- 1Unitat de Farmacologia, Facultat de Medicina i Ciències de la Salut, Institut de Neurociències, Universitat de Barcelona, L’Hospitalet de Llobregat, Catalonia, Spain
- 2Neuropharmacology and Pain Group, Neuroscience Program, IDIBELL-Institut d’Investigació Biomèdica de Bellvitge, L’Hospitalet de Llobregat, Catalonia, Spain
Chronic pain and depression are highly prevalent pathologies and cause a major socioeconomic burden to society. Chronic pain affects the emotional state of the individuals suffering from it, while depression worsens the prognosis of chronic pain patients and may diminish the effectiveness of pain treatments. There is a high comorbidity rate between both pathologies, which might share overlapping mechanisms. This review explores the evidence pinpointing a role for the ventral tegmental area (VTA) as a hub where both pain and emotional processing might converge. In addition, the feasibility of using the VTA as a possible therapeutic target is discussed. The role of the VTA, and the dopaminergic system in general, is highly studied in mood disorders, especially in deficits in reward-processing and motivation. Conversely, the VTA is less regarded where it concerns the study of central mechanisms of pain and its mood-associated consequences. Here, we first outline the brain circuits involving central processing of pain and mood disorders, focusing on the often-understudied role of the dopaminergic system and the VTA. Next, we highlight the state-of-the-art findings supporting the emergence of the VTA as a link where both pathways converge. Thus, we envision a promising part for the VTA as a putative target for innovative therapeutic approaches to treat chronic pain and its effects on mood. Finally, we emphasize the urge to develop and use animal models where both pain and depression-like symptoms are considered in conjunction.
Introduction
Chronic pain, with a prevalence that ranges from 10% to 40% worldwide, is a major concern in public health. It is the main reason for seeking medical care and represents a major societal burden due to its high socioeconomic impact (Goldberg and McGee, 2011; Breivik et al., 2013; Cohen and Mao, 2014; Cohen et al., 2021; De Ridder et al., 2021). Chronic pain significantly affects the quality of life and psychological wellbeing of patients. It affects not only the physical condition but also the emotional and psychological state of patients, disturbing mood, sleep, or cognitive processes (Baliki et al., 2006; Timmers et al., 2019). Neuropsychiatric disorders (e.g., depression) are often comorbid to chronic pain (Bassols et al., 1999; Mäntyselkä et al., 2001; Frießem et al., 2009; Goldberg and McGee, 2011; Cherif et al., 2020; Cohen et al., 2021). Several studies support the idea that persistent pain increases the likelihood of depression, together with exacerbating its symptoms (Humo et al., 2019). In addition, there are other studies showing fewer functional benefits for antidepressants in patients with comorbid chronic pain (Roughan et al., 2021). Similarly, depression worsens the prognosis of chronic pain patients and diminishes the effectiveness of analgesic treatments (Fishbain et al., 1997; Ren et al., 2016; Sheng et al., 2017). Altogether, it appears probable that there is an association between chronic pain and depression and that their treatment requires a comprehensive approach addressing both the physical and psychological aspects of both conditions.
Chronic pain and depression might share common or overlapping mechanisms. From a pharmacological point of view, antidepressant drugs can be used as analgesics, especially in neuropathic pain and other types of pain that are not well managed with first in line analgesic drugs (e.g., NSAIDs, opioids) (Bonilla-Jaime et al., 2022). Some of these effects may be explained by the activation of the descending pain modulatory system, which consists of neuronal projections from midbrain areas, including the periaqueductal gray (PAG) and the rostral ventral medulla (RVM), to the spinal cord (Obata, 2017). These projections are primarily formed by monoaminergic neurons (releasing noradrenaline and serotonin) that also release endogenous opioid peptides (Hokfelt et al., 1977). Nevertheless, the processing of pain is a complex mechanism and involves other areas, such as some thalamic nuclei, especially in the mediodorsal thalamus, which receive and process sensory information from the periphery (Willis and Westlund, 1997), and in addition have the highest density of opioid receptors (Tempel and Zukin, 1987; Ko et al., 2003). Other relevant areas are the amygdala or the insula, which are involved in the processing of emotional responses to pain (Corder et al., 2019; Takahashi et al., 2019).
Similarly, some analgesics have been proposed as possible antidepressants based on 1) the presence of endogenous opioid peptides in brain areas playing a major role in affective disorders (Jelen et al., 2022), and 2) the affinity for opioid receptors exhibited by some antidepressant drugs (Berrocoso et al., 2009; McHugh and Kelly, 2018). The recent development and approval of the well-established anesthetic and analgesic drug ketamine as a novel antidepressant is another example sustaining that pain and depression may share overlapping mechanisms. While the anesthetic effects of ketamine (and esketamine) are parsimoniously explained by its action as a glutamate N-methyl-D-aspartate receptor (NMDAR) non-competitive antagonist, the molecular mechanisms for its antidepressant effects are more controverted (Zanos et al., 2018). Indeed, we and others have shown that some of the rewarding and antidepressant effects of these drugs are mediated via opioid receptors (Bonaventura et al., 2021; Levinstein et al., 2023).
Taken from a neurochemical perspective, the interplay between the opioidergic and monoaminergic systems and the crossover effects for some analgesic and antidepressant drugs may explain the bidirectional connection between chronic pain and depression. However, from a circuit perspective, other neurotransmitter systems and brain areas might also be involved. One of these areas is the ventral tegmental area (VTA). The VTA is a midbrain region critical for motivation and reward via the projection of dopaminergic neurons to the nucleus accumbens (NAcc) and the prefrontal cortex (PFC) (Koob, 2009; Russo and Nestler, 2013; Cai and Tong, 2022), However, the VTA also projects to other nuclei including the anterior cingulate cortex (ACC), the olfactory bulb, the amygdala, and the hippocampus (Nair-Roberts et al., 2008; Sesack and Grace, 2010; Qi et al., 2016; Montardy et al., 2019). Accordingly, it could play a role both in the processing of pain and emotion. Here, we will review clinical and preclinical evidence supporting the role of the VTA as a possible hub where both pain and emotional processing converge.
Involvement of the VTA in pain processing
The perception of pain is a highly coordinated and dynamic process that involves interactions between multiple brain areas. As briefly discussed above, some thalamic nuclei are major hubs in the processing of pain (Willis and Westlund, 1997), since they receive information from the periphery and filter and direct the signal to other areas for further processing (Basbaum et al., 2009). The somatosensory cortex plays a crucial role in localizing where pain occurs, while the ACC and the PFC are responsible for processing its emotional and cognitive aspects and for other higher-order cognitive functions, such as decision-making. Other important areas involved in this process are the insula, which integrates sensory, emotional, and cognitive information related to pain; the periaqueductal gray (PAG), modulating the descending pain modulatory system; the hypothalamus, involved in autonomic nervous system responses; and the amygdala, which is part of the limbic system and is mainly associated with its emotional processing (Wiech et al., 2008; Basbaum et al., 2009; Garland, 2012; Moradi et al., 2015; Corder et al., 2019; Huang et al., 2022; Ma et al., 2023).
The VTA is not often considered as a primary area involved in pain processing. However, several studies support a putative role for the VTA in pain perception and processing. For instance, a recent study in rats showed that chronic pain decreases dopaminergic activity due to increased inhibition from the bed nucleus of the stria terminalis (BNST) (Takahashi et al., 2019), a region that mediates aversive experiences (Davis et al., 2010; Minami, 2019) (Figure 1). Conversely, in a rat model of neuropathic pain, VTA neurons showed increased burst firing 2 weeks after peripheral nerve injury, which suggested that the increased dopaminergic activity could be an early result of chronic maladaptation to persistent pain (Sagheddu et al., 2015). Of note, these dopaminergic neurons in the VTA are modulated by GABAergic neurons projected from the rostromedial tegmental nucleus (RMTg). These inhibitory projections have been shown to reduce VTA excitability during inflammatory pain (Markovic et al., 2021). Similarly, optogenetic stimulation of VTA reversed allodynia caused by nerve injury in mice (Watanabe et al., 2018). On the other hand, RMTg GABAergic neurons, which express opioid receptors, are thought to mediate the inhibitory effects of opioid drugs in regulating the VTA dopaminergic neurons (Johnson and North, 1992; Fields and Margolis, 2015; Taylor et al., 2019). Indeed, it has been shown that morphine elicits some of its antinociceptive effects through RMTg neurons, thus supporting a critical role of VTA activity not only on the rewarding effects of opioids but also on opioid-mediated analgesia (Taylor et al., 2019).
