduce cyclophosphamide (CyC)-induced cardiotoxicity by inhibiting ROS accumulation in cardiomyocytes and further attenuates cardiotoxicity caused by the combination of doxorubicin and cyclophosphamide (AC) by activating the ERK1/2 pathway (Zhang et al., 2022). QUE can upregulate the expression of AMPKα2, PPARα, and PGC-1α by regulating the AMPK pathway, improving myocardial energy metabolism and preventing Dox-induced myocardial injury in rats (Zakaria et al., 2018). Studies have shown that QUE reduces the effect of Dox on p53 expression, which may, to some extent, account for the attenuation of Dox-induced apoptosis by QUE (Dong et al., 2014). QUE reduces Dox-induced TNF-α, leading to a protective effect on rat cardiomyocytes (Matouk et al., 2013). QUE has bidirectional pharmacological effects on cardiomyocytes, as evidenced by the fact that low-dose QUE exerts cardioprotective effects by potentiating the activity of ERK1/2 and then modulating its target genes (Angeloni et al., 2007), whereas high-dose QUE exerts cardiotoxic effects by inhibiting the activity of ERK1/2 and then regulating its target genes to exert cardiotoxic effects (Daubney et al., 2015).
4.2 Chinese medicine Combination Formula
4.2.1 Qishen Huanwu Capsule
Qishen Huanwu Capsule (QSHWC) is a compound preparation mainly composed of Huangqi (Astragalus), Taizishen (Pseudostellaria heterophylla), Chuanxiong (Ligusticum chuanxiong), Danggui (Angelica sinensis), Chishao (Paeonia lactiflora), Taoren (Persica), Honghua (Carthamus tinctorius), Niuxi (Achyranthes bidentata), Banxia (Pinellia ternata), and Maidong (Ophiopogon japonicus). Its main components, quercetin (Chen et al., 2019), kaempferol (Xiao et al., 2012), and isorhamnetin (Sun et al., 2013) have significant anti-anthracycline cardiotoxicity effects. Kaempferol downregulates the expression of p53 and binds to the promoter of pro-apoptotic genes, thereby attenuating Dox-induced OS, apoptosis, and mitochondrial damage (Xiao et al., 2012). Baicalein, the main active substance in QSHWC, counteracts Dox-induced myocardial toxicity by enhancing the expression of Bcl-2, decreasing the expression of Bax, decreasing the ratio of Bax to Bcl-2, and inhibiting apoptosis in cardiomyocytes (Sahu et al., 2016). QSHWC effectively alleviated myocardial toxicity caused by pirarubicin (THP), which was mainly closely related to the initiation of the PI3K/Akt/mTOR pathway and the reduction of THP-induced excessive autophagy in cardiomyocytes (Wang et al., 2020). Qishen Huanwu Capsule regulates PI3K/Akt, MAPK and FOXO signaling pathways by regulating targets such as Akt1, MAPK1, MAPK8 and thereby inhibits oxidative stress and regulates apoptosis and autophagy to reduce the cardiotoxicity of anthracyclines. (Wang et al., 2021).
4.2.2 Tongmai Yangxin Pill
Tongmai Yangxin Pill (TMYXP) is a traditional Chinese medicine (TCM) that primarily contains ingredients such as Dihuang (Rehmannia glutinosa), Jixueteng (Millettia speciosa), Maidong (Ophiopogon japonicus), Gancao (Glycyrrhiza uralensis), Zhi Heshouwu (Polygonum multiflorum), Ejiao (Donkey-hide gelatin), Wuweizi (Schisandra chinensis), Dangshen (Codonopsis pilosula), Cuguojia (Testudinis Carapax Et Plastrum prepared with vinegar), Dazao (Ziziphus jujuba), and Guizhi (Cinnamomum cassia). After preliminary activity screening through various pharmacological studies, 80 compounds were identified from TMYX pills, including flavonoids, coumarins, iridoid glycosides, saponins and lignans, which had significant anti-inflammatory and antioxidant activities (Fan et al., 2016). OS response is an important biological process in which TMYXP plays a role, and its mechanism of action may be related to the Nrf2 and MAPK pathways. It was found that TMYXP could enhance anti-OS ability and reduce apoptosis by regulating signaling pathways such as Nrf2/HO-1 and p38-MAPK(Cui et al., 2018). Network pharmacology and metabolomics studies suggest that TMYXP can attenuate Dox-induced myocardial injury by regulating the upstream protein signaling pathway of insulin, MAPK, p53, and other signaling pathways, as well as regulating energy metabolism (Shu et al., 2023).
4.2.3 Compound Danshen Dropping Pill
Compound Danshen Dropping Pill (CDDP) is a well-known formula commonly used clinically against cardiovascular diseases (Feng et al., 2021). The three herbal ingredients include Danshen (Salvia miltiorrhiza), Sanqi (Panax notoginseng), and Bingpian (Borneol) (Guo et al., 2016; Hu et al., 2022), and it was found that its main active ingredients are danshenolic acids (e.g., danshenolic, etc.), saponins (e.g., ginsenoside Re), flavonoids (e.g., Tan IIA), and icariin, etc., CDDP treatment of rabbits with surgically induced acute myocardial infarction significantly inhibits cardiac apoptosis, reduces oxidative stress and inflammation, and improves cardiac function (Jun et al., 2014). CDDP can effectively reduce the level of Dox-induced serum TNFα and its expression in the heart (Feng et al., 2021). Experiments have demonstrated that CDDP can effectively reverse the inhibitory effect of Dox on p-AMPK (the active form of AMPK) and downregulate the expression of Nrf2, which in turn reduces ROS and FFA production, counteracts Dox-induced OS, and attenuates myocardial injury. Expression of transforming growth factor β1 (TGFβ1) and αsmooth muscle actin (αSMA), key mediators of fibrosis, was significantly increased in Dox-treated mice, and CDDP could significantly ameliorate Dox-induced myocardial fibrosis and inflammation and reduce the risk of cardiovascular injury by suppressing the expression of pro-fibrotic and pro-inflammatory molecules (Feng et al., 2021). CDDP was able to counteract Dox-induced cardiomyocyte apoptosis in vivo by modulating the expression of Bax protein (Feng et al., 2021).
4.3 Chinese medicine injection
4.3.1 Shenqifuzheng injection
The main components of Shenqi Fuzheng Injection (SQFZI) are Radix et Rhizoma Ginseng and Radix Astragali (Chen et al., 2019), which have the efficacy of benefiting qi and strengthening qi. SQFZI may play a protective role by increasing myocardial energy metabolism, inhibiting cell adhesion, suppressing inflammatory responses, reducing cardiomyocyte apoptosis, and preventing myocardial remodeling (Liao et al., 2018). Among the anti-cardiotoxic components of SQFZI, several members have been clarified in published studies to date. For example, ononin (a natural isoflavone glycoside), ononin has a significant antimyocardial injury effect, primarily by attenuating the effects of Dox treatment on cardiomyocyte apoptosis through activation of SIRT3 and inhibition of ER stress (Zhang et al., 2022). The role of SQFZI in combating cardiac injury is also related to microRNAs, MAPK and other signaling pathways (Rao et al., 2023). microRNAs (miRNAs) play a key regulatory role in cell proliferation, cell death, apoptosis and cell differentiation, and their dysregulation is closely related to cardiotoxicity (Pellegrini et al., 2020). It has been shown that blocking the MAPKDEBE signaling pathway modulates apoptosis, thereby attenuating myocardial toxicity (Zhang et al., 2021).
4.3.2 ShenMai injection
ShenMai injection (SMI) consists of Renshen (Ginseng Radix) and Maidong (Ophiopogonis Radix), which is derived from Shengmaisan in the Thousand Golden Essentials (Li et al., 2020). AMPK is central to Dox-induced cardiotoxicity events (Timm and Tyler, 2020). In vitro, SMI rescued Dox-injured H9c2 cardiomyocytes from apoptosis, mitochondrial ROS overproduction, and mitochondrial membrane potential depletion. In addition, SMI prevents Dox-induced cardiotoxicity by inhibiting mitochondrial oxidative stress and fragmentation through activation of AMPK and PI3K/Akt/GSK-3β signaling pathways (Li et al., 2020). SMI increased the viability of Dox-injured H9c2 cardiomyocytes and prevented apoptosis, and its protective effect against cardiotoxicity may be related to the activation of the PI3K/Akt pathway (Li et al., 2020; Zhang et al., 2021). SMI regulates cardiomyocyte apoptosis and autophagy by controlling the JNK (a unique autophagy-activating signal)-mTOR signaling pathway and blocking the Dox-induced apoptotic pathway and autophagy formation (Wu et al., 2021).
4.3.3 ShengMai injection
Shengmai injection (SgMI), a traditional Chinese medicine extracted from shanghai san including Renshen (Ginseng Radix), Maidong (Ophiopogonis Radix), and Wuweizi (Schisandra Chinensis Fructus) (Wang et al., 2020). It prevents cardiotoxicity of chemotherapy drugs by enhancing myocardial contractile function, reducing afterload and arrhythmia (Mao and Xu, 2007; Zhang and Zhang, 2007). SgMI alleviates myocardial damage and cardiac dysfunction in Dox-treated patients (Yang, 2008). SgMI alleviates cardiac damage and cardiac dysfunction by regulating TLR4, NF-κB, and other protein expression to reduce downstream inflammatory factor expression and attenuate cardiac damage (Qing-min et al., 2018). Studies have shown that SgMI attenuates OS-induced cardiomyocyte injury through Akt and ERK1/2 pathways (Zhu et al., 2019). SgMI can help ameliorate Dox-induced cardiomyocyte injury in rats by alleviating myocardial ER stress and ER stress specific apoptosis through inhibition of caspase-12-dependent pathways (Chen et al., 2015).
4.3.4 Xinmailong Injection
Xinmailong Injection (XMLI) is a bioactive compound extracted from the cockroach (a species of cockroach), which has been found to have favorable anti-cardiovascular damaging effects (Li et al., 2016). XMLI protects against Dox-induced myocardial injury by a mechanism that is mediated by HO-1 regulation of lysosomal function and improvement of autophagic flux, and reduces OS(Jiang et al., 2021). Findings have demonstrated that XML also inhibits cellular autophagy and attenuates Dox-induced cardiac damage through signaling pathways such as activation of PI3K/Akt, inhibition of ERK1/2, P38 MAPK, etc .,(Li et al., 2016). Beclin 1 has been identified as a Bcl-2-interacting protein and is significant for autophagy. XML attenuates the accumulation of Beclin1 with Atg7, increases protein kinase B (PKB)/Akt, PI3K, and Bcl-2 expression, inhibiting autophagy and ameliorating myocardial injury (Li et al., 2016).
5 Conclusion
In summary, based on the current literature review, the main types of secondary CDIA are atrial fibrillation (paroxysmal, persistent), ventricular arrhythmias (premature ventricular contraction, ventricular tachycardia, ventricular fibrillation) and sinus bradycardia (atrioventricular block). The mechanism of arrhythmia induced by chemotherapy drugs is very complex, mainly including oxidative stress, myocardial energy metabolism, endoplasmic reticulum stress, apoptosis, autophagy and inflammation. Chemotherapy drugs have the potential to induce cardiac toxicity, leading to conditions such as heart failure, cardiomyopathy, and myocardial injury through the above various pathways. Substances generated from myocardial damage or cell death caused by chemotherapy-related cytotoxicity serve as substrates for arrhythmias, contributing to the initiation and progression of arrhythmias. Chinese herbal medicine has been identified as having the capability to mitigate the adverse effects of chemotherapy drugs through diverse mechanisms. Specifically, certain Chinese herbal medicines, including CUR, DSS, QUE, QSHWC, CDDP, SMI, SgMI, and XMLI, demonstrate anti-oxidative stress properties. Additionally, compounds such as CUR, DSS, CAR, QUE, and CDDP exhibit anti-inflammatory effects, while CUR, DSS, CAR, QUE, QSHWC, TMYXP, CDDP, SQFZI, SMI, and SgMI are associated with anti-apoptotic properties. Some herbal medicines, including QSHWC, SMI, and XMLI, play a role in regulating autophagy, and others, such as SQFZI, and SgMI, contribute to the regulation of endoplasmic reticulum stress. Moreover, QUE has been recognized for its ability to enhance myocardial energy metabolism. Collectively, these herbal medicines function through diverse pathways to attenuate the arrhythmogenic toxicity induced by chemotherapy drugs. These pathways encompass the regulation of Keap1-Nrf2/NQO1 expression, prevention of the upregulation of Bax and downregulation of BCL-2 in mitochondrial apoptosis procedures. They also involve the promotion of the expression of AMPKα2, PPARα, and PGC-1α, activation of the PI3K/Akt signaling pathway, inhibition of the ERK1/2 and P38MAPK signaling pathways, and enhancement of Nrf2 expression. More importantly, Chinese medicine can play the role of anti-arrhythmia caused by chemotherapy drugs without affecting the anti-cancer effect of chemotherapy drugs. The limitations of this article are: up to now, there are few literature on the treatment of specific types of CDIA by certain Chinese medicine compounds or injections. This review first summarizes common types of CDIA, such as atrial fibrillation, ventricular arrhythmia, and sinus bradycardia. The potential intervention of CDIA with TCMs may not be limited to the above mentioned three arrhythmia. Prevention and/or treatment of specific type of CDIA with TCMs will be reviewed in the future once the dataset from clinical studies and basic research becomes available.
