- Experimental Medicine, Department of Medicine, Faculty of Medicine and Health Sciences, Stellenbosch University, Cape Town, South Africa
All disease, but especially non-communicable diseases, are related to dysfunction of one or more regulatory systems. In developing countries, long-term management of patients with chronic diseases has many challenges and is generally not financially viable, but Africa in particular, which is rich in diverse ethnomedicines presents a more feasible long-term therapeutic approach in this niche. However, despite comprehensive preclinical investigations on numerous plant-derived candidate medicines, only a small portion of these reach the patient as recognised medicines. In this review, we use the example of rooibos (Aspalathus linearis (Burm.f.) R. Dahlgren)–which is globally consumed as aromatic, caffeine-free tea–to illustrate the hurdles that need to be overcome in the low-to middle-income countries, before progression of ethnomedicines to official treatment regimens can be achieved. In terms of methodology, regulatory system focused rooibos papers indexed on PubMed for the past three decades (n = 112) were accessed. Papers reporting duplication of previous results were excluded, as well as review papers. Topics covered includes the high standard of ethnomedicine drug discovery and efficacy testing research performed in Africa (and South Africa in particular in the case of rooibos), the potential bias in terms of preclinical research focus, ethnomedicine ownership and the requirement for independent clinical trial coordination and/or management.
Introduction
A recent fact sheet by the World Health Organisation reported 37% of deaths in Africa to result from non-communicable diseases, with 63% of deaths classified as premature (i.e., before the age of 70) (Wangou et al., 2022). Even more alarmingly in terms of burden on public health systems, is that the International Diabetes Federation reported a diabetes incidence in Africa of 1 in 22 (IDF, 2021). Furthermore, in countries with increased rates of urbanisation and sedentary lifestyles, such as South Africa, incidence may be as high as 1 in 10 (Erzse et al., 2019). In 2015, the National Institute of Health estimated the cost of diabetes alone in Sub-Saharan Africa at USD 19.45 billion annually–1.2% of gross domestic product (Erzse et al., 2019). From these statistics, it is clear that long-term management of chronic patients using mainstream pharmaceuticals is neither financially viable, nor entirely successful.
Africa is rich in candidate medicines that were initially identified largely via indigenous knowledge systems (Sprent et al., 2010). Thus, Africa should be strategically poised at the frontier of medicine development to address the plight of the chronically ill in Africa and other low-to middle-income countries (LMICs) globally. However, despite African ethnomedicine riches, only a fraction of worthy candidate medicines reaches the point where they are officially recognised and registered as mainstream medicines, which would allow their incorporation into policy documents and widespread application in public sector medical centres.
With this mini-review, using the example of Rooibos (a globally consumed tea, prepared from Aspalathus linearis (Burm.f.) R. Dahlgren), we unpack some of the strengths and weaknesses of ethnomedicine research practices such as the research group bias and the relative lack of clinical trials. We also make suggestions on how the more unique challenges faced by African drug discovery and medicines development scientists, on their road to recognition of ethnomedicines as equivalent treatment regimes in disease, may be addressed. These include safety concerns, ethnomedicine ownership, stagnation of medicines development at the preclinical phase.
Rooibos: doomed to fail or on the road to success?
Rooibos is a herbal plant indigenous to South Africa and is globally consumed as tea, for its aromatic taste and health benefits. Rooibos is commercially available in two forms, unfermented (green) and fermented. Although the unfermented rooibos generally exhibits higher potency–likely due to its higher polyphenol content–similar health promoting attributes have been reported for both. Thus, distinction between the two types was not relevant for the purpose of this review.
Rooibos was chosen as example ethnomedicine, as it has been assessed in the context of modulation of many regulatory systems, which are central to the physiological dysregulation resulting in chronic disease. Furthermore, a robust body of literature generated by many different research groups exist on its potential as medicine in both a preventative and therapeutic approaches across various models and diseases, and it has been thoroughly characterised in terms of active constituents.
Evidence of general antioxidant and anti-inflammatory efficacy
Rooibos is widely known and consumed for its antioxidant activity. The antioxidant mechanism of rooibos seems multi-pronged: firstly, it has demonstrated ability to scavenge free radicals in a manner similar to vitamin C and E (Snijman et al., 2009; Lopez et al., 2022), a mechanism ascribed to mainly two active ingredients–aspalathin and nothofagin (Bramati et al., 2003; Joubert et al., 2005; Chen et al., 2013; Canda et al., 2014). In fact, rooibos has such high potency that pro-oxidant outcome have been reported for extracted (i.e., concentrated) rooibos when administered in the absence of disease or conditions of oxidative stress (Joubert et al., 2005). Secondly, rooibos constituents quercetin, aspalathin and catechin inhibit lipid peroxidation (Snijman et al., 2009), a mechanism likely responsible for its reported membrane-protective effects of rooibos (Ulicna et al., 2019; Pretorius and Smith, 2022). A multitude of studies in cell-free systems and in cell culture have also demonstrated improved antioxidant enzyme activities (particularly for catalase and superoxide dismutase (SOD)) (Waisundara and Hoon, 2015) and mitochondrial bioenergetics (Mthembu et al., 2021).
Redox benefits of rooibos administration have also been successfully translated into various in vivo settings. In rodent models, these benefits included increased rodent serum SOD and decreased urinary 8-Oxo-dG levels (Baba et al., 2009), attenuation of increases in plasma and hepatic MDA and decreases in whole blood and liver GSH:GSSH ratios (Ajuwon et al., 2014; van der Merwe et al., 2015), restoration of t-BHP- and CCl4-induced reduction of liver antioxidant status (Canda et al., 2014; Ulicna et al., 2019) and improved mitochondrial bioenergetics (elevated mitochondrial enzymes, improved capacity of the electron transport chain and increased energy production) (Ulicna et al., 2019). Also in invertebrate models such as C. elegans, rooibos supplementation decreased juglone-induced acute oxidative damage and extended the lifespan of Caenorhabditis elegans in a high glucose environment (Chen et al., 2013). Using quantitative real-time PCR, the authors demonstrated that aspalathin targeted stress and aging related genes as a potential mechanism of action.
