- 1Department of Pharmacy, Hangzhou Women’s Hospital (Hangzhou Maternity and Child Health Care Hospital), Hangzhou, China
- 2Guangzhou Institute of Respiratory Disease and China State Key Laboratory of Respiratory Disease, The First Affiliated Hospital of Guangzhou Medical University, Guangzhou, China
- 3Department of Pharmacy, Zhujiang Hospital, Southern Medical University, Guangzhou, China
- 4Department of Pharmacy, The Affiliated Changsha Central Hospital, Hengyang Medical School, University of South China, Changsha, China
Abstract
Doxorubicin is one of the most classical chemotherapeutic drugs for the treatment of cancer. However, resistance to the cytotoxic effects of doxorubicin in tumor cells remains a major obstacle. Aberrant expression of long non-coding RNAs (lncRNAs) has been associated with tumorigenesis and development via regulation of chromatin remodeling, transcription, and post-transcriptional processing. Emerging studies have also revealed that dysregulation of lncRNAs mediates the development of drug resistance through multiple molecules and pathways. In this review, we focus on the role and mechanism of lncRNAs in the progress of doxorubicin resistance in various cancers, which mainly include cellular drug transport, cell cycle disorder, anti-apoptosis, epithelial-mesenchymal transition, cancer stem cells, autophagy, tumor microenvironment, metabolic reprogramming and signaling pathways. This review is aimed to provide potential therapeutic targets for future cancer therapy, especially for the reversal of chemoresistance.
Introduction
Cancer has become one of the most common diseases and a leading cause of death. It is estimated that 19.3 million new cancer cases occurred worldwide in 2020 with almost 10.0 million cancer deaths (Sung et al., 2021). In 2040, the global cancer burden is even expected to reach 28.4 million cases, representing a 47% rise compared to 2020 (Sung et al., 2021). Research on cancer has drawn extensive attention and great progress has been made regarding to cancer screening, early diagnosis and effective treatment. For example, the mortality rate of breast cancer (BRCA) is shown to fall steadily, with an about 35% decline over the past three decades (Malvezzi et al., 2019). Chemotherapy alongside surgery and radiotherapy, usually constitutes the standard regimen of cancer therapy (Mariette et al., 2007). However, when cancer is advanced or patients cannot suffer surgery, chemotherapy then becomes the last strategy.
Doxorubicin (DOX) is an anthracycline antibiotic which was isolated from the pigment-producing Streptomyces peucetius early in the 1960s (A et al., 2000). It is one of the most widely employed chemotherapeutic agents for the treatment of both hematological and solid tumors, including breast cancer, ovarian cancer (OC), bladder cancer (BLCA), lung cancer (LC), and acute myeloblastic leukemia (AML) (Blum and Carter, 1974; Young et al., 1981; Hulst et al., 2022). Doxorubicin stabilizes a reaction intermediate in which DNA strands are cut and covalently linked to tyrosine residues of topoisomerase II (Top2), eventually blocking DNA relegation (Minotti et al., 2004; Pommier et al., 2010). In addition, doxorubicin generates free radicals, leading to DNA damage or lipid peroxidation; interferes with DNA unwinding or DNA strand separation and helicase activity; induces apoptosis in response to Top2 inhibition (Gewirtz, 1999; Minotti et al., 2004; Pommier et al., 2010). DOX can also induce histone eviction from open chromatin, which attenuates the DNA damage response, triggers epigenetic alterations and induces apoptosis (Pang et al., 2013).
Since its discovery, DOX has brought a substantial improvement in cancer therapy. The introduction of DOX into the adjuvant therapy of BRCA demonstrated definite benefit in disease-free survival and overall survival (Hortobágyi and Buzdar, 1993). Gastric cancer (GC) was ever considered refractory to chemotherapy, whereas the addition of DOX produced encouraging response rates over 40% and increased median overall survival (Wadler et al., 1985). Along with the wide application, intrinsic and acquired resistance to DOX remains a major clinical problem. Some studies revealed resistance to DOX due to increase of drug efflux and reduction in drug accumulation, mediated by members of the ATP-binding cassette (ABC) superfamily (Grant et al., 1994; Velamakanni et al., 2007; Broxterman et al., 2009). The members of ABC transporters regulate the absorption, distribution, and clearance of pharmacological agents (Vasiliou et al., 2009). However, though many investigations are devoted to the development of transporter inhibitors for reversal of resistance, it has not been successful in improving the clinical response to chemotherapy, causing our consideration on the real nature of chemoresistance (Abraham et al., 2009). Undoubtedly, it will be of key importance for clinical studies to define the exact mechanisms mediating doxorubicin resistance.
Long non-coding RNAs (lncRNAs) are a kind of transcriptional products with a length longer than 200 nucleotides and no or low protein-encoding ability (Alexander et al., 2010; Uchida and Dimmeler, 2015; Yang L et al., 2014). Similar to coding genes, lncRNAs are usually transcribed by RNA polymerase II and have a poly-A tail (Gibb et al., 2011), but their sequence are less conserved than that of mRNAs (Pang et al., 2006). LncRNA has only been regarded as the “transcriptional noise” of the genome, rather than having biological functions, for a long time after its discovery (Ponjavic et al., 2007; Struhl, 2007). In recent years, more and more studies have shown that lncRNAs are widely involved in the regulation of gene expression at epigenetic, transcriptional and posttranscriptional levels (Yang Get al., 2014), playing an important role in cell differentiation, organogenesis, tissue homeostasis and other critical life activities (Mercer et al., 2009; Hung and Chang, 2010; Schmitz et al., 2016). In addition, the abnormal expression of lncRNAs is also closely related to the occurrence and development of cancer and chemoresistance (Batista and Chang, 2013; Evans et al., 2016). Insights into the role of lncRNAs in DOX resistance will help to deepen our understanding of chemoresistance formation and provide potentially targetable or predictive biomarkers of chemotherapy, which is the point of our review.
lncRNA and doxorubicin resistance
LncRNAs have received extensive attention for its modulation in cancer progress as well as therapeutic response. Aiming to uncover the role and mechanism of lncRNAs in DOX resistance, databases were searched for published reports focused on “lncRNA and doxorubicin resistance”. The qualified articles were further straightened out and categorized in this section with the main mechanisms and implicated lncRNAs summarized in Figure 1.
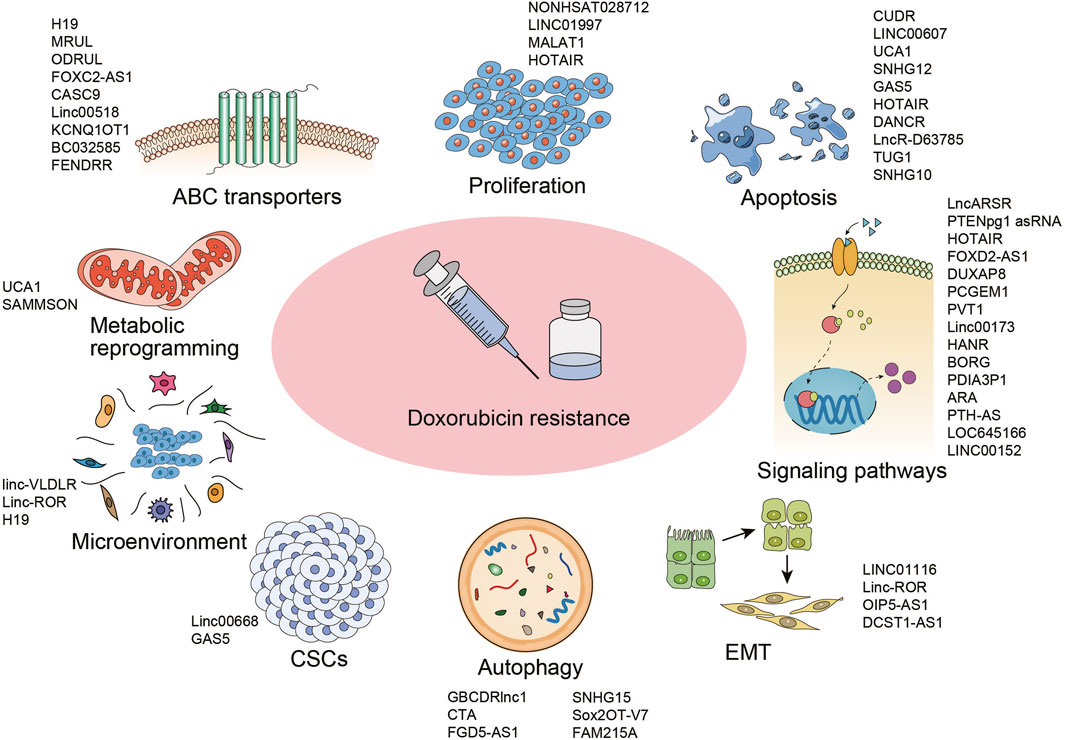
FIGURE 1. Mechanisms underlying doxorubicin resistance in cancer and the implicated lncRNAs. Abbreviations: ABC, ATP-binding cassette; EMT, epithelial to mesenchymal transition; CSCs, cancer stem cells.
ABC transporters
The ATP-binding cassette (ABC) transporter family is a big family regulating cellular levels of hormones, lipids, ions, xenobiotics and other small molecules (Robey et al., 2018). Altered membrane transport and enhanced drug efflux mediated by over-expression of ABC superfamily, including ABCB1 and ABCC1, is one of the main and most studied mechanisms of doxorubicin resistance (Lehne, 2000). Known LncRNAs mediating the regulation of transporter expression were summarized in Figure 2.
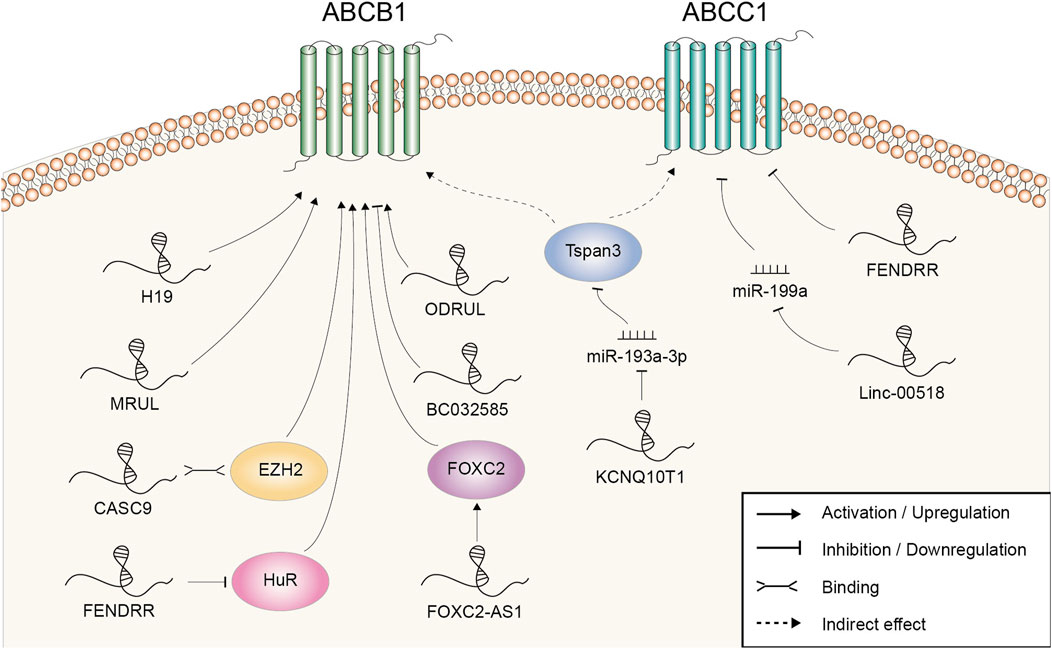
FIGURE 2. Doxorubicin resistance-related lncRNAs involved in the regulation of ABCB1 and ABCC1 and the underlying mechanisms.
H19 was the first lncRNA found to be implicated in ABCB1 regulation in human hepatocellular carcinoma (HCC). Reduced expression of H19 could suppress ABCB1 expression, which led to the increase of cellular DOX concentration and DOX sensitivity (Tsang and Kwok, 2007). Mechanistically, ABCB1 gene promoter was hypomethylated in resistant HCC cells, while H19 silencing induced a marked increase in ABCB1 promoter methylation and decrease in ABCB1 expression (Tsang and Kwok, 2007). There was also evidence that lncRNA MRUL could contribute to DOX resistance by playing an enhancer-like role in promoting ABCB1 expression in GC (Wang et al., 2014). MRUL knockdown led to increased drug accumulation and apoptosis in DOX-resistant SGC7901 cell (Wang et al., 2014). LncRNA microarray revealed that the expression levels of over 3,000 lncRNAs were altered in the DOX-resistant osteosarcoma (OSA) cell line MG63/DXR compared with the parental MG63 cell and ODRUL was the most upregulated one (Zhang et al., 2016). ODRUL might participate in DOX resistance by targeting ABCB1. In addition, the clinical results showed that high expression of ODRUL was correlated with poor chemotherapy response and prognosis (Zhang et al., 2016). Recently, Zhang et al. found that lncRNA FOXC2-AS1 expression was significantly higher in DOX-resistant OSA cell lines and tissues, and correlated with poor prognosis (Zhang C. L. et al., 2017). Functional studies revealed that silencing of FOXC2-AS1 abolished the growth of DOX-resistant OSA cell and improved the sensitivity to DOX in vitro and in vivo. Further mechanistic studies demonstrated that FOXC2-AS1 promoted the expression of transcription factor FOXC2 at both the transcription and post-transcription levels, further stimulating the expression of downstream ABCB1. CASC9, a lncRNA upregulated in doxorubicin-resistant BRCA cell, might regulate the expression of ABCB1 through EZH2. EZH2 was demonstrated to be a binding protein of CASC9. Meanwhile, EZH2 depletion resulted in suppressed ABCB1 expression (Jiang et al., 2018). Linc00518 and ABCC1 expression were both upregulated in DOX-resistant BRCA cell. Linc00518 could act as a molecular sponge of miR-199a to upregulate ABCC1 expression, thus conferring chemoresistance to DOX (Chang et al., 2018). LncRNA KCNQ1OT1 was upregulated in DOX resistant AML samples and cells. Through adsorbing miR-193a-3p, KCNQ1OT1 induced the expression of Tspan3. Unfortunately, the underlying mechanism of Tspan3 in chemoresistance was not revealed by the authors or reported elsewhere. However, the expression of ABCC1 and ABCB1 was found to be strictly regulated by Tspan3 (Sun et al., 2020).
