- 1Zhanjiang Key Laboratory of Orthopaedic Technology and Trauma Treatment, Zhanjiang Central Hospital, Guangdong Medical University, Zhanjiang, China
- 2Guangdong Provincial Key Laboratory for Research and Development of Natural Drug, School of Pharmacy, Guangdong Medical University, Zhanjiang, China
- 3Key Laboratory of Traditional Chinese Medicine for the Prevention and Treatment of Infectious Diseases, Guangdong Provincial Administration of Traditional Chinese Medicine (Central People’s Hospital of Zhanjiang), Zhanjiang, China
- 4Marine Medical Research Institute of Zhanjiang, Zhanjiang, China
Salvia miltiorrhiz, commonly known as “Danshen” in Chinese medicine, has longstanding history of application in cardiovascular and cerebrovascular diseases. Renowned for its diverse therapeutic properties, including promoting blood circulation, removing blood stasis, calming the mind, tonifying the blood, and benefiting the “Qi”, recent studies have revealed its significant positive effects on bone metabolism. This potential has garnered attention for its promising role in treating musculoskeletal disorders. Consequently, there is a high anticipation for a comprehensive review of the potential of Salvia miltiorrhiza in the treatment of various musculoskeletal diseases, effectively introducing an established traditional Chinese medicine into a burgeoning field. Aim of the review: Musculoskeletal diseases (MSDs) present significant challenges to healthcare systems worldwide. Previous studies have demonstrated the high efficacy and prospects of Salvia miltiorrhiza and its active ingredients for treatment of MSDs. This review aims to illuminate the newfound applications of Salvia miltiorrhiza and its active ingredients in the treatment of various MSDs, effectively bridging the gap between an established medicine and an emerging field. Methods: In this review, previous studies related to Salvia miltiorrhiza and its active ingredients on the treatment of MSD were collected, the specific active ingredients of Salvia miltiorrhiza were summarized, the effects of Salvia miltiorrhiza and its active ingredients for the treatment of MSDs, as well as their potential molecular mechanisms were reviewed and discussed. Results: Based on previous publications, Salvianolic acid A, salvianolic acid B, tanshinone IIA are the representative active ingredients of Salvia miltiorrhiza. Their application has shown significant beneficial outcomes in osteoporosis, fractures, and arthritis. Salvia miltiorrhiza and its active ingredients protect against MSDs by regulating different signaling pathways, including ROS, Wnt, MAPK, and NF-κB signaling. Conclusion: Salvia miltiorrhiza and its active ingredients demonstrate promising potential for bone diseases and have been explored across a wide variety of MSDs. Further exploration of Salvia miltiorrhiza’s pharmacological applications in MSDs holds great promise for advancing therapeutic interventions and improving the lives of patients suffering from these diseases.
1 Introduction
Musculoskeletal diseases (MSDs) encompass a wide range of disorders that includes muscles, bones, joints, tendons, and ligaments. MSDs take various forms that affect the human locomotor system. Common representative diseases include arthritis (osteoarthritis, OA; rheumatoid arthritis, RA), osteoporosis, fractures, sarcopenia, and tendonitis (World Health Organization, 2022). They particularly represent a range of conditions characterized by aging inflammation, degradation, and destruction of connective tissue in various sites of the locomotor system (Adami et al., 2019; Kaneko et al., 2022). These diseases not only cause chronic pain and limited mobility and flexibility for individuals, but also induce high disability and even mortality (Safiri et al., 2021). The direct costs associated with medical treatments, rehabilitation, and long-term care for individuals with MSDs are substantial. Moreover, there are indirect costs related to lost productivity and absenteeism from work (Blyth et al., 2019). The burden due to MSDs is growing worldwide along with the increasing aging population. Some musculoskeletal disorders, like osteoporosis (Langdahl, 2021) and rheumatoid arthritis (Fraenkel et al., 2021), have seen the development of several effective medicines to manage disease progression. For instance, in the case of osteoporosis, a range of medications has been developed, each with its own specific mode of operation. These include anti-resorptive agents (which inhibit osteoclast activity, such as Bisphosphonates and Denosumab), bone-forming agents (which stimulate osteoblast activity, such as Teriparatide), and dual-acting agents (which simultaneously stimulate osteoblasts and inhibit osteoclasts, such as Romosozumab). However, it is essential to recognize that most of MSDs are chronic and degenerative in nature. This implies that achieving complete recovery or a definitive cure may not be possible with the current treatment modalities (Conigliaro et al., 2019). Moving forward, it is imperative to continue research efforts aimed at developing novel therapeutic approaches. Early intervention and preventive strategies can play a pivotal role in mitigating the impact of MSDs on individuals’ quality of life (Williams et al., 2018; Cieza et al., 2021).
Salvia miltiorrhiza (SM) is a perennial herb belonging to the labiatae family, and its dried roots and rhizomes are commonly referred to as Danshen, regarded as “top-grade” herb in China. Its use dates back over 2000 years and was first recorded in the ancient Chinese pharmacological monograph <Shennong Ben Cao Jing>. SM is well-known for its diverse therapeutic properties, including promoting blood circulation, removing blood stasis (Wei et al., 2023), calming the mind, tonifying the blood, and enhancing the “Qi.” Over the centuries, the utilization of SM has exhibited remarkable efficacy in addressing a wide spectrum of ailments, including cardiovascular, neurological, cancer, osteoporosis, liver, gynecological, and chronic kidney diseases. It is utilized either as a standalone therapy or in combination with other traditional Chinese medicines. For instance, the combined administration of SM and astragalus membranaceus has been shown to ameliorate cyclosporin A-induced chronic nephrotoxicity through modulation of the gut-kidney axis. Danshen Zexie Decoction has exhibited effectiveness against non-alcoholic fatty liver disease by inhibiting the ROS/NLRP3/IL-1β pathway; Furthermore the combination of Danshen and gegen decoction has demonstrated a protective effect on hearts and cardiomyocytes in the model of post-ischemia reperfusion injury (Hu et al., 2012; Han et al., 2021; Biao et al., 2022).
Currently, most chemical ingredients of SM have been identified. Its main active ingredients consist of over 30 lipophilic compounds with diterpene groups and more than 50 hydrophilic compounds with phenolic acid groups. The lipophilic ingredients include tanshinone I-VI, cryptotanshinone, Isotanshinone I-II, tanshinol A, hydroxytanshinone, methyltanshinonate, and methylinitanshingwinoone. On the other hand, the hydrophilic ingredients mainly comprise salvianolic acid A, salvianolic acid B, protocatechuic aldehyde, dihydroisotanshione Ⅰ, tanshinlactone, tanshindiol A, and miltironel I (Zhou et al., 2005; Wang et al., 2007; Chen et al., 2014; Zhao et al., 2017) (Table 1). Previous studies have demonstrated that SM and its active ingredients possess anti-inflammatory, anti-oxidative, microcirculation-enhancing, and thrombosis-preventing effects (Cai et al., 2017; Liu C. D. et al., 2021; Liu Q. Y. et al., 2021). Tanshinone and cryptotanshinone have shown significant antibacterial effects (Li et al., 2023); while tanshinone IIA sulfonate and tanshinone have beneficial effects on the cardiovascular system. Tanshinone IIA sulfonate has been found to increase coronary blood flow, improve myocardial blood supply, alleviate the hypoxia and ischemia of myocardial tissue, and provide antithrombotic effect (Zhu et al., 2023). Additionally, tanshinol can dilate coronary artery and effectively improve the hypoxia and ischemia of myocardial tissue, and enhance ventricular diastolic function (Yin et al., 2017) The ingredients of SM demonstrate promising therapeutic effects in the treatment of cardiovascular diseases. Numerous formulations made from SM extracts have been approved as commercial medicines in China, including Danshen injection, tanshinone IIA sodium sulfonate injection, salvia polyphenolic acid salt injection, compound Danshen tablets, and compound Danshen dripping pills, which are used for the treatment of coronary heart disease, angina pectoris, myocardial infarction, and other related conditions. Additionally, Danshensu sodium injection and salvianolic acid B injection are being evaluated in clinical trials for the treatment of angina pectoris of coronary heart disease (Table 2).
Due to SM excellent effect of promoting angiogenesis, it is also used as an important ingredient in traditional Chinese herbal compound prescription for promoting tissue repair. In 1984, Zhang (1984) reported that SM root could enhance calcium deposition in the fracture healing process in mice, thereby promoting fracture healing. Subsequently, there has been an increasing focus on studying the protective effects of SM against MSDs, including osteoporosis, arthritis, fractures, and osteonecrosis, which pose significant health concerns. To date, active ingredients of SM such as tanshinol, salvianolic acid B, and tanshinone IIA have shown substantial protective effects on the musculoskeletal system (Figure 1). Liao Cui and other researchers (Cui et al., 2004; Luo et al., 2016; Zhang et al., 2016) found that salvianolic acid B and tanshinol induce osteogenic differentiation of bone marrow stromal stem cells (BMSCs) by upregulating the nitric oxide pathway. Additionally, they found that the water-soluble ingredients of SM had a multi-targeted effect on regulating bone metabolism and preventing osteoporosis, potentially through the regulation of oxidative stress (Yang et al., 2013). In a recent study, Gao et al. (2021) suggested that salvianolate increased bone mass in prednisone treated rheumatoid arthritis (RA) rats by modulating the RANKL/RANK/OPG signaling pathway. The skeletal protective effects of the active ingredients of SM and their therapeutic effects on skeletal diseases are increasingly being studied and extended to various musculoskeletal diseases.
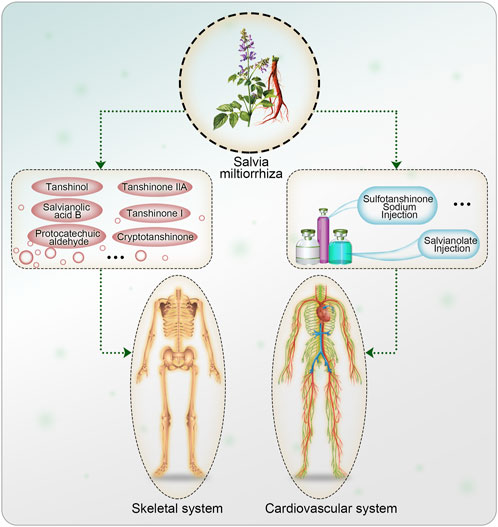
FIGURE 1. Active ingredients and formulations of Salvia miltiorrhiza on cardiovascular system and skeletal system.
