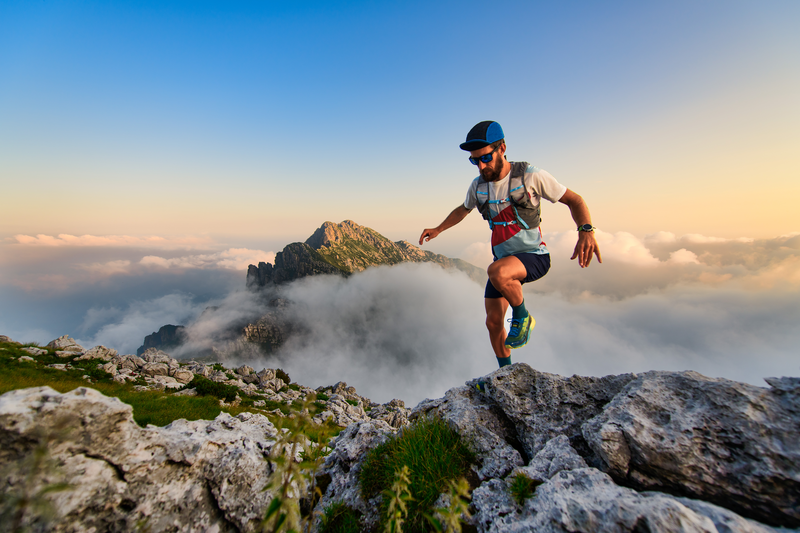
94% of researchers rate our articles as excellent or good
Learn more about the work of our research integrity team to safeguard the quality of each article we publish.
Find out more
ORIGINAL RESEARCH article
Front. Pharmacol. , 02 November 2023
Sec. Pharmacology of Infectious Diseases
Volume 14 - 2023 | https://doi.org/10.3389/fphar.2023.1278451
This article is part of the Research Topic Treatment of tick-borne diseases: Current status, challenges, and global perspectives View all 5 articles
Introduction: FLLL-32, a synthetic analog of curcumin, is a potent inhibitor of STAT3’s constitutive activation in a variety of cancer cells, and its anticancer properties have been demonstrated both in vitro and in vivo. It is also suggested that it might have other pharmacological activities including activity against different parasites.
Aim: This study therefore investigated the in vitro antiparasitic activity of FLLL-32 against four pathogenic Babesia species, B. bovis, B. bigemina, B. divergens, and B. caballi, and one Theileria species, Theileria equi. In vivo anti-Babesia microti activity of FLLL-32 was also evaluated in mice.
Methods: The FLLL-32, in the growth inhibition assay with a concentration range (0.005–50 μM), was tested for it’s activity against these pathogens. The reverse transcription PCR (RT-PCR) assay was used to evaluate the possible effects of FLLL-32 treatment on the mRNA transcription of the target B. bovis genes including S-adenosylhomocysteine hydrolase and histone deacetylase.
Results: The in vitro growth of B. bovis, B. bigemina, B. divergens, B. caballi, and T. equi was significantly inhibited in a dose-dependent manner (in all cases, p < 0.05). FLLL-32 exhibits the highest inhibitory effects on B. bovis growth in vitro, and it’s IC50 value against this species was 9.57 μM. The RT-PCR results showed that FLLL-32 inhibited the transcription of the B. bovis S-adenosylhomocysteine hydrolase gene. In vivo, the FLLL-32 showed significant inhibition (p < 0.05) of B. microti parasitemia in infected mice with results comparable to that of diminazene aceturate. Parasitemia level in B. microti-infected mice treated with FLLL-32 from day 12 post infection (pi) was reduced to reach zero level at day 16 pi when compared to the infected non-treated mice.
Conclusion: The present study demonstrated the antibabesial properties of FLLL-32 and suggested it’s usage in the treatment of babesiosis especially when utilized in combination therapy with other antibabesial drugs.
The most often used babesiacides for treating babesiosis in animals are diminazene aceturate (DA) and imidocarb dipropionate (ID) (Vial and Gorenflot, 2006). Studies have revealed that ID can remain in the products of treated animals for a long time after stopping the treatment (Mosqueda et al., 2012). Therefore, there is a paucity of it’s supply in some nations (Vial and Gorenflot, 2006; Mosqueda et al., 2012). Because of the effectiveness of multiple in vitro and in vivo culture methods, it is possible to used them to discover novel, and effective anti-piroplasmid compounds (Keroack et al., 2019). In this regard, several compounds recently demonstrated anti-piroplasm effects both in vitro and in vivo. For instance, the Malaria Box was used to screen for new potent antipiroplasm medications, such as 3-[(2-Hydroxyethyl)amino]-5-methylphenazin-5-ium “MMV396693,” by testing it against the growth of several bovine Babesia and equine piroplasms in vitro and the B. microti parasite in vivo (Rizk et al., 2019; Rizk et al., 2022). The mode of action for the antibabesial efficacy of MMV396693 is still unknown. However, our previous study (Rizk et al., 2022) suggested that both MMV396693 and ID might have a similar mode of action for inhibition Babesia parasite. The effectiveness of such compounds in combination therapy with other medication candidates can therefore be tested. In this concern, FLLL-32 is a synthetic analog of curcumin, which replaces the two hydrogens on the middle carbon with spiro-cycloalkyl rings to generate a diketo form. Such a chemical modification can prevent the enolization of FLLL32, thereby making it more stable (Lin et al., 2010b). Moreover, FLLL-32 can overcome limitations associated with using curcumin itself as poor bioavailability, low aqueous solubility, and rapid metabolism (Esatbeyoglu et al., 2012; Stanić, 2017; Morshedi et al., 2021).
FLLL-32 attracted a lot of attention due to its anti-infective, anti-mutagenic, anti-cancer, natural antioxidant, antimicrobial, and anti-inflammatory properties (Hussain et al., 2017; Huang et al., 2022). In addition, it specifically inhibits STAT3 and retains the cellular response to cytokines with anti-tumor activity on different types of cancer cells including colorectal cancer, glioblastoma, multiple myeloma, and liver cancer cells (Lin et al., 2010a). Despite the wide use of FLLL-32 as an anticancer, its antiprotozoal activity has not been determined yet. For that, the objective of this study was to evaluate the in vitro antiprotozoal activity of FLLL-32 against B. bovis, B. bigemina, B. divergens, B. caballi, and T. equi and to assess it’s possible synergistic interaction with two antibabesial drugs, DA and ID, and MMV396693 against these species. Additionally, its in vivo inhibitory efficacy on B. microti growth in mice was assessed. Eventually, this study also aimed for to provide a preliminary investigation of the underlying molecular mechanism of FLLL-32 action.
