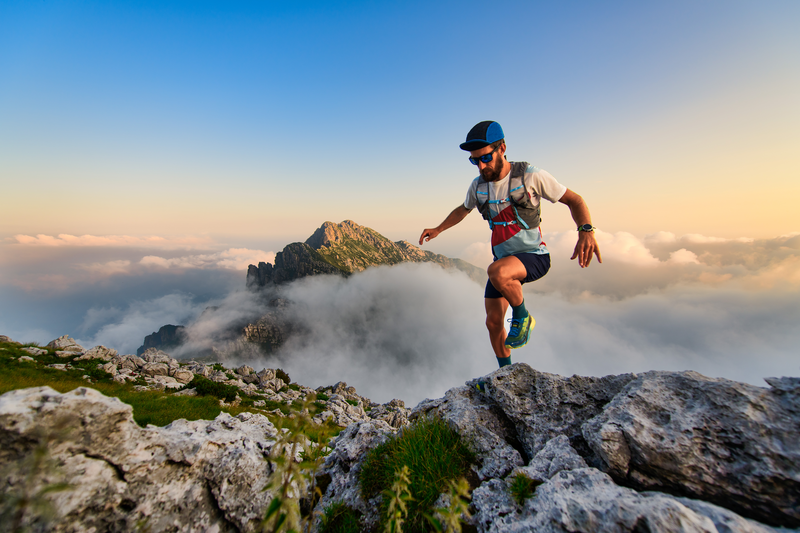
95% of researchers rate our articles as excellent or good
Learn more about the work of our research integrity team to safeguard the quality of each article we publish.
Find out more
REVIEW article
Front. Pharmacol. , 05 September 2023
Sec. Pharmacology of Infectious Diseases
Volume 14 - 2023 | https://doi.org/10.3389/fphar.2023.1242156
This article is part of the Research Topic The Evolution in Pharmacology of Infectious Diseases: 2022 View all 16 articles
Non-tuberculosis mycobacteria (NTM) skin infections have become increasingly prevalent in recent years, presenting a unique challenge in clinical management. This review explored the complexities of NTM infections localized to the superficial tissues and provided valuable insights into the optimal therapeutic strategies. The antibiotic selection should base on NTM species and their susceptibility profiles. It is recommended to adopt a comprehensive approach that considers the unique characteristics of superficial tissues to improve treatment effectiveness and reduce the incidence of adverse reactions, infection recurrence, and treatment failure. Infection control measures, patient education, and close monitoring should complement the treatment strategies to achieve favorable outcomes in managing NTM skin infections. Further efforts are warranted to elucidate factors and mechanisms contributing to treatment resistance and relapse. Future research should focus on exploring novel treatment options, innovative drug development/delivery platforms, and precise methodologies for determining therapeutic duration. Longitudinal studies are also needed to assess the long-term safety profiles of the integrated approaches.
Non-tuberculosis mycobacteria (NTM) (Sharma and Upadhyay, 2020; Pavlik et al., 2022), mycobacteria other than Mycobacterium tuberculosis and Mycobacterium leprae, have emerged as a significant source of infections (Kumar et al., 2021; Nogueira et al., 2021). Among the diverse manifestations of NTM infections, skin and soft tissue involvements are prevalent clinical presentations. Although not posing an immediate life-threatening risk, these infections can result in significant morbidity and adversely affect the quality of life for affected individuals. Notably, there has been a global increase in reported cases of superficial NTM infections (Mei et al., 2019; Philips et al., 2019; Gopalaswamy et al., 2020) with contributing factors including the expanding population of immunocompromised individuals (Blakney et al., 2022; Toth et al., 2022) who face heightened susceptibility during injury or cosmetic procedures (Ahmed et al., 2020; Wang C. J. et al., 2022; Ni et al., 2023). Furthermore, the rise in NTM infections can be attributed, in part, to the ongoing adaptation of NTM to the human host. NTM’s remarkable capacity to thrive within the diverse skin environment, while effectively evading immune responses, also plays a role in the escalating incidence of these infections (Luo et al., 2021).
In light of these observations, understanding and effectively managing NTM skin infections are crucial for public health and patient wellbeing. Regarding treatment choices for NTM infections, various factors come into play due to the unique nature of these infections. Generally, the choice of treatment for NTM infections depends on the specific species and susceptibility pattern of the isolated organisms, as well as the severity and extent of the infection (Pennington et al., 2021). Current treatment strategies often reference the guidelines (Daley et al., 2020) of the Infectious Disease Society of America (IDSA) and American Thoracic Society (ATS) on pulmonary NTM-infected diseases. However, it should be noted that the ATS/IDSA guidelines for pulmonary diseases may not be directly applied to all skin-involved cases due to the unique characteristics of cutaneous NTM infections. The invasive or disseminated NTM infections may require a greater variety of drugs and a more extended treatment duration (Liu et al., 2023). When it comes to superficial involved cases, consideration must be given to the potential variations in pathogen types resulting from diverse infection pathways. The unique physiological structures and functions of the skin (Gravitz, 2018), compared to other anatomical sites, may also influence drug absorption and distribution, warranting tailored treatment approaches. In addition, the influence of local microbiota (Grice and Segre, 2011) and differences in host immune responses (Nguyen and Soulika, 2019) should not be underestimated, as they significantly impact treatment outcomes. Surgical interventions, phototherapy (Yang et al., 2022), and heat application (Lee and Lee, 2017) should also be considered as viable alternative treatment options due to anatomical variations in the infected sites. Furthermore, the emergence of newly invented antimycobacterial agents, such as MmpL3 inhibitors and Efflux Pump inhibitors (Rindi, 2020; North et al., 2023), are believed to have potent against slow-growing mycobacteria (SGM) and rapid-growing mycobacteria (RGM), also highlights the need for timely revision of treatment approaches. Finally, although NTM and M. tuberculosis shares similar physiological characteristics, virulence factors, and genetic drug targets (Rifat et al., 2021; Mei et al., 2023), it is still not advisable to fully copy the treatment regimens of TB. Many drugs being developed for treating TB do not exhibit any antimicrobial activity against NTM (Saxena et al., 2021). In summary, those factors highlight the need for updating of targeted treatment approaches to enhance skin-involved patient outcomes.
The management of cutaneous infections caused by various NTM subtypes poses significant challenges, necessitating a careful balance between therapeutic benefits and potential risks. The optimization of diverse treatment approaches, as well as the mitigation of adverse effects and infection recurrence, remain critical objectives. Through this comprehensive review, our aim is to provide an in-depth analysis of the treatment strategies for NTM skin infections and shed light on the complexities involved in addressing these clinical aspects.
SGM (>7 days for mature colony formation in solid media) mainly includes Mycobacterium marinum (M. marinum), M. kansasii, Mycobacterium avium complex (MAC), and many others (Yan et al., 2023). Common risk factors for SGM skin infections include exposure to contaminated water sources, such as swimming in contaminated water bodies or handling fish tanks, and skin injuries like cuts or scrapes that serve as entry points for the bacteria. Clinical presentations of SGM infections typically involve the development of nodules or raised skin lesions at the site of entry, which gradually enlarge and may lead to non-healing wounds or abscess formation (Riccardi et al., 2022). Infected areas may become painful and swollen, and the infection can spread along lymphatic vessels in a linear fashion. The final goal of the targeted treatment of SGM infection is to facilitate rehabilitation, shorten the treatment course, and prevent the pathogen from further progressing to deeper tissues to avoid multiple distributions.
M. marinum is the predominant pathogen responsible for SGM, often leading to skin and soft tissue infections (Hashish et al., 2018). Early diagnosis and prompt treatment of M. marinum infections pose significant challenges, especially during the atypical stage, potentially complicating the subsequent course of medication (Trčko et al., 2021). Presently, there is a lack of standardized norms concerning the selection, dosages, and treatment duration of drugs, as well as the consideration of surgery as an adjunct to treatment options (Seidel et al., 2022). According to the recommendations of IDSA/ATS guidelines, treatment typically involves using a combination of two active drugs, such as ethambutol-macrolide combinations, and continuing therapy until 1–2 months after symptom resolution. However, it is essential to acknowledge that no randomized controlled trials have been conducted in this domain, and the available data are insufficient to establish statistically significant evidence on drug efficacy and tolerability. The scarcity of verified data necessitates further research to comprehensively evaluate the effectiveness and safety of different treatment regimens for M. marinum infections.
