- 1Department of Basic and Laboratory Sciences, Khomein University of Medical Sciences, Khomein, Iran
- 2Autoimmune Diseases Research Center, Shahid Beheshti Hospital, Kashan University of Medical Sciences, Kashan, Iran
- 3Physiology Research Center, Institute for Basic Sciences, Kashan University of Medical Sciences, Kashan, Iran
- 4Department of Health and Community Medicine, School of Medicine, Arak University of Medical Sciences, Arak, Iran
- 5Department of Biochemistry and Hematology, Faculty of Medicine, Semnan University of Medical Sciences, Semnan, Iran
- 6Cancer Research Center, Semnan University of Medical Sciences, Semnan, Iran
Exosomes are small membrane vesicles of endocytic origin that are produced by both tumor and normal cells and can be found in physiological fluids like plasma and cell culture supernatants. They include cytokines, growth factors, proteins, lipids, RNAs, and metabolites and are important intercellular communication controllers in several disorders. According to a vast amount of research, exosomes could support or inhibit tumor start and diffusion in a variety of solid and hematological malignancies by paracrine signaling. Exosomes are crucial therapeutic agents for a variety of illnesses, such as cancer and autoimmune diseases. This review discusses the most current and encouraging findings from in vitro and experimental in vivo research, as well as the scant number of ongoing clinical trials, with a focus on the impact of exosomes in the treatment of malignancies. Exosomes have great promise as carriers of medications, antagonists, genes, and other therapeutic materials that can be incorporated into their core in a variety of ways. Exosomes can also alter the metabolism of cancer cells, alter the activity of immunologic effectors, and alter non-coding RNAs, all of which can alter the tumor microenvironment and turn it from a pro-tumor to an anti-tumor milieu. This subject is covered in the current review, which also looks at how exosomes contribute to the onset and progression of hematological malignancies, as well as their importance in diagnosing and treating these conditions.
Introduction
Hematological malignancies pose significant challenges in terms of treatment options and patient outcomes (Mohseni Afshar et al., 2022). Current therapeutic approaches often have limitations, including systemic toxicity and the inability to specifically target malignant cells (Belfiore et al., 2018). The application of cell-derived exosomes in hematological malignancies therapy holds great promise and significance. Exosomes, as natural carriers of bioactive molecules, offer several advantages, such as targeted delivery, reduced off-target effects, and potential immunomodulatory effects (Dimik et al., 2023). By utilizing exosomes as therapeutic vehicles, it may be possible to enhance the efficacy of drug delivery, overcome treatment resistance, and modulate the tumor microenvironment in hematological malignancies (Xia et al., 2020). Therefore, understanding the potential of exosomes in this context is of immense importance, as it may contribute to the development of novel and more effective treatment strategies with improved outcomes for patients with hematological malignancies.
Exosomes are small extracellular vesicles that play a crucial role in intercellular communication and have garnered significant attention in the fields of tumor biology and therapeutics (Yokoi and Ochiya, 2021). These nanosized vesicles are secreted by various cell types and are enriched with proteins, nucleic acids, and lipids, reflecting the molecular composition of their parent cells. Their ability to transport and deliver cargo molecules, including proteins, RNA, and miRNAs, to recipient cells has highlighted their importance in cellular signaling and disease progression (Veerman et al., 2019; Tenchov et al., 2022).
In recent years, exosomes have emerged as key mediators in the tumor microenvironment, facilitating the transfer of bioactive molecules between cancer cells and nearby or distant cells. Through their cargo, exosomes can modulate cellular processes such as proliferation, angiogenesis, immune response, and metastasis, thereby influencing tumor development and progression (Jia et al., 2017; Jena and Mandal, 2021).
The unique characteristics of exosomes, including their stability in various body fluids, protection against degradation, and ability to cross biological barriers, make them attractive candidates for therapeutic applications (Alzhrani et al., 2021). Researchers have explored the potential of exosomes as natural drug delivery vehicles, harnessing their ability to target specific cells or tissues. By engineering exosomes or loading them with therapeutic agents, it is possible to enhance drug efficacy, reduce off-target effects, and overcome drug resistance (Kibria et al., 2018; He et al., 2022). Moreover, exosomes have shown promise as diagnostic and prognostic biomarkers for various diseases, including cancer. Their presence in bodily fluids, such as blood, urine, and saliva, allows for non-invasive and readily accessible sampling, making them valuable tools for disease detection, monitoring, and personalized medicine (Bei et al., 2017; Huang and Deng, 2019).
More details on the significance of exosomes in intercellular communication, tumor biology, and potential therapeutic applications are given below.
1. Intercellular communication: exosomes are involved in a wide range of cellular communication processes. They can transfer various biomolecules, including proteins, lipids, and nucleic acids, between cells. These cargo molecules can exert functional effects on recipient cells by altering gene expression, signaling pathways, and cellular behavior. Exosomes have been found to participate in intercellular communication in diverse physiological contexts, such as immune response modulation, neuronal signaling, and tissue repair (Ludwig and Giebel, 2012; Ahmadi and Rezaie, 2020; Huo et al., 2021).
2. Tumor biology: in the context of cancer, exosomes have emerged as key players in tumor progression and metastasis. Tumor-derived exosomes can promote tumor growth by enhancing angiogenesis, and the formation of new blood vessels that supply nutrients to the tumor. They can also suppress the immune system’s response to cancer cells, allowing tumors to evade immune surveillance. Moreover, exosomes can prepare distant sites for tumor metastasis by facilitating the recruitment and reprogramming of cells in pre-metastatic niches. These processes contribute to the aggressiveness and spread of cancer (Aslan et al., 2019; Li et al., 2019; Zhou et al., 2021).
3. Therapeutic applications: the unique properties of exosomes make them attractive for therapeutic applications. One promising approach is to use exosomes as natural carriers for delivering therapeutic molecules, such as drugs or nucleic acids, to target cells. Exosomes can be engineered to express specific surface proteins or loaded with therapeutic cargo, enabling targeted delivery to diseased tissues while minimizing off-target effects. Furthermore, exosomes have inherent stability and biocompatibility, making them suitable for drug delivery systems. Research is ongoing to optimize exosome-based therapies and improve their efficacy in treating various diseases, including cancer, neurodegenerative disorders, and cardiovascular diseases (Sun et al., 2020; Ke and Afonin, 2021; Bashyal et al., 2022).
4. Diagnostic and prognostic biomarkers: exosomes have garnered significant interest as potential biomarkers for disease diagnosis and prognosis. Their presence in various bodily fluids, including blood, urine, and cerebrospinal fluid, allows for non-invasive sample collection. The molecular cargo within exosomes reflects the physiological and pathological states of their parent cells, making them valuable for disease detection and monitoring. Analyzing the content of exosomes, such as specific proteins, RNA molecules, or DNA mutations, can provide insights into disease subtypes, treatment response, and disease progression. This information can aid in personalized medicine approaches, guiding treatment decisions, and monitoring patient outcomes (Li et al., 2015; Alipoor et al., 2018; Yu et al., 2022).
5. Future directions: the field of exosome research is still evolving, and there are several exciting areas for future exploration. Researchers are actively investigating the mechanisms of exosome biogenesis, cargo sorting, and release, as well as the specific signaling pathways involved in exosome-mediated communication (Zhang et al., 2019). Additionally, efforts are being made to standardize exosome isolation and characterization methods to ensure reliable and reproducible results. Further advancements in exosome engineering, cargo loading techniques, and targeted delivery strategies will contribute to the development of innovative therapeutic interventions.
In overall, exosomes have far-reaching implications in intercellular communication, tumor biology, and therapeutic applications. Their ability to transfer signaling molecules, influence cellular behavior, and serve as diagnostic biomarkers make them a fascinating area of research with broad implications for various fields of medicine. Continued investigations into exosome biology and engineering hold great promise for advancing our understanding and harnessing the potential of these remarkable nanovesicles.
Exosomes have emerged as an attractive area of research due to several gaps in knowledge and limitations in existing treatments in various fields of medicine. These include.
1. Limited drug delivery systems: current drug delivery systems often face challenges such as poor bioavailability, off-target effects, and limited ability to cross biological barriers (Adepu and Ramakrishna, 2021). Exosomes, with their inherent ability to transport cargo molecules, including therapeutic agents, offer a potential solution to overcome these limitations (O'Loughlin et al., 2012). By engineering exosomes or loading them with specific therapeutic agents, it is possible to enhance drug delivery to target cells or tissues, thereby improving treatment efficacy and reducing side effects (Liang et al., 2021).
2. Incomplete understanding of intercellular communication: the complex mechanisms of intercellular communication, particularly in diseases like cancer, are not fully understood. Exosomes play a crucial role in this communication process by transferring bioactive molecules between cells. Investigating the cargo and signaling pathways within exosomes can provide valuable insights into disease progression, resistance mechanisms, and potential therapeutic targets (Xie et al., 2017).
3. Diagnostic challenges: traditional diagnostic methods often require invasive procedures or may lack sensitivity and specificity. Exosomes, being present in various bodily fluids, offer a non-invasive and readily accessible source of biomarkers (Zhou et al., 2020). However, further research is needed to identify specific exosomal biomarkers and develop standardized diagnostic assays to improve disease detection, monitoring, and personalized medicine approaches.
4. Limited treatment options for neurodegenerative diseases: neurodegenerative diseases, such as Alzheimer’s and Parkinson’s, currently lack effective treatments that can halt or reverse disease progression (Neef et al., 2011). The unique properties of exosomes, including their ability to cross the blood-brain barrier and transport therapeutic molecules, make them a promising avenue for developing novel treatments for these diseases (Li et al., 2021). However, extensive research is still required to understand the intricacies of exosome-mediated delivery and to optimize therapeutic strategies.
5. Tumor microenvironment complexity: tumors are highly heterogeneous and consist of cells with distinct characteristics (Marusyk et al., 2012). The tumor microenvironment, which includes interactions between cancer cells, immune cells, and stromal cells, plays a crucial role in tumor development and response to treatment (Anderson and Simon, 2020). Exosomes act as mediators in this complex microenvironment, facilitating communication and modulating cellular processes (Maia et al., 2018). Understanding the specific roles of exosomes in tumor progression and therapy resistance can provide new insights and potential targets for more effective anticancer strategies.
Overall, exosome research addresses the current gaps in knowledge and limitations in existing treatments by offering a promising platform for drug delivery, understanding intercellular communication, improving diagnostics, exploring neurodegenerative disease treatments, and deciphering the complexities of the tumor microenvironment. Continued research in these areas will contribute to advancements in medicine and potentially lead to improved patient outcomes.
Due to this issue, this study aims to explore and evaluate the potential application of cell-derived exosomes in the therapy of hematological malignancies, such as leukemia, lymphoma, and multiple myeloma. The aim is to investigate the unique properties of exosomes, including their ability to deliver therapeutic agents and modulate immune responses, and assess their effectiveness in targeted drug delivery and immunomodulation within the context of hematological malignancies. By critically analyzing the existing literature and synthesizing the findings, this study aims to provide a comprehensive academic overview of the current state of research, identify gaps in knowledge, and propose future directions for the development and optimization of exosome-based therapies in hematological malignancies.
Biogenesis of exosome
Exosome biogenesis is a complex process involving multiple steps within the cell. This process includes the following steps.
1. Formation of early endosomes: the biogenesis of exosomes begins with the formation of early endosomes, which are membrane-bound compartments derived from the plasma membrane (Mincheva-Nilsson and Baranov, 2014). This process can be triggered by various stimuli, such as cellular activation, stress, or signaling pathways. The early endosomes internalize extracellular materials, including proteins, lipids, and nucleic acids, through processes like endocytosis and phagocytosis (Abels et al., 2016; Gurung et al., 2021).
2. Maturation into late endosomes: early endosomes mature into late endosomes through a process called maturation or maturation transition (van Weering et al., 2012). This maturation involves changes in the endosomal membrane composition and the inward budding of intraluminal vesicles (ILVs) within the late endosomal compartment (Nour and Modis, 2014; Van Niel et al., 2018). ILVs are formed by the invagination of the endosomal membrane, resulting in the encapsulation of specific cargoes within vesicles (Xu et al., 2018).
3. Formation of Multivesicular Bodies (MVBs): late endosomes containing ILVs are referred to as MVBs. MVBs can further undergo two distinct pathways: the recycling pathway or the pathway leading to exosome release (Rainero and Norman, 2013; Raudenska et al., 2021). In the recycling pathway, MVBs can fuse with the plasma membrane, releasing ILVs back into the extracellular space as exosomes (Van Niel et al., 2006; Cocucci and Meldolesi, 2015). In contrast, the pathway leading to exosome release involves the fusion of MVBs with lysosomes, resulting in the degradation of their content (Buschow et al., 2005; Villarroya-Beltri et al., 2016).
4. Exosome release: when MVBs fuse with the plasma membrane, the ILVs within them are released into the extracellular space as exosomes (Janas et al., 2015). This process of exosome release allows the transfer of bioactive molecules, including proteins, lipids, and nucleic acids, from the originating cell to recipient cells (Wang W. et al., 2020). Exosomes can then travel through body fluids, such as blood or cerebrospinal fluid, to reach target cells in local or distant locations (Zhang and Grizzle, 2014; Bátiz et al., 2016).