The compound FLLL-32 was obtained from (Merck KGaA 64271 Darmstadt, Germany) and dissolved in (0.1%) dimethyl sulfoxide (DMSO) for stock concentration and kept at −30°C until use. The antibabesial drug, DA (Ganaseg, Ciba-Geigy Japan Ltd., Tokyo, Japan) was employed as a control drug. DA, ID (Sigma-Aldrich, Tokyo, Japan), and MMV396693 (MolPort, Latvia) were used for the in vitro combination inhibition assay. The nucleic acid stain SYBR Green I (SGI) (Lonza, Rockland, United States; 10,000x) was stored at −20°C and thawed before use. A lysis solution comprising Tris (130 mM; pH 7.5), EDTA (10 mM), saponin (0.016%; W/V), and TritonX-100 (1.6%; V/V) had been prepared in advance and stored at 4°C. Both SGI and lysis buffer were used for inhibition assay either in vitro or in vivo.
Using a microaerophilic stationary-phase culture technique, B. bovis (Texas strain), B. bigemina (Argentina strain), B. divergens (German strain), B. Caballi (USDA strain), and Theileria equi (USDA strain) were grown and maintained in purified bovine or equine red blood cells (RBCs). Babesia caballi was cultured in RPMI 1640 medium, whereas B. bovis, B. bigemina, and T. equi were cultured in Medium 199 (both media were purchased from Sigma-Aldrich). For equine Babesia and Theileria parasites, 40% normal horse serum was added to the media, whereas media was supplemented with 40% normal bovine serum for bovine Babesia parasites, along with penicillin G at 60 units per mL, streptomycin at 60 mg/mL, and amphotericin B at 0.15 mg/mL (all from Sigma-Aldrich). Theileria equi cultures were supplied with 13.6 g of hypoxanthine (ICN Biomedicals, Inc., United States) per mL. All parasite cultures were grown at 37°C in a 5% CO2, 5% O2, and 90% N2 environment.
The fluorescence assay using an SGI stain was employed to study the effect of FLLL-32 on Babesia/Theileria growth (Rizk et al., 2015; Rizk et al., 2016). Double 96-well plates (Nunc, Roskilde, Denmark) were utilized to culture bovine Babesia as well as equine Babesia and Theileria in the infected pRBCs with either media only (blank wells) or a medium containing 0.005–200 μM FLLL-32. Positive control cultures, on the other hand, were treated with DA concentration ranging from 0.25 to 10 μM. Negative experimental controls included wells containing only the pRBCs with media containing the used solvent (0.1% DMSO). The plates were then incubated for 4 days at 37°C, and the IC50 values for FLLL-32 and DA were calculated on the 4th day based on growth inhibition in three separate experiments. On the fourth day of treatment, the viability assay was carried out by mixing 1.5 μL of the control or FLLL32-treated infected RBCs with 3.5 μL of parasite-free RBCs, suspending the mixture in fresh growth medium without the addition of drugs, and incubating the mixture at 37°C for the following 4 days without changing the medium (Rizk et al., 2016). The IC50 values of FLLL32 were calculated using the non-linear regression curve fit in GraphPad Prism 5.0 (GraphPad Software, Inc., San Diego, CA, United States) (Rizk et al., 2016; Rizk et al., 2017; Rizk et al., 2019; Rizk et al., 2021a; Rizk et al., 2021b; El-Sayed et al., 2023).
Combination therapies of FLLL-32 with the commonly used (DA, and ID), and the recently identified antibabesial drugs (MMV396693) were evaluated. The combination ratios ranged from 0.50 to 0.75 IC50s of the selected drugs (Supplementary Table S1) were prepared as previously described (Rizk et al., 2023). A 96-well plate containing B. bovis, B. bigemina, B. caballi, and T. equi pRBCs was treated with a two-drug combination; FLLL-32+DA, FLLL-32+ID, and FLLL-32+MMV396693 at concentrations of 0.5 x IC50 and 0.75 x IC50 in triplicates.
All in vitro tests were conducted at 1% parasitemia, and either 2.5% hematocrit (HCT) for B. bigemina and B. bovis, or 5% HCT for B. divergens, B. caballi, and T. equi parasites (Rizk et al., 2017). Next, 100 μL lysis buffer mixed with a 2× SGI was added to each well in the 96-wells plates after 4 days of incubation. The mean fluorescence values were then plotted against the logarithm of drug concentrations. Each drug concentration was tested in triplicate in each experiment and values of fluorescence assays were calculated from three separate experiments. The parasite survival was evaluated using non-linear expression analyses (Schadich et al., 2022).
Reverse transcription-PCR (RT-PCR) was used to assess the effect of FLLL-32 treatment on the mRNA transcription of the target B. bovis genes including S-adenosylhomocysteine hydrolase (BbSAHH) and the Histone deacetylase (BbHDAC3) (El-Sayed et al., 2023). Babesia bovis was grown in bovine RBCs on 24-well culture plates (Nunc, Roskilde) as previously described and was treated for 8 h with FLLL-32 at the 99% inhibitory concentration (IC99) (18.94 μM) and 0.1% DMSO as a negative control. After that, pRBCs were collected and washed with phosphate buffer saline. Following the manufacturer’s guidelines, total RNA was extracted using a commercial RNeasy mini kit (QIAGEN, Germantown Rd, Germantown, MD, United States). A Nanodrop 2000 spectrophotometer (Thermo Fisher Scientific Inc., Tokyo, Japan) was used to measure the RNA concentration, and one Step RNA Kit (AMV) (Takara, Japan) was used for conducting the RT-PCR following the manufacturer’s instructions. The B. bovis profilin (BbPROF) gene is used as the reference control gene. The BbSAHH, BbHDAC3, and BbPROF genes were amplified using total RNA (150 ng) from the treatment cultures and the control. The specific forward and reverse primers used are listed in Table 1, and the PCR conditions were 30 min at 50°C for reverse transcription, followed by 2 min of 94°C denaturation, 30 cycles of 94°C for 30 s denaturation, 55°C for 30 s annealing for BbHDAC3, BbPROF, and 57°C for BbSAHH, 1 min of 72°C extension, and 5 min of 72°C final extension (Mukhjargal et al., 2012). After staining with ethidium bromide, the amplified products were electrophoresed on 2.0% (w/v) agarose gels and visualized with a UV transilluminator (Nippon Gene, Tokyo, Japan). Gel electrophoresis bands were analyzed using ImageJ software (Schadich et al., 2012; El-Sayed et al., 2023).
