- 1School of Pharmacy, Shandong University of Traditional Chinese Medicine, Jinan, Shandong, China
- 2School of Pharmacy, Jining Medical University, Rizhao, Shandong, China
- 3Department of Nasopharyngeal Carcinoma, Sun Yat-sen University Cancer Center, Guangzhou, Guangdong, China
- 4School of Pharmacy, Weifang Medical University, Weifang, Shandong, China
- 5School of Pharmacy, Binzhou Medical University, Yantai, Shandong, China
Atherosclerosis is the leading cause of numerous cardiovascular diseases with a high mortality rate. Non-coding RNAs (ncRNAs), RNA molecules that do not encode proteins in human genome transcripts, are known to play crucial roles in various physiological and pathological processes. Recently, researches on the regulation of atherosclerosis by ncRNAs, mainly including microRNAs, long non-coding RNAs, and circular RNAs, have gradually become a hot topic. Traditional Chinese medicine has been proved to be effective in treating cardiovascular diseases in China for a long time, and its active monomers have been found to target a variety of atherosclerosis-related ncRNAs. These active monomers of traditional Chinese medicine hold great potential as drugs for the treatment of atherosclerosis. Here, we summarized current advancement of the molecular pathways by which ncRNAs regulate atherosclerosis and mainly highlighted the mechanisms of traditional Chinese medicine monomers in regulating atherosclerosis through targeting ncRNAs.
1 Introduction
Atherosclerosis is characterized by fibro-fat lesions on the walls of arteries with extremely high morbidity and mortality rate (Libby et al., 2019). Atherosclerosis is considered to be an important pathological basis for cerebrovascular and cardiovascular diseases such as cerebral infarction, coronary heart disease and myocardial infarction (Libby, 2021). There are many causes of atherosclerosis, such as inflammatory reactions (Zhu et al., 2018), cell death and aging (Bazioti et al., 2022), and endothelial to mesenchymal transition (Liang et al., 2022), among which chronic inflammation is the reason that has been frequently studied in the past few years. At the molecular level, telomere damage, genomic DNA damage, and mitochondrial DNA damage accumulate in vascular endothelial cells, which induce endothelial cell aging and chronic inflammation. Persistent inflammation results in increased accumulation of lymphocytes and macrophages, leading to atherosclerosis (Wang and Bennett, 2012; Okuyama et al., 2015; Ruparelia and Choudhury, 2020). The pathogenesis and therapeutic targets of atherosclerosis have long been the focus in the field of cardiovascular researches. Statins, inhibitors of the hydroxymethylglutaryl-CoA (HMG-CoA) reductase enzyme, are powerful cholesterol lowering medications and the most commonly used clinical drugs for the prevention and treatment of atherosclerosis (Okuyama et al., 2015). Statins can reduce morbidity and mortality in patients with cardiovascular diseases. However, statins may affect the drug-drug interactions because different safety and tolerability, especially when in combination with other cardiovascular drugs, which will lead to increased risk of statin-associated hepatotoxicity and myopathy (Bellosta and Corsini, 2018). Therefore, it is urgent to discover new therapeutic targets and new drugs for atherosclerosis.
RNAs in mammalian cells are complex, some of which have the function of encoding proteins, but some of which lack this function. At present, the RNAs that lack the function of encoding proteins are named as non-coding RNAs (ncRNAs), of which the most studied are microRNAs (miRNAs), long non-coding RNAs (lncRNAs), and circular RNAs (circRNAs) (Lu and Rothenberg, 2018; Chen et al., 2021a; Bridges et al., 2021). NcRNAs have been proved to play important regulatory roles in pathogenesis of atherosclerosis through affecting inflammatory reaction, cell activation and proliferation, and lipid metabolism (Feinberg and Moore, 2016; Aryal and Suarez, 2019). Nowadays, therapeutic strategies targeting ncRNAs have entered the clinical testing phase for the treatment of cancers and have been considered as an attractive approach for the treatment of atherosclerosis.
Since ancient times, numerous herbal medicines have been used for the treatment of atherosclerosis-related diseases and decoction is the main form of traditional Chinese medicine used in clinic. With the development of separation technology, it has become possible to separate more pharmacologically active monomers from traditional Chinese medicine. This allows researchers to conduct pharmacological studies on the specific monomers rather than the whole of medicinal plants. At present, studies have found that many active monomers of traditional Chinese medicine have positive effects on atherosclerosis, such as saponins (Luo et al., 2022), flavonoids (Park et al., 2006) and alkaloids (Li et al., 2021d). For example, berberine, an active ingredient extracted from Berberis aristata DC., can activate PPAR-γ pathway in macrophages, resulting in decreased expressions of inflammatory factors like monocyte chemoattractant protein-1 (MCP-1) and tumor necrosis factor-α (TNF-α) (Chen et al., 2008). Another study found that hydroxysafflor yellow A, a natural compound from Carthamus tinctorius L., exerts protective effects on atherosclerosis by suppressing vascular endothelial cell dysfunction, vascular smooth muscle cell proliferation and migration, foam cell formation, and platelet activation (Xue et al., 2021). These active monomers of traditional Chinese medicine are predicted to have high therapeutic potential in atherosclerosis treatment. However, the specific drug targets of these active ingredient are not fully understood, which limits clinical application.
Recently, more and more studies have found that ncRNAs are the key mediators of the pharmacological effect of traditional Chinese medicine. Here, we summarized current advances of mechanisms of ncRNAs in regulating atherosclerosis. Furthermore, we highlighted the current advances in the active monomers of traditional Chinese medicine which have atheroprotective effects by regulating ncRNAs.
2 The role of ncRNAs in regulating atherosclerosis
2.1 miRNAs and atherosclerosis
MiRNAs (typically 20–25 nucleotides) are single-stranded RNA molecules that can bind to complementary sequences within the 3′ untranslated region of mRNA targets. Once the miRNA binds to the mRNA, it can degrade the mRNA via cleavage or inhibit the translation of mRNAs into proteins (Winter et al., 2009; Thum and Mayr, 2012). MiRNAs are the most studied ncRNAs in atherosclerosis and have been shown to regulate the fate and function of atherosclerosis associated cells, including endothelial cells, inflammatory cells, and vascular smooth muscle cells (VSMCs). MiRNAs can affect endothelial cell function by exacerbating senescence of endothelial cells, which is considered as a key mechanism of atherosclerosis (Menghini et al., 2009; Fiedler and Thum, 2016). There are many miRNAs involved in the regulation of endothelial cell senescence, such as miR-146a and miR-217 (Wang et al., 2021; Xiao et al., 2021). Studies have found that mesenchymal stem cell-derived extracellular vesicles attenuate endothelial cell senescence by regulating miR-146a/Src signaling (Xiao et al., 2021). MiR-217 can also promote endothelial cell senescence through the SIRT1/p53 signaling pathway (Wang et al., 2021). In addition, miRNAs can control the inflammatory state of the vasculature by affecting leukocyte activation and infiltration (Perez-Sanchez et al., 2017; Pankratz et al., 2018). In the setting of atherosclerosis, miR-126 promotes macrophage polarization to the M2 phenotype by downregulating VEGFA and krüppel-like factor 4 (KLF4) (Shou et al., 2023). MiRNAs have also been shown to affect foam cell formation and subsequent plaque formation (Eken et al., 2017; Maitrias et al., 2017). MiR-302a has been shown to promote the formation of foam cells and increase the outflow of cholesterol in macrophage by increasing ATP-binding cassette transporter A1 (ABCA1) activity (Meiler et al., 2015). In addition, the function of VSMCs can also be regulated by miRNAs. For example, miR-146b-5p reduces the expression of its target genes Bag1 and Mmp16, thereby affecting the proliferation and migration of VSMCs during atherosclerosis (Sun et al., 2020). A study also found that miR-374 may be a potential biomarker for the diagnosis of atherosclerosis, and overexpression of miR-374 promotes the proliferation and migration of VSMCs (Wang et al., 2020b). MiR-663 can target high mobility group AT-hook 2 (HMGA2) to inhibit the proliferation of VSMCs, thereby delaying the development of atherosclerosis (Deng and Li, 2022). In conclusion, miRNAs regulate atherosclerosis through affecting the function of endothelial cells, macrophages, and VSMCs.
2.2 lncRNAs and atherosclerosis
LncRNAs are ncRNAs longer than 200 nucleotides (Di Mauro et al., 2018), which are abnormally expressed in many pathological tissues (Li et al., 2020; Zang et al., 2020). Unlike miRNAs, the actions of lncRNAs are relatively complex. LncRNA can be a source of miRNA. For example, miR-31 gene is embedded within an intron of the lncRNA LOC554202 and its transcription is regulated by the methylation state of the host gene promoter (Augoff et al., 2012). Morover, lncRNAs can bind to DNA, mRNA and proteins to regulate their expressions or functions (Guttman and Rinn, 2012). The most widely known mechanism is the competitive endogenous RNA (ceRNA), in which way lncRNA acts as a negative regulator of miRNA (Salmena et al., 2011). In recent years, studies have shown that lncRNAs are dynamically expressed in developing and diseased blood vessels, suggesting that lncRNAs have profound biological functions in atherosclerosis (Guo et al., 2019; Simion et al., 2020). LncRNAs can regulate atherosclerosis by influencing the function of vascular cells. For example, lncRNA HOXA11-AS is significantly upregulated in aortic tissue of atherosclerotic mice and oxidized low-density lipoprotein (ox-LDL)-induced endothelial cells. HOXA11-AS knockdown attenuates endothelial injuries by directly regulating the miR-515-5p/ROCK1/eNOS axis (Gao et al., 2022). In addition to endothelial cells, lncRNA also plays a role in atherosclerosis by affecting VSMCs and macrophages. For example, lncRNA TUG1 can promote the proliferation of VSMCs by regulating the miRNA-21/PTEN axis (Li et al., 2018b). LncRNA MAARS interacts with HuR to increase macrophage apoptosis in the blood vessels (Simion et al., 2020). What’s more, lncRNA kcnq1ot1 can compete with miR-452-3p to promote macrophage lipid accumulation and accelerate the development of atherosclerosis (Yu et al., 2020a).
2.3 circRNAs and atherosclerosis
CircRNAs are closed circular molecules, which distinguishes them from other linear RNA molecules. CircRNAs were originally considered as by-products of mRNA cleavage, but now they are thought to be stable and functional ncRNAs (Chen, 2016). Compared to miRNAs, circRNAs are less studied ncRNAs in atherosclerosis. Still, there are studies that have shown circRNAs can regulate the fate and function of atherosclerosis-associated cells, including endothelial cells, macrophages, and VSMCs. As with lncRNAs, circRNAs can also compete with miRNAs as ceRNAs, which is the mostly investigated mechanism (Ren et al., 2021). In endothelial cells, a study demonstrated that circ-RELL1 plays a pro-inflammatory role in endothelial cells by directly binding to miR-6873-3p and subsequently activating NF-κB signaling pathway (Huang et al., 2020). Circ_0086296 induces aberrant endothelial cell phenotypes by spongesizing miR-576-3p, resulting in severe atherosclerotic lesions (Zhang et al., 2022). In VSMCs, circRNA-0044073 promotes the proliferation and invasion of VSMCs by targeting miR-107 and activating the JAK/STAT signaling pathway (Shen et al., 2019). In macrophages, overexpression of circ_0004104 results in dysregulation of atherosclerosis-related genes in THP-1-derived macrophages (Wang et al., 2019). It is noticed that the role of circRNAs in atherosclerosis has rarely been studied, which may become a research hotspot in the future.
Since the role of ncRNAs in atherosclerosis is emerging, they have been considered as potential drug targets in developing therapeutic agents. As we know, traditional Chinese medicine has a long history of treating atherosclerosis in China. In particular, studies have shown that the monomers extracted from traditional Chinese medicine are the main functional components that possess anti-atherosclerotic activity, and these activities can be mediated by ncRNAs.
