- 1Center for Traditional Medicine and Drug Research, Kenya Medical Research Institute, Nairobi, Kenya
- 2Physcial Sciences Department, South Eastern Kenya University, Kitui, Kenya
Background: Medicinal plants have traditionally been used as remedies against malaria. The present review attempted to compile data on scientific research evidence on antimalarial medicinal plants screened at Kenya Medical Research Institute (KEMRI), Center for Traditional Medicine and Drug (CTMDR) Research from January 2003 to December 2021.
Methods: A systematic review was conducted using a predefined protocol based on PRISMA. Search was performed in Google Scholar and PubMed. One hundred and eight journal articles were identified 37 of which published on antimalarial/antiplasmodial work. Thirty journal articles with at least one author from KEMRI-CTMDR and accessible in full were selected for analysis. Relevant data was captured in MS Excel format and descriptive statistics, percentages and tables used to summarize the findings.
Results: Assessment of individual plant species was considered as an independent study resulting in 1170 antiplasmodial/antimalarial tests done from 197 plant species. One hundred and fifty plant species were screened in vitro, one in vivo and 46 were both in vivo and in vitro. Three hundred and forty-four of tests reported good activity (IC50 < 10 μg/mL or parasite suppression rate of ≥50%), 414 moderate activity (IC50 values of 10–49 μg/mL or parasite suppression rate of 30%–49%) and 412 were reports of inactivity (IC50 ˃ 50 μg/mL or parasite suppression rate of <30%). Fuerstia africana and Ludwigia erecta were reported to have the highest activities, with IC50 < 1 μg/mL against Plasmodium falciparum D6 strain and chemosuppression in mice at an oral dose of 100 mg/kg, was reported as 61.9% and 65.3% respectively. Fifty five antimalarial/antiplasmodial active compounds isolated from eight plant species were reported with resinone (39) having the best activity (IC50 < 1 μg/mL).
Conclusion: Though 344 of tests reported promising antimalarial activity, it was noted that there was limited evaluation of these plants in animal models, with only 9.0% (105/1170) studies and no clinical trials. This highlights an important research gap emphasizing the need for drug development studies that aim to progress study findings from preclinical to clinical studies. There is still need for extensive research on promising plant species aimed at developing new plant based antimalarial drugs.
1 Introduction
Morbidity and mortality caused by malaria is still a public health concern despite the fact that it is a curable and preventable disease. World Malaria Report of 2022 reveals that between 2019 and 2020, estimated malaria cases increased from 218 to 232 million, and deaths from 544 000 to 599 000 in the World Health Organization (WHO) African Region WHO (2022). In Kenya, malaria remains a major public health problem accounting for an estimated 13%–15% of outpatient consultations. The Plasmodium falciparum parasite, which causes the most severe form of the disease, accounts for more than 99% of infections. Malaria prevalence in Kenya varies considerably by season and across different geographic zones. This is because transmission and infection risks are mainly determined by altitude, rainfall patterns and temperature (Division of National Malaria Programme DNMP [Kenya] and International Classification of Functioning, Disability, and Health ICF, 2021). Key malaria control and prevention strategies that have been employed in Kenya include use of insecticide treated nets, intermittent preventive treatment during pregnancy (IPTp) using sulfadoxine pyrimethamine, indoor residual spraying and adoption of artemisinin combination therapy (ACT). However, the adaptation of the mosquitoes to insecticides and emergence and spread of drug resistant parasites, especially P. falciparum, is a drawback to these interventions. The WHO (2022) confirmed emergency of partial resistance to artemisinin drugs in some African countries, namely,: Rwanda, Eritrea, and Uganda. The possibility of the spread of artemisinin resistant parasites to other malaria endemic regions in Africa is inevitable. Therefore, the challenge to eliminate malaria remains significant hence the need for new agents that are cheap, safe, readily available, active against sensitive and drug resistant Plasmodium parasites or act in combination with existing drugs.
Medicinal plants have played a major role in discovery and development of antimalarial drugs. It is expected that medicinal plants would still serve as a source of new drug leads given their chemodiversity (Batista et al., 2009). Several studies have documented medicinal plants used in management of malaria by various local communities in Kenya (Muthaura et al., 2007a; Njoroge and Bussmann, 2007; Gathirwa et al., 2011; Mukungu et al., 2016). Continued research on Kenyan medicinal plants has offered plants extracts and purified secondary metabolites with potent antimalarialantiplasmodial activities (Muiva et al., 2009; Irungu et al., 2014; 2015; Muthaura et al., 2015b).
In this review we summarize research evidence on toxicity, cytotoxicity, antimalarial and antiplasmodial properties of medicinal plant extracts and secondary metabolites evaluated at Kenya Medical Research Institute, Center for Traditional Medicine and Drug Research (KEMRI-CTMDR) between January 2003 and December 2021. This review covers a period within which there was increased research activities on screening medicinal plants for antimalarial properties providing a recent outlook of our drug discovery efforts. We acknowledge that other Kenyan institution have documented medicinal plants with antimalarial activity. However, this review chose to exclusively focus on work done at KEMRI due to its renowned expertise in human health research, including rationalization of traditional medicine in Kenya.
2 Methods
2.1 Systematic review
A systematic review was conducted using a predefined protocol based on Preferred Reporting Items for Systematic Reviews and Meta-Analyses (PRISMA) (Page et al., 2021) guidelines including; literature search to identify potential articles, assessing the relevance of the articles quality and data extraction. The search was performed in Google Scholar and PubMed covering the period January 2003 to December 2021 and was limited to original English journal articles whose full text were accessible. Literature search was performed using key terms such as: Kenyan medicinal plants with antiplasmodial/antimalarial activities, antimalarial studies at Kenya Medical Research Institute (KEMRI), Center for Traditional Medicine and Drug Research (CTMDR), Kenyan antimalarial herbal remedies. We also searched with individual names of past and present Research Scientists working at KEMRI-CTMDR.
2.2 Inclusion and exclusion criteria
After a web search on pharmacological activities of medicinal plants screened at KEMRI-CTMDR, 108 journal articles were identified, 37 of which reported on antimalarial/antiplasmodial activities. Seven articles, did not meet our inclusion criteria since they either did not have an author from CTMDR, reported on synthetic compounds, were non-open access or were partially accessed (abstract only) as shown in Figure 1. Thirty journal articles that met our selection criteria were selected for analysis.
