- 1Laboratorio de Genética Molecular Humana, Departamento de Ciencias Biológicas, CENUR Litoral Norte-Sede Salto, Universidad de la República, Salto, Uruguay
- 2Red Latinoamericana de Implementación y Validación de Guías Clínicas Farmacogenómicas (RELIVAF-CYTED), Santiago, Chile
- 3Servicio Hemato Oncológico Pediátrico (SHOP), Centro Hospitalario Pereira Rossell (CHPR), Montevideo, Uruguay
In Uruguay, the pediatric acute lymphoblastic leukemia (ALL) cure rate is 82.2%, similar to those reported in developed countries. However, many patients suffer adverse effects that could be attributed, in part, to genetic variability. This study aims to identify genetic variants related to drugs administered during the induction phase and analyze their contribution to adverse effects, considering individual genetic ancestry. Ten polymorphisms in five genes (ABCB1, CYP3A5, CEP72, ASNS, and GRIA1) related to prednisone, vincristine, and L-asparaginase were genotyped in 200 patients. Ancestry was determined using 45 ancestry informative markers (AIMs). The sample ancestry was 69.2% European, 20.1% Native American, and 10.7% African, but with high heterogeneity. Mucositis, Cushing syndrome, and neurotoxicity were the only adverse effects linked with genetic variants and ancestry. Mucositis was significantly associated with ASNS (rs3832526; 3R/3R vs. 2R carriers; OR: = 6.88 [1.88–25.14], p = 0.004) and CYP3A5 (non-expressors vs. expressors; OR: 4.55 [1.01–20.15], p = 0.049) genes. Regarding Cushing syndrome, patients with the TA genotype (rs1049674, ASNS) had a higher risk of developing Cushing syndrome than those with the TT genotype (OR: 2.60 [1.23–5.51], p = 0.012). Neurotoxicity was significantly associated with ABCB1 (rs9282564; TC vs. TT; OR: 4.25 [1.47–12.29], p = 0.007). Moreover, patients with <20% Native American ancestry had a lower risk of developing neurotoxicity than those with ≥20% (OR: 0.312 [0.120–0.812], p = 0.017). This study shows the importance of knowing individual genetics to improve the efficacy and safety of acute lymphoblastic leukemia.
Introduction
In Uruguay, as in the rest of the world, leukemias are the most common pediatric cancer, constituting 30.2% (Kato, 2020; Dufort and Álvarez, 2021). Acute lymphoblastic leukemia (ALL) is the most common childhood leukemia (Maamari et al., 2020). Its incidence rate varies among populations. For instance, it is higher in the Hispanic and European-derived populations of the United States of America (USA) than in the African American and Asian American populations of this country (Fujita et al., 2021). Furthermore, compared with other populations, the incidence rate in Latin American population has increased more rapidly over time (Quiroz et al., 2019; Mejia-Arangure et al., 2021). Overall survival of ALL has remarkably improved, being 10% in the 1960s and reaching up to 90% nowadays (Al-Mahayri et al., 2017). In Uruguay, the ALL survival between 2008 and 2012 was 82.2%, similar to those reported for developed countries such as the United States, some East European countries, Japan, South Korea, and Australia (Castillo et al., 2012; Dufort and Álvarez, 2021).
In Uruguay, the Berlin–Frankfurt–Münster (IC-BFM) protocol is used to treat ALL pediatric patients, who are classified into three risk groups (standard, intermediate, and high), according to the age of diagnosis, white blood cell (WBC) count, presence of translocations, peripheral blood blast number on day +8 (PBB+8), and minimal residual disease (MRD) on days +15 and +33. The overall treatment consists in a 2-year chemotherapy divided into five phases. The first phase, induction to remission, consists of the administration of prednisone (PRED), vincristine (VCR), L-asparaginase (L-ASP), daunorubicin, and methotrexate (MTX). Although these drugs have shown high efficiency, their non-specific action and high administered doses make them potentially toxic (Gervasini and Vagace, 2012). The inter-individual variability in the treatment response can be explained by non-genetic (age, sex, concomitant diseases, and diet) and genetic factors (Arribas and Pérez, 2010). One of the most common adverse events is oral mucositis, occurring in 52%–80% of children treated with chemotherapeutic regimens (Cheng et al., 2008). Although some studies have reported an association between oral mucositis and genetic polymorphisms in different genes such as ABCB1, ABCC2, ABCG2, and MTHFR (Tantawy et al., 2010; Bektaş-Kayhan et al., 2012; Liu et al., 2014; Viana Filfo et al., 2021), these studies have not been able to be replicated.
PRED and dexamethasone are two glucocorticoids (GCs) administered in ALL therapy that increase therapeutic efficacy (Kawedia et al., 2011). However, GC administration has been associated with a variety of unwanted adverse effects, such as skin fragility, weight gain, increased infection risk, metabolic and cardiovascular impact, hypertension, hyperglycemia, Cushing syndrome, dyslipidemia, and bone toxicity (Schijvens et al., 2019). ABCB1 protein is a drug efflux pump with broad substrate specificity that can expulse PRED, VCR, MTX, and anthracyclines to the extracellular space (Gregers et al., 2015; Song et al., 2017). Therefore, any variation in the expression or activity levels of this protein could affect the response to the ALL treatment (Fletcher et al., 2010; Song et al., 2017; Mohammad et al., 2018). The relationship between rs2032582 (A>C/T) in the ABCB1 gene and the response to ALL treatment has been reported. In a study conducted in a Malay, Chinese, and Indian children cohort, the AA genotype was associated with lower event-free survival and a higher risk of relapse (Lu et al., 2014). On the other hand, a study in a Chinese population reported that individuals with CC, CT, and TT genotypes had a higher risk of relapse or death compared with CA, AA, or AT genotypes. However, no relationship was found between this variant and WBC at debut, PBB+8 (Zhai et al., 2012; Gasic et al., 2018), and MRD on days +15 and +33 (Zhai et al., 2012).
VCR is a plant alkaloid that exerts cytotoxic effects by interfering with the microtubule assembly and mitotic spindle formation. This inhibition leads to apoptosis (Jordan and Wilson, 2004; Echebarria Barona, 2016; Al-Mahayri et al., 2017). In the nervous system, VCR disrupts axonal microtubules, causing axonal inflammation in both myelinated and unmyelinated fibers. Consequently, sensory and motor functions are affected, thus influencing the patient’s life quality during or even after treatment (Carozzi et al., 2015; Martin-Guerrero et al., 2019). Several variables could shape the incidence of VCR-induced neurotoxicity: dose, cumulative dose, administration frequency, interaction with other drugs, genetic ancestry, gene variants, and even the methods used to determine this incidence (Sims, 2016; Stock et al., 2017; McClain et al., 2018; Laushke et al., 2019). In the liver, the CYP3A5 enzyme is the main VCR metabolizing enzyme, generating three inactive metabolites (Dennison et al., 2006; Lamba et al., 2012). CYP3A5 genetic variability has been associated with VCR-induced neurotoxicity both in vitro and in vivo (Aplenc et al., 2003; Egbelakin et al., 2011; Lamba et al., 2012). Homozygous or compound heterozygous individuals for CYP3A5 *3, *6, and *7 alleles present a higher risk of developing VCR-induced peripheral neuropathy events (Egbelakin et al., 2011). However, some studies did not find an association between the CYP3A5 genotype and neurotoxicity (Ceppi et al., 2014). Additionally, the CEP72 gene (which encodes for an essential centrosomal protein in the microtubule construction) contains a polymorphism (rs924607) whose role in VCR-induced neurotoxicity has been discussed (Diouf et al., 2015; Gutiérrez-Camino et al., 2016; Stock et al., 2017). Concerning genetic ancestry, a higher frequency of VCR-induced neuropathies has been reported in European-derived children than in African American and Hispanic children (Renberger et al., 2008; Sims, 2016; McClain et al., 2018).
