- 1Department of Forensic Medicine and Toxicology, Faculty of Veterinary Medicine, Benha University, Toukh, Egypt
- 2Department of Food Hygiene and Control, Faculty of Veterinary Medicine, Benha University, Toukh, Egypt
- 3Department of Avian and Rabbit Diseases, Faculty of Veterinary Medicine, Benha University, Toukh, Egypt
- 4Department of Biochemistry, Faculty of Veterinary Medicine, Benha University, Toukh, Egypt
- 5Department of Forensic Medicine and Clinical Toxicology, Faculty of Medicine, Benha University, Benha, Egypt
- 6Department of Biochemistry, Faculty of Veterinary Medicine, Damanhour University, Damanhour, Egypt
- 7Department of Clinical Sciences, College of Medicine, Princess Nourah bint Abdulrahman University, Riyadh, Saudi Arabia
- 8Department of Biology and Plant Protection, Faculty of Agriculture. University of Life Sciences “King Michael I" from Timișoara, Timișoara, Romania
- 9Department of Clinical Pathology, Faculty of Veterinary Medicine, University of Sadat City, Sadat City, Egypt
- 10Department of Pharmacology, Faculty of Veterinary Medicine, Menoufia University, Shebin Elkoum, Egypt
- 11Department of Food Hygiene, Faculty of Veterinary Medicine, Aswan University, Aswan, Egypt
- 12Department of Physiology, Faculty of Veterinary Medicine, Benha University, Toukh, Egypt
- 13Department of Zoology, College of Science, King Saud University, Riyadh, Saudi Arabia
Introduction: Aflatoxins (AFT) are ubiquitous environmental pollutants that are extremely dangerous for both human beings as well as animals. A safe, effective, and considerate strategy is therefore credited with controlling AFT intoxication. Therefore, our study aimed to evaluate the mitigating properties of Chlorella vulgaris (ChV) against AFT-induced nephrotoxicity and altered egg quality.
Methods: Quails were randomized into Control group (receiving a normal diet); ChV group (1 g/kg diet); AFT group (receiving an AFT-containing diet); and the AFT-ChV group were given both treatments.
Results and discussion: AFT provoked kidney injury, exhibited by increased renal biochemical parameters and reduced protein levels. Malondialdehyde (MDA) levels dramatically increased as a consequence of AFT exposure, and glutathione (GSH) levels, superoxide dismutase (SOD), and glutathione peroxidase (GPx) activities were also decreased. Substantial up-modulation of the mRNA expression of the inflammatory cytokines (TNF-α, IL-1β, and IL-6) was additionally reported. Furthermore, AFT residues were detected in the egg compromising its quality and nutritional value. Contrarily, ChV supplemented diet suppressed the AFT-prompted oxidative stress and inflammation, together with enhancing the nutritional value and quality of eggs and decreasing AFT residues. These beneficial impacts are proposed to be attributed to its antioxidant and nutritional ingredients. The molecular docking dynamics confirmed the inflammatory and apoptotic protein targets for ChV. Our findings recommend that adding ChV supplements to foods might guard against nephrotoxicity brought on by AFT exposure.
1 Introduction
Aflatoxins (AFTs), di-furanocoumarin metabolites, accumulate in food and fodders due to contamination with Aspergillus flavus and Aspergillus parasiticus strains (Zhang et al., 2022). The global climatic shift caused by global warming offers favorable conditions for fungi to grow and generate AFTs in contaminated crops, which can build up to lethal concentrations (Medina et al., 2017). The Food and Drug Administration (FDA) has classified AFTs as ineluctable contaminants due to their pervasiveness along with their potential jeopardy to the health of humans and animals (Abdel-Daim et al., 2021). AFT intoxication has genotoxic, mutagenic, and carcinogenic impacts and is designated by the International Agency for Research as a class-I carcinogen in various organs (Hebels et al., 2009; Ahmed et al., 2022).
The toxicity of AFTs is principally due to their metabolism to a more toxic metabolite, namely, aflatoxin-exo-8,9-epoxide (AFTO) through hepatic cytochrome-P450 biotransformation (Zhu et al., 2020; Yilmaz and Bag, 2022). AFTO is an extremely reactive intermediate that targets DNA’s guanine residues, resulting in DNA adducts and mutations. Thereafter, AFTO is detoxified by the phase-II glutathione (GSH) system to a minimally toxic GSH complex, which is then excreted from the cells via the mercapturic acid pathway (Dlamini et al., 2021). It is intriguing that in addition to the liver (Ahmed et al., 2022), the kidney is a probable target since AFT and AFTO are preferentially absorbed and concentrated by tubular cells, and their accumulation in the renal medulla before being expelled in the urine causes direct renal tubular damage (Wang et al., 2022).
There is ample proof indicating the embroilment of oxidative stress, inflammatory responses, and apoptotic pathway in AFT-induced nephrotoxicity (Owumi et al., 2020; Wang et al., 2022). Thus, excess generation of reactive oxygen species (ROS), with consumption of antioxidant enzymes after AFT intoxication, provokes tissue injuries such as DNA mutations, mitochondrial disruption, improper folding of protein, and ultimately necroptosis (Dlamini et al., 2021; Elgazzar et al., 2022; Li et al., 2022). Moreover, the stability of AFTs and their metabolites highlights the necessity for developing secure, feasible, and efficient strategies for reducing their damaging impacts (Kolosova and Stroka, 2012). Therefore, supplementation with natural antioxidants could be an appropriate therapeutic approach to counteract AFT-induced kidney damage.
Chlorella vulgaris [Chlorellaceae; Chlorella vulgaris var. vulgaris], ChV, the most renowned microalgae that pertain to the Chlorophyta class, has outstanding nutritional value, owing to their enrichment in bioactive compounds such as long-chain polyunsaturated fatty acids (PUFAs), phenolic compounds, proteins, amino acids, peptides, and vitamins (Andrade et al., 2018; Elbasuni et al., 2022), which play a beneficial role in animal and human health (Chen et al., 2023). Plenty of studies have been published about ChV as a splendid natural antioxidant, anti-inflammatory, and antiapoptotic agent (Abdelnour et al., 2019b; Abdelhamid et al., 2020). Accordingly, Accumulating literature has emphasized the effectiveness of ChV against a variety of nephrotoxic agents, including, mercury chloride (Blas-Valdivia et al., 2011), cadmium (Shim et al., 2009), and gentamicin (Al-Halaseh et al., 2022).
In accordance with this assertion, we speculated that ChV supplementation might mitigate oxidative stress and inflammation induced AFT. Consequently, the present study was intended to evaluate the modulatory impact of ChV as a feed supplement against AFT bioaccumulation and nephrotoxicity. Biochemical and oxidative stress markers, as well as pro-inflammatory-related gene expression, were evaluated in this study. In addition, quail production and egg quality were evaluated.
2 Materials and methods
2.1 Experimental approach
Japanese quails (Coturnix japonica; 10 weeks old with an average body weight of 200–250 g) were bought from the Faculty of Veterinary Medicine at Benha University in Egypt. Quails received a commercial maize and soybean meal baseline diet that satisfied all their nutritional needs according to the guidelines set forth by the NRC (1994) and free access to water as needed during the trial. They were reared in a well-ventilated room (25°C ± 2°C, humidity 55%–60%, and 17 h of light/day). Quails were allotted randomly over the deep litter floor pen compartments each consisting of eight females and two males. Following 2 weeks of acclimation, the quails were randomized and assigned into four groups, each group consisting of three replicates of five birds: the control group—fed on a basal diet; the ChV group—fed on a basal diet supplemented with a dried powder of whole ChV (1 g/kg) (Marine Toxins Lab, National Research Centre, Egypt) (Abdelnour et al., 2019b); the AFT group—fed on an AFT-contaminated diet (50 ppb; AFT mix, purity >98%, Merck, Darmstadt, Germany) as described by Elbasuni et al. (2022); and the AF + ChV group—fed on an AFT-contaminated diet along with ChV for 3 weeks. The same management, sanitary, and environmental standards were followed in the rearing of birds in each experimental group.
2.2 Productive performance assessment
Eggs were gathered and checked for deformity to calculate the proportion of cracked eggs. Egg mass was calculated by multiplying the number of eggs by their average weight.
2.3 Evaluation of egg nutritional values
Randomly selected eggs from each group were gathered, homogenized, and stored at 80°C for later analysis. Egg protein, fat, cholesterol, and triacylglycerol levels were assessed spectrophotometrically.
2.4 Biochemical analyses
Blood samples were drawn from the jugular vein, and sera were harvested and stored at −20°C for further biochemical analysis [urea (Cat# UR2110), creatinine (Cat# CR1250), uric acid (Cat# UA2120), total protein (Cat# TP2020), and albumin (Cat# AB1010) levels] at the end of the trial. All methods were conducted following the manufacturers’ instructions (Biodiagnostic, Cairo, Egypt).
2.5 Determination of renal tissue LPO and antioxidant enzyme activities
At the end of the trial, the quails were euthanized in the absence of other birds to reduce the distress. The birds were first anesthetized using 2% isoflurane inhalation followed by cervical dislocation. Kidney specimens from the humanely sacrificed quails were obtained and stored at −80°C until evaluation of tissue oxidation indices. Renal tissues were discretely homogenized in phosphate buffer, pH 7.4, and the homogenate was used to determine the MDA (Cat# MD2529) and GSH (Cat# GR2511) levels, as well as the SOD (Cat# SD2521) and GPx (Cat# GP2524) activities, according to the manufacturer’s recommendations (Biodiagnostic).