The in vitro drug sensitivity test (Hendrikx et al., 2022; Seidel et al., 2022) shows that M. marinum is moderately sensitive to streptomycin and resistant to azithromycin, isoniazid, and pyrazinamide. The minimum inhibitory concentrations (MICs) of levofloxacin, ciprofloxacin, and quinolones about M. marinum are high while keeping lower for rifampin, moxifloxacin, ethambutol, clarithromycin, linezolid, and tetracyclines. The results (Koushk-Jalali et al., 2019; Yeo et al., 2019; Castillo et al., 2020; Strobel et al., 2022) show that rifampicin, clarithromycin, sulfonamides, doxycycline, minocycline, and ethambutol are more suitable choices. Hence, given M. marinum’s susceptibility to numerous antibiotics, empirical treatment can be initiated at first, especially when susceptibility tests are unavailable (Oh et al., 2018). However, a test is needed when the condition is not improved after adequate treatment, or the mycobacterial culture is still positive after several months.
Several studies (Rallis et al., 2012; Chung et al., 2018; Franco-Paredes et al., 2018) indicated that oral monotherapy (single antibiotics such as clarithromycin, trimethoprim, and ciprofloxacin) is effective in immunocompetent patients only with superficial cutaneous M. marinum infections in the early stage, which also recommends that a suitable course of treatment need to last up 3–6 months, or the focus is limited and then proceed for 1–2 months (Aubry et al., 2017). Combined use of various active antimycobacterial agents is recommended under the involvement of deeper tissues, disseminated extracutaneous infection, and immunosuppressive status of hosts (Holden et al., 2018). Combinations, such as clarithromycin combined with rifampicin, clarithromycin combined with ethambutol, ethambutol combined with rifampicin, or three, are preferred (Strobel et al., 2022). The duration of therapy depends on the infection’s severity and treatment effects and could be extended moderately according to the host-drug interactions.
Surgeries (incision and drainage) are needed when the M. marinum goes deeper or poor curative effect for a long time. This may involve drainage of abscesses, removal of infected tissue, or excision of nodules or lesions that are unresponsive to antimicrobial therapy. Keeping the affected area clean and dry, avoiding activities that may traumatize the skin, and using appropriate dressings or bandages to protect the affected skin from further irritation or contamination are necessary. It is also recommended that amputation (Hendrikx et al., 2022) might be considered in case of severe cutaneous infections caused by multidrug-resistant isolates. Other than that, hot compress therapy (Strobel et al., 2022) might be a choice due to its high-temperature intolerance. (The optimal temperature is 30 Degrees Celsius).
Cutaneous infections caused by M. kansasii predominantly affect immunocompromised hosts, including individuals with conditions such as diabetes or those who have undergone renal transplantation (Zhang et al., 2017; Okuno et al., 2020). Often, these cutaneous infections are concomitant with pulmonary involvement. As a result, when managing M. kansasii infections in superficial tissues, it is reasonable to refer to the guidelines established for the treatment of pulmonary NTM infections. According to the official ATS/ERS/ESCMID/IDSA clinical practice guidelines, for patients with rifampin-susceptible M. kansasii, a treatment course lasting over 12 months, comprising rifampicin, ethambutol, and either isoniazid or a macrolide, is advised.
There are a few differences between the guidelines of ATS/IDSA and the consensus of the British Thoracic Society (BTS) (Haworth et al., 2017). Rifampin and ethambutol are the same, while the part combined with isoniazid or clarithromycin differs. Both regimes mentioned above need years of treatment duration. A recent study (Chapagain et al., 2020) indicated that the regime of BTS and a novel one (rifapentine + tedizolid + minocycline) show better efficacy on M. kansasii of pulmonary diseases. Another study (Moon et al., 2019) found that the macrolide-containing regimen is as effective as the isoniazid-containing regimen, which might reduce the cumulative side effects of long-term use of anti-tuberculosis drugs. Clofazimine only shows a modest to poor impact on M. kansasii in a clinic in an observational study (Srivastava and Gumbo, 2018) on the antimicrobial effect of clofazimine monotherapy in the intracellular-infection hollow fiber model of M. kansasii.
Although the result of in vitro susceptibility testing of M. kansasii correlates little with clinical outcomes generally, it is still something to be learned from this. According to the effects of susceptibility tests (Zhang et al., 2017; Bakuła et al., 2018; Wang et al., 2019), M. kansasii is susceptible to ethambutol, rifampin, ethambutol, clarithromycin, aminoglycosides, and fluoroquinolones, which might guide clinical work of complicated cases. M. kansasii is resistant to pyrazinamide, and its potential resistance mechanism is not necessarily related to gene mutation but to great genetic diversity globally (Guo et al., 2022).
MAC (Daley, 2017), such as M. avium, M. Intracellulare, M. Chimaera, M. indicuspranii, is a group of SGM commonly identified in the respiratory system of patients with severely immunocompromised statuses (To et al., 2020). Given the circumstances, MAC infections often exhibit a propensity to disseminate from the initial infected site to involve other organs and tissues. Thus, the management of MAC infections affecting the skin and soft tissues warrants a comprehensive approach akin to treating invasive or disseminated cases (Chen et al., 2020; Crilly et al., 2020). A 3 year cross-sectional study (Akrami et al., 2023) found that MAC was susceptible to amikacin, moxifloxacin, and clarithromycin, while resistant to linezolid, rifampin, isoniazid, and clofazimine. In a cases report (Fukushi et al., 2022) of pulmonary and disseminated MAC patients confirmed by tissue-direct polymerase chain reaction-based nucleic acid lateral flow immunoassay, clinicians treated two patients with clarithromycin (CAM, 800 mg/day), rifampicin (RIP, 600 mg/day), and ethambutol (EB, 700 mg/day) for a year. No adverse side effects or recurrence were founded during the treatment. Omadacycline was tested as a potential treatment option for pulmonary MAC in hollow fibre system model, possibly as an alternative treatment for a new MAC regimen. The results of susceptibility testing in a retrospective study (Mok et al., 2019) of 88 isolates showed that M. chimaera is susceptible to clarithromycin, amikacin, rifabutin, and streptomycin while resistant to moxifloxacin and linezolid with a high probability, which might influence the overall therapeutic strategy. Several studies (Maurer et al., 2019; Li et al., 2022; Schulthess et al., 2023) on susceptibility testing found that M. chimaera and other members of the MAC generally have similar susceptibility (clarithromycin, amikacin, and rifabutin). In summary, for MAC infections that are susceptible to macrolides, a regimen of at least three drugs, including a macrolide and ethambutol, is preferred over a monotherapy of just a macrolide or ethambutol.
Less common organisms include M. xenopi, M. malmoense, M. simiae, and M. szulgai. Despite the close phylogenetic relationship among these organisms, they exhibit discrete epidemiological characteristics and pathogenic behaviors. Therefore, the management of these SGM demand a nuanced approach that meticulously considers the distinctive attributes of each individual species. According to the established consensus, the recommended treatment approach involves a combination of two to three types of antibiotics, administered for a duration of at least 12 months beyond the point of culture conversion (Yan et al., 2023). For example, in the case of M. xenopi infections, a daily regimen that consists of at least three drugs: rifampicin, ethambutol, and either a macrolide or a fluoroquinolone (e.g., moxifloxacin) is recommended according to the official ATS/ERS/ESCMID/IDSA clinical practice guidelines. Nevertheless, the consensus regarding treatment recommendations (such as azithromycin, clarithromycin, rifampicin, ethambutol, amikacin, and moxifloxacin) for less common NTM species is largely based on low-quality evidence derived from published scientific literature.
Similar to SGM infections, direct inoculation of RGM can occur through various routines, including trauma, surgical procedures, injections, tattoos, and other operations that involve the disruption of the skin barrier. In such instances, RGM can potentially spread to deeper tissues and cause infections beyond the initial site of entry. Clinical presentations of RGM skin infections often involve the development of nodules, abscesses, or ulcers at the site of entry. These skin lesions can be painful, red, and may contain pus. In immunocompromised individuals or those with underlying medical conditions, RGM skin infections can be more severe.
Treatment of RGM infections includes various regimens with different response rates (Kasperbauer and De Groote, 2015). The selection of antibiotics is mainly based on the results of drug susceptibility tests. According to the results (Chang and Whipps, 2015; Dumic and Lutwick, 2021), RGM is more sensitive to tigecycline, tobramycin, clarithromycin, and amikacin. However, the susceptibility profile varies from species to species. Drug resistance (Forbes et al., 2018; Shrivastava et al., 2020) still poses a significant challenge to a successful outcome due to the presence of the erm41 gene, which could lead to inducible resistance to macrolide, prolong the therapy, and increase the incidence of drug-induced toxicity. Below are details of the most common RGM species.
