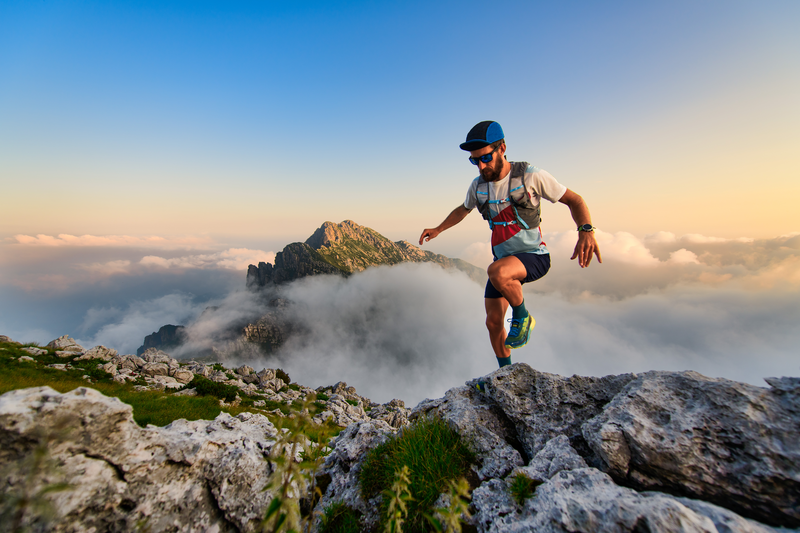
94% of researchers rate our articles as excellent or good
Learn more about the work of our research integrity team to safeguard the quality of each article we publish.
Find out more
REVIEW article
Front. Pharmacol. , 31 October 2023
Sec. Renal Pharmacology
Volume 14 - 2023 | https://doi.org/10.3389/fphar.2023.1276488
ELABELA (ELA), also known as Toddler or Apela, is a novel endogenous ligand of the angiotensin receptor AT1-related receptor protein (APJ). ELA is highly expressed in human embryonic, cardiac, and renal tissues and involves various biological functions, such as embryonic development, blood circulation regulation, and maintaining body fluid homeostasis. ELA is also closely related to the occurrence and development of acute kidney injury, hypertensive kidney damage, diabetic nephropathy, renal tumors, and other diseases. Understanding the physiological role of ELA and its mechanism of action in kidney-related diseases would provide new targets and directions for the clinical treatment of kidney diseases.
In 1993, O'Dowd et al. first identified the APJ receptor (putative receptor protein related to the angiotensin receptor AT1) from the human genome (O'Dowd et al., 1993). It is a G protein-coupled receptor (GPCR) with a seven α-transmembrane helices and is also known as an orphan G protein-coupled receptor (oGPCRs) because no endogenous ligands have been identified. The gene sequences of APJ and AT1 receptors share about 35% homology but do not bind to angiotensin II (Read et al., 2019).In 1998, Tatemoto et al. extracted and purified a new neurocardiovascular active peptide, Apelin, from bovine gastric secretions using a reverse pharmacological approach and established it as an endogenous ligand for the APJ receptor (Tatemoto et al., 1998). Apelin and its receptor are distributed in various tissues and organs of the body and are involved in the regulation of cardiovascular activity, angiogenesis, and the adipose islet axis, and play a critical role in the maintenance of body fluid homeostasis (Galanth et al., 2012; Chapman et al., 2014).
In 2013, Chng et al., for the first time, identified another novel endogenous ligand of APJ, ELABELA (ELA), in zebrafish embryos (Chng et al., 2013); also, Pauli et al. reported the same peptide structure and named it Toddler (Pauli et al., 2015). ELA is highly expressed in human embryonic, heart, and kidney tissues, and its role in promoting embryonic development, regulating blood circulation, and maintaining fluid homeostasis is being discovered gradually (Deng et al., 2015; Freyer et al., 2017; Sato et al., 2017). A current study showed that ELA is closely related to the pathophysiological function of the kidney and exerts a protective role in various kidney diseases (Chen et al., 2020a). This article reviews the structure, physiological function, and role of ELA in the development of kidney diseases.
The ELA gene of zebrafish is located on chromosome 1 and consists of three exons. ELA is expressed during zebrafish embryogenesis, from the middle blastocyst to 3 days after fertilization (Pauli et al., 2014). The human gene encoding ELA, AK092578, is located on chromosome 4 and contains three exons and a non-coding RNA transcript gene (Xie et al., 2014).The ELA mRNA consists a conserved open reading frame (ORF) and encodes a precursor protein composed of 54 amino acids. The N-terminal signal sequence of ELA consists 22 amino acid residues and a mature peptide ELA-32 comprising 22 amino acid residues, of which 13 amino acids at the C-terminal are conserved across vertebrates Under the action of endoplasmic reticulum and Golgi apparatus, ELA-32 is then disassembled into small molecular isoforms, such as ELA-21 and ELA-11(Ma et al., 2021). The specific amino acid sequences of the different isoforms are illustrated in Figure 1.
FIGURE 1. Amino acid sequences of ELA-32, ELA-21, and ELA-11 isoforms. The signal sequence of ELA, a precursor protein consisting of 54 amino acids, is cleaved to produce a mature 32 amino acid peptide (ELA-32). ELA-32 contains two cleavage sites that are cleaved to produce two additional amino acid fragments, ELA-21 and ELA-11, where in the N-terminal Gln of ELA-32 will be converted to pyroglutamic acid. ELA- 32 and ELA-21 form a bridge between Cys residues.
ELA was first identified in zebrafish embryogenesis, highly expressed in the neural tube (Stock et al., 2022). It mediates endodermal differentiation and cell migration, thereby inducing angiogenesis to promote early cardiac development during zebrafish embryogenesis (Pauli et al., 2014). Thus, ELA plays a critical role in the development of the embryonic cardiovascular system (Eberlé et al., 2019). Human embryonic stem cells (hESCs) also express and secrete ELA. Although ELA maintains self-renewal capacity in hESCs in a paracrine manner, its expression is rapidly downregulated during hESC differentiation (Miura et al., 2004; Ho et al., 2015). In addition to expression during embryonic development, ELA was highly expressed in adult kidney and prostate tissues (Wang et al., 2015; Coquerel et al., 2017; Xie et al., 2022; Xiong et al., 2023). In human vasculature, ELA is more highly expressed in the arteries than in veins but at low levels in the human heart and lung tissues (Yang et al., 2017a).