This review summarizes the effects and molecular mechanisms of SM and its active ingredients on MSD. The aim of this review is to provide evidence for the future application of SM in the prevention and treatment of MSD (Figure 2).
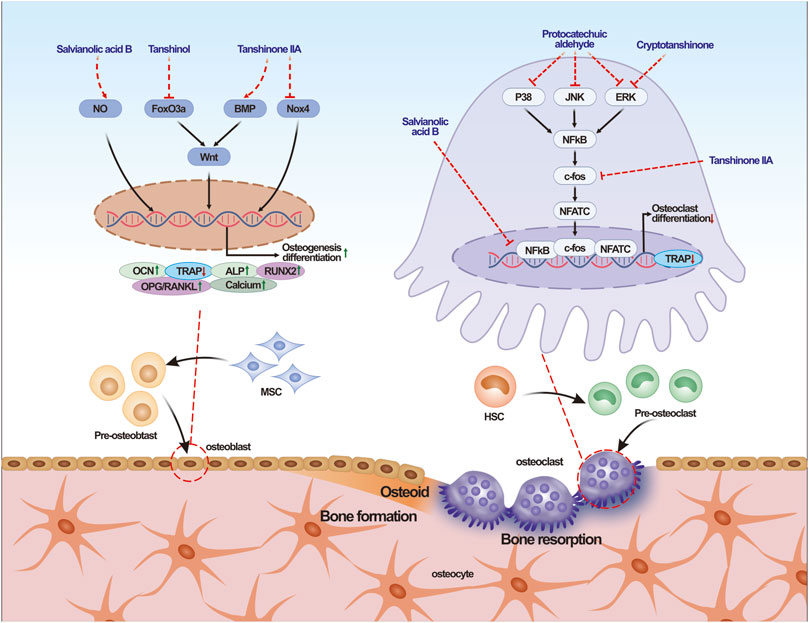
FIGURE 2. Effects and mechanism of Salvia miltiorrhiza and its effective ingredients on bone metabolism. Note: NO, Nitric Oxide; BMP, bone morphogenetic protein; Nox4, NADPH Oxidase 4; ALP, alkaline phosphatase; Runx2, Runt-related transcription factor 2; OCN, Ostecalcin; TRAP, Tartrate resistant acid phosphatase; RANKL, Receptor activator of nuclear factor kappa-Β ligand; OPG, Osteoprotegerin; MSC, mesenchymal stem cells; JNK, c-Jun-N-terminal-kinase; ERK, Extracellular regulated protein kinases; NF-κB, Nuclear factor kappa-B; NFATc, Nuclear Factor Of Activated T Cells; HSC, Hemapietic stem cell.
2 Effects and potential mechanism of SM and its effective ingredients on osteoporosis (OP)
2.1 Effects of SM and its active ingredients on osteogenesis
Osteoblasts are responsible for bone formation and play a critical role in regulating the metabolic homeostasis of bone regeneration (Abdallah et al., 2019). Previous studies have shown that the active ingredients of SM, including salvianolic acid B and tanshinone IIA, promote the proliferation and differentiation of osteoblasts, especially in glucocorticoids (GCs) induced osteoporosis (Lukert and Raisz, 1990). Salvianolic acid B has a protective effect on osteoblasts against prednisolone induced bone formation inhibition by stimulating osteoblast activity and differentiation, increasing osteogenesis marker Runx2, Osx, OCN, IGF-I, Col-I and HO-I (Qiao et al., 2019). Reactive oxygen species (ROS) has been reported to induce osteoblast apoptosis (Lee et al., 2006). MC3T3-E1 pre-osteoblasts treated with tanshinone IIA (1 µm) for 24 h reduced dexamethasone-induced apoptosis by inhibiting NADPH oxidase 4 (Nox4) expression (Li et al., 2015). Nox4 is an enzyme involved in ROS related-products (Goettsch et al., 2013). In vivo experiments showed that the treatment of tanshinone IIA 22 mg/kg/day in rats for 8 weeks significantly increased femoral bone mineral density, serum OCN, femoral biomachanical properties, and bone histomorphometric parameters in healthy female Wistar rats, while decreased TRAP expression (Yang et al., 2018).
Yang et al. (2013) demonstrated that salvianolic acid A (tanshinol) can attenuate oxidative stress by downregulating the FoxO3a signaling pathway and reducing oxidative stress, while upregulate the Wnt signaling pathway to counteract the inhibitory effect of H2O2 on osteogenic differentiation. Both classical and non-classical Wnt signaling pathways play important roles in bone remodeling, but the classical pathway mainly affects bone mass, while the non-classical pathway has a significant impact on bone homeostasis (Molagoda et al., 2019).
Nitric oxide (NO) is a free radical that exerts significant effects on osteoblast function. Studies have demonstrated that NO inhibits both bone resorption and bone formation, leading to reduced bone turnover in severe inflammatory conditions (van’t Hof and Ralston, 2001). Zhang X. et al. (2017) demonstrated that salvianolic acid B and tanshinol reversed the inhibitory effect of N-nitro-l-arginine methyl ester on osteogenic differentiation of bone marrow mesenchymal stem cells (BMSCs). They found that these compounds reversed the inhibition by reducing the expression of RANKL, increasing ALP, OCN, and the OPG/RANKL ratio. This modulation of the nitric oxide pathway stimulated the osteogenic differentiation of BMSCs. Oral administration of salvia miltiorrhiza aqueous extract 600 mg/kg/d in ovarectomized rats showed that salvia miltiorrhiza extract could significantly increase the bone density of trabecular bone in rats and reduce the degree of trabecular separation. In the liver of OVX rats, salvia miltiorrhiza could significantly reduce lipid deposition and malonaldehyde. By improving the activities of peroxidase dismutase, peroxidase, and glutathione peroxidase, salvia miltiorrhiza can directly improve cell proliferation and differentiation in H2O2-induced osteoblasts by reducing ROS (Dong et al., 2018).
In summary, previous studies revealed that the active ingredients of SM may reduce oxidative stress by down-regulating the FoxO3a signaling pathway while up-regulating the Wnt signaling pathway and nitric oxide pathway to promote osteoblast function and bone formation (Table 3).
2.2 Effects of SM and its active ingredients on osteoclastogenesis
Osteoporosis is primarily caused by an imbalance between bone resorption and bone formation, and excessive activation of osteoclasts significantly contributes to bone loss (Kikuta and Ishii, 2018). Studies demonstrated that active ingredients of SM, such as tanshinone IIA, tanshinone I, and cryptotanshinone, can reduce the formation of TRAP positive osteoclasts and suppress bone resorption (Lee et al., 2005). Kwak HB et al. found that tanshinone IIA downregulates RANKL-induced C-Fos and NFATc1, thereby inhibiting osteoblast differentiation. In the early stage of RANK signaling, activation of NF-κB and MAPKs can induce the production of c-Fos, while NFATc1 is an important regulator of RANKL-induced osteoclastogenesis. NFATc1 expression in osteoclast precursors can be upregulated by activating transcription factors such as NF-κB and activator protein-1 (AP-1) (Grigoriadis et al., 1994; Park et al., 2017; Liu et al., 2020). Wang W demonstrated that cryptotanshinone inhibited RANKL-induced osteoclastogenesis and NFATc1 expression in bone marrow macrophages (BMM) by regulating ERK and NF-κB signaling pathways (Wang W. et al., 2019). Subsequently, in a study of RAW264.7 and BMM cells, it was found that the water-soluble active ingredient of SM, protocatechuic aldehyde, reduced osteoclast formation and bone resorption, and inhibited osteoclast differentiation by inhibiting NF-κB and MAPK signaling pathways (Qu et al., 2021). However, further investigation is needed to clarify whether cryptotanshinone binds with procatechaldehyde and other active ingredients through homologous receptors on the surface of precursor cells, or directly enters the cells and inhibits the activation of NF-κB and MAPK pathways (Table 3).
2.3 Effects of SM and its active ingredients on bone marrow mesenchymal stem cell differentiation
BMSCs as one of the most critical cells in the MSC lineage, possessing a robust self-renewal capacity and the ability to differentiate into various cell types including osteoblasts, chondrocytes, and adipocytes. These cells play a pivotal role in bone development and remodeling. Imbalance in BMSC differentiation towards osteogenesis and adipogenesis is implicated in the pathogenesis of osteoporosis (Pittenger et al., 1999; Corrado et al., 2017; Qu et al., 2021). Ovariectomy (OVX) is a widely used model to induce osteoporosis in adult female rats. Wang L. et al. (2019) reported that OVX can induce hypermethylation of the promoter region of glycerol phosphate dehydrogenase (PHGDH), while treatment with tanshinone effectively inhibits OVX-induced osteoporosis and senescence of BMSCs by inhibiting PHGDH methylation and upregulating PHGDH expression. Bone morphogenetic proteins (BMPs) are multifunctional cytokines belonging to the transforming growth factor-β superfamily, and the BMP signaling pathway plays a crucial role in embryonic skeletal development and postnatal bone homeostasis (Wu et al., 2016). Studies demonstrated that treatment with tanshinone IIA at concentrations of 1 and 5 μM can upregulate alkaline phosphatase (ALP) activity and calcium content during osteogenic differentiation of BMSCs. Further experiments revealed that the upregulation of ALP activity and calcium content was primarily mediated by the activation of BMP and Wnt signaling pathways. However, co-treatment with Wnt inhibitor DKK-1 or BMP inhibitor noggin significantly attenuated the osteogenic differentiation effect of tanshinone IIA, indicating that tanshinone IIA promoted osteogenic differentiation primarily through upregulation of BMP and Wnt signaling pathways (Qian et al., 2015) (Table 3).