L-ASP is an ASNase that catalyzes the L-asparagine hydrolysis into L-aspartic acid and ammonia, depriving tumor cells of an essential growth factor (asparagine), resulting in their death (Verma et al., 2007; Shrivastava et al., 2016; Burke and Zalewska-Szewczyk, 2022). Although L-ASP administration has been one of the main contributions to pediatric ALL treatment in the last 50 years, it has also been associated with different adverse effects, among which hypersensitivity to the drug is prominent. Some studies have linked the occurrence of L-ASP allergy with the patient risk group (Chen et al., 2010; Kutszegi et al., 2015; Rajic et al., 2015). Furthermore, there are several investigations about variants in ASNS and GRIA1 genes and their influence on this adverse effect (Akagi et al., 2009; Chen et al., 2010; Pastorczak et al., 2014; Ben Tanfous et al., 2015; Kutszegi et al., 2015; Rajic et al., 2015). Moreover, Wacker et al. (2007) reported a higher frequency of L-ASP allergic reactions in pediatric ALL European-derived populations than in Hispanic or Afro-descendant populations. In agreement, Chen et al. (2010) reported that American–Indian ancestry is associated with a lower risk of developing L-ASP hypersensitivity. However, Kahn et al. (2018) did not find a significant difference in the incidence of L-ASP-associated toxicities between Hispanic and non-Hispanic children.
From a historical and genetic perspective, the Uruguayan population is considered an admixed population with at least three ancestries (Sans et al., 1997; Bonilla et al., 2004; Hidalgo et al., 2005; Bonilla et al., 2015). According to history, the Uruguayan territory prior to the European conquest was occupied by the Charrúas, Minuanes, Arachanes, Chanás, and Guaraníes Native American groups (Cabrera, 1992). The colonization of Uruguay occurred mainly by the Spanish (from Asturias, the Canary Islands, and Galicia) and Portuguese (from Portugal and Brazil) (Pi and Vidart, 1969; Sans et al., 1997; Sans et al., 2021). Due to the slave trade, Uruguay also received African populations of diverse origins, especially of Bantu ethnicity (Isola, 1975). Although recently Uruguay does not have Afro-Uruguayan or Native American isolated communities, the contribution of these populations is observed in the Uruguayans’ genetic background. The European genetic contribution varies between 70% and 80%, whereas the Native American and African contributions vary between 10%–14% and 6%–9.5%, respectively (Sans et al., 2021). This genetic structure is very enthralling for carrying out pharmacogenetics studies.
This investigation aims to identify genetic variants related to the drugs administered during the induction phase of pediatric ALL therapy (PDR, VCR, and L-ASP) and analyze their contribution to response and adverse effects, considering individual genetic ancestry.
Methodology
The protocol and procedure employed were in accordance with the principles of the Declaration of Helsinki and approved by the CENUR Litoral Norte, Universidad de la República, institutional ethics committee. Informed consent was obtained from parents, guardians, and patients, as required (Exp. 311170-001142-19, www.expe.edu.uy).
Patient samples
This investigation is a retrospective molecular epidemiology study. A total of 200 pediatric patients diagnosed with ALL (between 1 and 19 years old) were analyzed. Patients were treated according to the IC-BFM protocol at the Servicio Hemato Oncológico Pediátrico—Centro Hospitalario Pereira Rossell (SHOP-CHPR), Montevideo, Uruguay, and recruited between 2010 and 2020. SHOP-CHPR is the national reference center for pediatric ALL and treats children from the entire county. Patients’ clinical and demographic data are detailed in Supplementary Table S1. DNA was extracted from peripheral blood white cells using the salting-out method (Miller et al., 1988).
Genotyping
Ten polymorphisms in ABCB1, CYP3A5, CEP72, ASNS, and GRIA1 genes were genotyped by different molecular approaches such as PCR, PCR-RFLP, PCR-HRM, and TaqMan probes (Supplementary Table S2). For validation purposes, all patients carrying a rare variant allele, as well as a similar number of non-carriers, were subjected to Sanger sequencing. For frequent variants, 10% of the samples were selected to be sequenced, considering the genotype proportions. Individual and global ancestry were determined by genotyping 45 ancestry informative markers (AIMs), selected from the SNP panel published by Yaeger et al. (2008). The eligibility criteria were that they were adequately distributed across the genome and that at least one was in each of the autosomes. Moreover, the selection tried to contemplate a similar proportion of AIMs that can distinguish between the three possible pairs of ancestral populations. Nineteen AIMs were analyzed using SNaPshot multiplex (Thermo Fisher Scientific, Waltham, Massachusetts, United States), and 26 AIMs were analyzed by MassARRAY SNP genotyping (Agena Bioscience Inc., San Diego, United States).
Clinical and paraclinical data
From medical records, several clinical and paraclinical data were obtained: the number of blasts on days +1, +8, +15, and +33 and MRD on days +15 and +33, and relapses or deaths. Moreover, for each of the 33 days of the induction phase, a complete blood count and clinical symptoms such as infection, mucositis, erythema, gastrointestinal disorders, allergies, Cushing syndrome, and hyperglycemia were collected. Furthermore, neurological events and L-ASP allergy were checked throughout the treatment. These data were collected blinded to genotypes. The current investigation focused only on PRED response on day +8, mucositis, Cushing syndrome, L-ASP hypersensitivity, and neurotoxicity. Mucositis and Cushing syndrome were classified into severity grades, according to NIH-NCI Common Terminology Criteria for Adverse Events (CTCAE). Neurological toxicity was defined following the Spanish Society of Medical Oncology criteria (Blasco and Caballero, 2019), according to the presence of any of the following symptoms: progressive confusion, hallucinations, aphasia, speech disturbance, lethargy, drowsiness, seizures, ataxia, dysmetria, dysarthria, nystagmus, facial droop, rapid eye movement, loss of sensation in extremities, and paresthesia.
Statistical analysis
Genotypic and allelic frequencies were calculated by gene counting. The Hardy–Weinberg equilibrium (HWE) was estimated using Fisher’s exact test, and genotypic frequencies were compared to 1000 Genomes Project Consortium populations using the population differentiation test in Arlequin software v3.5.2 (Excoffier and Lischer, 2010). Moreover, the F-statistic FIS was calculated, according to Hedrick (2011). Individual and global genetic ancestry were calculated using STRUCTURE 2.3.4 software (Pritchard et al., 2000) using the following parameters: K = 3 (European, Amerindian, and African), 100,000 iterations for the burn-in period, and 1,000,000 additional iterations. The parental populations included 42 Europeans (Coriell’s North American panel), 37 West Africans (non-admixed Africans living in London, United Kingdom, and South Carolina, United States), and 30 Native Americans (15 Mayans and 15 Nahuas), who were genotyped on an Affymetrix 100 K SNP chip (data were kindly provided by Dr. Fejerman, University of California, San Francisco).
The relationships between ancestry and PRED response, and ancestry and toxicities were analyzed using the Mann–Whitney (MW) test, considering each ancestry separately. In some analyses, these components were dichotomized using a cut-off value based on their mean. The PRED response was measured as PBB+8 and dichotomized into two groups: <1000 and ≥1000 blasts. Toxicities were analyzed as presence/absence. Regarding CYP3A5, patients were classified as expressors or non-expressors. The formers carry at least one functional allele (*1), and the latter are either homozygous for one variant or compound heterozygous. The analysis between categorical variables was performed using the chi-squared test. When the chi-squared test showed significant differences, odd ratio (OR) risk analyses were performed. Additionally, the relationship between the number of events of each toxicity and genetic variants was analyzed using the MW test. Finally, classification and regression trees were performed using the Chi-squared Automatic Interaction Detector (CHAID) algorithm to analyze the toxicities, including genetic variants and ancestry. Statistical analyses were carried out using SPSS 22.0 software (IBM Corp Resealsed, 2013) with a significance p-value of 0.05.
