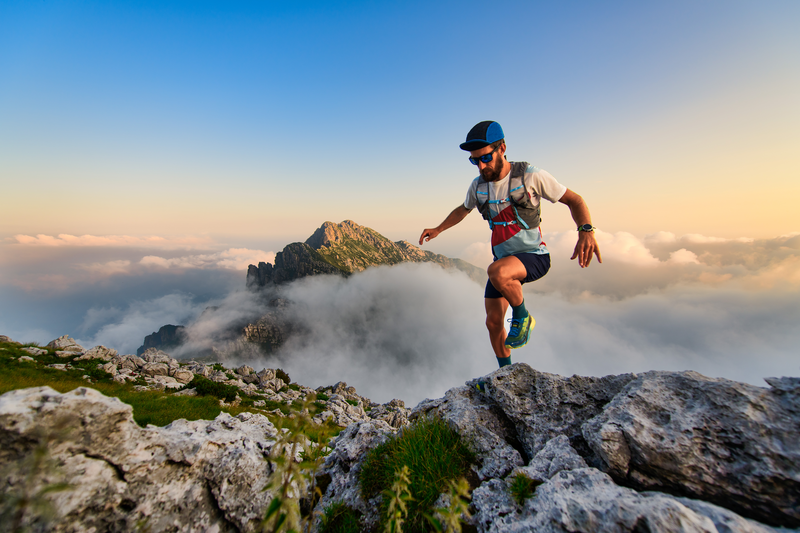
94% of researchers rate our articles as excellent or good
Learn more about the work of our research integrity team to safeguard the quality of each article we publish.
Find out more
REVIEW article
Front. Pharmacol. , 05 December 2023
Sec. Ethnopharmacology
Volume 14 - 2023 | https://doi.org/10.3389/fphar.2023.1273786
This article is part of the Research Topic Medicinal and Food Plants, Epigenetic regulation and Their Implications on the Microbiome in Disease View all 7 articles
Inonotus obliquus (Chaga mushroom) is an inexpensive fungus with a broad range of traditional and medicinal applications. These applications include therapy for breast, cervix, and skin cancers, as well as treating diabetes. However, its benefits are virtually untapped due to a limited understanding of its mycochemical composition and bioactivities. In this article, we explore the ethnobotany, mycochemistry, pharmacology, traditional therapeutic, cosmetic, and prospective agricultural uses. The review establishes that several secondary metabolites, such as steroids, terpenoids, and other compounds exist in chaga. Findings on its bioactivity have demonstrated its ability as an antioxidant, anti-inflammatory, antiviral, and antitumor agent. The study also demonstrates that Chaga powder has a long history of traditional use for medicinal purposes, pipe smoking rituals, and mystical future forecasts. The study further reveals that the applications of Chaga powder can be extended to industries such as pharmaceuticals, food, cosmetics, and agriculture. However numerous publications focused on the pharmaceutical benefits of Chaga with few publications on other applications. Overall, chaga is a promising natural resource with a wide range of potential applications and therefore the diverse array of therapeutic compounds makes it an attractive candidate for various applications such as plant biofertilizers and active ingredients in cosmetics and pharmaceutical products. Thus, further exploration of Chaga’s potential benefits in agriculture and other industries could lead to exciting new developments and innovations.
Inonotus obliquus (Chaga mushroom) is a sterile tree-destroying fungus that parasitizes the trunks of living birches. It belongs to the family Hymenochaetaceae and thrives in humid parts of Europe, Asia, and North America (Razumov et al., 2020; Abu-Reidah et al., 2021). The genus Inonotus includes about 100 species in a broad sense all of which have been identified as plant pathogens (Zhou and Wang, 2015). The distinction reflects changes in different groupings of the species over time as taxonomists have gained a better understanding of the relationships between different fungal species (May et al., 2019). The species obliquus is well-known for its medicinal and pharmacological properties (Balandaykin and Zmitrovich, 2015).
The preparation methods for indigenous herbal and non-medicinal uses of Chaga vary according to tribes and cultures. In China, Chaga mushrooms are processed for multiple applications by heating and crushing in water (Hwang et al., 2019). The same extraction method is applied in South Korea for similar purposes (Hong et al., 2015). In some tribes, Chaga mushrooms are used to boost the immune system and treat various diseases including diabetes and tumors (Abu-Reidah et al., 2021). In folk medicine, chaga is commonly consumed as a dietary mushroom as studies show it contains high levels of antioxidants, proteins, minerals, fiber, and vitamins. Apart from diet, it also plays a role as a functional food ingredient with several reported health-promoting activities including anti-cancer, anti-inflammatory, antioxidant, and antithrombotic (Lindequist et al., 2005; Rhee et al., 2008; Abu-Reidah et al., 2021). Other studies report the mushrooms’ general impact on the immune system and its potential in improving insulin resistance in type 2 diabetes (Géry et al., 2018; Abu-Reidah et al., 2021).
A comprehensive literature review (1900–2022) was conducted to assess the biological activities, mycochemical constituents, medicinal, and some nutritional potential of Chaga mushroom to supplement prior attempts focused on medicinal value (Milyuhina et al., 2022; Teplyakova et al., 2022). Globally, Chaga mushrooms are known for their therapeutic benefits, and novel bioactive metabolites in pharmaceutical, chemical, and cosmetic products (Peng and Shahidi, 2022). The application of Chaga is not limited to the medical field alone. The antioxidant properties of the fungus have demonstrated potential in animal production (Li et al., 2022). The addition of the mushroom to a nutrient matrix for livestock has been shown to increase the bioavailability of vitamin A in the digestive tract of livestock (Li et al., 2022). With these demonstrated benefits, the mushroom can be harnessed for use in other fields. Therefore, exploring the use of chaga in production of fertilizer can reduce environmental contamination that pose threat to both animal and plant and the overreliance on chemical fertilizer. This article therefore presents a summary of the biological functions of the Chaga mushroom linked to medical and agricultural applications.
A systematic and comprehensive search strategy was employed to source all relevant materials. This review covers material published between the years 1900 and Nov. 2022, including periodicals, books and/or chapters, essays, research articles, review papers, theses, and minutes from meetings. Only information written in the English language was located using web search engines such as Google, Google Scholar, Pub Med, Google Books, Semantic Scholar, Science Direct, Scopus, Worldwide Science, Web of Science, JSTOR, and ResearchGate. Approximately 90 scientific documents were used for this review. The word “Chaga” was also cross-referenced with related phrases, such as medicinal mushrooms, phytochemistry, biological qualities, and nutritional properties using Boolean operators such as ‘AND’, ‘OR’, ‘NOT’, or ‘AND NOT’.
According to Federhen (2012) scientific nomenclature “INOS” denotes fibre, “NOTON” denotes back, and “OBLIQUE” denotes unevenness of the sides (Figure 1) (Federhen, 2021).
Common names include Chaga, “Tschaga, Tschagapilz” (Russian), black birch touchwood, malalon mushroom, sterile conk trunk (North America/Europe), “Kreftjuilce” (Cancer polypore) (Norway), “Tikkatee” (Finland) and “Kabanoanatake” (Japan) (Zabel, 1976; Lee et al., 2008; Zhong et al., 2009; Beltrame et al., 2021). Chaga has several common names: in Russia, the fungus is called Chaga, which is derived from “Komi-Permyak,” the language of the Kama Basin, west of the Ural Mountains, but in England and Canada, Chaga is referred to as the birch’s sterile conk trunk (Zabel, 1976; Lee et al., 2008; Zhong et al., 2009; Beltrame et al., 2021). Owing to its therapeutic uses in modern medicine, Chaga is also known as the “Mushroom of Immortality”, in Japan, it is known as the “Diamond of the Forest” and the “King of Plants” in China (Nakajima et al., 2007; Zyryanova et al., 2010).
Chaga is commonly found in cooler climates, extending from the meridian zone in the mountains to the Northern hemisphere in subarctic regions (Figure 2). This mushroom is well known on three continents, including the cold climate regions of North America at latitudes of 45⁰ N– 50⁰ N (Canada, the United States of America), Asia (Russia, Kazakhstan, Siberia, South Korea, Japan), and Central and Northern Europe. Although Western and Southern Europe have cold climates, Chaga is rare in these regions perhaps owing to the length of unfavorable climatic conditions (Zhong et al., 2009; Abu-Reidah et al., 2021; Wasser, 2021).
In addition to their nutritional value, Chaga mushrooms are featured in various myths and were used by indigenous peoples in the management and treatment of various ailments. These myths and their uses vary among tribes, countries, and localities. In Canada, the Métis, Cree, Ojibway, Denesuline peoples of Northern Saskatchewan, and the Gitksan peoples of British Columbia all have myths about Chaga’s creation (Zmitrovich et al., 2020). It is said that “Wisakecak,” (a mythological character), was responsible for hurling the scab of Chaga onto the birch tree (Rogers, 2016; Peng and Shahidi, 2022). Some historians argue that Wisakecak discovered a birch tree while taking a stroll in the forest. He had been eating a piece of dried meat while wandering through the forest, and as he wandered, he became sleepy and absentmindedly threw the meat onto the tree trunk before settling under the tree and sleeping. While he slept, the meat began to rot, and the spores of the Chaga fungus began to grow on it (Il’ina & Uljašev, 2012). When Wisakecak awoke and saw the Chaga on the tree, he mistook it for the dried meat he had thrown and attempted to consume it. However, he quickly realized his mistake and spat it out, but not before some of the spores had entered his body. He then realized that the Chaga had healing properties and decided to leave it on the tree for the benefit of humanity (Rogers, 2012). In Anishinaabe culture, Chaga is considered a powerful medicinal mushroom and is often used in traditional medicine. Indigenous people also used Chaga mushrooms in pipe rituals because they produce a sweet smell (Zmitrovich et al., 2020). The legend of Wisakecak and the Chaga mushroom is a reminder of the importance of respecting the natural world and the role that traditional knowledge plays in understanding the benefits of natural remedies (Wasser and Volz, 2017).
In Northern Saskatchewan, the Denesuline people use two long strands of Chaga powder to predict the future. The Denesuline would sometimes lay out two rows of powdered Chaga, one for each possible future event. Each line was set on fire at the same time, and whichever one finished first was thought to determine what would happen next (Chung et al., 2010; Peng and Shahidi, 2022). Again, the Denesuline of Saskatchewan used a divination procedure with finely split intestinal fungus (Rogers, 2006). Each pile represented a separate event that occurred at the same time and is illuminated from different ends. The pile that burns through first determines what action takes place initially. “ETSEN DEK, ON”, or “it stinks while it is burning,” is the name given to this occurrence (Rogers, 2006).
According to historical records (Figure 3), the Khanty people of Western Siberia were the first to use Chaga medicinally, perhaps in the 12th century (Saar, 1991). The native Siberians would grind it up and add it to their everyday beverages, soups, and stews. Despite living in a difficult environment, the Siberians discovered that consuming chaga regularly protected against the beginning of degenerative diseases. They used it to increase vitality and live a long, healthy life. Modern Russians have noticed that cancer is not prevalent where the Chaga was traditionally utilized (Pilz, 2004). According to Moss, (2016), the Khanty tribe regularly consumed Chaga in several forms, including tea, “soap water,” and smoke (Moss, 2016). The tea was produced by slicing Chaga into tiny pieces with a knife, and the pieces were placed into boiling water and simmered for a few minutes. Chaga tea was consumed to aid in digestion, satiate appetite, and detoxify. In the 16th century, the Russian First Nations used Chaga to treat tumors related to angiogenesis. In Siberia, indigenous people used Chaga mushrooms to treat tuberculosis, liver conditions, and stomach diseases (gastritis and ulcers) (Shikov et al., 2014). In modern-day Russia, Chaga is used by hunters and foragers to increase their capacity to work, and to promote endurance (Shikov et al., 2014).
To make “soap water”, the fungus was first placed in the fire. After it becomes charred like smoldering charcoal, it was placed in a bucket of boiling water and swirled until it was broken up into little chunks. The black water that was produced because of this process has excellent cleansing and disinfecting properties (Lukina, 1975). Women who bathed themselves with such water were never sick, and the water was also used to cleanse the vaginal area after menstruation and childbirth. In earlier times, it was also used to wash the hands, feet, and sometimes the whole body in place of soap (Lukina, 1975). Saar (1991) reported that Chaga was also used for ceremonial washing and cleansing after menstruation. It was possible that a newly born child might also be washed as part of this ceremony (Shikov et al., 2014).
In northern America, Chaga is consumed as a tea or powder, inhaled, and smoked by the Indigenous people. “Posahkan” and “Wiskakecak” are the preferred names given by Cree healers due to Chaga’s healing virtues. The Métis also use Chaga for treating cancers of the breast, liver, colon, skin, cervix, and lungs, as well as for diabetes (Barkwell, 2018). Notably, in North America and especially in Canadian indigenous tribes including the Cree, Ojibway, Denesuline, and Gitksan, Chaga was used for the treatment and management of several diseases, including rheumatic and joint pain, infections, and tooth pain (Rogers, 2012). Additionally, Chaga mushroom tea was used by both the Ojibway and the Denesuline people as an antiviral agent. The Tanaina tribe in south-central Alaska in the United States of America also uses Chaga to treat toothaches (Turner and Cuerrier, 2022). The various uses are summarised in Table 1.
In China and Korea, empirical evidence suggests that for centuries, Chaga was traditionally taken as a tea to treat several ailments including pathogenic infections, gastrointestinal disorders, cancers, and liver disorders (Park et al., 2004; Géry et al., 2018; Gründemann et al., 2020). Similarly in Japan, Greece, and parts of eastern Europe, the mushroom has a history of use in folk medicine as a treatment for ulcers, gastritis, and tuberculosis (Cha et al., 2006a; Pan et al., 2013). It was recorded that the Grand Duke of Kievan Rus, Tsar Vladimir Monomakh, used Chaga to heal his lip tumors. Finnish soldiers used Chaga as a coffee substitute during World War II (Baek et al., 2018). When the troops’ supply of coffee ran short, they turned to the nearby woodlands for sclerotia, which they used to make Chaga tea.
According to Gorbunova et al. (2005), inhabitants of Siberia have long relied on Chaga infusions for medicinal purposes. Indeed, the fact that Befungin was the first Chaga product to be isolated and used in clinical therapy has been proven by Russian experts and is now known to be an effective treatment for psoriasis. It is worth mentioning that in the middle ages, the famed physician Avicenna experimented with the use of Chaga as a source of medicinal compounds (Ryzhova et al., 1997; Safin et al., 2018). Various traditional medicinal uses of Chaga around the world are summarized below (Figure 4).
Chaga mushroom usually grows on the bark of birch trees. This association matches the Cree legend that describes a type of scab growing along the trunk of birch trees (Rogers, 2012). Chaga has a texture similar to loose, rubbery wood or porous, crumbly rocks (Hobbs, 2003). The Chaga is not the fruiting body as seen in other mushrooms, it is the sclerotia that contains the mycelium. Chaga is a parasitic fungus that infects birch trees and looks like hard rock protrusions (Kim, 2005). Close examination using a microscope shows a brown hypha separated without pores as well as a wide partition with 2.five to seven um in diameter. Chaga arises through the action of basidiospores contaminating the duramen of birch trees through unhealed wounds (Brydon-Williams, 2019). Basidiospores, which are reproductive structures produced by the Chaga fungus, can enter the tree through these wounds and then grow and spread within the tree’s inner tissues, or duramen (Figure 5). Over time, the Chaga fungus can form large, woody growths or cankers on the tree’s surface, which are then harvested and processed for various uses (Thomas et al., 2020). The large woody growth is supported by root-like structures of the mushroom known as the mycelium. This structure grows inside the tissues of the tree and as it grows degrades the cell wall of the tree while simultaneously producing decay known as ‘white rot’ (Figure 5). The white rot degrades cellulose, hemicellulose, and lignin the major components found in the cell wall. By breaking down these materials, the Chaga fungus can extract nutrients from the tree and continue to grow and spread throughout its life (Deshpande and Arya, 2022).