Types of exosome
Different types of extracellular vesicles (EVs), including microvesicles (MVs), apoptotic bodies, and exosomes, have been categorized based on their origin, biogenesis, and functions (Minciacchi et al., 2015). The term “exosome” specifically refers to a group of nanovesicles with a diameter of 30–150 nm. These nanovesicles are generated within endosomes and released into the extracellular environment (Belfiore et al., 2018). The biogenesis of exosomes involves three distinct endosomal processes: the formation of endocytic vesicles, the generation of multivesicular bodies (MVBs), and the release of exosomes (Abels et al., 2016; Boriachek et al., 2018). Another mechanism to cease intracellular biogenesis is the degradation of exosomes in lysosomes. During the initial stage of exosome biogenesis, a portion of the cell membrane invaginates and forms an early endosome. Subsequently, early endosomes mature into late endosomes, which contain intraluminal vesicles (ILVs) such as MVBs. The formation of ILVs is regulated by endocytosis-associated proteins, the lipid raft complex, and the Rab and Ras GTPase families (Abels et al., 2016; Kim et al., 2018). MVBs serve as precursors for exosomes, as the composition of exosomes is derived from ILVs. Thus, exosome biogenesis is closely linked to endosomal maturation (Huotari and Helenius, 2011; Kim et al., 2018). Eventually, ILVs are released from MVBs, giving rise to exosomes in the extracellular milieu. The production of exosomes can occur independently or rely on the sorting machinery of the endosomal complex, which is essential for the transportation of proteins (Huotari and Helenius, 2011; Kim et al., 2018).
Exosomes are classified as either artificial or natural exosomes depending on whether they have undergone artificial modification. Animal- and plant-derived exosomes can be found in natural exosomes. Animal-derived exosomes can be classified into normal and tumoral exosomes since exosomes are produced in both normal and tumorous conditions (Zhang Y. et al., 2020; Song et al., 2021).
Exosomes are released by various cell types and have an immune-modulating role (Song et al., 2021). The majority of healthy cells, including B-cell, T cells, human umbilical cord, macrophages, natural killer (NK) cells, vein endothelial cells, dendritic cells (DC), and mesenchymal stem cells (MSC), can produce exosomes (Wang J-H. et al., 2020). They are composed of lipid bilayers and are identified by their hallmarks such as different types of lipids including cholesterol, ceramide, phosphatidylserine, and sphingomyelin, protein families such as β-galactosidase, glycoproteins N-linked glycans and O-linked glycans, signaling receptors such as FasL, TNF receptor and TfR, adhesion molecules such as P-selectin, α and β-integrin, cytoskeletal proteins include actin, cofilin and tubulin, growth factors and cytokines such as TGF-β, TNF-α, and TRAIL), ESCRT machinery such as TSG101 and ALIX), tetraspanins include CD82, CD81, CD63 and CD9, heat shock proteins (HSP) such as HSP-60, 70, and 80)), and nucleic acids includes DNA, miRNA, non-coding RNA and mRNA (Wang J-H. et al., 2020; Gurung et al., 2021). They play a crucial part in intercellular signaling, which is engaged in a variety of physiologic and pathological processes. Additionally, adipocytes, fibroblasts, tumor cells, immune cells, and other components create the tumor microenvironment (TME), which causes tumor homeostasis. Exosomes’ composition can serve as prognostic indicators or the foundation for a system of grading the progression of cancer. Angiogenesis, oncogenesis, metastasis, tumor growth, and treatment resistance are all impacted by exosomes that are released by tumor cells (Figure 1) (Li et al., 2020; Gurung et al., 2021).
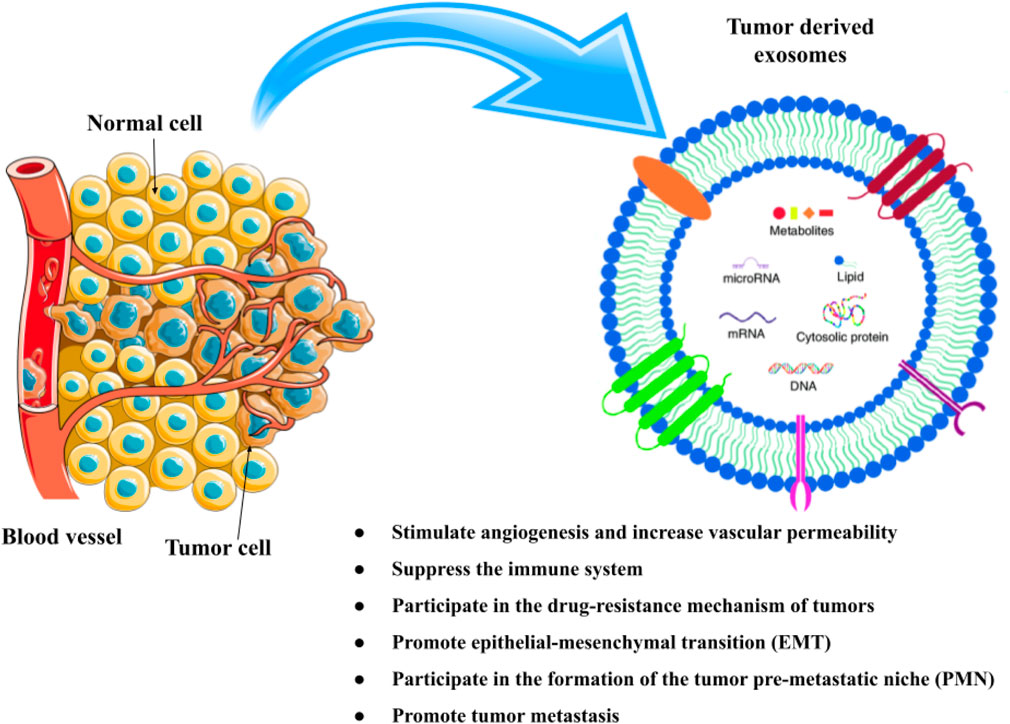
FIGURE 1. The composition and function of exosomes in cancer. Different kinds of proteins, nucleic acids, lipids, and metabolites can be found in exosomes.
Nine million cancer-related deaths and 18 million new cases are recorded each year. Of these, more than 400,000 cases—or roughly 90% of them—are connected to childhood cancer and occur in developing nations. While this percentage is over 80% in European countries, survival rates in African nations are as low as 20% (Galanello et al., 2003). Chemotherapy, surgery, targeted therapy, and radiotherapy are common cancer therapies (Debela et al., 2021; Moha et al., 2021). The most efficient forms of cancer treatment include radiotherapy and chemotherapy, but they can have side effects like long-term issues and medication resistance (Debela et al., 2021; Keyvani et al., 2022). By influencing the immune system to reactivate the anti-cancer immune response, cancer immunotherapy can control and remove tumors (Ghasemi et al., 2020; Ghasemi et al., 2021; Hasan-Abad et al., 2022; Chiang et al., 2023). The Food and Drug Administration (FDA) of the United States has given its approval. There are many immunotherapy medications available for use in therapeutic settings, including inhibitors of the protein cytotoxic T-lymphocyte-associated protein 4 (CTLA-4) and programmed cell death 1 (PD-1) ligand 1 (Chiang et al., 2023). Exosomes have been found to have the dual properties of inhibiting and promoting cancer in recent investigations on the various functions they play in the development of the disease. Exosomes, also known as cell-derived nanovesicles, may be used in cancer immunotherapy due to their molecular transfer and immunogenicity properties (Xu et al., 2020).
Exosomes’ ability to convey information and their high loading efficiency is being increasingly used in research that aims to deliver functional RNAs or medicine. Moreover, by altering their surface indicators, exosomes can become capable of targeting tumors (Li et al., 2020). Consequently, exosome-based therapy is viewed as a viable approach to treating cancer. We concentrated on the exosome-based therapeutic approach in the current review to treat hematological malignancies.
Exosome isolation methods
Exosome separation is essential for comprehending their mechanisms. Ultrafiltration, centrifugation, Immunocapture, size exclusion chromatography (SEC), microfluidics-based technologies, and affinity capture on antibody-coupled beads are examples of frequently used isolation techniques (Yang et al., 2020). The principles and characteristics of each method are explained below.
1. Ultracentrifugation: ultracentrifugation is a widely used method for exosome isolation. It involves sequential centrifugation steps to pellet and purify exosomes based on their size and density (Coughlan et al., 2020). The principle is to centrifuge the sample at high speeds to larger sediment particles, followed by ultracentrifugation to pellet exosomes. Advantages include relatively simple and cost-effective implementation. However, it can be time-consuming, requires specialized equipment, and may lead to co-isolation of other extracellular vesicles or contaminants (Taylor and Shah, 2015).
2. Density gradient centrifugation: this method separates exosomes based on their buoyant density (Yang et al., 2020). The sample is layered onto a density gradient medium and centrifuged, resulting in the formation of distinct bands corresponding to different vesicle populations. Exosomes can be collected from the appropriate density fraction. The principle is that exosomes with similar densities will migrate to specific regions in the gradient. Advantages include improved purity compared to ultracentrifugation. However, it requires longer processing time, gradient optimization, and can still result in the co-isolation of other vesicles (Li et al., 2017).
3. Size exclusion chromatography (SEC): SEC separates exosomes based on their size. The sample is loaded onto a column with porous beads, and smaller particles, including exosomes, elute later than larger particles. The principle is that exosomes can be separated from contaminants by their size exclusion. Advantages include simplicity, reproducibility, and minimal damage to exosomes. However, it may not provide high purity and can result in the loss of small-sized exosomes (Zhang and Lyden, 2019).
4. Immunocapture: this technique utilizes specific antibodies or affinity ligands immobilized on beads or surfaces to capture exosomes expressing specific surface markers. The principle is the selective binding of exosomes to the capture agent, allowing their isolation. Advantages include targeted isolation of specific exosomes and potential enrichment of subpopulations of interest. However, it relies on the availability of suitable antibodies, may result in co-isolation of non-exosomal material, and can have limited yield (Yang et al., 2022).
5. Microfluidics-based techniques: microfluidics platforms, such as microfluidic chips or nanostructures, enable precise manipulation and isolation of exosomes based on their size, surface markers, or other physical properties. The principle involves the controlled flow of samples through microchannels or nanostructures, allowing selective trapping or sorting of exosomes. Advantages include high specificity, minimal sample volume requirement, and potential for integration with downstream analysis. However, these techniques can be expensive, require specialized equipment, and have limited throughput (Lin et al., 2021).
The choice of isolation technique in clinical settings depends on various factors, including the type of bio fluid, required purity, sample volume, and downstream applications. Different techniques may be employed depending on the specific research objectives and available resources. It is worth noting that the field of exosome isolation is rapidly evolving, and new techniques are continually being developed and evaluated for their applicability in clinical settings. The most effective method for isolating exosomes is a centrifugation-based test. One of the greatest techniques for separating exosomes based on size, in addition to centrifugation, is ultrafiltration, which is now utilized in conjunction with ultracentrifuges (Yang et al., 2020). It has been demonstrated that exosomes can be found in the serum, urine, plasma, cerebrospinal fluid, and lymph of both healthy people and cancer patients (Mimeault and Batra, 2014).
Role of exosomes in the development of cancer
The maintenance of physiological homeostasis and the development of disease symptoms depend on cell-to-cell communication. By using paracrine and autocrine signaling, exosome-associated proteins, DNAs, RNAs, miRNAs, and metabolites can change the fate of recipient cells. Exosomes secreted by tumors have been thoroughly investigated in a variety of cancer types, including melanoma, breast cancer, renal cancer, and hematological cancer. For instance, TGF-1, which is linked to the TGF-1 receptor on the surface of the leukemia cells, is present in exosomes released by chronic myeloid leukemia cells. This causes the exosomes to activate the AKT, ERK, and anti-apoptotic pathways in the producer cells, which in turn causes the tumor to proliferate (Raimondo et al., 2015). Other reports on glioma cancer cells showed the paracrine mechanism of tumor-released exosomes in the development and metastasis of cancer. They found that glioma cells can transfer extracellular vesicles (EVs) with the EGFRvIII (oncogenic receptor) to adjacent glioma cells that lack this receptor, therefore leading to activation of the AKT pathway in neighboring glioma cells. Therefore, it leads to the activation of the AKT pathway in the adjacent glioma cells and causes anchor-independent growth of these cells (Al-Nedawi et al., 2008; Hasan-Abad et al., 2019).
So far, researchers have discovered that in pediatric and adult patients with acute myeloid leukemia (AML), the level of dsDNA in EVs in plasma decreases after treatment but increases after relapse (Bernardi et al., 2021). It was recently shown that cancer stem cell exosomes transported miR-19b-3p to renal carcinoma cells, which then triggered epithelial-mesenchymal transition (EMT) to drive tumor metastasis (Wang L. et al., 2019). p120-catenin in exosomes released from hepatocellular carcinoma (HCC) cells, on the other hand, inhibited the expansion, metastasis, and proliferation of HCC CSCs (Cheng et al., 2019). Table 1 summarizes examples of exosomes involved in the advancement of hematological malignancies. These findings may aid in the understanding of the role of exosomes in the development of cancer and hematological malignancies, leading to improved therapeutic methods (Figure 2).
