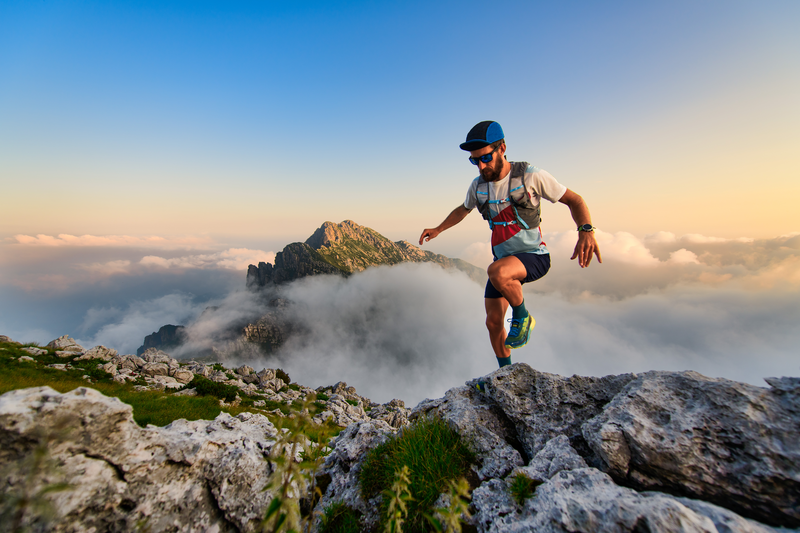
95% of researchers rate our articles as excellent or good
Learn more about the work of our research integrity team to safeguard the quality of each article we publish.
Find out more
REVIEW article
Front. Pharmacol. , 14 December 2023
Sec. Ethnopharmacology
Volume 14 - 2023 | https://doi.org/10.3389/fphar.2023.1303902
This article is part of the Research Topic Herbal Medical Products and Natural Products Targeting Aging and Age-Related Disorders – Ethnopharmacological Perspectives View all 7 articles
“Shengdeng”, a group of Tibetan medicines with diverse biological origins, has long been utilized in Tibet for the treatment of rheumatoid arthritis. It showcases remarkable efficacy in alleviating rheumatism, reducing swelling, and relieving pain. This study aimed to clarify the plant species used as “Shengdeng” and summarize their botanical distribution, traditional uses, phytochemistry, and pharmacology to promote its utilization and development. “Shengdeng” is derived from a remarkable collection of 14 plant species belonging to six distinct families. Extensive phytochemical investigations have led to the identification of 355 chemical constituents within “Shengdeng”. Pharmacological studies conducted on “Shengdeng” have revealed a wide range of beneficial properties, including antioxidant, anticancer, antimicrobial, antiviral, antiparasitic, anti-inflammatory, and anti-arthritic activities. Notably, flavonoids and triterpenoids emerge as the predominant groups among these constituents, contributing to the therapeutic potential and diverse applications of “Shengdeng”. The present review provides a concise summary of the recent advancements in textual research concerning the herbal and botanical distribution, traditional uses, phytochemistry, and pharmacological activities of “Shengdeng”. It is crucial to note that future research on “Shengdeng” should prioritize the analysis of its active ingredients and the establishment of rigorous quality standards. These aspects are essential for ensuring consistency, efficacy, and safety in its clinical application.
Tibetan medicine stands out for its distinctive theories and treatment methods, which have been refined and perfected through extensive clinical practice by the Tibetan people over centuries. Due to the unique Tibetan medical theory, diseases such as rheumatoid arthritis are considered areas of expertise in Tibetan medicine. In particular, the Tibetan medicine “Shengdeng” has shown significant efficacy in treating rheumatoid arthritis. However, there are numerous alternative names for “Shengdeng”, and its origin is complex, leading to a significant issue of adulteration and improper usage. Therefore, it is necessary to conduct a systematic review and study on the varieties of “Shengdeng” and summarize the current research status.
“Shengdeng”, a collection of herbal medicines with diverse biological origins, holds a significant place in traditional Tibetan medicine. The records of “Shengdeng” can be found in the Crystal Beads. Renowned for its efficacy in treating rheumatoid arthritis, “Shengdeng” has attracted considerable attention. The wide distribution of “Shengdeng” in Tibetan regions, coupled with the varying descriptions found in different Tibetan materia medica texts throughout history, has led to confusion regarding its origin. Additionally, the abundance of alternative substitutes further complicates the understanding of Shengdeng’s true source (Zou et al., 2020). In this comprehensive review, we present a meticulous exploration of the herbal and botanical distribution, traditional applications, phytochemistry, and pharmacology of “Shengdeng”. By analyzing the therapeutic potential of this remarkable material in improving human health, our findings contribute valuable insights to guide future research endeavors. This review aims to facilitate a deeper understanding of “Shengdeng” and its multifaceted role in traditional Tibetan medicine, serving as a valuable resource for both researchers and practitioners in the field.
Through meticulous textual analysis of historical Tibetan botanical drug records, a fascinating revelation emerges—the authentic Tibetan botanical drug products “Shengdeng” primarily comprise leguminous plant catechins cultivated in subtropical regions encompassing India, Myanmar, Africa, Guangdong, Yunnan, Zhejiang, Guangxi, and Taiwan. Remarkably, the origin, botanical morphology, taste, nature, and therapeutic efficacy of these medicinal materials harmonize impeccably with the corresponding descriptions elucidated in the “Tara Materia Medica”. According to the descriptions, the Crystal beads can be categorized into three distinct groups based on their colors: “Tanhong Shengdeng”, “Bihuang Shengdeng” and “Songbai Shengdeng”. These categories encompass a total of 14 plant species (Li, 2020). In the present review, a series of surveys of herbal texts and research literature was conducted to explore the source of “Shengdeng” and associated plants. The results are summarized in Table 1. The original plant pictures are in Figure 1. (A is quoted from Tibetan Medicine Records. B is quoted from Chinese Tibetan medicine. C, L, and M are quoted from Chinese Materia Medica. D and F are quoted from Atlas of Chinese Higher Plants. E, H, I, J, K, and N are quoted from Flora Reipublicae Popularis Sinicae. G is quoted from Chinese Union of Botanical Gardens.) (The full botanical plant names have been checked with http://www.theplantlist.org).
FIGURE 1. The botanical distribution of “Shengdeng”, (A) Xanthoceras sorbifolium Bunge. (B) Caesalpinia sappan L. (C) Rhamnella gilgitica Mansf. and Melch. (D) Rhamnus parvifolia Bunge. (E) Rhamnella forrestii W. W. Sm. (F) Rhamnella martinii (H. Lév.) C. K. Schneid. (G) Frangula crenata (Siebold & Zucc.) Miq. (H) Rhamnus sargentiana C. K. Schneid. (I) Rhamnus xizangensis Y.L. Chen and P.K. Chou. (J) Rhamnus dumetorum C. K. Schneid. (K) Rhamnus tangutica J. J. Vassil. (L) Cephalotaxus sinensis (Rehder and E.H.Wilson) H. L. Li. (M) Acacia catechu (L.f.) Willd. (N) Taxus yunnanensis W. C. Cheng and L. K. Fu. (C,L,M) are quoted from Chinese Materia Medica. (D,F) are quoted from Atlas of Chinese Higher Plants. (E,H,I,J,K,N) are quoted from Flora Reipublicae Popularis Sinicae. G is quoted from Chinese Union of Botanical Gardens.).
The “Shengdeng” species primarily inhabit the middle and lower altitude regions of the Qinghai-Tibet Plateau in China. The “Tanhong Shengdeng” variety is primarily distributed in high-altitude regions, notably Tibet, Sichuan, Yunnan, and other areas. In contrast, the “Bihuang Shengdeng” type exhibits a wide distribution and is commonly found along the hillside edges of forests. It predominantly flourishes in sub-montane to montane regions. Lastly, the “Songbai Shengdeng” variety thrives at relatively lower altitudes and can be found throughout the entirety of the country (Zou et al., 2020). This group of herbal medicines can be found in up to 12 countries and regions worldwide. In China specifically, the resources of “Shengdeng” are abundant, with presence documented in at least 20 provinces. Notably, the provinces of Sichuan and Yunnan exhibit significant concentrations of these valuable resources (Table 2).
In accordance with traditional Tibetan medicine theory, “Shengdeng” holds significant therapeutic value in the treatment of various conditions. It is commonly employed for addressing ailments such as rheumatoid arthritis, high-altitude polycythemia, and “Huangshui disease” in Tibetan medicine (Zou et al., 2020). Some prescriptions containing “Shengdeng” have been clinically tested and modern pharmacological studies have demonstrated their significant anti-inflammatory effects. Several clinical studies have reported the effectiveness of Ershiwuwei ErCha Wan in treating rheumatoid arthritis, highlighting its high application value (Huang et al., 2001; Zha et al., 2017; Liu et al., 2023). These medicinal properties have made “Shengdeng” a prominent ingredient in traditional Tibetan healing practices. We organize the details in Tables 3, 4.
In the last few decades, extensive research has led to the isolation and identification of approximately 355 chemical constituents found in the 14 plant species used as “Shengdeng”. These constituents encompass various compound types, including flavonoids, triterpenoids, protosappanin, brazilin, and taxanes. For further details, including the names of the metabolites, their corresponding plant sources, and the references, please refer to Supplementary Table S1.
Flavonoids are a ubiquitous group of naturally occurring polyphenolic metabolites characterized by the flavan nucleus (Peluso et al., 2015). A total of 69 flavonoids (1–69) have been reported. 19 flavonoids (1–19) have been isolated from Lignum X. sorbifolium Bunge (Figures 2–6) (Ni et al., 2009; Yang et al., 2020). Luteolin (20) was isolated from the husks of X. sorbifolium (Wan et al., 2015). (−)-epiafzelechin (21) was isolated from the wood of X. sorbifolium (Ma et al., 2004). Flavonoids (22–26) were isolated from Cephalotaxus sinensis (Rehder and E.H.Wilson) H.L.Li (Li et al., 2007a; Jiang et al., 2013). Flavonoids (27–68) were identified from Acacia catechu (L.f.) Willd (Li et al., 2010b; Negi et al., 2010; Li et al., 2011; Hong et al., 2015; Adhikari et al., 2021). Kaempferol-7-O-β-D-glucoside (69), Kaempferol (1), Quercetin (2), Naringenin (4), Aromadendrin (33), and Taxifolin (62) were also isolated from Rhamnella gilgitica Mansf. and Melch. (Figure 5) (Pan et al., 1998). Homoisoflavones (70–120) were identified from Caesalpinia sappan L. (Namikoshi et al., 1987; Wang et al., 2003; Nguyen et al., 2005; Wang, 2006; Shu, 2007; Chen et al., 2008; Wang et al., 2010; Zhao et al., 2010; Cai, 2012; Chen et al., 2012; Tang et al., 2012; Wang, 2013; Zhao et al., 2014; Sheng, 2016; Wang, 2016; Zhou, 2017).
