- 1Department of Neural Development and Disease, Korea Brain Research Institute (KBRI), Daegu, Republic of Korea
- 2Department of Brain and Cognitive Sciences, Daegu Gyeongbuk Institute of Science and Technology, Daegu, Republic of Korea
- 3Department of Bio-Health Technology, College of Biomedical Science, Kangwon National University, Chuncheon, Republic of Korea
Many researchers are attempting to identify drugs that can be repurposed as effective therapies for Alzheimer’s disease (AD). Several recent studies have highlighted epidermal growth factor receptor (EGFR) inhibitors approved for use as anti-cancer drugs as potential candidates for repurposing as AD therapeutics. In cancer, EGFR inhibitors target cell proliferation and angiogenesis, and studies in AD mouse models have shown that EGFR inhibitors can attenuate amyloid-beta (Aβ) pathology and improve cognitive function. In this review, we discuss the different functions of EGFR in cancer and AD and the potential of EGFR as a dual molecular target for AD diseases. In addition, we describe the effects of anti-cancer EGFR tyrosine kinase inhibitors (TKIs) on AD pathology and their prospects as therapeutic interventions for AD. By summarizing the physiological functions of EGFR in cancer and AD, this review emphasizes the significance of EGFR as an important molecular target for these diseases.
1 Introduction
Heredity and aging are common risk factors for cancer and Alzheimer’s disease (AD), which are the leading causes of death worldwide (White et al., 2014; Guerreiro and Bras, 2015; Majd et al., 2019). Numerous anti-cancer therapies are available, but therapeutic drugs for AD are scarce. AD is the most common neurodegenerative disease characterized by amyloid and tau protein aggregation and cognitive decline (Ates et al., 2016). Amyloid and tau protein aggregates are not only pathophysiological biomarkers of AD, but also cause neuronal loss, synapse destruction, and neuroinflammation (Serrano-Pozo et al., 2011; Krstic and Knuesel, 2013). Other potential mechanisms of AD progression are oxidative stress and epigenetic dysfunction.
Several studies have found an inverse association between cancer and AD, but others have argued for a parallel relationship (Majd et al., 2019; Nudelman et al., 2019; Branigan et al., 2021; Zhang et al., 2022). Although the underlying mechanism of the relationship between cancer and AD has not been thoroughly investigated, the diseases share hallmarks and risk factors. Risk factors for both cancer and AD include aging, smoking, obesity, and type 2 diabetes (Cannata et al., 2010; Cataldo et al., 2010; Mayeux and Stern, 2012; White et al., 2014; Emmerzaal et al., 2015). Strikingly, cell-cycle entry, which is required for cancer pathogenesis, is high in patients with AD (Majd et al., 2019). At the cellular level, the pathogenesis of AD and cancer both involve the phosphoinositide 3-kinase/protein kinase B/mammalian target of rapamycin (PI3K/AKT/mTOR) signaling pathway, which regulates cell proliferation, metabolism, growth, and autophagy (Pei and Hugon, 2008; Morgan et al., 2009; Advani, 2010; Talbot et al., 2012; Fumarola et al., 2014; Porta et al., 2014). Abnormal growth suppressor evasion is also observed in both cancer and AD. Specifically, cell growth and division in cancer often occur through inactivating mutations of tumor suppressors such as retinoblastoma transcriptional corepressor 1 (RB1) and TP53 (Hanahan and Weinberg, 2011; Fischer et al., 2016; Robinson et al., 2017). In patients with AD, levels of p27, a critical negative cell cycle regulator, are significantly reduced (Ogawa et al., 2003; Munoz et al., 2008). The systemic dysregulation of the cell cycle in both cancer and AD supports a correlation between these two diseases. Angiogenesis, cell adhesion inhibition, and inflammation are also shared by cancer and AD (Nudelman et al., 2019). Therefore, verifying the commonalities between cancer and AD might contribute to the development of effective therapeutic strategies.