ELA and apelin also act as endogenous ligands for APJ receptors, but their sequence similarity is minimal (Murza et al., 2016). Various isoforms of ELA can bind to APJ; ELA-32 and ELA-21 bind to APJ with subnanomolar and nanomolar affinities significantly better than that of the short peptide ELA-11 interaction with APJ (Yang et al., 2017a). The experiments of alanine scanning and mutational analysis suggest that ELA and apelin bind to different residues in the APJ receptor (Couvineau et al., 2020), resulting in distinct binding patterns between these endogenous ligands and the APJ receptor (Shin et al., 2017). Apelin or ELA binding to APJ initiates intracellular signaling by coupling different G proteins and exerting a wide range of biological effects (Masri et al., 2006; Zhang et al., 2017). On one hand, APJ mediates the vasodilation response of blood vessels through the coupling of Gαi/o to activate downstream ERK and PI3K-AKT signaling cascades (Zhang et al., 2017). On the other hand, APJ activates phospholipase C (PLC) and AMPK signaling by coupling Gαq/11 to enhance myocardial contractility and glucose uptake in skeletal muscle cells (Szokodi et al., 2002; Dray et al., 2008; Murza et al., 2016). Subsequently, the activated APJ receptor is uncoupled from G proteins, following which it mediates receptor endocytosis by recruiting β-arrestin and initiating β-arrestin-dependent intracellular signaling pathways that are involved in a key physiological regulatory processes in the body, including transcription, cell division, and apoptosis (Ceraudo et al., 2014; Ma et al., 2021). In addition to that, ELA can also bind to an unknown receptor and plays a crucial role in cell growth, survival, and self-renewal by activating the PI3K/AKT phosphorylation signaling pathway or inhibiting the p53 signaling pathway(Ho et al., 2017; Sato et al., 2017). (Figure 2)
FIGURE 2. Signaling pathways associated with the Elabela-Apelin-APJ systemNote. After binding with the APJ receptor, ELA/Apelin exerts different biological effects through G-protein-dependent and/or β-arrestin-dependent signaling pathways. ELA, Elabela; X receptor, unknown receptor; PI3K, phosphatidylinositol 3-kinase; pAKT, phosphorylated serine/threonine protein kinase; eNOS, endothelial nitric oxide synthase; NO, nitric oxide; mTOR, rapamycin target protein; MEK1/2, mitogen-activated protein kinase; ERK1/2, extracellular regulatory protein kinase; p70S6, p70 ribosomal S6 kinase; AMPK, AMP-dependent protein kinase; PIP2, phosphatidylinositol diphosphate; IP3, inositol triphosphate; PLC, phospholipase C; PKC, protein kinase C; DAG, diacylglycerol; CCND1, cell cycle protein.
Compared to [Pyr1]apelin-13, longer ligand isoforms such as apelin-17, apelin-36, ELA-21, and ELA-32 demonstrate a stronger bias towards the β-arrestin signaling pathway (Yang et al., 2017a; Yang et al., 2017b; Nyimanu et al., 2019). Our team’s previous research has also confirmed that apelin and ELA form a spatiotemporal dual-ligand system, exhibiting distinct signaling profiles upon binding to the APJ receptor (Nyimanu et al., 2019). ELA-32 and apelin-17 display a stronger preference for β-arrestin-dependent signaling pathways, while ELA-21 and pGlu1-apelin-13 exhibit more pronounced activity in G-protein-dependent signaling pathways (Chen J. et al., 2020). The involvement of this bias in the physiological functions and pathophysiology of APJ requires further investigation. In conclusion, the ligand activity and signaling bias demonstrated by different apelin and ELA isoforms contribute to a better understanding of the functionality of the APJ receptor and provide more options for drug development targeting this receptor.
Apelin and its receptor APJ are widely distributed in various tissues and highly expressed in glomerular and tubular structures in humans and rats (Hosoya et al., 2000). While ELA is mainly distributed in the kidney, RNAscope and immunofluorescence detected that these are concentrated on the apical membrane of the main cells of the outer to inner medullary collecting ducts in rats and co-localized with the AQP-2 marker of the main cells (Xu C. et al., 2020). A previous study showed that either lateral ventricular or intravenous administration of apelin or ELA significantly increases the urine output of the animals (Deng et al., 2015; Jiang et al., 2021). These findings suggested that ELA may directly affect the kidney, regulating renal hemodynamics and the substance transport function of the renal tubules and collecting ducts.
Renal blood flow is tightly regulated by renin-angiotensin system (RAS), which in turn affects glomerular blood flow and glomerular filtration rate (Gerbier et al., 2017). Apelin inhibits angiotensin II-induced contractile activity of the small glomerular inlet and outlet arteries (Hus-Citharel et al., 2008), regulates renal hemodynamics, and increases renal blood flow. This might be one of the mechanisms by which apelin produces its diuretic effect, but it is dependent on intact vascular endothelium and nitric oxide (NO) production (Hus-Citharel et al., 2008; Wang et al., 2015; Yang et al., 2015). On the other hand, since the straight small vessels receive blood supply from the small outflowing arteries, the apelin-induced vasodilatory response may promote diuresis by increasing renal medullary blood flow. Studies have demonstrated that ELA can also counteract the action of the renin-angiotensin system (RAS) in the distal renal tubules, exerting physiological effects similar to apelin (Chapman et al., 2021). (Figure 3)
FIGURE 3. Physiological role of ELA in the kidney. Apelin acts on the afferent and efferent arterioles, promotes vasodilation by producing nitric oxide (NO), and counteracts the effect of Ang II. The action of apelin antagonizes vasopressin signaling in renal tubules. In the principal cells of the collecting duct, apelin or elabela prevents pressor-induced translocation of aquaporin 2 (AQP2) to the apical membrane, thereby preventing water reabsorption. Afferent arterioles, small inlet arterioles; efferent arteriole, small outlet arterioles; AT1 receptor, angiotensin II-1 type 1 receptor; AVP, arginine vasopressin; V2R, arginine vasopressin type II receptor; AQP2, aquaporin 2.
In addition, APJ expression was progressively increased along the rat cortex to the inner medullary collecting duct (Hus-Citharel et al., 2014). Apelin acts directly on the renal tubules, exerting a diuretic effect in a dose-dependent manner and reducing urine osmolality (Hus-Citharel et al., 2014; 2008). In vivo and in vitro studies have shown that apelin affects water reabsorption and regulates urine output by inhibiting the translocation of water channel protein-2 to the apical membrane (Boulkeroua et al., 2019). In rodent kidneys, ELA is more abundantly expressed than apelin and is predominant in the medullary collecting ducts (O'Carroll et al., 2017). In adult rats, urinary flow rate and water uptake were significantly increased after intravenous administration of ELA or apelin. Interestingly, the effect of ELA was five times greater than that of apelin, suggesting that ELA is involved in the regulation of renal function (Deng et al., 2015). The urine flow rate and water intake in rats were inhibited by the use of ELA antagonist (ELA-PA). In addition, under pathological conditions of hypertension, long-term use of ELA can reduce the excretion of electrolyte sodium and chlorine, possibly through the regulation of osmotic pressure through reabsorption of sodium chloride (Schreiber et al., 2017). Since ELA expression is confined to the vascular endothelium and kidney in adults, it may play a critical role in the regulation of fluid homeostasis.
