- 1Institute of Liver Diseases, Shuguang Hospital Affiliated with Shanghai University of Traditional Chinese Medicine, Shanghai, China
- 2Department of Biology, School of Integrative Medicine, Shanghai University of Traditional Chinese Medicine, Shanghai, China
- 3Shanghai Key Laboratory of Traditional Chinese Clinical Medicine, Shanghai, China
- 4Key Laboratory of Liver and Kidney Diseases, Ministry of Education, Shanghai, China
Macrophages, an essential cell population involved in mediating innate immunity in the host, play a crucial role on the development of hepatic cirrhosis. Extensive studies have highlighted the potential therapeutic benefits of macrophage therapy in treating hepatic cirrhosis. This review aims to provide a comprehensive overview of the various effects and underlying mechanisms associated with macrophage therapy in the context of hepatic cirrhosis.
1 Introduction
Liver cirrhosis is a serious health concern that affects people worldwide, with over one million deaths occurring annually due to end-stage liver disease. China alone accounts for 50% of the cases (Asrani et al., 2019). Given the high mortality rate associated with this disease, researchers have been focusing on inhibiting or reversing cirrhosis. In recent years, macrophage infusion therapy has emerged as a promising treatment modality for liver disease. Macrophages are immune cells that are highly abundant in the liver, and there is growing evidence to suggest that macrophage infusion therapy can help reverse cirrhosis. This review provides an overview of the latest research on macrophage infusion therapy, which has shown great potential for treating liver fibrosis and improving development prospects.
2 Diversity and plasticity of hepatic macrophages
Hepatic macrophages make up a significant portion of all macrophages in the body and are composed of both liver-resident and various infiltrated macrophages. Normally, liver-resident Kupffer cells (KCs), which originate from the yolk sac, comprise the primary population of hepatic macrophages. When the liver is injured, bone marrow monocytes are recruited to the liver and acquire the function of KCs (Borst et al., 2018; Peiseler et al., 2023).
Macrophages are a highly versatile and diverse group of immune cells that are widely distributed throughout the body. Traditionally, macrophages can be classified into two primary types based on their activation pathways, biomarkers, and cytokine release: classically activated type 1 macrophages (M1) and alternatively activated type 2 macrophages (M2). M1 macrophages are activated through lipopolysaccharides and IFN-gamma, producing an abundance of proinflammatory cytokines such as IL-1β, IL-6, and TNF-α to mediate inflammation. In contrast, M2 macrophage formation is stimulated by cytokines such as IL-4, IL-10, and IL-13, which promote tissue remodeling while suppressing inflammation (Mosser and Edwards, 2008; Murray and Wynn, 2011). Given the enormous heterogeneity of macrophages, a new naming approach is proposed that describes the stimulus scenario and adopts nomenclature related to activation standards, which can to some extent alleviate the complexity of macrophages, i.e., M (IL-4), M (Ig), M (IL-10), M (GC), M (IFN-γ), M (LPS) and so forth (Murray et al., 2014).
In mouse experiments, bone marrow-derived macrophages express high levels of lymphocyte antigen 6 complex locus C (Ly-6C) (Mossanen et al., 2016) These macrophages also have the potential to differentiate into Ly-6C low expression cells, which demonstrate high levels of matrix metalloproteinases (MMPs) capable of degrading collagen (Lu et al., 2020). However, Ly-6Chigh and Ly-6Clow cells do not correspond one-to-one with M1 and M2 macrophages, they may represent a phenotype that is intermediate between the two (Ramachandran et al., 2012). There are no antigens in the human body that correspond to Ly-6C, but it is often suggested that Ly-6Chigh and Ly-6Clow monocytes are functionally analogous to human CD14+CD16− and CD14+CD16+ monocytes, respectively (Liaskou et al., 2013). However, CD14+CD16+ monocytes exhibit both pro-inflammatory and phagocytic activities. The former has opposing effects compared to Ly-6Clow cells, while the latter exhibits similar characteristics (Ramachandran et al., 2012; Liaskou et al., 2013).
3 Macrophage therapy on liver cirrhosis
The wide-ranging adaptability of macrophages underscores their significant contribution to hepatic fibrosis. In mice, Kupffer cell levels experienced rapid depletion during early-stage partial hepatectomy but recovered gradually thanks to local proliferation and infiltrating monocyte-derived macrophage replenishment (Ma et al., 2022). The restoration of macrophage function varied significantly at different stages of liver regeneration (Elchaninov et al., 2021). Furthermore, the absence of hepatic macrophages significantly hindered liver recovery from acetaminophen-induced liver injury (You et al., 2013). Studies with CD11b-DTR transgenic mice showed that deleting infiltrating macrophages during fibrosis regression impeded extracellular matrix (ECM) degradation, which worsened fibrosis (Duffield et al., 2005). Past research with Ccr2−/− transgenic mice affirmed the findings and strongly suggested that infiltrating macrophages play a pivotal role in resolving hepatic damage (Hogaboam et al., 2000; Dambach et al., 2002).
Liver cirrhosis was previously considered a troublesome disease, but recent studies have suggested that mesenchymal stem cell therapy may be able to induce cirrhosis reverse (Shi et al., 2021). Additionally, exogenous macrophage transplantation therapy, which can be autologous, allogeneic, or syngeneic, represents an alternative technique for regulating the hepatic microenvironment and shows promise in the treatment of liver fibrosis. Table 1 provides an overview of some of the promising studies that have been conducted on macrophage infusion therapy.
