- 1Key Laboratory of Ecology and Environment in Minority Areas (Minzu University of China), National Ethnic Affairs Commission, Beijing, China
- 2College of Life and Environmental Sciences, Minzu University of China, Beijing, China
- 3Xianggelila Bureau of Forestry and Grassland, Beijing, China
- 4Baoshan Administrative of Gaoligongshan National Nature Reserve, Baoshan, China
- 5College of Chemistry and Materials Engineering, Beijing Technology and Business University, Beijing, China
- 6BTBU-TANGYI Innovation Center for the Evaluation of the Safety and Efficacy of Bioengineering Raw Materials, Beijing, China
- 7Key Laboratory of Ethnomedicine (Minzu University of China), Ministry of Education, Beijing, China
- 8Institute of National Security Studies, Minzu University of China, Beijing, China
Cynoglossum amabile, a member of the Boraginaceae family, is a well-known traditional Chinese medicine and ethnomedicine known as Daotihu. Despite several studies confirming the presence of bioactive pyrrolizidine alkaloids such as amabiline, ambelline, echinatine, europine, and others in C. amabile, there has been no comprehensive review of its traditional uses, phytochemistry, and pharmacology thus far. This review was conducted by thoroughly examining the literature and analyzing network databases. It covers various aspects of C. amabile, including botanical characteristics, geographical distribution, traditional applications, phytochemistry, pharmacological activities, toxicology, and clinical applications. The results have shown that C. amabile has been traditionally used for medicinal, edible, and ornamental purposes in China for many centuries. The whole plant, root, and leaf of C. amabile are used by different ethnic groups, such as Lisu, Bai, Naxi, Yi, Jinuo, and Han, to treat malaria, hepatitis, dysentery, leucorrhea, tuberculosis cough, fracture, joint dislocation, trauma bleeding, and skin carbuncle abscess. A total of 47 chemical components, including alkaloids (pyrrolizidine alkaloids, PAs), sterols, organic acids, and saccharides, were isolated from C. amabile. Pharmacological studies show that the chemical extracts of C. amabile possess various biological activities, such as anti-inflammatory, anti-tumor, anti-microbial, cardiovascular effects, ganglionic action, and acetylcholinesterase inhibition. However, it is important to note that C. amabile exhibits hepatotoxicity, with its toxicity being linked to its primary PAs components. Although preliminary studies suggest potential applications in the treatment of prostate diseases and alopecia, further research is needed to validate these clinical uses. Our review highlights the traditional uses, phytochemistry, biological activity, toxicity, and clinical applications of C. amabile. It emphasizes the essential guiding role of the indigenous medicinal knowledge system in developing new drugs. Previous studies have shown that the phytochemical and pharmacological characteristics of C. amabile are significantly related to its traditional medicinal practices. Cynoglossum amabile has excellent market potential and can be further analyzed in terms of phytochemistry, pharmacology, and toxicology, which are critical for its clinical drug safety, quality evaluation, and resource development.
1 Introduction
Cynoglossum L. (Boraginaceae) is a cosmopolitan genus comprising 75 species. In China, there are 10 species and two varieties of Cynoglossum, primarily distributed in regions such as Yunnan, western Guizhou, southwestern to southeastern Tibet, Western Sichuan, and southern Gansu. Cynoglossum is commonly found in hillsides, grasslands, marginal lands, roadsides, and the edges of coniferous forests, typically at altitudes ranging from 1250 to 4565 m above sea level (https://powo.science.kew.org/taxon/urn:lsid:ipni.org:names:30003351-2, accessed on 25 November 2023). Most of the species within the Cynoglossum genus are known for their richness in pyrrolizidine alkaloids (PAs), some of which are recognized as important plant hepatotoxic components (Stegelmeier, 2011; Damianakos et al., 2013; Damianakos et al., 2016). PAs also constitute the primary sources of Cynoglossum’s pharmacological activities (Fayed, 2021; Vrieling, 2021). Despite its medicinal potential, the value of Cynoglossum in traditional medicine has been largely overlooked. This is due to incidents of poisoning resulting from accidental ingestion of Cynoglossum or its related components (Mudge et al., 2015; Lorena et al., 2016), which have led to its classification as a harmful weed in many countries (Stegelmeier et al., 2009). However, in China, Cynoglossum plants hold significant ethnobotanical value and have been utilized for food, ornaments, and medicinal purposes (Xu and Zhang, 2009; Zhang et al., 2014). China boasts a wealth of Cynoglossum resources, with many species having a long history of use in traditional medicines among ethnic communities. A notable example is Cynoglossum amabile Stapf et Drumm.
Cynoglossum amabile, known by various names in Mandarin, such as Daotihu (倒提壶), Lanbuqun (蓝布裙), Jizhuashen (鸡爪参), Lanhuashen (蓝花参), Goushihua (狗屎花), Lanluhu (拦路虎), Yibazhua (一把抓), is a traditional medicine employed by the Buyi, Achang, Bai, Jinuo, and other ethnic groups in China (Jia and Li, 2005). According to records in Chinese Materia Medica, An Illustrated Book of Plants, Tianbao Materia Medica, and Materia Medica of Southern Yunnan, C. amabile is characterized by its bitter taste and cool nature. It is credited with properties such as clearing heat and dampness, alleviating fatigue, staunching bleeding, relieving cough, and providing analgesic effects. It can be administered either alone or as a component in folk traditional medical practices. When taken orally, C. amabile is utilized in the treatment of conditions like acute nephritis, periodontitis, hepatitis, irregular menstruation, abnormal leucorrhea, edema, acute mandibular lymphadenitis, and angina pectoris. For external applications, it is effective in the treatment of sores, boils, carbuncles, poisonous snake bites, and bruise injuries (Wu, 1963; Lan, 1975; Editorial Board of Chinese Materia Medica, Traditional Chinese Medicine Administration of China, 1998; Gong et al., 2001).
However, there is currently no up-to-date review available on the traditional utilization, phytochemistry, pharmacology, toxicology, or clinical applications of C. amabile. This gap in knowledge restricts the safe use of C. amabile in clinical medication, quality assessment, and resource development. To further promote comprehensive research, development, and utilization of C. amabile, this paper aims to consolidate information on its traditional use, chemical composition, pharmacological activities, toxicological effects, and clinical applications. This endeavor is intended to enhance the safety of related medications and provide a scientific foundation for the rational and correct utilization of plants with potential liver toxicity.
2 Methodology
Keywords, including Cynoglossum amabile, Daotihu, Goushihua, Lanbuqun, and Jizhuashen, were used to search related literature and data through digital resources and paper-based materials. The earliest available documents were published in 1960, while the latest was published in August 2023. These documents discussed the traditional utilization, phytochemical constituents, pharmacological activities, and safety of C. amabile. Much of the literature research was conducted through the following nine online scientific databases: Google Scholar, SciFinder, Web of Science, Springer Link, PubMed, Wiley, CNKI, Baidu Scholar, China Science, and Technology Journal Database. The review also included results from Ph.D. theses, M. Sc. Dissertations, online botanical databases, and relevant conference proceedings written in English and Chinese.
3 Botanical description and geographic distribution
Cynoglossum amabile is a perennial herb that reaches a height of 15–60 cm. Its stems are either single or form several clusters, densely covered in appressed pubescence. Basal leaves have long petioles and are oblong-lanceolate or lanceolate, measuring 5–20 cm in length (including petioles) and 1.5–4 cm in width, occasionally reaching 5 cm, with dense pubescence on both surfaces. Stem leaves are oblong or lanceolate, sessile, 2–7 cm long, and exhibit very prominent lateral veins. The inflorescence is acutely branched, densely branched, extending straight upward and clustered into a panicle, without bracts. Pedicels are 2–3 mm long, slightly longer in fruit. The calyx is 2.5–3.5 mm long, densely pubescent on the outside, with ovate or oblong lobes ending in pointed tips. The corolla is typically blue with sparse white markings, measuring 5–6 mm in length and 8–10 mm in diameter at the base. Its lobes are round, about 2.5 mm long, with distinct reticulate veins, and the throat features five trapezoidal appendages, each around 1 mm in length. Filaments are approximately 0.5 mm long, inserted in the middle of the corolla tube, and the anthers are oblong, roughly 1 mm long. The style is linear-cylindrical, nearly as long as the calyx or shorter. The nutlets are ovate, 3–4 mm long, slightly concave on the back, densely covered with anchor-like spines, and have anchor-like spines along the margin at the base. They may have narrow or wide wing-like edges and a triangular insertion surface above the middle of the ventral surface (Figure 1). The flowering and fruiting period spans from May to September (source: http://www.iplant.cn/info/Cynoglossum%20amabile?t=foc, accessed on 20 July 2023).
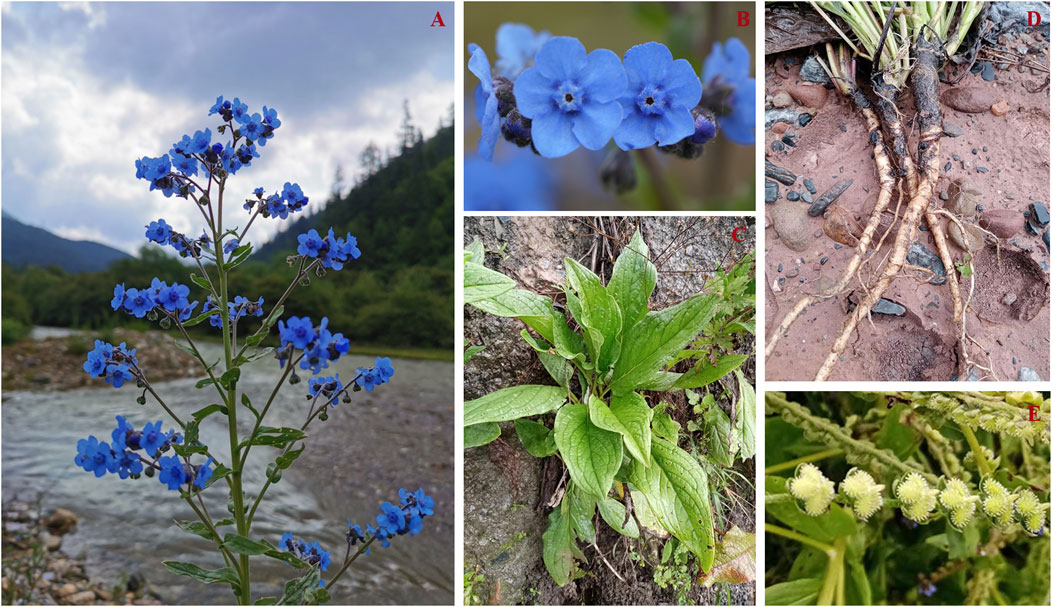
Figure 1. (A) Wild growth environment and plant morphology of Cynoglossum amabile. (B) Flowers. (C) Leaves. (D) Roots. (E) Seeds.