A genome-wide association study found a significant positive genetic correlation between cancer and AD, implying that the pathophysiology of cancer and AD share common genetic variants (Feng et al., 2017). Specifically, super-enhancer, a broad enhancer domain affecting cell type identification and function, exhibits a significant positive genetic correlation with cancer and AD, indicating a potential role of gene expression regulation in the common genetic etiologies of cancer and AD (Feng et al., 2017; Zhao et al., 2022). Several genes [e.g., epidermal growth factor receptor (EGFR) and amyloid precursor protein (APP)] are associated with both cancer and AD, and we and others have recently found that anti-cancer drugs can penetrate the blood-brain barrier (BBB) and modulate AD pathology (Ryu and McLarnon, 2008; Cramer et al., 2012). Specifically, inhibitors of EGFR and other tyrosine kinases, which are multitarget enzymes, may have practical value for treating cancer and AD (Mansour et al., 2021c). This review provides insights into the potential roles of EGFR and EGFR inhibitors in cancer and AD and related therapeutic strategies.
2 EGFR
EGFR is a cell surface growth factor receptor that regulates cell proliferation, differentiation, and survival (Yewale et al., 2013). EGFR was the first receptor tyrosine kinase (RTK) to be discovered and is a member of the ErbB family of RTKs (Wong and Guillaud, 2004; Roskoski, 2019). The EGFR gene contains 31 exons and encodes a 170-kDa transmembrane glycoprotein (Mitsudomi and Yatabe, 2010; Sabbah et al., 2020). EGFR is stimulated by ligands such as epidermal growth factor (EGF) and transforming growth factor-alpha (TGF-α) (Purba et al., 2017). Upon binding to the extracellular domain of EGFR, these ligands induce conformational changes in the receptor that facilitate the formation of receptor dimers or oligomers (Schlessinger, 2002). EGFR dimerization triggers the activation of its intrinsic tyrosine kinase activity and subsequent autophosphorylation of several tyrosine residues in the EGFR C-terminal domain (Yu et al., 2002). These phosphorylated tyrosine residues serve as docking sites for various signaling molecules and initiate canonical EGFR signaling pathways (Lemmon and Schlessinger, 2010). Although numerous reviews have discussed EGFR as a target in cancer, inflammatory diseases, and monogenic diseases, the regulation of EGFR expression or signaling as a multi-disease target requires further investigation.
3 EGFR in various cancers
EGFR plays an essential physiological role in regulating the development of epithelial tissue and homeostasis and hence is also linked to tumorigenesis, including lung cancer, breast cancer, and glioblastoma (Sigismund et al., 2018). EGFR is a critical modulator and a target for developing novel therapeutic strategies in various cancers. EGFR signaling modulates cancer cell proliferation through several metabolic processes (Sigismund et al., 2018). For example, Srivatsa et al. (2017) found that EGFR-expressing myeloid cells are abundant in the colorectal tumor stroma, indicating that EGFR in tumor-associated myeloid cells may be a diagnostic biomarker for colorectal cancer (CRC). CRISPR/Cas9-mediated elimination of EGFR significantly inhibits tumor cell growth and activates the mitogen-activated protein kinase (MAPK) (p-ERK1/2) pathway (Liu et al., 2020). Moreover, Song et al. (2020) identified upregulation of EGFR and phosphorylated signal transducer and activator of transcription 3 (p-STAT3) in breast cancer tissues.