3.1 Bone marrow-derived macrophages
Due to the ease of obtaining a large quantity of marrow stem cells, bone marrow-derived macrophages (BMDMs) are a promising candidate. Myelomonocytic cells can differentiate into non-activated macrophages, which can be activated under certain conditions. BMDMs exhibit characteristic macrophage cell surface markers F4/80 and CD11b, and express various mediators including anti-inflammatory, antifibrotic, and chemotactic. In a study using a fibrosis-induced model with carbon tetrachloride (CCl4), each mouse was administered 1 × 106 nonpolarized BMDMs through the portal vein. Following cell infusion, a 30% reduction in fibrotic areas was observed after 4 weeks (Thomas et al., 2011). BMDMs activated with lipopolysaccharides and interferon-γ (LPS/IFN-γ) demonstrate increased expression levels of isoform nitric oxide synthase (iNOS), interleukin (IL)-6, IL-12, tumor necrosis factor-alpha (TNF-α), matrix metalloproteinases (MMPs), and macrophage chemokine ligands CCL-2 and CXCL9. In contrast, BMDMs (IL-4) exhibit higher expression levels of arginase-1 and mannose receptor 1 (MRC1), as well as cytokines and chemokines including IL-10, CCL1, and CCL17 (Ma et al., 2017). Macrophages were injected through the tail vein into two different mouse models of chronic liver fibrosis - one induced by toxicity from CCl4 and the other from bile duct ligation (BDL) injury. Both BMDMs and BMDMs (LPS/IFN-γ) showed significant improvement in liver fibrosis, and BMDMs (LPS/IFN-γ) exhibited a better therapeutic effect (Ma et al., 2017). Interestingly, BMDMs (IL-4) were found to be ineffective in treating liver fibrosis in this study. However, the adoptive transfer of macrophages stimulated with dexamethasome reduced hepatic markers such as ALT and AST, ameliorating acute rejection in liver transplantation (Yang et al., 2020). This suggests that a specific subpopulation of M2-like macrophages has therapeutic potential for hepatic fibrosis. Additionally, a recent study identified key bioactive lipids that mediate the cytotherapeutic potential of polarized macrophages in post-hepatectomy liver dysfunction. It was found that infusion of BMDMs (IL-4) may have therapeutic potential for post-hepatectomy liver dysfunction (Sun et al., 2021). Overall, BMDMs represent a promising candidate for treating liver fibrosis due to their ability to differentiate into different phenotypes and express various mediators that can regulate the hepatic microenvironment.
3.2 Kupffer cells
Kupffer cells (KCs), which are liver-resident macrophages, play a crucial role in regulating liver homeostasis under normal and pathological conditions. In order to investigate the impact of KCs infusion on liver fibrosis, researchers isolated cells directly from the liver. These KCs were found to express CD11b, F4/80, and CD11c, which are markers for monocytes/macrophages (Merlin et al., 2016). It was discovered that the purity of cultured KCs could be improved by using macrophage colony stimulating factor (M-CSF), which also significantly promoted KCs proliferation in vitro (Li and He, 2021). Even when expanded in vitro, KCs retained their potential polarization and phagocytosis. After 4 weeks of treatment, infusion of KCs through the tail vein was shown to ameliorate liver fibrosis induced by CCl4 in mice, indicating that KCs may be another source for macrophage therapy (Li and He, 2021). These findings suggest that KCs may represent a promising target for macrophage therapy in liver fibrosis. By isolating and expanding these cells in vitro, it may be possible to generate a large number of high-purity KCs for use in therapeutic applications.
3.3 Other specialized macrophages
Large-scale production of therapeutic macrophages is a formidable challenge, but research suggests that induced pluripotent stem cells (iPSCs) could provide a solution (Zhang and Reilly, 2017). Macrophages derived from iPSCs closely resemble monocyte-derived cells in function. Embryonic stem cell-derived macrophages (ESDMs) and bone marrow-derived macrophages (BMDMs) have comparable morphology, with ESDMs appearing slightly larger in size but having lower phagocytic activity than BMDMs (Haideri et al., 2017). Furthermore, ESDMs exhibit lower expression of M1-related genes than BMDMs under conventional polarization regimes, while the expression of M2-related genes is increased. MMP-12 and MMP-13 are also lower in ESDMs compared to BMDMs, while MMP-9 is expressed at a higher level (Haideri et al., 2017). In a study assessing the antifibrotic capacity of ESDMs, cells were infused via intravenous injection 1 week after CCl4 injections, and analysis was conducted 21 days later. 50% reduction of fibrosis level was achieved when injecting 20 × 106 ESDMs, but there was no significant effect on fibrosis levels when using half the number of ESDMs. Notably, ESDMs were found to be more effective than BMDMs in repopulating Kupffer cell-depleted livers due to their phenotype being more similar to tissue resident macrophages (Haideri et al., 2017). These results highlight the importance of carefully considering the dosage and timing of ESDM delivery in order to maximize its potential therapeutic benefits. Despite requiring a larger number of ESDMs to achieve efficacy, they were still able to replicate the regression of fibrosis induced by primary macrophage infusion.
