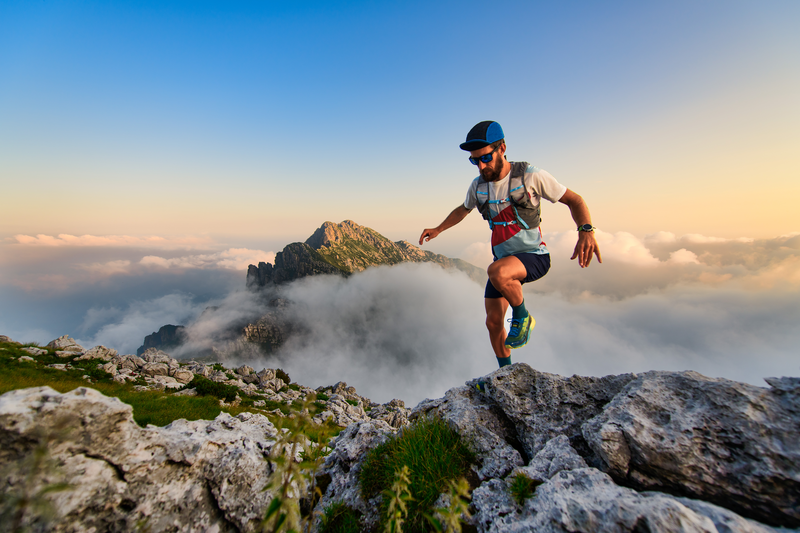
94% of researchers rate our articles as excellent or good
Learn more about the work of our research integrity team to safeguard the quality of each article we publish.
Find out more
REVIEW article
Front. Pharmacol. , 17 October 2023
Sec. Neuropharmacology
Volume 14 - 2023 | https://doi.org/10.3389/fphar.2023.1249644
Ischemic-induced neuronal injury arises due to low oxygen/nutrient levels and an inflammatory response that exacerbates neuronal loss. NOD-like receptor family pyrin domain-containing 3 (NLRP3) is an important regulator of inflammation after ischemic stroke, with its inhibition being involved in nerve regeneration. Curcumin, a main active ingredient in Chinese herbs, plays a positive role in neuronal repair and neuroprotection by regulating the NLRP3 signaling pathway. Nevertheless, the signaling mechanisms relating to how curcumin regulates NLRP3 inflammasome in inflammation and neural restoration following ischemic stroke are unknown. In this report, we summarize the main biological functions of the NLRP3 inflammasome along with the neuroprotective effects and underlying mechanisms of curcumin via impairment of the NLRP3 pathway in ischemic brain injury. We also discuss the role of medicinal interventions that target the NLRP3 and potential pathways, as well as possible directions for curcumin therapy to penetrate the blood–brain barrier (BBB) and hinder inflammation in ischemic stroke. This report conclusively demonstrates that curcumin has neuroprotective properties that inhibit inflammation and prevent nerve cell loss, thereby delaying the progression of ischemic brain damage.
Cerebral ischemia/stroke (CIS) causes severe tissue hypoxia and brain disorders due to endothelial cell dysfunction, over-activated inflammation, and neuronal loss instigated by insufficient or no oxygen and glucose supply to the brain. According to the World Stroke Organization, more than 12.2 million new stroke cases are reported annually, of which 62% are ischemic strokes. This can cost up to $860 billion annually. CIS is detrimental and may result in death and disability due to risk factors such as high systolic blood pressure, high fasting plasma glucose, and smoking; these induce hypertension, impaired glucose tolerance, and abnormal lipid metabolism (Feigin et al., 2022).
Currently, the main therapeutic strategy for CIS in clinical settings is thrombolytic surgeries such as intravenous thrombolysis and mechanical clot thrombectomy to restore blood flow as quickly as possible, while anti-coagulant and vasoprotective drugs are also employed. However, less than 50% of thrombolytic surgeries globally are statistically effective, and affected individuals lose nearly 72% of their healthy lives to stroke-related disability and death (Saini et al., 2021). The main reason for these could be inflammatory storm and neuronal injury in CIS and reperfusion after surgery. Once cerebral infarcts occur, the loss of neurons aggravates neuronal injuries by downregulating processes related to neuroprotective and regenerative biomarkers and pathways (Datta et al., 2011). In ischemic-induced vascular obstruction, oxidative stress causes the excessive production of reactive oxygen species (ROS), exacerbating neuronal injury and resulting in severe deficits (Zhao et al., 2022). Furthermore, the breakdown of the blood–brain barrier (BBB) leads to calcium overload and mitochondrial dysfunction due to ionic imbalance and glutamate excitotoxicity. This augments the risk of oxidative stress, neuronal apoptosis, and inflammation (Minutoli et al., 2016; Subedi and Gaire, 2021). It is therefore not surprising that pathways relating to oxidative stress have been extensively investigated for mitigating neuronal injuries (Chen et al., 2020). Effective modulation of the immune responses may also minimize neuronal injuries.
The NLRP inflammasome is a key tripartite multi-protein composite that contains the nucleotide-binding oligomerization domain (NOD), C-terminal caspase-recruitment domain (CARD), and activated caspase-1 that regulates inflammatory response and neuronal pyroptosis in ischemic stroke (Sarmah et al., 2020). When the inflammatory cascade begins, the NOD-like receptor (NLR) receives the inflammatory and DAMPs danger signals to trigger the mutation and release of IL-18 and IL-1β, thus accelerating neuronal dysfunction and causing BBB breakdown, cerebral edema, and eventual neuronal death (Kim et al., 2016). There are more than 20 members of the NLRP inflammasome family, including NLRP1, NLRP3, NLRP4, and NLRP6. NLRP3, one of the most investigated, is found in microglia and neurons in the brain and has been linked to neurogenesis, angiogenesis, neuroinflammation, and neuronal recovery in Alzheimer’s disease (AD), Parkinson’s disease (PD), and ischemic stroke (Shen et al., 2022). NLRP3 dysfunction leads to the activation of pro-inflammatory cytokines and ROS overproduction, as well as autophagic and mitochondrial impairments (Cicolari et al., 2021; Holbrook et al., 2021; Anderson et al., 2023). In contrast, inhibited NLRP3 showed neuroprotective properties in cognitive functional recovery and vasoneuronal remodeling after ischemia (Ward et al., 2019). Therefore, the NLRP3 inflammasome may be a promising therapeutic approach for ischemic stroke.
In recent years, significant investigation of curcumin has demonstrated its anti-tumorigenic, anti-inflammatory, anti-neuronal apoptosis, and blood vessel protective features (Jiang et al., 2007; Menon and Sudheer, 2007). Curcumin is the primary component of turmeric rhizome and a well-known polyphenolic agent. Its poor bioavailability and solubility have resulted in its limited clinical employment. However, studies on the nanoparticles and liposomes of curcumin in drug delivery systems have attempted to significantly improve its solubility, stability, and safety (Mahmood et al., 2015). Based on its pathomechanism, curcumin has been demonstrated to alleviate various acute and chronic neuronal disorders including depression, ischemic stroke, AD, brain injury, and spinal cord injury by regulating neuroprotective pathways and downregulating the inflammatory storm, thereby enhancing neurological functions (Bhat et al., 2019; Ramaholimihaso et al., 2020; Saleh et al., 2022; Gu et al., 2023). In ischemic stroke, curcumin has upregulated the expression of synaptic remodeling proteins, decreased brain infarct size, and enhanced BBB permeability (Wu et al., 2021), as well as the release of neurotransmitters and neurotrophic substances by curcumin-upregulated signal pathways for neuronal regeneration (Lan et al., 2018). Improving ischemic brain curcumin-inhibited cell apoptosis, ROS-induced inflammation, and modulated mitochondrial functions are key factors in triggering the NLRP3 inflammasome (Subedi and Gaire, 2021). When NLRP3 inflammasome activation was impaired by curcumin, mitigated inflammation and improved neuronal reparative effects were observed (Patel et al., 2020; Jin et al., 2022). Such evidence demonstrates curcumin's potential to inhibit the NLRP3 inflammasome. It remains to be determined how curcumin modulates the NLRP3 pathway to safeguard neurons and minimize inflammatory response. Furthermore, the anti-oxidative, anti-apoptotic, and neuroprotective regulation of curcumin in NLRP3 inflammasome, especially in ischemic stroke, is yet to be explored. In this review, the PubMed, China National Knowledge Infrastructure (CNKI), and Web of Science databases were searched until to August 2023 using the keywords “curcumin,” “ischemic stroke” OR “stroke,” and “NLRP3” OR “NLRP3 inflammasome” to demonstrate the pathways network between NLRP3 and curcumin therapy in ischemic stroke.
NLRP3 is a 115 kDa cytosolic protein and combines with caspase-1 and apoptotic-associated speck-like protein (ASC) to form the NLRP3 inflammatory complex. This complex can be activated by the innate immune system, including damage-associated molecular patterns (DAMPs), to initiate caspase-1 and IL-1β/IL-18 mutation and release and aggravate inflammatory reaction (Wang et al., 2022c). In the wake of ischemic stroke, increased DAMPs from injury cells and stimulated NLRP3 protein bind to the adapter protein ASC and pro-caspase-1, subsequently triggering the maturation of precursors IL-1β and IL-18 to induce neuroinflammation. Proinflammatory GSDMD works with the NLRP3 inflammasome to activate caspase-1 and cause pyroptotic cell death. This is exacerbated by intracellular Ca2+, high mitochondrial ROS production, and leukocyte recruitment that aggravate neuronal death (Yang et al., 2019). The NLRP3 inflammasome is expressed in immune cells such as neutrophils, dendritic cells, lymphocytes, epithelial cells, microglia, and neurons (Zahid et al., 2019). Both NLRP3 and NLRP3-dependent genes of the inflammasome are significantly elevated during the first phase of cerebral ischemia, intensifying cerebral ischemic injuries (Wang et al., 2022b). Interestingly, the suppression of NLRP3 has improved ischemic insult and neurovascular complications (Dodd et al., 2021).
The TLR4 is a pathogen recognition receptor. It is expressed mainly on microglia and, to a lesser extent, on astrocytes and neurons in both the central nervous system and ligands exogenous (PAMPs) or DAMPs that activate inflammation (Li et al., 2022a). TLR4 is stimulated by DAMPs in ischemic stroke, which then leads to the activation of NF-κB and AP1 via the MyD88-dependent signaling pathway. TLR4 signal activation causes the NLRP3 protein to trigger the NLRP3 inflammasome. This mechanism has been connected to NLRP3 dysfunction, including augmented release of pro-inflammatory cytokines (Luo et al., 2022b). The NF-κB transcription receives the signal from TLR4 and targets surface PPRs, leading to NLRP3 activation (Jin et al., 2019). Hence, TLR4/NF-κB is an upstream for NLRP3 to induce inflammation. TAK-242, a specific inhibitor of TLR4, inhibits NLRP3 inflammasome by curtailing NLRP3 and caspase-1 expression in oxygen–glucose deprivation reperfusion (OGD/R) BV2 cells (Liu et al., 2021a). Meisoindigo, an anti-inflammatory drug, has suppressed TLR4 and NF-κB proteins in ischemic stroke in a dose-dependent manner to alleviate brain damage. In the aforementioned study, meisoindigo prevented the alterations in the ischemic hemisphere 3 days after MCAO and OGD/R BV2 cells by downregulating the expression of TLR4/NF-κB, NLRP3, and M1 microglia. Meanwhile, the upregulation of NLRP3 and M1 microglia-related proteins could be improved by meisoindigo treatment in LPS-induced TLR4 activation (Ye et al., 2019). In addition, the inhibition of the TLR3/NF-κB pathway by Renshen Shouwu extract increased the newly developed neurons, thus improving neurological deficit after ischemic stroke while NLRP3 inflammasome expression was downregulated (Li et al., 2020c).