Mycobacterium fortuitum complex consists of M. peregrinum, M. porcinum, M. fortuitum and many others. Combined antibiotics treatment is often required, and surgical therapy may be needed optionally (Philips et al., 2019). After reviewing several in vitro antimicrobial susceptibility research (Forbes et al., 2018; Yeo et al., 2019; Da et al., 2020; Kumar et al., 2021; Das et al., 2022), we found that M. fortuitum strains were susceptible to many antibiotics. The isolates are susceptible to amikacin (intermediate to highly sensitive), ciprofloxacin (highly susceptible), doxycycline (intermediate susceptible), clofazimine, trimethoprim-sulfamethoxazole (TMP-SMX) and linezolid, resistance to all the antituberculosis agents, while different to macrolides (decreased sensitivity due to inducible susceptibility) and imipenem. A study (Chew et al., 2021) of 86 isolates showed that M. fortuitum is resistant to clarithromycin and tobramycin but susceptible to tetracyclines and quinolones. Similarly, a retrospective case series (Wang J. et al., 2022) of 18 patients with cutaneous M. fortuitum complex infections found that five uncomplicated infection cases showed an excellent response to the treatments. One patient received monotherapy of doxycycline for 8 weeks with no recurrence; the other four patients were treated with combined antibiotics, clarithromycin-minocycline, clarithromycin-ciprofloxacin, clarithromycin-TMP-SMX, and ciprofloxacin-TMP/SMX. Treatment courses range from 10 weeks to 40 weeks. While only three complicated infection patients with a prolonged period of the same therapy showed satisfactory clinical consequences, which meant immunosuppressed hosts were at higher risk of having persistent SGM infection than the immunocompetent population. Moreover, the findings from an in vitro and in vivo experiments (Ahmad et al., 2022) have demonstrated that gepotidacin, a first-in-class triazaacenapthylene topoisomerase inhibitor, exhibits a promising and potentially novel mechanism of action, allowing it to evade prevailing resistance mechanisms. These results underscore the potential of gepotidacin as a valuable therapeutic candidate with the ability to overcome resistance challenges commonly encountered with existing antimicrobial agents.
The results of susceptibility testing (Franco-Paredes et al., 2018; Uslu et al., 2019; Watanabe et al., 2022) indicated that M. chelonae is often susceptible to macrolides, cefoxitin, fluoroquinolones, and tobramycin. The monotherapy (clarithromycin) can be sufficient for localized or superficial infections but not enough for patients who develop potential resistance. At least two antibiotic agents (oral macrolide combined with cefoxitin, amikacin, or imipenem) and 4–6 months of systemic treatment are recommended for these complicated cases. A biologics side-effects induced case (Frizzell et al., 2020) showed that omadacycline monotherapy at a dose of 300 mg orally daily for 4 months was efficient against M. chelonae skin and skin structure infections without recurrence in a 1-year follow-up. Surgical debridement, incising, draining, and source control are recommended in treatment if there is extensive involvement of extra-pulmonary M. chelonae infection (Dumic and Lutwick, 2021). Like M. marinum, thermal therapy was efficacious due to its thermal sensitivity. In addition, routine treatment (antimicrobial and surgical therapies) added a single bacteriophage (Little et al., 2022) showed stable disease improvement with no evidence of bacterial resistance to the phage. Bacteriophage therapy involves using viruses to infect and target specific bacteria, leading to the destruction and elimination of the bacterial population. Given the current challenges posed by antimicrobial resistance, bacteriophage therapy has emerged as a promising and attractive therapeutic option.
The M. abscessus group (M. abscessus, M. massiliense, and M. bolletii) is the primary source of cutaneous involvement of RGM (Jeong et al., 2017; Franco-Paredes et al., 2018). M. abscessus has an irregular resistance pattern to numerous anti-NTM agents (Lee et al., 2015; To et al., 2020; Kumar et al., 2021). Compared to M. massiliense, some M. abscessus and M. bolletii isolates (not all) have inducible macrolide resistance due to the functional erm41 gene, which could lead to inadequate response to a macrolides-dominant therapeutic schedule. Hence, antimicrobial susceptibility testing on all clinically significant isolates is strongly recommended before starting the therapy. The susceptibility list should include at least amikacin, cefoxitin, imipenem, clarithromycin, linezolid, doxycycline, tigecycline, ciprofloxacin, and moxifloxacin. Per the official ATS/ERS/ESCMID/IDSA clinical practice guidelines, for M. abscessus infections, whether the strains possess inducible or mutational macrolide resistance or not, it is recommended to initiate with a macrolide-inclusive multidrug regimen, which should encompass at least three drugs proven effective in vitro. An observational study (Da et al., 2020) showed that all strains of the M. abscessus group were susceptible to amikacin, linezolid, clofazimine, and tigecycline and suggested a prolonged drug resistance testing of 14 days to determine the presence of inducible resistance to macrolides is necessary. Monotherapy (clarithromycin) has shown promising efficacy in uncomplicated non-pulmonary disease, probably because its hand and foot lesions may represent a self-limited characteristic (Lee et al., 2015). However, invasive or disseminated M. abscessus and M. bolletii infections are complicated to treat; a combination of medication and a more comprehensive treatment course are necessary (Comba et al., 2021). Surgical resection of the infected tissues following chemotherapy to lessen the extensive progress might be a possible curative treatment for complex cases.
In the context of treating M. abscessus infection, the preclinical and clinical data derived from a study (Singh et al., 2023) suggest that the inclusion of omadacycline at a dosage of 300 mg per day in combination regimens holds promise for potential evaluation in Phase III trials involving patients with pulmonary involvement of M. abscessus. Such investigations could potentially bear significant guiding implications for addressing skin-related issues as well. Moreover, bacteriophages have also been explored as a potential therapeutic option. A study (Gorzynski et al., 2023) revealed that the lytic efficiency of phages is influenced by environmental factors, particularly when dealing with biofilm and intracellular states of M. abscessus. This observation has important implications, as it aids in the identification of therapeutic phages capable of reducing bacterial fitness by hindering antibiotic efflux function and attenuating the intrinsic resistance mechanisms of M. abscessus through targeted therapeutic interventions. Thiostrepton, a promising novel therapeutic drug candidate, has demonstrated substantial inhibition of M. abscessus growth in various contexts, including wild-type strains, subspecies, clinical isolates, and drug-resistant mutants, as evidenced by in vitro experiments and macrophage models. Additionally, it exhibited a dose-dependent reduction in proinflammatory cytokine production, suggesting its potential as an anti-inflammatory agent in the context of M. abscessus infection (Kim et al., 2019).
According to the in vitro antimicrobial drug susceptibility testing, A study (Cantillon et al., 2022) using an open drug discovery approach found that oxazolidinones such as linezolid and doxycycline have excellent tissue penetration properties and are actively potent against M. chimaera. Two case reports (Shimizu et al., 2012; Wang C. J. et al., 2022) recommend combined therapy with adequate debridement and sensitive antibiotic administration for soft tissues in patients infected with M. smegmatis.
Since more and more extrapulmonary NTM-infected cases have been reported recently and no unified treatment proposal could be referred to, a safer, more effective, higher adherent, a broader spectrum of anti-NTM activities, and more cutaneous-specific treatment strategy is needed. Thus, we reviewed the treatment of NTM infections involving skin or soft tissues in recent years to give some suggestions on this topic.