Cynoglossum amabile is shade-tolerant and often blooms under conditions of sufficient sunshine. It thrives in environments with fertile soil and good drainage. The suitable temperature range for its growth is 18°C–20°C, and it is not tolerant of high temperatures. While native to China, C. amabile has been widely introduced in Central America and has reports of growth in northeastern North America, Kenya, and Tanzania. Nowadays, it is cultivated and naturalized in many parts of the world (source: http://www.iplant.cn/info/Cynoglossum%20amabile?t=foc, accessed on 7 August 2023).
4 Traditional uses
The flowers of Cynoglossum amabile bloom from late spring to early summer. They are blue in color and have an elegant and pleasing appearance. These flowers bear a resemblance to the “forget-me-not” (Myosotis alpestris F. W. Schmidt) and hold high ornamental value. They are suitable not only for combined potted plants and garden settings but also for enhancing the aesthetics of rocks, lawns, and garden pathways. In Yunnan, China, some ethnic communities cultivate C. amabile along fences for ornamental purposes.
In Liangshan Prefecture, Sichuan Province, China, the Yi people regard C. amabile as a wild edible plant and harvest its roots for food. According to Sichuan Zhong Yao Zhi, C. amabile possesses significant dietary therapy value. Residents in Sichuan often stew meat with the entire plant, which has a positive effect on treating conditions such as cough-induced aphonia and spitting blood (Chinese Academy of Sciences, 1960; Wang et al., 2020).
The primary value of C. amabile lies in its medicinal applications. The earliest records of its medicinal value can be found in “Dian Nan Ben Cao” (AD 1436), followed by mentions in ancient texts such as “Ben Cao Gang Mu Shi Yi” (AD 1765) and “Zhi Wu Ming Shi Tu Kao” (AD 1848) (Lan, 1975; Jia and Li, 2005). “Dian Nan Ben Cao” states that one name for C. amabile is Daotihu, also known as Yibazhua, Lanbuqun, Lanhuashen, and Goushihua, among others. It promotes urination, reduces edema, clears stomach heat, treats jaundice, relieves liver pain, and addresses seven types of hernia pain. Apart from the common blue-flowered C. amabile, the book mentions two other varieties with white and red flowers. The white-flowered variety was later identified as Cynoglossum amabile Stapf et Drumm f. eucanthum, and the red-flowered one as Cynoglossum amabile Stapf et Drumm f. ruberum. All three varieties can be used medicinally, with the blue-flowered one being the most commonly utilized (Lan, 1975).
Currently, many ethnic groups in China still maintain the traditional practice of using C. amabile for medicinal purposes (Geng et al., 2016; Gao et al., 2019; Wang et al., 2020; Zhang et al., 2022). As depicted in Table 1, different parts of C. amabile, including the whole plant, roots, and leaves, are utilized for various health conditions. The entire plant is typically collected in summer, then washed and dried or used fresh. When mashed and applied externally, the fresh plant can treat traumatic bleeding, fractures, joint dislocations, carbuncle, and abscess. When the sun-dried whole plant is decocted and ingested, it has effects such as clearing heat, removing dampness, dispersing blood stasis, stopping bleeding, and relieving cough. Roots are typically gathered in autumn, washed, sliced, and dried. They are commonly employed to address conditions like bleeding due to external injury, hepatitis, hernia, dysentery, malaria, and stranguria. Leaves are effective against a range of ailments, including various types of hernia pain, small bowel pain, bladder pain, and renal cysts (Jia and Li, 2005).
5 Phytochemistry
Research on the chemical components of Cynoglossum amabile can be primarily divided into two aspects: isolation and identification. The methods for isolating and identifying these components include ethanol extraction, PLC-MS/MS, DOLPA/AOT-UPLC, and IR/MS/NMR (Culvenor and Smith, 1967; Kedzierski and Buhler, 1986; Zhang et al., 2007; Fan et al., 2016; Fan et al., 2018). Presently, a total of 47 compounds have been identified in the leaves, flowers, seeds, and whole plants of C. amabile (Table 2). These 47 compounds encompass alkaloids, sterols, organic acids, amino acids, saccharides, triterpenoids, fatty acids, furans, and other categories.
5.1 Alkaloids
Alkaloids are the primary constituents of Cynoglossum, known for their diverse pharmacological and toxicological activities, such as anti-tumor, antibacterial, anti-diabetic, and hepatotoxic effects (Berkov et al., 2020; Rasouli et al., 2020; Zhou et al., 2021). Similar to other Cynoglossum plants, C. amabile employs alkaloid accumulation as its primary chemical defense mechanism (El-Shazly et al., 2001; Frölich et al., 2007; Damianakos et al., 2016).
A total of 12 alkaloids have been isolated and identified from C. amabile. For instance, Culvenor and Smith utilized paper chromatography, thin-layer chromatography (TLC), and gas chromatography to analyze dried C. amabile (163 g). They determined that it contained 0.53% N-oxide and 0.35% free base. The total crude base (380 mg) exhibited RF (paper) of 0.36, 0.40; RF (TLC) of 0.10, 0.30; and RT of 3.6. Subsequently, the crude base underwent countercurrent distribution between 20 mL phases of CHCl3 and potassium phosphate buffer at pH 7.7. After 50 transfers, base (184 mg) was isolated from tubes 20–32, with an RF (TLC) of 0.10, yielding amabiline with RF (paper) of 0.40 and an RF (TLC) of 0.10. From tubes 38–50, base (115 mg) with an RF (TLC) of 0.28 was isolated, yielding echinatine. Echinatine formed picrolonate yellow rosettes from ethanol, with a melting point of 209°C–209.5 °C (decomposition), remaining unchanged upon admixture with echinatine picrolonate (Found: C, 52.9; H, 6.0; N, 12.5. C15H25N05, C10HgN405 requires C, 53.3; H, 5.9; N, 12.4%) (Culvenor and Smith, 1967). Amabiline and Echinatine are both PAs (pyrrolizidine alkaloids).
Additionally, the PAs in C. amabile include heliosupine, supinine, and lasiocarpine. These alkaloids share a fundamental structure of 7-hydroxy-1-hydroxymethyl-6,7-dihydro-5h-pyrrolizidine, as depicted in Figure 2 (Schenk et al., 2015; Hama and Strobel, 2019). Other alkaloids found in C. amabile comprise heliotridine, heliotrine, rindeline, supinidine, and viridiflorine (Culvenor and Smith, 1967; El-Shazly et al., 1996; Fan et al., 2018).
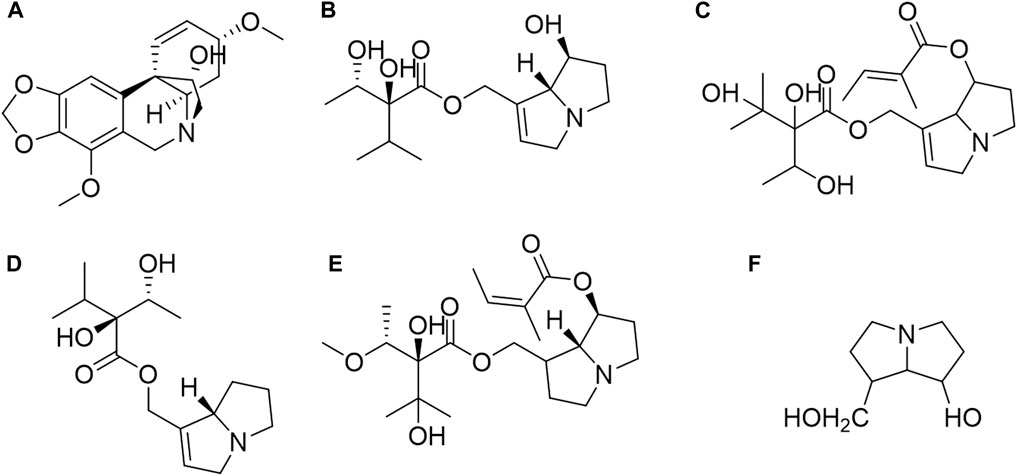
Figure 2. Structures of main PAs from Cynoglossum amabile and their basic structure. (A) Ambelline, (B) Echinatine, (C) Heliosupine, (D) Supinine, (E) Lasiocarpine, (F) The basic structure of PAs.
5.2 Sterols
Sterols are steroids containing hydroxyl groups. Their structure is akin to cholesterol but with a different side chain (Zhu et al., 2009; Melo et al., 2015; He et al., 2018). Most sterols possess double bonds at C-5, with the hydroxyl group at C-3 serving as an important functional group, enabling the formation of various derivatives (Das and Kumar, 2020; Xiong et al., 2020; Yan et al., 2022). When the double bond in a sterol is saturated, it is referred to as a stanol, and when esterified, it becomes a sterol ester (Ketomäki et al., 2003; O’Neill et al., 2005). Sterols can be categorized as phytosterols, animal sterols, and fungal sterols based on their sources. Common phytosterols include sitosterol, stigmasterol, and campesterol. Cholesterol is the primary animal sterol, while ergosterol is prevalent in fungi, primarily in molds and mushrooms (Martini et al., 2021; Shi et al., 2021; Zhang et al., 2021). The diverse number of methyl groups attached to C-4, the length of the side chain on C-11, and the number and location of double bonds contribute to the greater diversity of phytosterols compared to the other two sterol types (Kritchevsky and Chen, 2005; Marangoni and Poli, 2010). Sterols have many different biological functions. For example, animal sterols can be used as components of cell membranes and constitute adrenocortical hormones and sex hormones (Goldstein et al., 2006; Matić et al., 2014). Many phytosterols also have strong pharmacological or toxicological effects, such as digitalis and ouabain, which can enhance the contraction of the myocardium and be good drugs for the treatment of heart failure (Marangoni and Poli, 2010; Garcia et al., 2015; Ravi et al., 2020).