Mitochondrial dysfunction, including the overproduction of ROS, uncontrolled mitochondrial autophagy, and abnormal fission and fusion have been implicated in ischemic stroke (Yang et al., 2018; Lei et al., 2021; Shi et al., 2022b). In addition, apoptosis, ATP disruption, calcium buildup, and faulty mitochondrial biogenesis contribute to aberrant ROS production during ischemic stroke (Bai et al., 2021). ROS release from mitochondria and mitochondrial DNA (mtDNA) damage leads to the activation of the NLRP3 inflammasome and NF-κB pathway (Li et al., 2021a). Furthermore, mitochondrial malfunction aggravates ROS over-production to further promote NLRP3 inflammasome activation. It is notable that ROS and mitochondrial function are connected and controlled by the NLRP3 inflammasome (Wang et al., 2019b). Idebenone, a mitochondrial protectant, has suppressed NLRP3 inflammation by mitigating mitochondrial dysfunction induced by cerebral ischemia/stroke and microglia overactivation to alleviate infarct volume and neurological deficit (Peng et al., 2020). Mitochondrial depolarization and mtDNA damage occur in OGD/R BV2 cells. Diazoxide reverses mtDNA damage and NLRP3 inflammasome assembly in primary microglia, indicating that mitochondrial dysfunction is fundamental to NLRP3 inflammasome activation (Gong et al., 2018). Furthermore, increased ROS and uncontrolled cell mitochondrial autophagy by a variety of molecular signaling pathways have been related to the stimulation of NLRP3 inflammasome (Zhou et al., 2011; Zeng et al., 2022). In the OGD/R PC12 cell, Taohong Siwu decoction, a traditional Chinese medicine, inhibits ROS and NLRP3 activation while upregulating mitophagy-related proteins including Parkin and PINK1. These were reversed by mitochondrial division inhibitory factor 1, indicating that mitophagy is a negative regulator of NLRP3 inflammasome activation (Shi et al., 2023a). Electroacupuncture also upregulates the expression of mitophagy-associated proteins while suppressing ROS-induced NLRP3 expression, leading to improvement in cognitive and neuronal impairments (Zhong et al., 2022). Furthermore, ketogenic diets inhibit ROS and TXNIP/NLRP3 inflammasome activation by suppressing mitochondrial fission and downregulating mitochondrial translocation-related proteins to improve middle cerebral artery occlusion/reperfusion (MCAO/R)-injury (Guo et al., 2018). Dynamin-related protein 1 (Drp1) is a key regulator of mitochondrial fission. The pharmacological inhibition of Drp1 translocation prevents mitochondrial fragmentation and protects neurons from oxygen–glucose deprivation (OGD)-induced injury (Zhao et al., 2013). The suppression of ER stress and/or ROS generation has also been shown to alleviate NLRP3-mediated inflammation in stroke cases by inhibiting Drp1-related mitochondrial function (Guo et al., 2018). Moreover, oxytocin reduces mitochondrial fission and oxidative stress within 3 days of intracerebral hemorrhage by downregulating NLRP3, ASC, and caspase-1 expressions and upregulating p-PKA and p-DRP1 expressions to alleviate neurological dysfunction (Yang et al., 2023b). These were revised by oxytocin or PKA inhibitors (Yang et al., 2023b), indicating that mitochondrial fission is related to the activation of NLRP3 inflammasome.
The crosstalk between NLRP3 and autophagy reveals autophagy-regulated NLRP3 activation through the removal of ROS-producing damaged mitochondria and inflammasome cytokines (Saitoh and Akira, 2016; Biasizzo and Kopitar-Jerala, 2020). The inhibition of glycogen synthase kinase 3β (GSK-3β) could decrease NLRP3 inflammasome activation and upregulate LC3-II and p62 expression to reduce cerebral infarct volume, while 3-MA (autophagy inhibitor) could rescind the neuroprotective effect (Wang et al., 2019a). Autophagy intervention in an early stage of MCAO could ameliorate cerebral I/R injury through NLRP3-induced inflammation (Fu et al., 2022). NLRP3 inflammasome activation was impaired, and LC3-autophagy activation was increased, by 6-Gingerol, an anti-autophagic and anti-inflammatory medicinal agent. More importantly, this was reversed by the autophagy inhibitor 3-MA, implying that the inhibition of autophagy could increase NLRP3 expression and cell apoptosis (Luo et al., 2021). In addition, sinomenine inhibits NLRP3 and ASC expression in MACO mice and OGD/R cell models by inhibiting LC3-II-related autophagy and inflammation (Qiu et al., 2016). All these studies suggest that autophagy dysfunction has a significant impact on inflammation through NLRP3 activation. Autophagy inhibition could be significant in ameliorating inflammation in ischemic stroke. Conversely, autophagy activation in stroke may be beneficial for ischemic-induced neuronal injury. Geniposide in particular has been found to activate autophagy by increasing LC3 and beclin1 expressions and decreasing P62 expression while inhibiting the NLRP3 inflammasome (Fu et al., 2020). By preventing NLRP3 inflammasome activation-induced pyroptosis, moderate hypothermia therapy triggers autophagy to mitigate cerebral ischemic injury (Tu et al., 2019). Hence, autophagy may be advantageous or harmful, depending on the various stages of ischemic stroke and the interactions between signals and drug administration (Zhang et al., 2020). Silent information regulator family protein 1 (SIRT1) is essential for autophagy initiation and has been implicated as a regulator of autophagy in ischemic stroke (Teertam and Phanithi, 2022). It is notable that SIRT1 impairment after MCAO treatment activates the NLRP3 inflammasome and is reversed by SIRT1 inhibitors (i.e., EX527 and arctigenin) to exert neuroprotection by inhibiting SIRT1-dependent NLRP3 inflammasome (Zhang et al., 2017). Moreover, resveratrol (a SIRT1 agonist) has been demonstrated to inhibit MCAO-induced NLRP3 inflammasome activation by upregulating autophagy-related proteins including LC3B-II/LC3B-I and p62 to exert neuroprotection (He et al., 2017). The aforementioned studies indicate that SIRT1 is crucial for anti-NLRP3 inflammasome and regulating autophagy in ischemic stroke. Moreover, AMPK, an upstream of autophagy, has been linked to inflammation and NLRP3 expression in OGD/R and MCAO models (Huang et al., 2021). Aldolase A (ALDOA), the key protein in glycolysis flux and the mitochondrial damage process, is indispensable for NLRP3 activation. The inhibition of ALDOA regulated the clearance of damaged mitochondria depending on the AMPK activation and the SQSTM1/p62 transcription to control NLRP3 inflammasome activation (Bai et al., 2022). This could mean that ALDOA is a target of NLRP3 inflammasome activation through the AMPK–autophagy pathway (Bai et al., 2022). In ischemic stroke, the NLRP3-related protein expression that mitigates autophagy activation includes AMPK, mTOR, and ULK1. They have improved cognitive impairment and attenuated inflammation (Huang et al., 2021; Zhai et al., 2023). SINO, an anti-inflammatory drug, inhibits AMPK-mediated NLRP3 inflammasome activation against ischemic-induced brain injury (Qiu et al., 2016). In summary, autophagy regulators are potential targets for modulating NLRP3 inflammasome in ischemic stroke.
NLRP3 inflammasome could be regulated and activated by microglia receptors and molecules, along with chemoattractant and chemokines after ischemic stroke (Franke et al., 2021). NLRP3 inflammasome activation is bound up with microglia phenotypes including proinflammatory M1 and anti-inflammatory M2 (Yang et al., 2023a). CX3CR1 is highly expressed in microglia. The inhibition of chemokine CX3CR1 improves neurologic function and microglia inflammation in ischemic stroke (Tang et al., 2014). Furthermore, exogenous rCX3CL1 had a neuroprotective effect in MCAO mice by decreasing NLRP3 inflammasome-induced pyroptosis and NF-κB expression (Ge et al., 2022). In addition, microglia M1 polarization in BV2 cells has upregulated both CX3CR1 and CX3CL1 expressions in chronic brain hypoperfusion rats (Mao et al., 2020). This was negated by miR-195 that mimics downregulated M1 phenotypic expression (Mao et al., 2020). This indicates that CX3CR1/CX3CL1 regulation may relate to microglia M1/M2 cytokine and NLRP3 inflammasome. One of the key proteins that trigger the NLRP3 inflammasome to release inflammatory factors is receptor-interacting protein kinase 1 (RIPK1). This can initiate necroptosis by activating RIPK3. It is notable that inhibited RIPK1 did reduce the volume of cerebral infarction (Deng et al., 2019). RhTrx-1, a RIPK1 inhibitor, can minimize neuronal injury by inhibiting NLRP3 activation, ROS-induced mitochondrial damage, and altering microglial M1/M2 phenotype (Jiao et al., 2020). The triggering receptor expressed on myeloid cells 1 (TREM-1) is a surface molecule on macrophages and microglia that increases pro-inflammatory mediator secretion and release in ischemic stroke. LP17, as the TREM-1 inhibitor, ameliorates neurological deficit and ischemic brain damage by decreasing NLRP3, caspase-1 and GSDMD mutation and release, and by decreasing microglia M1 expression (Liang et al., 2020a). The purinergic 2X7 receptor (P2X7R) has been reported to be activated with TLR4 and NLRP3 formation, as well as M1/M2 phenotypes in inflammatory disease (Ren et al., 2021). The inhibition of NLRP3 along with shifting microglia polarization toward the protective M2 phenotype by ketogenic diet regulates the activation of the P2X7R and TLR4/MyD88/NF-κB/NLRP3 pathways in multiple sclerosis (Sun et al., 2023). P2X7R was inhibited by dexmedetomidine (Dex) to reduce MCAO brain infarct size along with the downregulation of caspase-1 p10 expression (Sun et al., 2021). Several medications, including midazolam, d-Carvone, the inhibitor of the Takeda G-protein-receptor-5 (TGR5), INT777, and JLX001, could prevent and suppress cell pyroptosis to downregulate the expression of inflammatory factors and NLRP3-related proteins to protect cortical neurons from ischemic stroke (Dai et al., 2020; Bian et al., 2021; Liang et al., 2021; Shi et al., 2022a). In addition to medicinal intervention, moderate-intensity continuous exercise training deactivates NLRP1/NLRP3 and inhibits NF-κB p65 to switch M1 microglia to the anti-inflammatory M2 phenotype and promote functional recovery after ischemia/reperfusion (I/R) injury (Liu et al., 2022).
Neuronal pyroptosis, a determining factor in neuronal death in cerebral ischemic-induced brain injury, is attenuated by NLRP3 inhibition. NLRP3 can also recruit apoptotic-associated proteins containing the caspase domains, which can then activate caspase-1 to cleave pro-IL-1β and pro-IL-18 trigger releases to initiate neuronal death (Feng et al., 2020). The administration of NLRP3 inhibitor MCC950 decreased infarct volumes to protect BBB integrity in ischemic stroke (Franke et al., 2021). The low-density lipoprotein receptor (LDLR) has been shown to be a regulator of NLRP3-induced neuronal pyroptosis. The NLRP3 inflammasome overactivation and long-term impairments in cognition and memory were found in LDLR-deficient MCAO mice (Sun et al., 2020a). NLRP3 inhibitor treatment in LDLR-deficient mice mitigated NLRP3-related neuroinflammation following ischemic stroke (Sun et al., 2020b). TLRs interact with NLRP3 to increase tissue inflammation and damage (Tajalli-Nezhad et al., 2019). The pathogenic-synergistic TLRs/NF-κB/NLRP3 pathway did cause excessive microglial activation and synaptic dysfunction. However, this was reversed by NLRP3 inhibitors to improve dopaminergic neuronal loss and motor deficit (Lee et al., 2019; Li et al., 2021c). In MCAO mice, intermittent theta-burst rTMS protected against neuronal damage and neurobehavior improvement by inhibiting the expression of neuronal pyroptosis-associated proteins, including caspase-1 and GSDMD and TLR4/NF-κB/NLRP3 signaling pathway in the peri-infarcted area (Luo et al., 2022b). Caspase-1 was also blocked by VX-765 to preserve the BBB integrity in the MCAO model and downregulate the pyroptotic protein expression, including NLRP3, GSDMD, and inflammatory-related factors, subsequently ameliorating ischemic-induced infarction and neuronal injury (Liang et al., 2020b; Franke et al., 2021). Moreover, the absence of IL-1β significantly reduced infarct volume and cell death in MCAO mice by downregulating IL-6, TNF-α, and pyroptotic-related molecule expression (Li et al., 2020a). These findings demonstrate that pyroptosis-related proteins may be a therapeutic target for ischemic stroke (Tuo et al., 2022).
MicroRNAs are important post-transcriptional regulators and are involved in various neurological disorders, including ischemic stroke. As one of the important inflammation-regulated microRNAs, miR-139 targets c-Jun, a part of activation protein −1 (AP-1), and modulates NLRP3 activation to reduce inflammation in OGD/R BV-2 and SH-SY5Y cells (Wang-S. et al., 2020a). Furthermore, miR- 423-5p inhibitor could inhibit NLRP3 inflammasome activation to alleviate cerebral ischemic/reperfusion injury (Luo et al., 2022a). miR-203a-3p and miR-153-3p as AMPK upstream targets inhibit apoptosis and oxidative stress by regulating NLRP3 inflammasome activation (Li et al., 2022b). The upregulation of miR-139-5p contributes to NLRP3-induced pyroptosis via the downregulation of FOXO1 and TXNIP expression (Yao et al., 2022). MiR-668 inhibitors also impair NLRP3 inflammasome activation and inflammatory cytokine expression, resulting in a reduction in the infarct area of ischemic brains (He and Zhang, 2020). Moreover, long noncoding RNAs (lncRNAs) are endogenous regulatory RNA molecules that are essential regulators in cerebral ischemic injury. lncRNA NEAT1, as one key lncRNA, regulates miR-22-3p and participates in neuronal pyroptosis inhibition (Zhang et al., 2021). Gastrodin, from the Chinese herb Tianma, could inhibit NLRP3 inflammasome activation and antioxidant by regulating the lncRNA NEAT1/miR-22-3p axis (Zhang et al., 2021).
Based on decreased risk, reduced immunogenicity, and anti-inflammatory function, stem cell therapies have shown considerable clinical promise in the treatment of stroke. In particular, human cord blood-derived multipotent stem cell (HCB-SC) therapy minimizes brain infarct size, infarct volume, improves neurobehavioral functioning, and prolong stroke survival. Furthermore, lymphocytes co-cultured with HCB-SCs in tMCAO mice augment CD4 + CD25 + Foxp3 + Tregs in peripheral blood and reduce inflammation by suppressing NLRP3 inflammasome (Zhao et al., 2019). A cell graft with fresh human umbilical cord blood mononuclear cells has been used in MCAO rats to enhance neovascularization and inhibit NF-κB, NLRP3, cleaved caspase-1, and IL-1β expressions (Liu et al., 2018a). Furthermore, neural stem cell therapy has downregulated the expressions of TLR4- and NLRP3-related proteins in microglia to show neuroprotective and anti-inflammatory effects (Wang et al., 2023). Moreover, exosomes secreted from stem cells have exerted anti-inflammatory, angiogenesis, and neurogenesis effects by inhibiting NLRP3-induced neuronal pyroptosis including NLRP3, ACS, caspase-1, and mature IL-1β and IL-18 expression that were activated by MCAO (Liu et al., 2021b).
