- 1Faculty of Pharmacy, Université de Montréal, Montréal, QC, Canada
- 2Department of Microbiology, Infectious Diseases and and Immunology, Université Laval, Québec, QC, Canada
- 3Institut Universitaire de Cardiologie et de Pneumologie de Québec, Département de Médecine, Faculté de Médecine, Université Laval, Québec, QC, Canada
- 4Department of Chemistry, Université de Montréal, Montréal, QC, Canada
The scientific and medical community faced an unprecedented global health hazard that led to nearly 7 million deaths attributable to the rapid spread of severe acute respiratory syndrome coronavirus 2 (SARS-CoV-2) infection. In spite of the development of efficient vaccines against SARS-CoV-2, many people remain at risk of developing severe symptoms as the virus continues to spread without beneficial patient therapy. The hyper-inflammatory response to SARS-CoV-2 infection progressing to acute respiratory distress syndrome remains an unmet medical need for improving patient care. The viral infection stimulates alveolar macrophages to adopt an inflammatory phenotype regulated, at least in part, by the cluster of differentiation 36 receptor (CD36) to produce unrestrained inflammatory cytokine secretions. We suggest herein that the modulation of the macrophage response using the synthetic CD36 ligand hexarelin offers potential as therapy for halting respiratory failure in SARS-CoV-2-infected patients.
Introduction
Severe acute respiratory syndrome coronavirus 2 (SARS-CoV-2) pandemic caused over 773 million infections and nearly 7 million deaths globally (WHO, 2023) between 2019 and 2023. Most infected patients were either asymptomatic or manifested mild to moderate flu-like symptoms common among viral respiratory tract infections. Some individuals, notably the elderly and those presenting comorbidities, often developed a rapid deterioration of respiratory functions and lower airway infection progressing to acute respiratory distress syndrome (ARDS) and in many instances, death (Ramadori, 2022; Zheng et al., 2022). In severe cases, COVID-19 (coronavirus disease 2019)-related ARDS has been typically associated with hyper-cytokinemia, so-called “cytokine storm,” featuring macrophage activation (Tao et al., 2023). Hyper-cytokinemia is initiated by different macrophage subsets in the lungs including interstitial, alveolar, and monocyte-derived macrophages, in particular (Merad and Martin, 2020; Gustine and Jones, 2021), which contribute to ARDS development in SARS-CoV-2-infected patients (Kosyreva et al., 2021).
The cluster of differentiation 36 receptor (CD36) is an extensively glycosylated class B2 scavenger receptor, that sits at the crossroad between lipid metabolism and innate immune response. Largely expressed in immune and non-immune cells, CD36 plays important immunomodulatory roles in health and disease (Silverstein and Febbraio, 2009). First identified as a long chain fatty acid transporter, CD36 has been later shown to mediate inflammatory signaling in response to damage-associated molecular pattern signals (DAMPs), such as oxidized phospholipids (oxPLs) (Miller et al., 2011; Chen et al., 2022; Dunigan-Russell et al., 2023). The binding domain for oxPLs has been identified in the CD36 protein (Ashraf et al., 2009). As a co-receptor of Toll-like receptor (TLR) heterodimer complexes TLR2/6 (Mellal et al., 2019) and TLR4/6 (Stewart et al., 2010), CD36 is implicated in triggering nuclear factor-kappa B (NF-κB) inflammatory signaling following exposure to oxPLs (Park, 2014). Moreover, CD36 was found to modulate pro-inflammatory macrophage phenotype (Sun et al., 2022). Appropriately, the pulmonary hyper-inflammation associated with SARS-CoV-2 infection was shown to rely, in part, upon the stimulation of the pro-inflammatory NF-κB signaling pathway by oxPL accumulation in the lungs and activation of monocyte-derived macrophage TLRs (Merad and Martin, 2020). Furthermore, CD36 was found to bind SARS-CoV-2-E (envelope) protein, which is a major viral structural protein having central roles in cytokine secretion, progression to ARDS-like symptoms and thrombosis in mice (Tang et al., 2023). Accordingly, CD36 has been proposed as target for severe COVID-19 patients (Vlasov et al., 2021; Alghanim et al., 2022).