3 Active monomers of traditional Chinese medicine relieve atherosclerosis by regulating ncRNAs
Nowadays, the researches about the regulation of atherosclerosis by active monomers of traditional Chinese medicine are tremendous. However, the drug targets of traditional Chinese medicine remain unclear, which affects the clinical application of these medicine. It is clear that ncRNAs appear to be important players during atherosclerosis and important targets of traditional Chinese medicine. Therefore, it is particularly important to discover the mechanism by which the active monomers of traditional Chinese medicine relieve atherosclerosis through ncRNAs.
3.1 Geniposide
Geniposide, an iridoid glucoside, is the main active ingredient of Gardenia jasminoides J. Ellis. Geniposide exhibits a variety of anti-inflammatory and anti-oxidative functions and has good therapeutic effects on cardiovascular diseases (Fu et al., 2012b). A study has found that geniposide treatment reduces lipid levels and plaque size in the mouse model of atherosclerosis. Mechanistically, geniposide downregulates miR-101 to upregulate mitogen-activated protein kinase phosphatase-1 (MKP-1) and suppresses the production of inflammatory factors in macrophages (Cheng et al., 2019). MiR-21 has been shown to play an important role in regulating inflammatory responses by targeting phosphatase and tensin homolog (PTEN) (Sheedy, 2015; Li et al., 2022). A study established a endothelial cell injury model by using ox-LDL and found geniposide protects endothelial cells from ox-LDL-induced injury by inhibiting oxidative stress and inflammation, and these effects are partly due to the enhancement of the miR-21/PTEN pathway (Zhou et al., 2020). Taken together, miR-101 and miR-21 are involved in the anti-inflammatory effect of geniposide in the setting of atherosclerosis.
3.2 Astragaloside IV
Astragaloside IV is a saponin isolated from Astragalus membranaceus (Fisch.) Bunge, which has excellent cardioprotective effects (Tan et al., 2020). Astragaloside IV has been reported to protect endothelial cells from oxidative damage caused by ox-LDL through regulating the LOX-1/NLRP3 signaling pathway (Qian et al., 2019a). Recently, a study found that circ_0000231 is the key downstream target of astragaloside IV, which regulates miR-135a-5p to target chloride intracellular channel 4 (CLIC4), and contributes to the protective role of astragaloside IV in ox-LDL-induced endothelial cell injury (Shao et al., 2021). CLIC4 is also a protein associated with endothelial cell apoptosis (Zhang et al., 2020b), indicating astragaloside IV may also inhibit endothelial cell apoptosis by regulating CLIC4 through circ_0000231. Several miRNAs have been shown to be the targets of astragaloside IV. For example, astragaloside IV can protect cardiomyocytes from hypoxia-induced injury by downregulating miR-23a and miR-92a (Gong et al., 2018). ABCA1, a membrane transporter that mediates cholesterol efflux (Chen et al., 2022), has been proved to be a target of miR-33a (Gao et al., 2018). A study has found that astragaloside IV can promote cholesterol efflux in macrophages and inhibit atherosclerosis through regulating miR-33a/ABCA1 pathway (Qin et al., 2018). The serum miR-17-5p is elevated in patients with atherosclerosis and miR-17-5p knockdown can alleviate atherosclerotic lesions and inhibit the proliferation and migration of VSMCs by directly up-regulating very low density lipoprotein receptor (VLDLR), or indirectly regulate VLDLR by affecting proprotein convertase subtilisin kexin 9 (PCSK9) (Tan et al., 2017). Astragaloside IV has been shown to downregulate miR-17-5p and further affect VLDLR expression, thus inhibiting vascular inflammation (Qin et al., 2022). In addition, lncRNA H19 has also been reported to mediate astragaloside IV’s anti-atherosclerotic effect. H19 negatively regulates dual-specificity phosphatase 5 (DUSP5) expression and represses DUSP5/ERK1/2 axis (Tao et al., 2016). Astragaloside IV could attenuate autophagy and mineralization of VSMCs in atherosclerosis by up-regulating H19 and inhibiting DUSP5 (Song et al., 2019). In summary, astragaloside IV can regulate the function of endothelial cells, VSMCs, and macrophages in atherosclerosis by targeting multiple miRNAs, lncRNAs and circRNAs. Therefore, it can be expected that astragaloside IV can exert an excellent anti-atherosclerotic effect through ncRNAs in the clinic.
3.3 Notoginsenoside R1
Notoginsenoside R1, the monomer extracted from Panax notoginseng (Burkill) F.H.Chen, has a unique effect of promoting blood circulation and has been used on clinical treatment of cardiovascular diseases (Lei et al., 2022). Myeloid differentiation primary response gene 88 (MyD88) is an important immunoregulatory factor, and studies have found that inhibiting MyD88 has a good effect on diabetes (Androulidaki et al., 2018). Notoginsenoside R1 was found to relieve high glucose-induced endothelial cell inflammation and oxidative stress by downregulating the MyD88 via up-regulating miR-147a (Li and Huang, 2021). The Toll-like receptor 4 (TLR4)/Nuclear factor-κB (NF-κB) pathway participates in oxidative stress and induces atherosclerosis in ApoE−/− mice by up-regulating inflammatory cytokines (Tang et al., 2015). A study revealed that notoginsenoside R1 could upregulate the expression of miR-221-3p to target TLR4/NF-κB pathway, thereby inhibiting ox-LDL-induced endothelial cell apoptosis, oxidative stress, and inflammation (Zhu et al., 2020). Notoginsenoside R1 may also play a role in delaying senescence of endothelial cells. Notoginsenoside R1 can decrease the expressions of miR-34a and p53, while increase the expression of SIRT1, thus enhancing the intracellular superoxide dismutase (SOD) activity and cell proliferation capacity in hydrogen peroxide-induced endothelial cell aging model (Lai et al., 2018). These studies suggest that notoginsenoside R1 has a strong and multifaceted endothelial protective effect through regulating ncRNAs.
3.4 Tanshinone IIA, salvianolic acid B, tanshinol
Tanshinone, extracted from the traditional Chinese medicine Salvia miltiorrhiza Bunge, is a fat-soluble phenanthrene quinone compound with bacteriostatic effect (Wang et al., 2017). Among tanshinone, tanshinone IIA has been clinically proved to have a more significant effect on cardiovascular diseases, especially its anti-inflammatory effect on macrophages. Tanshinone IIA reduces the production of inflammatory factors and adipogenesis in macrophages by up-regulating miR-130b and downregulating WNT5A, thereby relieving the development of atherosclerosis (Yuan et al., 2020). Previous studies have demonstrated that miR-712 is involved in atherosclerosis-related pathological processes, such as VSMCs calcification and endothelial cell inflammation (Son et al., 2013). Tanshinone IIA can inhibit VSMCs inflammation and proliferation by inhibiting miR-712-5p (Qin et al., 2020). KLF4, an evolutionarily conserved zinc-finger-containing transcription factor, is thought to induce M2 and inhibit M1 macrophage polarization (Liao et al., 2011). A study found that the miR-375/KLF4 pathway plays a dominant role in macrophage polarization and autophagy, and tanshinone IIA could activate KLF4 by inhibiting miR-375, leading to enhanced autophagy as well as M2 polarization of macrophages (Chen et al., 2019a). Tropomyosin 1 (TPM1), as a target gene for miR-21-5p (Baker, 2011), is involved in the formation, stabilization and regulation of cytoskeletal actin fibers (Gunning et al., 2015). It was found that tanshinone IIA could downregulate miR-21-5p and then target TPM1, which helps to inhibit the proliferation and migration of VSMCs (Jia et al., 2019).
Salvianolic acid B, a water-soluble compound extracted from S. miltiorrhiza Bunge, has been used to treat cardiovascular diseases for hundreds of years. MiR-146a is involved in the regulation of cell proliferation, migration, differentiation, and apoptosis (Cheng et al., 2013). A study has found that salvianolic acid B can inhibit angiotensin II-induced VSMCs proliferation and improve carotid artery ligation-induced neointimal hyperplasia by downregulating miR-146a (Zhao et al., 2019).
Tanshinol is also an active ingredient isolated from S. miltiorrhiza Bunge which has the effect of protecting vascular endothelium and reducing atherosclerosis (Song et al., 2014). MiR-26a has been proved to have anti-apoptotic effect on endothelial cells (Zhang et al., 2015). A study found that tanshinol inhibits apoptosis of endothelial cells and reduces atherosclerotic lesions via decreasing lncRNA TUG1 and increasing miR-26a in endothelial cells (Chen et al., 2016).
3.5 Genkwanin
Genkwanin is one of the major non-glycosylated flavonoids extracted from Daphne genkwa Siebold & Zucc. (Bao et al., 2019). MKP-1 is a key negative regulator of macrophage signaling in response to inflammatory stimulis and is responsible for shutting down the production of pro-inflammatory cytokines (Chen et al., 2002; Chi et al., 2006). Genkwanin was proved to potently decrease the production of proinflammatory mediators through downregulating miR-101 and increasing MKP-1 (Gao et al., 2014).
3.6 Dihydromyricetin
Dihydromyricetin, a bioactive flavonoid isolated from Ampelopsis cantoniensis var. grossedentata Hand. -Mazz. and Ziziphus jujuba Mill., has been found to have a wide range of pharmacological activities, such as anti-inflammatory (Sun et al., 2021), analgesic (Guan et al., 2019), anti-tumor (Chen et al., 2020) and hepatoprotective effects (Silva et al., 2020). Nitric oxide (NO), produced by endothelial nitric oxide synthase (eNOS), plays a key role in maintaining endothelial function, and impaired NO biosynthesis is a hallmark of atherosclerosis (Tousoulis et al., 2012; Cyr et al., 2020). There is evidence that overexpression of dimethylarginine dimethylaminohydrolase-1 (DDAH1) increases NO production through an asymmetric dimethylarginine (ADMA) manner (Pope et al., 2009). Studies suggested that dihydromyricetin treatment inhibits atherosclerotic lesion formation by increasing NO production by endothelial cells. MiR-21 expression can be reduced by dihydromyricetin in endothelial cells, which increases DDAH1 and reduces ADMA levels (Yang et al., 2018; Yang et al., 2020). Taken together, dihydromyricetin activates endothelial DDAH1/ADMA/eNOS/NO pathway by reducing miR-21, which relieves the pathogenesis of atherosclerosis.
3.7 Sulforaphane
Sulforaphane is an isothiocyanate, which is produced by the conversion of glucoraphanin through the myrosinase (Vanduchova et al., 2019). Sulforaphane, a potent antioxidant, is primarily found in several Brassicaceae vegetables, such as broccoli, cauliflower, cabbage, and Brussels sprouts. Sulforaphane has often been shown to protect cells from oxidative stress in cardiomyocytes and neural cells (Guerrero-Beltran et al., 2012). The nuclear factor erythroid-2-related factor 2 (Nrf2), a basic leucine zipper transcription factor that serves as a defense mechanism against oxidative stress, has been shown to be activated by sulforaphane (Bai et al., 2015; Houghton et al., 2016). SIRT1 is a potential target gene of miR-34a (Yamakuchi et al., 2008) and the role of the miR-34a/SIRT1 axis in oxidative stress-induced cellular damage has been demonstrated (Guo et al., 2017). Sulforaphane was found to protect endothelial cells from oxidative stress by regulating the miR-34a/SIRT1 axis through upregulation of Nrf2 (Li et al., 2021c). In addition, a study found that sulforaphane can reduce lipopolysaccharide-induced cell damage and oxidative stress by inhibiting miR-155 in microglia (Eren et al., 2018). MiR-155 was proved to aggravate the carotid atherosclerotic lesion through induction of endothelial cell apoptosis and activation of inflammasome in macrophages (Yin et al., 2019b). Therefore, it is possible that sulforaphane may limit the formation of atherosclerotic lesions by inhibiting miR-155, but clearly, more studies are needed to confirm this hypothesis.
3.8 Cyanidin-3-O-glucoside
Anthocyanins are abundant natural water-soluble pigments, which are relatively rich in the skin of Glycine max (L.) Merr. These compounds have been shown to exert antioxidant and anti-inflammatory properties (Zhang et al., 2020a). Cyanidin-3-O-glucoside is one of the most abundant anthocyanins in nature. A study found that cyanidin-3-O-glucoside treatment not only suppresses blood lipids, but also improves endothelial cell function in a rabbit atherosclerotic model. Mechanistically, these effects are due to decreased expression of miR-204-5p, which leads to the increased expression of SIRT1 and enhanced endothelial cell function (Wang et al., 2020c).