NTM skin involvements exhibit distinctive therapeutic disparities compared to other NTM-infected manifestations, owing to the unique structural characteristics of the skin, variations in drug distribution patterns, diverse modes of infection, relatively confined lesion distribution, milder disease severity, and a greater array of treatment modalities available. The management of NTM infections frequently entails the administration of multiple antimicrobial agents over extended durations, requiring vigilant clinical and laboratory monitoring. Nevertheless, the dearth of well-structured controlled trials investigating first-line treatment regimens, including optimal drug selection, dosage, and duration, poses challenges in formulating evidence-based guidelines for effectively managing a wide array of NTM species and associated diseases. Consequently, regimen selection should generally be guided by drug susceptibility testing. This testing involves assessing the susceptibility of the NTM isolate to various antimicrobial drugs, allowing for informed decisions on the most appropriate therapeutic approach. According to the results of drug sensitivity tests, our recommended treatment choices were summarized in Table 1. The establishment of rigorous clinical trials will be instrumental in addressing these knowledge gaps and facilitating the development of more effective and targeted treatment strategies for NTM skin infections. Waiting for species identification and susceptibility before treatment is reasonable without any delay for most superficial cases. However, the correlation between clinical outcomes and in vitro susceptibility thresholds remains undefined for the majority of NTM species (van Ingen et al., 2012a; Timmins, 2020). Different NTM subspecies have other susceptibility profiles to antimicrobial agents. The susceptible antibiotics against SGM differ from that of RGM (Alffenaar et al., 2021; Sharma et al., 2021). Therefore, subspecies level identification (no higher than the species level) and sensitivity testing of NTM, especially RGM, is recommended. For example, the Clinical and Laboratory Standards Institute (CLSI) recommends (Schoutrop et al., 2018) clarithromycin and amikacin susceptibility testing only for MAC, clarithromycin, and rifampicin for M. kansasii, and clarithromycin for M. abscessus complex. In addition, susceptibility testing should be prolonged as long as 6 weeks for SGM and 2 weeks for macrolides (Dartois and Dick, 2022). Recent advancements in molecular diagnostic techniques have improved the accuracy and speed of identifying NTM species and their drug susceptibilities, allowing for more precise and targeted treatment. However, over time, the stability of some antimycobacterial drugs is gradually affected by the pathogen, leading to a variable minimum inhibitory concentration (MIC), which then affects the final interpretation of the DST result. This partly explains why drug susceptibility testing results do not necessarily translate to a positive clinical response. The local microenvironments (Dey et al., 2010; Dartois and Dick, 2022), which can decrease therapeutic concentrations of drugs at the anatomical sites, might be another reason.
In addition, there is no well-defined treatment endpoint for superficial NTM infections. In contrast to TB or NTM pulmonary diseases, where the treatment endpoint can be determined by sputum specimen culture conversion and imaging results, defining the endpoint of treatment for superficial NTM infections remains uncertain. Typically, a treatment duration of 2–4 months is recommended for skin and soft-tissue NTM infections, while NTM pulmonary diseases often require at least 12 months of therapy after sputum culture reversion. To improve treatment efficacy while minimizing the risk of drug resistance, long-term and multidrug therapy is often necessary for NTM infections. However, this approach may lead to challenges such as drug interactions, drug-related adverse reactions (AEs), and high medication costs, potentially compromising treatment efficacy and patient compliance. Another method for determining the endpoint of treatment involves obtaining post-treatment specimens for culture to assess treatment efficacy, but this invasive procedure carries a heightened risk of reinfection, particularly in individuals with compromised immune systems. Finding a consensus on a specific and effective treatment endpoint for superficial NTM infections is imperative and demands further research and clinical investigation to ensure optimal patient outcomes and successful management of these challenging infections (Haworth et al., 2017; Wi, 2019). Currently, the determination of the treatment endpoint for skin NTM infection primarily relies on the assessment of changes in the patient’s clinical manifestations. These assessments typically involve evaluating the complete or substantial disappearance of preexisting skin lesions, the absence of new skin lesions, and the persistence of unchanged skin lesions after a specific duration of treatment. However, it is important to note that this criterion is subjective and lacks well-defined objective measures. To establish a more standardized and evidence-based approach for defining the treatment endpoint of skin NTM infection, further research and clinical investigations are necessary.
The low susceptibility of NTM to a wide range of antibiotics is attributed to their several characteristics. 1. Intrinsic resistance mechanisms: the first barrier is the unique metabolic condition [hydrophobicity of cell wall, and thereby low permeability (van Ingen et al., 2012a)] and the absence of porin or ABC transporter superfamily of the cell wall, which weakens the uptake and biotransformation of drugs and decreases the affinity with the drug target. 2. Inducible resistance mechanisms (Alffenaar et al., 2021): the second barrier is the genomic mutations of NTM, which could confer high-level resistance. Resistant strains are due to mutations at nucleotides. For instance, the changes of the 23S rRNA (functional erm genes) in M. abscessus isolates and of the RNA polymerase binding protein A (RbpA) in M. smegmatis are linked to the resistance to the macrolides and rifampicin (Dey et al., 2010). Comparative genomics and population genetics studies can provide insights into the genetic variability, evolution, and adaptation of NTM species. 3. Adaptative resistance mechanisms: he adaptability of NTM is the third barrier. NTM has extraordinary abilities in generation-upgrade time, and metabolic capabilities, which means they can adapt to stress before the cells are killed. They can form biofilms on the skin, which are complex microbial communities encased in an extracellular matrix. For example, one of the persistence strategies of NTM is hidden in biofilms (Slany et al., 2016), which generally leads to ten times less susceptibility to antibiotics than their counterparts. Understanding the mechanisms and dynamics of NTM biofilm formation on the skin is an active area of research, aiming to develop strategies to disrupt biofilms for improved treatment outcomes, including the use of biofilm-targeting agents and biofilm-disrupting techniques (such as enzymes, peptides, nanoparticles, and ultrasound). In addition, some studies (Huh et al., 2019) believe that NTM can enter a nonreplicating state and exhibit phenotypic drug resistance. However, up to now, the survey of resistance mechanisms associated with NTM still needs to be completed. Except for macrolides, the resistance mechanisms of many drugs still need to be clarified. It is essential to understand the basis for resistance and, more importantly, how to revise treatment choices to prevent the development of resistance.
For uncomplicated skin-involved cases, primary empirical treatments and antibiotic monotherapy could respond well in most patients. Single-drug or combined (clarithromycin, rifampin, and ethambutol) treatments depend on the specific characteristics of the hosts, location, and identification of species. When a poor response to treatment or rapid progression is found, in vitro susceptibility testing should be addressed throughout the treatment. Compared to antimicrobial agents’ therapy alone, additional surgical operation of the localized infection with medication has proven to have better outcomes for extracutaneous involvement. Regular monitoring of the patient’s clinical response to treatment, as well as laboratory testing to assess the effectiveness of antimicrobial therapy, is important in the management of NTM skin infections. Follow-up appointments with the treating physician should be scheduled as recommended to monitor progress and make any necessary adjustments to the treatment plan. Our recommendations for treating NTM skin infections and recommended procedures are summarized in Table 2 and Figure 1.
FIGURE 1. Procedure of NTM skin infection treatment. *: NTM, non-tuberculosis mycobacteria 1: The treatment should distinguish between mild and severe, drug resistance and non-resistance, initial and continuous stages, drug composition and dosage, children and adults, and HIV and non-HIV, etc. 3: Anti-NTM drugs include clarithromycin, azithromycin, ethambutol, amikacin, ciprofloxacin, moxifloxacin, rifampicin, rifampicin, isoniazid, cefoxitin, linezolid, chlorfazimine, tegacycline, imipenem/cilastatin, doxycycline, minocycline and compound sulfamethoxazole, etc. 4: Formulate the chemotherapy plan for NTM skin infections, the drugs should be selected according to the above-mentioned results. The type of medication and course of treatment are different for different NTM species. Experimental treatment of suspected NTM infections is not recommended. 5. Other treatment modalities are added depending on the patient’s condition. For patients with extensive lesions, abscess formation and poor drug efficacy, surgical debridement or foreign body removal can be actively used. 6. Monitor blood routine, liver and kidney function, blood electrolyte, urine routine, body mass, mycobacterium culture, hearing, visual field and color vision, electrocardiogram, etc. 7. Provide good patient education and explanation of the condition. For example, reduce contact with patients with NTM disease, and protect against human-to-human transmission. 8. Treatment outcome includes bacteriological negative conversion, bacteriological cure, clinical cure, cure, treatment failure, bacteriological recurrence, and death.