Table 1 summarizes the studies that evidence the beneficial effects that follow NLRP3 inhibition in cerebral ischemic stroke through various molecular pathways.
Curcumin has been shown to be effective in improving pathological features and preventing the development of various diseases. It also modulates inflammatory and metabolic processes to protect cells from oxidative stress (Lin et al., 2022). This section elaborates on several pathways and factors that enhance cell synaptic plasticity, microglia phenotype, and gut microbiota involving curcumin.
Curcumin has been shown to significantly enhance cognitive dysfunction and motor function in the central nervous system (Lo Cascio et al., 2021). Improvements in cognition are facilitated by reduced neuronal loss and hippocampal synaptic repair. The hippocampus is a significant part of the brain and involves several neuronal connections that control memory, learning, and emotional behavior (González-Granillo et al., 2022). A study showed that curcumin pre-treatment decreases neuronal death within the CA1 area of the hippocampi of LPS-induced depression rats, coinciding with improved synaptic function (Fan et al., 2021). In another study, curcumin inhibited long-term potentiation (LTP) in the synaptosomes of the hippocampal CA1 region and lowered Ca2+ concentration to improve synaptic plasticity (Shen et al., 2015). Additionally, curcumin reduced spontaneous neuronal activity, including changes in LFP phase coherence, to increase neuronal survival and the upregulation of synaptic proteins such as phospho-CaMKII and phospho-synapsin I in hippocampal slices exposed to Aβ-42 (Hoppe et al., 2013). In the dentate gyrus of the hippocampus, PSD thickness and synaptic width are increased by curcumin administration to enhance the number of newly developed neurons (Du et al., 2021). Furthermore, curcumin ameliorates synaptic mitochondrial dysfunction against age-related cognitive decline (Olesen et al., 2020). It also reduces synuclein formation and mitochondrial respiratory dysfunction in PD which has been exacerbated by ROS generated by chronic inflammation and severe motor and cognitive dysfunctions (Abdul-Latif et al., 2021). In cerebral ischemia/reperfusion, curcumin has minimized nerve damage and cognitive dysfunction, based on behavioral and immunohistochemical test results (Xu et al., 2019). In addition, the proliferation, differentiation, and maturation of neural stem cells were strengthened in the dentate gyrus area of the hippocampus after cerebral ischemia (Yang et al., 2021b). This followed the administration of curcumin.
ROS in astrocytes and microglia could be generated by nicotinamide adenine dinucleotide phosphate (NADPH) oxidase to drive the process of oxidative stress-induced brain damage after cerebral ischemia (Kahles et al., 2007; Orellana-Urzúa et al., 2020). Previous studies have demonstrated the anti-inflammatory and antioxidant properties of curcumin against neurodegeneration by its targeting of the NLRP3 inflammasome, PI3K/Akt/mTOR, and Nrf2 (Patel et al., 2020; Peng et al., 2021). By activating MAPK/Akt and PI3K/Akt signals, curcumin enhances the activity of antioxidant enzymes such as superoxide dismutase (SOD) and catalase against lipid and protein oxidation (Shah et al., 2007; Abrahams et al., 2019; Di Meo et al., 2019). Curcumin-induced Nrf2 upregulation in neurons curtailed ROS formation while increasing NO bioavailability (Shah et al., 2007). Heme oxygenase-1 (HO-1), a key protein, can interact with Nrf2 and be activated by curcumin to regulate inflammatory factors such as HIF-1α and NF-κB (Mhillaj et al., 2019). In OGD/R neurons and MCAO rats, curcumin attenuated OGD/R-induced NADPH upregulation to reduce oxidative stress by increasing the Akt/Nrf2 pathway to improve infarct size that could be reversed by Akt inhibitor (LY294002) (Wu et al., 2013).
Several studies have suggested that microglia activation may prevent chronic inflammation (Qin et al., 2019). Curcumin may alter microglia from M1 to M2, which then protects against neurological damage by blocking the ERK1/2 pathway and attenuating the formation of TNF-β, IL-1β, and IL-6 and NF-κB pathway activation in microglia (De Lorenzi et al., 2022). Microglia, as the immune cell in the brain, participates in the inflammation and release factors that determine the microglia phenotype (Ramírez-Carreto et al., 2023). Curcumin increased CD206 + Iba1 + microglia M2 phenotypic markers while decreasing M1 marker expression after 3 days in MCAO mice (Liu et al., 2017). Curcumin treatment also downregulated TLR4/NF-κB expression while upregulating TREM-2 expression, allowing the M1 pro-inflammatory phenotype (iNOS, IL-1, IL-6, and CD16/32) to be switched to the M2 anti-inflammatory phenotype (arginase 1, IL-4, IL-10, and CD206) (Zhang et al., 2019). By reducing pro-inflammation cytokines in subarachnoid hemorrhage, impaired TLR4 facilitates the decrement of brain water content and neurological deficits while curcumin treatment enhances these effects (Gao et al., 2019). Calmodulin-dependent protein kinase β (CaMKKβ) could activate the AMPK pathway. Its inhibition suppresses the effect of curcumin on LPS-induced BV2 microglia M1 phenotype gene expression (Qiao et al., 2020). It is noteworthy that interactions with neurons as well as changes in pathological stages and the release of numerous substances after brain injury may have an impact on curcumin-regulated microglia activation (Choi et al., 2011).
Gut microbiota dysbiosis (GMD) is involved in the inflammatory response in neurodegenerative diseases such as depression and ischemic stroke (Fan and Pedersen, 2021). Curcumin acts on the microbiota–gut–brain axis to directly protect damaged neurons by regulating gut microbiota (GM) (Pluta et al., 2022). The gastrointestinal tract’s GM is composed of various bacterial species that regulate gut digestion and metabolism to maintain homeostasis (Di Meo et al., 2019). The GMD decreases intestinal barrier and BBB permeability, which may exacerbate brain neuroinflammation and edema and complicate post-stroke neuronal damage (Denes et al., 2011). In addition, the abundance of harmful substances, such as lipopolysaccharides released by abnormal bacteria, could activate microglia and astrocytes, leading to accumulated inflammatory cytokines that may worsen the tissue microenvironment (Jiang et al., 2017; Chen et al., 2019c). Curcumin alters microbiota composition, increases bacteria’s anti-inflammatory capacity, and decreases serum LPS levels in both PD and obesity (Wang et al., 2020b; Syeda et al., 2021). In a recent study, curcumin increased phosphatidylcholine in the prefrontal cortex and modulated specific gut microbiota, especially Muribaculaceae, which was key to alleviating anxiety-like behaviors (Zhang et al., 2022). Furthermore, curcumin improves the microbial richness, diversity, and composition of GM including Bacteroidaceae at the family level and Prevotella at the genus level—key bacterial species in AD development (Sun et al., 2020b). In ischemic stroke, gastrointestinal complications affect post-stroke outcomes in up to 50% of patients. In addition, intestinal microorganisms and neuroactive compounds from GM have an impact on the metabolism and immune condition that also affect neuronal behavior pattern after ischemic stroke (Pluta et al., 2021; Wang et al., 2022a). Ischemic stroke induces GMD with increased Enterobacteriaceae that exacerbate cerebral infarction (Yin et al., 2015). Moreover, curcumin balances both beneficial and harmful bacteria in the GM to reduce the growth of pathogens and the production of harmful substances (Di Meo et al., 2019). Short-chain fatty acids, which are metabolites of microflora, could reduce pro-inflammatory factors and NF-κB expression. In CIS, butyric acid, a short-chain fatty acid, improves GM, enriches the beneficial microbiota Lactobacillus, and repairs leaky gut to reduce neurological impairment (Chen et al., 2019b). Curcumin also restores short-chain fatty acid profiles and ameliorates intestinal barrier (Cai et al., 2023). Furthermore, it ameliorates intestinal epithelial barrier injury induced by oxidative stress by regulating Parkin-mitophagy and AMPK pathways (Cao et al., 2020). Curcumin reduces Escherichia coli growth and reduces gut inflammation by suppressing the TLR4/NF-κB (Gan et al., 2019). Thus, the regulation of curcumin in the GM of ischemic stroke is closely associated with the inflammatory pathways.
In summary, several pathways are employed by curcumin to minimize inflammation. In addition, curcumin modulates microglia polarization and GM. These help curtail neuronal loss.
As the vital active ingredient in turmeric, curcumin has beneficial pharmacological functions such as anti-inflammation, antioxidation, and neuroprotection in brain injury (Fan and Lei, 2022). The NLRP3 inflammasome inhibition of curcumin has the potential to restrict inflammation and restore injured neurons (He et al., 2018). Curcumin can control NLRP3 inflammasome activation that may be mediated by the downregulation of inflammatory pathways, reduction of cell ROS, or restoration of mitochondrial function (Patel et al., 2020). New therapeutic approaches and direct clinical translational studies for ischemic stroke and other diseases through curcumin's inhibition of NLRP3 and NLRP3-related inflammatory pathways according to recent investigations are illustrated in Table 2.
Curcumin specifically impairs NLRP3 inflammasome activation without affecting either NLRC4 or AIM2 inflammasomes in LPS-induced bone marrow-derived macrophages (Yin et al., 2018). Moreover, in an LPS-induced disease, curcumin attenuates IL-1β secretion and regulates autophagy, sirtuin-2, and ROS to inhibit inflammation (Yin et al., 2018). In particular, curcumin suppresses DAB-induced TREM-1 and NLRP3 activation to alleviate cognitive impairment triggered by TLR4 and NF-κB upregulation (Nguyen et al., 2022). AI44 as a curcumin analog activates caspase-1 mutation, IL-1β, and pro–IL-18 by binding to peroxiredoxin 1 (PRDX1) but not to the TLR4/NF-κB pathway (Liu et al., 2018b). In THP-1 and murine RAW264.7 macrophages, monosodium urate induces NLRP3 inflammasome and NF-κB signaling upregulation that is reversed by curcumin, improving mitochondrial function to reduce ROS release and downregulate pro-inflammatory factors like Ik-Bα, IL-1β, and IL-6 (Chen et al., 2019a). Demethoxycurcumin prevents nanoparticle-induced NLRP3 activation in macrophages by inhibiting NF-κB (Nam et al., 2022). Moreover, curcumin decreases TNF-induced oxidant formation and NLRP3 inflammasome by downregulating the NF-κB, ERK1/2, and JNK pathways (Iglesias et al., 2022).
In neurological disease, neuronal injury and chronic stress could aggravate the inflammation accompanied by upregulated NLRP3 inflammasome (O'Brien et al., 2020). Curcumin has been shown to improve neuronal injury by regulating the NLRP3-related pathway and NLRP3-related inflammation (Ran et al., 2021). In depression, NF-κB and pro-inflammatory cytokine upregulation could be reversed by curcumin by decreasing IL-1β, TNF-α, and NLRP3 inflammasome expression (Ramaholimihaso et al., 2020). In ischemic-induced brain injury, NLRP3 inhibition through curcumin administration has been confirmed in several studies (Palomino-Antolin et al., 2022). MCC950 administration in NLRP3 knock-down mice increased ZO-1 and claudin-5 protein expression and decreased CCL-2/IL-1β expression to protect the BBB, improve neurological outcomes, and decrease infarct volume (Palomino-Antolin et al., 2022). In addition, α5β1 integrin expressed in endothelial cells showed that its knock-out could reduce BBB stabilization and significantly lower mitochondrial-induced oxidative stress and NLRP3 inflammasome, as well as claudin-5 and ZO-1 expression (Amruta and Bix, 2021). Curcumin protected the BBB stability of brain microvascular endothelial cells from OGD-induced permeability disruption by increasing HO-1 expression (Wang et al., 2013). Excessive glutamate is released after ischemic stroke along with ER and oxidative stresses. Curcumin regulates AMPK activity to rescue energy metabolic dysfunction and ER stress levels. Furthermore, curcumin protects hippocampal neurons from glutamate neurotoxicity by inhibiting TXNIP-NLRP3 inflammasome activity in an AMPK-dependent manner (Li et al., 2015). Moreover, NLRP3 activation in PC12 cells was inhibited by curcumin and NLRP3 inhibitor, MCC950, to decrease cell death and apoptosis (Li et al., 2020b). Curcumin has been shown to reduce neuroinflammation following ischemic stroke (Ran et al., 2021). It has also been observed to reduce pyroptotic proteins such as cleaved-caspase-1, GSDMS-N, and IL-1β following ischemic stroke (Ran et al., 2021). Additionally, curcumin has been shown to hinder the binding and activation of the NLRP3 inflammasome by inhibiting K+ and Ca2+ efflux, downregulating NF-κB, and diminishing the association between the ASC and NLRP3 (Yin et al., 2018; Hasanzadeh et al., 2020). Previous investigations have shown that curcumin and some of its analogs modulate magnesium ions to disrupt LPS-induced TLR4/MD activation (Zusso et al., 2019).
