- 1Department of Veterinary Pathology, Sindh Agriculture University Tandojam, Hyderabad, Pakistan
- 2Department of Pathobiology, University of Poonch Rawalakot, Rawalakot, Pakistan
- 3Department of Botany, University of Poonch Rawalakot, Rawalakot, Pakistan
Background: Kashmir Himalaya hosts the most diverse and rich flora in the world, which serves as grazing land for millions of small ruminants in the area. While most plant species are beneficial, some can be poisonous, causing economic losses and animal health issues for livestock. Consequently, this study is the first comprehensive report on the traditional phyto-toxicological knowledge in District Muzaffarabad and the assessment of its authenticity through experimental studies in rats.
Methods: The data regarding traditional knowledge was gathered from 70 key respondents through semi-structured interviews, which was quantitatively analyzed and authenticated through plant extract testing on Wistar female rats and comparison with published resources.
Results: A total of 46 poisonous plant species belonging to 23 families and 38 genera were reported to be poisonous in the study area. Results revealed that leaves were the most toxic plant parts (24 species, 52.1%), followed by the whole plant (18 species, 39.1%), stem (17 species, 36.9%), and seeds (10 species, 21.7%). At the organ level, liver as most susceptible affected by 13 species (28.2%), followed by the gastrointestinal tract (15 species, 32.6%), nervous system (13 species, 8.2%), dermis (8 species, 17.3%), renal (7 species, 15.2%), respiratory (4 species, 8.7%), cardiovascular system (3 species, 6.5%), and reproductive system (2 species, 4.3%). The poisonous plant species with high Relative frequency citation (RFC) and fidelity level (FL) were Nerium oleander (RFC, 0.6; FL, 100), Lantana camara (RFC, 0.6; FL, 100), and Ricinus communis (RFC, 0.6; FL, 100). Experimental assessment of acute toxicity assay in rats revealed that Nerium oleander was the most toxic plant with LD50 of (4,000 mg/kg), trailed by Ricinus communis (4,200 mg/kg), L. camara (4,500 mg/kg), and Datura stramonium (4,700 mg/kg); however, other plants showed moderate to mild toxicity. The major clinical observations were anorexia, piloerection, dyspnea, salivation, tachypnea, constipation, diarrhea, tremor, itchiness, and dullness.
Conclusion: This study showed that numerous poisonous plants pose a significant risk to the livestock industry within Himalayan territory, leading to substantial economic losses. Consequently, it is of utmost importance to conduct further comprehensive studies on the phytotoxicity of plants.
Introduction
Plants serve as the primary food source for herbivores and are also used for the treatment of many diseases (Elshafie et al., 2023). Nonetheless, some plants are also known for their toxicity in ruminants due to the presence of toxic compounds (Dubey et al., 2018). These toxins can have detrimental effects on the ruminants, leading to economic losses such as health deterioration, low productivity, deformed offspring, and even death (Kebede et al., 2015; Paim et al., 2023).
Ethnoveterinary studies involve the exploration and documentation of traditional knowledge and practices related to using plants, minerals, and other natural resources for managing animal health and wellbeing (Teuscher and Lindequist, 2023). These studies have gained significance due to their potential to offer sustainable and cost-effective solutions for livestock healthcare in rural and resource-limited areas. However, alongside the benefits, it is essential to address the potential risks associated with the use of plant-based remedies, including the risk of poisoning (Uprety et al., 2022).
Although the livestock are selective feeders while grazing or browzing in rangelands, occasional livestock poisonings are widespread worldwide in free-range systems due to poisonous plants (McKenzie, 2020). These cases may occur when preferred plant species are scarce during dry seasons, forcing animals to browse perennial shrubs and bushes rich in toxic secondary metabolites (Oladosu et al., 2016), causing production loss, morbidity, and mortality in animals (Dubey et al., 2018). The poisonous plants may sometimes be eaten accidentally (Nielsen et al., 2019). In addition, nomads and villagers may not be familiar with potentially toxic plants during hay making (Majeed et al., 2020). Thus, the most important factors that expose ruminants to plant poisoning are feed shortage, nutritional deficiency, and sudden exposure to plants (Stegelmeier et al., 2013).
Plant toxins might be synthesized within the plant itself, produced by microorganisms associated with animals, or simply incorporated and accumulated by the plant (Onyeyili et al., 2018). Plants can be categorized into different groups based on their poisonous principle (Oladosu et al., 2016). These toxic properties tend to be chemically identical or even similar within a single group of related plant species (Botha and Penrith, 2008), the nature of these toxins changes with respect to their origin and surrounding environmental conditions (Desta, 2019). The primary toxic compounds that serve as defense mechanisms in plants against herbivores include tannins (Kara and Sürmen, 2019), phenolics (Stevenson et al., 2017), alkaloids (Matsuura and Fett-Neto, 2015), phytohemagglutinins (Dubey et al., 2018), terpenes (Dearing and Weinstein, 2022), cyanogenic glycosides (Gershenzon, 2017), and oxalates (Karabourniotis et al., 2020).
Plant poisoning has the potential to impact a wide range of body systems. Numerous factors contribute to the toxicity of plants in livestock (Botha and Penrith, 2008). Among ruminants, the severity of plant toxicity can differ based on the animal species or breed, age, gender, and overall health status (Leong et al., 2017). Further, plant growth conditions, developmental stages, specific parts of the plant, and consumption amounts are all critical factors that influence the outcome (Dixit, 2007). The poisoning is also influenced by various chemical factors like size of particles, solubility, toxicity, rate of absorption and excretion, tendency to bind with body tissues or fluids, and absence of established metabolic processes (Khan et al., 2018). Diagnosis of plant-induced livestock poisoning involves considering the animals’ history, observed clinical symptoms, postmortem findings, signs of plant consumption through grazing or browsing, and the presence of toxic plants in the gastrointestinal tract (Botha and Penrith, 2008).
The harmful effects of poisoning can appear in ways, such as through contact, ingestion, absorption, and inhalation (Khan et al., 2018). Animals that encounter plants may experience symptoms. For example, they might suffer from skin irritation if they touch the plants (Modi et al., 2009), or they could be internally poisoned if they consume them (Crosera et al., 2009). There is a large diversity of plant toxins that can harm body systems in myriad ways. Aristolochic acid found in Aristolochia fangchi S.M.Hwang (Nortier et al., 2000) and Aristolochia clematitis L. has carcinogenic properties (Gluhovschi et al., 2010). Those who consume herbal remedies laced with A. fangchi or animals that consume crops laced with A. clematitis suffer from fatal urothelial neoplasms (Nortier et al., 2000). Ricinol, which is derived from Ricinus communis L. is responsible for a variety of symptoms, including gastrointestinal irritation, anorexia, apathy, dyspnea, piloerection, abortion, and acute purgation (Khan et al., 2018). Lantadenes from Lantana. camara L. cause hepatotoxicity, chronic cholestasis, piloerection, and photosensitivity (Khan et al., 2018). Leptopus cordifolius Decne. has a major toxin known as cicutoxin that causes muscle weakness, piloerection, bone lesions, aneurysms, and burning sensations (Rahman et al., 2021). The Datura stramonium L. contains sopolamine and hyoscyamine, which cause dermatitis, polydipsia, mydriasis, anorexia, abdominal pain, stupefaction, and restlessness (Murtala et al., 2023). Oleandrin from Nerium oleander L. causes a variety of effects, including piloerection, stomach salivation, vomiting, diarrhea, irregular heartbeats, drowsiness, tremors, seizures, seizures, coma, hepatotoxicity, and nephrotoxicity (Abdou et al., 2019). The cardiotoxic effect of Nerium oleander, for example, may cause death by severe cardiac arrhythmias (Shridhar, 2022).
The Muzaffarabad division in Azad Kashmir is rich in plant diversity, including a wide variety of potentially toxic plant species. These plants can be a threat to both animal health and the livestock industry in the region. Prior to our research, no comprehensive study had been conducted to identify and describe the native poisonous plants in Azad Jammu and Kashmir. Therefore, this study represents a pioneering effort to bridge this research gap by identifying these poisonous plants and thoroughly examining the harmful impacts of plant poisoning on animal wellbeing. The information regarding indigenous poisonous plants was collected from livestock holders through periodic surveys and validated through existing literature. Additionally, the accuracy of knowledge held by livestock keepers and available in published reports was assessed by testing the toxicity of selected poisonous plants through acute toxicity assay in Wistar female rats.
Materials and methods
Ethics approval and consent to participate
Formal consent was received from informants regarding data collection and publication, and the Participatory Rural Appraisal (PRA) approach, as mentioned in the Kyoto Protocol, was applied with the consent of the informant. Additionally, the study was approved by the Ethical committee and Board of Advance Studies and Research, Sindh Agriculture University Tandojam, in its 144th meeting under serial number DAS/937/2022.
Study area
Muzaffarabad is the capital of Azad Jammu and Kashmir, Pakistan (Figure 1). It is located in the western Himalayan range, between latitude 34º03′-34º35′N and longitude 73º23′-73º45′E, with a land area of around 1,642 km2. The study area exhibits a wide range of elevations, with the lowest point located in the southern Kohala locality at 582 m above sea level (m. a.s.l.) The highest points in the region are found at the summits of Makra Mountain and Neela Ganja Mountain, which reach heights of 3,819 m and 4,473 m, respectively. The variation in elevation across the study area has important implications for a range of ecological processes, including temperature gradients, precipitation patterns, and vegetation distribution. It also significantly impacts human activities such as agriculture and transportation infrastructure.
The bordered by the Muzaffarabad district with Hattian Bala district to the east, Neelum district to the northeast, Hazara division in Khyber Pakhtunkhwa (KPK) to the north and northwest, Bagh district to the south, and Murree hills in Punjab to the southwest. The topography is distinguished by a scenic landscape, with mountains ranging from subtropical valleys to the fascinating alpine zones of the Himalayas. Natural beauty abounds in the region, with dense forests, swiftly flowing rivers, and meandering streams. The Jhelum and the Neelum are the two notable rivers in the area (Khan et al., 2018).
The climate in Muzaffarabad district is subtropical highland. Temperatures range from −2–40°C between January and August. The average annual rainfall ranges between 1,000 and 1,300 mm, with the majority falling between May and August (around 680 mm). Winds blow from west to east during the day and from southeast to north at night. The wind speed is higher in the afternoon than in the morning. The snow line is approximately 1,200 m above sea level in winter and approximately 3,300 m. a.s.l. in summer (Qasim et al., 2010; AJK, 2015).
Survey for ethnoveterinary data collection and preservation
A thorough survey was conducted between August 2018 and January 2021 to gather data on livestock species, including goats, cows, sheep, buffaloes, and camels. The primary goal of the survey was to catalog and gather information on poisonous species and the risks involved. In 20 different places throughout the Muzaffarabad division, a total of 70 interviews with local herders and farmers were conducted. An observational field walk was included in the interviews, which used a semi-structured questionnaire. 40 skilled women and 30 highly experienced men, all between the ages of 30 and 65 years, participated in the study and had in-depth knowledge of raising livestock. The interviewers were an important part of the team that helped collect and identify plant specimens. The informants were chosen using the snowball method, and they came from various backgrounds and occupations, including farmers, livestock owners, veterinary professionals, and nomads (Bakarwals). Each subject gave informed consent prior to the interview, and the study complied with the International Society of Ethnobiology’s Code of Ethics (Mattalia et al., 2020).
