- 1Department of Respiratory and Critical Care Medicine, Aerospace Center Hospital, Peking University Aerospace School of Clinical Medicine, Beijing, China
- 2Department of Pharmaceutical Sciences, College of Pharmacy and Health Sciences, St. John’s University, Queens, NY, United States
- 3Department of Traditional Chinese Medicine, Beijing Geriatric Hospital, Beijing, China
- 4Department of Pharmacy, Aerospace Center Hospital, Peking University Aerospace School of Clinical Medicine, Beijing, China
- 5Department of Peripheral Vascular Intervention, Aerospace Center Hospital, Peking University Aerospace School of Clinical Medicine, Beijing, China
In light of a global rise in the number of patients with type 2 diabetes mellitus (T2DM) and obesity, non-alcoholic fatty liver disease (NAFLD), now known as metabolic dysfunction-associated fatty liver disease (MAFLD) or metabolic dysfunction-associated steatotic liver disease (MASLD), has become the leading cause of hepatocellular carcinoma (HCC), with the annual occurrence of MASLD-driven HCC expected to increase by 45%–130% by 2030. Although MASLD has become a serious major public health threat globally, the exact molecular mechanisms mediating MASLD-driven HCC remain an open problem, necessitating future investigation. Meanwhile, emerging studies are focusing on the utility of bioactive compounds to halt the progression of MASLD to MASLD-driven HCC. In this review, we first briefly review the recent progress of the possible mechanisms of pathogenesis and progression for MASLD-driven HCC. We then discuss the application of bioactive compounds to mitigate MASLD-driven HCC through different modulatory mechanisms encompassing anti-inflammatory, lipid metabolic, and gut microbial pathways, providing valuable information for future treatment and prevention of MASLD-driven HCC. Nonetheless, clinical research exploring the effectiveness of herbal medicines in the treatment of MASLD-driven HCC is still warranted.
Introduction
As the most common type of primary liver cancer, hepatocellular carcinoma (HCC) represents the fifth most common cancer worldwide (Akinyemiju et al., 2017). Currently, HCC is on the path to globally becoming the second most common cause of cancer-related death. Historically, cirrhosis induced by chronic infections (such as hepatitis B/C virus) and alcoholic hepatotoxicity are the two major causes of HCC (Villanueva, 2019). However, the incidence of chronic infection-associated HCC is decreasing with the development of anti-HCV drugs and anti-HBV vaccines (Akinyemiju et al., 2017). Accumulating evidence suggests that HCC-associated mortality is increasing steadily, indicating that other risk factors besides alcohol and viral hepatitis drive HCC pathogenesis.
With a global rise in the number of patients with type 2 diabetes mellitus (T2DM) and obesity, non-alcoholic fatty liver disease (NAFLD), now named metabolic dysfunction-associated fatty liver disease (MAFLD) (Eslam et al., 2020a; Eslam et al., 2020b; Lim et al., 2021; Pirola and Sookoian, 2022; Sangro et al., 2023) or metabolic dysfunction-associated steatotic liver disease (MASLD) (Yang et al., 2023; Rinella et al., 2023a; Rinella et al., 2023b; Rinella et al., 2023c; Noureddin et al., 2023; He et al., 2023; Chan et al., 2023; Hagström et al., 2023), has been postulated to become the leading etiology of HCC, leading to MASLD-associated hepatocellular carcinoma (MASLD-HCC) (Younossi et al., 2018; Zhou et al., 2019; Sarin et al., 2020; Talamantes et al., 2023). The prevalence of MASLD-HCC is increasing in most countries worldwide and presents a major healthcare burden (Koh et al., 2024). However, the underlying molecular mechanism of MASLD progression to HCC remains largely unknown (Yu et al., 2020; Di Maira et al., 2022). As a disease continuum progressing from simple steatosis to non-alcoholic steatohepatitis (NASH), now named as metabolic dysfunction-associated steatohepatitis (MASH) and fibrosis, MASLD affects 25% of adults and is one of the most common chronic liver diseases globally (Bence and Birnbaum, 2021; Powell et al., 2021; Kalligeros et al., 2023). Approximately 27% of cirrhosis induced by MASH could progress to MASLD-HCC (Ioannou, 2021) (Figure 1). Although several drugs are being tested for MASLD/MASH treatment, no curative agents are effective against MASLD-HCC (Wong, 2018). Therefore, there is an unmet need for the discovery of novel and safe drugs for MASLD-HCC. Meanwhile, emerging bioactive compounds have been shown to halt the progression of MASLD to MASLD-driven HCC (Khairnar et al., 2023).
This article aims to assess the recent advancements in pharmacological therapy against MASLD-HCC. In this review, we first briefly review the possible main mechanisms of the progression and pathogenesis of MASLD-HCC. Then, we overview the application of bioactive compounds in mitigating MASLD-HCC. We categorize drugs that treat MASLD-HCC by their mechanisms (anti-inflammatory, lipid metabolism, and gut microbiota), providing valuable information for future treatment and prevention of MASLD-HCC.
Distinctive features of MASLD-HCC
Patient demographics have revealed that patients with MASLD and HCC are mainly White, male, and older than patients with HCC from other origins (Degasperi and Colombo, 2016). A prospective multicenter study in Italy indicates that compared to patients with hepatitis C-related tumors, 54% of MASLD-HCC patients had no evidence of cirrhosis histologically or clinically, were younger, and were less frequently diagnosed during surveillance (Piscaglia et al., 2016). MASLD-HCC tumors tended to be larger and fell less frequently within the Milan criteria or in BCLC stage 0. Compared to HCV-related HCC, MASLD-HCC manifested an infiltrative pattern (Piscaglia et al., 2016). This observation was corroborated by a retrospective cohort HCC study at the Veterans Affairs Hospitals in the United States, in which patients with hepatitis C HCC were younger than those with MASLD-HCC (Mittal et al., 2015). HCC in veterans with MASLD was distinctively characterized by diabetes and dysmetabolism, including peripheral arteriopathy, myocardial infarction, and congestive heart failure. Owing to the burdens of age and comorbidities, patients in the hepatitis C group had a higher likelihood of curative treatment than those with MASLD-HCC (22% VS 11%) (Mittal et al., 2015). A more recent study provided compelling evidence that HCC may also develop in MASLD patients with persistently normal values of transaminase activity (Natarajan et al., 2020).
It has been shown in both population-based and large cohort studies that MASLD patients have an increased risk for metabolic syndrome and diabetes complication-associated cardiovascular comorbidities compared with patients with HCC without MASLD. The median age was 73 years and 66 years in patients with MASLD and hepatitis C, respectively, as per the SEER Registry (Younossi et al., 2015). White patients accounted for 57% (1,560/2,536) of 2,536 patients with hepatitis C and 76% (532/701) of 701 cases with MASLD (Younossi et al., 2015). These results aligned well with the findings of the cohort study of 1,500 American veterans with HCC, where it was reported that the prevalence of arterial hypertension (95% vs 70%) and diabetes (89% vs 33%) was higher in the MASLD patient group than in patients with hepatitis C (Mittal et al., 2015). MASLD patients had significantly higher rates of cardiovascular disease and peripheral vascular disease. Mittal’s study uncovered that a poor prognosis in patients with MASLD results from the burden of comorbidities, leading to limited access to more radical treatments, such as hepatic resection or transplantation (Mittal et al., 2015). A recent retrospective study replicated these findings, which compared the clinicopathological characteristics and prognosis of patients undergoing surgical resection in MASLD/MASH-associated HCC and other HCC etiologies (Pal Chaudhary et al., 2023). A total of 110 HCC patients had MASLD/MASH, and 150 patients had other etiologies. The median age at diagnosis was lower in the other etiology cohort than in the MASLD/MASH-HCC cohort, with a decreased number of female patients (Pal Chaudhary et al., 2023). The diameter of tumors induced by MASLD/MASH was more commonly >5 cm. There were no significant differences in rates of lymphovascular or perineural invasion, histologic grade, or serum AFP levels. There was a lower rate of background liver fibrosis, lower aspartate transaminase, lower alanine aminotransferase, and higher platelet counts in the MASLD/MASH cohort (Pal Chaudhary et al., 2023). These findings suggest that patients with MASLD/MASH-HCC more commonly presented with larger HCC tumors and lacked liver fibrosis than those with HCC due to other etiologies.
Pathogenesis of MASLD-driven HCC
Many studies have investigated the specific mechanisms of transition from MASLD/MASH to HCC. It is thought that this process involves multiple factors including oxidative stress, lipotoxicity, gut dysbiosis, metabolic imbalances, chronic injury, and hypoxia, which, in turn, stimulate chronic inflammation, tissue scarring, and HCC pathogenesis (Cannito et al., 2023). In this section, we will summarize the current knowledge about these factors and how they may be involved in the transition from MASLD/MASH to HCC (Figure 2).
Chronic inflammation in MASLD-HCC
Many variables play a role in the MASLD to MASH transition, including metabolic dysfunction, oxidative stress, gut dysbiosis, lipotoxicity, and hepatocellular necrosis, all of which induce chronic inflammation, leading to perpetual tissue injury, parenchymal cell regeneration, mutagenesis, and HCC tumorigenesis. Hepatic oxidative stress, inflammation, and insulin resistance (IR) are important features and hallmarks of MASLD-HCC (Talamantes et al., 2023). Enhanced inflammation and IR are found in patients with HCC, evidenced by elevated levels of pro-inflammatory cytokines such as interleukin-6 (IL-6), tumor necrosis factor-α (TNF-α), and C-reactive protein (Aleksandrova et al., 2014; Duan et al., 2022). Both obesity and IR systemically are responsible for HCC tumorigenesis as they induce inflammation and activate oncogenic pathways (Margini and Dufour, 2016). The chronic low-grade inflammation that has been linked to HCC development is a distinctive feature of MASLD (Talamantes et al., 2023). Hepatocellular injury due to persistent stimulation of innate and adaptive immune pathways, gut dysbiosis, and low-grade chronic systemic inflammation in MASLD (Cannito et al., 2023) all contribute to the pathogenesis of MASLD-HCC (Marengo et al., 2016; Polyzos et al., 2023; Talamantes et al., 2023). The liver is chronically exposed to TNF-α and IL-6, the two major obesity-associated adipose-derived pro-inflammatory cytokines (Avgerinos et al., 2019; Potoupni et al., 2021). Cytokines facilitate inflammatory and immune tolerance in the liver microenvironment. Chemokines have also been associated with the pathogenesis of MASLD-HCC. It was found that MASLD-HCC patients have higher plasma levels of IL-8, IL-13, chemokine (C–C motif) ligand (CCL-3), CCL-4, and CCL-5, which are correlated with activated circulating monocytes (Ponziani et al., 2019). Several signaling pathways linked to inflammation, steatosis, and oncogenes can be activated by IL-6 and TNF-α in hepatocytes. Upregulation of TNF-α and IL-6 promoted hepatic steatosis and inflammation in a mouse obesity model (Park et al., 2010). Of note, Janus kinase/signal transducer and activator of transcription 3 (JAK/STAT-3) and c-Jun N-terminal kinase/nuclear factor kappa B (JNK/NF-κB) pathways are commonly activated in HCC (Llovet et al., 2021). TNF-α activates the NF-κB and JNK pathways, inducing the transcription of genes involved in hepatic cell proliferation, inflammation, and apoptosis (Yu et al., 2013). IL-6 activates the JAK/STAT-3 pathway, which is linked to cell differentiation and cell growth (Grohmann et al., 2018). Furthermore, oncogenic STAT3 activity associated with HCC pathogenesis is induced by both the inflammatory response and steatosis (Park et al., 2010). The detailed role of intracellular mitogenic, anti-apoptotic, and other signaling pathways involved in the pathogenesis of MASLD-HCC has been recently reviewed [see (Polyzos et al., 2023; Talamantes et al., 2023) for a recent review].