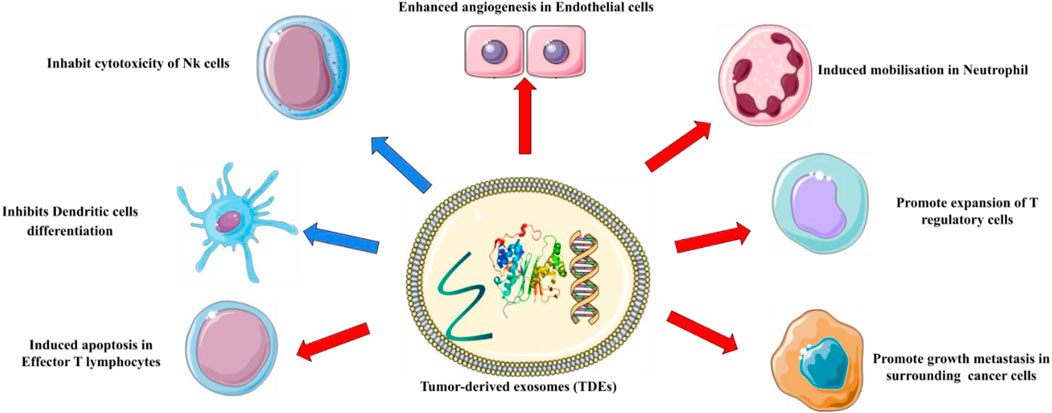
FIGURE 2. The roles of exosomes in cancer. Exosomes have been found to play a pivotal role in tumor initiation, growth, progression, and metastasis.
Exosome-based tumor suppression strategies
Exosomes play important roles in intercellular contact in a variety of clinical disorders, including cancer, diabetes, and Alzheimer’s disease. Exosome cargoes produced from tumors are linked to cancer development. As a result, novel and safe cancer treatment techniques such as regulation of exosome production and secretion, exosome-mediated cell communication and employing exosomes as carriers, and removal of active exosomal cargos have been proposed. Furthermore, exosomes can be used as a diagnostic tool (Tai et al., 2018). A study found that modulating intracellular Ca2+ levels with dimethyl amiloride (DMA), an inhibitor of Ca2+/Na + exchange, reduced exosome secretion in mice with EL4 lymphoma (Savina et al., 2003). Koch et al. did another trial to inhibit exosome realization. They found that preventing exosomal drug resistance with indomethacin improved the effect of anthracene-dione pixantrone and anthracycline doxorubicin in DLBCL cell lines (Koch et al., 2016).
The use of exosomes as carriers in the treatment of hematological malignancies
Exosomes can transport therapeutic molecules to cancer cells via acquired or innate ligands produced on their surfaces, such as proteins, nucleic acids, and other medicines. Recently, anticancer medicines doxorubicin and paclitaxel were successfully loaded into exosomes (Tian et al., 2014). Exosome drug delivery enhanced cancer targeting via an external magnetic field and reduced tumor growth, overcoming major challenges to the utilization of exosomes for cancer treatment. Exosomes containing the TRAIL (TNF-related apoptosis-inducing ligand) protein were found to increase apoptosis and prevent tumor growth in myeloid leukemia both in vitro (KMS11 multiple myeloma, INT12 melanoma, and B-cell lymphoma) and in vivo (mice) (Rivoltini et al., 2016a). Another study examined the anti-cancer effect of imatinib or BCR-ABL siRNA cargo with exosome on chronic myeloid leukemia (CML) in vitro (CML cell lines included: K562, imatinib K562R, and LAMA84 cells) and in vivo (mice). Exosomes target CML cells selectively with Lamp2b-IL3 and limit proliferation when loaded with BCR-ABL siRNA or imatinib by lowering the expression of the Bcr-Abl oncoprotein (Bellavia et al., 2017). Bellavia et al. employed HEK293 T cells that had been engineered to express the exosomal Lamp2b, which was attached to an Interleukin 3 (IL3) receptor. In contrast, despite normal cells, the IL3 receptor is overexpressed in CML blasts and acts as a therapeutic target in the cancer drug delivery system. They demonstrated that IL3L exosomes laden with the drugs BCR-ABL siRNA or Imatinib can target CML cells and limit cancer cell proliferation in vivo and in vitro (Bellavia et al., 2017).
Exosomes as biomarkers in hematological malignancies
Exosomes can be extracted from a variety of bodily fluids, including urine, plasma, serum, saliva, breast milk, malignant ascites fluid, and amniotic fluid (Keller et al., 2011). Furthermore, biologically active EVS extracted from plasma could be employed as novel biological indicators. In the case of cancer, a suitable biomarker should be able to detect possibly metastatic tumors during the primary stage, while the cancer can still be treated (Testa et al., 2021). EVs produced by cancer cells contain tumor-specific components that can shield their contents from proteases and nucleases while also increasing biomarker half-life. Recently, it was shown that miR-155 can be used as a biomarker in patients with monoclonal B-cell lymphocytosis and B-cell leukemia (Ferrajoli et al., 2013). Several investigations have proven the use of exosomal bioactive compounds as prognostic and diagnostic markers in other forms of cancer. The RNA biomarker AR-V7 RNA expression level, for example, increases during prostate cancer and can be detected using the PCR method. The level of the protein biomarker MIF in the plasma increases in pancreatic cancer, which may be identified using the ELISA method. Other biomarkers, such as EGFR vIII mRNA in glioblastoma (in plasma), integrin in breast cancer (in plasma), and ZFAS1 long noncoding RNA in gastric cancer (in serum), are examples of how exosomes can be used to diagnose cancer (Tai et al., 2018).
Except for CML (Philadelphia chromosome as a biomarker), the lack of particular molecular markers capable of being exploited has had a significant impact on the early diagnosis and treatment of these cancers. Several studies have found that mutations or aberrant expression of certain mRNAs or miRNAs are linked to hematological malignancies (Ferrajoli et al., 2013). Exosomal miR150, miR155, and miR1246 levels have been found to rise in AML patients (Hornick et al., 2015). The differential centrifugation method or the miRNeasy kit can be used to assess the serum or plasma level of certain miRNAs. In MM patients, lowering serum levels of exosomal miR20a-5p, miR16-5p, miR15a-5p, and miR16-5p has the potential to be used in the separation or construction of prognostic and diagnostic panels (Zhang et al., 2016).
Cell-derived exosomes as therapeutic agents
MSC-derived exosomes
In malignancies or diseases, MSC-exosomes can carry complicated payloads and affect body homeostasis (Lou et al., 2017). In the previous decade, MSC-exosomes have been used to treat a variety of hematological disorders including multiple myeloma (MM), myelodysplastic syndrome (MDS), lymphoma, GVHD, CLL, AML, and CML (Shen and Chen, 2021). They have a role in tumor growth by inducing matrix remodeling and angiogenesis in a pro-tumor niche, inhibiting apoptosis and the activity of anti-tumor immune cells such as CTL and NK cells, and delivering pro-survival cytokines (Shen and Chen, 2021). For example, there is evidence that bone marrow MSCs promote the formation of MM by secreting interleukin-6 (IL-6) as a tumor cell growth factor (Lou et al., 2017; Shen and Chen, 2021). MSCs are pluripotent cells with very diverse characteristics that play a dual function in cancer formation. This topic explains why clinical trials examining the effect of MSCs on cancer have been equivocal and have not progressed beyond phase 1. Approximately 42 clinical trials were registered on www.clinicaltrials.gov until 2019, with a focus on the efficacy of MSCs on malignancies, with just 13 focusing on hematologic malignancy. Only one of the 13 clinical trials looked at the anticancer effects of MSCs. Other investigations were linked to the immunomodulatory function of MSCs after stem cell transplantation (Rappa et al., 2012; Lou et al., 2017; Lee et al., 2019). As a result, more research is needed to comprehend the anti-cancer applicability of MSCs.
MSC anticancer mechanisms are still unknown. However, some of them are desirable for use in the prevention of hematological malignancies. Exosome-derived cancer cells are being studied for ways to silence or eliminate them (Lee et al., 2019). Exosome removal from the circulation (via plasmapheresis), prevention of exosome production (via DMA), exosome suppression (via silencing of targeting ESCRT proteins or GTP-ase Rab27A), prevention of endolysosomal contents functions (via Proton pump inhibitors (PPI), and prevention of exosome uptake or fusion by target cells (via blocking phosphatidyl serine with diannexin) are all under investigation strategies for (Vader et al., 2014). However, because of the essential and dual functions of exosomes, more research is required to develop an authorized safe cancer therapeutic technique.
According to ongoing research, MSC exosomes can infiltrate tumor locations due to an increased retention (EPR) impact and permeability, making them suitable delivery vehicles for suppressing cell proliferation and vascularization (Naseri et al., 2018). For example, combining modified MSC with medication-loaded nanoparticles containing the anticancer agent paclitaxel results in a significant reduction of tumor growth and survival in vivo (Naseri et al., 2018). Furthermore, the findings of this study revealed that using nano-engineered MSCs as a drug vehicle considerably increased the anticancer efficiency of traditional chemotherapy medicines (Layek et al., 2018). A study found that combining MSCs expressing exosomal siGRP78 with sorafenib could reduce proliferation, invasion, and reverse drug resistance in hepatocellular carcinoma cells (Li H. et al., 2018). There are now multiple clinical trials using exosome-based treatments for solid tumors, including lung cancer (23 studies), breast cancer (12 studies), renal cancer (three studies), and ovarian cancer (three studies) (www.clinicaltrials.gov website, keyword exosome). However, just two studies on the use of exosomes for non-Hodgkin B-cell lymphoma therapy were found in the field of hematologic malignancies (Fernandes et al., 2019). Several studies have found that MSCs inhibit both apoptosis and proliferation, enhance cell proliferation, and aid in tumor vasculature (Layek et al., 2018; Lee et al., 2019; Shen and Chen, 2021). Furthermore, because of the immunomodulatory properties of MSCs, which increase the rate of recurrence and metastasis, leading to chemo-resistance, and tending to shield tumor cells from drug-induced death, MSCs are not suitable for therapeutic usage (Chen et al., 2018; Lee et al., 2019).
Dendritic cell-derived exosomes
DCs have emerged as the most potent antigen-presenting cells (APCs) for cancer vaccinations in recent years. Several studies and phase I or II clinical trials have been done to monitor the anticancer effects of DCs (Pyzer et al., 2014). WT1 (Wilm’s tumor 1 protein) mRNA-electroporated DC vaccination was created by Van Tendeloo et al. They vaccinated ten AML patients in a phase I/II experiment. Vaccinated patients had an increase in WT1-specific T cells as well as an increase in WT1-specific IFN-producing CD8+ T cells (Van Tendeloo et al., 2010).
Although the DC-based vaccine provided benefits such as activation of T-helper cells, killer T cells, and B-cell, as well as induction of cancer-specific T-cell response, there were numerous disadvantages. Tumor cells, for example, may produce immunosuppressive soluble cytokines that transform DCs into tolerogenic DCs, resulting in T-reg cell activation (Palucka and Banchereau, 2012; Moradi Hasan-Abad et al., 2022). The use of exosomes produced from DCs (Dex) has been proposed as a remedy to the technical hurdles associated with DC-based immunotherapy (Andre et al., 2004). For example, in comparison to DCs, Dex has exceptional stability at −80°C for 6 months due to its high lipid makeup. Furthermore, Dex expresses adequate ligands for NK cell receptors and is resistant to immunosuppressive compounds and TME immunoregulation (Pitt et al., 2016).
Recently, a novel CML-RAE-1-Dex vaccination with RAE-1 expression was developed. RAE-1 is a key ligand of NK group 2 D (NKG2D), which is required for T- and NK-cell responses. Their discovery demonstrated an anticancer effect both in vitro and in vivo. Their Dex vaccination boosted the effector functions and proliferation of NK cells, CD8+ T cells, and CD4+ T cells, resulting in a strong anti-CML impact in vitro. Furthermore, in CML animal models, immunotherapy with CML-RAE-1-Dex reduced leukemogenesis and established long-lasting immunological memory (Du et al., 2022). Immune responses were similar in imatinib-resistant CML cells with the T315I mutation (Du et al., 2022).
Yao et al. employed an LEXs-pulsed DCs vaccination against leukemia in 2014. Exosomes produced from K562 leukemia cells (LEXK562) include immunologically related molecules (hsp70) as well as membrane-bound vesicles containing adhesion molecules (intercellular adhesion molecule-1). In comparison to LEXs and non-pulsed DCs, LEXs-pulsed DCs elicited a stronger anti-leukemic cytotoxic T-cell response (Yao et al., 2014). Dex-based vaccination technology is one of the areas of interest for researchers as the number of preclinical and clinical investigations on hematological malignancies continues to rise.
Natural killer cell-derived exosomes
Patients with AML exhibit a low NK-cell frequency and significantly lower NK cell activity in the peripheral blood, as well as lower expression of NCR, NKp46, NKp44, NKp30, and C-type lectin receptors, NKG2C and NKG2D (Szczepanski et al., 2011). In AML, NK cell functions (intracellular signaling, cytokine generation, and cytotoxicity) are reduced; NK cell dysfunction is probably associated with the disease’s initial expansion, progression, or recurrence (Shimasaki et al., 2020).
Exosomes containing essential cytotoxic chemicals such as LFA-1, IFN-, DNAM1, and PD-1 are produced in large amounts by stimulated NK cells (Shimasaki et al., 2020).