Primary human monocyte-derived macrophages (HMDMs) obtained from healthy donors exhibit a notable increase in the phagocytosis and repair markers CD163, CD169, and CD206, while showing a decrease in the inflammatory cytokine receptor CCR2. The transplantation of HMDMs via the spleen into an immunocompromised mouse model of liver fibrosis resulted in a regression of collagen and a reduction in markers of liver injury. These findings provide good manufacturing practice (GMP) -compatible protocols for large-scale manufacturing to produce HMDMs of clinical grade, which may be a promising source for macrophage therapy in liver fibrosis (Moore et al., 2015).
4 The mechanism underlying macrophage therapy on mouse liver fibrosis
The infusion of macrophages has been shown to play a critical role in ameliorating liver fibrosis through crosstalk with various cell types in the liver, including hepatocytes, hepatic stellate cells (HSCs), natural killer (NK) cells, endogenous macrophages, neutrophils, and hepatic progenitor cells (HPCs). This inter-population communication serves to facilitate liver inflammation mediation, promote collagen degradation, and enhance liver regeneration, ultimately contributing to the regression of hepatic fibrosis (as illustrated in Figure 1).
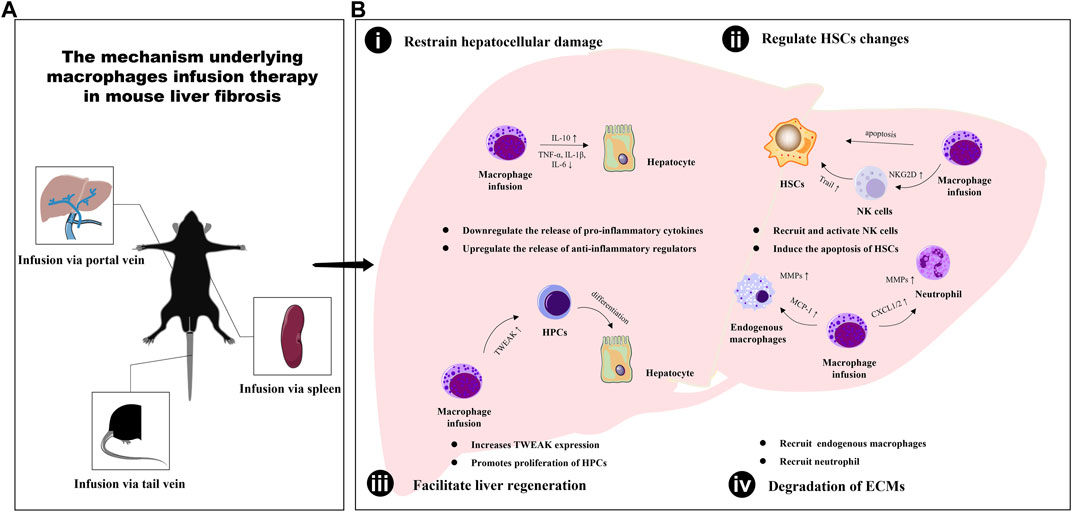
FIGURE 1. The mechanism underlying macrophages infusion therapy in mouse liver fibrosis. (A) The different types of macrophages are transfused into mice in three ways: tail vein injection, portal vein injection and spleen injection. (B) The infusion of macrophages has been shown to play a critical role in ameliorating liver fibrosis through enabling inter-population communication serves to restrain hepatocellular damage (i), regulate HSCs changes (ii), facilitate liver regeneration (iii) and promote extracellular matrix degradation (iv).
4.1 Inhibition of hepatocellular injury
The liver is mainly comprised of hepatocytes, which make up around 80% of its volume and perform crucial functions such as protein synthesis and energy metabolism regulation (Blouin et al., 1977). Liver fibrosis is a consequence of liver cell damage and repair. To effectively treat liver fibrosis, it is imperative to prevent hepatocyte death by addressing the root cause of injury. Various studies have suggested that there is communication between hepatic macrophages and hepatocytes. Macrophages can help alleviate liver fibrosis by eliminating dead hepatocytes and reducing inflammation in the liver (Triantafyllou et al., 2018; Shi et al., 2022). In a liver fibrosis mouse model, the transfer of differentiated macrophages from bone marrow via tail vein infusion promoted increased levels of anti-inflammatory factors such as IL-10, which protected against liver damage caused by inflammation (Thomas et al., 2011). The infused KCs also contributed to a reduction in inflammatory cells in the portal areas of the liver, while levels of pro-inflammatory factors IL-1β, IL-6, and TNF-α were decreased. An increase in serum albumin levels indicated an improvement in liver function (Li and He, 2021). Notch signaling has been found to regulate inflammatory responses. Furthermore, genetic modification of BMDMs via tail vein infusion protected hepatocytes from damage in a hepatic ischemia-reperfusion injury model, with an upregulation of hepatic expression of Notch1 and a downregulation of IL-1β and TNF-α (Ke et al., 2010; Huang et al., 2014). Additionally, macrophage infusion was found to protect mice from acetaminophen-induced acute liver injury (Merlin et al., 2016; Starkey Lewis et al., 2020). These findings highlight the potential of macrophage therapy in protecting hepatocytes from damage and reducing inflammation in the liver, which could ultimately lead to the prevention and treatment of liver fibrosis.