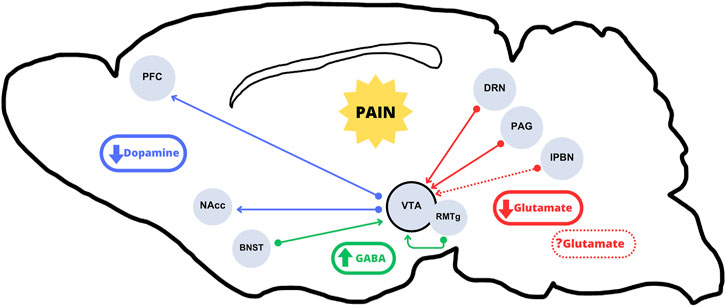
FIGURE 1. Incoming and arising pathways to and from the VTA subject to alterations under chronic pain conditions. The literature mainly suggests an overall hypoactivity of the VTA dopaminergic outputs in the mesolimbic and mesocortical pathways (blue arrows) in chronic pain conditions. Regarding VTA inputs, there would be a decrease in excitatory glutamate release from projections originating in the DRN and the PAG (solid red arrows). The role of lPBN excitatory neurons, which have been shown to be either activated or inhibited (dotted red arrows), is not clear. The resulting hypoglutamatergia, together with increased GABA release from the BNST and the RMTg (green arrows) would result in an overall VTA dopaminergic output inhibition.
The activity in the VTA can lead to multifaceted, even contrasting, effects in its projection areas (Lammel et al., 2014) and therefore, the outcomes of its activation are highly context dependent (Belujon and Grace, 2015). The mesolimbic dopaminergic circuit, which consists of the projection of dopaminergic neurons from the VTA to the NAcc, has been shown to be altered in chronic pain conditions (Ren et al., 2016; Campos-Jurado et al., 2019; Yang et al., 2020; Wang et al., 2023). These alterations often involve changes in neurochemistry or neurotransmitter receptors of both the dopaminergic and opioidergic systems [for review see (Taylor et al., 2016; Lin et al., 2023)]. For instance, in different neuropathic pain models, it was observed that an increase in the activity of the VTA dopaminergic neurons had positive effects on pain regulation and its associated mood effects (Abdul et al., 2022; Huang et al., 2022). Similarly, a recent study showed diminished firing and intrinsic excitability of VTA dopaminergic neurons under chronic pain conditions that was due to reduced glutamatergic input from the dorsal raphe nucleus, and that optogenetic modulation of this pathway produced analgesic effects (Wang et al., 2023). Nevertheless, few reports have focused on assessing whether modulating the reward system can exert analgesic effects. For instance, in neuropathic or cancer pain, activation of dopamine neurons projecting to the NAcc restore the allodynia (Watanabe et al., 2018). Similarly, microinjecting dopamine receptor ligands into the NAcc led to reduced injury-induced thermal allodynia (Sato et al., 2022). Particularly, they showed that the specific facilitation of dopamine D1 receptor-expressing medium spiny neurons (MSN) in the NAcc projecting to the VTA led to pain relief, while the inhibition of dopamine D2 receptor-expressing MSNs led to significant antinociceptive effects (Sato et al., 2022). Interestingly, the increased excitability of the D2-containing indirect pathway MSN of the medial shell of the NAcc worsens tactile allodynia following peripheral nerve injury (Ren et al., 2016). Finally, it has been also shown that, in chronic pain conditions, extracellular dopamine release evoked by morphine or cocaine administration is decreased suggesting that a hyporeactive state of the mesolimbic dopaminergic system under chronic pain conditions (Taylor et al., 2015).
Similarly, VTA projections to the PFC are altered upon chronic pain conditions. An example of this, and of the diverse effects of opioid drugs within the brain, is shown in the work by Zhao et al. (2007), in which the injection of morphine in the PFC alleviated mechanical allodynia in a mouse model of neuropathic pain. Another study in mice demonstrated that not necessarily opioid-mediated effects but just increased dopaminergic activity in the PFC, elicited by selective activation of VTA’s dopaminergic neurons, reduced mechanical hypersensitivity (Huang et al., 2020). This effect was explained by an increased PFC-mediated activity in the PAG (Huang et al., 2020). In fact, by using optogenetic manipulations, they demonstrated that the PFC-PAG circuit altered pain behavior by reducing the descending noradrenergic and serotoninergic modulation of spinal pain signals (Huang et al., 2019). The capacity of the PFC to receive nociceptive inputs but also to exert control over the pain sensation can be found elsewhere [for example, see (Apkarian et al., 2005; Ong et al., 2019; Kummer et al., 2020)]. Therefore, there is compelling evidence to support that areas other than the thalamus and canonical pain-related areas play a role in the processing of pain. Another area that might suffer alterations during chronic pain is the claustrum (Atilgan et al., 2022; Ntamati et al., 2023), a multimodal node with brain-wide connectivity and involved in several networks (Atilgan et al., 2022). Interestingly, the claustrum also receives dopaminergic inputs from the VTA (Wong et al., 2021), with dopamine causing a mostly inhibitory response of this structure (Wong et al., 2021), although the precise mechanisms of this inhibition are not yet well understood. However, it is interesting to note that an hypodopaminergic state could explain the reduced excitatory drive of the claustrum onto cortical structures like the ACC (Ntamati et al., 2023). In summary, speculatively, the VTA might have a central role as a flow regulator of the information integrated and processed in areas such as the NAcc, PFC, claustrum, thalamus and PAG. Altogether, these results suggest that the effects elicited by chronic pain through the limbic system could also be viewed as a promising target to reach effective analgesia.
The VTA as an emerging therapeutic target to treat depression
Depression is a complex, multifaceted, and disabling disorder affecting an estimate of 5% of the world’s adult population (World Health Organization, 2023). Decades of evidence showed the link between depression and the monoaminergic systems, especially the serotoninergic and noradrenergic (Coppen, 1967; Yohn et al., 2017). Although highly controversial and failing to reconcile the diverse symptomatology of depression (see Moncrieff et al., 2022) and its associated correspondence, the serotonergic hypothesis of depression supports the principal therapeutic treatments. The first-line class of antidepressant drugs are selective serotonin re-uptake inhibitors, which increase extracellular serotonin concentrations by blocking presynaptic serotonin transporters. Similarly, other popular antidepressant drug classes include both serotonin-noradrenaline reuptake inhibitors, tricyclic antidepressants, or monoamine-oxidase inhibitors (Cipriani et al., 2018; Lin et al., 2023), which also lead to increased extracellular levels of monoamines. However, all these medications have a delayed onset of action, and around 30%–40% of patients do not show an adequate response (Rush et al., 2006). More recently, psychedelic drugs, which may also act through the serotonergic system but targeting serotonin receptors instead of serotonin re-uptake or degradation, have been proposed as novel treatments for depression (Carhart-Harris et al., 2016; Griffiths et al., 2016; Reiff et al., 2020; Carhart-Harris et al., 2021; Daws et al., 2022). Of note, while their efficacy and safety are still a matter of debate, the role of the serotonergic system in their antidepressant action is also questioned (Hesselgrave et al., 2021; Moncrieff et al., 2022; Moliner et al., 2023).
Despite being the most studied, serotonin and noradrenaline are not the only monoamines implicated in depression. Several studies have shown that changes in the dopaminergic system are associated with both the pathogenesis and treatment of depression (Pani et al., 2000; Nestler and Carlezon, 2006; Yadid and Friedman, 2008; Tye et al., 2013; Belujon and Grace, 2017). Positron emission tomography (PET) imaging studies in humans reported a decrease in dopamine transporter binding potential in depressed patients, which is usually associated with an hypodopaminergic state (Meyer et al., 2001). Accordingly, pharmacological depletions in dopamine led to an increase of depressive symptoms in depressed patients or in subjects with a family history of depression but not in healthy subjects (Ruhé et al., 2007; Hasler et al., 2008). On the other hand, dopamine agonists such as the Parkinson’s disease (PD) medications pramipexole or aripiprazole have antidepressant properties in PD patients with concurrent depression or anhedonia (Lemke et al., 2006).
