- 1The First Clinical Medical College, Gansu University of Chinese Medicine, Lanzhou, China
- 2Department of Anesthesiology, Xianning Central Hospital, The First Affiliated Hospital of Hubei University of Science and Technology, Xianning, Hubei, China
- 3Department of Anesthesiology, Gansu Provincial Hospital, Lanzhou, Gansu, China
(R, S)- and (S)-ketamine have made significant progress in the treatment of treatment-resistant depression (TRD) and have become a research focus in recent years. However, they both have risks of psychomimetic effects, dissociative effects, and abuse liability, which limit their clinical use. Recent preclinical and clinical studies have shown that (R)-ketamine has a more efficient and lasting antidepressant effect with fewer side effects compared to (R, S)- and (S)-ketamine. However, a recent small-sample randomized controlled trial found that although (R)-ketamine has a lower incidence of adverse reactions in adult TRD treatment, its antidepressant efficacy is not superior to the placebo group, indicating its antidepressant advantage still needs further verification and clarification. Moreover, an increasing body of research suggests that (R)-ketamine might also have significant applications in the prevention and treatment of medical fields or diseases such as cognitive disorders, perioperative anesthesia, ischemic stroke, Parkinson’s disease, multiple sclerosis, osteoporosis, substance use disorders, inflammatory diseases, COVID-19, and organophosphate poisoning. This article briefly reviews the mechanism of action and research on antidepressants related to (R)-ketamine, fully revealing its application potential and development prospects, and providing some references and assistance for subsequent expanded research.
1 Introduction
In 1964, the short-acting anesthetic, analgesic, sympathomimetic, and dissociative effects of (R, S)-ketamine (ketamine) were first discovered in human trials (Domino et al., 1965). This was followed by immeasurable contributions in numerous clinical practices and scientific studies (Conahan, 1975; Domino, 2010; Li and Vlisides, 2016; Tyler et al., 2017). With its combined analgesic and sedative effects, it had been widely used in surgical anaesthesia and adjuvant analgesic therapy (Gao et al., 2016; Mion, 2017; Barrett et al., 2020), and especially exerted unique advantages in the relief of various acute and chronic pains (Schwenk et al., 2018; Barrett et al., 2020; Yang et al., 2020). However, due to its psychedelic and psychomimetic effects, induction of postoperative nightmares, and abuse liability, it experienced a period of decline in clinical use (Mion, 2017; Wei et al., 2020). In 2000, Berman et al. (Berman et al., 2000) first showed in seven patients with major depressive disorder (MDD) that (R, S)-ketamine had rapid and sustainable antidepressant effects. This discovery was considered a major breakthrough in over 50 years of depression research and reignited the interest of the medical community in (R, S)-ketamine and its two enantiomers [(S)-ketamine (esketamine) and (R)-ketamine (arketamine)]. In 2019, the (S)-ketamine nasal spray (Spravato), one of the enantiomers of (R, S)-ketamine, was licensed in the US and Europe to treat treatment-resistant depression (TRD). However, due to the need for further validation of its efficacy, safety, and abuse risk, its widespread use is limited, primarily distributed by a controlled system with clear risk assessment and mitigation strategies (Turner, 2019; Jelen et al., 2021).
Given the issues of (R, S)- and (S)-ketamine in the therapy of depression, researchers began to focus on the other enantiomer of ketamine, (R)-ketamine. They found that in contrast to (R, S)- and (S)-ketamine, (R)-ketamine not only did not induce psychomimetic-like symptoms but also produced a state of relaxation and a feeling of wellbeing in healthy subjects (Vollenweider et al., 1997), while at the same time having potent, long-lasting antidepressant effects with fewer side effects (Hashimoto, 2020; Wei et al., 2021b; Scotton et al., 2022). Chang et al. (Chang et al., 2019) showed in their comparative study that in the mouse chronic social defeat stress (CSDS) model, the antidepressant potency of ketamine and its two enantiomers was in the order of (R)-ketamine > (R, S)-ketamine > (S)-ketamine. What’s more, in other animal models of depression, researchers similarly confirmed that the antidepressant effect of (R)-ketamine was observed to be more effective and prolonged than that of (R, S)-ketamine and its other metabolites, such as (S)-ketamine and (2R,6R)-hydroxynorketamine (HNK) (Zhang et al., 2014; Yang et al., 2015; Fukumoto et al., 2017; Shirayama and Hashimoto, 2018; Yang et al., 2018; Johnston et al., 2023). However, a recent small-scale clinical trial indicated that the effects of (R)-ketamine on TRD patients were not superior to those of the placebo group (Leal et al., 2023). This contrasting result has drawn widespread attention and interest in the industry, and also raised questions about the actual antidepressant efficacy of (R)-ketamine.
Moreover (Wang et al., 2022b), previously reviewed the potential preventive and therapeutic effects of (R)-ketamine in neurological conditions like Alzheimer’s disease (AD), other dementias, Parkinson’s disease (PD), multiple sclerosis (MS), and ischemic stroke. However, we have found through further reading of the literature on (R)-ketamine that the extended research on (R)-ketamine is not limited to depression and neurological diseases. It also shows potential applications in perioperative anesthesia, osteoporosis, substance use disorders, inflammatory diseases, COVID-19, and organophosphate poisoning, and most of the mechanisms of action are inextricably linked to their antidepressant mechanisms. In this review, we briefly overview the current status and mechanisms of action of (R)-ketamine in antidepressant research. We also specifically review preclinical and clinical studies comparing the efficacy of (R)-ketamine with other antidepressants, metabolites, and placebos. This aims to resolve controversies over the antidepressant efficacy of (R)-ketamine and to guide further research in TRD and MDD patients. Additionally, we have compiled the latest research on (R)-ketamine in non-depressive treatments to guide its other potential applications and future research directions.
2 Research on the antidepressant effects of (R)-ketamine
2.1 Preclinical studies
Although the affinity of (R)-ketamine for the N-methyl-D-aspartate receptor (NMDAR) (inhibition constant Ki = 1.40 μmol/L) is 4-fold lower than that of (S)-ketamine (Ki = 0.30 μmol/L), in rodents, (R)-ketamine exhibits a stronger and more long-lasting antidepressant effect than (S)-ketamine, with fewer psychomotor side effects and a lower risk of abuse (Zhang et al., 2014; Yang et al., 2017a; Fukumoto et al., 2017; Chang et al., 2019). Additionally, (R)-ketamine shows a stronger and longer-lasting antidepressant effect than (R, S)-ketamine and the NMDAR antagonist Lanicemine (Ebert et al., 1997; Yang et al., 2015; Zanos et al., 2016; Yang et al., 2018). Moreover, this effect does not lead to a significant rise in the medial prefrontal cortex’s (mPFC) dopamine (DA) release (Ago et al., 2019; Zhou et al., 2021) reported that the bilateral lateral habenular nucleus (LHb) administration of 25 μg/μL (R)-ketamine and (2R, 6R)-HNK in rats (1 µL/side) did not significantly produce an antidepressant-like effect at 1 or 24 h. Thus, we can speculate that (R)-ketamine’s antidepressant effects might not be related to mechanisms such as NMDAR blockade, LHb neuronal firing, or DA receptor activation.
Further research indicated that α-amino-3-hydroxy-5-methyl-4-isoxazolepropionic acid receptor (AMPAR, one of the ionotropic glutamate receptors) antagonist NBQX, the transforming growth factor-β1 (TGF-β1) inhibitors RepSox and SB431542, the colony-stimulating factor 1 receptor (CSF1R) inhibitor PLX3397, and the γ-aminobutyric acid type A receptor (GABAAR) agonist muscimol can all inhibit the antidepressant effects of (R)-ketamine in animal models of depression. This suggests that the activation of TGF-β1 signaling pathway, CSF1R, AMPAR, and the inhibition of GABAAR are crucial for the rapid and long-lasting antidepressant effects of (R)-ketamine (Yang et al., 2015; Yang et al., 2018; Zhang et al., 2020; Rafało-Ulińska et al., 2022; Tang et al., 2023). Additionally, when compared to (S)-ketamine, the sustained antidepressant effects of (R)-ketamine may be primarily mediated through the nuclear receptor binding protein 1 (NRBP1), which is found in the microglial cells of the mPFC in adult mice. This is because (R)-ketamine was reported to activate ERK in primary microglial cells, thereby increasing the expression of NRBP1, brain-derived neurotrophic factor (BDNF), and the phosphorylated cAMP response element binding protein (p-CREB)/CREB, leading to its long-lasting antidepressant effects (Yao et al., 2022).
On the other hand, (R)-ketamine, through the BDNF-tropomyosin receptor kinase B (TrkB) signaling pathway, helps to restore the decreased BDNF levels in the prefrontal cortex (PFC), hippocampal CA3, and dentate gyrus (DG) regions of rodents (Yang et al., 2015; Yang et al., 2018; Fujita et al., 2020; Tan et al., 2020; Fujita et al., 2021; Lin et al., 2021; Qu et al., 2021). Simultaneously, (R)-ketamine can elevate the release of 5-hydroxytryptamine (5-HT) in the mPFC (Ago et al., 2019), and significantly inhibit the overexpression of the nuclear factor of activated T-cells 4 (NFATc4) signaling gene in the PFC (Ma et al., 2022c). These findings indicate that activating the BDNF-TrkB, NFATc4 signaling pathways, and endogenous 5-HT receptors might be key mechanisms for the antidepressant effects of (R)-ketamine.
In addition, activation of mammalian target of rapamycin (mTOR) and extracellular signal-regulated kinase (ERK) has been suggested as a hypothetical molecular mechanism for the antidepressant effects of ketamine (Tang et al., 2015; Zanos et al., 2018; Johnston et al., 2020). However, in CSDS model, (R)-ketamine significantly reversed the reduction of ERK and upstream effector mitogen-activated protein kinase/ERK kinase phosphorylation in the PFC and hippocampal DG of susceptible mice after CSDS, but not mTOR and its downstream effector ribosomal protein S6 kinase phosphorylation in the PFC. Meanwhile, mTOR inhibitors (rapamycin or AZD8055) also failed to block the antidepressant effects of (R)-ketamine (Yang et al., 2018).
However, in a chronic unpredictable mild stress (CUMS) model, (R)-ketamine was found to increase protein expression levels of phosphorylated mTOR (pmTOR) in the mouse PFC, reversing the CUMS-induced decrease in the pmTOR/mTOR ratio, but had no effect on ERK phosphorylation levels (Rafało-Ulińska and Pałucha-Poniewiera, 2022). These two diametrically opposed results may stem from different models of depression, study methods, dosing regimens, and timing of tissue collection, all of which may have a differential impact on the test results, and more in-depth studies will be needed in the future to arrive at more definitive answers.
Considering that the pathophysiology of MDD involves microRNAs (miRNAs) as a critical regulatory component of synaptic plasticity, this suggests that directly targeting miRNAs might be a potential therapeutic strategy for MDD (Zhou L. et al., 2021; Ma et al., 2022b) found that chronic restraint stress (CRS)-exposed mice could have their body weight loss, forced swimming test immobility duration, and sucrose preference greatly improved by giving (R)-ketamine (10 mg/kg) as a pretreatment 1 day prior to CRS. It also markedly attenuated the expression of miR-132-5p and its associated regulatory genes [BDNF, Methyl CpG binding protein 2 (MeCP2), Tgfb1, TGF-β receptor II (Tgfbr2)] in the PFC of mice given CRS. Additionally, the onset and progression of depression are closely associated with the upregulation of endoplasmic reticulum stress-related genes (Nevell et al., 2014). Therefore (Jóźwiak-Bębenista et al., 2022), compared the effects of two enantiomers of ketamine on the expression of endoplasmic reticulum stress-responsive genes in a human astrocyte cell line. The study found that (R)-ketamine has a relatively mild effect on the expression of genes in the unfolded protein response (UPR) pathway (Jóźwiak-Bębenista et al., 2022). Furthermore, it can also increase the expression of CREB3L1 in old astrocyte specifically induced substance (OASIS) encoded in astrocytes and CREB3/LUMAN mRNA in astrocytes, suggesting that the antidepressant effects of (R)-ketamine may be related to members of the OASIS family (Jóźwiak-Bębenista et al., 2022).
In addition, (R)-ketamine has anti-inflammatory effects (Zhang et al., 2021a; Zhang et al., 2021b), and significantly ameliorates increased spleen weight in CSDS-susceptible mice (Zhang et al., 2020; Wei et al., 2021a), resulting in amelioration of increased expression of the natural killer cell-activated receptor (NKG2D) in the spleen (Zhang et al., 2021). It can also partially repairs alterations in the gut microbiota that may be related to the onset and progression of certain diseases (Yang et al., 2017b; Qu et al., 2017; Wang et al., 2021; Wang et al., 2022a). This implies that the antidepressant mechanism of (R)-ketamine might be related to the brain-spleen axis and the microbiota-gut-brain axis (Getachew et al., 2018; Huang et al., 2019; Wilkowska et al., 2021; Wei et al., 2022).
Although the indirect metabolite of (R)-ketamine, (2R, 6R)-HNK, has shown rapid and/or long-lasting antidepressant effects in various animal models of depression (Zanos et al., 2016; Chou et al., 2018; Pham et al., 2018; Zanos et al., 2019a; Zanos et al., 2019b; Fukumoto et al., 2019; Highland et al., 2019). Interestingly, some researchers did not observe the sustained antidepressant effects of (2R, 6R)-HNK similar to (R)-ketamine (Yang et al., 2017a; Zhang et al., 2018a; Zhang et al., 2018c; Shirayama and Hashimoto, 2018; Yamaguchi et al., 2018; Xiong et al., 2019). Instead, they believe that (R)-ketamine produces its own antidepressant effects rather than being a result of (2R, 6R)-HNK (Shirayama and Hashimoto, 2017; Yamaguchi et al., 2018). A study pointed out that (2R, 6R)-HNK is an inert molecule that differs from ketamine pharmacologically and does not bind to specific high-affinity sites in the brain (Bonaventura et al., 2022). Thus, it might produce antidepressant effects indirectly by interacting with physical or chemical processes or by weakly interacting with many different molecular targets in various biological systems (Bonaventura et al., 2022). Therefore, the precise antidepressant effects, mechanisms, and specific functions of (2R, 6R)-HNK in the antidepressant effects of (R)-ketamine still require further research for confirmation.