Local names, usage, availability, and other valuable information about the plants, including toxic plant parts and plant toxicity levels, the type of animal affected, the nature of the disease, the type of symptoms that manifested, etc., were recorded on-site during the interviews. The plant species were collected, identified, noted, dried, pressed, and mounted on typical herbarium sheets. Plant identification was done by Dr. Sajjad Hussain, Assistant Professor and curator of the Poonch Herbarium, Department fo Botany, University of Poonch Rawalakot. Additionally, the Flora of India (https://sites.google.com/site/efloraofindia/), the Flora of Pakistan (Ali and Nasir, 1980), as well as the World Flora Online (https://www.worldfloraonline.org/) were used in the identification process. For the voucher specimens, standard herbarium processes were strictly followed. The voucher samples were kept in the University of Poonch Herbarium in the Department of Botany, University of Poonch Rawalakot, for future use.
Quantitative analyses of survey data
Relative frequency citation (RFC)
The data gathered was subjected to quantitative analysis using the Index of RFC, employing the formula provided below:
The RFC functions as a gauge for the particular importance of each species, gauged by how often they are mentioned in citations. The FC represents the proportion of survey participants who brought up the use of a specific species, divided by the total number of participants (N). It is worth mentioning that the analysis does not consider the various ways the species are used (Majeed et al., 2020).
Fidelity level (FL)
FL was assessed for frequently mentioned diseases or conditions using the approach outlined by Hoffman and Gallaher (2007). This indicates the percentage of individuals who identified a particular species as the cause of poisoning compared to the overall number of individuals who acknowledged the role of plant poisoning in affecting livestock (Khunoana et al., 2019).
In this context, Np is the count of individuals who associated a particular livestock ailment with plant poisoning, whereas N represents the overall count of individuals who witnessed disease symptoms or indicators in their livestock due to plant poisoning.
Priority ranking test
A priority ranking test was used to rank the top poisonous plants. In this test, 15 selected key respondents were asked to rank the plants based on the toxicity in the study area by following a method of Martin (1995).
Validation of folk knowledge through published scientific literature
For authenticity of the data obtained from informants on poisonous plants, a comprehensive literature review was conducted by systematically analyzing research papers from various electronic-based journals and books reporting information related to poisonous plants, their toxicity, and the symptoms of toxicity (Figure 2). Multiple search engines were utilized for a literature search, including Web of Science, Google Scholar, ResearchGate, PubMed, SciFinder, Scopus, Chemical Abstracts Services, books, dissertations, and technical reports. The search was performed using specific keywords, such as “poisonous plants,” “lethal dose LD50,” and “toxic compounds."
In every research article, we gathered information about various characteristics of each plant. These included their scientific names, family classification, names used locally, lifeform, the specific toxic plant parts, type of disease and animal affected, recommended dosage and levels of toxicity, specific toxin, and mechanism of action, clinical symptoms, susceptible organ and species.
Only those articles were selected that presented original research, were written in English, had undergone a peer-review process, and had full text available online through various scientific search engines. A total of 129 documents were ultimately selected for inclusion in this review, covering the period from 1981 to March 2023. In addition to academic research articles, this review’s basic framework was put together by consulting a range of books about local plants, broadcast news sources, and print media. Online botanical resources such as www.theplantlist.org, www.plantoftheworldonline.org, www.gbif.org, www.jstor.org, and www.tropics.org (accessed on 18 June 2023) were also used to gather data on other names for plants and their geographic distribution.
Assessment of acute toxicity of selected poisonous plants
Poisonous plant selection and preparation of extracts for acute toxicity assays
The 12 poisonous plants for toxicity assays were selected on the basis of a ranking based on a literature review, a survey from stakeholders, and the abundance of plants in the selected area. Poisonous plant leaves were collected from various areas in Muzaffarabad. Leaves were shade-dried at room temperature, and an electrical homogenizer was used to grind them to a powder. The ratio of the dried part’s (1.5 kg) weight to the original weight (4 kg) was calculated and then kept in glass bottles for further use in the experiment. The 120 g powder material was poured into a transparent glass bottle with 800 mL of 95% ethanol and soaked for 3 days. The glass bottle was labeled with a plant name. Further, the soaked material was filtered using a muslin cloth or filter paper. A rotary evaporator was used to remove the solvent (ethanol) from the flask. However, the extract was dried more thoroughly in the hot air oven at 65°C and stored at 40°C.
Selection and housing of animals (rats) for the experiment
Wistar female rats, aged 8–12 weeks, weighing 125–150 g, were obtained from DOW University of Health Sciences (DUHS), Karachi. These rats were maintained in the animal house of the Department of Veterinary Parasitology, Sindh Agriculture University, Tandojam. The animals were maintained under standard environmental conditions of a temperature of 24°C ± 10°C, a 12 h dark-light cycle, free access to drinking water, and a standard pelleted diet.
Screening of selected plants for toxicity by limit test
The limit test was performed as per guidelines of the Organisation for Economic Cooperation and Development (OECD) 423. The purpose of the limit test was to determine the toxicity of themostly reported plants, selected on the basis of the survey and literature review, by initially testing a dose of 2,000 mg/kg body weight and, as no deaths occurred in rats, finally a higher dose of 5,000 mg/kg body weight was tested showing higher signs of toxicity.
Preparation of doses and administration of treatments
The animals were weighed before the experiment (Table 1), and doses were calculated as per body weight. The ethanol extract of each plant was weighed, dissolved in 0.1 mL dimethylsulfoxide (DMSO), and then water was added to make a final volume of 1 mL. Rats were deprived of food and water 2 h before the experiment. The doses were orally administered to rats with the help of a 16-gauge gavage needle. The animals were examined for mortality and apparent changes at specific intervals, including the first, sixth, 12th, and 24th hour. Subsequently, the animals were monitored once a day for 2 days.
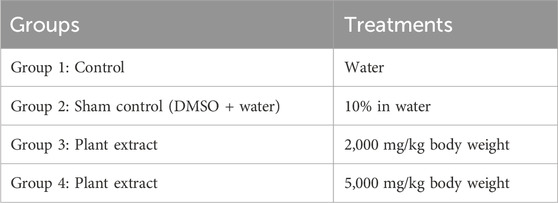
TABLE 1. Experimental design for determination of plant toxicity by Limit test showing different groups of animals orally administrated with different concentrations of extract (treatments).
Initially, for testing the dose of 2,000 mg/kg body weight, 36 rats were divided into 12 groups, each having three rats. Regular tap water was given to the control group, while 10% DMSO was added to water for the sham control group. The remaining treatment groups received a single oral dosage of various plant extracts (2,000 mg/kg body weight), as shown in Table 1. Following the OECD’s recommendations, the experiment was repeated using a new set of three rats for every different plant extract because no fatalities were observed, as outlined in Table 2.
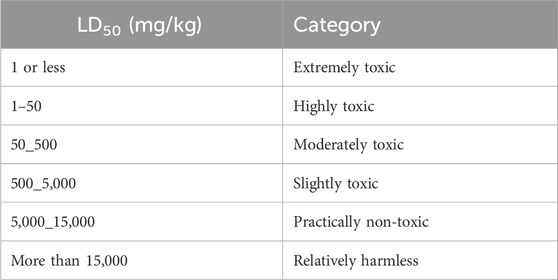
TABLE 2. Classification of toxicity of substances based on LD50 and dose ranges according to Organization for Economic Cooperation and Development (OECD) guidelines (Teke and Kuete, 2014).
Next, the 5,000 mg/kg body weight dose was tested. For this experiment, 30 rats were divided into 10 groups, each having three rats. The control group received regular tap water, while the sham control group was given 10% DMSO in water. Plant extracts (5,000 mg/kg body weight) were administered as a single oral dose for the remaining treatment groups. If the first animal survived, two further animals were dosed. If only one of the three animals died, the LD50 value was expected to exceed 5,000 mg/kg. If both animals died, then dosing proceeded at 2,000 mg/kg. If all three animals survived, the maximum dose administered was considered to be the LD50 value.
Determination of lethal dose 50 (LD50) through up-and-down method
Plant extracts causing mortality in rats at the dose of 5,000 mg/kg body weight were further tested using the up-and-down procedure following OECD test guidelines 425 (OECD, 2001) to determine the LD50. For this purpose, doses of 2,500, 3,000, 3,500, 4,000, 4,500, and 5,000 mg/kg were applied, respectively. The plants were classified into various toxicity grades as per the toxicity standards of OECD (Table 2). LD50 below 5,000 mg/kg is classified as highly toxic, while LD50 above 5,000 mg/kg is considered harmless (Ea et al., 2021).
The LD50 was calculated using the arithmetic method by (Alui and Nwude, 1982) using the formula LD50 = LD100-(Dd × Md)/N; where LD100 = dose that caused 100% mortality; N = number of animals per group; Dd × Md = dose difference (Dd) multiplied by mean death (Ea et al., 2021). LD50 is considered highly toxic if it is below 2,000 mg/kg, whereas it is considered safe if it exceeds 5,000 mg/kg (Hayes and Loomis, 1996).
Data analysis
The results were presented as the averages and the standard deviation (SD). The statistical significance was assessed through a one-way analysis of variance (ANOVA) followed by the post hoc least-significant difference (LSD) test. Results with p-values lower than 0.05 were regarded as significant.
Results
Demography
To collect data on plant poisoning affecting livestock across different areas of Muzaffarabad district, 70 participants were interviewed, consisting of 30 males and 40 females. This group encompassed various roles, including Gujjars (farmers), Bakkarwals (nomads), laboratory professionals, veterinarians, livestock holders, artificial inseminators, dairy owners, milk vendors, traditional healers, and agricultural experts. Notably, all traditional healers and veterinarians were of the male gender. The age spectrum of the respondents ranged from 20 to 80 years. Among the respondents, the predominant proportion, approximately 67.14%, lacked formal education, while the remaining individuals held masters or Ph.D. degrees (Table 3).
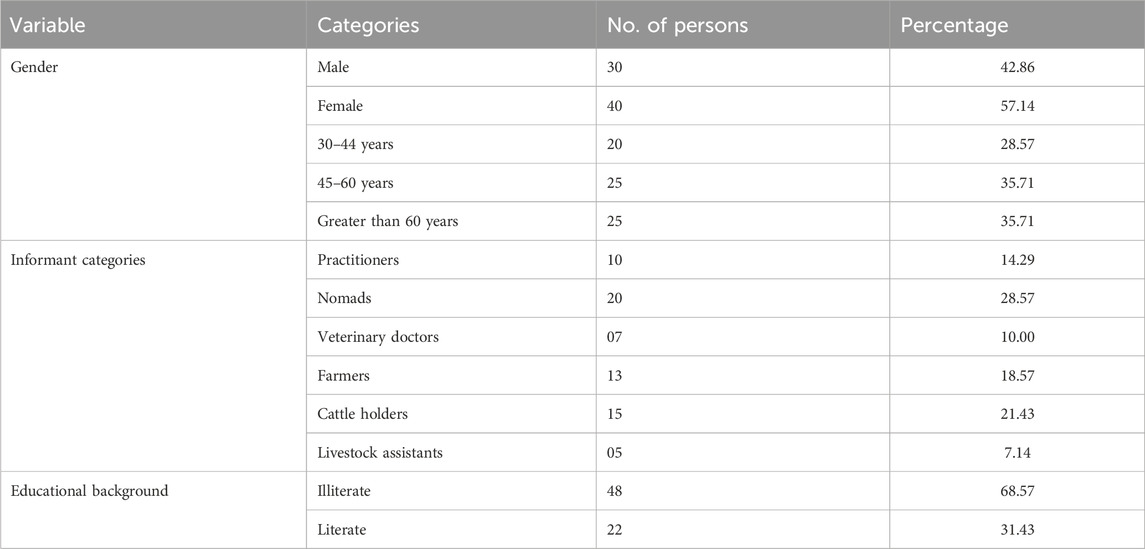
TABLE 3. Demographic profile of the selected informants from the study area showing their gender, categories, and educational background percentages.
