- 1Department of Pharmaceutical Sciences, Collegium Medicum, Jan Kochanowski University, Kielce, Poland
- 2Department of Cosmetology, University of Information Technology and Management in Rzeszów, Rzeszów, Poland
- 3Chair and Department of Pharmaceutical Botany, Faculty of Pharmacy, Medical College, Jagiellonian University, Cracow, Poland
- 4Department of Pathobiochemistry and Interdisciplinary Applications of Ion Chromatography, Biomedical Sciences, Medical University of Lublin, Lublin, Poland
- 5Department of Pharmacognosy with Medicinal Plants Garden, Medical University of Lublin, Lublin, Poland
- 6Department of Organic Chemistry and Technology, Faculty of Chemical Engineering and Technology, Cracow University of Technology, Cracow, Poland
Moringa oleifera Lam. (Moringaceae) is a species of tree with an increasing utility, occurring naturally mainly in Pakistan and northern India. M. oleifera is currently cultivated in Africa, South America, Asia and the Middle East. The usage of its leaves, seed oil, bark, fruits, flowers and roots has positive opinions of FDA (American Food and Drug Administration), EFSA (European Food Safety Authority) and CosIng (Cosmetic Ingredients database). The chemical composition of M. oleifera is dominated by: proteins (consisting mainly of amino acids such as arginine or serine), fatty acids (omega-3 and omega-6), vitamins (vitamin A, B and C and tocopherols), mineral salts (including several bioelements, such as calcium, magnesium, sodium, and potassium), valuable polyphenolic compounds from the group of phenolic acids (e.g., gallic acid, ferulic acid) and flavonoids (e.g., myricetin, rutoside, and kaempferol). The raw materials show antioxidant, hepatoprotective, anti-inflammatory and antimicrobial properties. Dietary supplements and alimentary products containing M. oleifera are recommended as health-promoting and “novel food” preparations. The main purpose of this work was a review of the latest scientific literature on M. oleifera, with particular emphasis on the studies focusing on its chemical composition, biological activity and safety. Moreover, the review tends to discuss the results of biotechnological studies using this material and the agronomical significance.
1 Introduction
Moringa oleifera Lam. (drumstick tree) is a tropical tree from the family Moringaceae. M. oleifera occurs naturally mainly in Pakistan and northwest India. Currently, this species is widely cultivated, primarily in India and several countries in Africa, South America, Asia and the Middle East (Nelson and Rau, 2011). Thanks to its health-promoting and nutritional properties, M. oleifera is called a “miracle tree”, “the tree of long life” or “the horseradish tree” because its roots are bearing a similar taste to horseradish. Due to the content of valuable bioactive compounds, M. oleifera combines nutritional and pharmaceutical values. Nutraceuticals are used to supplement the diet with substances of natural origin, which are present in food in limited amounts, and show properties conducive to maintaining health and maintaining the proper functioning of the body (Himanshu et al., 2018).
The leaves of M. oleifera (Moringae folium) are the main raw material used in the food and cosmetics industries. The drumstick tree belongs to the group of highly useful species that easy to grow and occur naturally in developing countries. This is a species which has been grown in many countries especially in Asia and Africa for centuries, and widely used by local communities for many purposes including as a food and local medication. The growing demand for this species has contributed to its intensive exploitation. Currently, not only the leaves are used as a raw material, but also its fruit, flower, bark, seed and root (Vergara-Jimenez et al., 2017).
M. oleifera provides products of high nutritional value, which is why the species is also referred to as “super foods”, “novel foods” and/or “healthy foods” (Joshi and Mehta, 2010). The species of interest contains, among others: proteins (amino acids), fatty acids (omega-3 and omega-6), vitamins (tocopherols), mineral salts (calcium, magnesium), phenolic acids (like gallic acid, ferulic acid) and flavonoids (myricetin) (Bennett et al., 2003; Anwar and Rashid, 2007; Sultana and Anwar, 2008; Sreelatha and Padma, 2009; Joshi and Mehta, 2010; Zhang, 2011; El-Massary et al., 2013; Ijarotimi et al., 2013; Wu et al., 2020). The latest scientific research confirms the health-promoting properties of M. oleifera known from the traditional medicine of India, China and Africa. Among the proved pharmacological properties of the tree its antiviral (Florence, 2014), antiinflammatory (Ezeamuzie et al., 1996; Maurya and Singh, 2014), hepatoprotective (Toppo et al., 2015; Sun et al., 2019) and antidepressant (Kaur et al., 2015) properties are confirmed.
M. oleifera is listed in the Ayurvedic pharmacopoeia of India only (Government of India Ministry of Ayush, 2016), as it does not have any WHO (World Health Organization) (WHO, 2023) nor EMA—HMPC (European Medicines Agency Committee on Herbal Medicinal Products) (EMA, 2023) monograph. This species, as a food plant, is mentioned in the documents of the FDA (American Food and Drug Administration) (FDA, 2023) and EFSA (European Food Safety Authority) (EFSA, 2023). In the food industry M. oleifera is used in the production of cakes, cereal gruels, bread, biscuits, dairy products, soups, salads and teas (Stevens et al., 2013; Hekmat et al., 2015; Karim et al., 2015). It is used not only in cuisine, food industry and dietetics, but also due to its valuable properties, is of high importance to herbal medicines and cosmetology. The tree can also be used to produce biofuels, whereas the plant’s residues are applied as an ecological fertilizer (in line with the “zero waste” trend) (Joshi and Mehta, 2010; Ali et al., 2013; El-Massary et al., 2013).
The review summarizes the information on the botanical, ecological and chemical characteristics of M. oleifera. The tradition of use and the current place of the species in agronomy, medicine and cosmetology are presented. Also, scientific research on its biological activities was reviewed and the safety of M. oleifera was discussed. In addition, biotechnological studies conducted using in vitro cultures of the drumstick tree are also described.
2 Botanical and ecological characteristics
M. oleifera is a tree with low environmental requirements, characterized by a rapid growth (Vergara-Jimenez et al., 2017). The tree reaches an average height of about 5–10 m. Some sources mention its height as of 1–12 m. Moringa leaves are composed of an odd number of leaflets 15–35 cm long. The leaflets are hairy, obovate, 4–24 mm in size. The flowers of M. oleifera are fragrant, 1–3.3 cm in diameter. They have white petals, 1–1.4 cm in size, and larger petals, which are also white or often colored red, and greenish at the base, measuring 1.2–3.3 cm. The inflorescence is a wide, spreading panicle. The fruit is a pod with 3 ribbed lobes. The pods are 18–32 cm long and 0.9–2.2 cm wide and contain about 26 seeds. The seeds are spherical, three-winged, 6–7 cm in size and about 1 cm in diameter. One tree produces between 3,000 and 9,000 seeds per season, and some sources give numbers as high as 15,000 to 25,000 (Vergara-Jimenez et al., 2017).
The Moringa species is drought tolerant, which makes it successful in growing in subtropical and tropical countries and in dry to humid tropical or subtropical climates at altitudes from 0 to 1,400 m above sea level (or up to 2,000 m above sea level depending on the source). The annual irrigation requirement for M. oleifera is low and varies between 760–2,500 mm of precipitation. The temperature suitable for the development of M. oleifera is 18°C–28°C (Nouman et al., 2014).
The natural (primary) sites of origin of M. oleifera are located in South Asia. It grows at the foot of the Himalayas in the area from north-eastern Pakistan to the northern state of West Bengal in India and north-eastern Bangladesh. M. oleifera is common in countries such as Nepal, Afghanistan, Sri Lanka, Bangladesh, India, Cambodia, Philippines, Hawaii (Nouman et al., 2014). M. oleifera has many names in different languages (Ramachandran et al., 1980; Fuglie, 1999; Parrotta, 2009; Paikra et al., 2017; Sujatha and Patel, 2017; World Flora Online, 2022; Trees for Life, 2023). A summary of M. oleifera nomenclature is provided in Supplementary Materials. M. oleifera is currently intensively cultivated on almost all continents in the equatorial area of Asia, Central America, South America, from Mexico to Peru, in Paraguay, Brazil and South Florida. The production of M. oleifera is of particular importance in tropical developing countries as it is an efficient source of food for both humans and animals (Mouchili et al., 2019).
3 Crops and cultivars
The traditional cultivation of M. oleifera, by seed, takes place in Pakistan, northwest India and Sudan. Vegetative propagation is used in Indonesia and West Africa and finally commercial cultivation of M. oleifera is conducted primarily in the other regions of India and Africa as well as Asia, South America and the Middle East (Nouman et al., 2014).
M. oleifera seeds germinate within 2 weeks of sowing (up to 2 cm). In nurseries, they are repotted 3–6 weeks after germination, when they reach a height of about 30 cm. Depending on the M. oleifera ecotype, the number of germinating seeds per kilogram is from 3 to 9 thousand. Ideal conditions for seed storage are around 3°C with a humidity of 5%–8% (Mouchili et al., 2019).
Cutting is another method used to propagate M. oleifera, by planting hard branches from adult trees (4–16 cm in diameter, and 1–2 m long) during the rainy season. After burying a third of the branches in the soil, they easily develop roots which grow to a considerable size within a few months and the plant develops (Nouman et al., 2014). In the literature, there are differences in the benefits of seedlings and seeds. According to Ramachandran et al. (Ramachandran et al., 1980) plants grown from seeds produce lower quality fruit, while Animashaun et al. (Animashaun et al., 2016) state that growing from seed brings benefits for plant stability and access to water by developing longer roots than the trees grown from the cuttings. M. oleifera is classified as a very fast growing tree. During the first 3–4 years, its annual growth is 1–2 m per year, and after a few years it reaches 12 m. Topping or pruning is standard practice to support side branches and to shape for easy harvesting (Nouman et al., 2014).
There are several types of M. oleifera leaf production. The most intensive production at which fertilization and irrigation is necessary is a dense spacing of 10 by 10 cm–20 by 20 cm, then the leaf harvest period is reached after 35–45 days. The second type is planting at a spacing of about 50 by 100 cm, this is referred to as semi-intensive production. In this type, only irrigation and fertilizer use is suggested, and the period between harvests is between 50 and 60 days. The last type is the largest spaced plant spacing in the agro-forestry system. Irrigation and fertilization of this type of crop is not necessary. The distances between the rows are quite large, between 2 and 4 m, and harvesting is carried out approximately every 60 days. There is a variability in yield and harvest frequency between the described types of M. oleifera crops (Oliveira et al., 1999), the time of year and the rainy season play a major role in both. More research is needed to assess the optimal spacing and harvest time of M. oleifera (Nouman et al., 2014).
M. oleifera leaves can be harvested mechanically and manually. They consist in cutting shoots at a height of 0.5–1 m above the ground. You can also pluck the leaves directly from the tree, this is a less effective technique as it leads to slower regrowth (Ahmed et al., 2016).
The tree bears fruit in the first year after its planting. Between 6 months and 3 years after planting, M. oleifera gives a small yield of fruit. After 3 years of planting, the tree can produce from 600 to 1,600 fruits per year. Depending on the cultivar, the plants show differences, and the pod production time varies from 6 months to over a year. Within 6 months new pods develop from pruning the branches (Ahmed et al., 2016).
Seed production is based on the collection of fruit (pods). Each pod contains approximately 26 seeds. After about 3 months become dry and should be harvested as soon as possible. One tree produces about 3,000 to 9,000 seeds (some sources even say 15,000 to 25,000) (Ahmed et al., 2016).
Different ecotypes of M. oleifera are cultivated in India, producing fruit with different characteristics; Chemmurungai (with distinctive red-tipped pods), Jaffna and Chavakacheri (with soft fruits), Palmurungai and Punmurungai (with bitter-tasting fruits), Kadumurungai (with small fruits), Kodikalmurungai (with short fruits). Ecotypes Puna Murungai, Palmurungai, Kodikkal Murungai are characterized by a high yield of pods. Unfortunately, despite the high variability in breeding databases, there is a lack of precise information on the genetic variability of the plant (Mouchili et al., 2019).
Low soil requirements and resistance to drought are of particular importance in the cultivation of M. oleifera. The plant can be grown in diverse soils, except those that are waterlogged. Slightly alkaline clay and sandy loam soils are considered the best media for this species due to their good drainage. For clay soils and high altitudes, it is necessary to use chemical (urea and nitrates) and organic (cattle, poultry, rabbit manure) fertilizers. Such intensification of production takes place, for example, in Western Cameroon (Mouchili et al., 2019).
4 Industrial scale production
Currently, the main producer of M. oleifera is India where the fruit is produced at the quantity of 1.1–1.3 million tons per year. The cultivation area of M. oleifera in India covers about 380 km2. The interest in plant breeding is related to its usefulness, ease, speed of growth and fertility, which is important in the countries most exposed to malnutrition (Gandji et al., 2018). According to the Trade Promotion Council of India (TPC) reports (Trade Promotion Council of India, 2021) M. oleifera is becoming more and more popular. The main countries importing leaves and seeds of M. oleifera are: United States of America, China, Canada, South Korea, Germany and other European countries. In 2018, the value of the global market for M. oleifera products was amounted for 5.5 billion USD, and by 2025 it is planned to exceed 10 billion USD. India is the dominant force in the M. oleifera export market, sharing about 80% of the total production volume in the world (Mouchili et al., 2019). The Indian states of Andhra Pradesh, Tamil Nadu and Odisha are of particular importance, as their export growth rate increases by about 26%–28% annually (Mouchili et al., 2019; Trade Promotion Council of India, 2021).
Due to its high nutrient content, M. oleifera is described in many sources as an alternative food in developing countries and is therefore widely cultivated there. Nigeria and Cameroon, both of which are considered developing countries, produce significant amounts of raw materials from M. oleifera. Trade of M. oleifera in Cameroon is twice as profitable as of other leafy vegetables (Gandji et al., 2018). In Nigeria, organic fertilizers derived from M. oleifera seeds are used. In addition, M. oleifera serves as a vegetable eaten by local people and animals (Ojo et al., 2016).
M. oleifera is also grown in Florida, Cuba, Jamaica and commercially in Hawaii and the volcanic island of O’ahu, the fruit production in these areas can be year-round. Fruit varieties of M. oleifera such as Chavakacheri, Jaffana and Chemmuungai are particularly popular there (Lynch, 2014).
5 Phytochemical characteristic
M. oleifera is a species rich in valuable phytocompounds. The most important groups of active compounds in the composition of M. oleifera are: carotenoids, tocopherols, flavonoids, phenolic acids, folates, polyunsaturated fatty acids, mineral salts, vitamins (Table 1). Dried leaves are the richest sources of polyphenols in the plant. The polyphenolic content depends however on the environmental conditions, harvesting season, the genetics of the plant, the stage of leaves maturity at the time of harvesting and the applied methods of drying and extraction. The main polyphenols of M. oleifera are flavonoids, phenolic acids and catechins (Table 1) (Bennett et al., 2003; Richter et al., 2003; Yang et al., 2008; Moyo et al., 2011).
Flavonoids are present mainly in the leaves of M. oleifera. Their estimated values depend on the process to which the leaves were subjected. The main flavonoids found in M. oleifera leaves are myricetin, rutin, apigenin, quercetin and kaempferol (Figure 1; Table 2) (Bennett et al., 2003; Richter et al., 2003; Yang et al., 2008; Moyo et al., 2011).
