- 1General Pathology Laboratory, Department of Experimental and Clinical Medicine, Internal Medicine Section, University of Florence, Florence, Italy
- 2Department of Medical Biotechnologies, University of Siena, Siena, Italy
Despite improvements in treatment, lung cancer is still a major health problem worldwide. Among lung cancer subtypes, the most frequent is represented by adenocarcinoma (belonging to the Non-Small Cell Lung Cancer class) although the most challenging and harder to treat is represented by Small Cell Lung Cancer, that occurs at lower frequency but has the worst prognosis. For these reasons, the standard of care for these patients is represented by a combination of surgery, radiation therapy and chemotherapy. In this view, searching for novel biomarkers that might help both in diagnosis and therapy is mandatory. In the last 30 years it was demonstrated that different families of ion channels are overexpressed in both lung cancer cell lines and primary tumours. The altered ion channel profile may be advantageous for diagnostic and therapeutic purposes since most of them are localised on the plasma membrane thus their detection is quite easy, as well as their block with specific drugs and antibodies. This review focuses on ion channels (Potassium, Sodium, Calcium, Chloride, Anion and Nicotinic Acetylcholine receptors) in lung cancer (both Non-Small Cell Lung Cancer and Small Cell Lung Cancer) and recapitulate the up-to-date knowledge about their role and clinical relevance for a potential use in the clinical setting, for lung cancer diagnosis and therapy.
1 Lung cancer epidemiology
According to the most recent estimates, lung cancer (LC) is still the most lethal cancer worldwide, responsible of 1.8 million deaths, and the second most frequent in both sexes, with 2.2 million new cases diagnosed in 2020 (Source: Globocan, https://gco.iarc.fr accessed on 10 August 2023). LC is more frequent in high-income countries, mainly due to smoking habits that represent the main risk factor for this malignancy (McIntyre and Ganti, 2017; Sung et al., 2021). Other common risk factors are the exposure to some chemicals such as asbestos (Markowitz, 2022), mustard gas (Ghanei and Harandi, 2010), radon (Lorenzo-González et al., 2019), arsenic (Soza-Ried et al., 2019), chromium (Kouokam et al., 2022), nickel (Shen and Zhang, 1994), uranium (Zhang et al., 2022), vinyl chloride (Girardi et al., 2022) and high dose ionizing radiations (Yan et al., 2022).
2 Histopathology
LC is generally divided into four major histological types (adenocarcinoma, squamous cell carcinoma, large cell carcinoma and small cell carcinoma) although combinations may also be present. Among the four histological types, the most represented is adenocarcinoma, accounting for 90%–95% of the LCs and the first three histotypes are collectively known as “Non-Small Cell Lung Carcinoma” (NSCLC).
2.1 Non-small cell lung carcinoma (NSCLC)
Adenocarcinoma is the most frequent LC type in women and non-smokers, accounting for 40% of LC (Travis et al., 2011; Ricotti et al., 2021). Unlike squamous cell carcinoma, adenocarcinomas are usually smaller and peripherical in location. Positivity for thyroid transcription factor-1 (TTF-1) is extremely frequent as well as mucin content that is detected in roughly 80% adenocarcinomas. The growing rate is lower compared to squamous cell carcinomas, although adenocarcinomas metastasize earlier and extensively.
Squamous cell carcinomas account for 21% of LC (AIOM, 2019; Ricotti et al., 2021), are more frequent in men and are highly related to smoking habits. From the histological point of view, such histotype is characterized by keratinization.
The main clinical and molecular features of NSCLC are reported in Table 1.
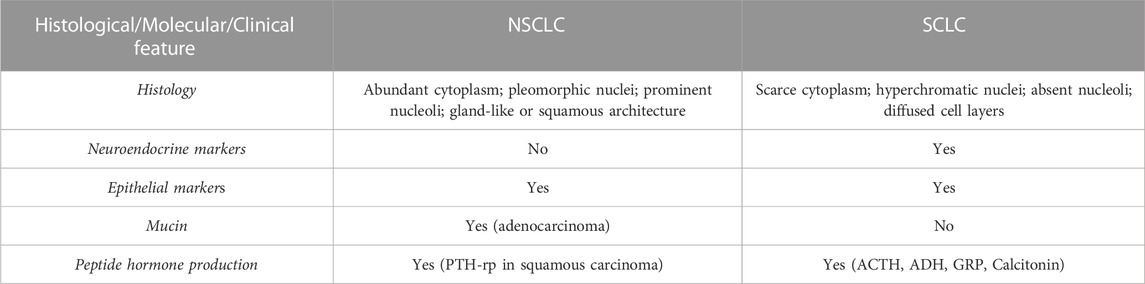
TABLE 1. Histological, clinical and molecular features of NSCLC and SCLC. PTH-rp, Parathormone related peptide; ACTH, Adrenocorticotropic hormone; ADH, antidiuretic hormone; GRP, Gastrin releasing peptide.
2.2 Small cell lung carcinoma (SCLC)
This tumour type accounts for approximately 15% of all lung cancers (Travis et al., 2011; Ettinger et al., 2022) and it is a highly malignant neoplasia characterised by peculiar small round or oval cells with scarce cytoplasm, little or no nucleoli and “salt and pepper” chromatin pattern (Nicholson et al., 2002). Mitotic figures are a frequent finding and necrotic areas are also quite common and extensive. For SCLC, the diagnosis is determined by both light and electron microscopy (to detect neuroendocrine granules) complemented by immunohistochemistry for neuroendocrine markers (chromogranin and synaptophysin) (Righi et al., 2022). The presence of neuroendocrine markers highlights the neuroendocrine origin of SCLC. The main clinical and molecular features of SCLC are reported in Table 1.
SCLC are the most aggressive LC, extensively metastasize, are virtually incurable by surgery and show a close relationship to smoking.
3 LC clinical features and treatment
LCs are invasive and silent lesions and represent one of the most insidious and aggressive forms of cancer. LC arises more frequently in people older than 50 years and the main clinical symptoms comprise cough (75%), thoracic pain (40%), weight loss (40%) and dyspnoea (20%).
NSCLC have generally a better prognosis than SCLC. The most important prognostic factor for NSCLC patients is represented by tumour stage and patients are treated according to their stage (Naruke et al., 1997; Schabath and Cote, 2019): surgery is the gold standard for stage I and II patients and for some stage III tumours; stage IV patients are treated with chemotherapy, palliative radiation or supportive therapy.
SCLC are generally diffused also at early stages therefore surgery is not a viable choice for treatment and systemic therapies (such as chemotherapy) are used (Waqar and Morgensztern, 2017).
In addition, in the last decade novel therapeutic targeted agents have been developed thanks to the knowledge of the genetic and molecular alterations carried by LC cells (Table 2).
A well-known and frequent side-effect of LC treatment is represented by cardiotoxicity (Pérez-Callejo et al., 2017) and also new strategies such as immune checkpoint or tyrosine kinase inhibitors are associated to cardiac toxic effects, ranging from asymptomatic QT prolongation to acute coronary syndromes, myocardial infarction, reduction in left ventricular ejection fraction, hypertension, symptomatic congestive heart failure and sudden death (Heinzerling et al., 2016). In order to prevent the cardiotoxicity induced by anticancer treatment different adjustments could be applied in the early stages (Cardinale et al., 2016; Pérez-Callejo et al., 2017): healthy lifestyle, modification of anticancer treatment schedules, use of cardioprotective drugs, control of cardiovascular risk factors, periodic evaluation of cardiac function, use of biomarkers in patients with high cardiovascular risk or subjected to highly cardiotoxic anticancer treatment (Pérez-Callejo et al., 2017).
Due to the pivotal role played by certain ion channels (ICs) in heart function, their potential relevance in LC treatment-induced cardiotoxicity has been evaluated (Uchikawa et al., 2022) also because of the modulation exerted by several cytotoxic agents on different ICs (Table 3). For example, crizotinib that has been approved for the NSCLC treatment, inhibits Kv11.1 the ion channel responsible for the delayed-rectifier potassium current in the heart. Such inhibition causes the prolongation of the QT interval in the electrocardiogram, leading to potentially fatal polymorphic ventricular tachycardia, the so-called torsades de pointes (Yap and Camm, 2003; Sanguinetti and Tristani-Firouzi, 2006; Shopp et al., 2014; Uchikawa et al., 2022). Interestingly, liposomes administration ameliorates drug-induced effects on Kv11.1 and if they were given prior to crizotinib the effects on the QT interval were decreased (Shopp et al., 2014).
4 LC genetics
In the last years, several genetic alterations have been described to occur in LC pathogenesis and some of them have been exploited as novel targets for therapy especially in NSCLC (Table 2). Among them, KRAS mutations have been shown to occur primarily in the adenocarcinoma histotype at higher frequency in smokers with respect to non-smokers (30% vs. 5%) (Judd et al., 2021). As in other tumours, KRAS mutations correlate with worse outcome due to the acquired resistance to epidermal growth factor receptor (EGFR) inhibitors (Cascetta et al., 2022). Moreover, mutations and amplifications of the EGFR gene are more frequently observed in women, nonsmokers, and people of Asian origin bearing an adenocarcinoma (Herbst et al., 2008). Another gene frequently amplified or mutated in LC is c-MET and also in this case targeted therapies have been developed (Herbst et al., 2008). As concerning tumour suppressor genes, the most important (i.e., Tp53, RB1 and p16) are inactivated or mutated with similar frequencies in adenocarcinoma and squamous cell carcinoma (roughly 50%, 15% and 65%) (Herbst et al., 2008). Moreover, tumour suppressor genes are also frequently deleted, especially in squamous cell carcinoma and the most frequently involved chromosomes are 3p, 9p, and 17p (Herbst et al., 2008). EGFR is overexpressed in NSCLC and similarly, HER-2/NEU is highly expressed in a small percentage of cases and gene amplification has also been detected (Testa et al., 2018).
SCLC shows a quite high mutation rate related to tobacco carcinogens (Peifer et al., 2012). Several abnormalities have been detected in SCLC, although none of them is specific for this tumour: frequent inactivation of TP53, RB1 and PTEN, 3p deletion in the region where the tumour suppressor gene FHIT is located, copy number gain in 7p 22.3, MYC amplification (involving several genes of the MYC family) (Voortman et al., 2010; George et al., 2015), low frequency of activating mutations in KRAS, EGFR and PI3KCA (Testa et al., 2018). Overall, a list of potential driver genes in SCLC has been identified: TP53, RB1, PTEN, SLIT2, EP300, CREBBP, MLL, EPHA7 and COBL (Peifer et al., 2012).
5 Ion channels in human cancer
It has been shown more than 30 years ago that cancer cells have a more depolarized membrane potential compared to healthy cells (Binggeli and Weinstein, 1986) and mounting evidences pointed out that ion channels and transporters might represent novel potential biomarkers in human cancers of different histogenesis, since their expression is frequently dysregulated and association with clinico-pathological features and outcome have been shown.