Floristic contribution
A total of 46 poisonous plant species belonging to 23 distinct families and 38 genera were documented (Table 4). The most prevalent life forms were herbs (26 species, 57.8%), followed by shrubs (12 species, 25.5%) and trees (8 species, 17.0%), as illustrated in Figure 3. Among these species, the most common family was Asteraceae (5 species, 10.8%), followed by Ranunculaceae (4 species, 8.7%), Solanaceae (4 species, 8.7%), Fabaceae (4 species, 8.7%), Euphorbiaceae (4 species, 8.7%), and Convolvulaceae (3 species, 6.5%). The remaining families were represented by either one or two species each (Figure 4).
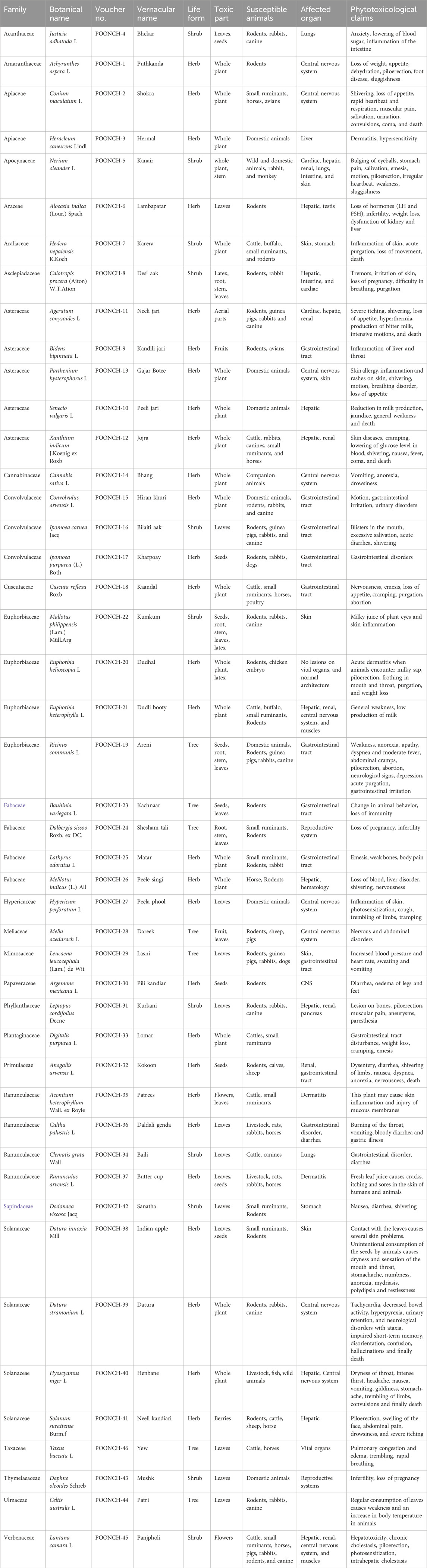
TABLE 4. Enlisted poisonous plants with their toxic parts, susceptible animals, affected organs, and their phytotoxicity reports by informants.
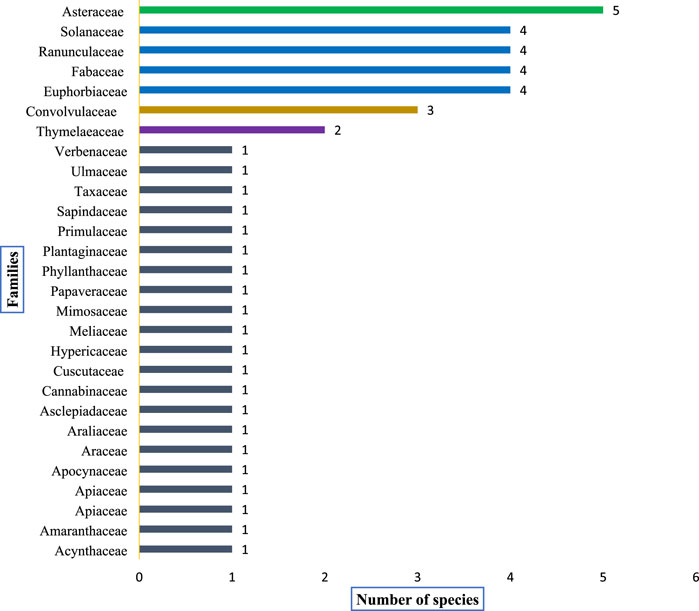
FIGURE 4. Shows dominant plant families and the distribution (number) of poisonous plants within each family recorded from the study area.
Toxic plant part (s) and most susceptible organs/systems
Plant toxicity in livestock was observed either in the entire plant or limited to some plant parts (Figure 5). The most toxic plant parts were leaves (24 species, 52.1%), followed by the whole plant (18 species, 39.1%), stem (17 species, 36.9%), seeds (10 species, 21.7%), roots (4 species, 8.7%), latex (3 species, 6.5%), fruit (2 species, 4.3%), flower (2 species, 4.3%), latex (2 species, 5.2%), flowers toxicity (1 species, 2.6%) and berries and aerial parts (1 species each, 2.1%). The most susceptible organ (Figure 6) was the liver, affected by 13 species (28.2%), followed by the gastrointestinal tract, affected by (15 species 32.6%), nervous system (13 species, 28.2%), dermis (8 species 17.3%), renal (7 species, 15.2%), respiratory (4 species, 8.7%), cardiovascular system (3 species, 6.5%), reproductive system (2 species, 4.3%).
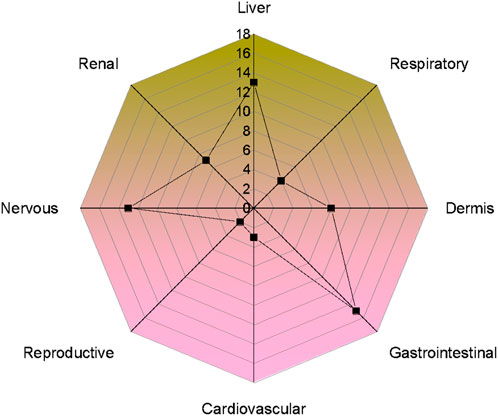
FIGURE 6. Number of poisonous plants causing different system/organ disorders in livestock of the study area.
Relative frequency of citations
N. oleander showed maximum RFC values (0.66), followed by L. camara (0.63), R. communis (0.60), D. stramonium (0.59), S. surattense (0.57), A. indica (0.54), L. cordifolius (0.53), P. hysterophorus (0.50), E. helioscopa (0.49), M. philippinensis (0.46), J. adhatoda (0.43), D. viscosa (0.39), and B. variegata (0.39) (Table 5).
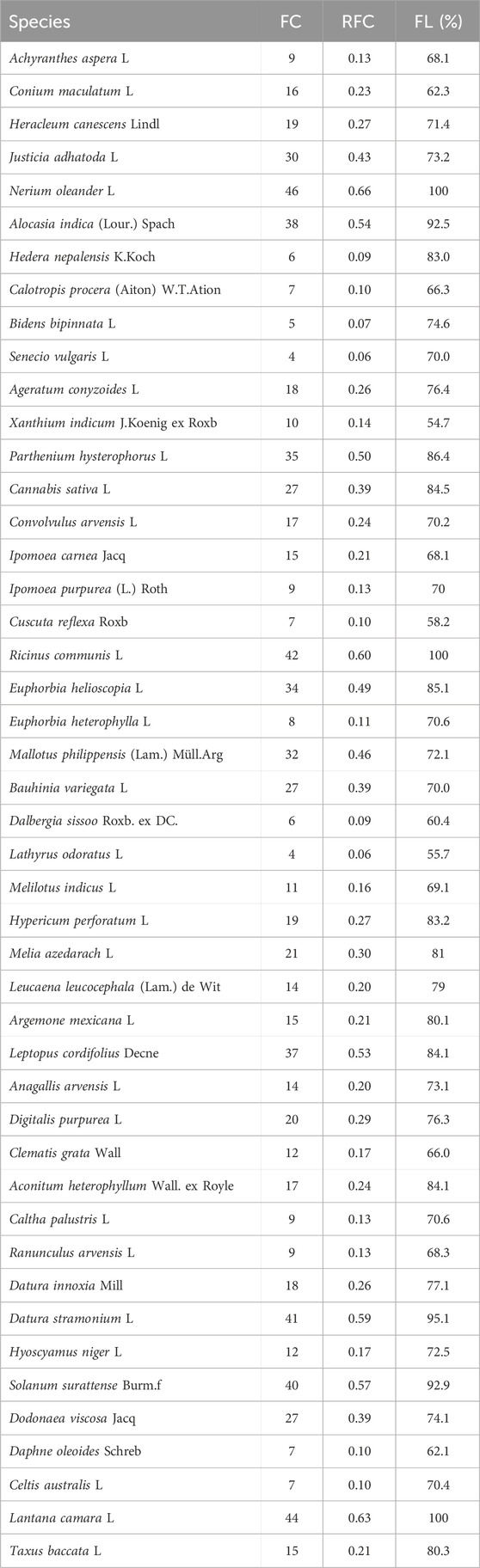
TABLE 5. List of poisonous plants showing Relative Frequency Citation (RFC) and Fidelity Level (FL). RFC shows the citing percentage (FC) of informants for a particular plant species while FL shows the percentage of informants who mentioned a certain poisonous plant for toxicity in livestock in the study area.
Fidelity level (FL)
The highly poisoned species were N. oleander (Fl, 100), L. camara (Fl, 100), R. communis (Fl, 100), D. stramonium (Fl, 95.1), S. surattense (Fl, 0.9), A. indica (Fl, 92.5), P. hysterophorus (Fl, 86.4), E. helioscopa (Fl, 85.1), L. cordifolius (Fl, 84.1), D. viscosa (Fl, 74.1), M. philippinensis (Fl, 72.1), J. adhatoda (Fl, 73.2), and B. variegata (Fl, 70.0) (Table 5).
Priority ranking test
In this test (Table 6), 15 selected key informants were asked to rank the plants based on their toxicity in the study area, following Martin (1995) and Alexiades (1996). The top poisonous plants were selected based on informant scoring, which depends on the toxicity of the plants, and their toxicity (LD50) was later confirmed from the literature survey and by performing acute toxicity assays. The total number of informants used was 15, and informants were asked to assign a score out of 100 according to their preference for toxicity. The top-ranked poisonous plant was L. camara (91), followed by R. communis (86), N. oleander (82), P. hysterophorus (79), D. stramonium (75), S. surattense (68), L. cordifolius (65), M. philippinensis (68), E. helioscopa (61), A. indica (55), J. adhatoda (44), D. viscosa (41), and B. variegata (39).
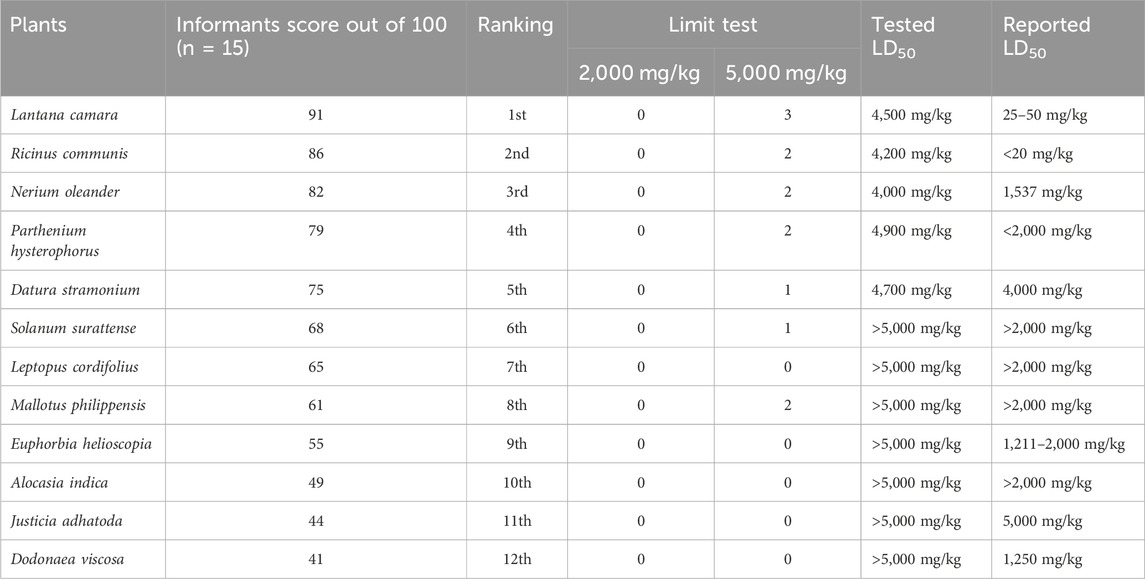
TABLE 6. List of highly poisonous plants showing informant scores and their ranking obtained from priority ranking test and acute toxicity assessment of these plants through a Limit test. In the Limit test, 0, 1, 2, and 3 show the number of mortalities in rats. Tested LD50 shows the lethality of these plants in rats obtained through the current experimental study, which is further compared with LD50 reported in different literature.
