- 1State Key Laboratory of Pharmaceutical Biotechnology, Medical School, Nanjing University, Nanjing, China
- 2Shanghai Shujing Biopharma Co., Ltd., Shanghai, China
- 3Jiangsu Nhwa Pharmaceutical Co., Ltd. & Jiangsu Key Laboratory of Central Nervous System Drug Research and Development, Xuzhou, China
Background: Schizophrenia is a serious psychiatric disorder that significantly affects the quality of life of patients. The objective of this study is to discover a novel antipsychotic candidate with highly antagonistic activity against both serotonin and dopamine receptors, demonstrating robust efficacy in animal models of positive, negative, and cognitive symptoms of schizophrenia.
Methods: In the present study, we examined the activity of antipsychotic drug (NH300094) on 5-HT2A, 5-HT2C, 5-HT1A, 5-HT1B, 5-HT7, H1, M1, Alpha1A, D2L, D2S, Alpha2A, D3 receptor functional assay in vitro. In addition, multiple animal models, including dizocilpine (MK-801) induced hyper-locomotion; APO induced climbing; Conditioned Avoidance Response (CAR); DOI-Induced Head Twitch; Forced swimming test; Scopolamine induced cognitive impairment model, were used to verify the antipsychotic activity of NH300094 in preclinical.
Results: In vitro functional assays have indicated that NH300094 is a potent antagonist of 5-HT receptors and dopamine receptors, with higher relative antagonistic activity against 5-HT2A receptor (5-HT2A IC50 = 0.47 nM) than dopamine receptors (D2L IC50 = 1.04 nM; D2S IC50 = 11.71 nM; D3 IC50 = 31.55 nM). Preclinical in vivo pharmacological study results showed that NH300094 was effective in multiple models, which is more extensive than the clinic drug Risperidone. Furthermore, the safety window for extrapyramidal side effects of NH300094 is significantly wider than that of Risperidone (For NH300094, mice catalepsy model ED50/ Mice MK-801 model ED50 = 104.6-fold; for Risperidone, mice catalepsy model ED50/ Mice MK-801 model ED50 = 12.9-fold), which suggests a potentially better clinical safety profile for NH300094.
Conclusion: NH300094 is a novel potent serotonin and dopamine receptors modulator, which has good safety profile and therapeutic potential for the treatment of schizophrenia with cognition disorders.
1 Introduction
Schizophrenia is a serious psychiatric disorder that significantly affects the quality of life of patients. It has a variety of psychopathological features including positive symptoms (hallucinations and delusions), negative symptoms (social withdrawal, spontaneous speech reduction, impaired motivation), and neurocognitive disorders (Owen et al., 2016). The global incidence of schizophrenia is about 1%, and the lifetime prevalence of patients is 0.7%–0.8% (Schultz et al., 2007; Lohrs and Hasan, 2019). It is estimated that there are more than 21 million schizophrenics worldwide (Kane et al., 2019). Antipsychotics are still the main treatment for schizophrenia. Typical and atypical antipsychotic drugs are two main categories of clinical drugs to control schizophrenia. However, these current drugs have many side effects and can only control part of the symptoms of patients. For example, typical antipsychotic drugs have limited efficacy in treating negative symptoms of schizophrenia, but cause extrapyramidal reactions (EPS), tardive dyskinesia and other adverse effects. Atypical antipsychotic drugs, e.g., risperidone, can prolonged the time interval from the beginning of the QRS complex to the end of the T-wave (QT interval), prolactin elevation and other adverse effects (Bhana et al., 2001; Lin et al., 2010; Adams et al., 2013). Additionally, current antipsychotic drugs are ineffective in about 30% of patients with treatment-resistant schizophrenia (Kane et al., 2019; Chakrabarti, 2021). Clozapine is the only drug currently recommended for refractory schizophrenia, but it is susceptible to obesity and fatal agranulocytosis, which limit its clinical use (Mijovic and MacCabe, 2020). Therefore, there are significant unmet medical needs for new antipsychotic drugs with more efficacious but less side effects (Kantrowitz et al., 2023).
Modulation of serotonin and dopamine receptors in the central nervous system has proven to be an effective way to treat psychiatric disorders (Malik et al., 2023; Stelmach et al., 2023). The dopamine receptor is the crucial target of all existing antipsychotics. Dopamine D2 receptor antagonists are thought to control positive symptoms in patients with schizophrenia (Farde et al., 1988; Casey and Canal, 2017; Juza et al., 2022). Schizophrenia may be controlled by the antagonism of the 5-HT2A receptor in synergy with the antagonism of the dopamine D2 receptor (Andree et al., 1997). However, many atypical antipsychotics have more selectivity for dopamine D2 receptor than 5-HT2A receptor at therapeutic doses in clinical. These atypical antipsychotic drugs with relatively high D2 receptor occupancy in the striatum and presumably other D2 expressing tissues such as pituitary gland, elevate prolactin levels and can induce extrapyramidal motor side effects at therapeutic doses. Selective 5-HT2A receptor antagonist has been proved not only to enhance dopamine D2 receptor antagonist-mediated antipsychotic efficacy but also to reduce hyperprolactinemia and motor side effects (Wadenberg et al., 2001; Gardell et al., 2007).
Furthermore, the dopamine D3 receptor may represent an important target for antipsychotic drugs (Schwartz et al., 2000; Gross et al., 2013). The dopamine D3 receptor has been recognized to have several central nervous system (CNS) functions, such as social behavior, movement control, emotional regulation, reward, learning, and cognition function (Kiss et al., 2021). Dopamine D3 receptor antagonists possess improving cognitive impairment activity, which may benefit the treatment of cognitive dysfunction associated with several psychiatric disorders (Laszy et al., 2005). Therefore, a medication that combines potent 5-HT2A receptor antagonism with optimal dopamine D2 receptor modulation, and the dopamine D3 receptor antagonism activity may present an ideal balance of dopaminergic and serotonergic neurotransmitter for the treatment of schizophrenia.
Taken together, we proposed the hypothesis that compounds acting synergistically on serotonin and dopamine receptors might be able to address schizophrenic symptoms with less or without inducing extrapyramidal symptoms (EPS) and other side effects. A series of fused heterocyclic derivatives were synthesized, which have potent activity in serotonin and dopamine receptors. The patent for the synthesis of the compounds has been published (Jing et al., 2021). NH300094 (8-(3-(4-(6-fluorobenzo [d]isoxazol-3-yl) piperidin-1-yl) propoxy)-1,2,5,6-tetrahydro-4H-pyrrolo [3,2,1-ij] quinolin-4-one) was characterized as a preclinical candidate compound based on the good preclinical profiles, which is a triple antagonist of 5-HT2A receptor, dopamine D2 receptor and dopamine D3 receptor. Additionally, it has strong inverse agonist activity of 5-HT1B and antagonistic activity of 5-HT1A receptor. NH300094 is being developed and clinically intended for the treatment of positive symptoms, negative symptoms and cognitive disorders of schizophrenia.
2 Materials and methods
2.1 Experiment cells
CHO-K1/5-HT2A and CHO-K1/D3 cells were purchased from Shanghai PerkinElmer Biotechnology Co., Ltd. CHO-K1/M1, HEK293/H1, CHO-K1/D2, HEK293/5-HT7 and CHO-K1/5-HT1A cells were purchased from Nanjing GenScript Biotechnology Co., Ltd. HEK293/5-HT2C, HEK293/Alpha1A and HEK293/Alpha2A cells were constructed by biology laboratory of Shanghai shujing Biopharma Co., Ltd. CHO-K1/5-HT1B cells were purchased from Wuhan Creater Biotechnology Co., Ltd.
2.2 Experimental animals
Male Wistar rats (weight, 200–230 g) and ICR mice (weight, 20–28 g) were purchased from SPF (Beijing) Biotechnology Co., Ltd. Male Sprague-Dawley (SD) rats (weight, 180–220 g) were purchased from Beijing Vital River Laboratory Animal Technology Co., Ltd. The animals were group feeding under standard conditions (temperature:20°C–26°C, humidity: 40%–70%, 12-h dark/light cycle). Before the testing, the animals were acclimated to the laboratory environment for 3 days, and the animal food and drinking water were freely provided. The animal experiment protocols were approved by the Institutional Animal Care and Use Committee of Jiangsu Nhwa Pharmaceutical Co., Ltd.
2.3 Drugs
NH300094 hydrochloride, risperidone and duloxetine hydrochloride were synthesized at Jiangsu Nhwa Pharmaceutical Co., Ltd. The molecular structure of NH300094 (PCT/CN 2020/129850) is shown in Figure 1. Other compounds such as (+)-MK-801 hydrogen maleate (M107-250MG, Sigma), rivastigmine hydrogen tartrate (LRAB1259, Sigma), R (2)-2,5-dimethoxy-4-iodoamphetamine (DOI) (D101-100MG, Sigma), R-(−)-Apomorphine (APO) hydrochloride (A4393, Sigma), L-Ascorbic acid Vc (A5960-25G, Sigma), (+)-Butaclamol (D033, Sigma) were purchased from Sigma-Aldrich (St. Louis, MO). Scopolamine hydrobromide (S107418-5g, Aladdin) was purchased from Aladdin. WAY-00635 (T2631, Targetmol), Ketanserin (T1066, Targetmol), Yohimbine (T2142, Targetmol), Pyrilamine (T1232, Targetmol), Atropine (T0375, Targetmol), Prazosin (T1050, Targetmol) were purchased from Targetmol. Methiothepin (HY-107836, MCE) was purchased from MCE. All drugs were dissolved in normal saline or deionized water and administered orally at 10 mL/kg (volume/body weight), unless otherwise indicated.