The antioxidant capacity of rooibos also links closely to an anti-inflammatory outcome. In this context, data from in vitro models of inflammation associate rooibos administration with improved tight junction protein expression and interaction status to maintain endothelial cellular structure and barrier integrity (Ku et al., 2015; Lee and Bae, 2015; Pretorius and Smith, 2022), modulation of PGE2 synthesis (Hedbrant et al., 2022), inhibition of basophil activation (Pedretti and Peter, 2020) and an inhibitory effect on concentrations of pro-inflammatory mediators–such as IL-1α, IL-6, IL-8, VCAM-1 and ATF4 gene expression (Lawal et al., 2019b), as well as IFN-γ, IL-12, IL-2, IL-17a, TNF-α, IL-8, IL-6, IL-1β and CXCL10 (Nehme et al., 2023)–and increased anti-inflammatory cytokine, IL-10 (Ichiyama et al., 2007). In addition, the flavonoid actives aspalathin and nothofagin were reported to prevent the expression of cell adhesion molecules and transendothelial migration of neutrophils in LPS- and high glucose-exposed cells (Ku et al., 2015; Lee and Bae, 2015), while other rooibos constituents quercetin, luteolin and chrysoeriol reportedly inhibited antigen- and calcium ionophore-stimulated degranulation in basophils (Morishita et al., 2019).
In the inflammation context, data from in vivo studies again corroborates in vitro data. Here, rooibos-treated rodents exposed to LPS exhibited blunted TNF-α and IL-6 responses (Ajuwon et al., 2014), while rooibos pre-treatment reversed DEP-induced increases in IL-8, TNF-α, NF-κB and IL-1β and decreases in IL-10 gene expression (Lawal et al., 2019a). Also in response to the hepatotoxic challenge CCl4, a reduced NF-κB expression was reported in rooibos-administered rats (Gabuza et al., 2022). In terms of specific flavonoids, mice treated with aspalathin and nothofagin showed blunted inflammatory responses (TNF-α, IL-6, NF-κB activation, endothelial hyperpermeability and leukocyte migration) to both LPS- and high glucose challenge (Ku et al., 2015; Lee and Bae, 2015).
In addition to general demonstrations of antioxidant and anti-inflammatory actions of rooibos, potential for therapeutic effect in specific disease states have also been reported. A large number of rodent studies have linked antioxidant and anti-inflammatory effects of rooibos to cardiovascular health in particular, including benefits in terms of both vasculature and myocardial tissue (Katengua-Thamahane et al., 2014; Ku et al., 2015; Lee and Bae, 2015; Obasa et al., 2020; Smit-van Schalkwyk et al., 2020; Smit et al., 2022).
Despite the solid pre-clinical database, we could find only one clinical trial that focused on elucidating benefits of rooibos supplementation in a vulnerable population - in subjects at risk for coronary heart disease, rooibos consumption improved plasma lipid profiles (decreased LDL-cholesterol and triacylglycerols; increased HDL-cholesterol) and redox status (decreases in lipid peroxidation (conjugated dienes); increases in glutathione and GSH:GSSH ratio), suggesting a beneficial effect of rooibos to reduce risk factors implicated in developing cardiovascular disease (Marnewick et al., 2010).
Endocrine (metabolic) efficacy
Given the high incidence of diabetes in developing countries, it is no surprise that a robust body of rooibos research exist in this context. One of the first reports on rooibos illustrated it to ameliorate diet-induced metabolic disturbances (including lipid profile, adipocyte size and hepatic steatosis) in hyperlipidemic mice (Beltran-Debon et al., 2011), suggesting rooibos to have a role as preventative modality in metabolic disease. Aspalathin was named one of the most important active ingredients in rooibos, facilitating a beneficial outcome in terms of glucose levels and insulin sensitivity in db/db mice (Kawano et al., 2009).
These positive reports sparked research focused on assessing the anti-diabetic potential of rooibos, which were largely led by researchers in South Africa, where rooibos grows naturally. Research expanded on the first studies, illustrating rooibos to enhance glucose uptake into (C2C12) murine skeletal muscle myotubules and to lower blood glucose with a potency similar to that of metformin (Muller et al., 2012) in streptozotocin (STZ)-induced diabetic rats, with the constituent polyphenols aspalathin and rutin demonstrated to act in synergy to achieve these effects. In addition, the constituent phenylpyruvic acid-2-O-glycoside (PPAG) was illustrated to play a major role in improving glucose metabolism in Chang (human hepatic) cells (Muller et al., 2013). Follow-up studies by the same group expanded on the antidiabetic potential of rooibos by demonstrating its ability to prevent experimentally induced insulin resistance and glucose-associated detrimental effects in several different cell types, including C2C12 murine skeletal muscle myotubules (Mazibuko et al., 2013), H9c2 murine embryonic cardiomyocytes (Dludla et al., 2016), 3T3-L1 murine adipocytes (Mazibuko et al., 2015) and C3A human liver carcinoma cells (Mazibuko-Mbeje et al., 2019) via antioxidant-dependent and independent mechanisms (Mazibuko-Mbeje et al., 2019; Mazibuko-Mbeje et al., 2022). In addition, rooibos was shown to reduce lipid accumulation in 3T3-L1 liver cells (Sanderson et al., 2014), suggesting a further beneficial anti-diabetic mechanism, while aspalathin specifically was demonstrated to protect pancreatic β-cells from in vitro glucotoxicity (Moens et al., 2020) and lipotoxicity (Moens et al., 2022).