The LD50 of various plants, according to literature survey
As depicted in Table 6, R. communis, was the most toxic plant in the light of literature, out of 12 plants that were also tested for toxicity in the current study, with LD50 of less than 20 mg/kg. This plant showed different symptoms in livestock, such as general body weakness, loss of appetite, difficulty in breathing, mild fever, abdominal pain, cramps, piloerection, miscarriage, neurological issues, depression, acute purgation, and sudden diarrhea. L. camara, on the other side, with LD50 157.37 mg/kg, caused liver damage, bile flow obstruction, hair erection, light sensitivity, and accumulation of bile in the liver. N. oleander with an LD50 157.37 mg/kg causes bulging of the eyeballs, stomach pain, salivation, emesis, motion, piloerection, irregular heartbeat, weakness, and sluggishness. E. helioscopa, with LD50 of 1,211 mg/kg, caused acute dermatitis when animals encounter milky sap, piloerection, frothing in the mouth and throat, purgation, weight loss, and D. viscosa LD50 1,250 mg/kg caused nausea, vomiting, and shivering in animals. The rest of the plants showed LD50 > 2,000 mg/kg, which, according to OECD 423, is less toxic.
Limit test for determination of plant LD50
The female Wistar rats orally administered ethanol extract at a dose of 2,000 mg/kg body weight showed no signs of toxicity or mortality for all 12 plants tested for toxicity (Table 6; Figure 7). However, when a 5,000 mg/kg body weight dose was applied, signs of toxicity, mortality, and a significant reduction in body weight were found in various selected poisonous plants, namely, N. oleander, R. communis, L. camara, and D. stramonium. Moreover, the mortality of animals was observed within 10–24 h with severe clinical manifestations, i.e., anorexia, water intake, piloerection, dyspnea, salivation, tachypnea, constipation, diarrhea, tremors, itchy nose, and dullness. The severe toxic effect caused by N. oleander was followed by R. communis, L. camara, and D. stramonium, but other plants showed moderate to mild clinical manifestations. For the determination of the exact LD50 of each plant, extracts were tested through the Up-and-Down method. The LD50 for poisonous plants was found to be 4,000, 4,200, 4,500, and 4,700 mg/kg for N. oleander, R. communis, L. camara, and D. stramonium, respectively (Table 7).
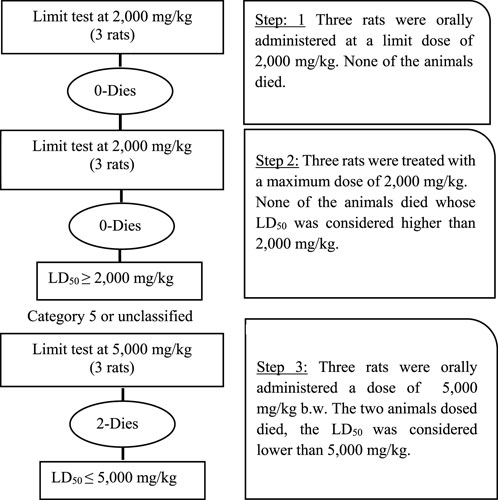
FIGURE 7. Systematic overview of acute toxicity in rats determined through limit test showing number of rats, dose concentration, and lethalities.
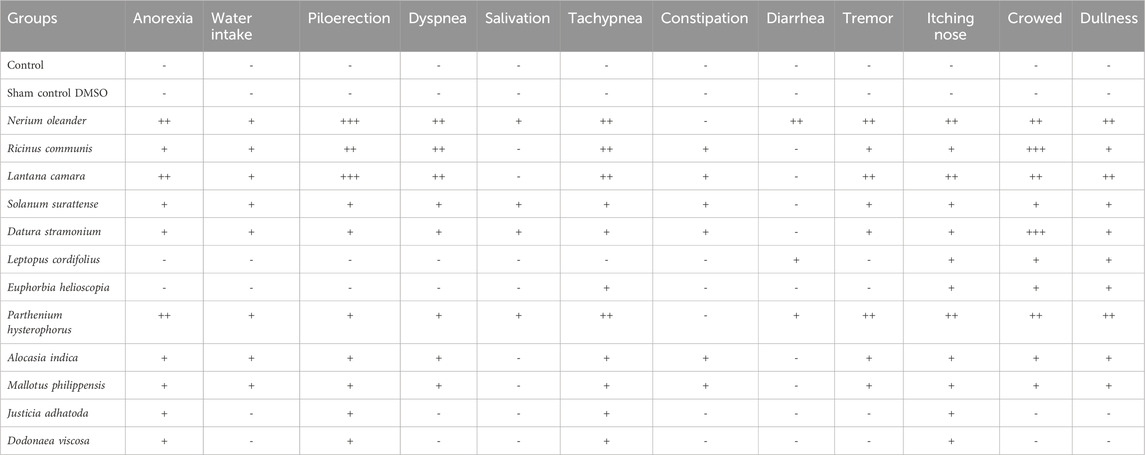
TABLE 7. Clinical symptoms of toxicity in different groups of rats treated with control, sham control, and poisonous plant extract. Where (−) shows no symptoms, (+) shows mild symptoms, (++) shows moderate symptoms, and (+++) shows severe symptoms.
Pearson’s correlation
Pearson’s correlation coefficient was computed to assess the relationship between the level of reported fidelity and RFC. The outcome reveals a substantial correlation between the FL and RFC variables, with a correlation coefficient of r = 0.9 (Figure 8).
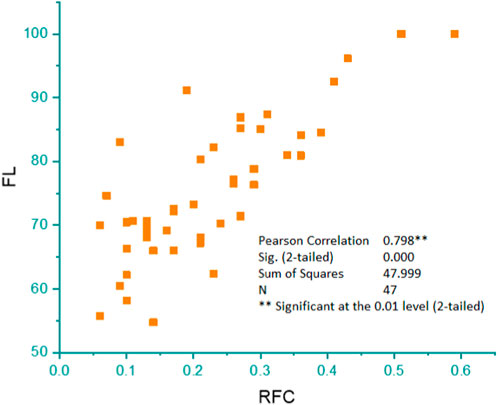
FIGURE 8. Pearson’s correlation showing the linear relationship between the percentage of informants who mentioned a certain poisonous plant species (FL) and the citing percentage of informants for a particular plant species (RFC).
Discussion
In our ecosystem, plants are essential for food, shelter, and aesthetic beauty (Saha et al., 2022). Nevertheless, not all plants are harmless when they interact with animals. Certainly, many plant species contain toxic compounds that can cause animals from mild irritation to severe illness or even death (Koshariya et al., 2023). Understanding plant toxicity in animals is critical for veterinarians, cattle holders, nomads, and wildlife managers to ensure the health and safety of both domestic and wild animals (Gupta, 2012; McKenzie, 2020). Our study area selection was motivated by the rich plant biodiversity and the lack of research on poisonous plants and their toxicity to livestock. As far as we know, no research has been conducted on plant phytotoxicity in District Muzaffarabad and its surroundings. A comprehensive study on the phytotoxicity of plants in this region is extremely important because this area has a significant number of small ruminants compared to other parts of Azad Jammu and Kashmir. It is crucial for farmers to be able to identify which plants are toxic for livestock and to take the necessary precautions to avoid them in order to prevent livestock from consuming them.
Demographic dynamics
In this study, 70 key informants interviewed were selected from both genders. Our informant selection is counter to the majority of ethno-veterinary studies, where men predominate. In the study area, women typically keep and care for animal herds, which can be explained by the ethnotoxicology study’s nature, cultural differences, and traditional roles in which women are responsible for household wellbeing and health. (Kefifa et al., 2020). The majority of informants were between the ages of 45 and 60 years. Age thus guarantees both a wealth of knowledge and expertise in such cases. These findings are consistent with those of Dansou et al. (2021) and Ouachinou et al. (2017), who reported that informants over the age of 40 are much more experienced. Kefifa et al. (2020) and Houndje et al. (2016) also revealed that most respondents were between 41 and 50–59 years old. This indicates that older people serve as sources of ethnomedical or ethno-veterinary wisdom, preserving knowledge of veterinary herbal medicine or toxicology over the years.
Plant diversity
This study reported 46 poisonous plants from 23 families and 38 genera. Asteraceae, Fabaceae, Ranunculaceae, Solanaceae, Euphorbiaceae, and Convolvulaceae were the most dominant families. Herbs were the most prevalent form of life, followed by shrubs and trees. Asteraceae has many species, including Bidens bipinnata, Senecio vulgaris, Ageratum conyzoides, Xanthium indicum, and P. hysterophorus. Asteraceae has been reported as a dominant plant family by many researches (Sema et al., 2018; Kefifa et al., 2020). There is a significant variation in floristic diversity within plant families depending on climate and study area (Dansou et al., 2021). This difference could also be explained by the diversity of socio-cultural clusters that differ from one nation to another since the utilization of natural plant resources for healing intentions might be linked with the knowledge of these socio-cultural groups. The Asteraceae, Solanaceae, Fabaceae, and Euphorbiaceae stand out as some of the most significant families of poisonous plants. Many researchers have previously documented the plants in these families as primarily responsible for plant poisoning (Wink, 2013; Parihaar et al., 2014; Diaz, 2015). Toxins such as alkaloids such as atropine, hyoscyamine, and scopolamine (Baloch et al., 2017), cynogenic glycosides like anthocyanins and anthrachinone (Randrianarivo et al., 2014; Serrano, 2018), saponin including both terpenoid (Randrianarivo et al., 2014) and steroids (Umair et al., 2017; Dusemund et al., 2018), and cyanogenic glucosides (Junior et al., 2022) make these families toxic.
Toxic plant parts and their toxicity
In the present study, phytotoxicity was confined to the entire plant or limited to some parts. The most toxic plant parts were the leaves, followed by the whole plant. The most susceptible systems to plant toxicity were the gastrointestinal tract, affected by 15 species; the liver affected by 13 species, and the nervous system affected by 13 species. It has been widely shown that leaves can be used for both therapeutic and grazing reasons. Leaves are metabolically dynamic plant parts (Alexiades, 1996). This is due to the fact that leaves are extremely nutrient-rich plant components that animals can readily chew through or swallow, unlike seeds and fruits (Matsuura et al., 2016). According to our research, most of the toxicity in livestock was produced by plants rich in cyanogenic chemicals, glycosides, saponins, and alkaloids. Animals exposed to alkaloids experience severe toxicity, which damages their liver and affects their neurological system (Adibah and Azzreena, 2019). In the majority of cases, they are lethal and cause depression, miscarriage, shivering, nausea, and immobility (Langford and Boor, 1996; Vetter, 2000; Baloch et al., 2017). Conversely, glycosides can induce paralysis, agitation, weakness, burning, tremors, and vision loss (Nguyen et al., 2020; Kharchoufa et al., 2021; Chen et al., 2023). Saponins can cause skin inflammation, liver issues, agitation, burning of skin, and necrosis, leading to kidney failure in livestock (Tadesse et al., 2017). Poisonous herbs and shrubs pose the greatest threat to grazing animals (Baloch et al., 2017; Bricarello et al., 2023). As in our case, both life forms were commonly available in the study area for cattle, whereas tree forage was typically offered to them by herders under food shortage.