Four groups of BALB/c mice (n = 5 per group) aged 8 weeks (CLEA, Tokyo, Japan) were injected intraperitoneally with 1 × 107 B. microti (Munich strain) iRBCs except for the mice in the first group which remained uninfected and served as a negative control. When infected mice demonstrated 1% parasitemia, mice in two experimental groups were given daily injections of tested drugs (FLLL-32 and DA) for 5 days whereas, one group was non-treated and served as a control group. One group was treated with FLLL-32 intraperitoneal at a dosage of 50 mg/kg while, the other group was given DA intraperitoneal at a dosage of 25 mg/kg (positive control). A venous tail blood sample (2.5 μL) was collected from each mouse and transferred to a 96-well plate with RPMI 1640 Medium previously mixed with 50 μl of lysis solution. Following that, 50 μL of lysis buffer with 2x SGI nucleic acid stain was mixed into each well. Eventually, the plate was incubated in the dark for 1 h. The inhibitory effects of FLLL-32 and DA on the growth of B. microti were evaluated using a fluorescence spectrophotometer every 48 h until 30 days post-inoculation. Following the completion of the study, all of the mice were euthanized humanely via inhalation of the chemical chloroform, which was followed by neck dislocation (physical euthanasia).
The obtained data were analyzed using GraphPad Prism. Differences between the control and treated groups were determined by one-way analysis of variance (ANOVA) and unpaired t-tests. The statistical significance was defined as p-value < 0.05. The statistically significant differences between the drug-treated and positive-control groups were used in the viability test as an indication of parasite regrowth (Rizk et al., 2016).
All experimental protocols in this work were approved by the Animal Care and Use Committee at Obihiro University of Agriculture and Veterinary Medicine (Approval No. 27-65). All experiments were carried out following the Fundamental Guidelines for the Proper Conduct of Animal Experiment and Related Activities at Academic Research Institutions issued by Japan’s Ministry of Education, Culture, Sports, Science, and Technology. The pathogen experiment’s IDs were as follows: Babesia microti: 20170905; equine piroplasm parasites: 201910-2; and bovine Babesia: 201708-4.
FLLL-32 treatments of 0.005-, 5, and 50 μM, respectively, significantly inhibited (p < 0.05) the in vitro growth of B. bovis, B. bigemina, and B. divergens (Figure 1). Meanwhile, 50, and 5 μM FLLL-32 treatments significantly inhibited (p < 0.05) the growth of B. caballi, and T. Equi, respectively (Figure 2). FLLL-32 exhibited the highest inhibitory effects on B. bovis growth, with an IC50 value of 9.57 ± 1.18 µM (Table 2). The estimated IC50 for B. divergens, B. bigemina, B. caballi, and T. equi were 26.46 ± 3.67, 28.14 ± 4.38, 28.95 ± 3.13, and 30.42 ± 1.54 µM, respectively (Table 1). After that, the regrowth of the parasites was assessed after stopping the treatment using the viability test. The results demonstrated that all tested parasites did not regrow at a dosage of ≥50 μM FLLL-32 (Supplementary Table S2). These findings suggest that FLLL-32 inhibits B. bovis growth more effectively than other bovine and equine piroplasmids in vitro.
FIGURE 1. Correlation between relative fluorescence units (RFUs) and the log concentrations of FLLL-32 (µm) on bovine Babesia parasites. (A) B. bovis. (B) B. bigemina. (C) B. divergens. Each value represents the mean of triplicate wells after subtraction of the background fluorescence for non-parasitized RBCs. The logarithmic values of drug concentration are of the base 10.
FIGURE 2. Correlation between relative fluorescence units (RFUs) and the log concentrations of FLLL-32 (µm) on equine piroplasmids. (A) T. equi. (B) B. caballi. Each value represents the mean of triplicate wells after subtraction of the background fluorescence for non-parasitized RBCs. The logarithmic values of drug concentration are of the base 10.
TABLE 2. IC50 values of FLLL32 and diminazene aceturate evaluated for bovine Babesia and equine Babesia and Theileria parasites.
To evaluate whether the in vitro inhibitory efficacy of FLLL-32 will increase when used in combination therapy, a combination consisting of FLLL-32 with either DA, ID, or MMV396693 was used. FLLL32 at a concentration of 0.75 x IC50 in combination with 0.5 x IC50 and 0.75 x IC50 DA showed additive effects against B. bovis on the fourth day of treatment (Table 3). FLLL-32 and MMV396693 combination at a concentration of 0.75 x IC50 exhibited an additive effect on B. bigemina (Table 4), meanwhile, the same combination showed synergistic interaction on the growth of B. Caballi (Table 5). FLLL-32 and ID combination at a concentration of 0.75xIC50 showed an additive effect on B. caballi (Table 5). Similarly, this drug combination (0.75 x IC50 FLLL32 and 0.75 x IC50 ID) showed synergistic interaction on the growth of T. equi (Table 6).
TABLE 3. Drug interactions of FLLL-32 in combination with diminazene aceturate (DA), imidocarb dipropionate (ID), and MMV396693 (MMV) on B. bovis.
TABLE 4. Drug interactions of FLLL-32 in combination with diminazene aceturate (DA), imidocarb dipropionate (ID), and MMV396693 (MMV) on B. bigemina.
TABLE 5. Drug interactions of FLLL-32 in combination with diminazene aceturate (DA), imidocarb dipropionate (ID), and MMV396693 (MMV) on B. caballi.
TABLE 6. Drug interactions of FLLL-32 in combination with diminazene aceturate (DA), imidocarb dipropionate (ID), and MMV396693 (MMV) on T. equi.
In the B. bovis culture, at the IC99 concentration, the FLLL-32 doesn’t affect the transcription of the BbHDAC3 gene in 8 h treatments as it was shown by comparison of the level of mRNA transcript of this gene in treated cells with those of controls (cells treated with 0.1% DMSO) (Figure 3). Interestingly, in these treatments, the expression of the other B. bovis gene, BbSAHH in the cells treated by FLLL-32 was completely inhibited while its level in controls was not changed (Figure 3). The expression level of BbPROF gene did not differ between treated cells and controls (data not shown).