Although researchers have studied the antidepressant effects of (R)-ketamine from multiple perspectives, its specific mechanisms of action and exact target sites remain unclear. Clearly, these preclinical research findings provide guidance for subsequent studies and also offer theoretical support for clinical treatments and new drug development. (Figure 1 briefly illustrates some of the antidepressant mechanisms of (R)-ketamine).
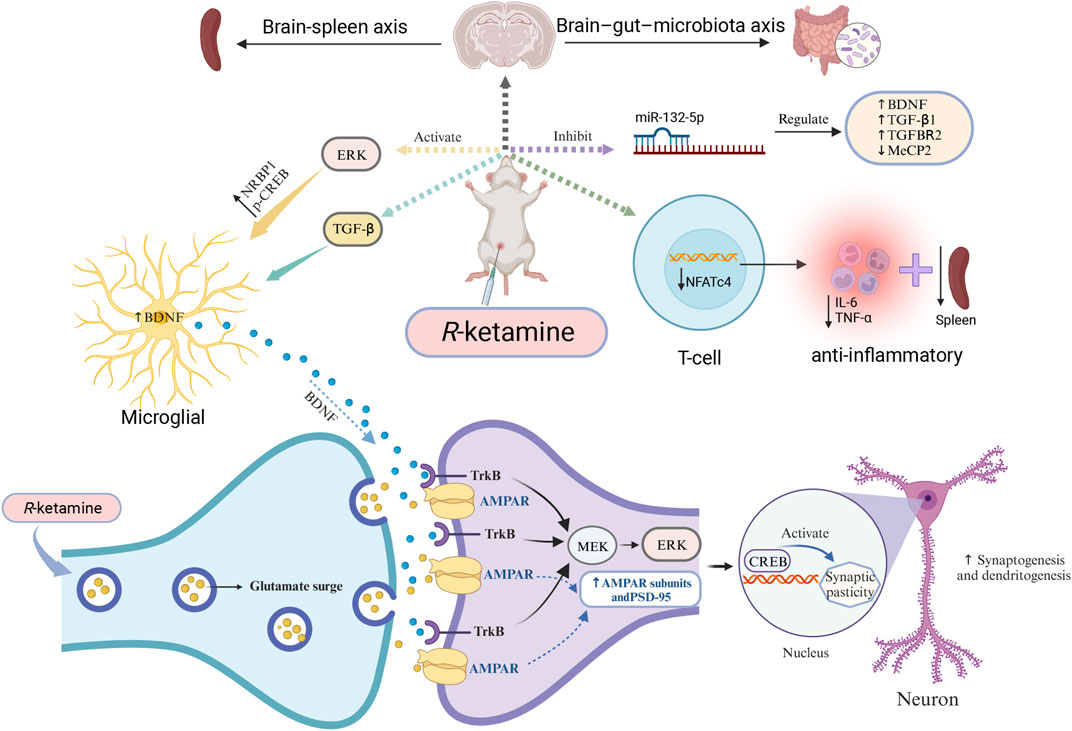
Figure 1. Mechanisms of (R)-ketamine antidepressant (partial) (R)-ketamine can activate ERK, thus increasing the expression of NRBP1, BDNF, and p-CREB in primary microglial cells. At the same time, it promotes the release of glutamate into the synaptic cleft, enhances AMPAR flux, activates cellular signaling, and increases the synaptic protein translation of AMPAR subunits and PSD-95, thereby promoting the formation of synapses and dendrites. Additionally, (R)-ketamine can regulate TGF-β signaling in microglial cells, increase the release of BDNF, and subsequently promote binding with the TrkB receptor. Through the MEK-ERK-CREB signaling pathway, it can enhance synaptogenesis and dendritogenesis, thus exhibiting its antidepressant effects. Furthermore, the antidepressant mechanism of (R)-ketamine may also involve the microbiome-gut-brain axis, the brain-spleen axis pathway, and the regulation of miR-132-5p and NFTc4. Abbreviations: AMPAR: α-amino-3-hydroxy-5-methyl-4-isoxazolepropionic acid receptor; BDNF: brain-derived neurotrophic factor; CREB: cyclic adenosine monophosphate response element-binding protein; ERK: extracellular signal-related kinase; MEK: mitogen-activated protein kinase; MeCP2: Methyl CpG binding protein 2. NFATc4: nuclear factor of activated T cells 4; PSD-95: postsynaptic density protein 95; TGF-β: transforming growth factor β; TrkB: tropomyosin receptor kinase B.
2.2 Clinical research
Currently, all drug formulations of (R)-ketamine have not been approved for the market, but researchers continue to explore its antidepressant efficacy and side effects in clinical settings. In 2020, (Leal et al., 2021), initially documented a 40-min single intravenous infusion of (R)-ketamine (0.5 mg/kg) in 7 TRD subjects. By comparing the Montgomery-Åsberg Depression Rating Scale (MADRS) scores before and after the infusion, they observed that (R)-ketamine produced a rapid and significant antidepressant effect in TRD participants. This effect began to appear 60 min after the infusion, and peaked at 240 min, and 43% of the participants still showed antidepressant effects on the 7th day (Leal et al., 2021). Regarding side effects, only a portion of the participants briefly experienced mild blurred vision and dizziness, with no occurrences of dissociation or hemodynamic changes, indicating good safety of (R)-ketamine (Leal et al., 2021). Although this study yielded surprising antidepressant efficacy at the time, certain limitations in terms of the level of evidence for open-label design need to be noted. Therefore, the team redesigned a randomized, double-blind, crossover, pilot trial with a total sample size of 10 cases at 1-week intervals and treated all TRD patients with 0.5 mg/kg (R)-ketamine and saline intravenously (Leal et al., 2023). The results showed an improvement in depressive symptoms over time (2 weeks of observational analysis) and a lower incidence of adverse events in all TRD patients, but there was no significant difference between the (R)-ketamine group and the saline control group (Leal et al., 2023). This unexpected finding has once again raised questions about the actual antidepressant efficacy of (R)-ketamine. Compared to a previous open-label trial (Leal et al., 2021), subjects in this study had a longer duration of depression and more psychiatric co-morbidities such as obsessive-compulsive disorder, social anxiety disorder and generalized anxiety disorder (Leal et al., 2023). Based on the experience of previous ketamine antidepressant studies, some patients need to receive two or more doses of the medication in order to produce a response (Phillips et al., 2019; Phillips et al., 2020). Thus, a single (R)-ketamine administration may not achieve a level of depression treatment that would normally require a cumulative effect of the drug. In addition, a crossover design trial may not be optimal given the current uncertainty about the ideal therapeutic dose and frequency of administration of (R)-ketamine. Considering that the assessment of depression efficacy is largely dependent on MADRS scores, it is difficult to achieve significant between-group differences with a small sample size of 10 patients. Thus, although this small pilot study by LEAL et al. (Leal et al., 2023) failed to demonstrate that a single intravenous infusion of (R)-ketamine was superior to placebo in improving depression, the possibility that (R)-ketamine has antidepressant effects in humans cannot be completely discounted (R)-ketamine may provide positive clinical benefits, at least in terms of medication safety and adverse effects. Recent studies on (R)-ketamine in the treatment of bi-directional depression have again demonstrated its favorable antidepressant effects (Bandeira et al., 2023). In the study, six subjects with type I and type II bipolar disorders were treated with (R)-ketamine intravenously on two separate occasions (1 week apart) at doses of 0.5 mg/kg and 1 mg/kg, respectively). Before and after treatment, the subjects’ mean total MADRS scores were reduced by more than 50% and little dissociative and manic symptoms were observed at both doses, demonstrating the feasibility and safety of (R)-ketamine for its rapid antidepressant effect in the treatment of bipolar depression (Bandeira et al., 2023). This further emphasizes that (R)-ketamine, as a novel antidepressant with good development potential, may in the future require larger sample sizes, more flexibility in dosage and frequency of administration, as well as more study design options (such as parallel subgroup design, etc.) to gain insights into the actual antidepressant efficacy of (R)-ketamine in clinical practice.
In 2018, China registered and approved a large randomized controlled trial that will compare the safety and effectiveness of (R)-ketamine with (S)-ketamine and (R, S)-ketamine in TRD treatment (ChiCTR1800015879) (Chinese Clinical Trial Registry, 2019). The following year, China’s Hengrui Medicine Co., Ltd. received approval from the National Medical Products Administration and began clinical trials to treat refractory depression with hydrochloride (R)-ketamine nasal spray (Netease, 2019). On 19 February 2021, the American company, Perception Neuroscience, released its Phase I clinical research data on (R)-ketamine, showing that the dose of (R)-ketamine (PCN-101) required to produce similar perceptual changes is much higher than that of (S)-ketamine, and the total dose below 150 mg is safe and well-tolerated (Cision PR Newswire, 2021). At the same time, the company subsequently initiated a Phase II confirmatory trial for TDR patients, which will be a key study to evaluate the therapeutic effects and related side effects of (R)-ketamine (Cision PR Newswire, 2021).
Moreover, regarding the controversy in preclinical studies about the antidepressant efficacy of (R)-ketamine’s direct metabolite (2R, 6R)-HNK, Grunebaum et al. (Grunebaum et al., 2019) found that 24 h after administering ketamine (0.5 mg/kg) intravenously to MDD patients with significant suicidal ideation, the patients had higher plasma concentrations of (2R, 6R)-HNK, but this was not significantly related to clinical improvement in depression. The result suggests that we should be more cautious when evaluating the antidepressant effects of (2R, 6R)-HNK (Grunebaum et al., 2019). Currently, a Phase I clinical trial for (2R, 6R)-HNK is being conducted, which will provide an objective assessment of the actual antidepressant effects of (2R, 6R)-HNK (ClinicalTrials, 2021).
Taken together, although (R)-ketamine has shown its distinct advantages in various animal models of depression, some clinical studies still have doubts about its actual antidepressant effects. To determine the specific antidepressant effects of (R)-ketamine and its metabolites, and to assess the safety, drug resistance, side effects, and abuse risks of medium-to long-term, high-dose use, we still need to rely on large-sample, multi-center, blind clinical randomized controlled trials, and compare various regimens in terms of dosing, frequency, and timing.
3 Research on non-depressive conditions
3.1 Cognitive impairments
There is growing evidence that cognitive deficits, especially mild cognitive deficits, are significantly present in childhood and adolescence prior to psychotic episodes (Mollon and Reichenberg, 2018). Cognitive deficits in the offspring of maternal immune activation (MIA) model mice in adulthood can be prevented by repeated intermittent use of (R)-ketamine (10 mg/kg/day, twice a week for 4 weeks) during adolescent and juvenile stages (P28-P56) and may reduce the risk of conversion to psychosis in adulthood through activation of the BDNF-TrkB signaling pathway in the brain (Tan et al., 2022). However, given the multiple concerns regarding the use of ketamine and its metabolites in adolescents, especially in infants and young children, translating the results of this study into clinical practice may take longer. In addition, long-term social isolation has been shown to potentially lead to social cognitive deficits (Beutel et al., 2017), and maintaining an individual’s social cognitive functioning typically involves two brain regions, the insula and prefrontal cortex (Menon and Uddin, 2010; Bicks et al., 2015). The unique activation of the anterior insula cortex (aIC) induced by (R)-ketamine (20 mg/kg, i.p.) restores the aIC function, which promotes the formation of social memories and ameliorates social cognitive impairment deficits in socially isolated reared mice (Yokoyama et al., 2024).
On the other hand, ketamine and its enantiomers have been shown to produce different effects on memory and cognitive function in individuals at different doses (Gill et al., 2021; Zhornitsky et al., 2022). It was shown that at a higher subanesthetic dose (20 mg/kg), both ketamine and its two enantiomers significantly impaired recognition memory in mice in the novel object recognition (NOR) test. Whereas at a lower dose (10 mg/kg), (S)-ketamine still produced significant impairment of recognition memory, but not (R)-ketamine (Ide et al., 2019). Furthermore, both ketamine and (S)-ketamine at doses of 10–20 mg/kg induced cognitive deficits in NMDA receptor subunit GluN2D knockout (GluN2D-KO) mice, but the same dose of (R)-ketamine did not inhibit cognitive functioning in GluN2D-KO mice (Ide et al., 2019), suggesting that, in terms of decreasing the impact on individual cognitive effects on memory, (R)-ketamine appears to have an advantage over ketamine and (S)-ketamine. In the future, there is still a need to further substantiate and explore the quantitative effects of various ketamine compounds on cognitive effects in human clinical studies.
The NMDAR antagonist phencyclidine (PCP) can cause schizophrenia-like symptoms, including cognitive impairments, in both healthy individuals and rodents. However, these cognitive impairments can be alleviated by intermittent use of (R)-ketamine twice a week for 2 weeks at a dose of 10 mg/kg/day rather than (S)-ketamine (Tan et al., 2020). Further, using (R)-ketamine in prevention and treatment can alleviate systemic and neuroinflammation in mice induced by lipopolysaccharide (LPS) and has preventive and therapeutic effects on delirium and cognitive impairments (Zhang et al., 2021b). Additionally, metabotropic glutamate receptors 2 and 3 (mGluR2/3) are considered potential drug targets for treating various neurological diseases (Li et al., 2022). Its selective inhibitors have demonstrated significant antidepressant effects with reduced side effects in research in preclinical and clinical settings (Jiang et al., 2023). Recent research demonstrated that when the mGlu2/3 receptor antagonist LY341495 was combined with (R)-ketamine, it not only reduced the side effects of (R)-ketamine, but was also effective against depression symptoms induced by CUMS. Combined treatment reversed CUMS-induced PFC-dependent memory deficits and restored the diminished strength of longterm potentiation (LTP), exerting characteristic antidepressant and cognitive-enhancing effects (Pałucha-Poniewiera et al., 2023). In addition, (R)-ketamine (7.5, 15, and 30 mg/kg, i.p.) dose-dependently increased electroencephalogram (EEG) theta power at 23 h of wakefulness and rapid eye movement (REM) sleep, further supporting its potential use in cognitive impairment (Pothorszki et al., 2024).