Relative frequency of citation and fidelity level of plants
N. oleander showed maximum relative frequency of citation (RFC) values (0.6), followed by L. camara (0.6), D. stramonium (0.4), R. communis (0.6), S. surattense (0.5), A. indica (0.5), L. cordifolius (0.5), P. hysterophorus (0.5), E. helioscopa (0.4), M. philippinensis (0.4), J. adhatoda (0.4), Dodonaea viscosa (0.3), and B. variegata (0.3). For instance, if a researcher wanted to assess the phytotoxicity potential of these plants, they might choose to plant N. oleander, L. camara, or R. communis due to their high RFC values. Alternatively, a gardener looking for low-maintenance landscaping options might choose A. indica or L. cordifolius, as they still have relatively high RFC values. However, it is important to note that high RFC values do not always indicate desirable qualities. For example, P. hysterophorus has a high RFC value but is considered an invasive species in many parts of the world, causing harm to native plants and ecosystems. Similarly, D. stramonium is highly toxic and can be dangerous if ingested or handled improperly. Plants with high RFC value had a reputation for being poisonous among the local inhabitants of the region. These plants have the potential to serve as the foundation for subsequent evaluation of the assessment of phytochemical profiles and further toxicological studies (Panter et al., 2011). It is crucial to prioritize the conservation of these plants to ensure the sustainable use of these resources, as they also have therapeutic properties (Mukheriee and Wahile, 2006). Notably, N. oleander, L. camara, R. communis, D. stramonium, Solanum surattense, and A. indica were among the highly toxic species. Plants exhibiting higher FL are considered suitable for further ethno-toxicological research (Bibi et al., 2014). Plant fidelity level shows the degree of reliance and consistency of local communities on specific plant species for various purposes (Chaachouay et al., 2022). It also highlights the cultural, medicinal, and economic significance of plants within a given society (Leonti, 2022). The level of plant fidelity can vary widely among different cultures and regions, depending on factors such as traditional knowledge, the availability of resources, and environmental conditions (Pratama et al., 2021).
Experimental acute toxicity
The current study aimed to assess the toxicities of poisonous plants in District Muzaffarabad, AJK. To achieve this, Wistar rats were subjected to a limit test following OECD guidelines to determine the toxicity of the plant extracts. At a dose of 5,000 mg/kg body weight, N. oleander, R. communis, L. camara, and D. stramonium caused toxicity and mortality. In contrast, other plants showed moderate to mild clinical manifestations. Additionally, mortality of animals was observed within 10–24 h with severe clinical manifestations, i.e., anorexia, water intake, piloerection, dyspnea, salivation, tachypnea, constipation, diarrhea, tremor, itching nose, and dullness.
There is a large diversity in methodology and purposes of various experimental studies whereby the toxicity of plants has been studied. Studies use diverse methods of preparing plant extracts, doses, routes of administration, animal species, etc., which result in variable toxicity reported as LD50 of various plants. Bandara et al. (2010) and Ceci et al. (2020) reported that the clinical signs of oleander toxicity are basically the same in all species. For the determination of the exact LD50 of each plant through the fine-tuning method, LD50 was considered poisonous plants. N. oleander with 4,000 mg/kg, followed by R. communis, L. camara, and D. stramonium, were found highly poisonous, indicating that their LD50 is less than 5,000 mg/kg body weight for four plants. In the case of the N. oleander plant extract, different researchers have reported varying LD50 values (Ceci et al., 2020; Shridhar, 2022). The lethal doses of various dried N. oleander leaves vary depending on the animal species (LD50). Cattle are more susceptible than small ruminants, according to the LD50, which is 50 mg/kg for cattle, 110 mg/kg for goats, and 250 mg/kg for sheep (Oryan et al., 1996). The LD50 of oleander for mice is 4,000 mg/kg, according to research conducted by others. While several studies came to the semi-quantitative conclusion that 3–10 oleander leaves could be deadly, the LD50 of oleander leaves for cattle is 50 mg/kg. According to reports, the LD50 of oleander for sheep is 250 mg/kg. However, there is disagreement on the lethal dose of oleander (Shridhar, 2022). According to the toxicity and lethality indicators associated with the type of toxin present in the plant’s aerial parts, the lethal dose of Thevetia peruviana for rats and mice is 447 mg/kg body weight (Ea et al., 2021).
Different susceptibilities may be due to experimental conditions and methodological differences. In the case of N. oleander plant extract, the LD50 values reported by different researchers may have resulted from differences in the experimental conditions and methodology used. For example, the use of different animal species, routes of administration, and types of extracts can affect the LD50 values reported. Additionally, differences in the preparation of an extract, like the concentration and purity of the extract, can also contribute to differences in LD50 values. Various clinical manifestations, such as diarrhea, abdominal pain, irregular heartbeat, seizures, coma, and death, were observed in rats receiving treatments. According to Prasad et al. (2016), the signs of toxicity in rats that appeared after oral administration of the ethanol extract of the plant’s aerial parts included tremor, dyspnea, tachypnea, severe hypothermia, muscular relaxation, urinary retention, generalized seizures, and death.
In the current study, the LD50 of the R. communis plant was determined to be 4,200 mg/kg. Similarly, Tewari et al. (2005) reported a rat oral LD50 of 4,560 mg/kg for R. communis. According to Das et al. (2000), the LD50 of R. communis was found to be 4,750 mg/kg body weight in rats. Additionally, in disagreement with the current study, Polito et al. (2019) reported LD50 value of 2,000 mg/kg for an intraperitoneal administration of a ricin-containing fraction of R. communis seed extract in mice. Murade et al. (2021) determined the oral LD50 of R. communis leaves in mice to be 89.3 mg/kg. The oral LD50 of R. communis leaves in rats was reported to be 131.1 mg/kg by Riaz et al. (2012).
When administered intravenously, the LD50 of ricin in rats is roughly 1.78 mg/kg, and when administered orally, it is roughly 21.8 mg/kg (Worbs et al., 2011). The authors further reported that the rats are particularly susceptible to the toxic effects of ricin, and even small doses can result in severe illness or death. Lethargy, weakness, loss of appetite, diarrhea, seizures, and respiratory failure are all possible signs of ricin poisoning in rats. On the other hand, Sadashiv (2011) reported that mice given an ethanol extract of the aerial parts of the R. communis plant did not exhibit significantly different behaviors.
In the present study, the LD50 (4,500 mg/kg) was observed for L. camara, which is in line with the study of Sharma et al. (2007). On the other hand, Jawonisi and Adoga, (2017) also reported LD50 greater than 5,000 mg/kg body weight in the rats. Kumar et al. (2016) reported an LD50 value of 2,526 mg/kg for an aqueous extract of L. camara leaves administered intraperitoneally in rats. However, Pandeya et al. (2022) reported an LD50 value of 2,891 mg/kg for an ethanolic extract of L. camara leaves administered orally to mice. In the current study, we observed some signs of toxicity such as loss of body weight, tremors, piloerection, diarrhea, and food and water consumption in rats administrated with L. camara. Mukherjee et al. (2010) tested the toxicity of L. camara leaves in rats and found symptoms of toxicity, such as weight loss, decreased food intake, and altered liver function. The rats also exhibited neurological symptoms like tremors, convulsions, and paralysis. Lantadenes is the toxic principle in L. camara leaves (Bevilacqua et al., 2011; Pour et al., 2011). Similarly, Sharma et al. (2007) observed that the main hepatotoxic compound is lantadene A, a pentacyclic triterpenoid.
The LD50 for D. stramonium was found to be 4,700 mg/kg in the present study. Murtala et al. (2023) determined that the LD50 was greater than 2,000 mg/kg. According to Lian et al. (2022), typical lethal doses of Datura seeds ranging from 50 to 100 may have atropine. In the experiment, clinical manifestations such as decreased activity and lethargy, ataxia (loss of coordination), tremors or seizures, salivation and lacrimation (excessive salivation and tearing), mydriasis (dilated pupils), respiratory depression or difficulty breathing, diarrhea, coma, or death were observed (Friedman, 2004). D. stramonium could trigger tachycardia, difficulty breathing, convulsions, and a decrease in locomotor activity in the presence of high levels of alkaloids such as atropine, hyoscamine, and scopolamine (Ogunmoyole et al., 2019). S. surratense is known to contain toxic compounds such as chaconine and solanine (Parvez et al., 2019). In the current study, symptoms of toxicity, such as gastrointestinal upset, vomiting, diarrhea, and even more severe symptoms, such as seizures and respiratory depression, were observed in our experiment.
In the present study, the LD50 of L. cordifolius was determined through a limit test and is greater than 5,000 mg/kg. Khan et al. (2018) reported several bioactive compounds from L. cordifolius, including cicutoxin, chlorogenic acid, rutin, and quercetin. The limit test results showed that LD50 exceeded 5,000 mg/kg. These findings imply that L. cordifolius is reasonably safe because it takes a high dose to be fatal. Several toxic substances, including diterpenes, triterpenes, phorbol esters, and ingenol esters, are known to be present in the plant species Euphorbia helioscopia, which is a member of the Euphorbiaceae family. The milky sap of the plant contains the diterpene ester known as helioscopinolide, which is the most notable toxic substance in this plant (Morita et al., 2014). According to the study of Aleksandrov et al. (2019), rats at an oral dose of 1,000 mg/kg showed signs of toxicity such as lethargy, decreased appetite, and diarrhea. Our results depicted that P. hysterophorus extract administrated in to rats resulted in an LD50 of 4,900 mg/kg. According to Narasimhan et al. (1984), P. hysterophorus extract has a toxic LD50 of 1,500 mg/kg in mice. It contains the active ingredient in Parthenium, a sesquiterpene lactone called parthenin. Parthenin causes animal toxicity, including hepatotoxicity, nephrotoxicity, and neurotoxicity (Khaket et al., 2015). We also reported LD50 of A. indica greater than 5,000 mg/kg. According to reports, these plants contain glycosides such as arisaematoside, oxalate crystals that can cause mouth pain and diarrhea (Basu et al., 2014). Desai et al. (1988) observed restlessness and hyperactivity, convulsions and tremors, lethargy, depression, reduced food intake, weight loss, diarrhea, and impaired coordination and balance caused by A. indica.
The current study also reported Mallotus philippensis with LD50 greater than 5,000 mg/kg. According to Thakur et al. (2005), this plant at a 2,000 mg/kg dose caused mortality in all the animals within 24 h. In addition, Gangwar et al. (2014) reported that M. philippinensis administered orally to mice at a dose of 1,000 mg/kg caused death in 50% of the animals within 24 h.
The different behavior of plant poisoning reported in our experimental and already reported studies can be attributed to plant nature, growth stages, geographic location, environmental conditions, the abundance of species, and the species and breed of livestock are just a few of the variables that can affect livestock variation (Mensah et al., 2019; Griffiths et al., 2021). The complex interactions between the chemical makeup of plants and the metabolic function of animals cause variations in plant toxicity (Jung et al., 2020). Plant species have different levels of toxins that can affect livestock differently. Poisonous plants can be classified by active ingredients (cyanide, nitrate, oxalate, pyrrolizidine alkaloids, glycosides, etc.), target organs and systems (cardiotoxic, hepatotoxic, neurotoxic, teratogenic plants, etc.), or family and genus (Ohlsen et al., 2022).