Among the leading phenolic acids that are spread mainly in the dried leaves, pods and seeds of M. oleifera; gallic acid, ellagic acid, ferulic acid, o-coumaric acid, sinapic acid, chlorogenic acid, caffeic acid and syringic acid are listed by the scientists (Figure 2; Table 3) (Yang et al., 2008; Moyo et al., 2011).
Catechins, namely, epicatechin, catechin and pyrocatechin are mainly found in the leaves of M. oleifera (Yang et al., 2008; Moyo et al., 2011; Florence, 2014; El-Harairy et al., 2016). Nevertheless, catechin was also detected in its pods and seeds (Table 4) (Yang et al., 2008).
The presence of pyrogallol has been demonstrated in ethanol extracts from the leaves (0.284 mg/100 g) (El-Harairy et al., 2016).
Also, the presence of glucosinolates and their breakdown products, like isothiocyanates, was identified in the roots, seeds and young leaves of M. oleifera (Table 5). Large amounts of glucosinolates were found especially in the extracts from vegetatively young leaves. The dominant glucosinolane of M. oleifera leaves is 4-O-(α-L-rhamnopyranosyloxy)-benzyl glucosinolate, also known as glucomoringin (Figure 3). Moreover, high concentrations of 4-(α-1-rhamnopyranosyloxy)-benzylglucosinolate has been isolated from the seeds, whereas high content of benzylglucosinolate and 4-(α-1-rhamnopyranosyloxy)-benzylglucosinolate was reported in the roots. The concentration of glucosinolanes and isothiocyanins in the leaves of M. oleifera is influenced by the maturity and physiological state of the plant (Bennett et al., 2003; Ahmed et al., 2016). Among the isothiocyanates present in the leaves, there is 4-[4′O-acetylalpha-L-rhamnosyloxy) benzyl] isothiocyanate, while in seeds 4 (α-L-rhamnosyloxy) isothiocyanate was identified (Figure 4; Table 5) (Eilert et al., 1981; Bennett et al., 2003).
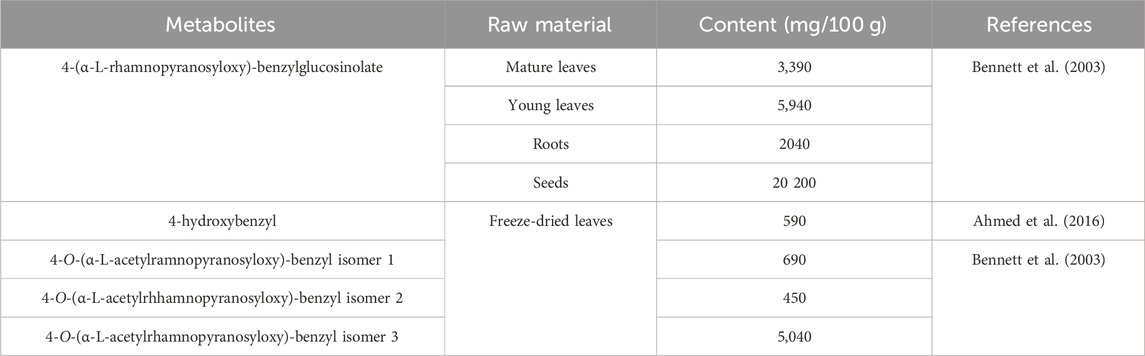
TABLE 5. The content of glucosinolates and isothiocyanins in different morphological organs of M. oleifera plant.
The presence of alkaloids has been demonstrated in the leaves of M. oleifera (Figure 5) such as N,α-L-rhamnopyranosyl vincosidamide, pyrrolemarumin 4″-O-α-L-rhamnopyranoside, 4-(α-L-rhamnopyranosyloxy) phenylacetonitrile (niazirin). 4′-Hydroxyphenylethanamide-α-L-rhamnopyranoside (marumoside A) and its 3-O-α-D-glucopyranosyl derivative (marmumoside B) and methyl 4-(α-L-rhamnopyranosyloxy)-benzylcarbamate (Ahmed et al., 2016).
Leaves, flowers, pods and seeds of drumstick tree are sources of vitamins. The former showed the presence of vitamins A, B1, B2, B3, C and E (Joshi and Mehta, 2010; Moyo et al., 2011; Zhang, 2011; Saini et al., 2014; Ahmed et al., 2016), the flowers and pods contain vitamin C, and the seeds contain tocopherols (α-tocopherols, γ-tocopherols, δ-tocopherols) (Ijarotimi et al., 2013; Vergara-Jimenez et al., 2017). In addition, the leaves contain carotenoids such β-carotene, 13-cis-lutein, 15-cis-β-carotene, trans-lutein, trans-luteoxin i trans-zeaxanthin (Figure 6; Table 6) (Joshi and Mehta, 2010; Moyo et al., 2011; Zhang, 2011; Saini et al., 2014).
Studies have also shown the presence of minerals in the leaves, roots, bark, pods and seeds. The leaves and pods contain zinc, phosphorus, magnesium, manganese, copper, potassium, selenium, sulfur, sodium, calcium and iron. Magnesium, potassium, calcium and iron were found in the root and bark, while zinc, phosphorus, magnesium, potassium, calcium and iron were found in the seeds (Table 7) (Sreelatha and Padma, 2009).
The leaves of drumstick tree contain arachidic acid, α-linolenic acid, γ-linolenic acid, linolenic acid, myristic acid, oleic acid and palmitic acid (Dai et al., 2020). The seeds were found to contain also other fatty acids, like eicosenoic acid, erucic acid and palmitic acid (Ijarotimi et al., 2013). In addition, the seeds of M. oleifera also contain sterols, e.g., campesterol,
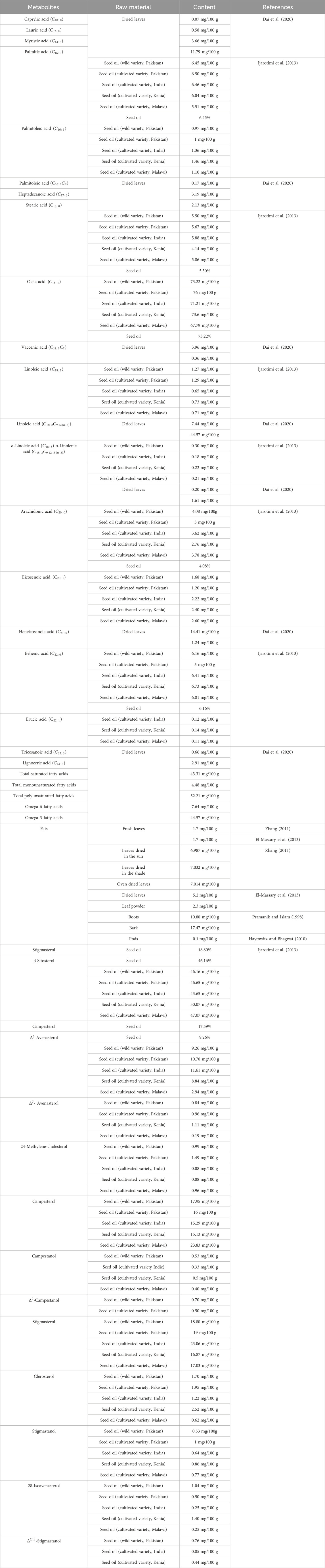
TABLE 8. The content of sterols/oils/fatty acids in different morphological organs of M. oleifera plant.
M. oleifera seeds are rich sources of high quality protein that contains essential amino acids. The protein content of M. oleifera seeds is about 52% (Tang et al., 2021). Similarly, essential amino acids are also found in the leaves and pods of the plant (Yang et al., 2008; Moyo et al., 2011; Dai et al., 2020). They are recommended as a valuable supplement of the diet. The essential amino acids identified in M. oleifera include arginine (Arg), phenylalanine (Phe), histidine (His), isoleucine (Ile), leucine (Leu), lysine (Lys), methionine (Met), threonine (Thr), tryptophan (Trp) and valine (Val). Endogenous amino acids include: aspartic acid (Asp), glutamic acid (Glu), alanine (Ala), tyrosine (Tyr), proline (Pro), cysteine (Cys), glycine (Gly), serine (Ser) and hydroxyproline (Hyp) (Figure 8; Table 9) (Yang et al., 2008; Moyo et al., 2011; Dai et al., 2020).
6 Ethnopharmacology
Available sources describe the wide use of M. oleifera in traditional Ayurvedic, Chinese (TCM) and African medicine. According to the Ayurvedic pharmacopoeia of India, dried bark and dried seeds of M. oleifera have been used in glycosuria and lipid disorders (Government of India Ministry of Ayush, 2016). Among the traditional uses of M. oleifera in the Ayurvedic Pharmacopoeia, one can distinguish the following actions: expectorant, antispasmodic, diuretic, stimulant, antiviral, choleretic, tonic, antiseptic, antipyretic, anthelmintic, antiparalytic and analgesic (for sore throat) properties. M. oleifera in India was also used in the treatment of asthma and diseases of the liver and spleen. In addition, attention is also drawn to recommending it for the administration in emotional problems or in venereal diseases (Khare, 2007).
M. oleifera is listed in the Chinese dictionary of herbal medicine, while in the classical herbal books it is not considered a commonly used herb. In Chinese medicine, the greatest health-promoting properties were attributed to the leaves of the plant that were used in colds (Ross, 2003). The leaves were also attributed to reverse severe malnutrition, rebuild the bone marrow and restore energy by influencing the behavior and maintenance of a positive attitude towards life (Nouman et al., 2014). The leaves were also used for gynecological problems such as vaginal discharge and vaginitis, and in the treatment of anemia (Government of India, Ministry of Ayush, 2016). M. oleifera seeds and fruits were recommended in patients with liver diseases, low appetite and flatulence. The root and bark were used to strengthen the functions of the stomach and spleen, aid urination, reduce headaches and dizziness. In addition, they were used in gastrointestinal problems, hypertension, flatulence and skin problems (Government of India, Ministryof Ayush, 2016).
In traditional African medicine, M. oleifera species was credited with anti-fatigue action, was used to treat anemia and malnutrition, and for its anthelmintic properties. In Malaysia, poultices of young M. oleifera leaves were administered to remove tapeworms. In African countries, the pods are used as an anti-diarrheal agent, in the treatment of dysentery, colitis and gonorrhea, the leaves—to treat inflammation of the glands, headache, and infections, whereas the root, bark and stem—to treat wounds and skin infections. In addition, several examples for the treatment of scurvy, eye pain, digestive ailments were described together with anti-hyaluronidase activity that was useful in the prevention of snake venom from spreading in the bloodstream. Other applications of the bark included the treatment of the upper respiratory tract infections, abortifacient and aphrodisiac properties (Posmontier, 2011).
7 Biological activities confirmed by scientific research
The review of the latest studies on the M. oleifera biological activity showed that extracts from different parts of the plant and isolated compounds may have different biological activities. A widely tested extract is M. oleifera leaf extract, which has both hepatoprotective effects in in vitro and in vivo tests, as well as antioxidant potential, neuroprotective (in vivo and in vitro tests), antidepressant (in vivo tests) as well as anticancer (in vivo and in vitro tests) effects. Recent studies have also shown its blood glucose lowering properties (in vivo studies), anti-inflammatory properties (in vitro studies), wound healing potential (in vitro studies), antibacterial and antifungal properties. Research has shown that M. oleiffera seed extracts and oil have the potential to be used in cosmetics through anti-aging, photoprotective and anti-psoriasis properties. Plentitude of metabolites of pharmacological significance synthesized by the M. oleifera may be used in the treatment of diseases of diverse etymology.
While the research on M. oleifera presents promising findings regarding its biological and therapeutic properties, a critical perspective warrants consideration. Firstly, the need for standardized methods across studies could enhance the comparability of results and strengthen the overall reliability of the findings. Additionally, exploring potential side effects or unintended consequences of prolonged use should be a focal point, especially when considering the application of M. oleifera extracts in skincare products. Furthermore, it would be valuable for future research to delve deeper into the mechanisms underlying the observed effects. Understanding the specific pathways through which M. oleifera components exert their antioxidant influence could provide valuable insights for targeted therapeutic interventions. Moreover, investigating the long-term impact of M. oleifera supplementation or application could shed light on its sustainability and safety in extended use. In the context of the in silico approach employed in some of the studies, it is crucial to acknowledge the inherent limitations of computational modeling. While molecular docking provides valuable predictions, experimental validation remains paramount to substantiate the proposed mechanisms of action.
Overall, while the current results of research suggest promising possibilities for M. oleifera in nutraceutical, cosmetological and pharmaceutical applications, addressing these critical considerations could enhance the scientific robustness and practical implications of these findings.
The biological activities of M. oleifera described so far are summarized in the following sections. Table 10 summarizes the latest scientific reports on this issue.
7.1 Anticancer activity
Recent studies have shown that a derivative of the main compound from M. oleifera 4-[(α-L-rhamnose oxy) benzyl] isothiocyanate (MITC) called MITC-12 significantly inhibited the growth of U251 (human glioblastoma astrocytoma), A375 (human malignant melanoma), A431 (human squamous carcinoma), HCT-116 (human colon cancer), HeLa (human cervix epitheloid carcinoma) and MDA-MB-231 (human Caucasian breast adenocarcinoma). MITC-12 significantly inhibited U251 cell proliferation in a time- and dose-dependent manner and reduced the number of EdU-positive cells, but was not toxic to normal human gastric mucosa cells (GES-1). MITC-12 induced apoptosis of U251 cells and increased the expression levels of caspase-3 and the Bax:Bcl-2 ratio. These results indicate that MITC-12 inhibits the proliferation of U251 cells, activating JNK, and regulating cell cycle-related proteins (Xie et al., 2023).
Glucomoringin isothiocyanate from M. oleifera seeds has an inhibitory effect on cell proliferation in the PC-3 prostate cancer cell line. Glucomoringin isothiocyanate induced time-dependent G2/M phase arrest. Glucomoringin isothiocyanate also activates apoptotic genes, including caspase, the tumor suppressor gene (p53), Akt/MAPK, and Bax from the pro-apoptotic Bcl family (Karim et al., 2023).
Stem and leaves extracts of M. oleifera significantly reduced the proliferation and induced apoptosis of 4T1 breast cancer cell line derived from the mammary gland tissue of a mouse BALB/c strain. The results indicated that the apoptosis pathway was likely induced by the activation of caspases 9 and -3 and the increase in the Bax/Bcl-2 ratio (Yousefirad et al., 2023).
Studies of M. oleifera leaf extracts in rats with a urethane model of lung cancer showed improved induced changes in the concentrations of glutathione, superoxide dismutase and malondialdehyde. Compared with the control group, rats treated with the extract showed a moderate amount of collagen fibers and tumor atrophy and approximately restored normal lung histology (Ibrahim et al., 2023).
The anticancer effect of M. oleifera leaf extracts were tested on a human myeloma cell line (U266B1). Methanol, ethanol, ethyl acetate and chloroform extracts from M. oleifera leaves were used in the study. Antitumor activity was measured by the neutral red uptake assay. The methanol extract showed the highest anticancer activity against U226B1 cells (IC50 = 0.32 μg/mL) (Table 10) (Parvathy and Umamaheshwari, 2007).