2.6 Quantitative real-time PCR
Using the RNeasy Mini Kit (Cat# 74104, QIAGEN Sciences Inc., Germantown, MD, United States), the total RNA was extracted in accordance with the manufacturer’s instructions. The primer sequences for the targeted genes (housekeeping gene 28S rRNA and the inflammatory cytokines TNF-α, IL-1β, and IL-6) are presented in Supplementary Table S1. The QuantiTect probe RT-PCR kit (Cat#204443, QIAGEN Sciences Inc.) was employed to carry out quantitative real-time PCR (qRT-PCR). Real-time PCR equipment (Applied Biosystems, Waltham, CA, United States) was used to capture signals. The cycling conditions for PCR were as follows: 50°C for 30 min, 94°C for 10 min, 40 cycles at 94°C for 15 s, and 60°C for 1 min. The cycle threshold (Ct) values and amplification curves were calculated using Stratagene Mx3005P software. Following normalization using the housekeeping gene, the 2Ct technique was used to calculate the fold changes in the expression levels, as described in Habotta et al. (2023).
2.7 Detection of aflatoxin residues in eggs
The egg was collected after the completion of the trial to detect the residual AFT using high-performance liquid chromatography (HPLC). Aflatoxin M1 reference materials (Sigma-Aldrich, St. Louis, MO, United States) were used in the analyses.
2.8 Molecular docking assessment
The three-dimensional structures of Coturnix japonica’s extracellular SOD1, SOD2, GPx, GR, GCLC, GSH synthase, IL1RAP, IL6RA, TNFRSF1A, and TRAF1 were generated using the Robetta server (Baek et al., 2021). Proteins were prepared for docking using Molecular Operating Environment (MOE 2015.10, Chemical Computing Group, Montreal, QC, Canada) software. The three-dimensional structures of AFB1, AFB2, AFG1, and AFG2 were retrieved from the PubChem (https://pubchem.ncbi.nlm.nih.gov/) database. In addition, bioactive compounds of ChV were retrieved from PubChem and LOTUS: Natural Products Online (https://lotus.naturalproducts.net/) databases. Furthermore, MOE software was used for molecular docking, protein–ligand interaction analysis, and visualization.
2.9 Statistical analyses
One-way analysis of variance (ANOVA) with the LSD post hoc test was used to analyze the collected data. Prior to performing ANOVA, all data were tested for normality (Shapiro–Wilk and Kolmogorov–Smirnov tests; p > 0.05) and homogeneity of variances (Levene’s test; p > 0.05). All analyses were performed using SPSS 25 software for Windows (SPSS Inc., Chicago, IL, United States). Data were represented as the mean ± SE. The data were considered statistically significant at p-values <0.05. Additionally, RStudio’s R version 4.0.2 was used to create a debiased sparse partial correlation (DSPC) algorithm network, clustering heatmap, and variable importance projection (VIP) score.
3 Results
3.1 Egg productive performance
The effect of ChV supplement on the productivity of quails that received a diet contaminated with AFT is shown in Table 1. The egg number, weight, and mass decreased significantly, while cracked eggs increased dramatically in AFT-intoxicated birds versus all other treated groups. With daily observation, the birds that fed the AFT diet showed watery droppings, abnormal gait, and ruffled feathers. On the other hand, the co-exposure to AFT and ChV caused marked betterment of the production performance of birds. Intriguingly, the ChV group surpassed the control group in terms of egg number, weight, and mass.
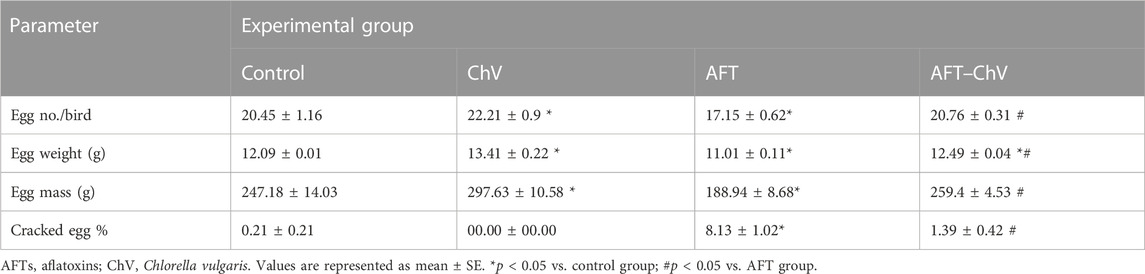
TABLE 1. Egg productive performance parameters of laying Japanese quails following ChV supplementation and/or AFT exposure.
3.2 Changes in the egg nutritional value following exposure to ChV and/or AFT
Table 2 shows that compared to controls, the ChV group showed a significant increase in the egg protein content while a significant decrease in egg fat, cholesterol, and triacylglycerol levels. ChV supplementation to quails that fed on an AFT-contaminated diet resulted in a noticeable increase in egg protein and a decrease in fat and cholesterol contents compared to the AFT group.
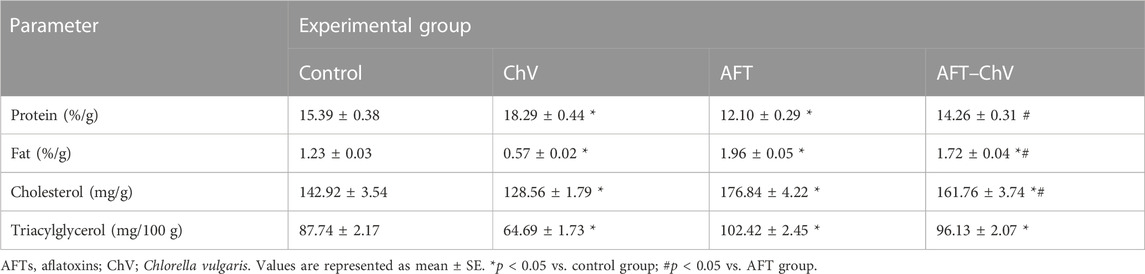
TABLE 2. Effect of ChV supplement on egg nutritive value of laying Japanese quails that fed on an AFT-contaminated diet.
3.3 Serum biochemical indices
In accordance with the findings depicted in Figure 1, quails that fed on an AFT-contaminated diet demonstrated a substantial increase in the kidney function test, including creatinine, urea, and uric acid, alongside a decrease in total protein and albumin levels, when confronted with those fed on a basal diet. In contrast, concurrent consumption of a diet containing ChV and AFT demonstrated amelioration in the renal function test and protein levels compared to those exposed to AFT solely.
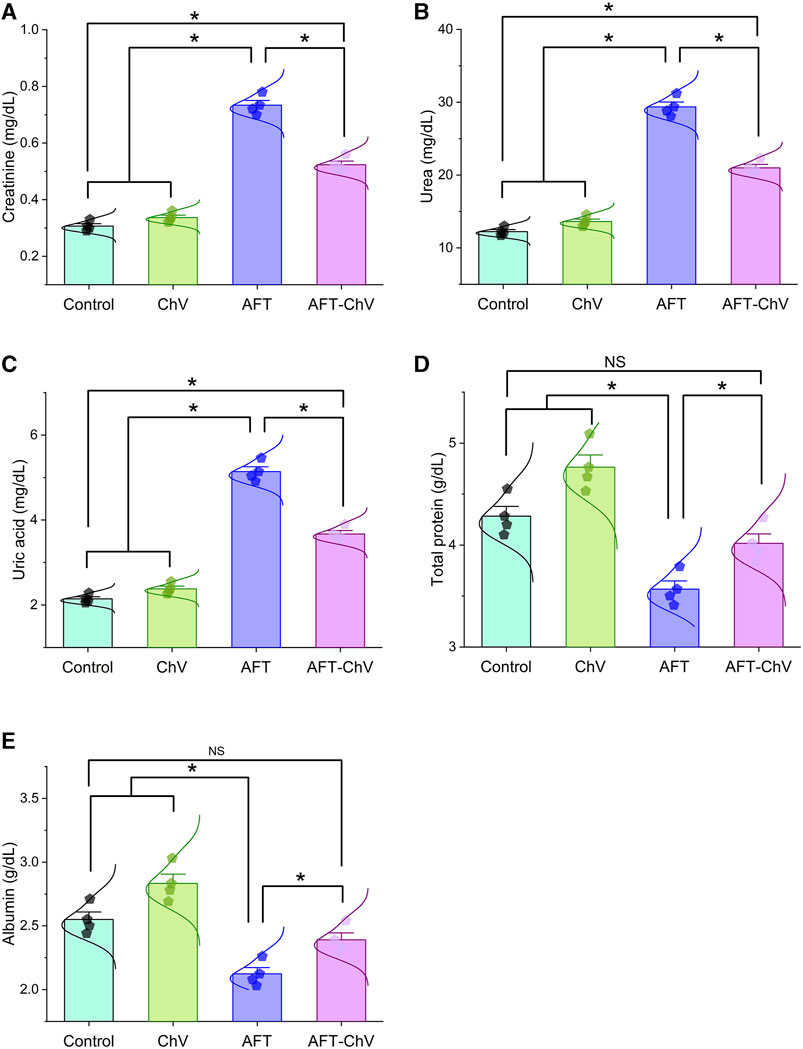
FIGURE 1. Bar-dot plot panel of serum biochemical tests upon ChV and/or AFT exposure. (A) Creatinine, (B) Urea, (C) Uric acid, (D) Total protein, and (E) Albumin. AFT, aflatoxins; ChV, Chlorella vulgaris. Values are represented as mean ± SE (*p < 0.05).
3.4 Lipid peroxidation and antioxidant parameter assay
AFT induced an obvious state of oxidative damage and lipid peroxidation, as shown in Figure 2. A substantial increase in the renal malondialdehyde (MDA) level together with a discernible reduction in the reduced-glutathione (GSH) level and the enzyme activities of superoxide dismutase (SOD) and glutathione peroxidase (GPx) was observed in birds that fed on an AFT-contaminated diet when compared to other groups. Notably, AFT-triggered oxidative stress was substantially hampered by ChV supplementation exhibited by a dramatic reduction in the MDA level and drastic increases in GSH, SOD, and GPx indices.