Ligands of CD36 derived from growth hormone-releasing peptides (GHRPs) such as hexarelin [H-His-2-methyl-D-Trp-Ala-Trp-D-Phe-Lys-NH2] and azapeptide analogs were reported to exhibit high binding affinity to CD36 in murine cardiac membranes (Bodart et al., 2002) and to exert anti-inflammatory effects (Bodart et al., 2002; Bessi et al., 2012; Huynh et al., 2017; Proulx et al., 2020). For example, azapeptide MPE-001 [H-His-D-Trp-Ala-azaTyr-D-Phe-Lys-NH2] was shown to palliate inflammation in response to photo-oxidative stress in mice by attenuating NF-κB and NOD-like receptor family, pyrin domain containing 3 (NLRP3) inflammasome pathways and concomitantly, by activating peroxisome proliferator-activated receptor gamma coactivator 1-alpha (PPAR-γ-PGC1α) signaling, to promote macrophage polarization towards an anti-inflammatory phenotype (Mellal et al., 2019). Various cellular responses to stimuli have been modulated by such peptide-based CD36 ligands (Proulx et al., 2020). For example, hexarelin has demonstrated cardioprotective effects in rodent models of cardiovascular dysfunction after daily subcutaneous administrations for up to 5 weeks (Ghigo et al., 1999; Locatelli et al., 1999). Cardiotropic activity has also been observed after acute administration of hexarelin to normal and growth hormone-deficient humans as well as during by-pass surgery (Bisi et al., 1999; Ghigo et al., 1999; Mao et al., 2014). In this perspective, evidence is provided to suggest that the pharmacologic modulation of CD36 response could attenuate the macrophage-driven hyper-inflammatory response observed in SARS-CoV-2-infected patients.
Pro-inflammatory macrophage phenotype and COVID-19
Activated macrophages release cytokines [e.g., interleukin (IL)-1β, IL-6, and tumor necrosis factor (TNF)-α] and chemokines [e.g., C-X-C ligand 8 (CXCL8) and C-C ligand 2 (CCL2)] (Atri et al., 2018; Knoll et al., 2021). Cytokines and chemokines recruit leukocytes to the lungs, leading to injury of endothelial and epithelial tissues with heightened inflammation in COVID-19 patients (Ragab et al., 2020). Moreover, COVID-19 induces endothelial and epithelial alveolar damage coupled to enhanced interstitial and alveolar permeability to proteins and fluids, reduced endothelial nitric oxide, and increased reactive oxygen species (ROS) production (Chernyak et al., 2020; Yuan et al., 2021; Otifi and Adiga, 2022). Prevention of the cytokine storm in SARS-CoV-2-infected patients remains relevant and of high priority (Kosyreva et al., 2021).
Current therapies for SARS-CoV-2-infected patients
Vaccination programs against SARS-CoV-2 have been effective but have suffered from inequality in global distribution and administration (Bayati et al., 2022). Furthermore, vaccination rates against COVID-19 are decreasing globally (Mathieu et al., 2020). In spite an apparent decline in viral burden, the risk of future coronavirus outbreaks is a major healthcare concern (Cui et al., 2023). Current COVID-19 therapeutics function typically as antiviral and anti-inflammatory agents (Niknam et al., 2022; Yuan et al., 2023). For example, patients presenting mild to moderate COVID-19 symptoms are treated with Paxlovid, which features the viral 3C-like protease inhibitor nirmatrelvir boosted by ritonavir, an inhibitor of cytochrome P450 CYP3A4 to prolong activity (Shah et al., 2022). Paxlovid should however be initiated within 5 days of onset of symptoms in patients at risk of progressing to a severe state, but not to those requiring hospitalization due to severe COVID-19 (Komorowski et al., 2022) nor to those with severe hepatic impairment (Chaplin, 2022; Chen et al., 2023). Anti-inflammatory therapy includes timely administration of steroidal and nonsteroidal agents such as dexamethasone which, despite reducing mortality by ∼30% in ventilated patients, was associated with severe side effects (Noreen et al., 2021) and with worsened clinical outcomes (De Stefano et al., 2020; Noreen et al., 2021). Inhibitors of Janus kinase (JAK1 and 2), such as baricitinib, have been approved for use against SARS-CoV-2 in emergency situations in the European Union and the USA (Bellino, 2022; Huang et al., 2022; Rubin, 2022). The recovery collaborative group (2022) reported that baricitinib reduced mortality in severe COVID-19 by about one-fifth (Abani et al., 2022). The humanized monoclonal antibody against IL-6, tocilizumab has been approved in Canada, Europe, and the USA (Bellino, 2022; Canada, 2022; FDA, 2022; Mohseni Afshar et al., 2023). Despite elevation of IL-6 in the cytokine storm of SARS-CoV-2-infected patients, caution has been expressed on the use of anti-IL-6 therapy because conditions worsened in some patients (Montazersaheb et al., 2022). Moreover, IL-6 blockade may not be sufficient in critically ill COVID-19 patients and may increase the risks for severe infection (Montazersaheb et al., 2022). In addition, cell-based strategies have been proposed to mitigate the abundance of infection fighting neutrophils and neutrophil extracellular traps during the progressive pulmonary dysfunction of critically ill COVID-19 patients (Mozzini and Girelli, 2020; Narasaraju et al., 2020; Veras et al., 2023). New therapeutic avenues are needed to safeguard vulnerable populations against a persistent incidence of COVID-19 infection.