The EGFR pathway is a widely recognized oncogenic pathway for non-small cell lung cancer (NSCLC), which represents approximately 75% of lung cancers (Bethune et al., 2010; Hsu et al., 2019). The EGFR pathway regulates the Bax/B-cell lymphoma 2 (Bcl-2) cascade, which is associated with apoptosis in NSCLC (Alam et al., 2022). Interestingly, a study identified EGFR overexpression or mutations in intracellular EGFR in 43%–89% of NSCLC cases (Gupta et al., 2009). Exon 19 deletion and L858R point mutation are the most frequent EGFR mutations in NSCLC (Khaddour et al., 2021). Activating somatic mutations in exons 18–21 of EGFR in NSCLC can continuously activate the EGFR kinase domain regardless of ligand binding and result in sustained downstream signaling. Several studies have reported that EGFR expression is increased by 40%–89% in NSCLC (Lu et al., 2001; Lynch et al., 2004; Al Olayan et al., 2012). Shao et al. (2022) found that upregulated EGFR signaling induces increased levels of the membrane-bound complement regulatory proteins (mCRPs) CD55 and CD59, thereby promoting tumor immune evasion in lung cancer cells (CD8+T). In addition, Ohsaki et al. (2000) observed shorter survival of NSCLC patients with EGFR overexpression. Selvaggi et al. (2004) found that EGFR expression is significantly increased in stage III of NSCLC, implying that EGFR levels are a potential prognostic factor. Merrick et al. (2006) observed a significantly positive relationship between EGFR expression and the progression of bronchial dysplasia, a precursor of lung carcinoma, indicating a role of EGFR upregulation in lung cancer development and progression. Yang et al. (2015) reported that EGFR mutation-mediated lung cancer is associated with downregulation of cluster of differentiation 82 (CD82), which promotes EGFR expression. Shien et al. (2012) discovered that EGFR silencing by siRNA significantly reduces the cell viability of EGFR-mutant cell lines (PC-9, HCC827, NCI-H820, and NCI-1975), further supporting EGFR as a promising therapeutic target in NSCLC. This critical role of EGFR upregulation in the development, progression, and longevity of lung cancer has led to the development of drugs that control EGFR activity and expression.
4 EGFR in Alzheimer’s disease (AD)
The general functions of EGFR in the central nervous system (CNS) include neural stem cell pool maintenance, astrocyte differentiation and maturation, oligodendrocyte maturation, and neurite outgrowth (Romano and Bucci, 2020). EGFR isoforms are expressed in neurons in the hippocampus, cerebellum, and cerebral cortex (Wong and Guillaud, 2004). Several recent studies have demonstrated that EGFR and its related signaling pathways that it mediates are crucial targets for modulating AD pathology. For instance, Wang et al. (2013) found that EGFR activation (p-EGFR/EGFR) is significantly increased in 8-month-old APP/PS1 mice (a model of AD) compared with wild-type mice. Excessive EGFR expression induces memory impairment in Aβ-overexpressing Drosophila (Prüßing et al., 2013). More importantly, dual overexpression of EGFR and Aβ42 synergistically promotes memory loss, implying that EGFR is upregulated in AD (Chiang et al., 2010). Notably, EGFR upregulation was recently shown to induce Aβ42 neurotoxicity and neuroinflammation and activate astrocytes (Ozbeyli et al., 2017; Chen et al., 2019b). AD patients exhibit neuritic plaques with EGFR expression in the cerebral cortex and hippocampus (Birecree et al., 1988). However, EGF treatment does not alter EGFR or Aβ levels in the brain and prevents cognitive dysfunction in E4FAD mice (Thomas et al., 2016). Taken together, the literature suggests that inhibition of EGFR modulates Aβ plaque accumulation, neuroinflammation, and cognitive function in mouse models of AD. Although there are conflicting reports regarding the role of EGFR in Aβ pathology, there is strong evidence that EGFR is a dual molecular target for cancer and AD (Figure 1A).