Emerging strategies are being explored to overcome drug resistance and improve treatment efficacy of complicated cases. 1. Screen existing drugs and new drugs: Novel antimicrobial drugs have shown promising activity against NTM species and may be considered in the treatment of NTM skin infections, particularly in cases where standard treatment regimens have failed or in the presence of drug-resistant strains. A study (Kaushik et al., 2019) showed that the new β-lactamase inhibitors relebactam and vaborbactam in combination with β-lactams have potent against M. abscessus complex clinical isolates in vitro. Clofazimine (Meir and Barkan, 2020), used for treating leprosy, is repurposed against M. abscessus. Besides, delamanid, pretomanid, and PIPD1 were also tested against M. abscessus. Telacebec is a promising novel drug with the potency of shorter duration and better tolerability (Lee and Pethe, 2022). However, the use of newer drugs may be limited by their potential side effects, higher costs, and availability. 2. Recombination of existing drugs: this is a very economical and efficient option. Previous studies (van Ingen et al., 2012b; Lee and Pethe, 2022) found that some antibiotics could increase cell wall permeability for the uptake of the second drug and accelerate durable cure, which indicates that the synergistic drug interactions could provide additional support in treating NTM infections. 3. Find new drugs according to new targets (Dartois and Dick, 2022): RNA polymerase, DNA gyrase, the ribosome, F-ATP synthase, and several enzymes. For instance, antibiotics that target oxidative phosphorylation energy-generate pathways could be a new choice. Alternatively, MmpL3 (Sethiya et al., 2020), a transporter crucial for exporting trehalose monomycolates to the periplasmic space and outer membrane, could also be a novel target in treating NTM. A study (Swain et al., 2021) found 15 new targets through screening 537 core proteins that researchers could further utilize to design inhibitors for discovering antimicrobial agents. In addition, some new drug research approaches are equally exciting. Macrophage infection assays, persister-specific assays, nonreplicating assays, biofilm assays, animal models, and lesion- or infection-site-specific pharmacokinetic assays, which can help us focus on skin and soft tissue, are instrumental methods to measure and evaluate effects when developing new anti-NTM drugs (Wu et al., 2018). For example, interferon-gamma, a cytokine that plays a role in the immune response against mycobacterial infections, has been used as an adjunct to antimicrobial therapy in some cases of NTM skin infections, particularly in patients with underlying immunosuppressive conditions. Other immune-enhancing agents, such as granulocyte-macrophage colony-stimulating factor (GM-CSF), have also been studied in the management of NTM infections. Fragment-based drug discovery (Togre et al., 2022) (FBDD) can concentrate on designing optimal inhibitors against potential therapeutic targets of NTM. A rabbit model could provide an acceptable surrogate model to study antibiotic penetration and simulate pharmacokinetic-pharmacodynamic tracks in vivo (Kaya et al., 2022).
Our article also has many limitations. First, randomized studies need to be added, and data regarding optimal treatment are limited. Clinical data on the efficacy of different treatment of NTM skin diseases in humans is limited, and further research is needed to determine its safety and effectiveness in clinical practice. Second, the resistance mechanism of NTM (genetic and pathogenic variations among species) infections needs to be understood more.
In summary, a comprehensive understanding of the various aspects discussed in this study is crucial for the effective management of cutaneous involvement caused by NTM. The ideal therapeutic approach should encompass a broader spectrum of anti-NTM activities while simultaneously considering the specific characteristics of cutaneous infections. The optimal treatment approach for NTM infections is still evolving, continuous research, clinical trials, and innovative therapeutic strategies are essential in the quest for safer, more effective, and tailored treatment options to combat NTM cutaneous involvement effectively. By addressing these aspects, clinicians can enhance patient outcomes and reduce the burden of NTM infections in affected populations.
Conceptualization and design: JL, literature search and writing (original draft): X-YW, methodology and writing (review and editing): Q-NJ. All authors contributed to the article and approved the submitted version.
This research was funded by the National high level hospital clinical research funding, 2022-PUMCH-A-163 and the National high level hospital clinical research funding, 2022-PUMCH-B-092.
The authors declare that the research was conducted in the absence of any commercial or financial relationships that could be construed as a potential conflict of interest.
All claims expressed in this article are solely those of the authors and do not necessarily represent those of their affiliated organizations, or those of the publisher, the editors and the reviewers. Any product that may be evaluated in this article, or claim that may be made by its manufacturer, is not guaranteed or endorsed by the publisher.
Ahmad, M. N., Garg, T., Singh, S., Shukla, R., Malik, P., Krishnamurthy, R. V., et al. (2022). In vitro and in vivo activity of gepotidacin against drug-resistant mycobacterial infections. Antimicrob. Agents Ch. 66, e0056422. doi:10.1128/aac.00564-22
Ahmed, I., Tiberi, S., Farooqi, J., Jabeen, K., Yeboah-Manu, D., Migliori, G. B., et al. (2020). Non-tuberculous mycobacterial infections-A neglected and emerging problem. Int. J. Infect. Dis. 92S, S46–S50. doi:10.1016/j.ijid.2020.02.022
Akrami, S., Dokht, K. A., and Hashemzadeh, M. (2023). Drug resistance profiles and related gene mutations in slow-growing non-tuberculous mycobacteria isolated in regional tuberculosis reference laboratories of Iran: a three year cross-sectional study. Pathog. Glob. Health 117, 52–62. doi:10.1080/20477724.2022.2049029
Alffenaar, J. W., Märtson, A. G., Heysell, S. K., Cho, J. G., Patanwala, A., Burch, G., et al. (2021). Therapeutic drug monitoring in non-tuberculosis mycobacteria infections. Clin. Pharmacokinet. 60, 711–725. doi:10.1007/s40262-021-01000-6
Aubry, A., Mougari, F., Reibel, F., and Cambau, E. (2017). Mycobacterium marinum. Mycobacterium Mar. Microbiol. Spectr. 5. doi:10.1128/microbiolspec.TNMI7-0038-2016
Bakuła, Z., Modrzejewska, M., Pennings, L., Proboszcz, M., Safianowska, A., Bielecki, J., et al. (2018). Drug susceptibility profiling and genetic determinants of drug resistance in Mycobacterium kansasii. Antimicrob. Agents Ch. 62, e01788-17. doi:10.1128/AAC.01788-17
Blakney, R. A., Ricotta, E. E., Frankland, T. B., Honda, S., Zelazny, A., Mayer-Barber, K. D., et al. (2022). Incidence of nontuberculous mycobacterial pulmonary infection, by ethnic group, Hawaii, USA, 2005-2019. Emerg. Infect. Dis. 28, 1543–1550. doi:10.3201/eid2808.212375