2.3 Data screening and extraction
The articles were further analyzed based on the originality of reported data determined by the description of the study design. Data was captured in excel format and the following information from each eligible journal article was extracted; article title, plant botanical name, family, plant collection site, part(s) of the plant used, type of study (in vitro or in vivo), Plasmodium strain tested, IC50 values,% chemosuppression, isolated compound (s), cytotoxicity (CC50 or IC50), toxicity (LD50) and extraction solvent used. Descriptive statistics was used to summarize the findings.
3 Results
3.1 Medicinal plants screened for antimalarial/antiplasmodial activity
KEMRI-CTMDR Research Scientists have published data on 197 plant species and their potential antimalarial/antiplasmodial activities within a period of 18 years (January 2003 to December 2021). An extensive search and abstract screening within this period revealed 30 articles with at least one researcher from KEMRI-CTMDR as the main/co-author. Out of the 30 journal articles considered in this review, 66.7% (20/30) of the studies were done by local collaborators compared to international collaborators at 33.3% (10/30). The most preferred journal was Journal of Ethnopharmacology with 11 publications out of the 30 articles analyzed (Table 1). Twenty six out of the 30 articles, focused on plants that were collected from within Kenya while four articles investigated plant materials that were collected from outside Kenya (Table 1).
In this paper, each assessment of a plant species was treated as a separate study, which means that depending on the number of plant species examined, an article could encompass multiple studies. In total there were 1170 antiplasmodial/antimalarial tests done from 197 plant species. One hundred and fifty (76.1%) plant species were screened in vitro, one (0.5%) in vivo and 46 (23.4%) were both in vivo and in vitro. Majority of studies reported crude extracts except three, where fraction blends obtained separately from Gongronema latifolium (Benth.) K. Schum, Artemisia annua L. and Lippia kituiensis Vatke were evaluated (Adebajo et al., 2013; Kangethe et al., 2016; Ng’etich et al., 2020).
3.2 Diversity of plants evaluated
Of the 197 plants species, the most studied plant families were Asteraceae 16 (8.1%), Verbenaceae, 9 (4.6%), Rubiaceae, 8 (4%), Fabaceae, 7 (3.6%) and Leguminosae, 7 (3.6%). The most investigated plant species were; Rotheca myricoides (Hochst.) Steane and Mabb Azadirachta indica A. Juss., Rhus natalensis Bernh. ex Krauss, Turraea robusta (Hochst.) Benth., Ximenia americana L., Vernonia auriculifera Hiern, Toddalia asiatica (L.) Lam., Maytenus undata (Thunb.) Blakelock, Lannea schweinfurthii (Engl.) Engl., Zanthoxylum chalybeum Engl., Harrisonia abyssinica Oliv. Fuerstia africana Oliv. and Asparagus racemosus Willd. Leaves, 85 (27%), stem barks, 87 (28%), root barks, 83 (26%) and whole plant 28 (9%) were the most common parts of the plants used to prepare extracts (Figure 2). Crude extracts dominated in the tests compared to tests done using isolated compounds at 1072 (91.6%) and 98 (8.4%), respectively. Moreover, a majority of the extracts were organic 401 (67.3%) compared to aqueous extracts 195 (32.7%). In ascending order: 1:1 mixture dichloromethane: methanol, 9 (1.5%), hexane, 13 (2.2%), chloroform, 13 (2.2%), petroleum ether, 17 (2.9%), ethyl acetate, 18 (3%), dichloromethane, 19 (3.2%), water, 195 (32.7%) and methanol 309 (51.8%) were the most frequent extraction solvents used.
3.3 In vitro and in vivo activities of plant extracts
The activities were divided into three categories; good (IC50 values <10 μg/mL or suppression rate of ≥50%), moderate (IC50 values of 10 μg/mL–49 μg/mL or suppression rate of 30%–49%) and inactive (IC50 values ˃ 50 μg/mL or suppression rate of <30%) (Waiganjo et al., 2020). In general, 344 (29.4%) of the antiplasmodial tests reported good activity, 414 (35.4%) moderate activity and 412 (35.2%) were reports of inactivity. For the in vitro tests (Supplementary Table S1), inactive reports were the majority 386 (33%) followed by moderate activity 379 (32.4%) and good activity 300 (25.6%). Of the 300 in vitro studies with good activity, 177 (59%) were active with IC50 between 5 and 10 μg/mL while 123 (41%) were highly active with IC50 < 5 μg/mL (Table 2). On the other hand, a majority of in vivo tests reported good activity 44 (41.9%) (Table 3) followed by moderate activity 35 (33.3%) and 26 (24.8%) reported inactivity (Supplementary Table S2). Plant species that were commonly reported to display promising antiplasmodial activities in different studies included; T. robusta which exhibited good antiplasmodial activity in 8 out of 13 tests (61.5%), T. asiatica, 14 out of 26 tests (53.8%), Erythrina burttii Baker f., 12 out of 22 tests (54.5%) and M. undata, 10 out of 14 tests (71.4%).
3.4 In vitro and in vivo activities of isolated compounds
Fifty five antimalarial/antiplasmodial active compounds isolated from eight plant species were reported. Of the 55 compounds, 7 (12.7%) and 48 (87.3%) were evaluated in vivo and in vitro, respectively. Twenty two of 55 (40%) compounds exhibited moderate activity while 16 (29%) were inactive. The most active compounds (IC50 values ≤10 μg/mL) were 17 (i.e., 5, 25, 26, 27, 28, 29, 31, 32, 34, 37, 40, 41, 42, 44, 46, 48) (Table 4) with resinone (39) having the best activity (IC50 < 1 μg/mL).