Autophagy and NLRP3 inflammasome have been identified as key players in cerebral I/R injury. Curcumin reduces early brain injury and improves neurological outcomes by suppressing NLRP3 inflammasome after ischemic stroke (Ran et al., 2021). Decreased LC3-II and HIF-1α expressions and increased p62 autophagy-related protein expression were observed following curcumin administration after an ischemic stroke (Forouzanfar et al., 2020). This implies that curcumin as an NLRP3 inflammasome inhibitor can regulate autophagy. More importantly, autophagy mediators could regulate NLRP3 inflammasome activation following curcumin administration in the wake of ischemic stroke. HIF-1α may be an NLRP3 inflammasome regulator in curcumin administration during stroke cases. A previous study showed both HIF-1α and NLRP3 to be upregulated after 6 h of brain ischemic reperfusion, continuing for 24 h (Jiang et al., 2020). A HIF-1α inhibitor (YC-1) significantly reduced NLRP3 inflammasome, resulting in the downregulation of cell apoptosis and pyroptosis (Jiang et al., 2020). Curcumin can also inhibit HIF-1α to alleviate brain injury by decreasing inflammation and TLR4/NF-κB/TNF-α and NO expression (Safdari et al., 2021). It can thus potentially regulate HIF-1α expression to influence NLRP3 and autophagy in ischemic stroke. Nonetheless, studies relating to curcumin in HIF-1α and autophagy are needed. The elevation of p-Akt/p-mTOR and the reduction of IL-1β, TLR4, p-38, and p-p38 levels have also been observed after curcumin administration in ischemic stroke and were concomitant with curtailed LC-3-II and NLRP3 markers (Huang et al., 2018). The PI3K/AKT/mTOR pathway plays a critical neuro-regulatory function through autophagy to support essential cellular function (Yang et al., 2021a). Curcumin ameliorates ischemic-induced brain impairment by upregulating the PI3K/AKT/mTOR pathway and downregulating autophagy and the TLR4/NF-κB pathway. However, the PI3K/AKT/mTOR (LY294002) or TLR4 (anisomycin) inhibitors suppressed these protective effects (Huang et al., 2018). Furthermore, curcumin inhibited NLRP3-related cell pyroptosis through autophagy, which was revised by the autophagy activator rapamycin (Yu et al., 2020). Autophagy is an important target of curcumin to regulate NLRP3 pathway. The PI3K/AKT/mTOR pathway interacts with autophagy and TLR4/NF-κB/NLRP3 pathways and could be indispensable to the anti-inflammatory and neuroprotective effects of curcumin. However, its effect on autophagy in NLRP3-driven neuroinflammation in ischemic stroke has not been evaluated.
Curcumin at 200 mg/kg and 300 mg/kg at 4 h post-MCAO significantly reduces the infarct area (Dohare et al., 2008). Curcumin crossed the BBB to exert maximum effect within 1 h and returned to normal levels in 2 days (Wang et al., 2005). It should be noted that curcumin has not been the subject of clinical trials due to its poor solubility and low absorption (Pan-On et al., 2022). Recently, better delivery systems such as polyester-based, nanomaterial, and arginine–glycine–aspartic acid (RGD)-loaded curcumin have attempted to improve its absorption and permeability. Notably, PLGA nanoparticles loaded with curcumin significantly enhance the oral absorption of curcumin (Xie et al., 2011). Additionally, curcumin-based nanotherapeutics inhibit NLRP3 inflammasome activation (Ruan et al., 2022). Furthermore, curcumin nanomaterial significantly improves memory deficit and the BBB to protect neurons from β-amyloid-induced activation of the NLRP3 related neuroinflammatory pathway (Ruan et al., 2022). Oral polymer nanoparticle-encapsulated curcumin has significantly enhanced synaptophysin puncta in the hippocampus of patients with chronic Gulf War illness to improve cognitive dysfunction by reducing ROS release and NF-κB/NLRP3 activation in microglia (Attaluri et al., 2022). Moreover, nanosystems containing curcumin (nCUR) have significantly decreased TUNEL-positive cells and mRNA expression of NLRP3, IL-1β, and macrophage infiltration to protect neurons from diabetic peripheral neuropathy (Dwivedi et al., 2022). In RGD-loaded curcumin liposome treatment, NLRP3, cleaved-caspase-1, and IL-1β remarkably decreased in LPS-induced macrophages (Shi et al., 2023b). PLGA-GA2 coupling nanoparticulate curcumin has good bioavailability and safety and has been demonstrated to exert anti-inflammatory effects in the livers and kidneys of diabetic kidney disease mice by inhibiting the P38 (MAPK) and P53 deactivation to decrease NLRP3 inflammasome (Ganugula et al., 2023). In ischemic stroke, curcumin nanoparticles have had better neuroprotective effects by downregulating NF-κB and pro-inflammatory expressions compared to using curcumin alone (Li et al., 2021b). Therefore, in ischemic stroke, investigations relating to cur–nanoparticles affecting NLRP3 inflammasome activation are warranted.
The inhibition of NLRP3 inflammasome is a new therapeutic approach to ischemic stroke for protecting injured neurons. By controlling effector molecules in the brain, curcumin, a strong inhibitor of NLRP3 inflammasome, has been proven to protect neurons from damage caused by ischemic stroke and neurological conditions. In this review, we evaluated how NLRP3 is activated by several pathways after ischemic stroke. Curcumin as a neuroprotective drug inhibits ROS formation and regulates microglia M1/M2 and gut microbiota to mitigate inflammation. The upstream and downstream pathways were clarified to show how curcumin regulates NLRP3 inflammasome to affect neuroinflammation and BBB integrity following ischemic stroke. The TLR4/NF-κB autophagy-related mediators and cell energy metabolism pathways were also clarified and the precise mechanisms shown by which curcumin affects the NLRP3 inflammasome (Figure 1). In conclusion, curcumin slows the development of ischemic stroke.
FIGURE 1. Curcumin is the primary component of turmeric’s rhizome and is currently under investigation for several clinical diseases. This review summarizes curcumin’s direct and indirect inhibition of NLRP3 inflammation activation in ischemic stroke. First, curcumin targets the NLRP3 to reduce caspase-1 and GSDMD cleavage, as well as IL-1β/IL-18 release and cell pyroptosis inhibition. Curcumin also regulates the imbalance of Ca2+ and K+ induced by ischemic stroke. In addition, curcumin modulates the AMPK/TXNIP/NLRP3 and P2x7R/NLRP3 pathways to rescue energy metabolism and reduce ROS formation. The BBB protection of curcumin has been demonstrated through regulation of ZO-1/claudin-5/NLRP3 pathways to downregulate TNF-α, IL-6, IL-1β, and CCL2 expressions. Furthermore, the TLR4/NF-κB/NLRP3 and autophagy-NLRP3 pathways play an important role in microglia alteration and inflammatory inhibition.
However, there are several challenges or limitations concerning the employment of curcumin. For instance, several mechanisms regarding the role of autophagy and NLRP3 in curcumin treatment after ischemic stroke remain to be investigated. Furthermore, specific mechanisms and targets of curcumin based NLRP3 inhibitors or NLRP3 knockout mice need to be explored in ischemic stroke treatment. Moreover, the effect of curcumin on gut microbiota in different stages after ischemic stroke along with the specific mechanisms involved needs to be assessed. This could facilitate understanding of the anti-inflammatory and protective effects of curcumin through the brain–gut axis. There are increasing reports relating to the protective effects of delivery systems-loaded curcumin, including RGD-load curcumin, PLGA-curcumin, and nano-curcumin. However, the bio-absorbability of these delivery systems-loaded curcumins are very different in ischemic stroke treatment. Therefore, more studies are needed to explore the recommended dose and therapeutic effect following the administration of curcumin, particularly nano-curcumin, for ischemic stroke in both preclinical and clinical settings. Meanwhile, to overcome the pharmacological limitations due to poor bioavailability, more analogs and derivatives of curcumin need to be developed to achieve high bioavailable and low toxic effects in future studies.
XD prepared the first draft of the manuscript. XD, NA, LX, and BB revised the manuscript. BZ conceptualized, and MF conceptualized and guided, this manuscript. All authors approved the final paper.
This study was supported by the Zhejiang Provincial Natural Science Foundation of China under grant no. LQ21H090006 and Zhejiang Province Traditional Chinese Medicine Science and Technology Projection under grant no. 2021ZQ071.
The authors declare that the research was conducted in the absence of any commercial or financial relationships that could be construed as a potential conflict of interest.
All claims expressed in this article are solely those of the authors and do not necessarily represent those of their affiliated organizations, or those of the publisher, the editors, and the reviewers. Any product that may be evaluated in this article, or claim that may be made by its manufacturer, is not guaranteed or endorsed by the publisher.
Abdul-Latif, R., Stupans, I., Allahham, A., Adhikari, B., and Thrimawithana, T. (2021). Natural antioxidants in the management of Parkinson's disease: Review of evidence from cell line and animal models. J. Integr. Med. 19 (4), 300–310. doi:10.1016/j.joim.2021.03.007
Abrahams, S., Haylett, W. L., Johnson, G., Carr, J. A., and Bardien, S. (2019). Antioxidant effects of curcumin in models of neurodegeneration, aging, oxidative and nitrosative stress: A review. Neuroscience 406, 1–21. doi:10.1016/j.neuroscience.2019.02.020
Amruta, N., and Bix, G. (2021). ATN-161 ameliorates ischemia/reperfusion-induced oxidative stress, fibro-inflammation, mitochondrial damage, and apoptosis-mediated tight junction disruption in bEnd.3 cells. Inflammation 44 (6), 2377–2394. doi:10.1007/s10753-021-01509-9
Anderson, F. L., Biggs, K. E., Rankin, B. E., and Havrda, M. C. (2023). NLRP3 inflammasome in neurodegenerative disease. Transl. Res. 252, 21–33. doi:10.1016/j.trsl.2022.08.006
Attaluri, S., Arora, M., Madhu, L. N., Kodali, M., Shuai, B., Melissari, L., et al. (2022). Oral nano-curcumin in a model of chronic Gulf war illness alleviates brain dysfunction with modulation of oxidative stress, mitochondrial function, neuroinflammation, neurogenesis, and gene expression. Aging Dis. 13 (2), 583–613. doi:10.14336/AD.2021.0829
Bai, D., Du, J., Bu, X., Cao, W., Sun, T., Zhao, J., et al. (2022). ALDOA maintains NLRP3 inflammasome activation by controlling AMPK activation. Autophagy 18 (7), 1673–1693. doi:10.1080/15548627.2021.1997051
Bai, R., Lang, Y., Shao, J., Deng, Y., Refuhati, R., and Cui, L. (2021). The role of NLRP3 inflammasome in cerebrovascular diseases pathology and possible therapeutic targets. ASN Neuro 13, 17590914211018100. doi:10.1177/17590914211018100
Bhat, A., Mahalakshmi, A. M., Ray, B., Tuladhar, S., Hediyal, T. A., Manthiannem, E., et al. (2019). Benefits of curcumin in brain disorders. BioFactors Oxf. Engl. 45 (5), 666–689. doi:10.1002/biof.1533