The specific mechanism by which ELA maintains fluid balance is not yet fully elucidated. In the central nervous system, apelin and arginine vasopressin (AVP) coexist in the same hypothalamic neuroendocrine neurons (Reaux et al., 2001; De Mota et al., 2004). Apelin, as a potent neurodiuretic peptide, directly inhibits the periodic firing activity of AVP neurons and the release of AVP through the APJ receptor, antagonizing the effects of AVP (Reaux-Le Goazigo et al., 2004; Azizi et al., 2008). Studies have shown that neuroendocrine neurons in the hypothalamus of humans and other mammals regulate the secretion of apelin and AVP in opposite directions via a “Yin-Yang” pattern in response to stimuli of different osmotic pressures. This control of AVP release and antidiuretic effect at the “optimal level” is crucial for maintaining body fluid balance (Girault-Sotias et al., 2021). It has been found that intracerebroventricular injection of ELA in mice can activate AVP neurons in the paraventricular nucleus (PVN), reduce food intake, and exert anorectic effects (Girault-Sotias et al., 2021). Microinjection of ELA-21 into the PVN increased renal sympathetic nerve activity and AVP levels in spontaneously hypertensive rats (Geng et al., 2020). However, it remains to be further studied whether ELA and AVP also maintain fluid balance through opposing regulatory effects.
Recent studies have demonstrated a protective role of ELA in kidney-related diseases. ELA treatment preserves glomerular structure, inhibits the expression of pro-fibrosis-related genes in rat kidneys, and improves renal fibrosis in rats (Schreiber et al., 2017; Xu C. et al., 2020; Chen et al., 2020c). When ELA levels in serum were reduced, renal function was impaired and the progression of renal disease was accelerated (Lu et al., 2020). These findings suggested that ELA is involved in the pathophysiological process of renal disease development and is a novel therapeutic target for renal disease.
Acute kidney injury, mainly caused by ischemia/reperfusion injury (IRI) and nephrotoxins, is characterized by rapid deterioration of renal function and high mortality; however, there is a lack of effective clinical treatment(Sharma et al., 2019). The pathological process of IRI includes inflammation and apoptosis(Bonventre and Zuk, 2004; Wang et al., 2020). In vitro studies found that the overexpression of ELA-32 and ELA-11 in renal tubular epithelial cells (NRK-52E) significantly inhibits cellular DNA damage, apoptosis, and inflammatory response induced by I/R or adriamycin treatment (Chen et al., 2017). Further in vivo experiments revealed that ELA expression levels were significantly reduced in mouse kidneys in the renal I/R injury model. The expression levels of inflammatory factors (IL-6, IL-8, and MCP1), kidney injury factor (KIM-1), fibrotic factors (vimentin, TGF-β1, fibronectin, collagen1a), DNA damage markers, and apoptotic factors were significantly elevated. At the same time, ELA-32 and ELA-11 inhibited I/R injury-induced renal fibrosis, inflammation, apoptosis, DNA damage response, and macrophage infiltration and attenuated tubular lesions and pathological scores of renal function (Chen et al., 2015; Chen et al., 2017). Furthermore, ELA improved the levels of blood creatinine, 24-h urine volume, proteinuria, urinary creatinine, and urea nitrogen in mice. Compared to ELA-32, ELA-11 showed better protection against I/R injury-induced DNA damage response, apoptosis, and cell viability (Chen et al., 2017). In addition, only ELA-11 showed significant inhibition of renal I/R injury-induced autophagy, although the mechanism of action is unclear(Chen et al., 2017). To further clarify whether ELA is involved in the protective effect against renal I/R injury through APJ receptor, Chen et al. knocked down APJ using siRNA in I/R-induced NRK-52E cells. This significantly enhanced cell viability and inhibited inflammatory responses and DNA damage effects, suggesting that the protective role of ELA in I/R is independent of the presence of APJ, and that ELA exerts nephroprotective effects through other unknown receptors (Chen et al., 2017).
Acute kidney injury caused by sepsis is a common clinical condition, and ELA has been reported to ameliorate cardiorenal injury in sepsis (Singbartl and Kellum, 2012). ELA is a small molecule peptide with short half-life in vivo, rendering it unsuitable for clinical treatment. Xu et al. fused the Fc structural domain of human immunoglobulin IgG with ELA-21 to produce Fc-ELA, which prolonged the plasma half-life of ELA and made it biologically active(Xu F. et al., 2020). In a mouse model of liposaccharide (LPS)-induced acute kidney injury, Fc-ELA fusion protein significantly ameliorated LPS-induced kidney injury and attenuated macrophage infiltration, renal inflammation, and apoptotic response(Wang, 2020). Therefore, Fc-ELA fusion protein has a significant nephroprotective effect on LPS-induced acute kidney injury and could be used in the treatment of the disease.
Hypertensive renal damage is the structural and functional damage to the kidney caused by primary hypertension, which has become the third cause of end-stage renal disease (ESRD) after primary glomerular disease and diabetic nephropathy (Bidani and Griffin, 2004). Hypertension-triggered RAS is involved in the pathophysiology of hypertensive renal damage (Yang and Xu, 2017; Xu et al., 2021). Sato et al. found that exogenous ELA inhibited Ang Ⅱ-induced hypertension in C57/BL6J wild-type mice and significantly reduced Ang Ⅱ-induced myocardial fibrosis, suggesting that ELA antagonizes the RAS (Sato et al., 2017). In high salt-loaded Dahl salt-sensitive rats, exogenous administration of ELA-32 significantly reduced blood pressure and proteinuria levels and was accompanied by a decline in the levels of soluble (pro) renin receptor (sPRR), Angiotensin converting enzyme (ACE), Ang Ⅱ, and reninogen/renin expression in the renal medulla of rats (Xu C. et al., 2020). ELA also significantly reduced the expression of inflammatory factors, renal fibrosis markers, and renal injury markers in high salt-induced hypertensive rats, confirming its role in regulating blood pressure and ameliorating hypertensive renal damage by antagonizing intrarenal RAS (Xu C. et al., 2020).