The robustness of the comprehensive preclinical data set generated by this group, is evident from the similarly positive data by other research groups illustrating benefits of rooibos in other rodent models of diabetes (Son et al., 2013; Kamakura et al., 2015), as well as in L6 myoblasts and pancreatic β-cells (Kamakura et al., 2015; Himpe et al., 2016). New technology was recently used to confirm known effects and mechanisms, e.g., via targeted cellomics screening (Pringle et al., 2021) and network pharmacology- and molecular dynamics simulation-based bioprospecting (Akoonjee et al., 2022). However, despite the robust preclinical data, translation into human models are still lacking.
Rooibos and its constituents have not yet been investigated for other potential endocrine effects to the same extent. Only one group has reported on the effect of rooibos and some constituents on adrenal steroidogenesis. Indeed, an inhibitory effect of rooibos on specific P450 enzymes was demonstrated in fibroblast-like primate kidney (COS-1) cells, as well as a general downregulation of synthesis of corticosteroid and aldosterone precursors in H295r human adrenocarcinoma cells (Schloms et al., 2012; Schloms and Swart, 2014). This data aligns with the “calming” effect anecdotally ascribed to rooibos. Encouragingly, this group also reported on parallel supplementation studies in humans (the same cohort study reported on in Marnewick et al. (2010) and rats. These in vivo studies jointly provided evidence of decreased glucocorticoid synthesis after rooibos consumption–most notably a lower corticosterone:testosterone ratio in rodents, and a lower cortisol:cortisone in humans (Schloms and Swart, 2014).
Neglected regulatory systems
Although rooibos is anecdotally acclaimed for its calming effect few studies have investigated the effect of rooibos on the brain and nervous system and associated behavioural outcomes. Somewhat disappointingly, most in vitro studies in this context–albeit with positive results–seem largely focused on antioxidant effects (Hong et al., 2014; Fisher et al., 2020; Ma et al., 2020; Lopez et al., 2022; Brasil et al., 2023). Only two of these expanded their investigation and also reported different modulatory mechanisms in the context of serotonin metabolism (Hong et al., 2014; Lopez et al., 2022), which provides some additional mechanistic support for an anxiolytic or calming effect.
However, the limited scope of these studies may be a limitation of neuronal cell models, as in vivo studies in rodents reported a larger variety of beneficial functional outcomes for rooibos, such as improved long-term spatial memory that was associated with increased striatal dopamine and 3-methoxytyramine (dopamine metabolite) levels, but not excessive motor activity (Pyrzanowska et al., 2019), as well as reduced anxiety and increased exploratory behaviour (Pyrzanowska et al., 2021). These findings have also been corroborated in zebrafish larvae (Lopez et al., 2022), in which a significant GABA agonist effect of rooibos was elucidated.
Collectively, available literature shows neuroprotective potential mediated by an increase in antioxidant enzyme activity (Akinrinmade et al., 2017) and/or modulation of neurotransmission (Lopez et al., 2022), which translates to improved behavioural outcome (Pyrzanowska et al., 2019; Pyrzanowska et al., 2021; Lopez et al., 2022). However, in this context, literature is still relatively sparse.
Somewhat related to this, when considering the known signalling between the brain and the gut, the tradition route of rooibos consumption (orally as a tea), as well as the recognition of the microbiome as additional regulatory system, it seems logical to also consider effects of rooibos in the gut. Indeed, in the rooibos context, emerging evidence suggests a prebiotic-like modulation of the gut microbiome. In vervet monkeys, rooibos normalised Firmicutes to Bacteroidetes ratio to rescue high-fat high-sugar diet-induced metabolic dysregulation (Mangwana, 2020), and increased relative abundance of beneficial bacterial species (Faecalibacterium prausnitzii, Prevotella stercorea and P. copri, Akkermansia muciniphia, Bacteroides intestinalis, Desulfovibrio piger and Bifidobacterium adolescentis). More recent in vitro studies confirmed increased probiotic microbial growth and favourably modulated secretome trace amine content to promote gut health (Pretorius et al., 2022; Pretorius and Smith, 2022). Taken together, changes in the gut microbiome are likely to influence host regulation and homeostasis and as such, the potential benefit conferred by rooibos supplementation necessitates additional robust investigation.
Requirements for transformation from tea to treatment
A few facts are clear from the rooibos context, which may be extrapolated more broadly to ethnomedicine in Africa. Firstly, existing data on rooibos is largely positive and reproducible across various experimental models and research groups, suggesting that rooibos has significant merit as candidate ethnomedicine. Secondly, perhaps due to limited scope of interest or specific expertise of isolated research groups, data on specific systems (such as redox) are almost excessive, while relatively lacking for others. Thirdly, despite almost two decades of preclinical research, translation into human trials is near absent (Figure 1), suggesting absence of a coordinated, systematic approach to medicine development. Moreover, the human studies that have been performed are often limited (few parameters assessed on available samples) and self-serving to the research group interest. We provide some suggestions on how these issues may be addressed going forward.
Addressing safety concerns
A potential obstacle to human testing of ethnomedicines in general, is concerns around safety. As would be the case for any mainstream candidate pharmaceutical, specific pharmacological and pharmacovigilance data on toxicity, risk for drug-interaction, ADME profile, risk of off-target adverse events, etc. would be required for any ethnomedicine to be registered as a mainstream pharmaceutical. Unfortunately, it seems as if this approach is deemed financially unfeasible in the African ethnomedicine environment, where marketing and exporting plant-derived products as food supplements are already generating substantial revenue.
In the case of rooibos, significant safety data has been generated mostly by preclinical researchers using in vitro models to address this. In terms of cytochrome p450 (CYP450) and other enzymes related to enzymes related to drug metabolism, data consistently suggest that rooibos may affect drug bioavailability (Marnewick et al., 2003; Matsuda et al., 2007; Fantoukh et al., 2019; Patel et al., 2019). This data has been confirmed in rodents in the context of hypoglycemic and hypolipidemic treatments (including metformin and atorvastatin) (Patel et al., 2019), of which atorvastatin is metabolised by CYP450 enzymes. Importantly, no herb-drug interaction was reported for metformin and rooibos co-treatment (Patel et al., 2019), suggesting this may be an avenue in to pursue in humans (Dludla et al., 2018). All in vivo studies seem to indicate potentiation of drug action by rooibos, especially that of atorvastatin, i.e., lower doses of pharmaceuticals may be required for therapeutic effect when used in combination with rooibos. A compounding approach may therefore limit incidence of adverse drug effects, as well as lowering treatment costs, illustrating both the importance of safety testing and the significant role that ethnomedicines could play in patient management, especially in poor countries. However, pre-clinical researchers rarely have the means or intent of progressing to clinical trials, which halts the transition to clinical trials at this point.