At present, there are four sterols identified from C. amabile, as shown in Figure 3, which are eleutheroside A, lupeol, stigmasterol, and β-sitosterol (Xu et al., 2008; Xu et al., 2009; Zhang, 2013). Xu et al. processed 3 kg of dried C. amabile by grinding them into powder. The coarse powder underwent three reflux extractions with 95% ethanol, each lasting 2 h. The resulting extracts were combined, concentrated, and sequentially extracted with petroleum ether (PE), chloroform, and ethyl acetate (EA). The PE fraction (19 g) was mixed with 200–300 mesh silica gel and applied to a column. Elution was performed using different ratios of PE and EA in TLC. Fractions with similar characteristics were combined, underwent recrystallization purification, and Eleutheroside A (50 mg) was obtained through EA elution. Stigmasterol (40 mg) was obtained through gradient elution with PE: EA (100:3) (Xu et al., 2008). Zhang conducted a similar process involving 30 kg of dried C. amabile. The material was ground into powder, and 3 kg of the resulting powder was extracted using 95% ethanol reflux. The extract was dispersed in water and successively extracted with PE, chloroform, EA, and n-butanol. The PE, chloroform, and n-butanol fractions were separated and identified using various chromatographic methods, including silica gel, MCI, glucose gel LH-20, and reverse-phase silica gel Rp-18, supplemented by recrystallization for purification. In total, 12 monomeric compounds were isolated and identified. Through physical and spectral analyses, as well as TLC comparison with standard substances, compounds seven and eight were identified as lupeol (77 mg) and β-sitosterol (254 mg), respectively (Zhang, 2013).
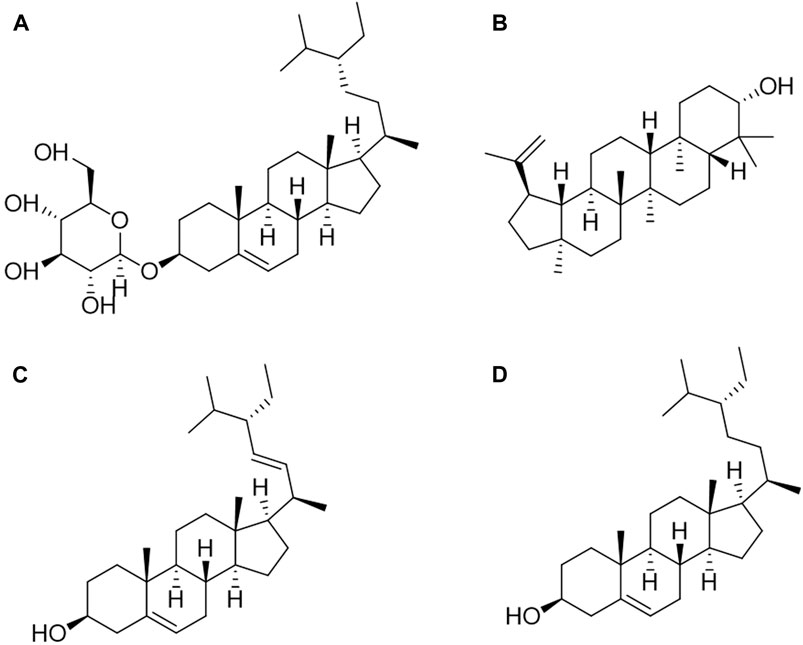
Figure 3. Structures of sterols from Cynoglossum amabile. (A) Eleutheroside A, (B) Lupeol, (C) Stigmasterol, (D) β-sitosterol.
5.3 Organic acids
Organic acids are compounds containing carboxyl groups within their molecules, encompassing carboxylic acids and their substituted counterparts. They naturally exist in animals and plants as free forms, salts, or esters (Bae and Lee, 2015; Ghislain et al., 2021; Huang et al., 2021). In a broader context, organic acids cover a wide range of compounds, including fatty acids or esters, phenolic acids or esters, amino acids or esters, and more. According to reported compounds, C. amabile contains 13 types of organic acids, including amino acids, phenolic acids, and fatty acids (Figure 4).
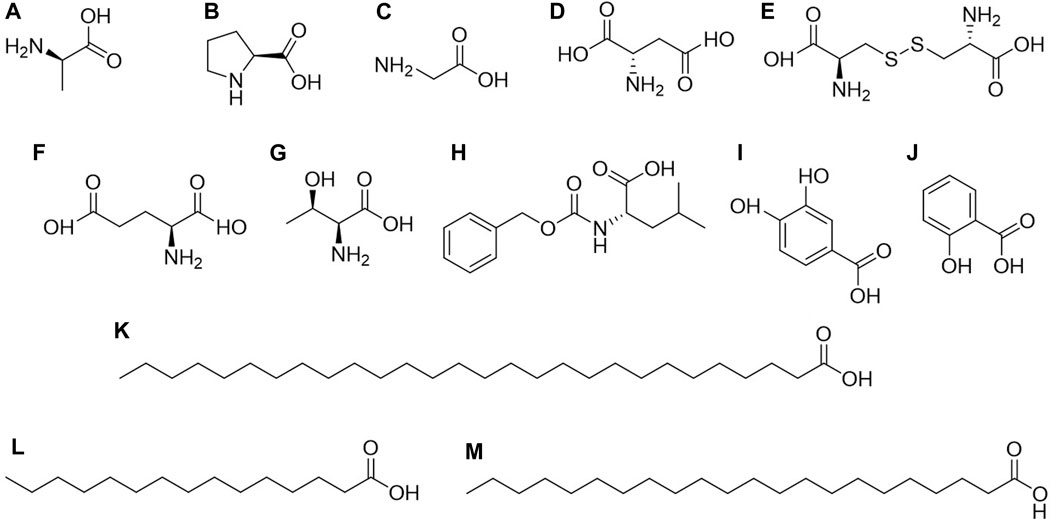
Figure 4. Structures of organic acids or esters from Cynoglossum amabile. (A) D-alanine, (B) DL proline, (C) Glycine, (D) L-aspartic acid, (E) L-cystine, (F) L-glutamic acid, (G) L-threonine, (H) N-carbobenzoxy-dl-leucine, (I) Protocatechuic acid, (J) Salicylic acid, (K) Octacosanoic acid, (L) Pentadecanoic acid, (M) Docosanoic acid.
Amino acids are primarily found in the seeds of C. amabile, including D-alanine, DL proline, Glycine, L-aspartic acid, L-cystine, L-glutamic acid, L-threonine, and N-carbobenzoxy-dl-leucine (Editorial Committee of Chinese Materia Medica, Traditional Chinese Medicine Administration of China, 1998), while phenolic acids and fatty acids are present throughout the entire plant. Among fatty acids, three types are identified: docosanoic acid, pentadecanoic acid, and octacosanoic acid. Salicylic acid and protocatechuic acid are the primary phenolic acids. As mentioned above, the components found in C. amabile by Xu et al. also include octacosanoic acid (200 mg). The 12 monomeric compounds separated and extracted from C. amabile by Zhang include docosanoic acid (11 mg), pentadecanoic acid (23 mg), and protocatechuic acid (18 mg) (Xu et al., 2008; Zhang, 2013). It is worth noting that these compounds are essential in organic synthesis and have widespread applications in pharmaceuticals, pesticides, rubber, dyes, food, and the fragrance industry, particularly in daily chemical products (Spepi et al., 2016; Tan et al., 2020).
5.4 Saccharides and others
Structurally, saccharides are polyhydroxy aldehydes and ketones and their polymers. They are widely present in plants, serving as vital components with significant roles in plant physiological and biochemical processes (Kapaev and Toukach, 2018; Xue et al., 2018; Li et al., 2019). Based on their structural differences, saccharides can be categorized into monosaccharides (such as glucose, fructose, and galactose), disaccharides (including sucrose, maltose, and lactose), and polysaccharides (such as starch and glycogen). Currently, nine saccharides have been isolated and identified from C. amabile seeds, encompassing both monosaccharides and polysaccharides (Editorial Board of Chinese Materia Medica, Traditional Chinese Medicine Administration of China, 1998; Alee et al., 2021; Debras et al., 2021) (Figure 5).
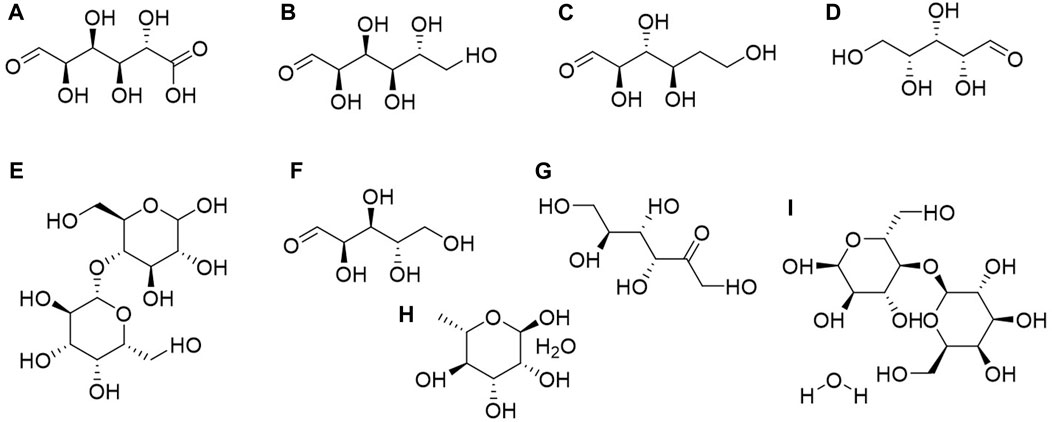
Figure 5. Structures of saccharides from the seeds of Cynoglossum amabile. (A) D-Glucuronic acid, (B) D (+)-Glucose, (C) D-Ribose, (D) D (+)-Xylose, (E) Lactose, (F) L-Arabinose, (G) L-fructose, (H) L (+)-Rhamnose monohydrate, (I) α-D-Lactose monohydrate.
Furthermore, C. amabile contains triterpenoid components, such as oleanolic acid, furan components [5- (hydroxymethyl) furan-2-yl] methanol in the entire plant, anthocyanin components like delphinidin 3,5-diglucoside in its flowers, and various other components, including viridifloric acid, amabilic acid, and 2α,3β,19α,23-tetrahydroxyolean-12-en-28-oic acid. Among these, oleanolic acid stands out as an intermediate compound used in medicine, pesticides, and dyes, with wide applications in the pharmaceutical and cosmetic industries (Culvenor and Smith, 1967; El-Shazly et al., 1996; Xu et al., 2009; Zhang, 2013).