Triterpenoids (121–185), also known as ganoderic acid, are widely distributed in nature, which are composed of several isoprene molecules linked end to end without a hydroxyl group. Most triterpenoids contain 30 carbon atoms, and a few with 27 carbon atoms (Gao et al., 2018). X. sorbifolium is known to contain triterpenoids as a frequently reported component. Among these triterpenoids, barrigenol-like triterpenoids (A, B) serve as the structural parent cores. Additionally, X. sorbifolium also contains lupane triterpenoids (C) and tirucallane triterpenoids (D). Barrigtogenol C, 16-deoxybarrigtogenol C, oleanolic acid, and protoaescigenin were also isolated from X. sorbifolium (Figures 7–9; Supplementary Tables S2–S4) (Wan et al., 2013).
FIGURE 8. The structure of the code names in the Supplementary Table S1.
Nine protosappanin (186–194) were isolated from C. sappan (Wang et al., 2003; Shu, 2007; Li et al., 2012; Wang, 2016). Among them, 10-omethylprotosappanin B, isoprotosappanin B, and 10-omethylisoprotosappanin B are derivatives derived from protosappanin B. Protosappanin D, on the other hand, is a dimer formed from protosappanin C. Additionally, protosappanin E1 or protosappanin E2 is produced through the polymerization of proto hematoxylin and hematoxylin metabolites. These metabolites represent various structural modifications and polymerizations of protosappanin metabolites, expanding the chemical diversity within this group (Figure 10).
The molecular formula of brazilin is C16H14O5, which is the main active component in C. sappan. A total of 13 brazilin metabolites (195–206) have been isolated and identified (Xu et al., 1994; Shu et al., 2007; Hung et al., 2009; Cooksey, 2010; Wang and Liang, 2016; Kim and Kim, 2018). Brizilide is not a component naturally present in hematoxylin itself but rather a newly derived component that emerges during the isolation process from brazilin hematoxylin (Supplementary Figure S1).
The taxanes or taxoids are a closely related group of antineoplastic agents that have a unique mechanism of action as inhibitors of mitosis, which are widely used in the therapy of ovarian, breast, lung, esophageal, prostate, bladder, and head and neck cancers. Many taxanes (208–271) were isolated from Taxus yunnanensis W.C.Cheng and L.K.Fu (Supplementary Figures S2–S5) (Yue et al., 1995; Zhang et al., 1995; Zhong et al., 1996; Zhang et al., 1997; Zhou et al., 1998; Shi et al., 1999; Li et al., 2000; Li et al., 2001; Shinozaki et al., 2001; Li et al., 2002a; Li et al., 2002b; Shinozaki et al., 2002; Li et al., 2003a; Nguyen et al., 2003; Tezuka et al., 2011; Hai et al., 2014).
In addition to the abovementioned chemical metabolites, several other metabolites have been identified, such as sappanols, flavonoid diglycoside, abietane diterpenoid, and norditerpenoids. Seven sappanols (272–278) were isolated from C. sappan (Supplementary Figure S6) (Xu et al., 2016; Ahmed et al., 2018; Zhao et al., 2019). A total of 20 metabolites (279–298) were isolated from C. sinensis, including flavonoid diglycoside, abietane diterpenoid, and norditerpenoids (Supplementary Figures S7, S8). Esters and fatty acid metabolites (299–313) were isolated from A. catechu (Supplementary Figures S8, S9) (Negi and Dave, 2010; Li et al., 2011; Thakur et al., 2018; Adhikari et al., 2021). Three lignans, seven steroids (314–323) and a Polyphenolic (350) were isolated from T. yunnanensis (Li et al., 2002a; Li et al., 2003b; Hafezi et al., 2020). Twenty cassane diterpenoids (324–337, 346, 351–355) and three lignans (347–349) were isolated from C. sappan (Ma et al., 2015; Tran et al., 2015; Zhu et al., 2017). Alkaloids (338–345) were isolated from C. sinensis (Supplementary Figure S10) (Ma et al., 2016).
The pharmacological studies conducted on “Shengdeng” have consistently shown its remarkable properties, including antioxidant, anticancer, antimicrobial, antiviral, antiparasitic, anti-inflammatory, and anti-arthritic activities, alongside other beneficial characteristics (Figure 11).
Among the metabolites isolated from C. sappan, including Brazilein (203), Sappanchalcone (272), Protosappanin A (186), Protosappanin B (187), and Protosappanin C (188), Brazilin (195) displayed the highest 2,2-diphenyl-1-trinitrophenylhydrazine (DPPH) free radical scavenging activity (Wetwitayaklung et al., 2005; Sasaki et al., 2007; Batubara et al., 2009). Some findings highlight the antioxidant potential of X. sorbifolium and its potential applications in combating oxidative stress (Li et al., 2010a; Zhang et al., 2010). The in vitro scavenging activity of the ethanol extract of R. gilgitica was assessed, revealing a robust capability to scavenge free radicals and efficiently reduce Fe3+ ions (Zhang et al., 2014b; Zhou et al., 2021). The antioxidant activity of various fractions of C. sinensis was assessed using DPPH and reducing power assays. The findings demonstrated that C. sinensis exhibits significant antioxidant activity (Saeed et al., 2007). Some findings collectively emphasize the exceptional antioxidant properties of A. catechu. The regulation of reactive oxygen species and the control of oxidative stress are vital for maintaining cellular balance. Plant extracts, rich in metabolites such as polyphenols, play a significant role in mitigating oxidative stress by demonstrating potent antioxidant activity (Huang et al., 2005; Cai et al., 2006; Hiraganahalli et al., 2012; Saha et al., 2016; Patil and Modak, 2017; Kumar et al., 2018; Babita, 2021a; Adhikari et al., 2021; Babita, 2021b; Shresta et al., 2021). Similarly, the methanol, ethanol, butanol, and water metabolites of A. catechu have IC50 values ranging from 92.48 to 529.30 μg/mL for the determination of DPPH radicals, ABTS radicals, and superoxide scavengers as well as for the reduction of copper and iron ions, which is primarily due to the presence of Quercetin (2), Kaempferol (1), and Chlorogenic acid (306) (Kumar et al., 2019).
Natural molecules have shown promise in providing potential solutions for combating cancer (Greenwell and Rahman, 2015).
Study demonstrated that C. sappan extract can inhibit cancer cell growth by inducing apoptosis and mitochondrial dysfunction in A549 cells (Widodo et al., 2022). The effect of 3-deoxysappanchalcone (273) on colon cancer cell growth revealed its inhibitory potential on the activity of T-lymphokine-activated killer cell-originated protein kinase (TOPK). The compound inhibited colon cancer cell proliferation and anchorage-independent cell growth, and it promoted G2/M cell phase arrest and programmed cell death (Zhao et al., 2019b). The apoptotic effect of brazilin (195) was confirmed in an in vitro model of breast cancer using the MCF-7 cell line. The automated docking tool also demonstrated the therapeutic effect of the brazilin A molecule on the apoptosis inhibitor B-cell lymphoma 2 (BCL-2) protein. This calcium-dependent pathway was mediated through the upregulation of microtubule-associated protein 1A/1B-light chain 2 (LC3-II) and downregulation of P62/SQSTM1 in osteosarcoma cells (Bukke et al., 2018). Using the MG-63 cell line, Kang examined the induction of autophagy by basilicin in osteosarcoma cell cultures and found that this effect was mediated through the Ca2+ forkhead box O3A protein (FOXO3A) pathway. In addition, brazilin (195) caused autophagic cell death in MG-63 cells by activating phosphorylation at the FOXO3A Ser7 site, initiating nuclear translocation of FOXO3A, and increasing its reporter gene activity, which results in the expression of autophagy-related genes and cell death (Kang et al., 2018). The IC50 value of Phanginin R (346) from C. sappan was detected in the range of 5.3 ± 1.9 to 12.3 ± 3.1 μM, indicating that it has evident cytotoxic effects on lung cancer A549 cells and ovarian cancer cells, as well as A2780 cells. Furthermore, phaginins exhibited the expression of the tumor suppressor protein p53, arrest of the cell cycle in the G1 phase, and initiation of apoptosis in A2780 cells, reflecting their anti-cancer properties (Bao et al., 2016).
Metabolites (351–354) exhibited significant inhibition against HL-60 cells (Tran et al., 2015). Studies have shown that brazilin (195) isolated from C. sappan inhibits BAF phosphorylation in vitro and in vivo. The results show that brazilin (195) is directly related to BAF. The inhibition of BAF phosphorylation leads to abnormal nuclear envelope re-organization and cell death, indicating that the disruption of nuclear envelope re-organization may be a novel anti-cancer therapy. Brazilin (195) could be a new cancer drug (Kim et al., 2015b). In another study, various botanical metabolites obtained from ethyl acetate extracts of C. sappan showed better anti-cancer activity than the isolated metabolites, indicating that the crude extract was more effective in relieving cancer than molecular extracts (Zhang et al, 2014a).
Recent research has shed light on the potential of metabolites derived from T. yunnanensis, such as AgNPs, heteropolysaccharide, and α-conidendrin, in the development of novel anti-cancer therapies (Yan et al., 2013; Xia et al., 2016; Hafezi et al., 2020).
Some findings suggest that C. sappan possesses diverse pharmacological properties, including antibacterial, antiviral, antimalarial, and iron-chelating activities, indicating its potential use in various therapeutic applications (Bukke et al., 2015; Bukke et al., 2015; Puttipan et al., 2018; Safitri et al., 2022).
In another study, from the heartwood of C. sappan, numerous neuraminidase inhibitory molecules were isolated, and the maximum inhibitory activity against three types of viral NAs (H1N1, H3N2, and H9N2) was exhibited by a homoisoflavonoid, namely, sappanone A (70), with IC50 values of 0.7, 1.1, and 1.0 mM, respectively. The viral neuraminidase (H1N1, H3N2, and H9N2) inhibitory activity of isolated sappanone A (70) did not significantly differ from than that of the standard drug oseltamivir with IC50 values of 5.8, 5.6, and 1.2 nM, respectively (Jeong et al., 2012). Caesalsappanin G (332) and Caesalsappanin H (333) isolated from C. sappan had a potent antimalarial activity with selectivity indices of 17.6 and 16.4, as well IC50 values of 0.78 and 0.52 mM, respectively, which are comparable to the standard compound chloroquine, with IC50 value of 0.37 ± 0.02 (Ma et al., 2015). In another study, two novel cassane diterpenes isolated from the seeds of mulberry C. sappan extracts were screened for their anti-plasmid activity against the chloroquine-resistant strain K1 of P. falciparum. The extracted metabolites Caesalsappanin R (324) and Caesalsappanin S (325) had a potent antimalarial activity with IC50 values of 3.6 and 25.1 mM, respectively, and the standard drug chloroquine has an IC50 value of 0.19 ± 0.05. However, the difference was not significant (Zhu et al., 2017).