Oxidative stress in MASLD-HCC
Accumulating evidence reveals that the role of oxidative stress in the pathogenesis of MASLD-HCC is well-elucidated and established, with precise mechanistic exploration recently published (Brahma et al., 2021; Gabbia et al., 2021). Excessive hepatic lipid exposure triggers oxidative stress-dependent cell damage through different mechanisms (Bessone et al., 2019). Oxidative stress and ensuing hepatocyte death substantially promote cell proliferation and activate hepatic resident Kupffer cells simultaneously, which secrete chemokines and cytokines to recruit other immune cells, thereby intensifying inflammation and further facilitating the production of reactive oxygen species (ROS) (Wen et al., 2021). At the same time, chronic oxidative stress promotes genomic DNA mutations and upregulates HCC development-related genes (Wen et al., 2021). Increased lipid accumulation can generate pathogenic drivers of carcinogenesis in hepatocytes, in particular oxidative DNA damage (Tanaka et al., 2013; Masarone et al., 2018), which has been found higher in the hepatocytes of MASH-HCC patients than in the hepatocytes of MASH patients without HCC(Tanaka et al., 2013). Inadequate mitochondrial respiratory chain activity and elevated mitochondrial fatty acid β-oxidation (FAO) lead to production of ROS in hepatocytes that can damage DNA in MASLD (Begriche et al., 2013). Dysfunction of antioxidant cellular mechanisms can result in an elevation in fatty acid (FA) metabolism-associated pathways, which causes fatty acid accumulation, steatosis, and metabolic stress (Masarone et al., 2018). Enhanced FA accumulation leads to de novo lipogenesis (DNL) and FAO, which promotes ROS generation (Perla et al., 2017). Hepatic steatosis functions as a precursor for ROS generation and can promote STAT-1 and STAT-3 activity through oxidizing constituently active phosphatases, driving MASH, fibrosis, and HCC pathogenesis (Grohmann et al., 2018). Since increased oxidative stress and ROS have been linked to the transition from MASLD to HCC, emerging studies have focused on uncovering the role of NADPH oxidases (NOXs) in MASLD-HCC. Wang et al. (2022) have shown that the transducer of the Hippo pathway, transcriptional co-activator with PDZ binding motif (TAZ), contributes to MASH-associated HCC in pre-tumor MASH hepatocytes via induction of Cybb and NOX2-mediated DNA damage. This interesting study provided compelling evidence that TAZ plays a role in the progression of MASH to HCC and suggested a future therapeutic option for treating MASH-HCC by targeting MASH through TAZ. Wang et al. (2022) provided a new research paradigm to assess the molecular mechanisms related to MASLD/MASH-HCC, observing pathogenesis by modulating TAZ-mediated NOX2-derived oxidative damage to the DNA of hepatocytes. The author suggested that TAZ-mediated therapies may be a potential avenue for MASH-HCC treatment.
Immune dysregulation in MASLD-HCC
The role of the immune response in the pathogenesis of MASLD-HCC has been comprehensively revealed and summarized elsewhere [see (Anstee et al., 2019; Hirsova et al., 2021; Zhang and Yang, 2021; Riaz et al., 2022; Yahoo et al., 2023) for a recent review]. In short, chronic inflammation induced by pathogen-associated molecular patterns (PAMPs) and damage-associated molecular patterns (DAMPs) serves as a significant driver of hepatocarcinogenesis (Park et al., 2010; Arrese et al., 2016). Vital immunosuppression by T regulatory cells (Tregs) and myeloid-derived suppressor cells (MDSCs) on cytotoxic CD8+ T cells and natural killer (NK) cells mediates immune escape from antitumor responses. Targeting the recruitment of MDSCs and Tregs into the liver is a potentially druggable step in the pathogenesis of MASH-HCC, especially after observing that platelet-derived platelet glycoprotein Ibα (GPIbα) proved critical for the development of MASH-HCC (Malehmir et al., 2019). The role of adaptive immunity in the pathogenesis of MASLD-HCC has also been revealed, showing that IgA+ plasma cells accumulate in MASH fibrosis and lead to MASLD-HCC by inhibiting CD8+ T cells via IL-10 and programmed cell death ligand 1 (PD-L1) expression (Shalapour et al., 2017). These immunosuppressive IgA+ plasma cells may play a key role in the gut microbiome–liver axis, contributing to HCC carcinogenesis by providing mucosal IgA exposure.
Gut microbiome dysbiosis in MASLD-HCC
Recent studies have shown that gut microbiota dysbiosis contributes to MASLD-HCC formation, even in the absence of cirrhosis (Xie et al., 2016; Sydor et al., 2020; Yahoo et al., 2023). Emerging studies demonstrate that the intestinal microbiota play a role in stimulating and maintaining liver inflammation, which becomes more pro-inflammatory as the disease progresses toward HCC (Xie et al., 2016; Sydor et al., 2020). This initial animal study was corroborated by a clinical study, which reported a decreased abundance of Bifidobacterium and an elevated abundance of Ruminococcaceae and Bacteroides in MASLD-HCC patients compared to patients with cirrhosis, which did not progress to MASLD-HCC (Ponziani et al., 2019). Decreased α-diversity (a measure of microbiome diversity applicable to a single sample) was found in patients with MASLD-HCC (Ponziani et al., 2019). Later work elaborated on this finding, demonstrating that a decreased α-diversity and the Chao-1 richness index were found in patients with MASLD-HCC (Behary et al., 2021). In addition, there is a relationship between the gut microbiota and several inflammatory cytokines such as higher levels of IL-8 and CCL-3 in patients with MASLD-HCC (Ponziani et al., 2019). This suggests that inflammation driven by gut microbiota may aggravate the progression of MASLD-HCC. This dysbiosis was confirmed by Behary et al. (2021) in MASLD-HCC and MASLD-cirrhosis patients. An increased rarity index was found in patients with MASH-HCC with cirrhosis (Sydor et al., 2020). Gut microbiota also function as cofactors in the pathogenesis of MASLD-HCC via interaction with immune cells. A recent study on 32 MASLD-HCC patients indicates that gut microbiota augment the expansion of IL-10+ Treg cells but reduce the expansion of CD8+ T cells (Behary et al., 2021). Gut microbiota dysbiosis contributes to the pathogenesis of MASLD-HCC in a spontaneous MASLD-HCC mouse model. Dietary cholesterol can trigger MASLD-HCC tumorigenesis by enhancing the abundance of Desulfovibrio, Mucispirillum, Desulfovibrionaceae, and Anaerotruncus and decreasing levels of Bacteroides and Bifidobacterium (Odenwald and Turner, 2017). Gut microbiota dysbiosis and alteration of gut bacterial metabolites contribute to the pathogenesis of MASLD-HCC in mice, and several probiotics including Lactobacillus and Bifidobacterium strains were identified as depleted in MASLD-HCC (Zhang X. et al., 2021).
Role of lipotoxicity and glucotoxicity in MASH and HCC development
Hepatocytes function as a major site for fat accumulation, making them a main target of lipotoxicity (Rao et al., 2023; Yahoo et al., 2023). IR in adipose tissue results in enhanced release of free FAs and delivery to the liver, allowing for excessive lipid accumulation and toxic metabolite-induced lipotoxicity, causing mitochondrial dysfunction and endoplasmic reticulum stress (ERS) (Venkatesan et al., 2023; Yahoo et al., 2023). Increased free FA flux to the mitochondria enhances the rates of FAO, leading to increased ROS production (Serviddio et al., 2013). In MASLD, the damage in balance between antioxidant mechanisms and ROS production results in oxidative stress and thereby further induces mitochondrial dysfunction. Uncontrolled ROS production and the ensuing oxidative stress directly cause damage to cellular macromolecules such as DNA, proteins, and lipids (Chen et al., 2020). Lipotoxicity mediated by diacylglycerol and non-esterified FAs facilitates hepatic IR and ERS, which results in chronic inflammation, hepatic fibrosis, hepatic cirrhosis, and ultimately HCC (Samuel et al., 2010; Hardy et al., 2016; Piscaglia et al., 2016; Foerster et al., 2022). A recent study has revealed that decreased lysine-specific demethylase 6B (KDM6B), a key mediator of gene transcription, contributes to the development of MASLD-related HCC. This is accomplished by acquiring resistance to lipotoxicity via epigenetic downregulation of G0S2 expression-mediated activation of adipose triglyceride lipase/patatin-like phospholipase domain containing 2 (ATGL/PNPLA2) (Hatano et al., 2023). KDM6B loss may promote cell survival through activation of ATGL/PNPLA2 in NASH-related HCC. A high ATGL/PNPLA2 activation level was found in KDM6B-KO cells. Genetic or pharmacological inhibition of ATGL/PNPLA2 increased lipid accumulation and decreased cell proliferation in KDM6B-KO cells. ATGL/PNPLA2 overexpression facilitates the growth of HCC cells (Liu et al., 2019). Silencing G0S2 conferred lipotoxicity resistance in KDM6B-expressed HCC cells, whereas ATGL/PNPLA2 inhibition in the KDM6B-KO cells reduced these effects. These results indicate that targeting the KDM6B–G0S2–ATGL/PNPLA2 pathway may be a useful therapeutic strategy for MASLD-related HCC. Pharmacologically induced lipotoxicity comprising LXR agonists and Raf inhibitors represents a promising therapeutic strategy for the treatment of MASLD-related HCC (Rudalska et al., 2021).
Current pharmacological therapies for MASH-driven HCC
Following a better understanding of the molecular mechanisms behind MASLD/MASH-HCC progression, research on anti-MASLD/MASH-HCC small molecules has intensified, resulting in an exponentially increasing number of published articles on this field. We present newly identified small molecules for the inhibition of MASLD-HCC disease progression (Table 1; Figure 3), describing drugs targeting anti-inflammatory, antioxidant, anti-fibrotic, anti-lipid metabolic, microbiota-pertaining, and immunomodulatory mechanisms.