Using the lncRNA expression profiling of BRCA patients from Gene Expression Omnibus datasets, our group screened out three lncRNAs (AK291479, U79293, and BC032585) to be significantly associated with anthracycline-based chemotherapeutic response (Zeng et al., 2019). BC032585 was further chosen to figure out its molecular function in vitro. It was observed that knockdown of BC032585 resulted in a stronger resistance to DOX as accessed by cell viability and this function was at least partly mediated by the upregulation of ABCB1. Collectively, this study had opened out a new approach for the identification of clinically useful lncRNA markers.
LncRNA could also negatively regulated ABCB1 expression and acted as a chemosensitivity mediator. LncRNA microarray found that FENDRR was the most downregulated lncRNA with a 22-fold change in the paired DOX-resistant and sensitive human OSA cell lines (Kun-Peng et al., 2017a). Functional studies revealed that FENDRR suppressed cell cycle, promoted apoptosis and increased DOX sensitivity of OSA cells in vitro. Moreover, further studies demonstrated that FENDRR inhibited DOX resistance through negatively affecting posttranscriptional expression of ABCB1 and ABCC1 (Kun-Peng et al., 2017b). FENDRR was also downregulated in resistant chronic myeloid leukaemia (CML) cells. The overexpression of FENDRR attenuated DOX resistance, as shown by increased DOX accumulation and enhanced cell apoptosis in vitro and in vivo. Both FENDRR and ABCB1 mRNA contained several AU-rich elements and competitively bound to the RNA-binding protein HuR (Zhang et al., 2019). Previous studies indicated that this interaction with RNA-binding protein was beneficial to keeping the mRNA stabilization and/or regulating the translation (Bish and Vogel, 2014). As a result, aberrations in FENDRR expression led to the opposite change of ABCB1 level.
Apoptosis
In addition to targeting the multidrug transporter proteins, a part of lncRNAs involved in DOX resistance have been shown to regulate apoptosis-related genes (Figure 3). It is unsurprising because triggering apoptosis induction to eliminate malignant cells is exactly the way how most chemotherapeutic drugs work (Mohammad et al., 2015).
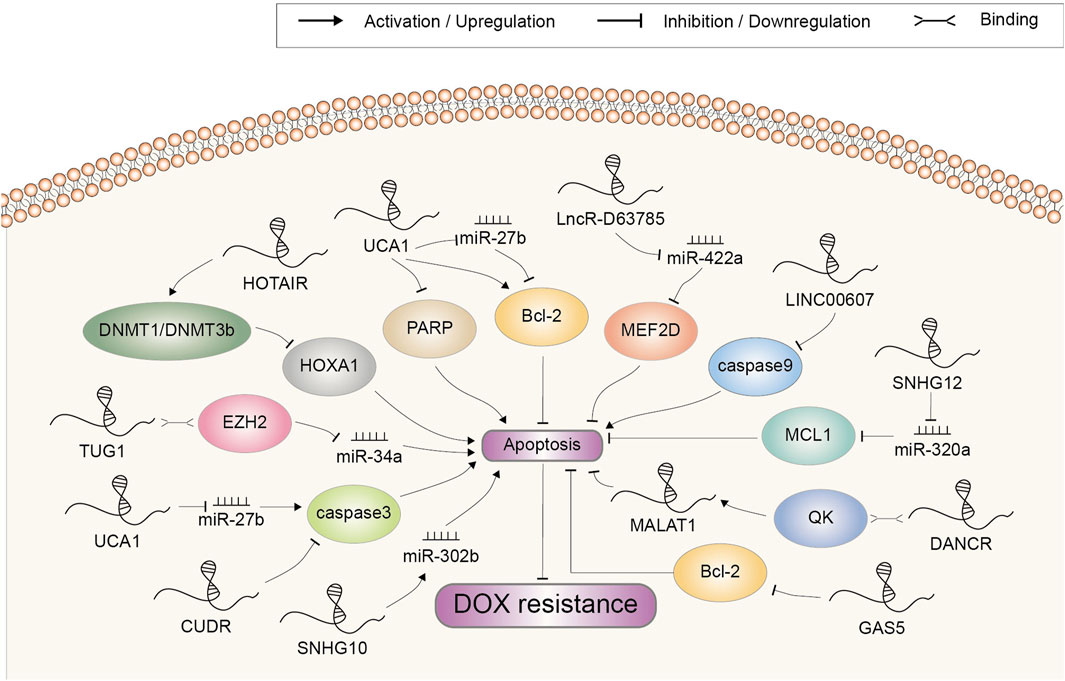
FIGURE 3. Doxorubicin resistance-related lncRNAs involved in the regulation of cell apoptosis and the underlying mechanisms. Abbreviations: DOX, doxorubicin.
Caspases are evolutionarily conserved cysteine proteases with a well-defined role in apoptosis. Mammalian apoptotic caspases are generally divided into the initiators (caspase 2, 8, 9, and 10) and the effectors (caspase 3, 6, and 7), all of which must undergo proteolytic activation to execute their function (Shi, 2002; Van Opdenbosch and Lamkanfi, 2019). CUDR was a novel gene found to be overexpressed in A10A cell, a DOX-resistant subline of human squamous carcinoma (HSC) A431 cell (Tsang et al., 2007). Since the CUDR cDNA sequence contained no distinct open reading frames, it was inferred that CUDR possibly exerted its function as a long non-coding RNA. Further study indicated that the CUDR-inhibited apoptosis was at least dependent on downregulation of caspase 3 (Tsang et al., 2007). LINC00607 was upregulated in DOX-resistant thyroid cancer (TC) cell. It decreased caspase 9 expression by promoting the methylation of caspase 9 promoter, thereby inhibiting the apoptosis induction and augmenting DOX resistance (Li L. et al., 2021).
Above-mentioned initiator caspases activation can be mediated by anti-apoptosis protein Bcl-2-regulated pathway under cytotoxic drugs-induced cellular stress (Shalini et al., 2015). Shang et al. found that UCA1 silencing advanced cell apoptosis induced by DOX in GC cell through promoting cleaved PARP protein expression and depressing the expression of Bcl-2, indicating a promoting role in resistance development (Shang et al., 2016). Another study also demonstrated that UCA1 increased chemoresistance of GC cell via negatively regulating miR-27b. Mechanistically, UCA1 knockdown or miR-27b overexpression increased DOX-induced cell apoptosis by decreasing Bcl-2 protein expression and increasing cleaved caspase 3 (Fang Q. et al., 2016). High expression of SNHG12 was correlated with chemoresistance to DOX and a poor overall survival in OSA. In addition, a higher expression of SNHG12 was revealed in DOX-resistant cells compared to parental sensitive cells. SNHG12 mainly targeted miR-320a to upregulate MCL1, which has been reported to be a Bcl-2 family apoptosis regulator and exhibit a crucial function in suppressing cell apoptosis (Zhao et al., 2017; Zhou B. et al., 2018). Notably, lncRNA GAS5 was reported to inhibit rather than promote chemoresistance in bladder transitional cell carcinoma (BTCC). Overexpression of GAS5 promoted the induction of apoptosis by DOX and depressed Bcl-2 expression, whereas upregulated Bcl-2 largely reversed GAS5-induced sensitivity to DOX. Clinically, BTCC patients with lower level of GAS5 had a significantly worse disease free survival (Zhang H. et al., 2017). Altogether, these data confirmed that lncRNAs could affect the response of cancer to DOX according to their regulation pattern in Bcl-2 expression.
HOTAIR was upregulated in the DOX-resistant small cell lung cancer cell (SCLC). Depletion of HOTAIR increased drug sensitivity by enhancing cell apoptosis and decelerating cell cycle progression. Moreover, HOTAIR knockdown reduced HOXA1 methylation by decreasing DNMT1 and DNMT3b expression. Summarily, HOTAIR modulated chemotherapy resistance in SCLC by regulating HOXA1 methylation (Fang S. et al., 2016). DANCR was found to be suppressed by DOX in a high throughput screening in colorectal cancer (CC) cell. Via interacting with the RNA-binding protein QK, DANCR enhanced the RNA stability of MALAT1, which further mediate the suppressive function of DANCR on DOX-induced apoptosis (Xiong et al., 2021). This study established DANCR as an important repressor of apoptosis in CC. LncR-D63785 was highly expressed in GC tissues and cells. Knockdown of lncR-D63785 fostered the apoptosis of GC cells treated with DOX. It functioned as a sponge of miR-422a and promoted chemoresistance by blocking miR-422-dependent suppression of MEF2D (Zhou Z. et al., 2018). TUG1, a lncRNA upregulated in DOX-resistant AML tissues and cells, could epigenetically suppress miR-34a expression via recruiting EZH2 to its promoter. Either TUG1 knockdown or miR-34a overexpression remarkably facilitated cell chemosensitivity by enhancing DOX-induced apoptosis (Li Q. et al., 2019). LncRNA SNHG10 was downregulated in triple negative breast cancer (TNBC) cells after DOX treatment, and overexpression of SNHG10 significantly promoted DOX-induced apoptosis. Mechanism research showed that SNHG10 could inhibit the development of resistance to DOX by upregulating miR-302b through methylation modulation (Aini et al., 2022).
Cell proliferation
Uncontrolled proliferation is a hallmark of cancer, typically utilized by cancer cells to resist chemotherapeutic agent-induced growth suppression (Zheng et al., 2019). LncRNAs were also found to participate in aberrant cell proliferation and DOX resistance (Figure 4). Microarray analysis revealed that NONHSAT028712 was significantly increased in DOX-resistant BRCA cells. Further study indicated that NONHSAT028712 mediated the development of chemoresistance through cis-regulating nearby CDK2 gene, which was required for the transition of cell cycle from G1 to S phase (He et al., 2016). LINC01977 could significantly promote BRCA cell proliferation and chemoresistance to DOX in vitro assays (Li Z. et al., 2021). It sponged miR-212-3p to prevent miRNA-mediated repression of GOLM1, which was reported to function as a key promoter of cell proliferation in several cancer types (Chen et al., 2015; Xu et al., 2017). MALAT1 was reported to be highly expressed in DOX-resistant BRCA cells. It could promote cell proliferation and colony formation to increase DOX resistance, mechanistically through recruiting E2F1 and activating downstream AGR2 expression (Li S. et al., 2022). MiR-570-3p was another target of MALAT1, which could inhibit the proliferation of BRCA cells and mediate the regulatory role of MALAT1 on DOX resistance (Yue et al., 2021). In HCC, elevation of MALAT1 also mediated tumor growth and DOX resistance via a MALAT1/miR-3129-5p/Nova1 axis (Cao et al., 2021). Other lncRNAs enhancing DOX resistance through increasing cell proliferation included HOTAIR (Wang H. et al., 2018). In GC cells, HOTAIR mainly counteracted with miR-217 to inhibit its suppressing effect in DOX resistance (Wang H. et al., 2018).
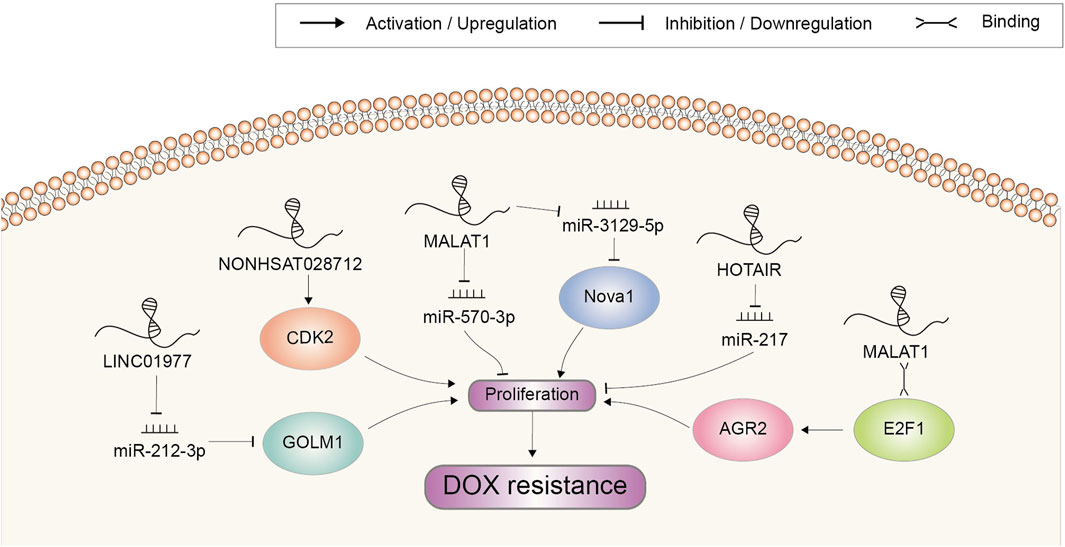
FIGURE 4. Doxorubicin resistance-related lncRNAs involved in the regulation of cell proliferation and the underlying mechanisms. Abbreviations: DOX, doxorubicin.