Several studies carried out in different solid tumours demonstrated that ICs are frequently mis-expressed and play important roles in the regulation of cancer cell behaviour. ICs regulate several cellular processes, and some of them represent hallmarks of cancer (Hanahan and Weinberg, 2000; Hanahan and Weinberg, 2011). The contribute of ICs to cancer development is complex and variegated as the ICs themselves (Figure 1) and the interplay between different members has been described. In fact, the occurrence and functional relevance of ICs within macromolecular complexes occurs in normal and pathological conditions (Heijman and Dobrev, 2018; Ponce-Balbuena et al., 2018; Eichel et al., 2019). It has been shown that in cancer cells ICs interact with different proteins with respect to normal cells (Becchetti et al., 2022). Examples for that are the Kv11.1/β1 integrin complex, selectively expressed in cancer cells (Becchetti et al., 2017), Kv10.1/Orai1/SPCA2 (Badaoui et al., 2018; Peretti et al., 2019), Kv10.1/Calmodulin (Marques-Carvalho et al., 2016), Orai1/TRPC1/SK3 (Potier-Cartereau et al., 2022).
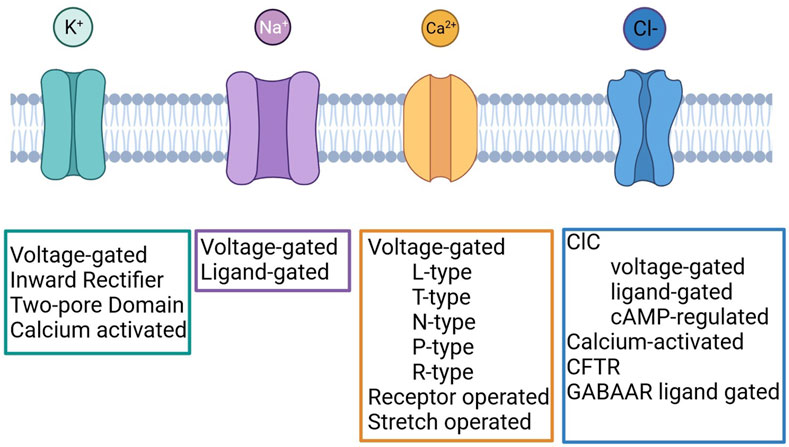
FIGURE 1. Classification of Potassium, Sodium, Calcium and Chloride channels. Created with BioRender.com.
In this context, after proper validation, ICs could represent novel cancer biomarkers (Lastraioli et al., 2015; Anderson et al., 2019). Moreover, since they are located on the cell membranes ICs represent potential targets to be exploited for diagnostic and therapeutic purposes (Arcangeli et al., 2009).
5.1 Ion channels in LC
A transcriptomic analysis was carried out to compare the expression of ion channel encoding genes in normal lung tissue and LC (Ko et al., 2014a). Overall, 37 differentially expressed genes were identified: ANO1, CACNA1C, CACNA1D, CACNA2D2, CACNB3, CLCC1, CLCN3, CLCN7, CLIC3, CLIC4, CLIC5, CLIC6, KCNAB1, KCNAB2, KCNJ2, KCNJ8, KCNE4, KCNK1, KCNK3, KCNK5, KCNQ3, KCNT2, MCOLN1, MCOLN2, MCOLN3, PKD1, PKD2, SCN4B, SCN7A, SCNN1B, SCNN1G, TPCN1, TRPC1, TRPC6, TRPM2, TRPV2, and VDAC1. It should be pointed out that, although included by Ko and others among the IC encoding genes, some ofthem (namely, CLCC1, CLCN3, CLCN7, CLIC3, CLIC4, CLIC5, and CLIC6) are not proper ion channel genes. In order to investigate the prognostic relevance of the above-mentioned genes, a risk score, based on the expression of the differentially expressed genes, was calculated for each patient to predict overall survival and recurrence-free survival in NSCLC. Overall, 31 genes were differentially expressed between adenocarcinoma and squamous cell carcinoma samples (Ko et al., 2014a).
In both NSCLC and SCLC several ICs have been proven to exert a biological role and, in some cases, also to have clinical relevance (Figure 2; Tables 4, 5) [see also the review by Bulk and others (Bulk et al., 2021)].
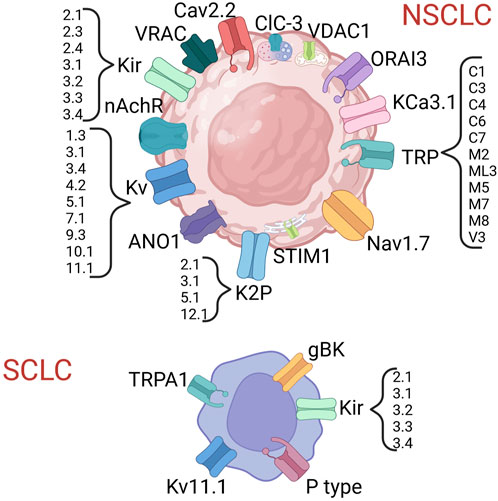
FIGURE 2. Ion channels shown to be expressed in lung cancer. Created with BioRender.com.
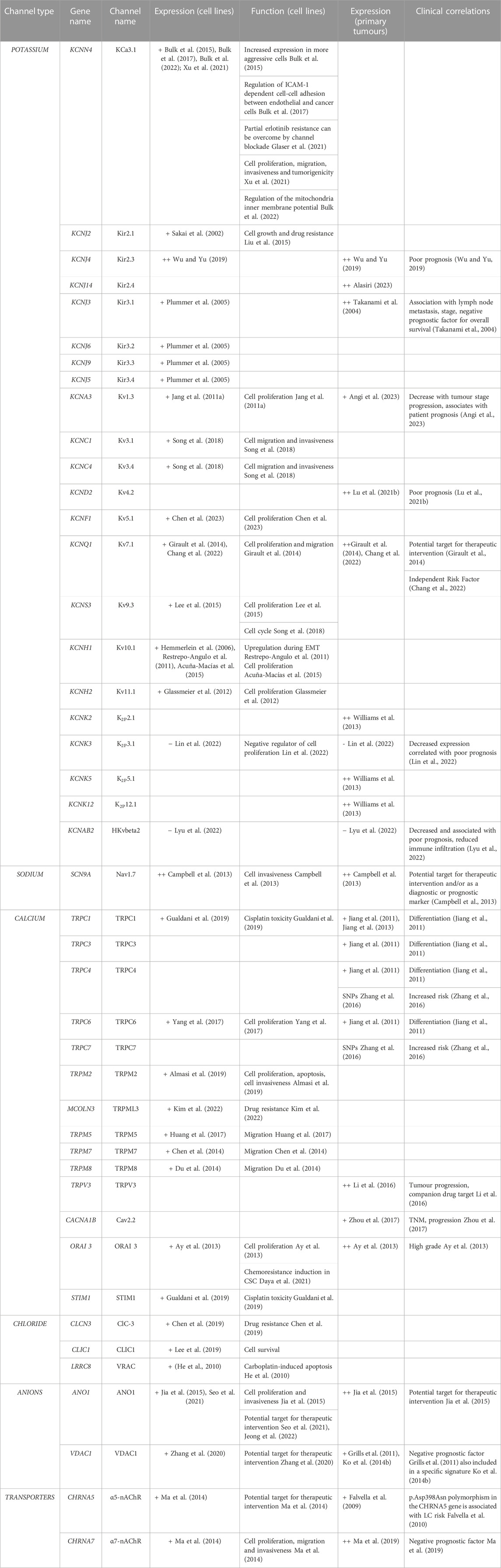
TABLE 4. Ion channels expressed in NSCLC. + = expressed, ++ = overexpressed. EMT, epithelial-mesenchymal transition; TNM, Tumour Node Metastasis.
5.1.1 Ion channels in lung carcinogenesis
Lu and others performed an in silico analysis showing that six ion channel genes (GJB2, CACNA1D, KCNQ1, SCNN1B, SCNN1G, and TRPV6) were differentially expressed in lung tumorigenesis (Lu A. et al., 2021). Among the six genes, lower expression of SCNN1B (through the hypermethylation of the promoter region) was associated with shorter overall survival. Also, the inactivation of FXYD3, an IC-related protein, was proven long ago to play a role in LC progression (Okudela et al., 2009). Among TRP channels, it was demonstrated that TRPM7 mRNA and protein levels are regulated by EGF, an activator of migration in LC (Gao et al., 2011). In general, ICs modulate different processes within the LC cell (proliferation, migration, invasiveness, drug resistance) thus their expression appears to be relevant in all the phases of LC carcinogenesis (Tables 4, 5). A scheme showing the main ICs involved in the different cell processes is reported in Figure 3.
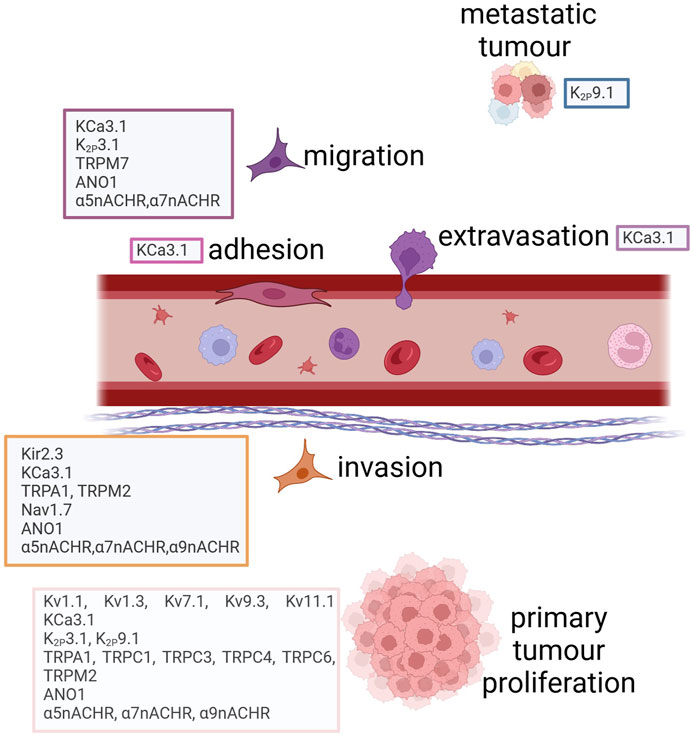
FIGURE 3. Ion channels involved in lung cancer progression. Created with BioRender.com.
