- 1Department of Clinical Korean Medicine, Graduate School of Kyung Hee University, Seoul, Republic of Korea
- 2Department of Internal Korean Medicine, Kyung Hee University Hospital at Gangdong, Seoul, Republic of Korea
- 3Department of Gastroenterology, College of Korean Medicine, Kyung Hee University, Seoul, Republic of Korea
Introduction: Motilin (MLN) is a gastrointestinal (GI) hormone produced in the upper small intestine. Its most well understood function is to participate in Phase III of the migrating myoelectric complex component of GI motility. Changes in MLN availability are associated with GI diseases such as gastroesophageal reflux disease and functional dyspepsia. Furthermore, herbal medicines have been used for several years to treat various GI disorders. We systematically reviewed clinical and animal studies on how herbal medicine affects the modulation of MLN and subsequently brings the therapeutic effects mainly focused on GI function.
Methods: We searched the PubMed, Embase, Cochrane, and Web of Science databases to collect all articles published until 30 July 2023, that reported the measurement of plasma MLN levels in human randomized controlled trials and in vivo herbal medicine studies. The collected characteristics of the articles included the name and ingredients of the herbal medicine, physiological and symptomatic changes after administering the herbal medicine, changes in plasma MLN levels, key findings, and mechanisms of action. The frequency patterns (FPs) of botanical drug use and their correlations were investigated using an FP growth algorithm.
Results: Nine clinical studies with 1,308 participants and 20 animal studies were included in the final analyses. Herbal medicines in clinical studies have shown therapeutic effects in association with increased levels of MLN, including GI motility regulation and symptom improvement. Herbal medicines have also shown anti-stress, anti-tumor, and anti-inflammatory effects in vivo. Various biochemical markers may correlate with MLN levels. Markers may have a positive correlation with plasma MLN levels included ghrelin, acetylcholine, and secretin, whereas a negative correlation included triglycerides and prostaglandin E2. Markers, such as gastrin and somatostatin, did not show any correlation with plasma MLN levels. Based on the FP growth algorithm, Glycyrrhiza uralensis and Paeonia japonica were the most frequently used species.
Conclusion: Herbal medicine may have therapeutic effects mainly on GI symptoms with involvement of MLN regulation and may be considered as an alternative option for the treatment of GI diseases. Further studies with more solid evidence are needed to confirm the efficacy and mechanisms of action of herbal medicines.
Systematic Review Registration: https://www.crd.york.ac.uk/prospero/display_record.php?RecordID=443244, identifier CRD42023443244.
1 Introduction
Motilin (MLN) is a gastric peptide hormone that was first isolated in the early 1970s and is known to control gastrointestinal (GI) tract movement (Brown, 1967; Brown et al., 1972). M cells, which prevail in the proximal region of the duodenum, secrete MLN in humans (Walsh et al., 1994). Notably, increased serum MLN levels accelerate bowel movements, and MLN exerts its effects by binding to MLN receptors (Itoh, 1997). Erythromycin (ER) was the first known MLN receptor agonist (Feighner et al., 1999) and was first used as a macrolide antibiotic in 1952. Its side effects include vomiting and diarrhea, which are two of the major effects of MLN in the GI tract (Putzi et al., 1983; Berthet et al., 2010). In one study, researchers found that ER mimics the effects of MLN on GI contractions in dogs (Itoh et al., 1984). Subsequent studies have supported the prokinetic activity of ER (Annese et al., 1992). Since it is known that MLN receptor agonists, such as ER, can target GI motility disorders, there have been numerous trials to create or identify other MLN receptor agonists; however, none of them have been successful either clinically or commercially (Omura et al., 1987; Tsuzuki et al., 1989). For example, the effect of the motilide ABT-229, an MLN receptor agonist, was assessed in randomized controlled trials (RCTs) involving 612 patients with functional dyspepsia, but it failed to provide symptomatic relief in patients with delayed gastric emptying (Talley et al., 2000). This disappointing outcome was attributed to receptor desensitization, which caused the receptor to react less strongly to the ligand (Tack and Peeters, 2001), due in part to the use of an inappropriate dosing regime and potential non-selectivity of action (Sanger et al., 2013).
Herbal formulas have been used as alternatives to Western medicine for treating GI symptoms such as constipation, diarrhea, and dyspepsia (Zhang et al., 2013; Zhang and Guo, 2015; Ren et al., 2021). Furthermore, several herbal medicines have been reported to affect plasma MLN levels. Daikenchuto, one of Japan’s most frequently prescribed traditional medicines, increases plasma MLN levels, enhances GI motility, and improves gastric dysrhythmia and postoperative gastroparesis (Mochiki et al., 2010). Notably, previous systematic reviews have reported that Rikkunshito and Banxia-xiexin tang, which are traditional Asian herbal medicines, are effective at improving the symptoms of functional dyspepsia by promoting MLN secretion (Ko et al., 2021; Kim et al., 2023).
Here, we conducted a systematic review to investigate the influence of herbal medicine on serum MLN and its effect on human and animal model. We assessed the effect of herbal medicine on the various symptoms including GI symptoms, and the direct or indirect correlation between symptoms and changes in MLN. The possible relationship between biochemical findings and plasma MLN was also investigated. In addition, we checked the most used botanical drugs and their combinations that increased or lowered plasma MLN using an association rule algorithm.
2 Methods
2.1 Objectives and registration
The objectives of this review were (1) to systematically review RCTs and in vivo studies investigating the effects of herbal medicine on various disorders mainly focused on GI function by regulating serum MLN levels and (2) to elucidate the mechanisms responsible for the change in GI function by MLN after the administration of herbal medicine. This systematic review followed the Preferred Reporting Items for Systematic Reviews and Meta-Analysis 2020 guidelines (Page et al., 2021) and provided its checklist as a Supplementary Material S1. This systematic review was registered in The International Prospective Register of Systematic Reviews under the identifier CRD42023443244.
2.2 Inclusion and exclusion criteria
This systematic review included RCTs and in vivo studies. In vitro studies, case reports, case series, and reviews were excluded. Studies that evaluated the effects of herbal medicines on serum MLN levels were included. To be eligible for inclusion, the herbal medicines had to consist of multiple botanical drugs (two minimum), but they could be administered in various forms such as decoctions, powders, granules, pills, and capsules. Additionally, patented drugs or over-the-counter drugs composed of botanical drugs, such as Dalitong granules (Nanchang Hongyi Pharmaceutical Co., Ltd., Shanghai, China), were included. Studies combining traditional therapies other than herbal medicines, such as acupuncture, moxibustion, and cupping therapy, were excluded.
2.3 Search strategy
RCTs and in vivo studies were searched separately.
2.3.1 Search strategy for RCTs
Patients who received herbal medicine as an intervention and whose serum MLN levels were measured were included. All types of dosage forms of oral herbal medicines were used as keywords. Data were extracted from PubMed, Embase, Web of Science, and Cochrane Library databases until July 2023. The search strategy for RCTs is shown in Table 1.
2.3.2 Search strategy for in vivo studies
The search keywords included all animals, such as rats, mice, dogs, rabbits, and monkeys, as well as MLN and different dosage forms of herbal medicine. Data were extracted from PubMed, Embase, Web of Science, and Cochrane until July 2023. The search strategy used for in vivo studies is shown in Table 2.
2.4 Selection and data extraction
Two authors (M-SC and J-WP) independently screened the studies to assess their eligibility for inclusion. Eligibility was evaluated by sequentially screening the articles’ titles, abstracts, and full texts. Endnote X9 (Clarivate Analytics, Philadelphia, PA, United States) was used to manage the search results. The independently extracted data from the studies was entered in a standard data extraction form. The form for the RCTs included information on the studies, such as the intervention, disease, sample size, treatment duration, change in plasma MLN levels, publication year, main outcome, and efficacy. The form for in vivo studies included information such as the intervention, animal breed, disease model, administration method, mechanisms, changes in MLN levels, main outcome, and efficacy. Discrepancies between the two authors (M-SC and J-WP) were resolved through discussion. If agreement was not reached, an arbiter (S-JK) intervened.