Author contributions
WL: Writing–original draft. XC: Writing–original draft. GZ: Writing–review and editing. YH: Writing–review and editing. YW: Writing–review and editing. YN: Writing–review and editing. HL: Writing–review and editing. AA: Writing–review and editing. JL: Methodology, Supervision, Validation, Writing–review and editing. LF: Methodology, Supervision, Validation, Writing–review and editing. GL: Methodology, Supervision, Validation, Writing–review and editing.
Funding
The author(s) declare that financial support was received for the research, authorship, and/or publication of this article. The work was supported by the Healthcare Special Project (No. 2022YB73), the Science and Technology Innovation Project of the China Academy of Traditional Chinese Medicine (No. CI 2021A03001), and the National Key Research and Development Program (No. 2019YFC1708404).
Conflict of interest
The authors declare that the research was conducted in the absence of any commercial or financial relationships that could be construed as a potential conflict of interest.
Publisher’s note
All claims expressed in this article are solely those of the authors and do not necessarily represent those of their affiliated organizations, or those of the publisher, the editors and the reviewers. Any product that may be evaluated in this article, or claim that may be made by its manufacturer, is not guaranteed or endorsed by the publisher.
References
Abd El-Aziz, T. A., Mohamed, R. H., Pasha, H. F., and Abdel-Aziz, H. R. (2012). Catechin protects against oxidative stress and inflammatory-mediated cardiotoxicity in adriamycin-treated rats. Clin. Exp. Med. 12 (4), 233–240. doi:10.1007/s10238-011-0165-2
Abdel-Daim, M. M., Kilany, O. E., Khalifa, H. A., and Ahmed, A. A. M. (2017). Allicin ameliorates doxorubicin-induced cardiotoxicity in rats via suppression of oxidative stress, inflammation and apoptosis. Cancer Chemother. Pharmacol. 80 (4), 745–753. doi:10.1007/s00280-017-3413-7
Abu Gazia, M., and El-Magd, M. A. (2018). Ameliorative effect of cardamom aqueous extract on doxorubicin-induced cardiotoxicity in rats. Cells Tissues Organs 206 (1-2), 62–72. doi:10.1159/000496109
Akolkar, G., da Silva Dias, D., Ayyappan, P., Bagchi, A. K., Jassal, D. S., Salemi, V. M. C., et al. (2017). Vitamin C mitigates oxidative/nitrosative stress and inflammation in doxorubicin-induced cardiomyopathy. Am. J. Physiol. Heart Circ. Physiol. 313 (4), H795–h809. doi:10.1152/ajpheart.00253.2017
Alexandre, J., Salem, J. E., Moslehi, J., Sassier, M., Ropert, C., Cautela, J., et al. (2021). Identification of anticancer drugs associated with atrial fibrillation: analysis of the WHO pharmacovigilance database. Eur. Heart J. Cardiovasc Pharmacother. 7 (4), 312–320. doi:10.1093/ehjcvp/pvaa037
Amaravadi, R., Kimmelman, A. C., and White, E. (2016). Recent insights into the function of autophagy in cancer. Genes Dev. 30 (17), 1913–1930. doi:10.1101/gad.287524.116
Amin, M. N., Siddiqui, S. A., Ibrahim, M., Hakim, M. L., Ahammed, M. S., Kabir., A., et al. (2020). Inflammatory cytokines in the pathogenesis of cardiovascular disease and cancer. SAGE Open Med. 8, 2050312120965752. doi:10.1177/2050312120965752
Amioka, M., Sairaku, A., Ochi, T., Okada, T., Asaoku, H., Kyo, T., et al. (2016). Prognostic significance of new-onset atrial fibrillation in patients with non-hodgkin's lymphoma treated with anthracyclines. Am. J. Cardiol. 118 (9), 1386–1389. doi:10.1016/j.amjcard.2016.07.049
Ando, M., Yokozawa, T., Sawada, J., Takaue, Y., Togitani, K., Kawahigashi, N., et al. (2000). Cardiac conduction abnormalities in patients with breast cancer undergoing high-dose chemotherapy and stem cell transplantation. Bone Marrow Transpl. 25 (2), 185–189. doi:10.1038/sj.bmt.1702106
Angeloni, C., Spencer, J. P., Leoncini, E., Biagi, P. L., and Hrelia, S. (2007). Role of quercetin and its in vivo metabolites in protecting H9c2 cells against oxidative stress. Biochimie 89 (1), 73–82. doi:10.1016/j.biochi.2006.09.006
Arbuck, S. G., Strauss., H., Rowinsky, E., Christian., M., Suffness, M., Adams., J., et al. (1993). A reassessment of cardiac toxicity associated with Taxol. J. Natl. Cancer Inst. Monogr. (15), 117–130.
Armandeh, M., Bameri, B., Samadi, M., Heidari, S., Foroumadi, R., and Abdollahi, M. (2022). A systematic review of nonclinical studies on the effect of curcumin in chemotherapy-induced cardiotoxicity. Curr. Pharm. Des. 28 (22), 1843–1853. doi:10.2174/1381612828666220513125312
Arunachalam, S., Nagoor Meeran, M. F., Azimullah, S., Sharma, C., Goyal, S. N., and Ojha, S. (2021). Nerolidol attenuates oxidative stress, inflammation, and apoptosis by modulating Nrf2/MAPK signaling pathways in doxorubicin-induced acute cardiotoxicity in rats. Antioxidants (Basel) 10 (6). doi:10.3390/antiox10060984
Attanasio, U., Pirozzi, F., Poto, R., Cuomo, A., Carannante, A., Russo, M., et al. (2021). Oxidative stress in anticancer therapies-related cardiac dysfunction. Free Radic. Biol. Med. 169, 410–415. doi:10.1016/j.freeradbiomed.2021.04.021
Bahadır, A., Ceyhan., A., Gergin, O., Yalçın, B., Ülger, M., Özyazgan, T. M., et al. (2018). Protective effects of curcumin and beta-carotene on cisplatin-induced cardiotoxicity: an experimental rat model. Anatol. J. Cardiol. 19 (3), 213–221. doi:10.14744/AnatolJCardiol.2018.53059
Bajgai, S. P., Prachyawarakorn, V., Mahidol, C., Ruchirawat, S., and Kittakoop, P. (2011). Hybrid flavan-chalcones, aromatase and lipoxygenase inhibitors, from Desmos cochinchinensis. Phytochemistry 72 (16), 2062–2067. doi:10.1016/j.phytochem.2011.07.002
Bao, X. Y., Zheng., Q., Tong, Q., Zhu, P. C., Zhuang, Z., Zheng, G. Q., et al. (2018). Danshensu for myocardial ischemic injury: preclinical evidence and novel methodology of quality assessment tool. Front. Pharmacol. 9, 1445. doi:10.3389/fphar.2018.01445
Barangi, S., Hayes, A. W., and Karimi, G. (2018). The more effective treatment of atrial fibrillation applying the natural compounds; as NADPH oxidase and ion channel inhibitors. Crit. Rev. Food Sci. Nutr. 58 (7), 1230–1241. doi:10.1080/10408398.2017.1379000
Barlogie, B., Tricot, G., Anaissie, E., Shaughnessy, J., Rasmussen, E., van Rhee., F., et al. (2006). Thalidomide and hematopoietic-cell transplantation for multiple myeloma. N. Engl. J. Med. 354 (10), 1021–1030. doi:10.1056/NEJMoa053583
Bartlett, J. J., Trivedi, P. C., and Pulinilkunnil, T. (2017). Autophagic dysregulation in doxorubicin cardiomyopathy. J. Mol. Cell Cardiol. 104, 1–8. doi:10.1016/j.yjmcc.2017.01.007
Baumann, P., Mandl-Weber, S., Oduncu, F., and Schmidmaier, R. (2008). Alkylating agents induce activation of NFkappaB in multiple myeloma cells. Leuk. Res. 32 (7), 1144–1147. doi:10.1016/j.leukres.2007.11.015
Beccafico, S., Morozzi., G., Marchetti, M. C., Riccardi., C., Sidoni, A., Donato, R., et al. (2015). Artesunate induces ROS- and p38 MAPK-mediated apoptosis and counteracts tumor growth in vivo in embryonal rhabdomyosarcoma cells. Carcinogenesis 36 (9), 1071–1083. doi:10.1093/carcin/bgv098
Behling, J., Kaes, J., Münzel, T., Grabbe, S., and Loquai, C. (2017). New-onset third-degree atrioventricular block because of autoimmune-induced myositis under treatment with anti-programmed cell death-1 (nivolumab) for metastatic melanoma. Melanoma Res. 27 (2), 155–158. doi:10.1097/cmr.0000000000000314
Bellezza, I., Giambanco, I., Minelli, A., and Donato, R. (2018). Nrf2-Keap1 signaling in oxidative and reductive stress. Biochim. Biophys. Acta Mol. Cell Res. 1865 (5), 721–733. doi:10.1016/j.bbamcr.2018.02.010
Bestion, E., Raymond, E., Mezouar, S., and Halfon, P. (2023). Update on autophagy inhibitors in cancer: opening up to a therapeutic combination with immune checkpoint inhibitors. Cells 12 (13). doi:10.3390/cells12131702
Bischiniotis, T. S., Lafaras, C. T., Platogiannis, D. N., Moldovan, L., Barbetakis, N. G., and Katseas, G. P. (2005). Intrapericardial cisplatin administration after pericardiocentesis in patients with lung adenocarcinoma and malignant cardiac tamponade. Hell. J. Cardiol. 46 (5), 324–329.