Signaling pathways
A fine-tuned regulation of signal transduction pathways is crucial for maintaining cellular and tissue homeostasis (SMA, 2020). Aberrant activation of oncogenic signaling pathways often lead to the transformation of normal cells to cancer cells with the acquirement of malignant phenotype (Palla et al., 2022). Many drugs with the ability of blocking dysregulated signaling pathways have been developed for cancer treatment. However, due to the crosstalk inside signaling network, awakening of alternative survival signaling pathways have become one dominating mechanism of chemoresistance (Dent et al., 2009). Similarly, any survival signaling pathway activated in response to toxic stress might also help cancer cells to escape DOX-based chemotherapy (Table 1).
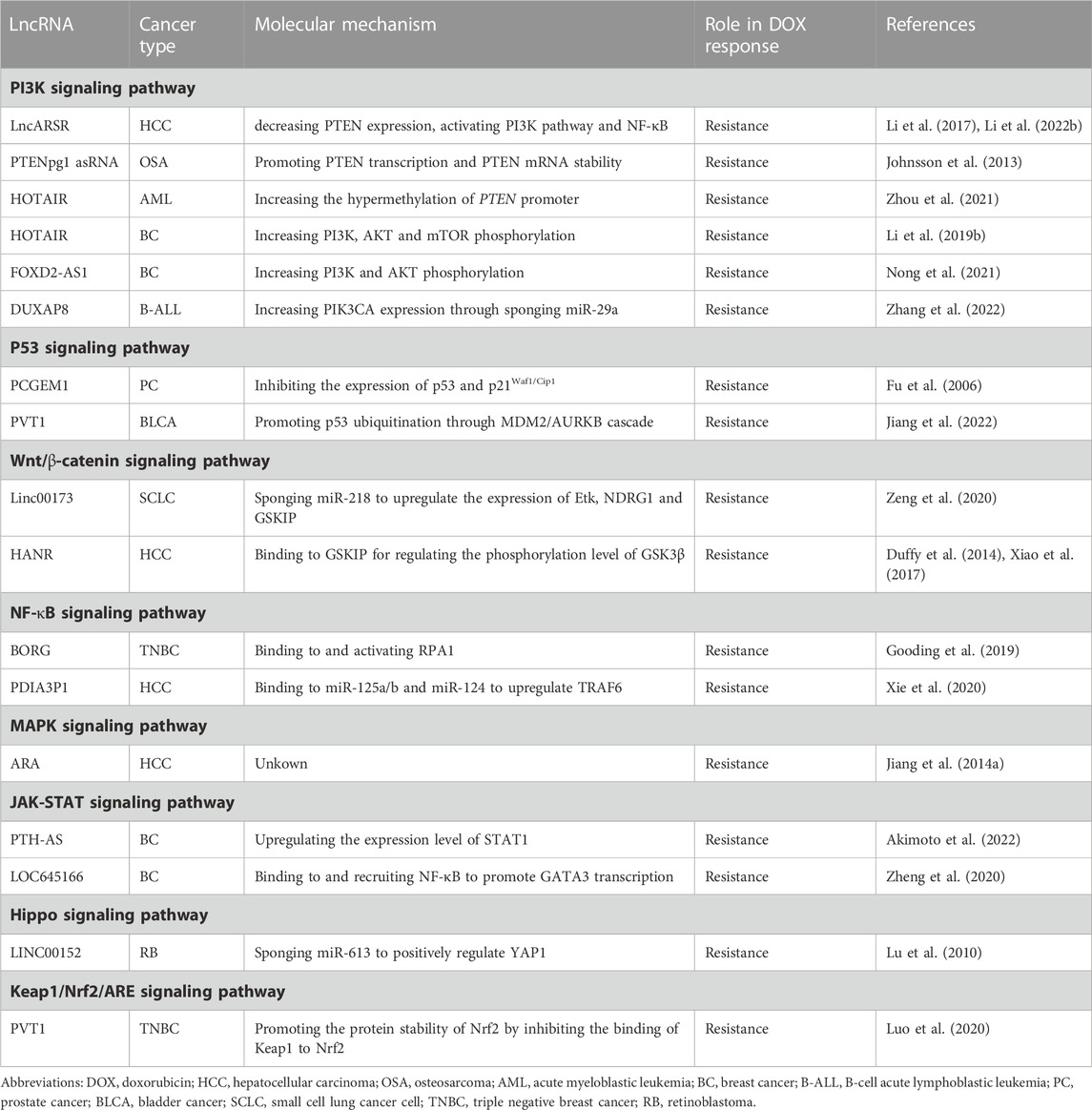
TABLE 1. LncRNAs implicated in doxorubicin resistance of cancer through multiple signaling pathways.
PI3K signaling pathway
PTEN tumor suppressor is a negative regulator of the PI3K/Akt pathway and is epigenetically silenced in multiple cancers (Álvarez-G et al., 2019). LncARSR overexpression inhibited DOX-induced cell apoptosis and enhanced DOX resistance in HCC while knockdown of lncARSR showed the opposite effects (Li et al., 2017). LncARSR decreased PTEN expression and activated the PI3K/Akt pathway. Furthermore, the effects of lncARSR on DOX resistance could be reversed by PTEN depletion or PI3K/Akt pathway inhibitors. Taken together, upregulated lncARSR promoted DOX resistance through activating the PTEN/PI3K pathway (Li et al., 2017). Subsequent study by Li et al. revealed that lncARSR further activated NF-κB in a PI3K pathway-dependent manner. NF-κB transactivated lncARSR through direct binding and activation of lncARSR promoter, forming a positive feedback regulatory loop among lncARSR, Akt and NF-κB. And this regulatory loop together promoted DOX resistance (Li Y. et al., 2022). LncRNA PTENpg1 regulated PTEN expression through sequestering numerous PTEN-targeting miRNAs. Moreover, two antisense RNA (asRNA) transcripts isoforms (α and β) were encoded from the PTENpg1 locus (Johnsson et al., 2013). The α isoform epigenetically regulated PTEN transcription via localizing to the PTEN promoter and catalyzing the formation of H3K27me3, while the β isoform interacted with PTENpg1 as an RNA:RNA pairing and post transcriptionally affected PTEN production. Suppression of this asRNA isoforms-regulated network led to a clear induction of PTEN protein level and a concomitant downregulation of pAKT. As a result, the OSA cells were significantly sensitized to DOX (Johnsson et al., 2013). Unlike PTENpg1 asRNA transcripts, HOTAIR was reported to modulate PTEN expression by increasing the hypermethylation of its promoter locus, thus suppressing PTEN expression and conferring DOX resistance in AML (Zhou et al., 2021). HOTAIR was also reported to reinforce DOX resistance by promoting the phosphorylation of AKT and activating AKT/mTOR signaling pathway in BC (Li Z. et al., 2019). Other lncRNAs implicated in PI3K signaling pathway and DOX resistance included FOXD2-AS1 in BC and DUXAP8 in B-cell acute lymphoblastic leukemia (B-ALL), further uncovering the central role of PI3K pathway in cancer DOX resistance (Nong et al., 2021; Zhang et al., 2022).
P53 signaling pathway
LncRNA PCGEM1 was specifically expressed in prostate tissue, and associated with prostate cancer (PC). The overexpression of PCGEM1 attenuated DOX-induced apoptosis in LNCaP cells (Fu et al., 2006). Moreover, the induction of p53 and p21Waf1/Cip1 due to DOX treatment was attenuated by PCGEM1 overexpression, as well as the protein levels of cleaved caspase 7 and cleaved PARP. These implied that PCGEM1 induced DOX resistance by inhibiting the function of p53-dependent apoptotic machinery (Fu et al., 2006). In BLCA cells, lncRNA PVT1 could interact with MDM2, promoting its expression and cascaded MDM2/AURKB-mediated p53 ubiquitination. Thus, p53 pathway-mediated tumor suppressor genes were suppressed, leading to elevated proliferation, invasion, and DOX resistance. Furthermore, addition of the MDM2 inhibitor Nutlin-3 could offset the increased DOX resistance induced by PVT1 overexpression, while overexpression of MDM2 or AURKB reversed PVT1 knockdown-induced sensitivity to DOX (Jiang et al., 2022).
Wnt/β-catenin signaling pathway
Linc00173 was first shown to be associated with the clinical stages and chemotherapeutic responses in SCLC. Elevated Linc00173 enhanced chemoresistance and cancer progression by sponging miR-218 to upregulate Etk expression. NDRG1 and GSKIP were positively regulated by Etk, which further induced the accumulation of β-catenin in the nucleus and activated Wnt/β-catenin pathway (Zeng et al., 2020). LncRNA HANR was demonstrated to be upregulated in HCC patients and predict a poor survival. Knockdown of HANR markedly enhanced the chemosensitivity of HCC cell lines to DOX, while overexpression of HANR showed the opposite effects. It was found that HANR bound to GSKIP for regulating the phosphorylation level and activity of GSK3β (Xiao et al., 2017). As a downstream target of GSK3β, Wnt/β-catenin pathway was thought to correspondingly perform its oncogenic function and impair the therapeutic outcome of DOX (Duffy et al., 2014).
NF-κB signaling pathway
NF-κB signaling pathway could be provoked by genotoxic agents-induced DNA damage, augmenting the transactivation of varieties of anti-apoptosis genes and subsequent chemoresistance of cancer cells (Taniguchi and Karin, 2018). LncRNA BORG was greatly induced within TNBC cells when subjected to chemotherapeutic stresses. It fostered the cell survival and rendered them resistant to the cytotoxic effects of DOX both in vitro and in vivo. This chemoresistant activity of BORG was contingent upon its binding to RPA1, as well as the concomitant stimulation of NF-κB signaling. Interestingly, the activation of NF-κB amplifies BORG expression, which further enhances NF-κB activation, forming a novel feed-forward NF-κB signaling loop (Gooding et al., 2019). LncRNA PDIA3P1 was upregulated in human HCC and associated with poorer recurrence-free survival. DOX treatment could also upregulate PDIA3P1 level by disrupting the binding of hMTR4 to PDIA3P1 and abrogating the subsequent hMTR4-mediated degradation. TRAF6 was ordinarily suppressed by miR-125a/b and miR-124, while upregulated PDIA3P1 could bind to miR-125a/b and miR-124 to relieve their repression on TRAF6, leading to the activation of NF-κB pathway and reduced DOX-triggered apoptosis (Xie et al., 2020).
MAPK signaling pathway
Jiang et al. discovered a new upregulated lncRNA named ARA in DOX-resistant BC cells, the expression of which was further found to be significantly associated with DOX sensitivity in a panel of BC cells as well as HCC cells. Knockdown of ARA inhibited cell proliferation and migration, induced G2/M cell cycle arrest and cell death, which together contributed to DOX resistance reverse. To investigate the functional role of ARA, microarray transcriptomic analysis was performed and genes regulated by ARA were enriched in multiple KEGG pathways, among which MAPK signaling pathway was the most outstanding (Jiang M. et al., 2014).
JAK-STAT signaling pathway
Emerging data indicate that JAK-STAT pathway confers cellular resistance to antitumor treatment with DNA-damaging agents, including DOX (Khodarev et al., 2012). Akimoto et al. reported that ectopic expression of lncRNA PTH-AS in BC cell T47D markedly upregulated the level of STAT1 and its downstream interferon-related DNA damage resistance signature (IRDS) genes (Akimoto et al., 2022). As expected, when treated with DOX at a relatively high concentration, T47D cells with forced PTH-AS expression exhibited a significant resistance to drug-induced inhibition. LncRNA LOC645166 was identified to be upregulated in DOX-resistant BC cells as well as tissues of nonresponsive patients. It strengthened the tolerance of breast cancer to DOX via binding and recruiting NF-κB to promote GATA3 transcription, further leading to the activation of STAT3 (Zheng et al., 2020). The NF-κB/GATA3/STAT3 signaling pathway provided a promising target for overcoming DOX resistance in breast cancer.
Hippo signaling pathway
In human retinoblastoma (RB), LINC00152 was reported to boost DOX resistance by sponging miR-613 to positively regulate YAP1 (Wan et al., 2020). YAP1 is a downstream effector of the Hippo signaling pathway, which is widely recognized as an important regulator in both organ size control and tumorigenesis (Lu et al., 2010). In previous studies, YAP1 had also been reported to have an effect on cell sensitivity to 5-fluorouracil and docetaxcel in esophageal cancer (Song et al., 2015). Thus, Hippo signaling pathway might be another molecular cascade responsible for lncRNA-mediated chemoresistance in cancer cells.