The aqueous, 50% ethanolic, and butanol extracts of A. catechu demonstrated antimicrobial activity against various bacteria, such as Staphylococcus aureus, Pseudomonas aeruginosa, Proteus mirabilis, E. coli, B. and subtilis (Patel et al., 2009; Negi and Dave, 2010; Joshi et al., 2011; Dashtdar et al., 2013; Modi et al., 2013; Panya et al., 2019; Shresta et al., 2021). In addition, a study confirmed that drupacine (338), 11-hydroxycephalotaxine (339), cephalancetine A (340), hsocephalotaxine (341), cephalotaxine β-N-oxide (342), 4-hydroxycephalotaxine (343), wilsonine (344), and cephalotaxine (345) isolated from C. sinensis have excellent activities against tobacco mosaic virus (TMV) and cucumber mosaic virus indoors and outdoors. Its control of TMV is comparable to that of a commercialized anti-viral agent (VA, active ingredient = a mixture of moroxydine hydrochloride and copper acetate). At the same dosage, the inactivation activities against TMV were not significantly different among drupacine (338), 11-hydroxycephalotaxine (339), cephalotaxine (345), and VA (inhibition ratio = 50.76%–53.41%). The inhibited TMV replications of all tested metabolites were inferior to that of VA, but the inhibition ratios of drupacine and cephalotaxine remained greater than 50% (Ma et al., 2016).
In a study, it was demonstrated that the heat-70% EtOH and microwave-70% EtOH extracts of C. sappan exhibited significant anti-inflammatory effects. Metabolites derived from C. sappan, such as episappanol (105), brazilin (195), prosapogenin B (348), sappanol (349), and protosappanin C (347), have shown promising potential for treating inflammation (Mueller et al., 2016; Chowdhury et al., 2019). Hematoxylin A, a isoflavonoid derived from C. sappan, induces anti-inflammatory effects by inhibiting the production of IL-6, prostaglandin E2 (PGE2), and NO in mouse macrophages. Saponin A inhibits the expression of iNOS and COX-2 in LPS-treated RAW264.7 cells. Furthermore, saponin A exhibits anti-inflammatory effects in vivo and protects c57bl/6 mice from LPS-induced death by modulating nuclear factor erythroid 2-related factor 2 (Nrf2) and NF-κB signaling pathways (Lee et al., 2015). In a related study, brazilin could induce an anti-inhibitor of the active groove of NF-κB (IkB) kinase, which targets upstream signaling elements of IkB kinase, thereby promoting formation by disrupting NF-κB activation and signaling complexes at the proximal IL-1 receptor (Jeon et al., 2014). Another study found that NO produced in LPS-induced RAW264.7 cells was scavenged by brazilin and sabanchalcone with IC50 values of 10.3 and 31.0 mM, respectively. The results showed that the inhibitory effect of brazilin and saponins on NO production was better than that of indomethacin (IC50 value of 46.5). The production of TNF-α and PGE2 was also inhibited by brazilin with IC50 values of 87.2 and 12.6 mM, respectively. Hence, the downregulation of the mRNA expression level of TNF-α, iNOS, and COX-2 genes was involved in the anti-inflammatory mechanism of brazilin (Tewtrakul et al., 2015). The anti-inflammatory potential of 3-deoxysappanchalcone (273) (3-DSC) in RAW264.7 cells was confirmed. 3-DSC enhances the expression of hemooxygenase-1 (HO-1) at the translational level, thereby activating the phosphatidylinositol 3-kinase mammalian target of the rapamycin (AKT/mTOR) pathway, which contributes to its anti-inflammatory properties. Based on the concept that HO-1 has anti-inflammatory properties, 3-DSC inhibited NO and IL-6 production in LPS-stimulated RAW264.7 cells (Kim et al., 2014).
A. catechu extract demonstrates the ability to inhibit the production of important inflammatory cytokines (Burnett et al., 2007; Tseng, et al., 2010; Yimam et al., 2010; Yimam et al., 2012; Sunil et al., 2019). A recent study investigated the anti-inflammatory activities of the 70% ethanol extract of R. gilgitica in both RAW264.7 macrophages and rats with complete Freund’s adjuvant (CFA)-induced arthritis (Huang et al., 2016).
Rheumatoid arthritis is a prevalent human health problem worldwide. Traditional medicines for RA worldwide have yielded some positive results. Overcoming osteoclastogenesis is considered as an active strategy for the treatment of bone-destroying diseases, RA, and osteoporosis. Brazilin could exhibit anti-inflammatory and chondroprotective effects in chondrocytes and human osteoarthritis cartilage. The antiarthritic effect of brazilin (195) was assessed using IL-1b-treated primary chondrocytes, TNF-α, and IL-1b-treated cartilage explants. The loss of glycosaminoglycan from cartilage explants stimulated with IL-1b and TNF-α reduced after Brazilin (195) treatment, and the anti-inflammatory activity was evident through the regulation of NFKB1/p50. In chondrocytes, basilicin inhibited the IL-1b-induced inhibition of osteoarthritis markers by inducing NFKB1/p50, indicating a chondroprotective effect (Weinmann et al., 2018). The acute inflammatory paw edema and arthritis index were found to be reduced by brazilin (195) in an in vivo model of arthritis using CIA mice (Jung et al., 2015a). Microstructural studies have shown that brazilin (195) treatment significantly increases bone density, prevents joint destruction and surface wear, and improves bone formation. In addition, serum concentrations of inflammatory cytokines such as IL-6, IL-1b, and TNF-α were attenuated by brazilin (195) treatment. In another study, the anti-inflammatory, bone-protective, and anti-RA activities were proven by evaluating the effect of Sappanchalcone (272) in CIA-presented male DBA/1J mice. Based on the aforementioned studies, the anti-arthritic activity of brazilin (195) (10 mg/kg) and Sappanchalcone (272) (10 mg/kg) was similar to that of standard drug methotrexate (3 mg/kg) (Jung et al., 2015a; Jung et al., 2015b). The opposite effect of brazilin (195) on osteoclast differentiation confirmed that brazilin (195) dose-dependently inhibited the receptor activator of nuclear factor Kpa-B ligand (RANKL) to promote osteoclast differentiation of RAW264.7 cells without signs of cytotoxicity. Brazilin (195) reduced RANKL-induced NF-κB p65 phosphorylation, extracellular signal-regulated kinases, and the appearance of inflammatory negotiator genes (TNF-α, INOS, IL-6, and COX-2) in RAW264.7 cells, indicating its therapeutic effect in avoiding bone loss (Kim et al., 2015a). Another study has proposed that the anti-rheumatoid arthritis effect of the ethyl acetate extract of R. gilgitica (RGEA) may be linked to its capacity to promote apoptosis and inhibit the inflammatory response, potentially by modulating the JAK-STAT pathway (Su et al., 2021). A study showed that the Tibetan medicine Qi-Sai-Er-Sang-Dang-Song Decoction inhibits TNF-α-induced rheumatoid arthritis in human fibroblast-like synoviocytes via regulating NOTCH1/NF-κB/NLRP3 pathway (Su et al., 2023).
Neurodegenerative diseases, including Alzheimer’s disease and Parkinson’s disease, present a significant health challenge in industrialized countries. These diseases are characterized by microglial activation and subsequent neuroinflammatory responses (Rajput et al., 2020; Singh and Devasahayam, 2020). The ethanolic extract of C. sappan has demonstrated neuroprotective and anti-cerebral ischemic effects in an experimental model (Wan et al., 2019). Protosappanin A (186) reversed the neuroinflammatory effect of BV2 cells under the action of LPS by significantly inhibiting the production of IL-1β and TNF-α. In addition, Protosappanin A (186) dose-dependently decreased the messenger ribonucleic acid (mRNA) expression of monocyte chemoattractant protein 1 (MCP-1), IL-1β, and IL-6. Moreover, Protosappanin A (186) inhibited the inflammatory pathway of LPS treatment by downregulating JAK2 and STAT3 phosphorylation and STAT3 nuclear translocation (Wang et al., 2017a). In vitro studies using the thioflavin-T fluorescence assay and transmission electron microscopy demonstrated that hematoxylin significantly reduced the cytotoxicity induced by Aβ42 by inhibiting the formation of Aβ42 fibrils (Tu et al., 2016). Brazilein (203) has been shown to reverse the elevated expression of TNF-α and nucleotide-binding oligomerization domain-containing protein 2 (NOD2) induced by cerebral ischemia and reperfusion in mice (Xiao et al., 2016).
Study showed that ethyl acetate extract of A. catechu (250 mg/kg) can inhibit the toxicity of liver injury in tetrachloride-induced albino rat using biochemical (measurement of serum transaminases, serum alkaline phosphatase, and serum bilirubin) and histopathological assessment (Jayasekhar et al., 1997). A. catechu herbal extracts were demonstrated as hepatoprotective with IC50 of 114.8 μg/mL on HepG2 cells toxified with tert-butyl hydroperoxide (t-BH). The anti-oxidant potential of this plant is attributed to its hepatoprotective activity by reducing lipid peroxidation and cell damage (Hiraganahalli et al., 2012). Similarly, plant ethyl acetate extract showed significant hepatoprotective ability in an in vivo model (Ray et al., 2006). Moreover, in a Wistar rat model experiment, A. catechu seed and bark extracts exhibited hepatoprotective effects, which were related to the decrease of the activity of liver enzymes (alanine aminotransferase, alkaline phosphatase, and aspartate aminotransferase) by reducing lipid peroxidation, and enhanced anti-oxidant activity by increasing glutathione and increasing the activity of peptides and superoxide dismutase (Lakshmi et al., 2018).
The extracts of A. catechu has shown an anti-diabetic activity with IC50 of 49.9 μg/mL toward porcine pancreatic α-amylase and 0.4977 mg/mL against α-glucosidase (Khadayat et al., 2020). Another study found that the methanol extract of A. catechu inhibited α-amylase with IC50 of 49.9 ± 0.4 μg/mL, and kinetic studies indicated that the extract exhibited mixed-type inhibition (Khadayat et al., 2020). A study showed that feeding with an ethanolic extract of catechins on streptozotocin (STZ-)-induced diabetic rats increased their glucose tolerance by 22% and 27% after 7 and 14 days, respectively, whereas those fed a low-dose STZ showed significantly increased glucose tolerance (Swayam, 2011). Catechin (27), Epicatechin (28), Gallocatechin (47), Epigallocatechin (48), and Procyanidin B1 (49)were identified from A. catechu, which exerted anti-diabetic effects by reducing the activities of α-glucosidase, α-amylase, and aldose reductase (Adhikari et al., 2021).
The hypoglycemic effect of C. sinensis leaves was studied in STZ-induced diabetic rats. The results show that C. sinensis leaf extract is a potential drug for treating diabetes, and its active ingredients include flavonoids (Li et al., 2007c) Furthermore, previous investigation suggested that C. sinensis extract has good hypoglycemic and hypolipidemic effects, which may be beneficial to hyperglycemia and may decrease HDL (Muhammad et al., 2013).
The historical use of “Shengdeng” as a traditional medicine for thousands of years indicates its long-standing reputation as a safe therapeutic option. Ancient texts documenting the use of “Shengdeng” do not mention any instances of toxicity associated with its usage.
A study has found the phenols from the leaves of X. sorbifolia could be used as natural neuroinflammation inhibitors (Li et al., 2016). Another study has shown that barrigenol-like triterpenoids derived from X. sorbifolia husks exhibited s significant inhibitory activity against the proliferation of three human tumor cell lines, namely, HepG2, HCT-116, and U87-MG (Wang et al., 2017b). Furthermore, a study indicated that the husk of X. sorbifolia might prevent inflammation-related neurodegenerative disorders by controlling the expression of the nuclear NF-κB signaling pathway, which clearly inhibited LPS-induced NO production in BV-2 cells (Zhao et al., 2022).