2.5 Quality assessment
Risk of bias evaluation was conducted for the RCTs. Review Manager (V5.3; The Nordic Cochrane Center, The Cochrane Collaboration, 2014; Copenhagen, Denmark) was used to manage the data. Two authors (M-SC and J-WP) independently assessed the risk of bias using the Cochrane Risk of Bias Tool (RoB 2) with the following items: (1) bias arising from the randomization process, (2) bias due to deviations from intended interventions, (3) bias due to missing outcome data, (4) bias in the measurement of the outcome, (5) bias in the selection of the reported results, and (6) overall bias. The results were categorized into three groups: low, high, or unclear risk of bias. All discrepancies between the two evaluators (M-SC and J-WP) were discussed. An arbiter (SK) intervened when needed.
2.6 Frequent pattern growth algorithm for data analysis
Frequent pattern (FP) growth algorithm analysis is a data-mining technique that has been widely used in healthcare, with the aim of discovering valuable correlations implicit in large data sets (Ait-Mlouk et al., 2017; Rauch, 2019). The FP growth algorithm produces frequent item sets by compressing them into an FP tree and retaining related information about the frequent items (Li et al., 2022). In recent years, the FP growth algorithm has been used in the field of herbal medicine and has achieved many gratifying research results (Leem et al., 2018; Chen et al., 2020). Therefore, an FP growth algorithm was used to determine the frequency patterns of botanical drug use and their correlations. After mining the botanical drugs used more than four times for all included studies, we listed them in order of frequency. Botanical drugs used in combination with other botanical drug were connected individually. Each node represents the items of botanical drug and the most frequently used item set was identified.
3 Results
3.1 Search process for included studies
3.1.1 Searching and narrowing down RCT studies
One hundred twenty-two records were identified in the database. Of the 122 studies, 34 were duplicates. Of the 88 remaining studies, 13 were not original studies, 27 did not use herbal medicine, four were in vitro studies, 17 were animal studies, and two were on unrelated topics. After reviewing 25 studies, nine were included in this review. This process is shown in Figure 1.
3.1.2 Searching and narrowing down in vivo studies
Two hundred ninety-five studies were identified from these databases. One hundred and forty duplicate records were removed. Of the 155 remaining studies, 11 were not written in English, seven were not original studies, 56 did not use herbal medicine, 51 were in vitro studies, and three were RCTs. After excluding these studies, 27 studies remained. Of the 27 studies, seven were on unrelated topics. Finally, 20 in vivo studies were included in this review (Figure 2).
3.2 Characteristics of included studies
3.2.1 Description of the RCT studies
In eight of the nine studies, herbal medicines were used alone, and in the remaining study (Ren et al., 2021), herbal medicines and an antibiotic (cefuroxime) were used in combination. Seven studies were conducted on patients with GI diseases or symptoms, one included patients with psychiatric symptoms, and the remaining included healthy individuals. The number of patients varied from 33 to 635, and the positive control groups were placebo (five studies), a gastroprokinetic agent (two studies: mosapride), a health functional food (one study), and a combination of Western medicines (one study: probiotics, antiviral, and nonsteroidal anti-inflammatory drugs). Eight studies were conducted with two groups (treatment and control), whereas one study (Zhang and Guo, 2015) had four groups: one control group and three different treatment groups. In seven studies, herbal medicines increased serum MLN levels, whereas in two studies, herbal medicines decreased MLN levels. Notably, herbal medicines showed various therapeutic effects through increased MLN levels, including GI symptom improvement, an increase in duodenal and jejunal motility, and the alleviation of depression. Herbal medicines also shortened the treatment time for diarrhea in patients with upper respiratory tract infections and recovered GI function in patients after abdominal surgery through a decrease in MLN levels. The characteristics of the included RCTs are summarized in Table 3. Additional information on the herbal medicines used in the included RCTs, such as the extraction type, ingredients, and daily administration dose, is shown in Table 4. The value of MLN level, concentration unit and p-value are provided as Supplementary Material S2.
3.2.2 Description of the in vivo studies
Of the 20 studies, 12, five, two, and one were conducted in rats, mice, pigs, and dogs, respectively. In 19 studies, herbal medicine was used alone, while in the remaining study (Liu et al., 2020), herbal medicine and an antidiarrheal agent (diphenoxylate) were combined. Furthermore, in most studies (16 studies), herbal medicines increased serum MLN levels, whereas three studies reported a decrease, and one study found no significant change. Most studies (13 studies) observed a laxative effect or increased GI motility due to the herbal medicine. Other reported effects included anti-stress, anti-tumor, and anti-inflammatory effects, and liver and gastric mucosa protection. The characteristics of the included in vivo studies are summarized in Table 5, while additional details about the herbal medicines, such as the extraction type, ingredients, and daily dose, are described in Table 6. The value of MLN level, concentration unit and p-value are provided as Supplementary Material S2.
3.3 Assessment of risk of bias
All RCTs included in this review were assessed for risk of bias using the Cochrane risk of bias tool. The results of the risk of bias assessment are shown in Figures 3,4. The quality of animal studies was assessed using ARRIVE checklist and provided as Supplementary Material S3.
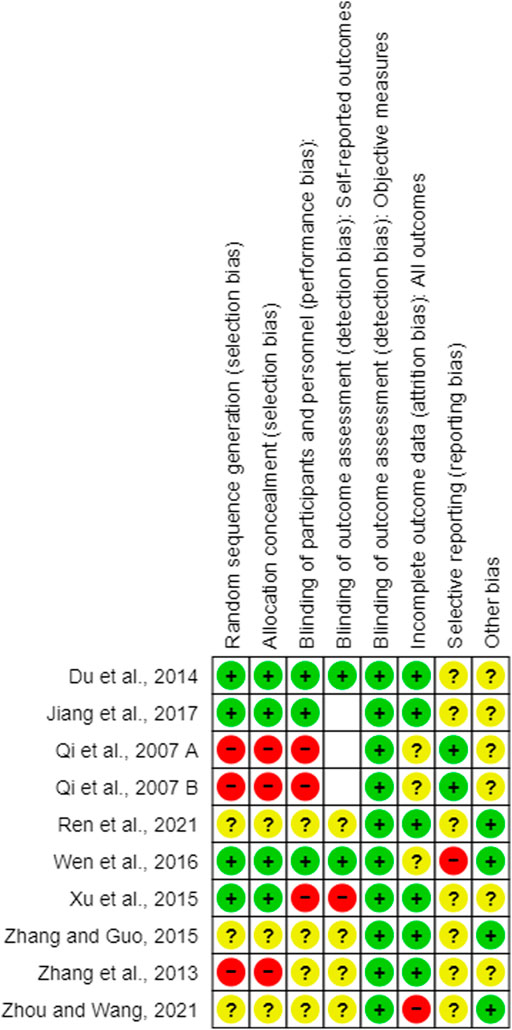
FIGURE 3. Summary of the risk of bias. +: low risk of bias; ?: unclear risk of bias; -: high risk of bias, empty slot: not applicable.
3.3.1 Random sequence generation
Four of the 10 studies (Du et al., 2014; Xu et al., 2015; Wen et al., 2016; Jiang et al., 2017) used the random sequence generation method. Three studies (Qi et al., 2007; Qi et al., 2007; Zhang et al., 2013) had a high level of selection bias. Qi et al. (2007A, B) generated the sequence according to the patients’ admission time, and Zhang et al. (2013) generated the sequence using the visiting order. The other three studies did not report the random sequencing method used (Zhang and Guo, 2015; Ren et al., 2021; Zhou and Wang, 2021).