Schreiber et al. mediated ELA gene expression in Dah1 salt-sensitive rats by injection of adeno-associated virus type 9 (AAV9) vector. The study also used a high-salt diet to establish a hypertension rat model and found that ELA delayed the development of elevated blood pressure in animals (Schreiber et al., 2017). After 12 weeks of ELA treatment, the excretion of sodium and chloride gradually decreased in rats, glomerulosclerosis and tubular injury were reduced, and the transcript levels of transforming growth factor β (TGF-β), type 1 collagen and fibronectin, and marker genes of renal fibrosis were decreased, suggesting that the sustained expression of ELA significantly reduces the blood pressure. Also, inhibition of the gene expression of fibrotic factors decreases interstitial fibrosis in the kidney(Schreiber et al., 2017). Thus, ELA may improve renal damage caused by long-term hypertension by inhibiting the activity of RAS and the development of interstitial fibrosis. These findings indicate that ELA provides a potential long-term treatment for hypertension and renal remodeling.
Diabetic kidney disease (DKD) is one of the most common and serious chronic microvascular complications of diabetes mellitus. It has become a major cause of ESRD and is considered an independent risk factor for many cardiovascular diseases (Umanath and Lewis, 2018; Zheng et al., 2018). DKD is clinically characterized by increased urinary protein excretion and loss of renal function, manifested by glomerular hypertrophy, hypofiltration, and renal fibrosis (Oshima et al., 2021). Clinical studies have found that serum ELA levels are reduced in patients with DKD and are significantly negatively correlated with urinary albumin/creatinine ratio (ACR) and serum creatinine, suggesting that the molecules is involved in the progression of DKD (Zhang et al., 2018).Therefore, the decrease in serum ELA levels may be a clinical predictive indicator for DKD patients, and may also become a new therapeutic drug for preventing or delaying the progression of DKD. However, currently we need to conduct more clinical studies to evaluate its safety and effectiveness in greater depth.
As a key regulator of diabetic glomerular injury, damage and a reduced number of podocytes are closely associated with the production of DKD proteinuria (Guo et al., 2015). In an STZ-induced type 1 diabetic mouse model, Zhang et al. observed decreased expression of the podocyte-specific associated proteins, synaptopodin and podocin (Zhang et al., 2018). ELA upregulates synaptopodin and podocin expression through PI3K/AKT/mTOR signaling pathway and reduces the apoptosis of foot cells (Zhang et al., 2019). In addition, ELA treatment for 6 months inhibited the renal inflammatory and fibrotic responses in diabetic mice and significantly improved renal dysfunction, thus exhibiting a protective effect against diabetic nephropathy.
Oxidative stress leads to excessive accumulation of reactive oxygen species (ROS) and causes kidney damage, which is crucial in the pathogenesis of DKD. Xu et al. confirmed that ELA reduces LPS-induced ROS production in kidney and renal tubular epithelial cells, prevents cell apoptosis, and participates in ELA-mediated cell protection through the PI3K/AKT signaling pathway(Xu F. et al., 2020). Furthermore, overexpression of renal ELA blocked the NADPH oxidase/ROS/NLRP3 inflammasome pathway and reduced ROS production in DOCA/salt-induced hypertensive rat kidneys. Thus, it could be deduced that ELA improves oxidative stress during DKD by inhibiting this pathway and exerts a protective effect on the kidneys (Chen et al., 2020a).
As one of the most common malignant tumors worldwide, kidney cancer has become a critical disease that threatens human health and life safety (Motzer et al., 1996; Curti, 2004). ELA promotes tumor cell proliferation, reduces apoptosis, and is closely related to tumor growth (Acik et al., 2019). A previous study examined ELA’s immunoreactivity in different Fuhrman renal cell carcinoma grades. No immunoreactivity to ELA was detected in Fuhrman grade 1 and 2 clear cell renal cell carcinoma, while that in Fuhrman grade 3 and 4 clear cell renal cell carcinoma was significantly lower than that in normal kidney tissue and that in grade 4 clear cell renal cell carcinoma was significantly higher than that in grade 1, 2, and 3 (Artas et al., 2019). Also, differences in ELA immunoreactivity were observed while comparing benign eosinophiloma and renal chromocytocarcinoma, and ELA expression in renal chromocytoma was significantly higher than in benign eosinophiloma of the kidney (Artas et al., 2019). This phenomenon suggested that ELA is critical in the differential diagnosis of renal tumor pathology.
The Cancer Genome Atlas (TCGA) data showed that the expression of ELA gene (APELA) was upregulated in the colon, lung, gastric, and thymoma cancers; however, APELA was systematically downregulated in all kidney cancer types, including renal chromocytocarcinoma, papillary kidney cancer, and clear cell renal cell carcinoma.Moreover, APELA is negatively correlated with the expression of the cell proliferation marker Ki-67 in the above kidney cancer. These data suggested the role of APELA in human kidney cancer and its negative association with the development of kidney cancer (Artas et al., 2019; Linehan and Ricketts, 2019; Liu et al., 2021). In the in vivo animal experiments, subcutaneous injection of ELA into kidney cancer model mice decreased the tumor growth in mice bearing ELA tumors (Soulet et al., 2020; Liet et al., 2021) ELA-11, ELA-32, and mut ELA-32 induced mTORC1 activation, inhibited ERK and AKT activation, and promoted apoptosis of renal cancer cells to exert anti-tumor effects. However, the combination of mut ELA and the angiogenesis inhibitor sunitinib (Sutent) enhances the effect of ELA in inhibiting tumor growth (Soulet et al., 2020). The replacement of arginine with serine residues in the protopeptide ELA-32 blocked the conversion of ELA32 to ELA11, allowing ELA-32 to mediate APJ endocytosis and recirculation rapidly, providing it a high affinity for APJ, facilitating better antitumor effects than the less stable protopeptide ELA (Soulet et al., 2020). Therefore, ELA or its derivatives have potential applications in the treatment of kidney cancer.
Apelin and its receptor APJ are widely distributed in the body and are involved in various physiological functions, including cardiovascular function regulation, neuroendocrinology, glucose metabolism, and angiogenesis. ELA is a novel endogenous agonist of the Apelin receptor with a similar role. In addition to its critical role in embryonic cardiovascular development, ELA is highly expressed in the adult kidney, where it is involved in renal excretory functions and maintains body fluid homeostasis. In addition, ELA is closely associated with the development of various renal diseases, including acute kidney injury, hypertensive kidney damage, diabetic nephropathy, and renal tumors (Figure 4), thereby deeming it a promising biomarker to identify different types of renal tumors. The discovery and exploration of ELA has been expanded in the pathogenesis of renal diseases, providing new targets and directions for treating related diseases. Several studies have found that the protective effects of ELA in kidney-related diseases are independent of APJ occurrence. The other receptors that bind to and act on ELA are yet to be identified, and the specific signaling mechanisms are to be elucidated. The current studies on ELA in kidney-related diseases are mainly focused on the cellular, molecular level and animal models, lacking large-scale clinical trials that need to be conducted in the future.