Another study evaluated the effectiveness of M. oleifera against the BRCA-1 breast cancer cell line. Molecular docking (computer method), studies of pharmacokinetic parameters and properties similar to other anti-cancer drugs were used to determine the anti-cancer potential. In addition, in silico analysis was used using Schrödinger: Molecular and Drug Discovery Suite and SWISS ADME. The results showed that compounds contained in M. oleifera such as rutoside, vicenin-2 and glucoside-3-O-quercetin have the highest binding energy in the active site of BRCA-1. The essential amino acids involved in the protein-ligand interaction in the active site are: glycine, leucine, lysine, arginine and valine (Table 10) (Balogun et al., 2021).
As shown above, the studies on M. oleifera have demonstrated the anticancer potential of this species. Despite the promising results, in vitro studies and in vivo models require further analysis whether the extracts affect the development of these tumors. Moreover, the molecular docking are the interesting starting point to consider the transmission research into in vivo studies.
7.2 Hepatoprotective activity
Recent studies have demonstrated the hepatoprotective effects of M. oleifera leaf extracts. The effect of an ethanolic extract of M. oleifera seed oil on liver markers was evaluated in Wistar rats exposed to dimethyl 2,2-dichlorovinylphosphate (DDVP, known as dichlorvos). M. oleifera seed oil regulated and increased plasma protein levels, reduced elevated levels of liver markers and inflammatory markers. Histopathological studies demonstrated the regenerative effect of M. oleifera seed oil on dichlorvos-induced liver damage (Saka et al., 2022).
Studies have shown that M. oleifera leaf extract reduced the elevation of serum alanine aminotransferase (ALT) and aspartate aminotransferase (AST) levels in mice with CCl4-induced liver injury. CCl4-induced apoptosis and genotoxicity were significantly alleviated by M. oleifera leaf extract. Co-administration of the preparation with M. oleifera leaf extract modulated the TLR4/NF-κB pathway, which was demonstrated by suppressed expression of TLR4 and NF-κB genes, as well as reduced expression of TLR4 and NF-κB-p65 proteins (Fathy and Mohammed, 2021).
A study on the effect of a hydroalcoholic M. oleifera leaf extract on the liver enzymes: alanine aminotransferase (ALT), aspartate aminotransferase (AST) and an enzyme related to the poultry biliary epithelium, alkaline phosphatase (ALP), was performed on rats. The extract was administered for 28 days. Liver damage was induced by administration of cadmium. In the group of animals receiving M. oleifera extract, a decrease in the activity of ALT, AST and ALP was demonstrated. Researchers found that the observed decrease in ALT, AST, ALP could be due to the hepatoprotective effect of M. oleifera leaf extract, resulting from the presence of quercetin and kaempferol (Table 10) (Toppo et al., 2015).
Another study assessed hepatoprotective properties of 1-O-(4-hydroxymethylphenyl)-α-L-rhamnopyranoside (MPG) found in M. oleifera. The studies were performed on L02 (human normal hepatocytes) and ICR (mouse inactivated embryonic fibroblasts) with tetrachloromethane-induced hepatotoxicity. M. oleifera leaf extracts (ethanol, methanol, aqueous and hexane) were also tested. Purified 1-O-(4-hydroxymethylphenyl)-α-L-rhamnopyranoside from the seed extract showed high efficiency. In addition, in vitro and in vivo antihepatotoxicity studies in mouse L02 and ICR cells showed that this compound significantly protected the liver against acute tetrachloromethane-induced hepatotoxicity. This compound mitigated oxidative stress, regulated the concentration of inflammatory mediators and counteracted apoptosis (Table 10) (Sun et al., 2019).
The exploration of hepatoprotective effects of M. oleifera leaf extracts, seed oil, and specific compounds like 1-O-(4-hydroxymethylphenyl)-α-L-rhamnopyranoside is intriguing. However, to establish their clinical significance, further research is imperative to validate these findings through comprehensive clinical trials, considering factors such as dosage, long-term effects, and potential side effects in diverse populations. Additionally, a more nuanced understanding of the underlying mechanisms and pathways involved in the hepatoprotective effects of M. oleifera compounds would contribute to a more robust assessment of their therapeutic potential in liver-related conditions.
7.3 Antioxidant potential
Recent research was conducted on Se-enriched M. oleifera seed protein hydrolysate. Peptides showed very good cellular protection and increased cell viability. Moreover, studies have shown effective reduction of the accumulation of reactive oxygen species and increasing the activity of superoxide dismutase and catalase in damaged HepG2 cells (Chen et al., 2023).
Studies have shown that methanol extracts from M. oleifera leaves have a protective effect against H2O2-induced oxidative damage by increasing cell viability and reducing free radicals. In in vitro tests, the extract reduced lipid peroxidation and increased the level of glutathione and the activity of antioxidant enzymes. Methanolic extracts from M. oleifera leaves also prevented mitochondrial dysfunction by regulating calcium levels and increasing the mitochondrial membrane potential (Gonzalez-Burgos et al., 2021).
The antioxidant activity of M. oleifera extracts was evaluated in vivo in a mouse model. The study aimed to analyze the effect of ethanol extract from M. oleifera stems on skin damage caused by oxidative stress of hydrogen peroxide and UVB radiation. In addition, the effect of the ethanol stem extract on the strengthening of the antioxidant system and the activation of the PPARα receptor in vitro and in vivo was investigated. It was stated that the extract protected the epidermal cells (keratinocytes) against oxidative stress in vitro. Topical treatment of mice with a cream containing M. oleifera stem extract inhibited the damage to the epidermis caused by UVB-induced oxidative stress. Then, the phytochemical composition of the extract was analyzed by HPLC and the mechanisms of action were searched using an in silico approach - molecular docking. The authors showed that rutoside may be the main component of the extract responsible for the activation of the PPARα receptor and the observed antioxidant effect (Table 10) (Zhou et al., 2018).
The antioxidant power of M. oleifera seed oil cream was assessed by Athikomkulchai et al. by the simple DPPH radical scavenging test. The results confirmed that antioxidant properties of the cream dependent on the presence of M. oleifera seed oil (Table 10) (Athikomkulchai et al., 2021).
Overall, the collective evidence from these studies suggests that M. oleifera, particularly its seed protein hydrolysate, leaf extracts, stem extracts, and seed oil, possesses notable antioxidant potential. These properties could be harnessed for potential therapeutic applications, emphasizing the need for further exploration of M. oleifera health-promoting effects.
7.4 Neuroprotective activity
In the studies in mice administered with M. oleifera seed oil and then intraperitoneally injected with scopolamine have showed that scopolamine increased protein expression of both tropomyosin receptor kinase B (TrkB), a brain-derived neurotrophic factor receptor, and nuclear factor kappa light chain enhancer of activated B cells (NF-κB). However, pretreatment with M. oleifera seed oil improved the increased levels of TrkB and NF-κB protein expression (Arozal et al., 2022).
Studies by Onasanwo et al. demonstrated the effect of a diet supplemented with M. oleifera on the spatial memory deficit induced by scopolamine. Scopolamine treatment led to loss of spatial memory function during spatial escape platform exploration in mice in the Morris water maze test. Meanwhile, M. oleifera treatment attenuated the loss of spatial memory function by significantly reducing escape latency and significantly increasing crossover frequency with time spent in the platform quadrant. Moreover, M. oleifera administration alleviated oxidative stress, restored cholinergic transmission by inhibiting acetylcholinesterase, and maintained neuronal integrity in mouse brain (Onasanwo et al., 2021).
Interestingly, M. oleifera leaf extract was administered to rats to evaluate its effects on memory mechanisms, neuronal density, malondialdehyde (MDA) levels, superoxide dismutase (SOD) activity, catalase (CAT), glutathione peroxidase (GPx) and acetylcholinesterase (AChE) levels in the hippocampus. The study showed that the treatment improved the spatial memory of the animals and reduced the symptoms of neurodegeneration within the hippocampus. The level of MDA and AChE was reduced, while the expression of SOD and CAT increased. Research results suggest that M. oleifera leaf extract, rich in polyphenols, may be a potential drug enhancing cognitive functions and protecting the neuronal cells (Table 10) (Sutalangka et al., 2013).
In another study on rats, the animals were given an extract from dried M. oleifera leaves to evaluate neuroprotective effects. When M. oleifera extract was administered, oxidative damage to the brain was significantly reduced. The authors of the study attributed this activity to the flavonoids present in the M. oleifera extract (Table 10) (Idoga et al., 2018).
The studies Oboh et al. focused on the analysis of the modulating effect of a diet containing M. oleifera seeds and leaves on the brains of streptozotocin-induced diabetic rats treated with acarbose. The study showed that M. oleifera has an effect on the increase in the concentration of compounds with antioxidant potential, decreases AChE and butyrylcholinesterase levels, as well as angiotensin I converting enzyme. Studies suggest the effect of a diet including M. oleifera on the prevention of cognitive dysfunction caused by hyperglycemia (Table 10) (Oboh et al., 2018).
The studies investigating the neuroprotective effects of M. oleifera in various experimental models provide valuable insights into its potential cognitive benefits. However, critical considerations include the need for more standardized protocols and diverse experimental settings to establish the consistency and generalizability of the observed effects across different neurological conditions. Additionally, future research should delve into the specific mechanisms through which M. oleifera influences neurobiology, ensuring a more comprehensive understanding of its potential therapeutic applications in cognitive health.
7.5 Antidepresant activity
Ethanol extracts from M. oleifera leaves were tested on mice using behavioral models of depression: in the forced swim test (FST), tail suspension test (TST) and locomotor activity test (LAT). The study showed significant changes in all tested activities, especially in the group receiving a combined dose of 200 mg/kg M. oleifera ethanol extract and 10 mg/kg fluoxetine. The antidepressant effect may be related to the effect on the noradrenergic-serotonergic neurotransmission pathway. The exact mechanism by which the effect of fluoxetine is enhanced by M. oleifera extract has not been indicated yet but may be related to its anti-inflammatory properties (Table 10) (Kaur et al., 2015).
While the study on the antidepressant effects of M. oleifera leaves is intriguing, the absence of a clear elucidation of the synergistic mechanism between the extract and fluoxetine raises questions about the precise interactions that enhance the antidepressant effect. Further research elucidating the specific pathways and molecular targets influenced by M. oleifera in conjunction with fluoxetine would provide a more comprehensive understanding of its potential role in augmenting antidepressant treatment.
7.6 Antidiabetic activity
Recent studies have demonstrated the antidiabetic nephropathy effect of M. oleifera leaf extract administered to rats with streptozotocin-induced diabetic nephropathy. Administration of the extract reduced high blood glucose levels, impaired kidney function and oxidative stress parameters in rats. Histological examination of the kidneys showed thickening of the glomerular basement membrane and alleviation of pathological changes. Studies have shown that M. oleifera leaf extract alleviates changes due to the reduction of blood glucose levels, oxidative stress and fibrosis formation by downregulating the expression of TGF-β1 and type IV collagen genes (Thongrung et al., 2023). Aljazzaf et al. investigated the effect of methanolic extract of M. oleifera leaves, methanol extract of M. oleifera seeds, and combined leaf and seed extract on antidiabetic and antioxidant activities in alloxan-induced diabetes-induced mice. Oral administration of the combined extracts significantly reduced fasting blood glucose levels to normal levels compared to the individual extracts; in addition, a significant decrease in cholesterol, triglycerides, creatinine, liver enzymes and oxidative markers was observed, with a simultaneous increase in antioxidant biomarkers (Aljazzaf et al., 2023).
The model of alloxan-induced diabetes in mice was used to study the anti-diabetic properties of M. oleifera extracts. The level of TAC (Total Antioxidant Capacity) in diabetic mice increased 1.94-fold, and in those taking the extract it decreased 1.64-fold. In mice treated with M. oleifera, the levels of creatinine and urea nitrogen in the blood were significantly lower than in animals that did not take the extract. Based on the presented data, the authors conclude that M. oleifera leaf extract may alleviate insulin resistance, increase TAC and improve immune tolerance in people with diabetes (Table 10) (Sreelatha et al., 2011).
The ethanol leaf extract of M. oleifera was also evaluated in the pancreatic α-amylase enzymatic inhibition test (Table 10) (Chigurupati et al., 2022).
While the studies highlight the potential antidiabetic effects of drumstick tree leaf extract in various experimental models, critical considerations include the need for more standardized protocols and diverse experimental settings to establish the consistency and generalizability of the observed effects across different diabetes-induced conditions. Additionally, a comprehensive understanding of the underlying mechanisms and pathways through which M. oleifera influences antidiabetic outcomes is crucial for validating its therapeutic potential in managing diabetic nephropathy and insulin resistance.
7.7 Anti-inflammatory activity
A study of the anti-inflammatory effect of M. oleifera root extracts was performed on rats in which the left paw was inflamed by injecting 0.1 mL of 1% carrageenan in saline. M. oleifera extract, depending on the concentration, reduced swelling by 35%–45% (Table 10) (Ezeamuzie et al., 1996).
Another group evaluated the anti-inflammatory effects of M. oleifera and its potential use in treating urinary tract infections in a human study. The first group of study participants received M. oleifera bark extract and medications and the other group was given only modern drugs. The study was conducted for a period of 12 weeks. In the group treated with drugs and M. oleifera, 66.67% of patients were cured. In the case of drugs administered alone the rate of 46.67% only was achieved. The authors of the study emphasize that the study needs to be replicated on a larger scale and over a longer period of time. In addition, they pointed out that polyphenolic compounds are responsible for the anti-inflammatory effect of M. oleifera (Table 10) (Maurya and Singh, 2014).
In another study, four types of M. oleifera leaf extracts were analyzed: chloroform, hexane, butanol and ethyl acetate. The study aimed to evaluate the anti-inflammatory potential and mechanism of cellular action in RAW264.7 cells induced by lipopolysaccharide (LPS). In the MTT (3-(4,5-dimethylthiazol-2-yl)-2,5-diphenulotetrazolium) assay to measure cell viability after 24 h of treating cells with extracts. Regardless of the dose, their viability decreased. All fractions were non-toxic at lower concentrations. A cellular cytotoxicity test was performed, which showed that the extract was not cytotoxic to macrophages at a concentration of up to 200 μg/mL. Acetate extract inhibited LPS-induced production of nitric oxide and pro-inflammatory cytokines in macrophages, regardless of concentration. It was the most effective extract. Immunoblotting showed that the acetate extract effectively inhibited the expression of inflammatory mediators, including inducible nitric oxide synthase (NOS), cyclooxygenase-2 (COX-2) and nuclear factor (NF)-κB p65 by suppressing the NF-κB signaling pathway (Table 10) (Arulselvan et al., 2016). While the anti-inflammatory effects of M. oleifera root extracts in the carrageenan-induced rat paw model are promising, the study lacks detailed insights into the specific mechanisms underlying this reduction in swelling, leaving questions about the precise pathways and mediators involved. Similarly, the human study on M. oleifera bark extract’s potential for treating urinary tract infections provides interesting preliminary results, but the short duration and limited scale of the study warrant caution in drawing definitive conclusions, emphasizing the need for more extensive and prolonged investigations. Table 10 presents a comparison of the key research findings regarding the anti-inflammatory properties of this plant.