6 Pharmacology
Cynoglossum amabile is a well-known traditional Chinese medicine often utilized in the treatment of hepatitis, dysentery, cough caused by tuberculosis, bleeding due to trauma, abnormal leucorrhea, and other diseases. As previously mentioned, C. amabile contains alkaloids, sterols, organic acids, saccharides, triterpenoids, and various other chemical components. Its primary component is PAs, indicating that this plant holds potential as an ethnomedicine deserving of significant research. A summary of the biological and pharmacological activities associated with C. amabile is presented in Table 3. Detailed information can be found below.
6.1 Anti-inflammatory
To assess the anti-inflammatory activity of amabilic acid extracted from C. amabile, SD mice were used as the test subjects. Aspirin served as the positive control, with a dosage of 60 mg/kg in the high-dose amabilic acid group and 30 mg/kg in the low-dose group. After 5 days of continuous intragastric administration, it was observed that amabilic acid significantly inhibited mouse auricle swelling caused by xylene (p < 0.01), surpassing the performance of aspirin, even when administered at half the dosage of aspirin (Xu et al., 2009). Therefore, it is essential to select an appropriate pharmacological model for further compound studies.
Echinatine, a primary component of PAs in C. amabile, was employed to test its inhibitory effect on NLRP3 inflammasome activation in mouse BM-derived macrophages (BMDMs) and LPS-primed human peripheral blood mononuclear cells (hPBMCs). The study revealed that echinatin exhibited no cytotoxicity at doses below 100 μM in BMDMs but effectively inhibited NLRP3 inflammasome by binding to heat-shock protein 90 (HSP90), thereby inhibiting its ATPase activity and disrupting the association between the cochaperone SGT1 and HSP90-NLRP3. Additionally, echinatin potently suppressed caspase-1 activation and IL-1β secretion in response to canonical NLRP3 stimuli. Furthermore, it demonstrated favorable pharmacological effects on liver inflammation and fibrosis in a mouse model of nonalcoholic steatohepatitis (NASH). These findings suggest that echinatin is a broad inhibitor of NLRP3 inflammasome, capable of suppressing both canonical and noncanonical NLRP3 inflammasome activation (Xu et al., 2021). This implies that C. amabile may serve as a promising candidate for therapeutic interventions in NLRP3 inflammasome-driven diseases.
6.2 Anti-tumor
Following the Cancer Chemotherapy National Service Center (CCNSC) protocols, the tumor-inhibitory properties of echinatine, europine, heliotrine, lasiocarpine, and supinine, the primary components in C. amabile, were assessed. Several tumor models including adenocarcinoma 755 in mice, lymphoid leukemia L1210, sarcoma 180, Walker 256 (intramuscular) in rats, Walker 256 (subcutaneous) in rats, and plasmacytoma one in hamsters were employed. Various dosing regimens were applied, ranging from one dose daily for 11 days to one dose daily for 15 days.
The results revealed widespread tumor-inhibitory activity among PAs. The tumors most frequently inhibited were Walker 256 (intramuscular) and adenocarcinoma 755. In some cases, the activity level against the former was very high, requiring heliotrine at a dose level of 125 mg/kg for complete or near-complete tumor destruction. Both heliotrine and lasiocarpine displayed activity against sarcoma 180. Lasiocarpine was effective against Walker 256 (subcutaneous) tumors but showed limited activity against leukemia 1210 and kB cell culture. Heliotrine exhibited strong activity against Walker 256 (intramuscular), while lasiocarpine showed moderate activity, and echinatine and supinine were essentially inactive. Although the known acute and chronic effects of PAs do not favor the development of tumor-suppressive drugs in analogs, these results suggest that for tumor-inhibitory activity, an optimum level or set of levels exists for one or more of the interrelated properties, such as water solubility, lipid-water partition coefficient, and base strength (Culvenor and Smith, 1967). Therefore, it may be possible to use these components to inhibit mitosis selectively, durably, and completely in parenchymal hepatocytes. If this activity can extend to liver cell-derived tumor cells, it holds promise for complete liver cancer inhibition and benefits the liver.
6.3 Antimicrobial
Four different polar fractions of the ethanol extract of C. amabile’s aerial parts were used to investigate their inhibitory effects on five bacteria, including Escherichia coli, Bacillus subtilis, Proteus vulgaris, Staphylococcus aureus, and Pseudomonas aeruginosa, as well as five plant pathogenic fungi, including Botryosphaeria rhodina, Fusarium oxysporum, Fusarium solani, Corynespora cassiicola, and Colletotrichum acutatum. The results demonstrated that the chloroform fraction had an inhibitory effect on E. coli and P. vulgaris, with a minimum inhibitory concentration (MIC) of 3 mg/mL. The EA fraction displayed inhibitory effects on E. coli, B. subtilis, and P. vulgaris, with an MIC of 2 mg/mL. The aqueous fraction only exhibited an inhibitory effect on E. coli, with an MIC of 20 mg/mL. The chloroform fraction displayed the most potent inhibitory effect on Fusarium solani, with a concentration of 50% of maximal effect (EC50) of 1.48 mg/mL. The EA fraction demonstrated the most potent inhibitory effects on B. rhodina and C. cassiicola, with EC50 values of 1.78 mg/mL and 0.63 mg/mL, respectively (Wang et al., 2014). These findings indicate that C. amabile possesses certain antibacterial activity, although its inhibitory effect varies among different strains.
However, this experiment only preliminarily conducted in vitro testing of C. amabile’s whole plant. Further research is needed to understand the mechanism of action on bacteria and plant pathogenic fungi through in vivo testing, separation and purification of effective antibacterial components from different parts of the plant, and the assessment of changes in the proportion of antibacterial and bacteria-promoting active substances.
6.4 Cardiovascular effect
To investigate the protective effect of echinatine on myocardial ischemia/reperfusion (I/R) injury in rats, adult Sprague-Dawley rats were randomly divided into five groups. Myocardial infarct size was estimated through 2,3,5-triphenyltetrazolium chloride staining. The coronary effluent was analyzed for the release of lactate dehydrogenase (LDH) and creatine kinase (CK) to assess cardiac injury. Concentrations of malondialdehyde (MDA), interleukin-6 (IL-6), and tumor necrosis factor-α (TNF-α) were determined, along with superoxide dismutase (SOD) activity using ELISA. Additionally, cardiomyocyte apoptosis analysis was conducted with POD, an in-situ cell death detection kit. The results indicated that echinatin (0.5 and 2.5 μg/mL) pretreatment enhanced the maximum up/down rate of the left ventricular pressure (±dp/dtmax), improved heart rate, increased left ventricular developed pressure (LVDP), enhanced coronary flow, and reduced CK and LDH levels in the coronary flow of the treated group compared to the I/R group. Echinatine limited CK and LDH levels, improved LVDP, reduced MDA, IL-6, and TNF-α levels, and increased SOD activity. The infarct size and cell apoptosis in the hearts of the rats in the echinatin-treated group were smaller and lower, respectively, than those in the hearts of the rats in the I/R control group. Therefore, echinatin exhibits a protective effect against I/R-induced myocardial injury (Tian, et al., 2016).
This effect of echinatine may be attributed to its antioxidant and anti-inflammatory activities. Researchers have reached a consensus that oxidative stress can trigger significant myocardial injury after I/R through certain pathways (Nazari et al., 2015; Sun et al., 2015). In this experiment, echinatine treatment significantly reduced the MDA content in I/R rats and improved the I/R-induced deterioration of total antioxidant capacity (SOD activity). Inflammation, which plays a crucial role in many disease states, is associated with enhanced expression of adhesive molecules in the vasculature, leading to the infiltration of larger populations of neutrophils and monocytes/macrophages. The release of pro-inflammatory cytokines from these activated leukocytes can cause tissue damage (Varela et al., 2013). The results of this experiment showed that I/R increased the production of these cytokines, while echinatine treatment reduced their concentrations. Overall, this extract may inhibit myocardial I/R injury through its anti-inflammatory activities.
6.5 Ganglionic blocking action
Heliotrine is one of the primary PAs found in C. amabile. To test its ganglionic blocking action, adult mongrel dogs (8–15 kg) of either sex were anesthetized with chloralose (70 mg/kg, i. v.). Drugs were administered through the cannulated femoral vein. The effect of graded doses of the test drug on blood pressure and nictitating membrane responses to nicotine (25 μg/kg) and adrenaline (10 μg/kg) was recorded. An interval of 5–10 min was maintained between two successive drug administrations. Meanwhile, the ED50 of the test drug against nicotine-induced spasmogenic response was determined on isolated guinea pig ileum. The results were compared with pentolinium, a known ganglion blocker. In vivo and in vitro experiments with heliotrine revealed that it selectively reduces the autonomic ganglion stimulant effects of nicotine, suggesting that it possesses ganglion blocking activity. In vitro studies suggest that heliotrine is approximately 13 times less potent than pentolinium. Additionally, heliotrine has neither central nervous system activity nor central muscle blocking effects and does not improve spasms caused by acetylcholine and barium chloride. The potentiation of adrenaline-induced vasopressor and nictitating membrane responses by the test compound may be caused by decentralization supersensitivity, a phenomenon known to occur after the administration of ganglion-blocking agents (Pandey et al., 1982).
6.6 Other crucial activities
Studies have indicated that cholinergic neurons in the basal forebrain of patients with Alzheimer’s disease (AD) are absent, the activity of acetylcholinesterase (AChE) is increased, and the content of acetylcholine, a neurotransmitter, is decreased. Consequently, targeting AChE and inhibiting its activity is a classic strategy for treating AD (Ibach and Haen, 2004; Kou et al., 2021). To analyze the interaction of PAs, such as heliosupine and heliotrine, with acetylcholine-related enzymes (acetylcholinesterase and butyrylcholinesterase), choline acetyltransferase (ChAT), and neuroreceptors (α1-and α2-adrenergic, nicotinergic [nACh], muscarinergic [mACh], and serotonin2 [5-HT2] receptors), testing was conducted. The results indicated that heliosupine and heliotrine displayed significant binding activities to mACh and 5-HT2 receptors, with IC50 values of 390.0 μM and 52.2 μM, 77.1 μM and 535.4 μM, respectively. This suggests that C. amabile may hold potential as a therapeutic agent for AD.