2.4 Receptor functional activity
2.4.1 5-HT2A, 5-HT2C, H1, M1, Alpha1A receptor functional assay
A calcium flow assay was used to test the activity of compounds on 5-HT2A, 5-HT2C, H1, M1, Alpha1A receptor. After the CHO-K1/5-HT2A, CHO-K1/M1, HEK293/5-HT2C, HEK293/H1 and HEK293/Alpha1A cells were lightly trypsinized, a density of 2×104 cells/well were inoculated in 384-well plates (Greiner-781946) which containing 20 μL cell medium in each well. The cells were routinely cultured at 37°C with 5% CO2 for 16–24 h prior to testing.
In agonist experiments, 20 μL of experimental buffer was added to each well of the assay plate after removing the medium. Then, 20 μL of fluorescent probe solution was added to each well. The assay plate was incubated in incubator at 37°C for 50 min, let it stand at room temperature for 10 min, then transferred to the reading position of the Fluorescent Image Plate Reader (FLIPR). The compound (10 μL) was added to the assay plate and the fluorescence signal was read for 210 s.
For the antagonist tests, 20 μL of experimental buffer and fluorescent probe solution was added to each well of the assay plate after removing of the culture medium. The assay plate was incubated in incubator at 37°C for 50 min, let it stand at room temperature for 10 min, then transferred to the reading position of the Fluorescent Image Plate Reader (FLIPR). The compound and control agonist serotonin (10 μL) was added to the assay plate and the fluorescence signal was read for 210 s. After reading the raw data from FLIPR, the EC50 and IC50 were calculated, respectively.
For both agonist and antagonist tests, the difference between the maximum value and the minimum value of fluorescence signal readings (rang, 1–210 s) was regarded as the change of relative fluorescent unit intensity (△RFU). The agonistic or antagonistic activity of drug was analyzed using the following equation:
Dose-response curves for agonist/antagonist were fitted using the software GraphPad Prism (version 8.0.2) with four parameter logistic equation.
2.4.2 D2L, D2S, 5-HT1A, 5-HT1B, Alpha2A receptor functional assay
The activity of the compounds on D2L, D2S, 5-HT1A, 5-HT1B and Alpha2A receptors was detected using cAMP assay. The CHO-K1/D2, CHO-K1/5-HT1A, HEK293/Alpha2A and CHO-K1/5-HT1B cells were diluted to the appropriate concentration with experimental buffer, and 10 μL of the cell solution was transferred to each well of the assay plate. The compound was then transferred to the assay plate using Tecan-D300e. After centrifuging at 1,000 rpm for 1 min, the assay plate was incubated for 15 min at room temperature. The appropriate amount of Forskolin solution was added to the cell plate, where the antagonist test assay requires an additional positive compound (dopamine for D2L, D2S; serotonin for 5-HT1A, 5-HT1B; DL-Adrenaline for Alpha2A), centrifuged at 1,000 rpm for 1 min. The plate was incubated for 45 min at room temperature before adding 10 μL cAMP-d2 solution and anti-cAMP-Cryptate solution to the assay plate, which was then centrifuged at 1,000 rpm for 1 min. The plate was incubated at room temperature for 1 h. And then read using Envision (PerkinElmer) with parameters set to excitation 340 nm and emission 620 nm/665 nm.
2.4.3 D3 receptor functional assay
For the D3 receptor functional assay, the Nano-Glo® luciferase assay system was used to detect the activity of the compounds. The CHO-K1/D3 cells were diluted to a final concentration of 5×105 cells/mL, and 20 μL of cell suspension (cell density of 10,000 cells/well) was added to each well of a 384-cell plate and incubated for 16–24 h in a 5% CO2 and 37°C incubator. The compounds were transferred to the assay plate using a Tecan-D300e, centrifuged at 1,000 rpm for 1 min, and incubated at 37°C for 30 min. After adding the Forskolin solution, the assay plate was centrifuged at 1,000 rpm for 1 min and incubated at 37°C for 4 h. The substrate and assay buffer (v/v, 1/50) were added to the assay plate, and then incubated at room temperature for 5 min after centrifuging at 1,000 rpm for 1 min. Finally, the assay plate was read using enzyme-labelling measuring instrument and the IC50 of compounds was calculated.
2.4.4 5-HT7 receptor functional assay
The activity of the compounds on 5-HT7 receptors was detected using Bright-Glo™ Luciferase assay system. The HEK293/5-HT7 cells were diluted to the 20000 cells/well with assay buffer, and 20 μL of the cell solution was transferred to each well of the assay plate (384-well plate). The test compound and positive compound were then transferred to the assay plate using Tecan-D300e. After centrifuging at 1,000 rpm for 1 min, the assay plate was incubated for 30 min at 37°C. The serotonin solution was transferred into assay plate, centrifuge at 1,000 rpm for 1 min, and incubated for 4 h at 37°C. After that the 30 μL of detection reagent was added to the cell plate, centrifuge at 1,000 rpm for 1 min, and read using Envision with the HTRF compatible reader.
2.5 In vivo pharmacological study
2.5.1 MK-801-induced hyperactivity in mice
According to the body weight, one hundred and twenty male ICR mice (5 weeks of age) were randomly divided into ten groups with twelve mice per group. The mice were then dosed with vehicle (p.o.), NH300094 (0.1, 0.3, 1 and 3 mg/kg, p.o.) or risperidone (0.1, 0.3, 1 and 3 mg/kg, p.o.) and placed back into their home-cage for 30 min. Immediately after injection with either saline or MK-801 (0.3 mg/kg, i.p.), the mice were placed into the test chambers (29 cm × 29 cm × 30 cm) for 60 min of locomotion recording using a tracking and computerized analysis system (TopScan Version 3.00, Clever Sys Inc., Leesburg, VA). After each test, the test chamber should be cleaned and wiped with 75% alcohol solution. The detailed flow chart of the test method is presented in Supplementary Figure S1A.
2.5.2 APO-induced climbing in mice
According to the body weight, one hundred and eight male ICR mice (5 weeks of age) were randomly divided into nine groups with twelve mice per group. The mice were treated with either vehicle (p.o.), NH300094 (0.03, 0.1, 0.3 and 1 mg/kg, p.o.) or risperidone (0.03, 0.1, 0.3 and 1 mg/kg, p.o.) and placed back into their home-cage for 60 min. Afterward, mice were injected with apomorphine (APO, 1 mg/kg, s.c.) and immediately placed individually into cylindrical cages (13 cm diameter, 15 cm high, with walls of vertical bars, 1 cm diameter) for behavior observation. The behavior of the mice was observed and scored at 10–11, 20–21, 30–31 min post injection of APO as follows: 0 = four paws on the cage floor; 1 = two paws holding the vertical bars of the cage; 2 = four paws holding the vertical bars of the cage. After each test, the test chamber should be cleaned and wiped with 75% alcohol solution. The detailed flow chart of the test method is presented in Supplementary Figure S1B.
2.5.3 DOI-induced head twitch in mice
The test referred to the previously description of the DOI-induced head twitch test in mice (Fantegrossi et al., 2010). One hundred and eight male ICR mice (5 weeks of age) were randomly divided into nine groups with twelve mice per group: control, NH300094 (0.001, 0.003, 0.01, and 0.03 mg/kg, p.o.), risperidone (0.001, 0.003, 0.01 and 0.03 mg/kg, p.o.). The mice were administered intragastrically with vehicle or compounds 60 min before the DOI (1 mg/kg, i.p.) injection. After that, the mice were immediately placed into the plexiglass box individually. The number of head twitches in the mice was counted by the blind observer over a 20-min period. The detailed flow chart of the test method is presented in Supplementary Figure S1C.
2.5.4 Conditioned avoidance response test in rats
The experiments were conducted in two phases: Phase I, conditioned avoidance response (CAR) training (112 male Wistar rats aged 7 weeks which were used when study started); Phase II, grouping the qualified rats and testing the efficacy of compounds in CAR test. Shuttle-box Avoidance Test Video Analysis System (DigBehv-SBG, Shanghai Jiliang Software Technology Co. Ltd.) were used to assess the rats conditioned avoidance response.
Phase I: The rats responded to the conditioned stimulus (auditory and visual) by training with foot shock reinforcement. Briefly, rats were placed into the CAR shuttle boxes for a 5-min habituation followed by 30 trials presented on a 20-s variable interval (20–30 s) stimulus. Each rats were subjected to a conditioned stimulus which consisted of 10s presentation of light and white noise, and then followed by a scrambled 1.5 mA foot shock for 10 s. Rats were recorded as “avoidance” if they had successfully moved to the other compartment during the stimulus process; Rats that ran to the other compartment during the shock was recorded as “escape”; Rats that failed to move to the other compartment during the shock period were recorded as “escape failure”. Rats with avoidance rates greater than 70% for 3 consecutive days were included in this study post-training.
Phase II: The qualified rats were randomly divided into 7 groups. Each rats were individually placed into a shuttle box for CAR testing 1 h post oral administration of vehicle, NH300094 (0.3, 1 and 3 mg/kg, p.o.) or risperidone (0.3, 1 and 3 mg/kg, p.o.). The procedure in the testing phase was the same as the Phase I described. The number of avoidances, escapes, and escape failures were recorded. The detailed flow chart of the test method is presented in Supplementary Figure S1D.
2.5.5 Novel object recognition in mice
The procedure was modified according to Bevins and Besheer (Bevins and Besheer, 2006). According to the body weight, one hundred and sixty male ICR mice (5 weeks of age) were randomly divided into ten groups with sixteen mice per group. The tests were conducted in a 50 cm × 35 cm × 20 cm chamber and the mice behavior was recording using a Hikvision video recording system (H.265, Hikvision Digtial Technology Co., Ltd.). All mice were allowed to freely explore the chamber environment for 10 min, and there were no objects placed in the chamber during acclimatization period. About 24 h after habituation, mice were dosed with vehicle (p.o.), NH300094 (0.04, 0.08 and 0.16 mg/kg, p.o.) or risperidone (0.04, 0.08 and 0.16 mg/kg, p.o.) 30 min before injected with scopolamine hydrobromide (3 mg/kg, i.p.). Rivastigmine hydrogen tartrate group (0.1, 0.3 and 1 mg/kg, i.p.) were dosed simultaneously with scopolamine hydrobromide (3 mg/kg, i.p.). Training was conducted 30 min post scopolamine hydrobromide administration by placing a single mouse into a chamber for 10 min with two exactly same objects positioned in the center of chamber. (The distance between the two objects was more than 20 cm). The short-term memory of mice was tested 1 h post training by exploring the chamber for 10 min in the presence of a new and familiar object. The 10-min testing was videotaped. After each test, the test chamber should be cleaned and wiped with 75% alcohol solution. All of the objects presented had similar sizes, colors and textures.