Keap1/Nrf2/ARE signaling pathway
Keap1/Nrf2 signaling pathway plays an important role in maintaining cellular redox balance (Buti et al., 2013). Aberrant activation of this pathway has frequently been detected in human cancers and is also related to resistance to chemotherapies in established cancers (Ge et al., 2017). It was revealed that PVT1 promoted the protein stability of Nrf2 by inhibiting the binding of Keap1 to Nrf2, which potentiated the resistance of TNBC cells to DOX (Luo et al., 2020).
Epithelial to mesenchymal transition (EMT)
EMT is a biological process in which epithelial cells transform into mesenchymal cells acquiring a motile phenotype (Pastushenko and Blanpain, 2019). Researches have revealed that EMT is not only closely related to tumor metastasis but also affects chemotherapy resistance. DOX resistance-related lncRNAs implicated in EMT regulation were summarized in Figure 5. In OSA, inhibition of LINC01116 suppressed cell viability, migration, and invasion, along with upregulated E-cadherin and downregulated vimentin. Accordingly, DOX resistance was attenuated. Further investigations indicated that LINC01116 regulated HMGA2 expression via EZH2-associated silencing of miR-424–5p and induced EMT (Li R. et al., 2021). Long intergenic non-protein coding RNA (linc)-regulator of reprogramming (ROR) was reported to promote invasion and metastasis in HCC. Knockdown of it notably suppressed EMT by downregulating TWIST1, increasing sensitivity of HCx`x`C cell to DOX (Zhang et al., 2021). FN1, is a glycoprotein present at the cell surface and in extracellular matrix tightly related to cellular adhesion and migration (Topalovski and Brekken, 2016). It was found to be significantly upregulated in the chemoresistant OSA cell lines and tissues and was related to unfavourable prognosis. LncRNA OIP5-AS1 acted as an upstream regulator of FN1 through sponging miR-200b-3p. Therefore, OIP5-AS1/miR-200b-3p/FN1 axis might be a promising target in treatment of OSA resistance to DOX (Kun-Peng et al., 2019). LncRNA DCST1-AS1 enhanced TGF-β/Smad signaling in TNBC cells through binding to ANXA1 and increasing its expression. Subsequently, the expression or secretion of proteins such as E-cadherin, SNAI1 and vimentin were coordinated to promote EMT and chemoresistance to DOX. Therefore, DCST1-AS1 represented a potentially promising therapy target for metastatic breast cancer (Tang et al., 2020).
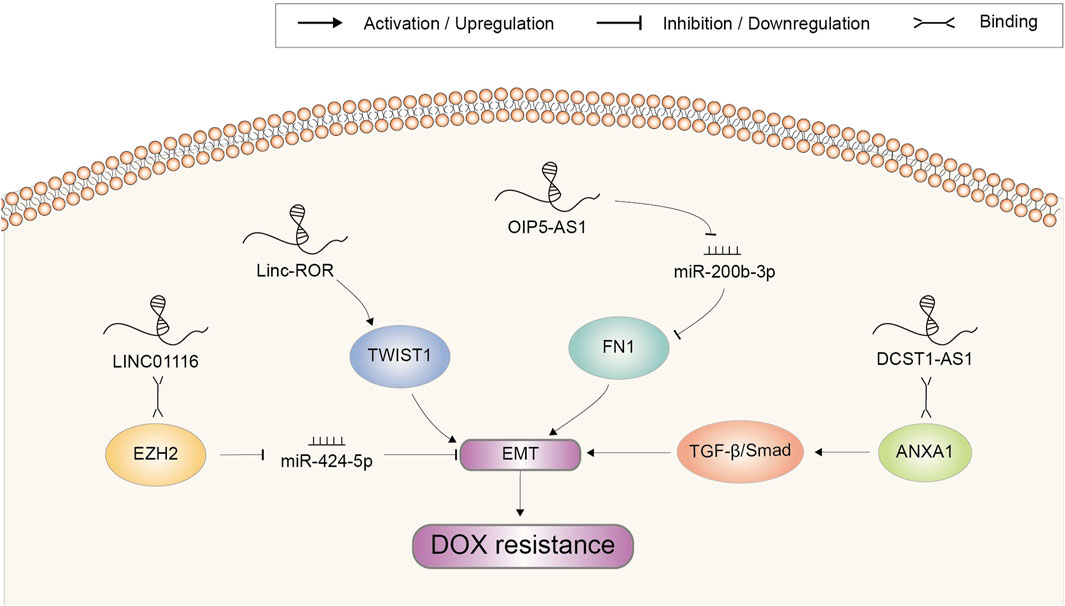
FIGURE 5. Doxorubicin resistance-related lncRNAs involved in the regulation of epithelial to mesenchymal transition and the underlying mechanisms. Abbreviations: DOX, doxorubicin; EMT, epithelial to mesenchymal transition.
Autophagy
Accumulating evidence supported the cytoprotective role of autophagy in drug resistance of cancer. When under cytotoxic effects of chemotherapeutic drugs, autophagy could contribute to maintaining the intracellular homeostasis and prolonging the survival of cancer cells through autophagosomes (Carew et al., 2007). Recent researches have suggested that dysregulated lncRNA play a role in the development of chemoresistance via autophagy (Figure 6). For example, lncRNA GBCDRlnc1 served as a critical regulator of the autophagic activity and DOX-resistant property of gallbladder cancer. Through direct molecular interaction, GBCDRlnc1 prevented the ubiquitination of PGK1, leading to the upregulation of PGK1 protein level. The ATG5-ATG12 conjugate, an essential complex for autophagy initiation, might be a downstream target of the GBCDRlnc1/PGK1 axis. Knockdown of GBCDRlnc1 dramatically downregulated PGK1, ATG5 and ATG12, suppressed autophagy and improved the sensitivity of gallbladder cancer cells to DOX (Cai et al., 2019).
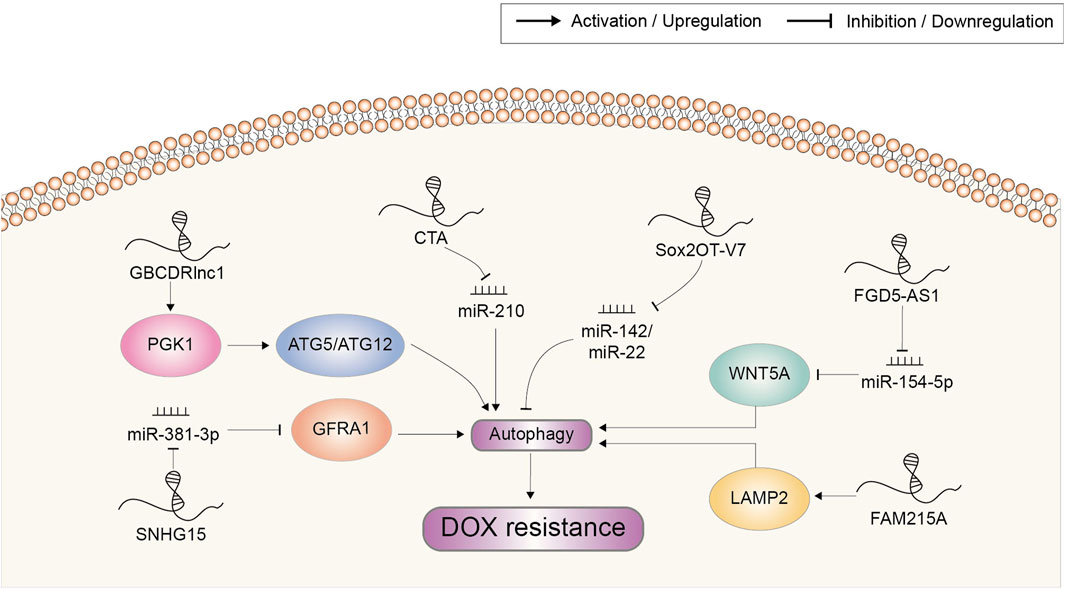
FIGURE 6. Doxorubicin resistance-related lncRNAs involved in the regulation of autophagy and the underlying mechanisms. Abbreviations: DOX, doxorubicin.
Wang et al. found that lncRNA CTA could be activated by DOX but was downregulated in DOX-resistant OSA cells. Overexpression of CTA could inhibit autophagy to overcome DOX resistance and promote apoptosis by competitively binding miR-210 (Wang et al., 2017). On the contrary, lncRNA FGD5-AS1 was upregulated in DOX-resistant OSA cells. It was reported to regulate the miR-154-5p/WNT5A axis by sponging miR-154-5p and thus potentiate autophagy-associated DOX resistance (Fei et al., 2022). Small nucleolar RNA host gene 15 (SNHG15) was also upregulated in DOX-resistant OSA cell lines. It elevated autophagy via targeting the miR-381-3p/GFRA1 axis to enhance DOX resistance (Zhang et al., 2020). Sox2OT-V7, another lncRNA involved in DOX resistance of OSA cells, could modulate autophagy through directly targeting miR-142/miR-22 (Zhu et al., 2020).
Lysosomes could sequester macromolecules generated from autophagy for degradation and recycling, and mediate multiple drug resistance in cancer (Piao and Amaravadi, 2016). In HCC, FAM215A was overexpressed and increased the resistance of cells to DOX-induced inhibition. This cell protective effect was proved to be achieved by stabilizing LAMP2, which constitutively contributed to lysosome formation and the maintenance of lysosomal content (Huang et al., 2020).
Cancer stem cells (CSCs)
CSCs represent a small fraction of cells in the tumor featured by their potential of self-renewal and initiating tumors. Studies demonstrated that CSCs were responsible for chemoresistance and tumor recurrence following chemotherapy (Wicha et al., 2006).
Linc00668 was observed to be increased in BC compared to normal tissues. Forced expression of Linc00668 enhanced self-renewal capacity of BC cells as well as DOX resistance. Mechanistically, Linc00668 interacted with SND1 to augment its transcriptional activity and the expression of target genes, including Nanog, Sox2, and Oct4, which were critical regulators of stem cell-like properties (Qian et al., 2020). GAS5 was reported to function in maintaining stemness in human CC cell line HCT116-derived CSCs. GAS5 knockdown suppressed the self-renewal capacity of CSCs and sensitized them to DOX by inducing apoptosis. Moreover, inhibition of Nodal growth differentiation factor (NODAL) signaling presented the similar results. Therefore, it was hypothesized that GAS5 exerted protective effects in CSCs under DOX treatment in a NODAL signaling-dependent manner (Zhou and Xiao, 2020).
Tumor microenvironment
Extracellular vesicles (EVs), mainly comprised of exosomes and microvesicles, are an important component of the tumor microenvironment (van Niel et al., 2018). EVs are a group of membrane-derived structures released by donor cell into the interstitial fluid. These EVs carry biological macromolecules such as protein, lipids and RNA, and can be taken up by recipient cells to achieve intercellular communication (Tkach and Théry, 2016). EVs are now considered as an additional mechanism for modulation of multiple physiological and pathological processes including chemoresistance (Mashouri et al., 2019).
Takahashi et al. (Takahashi et al., 2014a) identified a subset of lncRNAs in HCC that could be detected in EVs with at least 2-fold enrichment compared to donor cells. Among these lncRNAs, linc-VLDLR was also found to be significantly upregulated in malignant hepatocytes compared to non-malignant hepatocytes. Exposure of HCC cells to DOX increased linc-VLDLR expression within both cells and released EVs, and incubation with such EVs could reduce DOX-induced cell death in recipient cells. Further studies revealed that knockdown of linc-VLDLR suppressed cell viability, blocked cell-cycle progression and reduced the expression of ABCG2, leading to increased sensitivity of HCC cells to DOX (Takahashi et al., 2014a). Linc-ROR was another EV-transferred lncRNA in HCC (Takahashi et al., 2014b). Incubation with EVs originating from HCC cells increased linc-ROR expression and reduced DOX-induced cell death in recipient cells, whereas knockdown of linc-ROR augmented DOX-induced cytotoxicity. Besides, linc-ROR might mediate TGFβ-dependent chemoresistance in HCC, as TGFβ-increased expression of CD133+ tumor-initiating cells and colony growth were attenuated by linc-ROR knockdown. These findings all suggested an important role for linc-ROR in chemotherapeutic response of HCC (Takahashi et al., 2014b). LncRNA H19 had been proved to mediate the resistance of BC cells to DOX. Moreover, extracellular H19 could be incorporated into exosomes and delivered to sensitive cells, leading to the dissemination of DOX resistance. Therefore, exosomal H19 might be a potential target to reduce DOX resistance in BC (Wang et al., 2020).
Metabolic reprogramming
Cancers have been shown to evade chemotherapy by switching to alternate metabolism. Aerobic glycolysis is recognized as an emerging hallmark of malignant tumors. Normal cells process glucose through mitochondrial oxidative phosphorylation, whereas glycolysis is preferred in most cancer cells for energy production, even under aerobic conditions (Hanahan and Weinberg, 2011). Moreover, emerging evidence has revealed that augmented glycolysis might also contribute to the development of acquired chemoresistance (Tamada et al., 2012). For example, the effect of UCA1 on DOX resistance in AML cell centered around its regulation of HIF-1α-dependent glycolysis. Ectopic expression of UCA1 exhibited a remarkable increase of glucose consumption and effectively enhanced HIF-1α level (Zhang et al., 2018). As a pivotal transcription factor, HIF-1α has been documented to play a critical role in metabolic reprogramming and chemoresistance in various tumor cells (Warfel and El-Deiry, 2014). LncRNA SAMMSON was overexpressed in DOX-resistant BC cell (Orre et al., 2021). Silencing of SAMMSON revealed a decreased glycolytic metabolism and an increased oxidative metabolism. Concomitantly, less ROS were produced from the mitochondrial respiratory chain, while mitochondrial replication, transcription and translation were enhanced. These results highlighted the role of SAMMSON in the metabolic rewiring and development of chemoresistance in BC.