In addition, among healthy volunteers, (R)-ketamine has milder impacts on psychopathology and neurocognition to (S)-ketamine, which might produce a “negative experience”. Its potential “protective effect” can partially offset the adverse effects of (S)-ketamine (Passie et al., 2021). Patients who suffer from depression often encounter intense psychological distress and may have a distorted perception that “time is slowing or dilating”, and this perception is positively correlated with the intensity of suicidal ideation (Cáceda et al., 2020). However, unlike (S)-ketamine, the antidepressant effects of (R)-ketamine are not based on the underestimation of time or behavioral disorders, but might help enhance cognitive abilities (Popik et al., 2022). Considering that geriatric depression is closely related to all-cause dementia, AD, and vascular dementia (Zhao et al., 2022), and that dementia patients often exhibit anxiety, depression, and other neuropsychiatric symptoms (NPS) significantly associated with their overall cognitive abilities (Sabates et al., 2023), it is hypothesized that (R)-ketamine may have a useful preventive or delaying effect on the progression of late-life depression to dementia in patients. Furthermore, it may also have beneficial effects on the emotional and cognitive aspects of various types of dementia patients.
Therefore, synthesizing the above studies we can easily find that (R)-ketamine has a weak inhibitory effect on cognition, may improve cognitive dysfunction caused by other causes (such as psychiatric disorders) to a certain extent (Hashimoto, 2023), and may play a positive role in preventing or delaying the disease progression of AD, and it is expected to be a potential medication for preventing and treating cognitive deficits in patients in the future.
3.2 Perioperative anesthesia
Perioperative anaesthesia is one of the most prominent areas of clinical application of (R, S)-ketamine and (S)-ketamine in addition to the treatment of depression (Barrett et al., 2020). However, the use of (R)-ketamine in this area deserves deeper reflection, exploration and research. Indeed, (R)-ketamine was used in clinical trials as early as the 1980s, however, unfortunately, researchers at that time did not observe that (R)-ketamine exhibited stronger effects than (R, S)- and (S)-ketamine in hypnosis and analgesia (White et al., 1980; White et al., 1985). More recent studies, by administering an intravenous infusion of ketamine to healthy subjects and subsequently examining plasma metabolite concentrations at various time points, found that the plasma metabolite (R)-ketamine did not have any effect on the pain-relieving or dissociative effects of the racemate ketamine (Olofsen et al., 2022). The researchers thus hypothesized that (R)-ketamine may lack efficacy in pain relief (Olofsen et al., 2022). Considering these limited studies, the actual analgesic and sedative efficacy of (R)-ketamine has not been evaluated and supported by strong evidence due to limitations in trial design, original drug class, pain detection methodology, and subject population, and thus further confirmation from subsequent studies is still needed. In addition, unlike (R, S)- and (S)-ketamine, which induce cardiovascular side effects such as tachycardia, elevated blood pressure, and increased cardiac output to varying degrees (Geisslinger et al., 1993; Canuso et al., 2018; Zanos et al., 2018; Kamp et al., 2021), (R)- Ketamine is essentially unaltered in hemodynamic parameters in healthy subjects and is more advantageous in myocardial protection (Geisslinger et al., 1993; Leal et al., 2021; Olofsen et al., 2022). In studies of ketamine-related psychedelic side effects, elevated plasma concentrations of (R)-ketamine in humans do not significantly alter measured effects such as anti-injury perception and psychedelic symptoms originally induced by (S)- and (R, S)-ketamine (Olofsen et al., 2022). Instead, this may be synergistic with the nitric oxide (NO) donor sodium nitroprusside in reducing (R, S)-ketamine-induced psychedelic symptoms and internal and external perceptual deficits (Jonkman et al., 2018).
In addition, (R)-ketamine has shown elimination of anxiety-related behaviors and social interaction disorders induced by MIA (de Oliveira et al., 2021), as well as the ability to rapidly improve depression-like behaviors in rodents (Yang et al., 2018; Yao et al., 2022). This suggests that (R)-ketamine may also have some potential for development and application in alleviating preoperative anxiety and postoperative postpartum depression. Given the lower affinity of (R)-ketamine for the cytochrome P450 enzyme system, its drug clearance is 50% lower than that of (S)-ketamine, resulting in a longer retention and duration of action in the body (Kamp et al., 2020). Once the positive clinical efficacy and indications regarding the positive effects of (R)-ketamine in anxiolytic, antipsychedelic, cardioprotective, neuroprotective, and PND prevention and amelioration have been further demonstrated, its prolonged in vivo retention properties will be more conducive to a lasting perioperative effect.
3.3 Ischemic stroke
Ischemic stroke, also known as a stroke, is a frequent acute cerebrovascular illness that has high rates of morbidity and mortality (Collaborators, 2020). Very effective therapeutic drugs and methods are still limited currently (Oh et al., 2022). Research has shown that depression and anxiety were found to increase the risk of stroke (Nakada et al., 2023). Conversely, a stroke could also trigger and exacerbate symptoms of depression and anxiety (Chen et al., 2023). Early identification and prevention of post-stroke depression (PSD) can significantly improve patients’ depressive symptoms, promote the recovery of physical and cognitive functions, and thereby enhance the prognosis of stroke and the 10-year survival rate (Robinson and Jorge, 2016; Sun et al., 2023). It was reported that, in middle cerebral artery occlusion (MCAO) and chronic CUMS model rats, a single local injection of ketamine in the dentate gyrus region could enhance synaptic plasticity by regulating NMDAR/calcium/calcium-calmodulin-dependent protein kinase II (CaMKII), thereby producing significant and long-lasting antidepressant effects (Abdoulaye et al., 2021). Furthermore, ketamine, administered through intraperitoneal injection 30 min after MCAO, demonstrated significant reductions in infarct volume, edema ratio, and neurological deficit, providing neuroprotection against ischemic brain injury (Shu et al., 2012). Given that both ketamine and (S)-ketamine have shown improvements and neuroprotective effects against ischemic stroke (Shu et al., 2012; Zhang et al., 2023), (R)-ketamine, compared to ketamine and (S)-ketamine, has a stronger, more lasting antidepressant effect with fewer side effects (Johnston et al., 2023). Thus (Xiong et al., 2020), further confirmed that (R)-ketamine is also significant in ischemic stroke through animal experiments. The study demonstrated that after administering mice with (R)-ketamine (10 mg/kg) 30 min before (or 1 h after) MCAO and 24 h post-MCAO, it was observed that (R)-ketamine not only significantly alleviated brain injuries and behavioral abnormalities caused by MCAO but also showed stronger neuroprotective effects than (S)-Ketamine (Xiong et al., 2020). This suggests that (R)-ketamine may develop into a novel medication for the prevention and treatment of ischemic stroke or PSD.
3.4 Multiple sclerosis
MS is an immune-mediated disease that leads to inflammatory demyelinating lesions in the central nervous system, including the spinal cord and brain (Wang et al., 2021). Affected by early inflammation and delayed neurodegenerative lesions, the disability rate is extremely high (Dobson and Giovannoni, 2019). In MS patients, MDD and its severe symptoms are very common and are closely related to a significant reduction in quality of life and an increased risk of suicide (Jones et al., 2021). Wang et al.'s study (Wang et al., 2021) showed that after continuously injecting (R)-ketamine (10 mg/kg/day) into MS model mice for 15 days, the weight loss of the mice was significantly alleviated. Furthermore, unlike the saline, (R)-ketamine improved the clinical experimental autoimmune encephalitis (EAE) score of mice, attenuated their pathology scores, microglia activation, and the integrity of the blood-brain barrier in the spinal cord. To sum up, these findings indicate that there is a preventive effect of (R)-ketamine. On the other hand, cuprizone (CPZ) is often used to establish a mouse model that mimics the demyelination in MS patients due to its demyelinating effect. A recent study found that after continuously injecting mice with (R)-ketamine (10 mg/kg/day) twice a week for 6 weeks, it could improve the CPZ-induced corpus callosum demyelination and microglial cell activation by activating TrkB, promote myelin regeneration after discontinuing CPZ, and partially restored the abnormal β-diversity of the gut microbiota in mice treated with CPZ (Wang et al., 2022a). Therefore, these results imply that (R)-ketamine may be a potential drug for the prevention and treatment of MS, and the gut-microbiota-microglial crosstalk may significantly influence the effects of (R)-ketamine in the CPZ-treated MS model mice.
3.5 Parkinson’s disease
PD is a chronic progressive neurodegenerative disorder that primarily damages the neurons in the substantia nigra (SNr) responsible for producing dopamine. Although most of the current drugs can alleviate the motor symptoms of patients, they cannot provide adequate neuroprotection or halt the disease’s progression(Kieburtz et al., 2018). While the primary clinical manifestation of PD is motor symptoms, non-motor symptoms like anxiety and depression are also the most common and significant psychiatric features of PD (Landau et al., 2016). Moreover, these two symptoms often accompany PD patients and may persist throughout the disease course (Zhang et al., 2023). In addition, cognitive deficits related to learning and memory abilities have been observed in more than 15% of PD patients (Svenningsson et al., 2012). Subanesthetic doses (5, 10, and 15 mg/kg) of ketamine (i.p., once weekly) have all been shown to reverse depressive-like behavior, short-term memory impairment, pleasure deficits, and to improve gait deficits in rats with PD model induced by bilateral lesions in SNc (Vecchia et al., 2018; Vecchia et al., 2021). Meanwhile, ketamine at 8 mg/kg also exerted neuroprotective effects through the activation of cellular autophagy in PD model mice, which increased the number of nigrostriatal dopaminergic neurons (Fan et al., 2017). However, the potential risk of drug abuse and possible psychiatric side effects of ketamine are issues that need to be focused on in its future clinical treatment of PD. Fujita et al.’s study (Fujita et al., 2020) observed whether the two enantiomers of ketamine had neuroprotective effects in PD mice by 1-methyl-4-phenyl-1, 2, 3, 6-tetrahydropyridine (MPTP)-induced PD mouse model. The results showed that repeated intranasal administration of both (R)- and (S)-ketamine could effectively reduce the decline of dopamine transporter (DAT) in the striatum of mice caused by MPTP, with (R)-ketamine showing a more pronounced effect than (S)-ketamine (Fujita et al., 2020). Further, by continuously administering (R)-ketamine intranasally, tyrosine hydroxylase (TH) in the striatum and SNr decreased significantly as a result of MPTP, while (S)-ketamine did not have this effect (Fujita et al., 2020). In addition, BDNF provides trophic support through the TrkB signaling pathway to increase dendritic and axonal branching and synaptogenesis. This signaling pathway plays an important role in the development of neurodegenerative diseases such as PD and AD and psychiatric disorders such as depression, and has become a key target for the development of related therapeutic drugs (Hashimoto, 2010; 2013; Mitre et al., 2017). It has been shown that (R)-ketamine normalizes the protein levels of BDNF and p-TrkB in the PFC and hippocampal CA3 and DG regions of mice modeled for depression, promotes synaptogenesis, and ameliorates the reduction of dendritic spine densities in the prelimbic (PrL), CA3 and DG of the mPFC (Yang et al., 2015). Also, (R)-ketamine significantly attenuated MPTP-induced reduction of DAT in mouse striatum, and these ameliorative effects could be blocked by pretreatment with a TrkB antagonist (ANA-12) (Fujita et al., 2020). It is speculated that (R)-ketamine may play a neuroprotective role in counteracting MPTP-induced neurotoxic effects in the PD brain by activating the BDNF-TrkB signaling pathway in the striatum and SNr (Fujita et al., 2020), suggesting that it may be a possible new drug in the future for the prevention or treatment of neurodegenerative diseases such as Parkinson’s disease.
3.6 Osteoporosis
Osteoporosis is characterized by reduced bone density, deterioration of microarchitecture, and susceptibility to fragility fractures (Lane et al., 2000). MDD is one of the important risk factors for osteoporosis, and bone mineral density (BMD) is lower in both adults and women when combined with depression. Therefore, assessing and treating depression in these high-risk patients is crucial for preventing osteoporosis (Yuan et al., 2021). It was reported that the osteoprotegerin/nuclear factor κB receptor activator/nuclear factor κB receptor activator ligand (OPG/RANK/RANKL) signaling pathway and the osteopontin (OPN) system had essential involvement in bone metabolic abnormalities caused by MDD. Moreover, the antidepressant effects of (R, S)-ketamine might be associated with the improvement of inflammatory bone markers (Kadriu et al., 2018). Further research found that instead of (S)-ketamine and (2R, 6R)-HNK, (R)-ketamine dramatically reduced the elevated plasma levels of RANKL and ameliorated the reduction in the OPG/RANKL ratio in CSDS susceptible mice (Zhang et al., 2018b; Xiong et al., 2019). Additionally, it could also significantly increase the BMD of the femur in CSDS susceptible mice (Xiong et al., 2019). In conclusion, it could be inferred that the OPG/RANKL ratio in the blood of MDD patients might be a potential biomarker for assessing the antidepressant effects of (R)- and (R, S)-ketamine, which is crucial for diagnosis (Zhang et al., 2018b). Wan et al. (Wan et al., 2022) reported that administering (R)-ketamine (10 mg/kg/day, twice a week) continuously for 6 weeks could dramatically enhance the cortical bone density and overall density of ovariectomized (OVX) mice by modulating the anti-inflammatory effects of gut microbiota. Similarly, one dosage of 10 mg/kg of (R)-ketamine improved anhedonia-like behavior, as well as reduced femoral neck cortical and total BMD. Furthermore, one dose of (R)-ketamine altered gut microbiota composition, resulting in changes in thirteen metabolic pathways and six metabolites in CSDS susceptible mice. The findings suggest that by acting on the gut–microbiota–bone–brain axis, (R)-ketamine can ameliorate the anhedonia-like phenotype and decrease BMD in CSDS susceptible mice (Wan et al., 2023). As a result, (R)-ketamine might emerge as a promising drug for treating reduced bone density or osteoporosis in patients with depression in the future.