FIGURE 4. Role of ELA in kidney-related diseases.Acute kidney injury. ELA improves kidney function and attenuates DNA damage, apoptosis, and renal fibrosis in mice with kidney injury. Hypertensive kidney damage: ELA antagonizes the effect of RAS, reduces blood pressure, and attenuates glomerular, tubular injury, and interstitial fibrosis. Diabetic nephropathy: upregulation of synaptopodin and podocin expression decreases the death of podocytes, reduces LPS-induced ROS production in kidney and tubular epithelial cells, improves oxidative stress, and exerts renal protective effects. Renal tumors: ELA was systematically downregulated in renal suspensory cell carcinoma, papillary renal carcinoma, and clear cell renal cell carcinoma, and ELA was negatively correlated with the expression of Ki-67, a cell proliferation marker.
YrL: Writing–original draft. MJ: Writing–review and editing. YeL: Writing–review and editing. PC: Writing–review and editing. XC: Writing–review and editing.
The author(s) declare financial support was received for the research, authorship, and/or publication of this article. This study was financially supported by the National Natural Science Foundation of China (grant no. 31600949), Natural Science Foundation of Shandong Province (grant no. ZR2020MH151, ZR2021MH202), and Academic Promotion Programme of Shandong First Medical University (grant no. 2019QL013).
We would like to express our heartfelt gratitude to XC for their valuable suggestions on the reviews, We also hope to express our appreciation to the experts for editing and proofreading this manuscript.
The authors declare that the research was conducted in the absence of any commercial or financial relationships that could be construed as a potential conflict of interest.
All claims expressed in this article are solely those of the authors and do not necessarily represent those of their affiliated organizations, or those of the publisher, the editors and the reviewers. Any product that may be evaluated in this article, or claim that may be made by its manufacturer, is not guaranteed or endorsed by the publisher.
Acik, D. Y., Bankir, M., Baylan, F. A., and Aygun, B. (2019). Can ELABELA be a novel target in the treatment of chronic lymphocytic leukaemia. BMC cancer 19 (1), 1086. doi:10.1186/s12885-019-6325-6
Artas, G., Kuloglu, T., Dagli, A. F., Ugur, K., Yardim, M., Aydin, S., et al. (2019). A promising biomarker to distinguish benign and malignant renal tumors: ELABELA. Niger. J. Clin. Pract. 22 (3), 386–392. doi:10.4103/njcp.njcp_105_18
Azizi, M., Iturrioz, X., Blanchard, A., Peyrard, S., De Mota, N., Chartrel, N., et al. (2008). Reciprocal regulation of plasma apelin and vasopressin by osmotic stimuli. J. Am. Soc. Nephrol. 19 (5), 1015–1024. doi:10.1681/ASN.2007070816
Bidani, A. K., and Griffin, K. A. (2004). Pathophysiology of hypertensive renal damage: implications for therapy. Hypertension 44 (5), 595–601. doi:10.1161/01.HYP.0000145180.38707.84
Bonventre, J. V., and Zuk, A. (2004). Ischemic acute renal failure: an inflammatory disease. Kidney Int. 66 (2), 480–485. doi:10.1111/j.1523-1755.2004.761_2.x
Boulkeroua, C., Ayari, H., Khalfaoui, T., Lafrance, M., Besserer-Offroy, É., Ekindi, N., et al. (2019). Apelin-13 regulates vasopressin-induced aquaporin-2 expression and trafficking in kidney collecting duct cells. Cell. physiology Biochem. 53 (4), 687–700. doi:10.33594/000000165
Ceraudo, E., Galanth, C., Carpentier, E., Banegas-Font, I., Schonegge, A. M., Alvear-Perez, R., et al. (2014). Biased signaling favoring gi over β-arrestin promoted by an apelin fragment lacking the C-terminal phenylalanine. J. Biol. Chem. 289 (35), 24599–24610. doi:10.1074/jbc.M113.541698
Chapman, F. A., Nyimanu, D., Maguire, J. J., Davenport, A. P., Newby, D. E., and Dhaun, N. (2021). The therapeutic potential of apelin in kidney disease. Nat. Rev. Nephrol. 17 (12), 840–853. doi:10.1038/s41581-021-00461-z
Chapman, N. A., Dupré, D. J., and Rainey, J. K. (2014). The apelin receptor: physiology, pathology, cell signalling, and ligand modulation of a peptide-activated class A GPCR. Biochem. Cell Biol. = Biochimie Biol. Cell. 92 (6), 431–440. doi:10.1139/bcb-2014-0072
Chen, H., Wan, D., Wang, L., Peng, A., Xiao, H., Petersen, R. B., et al. (2015). Apelin protects against acute renal injury by inhibiting TGF-β1. Biochimica biophysica acta 1852 (7), 1278–1287. doi:10.1016/j.bbadis.2015.02.013
Chen, H., Wang, L., Wang, W., Cheng, C., Zhang, Y., Zhou, Y., et al. (2017). ELABELA and an ELABELA fragment protect against AKI. J. Am. Soc. Nephrol. JASN 28 (9), 2694–2707. doi:10.1681/ASN.2016111210
Chen, J., Chen, X., Li, S., Jiang, Y., Mao, H., Zhang, R., et al. (2020b). Individual phosphorylation sites at the C-terminus of the apelin receptor play different roles in signal transduction. Redox Biol. 36, 101629. doi:10.1016/j.redox.2020.101629
Chen, Z., Wu, C., Liu, Y., Li, H., Zhu, Y., Huang, C., et al. (2020c). ELABELA attenuates deoxycorticosterone acetate/salt-induced hypertension and renal injury by inhibition of NADPH oxidase/ROS/NLRP3 inflammasome pathway. Cell death Dis. 11 (8), 698. doi:10.1038/s41419-020-02912-0
Chen, Z., Wu, C., Liu, Y., Li, H., Zhu, Y., Huang, C., et al. (2020a). Correction: ELABELA attenuates deoxycorticosterone acetate/salt-induced hypertension and renal injury by inhibition of NADPH oxidase/ROS/NLRP3 inflammasome pathway. Cell death Dis. 11 (10), 905. doi:10.1038/s41419-020-02933-9
Chng, S. C., Ho, L., Tian, J., and Reversade, B. (2013). ELABELA: a hormone essential for heart development signals via the apelin receptor. Dev. Cell 27 (6), 672–680. doi:10.1016/j.devcel.2013.11.002