The experimental video was analyzed to record the time of mice exploring the new and old objects, respectively, and the differentiation index (DI) was calculated as follows: DI = new object exploration time/(new object exploration time + old object exploration time), which was used as the main evaluation index of discrimination ability. Analysis stopped when the total exploration time of the new and old objects reaches 20 s. If the total exploration time of the new and old objects was less than 20 s, the total 10 min of video would be analyzed. Exploration was defined as the distance between the nose of mouse and the object being less than 1 cm when the mouse actively explored the object. The movement of circling without sniffing or sitting on the object was not recorded as exploration behavior. The detailed flow chart of the test method is presented in Supplementary Figure S1E.
2.5.6 Forced swimming test in mice
The test referred to the previously description of the forced swimming test (FST) in mice (Porsolt et al., 1978). One hundred and sixty male ICR mice (5 weeks of age) were randomly divided into ten groups with sixteen mice per group: vehicle control, duloxetine (20 mg/kg, p.o.), risperidone (0.01, 0.03 and 0.1 mg/kg, p.o.) and NH300094 (0.003, 0.01 and 0.03 mg/kg, p.o.), the dosage of duloxetine was referred to the previous study (Xu et al., 2018). Each mouse was required to swim in an open cylindrical container (height of 25 cm, diameter of 10 cm) after intragastric administration of compounds 1 h. The container contained 1.2 L of water with temperature maintained at 24°C ± 1°C. The test used a computerized analysis and tracking system (Clever Sys Inc., Leesburg, VA) to recorded the duration of immobility (last 4 min of a total time of 6 min) about the testing mice. The detailed flow chart of the test method is presented in Supplementary Figure S1F.
2.5.7 Spontaneous locomotor activity test in mice
One hundred and eight male ICR mice (5 weeks of age) were randomly divided into nine groups with twelve mice per group. One hour after oral administration of vehicle (p.o.), NH300094 (0.1, 0.3, 1 and 3 mg/kg, p.o.), risperidone (0.1, 0.3, 1 and 3 mg/kg, p.o.), mice were individually placed into test chamber (29 cm × 29 cm × 30 cm) for locomotion recording for 60 min using a computerized analysis and tracking system (Clever Sys Inc., Leesburg, VA). After each test, the test chamber should be cleaned and wiped with 75% alcohol solution. The detailed flow chart of the test method is presented in Supplementary Figure S1G.
2.5.8 Catalepsy test in mice
The test referred to the previously description of the catalepsy test in mice (Kuschinsky and Hornykiewicz, 1972). According to the body weight, one hundred and eight male ICR mice (5 weeks of age) were randomly divided into nine groups with twelve mice per group. Catalepsy was assessed at 30, 60 and 90 min post oral administration of vehicle (p.o.), NH300094 (1, 3, 10 and 30 mg/kg, p.o.) or risperidone (0.1, 0.3, 1 and 3 mg/kg, p.o.). The front paws of mice were placed on a horizontal stainless bar (length: 20 cm; diameter: 0.3 cm; height: 5.5 cm). If this behavior of mouse lasted for 30 s or longer, catalepsy would be considered as positive, and 60 s was used as cut-off. After each test, the test area should be cleaned and wiped with 75% alcohol solution. The detailed flow chart of the test method is presented in Supplementary Figure S1H.
2.6 Pharmacokinetics assay
Six male SD rats (7 weeks of age) were randomly divided into two groups with three rats per group. Animals were fasted overnight and had free access to water before dosing. For the intravenous group, male SD rats were administered NH300094 by single intravenous bolus administration at a dose of 2 mg/kg. For the oral group, male SD rats were dosed orally with NH300094 at a dose of 10 mg/kg. Serial blood samples were collected at different time points (Pre-dose, 0.033, 0.083, 0.25, 0.5, 1, 2, 4, 6, 8, 12 and 24 h for the intravenous group; Pre-dose, 0.167, 0.333, 0.5, 1, 2, 4, 6, 8, 10, 12 and 24 h for the oral group) via jugular vein puncture from each study animal. All blood samples were transferred into commercial tube containing K2-EDTA. Plasma samples were prepared by centrifuging the blood samples at approximately 4°C, 3,200×g for 15 min, and then stored at −70°C until analysis (Supplementary Materials). The pharmacokinetic parameters were calculated using WinNonlin software (Version 6.3) according to non-compartmental model.
2.7 Statistical analysis
All raw data were calculated as the mean ± standard deviation (S.D.). Statistical analyses were conducted using GraphPad Prism version 8.0.2 (GraphPad Software). For in vitro assays, the IC50 and EC50 values were calculated by nonlinear regression analysis. For in vivo experiments, the data were analyzed statistically by one-way ANOVA followed by Dunnett’s multiple comparison test (p < 0.05).
3 Results
3.1 In vitro pharmacology
The results of tests in vitro showed that NH300094 has pharmacological activity against various targets, including antagonist activity against D2LR, D2SR, D3R, 5-HT1AR and 5-HT2AR. Additionally, inverse agonist activity was observed at 5-HT1BR (Table 1; Figure 2). The antagonistic activity to D2LR (IC50 = 1.04 ± 0.59 nM) and 5-HT2AR (IC50 = 0.47 ± 0.79 nM) was the most significant activity of NH300094, which indicates its potential anti-schizophrenia activity. NH300094 had lower antagonistic activities ratio of 5-HT2AR and D2R than risperidone (IC50 ratio = 0.45 for NH300094, IC50 ratio = 1.0 for risperidone), which is speculated lower extrapyramidal side effects (Meltzer et al., 1989). The high inverse agonist activity of 5-HT1BR (EC50 = 28.36 ± 12.52 nM) and antagonistic activities of 5-HT1AR (IC50 = 85.59 ± 61.53 nM) and D3R (IC50 = 31.55 ± 23.08 nM) suggesting that NH300094 has potential to improve not only the positive symptoms but also the cognitive dysfunctions (Laszy et al., 2005; Meneses, 2007; Ohno, 2011). NH300094 has no significant pharmacological activity against other targets in the study (Supplementary Table S1).
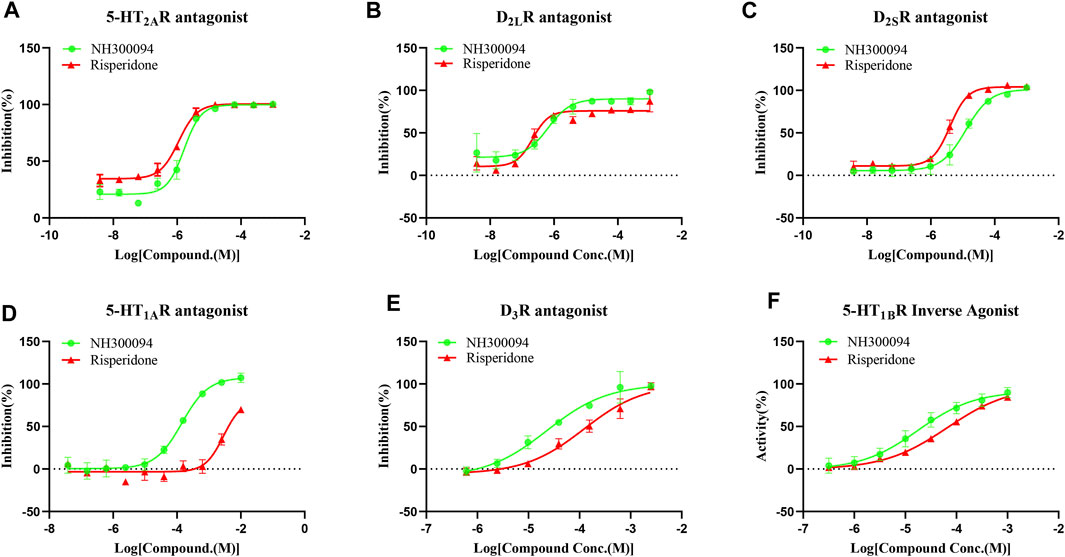
FIGURE 2. The in vitro pharmacological activity of NH300094 and risperidone at 5-HT2AR (A), D2LR (B), D2SR (C), 5-HT1AR (D), D3R (E), 5-HT1BR (F), which presented using concentration-dependence curves. The effects are determined by cAMP production or intracellular Ca2+ concentrations. Data are presented as means ± S.D. of three independent test.
3.2 Effects of NH300094 on MK-801-induced hyperactivity in mice
Single oral dose administrations of NH300094 (0.01, 0.03, 0.1 and 0.3 mg/kg) resulted in a dose-dependent inhibition of MK-801-induced hyperactivity in male ICR mice with an ED50 of approximately 0.07 mg/kg and Minimum effective dose (MED) of 0.03 mg/kg. Risperidone (0.01, 0.03, 0.1 and 0.3 mg/kg) also significantly reduced MK-801-induced hyperactivity with an ED50 of approximately 0.08 mg/kg and MED of 0.1 mg/kg (Figure 3A).