In neuroblastoma tumor-bearing animals, NK-92 cell-exosomes used as a drug delivery vehicle had good targeting capacity, with robust fluorescence observed 6 h after injection; in a subcutaneous tumor-bearing mouse model, exosomes were found in tumor cells 20 min after injection (Wang G. et al., 2019). Furthermore, exosomes released by NK cells with IL-15 had a greater targeting ability, were faster acting, and persisted in the tumor site and blood circulation for a longer period, with a half-life of up to 24 h (Zhu et al., 2019).
Another ability of NK cells to fight cancer is the production of exosomes or MVs containing cytotoxic proteins and miRNA. Granzyme A and B, Perforin, and granulysin have antitumor effects on NK cells by activating apoptotic pathways via caspase-dependent and -independent apoptotic pathways, single-stranded DNA damage, mitochondrial disruption resulting in cytochrome c release and activation of the apoptotic cascade, induces ER stress-mediated apoptosis, and damages the cell membrane. The anti-cancer action of miR-186 released by NK cells was demonstrated by reducing neuroblastoma cell proliferation and causing apoptosis (Wu et al., 2021).
Furthermore, when NK cells are pre-exposed to neuroblastoma cells, they release exosomes with greater expression of different activating receptors such as NKp46, NKp44, NKp30, and NKG2D, as well as enhanced cytotoxicity when compared to untreated NK cells. This work shows that, in addition to their cytotoxic role, NK cells can be positively impacted by neuroblastoma cells, resulting in efficient cytotoxicity against neuroblastoma tumors (Shoae-Hassani et al., 2017). These findings provide hope for NK cell-based cancer therapy. More research is needed, however, to extrapolate these findings to other forms of cancer and hematological malignancies. Boyiadzis et al. investigated the efficacy of NK cell exosomes on AML cell lines (Kasumi, MLL-1), K562 targets, and primary leukemia blasts. They discovered that NK cell-derived exosomes containing killer-cell immunoglobulin-like receptors, TGF, perforin, NKG2D, granzyme B, and PD-1 increase blast cell lysis and have anti-leukemia action against K562 targets and AML cell lines (Boyiadzis et al., 2018).
Di Pace and others discovered that a novel marker called DNAX Accessory Molecule-1 (DNAM1) is involved in the exosome-mediated cytotoxicity of NK cells in a children B acute lymphoblastic leukemia cell line. Furthermore, they report that the cytotoxic action of NK cell-derived exosomes was dose-dependent, with the percentage of killed cells increasing as exosome concentrations increased, reaching a maximum of 50. This feature suggests that they could be used in immunotherapy (Di Pace et al., 2020).
Despite their vital role in cancer defenses, NK cells’ application in cancer treatment is limited due to their diminished ability to reach tumor sites and the inhibitory effects of TME on their function or diminution of anti-cancer action within a few hours (Labani-Motlagh et al., 2016; Li Q. et al., 2018; Di Pace et al., 2020).
Future studies should focus on increasing the site specificity and durability of the anti-cancer response mediated by NK cell-generated exosomes via genetic engineering to better exploit these potent cells in cancer therapy. Exosome-mediated treatment approaches for hematological Cancers are shown in Table 2.
Challenges and limitations of the therapeutic use of exosomes
The unique properties of exosomes, such as stability, biocompatibility, and ability to transport bioactive molecules, make them promising candidates for therapeutic interventions. The therapeutic use of exosomes holds great promise, but several challenges and limitations need to be addressed for successful clinical translation. Overcoming these challenges requires interdisciplinary collaboration, innovative technologies, and a deep understanding of the complex biology of exosomes. The most important challenges and limitations of the therapeutic use of exosomes are explained below.
1. Isolation and purification: one of the key challenges is the development of standardized and reproducible methods for isolating and purifying exosomes (Ayala-Mar et al., 2019). Current isolation techniques, such as ultracentrifugation and immunocapture, have limitations in terms of scalability, purity, and yield. There is a need for robust and efficient isolation methods that can provide highly purified exosome populations suitable for clinical applications (Ayala-Mar et al., 2019; Liangsupree et al., 2021).
2. Heterogeneity: exosomes derived from different cell types or even from the same cell type can exhibit significant heterogeneity in terms of cargo composition and functional properties (O’Brien et al., 2020). Understanding and controlling this heterogeneity is crucial for ensuring consistent therapeutic outcomes. Characterization techniques and quality control measures need to be improved to assess the heterogeneity of exosomes and establish standardized criteria for their therapeutic use (Willis et al., 2017).
3. Scalability and manufacturing: the clinical translation of exosome-based therapies requires scalable and cost-effective manufacturing processes (Amondarain et al., 2023). Currently, the production of exosomes in large quantities remains a challenge. Developing scalable manufacturing platforms, including bioreactors and microfluidic systems, is necessary to meet the demands of clinical applications (Colao et al., 2018; Pan et al., 2023).
4. Targeted delivery: efficient and targeted delivery of exosomes to specific tissues or cells is essential for achieving desired therapeutic effects (Rehman et al., 2022). Overcoming biological barriers, such as clearance by the immune system and off-target effects, is critical for successful targeted delivery. Strategies like surface modification and engineering approaches need to be explored to enhance the specificity and efficiency of exosome delivery (Mitchell et al., 2021; Waheed et al., 2022).
5. Regulatory considerations: the therapeutic use of exosomes falls under regulatory frameworks that govern biological products and drug delivery systems (Bunggulawa et al., 2018). Understanding and complying with these regulations is crucial for the successful translation of exosome-based therapies from preclinical studies to clinical trials and commercialization (Willis et al., 2017).
6. Clinical translation and efficacy: although preclinical studies have shown promising results, the efficacy of exosome-based therapies in clinical settings is still being explored. The development of well-designed clinical trials and rigorous evaluation of therapeutic outcomes are necessary to determine the safety, efficacy, and long-term effects of exosome-based interventions.
Future of the therapeutic use of exosomes
The advancements in exosome research have the potential to transform cancer treatment by providing novel therapeutic strategies. Further investigation in these research directions will not only expand our knowledge of exosome biology but also pave the way for personalized, targeted, and more effective cancer therapies, ultimately improving patient outcomes and quality of life. Several areas warrant further investigation to harness the full potential of exosomes and improve patient outcomes.
Cargo and Functional Analysis: Future research should focus on elucidating the specific cargo components carried by exosomes and their functional relevance in cancer treatment (Zhang et al., 2019). Identifying the key molecules, such as miRNAs, proteins, and lipids, involved in modulating tumor growth, metastasis, immune response, and drug resistance will enhance our understanding of exosome-mediated intercellular communication and facilitate the development of targeted therapies (Mashouri et al., 2019).
Engineering and Modification: Investigating exosome engineering strategies, such as surface modification and cargo loading techniques, can enhance their targeting ability and therapeutic efficacy (Salunkhe et al., 2020). Further exploration of bioengineering approaches, including genetic engineering and synthetic biology, can enable the customization of exosomes for specific cancer types and individual patient needs.
Delivery Optimization: Improving the efficiency and specificity of exosome delivery to tumor sites remains a critical area of investigation (Subhan and Torchilin, 2019). Developing novel delivery methods, such as exosome-based drug carriers or nanoscale delivery systems, can enhance the stability, biodistribution, and cellular uptake of exosomes, leading to improved therapeutic outcomes and reduced off-target effects (Subhan and Torchilin, 2020).
Biomarker Discovery: Identifying reliable exosomal biomarkers for cancer diagnosis, prognosis, and treatment response prediction is an important research direction (Li et al., 2022). Comprehensive profiling of exosomal molecular signatures in large patient cohorts, combined with advanced bioinformatics approaches, can aid in the identification of biomarkers that can guide personalized treatment decisions and improve patient stratification.
Clinical Translation: Conducting well-designed clinical trials to evaluate the safety and efficacy of exosome-based therapies in cancer patients is crucial. Longitudinal studies assessing the long-term effects and monitoring potential adverse events associated with exosome treatments are needed. Collaboration between academia, industry, and regulatory bodies is essential to navigate the regulatory landscape and facilitate the translation of exosome research into clinical practice (Table 3).
Conclusion
The advancements in exosome research have the potential to transform cancer treatment by providing novel therapeutic strategies. Further investigation in these research directions will not only expand our knowledge of exosome biology but also pave the way for personalized, targeted, and more effective cancer therapies, ultimately improving patient outcomes and quality of life.
Exosomes are a relatively young field of study. Exosomes have sparked great attention in the realm of cancer therapy due to their potential use as new low-toxicity inhibitors in immunotherapy and diagnostic biomarkers, as well as a safer and more efficient method of delivering anti-cancer medications. However, there are various obstacles to their optimal utilization. Methods for large-scale manufacturing, isolation, and storage, in particular, should be carefully tuned. Furthermore, the therapeutic potential and delivery efficiency of exosomes, the route of administration, and the immunologic influence of exosomes on cancer cell suppression must all be carefully examined. In the case of hematological malignancies, concentrating on the use of exosomes for drug delivery, developing protocols for the prevention of drug resistance mediated by exosomes (such as using monoclonal antibodies, aptamers, nanoparticles, engineered and modified exosomes, etc.), and developing methods for blocking exosomes associated with the progression or metastasis of hematological malignancies can all contribute to better cancer therapy management in the future.
Author contributions
KG: Writing–review and editing, Investigation. AM-H: Writing–review and editing, Methodology, Validation, Writing–original draft. AS-N: Writing–review and editing, Software, Visualization. JJ: Writing–review and editing, Supervision. AG: Supervision, Writing–review and editing.
Conflict of interest
The authors declare that the research was conducted in the absence of any commercial or financial relationships that could be construed as a potential conflict of interest.
Publisher’s note
All claims expressed in this article are solely those of the authors and do not necessarily represent those of their affiliated organizations, or those of the publisher, the editors and the reviewers. Any product that may be evaluated in this article, or claim that may be made by its manufacturer, is not guaranteed or endorsed by the publisher.