3.3.2 Allocation
Four studies (Du et al., 2014; Xu et al., 2015; Wen et al., 2016; Jiang et al., 2017) showed a low risk of selection bias using a sealed envelope (Du et al., 2014; Jiang et al., 2017), PEMS 3.1 software (Wen et al., 2016), or a random number table (Xu et al., 2015) as the allocation method. Three studies had a high risk of bias using an open random allocation schedule (Qi et al., 2007 A; Qi et al., 2007 B) or the patients’ visiting order (Zhang et al., 2013) as allocation methods. Three studies (Zhang and Guo, 2015; Ren et al., 2021; Zhou and Wang, 2021) did not provide sufficient information to judge allocation bias.
3.3.3 Blinding of participants and personnel
Three studies (Du et al., 2014; Wen et al., 2016; Jiang et al., 2017) performed clinical experiments in a double-blind manner. Three studies (Qi et al., 2007A; Qi et al., 2007 B; Xu et al., 2015) used placebos that patients could easily recognize as a control drug (normal saline in one study (Xu et al., 2015) and a normal solution in two studies [Qi et al., 2007A; Qi et al., 2007B]); therefore, they were judged to have a high risk of performance bias. Finally, four studies (Zhang et al., 2013; Zhang and Guo, 2015; Ren et al., 2021; Zhou and Wang, 2021) did not provide sufficient information to judge bias.
3.3.4 Blinding of outcome assessment
3.3.4.1 Blinding of outcome assessment of self-reported measures
Three studies (Jiang et al., 2017; Qi et al., 2007 A; Qi et al., 2007 B) did not use subjective self-reported outcomes. Moreover, two studies (Du et al., 2014; Wen et al., 2016) were double-blinded, and the patient-reported outcomes had a low risk of bias, as the patients were unaware of their treatment allocation. In one study (Xu et al., 2015), the patients could not be blinded to the allocation; therefore, self-reported measures were used, which have a high risk of bias. The other four studies (Zhang et al., 2013; Zhang and Guo, 2015; Ren et al., 2021; Zhou and Wang, 2021) did not have sufficient information to confirm whether self-reported outcomes were assessed in a blinded manner.
3.3.4.2 Blinding of the outcome assessment of objective measures
All included RCTs used objective biochemical findings that were automatically recorded without intervention by the assessors; therefore, we judged them as having a low risk of detection bias.
3.3.5 Attrition bias
The difference in the number of dropouts between the treatment and control groups in one study (Zhou and Wang, 2021) was statistically significant; therefore, we judged it to have a high risk of attrition bias. Three studies (Qi et al., 2007A; Qi et al., 2007B; Wen et al., 2016) did not report patient dropouts. The other six studies (Zhang et al., 2013; Du et al., 2014; Xu et al., 2015; Zhang and Guo, 2015; Jiang et al., 2017; Ren et al., 2021) reported that the difference in the number of patients who dropped out between the treatment and control groups was not statistically significant (low risk of bias).
3.3.6 Selective bias
Two studies (Qi et al., 2007A; Qi et al., 2007B) reported the existence of an experimental protocol; therefore, they had a low risk of bias. Furthermore, in one study (Wen et al., 2016), one variable (bowel sounds) in the Methods section was not described in the Results section; therefore, it was judged as a high risk. The other seven studies (Zhang et al., 2013; Du et al., 2014; Xu et al., 2015; Zhang and Guo, 2015; Jiang et al., 2017; Ren et al., 2021; Zhang et al., 2021) were judged to have an unclear risk of bias due to insufficient reporting of the outcomes.
3.3.7 Others
Four studies (Zhang and Guo, 2015; Wen et al., 2016; Ren et al., 2021; Zhou and Wang, 2021) reported that they were free of conflicts of interest, whereas the other six studies (Qi et al., 2007; Qi et al., 2007; Zhang et al., 2013; Du et al., 2014; Xu et al., 2015; Jiang et al., 2017) did not report any conflicts of interest.
3.4 FP growth algorithm
The ingredients of the herbal medicines were extracted, mined, and analyzed, and the FP growth algorithm identified the most frequently used botanical drugs and their combinations (Figure 5). Among the included studies, Glycyrrhiza uralensis was most frequently used, followed by Paeonia japonica, Atractylodes macrocephala, Citrus aurantium, and Astragalus membranaceus. Combinations of three botanical drugs (Glycyrrhiza uralensis, Paeonia japonica, and Astragalus membranaceus), two botanical drugs (Glycyrrhiza uralensis and Atractylodes macrocephala), and two other botanical drugs (Glycyrrhiza uralensis and Angelicae sinensis) were most frequently used.
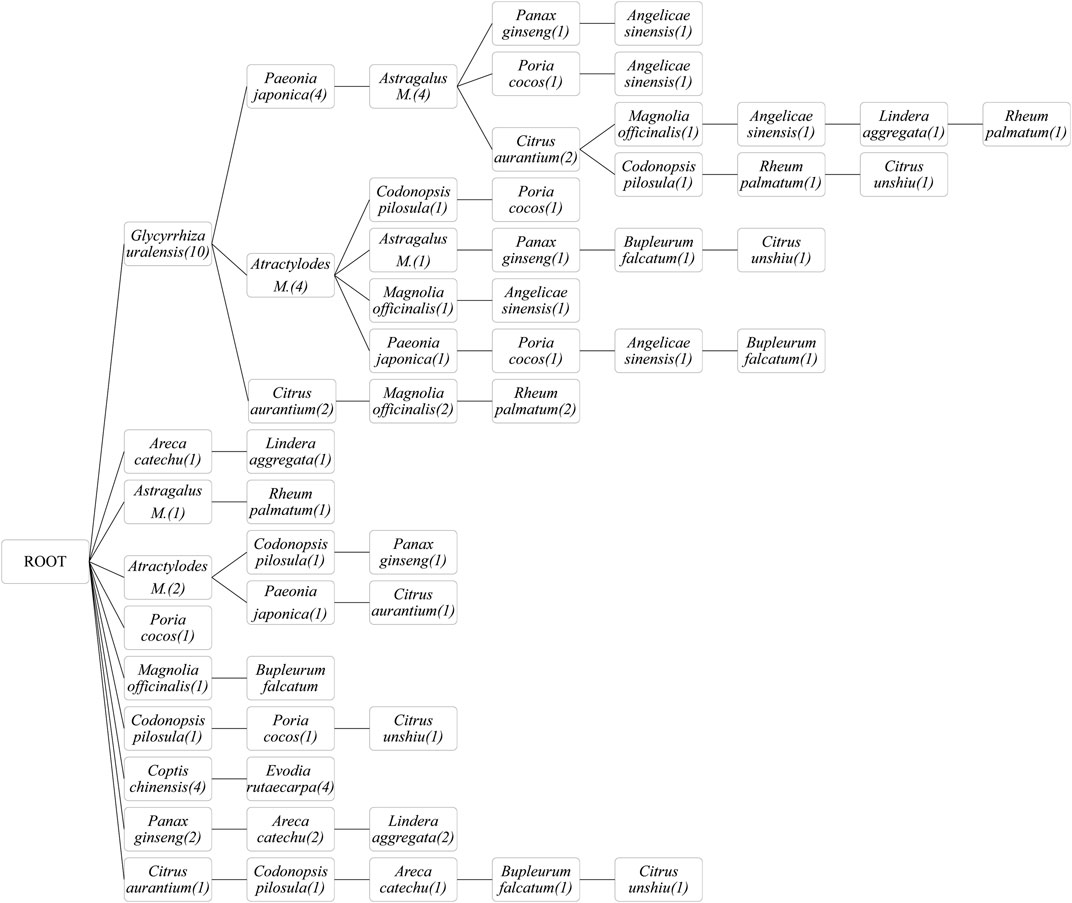
FIGURE 5. Frequent pattern growth algorithm of herbal medicines used. Astragalus M.: Astragalus membranaceus; Atractylodes M.: Atractylodes macrocephala.
