- 1Endocrine and Metabolic Diseases Hospital of Shandong First Medical University, Shandong First Medical University and Shandong Academy of Medical Sciences, Jinan, China
- 2Shandong First Medical University and Shandong Academy of Medical Sciences, Jinan, China
Insulin resistance presents a formidable public health challenge that is intricately linked to the onset and progression of various chronic ailments, including diabetes, cardiovascular disease, hypertension, metabolic syndrome, nonalcoholic fatty liver disease, and cancer. Effectively addressing insulin resistance is paramount in preventing and managing these metabolic disorders. Natural herbal remedies show promise in combating insulin resistance, with anthraquinone extracts garnering attention for their role in enhancing insulin sensitivity and treating diabetes. Anthraquinones are believed to ameliorate insulin resistance through diverse pathways, encompassing activation of the AMP-activated protein kinase (AMPK) signaling pathway, restoration of insulin signal transduction, attenuation of inflammatory pathways, and modulation of gut microbiota. This comprehensive review aims to consolidate the potential anthraquinone compounds that exert beneficial effects on insulin resistance, elucidating the underlying mechanisms responsible for their therapeutic impact. The evidence discussed in this review points toward the potential utilization of anthraquinones as a promising therapeutic strategy to combat insulin resistance and its associated metabolic diseases.
1 Introduction
Insulin resistance is characterized by a persistent loss of insulin sensitivity and is a prevalent risk factor contributing to obesity, hypertension, cardiovascular diseases, and type 2 diabetes (James et al., 2021; Wang et al., 2022; Sasaki et al., 2022). Additionally, insulin resistance increases the susceptibility to heart failure and fuels tumor growth, posing a substantial threat to human health and imposing a considerable economic burden on society and families. Notably, its prevalence is on the rise, reaching 20%–40% among young populations in developing countries (Artunc et al., 2016). Consequently, the implementation of effective strategies to ameliorate insulin resistance has become indispensable.
While no medication specifically targets insulin resistance, several antidiabetic drugs, including insulin sensitizers, insulin secretagogues, and alpha-glucosidase inhibitors, have been utilized to improve insulin resistance. However, these treatments often have some adverse effects and limitations. For instance, insulin sensitizers may lead to heart failure (Arnold et al., 2019) and weight gain (Dutta et al., 2023), insulin secretagogues may cause excessive insulin secretion and damage to pancreatic beta cells (Rustenbeck et al., 2004), and alpha-glucosidase inhibitors may result in diarrhea and gastrointestinal discomfort (Taylor et al., 2019).
Recently, a plethora of studies have indicated that natural products possessing mild pharmaceutical properties can significantly augment insulin sensitivity, suggesting that natural products may be a new strategy for the treatment of insulin resistance (Zhang et al., 2020; Zhang et al., 2020; Wang et al., 2022; Zhou et al., 2022). These findings underscore the considerable potential of natural products as promising alternatives to conventional treatments for metabolic disorders. Notably, a substantial proportion of these natural products known for their efficacy in combating obesity and ameliorating insulin resistance are rich in anthraquinones (Kumar et al., 2019). For instance, Cassiae semen (Ko et al., 2020), Rheum palmatum L. (Cui et al., 2019) and Aloe vera (Deora et al., 2021), all of which boast anthraquinones, are prominent examples of natural products that are widely employed for the amelioration of metabolic diseases. Anthraquinones, distinguished by their tricyclic diketone pharmacophoric structure (Alam et al., 2019) (Figure 1), constitute a class of plant secondary metabolites. Several studies have substantiated the capacity of anthraquinones to enhance insulin resistance, thereby signifying their promising candidacy as pharmacological agents for mitigating insulin resistance and associated metabolic disorders.
To date, no comprehensive review has been conducted to explore the mechanisms by which anthraquinones ameliorate insulin resistance. This review endeavors to bridge this knowledge gap by providing a systematic assessment of identified anthraquinones and elucidating their respective mechanisms for improving insulin resistance.
2 Characteristics of anthraquinones
Anthraquinones (9,10-dioxoanthracenes) are plant secondary metabolites containing a tricyclic dione pharmacophore structure. The anthraquinone ring is the fundamental parent structure of anthraquinones. Anthraquinone monomer refers to chemical compounds containing a single anthraquinone ring, whereas two monomeric anthraquinone units can undergo dehydration and condensation reactions via two distinct pathways to form dimeric anthraquinones (Malik and Müller, 2016). Some studies have revealed that anthraquinones can be substituted with various functional groups, including hydroxyl, alkyl, alkoxy, and sugar units. The specific type, number, and position of substituents on the parent nucleus are critical determinants of natural product bioactivity in this chemical class. For instance, rhein, which has a carboxylic acid group at the sixth substitution position, exhibits significantly greater lipid-lowering activity than aloe-emodin, which has a hydroxyl substitution at the same position (Fang et al., 2022).
Anthraquinones are commonly found in higher plants, such as Polygonaceae, Fabaceae, Rhamnaceae, Rubiaceae, and Liliaceae, either in the form of free anthraquinones or anthraquinone glycosides. Additionally, they are also found in the metabolites of lichens and fungi (Li and Jiang, 2018). It is now well established that anthraquinones exhibit a wide range of biological activities, such as anticancer (Zhang et al., 2021), anti-inflammatory (Xie et al., 2022), antibacterial (Qi et al., 2022), anti-oxidant (Yin et al., 2022), and antiviral effects (Dai et al., 2017). They have also been shown to have great potential in the prevention and treatment of various diseases, including cancer and diabetes.
Emodin, rhein, chrysophanol, aloe-emodin, and physcion are among the most common and representative anthraquinones found in traditional Chinese medicine. These compounds have been identified as the major bioactive components of R. palmatum L., Polygonum multiflorum, Cassiae semen, Aloe vera, and Senna (Khurm et al., 2020; Ma et al., 2022). Research has demonstrated the potential of these natural products to improve insulin resistance, making them promising agents for obesity prevention and treatment. Rumex dentatus L. is a natural medicinal plant rich in anthraquinones, including emodin. The study demonstrated its significant potential in reducing homeostatic model assessment of insulin resistance (HOMA-IR) and improving insulin resistance in diabetic rats (Elsayed et al., 2020). Aloe vera is rich in anthraquinones, including aloe-emodin and aloin. Some studies indicate that these bioactive molecules possess the potential to regulate pancreatic β cell function, suppress fat accumulation, and lower fasting blood glucose (FBG) levels, thus offering an effective therapeutic approach for alleviating obesity (Deora et al., 2021; Fu et al., 2022). Administration of Aloe vera extract in obese mice significantly reduced fasting blood glucose levels, improved glucose tolerance, mitigated adipose tissue inflammation, and subsequently ameliorated insulin resistance (Shin et al., 2011; An et al., 2021). A randomized controlled trial with obese individuals demonstrated that Aloe vera extracts significantly reduced body weight and HOMA-IR (Zhang et al., 2016). Anthraquinones are also widely distributed among other traditional herbals. Rheum palmatum L. is an herbal medicine rich in anthraquinones, including emodin, rhein, and chrysophanol, that has exhibited significant potential in attenuating adipose tissue inflammation and hepatic accumulation of triglycerides in mice. These findings suggest that R. palmatum L. may be a potential preventive and therapeutic strategy for obesity (Régnier et al., 2020). Oral administration of R. palmatum L. extracts significantly inhibited ectopic fat accumulation and was shown to improve insulin resistance in obese rats (Yang et al., 2016). Senna, a natural medicine rich in the anthraquinone-derived natural product sennoside A, has been shown to improve the oxidative stress response and alleviate the inflammatory reaction of adipose tissue, resulting in weight loss in rats (Nayan et al., 2021). Additionally, Cassiae semen, a natural medicine rich in anthraquinone-derived natural products such as aurantio-obtusin and alaternin, has been shown to lower FBG and insulin levels and enhance glucose uptake in skeletal muscle in obese mice, thus restoring insulin sensitivity (Wang et al., 2019). Additionally, we have summarized more than ten anthraquinones that can improve insulin resistance through various mechanisms (Table 1).
3 Mechanism of insulin resistance improvement by anthraquinones
3.1 Anthraquinones improve insulin resistance by attenuating impaired insulin signaling pathways
Upon engagement with its receptor, insulin sets in motion a myriad of intricate signaling cascades. Insulin resistance manifests when this finely tuned pathway falters, impeding the profound physiological effects of insulin. Remarkably, anthraquinones have demonstrated the ability to alleviate insulin resistance through diverse mechanisms. These include the inhibition of protein tyrosine phosphatase 1B (PTP1B) activity and facilitation of glucose transporter type 4 (GLUT4) expression and translocation, thereby potentially restoring the proper conduction of the insulin signaling pathway (Figure 2).
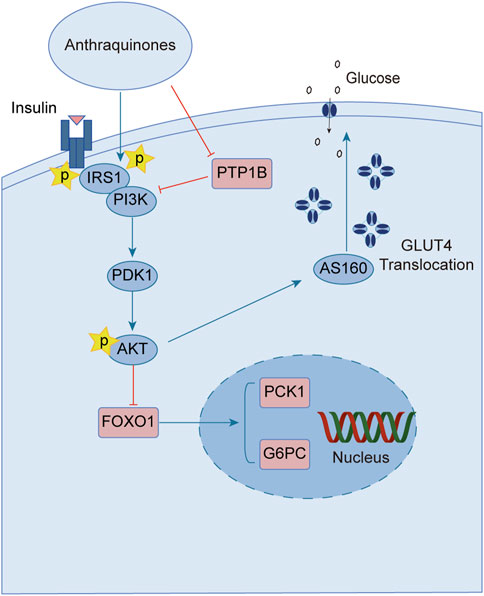
FIGURE 2. Anthraquinones exert a positive influence on insulin resistance by facilitating the transduction of insulin signaling. These bioactive compounds possess the capability to inhibit the activity of PTP-1B and enhance the expression of PI3K, thereby reinstating the effective conduction of the proximal insulin signaling pathway. Moreover, they elevate the levels of AKT phosphorylation, suppress the expression of FOXO1, and attenuate gluconeogenesis. Furthermore, they promote the expression and translocation of GLUT4, consequently augmenting cellular glucose uptake and fortifying the distal signaling pathways associated with insulin signaling.
Insulin binding to its receptor initiates a wide range of signaling cascades that ultimately lead to the uptake and utilization of glucose in insulin target tissues, including skeletal muscles, adipose tissue, and liver. PTP-1B, as a downstream regulatory factor, is a crucial negative regulator of insulin signal transduction. Overexpression of PTP-1B in adipose tissue can lead to dephosphorylation of insulin receptors and inhibit insulin signaling (Venable et al., 2000). Conversely, PTP-1B−/- mice show enhanced glucose tolerance and increased systemic insulin sensitivity (Elchebly et al., 1999; Behl et al., 2022), indicating that PTP-1B may serve as a potential therapeutic target for improving insulin resistance. Several studies indicate that anthraquinone compounds could inhibit the activity of PTP1B. Studies have demonstrated that (trans)-emodin-physcion bianthrone and (cis)-emodin-physcion bianthrone isolated from Polygonum cuspidatum show potent inhibitory effects against PTP-1B, with corresponding IC50 values of 2.77 and 7.29 μM, respectively (Zhao et al., 2017). Furthermore, enzyme kinetic analysis revealed that alaternin extracted from Cassiae semen could competitively inhibit PTP-1B activity, with a corresponding inhibition constant (Ki) value of 1.70 μM (Jung et al., 2016). Additionally, molecular docking simulations indicated that the interaction between alaternin and PTP-1B was primarily driven by hydrogen bonding and hydrophobic interactions. A study unveiled the steadfast binding of chrysophanol and emodin to the allosteric site of PTP-1B, shedding light on their intricate association. This site acts as a metastable inhibitor and inactivates the enzyme by blocking the mobility of the catalytic ring of the enzyme (Martínez-Aldino et al., 2021). Anthraquinones have been widely studied, and many of these natural products exhibit inhibitory effects against PTP-1B. Currently, most relevant studies have employed enzyme kinetic analysis or molecular docking simulations to explore their underlying mechanisms. Nevertheless, the cellular and animal realms remain relatively uncharted territories in this domain. Consequently, additional endeavors are imperative to unravel the labyrinthine interplay between anthraquinones and PTP-1B, unmasking their manifold effects across diverse model systems.