References
Abbaszade Dibavar, M., Soleimani, M., Atashi, A., Rassaei, N., and Amiri, S. (2018). The effect of simultaneous administration of arsenic trioxide and microvesicles derived from human bone marrow mesenchymal stem cells on cell proliferation and apoptosis of acute myeloid leukemia cell line. Artif. Cells, Nanomedicine, Biotechnol. 46 (3), S138–S146. doi:10.1080/21691401.2018.1489821
Abels, E. R., and Breakefield, X. O. (2016). Introduction to extracellular vesicles: biogenesis, RNA cargo selection, content, release, and uptake. Cell. Mol. Neurobiol. 36, 301–312. doi:10.1007/s10571-016-0366-z
Adepu, S., and Ramakrishna, S. (2021). Controlled drug delivery systems: current status and future directions. Molecules 26 (19), 5905. doi:10.3390/molecules26195905
Ahmadi, M., and Rezaie, J. (2020). Tumor cells derived-exosomes as angiogenenic agents: possible therapeutic implications. J. Transl. Med. 18 (1), 249–317. doi:10.1186/s12967-020-02426-5
Al-Nedawi, K., Meehan, B., Micallef, J., Lhotak, V., May, L., Guha, A., et al. (2008). Intercellular transfer of the oncogenic receptor EGFRvIII by microvesicles derived from tumour cells. Nat. Cell Biol. 10 (5), 619–624. doi:10.1038/ncb1725
Alipoor, S. D., Mortaz, E., Varahram, M., Movassaghi, M., Kraneveld, A. D., Garssen, J., et al. (2018). The potential biomarkers and immunological effects of tumor-derived exosomes in lung cancer. Front. Immunol. 9, 819. doi:10.3389/fimmu.2018.00819
Alzhrani, G. N., Alanazi, S. T., Alsharif, S. Y., Albalawi, A. M., Alsharif, A. A., Abdel-Maksoud, M. S., et al. (2021). Exosomes: isolation, characterization, and biomedical applications. Cell Biol. Int. 45 (9), 1807–1831. doi:10.1002/cbin.11620
Amondarain, M., Gallego, I., Puras, G., Saenz-del-Burgo, L., Luzzani, C., and Pedraz, J. L. (2023). The role of microfluidics and 3D-bioprinting in the future of exosome therapy. Trends Biotechnol. 2023, S0167. doi:10.1016/j.tibtech.2023.05.006
Anderson, N. M., and Simon, M. C. (2020). The tumor microenvironment. Curr. Biol. 30 (16), R921–R925. doi:10.1016/j.cub.2020.06.081
Andre, F., Escudier, B., Angevin, E., Tursz, T., and Zitvogel, L. (2004). Exosomes for cancer immunotherapy. Ann. Oncol. 15, iv141–iv144. doi:10.1093/annonc/mdh918
Aslan, C., Maralbashi, S., Salari, F., Kahroba, H., Sigaroodi, F., Kazemi, T., et al. (2019). Tumor-derived exosomes: implication in angiogenesis and antiangiogenesis cancer therapy. J. Cell. physiology 234 (10), 16885–16903. doi:10.1002/jcp.28374
Ayala-Mar, S., Donoso-Quezada, J., Gallo-Villanueva, R. C., Perez-Gonzalez, V. H., and González-Valdez, J. (2019). Recent advances and challenges in the recovery and purification of cellular exosomes. Electrophoresis 40 (23-24), 3036–3049. doi:10.1002/elps.201800526
Bashyal, S., Thapa, C., and Lee, S. (2022). Recent progresses in exosome-based systems for targeted drug delivery to the brain. J. Control. Release 348, 723–744. doi:10.1016/j.jconrel.2022.06.011
Bátiz, L. F., Castro, M. A., Burgos, P. V., Velásquez, Z. D., Muñoz, R. I., Lafourcade, C. A., et al. (2016). Exosomes as novel regulators of adult neurogenic niches. Front. Cell. Neurosci. 9, 501. doi:10.3389/fncel.2015.00501
Bei, Y., Yu, P., Cretoiu, D., Cretoiu, S. M., and Xiao, J. (2017). Exosomes-based biomarkers for the prognosis of cardiovascular diseases. Exosomes Cardiovasc. Dis. Biomarkers, Pathological Ther. Eff. 998, 71–88. doi:10.1007/978-981-10-4397-0_5
Belfiore, L., Saunders, D. N., Ranson, M., Thurecht, K. J., Storm, G., and Vine, K. L. (2018). Towards clinical translation of ligand-functionalized liposomes in targeted cancer therapy: challenges and opportunities. J. Control. release 277, 1–13. doi:10.1016/j.jconrel.2018.02.040
Bellavia, D., Raimondo, S., Calabrese, G., Forte, S., Cristaldi, M., Patinella, A., et al. (2017). Interleukin 3-receptor targeted exosomes inhibit in vitro and in vivo Chronic Myelogenous Leukemia cell growth. Theranostics 7 (5), 1333–1345. doi:10.7150/thno.17092
Bernardi, S., Zanaglio, C., Farina, M., Polverelli, N., Malagola, M., and Russo, D. (2021). dsDNA from extracellular vesicles (EVs) in adult AML. Ann. Hematol. 100 (5), 1355–1356. doi:10.1007/s00277-020-04109-z
Boriachek, K., Islam, M. N., Möller, A., Salomon, C., Nguyen, N. T., Hossain, M. S. A., et al. (2018). Biological functions and current advances in isolation and detection strategies for exosome nanovesicles. Small 14 (6), 1702153. doi:10.1002/smll.201702153
Boyiadzis, M., Hong, C. S., and Whiteside, T. L. (2018). Natural killer cell derived exosomes as a novel therapeutic for acute myeloid leukemia. Blood 132, 5226. doi:10.1182/blood-2018-99-115385
Bunggulawa, E. J., Wang, W., Yin, T., Wang, N., Durkan, C., Wang, Y., et al. (2018). Recent advancements in the use of exosomes as drug delivery systems. J. Nanobiotechnology 16 (1), 81–13. doi:10.1186/s12951-018-0403-9
Buschow, S. I., Liefhebber, J. M., Wubbolts, R., and Stoorvogel, W. (2005). Exosomes contain ubiquitinated proteins. Blood Cells, Mol. Dis. 35 (3), 398–403. doi:10.1016/j.bcmd.2005.08.005
Chapuy, B., Koch, R., Radunski, U., Corsham, S., Cheong, N., Inagaki, N., et al. (2008). Intracellular ABC transporter A3 confers multidrug resistance in leukemia cells by lysosomal drug sequestration. Leukemia 22 (8), 1576–1586. doi:10.1038/leu.2008.103
Chen, T., Zhang, G., Kong, L., Xu, S., Wang, Y., and Dong, M. (2019). Leukemia-derived exosomes induced IL-8 production in bone marrow stromal cells to protect the leukemia cells against chemotherapy. Life Sci. 221, 187–195. doi:10.1016/j.lfs.2019.02.003
Chen, W., Qin, Y., and Liu, S. (2018). Cytokines, breast cancer stem cells (BCSCs) and chemoresistance. Clin. Transl. Med. 7 (1), 27–13. doi:10.1186/s40169-018-0205-6
Cheng, M., Chen, Y., Xiao, W., Sun, R., and Tian, Z. (2013). NK cell-based immunotherapy for malignant diseases. Cell. Mol. Immunol. 10 (3), 230–252. doi:10.1038/cmi.2013.10
Cheng, Z., Lei, Z., Yang, P., Si, A., Xiang, D., Tang, X., et al. (2019). Exosome-transmitted p120-catenin suppresses hepatocellular carcinoma progression via STAT3 pathways. Mol. Carcinog. 58 (8), 1389–1399. doi:10.1002/mc.23022
Chiang, R. S., Desai, A., Glover, M. J., Hui, G., Ramchandran, K. J., Wakelee, H., et al. (2023). Racial diversity and reporting in United States food and drug administration registration trials for thoracic malignancies from 2006 to 2020. Cancer Investig. 41, 43–47. (just-accepted). doi:10.1080/07357907.2022.2131808
Cocucci, E., and Meldolesi, J. (2015). Ectosomes and exosomes: shedding the confusion between extracellular vesicles. Trends Cell Biol. 25 (6), 364–372. doi:10.1016/j.tcb.2015.01.004
Colao, I. L., Corteling, R., Bracewell, D., and Wall, I. (2018). Manufacturing exosomes: a promising therapeutic platform. Trends Mol. Med. 24 (3), 242–256. doi:10.1016/j.molmed.2018.01.006
Corrado, C., Raimondo, S., Saieva, L., Flugy, A. M., De Leo, G., and Alessandro, R. (2014). Exosome-mediated crosstalk between chronic myelogenous leukemia cells and human bone marrow stromal cells triggers an interleukin 8-dependent survival of leukemia cells. Cancer Lett. 348 (1-2), 71–76. doi:10.1016/j.canlet.2014.03.009
Corrado, C., Saieva, L., Raimondo, S., Santoro, A., De Leo, G., and Alessandro, R. (2016). Chronic myelogenous leukaemia exosomes modulate bone marrow microenvironment through activation of epidermal growth factor receptor. J. Cell. Mol. Med. 20 (10), 1829–1839. doi:10.1111/jcmm.12873
Coughlan, C., Bruce, K. D., Burgy, O., Boyd, T. D., Michel, C. R., Garcia-Perez, J. E., et al. (2020). Exosome isolation by ultracentrifugation and precipitation and techniques for downstream analyses. Curr. Protoc. Cell Biol. 88 (1), e110. doi:10.1002/cpcb.110
De Veirman, K., Wang, J., Xu, S., Leleu, X., Himpe, E., Maes, K., et al. (2016). Induction of miR-146a by multiple myeloma cells in mesenchymal stromal cells stimulates their pro-tumoral activity. Cancer Lett. 377 (1), 17–24. doi:10.1016/j.canlet.2016.04.024
Debela, D. T., Muzazu, S. G., Heraro, K. D., Ndalama, M. T., Mesele, B. W., Haile, D. C., et al. (2021). New approaches and procedures for cancer treatment: current perspectives. SAGE open Med. 9, 20503121211034366. doi:10.1177/20503121211034366
Di Pace, A. L., Tumino, N., Besi, F., Alicata, C., Conti, L. A., Munari, E., et al. (2020). Characterization of human NK cell-derived exosomes: role of DNAM1 receptor in exosome-mediated cytotoxicity against tumor. Cancers 12 (3), 661. doi:10.3390/cancers12030661
Dimik, M., Abeysinghe, P., Logan, J., and Mitchell, M. (2023). The exosome: a review of current therapeutic roles and capabilities in human reproduction. Drug Deliv. Transl. Res. 13 (2), 473–502. doi:10.1007/s13346-022-01225-3
Du, Z., Huang, Z., Chen, X., Jiang, G., Peng, Y., Feng, W., et al. (2022). Modified dendritic cell-derived exosomes activate both NK cells and T cells through the NKG2D/NKG2D-L pathway to kill CML cells with or without T315I mutation. Exp. Hematol. Oncol. 11 (1), 36–20. doi:10.1186/s40164-022-00289-8
Fernandes, M., Teixeira, A. L., and Medeiros, R. (2019). The opportunistic effect of exosomes on Non-Hodgkin Lymphoma microenvironment modulation. Crit. Rev. Oncology/Hematology 144, 102825. doi:10.1016/j.critrevonc.2019.102825
Ferrajoli, A., Shanafelt, T. D., Ivan, C., Shimizu, M., Rabe, K. G., Nouraee, N., et al. (2013). Prognostic value of miR-155 in individuals with monoclonal B-cell lymphocytosis and patients with B chronic lymphocytic leukemia. Blood, J. Am. Soc. Hematol. 122 (11), 1891–1899. doi:10.1182/blood-2013-01-478222
Fu, F-f., Zhu, X-j., Wang, H-x., Zhang, L-m., Yuan, G-l., Chen, Z-c., et al. (2017). BCR-ABL1-positive microvesicles malignantly transform human bone marrow mesenchymal stem cells in vitro. Acta Pharmacol. Sin. 38 (11), 1475–1485. doi:10.1038/aps.2017.116
Galanello, R., Eleftheriou, A., Traeger-Synodinos, J., Old, J., Petrou, M., and Angastiniotis, M. (2003). Prevention of thalassaemias and other haemoglobin disorders. Nicosia: Thalassaemia International Federation Publication.
Ghasemi, A., Falak, R., Mohammadi, M., Maleki, S. J., Assarezadegan, M-A., and Jafary, M. (2020). Incorporation of T-cell epitopes from tetanus and diphtheria toxoids into in-silico-designed hypoallergenic vaccine may enhance the protective immune response against allergens. Iran. J. Basic Med. Sci. 23 (5), 636–644. doi:10.22038/ijbms.2020.39749.9422
Ghasemi, A., Ghavimi, R., Momenzadeh, N., Hajian, S., and Mohammadi, M. (2021). Characterization of antitumor activity of a synthetic moronecidin-like peptide computationally predicted from the tiger tail seahorse hippocampus comes in tumor-bearing mice. Int. J. Peptide Res. Ther. 27 (4), 2391–2401. doi:10.1007/s10989-021-10260-6
Gong, J., Luk, F., Jaiswal, R., George, A. M., Grau, G. E. R., and Bebawy, M. (2013). Microparticle drug sequestration provides a parallel pathway in the acquisition of cancer drug resistance. Eur. J. Pharmacol. 721 (1-3), 116–125. doi:10.1016/j.ejphar.2013.09.044
Gross, J. C., Chaudhary, V., Bartscherer, K., and Boutros, M. (2012). Active Wnt proteins are secreted on exosomes. Nat. Cell Biol. 14 (10), 1036–1045. doi:10.1038/ncb2574
Gurung, S., Perocheau, D., Touramanidou, L., and Baruteau, J. (2021). The exosome journey: from biogenesis to uptake and intracellular signalling. Cell Commun. Signal. 19 (1), 47–19. doi:10.1186/s12964-021-00730-1
Haderk, F., Schulz, R., Iskar, M., Cid, L. L., Worst, T., Willmund, K. V., et al. (2017). Tumor-derived exosomes modulate PD-L1 expression in monocytes. Sci. Immunol. 2 (13), eaah5509. doi:10.1126/sciimmunol.aah5509
Haque, S., and Vaiselbuh, S. R. (2021). Cd19 chimeric antigen receptor-exosome targets cd19 positive b-lineage acute lymphocytic leukemia and induces cytotoxicity. Cancers 13 (6), 1401. doi:10.3390/cancers13061401
Haque, S., and Vaiselbuh, S. R. (2020). Silencing of exosomal miR-181a reverses pediatric acute lymphocytic leukemia cell proliferation. Pharmaceuticals 13 (9), 241. doi:10.3390/ph13090241
Hasan-Abad, A. M., Adabi, E., Sadroddiny, E., Khorramizadeh, M. R., Mazlomi, M. A., Mehravar, S., et al. (2019). Functional deimmunization of interferon beta-1b by identifying and silencing human T cells epitopes. Iran. J. Allergy, Asthma Immunol. 18, 427–440. doi:10.18502/ijaai.v18i4.1421