4 Discussion
MLN was first identified due to its prokinetic effects. In 1966, Brown et al. found that duodenal alkalinization increased the motility of denervated gastric pouches (Brown et al., 1966). In 1975, MLN was found to regulate the migrating motor complex in dogs, and this function was identified in 1979 in humans (Code and Marlett, 1975; Vantrappen et al., 1979). Furthermore, in 1989, the antibiotic ER was discovered as an MLN receptor agonist (Peeters et al., 1989). Subsequently, the receptor for MLN, the G protein-coupled receptor 38, was discovered in 1999 (Feighner et al., 1999). Notably, the scope of MLN studies has expanded to the potential involvement of MLN in signaling hunger in 2016 (Tack et al., 2016). A summary of the history of MLN is reported in Figure 6.
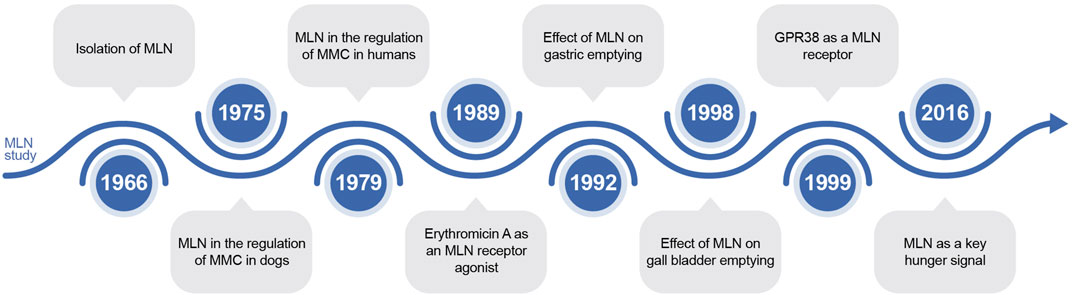
FIGURE 6. The timeline of discoveries related to motilin. MLN: motilin; GPR 38: G protein-coupled receptor 38.
MLN can influence the functioning of various parts of the body, including the GI tract, gallbladder, pancreas, rectum, and brain (Depoortere et al., 1997; Thielemans et al., 2001; Luiking et al., 2002; Kamerling et al., 2003; Sanger et al., 2011). The distribution of MLN and its receptors varies in mammals. In humans, MLN receptors are widespread. Moreover, although MLN is most abundantly expressed in the human gastroduodenal region, their actions might be related to the part of brain function (Depoortere et al., 1997; Thielemans et al., 2001; Sanger et al., 2013; Zhang et al., 2023). Although MLN is widely distributed in mammals, it is not active in all species. For example, in most rodents, due to genetic variations in the genes encoding MLN or its receptors during evolution, the gene structures have changed, rendering them functionally inactive (Sanger, 2022). There are studies that have measured MLN in rodent models that have developed specific genetic mutations (Delinsky et al., 2004; Sanger, 2022), and there is a report that the ghrelin receptor responds to extremely high concentration of MLN leading to improvement of gastrointestinal motility in rodents (Kim and Kim, 2019). The secretion and activity of motilin in rodent are still controversial.
4.1 Main findings
In most of the studies in this review (23 of 29), MLN levels were increased by herbal medicines. Furthermore, when serum MLN levels increase due to herbal medicines, the human body reacts by accelerating GI movement. For example, duodenal and jejunal motility increased, and indigestion and constipation were alleviated.
The effects of herbal medicines in animal studies were diverse, extending beyond their therapeutic impacts on GI motility and digestive function. For instance, platelet activation was reduced when MLN levels increased; an inhibitory effect on cancer was demonstrated in a chemotherapy model using Kunming mice; endoplasmic reticulum stress was reduced; and depression alleviated.
Notably, the change in serum MLN was statistically significant in all studies except for 1 study (Jin et al., 2001), and herbal medicine acted on the GI tract. Furthermore, the effects on the digestive system were consistent with the function of MLN, which increases GI motility, suggesting that MLN might be a powerful mediator of the actions of herbal medicine on GI motility. These data may provide evidence for the effect of herbal medicines on digestive system dysfunction.
4.2 Correlation between biochemical findings and MLN levels
In the present review, laboratory data, including serum levels of endogenous hormones, enzymes, neurotransmitters, inflammatory factors, gene expression markers, nutrient factors, and carrier proteins, may be correlated with changes in serum MLN levels. As shown in Tables 3 and 5, the biochemical data showed positive and negative trend, or no correlation with changes in plasma MLN levels. The relationship between various biochemical substances and plasma MLN levels are summarized in Table 7.
The correlation between certain biochemical findings and MLN levels in the present study was not in accordance with the findings of previous studies. For example, somatostatin was reported to be negatively correlated with MLN levels in studies by Deloose et al. (2019) and Kitazawa and Kaiya (2021), whereas the present review showed no such correlation. Furthermore, here, ghrelin showed a positive correlation with MLN levels; however, Kitazawa and Kaiya (2021) reported a negative correlation and Deloose et al. (2019) reported no correlation. Other biochemical findings, such as acetylcholine, triglyceride, prostaglandin E2, and secretin levels, also showed different correlations among the three studies (the present review, Deloose et al., 2019; Kitazawa and Kaiya, 2021). The comparison of correlations between the biochemical findings and MLN levels in 3 reviews (Deloose et al., 2019; Kitazawa and Kaiya, 2021; current review) are shown in Table 8.
The different results of biomarkers affecting serum MLN levels may be attributed to variations in studies conducted using different animals. MLN and its receptor have undergone variation across species during evolution, and the relationships between biomarkers and serum MLN levels in various animals are not fully understood (Kitazawa and Kaiya, 2021). Notably, previous studies have reported that secretin does not affect serum MLN levels in dogs (Lee et al., 1980; Poitras et al., 1993). In this review and in Deloose et al. (2019), however, secretin showed a negative correlation with serum MLN levels, and this result has been replicated in a study using pigs and in human clinical studies (Mitznegg et al., 1977; Jenssen et al., 1986; Dong et al., 2012).
Among macronutrients, lipids, such as triglycerides, are known to have contradictory effects on GI motility. Miedzybrodzka et al. (2021) reported that the long-chain fatty acid receptor FFA1 and the monoacylglycerol receptor GPR119 stimulated MLN secretion. However, a chronic high-fat diet increases glucose-dependent insulinotropic polypeptide (GIP) and glucagon-like peptide-1 (GLP-1) secretion (Wang et al., 2015). Notably, GIP and GLP-1 decrease GI motility (Thor et al., 1987; Hellström et al., 2008).
4.3 Botanical drugs and their combinations according to the FP growth algorithm
We analyzed the most commonly used botanical drugs and their combinations according to the FP growth algorithm and found that Glycyrrhiza uralensis, Paeonia japonica, Atractylodes macrocephala, Citrus aurantium, and Astragalus membranaceus were most frequently used. When analyzing frequently combined botanical drugs, the combinations of Glycyrrhiza uralensis with Paeonia japonica and Astragalus membranaceus, Glycyrrhiza uralensis with Atractylodes macrocephala, and Glycyrrhiza uralensis with Angelicae sinensis were most common. Furthermore, Glycyrrhiza uralensis, also known as licorice, is the most widely used botanical drug that harmonizes with the characteristics of other botanical drugs in traditional Chinese medicine (Jiang et al., 2020). A traditional Chinese quote states that “nine out of ten formulas contain licorice.” Licorice is usually combined with other botanical drugs owing to its balancing effect (Wang and Su, 2002). Moreover, Paeonia japonica has been shown to exert prokinetic effects by increasing gastric emptying and intestinal transit due to increased MLN levels in a rat model (Mu et al., 2020). Further, Paeonia japonica and Glycyrrhiza uralensis have been shown to inhibit the pacemaker potential of interstitial cells of Cajal, regulating GI motility, and this is associated with MLN (Choi et al., 2022). Atractylodes macrocephala increases the levels of MLN, resulting in improved gastric emptying with the activation of the vagal pathway (Zhang et al., 2021). Furthermore, the main active ingredients of Citrus aurantium (hesperidin or Fructus aurantii) promotes GI movement and gastric motility by regulating the secretion of MLN in a rat model of functional dyspepsia (Zhu et al., 2020; Jia et al., 2022). Finally, Yan et al. showed elevated MLN levels in patients with gastric cancer (Yan et al., 2009), and Astragalus membranaceus has been shown to reduce MLN levels and inactivate the NF-κB signaling pathway, indicating its protective effect on chronic atrophic gastritis (Tang et al., 2022).