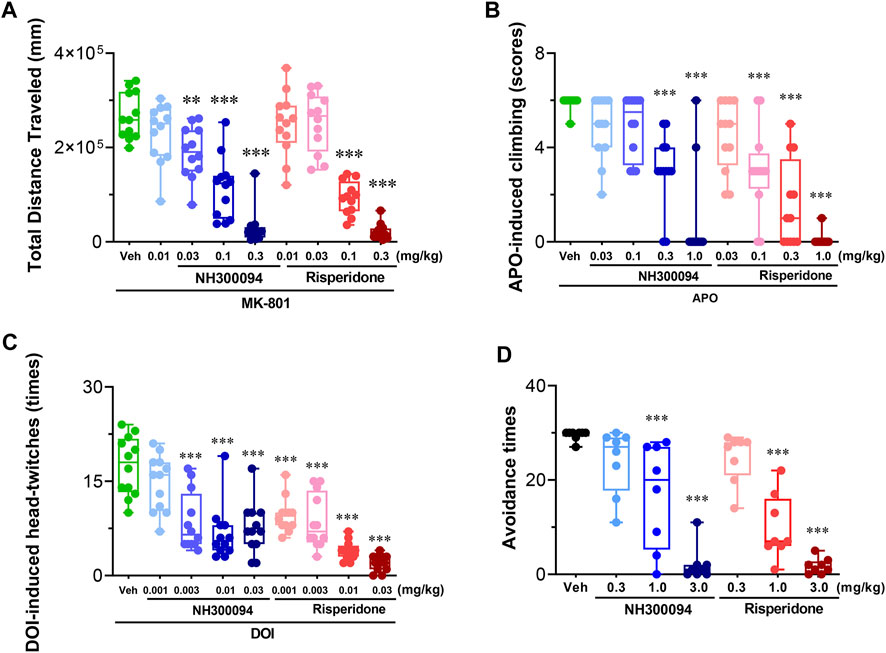
FIGURE 3. Effects of different doses of NH300094 on schizophrenia-like model compared with antipsychotic drugs risperidone in rodents. (A) Effects of NH300094 and risperidone on hyperactivity model induced by MK-801 (0.3 mg/kg, i.p.) in mice (n = 12). (B) Effects of NH300094 and risperidone on climbing behavior induced by APO (1.0 mg/kg, s.c.) in mice (n = 12). (C) Effects of NH300094 and risperidone on head-twitches behavior induced by DOI (1.0 mg/kg, s.c.) in mice (n = 12). (D) Effects of NH300094 and risperidone on the avoidance time of rats in conditional avoidance test (n = 8). Data are presented as box-and-whisker plot (min to max with all points) and are analyzed one-way ANOVA with Dunnett’s multiple comparisons tests. **p < 0.01, ***p < 0.001 compared with veh group. Veh: Vehicle.
3.3 Effects of NH300094 on APO-induced climbing in mice
Single oral dose administrations of NH300094 (0.03, 0.1, 0.3 and 1 mg/kg) resulted in a dose-dependent inhibition of APO-induced climbing in male ICR mice with an ED50 of approximately 0.29 mg/kg and MED of 0.3 mg/kg. Risperidone (0.03, 0.1, 0.3 and 1 mg/kg) also significantly reduced APO-induced climbing behavior, with an ED50 of approximately 0.1 mg/kg and MED of 0.1 mg/kg. These results indicate that NH300094 has potential antipsychotic effects in clinic (Figure 3B).
3.4 Effects of NH300094 on DOI-induced head twitch in mice
Single oral dose administrations of NH300094 (0.001, 0.003, 0.01 and 0.03 mg/kg) resulted in a dose-dependent inhibition of DOI-induced head twitch in male ICR mice with an ED50 of approximately 0.007 mg/kg and MED of 0.003 mg/kg. Risperidone (0.001, 0.003, 0.01, 0.03 mg/kg) also significantly reduced DOI-induced head twitch with an ED50 of approximately 0.002 mg/kg and MED of 0.001 mg/kg. The data from this study reveal that both NH300094 has potential antipsychotic effects in clinic. (Figure 3C).
3.5 Effects of NH300094 on conditioned avoidance response
Single oral dose administrations of NH300094 (0.3, 1 and 3 mg/kg) resulted in a dose-dependent inhibition of conditioned avoidance response of rats with an ED50 of approximately 1.02 mg/kg. Risperidone (0.3, 1 and 3 mg/kg) also significantly reduced conditioned avoidance response of rats with an ED50 of approximately 0.70 mg/kg. The results showed that NH300094 has potential antipsychotic effects in clinic (Figure 3D).
3.6 Effects of NH300094 on novel object recognition in mice
The cognitive deficits model of mice treated by scopolamine hydrobromide was established, and the effects of NH300094 on the model was examined using novel object recognition (NOR). In the 10-min test experiment, mice dosed with vehicle spent 12.94 s on a novel object and 7.06 s on a familiar object, with a differentiation index (DI) of 0.65. Mice treated with scopolamine hydrobromide showed significant new object recognition impairment by spending roughly equal time exploring an acquainted object and a novel object with a DI of 0.46. Compared with the DI of scopolamine hydrobromide-treated mice, the positive control, rivastigmine bitartrate-treated (0.3 and 1 mg/kg, i.p.) mice also significantly enhanced learning and memory ability, indicating that the testing system worked well for compound testing. In this study, we found that at doses of 0.01–0.16 mg/kg, NH300094 increased DI index of scopolamine hydrobromide-treated mice, while risperidone (0.04, 0.08 and 0.16 mg/kg, p.o.) did not affect the DI of scopolamine hydrobromide-treated mice. These results suggest that NH300094 may have the potential to improve cognitive deficits of schizophrenia (Figure 4A).
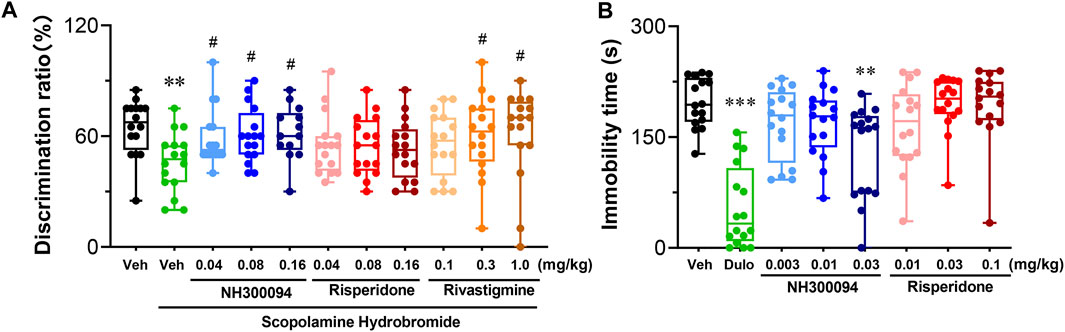
FIGURE 4. Effects of different doses of NH300094 on cognitive memory and depression-like behavior compared with antipsychotic drugs risperidone in mice. (A) The influence of different doses of NH300094 on the discrimination ratio compared to model group (n = 16). (B) The influence of NH300094 on mobility in FST (n = 16). Data are presented as box-and-whisker plot (min to max with all points) and are analyzed one-way ANOVA with Dunnett’s multiple comparisons tests. **p < 0.01, ***p < 0.001 compared with veh group. #p < 0.05 compared with model group. Veh: Vehicle.
3.7 Effects of NH300094 on forced swimming test in mice
A single oral dose of administrations of NH300094 (0.03 mg/kg) decreased immobility time in the FST, but single oral dose of administrations of risperidone (0.01, 0.03 and 0.1 mg/kg) did not affect the immobility time in the FST. The results indicate that NH300094 may have potential effects in improving the negative symptoms of schizophrenia, which is different from risperidone (Figure 4B).
3.8 Effects of NH300094 on spontaneous locomotor activity
A single oral dose of administrations of NH300094 (0.1, 0.3, 1 and 3 mg/kg) resulted in a dose-dependent inhibition of spontaneous locomotor activity in male ICR mice with an ED50 of approximately 0.49 mg/kg. Risperidone (0.1, 0.3, 1 and 3 mg/kg) also reduced spontaneous locomotor activity significantly with an ED50 of approximately 0.52 mg/kg. The ED50 of NH300094 in spontaneous locomotor activity is much higher than that of MK-801 induced hyper-locomotor activity, indicating the good safety margin of NH300094 (Figure 5A).
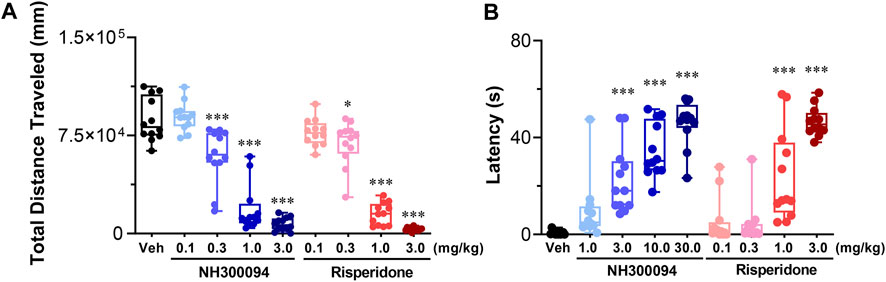
FIGURE 5. Side-effects of different doses of NH300094 compared with antipsychotic drugs risperidone in mice. (A) The influence of single acute treatment with NH300094 and risperidone on the locomotion activity in mice. (n = 12). (B) The influence of single acute treatment with NH300094 and risperidone on the catalepsy time in mice. (n = 12). Data are presented as box-and-whisker plot (min to max with all points) and are analyzed one-way ANOVA with Dunnett’s multiple comparisons tests. *p < 0.05, **p < 0.01, ***p < 0.001 compared with veh group. Veh: Vehicle.