GLUT4 is a protein that facilitates the translocation of glucose across cell membranes and is primarily expressed in adipose and muscle tissues. In these tissues, insulin resistance is associated with impaired insulin-dependent translocation of GLUT4, resulting in decreased glucose uptake (Leto and Saltiel, 2012; Zumbaugh et al., 2022). Aloe-emodin-8-O-β-D-glucoside, derived from Cassiae semen, exerts its influence by fostering the cellular uptake of glucose through the activation of the phosphatidylinositol pathway and the upregulation of GLUT4 expression (Anand et al., 2010). Upon subjecting insulin-resistant 3T3-L1 cells to the therapeutic influence of emodin, a marked enhancement in cellular glucose uptake was observed. Notably, this effect was found to be partially attenuated by wortmannin, an inhibitor of phosphatidylinositol 3-kinase (PI3K), thereby implicating the PI3K pathway as a crucial route through which emodin stimulates the facilitation of glucose uptake (Yang et al., 2007). The anthraquinone extracts derived from Cassiae semen elicited a substantial elevation in the phosphorylation states of Akt substrate of 160 kDa (AS160), Akt, and PI3K within the skeletal muscle of diabetic rats. This intricate cascade of events fosters the activation of the PI3K-AKT-AS160 signaling pathway, facilitating the translocation of GLUT4 and concomitantly resulting in a commendable reduction in FBG levels as well as fasting serum insulin (FSI) concentrations (Zhang et al., 2018). Aurantio-obtusin propels the activation of the important PI3K-AKT signaling pathway in both hepatic and adipose tissues. This remarkable orchestration induces a discernible reduction in fasting blood glucose levels while elevating glucose tolerance (Guo et al., 2021). Alizarin exerts a profound influence, effectively lowering fasting and postprandial blood glucose levels in diabetic mice. This versatile compound orchestrates a cascade of molecular events, stimulating the phosphorylation of insulin receptor substrate-1 (IRS-1) and Akt proteins while simultaneously enhancing the expression levels of GLUT4. Collectively, these molecular phenomena synergistically contribute to the amelioration of insulin resistance in mice afflicted with diabetes (Xu et al., 2019).
3.2 Anthraquinones improve insulin resistance by activating the AMPK signaling pathway
The AMPK signaling pathway plays a pivotal role in governing energy metabolism and upholding metabolic equilibrium. Robust evidence supports that AMPK activation enhances insulin sensitivity, promotes glucose uptake, and augments fatty acid oxidation across adipocytes, hepatocytes, and myocytes. Consequently, harnessing the power of AMPK activation has emerged as a potent therapeutic strategy for combating insulin resistance and type 2 diabetes. Notably, several investigations have revealed the ability of anthraquinones, including emodin, aloe emodin, and rhein, to activate the AMPK pathway. These compounds achieve this by increasing the expression and phosphorylation of vital upstream kinases of AMPK, such as protein kinase A (PKA), Ca2+/calmodulin-dependent protein kinase kinases (CaMKKs), and adiponectin. Furthermore, these remarkable agents demonstrate the capacity to ameliorate insulin resistance by orchestrating the AMPK pathway, thereby impeding lipid and cholesterol synthesis, enhancing fatty acid oxidation, and fostering glucose uptake (Figure 3).
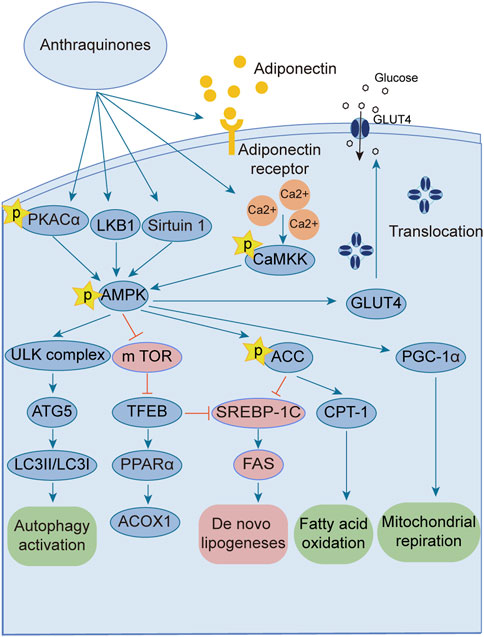
FIGURE 3. Anthraquinones activate the AMPK signaling pathway through various intricate pathways and mechanisms. This activation subsequently enhances lipid metabolism, culminating in a reduction in lipid synthesis and an increase in fatty acid oxidation. Ultimately, these profound effects contribute to the amelioration of insulin resistance.
The AMPK signaling pathway has emerged as a captivating avenue for the prevention and amelioration of insulin resistance (Towler and Hardie, 2007; Lin and Hardie, 2018; Zhang et al., 2022). Positioned as a pivotal kinase regulating energy homeostasis (Diniz et al., 2021), AMPK receives activation signals from an array of influential upstream regulators, including liver kinase B1 (LKB1) (Zhang et al., 2013), CaMKKs (Mungai et al., 2011), transforming growth factor beta-activated kinase1 (TAK1) (Momcilovic et al., 2006), PKA (Hurtado de Llera et al., 2014), and adiponectin (Iwabu et al., 2010; Li et al., 2020). Anthraquinones, including hypericin, danthrone, rhein, and emodin, exhibit the remarkable capacity to augment the expression of key kinases situated upstream of the AMPK signaling pathway, thereby promoting its activation. Through this activation, anthraquinones effectively curtail lipid synthesis, amplify fatty acid oxidation, enhance glucose uptake, and consequently alleviate insulin resistance. Hypericin has garnered attention as a potent agonist of PKA catalytic subunit alpha (PKACα), manifesting its capability to directly bind to PKACα. This direct engagement, in turn, sets forth a cascade of events that activate the PKA/AMPK signaling pathway, thereby effectively impeding the detrimental accumulation of ectopic lipids within the hepatic milieu (Liang et al., 2020). Danthron stimulates the AMPK signaling pathway by augmenting the heterodimerization of retinoid X receptor-alpha (RXRα) and peroxisome proliferator-activated receptor alpha (PPARα) with the adipoR2 promoter (Ma et al., 2021). Rhein exhibits the capacity to increase both the expression and phosphorylation of AMPK protein, thereby effectively activating the AMPK pathway (Lu et al., 2022). Within the composition of R. palmatum L., emodin has been validated as an activator of the AMPK signaling pathway. Its mode of action involves the facilitation of adiponectin expression and the mitigation of oxygen consumption in insulin-resistant C2C12 and 3T3-L1 cells (Chen et al., 2012; Zhang et al., 2015). Moreover, emodin elicits the activation of CaMKK2, a crucial upstream kinase, through the augmentation of intracellular Ca2+ concentration within L6 myotubular cells. This consequential event subsequently triggers the activation of the AMPK signaling pathway (Song et al., 2013).
Excessive energy availability promotes heightened flux of free fatty acids (FFAs) and aberrant lipid deposition, thereby contributing to insulin resistance (Nguyen et al., 2005; Jiao et al., 2011). The AMPK signaling pathway assumes a pivotal role in governing lipid metabolism and preventing undue lipid accumulation. Activation of AMPK effectively curtails the activity of acetyl-CoA carboxylase (ACC), alleviating its inhibitory influence on carnitine palmitoyltransferase 1 (CPT-1) and potentiating fatty acid oxidation (Monsénégo et al., 2012; Sheng et al., 2019). Moreover, AMPK activation downregulates the expression of sterol regulatory element-binding protein-1c (SREBP-1c) (Li et al., 2011) and CCAAT enhancer-binding protein alpha (C/EBPα) (Kawaguchi et al., 2002), transcription factors that orchestrate lipogenic gene expression, thereby diminishing lipid synthesis. Therefore, harnessing the AMPK signaling pathway represents an efficacious strategy to counter insulin resistance, as it heightens fat oxidation, suppresses lipogenesis, and fosters lipid homeostasis. Emodin, a naturally occurring compound found in medicinal plants, has demonstrated the capacity to augment fatty acid oxidation through the activation of the AMPK pathway in rats subjected to a high-fat diet (HFD). Its activation of AMPK leads to the upregulation of CPT-1 expression, concomitant with the downregulation of SREBP-1c and fatty acid synthase (FAS) expression, effectively inhibiting lipogenesis and curtailing lipid accumulation. As a result, emodin exerts a beneficial effect on insulin resistance (Tzeng et al., 2012b). Furthermore, intravenous administration of emodin has been observed to stimulate AMPK and ACC phosphorylation in skeletal muscle and liver tissue of HFD-fed mice, leading to reduced fasting blood glucose and fasting insulin levels, as well as improved insulin sensitivity (Song et al., 2013). Chrysophanic acid effectively attenuated weight gain in mice with diet-induced obesity. It also mitigated lipid accumulation and downregulated the expression of adipogenesis-associated factors, such as peroxisome proliferator-activated receptor gamma (PPARγ) and C/EBPα, in 3T3-L1 adipocytes (Lim et al., 2016). In a dose-dependent manner, danthrone exhibited a remarkable capacity to induce the phosphorylation of AMPK and ACC in both HepG2 and C2C12 cells. Furthermore, danthron treatment demonstrated significant efficacy in suppressing lipid synthesis by downregulating the expression of SREBP1c and FAS, thereby leading to reduced levels of total cholesterol (TC) and triglycerides (TGs). Intriguingly, the effects of danthrone on lipid and glucose metabolism were attenuated or reversed when coadministered with the AMPK inhibitor compound C (Zhou et al., 2013). Furthermore, aurantio-obtusin has been shown to induce the phosphorylation of transcription factor EB (TFEB) and bolster autophagic flux within hepatocytes by eliciting AMPK activation. This consequential activation subsequently upregulates the expression of PPARα and acyl-CoA oxidase 1 (ACOX1), thereby stimulating the oxidation of fatty acids. Concurrently, it inhibits the expression of SREBP-1 and FAS, thus culminating in a reduction in lipid synthesis and a decline in the accumulation of lipids in nonadipose tissues (Zhou et al., 2021). TFEB serves as a crucial regulator of autophagy and lysosomal function. Remarkably, TFEB overexpression has been demonstrated to effectively impede weight gain, curtail lipid accumulation, and ameliorate insulin resistance in mouse models of diet-induced obesity (Settembre et al., 2013). The addition of the Physcion supplement increased energy expenditure, contributing to improvements in plasma lipids, adipokines, cytokines, and fecal lipids. Notably, there was a reduction in hepatic FFA synthesis and an increase in FFA oxidation. A significant decrease in lipid synthesis was observed, while lipolysis and oxidation were enhanced.