In some cases, ICs are also regulated by LC risk factors. For example, nicotinic acetylcholine receptors are activated by compounds present in tobacco, such as nicotine and 4-(methylnitrosamino)-1-(3-pyridyl)-1-butanone (Grando, 2014) (see also paragraph 5.1.6). Similarly, miners are exposed to radon that represents a known risk factor for LC and it was demonstrated through a genome wide association study (GWAS) that six markers within the CHRNA5 and CHRNB4 genes, encoding the nicotinic cholinergic receptor alpha 5 and beta 4 subunits respectively, are associated with higher LC risk (Rosenberger et al., 2018). Arsenic, another well-known LC risk factor, modulates several potassium channels, namely, KCNA5 (positively associated with arsenic levels), CACNA1, KCNH2, KCNQ1, and KCNE1 (downregulated by arsenic) (Mo et al., 2011). Ionizing radiations are also an example of a LC risk factor that is associated with ICs. In particular, it was demonstrated that TRPM2 and TRPV1 channels are involved in the responses to γ- and UVB- irradiation DNA damage (Masumoto et al., 2013).
5.1.2 Potassium channels in LC
Recently, an in silico study addressing the clinical relevance of potassium channels in LC was published (Ko et al., 2019). The Authors identified 10 deregulated genes (5 up- and 5 downregulated) comparing LC and healthy lung tissue. A risk scoring was defined taking into account this 10 gene signature in order to predict clinical outcome independently from standard clinical prognostic factors, that might therefore be used along with conventional factors.
Potassium channels are a multi-gene family composed of four different subfamilies: voltage-gated potassium channels (VGKCs), inward rectifiers (IRK), two-pore domains (K2P) and calcium-activated channels (KCa) (Figure 1). In LC, several members of each subfamily have been detected and their role was investigated.
Among VGKC, different members (Kv1.3, Kv3.1, Kv3.4, Kv4.2, Kv5.1, Kv7.1, Kv9.3, Kv10.1, and Kv11.1) are involved in LC cell proliferation, migration and invasion (Jang et al., 2011a; Restrepo-Angulo et al., 2011; Glassmeier et al., 2012; Girault et al., 2014; Acuña-Macías et al., 2015; Lee et al., 2015; Chen et al., 2023) and some of them (Kv1.3, Kv4.2, and Kv7.1) have clinical relevance in NSCLC (Girault et al., 2014; Lu X. et al., 2021; Chang et al., 2022; Angi et al., 2023) (Table 4).
Kv11.1 channels are expressed in SCLC cell lines and they were shown to regulate cell proliferation (Glassmeier et al., 2012). Glioma Big Potassium Channels (gBK) are expressed in advanced SCLC thus representing a late-stage marker for this condition (Hoa et al., 2014) (Table 5).
Members of the IRK family were also shown to be involved in LC. In particular, Kir2.1, Kir2.4, Kir3.2, Kir3.3, and Kir3.4 are expressed in NSCLC (Sakai et al., 2002; Plummer et al., 2005; Wu and Yu, 2019) and SCLC (Plummer et al., 2005; Liu et al., 2015) cell lines (Tables 4, 5). Other members of the same family (Kir2.3 and Kir2.4) are expressed in primary LC (Takanami et al., 2004; Wu and Yu, 2019; Alasiri, 2023) and are associated with poor prognosis (Takanami et al., 2004; Wu and Yu, 2019) and lymph node involvement (Takanami et al., 2004) (Tables 4, 5). Interestingly, Kir2.1 regulates cell proliferation and multidrug resistance (MDR) in both NSCLC and SCLC cells (Liu et al., 2015; Wu and Yu, 2019) and also represents a prognostic factor, being overexpressed in primary SCLC and correlating with stage and response to chemotherapy (Liu et al., 2015) (Tables 4, 5).
The K2P subfamily is also involved in LC pathogenesis and it was shown that K2P3.1 is downregulated in NSCLC cells (where it negatively regulates cell proliferation) and in primary tumours where it is associated with poor prognosis (Lin et al., 2022). On the other hand, K2P2.1, K2P5.1, and K2P12.1 are over-expressed in primary NSCLC (Williams et al., 2013) (Table 4).
The only member of the Calcium activated subfamily that has been shown to be expressed in NSCLC is KCa3.1 (Bulk et al., 2015; 2017; 2022; Xu et al., 2021) (Table 4). In particular, increased expression of the channel was detected in more aggressive cells (Bulk et al., 2015) where it also regulates ICAM-1 dependent cell-cell adhesion between endothelial and cancer cells (Bulk et al., 2017). Moreover, KCa3.1 regulates cell proliferation, migration, invasiveness and tumorigenicity (Xu et al., 2021) and the mitochondria inner membrane potential (Bulk et al., 2022) (Table 4). Interestingly, it was shown that blocking the channel results in partial overcome of erlotinib resistance (Glaser et al., 2021) (Table 4).
5.1.3 Sodium channels in LC
Voltage Gated Sodium Channels of the Voltage-gated subfamily (Figure 1) have been shown to be expressed in NSCLC cells, where they possibly regulate tumour cell invasiveness, as in other tumour types (Onganer and Djamgoz, 2005; Fraser et al., 2014; Nelson et al., 2014; Djamgoz et al., 2019; Haworth and Brackenbury, 2019). Interestingly, the Nav blocker tetrodotoxin reduces the invasiveness of NSCLC cell lines (Roger et al., 2007; Campbell et al., 2013). In addition, the role of Nav1.7 channel, encoded by SCN9A gene, was investigated (Campbell et al., 2013). The Authors showed an EGFR-mediated transcriptional regulation of the channel expression, responsible of the invasive behaviour of NSCLC cells. The administration of the EGFR blocker gefitinib also affects Nav1.7 at the mRNA and protein level, as well as the sodium current (Campbell et al., 2013). Moreover, the immunohistochemical evaluation of primary samples suggested that Nav1.7 expression could have clinical relevance in NSCLC.
5.1.4 Calcium channels in LC
The expression of both voltage-gated and ligand-gated calcium channels (Figure 1) has been described in LC (Tables 4, 5). The most represented are TRP (Transient Receptor Potential) channels. In particular, TRPC1, TRPC6 TRPM2, TRPML3, TRPM5, TRPM7, and TRPM8 were found to be expressed in NSCLC cells (Chen et al., 2014; Du et al., 2014; Huang et al., 2017; Yang et al., 2017; Almasi et al., 2019; Gualdani et al., 2019; Kim et al., 2022). TRPC1 mediates cisplatin toxicity (Gualdani et al., 2019), TRPC6 and TRPM2 are involved in cell proliferation (Yang et al., 2017; Almasi et al., 2019), TRPM5, TRPM7 and TRPM8 modulate cell migration (Chen et al., 2014; Du et al., 2014; Huang et al., 2017) and TRPML3 is involved in MDR (Kim et al., 2022).
Members of the TRP family are also expressed in primary NSCLC: TRPC3 and TRPC6 (associated with differentiation) (Jiang et al., 2011), TRPC4 and TRPC7 (whose single nucleotide polymorphisms, SNPs) are associated with increased risk of LC) (Zhang et al., 2016). TRPV3 are overexpressed in primary NSCLC and have been proposed as drug companion (Li et al., 2016). In SCLC TRPA1 plays a pivotal role in cell survival (Schaefer et al., 2013) (Table 5).
The only member of the voltage-gated subfamily that has been detected in NSCLC is Cav2.2, overexpressed in primary tumours and associated with TNM stage and tumour progression (Zhou et al., 2017). In SCLC cell lines P-type calcium channels have been detected (Barry et al., 1995) (Table 5).
Two members of the Store-Operated subfamily of ligand-gated channels (ORAI 3 and STIM1) were also found to be expressed in NSCLC cells regulating cell proliferation (ORAI 3) (Ay et al., 2013) and cisplatin toxicity (STIM1) (Gualdani et al., 2019) (Table 4). Moreover, ORAI3 is involved in the chemoresistance induction of Cancer Stem Cells (CSC) (Daya et al., 2021) and is associated with high grade primary lesions (Ay et al., 2013).
5.1.5 Chloride and anion channels in LC
Among Chloride channels (Figure 1), three members have been shown to be expressed in NSCLC cells: the Chloride Voltage-gated channel 3 (ClC-3), functioning as a Chloride-Hydrogen antiporter (associated to MDR) (Chen et al., 2019), CLIC1 (that mediates cell survival) (Lee et al., 2019) and VRAC (involved in Carboplatin-induced apoptosis) (He et al., 2010) (Table 4).
The voltage-gated calcium-activated anion channel Anoctamin-1 (ANO1) is expressed in both NSCLC cell lines and primary tumours, mediating cell proliferation and invasiveness (Jia et al., 2015) and representing a potential therapeutic target (Jia et al., 2015; Seo et al., 2021; Jeong et al., 2022).
The voltage-dependent anion channel type 1 (VDAC1) was found to be expressed in NSCLC cells and was proposed as a therapeutic target (Zhang et al., 2020). Accordingly, a meta-analysis of surgically resected NSCLC led to identify VDAC1 as one of the most relevant genes. In particular, the channel was associated with poor overall survival and was an independent prognostic factor (Grills et al., 2011). Moreover, VDAC1 expression levels were upregulated in tumours compared with normal tissue including lung (Ko et al., 2014b). 44 VDAC1 interacting genes were identified and included (along with VDAC1) into a gene signature that turned out to be a prognostic biomarker to predict recurrence-free survival (Ko et al., 2014b).
5.1.6 Nicotinic acetylcholine receptors in LC
The nicotinic acetylcholine receptors (nAChRs) are the most studied channel type in LC (Table 4) (Falvella et al., 2009) and their relevance in LC carcinogenesis was hypothesized long ago, since they are activated by compounds present in tobacco, such as nicotine and 4-(methylnitrosamino)-1-(3-pyridyl)-1-butanone (Grando, 2014). nAChRs are a heterogeneous IC family comprising α and β subunits expressed in neurons, bronchial cells and keratinocytes (Egleton et al., 2008).
GWAS showed that the 15q25 nAChR gene cluster CHRNA5-A3-B4 is associated with nicotine dependence and LC (Amos et al., 2008). Moreover, the expression of the CHRNA5 gene encoding α5-nAchR was found to be increased in LC tissue and the p.Asp398Asn polymorphism was associated with LC risk (Falvella et al., 2010). Interestingly, the expression of α5-nAchR correlates with the hypoxia inducible factor (HIF) 1α in NSCLC (Ma et al., 2014) and since a α5-nAChR/HIF-1α/VEGF axis is involved in nicotine-induced tumor cell proliferation, α5-nAChR might represent a potential anticancer target in LC (Ma et al., 2014). The activation of nAChR by nicotine increases the migration and invasiveness of A549 LC cells (Sun and Ma, 2015) while silencing reverses the process (Zhang et al., 2017). The α7-nAChR subunit is upregulated in NSCLC tissue samples (Ma et al., 2019) and an in silico analysis indicated that this correlates with poor outcome.