3.9 Effects of NH300094 on catalepsy test
The catalepsy test is widely used for evaluating extrapyramidal side effects of dopamine antagonists (Adams et al., 2013). In this study, the minimal dose of NH300094 that induced catalepsy in mice is 3 mg/kg with an ED50 of 6.73 mg/kg. On the other hand, the minimal dose of risperidone that induced catalepsy in mice was 1 mg/kg with an ED50 of 1.35 mg/kg. These results indicate that NH300094 may have lower EPS side effects compared to risperidone (Figure 5B).
3.10 Pharmacokinetics study
Good pharmacokinetic characteristics are an important factor for clinical efficacy of drugs. The PK parameters of NH300094 were acquired by intravenous and intragastric administration in rats. The mean plasma concentration of NH300094 over 24 h is shown in Figure 6. The oral administration of NH300094 to rats resulted in a half-life of 1.28 h, and the area under the concentration time-curves for time zero to infinity was 14800 ng/mL*h. By comparing the exposure of NH300094 after oral and intravenous administrations, the absolute oral bioavailability of NH300094 was calculated as about 87.6% in rats. The other major pharmacokinetics parameters for different administration routes are presented in Table 2, demonstrating the excellent pharmacokinetic characteristics of NH300094 for further development.
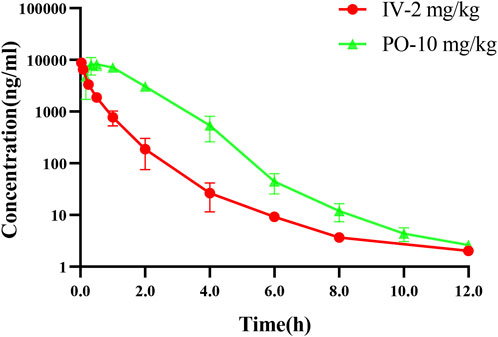
FIGURE 6. Mean plasma concentration-time profiles of NH300094 in rats after an oral or intravenous administration.
4 Discussion
Today, many antipsychotics have been developed, but these drugs have not been able to dissociate efficacy from side effects (Paul et al., 2022; Schneider-Thoma et al., 2022). Additionally, negative symptoms and cognitive dysfunctions of schizophrenia are difficult to manage, which impairs the patient’s ability to return to normal life (Kahn et al., 2015; Xu et al., 2022; Veleva et al., 2023). NH300094 is a novel anti-schizophrenia candidate with unique characteristics of D2 receptor, D3 receptor antagonism, 5-HT1A,5-HT2A receptor antagonism and 5-HT1B inverse agonism. Its antagonistic activity against 5-HT2A receptor is significantly higher than that against the dopamine receptor, which is in line with the characteristics of atypical anti-schizophrenia drugs. Preclinical studies results suggest that NH300094 has the potential to treat positive symptoms of schizophrenia as well as improve negative symptoms and cognitive impairment.
At present, the primary mechanism action of traditional antipsychotics is still to block the signaling of postsynaptic dopaminergic in the brain (Behr et al., 2000; Ichikawa et al., 2001; Muller-Spahn, 2002). Preclinical and clinical studies have clearly indicated that fronto-cortical dopamine system hypoactivity and striatal dopamine system hyperactivity associated with the occurrence of psychotics (McCutcheon et al., 2019; Rao et al., 2019). It is suggested that simultaneous blocking of dopamine D2 and 5-HT2A receptors improves the efficacy of antipsychotic drugs in patients with schizophrenia and reduces the risk of extrapyramidal symptoms (EPS) (Andree et al., 1997; Kusumi et al., 2015). The conditioned avoidance response study is a well-established preclinical antipsychotic animal model (Wadenberg et al., 2000; Gao et al., 2015). Antipsychotic drugs can selectively suppress the conditioned avoidance response of rats. PK/PD (pharmacokinetics/pharmacodynamics) studies have suggested that the relationship between the suppression of conditioned avoidance response and dopamine D2 receptor occupancy of rats correlates well with the relationship between human clinical effects and dopamine D2 receptor occupancy (Wadenberg et al., 2001; Olsen et al., 2008). The present data indicate that NH300094 has good antagonism effects with D2 receptor (IC50 = 1.04 nM), and showed good efficacy in CAR study of rats. It predicts good clinic efficacy for NH300094. Apomorphine is a potent dopamine agonist, challenged with apomorphine can induce specific climbing behaviors in mice on subsequent occasions (Costall et al., 1978; Davis et al., 1986). Dopamine antagonists inhibit climbing behaviors of mice dose-dependently (Kafka and Corbett, 1996). NH300094 significantly inhibits apomorphine induced climbing, indicating strong dopamine antagonism effects. Taken together with the CAR results, the antipsychotic effects of NH300094 in animal studies correlate well with the in vitro antagonism activity of Dopamine D2 and D3 receptor.
Serotonin receptor, particularly the 5-HT1A and 5-HT2A receptors, are useful targets for the treatment of schizophrenia (Poyurovsky et al., 2003; Meltzer et al., 2012). In the prefrontal cortex, 5-HT1A receptor and 5-HT2A receptor are mostly expressed in pyramidal neurons and are involved in the regulation of excitatory and inhibitory transmission in these neurons, which accounts for the antipsychotic effects (Burnet et al., 1996; Amargos-Bosch et al., 2004; Santana et al., 2004). The DOI induced head-twitch behavior is a useful model for studying the activation of 5-HT2A receptors in mice (Fantegrossi et al., 2010; Canal and Morgan, 2012). In 5-HT2A receptor null-mutant mice, the DOI-induced head twitches are completely abolished (Gonzalez-Maeso et al., 2003). In our study, the ED50 value of NH300094 in mice DOI model was 0.006 mg/kg post p.o. administration. NH300094 dose-dependently inhibits DOI induced head-twitch behaviors, which is consistent with the in vitro data. The FLIPR assay shows that NH300094 is a potent 5-HT2A receptor antagonist (IC50 = 0.47 nM). The density of 5-HT1A receptor is increased in the brains of chronic schizophrenia patients, implying an important role of 5-HT1A receptor in the pathogenesis of schizophrenia (Hashimoto et al., 1991; Millan, 2000). It is reported that antagonism of 5-HT1A receptor can improve cognitive impairment in schizophrenia (Meltzer and Sumiyoshi, 2008). The stimulation of the 5-HT1A receptor often interferes with memory-encoding mechanisms in brain, which leading to learning disabilities. However, antagonists of 5-HT1A receptor can enhance cortical cholinergic/hippocampal and/or glutamatergic neurotransmission, which promoting certain types of memory (Ogren et al., 2008; Yamada et al., 2023). 5-HT1A antagonists reversed the cognitive impairment induced by NMDA receptor antagonists or mACh receptor antagonists (Luttgen et al., 2005; Madjid et al., 2006). Lurasidone, a antipsychotics with potent 5-HT1A antagonistic activity, has been shown to improve the learning and memory deficits induced by MK-801 in rats (Ishiyama et al., 2007; Horisawa et al., 2011) and to improve the cognitive impairment in schizophrenia in the clinic (Samalin et al., 2014; Meltzer et al., 2020). In our study, NH300094 but not risperidone shows good 5-HT1A antagonistic activity in the c-AMP assay (NH300094 IC50 = 85.59 nM; risperidone IC50 = 4964.67 nM), and it has a very good in vivo efficacy in the NOR test in the scopolamine induced memory deficits model of mice. The minimal effects dose is lower than that of rivastigmine, a dementia disorders drug widely used for the treatment of Alzheimer’s disease (Marucci et al., 2021). Our data indicate that the 5-HT1A antagonism activity of NH300094 might be one of the mechanisms of memory improvement effects.
Glutamatergic dysfunction is considered another mechanism of schizophrenia (Kruse and Bustillo, 2022). Studies have shown that the extracellular concentrations of dopamine and serotonin increase in the nucleus accumbens (NAC) and prefrontal cortex (PFC) after systemic administration of N-methyl-D-aspartic acid receptor (NMDA) antagonists such as MK-801, indicating that modulation of dopamine and serotonin receptors could potentially help restore the glutamatergic dysfunction in schizophrenia (Marcus et al., 2001; Lopez-Gil et al., 2007; DelArco et al., 2008). In rodents, MK-801, a NMDA non-competitive antagonist, induces complex behavioral syndromes that include locomotor hyperactivity, stereotypy, disruption of sensorimotor gating, and social deficit (Jentsch and Roth, 1999). Hyperlocomotion induced by acute MK-801 treatment in mice is a reliable and robust model for antipsychotic drugs testing (Ninan and Kulkarni, 1999). In our study, NH300094 effectively attenuated hyperlocomotion produced by MK-801, indicating its potential role in restoring the function of NMDA receptors in schizophrenia patients.
Cognitive impairment is one of the main obstacles to clinical and functional recovery in schizophrenia (Harvey et al., 2022). In patients with schizophrenia, D3 receptor levels are elevated in the limbic striatum, suggesting that D3 receptor antagonists might be effective in treating schizophrenia (Gurevich et al., 1997). The studies have suggested that dopamine D3 receptor antagonists could improve cognitive function of rats, which may be helpful in the clinical treatment of cognitive dysfunction associated with psychiatric disorders (Laszy et al., 2005; Watson et al., 2012). Huang et al. found that cariprazine could increase dopamine, norepinephrine, and serotonin efflux in both rat nucleus accumbens (NAC) and ventral hippocampus (HIP) via the antagonism of D3 activity (Huang et al., 2019). Selective dopamine D3 receptor antagonists (SB-277011A and SB-414796A) could enhance the extracellular levels of acetylcholine (ACh) in the rat medial prefrontal cortex (mPFC), which may be beneficial in the treatment of cognitive dysfunction (Lacroix et al., 2006). NH300094 has potent dual dopamine D3 receptor and 5-HT1A receptor antagonism activity, which might account for its in vivo efficacy in improving cognitive function. Antagonism of 5-HT1A receptor has been shown to ameliorate cognitive impairment in AD and schizophrenia (Meltzer and Sumiyoshi, 2008; Ogren et al., 2008; Shimizu et al., 2013). It is suggested that 5-HT1A antagonists could improve cognitive function which mediated by postsynaptic 5-HT1A receptor; However, full 5-HT1A agonists impairs cognition by inhibiting the release of glutamate and acetylcholine in various regions of the brain (Jeltsch et al., 2004; Madjid et al., 2006). In addition, antagonism of dopamine D3 and 5-HT1A receptor could increase the efficacy but decrease the side effects of antipsychotics. Unlike the typical motor side effects caused by D2 antagonists, the low brain abundance and peculiar distribution of D3 receptors become valuable targets for the development of drug (Maramai et al., 2016). KKHA-761, a potent dopamine D3 receptor antagonist, has antipsychotic activity with low risk of EPS (Park et al., 2005). The distinctive functional profile of clozapine may be related to its partial agonist activity against 5-HT1A receptor (Millan., 2000). In our study, the unique profiles of dopamine D3 and 5-HT1A receptor antagonism of NH300094 might account for its cognitive improvement activity and better safety profiles.