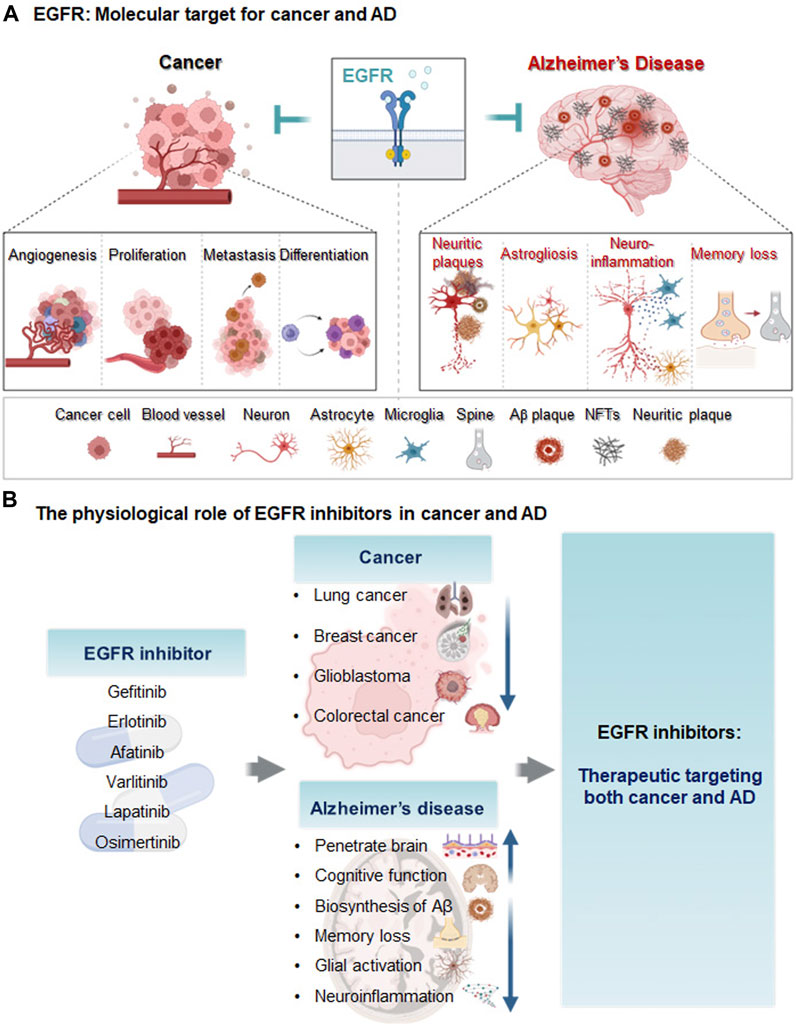
FIGURE 1. Diagram of EGFR as a molecular target and the effects of EGFR inhibitors on cancer and AD. (A) Epidermal growth factor receptor (EGFR) is a transmembrane protein receptor for the epidermal growth factor family that regulates cell growth and proliferation. In cancer, EGFR upregulation increases metastasis, angiogenesis, cancer cell proliferation, differentiation, and cancer viability. In animal models of AD and/or AD patients, EGFR levels are increased, leading to memory loss, astrogliosis, neuroinflammation and Aβ plaque formation. (B) The EGFR inhibitors gefitinib, afatinib, varlitinib, erlotinib, osimertinib, and lapatinib are anti-cancer drugs targeting EGFR. These EGFR inhibitors reduce AD pathology and improve cognitive function and thus may be potential therapeutic agents for cancer and AD.
5 Therapeutic applications of EGFR inhibitors
Despite substantial investments of resources and time in identifying new drugs for AD, clinical trials have produced disappointing results. Thus, the effects of EGFR on Aβ, neuroinflammation, and cognitive function have spurred growing interest in the potential repurposing of EGFR inhibitors used as anti-cancer drugs for the treatment of AD (Mansour et al., 2021c). The molecular mechanisms of EGFR in cancer and AD are also being investigated to develop disease-modifying drugs. Chen et al. (2019b) found that oxygen-glucose deprivation (OGD) increases EGFR phosphorylation and triggers downstream protein kinase B (AKT) and extracellular signal-regulated kinase (ERK) signaling pathways in primary cultured astrocytes and CTX-TNA2 cells. Characterization of EGFR signaling pathways and downstream cascades may reveal promising strategies for utilizing tyrosine kinase inhibitors (TKIs) as disease-modifying therapies in cancer and AD. In addition, EGFR TKIs have greater BBB penetration potential than most intravenous chemotherapies (Ahluwalia et al., 2018). A recent high-performance liquid chromatography (HPLC) analysis showed that ibrutinib can cross the BBB in WT mice (Lee et al., 2021a), and gefitinib, erlotinib, afatinib, varlitinib, lapatinib, and osimertinib are all known to cross the BBB (Lin et al., 2008; Babu et al., 2015; Mansour et al., 2021b; Colclough et al., 2021). Colclough et al. (2021) compared the BBB permeability of EGFR TKIs and found that osimertinib has the highest BBB penetration, with a Kpuu of 0.21, followed by Kpuu values of 0.084 for erlotinib, 0.0092 for gefitinib, and 0.0046 for afatinib. The low BBB permeability of erlotinib and gefitinib means that these drugs do not exhibit significant or persistent effects in the brain (Ahluwalia et al., 2018). Although the abilities of lapatinib, osimertinib, and CL-387,785 to penetrate the BBB have not been determined, lapatinib is expected to cross the BBB due to its low molecular weight and lipophilicity (Mansour et al., 2021b). However, studies of the use of BBB-penetrating EGFR inhibitors to treat AD remain scarce, and the mechanisms of BBB-permeable EGFR TKIs in AD remain to be elucidated. Several anti-cancer EGFR inhibitors that are candidates for AD therapy are described below, and the therapeutic effects, safety, and toxicity profiles of EGFR TKIs in cancer and AD are shown in Tables 1, 2; Figure 1B.