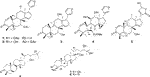
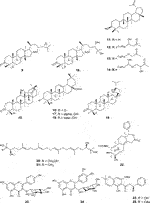
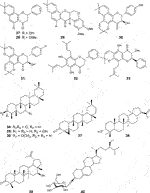
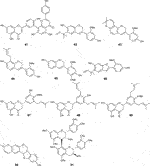
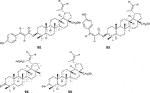
3.5 Cytotoxicity of plant extracts and compounds evaluated for antimalarial and antiplasmodial activity
In this review, a promising antimalarial extract was classified as lacking cytotoxicity to the mammalian cells by displaying an IC50 value greater than 90 μg/mL (Irungu et al., 2007). In general, there were 210 cytotoxicity tests from 40 plants. Out of the 40 plant species 14 (35%) had some degree of cytotoxicity across different studies. Plant families with the most cytotoxic (CC50 < 10 μg/mL) plant species were Meliaceae, Cucurbitaceae, Canellaceae, Asclepiadaceae, Asparagaceae and Lamiaceae. Fourteen (35%) of the plants tested were cytotoxic (CC50 < 10 μg/mL) and 8 (20%) demonstrated some toxicity levels (LD50 100 mg/kg) in mice. The plants with good and moderate antiplasmodial activity demonstrated some degree of cytotoxicity of 10% and 7.5%, respectively. Organic extracts especially methanol, petroleum ether, dichloromethane: 1; 1 mixture of methanol and dichloromethane were reported to have the highest degree of cytotoxicity (CC50 < 10 μg/mL). The most cytotoxic was dichloromethane extract from Warburgia ugandensis Sprague with CC50 0.34 μg/mL against L6, rat skeletal myoblast cells (Irungu et al., 2007). The most cytotoxic compounds were azadironolide (5) with CC50 of 8.5 μg/mL (HEp2 cells), oleanonic acid (16) with CC50 of 1.4 µM (HEp2 cells), 12α-acetoxy-7-deacetylazadirone (2) with CC50 of 4.3 µM (HEp2 cells), niloticin (6) with CC50 of 6.9 µM (HEp2 cells), hispidol B (7) with CC50 of 7.4 µM (HEp2 cells), amentoflavone (41) with CC50 of 0.34 μg/mL (L6 cells) and piscidinol A (8) with CC50 of 8.4 µM (HEp2 cells). The mentioned compounds had promising antiplasmodial activity, with azadironolide (5) being the most active. The most frequently used cells for cytotoxicity determination were Vero cells and HEp-2 cells.
4 Discussion
The global concern over the increasing resistance to primary antimalarial medications necessitates a boost in research efforts to discover and develop new drugs for malaria. The escalating resistance rates emphasize the urgency of accelerating the exploration and development of novel antimalarial drugs. It is evident from this review that a lot needs to be done towards the discovery of new antimalarial drugs. While numerous plant species have shown promising antiplasmodial effects, there has been limited evaluation of these plants in animal models, with only 9% (105/1170) in vivo studies and no clinical trial conducted. This highlights the importance of conducting comprehensive preclinical and clinical research. Pre-clinical and clinical research are a significant next step to determine the prospects of these promising medicinal plants (Al Rashid et al., 2020).
The majority of studies (91.6%) included in the analysis utilized crude plant extracts rather than pure compounds for their investigations. Such preference for crude extracts can be attributed to insufficient infrastructure required to process plant materials and extract pure compounds as well as an attempt to mimic the traditional preparation of plant remedies using alcoholic beverages. Preference for leaves, stem barks and root barks (Figure 2), can be attributed to their abundance and the local communities’ indigenous knowledge and skills on their uses (Umair et al., 2019). Additionally, the preference for harvesting these plant parts is influenced by their lower impact on the overall health and sustainability of medicinal plant populations (Araya et al., 2015).
In this review, the IC50 values below 10 μg/mL were regarded as the threshold for significant antimalarial activity. This cutoff is considered as the minimum requirement for preliminary positive result in screening of potential antimalarial plant extracts (Mohammed et al., 2014). A total of 151 plant species belonging to 48 families exhibited moderate to good antiplasmodial activity. Among the most extensively studied plant families were Asteraceae, Verbenaceae, Fabaceae, Euphorbiaceae, Rubiaceae, and Leguminosae while families with the highest number of active plants were Apocynaceae, Celestraceae, Euphorbiaceae and Rutaceae. These findings suggest that greater attention should be given to plants whose extracts were promising for the discovery of antimalarial drug leads. Regarding individual plant species, notable ones that have received significant research attention include R. myricoides, A. indica, R. natalensis, T. robusta, X. americana, T. asiatica, M. undata, L. schweinfurthii, Z. chalybeum, H. abyssinica, F. africana, A. racemosus and T. robusta. Their extracts have consistently demonstrated significant antiplasmodial activities in multiple studies (Gathirwa et al., 2007; 2008; 2011; Orwa et al., 2013; Muthaura et al., 2015a; Jeruto et al., 2015; Rotich et al., 2015). Therefore, further preclinical evaluation of these plant species is recommended. This review has identified most potent plant extracts with significant activity against P. falciparum, exhibiting an IC50 value of ≤1 μg/mL and/or a parasite suppression rate above 90%. The plant species whose extracts were classified as most potent include Combretum padoides Engl. and Diels, L. schweinfurthii, Clerodendrum eriophyllum (Hochst.) Vatke, Holarrhena floribunda (G. Don) T. Durand and Schinz, M. undata, E. burtii, Vernonia lasiopus O. Hoffm., F. africana, Ludwigia erecta (L.) H. Hara, Boscia salicifolia Oliv., and Premna chrysoclada (Bojer) Gürke. Furthermore, studies conducted by other researchers (Machumi, 2010; Machumi et al., 2010; Muganga et al., 2010; Zofou et al., 2013; Hoekou et al., 2017; Elhaj et al., 2021) have also reported good antiplasmodial activities of C. eriophyllum, H. floribunda, V. lasiopus, F. africana, and L. erecta.
In this review, we documented compounds that are reported to possess other pharmacological activities such as obovatin (27) which has shown great potential as an antibacterial agent (Akter et al., 2016). Other studies have demonstrated antiplasmodial and anticancer activities of compounds captured in this review, deguelin (29), (Varughese et al., 2019; Buyinza, 2020), friedelin (34) (Prabhu et al., 2011; Emsen et al., 2018; Joshi et al., 2022; Wuttikit and Thanakijcharoenpath, 2023), and epifriedelanol (35) (Kundu et al., 2000; Gashu, 2022; Wuttikit and Thanakijcharoenpath, 2023). The antiplasmodial activity of friedelin (34) was found to be lower in a study by Sadeghpour et al. (2006) compared to other research cited in the current review (Sadeghpour et al., 2006). In summary, these compounds have demonstrated promising antiplasmodial activity and are thus valuable candidates for further antimalarial drug development.