2.4 Effects of SM and its active ingredients on skeletal microcirculation
Under both physiological and pathological conditions, the skeletal vasculature plays a crucial role in maintaining bone homeostasis. The bone microvasculature functions as a vital supplier of oxygen, nutrients, and cytokines to bone tissue and associated cells, driving bone formation during bone development, repair, and regeneration (Chen et al., 2020). Glucocorticoids (GC) have been known to reduced blood vessels and blood flow in bone. Among the regulators of GC-induced bone remodeling in osteoporotic bone, vascular endothelial growth factor (VEGF) is considered to be of particular importance (Pufe et al., 2003; Jiang et al., 2015). Salvianolic acid B has been found to effectively treat GC-induced bone loss in rats by stimulating osteogenesis, bone marrow angiogenesis and inhibiting adipogenesis (Cui et al., 2012). Additionally, tanshinol has shown the potential to inhibit the TXNIP signaling pathway in rats with glucocorticoid-induced osteoporosis (GIO), thereby reversing the downregulation of Wnt and VEGF signaling pathways. Consequently, this process reduces microcirculation impairment and promotes bone formation (Lai et al., 2021).
In the treatment of the rabbit ischemic necrosis of the femoral head (ANFH) model, combined therapy involving SM and BMSCs has demonstrated promising results. This combined approach promotes re-ossification and hematologic reconstruction by increasing the expression of VEGF and BMP-2 in the femoral head (Wu et al., 2019). Furthermore, in a rat spinal fusion model, the incorporation of salvianolic acid B into a PLGA/β-TCP composite scaffold has shown potential in enhancing osteointegration. The scaffold promotes osteogenesis and angiogenesis, leading to improved outcomes (Lin et al., 2019) (Table 3).
3 Effects and potential mechanism of SM and its active ingredients on rheumatoid arthritis
Rheumatoid arthritis (RA) is a chronic, systemic, and autoimmune disease characterized by synovial inflammation, synovial hyperplasia, and progressive destruction of cartilage and joints (Huber et al., 2006; Lefevre et al., 2009; Zhai et al., 2017). Apoptosis of synovial fibroblasts (FLS) and inflammation are important in the pathogenesis of RA (Nygaard and Firestein, 2020). Inflammatory FLS in RA, which secrete a large amount of inflammatory cytokines, exacerbate the disease progression. Abnormal proliferation and insufficient apoptosis of FLS establish a vicious cycle constituting a significant process of RA development. Previous studies have shown that protocatechualdehyde, the metabolite of 3,4-Diacetoxy benzylidene diacetate (ACP), inhibited the production of IL-1α or PMA-induced IL-1β without inhibiting total protein synthesis in synovial cells of RA patients. This may be attributed to the inhibition of the PKC signaling pathway, suggesting that protocatechualdehyde may have a restorative effect on cartilage destruction (Watanabe et al., 1993). Liu et al. (2013) found that salvinorin injection inhibited the proliferation of RA fibroblastic synovial cells (RA FLSs) by promoting apoptosis. Subsequently, it was revealed that tanshinone IIA could induce apoptosis of fibroblast-like synoviocytes in RA by blocking the G2/M phase cell cycle and mitochondrial pathway (Jie et al., 2014). Moreover, it has also been shown that tanshinone IIA promotes apoptosis of RA FLS by upregulating the expression of long-stranded non-coding RNA (lncRNA) GAS5, while enhancing the expression of cleaved caspase-3/caspase-9 and inhibiting the PI3K/AKT signaling pathway (Li et al., 2018). Liu et al. found that the pro-apoptotic genes B-cell lymphoma 2 (Bcl-2) associated X protein (Bax) and Fas protein, were shown to be upregulated, whereas the expression levels of the anti-apoptotic gene Bcl-2 were downregulated following salvia miltiorrhiza treatment on RA FLSs (Liu et al., 2015).
TNF-α is a crucial cytokine implicated in bone destruction and osteoclast formation in RA, along with IL-1, IL-6, and IL-17, which are highly expressed in RA patients. TNF-α is considered as a key cytokine in the pathogenesis of RA leading to tissue damage, meanwhile it also presents a potential target for anti-RA drug development (Uttra et al., 2019). Tanshinone IIA, known for its potent antioxidant and anti-inflammatory properties, has been shown to effectively mitigate cartilage erosion and neutrophil infiltration in the ankle joint of adjuvant-induced arthritis (AIA) mice, while reducing the expression of pro-inflammatory cytokines in serum (Zhang S. et al., 2017). Tanshinone IIA inhibited the expression and release of IL-6 and TNF-α in neutrophils and promoted the apoptosis of neutrophils, suggesting its potential in improving RA by targeting neutrophils. Additionally, Tanshinone IIA sulfonate has been found to reduce the production of IL-1β, IL-6, MMP-1, and MMP-3 in TNF-α-induced RA FLS and inhibit their proliferation, migration, invasion, and inflammation by regulating the MAPK/NF-κB pathway (Wang Z. et al., 2019). Similar findings have been reported in AIA mice, where tanshinone IIA treatment significantly reduced synovial hyperplasia, inflammatory cell infiltration, and synovial tissue erosion (Du et al., 2020). Tanshinone IIA has also been found to inhibit the proliferation, migration, and invasion of RA FLS in a time- and dose-dependent manner, effectively suppressing the expression of MMP-2, MMP-3, MMP-8, MMP-9, IL-6, IL-1β, and IL-8 in TNF-α-induced RA FLS, primarily through the regulation of MAPK, AKT/mTOR, HIF-1, and NF-kB signaling pathways. Another compound, cryptotanshinone, has been shown to effectively improve inflammation and joint destruction in type II collagen-induced arthritis (CIA) rats by inhibiting the production of RANKL-induced pro-inflammatory cytokines such as IL-1, TNF-α, and IL-17 in bone marrow macrophages, reducing MMP-9 activity, and inhibiting osteoclast differentiation. Further studies have demonstrated that cryptotanshinone inhibits the degradation of NF-κB(IκB) inhibitors in vivo and in vitro, prevents lipopolysaccharide-induced nuclear translocation of NF-κB p65 in a time- and dose-dependent manner, and significantly inhibits the DNA-binding activity of NF-κB and NF-κB-dependent luciferase activity (Wang et al., 2015).
In a CIA rat model, salvianolic acid B has been shown to attenuate oxidative stress and inflammatory responses (Xia et al., 2018). In MH7A rheumatoid arthritis fibroblasts cell line, 10 μM salvianolic acid B protects and reverses damage induced by lipopolysaccharide (LPS) by inhibiting the expression of p53 and p21, suppressing cell apoptosis, and reducing the release of MCP-1, IL-6, and TNF-α. Salvianolic acid B has also been found to upregulate miR-142-3p expression and further suppress NF-κB and JNK pathways, suggesting its anti-RA effect may mediate by miR-142-3p (Meng et al., 2019).
In conclusion, the active ingredients of SM provide beneficial effects in RA mainly due to promoting RA FLS apoptosis and inhibiting inflammation. The potential mechanism may attribute to the regulation of MAPK, and NF-κB signaling pathways. There are some reports focus on the role and mechanism of SM in secondary osteoporosis induced by RA, which is worthy of further investigation (Table 4).
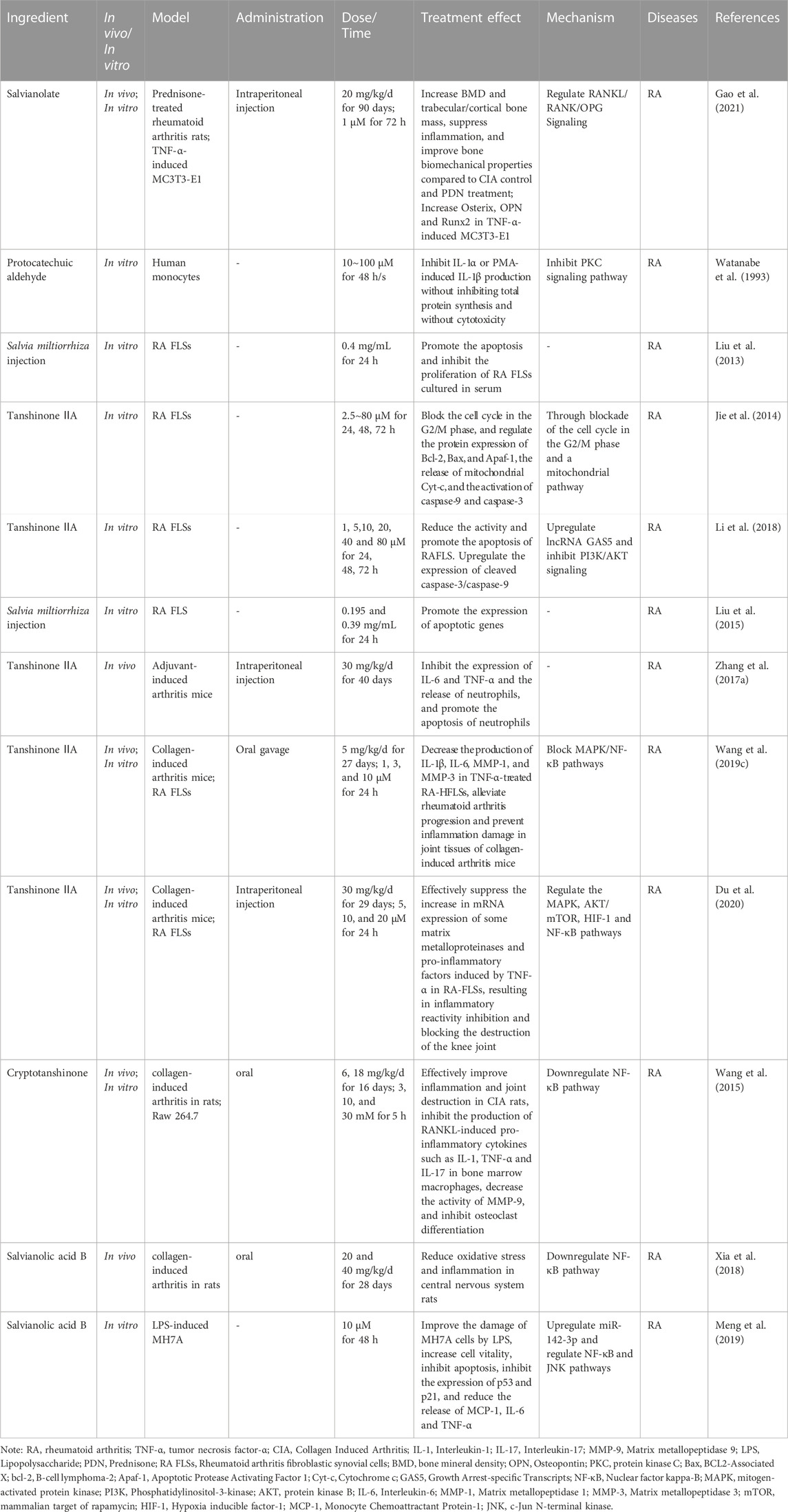
TABLE 4. Effects and mechanism of Salvia miltiorrhiza and its active ingredients on rheumatoid arthritis.