Brouty-Boye, D., Kolonias, D., and Lampidis, T. J. (1995). Antiproliferative activity of taxol on human tumor and normal breast cells vs effects on cardiac cells. Int. J. Cancer 60 (4), 571–575. doi:10.1002/ijc.2910600424
Brown, J. R., Moslehi, J., O'Brien, S., Ghia, P., Hillmen, P., Cymbalista, F., et al. (2017). Characterization of atrial fibrillation adverse events reported in ibrutinib randomized controlled registration trials. Haematologica 102 (10), 1796–1805. doi:10.3324/haematol.2017.171041
Burger, J. A., Tedeschi., A., Barr., P. M., Robak, T., Owen, C., Ghia, P., et al. (2015). Ibrutinib as initial therapy for patients with chronic lymphocytic leukemia. N. Engl. J. Med. 373 (25), 2425–2437. doi:10.1056/NEJMoa1509388
Buza, V., Rajagopalan, B., and Curtis, A. B. (2017). Cancer treatment-induced arrhythmias: focus on chemotherapy and targeted therapies. Circ. Arrhythm. Electrophysiol. 10 (8). doi:10.1161/circep.117.005443
Byrd, J. C., Furman, R. R., Coutre, S. E., Burger, J. A., Blum, K. A., Coleman., M., et al. (2015). Three-year follow-up of treatment-naïve and previously treated patients with CLL and SLL receiving single-agent ibrutinib. Blood 125 (16), 2497–2506. doi:10.1182/blood-2014-10-606038
Cai, J., Chen, X., Liu, X., Li, Z., Shi, A., Tang, X., et al. (2022). AMPK: the key to ischemia-reperfusion injury. J. Cell Physiol. 237 (11), 4079–4096. doi:10.1002/jcp.30875
Carresi, C., Musolino, V., Gliozzi, M., Maiuolo, J., Mollace, R., Nucera, S., et al. (2018). Anti-oxidant effect of bergamot polyphenolic fraction counteracts doxorubicin-induced cardiomyopathy: role of autophagy and c-kit(pos)CD45(neg)CD31(neg) cardiac stem cell activation. J. Mol. Cell Cardiol. 119, 10–18. doi:10.1016/j.yjmcc.2018.04.007
Chen, L., Zhu, H. M., Li, Y., Liu, Q. F., Hu., Y., Zhou, J. F., et al. (2021). Arsenic trioxide replacing or reducing chemotherapy in consolidation therapy for acute promyelocytic leukemia (APL2012 trial). Proc. Natl. Acad. Sci. U. S. A. 118 (6). doi:10.1073/pnas.2020382118
Chen, X., Peng, X., Luo., Y., You, J., Yin, D., Xu, Q., et al. (2019). Quercetin protects cardiomyocytes against doxorubicin-induced toxicity by suppressing oxidative stress and improving mitochondrial function via 14-3-3γ. Toxicol. Mech. Methods 29 (5), 344–354. doi:10.1080/15376516.2018.1564948
Chen, X., Qi., S., Li, Z., He, B., Li, H. L., Fu, J., et al. (2019). Shenqi Fuzheng injection (SFI) enhances IFN-α inhibitory effect on hepatocellular carcinoma cells by reducing VEGF expression: validation by gene silencing technique. Biomed. Res. Int. 2019, 8084109. doi:10.1155/2019/8084109
Chen, Y., Tang., Y., Xiang, Y., Xie, Y. Q., Huang, X. H., and Zhang, Y. C. (2015). Shengmai injection improved doxorubicin-induced cardiomyopathy by alleviating myocardial endoplasmic reticulum stress and caspase-12 dependent apoptosis. Biomed. Res. Int. 2015, 952671. doi:10.1155/2015/952671
Chowdhury, S., Sinha., K., Banerjee, S., and Sil, P. C. (2016). Taurine protects cisplatin induced cardiotoxicity by modulating inflammatory and endoplasmic reticulum stress responses. Biofactors 42 (6), 647–664. doi:10.1002/biof.1301
Christidi, E., and Brunham, L. R. (2021). Regulated cell death pathways in doxorubicin-induced cardiotoxicity. Cell Death Dis. 12 (4), 339. doi:10.1038/s41419-021-03614-x
Coromilas, E. J., Kochav, S., Goldenthal, I., Biviano, A., Garan, H., Goldbarg, S., et al. (2021). Worldwide survey of COVID-19-associated arrhythmias. Circ. Arrhythm. Electrophysiol. 14 (3), e009458. doi:10.1161/circep.120.009458
Cuadrado, A., Manda, G., Hassan, A., Alcaraz, M. J., Barbas, C., Daiber, A., et al. (2018). Transcription factor NRF2 as a therapeutic target for chronic diseases: a systems medicine approach. Pharmacol. Rev. 70 (2), 348–383. doi:10.1124/pr.117.014753
Cui, Y., Li, C., Zeng, C., Li, J., Zhu, Z., Chen, W., et al. (2018). Tongmai Yangxin pills anti-oxidative stress alleviates cisplatin-induced cardiotoxicity: network pharmacology analysis and experimental evidence. Biomed. Pharmacother. 108, 1081–1089. doi:10.1016/j.biopha.2018.09.095
Curigliano, G., Cardinale, D., Dent, S., Criscitiello, C., Aseyev, O., Lenihan, D., et al. (2016). Cardiotoxicity of anticancer treatments: epidemiology, detection, and management. CA Cancer J. Clin. 66 (4), 309–325. doi:10.3322/caac.21341
Das, J., Ghosh, J., Manna, P., and Sil, P. C. (2011). Taurine suppresses doxorubicin-triggered oxidative stress and cardiac apoptosis in rat via up-regulation of PI3-K/Akt and inhibition of p53, p38-JNK. Biochem. Pharmacol. 81 (7), 891–909. doi:10.1016/j.bcp.2011.01.008
Daubney, J., Bonner, P. L., Hargreaves, A. J., and Dickenson, J. M. (2015). Cardioprotective and cardiotoxic effects of quercetin and two of its in vivo metabolites on differentiated h9c2 cardiomyocytes. Basic Clin. Pharmacol. Toxicol. 116 (2), 96–109. doi:10.1111/bcpt.12319
Dobrev, D., Heijman, J., Hiram, R., Li, N., and Nattel, S. (2023). Inflammatory signalling in atrial cardiomyocytes: a novel unifying principle in atrial fibrillation pathophysiology. Nat. Rev. Cardiol. 20 (3), 145–167. doi:10.1038/s41569-022-00759-w
Doenst, T., Nguyen, T. D., and Abel, E. D. (2013). Cardiac metabolism in heart failure: implications beyond ATP production. Circ. Res. 113 (6), 709–724. doi:10.1161/circresaha.113.300376
Dong, Q., Chen, L., Lu, Q., Sharma, S., Li, L., Morimoto, S., et al. (2014). Quercetin attenuates doxorubicin cardiotoxicity by modulating Bmi-1 expression. Br. J. Pharmacol. 171 (19), 4440–4454. doi:10.1111/bph.12795
Drugs FDA (2023). FDA approved drug products. Available at: https://www.accessdata.fda.gov/scripts/cder/daf/.
Durak, I., Karaayvaz, M., Kavutcu, M., Cimen, M. Y., Kaçmaz, M., Büyükkoçak, S., et al. (2000). Reduced antioxidant defense capacity in myocardial tissue from Guinea pigs treated with 5-fluorouracil. J. Toxicol. Environ. Health A 59 (7), 585–589. doi:10.1080/009841000156709
Edwardson, D. W., Narendrula, R., Chewchuk, S., Mispel-Beyer, K., Mapletoft, J. P., and Parissenti, A. M. (2015). Role of drug metabolism in the cytotoxicity and clinical efficacy of anthracyclines. Curr. Drug Metab. 16 (6), 412–426. doi:10.2174/1389200216888150915112039
Elahi, M. M., Flatman, S., and Matata, B. M. (2008). Tracing the origins of postoperative atrial fibrillation: the concept of oxidative stress-mediated myocardial injury phenomenon. Eur. J. Cardiovasc Prev. Rehabil. 15 (6), 735–741. doi:10.1097/HJR.0b013e328317f38a
Elshazly, A. M., Nguyen, T. V. V., and Gewirtz, D. A. (2022). Is autophagy induction by PARP inhibitors a target for therapeutic benefit? Oncol. Res. 30 (1), 1–12. doi:10.32604/or.2022.026459
Eskandari, M. R., Moghaddam, F., Shahraki, J., and Pourahmad, J. (2015). A comparison of cardiomyocyte cytotoxic mechanisms for 5-fluorouracil and its pro-drug capecitabine. Xenobiotica 45 (1), 79–87. doi:10.3109/00498254.2014.942809
Fahdi, I. E., Gaddam, V., Saucedo, J. F., Kishan, C. V., Vyas., K., Deneke, M. G., et al. (2004). Bradycardia during therapy for multiple myeloma with thalidomide. Am. J. Cardiol. 93 (8), 1052–1055. doi:10.1016/j.amjcard.2003.12.061
Fan, Y., Man., S., Li, H., Liu, Y., Liu, Z., and Gao, W. (2016). Analysis of bioactive components and pharmacokinetic study of herb-herb interactions in the traditional Chinese patent medicine Tongmai Yangxin Pill. J. Pharm. Biomed. Anal. 120, 364–373. doi:10.1016/j.jpba.2015.12.032
Farhood, B., Mortezaee, K., Goradel, N. H., Khanlarkhani, N., Salehi., E., Nashtaei, M. S., et al. (2019). Curcumin as an anti-inflammatory agent: implications to radiotherapy and chemotherapy. J. Cell Physiol. 234 (5), 5728–5740. doi:10.1002/jcp.27442
Fazzini, L., Caggiari, L., Deidda, M., Onnis, C., Saba, L., Mercuro, G., et al. (2022). Metabolomic profiles on antiblastic cardiotoxicity: new perspectives for early diagnosis and cardioprotection. J. Clin. Med. 11 (22). doi:10.3390/jcm11226745
Feliz, V., Saiyad, S., Ramarao, S. M., Khan, H., Leonelli, F., and Guglin, M. (2011). Melphalan-induced supraventricular tachycardia: incidence and risk factors. Clin. Cardiol. 34 (6), 356–359. doi:10.1002/clc.20904
Feng, K., Liu, Y., Sun., J., Zhao, C., Duan, Y., Wang., W., et al. (2021). Compound Danshen Dripping Pill inhibits doxorubicin or isoproterenol-induced cardiotoxicity. Biomed. Pharmacother. 138, 111531. doi:10.1016/j.biopha.2021.111531
Focaccetti, C., Bruno., A., Magnani, E., Bartolini, D., Principi, E., Dallaglio, K., et al. (2015). Effects of 5-fluorouracil on morphology, cell cycle, proliferation, apoptosis, autophagy and ROS production in endothelial cells and cardiomyocytes. PLoS One 10 (2), e0115686. doi:10.1371/journal.pone.0115686
Font, J., Milliez, P., Ouazar, A. B., Klok, F. A., and Alexandre, J. (2023). Atrial fibrillation, cancer and anticancer drugs. Arch. Cardiovasc Dis. 116 (4), 219–226. doi:10.1016/j.acvd.2023.02.005
Garg, L., and Fradley, M. G. (2021). QT prolongation and cancer therapeutics: a coming Tempest or Much Ado about Nothing? Eur. Heart J. 42 (38), 3929–3931. doi:10.1093/eurheartj/ehab483
Gatica, D., Chiong, M., Lavandero, S., and Klionsky, D. J. (2015). Molecular mechanisms of autophagy in the cardiovascular system. Circ. Res. 116 (3), 456–467. doi:10.1161/circresaha.114.303788
Gawałko, M., Kapłon-Cieślicka, A., Hohl., M., Dobrev, D., and Linz, D. (2020). COVID-19 associated atrial fibrillation: incidence, putative mechanisms and potential clinical implications. Int. J. Cardiol. Heart Vasc. 30, 100631. doi:10.1016/j.ijcha.2020.100631
Ghibu, S., Delemasure, S., Richard., C., Guilland, J. C., Martin, L., Gambert, S., et al. (2012). General oxidative stress during doxorubicin-induced cardiotoxicity in rats: absence of cardioprotection and low antioxidant efficiency of alpha-lipoic acid. Biochimie 94 (4), 932–939. doi:10.1016/j.biochi.2011.02.015
Gottlieb, R. A., and Mentzer, R. M. (2013). Autophagy: an affair of the heart. Heart Fail Rev. 18 (5), 575–584. doi:10.1007/s10741-012-9367-2
Guglin, M., Aljayeh, M., Saiyad, S., Ali, R., and Curtis, A. B. (2009). Introducing a new entity: chemotherapy-induced arrhythmia. Europace 11 (12), 1579–1586. doi:10.1093/europace/eup300
Guo, J., Yong, Y., Cao., B., Sun., R., and Yu., X. (2016). Compound danshen dripping pills modulate the perturbed energy metabolism in a rat model of acute myocardial ischemia. Sci. Rep. 6, 37919. doi:10.1038/srep37919
Guo, Y., Tang., Y., Lu, G., and Gu, J. (2023). p53 at the crossroads between doxorubicin-induced cardiotoxicity and resistance: a nutritional balancing act. Nutrients 15 (10). doi:10.3390/nu15102259
Hamilton, S., and Terentyev, D. (2022). ER stress and calcium-dependent arrhythmias. Front. Physiol. 13, 1041940. doi:10.3389/fphys.2022.1041940
Han, X., Ren., D., Fan, P., Shen, T., and Lou, H. (2008). Protective effects of naringenin-7-O-glucoside on doxorubicin-induced apoptosis in H9C2 cells. Eur. J. Pharmacol. 581 (1-2), 47–53. doi:10.1016/j.ejphar.2007.11.048
Hardie, D. G., and Carling, D. (1997). The AMP-activated protein kinase--fuel gauge of the mammalian cell? Eur. J. Biochem. 246 (2), 259–273. doi:10.1111/j.1432-1033.1997.00259.x