Targeting lncRNAs for reversing doxorubicin resistance
Natural compounds including DOX occupy an important position in cancer therapy because of their diversity in structure and biological activity (Newman and Cragg, 2012). Owe to the multi-targeting capability, lncRNA might also be one of the targets of natural compounds. The recent reports regarding lncRNA-targeting natural compounds involved in DOX resistance are shown in Figure 7. Curcumin is a main active flavonoid component existing in Chinese herb Curcuma longa with the anti-tumor property (Moradi-Marjaneh et al., 2018). It had also been proved to suppress the resistance to DOX in acute myeloid leukemia. Mechanism study showed that lncRNA HOTAIR was inhibited by curcumin, which further mediated the sensitization effect of curcumin through the miR-20a-5p/WT1 axis (Liu et al., 2021). Epigallocatechin gallate is the highest content of polyphenol in green tea, which was reported to exert significant inhibitory effect on osteosarcoma cells including induce apoptosis, inhibit cell proliferation and invasion (Jiang L. et al., 2014). Moreover, Wang et al. reported that epigallocatechin gallate could produce synergistic effects with DOX on osteosarcoma cells by targeting lncRNA SOX2OT variant 7. On the one hand, epigallocatechin gallate decreased SOX2OT variant 7 to reduce DOX-induced autophagy, which played a pro-survival role in protecting cells from the growth inhibition of DOX. On the other hand, epigallocatechin gallate targeting SOX2OT variant 7 could partially inactivate the Notch3/DLL3 signaling cascade to reduce cell stemness then abate DOX resistance (Wang W. et al., 2018). Bruceine D (BD) is a quassinoid extracted from Brucea javanica which has an anti-tumor activity in various cancers (Lau et al., 2009). BD treatment in GC cells significantly downregulated the expression of LINC01667, further inhibiting the expression of Cyclin E1 by releasing miR-138-5p from LINC01667 sponge (Li et al., 2020). Thus, BD could inhibit the growth of GC cells and enhance the chemosensitivity of GC cells to DOX. Ursolic acid (UA), a pentacyclic triterpenoid compound, was reported to reverse DOX resistance in TNBC. It could inhibit the expression of ZEB1-AS1, which sponged miR-186-5p to upregulate ABCC1. Hence, UA treatment led to the decrease in ABCC1 expression (Lu et al., 2022). Together, combined therapy of above natural compounds with DOX might serve as an effective strategy to reduce the occurrence of chemoresistance and improve the curative effect in certain cancer, which needs further verification in the clinical practice.
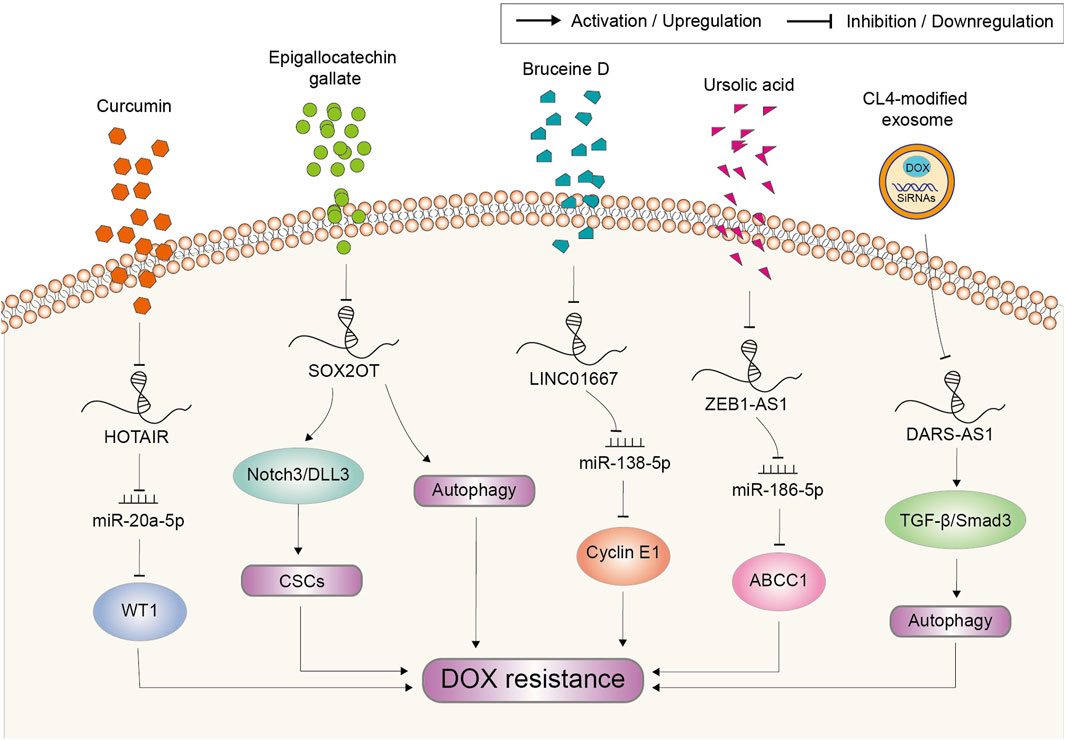
FIGURE 7. Potential strategies based on natural compounds and genetic tools to target lncRNAs for the reverse of doxorubicin resistance in cancer. Abbreviations: DOX, doxorubicin; CSCs, cancer stem cells.
Cancer occurs as a result of loss function of suppressor genes and activation of oncogenes (Caspi et al., 2021; Megyesfalvi et al., 2023). However, the conventional therapeutic using natural compounds or their analogs always lacks specific targets and induces serious side effects. Consequently, much attention has been directed towards the application of genetic tools in anticancer therapy. Small interfering RNA (siRNA) is the most extensively used tool applied in cancer therapy in the in vitro and in vivo study because of its potential in suppressing oncogenes (Mirzaei et al., 2021). As such tumor-promoting factors account for chemoresistance, targeting them through siRNA also provides an important strategy to reverse DOX resistance. However, the translational application of siRNA is still at its initial stage. There exist multiple limitations that challenge its efficacy, mainly including instability in blood circulation and incapability to enter cells (Ashrafizadeh et al., 2020). To overcome these difficulties, a variety of platforms have been developed for siRNAs delivery, which consist of lipid nanoparticles, liposomal nanoparticles, polymeric nanoparticles, silicon dioxide nanoparticles, carbon nanotubes, gold nanoparticles, iron oxide nanoparticles, aptamers and so on (Acharya et al., 2017). Nowadays, attempts based on these delivery platforms to target lncRNAs is still quite rare. A most recent study employed aptamer CL4-modified exosomes for the targeted delivery of DARS-AS1 siRNA and DOX to TNBC cells (Liu et al., 2023). The tumor growth was synergistically suppressed in vivo, while the delivery system did not induce any observed safety issues in mice. Meanwhile, in vitro experiments revealed that silencing DARS-AS1 decreased DOX resistance by suppressing autophagy via inhibition of the TGF-β/Smad3 signaling pathway (Figure 7). This study shows the outstanding application potential of genetic tool represented by siRNA in anti-cancer therapy and chemoresistance reverse.
Conclusion and perspectives
Resistance to therapeutic drugs represented by DOX is a major burden for successful cancer treatments. However, the underlying mechanisms of chemoresistance are not yet fully elucidated. Multiple reasons for DOX resistance have been summarized and listed here, mainly including cellular drug transport, cell cycle disorders, anti-apoptosis, epithelial-mesenchymal transition, cancer stem cells, autophagy, tumor microenvironment, metabolic reprogramming and oncogenic signaling pathways. It should be noticed that cancer might develop resistance to DOX through more than one mechanism. What’s more, recently found hallmarks, such as altered metabolic reprogramming and tumor microenvironment (Hanahan and Weinberg, 2011), have not only affected the development of new means to treat human cancer, but also enriched the connotation of chemoresistance. Future study on the nature of cancer is still in urgent need and will undoubtedly provide direction for deepening our understanding of how chemoresistance develops.
At present, most of the reported lncRNAs associated with DOX resistance were identified from laboratory-based results, which were far away from clinical status. This might explain why the clinical translation of chemoresistance reversal is difficult. For example, after the discovery of ABCB1, a number of inhibitors were identified and added to chemotherapy regimens. However, they all failed in clinical trials due to the inefficiency or unbearable toxicity (Gottesman et al., 2002; Binkhathlan and Lavasanifar, 2013). Therefore, future work should be focused on identifying target lncRNAs through well-designed clinical approaches. Obtainment of matched pre- and post-progression tumor biopsies from patients with acquired DOX resistance would be of great importance.
Although the study of lncRNAs on chemoresistance is in its infancy, growing evidence suggests that lncRNAs may serve as potential molecular targets for cancer therapy as well as reversal of chemoresistance. Still, the method to target lncRNAs in vivo remains an unsolved problem. Compounds such as curcumin and epigallocatechin gallate can regulate lncRNA expression, but they are lack of specificity. To take lncRNAs as novel therapy targets, there is still a long way to go. Nevertheless, studies over the last decades have established a solid foundation to warrant further investigation of lncRNAs on reversing chemoresistance.
Author contributions
Conceptualization, H-BZ, YH, and YZ; resources, H-BZ and YZ; writing—original draft prepa-ration, H-BZ and YH; writing—review and editing, H-BZ, YH, and J-LD; visualization, J-LD; supervision, G-YF and YZ; project administration, G-YF and YZ. All authors contributed to the article and approved the submitted version.
Funding
This research was funded by the National Natural Science Foundation of China (No. 82003870), Natural Science Foundation of Changsha (No. kq2014012), and the Key Research Project of Changsha Central Hospital (No. YNKY202204).
Conflict of interest
The authors declare that the research was conducted in the absence of any commercial or financial relationships that could be construed as a potential conflict of interest.
Publisher’s note
All claims expressed in this article are solely those of the authors and do not necessarily represent those of their affiliated organizations, or those of the publisher, the editors and the reviewers. Any product that may be evaluated in this article, or claim that may be made by its manufacturer, is not guaranteed or endorsed by the publisher.