4 Effects and potential mechanism of SM and its active ingredients on osteoarthritis (OA)
Osteoarthritis (OA) is one of the most common forms of arthritis, affecting over 25% of the global population over the age of 18 (Noth et al., 2008; Martel-Pelletier et al., 2016; Chen et al., 2017). Its main pathological features include degradation of articular cartilage, thickening of subchondral bone, formation of osteophytes, synovial inflammation, degradation of knee ligament and meniscus as well as hypertrophy of joint capsule (Pountos and Giannoudis, 2017). The NF-κB pathway is a common intracellular signaling pathway for multiple inflammatory factors in chondrocytes, and acts as a sensor of oxidative stress, involving in abnormal cartilage metabolism and promoting the progression of OA (Lepetsos et al., 2019). Chondrocytes have weak proliferative ability and lack the ability to renew themselves. Inflammatory processes and the ensuing apoptosis of chondrocytes are crucial in the initiation and development of OA (Wojdasiewicz et al., 2014). Jia et al. (2017) found that treatment with 0.25–0.5 mg/kg tanshinone IIA for 28 days in an OA rat model with anterior cruciate ligament resection (ACLT) and medial meniscus (MMx) resulted in significant histopathological changes in knee cartilage in the ACLT + MMx group. Tanshinone IIA at 0.5 mg/kg significantly inhibited cartilage degradation, improved Mankin score in OA model rats, and inhibited inflammatory cell accumulation and intima synovium rupture induced by ACLT + MMX in rats. Tanshinone IIA at 0.25–0.5 mg/kg dose-dependently inhibited chondrocytes apoptosis. In the ACLT + MMx group, 0.5 mg/kg of tanshinone IIA significantly inhibited the expression of matrix metalloproteinase in articular cartilage, increased the expression of tissue metalloproteinase inhibitor, and significantly decreased IL-1β, TNF-α, and iNOS inflammatory cytokines in serum. Similarly, in the study of OA rabbit model established by ACLT, it was found that after treatment with salvia miltiorrhiza injection, the levels of glutamate glycoside peptide (GSH) in synovial and articular cartilage of rabbits were significantly increased than those in OA group, and malondialdehyde (MDA) in synovial and articular cartilage of rabbits were significantly decreased. It is suggested that salvia miltiorrhiza may prevent OA joint cartilage degeneration through anti-oxidative stress (Bai and Li, 2016). Xu et al. (2018) found that salvia miltiorrhiza mitigated the destruction of OA cartilage by regulating JAK2/STAT3 and AKT signaling pathways. Feng et al. (2017) found that cryptotanshinone could prevent cartilage deterioration and subchondral bone thickening in OA mice. Cryptotanshinone significantly inhibited the production of NO and PGE2 as well as the expression of COX-2, iNOS, MMP-3, MMP-13, and ADAMTS-5 induced by IL-1β in vitro. Additionally, cryptanshinone significantly inhibited the activation of NF-κB and MAPK stimulated by IL-1β, suggesting that the therapeutic effect of cryptanshinone on OA is based on the inhibition of NF-κB and MAPK signaling pathways. LPS can significantly induce inflammatory damage in mouse chondrogenic cells (ATDC5 cells), including suppressed cell viability, enhanced apoptosis, and increased expression of pro-inflammatory factors. Tanshinone IIA protects ATDC5 cells from LPS-induced inflammatory damage by down-regulating the expression of miR-203a and inhibiting JAK/STAT and JNK pathways (Luan and Liang, 2018). According to the study of Zhou et al. (2021), tanshinone IIA can inhibit LPS-induced chondrocyte inflammation and apoptosis by regulating the expression of miR-155 and FOXO3 signaling.
In conclusion, the active ingredients of SM have been shown to alleviate the symptoms of OA by inhibiting the apoptosis of chondrocytes and down-regulating the expression of inflammatory markers. The potential underlying mechanism of this effects may relate to the inhibition of NF-κB and MAPK signaling pathways (Table 5).
5 Effects and potential mechanism of SM and its active ingredients on osteonecrosis of the femoral head (ON-FH)
Osteonecrosis of the femoral head (ON-FH) is a refractory and disabling hip disease caused by reduced blood supply to the femoral head and impaired bone marrow cells (Zhang et al., 2016; Mont et al., 2020). In recent years, traditional Chinese medicine demonstrated significant advantages in the treatment of femoral head necrosis. Salvia miltiorrhiza has been widely used in clinical non-surgical treatment of early and middle stage femoral head necrosis, with therapeutic effect that can improve ON-FH symptoms in patients and delay disease progression (Wang et al., 2010).
Salvianolic acid B has been found to have a significant anti-ischemic injury function, which is closely related to its antioxidant, free radical scavenging, and neuroprotection properties. Li and Wang (2017) found that salvianolic acid B could improve histopathological scores and inhibit osteoclast differentiation in a rat model of steroid-induced ON-FH. Salvianolic acid B also inhibited the expression of peroxisome proliferator-activated receptor gamma (PPARγ) and AP2 protein, while increasing the content of osteocalcin and alkaline phosphatase in steroid-induced ON-FH rats, suggesting that Salvianolic acid B prevented steroid-induced femoral head necrosis by inhibiting the expression of PPARγ. Subsequent studies by Xu et al. (2021) established a steroid-induced femoral head necrosis model (SIONFH) by intramuscular injection of methyl prednisone. Osteonecrosis of the femoral head was significantly alleviated after intraperitoneal injection of tanshinone I. In addition, tanshinone I increased the activity of alkaline phosphatase and the expression of osteoblast markers such as osteocalcin, type I collagen and osteopontin, while decreasing osteoclast markers, such as cathepsin K and tartrate-resistant acid phosphatase. Tanshinone I also reduced inflammation and oxidative stress, and activated the nuclear factor-erythrocyte 2-associated factor-2 (Nrf2) signaling pathway in the femoral head of ON-FH model (Table 6).
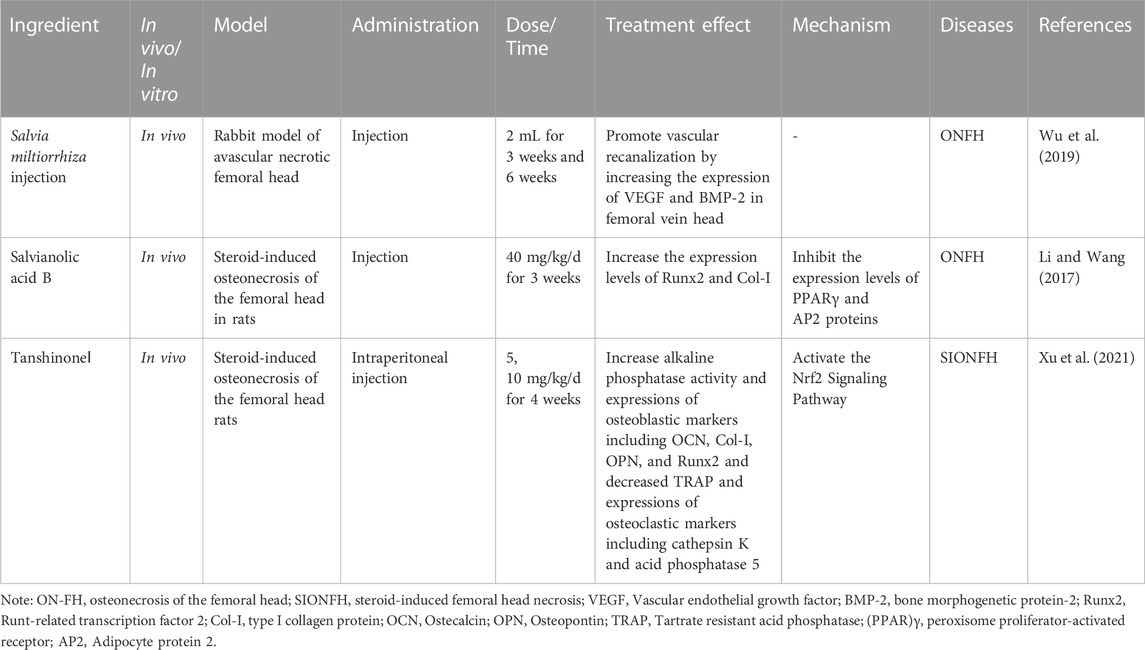
TABLE 6. Effects and mechanism of Salvia miltiorrhiza and its active ingredients on osteonecrosis of the femoral head.
6 Effects and potential mechanism of SM and its active ingredients on fracture healing
Fracture is one of the most common injuries worldwide, and its high incidence imposes a significant economic burden on society (Amin et al., 2014). Clinically, a fracture that has not healed completely for more than 3 months is referred to as “delayed healing,” while the absence of any healing signs after more than 9 months, it is considered “nonunion.” Studies have shown that approximately 5%–10% of fractures result in delayed or nonunion healing (Einhorn, 1998). Fracture healing is a complex pathophysiological process, typically divided into 4 phases of inflammatory response, cartilage formation, woven bone formation, and bone remodeling (Annamalai et al., 2018; Tian et al., 2019). SM had been used as one of the bioactive ingredients in the clinical fracture treatment of traditional Chinese medicine for many years. In a study of He and Shen (2014), salvianolic acid B was found to increase the activity of ALP and the secretion of osteocalcin in a time-and dose-dependent manner, thus accelerating the early fracture healing of tibia. In Liu’s study (Liu et al., 2018), tanshinol bone-targeted liposomes were formulated using pyrophosphorylated cholesterol as a bone targeting ligand for the treatment of delayed fracture. Local application of tanshinol bone-targeted liposomes to normal mouse fracture models and glucocorticoid-induced delayed fracture healing mice models significantly improved the formation and microstructure of fracture callus, accelerated the mineralization of the callus, shortened the fracture healing duration in mice, and significantly improved the biomechanical properties of the heal bone. Subsequent study on rabbit nonunion critical size defect model revealed that tanshinol bone-targeted liposomes incorporated with collagen sponge can significantly increase the expression of type II collagen, Runx2, VEGFA and osteocalcin in vivo, and stimulate nonunion healing by regulating histone deacetylase 3 (HDAC3) -mediated endochondral ossification (Zhou et al., 2020) (Table 7).