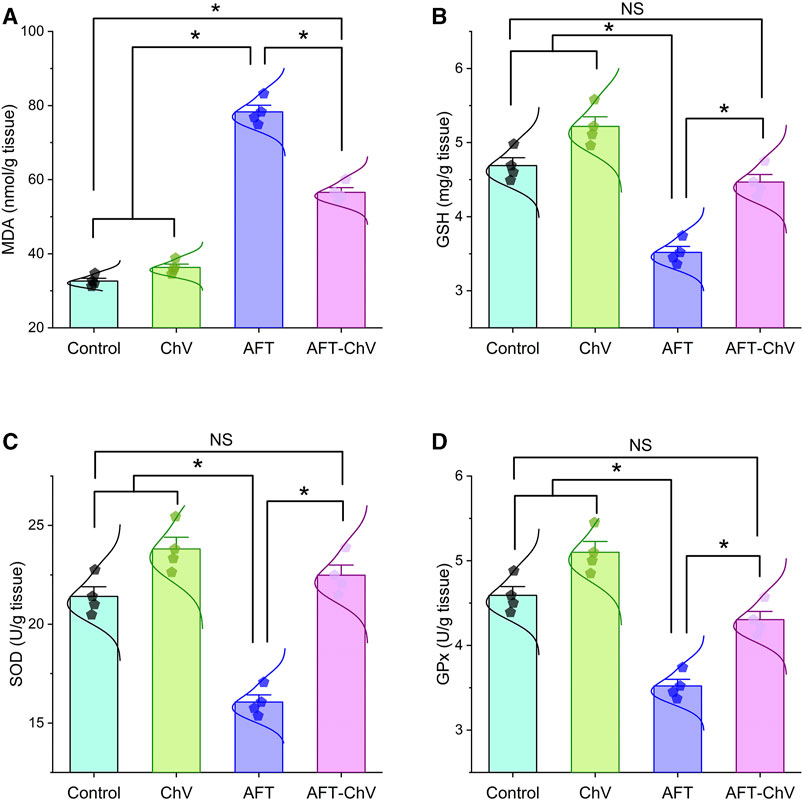
FIGURE 2. Bar-dot plot panel of oxidant/antioxidant indices following ChV and/or AFT treatment in the kidney tissue. (A) MDA, (B) GSH, (C) SOD, and (D) GPx. AFTs, aflatoxins; ChV, Chlorella vulgaris; GPx, glutathione peroxidase; GSH, reduced glutathione; MDA, malondialdehyde; SOD, superoxide dismutase. Values are represented as mean ± SE (*p < 0.05).
3.5 Expression levels of pro-inflammatory genes in the kidney tissue
As depicted in Figure 3, AFT could trigger renal inflammation indicated by considerable upregulation of mRNA expression levels of the pro-inflammatory cytokines (TNF-α, IL-1β, and IL-6) compared with controls. Nevertheless, birds that fed on a diet containing both ChV and AFT had a dampened inflammatory reaction in their kidneys, elucidated by the downregulation of the expression levels of the targeted pro-inflammatory genes.
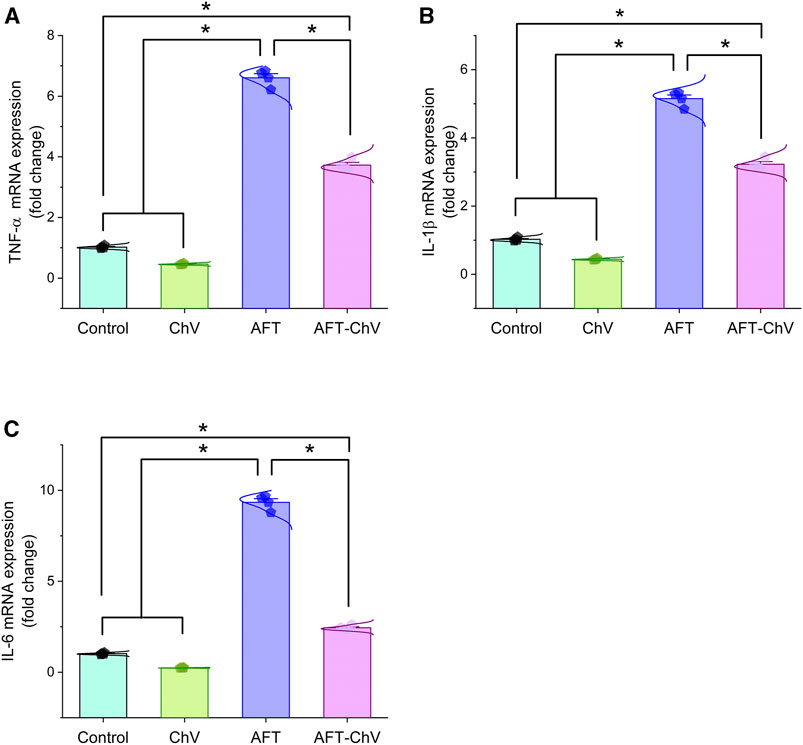
FIGURE 3. Bar-dot plot panel of mRNA expression of pro-inflammatory cytokines following ChV and/or AFT exposure in the kidney tissue. (A) TNF-α mRNA, (B) IL-1β mRNA, and (C) IL-6 mRNA. AFTs, aflatoxins; ChV, Chlorella vulgaris; IL-1β; interleukin-1β, IL-6, interleukin-6; TNF-α, tumor necrosis factor-α. Values are represented as mean ± SE (*p < 0.05).
3.6 Molecular docking
Data in Table 3 reveal the binding energy of AFT (B1, B2, G1, and G2) to the SOD1, SOD2, GPx, GR, GCLC, and GSH synthase binding sites of Japanese quails. AFB1 is the most abundant member of AFT in the naturally contaminated diet. The molecular docking dynamics revealed the great affinity of AFB1 to the SOD1, SOD2, GPx, GR, GCLC, and GSH synthase binding sites, with binding energy values of −5.34, −4.85, −5.57, −5.71, −7.26, and −6.69 kcal/mol, respectively (Figures 4A–F).
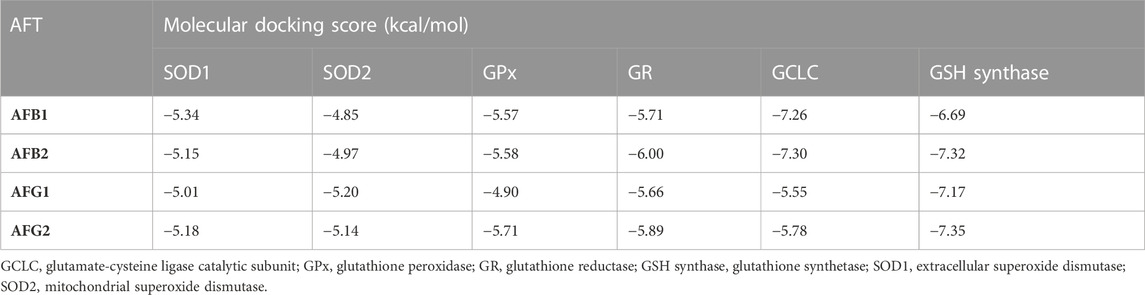
TABLE 3. Molecular docking scores of AFT on Japanese quails’ SOD1, SOD2, GPx, GR, GCLC, and GSH synthase.
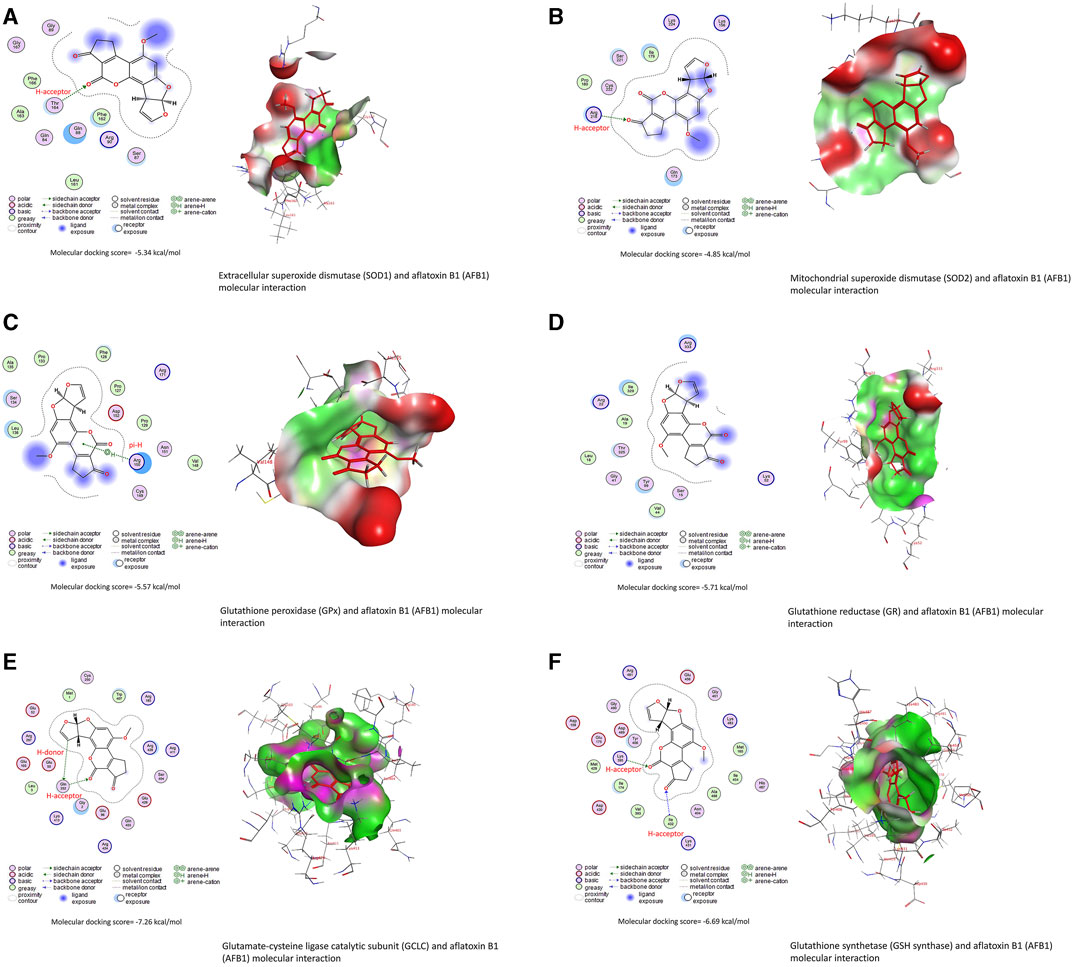
FIGURE 4. Molecular docking interactions of AFB1, AFB2, AFG1, and AFG2 with Japanese quails’ (A) extracellular superoxide dismutase (SOD1), (B) mitochondrial superoxide dismutase (SOD2), (C) glutathione peroxidase (GPx), (D) glutathione reductase (GR), (E) glutamate-cysteine ligase catalytic (GCLC) subunit, and (F) glutathione synthetase.