C. sinensis extracts have the potential to be developed into herbal products with hepatoprotective and nephroprotective properties (Saeed et al., 2008). Some fundings suggest that the aqueous extract of A. catechu has immunomodulatory effects on both cell-mediated and humoral immunity (Ismail and Asad, 2009).
According to Tibetan medical documents, a total of 14 species were used as “Shengdeng”. Extensive phytochemical investigations have resulted in the identification of 355 chemical constituents within “Shengdeng”. The pharmacological studies conducted on “Shengdeng” have unveiled a diverse array of beneficial properties, including potent antioxidant, anticancer, antimicrobial, antiviral, antiparasitic, anti-inflammatory, and anti-arthritic activities. However, it is important to acknowledge that there are still several unresolved issues that require further investigation and clarification in future research endeavors. These areas of focus will contribute to a more comprehensive understanding of the therapeutic potential and mechanisms of action of “Shengdeng”.
Firstly, as a representative multi-origin Tibetan medicine, “Shengdeng” exhibits inherent variations in chemical composition among different plant sources, leading to heterogeneity in the content of active constituents. This inherent variability may significantly impact the therapeutic efficacy and safety profiles of the medication (Kelsang et al., 2023). Secondly, although “Shengdeng” derived from different plant sources may share similar pharmacological effects, the inherent variations in botanical origin and growth conditions can give rise to nuanced pharmacodynamic profiles or even distinct therapeutic outcomes. Consequently, the evaluation of Shengdeng’s therapeutic efficacy and the design of its optimal formulations are confronted with intricate challenges. Thirdly, the research on multi-origin “Shengdeng” confronts the critical issue of establishing harmonized and comprehensive standards. The diverse array of plant species and their inherent differences necessitate the development of standardized methodologies and evaluation frameworks that can accommodate the unique characteristics of multi-origin plants. Achieving such standardization is pivotal to ensure consistent quality control and efficacy assessment. Lastly, Ancient texts documenting the use of “Shengdeng” do not mention any instances of toxicity associated with its usage. Modern pharmacological studies have not reported any side effects or toxicity at present. Therefore, further research on “Shengdeng” in this direction would be worthwhile.
Given the aforementioned challenges in the research of multi-origin Tibetan medicine “Shengdeng”, future investigations should focus on the following key areas. Firstly, it is crucial to conduct comprehensive chemical analysis and comparative studies on “Shengdeng” derived from different plant sources to identify the major bioactive metabolites and assess their quantitative variations. Innovative approaches such as multidimensional separation techniques like comprehensive two-dimensional liquid chromatography (2D-LC) should be employed to achieve improved separation and qualitative-quantitative analysis of the complex constituents in multi-origin “Shengdeng” (Bo et al., 2023). Secondly, establishing scientific quality control methods for “Shengdeng” is essential to ensure the herbal material’s quality. This involves developing standards and protocols for collection, storage, processing, and strengthening regulatory oversight and management of the entire herbal production process to ensure the stability of Shengdeng’s quality (Peng et al., 2022). Thirdly, conducting clinical research and experimental pharmacological evaluations are necessary to gain a deeper understanding of the variations and consistency in the pharmacological effects of multi-origin plants. Based on the research findings, suitable dosage forms and treatment regimens can be designed for different plant sources to enhance the consistency and controllability of therapeutic efficacy (Wang et al., 2022). Fourthly, it is imperative to establish standardized methods and evaluation systems specific to multi-origin plants like “Shengdeng” to ensure comparability and reproducibility in research and application. Developing a unified set of standards and guidelines encompassing plant collection, quality assessment, component analysis, and pharmacological evaluation is essential (Zhong et al., 2022). Lastly, Toxicity and side effects were evaluated using modern pharmacological methods in relevant animal models. These include safety pharmacology, genetic toxicology, acute and subchronic toxicology, absorption, distribution, metabolism and excretion (ADME) studies, reproductive and developmental toxicity, and carcinogenic potential assessments (Cai and Suo, 2023).
This review presents a comprehensive overview of the latest advancements in the textual research of “Shengdeng”, encompassing its herbal and botanical distribution, traditional uses, phytochemistry, and pharmacological activities. As an integral component of Tibetan medicine, “Shengdeng” holds significant medicinal value and is widely employed in clinical settings. By providing a comprehensive understanding of the plant species utilized as “Shengdeng” and their applications, this review contributes to the existing knowledge in the field and serves as a valuable resource for researchers and practitioners alike.
JM: Writing–original draft, Writing–review and editing. QL: Writing–original draft, Writing–review and editing. TW: Writing–original draft. HL: Writing–review and editing. JL: Writing–review and editing. RC: Writing–original draft. YZ: Writing–review and editing. JZ: Writing–review and editing. XX: Writing–review and editing. JS: Writing–original draft, Writing–review and editing.
This work was funded by the National Natural Science Foundation of China (82204765), the Nature Science Foundation of Sichuan (2022NSFSC1470), Sichuan Provincial Postdoctoral Special Funding Project (TB2023020) and the Xinglin Scholars Research Promotion Program of Chengdu University of Traditional Chinese Medicine (BSH2021030).
The authors declare that the research was conducted in the absence of any commercial or financial relationships that could be construed as a potential conflict of interest.
All claims expressed in this article are solely those of the authors and do not necessarily represent those of their affiliated organizations, or those of the publisher, the editors and the reviewers. Any product that may be evaluated in this article, or claim that may be made by its manufacturer, is not guaranteed or endorsed by the publisher.
The Supplementary Material for this article can be found online at: https://www.frontiersin.org/articles/10.3389/fphar.2023.1303902/full#supplementary-material
Adhikari, B., Aryal, B., and Bhattarai, B. R. (2021). A comprehensive review on the chemical composition and pharmacological activities of Acacia catechu (lf) willd. J. Chem-Ny 2021, 1–11. doi:10.1155/2021/2575598
Ahmed, A., Li, W., Zhang, J. S., Sam, P. H., Zou, Y. H., Tang, G. H., et al. (2018). A new bisabolane sesquiterpenoid and a new abietane diterpenoid from Cephalotaxus sinensis. Nat. Prod. Res. 175, 175–181. doi:10.1080/14786419.2017.1343323
Babita, A., Bikash, A., Niraj, A., Bibek, R. B., Karan, K., and Niranjan, P. (2021a). LC-HRMS profiling and antidiabetic, antioxidant, and antibacterial activities of Acacia catechu (Lf) willd. Biomed. Res. Int. 7588711. doi:10.1155/2021/7588711
Babita, A., Purushottam, N., Karan, K., Bikash, A., Dadhiram, K. C., Basanta, K. S., et al. (2021b). Antidiabetic, antimicrobial, and molecular profiling of selected medicinal plants. J. Evidence-Based Complement. Altern. Med. 5510099. doi:10.1155/2021/5510099
Bao, H., Zhang, L. L., Liu, Q. Y., Feng, L., Ye, Y., Lu, J. J., et al. (2016). Cytotoxic and pro-apoptotic effects of cassane diterpenoids from the seeds of Caesalpinia sappan in cancer cells. Molecules 791, 791. doi:10.3390/molecules21060791
Batubara, I., Mitsunaga, T., and Ohashi, H. (2009). Screening antiacne potency of Indonesian medicinal plants: antibacterial, lipase inhibition, and antioxidant activities. J. Wood Sci. 230, 230–235. doi:10.1007/s10086-008-1021-1
Bo, E., Peng, M., Leng, B., and Cai, R. N. (2023). Textual research on Tibetan medicine "gong Baga ji. Asia-Pacific Tradit. Med., 178–182. doi:10.11954/ytctyy.202301041
Bu, R. (2007). Research, development and utilization of Mongolian medicine. J. Med. Pharm. Chin. Minorities 78. doi:10.16041/j.cnki.cn15-1175.2007.01.040
Bukke, A. N., Hadi, F. N., Babu, K. S., and Shankar, P. C. (2018). In vitro studies data on anticancer activity of Caesalpinia sappan L. heartwood and leaf extracts on MCF7 and A549 cell lines. Data Brief. 19, 868–877. doi:10.1016/j.dib.2018.05.050
Bukke, A. N., Hadi, F. N., and Produtur, C. S. (2015). Comparative study of in vitro antibacterial activity of leaves, bark, heart wood and seed extracts of Caesalpinia sappan L. Asian pac. J. Trop. Dis. 903, 903–907. doi:10.1016/s2222-1808(15)60954-9
Burnett, B. P., Jia, Q., Zhao, Y., and Levy, R. M. (2007). A medicinal extract of Scutellaria baicalensis and Acacia catechu acts as a dual inhibitor of cyclooxygenase and 5-lipoxygenase to reduce inflammation. J. Med. Food. 10, 442–451. doi:10.1089/jmf.2006.255
Cai, Y. Z., Sun, M., Xing, J., Luo, Q., and Corke, H. (2006). Structure–radical scavenging activity relationships of phenolic compounds from traditional Chinese medicinal plants. Life Sci. 78, 2872–2888. doi:10.1016/j.lfs.2005.11.004
Cai, R. C., Suo, L. L. Z., Se, Z., Chen, J., and Dun, Z. (2023). Textual research on the name, variety and efficacy of multi-base original Tibetan medicine Bega. Mod. Traditional Chin. Med. Materia Medica-World Sci. Technol., 1–11. doi:10.3969/j.issn.1006-3773.2023.04.038
Cai, C. Q. (2012). Study on chemical constituents from heartwood of Caesalpinia sappan. Beijing, China: Minzu University of China.
Chan, P. K., Zhao, M., Che, C. T., and Mak, E. (2008). Cytotoxic acylated triterpene saponins from the husks of Xanthoceras sorbifolia. J. Nat. Prod. 71, 1247–1250. doi:10.1021/np070577v
Chen, Y. J., Takeda, T., Ogihara, Y., and Iitaka, Y. (1984). Studies on the constituents of Xanthoceras sorbifolia Bunge. II. Major sapogenol and a prosapogenin from the fruits of Xanthoceras sorbifolia Bunge. Chem. Pharm. Bull. 32, 3378–3383. doi:10.1248/cpb.32.3378
Chen, Y. j., Takeda, T., and Ogihara, Y. (1985a). Studies on the constituents of Xanthoceras sorbifolia Bunge. III. Minor prosapogenins from the fruits of Xanthoceras sorbifolia Bunge. Chem. Pharm. Bull. 33, 127–134. doi:10.1248/cpb.33.127
Chen, Y. j., Takeda, T., and Ogihara, Y. (1985b). Studies on the constituents of Xanthoceras sorbifolia Bunge. IV. Structures of the minor prosapogenins. Chem. Pharm. Bull. 33, 1043–1048. doi:10.1248/cpb.33.1043
Chen, Y. j., Takeda, T., and Ogihara, Y. (1985c). Studies on the constituents of Xanthoceras sorbifolia Bunge. V. Major saponins from the fruits of Xanthoceras sorbifolia Bunge. Chem. Pharm. Bull. 33, 1387–1394. doi:10.1248/cpb.33.1387
Chen, Y. P., Liu, L., Zhou, Y. H., Wen, J., Jiang, Y., and Tu, P. F. (2008). Chemical constituents from sappan Lignum. J. Chin. Pharm. Sci., 82–86.