5.1 Gefitinib
The EGFR/HER2 inhibitor Gefitinib is a first-generation EGFR TKI approved for lung cancer treatment (Arrieta et al., 2020). Gefitinib inhibits the binding of EGFR and adenosine triphosphate (ATP), thereby blocking EGFR autophosphorylation and downstream signaling cascades that control cell growth and trigger apoptosis (Jiang et al., 2005). Compared with erlotinib, gefitinib appears to be more effective and safer for treating NSCLC (Zhang et al., 2018).
Several studies have shown that gefitinib can ameliorate AD pathology. Gefitinib can penetrate the brain and thus positively affects non-EGFR targets that participate in AD pathology in E4FAD (APOE4-expressing) AD transgenic (Tg) mice (Thomas et al., 2016). A computational analysis indicated that gefitinib and the hydrophobic pocket of β-site APP cleaving enzyme (BACE) have complementary shapes and binding interactions, suggesting that gefitinib suppresses Aβ40/42 through BACE (Niu et al., 2014). In addition, gefitinib prevents memory loss in an Aβ42-overexpressing Drosophila model and rescues memory impairment in APP/PS1 Tg mice, a model of AD, indicating that EGFR inhibition can potentially improve cognitive function (Wang et al., 2012). In AD-induced Swiss albino mice, gefitinib attenuates hippocampal-dependent memory impairment as assessed by the Morris water maze (MWM) test and reduces acetylcholinesterase (AChE) activity (Dhamodharan et al., 2022). The ability of gefitinib to reduce Aβ-mediated AChE levels and attenuate cognitive impairments supports its potential as an AD treatment, but whether gefitinib directly affects other AD-associated factors (e.g., tau pathology) and its molecular mechanisms of action on AD pathology remain to be clarified.
5.2 Erlotinib
The EGFR-TKI erlotinib is an FDA-approved drug used to treat patients with NSCLC with mutations in the ATP-binding pocket of EGFR (Cohen et al., 2005; Smith, 2005; Nishimura et al., 2015; Lee et al., 2021b). Erlotinib suppresses the tumor growth of the human endometrial adenocarcinoma cell line HEC-1A, which expresses high levels of EGFR (Nishimura et al., 2015). Deep learning and machine learning algorithms predict that erlotinib is a BBB-permeable compound (Jang et al., 2022). Erlotinib blocks lipopolysaccharide (LPS)-induced nuclear factor kappa-light-chain-enhancer of activated B cells (NF-κB)-dependent cytokine production in C57BL/6J mice, implying that erlotinib modulates neuroinflammatory responses in the brain (De et al., 2015). However, due to their low BBB penetration, the effects of erlotinib and gefitinib on brain metastasis are neither significant nor persistent (Ahluwalia et al., 2018).