Hexarelin attenuates lung cytokine levels and improves survival
Transgenic mice possessing the human ACE2 protein, which is expressed under regulation of the human cytokeratin-18 promoter in epithelial cells (K18-hACE2), were treated with a subcutaneous injection of hexarelin (10 μmol/kg) or 0.9% NaCl vehicle 30 min prior to intranasal infection with 250 TCID50 of SARS-CoV-2 (strain Delta, B.1.617.2, National Microbiology Laboratory, Winnipeg, Manitoba, Canada). Thereafter, mice were treated daily with 0.9% NaCl or hexarelin for 9 days. The experimental protocol was approved by the institutional animal care committee of Université Laval (CPAUL, 2020-594), in accordance with the guidelines for the care and use of laboratory animals of the Canadian Council on Animal Care and the US National Institute of Health.
Daily treatment with the CD36 ligand increased survival of the infected mice relative to vehicle-treated mice (Figure 1A). Hexarelin treatment also reduced the accompanied weight loss of infected mice relative to the placebo-treated group (Figure 1B). Moreover, lung homogenates from the hexarelin-treated infected mice exhibited decreased cytokine levels relative to placebo-treated mice: e.g., CCL2, CCL4, interferon (IFN) α and IFN γ, were significantly reduced by 44%, 59%, 43% and 35%, respectively (Table 1).
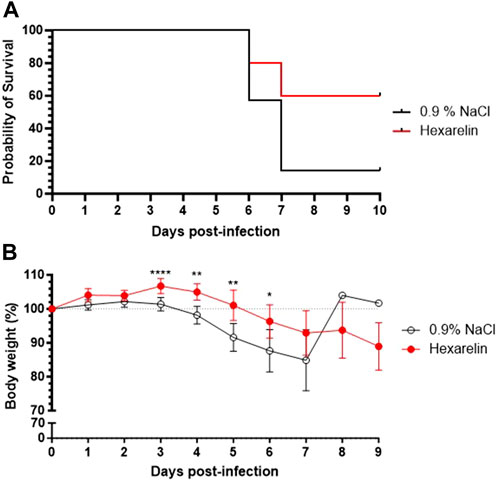
FIGURE 1. Treatment with hexarelin improves the survival of SARS-CoV-2-infected mice. (A) Kaplan Meier curve of K18-hACE2 mice infected with SARS-CoV-2. (B) Daily mean body weight of SARS-CoV-2-infected mice expressed as a percentage of body weight at day 0 (n = 7 mice per group).
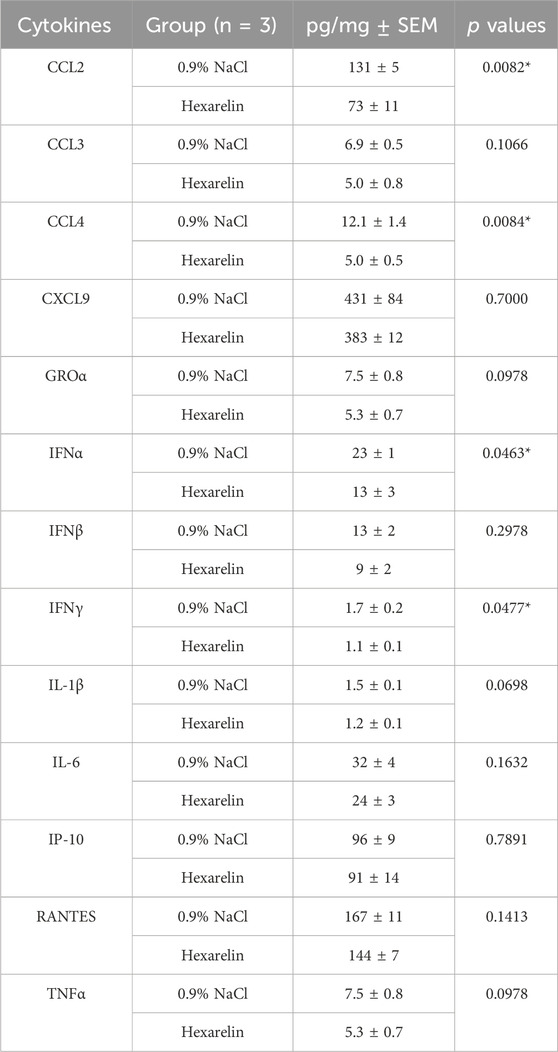
TABLE 1. Hexarelin decreased cytokine levels in lung homogenates at day 3 after SARS-CoV-2 infection.