3.7 Inflammatory disease
LPS can induce a phenotype similar to depression, causing systemic inflammation and increased spleen weight, so it is often used to establish inflammation-related rodent models of depression (Zhang et al., 2020). However, (R, S)-ketamine can attenuate or even reverse the inflammatory response of BV2 microglial cells caused by LPS (Lu et al., 2020) and reduce the levels of high mobility group protein B1 (HMGB1) in plasma and vital organs such as the heart, liver, and kidney, thereby increasing the 7-day survival rate of rats with sepsis caused by cecal ligation and puncture (CLP) (Zhang et al., 2014; Zhang et al., 2021a) further reported that the combined use of (R)-ketamine at 15 mg/kg for prevention and treatment significantly improved the 14-day survival rate of mice after CLP. Additionally, it can also improve the reduction in rectal temperature caused by sepsis 12 h after CLP, the elevated levels of plasma inflammatory cytokines, and the increase in injury markers of vital organs such as the heart, lungs, kidneys, and liver. All these point to (R)-ketamine’s possible effectiveness in the prevention and treatment of sepsis. On the other hand, pretreatment with 10 mg/kg of (R)-ketamine (6 days before LPS injection) or combined with treatments 24 h before and 10 min after LPS injection can effectively prevent inflammation and significantly reduce spleen enlargement, central and systemic inflammation, and cognitive dysfunction in mice caused by LPS (Zhang et al., 2021b; Ma et al., 2022c). Furthermore, pretreatment with (R)-ketamine at 10 mg/kg 6 days before LPS administration can effectively correct the aberrant expression of two miRNAs (miR-149 and miR-7688-5p) and NFATc4 mRNA in the PFC of mice induced by LPS, and partially restore the effects of LPS on the mouse gut microbiota, thereby continuously preventing depressive-like behavior in inflammation model mice (Ma et al., 2022a; Yao et al., 2022). Additionally, depression might increase the risk of inflammatory bowel disease, but using antidepressants to treat depression can reduce this risk (Frolkis et al., 2019). Fujita et al. (Fujita et al., 2021) found that repeated injection of (R)-ketamine (10 mg/kg/day, for 14 or 7 days) had positive effects on the ulcerative colitis (UC) model induced by dextran sulfate sodium (DSS) through the activation of TrkB. Together, these findings indicate that (R)-ketamine has significant anti-inflammatory effects, especially when administered multiple times or for prevention and treatment. Thus, it holds promise as a new drug choice for treating inflammatory diseases such as sepsis and UC.
3.8 COVID-19
Increasing evidence suggests that the novel coronavirus infection may have adverse effects on the central nervous system (CNS), causing psychiatric and neurological symptoms in those infected (Chen et al., 2022). The endoplasmic reticulum chaperone protein σ-1 receptor is crucial for the replication of the novel coronavirus in host cells and is considered a potential therapeutic target for COVID-19 patients (Gordon et al., 2020a; Gordon et al., 2020b). Research has found that (R, S)-ketamine not only has sedative, analgesic, and antidepressant effects but also has a minimal impact on respiration. It can interact with σ receptors (including σ-1 and σ-2) (Robson et al., 2012) and is believed to have potential benefits for the treatment of COVID-19 patients (Ortoleva, 2020; Akinosoglou et al., 2021). Moreover, compared to two other ketamine compounds, (R)-ketamine has a more pronounced effect on the σ-1 receptor (Hashimoto, 2021). It has significant advantages in improving inflammatory diseases of the central nervous system (e.g., the spinal cord and brain) as well as lung inflammation (Zhang et al., 2021a; Wang et al., 2021), and can help COVID-19 patients feel more comfortable (Vollenweider et al., 1997). Therefore, (R)-ketamine, with its dual anti-inflammatory and antidepressant effects, may benefit such patients and holds promise as a new therapeutic candidate for the future treatment of COVID-19.
3.9 Substance use disorder
The consumption of alcohol and illicit drugs represents a growing and intricate worldwide public health issue. Even though current behavioral interventions and drug treatments have achieved some results, the outcomes for treating substance use disorders (SUDs) are still not ideal (Jones et al., 2018). A systematic review found that using (R, S)-ketamine to treat SUDs can reduce patients’ craving, motivation, and use of cocaine. It can also increase the success rate of withdrawal from alcohol and opioids and maintain a significant difference from the control group for up to 2 years after a single dose (Jones et al., 2018; Witkin et al., 2020) demonstrated that on its own, (R)-ketamine did not cause conditioned place preference (CPP). In contrast, it blocked morphine-induced CPP and reduced overall morphine withdrawal scores in rats following naloxone-precipitation. Furthermore, it was reported that (R)-ketamine (20 mg/kg) could significantly alleviate morphine withdrawal signs, with its efficacy comparable to that of the commonly used opioid withdrawal drug, Lofexidine (Witkin et al., 2020). Pretreatment with (R)-ketamine at 3 mg/kg in rats showed a significant inhibitory trend towards tolerance induced by ethanol (ETOH), and it did not affect ETOH’s dependence or its effects (Shafique et al., 2021). Therefore, it can be seen that (R)-ketamine not only does not induce or exacerbate substance dependence but, on the contrary, can play a potential therapeutic role in ethanol- and opioid-induced SUDs. Future clinical randomized controlled trials will further validate its effects in treating substance use disorders.
3.10 Organophosphate poisoning
The repeated use of various low-dose nerve agent substitutes and organophosphate agents [diisopropyl fluorophosphate (DFP)] can simulate the chronic depressive state of Gulf War Illness (GWI) in rats, mainly manifesting as emotional and cognitive impairments (Phillips and Deshpande, 2016). Using this model, researchers administered a single dose of (R, S)-ketamine (10 mg/kg) to rats 3 months after exposure to DFP. The results showed that rats displayed antidepressant effects in the forced swim test 1 h after treatment, and this effect remained significant 24 h posttreatment (Ribeiro et al., 2020). Meanwhile, the reduced expression of BDNF in the rat brain due to DFP exposure did not show significant improvement 1 h after (R, S)-ketamine treatment, but 24 h later, the level of BDNF expression dramatically rose (Ribeiro et al., 2020). Additionally, in another experiment by the team, they found that (R, S)-ketamine and its two enantiomers could significantly ameliorate the GWI characteristic behaviors of DFP rats (Zhu et al., 2020). Furthermore, compared to (R, S)- and (S)-ketamine, the ameliorative effects of (R)-ketamine were more powerful, prolonged, and associated with less severe CNS adverse effects. (Zhu et al., 2020). Acetylcholinesterase inhibitors in organophosphate pesticides can affect cholinergic signaling modulation, and this cholinergic signal also involves the regulation of glutamatergic transmission (Picciotto et al., 2012). At sub-anesthetic doses, (R, S)-ketamine can elevate extracellular glutamate levels and glutamate cycling in rats (Zanos and Gould, 2018). Considering that the NMDA receptor family includes seven subunits: GluN1, GluN2A-D, and GluN3A-B, of which GluN2D is only associated with cognitive impairments and motor sensitization, as well as persistent antidepressant effects induced by (R)-ketamine, it is essentially unrelated to the effects of (R, S)-ketamine and (S)-ketamine (Ide et al., 2017; Ide and Ikeda, 2018; Ide et al., 2019). Therefore, (R)-ketamine may demonstrate its multifunctional effects in GWI by modulating glutamatergic signal transmission and is expected to become a novel choice for treating organophosphate poisoning.
4 Side effects and abuse potential of (R)-ketamine
(R)-ketamine demonstrates greater advantages in its antidepressant effects compared to ketamine and (S)-ketamine (Zhang et al., 2014; Fukumoto et al., 2017; Chang et al., 2019). However, in recent years, researchers have remained concerned about its potential for abuse and common side effects, such as psychotomimetic and dissociative effects, that are shared by ketamine and (S)-ketamine (Chang et al., 2019). studied the effects of ketamine and its enantiomers on spontaneous movement and pre-pulse inhibition (PPI) in the mouse CSDS model, finding the strength of these effects was ranked as follow: (S)-ketamine > (R, S)-ketamine > (R)-ketamine. At the same time, (R)-ketamine does not induce anhedonia-like effects (Witkin et al., 2020), nor does it cause acute hypermobility effects or significant PPI deficits (Yang et al., 2015). When administered at a dose of 20 mg/kg, it not only does not induce CPP in mice or increase their scores, but it also alleviates the dissociative and psychomimetic effects caused by (R, S)- or (S)-ketamine (Yang et al., 2015; Chang et al., 2019). In addition, in drug discrimination tests in rats, when the aim was to induce cognitive deficits, (R)-ketamine showed a specific discriminative stimulus effect, making drug discrimination challenging. Compared to (S)- and (R, S)-ketamine, the cognitive deficits it induced were milder (Popik et al., 2020). Nonetheless, Zanos et al. (Zanos et al., 2019a) pointed out that some previous conclusions might not be accurate, as many studies did not use a full range of relevant subanesthetic doses for testing. Zanos and his colleagues found that for CD-1 mice, the minimum effective anesthetic dose of (R)-ketamine was 120 mg/kg, while the subanesthetic dose was 90 mg/kg (Zanos et al., 2019a). Simultaneously, when the dose exceeded 20 mg/kg, (R)-ketamine caused motor discoordination in mice; at 40 mg/kg, it induced acute hyperlocomotion in mice and produced evident CPP; and at 90 mg/kg, it interfered with PPI. In conclusion, these suggest that (R)-ketamine may still induce various side effects and carry a risk of abuse when used in higher-than-antidepressant dosages (Zanos et al., 2019a).
Given that the addictive potential of ketamine and its enantiomers in different individuals seems to be closely related to its pharmacological properties and its impact on individual psychology (Shim, 2022), Bonaventura et al. (Bonaventura et al., 2021) analyzed the pharmacological and behavioral characteristics of (R)-ketamine and (S)-ketamine, finding that the two differ in the physiological mechanisms of drug dependence. Compared to (R)-ketamine, (S)-ketamine had a greater affinity and potency for opioid receptors (Bonaventura et al., 2021). However, repeated injections of a subanesthetic dose of (S)-ketamine (20 mg/kg, IP) led to significant psychomotor sensitization and CPP, while the behavioral changes caused by the same dose of (R)-ketamine were milder or almost negligible (Bonaventura et al., 2021). Thus, the pharmacological effects of (S)-ketamine might be the primary factor in the human abuse liability of racemic ketamine, whereas (R)-ketamine may not influence it (Bonaventura et al., 2021).
Recently (Shim, 2022), expressed doubts about the conclusion mentioned above, as a previous study reported that 10–30 mg/kg of (R)-ketamine significantly enhanced spontaneous motor activity in mice, surpassing the effects of 30 mg/kg of (S)-ketamine (Fukumoto et al., 2017). However, in their study (Bonaventura et al., 2021), administered acute injections of (R)-ketamine at 5, 10, and 20 mg/kg to mice, observing increased motor activity only at the 20 mg/kg dose, and its effect was weaker than that of (S)-ketamine at the same dose. Therefore, subsequent studies should delve into factors such as procedures, measurement timing, and species differences to explain the discrepancies in the studies above (Shim, 2022). On the other hand, in the mouse behavioral sensitization model, a mere 3-day observation period might be insufficient to conclusively determine whether low-dose repeated injections of (R)-ketamine lead to psychomotor sensitization. Thus, a longer treatment duration and lower doses should be used to accurately assess the effects of the two enantiomers on mice (Shim, 2022).
In summary, most current preclinical studies and some clinical research indicate that compared to (R, S)- and (S)-ketamine, (R)-ketamine exhibits milder effects in terms of psychosis-like symptoms, dissociative side effects, and abuse potential, with a higher safety profile (Geisslinger et al., 1993; Hashimoto, 2020; Wei et al., 2021b; Olofsen et al., 2022). However, when considering the abuse potential and psychomotor sensitization, the conclusions of existing research remain contentious. For this reason, future studies should involve large-sample, randomized, double-blind, placebo-controlled trials. These trials should encompass subjective evaluations of drug craving or preference, observations of behavioral sensitization from repeated dosing, and long-term follow-ups of patients with TRD and potential co-morbid SUDs to assess misuse; addiction; and psychomotor sensitization.
5 Conclusion
Due to the significant effects of ketamine in treating TRD, researchers have intensively studied its antidepressant molecular mechanisms over the past two decades, especially its two enantiomers, metabolites, functional characteristics, and selective targets. In spite of the fact that the etiology and mechanisms of MDD are not fully understood, the efficacy of antidepressants remains variable due to the complexity of the disease and individual differences in symptoms and gene expression profiles in patients with MDD (Xia et al., 2022). Additionally, although ketamine’s and its enantiomers’ benefits for treating MDD are widely recognized, the specific molecular mechanisms of its anti-depressant action remain unclear (Heifets et al., 2021). Furthermore, existing studies show that (R)-ketamine has significant advantages over other drugs in terms of antidepressant efficacy and side effects, but knowledge of its antidepressant mechanisms and targets of intervention remains limited. In the future, we need to integrate chemistry, biology, and genetic technology to further explore these mechanisms.