Results
Of the 200 patients, only 184 had medical records available. Furthermore, ancestry was successfully determined in 197 samples. Since not all patients were genotyped for all variants (due to the lack of DNA), the number of patients included in each analysis may differ. Genotypic, allelic frequencies, HWE, and FIS for the 10 variants are detailed in Table 1. With the exception of rs1049674 (ASNS), the others were found in HWE. For most polymorphisms, the patient sample differs from the African populations, followed by the Asians, and, to a lesser extent, from the Europeans and the Latin American populations (Supplementary Table S3).
The study population showed a tri-hybrid structure, with the European ancestral proportion being the main one (69.2% ± 14.0%), followed by the Native American (20.1% ± 12.3%) and the African (10.7% ± 7.4%). Regarding individual ancestry, the distribution of the three ancestral components presented high heterogeneity (Supplementary Figure S1).
Concerning ancestry and PRED response, patients with <1000 PBB+8 had a higher proportion of Native American ancestry (p = 0.034; Supplementary Figure S2). It was not possible to perform an OR analysis with a Native American cut-off value of 20% since all patients with more than 20% had <1000 PBB+8. None of the two variants analyzed in ABCB1 (rs2032582 and rs9282564) showed statistically significant differences concerning PRED response.
The relationship between gene variants and toxicities is shown in Supplementary Table S4. Twenty-four percent (N = 44) of the patients developed mucositis during the induction phase (32% were in grades two or three). Patients who do not express CYP3A5, as well as those with the 3R/3R genotype (rs3832526, ASNS), had a higher risk of developing mucositis (OR = 4.55 [1.01–20.15], p = 0.049 and OR = 6.88 [1.88–25.14], p = 0.004) (Supplementary Table S5). Moreover, the CYP3A5 non-expressors or the 3R/3R patients present a higher number of mucositis events (p = 0.034 and 0.001, respectively). Likewise, the mucositis classification tree shows both CYP3A5 and rs3832526 as the only variables that explain the presence of this toxicity (Figure 1). Ancestry was not associated with this toxicity either (Supplementary Table S6).
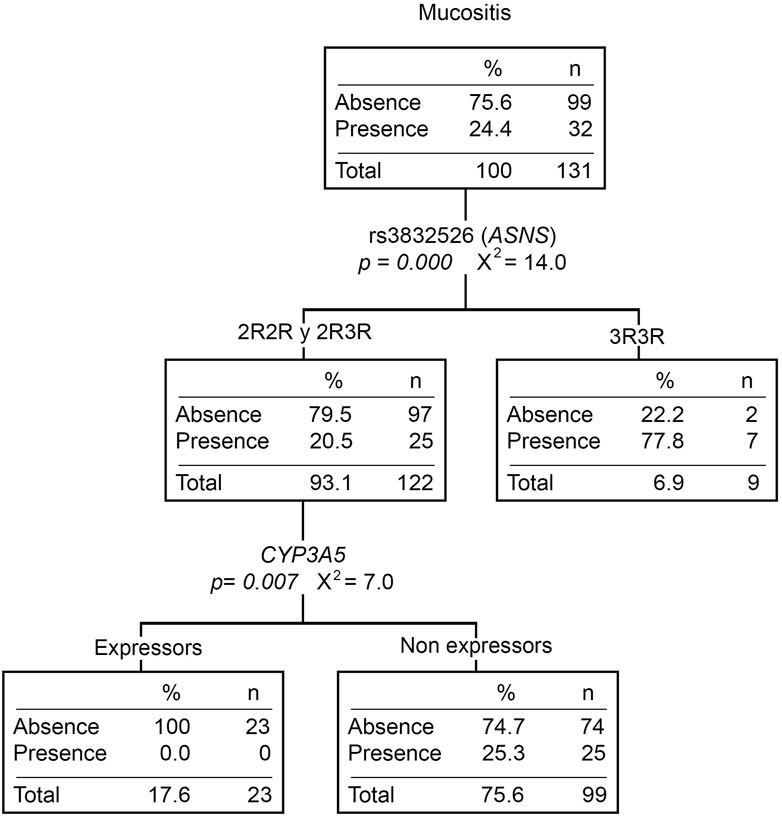
FIGURE 1. Mucositis classification tree. The presence/absence of mucositis was considered the dependent variable. ABCB1 and ASNS genotypes, the CYP3A5 phenotype (expressors and non-expressors), and Native American ancestry (greater or less than 20%) were considered independent variables. n, number of patients; p, p-value; X2, chi-squared coefficient.
Twenty-eight percent (N = 51) of the patients presented at least one Cushing event during the induction phase. Patients with the TA genotype for rs1049674 (ASNS) had a higher risk of developing Cushing syndrome compared to those with the TT genotype (OR = 2.60 [1.23–5.51], p = 0.012) (Supplementary Table S5). Moreover, patients with the TA genotype presented more Cushing events than those with the TT genotype (p = 0.005). Even though Cushing syndrome did not show statistically significant differences with any of the ancestries (Supplementary Table S6), the classification tree divided the TA genotype patients by the Native American ancestry. The percentage of patients with Cushing syndrome was higher in those with <20% Native American ancestry (Figure 2).
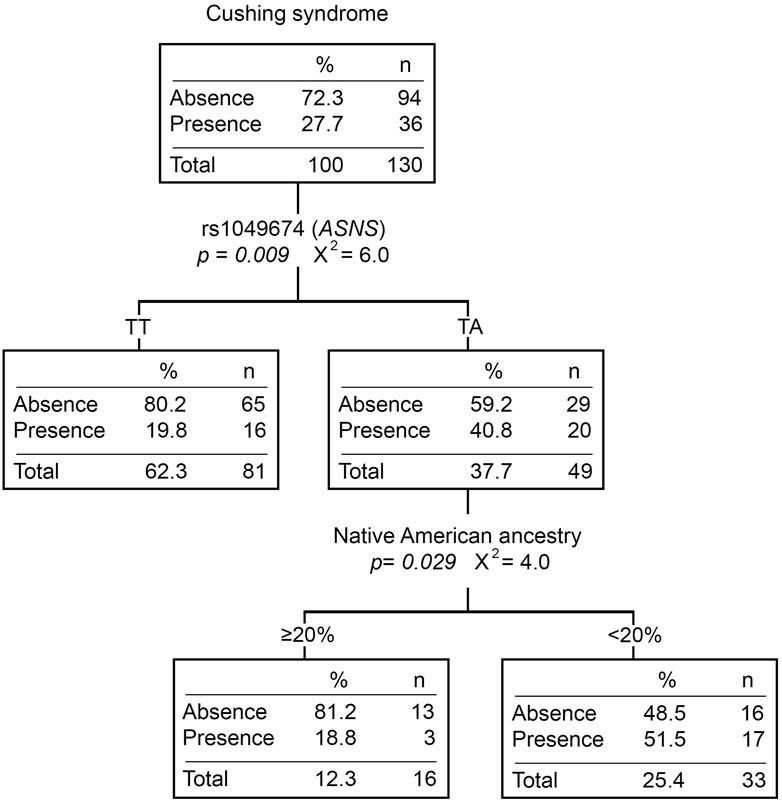
FIGURE 2. Cushing syndrome classification tree. The presence/absence of Cushing syndrome was considered the dependent variable. ABCB1 and ASNS genotypes, and Native American ancestry (greater or less than 20%) were considered independent variables. n, number of patients; p, p-value; X2, chi-square coefficient.