References
Arcamone, F., Cassinelli, G., Fantini, G., Grein, A., Orezzi, P., Pol, C., et al. (2000). Adriamycin, 14-hydroxydaunomycin, a new antitumor antibiotic from S. peucetius var. caesius. Reprinted from Biotechnology and Bioengineering, Vol. XI, Issue 6, Pages 1101-1110 (1969). Biotechnol. Bioeng. 67 (6), 704–713. doi:10.1002/(sici)1097-0290(20000320)67:6<704::aid-bit8>3.0.co;2-l
Abraham, J., Edgerly, M., Wilson, R., Chen, C., Rutt, A., Bakke, S., et al. (2009). A phase I study of the P-glycoprotein antagonist tariquidar in combination with vinorelbine. Clin. Cancer Res. 15 (10), 3574–3582. doi:10.1158/1078-0432.CCR-08-0938
Acharya, R., Saha, S., Ray, S., Hazra, S., Mitra, M. K., and Chakraborty, J. (2017). siRNA-nanoparticle conjugate in gene silencing: A future cure to deadly diseases? Mater Sci. Eng. C Mater Biol. Appl. 76, 1378–1400. doi:10.1016/j.msec.2017.03.009
Aini, S., Bolati, S., Ding, W., Liu, S., and Aili, S. (2022). LncRNA SNHG10 suppresses the development of doxorubicin resistance by downregulating miR-302b in triple-negative breast cancer. Bioengineered 13 (5), 11430–11439. doi:10.1080/21655979.2022.2063592
Akimoto, M., Susa, T., Okudaira, N., Hisaki, H., Iizuka, M., Okinaga, H., et al. (2022). A novel LncRNA PTH-AS upregulates interferon-related DNA damage resistance signature genes and promotes metastasis in human breast cancer xenografts. J. Biol. Chem. 298 (7), 102065. doi:10.1016/j.jbc.2022.102065
Alexander, R. P., Fang, G., Rozowsky, J., Snyder, M., and Gerstein, M. B. (2010). Annotating non-coding regions of the genome. Nat. Rev. Genet. 11 (8), 559–571. doi:10.1038/nrg2814
Álvarez-Garcia, V., Tawil, Y., Wise, H. M., and Leslie, N. R. (2019). Mechanisms of PTEN loss in cancer: It's all about diversity. Semin. Cancer Biol. 59, 66–79. doi:10.1016/j.semcancer.2019.02.001
Ashrafizadeh, M., Zarrabi, A., Hushmandi, K., Hashemi, F., Rahmani Moghadam, E., Raei, M., et al. (2020). Progress in natural compounds/siRNA Co-delivery employing nanovehicles for cancer therapy. ACS Comb. Sci. 22 (12), 669–700. doi:10.1021/acscombsci.0c00099
Batista, P. J., and Chang, H. Y. (2013). Long noncoding RNAs: cellular address codes in development and disease. Cell 152 (6), 1298–1307. doi:10.1016/j.cell.2013.02.012
Binkhathlan, Z., and Lavasanifar, A. (2013). P-Glycoprotein inhibition as a therapeutic approach for overcoming multidrug resistance in cancer: current status and future perspectives. Curr. Cancer Drug Targets 13 (3), 326–346. doi:10.2174/15680096113139990076
Bish, R., and Vogel, C. (2014). RNA binding protein-mediated post-transcriptional gene regulation in medulloblastoma. Mol. Cells 37 (5), 357–364. doi:10.14348/molcells.2014.0008
Blum, R. H., and Carter, S. K. (1974). Adriamycin. A new anticancer drug with significant clinical activity. Ann. Intern Med. 80 (2), 249–259. doi:10.7326/0003-4819-80-2-249
Broxterman, H. J., Gotink, K. J., and Verheul, H. M. (2009). Understanding the causes of multidrug resistance in cancer: a comparison of doxorubicin and sunitinib. Drug Resist Updat 12 (4-5), 114–126. doi:10.1016/j.drup.2009.07.001
Buti, S., Bersanelli, M., Sikokis, A., Maines, F., Facchinetti, F., Bria, E., et al. (2013). Chemotherapy in metastatic renal cell carcinoma today? A systematic review. Anticancer Drugs 24 (6), 535–554. doi:10.1097/CAD.0b013e3283609ec1
Cai, Q., Wang, S., Jin, L., Weng, M., Zhou, D., Wang, J., et al. (2019). Long non-coding RNA GBCDRlnc1 induces chemoresistance of gallbladder cancer cells by activating autophagy. Mol. Cancer 18 (1), 82. doi:10.1186/s12943-019-1016-0
Cao, Y., Zhang, F., Wang, H., Cui, J., and Liu, F. (2021). LncRNA MALAT1 mediates doxorubicin resistance of hepatocellular carcinoma by regulating miR-3129-5p/Nova1 axis. Mol. Cell Biochem. 476 (1), 279–292. doi:10.1007/s11010-020-03904-6
Carew, J. S., Nawrocki, S. T., and Cleveland, J. L. (2007). Modulating autophagy for therapeutic benefit. Autophagy 3 (5), 464–467. doi:10.4161/auto.4311
Caspi, M., Wittenstein, A., Kazelnik, M., Shor-Nareznoy, Y., and Rosin-Arbesfeld, R. (2021). Therapeutic targeting of the oncogenic Wnt signaling pathway for treating colorectal cancer and other colonic disorders. Adv. Drug Deliv. Rev. 169, 118–136. doi:10.1016/j.addr.2020.12.010
Chang, L., Hu, Z., Zhou, Z., and Zhang, H. (2018). Linc00518 contributes to multidrug resistance through regulating the MiR-199a/MRP1 Axis in breast cancer. Cell Physiol. Biochem. 48 (1), 16–28. doi:10.1159/000491659
Chen, X., Wang, Y., Tao, J., Shi, Y., Gai, X., Huang, F., et al. (2015). mTORC1 up-regulates GP73 to promote proliferation and migration of hepatocellular carcinoma cells and growth of xenograft tumors in mice. Gastroenterology 149 (3), 741–752. doi:10.1053/j.gastro.2015.05.005
Dent, P., Curiel, D. T., Fisher, P. B., and Grant, S. (2009). Synergistic combinations of signaling pathway inhibitors: mechanisms for improved cancer therapy. Drug Resist Updat 12 (3), 65–73. doi:10.1016/j.drup.2009.03.001
Duffy, D. J., Krstic, A., Schwarzl, T., Higgins, D. G., and Kolch, W. (2014). GSK3 inhibitors regulate MYCN mRNA levels and reduce neuroblastoma cell viability through multiple mechanisms, including p53 and Wnt signaling. Mol. Cancer Ther. 13 (2), 454–467. doi:10.1158/1535-7163.MCT-13-0560-T
Evans, J. R., Feng, F. Y., and Chinnaiyan, A. M. (2016). The bright side of dark matter: lncRNAs in cancer. J. Clin. Invest. 126 (8), 2775–2782. doi:10.1172/JCI84421
Fang, Q., Chen, X., and Zhi, X. (2016a). Long non-coding RNA (LncRNA) urothelial carcinoma associated 1 (UCA1) increases multi-drug resistance of gastric cancer via downregulating miR-27b. Med. Sci. Monit. 22, 3506–3513. doi:10.12659/msm.900688
Fang, S., Gao, H., Tong, Y., Yang, J., Tang, R., Niu, Y., et al. (2016b). Long noncoding RNA-HOTAIR affects chemoresistance by regulating HOXA1 methylation in small cell lung cancer cells. Lab. Invest. 96 (1), 60–68. doi:10.1038/labinvest.2015.123
Fei, D., Yuan, H., Zhao, M., and Zhao, D. (2022). LncRNA FGD5-AS1 potentiates autophagy-associated doxorubicin resistance by regulating the miR-154-5p/WNT5A axis in osteosarcoma. Cell Biol. Int. 46 (11), 1937–1946. doi:10.1002/cbin.11889
Fu, X., Ravindranath, L., Tran, N., Petrovics, G., and Srivastava, S. (2006). Regulation of apoptosis by a prostate-specific and prostate cancer-associated noncoding gene, PCGEM1. DNA Cell Biol. 25 (3), 135–141. doi:10.1089/dna.2006.25.135
Ge, W., Zhao, K., Wang, X., Li, H., Yu, M., He, M., et al. (2017). iASPP is an antioxidative factor and drives cancer growth and drug resistance by competing with Nrf2 for Keap1 binding. Cancer Cell 32 (5), 561–573. doi:10.1016/j.ccell.2017.09.008
Gewirtz, D. A. (1999). A critical evaluation of the mechanisms of action proposed for the antitumor effects of the anthracycline antibiotics adriamycin and daunorubicin. Biochem. Pharmacol. 57 (7), 727–741. doi:10.1016/s0006-2952(98)00307-4
Gibb, E. A., Brown, C. J., and Lam, W. L. (2011). The functional role of long non-coding RNA in human carcinomas. Mol. Cancer 10, 38. doi:10.1186/1476-4598-10-38
Gooding, A. J., Zhang, B., Gunawardane, L., Beard, A., Valadkhan, S., and Schiemann, W. P. (2019). The lncRNA BORG facilitates the survival and chemoresistance of triple-negative breast cancers. Oncogene 38 (12), 2020–2041. doi:10.1038/s41388-018-0586-4
Gottesman, M. M., Fojo, T., and Bates, S. E. (2002). Multidrug resistance in cancer: Role of ATP-dependent transporters. Nat. Rev. Cancer 2 (1), 48–58. doi:10.1038/nrc706
Grant, C. E., Valdimarsson, G., Hipfner, D. R., Almquist, K. C., Cole, S. P., and Deeley, R. G. (1994). Overexpression of multidrug resistance-associated protein (MRP) increases resistance to natural product drugs. Cancer Res. 54 (2), 357–361.
Hanahan, D., and Weinberg, R. A. (2011). Hallmarks of cancer: The next generation. Cell 144 (5), 646–674. doi:10.1016/j.cell.2011.02.013
He, D. X., Zhang, G. Y., Gu, X. T., Mao, A. Q., Lu, C. X., Jin, J., et al. (2016). Genome-wide profiling of long non-coding RNA expression patterns in anthracycline-resistant breast cancer cells. Int. J. Oncol. 49 (4), 1695–1703. doi:10.3892/ijo.2016.3665
Hortobágyi, G. N., and Buzdar, A. U. (1993). Present status of anthracyclines in the adjuvant treatment of breast cancer. Drugs 45 (2), 10–19. discussion 18-9. doi:10.2165/00003495-199300452-00004
Huang, P. S., Lin, Y. H., Chi, H. C., Tseng, Y. H., Chen, C. Y., Lin, T. K., et al. (2020). Dysregulated FAM215A stimulates LAMP2 expression to confer drug-resistant and malignant in human liver cancer. Cells 9 (4), 961. doi:10.3390/cells9040961
Hulst, M. B., Grocholski, T., Neefjes, J. J. C., van Wezel, G. P., and Metsä-Ketelä, M. (2022). Anthracyclines: biosynthesis, engineering and clinical applications. Nat. Prod. Rep. 39 (4), 814–841. doi:10.1039/d1np00059d
Hung, T., and Chang, H. Y. (2010). Long noncoding RNA in genome regulation: prospects and mechanisms. RNA Biol. 7 (5), 582–585. doi:10.4161/rna.7.5.13216
Jiang, B., Li, Y., Qu, X., Zhu, H., Tan, Y., Fan, Q., et al. (2018). Long noncoding RNA cancer susceptibility candidate 9 promotes doxorubicin-resistant breast cancer by binding to enhancer of zeste homolog 2. Int. J. Mol. Med. 42 (5), 2801–2810. doi:10.3892/ijmm.2018.3812
Jiang, L., Tao, C., He, A., and He, X. (2014b). Overexpression of miR-126 sensitizes osteosarcoma cells to apoptosis induced by epigallocatechin-3-gallate. World J. Surg. Oncol. 12, 383. doi:10.1186/1477-7819-12-383
Jiang, M., Huang, O., Xie, Z., Wu, S., Zhang, X., Shen, A., et al. (2014a). A novel long non-coding RNA-ARA: adriamycin resistance-associated. Biochem. Pharmacol. 87 (2), 254–283. doi:10.1016/j.bcp.2013.10.020
Jiang, X., Li, H., Fang, Y., and Xu, C. (2022). LncRNA PVT1 contributes to invasion and doxorubicin resistance of bladder cancer cells through promoting MDM2 expression and AURKB-mediated p53 ubiquitination. Environ. Toxicol. 37 (6), 1495–1508. doi:10.1002/tox.23501
Johnsson, P., Ackley, A., Vidarsdottir, L., Lui, W. O., Corcoran, M., Grandér, D., et al. (2013). A pseudogene long-noncoding-RNA network regulates PTEN transcription and translation in human cells. Nat. Struct. Mol. Biol. 20 (4), 440–446. doi:10.1038/nsmb.2516
Khodarev, N. N., Roizman, B., and Weichselbaum, R. R. (2012). Molecular pathways: interferon/stat1 pathway: Role in the tumor resistance to genotoxic stress and aggressive growth. Clin. Cancer Res. 18 (11), 3015–3021. doi:10.1158/1078-0432.CCR-11-3225
Kun-Peng, Z., Xiao-Long, M., and Chun-Lin, Z. (2017b). LncRNA FENDRR sensitizes doxorubicin-resistance of osteosarcoma cells through down-regulating ABCB1 and ABCC1. Oncotarget 42 (1), 71881–71893. doi:10.18632/oncotarget.17985
Kun-Peng, Z., Chun-Lin, Z., Xiao-Long, M., and Lei, Z. (2019). Fibronectin-1 modulated by the long noncoding RNA OIP5-AS1/miR-200b-3p axis contributes to doxorubicin resistance of osteosarcoma cells. J. Cell Physiol. 234 (5), 6927–6939. doi:10.1002/jcp.27435
Kun-Peng, Z., Xiao-Long, M., and Chun-Lin, Z. (2017a). LncRNA FENDRR sensitizes doxorubicin-resistance of osteosarcoma cells through down-regulating ABCB1 and ABCC1. Oncotarget 8 (42), 71881–71893. doi:10.18632/oncotarget.17985