Bian, H.-J., Xu, S.-Y., Li, H.-Q., Jia, J.-Q., Ye, L., Shu, S., et al. (2021). JLX001 ameliorates cerebral ischemia injury by modulating microglial polarization and compromising NLRP3 inflammasome activation via the NF-κB signaling pathway. Int. Immunopharmacol. 101, 108325. doi:10.1016/j.intimp.2021.108325
Biasizzo, M., and Kopitar-Jerala, N. (2020). Interplay between NLRP3 inflammasome and autophagy. Front. Immunol. 11, 591803. doi:10.3389/fimmu.2020.591803
Cai, B., Zhong, L., Wang, Q., Xu, W., Li, X., and Chen, T. (2023). Curcumin alleviates 1-methyl- 4-phenyl- 1,2,3,6-tetrahydropyridine- induced Parkinson's disease in mice via modulating gut microbiota and short-chain fatty acids. Front. Pharmacol. 14, 1198335. doi:10.3389/fphar.2023.1198335
Cao, S., Wang, C., Yan, J., Li, X., Wen, J., and Hu, C. (2020). Curcumin ameliorates oxidative stress-induced intestinal barrier injury and mitochondrial damage by promoting Parkin dependent mitophagy through AMPK-TFEB signal pathway. Free Radic. Biol. Med. 147, 8–22. doi:10.1016/j.freeradbiomed.2019.12.004
Chen, B., Li, H., Ou, G., Ren, L., Yang, X., and Zeng, M. (2019a). Curcumin attenuates MSU crystal-induced inflammation by inhibiting the degradation of IκBα and blocking mitochondrial damage. Arthritis Res. Ther. 21 (1), 193. doi:10.1186/s13075-019-1974-z
Chen, H., He, Y., Chen, S., Qi, S., and Shen, J. (2020). Therapeutic targets of oxidative/nitrosative stress and neuroinflammation in ischemic stroke: Applications for natural product efficacy with omics and systemic biology. Pharmacol. Res. 158, 104877. doi:10.1016/j.phrs.2020.104877
Chen, R., Xu, Y., Wu, P., Zhou, H., Lasanajak, Y., Fang, Y., et al. (2019b). Transplantation of fecal microbiota rich in short chain fatty acids and butyric acid treat cerebral ischemic stroke by regulating gut microbiota. Pharmacol. Res. 148, 104403. doi:10.1016/j.phrs.2019.104403
Chen, Y., Liang, J., Ouyang, F., Chen, X., Lu, T., Jiang, Z., et al. (2019c). Persistence of gut microbiota dysbiosis and chronic systemic inflammation after cerebral infarction in cynomolgus monkeys. Front. Neurol. 10, 661. doi:10.3389/fneur.2019.00661
Choi, D. K., Koppula, S., and Suk, K. (2011). Inhibitors of microglial neurotoxicity: Focus on natural products. Mol. (Basel, Switz. 16 (2), 1021–1043. doi:10.3390/molecules16021021
Cicolari, S., Catapano, A. L., and Magni, P. (2021). Inflammaging and neurodegenerative diseases: Role of NLRP3 inflammasome activation in brain atherosclerotic vascular disease. Mech. Ageing Dev. 195, 111467. doi:10.1016/j.mad.2021.111467
Dai, M., Wu, L., Yu, K., Xu, R., Wei, Y., Chinnathambi, A., et al. (2020). D-Carvone inhibit cerebral ischemia/reperfusion induced inflammatory response TLR4/NLRP3 signaling pathway. Biomed. Pharmacother. 132, 110870. doi:10.1016/j.biopha.2020.110870
Datta, A., Jingru, Q., Khor, T. H., Teo, M. T., Heese, K., and Sze, S. K. (2011). Quantitative neuroproteomics of an in vivo rodent model of focal cerebral ischemia/reperfusion injury reveals a temporal regulation of novel pathophysiological molecular markers. J. Proteome Res. 10 (11), 5199–5213. doi:10.1021/pr200673y
De Lorenzi, E., Franceschini, D., Contardi, C., Di Martino, R. M. C., Seghetti, F., Serra, M., et al. (2022). Modulation of amyloid beta-induced microglia activation and neuronal cell death by curcumin and analogues. Int. J. Mol. Sci. 23 (8), 4381. doi:10.3390/ijms23084381
Denes, A., Ferenczi, S., and Kovacs, K. J. (2011). Systemic inflammatory challenges compromise survival after experimental stroke via augmenting brain inflammation, blood-brain barrier damage and brain oedema independently of infarct size. J. Neuroinflammation 8, 164. doi:10.1186/1742-2094-8-164
Deng, X.-X., Li, S.-S., and Sun, F.-Y. (2019). Necrostatin-1 prevents necroptosis in brains after ischemic stroke via inhibition of RIPK1-mediated RIPK3/MLKL signaling. Aging Dis 10 (4), 807–817. doi:10.14336/AD.2018.0728
Di Meo, F., Margarucci, S., Galderisi, U., Crispi, S., and Peluso, G. (2019). Curcumin, gut microbiota, and neuroprotection. Nutrients 11 (10), 2426. doi:10.3390/nu11102426
Dodd, W. S., Noda, I., Martinez, M., Hosaka, K., and Hoh, B. L. (2021). NLRP3 inhibition attenuates early brain injury and delayed cerebral vasospasm after subarachnoid hemorrhage. J. Neuroinflammation 18 (1), 163. doi:10.1186/s12974-021-02207-x
Dohare, P., Garg, P., Jain, V., Nath, C., and Ray, M. (2008). Dose dependence and therapeutic window for the neuroprotective effects of curcumin in thromboembolic model of rat. Behav. Brain Res. 193 (2), 289–297. doi:10.1016/j.bbr.2008.06.012
Du, J., Deng, Y., Qiu, Z., Sun, G., Guo, Y., Hei, Z., et al. (2021). Curcumin alleviates chronic pain and improves cognitive impairment via enhancing hippocampal neurogenesis in sciatic nerve constriction rats. J. Pain Res. 14, 1061–1070. doi:10.2147/JPR.S299604
Dwivedi, S., Gottipati, A., Ganugula, R., Arora, M., Friend, R., Osburne, R., et al. (2022). Oral nanocurcumin alone or in combination with insulin alleviates STZ-induced diabetic neuropathy in rats. Mol. Pharm. 19 (12), 4612–4624. doi:10.1021/acs.molpharmaceut.2c00465
Fan, C., Li, Y., Lan, T., Wang, W., Mao, X., and Yu, S. Y. (2021). Prophylactic treatment of curcumin in a rat model of depression by attenuating hippocampal synaptic loss. Food Funct. 12 (22), 11202–11213. doi:10.1039/d1fo02676c
Fan, F., and Lei, M. (2022). Mechanisms underlying curcumin-induced neuroprotection in cerebral ischemia. Front. Pharmacol. 13, 893118. doi:10.3389/fphar.2022.893118
Fan, Y., and Pedersen, O. (2021). Gut microbiota in human metabolic health and disease. Nat. Rev. Microbiol. 19 (1), 55–71. doi:10.1038/s41579-020-0433-9
Feigin, V. L., Brainin, M., Norrving, B., Martins, S., Sacco, R. L., Hacke, W., et al. (2022). World stroke organization (WSO): Global stroke fact sheet 2022. Int. J. Stroke 17 (1), 18–29. doi:10.1177/17474930211065917
Feng, Y.-S., Tan, Z.-X., Wang, M.-M., Xing, Y., Dong, F., and Zhang, F. (2020). Inhibition of NLRP3 inflammasome: A prospective target for the treatment of ischemic stroke. Front. Cell. Neurosci. 14, 155. doi:10.3389/fncel.2020.00155
Forouzanfar, F., Read, M. I., Barreto, G. E., and Sahebkar, A. (2020). Neuroprotective effects of curcumin through autophagy modulation. IUBMB Life 72 (4), 652–664. doi:10.1002/iub.2209
Franke, M., Bieber, M., Kraft, P., Weber, A. N. R., Stoll, G., and Schuhmann, M. K. (2021). The NLRP3 inflammasome drives inflammation in ischemia/reperfusion injury after transient middle cerebral artery occlusion in mice. Brain Behav. Immun. 92, 223–233. doi:10.1016/j.bbi.2020.12.009
Fu, C., Zhang, X., Lu, Y., Wang, F., Xu, Z., Liu, S., et al. (2020). Geniposide inhibits NLRP3 inflammasome activation via autophagy in BV-2 microglial cells exposed to oxygen-glucose deprivation/reoxygenation. Int. Immunopharmacol. 84, 106547. doi:10.1016/j.intimp.2020.106547
Fu, K., Xu, W., Lenahan, C., Mo, Y., Wen, J., Deng, T., et al. (2022). Autophagy regulates inflammation in intracerebral hemorrhage: Enemy or friend? Front. Cell. Neurosci. 16, 1036313. doi:10.3389/fncel.2022.1036313
Gan, Z., Wei, W., Li, Y., Wu, J., Zhao, Y., Zhang, L., et al. (2019). Curcumin and resveratrol regulate intestinal bacteria and alleviate intestinal inflammation in weaned piglets. Molecules 24 (7), 1220. doi:10.3390/molecules24071220
Ganugula, R., Nuthalapati, N. K., Dwivedi, S., Zou, D., Arora, M., Friend, R., et al. (2023). Nanocurcumin combined with insulin alleviates diabetic kidney disease through P38/P53 signaling axis. J. Control Release 353, 621–633. doi:10.1016/j.jconrel.2022.12.012
Gao, Y., Zhuang, Z., Lu, Y., Tao, T., Zhou, Y., Liu, G., et al. (2019). Curcumin mitigates neuro-inflammation by modulating microglia polarization through inhibiting TLR4 Axis signaling pathway following experimental subarachnoid hemorrhage. Front. Neurosci. 13, 1223. doi:10.3389/fnins.2019.01223
Ge, Y., Wang, L., Wang, C., Chen, J., Dai, M., Yao, S., et al. (2022). CX3CL1 inhibits NLRP3 inflammasome-induced microglial pyroptosis and improves neuronal function in mice with experimentally-induced ischemic stroke. Life Sci. 300, 120564. doi:10.1016/j.lfs.2022.120564
Global, regional (2021). Global, regional, and national burden of stroke and its risk factors, 1990-2019: A systematic analysis for the global burden of disease study 2019. Lancet Neurol. 20 (10), 795–820. doi:10.1016/S1474-4422(21)00252-0
Gong, Z., Pan, J., Shen, Q., Li, M., and Peng, Y. (2018). Mitochondrial dysfunction induces NLRP3 inflammasome activation during cerebral ischemia/reperfusion injury. J. Neuroinflammation 15 (1), 242. doi:10.1186/s12974-018-1282-6
González-Granillo, A. E., Gnecco, D., Díaz, A., Garcés-Ramírez, L., de la Cruz, F., Juarez, I., et al. (2022). Curcumin induces cortico-hippocampal neuronal reshaping and memory improvements in aged mice. J. Chem. Neuroanat. 121, 102091. doi:10.1016/j.jchemneu.2022.102091
Gu, G., Ren, J., Zhu, B., Shi, Z., Feng, S., and Wei, Z. (2023). Multiple mechanisms of curcumin targeting spinal cord injury. Biomed. Pharmacother. 159, 114224. doi:10.1016/j.biopha.2023.114224
Guo, M., Wang, X., Zhao, Y., Yang, Q., Ding, H., Dong, Q., et al. (2018). Ketogenic diet improves brain ischemic tolerance and inhibits NLRP3 inflammasome activation by preventing drp1-mediated mitochondrial fission and endoplasmic reticulum stress. Front. Mol. Neurosci. 11, 86. doi:10.3389/fnmol.2018.00086
Hasanzadeh, S., Read, M. I., Bland, A. R., Majeed, M., Jamialahmadi, T., and Sahebkar, A. (2020). Curcumin: An inflammasome silencer. Pharmacol. Res. 159, 104921. doi:10.1016/j.phrs.2020.104921
He, J., and Zhang, X. (2020). miR-668 inhibitor attenuates mitochondrial membrane potential and protects against neuronal apoptosis in cerebral ischemic stroke. Folia Neuropathol. 58 (1), 22–29. doi:10.5114/fn.2020.94003
He, Q., Jiang, L., Man, S., Wu, L., Hu, Y., and Chen, W. (2018). Curcumin reduces neuronal loss and inhibits the NLRP3 inflammasome activation in an epileptic rat model. Curr. Neurovasc Res. 15 (3), 186–192. doi:10.2174/1567202615666180731100224
He, Q., Li, Z., Wang, Y., Hou, Y., Li, L., and Zhao, J. (2017). Resveratrol alleviates cerebral ischemia/reperfusion injury in rats by inhibiting NLRP3 inflammasome activation through Sirt1-dependent autophagy induction. Int. Immunopharmacol. 50, 208–215. doi:10.1016/j.intimp.2017.06.029
Holbrook, J. A., Jarosz-Griffiths, H. H., Caseley, E., Lara-Reyna, S., Poulter, J. A., Williams-Gray, C. H., et al. (2021). Neurodegenerative disease and the NLRP3 inflammasome. Front. Pharmacol. 12, 643254. doi:10.3389/fphar.2021.643254
Hoppe, J. B., Haag, M., Whalley, B. J., Salbego, C. G., and Cimarosti, H. (2013). Curcumin protects organotypic hippocampal slice cultures from Aβ1-42-induced synaptic toxicity. Toxicol Vitro 27 (8), 2325–2330. doi:10.1016/j.tiv.2013.10.002
Huang, L., Chen, C., Zhang, X., Li, X., Chen, Z., Yang, C., et al. (2018). Neuroprotective effect of curcumin against cerebral ischemia-reperfusion via mediating autophagy and inflammation. J. Mol. Neurosci. 64 (1), 129–139. doi:10.1007/s12031-017-1006-x