Cantillon, D., Goff, A., Taylor, S., Salehi, E., Fidler, K., Stoneham, S., et al. (2022). Searching for new therapeutic options for the uncommon pathogen Mycobacterium chimaera: an open drug discovery approach. Lancet Microbe 3, e382–e391. doi:10.1016/S2666-5247(21)00326-8
Castillo, N. E., Gurram, P., Sohail, M. R., Fida, M., and Abu, S. O. (2020). Fishing for a diagnosis, the impact of delayed diagnosis on the course of Mycobacterium marinum infection: 21 Years of experience at a tertiary care hospital. Open Forum Infect. Di 7, ofz550. doi:10.1093/ofid/ofz550
Chang, C. T., and Whipps, C. M. (2015). Activity of antibiotics against Mycobacterium species commonly found in laboratory zebrafish. J. Aquat. Anim. Health 27, 88–95. doi:10.1080/08997659.2015.1007176
Chapagain, M., Gumbo, T., Heysell, S. K., and Srivastava, S. (2020). Comparison of a novel regimen of rifapentine, tedizolid, and minocycline with standard regimens for treatment of pulmonary Mycobacterium kansasii. Antimicrob. Agents Ch. 64, e00810-20. doi:10.1128/AAC.00810-20
Chen, L. C., Huang, H. N., Yu, C. J., Chien, J. Y., and Hsueh, P. R. (2020). Clinical features and treatment outcomes of Mycobacterium chimaera lung disease and antimicrobial susceptibility of the mycobacterial isolates. J. Infect. 80, 437–443. doi:10.1016/j.jinf.2020.01.005
Chew, K. L., Octavia, S., Go, J., Yeoh, S. F., and Teo, J. (2021). MIC distributions of routinely tested antimicrobials and of rifabutin, eravacycline, delafloxacin, clofazimine, and bedaquiline for Mycobacterium fortuitum. Antimicrob. Agents Ch. 65, e0059321. doi:10.1128/AAC.00593-21
Chung, J., Ince, D., Ford, B. A., and Wanat, K. A. (2018). Cutaneous infections due to nontuberculosis Mycobacterium: recognition and management. Am. J. Clin. Dermatol 19, 867–878. doi:10.1007/s40257-018-0382-5
Comba, I. Y., Tabaja, H., Almeida, N., Fida, M., and Saleh, O. A. (2021). Bloodstream infections with rapidly growing nontuberculous mycobacteria. J. Clin. Tuber. Oth Myc 25, 100288. doi:10.1016/j.jctube.2021.100288
Crilly, N. P., Ayeh, S. K., and Karakousis, P. C. (2020). The new frontier of host-directed therapies for Mycobacterium avium complex. Front. Immunol. 11, 623119. doi:10.3389/fimmu.2020.623119
Da, M. O., Angulo, A., Rodríguez, M., Fernández-Figueiras, S., and de Waard, J. H. (2020). Drug susceptibility patterns of rapidly growing mycobacteria isolated from skin and soft tissue infections in Venezuela. Eur. J. Clin. Microbiol. 39, 433–441. doi:10.1007/s10096-019-03740-7
Daley, C. L., Iaccarino, J. M., Lange, C., Cambau, E., Wallace, R. J., Andrejak, C., et al. (2020). Treatment of nontuberculous mycobacterial pulmonary disease: an official ATS/ERS/ESCMID/IDSA clinical practice guideline: executive summary. Clin. Infect. Dis. 71, e1–e36. doi:10.1093/cid/ciaa241
Daley, C. L. (2017). Mycobacterium avium complex disease. Microbiol. Spectr. 5. doi:10.1128/microbiolspec.TNMI7-0045-2017
Dartois, V., and Dick, T. (2022). Drug development challenges in nontuberculous mycobacterial lung disease: tb to the rescue. J. Exp. Med. 219, e20220445. doi:10.1084/jem.20220445
Das, S., Mishra, B., Mohapatra, P. R., Preetam, C., and Rath, S. (2022). Clinical presentations of nontuberculous mycobacteria as suspected and drug-resistant tuberculosis: experience from a tertiary care center in Eastern India. Int. J. Mycobact. 11, 167–174. doi:10.4103/ijmy.ijmy_68_22
Dey, A., Verma, A. K., and Chatterji, D. (2010). Role of an RNA polymerase interacting protein, MsRbpA, from Mycobacterium smegmatis in phenotypic tolerance to rifampicin. Microbiol-Sgm 156, 873–883. doi:10.1099/mic.0.033670-0
Dumic, I., and Lutwick, L. (2021). Successful treatment of rapid growing mycobacterial infections with source control alone: case series. Idcases 26, e01332. doi:10.1016/j.idcr.2021.e01332
Forbes, B. A., Hall, G. S., Miller, M. B., Novak, S. M., Rowlinson, M. C., Salfinger, M., et al. (2018). Practical guidance for clinical microbiology laboratories: mycobacteria. Clin. Microbiol. Rev. 31, e00038-17. doi:10.1128/CMR.00038-17
Franco-Paredes, C., Marcos, L. A., Henao-Martínez, A. F., Rodríguez-Morales, A. J., Villamil-Gómez, W. E., Gotuzzo, E., et al. (2018). Cutaneous mycobacterial infections. Clin. Microbiol. Rev. 32, e00069-18. doi:10.1128/CMR.00069-18
Frizzell, M., Carr, E., and Brust, K. (2020). Omadacycline for treatment of Mycobacterium chelonae skin infection. Proc. (Bayl Univ. Med. Cent. 33, 610–611. doi:10.1080/08998280.2020.1792748
Fukushi, D., Murakami, K., Watanabe, Y., Sugimoto, N., Uehara, H., and Seki, M. (2022). Pulmonary and disseminated Mycobacterium avium complex cases confirmed by tissue-direct polymerase chain reaction-based nucleic acid lateral flow immunoassay of formalin-fixed paraffin-embedded tissues. Infect. Drug Resist 15, 1049–1054. doi:10.2147/IDR.S358112
Gopalaswamy, R., Shanmugam, S., Mondal, R., and Subbian, S. (2020). Of tuberculosis and non-tuberculous mycobacterial infections - a comparative analysis of epidemiology, diagnosis and treatment. J. Biomed. Sci. 27, 74. doi:10.1186/s12929-020-00667-6
Gorzynski, M., De Ville, K., Week, T., Jaramillo, T., and Danelishvili, L. (2023). Understanding the phage-host interaction mechanism toward improving the efficacy of current antibiotics in Mycobacterium abscessus. Biomedicines 11, 1379. doi:10.3390/biomedicines11051379
Grice, E. A., and Segre, J. A. (2011). The skin microbiome. Nat. Rev. Microbiol. 9, 244–253. doi:10.1038/nrmicro2537
Guo, Y., Cao, Y., Liu, H., Yang, J., Wang, W., Wang, B., et al. (2022). Clinical and microbiological characteristics of Mycobacterium kansasii pulmonary infections in China. Microbiol. Spectr. 10, e0147521. doi:10.1128/spectrum.01475-21
Hashish, E., Merwad, A., Elgaml, S., Amer, A., Kamal, H., Elsadek, A., et al. (2018). Mycobacterium marinum infection in fish and man: epidemiology, pathophysiology and management; a review. Vet. Quart. 38, 35–46. doi:10.1080/01652176.2018.1447171
Haworth, C. S., Banks, J., Capstick, T., Fisher, A. J., Gorsuch, T., Laurenson, I. F., et al. (2017). British Thoracic Society guidelines for the management of non-tuberculous mycobacterial pulmonary disease (NTM-PD). Thorax 72, ii1–ii64. doi:10.1136/thoraxjnl-2017-210927
Hendrikx, L., van Hees, C., de Steenwinkel, J., Bax, H. I., Sprong, T., Mulder, B., et al. (2022). Treatment and outcome of culture-confirmed Mycobacterium marinum disease. Open Forum Infect. Di 9, ofac077. doi:10.1093/ofid/ofac077
Holden, I. K., Kehrer, M., Andersen, A. B., Wejse, C., Svensson, E., and Johansen, I. S. (2018). Mycobacterium marinum infections in Denmark from 2004 to 2017: a retrospective study of incidence, patient characteristics, treatment regimens and outcome. Sci. Rep-Uk 8, 6738. doi:10.1038/s41598-018-24702-7
Huh, H. J., Kim, S. Y., Jhun, B. W., Shin, S. J., and Koh, W. J. (2019). Recent advances in molecular diagnostics and understanding mechanisms of drug resistance in nontuberculous mycobacterial diseases. Infect. Genet. Evol. 72, 169–182. doi:10.1016/j.meegid.2018.10.003