Discussion
The SARS-CoV-2 epidemic represents an unprecedented medical challenge worldwide (WHO, 2020). Disease course during SARS-CoV-2 infection features a similar profile to that of other highly pathogenic coronaviruses such as SARS-CoV and MERS-CoV, characterized by extensive leukocyte infiltration dominated by macrophages in post-mortem lungs of infected patients (Zhang et al., 2022). Mortality from SARS-CoV-2 has been mainly associated with the so-called cytokine storm creating a hyper-inflammatory state in infected patient lungs, leading to ARDS, compromised respiratory function, and multiple organ failure. The CD36 receptor has been associated with acute lung injury, contributing to the hyper-inflammatory response of lung macrophages after animal exposure to hydrogen peroxide, lipopolysaccharide, and malaria (Alghanim et al., 2022; Sun et al., 2022). As a co-receptor, CD36 regulates the assembly of TLR2/6 and TLR4/6 heterodimers for activating the transcription of pro-inflammatory cytokines, inflammasome priming, and production of reactive oxygen species in response to endogenous oxidized lipids (Sheedy et al., 2013; Mellal et al., 2019). Moreover, the E protein of SARS-CoV-2, which plays a central role in cytokine secretion and progression towards ARDS, was found to bind to CD36 and shown to be involved in COVID-19-induced thrombosis (Tang et al., 2023).
Hexarelin was previously shown to mitigate the development of HCl-induced ARDS-like symptoms in the bronchus and hypothesized to be able to attenuate lung inflammation and cytokine release in SARS-CoV-2-infected mice (Zambelli et al., 2021). We theorized that CD36 ligands, which modulate macrophage-driven inflammation, may curb the overreactive inflammatory response in a SARS-CoV-2-infected transgenic mouse model. We have found that the CD36 ligand hexarelin dampened many pro-inflammatory pathways, reducing type I interferon activity, chemokine levels and NLRP3 inflammasome priming. A limitation of our study is the unselective, dual binding of hexarelin towards CD36 and the ghrelin receptor (GHS-R1), both of which are expressed on macrophages. Future studies using selective azapeptide ligands are mandatory; nonetheless, the promise of modulating monocyte- and macrophage-driven hyper-cytokinemia by targeting CD36 suggests potential for mitigating SARS-CoV-2-mediated activation of inflammatory responses responsible for disease severity.
Data availability statement
The original contributions presented in the study are included in the article/supplementary material, further inquiries can be directed to the corresponding author.
Ethics statement
The animal study was approved by comité déthique du CHUL (CPAUL). The study was conducted in accordance with the local legislation and institutional requirements.
Author contributions
JG: Investigation, Project administration, Validation, Writing–original draft, Writing–review and editing. DH: Formal Analysis, Investigation, Validation, Writing–review and editing. ID: Formal Analysis, Investigation, Validation, Writing–review and editing. CL: Investigation, Writing–review and editing. RT: Investigation, Writing–review and editing. NF: Conceptualization, Funding acquisition, Resources, Supervision, Writing–review and editing. LF: Conceptualization, Funding acquisition, Resources, Supervision, Writing–review and editing. WL: Funding acquisition, Resources, Writing–review and editing. HO: Conceptualization, Funding acquisition, Resources, Supervision, Writing–review and editing. SM: Conceptualization, Funding acquisition, Methodology, Project administration, Resources, Supervision, Validation, Writing–original draft, Writing–review and editing.
Funding
The author(s) declare financial support was received for the research, authorship, and/or publication of this article. This work was supported by a grant from the Réseau québécois de recherche sur le médicament (RQRM), Université de Montréal to SM, HO, LF, and NF and by the Faculty of Pharmacy, Université de Montréal.
Acknowledgments
The authors wish to thank Cosmina Dabula and Mounia Lammali for constructive discussions of the manuscript.
Conflict of interest
The authors declare that the research was conducted in the absence of any commercial or financial relationships that could be construed as a potential conflict of interest.