In addition to its unique role in the treatment of depression, (R)-ketamine has been shown to have preventive, therapeutic and developmental potential in cognitive disorders, perioperative anaesthesia, cerebral ischaemic stroke, Parkinson’s disease, multiple sclerosis, osteoporosis, substance use disorders, inflammatory disorders, COVID-19, organophosphorus poisoning, etc. (Figure 2), and the related mechanism of action may be more similar to the antidepressant mechanism of (R)-ketamine. The mechanism of action may be more similar to the antidepressant mechanism of (R)-ketamine, while the mechanism other than the antidepressant effect needs to be explored by further studies due to the limitation of fewer studies at present. In addition, with further research, we believe that (R)-ketamine will have more new indications and application potentials to be explored by researchers around the world in the future.
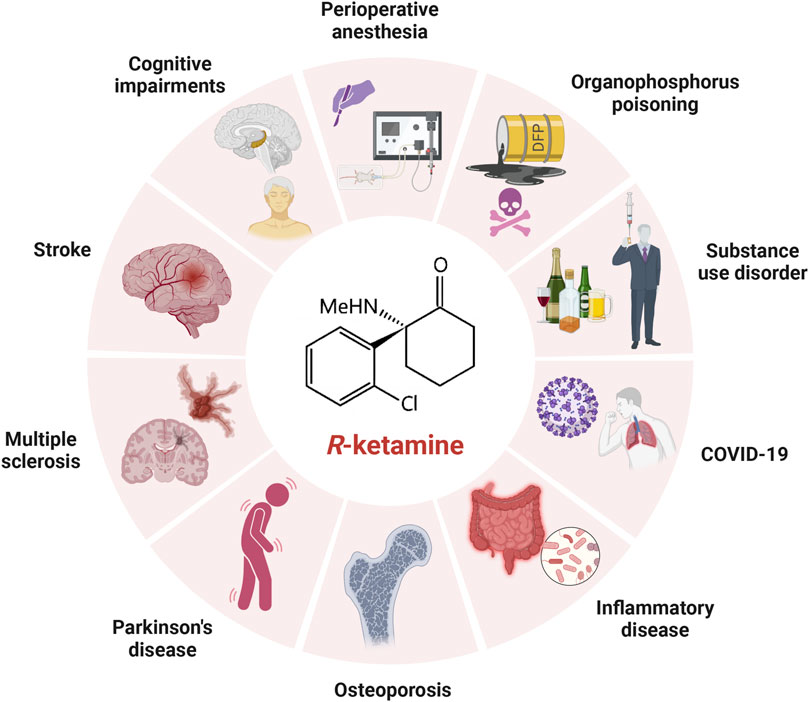
Figure 2. Applications of (R)-ketamine in treatments other than depression. Besides its use in treating depression, (R)-ketamine also holds significant potential in the prevention and treatment of perioperative anesthesia, ischemic stroke, cognitive disorders, Parkinson’s disease, multiple sclerosis, osteoporosis, substance use disorders, inflammatory diseases, COVID-19, and organophosphate poisoning.
Finally, despite the superior safety profile and fewer side effects of (R)-ketamine compared to (S)- and (R, S)-ketamine, it is essential to focus on potential negative issues arising from prolonged use or excessive dosage. For example, it remains to be investigated whether (R)-ketamine may present analogous problems to those associated with (R, S)-ketamine, such as cognitive impairments induced by neurotoxicity, diminished therapeutic efficacy due to drug tolerance, drug addiction and abuse resulting from psychological dependence, and the potential link between ulcerative cystitis and bladder cancer. These questions need to be systematically addressed in subsequent research studies.
Author contributions
SZ: Conceptualization, Funding acquisition, Writing–original draft, Writing–review and editing. YP: Investigation, Writing–original draft. JL: Data curation, Writing–original draft. LL: Data curation, Investigation, Writing–original draft. CA: Data curation, Writing–original draft. YW: Methodology, Writing–original draft. WjZ: Investigation, Software, Writing – original draft. WxZ: Supervision, Writing–original draft. SQ: Formal Analysis, Writing–original draft. WY: Funding acquisition, Project administration, Resources, Supervision, Writing–review and editing.
Funding
The author(s) declare financial support was received for the research, authorship, and/or publication of this article. This study was supported by the National Natural Science Foundation of China (81860233), Gansu Provincial Clinical Medical Research Center of Anesthesia and Brain Function (21JR7RA675), Gansu Provincial Natural Science Foundation (21JR7RA621), Gansu Provincial Key Research and Development Program Project (23YFFA0046), and the hospital-level project of Xianning Central Hospital / The First Affiliated Hospital of Hubei University of Science and Technology (2022XYB032).
Conflict of interest
The authors declare that the research was conducted in the absence of any commercial or financial relationships that could be construed as a potential conflict of interest.
Publisher’s note
All claims expressed in this article are solely those of the authors and do not necessarily represent those of their affiliated organizations, or those of the publisher, the editors and the reviewers. Any product that may be evaluated in this article, or claim that may be made by its manufacturer, is not guaranteed or endorsed by the publisher.
References
Abdoulaye, I. A., Wu, S. S., Chibaatar, E., Yu, D. F., Le, K., Cao, X. J., et al. (2021). Ketamine induces lasting antidepressant effects by modulating the NMDAR/CaMKII-mediated synaptic plasticity of the hippocampal dentate gyrus in depressive stroke model. Neural Plast. 2021, 6635084. doi:10.1155/2021/6635084
Ago, Y., Tanabe, W., Higuchi, M., Tsukada, S., Tanaka, T., Yamaguchi, T., et al. (2019). (R)-ketamine induces a greater increase in prefrontal 5-HT release than (S)-ketamine and ketamine metabolites via an AMPA receptor-independent mechanism. Int. J. Neuropsychopharmacol. 22 (10), 665–674. doi:10.1093/ijnp/pyz041
Akinosoglou, K., Gogos, A., Papageorgiou, C., Angelopoulos, E., and Gogos, C. (2021). Ketamine in COVID-19 patients: thinking out of the box. J. Med. Virol. 93 (7), 4069–4070. doi:10.1002/jmv.26681
Bandeira, I. D., Leal, G. C., Correia-Melo, F. S., Souza-Marques, B., Silva, S. S., Lins-Silva, D. H., et al. (2023). Arketamine for bipolar depression: open-label, dose-escalation, pilot study. J. Psychiatr. Res. 164, 229–234. doi:10.1016/j.jpsychires.2023.06.028
Barrett, W., Buxhoeveden, M., and Dhillon, S. (2020). Ketamine: a versatile tool for anesthesia and analgesia. Curr. Opin. Anaesthesiol. 33 (5), 633–638. doi:10.1097/aco.0000000000000916
Berman, R., Cappiello, A., Anand, A., Oren, D., Heninger, G., Charney, D., et al. (2000). Antidepressant effects of ketamine in depressed patients. Biol. Psychiatry 47 (4), 351–354. doi:10.1016/s0006-3223(99)00230-9
Beutel, M. E., Klein, E. M., Brähler, E., Reiner, I., Jünger, C., Michal, M., et al. (2017). Loneliness in the general population: prevalence, determinants and relations to mental health. BMC Psychiatry 17 (1), 97. doi:10.1186/s12888-017-1262-x
Bicks, L. K., Koike, H., Akbarian, S., and Morishita, H. (2015). Prefrontal cortex and social cognition in mouse and man. Front. Psychol. 6, 1805. doi:10.3389/fpsyg.2015.01805
Bonaventura, J., Gomez, J. L., Carlton, M. L., Lam, S., Sanchez-Soto, M., Morris, P. J., et al. (2022). Target deconvolution studies of (2R,6R)-hydroxynorketamine: an elusive search. Mol. Psychiatry 27 (10), 4144–4156. doi:10.1038/s41380-022-01673-w
Bonaventura, J., Lam, S., Carlton, M., Boehm, M. A., Gomez, J. L., Solís, O., et al. (2021). Pharmacological and behavioral divergence of ketamine enantiomers: implications for abuse liability. Mol. Psychiatry 26 (11), 6704–6722. doi:10.1038/s41380-021-01093-2
Cáceda, R., Carbajal, J., Salomon, R., Moore, J., Perlman, G., Padala, P., et al. (2020). Slower perception of time in depressed and suicidal patients. Eur. Neuropsychopharmacol. 40, 4–16. doi:10.1016/j.euroneuro.2020.09.004
Canuso, C., Singh, J., Fedgchin, M., Alphs, L., Lane, R., Lim, P., et al. (2018). Efficacy and safety of intranasal esketamine for the rapid reduction of symptoms of depression and suicidality in patients at imminent risk for suicide: results of a double-blind, randomized, placebo-controlled study. Am. J. Psychiatry 175 (7), 620–630. doi:10.1176/appi.ajp.2018.17060720
Chang, L., Zhang, K., Pu, Y., Qu, Y., Wang, S., Xiong, Z., et al. (2019). Comparison of antidepressant and side effects in mice after intranasal administration of (R,S)-ketamine, (R)-ketamine, and (S)-ketamine. Pharmacol. Biochem. Behav. 181, 53–59. doi:10.1016/j.pbb.2019.04.008
Chen, F., Cao, P., Liu, H., and Cai, D. (2022). The impact of COVID-19 and vaccine on the human nervous system. Neuroendocrinology 112, 1046–1057. doi:10.1159/000524234
Chen, Y. M., Chen, P. C., Lin, W. C., Hung, K. C., Chen, Y. B., Hung, C. F., et al. (2023). Predicting new-onset post-stroke depression from real-world data using machine learning algorithm. Front. Psychiatry 14, 1195586. doi:10.3389/fpsyt.2023.1195586
Chinese Clinical Trial Registry (2019). Efficacy and safety of ketamine, S-ketamine and R-ketamine in treatment resistant depression: a randomized controlled trial. Available at: https://www.chictr.org.cn/showproj.html?proj=26844 (Accessed May 17, 2019).
Chou, D., Peng, H. Y., Lin, T. B., Lai, C. Y., Hsieh, M. C., Wen, Y. C., et al. (2018). (2R,6R)-hydroxynorketamine rescues chronic stress-induced depression-like behavior through its actions in the midbrain periaqueductal gray. Neuropharmacology 139, 1–12. doi:10.1016/j.neuropharm.2018.06.033
Cision PR Newswire (2021). Perception Neuroscience’s PCN-101 (R-ketamine) demonstrates tolerability in phase 1 single ascending dose study. Available at: https://www.prnewswire.com/news-releases/perception-neurosciences-pcn-101-r-ketamine-demonstrates-tolerability-in-phase-1-single-ascending-dose-study-301231491.html (Accessed February 19, 2021).