Our review has demonstrated that majority of investigated plants have promising antiplasmodial activity. However, when the same plants were tested in a mouse model, their activity against malaria parasites decreased in most cases, with many plants showing no activity at all. For instance, Rotich et al. (2015) and Gathirwa et al. (2011) reported that Uvaria acuminate Oliv. and F. africana, displayed good antiplasmodial activity (IC50 < 10 μg/mL) but were inactive in vivo (chemosupressiom at 27.0% and 27.9%, respectively). The observed variations could be explained by the fact that in vitro studies involved direct contact between the extracts and the parasite, while for in vivo studies activity of the extracts/compounds might have been altered by metabolism. Nevertheless, a few studies have shown that plant activity can actually increase from in vitro to in vivo. For example, Muthaura et al. (2007b) demonstrated that Pittosporum viridiflorum Sims exhibited moderate activity in vitro [IC50 18.9 μg/mL and 17.7 μg/ml against D6 and W2 strains, respectively] but showed good activity in vivo with chemosuppression of 54.8% (Muthaura et al., 2007b). These findings suggest that plants could still possess significant antimalarial properties in animal models even if they do not show activity in vitro. Apparently, researchers proceed to in vivo studies only when they observe substantial antiplasmodial activity. This may explain the limited number of in vivo studies documented in this review. Despite the unsatisfactory outcomes observed in vitro, it still remains crucial to examine the antimalarial properties of plants through in vivo studies.
The present study identified significant inter study variations in the antiplasmodial activity of various plant species. Notably, considerable variation was observed for species such as P. chrysoclada, F. virosa, Grewia plagiophylla Burret, T. robusta, R. myricoides, A. racemosus, Vangueria acutiloba K. Schum., C. eriophyllum, H. abyssinica, V. lasiopus, W. ugandensis, Ajuga remota Benth., Tabernaemontana pachysiphon Stapf, Uvaria lucida Benth., Uvaria scheffleri Engl. and Diels, Vitex strickeri Moldenke, Warburgia stuhlmannii Engl. and Cyperus articulatus L. (Muthaura et al., 2007a; Muthaura et al., 2015a; Muthaura et al., 2015b; Irungu et al., 2007; Gathirwa et al., 2008; Gathirwa et al., 2011; Rukunga et al., 2008; Jeruto et al., 2015). Several factors may account for these differences, including variations in the extraction solvent used, which affects the yield and composition of extracted metabolites. Dichloromethane, for instance, primarily extracts apolar metabolites, while methanol extracts a range of polar to moderately apolar metabolites and water extracts polar metabolites. The choice of plant parts used in the studies also contributed to the observed variations, as certain parts may contain higher concentrations of specific active metabolites. Additionally, differences in extraction yields can arise due to the varying accumulation of active metabolites in different plant parts. Also, the location, environmental factors and season (dry and rainy seasons) have significant effect on the accumulation of various phytochemicals present in medicinal plants. During the dry season, there is a decrease in water and nutrient supply to plants. Nutritional stress can result in the accumulation of osmo-protectants to stabilize proteins structure and maintain membrane integrity and scavenge reactive oxygen species (ROS), with biomass and secondary metabolites production (Niinemets, 2016). Phenolic compounds including coumarins, flavonoids, cinnamic acids and lignans, as well as plant hormones such as auxins, salicylic acid, cytokinin, ethylene, gibberellic acid and jasmonic acid are involved in modulation of developmental processes in plants and determine plant responses to environmental stresses (Fang et al., 2011; Fayez and Bazaid, 2014; Giménez et al., 2014). On the other hand, plants growing in lower temperatures develop significant adjustments in several physiological and biochemical processes that enable them to survive under low temperature stress, and this causes inhibition in the synthesis and storage of secondary metabolites (Verma and Shukla, 2015).
Another factor that may contribute to the observed inter study variation is the strain of Plasmodium used in the experiments. Studies employing chloroquine-sensitive strains of the parasite, such as P. falciparum 3D7, D6, and NF54, tend to report higher antiplasmodial activity compared to studies utilizing chloroquine-resistant strains like W2, K39, ENT30, or K1. This variation in strain susceptibility to the tested extract/compound can influence the reported outcomes and contribute to the differences observed across studies. It is worth noting that the variation in the antiplasmodial activity of Turraea nilotica Hochst. ex Benth (Irungu et al., 2015). observed with pure compounds highlights an important issue. Even extracts that initially show low potency and might be disregarded during the initial screening process for further development may still contain active components with therapeutic potential, as mentioned by Kuria et al. (2001). In the given example, the preliminary analysis of the crude extract demonstrates an IC50 value of 59 μg/mL for the D6 strain and 47.4 μg/mL for the W2 strain, as indicated in Supplementary Table S1. However, within the same extract, there is a highly activeepimeric mixture, azadironolide (51) that exhibited an IC50 value of less than 5 μg/mL.
Data collated in this review showed 14 out of 40 (35%) plant species, exhibited high level of cytotoxicity (CC50 < 10 μg/mL). The plant families Meliaceae, Cucurbitaceae, Asclepiadaceae, Asparagaceae, Canellaceae and Lamiaceae were found to have the highest number of cytotoxic plant species. The most cytotoxic plants identified were W. ugandensis, X. americana and Khaya anthotheca (Welw.). Interestingly, W. ugandensis and X. americana have shown promising antiplasmodial/antimalarial activity in certain studies (Irungu et al., 2007; Muthaura et al., 2015b). This suggests that the observed strong antiplasmodial effects could probably be as a result of cytotoxicity rather than direct activity against the parasites themselves (Irungu et al., 2007). Other plants with significant cytotoxicity but also exhibiting moderate to good antiplasmodial/antimalarial properties include Vernonia amygdalina Delile, Baccharoides adoensis (Sch.Bip. ex A. Rich.) Hochr., Schkuhria pinnata (Lam.) Kuntze, Momordica foetida Schumach. and Thonn., Entada abyssinica Steud. ex A. Rich., Entandrophragma utile (Dawe and Sprague) Sprague (Obbo et al., 2019), C. eriophyllum (Irungu et al., 2007), Ekebergia capensis Sparrm (Irungu et al., 2014), T. robusta (Irungu et al., 2015) and F. africana (Rotich et al., 2015). The toxicity levels of most plant extracts in animal models were found to be minimal, even at dosages above 1000 mg/kg body weight. Aqueous extracts showed no adverse effects even at a dosage of 5000 mg/kg body weight. It is important to note that toxicity/cytotoxicity levels varied considerably, even within the same plant species. This variation could be attributed not only to the extraction solvent but also to differences in study design (in vivo or in vitro) and the specific plant parts tested.