Publisher’s note
All claims expressed in this article are solely those of the authors and do not necessarily represent those of their affiliated organizations, or those of the publisher, the editors and the reviewers. Any product that may be evaluated in this article, or claim that may be made by its manufacturer, is not guaranteed or endorsed by the publisher.
References
Abani, O., Abbas, A., Abbas, F., Abbas, J., Abbas, K., Abbas, M., et al. (2022). Baricitinib in patients admitted to hospital with COVID-19 (RECOVERY): a randomised, controlled, open-label, platform trial and updated meta-analysis. Lancet 400 (10349), 359–368. doi:10.1016/S0140-6736(22)01109-6
Alghanim, F., Mohan, T., Hasday, J. D., Shanholtz, C., and Birukov, K. (2022). “Soluble cluster of differentiation 36 (sCD36) as a biomarker for outcomes in coronavirus disease (COVID-19) acute respiratory distress syndrome (ARDS),” in A33. BIOMARKERS, allergy and immune responses in COVID-19 (China: ARDS), A1284.
Ashraf, M. Z., Kar, N. S., and Podrez, E. A. (2009). Oxidized phospholipids: biomarker for cardiovascular diseases. Int. J. Biochem. Cell. Biol. 41 (6), 1241–1244. doi:10.1016/j.biocel.2008.11.002
Atri, C., Guerfali, F. Z., and Laouini, D. (2018). Role of human macrophage polarization in inflammation during infectious diseases. Int. J. Mol. Sci. 19 (6), 1801. doi:10.3390/ijms19061801
Bayati, M., Noroozi, R., Ghanbari-Jahromi, M., and Jalali, F. S. (2022). Inequality in the distribution of Covid-19 vaccine: a systematic review. Int. J. Equity Health 21 (1), 122. doi:10.1186/s12939-022-01729-x
Bellino, S. (2022). COVID-19 treatments approved in the European Union and clinical recommendations for the management of non-hospitalized and hospitalized patients. Ann. Med. 54 (1), 2856–2860. doi:10.1080/07853890.2022.2133162
Bessi, V. L., Labbé, S. M., Huynh, D. N., Ménard, L., Jossart, C., Febbraio, M., et al. (2012). EP 80317, a selective CD36 ligand, shows cardioprotective effects against post-ischaemic myocardial damage in mice. Cardiovasc. Res. 96 (1), 99–108. doi:10.1093/cvr/cvs225
Bisi, G., Podio, V., Valetto, M. R., Broglio, F., Bertuccio, G., Del Rio, G., et al. (1999). Acute cardiovascular and hormonal effects of GH and hexarelin, a synthetic GH-releasing peptide, in humans. J. Endocrinol. Investig. 22 (4), 266–272. doi:10.1007/bf03343555
Bodart, V., Febbraio, M., Demers, A., McNicoll, N., Pohankova, P., Perreault, A., et al. (2002). CD36 mediates the cardiovascular action of growth hormone-releasing peptides in the heart. Circ. Res., 90(8), 844–849. doi:10.1161/01.RES.0000016164.02525.B4
Canada (2022). COVID-19 treatments [Online]. Government of Canada. Available at: https://www.canada.ca/en/health-canada/services/drugs-health-products/covid19-industry/drugs-vaccines-treatments/treatments.html (Accessed 2023).
Chaplin, S. (2022). Paxlovid: antiviral combination for the treatment of COVID-19. Prescriber 33 (3-4), 31–33. doi:10.1002/psb.1979
Chen, W., Liang, B., Wu, X., Li, L., Wang, C., and Xing, D. (2023). Advances and challenges in using nirmatrelvir and its derivatives against SARS-CoV-2 infection. J. Pharm. Anal. 13 (3), 255–261. doi:10.1016/j.jpha.2022.10.005
Chen, Y., Zhang, J., Cui, W., and Silverstein, R. L. (2022). CD36, a signaling receptor and fatty acid transporter that regulates immune cell metabolism and fate. J. Exp. Med. 219 (6), e20211314. doi:10.1084/jem.20211314
Chernyak, B. V., Popova, E. N., Prikhodko, A. S., Grebenchikov, O. A., Zinovkina, L. A., and Zinovkin, R. A. (2020). COVID-19 and oxidative stress. Biochem. (Mosc) 85 (12), 1543–1553. doi:10.1134/s0006297920120068
Cui, X., Wang, Y., Zhai, J., Xue, M., Zheng, C., and Yu, L. (2023). Future trajectory of SARS-CoV-2: constant spillover back and forth between humans and animals. Virus Res. 328, 199075. doi:10.1016/j.virusres.2023.199075
De Stefano, L., Bobbio-Pallavicini, F., Manzo, A., Montecucco, C., and Bugatti, S. (2020). A "window of therapeutic opportunity" for anti-cytokine therapy in patients with coronavirus disease 2019. Front. Immunol. 11, 572635. doi:10.3389/fimmu.2020.572635
Dunigan-Russell, K., Yaeger, M. J., Hodge, M. X., Kilburg-Basnyat, B., Reece, S. W., Birukova, A., et al. (2023). Scavenger receptor BI attenuates oxidized phospholipid-induced pulmonary inflammation. Toxicol. Appl. Pharmacol. 462, 116381. doi:10.1016/j.taap.2023.116381
FDA (2022). Frequently asked questions on the emergency use authorization for actemra (tocilizumab) for treatment of COVID-19 [Online]. Health, National Institutes of. Available at: https://www.fda.gov/media/150345/download (Accessed 2023).