3.9 Baicalin
Baicalin, one of the flavonoid compounds, is the main active component of traditional Chinese medicine Scutellaria baicalensis Georgi (Li et al., 2009). It has been shown that baicalin can alleviate the development of atherosclerosis through its anti-adipogenic, anti-inflammatory and antioxidant effects (Wu et al., 2018). The expression of miR-126 was found to be reduced in the peripheral blood of atherosclerotic patients (Jiang et al., 2014). High mobility group box 1 protein (HMGB1) is an essential facilitator of atherosclerosis by enhancing inflammation (Boteanu et al., 2017). It has been found that baicalin induces the upregulation of miR-126-5p and the downregulation of HMGB1, inhibiting ox-LDL-induced proliferation and migration of VSMCs (Chen et al., 2019b).
3.10 Curcumin
Curcumin is the main active ingredient of Curcuma longa L. and is mainly extracted from dried powdered turmeric. There is evidence that curcumin can modulate the inflammatory response and alleviate inflammatory diseases like atherosclerosis (Hasan et al., 2014; Chen et al., 2015). Studies found that the activated miR-126-3p from endothelial cells and VSMCs plays a key role in reducing vascular calcification (Zeng et al., 2021) and curcumin upregulates miR-126-3p expression (Li et al., 2021b). Therefore, we infer that miR-126-3p may be one of the targets of curcumin in the treatment of atherosclerosis. LncRNA MIAT has been shown to aggravate the atherosclerotic damage through the activation of PI3K/Akt signaling pathway (Sun et al., 2019). A study found that reduced expression of MIAT contributes to the protective effect of curcumin on atherosclerosis. MIAT regulates miR-124 by interacting with enhancer of zeste homolog 2 (EZH2), thereby relieving inflammation in endothelial cells (Ouyang et al., 2022). In addition, curcumin markedly suppresses miR-125a-5p and upregulates SIRT6 in macrophages, thereby regulating the ABCA1 expression and promoting cholesterol efflux of macrophages (Tan et al., 2021).
3.11 Epigallocatechin gallate
Epigallocatechin gallate (EGCG) is the most abundant catechin in green tea. EGCG has been shown to has various pharmacological effects including the anti-atherosclerotic effect, which is primarily achieved by promoting intracellular cholesterol efflux in macrophages (Jiang et al., 2012). Recent studies showed that miR-33a is an upstream regulator of ABCA1 (Wijesekara et al., 2012) and EGCG exerts anti-atherosclerotic effect by reducing miR-33a, thereby upregulating ABCA1 and promoting the efflux of cholesterol in macrophages (Yang et al., 2016).
3.12 Ginsenoside Rb2
Ginsenoside Rb2, extracted from Panax ginseng C.A. Mey., is a commonly used traditional Chinese medicine with antioxidant (Huang et al., 2014), anti-inflammatory (Huang et al., 2017) and anti-apoptotic activities (Gao et al., 2015). In macrophages, ginsenoside Rb2 has been found to exert anti-inflammatory effects by upregulating the expression of an ω-3 fatty acid receptor GPR120 (Huang et al., 2017). A recent study showed that ginsenoside Rb2 can also inhibit endothelial senescence and inflammation. Mechanistically, ginsenoside Rb2 has a specific binding affinity for miR-216a and further attenuates miR-216a-induced inflammatory processes and aging states through the Smad3/IκBα signaling pathway (Chen et al., 2021b).
3.13 Paeonol
Paeonol is one of the main active compounds in Tree Peony Bark, which has been found to have anti-inflammatory, anti-thrombotic and antioxidant properties (Fu et al., 2012a; Bao et al., 2013). Paeonol could increase the expression of miR-223 in macrophage-derived exosomes, and after the uptaking of exosomes by endothelial cells, the STAT3 signaling and the related inflammatory response in endothelial cells can be attenuated (Liu et al., 2018). Another study also found similarly protective results of paeonol on endothelial cells in hyperlipidemia-induced atherosclerosis, which is also attributed to cellular uptake of exosomal miR-223 (Shi et al., 2020). Additionally, paeonol also promotes miR-126 expression to inhibit monocyte adhesion to endothelial cells and block the activation of the PI3K/Akt/NF-κB signaling pathway (Yuan et al., 2016). Moreover, miR-21 and its target PTEN also contribute to the protective effects of paeonol on ox-LDL-induced endothelial injury (Liu et al., 2014). MiR-338-3p was proved to be increased in atherosclerotic lesions, and paeonol treatment could downregulates the expression of miR-338-3p and upregulates the expression of Tet methylcytosine dioxygenase 2 (TET2), thereby relieving ox-LDL-induced endothelial cell damage (Yin et al., 2019a; Yu et al., 2020b). Paeonol can also weaken ox-LDL-induced endothelial autophagy through regualting miR-30a/beclin-1 signaling (Li et al., 2018a). Overall, these studies indicate that paeonol has strong endothelial protective effects, which is associated with regulation of various miRNAs and their targets.
3.14 Puerarin
Pueraria lobata is the dried roots of legumes P. lobata (Willd.) Ohwi and Pueraria thunbergiana (Siebold & Zucc.) Benth. It is clinically used in the treatment of cardiovascular and cerebrovascular diseases (Wang et al., 2020a). Puerarin, an active monomer in Pueraria lobata, was reported to inhibit the proliferation and inflammation of VSMCs in atherosclerosis by reducing the expression of miR-29b-3p, thereby increasing the expression of insulin-like growth factor 1 (IGF1) (Li et al., 2023). Therefore, puerarin may have a beneficial effect in the treatment of atherosclerosis by regulating miRNA.
4 Conclusion and prospects
Atherosclerosis is a major cause of coronary heart disease, cerebral infarction, and some peripheral vascular diseases (Figure 1). With the improvement of living standards, the incidence and mortality of atherosclerosis-induced cardiovascular diseases have increased rapidly in recent years. During the development of atherosclerosis, abnormal expressions of ncRNAs affect the physiological functions of endothelial cells, macrophages, and VSMCs by regulating related signaling pathways or specific proteins. China has a long history of using herbal medicine to treat cardiovascular diseases and the anti-atheroscleroic effects of several herbal medicine are also demonstrated in animal experiments and human studies. The traditional Chinese medicine monomers have recently attracted more attention in the treatment of diseases because they have certain molecular structures, predicted pharmacological effects, less drug-drug interactions, and clear mechanisms of action. Many active monomers derived from traditional Chinese medicines have been evaluated in vivo and in vitro to ameliorate the development of atherosclerosis by targeting ncRNAs. This article reviews 16 active monomers in traditional Chinese medicine that can improve the development of atherosclerosis by targeting ncRNAs in endothelial cells, macrophages, and VSMCs (Table 1; Figures 2–4). Their structures are shown in Figure 5. Besides monomeric Chinese herbal extracts, Chinese herbal formulas and decoctions have also been proved to treat atherosclerosis by targeting ncRNAs. For example, Tongxinluo Capsule inhibits vascular inflammation and neointimal hyperplasia by inhibiting the expression of miR-155, thereby blocking the feedback loop between miR-155 and TNF-α (Zhang et al., 2014). Alismatis rhizoma decoction, a classic traditional Chinese Medicinal formula used for the treatment of cardiovascular and cerebrovascular diseases, can inhibit the expression of ERK1/2 and miR-17∼92a to inhibit ox-LDL-stimulated VSMCs proliferation (Shen et al., 2020). Among the ncRNAs regulated by active monomers of traditional Chinese medicine, miRNAs are the most studied. However, whether traditional Chinese medicine can exert functions via regulating lncRNAs, circRNAs or other ncRNAs is not well studied and requires more research.
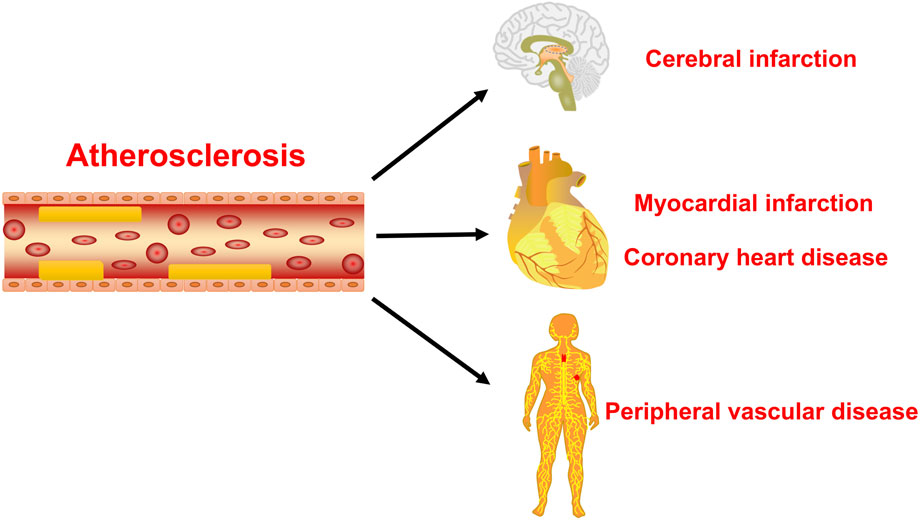
FIGURE 1. The main hazards of atherosclerosis. The atherosclerosis is the main cause of coronary heart disease, cerebral infarction, and peripheral vascular disease.
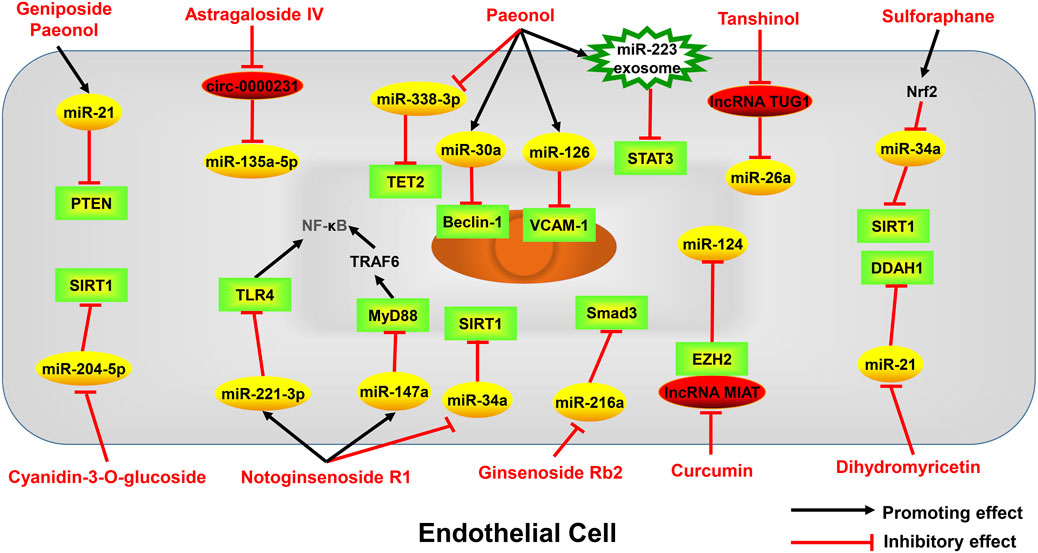
FIGURE 2. Active monomers of traditional Chinese medicine improve the occurrence and development of atherosclerosis through regulating ncRNAs in endothelial cells. PTEN phosphatase and tensin homolog deleted on chromosome ten, VCAM-1 Vascular cell adhesion molecule-1, TLR4 toll-like receptor 4, MyD88 myeloid differentiation primary response 88, TRAF6 TNF receptor-associated factor 6, NF-κB nuclear factor kappa-B, SIRT1 sirtuin-1, DDAH1 dimethylarginine dimethylaminohydrolase 1, EZH2 enhancer of zeste homolog 2, p53 tumor protein p53, Smad3 sma- and mad-related protein 3, STAT3 signal transducer and activator of transcription 3, TET2 tet methylcytosine dioxygenase 2.