5 Conclusion
This review has collated valuable foundational data that researchers in the field can utilize for the exploration and development of new antimalarial drug leads. Among the plant species studied, F. africana and L. erecta were found to have the highest activity, with IC50 values below 1 μg/mL against P. falciparum (D6), a chloroquine-sensitive strain. These plants also demonstrated significant parasite suppression at an oral dose of 100 mg/kg, with 61.9% and 65.3% for F. africana and L. erecta, respectively. Their LD50 values were above 3000 mg/kg, indicating low toxicity. Additionally, resinone (39) a compound isolated from the Drypetes gerrardii (Baill.) Hutch showed good activity against P. falciparum K1 multidrug-resistant strain, with an IC50 below 1 μg/mL. However, no information was provided regarding in vivo testing or toxicity assessments of this compound. While the in vitro results demonstrated promising activities of some plant extracts and their compounds, there has been limited evaluation of active plants extracts in vivo, and no clinical trials have been conducted yet. To address the research gap, preclinical studies should progress beyond in vitro and in vivo screening for antimalarial properties to include comprehensive studies on efficacy, safety and quality of promising extracts in animal models. Additionally, future studies geared towards product development should factor in intellectual property rights through local bodies such as Kenya Industrial Property Institute to address barriers that may arise and hinder development of lead compounds/phytomedicines from medicinal plants. Furthermore, the study revealed significant variations in the antiplasmodial activities of the plants across different studies. Notably, only a small number of plants had their active compounds identified. Furthermore, it is worth emphasizing the significance of assessing ethnomedical preparation procedures and establishing a correlation with laboratory extraction methods. This correlation is essential as it justifies the process of plant selection and, in turn, contributes to the validation of ethnomedicine. Hence, there is still need for further and extensive research with the aid of a stable strategy in the exploration and advancement of novel antimalarial compounds to tackle the escalating resistance observed in current primary antimalarial drugs across the globe.
Author contributions
BI: Conceptualization, Validation, Writing–original draft, Writing–review and editing. EO: Data curation, Validation, Writing–original draft, Writing–review and editing. MN: Methodology, Validation, Writing–review and editing. SN: Validation, Writing–review and editing. LK: Formal Analysis, Methodology, Validation, Writing–review and editing.
Funding
The author(s) declare that no financial support was received for the research, authorship, and/or publication of this article.
Acknowledgments
The authors would like to thank Director General, Kenya Medical Research Institute for providing a conducive working environment.
Conflict of interest
The authors declare that the research was conducted in the absence of any commercial or financial relationships that could be construed as a potential conflict of interest.
Publisher’s note
All claims expressed in this article are solely those of the authors and do not necessarily represent those of their affiliated organizations, or those of the publisher, the editors and the reviewers. Any product that may be evaluated in this article, or claim that may be made by its manufacturer, is not guaranteed or endorsed by the publisher.
Supplementary material
The Supplementary Material for this article can be found online at: https://www.frontiersin.org/articles/10.3389/fphar.2023.1268924/full#supplementary-material
Abbreviations
KEMRI, Kenya Medical Research Institute; CTMDR, Center for Traditional Medicine and Drug Research; PRISMA, Preferred Reporting Items for Systematic Reviews and Meta-Analysis; WHO, World Health Organization; DNMP, Division of National Malaria Program; ICF, International Classification of Functioning disability, and health; IPTp, Intermittent Preventive Treatment during pregnancy; ACT, Artemisinin Combination Therapy; DCM, Dichloromethane; Pet ether, Petroleum ether; EtOAc, ethyl acetate.
References
Adebajo, A. C., Odediran, S. A., Nneji, C. M., Iwalewa, E. O., Rukunga, G. M., Aladesanmi, A. J., et al. (2013). Evaluation of ethnomedical claims II: antimalarial activities of Gongronema latifolium root and stem. J. Herbs Spices Med. Plants 19, 97–118. doi:10.1080/10496475.2012.734012
Akter, K., Barnes, E. C., Loa-Kum-Cheung, W. L., Yin, P., Kichu, M., Brophy, J. J., et al. (2016). Antimicrobial and antioxidant activity and chemical characterisation of Erythrina stricta Roxb. (Fabaceae). J. Ethnopharmacol. 185, 171–181. doi:10.1016/j.jep.2016.03.011
Al Rashid, M. H., Kundu, A., Mandal, V., Wangchuk, P., and Mandal, S. C. (2020). Preclinical and clinical trials of Indian medicinal plants in disease control. Herb. Med. India Indig. Knowl. Pract. Innov. Its Value, 119–142. doi:10.1007/978-981-13-7248-3_9
Araya, S., Abera, B., and Giday, M. (2015). Study of plants traditionally used in public and animal health management in Seharti Samre District, Southern Tigray, Ethiopia. J. Ethnobiol. Ethnomedicine 11, 22–25. doi:10.1186/s13002-015-0015-5
Batista, R., De Jesus Silva Júnior, A., and De Oliveira, A. B. (2009). Plant-derived antimalarial agents: new leads and efficient phytomedicines. Part II. Non-alkaloidal natural products. Molecules 14, 3037–3072. doi:10.3390/molecules14083037
Buyinza, D. (2020). Antiplasmodial and anticancer principles from Millettia dura, Millettia leucantha and. Millettia lasiantha.
Division of National Malaria Programme (DNMP) [Kenya] and ICF (2021). Kenya malaria indicator survey 2020.
Elhaj, M. K., Yagi, S. M., Qahtan, A. A., Alshameri, A., Hodhod, M., Almunqedhi, B., et al. (2021). Screening of phytochemicals and bioactivities of different parts of Ludwigia erecta (L.) H. Hara. Biotechnol. Biotechnol. Equip. 35, 283–289. doi:10.1080/13102818.2021.1875875
Emsen, B., Engin, T., and Turkez, H. (2018). In vitro investigation of the anticancer activity of friedelin in glioblastoma multiforme. Afyon Kocatepe Üniversitesi Fen Ve Mühendis. Bilim. Derg. 18, 763–773. doi:10.5578/fmbd.67733
Fang, X., Yang, C., Wei, Y., Ma, Q., Yang, L., and Chen, X. (2011). Genomics grand for diversified plant secondary metabolites. Plant divers. Resour. 33, 53–64.