The fruiting bodies of Chaga, which appear in the form of black, irregularly shaped masses, develop between the bark and the sapwood of the tree and turn yellow to brown over time. This transformation usually takes between 2 and 12 years after the death of the birch (Bernicchia, 2005; Géry et al., 2018). Although the fruiting body of Chaga is usually harvested, the vegetative component rather contains more proteins as compared to the fruiting body (Rogers, 2006). The sclerotia of Chaga contains up to 30% betulin, a compound that is also found in birch trees. This betulin is believed to be absorbed by the Chaga fungus from the birch tree, where it is present in the form of betulinic acid. In addition to betulin, the Chaga core contains a greater amount of lanostanes, which are triterpenoids that have been shown to have a variety of potential health benefits (Wold et al., 2018). Betulinic acid possesses biological activities such as anti-cancer, anti-inflammatory, antiviral, and antioxidant (Hordyjewska et al., 2019).
The high concentration of melanin in the sclerotia (the hard, black outer layer of the Chaga mass) gives it its characteristic black color (Stamets, 2005). The sclerotia contain up to 30% betulin, while the core contains a greater amount of lanostanes. However, the harvested part of the Chaga is bicolored, consisting of the sclerotia and a golden, cork-like interior. As the Chaga mass ages, a greater proportion of its mass becomes associated with the interior rather than the sclerotia (Figure 5) (Thawthong et al., 2014).
Mycochemical analysis of Chaga extracts revealed several essential primary metabolites including polysaccharides, proteins, and other compounds (Kim et al., 2011; Peng and Shahidi, 2022). As of 2022, more than 250 secondary metabolites have been identified in chaga including betulin, vanillic acid, terpenoids, and lanosterol. Rogers (2006) further reports that the fungus is made up of primary metabolites including minerals, proteins, and polysaccharides. Several macro and micro nutrients have been identified from Chaga extracts which include carbohydrates (β-glucans, xylogalactoglucose) (Joo et al., 2010; Jayachandran et al., 2017; Wold et al., 2018), lipids (fecostrol, episterol, β-sitosterol) (Cha et al., 2005; Cha et al., 2006b), polyphenols (inonoblins A, phelligridins D, ferulic acid, foscoperianol D, vanillic acid) (Lee et al., 2007; Seo and Lee, 2010).Some of these compounds are presented below:
Huang et al. (2012) extracted and purified five Inonotus obliquus polysaccharides (IOPS) by column chromatography, namely,; IOP1b, IOP2a, IOP2c, IOP4 and IOP3a (Huang et al., 2012). In one study, the authors suggest that the sugar, -(1.3)- β-D-mannan influences β-glucan properties (Baek et al., 2012). Recently, both β-glucan has been found to have a variety of health-promoting properties, including immune modulation, anti-inflammatory effects, and antioxidant activity (Zhu et al., 2015). Yeast, mushrooms, bacteria, and algae are all sources of a dietary fiber compound called β glucan. This compound is widely recognized as a polysaccharide found in Chaga mushrooms along, with galactomannan (Basal et al., 2021; Eid et al., 2021; Peng and Shahidi, 2022). Chaga contains 8.57% β-glucan (Song 2020).
According to a study, by Razumov et al. (2020), they found that chaga hydrolyzed products contain many amino acids with aspartic acid, glycine, and glutamic acid making up, around 40% of the amino acids. The remaining 60% consists of lysine, threonine, methionine, alanine, tyrosine, serine, histidine, proline, tryptophan, arginine, and cysteine (Razumov et al., 2020). Other forms of amino acids such as peptides have also been reported by Hyun et al. (2006). In the study, the author identified a new peptide that prevents platelet aggression. In the study, analysis of the peptide using LC/MS revealed a molecular mass of 365 Da (Hyun et al., 2006).
Chaga has been observed to have a high concentration of elements, with potassium accounting for 50% of the total ash content, sodium accounting for 9%–13%, and manganese accounting for 1.2% (Shashkina et al., 2006). Other minerals such as copper, zinc, aluminum, sulfur, magnesium, phosphorus, and calcium have all been identified in chaga (Razumov et al., 2020).
Chaga also contains a significant number of mineral microelements. In their research, the scientists used x-ray fluorescence and atomic absorption spectroscopy to analyze chaga and determine its composition of macro and micro elements. Their findings revealed that chaga contains amounts of iodine, vanadium, zinc, copper, selenium, manganese, and sulphur (0.02%) rubidium (approximately 0.04%) sodium (approximately 0.05%) phosphorus (approximately 0.23%) chlorine (approximately 0.33%) calcium (approximately 0.37%) nitrogen (approximately 0.4%) magnesium (approximately 0.64%) hydrogen (around 3.6%) potassium (around 9 10%) and carbon constituting approximately 39%. (Razumov et al., 2020). These findings suggest that Chaga mushrooms and their derived products possess essential nutrients that are beneficial for crop cultivation (Peng and Shahidi, 2022).
Based on several studies of crude Chaga extracts, numerous bioactive compounds have been isolated and purified (Table 2).
Six polyphenols were recovered from Chaga mushrooms following extraction with methanol: inonoblins A, B, C, and phelligridins D, E, and G (Table 1) (Lee et al., 2007). Particularly, chaga contains a variety of phenols, including 4-hydroxy 3,5 dimethoxy benzoic acid, 2-hydroxy-1-hydroxymethyl ethyl ester, protocatechuic acid, caffeic acid, 3,4 dihydroxy benzene formaldehyde, 2,5-dihydroxyterephthalic acid, syringic acid and 3,4-dihydroxybenzalacetone (Nakajima et al., 2007; Nakajima et al., 2007). Additionally, gallic acid and dihydroxy benzoic acid ketones (DHBAs) have been found in Chaga. Show potential in their ability to combat cancer (Kim et al., 2011). Melanins are also generated by Chaga, which exhibit antioxidant and genoprotective properties (Shashkina et al., 2006). Babitskaya et al. (2000) demonstrated that copper ions, catechol, and tyrosine effectively promote the production of melanin, and o- and p-dephenoloxidase. The acid composition of Chaga includes acetic acid, butyric acid, oxalic acid, and formic acid (Razumov et al., 2020). Chaga extract also contains triterin, sterols (6%–8%), acid-resistant lignin (25%–30%), dietary fiber (2%), hemicellulose (12.5%), and folic acid (Shashkina et al., 2006).
Zheng et al. (2009) conducted a study to extract phenolic components from I. Obliquus by using fermentation. They used continuously stirred reactors to investigate how phenolic compounds accumulated in various cultures and their antioxidant properties. According to the study, Chaga demonstrated the production of melanin and polyphenols in the control medium. The introduction of H2O2 did not have an impact on the levels of phenols. It did increase the amount of phenol and melanin inside the cell. When Chaga was exposed to both H2O2 and arbutin there was an increase in phenol synthesis while extracellular phenol accumulation decreased. These findings suggest that incorporating an oxidizing agent into Chaga mycelia could boost the production of compounds such, as vanillin, salicylic acid, pyrocatechol, hydroquinone, and eugenol. This makes Chaga a more dependable source of pharmaceutically essential phenolic compounds.
Chaga contains numerous flavonoids, such as flavones, flavanones, anthocyanins, and catechins (apigenin, mariningin, corin, and quercetin) (Shashkina et al., 2006). As of 2022, a total of 31 flavanoids were detected in a Chaga sample (Peng and Shahidi, 2022). When the phenolic contents of mycelia and wild chaga culture were compared, flavonoids were detected in small quantities in wild Chaga, while other phenols such as melanins and styrylpyrones were present in larger quantities. In contrast, the mycelia cultures contained more flavonoids with trace quantities of melanin and styrylpyrones (Zheng et al., 2008). The Chaga mushroom contains a unique class of phenolics known as styrylpyrones (Peng and Shahidi, 2022). A total of 16 styrylpyrones have been detected previously, including phelligridin C, inoscavin C, hispidin, methylinoscavin C, davallialactone, inonoblin C, davallialactone, inonoblin B, phelligridin E, phelligridin D. Newly detected compounds included: inoscavin A, inoscavin D, phelligridin J, phelligridin A, phelliribsin A, methylinoscavin D.
Peng and Shahidi (2022) further detected several flavonoid derivatives such as apigenin, eriocitrin, rhoifolin, isorhamnetin-3-O-rutinoside, epigallocatechin, and epigallocatechin. However, other non-flavanoid phenolics such as inonoblin A, phelligridin, resveratrol, and phellxinye A were absent. In the same study, the 31 detected flavonoid derivatives included 18 flavones (ols), 5 flavanones (ols), 3 flavans (ols), aurones, isoflavones, ligniflvanoids and chalcones (Razumov et al., 2020).
Steroids are another bioactive component of Chaga (Wang et al., 2014), including fungal sterols (Kirk et al., 2008; Chen and Wang, 2014), ergosterol (Shin et al., 2000; Huynh, 2019), ergosterol peroxide (Zheng et al., 2011a; Kim et al., 2011), and β–sitosterol (Table 1). Wang et al. (2014) found that (0.01 and 0.1 g/L) dosages of extracts from birch bark and birch core had a substantial stimulatory impact on the generation of I. obliquus steroids in submerged Chaga cultures (p = 0.05). The aqueous extract (0.01 gL) of birch bark stimulated 97.3 percent greater production than the control (i.e., 225.8 mg/L). Although these extracts promoted both mycelial growth and steroid content, only methanol extracts enhanced the synthesis of betulin, cholesterol, lanosterol, stigmasterol, and sitosterol. Another study also demonstrated that birch bark extract may stimulate the synthesis of steroids in Chaga, which have anti-tumor and anti-inflammatory properties (Debnath et al., 2013).
In the study by Peng and Shahidi (2022), a total of 108 terpenoids were identified in chaga. Seventy-eight of these terpenoids were newly detected. Apart from terpenoid compound T108, all terpenoids included substituent groups with multiple oxygens, non-oxygenated derivatives. Apart from sesquiterpenoids, monoterpenoids, diterpenoids, tetraterpenoids, pentaterpenoids, the remaining 103 terpenoids were steroids and triterpenoids. Chaga contains esters of triterpenoids including hydroxycinnamoyl indicating the presence of impure terpenoids. However, in contrast to phenolic compounds, the high molecular weight of terpenoid compounds makes classification by structure challenging due to the possibility of having several isomers (Peng and Shahidi, 2022).
According to several research studies (Figure 6), Chaga mushrooms possess around 130 pharmacological properties including anticancer, immunomodulating, gene-protecting, and antimicrobial capabilities (Chung et al., 2010; Thomas et al., 2020; Kou et al., 2021). The details of some pharmacological activities are discussed below.
Chaga extract is widely recognized for its properties and multiple studies have shown its ability to counteract the effects of free radicals (Zheng et al., 2011b; Haines, 2013). In one study, to examine Chaga’s ability to operate as a superoxide dismutase (SOD) in scavenging radicals, the researchers utilized alcohol and three distinct hot-water extractions (each with a different temperature). According to Hu et al. (2009), their research revealed that the alcohol extract exhibited SOD activity, among all their investigations. On the hand, hot water extracts also showed SOD activity with higher temperatures resulting in stronger antioxidant abilities (Hu et al., 2009). Additionally, these researchers evaluated the four extracts’ capacity to scavenge 2,2 diphenyl 1 picrylhydrazyl (DPPH). Interestingly the alcohol extract displayed a higher DPPH scavenging ability as compared to hot water extracts (Hu et al., 2009).
Notably present in Chaga extracts is hispidin—a compound, with antioxidant and inflammatory properties. However, Chaga extracts’ composition can vary based on the source of the fungus, the extraction procedure, and the section extracted (Lee and Yun, 2006; Yusoo et al., 2002). Among the hispidin analogs are phelligridins, inoscavins, inonoblins, and davalialactone and its derivatives (Zheng et al., 2011b). These analogs contain the active ingredient that confers antioxidant action on the drug and is used to treat disorders associated with oxidative stress. In other studies, Giridharan et al. (2011) investigated the impact of the antioxidant activity of Chaga extract on the cognitive function of mice with amnesia. Researchers studied its effects on the brain and cognition after using the alkaloid scopolamine to induce cognitive impairment. In both tests, Chaga increased glutathione and superoxide dismutase levels (endogenous antioxidants), and improved learning and memory. The antioxidant properties of Chaga have gained recognition, for their ability to combat cancer promote heart health and help manage diabetes (Zhong et al., 2009; Song et al., 2013; Jiang et al., 2020). Moreover, findings demonstrate that Chaga extracts possess antioxidants that can effectively counteract radicals suggesting its potential in preventive and healing capacities, for various diseases (Mu et al., 2012). Song et al. (2004) examined NF-kB and antioxidant activity in malignant human keratinocytes (SCC-13) to determine whether pine bark extract, Chaga (Inonotus obliquus), and Chaga mycelium were effective. The activity of NF-BNF-B was downregulated in every substance that was evaluated using a cell-based NF-BNF-B monitoring assay. Using AGI-1120 (one to two mg) as the positive control and Chaga mushroom extract (0.05–0.1 mg), demonstrated a significant inhibitory effect on NF-BNF-B activity which implies that the Chaga mushroom extract was successful in scavenging DPPH radicals.
The extracts, from Inonotus obliquus known for their inflammatory properties, have gained popularity in countries like China, Korea, Japan, Russia, and the Baltics. When it was initially discovered in the mid-20th century it was utilized in the treatment of malignancies and digestive issues with no effects (Szychowski et al., 2021). Research has indicated that Chaga extract possesses inflammatory properties. Macrophages can release substances such, as nitric oxide, prostaglandin mediators, and pro-inflammatory cytokines (TNF α, IL 1 β IL 6) (Im et al., 2016). A study on the methanol and ethanol extracts of Chaga has shown that they inhibit macrophage activity by reducing the production of inflammatory mediators such as nitrogen oxides, prostaglandins (PGE2), and certain cytokines (Softa et al., 2019). According to a study conducted by Mishra et al. (2012), it was found that aqueous Chaga extracts have the potential to greatly alleviate effects caused by dextran sodium sulfate (DSS). These effects include reducing edema and mucosal damage and minimizing crypt loss. Additionally, the study revealed that Chaga extracts can effectively suppress the expression of inflammatory cytokines lower the levels of iNOS induced by DSS, and reduce the accumulation of myeloperoxidase in the colon (Mishra et al., 2012). The anti-inflammatory properties of Chaga associated with isolated ergosterol, ergosterol peroxide, and trametenolic acid were further confirmed by Kou et al. (2021). Ishfaq et al. (2022), demonstrated that aqueous extract of chaga possess anti-inflammatory chaacteristics. The study further demonstrated the ability of the extract to suppress expression of p53, caspace-3 and microcystin-LR known to cause inflammation and toxicity to the liver in mice (Ishfaq et al., 2022). In another study, Ishfaq et al. (2021), demonstrated that aqueous extract of chaga suppressed carbon tetrachloride (CCl4) induced damage in liver tissues in mice. The study also showed that ergosterol peroxide isolated from chaga has the ability to bind and inhibit activity of pro-inflammatory proteins (Ishfaq et al., 2021).
Betulin and betulinic acid have shown promising potential as antimicrobial and anti-inflammatory agents. With anti-inflammatory activities, these compounds are reported to modulate the activities of immune cells and prevent pro-inflammatory production (Oliveira-Costa et al., 2022). This, therefore, demonstrates its potential ability for the treatment of inflammatory ailments.