NH300094 is a novel antipsychotic with antagonist activity against 5-HT2AR > D2LR > D3R > 5-HT1AR receptors, showing powerful efficacy in positive, negative, and cognitive impairment animal models. In vitro mechanism studies showed that NH300094 could antagonize both dopamine receptor and 5-HT receptors, but its relative antagonistic potency against 5-HT2A receptor was higher than that of dopamine receptors. Its strong agonistic activity against 5-HT2A receptor may contribute to higher efficacy, overcome the limitations of current antipsychotics, and a better safety profile. Preclinical animal results showed that NH300094 was effective in multiple models, which is more extensive than the clinic drug Risperidone. The better safety margin of NH300094 may translate into a better clinical safety profile. In conclusion, NH300094 is a novel potent serotonin and dopamine receptors modulator, possessing potential for the treatment of schizophrenia with cognition disorder.
Data availability statement
The original contributions presented in the study are included in the article/Supplementary Material, further inquiries can be directed to the corresponding authors.
Ethics statement
The animal study was approved by the Institutional Animal Care and Use Committee (IACUC) of Jiangsu Nhwa Pharmaceutical Co., Ltd. The study was conducted in accordance with the local legislation and institutional requirements.
Author contributions
ZF: Writing–original draft. ZH: Writing–original draft. LL: Writing–original draft. MY: Data curation, Writing–review and editing. YZ: Methodology, Writing–original draft. PJ: Data curation, Writing–review and editing. XqX: Conceptualization, Writing–review and editing. JW: Writing–review and editing. YH: Writing–review and editing. XyX: Conceptualization, Writing–review and editing.
Funding
The author(s) declare that no financial support was received for the research, authorship, and/or publication of this article.
Conflict of interest
Authors ZF, ZH, LL, and YZ were employed by Shanghai Shujing Biopharma Co., Ltd. Authors ZF, ZH, MY, PJ, XqX, and XyX were employed by Jiangsu Nhwa Pharmaceutical Co., Ltd. & Jiangsu Key Laboratory of Central Nervous System Drug Research and Development.
The remaining authors declare that the research was conducted in the absence of any commercial or financial relationships that could be construed as a potential conflict of interest.
Publisher’s note
All claims expressed in this article are solely those of the authors and do not necessarily represent those of their affiliated organizations, or those of the publisher, the editors and the reviewers. Any product that may be evaluated in this article, or claim that may be made by its manufacturer, is not guaranteed or endorsed by the publisher.
Supplementary material
The Supplementary Material for this article can be found online at: https://www.frontiersin.org/articles/10.3389/fphar.2024.1298061/full#supplementary-material
References
Adams, C. E., Bergman, H., Irving, C. B., and Lawrie, S. (2013). Haloperidol versus placebo for schizophrenia. Cochrane Database Syst. Rev., CD003082. doi:10.1002/14651858.CD003082.pub3
Amargos-Bosch, M., Bortolozzi, A., Puig, M. V., Serrats, J., Adell, A., Celada, P., et al. (2004). Co-expression and in vivo interaction of serotonin1A and serotonin2A receptors in pyramidal neurons of prefrontal cortex. Cereb. Cortex 14, 281–299. doi:10.1093/cercor/bhg128
Andree, B., Halldin, C., Vrijmoed-de Vries, M., and Farde, L. (1997). Central 5-HT2A and D2 dopamine receptor occupancy after sublingual administration of ORG 5222 in healthy men. Psychopharmacol. Berl. 131, 339–345. doi:10.1007/s002130050301
Behr, J., Gloveli, T., Schmitz, D., and Heinemann, U. (2000). Dopamine depresses excitatory synaptic transmission onto rat subicular neurons via presynaptic D1-like dopamine receptors. J. Neurophysiol. 84, 112–119. doi:10.1152/jn.2000.84.1.112
Bevins, R. A., and Besheer, J. (2006). Object recognition in rats and mice: a one-trial nonmatching-to-sample learning task to study recognition memory. Nat. Protoc. 1, 1306–1311. doi:10.1038/nprot.2006.205
Bhana, N., Foster, R. H., Olney, R., and Plosker, G. L. (2001). Olanzapine: an updated review of its use in the management of schizophrenia. Drugs 61, 111–161. doi:10.2165/00003495-200161010-00011
Burnet, P. W., Eastwood, S. L., and Harrison, P. J. (1996). 5-HT1A and 5-HT2A receptor mRNAs and binding site densities are differentially altered in schizophrenia. Neuropsychopharmacology 15, 442–455. doi:10.1016/S0893-133X(96)00053-X
Canal, C. E., and Morgan, D. (2012). Head-twitch response in rodents induced by the hallucinogen 2,5-dimethoxy-4-iodoamphetamine: a comprehensive history, a re-evaluation of mechanisms, and its utility as a model. Drug Test. Anal. 4, 556–576. doi:10.1002/dta.1333
Casey, A. B., and Canal, C. E. (2017). Classics in chemical neuroscience: aripiprazole. ACS Chem. Neurosci. 8, 1135–1146. doi:10.1021/acschemneuro.7b00087
Chakrabarti, S. (2021). Clozapine resistant schizophrenia: newer avenues of management. World J. Psychiatry 11, 429–448. doi:10.5498/wjp.v11.i8.429
Costall, B., Naylor, R. J., and Nohria, V. (1978). Climbing behaviour induced by apomorphine in mice: a potential model for the detection of neuroleptic activity. Eur. J. Pharmacol. 50, 39–50. doi:10.1016/0014-2999(78)90251-0
Davis, A. S., Jenner, P., and Marsden, C. D. (1986). A comparison of motor behaviours in groups of rats distinguished by their climbing response to apomorphine. Br. J. Pharmacol. 87, 129–137. doi:10.1111/j.1476-5381.1986.tb10164.x
DelArco, A., Segovia, G., and Mora, F. (2008). Blockade of NMDA receptors in the prefrontal cortex increases dopamine and acetylcholine release in the nucleus accumbens and motor activity. Psychopharmacol. Berl. 201, 325–338. doi:10.1007/s00213-008-1288-3
Fantegrossi, W. E., Simoneau, J., Cohen, M. S., Zimmerman, S. M., Henson, C. M., Rice, K. C., et al. (2010). Interaction of 5-ht2a and 5-HT2C receptors in R(−)-2,5-Dimethoxy-4-iodoamphetamine-Elicited head twitch behavior in mice. J. Pharmacol. Exp. Ther. 335, 728–734. doi:10.1124/jpet.110.172247
Farde, L., Wiesel, F. A., Halldin, C., and Sedvall, G. (1988). Central D2-dopamine receptor occupancy in schizophrenic patients treated with antipsychotic drugs. Arch. Gen. Psychiatry 45, 71–76. doi:10.1001/archpsyc.1988.01800250087012
Gao, J., Feng, M., Swalve, N., Davis, C., Sui, N., and Li, M. (2015). Effects of repeated quetiapine treatment on conditioned avoidance responding in rats. Eur. J. Pharmacol. 769, 154–161. doi:10.1016/j.ejphar.2015.11.011
Gardell, L. R., Vanover, K. E., Pounds, L., Johnson, R. W., Barido, R., Anderson, G. T., et al. (2007). ACP-103, a 5-hydroxytryptamine 2A receptor inverse agonist, improves the antipsychotic efficacy and side-effect profile of haloperidol and risperidone in experimental models. J. Pharmacol. Exp. Ther. 322, 862–870. doi:10.1124/jpet.107.121715
Gonzalez-Maeso, J., Yuen, T., Ebersole, B. J., Wurmbach, E., Lira, A., Zhou, M., et al. (2003). Transcriptome fingerprints distinguish hallucinogenic and nonhallucinogenic 5-hydroxytryptamine 2A receptor agonist effects in mouse somatosensory cortex. J. Neurosci. 23, 8836–8843. doi:10.1523/JNEUROSCI.23-26-08836.2003
Gross, G., Wicke, K., and Drescher, K. U. (2013). Dopamine D3 receptor antagonism--still a therapeutic option for the treatment of schizophrenia. Naunyn Schmiedeb. Arch. Pharmacol. 386, 155–166. doi:10.1007/s00210-012-0806-3
Gurevich, E. V., Bordelon, Y., Shapiro, R. M., Arnold, S. E., Gur, R. E., and Joyce, J. N. (1997). Mesolimbic dopamine D3 receptors and use of antipsychotics in patients with schizophrenia. A postmortem study. Arch. Gen. Psychiatry 54, 225–232. doi:10.1001/archpsyc.1997.01830150047009
Harvey, P. D., Bosia, M., Cavallaro, R., Howes, O. D., Kahn, R. S., Leucht, S., et al. (2022). Cognitive dysfunction in schizophrenia: an expert group paper on the current state of the art. Schizophr. Res. Cogn. 29, 100249. doi:10.1016/j.scog.2022.100249
Hashimoto, T., Nishino, N., Nakai, H., and Tanaka, C. (1991). Increase in serotonin 5-HT1A receptors in prefrontal and temporal cortices of brains from patients with chronic schizophrenia. Life Sci. 48, 355–363. doi:10.1016/0024-3205(91)90556-q
Horisawa, T., Ishibashi, T., Nishikawa, H., Enomoto, T., Toma, S., Ishiyama, T., et al. (2011). The effects of selective antagonists of serotonin 5-HT7 and 5-HT1A receptors on MK-801-induced impairment of learning and memory in the passive avoidance and Morris water maze tests in rats: mechanistic implications for the beneficial effects of the novel atypical antipsychotic lurasidone. Behav. Brain Res. 220, 83–90. doi:10.1016/j.bbr.2011.01.034
Huang, M., He, W., Kiss, B., Farkas, B., Adham, N., and Meltzer, H. Y. (2019). The role of dopamine D(3) receptor partial agonism in cariprazine-induced neurotransmitter efflux in rat Hippocampus and nucleus accumbens. J. Pharmacol. Exp. Ther. 371, 517–525. doi:10.1124/jpet.119.259879
Ichikawa, J., Ishii, H., Bonaccorso, S., Fowler, W. L., O'Laughlin, I. A., and Meltzer, H. Y. (2001). 5-HT(2A) and D(2) receptor blockade increases cortical DA release via 5-HT(1A) receptor activation: a possible mechanism of atypical antipsychotic-induced cortical dopamine release. J. Neurochem. 76, 1521–1531. doi:10.1046/j.1471-4159.2001.00154.x
Ishiyama, T., Tokuda, K., Ishibashi, T., Ito, A., Toma, S., and Ohno, Y. (2007). Lurasidone (SM-13496), a novel atypical antipsychotic drug, reverses MK-801-induced impairment of learning and memory in the rat passive-avoidance test. Eur. J. Pharmacol. 572, 160–170. doi:10.1016/j.ejphar.2007.06.058
Jeltsch, H., Bertrand, F., Galani, R., Lazarus, C., Schimchowitsch, S., and Cassel, J. C. (2004). Intraseptal injection of the 5-HT1A/5-HT7 agonist 8-OH-DPAT and working memory in rats. Psychopharmacol. Berl. 175, 37–46. doi:10.1007/s00213-004-1783-0
Jentsch, J. D., and Roth, R. H. (1999). The neuropsychopharmacology of phencyclidine: from NMDA receptor hypofunction to the dopamine hypothesis of schizophrenia. Neuropsychopharmacology 20, 201–225. doi:10.1016/S0893-133X(98)00060-8
Jing, P., Guo, Q., Dou, F., Wan, Z. H., and Hu, Z. J. (2021). Fused heterocyclic derivative and use thereof. PCT/CN2020/129850. The World Intellectual Property Organization (WIPO), 05–27.