7.8 Wound healing potential
A study by Chin et al. aimed to develop and formulate a standardized foil dressing with M. oleifera leaf extract for use accelerating wound healing. For the purpose of the study, an extract of M. oleifera leaves was prepared using absolute ethanol (99.8%) and lower concentrations of ethanol and water. M. oleifera leaf extract was subjected to cold maceration in various solvents. After cold extraction, the extract was analyzed (by UHPLC) for the content of bioactive compounds. It was found that the richest was the aqueous extract. Subsequently, tests were carried out on scratched skin. The best healing properties were shown by the aqueous extract of M. oleifera leaves. According to the authors of the study, the healing properties of M. oleifera were probably due to the high content of bioactive compounds: chlorogenic acid, gallic acid, quercetin, kaempferol, rutoside and vicenin-2 (Table 10) (Chin et al., 2018).
In a study by Rodríguez-García et al. methanol extracts from M. oleifera leaves were tested. The research was carried out using human skin fibroblasts. A significant increase in the rate of fibroblast proliferation from 17% to 21% was observed. When cells were treated with low concentrations (0.01 and 0.1 μg/mL) of methanolic extract from M. oleifera leaves. Cell proliferation was dependent on the concentration of the extract; for the concentration of 500 μg/mL, the best effects of inhibition of proliferation were found. In the next step, a 1.5 mm wound was made in the fibroblast cell monolayer and incubated for 48 h at a specific concentration of the extract. Cells incubated with M. oleifera extract at concentrations of 0.01 and 0.1 μg/mL showed time (0, 4, 24, 48 h) and dose-dependent increase in migration. The extract with a concentration of 0.01 μg/mL turned out to be the most effective and closed the wound within 48 h. The study showed that 0.01 μg/mL methanol extract from M. oleifera leaves determines the highest proliferation and migration (70%) of human skin fibroblasts in vitro. In the studies, no toxicity of the extract was observed on fibroblasts. According to the researchers, flavonoids and other biologically active compounds were responsible for the obtained effects. Chromatographic analysis of the extract showed the presence of chlorogenic acid, myricetin 3-O-xylopyranoside, neohesperidin, rutoside, isoquercetin, kaempferol, quercetin and vicenin-2 (Table 10) (Rodríguez-García et al., 2021). The efforts by Chin et al. (2018) to develop a standardized foil dressing with M. oleifera leaf extract for wound healing show promise, especially with the identification of bioactive compounds like chlorogenic acid, gallic acid, quercetin, kaempferol, rutoside, and vicenin-2. However, the clinical relevance of these findings requires further validation through comprehensive clinical trials to establish the efficacy and safety of the proposed dressing in accelerating wound healing in real-world scenarios. Similarly, Rodríguez-García et al. (2021) study on methanol extracts from Moringa leaves demonstrates increased fibroblast proliferation and migration, yet the concentration-dependent effects and mechanisms underlying these observations warrant thorough exploration. The absence of observed toxicity is encouraging, but the translatability of these in vitro findings to human wound healing processes necessitates additional research, emphasizing the need for rigorous clinical investigations to ascertain the practical applicability of M. oleifera in wound healing.
7.9 Antipsoriatic activity
Ma et al. investigated the potential use of M. oleifera in the treatment of psoriasis. In the pathogenesis of psoriasis, Th17 lymphocytes with the participation of cytokines IL-23 and p19 play a key role. The anti-inflammatory effects of the bioactive components of M. oleifera have not been well elucidated and therefore limited information is available on its potential clinical use. Researchers have identified in the seed three compounds potentially important in the treatment of psoriasis, namely, niazirin, marmumoside A and D-glucoside-3-O-β-sitosterol. Subsequently, their effect on the expression of Th17-related cytokines was investigated. i.e., IL-12/IL-23 p40. IL-17A. IL-22, and IL-23 p19 using the THP-1 cell line stimulated with LPS (lipopolysaccharide). Data were collected at 0.5 h and 2 h for quantification of gene expression. The study used 12-O-tetradecanoyl phorbol-13-acetate (TPA) to induce skin lesions mimicking those caused by psoriasis in mice. Increased expression of pro-inflammatory cytokines associated with psoriasis was found. In the study, niazirin, marumoside A and D-glucoside-3-O-β-sitosterol, isolated from M. oleifera, attenuated the expression of cytokines essential for Th17. They also alleviated TPA-induced skin lesions in mice. This is the first report on the effectiveness of M. oleifera in psoriasis-like lesions in vivo (Table 10) (Ma et al., 2018). While Ma et al.'s investigation into the potential use of drumstick tree in treating psoriasis is promising, the limited understanding of the anti-inflammatory effects of its bioactive components raises questions about the depth of knowledge in this area. The quantitative analysis of the plant is noteworthy, but further research is crucial to elucidate the specific mechanisms and clinical implications of these components in psoriasis treatment. The study’s use of a mouse model to mimic psoriasis-like lesions is a significant step, but the translatability of these findings to human psoriasis requires careful consideration, emphasizing the need for more comprehensive clinical investigations to establish the potential clinical utility of M. oleifera in psoriasis management.
7.10 Antibacterial activity
The antibacterial activity of M. oleifera was assessed using aqueous and ethanol leaf extracts and the Gram-positive (Enterococcus faecalis and Staphylococcus aureus) and Gram-negative (Aeromonas caviae, E. coli, Pseudomonas aeruginosa, Salmonella enteritidis and Vibrio parahaemolyticus) bacterial strains. E. coli, P. aeruginosa and S. enteritidis were resistant to tested M. oleifera extracts. The most promising results were obtained in an assay where the extracts were used against S. aureus (inhibition zones: 23.3 mm; 25.4 mm), E. faecalis (inhibition zones: 19.4 mm; 17.8 mm), A. caviae (inhibition zones: 23.8 mm; 22.3 mm) and V. parahemolyticus (inhibition zones: 21.9 mm; 20.7 mm), for ethanol and aqueous extracts, respectively (Table 10) (Peixoto et al., 2011).
M. oleifera seed powder was subjected to an antibacterial test against Salmonella sp., S. aureus and E. coli strains. In the antibacterial test, M. oleifera powder was effective against each strain. The MIC values were 99.4% for Salmonella sp., 78.8% for E. coli and 57.3% for S. aureus, respectively (Table 10) (Aboagye et al., 2021).
The assessment of M. oleiferaantibacterial activity is valuable, yet the observed resistance of E. coli, P. aeruginosa, and S. enteritidis to the tested extracts raises questions about the broad-spectrum efficacy. Further exploration into the specific mechanisms underlying the observed antibacterial effects and potential variations in response among bacterial strains would contribute to a more nuanced understanding of M. oleifera applicability in combating bacterial infections. Additionally, the antibacterial test using M. oleifera seed powder presents promising results, but further investigations should address the concentration-dependent effects and consider potential variations in efficacy against different bacterial strains to ensure a comprehensive evaluation of its antibacterial potential.
7.11 Antifungal activity
The antifungal effect of aqueous and 90% ethanol extracts from dried leaves of M. oleifera was carried out against the following fungal strains: Saccharomyces cerevisiae, Candida albicans and Candida tropicalis. The extracts showed the strongest activity against S. cerevisiae, good antifungal activity against C. tropicalis and no activity against C. albicans (Table 10) (Patel et al., 2014). While the investigation into the antifungal effect of M. oleifera leaf extracts against various strains is noteworthy, critical considerations should address the specificity of the observed activity. Understanding the underlying mechanisms and potential variations in response among different fungal strains is crucial for assessing the practical applicability of these extracts. Additionally, further research should explore the safety and potential side effects of M. oleifera extracts, especially when considering their use as antifungal agents, to ensure a comprehensive evaluation of their efficacy and potential limitations.
7.12 Skin lightening and photoprotective potential
M. oleifera seed oil, due to the presence of essential fatty acids, has become the subject of research to create a nourishing herbal cream that also has ultraviolet radiation protection and skin brightening properties. In order to develop the cream, in addition to seed oil, M. oleifera leaf powder and red rice extract were also used. The tests showed that the prepared cream contained 36.4% oleic acid and 0.35% linoleic acid, and also contained essential amino acids. Fortification of red rice showed the presence of ZnO in the cosmetic, which has a protective effect against UV radiation. On the basis of the organoleptic assessment of the cream’s stability at room temperature, it was found that within 1 month the cream still has the correct consistency, smell and color. Studies show that environmental factors such as the Sun do not adversely affect the consistency of cosmetics with M. oleifera seed oil (Table 10) (Kumalaningsih and Arwani, 2018).
Skin lightening properties of M. oleifera seed extract were also proven using reconstructed human epidermis model. M. oleifera alone or in combination of Adansonia digitata seed oil reduced the melanin content by 21%–27% following 6 days of treatment. Obtained results was comparable with kojic acid, a substance currently used in skin lightening cosmetics (Zeitoun et al., 2020).
Skin-lightening potential was also shown for M. oleifera leaf extract which effectively inhibited diphenolase activity of tyrosinase in vitro (IC50 = 121.3 μg/mL). Kinetic studies indicated uncompetitive mechanism of tyrosinase inhibitor by M. oleifera leaf extract and HPLC analysis combined with computational studies suggested luteolin as the most probable and potent tyrosinase inhibitor (Hashim et al., 2021).
However, the critical evaluation should extend to long-term stability assessments and potential interactions between ingredients, especially under varied environmental conditions. Additionally, while promising, the skin-lightening properties demonstrated by M. oleifera seed and leaf extracts should prompt further investigations into their safety and long-term effects on skin health, addressing concerns related to prolonged use and potential side effects in diverse skin types.
7.13 Anti-aging activity
Isothiocyanates obtained from M. oleifera have anti-aging properties (Athira Nair et al., 2020). However, due to low solubility in water, easy degradation and difficult bioavailability, the use of preparations consisting of M. oleifera solely does not bring the expected results. Wang et al. investigated the combination of M. oleifera seed extract with nanoliposomes. Flexible nanoliposomes are effectively used as biocompatible polymers used, among others, for transdermal drug delivery. The study consisted of the preparation of amphiphilic hyaluronic acid conjugated with ceramide in order to provide an extract from M. oleifera seeds. The combined nanoparticles of M. oleifera extract, hyaluronic acid and ceramide have been tested in many aspects, such as polydispersity index, determination of particle size, in vitro release and in vivo skin penetration and in vitro photoaging protective effect. Due to the use of nanoparticles, the effectiveness of M. oleifera has significantly increased. The effectiveness of introducing the M. oleifera seed extract into the liposomes increased the effectiveness of the extract from 62.54% to 70.67%. and the size of the nanoparticles decreased from 266.1 nm to 192.8 nm. Good results were also observed in an in vivo skin permeation study, which showed an increase in permeability from 49.42% to 71.40%. In addition, the stability of the M. oleifera extract was improved (Wang et al., 2022). The nanoparticles also improved the action of antioxidant enzymes in the skin by scavenging free radicals that are induced by UVB. The result is skin protection and a reduction in the expression of matrix metalloproteases MMP-1, MMP-3 and MMP-9 activated in the photoaging process. Flexible nanoliposomes effectively improved the penetration of M. oleifera seed extract, however, the authors of the study concluded that more research should be conducted to confirm the thesis about their use in the prevention and therapy of photoaging (Table 10) (Athira Nair et al., 2020).
Despite the promising, anti-aging potential of M. oleifera, there are inherent challenges of low solubility, degradation, and poor bioavailability of the extract components necessitate innovative approaches. The strategy by combining M. oleifera seed extract with nanoliposomes seems to be a good solution for this issue. The nanoparticles also enhanced antioxidant enzyme activity, offering protection against UVB-induced free radicals and reducing the expression of matrix metalloproteases linked to photoaging. While these results are promising, the authors rightly call for further research to validate the application of these nanoliposomes in the prevention and therapy of photoaging.
8 M. oleifera as dietary supplement
The chemical composition of M. oleifera, and especially extracts from the leaves of the plant, determines their use in medicine. This plant has anti-inflammatory properties, antioxidant, hepatoprotective, neuroprotective and anticancer. Studies also show an anti-aging and wound healing effects. In addition, it can be used to support the treatment of pain, depression, psoriasis and diabetes. The bactericidal properties of the plant are also of great importance. A number of studies prove the antimicrobial activity of M. oleifera (Peixoto et al., 2011; Aboagye et al., 2021). There are many M. oleifera supplements on the market today (e.g., Swanson Moringa oleifera, Yango Moringa, Vitama Nature Moringa, Jiva Botanicals Moringa, Natgrown Moringa oleifera leaf).
9 Application in cosmetic industry
A summary description of the functions of individual ingredients and raw materials obtained from M. oleifera, approved for the production of cosmetics for the indicated purposes according to CosIng (Cosmetic Ingredient Database) (European Commission Cosing, 2020) is presented in Table 11. The oil and extracts obtained from M. oleifera are present in preparations produced, in Poland, Great Britain, Germany, United States of America, Italy, France, Bulgaria and Cambodia. They include face creams, eye creams, body and hair care products. In cosmetics, they provide antioxidant, regenerating or anti-aging properties (Athira Nair et al., 2020; Wang et al., 2022).
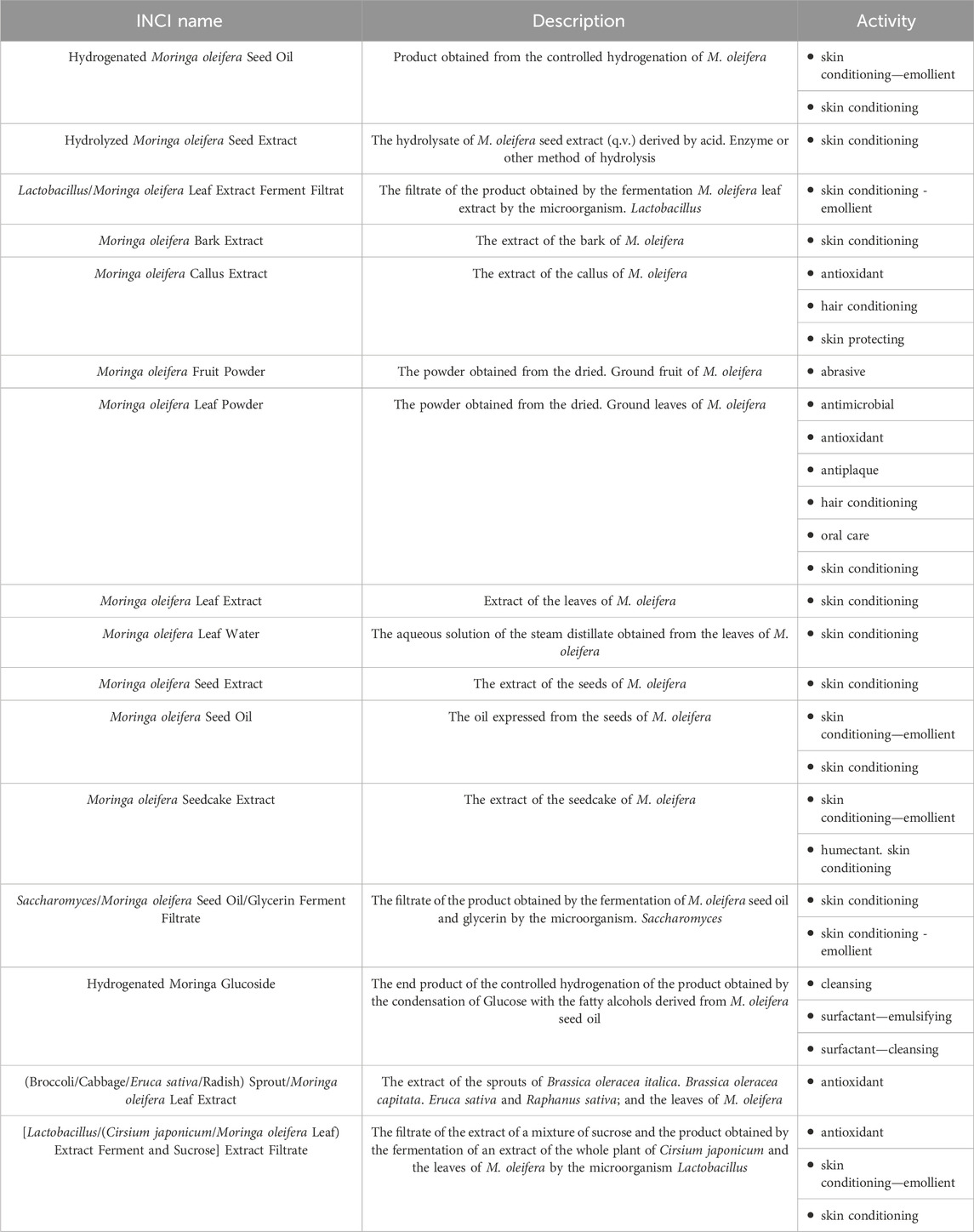
TABLE 11. The use of M. oleifera according to CosIng (Cosmetic Ingredient Database CosIng, 2023).