Ma et al. (2013) evaluated the anticancer properties of chaga using bioassay-guided preparative isolation. Acetate and petroleum ether extracts significantly reduced NO generation and NF-kB luciferase activity in macrophage RAW 264.7 cells and induced cytotoxicity in vitro in human prostate cancer PC3 and breast cancer MDA-MB-231 cells. Inotodiol, ergosterol peroxide, and trametenolic acid were recovered from these two fractions. Both ergosterol peroxide and trametenolic acid were cytotoxic to the human prostatic carcinoma cell PC3 and the human breast cancer MDA-MB-231. These findings may help in understanding Chaga’s anti-cancer activity. Different cancers, including Walker 256 carcinosarcoma, MCF-7 human breast adenocarcinoma, sarcoma 180, and carcinoma 755, are sensitive to the effects of lanolin triterpenoids isolated from Chaga (Shin et al., 2000). Additionally, hot water extracts of Chaga were shown to have anticancer activity against human colon cancer cells HT-29. The main mechanisms involved in this process were increasing the levels of proteins that promote cell death and decreasing the levels of proteins that prevent cell death. This was shown in a study conducted by (Heo et al., 2017). Another study by Mizuno et al. (1999), found that purified endopolysaccharide from cultivated mycelia of Chaga suppressed tumor cell proliferation and activated B cells and macrophages. Moreover, Chaga mycelium, had an effect, on cdc25 phosphatase, an enzyme that regulates the cycle of cancer cells. In a study using Sepharose, researchers found that the drug contains a water-soluble polysaccharide (ISP2a), which showed antitumor activity in vivo and significantly enhanced the immune response in tumor mice. As an added benefit, ISP2a enhanced lymphocyte proliferation and increased the production of TNF-α (Liuping et al., 2012). Satoru et al. (2016) sought to explore whether Chaga water extract might be utilized as a long-term cancer therapy. Chaga water extracts were extremely anti-blastema active in the treatment of cancer. A study published in 2008 by Youn et al. (2008) indicated that Chaga water extract inhibits the growth of cancer cells in vitro and triggers apoptosis (programmed cell death, in which the cell breaks down into distinct apoptotic bodies) in various carcinoma cells.
Particularly betulinic acid has demonstrated promise in cancer research as it has demonstrated potential in shrinking cancer growth cells, inhibiting apoptosis, and shrinking tumors (Zhang et al., 2015). Other researchers, attest to its anticancer activity and demonstrate its selective antagonistic activity towards cancer cells (Saha et al., 2015; Kumar et al., 2018).
In 2015, a study examined the anti-cancer activity of ergosterol peroxide isolated from chaga on colon cancer cells. The study demonstrated that the compound increased apoptosis and suppressed cell growth in colon cell lines. The compound further inhibited the β-catenin signaling pathway, a major player in colon cancer development (Kang et al., 2015). Similarly, Mishra et al. (2013) found that aqueous extract of chaga expresses anti-proliferative and anti-inflammatory activity on colon cancer cells and tumors in mice. The study also demonstrated that extracts suppresses the Wnt/β-catenin and NF-κB signaling pathways that are responsible for colon cancer onset and development (Mishra et al., 2013).
For anti-viral properties, studies report the inhibitory properties of bioactive compounds in Chaga against a host of viruses including hepatitis C, HIV, and herpes simplex virus make them attractive candidates for antiviral therapies (Navid et al., 2014; Paduch and Kandefer-Szerszen, 2014; Xiao et al., 2018).
Satoru et al. (2016), shows that Chaga extracts have been found to impede the replication of both hepatitis C virus and human immunodeficiency virus (HIV). The intriguing potential benefits of Chaga, in treating diseases have captured the attention of researchers. Jin et al. (2017) investigated the antiviral effects of Chaga extracts on feline viruses. They proved that Chaga therapy was effective in cell assays and had minimal cytotoxicity by using feline calicivirus cell models. According to research on adhesion’s mode of action, the treatment of calicivirus causes an inhibitory effect on viral particles by preventing viral binding and absorption. Research has shown that cats may experience gastrointestinal issues due, to the herpes virus panleukopenia virus, and infectious peritonitis virus. These viruses have a range of activities. In this study, scientists discovered that Chaga polysaccharides could potentially serve as an antiviral treatment, for both feline and human pathogens. Moreover, researchers from Poland have shown in 1998 that botulin and betulinic acid found in Chaga can stop blastema development (Liang et al., 2009). They discovered that betulin and betulinic acid are now potential anti-HIV medicines that inhibit HIV reverse transcriptase, in turn inhibiting HIV type 1 (Gogineni et al., 2015). Human influenza A and B and horse influenza A are also inhibited by constituents of the black exterior surface of Chaga (Zheng et al., 2010). Betulin, mycosterol, and lupeol, which are all found in mushrooms, are thought to be the primary antiviral agents (Pan et al., 2013). Polkovnikova et al. (2014), examined the antiviral efficacy of Chaga extract on Vero cells using Vero cell cultures and HSV (herpes simplex virus) type 1 infected Vero cells. Most of the HSV-infected Vero cells were protected by non-toxic sub-components. Deletion of viral DNA in Chaga-infected cells suggests that it protects Vero cells from HSV cytotoxicity.
More recent research by Eid et al. (2021) has shed light on the impact of Chaga on the novel coronavirus (SARS-CoV-2) that has been responsible for widespread outbreaks around the globe. In this study, the S1-carboxy-terminal domain of the SARS-receptor-binding CoV-2 domain was discovered to be closely related to -glucan, galactomannan, and betulinic acid. At the TRP-436, ASN-437, and ASN-440 sites, this interaction was substantial. The receptor binding domain of the most recent SARS-CoV-2 isolates had a furin cleavage site that was not seen in prior isolates. Since this response interacted with ACE-2 more often, this virus was able to infect more people. Chaga, according to those who took part in the research, might be used in conjunction with other medications to combat SARS-CoV-2. Hence, future anti-SARS-CoV-2 therapeutic development may be aided by the development of naturally derived anti-coronavirus therapies that include Chaga (Eid et al., 2021).
Platelet aggregation is a complicated event that is most likely the product of several metabolic pathways working in concert. The use of platelet inhibition in the prevention of thrombosis is a potential new treatment. According to a report by Hyun et al. (2006), water and ethanol extracts from 55 different kinds of mushroom mycelium or fruiting bodies were tested for platelet aggregation inhibitory activities in vitro. The ethanol extracts of Chaga ASI 74006 mycelia demonstrated the highest platelet aggregation inhibitory activity (71.2%), whereas the impacts of extracts from its fruiting bodies had very low inhibitory activity. The isolated peptide was found to have a strong inhibitory activity of 91.6% and a low molecular mass of 365 Da. It is assumed that the peptide from Chaga which inhibits platelet aggregation is quickly absorbed in the gut, and is commonly employed in the creation of antithrombotic medications and nutritional supplements for human consumption (Huang et al., 2012).
Researchers have found that Chaga mushroom extract can lower blood sugar (Rogers, 2012). The effects of Chaga on diabetes were evaluated by Xu et al. (2010). Mice with diabetes were given an extract of the polysaccharide fraction of Chaga, which reduced maleic dialdehyde activity. After histological morphological examination, the study revealed that the Chaga extract significantly reduced damage to their injured pancreatic tissues (Xu et al., 2011). The polysaccharides derived from Chaga have shown properties in combating high blood sugar levels and lipid oxidation (Xu et al., 2011; Hu et al., 2012). Another study investigated the chemical composition and blood sugar-lowering effects of the ethyl acetate fraction extracted from Chaga revealing its hyperglycemic and anti-lipid peroxidative actions, in mice with diabetes induced by alloxan (Lu et al., 2010). Postprandial hyperglycemia is closely associated with type 2 diabetes mellitus and its complications. The research demonstrated that an acidic protein-bound polysaccharide called IOPS derived from Chaga effectively inhibits the activity of β glucosidase at a concentration of 93.3 g/mL. Additionally, Chen et al. (2010) discovered that it also suppresses stress and the generation of compounds reactive to acid during Fe2+/ascorbate induced lipid peroxidation in rat livers (Chen et al., 2010). Exploring substances as inhibitors of β glucosidase could potentially lead to the development of foods or promising compounds, for combating diabetes (Chen et al., 2010). These findings have the potential to contribute to the development of safe inhibitors of β glucosidase. These inhibitors can be derived from sources making them a promising choice, for creating foods or lead compounds in anti diabetic treatment.
In a study by Kyoung et al. (2004), human lymphocytes were subjected to oxidative DNA damage, and an aqueous extract of Chaga was tested for its protective effect. One-cell electrophoresis for DNA fragmentation was used to detect oxidative damage in the test subjects’ bodies (comet analysis). The DNA fragmentation in cells that were pre-treated with Chaga extract was reduced by more than 40% compared to cells that had been treated with the positive control (100 micron/mole H2O2).
It has been shown that Inonotus obliquus polysaccharides (IOPS) may boost the immune system and reduce oxidative stress throughout the growth process (Youn et al., 2008). However, more studies are required to understand the impact of IOP, on genotoxicity in model organisms. Liuping et al. (2012) conducted experiments where they exposed embryos (12 h post fertilization) to UVB radiation (12 J/m2/s, 310 nm) for 10 s and then administered IOP therapy (2.5 mg/L) after 24 h post fertilization continuing for a duration of, up to 7 days (Liuping et al., 2012). Crimson, orange staining, the alkaline comet test, and qRT-PCR screening of DNA repair genes were used to evaluate genotoxic effects. The IOP-treated zebrafish were exposed to UVB at 5 days post-fertilization and subsequently exhibited a substantial decrease in DNA damage and improvement of the distorted structures. The relative mRNA expressions of RAD51, P53, and GADD45 dramatically increased in IOP-treated UVB-exposed zebrafish. DNA repair genes were shown to be coordinated in their response to UVB exposure, indicating a communal response. Finally, the IOP therapy reduced UVB-induced genotoxic effects on zebrafish embryos, allowing them to grow normally (Liuping et al., 2012).
Because of the lack of information on probable interactions between various dietary supplements, there is rising concern about negative effects. For the first time, Živković et al. (2019) investigated the genotoxic effects of Chaga and dihydroquercetin in combination. When Chaga (250 g/mL) and dihydroquercetin at 100 g/mL, 250 g/mL, and 500 g/mL were administered alone or together, no genotoxic impact was found on whole blood cells. The comet test was used to investigate the antigenot1oxic effectiveness against DNA damage caused by hydrogen peroxide (H2O2) in whole blood cells. Dihydroquercetin alone did not affect H2O2-induced DNA damage in cells, whereas the combination of Chaga and 500 g/mL dihydroquercetin was most effective. Hydrogen peroxide-induced oxidative damage to genomic material may be prevented in vitro by taking Chaga and dihydroquercetin together. Table 3 is the summary of the established literature report on the pharmacological potentials of Chaga mushrooms.
Melanin, a pigment, in the skin plays a vital role in shielding the skin from harmful UV radiation (Kukulyanskaya et al., 2002; Tsatmali et al., 2002). However, when exposed to UV light and other triggers the skin naturally produces melanin as a protective response (Yan et al., 2014). In other studies, two naturally occurring chemicals, birch sap from Betula alba and organic extracts from Chaga, were investigated for their potential to block the Sun’s ultraviolet (UV) rays (Alhallaf, 2020). Both pure birch sap and Chaga extracts, as well as a combination containing 5% organic birch sap and 5% Chaga, were tested.
The birch tree sap derived from Betula alba and Chaga proved effective, in reducing the production of cytokines when the skin was exposed to UV light. Keratinocytes are shielded from the DNA damage caused by UV light by extracts of birch sap and Chaga mushrooms. These findings demonstrate for the very first time that extracts of Chaga and Betula alba mushrooms shield skin cells from the damaging effects of UVA and UVB radiation because of their antioxidant, anti-inflammatory, and DNA-preserving and repairing properties. Moreover, they have the potential to be used in anti-aging and sun-screening cosmetics (Softa et al., 2019).
Based on a study’s findings, Song et al. (2004) concluded that Chaga and Chaga mycelium may inhibit NF-BNF-B in human skin (Figure 7). Chaga possesses antioxidants, like melanin and hispidin analogues (polyphenols) along with dismutase and catalase. This makes it a promising ingredient in the field of dermatology for addressing signs of aging such as wrinkles, sagging skin, and graying hair. Oxidative stress is believed to be a contributing factor to these manifestations of aging. Excessive free radicals resulting from sunlight exposure and other oxidative damage can lead to accelerated skin deterioration due, to the body’s inability to effectively neutralize them (Song et al., 2013). More antioxidants in the body might theoretically delay or even halt the aging process. The efficiency of Chaga in combating other kinds of oxidative stress implies that it may also combat aging, although no clear study has connected it to anti-aging effects (Géry et al., 2018).
Chaga sulphur soap is manufactured in South Korea, where it is said to have anti-aging and hydrating effects, among others (Ţura et al., 2018). Sagayama et al. (2019) conducted cell proliferation experiments to assess the impacts of Mongolians using Chaga sclerotium to wash their hair traditionally. They isolated five lanostane-type triterpenes (1–5) from human hair follicle dermal papilla cells (HFDPCs). These triterpenes outperformed the positive control, minoxidil, for their pro-proliferative effects on HFDPCs.
Yan et al. (2014) investigated the potential of Chaga to reduce melanin pigmentation and explore its use as a skin whitening ingredient in cosmetics. Specifically, they focused on tyrosinase, an enzyme, for stimulating production. It was discovered that the tyrosinase enzyme was inhibited by betulin and trametenolic acid, but other compounds such as inotodiol and lanosterol activated tyrosinase and increase pigment synthesis in laboratory cells. Pigment reduction may assist people with darker patches to achieve a more even skin tone, whilst pigment activation may be beneficial for those who have lost or decreased their pigment. It seems that Chaga has great potential as a cosmetic agent, and further studies are needed to determine how beneficial the various components may be for the cosmeceutical industry.
Chaga produces ligninolytic enzymes that act as biocatalysts in oxidoreductase processes such as degrading lignin and other parts of the cell wall (Dashtban et al., 2010). Particularly, ligninolytic peroxidase degrades polymers and other complex components in an oxidative process, splitting the entire lignin structure (Silvat, 2013). In a similar fashion, the enzyme degrades polysaccharide compounds in hemicellulose and cellulose. By breaking down these materials, Chaga can access the nutrients present in the wood and utilize them for its growth and survival (Cajthaml and Svobodová, 2012).
This ability of Chaga’s enzymes to degrade lignin and other woody materials has attracted interest for potential applications in various industries. One such application is bioremediation, where these enzymes play a role in the cleanup of polluted environments. By breaking down lignin and other organic pollutants, such as pesticides, dyes, and industrial chemicals, these enzymes contribute to the degradation of toxic compounds, leading to the remediation of contaminated sites and a reduction in environmental pollution (Singh et al., 2021). Another notable application lies in biofuel production. In one study, lignocellulosic biomass, including wood and agricultural residues, was reported to be beneficial as a feedstock for biofuel production. Ligninolytic peroxidase enzyme hydrolyzed the lignocellulosic materials, breaking them down into simpler sugars. These sugars were then fermented to produce biofuels like ethanol. By facilitating the degradation of biomass, these enzymes present a promising avenue for the advancement of biofuel technologies (Ilić et al., 2023).
In the paper and pulp industry, these enzymes have been reported to provide a more environmentally friendly alternative to the conventional pulping and bleaching processes. The enzymes activate the bond between ink and paper particles through hydrolysis which is subsequently removed via a flotation technique (Lee et al., 2013).
Traditionally, these industries rely on harsh chemicals and energy-intensive methods (Kaur et al., 2020). However, the enzyme was found to selectively degrade lignin, increasing the efficiency of the pulping process while reducing the dependence on chemicals. Furthermore, these enzymes can be employed in the bleaching process, minimizing the use of chlorine-based bleaching agents that pose harm to the environment (Singh et al., 2021).
Chaga enzymes have also shown potential in the textile and detergent industries. As bio-catalysts, they can be utilized in the processing of natural fibers like cotton and hemp (Pavithra et al., 2023; Asemoloye et al., 2021 reported that by effectively removing lignin and impurities from these fibers, the ligninolytic peroxidase enzyme contributed to the production of cleaner and higher-quality textiles. In the detergent industry, Chaga enzymes were found to enhance the efficiency of laundry detergents by aiding in stain removal and facilitating the degradation of organic matter (Asemoloye et al., 2021).
Aside from the pharmacological advantages of Chaga, there have been a few discoveries regarding its use in crop and animal husbandry. However, despite Chaga’s popularity and potential for use in agriculture, this application of the fungus has not been subjected to a rigorous scientific review. Hence, this part of the review will focus on Chaga and its prospective uses in agriculture (Figure 8).
The use of these mushrooms as lignocellulosic materials were conceptualized more than a century ago by Falck (1902), who proposed their use for improving agricultural waste and subsequently animal nutrition. After almost a century, several newer studies also focus on improving lignocellulosic materials for fodder (Lindenfelser et al., 1979; Hadar and Cohen-Arazi, 1986). This includes investigating the underlying biochemical mechanisms of the fungal degradation of agricultural waste. Studies have also considered the lignocellulose complex in straw and plant residues, and the reaction mechanisms connecting lignin polymers and hydrolytic enzymes such as cellulases and hemicelluloses (Hadar et al., 1992; Honda et al., 2000).