Jeong, S. H., Kim, S. Y., Huh, H. J., Ki, C. S., Lee, N. Y., Kang, C. I., et al. (2017). Mycobacteriological characteristics and treatment outcomes in extrapulmonary Mycobacterium abscessus complex infections. Int. J. Infect. Dis. 60, 49–56. doi:10.1016/j.ijid.2017.05.007
Kasperbauer, S. H., and De Groote, M. A. (2015). The treatment of rapidly growing mycobacterial infections. Clin. Chest Med. 36, 67–78. doi:10.1016/j.ccm.2014.10.004
Kaushik, A., Ammerman, N. C., Lee, J., Martins, O., Kreiswirth, B. N., Lamichhane, G., et al. (2019). In vitro activity of the new β-lactamase inhibitors relebactam and vaborbactam in combination with β-lactams against Mycobacterium abscessus complex clinical isolates. Antimicrob. Agents Ch. 63, e02623-18. doi:10.1128/AAC.02623-18
Kaya, F., Ernest, J. P., LoMauro, K., Gengenbacher, M., Madani, A., Aragaw, W. W., et al. (2022). A rabbit model to study antibiotic penetration at the site of infection for nontuberculous mycobacterial lung disease: macrolide case study. Antimicrob. Agents Ch. 66, e0221221. doi:10.1128/aac.02212-21
Kim, T. H., Hanh, B., Kim, G., Lee, D. G., Park, J. W., Lee, S. E., et al. (2019). Thiostrepton: a novel therapeutic drug candidate for Mycobacterium abscessus infection. Molecules 24, 4511. doi:10.3390/molecules24244511
Koushk-Jalali, B., Freitag, A. P., Tigges, C., Oellig, F., Hillemann, D., and Kreuter, A. (2019). Sporotrichoid fish tank granuloma. Qjm-Int J. Med. 112, 147. doi:10.1093/qjmed/hcy203
Kumar, C., Shrivastava, K., Singh, A., Chauhan, V., and Varma-Basil, M. (2021). Skin and soft-tissue infections due to rapidly growing mycobacteria: an overview. Int. J. Mycobact. 10, 293–300. doi:10.4103/ijmy.ijmy_110_21
Lee, B. S., and Pethe, K. (2022). Telacebec: an investigational antibacterial for the treatment of tuberculosis (TB). Expert Opin. Inv Drug 31, 139–144. doi:10.1080/13543784.2022.2030309
Lee, C. N., and Lee, J. Y. (2017). Local hyperthermia therapy for refractory cutaneous Mycobacterium marinum infection. Dermatol Ther. 30, e12561. doi:10.1111/dth.12561
Lee, M. R., Sheng, W. H., Hung, C. C., Yu, C. J., Lee, L. N., and Hsueh, P. R. (2015). Mycobacterium abscessus complex infections in humans. Emerg. Infect. Dis. 21, 1638–1646. doi:10.3201/2109.141634
Li, Y., Liu, C., Ma, A., He, W., Qiu, Q., Zhao, Y., et al. (2022). Identification and drug susceptibility testing of the subspecies of Mycobacterium avium complex clinical isolates in mainland China. J. Glob. Antimicrob. Re 31, 90–97. doi:10.1016/j.jgar.2022.05.027
Little, J. S., Dedrick, R. M., Freeman, K. G., Cristinziano, M., Smith, B. E., Benson, C. A., et al. (2022). Bacteriophage treatment of disseminated cutaneous Mycobacterium chelonae infection. Nat. Commun. 13, 2313. doi:10.1038/s41467-022-29689-4
Liu, Q., Du, J., An, H., Li, X., Guo, D., Li, J., et al. (2023). Clinical characteristics of patients with non-tuberculous mycobacterial pulmonary disease: a seven-year follow-up study conducted in a certain tertiary hospital in beijing. Front. Cell Infect. Mi 13, 1205225. doi:10.3389/fcimb.2023.1205225
Luo, T., Xu, P., Zhang, Y., Porter, J. L., Ghanem, M., Liu, Q., et al. (2021). Population genomics provides insights into the evolution and adaptation to humans of the waterborne pathogen Mycobacterium kansasii. Nat. Commun. 12, 2491. doi:10.1038/s41467-021-22760-6
Maurer, F. P., Pohle, P., Kernbach, M., Sievert, D., Hillemann, D., Rupp, J., et al. (2019). Differential drug susceptibility patterns of Mycobacterium chimaera and other members of the Mycobacterium avium-intracellulare complex. Clin. Microbiol. Infec 25, 371–379. doi:10.1016/j.cmi.2018.06.010
Mei, Y., Zhang, W., Shi, Y., Jiang, H., Chen, Z., Chokkakula, S., et al. (2019). Cutaneous tuberculosis and nontuberculous mycobacterial infections at a national specialized hospital in China. Acta Derm-Venereol 99, 997–1003. doi:10.2340/00015555-3283
Mei, Y. M., Zhang, W. Y., Sun, J. Y., Jiang, H. Q., Shi, Y., Xiong, J. S., et al. (2023). Genomic characteristics of Mycobacterium tuberculosis isolates of cutaneous tuberculosis. Front. Microbiol. 14, 1165916. doi:10.3389/fmicb.2023.1165916
Meir, M., and Barkan, D. (2020). Alternative and experimental therapies of Mycobacterium abscessus infections. Int. J. Mol. Sci. 21, 6793. doi:10.3390/ijms21186793
Mok, S., Hannan, M. M., Nölke, L., Stapleton, P., O'Sullivan, N., Murphy, P., et al. (2019). Antimicrobial susceptibility of clinical and environmental Mycobacterium chimaera isolates. Antimicrob. Agents Ch. 63, e00755-19. doi:10.1128/AAC.00755-19
Moon, S. M., Choe, J., Jhun, B. W., Jeon, K., Kwon, O. J., Huh, H. J., et al. (2019). Treatment with a macrolide-containing regimen for Mycobacterium kansasii pulmonary disease. Resp. Med. 148, 37–42. doi:10.1016/j.rmed.2019.01.012
Nguyen, A. V., and Soulika, A. M. (2019). The dynamics of the skin's immune system. Int. J. Mol. Sci. 20, 1811. doi:10.3390/ijms20081811
Ni, L., Zou, D., Yang, H., Gao, Z., Yu, Q., and Yang, L. (2023). Case report: subcutaneous Mycobacterium haemophilum infection in an immunocompetent patient after lipolysis injections. Front. Med-Lausanne 10, 1098047. doi:10.3389/fmed.2023.1098047
Nogueira, L. B., Garcia, C. N., Costa, M., Moraes, M. B., Kurizky, P. S., and Gomes, C. M. (2021). Non-tuberculous cutaneous mycobacterioses. Bras Dermatol 96, 527–538. doi:10.1016/j.abd.2021.04.005
North, E. J., Schwartz, C. P., Zgurskaya, H. I., and Jackson, M. (2023). Recent advances in mycobacterial membrane protein large 3 inhibitor drug design for mycobacterial infections. Expert Opin. Drug Dis. 18, 707–724. doi:10.1080/17460441.2023.2218082
Oh, T. H., Kim, U. J., Kang, S. J., Jang, H. C., Park, K. H., Jung, S. I., et al. (2018). Disseminated invasive Mycobacterium marinum infection involving the lung of a patient with diabetes mellitus. Infect. Chemother. 50, 59–64. doi:10.3947/ic.2018.50.1.59
Okuno, H., Tanaka, H., Hagiya, H., Yoshida, H., Hamaguchi, S., Hori, Y., et al. (2020). Mycobacterium kansasii arthritis of the elbow in an immunocompetent patient with a suspected soft-tissue tumor. J. Infect. Chemother. 26, 261–264. doi:10.1016/j.jiac.2019.07.015
Pavlik, I., Ulmann, V., and Falkinham, J. R. (2022). Nontuberculous mycobacteria: ecology and impact on animal and human health. Microorganisms 10, 1516. doi:10.3390/microorganisms10081516
Pennington, K. M., Vu, A., Challener, D., Rivera, C. G., Shweta, F., Zeuli, J. D., et al. (2021). Approach to the diagnosis and treatment of non-tuberculous mycobacterial disease. J. Clin. Tuber. Oth Myc 24, 100244. doi:10.1016/j.jctube.2021.100244
Philips, R. C., Hoyer, P. E., White, S. M., Tinkey, K. T., Loeffelholz, M., Andersen, C. R., et al. (2019). Cutaneous nontuberculous mycobacteria infections: a retrospective case series of 78 patients from the Texas gulf coast region. J. Am. Acad. Dermatol 81, 730–739. doi:10.1016/j.jaad.2019.04.022
Rallis, E., Falidas, E., and Stavropoulos, P. (2012). Mycobacterium marinum cutaneous infection with sporotrichoid distribution treated with azithromycin monotherapy. Skinmed 10, 54–55.