Conclusion
It is evident from the present study that poisonous plants pose a serious threat to livestock in Muzaffarabad. Plants with active ingredients such as cyanide, nitrate, oxalate, pyrrolizidine alkaloids, and glycosides resulted in gastrointestinal, neurological, hepatotoxic, and dermatological disorders in livestock. N. oleander, L. camara, R. communis, D. stramonium, S. surattense, A. indica, L. cordifolius, and P. hysterophorus were the highly poisonous species reported from the study area. However, other species exhibited mild to moderate symptoms. The study also shows different plant poisonings in nature can be explained by the complex interactions between plants and animals, as well as their nature, growth stages, geographic location, environmental conditions, and livestock species. In order to gain a deeper understanding of traditional knowledge related to poisonous plants and their effects on animal health, future research needs to be conducted in appropriate experimental conditions involving relevant livestock species. Cattleholders should also be informed about toxic plant hazards to reduce the risk of poisoning and provided with appropriate management strategies. Establishing diagnostic laboratories equipped with advanced analytical techniques can help in the early diagnosis and treatment of plant-poisoned animals, reducing mortality and economic losses. The findings of this study emphasize the need for continuous plant research and monitoring.
Data availability statement
The original contributions presented in the study are included in the article/Supplementary material, further inquiries can be directed to the corresponding author.
Ethics statement
The animal study was approved by The study was approved by the Ethical committee and Board of Advance Study, and Research, Sindh Agriculture University Tandojam in its 144th meeting under serial number DAS/937/2022. The study was conducted in accordance with the local legislation and institutional requirements.
Author contributions
FR: Data curation, Formal Analysis, Investigation, Writing–original draft. KA: Conceptualization, Supervision, Writing–review and editing. ZN: Conceptualization, Methodology, Resources, Supervision, Writing–original draft, Writing–review and editing. FP: Project administration, Visualization, Writing–review and editing. SK: Project administration, Resources, Validation, Visualization, Writing–review and editing. NS: Resources, Software, Validation, Visualization, Writing–review and editing.
Funding
The author(s) declare that no financial support was received for the research, authorship, and/or publication of this article.
Acknowledgments
We acknowledge the University of Poonch Rawalakot and Sindh Agriculture University Tandojam for providing facilities for this project. This study is a part of PhD research project of the first author (FR).
Conflict of interest
The authors declare that the research was conducted in the absence of any commercial or financial relationships that could be construed as a potential conflict of interest.
Publisher’s note
All claims expressed in this article are solely those of the authors and do not necessarily represent those of their affiliated organizations, or those of the publisher, the editors and the reviewers. Any product that may be evaluated in this article, or claim that may be made by its manufacturer, is not guaranteed or endorsed by the publisher.
References
Abdou, R. H., Basha, W. A., and Khalil, W. F. (2019). Subacute toxicity of Nerium oleander ethanolic extract in mice. Toxicol. Res. 35, 233–239. doi:10.5487/TR.2019.35.3.233
Abdullahi, M. I., Uba, A., Yaro, A., Maxwell, O., Yusuf, A. J., Kabir, S., et al. (2015). Phytochemical screening, acute toxicity study and evaluation of antidiabetic properties of the methanolic leaf extract of Vernonia glaberrima (Asteraceae). J. Pharmacol. Chem. Biol. Sci. 3, 169–177.
Abou-El-Hamd, E. M., Mohamed-Elamir, F. H., and Moustafa, M. F. (2012). Euphorbia helioscopia: chemical constituents and biological activities. Int. J. Phytopharm. 3 (1), 78–90.
Adibah, K. Z. M., and Azzreena, M. A. (2019). Plant toxins: alkaloids and their toxicities. GSC Biol. Pharm. Sci. 6 (2). doi:10.30574/gscbps.2019.6.2.0003
Ahmed, S. M. (2017). Hematological and biochemical changes in rabbits exposed to castor oil (Ricinus communis) under experimental conditions. Mustansiriyah J. Sci. 28 (1), 19–24. doi:10.23851/mjs.v28i1.307
Aleksandrov, M., Maksimova, V., and Koleva Gudeva, L. (2019). Review of the anticancer and cytotoxic activity of some species from genus Euphorbia. Agric. Conspec. Sci. 84 (1), 1–5.
Alexiades, M. N. (1996). Collecting ethnobotanical data: an introduction to basic concepts and techniques. Econ. Bot. 10, 53–94.
Ali, S., and Nasir, E. (1980). Flora of Pakistan. No. 135, Balanophoraceae. Karachi, Pakistan: University of Karachi.
Alui, Y.O., and Nwude, N. (1982). Determination of median lethal dose (LD50). Vet. Pharmacol. Toxicol. Exp., 43–45.
AJK, (2015). AJK at a glance. Muzaffarabad, Pakistan: Planning and Development Department, Government of Azad Jammu and Kashmir.
Aslani, M. R., Movassaghi, A. R., Janati-Pirouz, H., and Karazma, M. (2007). Experimental oleander (Nerium oleander) poisoning in goats: a clinical and pathological study. Iran. J. Vet. 8 (1), 58–63.
Baloch, A. H., Baloch, I. A., Ahmed, I., and Ahmed, S. (2017). A study of poisonous plants of Balochistan, Pakistan. Pure Appl. Biol. 6 (3), 989–1001. doi:10.19045/bspab.2017.600105
Bandara, V., Weinstein, S. A., White, J., and Eddleston, M. (2010). A review of the natural history, toxinology, diagnosis and clinical management of Nerium oleander (common oleander) and Thevetia peruviana (yellow oleander) poisoning. Toxicon 56 (3), 273–281. doi:10.1016/j.toxicon.2010.03.026
Barla, A., Kultur, S., and Oksuz, S. (2006). Secondary metabolites from Euphorbia helioscopia and their vasodepressor activity. Turk. J. Chem. 30 (3), 325–332.
Basu, S., Das, M., Sen, A., Choudhury, U. R., and Datta, G. (2014). Analysis of complete nutritional profile and identification of bioactive components present in Alocasia indica tuber cultivated in Howrah District of West Bengal, India. Asian pac. J. Trop. Med. 7, 527–533. doi:10.1016/S1995-7645(14)60285-6
Bernhoft, A. (2010). Bioactive compounds in plants—benefits and risks for man and animals. Oslo: The Norwegian Academy of Science and Letters.
Bevilacqua, A. H. V., Suffredini, I. B., Romoff, P., Lago, J. H. G., and Bernardi, M. M. (2011). Toxicity of apolar and polar Lantana camara L. crude extracts in mice. Res. Vet. Sci. 90 (1), 106–115. doi:10.1016/j.rvsc.2010.05.001
Bibi, T., Ahmad, M., Tareen, R. B., Tareen, N. M., Jabeen, R., Rehman, S. U., et al. (2014). Ethnobotany of medicinal plants in district Mastung of Balochistan province-Pakistan. J. Ethnopharmacol. 157, 79–89. doi:10.1016/j.jep.2014.08.042
Botha, C. J., and Penrith, M. L. (2008). Poisonous plants of veterinary and human importance in southern Africa. J. Ethnopharmacol. 119 (3), 549–558. doi:10.1016/j.jep.2008.07.022
Bricarello, P. A., Longo, C., da Rocha, R. A., and Hötzel, M. J. (2023). Understanding animal-plant-parasite interactions to improve the management of gastrointestinal nematodes in grazing ruminants. Pathogens 12 (4), 531. doi:10.3390/pathogens12040531
Ceci, L., Girolami, F., Capucchio, M. T., Colombino, E., Nebbia, C., Gosetti, F., et al. (2020). Outbreak of oleander (Nerium oleander) poisoning in dairy cattle: clinical and food safety implications. Toxins 12 (8), 471. doi:10.3390/toxins12080471
Chaachouay, N., Azeroual, A., Douira, A., and Zidane, L. (2022). Ethnoveterinary practices of medicinal plants among the Zemmour and Zayane tribes, Middle Atlas, Morocco. S. Afr. J. Bot. 151, 826–840. doi:10.1016/j.sajb.2022.11.009
Chen, Y., Liu, Y., Chen, N., Jin, Y., Yang, R., Yao, H., et al. (2023). A chemoinformatic analysis on natural glycosides with respect to biological origin and structural class. Nat. Prod. Rep. 40, 1464–1478. doi:10.1039/d2np00089j
Childers, N. F., and Margoles, M. S. (1993). Authors’ reply. J. Orthop. Surg. 14, 227. doi:10.1177/230949900601400231
Chowański, S., Adamski, Z., Marciniak, P., Rosiński, G., Büyükgüzel, E., Büyükgüzel, K., et al. (2016). A review of bioinsecticidal activity of Solanaceae alkaloids. Toxins 8 (3), 60. doi:10.3390/toxins8030060
Crosera, M., Bovenzi, M., Maina, G., Adami, G., Zanette, C., Florio, C., et al. (2009). Nanoparticle dermal absorption and toxicity: a review of the literature. Int. Arch. Occup. Environ. Health 82, 1043–1055. doi:10.1007/s00420-009-0458-x
Dansou, C. C., Olounladé, P. A., Konmy, B. S. B., Songbé, O., Arigbo, K. B., Aboh, A. B., et al. (2021). Ethno-veterinary survey and quantitative study of medicinal plants with anthelmintic potential used by sheep and goat breeders in the Cotton zone of Central Benin (West Africa). J 4 (4), 544–563. doi:10.3390/j4040040
Das, S. C., Isichei, C. O., Okwuasaba, F. K., Uguru, V. E., Onoruvwe, O., Olayinka, A. O., et al. (2000). Chemical, pathological and toxicological studies of the effects of RICOM-1013-J of Ricinus communis var Minor on women volunteers and rodents. Phytother. Res. 14 (1), 15–19. doi:10.1002/(sici)1099-1573(200002)14:1<15::aid-ptr205>3.0.co;2-p
Dearing, M. D., and Weinstein, S. B. (2022). Metabolic enabling and detoxification by mammalian gut microbes. Ann. Rev. Microbiol. 76, 579–596. doi:10.1146/annurev-micro-111121-085333
Desai, A. R., Patel, K. S., and Shah, K. R. (1988). Toxicity studies on Alocasia indica in rats. Indian J. Physiol. Pharmacol. 32 (4), 272–277.
Desta, A. H. (2019). Livestock poisoning plants: identification and its veterinary importance in Afar region of Ethiopia. Open Agric. J. 13 (1), 107–115. doi:10.2174/1874331501913010107
Diaz, G. J. (2015). Toxicosis by plant alkaloids in humans and animals in Colombia. Toxins 7 (12), 5408–5416. doi:10.3390/toxins7124892
Dixit, R. (2007). Pharmacokinetics and toxico kinetics: fundamentals and applications in Toxicology. Vet. Toxicol. Basic Clin. Principl. 2, 132–137.
Dubey, N. K., Dwivedy, A. K., Chaudhari, A. K., and Das, S. (2018). “Common toxic plants and their forensic significance,” in Natural products and drug discovery (Elsevier), 349–374.
Dusemund, B., Nowak, N., Sommerfeld, C., Lindtner, O., Schäfer, B., and Lampen, A. (2018). Risk assessment of pyrrolizidine alkaloids in food of plant and animal origin. Food Chem. Toxicol. 115, 63–72. doi:10.1016/j.fct.2018.03.005
Ea, N., Padikkala, J., and Mathew, L. (2021). Acute and subacute studies of Thevetia peruviana seed methanol extracts on Wistar male albino rats. Indian J. Pharm. Sci. 83 (5), 1000–1006. doi:10.36468/pharmaceutical-sciences.853
Elshafie, H. S., Camele, I., and Mohamed, A. A. (2023). A Comprehensive review on the biological, agricultural and pharmaceutical properties of secondary metabolites based-plant origin. Int. J. Mol. Sci. 24 (4), 3266. doi:10.3390/ijms24043266
Friedman, M. (2004). Analysis of biologically active compounds in potatoes (Solanum tuberosum), tomatoes (Lycopersicon esculentum), and jimson weed (Datura stramonium) seeds. J. Chromatogr. A 1054 (1-2), 143–155. doi:10.1016/j.chroma.2004.04.049
Gangwar, M., Goel, R. K., and Nath, G. (2014). Mallotus philippinensis Muell. Arg (Euphorbiaceae): ethnopharmacology and phytochemistry review. Biomed. Res. Int. 2014, 213973. doi:10.1155/2014/213973
Gershenzon, J. (2017). “The cost of plant chemical defense against herbivory: a biochemical perspective,” in Insect-plant interactions (1993) (United States: CRC Press), 105–176.