Bioactive compounds of ChV interacted with the binding sites of IL1RAP, IL6RA, TNFRSF1A, and TRAF1, as shown in Table 4. The top five bioactive compounds, phytofluene, naringin, hesperidin, zeta-carotene, and cis-phytoene, bind to the binding site of IL1RAP, with binding energy values of −10.23 (Figure 5A), −10.21, −10.19, −9.74, and −9.71 kcal/mol, respectively. The binding site of IL6RA can interact with zeta-carotene (Figure 5B), rutin, phytofluene, kaempferol, and cis-phytoene, with binding energy values of −8.65, −8.48, −8.48, −8.46, and −8.46 kcal/mol, respectively. In addition, the binding site of TNFRSF1A can be targeted by kaempferol (Figure 5C), cis-phytoene, loroxanthin, rutin, and phytofluene, with binding energy values of −8.08, −7.80, −7.63, −7.55, and −7.36 kcal/mol, respectively. The binding site of TRAF can interact with cis-phytoene (Figure 5D), zeta-carotene, hesperidin, naringin, and rutin, with binding energy values of −7.30, −7.24, −7.11, −7.01, and −6.97 kcal/mol, respectively.
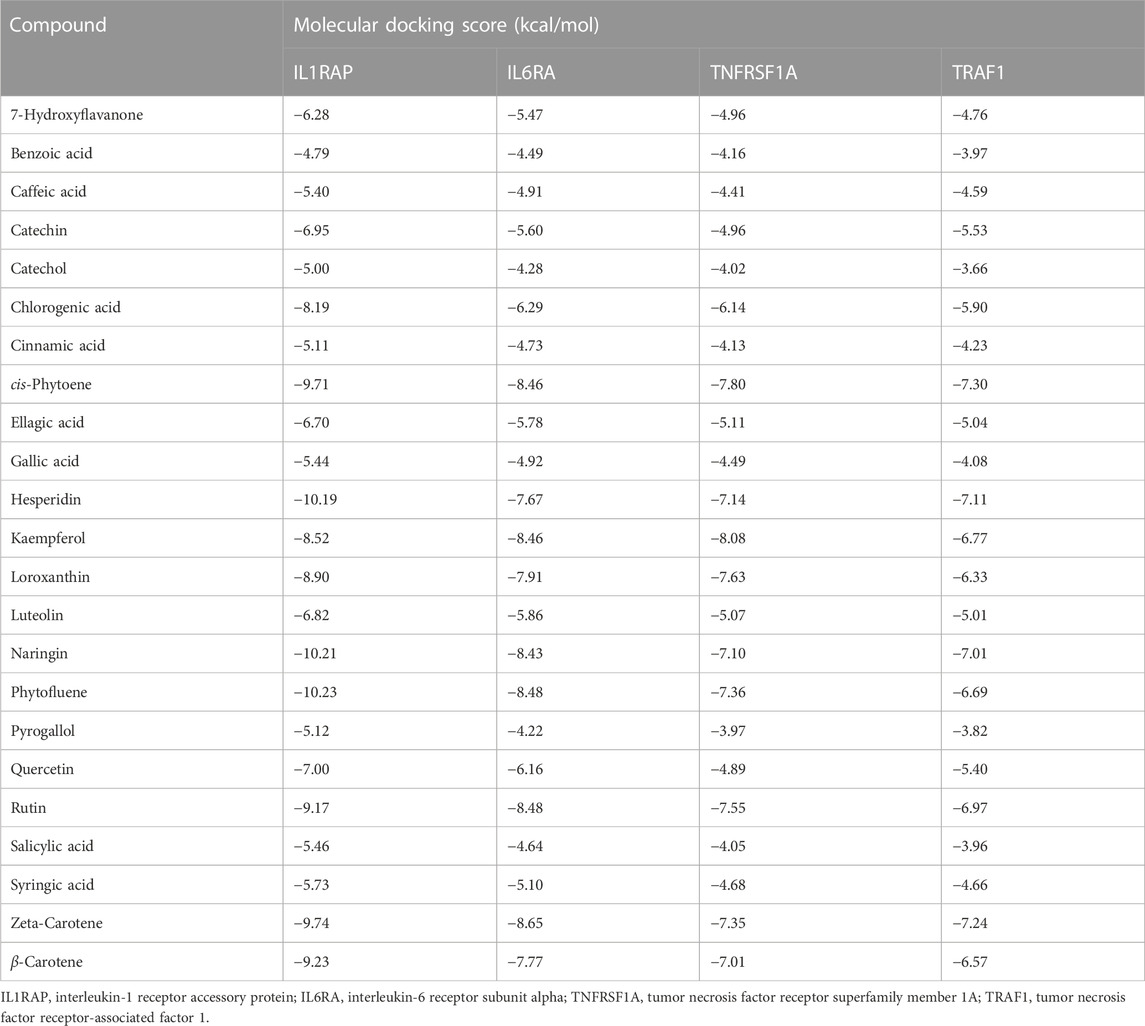
TABLE 4. Molecular docking scores of ChV’s bioactive compounds on Japanese quails’ IL1RAP, IL6RA, TNFRSF1A, and TRAF1.
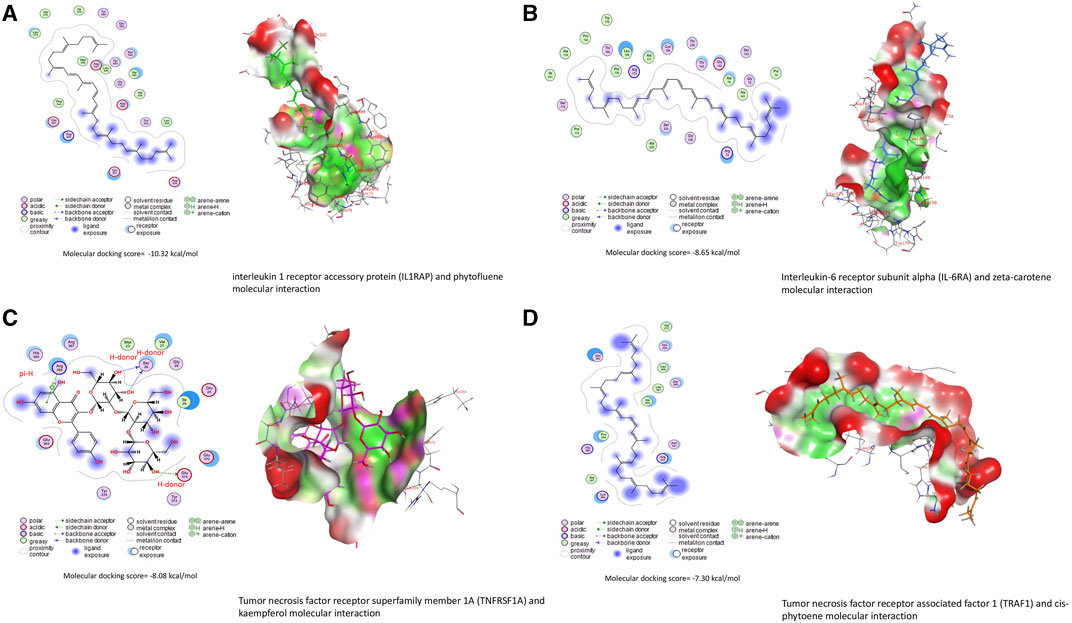
FIGURE 5. Molecular docking interactions of Chlorella vulgaris bioactive compounds with Japanese quails’ (A) interleukin-1 receptor accessory protein (IL1RAP), (B) interleukin-6 receptor subunit alpha (IL6RA), (C) tumor necrosis factor receptor superfamily member 1A (TNFRSF1A), and (D) tumor necrosis factor receptor-associated factor 1 (TRAF1).
3.7 Assessment of AFT residues in eggs
Figure 6 shows that birds that fed on the AFT-contaminated diet displayed a noticeable increase in the level of AFT residues in their eggs compared with birds that fed on a basal diet or supplemented with ChV solely. However, supplementation with ChV could lessen the accumulation of AFT in the eggs of birds that fed on an AFT-contaminated diet.
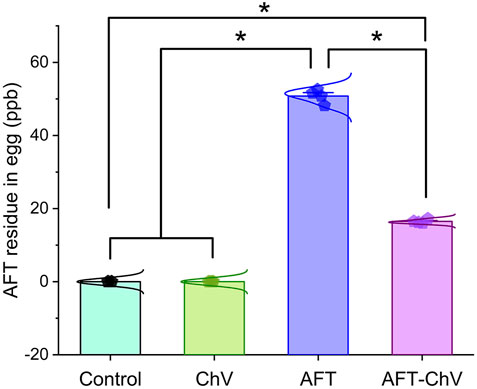
FIGURE 6. Bar-dot plot panel of the impact of ChV supplementation on egg total AFT residue. AFTs, aflatoxins; ChV, Chlorella vulgaris. Values are represented as mean ± SE (*p < 0.05).