Antibiotics have become a major aspect of livestock production, especially in developed countries (Rathgeber et al., 2008). The initial evidence, reported by Moore et al. (1946) showed the benefits of administering doses of antibiotics to chickens (Moore et al., 1946). Their study indicated that the inclusion of streptomycin improved the growth rate of the chicks (Moore et al., 1946). Chaga mushrooms contain a concentration (8.57%) of β glucan which is recognized for its immune system support properties and has been shown to improve the growth rate of fowls (Chandrasekaran et al., 2011). Currently, few studies conducted to confirm that incorporating β glucan, into animal feed has an overall impact, on the system and the growth of livestock.
Several studies have shown that the addition of β-glucan to animal feed positively affects the immune system and the growth of farm animals. One study, in particular, demonstrated that broiler feed supplemented with β glucan increased the weight of the broilers significantly as compared to broilers given feed without supplemented β glucan (Chae et al., 2006). Rathgeber et al. (2008) conducted studies that revealed β glucan to be equally effective, as virginiamycin, in promoting the growth of broiler chickens. Similarly, Dritz et al. (1995) demonstrated that the addition of β glucan improved the growth of nursery pigs while incorporating Chaga into their diet enhanced piglet growth and facilitated weight gain. Not are β glucans utilized in the food and pharmaceutical industries as anticancer and immune-modulating agents. They are also employed within the aquaculture and livestock sectors to enhance animals’ innate immunity (Jung et al., 2007; Zhu et al., 2016). According to (Jung et al., 2007), β-glucan from Paenibacillus polymyxa JB115 can be added to animal feed to enhance immunity and may act as an anticancer agent in livestock. Additionally, rainbow trout (Oncorhynchus mykiss) were fed β-glucan pellets, increasing the secretion of specific antibodies and Ig levels in serum (Skov et al., 2012). Owing to the emergence of new diseases and government efforts to prohibit antibiotics that promote growth while enhancing the efficacy of commercial agriculture, new opportunities for natural, highly effective, and inexpensive immunomodulators like Chaga mushrooms can compensate by inducing and enhancing disease resistance while reducing losses. Moreover, these mushrooms produce significant quantities of β-glucan that can be used in feed supplements (Cueno et al., 2004). These supplements can improve immunity, reducing the excessive use of antibiotics. This has been confirmed by the immunostimulation of fowl after being fed with Pleurotus ostreatus, which considerably improved without affecting the size or quality of the meat (Li et al., 2006; Zhang et al., 2008).
In addition, these medicinal mushrooms have been investigated extensively as prospective materials for improving the quality of agricultural lignocellulose waste (Bogan et al., 1999). In one study, Chaga mushroom helped to breakdown lignin, releasing cellulose that can be used by ruminants (Kamra and Zadražil, 1986). This breakdown mechanism was linked to the ligninolytic system of the mushroom made up of oxidative enzymes. In another study, the direct use of mushrooms as lignocellulosic residues in animal feed played an important role in the diet of ruminants (Streeter et al., 1982). Studies have also examined the use of Pleurotus spp in breaking down wheat straw under different conditions and substrate treatments (Zadrazil, 1997; Salmones et al., 2005; Khan et al., 2013; Muswati et al., 2021). A significant increase in digestibility of wheat straw was observed after treatment with the mushroom. Similarly, Hadar et al. (1992) found that the lignin constituents in wheat straw significantly decreased during 4 weeks of treatment with P. ostreatus. In vitro digestion also increased and the product was degraded by up to 40% in animals’ diets (Hadar et al., 1992).
A major barrier to the upgrade of straw to fibre is the expensive materials and equipment needed for the preparation of fungal substrates (Hüttermann et al., 2000). Hüttermann et al. (2000) described a new process for degrading agricultural wastes using Pleurotus spp. This included using solar heating for pasteurization, and treatment using detergents, tomato pomace, and potato pulp. These methods generated positive results in on-farm applications (Hüttermann et al., 2000). Moreover, in both in vitro and in vivo studies, Pleurotus spp. significantly improved the availability of roughage in animal diets due to its action on cellulose and lignin (Van Kuijk et al., 2015). In a feeding experiment by Hüttermann et al. (2000), rams fed with mushroom-treated straw increased in body weight. At the same time, the treated group demonstrated an increase in nutritive value (Hüttermann et al., 2000).
In a study by Nwafor et al. (2022), a significant increase in the mineral composition of animal feed produced from agro -waste was observed after treatment with extracts of mushrooms. Its amino acid and carbohydrate contents also increased significantly as compared to the positive control, confirming the potential for mushrooms to improve the nutritional composition of animal feed (Nwafor et al., 2022).
Boulet and Bussières (2018) mentioned that an arborist in Quebec employed Chaga powder and paste to treat beech tree blight caused by Cryphonectria parasitica. The incision healed over 2 years, and the trees became immune to blight. This means that Chaga might be used as an inoculation or crop-protection strategy for other tree species. Chaga is also used as a fertilizer to protect cultivated plants from Phytophthora, and as a plant growth stimulant to promote development (Shashkina et al., 2006). Some Russian researchers, especially P. A. Yakimov and others (Bulatov et al., 1959; Shashkina et al., 2006; Sysoeva et al., 2012), conducted detailed investigations of Chaga and its concentrated extracts along with those of other porous fungi about a century ago (Bulatov et al., 1959). The chemical composition of Chaga is significantly different from other polypores. An X-ray fluorescence approach, atomic absorption spectrum, and gravimetric analysis demonstrated that Chaga contains the following: carbon (39%), potassium (9%–10%), hydrogen (3.6%), nitrogen (0.4%), magnesium (0.64%), calcium (0.37%), chlorine (0.33%), phosphorus (0.23%), sodium (0.04%). The nitrogen content of Chaga is primarily part of proteins. The products of hydrolysis exposed 15 different amino acids, the most prevalent of which were glycine, aspartic, and glutamic acids (accounting for approximately 40%), as well as tyrosine, serine, threonine, leucine, methionine, lysine, and histidine (Shashkina et al., 2006).
Invitro studies have shown these mushrooms to be useful in the degradation of industrial dyes, phenols, soil decontamination, and wastewater treatment. Yet, no further analysis such as in situ tests has been done to further confirm these claims (Irie et al., 2001). Roy et al. (2015) investigated the improvement of plant health for Capsicum annuum L by treating it with spent mushroom substrate from button and oyster mushrooms. After several weeks of treatment, growth parameters such as height, branches, and yield improved significantly (Roy et al., 2015; Somnath et al., 2015). Apart from that, the substrate played a role in reducing soil phosphate and increasing root and leaf phosphate. The c9hlorophyll and carotenoid content of the leaves also significantly increased following soil treatment with the mushroom substrate (Roy et al., 2015).
Currently, mushroom substrates are recognized as inexpensive, ecologically friendly sources of organic fertilizers. Features such as high moisture, nutrient retention, high air permeability, loose texture, and rich pellet structure are known to improve soil structure and maintain a beneficial environment for soil microorganisms. Among the numerous applications of mushrooms to crop farming, the use of spent mushroom substrate remains the most effective and economical (Lou et al., 2017). The spent substrate, from mushrooms contains an amount of matter and essential nutrients like nitrogen, phosphorus, and potassium. These elements play a role in promoting plant growth. Through the utilization of mushroom substrate, as a soil amendment, farmers can enhance the quality and fertility of their soil without having to rely on synthetic fertilizers (Esmaielpour et al., 2017). Secondly, the use of spent mushroom substrate can help to reduce waste and save resources. After the mushrooms are harvested, the remaining substrate can be recycled and used as a soil amendment instead of being disposed of as waste (Cunha Zied et al., 2020). Third, the production of spent mushroom substrate is relatively low-cost compared to other types of organic amendments, such as compost or manure. This is because the substrate is already produced as a byproduct of mushroom cultivation, so there are no additional costs associated with its production (Meng et al., 2018).
Another study demonstrated that treatment with beneficial microorganisms improved the biological and nutritional activity of the substrate thereby improving soil structure, efficiency, quality, and ecological function (Mohd Hanafi et al., 2018). In another study, after composting, elements such as phosphorus, potassium, and nitrogen increased after treatment with the mushroom (Akdeniz, 2019; Sánchez, 2010). Moreover, during the composting process, mushroom substrates diminished the function of pathogenic microorganisms and by extension plant diseases, while supplying these essential nutrients.
Other studies have demonstrated the benefits of combining mushroom substrates with organic waste or sludge (Meng et al., 2018; Meng et al., 2019). In another study, substrate-compost mixes improved nitrogen content in plants by more slowly releasing nitrogen from the substrate, increasing overall uptake (Uzun, 2004). Grimm and Wosten (2018) also illustrated that mushroom substrates can be used to replace chemical fertilizers.
Field experiments by Courtney and Mullen (2008) compared a chemical fertilizer to an organic mushroom substrate. The application of mushroom substrate improved soil properties and the yield of barley by 50% relative to the 40% improvement from the chemical fertilizer (Courtney and Mullen, 2008). This improved performance was attributed to the increase of mineral contents by 3 times for the mushroom substrate, the chemical fertilizer yielded no increase. As mentioned earlier medicinal mushrooms like Chaga contain compounds such as polysaccharides, polyphenols, steroids, and terpenoids that have antibacterial and antioxidant properties (Glamočlija et al., 2015). Moreover, the application of mushroom-spent substrate to soils slowly releases these bioactive compounds into the soil. This can potentially benefit plants by acting as a defense against diseases. For example, a research study demonstrated that using this type of biofertilizer led to plant growth and increased resistance against the fungal pathogen Fusarium oxysporum (Awad-Allah et al., 2022). In another study focused on chili pepper plants affected by root rot disease scientists explored the potential of a biofertilizer derived from Bacillus subtilis bacteria. The findings revealed that applying this biofertilizer significantly reduced both disease incidence and severity while also promoting plant growth and higher yields (Sivasakthi et al., 2014). Similarly in a study involving tomato plants infected with Rhizoctonia solani fungus researchers investigated the effects of a biofertilizer derived from Trichoderma fungus, on growth and disease resistance levels (Sivasakthi et al., 2014). According to Awad-Allah et al. (2022) when the biofertilizer was applied it led to plant growth. Increased protection, against the pathogen (Awad-Allah et al., 2022). This claim is further supported by Othman et al. (2020) research, which showed that mushroom substrates have properties that can combat plant pathogens. Apart from agriculture, the antibacterial effect of these mushrooms has also been used to suppress food and clinical pathogens (Hearst et al., 2009).
Since antiquity, the use of Chaga and its crude extracts has been completely harmless. Although the useful bioactivities of Chaga have attracted a lot of attention in the last decade, the safety and toxicology of the fungus has not been extensively investigated. Moreover, few studies that have investigated the safety and toxicology present divergent views on the safety of the mushroom.
In one study, researchers claim that the fungus is safe for consumption, whether ingested by animals or plants. However, Cui et al., in their study showed that the ethanol extract of Chaga was found to be harmful to HaCaT keratinocytes cells when applied in concentrations ranging from 100 to 400 g/mL (Cui et al., 2005).
Another study has shown that the primary porcine hepatocytes (PLP2) were not significantly affected by alcohol-based and/or aqueous extracts of Chaga at a concentration of 400 ppm compared to those exposed to alcohol for the first time (Glamočlija et al., 2015). Furthermore, other studies and numerous investigations have concluded that the use of Chaga extract does not result in any impact, on weight fluctuations, or effects on liver and kidney functions in kumming mice and Sprague Dawley rats (Kim et al., 2006; Nakata et al., 2007; Yong et al., 2018). Yet varying concentrations of oxalic acid, a toxic substance found in the fungus are documented in several studies (Kikuchi et al., 2014; Glamočlija et al., 2015; Lee et al., 2020). Although the understanding of the exact mechanism between the concentration of oxalic acid and its excretion ability is unclear, it is possible that with time extended use of chaga in any form may lead to increased levels of oxalate in the blood. This increase in levels may pose a risk, for sensitive individuals and potentially lead to oxalate necrosis.
The overuse of natural fungi such as Chaga is a growing concern among medical experts around the world (Lumlertgul et al., 2018). It is currently widely accepted that conducting trials on every fungus worldwide is nearly impractical due to the high cost and workforce required. Moreover, the nutritional host largely influences the chemical composition and safety of mushroom. However, following strict safety and health regulations can safeguard the safety and efficacy of Chaga products. Moreover, more toxicology studies on chaga products are needed in the present literature to authenticate the safety of these products before their use in treating illnesses.
Inonotus obliquus (Chaga mushroom), just like other medicinal fungi has been acknowledged for its healing abilities in traditional, folk, and modern medicine. In the current review, the fungus has demonstrated its wide array of medicinal properties including antimicrobial, anti-inflammatory, anticancer, antioxidant, and anti-tumor evaluated in vitro and in vivo. Yet, current evidence on its biological activity is still to a large extent scanty. Although studies have established the benefits of Chaga’s bioactive properties, more rigorous research is needed to fully understand the fungus’s mechanisms of action and to harness its full potential for future use in modern medicine.
Extraction of bioactive components using water was found to be the most common technique of preparation (or as a decoction) by many researchers and indigenous people. Moreover, most claims about Chaga’s traditional medicinal properties have also been proven true, either by in vitro or in vivo testing. Further clinical trials and translational studies are however required to further acquire more information on the fungus that will serve as a basis for future research. This could provide the necessary data to support the development of innovative medicines or applications as well as present alternative use for this mushroom. Eventually, this could pave the way for harnessing the bioactive characteristics of Chaga for various treatments.
Most research on Chaga was conducted in Asia and North America, with no available literature related to Africa. This is understandable because of its preference for certain climatic conditions (e.g., cold-loving), its origins in Asia (particularly Russia), and its extensive distribution across Europe and North America. Despite the mushroom’s widespread availability and traditional uses, it may nevertheless be considered underutilized since its full potential has not yet been explored (for example, by confirming its use as a traditional medicine for diseases other than originally used, or by further applying the confirmed in vitro properties of pharmacology to in vivo research and human or clinical trials for drug development).
Furthermore, Chaga mushrooms have shown promise in agricultural applications. In animal farming, researchers have studied Chaga and its bioactive compound β glucan for their potential to stimulate the system and promote growth in livestock. Adding Chaga or β glucan to animal feed has been found to boost immunity enhance growth performance and lessen the reliance on antibiotics. Moreover, scientists have explored the use of Chaga mushrooms to enhance the quality and digestibility of waste which can serve as feed, for ruminant animals. Utilizing Chaga in animal farming holds the potential, for improving animal wellbeing, and productivity and minimize impact.
In crop farming, Chaga has shown potential as a crop protection strategy and plant growth stimulant. It has been used to treat tree blight caused by Cryphonectria parasitica and protect cultivated plants from Phytophthora. The application of Chaga or its extracts has been associated with improved plant growth parameters, increased nutrient content, and enhanced resistance to plant diseases. Furthermore, Chaga mushrooms and their spent substrate have been explored as organic fertilizers, rich in organic matter and nutrients, to improve soil quality and fertility. The use of spent mushroom substrate not only enhances soil structure but also reduces waste and saves resources in agricultural practices.
The most recent pharmaceutical importance of Chaga mushrooms has been reviewed to cater to the huge increase in demand for mushroom derivatives like polysaccharides and antibacterial agents and for its industrial and medicinal uses. Scientists spent many years investigating this fungus before its possible pharmacological effects could be validated, despite extensive Indigenous knowledge indicating that the mushroom has long been utilized medicinally. In summary, this review serves as a benchmark for researchers to further investigate this fungus, to better understand its medicinal value and benefits for crop and animal production, particularly as a potential candidate for biofertilization, and to further exploit its remarkable significance in the cosmeceutical and food industries.
EF: Investigation, Software, Writing–original draft, Writing–review and editing. CM: Supervision, Writing–review and editing. RJ: Writing–review and editing. LG: Writing–review and editing. CC: Writing–review and editing. MC: Supervision, Writing–review and editing. RT: Resources, Supervision, Writing–review and editing.