4.2 Regulate HSCs changes
Liver fibrosis is underpinned by the myofibroblastic trans-differentiation of hepatic stellate cells (HSCs) in response to liver injury, with α-SMA being a marker for activated HSCs. To prevent the progression of hepatic fibrosis, it is crucial to reduce HSC activation and transition or promote their senescence and apoptosis (Kong et al., 2012; Wang et al., 2020), which can occur following macrophage infusion. Remarkably, a significant decrease in the number of α-SMA-positive myofibroblast cells was observed in fibrotic mice treated with ESDMs, particularly those treated with the higher dose of 20 × 106 cells (Haideri et al., 2017). Thomas et al. (Thomas et al., 2011) previously demonstrated that transplantation of 1 × 106 BMDMs had a therapeutic effect, with α-SMA staining falling to 40% of control after 7 days. However, the decrease in myofibroblasts was no longer statistically significant after 1 month, indicating that the reduction in the myofibroblast population occurs soon after BMDMs delivery. Similar findings were reported in other studies, where BMDMs (LPS/IFN-γ) significantly reduced α-SMA and Desmin positive signals. α-SMA and TUNEL double staining further demonstrated that HSC apoptosis was an early event after macrophage infusion (Ma et al., 2017). Additionally, Li et al. (Li and He, 2021) found that KCs infusion similarly reduced the expression of α-SMA and decreased the expression of TGF-β, a master pro-fibrogenic cytokine associated with HSCs activation and liver fibrosis. It is worth noting that the changes in HSCs are influenced by other liver cells, including NK cells (Ping et al., 2023). It was observed that the infusion of BMDMs (LPS/IFN-γ) stimulated a significant increase in the number of NK cells, suggesting their recruitment. Moreover, NKG2D expression on NK cells in the liver was upregulated, along with high levels of TNF-related apoptosis inducing ligand (TRAIL)which mediated the induction of HSC apoptosis (Ma et al., 2017; Roehlen et al., 2020). This result indicated that the infused BMDMs (LPS/IFN-γ) increased the recruitment and activation of NK cells, ultimately contributing to the amelioration of fibrogenesis through TRAIL-mediated HSC apoptosis. These findings suggest that macrophage therapy may be a promising approach in reducing HSC activation and promoting their senescence and apoptosis, ultimately leading to the prevention and treatment of liver fibrosis.
4.3 Promote degradation of ECMs
The degradation of ECM components is controlled by the equilibrium between matrix metalloproteinases (MMPs) and their endogenous inhibitors, tissue inhibitors of MMPs (TIMPs). This balance is a predominant factor in the regression of liver fibrosis (Xu et al., 2004). It is widely acknowledged that MMPs are stored in macrophage and neutrophil granules. BMDMs therapy could stimulate the upregulation of monocyte chemotactic protein-1 (MCP-1), a macrophage chemokine, and CXCL1 and CXCL2, neutrophil chemokines, respectively (Thomas et al., 2011). MCP-1 belongs to the CC chemokine subfamily and binds to the CCR2 receptors of monocytes, while CXCL1 and CXCL2 may bind to the CXCR2 neutrophil surface receptor (Zlotnik and Yoshie, 2012). The interplay between these cytokines may play an instrumental role in the recruitment of endogenous macrophages and neutrophils. In this study, both cells contributed to the expression of MMP-9 and MMP-13 in the liver, leading to improved liver fibrosis, respectively. BMDMs (LPS/IFN-γ) infusion led to higher levels of endogenous macrophage infiltration than naïve BMDMs. This may be attributed to the elevated macrophage chemokines CCL2 and CCL3. In BMDMs (LPS/IFN-γ) treated fibrotic mouse livers, the expression of MMP-2, MMP-9, and MMP-13 was significantly increased, while the expression of TIMP-1 and TIMP-2 was decreased. Interestingly, BMDMs (LPS/IFN-γ)-recruited macrophages had a Ly6Clow restorative phenotype characterized by the authors as possessing high levels of MMPs and more phagocytic characteristics (Ma et al., 2017). Similar results were obtained through KCs infusion therapy, where the mRNA levels of MMP-2, MMP-9, and MMP-13 increased in endogenous F4/80+ cells from the liver after the infusion of KCs (Li and He, 2021). The above results approve that macrophage therapy may promote the expression of MMPs and contribute to the degradation of ECM components, ultimately leading to the regression of liver fibrosis.
4.4 Facilitate liver regeneration
In addition to relying on fiber-degrading, the reversal of hepatic fibrosis involves the restoration of hepatocyte numbers and function. Hepatic progenitor cells (HPCs) are stem cells that possess bipotentiality and can express markers of both hepatocytes and cholangiocytes. It was previously believed that HPCs were located at the level of Canals of Hering and differentiated into hepatocyte-like cells to aid in liver regeneration (Pu et al., 2023). Thomas et al. (Thomas et al., 2011) found that the mRNA levels of the HPCs marker cytokeratin-19 significantly increased in BMDMs recipients 3 days after delivery, with a 55% increase observed compared to control recipients. This suggests that BMM delivery may positively impact HPCs proliferation and differentiation. By day 7, there was a periportal expansion of pancytokeratin (PanCK)-positive HPCs in BMDMs recipients, indicating that BMDMs delivery may promote the differentiation of HPCs into mature hepatocytes (Thomas et al., 2011). Interestingly, a study reported an increase in PanCK+ cells after the infusion of BMDMs into normal mice via tail vein (Bird et al., 2013). In CCl4-injured animals who received both doses of ESDMs, there was a significantly higher number of PanCK-positive cells compared to control injured livers (Haideri et al., 2017). Moreover, the infusion of BMDM (LPS/IFN-γ) has been shown to improve hepatocyte proliferation and liver function in fibrotic liver disease (Ma et al., 2017). Taken together, these findings suggest that BMDM-based therapies may hold promise as a potential treatment for liver diseases by enhancing the proliferation and differentiation of HPCs and other hepatic progenitor cells.