Chen, P., Lei, J., Xu, X. D., and Yang, J. (2012). Chemical constituents and antibacterial activity contained in Caesalpinia millettii. China J. Chin. Mat. Med. 37, 2105–2107. doi:10.4268/cjcmm20121415
Chowdhury, M. A., Choi, M., Ko, W., Lee, H., Kim, S. C., Oh, H., et al. (2019). Standardized microwave extract of Sappan Lignum exerts anti-inflammatory effects through inhibition of NF-κB activation via regulation of heme oxygenase-1 expression. Mol. Med. Rep. 19, 1809–1816. doi:10.3892/mmr.2019.9811
Cooksey, C. (2010). Hematoxylin and related compounds–an annotated bibliography concerning their origin, properties, chemistry, and certain applications. Biotech. Histochem. 85, 65–82. doi:10.3109/10520290903048418
Cui, H., Xiao, H., Ran, X. K., Li, Y. Y., Dou, D. Q., and Kang, T. G. (2012). Two new oleanane-type pentacyclic triterpenoid saponins from the husks of Xanthoceras sorbifolia Bunge. J. Asian. Nat. Prod. Res. 14, 216–223. doi:10.1080/10286020.2011.641954
Dashtdar, M., Dashtdar, M. R., Dashtdar, B., Shirazi, M. K., and Khan, S. A. (2013). In-vitro, anti-bacterial activities of aqueous extracts of Acacia catechu (L.F.)Willd, castanea sativa, ephedra sinica stapf and shilajita mumiyo against gram positive and gram negative bacteria. J. Pharmacopunct 16, 15–22. doi:10.3831/KPI.2013.16.014
Fu, H., Guo, Y., Li, W., Dou, D., Kang, T., and Koike, K. (2010). A new angeloylated triterpenoid saponin from the husks of Xanthoceras sorbifolia Bunge. J. Nat. Med. 64, 80–84. doi:10.1007/s11418-009-0359-z
Gao, S. M., Liu, J. S., Wang, M., Cao, T. T., Qi, Y. D., Zhang, B. G., et al. (2018). Traditional uses, phytochemistry, pharmacology and toxicology of Codonopsis: a review. J. Ethnopharmacol. 219, 50–70. doi:10.1016/j.jep.2018.02.039
Greenwell, M., and Rahman, P. K. S. M. (2015). Medicinal plants: their use in anticancer treatment. Int. J. Pharm. Sci. Res. 4103, 4103–4112. doi:10.13040/IJPSR.0975-8232.6(10).4103-12
Guo, Y., Dou, D. Q., Kang, T. G., Wang, S. C., and Kuang, H. X. (2009). Structure elucidation and complete NMR spectral assignments of two new oleanane-type pentacyclic triterpenoid saponins from the husks of Xanthoceras sorbifolia Bunge. Magn. Reson. Chem. 47, 982–988. doi:10.1002/mrc.2479
Hafezi, K., Hemmati, A. A., Abbaszadeh, H., Valizadeh, A., and Makvandi, M. (2020). Anticancer activity and molecular mechanisms of α-conidendrin, a polyphenolic compound present in Taxus yunnanensis, on human breast cancer cell lines. Phytother. Res. 34, 1397–1408. doi:10.1002/ptr.6613
Hai, P., Wen, S. Z., Li, Y., Gao, Y., Jiang, X. J., and Wang, F. (2014). New taxane diterpenoids from Taxus yunnanensis. Nat. Prod. Bioprospect. 4, 47–51. doi:10.1007/s13659-014-0003-9
Hiraganahalli, D. B., Chandrasekaran, C. V., Dethe, S., Mundkinajeddu, D., Pandre, M. K., Balachandran, J., et al. (2012). Hepatoprotective and antioxidant activity of standardized herbal extracts. Pharmacogn. Mag. 116, 116. doi:10.4103/0973-1296.96553
Hong, S. S., Choi, Y. H., Suh, H. J., Kang, M. J., Shin, J. H., Kwon, O. O., et al. (2015). Flavonoid constituents of Acacia catechu. J. Appl. Biol. Chem. 58, 189–194. doi:10.3839/jabc.2015.030
Huang, D. J., Ou, B., and Prior, R. L. (2005). The chemistry behind antioxidant capacity assays. J. Agric. Food Chem. 53, 1841–1856. doi:10.1021/jf030723c
Huang, S., Liu, H. F., Quan, X. H., Jin, Y., Xuan, G. S., An, R. B., et al. (2016). Rhamnella gilgitica attenuates inflammatory responses in LPS-induced murine macrophages and complete Freund’s adjuvant-induced arthritis rats. Am. J. Chin. Med. 44, 1379–1392. doi:10.1142/S0192415X16500774
Huang, s.q. (2001). Clinical observation of 100 cases of Tibetan medicine Ershiwuwei ErCha Wan in the treatment of rheumatoid arthritis. J. Med. Pharm. Chin. (02), 11. doi:10.16041/j.cnki.cn15-1175.2001.02.007
Hung, M. S., Xu, Z. D., Lin, Y. C., Mao, J. H., Yang, C. T., Chang, P. J., et al. (2009). Identification of hematein as a novel inhibitor of protein kinase CK2 from a natural product library. BMC cancer 9, 1–10. doi:10.1186/1471-2407-9-135
Ismail, S., and Asad, M. (2009). Immunomodulatory activity of Acacia catechu. Indian. J. Physiol. Pharmacol. 53, 25–33.
Jayasekhar, P., Mohanan, P., and Rathinam, K. (1997). Hepatoprotective activity of ethyl acetate extract of Acacia catechu. Indian. J. Pharmacol. 426.
Jeon, J., Lee, J. H., Park, K. A., Byun, H. S., Lee, H., Lee, Y., et al. (2014). Brazilin selectively disrupts proximal IL-1 receptor signaling complex formation by targeting an IKK-upstream signaling components. Biochem. Pharmacol. 89, 515–525. doi:10.1016/j.bcp.2014.04.004
Jeong, H. J., Kim, Y. M., Kim, J. H., Kim, J. Y., Park, J. Y., Park, S. J., et al. (2012). Homoisoflavonoids from Caesalpinia sappan displaying viral neuraminidases inhibition. Biol. Pharm. Bull. 35, 786–790. doi:10.1248/bpb.35.786
Jiang, D., Dai, J. R., Deng, Y. L., Zhang, Y. Q., and Zhao, W. (2013). Study on the low quantity flavones of Cephalotaxus sinensis. J. Anhui Agric. Sci. 41 (09), 3848–3849+4015. doi:10.13989/j.cnki.0517-6611.2013.09.087
Joshi, S., Subedi, Y. P., and Paudel, S. K. (2011). Antibacterial and antifungal activity of heartwood of Acacia catechu of Nepal. J. Nepal Chem. Soc. 27, 94–99. doi:10.3126/jncs.v27i1.6667
Jung, E. G., Han, K. I., Hwang, S. G., Kwon, H. J., Patnaik, B. B., Kim, Y. H., et al. (2015a). Brazilin isolated from Caesalpinia sappan L. inhibits rheumatoid arthritis activity in a type-II collagen induced arthritis mouse model. BMC Complement. Altern. Med. 15 (1), 1–11. doi:10.1186/s12906-015-0648-x
Jung, E. G., Han, K. I., Kwon, H. J., Patnaik, B. B., Kim, W. J., Hur, G. M., et al. (2015b). Anti-inflammatory activity of sappanchalcone isolated from Caesalpinia sappan L. in a collagen-induced arthritis mouse model. Arch. Pharmacal Res. 38, 973–983. doi:10.1007/s12272-015-0557-z
Kang, Y., He, P. H., Wang, H., Ye, Y. B., Li, X., Xie, P. G., et al. (2018). Brazilin induces FOXO3A-dependent autophagic cell death by disturbing calcium homeostasis in osteosarcoma cells. Cancer Chemother. Pharmacol. 82, 479–491. doi:10.1007/s00280-018-3633-5
Kelsang, G. A., Zhao, Z. L., and Ni, L. H., (2023). Pharmacognostical research progress on Tibetan herb jie-ji. Chin. J. Ethnomedicine Ethnopharmacy, 52–57.
Khadayat, K., Marasini, B. P., Gautam, H., Ghaju, S., and Parajuli, N. (2020). Evaluation of the alpha-amylase inhibitory activity of Nepalese medicinal plants used in the treatment of diabetes mellitus. Clin. Phytosci. 1-8, 34. doi:10.1186/s40816-020-00179-8
Kim, J., and Kim, I. (2018). Design and synthesis of a hybrid framework of indanone and chromane: total synthesis of a homoisoflavanoid, brazilane. Org. Biomol. Chem. 16, 89–100. doi:10.1039/c7ob02758c
Kim, J. H., Choo, Y. Y., Tae, N., Min, B. S., and Lee, J. H. (2014). The anti-inflammatory effect of 3-deoxysappanchalcone is mediated by inducing heme oxygenase-1 via activating the AKT/mTOR pathway in murine macrophages. Int. Immunopharmacol. 22, 420–426. doi:10.1016/j.intimp.2014.07.025
Kim, J., Lee, H. K., Chang, T. S., Kang, K. S., and Hwang, G. S. (2015a). Inhibitory effect of brazilin on osteoclast differentiation and its mechanism of action. Int. Immunopharmacol. 29, 628–634. doi:10.1016/j.intimp.2015.09.018
Kim, S. H., Lyu, H. N., Kim, Y. S., Jeon, Y. H., Kim, W., Kim, S., et al. (2015b). Brazilin Isolated from Caesalpinia sappan suppresses nuclear envelope reassembly by inhibiting barrier-to-autointegration factor phosphorylation. J. Pharmacol. Exp. Ther. 352, 175–184. doi:10.1124/jpet.114.218792
Kumar, R., Arora, R., Mahajan, J., Mahey, S., and Arora, S. (2018). Polyphenols from cutch tree (Acacia catechu Willd.): normalize in vitro oxidative stress and exerts antiproliferative activity. Braz. Arch. Biol. Technol. 61. doi:10.1590/1678-4324-2017160728
Kumar, R., Mahey, S., Arora, R., Mahajan, J., Kumar, V., and Arora, S. (2019). Insights into biological properties of less explored bark of industrially important Acacia catechu Willd. Ind. Crops Prod. 111486, 111486. doi:10.1016/j.indcrop.2019.111486
Lakshmi, T., Renukadevi, B. S., Senthilkumar, S., Haribalan, P., Parameshwari, R., Vijayaraghavan, R., et al. (2018). Seed and bark extracts of Acacia catechu protects liver from acetaminophen induced hepatotoxicity by modulating oxidative stress, antioxidant enzymes and liver function enzymes in Wistar rat model. Pharmacother. 108, 838–844. doi:10.1016/j.biopha.2018.08.077
Lee, S., Choi, S. Y., Choo, Y. Y., Kim, O., Tran, P. T., Dao, C. T., et al. (2015). Sappanone A exhibits anti-inflammatory effects via modulation of Nrf2 and NF-κB. Int. Immunopharmacol. 28, 328–336. doi:10.1016/j.intimp.2015.06.015
Li, W., and Li, X. (2008). Chemical constituents in carpophore of Xanthoceras sorbifolia. Chin. Tradit. Herb. Drugs. 334-337. CNKI:SUN:ZCYO.0.2008-03-004.