4.4 Limitations
This study has several limitations. First, the studies included in this review were mostly conducted in China, which might have caused publication bias. Second, most in vivo studies were performed using mice and rats. Because of the pseudogenization of MLN genes in rodents, it is difficult to directly apply the results of in vivo studies to humans or other mammals in which MLN and its receptors have retained their function. Third, the heterogeneity between herbal medicines was high, which may be one of the reasons why a meta-analysis has not been conducted. Fourth, detailed information such as effective chemical profiles and quality control measures for defining the composition of the study material in original studies were lacking. Fifth, herbal medicines in this review were administered with the secondary variable for altering serum motilin, so there was lack of direct evidence for their causal relationship between the effect of herbal medicine and MLN. Finally, although we have described the herbal medicines in validated taxonomical way, we acknowledge that our efforts may not fully meet the requirements outlined in the ConPhyMP statement. In future studies, we are committed to strengthening our efforts to characterize the profile of herbal medicines used in clinical and animal studies investigations in line with the importance highlighted in the ConPhyMP statement to express our findings more comprehensively.
4.5 Strengths and future perspectives
To the best of our knowledge, this is the first systematic review investigating the effect of herbal medicine and its influence on MLN. We extensively reviewed various herbal medicines and diseases that can be affected by MLN. We also discussed how these herbal medicines may affect hormonal changes, thereby contributing to the scientific understanding of herbal medicine and facilitating its transition to evidence-based applications. Additionally, we used an FP growth pattern algorithm to identify the most used combinations of botanical drugs for managing plasma MLN levels.
In the future, more in-depth research is needed to determine the mechanism by which composition of herbal medicine and its metabolites affect MLN. For example, herbal medicine might be related to the action of MLN receptor. Furthermore, for clinical applications, MLN studies are needed in mammals without the pseudogenization of MLN genes, including humans. In addition, further research is needed to investigate the mutual relationships between the constituent botanical drugs.
Considering the antidepressant effects of herbal medicines, MLN is thought to be involved in serotonin release. Notably, through both vagus efferent neurons and serotonin pathways, MLN can facilitate GI tract movement (Takahashi, 2012). Thus, the in-depth mechanism responsible for these brain-gut connections requires further investigation. Furthermore, human ghrelin and MLN, the major gut hormones, act on structurally similar G-protein-coupled receptors and exhibit 50% overall identity with each other (Chen and Tsai, 2012; Sanger and Furness, 2016). Moreover, both hormones have functionally similar actions in initiating the migrating motor complex in the stomach, accelerating gastric emptying, and inducing “gastric hunger” (Chen and Tsai, 2012). Further studies analyzing the interrelationships between MLN and other gut hormones are required.
4.6 Conclusion
We found that most herbal medicines may be related to increase and decrease in serum MLN levels and bring various symptomatic improvement. Through the regulation of MLN, herbal medicines may exert a therapeutic effect on GI symptoms such as diarrhea, dyspepsia and gastroesophageal reflux disease, and various disorders including autonomic dysfunction and depression in human. Moreover, we found evidence of herbal medicines’ anti-cancer, anti-inflammatory, and anti-stress effects in animal models. This systematic review suggests that herbal medicine may be useful and beneficial in treating MLN-related disorders. Further studies are needed to investigate direct evidence of a therapeutically-relevant action of herbal medicine to MLN, and specify their metabolites in MLN regulation in animal models and humans.
Data availability statement
The original contributions presented in the study are included in the article/Supplementary Material, further inquiries can be directed to the corresponding author.
Author contributions
M-SC: Conceptualization, Formal Analysis, Investigation, Methodology, Writing–original draft. J-WP: Data curation, Resources, Writing–review and editing. JK: Data curation, Resources, Writing–review and editing. S-JK: Conceptualization, Data curation, Formal Analysis, Investigation, Methodology, Resources, Writing–review and editing.
Funding
The author(s) declare financial support was received for the research, authorship, and/or publication of this article. This research was supported by a National Research Foundation of Korea (NRF) grant funded by the Korean Government (MSIT) (No. 2022R1C1C1004937).
Conflict of interest
The authors declare that the research was conducted in the absence of any commercial or financial relationships that could be construed as a potential conflict of interest.
Publisher’s note
All claims expressed in this article are solely those of the authors and do not necessarily represent those of their affiliated organizations, or those of the publisher, the editors and the reviewers. Any product that may be evaluated in this article, or claim that may be made by its manufacturer, is not guaranteed or endorsed by the publisher.
Supplementary material
The Supplementary Material for this article can be found online at: https://www.frontiersin.org/articles/10.3389/fphar.2023.1286333/full#supplementary-material
References
Ait-Mlouk, A., Agouti, T., and Gharnati, F. (2017). Mining and prioritization of association rules for big data: multi-criteria decision analysis approach. J. Big Data 4, 42. doi:10.1186/s40537-017-0105-4
Annese, V., Janssens, J., Vantrappen, G., Tack, J., Peeters, T. L., Willemse, P., et al. (1992). Erythromycin accelerates gastric emptying by inducing antral contractions and improved gastroduodenal coordination. Gastroenterology 102 (3), 823–828. doi:10.1016/0016-5085(92)90164-t
Berthet, S., Charpiat, B., and Mabrut, J. Y. (2010). Erythromycin as a prokinetic agent: risk factors. J. Visc. Surg. 147 (2), e13–e18. doi:10.1016/j.jviscsurg.2010.06.001
Brown, J. C. (1967). Presence of a gastric motor-stimulating property in duodenal extracts. Gastroenterology 52 (2), 225–229. doi:10.1016/s0016-5085(67)80011-8
Brown, J. C., Cook, M. A., and Dryburgh, J. R. (1972). Motilin, a gastric motor activity-stimulating polypeptide: final purification, amino acid composition, and C-terminal residues. Gastroenterology 62 (3), 401–404. doi:10.1016/s0016-5085(72)80145-8
Brown, J. C., Johnson, L. P., and Magee, D. F. (1966). Effect of duodenal alkalinization on gastric motility. Gastroenterology 50 (3), 333–339. doi:10.1016/s0016-5085(66)80072-0
Cai, G. X., Liu, B. Y., Yi, J., Chen, X. M., and Liu, F. L. (2011). Simotang enhances gastrointestinal motility, motilin and cholecystokinin expression in chronically stressed mice. World J. Gastroenterol. 17 (12), 1594–1599. doi:10.3748/wjg.v17.i12.1594
Chen, C. W., Tsai, C. F., Tsai, Y. H., Wu, Y. C., and Chang, F. R. (2020). Association rule mining for the ordered placement of traditional Chinese medicine containers: an experimental study. Med. Baltim. 99 (18), e20090. doi:10.1097/MD.0000000000020090