Tumor necrosis factor-like weak inducer of apoptosis (TWEAK) is a member of the TNF superfamily that regulates mesenchymal progenitor cells through its receptor, Fibroblast growth factor-inducible 14 (Fn14). TWEAK usually stimulates the proliferation of hepatic progenitor cells (HPCs), which are stem cells that can differentiate into hepatocytes and aid in liver regeneration (Ko et al., 2019). Interestingly, endogenous macrophages have been identified as a major cell source of TWEAK during chronic liver injury (Bird et al., 2013). In studies involving BMDMs inoculated animals, the expression of TWEAK indicated significant proliferation of macrophage-mediated HPCs (Thomas et al., 2011). Additionally, Bird et al. (Bird et al., 2013) confirmed that the activation of ductular reaction following macrophage infusion therapy is dependent on TWEAK signaling. Furthermore, the infusion of HMDMs into a mouse hepatic fibrosis model also causes significant changes in TWEAK expression in the liver (Moore et al., 2015).
Other research has also confirmed the significance of macrophages in facilitating liver regeneration. For instance, in rats subjected to portal vein ligation, infusion of CD86+ macrophages of a specific type has been demonstrated to accelerate the liver regeneration response (Zhao et al., 2021). This discovery highlights the crucial role played by macrophages in promoting liver regeneration following injury. In a separate investigation, a mouse model was established to study liver injury and regeneration via partial hepatectomy, whereby BMDM (IL-4) were injected to aid in hepatocyte proliferation. The key lipid S1P was discovered to facilitate the infusion of BMDMs (IL-4), thereby promoting hepatocyte proliferation after hepatectomy. Notably, this study also revealed that infusion of BMDMs (LPS) led to hepatocyte apoptosis after hepatectomy (Sun et al., 2021). These results indicate that the functional phenotype of macrophages is crucial in regulating liver regeneration. To completely comprehend the mechanisms behind macrophage-mediated liver regeneration and to create novel therapies for liver injury and disease, more extensive research in this field is necessary.
5 Clinical trial of autologous macrophage therapy for liver cirrhosis
A common feature in patients with liver cirrhosis is a decrease or loss of liver macrophages (Ramachandran et al., 2019). However, the exact reasons for this are still unclear, and some hypotheses suggest that it may be due to the loss of essential cell-cell circuits, such as a loss of stellate cell genes leading to loss and inhibition of macrophages (Zhao et al., 2022). Despite the fact that there have been several large and well-conducted clinical trials exploring autologous cells therapy for liver cirrhosis, research on macrophages has been minimal. However, macrophages have been proven to be effective in treating liver fibrosis under Good Manufacturing Practice (GMP) compliant conditions, and this provides a new direction for the treatment of liver fibrosis in the future (Fraser et al., 2017). In 2019, a first-in-human, phase 1 dose-escalation trial of autologous macrophage therapy in nine adults with cirrhosis was conducted (Moroni et al., 2019). The results of this study showed that in 6 out of 9 patients, reductions in model for end-stage liver disease (MELD) score were observed. Moreover, several non-invasive measures of liver fibrosis improved following macrophage infusion, including transient elastography, serum ELF score (Friedrich-Rust et al., 2010) and the collagen turnover markers the N-terminal propeptide of type III collagen and type III collagen (Moroni et al., 2019). These findings highlight the potential antifibrotic effect of autologous monocyte-derived macrophage infusion in cirrhosis, confirming the safety, feasibility and maximum achievable dose of autologous macrophages. Currently, a randomized controlled phase 2 study is gradually being carried out to examine the efficacy of autologous macrophage therapy in improving liver function, noninvasive fibrosis markers and other clinical outcomes in patients with compensated cirrhosis (Brennan et al., 2021). This trial will provide the first high-quality examination of the efficacy of autologous macrophage therapy in treating liver cirrhosis. If successful, this therapy could offer a promising treatment option for patients suffering from liver cirrhosis.
6 Conclusion
In summary, recent studies have shown the potential of using exogenous macrophages to treat liver fibrosis, but there are still several challenges, which including identifying the most suitable source of macrophages, optimizing the dosage and frequency of macrophage transplantation, and improving the survival rate and function of macrophages, need to be addressed. To overcome these challenges, further research is needed to investigate the underlying mechanisms of exogenous macrophages in treating liver fibrosis and to optimize their use in clinical practice. By doing so, we can better understand how to harness the potential of exogenous macrophages as a promising treatment option for patients suffering from liver fibrosis.
Author contributions
DP: Writing–original draft. YP: Writing–review and editing. XH: Writing–review and editing. CL: Writing–review and editing.
Funding
The author(s) declare financial support was received for the research, authorship, and/or publication of this article. This work was supported by grants from the National Natural Science Foundation of China (No.81730109, 81874363 and 82174057); Shanghai Key Laboratory of Traditional Chinese Clinical Medicine (No. 20DZ2272200).
Conflict of interest
The authors declare that the research was conducted in the absence of any commercial or financial relationships that could be construed as a potential conflict of interest.