Importantly, erlotinib rescues memory deficits in APP/PS1 Tg mice as assessed by the MWM test, suggesting that erlotinib can modulate cognitive function (Wang et al., 2012). Although research has focused on the potential utility of erlotinib in treating AD, the effects of erlotinib on Aβ/tau pathology and its mechanisms of action in mouse models of AD require further study.
5.3 Afatinib
Afatinib is a second-generation EGFR-TKI with anti-inflammatory effects and is widely used to treat NSCLC (Chen et al., 2019b; Moosavi and Polineni, 2023). Afatinib also inhibits the migration, proliferation, and invasion of hepatocellular carcinoma (HCC) cells, implying anti-tumorigenic effects (Chen et al., 2019a). Treatment with a combination of afatinib and temozolomide suppresses brain tumor formation by inhibiting crosstalk between EGFRvIII, a constitutively active EGFR mutant, and the RTK cross-activation of tyrosine kinase receptor (cMet) (Vengoji et al., 2019). Interestingly, afatinib (1 or 10 nM) inhibits OGD-induced EGFR phosphorylation, astrocyte activation, and reduced proinflammatory cytokine levels in CTX-TNA2 cells (Chen et al., 2019b). These results suggest that afatinib has anti-inflammatory effects on OGD-induced neuroinflammation. However, whether afatinib regulates AD pathology and cognitive function in mouse models of AD is unknown. Although the direct effects of afatinib on AD pathology have not been comprehensively investigated, the anti-cancer and anti-inflammatory effects of afatinib indicate promising potential for repurposing as an AD therapy.
5.4 Varlitinib
Studies have examined the effects of varlitinib, an FDA-approved EGFR/HER2 inhibitor, on various cancers, including gastric, pancreatic, colorectal, and breast cancers (Dokduang et al., 2020). Varlitinib can penetrate the BBB and suppresses cell migration, invasion, and mammosphere formation through ERK/AKT signaling in triple-negative breast cancer (TNBC) cells (Babu et al., 2015; Liu et al., 2019). In addition, EGFR/HER2 inhibition by varlitinib has therapeutic effects on cholangiocarcinoma (CCA) (Dokduang et al., 2020). Whereas other EGFR inhibitors have side effects, varlitinib does not have significant toxicity in CCA-inoculated mice (Dokduang et al., 2020). Importantly, we recently demonstrated that varlitinib downregulates LPS-mediated neuroinflammation and tau pathology through dual specificity tyrosine phosphorylation regulated kinase 1A (DYRK1A), a tau kinase (Kim et al., 2022). In addition, we found that varlitinib significantly diminishes LPS-induced neuroinflammation in both BV2 microglial cells and primary astrocytes, suggesting that varlitinib has therapeutic effects on neuroinflammation and tau pathology (Kim et al., 2022). In contrast to its effects on cancer, the impact of varlitinib on AD pathology (including Aβ pathology and its mechanism of action) is poorly understood; thus, further studies are needed to evaluate the feasibility of using varlitinib for the treatment of AD.
5.5 Lapatinib
Like varlitinib, lapatinib is a dual TKI targeting both EGFR and HER2 and is currently used to treat cancer (Oakman et al., 2010). Lapatinib is a low-molecular-weight and lipophilic molecule and can penetrate the BBB (Mansour et al., 2021b). In HER2-positive breast cancer cells, a combination of lapatinib and capecitabine has synergistic anti-tumor effects (Matsumoto et al., 2018).