Chen, C. Y., and Tsai, C. Y. (2012). Ghrelin and motilin in the gastrointestinal system. Curr. Pharm. Des. 18 (31), 4755–4765. doi:10.2174/138161212803216915
Chen, S., Zhou, H., Lin, M., Mi, R., and Li, L. (2013). Decoction vs extracts-mixed solution: effect of yiqihuoxue formula on non-alcoholic fatty liver disease in rats. J. Tradit. Chin. Med. 33 (4), 513–517. doi:10.1016/s0254-6272(13)60157-0
Choi, N. R., Kim, J. N., Lee, M. J., and Kim, B. J. (2022). Inhibitory effects of jakyakgamcho-tang (Glycyrrhiza uralensis and Paeonia lactiflora) on the pacemaker potential of the interstitial cells of cajal in the murine small intestine. Appl. Sci. 12, 4175. doi:10.3390/app12094175
Code, C. F., and Marlett, J. A. (1975). The interdigestive myo-electric complex of the stomach and small bowel of dogs. J. Physiol. 246 (2), 289–309. doi:10.1113/jphysiol.1975.sp010891
Delinsky, D. C., Hill, K. T., White, C. A., and Bartlett, M. G. (2004). Quantitative determination of the polypeptide motilin in rat plasma by externally calibrated liquid chromatography/electrospray ionization mass spectrometry. Rapid Commun. Mass Spectrom. 18 (3), 293–298. doi:10.1002/rcm.1328
Deloose, E., Verbeure, W., Depoortere, I., and Tack, J. (2019). Motilin: from gastric motility stimulation to hunger signalling. Nat. Rev. Endocrinol. 15 (4), 238–250. doi:10.1038/s41574-019-0155-0
Deng, Z., Fu, Z., Yan, W., Nie, K., Ding, L., Ma, D., et al. (2021). The different effects of Chinese Herb Solid Drink and lactulose on gut microbiota in rats with slow transit constipation induced by compound diphenoxylate. Food Res. Int. 143, 110273. doi:10.1016/j.foodres.2021.110273
Depoortere, I., Van Assche, G., and Peeters, T. L. (1997). Distribution and subcellular localization of motilin binding sites in the rabbit brain. Brain Res. 777 (1-2), 103–109. doi:10.1016/s0006-8993(97)01094-9
Dong, H., Zhong, Y., Liu, F., Yang, K., Yu, J., and Xu, J. (2012). Regulating effects and mechanisms of Chinese medicine decoction on growth and gut hormone expression in heat stressed pigs. Livest. Sci. 143 (1), 77–84. doi:10.1016/j.livsci.2011.08.015
Du, H. G., Ming, L., Chen, S. J., and Li, C. D. (2014). Xiaoyao pill for treatment of functional dyspepsia in perimenopausal women with depression. World J. Gastroenterol. 20 (44), 16739–16744. doi:10.3748/wjg.v20.i44.16739
Feighner, S. D., Tan, C. P., McKee, K. K., Palyha, O. C., Hreniuk, D. L., Pong, S. S., et al. (1999). Receptor for motilin identified in the human gastrointestinal system. Science 284 (5423), 2184–2188. doi:10.1126/science.284.5423.2184
Geng, J., Jin, W., Hao, J., Huo, M., Zhang, Y., Xie, C., et al. (2021). Effects of dietary modified bazhen on reproductive performance, immunity, breast milk microbes, and metabolome characterization of sows. Front. Microbiol. 12, 758224. doi:10.3389/fmicb.2021.758224
Hellström, P. M., Näslund, E., Edholm, T., Schmidt, P. T., Kristensen, J., Theodorsson, E., et al. (2008). GLP-1 suppresses gastrointestinal motility and inhibits the migrating motorcomplex in healthy subjects and patients with irritable bowel syndrome. Neurogastroenterol. Motil. 20 (6), 649–659. doi:10.1111/j.1365-2982.2007.01079.x
Itoh, Z. (1997). Motilin and clinical application. Peptides 18 (4), 593–608. doi:10.1016/S0196-9781(96)00333-6
Itoh, Z., Nakaya, M., Suzuki, T., Arai, H., and Wakabayashi, K. (1984). Erythromycin mimics exogenous motilin in gastrointestinal contractile activity in the dog. Am. J. Physiol. 247 (6 Pt 1), G688–G694. doi:10.1152/ajpgi.1984.247.6.G688
Jenssen, T. G., Haukland, H. H., Florholmen, J., Jorde, R., and Burhol, P. G. (1986). Evidence of somatostatin as a humoral modulator of motilin release in man. A study of plasma motilin and somatostatin during intravenous infusion of somatostatin, secretin, cholecystokinin, and gastric inhibitory polypeptide. Scand. J. Gastroenterol. 21 (3), 273–280. doi:10.3109/00365528609003076
Jia, Q., Li, L., Wang, X., Wang, Y., Jiang, K., Yang, K., et al. (2022). Hesperidin promotes gastric motility in rats with functional dyspepsia by regulating Drp1-mediated ICC mitophagy. Front. Pharmacol. 13, 945624. doi:10.3389/fphar.2022.945624
Jiang, M., Zhao, S., Yang, S., Lin, X., He, X., Wei, X., et al. (2020). An "essential herbal medicine"-licorice: a review of phytochemicals and its effects in combination preparations. J. Ethnopharmacol. 249, 112439. doi:10.1016/j.jep.2019.112439
Jiang, Z., Cao, L. X., Liu, B., Chen, Q. C., Shang, W. F., Zhou, L., et al. (2017). Effects of Chinese herbal medicine Xiangbin prescription on gastrointestinal motility. World J. Gastroenterol. 23 (16), 2987–2994. doi:10.3748/wjg.v23.i16.2987
Jin, X. L., Shibata, C., Naito, H., Ueno, T., Funayama, Y., Fukushima, K., et al. (2001). Intraduodenal and intrajejunal administration of the herbal medicine, dai-kenchu-tou, stimulates small intestinal motility via cholinergic receptors in conscious dogs. Dig. Dis. Sci. 46 (6), 1171–1176. doi:10.1023/a:1010690624187
Ju, W. J., Zhao, Z. K., Chen, S. L., Zhou, D. D., Yang, W. N., Wen, X. P., et al. (2020). Buzhongyiqi decoction protects against loperamide-induced constipation by regulating the arachidonic acid pathway in rats. Front. Pharmacol. 11, 423. doi:10.3389/fphar.2020.00423
Kamerling, I. M., Burggraaf, J., van Haarst, A. D., Oppenhuizen-Duinker, M. F., Schoemaker, H. C., Biemond, I., et al. (2003). The effect of motilin on the rectum in healthy volunteers. Br. J. Clin. Pharmacol. 55 (6), 538–543. doi:10.1046/j.1365-2125.2003.01812.x
Kim, J. N., and Kim, B. J. (2019). The mechanism of action of ghrelin and motilin in the pacemaker potentials of interstitial cells of cajal from the murine small intestine. Mol. Cells 42 (6), 470–479. doi:10.14348/molcells.2019.0028
Kim, K., Ko, S. J., Cho, S. H., Kim, J., and Park, J. W. (2023). Herbal medicine, Banxia-xiexin tang, for functional dyspepsia: a systematic review and meta-analysis. Front. Pharmacol. 14, 1130257. doi:10.3389/fphar.2023.1130257
Kitazawa, T., and Kaiya, H. (2021). Motilin comparative study: structure, distribution, receptors, and gastrointestinal motility. Front. Endocrinol. (Lausanne) 12, 700884. doi:10.3389/fendo.2021.700884
Ko, S. J., Park, J., Kim, M. J., Kim, J., and Park, J. W. (2021). Effects of the herbal medicine Rikkunshito, for functional dyspepsia: a systematic review and meta-analysis. J. Gastroenterol. Hepatol. 36 (1), 64–74. doi:10.1111/jgh.15208
Kwon, H. E., Kim, J. N., Kwon, M. J., Lee, J. R., Kim, S. C., Nam, J. H., et al. (2021). The traditional medicine bojungikki-tang increases intestinal motility. Pharmacogn. Mag. 17, 1. doi:10.4103/pm.pm_507_20
Lee, K. Y., Kim, M. S., and Chey, W. Y. (1980). Effects of a meal and gut hormones on plasma motilin and duodenal motility in dog. Am. J. Physiol. 238 (4), G280–G283. doi:10.1152/ajpgi.1980.238.4.G280
Leem, J., Jung, W., Kim, Y., Kim, B., and Kim, K. (2018). Exploring the combination and modular characteristics of herbs for alopecia treatment in traditional Chinese medicine: an association rule mining and network analysis study. BMC Complement. Altern. Med. 18, 204. doi:10.1186/s12906-018-2269-7
Li, M., Lv, X., Liu, Y., Wang, L., and Song, J. (2022). TCM constitution analysis method based on parallel FP-growth algorithm in hadoop framework. J. Healthc. Eng. 2022, 9006096. doi:10.1155/2022/9006096
Li, T., Hu, M., Jiang, C., Zhang, D., Gao, M., Xia, J., et al. (2021). Laxative effect and mechanism of Tiantian Capsule on loperamide-induced constipation in rats. J. Ethnopharmacol. 266, 113411. doi:10.1016/j.jep.2020.113411