As a complex disorder, it is not possible to pinpoint a unique brain region or circuit responsible for all the symptoms of depression (Kempton et al., 2011; Spellman and Liston, 2020). Despite this, there are converging reports that observed alterations of the limbic corticostriatal circuitry (precisely, several subregions of the PFC and the NAcc) in anhedonia and reward processing in patients suffering from depression (Johnstone et al., 2007; Siegle et al., 2007; Pizzagalli et al., 2009; Mayberg et al., 1999). The activity of the PFC and the NAcc is heavily modulated by dopaminergic transmission originating in the VTA (Koob, 2009; Russo and Nestler, 2013). Hence, the putative role of the VTA as a therapeutic target for depression has been studied in several preclinical studies in rodents. Interestingly, it has been shown that optogenetic or electrical stimulation of the VTA to NAcc or PFC pathways can ameliorate depressive-like symptoms in animal models of depression (Gersner et al., 2010; Sesia et al., 2010; van der Plasse et al., 2012; Chaudhury et al., 2013; Tye et al., 2013; Bambico et al., 2015; Ferenczi et al., 2016). On the other hand, non-pharmacological treatments of depression (Schlaepfer et al., 2013; Bewernick et al., 2017), such as deep brain stimulation (DBS) or transcranial magnetic stimulation, have also successfully targeted the VTA or the medial forebrain bundle (the main pathway of fibers connecting the limbic midbrain and forebrain) to achieve antidepressant activity in treatment-resistant depressed patients (Schlaepfer et al., 2013; Fenoy et al., 2016; Bewernick et al., 2017). Similar results have been observed with peripheral stimulation: vagus nerve stimulation (VNS) has shown promising results in ameliorating depression scores in clinical trials with patients with mild or moderate symptoms (Fang et al., 2016; Rong et al., 2016). Interestingly, the mechanistic studies of VNS [reviewed in (Carron et al., 2023)] support the idea that its therapeutic effects are due to increased catecholamine release.
Lastly, it is important to highlight that emerging antidepressant therapies, distinct from those directly linked to monoaminergic function -such as ketamine- consistently pinpoint the PFC-NAcc-VTA pathways as crucial anatomical sites of their effects. (Kokkinou et al., 2018; Wu et al., 2021).
Converging mechanisms between pain and depression in the VTA
Taking the above into consideration, it becomes evident that the VTA is a key structure where both pathogenic and therapeutic mechanisms of pain and depression might converge. Therefore, the VTA might arise as a candidate to target the concurrence of both pathologies. However, despite the high comorbidity between both pathologies in humans (Goldberg and McGee, 2011; Cherif et al., 2020; Cohen et al., 2021) and the extensive clinical data that relate them (Lampl et al., 2016; Roughan et al., 2021; Zhou et al., 2021; Voute et al., 2023), only a few preclinical studies have addressed them together. Of note, these studies generally coincide in suggesting that afferents from the PAG or the parabrachial nucleus (PBN), the dorsal raphe nucleus (DRN) or the BNST directly or indirectly control dopaminergic activity in the VTA efferent regions, such as the NAcc and PFC (Waung et al., 2019; Yang et al., 2021; Zhang et al., 2021; Lee et al., 2023). Hence, the VTA seems to be critical in translating painful stimuli into aversive or depressive-like behaviors.
The work by Waung et al. (2019) demonstrated that excitatory neurons from the ventrolateral PAG (vlPAG) mainly project to VTA GABAergic neurons; however, it is important to highlight that they also detected both excitatory and inhibitory vlPAG inputs into a few dopaminergic neurons (Waung et al., 2019). Similarly, other studies described a parallel glutamatergic input from the lateral PBN (lPBN) to dopaminergic VTA neurons (Yang et al., 2021; Zhang et al., 2021). Therefore, VTA dopaminergic neurons may be relevant for relaying nociceptive signals from the spinal cord to midbrain nuclei. Indeed, when silencing these neurons pain sensation can be blocked. Thus, the ablation of lPBN to substantia nigra pars reticulata glutamatergic neurons was enough to reduce pain-mediated inhibition of dopamine release in mice (Coizet et al., 2010; Yang et al., 2021). Interestingly, one of the reports that addressed chronic pain and depression together (Lee et al., 2023) used a spinal nerve ligation (SNL) mouse model. This model resulted in a dysregulation of the glutamatergic transmission from PAG into the VTA. Then, by using chemogenetic tools to selectively recover the activity of the PAG-VTA pathway, they attenuated the SNL-induced depressive behavior of mice. Altogether, these studies showed that, in mice, pain reduced glutamatergic transmission to the VTA causing a decrease in dopamine release in its efferent areas (NAcc and PFC). The same hypodopaminergic state has been observed in chronic pain conditions, which might be due to the dampening of glutamatergic transmission from the DRN to the VTA, consequently decreasing dopamine release in the NAcc (Wang et al., 2023) (Figure 1). Accordingly, the activation of the DRN-VTA-NAcc pathway would be enough to decrease pain-like hypersensitivity and the concomitant anhedonic state. Another critical area could be the BNST. Several studies (Takahashi et al., 2019; Hara et al., 2020) have revealed the capacity of BNST to modulate the mesolimbic system, not only under chronic pain conditions but also in a depression model. In both cases (pain and depression), it seems likely that the inhibitory inputs to the VTA-projecting BNST neurons would led to neuroplastic changes, which would be a common mechanism between the two diseases (Takahashi et al., 2019; Hara et al., 2020).
As mentioned above, the opioidergic system plays an important role in controlling VTA-mediated dopamine release to the projecting areas (i.e., NAcc), an effect that is critical in regulating opioid reward and reinforcement. Nevertheless, some studies have shown that pain conditions can induce presynaptic MOR desensitization in the VTA, causing an increment in GABA release from RMTg neurons, and resulting in reduced dopamine release to dopaminergic projecting areas (Ozaki et al., 2002; Hipólito et al., 2015; Campos-Jurado et al., 2019). Similarly, chemogenetic activation of the NAcc-projecting VTA dopamine neurons allowed to overcome the pain-reduced motivated behaviors (Markovic et al., 2021). Altogether, pain-induced dysregulation of this circuit affects motivation and reward, supporting that it may act as a trigger to anhedonic-like behaviors. Additionally, apart from the neurochemical changes and the balance shift that leads to a hypodopaminergic state (Figure 1), chronic pain may cause other maladaptive effects in the VTA. For instance, chronic pain induced microglial activation in the VTA, which disrupted the homeostasis in GABAergic neurons and contributed to the decreased extracellular dopamine in the NAcc (Taylor et al., 2015). In conclusion, there are a number of studies supporting the idea that a hypodopaminergic state occurs upon chronic pain conditions, and that the decline in dopamine levels impairs motivated behavior (summarized in Figure 1).
On the other hand, other authors have proposed an alternative scenario. Thus, the development of depression-like behaviors in an animal model of chronic pain correlated with increased firing of the VTA dopaminergic neurons (Zhang et al., 2021). In this study, it was observed that blocking glutamatergic lPBN input to VTA dopamine neurons reversed the depressive-like behavior associated with chronic pain, however it did not affect the induced neuropathic pain sensitivity. In the opposite way, activation of this same circuit in naïve animals resulted in increased depressive-like behaviors, suggesting that the plastic changes induced by pain led to an increased firing neuronal rate that would be responsible for the comorbid emotional impaired state (Zhang et al., 2021).
Taken together, the evidence presented above suggests that the brain pathways incoming and arising from the VTA are part of a key, albeit understudied, circuit to explain the relationship between pain and depression. This hypothesis is further supported by the fact that pharmacological treatments for both pathologies not only overlap but they also directly or indirectly target the VTA. A paradigmatic example of this idea is represented by opioid drugs, which are used to treat pain but have ample actions on mood and have been proposed to treat depression (Browne et al., 2020). However, the abuse liability of opioid drugs makes them far from ideal to treat depression due to the higher vulnerability and comorbidity of addiction in patients with depressive disorders (Swendsen and Merikangas, 2000; Quello et al., 2005; McGrath et al., 2020). Alternatively, non-canonical drugs that target opioid receptors such as ketamine or methadone, which in addition show reduced abuse liability (Cai et al., 2019; Bonaventura et al., 2021), have become depression and pain medications.
Finally, as above-mentioned, a different, non-pharmacological, therapeutic approach to tackle neuropsychiatric disorders is electrical brain stimulation. This technique has been used for treating multiple conditions, such as movement disorders (e.g., Parkinson’s disease), epilepsy, pain and psychiatric conditions like addiction, schizophrenia, or depression, focusing on multiple areas (Lozano et al., 2019). For depression, most studies have proposed targeting areas like the lateral habenula, the NAcc, or the ACC [reviewed in (Schlaepfer and Bewernick, 2013)]. More recently, DBS into the VTA and/or the medial forebrain bundle has also emerged as a suitable treatment. Clinical studies have shown rapid and sustained antidepressant effects (Schlaepfer et al., 2013; Fenoy et al., 2018; Coenen et al., 2019), and they also support its safety as a chronic treatment for up to 6 weeks (Thiele et al., 2018). Regarding pain, DBS has been mostly investigated for cluster headache, a highly disabling pain that tremendously affects patients’ quality of life. Recent studies (Akram et al., 2016; Cappon et al., 2019) have demonstrated that this kind of treatment reduces the frequency and severity of migraine. Interestingly, Cappon et al. (2019) assessed the effects of DBS into the VTA in patients with cluster headache. They assessed the effects of the treatment on cognition, mood, behavior, and quality of life. Their findings suggest that the treatment induced a significant decrease in anxiety and better coping with pain, and an improvement in depression albeit it was not statistically significant (Akram et al., 2016; Cappon et al., 2019).