10 Application in the food industry
Due to the high content of nutrients, M. oleifera is described in many sources as an alternative food source, particularly in the fight against malnutrition in developing countries (Ijarotimi et al., 2013; Karim et al., 2015). Energy bars and oil cake containing M. olifera are also sold in India (Ijarotimi et al., 2013; Karim et al., 2015).
M. oleifera can be used for food fortification. It is about adding vitamins, minerals and other essential nutrients to food products for enrichment. Such an enriched product should still have consistent sensory properties (Allen et al., 2006). Kalowole et al. described the enrichment of wheat dough with a maximum of 8% M. oleifera leaf powder in order to maintain the sensory qualities of the dough. A more promising alternative is the use of seeds or flowers, as the leaf powder is too intensely colored (Kolawole et al., 2013; Karim et al., 2015).
“Amala” cake is a staple of nutrition in many parts of Africa. The use of powdered M. oleifera leaves in this dough at a concentration of 10% increased the protein content by about 48%. The content of calcium, potassium, magnesium, sodium and iron also increased. Unfortunately, the addition of M. oleifera affects the sensory attributes of the dough (Awoyale et al., 2010; Ramota and Ojo, 2013; Karim et al., 2015).
Cereal gruel with the addition of M. oleifera is used as a complementary food for infants and for the production of breakfast cereals. It was found that the addition of powdered leaves of M. oleifera can improve the nutritional value of the gruel. It increased the content of vitamin A and protein, iron, calcium and phosphorus. Importantly, the addition of 10% powdered leaves M. oleifera did not significantly change the sensory qualities of this food (Olorode and Idowu, 2013; Arise et al., 2014; Abioye and Mo, 2015).
Including powder from flowers, seeds or leaves of M. oleifera in bread recipes allows to make bread on its own or in combination with other flours. Moreover, the addition of M. oleifera increases the amount of protein and crude fiber. The only problem associated with the production of bread with M. oleifera are the changed sensory properties, changes in the color of the crust a decrease in the height of the loaf compared to breads made of wheat flour, or the herbal aroma of the bread. A taste-masking flavoring agent is used to change the herbal flavor (Chinma et al., 2014).
M. oleifera can also be used to increase the nutrient content in dairy products such as yogurt and cheese. The addition of 0.5% extract from M. oleifera leaves in yogurt is considered sensory acceptable. Another product that can be enriched by the addition of M. oleifera is cheese. The content of protein, carbohydrates and fat is increased when M. oleifera leaf powder is added to cheese made from buffalo milk. The authors of the research on this product suggest the addition of a maximum of 2% of M. oleifera leaves in order to maintain the sensory properties. It is worth emphasizing that some consumers would also accept a higher concentration due to the herbal flavor of the products (Salem et al., 2013; Hekmat et al., 2015; Lokapirnasari et al., 2021).
M. oleifera leaves have also found their application in the preparation of soups, teas and salads. However, this usage is not well understood and the studies on this type of application are still ongoing. For now, it is not possible to say exactly what sensory and nutritional values it would have (Stevens et al., 2013; Babayeju et al., 2014).
11 Biofuels
M. oleifera is one of the plants for which the oil is suitable for the production of biodiesel (Ofor and Nwufo, 2011). Jatropha and Biodiesel Promotion Center (CJP, India) (Jatropha Biodiesel, 2023) has developed a specific mass propagation technique for M. oleifera for the production of biodiesel by micro-cutting. This company is constantly striving to improve practices that enable high yields of oil. CJP scientists are working to perfect agronomy, horticulture and plant genetics to introduce new highly productive varieties. In 2021, the Advanced Biofuel Center (ABC), also referred to as Moringa India, released a brochure “Strategies for the Commercialization of Moringa”. It contains information on husbandry practices that are used to maintain efficient and effective production. It develops various cultivation technologies and cultivation techniques for: harvesting leaves, harvesting seeds for oil, and harvesting both leaves and seeds of the plant (Jatropha World, 2023).
The Center for Advanced Biofuels, based on the conducted scientific research, selected a high-yielding perennial variety (cultivar) of M. oleifera—cv. MOMAX3. This variety provides the highest yield in seed and oil cultivation. M. oleifera cv. MOMAX3 are about three times more productive in seeds compared to their average harvested from M. oleifera. This is possible due to the large number of seeds in one fruit, their relatively large mass (over 30 g in 100 seeds), early flowering and rapid germination of the plant. The annual seed production per hectare is 2–3 tons, but can be as high as 8 tons, whereas M. oleifera normally produces about a ton of seeds per hectare per year. It is worth noting that the seeds are not genetically modified. They have been developed by the scientific method of selection (Jatropha World, 2023). The Center for Advanced Biofuels also selects an annual variety—M. oleifera cv. PKM1, which is less popular but very productive and recommended for the leaf production (Jatropha World, 2023).
In addition, other studies have been conducted in Australia to demonstrate the environmental benefits of growing M. oleifera for biodiesel production. This analysis was aimed at evaluating the environmental performance of the production of 1,000 L of biodiesel obtained from M. oleifera oil seeds. The study was carried out both in dry and irrigated areas. It was found that approximately 1 kg of M. oleifera seeds should be used to produce 0.33 L of seed oil. To obtain 1,000 L of biodiesel, 1,000 L of oilseed oil and 100 L of methanol are used. It was therefore calculated that approximately 3,030 kg of seeds should be used to obtain the amount of biodiesel needed for the research. Studies have shown that the greenhouse gas emissions from M. oleifera seed biodiesel production are 13% higher under irrigated conditions than under dry conditions. This is due to the use of greenhouse gas-producing agricultural machinery, which is used more frequently during irrigation. Biodiesel from M. oleifera oilseeds can greatly help in the fight against global warming (Amouri et al., 2023).
12 Safety of use
With the popularization of M. oleifera as an ingredient in food and cosmetics and its possible use in many diseases, the issue of its safety has arisen. Safety studies focus mainly on the use of M. oleifera as a dietary supplement. Most often, they are carried out on M. oleifera leaf extracts, but more research is still needed on extracts from individual parts of the plant and their safety. It is also worth noting that cosmetics in which M. oleifera is used must undergo appropriate safety tests for their use on the skin so that they can also enter the cosmetics market. Adedapo et al. conducted a safety study of an aqueous extract of M. oleifera leaves. The extract was tested for oral toxicity and for subacute toxicity on histological, hematological and biochemical parameters. The studies were conducted on rats. The acute toxicity experiment did not cause death in animals even at a dose of 2000 mg/kg. Oral toxicity was tested at doses of 400, 800 and 1,600 mg/kg. Significant changes in many parameters were noted: hemoglobin percentage, packed cell volume, mean blood cell hemoglobin concentration, mean red cell volume, total and differential WBC, liver enzyme levels, total protein levels, and bilirubin levels. In the study of platelet levels, the water extract from M. oleifera leaves did not cause significant changes. In addition, the body weight of the experimental animals also changed. There were no changes in significant organs. The results proved that M. oleifera leaf extract is relatively safe to use for nutritional and medicinal purposes (Adedapo et al., 2009).
Another investigation of the toxicity of an aqueous extract from the plant’s leaves was a study by Awodele et al. (2012). In the acute toxicity study, the extract was administered to mice orally at a dose of up to 1,640 mg/kg and intraperitoneally at a dose of up to 2000 mg/kg. A 60-day chronic toxicity study was also performed. Mice received the extract at doses of 250, 500 and 1,500 mg/kg. The lethal dose LD50 (causing death in 50% of the tested individuals) was estimated at 1,585 mg/kg. In all tested haematological parameters and biochemical tests, administration of the extract did not cause significant differences. The authors of the study concluded that the aqueous extract of M. oleifera leaves is safe (Awodele et al., 2012). Asiedu-Gyekye et al. measured the content of microelements and macroelements in dried leaves of M. oleifera and assessed its toxic effects on animals. Experimental rats were given 40–5,000 mg/kg of leaf extract to determine toxicity. Animal blood samples were evaluated for biochemical and hematolological parameters. In both acute and subacute toxicity studies, no adverse effects were observed. However, the authors concluded that due to the high content of elements, the intake of M. oleifera extract should not exceed 70 g per day to prevent the accumulation of elements that could cause toxicity (Asiedu-Gyekye et al., 2014). Ali et al. conducted a study on the skin irritating potential of M. oleifera leaf extract. In vivo evaluation of skin irritation by patch test and long-term evaluation of erythema was performed for a cream with 3% M. oleifera leaf extract. The test product was safe for topical application, and the cream had soothing effect on erythema (Ali et al., 2013). The evaluation of the genotoxicity of M. oleifera seed powder extract was performed by Rolim et al. (2011). The tests showed no signs of genotoxicity of the extract. However, the authors of the study emphasize. that when used in high concentrations, it may pose a health risk (Rolim et al., 2011). Ajibade et al. conducted a safety study of methanol extracts from M. oleifera seeds. The experiment was performed on rats to determine acute and subacute toxicity. In the acute toxicity study, adverse effects were observed at a dose of 4,000 mg/100 g b. w. (body weight) mortality was found after administration of 5,000 mg/100 g b. w. methanolic extract of M. oleifera seeds. For concentrations lower than 3,000 mg/100 g b. w. no side effects were observed. In a subacute toxicity study, a decrease in body weight was noted in animals after administration of 1,600 mg/100 g. The authors stated that M. oleifera seed extract is safe as a nutritional product and it is possible to use it in medicine (Ajibade et al., 2013). Studies by Luqman et al. (2012) on leaves and fruits of M. oleifera showed that the fruit in doses of up to 100 mg/kg of b.w. of M. oleifera fruit extract are safe and should show only beneficial properties (Luqman et al., 2012). Athikomkulchai et al. tested the safety of M. oleifera seed oil on the skin. The developed cream had anti-radical properties and did not cause any adverse reactions (Athikomkulchai et al., 2021).
In the EFSA documents (EFSA, 2023) the information about the usage of dried M. oleifera extract used in India as an abortifacient can be found. However, there is not much information about this effect in scientific publications. EFSA officially states that M. oleifera is recognized as safe (EFSA, 2015). The FDA, (2023) only provides information on “the dangers of M. oleifera supplements purchased from certain manufacturers.” As is well known, dietary supplements do not have the obligation of such restrictive tests as in the case of drugs. Hence the information that the consumer is misled by some manufacturers of supplements containing M. oleifera.
In conclusion, studies conducted on M. oleifera confirm its safety. However, this species is not as common as other plants used in supplements due to the lack of enough safety research on extracts from each part of the plant.
13 Biotechnological research
Currently, there is an increasing demand for plant species important from the therapeutic point of view. To this end, researchers are increasingly developing micropropagation protocols for plants with valuable properties. Proper shoot regeneration is essential to initiate clonal propagation and/or genetic transformation. Plant biotechnology allows for the cultivation of plants under constant conditions, e.g., temperature, lighting, which may affect the content of biologically active compounds. Biotechnological research conducted on in vitro cultures of M. oleifera is aimed at developing plant micropropagation protocols (Smetanska, 2008; Karuppusamy, 2009).
In a study by Marfori et al. mature green M. oleifera pods were used. In the preparation phase, the pods were thoroughly washed and their surface sterilized. Seeds were applied on Murashige and Skoog (MS) medium with 30 g sucrose and 5 g agar. Cultures were grown for 1–2 weeks and kept in the dark at 27°C. After germination, the seedlings were transferred and grown under white light. After a month, the sprouted seedlings were harvested and transferred to a sprout-inducing medium. MS sprout induction medium contained 6-benzlaminopurine (BAP) and thidiazuron (TDZ) or kinetin (Kin) at a concentration of 0–10 mM. Shoot and roots were transferred to the MS inducing medium containing 1-naphthaleneacetic acid (NAA), indolebutyric acid (IBA) or indoleacetic acid (IAA) at concentrations of 0–5 µM. Rooted cuttings were transferred to fresh induction medium for continuous shoot production or to soil. Studies have shown that BAP at a concentration of 2.5 µM induced the largest number of shoots (4.6 shoots per explant). It was greater than with TDZ and Kin. For Kin, the highest score was recorded using a concentration of 5 µM (2.4 shoots per explant). TDZ was most effective at a concentration of 2.5 µM (2 shoots per explant). In the next step, the seedlings induced the highest number of roots after applying 0.25 µM NAA (6.8 roots per shoot after 1 week). In the literature there are noticeable differences in the proposed plant growth and development regulators (PGRs) used for M. oleifera micropropagation processes (Marfori, 2010). According to Fahey and Stephenson the best growth of M. oleifera microcuttings occurs with 4.44 µM BAP and 2.68 µM NAA (Stephenson and Fahey, 2004). On the other hand, Islam et al. found that the best results were obtained when using 4.44–6.66 µM BAP for shoot induction. and their rooting is best in a PGR-free culture. Differences in the plant source and type of explants used may be responsible for the discrepancies (Islam et al., 2021).