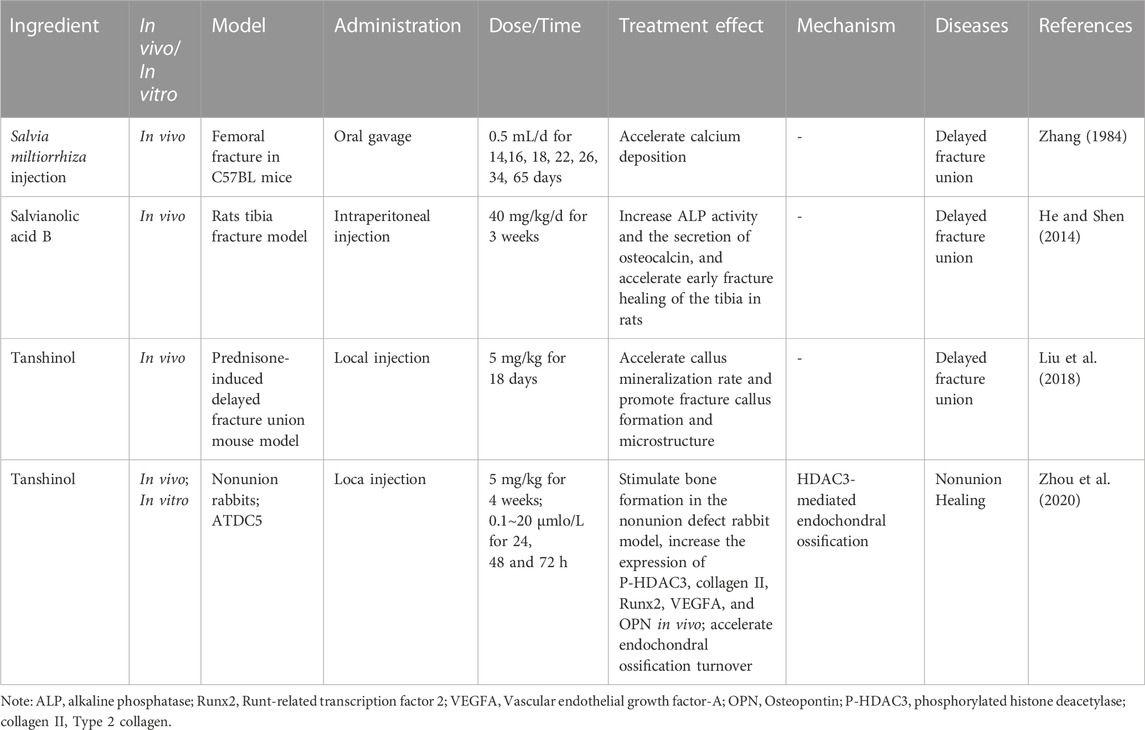
TABLE 7. Effects and mechanism of Salvia miltiorrhiza and its active ingredients on fracture healing.
7 Conclusion and perspectives
In this study, we conducted a comprehensive review of significant studies and demonstrated that the remarkable efficacy of SM and its active ingredients in treating MSD. Among the 38 publications collected for review, nearly half of the studies focus on osteoporosis (42.11%), followed by RA at 28.95%. Fracture repair and osteoarthritis studies account for 10.53% and 7.89%, respectively (Figure 3). It is important to highlight that the investigation of SM’s effects has expanded to cover a wide variety of MSD. Furthermore, the active ingredients found in SM show promising potential to be developed as new drugs for clinical treatment of MSD in the future.
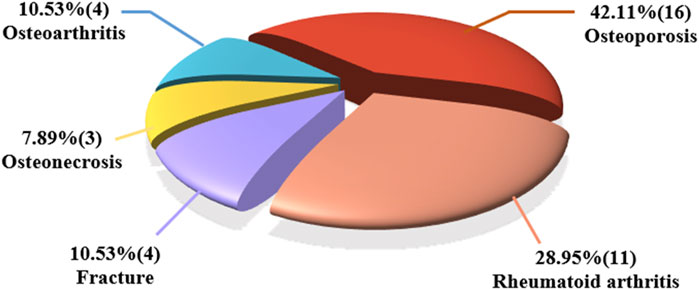
FIGURE 3. Proportion of the collected studies (38) related to Salvia miltiorrhiza and its active ingredients in the treatment of different types of MSD.
Based on the collected publications, it appears that one of the primary mechanisms underlying the therapeutic effects of SM in treating various skeletal disorders involves the inhibition of ROS signaling. ROS signaling is implicated in a wide range of cellular processes, including apoptosis, inflammation, and cellular differentiation. By targeting ROS signaling, SM may indirectly impact multiple cellular pathways, helping to mitigate the damaging effects of oxidative stress on bone cells and tissues. Inflammatory processes, often driven by ROS, are a common feature of many musculoskeletal diseases. SM’s ability to modulate inflammatory cytokines and pathways, as discussed in the text, may be linked to its impact on ROS signaling. By reducing ROS and oxidative stress, SM may simultaneously promote osteoblast function, inhibit osteoclastogenesis, and attenuate inflammatory processes. This multifaceted approach could enhance its overall therapeutic efficacy. Further research should focus on elucidating the precise mechanisms by which SM modulates ROS signaling in different cell types and tissues. Additionally, exploring potential crosstalk between ROS-related pathways and other signaling cascades will provide a more comprehensive understanding of SM’s therapeutic effects. Understanding the central role of ROS signaling in SM’s therapeutic effects has important clinical implications.
The biggest challenge for the clinical use of SM in treating MSDs lies in the need for rigorous scientific validation through well-designed clinical trials. While preclinical studies and experimental research have shown promising results regarding the efficacy of SM and its active ingredients in various MSDs, transitioning this knowledge to clinical practice still requires robust evidence of safety and effectiveness in human subjects. Rigorous clinical trials, dose optimization, mechanistic understanding, and adherence to regulatory standards are key steps in overcoming the challenges associated with the clinical use of SM for MSDs.
Author contributions
ZY: Data curation, Formal Analysis, Funding acquisition, Methodology, Project administration, Resources, Software, Visualization, Writing–original draft, Writing–review and editing. YuL: Conceptualization, Formal Analysis, Investigation, Supervision, Writing–original draft. JS: Data curation, Formal Analysis, Investigation, Methodology, Visualization, Writing–original draft. YG: Data curation, Formal Analysis, Investigation, Visualization, Writing–review and editing. HF: Formal Analysis, Investigation, Methodology, Visualization, Writing–review and editing. ZH: Formal Analysis, Investigation, Methodology, Writing–review and editing. MZ: Data curation, Methodology, Software, Validation, Writing–review and editing. WL: Investigation, Methodology, Validation, Writing–review and editing. LC: Conceptualization, Formal Analysis, Funding acquisition, Investigation, Project administration, Resources, Supervision, Validation, Writing–review and editing. YaL: Conceptualization, Data curation, Formal Analysis, Funding acquisition, Investigation, Methodology, Project administration, Supervision, Validation, Visualization, Writing–original draft, Writing–review and editing.
Funding
The author(s) declare financial support was received for the research, authorship, and/or publication of this article. This research was funded by grants from the National Natural Science Foundation of China (No. 81703584), The regional joint fund of natural science foundation of Guangdong province (No. 2020B1515120052), Guangdong Province Natural Science Foundation of China (No. 2022A1515220166, 2017A030310614, and 2021A1515010975), the Discipline Construction Fund of Central People’s Hospital of Zhanjiang (No. 2022A09), Discipline construction project of Guangdong Medical University (No. 4SG23002G, 4SG21156G, and CLP 2021B012), the Science and Technology Foundation of Zhanjiang (No. 2022A01099), Special Funds for Scientific Technological Innovation of Undergraduates in Guangdong Province (No. pdjh 2022a0214), Guangdong medical university research fund (No. FZZM05).
Conflict of interest
The authors declare that the research was conducted in the absence of any commercial or financial relationships that could be construed as a potential conflict of interest.
Publisher’s note
All claims expressed in this article are solely those of the authors and do not necessarily represent those of their affiliated organizations, or those of the publisher, the editors and the reviewers. Any product that may be evaluated in this article, or claim that may be made by its manufacturer, is not guaranteed or endorsed by the publisher.