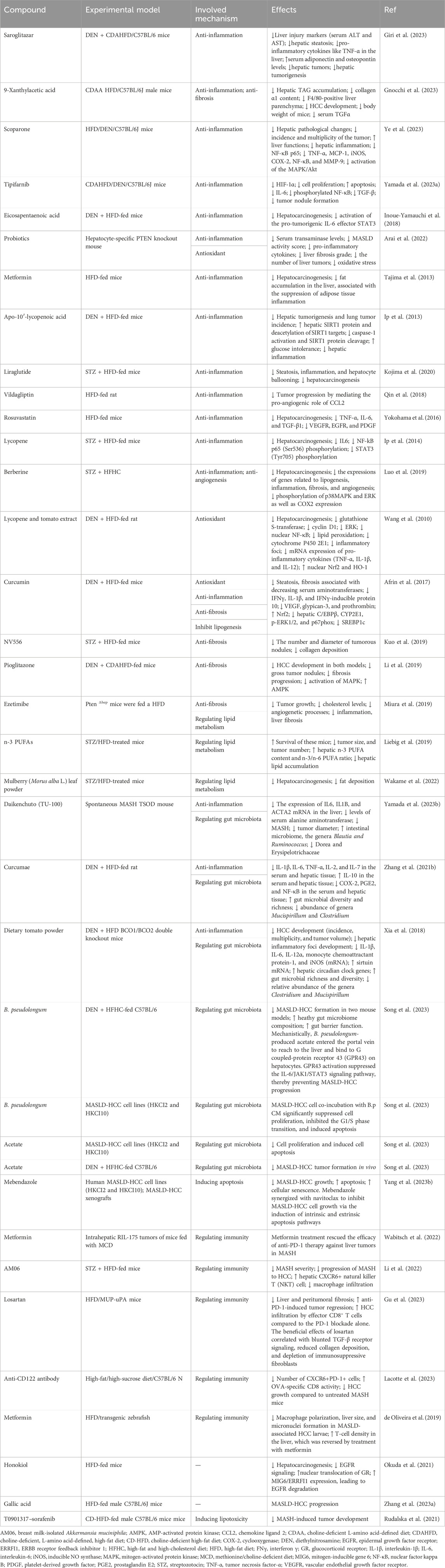
TABLE 1. List of available potential compounds targeting different mechanisms to treat MASLD-driven HCC.
Anti-inflammatory drugs
Saroglitazar, a novel PPAR-α/γ agonist with predominant PPAR-α activity, has been shown to inhibit symptoms of MASH. A recent study has shown that it prevents the development of MASLD-HCC in rodents (Giri et al., 2023). Saroglitazar significantly reversed hepatic steatosis, reduced hepatic injury, and decreased the levels of pro-inflammatory cytokines in the livers of diethylnitrosamine (DEN)-treated, choline-deficient, L-amino acid-defined, high-fat diet (CDAHFD)-fed C57BL/6 mice. Saroglitazar completely prevented hepatic tumorigenesis (Giri et al., 2023). The compound 9-xanthylacetic acid (XAA) inhibits the development of MASLD-HCC (Gnocchi et al., 2023). The choline-deficient l-amino acid-defined diet (CDAAD) induced metabolic imbalance by stimulating lysophosphatidic acid receptor 6 (LPAR6) expression in mice. XAA reverses CDAAD-induced increase in hepatic lipid accumulation, inflammation, fibrosis, and HCC development. These findings are corroborated by the results of gain- and loss-of-function of LPAR6 in HCC cells (Gnocchi et al., 2023). Scoparone (SCO), a compound originating from the leaves and stems of Artemisia capillaris, has many pharmacological effects such as anti-tumor, lipid-lowering, anti-hypotensive, anti-inflammatory, analgesic, anti-coagulant, and anti-asthmatic (Li et al., 2021; Jiang et al., 2022; Wei et al., 2022; Wu X. et al., 2023; Shen et al., 2023; Zhou et al., 2023). The most recent study showed that scoparone attenuates the pathological alterations observed in MASLD-HCC mouse models. Scoparone inhibits activation of mitogen-activated protein kinase (MAPK)/Akt signaling and reverses upregulation of NF-κB p65 and its target genes, including NF-κB, TNF-α, cyclooxygenase (COX-2), MCP-1, iNOS, and MMP-9, in MASLD-HCC models (Ye et al., 2023). These results suggest that scoparone is a potential therapeutic agent for MASLD-HCC as it inhibits MAPK/Akt/NF-κB-mediated inflammatory pathways. Tipifarnib is a farnesyltransferase inhibitor (FTI) possessing anti-inflammatory and anti-tumor effects (Untch et al., 2018; Egawa et al., 2021; Shu et al., 2021; Greenberg et al., 2022; Smith et al., 2023). Tipifarnib significantly reduced tumor nodule formation and exhibited anti-tumor and anti-inflammatory effects in a MASH-related HCC mouse model challenged with DEN and a high-fat diet (HFD), primarily by decreasing serum IL-6 (Yamada et al., 2023). Tipifarnib strongly inhibited cell proliferation, decreased the expression of HIF-1α, and induced apoptosis. Tipifarnib suppressed IL-6 secretion in vitro and in vivo (Yamada et al., 2023). Additionally, tipifarnib suppressed the expression of phosphorylated NF-κB and TGF-β (Yamada et al., 2023). The omega-3 polyunsaturated fatty acid eicosapentaenoic acid (EPA) inhibits the development of HCC induced by DEN and HFD, suggesting that EPA attenuates the development of obesity-related MASLD-HCC by suppressing STAT3 (Inoue-Yamauchi et al., 2018). An increasing number of studies have shown that probiotics can prevent and mitigate the development of cancer (Deng et al., 2023; Zhao et al., 2023). Probiotics suppressed HCC pathogenesis by inhibiting the gene expression of pro-inflammatory cytokines and reducing oxidative stress in a hepatocyte-specific PTEN knockout mouse MASLD model (Arai et al., 2022). Metformin, a promising antidiabetic medication for cancer treatment, inhibits long-term HFD-induced HCC tumorigenesis by inhibiting liver fat accumulation in the early stage (before the onset of MASLD) in C57Bl/6 mice (Tajima et al., 2013; Saengboonmee et al., 2021; Wu et al., 2023; Georgopoulos et al., 2023). Apo-10′-lycopenoic acid (APO10LA), a cleavage metabolite of lycopene, significantly reduced hepatic tumorigenesis and lung metastasis in C57Bl/6J mice challenged with DEN and HFD. This was accomplished by increasing hepatic SIRT1 protein activity, deacetylating SIRT1 targets, decreasing caspase-1 activation, and reducing hepatic inflammation, indicating APO10LA as having anti-inflammatory and anti-tumor effects in MASLD-HCC models (Ip et al., 2013). Liraglutide, a glucagon-like peptide-1 receptor agonist used for the treatment of T2DM, obesity, and chronic weight management, completely suppressed hepatic tumorigenesis in mice with streptozotocin (STZ) and HFD-induced MASH models through ameliorating steatosis, inflammation, and hepatocyte ballooning (Kojima et al., 2020). The dipeptidyl peptidase 4 (DPP4) inhibitor vildagliptin prevented MASLD-HCC in mouse models challenged with DEN and HFD by reversing HFD-induced CCL-2 production and angiogenesis, suggesting that the DPP4/CCL2/angiogenesis axis plays a key role in inhibiting MASLD-HCC. Targeting DPP4 may represent a novel therapeutic regimen for MASLD-HCC (Qin et al., 2018). As a 3-hydroxy-3-methyl-glutaryl-coenzyme-A (HMG-CoA) reductase inhibitor, rosuvastatin inhibited hepatic tumorigenesis in an STAM mouse model challenged with a HFD by decreasing the expression of pro-inflammatory cytokines, suggesting that rosuvastatin may function as a preventive drug against MASLD-HCC (Yokohama et al., 2016). Lycopene suppresses hepatic tumorigenesis in HFD-promoted HCC by inactivating hepatic pro-inflammatory signaling and inflammatory foci (Ip et al., 2014). Berberine reduced the incidence of tumors and mitigated MASH in a STZ high-fat high-cholesterol diet (HFHC) mice model, suppressing lipogenesis, inflammation, fibrosis, and angiogenesis (Luo et al., 2019). Meanwhile, berberine inhibited the phosphorylation of p38 MAPK, extracellular signal-regulated kinase (ERK), and suppressed expression of COX2, suggesting berberine attenuates MASH-HCC by halting inflammation and angiogenesis via p38 MAPK/ERK-COX2 pathways (Luo et al., 2019).
Antioxidant drugs
Dietary lycopene and tomato extract halt MASH-HCC in rats through antioxidant activity (Wang et al., 2010). Dietary lycopene from either tomato extract or pure compounds inhibited hepatic tumorigenesis in an MASLD-related HCC rat model challenged with DEN and a HFD. This was accomplished by inhibiting the activation of ERK and NF-κB, decreasing the expression of CYP2E1, reducing inflammatory foci, reducing pro-inflammatory cytokines, and increasing nuclear NF-E2-related factor-2 (Nrf2) and heme oxygenase-1 (HO-1) expression, suggesting that lycopene and tomato extract can inhibit MASH-HCC by reducing oxidative stress (Wang et al., 2010). Curcumin inhibits MASH-HCC pathogenesis in rats through antioxidant activity (Afrin et al., 2017). Curcumin inhibited hepatic tumorigenesis in a C57BL/6J male mice MASLD-related HCC model challenged with STZ and a HFD by inhibiting hepatic C/EBPβ, CYP2E1, p-ERK1/2, and p67phox, while upregulating Nrf2 (Afrin et al., 2017). Curcumin significantly reduced the translocation of high-mobility group box 1 (HMGB1) into the cytosol and decreased the protein expression of toll-like receptor 4 (TLR4). Curcumin reduced MASH-HCC by downregulating the protein expression of glypican-3, prothrombin, and vascular endothelial growth factor (VEGF) (Afrin et al., 2017).