Hasan-Abad, A. M., Mohammadi, M., Mirzaei, H., Mehrabi, M., Motedayyen, H., and Arefnezhad, R. (2022). Impact of oligomerization on the allergenicity of allergens. Clin. Mol. Allergy 20 (1), 5–10. doi:10.1186/s12948-022-00172-1
Hassanpour, M., Rezabakhsh, A., Rezaie, J., Nouri, M., and Rahbarghazi, R. (2020). Exosomal cargos modulate autophagy in recipient cells via different signaling pathways. Cell and Biosci. 10 (1), 92–16. doi:10.1186/s13578-020-00455-7
He, J., Ren, W., Wang, W., Han, W., Jiang, L., Zhang, D., et al. (2022). Exosomal targeting and its potential clinical application. Drug Deliv. Transl. Res. 12 (10), 2385–2402. doi:10.1007/s13346-021-01087-1
Hekmatirad, S., Moloudizargari, M., Moghadamnia, A. A., Kazemi, S., Mohammadnia-Afrouzi, M., Baeeri, M., et al. (2021). Inhibition of exosome release sensitizes U937 cells to PEGylated liposomal doxorubicin. Front. Immunol. 12, 692654. doi:10.3389/fimmu.2021.692654
Hong, C-S., Muller, L., Whiteside, T. L., and Boyiadzis, M. (2014). Plasma exosomes as markers of therapeutic response in patients with acute myeloid leukemia. Front. Immunol. 5, 160. doi:10.3389/fimmu.2014.00160
Hong, C-S., Sharma, P., Yerneni, S. S., Simms, P., Jackson, E. K., Whiteside, T. L., et al. (2017). Circulating exosomes carrying an immunosuppressive cargo interfere with cellular immunotherapy in acute myeloid leukemia. Sci. Rep. 7 (1), 14684. doi:10.1038/s41598-017-14661-w
Hornick, N. I., Huan, J., Doron, B., Goloviznina, N. A., Lapidus, J., Chang, B. H., et al. (2015). Serum exosome microRNA as a minimally-invasive early biomarker of AML. Sci. Rep. 5 (1), 11295–11312. doi:10.1038/srep11295
Houshmand, M., Garello, F., Stefania, R., Gaidano, V., Cignetti, A., Spinelli, M., et al. (2021). Targeting chronic myeloid leukemia stem/progenitor cells using venetoclax-loaded immunoliposome. Cancers 13 (6), 1311. doi:10.3390/cancers13061311
Huang, F., Wan, J., Hu, W., and Hao, S. (2018). Enhancement of anti-leukemia immunity by leukemia–derived exosomes via downregulation of TGF-β1 expression. Cell. Physiology Biochem. 44 (1), 240–254. doi:10.1159/000484677
Huang, T., and Deng, C-X. (2019). Current progresses of exosomes as cancer diagnostic and prognostic biomarkers. Int. J. Biol. Sci. 15 (1), 1–11. doi:10.7150/ijbs.27796
Huo, L., Du, X., Li, X., Liu, S., and Xu, Y. (2021). The emerging role of neural cell-derived exosomes in intercellular communication in health and neurodegenerative diseases. Front. Neurosci. 15, 738442. doi:10.3389/fnins.2021.738442
Huotari, J., and Helenius, A. (2011). Endosome maturation. EMBO J. 30 (17), 3481–3500. doi:10.1038/emboj.2011.286
Janas, T., Janas, M. M., Sapoń, K., and Janas, T. (2015). Mechanisms of RNA loading into exosomes. FEBS Lett. 589 (13), 1391–1398. doi:10.1016/j.febslet.2015.04.036
Jaworski, M., Marsland, B. J., Gehrig, J., Held, W., Favre, S., Luther, S. A., et al. (2014). Malt1 protease inactivation efficiently dampens immune responses but causes spontaneous autoimmunity. EMBO J. 33 (23), 2765–2781. doi:10.15252/embj.201488987
Jena, B. C., and Mandal, M. (2021). The emerging roles of exosomes in anti-cancer drug resistance and tumor progression: an insight towards tumor-microenvironment interaction. Biochimica Biophysica Acta (BBA)-Reviews Cancer 1875 (1), 188488. doi:10.1016/j.bbcan.2020.188488
Jia, Y., Chen, Y., Wang, Q., Jayasinghe, U., Luo, X., Wei, Q., et al. (2017). Exosome: emerging biomarker in breast cancer. Oncotarget 8 (25), 41717–41733. doi:10.18632/oncotarget.16684
Ke, W., and Afonin, K. A. (2021). Exosomes as natural delivery carriers for programmable therapeutic nucleic acid nanoparticles (NANPs). Adv. Drug Deliv. Rev. 176, 113835. doi:10.1016/j.addr.2021.113835
Keller, S., Ridinger, J., Rupp, A-K., Janssen, J. W., and Altevogt, P. (2011). Body fluid derived exosomes as a novel template for clinical diagnostics. J. Transl. Med. 9 (1), 86–89. doi:10.1186/1479-5876-9-86
Keyvani, V., Riahi, E., Yousefi, M., Esmaeili, S-A., Shafabakhsh, R., Moradi Hasan-Abad, A., et al. (2022). Gynecologic cancer, cancer stem cells, and possible targeted therapies. Front. Pharmacol. 13, 823572. doi:10.3389/fphar.2022.823572
Kibria, G., Ramos, E. K., Wan, Y., Gius, D. R., and Liu, H. (2018). Exosomes as a drug delivery system in cancer therapy: potential and challenges. Mol. Pharm. 15 (9), 3625–3633. doi:10.1021/acs.molpharmaceut.8b00277
Kim, Y-S., Ahn, J-S., Kim, S., Kim, H-J., Kim, S-H., and Kang, J-S. (2018). The potential theragnostic (diagnostic+ therapeutic) application of exosomes in diverse biomedical fields. Korean J. Physiology Pharmacol. 22 (2), 113–125. doi:10.4196/kjpp.2018.22.2.113
Koch, R., Aung, T., Vogel, D., Chapuy, B., Wenzel, D., Becker, S., et al. (2016). Nuclear trapping through inhibition of exosomal export by indomethacin increases cytostatic efficacy of doxorubicin and pixantrone. Clin. cancer Res. 22 (2), 395–404. doi:10.1158/1078-0432.CCR-15-0577
Kumar, B., Garcia, M., Weng, L., Jung, X., Murakami, J., Hu, X., et al. (2018). Acute myeloid leukemia transforms the bone marrow niche into a leukemia-permissive microenvironment through exosome secretion. Leukemia 32 (3), 575–587. doi:10.1038/leu.2017.259
Labani-Motlagh, A., Israelsson, P., Ottander, U., Lundin, E., Nagaev, I., Nagaeva, O., et al. (2016). Differential expression of ligands for NKG2D and DNAM-1 receptors by epithelial ovarian cancer-derived exosomes and its influence on NK cell cytotoxicity. Tumor Biol. 37 (4), 5455–5466. doi:10.1007/s13277-015-4313-2
Layek, B., Sadhukha, T., Panyam, J., and Prabha, S. (2018). Nano-engineered mesenchymal stem cells increase therapeutic efficacy of anticancer drug through true active tumor targeting. Mol. cancer Ther. 17 (6), 1196–1206. doi:10.1158/1535-7163.MCT-17-0682
Lee, M. W., Ryu, S., Kim, D. S., Lee, J. W., Sung, K. W., Koo, H. H., et al. (2019). Mesenchymal stem cells in suppression or progression of hematologic malignancy: current status and challenges. Leukemia 33 (3), 597–611. doi:10.1038/s41375-018-0373-9
Li, H., Yang, C., Shi, Y., and Zhao, L. (2018a). Exosomes derived from siRNA against GRP78 modified bone-marrow-derived mesenchymal stem cells suppress Sorafenib resistance in hepatocellular carcinoma. J. nanobiotechnology 16 (1), 103–113. doi:10.1186/s12951-018-0429-z
Li, J., Zheng, M., Shimoni, O., Banks, W. A., Bush, A. I., Gamble, J. R., et al. (2021). Development of novel therapeutics targeting the blood–brain barrier: from barrier to carrier. Adv. Sci. 8 (16), 2101090. doi:10.1002/advs.202101090
Li, K., Chen, Y., Li, A., Tan, C., and Liu, X. (2019). Exosomes play roles in sequential processes of tumor metastasis. Int. J. cancer 144 (7), 1486–1495. doi:10.1002/ijc.31774
Li, M., Rai, A. J., DeCastro, G. J., Zeringer, E., Barta, T., Magdaleno, S., et al. (2015). An optimized procedure for exosome isolation and analysis using serum samples: application to cancer biomarker discovery. Methods 87, 26–30. doi:10.1016/j.ymeth.2015.03.009
Li, P., Kaslan, M., Lee, S. H., Yao, J., and Gao, Z. (2017). Progress in exosome isolation techniques. Theranostics 7 (3), 789–804. doi:10.7150/thno.18133
Li, Q., Huang, Q., Huyan, T., Wang, Y., Huang, Q., and Shi, J. (2018b). Bifacial effects of engineering tumour cell-derived exosomes on human natural killer cells. Exp. Cell Res. 363 (2), 141–150. doi:10.1016/j.yexcr.2017.12.005
Li, S., Yi, M., Dong, B., Jiao, Y., Luo, S., and Wu, K. (2020). The roles of exosomes in cancer drug resistance and its therapeutic application. Clin. Transl. Med. 10 (8), e257. doi:10.1002/ctm2.257
Li, W., Liu, J-B., Hou, L-K., Yu, F., Zhang, J., Wu, W., et al. (2022). Liquid biopsy in lung cancer: significance in diagnostics, prediction, and treatment monitoring. Mol. cancer 21 (1), 25. doi:10.1186/s12943-022-01505-z
Liang, Y., Duan, L., Lu, J., and Xia, J. (2021). Engineering exosomes for targeted drug delivery. Theranostics 11 (7), 3183–3195. doi:10.7150/thno.52570
Liangsupree, T., Multia, E., and Riekkola, M-L. (2021). Modern isolation and separation techniques for extracellular vesicles. J. Chromatogr. A 1636, 461773. doi:10.1016/j.chroma.2020.461773
Lin, B., Lei, Y., Wang, J., Zhu, L., Wu, Y., Zhang, H., et al. (2021). Microfluidic-based exosome analysis for liquid biopsy. Small Methods 5 (3), 2001131. doi:10.1002/smtd.202001131
Liu, Y., Song, B., Wei, Y., Chen, F., Chi, Y., Fan, H., et al. (2018). Exosomes from mesenchymal stromal cells enhance imatinib-induced apoptosis in human leukemia cells via activation of caspase signaling pathway. Cytotherapy 20 (2), 181–188. doi:10.1016/j.jcyt.2017.11.006
Lou, G., Chen, Z., Zheng, M., and Liu, Y. (2017). Mesenchymal stem cell-derived exosomes as a new therapeutic strategy for liver diseases. Exp. Mol. Med. 49 (6), e346–e. doi:10.1038/emm.2017.63
Ludwig, A-K., and Giebel, B. (2012). Exosomes: small vesicles participating in intercellular communication. Int. J. Biochem. Cell Biol. 44 (1), 11–15. doi:10.1016/j.biocel.2011.10.005
Lunavat, T. R., Jang, S. C., Nilsson, L., Park, H. T., Repiska, G., Lässer, C., et al. (2016). RNAi delivery by exosome-mimetic nanovesicles–Implications for targeting c-Myc in cancer. Biomaterials 102, 231–238. doi:10.1016/j.biomaterials.2016.06.024
Lyu, T., Wang, Y., Li, D., Yang, H., Qin, B., Zhang, W., et al. (2021). Exosomes from BM-MSCs promote acute myeloid leukemia cell proliferation, invasion and chemoresistance via upregulation of S100A4. Exp. Hematol. Oncol. 10 (1), 24–13. doi:10.1186/s40164-021-00220-7
Maia, J., Caja, S., Strano Moraes, M. C., Couto, N., and Costa-Silva, B. (2018). Exosome-based cell-cell communication in the tumor microenvironment. Front. Cell Dev. Biol. 6, 18. doi:10.3389/fcell.2018.00018
Malavasi, F., Faini, A. C., Morandi, F., Castella, B., Incarnato, D., Oliviero, S., et al. (2021). Molecular dynamics of targeting CD38 in multiple myeloma. Br. J. Haematol. 193 (3), 581–591. doi:10.1111/bjh.17329
Marusyk, A., Almendro, V., and Polyak, K. (2012). Intra-tumour heterogeneity: a looking glass for cancer? Nat. Rev. cancer 12 (5), 323–334. doi:10.1038/nrc3261
Mashouri, L., Yousefi, H., Aref, A. R., Ahadi, A. M., Molaei, F., and Alahari, S. K. (2019). Exosomes: composition, biogenesis, and mechanisms in cancer metastasis and drug resistance. Mol. cancer 18, 75–14. doi:10.1186/s12943-019-0991-5
Mimeault, M., and Batra, S. K. (2014). Molecular biomarkers of cancer stem/progenitor cells associated with progression, metastases, and treatment resistance of aggressive cancers. Cancer Epidemiol. Biomarkers Prev. 23 (2), 234–254. doi:10.1158/1055-9965.EPI-13-0785
Mincheva-Nilsson, L., and Baranov, V. (2014). Placenta-derived exosomes and syncytiotrophoblast microparticles and their role in human reproduction: immune modulation for pregnancy success. Am. J. reproductive Immunol. 72 (5), 440–457. doi:10.1111/aji.12311
Minciacchi, V. R., Freeman, M. R., and Di Vizio, D. (2015). Extracellular vesicles in cancer: exosomes, microvesicles and the emerging role of large oncosomes. Seminars Cell and Dev. Biol. 40, 41–51. Elsevier. doi:10.1016/j.semcdb.2015.02.010
Mitchell, M. J., Billingsley, M. M., Haley, R. M., Wechsler, M. E., Peppas, N. A., and Langer, R. (2021). Engineering precision nanoparticles for drug delivery. Nat. Rev. drug Discov. 20 (2), 101–124. doi:10.1038/s41573-020-0090-8
Mohammadi, M., Moradi Hasan-Abad, A., Dehghani, P., Nabipour, I., Roozbehani, M., Hemphill, A., et al. (2021). Dicentracin-Like from Asian sea bass fish and Moronecidine-Like from Hippocampus comes: two candidate antimicrobial peptides against Leishmanina major infection. Int. J. Peptide Res. Ther. 27, 769–778. doi:10.1007/s10989-020-10125-4
Mohseni Afshar, Z., Hosseinzadeh, R., Barary, M., Ebrahimpour, S., Alijanpour, A., Sayad, B., et al. (2022). Challenges posed by COVID-19 in cancer patients: a narrative review. Cancer Med. 11 (4), 1119–1135. doi:10.1002/cam4.4519
Moradi Hasan-Abad, A., Esmaili, M. A., Ghotaslou, A., Atapour, A., Khoshroo, A., and Naghian, E. (2022). A review: electrochemical biosensors for testosterone detection. Anal. Bioanal. Electrochem. 14 (11), 1060–1077.