Juza, R., Vojtechova, I., Stefkova-Mazochova, K., Dehaen, W., Petrasek, T., Prchal, L., et al. (2022). Novel D(2)/5-HT receptor modulators related to cariprazine with potential implication to schizophrenia treatment. Eur. J. Med. Chem. 232, 114193. doi:10.1016/j.ejmech.2022.114193
Kafka, S. H., and Corbett, R. (1996). Selective adenosine A2A receptor/dopamine D2 receptor interactions in animal models of schizophrenia. Eur. J. Pharmacol. 295, 147–154. doi:10.1016/0014-2999(95)00668-0
Kahn, R. S., Sommer, I. E., Murray, R. M., Meyer-Lindenberg, A., Weinberger, D. R., Cannon, T. D., et al. (2015). Schizophrenia. Nat. Rev. Dis. Prim. 1, 15067. doi:10.1038/nrdp.2015.67
Kane, J. M., Agid, O., Baldwin, M. L., Howes, O., Lindenmayer, J. P., Marder, S., et al. (2019). Clinical guidance on the identification and management of treatment-resistant schizophrenia. J. Clin. Psychiatry 80, 18com12123. doi:10.4088/JCP.18com12123
Kantrowitz, J. T., Correll, C. U., Jain, R., and Cutler, A. J. (2023). New developments in the treatment of schizophrenia: an expert roundtable. Int. J. Neuropsychopharmacol. 26, 322–330. doi:10.1093/ijnp/pyad011
Kiss, B., Laszlovszky, I., Kramos, B., Visegrady, A., Bobok, A., Levay, G., et al. (2021). Neuronal dopamine D3 receptors: translational implications for preclinical research and CNS disorders. Biomolecules 11, 104. doi:10.3390/biom11010104
Kruse, A. O., and Bustillo, J. R. (2022). Glutamatergic dysfunction in schizophrenia. Transl. Psychiatry 12, 500. doi:10.1038/s41398-022-02253-w
Kuschinsky, K., and Hornykiewicz, O. (1972). Morphine catalepsy in the rat: relation to striatal dopamine metabolism. Eur. J. Pharmacol. 19, 119–122. doi:10.1016/0014-2999(72)90086-6
Kusumi, I., Boku, S., and Takahashi, Y. (2015). Psychopharmacology of atypical antipsychotic drugs: from the receptor binding profile to neuroprotection and neurogenesis. Psychiatry Clin. Neurosci. 69, 243–258. doi:10.1111/pcn.12242
Lacroix, L. P., Ceolin, L., Zocchi, A., Varnier, G., Garzotti, M., Curcuruto, O., et al. (2006). Selective dopamine D3 receptor antagonists enhance cortical acetylcholine levels measured with high-performance liquid chromatography/tandem mass spectrometry without anti-cholinesterases. J. Neurosci. Methods 157, 25–31. doi:10.1016/j.jneumeth.2006.03.017
Laszy, J., Laszlovszky, I., and Gyertyan, I. (2005). Dopamine D3 receptor antagonists improve the learning performance in memory-impaired rats. Psychopharmacol. Berl. 179, 567–575. doi:10.1007/s00213-004-2096-z
Lin, C. H., Kuo, C. C., Chou, L. S., Chen, Y. H., Chen, C. C., Huang, K. H., et al. (2010). A randomized, double-blind comparison of risperidone versus low-dose risperidone plus low-dose haloperidol in treating schizophrenia. J. Clin. Psychopharmacol. 30, 518–525. doi:10.1097/JCP.0b013e3181f28dff
Lohrs, L., and Hasan, A. (2019). Risk factors for the development of schizophrenia. Fortschr Neurol. Psychiatr. 87, 133–143. doi:10.1055/a-0836-7839
Lopez-Gil, X., Babot, Z., Amargos-Bosch, M., Sunol, C., Artigas, F., and Adell, A. (2007). Clozapine and haloperidol differently suppress the MK-801-increased glutamatergic and serotonergic transmission in the medial prefrontal cortex of the rat. Neuropsychopharmacology 32, 2087–2097. doi:10.1038/sj.npp.1301356
Luttgen, M., Elvander, E., Madjid, N., and Ogren, S. O. (2005). Analysis of the role of 5-HT1A receptors in spatial and aversive learning in the rat. Neuropharmacology 48, 830–852. doi:10.1016/j.neuropharm.2005.01.007
Madjid, N., Tottie, E. E., Luttgen, M., Meister, B., Sandin, J., Kuzmin, A., et al. (2006). 5-Hydroxytryptamine 1A receptor blockade facilitates aversive learning in mice: interactions with cholinergic and glutamatergic mechanisms. J. Pharmacol. Exp. Ther. 316, 581–591. doi:10.1124/jpet.105.092262
Malik, J. A., Yaseen, Z., Thotapalli, L., Ahmed, S., Shaikh, M. F., and Anwar, S. (2023). Understanding translational research in schizophrenia: a novel insight into animal models. Mol. Biol. Rep. 50, 3767–3785. doi:10.1007/s11033-023-08241-7
Maramai, S., Gemma, S., Brogi, S., Campiani, G., Butini, S., Stark, H., et al. (2016). Dopamine D3 receptor antagonists as potential therapeutics for the treatment of neurological diseases. Front. Neurosci. 10, 451. doi:10.3389/fnins.2016.00451
Marcus, M. M., Mathe, J. M., Nomikos, G. G., and Svensson, T. H. (2001). Effects of competitive and non-competitive NMDA receptor antagonists on dopamine output in the shell and core subdivisions of the nucleus accumbens. Neuropharmacology 40, 482–490. doi:10.1016/s0028-3908(00)00199-4
Marucci, G., Buccioni, M., Ben, D. D., Lambertucci, C., Volpini, R., and Amenta, F. (2021). Efficacy of acetylcholinesterase inhibitors in Alzheimer's disease. Neuropharmacology 190, 108352. doi:10.1016/j.neuropharm.2020.108352
McCutcheon, R. A., Abi-Dargham, A., and Howes, O. D. (2019). Schizophrenia, dopamine and the striatum: from biology to symptoms. Trends Neurosci. 42, 205–220. doi:10.1016/j.tins.2018.12.004
Meltzer, H. Y., Massey, B. W., and Horiguchi, M. (2012). Serotonin receptors as targets for drugs useful to treat psychosis and cognitive impairment in schizophrenia. Curr. Pharm. Biotechnol. 13, 1572–1586. doi:10.2174/138920112800784880
Meltzer, H. Y., Matsubara, S., and Lee, J. C. (1989). The ratios of serotonin2 and dopamine2 affinities differentiate atypical and typical antipsychotic drugs. Psychopharmacol. Bull. 25, 390–392.