In another study by Saini et al. worked on methods for rapid in vitro regeneration of M. oleifera and evaluated the yield of nutrient-enriched tissue cultures. The study was conducted on healthy, uniform M. oleifera seeds from India. These seeds were surface sterilized by immersing them in 0.1% Mercury chloride for 2 min and in 20% sodium hypochlorite for 10 min. The tested seeds were rinsed three times with distilled water, then the seed coats were removed and sterilized again for 5 min in 20% hypochlorite, then rinsed three times with distilled water. The sterilized seeds were placed on MS medium containing 30 g/L sucrose solidified with 5 g/L agar (pH was 5.8). The cultures were kept in the dark for 15 days at 27°C and then, after germination, were transferred to white fluorescent lamps. Shoots were induced by using sprouted cuttings of 3-4 nodes 3 or 4 weeks after inoculation. The explants were transferred to an MS induction medium containing tria-contanol at a concentration of 0–11.39 nmol and 0–8.88 nmol BAP and 0–5.37 μmol NAA to determine their effect on shoot formation. After 15 days, the number and length of shoots were assessed. Then, the obtained microshoots were repeatedly passaged on MS medium containing 4.44 nmol BAP. The next step was rooting the shoots. Segments with induced shoots were transferred to root-inducing MS medium containing IAA at a concentration of 0–5.71 nmol and optionally IBA at a concentration of 0–4.92 µmol. After 7 days, the number of roots per shoot and the length of roots were recorded. One-year-old M. oleifera rooted and transplanted in vitro was used to obtain field results. α-Tocopherol, carotenoids and chlorophyll were extracted. In the results of the study, the authors state that with the use of 4.44 μmol BAP, the largest number of shoots was induced (9 shoots per explant). Other substances used were less effective. The highest number of roots—15—was recorded after 7 days using IBA at a concentration of 4.92 nmol and IAA at a concentration of 2.85 nmol. In a plant nutrient study, it was determined that tissue culture increased the amount of nutrients from 5.5% to 14.7% compared to control culture. The high content of nutrients was due to the intensive vegetative growth of plants propagated in vitro (Saini et al., 2012).
M. oleifera is subjected to numerous scientific studies, but there is still a lack of research on the use of in vitro cultures in cosmetics. A study by Zanell et al. presents information on the induction of antioxidant metabolites in M. oleifera callus by abiotic stress. M. oleifera leaf callus was cultured in vitro and then various methods of inducing abiotic stress were tested. Exposure to high and low temperatures (4°C and 45°C) was applied, however, it did not cause any changes in terms of antioxidant metabolites. Then the callus was treated with salicylic acid at a concentration of 200 µmol and NaCl at a concentration of 50–100 µmol. The study lasted 30 days. The highest production of metabolites in callus from M. oleiferawas found after 30 days of using NaCl at a concentration of 100 μmol, this was equal to the dose measured in a fresh M. oleifera leaf. High concentrations of NaCl (200 µmol) were able to inhibit callus growth and reduce the amount and bioactivity of its secondary metabolites (Zanella et al., 2019).
Mathur et al. conducted a study on callus cultures in vitro M. oleifera. Cultures were initiated from M. oleifera seeds and nodal segments on MS medium. Various concentrations and combinations of auxins and cytokinins were used. The best callus induction was found with 3 mg BAP and 3 mg IBA. Callus rooting in terms of morphogenesis was observed in MS media with 2 mg/L BAP and 0.2 mg/L KIN and 3 mg/L IBA and 0.5 mg/L NAA. Rooted plants were transferred to greenhouse conditions, after transplanting the seedlings, as much as 95% of the seedlings survived (Mathur et al., 2014).
14 Potential application in agriculture
Water extracts from the leaves, roots and pods were tested against 7 phytopathogenic fungi: Fusarium oxysporum, Fusarium solani, A. solani, A. alternata, Rhizoctionia solani, Sclerotium rolfsii and Macrophomina phaseolina. An agar medium test was used for the study. M. oleifera root extracts were used at concentrations of 5, 10, 15, 20% and 25%. Leaf and pod extracts were used at concentrations of 10, 20, 30, 40% and 50%. On 6 mm agar discs with hyphae of each of the above mushrooms, 20 mL of extracts were added. The percent inhibition of mycelial growth was calculated. In addition, a test was performed using the dry weight of the mycelium. It consisted in mixing an aqueous extract of roots, leaves and pods with sterilized potato broth medium, obtaining concentrations of: 0.5%; 1%; 1.5%; 2%; 2.5%; 5%; 10%; 15%; 20%, and 25%. Oil and M. oleifera seed extract were dosed in portions from 50 to 250 mL in an Erlenmeyer flask. The agar disk with fungal hyphae was transferred to flasks with M. oleifera extracts and incubated. The pathogen reduction effect was different and depended on the concentration of extracts containing M. oleifera. Higher antifungal activity was obtained using higher concentrations of extracts, but in the case of testing with dry weight, the results were the most promising and reduced the mycelium by up to half. The results indicate that M. oleifera can be used as a natural fungicide, a powerful biofungicide. The minimum inhibitory concentration (MIC) for the root was 25% and was 100% inhibitory on F. oxysporum, F. solani, A. solani. A. alternata, more than 90% R. solani, S. rolfsii and 87.4% M. phaseolina. For the leaf and pod extract, the MIC was 50% and had an inhibitory effect of 100% on F. oxysporum, F. solani, over 90% on A. solani and over 80% on A. alternata, R. solani, S. rolfsii, M. phaseolina (El-Mohamedy and Abdalla, 2014).
The latest study aimed to determine the antifungal potential of M. oleifera against Botrytis cinerea. Methanol and ethanol extracts from dried leaves and seeds were tested. The extracts were plated with five-day-old cultures of B. cinerea mycelia on agar plates (100 mg/mL). The plates were incubated at 28°C for 5–7 days. The MIC was determined by the Broekaert method using a microdilution technique or a microtiter plate. Studies have shown a highly inhibitory effect (99%) on the growth of B. cinerea when using an ethanol extract of the leaves. The MIC was 5 mg/mL and inhibited 100% of the fungal embryos. The minimum fungicidal concentration (MFC) of the ethanol extract from the leaves was 10 mg/mL (Ahmadu et al., 2021).
M. oleifera seeds were tested for their antibacterial activity against the S. aureus strain. The potential effect of seeds as a magnetic coagulant in the elimination of S. aureus in the treatment of dairy wastewater was assessed. 4,990 mL of distilled water and 10 mL of UHT milk were placed in a sterile container. The solution was homogenized by hand and then 5 µL of active S. aureus culture was added. The following evaluations were made: pH, temperature, color, spectrophotometric evaluation of turbidity, microbiological load test. Iron sulfate and iron nitrate nanohydrate were homogenized in distilled water, then sodium hydroxide was added.
15 Summary
M. oleifera is a species of a tropical tree with an increasing importance in the food, pharmaceutical, cosmetic, and agricultural industries. Thanks to its outstanding health-promoting properties, it has earned a few nicknames, like ‘the miraculous tree’ and ‘the tree of long life’. The raw material most often obtained from M. oleifera is the leaf, however, other parts have been used as raw materials, including its fruit, flower, bark, seed and root.
M. oleifera provides raw materials with high nutritional value. M. oleifera seed oil used in addition to the food industry in the cosmetic industry due to its conditioning properties. The chemical profile is dominated by: proteins (amino acids such as arginine and serine), fatty acids (omega-3 and omega-6), vitamins (vitamin A, B and C and tocopherols), minerals (calcium, magnesium, sodium, potassium) and valuable polyphenolic compounds like phenolic acids (gallic acid, ferulic acid) and flavonoids (myricetin, rutoside, kaempferol).
The latest scientific research confirms the health-promoting properties of M. oleifera known from traditional medicine of India, China and African countries, such as: antioxidant, antibacterial, anti-inflammatory, hepatoprotective and antidepressant effects. In addition, research indicates other valuable properties of the raw material, such as neuroprotective, anti-diabetic, accelerating wound healing, anti-psoriatic or anti-cancer properties. M. oleifera as a food plant has descriptions in FDA and EFSA documents. The safety data indicate that M. oleifera is used in health, food and cosmetic products with high confidence.
The growing interest of the food and cosmetics industry as well as numerous studies proving the valuable biological properties of M. oleifera may result in greater interest in this species in the medicinal industry in the future.
Author contributions
MK-S: Data curation, Formal Analysis, Visualization, Writing–original draft, Writing–review and editing. KB-B: Funding acquisition, Writing–original draft. AR: Writing–original draft. EB: Formal Analysis, Writing–review and editing. MT-M: Formal Analysis, Writing–review and editing. KK-S: Formal Analysis, Writing–review and editing. WK-K: Formal Analysis, Writing–review and editing. MM: Formal Analysis, Writing–review and editing. AS: Conceptualization, Data curation, Formal Analysis, Investigation, Methodology, Project administration, Resources, Supervision, Visualization, Writing–original draft, Writing–review and editing.
Funding
The author(s) declare financial support was received for the research, authorship, and/or publication of this article. This study was funded by the Program of the Polish Ministry of Science and Higher Education no. N42/DBS/000273 and Statutory Project DS503-07-01-38 of the University of Information Technology and Management in Rzeszów.
Conflict of interest
The authors declare that the research was conducted in the absence of any commercial or financial relationships that could be construed as a potential conflict of interest.
The author(s) declared that they were an editorial board member of Frontiers, at the time of submission. This had no impact on the peer review process and the final decision.
Publisher’s note
All claims expressed in this article are solely those of the authors and do not necessarily represent those of their affiliated organizations, or those of the publisher, the editors and the reviewers. Any product that may be evaluated in this article, or claim that may be made by its manufacturer, is not guaranteed or endorsed by the publisher.
Supplementary material
The Supplementary Material for this article can be found online at: https://www.frontiersin.org/articles/10.3389/fphar.2024.1288382/full#supplementary-material
References
Abioye, V. F., and Mo, A. (2015). Proximate composition and sensory properties of Moringa fortified maize-ogi. J. Nutr. Food Sci. s12, 1–4. doi:10.4172/2155-9600.s12-001
Aboagye, G., Navele, M., and Essuman, E. (2021). Protocols for assessing antibacterial and water coagulation potential of Moringa oleifera seed powder. MethodsX 8, 101283. doi:10.1016/j.mex.2021.101283
Adedapo, A. A., Mogbojuri, O. M., and Emikpe, B. O. (2009). Safety evaluations of the aqueous extract of the leaves of Moringa oleifera in rats. J. Med. Plant Res. 3, 586–591.
Afuang, W., Siddhuraju, P., and Becker, K. (2003). Comparative nutritional evaluation of raw, methanol extracted residues and methanol extracts of moringa (Moringa oleifera Lam.) leaves on growth performance and feed utilization in Nile tilapia (Oreochromis niloticus L.). Aquac. Res. 34, 1147–1159. doi:10.1046/j.1365-2109.2003.00920.x
Ahmadu, T., Ahmad, K., Ismail, S. I., Rashed, O., Asib, N., and Omar, D. (2021). Antifungal efficacy of Moringa oleifera leaf and seed extracts against botrytis cinerea causing gray mold disease of tomato (Solanum lycopersicum l.). Braz. J. Biol. 81, 1007–1022. doi:10.1590/1519-6984.233173
Ahmed, K., Banik, R., Hossain, M., and Jahan, I. (2016). Vitamin C (L-ascorbic Acid) content in different parts of Moringa oleifera grown in Bangladesh. Am. Chem. Sci. J. 11, 1–6. doi:10.9734/acsj/2016/21119
Ajibade, T. O., Arowolo, R., and Olayemi, F. O. (2013). Phytochemical screening and toxicity studies on the methanol extract of the seeds of Moringa oleifera. J. Complement. Integr. Med. 10, 11–16. doi:10.1515/jcim-2012-0015
Ali, A., Akhtar, N., Mumtaz, A. M., and Khan, M. S. (2013). In vivo skin irritation potential of a cream containing Moringa oleifera leaf extract. Afr. J. Pharm. Pharmacol. 7, 289–293. doi:10.5897/AJPP12.890
Aljazzaf, B., Regeai, S., Elghmasi, S., Alghazir, N., Balgasim, A., Ismail, I. M. H., et al. (2023). Evaluation of antidiabetic effect of combined leaf and seed extracts of Moringa oleifera (Moringaceae) on alloxan-induced diabetes in mice: a biochemical and histological study. Oxid. Med. Cell. Longev. 2023, 9136217. doi:10.1155/2023/9136217
Allen, R. J., Baddeley, A. D., and Hitch, G. J. (2006). Is the binding of visual features in working memory resource-demanding? J. Exp. Psychol. Gen. 135, 298–313. doi:10.1037/0096-3445.135.2.298
Amouri, M., Ahmed-Zaid, T., Aziza, M. A., and Zandouche, O. (2023). Life cycle assessment of Moringa oleifera derived biodiesel: energy efficiency, CO2 intensity and environmental impacts. Environ. Prog. Sustain. Energy. 42 (4), e14079. doi:10.1002/ep.14079
Animashaun, J. O., Ibrahim, H. K., Omotesho, K. F., and Aderinoye-Abdulwahab, S. (2016). Determinants of the neglected and underutilized species’ cultivation by small-scale farmers in Nigerian Guinea Savannah. J. Agribus. Rural. Dev. 4, 493–499. doi:10.17306/JARD.2016.76
Anwar, F., and Rashid, U. (2007). Physicochemical characteristics of Moringa oleifera seeds and seed oil from a wild provenance of Pakistan. Pak. J. Bot. 39, 1443–1453.
Arise, A. K., Arise, R. O., Sanusi, M. O., Esan, O. T., and Oyeyinka, S. A. (2014). Effect of Moringa oleifera flower fortification on the nutritional quality and sensory properties of weaning food. Croat. J. Food Sci. Technol. 6, 65–71. doi:10.17508/cjfst.2014.6.2.01
Arozal, W., Purwoningsih, E., Lee, H. J., Barinda, A. J., and Munim, A. (2022). Effects of Moringa oleifera in two independents formulation and as neuroprotective agent against scopolamine-induced memory impairment in mice. Front. Nutr. 9, 799127–799213. doi:10.3389/fnut.2022.799127
Arulselvan, P., Tan, W. S., Gothai, S., Muniandy, K., Fakurazi, S., Esa, N. M., et al. (2016). Anti-inflammatory potential of ethyl acetate fraction of Moringa oleifera in downregulating the NF- κB signaling pathway in lipopolysaccharide-stimulated macrophages. Molecules 21, 1452. doi:10.3390/molecules21111452
Asiedu-Gyekye, I. J., Frimpong-Manso, S., Awortwe, C., Antwi, D. A., and Nyarko, A. K. (2014). Micro- and macroelemental composition and safety evaluation of the nutraceutical Moringa oleifera leaves. J. Toxicol. 2014, 786979–787013. doi:10.1155/2014/786979
Athikomkulchai, S., Tunit, P., Tadtong, S., Jantrawut, P., Sommano, S. R., and Chittasupho, C. (2021). Moringa oleifera seed oil formulation physical stability and chemical constituents for enhancing skin hydration and antioxidant activity. Cosmetics 8, 2. doi:10.3390/cosmetics8010002
Athira Nair, D., James, T. J., Sreelatha, S. L., Kariyil, B. J., and Nair, S. N. (2020). Moringa oleifera (Lam.): a natural remedy for ageing? Nat. Prod. Res. 35, 6216–6222. doi:10.1080/14786419.2020.1837815
Awodele, O., Adekunle, I., Odoma, S., Teixeira, J. A., and Oluseye, V. (2012). Toxicological evaluation of the aqueous leaf extract of Moringa oleifera Lam. (Moringaceae). J. Ethnopharmacol. 139, 330–336. doi:10.1016/j.jep.2011.10.008
Awoyale, W., Maziya-Dixon, B., Sanni, L., and Shittu, T. A. (2010). Nutritional and sensory properties of amala supplemented with distiller’s spent grain (DSG). J. Food, Agric. Environ. 8, 66–70.