Ghigo, E., Arvat, E., Broglio, F., Giordano, R., Gianotti, L., Muccioli, G., et al. (1999). Endocrine and non-endocrine activities of growth hormone secretagogues in humans. Hormone Res. 51 (Suppl. 3), 9–15. doi:10.1159/000053156
Gustine, J. N., and Jones, D. (2021). Immunopathology of hyperinflammation in COVID-19. Am. J. Pathology 191 (1), 4–17. doi:10.1016/j.ajpath.2020.08.009
Huang, J., Zhou, C., Deng, J., and Zhou, J. (2022). JAK inhibition as a new treatment strategy for patients with COVID-19. Biochem. Pharmacol. 202, 115162. doi:10.1016/j.bcp.2022.115162
Huynh, D. N., Bessi, V. L., Ménard, L., Piquereau, J., Proulx, C., Febbraio, M., et al. (2017). Adiponectin has a pivotal role in the cardioprotective effect of CP-3(iv), a selective CD36 azapeptide ligand, after transient coronary artery occlusion in mice. 32, 807–818. doi:10.1096/fj.201700505R
Knoll, R., Schultze, J. L., and Schulte-Schrepping, J. (2021). Monocytes and macrophages in COVID-19. Front. Immunol. 12, 720109. doi:10.3389/fimmu.2021.720109
Komorowski, A., Tseng, A., Vandersluis, S., Leung, E., Ciccotelli, W., Langford, B., et al. (2022). Evidence-based recommendations on the use of nirmatrelvir/ritonavir (Paxlovid) for adults in ontario. Infect. Dis. Clin. Care. doi:10.47326/ocsat.2022.03.57.1.0
Kosyreva, A., Dzhalilova, D., Lokhonina, A., Vishnyakova, P., and Fatkhudinov, T. (2021). The role of macrophages in the pathogenesis of SARS-CoV-2-associated acute respiratory distress syndrome. Front. Immunol. 12, 682871. doi:10.3389/fimmu.2021.682871
Locatelli, V., Rossoni, G., Schweiger, F., Torsello, A., De Gennaro Colonna, V., Bernareggi, M., et al. (1999). Growth hormone-independent cardioprotective effects of hexarelin in the rat. Endocrinology 140 (9), 4024–4031. doi:10.1210/endo.140.9.6948
Mao, Y., Tokudome, T., and Kishimoto, I. (2014). The cardiovascular action of hexarelin. J. Geriatr. Cardiol. 11 (3), 253–258. doi:10.11909/j.issn.1671-5411.2014.03.007
Mathieu, E., Ritchie, H., Rodés-Guirao, L., Appel, C., Giattino, C., Hasell, J., et al. (2020). Coronavirus pandemic (COVID-19). Available at: https://ourworldindata.org/coronavirus (Accessed September 5, 2023).