3.8 Biological networks, hierarchical clustering heatmap, and variable importance in projection (VIP) score
Multivariate analyses were conducted to determine the association between various parameters and treated groups, as shown in Figure 7. Biological networks of all variables were constructed. The nodes display various parameters, while the lines depict the relationships among these variables in the DSPC algorithm network (Figure 7A). During the data normalization stage, the data were changed to log or cubic roots for the best performance. The DSPC network is more suitable for creating biologically pertinent networks and discovering unidentified substances. The variable that is closer to the center exhibits a stronger association with these selected parameters and the most relevant position in the network, such as AFT residue. On the other hand, AFT residue was positively correlated with the majority of examined parameters while negatively correlated with egg protein%. Furthermore, the metabolic pathway network was also constructed to explore the relationships between the most disrupted pathways and various parameters induced by AFT exposure.
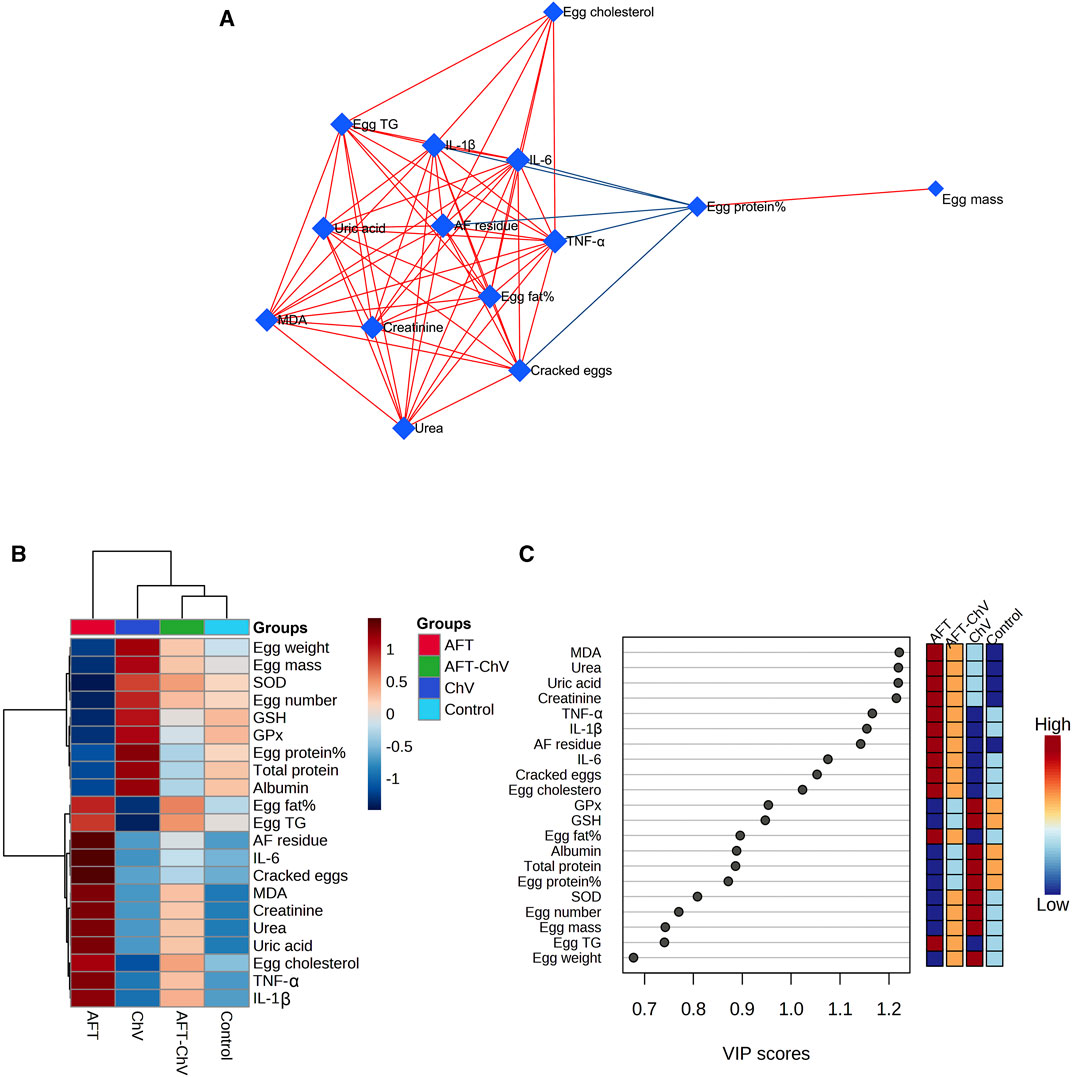
FIGURE 7. Clustering analysis of whole datasets after ChV and/or AFT exposure. (A) DSPC network of substantially distinct variables in the control and exposed groups. In the DSPC network, the nodes represent the measured variables, while the edges signify the correlation measures. Variables with the stronger correlation group cluster together and have wider edges between them. The blue lines display a negative correlation, while the red lines display a positive correlation with variables. (B) Heatmap and hierarchical clustering provide a visual summary of all the data. Each colored cell on the map represents a concentration value, and the rows and columns are made of different averages and treatment sets, respectively. Dark red has the highest value on the gradation scale, while blue has the lowest. (C) VIP score; the average concentrations of the measured variables are displayed for each study group in colored boxes on the right, and a colored scale from maximum (red) to least (blue) represents the contribution strength. AFTs, aflatoxins; ChV, Chlorella vulgaris; DSPC, debiased sparse partial correlation; VIP score, variable importance in projection score.
The clustering heatmap exemplifies an evident visual depiction of all datasets (Figure 7B) and reveals a notable discrepancy in the concentration of whole measured parameters in response to AFT toxicity compared to other groups. These findings posit that the quails that fed on an AFT-contaminated diet exhibited greater damage than those of other groups.
Additionally, according to the VIP score, the top influential factors in our study were MDA, urea, uric acid, creatinine, TNF-α, IL-1β, and AFT residue which were sensitive to various treatments and could distinguish AFT treatment from others (Figure 7C).
4 Discussion
AFT is the most hazardous toxin that frequently contaminates grains, food, and feedstuff and remains after food processing, raising serious health concerns (Wang et al., 2022). As its metabolites (AFTO) preferentially accumulate in the renal tissue during urinary excretion, it has been postulated in a number of studies as a potential contributor to renal disorders (Yilmaz et al., 2018; Abdel-Daim et al., 2021).
Ample literature, including our and others’ preceding studies, strongly indicate that excess oxidant production and cellular antioxidant depletion are fundamental pathways embroiled in AFT-induced nephrotoxicity (Owumi et al., 2020; Abdel-Daim et al., 2021; Wang et al., 2022). This, owing to the ability of AFT to directly attack the cellular macromolecules, notably, nucleic acid and proteins, results in immense ROS formation, including superoxide anions (O2•–), hydroxyl radicals (OH•), and hydrogen peroxide (H2O2). Interestingly, SOD is an antioxidant enzyme that promotes the breakdown of O2•− radical into O2 and H2O2, thereby annihilating oxidative damage (Wispriyono et al., 2021). Additionally, GSH plays a crucial role as a co-substrate for enzyme GPx, which is in charge of detoxifying the H2O2 and lipid hydroperoxides, as well as direct neutralization of AFT (by forming an AFT–GSH conjugation) (Kemal and Seker, 2023). However, Fenton’s reaction is initiated when antioxidants are depleted by AFT-initiated ROS; therefore, enormous amounts of OH• are built up, which aggressively attack the phospholipid membrane, leading to MDA accumulation (Aboubakr et al., 2021; Abdelnaby et al., 2022). MDA is the byproduct of lipid peroxidation (LPO) that represents the degree of membrane damage. It could be more detrimental to human health than AFT itself through engaging with essential intracellular molecules, thus expediting oxidative injury (Fouad et al., 2019).
Accordingly, oxidative damage is prominently emphasized in our study, expounded by an evident drop in GSH levels and the activities of SOD and GPx in renal tissues. The ongoing work has also further proven the detrimental impact of AFT-induced LPO on the tubular epithelial membrane expounded by the substantial elevation of the antioxidant enzymes such as MDA. As a consequence, tubular dysfunction occurs and is demonstrated by a significant upsurge in the serum levels of urea, creatinine, and uric acid. These results support our preceding research, which revealed a positive association between the MDA level and the increased serum levels of renal function parameters (Abdelnaby et al., 2022). Our findings are in agreement with those of Manafi (2018) who reported that the albumin level is negatively affected when Japanese quails are treated with AFT. Furthermore, as described in previous reports, the current study revealed evident decreases in serum albumin and total protein levels following AFT exposure. Such decreases might be attributed to impaired tubular reabsorption with increased urinary protein loss (Abdeen et al., 2020). In other words, the accumulated MDA may trigger further DNA and protein oxidation, resulting in the inhibition of the processes of translation and transcription of mRNA and thence protein synthesis (Abdeen et al., 2021; Aboubakr et al., 2021).
Furthermore, the elevated levels of ROS due to AFT exposure led to the deletion of antioxidant status in the renal tissue, as evidenced by significant reductions in GSH, SOD, and GPx in the current study. In addition, the molecular docking study revealed the binding interaction between AFT and SOD1, SOD2, GPx, GR, GCLC, and GSH synthase. In the same context, AFT significantly elevated MDA and reduced whole-blood GSH (Citil et al., 2005) and hepatic SOD and GPx (Elbasuni et al., 2022) in Japanese quails.
In conformity to accumulating evidence, inflammation and oxidative stress are remarkably correlated. Increased ROS production boosts the nuclear factor kappa B (NF-κB) signaling cascade and promotes the expression of pro-inflammatory cytokine genes, resulting in a profound inflammatory reaction (Ahmed et al., 2022). TNF-α is the most important pro-inflammatory cytokine that is implicated in the activation of NF-κB, stimulating the regulation of interleukins and other inflammatory mediators downstream (Rajendran et al., 2018). Our results support the aforementioned mechanism, exhibited by enhanced gene expression of inflammatory factors, TNF-α, IL-1β, and IL-6, in response to AFT intoxication. Thus, we propose that the inflammatory process is a possible pathway that involved AFT-induced nephropathy. These findings are consistent with those of Gao et al. (2021) who observed the overexpression of these inflammatory cytokines in chick kidneys upon AFT exposure.