Publisher’s note
All claims expressed in this article are solely those of the authors and do not necessarily represent those of their affiliated organizations, or those of the publisher, the editors and the reviewers. Any product that may be evaluated in this article, or claim that may be made by its manufacturer, is not guaranteed or endorsed by the publisher.
References
Asrani, S. K., Devarbhavi, H., Eaton, J., and Kamath, P. S. (2019). Burden of liver diseases in the world. J. Hepatol. 70, 151–171. doi:10.1016/j.jhep.2018.09.014
Bird, T. G., Lu, W. Y., Boulter, L., Gordon-Keylock, S., Ridgway, R. A., Williams, M. J., et al. (2013). Bone marrow injection stimulates hepatic ductular reactions in the absence of injury via macrophage-mediated TWEAK signaling. Proc. Natl. Acad. Sci. U. S. A. 110, 6542–6547. doi:10.1073/pnas.1302168110
Blouin, A., Bolender, R. P., and Weibel, E. R. (1977). Distribution of organelles and membranes between hepatocytes and nonhepatocytes in the rat liver parenchyma. A stereological study. J. Cell Biol. 72, 441–455. doi:10.1083/jcb.72.2.441
Borst, K., Frenz, T., Spanier, J., Tegtmeyer, P. K., Chhatbar, C., Skerra, J., et al. (2018). Type I interferon receptor signaling delays Kupffer cell replenishment during acute fulminant viral hepatitis. J. Hepatol. 68, 682–690. doi:10.1016/j.jhep.2017.11.029
Brennan, P. N., Macmillan, M., Manship, T., Moroni, F., Glover, A., Graham, C., et al. (2021). Study protocol: a multicentre, open-label, parallel-group, phase 2, randomised controlled trial of autologous macrophage therapy for liver cirrhosis (MATCH). BMJ Open 11, e053190. doi:10.1136/bmjopen-2021-053190
Dambach, D. M., Watson, L. M., Gray, K. R., Durham, S. K., and Laskin, D. L. (2002). Role of CCR2 in macrophage migration into the liver during acetaminophen-induced hepatotoxicity in the mouse. Hepatology 35, 1093–1103. doi:10.1053/jhep.2002.33162
Duffield, J. S., Forbes, S. J., Constandinou, C. M., Clay, S., Partolina, M., Vuthoori, S., et al. (2005). Selective depletion of macrophages reveals distinct, opposing roles during liver injury and repair. J. Clin. Invest. 115, 56–65. doi:10.1172/JCI22675
Elchaninov, A., Nikitina, M., Vishnyakova, P., Lokhonina, A., Makarov, A., Sukhikh, G., et al. (2021). Macro- and microtranscriptomic evidence of the monocyte recruitment to regenerating liver after partial hepatectomy in mouse model. Biomed. Pharmacother. 138, 111516. doi:10.1016/j.biopha.2021.111516
Fraser, A. R., Pass, C., Burgoyne, P., Atkinson, A., Bailey, L., Laurie, A., et al. (2017). Development, functional characterization and validation of methodology for GMP-compliant manufacture of phagocytic macrophages: a novel cellular therapeutic for liver cirrhosis. Cytotherapy 19, 1113–1124. doi:10.1016/j.jcyt.2017.05.009
Friedrich-Rust, M., Rosenberg, W., Parkes, J., Herrmann, E., Zeuzem, S., and Sarrazin, C. (2010). Comparison of ELF, FibroTest and FibroScan for the non-invasive assessment of liver fibrosis. BMC Gastroenterol. 10, 103. doi:10.1186/1471-230X-10-103
Haideri, S. S., Mckinnon, A. C., Taylor, A. H., Kirkwood, P., Starkey Lewis, P. J., O'duibhir, E., et al. (2017). Injection of embryonic stem cell derived macrophages ameliorates fibrosis in a murine model of liver injury. NPJ Regen. Med. 2, 14. doi:10.1038/s41536-017-0017-0
Hogaboam, C. M., Bone-Larson, C. L., Steinhauser, M. L., Matsukawa, A., Gosling, J., Boring, L., et al. (2000). Exaggerated hepatic injury due to acetaminophen challenge in mice lacking C-C chemokine receptor 2. Am. J. Pathol. 156, 1245–1252. doi:10.1016/S0002-9440(10)64995-4
Huang, J., Shen, X. D., Yue, S., Zhu, J., Gao, F., Zhai, Y., et al. (2014). Adoptive transfer of heme oxygenase-1 (HO-1)-modified macrophages rescues the nuclear factor erythroid 2-related factor (Nrf2) antiinflammatory phenotype in liver ischemia/reperfusion injury. Mol. Med. 20, 448–455. doi:10.2119/molmed.2014.00103
Ke, B., Shen, X. D., Gao, F., Ji, H., Qiao, B., Zhai, Y., et al. (2010). Adoptive transfer of ex vivo HO-1 modified bone marrow-derived macrophages prevents liver ischemia and reperfusion injury. Mol. Ther. 18, 1019–1025. doi:10.1038/mt.2009.285
Ko, S., Russell, J. O., Tian, J., Gao, C., Kobayashi, M., Feng, R., et al. (2019). Hdac1 regulates differentiation of bipotent liver progenitor cells during regeneration via Sox9b and Cdk8. Gastroenterology 156, 187–202. doi:10.1053/j.gastro.2018.09.039