Coquerel, D., Chagnon, F., Sainsily, X., Dumont, L., Murza, A., Côté, J., et al. (2017). ELABELA improves cardio-renal outcome in fatal experimental septic shock. Crit. care Med. 45 (11), e1139–e1148. doi:10.1097/CCM.0000000000002639
Couvineau, P., Llorens-Cortes, C., and Iturrioz, X. (2020). Elabela/Toddler and apelin bind differently to the apelin receptor. FASEB J. 34 (6), 7989–8000. doi:10.1096/fj.201903029R
De Mota, N., Reaux-Le Goazigo, A., El Messari, S., Chartrel, N., Roesch, D., Dujardin, C., et al. (2004). Apelin, a potent diuretic neuropeptide counteracting vasopressin actions through inhibition of vasopressin neuron activity and vasopressin release. Proc. Natl. Acad. Sci. U. S. A. 101 (28), 10464–10469. doi:10.1073/pnas.0403518101
Deng, C., Chen, H., Yang, N., Feng, Y., and Hsueh, A. J. (2015). Apela regulates fluid homeostasis by binding to the APJ receptor to activate gi signaling. J. Biol. Chem. 290 (30), 18261–18268. doi:10.1074/jbc.M115.648238
Dray, C., Knauf, C., Daviaud, D., Waget, A., Boucher, J., Buléon, M., et al. (2008). Apelin stimulates glucose utilization in normal and obese insulin-resistant mice. Cell metab. 8 (5), 437–445. doi:10.1016/j.cmet.2008.10.003
Eberlé, D., Marousez, L., Hanssens, S., Knauf, C., Breton, C., Deruelle, P., et al. (2019). Elabela and Apelin actions in healthy and pathological pregnancies. Cytokine & growth factor Rev. 46, 45–53. doi:10.1016/j.cytogfr.2019.03.003
Freyer, L., Hsu, C. W., Nowotschin, S., Pauli, A., Ishida, J., Kuba, K., et al. (2017). Loss of Apela peptide in mice causes low penetrance embryonic lethality and defects in early mesodermal derivatives. Cell Rep. 20 (9), 2116–2130. doi:10.1016/j.celrep.2017.08.014
Galanth, C., Hus-Citharel, A., Li, B., and Llorens-Cortès, C. (2012). Apelin in the control of body fluid homeostasis and cardiovascular functions. Curr. Pharm. Des. 18 (6), 789–798. doi:10.2174/138161212799277770
Geng, Z., Ye, C., Tong, Y., Zhang, F., Zhou, Y. B., and Xiong, X. Q. (2020). Exacerbated pressor and sympathoexcitatory effects of central Elabela in spontaneously hypertensive rats. Am. J. physiology. Heart circulatory physiology 318 (1), H124–H134. doi:10.1152/ajpheart.00449.2019
Gerbier, R., Alvear-Perez, R., Margathe, J. F., Flahault, A., Couvineau, P., Gao, J., et al. (2017). Development of original metabolically stable apelin-17 analogs with diuretic and cardiovascular effects. FASEB J. 31 (2), 687–700. doi:10.1096/fj.201600784R
Girault-Sotias, P. E., Gerbier, R., Flahault, A., de Mota, N., and Llorens-Cortes, C. (2021). Apelin and vasopressin: the yin and Yang of water balance. Front. Endocrinol. 12, 735515. doi:10.3389/fendo.2021.735515
Guo, C., Liu, Y., Zhao, W., Wei, S., Zhang, X., Wang, W., et al. (2015). Apelin promotes diabetic nephropathy by inducing podocyte dysfunction via inhibiting proteasome activities. J. Cell. Mol. Med. 19 (9), 2273–2285. doi:10.1111/jcmm.12619
Ho, L., Tan, S. Y., Wee, S., Wu, Y., Tan, S. J. C., Ramakrishna, N. B., et al. (2015). ELABELA is an endogenous growth factor that sustains hESC self-renewal via the PI3K/AKT pathway. Cell stem Cell 17 (4), 435–447. doi:10.1016/j.stem.2015.08.010
Ho, L., van Dijk, M., Chye, S., Messerschmidt, D. M., Chng, S. C., Ong, S., et al. (2017). ELABELA deficiency promotes preeclampsia and cardiovascular malformations in mice. Science 357 (6352), 707–713. doi:10.1126/science.aam6607
Hosoya, M., Kawamata, Y., Fukusumi, S., Fujii, R., Habata, Y., Hinuma, S., et al. (2000). Molecular and functional characteristics of APJ. Tissue distribution of mRNA and interaction with the endogenous ligand apelin. J. Biol. Chem. 275 (28), 21061–21067. doi:10.1074/jbc.M908417199
Hus-Citharel, A., Bodineau, L., Frugière, A., Joubert, F., Bouby, N., and Llorens-Cortes, C. (2014). Apelin counteracts vasopressin-induced water reabsorption via cross talk between apelin and vasopressin receptor signaling pathways in the rat collecting duct. Endocrinology 155 (11), 4483–4493. doi:10.1210/en.2014-1257
Hus-Citharel, A., Bouby, N., Frugière, A., Bodineau, L., Gasc, J. M., and Llorens-Cortes, C. (2008). Effect of apelin on glomerular hemodynamic function in the rat kidney. Kidney Int. 74 (4), 486–494. doi:10.1038/ki.2008.199
Jiang, Y., Yan, M., Wang, C., Wang, Q., Chen, X., Zhang, R., et al. (2021). The effects of apelin and elabela ligands on apelin receptor distinct signaling profiles. Front. Pharmacol. 12, 630548. doi:10.3389/fphar.2021.630548
Liet, B., Nys, N., and Siegfried, G. (2021). Elabela/toddler: new peptide with a promising future in cancer diagnostic and therapy. Biochimica biophysica acta. Mol. Cell Res. 1868 (9), 119065. doi:10.1016/j.bbamcr.2021.119065
Linehan, W. M., and Ricketts, C. J. (2019). The Cancer Genome Atlas of renal cell carcinoma: findings and clinical implications. Nat. Rev. Urol. 16 (9), 539–552. doi:10.1038/s41585-019-0211-5
Liu, L., Yi, X., Lu, C., Wang, Y., Xiao, Q., Zhang, L., et al. (2021). Study progression of apelin/APJ signaling and Apela in different types of cancer. Front. Oncol. 11, 658253. doi:10.3389/fonc.2021.658253
Lu, X., Liu, S., Luan, R., Cui, W., Chen, Y., Zhang, Y., et al. (2020). Serum elabela and apelin levels during different stages of chronic kidney disease. Ren. Fail. 42 (1), 667–672. doi:10.1080/0886022X.2020.1792926
Ma, Z., Song, J. J., Martin, S., Yang, X. C., and Zhong, J. C. (2021). The Elabela-APJ axis: a promising therapeutic target for heart failure. Heart Fail. Rev. 26 (5), 1249–1258. doi:10.1007/s10741-020-09957-5