Supplementary material
The Supplementary Material for this article can be found online at: https://www.frontiersin.org/articles/10.3389/fphar.2023.1276038/full#supplementary-material
References
Abdallah, D., Skafi, N., Hamade, E., Borel, M., Reibel, S., Vitale, N., et al. (2019). Effects of phospholipase D during cultured osteoblast mineralization and bone formation. J. Cell Biochem. 120, 5923–5935. doi:10.1002/jcb.27881
Adami, G., Fassio, A., Rossini, M., Caimmi, C., Giollo, A., Orsolini, G., et al. (2019). Osteoporosis in rheumatic diseases. Int. J. Mol. Sci. 20, 5867. doi:10.3390/ijms20235867
Amin, S., Achenbach, S. J., Atkinson, E. J., Khosla, S., and Melton, L. J. (2014). Trends in fracture incidence: a population-based study over 20 years. J. Bone Min. Res. 29, 581–589. doi:10.1002/jbmr.2072
Annamalai, R. T., Turner, P. A., Carson, W. F. T., Levi, B., Kunkel, S., and Stegemann, J. P. (2018). Harnessing macrophage-mediated degradation of gelatin microspheres for spatiotemporal control of BMP2 release. Biomaterials 161, 216–227. doi:10.1016/j.biomaterials.2018.01.040
Bai, B., and Li, Y. (2016). Danshen prevents articular cartilage degeneration via antioxidation in rabbits with osteoarthritis. Osteoarthr. Cartil. 24, 514–520. doi:10.1016/j.joca.2015.10.004
Biao, Y., Chen, J., Liu, C., Wang, R., Han, X., Li, L., et al. (2022). Protective effect of danshen zexie decoction against non-alcoholic fatty liver disease through inhibition of ROS/NLRP3/IL-1β pathway by Nrf2 signaling activation. Front. Pharmacol. 13, 877924. doi:10.3389/fphar.2022.877924
Blyth, F. M., Briggs, A. M., Schneider, C. H., Hoy, D. G., and March, L. M. (2019). The global burden of musculoskeletal pain-where to from here? Am. J. Public Health 109, 35–40. doi:10.2105/AJPH.2018.304747
Cai, M., Guo, Y., Wang, S., Wei, H., Sun, S., Zhao, G., et al. (2017). Tanshinone IIA elicits neuroprotective effect through activating the nuclear factor erythroid 2-related factor-dependent antioxidant response. Rejuvenation Res. 20, 286–297. doi:10.1089/rej.2016.1912
Chen, D., Shen, J., Zhao, W., Wang, T., Han, L., Hamilton, J. L., et al. (2017). Osteoarthritis: toward a comprehensive understanding of pathological mechanism. Bone Res. 5, 16044. doi:10.1038/boneres.2016.44
Chen, J., Hendriks, M., Chatzis, A., Ramasamy, S. K., and Kusumbe, A. P. (2020). Bone vasculature and bone marrow vascular niches in health and disease. J. Bone Min. Res. 35, 2103–2120. doi:10.1002/jbmr.4171
Chen, X., Guo, J., Bao, J., Lu, J., and Wang, Y. (2014). The anticancer properties of Salvia miltiorrhiza Bunge (Danshen): a systematic review. Med. Res. Rev. 34, 768–794. doi:10.1002/med.21304
Cieza, A., Causey, K., Kamenov, K., Hanson, S. W., Chatterji, S., and Vos, T. (2021). Global estimates of the need for rehabilitation based on the global burden of disease study 2019: a systematic analysis for the global burden of disease study 2019. Lancet 396, 2006–2017. doi:10.1016/S0140-6736(20)32340-0
Conigliaro, P., Triggianese, P., De Martino, E., Fonti, G. L., Chimenti, M. S., Sunzini, F., et al. (2019). Challenges in the treatment of rheumatoid arthritis. Autoimmun. Rev. 18, 706–713. doi:10.1016/j.autrev.2019.05.007
Corrado, A., Sanpaolo, E. R., Di Bello, S., and Cantatore, F. P. (2017). Osteoblast as a target of anti-osteoporotic treatment. Postgrad. Med. 129, 858–865. doi:10.1080/00325481.2017.1362312
Cui, L., Li, T., Liu, Y., Zhou, L., Li, P., Xu, B., et al. (2012). Salvianolic acid B prevents bone loss in prednisone-treated rats through stimulation of osteogenesis and bone marrow angiogenesis. PLoS One 7, e34647. doi:10.1371/journal.pone.0034647
Cui, L., Zhou, L., Liu, Y., Ai, C., Wu, T., and Wu, Y. (2004). Preventing cancellous bone loss in steroid-treated rats and stimulating bone formation by water extract of salvia miltiorrhixa and danshensu. Chin. Pharmacol. Bull., 286–291. doi:10.3321/j.issn:1001-1978.2004.03.013
Dong, X. L., Yu, W. X., Li, C. M., He, S., Zhou, L. P., Poon, C. W., et al. (2018). Danshen (Salvia miltiorrhiza) protects ovariectomized rats fed with high-saturated fat-sucrose diet from bone loss. Osteoporos. Int. 29, 223–235. doi:10.1007/s00198-017-4254-2
Du, H., Wang, Y., Zeng, Y., Huang, X., Liu, D., Ye, L., et al. (2020). Tanshinone IIA suppresses proliferation and inflammatory cytokine production of synovial fibroblasts from rheumatoid arthritis patients induced by TNF-alpha and attenuates the inflammatory response in AIA mice. Front. Pharmacol. 11, 568. doi:10.3389/fphar.2020.00568
Einhorn, T. A. (1998). The cell and molecular biology of fracture healing. Clin. Orthop. Relat. Res. S7-21, S7–S21. doi:10.1097/00003086-199810001-00003
Feng, Z., Zheng, W., Li, X., Lin, J., Xie, C., Li, H., et al. (2017). Cryptotanshinone protects against IL-1β-induced inflammation in human osteoarthritis chondrocytes and ameliorates the progression of osteoarthritis in mice. Int. Immunopharmacol. 50, 161–167. doi:10.1016/j.intimp.2017.06.017
Fraenkel, L., Bathon, J. M., England, B. R., St Clair, E. W., Arayssi, T., Carandang, K., et al. (2021). 2021 American college of rheumatology guideline for the treatment of rheumatoid arthritis. Arthritis Rheumatol. 73, 924–939. doi:10.1002/acr.24596
Gao, X., Wu, Q., Zhang, X., Tian, J., Liang, D., Min, Y., et al. (2021). Salvianolate ameliorates osteopenia and improves bone quality in prednisone-treated rheumatoid arthritis rats by regulating RANKL/RANK/OPG signaling. Front. Pharmacol. 12, 710169. doi:10.3389/fphar.2021.710169
Goettsch, C., Babelova, A., Trummer, O., Erben, R. G., Rauner, M., Rammelt, S., et al. (2013). NADPH oxidase 4 limits bone mass by promoting osteoclastogenesis. J. Clin. Invest. 123, 4731–4738. doi:10.1172/JCI67603
Grigoriadis, A. E., Wang, Z. Q., Cecchini, M. G., Hofstetter, W., Felix, R., Fleisch, H. A., et al. (1994). c-Fos: a key regulator of osteoclast-macrophage lineage determination and bone remodeling. Science 266, 443–448. doi:10.1126/science.7939685
Han, C., Jiang, Y. H., Li, W., and Liu, Y. (2021). Astragalus membranaceus and Salvia miltiorrhiza ameliorates cyclosporin A-induced chronic nephrotoxicity through the "gut-kidney axis. J. Ethnopharmacol. 269, 113768. doi:10.1016/j.jep.2020.113768
He, X., and Shen, Q. (2014). Salvianolic acid B promotes bone formation by increasing activity of alkaline phosphatase in a rat tibia fracture model: a pilot study. BMC Complement. Altern. Med. 14, 493. doi:10.1186/1472-6882-14-493
Hu, F., Koon, C. M., Chan, J. Y., Lau, K. M., and Fung, K. P. (2012). The cardioprotective effect of danshen and gegen decoction on rat hearts and cardiomyocytes with post-ischemia reperfusion injury. BMC Complement. Altern. Med. 12, 249. doi:10.1186/1472-6882-12-249
Huber, L. C., Distler, O., Tarner, I., Gay, R. E., Gay, S., and Pap, T. (2006). Synovial fibroblasts: key players in rheumatoid arthritis. Rheumatol. Oxf. 45, 669–675. doi:10.1093/rheumatology/kel065
Jia, P. T., Zhang, X. L., Zuo, H. N., Lu, X., and Li, L. (2017). Articular cartilage degradation is prevented by tanshinone IIA through inhibiting apoptosis and the expression of inflammatory cytokines. Mol. Med. Rep. 16, 6285–6289. doi:10.3892/mmr.2017.7340
Jiang, Y., Liu, C., Chen, W., Wang, H., Wang, C., and Lin, N. (2015). Tetramethylpyrazine enhances vascularization and prevents osteonecrosis in steroid-treated rats. Biomed. Res. Int. 2015, 315850. doi:10.1155/2015/315850
Jie, L., Du, H., Huang, Q., Wei, S., Huang, R., and Sun, W. (2014). Tanshinone IIA induces apoptosis in fibroblast-like synoviocytes in rheumatoid arthritis via blockade of the cell cycle in the G2/M phase and a mitochondrial pathway. Biol. Pharm. Bull. 37, 1366–1372. doi:10.1248/bpb.b14-00301
Kaneko, K., Shikano, K., Kawazoe, M., Kawai, S., and Nanki, T. (2022). Efficacy of Denosumab for osteoporosis in patients with rheumatic diseases. Intern Med. 61, 2405–2415. doi:10.2169/internalmedicine.8560-21
Kikuta, J., and Ishii, M. (2018). Bone imaging: osteoclast and osteoblast dynamics. Methods Mol. Biol. 1763, 1–9. doi:10.1007/978-1-4939-7762-8_1
Kwak, H. B., Yang, D., Ha, H., Lee, J. H., Kim, H. N., Woo, E. R., et al. (2006). Tanshinone IIA inhibits osteoclast differentiation through down-regulation of c-Fos and NFATc1. Exp. Mol. Med. 38, 256–264. doi:10.1038/emm.2006.31
Lai, W., Mo, Y., Wang, D., Zhong, Y., Lu, L., Wang, J., et al. (2021). Tanshinol alleviates microcirculation disturbance and impaired bone formation by attenuating TXNIP signaling in GIO rats. Front. Pharmacol. 12, 722175. doi:10.3389/fphar.2021.722175
Langdahl, B. L. (2021). Overview of treatment approaches to osteoporosis. Br. J. Pharmacol. 178, 1891–1906. doi:10.1111/bph.15024
Lee, D. H., Lim, B. S., Lee, Y. K., and Yang, H. C. (2006). Effects of hydrogen peroxide (H2O2) on alkaline phosphatase activity and matrix mineralization of odontoblast and osteoblast cell lines. Cell Biol. Toxicol. 22, 39–46. doi:10.1007/s10565-006-0018-z
Lee, S. Y., Choi, D. Y., and Woo, E. R. (2005). Inhibition of osteoclast differentiation by tanshinones from the root of Salvia miltiorrhiza bunge. Arch. Pharm. Res. 28, 909–913. doi:10.1007/BF02973876
Lefevre, S., Knedla, A., Tennie, C., Kampmann, A., Wunrau, C., Dinser, R., et al. (2009). Synovial fibroblasts spread rheumatoid arthritis to unaffected joints. Nat. Med. 15, 1414–1420. doi:10.1038/nm.2050
Lepetsos, P., Papavassiliou, K. A., and Papavassiliou, A. G. (2019). Redox and NF-κB signaling in osteoarthritis. Free Radic. Biol. Med. 132, 90–100. doi:10.1016/j.freeradbiomed.2018.09.025
Li, G., Liu, Y., Meng, F., Xia, Z., Wu, X., Fang, Y., et al. (2018). Tanshinone IIA promotes the apoptosis of fibroblast-like synoviocytes in rheumatoid arthritis by up-regulating lncRNA GAS5. Biosci. Rep. 38. doi:10.1042/BSR20180626
Li, J., He, C., Tong, W., Zou, Y., Li, D., Zhang, C., et al. (2015). Tanshinone IIA blocks dexamethasone-induced apoptosis in osteoblasts through inhibiting Nox4-derived ROS production. Int. J. Clin. Exp. Pathol. 8, 13695–13706.