Conclusion
About a third of patients suffering from chronic pain also present depressive symptoms (Cherif et al., 2020). In addition, patients with concurrent chronic pain and depression show poorer prognosis than those with chronic pain alone (Fishbain et al., 1997). Hence, individuals with comorbid chronic pain and depression could benefit from a comprehensive approach to address both conditions simultaneously and improve their overall wellbeing and quality of life. However, most of the preclinical research is focused on either the development of novel analgesic treatments or understanding depressive-like behaviors per separate. Here, we highlight the importance of studying pain and depression as coexisting pathologies rather than by themselves to learn more about the converging pathways and mechanisms that can explain their comorbidity and find new effective strategies to treat them in conjunction.
A potential approach could involve targeting, through either pharmacological means or non-pharmacological methods like several forms of brain stimulation, toward brain regions that function as a communication hub connecting both conditions. From the evidence presented in this review it becomes evident that one of such brain regions could be the VTA. Thus, we propose that modulating the activity of the VTA can be regarded as a novel therapeutic opportunity to treat the concurrence of pain and depression. Although further studies will be required to elucidate the precise actions needed to target the VTA with the desirable therapeutic effects, the growing range of neuromodulation technologies, which allow precise and cell-specific control of neural activity, present unprecedented possibilities to tackle these devastating disorders from a newer perspective.
Author contributions
MF-G: Conceptualization, Writing–original draft. AR: Conceptualization, Writing–original draft. MG-P: Conceptualization, Writing–original draft. VF-D: Conceptualization, Funding acquisition, Supervision, Writing–review and editing. JB: Conceptualization, Funding acquisition, Supervision, Writing–review and editing.
Funding
The authors declare financial support was received for the research, authorship, and/or publication of this article. This work was supported by Plan Nacional Sobre Drogas, Ministerio de Sanidad, Grant numbers 2021I068 (to VF-D) and 2021I070 (to JB), by Fundación Española del Dolor, Grant number BF2-19-09 (to VF-D), and by the Spanish Ministerio de Ciencia e Innovación/Agencia Estatal de Investigación, Grant numbers RYC-2019-027371-I (to JB) and PID 2020-117989RA-I00 (to JB).
Conflict of interest
The authors declare that the research was conducted in the absence of any commercial or financial relationships that could be construed as a potential conflict of interest.
The authors declared that they were an editorial board member of Frontiers, at the time of submission. This had no impact on the peer review process and the final decision.
Publisher’s note
All claims expressed in this article are solely those of the authors and do not necessarily represent those of their affiliated organizations, or those of the publisher, the editors and the reviewers. Any product that may be evaluated in this article, or claim that may be made by its manufacturer, is not guaranteed or endorsed by the publisher.
References
Abdul, M., Yan, H. Q., Zhao, W. N., Lyu, X. B., Xu, Z., Yu, X. L., et al. (2022). VTA-NAc glutaminergic projection involves in the regulation of pain and pain-related anxiety. Front. Mol. Neurosci. 15, 1083671. doi:10.3389/fnmol.2022.1083671
Akram, H., Miller, S., Lagrata, S., Hyam, J., Jahanshahi, M., Hariz, M., et al. (2016). Ventral tegmental area deep brain stimulation for refractory chronic cluster headache. Neurology 86, 1676–1682. doi:10.1212/WNL.0000000000002632
Apkarian, A. V., Bushnell, M. C., Treede, R. D., and Zubieta, J. K. (2005). Human brain mechanisms of pain perception and regulation in health and disease. Eur. J. Pain 9, 463–484. doi:10.1016/j.ejpain.2004.11.001
Atilgan, H., Doody, M., Oliver, D. K., McGrath, T. M., Shelton, A. M., Echeverria-Altuna, I., et al. (2022). Human lesions and animal studies link the claustrum to perception, salience, sleep and pain. Brain 145, 1610–1623. doi:10.1093/brain/awac114
Baliki, M. N., Chialvo, D. R., Geha, P. Y., Levy, R. M., Harden, R. N., Parrish, T. B., et al. (2006). Chronic pain and the emotional brain: Specific brain activity associated with spontaneous fluctuations of intensity of chronic back pain. J. Neurosci. 26, 12165–12173. doi:10.1523/JNEUROSCI.3576-06.2006
Bambico, F. R., Bregman, T., Diwan, M., Li, J., Darvish-Ghane, S., Li, Z., et al. (2015). Neuroplasticity-dependent and -independent mechanisms of chronic deep brain stimulation in stressed rats. Transl. Psychiatry 5, e674. doi:10.1038/tp.2015.166
Basbaum, A. I., Bautista, D. M., Scherrer, G., and Julius, D. (2009). Cellular and molecular mechanisms of pain. Cell 139, 267–284. Preprint at. doi:10.1016/j.cell.2009.09.028
Bassols, A., Bosch, F., Campillo, M., Cañellas, M., and Baños, J. E. (1999). An epidemiological comparison of pain complaints in the general population of Catalonia (Spain). Pain 83, 9–16. doi:10.1016/s0304-3959(99)00069-x
Belujon, P., and Grace, A. A. (2017). Dopamine system dysregulation in major depressive disorders. Int. J. Neuropsychopharmacol. 20, 1036–1046. doi:10.1093/ijnp/pyx056
Belujon, P., and Grace, A. A. (2015). Regulation of dopamine system responsivity and its adaptive and pathological response to stress. Proc. R. Soc. B Biol. Sci. 282, 20142516. doi:10.1098/rspb.2014.2516
Berrocoso, E., Sanchez-Blazquez, P., Garzon, J., and Mico, J. (2009). Opiates as antidepressants. Curr. Pharm. Des. 15, 1612–1622. doi:10.2174/138161209788168100
Bewernick, B. H., Kayser, S., Gippert, S. M., Switala, C., Coenen, V. A., and Schlaepfer, T. E. (2017). Deep brain stimulation to the medial forebrain bundle for depression-long-term outcomes and a novel data analysis strategy. Brain Stimul. 10, 664–671. doi:10.1016/j.brs.2017.01.581
Bonaventura, J., Lam, S., Carlton, M., Boehm, M. A., Gomez, J. L., Solís, O., et al. (2021). Pharmacological and behavioral divergence of ketamine enantiomers:implications for abuse liability. Mol. Psychiatry 26, 6704–6722. doi:10.1038/s41380-021-01093-2
Bonilla-Jaime, H., Sánchez-Salcedo, J. A., Estevez-Cabrera, M. M., Molina-Jiménez, T., Cortes-Altamirano, J. L., and Alfaro-Rodríguez, A. (2022). Depression and pain: Use of antidepressants. Curr. Neuropharmacol. 20, 384–402. doi:10.2174/1570159X19666210609161447
Breivik, H., Eisenberg, E., and O’Brien, T.OPENMinds (2013). The individual and societal burden of chronic pain in Europe: The case for strategic prioritisation and action to improve knowledge and availability of appropriate care. BMC Public Health 13, 1229. doi:10.1186/1471-2458-13-1229