Fayez, K. A., and Bazaid, S. A. (2014). Improving drought and salinity tolerance in barley by application of salicylic acid and potassium nitrate. J. Saudi Soc. Agric. Sci. 13, 45–55. doi:10.1016/j.jssas.2013.01.001
Fotie, J., Bohle, D. S., Leimanis, M. L., Georges, E., Rukunga, G., and Nkengfack, A. E. (2006). Lupeol long-chain fatty acid esters with antimalarial activity from Holarrhena floribunda. Holarrhena Floribunda. J. Nat. Prod. 69, 62–67. doi:10.1021/np050315y
Gashu, M. (2022). Epifriedelanol and dammara-20,24-dien-3-yl acetate from Ethiopian inula confertiflora root extract. J. Chem. 2022, 1–4. doi:10.1155/2022/2114595
Gathirwa, J. W., Rukunga, G. M., Mwitari, P. G., Mwikwabe, N. M., Kimani, C. W., Muthaura, C. N., et al. (2011). Traditional herbal antimalarial therapy in Kilifi district, Kenya. J. Ethnopharmacol. 134, 434–442. doi:10.1016/j.jep.2010.12.043
Gathirwa, J. W., Rukunga, G. M., Njagi, E. N. M., Omar, S. A., Mwitari, P. G., Guantai, A. N., et al. (2008). The in vitro anti-plasmodial and in vivo anti-malarial efficacy of combinations of some medicinal plants used traditionally for treatment of malaria by the Meru community in Kenya. J. Ethnopharmacol. 115, 223–231. doi:10.1016/j.jep.2007.09.021
Gathirwa, J. W., Rukunga, G. M., Njagi, E. N., Omar, S. A., Guantai, A. N., Muthaura, C. N., et al. (2007). In vitro anti-plasmodial and in vivo anti-malarial activity of some plants traditionally used for the treatment of malaria by the Meru community in Kenya. J. Nat. Med. 61, 261–268. doi:10.1007/s11418-007-0140-0
Giménez, M. J., Valverde, J. M., Valero, D., Guillén, F., Martínez-Romero, D., Serrano, M., et al. (2014). Quality and antioxidant properties on sweet cherries as affected by preharvest salicylic and acetylsalicylic acids treatments. Food Chem. 160, 226–232. doi:10.1016/j.foodchem.2014.03.107
Hoekou, Y. P., Tchacondo, T., Karou, S. D., Yerbanga, R. S., Achoribo, E., Da, O., et al. (2017). Therapeutic potentials of ethanolic extract of leaves of Holarrhena floribunda (G. Don) Dur. and Schinz (apocynaceae). Afr. J. Tradit. Complement. Altern. Med. 14, 227–233. doi:10.21010/ajtcam.v14i2.24
Irungu, B. N., Adipo, N., Orwa, J. A., Kimani, F., Heydenreich, M., Midiwo, J. O., et al. (2015). Antiplasmodial and cytotoxic activities of the constituents of Turraea robusta and. Turraea Nilotica. J. Ethnopharmacol. doi:10.1016/j.jep.2015.08.039
Irungu, B. N., Mbabub, M. J., Kiboi, D. M., Moindi, E., Kinyua, J., and Romano, M. (2012). In vivo antimalarial and acute toxicity properties of hexane and chloroform extracts from Clausena anisata (Willd.) Benth. Afr. J. Pharmacol. Ther. Vol. 1, 24–29.
Irungu, B. N., Rukunga, G. M., Mungai, G. M., and Muthaura, C. N. (2007). In vitro antiplasmodial and cytotoxicity activities of 14 medicinal plants from Kenya. South Afr. J. Bot. 73, 204–207. doi:10.1016/j.sajb.2006.11.004
Irungu, B., Orwa, J., Gruhonjic, A., Fitzpatrick, P., Landberg, G., Kimani, F., et al. (2014). Constituents of the roots and leaves of Ekebergia capensis and their potential antiplasmodial and cytotoxic activities. Molecules 19, 14235–14246. doi:10.3390/molecules190914235
Jeruto, P., Nyangacha, R., and Mutai, C. (2015). In vitro and in vivo antiplasmodial activity of extracts of selected Kenyan medicinal plants. Afr. J. Pharmacol. Ther. 9, 505. doi:10.5897/ajpp2013.3886
Joshi, B. P., Bhandare, V. V., Vankawala, M., Patel, P., Patel, R., Vyas, B., et al. (2022). Friedelin, a novel inhibitor of CYP17A1 in prostate cancer from Cassia tora. Cassia Tora. J. Biomol. Struct. Dyn., 1–26. doi:10.1080/07391102.2022.2145497
Kangethe, L. N., Ahmed, H., Omar, S., Gathirwa, J., Kirira, P., Kaniaru, S., et al. (2016). Synergistic antiplasmodial activity of Artemisia annua fractions against in vitro cultures of Plasmodium falciparum. Afr. J. Pharmacol. Ther.
Kigondu, E. V. M., Rukunga, G. M., Gathirwa, J. W., Irungu, B. N., Mwikwabe, N. M., Amalemba, G. M., et al. (2011). Antiplasmodial and cytotoxicity activities of some selected plants used by the Maasai community, Kenya. South Afr. J. Bot. 77, 725–729. doi:10.1016/j.sajb.2011.03.008
Kigondu, E. V., Rukunga, G. M., Keriko, J. M., Tonui, W. K., Gathirwa, J. W., Kirira, P. G., et al. (2009). Anti-parasitic activity and cytotoxicity of selected medicinal plants from Kenya. J. Ethnopharmacol. 123, 504–509. doi:10.1016/j.jep.2009.02.008
Kirira, P. G., Rukunga, G. M., Wanyonyi, A. W., Muregi, F. M., Gathirwa, J. W., Muthaura, C. N., et al. (2006). Anti-plasmodial activity and toxicity of extracts of plants used in traditional malaria therapy in Meru and Kilifi Districts of Kenya. J. Ethnopharmacol. 106, 403–407. doi:10.1016/j.jep.2006.01.017
kundu, J. K., Rouf, A., Hossain, M. N., Hasan, C. M., and Rashid, M. A. (2000). Antitumor activity of epifriedelanol from Vitis trifolia. Fitoterapia 71, 577–579. doi:10.1016/s0367-326x(00)00191-x
Kuria, K. A. M., De Coster, S., Muriuki, G., Masengo, W., Kibwage, I., Hoogmartens, J., et al. (2001). Antimalarial activity of Ajuga remota Benth (labiatae) and Caesalpinia volkensii harms (caesalpiniaceae): in vitro confirmation of ethnopharmacological use. J. Ethnopharmacol. 74, 141–148. doi:10.1016/s0378-8741(00)00367-6
Machumi, F. (2010). Phytochemical investigation of four Kenyan plants from families verbenaceae and asteraceae for their antiplasmodial antileishmanial and antioxidant activities. Available at: http://erepository.uonbi.ac.ke/handle/11295/6189 [Accessed April 24, 2015].