Progression to clinical trials
Although reproducibility of research data should be confirmed, the decision that pre-clinical data is sufficient to warrant clinical trials in humans, should be made timeously to prevent stagnation of medicines development at the preclinical stage. We believe that the cost, in terms of human resources, monetary expense, legislative compliance and administrative resources, is only one reason for the relative lack of throughput. In the case of rooibos, clear research “silos” are evident, although there is clearly global interest in rooibos (Figure 2), suggesting lack of a coordinated effort. Given the fact that identification of commercial ownership of an ethnomedicine is a complex negotiation, especially when no refinement or extraction process is required for therapeutic potency, the manufacturing pharmaceutical industry is understandably not interested in coordinating or funding research on any particular ethnomedicine. Although indigenous ownership has been acknowledged for rooibos via traditional knowledge and access-benefit sharing regulations leading to the Rooibos Benefit Sharing Agreement (UEBT, 2017; Schroeder et al., 2020; Meyer and Naicker, 2023), this resulted from a tedious 6-year administrative process. While the signing of this agreement has positively impacted the future of rooibos as potential medicine, this route is not the norm for the majority of ethnomedicines, where bioprospecting is neglected and ethnomedicines are sold as dietary supplements only. There thus exists a dire need for a knowledgeable and suitably equipped entity to take charge of the ethnomedicine pipeline from bench to bedside (as is currently attempted for rooibos by the South African Rooibos Council).
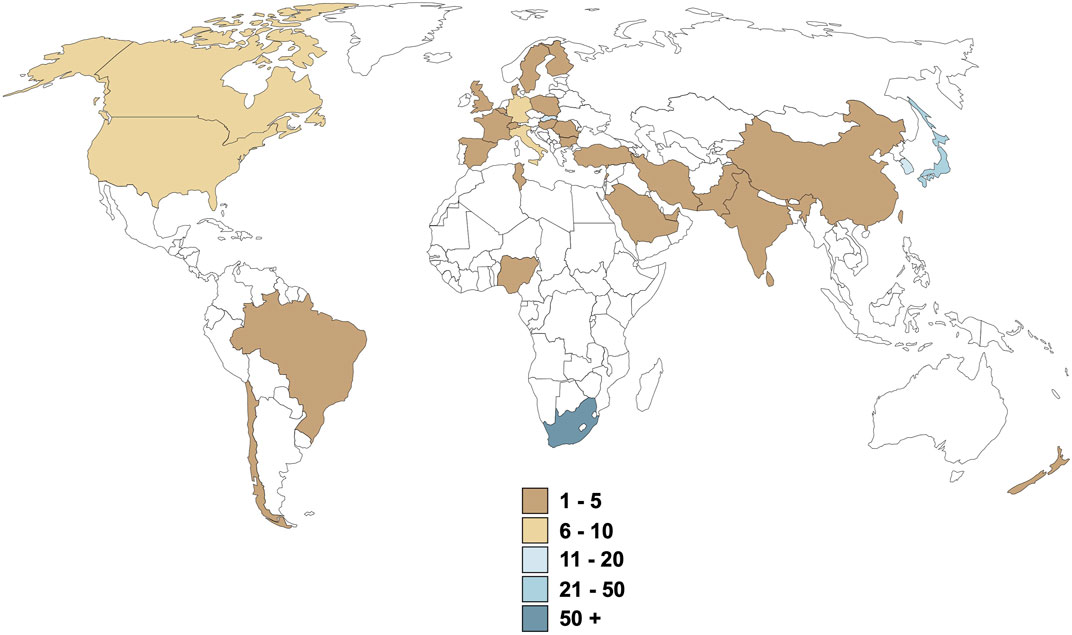
FIGURE 2. Origin of health-related rooibos research over the last 3 decades. Values are representative of the number of publications produced, excluding literature reviews and case reports.
The World Health Organization (WHO) seems to be taking the lead in recognition of traditional medicines, with the new WHO Global Centre for Traditional Medicine scheduled to open its doors in 2024 (WHO, 2023). While the priority of this centre is not yet clear, the expectation and hope is that this centre may play a role in “talent identification” of ethnomedicines, connecting role players with various expertise and backgrounds to construct multidisciplinary, equipped teams, as well as facilitating the progression of candidate medicines through a clinical trial process.
Conclusion
Clearly, preclinical researchers in Africa are doing high quality research to aid in identification of potential ethnomedicines and to provide scientific support for traditional practices which may contribute to mainstream medical care. However, given the dire state of long-term chronic patient management in poor countries, we believe that a centralised agency is required to improve the coherence of currently disjointed efforts, in order to achieve affordable medicine and improvement of quality of life for all.
Author contributions
LP: Conceptualization, Validation, Writing–original draft, Writing–review and editing. CS: Conceptualization, Supervision, Validation, Writing–original draft, Writing–review and editing.
Funding
The author(s) declare that financial support was received for the research, authorship, and/or publication of this article. The authors would like to acknowledge Professors Jean Millar and Robert Fenn for financial support. Stellenbosch University is acknowledged for financial support.
Conflict of interest
The authors declare that the research was conducted in the absence of any commercial or financial relationships that could be construed as a potential conflict of interest.
Publisher’s note
All claims expressed in this article are solely those of the authors and do not necessarily represent those of their affiliated organizations, or those of the publisher, the editors and the reviewers. Any product that may be evaluated in this article, or claim that may be made by its manufacturer, is not guaranteed or endorsed by the publisher.