The author(s) declare that no financial support was received for the research, authorship, and/or publication of this article.
We thank Rita Hall (CEO and President) of Beehive Vital Elements, Corner Brook, NL, Canada, for comments on the manuscripts.
The authors declare that the research was conducted in the absence of any commercial or financial relationships that could be construed as a potential conflict of interest.
All claims expressed in this article are solely those of the authors and do not necessarily represent those of their affiliated organizations, or those of the publisher, the editors and the reviewers. Any product that may be evaluated in this article, or claim that may be made by its manufacturer, is not guaranteed or endorsed by the publisher.
Abu-Reidah, I. M., Critch, A. L., Manful, C. F., Rajakaruna, A., Vidal, N. P., Pham, T. H., et al. (2021). Effects of pH and temperature on water under pressurized conditions in the extraction of nutraceuticals from chaga (Inonotus obliquus) mushroom. Antioxidants 10 (8), 1322. doi:10.3390/antiox10081322
Akdeniz, N. (2019). A systematic review of biochar use in animal waste composting. Waste Manag. 88, 291–300. doi:10.1016/j.wasman.2019.03.054
Alhallaf, W. A. A. (2020). Investigation of anti-inflammatory and antioxidants properties of phenolic compounds from Inonotus obliquus using different extraction methods. The University of Maine.
Arata, S., Watanabe, J., Maeda, M., Yamamoto, M., Matsuhashi, H., Mochizuki, M., et al. (2016). Continuous intake of the Chaga mushroom (Inonotus obliquus) aqueous extract suppresses cancer progression and maintains body temperature in mice. Heliyon 2 (5), e00111. doi:10.1016/j.heliyon.2016.e00111
Asemoloye, M. D., Marchisio, M. A., Gupta, V. K., and Pecoraro, L. (2021). Genome-based engineering of ligninolytic enzymes in fungi. Microb. cell factories 20, 20–18. doi:10.1186/s12934-021-01510-9
Awad-Allah, E. F., Shams, A. H., Helaly, A. A., and Ragheb, E. I. (2022). Effective applications of Trichoderma spp. as biofertilizers and biocontrol agents mitigate tomato Fusarium wilt disease. Agriculture 12 (11), 1950. doi:10.3390/agriculture12111950
Ayoub, N., Lass, D., and Schultze, W. (2009). Volatile constituents of the medicinal fungus chaga Inonotus obliquus (Pers: Fr.) Pilát (Aphyllophoromycetideae). Int. J. Med. Mushrooms. 11 (1).
Babitskaya, V., Shcherba, V., and Lkonnikova, N. (2000). Melanin complex of the fungus Inonotus obliquus. Appl. Biochem. Microbiol. 36 (4), 377–381. doi:10.1007/bf02738046
Baek, G.-H., Jeong, H.-S., Kim, H., Yoon, T.-J., Suh, H.-J., and Yu, K.-W. (2012). Pharmacological activity of chaga mushroom on extraction conditions and immunostimulating polysaccharide. J. Korean Soc. Food Sci. Nutr. 41 (10), 1378–1387. doi:10.3746/jkfn.2012.41.10.1378
Baek, J., Roh, H.-S., Baek, K.-H., Lee, S., Lee, S., Song, S.-S., et al. (2018). Bioactivity-based analysis and chemical characterization of cytotoxic constituents from Chaga mushroom (Inonotus obliquus) that induce apoptosis in human lung adenocarcinoma cells. J. Ethnopharmacol. 224, 63–75. doi:10.1016/j.jep.2018.05.025
Balandaykin, M. E., and Zmitrovich, I. V. (2015). Review on Chaga medicinal mushroom, Inonotus obliquus (Higher Basidiomycetes): realm of medicinal applications and approaches on estimating its resource potential. Int. J. Med. Mushrooms 17 (2), 95–104. doi:10.1615/intjmedmushrooms.v17.i2.10
Beltrame, G., Trygg, J., Hemming, J., Han, Z., and Yang, B. (2021). Comparison of polysaccharides extracted from cultivated mycelium of Inonotus obliquus with polysaccharide fractions obtained from sterile conk (chaga) and birch heart rot. J. Fungi 7 (3), 189. doi:10.3390/jof7030189
Barkwell, L. J. (2018). La michinn: traditional metis medicines and healing. Winnepeg: Louis Riel Institute Press.
Basal, W. T., Elfiky, A., and Eid, J. (2021). Chaga medicinal mushroom inonotus obliquus (agaricomycetes) terpenoids may interfere with SARS-CoV-2 spike protein recognition of the host cell: a molecular docking study. Int. J. Med. mushrooms, 23(3), 1–14. doi:10.1615/IntJMedMushrooms.2021037942
Courtney, R., and Mullen, G. (2008). Soil quality and barley growth as influenced by the land application of two compost types. Bioresour. Technol. 99(8), 2913–2918. doi:10.1016/j.biortech.2007.06.034
Bogan, B. W., Lamar, R., Burgos, W., and Tien, M. (1999). Extent of humification of anthracene, fluoranthene, and benzo[α]pyrene byPleurotus ostreatusduring growth in PAH-contaminated soils. Lett. Appl. Microbiol. 28 (4), 250–254. doi:10.1046/j.1365-2672.1999.00537.x
Boulet, B., and Bussières, G. (2018). Regard nouveau sur la biologie du polypore ponctué: fomitiporia punctata (P. Karsten) Murrill. Le. Nat. Can. 142 (3), 59–72. doi:10.7202/1050999ar
Brydon-Williams, R. (2019). Distribution, presence, Ecology, and harvest Dynamics of the chaga fungus (Inonotus obliquus) in the white mountain. National Forest University of New Hampshire.
Bulatov, P., Berezina, M. M., and Yakimov, P. (1959). The'chaga'and its therapeutical use in stage IV cancer. The'chaga'and its therapeutical use in stage IV cancer.
Burczyk, J., Gawron, A., Slotwinska, M., Smietana, B., and Terminska, K. (1996). Antimitotic activity of aqueous extracts of Inonotus obliquus. Boll. Chim. Farm. 135 (5), 306–309.
Cajthaml, T., and Svobodová, K. (2012). “Biodegradation of aromatic pollutants by ligninolytic fungal strains,” in Microbial degradation of Xenobiotics (Springer), I, 291–316.
Cha, J.-Y., Jun, B.-S., Yoo, K.-S., Hahm, J.-R., and Cho, Y.-S. (2006b). Fermented chaga mushroom (Inonotus obliquus) effects on hypolipidemia and hepatoprotection in Otsuka Long-Evans Tokushima fatty (OLETF) rats. Food Sci. Biotechnol. 15 (1), 122–127.
Cha, J.-Y., Jun, B.-S., Kim, J.-W., Park, S.-H., Lee, C.-H., and Cho, Y.-S. (2006a). Hypoglycemic effects of fermented chaga mushroom (Inonotus obliquus) in the diabetic otsuka long-evans tokushima fatty (OLETF) rat. Food Sci. Biotechnol. 15 (5), 89–95.
Cha, J.-Y., Jun, B.-S., Lee, C.-H., Yooi, K.-S., Moon, J.-C., and Cho, Y.-S. (2005). Hypoglycemic and antioxidative effects of fermented chaga mushroom (Inonotus obliquus) on streptozotocin-induced diabetic rats. J. Life Sci. 15 (5), 809–818.
Chae, B., Lohakare, J., Moon, W., Lee, S., Park, Y., and Hahn, T.-W. (2006). Effects of supplementation of β-glucan on the growth performance and immunity in broilers. Res. veterinary Sci. 80 (3), 291–298. doi:10.1016/j.rvsc.2005.07.008
Chandrasekaran, G., Oh, D.-S., and Shin, H.-J. (2011). Properties and potential applications of the culinary-medicinal cauliflower mushroom, Sparassis crispa Wulf.: Fr. (Aphyllophoromycetideae): a review. Int. J. Med. Mushrooms 13 (2), 177–183. doi:10.1615/intjmedmushr.v13.i2.100
Chen, H., Lu, X., Qu, Z., Wang, Z., and Zhang, L. (2010). Glycosidase inhibitory activity and antioxidant properties of a polysaccharide from the mushroom Inonotus obliquus. J. Food Biochem. 34, 178–191. doi:10.1111/j.1745-4514.2009.00322.x
Chen, H., and Wang, J. (2014). Phytochemistry, traditional uses and health benefits of the mushroom Inonotus obliquus (Chaga), I. Nova Science Publishers.
Chang, y., Bai, M., Xue, X. B., Zou, C. X., Huang, X. X., and Song, S. J. (2022). Isolation of chemical compositions as dietary antioxidant supplements and neuroprotectants from Chaga mushroom (Inonotus obliquus). Food Biosci. 47, 101623.
Chung, M. J., Chung, C.-K., Jeong, Y., and Ham, S.-S. (2010). Anticancer activity of subfractions containing pure compounds of Chaga mushroom (Inonotus obliquus) extract in human cancer cells and in Balbc/c mice bearing Sarcoma-180 cells. Nutr. Res. Pract. 4 (3), 177–182. doi:10.4162/nrp.2010.4.3.177
Cueno, R., Morillo, T., Carter, S., Lachmann, M., Park, J., and Schneider, J. (2004). Evaluation of Beta-glucan and antibiotics on growth performance and Carcass Traits of Weanling and finishing pigs. Copyright.
Cui, Y., Kim, D.-S., and Park, K.-C. (2005). Antioxidant effect of Inonotus obliquus. J. Ethnopharmacol. 96 (1-2), 79–85. doi:10.1016/j.jep.2004.08.037
Cunha Zied, D., Sánchez, J. E., Noble, R., and Pardo-Giménez, A. (2020). Use of spent mushroom substrate in new mushroom crops to promote the transition towards a circular economy. Agronomy 10 (9), 1239. doi:10.3390/agronomy10091239
Dashtban, M., Schraft, H., Syed, T. A., and Qin, W. (2010). Fungal biodegradation and enzymatic modification of lignin. Int. J. Biochem. Mol. Biol. 1 (1), 36–50.
Debnath, T., Park, S. R., Kim, D. H., Jo, J. E., and Lim, B. O. (2013). Anti-oxidant and anti-inflammatory activities of Inonotus obliquus and germinated brown rice extracts. Molecules 18 (8), 9293–9304. doi:10.3390/molecules18089293
Deshpande, A. G., and Arya, A. (2022). “Mushroom biotechnology: developing cultivation protocol for four different mushrooms and accessing their potential in pollution management,” in Biology, cultivation and applications of mushrooms (Springer), 457–485.
Dritz, S., Shi, J., Kielian, T., Goodband, R., Nelssen, J., Tokach, M., et al. (1995). Influence of dietary β-glucan on growth performance, nonspecific immunity, and resistance to Streptococcus suis infection in weanling pigs. J. Animal Sci. 73 (11), 3341–3350. doi:10.2527/1995.73113341x
Eid, J. I., Das, B., Al-Tuwaijri, M. M., and Basal, W. T. (2021). Targeting SARS-CoV-2 with Chaga mushroom: an in silico study toward developing a natural antiviral compound. Food Sci. Nutr. 9 (12), 6513–6523. doi:10.1002/fsn3.2576
Esmaielpour, B., Rahmanian, M., Heidarpour, O., and Shahriari, M. H. (2017). Effect of vermicompost and spent mushroom compost on the nutrient and essential oil composition of basil (Ocimum basilicum L.). J. Essent. Oil Bear. Plants 20 (5), 1283–1292. doi:10.1080/0972060x.2017.1396931
Falck, R. (1902). Die Cultur der Oidien und ihre Rückführung in die höhere Fruchtform bei den Basidiomyceten. Druck Nischkowsky.
Federhen, S. (2012). The NCBI taxonomy database. Nucleic acids Res. 40 (D1), D136–D143. doi:10.1093/nar/gkr1178
Geng, Y., Lu, Z.-M., Huang, W., Xu, H.-Y., Shi, J.-S., and Xu, Z.-H. (2013). Bioassay-guided isolation of DPP-4 inhibitory fractions from extracts of submerged cultured of Inonotus obliquus. Molecules 18 (1), 1150–1161. doi:10.3390/molecules18011150
Géry, A., Dubreule, C., André, V., Rioult, J.-P., Bouchart, V., Heutte, N., et al. (2018). Chaga (Inonotus obliquus), a future potential medicinal fungus in oncology? A chemical study and a comparison of the cytotoxicity against human lung adenocarcinoma cells (A549) and human bronchial epithelial cells (BEAS-2B). Integr. cancer Ther. 17 (3), 832–843. doi:10.1177/1534735418757912
Grimm, D., and Wösten, H. A. (2018). Mushroom cultivation in the circular economy. Appl. Microbiol. Biotechnol. 102, 7795–7803. doi:10.1007/s00253-018-9226-8
Giridharan, V. V., Thandavarayan, R. A., and Konishi, T. (2011). Amelioration of scopolamine induced cognitive dysfunction and oxidative stress by Inonotus obliquus–a medicinal mushroom. Food & Funct. 2 (6), 320–327. doi:10.1039/c1fo10037h
Glamočlija, J., Ćirić, A., Nikolić, M., Fernandes, Â., Barros, L., Calhelha, R. C., et al. (2015). Chemical characterization and biological activity of Chaga (Inonotus obliquus), a medicinal “mushroom”. J. Ethnopharmacol. 162, 323–332. doi:10.1016/j.jep.2014.12.069
Gogineni, V., Schinazi, R. F., and Hamann, M. T. (2015). Role of marine natural products in the genesis of antiviral agents. Chem. Rev. 115 (18), 9655–9706. doi:10.1021/cr4006318
Gorbunova, I. A., Perova, N., and Teplyakova, T. V. (2005). Medicinal mushrooms of southwest Siberia. Int. J. Med. Mushrooms 7 (3), 403–404. doi:10.1615/intjmedmushr.v7.i3.480
Gründemann, C., Reinhardt, J. K., and Lindequist, U. (2020). European medicinal mushrooms: do they have potential for modern medicine? An update. Phytomedicine 66, 153131. doi:10.1016/j.phymed.2019.153131
Hadar, Y., and Cohen-Arazi, E. (1986). Chemical composition of the edible mushroom Pleurotus ostreatus produced by fermentation. Appl. Environ. Microbiol. 51 (6), 1352–1354. doi:10.1128/AEM.51.6.1352-1354.1986
Hadar, Y., Kerem, Z., Gorodecki, B., and Ardon, O. (1992). “Utilization of lignocellulosic waste by the edible mushroom, Pleurotus,” in Microorganisms to combat pollution (Springer), 65–81.
Haines, A. (2013). Review of medical uses of chaga {Inonotus obliquus}. Available at: http://www.wildfeder.com/.
Ham, S.-S., Kim, S.-H., Moon, S.-Y., Chung, M. J., Cui, C.-B., Han, E.-K., et al. (2009). Antimutagenic effects of subfractions of Chaga mushroom (Inonotus obliquus) extract. Mutat. Research/Genetic Toxicol. Environ. Mutagen. 672 (1), 55–59. doi:10.1016/j.mrgentox.2008.10.002
Handa, N., Yamada, T., and Tanaka, R. (2010). An unusual lanostane-type triterpenoid, spiroinonotsuoxodiol, and other triterpenoids from Inonotus obliquus. Phytochemistry 71 (14-15), 1774–1779. doi:10.1016/j.phytochem.2010.07.005
He, J., Feng, X.-Z., Lu, Y., and Zhao, B. (2001). Three new triterpenoids from Fuscoporia obliqua. J. Asian Nat. Prod. Res. 3 (1), 55–61. doi:10.1080/10286020108042839
Hearst, R., Nelson, D., McCollum, G., Millar, B. C., Maeda, Y., Goldsmith, C. E., et al. (2009). An examination of antibacterial and antifungal properties of constituents of Shiitake (Lentinula edodes) and Oyster (Pleurotus ostreatus) mushrooms. Complementary Ther. Clin. Pract. 15 (1), 5–7. doi:10.1016/j.ctcp.2008.10.002
Heo, J.-W., No, M.-H., Park, D.-H., Kang, J.-H., Seo, D. Y., Han, J., et al. (2017). Effects of exercise on obesity-induced mitochondrial dysfunction in skeletal muscle. Korean J. Physiology Pharmacol. 21 (6), 567–577. doi:10.4196/kjpp.2017.21.6.567
Hobbs, C. (2003). Medicinal mushrooms: an exploration of tradition, health and culture. Botanica press.