Lau, S. T., Lin, Z. X., Liao, Y., Zhao, M., Cheng, C. H. K., and Leung, P. S. (2009). Bruceine D induces apoptosis in pancreatic adenocarcinoma cell line PANC-1 through the activation of p38-mitogen activated protein kinase. Cancer Lett. 281 (1), 42–52. doi:10.1016/j.canlet.2009.02.017
Lehne, G. (2000). P-glycoprotein as a drug target in the treatment of multidrug resistant cancer. Curr. Drug Targets 1 (1), 85–99. doi:10.2174/1389450003349443
Li, L., Dong, Z., Shi, P., Tan, L., Xu, J., Huang, P., et al. (2020). Bruceine D inhibits cell proliferation through downregulating LINC01667/MicroRNA-138-5p/cyclin E1 Axis in gastric cancer. Front. Pharmacol. 11, 584960. doi:10.3389/fphar.2020.584960
Li, L., Gao, Z., Zhao, L., Ren, P., and Shen, H. (2021a). Long non-coding RNA LINC00607 silencing exerts antioncogenic effects on thyroid cancer through the CASP9 Promoter methylation. J. Cell Mol. Med. 25 (16), 7608–7620. doi:10.1111/jcmm.16265
Li, Q., Song, W., and Wang, J. (2019a). TUG1 confers Adriamycin resistance in acute myeloid leukemia by epigenetically suppressing miR-34a expression via EZH2. Biomed. Pharmacother. 109, 1793–1801. doi:10.1016/j.biopha.2018.11.003
Li, R., Ruan, Q., Zheng, J., Zhang, B., and Yang, H. (2021c). LINC01116 promotes doxorubicin resistance in osteosarcoma by epigenetically silencing miR-424-5p and inducing epithelial-mesenchymal transition. Front. Pharmacol. 12, 632206. doi:10.3389/fphar.2021.632206
Li, S., Jiang, F., Chen, F., Deng, Y., and Pan, X. (2022a). Effect of m6A methyltransferase METTL3 -mediated MALAT1/E2F1/AGR2 axis on adriamycin resistance in breast cancer. J. Biochem. Mol. Toxicol. 36 (1), e22922. doi:10.1002/jbt.22922
Li, Y., Li, J., Chen, H., Wang, J., Jiang, L., and Tan, X. (2022b). The lncARSR/PTEN/Akt/nuclear factor-kappa B feedback regulatory loop contributes to doxorubicin resistance in hepatocellular carcinoma. J. Biochem. Mol. Toxicol. 36 (9), e23119. doi:10.1002/jbt.23119
Li, Y., Ye, Y., Feng, B., and Qi, Y. (2017). Long noncoding RNA lncARSR promotes doxorubicin resistance in hepatocellular carcinoma via modulating PTEN-PI3K/Akt pathway. J. Cell Biochem. 118, 4498–4507. doi:10.1002/jcb.26107
Li, Z., Li, Y., Wang, X., Liang, Y., Luo, D., Han, D., et al. (2021b). LINC01977 promotes breast cancer progression and chemoresistance to doxorubicin by targeting miR-212-3p/GOLM1 Axis. Front. Oncol. 11, 657094. doi:10.3389/fonc.2021.657094
Li, Z., Qian, J., Li, J., and Zhu, C. (2019b). Knockdown of lncRNA-HOTAIR downregulates the drug-resistance of breast cancer cells to doxorubicin via the PI3K/AKT/mTOR signaling pathway. Exp. Ther. Med. 18 (1), 435–442. doi:10.3892/etm.2019.7629
Liu, J. M., Li, M., Luo, W., and Sun, H. B. (2021). Curcumin attenuates Adriamycin-resistance of acute myeloid leukemia by inhibiting the lncRNA HOTAIR/miR-20a-5p/WT1 axis. Lab. Invest. 101 (10), 1308–1317. doi:10.1038/s41374-021-00640-3
Liu, X., Zhang, G., Yu, T., Liu, J., Chai, X., Yin, D., et al. (2023). CL4-modified exosomes deliver lncRNA DARS-AS1 siRNA to suppress triple-negative breast cancer progression and attenuate doxorubicin resistance by inhibiting autophagy. Int. J. Biol. Macromol. 250, 126147. doi:10.1016/j.ijbiomac.2023.126147
Lu, L., Li, Y., Kim, S. M., Bossuyt, W., Liu, P., Qiu, Q., et al. (2010). Hippo signaling is a potent in vivo growth and tumor suppressor pathway in the mammalian liver. Proc. Natl. Acad. Sci. U. S. A. 107 (4), 1437–1442. doi:10.1073/pnas.0911427107
Lu, Q., Chen, W., Ji, Y., Liu, Y., and Xue, X. (2022). Ursolic acid enhances cytotoxicity of doxorubicin-resistant triple-negative breast cancer cells via ZEB1-AS1/miR-186-5p/ABCC1 Axis. Cancer Biother Radiopharm. 37 (8), 673–683. doi:10.1089/cbr.2020.4147
Luo, Y., Zhang, W., Xu, L., Chen, Y., Xu, Y., and Yuan, L. (2020). Long non-coding RNA PVT1 regulates the resistance of the breast cancer cell line MDA-MB-231 to doxorubicin via Nrf2. Technol. Cancer Res. Treat. 19, 1533033820980763. doi:10.1177/1533033820980763
Malvezzi, M., Carioli, G., Bertuccio, P., Boffetta, P., Levi, F., La Vecchia, C., et al. (2019). European cancer mortality predictions for the year 2019 with focus on breast cancer. Ann. Oncol. 30 (5), 781–787. doi:10.1093/annonc/mdz051
Mariette, C., Piessen, G., and Triboulet, J. P. (2007). Therapeutic strategies in oesophageal carcinoma: Role of surgery and other modalities. Lancet Oncol. 8 (6), 545–553. doi:10.1016/S1470-2045(07)70172-9
Mashouri, L., Yousefi, H., Aref, A. R., Ahadi, A. M., Molaei, F., and Alahari, S. K. (2019). Exosomes: composition, biogenesis, and mechanisms in cancer metastasis and drug resistance. Mol. Cancer 18 (1), 75. doi:10.1186/s12943-019-0991-5
Megyesfalvi, Z., Gay, C. M., Popper, H., Pirker, R., Ostoros, G., Heeke, S., et al. (2023). Clinical insights into small cell lung cancer: Tumor heterogeneity, diagnosis, therapy, and future directions. CA Cancer J. Clin. 2023, 21785. doi:10.3322/caac.21785
Mercer, T. R., Dinger, M. E., and Mattick, J. S. (2009). Long non-coding RNAs: insights into functions. Nat. Rev. Genet. 10 (3), 155–159. doi:10.1038/nrg2521
Minotti, G., Menna, P., Salvatorelli, E., Cairo, G., and Gianni, L. (2004). Anthracyclines: molecular advances and pharmacologic developments in antitumor activity and cardiotoxicity. Pharmacol. Rev. 56 (2), 185–229. doi:10.1124/pr.56.2.6
Mirzaei, S., Gholami, M. H., Hashemi, F., Zabolian, A., Hushmandi, K., Rahmanian, V., et al. (2021). Employing siRNA tool and its delivery platforms in suppressing cisplatin resistance: Approaching to a new era of cancer chemotherapy. Life Sci. 277, 119430. doi:10.1016/j.lfs.2021.119430
Mohammad, R. M., Muqbil, I., Lowe, L., Yedjou, C., Hsu, H. Y., Lin, L. T., et al. (2015). Broad targeting of resistance to apoptosis in cancer. Semin. Cancer Biol. 35 (0), S78–s103. doi:10.1016/j.semcancer.2015.03.001
Moradi-Marjaneh, R., Hassanian, S. M., Rahmani, F., Aghaee-Bakhtiari, S. H., Avan, A., and Khazaei, M. (2018). Phytosomal curcumin elicits anti-tumor properties through suppression of angiogenesis, cell proliferation and induction of oxidative stress in colorectal cancer. Curr. Pharm. Des. 24 (39), 4626–4638. doi:10.2174/1381612825666190110145151
Newman, D. J., and Cragg, G. M. (2012). Natural products as sources of new drugs over the 30 years from 1981 to 2010. J. Nat. Prod. 75 (3), 311–335. doi:10.1021/np200906s
Nong, Q., Yu, S., Hu, H., and Hu, X. (2021). Knockdown of lncRNA FOXD2-AS1 inhibits proliferation, migration, and drug resistance of breast cancer cells. Comput. Math. Methods Med. 2021, 9674761. doi:10.1155/2021/9674761
Orre, C., Dieu, X., Guillon, J., Gueguen, N., Ahmadpour, S. T., Dumas, J. F., et al. (2021). The long non-coding RNA SAMMSON is a regulator of chemosensitivity and metabolic orientation in MCF-7 doxorubicin-resistant breast cancer cells. Biol. (Basel) 10 (11), 1156. doi:10.3390/biology10111156
Palla, M., Scarpato, L., Di Trolio, R., and Ascierto, P. A. (2022). Sonic hedgehog pathway for the treatment of inflammatory diseases: implications and opportunities for future research. J. Immunother. Cancer 10 (6), e004397. doi:10.1136/jitc-2021-004397
Pang, B., Qiao, X., Janssen, L., Velds, A., Groothuis, T., Kerkhoven, R., et al. (2013). Drug-induced histone eviction from open chromatin contributes to the chemotherapeutic effects of doxorubicin. Nat. Commun. 4, 1908. doi:10.1038/ncomms2921
Pang, K. C., Frith, M. C., and Mattick, J. S. (2006). Rapid evolution of noncoding RNAs: lack of conservation does not mean lack of function. Trends Genet. 22 (1), 1–5. doi:10.1016/j.tig.2005.10.003
Pastushenko, I., and Blanpain, C. (2019). EMT transition States during tumor progression and metastasis. Trends Cell Biol. 29 (3), 212–226. doi:10.1016/j.tcb.2018.12.001
Piao, S., and Amaravadi, R. K. (2016). Targeting the lysosome in cancer. Ann. N. Y. Acad. Sci. 1371 (1), 45–54. doi:10.1111/nyas.12953
Pommier, Y., Leo, E., Zhang, H., and Marchand, C. (2010). DNA topoisomerases and their poisoning by anticancer and antibacterial drugs. Chem. Biol. 17 (5), 421–433. doi:10.1016/j.chembiol.2010.04.012
Ponjavic, J., Ponting, C. P., and Lunter, G. (2007). Functionality or transcriptional noise? Evidence for selection within long noncoding RNAs. Genome Res. 17 (5), 556–565. doi:10.1101/gr.6036807
Qian, W., Zhu, Y., Wu, M., Guo, Q., Wu, Z., Lobie, P. E., et al. (2020). Linc00668 promotes invasion and stem cell-like properties of breast cancer cells by interaction with SND1. Front. Oncol. 10, 88. doi:10.3389/fonc.2020.00088
Robey, R. W., Pluchino, K. M., Hall, M. D., Fojo, A. T., Bates, S. E., and Gottesman, M. M. (2018). Revisiting the role of ABC transporters in multidrug-resistant cancer. Nat. Rev. Cancer 18 (7), 452–464. doi:10.1038/s41568-018-0005-8
Schmitz, S. U., Grote, P., and Herrmann, B. G. (2016). Mechanisms of long noncoding RNA function in development and disease. Cell Mol. Life Sci. 73 (13), 2491–2509. doi:10.1007/s00018-016-2174-5
Shalini, S., Dorstyn, L., Dawar, S., and Kumar, S. (2015). Old, new and emerging functions of caspases. Cell Death Differ. 22 (4), 526–539. doi:10.1038/cdd.2014.216
Shang, C., Guo, Y., Zhang, J., and Huang, B. (2016). Silence of long noncoding RNA UCA1 inhibits malignant proliferation and chemotherapy resistance to adriamycin in gastric cancer. Cancer Chemother. Pharmacol. 77 (5), 1061–1067. doi:10.1007/s00280-016-3029-3
Shi, Y. (2002). Mechanisms of caspase activation and inhibition during apoptosis. Mol. Cell 9 (3), 459–470. doi:10.1016/s1097-2765(02)00482-3
Sma, A. N. (2020). The canonical Wnt signaling (Wnt/β-Catenin pathway): A potential target for cancer prevention and therapy. Iran. Biomed. J. 24 (5), 269–280. doi:10.29252/ibj.24.5.264
Song, S., Honjo, S., Jin, J., Chang, S. S., Scott, A. W., Chen, Q., et al. (2015). The Hippo coactivator YAP1 mediates EGFR overexpression and confers chemoresistance in esophageal cancer. Clin. Cancer Res. 21 (11), 2580–2590. doi:10.1158/1078-0432.CCR-14-2191
Struhl, K. (2007). Transcriptional noise and the fidelity of initiation by RNA polymerase II. Nat. Struct. Mol. Biol. 14 (2), 103–105. doi:10.1038/nsmb0207-103
Sun, H., Sun, Y., Chen, Q., and Xu, Z. (2020). LncRNA KCNQ1OT1 contributes to the progression and chemoresistance in acute myeloid leukemia by modulating Tspan3 through suppressing miR-193a-3p. Life Sci. 241, 117161. doi:10.1016/j.lfs.2019.117161
Sung, H., Ferlay, J., Siegel, R. L., Laversanne, M., Soerjomataram, I., Jemal, A., et al. (2021). Global cancer statistics 2020: GLOBOCAN estimates of incidence and mortality worldwide for 36 cancers in 185 countries. CA Cancer J. Clin. 71 (3), 209–249. doi:10.3322/caac.21660
Takahashi, K., Yan, I. K., Kogure, T., Haga, H., and Patel, T. (2014b). Extracellular vesicle-mediated transfer of long non-coding RNA ROR modulates chemosensitivity in human hepatocellular cancer. FEBS Open Bio 4, 458–467. doi:10.1016/j.fob.2014.04.007
Takahashi, K., Yan, I. K., Wood, J., Haga, H., and Patel, T. (2014a). Involvement of extracellular vesicle long noncoding RNA (linc-VLDLR) in tumor cell responses to chemotherapy. Mol. Cancer Res. 12 (10), 1377–1387. doi:10.1158/1541-7786.MCR-13-0636