5.2 Ion channels as therapeutic targets
As previously stated, LC treatment nowadays can benefit from molecular targeting (including the application of small molecule tyrosine kinase inhibitors and monoclonal antibodies) that is generally well tolerated by patients (Bittner et al., 2014). Since ICs have been proven to play major roles in LC pathogenesis and progression their relevance as potential therapeutic targets was also evaluated. Several drugs acting as IC blocker exist and some of them have also been tested in clinical trials for other malignancies. For example, the opioid U50488H was shown to promote chemosensitivity, to inhibit proliferation and growth of NSCLC (Kuzumaki et al., 2012) and to block Kir3.1–4 channels (Plummer et al., 2005; D’Amico et al., 2013). Senicapoc, an inhibitor of KCa3.1 channels, was used in a phase III clinical trial for sickle cell anemia (Ataga et al., 2011), and it was also shown to reduce tumor growth in mice xenografted with A549 NSCLC cells (Bulk et al., 2015). The same occurs for another potassium channel blocker (dendrotoxin-κ) acting on Kv11.1 (Jang et al., 2011b). Margatoxin, an inhibitor of Kv1.3 potassium channel, exerts an antiproliferative effect on A549 cells (Jang et al., 2011a). The TRPV6 inhibitor SOR-C13 was used in a phase I clinical trial for the treatment of advanced solid tumors (Fu et al., 2017). IC inhibitors have also been used in combination therapy with chemotherapeutic agents as the Cav3.1 channel blocker in A549 cells (Byun et al., 2016). This aspect is particularly relevant since a combined therapy might overcome the resistance of tyrosine kinase inhibitors as gefitinib (Jeon et al., 2012), or reduce chemotherapy side effects. On the other hand, a general problem to be faced when using K+ channel blockers is represented by their relevance and role in excitable cells (Arcangeli and Becchetti, 2010). An example is represented by Kv11.1 that plays a pivotal role in the repolarization phase following the action potential in cardiomyocytes (Sanguinetti and Tristani-Firouzi, 2006). For this reason, blocking Kv11.1 causes the prolongation of the QT interval thus leading to ventricular arrhythmia and fibrillation with the generation of torsade de pointes (Witchel and Hancox, 2000). The main Kv11.1 blockers belong to class III antiarrhythmic drugs, nevertheless the channel is also blocked by other types of compounds, such as antibiotics (erythromycin), antihistaminics (terfenadine), antipsychotics (sertindole) and prokinetics (cisapride). Importantly, not all Kv11.1 blockers are arrhythmogenic (Wallis, 2010), examples are represented by Verapamil and sertindole (D’Amico et al., 2013). In order to overcome the cardiotoxicity induced by IC blockers different strategies can be applied (D’Amico et al., 2013): i) using non torsadogenic blockers; ii) using state-specific blockers such as R-roscovitine (Ganapathi et al., 2009); iii) using tumour-specific drugs such as CD 160130 (Gasparoli et al., 2015); iv) using monoclonal antibodies (Iorio et al., 2019) and v) using bispecific antibodies directed against tumour-specific macromolecular complexes such as the Kv11.1/β1 integrin complex (Duranti et al., 2021a; 2021b; Iorio et al., 2022; Lottini et al., 2023).
6 Concluding remarks
Lung cancer is an important health issue worldwide due to the high incidence and mortality, especially for SCLC, thus, searching for novel biomarkers and targets for LC is mandatory.
It is now known that ICs control several cancer hallmarks and could therefore serve as molecular markers in cancer. Moreover, blocking the activity of ICs impairs tumour growth, paving the road to the pharmaceutical exploitation of ICs. Due to their peculiar localization, ICs can be also easily detected and blocked by either drugs or antibodies. In the context of LC, the relevance of ICs is multiple (see the cartoon depicted in Figure 4) since some of them are activated by known risk factors and might be the molecular cause of cardiotoxicity induced by therapeutic agents commonly used in LC; on the other hand, ICs modulate key cell processes and contribute to LC progression. Finally, ICs might be exploited for diagnostic and prognostic purposes, as well as being used as novel therapeutic targets.
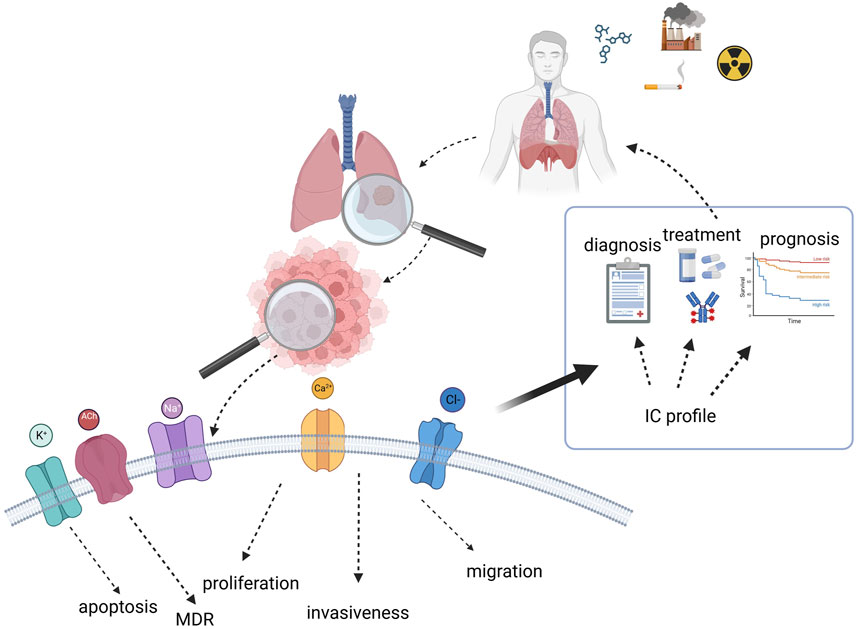
FIGURE 4. Cartoon summarizing the role and relevance of ion channels in lung cancer and their possible exploitation for clinical purposes. Created with BioRender.com.
Author contributions
CC: Investigation, Writing–original draft, Methodology, Resources. GC: Investigation, Methodology, Resources, Writing–original draft. MS: Investigation, Resources, Writing–original draft. EL: Investigation, Writing–original draft, Conceptualization, Funding acquisition, Project administration, Supervision, Visualization, Writing–review and editing.
Funding
The author(s) declare financial support was received for the research, authorship, and/or publication of this article. This research was funded by Fondazione Cassa di Risparmio di Pistoia e Pescia (grant CaPoS) and by Regione Toscana and Fondazione Cassa di Risparmio di Pistoia e Pescia (grant MutCoP).
Conflict of interest
The authors declare that the research was conducted in the absence of any commercial or financial relationships that could be construed as a potential conflict of interest.
Publisher’s note
All claims expressed in this article are solely those of the authors and do not necessarily represent those of their affiliated organizations, or those of the publisher, the editors and the reviewers. Any product that may be evaluated in this article, or claim that may be made by its manufacturer, is not guaranteed or endorsed by the publisher.
References
Acuña-Macías, I., Vera, E., Vázquez-Sánchez, A. Y., Mendoza-Garrido, M. E., and Camacho, J. (2015). Differential regulation of human Eag1 channel expression by serum and epidermal growth factor in lung and breast cancer cells. Onco. Targets. Ther. 8, 2959–2965. doi:10.2147/OTT.S85504
Agarwal, J. R., Griesinger, F., Stühmer, W., and Pardo, L. A. (2010). The potassium channel Ether à go-go is a novel prognostic factor with functional relevance in acute myeloid leukemia. Mol. Cancer 9, 18–16. doi:10.1186/1476-4598-9-18
Alasiri, G. (2023). Comprehensive analysis of KCNJ14 potassium channel as a biomarker for cancer progression and development. Int. J. Mol. Sci. 24, 2049. doi:10.3390/IJMS24032049
Almasi, S., Long, C. Y., Sterea, A., Clements, D. R., Gujar, S., and El Hiani, Y. (2019). TRPM2 silencing causes G2/M arrest and apoptosis in lung cancer cells via increasing intracellular ROS and RNS levels and activating the JNK pathway. Cell. Physiol. biochem. 52, 742–757. doi:10.33594/000000052
Amos, C. I., Wu, X., Broderick, P., Gorlov, I. P., Gu, J., Eisen, T., et al. (2008). Genome-wide association scan of tag SNPs identifies a susceptibility locus for lung cancer at 15q25.1. Nat. Genet. 40, 616–622. doi:10.1038/NG.109
Anderson, K. J., Cormier, R. T., and Scott, P. M. (2019). Role of ion channels in gastrointestinal cancer. World J. Gastroenterol. 25, 5732–5772. doi:10.3748/wjg.v25.i38.5732
Angi, B., Muccioli, S., Szabò, I., and Leanza, L. (2023). A meta-analysis study to infer voltage-gated K+ channels prognostic value in different cancer types. Antioxidants (Basel, Switz. 12, 573. doi:10.3390/ANTIOX12030573
Arcangeli, A., and Becchetti, A. (2010). New trends in cancer therapy: targeting ion channels and transporters. Pharm. (Basel) 3, 1202–1224. doi:10.3390/PH3041202
Arcangeli, A., Crociani, O., Lastraioli, E., Masi, A., Pillozzi, S., and Becchetti, A. (2009). Targeting ion channels in cancer: a novel frontier in antineoplastic therapy. Curr. Med. Chem. 16, 66–93. doi:10.2174/092986709787002835
Ataga, K. I., Reid, M., Ballas, S. K., Yasin, Z., Bigelow, C., James, L. S., et al. (2011). Improvements in haemolysis and indicators of erythrocyte survival do not correlate with acute vaso-occlusive crises in patients with sickle cell disease: a phase III randomized, placebo-controlled, double-blind study of the Gardos channel blocker senicapoc (ICA-17043). Br. J. Haematol. 153, 92–104. doi:10.1111/J.1365-2141.2010.08520.X
Ay, A.-S., Benzerdjerb, N., Sevestre, H., Ahidouch, A., and Ouadid-Ahidouch, H. (2013). Orai3 constitutes a native store-operated calcium entry that regulates non small cell lung adenocarcinoma cell proliferation. PLoS One 8, e72889. doi:10.1371/JOURNAL.PONE.0072889