Honda, Y., Matsuyama, T., Irie, T., Watanabe, T., and Kuwahara, M. (2000). Carboxin resistance transformation of the homobasidiomycete fungus Pleurotus ostreatus. Curr. Genet. 37 (3), 209–212. doi:10.1007/s002940050521
Hong, K. B., Noh, D. O., Park, Y., and Suh, H. J. (2015). Hepatoprotective activity of water extracts from chaga medicinal mushroom, Inonotus obliquus (higher basidiomycetes) against tert-butyl Hydroperoxide− induced oxidative liver injury in primary cultured rat hepatocytes. Int. J. Med. Mushrooms 17 (11), 1069–1076. doi:10.1615/intjmedmushrooms.v17.i11.70
Hordyjewska, A., Ostapiuk, A., Horecka, A., and Kurzepa, J. (2019). Betulin and betulinic acid: triterpenoids derivatives with a powerful biological potential. Phytochem. Rev. 18, 929–951. doi:10.1007/s11101-019-09623-1
Mohd Hanafi, F. H., Rezania, S., Mat Taib, S., Md Din, M. F., Yamauchi, M., Sakamoto, M., et al. (2018). Environmentally sustainable applications of agro-based spent mushroom substrate (SMS): an overview. J. Material Cycles Waste Manag. 20, 1383–1396. doi:10.1007/s10163-018-0739-0
Hu, H., Zhang, Z., Lei, Z., Yang, Y., and Sugiura, N. (2009). Comparative study of antioxidant activity and antiproliferative effect of hot water and ethanol extracts from the mushroom Inonotus obliquus. J. Biosci. Bioeng. 107 (1), 42–48. doi:10.1016/j.jbiosc.2008.09.004
Hu, T., Liu, P., Ni, Y., and Lu, C. (2012). Isolation, purification and effects of hypoglycemic functional polysaccharides from Inonotus obliquus. Afr. J. Biotechnol. 11 (30), 7738–7743. doi:10.5897/AJB11.3536
Huang, S.-q., Ding, S., and Fan, L. (2012). Antioxidant activities of five polysaccharides from Inonotus obliquus. Int. J. Biol. Macromol. 50 (5), 1183–1187. doi:10.1016/j.ijbiomac.2012.03.019
Hüttermann, A., Hamza, A., Chet, I., Majcherczyk, A., Fouad, T., Badr, A., et al. (2000). Recycling of agricultural wastes by white-rot fungi for the production of fodder for ruminants. Agro Food Ind. Hi-Tech 11 (6), 29–32.
Huynh, N. (2019). Supercritical CO2 extraction of triterpenoids from Inonotus obliquus University of Turku]. Finland.
Hwang, A. Y., Yang, S. C., Kim, J., Lim, T., Cho, H., and Hwang, K. T. (2019). Effects of non-traditional extraction methods on extracting bioactive compounds from chaga mushroom (Inonotus obliquus) compared with hot water extraction. LWT 110, 80–84. doi:10.1016/j.lwt.2019.04.073
Hyun, K. W., Jeong, S. C., Lee, D. H., Park, J. S., and Lee, J. S. (2006). Isolation and characterization of a novel platelet aggregation inhibitory peptide from the medicinal mushroom, Inonotus obliquus. Peptides 27 (6), 1173–1178. doi:10.1016/j.peptides.2005.10.005
Ilić, N., Milić, M., Beluhan, S., and Dimitrijević-Branković, S. (2023). Cellulases: from lignocellulosic biomass to improved production. Energies, 16(8), 3598. doi:10.3390/en16083598
Il’ina, I., and Uljašev, O. (2012). Gender and myth in traditional Komi-Zyrjan culture, 308. Mythic Discourses.
Im, K. H., Nguyen, T. K., Choi, J., and Lee, T. S. (2016). In vitro antioxidant, anti-diabetes, anti-dementia, and inflammation inhibitory effect of Trametes pubescens fruiting body extracts. Molecules 21 (5), 639. doi:10.3390/molecules21050639
Irie, T., Honda, Y., Watanabe, T., and Kuwahara, M. (2001). Efficient transformation of filamentous fungus Pleurotus ostreatus using single-strand carrier DNA. Appl. Microbiol. Biotechnol. 55 (5), 563–565. doi:10.1007/s002530000535
Ishfaq, P. M., Mishra, A., Mishra, S., Ahmad, Z., Gayen, S., Jain, S. K., et al. (2021). Inonotus obliquus aqueous extract suppresses carbon tetrachloride-induced hepatic injury through modulation of antioxidant enzyme system and anti-inflammatory mechanism. Clin. Cancer Drugs 8 (2), 122–136. doi:10.2174/2212697x08666211130130119
Ishfaq, P. M., Mishra, S., Mishra, A., Ahmad, Z., Gayen, S., Jain, S. K., et al. (2022). Inonotus obliquus aqueous extract prevents histopathological alterations in liver induced by environmental toxicant Microcystin. Curr. Res. Pharmacol. Drug Discov. 3, 100118. doi:10.1016/j.crphar.2022.100118
Jayachandran, M., Xiao, J., and Xu, B. (2017). A critical review on health promoting benefits of edible mushrooms through gut microbiota. Int. J. Mol. Sci. 18 (9), 1934. doi:10.3390/ijms18091934
Jiang, S., Shi, F., Lin, H., Ying, Y., Luo, L., Huang, D., et al. (2020). Inonotus obliquus polysaccharides induces apoptosis of lung cancer cells and alters energy metabolism via the LKB1/AMPK axis. Int. J. Biol. Macromol. 151, 1277–1286. doi:10.1016/j.ijbiomac.2019.10.174
Jin, T., Xiaoliang, H., Dafei, L., Hongxia, W., and Liandong, Q. (2017). Identification of Inonotus obliquus polysaccharide with broad-spectrum antiviral activity against multi-feline viruses. Int. J. Biol. Macromol. 95, 160–167. doi:10.1016/j.ijbiomac.2016.11.054
Joo, J. I., Kim, D. H., and Yun, J. W. (2010). Extract of Chaga mushroom (Inonotus obliquus) stimulates 3t3-l1 adipocyte differentiation. Phytotherapy Res. 24 (11), 1592–1599. doi:10.1002/ptr.3180
Jung, H.-K., Hong, J.-H., Park, S.-C., Park, B.-K., Nam, D.-H., and Kim, S.-D. (2007). Production and physicochemical characterization of β-glucan produced byPaenibacillus polymyxa JB115. Biotechnol. Bioprocess Eng. 12 (6), 713–719. doi:10.1007/bf02931090
Kahlos, K., Hiltunen, R., and v Schantz, M. (1984). 3beta-Hydroxy-lanosta-8,24-dien-21-al, a new triterpene from inontus obliquus. Planta medica. 50 (02), 197–198. doi:10.1055/s-2007-969674
Kamra, D., and Zadražil, F. (1986). Influence of gaseous phase, light and substrate pretreatment on fruit-body formation, lignin degradation and in vitro digestibility of wheat straw fermented with Pleurotus spp. Agric. wastes 18 (1), 1–17. doi:10.1016/0141-4607(86)90103-4
Kang, J.-H., Jang, J.-E., Mishra, S. K., Lee, H.-J., Nho, C. W., Shin, D., et al. (2015). Ergosterol peroxide from Chaga mushroom (Inonotus obliquus) exhibits anti-cancer activity by down-regulation of the β-catenin pathway in colorectal cancer. J. Ethnopharmacol. 173, 303–312. doi:10.1016/j.jep.2015.07.030
Kaur, R., Tyagi, R. D., and Zhang, X. (2020). Review on pulp and paper activated sludge pretreatment, inhibitory effects and detoxification strategies for biovalorization. Environ. Res. 182, 109094. doi:10.1016/j.envres.2019.109094
Khan, M. W., Ali, M. A., Khan, N. A., Khan, M. A., Rehman, A., and Javed, N. (2013). Effect of different levels of lime and pH on mycelial growth and production efficiency of oyster mushroom (Pleurotus spp.). Pak. J. Bot. 45 (1), 297–302.
Kikuchi, Y., Seta, K., Ogawa, Y., Takayama, T., Nagata, M., Taguchi, T., et al. (2014). Chaga mushroom-induced oxalate nephropathy. Clin. Nephrol. 81 (6), 440–444. doi:10.5414/CN107655
Kim, T. I., Choi, J.-G., Kim, J. H., Li, W., and Chung, H.-S. (2020). Blocking effect of chaga mushroom (Inonotus oliquus) extract for immune checkpoint CTLA-4/CD80 interaction. Appl. Sci. 10 (17), 5774. doi:10.3390/app10175774
Kim, Y. J., Park, J., Min, B. S., and Shim, S. H. (2011). Chemical constituents from the sclerotia of Inonotus obliquus. J. Korean Soc. Appl. Biol. Chem. 54 (2), 287–294. doi:10.3839/jksabc.2011.045
Kim, Y. O., Park, H. W., Kim, J. H., Lee, J. Y., Moon, S. H., and Shin, C. S. (2006). Anti-cancer effect and structural characterization of endo-polysaccharide from cultivated mycelia of Inonotus obliquus. Life Sci. 79 (1), 72–80. doi:10.1016/j.lfs.2005.12.047
Kim, Y.-R. (2005). Immunomodulatory activity of the water extract from medicinal mushroom Inonotus obliquus. Mycobiology 33 (3), 158–162. doi:10.4489/MYCO.2005.33.3.158
Kirk, P. M., Cannon, P. F., Minter, D., and Stalpers, J. (2008). Dictionary of the fungi. 10th edition. Wallingford, UK: CAB International.
Ko, S.-k., Jin, M., and Pyo, M.-y. (2011). Inonotus obliquus extracts suppress antigen-specific IgE production through the modulation of Th1/Th2 cytokines in ovalbumin-sensitized mice. J. Ethnopharmacol. 137 (3), 1077–1082. doi:10.1016/j.jep.2011.07.024
Kou, R.-W., Han, R., Gao, Y.-Q., Li, D., Yin, X., and Gao, J.-M. (2021). Anti-neuroinflammatory polyoxygenated lanostanoids from Chaga mushroom Inonotus obliquus. Phytochemistry 184, 112647. doi:10.1016/j.phytochem.2020.112647
Kukulyanskaya, T., Kurchenko, N., Kurchenko, V., and Babitskaya, V. (2002). Physicochemical properties of melanins produced by the sterile form of Inonotus obliquus (“Chagi”) in natural and cultivated fungus. Appl. Biochem. Microbiol. 38 (1), 58–61. doi:10.1023/a:1013204706055
Kumar, P., Bhadauria, A. S., Singh, A. K., and Saha, S. (2018). Betulinic acid as apoptosis activator: molecular mechanisms, mathematical modeling and chemical modifications. Life Sci. 209, 24–33. doi:10.1016/j.lfs.2018.07.056
Kyoung, P. Y., Burm, L. H., Eun-Jae, J., Sung, J. H., and Myung-Hee, K. (2004). Chaga mushroom extract inhibits oxidative DNA damage in human lymphocytes as assessed by comet assay. Biofactors 21 (1-4), 109–112. doi:10.1002/biof.552210120
Lee, C. K., Ibrahim, D., and Omar, I. C. (2013). Enzymatic deinking of various types of waste paper: efficiency and characteristics. Process Biochem. 48 (2), 299–305. doi:10.1016/j.procbio.2012.12.015
Lee, I.-K., Kim, Y.-S., Jang, Y.-W., Jung, J.-Y., and Yun, B.-S. (2007). New antioxidant polyphenols from the medicinal mushroom Inonotus obliquus. Bioorg. Med. Chem. Lett. 17 (24), 6678–6681. doi:10.1016/j.bmcl.2007.10.072
Lee, I.-K., and Yun, B.-S. (2006). Hispidin analogs from the mushroom Inonotus xeranticus and their free radical scavenging activity. Bioorg. Med. Chem. Lett. 16 (9), 2376–2379. doi:10.1016/j.bmcl.2006.01.121
Lee, K., Nagajyothi, P., Sreekanth, T., and Park, S. (2015). Eco-friendly synthesis of gold nanoparticles (AuNPs) using Inonotus obliquus and their antibacterial, antioxidant and cytotoxic activities. J. Industrial Eng. Chem. 26, 67–72. doi:10.1016/j.jiec.2014.11.016
Lee, M.-W., Hur, H., Chang, K.-C., Lee, T.-S., Ka, K.-H., and Jankovsky, L. (2008). Introduction to distribution and ecology of sterile conks of Inonotus obliquus. Mycobiology 36 (4), 199–202. doi:10.4489/MYCO.2008.36.4.199
Lee, S., Lee, H. Y., Park, Y., Ko, E. J., Ban, T. H., Chung, B. H., et al. (2020). Development of end stage renal disease after long-term ingestion of chaga mushroom: case report and review of literature. J. Korean Med. Sci. 35 (19), e122. doi:10.3346/jkms.2020.35.e122
Lee, S. H., Hwang, H. S., and Yun, J. W. (2009). Antitumor activity of water extract of a mushroom, Inonotus obliquus, against HT-29 human colon cancer cells. Phytotherapy Res. 23 (12), 1784–1789. doi:10.1002/ptr.2836
Lemieszek, M. K., Langner, E., Kaczor, J., Kandefer-Szerszen, M., Sanecka, B., Mazurkiewicz, W., et al. (2011). Anticancer effects of fraction isolated from fruiting bodies of Chaga medicinal mushroom, Inonotus obliquus (Pers.: Fr.) Pilát (Aphyllophoromycetideae): in vitro studies. Int. J. Med. Mushrooms 13 (2), 131–143. doi:10.1615/intjmedmushr.v13.i2.50
Li, J., Li, D., Xing, J., Cheng, Z., and Lai, C. (2006). Effects of β-glucan extracted from Saccharomyces cerevisiae on growth performance, and immunological and somatotropic responses of pigs challenged with Escherichia coli lipopolysaccharide. J. Animal Sci. 84 (9), 2374–2381. doi:10.2527/jas.2004-541
Li, N., Senotrusova, T., Son, O., Podvolotskaya, A., Ershova, T., and Levchuk, T. (2022). APPLICATION OF A NATURAL ANTIOXIDANT FOR PROTECT FEED VITAMIN A. J. Agric. Environ. 8 (28). doi:10.23649/jae.2022.28.8.008
Liang, L., Zhang, Z., and Wang, H. (2009). Antioxidant activities of extracts and subfractions from Inonotus Obliquus. Int. J. food Sci. Nutr. 60 (2), 175–184. doi:10.1080/09637480903042279
Lindenfelser, L., Detroy, R., Ramstack, J., and Worden, K. (1979). Biological modification of the lignin and cellulose components of wheat straw by Pleurotus ostreatus. Dev. Ind. Microbiol. 20, 541–551.
Lindequist, U., Niedermeyer, T. H., and Jülich, W.-D. (2005). The pharmacological potential of mushrooms. Evidence-based complementary Altern. Med. 2, 285–299. doi:10.1093/ecam/neh107
Liu, C., Zhao, C., Pan, H.-H., Kang, J., Yu, X.-T., Wang, H.-Q., et al. (2014). Chemical constituents from Inonotus obliquus and their biological activities. J. Nat. Prod. 77 (1), 35–41. doi:10.1021/np400552w
Liuping, F., Shaodong, D., Lianzhong, A., and Kequan, D. (2012). Antitumor and immunomodulatory activity of water-soluble polysaccharide from Inonotus obliquus. Carbohydr. Polym. 90 (2), 870–874. doi:10.1016/j.carbpol.2012.06.013
Lou, Z., Sun, Y., Bian, S., Baig, S. A., Hu, B., and Xu, X. (2017). Nutrient conservation during spent mushroom compost application using spent mushroom substrate derived biochar. Chemosphere 169, 23–31. doi:10.1016/j.chemosphere.2016.11.044
Lu, X., Chen, H., Dong, P., Fu, L., and Zhang, X. (2010). Phytochemical characteristics and hypoglycaemic activity of fraction from mushroom Inonotus obliquus. J. Sci. Food Agric. 90 (2), 276–280. doi:10.1002/jsfa.3809
Lukina, N. (1975). Narodnye sredstva po sohraneniyu zdorovya i zhizni u vostochnyh khantov, Etnograficheskiye aspekty izucheniya narodnoi meditsiny, Tezisy vsesoyuznoi nauchnoi konferentsii 1–12 marta 1975 g, 26–27. Avaiable at: https://www.scopus.com/inward/record.uri?eid=2-s2.0-84915375596&partnerID=40&md5=6b2ad12f9337c69396f449e54962ea96.