Furthermore, in an aphid resistance activity test experiment, amabiline was tested against Aphis citricola. The results demonstrated that amabiline exhibited considerable aphicidal activity, with LD50 values of 67.44 ng/aphid (Yan et al., 2018). Aphids are among the most destructive and economically significant plant pests globally, causing substantial financial losses. These results suggest that C. amabile has the potential to be developed as a biological pesticide to combat the harm caused by aphids.
7 Toxicity
Since the 1930s, there have been explosive cases or individual cases of human liver poisoning caused by plants containing PAs in Jamaica, India, Afghanistan, the United States, Europe, and other places, leading to global concern about its hepatotoxicity (Mathon et al., 2014; Yang et al., 2018; Xu et al., 2019). Modern research indicates that the most basic structural unit of PAs’s toxicity is when there are double bonds at one and 2, and at least one hydroxyl group at seven or nine is esterified. The toxicity of diesters is greater than that of monoesters, and macrocyclic diester PAs are more toxic than open-chain diesters (Hartmann et al., 1997; Fu et al., 2014). The pharmacological activity and toxicity of Cynoglossum amabile are derived from a large number of PAs (Yan et al., 2011; Vrieling, 2021).
7.1 Hepatic and pulmonary toxicity
Numerous experiments and clinical observations have revealed that the toxicity of PAs often occurs in the liver. Exposure to large amounts can lead to acute poisoning, characterized by hepatic veno-occlusive disease (HVOD) or hepatic sinusoidal obstruction syndrome (SOS). Long-term ingestion of small amounts of PAs results in chronic toxicity, manifested as hepatic giant cell formation and hepatic fibrosis (Tang et al., 2007; Tang and Hattori, 2011). PAs undergo metabolic activation by liver P450s, producing pyrrolic metabolites or “metabolic pyrroles.” These pyrrolic metabolites enter the cytoplasm, where they react with essential life substances such as thiol-containing amino acids, small peptides, proteins, enzymes, and RNA. Another portion degrades rapidly, forming a series of secondary toxic metabolites, including tissue-bound pyrroles, glutathione pyrrolic conjugates (e.g., 7-GSH-DHR), and dehydroretronecine (DHR). These reactions lead to continuous poisoning of liver cells during detoxification, resulting in irreversible liver cell dysfunction, necrosis, and tissue damage (Han et al., 2011; Yang et al., 2017; Suparmi et al., 2020).
The lungs are another important target organ for the toxicity of certain PAs, manifesting as pulmonary arterial hypertension and pulmonary veno-occlusive disease. The formation of pyrrolic metabolites is also implicated in lung toxicity. For example, in vitro experiments show that PAS in rats generates pyrrolic-protein adducts in the liver and lungs. Severe damage occurs in the pulmonary artery endothelial cells and abnormal proliferation of pulmonary artery smooth muscle cells when mild liver damage is present in rats. These results suggest that a significant portion of dehydropyrrolizidine alkaloids (DHPAs) transfers from the liver to the lungs, preferentially inducing lung toxicity (Kempf et al., 2010; Sun et al., 2019; Song et al., 2020). However, the mechanism behind pulmonary toxicity, whether it is related to liver metabolism, and how pyrrolic metabolites are transported from the liver to the lungs remain unclear (Tang and Hattori, 2011; Nakai et al., 2019).
7.2 Genotoxicity and carcinogenicity
Genotoxicity refers to the ability of a substance to damage cellular DNA directly or indirectly, causing gene mutations or mutations in the body, with the potential or tendency to induce mutations and carcinogenesis. Genotoxic substances have the characteristic of causing damage to genetic material even at low concentrations and are highly toxic (Tang and Hattori, 2011; Luckert et al., 2015). After metabolic activation, PAs exhibit various genetic toxicities. Long-term ingestion of PAs leads to changes in cell morphology, enlargement of cell nuclei, loss of cell metabolic function, mitotic inhibition, lipid degeneration, and cell carcinogenesis. This includes DNA binding, DNA cross-linking, DNA-protein cross-linking, and chromosomal aberrations (Xia et al., 2008; Brown et al., 2015). For example, treatment of P53 knockout mice with different concentrations of PAs shows dose-dependent promotion of tumor occurrence and development, primarily manifested as hepatic hemangiosarcoma with accompanying vascular dilation (hepatic peliosis) (Brown et al., 2015).
The carcinogenic effect of PAs is related to the formation of pyrrolic-DNA adducts. PAs and PANOs in rat livers produce pyrrolic derivatives that bind to DNA, generating pyrrolic-DNA adducts, potential biomarkers for PAs’ carcinogenicity. The levels of adduct formation differ for PAs with different structures, with the ranking as follows: diester PAs > monoester PAs - diester PANOs >> non-esterified (Xia et al., 2008).
7.3 Toxicity control methods
Due to the complex chemical structure of PAs, removing these components poses significant challenges, a problem faced by many herbal medicines containing PAs. Currently, there is no universally accepted and determined method to completely remove pyrrolizidine alkaloids (PAs) from plants. However, research indicates that PAs are primarily eliminated through hydrolysis reactions and N-oxidation excretion (Habs et al., 2018). When exposure to PAs is low, reduced glutathione can bind with the dehydrogenated metabolites of PAs, preventing the latter from forming toxic complexes with DNA or proteins, representing the main detoxification pathway for PAs (Yang et al., 2016). In addition, oxidative damage also contributes to the toxicity of PAs (Yang et al., 2016; Habs et al., 2018), so substances with antioxidant properties also have a certain protective and ameliorative effect on the toxicity of PAs.
Currently, many countries or organizations have established regulations regarding the widespread presence and harm of PAs in human food and natural medicines (Kempf et al., 2010). For example, the World Health Organization (WHO) has recommended monitoring the content of PAs in honey and dairy products and issued health and safety guidelines on pyrrolizidine alkaloids in 1989. Dutch authorities stipulate that the total PAs content in 1 kg or 1 L of herbal products or extracts must not exceed 1 μg (1 ppb) (Kempf et al., 2010). The U.S. Food and Drug Administration (FDA) prohibits the use of comfrey (Symphytum) plants from the Boraginaceae family in the food processing industry (Tang and Hattori, 2011). Recently, the European Commission has mandated that pyrrolizidine alkaloid components should not be detectable in borage oil (primarily seed oil from Echium plantagineum L. of the Boraginaceae family) used as a food ingredient or additive (with a detection limit of 4 ppb) (Kempf et al., 2010; Tang and Hattori, 2011; Luckert et al., 2015). These regulations are worth considering and learning from when applying and promoting the use of herbal materials containing PAs, such as C. amabile, Onosma paniculatum Bur. Et Franch, and Senecio scandens Buch.-Ham. ex D. Don.
8 Clinical application
In traditional medicine, Cynoglossum amabile is commonly used by people in Southwest China to treat malaria, hepatitis, dysentery, urinary pain, leucorrhea, tuberculosis, and cough. It is also applied externally to treat trauma, bleeding, fractures, and joint dislocations. The researchers are currently actively exploring the clinical applications of this herbal medicine.
In the treatment of cardiovascular diseases, Xinyihao, a compound preparation composed of C. amabile, possesses functions such as promoting blood circulation, removing blood stasis, strengthening the spleen and Qi, and reducing swelling and pain. It can increase coronary flow, resist coronary spasms caused by pituitrin, reduce oxygen consumption, and withstand atmospheric and low-pressure hypoxia (Dai, 2015; Li, 2017).
According to a Chinese patent report, using C. amabile and its extracts to treat prostate disease and sexual dysfunction caused by prostate disease offers fast and reliable effects. They can enhance and repair certain physiological functions of the human body, improving overall defense and quality of life (Zhao, 2007a).
Cynoglossum amabile extract is used to treat hemorrhoids, constipation, anal prolapse, and other anorectal diseases. It exhibits rapid onset, a stable effect, improvement and repair of human physiological functions, and increased resistance. It has been used by nearly 100 patients with an effective rate of about 90%, offering a new option for clinical treatment of these conditions (Zhao, 2016).
Additionally, preparations made from C. amabile’s original medicinal materials, processed products, or extracts have shown a positive effect in treating alopecia, with an effective rate of about 85%, especially in cases of androgenic alopecia. After 30 days of treatment, patients generally experience reduced hair loss. After 2–3 months of use, atrophic hair follicles begin to repair, new hair grows in bald areas, and the bald area is gradually covered by new hair. Hair growth and metabolism gradually return to normal (Zhao, 2007b).
However, as a toxic plant medicine, there is a far from sufficient understanding of the clinical research on the use of C. amabile. Limited patent reports only indicate the potential future applications of this medicine and provide basic formulations and preparation methods, without offering detailed clinical research results and relevant parameters. For the promotion and application of a medicine, specialized clinical studies are indispensable. This includes specifying the types of clinical studies conducted (open-label, blind, controlled trials, etc.), dosage administration (and whether the extract is standardized), duration, and study outcomes (the percentage of subjects showing a positive response to the treatment). Other crucial information involves the extract/compound used, parameters influenced by the extract/compound administration, types of compounds that may have therapeutic potential and their mechanisms of action, and whether registered subjects reported any side effects or toxicity.
9 Conclusion
Cynoglossum amabile is commonly used in ethnomedicine in Southwest China and has a long history of medicinal use. This paper provides a comprehensive review of the botanical description, geographic distribution, traditional utilization, phytochemistry, pharmacology, toxicology, and clinical applications of C. amabile. The whole plant, roots, and leaves of C. amabile are often utilized as medicine in folk practices, each exhibiting different efficacies. Phytochemical studies have identified the main chemical components in C. amabile as pyrrolizidine alkaloids (PAs), sterols, and organic acids or esters. Among these, PAs are the most abundant, and the pharmacological effects, including anti-inflammatory, anti-tumor, anti-microbial, cardiovascular effects, ganglionic blocking, acetylcholinesterase inhibition, and hepatotoxicity of C. amabile, are primarily attributed to them. The absorption, distribution, metabolism, and excretion of PAs are closely linked to their pharmacological effects and toxicity mechanisms.