ClinicalTrials (2021). Phase 1 evaluation of (2r,6r)-hydroxynorketamine. Available at: https://clinicaltrials.gov/study/NCT04711005 (Accessed January 15, 2021)
Collaborators, G. D. a. I. (2020). Global burden of 369 diseases and injuries in 204 countries and territories, 1990-2019: a systematic analysis for the Global Burden of Disease Study 2019. Lancet 396 (10258), 1204–1222. doi:10.1016/s0140-6736(20)30925-9
Conahan, T. J. (1975). New intravenous anesthetics. Surg. Clin. North Am. 55 (4), 851–859. doi:10.1016/s0039-6109(16)40686-9
de Carvalho, C., Lopes, M., Constantino, L., Hoeller, A., de Melo, H., Guarnieri, R., et al. (2021). The ERK phosphorylation levels in the amygdala predict anxiety symptoms in humans and MEK/ERK inhibition dissociates innate and learned defensive behaviors in rats. Mol. Psychiatry 26 (12), 7257–7269. doi:10.1038/s41380-021-01203-0
Dobson, R., and Giovannoni, G. (2019). Multiple sclerosis - a review. Eur. J. Neurol. 26 (1), 27–40. doi:10.1111/ene.13819
Domino, E. (2010). Taming the ketamine tiger. 1965. Anesthesiology 113 (3), 678–684. doi:10.1097/ALN.0b013e3181ed09a2
Domino, E., Chodoff, P., and Corssen, G. (1965). Pharmacologic effects of CI-581, a new dissociative anesthetic, in man. Clin. Pharmacol. Ther. 6, 279–291. doi:10.1002/cpt196563279
Ebert, B., Mikkelsen, S., Thorkildsen, C., and Borgbjerg, F. (1997). Norketamine, the main metabolite of ketamine, is a non-competitive NMDA receptor antagonist in the rat cortex and spinal cord. Eur. J. Pharmacol. 333 (1), 99–104. doi:10.1016/s0014-2999(97)01116-3
Fan, J. C., Song, J. J., Wang, Y., Chen, Y., and Hong, D. X. (2017). Neuron-protective effect of subanesthestic-dosage ketamine on mice of Parkinson's disease. Asian Pac J. Trop. Med. 10 (10), 1007–1010. doi:10.1016/j.apjtm.2017.09.014
Frolkis, A. D., Vallerand, I. A., Shaheen, A. A., Lowerison, M. W., Swain, M. G., Barnabe, C., et al. (2019). Depression increases the risk of inflammatory bowel disease, which may be mitigated by the use of antidepressants in the treatment of depression. Gut 68 (9), 1606–1612. doi:10.1136/gutjnl-2018-317182
Fujita, A., Fujita, Y., Pu, Y., Chang, L., and Hashimoto, K. (2020). MPTP-induced dopaminergic neurotoxicity in mouse brain is attenuated after subsequent intranasal administration of (R)-ketamine: a role of TrkB signaling. Psychopharmacology 237 (1), 83–92. doi:10.1007/s00213-019-05346-5
Fujita, Y., Hashimoto, Y., Hashimoto, H., Chang, L., and Hashimoto, K. (2021). Dextran sulfate sodium-induced inflammation and colitis in mice are ameliorated by (R)-ketamine, but not (S)-ketamine: a role of TrkB signaling. Eur. J. Pharmacol. 897, 173954. doi:10.1016/j.ejphar.2021.173954
Fukumoto, K., Fogaça, M. V., Liu, R. J., Duman, C., Kato, T., Li, X. Y., et al. (2019). Activity-dependent brain-derived neurotrophic factor signaling is required for the antidepressant actions of (2R,6R)-hydroxynorketamine. Proc. Natl. Acad. Sci. U. S. A. 116 (1), 297–302. doi:10.1073/pnas.1814709116
Fukumoto, K., Toki, H., Iijima, M., Hashihayata, T., Yamaguchi, J., Hashimoto, K., et al. (2017). Antidepressant potential of (R)-ketamine in rodent models: comparison with (S)-ketamine. J. Pharmacol. Exp. Ther. 361 (1), 9–16. doi:10.1124/jpet.116.239228
Gao, M., Rejaei, D., and Liu, H. (2016). Ketamine use in current clinical practice. Acta Pharmacol. Sin. 37 (7), 865–872. doi:10.1038/aps.2016.5
Geisslinger, G., Hering, W., Thomann, P., Knoll, R., Kamp, H., and Brune, K. (1993). Pharmacokinetics and pharmacodynamics of ketamine enantiomers in surgical patients using a stereoselective analytical method. Br. J. Anaesth. 70 (6), 666–671. doi:10.1093/bja/70.6.666
Getachew, B., Aubee, J. I., Schottenfeld, R. S., Csoka, A. B., Thompson, K. M., and Tizabi, Y. (2018). Ketamine interactions with gut-microbiota in rats: relevance to its antidepressant and anti-inflammatory properties. BMC Microbiol. 18 (1), 222. doi:10.1186/s12866-018-1373-7
Gill, H., Gill, B., Rodrigues, N. B., Lipsitz, O., Rosenblat, J. D., El-Halabi, S., et al. (2021). The effects of ketamine on cognition in treatment-resistant depression: a systematic review and priority avenues for future research. Neurosci. Biobehav Rev. 120, 78–85. doi:10.1016/j.neubiorev.2020.11.020
Gordon, D., Hiatt, J., Bouhaddou, M., Rezelj, V., Ulferts, S., Braberg, H., et al. (2020a). Comparative host-coronavirus protein interaction networks reveal pan-viral disease mechanisms. Science 370 (6521), eabe9403. doi:10.1126/science.abe9403
Gordon, D., Jang, G., Bouhaddou, M., Xu, J., Obernier, K., White, K., et al. (2020b). A SARS-CoV-2 protein interaction map reveals targets for drug repurposing. Nature 583 (7816), 459–468. doi:10.1038/s41586-020-2286-9
Grunebaum, M. F., Galfalvy, H. C., Choo, T. H., Parris, M. S., Burke, A. K., Suckow, R. F., et al. (2019). Ketamine metabolite pilot study in a suicidal depression trial. J. Psychiatr. Res. 117, 129–134. doi:10.1016/j.jpsychires.2019.08.005
Hashimoto, K. (2010). Brain-derived neurotrophic factor as a biomarker for mood disorders: an historical overview and future directions. Psychiatry Clin. Neurosci. 64 (4), 341–357. doi:10.1111/j.1440-1819.2010.02113.x
Hashimoto, K. (2013). Sigma-1 receptor chaperone and brain-derived neurotrophic factor: emerging links between cardiovascular disease and depression. Prog. Neurobiol. 100, 15–29. doi:10.1016/j.pneurobio.2012.09.001
Hashimoto, K. (2020). Molecular mechanisms of the rapid-acting and long-lasting antidepressant actions of (R)-ketamine. Biochem. Pharmacol. 177, 113935. doi:10.1016/j.bcp.2020.113935
Hashimoto, K. (2021). Repurposing of CNS drugs to treat COVID-19 infection: targeting the sigma-1 receptor. Eur. Arch. Psychiatry Clin. Neurosci. 271 (2), 249–258. doi:10.1007/s00406-020-01231-x
Hashimoto, K. (2023). Arketamine for cognitive impairment in psychiatric disorders. Eur. Arch. Psychiatry Clin. Neurosci. 273 (7), 1513–1525. doi:10.1007/s00406-023-01570-5
Heifets, B., Bentzley, B., Williams, N., and Schatzberg, A. (2021). Unraveling the opioid actions of S-ketamine and R-ketamine: comment on Bonaventura et al. Mol. Psychiatry 26 (11), 6104–6106. doi:10.1038/s41380-021-01167-1
Highland, J. N., Morris, P. J., Zanos, P., Lovett, J., Ghosh, S., Wang, A. Q., et al. (2019). Mouse, rat, and dog bioavailability and mouse oral antidepressant efficacy of (2R,6R)-hydroxynorketamine. J. Psychopharmacol. 33 (1), 12–24. doi:10.1177/0269881118812095
Huang, N., Hua, D., Zhan, G., Li, S., Zhu, B., Jiang, R., et al. (2019). Role of actinobacteria and coriobacteriia in the antidepressant effects of ketamine in an inflammation model of depression. Pharmacol. Biochem. Behav. 176, 93–100. doi:10.1016/j.pbb.2018.12.001
Ide, S., and Ikeda, K. (2018). Mechanisms of the antidepressant effects of ketamine enantiomers and their metabolites. Biol. Psychiatry 84 (8), 551–552. doi:10.1016/j.biopsych.2018.07.018
Ide, S., Ikekubo, Y., Mishina, M., Hashimoto, K., and Ikeda, K. (2017). Role of NMDA receptor GluN2D subunit in the antidepressant effects of enantiomers of ketamine. J. Pharmacol. Sci. 135 (3), 138–140. doi:10.1016/j.jphs.2017.11.001
Ide, S., Ikekubo, Y., Mishina, M., Hashimoto, K., and Ikeda, K. (2019). Cognitive impairment that is induced by (R)-ketamine is abolished in NMDA GluN2D receptor subunit knockout mice. Int. J. Neuropsychopharmacol. 22 (7), 449–452. doi:10.1093/ijnp/pyz025
Jelen, L., Young, A., and Stone, J. (2021). Ketamine: a tale of two enantiomers. J. Psychopharmacol. 35 (2), 109–123. doi:10.1177/0269881120959644
Jiang, Y., Zou, M., Ren, T., and Wang, Y. (2023). Are mGluR2/3 inhibitors potential compounds for novel antidepressants? Cell. Mol. Neurobiol. 43 (5), 1931–1940. doi:10.1007/s10571-022-01310-8
Johnston, J. N., Henter, I. D., and Zarate, C. A. (2023). The antidepressant actions of ketamine and its enantiomers. Pharmacol. Ther. 246, 108431. doi:10.1016/j.pharmthera.2023.108431
Johnston, J. N., Thacker, J. S., Desjardins, C., Kulyk, B. D., Romay-Tallon, R., Kalynchuk, L. E., et al. (2020). Ketamine rescues hippocampal reelin expression and synaptic markers in the repeated-corticosterone chronic stress paradigm. Front. Pharmacol. 11, 559627. doi:10.3389/fphar.2020.559627
Jones, C., Motl, R., and Sandroff, B. (2021). Depression in multiple sclerosis: is one approach for its management enough? Mult. Scler. Relat. Disord. 51, 102904. doi:10.1016/j.msard.2021.102904
Jones, J., Mateus, C., Malcolm, R., Brady, K., and Back, S. (2018). Efficacy of ketamine in the treatment of substance use disorders: a systematic review. Front. Psychiatry 9, 277. doi:10.3389/fpsyt.2018.00277
Jonkman, K., van der Schrier, R., van Velzen, M., Aarts, L., Olofsen, E., Sarton, E., et al. (2018). Differential role of nitric oxide in the psychedelic symptoms induced by racemic ketamine and esketamine in human volunteers. Br. J. Anaesth. 120 (5), 1009–1018. doi:10.1016/j.bja.2018.01.022
Jóźwiak-Bębenista, M., Sokołowska, P., Siatkowska, M., Panek, C. A., Komorowski, P., Kowalczyk, E., et al. (2022). The importance of endoplasmic reticulum stress as a novel antidepressant drug target and its potential impact on CNS disorders. Pharmaceutics 14 (4), 846. doi:10.3390/pharmaceutics14040846
Kadriu, B., Gold, P., Luckenbaugh, D., Lener, M., Ballard, E., Niciu, M., et al. (2018). Acute ketamine administration corrects abnormal inflammatory bone markers in major depressive disorder. Mol. Psychiatry 23 (7), 1626–1631. doi:10.1038/mp.2017.109
Kamp, J., Jonkman, K., van Velzen, M., Aarts, L., Niesters, M., Dahan, A., et al. (2020). Pharmacokinetics of ketamine and its major metabolites norketamine, hydroxynorketamine, and dehydronorketamine: a model-based analysis. Br. J. Anaesth. 125 (5), 750–761. doi:10.1016/j.bja.2020.06.067
Kamp, J., van Velzen, M., Aarts, L., Niesters, M., Dahan, A., and Olofsen, E. (2021). Stereoselective ketamine effect on cardiac output: a population pharmacokinetic/pharmacodynamic modelling study in healthy volunteers. Br. J. Anaesth. 127 (1), 23–31. doi:10.1016/j.bja.2021.02.034
Kieburtz, K., Katz, R., and Olanow, C. (2018). New drugs for Parkinson's disease: the regulatory and clinical development pathways in the United States. Mov. Disord. 33 (6), 920–927. doi:10.1002/mds.27220
Landau, S., Harris, V., Burn, D. J., Hindle, J. V., Hurt, C. S., Samuel, M., et al. (2016). Anxiety and anxious-depression in Parkinson's disease over a 4-year period: a latent transition analysis. Psychol. Med. 46 (3), 657–667. doi:10.1017/s0033291715002196
Lane, J. M., Russell, L., and Khan, S. N. (2000). Osteoporosis. Clin. Orthop. Relat. Res. 372, 139–150. doi:10.1097/00003086-200003000-00016
Leal, G., Bandeira, I., Correia-Melo, F., Telles, M., Mello, R., Vieira, F., et al. (2021). Intravenous arketamine for treatment-resistant depression: open-label pilot study. Eur. Arch. Psychiatry Clin. Neurosci. 271 (3), 577–582. doi:10.1007/s00406-020-01110-5
Leal, G. C., Souza-Marques, B., Mello, R. P., Bandeira, I. D., Caliman-Fontes, A. T., Carneiro, B. A., et al. (2023). Arketamine as adjunctive therapy for treatment-resistant depression: a placebo-controlled pilot study. J. Affect. Disord. 330, 7–15. doi:10.1016/j.jad.2023.02.151
Li, L., and Vlisides, P. (2016). Ketamine: 50 years of modulating the mind. Front. Hum. Neurosci. 10, 612. doi:10.3389/fnhum.2016.00612
Li, S. H., Abd-Elrahman, K. S., and Ferguson, S. S. G. (2022). Targeting mGluR2/3 for treatment of neurodegenerative and neuropsychiatric diseases. Pharmacol. Ther. 239, 108275. doi:10.1016/j.pharmthera.2022.108275
Lin, P., Ma, Z., Mahgoub, M., Kavalali, E., and Monteggia, L. (2021). A synaptic locus for TrkB signaling underlying ketamine rapid antidepressant action. Cell Rep. 36 (7), 109513. doi:10.1016/j.celrep.2021.109513
Lu, Y., Ding, X., Wu, X., and Huang, S. (2020). Ketamine inhibits LPS-mediated BV2 microglial inflammation via NMDA receptor blockage. Fundam. Clin. Pharmacol. 34 (2), 229–237. doi:10.1111/fcp.12508
Ma, L., Wang, L., Chang, L., Shan, J., Qu, Y., Wang, X., et al. (2022a). A role of microRNA-149 in the prefrontal cortex for prophylactic actions of (R)-ketamine in inflammation model. Neuropharmacology 219, 109250. doi:10.1016/j.neuropharm.2022.109250
Ma, L., Wang, L., Chang, L., Shan, J., Qu, Y., Wang, X., et al. (2022b). A key role of miR-132-5p in the prefrontal cortex for persistent prophylactic actions of (R)-ketamine in mice. Transl. Psychiatry 12 (1), 417. doi:10.1038/s41398-022-02192-6
Ma, L., Zhang, J., Fujita, Y., Qu, Y., Shan, J., Wan, X., et al. (2022c). Nuclear factor of activated T cells 4 in the prefrontal cortex is required for prophylactic actions of (R)-ketamine. Transl. Psychiatry 12 (1), 27. doi:10.1038/s41398-022-01803-6
Menon, V., and Uddin, L. Q. (2010). Saliency, switching, attention and control: a network model of insula function. Brain Struct. Funct. 214 (5-6), 655–667. doi:10.1007/s00429-010-0262-0
Mion, G. (2017). History of anaesthesia: the ketamine story - past, present and future. Eur. J. Anaesthesiol. 34 (9), 571–575. doi:10.1097/eja.0000000000000638
Mitre, M., Mariga, A., and Chao, M. V. (2017). Neurotrophin signalling: novel insights into mechanisms and pathophysiology. Clin. Sci. (Lond.) 131 (1), 13–23. doi:10.1042/cs20160044
Mollon, J., and Reichenberg, A. (2018). Cognitive development prior to onset of psychosis. Psychol. Med. 48 (3), 392–403. doi:10.1017/s0033291717001970
Nakada, S., Ho, F. K., Celis-Morales, C., Jackson, C. A., and Pell, J. P. (2023). Individual and joint associations of anxiety disorder and depression with cardiovascular disease: a UK Biobank prospective cohort study. Eur. Psychiatry 66 (1), e54. doi:10.1192/j.eurpsy.2023.2425
Netease (2019). Hengrui Medicine: (R)-ketamine hydrochloride nasal spray receives notice of clinical trial. Available at: https://www.163.com/dy/article/EF2UQAAL05387CG0.html (Accessed May 13, 2019).