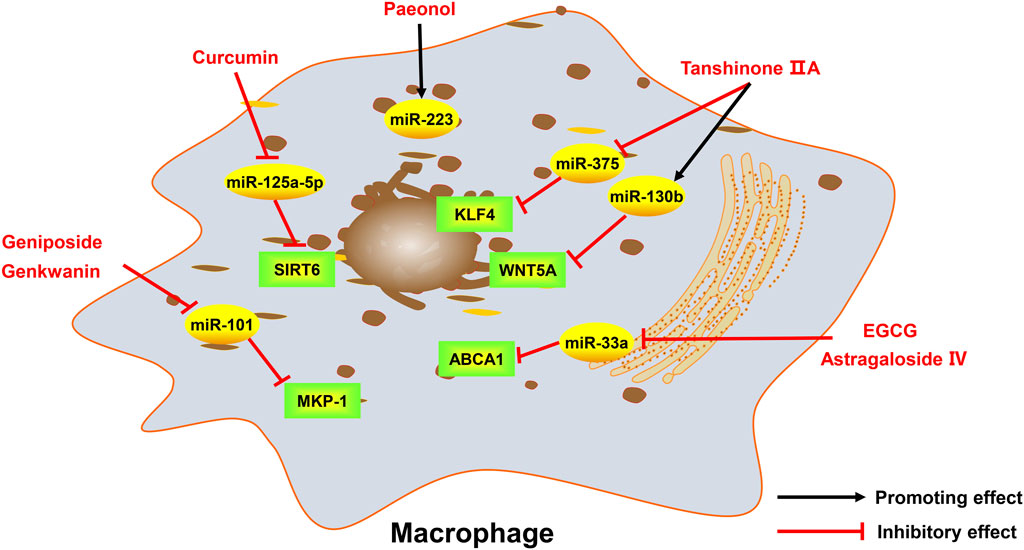
FIGURE 3. Active monomers of traditional Chinese medicine improve the occurrence and development of atherosclerosis through regulating ncRNAs in macrophages. MKP-1 mitogen-activated protein kinase phosphatase 1, ABCA1 ATP-binding cassette transporter A1, WNT5A wingless/integrated-5A, KLF4 krüppel-like factor 4, SIRT6 sirtuin 6.
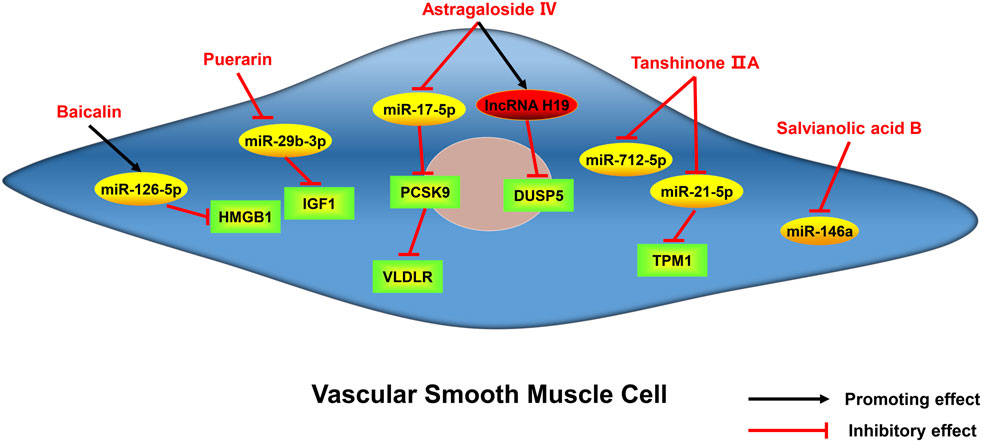
FIGURE 4. Active monomers of traditional Chinese medicine improve the occurrence and development of atherosclerosis through regulating ncRNAs in vascular smooth muscle cells. DUSP5 dual specificity phosphatase 5, PCSK9 proprotein convertase subtilisin/kexin type 9, TPM1 tropomyosin 1, IGF1 insulin-like growth factor 1, HMGB1 high mobility group box 1 protein, VLDLR very low-density lipoprotein receptor.
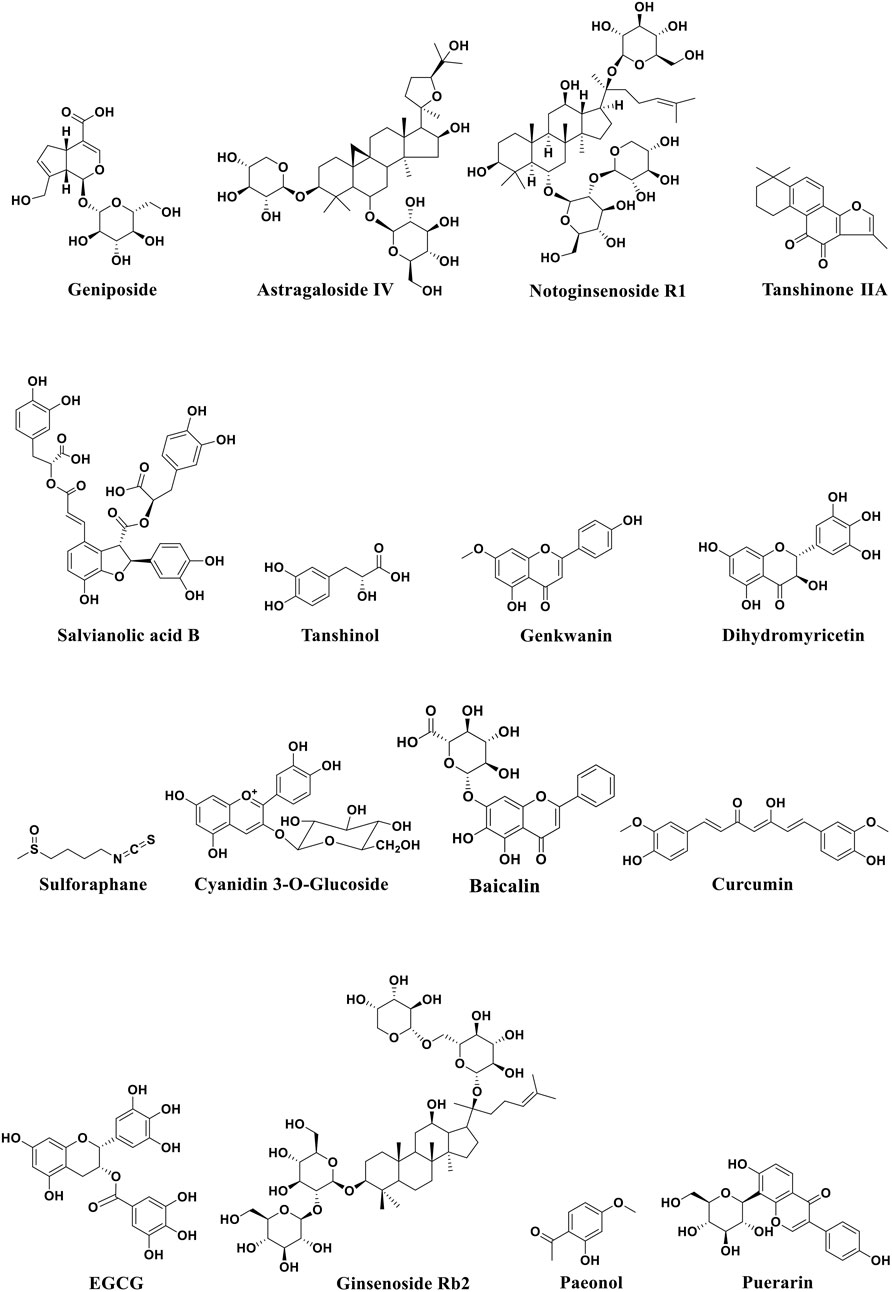
FIGURE 5. Structural formula of active monomers of traditional Chinese medicine that exhibit anti-atherosclerotic activities.
NcRNAs are the most abundant transcripts in cells. In addition to seaching ncRNAs from published papers, we can identify or screen new ncRNAs in the following ways: firsty, we can utilize publicly available genomic and transcriptomic databases, such as Ensembl, NCBI, and UCSC to identify regions of the genome that are transcribed but not coding for proteins, indicating potential ncRNAs; additionally, high-throughput sequencing like RNA-Seq can be used to identify novel transcripts, including potential ncRNAs; furthermore, we can also compare the genomic sequences across various species in order to find conserved non-coding regions, which may potentially serve as ncRNAs; besides, machine learning algorithms based on sequence features and structural properties of ncRNAs can also be used to predict potential novel ncRNAs. After discovering new ncRNAs, techniques like CRISPR/Cas9, RNA interference, qRT-PCR, Northern blotting, in situ hybridization and other functional assays can be used to identify the specific biological functions of the ncRNAs. It can be expected that future studies will find more and more ncRNAs that related to atherosclerosis and these ncRNAs can be used as drug targets for development of anti-atherosclerotic drugs.
Over the past decades, substantial effort has been made towards the clinical application of RNA-based therapeutics, such as small interfering RNAs and antisense oligonucleotides. However, since the hurdle of immunogenicity, specificity, and delivery, some studies demonstrated limited efficacy or toxicity of ncRNAs-based therapies. Therefore, traditional Chinese medicine may become alternative drugs by targeting ncRNAs to treat atherosclerosis. It is worth noting that most studies suggest that traditional Chinese medicine treats atherosclerosis by targeting a specific ncRNA. However, the mechanism of ceRNA suggests that ncRNAs may have complex interactions in cells. What’s more, a ncRNA may also have multiple targets. Therefore, we should further explore the anti-atherosclerotic mechanisms and clinical safety of these traditional Chinese medicine in more detail. It is hoped that by studying the regulation of ncRNAs by traditional Chinese medicine, it will provide theoretical support for the future research and clinical application of traditional Chinese medicine for treatment of atherosclerosis.
While many traditional Chinese medicines have therapeutic effects on atherosclerosis, some research has also identified potential side effects of certain Chinese herbs that can exacerbate atherosclerosis. For example, a moderate dosage of marijuana proves highly efficient in alleviating chronic pain (Carter et al., 2015), but marijuana can also cause cardiovascular side effects, such as endothelial dysfunction and atherosclerosis (Feng et al., 2022). Proanthocyanidin A1 can promotes the production of platelets to ameliorate chemotherapy-induced thrombocytopenia (Wang et al., 2022) and TMEA, a polyphenol in Sanguisorba officinalis L., can facilitate megakaryocyte differentiation and platelet production (Li et al., 2021a). However, the increased platelets can raise the risk of blood clot formation in patients with atherosclerosis (Barrett et al., 2019). Therefore, when patients have concurrent atherosclerosis, the use of these Chinese herbal medicines should be avoided. Furthermore, studying the ncRNAs that may mediate these effects is of significant importance, but this field is still lacking in research and requires further investigation.
Author contributions
GL: Conceptualization, Data curation, Methodology, Writing–original draft. LT: Writing–review and editing. XZ: Data curation, Writing–review and editing. MW: Software, Writing–review and editing. ZZ: Data curation, Writing–review and editing. JZ: Validation, Writing–review and editing. HG: Data curation, Writing–review and editing. ML: Writing–review and editing. WQ: Funding acquisition, Supervision, Writing–review and editing, Conceptualization.
Funding
The author(s) declare financial support was received for the research, authorship, and/or publication of this article. This work was supported by National Natural Science Foundation of China (82073846 and 81800399), Joint Fund of Shandong Natural Science Foundation (ZR2021LZY007), Shandong Province Universities’s Plan for Youth Innovation Teams (2022KJ103). Key R&D Program of Jining (2022YXNS153), and Rizhao Outstanding Youth Science Fund (RZ2021ZR22).
Conflict of interest
The authors declare that the research was conducted in the absence of any commercial or financial relationships that could be construed as a potential conflict of interest.