Harstad, E. B., and Klaassen, C. D. (2002). iNOS-null mice are not resistant to cadmium chloride-induced hepatotoxicity. Toxicology 175 (1-3), 83–90. doi:10.1016/s0300-483x(02)00068-9
Heinzerling, L., Ott, P. A., Hodi, F. S., Husain, A. N., Tajmir-Riahi, A., Tawbi, H., et al. (2016). Cardiotoxicity associated with CTLA4 and PD1 blocking immunotherapy. J. Immunother. Cancer 4, 50. doi:10.1186/s40425-016-0152-y
Herrmann, J. (2020). Adverse cardiac effects of cancer therapies: cardiotoxicity and arrhythmia. Nat. Rev. Cardiol. 17 (8), 474–502. doi:10.1038/s41569-020-0348-1
Hilmi, M., Ederhy, S., Waintraub, X., Funck-Brentano, C., Cohen, A., Vozy, A., et al. (2020). Cardiotoxicity associated with gemcitabine: literature review and a pharmacovigilance study. Pharm. (Basel) 13 (10). doi:10.3390/ph13100325
Hiram, R., Xiong, F., Naud, P., Xiao, J., Sirois, M., Tanguay, J. F., et al. (2021). The inflammation-resolution promoting molecule resolvin-D1 prevents atrial proarrhythmic remodelling in experimental right heart disease. Cardiovasc Res. 117 (7), 1776–1789. doi:10.1093/cvr/cvaa186
Hosseinzadeh, L., Behravan, J., Mosaffa, F., Bahrami, G., Bahrami, A., and Karimi, G. (2011). Curcumin potentiates doxorubicin-induced apoptosis in H9c2 cardiac muscle cells through generation of reactive oxygen species. Food Chem. Toxicol. 49 (5), 1102–1109. doi:10.1016/j.fct.2011.01.021
Hou, J., Yun., Y., Cui, C., and Kim, S. (2022). Ginsenoside Rh2 mitigates doxorubicin-induced cardiotoxicity by inhibiting apoptotic and inflammatory damage and weakening pathological remodelling in breast cancer-bearing mice. Cell Prolif. 55 (6), e13246. doi:10.1111/cpr.13246
Hu, Y. X., You, H. M., Ren., C. Z., Hu, B. W., Zhang, L. J., Zhang, Y. D., et al. (2022). Proangiogenesis effects of compound danshen dripping pills in zebrafish. BMC Complement. Med. Ther. 22 (1), 112. doi:10.1186/s12906-022-03589-y
Hussein, (2012). Ameliorating effect of DL-α-lipoic acid against cisplatin-induced nephrotoxicity and cardiotoxicity in experimental animals. Drug Discov. Ther. doi:10.5582/ddt.2012.v6.3.147
Jain, A., and Rani, V. (2018). Mode of treatment governs curcumin response on doxorubicin-induced toxicity in cardiomyoblasts. Mol. Cell Biochem. 442 (1-2), 81–96. doi:10.1007/s11010-017-3195-6
Jiang, Y., Liu., Y., Xiao, W., Zhang, D., Liu, X., Xiao, H., et al. (2021). Xinmailong attenuates doxorubicin-induced lysosomal dysfunction and oxidative stress in H9c2 cells via HO-1. Oxid. Med. Cell Longev. 2021, 5896931. doi:10.1155/2021/5896931
Jin, J., Qiu, S., Wang., P., Liang, X., Huang, F., Wu., H., et al. (2019). Cardamonin inhibits breast cancer growth by repressing HIF-1α-dependent metabolic reprogramming. J. Exp. Clin. Cancer Res. 38 (1), 377. doi:10.1186/s13046-019-1351-4
Jun, Y., Chunju, Y., Qi, A., Liuxia, D., and Guolong, Y. (2014). The effects of compound danshen dripping pills and human umbilical cord blood mononuclear cell transplant after acute myocardial infarction. Exp. Clin. Transpl. 12 (2), 123–128.
Kayser, S., Schlenk, R. F., Lebon, D., Carre, M., Götze, K. S., Stölzel, F., et al. (2021). Characteristics and outcome of patients with low-/intermediate-risk acute promyelocytic leukemia treated with arsenic trioxide: an international collaborative study. Haematologica 106 (12), 3100–3106. doi:10.3324/haematol.2021.278722
Khan, M. A., Masood, N., Husain, N., Ahmad, B., Aziz, T., and Naeem, A. (2012). A retrospective study of cardiotoxicities induced by 5-fluouracil (5-FU) and 5-FU based chemotherapy regimens in Pakistani adult cancer patients at Shaukat Khanum Memorial Cancer Hospital and Research Center. J. Pak Med. Assoc. 62 (5), 430–434.
Kilickap, S., Barista, I., Akgul, E., Aytemir, K., Aksoy, S., and Tekuzman, G. (2007). Early and late arrhythmogenic effects of doxorubicin. South Med. J. 100 (3), 262–265. doi:10.1097/01.smj.0000257382.89910.fe
Kim, E. K., and Choi, E. J. (2015). Compromised MAPK signaling in human diseases: an update. Arch. Toxicol. 89 (6), 867–882. doi:10.1007/s00204-015-1472-2
Kim, J. S., He, L., and Lemasters, J. J. (2003). Mitochondrial permeability transition: a common pathway to necrosis and apoptosis. Biochem. Biophys. Res. Commun. 304 (3), 463–470. doi:10.1016/s0006-291x(03)00618-1
Kitani, T., Ong, S.-G., Lam, C. K., Rhee., J.-W., Zhang, J. Z., Oikonomopoulos, A., et al. (2019). Human-induced pluripotent stem cell model of trastuzumab-induced cardiac dysfunction in patients with breast cancer. Circulation 139 (21), 2451–2465. doi:10.1161/circulationaha.118.037357
Koleini, N., and Kardami, E. (2017). Autophagy and mitophagy in the context of doxorubicin-induced cardiotoxicity. Oncotarget 8 (28), 46663–46680. doi:10.18632/oncotarget.16944
Kupari, M., Volin, L., Suokas, A., Timonen, T., Hekali, P., and Ruutu, T. (1990). Cardiac involvement in bone marrow transplantation: electrocardiographic changes, arrhythmias, heart failure and autopsy findings. Bone Marrow Transpl. 5 (2), 91–98.
Lamberti, M., Porto, S., Zappavigna, S., Addeo, E., Marra., M., Miraglia, N., et al. (2014). A mechanistic study on the cardiotoxicity of 5-fluorouracil in vitro and clinical and occupational perspectives. Toxicol. Lett. 227 (3), 151–156. doi:10.1016/j.toxlet.2014.03.018
Lan, Y., Wang., Y., Huang, K., and Zeng, Q. (2020). Heat shock protein 22 attenuates doxorubicin-induced cardiotoxicity via regulating inflammation and apoptosis. Front. Pharmacol. 11, 257. doi:10.3389/fphar.2020.00257
Lara, P. N., Mack, P. C., Synold, T., Frankel, P., Longmate, J., Gumerlock, P. H., et al. (2005). The cyclin-dependent kinase inhibitor UCN-01 plus cisplatin in advanced solid tumors: a California cancer consortium phase I pharmacokinetic and molecular correlative trial. Clin. Cancer Res. 11 (12), 4444–4450. doi:10.1158/1078-0432.Ccr-04-2602
Lee, J. H., Jung, H. S., Giang, P. M., Jin, X., Lee, S., Son, P. T., et al. (2006). Blockade of nuclear factor-kappaB signaling pathway and anti-inflammatory activity of cardamomin, a chalcone analog from Alpinia conchigera. J. Pharmacol. Exp. Ther. 316 (1), 271–278. doi:10.1124/jpet.105.092486
Lee, M. Y., Seo, C. S., Lee, J. A., Shin, I. S., Kim, S. J., Ha, H., et al. (2012). Alpinia katsumadai H(AYATA) seed extract inhibit LPS-induced inflammation by induction of heme oxygenase-1 in RAW264.7 cells. Inflammation 35 (2), 746–757. doi:10.1007/s10753-011-9370-0
Leong, D. P., Caron, F., Hillis, C., Duan, A., Healey, J. S., Fraser., G., et al. (2016). The risk of atrial fibrillation with ibrutinib use: a systematic review and meta-analysis. Blood 128 (1), 138–140. doi:10.1182/blood-2016-05-712828
Li, A. L., Lian, L., Chen, X. N., Cai, W. H., Fan, X. B., Fan, Y. J., et al. (2023). The role of mitochondria in myocardial damage caused by energy metabolism disorders: from mechanisms to therapeutics. Free Radic. Biol. Med. 208, 236–251. doi:10.1016/j.freeradbiomed.2023.08.009
Li, H., Mao., Y., Zhang, Q., Han, Q., Man., Z., Zhang, J., et al. (2016). Xinmailong mitigated epirubicin-induced cardiotoxicity via inhibiting autophagy. J. Ethnopharmacol. 192, 459–470. doi:10.1016/j.jep.2016.08.031
Li, L., Li., J., Wang., Q., Zhao, X., Yang, D., Niu, L., et al. (2020). Shenmai injection protects against doxorubicin-induced cardiotoxicity via maintaining mitochondrial homeostasis. Front. Pharmacol. 11, 815. doi:10.3389/fphar.2020.00815
Li, L., Ni., J., Li, M., Chen, J., Han, L., Zhu, Y., et al. (2017). Ginsenoside Rg3 micelles mitigate doxorubicin-induced cardiotoxicity and enhance its anticancer efficacy. Drug Deliv. 24 (1), 1617–1630. doi:10.1080/10717544.2017.1391893
Li, L., Yang, D., Li, J., Niu, L., Chen, Y., Zhao, X., et al. (2020). Investigation of cardiovascular protective effect of Shenmai injection by network pharmacology and pharmacological evaluation. BMC Complement. Med. Ther. 20 (1), 112. doi:10.1186/s12906-020-02905-8
Li, L. F., Ma., Z. C., Wang., Y. G., Tang, X. L., Tan, H. L., Xiao, C. R., et al. (2017). Protective effect of ginsenoside Rb-on doxorubicin-induced myocardial autophagy. Zhongguo Zhong Yao Za Zhi 42 (7), 1365–1369. doi:10.19540/j.cnki.cjcmm.20170222.009
Li, S., Wang., W., Niu, T., Wang., H., Li, B., Shao, L., et al. (2014). Nrf2 deficiency exaggerates doxorubicin-induced cardiotoxicity and cardiac dysfunction. Oxid. Med. Cell Longev. 2014, 748524. doi:10.1155/2014/748524
Li, W., Wu., X., Li, M., Wang., Z., Li, B., Qu, X., et al. (2016). Cardamonin alleviates pressure overload-induced cardiac remodeling and dysfunction through inhibition of oxidative stress. J. Cardiovasc Pharmacol. 68 (6), 441–451. doi:10.1097/fjc.0000000000000430
Li, Y. Y., Huang, S. S., Lee, M. M., Deng, J. S., and Huang, G. J. (2015). Anti-inflammatory activities of cardamonin from Alpinia katsumadai through heme oxygenase-1 induction and inhibition of NF-κB and MAPK signaling pathway in the carrageenan-induced paw edema. Int. Immunopharmacol. 25 (2), 332–339. doi:10.1016/j.intimp.2015.02.002
Liao, J., Hao, C., Huang, W., Shao, X., Song, Y., Liu, L., et al. (2018). Network pharmacology study reveals energy metabolism and apoptosis pathways-mediated cardioprotective effects of Shenqi Fuzheng. J. Ethnopharmacol. 227, 155–165. doi:10.1016/j.jep.2018.08.029
Liu, J., Chang, F., Li, F., Fu, H., Wang., J., Zhang, S., et al. (2015). Palmitate promotes autophagy and apoptosis through ROS-dependent JNK and p38 MAPK. Biochem. Biophys. Res. Commun. 463 (3), 262–267. doi:10.1016/j.bbrc.2015.05.042
Liu, J., Wang, H., and Li, J. (2016). Inflammation and inflammatory cells in myocardial infarction and reperfusion injury: a double-edged sword. Clin. Med. Insights Cardiol. 10, 79–84. doi:10.4137/cmc.S33164
Lopaschuk, G. D., Karwi, Q. G., Tian, R., Wende, A. R., and Abel, E. D. (2021). Cardiac energy metabolism in heart failure. Circ. Res. 128 (10), 1487–1513. doi:10.1161/circresaha.121.318241
Lu, M. C., Ji, J. A., Jiang, Z. Y., and You, Q. D. (2016). The keap1-nrf2-ARE pathway as a potential preventive and therapeutic target: an update. Med. Res. Rev. 36 (5), 924–963. doi:10.1002/med.21396
Luan, Y., Luan, Y., Feng, Q., Chen, X., Ren, K. D., and Yang, Y. (2021). Emerging role of mitophagy in the heart: therapeutic potentials to modulate mitophagy in cardiac diseases. Oxid. Med. Cell Longev. 2021, 3259963. doi:10.1155/2021/3259963
Lv, X., Zhu, Y., Deng., Y., Zhang, S., Zhang, Q., Zhao, B., et al. (2020). Glycyrrhizin improved autophagy flux via HMGB1-dependent Akt/mTOR signaling pathway to prevent Doxorubicin-induced cardiotoxicity. Toxicology 441, 152508. doi:10.1016/j.tox.2020.152508
Lv, X. F., Wen, R. Q., Liu, K., Zhao, X. K., Pan, C. L., Gao, X., et al. (2022). Role and molecular mechanism of traditional Chinese medicine in preventing cardiotoxicity associated with chemoradiotherapy. Front. Cardiovasc Med. 9, 1047700. doi:10.3389/fcvm.2022.1047700
Manohar, S., and Leung, N. (2018). Cisplatin nephrotoxicity: a review of the literature. J. Nephrol. 31 (1), 15–25. doi:10.1007/s40620-017-0392-z
Mao, Z. Z., and Xu, X. (2007). Overview and prospects of research on the mechanism of Shengmai injection in the treatment of heart failure. J. Emerg. Traditional Chin. Med. 16 (2), 216–217.