Naoyo, N., Hirohisa, Y., Takashi, N., Toshiharu, K., and Masamichi, K. (2006). Angiogenesis in cancer. Vasc. Health Risk Manag. 2 (3), 213–219. doi:10.2147/vhrm.2006.2.3.213
Naseri, Z., Oskuee, R. K., Jaafari, M. R., and Moghadam, M. F. (2018). Exosome-mediated delivery of functionally active miRNA-142-3p inhibitor reduces tumorigenicity of breast cancer in vitro and in vivo. Int. J. nanomedicine 13, 7727–7747. doi:10.2147/IJN.S182384
Neef, D. W., Jaeger, A. M., and Thiele, D. J. (2011). Heat shock transcription factor 1 as a therapeutic target in neurodegenerative diseases. Nat. Rev. Drug Discov. 10 (12), 930–944. doi:10.1038/nrd3453
Nour, A. M., and Modis, Y. (2014). Endosomal vesicles as vehicles for viral genomes. Trends Cell Biol. 24 (8), 449–454. doi:10.1016/j.tcb.2014.03.006
O'Loughlin, A. J., Woffindale, C. A., and Wood, M. A. J. (2012). Exosomes and the emerging field of exosome-based gene therapy. Curr. gene Ther. 12 (4), 262–274. doi:10.2174/156652312802083594
O’Brien, K., Breyne, K., Ughetto, S., Laurent, L. C., and Breakefield, X. O. (2020). RNA delivery by extracellular vesicles in mammalian cells and its applications. Nat. Rev. Mol. Cell Biol. 21 (10), 585–606. doi:10.1038/s41580-020-0251-y
Ohyashiki, J. H., Umezu, T., and Ohyashiki, K. (2016). Exosomes promote bone marrow angiogenesis in hematologic neoplasia: the role of hypoxia. Curr. Opin. Hematol. 23 (3), 268–273. doi:10.1097/MOH.0000000000000235
Paggetti, J., Haderk, F., Seiffert, M., Janji, B., Distler, U., Ammerlaan, W., et al. (2015). Exosomes released by chronic lymphocytic leukemia cells induce the transition of stromal cells into cancer-associated fibroblasts. J. Am. Soc. Hematol. 126 (9), 1106–1117. doi:10.1182/blood-2014-12-618025
Palucka, K., and Banchereau, J. (2012). Cancer immunotherapy via dendritic cells. Nat. Rev. Cancer 12 (4), 265–277. doi:10.1038/nrc3258
Pan, W., Chen, H., Wang, A., Wang, F., and Zhang, X. (2023). Challenges and strategies: scalable and efficient production of mesenchymal stem cells-derived exosomes for cell-free therapy. Life Sci. 319, 121524. doi:10.1016/j.lfs.2023.121524
Pilzer, D., and Fishelson, Z. (2005). Mortalin/GRP75 promotes release of membrane vesicles from immune attacked cells and protection from complement-mediated lysis. Int. Immunol. 17 (9), 1239–1248. doi:10.1093/intimm/dxh300
Pitt, J. M., André, F., Amigorena, S., Soria, J-C., Eggermont, A., Kroemer, G., et al. (2016). Dendritic cell–derived exosomes for cancer therapy. J. Clin. investigation 126 (4), 1224–1232. doi:10.1172/JCI81137
Prieto, D., Sotelo, N., Seija, N., Sernbo, S., Abreu, C., Durán, R., et al. (2017). S100-A9 protein in exosomes from chronic lymphocytic leukemia cells promotes NF-κB activity during disease progression. Blood, J. Am. Soc. Hematol. 130 (6), 777–788. doi:10.1182/blood-2017-02-769851
Pyzer, A. R., Avigan, D. E., and Rosenblatt, J. (2014). Clinical trials of dendritic cell-based cancer vaccines in hematologic malignancies. Hum. vaccines Immunother. 10 (11), 3125–3131. doi:10.4161/21645515.2014.982993
Raimondo, S., Saieva, L., Corrado, C., Fontana, S., Flugy, A., Rizzo, A., et al. (2015). Chronic myeloid leukemia-derived exosomes promote tumor growth through an autocrine mechanism. Cell Commun. Signal. 13 (1), 8–12. doi:10.1186/s12964-015-0086-x
Rainero, E., and Norman, J. C. (2013). Late endosomal and lysosomal trafficking during integrin-mediated cell migration and invasion: cell matrix receptors are trafficked through the late endosomal pathway in a way that dictates how cells migrate. Bioessays 35 (6), 523–532. doi:10.1002/bies.201200160
Rappa, G., Mercapide, J., and Lorico, A. (2012). Spontaneous formation of tumorigenic hybrids between breast cancer and multipotent stromal cells is a source of tumor heterogeneity. Am. J. pathology 180 (6), 2504–2515. doi:10.1016/j.ajpath.2012.02.020
Raudenska, M., Balvan, J., and Masarik, M. (2021). Crosstalk between autophagy inhibitors and endosome-related secretory pathways: a challenge for autophagy-based treatment of solid cancers. Mol. Cancer 20 (1), 140–227. doi:10.1186/s12943-021-01423-6
Rehman, F. U., Liu, Y., Zheng, M., and Shi, B. (2022). Exosomes based strategies for brain drug delivery. Biomaterials 239, 121949. doi:10.1016/j.biomaterials.2022.121949
Reiners, K. S., Topolar, D., Henke, A., Simhadri, V. R., Kessler, J., Sauer, M., et al. (2013). Soluble ligands for NK cell receptors promote evasion of chronic lymphocytic leukemia cells from NK cell anti-tumor activity. Blood, J. Am. Soc. Hematol. 121 (18), 3658–3665. doi:10.1182/blood-2013-01-476606
Rezaie, J., Ahmadi, M., Ravanbakhsh, R., Mojarad, B., Mahbubfam, S., Shaban, S. A., et al. (2022). Tumor-derived extracellular vesicles: the metastatic organotropism drivers. Life Sci. 289, 120216. doi:10.1016/j.lfs.2021.120216
Rezaie, J., Aslan, C., Ahmadi, M., Zolbanin, N. M., Kashanchi, F., and Jafari, R. (2021). The versatile role of exosomes in human retroviral infections: from immunopathogenesis to clinical application. Cell and Biosci. 11, 19–15. doi:10.1186/s13578-021-00537-0
Rivoltini, L., Chiodoni, C., Squarcina, P., Tortoreto, M., Villa, A., Vergani, B., et al. (2016a). TNF-related apoptosis-inducing ligand (TRAIL)-Armed exosomes deliver proapoptotic signals to tumor site. Clin. cancer Res. 22 (14), 3499–3512. doi:10.1158/1078-0432.CCR-15-2170
Rivoltini, L., Chiodoni, C., Squarcina, P., Tortoreto, M., Villa, A., Vergani, B., et al. (2016b). TNF-related apoptosis-inducing ligand (TRAIL)–armed exosomes deliver proapoptotic signals to tumor site. Clin. Cancer Res. 22 (14), 3499–3512. doi:10.1158/1078-0432.CCR-15-2170
Roccaro, A. M., Sacco, A., Maiso, P., Azab, A. K., Tai, Y-T., Reagan, M., et al. (2013). BM mesenchymal stromal cell–derived exosomes facilitate multiple myeloma progression. J. Clin. investigation 123 (4), 1542–1555. doi:10.1172/JCI66517
Ruiss, R., Jochum, S., Mocikat, R., Hammerschmidt, W., and Zeidler, R. (2011). EBV-gp350 confers B-cell tropism to tailored exosomes and is a neo-antigen in normal and malignant B cells—A new option for the treatment of B-CLL. PloS one 6 (10), e25294. doi:10.1371/journal.pone.0025294
Salunkhe, S., Basak, M., Chitkara, D., and Mittal, A. (2020). Surface functionalization of exosomes for target-specific delivery and in vivo imaging and tracking: strategies and significance. J. Control. Release 326, 599–614. doi:10.1016/j.jconrel.2020.07.042
Savina, A., Furlán, M., Vidal, M., and Colombo, M. I. (2003). Exosome release is regulated by a calcium-dependent mechanism in K562 cells. J. Biol. Chem. 278 (22), 20083–20090. doi:10.1074/jbc.M301642200
Sezer, O., Jakob, C., Eucker, J., Niemöller, K., Gatz, F., Wernecke, K. D., et al. (2001). Serum levels of the angiogenic cytokines basic fibroblast growth factor (bFGF), vascular endothelial growth factor (VEGF) and hepatocyte growth factor (HGF) in multiple myeloma. Eur. J. Haematol. 66 (2), 83–88. doi:10.1034/j.1600-0609.2001.00348.x
Shen, M., and Chen, T. (2021). Mesenchymal stem cell-derived exosomes and their potential agents in hematological diseases. Oxidative Med. Cell. Longev. 2021, 4539453. doi:10.1155/2021/4539453
Shimasaki, N., Jain, A., and Campana, D. (2020). NK cells for cancer immunotherapy. Nat. Rev. Drug Discov. 19 (3), 200–218. doi:10.1038/s41573-019-0052-1
Shoae-Hassani, A., Hamidieh, A. A., Behfar, M., Mohseni, R., Mortazavi-Tabatabaei, S. A., and Asgharzadeh, S. (2017). NK cell–derived exosomes from NK cells previously exposed to neuroblastoma cells augment the antitumor activity of cytokine-activated NK cells. J. Immunother. Hagerst. Md 1997) 40 (7), 265–276. doi:10.1097/CJI.0000000000000179
Song, Y., Kim, Y., Ha, S., Sheller-Miller, S., Yoo, J., Choi, C., et al. (2021). The emerging role of exosomes as novel therapeutics: biology, technologies, clinical applications, and the next. Am. J. Reproductive Immunol. 85 (2), e13329. doi:10.1111/aji.13329
Subhan, M. A., and Torchilin, V. (2019). Efficient nanocarriers of siRNA therapeutics for cancer treatment. Transl. Res. 214, 62–91. doi:10.1016/j.trsl.2019.07.006
Subhan, M. A., and Torchilin, V. (2020). siRNA based drug design, quality, delivery and clinical translation. Nanomedicine Nanotechnol. Biol. Med. 29, 102239. doi:10.1016/j.nano.2020.102239
Sun, D., Zhuang, X., Xiang, X., Liu, Y., Zhang, S., Liu, C., et al. (2010). A novel nanoparticle drug delivery system: the anti-inflammatory activity of curcumin is enhanced when encapsulated in exosomes. Mol. Ther. 18 (9), 1606–1614. doi:10.1038/mt.2010.105
Sun, R., Liu, W., Zhao, Y., Chen, H., Wang, Z., Zhang, Y., et al. (2021). Exosomal circRNA as a novel potential therapeutic target for multiple myeloma-related myocardial damage. Cancer Cell Int. 21 (1), 311–316. doi:10.1186/s12935-021-02011-w
Sun, W., Xing, C., Zhao, L., Zhao, P., Yang, G., and Yuan, L. (2020). Ultrasound assisted exosomal delivery of tissue responsive mRNA for enhanced efficacy and minimized off-target effects. Mol. Therapy-Nucleic Acids. 20, 558–567. doi:10.1016/j.omtn.2020.03.016
Szczepanski, M. J., Szajnik, M., Welsh, A., Whiteside, T. L., and Boyiadzis, M. (2011). Blast-derived microvesicles in sera from patients with acute myeloid leukemia suppress natural killer cell function via membrane-associated transforming growth factor-beta1. Haematologica 96 (9), 1302–1309. doi:10.3324/haematol.2010.039743
Tai, Y. L., Chen, K. C., Hsieh, J. T., and Shen, T. L. (2018). Exosomes in cancer development and clinical applications. Cancer Sci. 109 (8), 2364–2374. doi:10.1111/cas.13697
Taverna, S., Giallombardo, M., Pucci, M., Flugy, A., Manno, M., Raccosta, S., et al. (2015). Curcumin inhibits in vitro and in vivo chronic myelogenous leukemia cells growth: a possible role for exosomal disposal of miR-21. Oncotarget 6 (26), 21918–21933. doi:10.18632/oncotarget.4204
Taylor, D. D., and Shah, S. (2015). Methods of isolating extracellular vesicles impact down-stream analyses of their cargoes. Methods 87, 3–10. doi:10.1016/j.ymeth.2015.02.019
Tenchov, R., Sasso, J. M., Wang, X., Liaw, W-S., Chen, C-A., and Zhou, Q. A. (2022). Exosomes─ nature’s lipid nanoparticles, a rising star in drug delivery and diagnostics. ACS Nano 16 (11), 17802–17846. doi:10.1021/acsnano.2c08774
Testa, A., Venturelli, E., and Brizzi, M. F. (2021). Extracellular vesicles: new tools for early diagnosis of breast and genitourinary cancers. Int. J. Mol. Sci. 22 (16), 8430. doi:10.3390/ijms22168430
Tian, Y., Li, S., Song, J., Ji, T., Zhu, M., Anderson, G. J., et al. (2014). A doxorubicin delivery platform using engineered natural membrane vesicle exosomes for targeted tumor therapy. Biomaterials 35 (7), 2383–2390. doi:10.1016/j.biomaterials.2013.11.083
Torreggiani, E., Roncuzzi, L., Perut, F., Zini, N., and Baldini, N. (2016). Multimodal transfer of MDR by exosomes in human osteosarcoma. Int. J. Oncol. 49 (1), 189–196. doi:10.3892/ijo.2016.3509