Machumi, F., Samoylenko, V., Yenesew, A., Derese, S., Midiwo, J. O., Wiggers, F. T., et al. (2010). Antimicrobial and antiparasitic abietane diterpenoids from the roots of. Clerodendrum Eriophyllum. Nat. Prod. Commun. 5, 1934578X1000500605. doi:10.1177/1934578X1000500605
Mohammed, T., Erko, B., and Giday, M. (2014). Evaluation of antimalarial activity of leaves of Acokanthera schimperi and Croton macrostachyus against Plasmodium berghei in Swiss albino mice. BMC Complement. Altern. Med. 14, 1–10. doi:10.1186/1472-6882-14-314
Muganga, R., Angenot, L., Tits, M., and Frederich, M. (2010). Antiplasmodial and cytotoxic activities of Rwandan medicinal plants used in the treatment of malaria. J. Ethnopharmacol. 128, 52–57. doi:10.1016/j.jep.2009.12.023
Muiva, L. M., Yenesew, A., Derese, S., Heydenreich, M., Peter, M. G., Akala, H. M., et al. (2009). Antiplasmodial β-hydroxydihydrochalcone from seedpods of Tephrosia elata. Tephrosia Elata. Phytochem. Lett. 2, 99–102. doi:10.1016/j.phytol.2009.01.002
Muiva-Mutisya, L. M., Atilaw, Y., Heydenreich, M., Koch, A., Akala, H. M., Cheruiyot, A. C., et al. (2017). Antiplasmodial prenylated flavanonols from. Tephrosia Subtriflora. Nat. Prod. Res., 1–8.
Muiva-Mutisya, L. M., Atilaw, Y., Heydenreich, M., Koch, A., Akala, H. M., Cheruiyot, A. C., et al. (2018). Antiplasmodial prenylated flavanonols from Tephrosia subtriflora. Tephrosia Subtriflora. Nat. Prod. Res. 32, 1407–1414. doi:10.1080/14786419.2017.1353510
Mukungu, N., Abuga, K., Okalebo, F., Ingwela, R., and Mwangi, J. (2016). Medicinal plants used for management of malaria among the Luhya community of Kakamega East sub-County, Kenya. J. Ethnopharmacol. 194, 98–107. doi:10.1016/j.jep.2016.08.050
Muregi, F. W., Chhabra, S. C., Njagi, E. N. M., Lang’at-Thoruwa, C. C., Njue, W. M., Orago, A. S. S., et al. (2003). In vitro antiplasmodial activity of some plants used in Kisii, Kenya against malaria and their chloroquine potentiation effects. J. Ethnopharmacol. 84, 235–239. doi:10.1016/s0378-8741(02)00327-6
Mutai, C., Bii, C., Rukunga, G., Ondicho, J., Mwitari, P., Abatis, D., et al. (2008). Antimicrobial activity of pentacyclic triterpenes isolated from Acacia mellifera. Afr. J. Tradit. Complement. Altern. Med. AJTCAM 6, 42–48.
Muthaura, C. N., Keriko, J. M., Mutai, C., Yenesew, A., Gathirwa, J. W., Irungu, B. N., et al. (2015a). Antiplasmodial potential of traditional antimalarial phytotherapy remedies used by the Kwale community of the Kenyan Coast. J. Ethnopharmacol. 170, 148–157. doi:10.1016/j.jep.2015.05.024
Muthaura, C. N., Keriko, J. M., Mutai, C., Yenesew, A., Gathirwa, J. W., Irungu, B. N., et al. (2015b). Antiplasmodial potential of traditional phytotherapy of some remedies used in treatment of malaria in Meru-Tharaka Nithi County of Kenya. J. Ethnopharmacol. 175, 315–323. doi:10.1016/j.jep.2015.09.017
Muthaura, C. N., Rukunga, G. M., Chhabra, S. C., Mungai, G. M., and Njagi, E. N. M. (2007a). Traditional antimalarial phytotherapy remedies used by the Kwale community of the Kenyan Coast. J. Ethnopharmacol. 114, 377–386. doi:10.1016/j.jep.2007.08.033
Muthaura, C. N., Rukunga, G. M., Chhabra, S. C., Omar, S. A., Guantai, A. N., Gathirwa, J. W., et al. (2007b). Antimalarial activity of some plants traditionally used in Meru district of Kenya. Phytother. Res. 21, 860–867. doi:10.1002/ptr.2170
Ng’etich, J., Swai, H., Njokah, J., Wachira, S., Koech, L., and Gathirwa, J. (2020). Antimalarial potential and phytochemical composition of fractions of Lippia kituiensis Vatke (Verbenaceae) growing in Northern Tanzania.
Ng′ang′a, M. M., Hussain, H., Chhabra, S., Langat-Thoruwa, C., Irungu, B. N., Al-Harrasi, A., et al. (2012). Antiplasmodial activity of compounds from Drypetes gerrardii. Chem. Nat. Compd. 48, 339–340. doi:10.1007/s10600-012-0244-y
Niinemets, Ü. (2016). Uncovering the hidden facets of drought stress: secondary metabolites make the difference. Tree Physiol. 36, 129–132. doi:10.1093/treephys/tpv128
Njoroge, G. N., and Bussmann, R. W. (2007). Ethnotherapeautic management of skin diseases among the Kikuyus of Central Kenya. J. Ethnopharmacol. 111, 303–307. doi:10.1016/j.jep.2006.11.025
Nyangacha, R. M., Gathirwa, J. W., Muthaura, C. N., Mungai, G. M., Mwikwabe, N., Ondicho, J. M., et al. (2012). Antimalarial activity and toxicity evaluation of Kenyan Hugonia castaneifolia Engl. Teclea nobilis Del. and Turraea mombassana C. DC. Afr. J. Health Sci. 23, 305–315.