Babayeju, A., Gbadebo, C., Obalowu, M., Otunola, G., Nmom, I., Kayode, R., et al. (2014). Comparison of organoleptic properties of egusi and efo riro soup blends produced with moringa and spinach leaves. Food Sci. Qual. Manag. 28, 15–18.
Balogun, T. A., Buliaminu, K. D., Chukwudozie, O. S., Tiamiyu, Z. A., and Idowu, T. J. (2021). Anticancer potential of Moringa oleifera on BRCA-1 gene: systems biology. Bioinform. Biol. Insights 15, 11779322211010703. doi:10.1177/11779322211010703
Bennett, R. N., Mellon, F. A., Foidl, N., Pratt, J. H., Dupont, M. S., Perkins, L., et al. (2003). Profiling glucosinolates and phenolics in vegetative and reproductive tissues of the multi-purpose trees Moringa oleifera L. (Horseradish tree) and Moringa stenopetala L. J. Agric. Food Chem. 51, 3546–3553. doi:10.1021/jf0211480
Chen, B., Miao, J., Ye, H., Xia, Z., Huang, W., Guo, J., et al. (2023). Purification, identification, and mechanistic investigation of novel selenium-enriched antioxidant peptides from Moringa oleifera seeds. J. Agric. Food Chem. 71, 4625–4637. doi:10.1021/acs.jafc.2c08965
Chigurupati, S., Al-murikhy, A., Almahmoud, S. A., Almoshari, Y., Saber Ahmed, A., Vijayabalan, S., et al. (2022). Molecular docking of phenolic compounds and screening of antioxidant and antidiabetic potential of Moringa oleifera ethanolic leaves extract from Qassim region, Saudi Arabia. Saudi J. Biol. Sci. 29, 854–859. doi:10.1016/j.sjbs.2021.10.021
Chin, C. Y., Jalil, J., Ng, P. Y., and Ng, S. F. (2018). Development and formulation of Moringa oleifera standardised leaf extract film dressing for wound healing application. J. Ethnopharmacol. 212, 188–199. doi:10.1016/j.jep.2017.10.016
Chinma, C. E., Gbadamosi, K. B., Ogunsina, B. S., Oloyede, O. O., and Salami, S. O. (2014). Effect of addition of germinated moringa seed flour on the quality attributes of wheat-based cake. J. Food Process. Preserv. 38, 1737–1742. doi:10.1111/jfpp.12136
Cosmetic Ingredient Database (CosIng) (2023). Cosmetic ingredient database (CosIng). Available at: https://ec.europa.eu/growth/sectors/cosmetics/cosing_en (Accessed June 25, 2023).
Dai, J., Tao, L., Shi, C., Yang, S., Li, D., Sheng, J., et al. (2020). Fermentation improves calcium bioavailability in Moringa oleifera leaves and prevents bone loss in calcium-deficient rats. Food Sci. Nutr. 8, 3692–3703. doi:10.1002/fsn3.1653
Dhakad, A. K., Ikram, M., Sharma, S., Khan, S., Pandey, V. V., and Singh, A. (2019). Biological, nutritional, and therapeutic significance of Moringa oleifera Lam. Phyther. Res. 33, 2870–2903. doi:10.1002/ptr.6475
EFSA (2015). Technical Report on the notification of leaf powder of Moringa stenopetala as a traditional food from a third country pursuant to Article 14 of Regulation (EU) 2015/2283. EFSA J. 16. doi:10.2903/sp.efsa.2019.EN-1672
EFSA (2023). European food safety authority (EFSA). Available at: http://www.efsa.europa.eu/ (Accessed June 25, 2023).
Eilert, U., Wolters, B., and Nahrstedt, A. (1981). The antibiotic principle of seeds of Moringa oleifera and Moringa stenopetala. Planta Med. 42, 55–61. doi:10.1055/s-2007-971546
El-Harairy, M., Abdel-Khalek, A., Khalil, W., Khalifa, E., El-Khateeb, A., and Abdulrhmn, A. (2016). Effect of aqueous extracts of Moringa oleifera leaves or Arctium lappa roots on lipid peroxidation and membrane integrity of ram sperm preserved at cool temperature. J. Anim. Poult. Prod. 7, 467–473. doi:10.21608/jappmu.2016.48804
El-Massary, F. H. M., Mossa, M. E., and Youssef, S. M. (2013). Moringa oleifera plant "value and utilization in food processing. Egypt. J. Agric. Res. 91, 1597–1909. doi:10.21608/ejar.2013.166383
El-Mohamedy, R. S. R., and Abdalla, A. M. (2014). Evaluation of antifungal activity of Moringa oleifera extracts as natural fungicide against some plant pathogenic fungi in-vitro. J. Agric. Technol. 10, 963–982.
EMA (2023). European medicines agency. Available at: https://www.ema.europa.eu/ (Accessed June 18, 2023).
European Commission Cosing (2020). CosIng - cosmetic database. Available online: https://ec.europa.eu/growth/tools-databases/cosing/ (Accessed June 4, 2023).
Ezeamuzie, I. C., Ambakederemo, a W., Shode, F. O., Ekwebelem, S. C., and Harcourt, P. (1996). Antiinflammatory effects of Moringa oleifera. Int. J. Pharmacogn. 34, 207–212. doi:10.1076/phbi.34.3.207.13211
Fathy, S. M., and Mohammed, M. S. M. (2021). Moringa oleifera Lam. leaf extract mitigates carbon tetrachloride-mediated hepatic inflammation and apoptosis via targeting oxidative stress and toll-like receptor 4/nuclear factor kappa B pathway in mice. Food Sci. Hum. Wellness 10, 383–391. doi:10.1016/j.fshw.2021.02.030
FDA (2023). U. S. Food and drug administration. Available at: https://www.fda.gov/ (Accessed June 18, 2023).
Florence, I. (2014). Comparative evaluation of in vitro antioxidant properties of Cajanus cajan seed and Moringa oleifera leaf extracts. Int. J. Biochem. Res. Rev. 4, 163–172. doi:10.9734/ijbcrr/2014/6460
Fuglie, L. J. (1999). The miracle tree: Moringa oleifera, natural nutrition for the tropics. Church World Serv. Dakar 68.
Gandji, K., Chadare, F. J., Idohou, R., Salako, V. K., Assogbadjo, A. E., and Kakaï, R. G. (2018). Status and utilisation of Moringa oleifera Lam: a review. Afr. Crop Sci. J. 26, 137–156. doi:10.4314/acsj.v26i1.10
Gonzalez-Burgos, E., Ureña-Vacas, I., Sanchez, M., and Gomez-Serranillos, P. (2021). Nutritional value of Moringa oleifera Lam. leaf powder and mitochondrial regulation. Nutrients 13, 2203. doi:10.3390/nu13072203
Gopalakrishnan, L., Doriya, K., and Kumar, D. S. (2016). Moringa oleifera: a review on nutritive importance and its medicinal application. Food Sci. Hum. Wellness 5, 49–56. doi:10.1016/j.fshw.2016.04.001
Government of India, Ministry of Ayush (2016). The ayurvedic pharmacopoeia of India Part I volume IX. Ghaziabad: FPharmacopoeia Commission for Indian Medicine and Homeopathy.
Hashim, F. J., Vichitphan, S., Han, J., and Vichitphan, K. (2021). Alternative approach for specific tyrosinase inhibitor screening: uncompetitive inhibition of tyrosinase by Moringa oleifera. Molecules 26, 4576. doi:10.3390/molecules26154576
Haytowitz, D. B., and Bhagwat, S. (2010). USDA database for the oxygen radical absorbance capacity (ORAC) of selected foods, release 2. Beltsville, Maryland: US Deparntment Agric.
Hekmat, S., Morgan, K., Soltani, M., and Gough, R. (2015). Sensory evaluation of locally-grown fruit purees and inulin fibre on probiotic yogurt in Mwanza, Tanzania and the microbial analysis of probiotic yogurt fortified with Moringa oleifera. J. Heal. Popul. Nutr. 33, 60–67.
Himanshu, , Chauhan, M., Sonawane, S. K., and Arya, S. S. (2018). Nutritional and nutraceutical properties of millets: a review. Clin. J. Nutr. Diet. 1, 1–10.
Ibrahim, M. A., Mohamed, S. R., Dkhil, M. A., Thagfan, F. A., Abdel, R., and Soliman, D. (2023). The effect of Moringa oleifera leaf extracts against urethane - induced lung cancer in rat model. Environ. Sci. Pollut. Res. 30, 37280–37294. doi:10.1007/s11356-022-24813-9
Idoga, E. S., Ambali, S. F., Ayo, J. O., and Mohammed, A. (2018). Assessment of antioxidant and neuroprotective activities of methanol extract of Moringa oleifera Lam. leaves in subchronic chlorpyrifos-intoxicated rats. Comp. Clin. Path. 27, 917–925. doi:10.1007/s00580-018-2682-9
Ijarotimi, O. S., Adeoti, O. A., and Ariyo, O. (2013). Comparative study on nutrient composition, phytochemical, and functional characteristics of raw, germinated, and fermented Moringa oleifera seed flour. Food Sci. Nutr. 1, 452–463. doi:10.1002/fsn3.70
Islam, Z., Islam, S. M. R., Hossen, F., Mahtab-ul-islam, K., Hasan, R., and Karim, R. (2021). Moringa oleifera is a prominent source of nutrients with potential health benefits. Int. J. Food Sci. 10, 6627265, 6627265. doi:10.1155/2021/6627265
Jatropha Biodiesel (2023). Agronomy-Moringa plants cultivation Technology,Moringa tree, jatropa cultivation India. Available at: http://www.jatrophabiodiesel.org/moringa/agronomy.php (Accessed May 30, 2023).
Jatropha World (2023). Moringa oleifera. Available at: http://www.jatrophaworld.org/moringa_oleifera_86.html (Accessed May 30, 2023).
Joshi, P., and Mehta, D. (2010). Effect of dehydration on the nutritive value of drumstick leaves. J. Metabolomics Syst. Biol. 1, 5–9.
Karim, N. A. A., Adam, A. H. B., Jaafaru, M. S., Rukayadi, Y., and Razis, F. A. (2023). Apoptotic potential of glucomoringin isothiocyanate (GMG-ITC) isolated from Moringa oleifera Lam seeds on human prostate cancer cells (PC-3). Molecules 28, 3214. doi:10.3390/molecules28073214
Karim, O., Kayode, R., Oyeyinka, S., Oyeyinka, A., and Science, F. (2015). Physicochemical properties of stiff dough “amala” prepared from plantain. Hrana u Zdr. i Boles. Znan. časopis za Nutr. i dijetetiku 4, 48–58.
Karuppusamy, S. (2009). A review on trends in production of secondary metabolites from higher plants by in vitro tissue, organ and cell cultures. J. Med. Plants Res. 3, 1222–1239.
Kashyap, A. S., Manzar, N., Nebapure, S. M., Rajawat, M. V. S., Deo, M. M., Singh, J. P., et al. (2022). Recent advances in drumstick (Moringa oleifera) leaves bioactive compounds: composition, health benefits, bioaccessibility, and dietary applications. Antioxidants 11, 1–37. doi:10.3390/antiox11020404
Kaur, G., Invally, M., Sanzagiri, R., and Buttar, H. S. (2015). Evaluation of the antidepressant activity of Moringa oleifera alone and in combination with fluoxetine. J. Ayurveda Integr. Med. 6, 273–279. doi:10.4103/0975-9476.172384
Kolawole, F. L., Balogun, M. A., Opaleke, D. O., and Amali, H. E. (2013). An evaluation of nutritional and sensory qualities of wheat Moringa cake. Agrosearch 13, 87–93. doi:10.4314/agrosh.v13i1.9
Kumalaningsih, S., and Arwani, M. (2018). Nutritious pure herbal whitening sun cream processed from seed and leaf of Moringa oleifera fortified with Red Rice. Pharmacogn. J. 10, 77–80. doi:10.5530/pj.2018.1.15
Lokapirnasari, W. P., Maslachah, L., Sahidu, A. M., and Yulianto, A. B. (2021). The potency of Pediococcus pentosaceus incubated with Moringa oleifera in fermentation process to increase nutrient content of rice bran. IOP Conf. Ser. Earth Environ. Sci. 718, 012036. doi:10.1088/1755-1315/718/1/012036
Luqman, S., Srivastava, S., Kumar, R., Maurya, A. K., and Chanda, D. (2012). Experimental assessment of Moringa oleifera leaf and fruit for its antistress, antioxidant, and scavenging potential using in vitro and in vivo assays. Evidence-based Complement. Altern. Med. 2012, 519084–519112. doi:10.1155/2012/519084
Lynch, C. (2014). Determining fruit fly (Family Tephritidae) host status of moringa tree pods, Moringa oleifera Lam., in Hawai’i. Food Provid. 2014, 1–10.
Ma, N., Tang, Q., Wu, W. T., Huang, X. A., Xu, Q., Rong, G. L., et al. (2018). Three constituents of Moringa oleifera seeds regulate expression of Th17-relevant cytokines and ameliorate tpa-induced psoriasis-like skin lesions in mice. Molecules 23, 3256–3259. doi:10.3390/molecules23123256
Marfori, E. C. (2010). Clonal micropropagation of Moringa oleifera L. Philipp. Agric. Sci. 93, 454–457.
Mathur, M., Yadav, S., Katariya, P. K., and Kamal, R. (2014). In vitro propagation and biosynthesis of steroidal sapogenins from various morphogenetic stages of Moringa oleifera Lam., and their antioxidant potential. Acta Physiol. Plant. 36, 1749–1762. doi:10.1007/s11738-014-1549-1
Maurya, S. K., and Singh, A. K. (2014). Clinical efficacy of Moringa oleifera Lam. stems bark in urinary tract infections. ISRN Urol. 2014, 906843. doi:10.1155/2014/906843
Mhdawi, A. Q. M. Z. (2022). The Moringa tree and its many uses, especially in the agricultural field. Arab. J. Sci. Publ. 2, 31–38.
Mouchili, M., Tendonkeng, F., Miégoué, E., Wauffo, D. F., Watsop, H. M., Tedonkeng, E. P., et al. (2019). Effects of different poultry manure fertilization levels and cutting times on Moringa oleifera production. Cienc. Investig. Agrar. 46, 310–318. doi:10.7764/rcia.v46i3.2060
Moyo, B., Masika, P. J., Hugo, A., and Muchenje, V. (2011). Nutritional characterization of moringa (Moringa oleifera Lam.) leaves. Afr. J. Biotechnol. 10, 12925–12933. doi:10.5897/ajb10.1599
Nelson, S., and Rau, M. (2011). Moringa: the science behind the miracle tree. Sci. Sch. 2011, 21–26.