Anti-fibrosis drugs
The cyclophilin inhibitor derived from sanglifehrins NV556 reduces MASH-HCC by inhibiting fibrosis (Kuo et al., 2019). NV556 inhibited hepatic tumorigenesis in a mice model challenged with STZ and a HFD by inhibiting fibrosis, rather than altering inflammation, steatosis, and systemic cytokine generation, suggesting NV556 as a promising agent for the treatment of MASH-driven fibrosis and HCC (Kuo et al., 2019). Pioglitazone effectively reduced hepatic tumorigenesis in an MASH-related HCC mice model challenged with DEN and choline-deficient, L-amino acid-defined, high-fat diet (CDAHFD) by inhibiting fibrosis via inactivating MAPK and upregulating the hepatoprotective AMP-activated protein kinase (AMPK) pathway (Li et al., 2019). An inhibitor of cholesterol absorption, ezetimibe, reduces MASH-HCC by inhibiting fibrosis (Miura et al., 2019). Ezetimibe inhibited hepatic tumorigenesis in hepatocyte-specific phosphatase and tensin homolog (Pten)-deficient (PtenΔhep) mice challenged with a HFD by suppressing liver fibrosis and inflammation (Miura et al., 2019).
Drugs regulating lipid metabolism
Dietary n-3 polyunsaturated fatty acids (PUFAs) reduced hepatic tumorigenesis in an MASLD-related HCC mice model challenged with STZ and a HFD by regulating lipid metabolism, evidenced by decreased hepatic lipid accumulation and increased hepatic content of n-3 PUFAs and a higher n-3/n-6 PUFA ratio, suggesting n-3 PUFAs as a new therapeutic regimen for MASLD-HCC (Liebig et al., 2019). Dietary mulberry (Morus alba L.) leaf powder reduced hepatic tumorigenesis in an MASLD-related HCC C57L/6J mice model challenged with STZ and a HFD by regulating lipid metabolism, evidenced by reduced fat deposition and adenoma. This study suggests that the administration of mulberry leaf powder in STAM mice inhibits the progression of MASH-HCC, highlighting that it may be effective in preventing the development of MASH-HCC in humans (Wakame et al., 2022).
Regulating gut microbiota
Daikenchuto (TU-100) reduced hepatic tumorigenesis in Tsumura–Suzuki obese diabetes mice with the spontaneous onset of MASH and HCC by modulating the intestinal microbiome, evidenced by increased Blautia and Ruminococcus genera and decreased Dorea and Erysipelotrichaceae genera (Yamada et al., 2023). Curcumae reduced hepatic tumorigenesis in an MASLD-related HCC mice model challenged with DEN and a HFD by suppressing the levels of pro-inflammatory cytokines and inflammatory mediators including prostaglandin E2 (PGE2), COX-2, and NF-κB and additionally augmented the level of IL-10 in the hepatic tissue and serum. Furthermore, Curcumae enhanced the diversity and richness of gut microbiota and decreased the relative abundance of Clostridium and Mucispirillum (Zhang et al., 2021). This study suggests that Curcumae attenuates MASLD-HCC via regulating gut microbiota, along with inhibiting oxidative stress and its associated inflammation. Dietary tomato powder inhibits MASLD-HCC by regulating gut microbiota in mice with loss of carotenoid cleavage enzymes (Xia et al., 2018). Dietary tomato powder reduced hepatic tumorigenesis in a β-carotene-15, 15′-oxygenase (BCO1)/BCO2 double-knockout mice model challenged with DEN and HFD. This was accomplished by decreasing hepatic inflammatory foci development and expression of pro-inflammatory genes, increasing the expression of SIRT1, hepatic circadian clock genes, and nicotinamide phosphoribosyltransferases (Xia et al., 2018). Dietary tomato powder also increased gut microbial diversity and richness, significantly decreasing the relative abundance of the genera Clostridium and Mucispirillum (Xia et al., 2018). This study indicates that dietary tomato powder prevents MASLD-HCC by inhibiting inflammation and modulating gut microbiota independent of carotenoid cleavage enzymes (Xia et al., 2018). Recent studies have shown that Bifidobacterium pseudolongum is a potential novel probiotic for the prevention of MASLD-HCC. Mechanistically, it was found to suppress MASLD-HCC progression by secreting acetate, which by binding to the hepatic G-coupled-protein receptor 43 (GPR43) suppresses the activation of hepatic oncogenic IL-6/JAK1/STAT3 signaling pathways (Song et al., 2023).
Regulating immunity
MASH impaired the effect of anti-PD-1 therapy by inducing a pro-inflammatory phenotypic change and impairing the metabolism of hepatic CD8+ T cells in multiple murine MASH liver cancer models. Reduced motility of intratumoral CD8+ T cells was found through in vivo imaging analysis. Metformin was found to modulate the anti-PD-1 therapy efficacy against liver tumors in MASH models (Wabitsch et al., 2022). HFD alters macrophage polarization and promotes the liver inflammatory microenvironment, exacerbating cancer progression in MASLD/MASH-associated zebrafish HCC models. Metformin reversed MASLD/MASH-HCC by modulating the immune response via altering T-cell infiltration and macrophage polarization (de Oliveira et al., 2019). Gut Akkermansia muciniphila is reduced in mice and patients with MASH-related HCC (Li et al., 2022). Breast milk-isolated A. muciniphila (AM06) improved the severity of MASH, in addition to inhibiting the pathogenesis of MASH-HCC, indicated by decreased macrophage infiltration and an increased level of hepatic CXCR6+ natural killer T (NKT) cells (Li et al., 2022). The anti-tumor effects of A. muciniphila were attenuated by NKT cell deficiency in mice (CD1d−/− and CXCR6−/−). A. muciniphila enhanced the NKT cell-mediated killing of HepG2 cells (Li et al., 2022). Peritumoral fibrosis exerts an obstacle to T-cell-mediated tumor regression in mouse models of MASH-HCC. The antihypertensive drug angiotensin II receptor inhibitor losartan inhibited liver and peritumoral fibrosis, thereby substantially boosting tumor regression induced by anti-PD-1 therapy, mostly by facilitating effector CD8+ T-cell-mediated infiltration. The beneficial effects of losartan are associated with blunting of TGF-β receptor signaling, reduced fibrosis, and depletion of immunosuppressive fibroblasts (Gu et al., 2023).
Conclusion and future perspectives
This review summarizes the recent progress of research on the pathological pathways and underlying mechanisms of MASLD/MASH-HCC and reviews the application of compounds in the treatment of MASLD-HCC. This review is expected to improve our knowledge of the molecular mechanisms of MASLD/MASH-HCC and highlight strategies for targeting MASLD/MASH-HCC by pharmacological modulation as potential novel therapeutic targets. The progression of MASLD/MASH to HCC is still an international research hotspot, and there has been some progress in elucidating metabolic disorder/gut microbiota imbalance and immune factors in the progression of MASLD/MASH to HCC. However, the exploration of treatment for MASLD/MASH-HCC is still in its infancy. In this article, based on a brief review of the latest research on the pathogenesis and progress of MASLD-HCC, we further summarize and organize the latest results of compounds in the treatment of MASLD-HCC. Based on the main mechanisms of anti-inflammatory, antioxidant, gut microbiota regulation, lipid metabolism regulation, and liver fibrosis inhibition, we classified the drugs used to treat MASLD/MASH-HCC and elucidated their pharmacological effects and related mechanisms of action. Adding further complexity, the same drug may inhibit MASLD/MASH-HCC through one or more of the above mechanisms, adding new insights for future polyreceptor pharmacology. Although continuous exploratory experimental research is still ongoing, the exact molecular mechanisms of these drugs in treating MASLD/MASH-HCC still need to be further explored.
After our detailed discussion, it is important to recall limitations that still plague the field of MASLD/MASH-HCC research. The first limitation is that the etiology of MASLD/MASH-HCC remains largely unclear, and further research is still needed. Recent studies have shown that new mechanisms of regulated cell death, such as ferroptosis, can be involved in the development and progression of MASLD (Feng et al., 2022; Wang et al., 2022; Zhang et al., 2023b; Zou et al., 2023) to HCC (Cong et al., 2022; Wang et al., 2023a; Wang et al., 2023b; Xu et al., 2023), and modulating ferroptosis may be a potential novel therapeutic target for MASLD and HCC (Yin et al., 2022; Ajoolabady et al., 2023; Cheng et al., 2023). However, mechanistic details relating to ferroptosis and the molecular pathways concerning transition of MASLD to HCC have been lacking. It is of fundamental importance to understand the importance of ferroptosis in the pathogenesis of MASLD-HCC. The second limitation is that the mechanism of specific genetic factors in the progression of MASLD/MASH to HCC and the exact downstream molecules are currently unclear, and further research is needed. The third limitation is that there is a lack of large-scale clinical trials on targeted MASLD/MASH-HCC drugs. The fourth limitation is that it is still largely unclear how one may develop or repurpose existing drugs for new clinical indications.
Due to a significant increase in obesity and T2DM globally, MASLD has gradually become an important cause of HCC, leading to the occurrence of MASLD-HCC. To note, HCC is characterized by high heterogeneity and different genetic mutations that could damage the effectiveness of current treatments. Therefore, future clinical trials should consider the most representative genetic mutations and find new strategies to identify biomarkers to guide personalized and precision treatment for HCC. Currently, there are no approved effective drugs to treat MASLD/MASH, implying that the targeted inhibition of MASLD/MASH and preventing disease progression to HCC have important social significance and are worthy of further exploration.
Author contributions
YW: conceptualization, funding acquisition, and writing–original draft. JF: visualization and writing–review and editing. TL: conceptualization and writing–review and editing. YL: data curation and writing–review and editing. ZR: data curation and writing–review and editing. JC: funding acquisition, project administration, and writing–review and editing. MD: investigation and writing–review and editing.
Funding
The author(s) declare that financial support was received for the research, authorship, and/or publication of this article. This work was supported in part by the Science Foundation of CASIC (2020-LCYL-009), the Science Foundation of ASCH (YN202104), and the Hygiene and Health Development Scientific Research Fostering Plan of Haidian District, Beijing (HP2021-19-50701).
Conflict of interest
The authors declare that the research was conducted in the absence of any commercial or financial relationships that could be construed as a potential conflict of interest.
Publisher’s note
All claims expressed in this article are solely those of the authors and do not necessarily represent those of their affiliated organizations, or those of the publisher, the editors, and the reviewers. Any product that may be evaluated in this article, or claim that may be made by its manufacturer, is not guaranteed or endorsed by the publisher.