Forty percent of the patients (N = 74) developed L-ASP allergy during treatment (6.7% during the induction phase). Neither ASNS and GRIA1 variants nor ancestry was statistically significantly associated with L-ASP hypersensitivity (Supplementary Tables S4, S6).
Twenty-nine patients (15.7%) experienced at least one neurological event during treatment, mostly in post-induction phases (83%). Patients with the CT (rs9282564, ABCB1) genotype had a higher risk of developing neurological toxicity than those with the TT genotype (OR = 4.25 [1.47–12.29], p = 0.007) (Supplementary Table S5). Moreover, patients with neurotoxicity had lower Native American ancestry (p = 0.002). Those patients with ≥20% Native American ancestry had a lower risk of developing this toxicity compared to those with <20% (OR = 0.31 [0.12–0.81], p = 0.017). When analyzing neurotoxicity with the Native American ancestry and genotypic variants, the CT genotype of rs9282564 was the only explanatory variable (Figure 3).
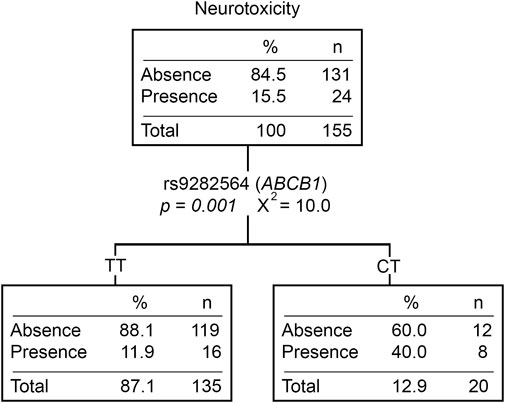
FIGURE 3. Neurotoxicity classification tree. The presence/absence of neurotoxicity was considered the dependent variable. ABCB1, CEP72, ASNS genotypes, CYP3A5 phenotype (expressors and non-expressors), and Native American ancestry (greater or less than 20%) were considered independent variables. n, number of patients; P, p-value; X2, chi-squared coefficient.
Discussion
Only rs1049674 (ASNS) was not found in HWE, showing an excess of heterozygotes (Table 1). This equilibrium deviation could be due to a genotyping error, the existence of evolutionary factors, population substructure, or admixture linkage disequilibrium (ALD), as reported by Bonilla et al. (2015). Since this polymorphism was also not found in HWE in a control sample (data not shown) and was validated by Sanger sequencing, a genotyping error was discarded as an explanation. As rs1049674 was in linkage equilibrium with most of the polymorphisms analyzed (data not shown), the rest of the polymorphisms were in HWE, and the FIS values were near zero (either positive or negative), a population substructure or ALD are not the most plausible explanations. This equilibrium deviation needs to be further addressed.
The ancestry heterogeneity observed and the global ancestry agree with genetic and sociodemographic data previously reported for Uruguay (Instituto Nacional de Estadística, 2011; Sans et al., 2021). However, the Native American and African ancestry are slightly higher than those previously published for Uruguay (Sans et al., 1997; Bonilla et al., 2004; Bonilla et al., 2015; Sans et al., 2021). This difference could be attributable to the fact that most of the patients belong to the public healthcare system. According to Bonilla et al. (2015) and Sans et al. (2021), Native American and African ancestries are slightly higher in the public healthcare system population, showing heterogeneity according to the socioeconomic level. Additionally, the association between the Native American ancestry and ALL risk (Walsh et al., 2013) could also explain the observed higher proportion of this ancestry.
Furthermore, patients with <1000 PBB+8 had a significantly higher Native American ancestry than those with ≥1000 (Supplementary Figure S2), suggesting a better PRED response. This result does not agree with other studies that associate a worse treatment response with the Native American ancestry (Bhatia et al., 2002; Kadan-Lottick et al., 2003; Bhatia, 2011; Yang et al., 2012; Quiroz et al., 2019; Lee et al., 2022). Therefore, there could be other genetic and non-genetic factors associated with the Native American component that should be studied.
Despite oral mucositis being one of the most frequent side effects of antineoplastic therapy, few studies analyze possible genetic risk factors. In this study, CYP3A5 non-expressors and patients with the 3R3R genotype (rs3832526, ASNS) showed a higher risk of developing mucositis and a higher number of mucositis events than those who express CYP3A5 and carry the 2R allele, respectively. The CYP3A5 enzyme converts VCR into inactive metabolites. A deficiency of this enzyme activity could lead to VCR accumulation, which could induce the development of toxicities (López-López et al., 2016). On the other hand, some studies associate mucositis with L-ASP administration, but none of them investigate the role of genetic variants (Müller et al., 2000; Ortiz et al., 2013). The 3R3R genotype has been associated with a higher risk of developing L-ASP hypersensitivity (Ben Tanfous et al., 2015). Although hypersensitivity generally manifests as anaphylaxis, edema, urticaria, and erythema, among others (Fonseca et al., 2021), we cannot rule out mucositis as a possible manifestation.
Moreover, Cushing syndrome was associated with rs1049674 (ASNS). Patients with the TA genotype for rs1049674 had a higher risk of developing Cushing syndrome and more events than those with the TT genotype. Although the impact of this variant is unknown, changes in ASNS expression could cause greater sensitivity to L-ASP and lead to the development of Cushing’s syndrome. Moreover, the percentage of TA patients that developed Cushing syndrome was higher among those with <20% Native American ancestry than those with ≥20% (Figure 2). Although we cannot rule out that the Native American ancestry protective effect is due to parental population frequencies of rs1049674, when a multidimensional scaling (MDS) is constructed from genetic distances (FST), the subpopulation without Cushing syndrome is clustered with Latin American populations and split from the one with Cushing syndrome (Supplementary Figure S3A).
Similar to previous reports, 40.2% of the patients developed L-ASP allergy during treatment, especially in the post-induction phases. Neither ASNS- or GRIA1- analyzed variants nor ancestry were significantly associated with L-ASP allergy. Since there are contradictory results on the role of ASNS and GRIA1 variants in this toxicity (Zalewska-Szewczyk et al., 2007; Chen et al., 2010; Pieters et al., 2011; Ben Tanfous et al., 2015; Kutszegi et al., 2015; Rajic et al., 2015; Youssef et al., 2021), the lack of association was not a surprise. Regarding ancestry, the difference between our result and that reported by Chen et al. (2010) could be explained by different admixture proportions or different Native American ancestral populations.
Given that 83% of the neurotoxicity observed was in post-induction phases, it cannot be affirmed that this toxicity is only due to VCR administration. Other drugs, such as MTX, could also influence this toxicity development (Vezmar et al., 2003; Bhojwani et al., 2014). Those patients with the CT genotype for rs9282564 (ABCB1) showed a higher risk of developing this adverse effect than those with the TT genotype. Until now, this variant has not been associated with adverse effects derived from ALL therapy. Since VCR and MTX are transported outside the cell by ABCB1 (Whirl-Carrillo et al., 2012), variants that modify the activity of this transporter could cause various toxicities, such as the neurological toxicity. On the other hand, those patients with neurological toxicity had a significantly lower Native American ancestry than those who did not. As with PRED and Cushing syndrome, a relationship between having a higher Native American ancestry and a lower risk of developing toxicity is depicted. Although some investigations report an association between neurotoxicity and the ancestry (Renbarger et al., 2008; Sims, 2016; McClain et al., 2018), they are mostly based on the high frequency of CYP3A5 expressors in Afro-descendent populations.