Mascolo, A., Sportiello, L., Rafaniello, C., Donniacuo, M., Ruggiero, D., Scisciola, L., et al. (2023). Do immune checkpoint inhibitors share the same pharmacological feature in the risk of cardiac arrhythmias? Biomed. Pharmacother. 164, 114912. doi:10.1016/j.biopha.2023.114912
Mason, F. E., Pronto, J. R. D., Alhussini, K., Maack, C., and Voigt, N. (2020). Cellular and mitochondrial mechanisms of atrial fibrillation. Basic Res. Cardiol. 115 (6), 72. doi:10.1007/s00395-020-00827-7
Mathur, K., Saini, A., Ellenbogen, K. A., and Shepard, R. K. (2017). Profound sinoatrial arrest associated with ibrutinib. Case Rep. Oncol. Med. 2017, 7304021. doi:10.1155/2017/7304021
Matouk, A. I., Taye, A., Heeba, G. H., and El-Moselhy, M. A. (2013). Quercetin augments the protective effect of losartan against chronic doxorubicin cardiotoxicity in rats. Environ. Toxicol. Pharmacol. 36 (2), 443–450. doi:10.1016/j.etap.2013.05.006
McSweeney, K. M., Bozza, W. P., Alterovitz, W. L., and Zhang, B. (2019). Transcriptomic profiling reveals p53 as a key regulator of doxorubicin-induced cardiotoxicity. Cell Death Discov. 5, 102. doi:10.1038/s41420-019-0182-6
Mei, M., Tang, F., Lu, M., He, X., Wang., H., Hou, X., et al. (2015). Astragaloside IV attenuates apoptosis of hypertrophic cardiomyocyte through inhibiting oxidative stress and calpain-1 activation. Environ. Toxicol. Pharmacol. 40 (3), 764–773. doi:10.1016/j.etap.2015.09.007
Mei, Y., Thompson, M. D., Cohen, R. A., and Tong, X. (2015). Autophagy and oxidative stress in cardiovascular diseases. Biochim. Biophys. Acta 1852 (2), 243–251. doi:10.1016/j.bbadis.2014.05.005
Michalson, K. T., Groban, L., Howard, T. D., Shively, C. A., Sophonsritsuk, A., Appt, S. E., et al. (2018). Estradiol treatment initiated early after ovariectomy regulates myocardial gene expression and inhibits diastolic dysfunction in female cynomolgus monkeys: potential roles for calcium homeostasis and extracellular matrix remodeling. J. Am. Heart Assoc. 7 (21), e009769. doi:10.1161/jaha.118.009769
Mileshkin, L. R., Seymour, J. F., Wolf, M. M., Gates., P., Januszewicz, E. H., Joyce, P., et al. (2005). Cardiovascular toxicity is increased, but manageable, during high-dose chemotherapy and autologous peripheral blood stem cell transplantation for patients aged 60 years and older. Leuk. Lymphoma 46 (11), 1575–1579. doi:10.1080/10428190500235884
Minamino, T., Komuro, I., and Kitakaze, M. (2010). Endoplasmic reticulum stress as a therapeutic target in cardiovascular disease. Circ. Res. 107 (9), 1071–1082. doi:10.1161/circresaha.110.227819
Mir, H., Alhussein, M., Alrashidi, S., Alzayer, H., Alshatti, A., Valettas, N., et al. (2018). Cardiac complications associated with checkpoint inhibition: a systematic review of the literature in an important emerging area. Can. J. Cardiol. 34 (8), 1059–1068. doi:10.1016/j.cjca.2018.03.012
Mohajeri, M., and Sahebkar, A. (2018). Protective effects of curcumin against doxorubicin-induced toxicity and resistance: a review. Crit. Rev. Oncol. Hematol. 122, 30–51. doi:10.1016/j.critrevonc.2017.12.005
Monkkonen, T., and Debnath, J. (2018). Inflammatory signaling cascades and autophagy in cancer. Autophagy 14 (2), 190–198. doi:10.1080/15548627.2017.1345412
Najafi, M., Mortezaee, K., Rahimifard, M., Farhood, B., and Haghi-Aminjan, H. (2020). The role of curcumin/curcuminoids during gastric cancer chemotherapy: a systematic review of non-clinical study. Life Sci. 257, 118051. doi:10.1016/j.lfs.2020.118051
Narezkina, A., Narayan, H. K., and Zemljic-Harpf, A. E. (2021). Molecular mechanisms of anthracycline cardiovascular toxicity. Clin. Sci. (Lond). 135 (10), 1311–1332. doi:10.1042/cs20200301
Necela, B. M., Axenfeld, B. C., Serie, D. J., Kachergus, J. M., Perez, E. A., Thompson, E. A., et al. (2017). The antineoplastic drug, trastuzumab, dysregulates metabolism in iPSC-derived cardiomyocytes. Clin. Transl. Med. 6 (1), 5. doi:10.1186/s40169-016-0133-2
Oakes, S. A., and Papa, F. R. (2015). The role of endoplasmic reticulum stress in human pathology. Annu. Rev. Pathol. 10, 173–194. doi:10.1146/annurev-pathol-012513-104649
Olivieri, A., Corvatta, L., Montanari, M., Brunori, M., Offidani, M., Ferretti, G. F., et al. (1998). Paroxysmal atrial fibrillation after high-dose melphalan in five patients autotransplanted with blood progenitor cells. Bone Marrow Transpl. 21 (10), 1049–1053. doi:10.1038/sj.bmt.1701217
Omidkhoda, N., Wallace Hayes., A., Reiter, R. J., and Karimi, G. (2019). The role of MicroRNAs on endoplasmic reticulum stress in myocardial ischemia and cardiac hypertrophy. Pharmacol. Res. 150, 104516. doi:10.1016/j.phrs.2019.104516
O'Neal, W. T., Lakoski, S. G., Qureshi, W., Judd, S. E., Howard, G., Howard, V. J., et al. (2015). Relation between cancer and atrial fibrillation (from the REasons for geographic and racial differences in stroke study). Am. J. Cardiol. 115 (8), 1090–1094. doi:10.1016/j.amjcard.2015.01.540
Ou, S. H., Tang., Y., Polli, A., Wilner, K. D., and Schnell, P. (2016). Factors associated with sinus bradycardia during crizotinib treatment: a retrospective analysis of two large-scale multinational trials (PROFILE 1005 and 1007). Cancer Med. 5 (4), 617–622. doi:10.1002/cam4.622
Pasini, E., Corsetti, G., and Dioguardi, F. S. (2023). Nutritional supplementation and exercise as essential allies in the treatment of chronic heart failure: the metabolic and molecular bases. Nutrients 15 (10). doi:10.3390/nu15102337
Passalia, C., Minetto, P., Arboscello, E., Balleari, E., Bellodi, A., Del Corso, L., et al. (2013). Cardiovascular adverse events complicating the administration of rituximab: report of two cases. Tumori 99 (6), 288e–292e. doi:10.1700/1390.15471
Pastori, D., Miyazawa, K., Li, Y., Shahid, F., Hado, H., and Lip, G. Y. H. (2018). Inflammation and the risk of atrial high-rate episodes (AHREs) in patients with cardiac implantable electronic devices. Clin. Res. Cardiol. 107 (9), 772–777. doi:10.1007/s00392-018-1244-0
Patel, P., Dokainish, H., Tsai, P., and Lakkis, N. (2010). Update on the association of inflammation and atrial fibrillation. J. Cardiovasc Electrophysiol. 21 (9), 1064–1070. doi:10.1111/j.1540-8167.2010.01774.x
Pellegrini, L., Sileno, S., D'Agostino., M., Foglio, E., Florio, M. C., Guzzanti, V., et al. (2020). MicroRNAs in cancer treatment-induced cardiotoxicity. Cancers (Basel) 12 (3). doi:10.3390/cancers12030704
Peng, S., Hou, Y., Yao, J., and Fang, J. (2017). Activation of Nrf2-driven antioxidant enzymes by cardamonin confers neuroprotection of PC12 cells against oxidative damage. Food Funct. 8 (3), 997–1007. doi:10.1039/c7fo00054e
Phillips, G. L., Meisenberg, B., Reece, D. E., Adams., V. R., Badros, A., Brunner, J., et al. (2004). Amifostine and autologous hematopoietic stem cell support of escalating-dose melphalan: a phase I study. Biol. Blood Marrow Transpl. 10 (7), 473–483. doi:10.1016/j.bbmt.2004.03.001
Piotrowski, G., Gawor, R., Słomka, R., Banasiak, M., Strzelecki, P., Gawor, Z., et al. (2012). Cardioverter-defibrillator in the treatment of arrhythmia induced by trastuzumab used in the adjuvant setting in a patient with positive human epidermal growth factor receptor type-2 breast cancer. Kardiol. Pol. 70 (7), 756–757.