Regarding the effect of AFT on the nutritional value of eggs, contrary to other treated groups, AFT had an impact on the nutritional value of eggs collected from quails subjected to AFT. That was exhibited in the current work by reduced protein content and elevated levels of total fat, cholesterol, and triacylglycerol contents. Since the ROS produced by AFT substantially induces DNA adducts and protein oxidation, protein synthesis and lipid metabolism are negatively affected (Abdel-Daim et al., 2020; 2021; Ahmed et al., 2022). As anticipated, the current trial proved the presence of substantial residuals of AFT in eggs, which has an impact on the safety and quality of edible components of birds that fed on an AFT-contaminated diet. Manafi (2018) reported that AFT metabolites are carried over from quail feed to eggs and thence to the consumer. This finding agrees with that of Oliveira et al. (2003) who identified AFT residues in the eggs of laying quails.
A plethora of research has investigated the economic implications of various levels of AFT in egg production. A reduction in egg number along with egg weight was observed by Oliveira et al. (2003) following dietary AFT inclusion in Japanese quails, similar to that reported in the current work. This reduction in egg production and quality may be due to the negative impact of AFT on egg development through the disruption mobilization of fat from the liver to the ovary (Manafi, 2018). Moreover, our data demonstrated poor eggshell quality in quails that fed on an AFT-contaminated diet, and this is due to reduced deposition of calcium in the bone after AFT exposure, which accounts for one-third of the calcium supply required for eggshell formation. AFT-induced malfunction of the kidney leads to decreased synthesis and activation of vitamin D and thus decreased calcium deposition in the bone (Fouad et al., 2019).
ChV is a green microalga frequently used as a prophylactic remedy to maintain renal health due to its renowned antioxidant, anti-inflammatory, and immune-modulating properties (Blas-Valdivia et al., 2011; Al-Halaseh et al., 2022). The antioxidant capability has been related to its phenolic constituents identified among other active phytoconstituents such as carotenoids, lutein, catechin, carotenoids, gallic acid, caffeic acids, benzoic acid, chlorogenic acid, and rutin (Blas-Valdivia et al., 2011; Andrade et al., 2018; Prabakaran et al., 2019; Sikiru et al., 2019). Additionally, it contains a variety of essential trace elements (Cu, Zn, Se, and Fe) that are required for the function of numerous antioxidant metalloenzymes (Abu-Serie et al., 2018).
Accumulating evidence corroborates that ChV consumption counteracts oxidative stress via modulating antioxidant enzymes, scavenging the free radicals, and mitigating LPO (Sikiru et al., 2019). This hypothesis is supported by our finding, indicated by improvements in renal function and oxidant/antioxidant status in birds concurrently treated with AFT and ChV. Our findings agree with the previous reports that ChV supplementation drastically decreased the generation of MDA along with increased antioxidant enzyme levels in a fish model (Abdelhamid et al., 2020). Another report documented increased antioxidative enzyme activities in rabbits fed on ChV supplements (Abdelnour et al., 2019a).
Along with its antioxidant activity, ChV exhibits a well-known potent anti-inflammatory property via regulating inflammatory cytokine release, including IL-6, TNF-α, and iNOS, as well as inhibiting proteinase and lipoxygenase activities, which are implicated in the inflammatory process (Prabakaran et al., 2019; Abdelhamid et al., 2020). Additionally, the molecular docking study revealed the powerful binding of ChV bioactive compounds to the binding sites of IL1RAP, IL6RA, TNFRSF1A, and TRAF1 in quails. Accumulating evidence indicates that ChV’s potential to mitigate inflammation is ascribed to its abundance in PUFA, which plays a crucial role in regulating the release of pro-inflammatory cytokines and relieving cellular inflammation (Abdelnour et al., 2019a; Remize et al., 2021; Yang et al., 2022). This is in agreement with Abdelhamid et al. (2020) who reported that ChV supplementation contributed to the downregulation of TNF-α in splenic fish. Another report elucidated that ChV’s ability to suppress inflammation might be attributed to its capacity to upmodulate cellular antioxidant indices while downregulating inflammatory mediators (Abu-Serie et al., 2018). In an endorsement of previous studies, the current study elucidates the anti-inflammatory action of ChV, as explained by the downregulation of inflammatory cytokines.
It is worth mentioning that the findings of our research highlight the economic benefits of ChV as an egg production enhancer and nutritional value promoter when compared to the AFT group. ChV contains a plethora of high-quality nutrients, such as vital amino acids, vitamins, and minerals, and functions as a growth enhancer and productive booster (Lamminen et al., 2019). Additionally, supplementation of ChV reduced the AFT residue compared to the AFT group in our trial. Such enhancement might be related to ChV’s antioxidant activity, improving renal function and, in turn, the metabolic processes. According to Zheng et al. (2012), feeding diets supplemented with ChV enhanced the eggs’ morphological traits, which concurs with the current study. Furthermore, the results indicate that ChV could enhance egg quality and laying performance of laying hens, which is in agreement with previous studies (Halle et al., 2009).
Additionally, multivariate statistical analyses using the DSPC network, clustering heatmap, and VIP score were carried out to analyze the variable contributions affected by different treatments on renal tissue and quail eggs. The DSPC network revealed that AFT residue exhibits the more relevant position in the network and positively correlated with the majority of examined parameters while negatively correlated with egg protein%. The clustering heatmap effectively epitomizes that AFT exposure induced significant alterations in all examined parameters compared to other treatment groups, indicating prospective improvements in those parameters when ChV was supplemented. MDA, urea, uric acid, creatinine, TNF-α, IL-1β, and AFT residue were also found to be the top impacting variables in our study according to the VIP score. The molecular pathways underpinning ChV’s potential to protect against kidney damage induced by AFT are shown in Figure 8.
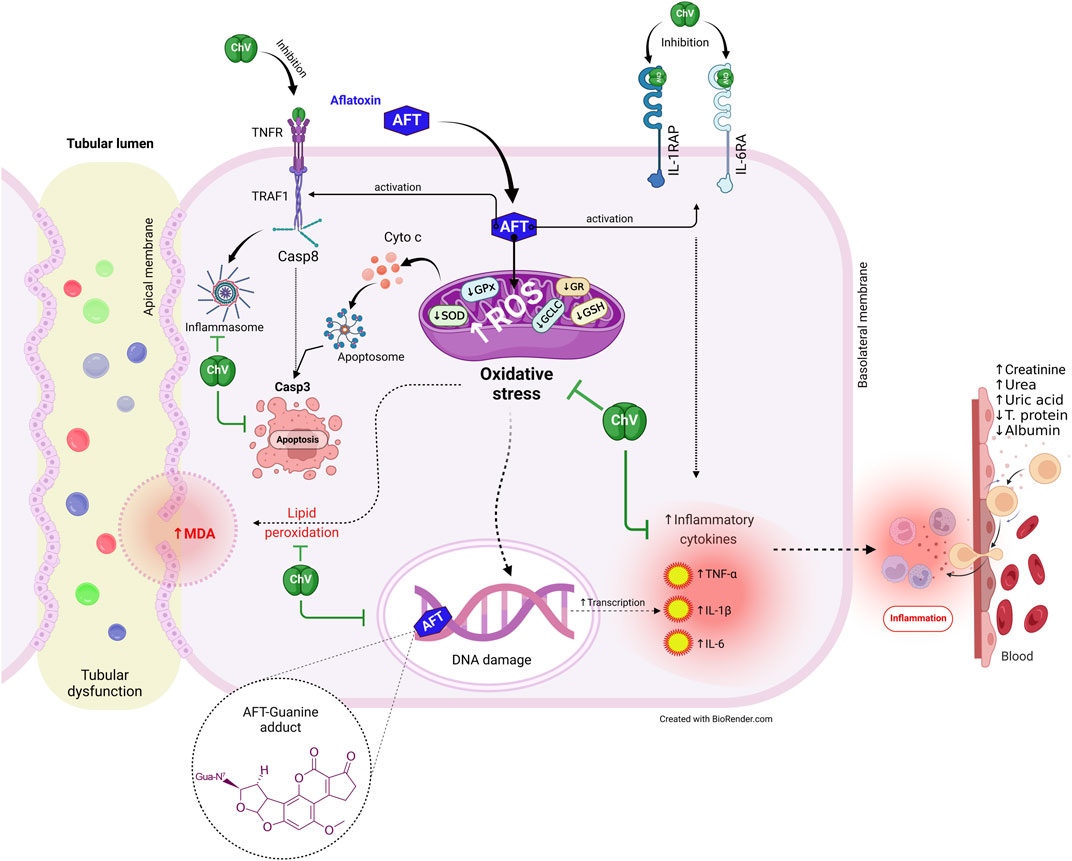
FIGURE 8. The protective effect of ChV against AFT-induced kidney injury is underpinned by molecular processes. AFTs, aflatoxins; ChV, Chlorella vulgaris; GCLC, glutamate-cysteine ligase catalytic subunit; GPx, glutathione peroxidase; GR, glutathione reductase; GSH synthetase, glutathione synthetase; IL1RAP, interleukin-1 receptor accessory protein; IL6RA, interleukin-6 receptor subunit alpha; MDA, malondialdehyde; ROS, reactive oxygen species; SOD, superoxide dismutase; TNFR, tumor necrosis factor receptor; TRAF1, tumor necrosis factor receptor-associated factor 1.
5 Conclusion
AFT induces renal dysfunction via the stimulation of oxidative stress, LPO, and inflammatory response, which leads to decreased egg nutritional value and increased AFT accumulation in the egg. ChV supplement has the capability to safeguard the kidney from the detrimental effects of AFT. This is likely due to ChV’s enriched nutritional constituents and antioxidant, ROS-scavenging, and anti-inflammatory attributes. The antioxidant, inflammatory, and apoptotic proteins targeted by ChV active constituents were validated by molecular docking dynamics. Thus, to mitigate the potentially harmful effects of AFT on both humans and animals, we strongly suggest that ChV supplementation may be a promising, secure, and economical biological approach.