Li, S. H., Zhang, H. J., Yao, P., Sun, H. D., and Fong, H. H. S. (2000). Rearranged taxanes from the bark of Taxus yunnanensis. J. Nat. Prod. 63, 1488–1491. doi:10.1021/np000118t
Li, S. H., Zhang, H. J., Yao, P., Sun, H. D., and Fong, H. H. S. (2001). Taxane diterpenoids from the bark of Taxus yunnanensis. Phytochemistry 58, 369–374. doi:10.1016/s0031-9422(01)00218-7
Li, S. H., Zhang, H. J., Niu, X. M., Yao, P., Sun, H. D., and Fong, H. H. S. (2002a). Taxuyunnanines SV, new taxoids from Taxus yunnanensis. Planta Med. 68, 253–257. doi:10.1055/s-2002-23136
Li, S. H., Zhang, H. J., Yao, P., Niu, X. M., Xiang, W., Sun, H. D., et al. (2002b). Non-taxane compounds from the bark of Taxus yunnanensis. J. Asian Nat. Prod. Res. 4, 147–154. doi:10.1080/10286020290027443
Li, S. H., Zhang, H. J., Niu, X. M., Yao, P., Sun, H. D., and Fong, H. H. S. (2003a). Novel taxoids from the Chinese yew Taxus yunnanensis. Tetrahedron 59, 37–45. doi:10.1016/s0040-4020(02)01483-7
Li, S. H., Zhang, H. J., Yao, P., Niu, X. M., Xiang, W., and Sun, H. D. (2003b). Two new lignans from Taxus yunnanensis. Chin. J. Chem. 21, 926–930. doi:10.1002/cjoc.20030210741
Li, Z. L., Li, X., and Zhang, P. (2004). Research progress in the chemical constituents and pharmacological activities of Xanthoceras sorbifolia Bunge. J. Shenyang Pharm. Univ., 472–475. doi:10.1016/s0898-6568(03)00096-2
Li, W., Li, X., Yang, J., Li, L. H., Li, N., and Meng, D. L. (2006). Two new triterpenoids from the carpophore of Xanthoceras sorbifolia Bunge. Pharmazie 61, 810–811.
Li, W., Dai, R. J., Yu, Y. H., Li, L., Meng, W. W., and Deng, Y. L. (2007a). Two new flavonoid diglycosides from Cephalotaxus sinensis. Chin. Chem. Lett. 18, 837–839. doi:10.1016/j.cclet.2007.05.018
Li, W., Dai, R. J., Yu, Y. H., Li, L., Wu, C. M., Luan, W. W., et al. (2007b). Antihyperglycemic effect of Cephalotaxus sinensis leaves and GLUT-4 translocation facilitating activity of its flavonoid constituents. Biol. Pharm. Bull. 30, 1123–1129. doi:10.1248/bpb.30.1123
Li, W., Li, X., Meng, D. L., Zhang, P., and Li, Z. L. (2007c). Two new triterpenoids from the carpophore of Xanthoceras sorbifolia Bunge. Pharmazie 9, 7–11. doi:10.1080/10286020500289386
Li, W., Li, X., Yang, J., Meng, D. L., and Li, N. (2008). Two new triterpenoid saponins from the carpophore of Xanthoceras sorbifolia Bunge. J. Asian Nat. Prod. Res. 10, 260–264. doi:10.1080/10286020701605224
Li, J., Zu, Y. G., Fu, Y. J., Yang, Y. C., Li, S. M., Li, Z. N., et al. (2010a). Optimization of microwave-assisted extraction of triterpene saponins from defatted residue of yellow horn (Xanthoceras sorbifolia Bunge.) kernel and evaluation of its antioxidant activity. Innov. Food Sci. Emerg. Technol. 11, 637–643. doi:10.1016/j.ifset.2010.06.004
Li, X. C., Wang, H. Q., Liu, C., and Chen, R. Y. (2010b). Chemical constituents of Acacia catechu. China J. Chin. Mat. Med. 35, 1425–1427. doi:10.4268/cjcmm20101114
Li, X. C., Liu, C., Yang, L. X., and Chen, R. Y. (2011). Phenolic compounds from the aqueous extract of Acacia catechu. J. Asian Nat. Prod. Res. 13, 826–830. doi:10.1080/10286020.2011.597384
Li, Q., Wang, R. P., and Zou, X. (2012). Chemical composition and anti-tumor research of Caesalpinia sappan. Hunan Zhongyiyao Daxue Xuebao, 76–78. doi:10.3969/j.issn.1674-070X.2012.04.033.076.03
Li, N., Wang, Y., Li, X. Z., Zhang, H., Zhou, D., Wang, W. L., et al. (2016). Bioactive phenols as potential neuroinflammation inhibitors from the leaves of Xanthoceras sorbifolia Bunge. Chem. Lett. 26, 5018–5023. doi:10.1016/j.bmcl.2016.08.094
Li, Z. L. (2006). Studies on the chemical constituents and biological activities of the husks of Xanthoceras sorbifolia bge (Shenyang: Shenyang Pharmaceutical University). Master.
Li, Q. Y. (2020). Study on material basis of anti-rheumatoid arthritis of Tibetan medicine Rhamnella gilgitica. China: Chengdu University of Traditional Chinese Medicine. doi:10.26988/d.cnki.gcdzu.2020.000521
Liu, J., Tao, Y., Zou, X., Liu, Q., Meng, X., Zhang, Y., et al. (2023). In vitro and in vivo exploration of the anti-atopic dermatitis mechanism of action of Tibetan medicine Qi-Sai-Er-Sang-Dang-Song decoction. J. Ethnopharmacol. 306, 116155. doi:10.1016/j.jep.2023.116155
Ma, C., Nakamura, N., Hattori, M., Kakuda, H., Qiao, J., and Yu, H. (2000). Inhibitory effects on HIV-1 protease of constituents from the wood of Xanthoceras sorbifolia. J. Nat. Prod. 63, 238–242. doi:10.1021/np9902441
Ma, C. M., Nakamura, N., Nawawi, A., Hattori, M., and Cai, S. Q. (2004). A novel protoilludane sesquiterpene from the wood of Xanthoceras sorbifolia. Chin. Chem. Lett. 65-67.
Ma, G. X., Wu, H. F., Chen, D. L., Zhu, N. L., Zhu, Y. D., Sun, Z. H., et al. (2015). Antimalarial and antiproliferative cassane diterpenes of Caesalpinia sappan. J. Nat. Prod. 78, 2364–2371. doi:10.1021/acs.jnatprod.5b00317
Ma, S. J., Shi, X. L., Yan, H., Ma, Z. Q., and Zhang, X. (2016). Antiphytoviral activity of alkaloids from Cephalotaxus sinensis. Ind. Crops Prod. 94, 658–664. doi:10.1016/j.indcrop.2016.09.047
Modi, M., Dezzutti, C. S., Kulshreshtha, S., Rawat, A. K. S., Srivastava, S. K., Malhotra, S., et al. (2013). Extracts from Acacia catechu suppress HIV-1 replication by inhibiting the activities of the viral protease and Tat. Virol. J. 1-17. doi:10.1186/1743-422X-10-309
Mueller, M., Weinmann, D., Toegel, S., Holzer, W., Unger, F., and Viernstein, H. (2016). Compounds from Caesalpinia sappan with anti-inflammatory properties in macrophages and chondrocytes. Food Funct. 7, 1671–1679. doi:10.1039/c5fo01256b
Muhammad, K. S., Rauf, A., Shabnam, J., Yulin, D., and Rongji, D. (2013). Hypoglycemic and hypolipidemic effects of Cephalotaxus sinensis in STZ-induced diabetic rats. J. Med. Plants Res., 1310–1316. doi:10.5897/JMPR12.844
Namikoshi, M., Nakata, H., and Saitoh, T. (1987). Homoisoflavonoids from Caesalpinia sappan. Phytochemistry 26, 1831–1833. doi:10.1016/s0031-9422(00)82298-0
Negi, B. S., and Dave, B. P. (2010). In vitro antimicrobial activity of Acacia catechu and its phytochemical analysis. Indian J. Microbiol. 50, 369–374. doi:10.1007/s12088-011-0061-1
Nguyen, N. T., Banskota, A. H., Tezuka, Y., Nobukawa, T., and Kadota, S. (2003). Diterpenes and sesquiterpenes from the bark of Taxus yunnanensis. Phytochemistry 64, 1141–1147. doi:10.1016/s0031-9422(03)00503-x
Nguyen, M. T. T., Awale, S., Tezuka, Y., Letran, Q., and Kadota, S. (2005). Xanthine oxidase inhibitors from the heartwood of Vietnamese Caesalpinia sappan. Pharm. Bull. 53, 984–988. doi:10.1248/cpb.53.984
Ni, H. Y., and Zhang, Z. H. (2009). Studies on the chemical constituents of Xanthoceras sorbifolia. J. Chin. Med. Mat. 32, 702–704. doi:10.13863/j.issn1001-4454.2009.05.039
Pan, Q., Yang, P. Q., Chen, G. H., and Liu, W. J. (1998). Studies on the chemical constituents of traditional Tibetan medicinal herb Shengdeng (Rhamnella gilgitica)(I). Chin. Tradit. Herb. Drugs., 76–79.
Panya, A., Yongpitakwattana, P., Budchart, P., Sawasdee, N., Krobthong, S., Paemanee, A., et al. (2019). Novel bioactive peptides demonstrating anti-dengue virus activity isolated from the Asian medicinal plant Acacia catechu. Chem. Biol. Drug Des. 93, 100–109. doi:10.1111/cbdd.13400
Patel, J. D., Kumar, V., and Bhatt, S. A. (2009). Antimicrobial screening and phytochemical analysis of the resin part of Acacia catechu. Pharm. Biol. 47, 34–37. doi:10.1080/13880200802400527
Patil, A., and Modak, M. (2017). Comparative evaluation of oxidative stress modulating and DNA protective activities of aqueous and methanolic extracts of Acacia catechu. Medicines 65, 65. doi:10.3390/medicines4030065
Peluso, I., Miglio, C., Morabito, G., Ioannone, F., and Serafini, M. (2015). Flavonoids and immune function in human: a systematic review. Crit. Rev. Food Sci. Nutr. 55, 383–395. doi:10.1080/10408398.2012.656770
Peng, M. J., Leng, B. J., Duo, J. Z. M., Bo, E. D., Duo, J. R. Q., and Cai, R. J. N. (2022). Textual research on Tibetan medicine rani. Guid. J. Traditional Chin. Med. Pharm. 129–132+136. doi:10.13862/j.cn43-1446/r.2022.11.029
Puttipan, R., Chansakaow, S., Khongkhunthian, S., and Okonogi, S. (2018). Caesalpinia sappan: a promising natural source of antimicrobial agent for inhibition of cariogenic bacteria. Drug Discov. Ther. 12, 197–205. doi:10.5582/ddt.2018.01035
Qi, G. Y. (2018). Studies on chemical constituents and biological activity of husk of Xanthoceras sorbifolia (Lanzhou: Lanzhou University of Technology). Master.