Huang, Z., Zhou, X., Zhang, X., Huang, L., Sun, Y., Cheng, Z., et al. (2021). Pien-Tze-Huang, a Chinese patent formula, attenuates NLRP3 inflammasome-related neuroinflammation by enhancing autophagy via the AMPK/mTOR/ULK1 signaling pathway. Biomed. Pharmacother. 141, 111814. doi:10.1016/j.biopha.2021.111814
Iglesias, D. E., Cremonini, E., Oteiza, P. I., and Fraga, C. G. (2022). Curcumin mitigates TNFα-induced caco-2 cell monolayer permeabilization through modulation of NF-κB, ERK1/2, and JNK pathways. Mol. Nutr. Food Res. 66 (21), e2101033. doi:10.1002/mnfr.202101033
Jiang, C., Li, G., Huang, P., Liu, Z., and Zhao, B. (2017). The gut microbiota and alzheimer's disease. J. Alzheimers Dis. 58 (1), 1–15. doi:10.3233/JAD-161141
Jiang, J., Wang, W., Sun, Y. J., Hu, M., Li, F., and Zhu, D. Y. (2007). Neuroprotective effect of curcumin on focal cerebral ischemic rats by preventing blood-brain barrier damage. Eur. J. Pharmacol. 561 (1-3), 54–62. doi:10.1016/j.ejphar.2006.12.028
Jiang, Q., Geng, X., Warren, J., Eugene Paul Cosky, E., Kaura, S., Stone, C., et al. (2020). Hypoxia inducible factor-1α (HIF-1α) mediates NLRP3 inflammasome-dependent-pyroptotic and apoptotic cell death following ischemic stroke. Neuroscience 448, 126–139. doi:10.1016/j.neuroscience.2020.09.036
Jiao, Y., Wang, J., Zhang, H., Cao, Y., Qu, Y., Huang, S., et al. (2020). Inhibition of microglial receptor-interacting protein kinase 1 ameliorates neuroinflammation following cerebral ischaemic stroke. J. Cell. Mol. Med. 24 (21), 12585–12598. doi:10.1111/jcmm.15820
Jin, T., Zhang, Y., Botchway, B. O. A., Zhang, J., Fan, R., Zhang, Y., et al. (2022). Curcumin can improve Parkinson's disease via activating BDNF/PI3k/Akt signaling pathways. Food Chem. Toxicol. 164, 113091. doi:10.1016/j.fct.2022.113091
Jin, X., Liu, M.-Y., Zhang, D.-F., Zhong, X., Du, K., Qian, P., et al. (2019). Baicalin mitigates cognitive impairment and protects neurons from microglia-mediated neuroinflammation via suppressing NLRP3 inflammasomes and TLR4/NF-κB signaling pathway. CNS Neurosci. Ther. 25 (5), 575–590. doi:10.1111/cns.13086
Kahles, T., Luedike, P., Endres, M., Galla, H.-J., Steinmetz, H., Busse, R., et al. (2007). NADPH oxidase plays a central role in blood-brain barrier damage in experimental stroke. Stroke 38 (11), 3000–3006. doi:10.1161/STROKEAHA.107.489765
Kim, Y. K., Shin, J. S., and Nahm, M. H. (2016). NOD-like receptors in infection, immunity, and diseases. Yonsei Med. J. 57 (1), 5–14. doi:10.3349/ymj.2016.57.1.5
Lan, C., Chen, X., Zhang, Y., Wang, W., Wang, W. E., Liu, Y., et al. (2018). Curcumin prevents strokes in stroke-prone spontaneously hypertensive rats by improving vascular endothelial function. BMC Cardiovasc Disord. 18 (1), 43. doi:10.1186/s12872-018-0768-6
Lee, E., Hwang, I., Park, S., Hong, S., Hwang, B., Cho, Y., et al. (2019). MPTP-driven NLRP3 inflammasome activation in microglia plays a central role in dopaminergic neurodegeneration. Cell. Death Differ. 26 (2), 213–228. doi:10.1038/s41418-018-0124-5
Lei, L., Yang, S., Lu, X., Zhang, Y., and Li, T. (2021). Research progress on the mechanism of mitochondrial autophagy in cerebral stroke. Front. Aging Neurosci. 13, 698601. doi:10.3389/fnagi.2021.698601
Li, D., Yang, S., Xing, Y., Pan, L., Zhao, R., Zhao, Y., et al. (2021a). Novel insights and current evidence for mechanisms of atherosclerosis: Mitochondrial dynamics as a potential therapeutic target. Front. Cell. Dev. Biol. 9, 673839. doi:10.3389/fcell.2021.673839
Li, F., Xu, Y., Li, X., Wang, X., Yang, Z., Li, W., et al. (2021b). Triblock copolymer nanomicelles loaded with curcumin attenuates inflammation via inhibiting the NF-κB pathway in the rat model of cerebral ischemia. Int. J. Nanomedicine 16, 3173–3183. doi:10.2147/IJN.S300379
Li, Q., Cao, Y., Dang, C., Han, B., Han, R., Ma, H., et al. (2020a). Inhibition of double-strand DNA-sensing cGAS ameliorates brain injury after ischemic stroke. EMBO Mol. Med. 12 (4), e11002. doi:10.15252/emmm.201911002
Li, R., Zhou, Y., Zhang, S., Li, J., Zheng, Y., and Fan, X. (2022a). The natural (poly)phenols as modulators of microglia polarization via TLR4/NF-κB pathway exert anti-inflammatory activity in ischemic stroke. Eur. J. Pharmacol. 914, 174660. doi:10.1016/j.ejphar.2021.174660
Li, X. N., Xu, J. J., Wu, J. B., Ji, L., Yuan, C. H., and Wang, Z. P. (2020b). Curcumin exerts protective effect on PC12 cells against lidocaine-induced cytotoxicity by suppressing the formation of NLRP3 inflammasome. Eur. Rev. Med. Pharmacol. Sci. 24 (12), 7092–7100. doi:10.26355/eurrev_202006_21703
Li, Y., Li, J., Li, S., Li, Y., Wang, X., Liu, B., et al. (2015). Curcumin attenuates glutamate neurotoxicity in the hippocampus by suppression of ER stress-associated TXNIP/NLRP3 inflammasome activation in a manner dependent on AMPK. Toxicol. Appl. Pharmacol. 286 (1), 53–63. doi:10.1016/j.taap.2015.03.010
Li, Y., Liang, W., Guo, C., Chen, X., Huang, Y., Wang, H., et al. (2020c). Renshen Shouwu extract enhances neurogenesis and angiogenesis via inhibition of TLR4/NF-κB/NLRP3 signaling pathway following ischemic stroke in rats. J. Ethnopharmacol. 253, 112616. doi:10.1016/j.jep.2020.112616
Li, Y., Peng, B., Li, Y., Huang, A., Peng, Y., Yu, Q., et al. (2022b). MiR-203a-3p/153-3p improves cognitive impairments induced by ischemia/reperfusion via blockade of SRC-mediated MAPK signaling pathway in ischemic stroke. Chem. Biol. Interact. 358, 109900. doi:10.1016/j.cbi.2022.109900
Li, Y., Xia, Y., Yin, S., Wan, F., Hu, J., Kou, L., et al. (2021c). Targeting microglial α-synuclein/TLRs/NF-kappaB/NLRP3 inflammasome Axis in Parkinson's disease. Front. Immunol. 12, 719807. doi:10.3389/fimmu.2021.719807
Liang, H., Matei, N., McBride, D. W., Xu, Y., Zhou, Z., Tang, J., et al. (2021). TGR5 activation attenuates neuroinflammation via Pellino3 inhibition of caspase-8/NLRP3 after middle cerebral artery occlusion in rats. J. Neuroinflammation 18 (1), 40. doi:10.1186/s12974-021-02087-1
Liang, Y.-B., Song, P.-P., Zhu, Y.-H., Xu, J.-M., Zhu, P.-Z., Liu, R.-R., et al. (2020a). TREM-1-targeting LP17 attenuates cerebral ischemia-induced neuronal injury by inhibiting oxidative stress and pyroptosis. Biochem. Biophys. Res. Commun. 529 (3), 554–561. doi:10.1016/j.bbrc.2020.05.056
Liang, Y., Song, P., Chen, W., Xie, X., Luo, R., Su, J., et al. (2020b). Inhibition of caspase-1 ameliorates ischemia-associated blood-brain barrier dysfunction and integrity by suppressing pyroptosis activation. Front. Cell. Neurosci. 14, 540669. doi:10.3389/fncel.2020.540669
Lin, H. W., Chen, T. C., Yeh, J. H., Tsou, S. C., Wang, I., Shen, T. J., et al. (2022). Suppressive effect of tetrahydrocurcumin on Pseudomonas aeruginosa lipopolysaccharide-induced inflammation by suppressing JAK/STAT and Nrf2/HO-1 pathways in microglial cells. Oxid. Med. Cell. Longev. 2022, 4978556. doi:10.1155/2022/4978556
Liu, J., Ma, W., Zang, C.-H., Wang, G.-D., Zhang, S.-J., Wu, H.-J., et al. (2021a). Salidroside inhibits NLRP3 inflammasome activation and apoptosis in microglia induced by cerebral ischemia/reperfusion injury by inhibiting the TLR4/NF-κB signaling pathway. Ann. Transl. Med. 9 (22), 1694. doi:10.21037/atm-21-5752
Liu, L., Cen, J., Man, Y., Li, J., Zhang, D., Wang, F., et al. (2018a). Transplantation of human umbilical cord blood mononuclear cells attenuated ischemic injury in MCAO rats via inhibition of NF-κB and NLRP3 inflammasome. Neuroscience 369, 314–324. doi:10.1016/j.neuroscience.2017.11.027
Liu, M. X., Luo, L., Fu, J. H., He, J. Y., Chen, M. Y., He, Z. J., et al. (2022). Exercise-induced neuroprotection against cerebral ischemia/reperfusion injury is mediated via alleviating inflammasome-induced pyroptosis. Exp. Neurol. 349, 113952. doi:10.1016/j.expneurol.2021.113952
Liu, W., Guo, W., Zhu, Y., Peng, S., Zheng, W., Zhang, C., et al. (2018b). Targeting peroxiredoxin 1 by a curcumin analogue, AI-44, inhibits NLRP3 inflammasome activation and attenuates lipopolysaccharide-induced sepsis in mice. J. Immunol. 201 (8), 2403–2413. doi:10.4049/jimmunol.1700796
Liu, X., Zhang, M., Liu, H., Zhu, R., He, H., Zhou, Y., et al. (2021b). Bone marrow mesenchymal stem cell-derived exosomes attenuate cerebral ischemia-reperfusion injury-induced neuroinflammation and pyroptosis by modulating microglia M1/M2 phenotypes. Exp. Neurol. 341, 113700. doi:10.1016/j.expneurol.2021.113700
Liu, Z., Ran, Y., Huang, S., Wen, S., Zhang, W., Liu, X., et al. (2017). Curcumin protects against ischemic stroke by titrating microglia/macrophage polarization. Front. Aging Neurosci. 9, 233. doi:10.3389/fnagi.2017.00233
Lo Cascio, F., Marzullo, P., Kayed, R., and Palumbo Piccionello, A. (2021). Curcumin as scaffold for drug discovery against neurodegenerative diseases. Biomedicines 9 (2), 173. doi:10.3390/biomedicines9020173
Luo, J., Chen, J., Yang, C., Tan, J., Zhao, J., Jiang, N., et al. (2021). 6-Gingerol protects against cerebral ischemia/reperfusion injury by inhibiting NLRP3 inflammasome and apoptosis via TRPV1/FAF1 complex dissociation-mediated autophagy. Int. Immunopharmacol. 100, 108146. doi:10.1016/j.intimp.2021.108146
Luo, J., Jiang, N., Chen, J., Yu, G., Zhao, J., Yang, C., et al. (2022a). Inhibition of miR-423-5p exerts neuroprotective effects in an experimental rat model of cerebral ischemia/reperfusion injury. Neuroscience 503, 95–106. doi:10.1016/j.neuroscience.2022.08.024
Luo, L., Liu, M., Fan, Y., Zhang, J., Liu, L., Li, Y., et al. (2022b). Intermittent theta-burst stimulation improves motor function by inhibiting neuronal pyroptosis and regulating microglial polarization via TLR4/NFκB/NLRP3 signaling pathway in cerebral ischemic mice. J. Neuroinflammation 19 (1), 141. doi:10.1186/s12974-022-02501-2
Mahmood, K., Zia, K. M., Zuber, M., Salman, M., and Anjum, M. N. (2015). Recent developments in curcumin and curcumin based polymeric materials for biomedical applications: A review. Int. J. Biol. Macromol. 81, 877–890. doi:10.1016/j.ijbiomac.2015.09.026
Mao, M., Xu, Y., Zhang, X.-Y., Yang, L., An, X.-B., Qu, Y., et al. (2020). MicroRNA-195 prevents hippocampal microglial/macrophage polarization towards the M1 phenotype induced by chronic brain hypoperfusion through regulating CX3CL1/CX3CR1 signaling. J. Neuroinflammation 17 (1), 244. doi:10.1186/s12974-020-01919-w
Menon, V. P., and Sudheer, A. R. (2007). Antioxidant and anti-inflammatory properties of curcumin. Adv. Exp. Med. Biol. 595, 105–125. doi:10.1007/978-0-387-46401-5_3
Mhillaj, E., Tarozzi, A., Pruccoli, L., Cuomo, V., Trabace, L., and Mancuso, C. (2019). Curcumin and heme oxygenase: Neuroprotection and beyond. Int. J. Mol. Sci. 20 (10), 2419. doi:10.3390/ijms20102419
Minutoli, L., Puzzolo, D., Rinaldi, M., Irrera, N., Marini, H., Arcoraci, V., et al. (2016). ROS-mediated NLRP3 inflammasome activation in brain, heart, kidney, and testis ischemia/reperfusion injury. Oxid. Med. Cell. Longev. 2016, 2183026. doi:10.1155/2016/2183026
Nam, Y.-J., Choi, J., Lee, J. S., Seo, C., Lee, G., Lee, Y., et al. (2022). Curcuma phaeocaulis inhibits NLRP3 inflammasome in macrophages and ameliorates nanoparticle-induced airway inflammation in mice. Mol. (Basel, Switz. 27 (7), 2101. doi:10.3390/molecules27072101