References
Afrin, R., Arumugam, S., Rahman, A., Wahed, M. I. I., Karuppagounder, V., Harima, M., et al. (2017). Curcumin ameliorates liver damage and progression of NASH in NASH-HCC mouse model possibly by modulating HMGB1-NF-κB translocation. Int. Immunopharmacol. 44, 174–182. doi:10.1016/j.intimp.2017.01.016
Ajoolabady, A., Tang, D., Kroemer, G., and Ren, J. (2023). Ferroptosis in hepatocellular carcinoma: mechanisms and targeted therapy. Br. J. Cancer. 128, 190–205. doi:10.1038/s41416-022-01998-x
Akinyemiju, T., Abera, S., Ahmed, M., Alam, N., Alemayohu, M. A., Allen, C., et al. (2017). The burden of primary liver cancer and underlying etiologies from 1990 to 2015 at the global, regional, and national level: results from the global burden of disease study 2015. JAMA Oncol. 3 (12), 1683–1691. doi:10.1001/jamaoncol.2017.3055
Aleksandrova, K., Boeing, H., Nöthlings, U., Jenab, M., Fedirko, V., Kaaks, R., et al. (2014). Inflammatory and metabolic biomarkers and risk of liver and biliary tract cancer. Hepatology 60, 858–871. doi:10.1002/hep.27016
Anstee, Q. M., Reeves, H. L., Kotsiliti, E., Govaere, O., and Heikenwalder, M. (2019). From NASH to HCC: current concepts and future challenges. Nat. Rev. Gastroenterol. Hepatol. 16, 411–428. doi:10.1038/s41575-019-0145-7
Arai, N., Miura, K., Aizawa, K., Sekiya, M., Nagayama, M., Sakamoto, H., et al. (2022). Probiotics suppress nonalcoholic steatohepatitis and carcinogenesis progression in hepatocyte-specific PTEN knockout mice. Sci. Rep. 12, 16206. doi:10.1038/s41598-022-20296-3
Arrese, M., Cabrera, D., Kalergis, A. M., and Feldstein, A. E. (2016). Innate immunity and inflammation in NAFLD/NASH. Dig. Dis. Sci. 61, 1294–1303. doi:10.1007/s10620-016-4049-x
Avgerinos, K. I., Spyrou, N., Mantzoros, C. S., and Dalamaga, M. (2019). Obesity and cancer risk: emerging biological mechanisms and perspectives. Metab. Clin. Exp. 92, 121–135. doi:10.1016/j.metabol.2018.11.001
Begriche, K., Massart, J., Robin, M. A., Bonnet, F., and Fromenty, B. (2013). Mitochondrial adaptations and dysfunctions in nonalcoholic fatty liver disease. Hepatology 58, 1497–1507. doi:10.1002/hep.26226
Behary, J., Amorim, N., Jiang, X. T., Raposo, A., Gong, L., McGovern, E., et al. (2021). Gut microbiota impact on the peripheral immune response in non-alcoholic fatty liver disease related hepatocellular carcinoma. Nat. Commun. 12 (1), 187. doi:10.1038/s41467-020-20422-7
Bence, K. K., and Birnbaum, M. J. (2021). Metabolic drivers of non-alcoholic fatty liver disease. Mol. Metab. 50, 50101143. doi:10.1016/j.molmet.2020.101143
Bessone, F., Razori, M. V., and Roma, M. G. (2019). Molecular pathways of nonalcoholic fatty liver disease development and progression. Cell. Mol. Life Sci. 76, 99–128. doi:10.1007/s00018-018-2947-0
Brahma, M. K., Gilglioni, E. H., Zhou, L., Trépo, E., Chen, P., and Gurzov, E. N. (2021). Oxidative stress in obesity-associated hepatocellular carcinoma: sources, signaling and therapeutic challenges. Oncogene 40, 5155–5167. doi:10.1038/s41388-021-01950-y
Cannito, S., Dianzani, U., Parola, M., Albano, E., and Sutti, S. (2023). Inflammatory processes involved in NASH-related hepatocellular carcinoma. Biosci. Rep. 43, BSR20221271. doi:10.1042/BSR20221271
Chan, W. K., Chuah, K. H., Rajaram, R. B., Lim, L. L., Ratnasingam, J., and Vethakkan, S. R. (2023). Metabolic dysfunction-associated steatotic liver disease (MASLD): a state-of-the-art review. J. Obes. Metab. Syndr. 32, 197–213. doi:10.7570/jomes23052
Chen, Z., Tian, R., She, Z., Cai, J., and Li, H. (2020). Corrigendum to "Role of oxidative stress in the pathogenesis of nonalcoholic fatty liver disease" [Free Radic. Biol. Med. 152 (2020) 116-141]. Free Radic. Biol. Med. 152, 174–141. doi:10.1016/j.freeradbiomed.2020.06.011
Cheng, Z., Chu, H., Zhu, Q., and Yang, L. (2023). Ferroptosis in non-alcoholic liver disease: molecular mechanisms and therapeutic implications. Front. Nutr. 10, 1090338. doi:10.3389/fnut.2023.1090338
Cong, T., Luo, Y., Fu, Y., Liu, Y., Li, Y., and Li, X. (2022). New perspectives on ferroptosis and its role in hepatocellular carcinoma. Chin. Med. J. 135, 2157–2166. doi:10.1097/CM9.0000000000002327
Degasperi, E., and Colombo, M. (2016). Distinctive features of hepatocellular carcinoma in non-alcoholic fatty liver disease. Lancet Gastroenterology Hepatology 1 (2), 156–164. doi:10.1016/S2468-1253(16)30018-8
Deng, X., Yang, J., Zhang, Y., Chen, X., Wang, C., Suo, H., et al. (2023). An update on the pivotal roles of probiotics, their components, and metabolites in preventing colon cancer. Foods 12, 3706. doi:10.3390/foods12193706
de Oliveira, S., Houseright, R. A., Graves, A. L., Golenberg, N., Korte, B. G., Miskolci, V., et al. (2019). Metformin modulates innate immune-mediated inflammation and early progression of NAFLD-associated hepatocellular carcinoma in zebrafish. J. Hepatol. 70 (4), 710–721. doi:10.1016/j.jhep.2018.11.034
Di Maira, G., Foglia, B., Napione, L., Turato, C., Maggiora, M., Sutti, S., et al. (2022). Oncostatin M is overexpressed in NASH-related hepatocellular carcinoma and promotes cancer cell invasiveness and angiogenesis. J. Pathol. 257, 82–95. doi:10.1002/path.5871
Duan, Y., Pan, X., Luo, J., Xiao, X., Li, J., Bestman, P. L., et al. (2022). Association of inflammatory cytokines with non-alcoholic fatty liver disease. Front. Immunol. 13, 880298. doi:10.3389/fimmu.2022.880298
Egawa, N., Tanaka, T., Matsufuji, S., Yamada, K., Ito, K., Kitagawa, H., et al. (2021). Antitumor effects of low-dose tipifarnib on the mTOR signaling pathway and reactive oxygen species production in HIF-1α-expressing gastric cancer cells. FEBS Open Bio 11, 1465–1475. doi:10.1002/2211-5463.13154
Eslam, M., Newsome, P. N., Sarin, S. K., Anstee, Q. M., Targher, G., Romero-Gomez, M., et al. (2020a). A new definition for metabolic dysfunction-associated fatty liver disease: an international expert consensus statement. J. Hepatol. 73 (1), 202–209. doi:10.1016/j.jhep.2020.03.039
Eslam, M., Sanyal, A. J., and George, J.International Consensus Panel (2020b). MAFLD: a consensus-driven proposed nomenclature for metabolic associated fatty liver disease. Gastroenterology 158 (7), 1999–2014.e1. doi:10.1053/j.gastro.2019.11.312
Feng, G., Byrne, C. D., Targher, G., Wang, F., and Zheng, M. H. (2022). Ferroptosis and metabolic dysfunction-associated fatty liver disease: is there a link. Liver Int. 42, 1496–1502. doi:10.1111/liv.15163
Foerster, F., Gairing, S. J., Müller, L., and Galle, P. R. (2022). NAFLD-driven HCC: safety and efficacy of current and emerging treatment options. J. Hepatol. 76, 446–457. doi:10.1016/j.jhep.2021.09.007
Gabbia, D., Cannella, L., and De Martin, S. (2021). The role of oxidative stress in NAFLD-NASH-HCC transition-focus on NADPH oxidases. Biomedicines 9, 687. doi:10.3390/biomedicines9060687
Georgopoulos, N. S., Tolia, M., Mauri, D., Kamposioras, K., Charalampakis, N., Tsoukalas, N., et al. (2023). Metformin: a promising radiosensitizer in neoadjuvant rectal cancer treatment. Rev. Recent Clin. Trials 18, 172–180. doi:10.2174/1574887118666230428114349
Giri, S. R., Bhoi, B., Trivedi, C., Rath, A., Rathod, R., Sharma, A., et al. (2023). Saroglitazar suppresses the hepatocellular carcinoma induced by intraperitoneal injection of diethylnitrosamine in C57BL/6 mice fed on choline deficient, l-amino acid-defined, high-fat diet. BMC Cancer 23, 59. doi:10.1186/s12885-023-10530-0
Gnocchi, D., Afonso, M. B., Cavalluzzi, M. M., Lentini, G., Ingravallo, G., Sabbà, C., et al. (2023). Inhibition of lysophosphatidic acid receptor 6 upregulated by the choline-deficient l-amino acid-defined diet prevents hepatocarcinogenesis in mice. Mol. Carcinog. 62 (5), 577–582. doi:10.1002/mc.23516
Greenberg, J. W., Kim, H., Ahn, M., Moustafa, A. A., Zhou, H., Barata, P. C., et al. (2022). Combination of tipifarnib and sunitinib overcomes renal cell carcinoma resistance to tyrosine kinase inhibitors via tumor-derived exosome and T cell modulation. Cancers (Basel) 14, 903. doi:10.3390/cancers14040903
Grohmann, M., Wiede, F., Dodd, G. T., Gurzov, E. N., Ooi, G. J., Butt, T., et al. (2018). Obesity drives STAT-1-dependent NASH and STAT-3-dependent HCC. Cell 175, 1289–1306. doi:10.1016/j.cell.2018.09.053