Polk, A., Vaage-Nilsen, M., Vistisen, K., and Nielsen, D. L. (2013). Cardiotoxicity in cancer patients treated with 5-fluorouracil or capecitabine: a systematic review of incidence, manifestations and predisposing factors. Cancer Treat. Rev. 39 (8), 974–984. doi:10.1016/j.ctrv.2013.03.005
Polk, A., Vistisen, K., Vaage-Nilsen, M., and Nielsen, D. L. (2014). A systematic review of the pathophysiology of 5-fluorouracil-induced cardiotoxicity. BMC Pharmacol. Toxicol. 15, 47. doi:10.1186/2050-6511-15-47
Qanungo, S., Uys, J. D., Manevich, Y., Distler, A. M., Shaner., B., Hill, E. G., et al. (2014). N-acetyl-L-cysteine sensitizes pancreatic cancers to gemcitabine by targeting the NFκB pathway. Biomed. Pharmacother. 68 (7), 855–864. doi:10.1016/j.biopha.2014.08.007
Qi, J. Y., Yang., Y. K., Jiang, C., Zhao, Y., Wu., Y. C., Han, X., et al. (2022). Exploring the mechanism of Danshensu in the treatment of doxorubicin-induced cardiotoxicity based on network pharmacology and experimental evaluation. Front. Cardiovasc Med. 9, 827975. doi:10.3389/fcvm.2022.827975
Qi, W., Boliang, W., Xiaoxi, T., Guoqiang, F., Jianbo, X., and Gang, W. (2020). Cardamonin protects against doxorubicin-induced cardiotoxicity in mice by restraining oxidative stress and inflammation associated with Nrf2 signaling. Biomed. Pharmacother. 122, 109547. doi:10.1016/j.biopha.2019.109547
Qin, Y., Sun., C. Y., Lu, F. R., Shu, X. R., Yang, D., Chen, L., et al. (2012). Cardamonin exerts potent activity against multiple myeloma through blockade of NF-κB pathway in vitro. Leuk. Res. 36 (4), 514–520. doi:10.1016/j.leukres.2011.11.014
Qing, G., Yan, P., and Xiao, G. (2006). Hsp90 inhibition results in autophagy-mediated proteasome-independent degradation of IkappaB kinase (IKK). Cell Res. 16 (11), 895–901. doi:10.1038/sj.cr.7310109
Qing-min, X., Shu, L., Yong-hua, Z., Lan, L., Chun-gang, Z., and Li-juan, S. (2018). Effect of shengmaisan on DCM rats and TLR-4/NF-κB inflammatory signal pathway. 24 (2), 128–134.
Quezado, Z. M., Wilson, W. H., Cunnion, R. E., Parker, M. M., Reda, D., Bryant, G., et al. (1993). High-dose ifosfamide is associated with severe, reversible cardiac dysfunction. Ann. Intern Med. 118 (1), 31–36. doi:10.7326/0003-4819-118-1-199301010-00006
Rahman, I. (2002). Oxidative stress, transcription factors and chromatin remodelling in lung inflammation. Biochem. Pharmacol. 64 (5-6), 935–942. doi:10.1016/s0006-2952(02)01153-x
Rajkumar, S. V., Gertz, M. A., Lacy, M. Q., Dispenzieri, A., Fonseca, R., Geyer, S. M., et al. (2003). Thalidomide as initial therapy for early-stage myeloma. Leukemia 17 (4), 775–779. doi:10.1038/sj.leu.2402866
Rao, Y., Wang., Y., Lin., Z., Zhang, X., Ding, X., Yang., Y., et al. (2023). Comparative efficacy and pharmacological mechanism of Chinese patent medicines against anthracycline-induced cardiotoxicity: an integrated study of network meta-analysis and network pharmacology approach. Front. Cardiovasc Med. 10, 1126110. doi:10.3389/fcvm.2023.1126110
Rapa, S. F., Magliocca, G., Pepe, G., Amodio, G., Autore, G., Campiglia, P., et al. (2021). Protective effect of pomegranate on oxidative stress and inflammatory response induced by 5-fluorouracil in human keratinocytes. Antioxidants (Basel) 10 (2). doi:10.3390/antiox10020203
Rawat, P. S., Jaiswal, A., Khurana., A., Bhatti, J. S., and Navik, U. (2021). Doxorubicin-induced cardiotoxicity: an update on the molecular mechanism and novel therapeutic strategies for effective management. Biomed. Pharmacother. 139, 111708. doi:10.1016/j.biopha.2021.111708
Ren, F. F., Xie, Z. Y., Jiang, Y. N., Guan, X., Chen, Q. Y., Lai, T. F., et al. (2022). Dapagliflozin attenuates pressure overload-induced myocardial remodeling in mice via activating SIRT1 and inhibiting endoplasmic reticulum stress. Acta Pharmacol. Sin. 43 (7), 1721–1732. doi:10.1038/s41401-021-00805-2
Ren, J., Bi., Y., Sowers, J. R., Hetz, C., and Zhang, Y. (2021). Endoplasmic reticulum stress and unfolded protein response in cardiovascular diseases. Nat. Rev. Cardiol. 18 (7), 499–521. doi:10.1038/s41569-021-00511-w
Richards, W. G., Zellos, L., Bueno, R., Jaklitsch, M. T., Jänne, P. A., Chirieac, L. R., et al. (2006). Phase I to II study of pleurectomy/decortication and intraoperative intracavitary hyperthermic cisplatin lavage for mesothelioma. J. Clin. Oncol. 24 (10), 1561–1567. doi:10.1200/jco.2005.04.6813
Roboz, G. J., Ritchie., E. K., Carlin, R. F., Samuel., M., Gale., L., Provenzano-Gober, J. L., et al. (2014). Prevalence, management, and clinical consequences of QT interval prolongation during treatment with arsenic trioxide. J. Clin. Oncol. 32 (33), 3723–3728. doi:10.1200/jco.2013.51.2913
Rochette, L., Guenancia, C., Gudjoncik, A., Hachet, O., Zeller, M., Cottin, Y., et al. (2015). Anthracyclines/trastuzumab: new aspects of cardiotoxicity and molecular mechanisms. Trends Pharmacol. Sci. 36 (6), 326–348. doi:10.1016/j.tips.2015.03.005
Roden, D. M. (2016). Predicting drug-induced QT prolongation and torsades de pointes. J. Physiol. 594 (9), 2459–2468. doi:10.1113/jp270526
Saha, S., Bhattacharjee, P., Mukherjee, S., Mazumdar, M., Chakraborty, S., Khurana., A., et al. (2014). Contribution of the ROS-p53 feedback loop in thuja-induced apoptosis of mammary epithelial carcinoma cells. Oncol. Rep. 31 (4), 1589–1598. doi:10.3892/or.2014.2993
Sahu, B. D., Kumar., J. M., Kuncha, M., Borkar, R. M., Srinivas, R., and Sistla, R. (2016). Baicalein alleviates doxorubicin-induced cardiotoxicity via suppression of myocardial oxidative stress and apoptosis in mice. Life Sci. 144, 8–18. doi:10.1016/j.lfs.2015.11.018
Sahu, R., Dua, T. K., Das, S., De Feo, V., and Dewanjee, S. (2019). Wheat phenolics suppress doxorubicin-induced cardiotoxicity via inhibition of oxidative stress, MAP kinase activation, NF-κB pathway, PI3K/Akt/mTOR impairment, and cardiac apoptosis. Food Chem. Toxicol. 125, 503–519. doi:10.1016/j.fct.2019.01.034
Salminen, A., Hyttinen, J. M., Kauppinen, A., and Kaarniranta, K. (2012). Context-dependent regulation of autophagy by IKK-NF-κB signaling: impact on the aging process. Int. J. Cell Biol. 2012, 849541. doi:10.1155/2012/849541
Sara, J. D., Kaur., J., Khodadadi, R., Rehman, M., Lobo, R., Chakrabarti, S., et al. (2018). 5-fluorouracil and cardiotoxicity: a review. Ther. Adv. Med. Oncol. 10, 1758835918780140. doi:10.1177/1758835918780140
Shabalala, S., Muller., C. J. F., Louw, J., and Johnson, R. (2017). Polyphenols, autophagy and doxorubicin-induced cardiotoxicity. Life Sci. 180, 160–170. doi:10.1016/j.lfs.2017.05.003
Shakeri, A., Zirak, M. R., Wallace Hayes., A., Reiter, R., and Karimi, G. (2019). Curcumin and its analogues protect from endoplasmic reticulum stress: mechanisms and pathways. Pharmacol. Res. 146, 104335. doi:10.1016/j.phrs.2019.104335
Sharma, A., McKeithan, W. L., Serrano., R., Kitani, T., Burridge, P. W., Del Álamo., J. C., et al. (2018). Use of human induced pluripotent stem cell-derived cardiomyocytes to assess drug cardiotoxicity. Nat. Protoc. 13 (12), 3018–3041. doi:10.1038/s41596-018-0076-8
Sharma, A., Parikh, M., Shah, H., and Gandhi, T. (2020). Modulation of Nrf2 by quercetin in doxorubicin-treated rats. Heliyon 6 (4), e03803. doi:10.1016/j.heliyon.2020.e03803
Sharma, R., Oni, O. A., Gupta., K., Sharma, M., Sharma, R., Singh, V., et al. (2017). Normalization of testosterone levels after testosterone replacement therapy is associated with decreased incidence of atrial fibrillation. J. Am. Heart Assoc. 6 (5). doi:10.1161/jaha.116.004880
Sheu, M. T., Jhan, H. J., Hsieh, C. M., Wang, C. J., and Ho, H. O. (2015). Efficacy of antioxidants as a Complementary and Alternative Medicine (CAM) in combination with the chemotherapeutic agent doxorubicin. Integr. Cancer Ther. 14 (2), 184–195. doi:10.1177/1534735414564425
Shi, Y., Nikulenkov, F., Zawacka-Pankau, J., Li, H., Gabdoulline, R., Xu, J., et al. (2014). ROS-dependent activation of JNK converts p53 into an efficient inhibitor of oncogenes leading to robust apoptosis. Cell Death Differ. 21 (4), 612–623. doi:10.1038/cdd.2013.186
Shu, L., Wang., Y., Huang, W., Fan, S., Pan, J., Lv., Q., et al. (2023). Integrating metabolomics and network pharmacology to explore the mechanism of Tongmai Yangxin pills in ameliorating doxorubicin-induced cardiotoxicity. ACS Omega 8 (20), 18128–18139. doi:10.1021/acsomega.3c01441
Sies, H. (2015). Oxidative stress: a concept in redox biology and medicine. Redox Biol. 4, 180–183. doi:10.1016/j.redox.2015.01.002
Singh, P., Sharma, R., McElhanon, K., Allen, C. D., Megyesi, J. K., Beneš, H., et al. (2015). Sulforaphane protects the heart from doxorubicin-induced toxicity. Free Radic. Biol. Med. 86, 90–101. doi:10.1016/j.freeradbiomed.2015.05.028
Sinha, N., and Dabla, P. K. (2015). Oxidative stress and antioxidants in hypertension-a current review. Curr. Hypertens. Rev. 11 (2), 132–142. doi:10.2174/1573402111666150529130922
Sirish, P., Diloretto, D. A., Thai, P. N., and Chiamvimonvat, N. (2021). The critical roles of proteostasis and endoplasmic reticulum stress in atrial fibrillation. Front. Physiol. 12, 793171. doi:10.3389/fphys.2021.793171
Steinberg, J. S., Cohen, A. J., Wasserman, A. G., Cohen, P., and Ross, A. M. (1987). Acute arrhythmogenicity of doxorubicin administration. Cancer 60 (6), 1213–1218. doi:10.1002/1097-0142(19870915)60:6<1213::aid-cncr2820600609>3.0.co;2-v
Sumneang, N., Tanajak, P., and Oo, T. T. (2023). Toll-like receptor 4 inflammatory perspective on doxorubicin-induced cardiotoxicity. Molecules 28 (11). doi:10.3390/molecules28114294
Sun, H., Wang, Z., and Yakisich, J. S. (2013). Natural products targeting autophagy via the PI3K/Akt/mTOR pathway as anticancer agents. Anticancer Agents Med. Chem. 13 (7), 1048–1056. doi:10.2174/18715206113139990130
Sun, J., Sun., G., Meng., X., Wang., H., Luo, Y., Qin, M., et al. (2013). Isorhamnetin protects against doxorubicin-induced cardiotoxicity in vivo and in vitro. PLoS One 8 (5), e64526. doi:10.1371/journal.pone.0064526
Sung, H., Ferlay, J., Siegel, R. L., Laversanne, M., Soerjomataram, I., Jemal, A., et al. (2021). Global cancer statistics 2020: GLOBOCAN estimates of incidence and mortality worldwide for 36 cancers in 185 countries. CA Cancer J. Clin. 71 (3), 209–249. doi:10.3322/caac.21660
Szyller, J., Jagielski, D., and Bil-Lula, I. (2022). Antioxidants in arrhythmia treatment—still a controversy? A review of selected clinical and laboratory research. Antioxidants 11 (6). doi:10.3390/antiox11061109
Tam, A. B., Mercado, E. L., Hoffmann, A., and Niwa, M. (2012). ER stress activates NF-κB by integrating functions of basal IKK activity, IRE1 and PERK. PLoS One 7 (10), e45078. doi:10.1371/journal.pone.0045078