Since the Native American ancestry and rs9282564 (ABCB1) were not associated (data not shown), these two variables would independently explain the presence of neurotoxicity. However, the classification and regression tree for neurotoxicity showed that the only explanatory variable was the rs9282564 genotype (Figure 3). It is possible that the loss of Native American ancestry association is due to the fact that not all the patients had the entire information for this analysis (toxicity, ancestry, and genetic variants). Although the Native American ancestry does not reach the desired significance, we cannot discard a possible effect of it on neurological toxicity. Moreover, MDS does not allow ruling out the implications of the Native American ancestry. Since rs9282564 does not properly discriminate between the 1000 genome populations, it is not possible to assign either of the two subpopulations (neuro and non-neuro) to a particular genetic background (Supplementary Figure S3B). It would be desirable to expand this analysis to determine the importance of Native American ancestry in neurotoxicity.
The associations hitherto observed between the ancestry and treatment response/toxicities show an apparent protection of the Native American ancestry. This could be due to several factors: a) the homogeneity in health access of these patients could reveal genetic factors that might not be observed in other studies, b) the Uruguayan Native American ancestry may not be the same reported for other admixed populations, and c) micro-evolutionary factors such as genetic drift or bottlenecks that have varied the frequency of variants within the Native American ancestry.
To the best of our knowledge, this is the first report that investigates side effects caused by drugs administered during the induction phase in Latin America. Even though 200 may be considered a small number, Uruguay has approximately 20 new pediatric patients with ALL per year. Hence, analyzing a 200-individual sample represents more than 10 years of ALL patients in our country. Moreover, the treatment response homogeneity among patients, regardless of patients’ socioeconomic levels, is another strength of this investigation. As for the weaknesses, although estimating an ancestry with 45 AIMs is a valid approach, some works recommend a minimum use of 50 markers (Russo et al., 2016). Moreover, having genomic data from ancestral populations more representative of the Uruguayan population would allow the ancestry estimation to be more precise. To conclude, this investigation highlights the importance of studying Latin American populations due to their differences with ancestral populations and their high heterogeneity.
Data availability statement
The raw data supporting the conclusion of this article will be made available by the authors, without undue reservation.
Ethics statement
The studies involving humans were approved by Comité de Ética en Investigación Institucional del CENUR Litoral Norte, Universidad de la República, Uruguay (Exp. 311170-001142-19). The studies were conducted in accordance with the local legislation and institutional requirements. Written informed consent for participation in this study was provided by the participants’ legal guardians/next of kin.
Author contributions
GB-R: project administration, writing–original draft, writing–review and editing, conceptualization, formal analysis, investigation, and methodology. YM: resources and writing–review and editing. NO: resources and writing–review and editing. MS: resources and writing–review and editing. LC: resources and writing–review and editing. AS: supervision, writing–review and editing, conceptualization, funding acquisition, investigation, and methodology. JL: supervision, writing–review and editing, conceptualization, funding acquisition, investigation, and methodology.
Funding
The author(s) declare that financial support was received for the research, authorship, and/or publication of this article. Financial support from Centro Universitario Regional (CENUR) Litoral Norte, Universidad de la República (UdelaR), Comisión Sectorial de Investigación Científica (CSIC), Agencia Nacional de Investigación e Innovación (ANII), and Programa de Desarrollo de las Ciencias Básicas (PEDECIBA).
Conflict of interest
The authors declare that the research was conducted in the absence of any commercial or financial relationships that could be construed as a potential conflict of interest.
Publisher’s note
All claims expressed in this article are solely those of the authors and do not necessarily represent those of their affiliated organizations, or those of the publisher, the editors, and the reviewers. Any product that may be evaluated in this article, or claim that may be made by its manufacturer, is not guaranteed or endorsed by the publisher.
Supplementary material
The Supplementary Material for this article can be found online at: https://www.frontiersin.org/articles/10.3389/fphar.2023.1278769/full#supplementary-material
References
Akagi, T., Yin, D., Kawamata, N., Bartram, C. R., Hofmann, W. K., Song, J. H., et al. (2009). Functional analysis of a novel DNA polymorphism of a tandem repeated sequence in the asparagine synthetase gene in acute lymphoblastic leukemia cells. Leuk. Res. 33 (7), 991–996. doi:10.1016/j.leukres.2008.10.022
Al-Mahayri, Z. N., Patrinos, G. P., and Ali, B. R. (2017). Pharmacogenomics in pediatric acutelymphoblastic leukemia: promises and limitations. Pharmacogenomics 18 (7), 687–699. doi:10.2217/pgs-2017-0005
Aplenc, R., Glatfelter, W., Han, P., Rappaport, E., La, M., Cnaan, A., et al. (2003). CYP3A genotypes and treatment response in paediatric acute lymphoblastic leukaemia. Br. J. Haematol. 122 (2), 240–244. doi:10.1046/j.1365-2141.2003.04430.x
Arribas, I. A., and Pérez, M. J. L. (2010). Farmacogenética y variabilidad interindividual en la respuesta a los medicamentos. Zaragoza, Spain: Colegio Oficial de Farmacéuticos de Zaragoza.
Bektas-Kayhan, K., Kucukhuseyin, O., Karagoz, G., Unur, M., Ozturk, O., Unuvar, A., et al. (2012). Is the MDR1 C3435T polymorphism responsible for oral mucositis in children with acute lymphoblastic leukemia? Asian Pac. J. Cancer Prev. 13 (10), 5251–5255. doi:10.7314/apjcp.2012.13.10.5251
Ben Tanfous, M., Sharif-Askari, B., Ceppi, F., Laaribi, H., Gagné, V., Rousseau, J., et al. (2015). Polymorphisms of asparaginase pathway and asparaginase-related complications in children with acute lymphoblastic leukemia. Clin. Cancer Res. 21 (2), 329–334. doi:10.1158/1078-0432.CCR-14-0508
Bhatia, S. (2011). Role of genetic susceptibility in development of treatment-related adverse outcomes in cancer survivors. Cancer Epidemiol. Prev. Biomarkers 20 (10), 2048–2067. doi:10.1158/1055-9965.EPI-11-0659
Bhatia, S., Sather, H. N., Heerema, N. A., Trigg, M. E., Gaynon, P. S., and Robison, L. L. (2002). Racial and ethnic differences in survival of children with acute lymphoblastic leukemia. Blood, J. Am. Soc. Hematol. 100 (6), 1957–1964. doi:10.1182/blood-2002-02-0395
Bhojwani, D., Sabin, N. D., Pei, D., Yang, J. J., Khan, R. B., Panetta, J. C., et al. (2014). Methotrexate-induced neurotoxicity and leukoencephalopathy in childhood acute lymphoblastic leukemia. J. Clin. Oncol. 32 (9), 949–959. doi:10.1200/JCO.2013.53.0808
Blasco, A., and Caballero, C. (2019). Toxicidad de los tratamientos oncológicos SEOM. Avaliable at: https://www.seom.org/guia-actualizada-de-tratamientos/toxicidad-de-los-tratamientos-oncologicos?showall=1&showall=1.
Bonilla, C., Bertoni, B., González, S., Cardoso, H., Brum-Zorrilla, N., and Sans, M. (2004). Substantial Native American female contribution to the population of Tacuarembó, Uruguay, reveals past episodes of sex-biased gene flow. Am. J. Hum. Biol. 16, 289–297. doi:10.1002/ajhb.20025
Bonilla, C., Bertoni, B., Hidalgo, P. C., Artagaveytia, N., Ackermann, E., Barreto, I., et al. (2015). Breast cancer risk and genetic ancestry: a case-control study in Uruguay. BMC women’s health 15 (1), 11–10. doi:10.1186/s12905-015-0171-8
Burke, M. J., and Zalewska-Szewczyk, B. (2022). Hypersensitivity reactions to asparaginase therapy in acute lymphoblastic leukemia: immunology and clinical consequences. Future Oncol. 18 (10), 1285–1299. doi:10.2217/fon-2021-1288
Cabrera, L. (1992). El Indígena y la conquista en la Cuenca de la Laguna Merím. Editor del V Centenario. Montevideo: Ed. Universidad de la República Vol. 1, 97–122.