Activation of the AMPK signaling pathway upholds energy homeostasis by efficiently dissipating surplus energy as heat (Yang et al., 2021). White adipose tissue (WAT) assumes the role of an energy reservoir, storing excess energy in the form of fat. Its accumulation has been closely associated with metabolic disorders (Maqdasy et al., 2022). Conversely, brown adipose tissue (BAT) expends energy through thermogenesis, exerting an inverse relationship with blood glucose levels, insulin resistance, and obesity (Anhê et al., 2019; Xu et al., 2020; Sugimoto et al., 2022; Villarroya and Gavaldà-Navarro, 2022). Certain stimuli, such as cold exposure, exercise, or specific hormonal cues, can instigate a process known as “browning” in WAT (Liu et al., 2022). The process of WAT browning entails the activation of uncoupling protein 1 (UCP-1), a pivotal factor associated with prompt and adaptive thermogenesis (Fedorenko et al., 2012). This process is governed by the transcriptional coactivator peroxisome proliferator-activated receptor-γ co-activator-1α (PGC-1α), which exerts crucial regulatory control over UCP-1 expression and thermogenesis in BAT (Boström et al., 2012). Using primary cultured brown adipocytes as in vitro models and HFD-induced obese mice as in vivo models, the administration of chrysophanol yielded a notable reduction in weight gain among obese mice. Furthermore, chrysophanol treatment substantially upregulated the expression of UCP1 and PGC1α. Interestingly, in brown adipocytes, the coadministration of Compound C, an inhibitor of AMPK, effectively nullified the impact of chrysophanol on AMPKα, thereby indicating the partial involvement of the AMPKα pathway in chrysophanol efficacy (Lim et al., 2016). Sirtuin 6 (SIRT6) is a multifunctional enzyme with ADP-ribosyltransferase and histone deacetylase activities that orchestrates the recruitment of phosphorylated transcription factor 2 (ATF2), leading to enhanced expression of PGC-1α (Yao et al., 2017). The upregulated expression of SIRT6 increases the phosphorylation of AMPK, thereby ameliorating insulin resistance (Luo et al., 2018; Fan et al., 2019). Chrysophanol administration in mice with HFD-induced obesity substantially elevates SIRT6 and UCP-1 expression within WAT. This finding was further supported by metabolic cage data, elucidating the augmentation of thermogenesis. Importantly, these effects were not observed in Sirt6-deficient mice (Sirt6−/−), underscoring the pivotal role of SIRT6 in mediating the impact of chrysophanol. Collectively, these discoveries highlight the therapeutic potential of chrysophanol in combating obesity and related metabolic disorders by virtue of its ability to upregulate SIRT6, subsequently promoting the upregulation of PGC-1α and UCP-1 in BAT (Liu et al., 2020). Aurantio-obtusin (AO) is a bioactive compound found in Cassiae semen that is a Chinese traditional medicinal herb. It demonstrated remarkable efficacy in enhancing hepatic lipid metabolism in a mouse model of hepatic steatosis. The administration of AO significantly increased mitochondrial metabolism and upregulated UCP1 expression. This effect was achieved through the activation of PPARα signaling, both in vivo and in primary brown adipocytes (Yi-Jie et al., 2023).
3.3 Anthraquinones ameliorate insulin resistance by inhibiting inflammatory pathways
Emerging research has illuminated the pivotal contribution of low-grade chronic inflammation in the development and advancement of insulin resistance. Notably, anthraquinones have demonstrated their potential in mitigating insulin resistance linked to inflammation. These compounds exhibit the ability to ameliorate adipocyte inflammatory infiltration, suppress the secretion of diverse inflammatory factors by adipocytes, induce macrophage M2 polarization, and enhance overall systemic inflammation regulation (Figure 4).
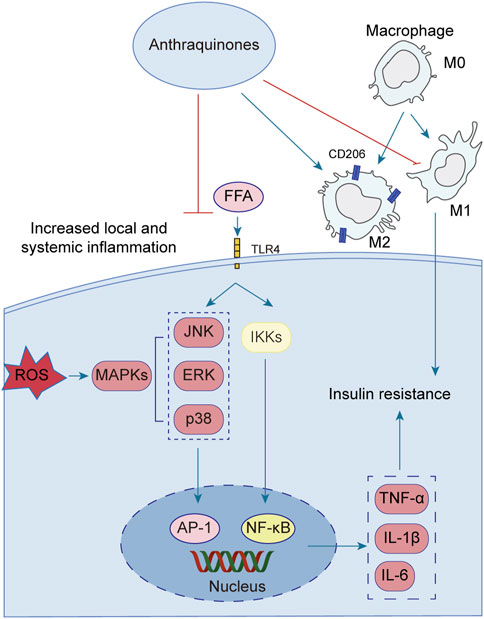
FIGURE 4. Low-grade chronic systemic inflammation is intricately linked to insulin resistance. Anthraquinones exert a direct inhibitory effect on the MAPK and NF-κB pathways, resulting in the suppression of pro-inflammatory cytokines such as TNF-α, IL-1β, IL-6, and IL-8. Moreover, anthraquinones effectively reduce ROS levels and foster the polarization of M2 macrophages. By mitigating low-grade chronic systemic inflammation through these mechanisms, anthraquinones contribute to the improvement of insulin resistance.
A growing body of evidence highlights the intimate association between low-grade chronic systemic inflammation and insulin resistance (Ahmed et al., 2021). The elevation of FFA triggers the activation of Toll-like receptor 4 (TLR4), initiating a cascade of signaling events involving key regulators such as nuclear factor kappaB (NF-κB) and c-Jun N-terminal kinase (JNK) (Wang et al., 2017; Li et al., 2020; Zhang et al., 2023). Activation of the NF-κB and JNK pathways increases the secretion of various inflammatory mediators, including tumor necrosis factor-alpha (TNF-α), interleukin-1beta (IL-1β), and interleukin-6 (IL-6) (Glass and Olefsky, 2012; Jiang et al., 2022; Park et al., 2022). These pro-inflammatory factors perpetuate systemic inflammation, thus contributing to the persistence of insulin resistance. Several anthraquinones have emerged as potential therapeutics for reducing pro-inflammatory mediators and improving insulin resistance. For instance, chrysophanol demonstrated efficacy by downregulating the expression of TNF-α, IL-1β, IL-6, and IL-8 in mice subjected to a HFD (Lian et al., 2017). Similarly, rhein showcased its beneficial effects by attenuating weight gain, lipid accumulation, and IL-6, IL-1β, and TNF-α levels in adipose tissue and serum of rats with HFD-induced obesity (Ji and Gu, 2021). Molecular docking studies further revealed that rhein effectively bound to TNF-α, IL-6, and NF-κB, with binding energies of −8.9, −7.1, and −7.6 kcal/mol, respectively, suggesting its potential as a modulator of the TNF signaling pathway (Jiang et al., 2022). Additionally, rhein exhibited inhibitory effects on mitogen-activated protein kinase (MAPK) signaling in macrophages, leading to reduced transcription of the proinflammatory mediators TNF-α and IL-1β (Chang et al., 2019). Aloe-emodin demonstrated its capability to diminish TNF-α and IL-6 production while suppressing the NF-κB pathway, thereby restoring insulin signaling and ameliorating insulin resistance (Dou et al., 2019; Quan et al., 2019). Furthermore, emodin was shown to inhibit the expression of adipokines, including TNF-α, IL-1β, and IL-6, in adipocytes, potentially through the inhibition of p38, extracellular-signal-regulated kinase (ERK), and JNK phosphorylation (Fang et al., 2022). In a dose-dependent manner, physcion exhibited the capacity to attenuate the gene expression levels of TNF-α, IL-6, and IL-1β in a hepatocellular carcinoma cell line (HepG2) upon induction of inflammation by lipopolysaccharide (LPS) (Selim et al., 2019). Aloe-emodin significantly attenuated the production of nitric oxide (NO), IL-6, and IL-1β in LPS-stimulated RAW264.7 cells. Western blot analysis revealed that aloe-emodin suppressed the LPS-induced expression of iNOS protein, degradation of IκBα, and phosphorylation of ERK, p38, JNK, and Akt. These findings elucidate the anti-inflammatory properties of aloe-emodin, which likely involve the attenuation of proinflammatory cytokine production in LPS-induced RAW264.7 macrophages through the inhibition of the NF-κB, MAPK, and PI3K signaling pathways (Hu et al., 2014). Furthermore, the nitrogen-containing derivatives of aloe-emodin demonstrated superior efficacy in inhibiting nitric oxide, with an IC50 value of 3.15 μM. Furthermore, these derivatives exhibited a significant reduction in the levels of the pro-inflammatory cytokines TNF-α, IL-1β, and IL-6, as well as iNOS and COX-2 enzymes (Shang et al., 2022).
Macrophages can be classified into two distinct subtypes based on their activation state: M1-polarized macrophages and M2-polarized macrophages. In the context of obesity, macrophages tend to favor the M1 phenotype, exacerbating adipose tissue inflammation and contributing to systemic insulin resistance (Li et al., 2018). Conversely, M2 polarization is associated with anti-inflammatory effects, lower body weight, and enhanced insulin sensitivity (Chen et al., 2019). In vitro and in vivo experiments have demonstrated the inhibitory effects of emodin on the p65-NF-κB complex, along with its ability to enhance the prevalence of M2 (anti-inflammatory)-like phenotype macrophages (Chen et al., 2022). Rhein possesses the ability to shift macrophages toward the M2 phenotype. This was achieved through the downregulation of the M1 marker inducible nitric oxide synthase (iNOS) in mouse colon tissue and the upregulation of CD206, Arg1, IL-10, and ChIL3, which are indicative of M2 macrophage activation (Zhou et al., 2022). The triggering receptor expressed on myeloid cell 2 (TREM2), a member of the immunoglobulin receptor superfamily, has been found to enhance the production of anti-inflammatory cytokines and the expression of M2 marker genes when overexpressed (Korvatska et al., 2020). Upregulation of TREM2 has been demonstrated to mitigate insulin resistance induced by obesity (Carrasco et al., 2019). In mice with HFD-induced obesity, emodin effectively induced the polarization of M2 macrophages through the upregulation of TREM2 expression. This intervention notably alleviated local and systemic inflammation, curbed weight gain and lipid accumulation, reduced fasting glucose and fasting insulin levels, and improved insulin sensitivity (Yu et al., 2021). Rhein exhibited notable efficacy in reducing tissue inflammation and facilitating the transition of macrophages toward an M2 polarization state in an LPS-induced model. In vitro experiments demonstrated that rhein effectively mitigated intracellular ROS levels, suppressed the activation of P65, and thereby hindered macrophage polarization toward an M1 phenotype. Mechanistically, the protective effects of rhein were attributed to its modulation of the nuclear factor of activated T cells c1 (NFATc1)/TREM2 axis, as evidenced by the substantial attenuation observed in blocking experiments targeting both TREM2 and NFATc1 (Li et al., 2023).
The interplay between oxidative stress and inflammation can mutually aggravate insulin resistance. Reactive oxygen species (ROS), such as superoxide dismutase (SOD) and malondialdehyde (MDA), can stimulate the production of inflammatory factors, while cellular inflammatory factors, in turn, promote the generation of free radicals (Kwak et al., 2017). Oxidative stress poses detrimental effects on pancreatic beta cell function, leading to apoptosis and exacerbating insulin resistance (Costes et al., 2006). Notably, aloe-emodin has demonstrated the ability to reduce ROS levels in RIN-5F cells exposed to high glucose conditions, thus safeguarding these cells (Alshatwi and Subash-Babu, 2016). Physcion, a bioactive compound derived from rhubarb, exhibits notable properties, such as antihypertensive, antibacterial, and antitumor activities. Remarkably, physcion demonstrated the capacity to reduce body weight and plasma TG levels in rats subjected to a HFD. Palmitic acid increased the levels of ROS and MDA and reduced the levels of NO, SOD and GSH-Px. These trends were reversed by physcion. In addition, physcion reversed PA-induced activation of the NF-κB/TNF-α pathway in HUVECs (Wang et al., 2023). The pancreatic and duodenal homeobox-1 (PDX1) protein plays a pivotal role in pancreatic development, maturation, and the functioning of β cells (McKinnon and Docherty, 2001). In the context of glycotoxicity and lipotoxicity, oxidative stress further hinders PDX1 expression, resulting in β cell dysfunction and apoptosis (Hong et al., 2012). Conversely, hypericin enhances PDX1 expression through ERK activation in mice subjected to high-fat and high-glucose diets, thereby ameliorating glucose intolerance and insulin resistance. This intervention also leads to reduced fasting blood glucose levels, attenuation of islet-β cell apoptosis, and inhibition of nitric oxide (NO) production induced by glucotoxicity and lipotoxicity (Liang et al., 2019). Furthermore, in vivo experimentation revealed that emodin impeded the manifestation of TNF-α, IL-6, and MDA within both the circulating serum and tissues while concurrently augmenting the concentrations of SOD and GSH (Shang et al., 2021). Rhein exhibited a potent inhibitory effect on LPS-induced intestinal inflammation and oxidative stress. This was evidenced by a significant reduction in serum and intestinal levels of TNF-α, IL-1β, IL-6, and nitric oxide. Additionally, it downregulated MDA levels in the small intestine. Remarkably, rhein also inhibited the phosphorylation of JNK and p38 MAPK while activating the nuclear factor E2-related factor 2 (Nrf2) pathway (Zhuang et al., 2019).