Lapatinib has been shown to ameliorate autoimmune encephalomyelitis, a functional disorder of the CNS (Akama-Garren et al., 2015). In addition, lapatinib has neuroprotective effects against neuronal ferroptosis, indicating the involvement of ferroptosis in AD pathologies (Jia et al., 2020; Chen et al., 2021). A study of the effects of lapatinib on cognitive function in vivo found that lapatinib rescues short/long-term recognition memory impairment by activating the PI3K/AKT/glycogen synthase kinase-3 beta (GSK-3β) pathway in D-galactose/ovariectomized (D-gal/OVX) rats (Mansour et al., 2021b). The same study demonstrated that lapatinib decreases Aβ1-42 and p-tau levels and suppresses HER2 expression in D-gal/OVX rats (Mansour et al., 2021b). Thus, inhibition of HER2 by lapatinib promotes autophagy and reduces Aβ1–42 and p-tau levels, consistent with the results of previous studies of the role of EGFR/HER2 in autophagy (Mansour et al., 2021a; Mansour et al., 2022). Overall, lapatinib is a promising candidate anti-cancer drug for repositioning as an AD therapeutic. However, further studies of the effects of lapatinib on AD pathophysiology are needed.
5.6 Osimertinib
Osimertinib is an irreversible EGFR inhibitor and a third-generation TKI with high brain penetration (Makhlin et al., 2019). Osimertinib targets EGFR T790, which is resistant to most first- and second-generation EGFR TKIs (Janjigian et al., 2014; Jiang and Zhou, 2014; Barbuti et al., 2019). Osimertinib is known for its clinical activities in glioblastoma and NSCLC (Makhlin et al., 2019; Gen et al., 2022). In vitro, osimertinib has higher affinity for EGFR L858R/T790M than for wild-type EGFR (Kuijper et al., 2015; Greig, 2016). Interestingly, a recent report indicated that osimertinib is also highly effective against CNS metastasis (Liam, 2019). Furthermore, Ge et al. (2017) observed a higher probability and frequency of EGFR mutations in NSCLC patients with brain metastasis than in NSCLC patients without brain metastasis. In addition, the incidence of brain metastasis is higher in NSCLC patients with mutated EGFR than in NSCLC patients with wild-type EGFR, implying a correlation between EGFR mutation and brain metastasis (Ge et al., 2017). Whether the potential effectiveness of osimertinib in the CNS extends to AD pathology is unknown.
The relationships between specific EGFR mutations (exon 19 deletions and exon 21 L858R) and AD have not been examined. Jolly et al. (2022) investigated the associations of the locations of mutations in EGF-like repeats (EGFr) with vascular cognitive impairment (VCI) in patients with cerebral autosomal dominant arteriopathy with subcortical infarcts and leukoencephalopathy (CADASIL), one of the most common forms of stroke and early-onset dementia, and found no significant relationship (Jolly et al., 2022). Although these results are not consistent with the mechanism of osimertinib, different results might be obtained for the relationships between EGFR mutations and ADs. Osimertinib may have utility as an EGFR inhibitor for treating AD pathology due to its high brain penetration, but the impact of osimertinib on AD has not been studied.
5.7 CL-387,785
CL-387,785 is an irreversible selective EGFR inhibitor designed to specifically inhibit EGFR autophosphorylation and tumor cell proliferation (Discafani et al., 1999). CL-387,785 inhibits not only wild-type EGFR but also EGFR T790M, which is resistant to EGFR TKIs such as erlotinib, gefitinib, and afatinib (Kobayashi et al., 2005; Yun et al., 2008; Mok et al., 2009; Rosell et al., 2012; Zhang et al., 2012; Chi et al., 2013; Sequist et al., 2013; Niu and Wu, 2014). Thus, CL-387,785 is expected to solve the cause of drug resistance. Although CL-387,785 is only approved for research purposes, several studies have indicated therapeutic effects of CL-387,785 on lung cancer. For instance, CL-387,785 inhibits colony formation by lung cancer cells expressing an EGFR missense or deletion mutant more effectively than gefitinib and erlotinib, suggesting that CL-387,785 may be a good therapeutic for lung cancer with exon 20 insertion mutations of EGFR (Greulich et al., 2005). In addition, CL-387,785 inhibits the proliferation and apoptosis of NSCLC H1975 cells expressing EGFR T790M, indicating that CL-387,785 can restrict the invasion and metastasis of NSCLC H1975 cells (Cai et al., 2023).