Kong, X., Feng, D., Wang, H., Hong, F., Bertola, A., Wang, F. S., et al. (2012). Interleukin-22 induces hepatic stellate cell senescence and restricts liver fibrosis in mice. Hepatology 56, 1150–1159. doi:10.1002/hep.25744
Li, W., and He, F. (2021). Infusion of kupffer cells expanded in vitro ameliorated liver fibrosis in a murine model of liver injury. Cell Transpl. 30, 9636897211004090. doi:10.1177/09636897211004090
Liaskou, E., Zimmermann, H. W., Li, K. K., Oo, Y. H., Suresh, S., Stamataki, Z., et al. (2013). Monocyte subsets in human liver disease show distinct phenotypic and functional characteristics. Hepatology 57, 385–398. doi:10.1002/hep.26016
Lu, W., Ma, G., Sheng, Z., Wang, Q., Chen, L., Qi, J., et al. (2020). MSCs contribute to the conversion of Ly6C(high) monocytes into Ly6C(low) subsets under AMI. Stem Cells Int. 2020, 2460158. doi:10.1155/2020/2460158
Ma, P., Zhao, W., Gao, C., Liang, Z., Zhao, L., Qin, H., et al. (2022). The contribution of hepatic macrophage heterogeneity during liver regeneration after partial hepatectomy in mice. J. Immunol. Res. 2022, 3353250. doi:10.1155/2022/3353250
Ma, P. F., Gao, C. C., Yi, J., Zhao, J. L., Liang, S. Q., Zhao, Y., et al. (2017). Cytotherapy with M1-polarized macrophages ameliorates liver fibrosis by modulating immune microenvironment in mice. J. Hepatol. 67, 770–779. doi:10.1016/j.jhep.2017.05.022
Merlin, S., Bhargava, K. K., Ranaldo, G., Zanolini, D., Palestro, C. J., Santambrogio, L., et al. (2016). Kupffer cell transplantation in mice for elucidating monocyte/macrophage biology and for potential in cell or gene therapy. Am. J. Pathol. 186, 539–551. doi:10.1016/j.ajpath.2015.11.002
Moore, J. K., Mackinnon, A. C., Wojtacha, D., Pope, C., Fraser, A. R., Burgoyne, P., et al. (2015). Phenotypic and functional characterization of macrophages with therapeutic potential generated from human cirrhotic monocytes in a cohort study. Cytotherapy 17, 1604–1616. doi:10.1016/j.jcyt.2015.07.016
Moroni, F., Dwyer, B. J., Graham, C., Pass, C., Bailey, L., Ritchie, L., et al. (2019). Safety profile of autologous macrophage therapy for liver cirrhosis. Nat. Med. 25, 1560–1565. doi:10.1038/s41591-019-0599-8
Mossanen, J. C., Krenkel, O., Ergen, C., Govaere, O., Liepelt, A., Puengel, T., et al. (2016). Chemokine (C-C motif) receptor 2-positive monocytes aggravate the early phase of acetaminophen-induced acute liver injury. Hepatology 64, 1667–1682. doi:10.1002/hep.28682
Mosser, D. M., and Edwards, J. P. (2008). Exploring the full spectrum of macrophage activation. Nat. Rev. Immunol. 8, 958–969. doi:10.1038/nri2448
Murray, P. J., Allen, J. E., Biswas, S. K., Fisher, E. A., Gilroy, D. W., Goerdt, S., et al. (2014). Macrophage activation and polarization: nomenclature and experimental guidelines. Immunity 41, 14–20. doi:10.1016/j.immuni.2014.06.008
Murray, P. J., and Wynn, T. A. (2011). Protective and pathogenic functions of macrophage subsets. Nat. Rev. Immunol. 11, 723–737. doi:10.1038/nri3073
Peiseler, M., Araujo David, B., Zindel, J., Surewaard, B. G. J., Lee, W. Y., Heymann, F., et al. (2023). Kupffer cell-like syncytia replenish resident macrophage function in the fibrotic liver. Science 381, eabq5202. doi:10.1126/science.abq5202
Ping, D. B., Sun, X., Peng, Y., and Liu, C. H. (2023). Cyp4a12-mediated retinol metabolism in stellate cells is the antihepatic fibrosis mechanism of the Chinese medicine Fuzheng Huayu recipe. Chin. Med. 18, 51. doi:10.1186/s13020-023-00754-4
Pu, W., Zhu, H., Zhang, M., Pikiolek, M., Ercan, C., Li, J., et al. (2023). Bipotent transitional liver progenitor cells contribute to liver regeneration. Nat. Genet. 55, 651–664. doi:10.1038/s41588-023-01335-9
Ramachandran, P., Dobie, R., Wilson-Kanamori, J. R., Dora, E. F., Henderson, B. E. P., Luu, N. T., et al. (2019). Resolving the fibrotic niche of human liver cirrhosis at single-cell level. Nature 575, 512–518. doi:10.1038/s41586-019-1631-3
Ramachandran, P., Pellicoro, A., Vernon, M. A., Boulter, L., Aucott, R. L., Ali, A., et al. (2012). Differential Ly-6C expression identifies the recruited macrophage phenotype, which orchestrates the regression of murine liver fibrosis. Proc. Natl. Acad. Sci. U. S. A. 109, E3186–E3195. doi:10.1073/pnas.1119964109
Roehlen, N., Crouchet, E., and Baumert, T. F. (2020). Liver fibrosis: mechanistic concepts and therapeutic perspectives. Cells 9, 875. doi:10.3390/cells9040875