Gu, L., Zhu, Y., Lee, M., Nguyen, A., Ryujin, N. T., Huang, J. Y., et al. (2023). Angiotensin II receptor inhibition ameliorates liver fibrosis and enhances hepatocellular carcinoma infiltration by effector T cells. Proc. Natl. Acad. Sci. U.S.A. 120 (19), e2300706120. doi:10.1073/pnas.2300706120
Hagström, H., Vessby, J., Ekstedt, M., and Shang, Y. (2023). 99% of patients with NAFLD meet MASLD criteria and natural history is therefore identical. J. Hepatol. 2023 (23), 05080–05088. [pii]. doi:10.1016/j.jhep.2023.08.026
Hardy, T., Oakley, F., Anstee, Q. M., and Day, C. P. (2016). Nonalcoholic fatty liver disease: pathogenesis and disease spectrum. Annu. Rev. Pathol. 11, 451–496. doi:10.1146/annurev-pathol-012615-044224
Hatano, M., Akiyama, Y., Shimada, S., Yagi, K., Akahoshi, K., Itoh, M., et al. (2023). Loss of KDM6B epigenetically confers resistance to lipotoxicity in nonalcoholic fatty liver disease-related HCC. Hepatol. Commun. 7, e0277. doi:10.1097/HC9.0000000000000277
He, L., Zheng, W., Qiu, K., Kong, W., and Zeng, T. (2023). Changing from NAFLD to MASLD: the new definition can more accurately identify individuals at higher risk for diabetes. J. Hepatol. S0168-8278 (23), 05164. [pii]. doi:10.1016/j.jhep.2023.09.035
Hirsova, P., Bamidele, A. O., Wang, H., Povero, D., and Revelo, X. S. (2021). Emerging roles of T cells in the pathogenesis of nonalcoholic steatohepatitis and hepatocellular carcinoma. Front. Endocrinol. (Lausanne) 12, 760860. doi:10.3389/fendo.2021.760860
Inoue-Yamauchi, A., Itagaki, H., and Oda, H. (2018). Eicosapentaenoic acid attenuates obesity-related hepatocellular carcinogenesis. Carcinogenesis 39 (1), 28–35. doi:10.1093/carcin/bgx112
Ioannou, G. N. (2021). Epidemiology and risk-stratification of NAFLD-associated HCC. J. Hepatol. 75 (6), 1476–1484. doi:10.1016/j.jhep.2021.08.012
Ip, B. C., Hu, K. Q., Liu, C., Smith, D. E., Obin, M. S., Ausman, L. M., et al. (2013). Lycopene metabolite, apo-10'-lycopenoic acid, inhibits diethylnitrosamine-initiated, high fat diet-promoted hepatic inflammation and tumorigenesis in mice. Cancer Prev. Res. (Phila) 6 (12), 1304–1316. doi:10.1158/1940-6207.CAPR-13-0178
Ip, B. C., Liu, C., Ausman, L. M., von Lintig, J., and Wang, X. D. (2014). Lycopene attenuated hepatic tumorigenesis via differential mechanisms depending on carotenoid cleavage enzyme in mice. Cancer Prev. Res. (Phila) 7 (12), 1219–1227. doi:10.1158/1940-6207.CAPR-14-0154
Jiang, Y., Xu, J., Huang, P., Yang, L., Liu, Y., Li, Y., et al. (2022). Scoparone improves nonalcoholic steatohepatitis through alleviating JNK/sab signaling pathway-mediated mitochondrial dysfunction. Front. Pharmacol. 13, 863756. doi:10.3389/fphar.2022.863756
Kalligeros, M., Vassilopoulos, A., Vassilopoulos, S., Victor, D. W., Mylonakis, E., and Noureddin, M. (2023). Prevalence of steatotic liver disease (MASLD, MetALD, and ALD) in the United States: NHANES 2017-2020. Clin. Gastroenterol. Hepatol. S1542-3565 (23), 00914–00914X. [pii]. doi:10.1016/j.cgh.2023.11.003
Khairnar, R., Islam, M. A., Fleishman, J., and Kumar, S. (2023). Shedding light on non-alcoholic fatty liver disease: pathogenesis, molecular mechanisms, models, and emerging therapeutics. LIFE Sci. 312, 121185. doi:10.1016/j.lfs.2022.121185
Koh, J. H., Wang, M., Suzuki, H., Muthiah, M., Ng, C. H., and Huang, D. Q. (2024). NAFLD and NAFLD-related HCC in asia: burden and surveillance. J. Clin. Exp. Hepatol. 14, 101213. doi:10.1016/j.jceh.2023.06.013
Kojima, M., Takahashi, H., Kuwashiro, T., Tanaka, K., Mori, H., Ozaki, I., et al. (2020). Glucagon-like peptide-1 receptor agonist prevented the progression of hepatocellular carcinoma in a mouse model of nonalcoholic steatohepatitis. Int. J. Mol. Sci. 21 (16), 5722. doi:10.3390/ijms21165722
Kuo, J., Serrano, S. S., Grönberg, A., Massoumi, R., Hansson, M. J., and Gallay, P. (2019). Cyclophilin inhibitor NV556 reduces fibrosis and hepatocellular carcinoma development in mice with non-alcoholic steatohepatitis. Front. Pharmacol. 10, 1129. doi:10.3389/fphar.2019.01129
Lacotte, S., Slits, F., Moeckli, B., Peloso, A., Koenig, S., Tihy, M., et al. (2023). Anti-CD122 antibody restores specific CD8(+) T cell response in nonalcoholic steatohepatitis and prevents hepatocellular carcinoma growth. Oncoimmunology 12 (1), 2184991. doi:10.1080/2162402X.2023.2184991
Li, N., Yang, F., Liu, D. Y., Guo, J. T., Ge, N., and Sun, S. Y. (2021). Scoparone inhibits pancreatic cancer through PI3K/Akt signaling pathway. World J. Gastrointest. Oncol. 13, 1164–1183. doi:10.4251/wjgo.v13.i9.1164
Li, S., Ghoshal, S., Sojoodi, M., Arora, G., Masia, R., Erstad, D. J., et al. (2019). Pioglitazone reduces hepatocellular carcinoma development in two rodent models of cirrhosis. J. Gastrointest. Surg. 23 (1), 101–111. doi:10.1007/s11605-018-4004-6
Li, T., Lin, X., Shen, B., Zhang, W., Liu, Y., Liu, H., et al. (2022). Akkermansia muciniphila suppressing nonalcoholic steatohepatitis associated tumorigenesis through CXCR6(+) natural killer T cells. Front. Immunol. 13, 1047570. doi:10.3389/fimmu.2022.1047570
Liebig, M., Dannenberger, D., Vollmar, B., and Abshagen, K. (2019). n-3 PUFAs reduce tumor load and improve survival in a NASH-tumor mouse model. Ther. Adv. Chronic Dis. 10, 2040622319872118. doi:10.1177/2040622319872118
Lim, S., Kim, J. W., and Targher, G. (2021). Links between metabolic syndrome and metabolic dysfunction-associated fatty liver disease. Trends Endocrinol. metabolism TEM 32, 500–514. doi:10.1016/j.tem.2021.04.008
Liu, M., Yu, X., Lin, L., Deng, J., Wang, K., Xia, Y., et al. (2019). ATGL promotes the proliferation of hepatocellular carcinoma cells via the p-AKT signaling pathway. J. Biochem. Mol. Toxicol. 33, e22391. doi:10.1002/jbt.22391
Llovet, J. M., Kelley, R. K., Villanueva, A., Singal, A. G., Pikarsky, E., Roayaie, S., et al. (2021). Hepatocellular carcinoma. Nat. Rev. Dis. Prim. 7, 6. doi:10.1038/s41572-020-00240-3
Luo, Y., Tian, G., Zhuang, Z., Chen, J., You, N., Zhuo, L., et al. (2019). Berberine prevents non-alcoholic steatohepatitis-derived hepatocellular carcinoma by inhibiting inflammation and angiogenesis in mice. Am. J. Transl. Res. 11 (5), 2668–2682.
Malehmir, M., Pfister, D., Gallage, S., Szydlowska, M., Inverso, D., Kotsiliti, E., et al. (2019). Platelet GPIbα is a mediator and potential interventional target for NASH and subsequent liver cancer. Nat. Med. 25, 641–655. doi:10.1038/s41591-019-0379-5
Marengo, A., Rosso, C., and Bugianesi, E. (2016). Liver cancer: connections with obesity, fatty liver, and cirrhosis. Annu. Rev. Med. 67, 103–117. doi:10.1146/annurev-med-090514-013832
Margini, C., and Dufour, J. F. (2016). The story of HCC in NAFLD: from epidemiology, across pathogenesis, to prevention and treatment. Liver Int. 36, 317–324. doi:10.1111/liv.13031
Masarone, M., Rosato, V., Dallio, M., Gravina, A. G., Aglitti, A., Loguercio, C., et al. (2018). Role of oxidative stress in pathophysiology of nonalcoholic fatty liver disease. Oxid. Med. Cell Longev. 2018, 9547613. doi:10.1155/2018/9547613
Mittal, S., Sada, Y. H., El-Serag, H. B., Kanwal, F., Duan, Z., Temple, S., et al. (2015). Temporal trends of nonalcoholic fatty liver disease-related hepatocellular carcinoma in the veteran affairs population. Clin. Gastroenterol. Hepatol. 13 (3), 594–601. doi:10.1016/j.cgh.2014.08.013
Miura, K., Ohnishi, H., Morimoto, N., Minami, S., Ishioka, M., Watanabe, S., et al. (2019). Ezetimibe suppresses development of liver tumors by inhibiting angiogenesis in mice fed a high-fat diet. Cancer Sci. 110 (2), 771–783. doi:10.1111/cas.13902
Mu, W., Jiang, Y., Liang, G., Feng, Y., and Qu, F. (2023). Metformin: a promising antidiabetic medication for cancer treatment. Curr. Drug Targets 24, 41–54. doi:10.2174/1389450124666221104094918
Natarajan, Y., Kramer, J. R., Yu, X., Li, L., Thrift, A. P., El-Serag, H. B., et al. (2020). Risk of cirrhosis and hepatocellular cancer in patients with NAFLD and normal liver enzymes. Hepatology 72 (4), 1242–1252. doi:10.1002/hep.31157
Noureddin, M., Wei, L., Castera, L., and Tsochatzis, E. A. (2023). Embracing change: from NAFLD to MASLD under the steatotic liver disease umbrella. Clin. Gastroenterol. Hepatol. S1542-3565 (23), 00833–00839. [pii].