Badaoui, M., Mimsy-Julienne, C., Saby, C., Gulick, L., Peretti, M., Jeannesson, P., et al. (2018). Collagen type 1 promotes survival of human breast cancer cells by overexpressing Kv10.1 potassium and Orai1 calcium channels through DDR1-dependent pathway. Oncotarget 9, 24653–24671. doi:10.18632/ONCOTARGET.19065
Barry, E. L. R., Viglione, M. P., Kim, Y. I., and Froehner, S. C. (1995). Expression and antibody inhibition of P-type calcium channels in human small-cell lung carcinoma cells. J. Neurosci. 15, 274–283. doi:10.1523/JNEUROSCI.15-01-00274.1995
Becchetti, A., Crescioli, S., Zanieri, F., Petroni, G., Mercatelli, R., Coppola, S., et al. (2017). The conformational state of hERG1 channels determines integrin association, downstream signaling, and cancer progression. Sci. Signal. 10, eaaf3236. doi:10.1126/SCISIGNAL.AAF3236
Becchetti, A., Duranti, C., and Arcangeli, A. (2022). Dynamics and physiological meaning of complexes between ion channels and integrin receptors: the case of Kv11.1. Am. J. Physiol. Cell Physiol. 322, C1138–C1150. doi:10.1152/AJPCELL.00107.2022
Binggeli, R., and Weinstein, R. C. (1986). Membrane potentials and sodium channels: hypotheses for growth regulation and cancer formation based on changes in sodium channels and gap junctions. J. Theor. Biol. 123, 377–401. doi:10.1016/S0022-5193(86)80209-0
Bittner, N., Ostoros, G., and Géczi, L. (2014). New treatment options for lung adenocarcinoma--in view of molecular background. Pathol. Oncol. Res. 20, 11–25. doi:10.1007/S12253-013-9719-9
Bulk, E., Ay, A. S., Hammadi, M., Ouadid-Ahidouch, H., Schelhaas, S., Hascher, A., et al. (2015). Epigenetic dysregulation of KCa 3.1 channels induces poor prognosis in lung cancer. Int. J. cancer 137, 1306–1317. doi:10.1002/IJC.29490
Bulk, E., Kramko, N., Liashkovich, I., Glaser, F., Schillers, H., Schnittler, H. J., et al. (2017). KCa3.1 channel inhibition leads to an ICAM-1 dependent increase of cell-cell adhesion between A549 lung cancer and HMEC-1 endothelial cells. Oncotarget 8, 112268–112282. doi:10.18632/ONCOTARGET.22735
Bulk, E., Todesca, L. M., Bachmann, M., Szabo, I., Rieke, M., and Schwab, A. (2022). Functional expression of mitochondrial KCa3.1 channels in non-small cell lung cancer cells. Pflugers Arch. 474, 1147–1157. doi:10.1007/S00424-022-02748-X
Bulk, E., Todesca, L. M., and Schwab, A. (2021). Ion channels in lung cancer. Rev. Physiol. Biochem. Pharmacol. 181, 57–79. doi:10.1007/112_2020_29
Byun, J. S., Sohn, J. M., Leem, D. G., Park, B., Nam, J. H., Shin, D. H., et al. (2016). In vitro synergistic anticancer activity of the combination of T-type calcium channel blocker and chemotherapeutic agent in A549 cells. Bioorg. Med. Chem. Lett. 26, 1073–1079. doi:10.1016/J.BMCL.2015.12.010
Campbell, T. M., Main, M. J., and Fitzgerald, E. M. (2013). Functional expression of the voltage-gated Na⁺-channel Nav1.7 is necessary for EGF-mediated invasion in human non-small cell lung cancer cells. J. Cell Sci. 126, 4939–4949. doi:10.1242/JCS.130013
Cardinale, D., Biasillo, G., and Cipolla, C. M. (2016). Curing cancer, saving the heart: a challenge that cardioncology should not miss. Curr. Cardiol. Rep. 18, 51. doi:10.1007/S11886-016-0731-Z
Cascetta, P., Marinello, A., Lazzari, C., Gregorc, V., Planchard, D., Bianco, R., et al. (2022). KRAS in NSCLC: state of the art and future perspectives. Cancers (Basel) 14, 5430. doi:10.3390/CANCERS14215430
Chang, K. T., Wu, H. J., Liu, C. W., Li, C. Y., and Lin, H. Y. (2022). A novel role of arrhythmia-related gene KCNQ1 revealed by multi-omic analysis: theragnostic value and potential mechanisms in lung adenocarcinoma. Int. J. Mol. Sci. 23, 2279. doi:10.3390/IJMS23042279
Chen, C. Y., Wu, P. Y., Van Scoyk, M., Simko, S. A., Chou, C. F., and Winn, R. A. (2023). KCNF1 promotes lung cancer by modulating ITGB4 expression. Cancer Gene Ther. 30, 414–423. doi:10.1038/S41417-022-00560-4
Chen, J., Luan, Y., Yu, R., Zhang, Z., Zhang, J., and Wang, W. (2014). Transient receptor potential (TRP) channels, promising potential diagnostic and therapeutic tools for cancer. Biosci. Trends 8, 1–10. doi:10.5582/BST.8.1
Chen, Q., Liu, X., Luo, Z., Wang, S., Lin, J., Xie, Z., et al. (2019). Chloride channel-3 mediates multidrug resistance of cancer by upregulating P-glycoprotein expression. J. Cell. Physiol. 234, 6611–6623. doi:10.1002/JCP.27402
Chen, S. Z., Jiang, M., and Zhen, Y. S. (2005). HERG K+ channel expression-related chemosensitivity in cancer cells and its modulation by erythromycin. Cancer Chemother. Pharmacol. 56, 212–220. doi:10.1007/s00280-004-0960-5
D’Amico, M., Gasparoli, L., and Arcangeli, A. (2013). Potassium channels: novel emerging biomarkers and targets for therapy in cancer. Recent Pat. anticancer. Drug Discov. 8, 53–65. doi:10.2174/15748928130106
Daya, H. A., Kouba, S., Ouled-Haddou, H., Benzerdjeb, N., Telliez, M. S., Dayen, C., et al. (2021). Orai3-Mediates cisplatin-resistance in non-small cell lung cancer cells by enriching cancer Stem cell population through PI3K/AKT pathway. Cancers (Basel) 13, 2314. doi:10.3390/CANCERS13102314
Djamgoz, M. B. A., Fraser, S. P., and Brackenbury, W. J. (2019). In vivo evidence for voltage-gated sodium channel expression in carcinomas and potentiation of metastasis. Cancers (Basel) 11, 1675. doi:10.3390/CANCERS11111675
Du, G. J., Li, J. H., Liu, W. J., Liu, Y. H., Zhao, B., Li, H. R., et al. (2014). The combination of TRPM8 and TRPA1 expression causes an invasive phenotype in lung cancer. Tumour Biol. 35, 1251–1261. doi:10.1007/S13277-013-1167-3
Duranti, C., Iorio, J., Lottini, T., Lastraioli, E., Crescioli, S., Bagni, G., et al. (2021a). Harnessing the hERG1/β1 integrin complex via a novel bispecific single-chain antibody: an effective strategy against solid cancers. Mol. Cancer Ther. 20, 1338–1349. doi:10.1158/1535-7163.MCT-20-1111
Duranti, C., Lastraioli, E., Iorio, J., Capitani, C., Carraresi, L., Gonnelli, L., et al. (2021b). Expression and purification of a novel single-chain diabody (scDb-hERG1/β1) from Pichia pastoris transformants. Protein Expr. Purif. 184, 105879. doi:10.1016/J.PEP.2021.105879
Egleton, R. D., Brown, K. C., and Dasgupta, P. (2008). Nicotinic acetylcholine receptors in cancer: multiple roles in proliferation and inhibition of apoptosis. Trends Pharmacol. Sci. 29, 151–158. doi:10.1016/J.TIPS.2007.12.006
Eichel, C. A., Ríos-Pérez, E. B., Liu, F., Jameson, M. B., Jones, D. K., Knickelbine, J. J., et al. (2019). A microtranslatome coordinately regulates sodium and potassium currents in the human heart. Elife 8, e52654. doi:10.7554/ELIFE.52654
Ettinger, D. S., Wood, D. E., Aisner, D. L., Akerley, W., Bauman, J. R., Bharat, A., et al. (2022). Non–small cell lung cancer, version 3.2022, NCCN clinical practice guidelines in oncology. J. Natl. Compr. Cancer Netw. 20, 497–530. doi:10.6004/JNCCN.2022.0025
Falvella, F. S., Galvan, A., Colombo, F., Frullanti, E., Pastorino, U., and Dragani, T. A. (2010). Promoter polymorphisms and transcript levels of nicotinic receptor CHRNA5. J. Natl. Cancer Inst. 102, 1366–1370. doi:10.1093/JNCI/DJQ264
Falvella, F. S., Galvan, A., Frullanti, E., Spinola, M., Calabrò, E., Carbone, A., et al. (2009). Transcription deregulation at the 15q25 locus in association with lung adenocarcinoma risk. Clin. Cancer Res. 15, 1837–1842. doi:10.1158/1078-0432.CCR-08-2107
Fraser, S. P., Ozerlat-Gunduz, I., Brackenbury, W. J., Fitzgerald, E. M., Campbell, T. M., Coombes, R. C., et al. (2014). Regulation of voltage-gated sodium channel expression in cancer: hormones, growth factors and auto-regulation. Philos. Trans. R. Soc. Lond. B. Biol. Sci. 369, 20130105. doi:10.1098/RSTB.2013.0105
Fu, S., Hirte, H., Welch, S., Ilenchuk, T. T., Lutes, T., Rice, C., et al. (2017). First-in-human phase I study of SOR-C13, a TRPV6 calcium channel inhibitor, in patients with advanced solid tumors. Invest. New Drugs 35, 324–333. doi:10.1007/S10637-017-0438-Z
Ganapathi, S. B., Kester, M., and Elmslie, K. S. (2009). State-dependent block of HERG potassium channels by R-roscovitine: implications for cancer therapy. Am. J. Physiol. Cell Physiol. 296, C701–C710. doi:10.1152/AJPCELL.00633.2008
Gao, H., Chen, X., Du, X., Guan, B., Liu, Y., and Zhang, H. (2011). EGF enhances the migration of cancer cells by up-regulation of TRPM7. Cell Calcium 50, 559–568. doi:10.1016/J.CECA.2011.09.003