Carozzi, V. A., Canta, A., and Chiorazzi, A. (2015). Chemotherapy-induced peripheral neuropathy: what do we know about mechanisms? Neurosci. Lett. 596, 90–107. doi:10.1016/j.neulet.2014.10.014
Castillo, L., Dabezies, A., Dufort, G., Carracedo, M., Castiglioni, M., Simón, E., et al. (2012). Evolucion del cáncer pediátrico en Uruguay (1992-2011). Arch. Pediatría del Urug. 83 (1), 26–30.
Ceppi, F., Langlois-Pelletier, C., Gagné, V., Rousseau, J., Ciolino, C., De Lorenzo, S., et al. (2014). Polymorphismsof the vincristine pathway and response to treatment in children with childhood acute lymphoblastic leukemia. Pharmacogenomics 15 (8), 1105–1116. doi:10.2217/pgs.14.68
Chambliss, A. B., Resnick, M., Petrides, A. K., Clarke, W. A., and Marzinke, M. A. (2017). Rapid screening for targeted genetic variants via high-resolution melting curve analysis. Clin. Chem. Laboratory Med. (CCLM) 55 (4), 507–516. doi:10.1515/cclm-2016-0603
Chen, S. H., Pei, D., Yang, W., Cheng, C., Jeha, S., Cox, N. J., et al. (2010). Genetic variations in GRIA1 on chromosome 5q33 related to asparaginase hypersensitivity. Clin. Pharmacol. Ther. 96 (5), 191–196. doi:10.1038/clpt.2010.94
Cheng, K. K., Goggins, W. B., Lee, V. W., and Thompson, D. R. (2008). Risk factors for oral mucositis in children undergoing chemotherapy: a matched case-control study. Oral Oncol. 44 (11), 1019–1025. doi:10.1016/j.oraloncology.2008.01.003
Dennison, J. B., Kulanthaivel, P., Barbuch, R. J., Renbarger, J. L., Ehlhardt, W. J., and Hall, S. D. (2006). Selective metabolism of vincristine in vitro by CYP3A5. Drug Metab. Dispos. 34 (8), 1317–1327. doi:10.1124/dmd.106.009902
Diouf, B., Crews, K. R., Lew, G., Pei, D., Cheng, C., Bao, J., et al. (2015). Association of an inherited genetic variant with vincristine-related peripheral neuropathy in children with acute lymphoblastic leukemia. JAMA 313 (8), 815–823. doi:10.1001/jama.2015.0894
Dufort, Y., and Álvarez, G. (2021). Epidemiología del cáncer en niños y adolescentes en Uruguay: 2008-2012. Un estudio de registro poblacional. Arch. Pediatría del Urug. 92 (1), 3. doi:10.31134/AP.92.1.3
Echebarria Barona, A. B. (2016). Implicación de los polimorfismos genéticos en la neurotoxicidad por Vincristina en pacientes pediátricos diagnosticados de Leucemia Linfoblástica Aguda. PhD Thesis from Facultad de Medicina y Odontología. Departamento de Genética, Antropología Física y Fisiología Animal. Universidad del País Vasco.
Egbelakin, A., Ferguson, M. J., MacGill, E. A., Lehmann, A. S., Topletz, A. R., Quinney, S. K., et al. (2011). Increased risk of vincristine neurotoxicity associated with low CYP3A5 expression genotype in children with acute lymphoblastic leukemia. Pediatr. Blood Cancer 56 (3), 361–367. doi:10.1002/pbc.22845
Excoffier, L., and Lischer, H. E. (2010). Arlequin suite ver 3.5: a new series of programs to perform population genetics analyses under Linux and Windows. Mol. Ecol. Resour. 10 (3), 564–567. doi:10.1111/j.1755-0998.2010.02847.x
Fletcher, J. I., Haber, M., Henderson, M. J., and Norris, M. D. (2010). ABC transporters in cancer: more than just drug efflux pumps. Nat. Rev. Cancer 10 (2), 147–156. doi:10.1038/nrc2789
Fonseca, M. H. G., da Silva Fiúza, T., de Morais, S. B., and Trevizani, R. (2021). Circumventing the side effects of L-asparaginase. Biomed. Pharmacother. 139, 111616. doi:10.1016/j.biopha.2021.111616
Fujita, T. C., Sousa-Pereira, N., Amarante, M. K., and Watanabe, M. A. E. (2021). Acute lymphoid leukemia etiopathogenesis. Mol. Biol. Rep. 48 (1), 817–822. doi:10.1007/s11033-020-06073-3
Gasic, V., Zukic, B., Stankovic, B., Janic, D., Dokmanovic, L., Lazic, J., et al. (2018). Pharmacogenomic markers of glucocorticoid response in the initial phase of remission induction therapy in childhood acute lymphoblastic leukemia. Radiology Oncol. 52 (3), 296–306. doi:10.2478/raon-2018-0034
Gervasini, G., and Vagace, J. M. (2012). Impact of genetic polymorphisms on chemotherapy toxicity in childhood acute lymphoblastic leukemia. Front. Genet. 3, 249. doi:10.3389/fgene.2012.00249
Gregers, J., Green, H., Christensen, I. J., Dalhoff, K., Schroeder, H., Carlsen, N., et al. (2015). Polymorphisms in the ABCB1 gene and effect on outcome and toxicity in childhood acute lymphoblastic leukemia. pharmacogenomics J. 15 (4), 372–379. doi:10.1038/tpj.2014.81
Gutierrez-Camino, A., Martin-Guerrero, I., Lopez-Lopez, E., Echebarria-Barona, A., Garcia-Orad, A., Zabalza, I., et al. (2016). Lack of association of the CEP72 rs924607 TT genotype with vincristine-related peripheral neuropathy during the early phase of pediatric acute lymphoblastic leukemia treatment in a Spanish population. Pharmacogenet Genomics 26 (2), 100–102. doi:10.1097/FPC.0000000000000191
Hidalgo, P. C., Bengochea, M., Abilleira, D., Cabrera, A., and Alvarez, I. (2005). Genetic admixture estimate in the Uruguayan population based on the loci LDLR, GYPA, HBGG, GC and D7S8. Int. J. Hum. Genet. 5 (3), 217–222. doi:10.31901/24566330.2005/05.03.10
Instituto Nacional de Estadística (2011). Ascendencia étnico-racial. Avaliable at: http://www.ine.gub.uy/censos2011/resultadosfinales/pais%20poblacion.html.
Isola (1975). “La esclavitud en el Uruguay desde sus comienzos hasta su extinción. (1743-1852),” in Comisión Nacional de Homenaje del Sesquicentenario de los hechos históricos de 1825 (Montevideo, Uruguay: Talleres Gráficos Monteverde y Cia).