Meltzer, H. Y., Share, D. B., Jayathilake, K., Salomon, R. M., and Lee, M. A. (2020). Lurasidone improves psychopathology and cognition in treatment-resistant schizophrenia. J. Clin. Psychopharmacol. 40, 240–249. doi:10.1097/JCP.0000000000001205
Meltzer, H. Y., and Sumiyoshi, T. (2008). Does stimulation of 5-HT(1A) receptors improve cognition in schizophrenia? Behav. Brain Res. 195, 98–102. doi:10.1016/j.bbr.2008.05.016
Meneses, A. (2007). Do serotonin(1-7) receptors modulate short and long-term memory? Neurobiol. Learn Mem. 87, 561–572. doi:10.1016/j.nlm.2006.12.005
Mijovic, A., and MacCabe, J. H. (2020). Clozapine-induced agranulocytosis. Ann. Hematol. 99, 2477–2482. doi:10.1007/s00277-020-04215-y
Millan, M. J. (2000). Improving the treatment of schizophrenia: focus on serotonin (5-HT)(1A) receptors. J. Pharmacol. Exp. Ther. 295, 853–861.
Muller-Spahn, F. (2002). Current use of atypical antipsychotics. Eur. Psychiatry 17 (Suppl. 4), 377s–384s. doi:10.1016/s0924-9338(03)00077-4
Ninan, I., and Kulkarni, S. K. (1999). Preferential inhibition of dizocilpine-induced hyperlocomotion by olanzapine. Eur. J. Pharmacol. 368, 1–7. doi:10.1016/s0014-2999(98)00982-0
Ogren, S. O., Eriksson, T. M., Elvander-Tottie, E., D'Addario, C., Ekstrom, J. C., Svenningsson, P., et al. (2008). The role of 5-HT(1A) receptors in learning and memory. Behav. Brain Res. 195, 54–77. doi:10.1016/j.bbr.2008.02.023
Ohno, Y. (2011). Therapeutic role of 5-HT1A receptors in the treatment of schizophrenia and Parkinson's disease. CNS Neurosci. Ther. 17, 58–65. doi:10.1111/j.1755-5949.2010.00211.x
Olsen, C. K., Brennum, L. T., and Kreilgaard, M. (2008). Using pharmacokinetic-pharmacodynamic modelling as a tool for prediction of therapeutic effective plasma levels of antipsychotics. Eur. J. Pharmacol. 584, 318–327. doi:10.1016/j.ejphar.2008.02.005
Owen, M. J., Sawa, A., and Mortensen, P. B. (2016). Schizophrenia. Lancet 388, 86–97. doi:10.1016/S0140-6736(15)01121-6
Park, W. K., Jeong, D., Cho, H., Lee, S. J., Cha, M. Y., Pae, A. N., et al. (2005). KKHA-761, a potent D3 receptor antagonist with high 5-HT1A receptor affinity, exhibits antipsychotic properties in animal models of schizophrenia. Pharmacol. Biochem. Behav. 82, 361–372. doi:10.1016/j.pbb.2005.09.006
Paul, S. M., Yohn, S. E., Popiolek, M., Miller, A. C., and Felder, C. C. (2022). Muscarinic acetylcholine receptor agonists as novel treatments for schizophrenia. Am. J. Psychiatry 179, 611–627. doi:10.1176/appi.ajp.21101083
Porsolt, R. D., Anton, G., Blavet, N., and Jalfre, M. (1978). Behavioural despair in rats: a new model sensitive to antidepressant treatments. Eur. J. Pharmacol. 47, 379–391. doi:10.1016/0014-2999(78)90118-8
Poyurovsky, M., Koren, D., Gonopolsky, I., Schneidman, M., Fuchs, C., Weizman, A., et al. (2003). Effect of the 5-HT2 antagonist mianserin on cognitive dysfunction in chronic schizophrenia patients: an add-on, double-blind placebo-controlled study. Eur. Neuropsychopharmacol. 13, 123–128. doi:10.1016/s0924-977x(02)00155-4
Rao, N., Northoff, G., Tagore, A., Rusjan, P., Kenk, M., Wilson, A., et al. (2019). Impaired prefrontal cortical dopamine release in schizophrenia during a cognitive task: a [11C]flb 457 positron emission tomography study. Schizophr. Bull. 45, 670–679. doi:10.1093/schbul/sby076
Samalin, L., BenGharbia, M., Garnier, M., and Llorca, P. M. (2014). Short-term efficacy and safety of lurasidone in the treatment of schizophrenia. Encephale 40, 507–517. doi:10.1016/j.encep.2014.10.009
Santana, N., Bortolozzi, A., Serrats, J., Mengod, G., and Artigas, F. (2004). Expression of serotonin1A and serotonin2A receptors in pyramidal and GABAergic neurons of the rat prefrontal cortex. Cereb. Cortex 14, 1100–1109. doi:10.1093/cercor/bhh070
Schneider-Thoma, J., Chalkou, K., Dorries, C., Bighelli, I., Ceraso, A., Huhn, M., et al. (2022). Comparative efficacy and tolerability of 32 oral and long-acting injectable antipsychotics for the maintenance treatment of adults with schizophrenia: a systematic review and network meta-analysis. Lancet 399, 824–836. doi:10.1016/S0140-6736(21)01997-8
Schultz, S. H., North, S. W., and Shields, C. G. (2007). Schizophrenia: a review. Am. Fam. Physician 75, 1821–1829.
Schwartz, J. C., Diaz, J., Pilon, C., and Sokoloff, P. (2000). Possible implications of the dopamine D(3) receptor in schizophrenia and in antipsychotic drug actions. Brain Res. Brain Res. Rev. 31, 277–287. doi:10.1016/s0165-0173(99)00043-0
Shimizu, S., Mizuguchi, Y., and Ohno, Y. (2013). Improving the treatment of schizophrenia: role of 5-HT receptors in modulating cognitive and extrapyramidal motor functions. CNS Neurol. Disord. Drug Targets 12, 861–869. doi:10.2174/18715273113129990088
Stelmach, A., Guzek, K., Roznowska, A., Najbar, I., and Sadakierska-Chudy, A. (2023). Antipsychotic drug-aripiprazole against schizophrenia, its therapeutic and metabolic effects associated with gene polymorphisms. Pharmacol. Rep. 75, 19–31. doi:10.1007/s43440-022-00440-6
Veleva, I., Stoychev, K., Stoimenova-Popova, M., and Mineva-Dimitrova, E. (2023). Impact of cognitive disturbances and clinical symptoms on disability in patients with paranoid schizophrenia: a study of a Bulgarian clinical sample. Int. J. Environ. Res. Public Health 20, 2459. doi:10.3390/ijerph20032459
Wadenberg, M. L., Kapur, S., Soliman, A., Jones, C., and Vaccarino, F. (2000). Dopamine D2 receptor occupancy predicts catalepsy and the suppression of conditioned avoidance response behavior in rats. Psychopharmacol. Berl. 150, 422–429. doi:10.1007/s002130000466
Wadenberg, M. L., Soliman, A., VanderSpek, S. C., and Kapur, S. (2001). Dopamine D(2) receptor occupancy is a common mechanism underlying animal models of antipsychotics and their clinical effects. Neuropsychopharmacology 25, 633–641. doi:10.1016/S0893-133X(01)00261-5
Watson, D. J., Loiseau, F., Ingallinesi, M., Millan, M. J., Marsden, C. A., and Fone, K. C. (2012). Selective blockade of dopamine D3 receptors enhances while D2 receptor antagonism impairs social novelty discrimination and novel object recognition in rats: a key role for the prefrontal cortex. Neuropsychopharmacology 37, 770–786. doi:10.1038/npp.2011.254
Xu, J., Li, Y., Tian, B., Liu, H., Wu, S., and Wang, W. (2022). The effects and mechanism of environmental enrichment on MK-801 induced cognitive impairment in rodents with schizophrenia. Front. Cell Neurosci. 16, 1024649. doi:10.3389/fncel.2022.1024649
Xu, X. Q., Wei, Y. W., Guo, Q., Zhao, S., Liu, Z. Q., Xiao, T., et al. (2018). Pharmacological characterization of H05, a novel serotonin and noradrenaline reuptake inhibitor with moderate 5-HT2A antagonist activity for the treatment of depressions. J. Pharmacol. Exp. Ther. 365, 624–635. doi:10.1124/jpet.118.248351
Yamada, R., Wada, A., Stickley, A., Yokoi, Y., and Sumiyoshi, T. (2023). Effect of 5-HT1A receptor partial agonists of the azapirone class as an add-on therapy on psychopathology and cognition in schizophrenia: a systematic review and meta-analysis. Int. J. Neuropsychopharmacol. 26, 249–258. doi:10.1093/ijnp/pyad004
Keywords: schizophrenia, antipsychotic, dopamine and serotonin receptors, cognitive improvement, risperidone
Citation: Feng Z, Hu Z, Li L, Yu M, Zhang Y, Jing P, Xu X, Wu J, Hu Y and Xu X (2024) Assessing NH300094, a novel dopamine and serotonin receptor modulator with cognitive enhancement property for treating schizophrenia. Front. Pharmacol. 15:1298061. doi: 10.3389/fphar.2024.1298061
Received: 21 September 2023; Accepted: 03 January 2024;
Published: 24 January 2024.
Edited by:
Rui Liu, Chinese Academy of Medical Sciences and Peking Union Medical College, ChinaReviewed by:
Patricio Ernesto Iturriaga-Vasquez, University of La Frontera, ChileMagdalena Kotańska, Jagiellonian University Medical College, Poland
Copyright © 2024 Feng, Hu, Li, Yu, Zhang, Jing, Xu, Wu, Hu and Xu. This is an open-access article distributed under the terms of the Creative Commons Attribution License (CC BY). The use, distribution or reproduction in other forums is permitted, provided the original author(s) and the copyright owner(s) are credited and that the original publication in this journal is cited, in accordance with accepted academic practice. No use, distribution or reproduction is permitted which does not comply with these terms.
*Correspondence: Xiangyang Xu, xuxiangyang@nhwa-group.com; Yiqiao Hu, huyiqiao@nju.edu.cn; Jinhui Wu, wuj@nju.edu.cn
†These authors have contributed equally to this work