Nguyen, H. D., Jo, W. H., Hoang, N. H. M., and Kim, M.-S. (2022). Curcumin-attenuated TREM-1/DAP12/NLRP3/caspase-1/IL1B, TLR4/NF-κB pathways, and tau hyperphosphorylation induced by 1,2-diacetyl benzene: An in vitro and in silico study. Neurotox. Res. 40 (5), 1272–1291. doi:10.1007/s12640-022-00535-1
O'Brien, W. T., Pham, L., Symons, G. F., Monif, M., Shultz, S. R., and McDonald, S. J. (2020). The NLRP3 inflammasome in traumatic brain injury: Potential as a biomarker and therapeutic target. J. Neuroinflammation 17 (1), 104. doi:10.1186/s12974-020-01778-5
Olesen, M. A., Torres, A. K., Jara, C., Murphy, M. P., and Tapia-Rojas, C. (2020). Premature synaptic mitochondrial dysfunction in the hippocampus during aging contributes to memory loss. Redox Biol. 34, 101558. doi:10.1016/j.redox.2020.101558
Orellana-Urzúa, S., Rojas, I., Líbano, L., and Rodrigo, R. (2020). Pathophysiology of ischemic stroke: Role of oxidative stress. Curr. Pharm. Des. 26 (34), 4246–4260. doi:10.2174/1381612826666200708133912
Palomino-Antolin, A., Narros-Fernández, P., Farré-Alins, V., Sevilla-Montero, J., Decouty-Pérez, C., Lopez-Rodriguez, A. B., et al. (2022). Time-dependent dual effect of NLRP3 inflammasome in brain ischaemia. Br. J. Pharmacol. 179 (7), 1395–1410. doi:10.1111/bph.15732
Pan-On, S., Dilokthornsakul, P., and Tiyaboonchai, W. (2022). Trends in advanced oral drug delivery system for curcumin: A systematic review. J. Control Release 348, 335–345. doi:10.1016/j.jconrel.2022.05.048
Patel, S. S., Acharya, A., Ray, R. S., Agrawal, R., Raghuwanshi, R., and Jain, P. (2020). Cellular and molecular mechanisms of curcumin in prevention and treatment of disease. Crit. Rev. Food Sci. Nutr. 60 (6), 887–939. doi:10.1080/10408398.2018.1552244
Peng, J., Wang, H., Gong, Z., Li, X., He, L., Shen, Q., et al. (2020). Idebenone attenuates cerebral inflammatory injury in ischemia and reperfusion via dampening NLRP3 inflammasome activity. Mol. Immunol. 123, 74–87. doi:10.1016/j.molimm.2020.04.013
Peng, Y., Ao, M., Dong, B., Jiang, Y., Yu, L., Chen, Z., et al. (2021). Anti-inflammatory effects of curcumin in the inflammatory diseases: Status, limitations and countermeasures. Drug Des. Devel Ther. 15, 4503–4525. doi:10.2147/DDDT.S327378
Pluta, R., Furmaga-Jabłońska, W., Januszewski, S., and Czuczwar, S. J. (2022). Post-ischemic brain neurodegeneration in the form of alzheimer's disease proteinopathy: Possible therapeutic role of curcumin. Nutrients 14 (2), 248. doi:10.3390/nu14020248
Pluta, R., Januszewski, S., and Czuczwar, S. J. (2021). The role of gut microbiota in an ischemic stroke. Int. J. Mol. Sci. 22 (2), 915. doi:10.3390/ijms22020915
Qiao, P., Ma, J., Wang, Y., Huang, Z., Zou, Q., Cai, Z., et al. (2020). Curcumin prevents neuroinflammation by inducing microglia to transform into the M2-phenotype via CaMKKβ-dependent activation of the AMP-activated protein kinase signal pathway. Curr. Alzheimer Res. 17 (8), 735–752. doi:10.2174/1567205017666201111120919
Qin, C., Zhou, L.-Q., Ma, X.-T., Hu, Z.-W., Yang, S., Chen, M., et al. (2019). Dual functions of microglia in ischemic stroke. Neurosci. Bull. 35 (5), 921–933. doi:10.1007/s12264-019-00388-3
Qiu, J., Wang, M., Zhang, J., Cai, Q., Lu, D., Li, Y., et al. (2016). The neuroprotection of Sinomenine against ischemic stroke in mice by suppressing NLRP3 inflammasome via AMPK signaling. Int. Immunopharmacol. 40, 492–500. doi:10.1016/j.intimp.2016.09.024
Ramaholimihaso, T., Bouazzaoui, F., and Kaladjian, A. (2020). Curcumin in depression: Potential mechanisms of action and current evidence-A narrative review. Front. Psychiatry 11, 572533. doi:10.3389/fpsyt.2020.572533
Ramírez-Carreto, R. J., Rodríguez-Cortés, Y. M., Torres-Guerrero, H., and Chavarría, A. (2023). Possible implications of obesity-primed microglia that could contribute to stroke-associated damage. Cell. Mol. Neurobiol.;43(6):2473–2490. doi:10.1007/s10571-023-01329-5
Ran, Y., Su, W., Gao, F., Ding, Z., Yang, S., Ye, L., et al. (2021). Curcumin ameliorates white matter injury after ischemic stroke by inhibiting microglia/macrophage pyroptosis through NF-κB suppression and NLRP3 inflammasome inhibition. Oxid. Med. Cell. Longev. 2021, 1552127. doi:10.1155/2021/1552127
Ren, W., Rubini, P., Tang, Y., Engel, T., and Illes, P. (2021). Inherent P2X7 receptors regulate macrophage functions during inflammatory diseases. Int. J. Mol. Sci. 23 (1), 232. doi:10.3390/ijms23010232
Ruan, Y., Xiong, Y., Fang, W., Yu, Q., Mai, Y., Cao, Z., et al. (2022). Highly sensitive Curcumin-conjugated nanotheranostic platform for detecting amyloid-beta plaques by magnetic resonance imaging and reversing cognitive deficits of Alzheimer's disease via NLRP3-inhibition. J. Nanobiotechnology 20 (1), 322. doi:10.1186/s12951-022-01524-4
Safdari, M. R., Shakeri, F., Mohammadi, A., Bibak, B., Alesheikh, P., Jamialahmadi, T., et al. (2021). Role of herbal medicines in the management of brain injury. Adv. Exp. Med. Biol. 1328, 287–305. doi:10.1007/978-3-030-73234-9_19
Saini, V., Guada, L., and Yavagal, D. R. (2021). Global epidemiology of stroke and access to acute ischemic stroke interventions. Neurology 97, S6–S16. doi:10.1212/WNL.0000000000012781
Saitoh, T., and Akira, S. (2016). Regulation of inflammasomes by autophagy. J. Allergy Clin. Immunol. 138 (1), 28–36. doi:10.1016/j.jaci.2016.05.009
Saleh, D. O., Nasr, M., Hassan, A., El-Awdan, S. A., and Abdel Jaleel, G. A. (2022). Curcumin nanoemulsion ameliorates brain injury in diabetic rats. J. Food Biochem. 46 (7), e14104. doi:10.1111/jfbc.14104
Sarmah, D., Datta, A., Raut, S., Sarkar, A., Shah, B., Bohra, M., et al. (2020). The role of inflammasomes in atherosclerosis and stroke pathogenesis. Curr. Pharm. Des. 26 (34), 4234–4245. doi:10.2174/1381612826666200427084949
Shah, Z. A., Li, R. C., Thimmulappa, R. K., Kensler, T. W., Yamamoto, M., Biswal, S., et al. (2007). Role of reactive oxygen species in modulation of Nrf2 following ischemic reperfusion injury. Neuroscience 147 (1), 53–59. doi:10.1016/j.neuroscience.2007.02.066
Shen, L.-L., Jiang, M.-L., Liu, S.-S., Cai, M.-C., Hong, Z.-Q., Lin, L.-Q., et al. (2015). Curcumin improves synaptic plasticity impairment induced by HIV-1gp120 V3 loop. Neural Regen. Res. 10 (6), 925–931. doi:10.4103/1673-5374.158358
Shen, Y., Qian, L., Luo, H., Li, X., Ruan, Y., Fan, R., et al. (2022). The significance of NLRP inflammasome in neuropsychiatric disorders. Brain Sci. 12 (8), 1057. doi:10.3390/brainsci12081057
Shi, M., Chen, J., Liu, T., Dai, W., Zhou, Z., Chen, L., et al. (2022a). Protective effects of remimazolam on cerebral ischemia/reperfusion injury in rats by inhibiting of NLRP3 inflammasome-dependent pyroptosis. Drug Des. Devel Ther. 16, 413–423. doi:10.2147/DDDT.S344240
Shi, Y., Han, L., Zhang, X., Xie, L., Pan, P., and Chen, F. (2022b). Selenium alleviates cerebral ischemia/reperfusion injury by regulating oxidative stress, mitochondrial fusion and ferroptosis. Neurochem. Res. 47 (10), 2992–3002. doi:10.1007/s11064-022-03643-8
Shi, Y., Liu, Q., Chen, W., Wang, R., Wang, L., Liu, Z.-Q., et al. (2023a). Protection of Taohong Siwu Decoction on PC12 cells injured by oxygen glucose deprivation/reperfusion via mitophagy-NLRP3 inflammasome pathway in vitro. J. Ethnopharmacol. 301, 115784. doi:10.1016/j.jep.2022.115784
Shi, Y., Wu, Q., Lu, Y., Meng, L.-P., Xu, X.-L., Wang, X.-J., et al. (2023b). Arginine-glycine-aspartic acid-anchored curcumin-based nanotherapeutics inhibit pyroptosis-induced cytokine release syndrome for in vivo and in vitro sepsis applications. Curr. Pharm. Des. 29, 283–294. doi:10.2174/1381612829666230201144029
Subedi, L., and Gaire, B. P. (2021). Neuroprotective effects of curcumin in cerebral ischemia: Cellular and molecular mechanisms. ACS Chem. Neurosci. 12 (14), 2562–2572. doi:10.1021/acschemneuro.1c00153
Sun, K., Zhang, J., Yang, Q., Zhu, J., Zhang, X., Wu, K., et al. (2021). Dexmedetomidine exerts a protective effect on ischemic brain injury by inhibiting the P2X7R/NLRP3/Caspase-1 signaling pathway. Brain Res. Bull. 174, 11–21. doi:10.1016/j.brainresbull.2021.05.006
Sun, R., Peng, M., Xu, P., Huang, F., Xie, Y., Li, J., et al. (2020a). Low-density lipoprotein receptor (LDLR) regulates NLRP3-mediated neuronal pyroptosis following cerebral ischemia/reperfusion injury. J. Neuroinflammation 17 (1), 330. doi:10.1186/s12974-020-01988-x
Sun, W., Wang, Q., Zhang, R., and Zhang, N. (2023). Ketogenic diet attenuates neuroinflammation and induces conversion of M1 microglia to M2 in an EAE model of multiple sclerosis by regulating the NF-κB/NLRP3 pathway and inhibiting HDAC3 and P2X7R activation. Food Funct. 14 (15), 7247–7269. doi:10.1039/d3fo00122a
Sun, Z. Z., Li, X. Y., Wang, S., Shen, L., and Ji, H. F. (2020b). Bidirectional interactions between curcumin and gut microbiota in transgenic mice with Alzheimer's disease. Appl. Microbiol. Biotechnol. 104 (8), 3507–3515. doi:10.1007/s00253-020-10461-x
Syeda, T., Sanchez-Tapia, M., Orta, I., Granados-Portillo, O., Perez-Jimenez, L., Rodriguez-Callejas, J. D., et al. (2021). Bioactive foods decrease liver and brain alterations induced by a high-fat-sucrose diet through restoration of gut microbiota and antioxidant enzymes. Nutrients 14 (1), 22. doi:10.3390/nu14010022
Tajalli-Nezhad, S., Karimian, M., Beyer, C., Atlasi, M. A., and Azami Tameh, A. (2019). The regulatory role of toll-like receptors after ischemic stroke: Neurosteroids as TLR modulators with the focus on TLR2/4. Cell. Mol. Life Sci. 76 (3), 523–537. doi:10.1007/s00018-018-2953-2
Tang, Z., Gan, Y., Liu, Q., Yin, J.-X., Liu, Q., Shi, J., et al. (2014). CX3CR1 deficiency suppresses activation and neurotoxicity of microglia/macrophage in experimental ischemic stroke. J. Neuroinflammation 11, 26. doi:10.1186/1742-2094-11-26
Teertam, S. K., and Phanithi, P. B. (2022). Up-regulation of sirtuin-1/autophagy signaling in human cerebral ischemia: Possible role in caspase-3 mediated apoptosis. Heliyon 8 (12), e12278. doi:10.1016/j.heliyon.2022.e12278
Tu, Y., Guo, C., Song, F., Huo, Y., Geng, Y., Guo, M., et al. (2019). Mild hypothermia alleviates diabetes aggravated cerebral ischemic injury via activating autophagy and inhibiting pyroptosis. Brain Res. Bull. 150, 1–12. doi:10.1016/j.brainresbull.2019.05.003
Tuo, Q.-Z., Zhang, S.-T., and Lei, P. (2022). Mechanisms of neuronal cell death in ischemic stroke and their therapeutic implications. Med. Res. Rev. 42 (1), 259–305. doi:10.1002/med.21817
Wang, J., Zhang, H., He, J., and Xiong, X. (2022a). The role of the gut microbiota in the development of ischemic stroke. Front. Immunol. 13, 845243. doi:10.3389/fimmu.2022.845243
Wang, L., Ren, W., Wu, Q., Liu, T., Wei, Y., Ding, J., et al. (2022b). NLRP3 inflammasome activation: A therapeutic target for cerebral ischemia-reperfusion injury. Front. Mol. Neurosci. 15, 847440. doi:10.3389/fnmol.2022.847440