Nevell, L., Zhang, K., Aiello, A. E., Koenen, K., Galea, S., Soliven, R., et al. (2014). Elevated systemic expression of ER stress related genes is associated with stress-related mental disorders in the Detroit Neighborhood Health Study. Psychoneuroendocrinology 43, 62–70. doi:10.1016/j.psyneuen.2014.01.013
Oh, B., Santhanam, S., Azadian, M., Swaminathan, V., Lee, A., McConnell, K., et al. (2022). Electrical modulation of transplanted stem cells improves functional recovery in a rodent model of stroke. Nat. Commun. 13 (1), 1366. doi:10.1038/s41467-022-29017-w
Olofsen, E., Kamp, J., Henthorn, T., van Velzen, M., Niesters, M., Sarton, E., et al. (2022). Ketamine psychedelic and antinociceptive effects are connected. Anesthesiology 136, 792–801. doi:10.1097/aln.0000000000004176
Ortoleva, J. (2020). Consider Adjunctive Ketamine in mechanically ventilated coronavirus disease-2019 Patients. J. Cardiothorac. Vasc. Anesth. 34 (10), 2580. doi:10.1053/j.jvca.2020.04.037
Pałucha-Poniewiera, A., Bobula, B., and Rafało-Ulińska, A. (2023). The antidepressant-like activity and cognitive enhancing effects of the combined administration of (R)-Ketamine and LY341495 in the CUMS model of depression in mice are related to the modulation of excitatory synaptic transmission and LTP in the PFC. Pharm. (Basel) 16 (2), 288. doi:10.3390/ph16020288
Passie, T., Adams, H., Logemann, F., Brandt, S., Wiese, B., and Karst, M. (2021). Comparative effects of (S)-ketamine and racemic (R/S)-ketamine on psychopathology, state of consciousness and neurocognitive performance in healthy volunteers. Eur. Neuropsychopharmacol. 44, 92–104. doi:10.1016/j.euroneuro.2021.01.005
Pham, T. H., Defaix, C., Xu, X., Deng, S. X., Fabresse, N., Alvarez, J. C., et al. (2018). Common neurotransmission recruited in (R,S)-ketamine and (2R,6R)-hydroxynorketamine-induced sustained antidepressant-like effects. Biol. Psychiatry 84 (1), e3–e6. doi:10.1016/j.biopsych.2017.10.020
Phillips, J. L., Norris, S., Talbot, J., Birmingham, M., Hatchard, T., Ortiz, A., et al. (2019). Single, repeated, and maintenance ketamine infusions for treatment-resistant depression: a Randomized Controlled Trial. Am. J. Psychiatry 176 (5), 401–409. doi:10.1176/appi.ajp.2018.18070834
Phillips, J. L., Norris, S., Talbot, J., Hatchard, T., Ortiz, A., Birmingham, M., et al. (2020). Single and repeated ketamine infusions for reduction of suicidal ideation in treatment-resistant depression. Neuropsychopharmacology 45 (4), 606–612. doi:10.1038/s41386-019-0570-x
Phillips, K. F., and Deshpande, L. S. (2016). Repeated low-dose organophosphate DFP exposure leads to the development of depression and cognitive impairment in a rat model of Gulf War Illness. Neurotoxicology 52, 127–133. doi:10.1016/j.neuro.2015.11.014
Picciotto, M., Higley, M., and Mineur, Y. (2012). Acetylcholine as a neuromodulator: cholinergic signaling shapes nervous system function and behavior. Neuron 76 (1), 116–129. doi:10.1016/j.neuron.2012.08.036
Popik, P., Hogendorf, A., Bugno, R., Khoo, S., Zajdel, P., Malikowska-Racia, N., et al. (2022). Effects of ketamine optical isomers, psilocybin, psilocin and norpsilocin on time estimation and cognition in rats. Psychopharmacology 239, 1689–1703. doi:10.1007/s00213-021-06020-5
Popik, P., Khoo, S., Kuziak, A., Golebiowska, J., Potasiewicz, A., Hogendorf, A., et al. (2020). Distinct cognitive and discriminative stimulus effects of ketamine enantiomers in rats. Pharmacol. Biochem. Behav. 197, 173011. doi:10.1016/j.pbb.2020.173011
Pothorszki, D., Koncz, S., Török, D., Papp, N., and Bagdy, G. (2024). Unique effects of (R)-ketamine compared to (S)-ketamine on EEG theta power in rats. Pharm. (Basel) 17 (2), 194. doi:10.3390/ph17020194
Qu, Y., Shan, J., Wang, S., Chang, L., Pu, Y., Wang, X., et al. (2021). Rapid-acting and long-lasting antidepressant-like action of (R)-ketamine in Nrf2 knock-out mice: a role of TrkB signaling. Eur. Arch. Psychiatry Clin. Neurosci. 271 (3), 439–446. doi:10.1007/s00406-020-01208-w
Qu, Y., Yang, C., Ren, Q., Ma, M., Dong, C., and Hashimoto, K. (2017). Comparison of (R)-ketamine and lanicemine on depression-like phenotype and abnormal composition of gut microbiota in a social defeat stress model. Sci. Rep. 7 (1), 15725. doi:10.1038/s41598-017-16060-7
Rafało-Ulińska, A., Brański, P., and Pałucha-Poniewiera, A. (2022). Combined administration of (R)-ketamine and the mGlu2/3 receptor antagonist LY341495 induces rapid and sustained effects in the CUMS model of depression via a TrkB/BDNF-dependent mechanism. Pharm. (Basel) 15 (2), 125. doi:10.3390/ph15020125
Rafało-Ulińska, A., and Pałucha-Poniewiera, A. (2022). The effectiveness of (R)-ketamine and its mechanism of action differ from those of (S)-ketamine in a chronic unpredictable mild stress model of depression in C57BL/6J mice. Behav. Brain Res. 418, 113633. doi:10.1016/j.bbr.2021.113633
Ribeiro, A., Zhu, J., Kronfol, M., Jahr, F., Younis, R., Hawkins, E., et al. (2020). Molecular mechanisms for the antidepressant-like effects of a low-dose ketamine treatment in a DFP-based rat model for Gulf War Illness. Neurotoxicology 80, 52–59. doi:10.1016/j.neuro.2020.06.011
Robinson, R., and Jorge, R. (2016). Post-stroke depression: a review. Am. J. Psychiatry 173 (3), 221–231. doi:10.1176/appi.ajp.2015.15030363
Robson, M. J., Elliott, M., Seminerio, M. J., and Matsumoto, R. R. (2012). Evaluation of sigma (σ) receptors in the antidepressant-like effects of ketamine in vitro and in vivo. Eur. Neuropsychopharmacol. 22 (4), 308–317. doi:10.1016/j.euroneuro.2011.08.002
Sabates, J., Chiu, W. H., Loi, S., Lampit, A., Gavelin, H. M., Chong, T., et al. (2023). The associations between neuropsychiatric symptoms and cognition in people with dementia: a systematic review and meta-analysis. Neuropsychol. Rev. doi:10.1007/s11065-023-09608-0
Schwenk, E. S., Viscusi, E. R., Buvanendran, A., Hurley, R. W., Wasan, A. D., Narouze, S., et al. (2018). Consensus guidelines on the use of intravenous ketamine infusions for acute pain management from the American society of regional anesthesia and pain medicine, the American Academy of pain medicine, and the American society of anesthesiologists. Reg. Anesth. Pain Med. 43 (5), 456–466. doi:10.1097/aap.0000000000000806
Scotton, E., Antqueviezc, B., Vasconcelos, M. F., Dalpiaz, G., Paul Géa, L., Ferraz Goularte, J., et al. (2022). Is (R)-ketamine a potential therapeutic agent for treatment-resistant depression with less detrimental side effects? A review of molecular mechanisms underlying ketamine and its enantiomers. Biochem. Pharmacol. 198, 114963. doi:10.1016/j.bcp.2022.114963
Shafique, H., Witkin, J., Smith, J., Kaniecki, K., Sporn, J., Holuj, M., et al. (2021). Rapid tolerance to behavioral effects of ethanol in rats: prevention by R-(-)-ketamine. Pharmacol. Biochem. Behav. 203, 173152. doi:10.1016/j.pbb.2021.173152
Shim, I. (2022). Distinct functions of S-ketamine and R-ketamine in mediating biobehavioral processes of drug dependency: comments on Bonaventura et al. Mol. Psychiatry. doi:10.1038/s41380-022-01629-0
Shirayama, Y., and Hashimoto, K. (2017). Effects of a single bilateral infusion of R-ketamine in the rat brain regions of a learned helplessness model of depression. Eur. Arch. Psychiatry Clin. Neurosci. 267 (2), 177–182. doi:10.1007/s00406-016-0718-1
Shirayama, Y., and Hashimoto, K. (2018). Lack of antidepressant effects of (2R,6R)-hydroxynorketamine in a rat learned helplessness model: comparison with (R)-ketamine. Int. J. Neuropsychopharmacol. 21 (1), 84–88. doi:10.1093/ijnp/pyx108
Shu, L., Li, T., Han, S., Ji, F., Pan, C., Zhang, B., et al. (2012). Inhibition of neuron-specific CREB dephosphorylation is involved in propofol and ketamine-induced neuroprotection against cerebral ischemic injuries of mice. Neurochem. Res. 37 (1), 49–58. doi:10.1007/s11064-011-0582-3
Sun, S., Li, Z., Xiao, Q., Tan, S., Hu, B., and Jin, H. (2023). An updated review on prediction and preventive treatment of post-stroke depression. Expert Rev. Neurother. 23, 721–739. doi:10.1080/14737175.2023.2234081
Svenningsson, P., Westman, E., Ballard, C., and Aarsland, D. (2012). Cognitive impairment in patients with Parkinson's disease: diagnosis, biomarkers, and treatment. Lancet Neurol. 11 (8), 697–707. doi:10.1016/s1474-4422(12)70152-7
Tan, Y., Fujita, Y., Pu, Y., Chang, L., Qu, Y., Wang, X., et al. (2022). Repeated intermittent administration of (R)-ketamine during juvenile and adolescent stages prevents schizophrenia-relevant phenotypes in adult offspring after maternal immune activation: a role of TrkB signaling. Eur. Arch. Psychiatry Clin. Neurosci. 272, 693–701. doi:10.1007/s00406-021-01365-6
Tan, Y., Fujita, Y., Qu, Y., Chang, L., Pu, Y., Wang, S., et al. (2020). Phencyclidine-induced cognitive deficits in mice are ameliorated by subsequent repeated intermittent administration of (R)-ketamine, but not (S)-ketamine: role of BDNF-TrkB signaling. Pharmacol. Biochem. Behav. 188, 172839. doi:10.1016/j.pbb.2019.172839
Tang, J., Xue, W., Xia, B., Ren, L., Tao, W., Chen, C., et al. (2015). Involvement of normalized NMDA receptor and mTOR-related signaling in rapid antidepressant effects of Yueju and ketamine on chronically stressed mice. Sci. Rep. 5, 13573. doi:10.1038/srep13573
Tang, X. H., Diao, Y. G., Ren, Z. Y., Zang, Y. Y., Zhang, G. F., Wang, X. M., et al. (2023). A role of GABAA receptor α1 subunit in the hippocampus for rapid-acting antidepressant-like effects of ketamine. Neuropharmacology 225, 109383. doi:10.1016/j.neuropharm.2022.109383
Turner, E. (2019). Esketamine for treatment-resistant depression: seven concerns about efficacy and FDA approval. Lancet Psychiatry 6 (12), 977–979. doi:10.1016/s2215-0366(19)30394-3
Tyler, M., Yourish, H., Ionescu, D., and Haggarty, S. (2017). Classics in chemical neuroscience: ketamine. ACS Chem. Neurosci. 8 (6), 1122–1134. doi:10.1021/acschemneuro.7b00074
Vecchia, D. D., Kanazawa, L. K. S., Wendler, E., de Almeida Soares Hocayen, P., Bruginski, E., Campos, F. R., et al. (2018). Effects of ketamine on vocal impairment, gait changes, and anhedonia induced by bilateral 6-OHDA infusion into the substantia nigra pars compacta in rats: therapeutic implications for Parkinson's disease. Behav. Brain Res. 342, 1–10. doi:10.1016/j.bbr.2017.12.041
Vecchia, D. D., Kanazawa, L. K. S., Wendler, E., Hocayen, P. A. S., Vital, M., Takahashi, R. N., et al. (2021). Ketamine reversed short-term memory impairment and depressive-like behavior in animal model of Parkinson's disease. Brain Res. Bull. 168, 63–73. doi:10.1016/j.brainresbull.2020.12.011
Vollenweider, F., Leenders, K., Oye, I., Hell, D., and Angst, J. (1997). Differential psychopathology and patterns of cerebral glucose utilisation produced by (S)- and (R)-ketamine in healthy volunteers using positron emission tomography (PET). Eur. Neuropsychopharmacol. 7 (1), 25–38. doi:10.1016/s0924-977x(96)00042-9
Wan, X., Eguchi, A., Chang, L., Mori, C., and Hashimoto, K. (2023). Beneficial effects of arketamine on the reduced bone mineral density in susceptible mice after chronic social defeat stress: role of the gut-microbiota-bone-brain axis. Neuropharmacology 228, 109466. doi:10.1016/j.neuropharm.2023.109466
Wan, X., Eguchi, A., Fujita, Y., Ma, L., Wang, X., Yang, Y., et al. (2022). Effects of (R)-ketamine on reduced bone mineral density in ovariectomized mice: a role of gut microbiota. Neuropharmacology 213, 109139. doi:10.1016/j.neuropharm.2022.109139
Wang, X., Chang, L., Tan, Y., Qu, Y., Shan, J., and Hashimoto, K. (2021). (R)-ketamine ameliorates the progression of experimental autoimmune encephalomyelitis in mice. Brain Res. Bull. 177, 316–323. doi:10.1016/j.brainresbull.2021.10.013