Despite its limited application in clinical settings, there are numerous research gaps concerning C. amabile. It is crucial to analyze this plant using innovative technologies and methodologies. For instance, there is insufficient research on the in vivo and ex vivo metabolism of PAs in C. amabile, particularly regarding their pharmacokinetics (absorption, distribution, and excretion) in animals and humans. Pharmacokinetic studies can unveil the metabolic pathways and biological activities of the identified components or differential metabolites in the root, stem, leaf, flower, seed, and other parts of C. amabile, thereby establishing the scientific foundation for its traditional folk use and offering guidance for further development and application. Moreover, the presence of salicylic acid, protocatechuic acid, and oleanolic acid in C. amabile suggests its significant potential in the daily chemical industry. However, it is essential to prioritize an in-depth analysis of the chemical components.
Author contributions
YF: Data curation, Formal Analysis, Investigation, Writing–original draft. MW: Investigation, Writing–review and editing. QZ: Investigation, Visualization, Writing–review and editing. SO: Writing–review and editing. WM: Investigation, Writing–review and editing. CX: Investigation, Writing–review and editing. MW: Conceptualization, Supervision, Writing–review and editing. CL: Conceptualization, Funding acquisition, Supervision, Writing–review and editing.
Funding
The author(s) declare financial support was received for the research, authorship, and/or publication of this article. This research was funded by the Yunnan Provincial Science and Technology Talent and Platform Plan (202305AF150121), Assessment of Edible and Medicinal Plant Diversity and Associated Traditional Knowledge in Gaoligong Mountains (GBP-2022-01), the National Natural Science Foundation of China (31761143001 and 31870316), China Scholarship Council (202206390021), the Minzu University of China (2020MDJC03, 2022ZDPY10, and 2022GJAQ04) and the China Scholarship Council (202206390021).
Acknowledgments
We thank the local people in Yunnan, who assisted us in accessing the valuable information associated with traditional use purposes of Cynoglossum amabile, as well as the members of the Ethnobotanical Laboratory at the Minzu University of China, who participated in the field surveys and supported the completion of this manuscript.
Conflict of interest
The authors declare that the research was conducted in the absence of any commercial or financial relationships that could be construed as a potential conflict of interest.
Publisher’s note
All claims expressed in this article are solely those of the authors and do not necessarily represent those of their affiliated organizations, or those of the publisher, the editors and the reviewers. Any product that may be evaluated in this article, or claim that may be made by its manufacturer, is not guaranteed or endorsed by the publisher.
References
Alee, M., Duan, Q. F., Chen, Y., Liu, H. S., Ali, A., Zhu, J., et al. (2021). Plasticization efficiency and characteristics of monosaccharides, disaccharides, and low-molecular-weight polysaccharides for starch-based materials. ACS Sustain Chem. Eng. 9, 11960–11969. doi:10.1021/acssuschemeng.1c04374
Bae, Y. M., and Lee, S. Y. (2015). Combined effects of organic acids and salt depending on type of acids and pathogens in laboratory media and acidified pickle. J. Appl. Microbiol. 119, 455–464. doi:10.1111/jam.12845
Berkov, S., Osorio, E., Viladomat, F., and Bastida, J. (2020). Chemodiversity, chemotaxonomy and chemoecology of Amaryllidaceae alkaloids. Alkaloids Chem. Biol. 83, 113–185. doi:10.1016/bs.alkal.2019.10.002
Brown, A. W., Stegelmeier, B. L., Colegate, S. M., Panter, K. E., Knoppel, E. L., and Hall, J. O. (2015). Heterozygous p53 knockout mouse model for dehydropyrrolizidine alkaloidinduced carcinogenesis. J. Appl. Toxicol. 35, 1557–1563. doi:10.1002/jat.3120
Chinese Academy of Sciences (1960). Si chuan Zhong Yao Zhi. People’s Publishing House of Sichuan. Sichuan.
Culvenor, C., and Smith, L. W. (1967). The alkaloids of Cynoglossum australe R.Br. and C. amabile Stapf and Drummond. Aust. J. Chem. 20, 2499–2503. doi:10.1071/CH9672499
Dai, W. J. (2015). A kind of medicinal preparation for nourishing the heart and soothing the mind. CN105147905A. Jiangsu: Google Patents.
Damianakos, H., Jeziorek, M., Pietrosiuk, A., Sykłowska-Baranek, K., and Chinou, I. (2013). Isolation of pyrrolizidine alkaloids from Cynoglossum columnae ten. (Boraginaceae). Planta Med. 79, 13. doi:10.1055/s-0033-1352119
Damianakos, H., Jeziorek, M., Sykłowska-Baranek, K., Buchwald, W., Pietrosiuk, A., and Chinou, L. (2016). Pyrrolizidine alkaloids from Cynoglossum columnae ten. (Boraginaceae). Phytochem. Lett. 15, 234–237. doi:10.1016/j.phytol.2016.02.005
Das, M., and Kumar, G. S. (2020). Potential role of mycosterols in hyperlipidemia-A review. Steroids 166, 108775. doi:10.1016/j.steroids.2020.108775
Debras, C., Chazelas, E., Srour, B., Julia, C., Schneider, E., Kesse-Guyot, E., et al. (2021). Fermentable oligosaccharides, disaccharides, monosaccharides, and polyols (FODMAPs) and cancer risk in the prospective NutriNet-santé cohort. Cohort J. Nutr. 152, 1059–1069. doi:10.1093/jn/nxab379
Edgar, J. A., Roeder, E., and Molyneux, R. J. (2002). Honey from plants containing pyrrolizidine alkaloids: a potential threat to health. J. Agric. Food Chem. 50, 2719–2730. doi:10.1021/jf0114482
Editorial Committee of Chinese Materia Medica, Traditional Chinese medicine administration of China (1998). Chinese Materia Medica. Shanghai: Shanghai Science and Technology Press.
El-Shazly, A., Sarg, T., Ateya, A., E.Abdel Aziz, E. A., Witte, L., and Wink, M. (1996). Pyrrolizidine alkaloids of Cynoglossum officinale and Cynoglossum amabile (family Boraginaceae). Biochem. Syst. Ecol. 24, 415–421. doi:10.1016/0305-1978(96)00035-X
El-Shazly, A., Sarg, T., Witte, L., and Winkc, M. (2001). Corrigendum to pyrrolizidine alkaloids from Cynoglossum creticum. Phytochemistry 57, 317. doi:10.1016/S0031-9422(00)00457-X
Fan, Q. Y., Wang, W. H., and Dai, C. M. (2018). Determination of four alkaloids in Cynoglossum amabile Stapf et Drumm by ultrahigh performance liquid chromatography with DOLPA/AOT-isooctane reverse micelles extraction. J. Instrum. Anal. 37, 1445–1450. doi:10.3969/j.issn.1004-4957.2018.12.007
Fan, Q. Y., Zhang, H. H., and Wang, W. H. (2016). Research on separation and purification of alkaloids in Cynoglossum amabile Stapf et Drumm with resin. J. Instrum. Anal. 35, 1338–1342. doi:10.3969/j.issn.1004-4957.2016.10.021
Fayed, M. (2021). Heliotropium, a genus rich in pyrrolizidine alkaloids: a systematic review following its phytochemistry and pharmacology. Phytomed Plus 1, 100036. doi:10.1016/j.phyplu.2021.100036
Frölich, C., Ober, D., and Hartmann, T. (2007). Tissue distribution, core biosynthesis and diversification of pyrrolizidine alkaloids of the lycopsamine type in three Boraginaceae species. Phytochemistry 68, 1026–1037. doi:10.1016/j.phytochem.2007.01.002
Fu, P. P., Xia, Q. S., Lin, G., and Chou, M. W. (2014). Pyrrolizidine alkaloids--genotoxicity, metabolism enzymes, metabolic activation, and mechanisms. Drug Metab. Rev. 36, 1–55. doi:10.1081/dmr-120028426
Gao, L. L., Wei, N., Yang, G. P., Zhang, Z. X., Liu, G. Z., and Cai, C. T. (2019). Ethnomedicine study on traditional medicinal plants in the wuliang Mountains of jingdong, yunnan, China. J. Ethnobiol. Ethnomed 15, 41. doi:10.1186/s13002-019-0316-1
Garcia, A., Liu, C., Hannam, W., Fry, N., and Rasmussen, H. (2015). Not all cardiac glycosides are equal: could ouabain prove to be better than digoxin for heart failure? Heart Lung Circ. 24, S213. doi:10.1016/j.hlc.2015.06.243
Geng, Y. F., Zhang, Y., Ranjitkar, S., Huai, H. Y., and Wang, Y. H. (2016). Traditional knowledge and its transmission of wild edibles used by the Naxi in baidi village, northwest yunnan Province. J. Ethnobiol. Ethnomed 12, 10. doi:10.1186/s13002-016-0082-2
Ghislain, M., Reyrolle, M., Sotiropoulos, J. M., Pigot, T., and Bechec, M. L. (2021). Chemical ionization of carboxylic acids and esters in negative mode selected ion flow tube-mass spectrometry (SIFT-MS). Microchem J. 169, 106609. doi:10.1016/j.microc.2021.106609
Goldstein, J. L., Debose-Boyd, R. A., and Brown, M. S. (2006). Protein sensors for membrane sterols. Cell 124, 35–46. doi:10.1016/j.cell.2005.12.022
Gong, X. L., Xie, Z. W., and Wu, J. L. (2001). Tianbao Materia Medica. Beijing: Traditional Chinese Medicine Classics Press.
Habs, M., Binder, K., Krauss, S., Müller, K., Ernst, B., Valentini, L., et al. (2018). A balanced risk-benefit analysis to determine human risks associated with pyrrolizidine alkaloids (PA)-The case of herbal medicinal products containing St. John's wort extracts (SJW). Nutrients 10, 804. doi:10.3390/nu10070804
Hama, J. R., and Strobel, B. W. (2019). Pyrrolizidine alkaloids quantified in soil and water using UPLC-MS/MS. RSC Adv. 9, 30350–30357. doi:10.1039/C9RA05301H
Han, H. L., Jiang, C. L., Wang, C., Wang, Z. Q., Chai, Y. F., Zhang, X. C., et al. (2022). Development, optimization, validation and application of ultra high performance liquid chromatography tandem mass spectrometry for the analysis of pyrrolizidine alkaloids and pyrrolizidine alkaloid N-oxides in teas and weeds. Food control. 132, 108518. doi:10.1016/j.foodcont.2021.108518
Han, J. Y., Liang, A. H., and Gao, S. R. (2011). Research progress of especial toxicity and of pyrrolizidine alkaloids. China J. Chin. Materia Medica 36, 1397–1401. doi:10.4268/cjcmm20111030
Hartmann, T., Witte, L., Ehmke, A., Theuring, C., Rowell-Rahier, M., and Pasteels, J. M. (1997). Selective sequestration and metabolism of plant derived pyrrolizidine alkaloids by chrysomelid leaf beetles. Phytochemistry 45, 489–497. doi:10.1016/S0031-9422(97)00009-5
He, W. S., Zhu, H., and Chen, Z. Y. (2018). Plant sterols: chemical and enzymatic structural modifications and effects on their cholesterol-lowering activity. J. Agric. Food Chem. 66, 3047–3062. doi:10.1021/acs.jafc.8b00059
Huang, Y., Ren, T. Y., Wang, H. Q., Xie, J., Lin, J., Jiang, Y. E., et al. (2021). Codon pair optimization (CPO): a software tool for synthetic gene design based on codon pair bias to improve the expression of recombinant proteins in Pichia pastoris. Food and Mach. 37, 209–214. doi:10.1186/s12934-021-01696-y
Ibach, B., and Haen, E. (2004). Acetylcholinesterase inhibition in Alzheimer’s disease. Curr. Pharm. Des. 10, 231–251. doi:10.2174/1381612043386509
Jia, M. R., and Li, X. R. (2005). Zhong guo min zu Yao Zhi Yao. Beijing: The Medicine Science and Technology Press of China.