Tamargo, J., Caballero, R., and Delpón, E. (2015). Cancer chemotherapy and cardiac arrhythmias: a review. Drug Saf. 38 (2), 129–152. doi:10.1007/s40264-014-0258-4
Tan, Y., Wan, H. H., Sun., M. M., Zhang, W. J., Dong, M., Ge, W., et al. (2021). Cardamonin protects against lipopolysaccharide-induced myocardial contractile dysfunction in mice through Nrf2-regulated mechanism. Acta Pharmacol. Sin. 42 (3), 404–413. doi:10.1038/s41401-020-0397-3
Taneike, M., Yamaguchi, O., Nakai, A., Hikoso, S., Takeda, T., Mizote, I., et al. (2010). Inhibition of autophagy in the heart induces age-related cardiomyopathy. Autophagy 6 (5), 600–606. doi:10.4161/auto.6.5.11947
Tang, Y., Wang., M., Le, X., Meng., J., Huang, L., Yu., P., et al. (2011). Antioxidant and cardioprotective effects of Danshensu (3-(3, 4-dihydroxyphenyl)-2-hydroxy-propanoic acid from Salvia miltiorrhiza) on isoproterenol-induced myocardial hypertrophy in rats. Phytomedicine 18 (12), 1024–1030. doi:10.1016/j.phymed.2011.05.007
Thandavarayan, R. A., Giridharan, V. V., Arumugam., S., Suzuki, K., Ko, K. M., Krishnamurthy, P., et al. (2015). Schisandrin B prevents doxorubicin induced cardiac dysfunction by modulation of DNA damage, oxidative stress and inflammation through inhibition of MAPK/p53 signaling. PLoS One 10 (3), e0119214. doi:10.1371/journal.pone.0119214
Thix, C. A., Königsrainer, I., Kind, R., Wied, P., and Schroeder, T. H. (2009). Ventricular tachycardia during hyperthermic intraperitoneal chemotherapy. Anaesthesia 64 (10), 1134–1136. doi:10.1111/j.1365-2044.2009.05993.x
Thompson, P. A., Lévy, V., Tam, C. S., Al Nawakil, C., Goudot, F. X., Quinquenel, A., et al. (2016). Atrial fibrillation in CLL patients treated with ibrutinib. An international retrospective study. Br. J. Haematol. 175 (3), 462–466. doi:10.1111/bjh.14324
Tilleman, T. R., Richards., W. G., Zellos, L., Johnson, B. E., Jaklitsch, M. T., Mueller., J., et al. (2009). Extrapleural pneumonectomy followed by intracavitary intraoperative hyperthermic cisplatin with pharmacologic cytoprotection for treatment of malignant pleural mesothelioma: a phase II prospective study. J. Thorac. Cardiovasc Surg. 138 (2), 405–411. doi:10.1016/j.jtcvs.2009.02.046
Timm, K. N., and Tyler, D. J. (2020). The role of AMPK activation for cardioprotection in doxorubicin-induced cardiotoxicity. Cardiovasc Drugs Ther. 34 (2), 255–269. doi:10.1007/s10557-020-06941-x
Tocchetti, C. G., Cadeddu, C., Di Lisi, D., Femminò, S., Madonna, R., Mele, D., et al. (2019). From molecular mechanisms to clinical management of antineoplastic drug-induced cardiovascular toxicity: a translational overview. Antioxid. Redox Signal 30 (18), 2110–2153. doi:10.1089/ars.2016.6930
Tomkowski, W. Z., and Filipecki, S. (1997). Intrapericardial cisplatin for the management of patients with large malignant pericardial effusion in the course of the lung cancer. Lung Cancer 16 (2-3), 215–222. doi:10.1016/s0169-5002(96)00631-9
Tomkowski, W. Z., Wiśniewska, J., Szturmowicz, M., Kuca, P., Burakowski, J., Kober, J., et al. (2004). Evaluation of intrapericardial cisplatin administration in cases with recurrent malignant pericardial effusion and cardiac tamponade. Support Care Cancer 12 (1), 53–57. doi:10.1007/s00520-003-0533-x
Udristioiu, A., and Nica-Badea, D. (2019). Autophagy dysfunctions associated with cancer cells and their therapeutic implications. Biomed. Pharmacother. 115, 108892. doi:10.1016/j.biopha.2019.108892
Vejpongsa, P., and Yeh, E. T. (2014). Prevention of anthracycline-induced cardiotoxicity: challenges and opportunities. J. Am. Coll. Cardiol. 64 (9), 938–945. doi:10.1016/j.jacc.2014.06.1167
Wallace, K. B., Sardão, V. A., and Oliveira, P. J. (2020). Mitochondrial determinants of doxorubicin-induced cardiomyopathy. Circ. Res. 126 (7), 926–941. doi:10.1161/circresaha.119.314681
Wallace, N., Wong., E., Cooper, D., and Chao, H. (2016). A case of new-onset cardiomyopathy and ventricular tachycardia in a patient receiving ibrutinib for relapsed mantle cell lymphoma. Clin. Case Rep. 4 (12), 1120–1121. doi:10.1002/ccr3.719
Wang, E. Y., Gang., H., Aviv., Y., Dhingra, R., Margulets, V., and Kirshenbaum, L. A. (2013). p53 mediates autophagy and cell death by a mechanism contingent on Bnip3. Hypertension 62 (1), 70–77. doi:10.1161/hypertensionaha.113.01028
Wang, F., He, Z., Dai, W., Li, Q., Liu, X., Zhang, Z., et al. (2015). The role of the vascular endothelial growth factor/vascular endothelial growth factor receptors axis mediated angiogenesis in curcumin-loaded nanostructured lipid carriers induced human HepG2 cells apoptosis. J. Cancer Res. Ther. 11 (3), 597–605. doi:10.4103/0973-1482.159086
Wang, F., Wang., L., Jiao, Y., and Wang, Z. (2020). Qishen Huanwu capsule reduces pirarubicin-induced cardiotoxicity in rats by activating the PI3K/Akt/mTOR pathway. Ann. Palliat. Med. 9 (5), 3453–3461. doi:10.21037/apm-20-1746
Wang, F., Wang., L., Liu, F., Meng., L., Zhao, N., Zhai, X., et al. (2021). Investigation of the mechanism of the reduction of anthracycline-induced cardiotoxicity by Qishen Huanwu Capsule based on network pharmacology. Ann. Palliat. Med. 10 (1), 16–28. doi:10.21037/apm-20-2204
Wang, J., Zhou, J., Ding, X., Zhu, L., Jiang, K., Fu, M., et al. (2015). Qiliqiangxin improves cardiac function and attenuates cardiac remodeling in rats with experimental myocardial infarction. Int. J. Clin. Exp. Pathol. 8 (6), 6596–6606.
Wang, K., Lv., Q., Miao, Y. M., Qiao, S. M., Dai, Y., and Wei, Z. F. (2018). Cardamonin, a natural flavone, alleviates inflammatory bowel disease by the inhibition of NLRP3 inflammasome activation via an AhR/Nrf2/NQO1 pathway. Biochem. Pharmacol. 155, 494–509. doi:10.1016/j.bcp.2018.07.039
Wang, L., Ma., R., Liu, C., Liu, H., Zhu, R., Guo, S., et al. (2017). Salvia miltiorrhiza: a potential red light to the development of cardiovascular diseases. Curr. Pharm. Des. 23 (7), 1077–1097. doi:10.2174/1381612822666161010105242
Wang, L. F., Su, S. W., Wang., L., Zhang, G. Q., Zhang, R., Niu, Y. J., et al. (2015). Tert-butylhydroquinone ameliorates doxorubicin-induced cardiotoxicity by activating Nrf2 and inducing the expression of its target genes. Am. J. Transl. Res. 7 (10), 1724–1735.
Wang, M. L., Blum, K. A., Martin, P., Goy, A., Auer, R., Kahl, B. S., et al. (2015). Long-term follow-up of MCL patients treated with single-agent ibrutinib: updated safety and efficacy results. Blood 126 (6), 739–745. doi:10.1182/blood-2015-03-635326
Wang, S., Kotamraju, S., Konorev, E., Kalivendi, S., Joseph, J., and Kalyanaraman, B. (2002). Activation of nuclear factor-kappaB during doxorubicin-induced apoptosis in endothelial cells and myocytes is pro-apoptotic: the role of hydrogen peroxide. Biochem. J. 367 (Pt 3), 729–740. doi:10.1042/bj20020752
Wang, S. H., Tsai, K. L., Chou, W. C., Cheng, H. C., Huang, Y. T., Ou, H. C., et al. (2022). Quercetin mitigates cisplatin-induced oxidative damage and apoptosis in cardiomyocytes through Nrf2/HO-1 signaling pathway. Am. J. Chin. Med. 50 (5), 1281–1298. doi:10.1142/s0192415x22500537
Wang, X., Chen, L., Wang., T., Jiang, X., Zhang, H., Li, P., et al. (2015). Ginsenoside Rg3 antagonizes adriamycin-induced cardiotoxicity by improving endothelial dysfunction from oxidative stress via upregulating the Nrf2-ARE pathway through the activation of akt. Phytomedicine 22 (10), 875–884. doi:10.1016/j.phymed.2015.06.010
Wang, X., Wang., X. L., Chen, H. L., Wu., D., Chen, J. X., Wang, X. X., et al. (2014). Ghrelin inhibits doxorubicin cardiotoxicity by inhibiting excessive autophagy through AMPK and p38-MAPK. Biochem. Pharmacol. 88 (3), 334–350. doi:10.1016/j.bcp.2014.01.040
Wang, Y., Zhou, X., Chen, X., Wang., F., Zhu, W., Yan., D., et al. (2020). Efficacy and safety of shengmai injection for chronic heart failure: a systematic review of randomized controlled trials. Evid. Based Complement. Altern. Med. 2020, 9571627. doi:10.1155/2020/9571627
Wang, Z., Wang., M., Liu, J., Ye., J., Jiang, H., Xu, Y., et al. (2018). Inhibition of TRPA1 attenuates doxorubicin-induced acute cardiotoxicity by suppressing oxidative stress, the inflammatory response, and endoplasmic reticulum stress. Oxid. Med. Cell Longev. 2018, 5179468. doi:10.1155/2018/5179468
Wei, X., Liu, L., Guo, X., Wang., Y., Zhao, J., and Zhou, S. (2018). Light-activated ROS-responsive nanoplatform codelivering apatinib and doxorubicin for enhanced chemo-photodynamic therapy of multidrug-resistant tumors. ACS Appl. Mater Interfaces 10 (21), 17672–17684. doi:10.1021/acsami.8b04163
Wenningmann, N., Knapp., M., Ande, A., Vaidya, T. R., and Ait-Oudhia, S. (2019). Insights into doxorubicin-induced cardiotoxicity: molecular mechanisms, preventive strategies, and early monitoring. Mol. Pharmacol. 96 (2), 219–232. doi:10.1124/mol.119.115725
Wu, Y. P., Zhang, S., Xin, Y. F., Gu, L. Q., Xu, X. Z., Zhang, C. D., et al. (2021). Evidences for the mechanism of Shenmai injection antagonizing doxorubicin-induced cardiotoxicity. Phytomedicine 88, 153597. doi:10.1016/j.phymed.2021.153597
Xiao, B., Hong, L., Cai, X., Mei, S., Zhang, P., and Shao, L. (2019). The true colors of autophagy in doxorubicin-induced cardiotoxicity. Oncol. Lett. 18 (3), 2165–2172. doi:10.3892/ol.2019.10576
Xiao, J., Sun., G. B., Sun., B., Wu., Y., He, L., Wang., X., et al. (2012). Kaempferol protects against doxorubicin-induced cardiotoxicity in vivo and in vitro. Toxicology 292 (1), 53–62. doi:10.1016/j.tox.2011.11.018
Xu, Z. M., Li, C. B., Liu, Q. L., Li, P., and Yang, H. (2018). Ginsenoside Rg1 prevents doxorubicin-induced cardiotoxicity through the inhibition of autophagy and endoplasmic reticulum stress in mice. Int. J. Mol. Sci. 19 (11). doi:10.3390/ijms19113658
Yang, X., An., N., Zhong, C., Guan, M., Jiang, Y., Li, X., et al. (2020). Enhanced cardiomyocyte reactive oxygen species signaling promotes ibrutinib-induced atrial fibrillation. Redox Biol. 30, 101432. doi:10.1016/j.redox.2020.101432
Yang, X., Li., G., Yang., T., Guan, M., An., N., Yang, F., et al. (2020). Possible susceptibility genes for intervention against chemotherapy-induced cardiotoxicity. Oxid. Med. Cell Longev. 2020, 4894625. doi:10.1155/2020/4894625
Yang, X., Li, X., Yuan, M., Tian, C., Yang., Y., Wang., X., et al. (2018). Anticancer therapy-induced atrial fibrillation: electrophysiology and related mechanisms. Front. Pharmacol. 9, 1058. doi:10.3389/fphar.2018.01058
Yang, X., Liu, N., Li, X., Yang., Y., Wang., X., Li, L., et al. (2018). A review on the effect of traditional Chinese medicine against anthracycline-induced cardiac toxicity. Front. Pharmacol. 9, 444. doi:10.3389/fphar.2018.00444
Yang, X. L. (2008). Clinical observation on the prevention of doxorubicin-associated cardiotoxicity by Shengmai injection. Guide China Med. 6 (15), 203–205.