Publisher’s note
All claims expressed in this article are solely those of the authors and do not necessarily represent those of their affiliated organizations, or those of the publisher, the editors and the reviewers. Any product that may be evaluated in this article, or claim that may be made by its manufacturer, is not guaranteed or endorsed by the publisher.
References
Androulidaki, A., Wachsmuth, L., Polykratis, A., and Pasparakis, M. (2018). Differential role of MyD88 and TRIF signaling in myeloid cells in the pathogenesis of autoimmune diabetes. PloS ONE 13 (3), e0194048. doi:10.1371/journal.pone.0194048
Aryal, B., and Suarez, Y. (2019). Non-coding RNA regulation of endothelial and macrophage functions during atherosclerosis. Vasc. Pharmacol. 114, 64–75. doi:10.1016/j.vph.2018.03.001
Augoff, K., McCue, B., Plow, E. F., and Sossey-Alaoui, K. (2012). miR-31 and its host gene lncRNA LOC554202 are regulated by promoter hypermethylation in triple-negative breast cancer. Mol. Cancer 11, 5. doi:10.1186/1476-4598-11-5
Bai, Y., Wang, X., Zhao, S., Ma, C., Cui, J., and Zheng, Y. (2015). Sulforaphane protects against cardiovascular disease via Nrf2 activation. Oxid. Med. Cell. Longev. 2015, 407580. doi:10.1155/2015/407580
Baker, A. H. (2011). MicroRNA 21 "shapes" vascular smooth muscle behavior through regulating tropomyosin 1. Arterioscler. Thromb. Vasc. Biol. 31 (9), 1941–1942. doi:10.1161/ATVBAHA.111.231985
Bao, M. H., Zhang, Y. W., and Zhou, H. H. (2013). Paeonol suppresses oxidized low-density lipoprotein induced endothelial cell apoptosis via activation of LOX-1/p38MAPK/NF-κB pathway. J. Ethnopharmacol. 146 (2), 543–551. doi:10.1016/j.jep.2013.01.019
Bao, Y., Sun, Y. W., Ji, J., Gan, L., Zhang, C. F., Wang, C. Z., et al. (2019). Genkwanin ameliorates adjuvant-induced arthritis in rats through inhibiting JAK/STAT and NF-κB signaling pathways. Phytomedicine 63, 153036. doi:10.1016/j.phymed.2019.153036
Barrett, T. J., Schlegel, M., Zhou, F., Gorenchtein, M., Bolstorff, J., Moore, K. J., et al. (2019). Platelet regulation of myeloid suppressor of cytokine signaling 3 accelerates atherosclerosis. Sci. Transl. Med. 11 (517), eaax0481. doi:10.1126/scitranslmed.aax0481
Bazioti, V., La Rose, A. M., Maassen, S., Bianchi, F., de Boer, R., Halmos, B., et al. (2022). T cell cholesterol efflux suppresses apoptosis and senescence and increases atherosclerosis in middle aged mice. Nat. Commun. 13 (1), 3799. doi:10.1038/s41467-022-31135-4
Bellosta, S., and Corsini, A. (2018). Statin drug interactions and related adverse reactions: an update. Expert Opin. Drug Saf. 17 (1), 25–37. doi:10.1080/14740338.2018.1394455
Boteanu, R. M., Suica, V. I., Uyy, E., Ivan, L., Dima, S. O., Popescu, I., et al. (2017). Alarmins in chronic noncommunicable diseases: atherosclerosis, diabetes and cancer. J. Proteomics 153, 21–29. doi:10.1016/j.jprot.2016.11.006
Bridges, M. C., Daulagala, A. C., and Kourtidis, A. (2021). LNCcation: lncRNA localization and function. J. Cell Biol. 220 (2), e202009045. doi:10.1083/jcb.202009045
Carter, G. T., Javaher, S. P., Nguyen, M. H. V., Garret, S., and Carlini, B. H. (2015). Re-branding cannabis: the next generation of chronic pain medicine? Pain Manag. 5 (1), 13–21. doi:10.2217/pmt.14.49
Chen, C., Cheng, G., Yang, X., Li, C., Shi, R., and Zhao, N. (2016). Tanshinol suppresses endothelial cells apoptosis in mice with atherosclerosis via lncRNA TUG1 up-regulating the expression of miR-26a. Am. J. Transl. Res. 8 (7), 2981–2991.
Chen, F. L., Yang, Z. H., Liu, Y., Li, L. X., Liang, W. C., Wang, X. C., et al. (2008). Berberine inhibits the expression of TNFalpha, MCP-1, and IL-6 in AcLDL-stimulated macrophages through PPARgamma pathway. Endocrine 33 (3), 331–337. doi:10.1007/s12020-008-9089-3
Chen, F. Y., Zhou, J., Guo, N., Ma, W. G., Huang, X., Wang, H., et al. (2015). Curcumin retunes cholesterol transport homeostasis and inflammation response in M1 macrophage to prevent atherosclerosis. Biochem. Biophys. Res. Commun. 467 (4), 872–878. doi:10.1016/j.bbrc.2015.10.051
Chen, L., Wang, C., Sun, H., Wang, J., Liang, Y., Wang, Y., et al. (2021a). The bioinformatics toolbox for circRNA discovery and analysis. Brief. Bioinform. 22 (2), 1706–1728. doi:10.1093/bib/bbaa001
Chen, L., Yang, Z. S., Zhou, Y. Z., Deng, Y., Jiang, P., and Tan, S. L. (2020). Dihydromyricetin inhibits cell proliferation, migration, invasion and promotes apoptosis via regulating miR-21 in human cholangiocarcinoma cells. J. Cancer 11 (19), 5689–5699. doi:10.7150/jca.45970
Chen, L., Zhao, Z. W., Zeng, P. H., Zhou, Y. J., and Yin, W. J. (2022). Molecular mechanisms for ABCA1-mediated cholesterol efflux. Cell Cycle 21 (11), 1121–1139. doi:10.1080/15384101.2022.2042777
Chen, L. L. (2016). The biogenesis and emerging roles of circular RNAs. Nat. Rev. Mol. Cell BIO 17 (4), 205–211. doi:10.1038/nrm.2015.32
Chen, P., Li, J., Barnes, J., Kokkonen, G. C., Lee, J. C., and Liu, Y. (2002). Restraint of proinflammatory cytokine biosynthesis by mitogen-activated protein kinase phosphatase-1 in lipopolysaccharide-stimulated macrophages. J. Immunol. 169 (11), 6408–6416. doi:10.4049/jimmunol.169.11.6408
Chen, W., Li, X., Guo, S., Song, N., Wang, J., Jia, L., et al. (2019a). Tanshinone IIA harmonizes the crosstalk of autophagy and polarization in macrophages via miR-375/KLF4 pathway to attenuate atherosclerosis. Int. Immunopharmacol. 70, 486–497. doi:10.1016/j.intimp.2019.02.054
Chen, Y., Wang, S., Yang, S., Li, R., Yang, Y., Chen, Y., et al. (2021b). Inhibitory role of ginsenoside Rb2 in endothelial senescence and inflammation mediated by microRNA-216a. Mol. Med. Rep. 23 (6), 415. doi:10.3892/mmr.2021.12054
Chen, Z., Pan, X., Sheng, Z., Yan, G., Chen, L., and Ma, G. (2019b). Baicalin suppresses the proliferation and migration of ox-LDL-VSMCs in atherosclerosis through upregulating miR-126-5p. Biol. Pharm. Bull. 42 (9), 1517–1523. doi:10.1248/bpb.b19-00196
Cheng, H. S., Sivachandran, N., Lau, A., Boudreau, E., Zhao, J. L., Baltimore, D., et al. (2013). MicroRNA-146 represses endothelial activation by inhibiting pro-inflammatory pathways. EMBO Mol. Med. 5 (7), 1017–1034. doi:10.1002/emmm.201202318
Cheng, S., Zhou, F., Xu, Y., Liu, X., Zhang, Y., Gu, M., et al. (2019). Geniposide regulates the miR-101/MKP-1/p38 pathway and alleviates atherosclerosis inflammatory injury in ApoE(-/-) mice. Immunobiology 224 (2), 296–306. doi:10.1016/j.imbio.2018.12.005
Chi, H., Barry, S. P., Roth, R. J., Wu, J. J., Jones, E. A., Bennett, A. M., et al. (2006). Dynamic regulation of pro- and anti-inflammatorycytokines by MAPK phosphatase 1 (MKP-1) in innate immune responses. Proc. Natl. Acad. Sci. U. S. A. 103 (7), 2274–2279. doi:10.1073/pnas.0510965103
Cyr, A. R., Huckaby, L. V., Shiva, S. S., and Zuckerbraun, B. S. (2020). Nitric Oxide and endothelial dysfunction. Crit. Care Clin. 36 (2), 307–321. doi:10.1016/j.ccc.2019.12.009
Deng, Z., and Li, L. (2022). Effect of miR-663 on atherosclerosis by regulating the proliferation of vascular smooth muscle cells in lipid plaques. Vascular 23, 170853812210988. doi:10.1177/17085381221098826
Di Mauro, V., Barandalla-Sobrados, M., and Catalucci, D. (2018). The noncoding-RNA landscape in cardiovascular health and disease. NCRNA 3 (1), 12–19. doi:10.1016/j.ncrna.2018.02.001
Eken, S. M., Jin, H., Chernogubova, E., Li, Y., Simon, N., Sun, C., et al. (2017). MicroRNA-210 enhances fibrous cap stability in advanced atherosclerotic lesions. Circ. Res. 120 (4), 633–644. doi:10.1161/CIRCRESAHA.116.309318
Eren, E., Tufekci, K. U., Isci, K. B., Tastan, B., Genc, K., and Genc, S. (2018). Sulforaphane inhibits lipopolysaccharide-induced inflammation, cytotoxicity, oxidative stress, and miR-155 expression and switches to mox phenotype through activating extracellular signal-regulated kinase 1/2-nuclear factor erythroid 2-related factor 2/antioxidant response element pathway in murine microglial cells. Front. Immunol. 9, 36. doi:10.3389/fimmu.2018.00036
Feinberg, M. W., and Moore, K. J. (2016). MicroRNA regulation of atherosclerosis. Circ. Res. 118 (4), 703–720. doi:10.1161/CIRCRESAHA.115.306300
Feng, X., Xu, S., and Weng, J. (2022). Marijuana and endothelial dysfunction: new mechanism and therapy. Trends Mol. Med. 28 (8), 613–615. doi:10.1016/j.molmed.2022.05.009
Fiedler, J., and Thum, T. (2016). New insights into miR-17-92 cluster regulation and angiogenesis. Circ. Res. 118 (1), 9–11. doi:10.1161/CIRCRESAHA.115.307935
Fu, P. K., Wu, C. L., Tsai, T. H., and Hsieh, C. L. (2012a). Anti-inflammatory and anticoagulative effects of paeonol on LPS-induced acute lung injury in rats. Evid. Based Complement. Altern. Med. 2012, 837513. doi:10.1155/2012/837513
Fu, Y., Liu, B., Liu, J., Liu, Z., Liang, D., Li, F., et al. (2012b). Geniposide, from Gardenia jasminoides Ellis, inhibits the inflammatory response in the primary mouse macrophages and mouse models. Int. Immunopharmacol. 14 (4), 792–798. doi:10.1016/j.intimp.2012.07.006
Gao, B., Huang, Q., Jie, Q., Zhang, H. Y., Wang, L., Guo, Y. S., et al. (2015). Ginsenoside-Rb2 inhibits dexamethasone-induced apoptosis through promotion of GPR120 induction in bone marrow-derived mesenchymal stem cells. Stem Cells Dev. 24 (6), 781–790. doi:10.1089/scd.2014.0367
Gao, F., Wang, X. C., Luo, Z. D., Hu, G. Q., Ma, M. Q., Liang, Y., et al. (2022). LncRNA HOXA11-AS promotes vascular endothelial cell injury in atherosclerosis by regulating the miR-515-5p/ROCK1 axis. Esc. heart Fail 9 (4), 2259–2271. doi:10.1002/ehf2.13815