Lin, Z., Guo, J., Chen, W., Deng, L., Zhang, X., Huang, W., et al. (2016). Chai-qin-cheng-qi decoction improves intestinal motility by regulating protein kinase C-and adenylate cyclase-mediated Ca2+ release in colonic smooth muscle cells in rats with acute necrotizing pancreatitis. PANCREAS 45 (10), 1519. doi:10.1016/j.pan.2016.05.066
Liu, C., He, Q., Zeng, L., Shen, L., Luo, Q., Zhang, W., et al. (2021). Digestion-promoting effects and mechanisms of dashanzha pill based on raw and charred crataegi Fructus. Chem. Biodivers. 18 (12), e2100705. doi:10.1002/cbdv.202100705
Liu, S., Sui, D., Fu, W., Yu, X., Li, Y., Wu, X., et al. (2020). Laxative effects of yangyin tongmi capsule on a model of diphenoxylate-induced constipation in mice. Evidence-Based Complementary Altern. Med. 2020, 1471824. doi:10.1155/2020/1471824
Luiking, Y. C., Akkermans, L. M., Peeters, T. L., Cnossen, P. J., Nieuwenhuijs, V. B., and Vanberge -Henegouwen, G. P. (2002). Effects of motilin on human interdigestive gastrointestinal and gallbladder motility, and involvement of 5HT3 receptors. Neurogastroenterol. Motil. 14 (2), 151–159. doi:10.1046/j.1365-2982.2002.00314.x
Miedzybrodzka, E. L., Foreman, R. E., Lu, V. B., George, A. L., Smith, C. A., Larraufie, P., et al. (2021). Stimulation of motilin secretion by bile, free fatty acids, and acidification in human duodenal organoids. Mol. Metab. 54, 101356. doi:10.1016/j.molmet.2021.101356
Mitznegg, P., Bloom, S. R., Domschke, W., Haecki, W. H., Domschke, S., Belohlavek, D., et al. (1977). Effect of secretin on plasma motilin in man. Gut 18 (6), 468–471. doi:10.1136/gut.18.6.468
Mochiki, E., Yanai, M., Ohno, T., and Kuwano, H. (2010). The effect of traditional Japanese medicine (Kampo) on gastrointestinal function. Surg. Today 40 (12), 1105–1111. doi:10.1007/s00595-010-4388-8
Mu, D. Z., Xue, M., Xu, J. J., Hu, Y., Chen, Y., Ren, P., et al. (2020). Antidepression and prokinetic effects of paeoniflorin on rats in the forced swimming test via polypharmacology. Evid. Based Complement. Altern. Med. 2020, 2153571. doi:10.1155/2020/2153571
Omura, S., Tsuzuki, K., Sunazuka, T., Marui, S., Toyoda, H., Inatomi, N., et al. (1987). Macrolides with gastrointestinal motor stimulating activity. J. Med. Chem. 30 (11), 1941–1943. doi:10.1021/jm00394a001
Page, M. J., McKenzie, J. E., Bossuyt, P. M., Boutron, I., Hoffmann, T. C., Mulrow, C. D., et al. (2021). The PRISMA 2020 statement: an updated guideline for reporting systematic reviews. BMJ 372, n71. doi:10.1136/bmj.n71
Peeters, T., Matthijs, G., Depoortere, I., Cachet, T., Hoogmartens, J., and Vantrappen, G. (1989). Erythromycin is a motilin receptor agonist. Am. J. Physiol. 257 (3 Pt 1), G470–G474. doi:10.1152/ajpgi.1989.257.3.G470
Poitras, P., Dumont, A., Cuber, J. C., and Trudel, L. (1993). Cholinergic regulation of motilin release from isolated canine intestinal cells. Peptides 14 (2), 207–213. doi:10.1016/0196-9781(93)90031-b
Putzi, R., Blaser, J., Lüthy, R., Wehrli, R., and Siegenthaler, W. (1983). Side-effects due to the intravenous infusion of erythromycin lactobionate. Infection 11 (3), 161–163. doi:10.1007/bf01641296
Qi, Q. H., Wang, J., Liang, G. G., and Wu, X. Z. (2007). Da-Cheng-Qi-Tang promotes the recovery of gastrointestinal motility after abdominal surgery in humans. Dig. Dis. Sci. 52 (6), 1562–1570. doi:10.1007/s10620-007-9751-2
Qiu, Y., Hu, J. L., Zhao, C. C., Zhang, J. Q., Wu, F., Ma, B. L., et al. (2019). Zhujie hewei granules ameliorated reflux esophagitis in rats. Evid. Based Complement. Altern. Med. 2019, 1392020. doi:10.1155/2019/1392020
Rauch, J. (2019). Expert deduction rules in data mining with association rules: a case study. Knowl. Inf. Syst. 59, 167–195. doi:10.1007/s10115-018-1206-x
Ren, X., Wang, Y., He, Z., Liu, H., and Xue, K. (2021). Effects of cefuroxime axetil combined with Xingpi Yanger granules on the serum gastrin, motilin, and somatostatin levels in children with upper respiratory tract infection accompanied by diarrhea: results of a randomized trial. Transl. Pediatr. 10 (8), 2106–2113. doi:10.21037/tp-21-314
Sanger, G. J. (2022). Why is motilin active in some studies with mice, rats, and Guinea pigs, but not in others? Implications for functional variability among rodents. Pharmacol. Res. Perspect. 10 (2), e00900. doi:10.1002/prp2.900
Sanger, G. J., and Furness, J. B. (2016). Ghrelin and motilin receptors as drug targets for gastrointestinal disorders. Nat. Rev. Gastroenterol. Hepatol. 13 (1), 38–48. doi:10.1038/nrgastro.2015.163
Sanger, G. J., Holbrook, J. D., and Andrews, P. L. (2011). The translational value of rodent gastrointestinal functions: a cautionary tale. Trends Pharmacol. Sci. 32 (7), 402–409. doi:10.1016/j.tips.2011.03.009
Sanger, G. J., Wang, Y., Hobson, A., and Broad, J. (2013). Motilin: towards a new understanding of the gastrointestinal neuropharmacology and therapeutic use of motilin receptor agonists. Br. J. Pharmacol. 170, 1323–1332. doi:10.1111/bph.12075
Sun, Y., Yan, C., Jin, S., Shi, C., Zhao, J., and Li, G. (2020). Curative effect and mechanism of guiren runchang granules on morphine-induced slow transit constipation in mice. Evidence-based Complementary Altern. Med. 2020, 5493192. doi:10.1155/2020/5493192
Tack, J., Deloose, E., Ang, D., Scarpellini, E., Vanuytsel, T., Van Oudenhove, L., et al. (2016). Motilin-induced gastric contractions signal hunger in man. Gut 65 (2), 214–224. doi:10.1136/gutjnl-2014-308472
Tack, J., and Peeters, T. (2001). What comes after macrolides and other motilin stimulants? Gut 49 (3), 317–318. doi:10.1136/gut.49.3.317
Takahashi, T. (2012). Mechanism of interdigestive migrating motor complex. J. Neurogastroenterol. Motil. 18 (3), 246–257. doi:10.5056/jnm.2012.18.3.246
Talley, N. J., Verlinden, M., Snape, W., Beker, J. A., Ducrotte, P., Dettmer, A., et al. (2000). Failure of a motilin receptor agonist (ABT-229) to relieve the symptoms of functional dyspepsia in patients with and without delayed gastric emptying: a randomized double-blind placebo-controlled trial. Aliment. Pharmacol. Ther. 14 (12), 1653–1661. doi:10.1046/j.1365-2036.2000.00868.x
Tang, Z., Wang, Y., and Huang, Y. (2022). Astragalus polysaccharide inhibits apoptosis and inflammation to alleviate chronic atrophic gastritis through NF-κB signaling pathway in rats. Food Sci. Technol. 2022, 42. doi:10.1590/fst.121921
Thielemans, L., Depoortere, I., Van Assche, G., Bender, E., and Peeters, T. L. (2001). Demonstration of a functional motilin receptor in TE671 cells from human cerebellum. Brain Res. 895 (1-2), 119–128. doi:10.1016/s0006-8993(01)02055-8
Thor, P., Laskiewicz, J., Konturek, J. W., Konturek, S. J., and Creutzfeldt, W. (1987). Role of GIP and insulin in glucose-induced changes in intestinal motility patterns. Am. J. Physiol. 252 (1 Pt 1), G8–G12. doi:10.1152/ajpgi.1987.252.1.G8
Tsuzuki, K., Sunazuka, T., Marui, S., Toyoda, H., Omura, S., Inatomi, N., et al. (1989). Motilides, macrolides with gastrointestinal motor stimulating activity. I. O-substituted and tertiary N-substituted derivatives of 8,9-anhydroerythromycin A 6,9-hemiacetal. Chem. Pharm. Bull. (Tokyo) 37 (10), 2687–2700. doi:10.1248/cpb.37.2687
Vantrappen, G., Janssens, J., Peeters, T. L., Bloom, S. R., Christofides, N. D., and Hellemans, J. (1979). Motilin and the interdigestive migrating motor complex in man. Dig. Dis. Sci. 24 (7), 497–500. doi:10.1007/bf01489315
Walsh, J. H., Dockray, G. J., and Mitty, R. D. (1994). Gut peptides: biochemistry and physiology. New York: Raven Press.