Shi, H., Wang, X., Li, F., Gerlach, B. D., Yurdagul, A., Moore, M. P., et al. (2022). CD47-SIRPα axis blockade in NASH promotes necroptotic hepatocyte clearance by liver macrophages and decreases hepatic fibrosis. Sci. Transl. Med. 14, eabp8309. doi:10.1126/scitranslmed.abp8309
Shi, M., Li, Y. Y., Xu, R. N., Meng, F. P., Yu, S. J., Fu, J. L., et al. (2021). Mesenchymal stem cell therapy in decompensated liver cirrhosis: a long-term follow-up analysis of the randomized controlled clinical trial. Hepatol. Int. 15, 1431–1441. doi:10.1007/s12072-021-10199-2
Starkey Lewis, P., Campana, L., Aleksieva, N., Cartwright, J. A., Mackinnon, A., O'duibhir, E., et al. (2020). Alternatively activated macrophages promote resolution of necrosis following acute liver injury. J. Hepatol. 73, 349–360. doi:10.1016/j.jhep.2020.02.031
Sun, H., Sun, S., Chen, G., Xie, H., Yu, S., Lin, X., et al. (2021). Ceramides and sphingosine-1-phosphate mediate the distinct effects of M1/M2-macrophage infusion on liver recovery after hepatectomy. Cell Death Dis. 12, 324. doi:10.1038/s41419-021-03616-9
Thomas, J. A., Pope, C., Wojtacha, D., Robson, A. J., Gordon-Walker, T. T., Hartland, S., et al. (2011). Macrophage therapy for murine liver fibrosis recruits host effector cells improving fibrosis, regeneration, and function. Hepatology 53, 2003–2015. doi:10.1002/hep.24315
Triantafyllou, E., Pop, O. T., Possamai, L. A., Wilhelm, A., Liaskou, E., Singanayagam, A., et al. (2018). MerTK expressing hepatic macrophages promote the resolution of inflammation in acute liver failure. Gut 67, 333–347. doi:10.1136/gutjnl-2016-313615
Wang, Q., Wei, S., Zhou, H., Li, L., Zhou, S., Shi, C., et al. (2020). MicroRNA-98 inhibits hepatic stellate cell activation and attenuates liver fibrosis by regulating HLF expression. Front. Cell Dev. Biol. 8, 513. doi:10.3389/fcell.2020.00513
Xu, G. F., Li, P. T., Wang, X. Y., Jia, X., Tian, D. L., Jiang, L. D., et al. (2004). Dynamic changes in the expression of matrix metalloproteinases and their inhibitors, TIMPs, during hepatic fibrosis induced by alcohol in rats. World J. Gastroenterol. 10, 3621–3627. doi:10.3748/wjg.v10.i24.3621
Yang, P., Zhang, X., Lin, Z., Wang, Q., Guo, D., Zhang, P., et al. (2020). Adoptive transfer of polarized M2c macrophages ameliorates acute rejection in rat liver transplantation. Am. J. Transl. Res. 12, 2614–2626.
You, Q., Holt, M., Yin, H., Li, G., Hu, C. J., and Ju, C. (2013). Role of hepatic resident and infiltrating macrophages in liver repair after acute injury. Biochem. Pharmacol. 86, 836–843. doi:10.1016/j.bcp.2013.07.006
Zhang, H., and Reilly, M. P. (2017). Human induced pluripotent stem cell-derived macrophages for unraveling human macrophage biology. Arterioscler. Thromb. Vasc. Biol. 37, 2000–2006. doi:10.1161/ATVBAHA.117.309195
Zhao, D., Yang, F., Wang, Y., Li, S., Li, Y., Hou, F., et al. (2022). ALK1 signaling is required for the homeostasis of Kupffer cells and prevention of bacterial infection. J. Clin. Invest. 132, e150489. doi:10.1172/JCI150489
Zhao, J., Zhao, W., Zhou, H., Xu, H., and Yu, L. (2021). Injection of CD86(+) macrophages instead of liver partition for the acceleration of liver regeneration after portal vein ligation in rats. Arch. Med. Sci. 17, 843–847. doi:10.5114/aoms/133361
Keywords: cell therapy, liver cirrhosis, macrophage cytotherapy, mechanism, transfusion
Citation: Ping D, Peng Y, Hu X and Liu C (2023) Macrophage cytotherapy on liver cirrhosis. Front. Pharmacol. 14:1265935. doi: 10.3389/fphar.2023.1265935
Received: 24 July 2023; Accepted: 04 December 2023;
Published: 15 December 2023.
Edited by:
Ramin Massoumi, Lund University, SwedenReviewed by:
Andrey Elchaninov, National Medical Research Center of Obstetrics, Gynecology and Perinatology Named After Academician V.I. Kulakova, RussiaZhaohui Bai, Peking University, China
Copyright © 2023 Ping, Peng, Hu and Liu. This is an open-access article distributed under the terms of the Creative Commons Attribution License (CC BY). The use, distribution or reproduction in other forums is permitted, provided the original author(s) and the copyright owner(s) are credited and that the original publication in this journal is cited, in accordance with accepted academic practice. No use, distribution or reproduction is permitted which does not comply with these terms.
*Correspondence: Xudong Hu, aHV4dWRvbmdzaEAxMjYuY29t; Chenghai Liu, Y2hlbmdoYWlsaXVAaG90bWFpbC5jb20=