Browne, C. A., Jacobson, M. L., and Lucki, I. (2020). Novel targets to treat depression: Opioid-based therapeutics. Harv Rev. Psychiatry 28, 40–59. doi:10.1097/HRP.0000000000000242
Cai, J., and Tong, Q. (2022). Anatomy and function of ventral tegmental area glutamate neurons. Front. Neural Circuits 16, 867053. doi:10.3389/fncir.2022.867053
Cai, N. S., Quiroz, C., Bonaventura, J., Bonifazi, A., Cole, T. O., Purks, J., et al. (2019). Opioid-galanin receptor heteromers mediate the dopaminergic effects of opioids. J. Clin. Invest. 129, 2730–2744. doi:10.1172/JCI126912
Campos-Jurado, Y., Igual-López, M., Padilla, F., Zornoza, T., Granero, L., Polache, A., et al. (2019). Activation of MORs in the VTA induces changes on cFos expression in different projecting regions: Effect of inflammatory pain. Neurochem. Int. 131, 104521. doi:10.1016/j.neuint.2019.104521
Cappon, D., Ryterska, A., Lagrata, S., Miller, S., Akram, H., Hyam, J., et al. (2019). Ventral tegmental area deep brain stimulation for chronic cluster headache: Effects on cognition, mood, pain report behaviour and quality of life. Cephalalgia 39, 1099–1110. doi:10.1177/0333102419839957
Carhart-Harris, R., Giribaldi, B., Watts, R., Baker-Jones, M., Murphy-Beiner, A., Murphy, R., et al. (2021). Trial of psilocybin versus escitalopram for depression. N. Engl. J. Med. 384, 1402–1411. doi:10.1056/NEJMoa2032994
Carhart-Harris, R. L., Bolstridge, M., Rucker, J., Day, C. M. J., Erritzoe, D., Kaelen, M., et al. (2016). Psilocybin with psychological support for treatment-resistant depression: An open-label feasibility study. Lancet Psychiatry 3, 619–627. doi:10.1016/S2215-0366(16)30065-7
Carron, R., Roncon, P., Lagarde, S., Dibué, M., Zanello, M., and Bartolomei, F. (2023). Latest views on the mechanisms of action of surgically implanted cervical vagal nerve stimulation in epilepsy. Neuromodulation 26, 498–506. doi:10.1016/j.neurom.2022.08.447
Chaudhury, D., Walsh, J. J., Friedman, A. K., Juarez, B., Ku, S. M., Koo, J. W., et al. (2013). Rapid regulation of depression-related behaviours by control of midbrain dopamine neurons. Nature 493, 532–536. doi:10.1038/nature11713
Cherif, F., Zouari, H. G., Cherif, W., Hadded, M., Cheour, M., and Damak, R. (2020). Depression prevalence in neuropathic pain and its impact on the quality of life. Pain Res. Manag. 2020, 7408508. doi:10.1155/2020/7408508
Cipriani, A., Furukawa, T. A., Salanti, G., Chaimani, A., Atkinson, L. Z., Ogawa, Y., et al. (2018). Comparative efficacy and acceptability of 21 antidepressant drugs for the acute treatment of adults with major depressive disorder: A systematic review and network meta-analysis. Lancet 391, 1357–1366. doi:10.1016/S0140-6736(17)32802-7
Coenen, V. A., Bewernick, B. H., Kayser, S., Kilian, H., Boström, J., Greschus, S., et al. (2019). Superolateral medial forebrain bundle deep brain stimulation in major depression: A gateway trial. Neuropsychopharmacology 44, 1224–1232. doi:10.1038/s41386-019-0369-9
Cohen, S. P., and Mao, J. (2014). Neuropathic pain: Mechanisms and their clinical implications. BMJ 348, f7656. doi:10.1136/bmj.f7656
Cohen, S. P., Vase, L., and Hooten, W. M. (2021). Chronic pain: An update on burden, best practices, and new advances. Lancet 397, 2082–2097. Preprint at. doi:10.1016/S0140-6736(21)00393-7
Coizet, V., Dommett, E. J., Klop, E. M., Redgrave, P., and Overton, P. G. (2010). The parabrachial nucleus is a critical link in the transmission of short latency nociceptive information to midbrain dopaminergic neurons. Neuroscience 168, 263–272. doi:10.1016/j.neuroscience.2010.03.049
Coppen, A. (1967). The biochemistry of affective disorders. Br. J. Psychiatry 113, 1237–1264. doi:10.1192/bjp.113.504.1237
Corder, G., Ahanonu, B., Grewe, B. F., Wang, D., Schnitzer, M. J., and Scherrer, G. (2019). An amygdalar neural ensemble that encodes the unpleasantness of pain. Sci. (1979) 363, 276–281. doi:10.1126/science.aap8586
Davis, M., Walker, D. L., Miles, L., and Grillon, C. (2010). Phasic vs sustained fear in rats and humans: Role of the extended amygdala in fear vs anxiety. Neuropsychopharmacology 35, 105–135. Preprint at. doi:10.1038/npp.2009.109
Daws, R. E., Timmermann, C., Giribaldi, B., Sexton, J. D., Wall, M. B., Erritzoe, D., et al. (2022). Increased global integration in the brain after psilocybin therapy for depression. Nat. Med. 28, 844–851. doi:10.1038/s41591-022-01744-z
De Ridder, D., Adhia, D., and Vanneste, S. (2021). The anatomy of pain and suffering in the brain and its clinical implications. Neurosci. Biobehav Rev. 130, 125–146. doi:10.1016/j.neubiorev.2021.08.013
Fang, J., Rong, P., Hong, Y., Fan, Y., Liu, J., Wang, H., et al. (2016). Transcutaneous vagus nerve stimulation modulates default mode network in major depressive disorder. Biol. Psychiatry 79, 266–273. doi:10.1016/j.biopsych.2015.03.025
Fenoy, A. J., Schulz, P. E., Selvaraj, S., Burrows, C. L., Zunta-Soares, G., Durkin, K., et al. (2018). A longitudinal study on deep brain stimulation of the medial forebrain bundle for treatment-resistant depression. Transl. Psychiatry 8, 111. doi:10.1038/s41398-018-0160-4
Fenoy, A. J., Schulz, P., Selvaraj, S., Burrows, C., Spiker, D., Cao, B., et al. (2016). Deep brain stimulation of the medial forebrain bundle: Distinctive responses in resistant depression. J. Affect Disord. 203, 143–151. doi:10.1016/j.jad.2016.05.064
Ferenczi, E. A., Zalocusky, K. A., Liston, C., Grosenick, L., Warden, M. R., Amatya, D., et al. (2016). Prefrontal cortical regulation of brainwide circuit dynamics and reward-related behavior. Sci. (1979) 351, aac9698. doi:10.1126/science.aac9698
Fields, H. L., and Margolis, E. B. (2015). Understanding opioid reward. Trends Neurosci. 38, 217–225. doi:10.1016/j.tins.2015.01.002
Fishbain, D. A., Cutler, R., Rosomoff, H. L., and Rosomoff, R. S. (1997). Chronic pain-associated depression: Antecedent or consequence of chronic pain? A review. Clin. J. Pain 13, 116–137. doi:10.1097/00002508-199706000-00006
Frießem, C. H., Willweber-Strumpf, A., and Zenz, M. W. (2009). Chronic pain in primary care. German figures from 1991 and 2006. BMC Public Health 9, 1–9. doi:10.1186/1471-2458-9-299
Garland, E. L. (2012). Pain processing in the human nervous system: A selective review of nociceptive and biobehavioral pathways. Prim. Care Clin. Office Pract. 39, 561–571. doi:10.1016/j.pop.2012.06.013
Gersner, R., Toth, E., Isserles, M., and Zangen, A. (2010). Site-specific antidepressant effects of repeated subconvulsive electrical stimulation: Potential role of brain-derived neurotrophic factor. Biol. Psychiatry 67, 125–132. doi:10.1016/j.biopsych.2009.09.015
Goldberg, D. S., and McGee, S. J. (2011). Pain as a global public health priority. BMC Public Health 11, 770. doi:10.1186/1471-2458-11-770