Odenwald, M. A., and Turner, J. R. (2017). The intestinal epithelial barrier: a therapeutic target. Nat. Rev. Gastroenterol. Hepatol. 14 (1), 9–21. doi:10.1038/nrgastro.2016.169
Okuda, K., Umemura, A., Umemura, S., Kataoka, S., Taketani, H., Seko, Y., et al. (2021). Honokiol prevents non-alcoholic steatohepatitis-induced liver cancer via EGFR degradation through the glucocorticoid receptor-MIG6 Axis. Cancers (Basel) 13 (7), 1515. doi:10.3390/cancers13071515
Pal Chaudhary, S., Reyes, S., Chase, M. L., Govindan, A., Zhao, L., Luther, J., et al. (2023). Resection of NAFLD/NASH-related hepatocellular carcinoma (HCC): clinical features and outcomes compared with HCC due to other etiologies. Oncologist 28 (4), 341–350. doi:10.1093/oncolo/oyac251
Park, E. J., Lee, J. H., Yu, G. Y., He, G., Ali, S. R., Holzer, R. G., et al. (2010). Dietary and genetic obesity promote liver inflammation and tumorigenesis by enhancing IL-6 and TNF expression. Cell 140, 197–208. doi:10.1016/j.cell.2009.12.052
Perla, F. M., Prelati, M., Lavorato, M., Visicchio, D., and Anania, C. (2017). The role of lipid and lipoprotein metabolism in non-alcoholic fatty liver disease. Child. (Basel, Switz. 4, 46. doi:10.3390/children4060046
Pirola, C. J., and Sookoian, S. (2022). Metabolic dysfunction-associated fatty liver disease: advances in genetic and epigenetic implications. Curr. Opin. Lipidol. 33, 95–102. doi:10.1097/MOL.0000000000000814
Piscaglia, F., Svegliati-Baroni, G., Barchetti, A., Pecorelli, A., Marinelli, S., Tiribelli, C., et al. (2016). Clinical patterns of hepatocellular carcinoma in nonalcoholic fatty liver disease: a multicenter prospective study. Hepatology 63 (3), 827–838. doi:10.1002/hep.28368
Polyzos, S. A., Chrysavgis, L., Vachliotis, I. D., Chartampilas, E., and Cholongitas, E. (2023). Nonalcoholic fatty liver disease and hepatocellular carcinoma:Insights in epidemiology, pathogenesis, imaging, prevention and therapy. Semin. Cancer Biol. 93, 20–35. doi:10.1016/j.semcancer.2023.04.010
Ponziani, F. R., Bhoori, S., Castelli, C., Putignani, L., Rivoltini, L., Del Chierico, F., et al. (2019). Hepatocellular carcinoma is associated with gut microbiota profile and inflammation in nonalcoholic fatty liver disease. Hepatology 69, 107–120. doi:10.1002/hep.30036
Potoupni, V., Georgiadou, M., Chatzigriva, E., Polychronidou, G., Markou, E., Zapantis Gakis, C., et al. (2021). Circulating tumor necrosis factor-α levels in non-alcoholic fatty liver disease: a systematic review and a meta-analysis. J. Gastroenterol. Hepatol. 36, 3002–3014. doi:10.1111/jgh.15631
Powell, E. E., Wong, V. W., and Rinella, M. (2021). Non-alcoholic fatty liver disease. Lancet 397 (10290), 2212–2224. doi:10.1016/S0140-6736(20)32511-3
Qin, C. J., Zhao, L. H., Zhou, X., Zhang, H. L., Wen, W., Tang, L., et al. (2018). Inhibition of dipeptidyl peptidase IV prevents high fat diet-induced liver cancer angiogenesis by downregulating chemokine ligand 2. Cancer Lett. 420, 26–37. doi:10.1016/j.canlet.2018.01.064
Rao, G., Peng, X., Li, X., An, K., He, H., Fu, X., et al. (2023). Unmasking the enigma of lipid metabolism in metabolic dysfunction-associated steatotic liver disease: from mechanism to the clinic. Front. Med. (Lausanne). 10, 1294267. doi:10.3389/fmed.2023.1294267
Riaz, F., Wei, P., and Pan, F. (2022). Fine-tuning of regulatory T cells is indispensable for the metabolic steatosis-related hepatocellular carcinoma: a review. Front. Cell Dev. Biol. 10, 949603. doi:10.3389/fcell.2022.949603
Rinella, M. E., Lazarus, J. V., Ratziu, V., Francque, S. M., Sanyal, A. J., Kanwal, F., et al. (2023a). A multi-society Delphi consensus statement on new fatty liver disease nomenclature. J. Hepatol. S0168-8278 (23), 1966–1986. [pii]. doi:10.1097/hep.0000000000000520
Rinella, M. E., Lazarus, J. V., Ratziu, V., Francque, S. M., Sanyal, A. J., Kanwal, F., et al. (2023b). A multi-society Delphi consensus statement on new fatty liver disease nomenclature. Ann. Hepatol. 29, 101133. doi:10.1016/j.aohep.2023.101133
Rinella, M. E., Lazarus, J. V., Ratziu, V., Francque, S. M., Sanyal, A. J., Kanwal, F., et al. (2023c). A multi-society Delphi consensus statement on new fatty liver disease nomenclature. Hepatology 79, 1542–1556. doi:10.1016/j.jhep.2023.06.003
Rudalska, R., Harbig, J., Snaebjornsson, M. T., Klotz, S., Zwirner, S., Taranets, L., et al. (2021). LXRα activation and Raf inhibition trigger lethal lipotoxicity in liver cancer. Nat. Cancer 2, 201–217. doi:10.1038/s43018-020-00168-3
Saengboonmee, C., Sanlung, T., and Wongkham, S. (2021). Repurposing metformin for cancer treatment: a great challenge of a promising drug. Anticancer Res. 41, 5913–5918. doi:10.21873/anticanres.15410
Samuel, V. T., Petersen, K. F., and Shulman, G. I. (2010). Lipid-induced insulin resistance: unravelling the mechanism. Lancet 375, 2267–2277. doi:10.1016/S0140-6736(10)60408-4
Sangro, P., de la Torre Aláez, M., Sangro, B., and D'Avola, D. (2023). Metabolic dysfunction-associated fatty liver disease (MAFLD): an update of the recent advances in pharmacological treatment. J. Physiol. Biochem. 79, 869–879. doi:10.1007/s13105-023-00954-4
Sarin, S. K., Kumar, M., Eslam, M., George, J., Al Mahtab, M., Akbar, S. M. F., et al. (2020). Liver diseases in the asia-pacific region: a lancet gastroenterology and hepatology commission. Lancet Gastroenterol. Hepatol. 5 (2), 167–228. doi:10.1016/S2468-1253(19)30342-5
Serviddio, G., Bellanti, F., and Vendemiale, G. (2013). Free radical biology for medicine: learning from nonalcoholic fatty liver disease. Free Radic. Biol. Med. 65, 952–968. doi:10.1016/j.freeradbiomed.2013.08.174
Shalapour, S., Lin, X. J., Bastian, I. N., Brain, J., Burt, A. D., Aksenov, A. A., et al. (2017). Inflammation-induced IgA+ cells dismantle anti-liver cancer immunity. Nature 551, 340–345. doi:10.1038/nature24302
Shen, H., Wei, Y., Yang, Q., Cai, Y., Zhu, K., and Chen, X. (2023). Scoparone induces both apoptosis and ferroptosis via multiple mechanisms in non-small-cell lung cancer cells. Toxicol Vitro 91, 105627. doi:10.1016/j.tiv.2023.105627
Shu, L., Wang, D., Nannapaneni, S., Sun, Y., Griffith, C. C., Wang, X., et al. (2021). Tipifarnib enhances anti-EGFR activity of cetuximab in non-HRas mutated head and neck squamous cell carcinoma cancer (HNSCC). Oral Oncol. 122, 105546. doi:10.1016/j.oraloncology.2021.105546
Smith, A. E., Chan, S., Wang, Z., McCloskey, A., Reilly, Q., Wang, J. Z., et al. (2023). Tipifarnib potentiates the antitumor effects of PI3Kα inhibition in PIK3CA- and HRAS-dysregulated HNSCC via convergent inhibition of mTOR activity. Cancer Res. 83, 3252–3263. doi:10.1158/0008-5472.CAN-23-0282
Song, Q., Zhang, X., Liu, W., Wei, H., Liang, W., Zhou, Y., et al. (2023). Bifidobacterium pseudolongum-generated acetate suppresses non-alcoholic fatty liver disease-associated hepatocellular carcinoma. J. Hepatol. S0168-8278 (23), 1352–1365. [pii]. doi:10.1016/j.jhep.2023.07.005
Sydor, S., Best, J., Messerschmidt, I., Manka, P., Vilchez-Vargas, R., Brodesser, S., et al. (2020). Altered microbiota diversity and bile acid signaling in cirrhotic and noncirrhotic NASH-HCC. Clin. Transl. Gastroenterol. 11 (3), e00131. doi:10.14309/ctg.0000000000000131
Tajima, K., Nakamura, A., Shirakawa, J., Togashi, Y., Orime, K., Sato, K., et al. (2013). Metformin prevents liver tumorigenesis induced by high-fat diet in C57Bl/6 mice. Am. J. Physiol. Endocrinol. Metab. 305 (8), E987–E998. doi:10.1152/ajpendo.00133.2013
Talamantes, S., Lisjak, M., Gilglioni, E. H., Llamoza-Torres, C. J., Ramos-Molina, B., and Gurzov, E. N. (2023). Non-alcoholic fatty liver disease and diabetes mellitus as growing aetiologies of hepatocellular carcinoma. JHEP Rep. 5, 100811. doi:10.1016/j.jhepr.2023.100811
Tanaka, S., Miyanishi, K., Kobune, M., Kawano, Y., Hoki, T., Kubo, T., et al. (2013). Increased hepatic oxidative DNA damage in patients with nonalcoholic steatohepatitis who develop hepatocellular carcinoma. J. Gastroenterol. 48, 1249–1258. doi:10.1007/s00535-012-0739-0
Untch, B. R., Dos Anjos, V., Garcia-Rendueles, M., Knauf, J. A., Krishnamoorthy, G. P., Saqcena, M., et al. (2018). Tipifarnib inhibits HRAS-driven dedifferentiated thyroid cancers. Cancer Res. 78, 4642–4657. doi:10.1158/0008-5472.CAN-17-1925
Venkatesan, N., Doskey, L. C., and Malhi, H. (2023). The role of endoplasmic reticulum in lipotoxicity during metabolic dysfunction-associated steatotic liver disease (MASLD) pathogenesis. Am. J. Pathol. 193, 1887–1899. doi:10.1016/j.ajpath.2023.08.007
Villanueva, A. (2019). Hepatocellular carcinoma. N. Engl. J. Med. 380 (15), 1450–1462. doi:10.1056/NEJMra1713263
Wabitsch, S., McCallen, J. D., Kamenyeva, O., Ruf, B., McVey, J. C., Kabat, J., et al. (2022). Metformin treatment rescues CD8(+) T-cell response to immune checkpoint inhibitor therapy in mice with NAFLD. J. Hepatol. 77 (3), 748–760. doi:10.1016/j.jhep.2022.03.010
Wakame, K., Sato, K., Kasai, M., Kikuchi, E., Shimizu, K., Kudo, A., et al. (2022). Oral administration of Mulberry (morus alba L.) Leaf powder prevents the development of hepatocellular carcinoma in stelic animal model (STAM) mice. Anticancer Res. 42 (8), 4055–4062. doi:10.21873/anticanres.15902