3.4 Anthraquinones mitigate insulin resistance by regulating the intestinal microbiota
Emerging studies have unveiled the close interconnection between metabolic disorders and the perturbations observed in the composition and functionality of the intestinal microbiota (Ussar et al., 2015; Zeng et al., 2020). Manipulating the gut microbiota has emerged as a promising therapeutic strategy to enhance insulin sensitivity in the host (Chambers et al., 2019; Naderpoor et al., 2019). Anthraquinones have been demonstrated to effectively modulate gut dysbiosis by promoting the proliferation of beneficial bacteria while concurrently suppressing the abundance of potentially pathogenic counterparts. Notably, these compounds have been shown to enhance insulin resistance through the preservation of gut mucosal integrity and the reduction in metabolic endotoxemia. As such, anthraquinones present a promising approach for restoring the gut microbiota (Figure 5).
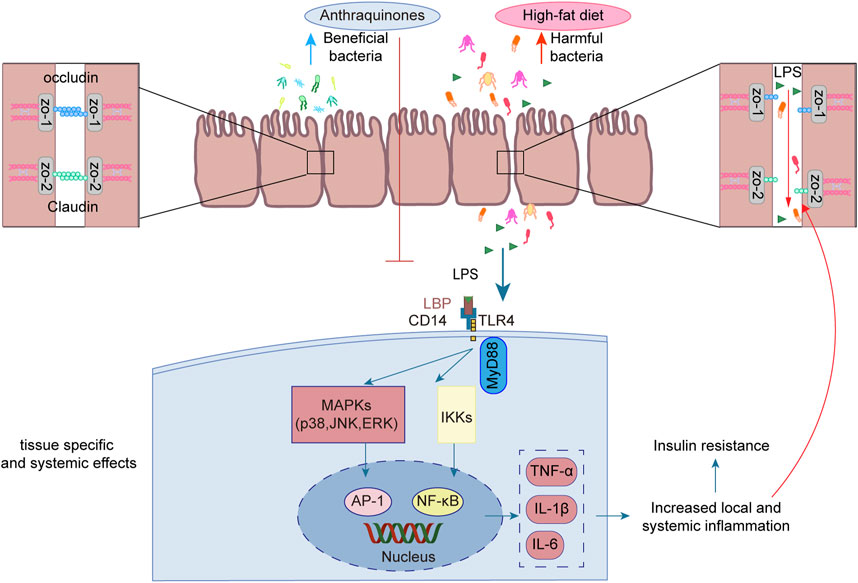
FIGURE 5. Anthraquinones efficiently attenuated the elevation in plasma LPS levels induced by a HFD while simultaneously dampening LPS-induced inflammatory responses in intestinal epithelial cells. Furthermore, these compounds demonstrated the ability to safeguard the continuity and integrity of colonic enterocytes through the upregulation of crucial tight junction proteins, including occludin, claudin, and ZO-1.
LPS, also referred to as endotoxins, is a sizable compound present in the outer membrane of Gram-negative bacteria (Simpson and Trent, 2019). Following the destruction of Gram-negative bacteria, the breakdown of their cell walls results in the release of LPS into the surrounding milieu (Tulkens et al., 2020). High-energy diets have been demonstrated to elicit heightened plasma LPS levels in murine experiments, as well as in a substantial cohort of healthy men drawn from a population-based sample (Amar et al., 2008). The translocation of LPS across the gut lining serves as a hallmark associated with insulin resistance, obesity, and diabetes. The leakage of LPS into the bloodstream initiates a state of low-grade inflammation, exerting profound effects on the liver, adipose tissue, and muscle metabolism (Cani et al., 2008). Long-term subcutaneous infusion of LPS in mice results in various alterations, including elevated weight gain, insulin resistance, WAT inflammation, heightened systemic LPS levels, and increased intestinal permeability (Cani et al., 2007). The regulation of tight junction permeability plays a pivotal role in maintaining the integrity of the intestinal barrier. Disruption of these tight junctions results in compromised barrier function, leading to “leakage” and subsequently causing an elevation in intestinal permeability (Ma et al., 2004). Additionally, an increase in LPS leads to its binding with TLR4, consequently activating the TLR4 signaling pathway. This signaling cascade involves downstream regulators such as NF-κB and JNK (Płóciennikowska et al., 2015). Rhein demonstrated a salutary influence by promoting body weight reduction and enhancing glucose tolerance in mice with diet-induced obesity. Moreover, it efficiently attenuated the surge in plasma LPS levels induced by a HFD while concurrently mitigating the accumulation of proinflammatory macrophages within the colon (Wang et al., 2016). Another investigation demonstrated the ameliorative effects of rhein on LPS-induced intestinal barrier injury, achieved through the modulation of Nrf2 and MAPK signaling pathways (Zhuang et al., 2019). Rhein restores the expression of claudin-1, E-cadherin, and mucus secretion to reduce intestinal permeability in chronic mouse colitis model induced by dextran sulfate sodium (Wu et al., 2020). Emodin demonstrated the capacity to suppress LPS-induced inflammatory responses in intestinal epithelial cells while simultaneously enhancing intestinal barrier function through the upregulation of ZO-1 and occludin expression (Zhang et al., 2020). Aurantio-obtusin exerts a favorable influence on intestinal barrier function by upregulating the expression of occludin and ZO-1 in HFD-fed mice. Moreover, it reduces serum LPS levels and attenuates the production of inflammatory mediators (Luo et al., 2021). Sennoside A was observed to safeguard the continuity and integrity of colonic enterocytes in mice with diet-induced obesity by upregulating the expression of tight junction proteins, namely, occludin, claudin-2, and ZO-1. This mechanism effectively restores colonic barrier function (Ma et al., 2020).
In murine models, it has been demonstrated that the consumption of a HFD induces notable modifications in the composition of the gut microbiota (Rabot et al., 2016; Tan et al., 2021). This perturbation is characterized by a decline in the abundance of beneficial bacteria, such as Bifidobacterium (Yang et al., 2022) and Lactobacillus (Lee et al., 2021), coupled with an elevation in the prevalence of potentially pathogenic microbes, including Bilophila wadsworthia (Natividad et al., 2018) and Ruminococcus gnavus (Grahnemo et al., 2022). The intervention of anthraquinones of Cassiae semen effectively increased Bacteroides, Lactobacillus, and Parabacteroides in HFD-fed rats (Mei et al., 2015). Another investigation demonstrated a significant abundance of Lactobacillus after rhein treatment (Wu et al., 2020). The modulation of gut microbiota contributed to the amelioration of metabolic syndrome in mice subjected to a HFD (Shi et al., 2019; Wang et al., 2020). In a double-blind randomized placebo-controlled pilot trial utilizing oral fecal microbiota transplantation (FMT) capsules, patients receiving FMT exhibited enduring alterations in their microbiomes linked to obesity, converging toward those characteristic of the lean donor (p < 0.001) (Allegretti et al., 2020). An increase in the abundance of potentially advantageous bacteria may ameliorate insulin resistance. Akkermansia muciniphila has been identified as a key regulator of energy metabolism, glucose tolerance, and the maturation and functionality of the immune system in human individuals (Yoon et al., 2021). Furthermore, the absence of A. muciniphila has been implicated in the disruption of gut barrier integrity, exerting consequential effects on other bacterial populations, ultimately precipitating the development of insulin resistance (Yang et al., 2019). A study demonstrated that aurantio-obtusin significantly augments the abundance of Akkermansia winderi in mice fed a HFD (Luo et al., 2021). Rhein effectively restrained the elevated plasma LPS levels induced by a HFD and modulated the gut microbiota by reducing Bacteroides-Prevotella spp. and Desulfovibrio spp. DNA while simultaneously increasing Bifidobacterium spp. and Lactobacillus spp. DNA (Wang et al., 2016).
4 Conclusion and future perspectives
In recent years, metabolic disorders have emerged as a pressing global health concern. Projections suggest that obesity will affect approximately one billion individuals by 2030, and diabetes cases will escalate to 783 million by 2045, posing significant challenges to healthcare systems worldwide. Numerous studies have indicated that anthraquinones hold promise in improving insulin resistance, a pivotal factor in preventing and treating various diseases, including diabetes, obesity, and other metabolic syndromes. Thus, this systematic review aims to comprehensively elucidate the mechanisms underlying the potential of anthraquinones in ameliorating insulin resistance, thereby fostering a deeper understanding of their therapeutic applications.
While numerous anthraquinones exhibit potential in ameliorating insulin resistance, the current research predominantly focuses on key compounds such as emodin, chrysophanol, rhein, and aloe-emodin. The precise mechanisms by which these compounds improve insulin resistance warrant further investigation. Moreover, existing studies primarily utilize animal models, cell culture models, or enzymatic methods to explore the potential of anthraquinone natural products in insulin resistance improvement. Therefore, well-designed, multicenter trials with large sample sizes are imperative to evaluate the effects of anthraquinones in human subjects with insulin resistance. It is hoped that upon integrating anthraquinones as treatment options for insulin resistance, they will prove to be both safer and more efficacious, offering innovative approaches to addressing metabolic disorders.
Overall, the potential of anthraquinones to improve insulin resistance through multiple pathways makes them a promising candidate for the treatment of insulin resistance and related metabolic disorders.
Author contributions
WX: Investigation, Writing–original draft. SL: Writing–original draft, Writing–review and editing. LL: Writing–review and editing. SZ: Writing–review and editing. XW: Writing–review and editing. WD: Writing–review and editing. LD: Writing–review and editing. XZ: Conceptualization, Supervision, Writing–review and editing. ZW: Conceptualization, Supervision, Writing–review and editing.
Funding
The author(s) declare financial support was received for the research, authorship, and/or publication of this article. This work was supported by the Shandong Province “Double-Hundred Talent Plan” on 100 Foreign Experts and 100 Foreign Expert Teams Introduction (WST2018004), the Natural Science Foundation of Shandong Province (ZR2023MH091), and the Innovation Project of Shandong Academy of Medical Sciences (2022).
Conflict of interest
The authors declare that the research was conducted in the absence of any commercial or financial relationships that could be construed as a potential conflict of interest.
Publisher’s note
All claims expressed in this article are solely those of the authors and do not necessarily represent those of their affiliated organizations, or those of the publisher, the editors and the reviewers. Any product that may be evaluated in this article, or claim that may be made by its manufacturer, is not guaranteed or endorsed by the publisher.