Wang, Q.-S., Luo, X.-Y., Fu, H., Luo, Q., Wang, M.-Q., and Zou, D.-Y. (2020a). MiR-139 protects against oxygen-glucose deprivation/reoxygenation (OGD/R)-induced nerve injury through targeting c-Jun to inhibit NLRP3 inflammasome activation. J. Stroke Cerebrovasc. Dis. 29 (9), 105037. doi:10.1016/j.jstrokecerebrovasdis.2020.105037
Wang, Q., Sun, A. Y., Simonyi, A., Jensen, M. D., Shelat, P. B., Rottinghaus, G. E., et al. (2005). Neuroprotective mechanisms of curcumin against cerebral ischemia-induced neuronal apoptosis and behavioral deficits. J. Neurosci. Res. 82 (1), 138–148. doi:10.1002/jnr.20610
Wang, Y.-f., Gu, Y.-t., Qin, G.-h., Zhong, L., and Meng, Y.-n. (2013). Curcumin ameliorates the permeability of the blood-brain barrier during hypoxia by upregulating heme oxygenase-1 expression in brain microvascular endothelial cells. J. Mol. Neurosci. MN 51 (2), 344–351. doi:10.1007/s12031-013-9989-4
Wang, Y., Meng, C., Zhang, J., Wu, J., and Zhao, J. (2019a). Inhibition of GSK-3β alleviates cerebral ischemia/reperfusion injury in rats by suppressing NLRP3 inflammasome activation through autophagy. Int. Immunopharmacol. 68, 234–241. doi:10.1016/j.intimp.2018.12.042
Wang, Y., Shi, P., Chen, Q., Huang, Z., Zou, D., Zhang, J., et al. (2019b). Mitochondrial ROS promote macrophage pyroptosis by inducing GSDMD oxidation. J. Mol. Cell. Biol. 11 (12), 1069–1082. doi:10.1093/jmcb/mjz020
Wang, Z., Xu, G., Li, Z., Xiao, X., Tang, J., and Bai, Z. (2022c). NLRP3 inflammasome pharmacological inhibitors in Glycyrrhiza for NLRP3-driven diseases treatment: Extinguishing the fire of inflammation. J. Inflamm. Res. 15, 409–422. doi:10.2147/JIR.S344071
Wang, Z., Yang, C., Liu, J., Chun-Kit Tong, B., Zhu, Z., Malampati, S., et al. (2020b). A curcumin derivative activates TFEB and protects against parkinsonian neurotoxicity in vitro. Int. J. Mol. Sci. 21 (4), 1515. doi:10.3390/ijms21041515
Wang, Z., Zhang, S., Du, J., Lachance, B. B., Chen, S., Polster, B. M., et al. (2023). Neuroprotection of NSC therapy is superior to glibenclamide in cardiac arrest-induced brain injury via neuroinflammation regulation. Transl. Stroke Res. 14 (5), 723–739. doi:10.1007/s12975-022-01047-y
Ward, R., Li, W., Abdul, Y., Jackson, L., Dong, G., Jamil, S., et al. (2019). NLRP3 inflammasome inhibition with MCC950 improves diabetes-mediated cognitive impairment and vasoneuronal remodeling after ischemia. Pharmacol. Res. 142, 237–250. doi:10.1016/j.phrs.2019.01.035
Wu, J., Li, Q., Wang, X., Yu, S., Li, L., Wu, X., et al. (2013). Neuroprotection by curcumin in ischemic brain injury involves the Akt/Nrf2 pathway. PloS One 8 (3), e59843. doi:10.1371/journal.pone.0059843
Wu, S., Guo, T., Qi, W., Li, Y., Gu, J., Liu, C., et al. (2021). Curcumin ameliorates ischemic stroke injury in rats by protecting the integrity of the blood-brain barrier. Exp. Ther. Med. 22 (1), 783. doi:10.3892/etm.2021.10215
Xie, X., Tao, Q., Zou, Y., Zhang, F., Guo, M., Wang, Y., et al. (2011). PLGA nanoparticles improve the oral bioavailability of curcumin in rats: Characterizations and mechanisms. J. Agric. Food Chem. 59 (17), 9280–9289. doi:10.1021/jf202135j
Xu, H., Nie, B., Liu, L., Zhang, C., Zhang, Z., Xu, M., et al. (2019). Curcumin prevents brain damage and cognitive dysfunction during ischemic-reperfusion through the regulation of miR-7-5p. Curr. Neurovasc Res. 16 (5), 441–454. doi:10.2174/1567202616666191029113633
Yang, B., Li, Y., Ma, Y., Zhang, X., Yang, L., Shen, X., et al. (2021a). Selenium attenuates ischemia/reperfusion injury-induced damage to the blood-brain barrier in hyperglycemia through PI3K/AKT/mTOR pathway-mediated autophagy inhibition. Int. J. Mol. Med. 48 (3), 178. doi:10.3892/ijmm.2021.5011
Yang, J.-L., Mukda, S., and Chen, S.-D. (2018). Diverse roles of mitochondria in ischemic stroke. Redox Biol. 16, 263–275. doi:10.1016/j.redox.2018.03.002
Yang, L., Zhang, Y., Yu, X., Li, D., Liu, N., Xue, X., et al. (2023a). Periventricular microglia polarization and morphological changes accompany NLRP3 inflammasome-mediated neuroinflammation after hypoxic-ischemic white matter damage in premature rats. J. Immunol. Res. 2023, 5149306. doi:10.1155/2023/5149306
Yang, M., Deng, S., Jiang, J., Tian, M., Xiao, L., and Gong, Y. (2023b). Oxytocin improves intracerebral hemorrhage outcomes by suppressing neuronal pyroptosis and mitochondrial fission. Stroke 54 (7), 1888–1900. doi:10.1161/STROKEAHA.123.043391
Yang, X., Song, D., Chen, L., Xiao, H., Ma, X., Jiang, Q., et al. (2021b). Curcumin promotes neurogenesis of hippocampal dentate gyrus via Wnt/β-catenin signal pathway following cerebral ischemia in mice. Brain Res. 1751, 147197. doi:10.1016/j.brainres.2020.147197
Yang, Y., Wang, H., Kouadir, M., Song, H., and Shi, F. (2019). Recent advances in the mechanisms of NLRP3 inflammasome activation and its inhibitors. Cell. Death Dis. 10 (2), 128. doi:10.1038/s41419-019-1413-8
Yao, Y., Hu, S., Zhang, C., Zhou, Q., Wang, H., Yang, Y., et al. (2022). Ginsenoside Rd attenuates cerebral ischemia/reperfusion injury by exerting an anti-pyroptotic effect via the miR-139-5p/FoxO1/Keap1/Nrf2 axis. Int. Immunopharmacol. 105, 108582. doi:10.1016/j.intimp.2022.108582
Ye, Y., Jin, T., Zhang, X., Zeng, Z., Ye, B., Wang, J., et al. (2019). Meisoindigo protects against focal cerebral ischemia-reperfusion injury by inhibiting NLRP3 inflammasome activation and regulating microglia/macrophage polarization via TLR4/NF-κB signaling pathway. Front. Cell. Neurosci. 13, 553. doi:10.3389/fncel.2019.00553
Yin, H., Guo, Q., Li, X., Tang, T., Li, C., Wang, H., et al. (2018). Curcumin suppresses IL-1β secretion and prevents inflammation through inhibition of the NLRP3 inflammasome. J. Immunol. 200 (8), 2835–2846. doi:10.4049/jimmunol.1701495
Yin, J., Liao, S.-X., He, Y., Wang, S., Xia, G.-H., Liu, F.-T., et al. (2015). Dysbiosis of gut microbiota with reduced trimethylamine-N-oxide level in patients with large-artery atherosclerotic stroke or transient ischemic attack. J. Am. Heart Assoc. 4 (11), e002699. doi:10.1161/JAHA.115.002699
Yu, W., Qin, X., Zhang, Y., Qiu, P., Wang, L., Zha, W., et al. (2020). Curcumin suppresses doxorubicin-induced cardiomyocyte pyroptosis via a PI3K/Akt/mTOR-dependent manner. Cardiovasc Diagn Ther. 10 (4), 752–769. doi:10.21037/cdt-19-707
Zahid, A., Li, B., Kombe, A. J. K., Jin, T., and Tao, J. (2019). Pharmacological inhibitors of the NLRP3 inflammasome. Front. Immunol. 10, 2538. doi:10.3389/fimmu.2019.02538
Zeng, X., Zhang, Y.-D., Ma, R.-Y., Chen, Y.-J., Xiang, X.-M., Hou, D.-Y., et al. (2022). Activated Drp1 regulates p62-mediated autophagic flux and aggravates inflammation in cerebral ischemia-reperfusion via the ROS-RIP1/RIP3-exosome axis. Mil. Med. Res. 9 (1), 25. doi:10.1186/s40779-022-00383-2
Zhai, J., Li, N., Zhang, X., Li, Y., Ma, K., Wang, R., et al. (2023). Isoflurane enhances autophagy by activating AMPK/ULK1, inhibits NLRP3, and reduces cognitive impairment after cerebral ischemia-reperfusion injury in rats. J. Mol. Neurosci. MN 73, 549–562. doi:10.1007/s12031-023-02135-w
Zhang, F., Zhou, Y., Chen, H., Jiang, H., Zhou, F., Lv, B., et al. (2022). Curcumin alleviates DSS-induced anxiety-like behaviors via the microbial-brain-gut Axis. Oxid. Med. Cell. Longev. 2022, 6244757. doi:10.1155/2022/6244757
Zhang, H.-S., Ouyang, B., Ji, X.-Y., and Liu, M.-F. (2021). Gastrodin alleviates cerebral ischaemia/reperfusion injury by inhibiting pyroptosis by regulating the lncRNA NEAT1/miR-22-3p Axis. Neurochem. Res. 46 (7), 1747–1758. doi:10.1007/s11064-021-03285-2
Zhang, J., Zheng, Y., Luo, Y., Du, Y., Zhang, X., and Fu, J. (2019). Curcumin inhibits LPS-induced neuroinflammation by promoting microglial M2 polarization via TREM2/TLR4/NF-κB pathways in BV2 cells. Mol. Immunol. 116, 29–37. doi:10.1016/j.molimm.2019.09.020
Zhang, S., Jiang, L., Che, F., Lu, Y., Xie, Z., and Wang, H. (2017). Arctigenin attenuates ischemic stroke via SIRT1-dependent inhibition of NLRP3 inflammasome. Biochem. Biophysical Res. Commun. 493 (1), 821–826. doi:10.1016/j.bbrc.2017.08.062
Zhang, Y., Cao, Y., and Liu, C. (2020). Autophagy and ischemic stroke. Adv. Exp. Med. Biol. 1207, 111–134. doi:10.1007/978-981-15-4272-5_7
Zhao, Y., Chen, F., Chen, S., Liu, X., Cui, M., and Dong, Q. (2013). The Parkinson's disease-associated gene PINK1 protects neurons from ischemic damage by decreasing mitochondrial translocation of the fission promoter Drp1. J. Neurochem. 127 (5), 711–722. doi:10.1111/jnc.12340
Zhao, Y., Zhang, X., Chen, X., and Wei, Y. (2022). Neuronal injuries in cerebral infarction and ischemic stroke: From mechanisms to treatment (Review). Int. J. Mol. Med. 49 (2), 15. doi:10.3892/ijmm.2021.5070
Zhao, Y., Zhu, T., Li, H., Zhao, J., and Li, X. (2019). Transplantation of lymphocytes Co-cultured with human cord blood-derived multipotent stem cells attenuates inflammasome activity in ischemic stroke. Clin. Interv. Aging 14, 2261–2271. doi:10.2147/CIA.S223595
Zhong, X., Chen, B., Li, Z., Lin, R., Ruan, S., Wang, F., et al. (2022). Electroacupuncture ameliorates cognitive impairment through the inhibition of NLRP3 inflammasome activation by regulating melatonin-mediated mitophagy in stroke rats. Neurochem. Res. 47 (7), 1917–1930. doi:10.1007/s11064-022-03575-3
Zhou, R., Yazdi, A. S., Menu, P., and Tschopp, J. (2011). A role for mitochondria in NLRP3 inflammasome activation. Nature 469 (7329), 221–225. doi:10.1038/nature09663
Zusso, M., Lunardi, V., Franceschini, D., Pagetta, A., Lo, R., Stifani, S., et al. (2019). Ciprofloxacin and levofloxacin attenuate microglia inflammatory response via TLR4/NF-kB pathway. J. Neuroinflammation 16 (1), 148. doi:10.1186/s12974-019-1538-9
Keywords: curcumin, ischemic stroke, NLRP3 inflammasome, inflammation, neuroprotection
Citation: Du X, Amin N, Xu L, Botchway BOA, Zhang B and Fang M (2023) Pharmacological intervention of curcumin via the NLRP3 inflammasome in ischemic stroke. Front. Pharmacol. 14:1249644. doi: 10.3389/fphar.2023.1249644
Received: 29 June 2023; Accepted: 26 September 2023;
Published: 17 October 2023.
Edited by:
Hisashi Shirakawa, Kyoto University, JapanReviewed by:
Macarena Hernández-Jiménez, Complutense University of Madrid, SpainCopyright © 2023 Du, Amin, Xu, Botchway, Zhang and Fang. This is an open-access article distributed under the terms of the Creative Commons Attribution License (CC BY). The use, distribution or reproduction in other forums is permitted, provided the original author(s) and the copyright owner(s) are credited and that the original publication in this journal is cited, in accordance with accepted academic practice. No use, distribution or reproduction is permitted which does not comply with these terms.
*Correspondence: Marong Fang, ZmFuZ21hcm9Aemp1LmVkdS5jbg==
Disclaimer: All claims expressed in this article are solely those of the authors and do not necessarily represent those of their affiliated organizations, or those of the publisher, the editors and the reviewers. Any product that may be evaluated in this article or claim that may be made by its manufacturer is not guaranteed or endorsed by the publisher.
Research integrity at Frontiers
Learn more about the work of our research integrity team to safeguard the quality of each article we publish.