Wang, S., Liu, Z., Geng, J., Li, L., and Feng, X. (2022). An overview of ferroptosis in non-alcoholic fatty liver disease. Biomed. Pharmacother. 153, 113374. doi:10.1016/j.biopha.2022.113374
Wang, X., Zeldin, S., Shi, H., Zhu, C., Saito, Y., Corey, K. E., et al. (2022). TAZ-induced Cybb contributes to liver tumor formation in non-alcoholic steatohepatitis. J. Hepatol. 76, 910–920. doi:10.1016/j.jhep.2021.11.031
Wang, Y., Ausman, L. M., Greenberg, A. S., Russell, R. M., and Wang, X. D. (2010). Dietary lycopene and tomato extract supplementations inhibit nonalcoholic steatohepatitis-promoted hepatocarcinogenesis in rats. Int. J. Cancer 126 (8), 1788–1796. doi:10.1002/ijc.24689
Wang, Y., Hu, J., Wu, S., Fleishman, J. S., Li, Y., Xu, Y., et al. (2023b). Targeting epigenetic and posttranslational modifications regulating ferroptosis for the treatment of diseases. Signal Transduct. Target Ther. 8, 449. doi:10.1038/s41392-023-01720-0
Wang, Y., Wu, X., Ren, Z., Li, Y., Zou, W., Chen, J., et al. (2023a). Overcoming cancer chemotherapy resistance by the induction of ferroptosis. Drug resist. updat. 66, 100916. doi:10.1016/j.drup.2022.100916
Wei, M., Li, T., Cao, H., He, H., Yang, C., Yin, Y., et al. (2022). The effects of scoparone on alcohol and high-fat diet-induced liver injury revealed by RNA sequencing. Biomed. Pharmacother. 155, 113770. doi:10.1016/j.biopha.2022.113770
Wen, Y., Lambrecht, J., Ju, C., and Tacke, F. (2021). Hepatic macrophages in liver homeostasis and diseases-diversity, plasticity and therapeutic opportunities. Cell. Mol. Immunol. 18, 45–56. doi:10.1038/s41423-020-00558-8
Wong, V. W. (2018). Current prevention and treatment options for NAFLD. Adv. Exp. Med. Biol. 157, 1061149–1061157. doi:10.1007/978-981-10-8684-7_12
Wu, X., Li, X., Li, J., Zhao, X., Cui, Y., and Eerdun, C. (2023a). Scoparone inhibits breast cancer cell viability through the NF-κB signaling pathway. Exp. Ther. Med. 26, 328. doi:10.3892/etm.2023.12027
Wu, Z., Wang, W., Wei, L., and Zhu, S. (2023b). Current status and frontier tracking of clinical trials on Metformin for cancer treatment. J. Cancer Res. Clin. Oncol. 149, 16931–16946. doi:10.1007/s00432-023-05391-w
Xia, H., Liu, C., Li, C. C., Fu, M., Takahashi, S., Hu, K. Q., et al. (2018). Dietary tomato powder inhibits high-fat diet-promoted hepatocellular carcinoma with alteration of gut microbiota in mice lacking carotenoid cleavage enzymes. Cancer Prev. Res. (Phila) 11 (12), 797–810. doi:10.1158/1940-6207.CAPR-18-0188
Xie, G., Wang, X., Liu, P., Wei, R., Chen, W., Rajani, C., et al. (2016). Distinctly altered gut microbiota in the progression of liver disease. Oncotarget 7 (15), 19355–19366. doi:10.18632/oncotarget.8466
Xu, Q., Ren, L., Ren, N., Yang, Y., Pan, J., Zheng, Y., et al. (2023). Ferroptosis: a new promising target for hepatocellular carcinoma therapy. Mol. Cell. Biochem. doi:10.1007/s11010-023-04893-y
Yahoo, N., Dudek, M., Knolle, P., and Heikenwälder, M. (2023). Role of immune responses in the development of NAFLD-associated liver cancer and prospects for therapeutic modulation. J. Hepatol. 79, 538–551. doi:10.1016/j.jhep.2023.02.033
Yamada, K., Tanaka, T., Kai, K., Matsufuji, S., Ito, K., Kitajima, Y., et al. (2023a). Suppression of NASH-related HCC by farnesyltransferase inhibitor through inhibition of inflammation and hypoxia-inducible factor-1α expression. Int. J. Mol. Sci. 24 (14), 11546. doi:10.3390/ijms241411546
Yamada, S., Morine, Y., Imura, S., Ikemoto, T., Saito, Y., Shimizu, M., et al. (2023b). Effect of daikenchuto (TU-100) on carcinogenesis in non-alcoholic steatohepatitis. J. Med. Invest. 70 (1.2), 66–73. doi:10.2152/jmi.70.66
Yang, A., Zhu, X., Zhang, L., and Ding, Y. (2023a). Transitioning from NAFLD to MAFLD and MASLD: consistent prevalence and risk factors in a Chinese cohort. J. Hepatol. S0168-8278 (23), 05161–05169. [pii]. doi:10.1016/j.jhep.2023.09.033
Yang, Z., Gao, S., Wong, C. C., Liu, W., Chen, H., Shang, H., et al. (2023b). TUBB4B is a novel therapeutic target in non-alcoholic fatty liver disease-associated hepatocellular carcinoma. J. Pathol. 260 (1), 71–83. doi:10.1002/path.6065
Ye, M., Liu, C., Liu, J., Lu, F., Xue, J., Li, F., et al. (2023). Scoparone inhibits the development of hepatocellular carcinoma by modulating the p38 MAPK/Akt/NF-κB signaling in nonalcoholic fatty liver disease mice. Environ. Toxicol. doi:10.1002/tox.23851
Yin, L., Liu, P., Jin, Y., Ning, Z., Yang, Y., and Gao, H. (2022). Ferroptosis-related small-molecule compounds in cancer therapy: strategies and applications. Eur. J. Med. Chem. 244, 114861. doi:10.1016/j.ejmech.2022.114861
Yokohama, K., Fukunishi, S., Ii, M., Nakamura, K., Ohama, H., Tsuchimoto, Y., et al. (2016). Rosuvastatin as a potential preventive drug for the development of hepatocellular carcinoma associated with non-alcoholic fatty liver disease in mice. Int. J. Mol. Med. 38 (5), 1499–1506. doi:10.3892/ijmm.2016.2766
Younossi, Z., Anstee, Q. M., Marietti, M., Hardy, T., Henry, L., Eslam, M., et al. (2018). Global burden of NAFLD and NASH: trends, predictions, risk factors and prevention. Nat. Rev. Gastroenterol. Hepatol. 15 (1), 11–20. doi:10.1038/nrgastro.2017.109
Younossi, Z. M., Otgonsuren, M., Henry, L., Venkatesan, C., Mishra, A., Erario, M., et al. (2015). Association of nonalcoholic fatty liver disease (NAFLD) with hepatocellular carcinoma (HCC) in the United States from 2004 to 2009. Hepatology 62 (6), 1723–1730. doi:10.1002/hep.28123
Yu, J., Shen, J., Sun, T. T., Zhang, X., and Wong, N. (2013). Obesity, insulin resistance, NASH and hepatocellular carcinoma. Semin. Cancer Biol. 23, 483–491. doi:10.1016/j.semcancer.2013.07.003
Yu, X., Lin, Q., Wu, Z., Zhang, Y., Wang, T., Zhao, S., et al. (2020). ZHX2 inhibits SREBP1c-mediated de novo lipogenesis in hepatocellular carcinoma via miR-24-3p. J. Pathol. 252, 358–370. doi:10.1002/path.5530
Zhang, C., and Yang, M. (2021). Targeting T cell subtypes for NAFLD and NAFLD-related HCC treatment: an opinion. Front. Med. (Lausanne) 8, 789859. doi:10.3389/fmed.2021.789859
Zhang, J., Xie, H., Yao, J., Jin, W., Pan, H., Pan, Z., et al. (2023b). TRIM59 promotes steatosis and ferroptosis in non-alcoholic fatty liver disease via enhancing GPX4 ubiquitination. Hum. Cell. 36, 209–222. doi:10.1007/s13577-022-00820-3
Zhang, J., Zhang, W., Yang, L., Zhao, W., Liu, Z., Wang, E., et al. (2023a). Phytochemical gallic acid alleviates nonalcoholic fatty liver disease via AMPK-ACC-PPARa axis through dual regulation of lipid metabolism and mitochondrial function. Phytomedicine 109, 154589. doi:10.1016/j.phymed.2022.154589
Zhang, X., Coker, O. O., Chu, E. S., Fu, K., Lau, H., Wang, Y. X., et al. (2021a). Dietary cholesterol drives fatty liver-associated liver cancer by modulating gut microbiota and metabolites. Gut 70 (4), 761–774. doi:10.1136/gutjnl-2019-319664
Zhang, Y., Li, X., and Li, X. (2021b). Curcumae ameliorates diethylnitrosamine-induced hepatocellular carcinoma via alteration of oxidative stress, inflammation and gut microbiota. J. Inflamm. Res. 14, 5551–5566. doi:10.2147/JIR.S330499
Zhao, J., Liao, Y., Wei, C., Ma, Y., Wang, F., Chen, Y., et al. (2023). Potential ability of probiotics in the prevention and treatment of colorectal cancer. Clin. Med. Insights-Oncology 17, 11795549231188225. doi:10.1177/11795549231188225
Zhou, F., Zhou, J., Wang, W., Zhang, X. J., Ji, Y. X., Zhang, P., et al. (2019). Unexpected rapid increase in the burden of NAFLD in China from 2008 to 2018: a systematic review and meta-analysis. Hepatology 70 (4), 1119–1133. doi:10.1002/hep.30702
Zhou, Y., Han, Z., Zhao, Z., and Zhang, J. (2023). Scoparone attenuates glioma progression and improves the toxicity of temozolomide by suppressing RhoA/ROCK1 signaling. Environ. Toxicol. doi:10.1002/tox.23882
Keywords: hepatocellular carcinoma, non-alcoholic fatty liver disease, metabolic dysfunction-associated steatotic liver disease, natural products, treatment
Citation: Wang Y, Fleishman JS, Li T, Li Y, Ren Z, Chen J and Ding M (2024) Pharmacological therapy of metabolic dysfunction-associated steatotic liver disease-driven hepatocellular carcinoma. Front. Pharmacol. 14:1336216. doi: 10.3389/fphar.2023.1336216
Received: 10 November 2023; Accepted: 31 December 2023;
Published: 19 January 2024.
Edited by:
Stalin Antony, University of Electronic Science and Technology of China, ChinaReviewed by:
Vineet Mahajan, University of Pittsburgh, United StatesEvangelos Cholongitas, National and Kapodistrian University of Athens, Greece
Copyright © 2024 Wang, Fleishman, Li, Li, Ren, Chen and Ding. This is an open-access article distributed under the terms of the Creative Commons Attribution License (CC BY). The use, distribution or reproduction in other forums is permitted, provided the original author(s) and the copyright owner(s) are credited and that the original publication in this journal is cited, in accordance with accepted academic practice. No use, distribution or reproduction is permitted which does not comply with these terms.
*Correspondence: Yumin Wang, NzIxd2FuZ3ltQGFsaXl1bi5jb20=; Jichao Chen, Y2hlbl9odHp4eXlAc2luYS5jb20=; Mingchao Ding, ZG1jX3p4bEB2aXAuc2luYS5jb20=
†These authors have contributed equally to this work