Kapaev, R. R., and Toukach, P. V. (2018). GRASS: semi-automated NMR-based structure elucidation of saccharides. Bioinformatics 15, 957–963. doi:10.1093/bioinformatics/btx696
Kedzierski, B., and Buhler, D. R. (1986). The formation of 6,7-dihydro-7-hydroxy-1-hydroxy-methyl-5h-pyrrolizine, a metabolite of pyrrolizidine alkaloids. Chem. Biol. Interact. 57, 217–222. doi:10.1016/0009-2797(86)90039-6
Kempf, M., Reinhard, A., and Beuerle, T. (2010). Pyrrolizidine alkaloids (PAs) in honey and pollen-legal regulation of PA levels in food and animal feed required. Mol. Nutr. Food Res. 54, 158–168. doi:10.1002/mnfr.200900529
Ketomäki, A. M., Gylling, H., Antikainen, M., Siimes, M. A., and Miettinen, T. A. (2003). Red cell and plasma plant sterols are related during consumption of plant stanol and sterol ester spreads in children with hypercholesterolemia. J. Pediatr. 142, 524–531. doi:10.1067/mpd.2003.193
Kou, X. D., Liu, J. J., Chen, Y. H., Yang, A. H., and Shen, R. (2021). Emodin derivatives with multi-factor anti-AD activities: AChE inhibitor, anti-oxidant and metal chelator. J. Mol. Struct. 1239, 130459. doi:10.1016/j.molstruc.2021.130459
Kritchevsky, D., and Chen, S. C. (2005). Phytosterols-health benefits and potential concerns: a review. Nutr. Res. 25, 413–428. doi:10.1016/j.nutres.2005.02.003
Li, G. L. (2017). A kind of traditional Chinese medicine preparation for nourishing the heart and calming the nerves and preparation method thereof. Zhejiang: CN106729017A.
Li, N., Yu, X., Yu, Q. H., and Wang, M. (2019). Research progress on stability of polysaccharides in traditional Chinese medicine. China J. Chin. Materia Medica 44, 4793–4799. doi:10.19540/j.cnki.cjcmm.20190916.309
Lorena, L., Roberta, M., Alessandra, R., Clara, M., and Francesca, C. (2016). Evaluation of Some pyrrolizidine alkaloids in honey samples from the Veneto Region (Italy) by LC-MS/MS. Food Anal. Methods 9, 1825–1836. doi:10.1007/s12161-015-0364-7
Luckert, C., Hessel, S., Lenze, D., and Lampen, A. (2015). Disturbance of gene expression in primary human hepatocytes by hepatotoxic pyrrolizidine alkaloids: a whole genome transcriptome analysis. Toxicol. vitro 29, 1669–1682. doi:10.1016/j.tiv.2015.06.021
Marangoni, F., and Poli, A. (2010). Phytosterols and cardiovascular health. Pharmacol. Res. 61, 193–199. doi:10.1016/j.phrs.2010.01.001
Martini, M., Salari, F., Buttau, L., and Altomonte, L. (2021). Natural content of animal and plant sterols, alpha-tocopherol and fatty acid profile in sheep milk and cheese from mountain farming. Small Rumin. Res. 201, 106419. doi:10.1016/j.smallrumres.2021.106419
Mathon, C., Edder, P., Bieri, S., and Christen, P. (2014). Survey of pyrrolizidine alkaloids in teas and herbal teas on the swiss market using HPLC-MS/MS. Anal. Bioanal. Chem. 406, 7345–7354. doi:10.1007/s00216-014-8142-8
Matić, I., Grujić, S., Jauković, Z., and Laušević, M. (2014). Trace analysis of selected hormones and sterols in river sediments by liquid chromatography-atmospheric pressure chemical ionization-tandem mass spectrometry. J. Chromatogr. A 1364, 117–127. doi:10.1016/j.chroma.2014.08.061
Melo, M. L. S., Silva, M. M. C., Iuliano, L., and Lizard, G. (2015). Oxysterols and related sterols: chemical, biochemical and biological aspects. Steroids 99, 117–118. doi:10.1016/j.steroids.2015.05.006
Mudge, E. M., Jones, A. M. P., and Brown, P. N. (2015). Quantification of pyrrolizidine alkaloids in North American plants and honey by LC-MS: single laboratory validation. Food Addit. Contam. A 32, 2068–2074. doi:10.1080/19440049.2015.1099743
Nakai, G., Shimura, D., Uesugi, K., Kajimura, I., Jiao, Q. B., Kusakari, Y., et al. (2019). Pyruvate dehydrogenase activation precedes the downregulation of fatty acid oxidation in monocrotaline-induced myocardial toxicity in mice. Heart Vessels 34, 545–555. doi:10.1007/s00380-018-1293-3
Nazari, A., Sadr, S. S., Faghihi, M., Azizi, Y., Hosseini, M. J., Mobarra, N., et al. (2015). Vasopressin attenuates ischemia-reperfusion injury via reduction of oxidative stress and inhibition of mitochondrial permeability transition pore opening in rat hearts. Eur. J. Pharmacol. 760, 96–102. doi:10.1016/j.ejphar.2015.04.006
O’Neill, F. H., Sanders, T. A. B., and Thompson, G. R. (2005). Comparison of efficacy of plant stanol ester and sterol ester: short-term and longer-term studies. Am. J. Cardiol. 96, 29D–36D. doi:10.1016/j.amjcard.2005.03.017
Pandey, V. B., Singh, J. P., Rao, Y. V., and Acharya, S. B. (1982). Isolation and pharmacological action of heliotrine, the major alkaloid of Heliotropium indicum seeds. Planta Med. 45, 229–233. doi:10.1055/s-2007-971378
Rasouli, H., Yarani, R., Pociot, F., and Popović-Djordjević, J. B. (2020). Anti-diabetic potential of plant alkaloids: revisiting current findings and future perspectives. Pharmacol. Res. 155, 104723. doi:10.1016/j.phrs.2020.104723
Ravi, B. G., Guardian, M., Dickman, R., and Wang, Z. Q. (2020). Profiling and structural analysis of cardenolides in two species of digitalis using liquid chromatography coupled with high-resolution mass spectrometry. J. Chromatogr. A 1618, 460903. doi:10.1016/j.chroma.2020.460903
Schenk, A., Siewert, B., Toff, S., and Drewe, J. (2015). UPLC-TOF-MS for sensitive quantification of naturally occurring pyrrolizidine alkaloids in Petasites hybridus extract (Ze 339). J. Chromatogr. B 997, 23–29. doi:10.1016/j.jchromb.2015.05.027
Schmeller, T., El-Shazly, A., and Wink, M. (1997). Allelochemical activities of pyrrolizidine alkaloids: interactions with neuroreceptors and acetylcholine related enzymes. J. Chem. Ecol. 23, 399–416. doi:10.1023/b:joec.0000006367.51215.88
Shi, T., Wu, G. C., Jin, Q. Z., and Wang, X. G. (2021). Detection of Camellia oil adulteration using chemometrics based on fatty acids gc fingerprints and phytosterols GC-MS fingerprints. Food Chem. 352, 129422. doi:10.1016/j.foodchem.2021.129422
Smadi, A., Bitam, F., Ciavatta, M. L., Carbone, M., Bertella, A., and Gavagnin, M. (2020). Chemical constituents of the aerial parts of Algerian Galium brunneum: isolation of new hydroperoxy sterol glucosyl derivatives. Phytochem. Lett. 38, 39–45. doi:10.1016/j.phytol.2020.05.002
Song, Z., He, Y., Ma, J., Fu, P. P., and Lin, G. (2020). Pulmonary toxicity is a common phenomenon of toxic pyrrolizidine alkaloids. J. Environ. Sci. Health C 38, 124–140. doi:10.1080/26896583.2020.1743608
Spepi, A., Duce, C., Pedone, A., Presti, D., Rivera, J. G., Ierardi, V., et al. (2016). Experimental and DFT characterization of halloysite nanotubes loaded with salicylic acid. J. Phys. Chem. C 120, 26759–26769. doi:10.1021/acs.jpcc.6b06964
Stegelmeier, B. L. (2011). Pyrrolizidine alkaloid-containing toxic plants (Senecio, Crotalaria, Cynoglossum, Amsinckia, Heliotropium, and Echium spp.). Vet. Clin. N. Am-Food. A 27, 419–428. doi:10.1016/j.cvfa.2011.02.013
Stegelmeier, B. L., Gardner, D. R., and Davis, T. Z. (2009). Livestock poisoning with pyrrolizidine-alkaloid-containing plants (Senecio, Crotalaria, Cynoglossum, Amsinckia, Heliotropium, and Echium spp.). Rangelands 31, 35–37. doi:10.2111/1551-501x-31.1.35
Sun, L. W., Fan, H., Yang, L. G., Shi, L. L., and Liu, Y. J. (2015). Tyrosol prevents ischemia/reperfusion-induced cardiac injury in H9c2 cells: involvement of ROS, Hsp70, JNK and ERK, and apoptosis. Molecules 20, 3758–3775. doi:10.3390/molecules20033758
Sun, X. X., Xiang, E., Qiu, S. K., Wang, H., and Guo, Y. (2019). Research progress on toxicity of pyrrolizidine alkaloids. Chin. J. Pharmacovigil. 16, 76–80. doi:10.3969/j.issn.1672-8629.2019.02.003
Suparmi, S., Wesseling, S., and Rietjens, I. (2020). Monocrotaline-induced liver toxicity in rat predicted by a combined in vitro physiologically based kinetic modeling approach. Arch. Toxicol. 94, 3281–3295. doi:10.1007/s00204-020-02798-z
Tan, X. M., Zhu, J. Y., and Wakisaka, M. (2020). Effect of protocatechuic acid on Euglena gracilis growth and accumulation of metabolites. Sustainability 12, 9158. doi:10.3390/su12219158