Yang, Y., Zhou, Q., Gao, A., Chen, L., and Li, L. (2020). Endoplasmic reticulum stress and focused drug discovery in cardiovascular disease. Clin. Chim. Acta 504, 125–137. doi:10.1016/j.cca.2020.01.031
Yarmohammadi, F., Rezaee, R., Haye, A. W., and Karimi, G. (2021). Endoplasmic reticulum stress in doxorubicin-induced cardiotoxicity may be therapeutically targeted by natural and chemical compounds: a review. Pharmacol. Res. 164, 105383. doi:10.1016/j.phrs.2020.105383
Yarmohammadi, F., Rezaee, R., and Karimi, G. (2021). Natural compounds against doxorubicin-induced cardiotoxicity: a review on the involvement of Nrf2/ARE signaling pathway. Phytother. Res. 35 (3), 1163–1175. doi:10.1002/ptr.6882
Ye, J. Z., Hansen., F. B., Mills, R. W., and Lundby, A. (2021). Oncotherapeutic protein kinase inhibitors associated with pro-arrhythmic liability. JACC CardioOncol 3 (1), 88–97. doi:10.1016/j.jaccao.2021.01.009
Yilmaz, U., Oztop, I., Ciloglu, A., Okan, T., Tekin, U., Yaren, A., et al. (2007). 5-fluorouracil increases the number and complexity of premature complexes in the heart: a prospective study using ambulatory ECG monitoring. Int. J. Clin. Pract. 61 (5), 795–801. doi:10.1111/j.1742-1241.2007.01323.x
Yin, J., Guo, J., Zhang, Q., Cui, L., Zhang, L., Zhang, T., et al. (2018). Doxorubicin-induced mitophagy and mitochondrial damage is associated with dysregulation of the PINK1/parkin pathway. Toxicol Vitro 51, 1–10. doi:10.1016/j.tiv.2018.05.001
Yin, Y., Guan, Y., Duan, J., Wei., G., Zhu, Y., Quan, W., et al. (2013). Cardioprotective effect of Danshensu against myocardial ischemia/reperfusion injury and inhibits apoptosis of H9c2 cardiomyocytes via Akt and ERK1/2 phosphorylation. Eur. J. Pharmacol. 699 (1-3), 219–226. doi:10.1016/j.ejphar.2012.11.005
Yu, Q., Zhao, G., Liu, J., Peng, Y., Xu, X., Zhao, F., et al. (2023). The role of histone deacetylases in cardiac energy metabolism in heart diseases. Metabolism 142, 155532. doi:10.1016/j.metabol.2023.155532
Yu, Y., Sun., J., Wang., R., Liu, J., Wang, P., and Wang, C. (2019). Curcumin management of myocardial fibrosis and its mechanisms of action: a review. Am. J. Chin. Med. 47 (8), 1675–1710. doi:10.1142/s0192415x19500861
Yun, S., Vincelette, N. D., Acharya, U., and Abraham, I. (2017). Risk of atrial fibrillation and bleeding diathesis associated with ibrutinib treatment: a systematic review and pooled analysis of four randomized controlled trials. Clin. Lymphoma Myeloma Leuk. 17 (1), 31–37.e13. doi:10.1016/j.clml.2016.09.010
Zakaria, N., Khalil, S. R., Awad, A., and Khairy, G. M. (2018). Quercetin reverses altered energy metabolism in the heart of rats receiving adriamycin chemotherapy. Cardiovasc Toxicol. 18 (2), 109–119. doi:10.1007/s12012-017-9420-4
Zamorano, J. L., Lancellotti, P., Rodriguez Muñoz, D., Aboyans, V., Asteggiano, R., Galderisi, M., et al. (2016). 2016 ESC Position Paper on cancer treatments and cardiovascular toxicity developed under the auspices of the ESC Committee for Practice Guidelines: the Task Force for cancer treatments and cardiovascular toxicity of the European Society of Cardiology (ESC). Eur. Heart J. 37 (36), 2768–2801. doi:10.1093/eurheartj/ehw211
Zellos, L., Richards, W. G., Capalbo, L., Jaklitsch, M. T., Chirieac, L. R., Johnson, B. E., et al. (2009). A phase I study of extrapleural pneumonectomy and intracavitary intraoperative hyperthermic cisplatin with amifostine cytoprotection for malignant pleural mesothelioma. J. Thorac. Cardiovasc Surg. 137 (2), 453–458. doi:10.1016/j.jtcvs.2008.07.055
Zhang, F., Liu., L., Xie, Y., Wang., J., Chen, X., Zheng, S., et al. (2022). Cardiac contractility modulation ameliorates myocardial metabolic remodeling in a rabbit model of chronic heart failure through activation of AMPK and PPAR-α pathway. Open Med. (Wars) 17 (1), 365–374. doi:10.1515/med-2022-0415
Zhang, H., Weng., J., Sun., S., Zhou, J., Yang, Q., Huang, X., et al. (2022). Ononin alleviates endoplasmic reticulum stress in doxorubicin-induced cardiotoxicity by activating SIRT3. Toxicol. Appl. Pharmacol. 452, 116179. doi:10.1016/j.taap.2022.116179
Zhang, H. W., and Zhang, H. (2007). Observation on the efficacy of Shengmai injection in preventing acute cardiotoxicity of adriamycin in 40 cases. J. Emerg. Traditional Chin. Med. 16 (9), 1082–1110.
Zhang, J., Xiang, Q., Wu., M., Lao., Y.-Z., Xian, Y.-F., Xu, H.-X., et al. (2023). Autophagy regulators in cancer. Int. J. Mol. Sci. 24 (13). doi:10.3390/ijms241310944
Zhang, J., Zhang, Q., Liu, G., and Zhang, N. (2019). Therapeutic potentials and mechanisms of the Chinese traditional medicine Danshensu. Eur. J. Pharmacol. 864, 172710. doi:10.1016/j.ejphar.2019.172710
Zhang, L., Feng, M., Wang., X., Zhang, H., Ding, J., Cheng, Z., et al. (2021). Peptide Szeto-Schiller 31 ameliorates doxorubicin-induced cardiotoxicity by inhibiting the activation of the p38 MAPK signaling pathway. Int. J. Mol. Med. 47 (4). doi:10.3892/ijmm.2021.4896
Zhang, P., Zhang, J., Zhao, L., Li, S., and Li, K. (2022). Quercetin attenuates the cardiotoxicity of doxorubicin-cyclophosphamide regimen and potentiates its chemotherapeutic effect against triple-negative breast cancer. Phytother. Res. 36 (1), 551–561. doi:10.1002/ptr.7342
Zhang, Q., and Wu, L. (2022). In vitro and in vivo cardioprotective effects of curcumin against doxorubicin-induced cardiotoxicity: a systematic review. J. Oncol. 2022, 7277562. doi:10.1155/2022/7277562
Zhang, X., Lv., S., Zhang, W., Jia, Q., Wang., L., Ding, Y., et al. (2021). Shenmai injection improves doxorubicin cardiotoxicity via miR-30a/Beclin 1. Biomed. Pharmacother. 139, 111582. doi:10.1016/j.biopha.2021.111582
Zhang, X., Zhu, Y., Dong, S., Zhang, A., Lu, Y., Li, Y., et al. (2019). Role of oxidative stress in cardiotoxicity of antineoplastic drugs. Life Sci. 232. doi:10.1016/j.lfs.2019.06.001
Zhang, Y., Wang., Y., Ma., Z., Liang, Q., Tang, X., Tan, H., et al. (2017). Ginsenoside Rb1 inhibits doxorubicin-triggered H9C2 cell apoptosis via aryl hydrocarbon receptor. Biomol. Ther. Seoul. 25 (2), 202–212. doi:10.4062/biomolther.2016.066
Zhao, X., Tian, Z., Sun, M., and Dong, D. (2023). Nrf2: a dark horse in doxorubicin-induced cardiotoxicity. Cell Death Discov. 9 (1), 261. doi:10.1038/s41420-023-01565-0
Zhao, Y. Y., Chen, L. H., Huang, L., Li, Y. Z., Yang., C., Zhu, Y., et al. (2022). Cardiovascular protective effects of GLP-1: a focus on the MAPK signaling pathway. Biochem. Cell Biol. 100 (1), 9–16. doi:10.1139/bcb-2021-0365
Zhou, Y., Suo, W., Zhang, X., Lv., J., Liu, Z., and Liu, R. (2022). Roles and mechanisms of quercetin on cardiac arrhythmia: a review. Biomed. Pharmacother. 153, 113447. doi:10.1016/j.biopha.2022.113447
Zhu, C., Wang., Y., Liu, H., Mu., H., Lu, Y., Zhang, J., et al. (2017). Oral administration of Ginsenoside Rg1 prevents cardiac toxicity induced by doxorubicin in mice through anti-apoptosis. Oncotarget 8 (48), 83792–83801. doi:10.18632/oncotarget.19698
Keywords: chemotherapeutic drugs, traditional Chinese medicine, arrhythmia, adverse effects, review
Citation: Li W, Cheng X, Zhu G, Hu Y, Wang Y, Niu Y, Li H, Aierken A, Li J, Feng L and Liu G (2024) A review of chemotherapeutic drugs-induced arrhythmia and potential intervention with traditional Chinese medicines. Front. Pharmacol. 15:1340855. doi: 10.3389/fphar.2024.1340855
Received: 19 November 2023; Accepted: 05 March 2024;
Published: 20 March 2024.
Edited by:
Ruiwen Zhang, University of Houston, United StatesReviewed by:
Liang Guo, Bristol Myers Squibb, United StatesOlufunke Olorundare, University of Ilorin, Nigeria
Copyright © 2024 Li, Cheng, Zhu, Hu, Wang, Niu, Li, Aierken, Li, Feng and Liu. This is an open-access article distributed under the terms of the Creative Commons Attribution License (CC BY). The use, distribution or reproduction in other forums is permitted, provided the original author(s) and the copyright owner(s) are credited and that the original publication in this journal is cited, in accordance with accepted academic practice. No use, distribution or reproduction is permitted which does not comply with these terms.
*Correspondence: Jie Li, qfm2020jieli@yeah.net; Ling Feng, flgamyy@163.com; Guifang Liu, lgf31@126.com
†These authors have contributed equally to this work and share first authorship