Data availability statement
The original contributions presented in the study are included in the article/Supplementary Material; further inquiries can be directed to the corresponding authors.
Ethics statement
The animal study was approved by the Ethics Committee of the Faculty of Veterinary Medicine at Benha University who endorsed the use of experimental animals and the experimental design for the animal study protocol (Approval No. BUFVTM 30-10-22). The study was conducted in accordance with the local legislation and institutional requirements.
Author contributions
AhA: Conceptualization, Formal Analysis, Investigation, Resources, Supervision, Validation, Visualization, Writing–original draft, Writing–review and editing. RE: Conceptualization, Formal Analysis, Investigation, Methodology, Writing–original draft. SE: Conceptualization, Data curation, Investigation, Methodology, Validation, Writing–original draft. AS: Conceptualization, Data curation, Formal Analysis, Investigation, Methodology, Writing–original draft. AfA: Data curation, Formal Analysis, Methodology, Software, Validation, Visualization, Writing–original draft, Writing–review and editing. AE-F: Data curation, Formal Analysis, Investigation, Methodology, Software, Visualization, Writing–original draft, Resources, SFI: Data curation, Formal Analysis, Funding acquisition, Resources, Software, Validation, Writing–original draft, Writing–review and editing. OM: Data curation, Formal Analysis, Funding acquisition, Resources, Software, Validation, Writing–original draft, Writing–review and editing. LF: Data curation, Formal Analysis, Software, Validation, Writing–original draft, Writing–review and editing. AMA: Conceptualization, Formal Analysis, Investigation, Methodology, Writing–original draft. ME-H: Data curation, Investigation, Methodology, Software, Validation, Writing–original draft. NE: Data curation, Investigation, Methodology, Software, Validation, Writing–original draft. SSI: Conceptualization, Data curation, Formal Analysis, Investigation, Methodology, Software, Validation, Writing–original draft.
Funding
This research was supported by the Princess Nourah bint Abdulrahman University Researchers Supporting Project number (PNURSP2023R127), Princess Nourah bint Ab-dulrahman University, Riyadh, Saudi Arabia. This research was funded by the Researchers Supporting Project number (RSPD2023R811), King Saud University, Riyadh, Saudi Arabia. Moreover, this paper is published from the project 6PFE of the University of Life Sciences “King Mihai I” from Timisoara and Research Institute for Biosecurity and Bioengineering from Timisoara.
Acknowledgments
The authors thank the STDF (Grant No. 31290) and ASRT (Grant No. 2020/D60) for offering all facilities for this study. The authors wish to express their gratitude to the Princess Nourah bint Abdulrahman University Researchers Supporting Project number (PNURSP2023R127), Princess Nourah bint Abdulrahman University, Riyadh, Saudi Arabia. This research was funded by the Researchers Supporting Project number (RSPD2023R811), King Saud University, Riyadh, Saudi Arabia.
Conflict of interest
The authors declare that the research was conducted in the absence of any commercial or financial relationships that could be construed as a potential conflict of interest.
Publisher’s note
All claims expressed in this article are solely those of the authors and do not necessarily represent those of their affiliated organizations, or those of the publisher, the editors, and the reviewers. Any product that may be evaluated in this article, or claim that may be made by its manufacturer, is not guaranteed or endorsed by the publisher.
Supplementary material
The Supplementary Material for this article can be found online at: https://www.frontiersin.org/articles/10.3389/fphar.2023.1291965/full#supplementary-material
References
Abdeen, A., Abdelkader, A., Elgazzar, D., Aboubakr, M., Abdulah, O. A., Shoghy, K., et al. (2020). Coenzyme Q10 supplementation mitigates piroxicam-induced oxidative injury and apoptotic pathways in the stomach, liver, and kidney. Biomed. Pharmacother. 130, 110627. doi:10.1016/j.biopha.2020.110627
Abdeen, A., Samir, A., Elkomy, A., Aboubaker, M., Habotta, O. A., Gaber, A., et al. (2021). The potential antioxidant bioactivity of date palm fruit against gentamicin-mediated hepato-renal injury in male albino rats. Biomed. Pharmacother. 143, 112154. doi:10.1016/j.biopha.2021.112154
Abdel-Daim, M., Dawood, M. A. O., AlKahtane, A. A., Abdeen, A., Abdel-Latif, H. M. R., Senousy, H. H., et al. (2020). Spirulina platensis mediated the biochemical indices and antioxidative function of Nile tilapia (Oreochromis niloticus) intoxicated with aflatoxin B1. Toxicon 184, 152–157. doi:10.1016/j.toxicon.2020.06.001
Abdel-Daim, M. M., Abdeen, A., Jalouli, M., Abdelkader, A., Megahed, A., Alkahtane, A., et al. (2021). Fucoidan supplementation modulates hepato-renal oxidative stress and DNA damage induced by aflatoxin B1 intoxication in rats. Sci. Total Environ. 768, 144781. doi:10.1016/j.scitotenv.2020.144781
Abdelhamid, F. M., Elshopakey, G. E., and Aziza, A. E. (2020). Ameliorative effects of dietary Chlorella vulgaris and β-glucan against diazinon-induced toxicity in Nile tilapia (Oreochromis niloticus). Fish. Shellfish Immunol. 96, 213–222. doi:10.1016/j.fsi.2019.12.009
Abdelnaby, A., Abdel-Aleem, N., Mansour, A., Abdelkader, A., Ibrahim, A. N., Sorour, S. M., et al. (2022). The combination of Tamarindus indica and coenzyme Q10 can be a potential therapy preference to attenuate cadmium-induced hepatorenal injury. Front. Pharmacol. 13, 954030–954114. doi:10.3389/fphar.2022.954030
Abdelnour, S. A., Abd El-Hack, M. E., Arif, M., Khafaga, A. F., and Taha, A. E. (2019a). The application of the microalgae Chlorella spp. as a supplement in broiler feed. Worlds. Poult. Sci. J. 75, 305–318. doi:10.1017/s0043933919000047
Abdelnour, S. A., Sheiha, A. M., Taha, A. E., Swelum, A. A., Alarifi, S., Alkahtani, S., et al. (2019b). Impacts of enriching growing rabbit diets with chlorella vulgaris microalgae on growth, blood variables, carcass traits, immunological and antioxidant indices. Animals 9, 788. doi:10.3390/ani9100788
Aboubakr, M., Abdelkader, A., Habotta, O. A., Adel, N., Emam, M. A., Abdelhiee, E. Y., et al. (2021). Cefepime and diclofenac sodium combined treatment-potentiated multiple organ injury: role of oxidative damage and disrupted lipid metabolism. J. Biochem. Mol. Toxicol. 35, e22929. doi:10.1002/jbt.22929
Abu-Serie, M. M., Habashy, N. H., and Attia, W. E. (2018). In vitro evaluation of the synergistic antioxidant and anti-inflammatory activities of the combined extracts from Malaysian Ganoderma lucidum and Egyptian Chlorella vulgaris. BMC Complement. Altern. Med. 18, 154–213. doi:10.1186/s12906-018-2218-5
Ahmed, N., El-Rayes, S. M., Khalil, W. F., Abdeen, A., Abdelkader, A., Youssef, M., et al. (2022). Arabic gum could alleviate the aflatoxin B1-provoked hepatic injury in rat: the involvement of oxidative stress, inflammatory, and apoptotic pathways. Toxins (Basel) 14, 605. doi:10.3390/toxins14090605
Al-Halaseh, L. K., Sweiss, M. A., Issa, R. A., AlKassasbeh, R., Abbas, M. A., Al-Jawabri, N. A., et al. (2022). Nephroprotective activity of green microalgae, chlorella sorokiniana isolated from Jordanian water. J. Pure Appl. Microbiol. 16, 2775–2782. doi:10.22207/JPAM.16.4.49
Andrade, L. M., Andrade, C. J., Dias, M., Nascimento, C., and Mendes, M. A. (2018). Chlorella and spirulina microalgae as sources of functional foods, nutraceuticals, and food supplements; an overview. Nutraceuticals, Food Suppl. 6, 45–58. doi:10.15406/mojfpt.2018.06.00144
Baek, M., DiMaio, F., Anishchenko, I., Dauparas, J., Ovchinnikov, S., Lee, G. R., et al. (2021). Accurate prediction of protein structures and interactions using a three-track neural network. Yearb. Paediatr. Endocrinol. 373, 871–876. doi:10.1126/science.abj8754
Blas-Valdivia, V., Ortiz-Butrón, R., Pineda-Reynoso, M., Hernández-Garcia, A., and Cano-Europa, E. (2011). Chlorella vulgaris administration prevents HgCl2-caused oxidative stress and cellular damage in the kidney. J. Appl. Phycol. 23, 53–58. doi:10.1007/s10811-010-9534-6
Chen, W., Li, T., Du, S., Chen, H., and Wang, Q. (2023). Microalgal polyunsaturated fatty acids: hotspots and production techniques. Front. Bioeng. Biotechnol. 11, 1146881–1146922. doi:10.3389/fbioe.2023.1146881
Citil, M., Gunes, V., Atakisi, O., Ozcan, A., Tuzcu, M., and Dogan, A. (2005). Protective effect of L-carnitine against oxidative damage caused by experimental chronic aflatoxicosis in quail (Coturnix coturnix). Acta Vet. Hung. 53, 319–324. doi:10.1556/AVET.53.2005.3.5
Dlamini, N. Z., Somboro, A. M., Amoako, D. G., Arhin, I., Khumalo, H. M., and Khan, R. B. (2021). Toxicogenicity and mechanistic pathways of aflatoxin B1 induced renal injury. Environ. Toxicol. 36, 1857–1872. doi:10.1002/tox.23306
Elbasuni, S. S., Ibrahim, S. S., Elsabagh, R., Nada, M. O., Elshemy, M. A., Ismail, A. K., et al. (2022). The preferential therapeutic potential of chlorella vulgaris against aflatoxin-induced hepatic injury in quail. Toxins (Basel). 14, 843–916. doi:10.3390/toxins14120843
Elgazzar, D., Aboubakr, M., Bayoumi, H., Ibrahim, A. N., Sorour, S. M., El-Hewaity, M., et al. (2022). Tigecycline and gentamicin-combined treatment enhances renal damage: oxidative stress, inflammatory reaction, and apoptosis interplay. Pharmaceuticals 15. doi:10.3390/ph15060736
Fouad, A. M., Ruan, D., El Senousey, H. A. K., Chen, W., Jiang, S., and Zheng, C. (2019). Harmful effects and control strategies of aflatoxin B₁ produced by Aspergillus flavus and Aspergillus parasiticus strains on poultry: review. Toxins (Basel). 11, 1–21. doi:10.3390/toxins11030176
Gao, X., Xu, J., Jiang, L., Liu, W., Hong, H., Qian, Y., et al. (2021). Morin alleviates aflatoxin B1-induced liver and kidney injury by inhibiting heterophil extracellular traps release, oxidative stress and inflammatory responses in chicks. Poult. Sci. 100, 101513. doi:10.1016/j.psj.2021.101513
Habotta, O. A., Abdeen, A., El-Hanafy, A. A., Yassin, N., Elgameel, D., Ibrahim, S. F., et al. (2023). Sesquiterpene nootkatone counteracted the melamine-induced neurotoxicity via repressing of oxidative stress, inflammatory, and apoptotic trajectories. Biomed. Pharmacother. 165, 115133. doi:10.1016/j.biopha.2023.115133
Halle, I., Janczyk, P., Freyer, G., and Souffrant, W. B. (2009). Effect of microalgae Chlorella vulgaris on laying hen performance. Arch. Zootech. 12, 5–13.