Gasparoli, L., D’Amico, M., Masselli, M., Pillozzi, S., Caves, R., Khuwaileh, R., et al. (2015). New pyrimido-indole compound CD-160130 preferentially inhibits the KV11.1B isoform and produces antileukemic effects without cardiotoxicity. Mol. Pharmacol. 87, 183–196. doi:10.1124/MOL.114.094920
George, J., Lim, J. S., Jang, S. J., Cun, Y., Ozretia, L., Kong, G., et al. (2015). Comprehensive genomic profiles of small cell lung cancer. Nature 524, 47–53. doi:10.1038/NATURE14664
Ghanei, M., and Harandi, A. A. (2010). Lung carcinogenicity of sulfur mustard. Clin. Lung Cancer 11, 13–17. doi:10.3816/CLC.2010.N.002
Girardi, P., Barbiero, F., Baccini, M., Comba, P., Pirastu, R., Mastrangelo, G., et al. (2022). Mortality for lung cancer among PVC baggers employed in the vinyl chloride industry. Int. J. Environ. Res. Public Health 19, 6246. doi:10.3390/IJERPH19106246
Girault, A., Privé, A., Thu Ngan Trinh, N., Bardou, O., Ferraro, P., Joubert, P., et al. (2014). Identification of KvLQT1 K+ channels as new regulators of non-small cell lung cancer cell proliferation and migration. Int. J. Oncol. 44, 838–848. doi:10.3892/IJO.2013.2228
Glaser, F., Hundehege, P., Bulk, E., Todesca, L. M., Schimmelpfennig, S., Nass, E., et al. (2021). KCa channel blockers increase effectiveness of the EGF receptor TK inhibitor erlotinib in non-small cell lung cancer cells (A549). Sci. Rep. 11, 18330. doi:10.1038/S41598-021-97406-0
Glassmeier, G., Hempel, K., Wulfsen, I., Bauer, C. K., Schumacher, U., and Schwarz, J. R. (2012). Inhibition of HERG1 K + channel protein expression decreases cell proliferation of human small cell lung cancer cells. Pflugers Arch. Eur. J. Physiol. 463, 365–376. doi:10.1007/s00424-011-1045-z
Grando, S. A. (2014). Connections of nicotine to cancer. Nat. Rev. Cancer 14, 419–429. doi:10.1038/NRC3725
Grills, C., Jithesh, P. V., Blayney, J., Zhang, S. D., and Fennell, D. A. (2011). Gene expression meta-analysis identifies VDAC1 as a predictor of poor outcome in early stage non-small cell lung cancer. PLoS One 6, e14635. doi:10.1371/JOURNAL.PONE.0014635
Gualdani, R., de Clippele, M., Ratbi, I., Gailly, P., and Tajeddine, N. (2019). Store-operated calcium entry contributes to cisplatin-induced cell death in non-small cell lung carcinoma. Cancers (Basel) 11, 430. doi:10.3390/CANCERS11030430
Han, Y., Shi, Y., Han, Z., Sun, L., and Fan, D. (2007). Detection of potassium currents and regulation of multidrug resistance by potassium channels in human gastric cancer cells. Cell Biol. Int. 31, 741–747. doi:10.1016/J.CELLBI.2007.01.008
Han, Y., Zhou, Y., Zhou, L., Jia, X., Yu, X., An, X., et al. (2022). Blockade of chloride channel-3 enhances cisplatin sensitivity of cholangiocarcinoma cells though inhibiting autophagy. Can. J. Physiol. Pharmacol. 100, 584–593. doi:10.1139/CJPP-2022-0058
Hanahan, D., and Weinberg, R. A. (2000). The hallmarks of cancer. Cell 100, 57–70. doi:10.1016/S0092-8674(00)81683-9
Hanahan, D., and Weinberg, R. A. (2011). Hallmarks of cancer: the next generation. Cell 144, 646–674. doi:10.1016/J.CELL.2011.02.013
Haworth, A. S., and Brackenbury, W. J. (2019). Emerging roles for multifunctional ion channel auxiliary subunits in cancer. Cell Calcium 80, 125–140. doi:10.1016/J.CECA.2019.04.005
He, W., Li, H., Min, X., Liu, J., Hu, B., Hou, S., et al. (2010). Activation of volume-sensitive Cl(-) channel is involved in carboplatin-induced apoptosis in human lung adenocarcinoma cells. Cancer Biol. Ther. 9, 885–891. doi:10.4161/CBT.9.11.11666
Heijman, J., and Dobrev, D. (2018). Ion channels as part of macromolecular multiprotein complexes: clinical significance. Herzschrittmacherther. Elektrophysiol. 29, 30–35. doi:10.1007/S00399-017-0542-Y
Heinzerling, L., Ott, P. A., Hodi, F. S., Husain, A. N., Tajmir-Riahi, A., Tawbi, H., et al. (2016). Cardiotoxicity associated with CTLA4 and PD1 blocking immunotherapy. J. Immunother. cancer 4, 50. doi:10.1186/S40425-016-0152-Y
Hemmerlein, B., Weseloh, R. M., de Queiroz, F. M., Knötgen, H., Sánchez, A., Rubio, M. E., et al. (2006). Overexpression of Eag1 potassium channels in clinical tumours. Mol. Cancer 5, 41. doi:10.1186/1476-4598-5-41
Herbst, R. S., Heymach, J. V., and Lippman, S. M. (2008). Lung cancer. N. Engl. J. Med. 359, 1367–1380. doi:10.1056/NEJMRA0802714
Hoa, N. T., Ge, L., Tajhya, R. B., Beeton, C., Cornforth, A. N., Abolhoda, A., et al. (2014). Small cell lung cancer cells express the late stage gBK tumor antigen: a possible immunotarget for the terminal disease. Am. J. Transl. Res. 6, 188–205. Available at: https://www.ncbi.nlm.nih.gov/pmc/articles/PMC4058303/. (Accessed. August. 22. 2023).
Huang, C., Qin, Y., Liu, H., Liang, N., Chen, Y., Ma, D., et al. (2017). Downregulation of a novel long noncoding RNA TRPM2-AS promotes apoptosis in non-small cell lung cancer. Tumour Biol. 39, 1010428317691191. doi:10.1177/1010428317691191
Hui, C., Lan, Z., Yue-Li, L., Li-Lin, H., and Li-Lin, H. (2015). Knockdown of Eag1 expression by RNA interference increases chemosensitivity to cisplatin in ovarian cancer cells. Reprod. Sci. 22, 1618–1626. doi:10.1177/1933719115590665
Iorio, J., Antonuzzo, L., Scarpi, E., D’Amico, M., Duranti, C., Messerini, L., et al. (2022). Prognostic role of hERG1 potassium channels in neuroendocrine tumours of the ileum and pancreas. Int. J. Mol. Sci. 23, 10623. doi:10.3390/IJMS231810623
Iorio, J., Duranti, C., Lastraioli, E., Iorio, J., Duranti, C., and Lastraioli, E. (2019). Ion channels and transporters as cancer biomarkers and targets for diagnostics with antibodies. Transl. Res. Cancer. doi:10.5772/INTECHOPEN.90401
Jang, S. H., Choi, S. Y., Ryu, P. D., and Lee, S. Y. (2011a). Anti-proliferative effect of Kv1.3 blockers in A549 human lung adenocarcinoma in vitro and in vivo. Eur. J. Pharmacol. 651, 26–32. doi:10.1016/J.EJPHAR.2010.10.066
Jang, S. H., Ryu, P. D., and Lee, S. Y. (2011b). Dendrotoxin-κ suppresses tumor growth induced by human lung adenocarcinoma A549 cells in nude mice. J. Vet. Sci. 12, 35–40. doi:10.4142/JVS.2011.12.1.35
Jeon, W.Il, Ryu, P. D., and Lee, S. Y. (2012). Effects of voltage-gated K+ channel blockers in gefitinib-resistant H460 non-small cell lung cancer cells. Anticancer Res. 32, 5279–5284.
Jeong, S. B., Das, R., Kim, D. H., Lee, S., Oh, H. I., Jo, S., et al. (2022). Anticancer effect of verteporfin on non-small cell lung cancer via downregulation of ANO1. Biomed. Pharmacother. 153, 113373. doi:10.1016/J.BIOPHA.2022.113373
Jia, L., Liu, W., Guan, L., Lu, M., and Wang, K. W. (2015). Inhibition of calcium-activated chloride channel ANO1/tmem16a suppresses tumor growth and invasion in human lung cancer. PLoS One 10, e0136584. doi:10.1371/JOURNAL.PONE.0136584
Jiang, H. N., Zeng, B., Zhang, Y., Daskoulidou, N., Fan, H., Qu, J. M., et al. (2013). Involvement of TRPC channels in lung cancer cell differentiation and the correlation analysis in human non-small cell lung cancer. PLoS One 8, e67637. doi:10.1371/JOURNAL.PONE.0067637
Jiang, L. H., Gamper, N., and Beech, D. J. (2011). Properties and therapeutic potential of transient receptor potential channels with putative roles in adversity: focus on TRPC5, TRPM2 and TRPA1. Curr. Drug Targets 12, 724–736. doi:10.2174/138945011795378568
Judd, J., Karim, N. A., Khan, H., Naqash, A. R., Baca, Y., Xiu, J., et al. (2021). Characterization of KRAS mutation subtypes in non-small cell lung cancer. Mol. Cancer Ther. 20, 2577–2584. doi:10.1158/1535-7163.MCT-21-0201
Kim, M. S., Yang, S. H., and Kim, M. S. (2022). TRPML3 enhances drug resistance in non-small cell lung cancer cells by promoting Ca2+-mediated lysosomal trafficking. Biochem. Biophys. Res. Commun. 627, 152–159. doi:10.1016/J.BBRC.2022.08.051
Ko, E. A., Kim, Y. W., Lee, D., Choi, J., Kim, S., Seo, Y., et al. (2019). Expression of potassium channel genes predicts clinical outcome in lung cancer. Korean J. Physiol. Pharmacol. 23, 529–537. doi:10.4196/KJPP.2019.23.6.529
Ko, J. H., Gu, W., Lim, I., Bang, H., Ko, E. A., and Zhou, T. (2014a). Ion channel gene expression in lung adenocarcinoma: potential role in prognosis and diagnosis. PLoS One 9, e86569. doi:10.1371/JOURNAL.PONE.0086569
Ko, J. H., Gu, W., Lim, I., Zhou, T., and Bang, H. (2014b). Expression profiling of mitochondrial voltage-dependent anion channel-1 associated genes predicts recurrence-free survival in human carcinomas. PLoS One 9, e110094. doi:10.1371/JOURNAL.PONE.0110094