References
Ahmed, B., Sultana, R., and Greene, M. W. (2021). Adipose tissue and insulin resistance in obese. Biomed. Pharmacother. 137, 111315. doi:10.1016/j.biopha.2021.111315
Alam, M. B., Bajpai, V. K., Ra, J.-S., Lim, J.-Y., An, H., Shukla, S., et al. (2019). Anthraquinone-type inhibitor of α-glucosidase enhances glucose uptake by activating an insulin-like signaling pathway in C2C12 myotubes. Food Chem. Toxicol. 129, 337–343. doi:10.1016/j.fct.2019.05.005
Allegretti, J. R., Kassam, Z., Mullish, B. H., Chiang, A., Carrellas, M., Hurtado, J., et al. (2020). Effects of fecal microbiota transplantation with oral capsules in obese patients. Clin. Gastroenterol. Hepatol. 18, 855–863. doi:10.1016/j.cgh.2019.07.006
Alshatwi, A. A., and Subash-Babu, P. (2016). Aloe-emodin protects RIN-5F (pancreatic β-cell) cell from glucotoxicity via regulation of pro-inflammatory cytokine and downregulation of bax and caspase 3. Biomol. Ther. Seoul. 24, 49–56. doi:10.4062/biomolther.2015.056
Amar, J., Burcelin, R., Ruidavets, J. B., Cani, P. D., Fauvel, J., Alessi, M. C., et al. (2008). Energy intake is associated with endotoxemia in apparently healthy men. Am. J. Clin. Nutr. 87, 1219–1223. doi:10.1093/ajcn/87.5.1219
An, J., Lee, H., Lee, S., Song, Y., Kim, J., Park, I. H., et al. (2021). Modulation of pro-inflammatory and anti-inflammatory cytokines in the fat by an aloe gel-based formula, QDMC, is correlated with altered gut microbiota. Immune Netw. 21, e15. doi:10.4110/in.2021.21.e15
Anand, S., Muthusamy, V. S., Sujatha, S., Sangeetha, K. N., Bharathi Raja, R., Sudhagar, S., et al. (2010). Aloe emodin glycosides stimulates glucose transport and glycogen storage through PI3K dependent mechanism in L6 myotubes and inhibits adipocyte differentiation in 3T3L1 adipocytes. FEBS Lett. 584, 3170–3178. doi:10.1016/j.febslet.2010.06.004
Anhê, F. F., Nachbar, R. T., Varin, T. V., Trottier, J., Dudonné, S., Le Barz, M., et al. (2019). Treatment with camu camu (Myrciaria dubia) prevents obesity by altering the gut microbiota and increasing energy expenditure in diet-induced obese mice. Gut 68, 453–464. doi:10.1136/gutjnl-2017-315565
Arnold, S. V., Inzucchi, S. E., Echouffo-Tcheugui, J. B., Tang, F., Lam, C. S. P., Sperling, L. S., et al. (2019). Understanding contemporary use of thiazolidinediones. Circ. Heart Fail 12, e005855. doi:10.1161/CIRCHEARTFAILURE.118.005855
Artunc, F., Schleicher, E., Weigert, C., Fritsche, A., Stefan, N., and Häring, H.-U. (2016). The impact of insulin resistance on the kidney and vasculature. Nat. Rev. Nephrol. 12, 721–737. doi:10.1038/nrneph.2016.145
Behl, T., Gupta, A., Sehgal, A., Albarrati, A., Albratty, M., Meraya, A. M., et al. (2022). Exploring protein tyrosine phosphatases (PTP) and PTP-1B inhibitors in management of diabetes mellitus. Biomed. Pharmacother. 153, 113405. doi:10.1016/j.biopha.2022.113405
Boström, P., Wu, J., Jedrychowski, M. P., Korde, A., Ye, L., Lo, J. C., et al. (2012). A PGC1-α-dependent myokine that drives brown-fat-like development of white fat and thermogenesis. Nature 481, 463–468. doi:10.1038/nature10777
Cani, P. D., Amar, J., Iglesias, M. A., Poggi, M., Knauf, C., Bastelica, D., et al. (2007). Metabolic endotoxemia initiates obesity and insulin resistance. Diabetes 56, 1761–1772. doi:10.2337/db06-1491
Cani, P. D., Bibiloni, R., Knauf, C., Waget, A., Neyrinck, A. M., Delzenne, N. M., et al. (2008). Changes in gut microbiota control metabolic endotoxemia-induced inflammation in high-fat diet-induced obesity and diabetes in mice. Diabetes 57, 1470–1481. doi:10.2337/db07-1403
Carrasco, K., Boufenzer, A., Jolly, L., Le Cordier, H., Wang, G., Heck, A. J., et al. (2019). TREM-1 multimerization is essential for its activation on monocytes and neutrophils. Cell Mol. Immunol. 16, 460–472. doi:10.1038/s41423-018-0003-5
Chambers, E. S., Byrne, C. S., Morrison, D. J., Murphy, K. G., Preston, T., Tedford, C., et al. (2019). Dietary supplementation with inulin-propionate ester or inulin improves insulin sensitivity in adults with overweight and obesity with distinct effects on the gut microbiota, plasma metabolome and systemic inflammatory responses: a randomised cross-over trial. Gut 68, 1430–1438. doi:10.1136/gutjnl-2019-318424
Chang, W.-C., Chu, M.-T., Hsu, C.-Y., Wu, Y.-J. J., Lee, J.-Y., Chen, T.-J., et al. (2019). Rhein, an anthraquinone drug, suppresses the NLRP3 inflammasome and macrophage activation in urate crystal-induced gouty inflammation. Am. J. Chin. Med. 47, 135–151. doi:10.1142/S0192415X19500071
Chen, C., Lin, Z., Liu, W., Hu, Q., Wang, J., Zhuang, X., et al. (2022). Emodin accelerates diabetic wound healing by promoting anti-inflammatory macrophage polarization. Eur. J. Pharmacol. 936, 175329. doi:10.1016/j.ejphar.2022.175329
Chen, G., Ni, Y., Nagata, N., Xu, L., Zhuge, F., Nagashimada, M., et al. (2019). Pirfenidone prevents and reverses hepatic insulin resistance and steatohepatitis by polarizing M2 macrophages. Lab. Invest. 99, 1335–1348. doi:10.1038/s41374-019-0255-4
Chen, Z., Zhang, L., Yi, J., Yang, Z., Zhang, Z., and Li, Z. (2012). Promotion of adiponectin multimerization by emodin: a novel AMPK activator with PPARγ-agonist activity. J. Cell Biochem. 113, 3547–3558. doi:10.1002/jcb.24232
Costes, S., Broca, C., Bertrand, G., Lajoix, A.-D., Bataille, D., Bockaert, J., et al. (2006). ERK1/2 control phosphorylation and protein level of cAMP-responsive element-binding protein: a key role in glucose-mediated pancreatic beta-cell survival. Diabetes 55, 2220–2230. doi:10.2337/db05-1618
Cui, H.-X., Zhang, L.-S., Luo, Y., Yuan, K., Huang, Z.-Y., and Guo, Y. (2019). A purified anthraquinone-glycoside preparation from rhubarb ameliorates type 2 diabetes mellitus by modulating the gut microbiota and reducing inflammation. Front. Microbiol. 10, 1423. doi:10.3389/fmicb.2019.01423
Dai, J.-P., Wang, Q.-W., Su, Y., Gu, L.-M., Zhao, Y., Chen, X.-X., et al. (2017). Emodin inhibition of influenza A virus replication and influenza viral pneumonia via the Nrf2, TLR4, p38/JNK and NF-kappaB pathways. Molecules 22, 1754. doi:10.3390/molecules22101754
Deora, N., Sunitha, M. M., Satyavani, M., Harishankar, N., Vijayalakshmi, M. A., Venkataraman, K., et al. (2021). Alleviation of diabetes mellitus through the restoration of β-cell function and lipid metabolism by Aloe vera (L.) Burm. f. extract in obesogenic WNIN/GR-Ob rats. J. Ethnopharmacol. 272, 113921. doi:10.1016/j.jep.2021.113921
Diniz, T. A., de Lima Junior, E. A., Teixeira, A. A., Biondo, L. A., da Rocha, L. A. F., Valadão, I. C., et al. (2021). Aerobic training improves NAFLD markers and insulin resistance through AMPK-PPAR-α signaling in obese mice. Life Sci. 266, 118868. doi:10.1016/j.lfs.2020.118868
Dou, F., Liu, Y., Liu, L., Wang, J., Sun, T., Mu, F., et al. (2019). Aloe-emodin ameliorates renal fibrosis via inhibiting PI3K/Akt/mTOR signaling pathway in vivo and in vitro. Rejuvenation Res. 22, 218–229. doi:10.1089/rej.2018.2104
Dutta, D., Bhattacharya, S., Kumar, M., Datta, P. K., Mohindra, R., and Sharma, M. (2023). Efficacy and safety of novel thiazolidinedione lobeglitazone for managing type-2 diabetes a meta-analysis. Diabetes Metab. Syndr. 17, 102697. doi:10.1016/j.dsx.2022.102697
Elchebly, M., Payette, P., Michaliszyn, E., Cromlish, W., Collins, S., Loy, A. L., et al. (1999). Increased insulin sensitivity and obesity resistance in mice lacking the protein tyrosine phosphatase-1B gene. Science 283, 1544–1548. doi:10.1126/science.283.5407.1544
Elsayed, R. H., Kamel, E. M., Mahmoud, A. M., El-Bassuony, A. A., Bin-Jumah, M., Lamsabhi, A. M., et al. (2020). Rumex dentatus L. phenolics ameliorate hyperglycemia by modulating hepatic key enzymes of carbohydrate metabolism, oxidative stress and PPARγ in diabetic rats. Food Chem. Toxicol. 138, 111202. doi:10.1016/j.fct.2020.111202
Fan, Y., Yang, Q., Yang, Y., Gao, Z., Ma, Y., Zhang, L., et al. (2019). Sirt6 suppresses high glucose-induced mitochondrial dysfunction and apoptosis in podocytes through AMPK activation. Int. J. Biol. Sci. 15, 701–713. doi:10.7150/ijbs.29323
Fang, J.-Y., Huang, T.-H., Chen, W.-J., Aljuffali, I. A., and Hsu, C.-Y. (2022). Rhubarb hydroxyanthraquinones act as antiobesity agents to inhibit adipogenesis and enhance lipolysis. Biomed. Pharmacother. 146, 112497. doi:10.1016/j.biopha.2021.112497
Fedorenko, A., Lishko, P. V., and Kirichok, Y. (2012). Mechanism of fatty-acid-dependent UCP1 uncoupling in brown fat mitochondria. Cell 151, 400–413. doi:10.1016/j.cell.2012.09.010
Fu, S., Dang, Y., Xu, H., Li, A., Zhou, X., Gao, X., et al. (2022). Aloe vera-fermented beverage ameliorates obesity and gut dysbiosis in high-fat-diet mice. Foods 11, 3728. doi:10.3390/foods11223728
Glass, C. K., and Olefsky, J. M. (2012). Inflammation and lipid signaling in the etiology of insulin resistance. Cell Metab. 15, 635–645. doi:10.1016/j.cmet.2012.04.001
Grahnemo, L., Nethander, M., Coward, E., Gabrielsen, M. E., Sree, S., Billod, J.-M., et al. (2022). Cross-sectional associations between the gut microbe Ruminococcus gnavus and features of the metabolic syndrome. Lancet Diabetes Endocrinol. 10, 481–483. doi:10.1016/S2213-8587(22)00113-9
Guo, C.-Y., Liao, W.-T., Qiu, R.-J., Zhou, D.-S., Ni, W.-J., Yu, C.-P., et al. (2021). Aurantio-obtusin improves obesity and insulin resistance induced by high-fat diet in obese mice. Phytother. Res. 35, 346–360. doi:10.1002/ptr.6805
Hong, S.-W., Lee, J., Park, S. E., Rhee, E.-J., Park, C.-Y., Oh, K.-W., et al. (2012). Repression of sterol regulatory element-binding protein 1-c is involved in the protective effects of exendin-4 in pancreatic β-cell line. Mol. Cell Endocrinol. 362, 242–252. doi:10.1016/j.mce.2012.07.004
Hu, B., Zhang, H., Meng, X., Wang, F., and Wang, P. (2014). Aloe-emodin from rhubarb (Rheum rhabarbarum) inhibits lipopolysaccharide-induced inflammatory responses in RAW264.7 macrophages. J. Ethnopharmacol. 153, 846–853. doi:10.1016/j.jep.2014.03.059
Huo, J., Wang, T., Wei, B., Shi, X., Yang, A., Chen, D., et al. (2022). Integrated network pharmacology and intestinal flora analysis to determine the protective effect of Xuanbai-Chengqi decoction on lung and gut injuries in influenza virus-infected mice. J. Ethnopharmacol. 298, 115649. doi:10.1016/j.jep.2022.115649