FIGURE 3. Reverse transcription-PCR analysis of B. bovis HDAC3 and B. bovis SAHH genes from B. bovis cultures treated with FLLL32 at IC99 concentration and DMSO (0.1%) used as a control for 8 h. (A) Percentages of gel electrophoresis band peak for B. bovis HDAC3. (B) Gel electrophoresis for B. bovis HDAC3 (lane 1, FLLL32 -treated culture; lane 2, control culture). (C) Percentages of gel electrophoresis band peak for (B) B. bovis SAHH. (D) Gel electrophoresis for B. bovis SAHH (lane 1, control culture; lane 2, FLLL32 -treated culture). M, molecular size marker. The percentages were determined by ImageJ software.
Parasitemia levels were significantly reduced (p < 0.05) in mice treated with FLLL-32 from day10 post infection (pi) to reach zero level at day 16 pi in comparison with the non-treated control group (Figure 4). Treatment with 50 mg/kg FLLL-32 resulted in 35% inhibition at day 10 p.i. (peak of parasitemia) (Figure 4). Peak fluorescence values in the treated groups with FLLL-32 50 mg/kg reached an average of 1213 at day 12 pi. Fluorescence readings were significantly reduced (p < 0.05) in mice treated with FLLL-32 from days 10–26 p.i. When compared to positive control mice (infected nontreated) which is similar to DA-treated mice (Figure 4). The obtained results suggested the hopeful antibabesial efficacy of FLLL-32 in an infected experimental animal model.
FIGURE 4. Inhibitory effect of FLLL-32 on the growth of Babesia microti. Each value represents the mean ± standard deviation of five mice per experimental group. Diminazene aceturate (DA) was used as the control drug and the non-treated group was used as negative control mice. Asymbol indicates significant differences (#p < 0.05) from day 10 to day 26 post-inoculation between the FLLL-32–treated and control groups.
Emerging data suggest that natural products may represent effective candidate molecules for drug discovery, however, their clinical utility is somewhat limited due to the poor bioavailability and target selectivity (Ćavar Zeljković et al., 2022). Therefore, efforts are underway to design and synthesize novel analogs with a higher bioavailability and target specificity (Bill et al., 2010a; Ćavar Zeljković et al., 2022). FLLL-32 is one of the curcumin analogs which modeled based on the diketone form of curcumin binding to the SH2 domain of STAT3 (Yang and Lesinski, 2012). Curcuma and its associated bioactive compounds showed some antiprotozoal activities against Plasmodium, Leishmania, Trypanosoma, Babesia, and Giardia (Haddad et al., 2011). These derivatives are shown to have several pharmacological effects such as anti-inflammatory, antioxidant, anticarcinogenic, antibacterial, antifungal, antiprotozoal, antiviral, and immunomodulatory (Abd El-Hack et al., 2021).
General talking, B. bovis, B. bigemina, and B. divergens are the most economically important bovine babesiosis (Rizk et al., 2020). However, B. bovis infection is associated with more severe disease and higher mortality and is considered the most virulent species of bovine babesiosis (Schnittger et al., 2012; Gohil et al., 2013; Suarez et al., 2019). Because of the limitations of chemoprophylaxis and acaricide control of transmitting vectors, live attenuated vaccine immunization against B. bovis has been used as an alternate control method (Rizk et al., 2020). However, several drawbacks associated with the production of these control methods have been identified (Cuy-Chaparro et al., 2023). Therefore, safer anti-babesial medications that either cure the infection or reduce the dosages of DA, and ID supplied are likely to be more effective methods.
In the present study, FLLL-32 showed potent in vitro inhibitory effects against the growth of various Babesia species and exhibited the highest inhibitory effects on B. bovis growth. Interestingly, the IC50 of FLLL-32 for B. bovis was lower than those previously obtained with other antibabesial drugs including gedunin (17.86 µM) (Rizk et al., 2015), N-acetyl-l-cysteine (332.11 µM) (Rizk et al., 2017), enoxacin (38.04 µM) (Rizk et al., 2018), and thymoquinone (35.41 µM) (El-Sayed et al., 2019; Rizk et al., 2020). In general, several factors related to the screening parasite, including parasite type, strain, and size, affect the effectiveness of the tested medications (Rizk et al., 2019; Rizk et al., 2020). The medium used, the HCT, and whether or not serum is present in the in vitro culture have an impact on the calculated IC50s of the medicine being tested (Rizk et al., 2015; Rizk et al., 2016). As a result, the inconsistent FLLL-32 IC50 values in the current investigation could be explained by variations in the parasite species or culture conditions between the several screened piroplasm.
Indeed, several antibabesial compounds obtained from natural herbal sources were evaluated against babesiosis in our laboratory. For example, allicin and fusidic acid (Salama et al., 2013; Salama et al., 2014) exhibited much higher IC50 values (µM) against B. bovis, B. bigemina, B. caballi, and T. equi than those calculated for FLLL-32. In the same way, turmeric methanolic extract showed in vitro inhibitory activity against B. divergens, B. caballi, and B. bovis higher than those estimated for FLLL-32 (Rizk et al., 2021b). Of note, the in vivo inhibition of FLLL-32 against B. microti in mice at day with peak parasitemia (day 10) was higher than those caused by 100 mg/kg enoxacin (21%), 150 mg/kg norfloxacin (15%), and 700 mg/kg ofloxacin (23%) (Rizk et al., 2018).
FLLL32 has exceptional biochemical properties, and in particular inhibits signal transducer and activator of transcription 3 (STAT3) phosphorylation, DNA-binding activity, and transactivation, and demonstrates significant growth suppressive activity in a variety of human cancer cells (Lin et al., 2010a; Bill et al., 2010b; Lin et al., 2010b; Fossey et al., 2011; Wei et al., 2011; Bill et al., 2012). Furthermore, FLLL32 can suppress IFNα and interleukin-6-induced STAT3 phosphorylation (Lin et al., 2010a; Onimoe et al., 2012). Curcuminoids have been identified as JMJD2 histone demethylase inhibitors, with FLLL-32 inhibiting only JMJD2D (Kim et al., 2014). It has been proposed that FLLL-32 reduces intestinal damage in necrotizing enterocolitis (Eckert et al., 2017). In this study, we investigated the effects of FLLL-32 treatment on two B. bovis genes BbHDAC3 and BbSAHH. However, the effect of apicidin which is an inhibitor of histone deacetylase (HDAC) on Babesia parasite growth has been previously reported (Munkhjargal et al., 2009; Munkhjargal et al., 2012) but FLLL-32 showed no effects on the mRNA transcription. On the contrary, the mRNA transcription of the BbSAHH of cultures treated with FLLL-32 was significantly inhibited (p < 0.05) but not the mRNA transcription of the control BbPROF gene in comparison to cultures treated with DMSO (0.1%) within 8 h of treatment.