Mellal, K., Omri, S., Mulumba, M., Tahiri, H., Fortin, C., Dorion, M.-F., et al. (2019). Immunometabolic modulation of retinal inflammation by CD36 ligand. Sci. Rep. 9 (1), 12903. doi:10.1038/s41598-019-49472-8
Merad, M., and Martin, J. C. (2020). Pathological inflammation in patients with COVID-19: a key role for monocytes and macrophages. Nat. Rev. Immunol. 20 (6), 355–362. doi:10.1038/s41577-020-0331-4
Miller, Y. I., Choi, S.-H., Wiesner, P., Fang, L., Harkewicz, R., Hartvigsen, K., et al. (2011). Oxidation-specific epitopes are danger-associated molecular patterns recognized by pattern recognition receptors of innate immunity Immunity. 108(2), 235–248. doi:10.1161/CIRCRESAHA.110.223875
Mohseni Afshar, Z., Barary, M., Babazadeh, A., Tavakoli Pirzaman, A., Hosseinzadeh, R., Alijanpour, A., et al. (2023). The role of cytokines and their antagonists in the treatment of COVID-19 patients. Rev. Med. Virol. 33 (1), e2372. doi:10.1002/rmv.2372
Montazersaheb, S., Hosseiniyan Khatibi, S. M., Hejazi, M. S., Tarhriz, V., Farjami, A., Ghasemian Sorbeni, F., et al. (2022). COVID-19 infection: an overview on cytokine storm and related interventions. Virology J. 19 (1), 92. doi:10.1186/s12985-022-01814-1
Mozzini, C., and Girelli, D. (2020). The role of Neutrophil Extracellular Traps in Covid-19: only an hypothesis or a potential new field of research? Thromb. Res. 191, 26–27. doi:10.1016/j.thromres.2020.04.031
Narasaraju, T., Tang, B. M., Herrmann, M., Muller, S., Chow, V. T. K., and Radic, M. (2020). Neutrophilia and NETopathy as key pathologic drivers of progressive lung impairment in patients with COVID-19. Front. Pharmacol. 11, 870. doi:10.3389/fphar.2020.00870
Niknam, Z., Jafari, A., Golchin, A., Danesh Pouya, F., Nemati, M., Rezaei-Tavirani, M., et al. (2022). Potential therapeutic options for COVID-19: an update on current evidence. Eur. J. Med. Res. 27 (1), 6. doi:10.1186/s40001-021-00626-3
Noreen, S., Maqbool, I., and Madni, A. (2021). Dexamethasone: therapeutic potential, risks, and future projection during COVID-19 pandemic. Eur. J. Pharmacol. 894, 173854. doi:10.1016/j.ejphar.2021.173854
Otifi, H. M., and Adiga, B. K. (2022). Endothelial dysfunction in covid-19 infection. Am. J. Med. Sci. 363 (4), 281–287. doi:10.1016/j.amjms.2021.12.010
Park, Y. M. (2014). CD36, a scavenger receptor implicated in atherosclerosis. Exp. Mol. Med. 46 (6), e99. doi:10.1038/emm.2014.38
Proulx, C., Zhang, J., Sabatino, D., Chemtob, S., Ong, H., and Lubell, W. D. (2020). Synthesis and biomedical potential of azapeptide modulators of the cluster of differentiation 36 receptor (CD36). Biomedicines 8 (8), 241. doi:10.3390/biomedicines8080241
Ragab, D., Salah Eldin, H., Taeimah, M., Khattab, R., and Salem, R. (2020). The COVID-19 cytokine storm; what we know so far. Front. Immunol. 11, 1446. doi:10.3389/fimmu.2020.01446
Ramadori, G. P. (2022). SARS-CoV-2-Infection (COVID-19): clinical course, viral acute respiratory distress syndrome (ARDS) and cause(s) of death. Med. Sci. (Basel) 10 (4), 58. doi:10.3390/medsci10040058
Rubin, R. (2022). Dietary supplement sellers receive warnings. JAMA 327 (23), 2281. doi:10.1001/jama.2022.9179
Shah, M. M., Joyce, B., Plumb, I. D., Sahakian, S., Feldstein, L. R., Barkley, E., et al. (2022). Paxlovid associated with decreased hospitalization rate among adults with COVID-19 - United States, april-september 2022. MMWR Morb. Mortal. Wkly. Rep. 71 (48), 1531–1537. doi:10.15585/mmwr.mm7148e2
Sheedy, F. J., Grebe, A., Rayner, K. J., Kalantari, P., Ramkhelawon, B., Carpenter, S. B., et al. (2013). CD36 coordinates NLRP3 inflammasome activation by facilitating intracellular nucleation of soluble ligands into particulate ligands in sterile inflammation. Nat. Immunol. 14 (8), 812–820. doi:10.1038/ni.2639
Silverstein, R. L., and Febbraio, M. (2009). CD36, a scavenger receptor involved in immunity, metabolism, angiogenesis, and behavior. Sci. Signal 2 (72), re3. doi:10.1126/scisignal.272re3