Tang, J., Akao, T., Nakamura, N., Wang, Z. T., Takagawa, K., Sasahara, M., et al. (2007). In vitro metabolism of isoline, a pyrrolizidine alkaloid from Ligularia duciformis, by rodent liver microsomal esterase and enhanced hepatotoxicity by esterase inhibitors. Drug Metab. Dispos. 35, 1832–1839. doi:10.1124/dmd.107.016311
Tang, J., and Hattori, M. (2011). Pyrrolizidine alkaloids-containing Chinese medicines in the Chinese Pharmacopoeia and related safety concerns. Acta Pharm. Sin. 46, 762–772. doi:10.16438/j.0513-4870.2011.07.002
Tian, X. H., Liu, C. L., Jiang, H. L., Zhang, Y., Han, J. C., Liu, J., et al. (2016). Cardioprotection provided by echinatin against ischemia/reperfusion in isolated rat hearts. BMC Cardiovasc Disord. 16, 119. doi:10.1186/s12872-016-0294-3
Varela, L. M., Ortega-Gomez, A., Lopez, S., Abia, R., Muriana, F. J., and Bermudez, B. (2013). The effects of dietary fatty acids on the postprandial triglyceride-rich lipoprotein/Apob48 receptor axis in human monocyte/macrophage cells. J. Nutr. Biochem. 24, 2031–2039. doi:10.1016/j.jnutbio.2013.07.004
Vrieling, K., and Ruan, W. (2021). Current knowledge and perspectives of pyrrolizidine alkaloids in pharmacological applications: a mini-review. Molecules 26, 1970. doi:10.3390/molecules26071970
Wang, F., Liang, Q., Yang, J. Z., and Wu, J. R. (2014). Anti-microbial activity of the extracts from Cynoglossum amabile. J. Northwest For. Univ. 29, 125–128. doi:10.3969/j.issn.1001-7461.2014.05.23
Wang, J., Seyler, B. C., Ticktin, T., Zeng, Y. G., and Ayu, K. (2020). An ethnobotanical survey of wild edible plants used by the Yi People of Liangshan Prefecture, Sichuan Province, China. J. Ethnobiol. Ethnomed 16, 10. doi:10.1186/s13002-019-0349-5
Xia, Q. S., Yan, J., Chou, M. W., and Fu, P. P. (2008). Formation of DHP-derived DNA adducts from metabolic activation of the prototype heliotridine-type pyrrolizidine alkaloid, heliotrine. Toxicol. Lett. 178, 77–82. doi:10.1016/j.toxlet.2008.02.008
Xiong, F., Jiang, K. Y., Chen, Y., Ju, Z. C., Yang, L., Xiong, A. Z., et al. (2020). Protein cross-linking in primary cultured mouse hepatocytes by dehydropyrrolizidine alkaloids: structure-toxicity relationship. Toxicon 186, 4–11. doi:10.1016/j.toxicon.2020.07.015
Xu, G., Fu, S. B., Zhan, X. Y., Wang, Z. L., Zhang, P., Shi, W., et al. (2021). Echinatin effectively protects against NLRP3 inflammasome-driven diseases by targeting HSP90. JCI Insight 6, e134601. doi:10.1172/jci.insight.134601
Xu, J., Wang, W. Q., Yang, X., Xiong, A. Z., Yang, L., and Wang, Z. T. (2019). Pyrrolizidine alkaloids: an update on their metabolism and hepatotoxicity mechanism. Liver Res. 3, 176–184. doi:10.1016/j.livres.2019.11.004
Xu, Q. J., and Zhang, D. Z. (2009). Advances in the study of the Cynoglossum. Lishizhen Med. Materia Medica Res. 20, 144–146. doi:10.3969/j.issn.1008-0805.2009.01.076
Xu, Q. J., Zhang, D. Z., Dang, L. J., and Li, K. P. (2008). Chemical constituents of Cynoglossum amabile. J. Guangdong Coll. Pharm. 24, 550–551. doi:10.16809/j.cnki.1006-8783.2008.06.004
Xu, Q. J., Zhang, D. Z., Dang, L. J., and Li, K. P. (2009). Triterpene acids from Cynoglossum amabile. Chem. Res. Chin. Univ. 25, 404–406. doi:10.1360/972009-470
Xue, S. J., Wang, L. L., Chen, S. Q., and Cheng, Y. X. (2018). Simultaneous analysis of saccharides between fresh and processed radix rehmanniae by HPLC and UHPLC-LTQ-orbitrap-MS with multivariate statistical analysis. Molecules 23, 541. doi:10.3390/molecules23030541
Yan, H., Xie, N., Zhong, C. Q., Su, A. Q., Hui, X. L., Zhang, X., et al. (2018). Aphicidal activities of Amaryllidaceae alkaloids from bulbs of Lycoris radiata against Aphis citricola. Ind. Crop Prod. 123, 372–378. doi:10.1016/j.indcrop.2018.06.082
Yan, Y., Lei, Z., Wang, Y. F., Chang, M. L., Huo, C. H., Gu, Y. C., et al. (2011). Chemical and pharmacological research on plants from the genus Senecio. Chem. Biodivers. 8, 13–72. doi:10.1002/cbdv.201000027
Yan, Y. P., Ma, C. G., and Jing, L. L. (2022). Progress on the effect of phytosterols in liposomes. China Oils Fats 47, 27–31. doi:10.19902/j.cnki.zgyz.1003-7969.210204
Yang, M., Ruan, J., Fu, P. P., and Lin, G. (2016). Cytotoxicity of pyrrolizidine alkaloid in human hepatic parenchymal and sinusoidal endothelial cells: firm evidence for the reactive metabolites mediated pyrrolizidine alkaloid-induced hepatotoxicity. Chem. Biol. Interact. 243, 119–126. doi:10.1016/j.cbi.2015.09.011
Yang, X. J., Li, W. W., Li, H., Wang, X., Chen, Y., Guo, X. C., et al. (2018). A difference in internal exposure makes newly weaned mice more susceptible to the hepatotoxicity of retrorsine than adult mice. Chem. Res. Toxicol. 31, 1348–1355. doi:10.1021/acs.chemrestox.8b00220
Yang, X. J., Li, W. W., Sun, Y., Guo, X. C., Huang, W. L., Peng, Y., et al. (2017). Comparative study of hepatotoxicity of pyrrolizidine alkaloids retrorsine and monocrotaline. Chem. Res. Toxicol. 30, 532–539. doi:10.1021/acs.chemrestox.6b00260
Zhang, F., Wang, C. H., Xiong, A. Z., Wang, W., Yang, L., Branford-White, C. J., et al. (2007). Quantitative analysis of total retronecine esters-type pyrrolizidine alkaloids in plant by high performance liquid chromatography. Anal. Chim. Acta 605, 94–101. doi:10.1016/j.aca.2007.10.021
Zhang, J. L., Zhang, L., Wu, Z. Z., Zhang, P., Liu, R. J., Chang, M., et al. (2021). The dopaminergic neuroprotective effects of different phytosterols identified in rice bran and rice bran oil. Food Funct. 12, 10538–10549. doi:10.1039/d1fo01509e
Zhang, M. S., Li, H. T., Wang, J. Q., Tang, M. H., Zhang, X. B., Yang, S. H., et al. (2022). Market survey on the traditional medicine of the Lijiang area in Yunnan Province, China. J. Ethnobiol. Ethnomed 18, 40. doi:10.1186/s13002-022-00532-w
Zhang, X. J. (2013). Study on the microscopic identification and chemical composition of Cynoglossum amabile. Yunnan: Yunnan University of Chinese Medicine.
Zhang, X. J., Zuo, A. X., and Hao, J. J. (2014). Study on the chemical constituents of medicinal Cynoglossum amabile in Western Yunnan. J. Med. Inf. 34, 38. doi:10.3969/j.issn.1006-1959.2014.34.051
Zhao, M. S. (2007a). Application of Cynoglossum amabile in preparation of drugs for treatment of prostate diseases. CN101011443. Sichuan: Google Patents.
Zhao, M. S. (2016). New uses of the plants of the Cynoglossum L. and their extracts. CN105395591A. Sichuan: Google Patents.
Zhou, S. Y., Huang, G. L., and Chen, G. Y. (2021). Synthesis and anti-tumor activity of marine alkaloids. Bioorg Med. Chem. Lett. 41, 128009. doi:10.1016/j.bmcl.2021.128009
Keywords: Cynoglossum amabile, ethnomedicine, traditional utilization, pyrrolizidine alkaloids, pharmacology, hepatotoxicity
Citation: Fan Y, Wang M, Zhang Q, Ouyang S, Mao W, Xu C, Wang M and Long C (2024) Traditional uses, phytochemistry, pharmacology, toxicity and clinical application of traditional Chinese medicine Cynoglossum amabile: a review. Front. Pharmacol. 15:1325283. doi: 10.3389/fphar.2024.1325283
Received: 20 October 2023; Accepted: 25 March 2024;
Published: 09 April 2024.
Edited by:
Adam Matkowski, Wroclaw Medical University, PolandReviewed by:
Katarzyna Syklowska-Baranek, Medical University of Warsaw, PolandRufeng Wang, Beijing University of Chinese Medicine, China
Copyright © 2024 Fan, Wang, Zhang, Ouyang, Mao, Xu, Wang and Long. This is an open-access article distributed under the terms of the Creative Commons Attribution License (CC BY). The use, distribution or reproduction in other forums is permitted, provided the original author(s) and the copyright owner(s) are credited and that the original publication in this journal is cited, in accordance with accepted academic practice. No use, distribution or reproduction is permitted which does not comply with these terms.
*Correspondence: Min Wang, wangmin_216@163.com; Chunlin Long, long.chunlin@muc.edu.cn