The enzyme S-adenosylhomocysteine hydrolase (SAHH) catalyzes the reversible breakdown of S-adenosylhomocysteine (SAH) to homocysteine and adenosine (De La Haba, and Cantoni, 1959). Homocysteine and adenosine product elimination are necessary for SAH hydrolysis. SAH accumulation can inhibit methyltransferase activity by having a high affinity binding to the enzyme active site in the absence of effective product elimination (Hu et al., 1999; Yi et al., 2000). Because it can alter the cellular methylation of phospholipids, proteins, small molecules, DNA, and RNA, SAHH has become an attractive pharmaceutical target (Chiang, 1998). Several nucleoside inhibitors of SAHH have therefore been synthesized, having pharmacological and biological effects such as antiviral effects (Chiang, 1998; Clerq, 1998). S-adenosylhomocysteine hydrolase was shown to be an interesting target for the development of novel anti-malarial agents (Bitonti et al., 1990; Kitade et al., 2003; Nakanishi et al., 2005; Chandra et al., 2021). SAHH gene from Plasmodium falciparum (PfSAHH) was well characterized (Creedon et al., 1994; Bujnicki et al., 2003; Tanaka et al., 2004) however, no studies have been conducted on B. bovis. High binding affinity with PfSAHH has been found for curcumin and its derivatives which curcumin as a potential candidate for the development of antimalarial drugs (Singh et al., 2013). Altogether, SAH hydrolase may be a promising pharmacological target for developing antibabesial drugs, particularly for the most virulent species, B. bovis. Although, the present study evaluated the in vitro and in vivo antibabesial efficacy of FLLL-32, future studies are required to assess the effect of this drug on the developmental growth arrest using the phenotypic assay.
The curcumin analog FLLL-32 showed potent inhibitory effects on the in vitro growth of B. bovis, B. bigemina, B. divergens, B. caballi, and T. equi with B. bovis being the most susceptible species. FLLL-32 was shown to inhibit the enzyme S-adenosylhomocysteine hydrolase (SAHH) of B. bovis that can cause increasing the SAH to S-adenosylmethionine (SAM) ratio and blocking SAM-dependent methyltransferase, which catalyzes the methylation process required for parasite growth. Moreover, the compound showed antibabesial activities against the in vivo growth of B. microti in infected mice. Further studies are required to characterize the SAHH enzyme of B. bovis and to investigate the inhibitor’s interaction especially FLLL-32 with this drug target.
The raw data supporting the conclusion of this article will be made available by the authors, without undue reservation.
All experimental protocols in this work were approved by the Animal Care and Use Committee at Obihiro University of Agriculture and Veterinary Medicine (Approval No. 27-65). All experiments were carried out following the Fundamental Guidelines for the Proper Conduct of Animal Experiment and Related Activities at Academic Research Institutions issued by Japan’s Ministry of Education, Culture, Sports, Science, and Technology. The pathogen experiment’s IDs were as follows: Babesia microti: 20170905; equine piroplasm parasites: 201910-2; and bovine Babesia: 201708-4. The study was conducted in accordance with the local legislation and institutional requirements.
SAE-SE-S: Conceptualisation, Data curation, Formal analysis, Funding acquisition, Methodology, Writing–original draft, Writing–review and editing. E-SE-A: Writing–original draft, Writing–review and editing. HB: Resources, Software, Writing–original draft, Writing–review and editing. MS-A: Formal analysis, Funding acquisition, Software, Writing–original draft, Writing–review and editing. SAl: Formal analysis. NA: Software, Writing–review and editing. SA: Software. MA: Formal analysis, Software, Writing–review and editing. II: Conceptualisation, Funding acquisition, Investigation, Project administration, Resources, Supervision, Validation, Visualisation, Writing–review and editing. MR: Conceptualisation, Data curation, Formal analysis, Investigation, Methodology, Resources, Software, Visualisation, Writing–original draft, Writing–review and editing. All authors contributed to the article and approved the submitted version.
The author(s) declare financial support was received for the research, authorship, and/or publication of this article. This study was supported by the Deputyship for Research and Innovation, Ministry of Education in Saudi Arabia for funding the publication fee of this research work through project number: ISP23-73.
The authors would like to thank Naoaki Yokoyama, National Research Center for Protozoan Diseases, Obihiro University of Agriculture and Veterinary Medicine, Inada-cho, Obihiro, Hokkaido, Japan for his scientific support and discussion. The authors also extend their appreciation to the Medicines for Malaria Venture Malaria Box Challenge Grant and the Ministry of Education, Culture, Sports, Science, and Technology of Japan.
The authors declare that the research was conducted in the absence of any commercial or financial relationships that could be construed as a potential conflict of interest.
The authors declared that they were an editorial board member of Frontiers, at the time of submission. This had no impact on the peer review process and the final decision.
All claims expressed in this article are solely those of the authors and do not necessarily represent those of their affiliated organizations, or those of the publisher, the editors and the reviewers. Any product that may be evaluated in this article, or claim that may be made by its manufacturer, is not guaranteed or endorsed by the publisher.
The Supplementary Material for this article can be found online at: https://www.frontiersin.org/articles/10.3389/fphar.2023.1278451/full#supplementary-material
Abd El-Hack, M. E., El-Saadony, M. T., Swelum, A. A., Arif, M., Abo Ghanima, M. M., Shukry, M., et al. (2021). Curcumin, the active substance of turmeric: its effects on health and ways to improve its bioavailability. J. Sci. Food Agric. 101 (14), 5747–5762. doi:10.1002/jsfa.11372
Bill, M. A., Fuchs, J. R., Li, C., Yui, J., Bakan, C., Benson, D. M., et al. 2010b. The small molecule curcumin analog FLLL32 induces apoptosis in melanoma cells via STAT3 inhibition and retains the cellular response to cytokines with anti-tumor activity. Mol. Cancer;9:165. doi:10.1186/1476-4598-9-165
Bill, M. A., Fuchs, J. R., Li, C., Yui, J., Bakan, C., Benson, D. M., et al. (2010a). The small molecule curcumin analog FLLL32 induces apoptosis in melanoma cells via STAT3 inhibition and retains the cellular response to cytokines with anti-tumor activity. Mol. cancer 9, 165–212. doi:10.1186/1476-4598-9-165
Bill, M. A., Nicholas, C., Mace, T. A., Etter, J. P., Li, C., Schwartz, E. B., et al. (2012). Structurally modified curcumin analogs inhibit STAT3 phosphorylation and promote apoptosis of human renal cell carcinoma and melanoma cell lines.