Hurtado de Llera, A., Martin-Hidalgo, D., Gil, M. C., Garcia-Marin, L. J., and Bragado, M. J. (2014). The calcium/CaMKKalpha/beta and the cAMP/PKA pathways are essential upstream regulators of AMPK activity in boar spermatozoa. Biol. Reprod. 90, 29. doi:10.1095/biolreprod.113.112797
Iwabu, M., Yamauchi, T., Okada-Iwabu, M., Sato, K., Nakagawa, T., Funata, M., et al. (2010). Adiponectin and AdipoR1 regulate PGC-1alpha and mitochondria by Ca(2+) and AMPK/SIRT1. Nature 464, 1313–1319. doi:10.1038/nature08991
James, D. E., Stöckli, J., and Birnbaum, M. J. (2021). The aetiology and molecular landscape of insulin resistance. Nat. Rev. Mol. Cell Biol. 22, 751–771. doi:10.1038/s41580-021-00390-6
Ji, L., and Gu, H. (2021). The anti-obesity effects of rhein on improving insulin resistance (IR) and blood lipid levels are involved in endoplasmic reticulum stress (ERs), inflammation, and oxidative stress in vivo and vitro. Bioengineered 12, 5797–5813. doi:10.1080/21655979.2021.1969196
Jiang, B., Wang, D., Hu, Y., Li, W., Liu, F., Zhu, X., et al. (2022a). Serum amyloid A1 exacerbates hepatic steatosis via TLR4-mediated NF-κB signaling pathway. Mol. Metab. 59, 101462. doi:10.1016/j.molmet.2022.101462
Jiang, H., Mao, T., Liu, Y., Tan, X., Sun, Z., Cheng, Y., et al. (2022b). Protective effects and mechanisms of yinchen linggui zhugan decoction in HFD-induced nonalcoholic fatty liver disease rats based on network pharmacology and experimental verification. Front. Pharmacol. 13, 908128. doi:10.3389/fphar.2022.908128
Jiao, P., Ma, J., Feng, B., Zhang, H., Diehl, J. A., Chin, Y. E., et al. (2011). FFA-induced adipocyte inflammation and insulin resistance: involvement of ER stress and IKKβ pathways. Obes. (Silver Spring) 19, 483–491. doi:10.1038/oby.2010.200
Jung, H. A., Ali, M. Y., and Choi, J. S. (2016). Promising inhibitory effects of anthraquinones, naphthopyrone, and naphthalene glycosides, from Cassia obtusifolia on α-glucosidase and human protein tyrosine phosphatases 1B. Molecules 22, 28. doi:10.3390/molecules22010028
Kawaguchi, T., Osatomi, K., Yamashita, H., Kabashima, T., and Uyeda, K. (2002). Mechanism for fatty acid “sparing” effect on glucose-induced transcription: regulation of carbohydrate-responsive element-binding protein by AMP-activated protein kinase. J. Biol. Chem. 277, 3829–3835. doi:10.1074/jbc.M107895200
Khurm, M., Wang, X., Zhang, H., Hussain, S. N., Qaisar, M. N., Hayat, K., et al. (2020). The genus Cassia L.: ethnopharmacological and phytochemical overview. Phytother. Res. 35, 2336–2385. doi:10.1002/ptr.6954
Ko, E., Um, M. Y., Choi, M., Han, T., Kim, I.-H., and Shin, S. (2020). Cassia tora seed improves pancreatic mitochondrial function leading to recovery of glucose metabolism. Am. J. Chin. Med. 48, 615–629. doi:10.1142/S0192415X20500317
Korvatska, O., Kiianitsa, K., Ratushny, A., Matsushita, M., Beeman, N., Chien, W.-M., et al. (2020). Triggering receptor expressed on myeloid cell 2 R47H exacerbates immune response in alzheimer’s disease brain. Front. Immunol. 11, 559342. doi:10.3389/fimmu.2020.559342
Kumar, R., Singh, A. K., Gupta, A., Bishayee, A., and Pandey, A. K. (2019). Therapeutic potential of Aloe vera-A miracle gift of nature. Phytomedicine 60, 152996. doi:10.1016/j.phymed.2019.152996
Kwak, H. J., Yang, D., Hwang, Y., Jun, H.-S., and Cheon, H. G. (2017). Baicalein protects rat insulinoma INS-1 cells from palmitate-induced lipotoxicity by inducing HO-1. PLoS One 12, e0176432. doi:10.1371/journal.pone.0176432
Lee, J., Park, S., Oh, N., Park, J., Kwon, M., Seo, J., et al. (2021). Oral intake of Lactobacillus plantarum L-14 extract alleviates TLR2-and AMPK-mediated obesity-associated disorders in high-fat-diet-induced obese C57BL/6J mice. Cell Prolif. 54, e13039. doi:10.1111/cpr.13039
Leto, D., and Saltiel, A. R. (2012). Regulation of glucose transport by insulin: traffic control of GLUT4. Nat. Rev. Mol. Cell Biol. 13, 383–396. doi:10.1038/nrm3351
Li, B., Leung, J. C. K., Chan, L. Y. Y., Yiu, W. H., and Tang, S. C. W. (2020a). A global perspective on the crosstalk between saturated fatty acids and Toll-like receptor 4 in the etiology of inflammation and insulin resistance. Prog. Lipid Res. 77, 101020. doi:10.1016/j.plipres.2019.101020
Li, C., Xu, M. M., Wang, K., Adler, A. J., Vella, A. T., and Zhou, B. (2018). Macrophage polarization and meta-inflammation. Transl. Res. 191, 29–44. doi:10.1016/j.trsl.2017.10.004
Li, X., Xiao, C., Yuan, J., Chen, X., Li, Q., and Shen, F. (2023). Rhein-attenuates LPS-induced acute lung injury via targeting NFATc1/Trem2 axis. Inflamm. Res. 72, 1237–1255. doi:10.1007/s00011-023-01746-8
Li, X., Zhang, D., Vatner, D. F., Goedeke, L., Hirabara, S. M., Zhang, Y., et al. (2020b). Mechanisms by which adiponectin reverses high fat diet-induced insulin resistance in mice. Proc. Natl. Acad. Sci. U. S. A. 117, 32584–32593. doi:10.1073/pnas.1922169117
Li, Y., and Jiang, J.-G. (2018). Health functions and structure-activity relationships of natural anthraquinones from plants. Food Funct. 9, 6063–6080. doi:10.1039/c8fo01569d
Li, Y., Xu, S., Mihaylova, M. M., Zheng, B., Hou, X., Jiang, B., et al. (2011). AMPK phosphorylates and inhibits SREBP activity to attenuate hepatic steatosis and atherosclerosis in diet-induced insulin-resistant mice. Cell Metab. 13, 376–388. doi:10.1016/j.cmet.2011.03.009
Li, Y., Yu, Z., Liu, Y., Wang, T., Liu, Y., Bai, Z., et al. (2022). Dietary α-linolenic acid-rich flaxseed oil ameliorates high-fat diet-induced atherosclerosis via gut microbiota-inflammation-artery Axis in ApoE -/- mice. Front. Cardiovasc Med. 9, 830781. doi:10.3389/fcvm.2022.830781
Lian, Y., Xia, X., Zhao, H., and Zhu, Y. (2017). The potential of chrysophanol in protecting against high fat-induced cardiac injury through Nrf2-regulated anti-inflammation, anti-oxidant and anti-fibrosis in Nrf2 knockout mice. Biomed. Pharmacother. 93, 1175–1189. doi:10.1016/j.biopha.2017.05.148
Liang, C., Hao, F., Yao, X., Qiu, Y., Liu, L., Wang, S., et al. (2019). Hypericin maintians PDX1 expression via the Erk pathway and protects islet β-cells against glucotoxicity and lipotoxicity. Int. J. Biol. Sci. 15, 1472–1487. doi:10.7150/ijbs.33817
Liang, C., Li, Y., Bai, M., Huang, Y., Yang, H., Liu, L., et al. (2020). Hypericin attenuates nonalcoholic fatty liver disease and abnormal lipid metabolism via the PKA-mediated AMPK signaling pathway in vitro and in vivo. Pharmacol. Res. 153, 104657. doi:10.1016/j.phrs.2020.104657
Lim, H., Park, J., Kim, H.-L., Kang, J., Jeong, M.-Y., Youn, D.-H., et al. (2016). Chrysophanic acid suppresses adipogenesis and induces thermogenesis by activating AMP-activated protein kinase alpha in vivo and in vitro. Front. Pharmacol. 7, 476. doi:10.3389/fphar.2016.00476
Lin, S.-C., and Hardie, D. G. (2018). AMPK: sensing glucose as well as cellular energy status. Cell Metab. 27, 299–313. doi:10.1016/j.cmet.2017.10.009
Liu, K., Liu, X., Deng, Y., Li, Z., and Tang, A. (2022). CircRNA-mediated regulation of brown adipose tissue adipogenesis. Front. Nutr. 9, 926024. doi:10.3389/fnut.2022.926024
Liu, X., Yang, Z., Li, H., Luo, W., Duan, W., Zhang, J., et al. (2020). Chrysophanol alleviates metabolic syndrome by activating the SIRT6/AMPK signaling pathway in Brown adipocytes. Oxid. Med. Cell Longev. 2020, 7374086. doi:10.1155/2020/7374086
Lu, W., Zhu, H., Wu, J., Liao, S., Cheng, G., and Li, X. (2022). Rhein attenuates angiotensin II-induced cardiac remodeling by modulating AMPK-FGF23 signaling. J. Transl. Med. 20, 305. doi:10.1186/s12967-022-03482-9
Luo, H., Wu, H., Wang, L., Xiao, S., Lu, Y., Liu, C., et al. (2021). Hepatoprotective effects of Cassiae Semen on mice with non-alcoholic fatty liver disease based on gut microbiota. Commun. Biol. 4, 1357. doi:10.1038/s42003-021-02883-8
Luo, P., Qin, C., Zhu, L., Fang, C., Zhang, Y., Zhang, H., et al. (2018). Ubiquitin-specific peptidase 10 (USP10) inhibits hepatic steatosis, insulin resistance, and inflammation through Sirt6. Hepatology 68, 1786–1803. doi:10.1002/hep.30062
Ma, C., Wang, Z., Xia, R., Wei, L., Zhang, C., Zhang, J., et al. (2021). Danthron ameliorates obesity and MAFLD through activating the interplay between PPARα/RXRα heterodimer and adiponectin receptor 2. Biomed. Pharmacother. 137, 111344. doi:10.1016/j.biopha.2021.111344
Ma, L., Cao, X., Ye, X., Qi, Y., Zhu, Y., Ye, J., et al. (2020). Sennoside A restores colonic barrier function through protecting colon enterocytes from ROS-induced mitochondrial damage in diet-induced obese mice. Biochem. Biophys. Res. Commun. 526, 519–524. doi:10.1016/j.bbrc.2020.03.117
Ma, Q., Wang, C.-Z., Sawadogo, W. R., Bian, Z.-X., and Yuan, C.-S. (2022). Herbal medicines for constipation and phytochemical comparison of active components. Am. J. Chin. Med. 50, 723–732. doi:10.1142/S0192415X2250029X
Ma, T. Y., Iwamoto, G. K., Hoa, N. T., Akotia, V., Pedram, A., Boivin, M. A., et al. (2004). TNF-alpha-induced increase in intestinal epithelial tight junction permeability requires NF-kappa B activation. Am. J. Physiol. Gastrointest. Liver Physiol. 286, G367–G376. doi:10.1152/ajpgi.00173.2003
Malik, E. M., and Müller, C. E. (2016). Anthraquinones as pharmacological tools and drugs. Med. Res. Rev. 36, 705–748. doi:10.1002/med.21391
Maqdasy, S., Lecoutre, S., Renzi, G., Frendo-Cumbo, S., Rizo-Roca, D., Moritz, T., et al. (2022). Impaired phosphocreatine metabolism in white adipocytes promotes inflammation. Nat. Metab. 4, 190–202. doi:10.1038/s42255-022-00525-9
Martínez-Aldino, I. Y., Villaseca-Murillo, M., Morales-Jiménez, J., and Rivera-Chávez, J. (2021). Absolute configuration and protein tyrosine phosphatase 1B inhibitory activity of xanthoepocin, a dimeric naphtopyrone from Penicillium sp. IQ-429. Bioorg Chem. 115, 105166. doi:10.1016/j.bioorg.2021.105166
McKinnon, C. M., and Docherty, K. (2001). Pancreatic duodenal homeobox-1, PDX-1, a major regulator of beta cell identity and function. Diabetologia 44, 1203–1214. doi:10.1007/s001250100628