Umezu, T., Tadokoro, H., Azuma, K., Yoshizawa, S., Ohyashiki, K., and Ohyashiki, J. H. (2014). Exosomal miR-135b shed from hypoxic multiple myeloma cells enhances angiogenesis by targeting factor-inhibiting HIF-1. J. Am. Soc. Hematol. 124 (25), 3748–3757. doi:10.1182/blood-2014-05-576116
Vader, P., Breakefield, X. O., and Wood, M. J. (2014). Extracellular vesicles: emerging targets for cancer therapy. Trends Mol. Med. 20 (7), 385–393. doi:10.1016/j.molmed.2014.03.002
Valenti, R., Huber, V., Filipazzi, P., Pilla, L., Sovena, G., Villa, A., et al. (2006). Human tumor-released microvesicles promote the differentiation of myeloid cells with transforming growth factor-β–mediated suppressive activity on T lymphocytes. Cancer Res. 66 (18), 9290–9298. doi:10.1158/0008-5472.CAN-06-1819
Van Niel, G., d'Angelo, G., and Raposo, G. (2018). Shedding light on the cell biology of extracellular vesicles. Nat. Rev. Mol. Cell Biol. 19 (4), 213–228. doi:10.1038/nrm.2017.125
Van Niel, G., Porto-Carreiro, I., Simoes, S., and Raposo, G. (2006). Exosomes: a common pathway for a specialized function. J. Biochem. 140 (1), 13–21. doi:10.1093/jb/mvj128
Van Tendeloo, V. F., Van de Velde, A., Van Driessche, A., Cools, N., Anguille, S., Ladell, K., et al. (2010). Induction of complete and molecular remissions in acute myeloid leukemia by Wilms’ tumor 1 antigen-targeted dendritic cell vaccination. Proc. Natl. Acad. Sci. 107 (31), 13824–13829. doi:10.1073/pnas.1008051107
van Weering, J. R., Verkade, P., and Cullen, P. J. (2012). SNX–BAR-Mediated endosome tubulation is Co-ordinated with endosome maturation. Traffic 13 (1), 94–107. doi:10.1111/j.1600-0854.2011.01297.x
Veerman, R. E., Akpinar, G. G., Eldh, M., and Gabrielsson, S. (2019). Immune cell-derived extracellular vesicles–functions and therapeutic applications. Trends Mol. Med. 25 (5), 382–394. doi:10.1016/j.molmed.2019.02.003
Villarroya-Beltri, C., Baixauli, F., Mittelbrunn, M., Fernández-Delgado, I., Torralba, D., Moreno-Gonzalo, O., et al. (2016). ISGylation controls exosome secretion by promoting lysosomal degradation of MVB proteins. Nat. Commun. 7 (1), 13588. doi:10.1038/ncomms13588
Waheed, S., Li, Z., Zhang, F., Chiarini, A., Armato, U., and Wu, J. (2022). Engineering nano-drug biointerface to overcome biological barriers toward precision drug delivery. J. Nanobiotechnology 20 (1), 395. doi:10.1186/s12951-022-01605-4
Wang, G., Hu, W., Chen, H., Shou, X., Ye, T., and Xu, Y. (2019b). Cocktail strategy based on NK cell-derived exosomes and their biomimetic nanoparticles for dual tumor therapy. Cancers 11 (10), 1560. doi:10.3390/cancers11101560
Wang, J., De Veirman, K., Faict, S., Frassanito, M. A., Ribatti, D., Vacca, A., et al. (2016). Multiple myeloma exosomes establish a favourable bone marrow microenvironment with enhanced angiogenesis and immunosuppression. J. pathology 239 (2), 162–173. doi:10.1002/path.4712
Wang, J., Hendrix, A., Hernot, S., Lemaire, M., De Bruyne, E., Van Valckenborgh, E., et al. (2014). Bone marrow stromal cell-derived exosomes as communicators in drug resistance in multiple myeloma cells. J. Am. Soc. Hematol. 124 (4), 555–566. doi:10.1182/blood-2014-03-562439
Wang, J-H., Liu, X-L., Sun, J-M., Yang, J-H., Xu, D-H., and Yan, S-S. (2020b). Role of mesenchymal stem cell derived extracellular vesicles in autoimmunity: a systematic review. World J. Stem Cells 12 (8), 879–896. doi:10.4252/wjsc.v12.i8.879
Wang, L., Yang, G., Zhao, D., Wang, J., Bai, Y., Peng, Q., et al. (2019a). CD103-positive CSC exosome promotes EMT of clear cell renal cell carcinoma: role of remote MiR-19b-3p. Mol. cancer 18 (1), 86–15. doi:10.1186/s12943-019-0997-z
Wang, W., Zhu, N., Yan, T., Shi, Y-N., Chen, J., Zhang, C-J., et al. (2020a). The crosstalk: exosomes and lipid metabolism. Cell Commun. Signal. 18 (1), 119–212. doi:10.1186/s12964-020-00581-2
Webber, J., Steadman, R., Mason, M. D., Tabi, Z., and Clayton, A. (2010). Cancer exosomes trigger fibroblast to myofibroblast differentiation. Cancer Res. 70 (23), 9621–9630. doi:10.1158/0008-5472.CAN-10-1722
Whiteside, T. L. (2013). Immune modulation of T-cell and NK (natural killer) cell activities by TEXs (tumour-derived exosomes). Biochem. Soc. Trans. 41 (1), 245–251. doi:10.1042/BST20120265
Whiteside, T. L. (2016). Tumor-derived exosomes and their role in cancer progression. Adv. Clin. Chem. 74, 103–141. doi:10.1016/bs.acc.2015.12.005
Wieckowski, E. U., Visus, C., Szajnik, M., Szczepanski, M. J., Storkus, W. J., and Whiteside, T. L. (2009). Tumor-derived microvesicles promote regulatory T cell expansion and induce apoptosis in tumor-reactive activated CD8+ T lymphocytes. J. Immunol. 183 (6), 3720–3730. doi:10.4049/jimmunol.0900970
Willis, G. R., Kourembanas, S., and Mitsialis, S. A. (2017). Toward exosome-based therapeutics: isolation, heterogeneity, and fit-for-purpose potency. Front. Cardiovasc. Med. 4, 63. doi:10.3389/fcvm.2017.00063
Wu, F., Xie, M., Hun, M., She, Z., Li, C., Luo, S., et al. (2021). Natural killer cell-derived extracellular vesicles: novel players in cancer immunotherapy. Front. Immunol. 12, 658698. doi:10.3389/fimmu.2021.658698
Xia, Y., Rao, L., Yao, H., Wang, Z., Ning, P., and Chen, X. (2020). Engineering macrophages for cancer immunotherapy and drug delivery. Adv. Mater. 32 (40), 2002054. doi:10.1002/adma.202002054
Xie, Y., Bai, O., Zhang, H., Yuan, J., Zong, S., Chibbar, R., et al. (2010). Membrane-bound HSP70-engineered myeloma cell-derived exosomes stimulate more efficient CD8+ CTL-and NK-mediated antitumour immunity than exosomes released from heat-shocked tumour cells expressing cytoplasmic HSP70. J. Cell. Mol. Med. 14 (11), 2655–2666. doi:10.1111/j.1582-4934.2009.00851.x
Xie, Y., Chen, Y., Zhang, L., Ge, W., and Tang, P. (2017). The roles of bone-derived exosomes and exosomal micro RNA s in regulating bone remodelling. J. Cell. Mol. Med. 21 (5), 1033–1041. doi:10.1111/jcmm.13039
Xu, R., Rai, A., Chen, M., Suwakulsiri, W., Greening, D. W., and Simpson, R. J. (2018). Extracellular vesicles in cancer—Implications for future improvements in cancer care. Nat. Rev. Clin. Oncol. 15 (10), 617–638. doi:10.1038/s41571-018-0036-9
Xu, Z., Zeng, S., Gong, Z., and Yan, Y. (2020). Exosome-based immunotherapy: a promising approach for cancer treatment. Mol. cancer 19 (1), 160–216. doi:10.1186/s12943-020-01278-3
Yang, D., Zhang, W., Zhang, H., Zhang, F., Chen, L., Ma, L., et al. (2020). Progress, opportunity, and perspective on exosome isolation-efforts for efficient exosome-based theranostics. Theranostics 10 (8), 3684–3707. doi:10.7150/thno.41580
Yang, K., Jia, M., Cheddah, S., Zhang, Z., Wang, W., Li, X., et al. (2022). Peptide ligand-SiO2 microspheres with specific affinity for phosphatidylserine as a new strategy to isolate exosomes and application in proteomics to differentiate hepatic cancer. Bioact. Mater. 15, 343–354. doi:10.1016/j.bioactmat.2021.12.017
Yao, Y., Wang, C., Wei, W., Shen, C., Deng, X., Chen, L., et al. (2014). Dendritic cells pulsed with leukemia cell-derived exosomes more efficiently induce antileukemic immunities. PloS one 9 (3), e91463. doi:10.1371/journal.pone.0091463
Yeh, Y-Y., Ozer, H. G., Lehman, A. M., Maddocks, K., Yu, L., Johnson, A. J., et al. (2015). Characterization of CLL exosomes reveals a distinct microRNA signature and enhanced secretion by activation of BCR signaling. Blood, J. Am. Soc. Hematol. 125 (21), 3297–3305. doi:10.1182/blood-2014-12-618470
Yokoi, A., and Ochiya, T. (2021). Exosomes and extracellular vesicles: rethinking the essential values in cancer biology. Seminars Cancer Biol. 74, 79–91. Elsevier. doi:10.1016/j.semcancer.2021.03.032
Yu, D., Li, Y., Wang, M., Gu, J., Xu, W., Cai, H., et al. (2022). Exosomes as a new frontier of cancer liquid biopsy. Mol. cancer 21 (1), 56. doi:10.1186/s12943-022-01509-9
Zhang, H., and Lyden, D. (2019). Asymmetric-flow field-flow fractionation technology for exomere and small extracellular vesicle separation and characterization. Nat. Protoc. 14 (4), 1027–1053. doi:10.1038/s41596-019-0126-x
Zhang, H-G., and Grizzle, W. E. (2014). Exosomes: a novel pathway of local and distant intercellular communication that facilitates the growth and metastasis of neoplastic lesions. Am. J. pathology 184 (1), 28–41. doi:10.1016/j.ajpath.2013.09.027
Zhang, L., Pan, L., Xiang, B., Zhu, H., Wu, Y., Chen, M., et al. (2016). Potential role of exosome-associated microRNA panels and in vivo environment to predict drug resistance for patients with multiple myeloma. Oncotarget 7 (21), 30876–30891. doi:10.18632/oncotarget.9021
Zhang, X., Yang, Y., Yang, Y., Chen, H., Tu, H., and Li, J. (2020b). Exosomes from bone marrow microenvironment-derived mesenchymal stem cells affect CML cells growth and promote drug resistance to tyrosine kinase inhibitors. Stem Cells Int. 2020, 8890201. doi:10.1155/2020/8890201
Zhang, Y., Bi, J., Huang, J., Tang, Y., Du, S., and Li, P. (2020a). Exosome: a review of its classification, isolation techniques, storage, diagnostic and targeted therapy applications. Int. J. nanomedicine 15, 6917–6934. doi:10.2147/IJN.S264498
Zhang, Y., Liu, Y., Liu, H., and Tang, W. H. (2019). Exosomes: biogenesis, biologic function and clinical potential. Cell and Biosci. 9 (1), 19–18. doi:10.1186/s13578-019-0282-2
Zhou, H., He, X., He, Y., Ou, C., and Cao, P. (2021). Exosomal circRNAs: emerging players in tumor metastasis. Front. Cell Dev. Biol. 9, 786224. doi:10.3389/fcell.2021.786224
Zhou, R., Wang, L., Zhao, G., Chen, D., Song, X., Momtazi-Borojeni, A. A., et al. (2020). Circulating exosomal microRNAs as emerging non-invasive clinical biomarkers in heart failure: mega bio-roles of a nano bio-particle. Iubmb Life 72 (12), 2546–2562. doi:10.1002/iub.2396
Keywords: exosomes, hematological, therapeutic agents, cancer, biogenesis
Citation: Ghaffari K, Moradi-Hasanabad A, Sobhani-Nasab A, Javaheri J and Ghasemi A (2023) Application of cell-derived exosomes in the hematological malignancies therapy. Front. Pharmacol. 14:1263834. doi: 10.3389/fphar.2023.1263834
Received: 20 July 2023; Accepted: 31 August 2023;
Published: 08 September 2023.
Edited by:
Hui Liu, The University of Hong Kong, Hong Kong SAR, ChinaReviewed by:
Sankha Bhattacharya, SVKM’s Narsee Moonjee Institute of Management and Studies (NMIMS), IndiaJennifer Speth, University of Michigan, United States
Copyright © 2023 Ghaffari, Moradi-Hasanabad, Sobhani-Nasab, Javaheri and Ghasemi. This is an open-access article distributed under the terms of the Creative Commons Attribution License (CC BY). The use, distribution or reproduction in other forums is permitted, provided the original author(s) and the copyright owner(s) are credited and that the original publication in this journal is cited, in accordance with accepted academic practice. No use, distribution or reproduction is permitted which does not comply with these terms.
*Correspondence: Ali Ghasemi, YS5xYXNlbWkyMDEyQHlhaG9vLmNvbQ==, YS5naGFzZW1pQHNlbXVtcy5hYy5pcg==