Gao, J. H., Zeng, M. Y., Yu, X. H., Zeng, G. F., He, L. H., Zheng, X. L., et al. (2018). Visceral adipose tissue-derived serine protease inhibitor accelerates cholesterol efflux by up-regulating ABCA1 expression via the NF-κB/miR-33a pathway in THP-1 macropahge-derived foam cells. Biochem. Biophys. Res. Commun. 500 (2), 318–324. doi:10.1016/j.bbrc.2018.04.066
Gao, Y., Liu, F., Fang, L., Cai, R., Zong, C., and Qi, Y. (2014). Genkwanin inhibits proinflammatory mediators mainly through the regulation of miR-101/MKP-1/MAPK pathway in LPS-activated macrophages. PloS ONE 9 (5), e96741. doi:10.1371/journal.pone.0096741
Gong, L., Chang, H., Zhang, J., Guo, G., Shi, J., and Xu, H. (2018). Astragaloside IV protects rat cardiomyocytes from hypoxia-induced injury by downregulation of miR-23a and miR-92a. Cell. Physiol. biochem. 49 (6), 2240–2253. doi:10.1159/000493827
Guan, S., Shen, Y., Ge, H., Xiong, W., He, L., Liu, L., et al. (2019). Dihydromyricetin alleviates diabetic neuropathic pain and depression comorbidity symptoms by inhibiting P2X(7) receptor. Front. Psychiatry 10, 770. doi:10.3389/fpsyt.2019.00770
Guerrero-Beltran, C. E., Calderon-Oliver, M., Pedraza-Chaverri, J., and Chirino, Y. I. (2012). Protective effect of sulforaphane against oxidative stress: recent advances. Exp. Toxicol. Pathol. 64 (5), 503–508. doi:10.1016/j.etp.2010.11.005
Gunning, P. W., Hardeman, E. C., Lappalainen, P., and Mulvihill, D. P. (2015). Tropomyosin - master regulator of actin filament function in the cytoskeleton. J. Cell Sci. 128 (16), 2965–2974. doi:10.1242/jcs.172502
Guo, F. X., Wu, Q., Li, P., Zheng, L., Ye, S., Dai, X. Y., et al. (2019). The role of the LncRNA-FA2H-2-MLKL pathway in atherosclerosis by regulation of autophagy flux and inflammation through mTOR-dependent signaling. Cell Death Differ. 26 (9), 1670–1687. doi:10.1038/s41418-018-0235-z
Guo, Y., Li, P., Gao, L., Zhang, J., Yang, Z., Bledsoe, G., et al. (2017). Kallistatin reduces vascular senescence and aging by regulating microRNA-34a-SIRT1 pathway. Aging Cell 16 (4), 837–846. doi:10.1111/acel.12615
Guttman, M., and Rinn, J. L. (2012). Modular regulatory principles of large non-coding RNAs. Nature 482 (7385), 339–346. doi:10.1038/nature10887
Hasan, S. T., Zingg, J. M., Kwan, P., Noble, T., Smith, D., and Meydani, M. (2014). Curcumin modulation of high fat diet-induced atherosclerosis and steatohepatosis in LDL receptor deficient mice. Atherosclerosis 232 (1), 40–51. doi:10.1016/j.atherosclerosis.2013.10.016
Houghton, C. A., Fassett, R. G., and Coombes, J. S. (2016). Sulforaphane and other nutrigenomic Nrf2 activators: can the clinician's expectation be matched by the reality? Oxid. Med. Cell. Longev. 2016, 7857186. doi:10.1155/2016/7857186
Huang, H. S., Huang, X. Y., Yu, H. Z., Xue, Y., and Zhu, P. L. (2020). Circular RNA circ-RELL1 regulates inflammatory response by miR-6873-3p/MyD88/NF-κB axis in endothelial cells. Biochem. Biophys. Res. Commun. 525 (2), 512–519. doi:10.1016/j.bbrc.2020.02.109
Huang, Q., Gao, B., Jie, Q., Wei, B. Y., Fan, J., Zhang, H. Y., et al. (2014). Ginsenoside-Rb2 displays anti-osteoporosis effects through reducing oxidative damage and bone-resorbing cytokines during osteogenesis. Bone 66, 306–314. doi:10.1016/j.bone.2014.06.010
Huang, Q., Wang, T., and Wang, H. Y. (2017). Ginsenoside Rb2 enhances the anti-inflammatory effect of omega-3 fatty acid in LPS-stimulated RAW264.7 macrophages by upregulating GPR120 expression. Acta Pharmacol. Sin. 38 (2), 192–200. doi:10.1038/aps.2016.135
Jia, S., Ma, W. D., Zhang, C. Y., Zhang, Y., Yao, Z. H., Quan, X. H., et al. (2019). Tanshinone IIA attenuates high glucose induced human VSMC proliferation and migration through miR-21-5p-mediated tropomyosin 1 downregulation. Arch. Biochem. Biophys. 677, 108154. doi:10.1016/j.abb.2019.108154
Jiang, J., Mo, Z. C., Yin, K., Zhao, G. J., Lv, Y. C., Ouyang, X. P., et al. (2012). Epigallocatechin-3-gallate prevents TNF-α-induced NF-κB activation thereby upregulating ABCA1 via the Nrf2/Keap1 pathway in macrophage foam cells. Int. J. Mol. Med. 29 (5), 946–956. doi:10.3892/ijmm.2012.924
Jiang, Y., Wang, H. Y., Li, Y., Guo, S. H., Zhang, L., Cai, J. H., et al. (2014). Peripheral blood miRNAs as a biomarker for chronic cardiovascular diseases. Sci. Rep. 4, 5026. doi:10.1038/srep05026
Lai, X. H., Lei, Y., Yang, J., and Xiu, C. K. (2018). Effect of microRNA-34a/SIRT1/p53 signal pathway on notoginsenoside R₁ delaying vascular endothelial cell senescence. China J. Chin. Mater. Med. 43 (3), 577–584. doi:10.19540/j.cnki.cjcmm.20180110.001
Lei, W., Yan, Y., Ma, Y., Jiang, M., Zhang, B., Zhang, H., et al. (2022). Notoginsenoside R1 regulates ischemic myocardial lipid metabolism by activating the AKT/mTOR signaling pathway. Front. Pharmacol. 13, 905092. doi:10.3389/fphar.2022.905092
Li, C., Yang, L., Wu, H., and Dai, M. (2018a). Paeonol inhibits oxidized low-density lipoprotein-induced vascular endothelial cells autophagy by upregulating the expression of miRNA-30a. Front. Pharmacol. 9, 95. doi:10.3389/fphar.2018.00095
Li, C., Zhou, L., Lin, G., and Zuo, Z. (2009). Contents of major bioactive flavones in proprietary traditional Chinese medicine products and reference herb of radix scutellariae. J. Pharm. Biomed. Anal. 50 (3), 298–306. doi:10.1016/j.jpba.2009.04.028
Li, F. P., Lin, D. Q., and Gao, L. Y. (2018b). LncRNA TUG1 promotes proliferation of vascular smooth muscle cell and atherosclerosis through regulating miRNA-21/PTEN axis. Eur. Rev. Med. Pharmacol. Sci. 22 (21), 7439–7447. doi:10.26355/eurrev_201811_16284
Li, H., Jiang, X., Shen, X., Sun, Y., Jiang, N., Zeng, J., et al. (2021a). TMEA, a polyphenol in Sanguisorba officinalis, promotes thrombocytopoiesis by upregulating PI3K/akt signaling. Front. Cell Dev. Biol. 9, 708331. doi:10.3389/fcell.2021.708331
Li, J., Li, Y., Yuan, X., Yao, D., Gao, Z., Niu, Z., et al. (2023). The effective constituent puerarin, from Pueraria lobata, inhibits the proliferation and inflammation of vascular smooth muscle in atherosclerosis through the miR-29b-3p/IGF1 pathway. Pharm. Biol. 61 (1), 1–11. doi:10.1080/13880209.2022.2099430
Li, R., Hu, Y., and Hou, S. (2022). An exploration of oral-gut pathogens mediating immune escape of pancreatic cancer via miR-21/PTEN axis. Front. Microbiol. 13, 928846. doi:10.3389/fmicb.2022.928846
Li, S., Stockl, S., Lukas, C., Herrmann, M., Brochhausen, C., Konig, M. A., et al. (2021b). Curcumin-primed human BMSC-derived extracellular vesicles reverse IL-1β-induced catabolic responses of OA chondrocytes by upregulating miR-126-3p. Stem Cell. Res. Ther. 12 (1), 252. doi:10.1186/s13287-021-02317-6
Li, T., Pang, Q., Liu, Y., Bai, M., Peng, Y., and Zhang, Z. (2021c). Sulforaphane protects human umbilical vein endothelial cells from oxidative stress via the miR-34a/SIRT1 axis by upregulating nuclear factor erythroid-2-related factor 2. Exp. Ther. Med. 21 (3), 186. doi:10.3892/etm.2021.9617
Li, X. Q., and Huang, T. Y. (2021). Notoginsenoside R1 alleviates high glucose-induced inflammation and oxidative stress in HUVECs via upregulating miR-147a. Kaohsiung J. Med. Sci. 37 (12), 1101–1112. doi:10.1002/kjm2.12433
Li, Y., Zhang, Y., Lu, J., Yin, Y., Xie, J., and Xu, B. (2021d). Anti-inflammatory mechanisms and research progress of colchicine in atherosclerotic therapy. J. Cell. Mol. Med. 25 (17), 8087–8094. doi:10.1111/jcmm.16798
Li, Z., Xie, X., Fan, X., and Li, X. (2020). Long Non-coding RNA MINCR regulates miR-876-5p/GSPT1 axis to aggravate glioma progression. Neurochem. Res. 45 (7), 1690–1699. doi:10.1007/s11064-020-03029-8
Liang, G., Wang, S., Shao, J., Jin, Y. J., Xu, L., Yan, Y., et al. (2022). Tenascin-X mediates flow-induced suppression of EndMT and atherosclerosis. Circ. Res. 130 (11), 1647–1659. doi:10.1161/CIRCRESAHA.121.320694
Liao, X., Sharma, N., Kapadia, F., Zhou, G., Lu, Y., Hong, H., et al. (2011). Kruppel-like factor 4 regulates macrophage polarization. J. Clin. Invest. 121 (7), 2736–2749. doi:10.1172/JCI45444
Libby, P. (2021). The changing landscape of atherosclerosis. Nature 592 (7855), 524–533. doi:10.1038/s41586-021-03392-8
Libby, P., Buring, J. E., Badimon, L., Hansson, G. K., Deanfield, J., Bittencourt, M. S., et al. (2019). Atherosclerosis. Nat. Rev. Dis. Prim. 5 (1), 56. doi:10.1038/s41572-019-0106-z
Liu, Y., Li, C., Wu, H., Xie, X., Sun, Y., and Dai, M. (2018). Paeonol attenuated inflammatory response of endothelial cells via stimulating monocytes-derived exosomal MicroRNA-223. Front. Pharmacol. 9, 1105. doi:10.3389/fphar.2018.01105
Liu, Y. R., Chen, J. J., and Dai, M. (2014). Paeonol protects rat vascular endothelial cells from ox-LDL-induced injury in vitro via downregulating microRNA-21 expression and TNF-alpha release. Acta Pharmacol. Sin. 35 (4), 483–488. doi:10.1038/aps.2013.190
Lu, T. X., and Rothenberg, M. E. (2018). MicroRNA. J. Allergy Clin. Immunol. 141 (4), 1202–1207. doi:10.1016/j.jaci.2017.08.034
Luo, H., Chen, J., Su, C., and Zha, L. (2022). Advances in the bioactivities of phytochemical saponins in the prevention and treatment of atherosclerosis. Nutrients 14 (23), 4998. doi:10.3390/nu14234998
Maitrias, P., Metzinger-Le Meuth, V., Nader, J., Reix, T., Caus, T., and Metzinger, L. (2017). The involvement of miRNA in carotid-related stroke. Arterioscler. Thromb. Vasc. Biol. 37 (9), 1608–1617. doi:10.1161/ATVBAHA.117.309233