Mei, L., Tang, Y., Li, M., Yang, P., Liu, Z., Yuan, J., et al. (2015). Co-administration of cholesterol-lowering probiotics and anthraquinone from Cassia obtusifolia L. Ameliorate non-alcoholic fatty liver. PLoS One 10, e0138078. doi:10.1371/journal.pone.0138078
Momcilovic, M., Hong, S.-P., and Carlson, M. (2006). Mammalian TAK1 activates Snf1 protein kinase in yeast and phosphorylates AMP-activated protein kinase in vitro. J. Biol. Chem. 281, 25336–25343. doi:10.1074/jbc.M604399200
Monsénégo, J., Mansouri, A., Akkaoui, M., Lenoir, V., Esnous, C., Fauveau, V., et al. (2012). Enhancing liver mitochondrial fatty acid oxidation capacity in obese mice improves insulin sensitivity independently of hepatic steatosis. J. Hepatol. 56, 632–639. doi:10.1016/j.jhep.2011.10.008
Mungai, P. T., Waypa, G. B., Jairaman, A., Prakriya, M., Dokic, D., Ball, M. K., et al. (2011). Hypoxia triggers AMPK activation through reactive oxygen species-mediated activation of calcium release-activated calcium channels. Mol. Cell Biol. 31, 3531–3545. doi:10.1128/MCB.05124-11
Naderpoor, N., Mousa, A., Gomez-Arango, L. F., Barrett, H. L., Dekker Nitert, M., and de Courten, B. (2019). Faecal microbiota are related to insulin sensitivity and secretion in overweight or obese adults. J. Clin. Med. 8, 452. doi:10.3390/jcm8040452
Natividad, J. M., Lamas, B., Pham, H. P., Michel, M.-L., Rainteau, D., Bridonneau, C., et al. (2018). Bilophila wadsworthia aggravates high fat diet induced metabolic dysfunctions in mice. Nat. Commun. 9, 2802. doi:10.1038/s41467-018-05249-7
Nayan, S. I., Chowdhury, F. I., Akter, N., Rahman, M. M., Selim, S., Saffoon, N., et al. (2021). Leaf powder supplementation of Senna alexandrina ameliorates oxidative stress, inflammation, and hepatic steatosis in high-fat diet-fed obese rats. PLoS One 16, e0250261. doi:10.1371/journal.pone.0250261
Nguyen, M. T. A., Satoh, H., Favelyukis, S., Babendure, J. L., Imamura, T., Sbodio, J. I., et al. (2005). JNK and tumor necrosis factor-alpha mediate free fatty acid-induced insulin resistance in 3T3-L1 adipocytes. J. Biol. Chem. 280, 35361–35371. doi:10.1074/jbc.M504611200
Park, J. E., Kang, E., and Han, J. S. (2022). HM-chromanone attenuates TNF-α-mediated inflammation and insulin resistance by controlling JNK activation and NF-κB pathway in 3T3-L1 adipocytes. Eur. J. Pharmacol. 921, 174884. doi:10.1016/j.ejphar.2022.174884
Płóciennikowska, A., Hromada-Judycka, A., Borzęcka, K., and Kwiatkowska, K. (2015). Co-operation of TLR4 and raft proteins in LPS-induced pro-inflammatory signaling. Cell Mol. Life Sci. 72, 557–581. doi:10.1007/s00018-014-1762-5
Qi, Y., Liu, Y., Zhang, B., Wang, M., Cao, L., Song, L., et al. (2022). Comparative antibacterial analysis of the anthraquinone compounds based on the AIM theory, molecular docking, and dynamics simulation analysis. J. Mol. Model 29, 16. doi:10.1007/s00894-022-05406-2
Quan, Y., Gong, L., He, J., Zhou, Y., Liu, M., Cao, Z., et al. (2019). Aloe emodin induces hepatotoxicity by activating NF-κB inflammatory pathway and P53 apoptosis pathway in zebrafish. Toxicol. Lett. 306, 66–79. doi:10.1016/j.toxlet.2019.02.007
Rabot, S., Membrez, M., Blancher, F., Berger, B., Moine, D., Krause, L., et al. (2016). High fat diet drives obesity regardless the composition of gut microbiota in mice. Sci. Rep. 6, 32484. doi:10.1038/srep32484
Régnier, M., Rastelli, M., Morissette, A., Suriano, F., Le Roy, T., Pilon, G., et al. (2020). Rhubarb supplementation prevents diet-induced obesity and diabetes in association with increased Akkermansia muciniphila in mice. Nutrients 12, 2932. doi:10.3390/nu12102932
Rustenbeck, I., Krautheim, A., Jörns, A., and Steinfelder, H. J. (2004). Beta-cell toxicity of ATP-sensitive K+ channel-blocking insulin secretagogues. Biochem. Pharmacol. 67, 1733–1741. doi:10.1016/j.bcp.2004.01.016
Sasaki, N., Maeda, R., Ozono, R., Yoshimura, K., Nakano, Y., and Higashi, Y. (2022). Adipose tissue insulin resistance predicts the incidence of hypertension: the Hiroshima Study on Glucose Metabolism and Cardiovascular Diseases. Hypertens. Res. 45, 1763–1771. doi:10.1038/s41440-022-00987-0
Selim, N. M., Elgazar, A. A., Abdel-Hamid, N. M., El-Magd, M. R. A., Yasri, A., Hefnawy, H. M. E., et al. (2019). Chrysophanol, physcion, hesperidin and curcumin modulate the gene expression of pro-inflammatory mediators induced by LPS in HepG2: in silico and molecular studies. Anti-oxidants (Basel) 8, 371. doi:10.3390/antiox8090371
Settembre, C., De Cegli, R., Mansueto, G., Saha, P. K., Vetrini, F., Visvikis, O., et al. (2013). TFEB controls cellular lipid metabolism through a starvation-induced autoregulatory loop. Nat. Cell Biol. 15, 647–658. doi:10.1038/ncb2718
Shang, H., Guo, J., Wang, P., Li, L., Tian, Y., Li, X., et al. (2022). Design, synthesis and anti-inflammatory evaluation of aloe-emodin derivatives as potential modulators of Akt, NF-κB and JNK signaling pathways. Eur. J. Med. Chem. 238, 114511. doi:10.1016/j.ejmech.2022.114511
Shang, L., Liu, Y., Li, J., Pan, G., Zhou, F., and Yang, S. (2021). Emodin protects sepsis associated damage to the intestinal mucosal barrier through the VDR/Nrf2/HO-1 pathway. Front. Pharmacol. 12, 724511. doi:10.3389/fphar.2021.724511
Sheng, D., Zhao, S., Gao, L., Zheng, H., Liu, W., Hou, J., et al. (2019). BabaoDan attenuates high-fat diet-induced non-alcoholic fatty liver disease via activation of AMPK signaling. Cell Biosci. 9, 77. doi:10.1186/s13578-019-0339-2
Shi, X., Zhou, X., Chu, X., Wang, J., Xie, B., Ge, J., et al. (2019). Allicin improves metabolism in high-fat diet-induced obese mice by modulating the gut microbiota. Nutrients 11, 2909. doi:10.3390/nu11122909
Shin, E., Shin, S., Kong, H., Lee, S., Do, S.-G., Jo, T. H., et al. (2011). Dietary aloe reduces adipogenesis via the activation of AMPK and suppresses obesity-related inflammation in obese mice. Immune Netw. 11, 107–113. doi:10.4110/in.2011.11.2.107
Simpson, B. W., and Trent, M. S. (2019). Pushing the envelope: LPS modifications and their consequences. Nat. Rev. Microbiol. 17, 403–416. doi:10.1038/s41579-019-0201-x
Song, P., Kim, J. H., Ghim, J., Yoon, J. H., Lee, A., Kwon, Y., et al. (2013). Emodin regulates glucose utilization by activating AMP-activated protein kinase. J. Biol. Chem. 288, 5732–5742. doi:10.1074/jbc.M112.441477
Sugimoto, S., Mena, H. A., Sansbury, B. E., Kobayashi, S., Tsuji, T., Wang, C.-H., et al. (2022). Brown adipose tissue-derived MaR2 contributes to cold-induced resolution of inflammation. Nat. Metab. 4, 775–790. doi:10.1038/s42255-022-00590-0
Tan, R., Dong, H., Chen, Z., Jin, M., Yin, J., Li, H., et al. (2021). Intestinal microbiota mediates high-fructose and high-fat diets to induce chronic intestinal inflammation. Front. Cell Infect. Microbiol. 11, 654074. doi:10.3389/fcimb.2021.654074
Taylor, S. I., Blau, J. E., Rother, K. I., and Beitelshees, A. L. (2019). SGLT2 inhibitors as adjunctive therapy for type 1 diabetes: balancing benefits and risks. Lancet Diabetes Endocrinol. 7, 949–958. doi:10.1016/S2213-8587(19)30154-8
Towler, M. C., and Hardie, D. G. (2007). AMP-activated protein kinase in metabolic control and insulin signaling. Circ. Res. 100, 328–341. doi:10.1161/01.RES.0000256090.42690.05
Tulkens, J., Vergauwen, G., Van Deun, J., Geeurickx, E., Dhondt, B., Lippens, L., et al. (2020). Increased levels of systemic LPS-positive bacterial extracellular vesicles in patients with intestinal barrier dysfunction. Gut 69, 191–193. doi:10.1136/gutjnl-2018-317726
Tzeng, T.-F., Lu, H.-J., Liou, S.-S., Chang, C. J., and Liu, I.-M. (2012a). Emodin, a naturally occurring anthraquinone derivative, ameliorates dyslipidemia by activating AMP-activated protein kinase in high-fat-diet-fed rats. Evid. Based Complement. Altern. Med. 2012, 781812. doi:10.1155/2012/781812
Tzeng, T.-F., Lu, H.-J., Liou, S.-S., Chang, C. J., and Liu, I.-M. (2012b). Emodin protects against high-fat diet-induced obesity via regulation of AMP-activated protein kinase pathways in white adipose tissue. Planta Med. 78, 943–950. doi:10.1055/s-0031-1298626
Ussar, S., Griffin, N. W., Bezy, O., Fujisaka, S., Vienberg, S., Softic, S., et al. (2015). Interactions between gut microbiota, host genetics and diet modulate the predisposition to obesity and metabolic syndrome. Cell Metab. 22, 516–530. doi:10.1016/j.cmet.2015.07.007
Venable, C. L., Frevert, E. U., Kim, Y. B., Fischer, B. M., Kamatkar, S., Neel, B. G., et al. (2000). Overexpression of protein-tyrosine phosphatase-1B in adipocytes inhibits insulin-stimulated phosphoinositide 3-kinase activity without altering glucose transport or Akt/Protein kinase B activation. J. Biol. Chem. 275, 18318–18326. doi:10.1074/jbc.M908392199
Villarroya, F., and Gavaldà-Navarro, A. (2022). Brown fat resolves hepatic inflammation in obesity. Nat. Metab. 4, 649–650. doi:10.1038/s42255-022-00596-8
Wang, C., Ha, X., Li, W., Xu, P., Gu, Y., Wang, T., et al. (2017). Correlation of TLR4 and KLF7 in inflammation induced by obesity. Inflammation 40, 42–51. doi:10.1007/s10753-016-0450-z
Wang, H., Zhang, H., Gao, Z., Zhang, Q., and Gu, C. (2022a). The mechanism of berberine alleviating metabolic disorder based on gut microbiome. Front. Cell Infect. Microbiol. 12, 854885. doi:10.3389/fcimb.2022.854885
Wang, P., Gao, J., Ke, W., Wang, J., Li, D., Liu, R., et al. (2020). Resveratrol reduces obesity in high-fat diet-fed mice via modulating the composition and metabolic function of the gut microbiota. Free Radic. Biol. Med. 156, 83–98. doi:10.1016/j.freeradbiomed.2020.04.013
Wang, Q.-Y., Tong, A.-H., Pan, Y.-Y., Zhang, X.-D., Ding, W.-Y., and Xiong, W. (2019). The effect of cassia seed extract on the regulation of the LKB1-AMPK-GLUT4 signaling pathway in the skeletal muscle of diabetic rats to improve the insulin sensitivity of the skeletal muscle. Diabetol. Metab. Syndr. 11, 108. doi:10.1186/s13098-019-0504-0
Wang, S., Huang, X.-F., Zhang, P., Wang, H., Zhang, Q., Yu, S., et al. (2016). Chronic rhein treatment improves recognition memory in high-fat diet-induced obese male mice. J. Nutr. Biochem. 36, 42–50. doi:10.1016/j.jnutbio.2016.07.008