Rajput, M. S., Nirmal, N. P., Rathore, D., and Dahima, R. (2020). Dimethyl fumarate exerts neuroprotection by modulating calcineurin/NFAT1 and NFκB dependent BACE1 activity in Aβ1-42 treated neuroblastoma SH-SY5Y cells. Brain Res. Bull. 97-107, 97–107. doi:10.1016/j.brainresbull.2020.08.024
Ray, D., Sharatchandra, K. H., and Thokchom, I. S. (2006). Antipyretic, antidiarrhoeal, hypoglycaemic and hepatoprotective activities of ethyl acetate extract of Acacia catechu Willd. in albino rats. Indian J. Pharmacol. 408. doi:10.4103/0253-7613.28207
Saeed, M. K., Deng, Y. L., Parveen, Z., Dai, R., Ahmad, W., and Yu, Y. H. (2007). “Radical scavenging activity of various fractions of Cephalotaxus sinensis (Rehd et Wile) Li,” in 2007 IEEE/ICME International Conference on Complex Medical Engineering, Beijing Jingfeng Hotel, China, May 23-27, 2007, 1651–1654. doi:10.1109/iccme.2007.4382027
Saeed, M. K., Deng, Y. L., and Dai, R. J. (2008). Attenuation of biochemical parameters in streptozotocin-induced diabetic rats by oral administration of extracts and fractions of Cephalotaxus sinensis. J. Clin. Biochem. Nutr. 42, 21–28. doi:10.3164/jcbn2008004
Safitri, R., Siahaan, M., and Ghozali, M. (2022). Antimicrobial activity of sappan wood extract (Caesalpinia sappan L.) against Streptococcus pneumoniae. Int. J. Health. Sci-Ijh., 7881–7896. doi:10.53730/ijhs.v6ns1.6797
Saha, M. R., Dey, P., Begum, S., De, B., Chaudhuri, T. K., Sarker, D. D., et al. (2016). Effect of Acacia catechu (Lf) Willd. on oxidative stress with possible implications in allev-iating selected cognitive disorders. PloS one 11, e0150574. doi:10.1371/journal.pone.0150574
Sasaki, Y., Hosokawa, T., Nagai, M., and Nagumo, S. (2007). In vitro study for inhibition of NO production about constituents of Sappan Lignum. Pharm. Bull. 30, 193–196. doi:10.1248/bpb.30.193
Sheng, F. W. (2016). Caesalpinia sappan linn pieces and its effect on tyrosinase activity. Henan: Henan University.
Shi, Q. W., Oritani, T., Sugiyama, T., Zhao, D., and Murakami, R. (1999). Three new taxane diterpenoids from seeds of the Chinese yew, Taxus yunnanensis and T. chinensis var. mairei. Planta Med. 65, 767–770. doi:10.1055/s-2006-960864
Shinozaki, Y., Fukamiya, N., Fukushima, M., Okano, M., Nehira, T., Tagahara, K., et al. (2001). Dantaxusins A and B, two new taxoids from Taxus yunnanensis. J. Nat. Prod. 1073, 1073–1076. doi:10.1021/np0100643
Shinozaki, Y., Fukamiya, N., Fukushima, M., Okano, M., Nehira, T., Tagahara, K., et al. (2002). Dantaxusins C and D, two novel taxoids from Taxus yunnanensis. J. Nat. Prod. 65, 371–374. doi:10.1021/np010435f
Shresta, S., Bhattarai, B. R., Adhikari, B., Rayamajhee, B., Poudel, P., Khanal, S., et al. (2021). Evaluation of phytochemical, antioxidant and antibacterial activities of selected medicinal plants. Nepal. J. Biotechnol. 9, 50–62. doi:10.3126/njb.v9i1.38667
Shu, S. H., Zhang, L., Du, G. H., and Qin, H. L. (2007). Study on the chemical constituents of Caesalpinia sappan. Nat. Prod. Res. Dev. 63-66. doi:10.16333/j.1001-6880.2007.01.016
Shu, S. H. (2007). Studies on the chemical constituents of Caesalpinia sappan and bridelia tomentosa. Beijing, China: PekingUnionMedicalCollege.
Singh, E., and Devasahayam, G. (2020). Neurodegeneration by oxidative stress: a review on prospective use of small molecules for neuroprotection. Mol. Biol. Rep. 47, 3133–3140. doi:10.1007/s11033-020-05354-1
Su, J., Li, Q., Liu, J., Wang, H., Li, X., Wuntrang, D., et al. (2021). Ethyl acetate extract of Tibetan medicine Rhamnella gilgitica ameliorated type II collagen-induced arthritis in rats via regulating JAK-STAT signaling pathway. J. Ethnopharmacol. 113514, 113514. doi:10.1016/j.jep.2020.113514
Su, J., Tao, Y., Liu, J., Sun, J., Zeng, Y., Meng, X., et al. (2023). Tibetan medicine Qi-Sai-Er-Sang-Dang-Song Decoction inhibits TNF-α-induced rheumatoid arthritis in human fibroblast-like synoviocytes via regulating NOTCH1/NF-κB/NLRP3 pathway. J. Ethnopharmacol. 310, 116402. doi:10.1016/j.jep.2023.116402
Sunil, M. A., Sunitha, V. S., Radhakrishnan, E. K., and Jyothis, M. (2019). Immunomodulatory activities of Acacia catechu, a traditional thirst quencher of South India. J. Ayurveda. Integr. Med. 10, 185–191. doi:10.1016/j.jaim.2017.10.010
Swayam, P. S., Akansha, M., Vikram, B. T., Narender, A. K. S., and Srivastava, A. K. (2011). Acacia catechu hard wood: potential anti-diabetic cum anti-dyslipidemic. Med. Chem. Res. 20, 1732–1739. doi:10.1007/s00044-010-9479-y
Tang, K. L., Yin, Z. Q., Zhang, J., Zhang, Q. W., and Cai, L. (2012). Chemical constituents from the ethyl acetate extract of Caesalpinia sappan. Pharm. Clin. Res., 196–198. doi:10.13664/j.cnki.pcr.2012.03.007
Tewtrakul, S., Tungcharoen, P., Sudsai, T., Karalai, C., Ponglimanont, C., and Yodsaoue, O. (2015). Antiinflammatory and wound healing effects of Caesalpinia sappan L. Phytother. Res. 29, 850–856. doi:10.1002/ptr.5321
Tezuka, Y., Morikawa, K., Li, F., Auw, L., Awale, S., Nobukawa, T., et al. (2011). Cytochrome P450 3A4 inhibitory constituents of the wood of Taxus yunnanensis. J. Nat. Prod. 74, 102–105. doi:10.1021/np100665j
Thakur, A. V., Ambwani, S., and Ambwani, T. K. (2018). Preliminary phytochemical screening and GC-MS analysis of leaf extract of Acacia catechu (Lf) Willd. Int. J. Tradit. Herb. Med. 81-85.
Tran, M. H., Nguyen, M. T. T., Nguyen, H. D., Nguyen, T. D., and Phuong, T. T. (2015). Cytotoxic constituents from the seeds of Vietnamese Caesalpinia sappan. Pharm. Biol. 53, 1549–1554. doi:10.3109/13880209.2014.986686
Tseng, C. J., Sung, S., Jia, Q., Zhao, Y., Burnett, B., Park, D. R., et al. (2010). A medicinal plant extract of scutellaria baicalensis and acacia catechu reduced LPS-stimulated gene expression in immune cells: a comprehensive genomic study using QPCR, ELISA, and microarray. J. Diet. Suppl. 7, 253–272. doi:10.3109/19390211.2010.493169
Tu, Y. L., Ma, S., Liu, F. F., Sun, Y., and Dong, X. Y. (2016). Hematoxylin inhibits amyloid β-protein fibrillation and alleviates amyloid-induced cytotoxicity. J. Phys. Chem. B 120, 11360–11368. doi:10.1021/acs.jpcb.6b06878
Wan, G. S., Wang, X. B., Wu, L. J., and Gao, H. Y. (2013). Advances in studies on chemical constituents of Xanthoceras sorbifolia and their pharmacological activities. Chin. Herb. Med., 1842–1851. doi:10.7501/j.issn.0253-2670.2013.13.027
Wan, G. S., Ren, Y. H., Gao, H. Y., Bai, S., Xi, R. G., and Wang, X. B. (2015). Isolation and identification of chemical constituents from the husks of Xanthoceras sorbifolium Bunge. J. Shenyang Pharm. Univ., 18–21. doi:10.14066/j.cnki.cn21-1349/r.2015.01.004
Wan, Y. J., Xu, L., Song, W. T., Liu, Y. Q., Wang, L. C., Zhao, M. B., et al. (2019). The ethanolic extract of caesalpinia sappan heartwood inhibits cerebral ischemia/reperfusion injury in a rat model through a multi-targeted pharmacological mechanism. Front. Pharmacol. 29, 29. doi:10.3389/fphar.2019.00029
Wang, Z., and Liang, J. Y. (2016). PTSD, a disorder with an immunological component. Herb. Drugs. 7, 219–222. doi:10.3389/fimmu.2016.00219
Wang, D., Chen, C., and Zhou, Y. (2003). Research progress on clinical pharmacology and chemical composition of Caesalpinia sappan. Inf. Traditional Chin. Med., 15–16. doi:10.3969/j.issn.1002-2406.2003.03.008
Wang, Z. Y., Wang, Z. Q., and Zhou, Y. B. (2010). Studies on the chemical constituents in herb of Caesalpinia sappan L.(Ⅰ). Nat. Prod. Res. Dev. 590-593. doi:10.16333/j.1001-6880.2010.04.040
Wang, Y., Jiang, S., Meng, D. L., and Li, N. (2011). Advances in study on chemical and biological activity of Xanthoceras sorbifolia. Drugs Clin., 269–273.
Wang, X., Zhao, H. X., and Mu, Y. L. (2013). Chemical constituents from Caesalpinia sappan L. Food Drug, 86–88.
Wang, D., Su, D., Yu, B., Chen, C. M., Cheng, L., Li, X. Z., et al. (2017a). Novel anti-tumour barringenol-like triterpenoids from the husks of Xanthoceras sorbifolia Bunge and their three dimensional quantitative structure activity relationships analysis. Fitoterapia 116, 51–60. doi:10.1016/j.fitote.2016.11.002
Wang, L. C., Liao, L. X., Zhao, M. B., Dong, X., Zeng, K. W., and Tu, P. F. (2017b). Protosappanin A exerts anti-neuroinflammatory effect by inhibiting JAK2-STAT3 pathway in lipopolysaccharide-induced BV2 microglia. Chin. J. Nat. Med. 15, 674–679. doi:10.1016/S1875-5364(17)30096-1
Wang, N. Y., Wu, X. Y., Yi, H., Li, H. Y., Kuang, T. T., Zhang, J., et al. (2022). Herbal textual research and modern research progress of the Tibetan medicine Herba Artemisia. Pharm. Clin. Chin. Materia Medica, 100–103.