Stewart, C. R., Stuart, L. M., Wilkinson, K., van Gils, J. M., Deng, J., Halle, A., et al. (2010). CD36 ligands promote sterile inflammation through assembly of a Toll-like receptor 4 and 6 heterodimer. Nat. Immunol. 11 (2), 155–161. doi:10.1038/ni.1836
Sun, S., Yao, Y., Huang, C., Xu, H., Zhao, Y., Wang, Y., et al. (2022). CD36 regulates LPS-induced acute lung injury by promoting macrophages M1 polarization. Cell. Immunol. 372, 104475. doi:10.1016/j.cellimm.2021.104475
Tang, Z., Xu, Y., Tan, Y., Shi, H., Jin, P., Li, Y., et al. (2023). CD36 mediates SARS-CoV-2-envelope-protein-induced platelet activation and thrombosis. Nat. Commun. 14 (1), 5077. doi:10.1038/s41467-023-40824-7
Tao, H., Xu, Y., and Zhang, S. (2023). The role of macrophages and alveolar epithelial cells in the development of ARDS. Inflammation 46 (1), 47–55. doi:10.1007/s10753-022-01726-w
Veras, F. P., Gomes, G. F., Silva, B. M. S., Caetité, D. B., Almeida, C. J. L. R., Silva, C. M. S., et al. (2023). Targeting neutrophils extracellular traps (NETs) reduces multiple organ injury in a COVID-19 mouse model. Respir. Res. 24 (1), 66. doi:10.1186/s12931-023-02336-2
Vlasov, I., Panteleeva, A., Usenko, T., Nikolaev, M., Izumchenko, A., Gavrilova, E., et al. (2021). Transcriptomic profiles reveal downregulation of low-density lipoprotein particle receptor pathway activity in patients surviving severe COVID-19. Cells 10 (12), 3495. doi:10.3390/cells10123495
WHO (2020). Coronavirus disease 2019 (COVID-19) situation report-209. Available at: https://www.who.int/docs/default-source/coronaviruse/situation-reports/20200816-covid-19-sitrep-209.pdf?sfvrsn=5dde1ca2_2 (Accessed August 17, 2020).
WHO (2023). WHO COVID-19 dashboard. Available at: https://data.who.int/dashboards/covid19/deaths?n=c (Accessed December 26, 2023).
Yuan, S., Jiang, S.-C., Zhang, Z.-W., Fu, Y.-F., Hu, J., and Li, Z.-L. (2021). The role of alveolar edema in COVID-19. Cells 10 (8), 1897. doi:10.3390/cells10081897
Yuan, Y., Jiao, B., Qu, L., Yang, D., and Liu, R. (2023). The development of COVID-19 treatment. Front. Immunol. 14, 1125246. doi:10.3389/fimmu.2023.1125246
Zambelli, V., Rizzi, L., Delvecchio, P., Bresciani, E., Rezoagli, E., Molteni, L., et al. (2021). Hexarelin modulates lung mechanics, inflammation, and fibrosis in acute lung injury. Drug Target Insights 15, 26–33. doi:10.33393/dti.2021.2347
Zhang, Z., Penn, R., Barclay, W. S., and Giotis, E. S. (2022). Naïve human macrophages are refractory to SARS-CoV-2 infection and exhibit a modest inflammatory response early in infection. Viruses 14 (2), 441. doi:10.3390/v14020441
Keywords: coronavirus, SARS-CoV-2, hyper-inflammation, cytokine storm, pneumonia, macrophages, azapeptides, CD36
Citation: Gauvin J, Huynh DN, Dubuc I, Lê C, Tugores R, Flamand N, Flamand L, Lubell WD, Ong H and Marleau S (2024) Pharmacological targeting of the hyper-inflammatory response to SARS-CoV-2-infected K18-hACE2 mice using a cluster of differentiation 36 receptor modulator. Front. Pharmacol. 15:1303342. doi: 10.3389/fphar.2024.1303342
Received: 27 September 2023; Accepted: 25 January 2024;
Published: 07 February 2024.
Edited by:
Olumayokun Olajide, University of Huddersfield, United KingdomReviewed by:
Lucia Signorini, University of Milan, ItalyIfeoluwa Oluleke Awogbindin, University of Victoria, Canada
Copyright © 2024 Gauvin, Huynh, Dubuc, Lê, Tugores, Flamand, Flamand, Lubell, Ong and Marleau. This is an open-access article distributed under the terms of the Creative Commons Attribution License (CC BY). The use, distribution or reproduction in other forums is permitted, provided the original author(s) and the copyright owner(s) are credited and that the original publication in this journal is cited, in accordance with accepted academic practice. No use, distribution or reproduction is permitted which does not comply with these terms.
*Correspondence: Sylvie Marleau, sylvie.marleau@umontreal.ca