References
Ajuwon, O. R., Oguntibeju, O. O., and Marnewick, J. L. (2014). Amelioration of lipopolysaccharide-induced liver injury by aqueous rooibos (Aspalathus linearis) extract via inhibition of pro-inflammatory cytokines and oxidative stress. BMC Complementary Altern. Med. 14, 392. doi:10.1186/1472-6882-14-392
Akinrinmade, O., Omoruyi, S., Dietrich, D., and Ekpo, O. (2017). Long-term consumption of fermented rooibos herbal tea offers neuroprotection against ischemic brain injury in rats. Acta Neurobiol. Exp. 77, 94–105. doi:10.21307/ane-2017-040
Akoonjee, A., Rampadarath, A., Aruwa, C. E., Ajiboye, T. A., Ajao, A. A., and Sabiu, S. (2022). Network pharmacology- and molecular dynamics simulation-based bioprospection of Aspalathus linearis for type-2 diabetes care. Metabolites 12, 1013. doi:10.3390/metabo12111013
Baba, H., Ohtsuka, Y., Haruna, H., Lee, T., Nagata, S., Maeda, M., et al. (2009). Studies of anti-inflammatory effects of Rooibos tea in rats. Pediatr. Int. 51, 700–704. doi:10.1111/j.1442-200X.2009.02835.x
Beltran-Debon, R., Rull, A., Rodriguez-Sanabria, F., Iswaldi, I., Herranz-Lopez, M., Aragones, G., et al. (2011). Continuous administration of polyphenols from aqueous rooibos (Aspalathus linearis) extract ameliorates dietary-induced metabolic disturbances in hyperlipidemic mice. Phytomedicine 18, 414–424. doi:10.1016/j.phymed.2010.11.008
Bramati, L., Aquilano, F., and Pietta, P. (2003). Unfermented rooibos tea: quantitative characterization of flavonoids by HPLC−UV and determination of the total antioxidant activity. J. Agric. Food Chem. 51, 7472–7474. doi:10.1021/jf0347721
Brasil, F. B., De Almeida, F. J. S., Luckachaki, M. D., Dall’oglio, E. L., and De Oliveira, M. R. (2023). The C-glucosyl flavone isoorientin pretreatment attenuates the methylglyoxal-induced mitochondrial dysfunction in the human neuroblastoma SH-SY5Y cells: role for the AMPK-PI3K/Akt/Nrf2/gamma-GCL/GSH axis. Metab. Brain Dis. 38, 437–452. doi:10.1007/s11011-022-00966-x
Canda, B. D., Oguntibeju, O. O., and Marnewick, J. L. (2014). Effects of consumption of rooibos (Aspalathus linearis) and a rooibos-derived commercial supplement on hepatic tissue injury by tert-butyl hydroperoxide in Wistar rats. Oxid. Med. Cell Longev. 2014, 716832, doi:10.1155/2014/716832
Chen, W., Sudji, I. R., Wang, E., Joubert, E., Van Wyk, B. E., and Wink, M. (2013). Ameliorative effect of aspalathin from rooibos (Aspalathus linearis) on acute oxidative stress in Caenorhabditis elegans. Phytomedicine 20, 380–386. doi:10.1016/j.phymed.2012.10.006
Dludla, P. V., Gabuza, K. B., Muller, C. J. F., Joubert, E., Louw, J., and Johnson, R. (2018). Aspalathin, a C-glucosyl dihydrochalcone from rooibos improves the hypoglycemic potential of metformin in type 2 diabetic (db/db) mice. Physiol. Res. 67, 813–818. doi:10.33549/physiolres.933891
Dludla, P. V., Muller, C. J., Joubert, E., Louw, J., Gabuza, K. B., Huisamen, B., et al. (2016). Phenylpyruvic acid-2-O-beta-D-Glucoside attenuates high glucose-induced apoptosis in H9c2 cardiomyocytes. Planta Med. 82, 1468–1474. doi:10.1055/s-0042-110856
Erzse, A., Stacey, N., Chola, L., Tugendhaft, A., Freeman, M., and Hofman, K. (2019). The direct medical cost of type 2 diabetes mellitus in South Africa: a cost of illness study. Glob. Health Action 12, 1636611. doi:10.1080/16549716.2019.1636611
Fantoukh, O. I., Dale, O. R., Parveen, A., Hawwal, M. F., Ali, Z., Manda, V. K., et al. (2019). Safety assessment of phytochemicals derived from the globalized South African rooibos tea (Aspalathus linearis) through interaction with CYP, PXR, and P-gp. J. Agric. Food Chem. 67, 4967–4975. doi:10.1021/acs.jafc.9b00846
Fisher, D., Thomas, K. A., and Abdul-Rasool, S. (2020). The synergistic and neuroprotective effects of alcohol-antioxidant treatment on blood-brain barrier endothelial cells. Alcohol Clin. Exp. Res. 44, 1997–2007. doi:10.1111/acer.14433
Gabuza, K. B., Buthelezi, N., Kappo, A. P., Mabuda, T. I., Mosa, R., Louw, J., et al. (2022). In vitro and in vivo hepatotoxicity study of Afriplex GRT through an inflammatory response. Toxicol. Rep. 9, 1920–1928. doi:10.1016/j.toxrep.2022.10.006
Hedbrant, A., Persson, I., Erlandsson, A., and Wijkander, J. (2022). Green, black and rooibos tea inhibit prostaglandin E2 formation in human monocytes by inhibiting expression of enzymes in the prostaglandin E2 pathway. Molecules 27, 397. doi:10.3390/molecules27020397
Himpe, E., Cunha, D. A., Song, I., Bugliani, M., Marchetti, P., Cnop, M., et al. (2016). Phenylpropenoic acid glucoside from rooibos protects pancreatic beta cells against cell death induced by acute injury. PLoS One 11, e0157604. doi:10.1371/journal.pone.0157604
Hong, I. S., Lee, H. Y., and Kim, H. P. (2014). Anti-oxidative effects of Rooibos tea (Aspalathus linearis) on immobilization-induced oxidative stress in rat brain. PLoS One 9, e87061. doi:10.1371/journal.pone.0087061
Ichiyama, K., Tai, A., and Yamamoto, I. (2007). Augmentation of antigen-specific antibody production and IL-10 generation with a fraction from Rooibos (Aspalathus linearis) tea. Biosci. Biotechnol. Biochem. 71, 598–602. doi:10.1271/bbb.60536
Idf (2021). “Diabetes in Africa,”. Editor I. D. Federation 10th ed. Available at: https://diabetesatlas.org/idfawp/resource-files/2022/01/IDF-Atlas-Factsheet-2021_AFR.pdf.