Jordan, M. A., and Wilson, L. (2004). Microtubules as a target for anticancer drugs. Nat. Rev. Cancer 4 (4), 253–265. doi:10.1038/nrc1317
Kadan-Lottick, N. S., Ness, K. K., Bhatia, S., and Gurney, J. G. (2003). Survival variability by race and ethnicity in childhood acute lymphoblastic leukemia. Jama 290 (15), 2008–2014. doi:10.1001/jama.290.15.2008
Kahn, J. M., Cole, P. D., Blonquist, T. M., Stevenson, K., Jin, Z., Barrera, S., et al. (2018). An investigation of toxicities and survival in hispanic children and adolescents with ALL: results from the dana-farber cancer Institute ALL Consortium protocol 05-001. Pediatr. blood cancer 65 (3), e26871. doi:10.1002/pbc.26871
Kato, M. (2020). Pediatric acute lymphoblastic leukemia. Tokyo, Japan. Department of Transplantation and Cell Therapy, Children’s Cancer Center National Center for Child Health and Development, Setagaya-ku. doi:10.1007/978-981-15-0548-5
Kawedia, J. D., Kaste, S. C., Pei, D., Panetta, J. C., Cai, X., Cheng, C., et al. (2011). Pharmacokinetic, pharmacodynamic, and pharmacogenetic determinants of osteonecrosis in children with acute lymphoblastic leukemia. Blood 117, 2340–2347. doi:10.1182/blood-2010-10-311969
Kutszegi, N., Semsei, Á. F., Gézsi, A., Sági, J. C., Nagy, V., Csordás, K., et al. (2015). Subgroups of paediatricacute lymphoblastic leukaemia might differ significantly in genetic predisposition to asparaginase hypersensitivity. PLoSONE 10 (10), e0140136. doi:10.1371/journal.pone.0140136
Lamba, J., Hebert, J. M., Schuetz, E. G., Klein, T. E., and Altman, R. B. (2012). PharmGKB summary: very important pharmacogene information for CYP3A5. Pharmacogenet Genomics 22 (7), 555–558. doi:10.1097/FPC.0b013e328351d47f
Lauschke, V. M., Zhou, Y., and Ingelman-Sundberg, M. (2019). Novel genetic and epigenetic factors of importance for inter-individual differences in drug disposition, response and toxicity. Pharmacol. Ther. 197, 122–152. doi:10.1016/j.pharmthera.2019.01.002
Lee, S. H., Antillon-Klussmann, F., Pei, D., Yang, W., Roberts, K. G., Li, Z., et al. (2022). Association of genetic ancestry with the molecular subtypes and prognosis of childhood acute lymphoblastic leukemia. JAMA Oncol. 8 (3), 354–363. doi:10.1001/jamaoncol.2021.6826
Liu, Y., Yin, Y., Sheng, Q., Lu, X., Wang, F., Lin, Z., et al. (2014). Association of ABCC2− 24C> T polymorphism with high-dose methotrexate plasma concentrations and toxicities in childhood acute lymphoblastic leukemia. PloS one 9 (1), e82681. doi:10.1371/journal.pone.0082681
Lopez-Lopez, E., Gutierrez-Camino, A., Astigarraga, I., Navajas, A., Echebarria-Barona, A., Garcia-Miguel, P., et al. (2016). Vincristine pharmacokinetics pathway and neurotoxicity during early phases of treatment in pediatric acute lymphoblastic leukemia. Pharmacogenomics 17 (7), 731–741. doi:10.2217/pgs-2016-0001
Lu, Y., Kham, S. K. Y., Ariffin, H., Oei, A. M. I., Lin, H. P., Tan, A. M., et al. (2014). Host genetic variants of ABCB1 and IL15 influence treatment outcome in paediatric acute lymphoblastic leukaemia. Br. J. cancer 110 (6), 1673–1680. doi:10.1038/bjc.2014.7
Maamari, D., El-Khoury, H., Saifi, O., Muwakkit, S. A., and Zgheib, N. K. (2020). Implementation of pharmacogenetics to individualize treatment regimens for children with acute lymphoblastic leukemia. Pharmacogenomics Personalized Med. 13, 295–317. doi:10.2147/PGPM.S239602
Martin-Guerrero, I., Gutierrez-Camino, A., Echebarria-Barona, A., Astigarraga, I., García de Andoind, N., Narvajas, A., et al. (2019). Variants in vincristine pharmacodynamic genes involved in neurotoxicity at induction phase in the therapy of pediatric acute lymphoblastic leukemia. Pharmacogenomics J. Feb 6, 564–569. doi:10.1038/s41397-019-0081-5
McClain, C. A., Bernhardt, M. B., Berger, A., Bernini, J. C., Marquez-Do, D., Winslow, R., et al. (2018). Pharmacogenetic association with neurotoxicity in Hispanic children with acute lymphoblastic leukaemia. Br. J. Haematol. 181 (5), 684–687. doi:10.1111/bjh.14678
Mejia-Arangure, J. M., McNally, R. J. Q., and Pombo-de-Oliveira, M. S. (2021). Editorial: prevention in acute leukemias in children. Front. Public Health 9, 700739. doi:10.3389/fpubh.2021.700739
Miller, S., Dykes, D., and Polesky, H. (1988). A simple salting out procedure for extracting DNA from human nucleated cells. Nucleic acids Res. 16 (3), 1215. doi:10.1093/nar/16.3.1215
Mohammad, I. S., He, W., and Yin, L. (2018). Understanding of human ATP binding cassette superfamily and novel multidrug resistance modulators to overcome MDR. Biomed. Pharmacother. Apr 100, 335–348. doi:10.1016/j.biopha.2018.02.038
Müller, H. J., Löning, L., Horn, A., Schwabe, D., Gunkel, M., Schrappe, M., et al. (2000). Pegylated asparaginase (Oncaspar) in children with ALL: drug monitoring in reinduction according to the ALL/NHL-BFM 95 protocols. Br. J. Haematol. 110 (2), 379–384. doi:10.1046/j.1365-2141.2000.02187.x
Ortiz, M. I., Rivera-Roldán, S., Escamilla-Acosta, M. A., Romo-Hernández, G., Ponce-Monter, H. A., and Escárcega, R. (2013). Side effects of vincristine and L-asparaginase in patients with acute lymphoblastic leukemia in a mexican pediatric hospital. Pharmacol. Pharm. 4, 8. doi:10.4236/pp.2013.43050
Pastorczak, A., Fendler, W., Zalewska-Szewczyk, B., Górniak, P., Lejman, M., Trelińska, J., et al. (2014). Asparagine synthetase (ASNS) gene polymorphism is associated with the outcome of childhood acute lymphoblastic leukemia by affecting early response to treatment. Leukemia Res. 38 (2), 180–183. doi:10.1016/j.leukres.2013.10.027
Pi, R., and Vidart, D. (1969). El legado de los inmigrantes. I. Montevideo: Nuestra Tierra 29, 1–60.
Pieters, R., Hunger, S. P., Boos, J., Rizzari, C., Silverman, L., Baruchel, A., et al. (2011). L-asparaginase treatment in acute lymphoblastic leukemia: a focus on Erwinia asparaginase. Cancer 117 (2), 238–249. doi:10.1002/cncr.25489
Pritchard, J. K., Stephens, M., and Donnelly, P. (2000). Inference of population structure using multilocus genotype data. Genetics 155 (2), 945–959. doi:10.1093/genetics/155.2.945
Quiroz, E., Aldoss, I., Pullarkat, V., Rego, E., Marcucci, G., and Douer, D. (2019). The emerging story of acute lymphoblastic leukemia among the Latin American population–biological and clinical implications. Blood Rev. 33, 98–105. doi:10.1016/j.blre.2018.08.002
Rajić, V., Debeljak, M., Goričar, K., and Jazbec, J. (2015). Polymorphisms in GRIA1 gene are a risk factor for asparaginase hypersensitivity during the treatment of childhood acute lymphoblastic leukemia. Leukemia Lymphoma 56, 3103–3108. doi:10.3109/10428194.2015.1020802
Renbarger, J. L., McCammack, K. C., Rouse, C. E., and Hall, S. D. (2008). Effect of race on vincristineassociated neurotoxicity in pediatric acute lymphoblastic leukemia patients. Pediatr. Blood Cancer 50 (4), 769–771. doi:10.1002/pbc.21435
Russo, M. G., Di Fabio Rocca, F., Doldán, P., Cardozo, D. G., Dejean, C. B., Seldes, V., et al. (2016). Evaluación del número mínimo de marcadores para estimar ancestría individual en una muestra de la población Argentina. Rev. del Mus. Antropol. 9 (1), 49–56. doi:10.31048/1852.4826.v9.n1.12579
Sans, M., Figueiro, G., Bonilla, C., Bertoni, B., Cappetta, M., Artagaveytia, N., et al. (2021). Ancestría genética y estratificación social en Montevideo, Uruguay. Rev. Argent. Antropol. biológica 23 (1), 029–110. doi:10.24215/18536387e029
Sans, M., Salzano, F. M., and Chakraborty, R. (1997). Historical genetics in Uruguay: estimates of biological origins and their problems. Hum. Biol. 69, 161–170.