Griffiths, R. R., Johnson, M. W., Carducci, M. A., Umbricht, A., Richards, W. A., Richards, B. D., et al. (2016). Psilocybin produces substantial and sustained decreases in depression and anxiety in patients with life-threatening cancer: A randomized double-blind trial. J. Psychopharmacol. 30, 1181–1197. doi:10.1177/0269881116675513
Hara, R., Takahashi, D., Takehara, T., Amano, T., and Minami, M. (2020). Inhibitory synaptic transmissions to the bed nucleus of the stria terminalis neurons projecting to the ventral tegmental area are enhanced in rats exposed to chronic mild stress. Mol. Brain 13, 139. doi:10.1186/s13041-020-00684-4
Hasler, G., Fromm, S., Carlson, P. J., Luckenbaugh, D. A., Waldeck, T., Geraci, M., et al. (2008). Neural response to catecholamine depletion in unmedicated subjects with major depressive disorder in remission and healthy subjects. Arch. Gen. Psychiatry 65, 521–531. doi:10.1001/archpsyc.65.5.521
Hesselgrave, N., Troppoli, T. A., Wulff, A. B., Cole, A. B., and Thompson, S. M. (2021). Harnessing psilocybin: Antidepressant-like behavioral and synaptic actions of psilocybin are independent of 5-HT2R activation in mice. Proc. Natl. Acad. Sci. U. S. A. 118, 20224891188–e2022489127. doi:10.1073/pnas.2022489118
Hipólito, L., Wilson-Poe, A., Campos-Jurado, Y., Zhong, E., Gonzalez-Romero, J., Virag, L., et al. (2015). Inflammatory pain promotes increased opioid self-administration: Role of dysregulated ventral tegmental area µ opioid receptors. J. Neurosci. 35, 12217–12231. doi:10.1523/JNEUROSCI.1053-15.2015
Hokfelt, T., Ljungdahl, A., Terenius, L., Elde, R., and Nilsson, G. (1977). Immunohistochemical analysis of peptide pathways possibly related to pain and analgesia: Enkephalin and substance P. Proc. Natl. Acad. Sci. U. S. A. 74, 3081–3085. doi:10.1073/pnas.74.7.3081
Huang, J., Gadotti, V. M., Chen, L., Souza, I. A., Huang, S., Wang, D., et al. (2019). A neuronal circuit for activating descending modulation of neuropathic pain. Nat. Neurosci. 22, 1659–1668. doi:10.1038/s41593-019-0481-5
Huang, M., Wang, G., Lin, Y., Guo, Y., Ren, X., Shao, J., et al. (2022). Dopamine receptor D2, but not D1, mediates the reward circuit from the ventral tegmental area to the central amygdala, which is involved in pain relief. Mol. Pain 18, 174480692211450–13. doi:10.1177/17448069221145096
Huang, S., Zhang, Z., Gambeta, E., Xu, S. C., Thomas, C., Godfrey, N., et al. (2020). Dopamine inputs from the ventral tegmental area into the medial prefrontal cortex modulate neuropathic pain-associated behaviors in mice. Cell Rep. 31, 107812. doi:10.1016/j.celrep.2020.107812
Humo, M., Lu, H., and Yalcin, I. (2019). The molecular neurobiology of chronic pain–induced depression. Cell Tissue Res. 377, 21–43. doi:10.1007/s00441-019-03003-z
Jelen, L. A., Stone, J. M., Young, A. H., and Mehta, M. A. (2022). The opioid system in depression. Neurosci. Biobehav Rev. 140, 104800. doi:10.1016/j.neubiorev.2022.104800
Johnson, S. W., and North, R. A. (1992). Opioids excite dopamine neurons by hyperpolarization of local interneurons. J. Neurosci. 12, 483–488. doi:10.1523/JNEUROSCI.12-02-00483.1992
Johnstone, T., Van Reekum, C. M., Urry, H. L., Kalin, N. H., and Davidson, R. J. (2007). Failure to regulate: Counterproductive recruitment of top-down prefrontal-subcortical circuitry in major depression. J. Neurosci. 27, 8877–8884. doi:10.1523/JNEUROSCI.2063-07.2007
Kempton, M. J., Salvador, Z., Munafò, M. R., Geddes, J. R., Simmons, A., Frangou, S., et al. (2011). Structural neuroimaging studies in major depressive disorder. Meta-analysis and comparison with bipolar disorder. Arch. Gen. Psychiatry 68, 675–690. doi:10.1001/archgenpsychiatry.2011.60
Ko, M. C. H., Lee, H., Harrison, C., Clark, M. J., Song, H. F., Naughton, N. N., et al. (2003). Studies of micro-kappa-and delta-opioid receptor density and G protein activation in the cortex and thalamus of monkeys. J. Pharmacol. Exp. Ther. 306, 179–186. doi:10.1124/jpet.103.050625
Kokkinou, M., Ashok, A. H., and Howes, O. D. (2018). The effects of ketamine on dopaminergic function: Meta-Analysis and review of the implications for neuropsychiatric disorders. Mol. Psychiatry 23, 59–69. Preprint at. doi:10.1038/mp.2017.190
Koob, G. F. (2009). Dynamics of neuronal circuits in addiction: Reward, antireward, and emotional memory. Pharmacopsychiatry 42 (1), S32–S41. doi:10.1055/s-0029-1216356
Kummer, K. K., Mitrić, M., Kalpachidou, T., and Kress, M. (2020). The medial prefrontal cortex as a central hub for mental comorbidities associated with chronic pain. Int. J. Mol. Sci. 21, 3440. Preprint at. doi:10.3390/ijms21103440
Lammel, S., Lim, B. K., and Malenka, R. C. (2014). Reward and aversion in a heterogeneous midbrain dopamine system. Neuropharmacology 76, 351–359. doi:10.1016/j.neuropharm.2013.03.019
Lampl, C., Thomas, H., Tassorelli, C., Katsarava, Z., Laínez, J. M., Lantéri-Minet, M., et al. (2016). Headache, depression and anxiety: Associations in the eurolight project. J. Headache Pain 17, 59. doi:10.1186/s10194-016-0649-2
Lee, M. T., Peng, W. H., Wu, C. C., Kan, H. W., Wang, D. W., Teng, Y. N., et al. (2023). Impaired ventrolateral periaqueductal gray-ventral tegmental area pathway contributes to chronic pain-induced depression-like behavior in mice. Mol. Neurobiol. 60, 5708–5724. doi:10.1007/s12035-023-03439-z
Lemke, M. R., Brecht, H. M., Koester, J., and Reichmann, H. (2006). Effects of the dopamine agonist pramipexole on depression, anhedonia and motor functioning in Parkinson’s disease. J. Neurol. Sci. 248, 266–270. doi:10.1016/j.jns.2006.05.024
Levinstein, M. R., Carlton, M. L., Di Ianni, T., Ventriglia, E. N., Rizzo, A., Gomez, J. L., et al. (2023). Mu opioid receptor activation mediates (S)-ketamine reinforcement in rats: Implications for abuse liability. Biol. Psychiatry 93, 1118–1126. doi:10.1016/j.biopsych.2022.12.019
Lin, J., Liu, W., Guan, J., Cui, J., Shi, R., Wang, L., et al. (2023). Latest updates on the serotonergic system in depression and anxiety. Front. Synaptic Neurosci. 15, 1124112. Preprint at. doi:10.3389/fnsyn.2023.1124112
Lozano, A. M., Lipsman, N., Bergman, H., Brown, P., Chabardes, S., Chang, J. W., et al. (2019). Deep brain stimulation: Current challenges and future directions. Nat. Rev. Neurol. 15, 148–160. Preprint at. doi:10.1038/s41582-018-0128-2
Ma, Y., Zhao, W., Chen, D., Zhou, D., Gao, Y., Bian, Y., et al. (2023). Disinhibition of mesolimbic dopamine circuit by the lateral hypothalamus regulates pain sensation. J. Neurosci. 43, 4525–4540. doi:10.1523/JNEUROSCI.2298-22.2023
Mäntyselkä, P., Kumpusalo, E., Ahonen, R., Kumpusalo, A., Kauhanen, J., Viinamäki, H., et al. (2001). Pain as a reason to visit the doctor: A study in Finnish primary health care. Pain 89, 175–180. doi:10.1016/s0304-3959(00)00361-4