Wang, F., and Su, Y. (2002). Pharmacological action and clinical application of licorice. LiShizhen Med. Materia Medica Res. 13, 303–304.
Wang, F., Yoder, S. M., Yang, Q., Kohan, A. B., Kindel, T. L., Wang, J., et al. (2015). Chronic high-fat feeding increases GIP and GLP-1 secretion without altering body weight. Am. J. Physiol. Gastrointest. Liver Physiol. 309 (10), G807–G815. doi:10.1152/ajpgi.00351.2013
Wang, T., Yan, Y. F., Yang, L., Huang, Y. Z., Duan, X. H., Su, K. H., et al. (2020). Effects of Zuojin pill on depressive behavior and gastrointestinal function in rats with chronic unpredictable mild stress: role of the brain-gut axis. J. Ethnopharmacol. 254, 112713. doi:10.1016/j.jep.2020.112713
Wen, S. L., Feng, X., Chen, Z. Q., Xiao, J., and Zhang, W. X. (2016). Effect of XiangBin granules on post-operative gastrointestinal function and brain-gut peptides after transabdominal gynecological surgery. Eur. J. Obstet. Gynecol. Reprod. Biol. 205, 1–6. doi:10.1016/j.ejogrb.2016.07.496
Xi, S., Zhai, X., Wang, Y., Gong, Y., Fu, B., Gao, C., et al. (2021). The ciji-hua'ai-baosheng II formula attenuates chemotherapy-induced anorexia in mice with H(22) hepatocellular carcinoma. Front. Pharmacol. 12, 715824. doi:10.3389/fphar.2021.715824
Xu, Z., Xu, C., Ge, H., Li, Y., Chu, L., Zhang, J., et al. (2015). Modified dachengqi tang improves decreased gastrointestinal motility in postoperative esophageal cancer patients. J. Tradit. Chin. Med. 35 (3), 249–254. doi:10.1016/s0254-6272(15)30093-5
Yan, F., Zhang, Q. Y., Jiao, L., Han, T., Zhang, H., Qin, L. P., et al. (2009). Synergistic hepatoprotective effect of Schisandrae lignans with Astragalus polysaccharides on chronic liver injury in rats. Phytomedicine 16 (9), 805–813. doi:10.1016/j.phymed.2009.02.004
Yan, S., Hao, M., Yang, H., Sun, M., Wu, B., Yue, Y., et al. (2020). Metabolomics study on the therapeutic effect of the Chinese herb pair Fructus Aurantii Immaturus and Rhizoma Atractylodis Macrocephalae in constipated rats based on UPLC-Q/TOF-MS analysis. Ann. Palliat. Med. 9 (5), 2837–2852. doi:10.21037/apm-20-280
Zhang, C., Guo, L., Guo, X., Li, G., and Guo, X. (2013). Short and long-term efficacy of combining Fuzhengliqi mixture with acupuncture in treatment of functional constipation. J. Tradit. Chin. Med. 33 (1), 51–59. doi:10.1016/s0254-6272(13)60100-4
Zhang, C. H., Su, X. L., Liu, Y. Y., Lin, L., Wang, Y. C., Li, X. D., et al. (2021). Study on the effect of antiplatelet and gastric mucosal protection of traditional Chinese medicine invigorating Qi and hemostasis. J. Inflamm. Res. 14, 3023–3037. doi:10.2147/jir.S316862
Zhang, C. X., and Guo, L. K. (2015). Dalitong granule combined with electroacupuncture in the treatment of functional dyspepsia: a randomized controlled trial. Chin. J. Integr. Med. 21 (10), 743–750. doi:10.1007/s11655-015-2175-5
Zhang, W. J., Zhao, Z. Y., Chang, L. K., Cao, Y., Wang, S., Kang, C. Z., et al. (2021). Atractylodis Rhizoma: a review of its traditional uses, phytochemistry, pharmacology, toxicology and quality control. J. Ethnopharmacol. 266, 113415. doi:10.1016/j.jep.2020.113415
Zhang, Y., Zhao, J., Hu, N., Wang, J., Chen, X., Wang, K., et al. (2023). Motilin and its receptor are expressed in the dorsal horn in a rat model of acute incisional pain: intrathecal motilin injection alleviates pain behaviors. Front. Neurosci. 17, 1104862. doi:10.3389/fnins.2023.1104862
Zhang, Z., Yang, S., Lin, X., Huang, Y., Wei, X., Zhou, J., et al. (2021). Metabolomics of Spleen-Yang deficiency syndrome and the therapeutic effect of Fuzi Lizhong pill on regulating endogenous metabolism. J. Ethnopharmacol. 278, 114281. doi:10.1016/j.jep.2021.114281
Zhao, Y. L., Shi, W. L., Shan, L. M., Wang, J. B., Zhao, H. P., and Xiao, X. H. (2009). Differences in effects of Zuojin Pills and its similar formulas on Wei cold model in rats. Chin. J. Integr. Med. 15 (4), 293–298. doi:10.1007/s11655-009-0293-7
Zhou, Y., and Wang, S. (2021). Efficacy of Jianpiyangxue granule on gastrointestinal autonomic nerve dysfunction and its impact on adverse reactions. Am. J. Transl. Res. 13 (11), 12929–12938.
Keywords: motilin, herbal medicine, gastrointestinal motility, gastrointestinal tract, systematic review
Citation: Cho M-S, Park J-W, Kim J and Ko S-J (2023) The influence of herbal medicine on serum motilin and its effect on human and animal model: a systematic review. Front. Pharmacol. 14:1286333. doi: 10.3389/fphar.2023.1286333
Received: 31 August 2023; Accepted: 04 December 2023;
Published: 14 December 2023.
Edited by:
Juei-Tang Cheng, Chang Jung Christian University, TaiwanReviewed by:
Gareth J. Sanger, Queen Mary University of London, United KingdomRavi Philip Rajkumar, Jawaharlal Institute of Postgraduate Medical Education and Research (JIPMER), India
Copyright © 2023 Cho, Park, Kim and Ko. This is an open-access article distributed under the terms of the Creative Commons Attribution License (CC BY). The use, distribution or reproduction in other forums is permitted, provided the original author(s) and the copyright owner(s) are credited and that the original publication in this journal is cited, in accordance with accepted academic practice. No use, distribution or reproduction is permitted which does not comply with these terms.
*Correspondence: Seok-Jae Ko, a29rb2tva28xMTlAZGF1bS5uZXQ=