Meiler, S., Baumer, Y., Toulmin, E., Seng, K., and Boisvert, W. A. (2015). MicroRNA 302a is a novel modulator of cholesterol homeostasis and atherosclerosis. Arterioscler. Thromb. Vasc. Biol. 35 (2), 323–331. doi:10.1161/ATVBAHA.114.304878
Menghini, R., Casagrande, V., Cardellini, M., Martelli, E., Terrinoni, A., Amati, F., et al. (2009). MicroRNA 217 modulates endothelial cell senescence via silent information regulator 1. Circulation 120 (15), 1524–1532. doi:10.1161/CIRCULATIONAHA.109.864629
Okuyama, H., Langsjoen, P. H., Hamazaki3, T., Ogushi, Y., Hama, R., Kobayashi, T., et al. (2015). Statins stimulate atherosclerosis and heart failure: pharmacological mechanisms. Expert Rev. Clin. Pharmacol. 8 (2), 189–199. doi:10.1586/17512433.2015.1011125
Ouyang, S., Zhang, O., Xiang, H., Yao, Y. H., and Fang, Z. Y. (2022). Curcumin improves atherosclerosis by inhibiting the epigenetic repression of lncRNA MIAT to miR-124. Vascular 30, 1213–1223. doi:10.1177/17085381211040974
Pankratz, F., Hohnloser, C., Bemtgen, X., Jaenich, C., Kreuzaler, S., Hoefer, I., et al. (2018). MicroRNA-100 suppresses chronic vascular inflammation by stimulation of endothelial autophagy. Circ. Res. 122 (3), 417–432. doi:10.1161/CIRCRESAHA.117.311428
Park, K. H., Park, Y. D., Han, J. M., Im, K. R., Lee, B. W., Jeong, I. Y., et al. (2006). Anti-atherosclerotic and anti-inflammatory activities of catecholic xanthones and flavonoids isolated from cudrania tricuspidata. Bioorg. Med. Chem. Lett. 16 (21), 5580–5583. doi:10.1016/j.bmcl.2006.08.032
Perez-Sanchez, C., Aguirre, M. A., Ruiz-Limon, P., Abalos-Aguilera, M. C., Jimenez-Gomez, Y., Arias-de la Rosa, I., et al. (2017). Ubiquinol effects on antiphospholipid syndrome prothrombotic profile: a randomized, placebo-controlled trial. Arterioscler. Thromb. Vasc. Biol. 37 (10), 1923–1932. doi:10.1161/ATVBAHA.117.309225
Pope, A. J., Karrupiah, K., Kearns, P. N., Xia, Y., and Cardounel, A. J. (2009). Role of dimethylarginine dimethylaminohydrolases in the regulation of endothelial nitric oxide production. J. Biol. Chem. 284 (51), 35338–35347. doi:10.1074/jbc.M109.037036
Qian, W., Cai, X., Qian, Q., Zhuang, Q., Yang, W., Zhang, X., et al. (2019a). Astragaloside IV protects endothelial progenitor cells from the damage of ox-LDL via the LOX-1/NLRP3 inflammasome pathway. Drug Des. devel. Ther. 13, 2579–2589. doi:10.2147/DDDT.S207774
Qin, H. W., Li, Y. J., Zhang, Z. X., Ren, K., Li, S. J., and Lu, Y. B. (2018). Anti-atherosclerosis effect of astragaloside is related to miR-33a/ABCA1 signaling. Chin. J. Pathophysiol. 34 (12), 2120–2126. doi:10.3969/j.issn.1000-4718.2018.12.002
Qin, H. W., Zhang, Q. S., Li, Y. J., Li, W. T., and Wang, Y. (2022). Molecular mechanism of astragaloside Ⅳ against atherosclerosis by regulating miR-17-5p and PCSK9/VLDLR signal pathway. China J. Chin. Mater. Med. 47 (2), 492–498. doi:10.19540/j.cnki.cjcmm.20210918.701
Qin, Y., Zheng, B., Yang, G. S., Zhou, J., Yang, H. J., Nie, Z. Y., et al. (2020). Tanshinone ⅡA inhibits VSMC inflammation and proliferation in vivo and in vitro by downregulating miR-712-5p expression. Eur. J. Pharmacol. 880, 173140. doi:10.1016/j.ejphar.2020.173140
Ren, P., Wang, J., Li, L., Lin, X., Wu, G., Chen, J., et al. (2021). Identification of key genes involved in the recurrence of glioblastoma multiforme using weighted gene co-expression network analysis and differential expression analysis. Bioengineered 12 (1), 3188–3200. doi:10.1080/21655979.2021.1943986
Ruparelia, N., and Choudhury, R. (2020). Inflammation and atherosclerosis: what is on the horizon? Heart 106 (1), 80–85. doi:10.1136/heartjnl-2018-314230
Salmena, L., Poliseno, L., Tay, Y., Kats, L., and Pandolfi, P. P. (2011). A ceRNA hypothesis: the rosetta stone of a hidden RNA language? Cell 146 (3), 353–358. doi:10.1016/j.cell.2011.07.014
Shao, X., Liu, Z., Liu, S., Lin, N., and Deng, Y. (2021). Astragaloside IV alleviates atherosclerosis through targeting circ_0000231/miR-135a-5p/CLIC4 axis in AS cell model in vitro. Mol. Cell. Biochem. 476 (4), 1783–1795. doi:10.1007/s11010-020-04035-8
Sheedy, F. J. (2015). Turning 21: induction of miR-21 as a key switch in the inflammatory response. Front. Immunol. 6, 19. doi:10.3389/fimmu.2015.00019
Shen, J., Wei, W., Wang, X., Yang, J., Lu, L., Lv, X., et al. (2020). Proliferation of vascular smooth muscle cells under ox-LDL is regulated by alismatis rhizoma decoction via InhibitingERK1/2 and miR-17∼92a cluster activation. Evid. Based Complement. Alternat. Med. 2020, 7275246. doi:10.1155/2020/7275246
Shen, L., Hu, Y., Lou, J., Yin, S., Wang, W., Wang, Y., et al. (2019). CircRNA-0044073 is upregulated in atherosclerosis and increases the proliferation and invasion of cells by targeting miR-107. Mol. Med. Rep. 19 (5), 3923–3932. doi:10.3892/mmr.2019.10011
Shi, X., Xie, X., Sun, Y., He, H., Huang, H., Liu, Y., et al. (2020). Paeonol inhibits NLRP3 mediated inflammation in rat endothelial cells by elevating hyperlipidemic rats plasma exosomal miRNA-223. Eur. J. Pharmacol. 885, 173473. doi:10.1016/j.ejphar.2020.173473
Shou, X., Wang, Y., Jiang, Q., Chen, J., and Liu, Q. (2023). miR-126 promotes M1 to M2 macrophage phenotype switching via VEGFA and KLF4. PeerJ 11, e15180. doi:10.7717/peerj.15180
Silva, J., Yu, X., Moradian, R., Folk, C., Spatz, M. H., Kim, P., et al. (2020). Dihydromyricetin protects the liver via changes in lipid metabolism and enhanced ethanol metabolism. Alcohol. Clin. Exp. Res. 44 (5), 1046–1060. doi:10.1111/acer.14326
Simion, V., Zhou, H., Haemmig, S., Pierce, J. B., Mendes, S., Tesmenitsky, Y., et al. (2020). A macrophage-specific lncRNA regulates apoptosis and atherosclerosis by tethering HuR in the nucleus. Nat. Commun. 11 (1), 6135. doi:10.1038/s41467-020-19664-2
Son, D. J., Kumar, S., Takabe, W., Kim, C. W., Ni, C. W., Alberts-Grill, N., et al. (2013). The atypical mechanosensitive microRNA-712 derived from pre-ribosomal RNA induces endothelial inflammation and atherosclerosis. Nat. Commun. 4, 3000. doi:10.1038/ncomms4000
Song, W., Pu, J., and He, B. (2014). Tanshinol protects human umbilical vein endothelial cells against hydrogen peroxide-induced apoptosis. Mol. Med. Rep. 10 (5), 2764–2770. doi:10.3892/mmr.2014.2541
Song, Z., Wei, D., Chen, Y., Chen, L., Bian, Y., Shen, Y., et al. (2019). Association of astragaloside IV-inhibited autophagy and mineralization in vascular smooth muscle cells with lncRNA H19 and DUSP5-mediated ERK signaling. Toxicol. Appl. Pharmacol. 364, 45–54. doi:10.1016/j.taap.2018.12.002
Sun, D., Xiang, G., Wang, J., Li, Y., Mei, S., Ding, H., et al. (2020). miRNA 146b-5p protects against atherosclerosis by inhibiting vascular smooth muscle cell proliferation and migration. Epigenomics 12 (24), 2189–2204. doi:10.2217/epi-2020-0155
Sun, G., Li, Y., and Ji, Z. (2019). Up-regulation of MIAT aggravates the atherosclerotic damage in atherosclerosis mice through the activation of PI3K/Akt signaling pathway. Drug Deliv. 26 (1), 641–649. doi:10.1080/10717544.2019.1628116
Sun, Y., Liu, S., Yang, S., Chen, C., Yang, Y., Lin, M., et al. (2021). Mechanism of dihydromyricetin on inflammatory diseases. Front. Pharmacol. 12, 794563. doi:10.3389/fphar.2021.794563
Tan, C., Zhou, L., Wen, W., and Xiao, N. (2021). Curcumin promotes cholesterol efflux by regulating ABCA1 expression through miR-125a-5p/SIRT6 axis in THP-1 macrophage to prevent atherosclerosis. J. Toxicol. Sci. 46 (5), 209–222. doi:10.2131/jts.46.209
Tan, L., Meng, L., Shi, X., and Yu, B. (2017). Knockdown of microRNA-17-5p ameliorates atherosclerotic lesions in ApoE(-/-) mice and restores the expression of very low density lipoprotein receptor. Biotechnol. Lett. 39 (7), 967–976. doi:10.1007/s10529-017-2337-y
Tan, Y. Q., Chen, H. W., and Li, J. (2020). Astragaloside IV: an effective drug for the treatment of cardiovascular diseases. Drug Des. devel. Ther. 14, 3731–3746. doi:10.2147/DDDT.S272355
Tang, Y. L., Jiang, J. H., Wang, S., Liu, Z., Tang, X. Q., Peng, J., et al. (2015). TLR4/NF-κB signaling contributes to chronic unpredictable mild stress-induced atherosclerosis in ApoE-/- mice. PloS ONE 10 (4), e0123685. doi:10.1371/journal.pone.0123685
Tao, H., Cao, W., Yang, J. J., Shi, K. H., Zhou, X., Liu, L. P., et al. (2016). Long noncoding RNA H19 controls DUSP5/ERK1/2 axis in cardiac fibroblast proliferation and fibrosis. Cardiovasc. Pathol. 25 (5), 381–389. doi:10.1016/j.carpath.2016.05.005
Thum, T., and Mayr, M. (2012). Review focus on the role of microRNA in cardiovascular biology and disease. Cardiovasc. Res. 93 (4), 543–544. doi:10.1093/cvr/cvs085
Tousoulis, D., Kampoli, A. M., Tentolouris, C., Papageorgiou, N., and Stefanadis, C. (2012). The role of nitric oxide on endothelial function. Curr. Vasc. Pharmacol. 10 (1), 4–18. doi:10.2174/157016112798829760
Vanduchova, A., Anzenbacher, P., and Anzenbacherova, E. (2019). Isothiocyanate from broccoli, sulforaphane, and its properties. J. Med. Food 22 (2), 121–126. doi:10.1089/jmf.2018.0024
Wang, D., Lu, C., Sun, F., Cui, M., Mu, H., Duan, J., et al. (2017). A tanshinone I derivative enhances the activities of antibiotics against Staphylococcus aureus in vitro and in vivo. Res. Microbiol. 168 (1), 46–54. doi:10.1016/j.resmic.2016.08.002
Wang, J. C., and Bennett, M. (2012). Aging and atherosclerosis: mechanisms, functional consequences, and potential therapeutics for cellular senescence. Circ. Res. 111 (2), 245–259. doi:10.1161/CIRCRESAHA.111.261388
Wang, L., Shen, C., Wang, Y., Zou, T., Zhu, H., Lu, X., et al. (2019). Identification of circular RNA Hsa_circ_0001879 and Hsa_circ_0004104 as novel biomarkers for coronary artery disease. Atherosclerosis 286, 88–96. doi:10.1016/j.atherosclerosis.2019.05.006