Joubert, E., Winterton, P., Britz, T. J., and Gelderblom, W. C. A. (2005). Antioxidant and pro-oxidant activities of aqueous extracts and crude polyphenolic fractions of rooibos (Aspalathus linearis). J. Agric. Food Chem. 53, 10260–10267. doi:10.1021/jf051355a
Kamakura, R., Son, M. J., De Beer, D., Joubert, E., Miura, Y., and Yagasaki, K. (2015). Antidiabetic effect of green rooibos (Aspalathus linearis) extract in cultured cells and type 2 diabetic model KK-A(y) mice. Cytotechnology 67, 699–710. doi:10.1007/s10616-014-9816-y
Katengua-Thamahane, E., Marnewick, J. L., Ajuwon, O. R., Chegou, N. N., Szucs, G., Ferdinandy, P., et al. (2014). The combination of red palm oil and rooibos show anti-inflammatory effects in rats. J. Inflamm. (Lond) 11, 41. doi:10.1186/s12950-014-0041-4
Kawano, A., Nakamura, H., Hata, S., Minakawa, M., Miura, Y., and Yagasaki, K. (2009). Hypoglycemic effect of aspalathin, a rooibos tea component from Aspalathus linearis, in type 2 diabetic model db/db mice. Phytomedicine 16, 437–443. doi:10.1016/j.phymed.2008.11.009
Ku, S. K., Kwak, S., Kim, Y., and Bae, J. S. (2015). Aspalathin and Nothofagin from Rooibos (Aspalathus linearis) inhibits high glucose-induced inflammation in vitro and in vivo. Inflammation 38, 445–455. doi:10.1007/s10753-014-0049-1
Lawal, A. O., Davids, L. M., and Marnewick, J. L. (2019a). Rooibos (Aspalathus linearis) and honeybush (Cyclopia species) modulate the oxidative stress associated injury of diesel exhaust particles in human umbilical vein endothelial cells. Phytomedicine 59, 152898. doi:10.1016/j.phymed.2019.152898
Lawal, A. O., Oluyede, D. M., Adebimpe, M. O., Olumegbon, L. T., Awolaja, O. O., Elekofehinti, O. O., et al. (2019b). The cardiovascular protective effects of rooibos (Aspalathus linearis) extract on diesel exhaust particles induced inflammation and oxidative stress involve NF-κB- and Nrf2-dependent pathways modulation. Heliyon 5, e01426. doi:10.1016/j.heliyon.2019.e01426
Lee, W., and Bae, J. S. (2015). Anti-inflammatory effects of aspalathin and nothofagin from rooibos (Aspalathus linearis) in vitro and in vivo. Inflammation 38, 1502–1516. doi:10.1007/s10753-015-0125-1
Lopez, V., Casedas, G., Petersen-Ross, K., Powrie, Y., and Smith, C. (2022). Neuroprotective and anxiolytic potential of green rooibos (Aspalathus linearis) polyphenolic extract. Food Funct. 13, 91–101. doi:10.1039/d1fo03178c
Ma, L., Zhang, B., Liu, J., Qiao, C., Liu, Y., Li, S., et al. (2020). Isoorientin exerts a protective effect against 6-OHDA-induced neurotoxicity by activating the AMPK/AKT/Nrf2 signalling pathway. Food Funct. 11, 10774–10785. doi:10.1039/d0fo02165b
Mangwana, N. (2020). The in vitro faecal evaluation of prebiotic effects of rooibos phenolic compounds on the gut microbiota of vervet monkeys (Chlorocebus pygerythrus). Cape Town, South Africa: MSc (Environmental Health), Cape Peninsula University of Technology.