Lumlertgul, N., Siribamrungwong, M., Jaber, B. L., and Susantitaphong, P. (2018). Secondary oxalate nephropathy: a systematic review. Kidney Int. Rep. 3 (6), 1363–1372. doi:10.1016/j.ekir.2018.07.020
Ma, L., Chen, H., Dong, P., and Lu, X. (2013). Anti-inflammatory and anticancer activities of extracts and compounds from the mushroom Inonotus obliquus. Food Chem. 139 (1-4), 503–508. doi:10.1016/j.foodchem.2013.01.030
May, T. W., Redhead, S. A., Bensch, K., Hawksworth, D. L., Lendemer, J., Lombard, L., et al. (2019). Chapter F of the international code of nomenclature for algae, fungi, and plants as approved by the 11th international mycological congress, san juan, Puerto Rico. IMA fungus 10 (1), 1–14. doi:10.1186/s43008-019-0019-1
Meng, X., Dai, J., Zhang, Y., Wang, X., Zhu, W., Yuan, X., et al. (2018). Composted biogas residue and spent mushroom substrate as a growth medium for tomato and pepper seedlings. J. Environ. Manag. 216, 62–69. doi:10.1016/j.jenvman.2017.09.056
Meng, X., Liu, B., Zhang, H., Wu, J., Yuan, X., and Cui, Z. (2019). Co-composting of the biogas residues and spent mushroom substrate: physicochemical properties and maturity assessment. Bioresour. Technol. 276, 281 –287. doi:10.1016/j.biortech.2018.12.097
Milyuhina, A., Kyzdarbek, U., and Romazyaeva, I. (2022). Assessment of antimicrobial activity of medicinal plants’ extracts. AIP Conf. Proc. doi:10.1063/5.0071327
Mishra, S., Kang, J., Song, K., Park, M., Kim, D., Park, Y., et al. (2013). Inonotus obliquus suppresses proliferation of colorectal cancer cells and tumor growth in mice models by downregulation of β-catenin/NF-κB-signaling pathways. Eur. J. Inflamm. 11 (3), 615–629. doi:10.1177/1721727x1301100306
Mishra, S. K., Kang, J.-H., Kim, D.-K., Oh, S. H., and Kim, M. K. (2012). Orally administered aqueous extract of Inonotus obliquus ameliorates acute inflammation in dextran sulfate sodium (DSS)-induced colitis in mice. J. Ethnopharmacol. 143 (2), 524–532. doi:10.1016/j.jep.2012.07.008
Mizuno, T., Zhuang, C., Abe, K., Okamoto, H., Kiho, T., Ukai, S., et al. (1999). Antitumor and hypoglycemic activities of polysaccharides from the sclerotia and mycelia of Inonotus obliquus (Pers.: Fr.) Pil. (Aphyllophoromycetideae). Int. J. Med. Mushrooms 1 (4), 301–316. doi:10.1615/intjmedmushr.v1.i4.20
Moon, B.-H., and Lee, W.-C. (2009). Studies on the anti-cancer activity of chaga mushroom extract. J. Korean Med. 30 (4), 1–12.
Moore, P., Evenson, A., Luckey, T., McCoy, E., Elvehjem, C., and Hart, E. (1946). Use of sulfasuxidine, streptothricin, and streptomycin in nutritional studies with the chick. J. Biol. Chem. 165 (2), 437–441. doi:10.1016/s0021-9258(17)41154-9
Moss, R. W. (2016). Chaga mushroom and cancer. Retrieved January, 16, 2023 from http://www.nrocrc.com/news/headline_news/2016/07/16/160.html.
Mu, H., Zhang, A., Zhang, W., Cui, G., Wang, S., and Duan, J. (2012). Antioxidative properties of crude polysaccharides from Inonotus obliquus. Int. J. Mol. Sci. 13 (7), 9194–9206. doi:10.3390/ijms13079194
Muswati, C., Simango, K., Tapfumaneyi, L., Mutetwa, M., and Ngezimana, W. (2021). The effects of different substrate combinations on growth and yield of oyster mushroom (Pleurotus ostreatus). Int. J. Agron. 2021, 1–10. doi:10.1155/2021/9962285
Najafzadeh, M., Reynolds, P. D., Baumgartner, A., Jerwood, D., and Anderson, D. (2007). Chaga mushroom extract inhibits oxidative DNA damage in lymphocytes of patients with inflammatory bowel disease. Biofactors 31 (3), 191–200. doi:10.1002/biof.5520310306
Nakajima, Y., Sato, Y., and Konishi, T. (2007). Antioxidant small phenolic ingredients in Inonotus obliquus (persoon) Pilat (Chaga). Chem. Pharm. Bull. 55 (8), 1222–1226. doi:10.1248/cpb.55.1222
Nakata, T., Yamada, T., Taji, S., Ohishi, H., Wada, S.-i., Tokuda, H., et al. (2007). Structure determination of inonotsuoxides A and B and in vivo anti-tumor promoting activity of inotodiol from the sclerotia of Inonotus obliquus. Bioorg. Med. Chem. 15 (1), 257–264. doi:10.1016/j.bmc.2006.09.064
Navid, M. H., Laszczyk-Lauer, M., Reichling, J., and Schnitzler, P. (2014). Pentacyclic triterpenes in birch bark extract inhibit early step of herpes simplex virus type 1 replication. Phytomedicine, 21(11), 1273–1280. doi:10.1016/j.phymed.2014.06.007
Ning, X., Luo, Q., Li, C., Ding, Z., Pang, J., and Zhao, C. (2014). Inhibitory effects of a polysaccharide extract from the Chaga medicinal mushroom, Inonotus obliquus (higher Basidiomycetes), on the proliferation of human neurogliocytoma cells. Int. J. Med. Mushrooms 16 (1), 29–36. doi:10.1615/intjmedmushr.v16.i1.30
Nwafor, S. O., Adenipekun, C. O., Aruwa, G., and Asemoloye, M. D. (2022). Biotreatment of selected agrowaste products with some culinary-medicinal mushrooms enhances their nutrient composition for use as animal feed in Nigeria. Int. J. Med. Mushrooms 24 (6), 57–68. doi:10.1615/IntJMedMushrooms.2022043952
Oliveira-Costa, J. F., Meira, C. S., Neves, M. V. G. d., Dos Reis, B. P. Z. C., and Soares, M. B. P. (2022). Anti-inflammatory activities of betulinic acid: a review. Front. Pharmacol., 13, 883857. doi:10.3389/fphar.2022.883857
Othman, N. Z., Sarjuni, M. N. H., Rosli, M. A., Nadri, M. H., Yeng, L. H., Ying, O. P., et al. (2020). Spent mushroom substrate as biofertilizer for agriculture application. Valoris. Agro-industrial Residues, 37–57. Volume I: Biological Approaches. doi:10.1007/978-3-030-39137-9_2
Paduch, R., and Kandefer-Szerszen, M. (2014). Antitumor and antiviral activity of pentacyclic triterpenes. Mini. Rev. Org. Chem. 11, 262–268. doi:10.2174/1570193x1103140915105240
Pan, H.-h., Yu, X.-t., Li, T., Wu, H.-l., Jiao, C.-w., Cai, M.-h., et al. (2013). Aqueous extract from a Chaga medicinal mushroom, Inonotus obliquus (higher Basidiomycetes), prevents herpes simplex virus entry through inhibition of viral-induced membrane fusion. Int. J. Med. Mushrooms 15 (1), 29–38. doi:10.1615/intjmedmushr.v15.i1.40
Park, S., Shin, H., Park, D., Kim, H., Byun, Y., and Lee, K. Y. (2021). Structure elucidation of a new triterpene from Inonotus obliquus. Magnetic Reson. Chem. 59 (4), 489–494. doi:10.1002/mrc.5102
Park, Y. K., Lee, H. B., Jeon, E.-J., Jung, H. S., and Kang, M.-H. (2004). Chaga mushroom extract inhibits oxidative DNA damage in human lymphocytes as assessed by comet assay. Biofactors 21 (1-4), 109–112. doi:10.1002/biof.552210120
Park, Y.-M., Won, J.-H., Kim, Y.-H., Choi, J.-W., Park, H.-J., and Lee, K.-T. (2005). In vivo and in vitro anti-inflammatory and anti-nociceptive effects of the methanol extract of Inonotus obliquus. J. Ethnopharmacol. 101 (1-3), 120–128. doi:10.1016/j.jep.2005.04.003
Pavithra, S., Jayaprakash, J., Gummadi, S. N., and Giri Dev, V. R. (2023). Assessment of process integration approach for coir biosoftening and lignin-modifying enzyme production from agro residues. Biofuels, Bioprod. Biorefining, 17, 921–932. doi:10.1002/bbb.2484
Peng, H., and Shahidi, F. (2022). Qualitative analysis of secondary metabolites of chaga mushroom (Inonotus Obliquus): phenolics, fatty acids, and terpenoids. J. Food Bioact. 17. doi:10.31665/jfb.2022.17304
Pilz, D. (2004). Chaga and other fungal resources: assessment of sustainable commercial harvesting in Khabarovsk and Primorsky Krais, Russia. Khabarovsk, Russia: Report prepared for Winrock International, Morrilton, Arkansas and the FOREST Project.
Polkovnikova, M., Nosik, N., Garaev, T., Kondrashina, N., Finogenova, M., and Shibnev, V. (2014). A study of the antiherpetic activity of the chaga mushroom (Inonotus obliquus) extracts in the Vero cells infected with the herpes simplex virus. Vopr. Virusol. 59 (2), 45–48.
Rathgeber, B., Budgell, K., MacIsaac, J., Mirza, M., and Doncaster, K. (2008). Growth performance and spleen and bursa weight of broilers fed yeast beta-glucan. Can. J. animal Sci. 88 (3), 469–473. doi:10.4141/cjas07101
Razumov, E. Y., Safin, R., Mukhametzyanov, S. R., Baigildeeva, E., Safina, A., and Lebedev, D. (2020). Studies of the composition of the cryogenic ground chaga. IOP Conf. Ser. Mater. Sci. Eng. 986, 012029. doi:10.1088/1757-899x/986/1/012029
Rhee, S. J., Cho, S. Y., Kim, K. M., Cha, D.-S., and Park, H.-J. (2008). A comparative study of analytical methods for alkali-soluble β-glucan in medicinal mushroom, Chaga (Inonotus obliquus). LWT-Food Sci. Technol. 41 (3), 545–549. doi:10.1016/j.lwt.2007.03.028
Rogers, R. (2016). Mushroom essences: vibrational healing from the kingdom fungi. North Atlantic Books.
Rogers, R. D. (2006). The fungal pharmacy: medicinal mushrooms of Western Canada. Prairie Deva Press. Retrieved January, 16, 2023 from Avaiable at: https://www.herbalgram.org/resources/herbalgram/issues/79/table-of-contents/article3297/.
Roy, S., Barman, S., Chakraborty, U., and Chakraborty, B. (2015). Evaluation of spent mushroom substrate as biofertilizer for growth improvement of Capsicum annuum L. J. Appl. Biol. Biotechnol. 3 (3), 0–2. doi:10.7324/JABB.2015.3305
Ryzhova, G., Kravtsova, S., Matasova, S., and Gribel, H. (1997). Chemical and Parmacuetical characteristics of dried Chaga extract. Chemistry-pharmaceutical J. 10, 44–47.
Saar, M. (1991). Fungi in Khanty folk medicine. J. Ethnopharmacol. 31 (2), 175–179. doi:10.1016/0378-8741(91)90003-v
Safin, R. R., Razumov, E. Y., Khasanshin, R. R., Gubernatorov, V. V., and Saerova, K. V. (2018). Effect of growth conditions on qualitative characteristics of chaga mushroom. Int. Multidiscip. Sci. GeoConference SGEM 18 (3.2), 781–788. doi:10.5593/sgem2018/3.2/S14.100
Sagayama, K., Tanaka, N., Fukumoto, T., and Kashiwada, Y. (2019). Lanostane-type triterpenes from the sclerotium of Inonotus obliquus (Chaga mushrooms) as proproliferative agents on human follicle dermal papilla cells. J. Nat. Med. 73 (3), 597–601. doi:10.1007/s11418-019-01280-0
Saha, S., Ghosh, M., and Dutta, S. K. (2015). A potent tumoricidal co-drug ‘Bet-CA’-an ester derivative of betulinic acid and dichloroacetate selectively and synergistically kills cancer cells. Sci. Rep. 5 (1), 7762. doi:10.1038/srep07762
Salmones, D., Mata, G., and Waliszewski, K. N. (2005). Comparative culturing of Pleurotus spp. on coffee pulp and wheat straw: biomass production and substrate biodegradation. Bioresour. Technol. 96 (5), 537–544. doi:10.1016/j.biortech.2004.06.019
Sánchez, C. (2010). Cultivation of Pleurotus ostreatus and other edible mushrooms. Appl. Microbiol. Biotechnol. 85, 1321–1337. doi:10.1007/s00253-009-2343-7
Satoru, A., Jun, W., Masako, M., Masato, Y., Hideto, M., Mamiko, M., et al. (2016). Continuous intake of the Chaga mushroom (Inonotus obliquus) aqueous extract suppresses cancer progression and maintains body temperature in mice. Heliyon 2 (5), e00111. doi:10.1016/j.heliyon.2016.e00111
Seo, H.-K., and Lee, S.-C. (2010). Antioxidant activity of subcritical water extracts from Chaga mushroom (Inonotus obliquus). Sep. Sci. Technol. 45 (2), 198–203. doi:10.1080/01496390903423899
Shashkina, M. Y., Shashkin, P., and Sergeev, A. (2006). Chemical and medicobiological properties of chaga (review). Pharm. Chem. J. 40 (10), 560–568. doi:10.1007/s11094-006-0194-4
Shikov, A. N., Pozharitskaya, O. N., Makarov, V. G., Wagner, H., Verpoorte, R., and Heinrich, M. (2014). Medicinal plants of the Russian Pharmacopoeia; their history and applications. J. Ethnopharmacol. 154 (3), 481–536. doi:10.1016/j.jep.2014.04.007
Shin, Y., Tamai, Y., and Terazawa, M. (2000). Chemical Constituents of Inonotus obliquus Ⅰ.: a new triterpene, 3β-hydroxy-8, 24-dien-lanosta-21, 23-lactone from sclerotium. Eurasian J. For. Res. 1, 43–50. doi:10.1615/IntJMedMushr.v2.i3.30
Silvat, D. (2013). Biodegradation of vinasse: fungal lignolytic enzymes and their application in the bioethanol industry, I. Taylor & Francis.