Gluhovschi, G., Margineanu, F., Kaycsa, A., Velciov, S., Gluhovschi, C., Bob, F., et al. (2010). Therapeutic remedies based on Aristolochia clematitis in the main foci of Balkan endemic nephropathy in Romania. Nephron Clin. Pract. 116 (1), 36–46. doi:10.1159/000314549
Griffiths, M. R., Strobel, B. W., Hama, J. R., and Cedergreen, N. (2021). Toxicity and risk of plant-produced alkaloids to Daphnia magna. Environ. Sci. Eur. 33, 10–12. doi:10.1186/s12302-020-00452-0
Hoffman, B., and Gallaher, T. (2007). Importance indices in ethnobotany. Ethnobot. Res. Appl. 5, 201–218. doi:10.17348/era.5.0.201-218
Houndje, E. M. B., Ogni, C. A., Noudeke, N., Farougou, S., Youssao, A. K. I., and Kpodekon, T. M. (2016). Recettes ethno-vétérinaire à base de plantes médicinales utilisées pour le traitement de la fièvre aphteuse au Bénin. Int. J. Biol. Chem. 10 (5), 2090–2107. doi:10.4314/ijbcs.v10i5.13
Jawonisi, I., and Adoga, G. (2017). Acute toxicity, haematological and histopathological assessment of the ethanolic extract of Lantana camara leaf. J. Pharma Biores 14 (1), 45–50. doi:10.4314/jpb.v14i1.6
Jung, S., Lauter, J., Hartung, N. M., These, A., Hamscher, G., and Wissemann, V. (2020). Genetic and chemical diversity of the toxic herb Jacobaea vulgaris Gaertn. (syn. Senecio jacobaea L.) in Northern Germany. Phytochem 172, 112235. doi:10.1016/j.phytochem.2019.112235
Junior, J. I. G., Ferreira, M. R. A., de Oliveira, A. M., and Soares, L. A. L. (2022). Croton sp.: a review about popular uses, biological activities and chemical composition. Res. Soc. Dev. 11 (2), 57311225306. doi:10.33448/rsd-v11i2.25306
Kamboj, A., and Saluja, A. K. (2010). Phytopharmacological review of Xanthium strumarium L. (Cocklebur). Int. J. Green Pharm. 4 (3), 129. doi:10.4103/0973-8258.69154
Kara, E., and Sürmen, M. (2019). The effects of secondary metabolites of rangeland and pasture plants on the animal health in Mediterranean ecological conditions. J.US-China Med. Sci. 16, 63–72. doi:10.17265/1548-6648/2019.02.003
Karabourniotis, G., Horner, H. T., Bresta, P., Nikolopoulos, D., and Liakopoulos, G. (2020). New insights into the functions of carbon–calcium inclusions in plants. New Phytol. 228 (3), 845–854. doi:10.1111/nph.16763
Kebede, A., Zenebe, T., Abera, D., and Kebede, G. (2015). Assessment of poisonous plants to livestock in and around Nekemte area, east Wollega zone of Oromia regional state, western Ethiopia. Nat. Sci. 13 (8), 8–13.
Keeler, R. F., Van Kampen, K. R., and James, L. F. (2013). Effects of poisonous plants on livestock. Elsevier.
Kefifa, A., Saidi, A., Hachem, K., and Mehalhal, O. (2020). An ethnobotanical survey and quantitative study of indigenous medicinal plants used in the Algerian semi-arid region. Phytothérapie 18 (3-4), 204–219. doi:10.3166/phyto-2018-0077
Khaket, T. P., Aggarwal, H., Jodha, D., Dhanda, S., and Singh, J. (2015). Parthenium hysterophorus in current scenario: a toxic weed with industrial, agricultural and medicinal applications. J. Plant Sci. 10 (2), 42–53. doi:10.3923/jps.2015.42.53
Khan, R. U., Mehmood, S., and Khan, S. U. (2018). Toxic effect of common poisonous plants of district Bannu, Khyber Pakhtunkhwa, Pakistan. Pak. J. Pharma. Sci. 31 (1), 57–67.
Kharchoufa, L., Bouhrim, M., Bencheikh, N., Addi, M., Hano, C., Mechchate, H., et al. (2021). Potential toxicity of medicinal plants inventoried in northeastern Morocco: an ethnobotanical approach. Plants 10 (6), 1108. doi:10.3390/plants10061108
Khunoana, E. T., Madikizela, B., Erhabor, J. O., Nkadimeng, S. M., Arnot, L. F., Van Wyk, I., et al. (2019). A survey of plants used to treat livestock diseases in the Mnisi community, Mpumalanga, South Africa, and investigation of their antimicrobial activity. South Afr. J. Bot. 126, 21–29. doi:10.1016/j.sajb.2019.07.026
Koshariya, A. K., Mahant, M. M., Afsana, C., and Reddypriya, P. (2023). Introduction to plants pathology. United States: AG Publishing House AGPH Books.
Kumar, R., Katiyar, R., Kumar, S., Kumar, T., and Singh, V. (2016). Lantana camara: an alien weed, its impact on animal health and strategies to control. J. Exp. Biol. 4 (3S), 321–337. doi:10.18006/2016.4(3s).321.337
Langford, S. D., and Boor, P. J. (1996). Oleander toxicity: an examination of human and animal toxic exposures. Toxicology 109 (1), 1–13. doi:10.1016/0300-483x(95)03296-r
Leong, L. E. X., Khan, S., Davis, C. K., Denman, S. E., and McSweeney, C. S. (2017). Fluoroacetate in plants-a review of its distribution, toxicity to livestock and microbial detoxification. J. Anim. Sci. Biotech. 8 (1), 55–11. doi:10.1186/s40104-017-0180-6
Leonti, M. (2022). The relevance of quantitative ethnobotanical indices for ethnopharmacology and ethnobotany. J. Ethnopharmacol. 288, 115008. doi:10.1016/j.jep.2022.115008
Lian, W., Wang, Y., Zhang, J., Yan, Y., Xia, C., Gan, H., et al. (2022). The genus Datura L. (Solanaceae): a systematic review of botany, traditional use, phytochemistry, pharmacology, and toxicology. Phytochem 204, 113446. doi:10.1016/j.phytochem.2022.113446
Majeed, M., Bhatti, K. H., Amjad, M. S., Abbasi, A. M., Bussmann, R. W., Nawaz, F., et al. (2020). Ethno-veterinary uses of poaceae in Punjab, Pakistan. PloS One 15 (11), e0241705. doi:10.1371/journal.pone.0241705
Matsuura, M., Kato, S., Saikawa, Y., Nakata, M., and Hashimoto, K. (2016). Identification of cyclopropylacetyl-(R)-carnitine, a unique chemical marker of the fatally toxic mushroom Russula subnigricans. Chem. Pharm. Bull. 64 (6), 602–608. doi:10.1248/cpb.c15-01033
Mattalia, G., Sõukand, R., Corvo, P., and Pieroni, A. (2020). Dissymmetry at the border: wild food and medicinal ethnobotany of Slovenes and Friulians in NE Italy. Econ. Bot. 74, 1–14. doi:10.1007/s12231-020-09488-y
McKenzie, R. (2020). Australia’s Poisonous Plants, Fungi and Cyanobacteria: a guide to species of medical and veterinary importance. Australia: Csiro Publishing.
Meen, M. L., Dudi, A., and Singh, D. (2020). Ethnoveterinary study of medicinal plants in a tribal society of Marwar region of Rajasthan. India. J. pharmacog. Phytochem. 9 (4), 549–554.
Mensah, M. L., Komlaga, G., Forkuo, A. D., Firempong, C., Anning, A. K., and Dickson, R. A. (2019). Toxicity and safety implications of herbal medicines used in Africa. Herb. Med. 63, 1992–0849.
Morita, H., Kawashima, N., Terasaki, Y., Iwashina, T., Kitajima, J., and Kogure, N. (2014). Identification of 12-deoxyphorbol diesters from Euphorbia helioscopia as helioscopinolides and their tumor-promoting activity. J. Nat. Prod. 77 (3), 670–676.
Mukheriee, P. K., and Wahile, A. (2006). Integrated approaches towards drug development from Ayurveda and other Indian system of medicines. J. Ethnopharmacol. 103 (1), 25–35. doi:10.1016/j.jep.2005.09.024
Mukherjee, A. K., Basak, B., and Saha, B. P. (2010). Lantana camara L. berry extract exerts antidiarrhoeal activity in rats through inhibition of the colonic motor function. J. Nat. Rem. 10 (1), 81–89.
Mukherjee, S., Sushma, V., Patra, S., Barui, A. K., Bhadra, M. P., Sreedhar, B., et al. (2012). Green chemistry approach for the synthesis and stabilization of biocompatible gold nanoparticles and their potential applications in cancer therapy. Nanotechnology 23 (45), 455103. doi:10.1088/0957-4484/23/45/455103
Mulla, W. A., Chopade, A. R., Bhise, S. B., Burade, K. B., and Khanwelkar, C. C. (2011). Evaluation of antidiarrheal and in vitro antiprotozoal activities of extracts of leaves of Alocasia indica. Pharm. Biol. 49 (4), 354–361. doi:10.3109/13880209.2010.517211
Murade, V., Waghmare, A., Pakhare, D., Dichayal, S., Patil, R., Wanjari, M., et al. (2021). A plausible involvement of GABAA/benzodiazepine receptor in the anxiolytic-like effect of ethyl acetate fraction and quercetin isolated from Ricinus communis Linn. leaves in mice. Phytomed. Plus 1 (3), 100041. doi:10.1016/j.phyplu.2021.100041
Murtala, A. A., Oladapo, O. E., Aderionla, A. A., Olooto, W. E., Soyinka, O. O., Folarin, R. O., et al. (2023). Sub-chronic (ninety days) toxicity study of hydroethanolic leaf extract of Datura stramonium L. in rodents. Clin. Comp. Med. Pharmacol. 100090.
Naiel, M. A., Shehata, A. M., Negm, S. S., Abd El-Hack, M. E., Amer, M. S., Khafaga, A. F., et al. (2020). The new aspects of using some safe feed additives on alleviated imidacloprid toxicity in farmed fish: a review. Rev. Aquac. 12 (4), 2250–2267. doi:10.1111/raq.12432
Narasimhan, T. R., Keshava Murthy, B. S., Harindranath, N., and Subba Rao, P. V. (1984). Characterization of a toxin from Parthenium hysterophorus and its mode of excretion in animals. J. Biosci. 6 (5), 729–738. doi:10.1007/bf02702716
Nguyen, L. T., Farcas, A. C., Socaci, S. A., Tofana, M., Diaconeasa, Z. M., Pop, O. L., et al. (2020). An overview of saponins–a bioactive group. Bull. UASVM Food Sci. Technol. 77 (1), 25–36. doi:10.15835/buasvmcn-fst:2019.0036
Nielsen, D. B., Rimbey, N. R., and James, L. F. (2019). “Economic considerations of poisonous plants on livestock,” in The ecology and economic impact of poisonous plants on livestock production (United States: CRC Press), 5–15.