Marnewick, J. L., Joubert, E., Swart, P., Van Der Westhuizen, F., and Gelderblom, W. (2003). Modulation of hepatic drug metabolizing enzymes and oxidative status by rooibos (Aspalathus linearis) and honeybush (Cyclopia intermedia), green and black (Camellia sinensis) teas in rats. J. Agric. Food Chem. 51, 8113–8119. doi:10.1021/jf0344643
Marnewick, J. L., Rautenbach, F., Venter, I., Neethling, H., Blackhurst, D. M., Wolmarans, P., et al. (2010). Effects of rooibos (Aspalathus linearis) on oxidative stress and biochemical parameters in adults at risk for cardiovascular disease. J. Ethnopharmacol. 133, 46–52. doi:10.1016/j.jep.2010.08.061
Matsuda, K., Nishimura, Y., Kurata, N., Iwase, M., and Yasuhara, H. (2007). Effects of continuous ingestion of herbal teas on intestinal CYP3A in the rat. J. Pharmacol. Sci. 103, 214–221. doi:10.1254/jphs.fp0061311
Mazibuko, S. E., Joubert, E., Johnson, R., Louw, J., Opoku, A. R., and Muller, C. J. (2015). Aspalathin improves glucose and lipid metabolism in 3T3-L1 adipocytes exposed to palmitate. Mol. Nutr. Food Res. 59, 2199–2208. doi:10.1002/mnfr.201500258
Mazibuko, S. E., Muller, C. J., Joubert, E., De Beer, D., Johnson, R., Opoku, A. R., et al. (2013). Amelioration of palmitate-induced insulin resistance in C2C12 muscle cells by rooibos (Aspalathus linearis). Phytomedicine 20, 813–819. doi:10.1016/j.phymed.2013.03.018
Mazibuko-Mbeje, S. E., Dludla, P. V., Johnson, R., Joubert, E., Louw, J., Ziqubu, K., et al. (2019). Aspalathin, a natural product with the potential to reverse hepatic insulin resistance by improving energy metabolism and mitochondrial respiration. PLoS One 14, e0216172. doi:10.1371/journal.pone.0216172
Mazibuko-Mbeje, S. E., Mthembu, S. X., Muller, C. J., Ziqubu, K., Muvhulawa, N., Modibedi, R. V., et al. (2022). Aspalathin alleviates skeletal muscle insulin resistance and mitochondrial dysfunction. Physiol. Res. 71, 643–656. doi:10.33549/physiolres.934844
Meyer, C., and Naicker, K. (2023). Collective intellectual property of Indigenous peoples and local communities: exploring power asymmetries in the rooibos geographical indication and industry-wide benefit-sharing agreement. Res. Policy 52, 104851. doi:10.1016/j.respol.2023.104851
Moens, C., Bensellam, M., Himpe, E., Muller, C. J. F., Jonas, J. C., and Bouwens, L. (2020). Aspalathin protects insulin-producing beta cells against glucotoxicity and oxidative stress-induced cell death. Mol. Nutr. Food Res. 64, e1901009. doi:10.1002/mnfr.201901009
Moens, C., Muller, C. J. F., and Bouwens, L. (2022). In vitro comparison of various antioxidants and flavonoids from Rooibos as beta cell protectants against lipotoxicity and oxidative stress-induced cell death. PLoS One 17, e0268551. doi:10.1371/journal.pone.0268551
Morishita, Y., Ikeda, K., Matsuno, H., Ito, H., and Tai, A. (2019). Identification of degranulation inhibitors from rooibos (Aspalathus linearis) tea in rat basophilic leukaemia cells. Nat. Prod. Res. 33, 1472–1476. doi:10.1080/14786419.2017.1416377
Mthembu, S. X. H., Muller, C. J. F., Dludla, P. V., Madoroba, E., Kappo, A. P., and Mazibuko-Mbeje, S. E. (2021). Rooibos flavonoids, aspalathin, isoorientin, and orientin ameliorate antimycin A-induced mitochondrial dysfunction by improving mitochondrial bioenergetics in cultured skeletal muscle cells. Molecules 26, 6289. doi:10.3390/molecules26206289
Muller, C. J., Joubert, E., De Beer, D., Sanderson, M., Malherbe, C. J., Fey, S. J., et al. (2012). Acute assessment of an aspalathin-enriched green rooibos (Aspalathus linearis) extract with hypoglycemic potential. Phytomedicine 20, 32–39. doi:10.1016/j.phymed.2012.09.010
Muller, C. J., Joubert, E., Pheiffer, C., Ghoor, S., Sanderson, M., Chellan, N., et al. (2013). Z-2-(beta-D-glucopyranosyloxy)-3-phenylpropenoic acid, an alpha-hydroxy acid from rooibos (Aspalathus linearis) with hypoglycemic activity. Mol. Nutr. Food Res. 57, 2216–2222. doi:10.1002/mnfr.201300294
Nehme, R., Chervet, A., Decombat, C., Longechamp, L., Rossary, A., Boutin, R., et al. (2023). Aspalathus linearis (rooibos) targets adipocytes and obesity-associated inflammation. Nutrients 15, 1751. doi:10.3390/nu15071751
Obasa, Z., Van Vuuren, M. A., Huisamen, B., and Windvogel, S. L. (2020). The modulating effects of green rooibos (Aspalathus linearis) extract on vascular function and antioxidant status in obese Wistar rats. Cardiovasc. J. Afr. 32, 87–97. doi:10.5830/CVJA-2020-048
Patel, O., Muller, C. J. F., Joubert, E., Rosenkranz, B., Taylor, M. J. C., Louw, J., et al. (2019). Pharmacokinetic interaction of green rooibos extract with atorvastatin and metformin in rats. Front. Pharmacol. 10, 1243. doi:10.3389/fphar.2019.01243
Pedretti, S., and Peter, J. (2020). Rooibos herbal tea reduces allergic basophil activation in adult atopic patients. J. Ethnopharmacol. 252, 112353. doi:10.1016/j.jep.2019.112353
Pretorius, L., and Smith, C. (2022). Aspalathus linearis (rooibos) and agmatine may act synergistically to beneficially modulate intestinal tight junction integrity and inflammatory profile. Pharm. (Basel) 15, 1097. doi:10.3390/ph15091097
Pretorius, L., Van Staden, A. D., Kellermann, T. A., Henning, N., and Smith, C. (2022). Rooibos (Aspalathus linearis) alters secretome trace amine profile of probiotic and commensal microbes in vitro. J. Ethnopharmacol. 297, 115548. doi:10.1016/j.jep.2022.115548
Pringle, N. A., Van De Venter, M., and Koekemoer, T. C. (2021). Comprehensive in vitro antidiabetic screening of Aspalathus linearis using a target-directed screening platform and cellomics. Food Funct. 12, 1020–1038. doi:10.1039/d0fo02611e