Wang, T., Li, M., Zeng, T., Hu, R., Xu, Y., Xu, M., et al. (2022b). Association between insulin resistance and cardiovascular disease risk varies according to glucose tolerance status: a nationwide prospective cohort study. Diabetes Care 45, 1863–1872. doi:10.2337/dc22-0202
Wang, Y.-H., Liu, Y.-P., Zhu, J.-Q., Zhou, G. H., Zhang, F., An, Q., et al. (2023). Physcion prevents high-fat diet-induced endothelial dysfunction by inhibiting oxidative stress and endoplasmic reticulum stress pathways. Eur. J. Pharmacol. 943, 175554. doi:10.1016/j.ejphar.2023.175554
Wei, Z., Shen, P., Cheng, P., Lu, Y., Wang, A., and Sun, Z. (2020). Gut bacteria selectively altered by sennoside A alleviate type 2 diabetes and obesity traits. Oxid. Med. Cell Longev. 2020, 2375676. doi:10.1155/2020/2375676
Wu, J., Wei, Z., Cheng, P., Qian, C., Xu, F., Yang, Y., et al. (2020). Rhein modulates host purine metabolism in intestine through gut microbiota and ameliorates experimental colitis. Theranostics 10, 10665–10679. doi:10.7150/thno.43528
Xie, P., Yan, L.-J., Zhou, H.-L., Cao, H.-H., Zheng, Y.-R., Lu, Z.-B., et al. (2022). Emodin protects against lipopolysaccharide-induced acute lung injury via the JNK/Nur77/c-Jun signaling pathway. Front. Pharmacol. 13, 717271. doi:10.3389/fphar.2022.717271
Xu, L., Xing, M., Xu, X., Saadeldeen, F. S., Liu, Z., Wei, J., et al. (2019). Alizarin increase glucose uptake through PI3K/Akt signaling and improve alloxan-induced diabetic mice. Future Med. Chem. 11, 395–406. doi:10.4155/fmc-2018-0515
Xu, Y., Wang, N., Tan, H.-Y., Li, S., Zhang, C., Zhang, Z., et al. (2020). Panax notoginseng saponins modulate the gut microbiota to promote thermogenesis and beige adipocyte reconstruction via leptin-mediated AMPKα/STAT3 signaling in diet-induced obesity. Theranostics 10, 11302–11323. doi:10.7150/thno.47746
Yang, B., Yu, Q., Chang, B., Guo, Q., Xu, S., Yi, X., et al. (2021). MOTS-c interacts synergistically with exercise intervention to regulate PGC-1α expression, attenuate insulin resistance and enhance glucose metabolism in mice via AMPK signaling pathway. Biochim. Biophys. Acta Mol. Basis Dis. 1867, 166126. doi:10.1016/j.bbadis.2021.166126
Yang, J., Ma, Y., Li, T., Pang, Y., Zhang, H., Xie, Y., et al. (2022). Ameliorative effects of Bifidobacterium animalis subsp. lactis J-12 on hyperglycemia in pregnancy and pregnancy outcomes in a high-fat-diet/streptozotocin-induced rat model. Nutrients 15, 170. doi:10.3390/nu15010170
Yang, M., Li, X., Zeng, X., Ou, Z., Xue, M., Gao, D., et al. (2016). Rheum palmatum L. Attenuates high fat diet-induced hepatosteatosis by activating AMP-activated protein kinase. Am. J. Chin. Med. 44, 551–564. doi:10.1142/S0192415X16500300
Yang, Y., Shang, W., Zhou, L., Jiang, B., Jin, H., and Chen, M. (2007). Emodin with PPARgamma ligand-binding activity promotes adipocyte differentiation and increases glucose uptake in 3T3-Ll cells. Biochem. Biophys. Res. Commun. 353, 225–230. doi:10.1016/j.bbrc.2006.11.134
Yang, Y., Zhong, Z., Wang, B., Xia, X., Yao, W., Huang, L., et al. (2019). Early-life high-fat diet-induced obesity programs hippocampal development and cognitive functions via regulation of gut commensal Akkermansia muciniphila. Neuropsychopharmacology 44, 2054–2064. doi:10.1038/s41386-019-0437-1
Yao, L., Cui, X., Chen, Q., Yang, X., Fang, F., Zhang, J., et al. (2017). Cold-inducible SIRT6 regulates thermogenesis of Brown and beige fat. Cell Rep. 20, 641–654. doi:10.1016/j.celrep.2017.06.069
Yi-Jie, L., Rui-Yu, W., Run-Ping, L., Kai-Yi, W., Ming-Ning, D., Rong, S., et al. (2023). Aurantio-obtusin ameliorates obesity by activating PPARα-dependent mitochondrial thermogenesis in brown adipose tissues. Acta Pharmacol. Sin. 44, 1826–1840. doi:10.1038/s41401-023-01089-4
Yin, Z., Gao, D., Du, K., Han, C., Liu, Y., Wang, Y., et al. (2022). Rhein ameliorates cognitive impairment in an APP/PS1 transgenic mouse model of alzheimer’s disease by relieving oxidative stress through activating the SIRT1/PGC-1α pathway. Oxid. Med. Cell Longev. 2022, 2524832. doi:10.1155/2022/2524832
Yoon, H. S., Cho, C. H., Yun, M. S., Jang, S. J., You, H. J., Kim, J.-H., et al. (2021). Akkermansia muciniphila secretes a glucagon-like peptide-1-inducing protein that improves glucose homeostasis and ameliorates metabolic disease in mice. Nat. Microbiol. 6, 563–573. doi:10.1038/s41564-021-00880-5
Yu, F., Yu, N., Peng, J., Zhao, Y., Zhang, L., Wang, X., et al. (2021). Emodin inhibits lipid accumulation and inflammation in adipose tissue of high-fat diet-fed mice by inducing M2 polarization of adipose tissue macrophages. FASEB J. 35, e21730. doi:10.1096/fj.202100157RR
Zeng, S.-L., Li, S.-Z., Xiao, P.-T., Cai, Y.-Y., Chu, C., Chen, B.-Z., et al. (2020). Citrus polymethoxyflavones attenuate metabolic syndrome by regulating gut microbiome and amino acid metabolism. Sci. Adv. 6, eaax6208. doi:10.1126/sciadv.aax6208
Zhang, C.-S., Li, M., Wang, Y., Li, X., Zong, Y., Long, S., et al. (2022). The aldolase inhibitor aldometanib mimics glucose starvation to activate lysosomal AMPK. Nat. Metab. 4, 1369–1401. doi:10.1038/s42255-022-00640-7
Zhang, H., Yi, J.-K., Huang, H., Park, S., Park, S., Kwon, W., et al. (2021). Rhein suppresses colorectal cancer cell growth by inhibiting the mTOR pathway in vitro and in vivo. Cancers (Basel) 13, 2176. doi:10.3390/cancers13092176
Zhang, K., Qin, X., Qiu, J., Sun, T., Qu, K., Din, A. U., et al. (2023). Desulfovibrio desulfuricans aggravates atherosclerosis by enhancing intestinal permeability and endothelial TLR4/NF-κB pathway in Apoe<i>-/-</i> mice. Genes Dis. 10, 239–253. doi:10.1016/j.gendis.2021.09.007
Zhang, L., Wu, X., Yang, R., Chen, F., Liao, Y., Zhu, Z., et al. (2020a). Effects of berberine on the gastrointestinal microbiota. Front. Cell Infect. Microbiol. 10, 588517. doi:10.3389/fcimb.2020.588517
Zhang, M., Li, X., Liang, H., Cai, H., Hu, X., Bian, Y., et al. (2018). Semen Cassiae extract improves glucose metabolism by promoting GlUT4 translocation in the skeletal muscle of diabetic rats. Front. Pharmacol. 9, 235. doi:10.3389/fphar.2018.00235
Zhang, X., Zhang, R., Lv, P., Yang, J., Deng, Y., Xu, J., et al. (2015). Emodin up-regulates glucose metabolism, decreases lipolysis, and attenuates inflammation in vitro. J. Diabetes 7, 360–368. doi:10.1111/1753-0407.12190
Zhang, X.-Y., Chen, J., Yi, K., Peng, L., Xie, J., Gou, X., et al. (2020b). Phlorizin ameliorates obesity-associated endotoxemia and insulin resistance in high-fat diet-fed mice by targeting the gut microbiota and intestinal barrier integrity. Gut Microbes 12, 1–18. doi:10.1080/19490976.2020.1842990
Zhang, Y., Liu, W., Liu, D., Zhao, T., and Tian, H. (2016). Efficacy of aloe vera supplementation on prediabetes and early non-treated diabetic patients: a systematic review and meta-analysis of randomized controlled trials. Nutrients 8, 388. doi:10.3390/nu8070388
Zhang, Y.-L., Guo, H., Zhang, C.-S., Lin, S.-Y., Yin, Z., Peng, Y., et al. (2013). AMP as a low-energy charge signal autonomously initiates assembly of AXIN-AMPK-LKB1 complex for AMPK activation. Cell Metab. 18, 546–555. doi:10.1016/j.cmet.2013.09.005
Zhao, Y., Chen, M. X., Kongstad, K. T., Jäger, A. K., and Staerk, D. (2017). Potential of Polygonum cuspidatum root as an antidiabetic food: dual high-resolution α-glucosidase and PTP1B inhibition profiling combined with HPLC-HRMS and NMR for identification of antidiabetic constituents. J. Agric. Food Chem. 65, 4421–4427. doi:10.1021/acs.jafc.7b01353
Zhou, F., Ding, M., Gu, Y., Fan, G., Liu, C., Li, Y., et al. (2021). Aurantio-obtusin attenuates non-alcoholic fatty liver disease through AMPK-mediated autophagy and fatty acid oxidation pathways. Front. Pharmacol. 12, 826628. doi:10.3389/fphar.2021.826628
Zhou, R., Wang, L., Xu, X., Chen, J., Hu, L., Chen, L., et al. (2013). Danthron activates AMP-activated protein kinase and regulates lipid and glucose metabolism in vitro. Acta Pharmacol. Sin. 34, 1061–1069. doi:10.1038/aps.2013.39
Zhou, W., Yang, T., Xu, W., Huang, Y., Ran, L., Yan, Y., et al. (2022a). The polysaccharides from the fruits of Lycium barbarum L. confer anti-diabetic effect by regulating gut microbiota and intestinal barrier. Carbohydr. Polym. 291, 119626. doi:10.1016/j.carbpol.2022.119626
Zhou, Y., Gao, C., Vong, C. T., Tao, H., Li, H., Wang, S., et al. (2022b). Rhein regulates redox-mediated activation of NLRP3 inflammasomes in intestinal inflammation through macrophage-activated crosstalk. Br. J. Pharmacol. 179, 1978–1997. doi:10.1111/bph.15773
Zhuang, S., Zhong, J., Bian, Y., Fan, Y., Chen, Q., Liu, P., et al. (2019). Rhein ameliorates lipopolysaccharide-induced intestinal barrier injury via modulation of Nrf2 and MAPKs. Life Sci. 216, 168–175. doi:10.1016/j.lfs.2018.11.048
Zumbaugh, M. D., Johnson, S. E., Shi, T. H., and Gerrard, D. E. (2022). Molecular and biochemical regulation of skeletal muscle metabolism. J. Anim. Sci. 100, skac035. skac035. doi:10.1093/jas/skac035
Glossary
Keywords: anthraquinones, insulin resistance, natural products, intestinal microbiome, antiinflammation
Citation: Xia W, Li S, Li L, Zhang S, Wang X, Ding W, Ding L, Zhang X and Wang Z (2023) Role of anthraquinones in combating insulin resistance. Front. Pharmacol. 14:1275430. doi: 10.3389/fphar.2023.1275430
Received: 10 August 2023; Accepted: 06 November 2023;
Published: 20 November 2023.
Edited by:
Abd El-Latif Hesham, Beni-Suef University, EgyptReviewed by:
Jia-Wen Shou, The Chinese University of Hong Kong, ChinaYasmina Esther Hernández-Santana, APC, Ireland
Copyright © 2023 Xia, Li, Li, Zhang, Wang, Ding, Ding, Zhang and Wang. This is an open-access article distributed under the terms of the Creative Commons Attribution License (CC BY). The use, distribution or reproduction in other forums is permitted, provided the original author(s) and the copyright owner(s) are credited and that the original publication in this journal is cited, in accordance with accepted academic practice. No use, distribution or reproduction is permitted which does not comply with these terms.
*Correspondence: Xiandang Zhang, eGlhbmRhbmd6aEAxNjMuY29t; Zhibin Wang, d2FuZ3poaWJpbkBzZGZtdS5lZHUuY24=