Tamada, M., Nagano, O., Tateyama, S., Ohmura, M., Yae, T., Ishimoto, T., et al. (2012). Modulation of glucose metabolism by CD44 contributes to antioxidant status and drug resistance in cancer cells. Cancer Res. 72 (6), 1438–1448. doi:10.1158/0008-5472.CAN-11-3024
Tang, L., Chen, Y., Chen, H., Jiang, P., Yan, L., Mo, D., et al. (2020). DCST1-AS1 promotes TGF-β-induced epithelial-mesenchymal transition and enhances chemoresistance in triple-negative breast cancer cells via ANXA1. Front. Oncol. 10, 280. doi:10.3389/fonc.2020.00280
Taniguchi, K., and Karin, M. (2018). NF-κB, inflammation, immunity and cancer: coming of age. Nat. Rev. Immunol. 18 (5), 309–324. doi:10.1038/nri.2017.142
Tkach, M., and Théry, C. (2016). Communication by extracellular vesicles: Where we are and where we need to go. Cell 164 (6), 1226–1232. doi:10.1016/j.cell.2016.01.043
Topalovski, M., and Brekken, R. A. (2016). Matrix control of pancreatic cancer: New insights into fibronectin signaling. Cancer Lett. 381 (1), 252–258. doi:10.1016/j.canlet.2015.12.027
Tsang, W. P., and Kwok, T. T. (2007). Riboregulator H19 induction of MDR1-associated drug resistance in human hepatocellular carcinoma cells. Oncogene 26 (33), 4877–4881. doi:10.1038/sj.onc.1210266
Tsang, W. P., Wong, T. W. L., Cheung, A. H. H., Co, C. N. N., and Kwok, T. T. (2007). Induction of drug resistance and transformation in human cancer cells by the noncoding RNA CUDR. RNA 13 (6), 890–898. doi:10.1261/rna.359007
Uchida, S., and Dimmeler, S. (2015). Long noncoding RNAs in cardiovascular diseases. Circ. Res. 116 (4), 737–750. doi:10.1161/CIRCRESAHA.116.302521
van Niel, G., D'Angelo, G., and Raposo, G. (2018). Shedding light on the cell biology of extracellular vesicles. Nat. Rev. Mol. Cell Biol. 19 (4), 213–228. doi:10.1038/nrm.2017.125
Van Opdenbosch, N., and Lamkanfi, M. (2019). Caspases in cell death, inflammation, and disease. Immunity 50 (6), 1352–1364. doi:10.1016/j.immuni.2019.05.020
Vasiliou, V., Vasiliou, K., and Nebert, D. W. (2009). Human ATP-binding cassette (ABC) transporter family. Hum. Genomics 3 (3), 281–290. doi:10.1186/1479-7364-3-3-281
Velamakanni, S., Wei, S. L., Janvilisri, T., and van Veen, H. W. (2007). ABCG transporters: structure, substrate specificities and physiological roles: a brief overview. J. Bioenerg. Biomembr. 39 (5-6), 465–471. doi:10.1007/s10863-007-9122-x
Wadler, S., Green, M., and Muggia, F. (1985). The role of anthracyclines in the treatment of gastric cancer. Cancer Treat. Rev. 12 (2), 105–132. doi:10.1016/0305-7372(85)90003-9
Wang, Y., Xin, D., and Zhou, L. (2020). LncRNA LINC00152 increases the aggressiveness of human retinoblastoma and enhances carboplatin and adriamycin resistance by regulating MiR-613/yes-associated protein 1 (YAP1) Axis. Med. Sci. Monit. 26, e920886. doi:10.12659/MSM.920886
Wang, H., Qin, R., Guan, A., Yao, Y., Huang, Y., Jia, H., et al. (2018a). HOTAIR enhanced paclitaxel and doxorubicin resistance in gastric cancer cells partly through inhibiting miR-217 expression. J. Cell Biochem. 119 (9), 7226–7234. doi:10.1002/jcb.26901
Wang, W., Chen, D., and Zhu, K. (2018b). SOX2OT variant 7 contributes to the synergistic interaction between EGCG and Doxorubicin to kill osteosarcoma via autophagy and stemness inhibition. J. Exp. Clin. Cancer Res. 37 (1), 37. doi:10.1186/s13046-018-0689-3
Wang, X., Pei, X., Guo, G., Qian, X., Dou, D., Zhang, Z., et al. (2020). Exosome-mediated transfer of long noncoding RNA H19 induces doxorubicin resistance in breast cancer. J. Cell Physiol. 235 (10), 6896–6904. doi:10.1002/jcp.29585
Wang, Y., Zhang, D., Wu, K., Zhao, Q., Nie, Y., and Fan, D. (2014). Long noncoding RNA MRUL promotes ABCB1 expression in multidrug-resistant gastric cancer cell sublines. Mol. Cell Biol. 34 (17), 3182–3193. doi:10.1128/MCB.01580-13
Wang, Z., Liu, Z., and Wu, S. (2017). Long non-coding RNA CTA sensitizes osteosarcoma cells to doxorubicin through inhibition of autophagy. Oncotarget 8 (19), 31465–31477. doi:10.18632/oncotarget.16356
Warfel, N. A., and El-Deiry, W. S. (2014). HIF-1 signaling in drug resistance to chemotherapy. Curr. Med. Chem. 21 (26), 3021–3028. doi:10.2174/0929867321666140414101056
Wicha, M. S., Liu, S., and Dontu, G. (2006). Cancer stem cells: an old idea--a paradigm shift. Cancer Res. 66 (4), 1883–1890. ; discussion 1895-6. doi:10.1158/0008-5472.CAN-05-3153
Xiao, J., Lv, Y., Jin, F., Liu, Y., Ma, Y., Xiong, Y., et al. (2017). LncRNA HANR promotes tumorigenesis and increase of chemoresistance in hepatocellular carcinoma. Cell Physiol. Biochem. 43 (5), 1926–1938. doi:10.1159/000484116
Xie, C., Zhang, L. Z., Chen, Z. L., Zhong, W. J., Fang, J. H., Zhu, Y., et al. (2020). A hMTR4-PDIA3P1-miR-125/124-TRAF6 regulatory Axis and its function in NF kappa B signaling and chemoresistance. Hepatology 71 (5), 1660–1677. doi:10.1002/hep.30931
Xiong, M., Wu, M., Dan Peng, H., Huang, W., Chen, Z., Ke, H., et al. (2021). LncRNA DANCR represses Doxorubicin-induced apoptosis through stabilizing MALAT1 expression in colorectal cancer cells. Cell Death Dis. 12 (1), 24. doi:10.1038/s41419-020-03318-8
Xu, R., Ji, J., Zhang, X., Han, M., Zhang, C., Xu, Y., et al. (2017). PDGFA/PDGFRα-regulated GOLM1 promotes human glioma progression through activation of AKT. J. Exp. Clin. Cancer Res. 36 (1), 193. doi:10.1186/s13046-017-0665-3
Yang, G., Lu, X., and Yuan, L. (2014). LncRNA: a link between RNA and cancer. Biochim. Biophys. Acta 1839 (11), 1097–1109. doi:10.1016/j.bbagrm.2014.08.012
Yang L, L., Froberg, J. E., and Lee, J. T. (2014). Long noncoding RNAs: fresh perspectives into the RNA world. Trends Biochem. Sci. 39 (1), 35–43. doi:10.1016/j.tibs.2013.10.002
Young, R. C., Ozols, R. F., and Myers, C. E. (1981). The anthracycline antineoplastic drugs. N. Engl. J. Med. 305 (3), 139–153. doi:10.1056/NEJM198107163050305
Yue, X., Wu, W. Y., Dong, M., and Guo, M. (2021). LncRNA MALAT1 promotes breast cancer progression and doxorubicin resistance via regulating miR-570-3p. Biomed. J. 44 (6), S296–s304. doi:10.1016/j.bj.2020.11.002
Zeng, F., Wang, Q., Wang, S., Liang, S., Huang, W., Guo, Y., et al. (2020). Linc00173 promotes chemoresistance and progression of small cell lung cancer by sponging miR-218 to regulate Etk expression. Oncogene 39 (2), 293–307. doi:10.1038/s41388-019-0984-2
Zeng, Y., Wang, G., Zhou, C. F., Zhang, H. B., Sun, H., Zhang, W., et al. (2019). LncRNA profile study reveals a three-LncRNA signature associated with the pathological complete response following neoadjuvant chemotherapy in breast cancer. Front. Pharmacol. 10, 574. doi:10.3389/fphar.2019.00574
Zhang, C. L., Zhu, K. P., and Ma, X. L. (2017a). Antisense lncRNA FOXC2-AS1 promotes doxorubicin resistance in osteosarcoma by increasing the expression of FOXC2. Cancer Lett. 396, 66–75. doi:10.1016/j.canlet.2017.03.018
Zhang, C. L., Zhu, K. P., Shen, G. Q., and Zhu, Z. S. (2016). A long non-coding RNA contributes to doxorubicin resistance of osteosarcoma. Tumour Biol. 37 (2), 2737–2748. doi:10.1007/s13277-015-4130-7
Zhang, F., Li, X., Liu, H., Xi, T., and Zheng, L. (2019). LncRNA FENDRR attenuates adriamycin resistance via suppressing MDR1 expression through sponging HuR and miR-184 in chronic myelogenous leukaemia cells. FEBS Lett. 593 (15), 1993–2007. doi:10.1002/1873-3468.13480
Zhang, H., Guo, Y., Song, Y., and Shang, C. (2017b). Long noncoding RNA GAS5 inhibits malignant proliferation and chemotherapy resistance to doxorubicin in bladder transitional cell carcinoma. Cancer Chemother. Pharmacol. 79 (1), 49–55. doi:10.1007/s00280-016-3194-4
Zhang, J., Rao, D., Ma, H., Kong, D., Xu, X., and Lu, H. (2020). LncRNA SNHG15 contributes to doxorubicin resistance of osteosarcoma cells through targeting the miR-381-3p/GFRA1 axis. Open Life Sci. 15 (1), 871–883. doi:10.1515/biol-2020-0086
Zhang, L., Zhou, S., Zhou, T., Li, X., and Tang, J. (2022). Targeting the lncRNA DUXAP8/miR-29a/PIK3CA network restores doxorubicin chemosensitivity via PI3K-AKT-mTOR signaling and synergizes with inotuzumab ozogamicin in chemotherapy-resistant B-cell acute lymphoblastic leukemia. Front. Oncol. 12, 773601. doi:10.3389/fonc.2022.773601
Zhang, Y., Liu, Y., and Xu, X. (2018). Knockdown of LncRNA-UCA1 suppresses chemoresistance of pediatric AML by inhibiting glycolysis through the microRNA-125a/hexokinase 2 pathway. J. Cell Biochem. 119 (7), 6296–6308. doi:10.1002/jcb.26899
Zhang, Y., Wu, W., Sun, Q., Ye, L., Zhou, D., and Wang, W. (2021). linc-ROR facilitates hepatocellular carcinoma resistance to doxorubicin by regulating TWIST1-mediated epithelial-mesenchymal transition. Mol. Med. Rep. 23 (5), 340. doi:10.3892/mmr.2021.11979
Zhao, Y., He, J., Li, J., Peng, X., Wang, X., Dong, Z., et al. (2017). Demethylzeylasteral inhibits cell proliferation and induces apoptosis through suppressing MCL1 in melanoma cells. Cell Death Dis. 8 (10), e3133. doi:10.1038/cddis.2017.529
Zheng, K., Kitazato, K., and Wang, Y. (2019). Selective autophagy regulates cell cycle in cancer therapy. Theranostics 9 (1), 104–125. doi:10.7150/thno.30308
Zheng, R., Jia, J., Guan, L., Yuan, H., Liu, K., Liu, C., et al. (2020). Long noncoding RNA lnc-LOC645166 promotes adriamycin resistance via NF-κB/GATA3 axis in breast cancer. Aging (Albany NY) 12 (10), 8893–8912. doi:10.18632/aging.103012
Zhou, B., Li, L., Li, Y., Sun, H., and Zeng, C. (2018a). Long noncoding RNA SNHG12 mediates doxorubicin resistance of osteosarcoma via miR-320a/MCL1 axis. Biomed. Pharmacother. 106, 850–857. doi:10.1016/j.biopha.2018.07.003
Zhou, W., Xu, S., Chen, X., and Wang, C. (2021). HOTAIR suppresses PTEN via DNMT3b and confers drug resistance in acute myeloid leukemia. Hematology 26 (1), 170–178. doi:10.1080/16078454.2021.1880733
Zhou, X., and Xiao, D. (2020). Long non-coding RNA GAS5 is critical for maintaining stemness and induces chemoresistance in cancer stem-like cells derived from HCT116. Oncol. Lett. 19 (5), 3431–3438. doi:10.3892/ol.2020.11471
Zhou, Z., Lin, Z., He, Y., Pang, X., Wang, Y., Ponnusamy, M., et al. (2018b). The long noncoding RNA D63785 regulates chemotherapy sensitivity in human gastric cancer by targeting miR-422a. Mol. Ther. Nucleic Acids 12, 405–419. doi:10.1016/j.omtn.2018.05.024
Keywords: long non-coding RNA, doxorubicin, drug resistance, cancer, molecular mechanisms
Citation: Zhang H-B, Hu Y, Deng J-L, Fang G-Y and Zeng Y (2023) Insights into the involvement of long non-coding RNAs in doxorubicin resistance of cancer. Front. Pharmacol. 14:1243934. doi: 10.3389/fphar.2023.1243934
Received: 21 June 2023; Accepted: 31 August 2023;
Published: 15 September 2023.
Edited by:
Sujit Nair, Viridis BioPharma Pvt. Ltd., IndiaReviewed by:
Mihir Khambete, Yale University, United StatesSaiprasad Ajgaonkar, University of Mumbai, India
Copyright © 2023 Zhang, Hu, Deng, Fang and Zeng. This is an open-access article distributed under the terms of the Creative Commons Attribution License (CC BY). The use, distribution or reproduction in other forums is permitted, provided the original author(s) and the copyright owner(s) are credited and that the original publication in this journal is cited, in accordance with accepted academic practice. No use, distribution or reproduction is permitted which does not comply with these terms.
*Correspondence: Ying Zeng, zengying2007@163.com; Guo-Ying Fang, fgy2020@sina.com