Masri, B., Morin, N., Pedebernade, L., Knibiehler, B., and Audigier, Y. (2006). The apelin receptor is coupled to Gi1 or Gi2 protein and is differentially desensitized by apelin fragments. J. Biol. Chem. 281 (27), 18317–18326. doi:10.1074/jbc.M600606200
Miura, T., Luo, Y., Khrebtukova, I., Brandenberger, R., Zhou, D., Thies, R. S., et al. (2004). Monitoring early differentiation events in human embryonic stem cells by massively parallel signature sequencing and expressed sequence tag scan. Stem cells Dev. 13 (6), 694–715. doi:10.1089/scd.2004.13.694
Motzer, R. J., Bander, N. H., and Nanus, D. M. (1996). Renal-cell carcinoma. N. Engl. J. Med. 335 (12), 865–875. doi:10.1056/NEJM199609193351207
Murza, A., Sainsily, X., Coquerel, D., Côté, J., Marx, P., Besserer-Offroy, É., et al. (2016). Discovery and structure-activity relationship of a bioactive fragment of ELABELA that modulates vascular and cardiac functions. J. Med. Chem. 59 (7), 2962–2972. doi:10.1021/acs.jmedchem.5b01549
Nyimanu, D., Kuc, R. E., Williams, T. L., Bednarek, M., Ambery, P., Jermutus, L., et al. (2019). Apelin-36-[L28A] and Apelin-36-[L28C(30kDa-PEG)] peptides that improve diet induced obesity are G protein biased ligands at the apelin receptor. Peptides 121, 170139. doi:10.1016/j.peptides.2019.170139
O'Carroll, A. M., Salih, S., Griffiths, P. R., Bijabhai, A., Knepper, M. A., and Lolait, S. J. (2017). Expression and functional implications of the renal apelinergic system in rodents. PloS one 12 (8), e0183094. doi:10.1371/journal.pone.0183094
O'Dowd, B. F., Heiber, M., Chan, A., Heng, H. H., Tsui, L. C., Kennedy, J. L., et al. (1993). A human gene that shows identity with the gene encoding the angiotensin receptor is located on chromosome 11. Gene 136 (1-2), 355–360. doi:10.1016/0378-1119(93)90495-o
Oshima, M., Shimizu, M., Yamanouchi, M., Toyama, T., Hara, A., Furuichi, K., et al. (2021). Trajectories of kidney function in diabetes: a clinicopathological update. Nat. Rev. Nephrol. 17 (11), 740–750. doi:10.1038/s41581-021-00462-y
Pauli, A., Norris, M. L., Valen, E., Chew, G. L., Gagnon, J. A., Zimmerman, S., et al. (2014). Toddler: an embryonic signal that promotes cell movement via Apelin receptors. Science 343 (6172), 1248636. doi:10.1126/science.1248636
Pauli, A., Valen, E., and Schier, A. F. (2015). Identifying (non-)coding RNAs and small peptides: challenges and opportunities. BioEssays 37 (1), 103–112. doi:10.1002/bies.201400103
Read, C., Nyimanu, D., Williams, T. L., Huggins, D. J., Sulentic, P., Macrae, R. G. C., et al. (2019). International union of basic and clinical Pharmacology. CVII. Structure and Pharmacology of the apelin receptor with a recommendation that elabela/toddler is a second endogenous peptide ligand. Pharmacol. Rev. 71 (4), 467–502. doi:10.1124/pr.119.017533
Reaux, A., De Mota, N., Skultetyova, I., Lenkei, Z., El Messari, S., Gallatz, K., et al. (2001). Physiological role of a novel neuropeptide, apelin, and its receptor in the rat brain. J. Neurochem. 77 (4), 1085–1096. doi:10.1046/j.1471-4159.2001.00320.x
Reaux-Le Goazigo, A., Morinville, A., Burlet, A., Llorens-Cortes, C., and Beaudet, A. (2004). Dehydration-induced cross-regulation of apelin and vasopressin immunoreactivity levels in magnocellular hypothalamic neurons. Endocrinology 145 (9), 4392–4400. doi:10.1210/en.2004-0384
Sato, T., Sato, C., Kadowaki, A., Watanabe, H., Ho, L., Ishida, J., et al. (2017). ELABELA-APJ axis protects from pressure overload heart failure and angiotensin II-induced cardiac damage. Cardiovasc. Res. 113 (7), 760–769. doi:10.1093/cvr/cvx061
Schreiber, C. A., Holditch, S. J., Generous, A., and Ikeda, Y. (2017). Sustained ELABELA gene therapy in high-salt diet-induced hypertensive rats. Curr. gene Ther. 16 (5), 349–360. doi:10.2174/1566523217666161121111906
Sharma, N., Anders, H. J., and Gaikwad, A. B. (2019). Fiend and friend in the renin angiotensin system: an insight on acute kidney injury. Biomed. Pharmacother. = Biomédecine pharmacothérapie 110, 764–774. doi:10.1016/j.biopha.2018.12.018
Shin, K., Chapman, N. A., Sarker, M., Kenward, C., Huang, S. K., Weatherbee-Martin, N., et al. (2017). Bioactivity of the putative apelin proprotein expands the repertoire of apelin receptor ligands. Biochimica biophysica acta. General Subj. 1861 (8), 1901–1912. doi:10.1016/j.bbagen.2017.05.017
Singbartl, K., and Kellum, J. A. (2012). AKI in the ICU: definition, epidemiology, risk stratification, and outcomes. Kidney Int. 81 (9), 819–825. doi:10.1038/ki.2011.339
Soulet, F., Bodineau, C., Hooks, K. B., Descarpentrie, J., Alves, I., Dubreuil, M., et al. (2020). ELA/APELA precursor cleaved by furin displays tumor suppressor function in renal cell carcinoma through mTORC1 activation. JCI insight 5 (14), e129070. doi:10.1172/jci.insight.129070
Stock, J., Kazmar, T., Schlumm, F., Hannezo, E., and Pauli, A. (2022). A self-generated Toddler gradient guides mesodermal cell migration. Sci. Adv. 8 (37), eadd2488. doi:10.1126/sciadv.add2488
Szokodi, I., Tavi, P., Földes, G., Voutilainen-Myllylä, S., Ilves, M., Tokola, H., et al. (2002). Apelin, the novel endogenous ligand of the orphan receptor APJ, regulates cardiac contractility. Circulation Res. 91 (5), 434–440. doi:10.1161/01.res.0000033522.37861.69
Tatemoto, K., Hosoya, M., Habata, Y., Fujii, R., Kakegawa, T., Zou, M. X., et al. (1998). Isolation and characterization of a novel endogenous peptide ligand for the human APJ receptor. Biochem. biophysical Res. Commun. 251 (2), 471–476. doi:10.1006/bbrc.1998.9489
Umanath, K., and Lewis, J. B. (2018). Update on diabetic nephropathy: core curriculum 2018. Am. J. kidney Dis. 71 (6), 884–895. doi:10.1053/j.ajkd.2017.10.026