Wang, X., Chang, L., Wan, X., Tan, Y., Qu, Y., Shan, J., et al. (2022a). (R)-ketamine ameliorates demyelination and facilitates remyelination in cuprizone-treated mice: a role of gut-microbiota-brain axis. Neurobiol. Dis. 165, 105635. doi:10.1016/j.nbd.2022.105635
Wang, X., Yang, J., and Hashimoto, K. (2022b). (R)-ketamine as prophylactic and therapeutic drug for neurological disorders: beyond depression. Neurosci. Biobehav. Rev. 139, 104762. doi:10.1016/j.neubiorev.2022.104762
Wei, Y., Chang, L., and Hashimoto, K. (2020). A historical review of antidepressant effects of ketamine and its enantiomers. Pharmacol. Biochem. Behav. 190, 172870. doi:10.1016/j.pbb.2020.172870
Wei, Y., Chang, L., and Hashimoto, K. (2021a). Intranasal administration of transforming growth factor-β1 elicits rapid-acting antidepressant-like effects in a chronic social defeat stress model: a role of TrkB signaling. Eur. Neuropsychopharmacol. 50, 55–63. doi:10.1016/j.euroneuro.2021.04.010
Wei, Y., Chang, L., and Hashimoto, K. (2021b). Molecular mechanisms underlying the antidepressant actions of arketamine: beyond the NMDA receptor. Mol. Psychiatry. 27, 559–573. doi:10.1038/s41380-021-01121-1
Wei, Y., Wang, T., Liao, L., Fan, X., Chang, L., and Hashimoto, K. (2022). Brain-spleen axis in health and diseases: a review and future perspective. Brain Res. Bull. 182, 130–140. doi:10.1016/j.brainresbull.2022.02.008
White, P., Ham, J., Way, W., and Trevor, A. (1980). Pharmacology of ketamine isomers in surgical patients. Anesthesiology 52 (3), 231–239. doi:10.1097/00000542-198003000-00008
White, P., Schüttler, J., Shafer, A., Stanski, D., Horai, Y., and Trevor, A. (1985). Comparative pharmacology of the ketamine isomers. Studies in volunteers. Br. J. Anaesth. 57 (2), 197–203. doi:10.1093/bja/57.2.197
Wilkowska, A., Szałach Ł, P., and Cubała, W. J. (2021). Gut microbiota in depression: a focus on ketamine. Front. Behav. Neurosci. 15, 693362. doi:10.3389/fnbeh.2021.693362
Witkin, J., Kranzler, J., Kaniecki, K., Popik, P., Smith, J., Hashimoto, K., et al. (2020). R-(-)-ketamine modifies behavioral effects of morphine predicting efficacy as a novel therapy for opioid use disorder. Pharmacol. Biochem. Behav. 194, 172927. doi:10.1016/j.pbb.2020.172927
Xia, M., Liu, J., Mechelli, A., Sun, X., Ma, Q., Wang, X., et al. (2022). Connectome gradient dysfunction in major depression and its association with gene expression profiles and treatment outcomes. Mol. Psychiatry. 27, 1384–1393. doi:10.1038/s41380-022-01519-5
Xiong, Z., Chang, L., Qu, Y., Pu, Y., Wang, S., Fujita, Y., et al. (2020). Neuronal brain injury after cerebral ischemic stroke is ameliorated after subsequent administration of (R)-ketamine, but not (S)-ketamine. Pharmacol. Biochem. Behav. 191, 172904. doi:10.1016/j.pbb.2020.172904
Xiong, Z., Fujita, Y., Zhang, K., Pu, Y., Chang, L., Ma, M., et al. (2019). Beneficial effects of (R)-ketamine, but not its metabolite (2R,6R)-hydroxynorketamine, in the depression-like phenotype, inflammatory bone markers, and bone mineral density in a chronic social defeat stress model. Behav. Brain Res. 368, 111904. doi:10.1016/j.bbr.2019.111904
Yamaguchi, J., Toki, H., Qu, Y., Yang, C., Koike, H., Hashimoto, K., et al. (2018). (2R,6R)-hydroxynorketamine is not essential for the antidepressant actions of (R)-ketamine in mice. Neuropsychopharmacology 43 (9), 1900–1907. doi:10.1038/s41386-018-0084-y
Yang, C., Qu, Y., Abe, M., Nozawa, D., Chaki, S., and Hashimoto, K. (2017a). (R)-ketamine shows greater potency and longer lasting antidepressant effects than its metabolite (2R,6R)-hydroxynorketamine. Biol. Psychiatry 82 (5), e43–e44. doi:10.1016/j.biopsych.2016.12.020
Yang, C., Qu, Y., Fujita, Y., Ren, Q., Ma, M., Dong, C., et al. (2017b). Possible role of the gut microbiota-brain axis in the antidepressant effects of (R)-ketamine in a social defeat stress model. Transl. Psychiatry 7 (12), 1294. doi:10.1038/s41398-017-0031-4
Yang, C., Ren, Q., Qu, Y., Zhang, J., Ma, M., Dong, C., et al. (2018). Mechanistic target of rapamycin-independent antidepressant effects of (R)-ketamine in a social defeat stress model. Biol. Psychiatry 83 (1), 18–28. doi:10.1016/j.biopsych.2017.05.016
Yang, C., Shirayama, Y., Zhang, J., Ren, Q., Yao, W., Ma, M., et al. (2015). R-ketamine: a rapid-onset and sustained antidepressant without psychotomimetic side effects. Transl. Psychiatry 5, e632. doi:10.1038/tp.2015.136
Yang, Y., Maher, D. P., and Cohen, S. P. (2020). Emerging concepts on the use of ketamine for chronic pain. Expert Rev. Clin. Pharmacol. 13 (2), 135–146. doi:10.1080/17512433.2020.1717947
Yao, W., Cao, Q., Luo, S., He, L., Yang, C., Chen, J., et al. (2022). Microglial ERK-NRBP1-CREB-BDNF signaling in sustained antidepressant actions of (R)-ketamine. Mol. Psychiatry 27 (3), 1618–1629. doi:10.1038/s41380-021-01377-7
Yokoyama, R., Ago, Y., Igarashi, H., Higuchi, M., Tanuma, M., Shimazaki, Y., et al. (2024). (R)-ketamine restores anterior insular cortex activity and cognitive deficits in social isolation-reared mice. Mol. Psychiatry. doi:10.1038/s41380-024-02419-6
Yuan, S., Chen, J., Zeng, L., Zhou, C., Yu, S., and Fang, L. (2021). Association of bone mineral density and depression in different bone sites and ages: a meta-analysis. Food Sci. Nutr. 9 (9), 4780–4792. doi:10.1002/fsn3.2379
Zanos, P., and Gould, T. (2018). Mechanisms of ketamine action as an antidepressant. Mol. Psychiatry 23 (4), 801–811. doi:10.1038/mp.2017.255
Zanos, P., Highland, J., Liu, X., Troppoli, T., Georgiou, P., Lovett, J., et al. (2019a). (R)-ketamine exerts antidepressant actions partly via conversion to (2R,6R)-hydroxynorketamine, while causing adverse effects at sub-anaesthetic doses. Br. J. Pharmacol. 176 (14), 2573–2592. doi:10.1111/bph.14683
Zanos, P., Highland, J., Stewart, B., Georgiou, P., Jenne, C., Lovett, J., et al. (2019b). (2R,6R)-hydroxynorketamine exerts mGlu2 receptor-dependent antidepressant actions. Proc. Natl. Acad. Sci. U. S. A. 116 (13), 6441–6450. doi:10.1073/pnas.1819540116
Zanos, P., Moaddel, R., Morris, P., Riggs, L., Highland, J., Georgiou, P., et al. (2018). Ketamine and ketamine metabolite pharmacology: insights into therapeutic mechanisms. Pharmacol. Rev. 70 (3), 621–660. doi:10.1124/pr.117.015198
Zanos, P., Moaddel, R., Morris, P. J., Georgiou, P., Fischell, J., Elmer, G. I., et al. (2016). NMDAR inhibition-independent antidepressant actions of ketamine metabolites. Nature 533 (7604), 481–486. doi:10.1038/nature17998
Zhang, J., Li, S., and Hashimoto, K. (2014a). R (-)-ketamine shows greater potency and longer lasting antidepressant effects than S (+)-ketamine. Pharmacol. Biochem. Behav. 116, 137–141. doi:10.1016/j.pbb.2013.11.033
Zhang, J., Ma, L., Chang, L., Pu, Y., Qu, Y., and Hashimoto, K. (2020a). A key role of the subdiaphragmatic vagus nerve in the depression-like phenotype and abnormal composition of gut microbiota in mice after lipopolysaccharide administration. Transl. psychiatry 10 (1), 186. doi:10.1038/s41398-020-00878-3
Zhang, J., Ma, L., Hashimoto, Y., Wan, X., Shan, J., Qu, Y., et al. (2021a). (R)-ketamine ameliorates lethal inflammatory responses and multi-organ injury in mice induced by cecum ligation and puncture. Life Sci. 284, 119882. doi:10.1016/j.lfs.2021.119882
Zhang, J., Ma, L., Wan, X., Shan, J., Qu, Y., and Hashimoto, K. (2021b). (R)-ketamine attenuates LPS-induced endotoxin-derived delirium through inhibition of neuroinflammation. Psychopharmacol. 238 (10), 2743–2753. doi:10.1007/s00213-021-05889-6
Zhang, K., Fujita, Y., and Hashimoto, K. (2018a). Lack of metabolism in (R)-ketamine's antidepressant actions in a chronic social defeat stress model. Sci. Rep. 8 (1), 4007. doi:10.1038/s41598-018-22449-9
Zhang, K., Ma, M., Dong, C., and Hashimoto, K. (2018b). Role of inflammatory bone markers in the antidepressant actions of (R)-ketamine in a chronic social defeat stress model. Int. J. Neuropsychopharmacol. 21 (11), 1025–1030. doi:10.1093/ijnp/pyy065
Zhang, K., Sakamoto, A., Chang, L., Qu, Y., Wang, S., Pu, Y., et al. (2021c). Splenic NKG2D confers resilience versus susceptibility in mice after chronic social defeat stress: beneficial effects of (R)-ketamine. Eur. Arch. Psychiatry Clin. Neurosci. 271 (3), 447–456. doi:10.1007/s00406-019-01092-z
Zhang, K., Toki, H., Fujita, Y., Ma, M., Chang, L., Qu, Y., et al. (2018c). Lack of deuterium isotope effects in the antidepressant effects of (R)-ketamine in a chronic social defeat stress model. Psychopharmacol. 235 (11), 3177–3185. doi:10.1007/s00213-018-5017-2
Zhang, K., Yang, C., Chang, L., Sakamoto, A., Suzuki, T., Fujita, Y., et al. (2020b). Essential role of microglial transforming growth factor-β1 in antidepressant actions of (R)-ketamine and the novel antidepressant TGF-β1. Transl. psychiatry 10 (1), 32. doi:10.1038/s41398-020-0733-x
Zhang, L. M., Wu, Z. Y., Liu, J. Z., Li, Y., Lv, J. M., Wang, L. Y., et al. (2023a). Subanesthetic dose of S-ketamine improved cognitive dysfunction via the inhibition of hippocampal astrocytosis in a mouse model of post-stroke chronic stress. J. Psychiatr. Res. 158, 1–14. doi:10.1016/j.jpsychires.2022.12.010
Zhang, T., Yang, R., Pan, J., and Huang, S. (2023b). Parkinson's disease related depression and anxiety: a 22-year bibliometric analysis (2000-2022). Neuropsychiatr. Dis. Treat. 19, 1477–1489. doi:10.2147/ndt.S403002
Zhang, Z., Zhang, L., Zhou, C., and Wu, H. (2014b). Ketamine inhibits LPS-induced HGMB1 release in vitro and in vivo. Int. Immunopharmacol. 23 (1), 14–26. doi:10.1016/j.intimp.2014.08.003
Zhao, Q., Xiang, H., Cai, Y., Meng, S. S., Zhang, Y., and Qiu, P. (2022). Systematic evaluation of the associations between mental disorders and dementia: an umbrella review of systematic reviews and meta-analyses. J. Affect. Disord. 307, 301–309. doi:10.1016/j.jad.2022.03.010
Zhornitsky, S., Tourjman, V., Pelletier, J., Assaf, R., Li, C. R., and Potvin, S. (2022). Acute effects of ketamine and esketamine on cognition in healthy subjects: a meta-analysis. Prog. Neuropsychopharmacol. Biol. Psychiatry 118, 110575. doi:10.1016/j.pnpbp.2022.110575
Zhou, L., Zhu, Y., Chen, W., and Tang, Y. (2021a). Emerging role of microRNAs in major depressive disorder and its implication on diagnosis and therapeutic response. J. Affect. Disord. 286, 80–86. doi:10.1016/j.jad.2021.02.063
Zhou, X., Zhang, C., Miao, J., Chen, Z., Dong, H., and Liu, C. (2021b). The sustained antidepressant effects of ketamine are independent of the lateral habenula. J. Neurosci. 41 (18), 4131–4140. doi:10.1523/jneurosci.2521-20.2021
Keywords: (R)-ketamine, antidepressant efficacy, treatment-resistant depression (TRD), side effects, ischemic stroke, cognitive disorders
Citation: Zhang S, Pu Y, Liu J, Li L, An C, Wu Y, Zhang W, Zhang W, Qu S and Yan W (2024) Exploring the multifaceted potential of (R)-ketamine beyond antidepressant applications. Front. Pharmacol. 15:1337749. doi: 10.3389/fphar.2024.1337749
Received: 13 November 2023; Accepted: 01 April 2024;
Published: 11 April 2024.
Edited by:
Rui Liu, Chinese Academy of Medical Sciences and Peking Union Medical College, ChinaReviewed by:
Xiaolei Zhu, Johns Hopkins University, United StatesRaquel Romay-Tallon, Rush University, United States
Copyright © 2024 Zhang, Pu, Liu, Li, An, Wu, Zhang, Zhang, Qu and Yan. This is an open-access article distributed under the terms of the Creative Commons Attribution License (CC BY). The use, distribution or reproduction in other forums is permitted, provided the original author(s) and the copyright owner(s) are credited and that the original publication in this journal is cited, in accordance with accepted academic practice. No use, distribution or reproduction is permitted which does not comply with these terms.
*Correspondence: Wenjun Yan, MTM4OTMzNzA3MDBAMTYzLmNvbQ==