Bitonti, A. J., Baumann, R. J., Jarvi, E. T., McCarthy, J. R., and Mccann, P. P. (1990). Antimalarial activity of a 4', 5'-unsaturated 5'-fluoroadenosine mechanism-based inhibitor of S-adenosyl-L-homocysteine hydrolase. Biochem. Pharmacol. 40 (3), 601–606. doi:10.1016/0006-2952(90)90562-y
Bujnicki, J. M., Prigge, S. T., Caridha, D., and Chiang, P. K. (2003). Structure, evolution, and inhibitor interaction of S-adenosyl-L-homocysteine hydrolase from Plasmodium falciparum. Proteins Struct. Funct. Bioinforma. 52 (4), 624–632. doi:10.1002/prot.10446
Ćavar Zeljković, S., Schadich, E., Dzubak, P., Hajdúch, M., and Tarkowski, P. (2022). Antiviral activity of selected Lamiaceae essential oils and their monoterpenes against SARS-Cov-2. Front. Pharmacol. 13, 1–13. doi:10.3389/fphar.2022.893634
Chandra, G., Patel, S., Panchal, M., and Singh, D. V. (2021). S-adenosyl-L-homocysteine hydrolase: its inhibitory activity against Plasmodium falciparum and development of malaria drugs. Mini Rev. Med. Chem. 21 (7), 833–846. doi:10.2174/1389557521666201218155321
Chiang, P. K. (1998). Biological effects of inhibitors of S-adenosylhomocysteine hydrolase. Pharmacol. Ther. 77 (2), 115–134. doi:10.1016/s0163-7258(97)00089-2
Clerq, E. D. (1998). Carbocyclic adenosine analogues as S-adenosylhomocysteine hydrolase inhibitors and antiviral agents: recent advances. Nucleosides Nucleotides 17 (1-3), 625–634. doi:10.1080/07328319808005205
Creedon, K. A., Rathod, P. K., and Wellems, T. E. (1994). Plasmodium falciparum S-adenosylhomocysteine hydrolase. cDNA identification, predicted protein sequence, and expression in Escherichia coli. J. Biol. Chem. 269 (23), 16364–16370. doi:10.1016/s0021-9258(17)34016-4
Cuy-Chaparro, L., Reyes, C., Díaz-Guiot, E. V., Moreno-Pérez, D. A., and Patarroyo, M. A. (2023). Developing anti-babesia bovis blood stage vaccines: a new perspective regarding synthetic vaccines. Int. J. Mol. Sci. 24 (6), 5219. doi:10.3390/ijms24065219
De La Haba, G., and Cantoni, G. L. (1959). The enzymatic synthesis of S-adenosyl-L-homocysteine from adenosine and homocysteine. J. Biol. Chem. 234 (3), 603–608. doi:10.1016/s0021-9258(18)70253-6
El-Sayed, S. A. E., Rizk, M. A., Yokoyama, N., and Igarashi, I. (2019). Evaluation of the in vitro and in vivo inhibitory effect of thymoquinone on piroplasm parasites. Parasit. Vectors 16 (12), 37. doi:10.1186/s13071-019-3296-z
El-Sayed, S. A. E.-S., El-Alfy, E.-S., Sayed-Ahmed, M. Z., Mohanta, U. K., Alqahtani, S. S., Alam, N., et al. (2023). Evaluating the inhibitory effect of resveratrol on the multiplication of several Babesia species and Theileria equi on in vitro cultures, and Babesia microti in mice. Front. Pharmacol. 14, 1192999. doi:10.3389/fphar.2023.1192999
Esatbeyoglu, T., Huebbe, P., Ernst, I. M., Chin, D., Wagner, A. E., and Rimbach, G. (2012). Curcumin—from molecule to biological function. Angew. Chem. Int. Ed. 51 (22), 5308–5332. doi:10.1002/anie.201107724
Fossey, S. L., Bear, M. D., Lin, J., Li, C., Schwartz, E. B., Li, P. K., et al. (2011). The novel curcumin analog FLLL32 decreases STAT3 DNA binding activity and expression, and induces apoptosis in osteosarcoma cell lines. BMC cancer 11 (1), 112–115. doi:10.1186/1471-2407-11-112
Gohil, S., Herrmann, S., Günther, S., and Cooke, B. M. (2013). Bovine babesiosis in the 21st century: advances in biology and functional genomics. Int. J. Parasitol. 43 (2), 125–132. doi:10.1016/j.ijpara.2012.09.008
Gupta, S. C., Patchva, S., and Aggarwal, B. B. (2013). Therapeutic roles of curcumin: lessons learned from clinical trials. AAPS J. 15, 195–218. doi:10.1208/s12248-012-9432-8
Gupta, S. C., Patchva, S., Koh, W., and Aggarwal, B. B. (2012). Discovery of curcumin, a component of golden spice, and its miraculous biological activities. Clin. Exp. Pharmacol. physiology 39 (3), 283–299. doi:10.1111/j.1440-1681.2011.05648.x
Haddad, M., Sauvain, M., and Deharo, E. (2011). Curcuma as a parasiticidal agent: a review. Planta medica. 77 (06), 672–678. doi:10.1055/s-0030-1250549
Hu, Y., Komoto, J., Huang, Y., Gomi, T., Ogawa, H., Takata, Y., et al. (1999). Crystal structure of S-adenosylhomocysteine hydrolase from rat liver. Biochemistry 38 (26), 8323–8333. doi:10.1021/bi990332k
Huang, Q., Zhang, Y., Zheng, Y., Yang, H., Yang, Y., Mo, Y., et al. (2022). Molecular mechanism of curcumin and its analogs as multifunctional compounds against pancreatic cancer. Nutr. Cancer 74 (9), 3096–3108. doi:10.1080/01635581.2022.2071451
Hussain, Z., Thu, H. E., Amjad, M. W., Hussain, F., Ahmed, T. A., and Khan, S. (2017). Exploring recent developments to improve antioxidant, anti-inflammatory and antimicrobial efficacy of curcumin: a review of new trends and future perspectives. Mater. Sci. Eng. C 77, 1316–1326. doi:10.1016/j.msec.2017.03.226
Keroack, C. D., Elsworth, B., and Duraisingh, M. T. (2019). To kill a piroplasm: genetic technologies to advance drug discovery and target identification in Babesia. Int. J. Parasitol. 49 (2), 153–163. doi:10.1016/j.ijpara.2018.09.005
Kim, T. D., Fuchs, J. R., Schwartz, E., Abdelhamid, D., Etter, J., Berry, W. L., et al. (2014). Pro-growth role of the JMJD2C histone demethylase in HCT-116 colon cancer cells and identification of curcuminoids as JMJD2 inhibitors. Am. J. Transl. Res. 6 (3), 236–247.