Nouman, W., Basra, S. M. A., Siddiqui, M. T., Yasmeen, A., Gull, T., and Alcayde, M. A. C. (2014). Potential of Moringa oleifera L. as livestock fodder crop: a review. Turkish J. Agric. For. 38, 1–14. doi:10.3906/tar-1211-66
Oboh, G., Oyeleye, S. I., Akintemi, O. A., and Olasehinde, T. A. (2018). Moringa oleifera supplemented diet modulates nootropic-related biomolecules in the brain of STZ-induced diabetic rats treated with acarbose. Metab. Brain Dis. 33, 457–466. doi:10.1007/s11011-018-0198-2
Ofor, M., and Nwufo, M. I. (2011). The search for alternative energy sources: jatropha and Moringa seeds for biofuel production. J. Agric. Soc. Res. 11, 87–94. doi:10.4314/jasr.v11i2
Ojo, T. O., Ogunleye, A. S., and Alimi, T. (2016). Factor affecting the profitability of Moringa oleifera production in Oyo State, Nigeria. Ife J. Agric. 28, 46–57.
Oliveira, A. M. de, Mateus, G. A. P., Santos, T. R. T., dos Filho, B. A. de A., Gomes, R. G., and Bergamasco, R. (2021). Functionalized magnetite nanoparticles with Moringa oleifera with potent antibacterial action in wastewater. Environ. Technol. (United Kingdom) 42, 4296–4305. doi:10.1080/09593330.2020.1754923
Oliveira, J. T. A., Silveira, S. B., Vasconcelos, I. M., Cavada, B. S., and Moreira, R. A. (1999). Compositional and nutritional attributes of seeds from the multiple purpose tree Moringa oleifera Lamarck. J. Sci. Food Agric. 79, 815–820. doi:10.1002/(SICI)1097-0010(19990501)79:6<815::AID-JSFA290>3.0.CO;2-P
Olorode, O. O., and Idowu, M. A. (2013). Effect of benoil (Moringa oleifera) leaf powder on the quality characteristics of ‘Ogi’. Am. J. Food Nutr. 3, 83–89. doi:10.5251/ajfn.2013.3.2.83.89
Onasanwo, S. A., Adamaigbo, V. O., Adebayo, O. G., and Eleazer, S. E. (2021). Moringa oleifera - supplemented diet protect against cortico - hippocampal neuronal degeneration in scopolamine - induced spatial memory deficit in mice: role of oxido - inflammatory and cholinergic neurotransmission pathway. Metab. Brain Dis. 36, 2445–2460. doi:10.1007/s11011-021-00855-9
Paikra, B. K., Dhongade, H. K. J., and Gidwani, B. (2017). Phytochemistry and pharmacology of Moringa oleifera Lam. J. Pharmacopuncture 20, 194–200. doi:10.3831/kpi.2017.20.022
Parrotta, A. (2009). “Moringa oleifera Lam., 1785,” in Enzyklopädie der Holzgewächse, Handb. und Atlas der Dendrologie, 1–8. doi:10.1089/jop.2010.0049
Parvathy, M. V. S., and Umamaheshwari, A. (2007). Cytotoxic effect of Moringa oleifera leaf extracts on human multiple myeloma cell lines. Trends Med. Res. 2, 44–50. doi:10.3923/tmr.2007.44.50
Patel, P., Patel, N., Patel, D., Desai, S., and Meshram, D. (2014). Phytochemical analysis and antifungal activity of Moringa oleifera. Int. J. Pharm. Pharm. Sci. 6, 144–147.
Patel, S., Thakur, A. S., Chandy, A., and Manigauha, A. (2010). Moringa oleifera: a review of their medicinal and economical importance to the health and nation. Drug Invent 2, 339–342.
Peixoto, J. R. O., Silva, G. C., Costa, R. A., de Sousa Fontenelle, , Fontenelle, J. R. L. S., Fernardes Vieira, G. H., et al. (2011). In vitro antibacterial effect of aqueous and ethanolic Moringa leaf extracts. Asian pac. J. Trop. Med. 4, 201–204. doi:10.1016/S1995-7645(11)60069-2
Posmontier, B. (2011). The Medicinal qualities of Moringa oleifera. Holist. Nurs. Pract. 25, 80–87. doi:10.1097/HNP.0b013e31820dbb27
Pramanik, A., and Islam, S. S. (1998). Chemical investigation of aqueous extract of the mature and premature flowers of Moringa oleifera (Sajina) and structural studies of a polysaccharide isolated from it’s premature flowers. Indian J. Chem. - Sect. B Org. Med. Chem. 37, 676–682.
Ramachandran, C., Peter, K. V., and Gopalakrishnan, P. K. (1980). Drumstick (Moringa oleifera): a multipurpose indian vegetable. Econ. Bot. 34, 276–283. doi:10.1007/bf02858648
Ramota, O., and Ojo, R. M. (2013). Proximate, mineral and sensory qualities of ‘Amala’ prepared from yam flour fortified with Moringa leaf powder. Food Sci. Qual. Manag. 12, 10–23.
Richter, N., Siddhuraju, P., and Becker, K. (2003). Evaluation of nutritional quality of Moringa (Moringa oleifera Lam.) leaves as an alternative protein source for Nile tilapia (Oreochromis niloticus L.). Aquaculture 217, 599–611. doi:10.1016/S0044-8486(02)00497-0
Rodríguez-García, T., Camacho-Díaz, B. H., Jiménez–Aparicio, A. R., Santaolalla-Tapia, J., Evangelista-Lozano, S., and Arenas-Ocampo, M. L. (2021). Cell proliferation and migration in human skin sibroblasts induced by Moringa oleifera. Rev. Bras. Farmacogn. 31, 302–309. doi:10.1007/s43450-021-00160-7
Rolim, L. A. D. M. M., Macedo, M. F. S., Sisenando, H. A., Napole, T. H., Felzenszwalb, I., Aiub, C. A. F., et al. (2011). Genotoxicity evaluation of Moringa oleifera seed extract and lectin. J. Food Sci. 76, T53–T58. doi:10.1111/j.1750-3841.2010.01990.x
Ross, I. A. (2003). “Moringa pterygosperma,” in Medicinal plant of the world (New York: Humana Press Inc.).
Saini, R. K., Shetty, N. P., Giridhar, P., and Ravishankar, G. A. (2012). Rapid in vitro regeneration method for Moringa oleifera and performance evaluation of field grown nutritionally enriched tissue cultured plants. 3 Biotech. 2, 187–192. doi:10.1007/s13205-012-0045-9
Saini, R. K., Shetty, N. P., Prakash, M., and Giridhar, P. (2014). Effect of dehydration methods on retention of carotenoids, tocopherols, ascorbic acid and antioxidant activity in Moringa oleifera leaves and preparation of a RTE product. J. Food Sci. Technol. 51, 2176–2182. doi:10.1007/s13197-014-1264-3
Saka, W. A., Adeshina, O. A., Yusuf, M. G., and Omole, A. I. (2022). Hepatoprotective and renoprotective effect of Moringa oleifera seed oil on dichlorvos-induced toxicity in male wistar rats. Niger. J. Physiol. Sci. 37, 119–126. doi:10.54548/njps.v37i1.15
Salem, A. S., Salama, W. M., Hassanein, A. M., and El-Ghandour, H. M. A. (2013). Enhancement of nutritional and biological values of labneh by adding dry leaves of Moringa oleifera as innovative dairy products. World Appl. Sci. J. 22, 1194–1602. doi:10.5829/idosi.wasj.2013.22.11.13024
Smetanska, I. (2008). Production of secondary metabolites using plant cell cultures. Adv. Biochem. Eng. Biotechnol. 111, 187–228. doi:10.1007/10_2008_103
Sreelatha, S., Jeyachitra, A., and Padma, P. R. (2011). Antiproliferation and induction of apoptosis by Moringa oleifera leaf extract on human cancer cells. Food Chem. Toxicol. 49, 1270–1275. doi:10.1016/j.fct.2011.03.006
Sreelatha, S., and Padma, P. R. (2009). Antioxidant activity and total phenolic content of Moringa oleifera leaves in two stages of maturity. Plant Foods Hum. Nutr. 64, 303–311. doi:10.1007/s11130-009-0141-0
Stephenson, K. K., and Fahey, J. W. (2004). Development of tissue culture methods for the rescue and propagation of endangered Moringa spp. germplasm. Economi 58, S116–S124. doi:10.1663/0013-0001(2004)58[s116:dotcmf]2.0.co;2
Stevens, G. C., Baiyeri, K. P., and Akinnnagbe, O. (2013). Ethno-medicinal and culinary uses of Moringa oleifera Lam. in Nigeria. J. Med. Plants Res. 7, 799–804. doi:10.5897/JMPR12.1221
Sujatha, B. K., and Patel, P. (2017). Moringa oleifera – nature ’ s gold. Imp. J. Interdiscip. Res. 3, 1175–1179.
Sultana, B., and Anwar, F. (2008). Flavonols (kaempeferol, quercetin, myricetin) contents of selected fruits, vegetables and medicinal plants. Food Chem. 108, 879–884. doi:10.1016/j.foodchem.2007.11.053
Sun, C., Li, W., Liu, Y., Deng, W., Adu-Frimpong, M., Zhang, H., et al. (2019). In vitro/in vivo hepatoprotective properties of 1-O-(4-hydroxymethylphenyl)-α-L-rhamnopyranoside from Moringa oleifera seeds against carbon tetrachloride-induced hepatic injury. Food Chem. Toxicol. 131, 110531. doi:10.1016/j.fct.2019.05.039
Sutalangka, C., Wattanathorn, J., Muchimapura, S., and Thukham-mee, W. (2013). Moringa oleifera mitigates memory impairment and neurodegeneration in animal model of age-related dementia. Oxid. Med. Cell. Longev. 2013, 695936. doi:10.1155/2013/695936
Taiwo Olagbemide, P., and Alikwe, P. C. N. (2015). Proximate analysis and chemical composition of raw and defatted Moringa oleifera kernel ecotoxicological assessment of fish in aquatic environment. Adv. Life Sci. Technol. 24, 92–100.
Tang, S. Q., Du, Q. H., and Fu, Z. (2021). Ultrasonic treatment on physicochemical properties of water-soluble protein from Moringa oleifera seed. Ultrason. Sonochem. 71, 105357. doi:10.1016/j.ultsonch.2020.105357
Thongrung, R., Pannangpetch, P., Senggunprai, L., Sangkhamanon, S., Boonloh, K., and Tangsucharit, P. (2023). Moringa oleifera leaf extract ameliorates early stages of diabetic nephropathy in streptozotocin-induced diabetic rats. J. Appl. Pharm. Sci. 13, 158–166. doi:10.7324/JAPS.2023.89429
Toppo, R., Roy, B. K., Gora, R. H., Baxla, S. L., and Kumar, P. (2015). Hepatoprotective activity of Moringa oleifera against cadmium toxicity: in rats. Vet. World 8, 537–540. doi:10.14202/vetworld.2015.537-540
Trade Promotion Council of India (2021). Moringa: on track for an export surge? – India business and trade, an initiative of trade promotion Council of India. Available at: https://www.tpci.in/indiabusinesstrade/blogs/moringa-on-track-for-an-export-surge/ (Accessed May 29, 2023).
Trees for Life (2023). Names of moringa. Available at: https://treesforlife.org (Accessed June 26, 2023).
Vergara-Jimenez, M., Almatrafi, M. M., and Fernandez, M. L. (2017). Bioactive components in Moringa oleifera leaves protect against chronic disease. Antioxidants 6, 91–13. doi:10.3390/antiox6040091
Wang, Y., Ouyang, Q., Chang, X., Yang, M., He, J., Tian, Y., et al. (2022). Anti-photoaging effects of flexible nanoliposomes encapsulated Moringa oleifera Lam. isothiocyanate in UVB-induced cell damage in HaCaT cells. Drug Deliv. 29, 871–881. doi:10.1080/10717544.2022.2039802
WHO (2023). World health organization. Available at: https://www.who.int/ (Accessed December 18, 2022).
World Flora Online (2022). World Flora online. Available at: http://www.worldfloraonline.org/ (Accessed August 9, 2023).
Wu, L., Li, L., Chen, S., Wang, L., and Lin, X. (2020). Deep eutectic solvent-based ultrasonic-assisted extraction of phenolic compounds from Moringa oleifera L. leaves: optimization, comparison and antioxidant activity. Sep. Purif. Technol. 247, 117014. doi:10.1016/j.seppur.2020.117014
Xie, J., Yang, M. R., Hu, X., Hong, Z. S., Bai, Y. Y., Sheng, J., et al. (2023). Moringa oleifera Lam. isothiocyanate quinazolinone derivatives inhibit U251 glioma cell proliferation through cell cycle regulation and apoptosis induction. Int. J. Mol. Sci. 24, 11376. doi:10.3390/ijms241411376
Yang, R. Y., Lin, S., and Kuo, G. (2008). Content and distribution of flavonoids among 91 edible plant species. Asia Pac. J. Clin. Nutr. 17, 275–279.
Yousefirad, A., Rastegari, A. A., Shahanipour, K., and Monajemi, R. (2023). Evaluation of Proliferative inhibition effect of Moringa oleifera total extract on breast cancer: an in vitro and in vivo study. Iran. J. Sci. 47, 653–662. doi:10.1007/s40995-023-01434-6
Zanella, L., Gismondi, A., Di Marco, G., Braglia, R., Scuderi, F., Redi, E. L., et al. (2019). Induction of antioxidant metabolites in Moringa oleifera callus by abiotic stresses. J. Nat. Prod. 82, 2379–2386. doi:10.1021/acs.jnatprod.8b00801
Zeitoun, H., Michael-Jubeli, R., El Khoury, R., Baillet-Guffroy, A., Tfayli, A., Salameh, D., et al. (2020). Skin lightening effect of natural extracts coming from Senegal botanical biodiversity. Int. J. Dermatol. 59, 178–183. doi:10.1111/ijd.14699
Zhang, M. (2011). Phytochemicals, antioxidant and antimicrobial activity of Hibiscus sabdariffa, Centella asiatica, Moringa oleifera and Murraya koenigii leaves. J. Med. Plants Res. 5 (30), 6672–6680. doi:10.5897/jmpr11.621
Keywords: Moringa oleifera, drumstick tree, chemical composition, nutritional properties, pharmacological activities, cosmetology applications, agronomic importance
Citation: Klimek-Szczykutowicz M, Gaweł-Bęben K, Rutka A, Blicharska E, Tatarczak-Michalewska M, Kulik-Siarek K, Kukula-Koch W, Malinowska MA and Szopa A (2024) Moringa oleifera (drumstick tree)—nutraceutical, cosmetological and medicinal importance: a review. Front. Pharmacol. 15:1288382. doi: 10.3389/fphar.2024.1288382
Received: 04 September 2023; Accepted: 05 January 2024;
Published: 02 February 2024.
Edited by:
Lei Zhao, Chinese Academy of Sciences (CAS), ChinaReviewed by:
Sapna Langyan, Indian Council of Agricultural Research (ICAR), IndiaAnoma Janaki Mohotti, University of Peradeniya, Sri Lanka
Copyright © 2024 Klimek-Szczykutowicz, Gaweł-Bęben, Rutka, Blicharska, Tatarczak-Michalewska, Kulik-Siarek, Kukula-Koch, Malinowska and Szopa. This is an open-access article distributed under the terms of the Creative Commons Attribution License (CC BY). The use, distribution or reproduction in other forums is permitted, provided the original author(s) and the copyright owner(s) are credited and that the original publication in this journal is cited, in accordance with accepted academic practice. No use, distribution or reproduction is permitted which does not comply with these terms.
*Correspondence: Agnieszka Szopa, YS5zem9wYUB1ai5lZHUucGw=