Obbo, C. J. D., Kariuki, S. T., Gathirwa, J. W., Olaho-Mukani, W., Cheplogoi, P. K., and Mwangi, E. M. (2019). In vitro antiplasmodial, antitrypanosomal and antileishmanial activities of selected medicinal plants from Ugandan flora: refocusing into multi-component potentials. J. Ethnopharmacol. 229, 127–136. doi:10.1016/j.jep.2018.09.029
Orwa, J. A., Ngeny, L., Mwikwabe, N. M., Ondicho, J., and Jondiko, I. J. O. (2013). Antimalarial and safety evaluation of extracts from Toddalia asiatica (L) Lam. (Rutaceae). J. Ethnopharmacol. 145, 587–590. doi:10.1016/j.jep.2012.11.034
Page, M. J., McKenzie, J. E., Bossuyt, P. M., Boutron, I., Hoffmann, T. C., Mulrow, C. D., et al. (2021). The PRISMA 2020 statement: an updated guideline for reporting systematic reviews. Syst. Rev. 10, 89. doi:10.1186/s13643-021-01626-4
Prabhu, A., Krishnamoorthy, M., Prasad, D. J., and Naik, P. (2011). Anticancer activity of friedelin isolated from ethanolic leaf extract of Cassia tora on HeLa and HSC-1 Cell Lines. Indian J. Appl. Res. 3, 1–4. doi:10.15373/2249555x/oct2013/121
Rotich, C. K., Nyangacha, R. M., Wachira, S. W., Ngeiywa, M. M., and Kigondu, E. V. (2015). Antimalarial activities and toxicity levels of selected medicinal plants used in Kenya. Afr. J. Pharmacol. Ther.
Rukunga, G. M., Gathirwa, J. W., Omar, S. A., Muregi, F. W., Muthaura, C. N., Kirira, P. G., et al. (2009). Anti-plasmodial activity of the extracts of some Kenyan medicinal plants. J. Ethnopharmacol. 121, 282–285. doi:10.1016/j.jep.2008.10.033
Rukunga, G. M., Muregi, F. W., Omar, S. A., Gathirwa, J. W., Muthaura, C. N., Peter, M. G., et al. (2008). Anti-plasmodial activity of the extracts and two sesquiterpenes from Cyperus articulatus. Cyperus Articul. Fitoter. 79, 188–190. doi:10.1016/j.fitote.2007.11.010
Sadeghpour, O., Asghari, G., Ardekani, M. R. S., and Jaroszewski, J. W. (2006). Phytochemical study of Artemisia persica Boiss. and evaluation of its antiplasmodial activity. Planta Med. 72, 136. doi:10.1055/s-2006-949936
Udu, R., Oyweri, J., and Gathirwa, J. (2021). Antimalarial activity of Nigella sativa L. Seed extracts and selection of resistance in Plasmodium berghei ANKA in a mouse model. J. Pathog. 2021, 1–10. doi:10.1155/2021/6165950
Umair, M., Altaf, M., Bussmann, R. W., and Abbasi, A. M. (2019). Ethnomedicinal uses of the local flora in Chenab riverine area, Punjab province Pakistan. J. Ethnobiol. Ethnomedicine 15, 7–31. doi:10.1186/s13002-019-0285-4
Varughese, R. S., Lam, W. S.-T., Marican, A. A., bin, H., Viganeshwari, S. H., Bhave, A. S., et al. (2019). Biopharmacological considerations for accelerating drug development of deguelin, a rotenoid with potent chemotherapeutic and chemopreventive potential. Cancer 125, 1789–1798. doi:10.1002/cncr.32069
Verma, N., and Shukla, S. (2015). Impact of various factors responsible for fluctuation in plant secondary metabolites. J. Appl. Res. Med. Aromat. Plants 2, 105–113. doi:10.1016/j.jarmap.2015.09.002
Wachira, G. K., Muregi, F. W., Kimani, F. T., Gathirwa, J. W., Mwitari, P. G., Tolo, F. M., et al. (2018). Evaluation of antiplasmodial activity and safety of flower extracts of Chrysanthemum cinerariaefolium, singly and in combination with chloroquine, lumefantrine and piperaquine. Eur. J. Med. Plants 22, 1–14. doi:10.9734/ejmp/2018/39246
Waiganjo, B., Moriasi, G., Onyancha, J., Elias, N., and Muregi, F. (2020). Antiplasmodial and cytotoxic activities of extracts of selected medicinal plants used to treat malaria in Embu County. Kenya. J. Parasitol. Res. doi:10.1155/2020/8871375
Wuttikit, S., and Thanakijcharoenpath, W. (2023). Isolation and characterization of triterpenoids from the stem of. Diospyros gracilis. IOP Conf. Ser. Earth Environ. Sci. 1139, 012001. doi:10.1088/1755-1315/1139/1/012001
Yenesew, A., Akala, H. M., Twinomuhwezi, H., Chepkirui, C., Irungu, B. N., Eyase, F. L., et al. (2012). The antiplasmodial and radical scavenging activities of flavonoids of Erythrina burttii. Acta Trop. 123, 123–127. doi:10.1016/j.actatropica.2012.04.011
Keywords: malaria, medicinal plants, antimalarial, antiplasmodial, Plasmodium falciparum, drug discovery, cytotoxicity
Citation: Irungu B, Okari E, Nyangi M, Njeru S and Koech L (2023) Potential of medicinal plants as antimalarial agents: a review of work done at Kenya Medical Research Institute. Front. Pharmacol. 14:1268924. doi: 10.3389/fphar.2023.1268924
Received: 28 July 2023; Accepted: 06 October 2023;
Published: 20 October 2023.
Edited by:
Hellen Oketch-Rabah, United States Pharmacopeial Convention, United StatesReviewed by:
Armando Caceres, Galileo University, GuatemalaAmy Roe, Procter and Gamble, United States
Edward Mberu Kamau, World Health Organization, Switzerland
Brinda Somanadhan, Hilleman Laboratories Singapore Pte. Ltd., Singapore
Copyright © 2023 Irungu, Okari, Nyangi, Njeru and Koech. This is an open-access article distributed under the terms of the Creative Commons Attribution License (CC BY). The use, distribution or reproduction in other forums is permitted, provided the original author(s) and the copyright owner(s) are credited and that the original publication in this journal is cited, in accordance with accepted academic practice. No use, distribution or reproduction is permitted which does not comply with these terms.
*Correspondence: Beatrice Irungu, birungu@kemri.go.ke
†These authors share first authorship