Kitade, Y., Kojima, H., Zulfiqur, F., Kim, H. S., and Wataya, Y. (2003). Synthesis of 2-fluoronoraristeromycin and its inhibitory activity against Plasmodium falciparum S-adenosyl-L-homocysteine hydrolase. Bioorg. Med. Chem. Lett. 13 (22), 3963–3965. doi:10.1016/j.bmcl.2003.08.074
Lin, L., Deangelis, S., Foust, E., Fuchs, J., Li, C., Li, P. K., et al. (2010b). A novel small molecule inhibits STAT3 phosphorylation and DNA binding activity and exhibits potent growth suppressive activity in human cancer cells. Mol. cancer 9 (1), 217–310. doi:10.1186/1476-4598-9-217
Lin, L., Hutzen, B., Zuo, M., Ball, S., Deangelis, S., Foust, E., et al. (2010a). Novel STAT3 phosphorylation inhibitors exhibit potent growth-suppressive activity in pancreatic and breast cancer cells. Cancer Res. 70 (6), 2445–2454. doi:10.1158/0008-5472.CAN-09-2468
Morshedi, K., Borran, S., Ebrahimi, M. S., Masoud khooy, M. J., Seyedi, Z. S., Amiri, A., et al. (2021). Therapeutic effect of curcumin in gastrointestinal cancers: a comprehensive review. Phytotherapy Res. 35 (9), 4834–4897. doi:10.1002/ptr.7119
Mosqueda, J., Olvera-Ramírez, A., Aguilar-Tipacamu, G., and J Canto, G. (2012). Current advances in detection and treatment of babesiosis. Curr. Med. Chem. 19 (10), 1504–1518. doi:10.2174/092986712799828355
Munkhjargal, T., AbouLaila, M., Ueno, A., Sivakumar, T., Nakano, Y., Yokoyama, M., et al. (2012). Cloning and characterization of histone deacetylase from Babesia bovis. Veterinary Parasitol. 190 (3-4), 423–433. doi:10.1016/j.vetpar.2012.06.026
Munkhjargal, T., Aboulaila, M. R. A., Sivakumar, T., Yokoyama, N., and Igarashi, I. (2009). Inhibitory effect of apicidin on in vitro and in vivo growth of Babesia parasites. J. protozoology Res. 19 (1), 42–49. doi:10.32268/jprotozoolres.19.1_42
Nakanishi, M., Yabe, S., Tanaka, N., Ito, Y., Nakamura, K. T., and Kitade, Y. (2005). Mutational analyses of Plasmodium falciparum and human S-adenosylhomocysteine hydrolases. Mol. Biochem. Parasitol. 143 (2), 146–151. doi:10.1016/j.molbiopara.2005.05.012
Onimoe, G. I., Liu, A., Lin, L., Wei, C. C., Schwartz, E. B., Bhasin, D., et al. (2012). Small molecules, LLL12 and FLLL32, inhibit STAT3 and exhibit potent growth suppressive activity in osteosarcoma cells and tumor growth in mice. Investig. new drugs 30, 916–926. doi:10.1007/s10637-011-9645-1
Rizk, M. A., AbouLaila, M., El-Sayed, S. A. E., Guswanto, A., Yokoyama, N., and Igarashi, I. (2018). Inhibitory effects of fluoroquinolone antibiotics on Babesia divergens and Babesia microti, blood parasites of veterinary and zoonotic importance. Infect. Drug Resist. 11, 1605–1615. doi:10.2147/IDR.S159519
Rizk, M. A., Baghdadi, H. B., El-Sayed, S. A. E. S., Eltaysh, R., and Igarashi, I. (2022). Repurposing of the Malaria Box for Babesia microti in mice identifies novel active scaffolds against piroplasmosis. Parasites Vectors 15 (1), 329–410. doi:10.1186/s13071-022-05430-4
Rizk, M. A., El-Sayed, S. A. E., El-Alfy, E. S., and Igarashi, I. (2023). Imidazo[1,2-a]pyridine: a highly potent therapeutic agent clears piroplasm infection in vitro. Acta Parasitol. Mar. 68 (1), 249–256. doi:10.1007/s11686-022-00655-w
Rizk, M. A., El-Sayed, S. A. E., Nassif, M., Mosqueda, J., Xuan, X., and Igarashi, I. (2020). Assay methods for in vitro and in vivo anti-Babesia drug efficacy testing: current progress, outlook, and challenges. Vet. Parasitol. 279, 109013. doi:10.1016/j.vetpar.2019.109013
Rizk, M. A., El-Sayed, S. A. E. S., AbouLaila, M., Tuvshintulga, B., Yokoyama, N., and Igarashi, I. (2016). Large-scale drug screening against Babesia divergens parasite using a fluorescence-based high-throughput screening assay. Veterinary Parasitol. 227, 93–97. doi:10.1016/j.vetpar.2016.07.032
Rizk, M. A., El-Sayed, S. A. E. S., AbouLaila, M., Yokoyama, N., and Igarashi, I. (2017). Evaluation of the inhibitory effect of N-acetyl-L-cysteine on Babesia and Theileria parasites. Exp. Parasitol. 179, 43–48. doi:10.1016/j.exppara.2017.06.003
Rizk, M. A., El-Sayed, S. A. E.-S., Alkhoudary, M. S., Alsharif, K. F., Abdel-Daim, M. M., and Igarashi, I. (2021a). Compounds from the medicines for malaria venture Box inhibit in vitro growth of Babesia divergens, a blood-borne parasite of veterinary and zoonotic importance. Molecules 26, 7118. doi:10.3390/molecules26237118
Rizk, M. A., El-Sayed, S. A. E. S., El-Khodery, S., Yokoyama, N., and Igarashi, I. (2019). Discovering the in vitro potent inhibitors against Babesia and Theileria parasites by repurposing the Malaria Box: a review. Veterinary Parasitol. 274, 108895. doi:10.1016/j.vetpar.2019.07.003
Rizk, M. A., El-Sayed, S. A. E. S., and Igarashi, I. (2021b). Effects of methanolic extract from turmeric (Curcuma longa) against the in vitro multiplication of several Babesia species and Theileria equi. Parasitologia 1 (4), 188–196. doi:10.3390/parasitologia1040020