Li, S., and Wang, J. (2017). Salvianolic acid B prevents steroid-induced osteonecrosis of the femoral head via PPARγ expression in rats. Exp. Ther. Med. 13, 651–656. doi:10.3892/etm.2016.4008
Li, Z., Wei, W., Zhang, M., Guo, X., Zhang, B., Wang, D., et al. (2023). Cryptotanshinone-doped photothermal synergistic MXene@PDA nanosheets with antibacterial and anti-inflammatory properties for wound healing. Adv. Healthc. Mater 12, e2301060. doi:10.1002/adhm.202301060
Lin, S., Cui, L., Chen, G., Huang, J., Yang, Y., Zou, K., et al. (2019). PLGA/β-TCP composite scaffold incorporating salvianolic acid B promotes bone fusion by angiogenesis and osteogenesis in a rat spinal fusion model. Biomaterials 196, 109–121. doi:10.1016/j.biomaterials.2018.04.004
Liu, C., He, Y., Xu, X., He, L., He, B., and Kong, L. (2020). The study of natural compounds targeting RANKL signaling pathway for the treatment of bone diseases. Curr. Drug Targets 21, 344–357. doi:10.2174/1389450120666190930145144
Liu, C. D., Liu, N. N., Zhang, S., Ma, G. D., Yang, H. G., Kong, L. L., et al. (2021a). Salvianolic acid A prevented cerebrovascular endothelial injury caused by acute ischemic stroke through inhibiting the Src signaling pathway. Acta Pharmacol. Sin. 42, 370–381. doi:10.1038/s41401-020-00568-2
Liu, Q. S., Luo, X. Y., Jiang, H., Xing, Y., Yang, M. H., Yuan, G. H., et al. (2015). Salvia miltiorrhiza injection restores apoptosis of fibroblast-like synoviocytes cultured with serum from patients with rheumatoid arthritis. Mol. Med. Rep. 11, 1476–1482. doi:10.3892/mmr.2014.2779
Liu, Q. S., Zhu, X. C., Li, J. A., Xing, Y., Jiang, H., Zhang, J., et al. (2013). Effects of danshen injection on the proliferation of rheumatoid arthritis fibroblast-like synoviocytes cultured with human serum. Zhongguo Zhong Xi Yi Jie He Za Zhi 33, 674–678. doi:10.7661/CJIM.2013.05.0674
Liu, Q. Y., Zhuang, Y., Song, X. R., Niu, Q., Sun, Q. S., Li, X. N., et al. (2021b). Tanshinone IIA prevents LPS-induced inflammatory responses in mice via inactivation of succinate dehydrogenase in macrophages. Acta Pharmacol. Sin. 42, 987–997. doi:10.1038/s41401-020-00535-x
Liu, Y., Jia, Z., Akhter, M. P., Gao, X., Wang, X., Wang, X., et al. (2018). Bone-targeting liposome formulation of Salvianic acid A accelerates the healing of delayed fracture Union in Mice. Nanomedicine 14, 2271–2282. doi:10.1016/j.nano.2018.07.011
Luan, L., and Liang, Z. (2018). Tanshinone IIA protects murine chondrogenic ATDC5 cells from lipopolysaccharide-induced inflammatory injury by down-regulating microRNA-203a. Biomed. Pharmacother. 103, 628–636. doi:10.1016/j.biopha.2018.04.051
Lukert, B. P., and Raisz, L. G. (1990). Glucocorticoid-induced osteoporosis: pathogenesis and management. Ann. Intern Med. 112, 352–364. doi:10.7326/0003-4819-112-5-352
Luo, S., Yang, Y., Chen, J., Zhong, Z., Huang, H., Zhang, J., et al. (2016). Tanshinol stimulates bone formation and attenuates dexamethasone-induced inhibition of osteogenesis in larval zebrafish. J. Orthop. Transl. 4, 35–45. doi:10.1016/j.jot.2015.07.002
Martel-Pelletier, J., Barr, A. J., Cicuttini, F. M., Conaghan, P. G., Cooper, C., Goldring, M. B., et al. (2016). Osteoarthritis. Nat. Rev. Dis. Prim. 2, 16072. doi:10.1038/nrdp.2016.72
Meng, D., Li, J., Li, H., and Wang, K. (2019). Salvianolic acid B remits LPS-induced injury by up-regulating miR-142-3p in MH7A cells. Biomed. Pharmacother. 115, 108876. doi:10.1016/j.biopha.2019.108876
Molagoda, I. M. N., Karunarathne, W., Choi, Y. H., Park, E. K., Jeon, Y. J., Lee, B. J., et al. (2019). Fermented oyster extract promotes osteoblast differentiation by activating the wnt/β-catenin signaling pathway, leading to bone formation. Biomolecules 9, 711. doi:10.3390/biom9110711
Mont, M. A., Salem, H. S., Piuzzi, N. S., Goodman, S. B., and Jones, L. C. (2020). Nontraumatic osteonecrosis of the femoral head: where do we stand today? a 5-year update. J. Bone Jt. Surg. Am. 102, 1084–1099. doi:10.2106/JBJS.19.01271
Noth, U., Steinert, A. F., and Tuan, R. S. (2008). Technology insight: adult mesenchymal stem cells for osteoarthritis therapy. Nat. Clin. Pract. Rheumatol. 4, 371–380. doi:10.1038/ncprheum0816
Nygaard, G., and Firestein, G. S. (2020). Restoring synovial homeostasis in rheumatoid arthritis by targeting fibroblast-like synoviocytes. Nat. Rev. Rheumatol. 16, 316–333. doi:10.1038/s41584-020-0413-5
Park, J. H., Lee, N. K., and Lee, S. Y. (2017). Current understanding of RANK signaling in osteoclast differentiation and maturation. Mol. Cells 40, 706–713. doi:10.14348/molcells.2017.0225
Pittenger, M. F., Mackay, A. M., Beck, S. C., Jaiswal, R. K., Douglas, R., Mosca, J. D., et al. (1999). Multilineage potential of adult human mesenchymal stem cells. Science 284, 143–147. doi:10.1126/science.284.5411.143
Pountos, I., and Giannoudis, P. V. (2017). Modulation of cartilage's response to injury: can chondrocyte apoptosis be reversed? Injury 48, 2657–2669. doi:10.1016/j.injury.2017.11.032
Pufe, T., Scholz-Ahrens, K. E., Franke, A. T., Petersen, W., Mentlein, R., Varoga, D., et al. (2003). The role of vascular endothelial growth factor in glucocorticoid-induced bone loss: evaluation in a minipig model. Bone 33, 869–876. doi:10.1016/j.bone.2003.08.002
Qian, K., Xu, H., Dai, T., and Shi, K. (2015). Effects of Tanshinone IIA on osteogenic differentiation of mouse bone marrow mesenchymal stem cells. Naunyn Schmiedeb. Arch. Pharmacol. 388, 1201–1209. doi:10.1007/s00210-015-1154-x
Qiao, J., Liu, A., Liu, J., Guan, D., and Chen, T. (2019). Salvianolic acid B (Sal B) alleviates the decreased activity induced by prednisolone acetate on osteoblasts by up-regulation of bone formation and differentiation genes. Food Funct. 10, 6184–6192. doi:10.1039/c9fo01246j
Qu, Y., Liu, X., Zong, S., Sun, H., Liu, S., and Zhao, Y. (2021). Protocatechualdehyde inhibits the osteoclast differentiation of RAW264.7 and BMM cells by regulating NF-κB and MAPK activity. Biomed. Res. Int. 2021, 6108999. doi:10.1155/2021/6108999
Safiri, S., Kolahi, A. A., Cross, M., Hill, C., Smith, E., Carson-Chahhoud, K., et al. (2021). Prevalence, deaths, and disability-adjusted life years due to musculoskeletal disorders for 195 countries and territories 1990-2017. Arthritis Rheumatol. 73, 702–714. doi:10.1002/art.41571
Tian, L., Tang, N., Ngai, T., Wu, C., Ruan, Y., Huang, L., et al. (2019). Hybrid fracture fixation systems developed for orthopaedic applications: a general review. J. Orthop. Transl. 16, 1–13. doi:10.1016/j.jot.2018.06.006
Uttra, A. M., Alamgeer, , Shahzad, M., Shabbir, A., Jahan, S., Bukhari, I. A., et al. (2019). Ribes orientale: a novel therapeutic approach targeting rheumatoid arthritis with reference to pro-inflammatory cytokines, inflammatory enzymes and anti-inflammatory cytokines. J. Ethnopharmacol. 237, 92–107. doi:10.1016/j.jep.2019.03.019
Van't Hof, R. J., and Ralston, S. H. (2001). Nitric oxide and bone. Immunology 103, 255–261. doi:10.1046/j.1365-2567.2001.01261.x
Wang, C. X., Shen, J. G., Jiang, T., Wu, J. M., Dong, L. Q., and Wang, W. D. (2010). Effective analysis of continous perfusion with Dandshen and heparin for treatment of femoral head necrosis. Zhongguo Gu Shang 23, 383–385. doi:10.3969/j.issn.1003-0034.2010.05.021
Wang, L., Cheng, L., Zhang, B., Wang, N., and Wang, F. (2019a). Tanshinone prevents alveolar bone loss in ovariectomized osteoporosis rats by up-regulating phosphoglycerate dehydrogenase. Toxicol. Appl. Pharmacol. 376, 9–16. doi:10.1016/j.taap.2019.05.014
Wang, W., Huang, M., Hui, Y., Yuan, P., Guo, X., and Wang, K. (2019b). Cryptotanshinone inhibits RANKL-induced osteoclastogenesis by regulating ERK and NF-κB signaling pathways. J. Cell Biochem. 120, 7333–7340. doi:10.1002/jcb.28008
Wang, X., Morris-Natschke, S. L., and Lee, K. H. (2007). New developments in the chemistry and biology of the bioactive constituents of Tanshen. Med. Res. Rev. 27, 133–148. doi:10.1002/med.20077
Wang, Y., Wang, S., Li, Y., Jiang, J., Zhou, C., Li, C., et al. (2015). Therapeutic effect of Cryptotanshinone on collagen-induced arthritis in rats via inhibiting nuclear factor kappa B signaling pathway. Transl. Res. 165, 704–716. doi:10.1016/j.trsl.2014.12.004
Wang, Z., Li, J., Zhang, J., and Xie, X. (2019c). Sodium tanshinone IIA sulfonate inhibits proliferation, migration, invasion and inflammation in rheumatoid arthritis fibroblast-like synoviocytes. Int. Immunopharmacol. 73, 370–378. doi:10.1016/j.intimp.2019.05.023
Watanabe, K., Hayashi, H., and Mori, Y. (1993). Effect of a benzylidene derivative, a novel antirheumatic agent, on IL-1 production. Pharmacol. Res. 28, 59–72. doi:10.1006/phrs.1993.1110
Wei, B., Sun, C., Wan, H., Shou, Q., Han, B., Sheng, M., et al. (2023). Bioactive components and molecular mechanisms of Salvia miltiorrhiza Bunge in promoting blood circulation to remove blood stasis. J. Ethnopharmacol. 317, 116697. doi:10.1016/j.jep.2023.116697
Williams, A., Kamper, S. J., Wiggers, J. H., O'brien, K. M., Lee, H., Wolfenden, L., et al. (2018). Musculoskeletal conditions may increase the risk of chronic disease: a systematic review and meta-analysis of cohort studies. BMC Med. 16, 167. doi:10.1186/s12916-018-1151-2
Wojdasiewicz, P., Poniatowski, L. A., and Szukiewicz, D. (2014). The role of inflammatory and anti-inflammatory cytokines in the pathogenesis of osteoarthritis. Mediat. Inflamm. 2014, 561459. doi:10.1155/2014/561459
World Health Organization (2022). Musculoskeletal health. Available at: https://www.who.int/news-room/fact-sheets/detail/musculoskeletal-conditions (Accessed November 21, 2019).