Wang, Z. Q. (2006). Screen of fractions with immunosuppressive activity and study on chemical components of Caesalpinia sappan L. Harbin, China: Heilongjiang University of Traditional Chinese Medicine.
Weinmann, D., Mueller, M., Walzer, S. M., Hobusch, G. M., Lass, R., Gahleitner, C., et al. (2018). Brazilin blocks catabolic processes in human osteoarthritic chondrocytes via inhibition of NFKB1/p50. J. Orthop. Res. 36, 2431–2438. doi:10.1002/jor.24013
Wetwitayaklung, P., Phaechamud, T., and Keokitichai, S. (2005). The antioxidant activity of Caesalpinia sappan L. Heartwood in various ages. Phitsanulok, Thailand: Naresuan University Journal, 43–52.
Widodo, N., Puspitarini, S., Widyananda, M. H., Alamsyah, A., Wicaksono, S. T., Masruri, M., et al. (2022). Anticancer activity of Caesalpinia sappan by downregulating mitochondrial genes in A549 lung cancer cell line. F1000Research 169, 169. doi:10.12688/f1000research.76187.2
Xia, Q. H., Ma, Y. J., and Wang, J. W. (2016). Biosynthesis of silver nanoparticles using Taxus yunnanensis callus and their antibacterial activity and cytotoxicity in human cancer cells. Nanomaterials-Basel 160, 160. doi:10.3390/nano6090160
Xiao, J. Y., Yu, S. C., Zhi, Y. Y., Xin, P. W., Jiang, J. F., Fan, L., et al. (2016). Brazilein inhibits neuronal inflammation induced by cerebral ischemia and oxygen-glucose deprivation through targeting NOD2 expression. Chin. J. Nat. Med. 14, 354–362. doi:10.3724/SP.J.1009.2016.00354
Xu, H., Zhou, Z. H., and Yang, J. S. (1994). Studies on compounds from caesaipinia sappan L. China J. Chin. Mat. Med., 485–486+511.
Xu, J. B., Fan, Y. Y., Gan, L. S., Zhou, Y. B., Li, J., and Yue, J. M. (2016). Cephalotanins A–D, four norditerpenoids represent three highly rigid carbon skeletons from Cephalotaxus sinensis. Chem. Eur. J. 22, 14648–14654. doi:10.1002/chem.201603373
Yan, C. Y., Yin, Y., Zhang, D. W., Yang, W., and Yu, R. M. (2013). Structural characterization and in vitro antitumor activity of a novel polysaccharide from Taxus yunnanensis. Carbohydr. Polym. 96, 389–395. doi:10.1016/j.carbpol.2013.04.012
Yang, Q., Wang, X. Q., and Ding, Y. X. (2020). Chemical constituents from the stems of Xanthoceras sorbifolia. Chin. Tradit. Pat. Med., 2062–2066. doi:10.3969/j.issn.1001-1528.2020.08.017
Yimam, M., Zhao, Y., Ma, W. W., Jia, Q., Do, S. G., and Shin, J. H. (2010). 90-day oral toxicity study of UP446, a combination of defined extracts of Scutellaria baicalensis and Acacia catechu, in rats. Food Chem. Toxicol. 48, 1202–1209. doi:10.1016/j.fct.2010.02.011
Yimam, M., Brownell, L., Hodges, M., and Jia, Q. (2012). Analgesic effects of a standardized bioflavonoid composition from Scutellaria baicalensis and Acacia catechu. J. Diet. Suppl. 9, 155–165. doi:10.3109/19390211.2012.708713
Yu, L., Tang, X., Chen, L., Wang, M., Jian, J., Cao, S., et al. (2012a). Oleanane-type triterpenoid saponins from Xanthoceras sorbifolia Bunge. Fitoterapia 83, 1636–1642. doi:10.1016/j.fitote.2012.09.015
Yu, L. L., Wang, X. B., Wei, X. C., Wang, M. M., Chen, L. X., Cao, S. J., et al. (2012b). Triterpenoid saponins from Xanthoceras sorbifolia Bunge and their inhibitory activity on human cancer cell lines. Bioorg Med. Chem. Lett. 22, 5232–5238. doi:10.1016/j.bmcl.2012.06.061
Yue, Q., Fang, Q. C., Liang, X. T., and He, C. H. (1995). Taxayuntin E and F: two taxanes from leaves and stems of Taxus yunnanensis. Phytochemistry 39, 871–873. doi:10.1016/0031-9422(95)00017-2
Zha, S. (2017). Ershiwuwei ErCha wan in the treatment of rheumatoid arthritis curative effect is discussed in this paper. Smart Healthc. 3 (05), 67–68. doi:10.19335/j.cnki.2096-1219.2017.05.28
Zhang, H., Sun, H., and Takeda, Y. (1995). Four new taxanes from the roots of Taxus yunnanensis. J. Nat. Prod. 58, 1153–1159. doi:10.1021/np50122a001
Zhang, H. J., Mu, Q., Xiang, W., Yao, P., Sun, H. D., and Yoshio, T. (1997). Intramolecular transesterified taxanes from Taxus yunnanensis. Phytochemistry 44, 911–915. doi:10.1016/s0031-9422(96)00658-9
Zhang, S., Zu, Y. G., Fu, Y. J., Luo, M., Liu, W., Li, J., et al. (2010). Supercritical carbon dioxide extraction of seed oil from yellow horn (Xanthoceras sorbifolia Bunge.) and its anti-oxidant activity. Bioresour. Technol. 101, 2537–2544. doi:10.1016/j.biortech.2009.11.082
Zhang, Q., Liu, L. J., Qi, X. M., Qi, C. T., and Yu, Q. (2014a). Inhibitory activities of Lignum Sappan extractives on growth and growth-related signaling of tumor cells. Chin. J. Nat. Med. 12, 607–612. doi:10.1016/S1875-5364(14)60092-3
Zhang, Y. Y., Zhao, L., Huang, S., Jin, Y., Pei, X. N., and Xu, D. (2014b). The Study on vitro Antioxidant Effect of Each Part of Ethanol Extrac of Rhamnella gilgitica Mansfeild et Melch. Med. Plant. 20.
Zhao, H. X., Bai, H., Li, W., Li, J., and Wang, Y. S. (2010). Study on chemical constituents ofCaesalpinia sappan L. Food Drug, 176–180. doi:10.3969/j.issn.1672-979X.2010.03.008
Zhao, H., Wang, X., Li, W., Koike, K., and Bai, H. (2014). A new minor homoisoflavonoid from Caesalpinia sappan. Nat. Prod. Res. 28, 102–105. doi:10.1080/14786419.2013.847439
Zhao, C. X., Li, B. Q., Shao, Z. X., Li, D. H., Jing, Y. K., Li, Z. L., et al. (2019). Cephasinenoside A, a new cephalotane diterpenoid glucoside from Cephalotaxus sinensis. Tetrahedron Lett. 60, 151154. doi:10.1016/j.tetlet.2019.151154
Zhao, R., Huang, H., Choi, B. Y., Liu, X. J., Zhang, M., Zhou, S. L., et al. (2019). Cell growth inhibition by 3-deoxysappanchalcone is mediated by directly targeting the TOPK signaling pathway in colon cancer. Phytomedicine 61, 152813. doi:10.1016/j.phymed.2018.12.036
Zhao, J. M., Xu, J. X., Zhang, Z. Q., Shao, Z. T., and Meng, D. L. (2022). Barrigenol-like triterpenoid saponins from the husks of Xanthoceras sorbifolia bunge and their anti-inflammatory activity by inhibiting COX-2 and iNOS expression. Phytochemistry 204, 113430. doi:10.1016/j.phytochem.2022.113430
Zhong, S. Z., Hua, Z. X., and Fan, J. S. (1996). A new taxane diterpene from Taxus yunnanensis. J. Nat. Prod. 59, 603–605. doi:10.1021/np9600863
Zhong, L., Zhou, X. M., and Zhang, J. (2022). “Research on varieties of Tibetan medicine “jijie”,” in Modernization of traditional Chinese medicine and materia medica-world science and technology, 3718–3727. doi:10.11842/wst.20210913008
Zhou, J. Y., Zhang, P. L., Chen, W. M., and Fang, Q. C. (1998). Taxayuntin H and J from Taxus yunnanensis. Phytochemistry 48, 1387–1389. doi:10.1016/s0031-9422(98)00078-8
Zhou, Z. H., Zhou, Z. Q., and Suo, L. O. Z. (2021). Purification of Total Flavonoids from Rhamnella gilgitica Mansf. et Melch. by Macroporous Adsorption Resin and Its Antioxidant Activity. Chin. J. Ethnomedicine Ethnopharmacy, 41–47+53.
Zhou, X. Z. (2017). Study on chemicals and absolute bioavailability of sappan Lignum,Heartwood of Caesalpinia sappan L. Guangzhou, China: Guangdong Pharmaceutical University.
Zhu, N. L., Sun, Z. H., Hu, M. G., Wu, T. Y., Yuan, J. Q., Wu, H. F., et al. (2017). New cassane diterpenoids from Caesalpinia sappan and their antiplasmodial activity. Molecules 1751, 1751. doi:10.3390/molecules22101751
Keywords: Tibetan medicine, Shengdeng, herbal textual research, phytochemistry, pharmacology
Citation: Ma J, Li Q, Wang T, Lu H, Liu J, Cai R, Zhang Y, Zhang J, Xie X and Su J (2023) A comprehensive review of Shengdeng in Tibetan medicine: textual research, herbal and botanical distribution, traditional uses, phytochemistry, and pharmacology. Front. Pharmacol. 14:1303902. doi: 10.3389/fphar.2023.1303902
Received: 28 September 2023; Accepted: 13 November 2023;
Published: 14 December 2023.
Edited by:
Miguel Angel Prieto Lage, University of Vigo, SpainReviewed by:
James David Adams, Independent Researcher, Benicia, CA, United StatesCopyright © 2023 Ma, Li, Wang, Lu, Liu, Cai, Zhang, Zhang, Xie and Su. This is an open-access article distributed under the terms of the Creative Commons Attribution License (CC BY). The use, distribution or reproduction in other forums is permitted, provided the original author(s) and the copyright owner(s) are credited and that the original publication in this journal is cited, in accordance with accepted academic practice. No use, distribution or reproduction is permitted which does not comply with these terms.
*Correspondence: Jing Zhang, MzE2NDkxMjNAcXEuY29t; Xiaolong Xie, eGlleGlhb2xvbmc3OEAxNjMuY29t; Jinsong Su, c3VqaW5zb25nQGNkdXRjbS5lZHUuY24=
†These authors have contributed equally to this work
Disclaimer: All claims expressed in this article are solely those of the authors and do not necessarily represent those of their affiliated organizations, or those of the publisher, the editors and the reviewers. Any product that may be evaluated in this article or claim that may be made by its manufacturer is not guaranteed or endorsed by the publisher.
Research integrity at Frontiers
Learn more about the work of our research integrity team to safeguard the quality of each article we publish.