Markovic, T., Pedersen, C. E., Massaly, N., Vachez, Y. M., Ruyle, B., Murphy, C. A., et al. (2021). Pain induces adaptations in ventral tegmental area dopamine neurons to drive anhedonia-like behavior. Nat. Neurosci. 24, 1601–1613. doi:10.1038/s41593-021-00924-3
Mayberg, H. S., et al. (1999). Reciprocal limbic-cortical function and negative mood: Converging PET findings in depression and normal sadness. Depress. Sci. Ment. Health 6, 245–253. doi:10.1176/ajp.156.5.675
McGrath, J., Lim, C. W., Plana-Ripoll, O., Holtz, Y., Agerbo, E., Momen, N. C., et al. (2020). Comorbidity within mental disorders: A comprehensive analysis based on 145 990 survey respondents from 27 countries. Epidemiol. Psychiatr. Sci. 29, e153. doi:10.1017/S2045796020000633
McHugh, K. L., and Kelly, J. P. (2018). Modulation of the central opioid system as an antidepressant target in rodent models. Prog. Brain Res. 239, 49–87. doi:10.1016/bs.pbr.2018.07.003
Meyer, J. H., Krüger, S., Wilson, A. A., Christensen, B. K., Goulding, V. S., Schaffer, A., et al. (2001). Lower dopamine transporter binding potential in striatum during depression. Neuroreport 12, 4121–4125. doi:10.1097/00001756-200112210-00052
Minami, M. (2019). The role of the bed nucleus of the stria terminalis in pain-induced aversive motivation. Curr. Opin. Behav. Sci. 26, 46–53. Preprint at. doi:10.1016/j.cobeha.2018.10.003
Moliner, R., Girych, M., Brunello, C. A., Kovaleva, V., Biojone, C., Enkavi, G., et al. (2023). Psychedelics promote plasticity by directly binding to BDNF receptor TrkB. Nat. Neurosci. 26, 1032–1041. doi:10.1038/s41593-023-01316-5
Moncrieff, J., Cooper, R. E., Stockmann, T., Amendola, S., Hengartner, M. P., and Horowitz, M. A. (2022). The serotonin theory of depression: A systematic umbrella review of the evidence. Mol. Psychiatry, 1–14. doi:10.1038/s41380-022-01661-0
Montardy, Q., Zhou, Z., Lei, Z., Liu, X., Zeng, P., Chen, C., et al. (2019). Characterization of glutamatergic VTA neural population responses to aversive and rewarding conditioning in freely-moving mice. Sci. Bull. (Beijing) 64, 1167–1178. doi:10.1016/j.scib.2019.05.005
Moradi, M., Yazdanian, M., and Haghparast, A. (2015). Role of dopamine D2-like receptors within the ventral tegmental area and nucleus accumbens in antinociception induced by lateral hypothalamus stimulation. Behav. Brain Res. 292, 508–514. doi:10.1016/j.bbr.2015.07.007
Nair-Roberts, R. G., Chatelain-Badie, S. D., Benson, E., White-Cooper, H., Bolam, J. P., and Ungless, M. A. (2008). Stereological estimates of dopaminergic, GABAergic and glutamatergic neurons in the ventral tegmental area, substantia nigra and retrorubral field in the rat. Neuroscience 152, 1024–1031. doi:10.1016/j.neuroscience.2008.01.046
Nestler, E. J., and Carlezon, W. A. (2006). The mesolimbic dopamine reward circuit in depression. Biol. Psychiatry 59, 1151–1159. doi:10.1016/j.biopsych.2005.09.018
Ntamati, N. R., Acuñ, M. A., and Nevian, T. (2023). Pain-induced adaptations in the claustro-cingulate pathway. Cell Rep. 42 (5), 112506. doi:10.1016/j.celrep.2023.112506
Obata, H. (2017). Analgesic mechanisms of antidepressants for neuropathic pain. Int. J. Mol. Sci. 18, 2483. doi:10.3390/ijms18112483
Ong, W. Y., Stohler, C. S., and Herr, D. R. (2019). Role of the prefrontal cortex in pain processing. Mol. Neurobiol. 56, 1137–1166. doi:10.1007/s12035-018-1130-9
Ozaki, S., Narita, M., Narita, M., Iino, M., Sugita, J., Matsumura, Y., et al. (2002). Suppression of the morphine-induced rewarding effect in the rat with neuropathic pain: Implication of the reduction in μ-opioid receptor functions in the ventral tegmental area. J. Neurochem. 82, 1192–1198. doi:10.1046/j.1471-4159.2002.01071.x
Pani, L., Porcella, A., and Gessa, G. L. (2000). The role of stress in the pathophysiology of the dopaminergic system. Mol. Psychiatry 5, 14–21. doi:10.1038/sj.mp.4000589
Pizzagalli, D. A., Holmes, A. J., Dillon, D. G., Goetz, E. L., Birk, J. L., Bogdan, R., et al. (2009). Reduced caudate and nucleus accumbens response to rewards in unmedicated individuals with major depressive disorder. Am. J. Psychiatry 166, 702–710. doi:10.1176/appi.ajp.2008.08081201
Qi, J., Zhang, S., Wang, H. L., Barker, D. J., Miranda-Barrientos, J., and Morales, M. (2016). VTA glutamatergic inputs to nucleus accumbens drive aversion by acting on GABAergic interneurons. Nat. Neurosci. 19, 725–733. doi:10.1038/nn.4281
Quello, S. B., Kathleen Brady, B. T., and Sonne, S. C. (2005). Mood disorders and substance use disorder: A complex comorbidity. Sci. Pract. Perspect. 3 (1), 13–21. doi:10.1151/spp053113
Reiff, C. M., Richman, E. E., Nemeroff, C. B., Carpenter, L. L., Widge, A. S., Rodriguez, C. I., et al. (2020). Psychedelics and psychedelic-assisted psychotherapy. Am. J. Psychiatry 177, 391–410. doi:10.1176/appi.ajp.2019.19010035
Ren, W., Centeno, M. V., Berger, S., Wu, Y., Na, X., Liu, X., et al. (2016). The indirect pathway of the nucleus accumbens shell amplifies neuropathic pain. Nat. Neurosci. 19, 220–222. doi:10.1038/nn.4199
Rong, P., Liu, J., Wang, L., Liu, R., Fang, J., Zhao, J., et al. (2016). Effect of transcutaneous auricular vagus nerve stimulation on major depressive disorder: A nonrandomized controlled pilot study. J. Affect Disord. 195, 172–179. doi:10.1016/j.jad.2016.02.031
Roughan, W. H., Campos, A. I., García-Marín, L. M., Cuéllar-Partida, G., Lupton, M. K., Hickie, I. B., et al. (2021). Comorbid chronic pain and depression: Shared risk factors and differential antidepressant effectiveness. Front. Psychiatry 12, 643609–13. doi:10.3389/fpsyt.2021.643609
Ruhé, H. G., Mason, N. S., and Schene, A. H. (2007). Mood is indirectly related to serotonin, norepinephrine and dopamine levels in humans: A meta-analysis of monoamine depletion studies. Mol. Psychiatry 12, 331–359. doi:10.1038/sj.mp.4001949
Rush, A. J., Trivedi, M. H., Wisniewski, S. R., Nierenberg, A. A., Stewart, J. W., Warden, D., et al. (2006). Acute and longer-term outcomes in depressed outpatients requiring one or several treatment steps: A STAR*D report. Am. J. Psychiatry 163, 1905–1917. doi:10.1176/ajp.2006.163.11.1905
Russo, S. J., and Nestler, E. J. (2013). The brain reward circuitry in mood disorders. Nat. Rev. Neurosci. 14, 609–625. doi:10.1038/nrn3381
Sagheddu, C., Aroni, S., De Felice, M., Lecca, S., Luchicchi, A., Melis, M., et al. (2015). Enhanced serotonin and mesolimbic dopamine transmissions in a rat model of neuropathic pain. Neuropharmacology 97, 383–393. doi:10.1016/j.neuropharm.2015.06.003
Sato, D., Narita, M., Hamada, Y., Mori, T., Tanaka, K., Tamura, H., et al. (2022). Relief of neuropathic pain by cell-specific manipulation of nucleus accumbens dopamine D1-and D2-receptor-expressing neurons. Mol. Brain 15, 10. doi:10.1186/s13041-021-00896-2
Schlaepfer, T. E., and Bewernick, B. H. (2013). Deep brain stimulation for major depression THE NEED FOR NEW TREATMENT OPTIONS IN THERAPY-RESISTANT DEPRESSION.