Kouokam, J. C., Meaza, I., and Wise, J. P. (2022). Inflammatory effects of hexavalent chromium in the lung: a comprehensive review. Toxicol. Appl. Pharmacol. 455, 116265. doi:10.1016/J.TAAP.2022.116265
Kuzumaki, N., Suzuki, A., Narita, M., Hosoya, T., Nagasawa, A., Imai, S., et al. (2012). Effect of κ-opioid receptor agonist on the growth of non-small cell lung cancer (NSCLC) cells. Br. J. Cancer 106, 1148–1152. doi:10.1038/BJC.2011.574
Lastraioli, E., Iorio, J., and Arcangeli, A. (2015). Ion channel expression as promising cancer biomarker. Biochim. Biophys. Acta - Biomembr. 1848, 2685–2702. doi:10.1016/j.bbamem.2014.12.016
Leanza, L., O’Reilly, P., Doyle, A., Venturini, E., Zoratti, M., Szegezdi, E., et al. (2014). Correlation between potassium channel expression and sensitivity to drug-induced cell death in tumor cell lines. Curr. Pharm. Des. 20, 189–200. doi:10.2174/13816128113199990032
Lee, J. H., Park, J. W., Byun, J. K., Kim, H. K., Ryu, P. D., Lee, S. Y., et al. (2015). Silencing of voltage-gated potassium channel KV9.3 inhibits proliferation in human colon and lung carcinoma cells. Oncotarget 6, 8132–8143. doi:10.18632/ONCOTARGET.3517
Lee, J. R., Lee, J. Y., Kim, H. J., Hahn, M. J., Kang, J. S., and Cho, H. (2019). The inhibition of chloride intracellular channel 1 enhances Ca2+ and reactive oxygen species signaling in A549 human lung cancer cells. Exp. Mol. Med. 51, 81. doi:10.1038/S12276-019-0279-2
Li, X., Zhang, Q., Fan, K., Li, B., Li, H., Qi, H., et al. (2016). Overexpression of TRPV3 correlates with tumor progression in non-small cell lung cancer. Int. J. Mol. Sci. 17, 437. doi:10.3390/IJMS17040437
Lin, G., Lin, L., Lin, H., Chen, W., Chen, L., Chen, X., et al. (2022). KCNK3 inhibits proliferation and glucose metabolism of lung adenocarcinoma via activation of AMPK-TXNIP pathway. Cell death Discov. 8, 360. doi:10.1038/S41420-022-01152-9
Liu, H., Huang, J., Peng, J., Wu, X., Zhang, Y., Zhu, W., et al. (2015). Upregulation of the inwardly rectifying potassium channel Kir2.1 (KCNJ2) modulates multidrug resistance of small-cell lung cancer under the regulation of miR-7 and the Ras/MAPK pathway. Mol. Cancer 14, 59. doi:10.1186/S12943-015-0298-0
Lorenzo-González, M., Torres-Durán, M., Barbosa-Lorenzo, R., Provencio-Pulla, M., Barros-Dios, J. M., and Ruano-Ravina, A. (2019). Radon exposure: a major cause of lung cancer. Expert Rev. Respir. Med. 13, 839–850. doi:10.1080/17476348.2019.1645599
Lottini, T., Duranti, C., Iorio, J., Martinelli, M., Colasurdo, R., D’Alessandro, F. N., et al. (2023). Combination therapy with a bispecific antibody targeting the hERG1/β1 integrin complex and gemcitabine in pancreatic ductal adenocarcinoma. Cancers (Basel) 15, 2013. doi:10.3390/CANCERS15072013
Lu, A., Shi, Y., Liu, Y., Lin, J., Zhang, H., Guo, Y., et al. (2021a). Integrative analyses identified ion channel genes GJB2 and SCNN1B as prognostic biomarkers and therapeutic targets for lung adenocarcinoma. Lung Cancer 158, 29–39. doi:10.1016/J.LUNGCAN.2021.06.001
Lu, X., Li, K., and Yang, J. (2021b). Potassium voltage-gated channel subfamily D member 2 induces an aggressive phenotype in lung adenocarcinoma. Neoplasma 68, 135–143. doi:10.4149/NEO_2020_200629N667
Lyu, Y., Wang, Q., Liang, J., Zhang, L., and Zhang, H. (2022). The ion channel gene KCNAB2 is associated with poor prognosis and loss of immune infiltration in lung adenocarcinoma. Cells 11, 3438. doi:10.3390/CELLS11213438
Ma, G., Ji, D., Qu, X., Liu, S., Yang, X., Wang, G., et al. (2019). Mining and validating the expression pattern and prognostic value of acetylcholine receptors in non-small cell lung cancer. Med. Baltim. 98, e15555. doi:10.1097/MD.0000000000015555
Ma, X., Jia, Y., Zu, S., Li, R., Jia, Y., Zhao, Y., et al. (2014). α5 Nicotinic acetylcholine receptor mediates nicotine-induced HIF-1α and VEGF expression in non-small cell lung cancer. Toxicol. Appl. Pharmacol. 278, 172–179. doi:10.1016/J.TAAP.2014.04.023
Markowitz, S. B. (2022). Lung cancer screening in asbestos-exposed populations. Int. J. Environ. Res. Public Health 19, 2688. doi:10.3390/IJERPH19052688
Marques-Carvalho, M. J., Oppermann, J., Muñoz, E., Fernandes, A. S., Gabant, G., Cadene, M., et al. (2016). Molecular insights into the mechanism of calmodulin inhibition of the EAG1 potassium channel. Structure 24, 1742–1754. doi:10.1016/J.STR.2016.07.020
Masumoto, K., Tsukimoto, M., and Kojima, S. (2013). Role of TRPM2 and TRPV1 cation channels in cellular responses to radiation-induced DNA damage. Biochim. Biophys. Acta 1830, 3382–3390. doi:10.1016/J.BBAGEN.2013.02.020
McIntyre, A., and Ganti, A. K. (2017). Lung cancer-A global perspective. J. Surg. Oncol. 115, 550–554. doi:10.1002/JSO.24532
Mo, J., Xia, Y., Wade, T. J., DeMarini, D. M., Davidson, M., and Mumford, J. (2011). Altered gene expression by low-dose arsenic exposure in humans and cultured cardiomyocytes: assessment by real-time PCR arrays. Int. J. Environ. Res. Public Health 8, 2090–2108. doi:10.3390/IJERPH8062090
Naruke, T., Tsuchiya, R., Kondo, H., Asamura, H., and Nakayama, H. (1997). Implications of staging in lung cancer. Chest 112, 242S–248S. doi:10.1378/CHEST.112.4_SUPPLEMENT.242S
Nelson, M., Millican-Slater, R., Forrest, L. C., and Brackenbury, W. J. (2014). The sodium channel β1 subunit mediates outgrowth of neurite-like processes on breast cancer cells and promotes tumour growth and metastasis. Int. J. cancer 135, 2338–2351. doi:10.1002/IJC.28890
Nicholson, S. A., Beasley, M. B., Brambilla, E., Hasleton, P. S., Colby, T. V., Sheppard, M. N., et al. (2002). Small cell lung carcinoma (SCLC): a clinicopathologic study of 100 cases with surgical specimens. Am. J. Surg. Pathol. 26, 1184–1197. doi:10.1097/00000478-200209000-00009
Okada, Y., Numata, T., Sato-Numata, K., Sabirov, R. Z., Liu, H., Mori, S., et al. (2019). Roles of volume-regulatory anion channels, VSOR and Maxi-Cl, in apoptosis, cisplatin resistance, necrosis, ischemic cell death, stroke and myocardial infarction. Curr. Top. Membr. 83, 205–283. doi:10.1016/bs.ctm.2019.03.001
Okudela, K., Yazawa, T., Ishii, J., Woo, T., Mitsui, H., Bunai, T., et al. (2009). Down-regulation of FXYD3 expression in human lung cancers: its mechanism and potential role in carcinogenesis. Am. J. Pathol. 175, 2646–2656. doi:10.2353/AJPATH.2009.080571
Onganer, P. U., and Djamgoz, M. B. A. (2005). Small-cell lung cancer (human): potentiation of endocytic membrane activity by voltage-gated Na+ channel expression in vitro. J. Membr. Biol. 204, 67–75. doi:10.1007/s00232-005-0747-6
Peifer, M., Fernández-Cuesta, L., Sos, M. L., George, J., Seidel, D., Kasper, L. H., et al. (2012). Integrative genome analyses identify key somatic driver mutations of small-cell lung cancer. Nat. Genet. 44, 1104–1110. doi:10.1038/NG.2396
Peretti, M., Badaoui, M., Girault, A., Van Gulick, L., Mabille, M. P., Tebbakha, R., et al. (2019). Original association of ion transporters mediates the ECM-induced breast cancer cell survival: Kv10.1-Orai1-SPCA2 partnership. Sci. Rep. 91 (9), 1175. doi:10.1038/s41598-018-37602-7
Pérez-Callejo, D., Torrente, M., Brenes, M. A., Núñez, B., and Provencio, M. (2017). Lung cancer as a cardiotoxic state: a review. Med. Oncol. 34, 159. doi:10.1007/S12032-017-1012-4
Pillozzi, S., D’Amico, M., Bartoli, G., Gasparoli, L., Petroni, G., Crociani, O., et al. (2017). The combined activation of KCa3.1 and inhibition of Kv11.1/hERG1 currents contribute to overcome Cisplatin resistance in colorectal cancer cells. Br. J. Cancer 118, 200–212. doi:10.1038/bjc.2017.392
Plummer, H. K., Dhar, M. S., Cekanova, M., and Schuller, H. M. (2005). Expression of G-protein inwardly rectifying potassium channels (GIRKs) in lung cancer cell lines. BMC Cancer 5, 104. doi:10.1186/1471-2407-5-104
Ponce-Balbuena, D., Guerrero-Serna, G., Valdivia, C. R., Caballero, R., Diez-Guerra, F. J., Jiménez-Vázquez, E. N., et al. (2018). Cardiac Kir2.1 and NaV1.5 channels traffic together to the sarcolemma to control excitability. Circ. Res. 122, 1501–1516. doi:10.1161/CIRCRESAHA.117.311872
Potier-Cartereau, M., Raoul, W., Weber, G., Mahéo, K., Rapetti-Mauss, R., Gueguinou, M., et al. (2022). Potassium and calcium channel complexes as novel targets for cancer research. Rev. Physiol. Biochem. Pharmacol. 183, 157–176. doi:10.1007/112_2020_24
Restrepo-Angulo, I., Sánchez-Torres, C., and Camacho, J. (2011). Human EAG1 potassium channels in the epithelial-to- mesenchymal transition in lung cancer cells. Anticancer Res. 31, 1265–1270.