Wang, Y. (2020). Recombinant Elabela-Fc fusion protein has extended plasma half-life and mitigates post-infarct heart dysfunction in rats. Int. J. Cardiol. 300, 217–218. doi:10.1016/j.ijcard.2019.06.043
Wang, Z., Sun, R., Wang, G., Chen, Z., Li, Y., Zhao, Y., et al. (2020). SIRT3-mediated deacetylation of PRDX3 alleviates mitochondrial oxidative damage and apoptosis induced by intestinal ischemia/reperfusion injury. Redox Biol. 28, 101343. doi:10.1016/j.redox.2019.101343
Wang, Z., Yu, D., Wang, M., Wang, Q., Kouznetsova, J., Yang, R., et al. (2015). Elabela-apelin receptor signaling pathway is functional in mammalian systems. Sci. Rep. 5, 8170. doi:10.1038/srep08170
Xie, F., Lv, D., and Chen, L. (2014). ELABELA: a novel hormone in cardiac development acting as a new endogenous ligand for the APJ receptor. Acta biochimica biophysica Sinica 46 (7), 620–622. doi:10.1093/abbs/gmu032
Xie, S., Xu, F., Lu, Y., Zhang, Y., Li, X., Yu, M., et al. (2022). Elabela attenuates the TGF-β1-induced epithelial-mesenchymal transition of peritoneal mesothelial cells in patients receiving peritoneal dialysis. Front. Pharmacol. 13, 890881. doi:10.3389/fphar.2022.890881
Xiong, M., Chen, H., Fan, Y., Jin, M., Yang, D., Chen, Y., et al. (2023). Tubular Elabela-APJ axis attenuates ischemia-reperfusion induced acute kidney injury and the following AKI-CKD transition by protecting renal microcirculation. Theranostics 13 (10), 3387–3401. doi:10.7150/thno.84308
Xu, C., Wang, F., Chen, Y., Xie, S., Sng, D., Reversade, B., et al. (2020a). ELABELA antagonizes intrarenal renin-angiotensin system to lower blood pressure and protects against renal injury. Am. J. physiology. Ren. physiology 318 (5), F1122–F1135. doi:10.1152/ajprenal.00606.2019
Xu, F., Zhou, H., Wu, M., Zhang, H., Zhang, Y., Zhao, Q., et al. (2020b). Fc-Elabela fusion protein attenuates lipopolysaccharide-induced kidney injury in mice. Biosci. Rep. 40 (9), BSR20192397. doi:10.1042/BSR20192397
Xu, L., Hu, G., Qiu, J., Fan, Y., Ma, Y., Miura, T., et al. (2021). High fructose-induced hypertension and renal damage are exaggerated in Dahl salt-sensitive rats via renal renin-angiotensin system activation. J. Am. Heart Assoc. 10 (14), e016543. doi:10.1161/JAHA.120.016543
Yang, P., Kuc, R. E., Brame, A. L., Dyson, A., Singer, M., Glen, R. C., et al. (2017b). [Pyr(1)]Apelin-13((1-12)) is a biologically active ACE2 metabolite of the endogenous cardiovascular peptide [Pyr(1)]Apelin-13. Front. Neurosci. 11, 92. doi:10.3389/fnins.2017.00092
Yang, P., Maguire, J. J., and Davenport, A. P. (2015). Apelin, Elabela/Toddler, and biased agonists as novel therapeutic agents in the cardiovascular system. Trends Pharmacol. Sci. 36 (9), 560–567. doi:10.1016/j.tips.2015.06.002
Yang, P., Read, C., Kuc, R. E., Buonincontri, G., Southwood, M., Torella, R., et al. (2017a). Elabela/Toddler is an endogenous agonist of the apelin APJ receptor in the adult cardiovascular system, and exogenous administration of the peptide compensates for the downregulation of its expression in pulmonary arterial hypertension. Circulation 135 (12), 1160–1173. doi:10.1161/CIRCULATIONAHA.116.023218
Yang, T., and Xu, C. (2017). Physiology and pathophysiology of the intrarenal renin-angiotensin system: an update. J. Am. Soc. Nephrol. 28 (4), 1040–1049. doi:10.1681/ASN.2016070734
Zhang, H., Gong, D., Ni, L., Shi, L., Xu, W., Shi, M., et al. (2018). Serum elabela/toddler levels are associated with albuminuria in patients with type 2 diabetes. Cell. physiology Biochem. 48 (3), 1347–1354. doi:10.1159/000492093
Zhang, Y., Wang, Y., Luo, M., Xu, F., Lu, Y., Zhou, X., et al. (2019). Elabela protects against podocyte injury in mice with streptozocin-induced diabetes by associating with the PI3K/Akt/mTOR pathway. Peptides 114, 29–37. doi:10.1016/j.peptides.2019.04.005
Zhang, Z. Z., Wang, W., Jin, H. Y., Chen, X., Cheng, Y. W., Xu, Y. L., et al. (2017). Apelin is a negative regulator of angiotensin II-mediated adverse myocardial remodeling and dysfunction. Hypertension 70 (6), 1165–1175. doi:10.1161/HYPERTENSIONAHA.117.10156
Keywords: ELABELA, apelin, APJ, acute kidney injury, hypertensive kidney damage, diabetic nephropathy, renal cancer
Citation: Liu Y, Jiang M, Li Y, Chen P and Chen X (2023) Advances in the study of ELABELA in renal physiological functions and related diseases. Front. Pharmacol. 14:1276488. doi: 10.3389/fphar.2023.1276488
Received: 12 August 2023; Accepted: 17 October 2023;
Published: 31 October 2023.
Edited by:
Elham Ahmadian, Tabriz University of Medical Sciences, IranReviewed by:
Diogo B. Peruchetti, Federal University of Minas Gerais, BrazilCopyright © 2023 Liu, Jiang, Li, Chen and Chen. This is an open-access article distributed under the terms of the Creative Commons Attribution License (CC BY). The use, distribution or reproduction in other forums is permitted, provided the original author(s) and the copyright owner(s) are credited and that the original publication in this journal is cited, in accordance with accepted academic practice. No use, distribution or reproduction is permitted which does not comply with these terms.
*Correspondence: XiaoYu Chen, eHk4MDEyNkAxNjMuY29t
Disclaimer: All claims expressed in this article are solely those of the authors and do not necessarily represent those of their affiliated organizations, or those of the publisher, the editors and the reviewers. Any product that may be evaluated in this article or claim that may be made by its manufacturer is not guaranteed or endorsed by the publisher.
Research integrity at Frontiers
Learn more about the work of our research integrity team to safeguard the quality of each article we publish.