Riccardi, N., Antonello, R. M., Canetti, D., and Polidori, M. (2022). An octopus gift: mycobacterium marinum multiple skin lesions. Infection 50, 1631–1632. doi:10.1007/s15010-022-01851-6
Rifat, D., Chen, L., Kreiswirth, B. N., and Nuermberger, E. L. (2021). Genome-wide essentiality analysis of Mycobacterium abscessus by saturated transposon mutagenesis and deep sequencing. Mbio 12, e0104921. doi:10.1128/mBio.01049-21
Rindi, L. (2020). Efflux Pump inhibitors against nontuberculous mycobacteria. Int. J. Mol. Sci. 21, 4191. doi:10.3390/ijms21124191
Saxena, S., Spaink, H. P., and Forn-Cuní, G. (2021). Drug resistance in nontuberculous mycobacteria: mechanisms and models. Biology-Basel 10, 96. doi:10.3390/biology10020096
Schoutrop, E., Brouwer, M., Jenniskens, J., Ferro, B. E., Mouton, J. W., Aarnoutse, R. E., et al. (2018). The stability of antimycobacterial drugs in media used for drug susceptibility testing. Diagn Micr Infec Dis. 92, 305–308. doi:10.1016/j.diagmicrobio.2018.06.015
Schulthess, B., Schäfle, D., Kälin, N., Widmer, T., and Sander, P. (2023). Drug susceptibility distributions of Mycobacterium chimaera and other non-tuberculous mycobacteria. Antimicrob. Agents Ch. 65, e02131-20. doi:10.1128/AAC.02131-20
Seidel, A., Nunes, D. H., Fernandes, C., and Funchal, G. (2022). Skin infection by Mycobacterium marinum - diagnostic and therapeutic challenge. Bras Dermatol 97, 366–368. doi:10.1016/j.abd.2021.03.013
Sethiya, J. P., Sowards, M. A., Jackson, M., and North, E. J. (2020). MmpL3 inhibition: a new approach to treat nontuberculous mycobacterial infections. Int. J. Mol. Sci. 21, 6202. doi:10.3390/ijms21176202
Sharma, M., Malhotra, B., and Khandelwal, S. (2021). Drug susceptibiity testing of nontuberculous mycobacteria by broth microdilution method. Indian J. Med. Microbi 39, 306–310. doi:10.1016/j.ijmmb.2021.03.015
Sharma, S. K., and Upadhyay, V. (2020). Epidemiology, diagnosis and treatment of non-tuberculous mycobacterial diseases. Indian J. Med. Res. 152, 185–226. doi:10.4103/ijmr.IJMR_902_20
Shimizu, F., Hatano, Y., Okamoto, O., Katagiri, K., Fujiwara, S., Sato, S., et al. (2012). Mycobacterium smegmatis soft tissue infection. Int. J. Dermatol 51, 1518–1520. doi:10.1111/j.1365-4632.2010.04835.x
Shrivastava, K., Kumar, C., Singh, A., Narang, A., Giri, A., Sharma, N. K., et al. (2020). An overview of pulmonary infections due to rapidly growing mycobacteria in South Asia and impressions from a subtropical region. Int. J. Mycobact. 9, 62–70. doi:10.4103/ijmy.ijmy_179_19
Singh, S., Wang, J. Y., Heysell, S. K., McShane, P. J., Wadle, C., Shankar, P., et al. (2023). Omadacycline pharmacokinetics/pharmacodynamics in the hollow fiber model and clinical validation of efficacy to treat pulmonary Mycobacterium abscessus disease. Int. J. Antimicrob. Ag. 62, 106847. doi:10.1016/j.ijantimicag.2023.106847
Slany, M., Ulmann, V., and Slana, I. (2016). Avian mycobacteriosis: still existing threat to humans. Biomed. Res. Int. 2016, 4387461. doi:10.1155/2016/4387461
Srivastava, S., and Gumbo, T. (2018). Clofazimine for the treatment of Mycobacterium kansasii. Antimicrob. Agents Ch. 62, e00248-18. doi:10.1128/AAC.00248-18
Strobel, K., Sickenberger, C., Schoen, C., Kneitz, H., Kolb-Mäurer, A., and Goebeler, M. (2022). Diagnosis and therapy of Mycobacterium marinum: a single-center 21-year retrospective analysis. J. Dtsch. Dermatol Ges. 20, 1211–1218. doi:10.1111/ddg.14847
Swain, A., Gnanasekar, P., Prava, J., Rajeev, A. C., Kesarwani, P., Lahiri, C., et al. (2021). A comparative genomics approach for shortlisting broad-spectrum drug targets in nontuberculous mycobacteria. Microb. Drug Resist 27, 212–226. doi:10.1089/mdr.2020.0161
Timmins, G. S. (2020). What are the challenges in commercial non-tuberculous mycobacteria (NTM) drug discovery and how should we move forward? Expert Opin. Drug Dis. 15, 7–9. doi:10.1080/17460441.2020.1673362
To, K., Cao, R., Yegiazaryan, A., Owens, J., and Venketaraman, V. (2020). General overview of nontuberculous mycobacteria opportunistic pathogens: mycobacterium avium and Mycobacterium abscessus. J. Clin. Med. 9, 2541. doi:10.3390/jcm9082541
Togre, N. S., Vargas, A. M., Bhargavi, G., Mallakuntla, M. K., and Tiwari, S. (2022). Fragment-based drug discovery against mycobacteria: the success and challenges. Int. J. Mol. Sci. 23, 10669. doi:10.3390/ijms231810669
Toth, E., Waller, J. L., Bollag, W. B., Siddiqui, B., Mohammed, A., Kheda, M., et al. (2022). Non-tuberculous mycobacterial infections in patients with end-stage renal disease: prevalence, risk factors, and mortality. J. Invest. Med. 2022, 002462–12022. doi:10.1136/jim-2022-002462
Trčko, K., Plaznik, J., and Miljković, J. (2021). Mycobacterium marinum hand infection masquerading as tinea manuum: a case report and literature review. Acta Dermatoven Alp. 30, 91–93. doi:10.15570/actaapa.2021.23
Uslu, U., Böhm, O., Heppt, F., and Sticherling, M. (2019). Skin and soft tissue infections caused by Mycobacterium chelonae: more common than expected? Acta Derm-Venereol 99, 889–893. doi:10.2340/00015555-3230
van Ingen, J., Boeree, M. J., van Soolingen, D., and Mouton, J. W. (2012a). Resistance mechanisms and drug susceptibility testing of nontuberculous mycobacteria. Drug Resist Update 15, 149–161. doi:10.1016/j.drup.2012.04.001
van Ingen, J., Totten, S. E., Helstrom, N. K., Heifets, L. B., Boeree, M. J., and Daley, C. L. (2012b). In vitro synergy between clofazimine and amikacin in treatment of nontuberculous mycobacterial disease. Antimicrob. Agents Ch. 56, 6324–6327. doi:10.1128/AAC.01505-12
Wang, C. J., Song, Y., Li, T., Hu, J., Chen, X., and Li, H. (2022a). Mycobacterium smegmatis skin infection following cosmetic procedures: report of two cases. Clin. Cosmet. Inv Derm. 15, 535–540. doi:10.2147/CCID.S359010
Wang, D. M., Liao, Y., Li, Q. F., Zhu, M., Wu, G. H., Xu, Y. H., et al. (2019). Drug resistance and pathogenic spectrum of patients coinfected with nontuberculous mycobacteria and human-immunodeficiency virus in Chengdu, China. Chin. Med. J-Peking 132, 1293–1297. doi:10.1097/CM9.0000000000000235
Wang, J., Huang, J., Peng, S., Li, L., Zhong, K., and Chen, T. (2022b). A clinical case and a review of Mycobacterium fortuitum infections direct diagnosis approach and treatment in a patient with leg fractures. J. Infect. Dev. Countr 16, 1650–1654. doi:10.3855/jidc.16635
Watanabe, Y., Fujita, H., Fukushima, S., and Nakamura, I. (2022). Disseminated Mycobacterium chelonae infection, including discitis. Intern. Med. 61, 2085–2086. doi:10.2169/internalmedicine.8539-21
Wi, Y. M. (2019). Treatment of extrapulmonary nontuberculous mycobacterial diseases. Infect. Chemother. 51, 245–255. doi:10.3947/ic.2019.51.3.245
Wu, M. L., Aziz, D. B., Dartois, V., and Dick, T. (2018). NTM drug discovery: status, gaps and the way forward. Drug Discov. Today 23, 1502–1519. doi:10.1016/j.drudis.2018.04.001
Yan, M., Brode, S. K., and Marras, T. K. (2023). The other nontuberculous mycobacteria: clinical aspects of lung disease caused by less common slowly growing nontuberculous mycobacteria species. Chest 163, 281–291. doi:10.1016/j.chest.2022.09.025
Yang, Z., Feng, Y., Li, D., Pang, Z., Wang, S., Chen, H., et al. (2022). 5-aminolevulinic acid-photodynamic therapy ameliorates cutaneous granuloma by killing drug-resistant Mycobacterium marinum. Photodiagn Photodyn. 38, 102839. doi:10.1016/j.pdpdt.2022.102839
Yeo, P. M., Lee, S. X., Tan, Y. E., Sng, L. H., and Ang, C. C. (2019). Epidemiology, risk factors, and outcomes of adult cutaneous non-tuberculous mycobacterial infection over a 10-year period in Singapore. Int. J. Dermatol 58, 679–687. doi:10.1111/ijd.14356
Keywords: non-tuberculosis mycobacteria (NTMs), skin diseases, infectious, anti-bacterial, mycobacterium infections, nontuberculous, atypical mycobacteria
Citation: Wang X-Y, Jia Q-N and Li J (2023) Treatment of non-tuberculosis mycobacteria skin infections. Front. Pharmacol. 14:1242156. doi: 10.3389/fphar.2023.1242156
Received: 18 June 2023; Accepted: 25 August 2023;
Published: 05 September 2023.
Edited by:
Exequiel Oscar Jesus Porta, Durham University, United KingdomReviewed by:
Esteban Panozzo Zenere, National University of Rosario, ArgentinaCopyright © 2023 Wang, Jia and Li. This is an open-access article distributed under the terms of the Creative Commons Attribution License (CC BY). The use, distribution or reproduction in other forums is permitted, provided the original author(s) and the copyright owner(s) are credited and that the original publication in this journal is cited, in accordance with accepted academic practice. No use, distribution or reproduction is permitted which does not comply with these terms.
*Correspondence: Jun Li, bGlqdW4wNjMyMUBwdW1jaC5jbg==
†These authors have contributed equally to this work and share first authorship
Disclaimer: All claims expressed in this article are solely those of the authors and do not necessarily represent those of their affiliated organizations, or those of the publisher, the editors and the reviewers. Any product that may be evaluated in this article or claim that may be made by its manufacturer is not guaranteed or endorsed by the publisher.
Research integrity at Frontiers
Learn more about the work of our research integrity team to safeguard the quality of each article we publish.