Ricotti, A., Sciannameo, V., Balzi, W., Roncadori, A., Canavese, P., Avitabile, A., et al. (2021). Incidence and prevalence analysis of non-small-cell and small-cell lung cancer using administrative data. Int. J. Environ. Res. Public Health 18, 9076. doi:10.3390/ijerph18179076
Righi, L., Volante, M., and Papotti, M. (2022). Small-cell carcinoma of the lung: what we learned about it? Acta Cytol. 66, 257–268. doi:10.1159/000519688
Roger, S., Rollin, J., Barascu, A., Besson, P., Raynal, P. I., Iochmann, S., et al. (2007). Voltage-gated sodium channels potentiate the invasive capacities of human non-small-cell lung cancer cell lines. Int. J. Biochem. Cell Biol. 39, 774–786. doi:10.1016/J.BIOCEL.2006.12.007
Rosa, P., Catacuzzeno, L., Sforna, L., Mangino, G., Carlomagno, S., Mincione, G., et al. (2018). BK channels blockage inhibits hypoxia-induced migration and chemoresistance to cisplatin in human glioblastoma cells. J. Cell. Physiol. 233, 6866–6877. doi:10.1002/JCP.26448
Rosenberger, A., Hung, R. J., Christiani, D. C., Caporaso, N. E., Liu, G., Bojesen, S. E., et al. (2018). Genetic modifiers of radon-induced lung cancer risk: a genome-wide interaction study in former uranium miners. Int. Arch. Occup. Environ. Health 91, 937–950. doi:10.1007/S00420-018-1334-3
Sakai, H., Shimizu, T., Hori, K., Ikari, A., Asano, S., and Takeguchi, N. (2002). Molecular and pharmacological properties of inwardly rectifying K+ channels of human lung cancer cells. Eur. J. Pharmacol. 435, 125–133. doi:10.1016/S0014-2999(01)01567-9
Samuel, P., Pink, R. C., Caley, D. P., Currie, J. M. S., Brooks, S. A., and Carter, D. R. F. (2016). Over-expression of miR-31 or loss of KCNMA1 leads to increased cisplatin resistance in ovarian cancer cells. Tumour Biol. 37, 2565–2573. doi:10.1007/S13277-015-4081-Z
Sanguinetti, M. C., and Tristani-Firouzi, M. (2006). hERG potassium channels and cardiac arrhythmia. Nature 440, 463–469. doi:10.1038/NATURE04710
Schabath, M. B., and Cote, M. L. (2019). Cancer progress and priorities: lung cancer. Cancer Epidemiol. Biomarkers Prev. 28, 1563–1579. doi:10.1158/1055-9965.EPI-19-0221
Schaefer, E. A. M., Stohr, S., Meister, M., Aigner, A., Gudermann, T., and Buech, T. R. H. (2013). Stimulation of the chemosensory TRPA1 cation channel by volatile toxic substances promotes cell survival of small cell lung cancer cells. Biochem. Pharmacol. 85, 426–438. doi:10.1016/J.BCP.2012.11.019
Seo, Y., Jeong, S. B., Woo, J. H., Kwon, O. B., Lee, S., Oh, H. I., et al. (2021). Diethylstilbestrol, a novel ANO1 inhibitor, exerts an anticancer effect on non-small cell lung cancer via inhibition of ANO1. Int. J. Mol. Sci. 22, 7100. doi:10.3390/IJMS22137100
Shen, H. M., and Zhang, Q. F. (1994). Risk assessment of nickel carcinogenicity and occupational lung cancer. Environ. Health Perspect. 102 (1), 275–282. doi:10.1289/EHP.94102S1275
Shopp, G. M., Helson, L., Bouchard, A., Salvail, D., and Majeed, M. (2014). Liposomes ameliorate crizotinib- and nilotinib-induced inhibition of the cardiac I Kr channel and QTc prolongation. Anticancer Res. 34, 4733–4740.
Song, M. S., Park, S. M., Park, J. S., Byun, J. H., Jin, H. J., Seo, S. H., et al. (2018). Kv3.1 and Kv3.4, are involved in cancer cell migration and invasion. Int. J. Mol. Sci. 19, 1061. doi:10.3390/IJMS19041061
Soza-Ried, C., Bustamante, E., Caglevic, C., Rolfo, C., Sirera, R., and Marsiglia, H. (2019). Oncogenic role of arsenic exposure in lung cancer: a forgotten risk factor. Crit. Rev. Oncol. Hematol. 139, 128–133. doi:10.1016/J.CRITREVONC.2019.01.012
Sun, H., and Ma, X. (2015). α5-nAChR modulates nicotine-induced cell migration and invasion in A549 lung cancer cells. Exp. Toxicol. Pathol. 67, 477–482. doi:10.1016/J.ETP.2015.07.001
Sung, H., Ferlay, J., Siegel, R. L., Laversanne, M., Soerjomataram, I., Jemal, A., et al. (2021). Global cancer statistics 2020: GLOBOCAN estimates of incidence and mortality worldwide for 36 cancers in 185 countries. Ca. Cancer J. Clin. 71, 209–249. doi:10.3322/CAAC.21660
Takanami, I., Inoue, Y., and Gika, M. (2004). G-protein inwardly rectifying potassium channel 1 (GIRK 1) gene expression correlates with tumor progression in non-small cell lung cancer. BMC Cancer 4, 79. doi:10.1186/1471-2407-4-79
Testa, U., Castelli, G., and Pelosi, E. (2018). Lung cancers: molecular characterization, clonal heterogeneity and evolution, and cancer Stem cells. Cancers (Basel) 10, 248. doi:10.3390/CANCERS10080248
Travis, W. D., Brambilla, E., Noguchi, M., Nicholson, A. G., Geisinger, K. R., Yatabe, Y., et al. (2011). International association for the study of lung cancer/american thoracic society/european respiratory society international multidisciplinary classification of lung adenocarcinoma. J. Thorac. Oncol. 6, 244–285. doi:10.1097/JTO.0B013E318206A221
Uchikawa, M., Hashiguchi, M., and Shiga, T. (2022). Drug-induced QT prolongation and torsade de Pointes in spontaneous adverse event reporting: a retrospective analysis using the Japanese adverse drug event report database (2004-2021). Drugs - real world outcomes 9, 551–559. doi:10.1007/S40801-022-00328-0
Voortman, J., Lee, J. H., Killian, J. K., Suuriniemi, M., Wang, Y., Lucchi, M., et al. (2010). Array comparative genomic hybridization-based characterization of genetic alterations in pulmonary neuroendocrine tumors. Proc. Natl. Acad. Sci. U. S. A. 107, 13040–13045. doi:10.1073/PNAS.1008132107
Wallis, R. M. (2010). Integrated risk assessment and predictive value to humans of non-clinical repolarization assays. Br. J. Pharmacol. 159, 115–121. doi:10.1111/J.1476-5381.2009.00395.X
Waqar, S. N., and Morgensztern, D. (2017). Treatment advances in small cell lung cancer (SCLC). Pharmacol. Ther. 180, 16–23. doi:10.1016/J.PHARMTHERA.2017.06.002
Williams, S., Bateman, A., and O’Kelly, I. (2013). Altered expression of two-pore domain potassium (K2P) channels in cancer. PLoS One 8, e74589. doi:10.1371/JOURNAL.PONE.0074589
Witchel, H. J., and Hancox, J. C. (2000). Familial and acquired long qt syndrome and the cardiac rapid delayed rectifier potassium current. Clin. Exp. Pharmacol. Physiol. 27, 753–766. doi:10.1046/J.1440-1681.2000.03337.X
Wu, X. Y., and Yu, X. Y. (2019). Overexpression of KCNJ4 correlates with cancer progression and unfavorable prognosis in lung adenocarcinoma. J. Biochem. Mol. Toxicol. 33, e22270. doi:10.1002/JBT.22270
Xu, P., Mo, X., Xia, R., Jiang, L., Zhang, C., Xu, H., et al. (2021). KCNN4 promotes the progression of lung adenocarcinoma by activating the AKT and ERK signaling pathways. Cancer Biomark. 31, 187–201. doi:10.3233/CBM-201045
Yan, Y., Fu, J., Kowalchuk, R. O., Wright, C. M., Zhang, R., Li, X., et al. (2022). Exploration of radiation-induced lung injury, from mechanism to treatment: a narrative review. Transl. Lung Cancer Res. 11, 307–322. doi:10.21037/tlcr-22-108
Yang, L. L., Liu, B. C., Lu, X. Y., Yan, Y., Zhai, Y. J., Bao, Q., et al. (2017). Inhibition of TRPC6 reduces non-small cell lung cancer cell proliferation and invasion. Oncotarget 8, 5123–5134. doi:10.18632/ONCOTARGET.14034
Yap, Y. G., and Camm, A. J. (2003). Drug induced QT prolongation and torsades de pointes. Heart 89, 1363–1372. doi:10.1136/HEART.89.11.1363
Zhang, L., Chu, J., Xia, B., Xiong, Z., Zhang, S., and Tang, W. (2022). Health effects of particulate uranium exposure. Toxics 10, 575. doi:10.3390/TOXICS10100575
Zhang, L., Townsend, D. M., Morris, M., Maldonado, E. N., Jiang, Y. L., Broome, A. M., et al. (2020). Voltage-dependent anion channels influence cytotoxicity of ME-344, a therapeutic isoflavone. J. Pharmacol. Exp. Ther. 374, 308–318. doi:10.1124/JPET.120.000009
Zhang, R., Tian, P., Chi, Q., Wang, J., Wang, Y., Sun, L., et al. (2012). Human ether-à-go-go-related gene expression is essential for cisplatin to induce apoptosis in human gastric cancer. Oncol. Rep. 27, 433–440. doi:10.3892/OR.2011.1515
Zhang, Y., Jia, Y., Li, P., li, H., Xiao, D., Wang, Y., et al. (2017). Reciprocal activation of α5-nAChR and STAT3 in nicotine-induced human lung cancer cell proliferation. J. Genet. Genomics 44, 355–362. doi:10.1016/J.JGG.2017.03.003
Zhang, Y., Zhou, L. E. I., Zhang, J., Zhang, L., Yan, X., and Su, J. (2018). Suppression of chloride voltage-gated channel 3 expression increases sensitivity of human glioma U251 cells to cisplatin through lysosomal dysfunction. Oncol. Lett. 16, 835–842. doi:10.3892/OL.2018.8736
Zhang, Z., Wang, J., He, J., Zeng, X., Chen, X., Xiong, M., et al. (2016). Identification of TRPCs genetic variants that modify risk for lung cancer based on the pathway and two-stage study. Meta gene 9, 191–196. doi:10.1016/J.MGENE.2016.07.005
Keywords: lung cancer, SCLC, NSCLC, potassium channels, sodium channels, calcium channels, chloride channels, anion channels
Citation: Capitani C, Chioccioli Altadonna G, Santillo M and Lastraioli E (2023) Ion channels in lung cancer: biological and clinical relevance. Front. Pharmacol. 14:1283623. doi: 10.3389/fphar.2023.1283623
Received: 26 August 2023; Accepted: 16 October 2023;
Published: 24 October 2023.
Edited by:
Oscar Moran, National Research Council (CNR), ItalyReviewed by:
Javier Camacho, National Polytechnic Institute of Mexico (CINVESTAV), MexicoMichael Pusch, National Research Council (CNR), Italy
Copyright © 2023 Capitani, Chioccioli Altadonna, Santillo and Lastraioli. This is an open-access article distributed under the terms of the Creative Commons Attribution License (CC BY). The use, distribution or reproduction in other forums is permitted, provided the original author(s) and the copyright owner(s) are credited and that the original publication in this journal is cited, in accordance with accepted academic practice. No use, distribution or reproduction is permitted which does not comply with these terms.
*Correspondence: Elena Lastraioli, ZWxlbmEubGFzdHJhaW9saUB1bmlmaS5pdA==
†These authors have contributed equally to this work