Schijvens, A. M., Ter Heine, R., De Wildt, S. N., and Schreuder, M. F. (2019). Pharmacology and pharmacogenetics of prednisone and prednisolone in patients with nephrotic syndrome. Pediatr. Nephrol. 34 (3), 389–403. doi:10.1007/s00467-018-3929-z
Shrivastava, A., Khan, A. A., Khurshid, M., Kalam, M. A., Jain, S. K., and Singhal, P. K. (2016). Recent developments in l-asparaginase discovery and its potential as anticancer agent. Crit. Rev. oncology/hematology 100, 1–10. doi:10.1016/j.critrevonc.2015.01.002
Sims, R. P. (2016). The effect of race on the CYP3A-mediated metabolism of vincristine in pediatric patients with acute lymphoblastic leukemia. J. Oncol. Pharm. Pract. 22, 76–81. doi:10.1177/1078155214553143
Song, Q. Q., Xie, W. Y., Tang, Y. J., Zhang, J., and Liu, J. (2017). Genetic variation in the glucocorticoid pathway involved in interindividual differences in the glucocorticoid treatment. Pharmacogenomics 18 (3), 293–316. doi:10.2217/pgs-2016-0151
Stock, W., Diouf, B., Crews, K. R., Pei, D., Cheng, C., Laumann, K., et al. (2017). An inherited genetic variant in CEP72 promoter predisposes to vincristine-induced peripheral neuropathy in adults with acute lymphoblastic leukemia. Clin. Pharmacol. Ther. 101 (3), 391–395. doi:10.1002/cpt.506
Tantawy, A. A., El-Bostany, E. A., Adly, A. A., Abou El Asrar, M., El-Ghouroury, E. A., and Abdulghaffar, E. E. (2010). Methylene tetrahydrofolate reductase gene polymorphism in Egyptian children with acute lymphoblastic leukemia. Blood coagulation fibrinolysis 21 (1), 28–34. doi:10.1097/MBC.0b013e32833135e9
Verma, N., Kumar, K., Kaur, G., and Anand, S. (2007). L-asparaginase: a promising chemotherapeutic agent. Crit. Rev. Biotechnol. 27, 45–62. doi:10.1080/07388550601173926
Vezmar, S., Becker, A., Bode, U., and Jaehde, U. (2003). Biochemical and clinical aspects of methotrexate neurotoxicity. Chemotherapy 49 (1-2), 92–104. doi:10.1159/000069773
Viana Filho, J. M. C., Coêlho, M. D. C., Ribeiro, I. L. A., Persuhn, D. C., Valença, A. M. G., and Oliveira, N. F. P. D. (2021). ABCG2 polymorphism, age and leukocyte count may contribute to oral mucositis in oncopediatric patients. Braz. Dent. J. 32, 14–26. doi:10.1590/0103-6440202103768
Wacker, P., Land, V. J., Camitta, B. M., Kurtzberg, J., Pullen, J., Harris, M. B., et al. (2007). Allergic reactions to E. coli L-asparaginase do not affect outcome in childhood B-precursor acute lymphoblastic leukemia: a Children's Oncology Group Study. J. Pediatr. hematology/oncology 29 (9), 627–632. doi:10.1097/MPH.0b013e3181483df1
Walsh, K. M., Chokkalingam, A. P., Hsu, L. I., Metayer, C., De Smith, A. J., Jacobs, D. I., et al. (2013). Associations between genome-wide Native American ancestry, known risk alleles and B-cell ALL risk in Hispanic children. Leukemia 27 (12), 2416–2419. doi:10.1038/leu.2013.130
Whirl-Carrillo, M., McDonagh, E. M., Hebert, J. M., Gong, L., Sangkuhl, K., Thorn, C. F., et al. (2012). Pharmacogenomics knowledge for personalized medicine. Clin. Pharmacol. Ther. 92 (4), 414–417. doi:10.1038/clpt.2012.96
Yaeger, R., Avila-Bront, A., Abdul, K., Nolan, P. C., Grann, V. R., Birchette, M. G., et al. (2008). Comparing genetic ancestry and self-described race in african americans born in the United States and in Africa. Cancer Epidemiol. Biomarkers Prev. 17 (6), 1329–1338. doi:10.1158/1055-9965.EPI-07-2505
Yan, R. J., Lou, T. T., Wu, Y. F., and Chen, W. S. (2017). Single nucleotide polymorphisms of ABCB1 gene and response to etanercept treatment in patients with ankylosing spondylitis in a Chinese Han population. Medicine 96 (5), e5929. doi:10.1097/MD.0000000000005929
Yang, J. J., Cheng, C., Devidas, M., Cao, X., Campana, D., Yang, W., et al. (2012). Genome-wide association study identifies germline polymorphisms associated with relapse of childhood acute lymphoblastic leukemia. Blood 120 (20), 4197–4204. doi:10.1182/blood-2012-07-440107
Youssef, Y. H., Makkeyah, S. M., Soliman, A. F., and Meky, N. H. (2021). Influence of genetic variants in asparaginase pathway on the susceptibility to asparaginase-related toxicity and patients' outcome in childhood acute lymphoblastic leukemia. Cancer Chemother. Pharmacol. 88 (2), 313–321. doi:10.1007/s00280-021-04290-6
Zalewska-Szewczyk, B., Andrzejewski, W., Młynarski, W., Jędrychowska-Dańska, K., Witas, H., and Bodalski, J. (2007). The anti-asparagines antibodies correlate with L-asparagines activity and may affect clinical outcome of childhood acute lymphoblastic leukemia. Leukemia lymphoma 48 (5), 931–936. doi:10.1080/10428190701292049
Keywords: acute lymphoblastic leukemia, ALL, toxicity, ancestry, pharmacogenetics, pharmacogenomics
Citation: Burgueño-Rodríguez G, Méndez Y, Olano N, Schelotto M, Castillo L, Soler AM and da Luz J (2023) Pharmacogenetics of pediatric acute lymphoblastic leukemia in Uruguay: adverse events related to induction phase drugs. Front. Pharmacol. 14:1278769. doi: 10.3389/fphar.2023.1278769
Received: 16 August 2023; Accepted: 30 October 2023;
Published: 17 November 2023.
Edited by:
Lucia Guadalupe Taja Chayeb, National Institute of Cancerology (INCAN), MexicoReviewed by:
Immaculate Mbongo Langmia, University of Tübingen, GermanyRocio Gomez, Center for Research and Advanced Studies (CINVESTAV), Mexico
Ingrid Fricke-Galindo, Instituto Nacional de Enfermedades Respiratorias-México (INER), Mexico
Copyright © 2023 Burgueño-Rodríguez, Méndez, Olano, Schelotto, Castillo, Soler and da Luz. This is an open-access article distributed under the terms of the Creative Commons Attribution License (CC BY). The use, distribution or reproduction in other forums is permitted, provided the original author(s) and the copyright owner(s) are credited and that the original publication in this journal is cited, in accordance with accepted academic practice. No use, distribution or reproduction is permitted which does not comply with these terms.
*Correspondence: Ana María Soler, anamasoler@gmail.com; Julio da Luz, jdal@fmed.edu.uy