Wu, M., Chen, G., and Li, Y. P. (2016). TGF-beta and BMP signaling in osteoblast, skeletal development, and bone formation, homeostasis and disease. Bone Res. 4, 16009. doi:10.1038/boneres.2016.9
Wu, Y., Zhang, C., Wu, J., Han, Y., and Wu, C. (2019). Angiogenesis and bone regeneration by mesenchymal stem cell transplantation with danshen in a rabbit model of avascular necrotic femoral head. Exp. Ther. Med. 18, 163–171. doi:10.3892/etm.2019.7556
Xia, Z. B., Yuan, Y. J., Zhang, Q. H., Li, H., Dai, J. L., and Min, J. K. (2018). Salvianolic acid B suppresses inflammatory mediator levels by downregulating NF-κB in a rat model of rheumatoid arthritis. Med. Sci. Monit. 24, 2524–2532. doi:10.12659/msm.907084
Xu, X., Lv, H., Li, X., Su, H., Zhang, X., and Yang, J. (2018). Danshen attenuates cartilage injuries in osteoarthritis in vivo and in vitro by activating JAK2/STAT3 and AKT pathways. Exp. Anim. 67, 127–137. doi:10.1538/expanim.17-0062
Xu, X., Shen, Y., Lv, H., Zhao, J., Li, X., Gao, L., et al. (2021). Tanshinone I mitigates steroid-induced osteonecrosis of the femoral head and activates the Nrf2 signaling pathway in rats. Evid. Based Complement. Altern. Med. 2021, 8002161. doi:10.1155/2021/8002161
Yang, F. F., Gao, Y. H., Xi, H. R., Li, W. Y., Ma, H. P., and Chen, K. M. (2018). Effect of compound medicine of tanshinone 2A and resveratrol on peak bone mass in growing rats. Zhongguo Yi Xue Ke Xue Yuan Xue Bao 40, 456–462. doi:10.3881/j.issn.1000-503X.9912
Yang, Y., Su, Y., Wang, D., Chen, Y., Wu, T., Li, G., et al. (2013). Tanshinol attenuates the deleterious effects of oxidative stress on osteoblastic differentiation via Wnt/FoxO3a signaling. Oxid. Med. Cell Longev. 2013, 351895. doi:10.1155/2013/351895
Yin, Y., Duan, J., Guo, C., Wei, G., Wang, Y., Guan, Y., et al. (2017). Danshensu accelerates angiogenesis after myocardial infarction in rats and promotes the functions of endothelial progenitor cells through SDF-1α/CXCR4 axis. Eur. J. Pharmacol. 814, 274–282. doi:10.1016/j.ejphar.2017.08.035
Zhai, K. F., Duan, H., Luo, L., Cao, W. G., Han, F. K., Shan, L. L., et al. (2017). Protective effects of paeonol on inflammatory response in IL-1β-induced human fibroblast-like synoviocytes and rheumatoid arthritis progression via modulating NF-κB pathway. Inflammopharmacology 25, 523–532. doi:10.1007/s10787-017-0385-5
Zhang, J. Y. (1984). Effect of Salvia miltiorrhiza root on calcium deposition in experimental fracture healing. Zhong Xi Yi Jie He Za Zhi 4, 536–539.
Zhang, S., Huang, G., Yuan, K., Zhu, Q., Sheng, H., Yu, R., et al. (2017a). Tanshinone IIA ameliorates chronic arthritis in mice by modulating neutrophil activities. Clin. Exp. Immunol. 190, 29–39. doi:10.1111/cei.12993
Zhang, X., Zou, L., Li, J., Xu, B., Wu, T., Fan, H., et al. (2017b). Salvianolic acid B and danshensu induce osteogenic differentiation of rat bone marrow stromal stem cells by upregulating the nitric oxide pathway. Exp. Ther. Med. 14, 2779–2788. doi:10.3892/etm.2017.4914
Zhang, Y. L., Chen, S., Ai, Z. S., Gao, Y. S., Mei, J., and Zhang, C. Q. (2016). Osteonecrosis of the femoral head, nonunion and potential risk factors in Pauwels grade-3 femoral neck fractures: a retrospective cohort study. Med. Baltim. 95, e3706. doi:10.1097/MD.0000000000003706
Zhao, W., Feng, H., Guo, S., Han, Y., and Chen, X. (2017). Danshenol A inhibits TNF-alpha-induced expression of intercellular adhesion molecule-1 (ICAM-1) mediated by NOX4 in endothelial cells. Sci. Rep. 7, 12953. doi:10.1038/s41598-017-13072-1
Zhou, B., Li, L. H., Tan, L. M., Luo, W. B., Xiong, H., Lu, X. L., et al. (2021). Tanshinone IIA ameliorates inflammation response in osteoarthritis via inhibition of miR-155/FOXO3 Axis. Pharmacology 106, 20–28. doi:10.1159/000505493
Zhou, L., Wu, H., Gao, X., Zheng, X., Chen, H., Li, H., et al. (2020). Bone-targeting liposome-encapsulated salvianic acid A improves nonunion healing through the regulation of HDAC3-mediated endochondral ossification. Drug Des. Devel Ther. 14, 3519–3533. doi:10.2147/DDDT.S263787
Zhou, L., Zuo, Z., and Chow, M. S. (2005). Danshen: an overview of its chemistry, pharmacology, pharmacokinetics, and clinical use. J. Clin. Pharmacol. 45, 1345–1359. doi:10.1177/0091270005282630
Zhu, P. C., Shen, J., Qian, R. Y., Xu, J., Liu, C., Hu, W. M., et al. (2023). Effect of tanshinone IIA for myocardial ischemia/reperfusion injury in animal model: preclinical evidence and possible mechanisms. Front. Pharmacol. 14, 1165212. doi:10.3389/fphar.2023.1165212
Glossary
Keywords: bone, joint, osteoarthritis, osteoporosis, Salvia miltiorrhiza, musculoskeletal diseases
Citation: Ye Z, Liu Y, Song J, Gao Y, Fang H, Hu Z, Zhang M, Liao W, Cui L and Liu Y (2023) Expanding the therapeutic potential of Salvia miltiorrhiza: a review of its pharmacological applications in musculoskeletal diseases. Front. Pharmacol. 14:1276038. doi: 10.3389/fphar.2023.1276038
Received: 11 August 2023; Accepted: 20 November 2023;
Published: 05 December 2023.
Edited by:
Guoju Hong, Guangzhou University of Chinese Medicine, ChinaReviewed by:
Jia Qing Yun, Linyi People’s Hospital, ChinaZiyi Wang, Johns Hopkins University, United States
Copyright © 2023 Ye, Liu, Song, Gao, Fang, Hu, Zhang, Liao, Cui and Liu. This is an open-access article distributed under the terms of the Creative Commons Attribution License (CC BY). The use, distribution or reproduction in other forums is permitted, provided the original author(s) and the copyright owner(s) are credited and that the original publication in this journal is cited, in accordance with accepted academic practice. No use, distribution or reproduction is permitted which does not comply with these terms.
*Correspondence: Liao Cui, cuiliao@163.com; Yanzhi Liu, liuyanzhi02@163.com
†These authors have contributed equally to this work