Nortier, J. L., Martinez, M. C. M., Schmeiser, H. H., Arlt, V. M., Bieler, C. A., Petein, M., et al. (2000). Urothelial carcinoma associated with the use of a Chinese herb (Aristolochia fangchi). N. Engl. J. Med. 342 (23), 1686–1692. doi:10.1056/NEJM200006083422301
OECD (2001). OECD guideline for testing of chemicals. Paris, France: Organisation for Economic Co-Operation and Development.
Ogunmoyole, T., Adeyeye, R. I., Olatilu, B. O., Akande, O. A., and Agunbiade, O. J. (2019). Multiple organ toxicity of Datura stramonium seed extracts. Toxicol. Rep. 6, 983–989. doi:10.1016/j.toxrep.2019.09.011
Ohlsen, S., Ganter, M., Wohlsein, P., Reckels, B., Huckauf, A., Lenzewski, N., et al. (2022). Grazing ecology of sheep and its impact on vegetation and animal health in pastures dominated by common ragwort (Senecio jacobaea L.) Part 1: vegetation. Animals 12 (8), 1000. doi:10.3390/ani12081000
Oladosu, Y., Rafii, M. Y., Abdullah, N., Hussin, G., Ramli, A., Rahim, H. A., et al. (2016). Principle and application of plant mutagenesis in crop improvement: a review. Biotech. Biotechnol. Equip. 30 (1), 1–16. doi:10.1080/13102818.2015.1087333
Onyeyili, P. A., Chibuzo, G. A., Brisaibe, F., and Egwu, G. O. (2018). Accidental plant poisoning of sheep in an arid zone of Nigeria. Roma (Italia): Organizacion de las Naciones Unidas para la Agricultura y la Alimentacion.
Oryan, A., Maham, M., Rezakhani, A., and Maleki, M. (1996). Morphological studies on experimental oleander poisoning in cattle. J. Vet. Med. 43 (1-10), 625–634. doi:10.1111/j.1439-0442.1996.tb00496.x
Ouachinou, J. M. S., Adomou, A. C., Dassou, G. H., Hounnankpon, Y., Tossou, G. M., and Akoegninou, A. (2017). Connaissances et pratiques ethnobotaniques en médecines traditionnelles vétérinaire et humaine au Bénin: similarité ou dissemblance? J. Appl. Biosci. Sci. 113, 11174–11183. doi:10.4314/jab.v113i1.6
Paim, R. D. C. S., de Paula, L. G. F., Soares, D. M., Rocha, T. F. G., Ribeiro, A. L., Barros, N., et al. (2023). Toxic plants from the perspective of a “Quilombola” community in the Cerrado region of Brazil. Toxicon 224, 107028. doi:10.1016/j.toxicon.2023.107028
Pandeya, S., Sharma, N., and Basyal, D. (2022). Phytochemical and biological screening of Lantana camara L. leaves extract. J. Nep. Chem. Soc. 43 (1), 43–53. doi:10.3126/jncs.v43i1.47029
Panter, K. E., Welch, K. D., and Gardner, D. R. (2011). “Toxic plants,” in Reproductive and developmental toxicology (Academic Press), 689–705.
Parihaar, R. S., Bargali, K., and Bargali, S. S. (2014). Diversity and uses of Ethno-medicinal plants associated with traditional agroforestry systems in Kumaun Himalaya. Indian J. Agric. Sci. 84 (12), 1470–1476. doi:10.56093/ijas.v84i12.45221
Parvez, M. K., Al-Dosari, M. S., Arbab, A. H., Alam, P., Alsaid, M. S., and Khan, A. A. (2019). Hepatoprotective effect of Solanum surattense leaf extract against chemical-induced oxidative and apoptotic injury in rats. BMC Complement. Altern. Med. 19 (1), 154–159. doi:10.1186/s12906-019-2553-1
Polito, L., Bortolotti, M., Battelli, M. G., Calafato, G., and Bolognesi, A. (2019). Ricin: an ancient story for a timeless plant toxin. Toxins 11 (6), 324. doi:10.3390/toxins11060324
Pour, B. M., Latha, L. Y., and Sasidharan, S. (2011). Cytotoxicity and oral acute toxicity studies of Lantana camara leaf extract. Molecules 16 (5), 3663–3674. doi:10.3390/molecules16053663
Prasad, A., Krishnaveni, K., Neha, K. A., Neethu, T. D., Shanmugasundaram, R., and Sambathkumar, R. (2016). A review on management of common oleander and yellow oleander poisoning. World J. Pharm. Sci. 5 (12), 493–503.
Pratama, A. M., Herawati, O., Nabila, A. N., Belinda, T. A., and Wijayanti, A. D. (2021). Ethnoveterinary study of medicinal plants used for cattle treatment in Bojonegoro District, East Java, Indonesia. Biodiversitas J. Bio. Div. 22 (10). doi:10.13057/biodiv/d221014
Qasim, M., Tayyab, H. M., and Ahmad, E. (2010). Use of salvage wood for reconstruction in earthquake-affected sites of Muzaffarabad, Azad Jammu and Kashmir. World Appl. Sci. J. 8 (7), 914–916.
Rahman, S., Jan, G., Jan, F. G., and Rahim, H. U. (2021). Phytochemical screening and antidiabetic, antihyperlipidemic, and antioxidant effects of Leptopus cordifolius Decne. in diabetic mice. Front. Pharmacol. 12, 643242. doi:10.3389/fphar.2021.643242
Randrianarivo, H. R., Razafindrakoto, A. R., Ratsimanohatra, H. C., Randriamampianina, L. J., Rajemiarimoelisoa, C. F., Ramamonjisoa, L., et al. (2014). Toxic effects of seed methanolic extracts of endemic Albizia species (Fabaceae) from Madagascar on animals. J. Sci. 8 (8), 676–689.
Riaz, T., Abbasi, M. A., Rehman, K. U., and Muhammad, N. (2012). Toxicity study of methanolic extract of Ricinus communis seeds on liver and kidney functions. J. Med. Plant Res. 6 (21), 3918–3922.
Saha, S., Saha, A., Saha, K., Sarker, K. K., and Chowdhury, Z. J. (2022). Review on plant biodiversity and conservation in Bangladesh: drawbacks and prospects. Am. J. Agri. Sci. Eng. Technol. 6 (2), 95–102. doi:10.54536/ajaset.v6i2.597
Sema, M., Atakpama, W., Kanda, M., Koumantiga, D., Batawila, K., and Akpagana, K. (2018). Une forme de spécialisation de la medicine traditionnelle au Togo: cas de la préfecture de doufelgou. J. Rech. Sci. Univ. Lomé. 20 (4), 29–43.
Serrano, R. (2018). Toxic plants: knowledge, medicinal uses and potential human health risks. Env. Ecol. Res. 6 (5), 487–492. doi:10.13189/eer.2018.060509
Sharma, O. P., Sharma, S., Pattabhi, V., Mahato, S. B., and Sharma, P. D. (2007). A review of the hepatotoxic plant Lantana camara. Crit. Rev. Toxicol. 37 (4), 313–352. doi:10.1080/10408440601177863
Shridhar, N. B. (2022). Nerium oleander toxicity: a review. Int. J. Adv. Acad. Stud. 4 (10), 23–32. doi:10.33545/27068919.2022.v4.i3a.813
Stegelmeier, B. L., Field, R., Panter, K. E., Hall, J. O., Welch, K. D., Pfister, J. A., et al. (2013). “Selected poisonous plants affecting animal and human health,” in Haschek and rousseaux’s handbook of toxicologic pathology (Academic Press), 1259–1314.
Stevenson, P. C., Nicolson, S. W., and Wright, G. A. (2017). Plant secondary metabolites in nectar: impacts on pollinators and ecological functions. Funct. Ecol. 31 (1), 65–75. doi:10.1111/1365-2435.12761
Tadesse, E., Engidawork, E., Nedi, T., and Mengistu, G. (2017). Evaluation of the anti-diarrheal activity of the aqueous stem extract of Lantana camara Linn (Verbenaceae) in mice. BMC Compl. Altern. Med. 17 (1), 190–198. doi:10.1186/s12906-017-1696-1
Teke, G. N., and Kuete, V. (2014). “Acute and subacute toxicities of African medicinal plants,” in Toxicological survey of African medicinal plants (Elsevier), 63–98.
Termote, C., Van Damme, P., and Dhed’a Djailo, B. (2011). Eating from the wild: turumbu, Mbole and Bali traditional knowledge on non-cultivated edible plants, District Tshopo, DRCongo. Genet. Resour. Crop Evol. 58, 585–618. doi:10.1007/s10722-010-9602-4
Teuscher, E., and Lindequist, U. (2023). Natural poisons and venoms: plant toxins: terpenes and steroids. Germany: Walter de Gruyter GmbH and Co KG.
Tewari, S. K., Gupta, A. K., Saha, S., Kumar, A., Pal, A., Tripathi, A., et al. (2005). Antidiabetic and toxicological evaluation of aqueous extract of seed of Ricinus communis Linn. in rats. J. Ethnopharmacol. 97 (2), 248–253.
Thakur, S. C., Thakur, S. S., Chaube, S. K., and Singh, S. P. (2005). An etheral extract of Kamala (Mallotus philippinensis (Moll. Arg) Lam.) seed induce adverse effects on reproductive parameters of female rats. Rep. Toxicol. 20 (1), 149–156. doi:10.1016/j.reprotox.2004.12.008
Umair, M., Altaf, M., and Abbasi, A. M. (2017). An ethnobotanical survey of indigenous medicinal plants in Hafizabad district, Punjab-Pakistan. PloS One 12 (6), e0177912. doi:10.1371/journal.pone.0177912
Uprety, Y., Karki, S., Poudel, R. C., and Kunwar, R. M. (2022). Ethnoveterinary use of plants and its implication for sustainable livestock management in Nepal. Front. Vet. Sci. 9, 930533. doi:10.3389/fvets.2022.930533
Vetter, J. (2000). Plant cyanogenic glycosides. Toxicon 38 (1), 11–36. doi:10.1016/s0041-0101(99)00128-2
Wink, M. (2013). Evolution of secondary metabolites in legumes (Fabaceae). S. Afr. J. Bot. 89, 164–175. doi:10.1016/j.sajb.2013.06.006
Worbs, S., Köhler, K., Pauly, D., Avondet, M. A., Schaer, M., Dorner, M. B., et al. (2011). Ricinus communis intoxications in human and veterinary medicine—a summary of real cases. Toxins 3 (10), 1332–1372. doi:10.3390/toxins3101332
Keywords: plant poisoning, traditional knowledge, relative frequency of citation, fidelity level, acute toxicity, LD50
Citation: Rasool F, Nizamani ZA, Ahmad KS, Parveen F, Khan SA and Sabir N (2024) An appraisal of traditional knowledge of plant poisoning of livestock and its validation through acute toxicity assay in rats. Front. Pharmacol. 15:1328133. doi: 10.3389/fphar.2024.1328133
Received: 26 October 2023; Accepted: 30 January 2024;
Published: 14 February 2024.
Edited by:
Mohan Lal, North East Institute of Science and Technology (CSIR), IndiaReviewed by:
Francis-Alfred Unuagbe Attah, University of Ilorin, NigeriaArmando Caceres, Galileo University, Guatemala
Copyright © 2024 Rasool, Nizamani, Ahmad, Parveen, Khan and Sabir. This is an open-access article distributed under the terms of the Creative Commons Attribution License (CC BY). The use, distribution or reproduction in other forums is permitted, provided the original author(s) and the copyright owner(s) are credited and that the original publication in this journal is cited, in accordance with accepted academic practice. No use, distribution or reproduction is permitted which does not comply with these terms.
*Correspondence: Khawaja Shafique Ahmad, YWhtYWRrc0B1cHIuZWR1LnBr