Wang, R., Hu, X., Wang, J., Zhou, L., Hong, Y., Zhang, Y., et al. (2022). Proanthocyanidin A1 promotes the production of platelets to ameliorate chemotherapy-induced thrombocytopenia through activating JAK2/STAT3 pathway. Phytomedicine 95, 153880. doi:10.1016/j.phymed.2021.153880
Wang, S., Zhang, S., Wang, S., Gao, P., and Dai, L. (2020a). A comprehensive review on pueraria: insights on its chemistry and medicinal value. Biomed. Pharmacother. 131, 110734. doi:10.1016/j.biopha.2020.110734
Wang, W., Ma, F., and Zhang, H. (2020b). MicroRNA-374 is a potential diagnostic biomarker for atherosclerosis and regulates the proliferation and migration of vascular smooth muscle cells. Cardiovasc Diagn Ther. 10 (4), 687–694. doi:10.21037/cdt-20-444
Wang, Z., Shi, D., Zhang, N., Yuan, T., and Tao, H. (2021). MiR-217 promotes endothelial cell senescence through the SIRT1/p53 signaling pathway. J. Mol. Histol. 52 (2), 257–267. doi:10.1007/s10735-020-09945-x
Wang, Z., Zhang, M., Wang, Z., Guo, Z., Wang, Z., and Chen, Q. (2020c). Cyanidin-3-O-glucoside attenuates endothelial cell dysfunction by modulating miR-204-5p/SIRT1-mediated inflammation and apoptosis. Biofactors 46 (5), 803–812. doi:10.1002/biof.1660
Wijesekara, N., Zhang, L. H., Kang, M. H., Abraham, T., Bhattacharjee, A., Warnock, G. L., et al. (2012). miR-33a modulates ABCA1 expression, cholesterol accumulation, and insulin secretion in pancreatic islets. Diabetes 61 (3), 653–658. doi:10.2337/db11-0944
Winter, J., Jung, S., Keller, S., Gregory, R. I., and Diederichs, S. (2009). Many roads to maturity: microRNA biogenesis pathways and their regulation. Nat. Cell Biol. 11 (3), 228–234. doi:10.1038/ncb0309-228
Wu, Y., Wang, F., Fan, L., Zhang, W., Wang, T., Du, Y., et al. (2018). Baicalin alleviates atherosclerosis by relieving oxidative stress and inflammatory responses via inactivating the NF-κB and p38 MAPK signaling pathways. Biomed. Pharmacother. 97, 1673–1679. doi:10.1016/j.biopha.2017.12.024
Xiao, X., Xu, M., Yu, H., Wang, L., Li, X., Rak, J., et al. (2021). Mesenchymal stem cell-derived small extracellular vesicles mitigate oxidative stress-induced senescence in endothelial cells via regulation of miR-146a/Src. Signal Transduct. Target Ther. 6 (1), 354. doi:10.1038/s41392-021-00765-3
Xue, X., Deng, Y., Wang, J., Zhou, M., Liao, L., Wang, C., et al. (2021). Hydroxysafflor yellow A, a natural compound from Carthamus tinctorius L with good effect of alleviating atherosclerosis. Phytomedicine 91, 153694. doi:10.1016/j.phymed.2021.153694
Yamakuchi, M., Ferlito, M., and Lowenstein, C. J. (2008). miR-34a repression of SIRT1 regulates apoptosis. Proc. Natl. Acad. Sci. U. S. A. 105 (36), 13421–13426. doi:10.1073/pnas.0801613105
Yang, D., Tan, S., Yang, Z., Jiang, P., Qin, C., Yuan, Q., et al. (2018). Dihydromyricetin attenuates TNF-alpha-Induced endothelial dysfunction through miR-21-mediated DDAH1/ADMA/NO signal pathway. Biomed. Res. Int. 2018, 1047810. doi:10.1155/2018/1047810
Yang, D., Yang, Z., Chen, L., Kuang, D., Zou, Y., Li, J., et al. (2020). Dihydromyricetin increases endothelial nitric oxide production and inhibits atherosclerosis through microRNA-21 in apolipoprotein E-deficient mice. J. Cell. Mol. Med. 24 (10), 5911–5925. doi:10.1111/jcmm.15278
Yang, H. X., Gao, Y., Jiang, H. B., and Liu, L. S. (2016). EGCG upregulated ABCA1 expression by decreasing miR-33a generation to reduce lipid accumulation of macrophage-derived foam cells. Chin. Pharmacol. Bull. 32, 1279–1284. doi:10.3969/j.issn.1001-1978.2016.09.018
Yin, J., Hou, X., and Yang, S. (2019a). microRNA-338-3p promotes ox-LDL-induced endothelial cell injury through targeting BAMBI and activating TGF-β/Smad pathway. J. Cell. Physiol. 234 (7), 11577–11586. doi:10.1002/jcp.27814
Yin, R., Zhu, X., Wang, J., Yang, S., Ma, A., Xiao, Q., et al. (2019b). MicroRNA-155 promotes the ox-LDL-induced activation of NLRP3 inflammasomes via the ERK1/2 pathway in THP-1 macrophages and aggravates atherosclerosis in ApoE-/- mice. Ann. Palliat. Med. 8 (5), 676–689. doi:10.21037/apm.2019.10.11
Yu, X. H., Deng, W. Y., Chen, J. J., Xu, X. D., Liu, X. X., Chen, L., et al. (2020a). LncRNA kcnq1ot1 promotes lipid accumulation and accelerates atherosclerosis via functioning as a ceRNA through the miR-452-3p/HDAC3/ABCA1 axis. Cell Death Dis. 11 (12), 1043. doi:10.1038/s41419-020-03263-6
Yu, Y., Yan, R., Chen, X., Sun, T., and Yan, J. (2020b). Paeonol suppresses the effect of ox-LDL on mice vascular endothelial cells by regulating miR-338-3p/TET2 axis in atherosclerosis. Mol. Cell. Biochem. 475 (1-2), 127–135. doi:10.1007/s11010-020-03865-w
Yuan, L., Li, Q., Zhang, Z., Liu, Q., Wang, X., and Fan, L. (2020). Tanshinone IIA inhibits the adipogenesis and inflammatory response in ox-LDL-challenged human monocyte-derived macrophages via regulating miR-130b/WNT5A. J. Cell. Biochem. 121 (2), 1400–1408. doi:10.1002/jcb.29375
Yuan, X., Chen, J., and Dai, M. (2016). Paeonol promotes microRNA-126 expression to inhibit monocyte adhesion to ox-LDL-injured vascular endothelial cells and block the activation of the PI3K/Akt/NF-κB pathway. Int. J. Mol. Med. 38 (6), 1871–1878. doi:10.3892/ijmm.2016.2778
Zang, H. L., Li, Y. H., and Huang, G. M. (2020). Long-chain non-coding RNA Linc00888 promotes the proliferation and migration of esophageal cancer cells by downregulating miR-34a expression. Eur. Rev. Med. Pharmacol. Sci. 24 (21), 11081–11089. doi:10.26355/eurrev_202011_23594
Zeng, P., Yang, J., Liu, L., Yang, X., Yao, Z., Ma, C., et al. (2021). ERK1/2 inhibition reduces vascular calcification by activating miR-126-3p-DKK1/LRP6 pathway. Theranostics 11 (3), 1129–1146. doi:10.7150/thno.49771
Zhang, H., Xu, Z., Zhao, H., Wang, X., Pang, J., Li, Q., et al. (2020a). Anthocyanin supplementation improves anti-oxidative and anti-inflammatory capacity in a dose-response manner in subjects with dyslipidemia. Redox Biol. 32, 101474. doi:10.1016/j.redox.2020.101474
Zhang, M., Zhu, Y., Zhu, J., Xie, Y., Wu, R., Zhong, J., et al. (2022). circ_0086296 induced atherosclerotic lesions via the IFIT1/STAT1 feedback loop by sponging miR-576-3p. Cell. Mol. Biol. Lett. 27 (1), 80. doi:10.1186/s11658-022-00372-2
Zhang, R. N., Zheng, B., Li, L. M., Zhang, J., Zhang, X. H., and Wen, J. K. (2014). Tongxinluo inhibits vascular inflammation and neointimal hyperplasia through blockade of the positive feedback loop between miR-155 and TNF-α. Am. J. Physiol. Heart Circ. Physiol. 307 (4), H552–H562. doi:10.1152/ajpheart.00936.2013
Zhang, X., Wang, Z., Li, W., Huang, R., Zheng, D., and Bi, G. (2020b). MicroRNA-217-5p ameliorates endothelial cell apoptosis induced by ox-LDL by targeting CLIC4. Nutr. Metab. Cardiovasc. Dis. 30 (3), 523–533. doi:10.1016/j.numecd.2019.09.027
Zhang, Y., Qin, W., Zhang, L., Wu, X., Du, N., Hu, Y., et al. (2015). MicroRNA-26a prevents endothelial cell apoptosis by directly targeting TRPC6 in the setting of atherosclerosis. Sci. Rep. 5, 9401. doi:10.1038/srep09401
Zhao, X. S., Zheng, B., Wen, Y., Sun, Y., Wen, J. K., and Zhang, X. H. (2019). Salvianolic acid B inhibits Ang II-induced VSMC proliferation in vitro and intimal hyperplasia in vivo by downregulating miR-146a expression. Phytomedicine 58, 152754. doi:10.1016/j.phymed.2018.11.014
Zhou, S., Sun, Y., Zhao, K., Gao, Y., Cui, J., Qi, L., et al. (2020). miR21/PTEN pathway mediates the cardioprotection of geniposide against oxidized lowdensity lipoproteininduced endothelial injury via suppressing oxidative stress and inflammatory response. Int. J. Mol. Med. 45 (5), 1305–1316. doi:10.3892/ijmm.2020.4520
Zhu, L., Gong, X., Gong, J., Xuan, Y., Fu, T., Ni, S., et al. (2020). Notoginsenoside R1 upregulates miR-221-3p expression to alleviate ox-LDL-induced apoptosis, inflammation, and oxidative stress by inhibiting the TLR4/NF-κB pathway in HUVECs. Braz. J. Med. Biol. Res. 53 (6), e9346. doi:10.1590/1414-431x20209346
Zhu, Y., Xian, X., Wang, Z., Bi, Y., Chen, Q., Han, X., et al. (2018). Research progress on the relationship between atherosclerosis and inflammation. Biomolecules 8 (3), 80. doi:10.3390/biom8030080
Glossary
Keywords: non-coding RNAs, traditional Chinese medicine, atherosclerosis, cardiovascular diseases, active monomer
Citation: Liu G, Tan L, Zhao X, Wang M, Zhang Z, Zhang J, Gao H, Liu M and Qin W (2023) Anti-atherosclerosis mechanisms associated with regulation of non-coding RNAs by active monomers of traditional Chinese medicine. Front. Pharmacol. 14:1283494. doi: 10.3389/fphar.2023.1283494
Received: 26 August 2023; Accepted: 23 October 2023;
Published: 06 November 2023.
Edited by:
Ismail Laher, University of British Columbia, CanadaReviewed by:
Yuan Xinxu, Virginia Commonwealth University, United StatesPiyushkumar R. Kapopara, Thermo Fisher Scientific, United States
Copyright © 2023 Liu, Tan, Zhao, Wang, Zhang, Zhang, Gao, Liu and Qin. This is an open-access article distributed under the terms of the Creative Commons Attribution License (CC BY). The use, distribution or reproduction in other forums is permitted, provided the original author(s) and the copyright owner(s) are credited and that the original publication in this journal is cited, in accordance with accepted academic practice. No use, distribution or reproduction is permitted which does not comply with these terms.
*Correspondence: Wei Qin, qinwei@mail.jnmc.edu.cn