Rizk, M. A., El-Sayed, S. A. E. S., Terkawi, M. A., Youssef, M. A., El Said, E. S. E. S., Elsayed, G., et al. (2015). Optimization of a fluorescence-based assay for large-scale drug screening against Babesia and Theileria parasites. PloS one 10 (4), e0125276. doi:10.1371/journal.pone.0125276
Salama, A. A., AbouLaila, M., Moussa, A. A., Nayel, M. A., El-Sify, A., Terkawi, M. A., et al. (2013). Evaluation of in vitro and in vivo inhibitory effects of fusidic acid on Babesia and Theileria parasites. Veterinary Parasitol. 191 (1-2), 1–10. doi:10.1016/j.vetpar.2012.08.022
Salama, A. A., AbouLaila, M., Terkawi, M. A., Mousa, A., El-Sify, A., Allaam, M., et al. (2014). Inhibitory effect of allicin on the growth of Babesia and Theileria equi parasites. Parasitol. Res. 113, 275–283. doi:10.1007/s00436-013-3654-2
Schadich, E., Mason, D., and Sin, F. (2012). Co-expressed peJK genes of lobster Jasus edwardsii. Aust. J. Zoology 60 (1), 10–17. doi:10.1071/zo11105
Schadich, E., Nylén, S., Gurská, S., Kotulová, J., Andronati, S., Pavlovsky, V., et al. (2022). Activity of 1-aryl-4-(naphthalimidoalkyl) piperazine derivatives against Leishmania major and Leishmania mexicana. Parasitol. Int. 91, 102647. doi:10.1016/j.parint.2022.102647
Schnittger, L., Rodriguez, A. E., Florin-Christensen, M., and Morrison, D. A. (2012). Babesia: a world emerging. Infect. Genet. Evol. 12 (8), 1788–1809. doi:10.1016/j.meegid.2012.07.004
Singh, D. B., Gupta, M. K., Singh, D. V., Singh, S. K., and Misra, K. (2013). Docking and in silico ADMET studies of noraristeromycin, curcumin and its derivatives with Plasmodium falciparum SAH hydrolase: a molecular drug target against malaria. Interdiscip. Sci. Comput. Life Sci. 5, 1–12. doi:10.1007/s12539-013-0147-z
Stanić, Z. (2017). Curcumin, a compound from natural sources, a true scientific challenge–a review. Plant foods Hum. Nutr. 72, 1–12. doi:10.1007/s11130-016-0590-1
Suarez, C. E., Alzan, H. F., Silva, M. G., Rathinasamy, V., Poole, W. A., and Cooke, B. M. (2019). Unravelling the cellular and molecular pathogenesis of bovine babesiosis: is the sky the limit? Int. J. Parasitol. 49 (2), 183–197. doi:10.1016/j.ijpara.2018.11.002
Tanaka, N., Nakanishi, M., Kusakabe, Y., Shiraiwa, K., Yabe, S., Ito, Y., et al. (2004). Crystal structure of S-adenosyl-L-homocysteine hydrolase from the human malaria parasite Plasmodium falciparum. J. Mol. Biol. 343 (4), 1007–1017. doi:10.1016/j.jmb.2004.08.104
Vial, H. J., and Gorenflot, A. (2006). Chemotherapy against babesiosis. Veterinary Parasitol. 138 (1-2), 147–160. doi:10.1016/j.vetpar.2006.01.048
Wei, C. C., Ball, S., Lin, L., Liu, A., Fuchs, J. R., Li, P. K., et al. (2011). Two small molecule compounds, LLL12 and FLLL32, exhibit potent inhibitory activity on STAT3 in human rhabdomyosarcoma cells. Int. J. Oncol. 38 (1), 279–285. doi:10.3892/ijo_00000848
Yang, J., and Lesinski, G. B. (2012). “Curcumin analogs as inhibitors of the jak-STAT signal transduction pathway,” in Novel apoptotic regulators in carcinogenesis (Dordrecht: Springer Netherlands), 247–266.
Keywords: FLLL-32, Babesia, Theileria equi, in vitro, in vivo
Citation: El-Sayed SAE-S, El-Alfy E-S, Baghdadi HB, Sayed-Ahmed MZ, Alqahtani SS, Alam N, Ahmad S, Ali MS, Igarashi I and Rizk MA (2023) Antiparasitic activity of FLLL-32 against four Babesia species, B. bovis, B. bigemina, B. divergens and B. caballi, and one Theileria species, Theileria equi in vitro, and Babesia microti in mice. Front. Pharmacol. 14:1278451. doi: 10.3389/fphar.2023.1278451
Received: 16 August 2023; Accepted: 23 October 2023;
Published: 02 November 2023.
Edited by:
Karunakaran Kalesh, Teesside University, United KingdomReviewed by:
Ermin Schadich, Palacký University, Olomouc, CzechiaCopyright © 2023 El-Sayed, El-Alfy, Baghdadi, Sayed-Ahmed, Alqahtani, Alam, Ahmad, Ali, Igarashi and Rizk. This is an open-access article distributed under the terms of the Creative Commons Attribution License (CC BY). The use, distribution or reproduction in other forums is permitted, provided the original author(s) and the copyright owner(s) are credited and that the original publication in this journal is cited, in accordance with accepted academic practice. No use, distribution or reproduction is permitted which does not comply with these terms.
*Correspondence: Shimaa Abd El-Salam El-Sayed, c2hpbWFhX2FAbWFucy5lZHUuZWc=; Mohamed Z. Sayed-Ahmed, bXpha2FyaWFAamF6YW51LmVkdS5zYQ==; Mohamed Abdo Rizk, ZHJfbW9oX2FiZG8yMDA4QG1hbnMuZWR1LmVn
Disclaimer: All claims expressed in this article are solely those of the authors and do not necessarily represent those of their affiliated organizations, or those of the publisher, the editors and the reviewers. Any product that may be evaluated in this article or claim that may be made by its manufacturer is not guaranteed or endorsed by the publisher.
Research integrity at Frontiers
Learn more about the work of our research integrity team to safeguard the quality of each article we publish.