Schlaepfer, T. E., Bewernick, B. H., Kayser, S., Mädler, B., and Coenen, V. A. (2013). Rapid effects of deep brain stimulation for treatment-resistant major depression. Biol. Psychiatry 73, 1204–1212. doi:10.1016/j.biopsych.2013.01.034
Sesack, S. R., and Grace, A. A. (2010). Cortico-basal ganglia reward network: Microcircuitry. Neuropsychopharmacology 35, 27–47. doi:10.1038/npp.2009.93
Sesia, T., Bulthuis, V., Tan, S., Lim, L. W., Vlamings, R., Blokland, A., et al. (2010). Deep brain stimulation of the nucleus accumbens shell increases impulsive behavior and tissue levels of dopamine and serotonin. Exp. Neurol. 225, 302–309. doi:10.1016/j.expneurol.2010.06.022
Sheng, J., Liu, S., Wang, Y., Cui, R., and Zhang, X. (2017). The link between depression and chronic pain: Neural mechanisms in the brain. Neural Plast. 2017, 9724371. doi:10.1155/2017/9724371
Siegle, G. J., Thompson, W., Carter, C. S., Steinhauer, S. R., and Thase, M. E. (2007). Increased amygdala and decreased dorsolateral prefrontal BOLD responses in unipolar depression: Related and independent features. Biol. Psychiatry 61, 198–209. doi:10.1016/j.biopsych.2006.05.048
Spellman, T., and Liston, C. (2020). Toward circuit mechanisms of pathophysiology in depression. Am. J. Psychiatry 177, 381–390. doi:10.1176/appi.ajp.2020.20030280
Swendsen, J. D., and Merikangas, K. R. (2000). The comorbidity of depression and substance use disorders. Clin. Psychol. Rev. 20, 173–189. doi:10.1016/s0272-7358(99)00026-4
Takahashi, D., Asaoka, Y., Kimura, K., Hara, R., Arakaki, S., Sakasai, K., et al. (2019). Tonic suppression of the mesolimbic dopaminergic system by enhanced corticotropin-releasing factor signaling within the bed nucleus of the stria terminalis in chronic pain model rats. J. Neurosci. 39, 8376–8385. doi:10.1523/JNEUROSCI.3047-18.2019
Taylor, A. M. W., Becker, S., Schweinhardt, P., and Cahill, C. (2016). Mesolimbic dopamine signaling in acute and chronic pain: Implications for motivation, analgesia, and addiction. Pain 157, 1194–1198. Preprint at. doi:10.1097/j.pain.0000000000000494
Taylor, A. M. W., Castonguay, A., Murphy, N. P., Ghogha, A., Cook, C., et al. (2015). Microglia disrupt mesolimbic reward circuitry in chronic pain. J. Neurosci. 35, 8442–8450. doi:10.1523/JNEUROSCI.4036-14.2015
Taylor, N. E., Long, H., Pei, J., Kukutla, P., Phero, A., Hadaegh, F., et al. (2019). The rostromedial tegmental nucleus: A key modulator of pain and opioid analgesia. Pain 160, 2524–2534. doi:10.1097/j.pain.0000000000001647
Tempel, A., and Zukin, R. S. (1987). Neuroanatomical patterns of the mu, delta, and kappa opioid receptors of rat brain as determined by quantitative in vitro autoradiography. Proc. Natl. Acad. Sci. U. S. A. 84, 4308–4312. doi:10.1073/pnas.84.12.4308
Thiele, S., Furlanetti, L., Pfeiffer, L. M., Coenen, V. A., and Döbrössy, M. D. (2018). The effects of bilateral, continuous, and chronic Deep Brain Stimulation of the medial forebrain bundle in a rodent model of depression. Exp. Neurol. 303, 153–161. doi:10.1016/j.expneurol.2018.02.002
Timmers, I., Quaedflieg, C. W. E. M., Hsu, C., Heathcote, L. C., Rovnaghi, C. R., and Simons, L. E. (2019). The interaction between stress and chronic pain through the lens of threat learning. Neurosci. Biobehav Rev. 107, 641–655. doi:10.1016/j.neubiorev.2019.10.007
Tye, K. M., Mirzabekov, J. J., Warden, M. R., Ferenczi, E. A., Tsai, H. C., Finkelstein, J., et al. (2013). Dopamine neurons modulate neural encoding and expression of depression-related behaviour. Nature 493, 537–541. doi:10.1038/nature11740
van der Plasse, G., Schrama, R., van Seters, S. P., Vanderschuren, L. J. M. J., and Westenberg, H. G. M. (2012). Deep brain stimulation reveals a dissociation of consummatory and motivated behaviour in the medial and lateral nucleus accumbens shell of the rat. PLoS One 7, e33455. doi:10.1371/journal.pone.0033455
Voute, M., Lambert, C., Pereira, B., and Pickering, G. (2023). Assessment of initial depressive state and pain relief with ketamine in patients with chronic refractory pain. JAMA Netw. Open 6, e2314406. doi:10.1001/jamanetworkopen.2023.14406
Wang, X. Y., Jia, W. B., Xu, X., Chen, R., Wang, L. B., Su, X. J., et al. (2023). A glutamatergic DRN–VTA pathway modulates neuropathic pain and comorbid anhedonia-like behavior in mice. Nat. Commun. 14, 5124. doi:10.1038/s41467-023-40860-3
Watanabe, M., Narita, M., Hamada, Y., Yamashita, A., Tamura, H., Ikegami, D., et al. (2018). Activation of ventral tegmental area dopaminergic neurons reverses pathological allodynia resulting from nerve injury or bone cancer. Mol. Pain 14, 1744806918756406. doi:10.1177/1744806918756406
Waung, M. W., Margolis, E. B., Charbit, A. R., and Fields, H. L. (2019). A midbrain circuit that mediates headache aversiveness in rats. Cell Rep. 28, 2739–2747. doi:10.1016/j.celrep.2019.08.009
Wiech, K., Ploner, M., and Tracey, I. (2008). Neurocognitive aspects of pain perception. Trends Cogn. Sci. 12, 306–313. doi:10.1016/j.tics.2008.05.005
Willis, W. D., and Westlund, K. N. (1997). Neuroanatomy of the pain system and of the pathways that modulate pain. J. Clin. Neurophysiology 14, 2–31. doi:10.1097/00004691-199701000-00002
Wong, K. L. L., Nair, A., and Augustine, G. J. (2021). Changing the cortical conductor’s tempo: Neuromodulation of the claustrum. Front. Neural Circuits 15, 658228. doi:10.3389/fncir.2021.658228
World Health Organization (2023). Depressive disorder (depression). Available at: https://www.who.int/news-room/fact-sheets/detail/depression.
Wu, M., Minkowicz, S., Dumrongprechachan, V., Hamilton, P., Xiao, L., and Kozorovitskiy, Y. (2021). Attenuated dopamine signaling after aversive learning is restored by ketamine to rescue escape actions. Elife 10, e64041. doi:10.7554/eLife.64041
Yadid, G., and Friedman, A. (2008). Dynamics of the dopaminergic system as a key component to the understanding of depression. Prog. Brain Res. 172, 265–286. doi:10.1016/S0079-6123(08)00913-8
Yang, H., de Jong, J. W., Cerniauskas, I., Peck, J. R., Lim, B. K., Gong, H., et al. (2021). Pain modulates dopamine neurons via a spinal–parabrachial–mesencephalic circuit. Nat. Neurosci. 24, 1402–1413. doi:10.1038/s41593-021-00903-8
Yang, S., Boudier-Revéret, M., Choo, Y. J., and Chang, M. C. (2020). Association between chronic pain and alterations in the mesolimbic dopaminergic system. Brain Sci. 10, 701–14. Preprint at. doi:10.3390/brainsci10100701
Yohn, C. N., Gergues, M. M., and Samuels, B. A. (2017). The role of 5-HT receptors in depression. Mol. Brain 10, 28. doi:10.1186/s13041-017-0306-y
Zanos, P., Moaddel, R., Morris, P. J., Riggs, L. M., Highland, J. N., Georgiou, P., et al. (2018). Ketamine and ketamine metabolite pharmacology: Insights into therapeutic mechanisms. Pharmacol. Rev. 70, 621–660. doi:10.1124/pr.117.015198
Zhang, L., Wang, J., Niu, C., Zhang, Y., Zhu, T., Huang, D., et al. (2021). Activation of parabrachial nucleus - ventral tegmental area pathway underlies the comorbid depression in chronic neuropathic pain in mice. Cell Rep. 37, 109936. doi:10.1016/j.celrep.2021.109936
Zhao, M., Wang, J. Y., Jia, H., and Tang, J. S. (2007). Roles of different subtypes of opioid receptors in mediating the ventrolateral orbital cortex opioid-induced inhibition of mirror-neuropathic pain in the rat. Neuroscience 144, 1486–1494. doi:10.1016/j.neuroscience.2006.11.009
Keywords: ventral tegmental area, dopamine, pain, depression, nociception, anhedonia, electrical brain stimulation
Citation: Flores-García M, Rizzo A, Garçon-Poca MZ, Fernández-Dueñas V and Bonaventura J (2023) Converging circuits between pain and depression: the ventral tegmental area as a therapeutic hub. Front. Pharmacol. 14:1278023. doi: 10.3389/fphar.2023.1278023
Received: 15 August 2023; Accepted: 25 September 2023;
Published: 02 October 2023.
Edited by:
Luca Posa, Cornell University, United StatesReviewed by:
Nicolas Massaly, University of California, Los Angeles, United StatesGlenn D. R. Watson, Duke University, United States
Copyright © 2023 Flores-García, Rizzo, Garçon-Poca, Fernández-Dueñas and Bonaventura. This is an open-access article distributed under the terms of the Creative Commons Attribution License (CC BY). The use, distribution or reproduction in other forums is permitted, provided the original author(s) and the copyright owner(s) are credited and that the original publication in this journal is cited, in accordance with accepted academic practice. No use, distribution or reproduction is permitted which does not comply with these terms.
*Correspondence: Víctor Fernández-Dueñas, vfernandez@ub.edu; Jordi Bonaventura, jbonaventura@ub.edu
†These authors have contributed equally to this work