Pyrzanowska, J., Fecka, I., Mirowska-Guzel, D., Joniec-Maciejak, I., Blecharz-Klin, K., Piechal, A., et al. (2019). Long-term administration of Aspalathus linearis infusion affects spatial memory of adult Sprague-Dawley male rats as well as increases their striatal dopamine content. J. Ethnopharmacol. 238, 111881. doi:10.1016/j.jep.2019.111881
Pyrzanowska, J., Joniec-Maciejak, I., Blecharz-Klin, K., Piechal, A., Mirowska-Guzel, D., Fecka, I., et al. (2021). Aspalathus linearis infusion affects hole-board test behaviour and amino acid concentration in the brain. Neurosci. Lett. 747, 135680. doi:10.1016/j.neulet.2021.135680
Sanderson, M., Mazibuko, S. E., Joubert, E., De Beer, D., Johnson, R., Pheiffer, C., et al. (2014). Effects of fermented rooibos (Aspalathus linearis) on adipocyte differentiation. Phytomedicine 21, 109–117. doi:10.1016/j.phymed.2013.08.011
Schloms, L., Storbeck, K. H., Swart, P., Gelderblom, W. C., and Swart, A. C. (2012). The influence of Aspalathus linearis (Rooibos) and dihydrochalcones on adrenal steroidogenesis: quantification of steroid intermediates and end products in H295R cells. J. Steroid Biochem. Mol. Biol. 128, 128–138. doi:10.1016/j.jsbmb.2011.11.003
Schloms, L., and Swart, A. C. (2014). Rooibos flavonoids inhibit the activity of key adrenal steroidogenic enzymes, modulating steroid hormone levels in H295R cells. Molecules 19, 3681–3695. doi:10.3390/molecules19033681
Schroeder, D., Chennells, R., Louw, C., Snyders, L., and Hodges, T. (2020). The rooibos benefit sharing agreement-breaking new ground with respect, honesty, fairness, and care. Camb Q. Healthc. Ethics 29, 285–301. doi:10.1017/S0963180119001075
Smit, S. E., Manirafasha, C., Marais, E., Johnson, R., and Huisamen, B. (2022). Cardioprotective function of green rooibos (Aspalathus linearis) extract supplementation in ex vivo ischemic prediabetic rat hearts. Planta Med. 88, 62–78. doi:10.1055/a-1239-9236
Smit-Van Schalkwyk, M., Windvogel, S. L., and Strijdom, H. (2020). Rooibos (Aspalathus linearis) protects against nicotine-induced vascular injury and oxidative stress in Wistar rats. Cardiovasc. J. Afr. 31, 81–90. doi:10.5830/CVJA-2019-052
Snijman, P. W., Joubert, E., Ferreira, D., Li, X. C., Ding, Y., Green, I. R., et al. (2009). Antioxidant activity of the dihydrochalcones aspalathin and nothofagin and their corresponding flavones in relation to other rooibos (Aspalathus linearis) flavonoids, epigallocatechin gallate, and trolox. J. Agric. Food Chem. 57, 6678–6684. doi:10.1021/jf901417k
Son, M. J., Minakawa, M., Miura, Y., and Yagasaki, K. (2013). Aspalathin improves hyperglycemia and glucose intolerance in obese diabetic ob/ob mice. Eur. J. Nutr. 52, 1607–1619. doi:10.1007/s00394-012-0466-6
Sprent, J. I., Odee, D. W., and Dakora, F. D. (2010). African legumes: a vital but under-utilized resource. J. Exp. Bot. 61, 1257–1265. doi:10.1093/jxb/erp342
Uebt (2017). “ABS in South Africa,” in U.F.E. Biotrade. Available at: https://www.abs-biotrade.info/fileadmin/Downloads/2.%20PARTNER%20COUNTRIES/SOUTH%20AFRICA/4.%20STATUS%20QUO%20ABS%20AND%20BIOTRADE/ABS_Regulations/Publication-UEBT-ABS-I-ABS-in-South-Africa-2017.pdf.
Ulicna, O., Vancova, O., Kucharska, J., Janega, P., and Waczulikova, I. (2019). Rooibos tea (Aspalathus linearis) ameliorates the CCl4-induced injury to mitochondrial respiratory function and energy production in rat liver. Gen. Physiol. Biophys. 38, 15–25. doi:10.4149/gpb_2018037
Van Der Merwe, J. D., De Beer, D., Joubert, E., and Gelderblom, W. C. (2015). Short-term and sub-chronic dietary exposure to aspalathin-enriched green rooibos (Aspalathus linearis) extract affects rat liver function and antioxidant status. Molecules 20, 22674–22690. doi:10.3390/molecules201219868
Waisundara, V. Y., and Hoon, L. Y. (2015). Free radical scavenging ability of Aspalathus linearis in two in vitro models of diabetes and cancer. J. Tradit. Complement. Med. 5, 174–178. doi:10.1016/j.jtcme.2014.11.009
Wangou, M. M., Bouesso, B. R. O., Sy, S., Bataliack, S. M., Barango, P., Bady, D. C., et al. (2022). "Noncommunicable diseases in Africa the invisible epidemic", in: aho.afro.who.int. (ed.) W.H.O.a.R. Integrated african health observatory, Brazzaville, Republic of Congo: Ncd Team. December, (ed.).
WHO (2023). WHO global centre for traditional medicine. Available at: https://www.who.int/initiatives/who-global-centre-for-traditional-medicine.
Keywords: rooibos, clinical trial, human, challenges, regulatory systems, gut-brain, inflammation
Citation: Pretorius L and Smith C (2023) Translation of preclinical ethnomedicine data in LMICs: the example of rooibos. Front. Pharmacol. 14:1328828. doi: 10.3389/fphar.2023.1328828
Received: 27 October 2023; Accepted: 07 December 2023;
Published: 19 December 2023.
Edited by:
Hellen Oketch-Rabah, United States Pharmacopeial Convention, United StatesReviewed by:
Phiwayinkosi V. Dludla, South African Medical Research Council, South AfricaThomas Brendler, University of Johannesburg, South Africa
Copyright © 2023 Pretorius and Smith. This is an open-access article distributed under the terms of the Creative Commons Attribution License (CC BY). The use, distribution or reproduction in other forums is permitted, provided the original author(s) and the copyright owner(s) are credited and that the original publication in this journal is cited, in accordance with accepted academic practice. No use, distribution or reproduction is permitted which does not comply with these terms.
*Correspondence: Carine Smith, csmith@sun.ac.za