Singh, A. K., Bilal, M., Iqbal, H. M., Meyer, A. S., and Raj, A. (2021). Bioremediation of lignin derivatives and phenolics in wastewater with lignin modifying enzymes: status, opportunities and challenges. Sci. Total Environ., 777, 145988–146021. doi:10.1016/j.scitotenv.2021.145988
Sivasakthi, S., Usharani, G., and Saranraj, P. (2014). Biocontrol potentiality of plant growth promoting bacteria (PGPR)-Pseudomonas fluorescens and Bacillus subtilis: a review. Afr. J. Agric. Res. 9 (16), 1265–1277. doi:10.5897/AJAR2013.7914
Skov, J., Kania, P. W., Holten-Andersen, L., Fouz, B., and Buchmann, K. (2012). Immunomodulatory effects of dietary β-1, 3-glucan from Euglena gracilis in rainbow trout (Oncorhynchus mykiss) immersion vaccinated against Yersinia ruckeri. Fish shellfish Immunol. 33 (1), 111–120. doi:10.1016/j.fsi.2012.04.009
Softa, M., Percoco, G., Lati, E., and Bony, P. (2019). Birch Sap (<i&gt;Betula alba&lt;/i&gt;) and Chaga Mushroom (<i&gt;Inonotus obliquus&lt;/i&gt;) Extracts Show Anti-Oxidant, Anti-Inflammatory and DNA Protection/Repair Activity <i&gt;in vitro&lt;/i&gt;. J. Cosmet. Dermatological Sci. Appl. 9 (02), 188–205. doi:10.4236/jcdsa.2019.92016
Somnath, R., Shibu, B., Usha, C., and Bishwanath, C. (2015). Evaluation of spent mushroom substrate as biofertilizer for growth improvement of Capsicum annuum L. J. Appl. Biol. Biotechnol. 3 (3), 22–27. doi:10.7324/JABB.2015.3305
Song, F.-Q., Liu, Y., Kong, X.-S., Chang, W., and Song, G. (2013). Progress on understanding the anticancer mechanisms of medicinal mushroom: Inonotus obliquus. Asian Pac. J. Cancer Prev. 14 (3), 1571–1578. doi:10.7314/apjcp.2013.14.3.1571
Song, H.-S., Lee, Y.-J., Kim, S.-K., Moon, W.-K., Kim, D.-W., Kim, Y.-S., et al. (2004). Downregulatory effect of AGI-1120$({\alpha}-glucosidase inhibitor) $ and chaga mushroom (Inonotus obliquus) on cellular $ NF-{\kappa} B $ activation and their antioxidant activity. Korean J. Pharmacogn. 35 (1), 92–97.
Stamets, P. (2005). Mycelium running: how mushrooms can help save the world. Berkeley: Ten speed Press.
Streeter, C., Conway, K., Horn, G., and Mader, T. (1982). Nutritional evaluation of wheat straw incubated with the edible mushroom, Pleurotus ostreatus. J. Animal Sci. 54 (1), 183–188. doi:10.2527/jas1982.541183x
Sun, J.-E., Ao, Z.-H., Lu, Z.-M., Xu, H.-Y., Zhang, X.-M., Dou, W.-F., et al. (2008). Antihyperglycemic and antilipidperoxidative effects of dry matter of culture broth of Inonotus obliquus in submerged culture on normal and alloxan-diabetes mice. J. Ethnopharmacol. 118 (1), 7–13. doi:10.1016/j.jep.2008.02.030
Sysoeva, M., Yumaeva, L., Kuznetsova, O. Y., Ziyatdinova, G., Budnikov, G., and Mel’nikova, N. (2012). Study of the composition of biologically active compounds in chaga meal. Perspectives of application of chaga meal in pharmaceutical industry. Russ. J. General Chem. 82 (3), 586–594. doi:10.1134/s1070363212030383
Szychowski, K. A., Skóra, B., Pomianek, T., and Gmiński, J. (2021). Inonotus obliquus–from folk medicine to clinical use. J. traditional complementary Med. 11 (4), 293–302. doi:10.1016/j.jtcme.2020.08.003
Taji, S., Yamada, T., Wada, S.-i., Tokuda, H., Sakuma, K., and Tanaka, R. (2008). Lanostane-type triterpenoids from the sclerotia of Inonotus obliquus possessing anti-tumor promoting activity. Eur. J. Med. Chem. 43 (11), 2373–2379. doi:10.1016/j.ejmech.2008.01.037
Tanaka, R., Toyoshima, M., and Yamada, T. (2011). New lanostane-type triterpenoids, inonotsutriols D, and E, from Inonotus obliquus. Phytochem. Lett. 4 (3), 328–332. doi:10.1016/j.phytol.2011.07.001
Teplyakova, T. V., Pyankov, O. V., Safatov, A. S., Ovchinnikova, A. S., Kosogova, T. A., Skarnovich, M. O., et al. (2022). Water extract of the chaga medicinal mushroom, Inonotus obliquus (agaricomycetes), inhibits SARS-CoV-2 replication in Vero E6 and Vero cell culture experiments. Int. J. Med. Mushrooms 24 (2), 23–30. doi:10.1615/IntJMedMushrooms.2021042012
Turner, N. J., and Cuerrier, A. (2022). ‘Frog’s umbrella’and ‘ghost’s face powder’: the cultural roles of mushrooms and other fungi for Canadian Indigenous Peoples. Botany 100 (2), 183–205. doi:10.1139/cjb-2021-0052
Thawthong, A., Karunarathna, S. C., Thongklang, N., Chukeatirote, E., Kakumyan, P., Chamyuang, S., et al. (2014). Discovering and domesticating wild tropical cultivatable mushrooms. Chiang Mai J. Sci. 41 (4), 731–764.
Thomas, P. W., Elkhateeb, W. A., and Daba, G. M. (2020). Chaga (Inonotus obliquus): a medical marvel but a conservation dilemma. Sydowia 72, 123–130. doi:10.12905/0380.sydowia72-2020-0123
Tsatmali, M., Ancans, J., and Thody, A. J. (2002). Melanocyte function and its control by melanocortin peptides. J. Histochem. Cytochem. 50 (2), 125–133. doi:10.1177/002215540205000201
Ţura, D., Wasser, S. P., and Zmitrovich, I. V. (2018). Wood-inhabiting fungi: applied aspects. CRC Press, 245–292. Fungi.
Uzun, I. (2004). Use of spent mushroom compost in sustainable fruit production. J. Fruit Ornam. Plant Res. 12, 157–165. Available at: http://www.inhort.pl/files/journal_pdf/journal_2004spec/full2004-18spec.pdf.
Van Kuijk, S., Sonnenberg, A., Baars, J., Hendriks, W., and Cone, J. (2015). Fungal treated lignocellulosic biomass as ruminant feed ingredient: a review. Biotechnol. Adv. 33 (1), 191–202. doi:10.1016/j.biotechadv.2014.10.014
Wang, L.-X., Lu, Z.-M., Geng, Y., Zhang, X.-M., Xu, G.-H., Shi, J.-S., et al. (2014). Stimulated production of steroids in Inonotus obliquus by host factors from birch. J. Biosci. Bioeng. 118 (6), 728–731. doi:10.1016/j.jbiosc.2014.05.022
Wasser, S. (2021). Book review: medicinal mushrooms: the essential guide. Int. J. Med. Mushrooms 23, 97–101. doi:10.1615/intjmedmushrooms.2021040287
Wasser, S. P., and Volz, P. A. (2017). Medicinal mushrooms in human clinical studies. Part I. Anticancer, oncoimmunological, and immunomodulatory activities: a review. Int. J. Med. Mushrooms 19 (6), 279–317. doi:10.1615/IntJMedMushrooms.v19.i4.10
Wold, C. W., Kjeldsen, C., Corthay, A., Rise, F., Christensen, B. E., Duus, J. Ø., et al. (2018). Structural characterization of bioactive heteropolysaccharides from the medicinal fungus Inonotus obliquus (Chaga). Carbohydr. Polym. 185, 27–40. doi:10.1016/j.carbpol.2017.12.041
Xiao, S., Tian, Z., Wang, Y., Si, L., Zhang, L., and Zhou, D. (2018). Recent progress in the antiviral activity and mechanism study of pentacyclic triterpenoids and their derivatives. Bentham Sci. 38, 951–976. doi:10.1002/med.21484
Xiuhong, Z., Yue, Z., Shuyan, Y., and Zhonghua, Z. (2015). Effect of Inonotus obliquus polysaccharides on physical fatigue in mice. J. Traditional Chin. Med. 35 (4), 468–472. doi:10.1016/s0254-6272(15)30126-6
Xu, X., Pang, C., Yang, C., Zheng, Y., Xu, H.-Y., Lu, Z., et al. (2010). Antihyperglycemic and antilipidperoxidative effects of polysaccharides extracted from medicinal mushroom Chaga, Inonotus obliquus (Pers.: Fr.) Pilat (Aphyllophoromycetideae) on alloxan-diabetes mice. Int. J. Med. Mushrooms 12 (3), 235–244. doi:10.1615/intjmedmushr.v12.i3.20
Xu, X., Wu, Y., and Chen, H. (2011). Comparative antioxidative characteristics of polysaccharide-enriched extracts from natural sclerotia and cultured mycelia in submerged fermentation of Inonotus obliquus. Food Chem. 127 (1), 74–79. doi:10.1016/j.foodchem.2010.12.090
Yan, Z.-F., Yang, Y., Tian, F.-H., Mao, X.-X., Li, Y., and Li, C.-T. (2014). Inhibitory and acceleratory effects of Inonotus obliquus on tyrosinase activity and melanin formation in B16 melanoma cells. Evidence-based complementary Altern. Med. 2014, 259836. doi:10.1155/2014/259836
Ying, Y.-M., Zhang, L.-Y., Zhang, X., Bai, H.-B., Liang, D.-E., Ma, L.-F., et al. (2014). Terpenoids with alpha-glucosidase inhibitory activity from the submerged culture of Inonotus obliquus. Phytochemistry 108, 171–176. doi:10.1016/j.phytochem.2014.09.022
Yong, T., Chen, S., Liang, D., Zuo, D., Diao, X., Deng, C., et al. (2018). Actions of Inonotus obliquus against hyperuricemia through XOD and bioactives screened by molecular modeling. Int. J. Mol. Sci. 19 (10), 3222. doi:10.3390/ijms19103222
Youn, M.-J., Kim, J.-K., Park, S.-Y., Kim, Y., Kim, S.-J., Lee, J. S., et al. (2008). Chaga mushroom (Inonotus obliquus) induces G0/G1 arrest and apoptosis in human hepatoma HepG2 cells. World J. gastroenterology WJG 14 (4), 511–517. doi:10.3748/wjg.14.511
Yusoo, S., Yutaka, T., and Minoru, T. (2002). Triterpenoids, steroids, and a new sesquiterpen from Inonotus obliquus (Pers.: Fr.) Pilat. Int. J. Med. Mushrooms 4 (2), 8. doi:10.1615/IntJMedMushr.v4.i2.10
Zabel, R. A. (1976). Basidiocarp development in Inonotus obliquus and its inhibition by stem treatments. For. Sci. 22 (4), 431–437. doi:10.1093/forestscience/22.4.431
Zadrazil, F. (1997). Changes in in vitro digestibility of wheat straw during fungal growth and after harvest of oyster mushrooms (Pleurotus spp.) on laboratory and industrial scale. J. Appl. animal Res. 11 (1), 37–48. doi:10.1080/09712119.1997.9706159
Zhang, B., Guo, Y., Wang, Z., Sun, J., Cheng, J. M., Liu, R., et al. (2008). Prognostic analysis of pulmonary metastases from hepatocellular carcinoma. Asian-Australasian J. Animal Sci. 21 (2), 237–243. doi:10.1007/s12072-008-9052-7
Zhang, D. M., Xu, H. G., Wang, L., Li, Y. J., Sun, P. H., Wu, X. M., et al. (2015). Betulinic acid and its derivatives as potential antitumor agents. Med. Res. Rev., 35(6), 1127–1155. doi:10.1002/med.21353
Zhao, F., Mai, Q., Ma, J., Xu, M., Wang, X., Cui, T., et al. (2015). Triterpenoids from Inonotus obliquus and their antitumor activities. Fitoterapia 101, 34–40. doi:10.1016/j.fitote.2014.12.005
Zhao, F., Xia, G., Chen, L., Zhao, J., Xie, Z., Qiu, F., et al. (2016). Chemical constituents from Inonotus obliquus and their antitumor activities. J. Nat. Med. 70 (4), 721–730. doi:10.1007/s11418-016-1002-4
Zheng, W., Miao, K., Liu, Y., Zhao, Y., Zhang, M., Pan, S., et al. (2010). Chemical diversity of biologically active metabolites in the sclerotia of Inonotus obliquus and submerged culture strategies for up-regulating their production. Appl. Microbiol. Biotechnol. 87, 1237–1254. doi:10.1007/s00253-010-2682-4
Zheng, W., Zhang, M., Zhao, Y., Miao, K., Pan, S., Cao, F., et al. (2011a). Analysis of antioxidant metabolites by solvent extraction from sclerotia of Inonotus obliquus (Chaga). Phytochem. Anal. 22 (2), 95–102. doi:10.1002/pca.1225
Zheng, W., Zhao, Y., Zhang, M., Wei, Z., Miao, K., and Sun, W. (2009). Oxidative stress response of Inonotus obliquus induced by hydrogen peroxide. Med. Mycol. 47 (8), 814–823. doi:10.3109/13693780802653933
Zheng, W., Zhao, Y., Zheng, X., Liu, Y., Pan, S., Dai, Y., et al. (2011b). Production of antioxidant and antitumor metabolites by submerged cultures of Inonotus obliquus cocultured with Phellinus punctatus. Appl. Microbiol. Biotechnol. 89 (1), 157–167. doi:10.1007/s00253-010-2846-2
Zheng, W.-F., Zhao, Y.-X., Zhang, M., Yin, Z., Chen, C., and Wei, Z. (2008). Phenolic compounds from Inonotus obliquus and their immune stimulating effects. Mycosystema 27 (4), 574–581.
Zhong, X.-h., Ren, K., Lu, S.-j., Yang, S.-y., and Sun, D.-z. (2009). Progress of research on Inonotus obliquus. Chin. J. Integr. Med. 15 (2), 156–160. doi:10.1007/s11655-009-0156-2
Zhou, L.-W., and Wang, X.-Y. (2015). <I>Inonotus griseus</I> sp. nov. from eastern China. Mycotaxon 130 (3), 661–669. doi:10.5248/130.661
Zhu, F., Du, B., Bian, Z., and Xu, B. (2015). Beta-glucans from edible and medicinal mushrooms: characteristics, physicochemical and biological activities. J. Food Compos. Analysis 41, 165–173. doi:10.1016/j.jfca.2015.01.019
Zhu, F., Du, B., and Xu, B. (2016). A critical review on production and industrial applications of beta-glucans. Food Hydrocoll. 52, 275–288. doi:10.1016/j.foodhyd.2015.07.003
Zmitrovich, I. V., Denisova, N. P., Balandaykin, M. E., Belova, N. V., Bondartseva, M. A., Perevedentseva, L. G., et al. (2020). Chaga and its bioactive complexes: history and perspectives. Pharm. Formulas 2 (2), 84–93. doi:10.17816/phf34803
Zyryanova, O. A., Terazawa, M., Koike, T., and Zyryanov, V. I. (2010). White birch trees as resource species of Russia: their distribution, ecophysiological features, multiple utilizations. Eurasian J. For. Res. 13 (1), 25–40.
Keywords: Chaga, mycochemistry, ethnomycology, pharmacology, agricultural uses, traditional uses
Citation: Fordjour E, Manful CF, Javed R, Galagedara LW, Cuss CW, Cheema M and Thomas R (2023) Chaga mushroom: a super-fungus with countless facets and untapped potential. Front. Pharmacol. 14:1273786. doi: 10.3389/fphar.2023.1273786
Received: 07 August 2023; Accepted: 16 October 2023;
Published: 05 December 2023.
Edited by:
Byung Hee Chun, Pukyong National University, Republic of KoreaReviewed by:
Shelini Surendran, University of Surrey, United KingdomCopyright © 2023 Fordjour, Manful, Javed, Galagedara, Cuss, Cheema and Thomas. This is an open-access article distributed under the terms of the Creative Commons Attribution License (CC BY). The use, distribution or reproduction in other forums is permitted, provided the original author(s) and the copyright owner(s) are credited and that the original publication in this journal is cited, in accordance with accepted academic practice. No use, distribution or reproduction is permitted which does not comply with these terms.
*Correspondence: Eric Fordjour, ZWZvcmRqb0B1d28uY2E=; Raymond Thomas, cnRob21hMkB1d28uY2E=
Disclaimer: All claims expressed in this article are solely those of the authors and do not necessarily represent those of their affiliated organizations, or those of the publisher, the editors and the reviewers. Any product that may be evaluated in this article or claim that may be made by its manufacturer is not guaranteed or endorsed by the publisher.
Research integrity at Frontiers
Learn more about the work of our research integrity team to safeguard the quality of each article we publish.