Hebels, D. G. A. J., Jennen, D. G. J., Kleinjans, J. C. S., and de Kok, T. M. C. M. (2009). Molecular signatures of N-nitroso compounds in Caco-2 cells: implications for colon carcinogenesis. Toxicol. Sci. 108, 290–300. doi:10.1093/toxsci/kfp035
Kemal, G., and Seker, D. (2023). Determination of glutathione peroxidase activity and oxidized/reduced glutathione ratio in patients with colon cancer. GSC Biol. Pharm. Sci. 22, 196–202. doi:10.30574/gscbps.2023.22.2.0067
Kolosova, A., and Stroka, J. (2012). Evaluation of the effect of mycotoxin binders in animal feed on the analytical performance of standardised methods for the determination of mycotoxins in feed. Food Addit. Contam. Part A 29, 1959–1971. doi:10.1080/19440049.2012.720035
Lamminen, M., Halmemies-Beauchet-Filleau, A., Kokkonen, T., Jaakkola, S., and Vanhatalo, A. (2019). Different microalgae species as a substitutive protein feed for soya bean meal in grass silage based dairy cow diets. Anim. Feed Sci. Technol. 247, 112–126. doi:10.1016/j.anifeedsci.2018.11.005
Li, S., Liu, R., Xia, S., Wei, G., Ishfaq, M., Zhang, Y., et al. (2022). Protective role of curcumin on aflatoxin B1-induced TLR4/RIPK pathway mediated-necroptosis and inflammation in chicken liver. Ecotoxicol. Environ. Saf. 233, 113319. doi:10.1016/j.ecoenv.2022.113319
Manafi, M. (2018). Toxicity of aflatoxin B1 on laying Japanese quails (Coturnix coturnix japonica). J. Appl. Anim. Res. 46, 953–959. doi:10.1080/09712119.2018.1436550
Medina, Á., González-Jartín, J. M., and Sainz, M. J. (2017). Impact of global warming on mycotoxins. Curr. Opin. Food Sci. 18, 76–81. doi:10.1016/j.cofs.2017.11.009
Oliveira, F., Rosmaninho, F. J., Castro, A. L., Butkeraitis, P., and Corrêa, B. (2003). Aflatoxin residues in eggs of laying Japanese quail after long-term administration of rations containing low levels of aflatoxin B1. Food Addit. Contam. 20, 648–653. doi:10.1080/0265203031000119025
Owumi, S., Najophe, E. S., Farombi, E. O., and Oyelere, A. K. (2020). Gallic acid protects against Aflatoxin B1 -induced oxidative and inflammatory stress damage in rats kidneys and liver. J. Food Biochem. 44, e13316. doi:10.1111/jfbc.13316
Prabakaran, G., Moovendhan, M., Arumugam, A., Matharasi, A., Dineshkumar, R., and Sampathkumar, P. (2019). Evaluation of chemical composition and in vitro antiinflammatory effect of marine microalgae chlorella vulgaris. Waste Biomass Valorization 10, 3263–3270. doi:10.1007/s12649-018-0370-2
Rajendran, P., Chen, Y. F., Chen, Y. F., Chung, L. C., Tamilselvi, S., Shen, C. Y., et al. (2018). The multifaceted link between inflammation and human diseases. J. Cell. Physiol. 233, 6458–6471. doi:10.1002/jcp.26479
Remize, M., Brunel, Y., Silva, J. L., Berthon, J. Y., and Filaire, E. (2021). Microalgae n-3 PUFAs production and use in food and feed industries. Mar. Drugs 19, 113–129. doi:10.3390/MD19020113
Shim, J. A., Son, Y. A., Park, J. M., and Kim, M. K. (2009). Effect of Chlorella intake on Cadmium metabolism in rats. Nutr. Res. Pract. 3, 15–22. doi:10.4162/nrp.2009.3.1.15
Sikiru, A. B., Arangasamy, A., Alemede, I. C., Guvvala, P. R., Egena, S. S. A., Ippala, J. R., et al. (2019). Chlorella vulgaris supplementation effects on performances, oxidative stress and antioxidant genes expression in liver and ovaries of New Zealand White rabbits. Heliyon 5, e02470. doi:10.1016/j.heliyon.2019.e02470
Wang, Y., Liu, F., Zhou, X., Liu, M., Zang, H., Liu, X., et al. (2022). Alleviation of oral exposure to aflatoxin B1-induced renal dysfunction, oxidative stress, and cell apoptosis in mice kidney by curcumin. Antioxidants 11, 1082–1117. doi:10.3390/antiox11061082
Wispriyono, B., Jalaludin, J., Kusnoputranto, H., Pakpahan, S., Permata Aryati, G., Pratama, S., et al. (2021). Glutathione (GSH) and superoxide dismutase (SOD) levels among junior high school students induced by indoor particulate matter 2.5 (PM2.5) and nitrogen dioxide (NO2) exposure. J. Public Health Res. 10, 2372. doi:10.4081/jphr.2021.2372
Yang, L., Hu, F., Yan, Y., Yu, S., Chen, T., and Wang, Z. (2022). Anti-inflammatory effect of acetone extracts from microalgae chlorella sp. WZ13 on RAW264.7 cells and TPA-induced ear edema in mice. Front. Mar. Sci. 9, 1–11. doi:10.3389/fmars.2022.920082
Yilmaz, S., and Bag, H. (2022). Afl atoxin B1: mechanism, oxidative stress, and eff ects on animal health. J. Animal Biol. Veterinary Med. 2022, 17–24. doi:10.29328/journal.ivs.1001037
Yilmaz, S., Kaya, E., Karaca, A., and Karatas, O. (2018). Aflatoxin B1 induced renal and cardiac damage in rats: protective effect of lycopene. Res. Vet. Sci. 119, 268–275. doi:10.1016/j.rvsc.2018.07.007
Zhang, J., Hu, S., Zhao, C., Zhou, Y., Zhang, L., Liu, H., et al. (2022). Genome-scale CRISPR knockout screening identifies BACH1 as a key regulator of aflatoxin B1-induced oxidative damage. Antioxidants 11, 1787. doi:10.3390/antiox11091787
Zheng, L., Oh, S. T., Jeon, J. Y., Moon, B. H., Kwon, H. S., Lim, S. U., et al. (2012). The dietary effects of fermented chlorella vulgaris (CBT(®)) on production performance, liver lipids and intestinal microflora in laying hens. Asian-Australasian J. Anim. Sci. 25, 261–266. doi:10.5713/ajas.2011.11273
Keywords: oxidative stress, inflammatory cytokines, apoptosis, residues, Japanese quail, computational modeling
Citation: Abdeen A, Elsabagh R, Elbasuni SS, Said AM, Abdelkader A, El-Far AH, Ibrahim SF, Mihaela O, Fericean L, Abdelfattah AM, El-Hewaity M, Elbarbary N, Kadah AY and Ibrahim SS (2023) Microalgae (Chlorella vulgaris) attenuates aflatoxin-associated renal injury. Front. Pharmacol. 14:1291965. doi: 10.3389/fphar.2023.1291965
Received: 10 September 2023; Accepted: 30 October 2023;
Published: 27 December 2023.
Edited by:
Patrícia Mendonça Rijo, Lusofona University, PortugalReviewed by:
Taghred M. Saber, Zagazig University, EgyptMohamed L. Ashour, Ain Shams University, Egypt
Copyright © 2023 Abdeen, Elsabagh, Elbasuni, Said, Abdelkader, El-Far, Ibrahim, Mihaela, Fericean, Abdelfattah, El-Hewaity, Elbarbary, Kadah and Ibrahim. This is an open-access article distributed under the terms of the Creative Commons Attribution License (CC BY). The use, distribution or reproduction in other forums is permitted, provided the original author(s) and the copyright owner(s) are credited and that the original publication in this journal is cited, in accordance with accepted academic practice. No use, distribution or reproduction is permitted which does not comply with these terms.
*Correspondence: Ahmed Abdeen, YWhtZWQuYWJkZWVuQGZ2dG0uYnUuZWR1LmVn; Samah F. Ibrahim, c2ZpYnJhaGltQHBudS5lZHUuc2E=; Ostan Mihaela, bWloYWVsYV9vc3RhbkB1c3Z0LnJvJiN4MDIwMGE7