With respect to potential effects on AD, CL-387,785 significantly reduces C99-CTF (c-terminal fragment) and APP intracellular domain (AICD) levels in C99-YFP–overexpressing HEK293 cells and C99 CTF-expressing zebrafish (Wang et al., 2017). More importantly, CL-387,785 rescues spatial learning and memory and reduces Aβ levels in APP/PS1 Tg mice (Wang et al., 2017). CL-387,785 also reduces the LC3-II/LC3-I ratio, which is crucial for activating autophagy, promoting the clearance of Aβ40 and Aβ42, and improving memory (Wang et al., 2017). Although the effects of CL-387,785 on AD pathology (i.e., tau) and its mechanism of action require further investigation, CL-387,785 can be considered a potential EGFR TKI for both cancer and AD.
Conclusion and future directions
Several recent studies have revealed associations of EGFR with cancer and AD; thus, regulating EGFR expression may be a strategy for treating both diseases. However, comprehensive studies of the roles of EGFR and EGFR inhibitors (TKIs) in cancer and AD are not available. In addition, although EGFR is a potential target for AD treatment, the effectiveness of major anti-cancer EGFR TKIs as AD therapeutics has received little attention. This review highlights the functional roles of EGFR and EGFR TKIs in cancer and AD. Specifically, EGFR upregulation induces various types of cancer and promotes Aβ pathology. EGFR inhibition has promising effects on both diseases, including inhibiting cancer cell migration and AD pathology (e.g., Aβ, neuroinflammation, and cognitive function). The literature and our findings suggest that anti-cancer drugs can be regarded as candidates for repurposing as AD treatments. However, the direct relationship between EGFR and AD, the effects of EGFR on tau pathology in mouse models of AD, and the mechanisms of action of EGFR in the brain are still unclear. Moreover, the effects of EGFR TKIs on AD pathology have not been well examined. Further studies are required to address these issues and may provide significant insights into cancer therapy and AD progression.
Author contributions
H-JC, YJJ, JK, and H-SH wrote the manuscript. JK and H-SH conceived the study. All authors contributed to the article and approved the submitted version.
Funding
This work was supported by the National Research Foundation of Korea (grant number 2021R1F1A1057865, JK) and the KBRI basic research program through KBRI funded by the Ministry of Science, ICT and Future Planning (grant numbers 23-BR-02-03, 23-BR-02-12, 23-BR-03-07, 23-BR-03-01, 23-BR-05-02, H-SH) and a National Research Council of Science and Technology (NST) grant funded by the Korean government (CCL22061-100, H-SH).
Acknowledgments
We thank neurodegenerative diseases lab members for editing and valuable comments on our manuscript. The figures were created in BioRender.com.
Conflict of interest
The authors declare that the research was conducted in the absence of any commercial or financial relationships that could be construed as a potential conflict of interest.
Publisher’s note
All claims expressed in this article are solely those of the authors and do not necessarily represent those of their affiliated organizations, or those of the publisher, the editors and the reviewers. Any product that may be evaluated in this article, or claim that may be made by its manufacturer, is not guaranteed or endorsed by the publisher.
References
Advani, S. (2010). Targeting mTOR pathway: A new concept in cancer therapy. Indian J. Med. Paediatr. Oncol. 31, 132–136. doi:10.4103/0971-5851.76197
Agema, B. C., Veerman, G. D. M., Steendam, C. M. J., Lanser, D. a. C., Preijers, T., Van Der Leest, C., et al. (2022). Improving the tolerability of osimertinib by identifying its toxic limit. Ther. Adv. Med. Oncol. 14, 17588359221103212. doi:10.1177/17588359221103212
Ahluwalia, M. S., Becker, K., and Levy, B. P. (2018). Epidermal growth factor receptor tyrosine kinase inhibitors for central nervous system metastases from non-small cell lung cancer. Oncologist 23, 1199–1209. doi:10.1634/theoncologist.2017-0572
Akama-Garren, E., Swanson, C., and Robinson, W. H. (2015). Epidermal growth factor receptor inhibition treats experimental autoimmune Encephalomyelitis. MURJ 22, 20–27.
Al Olayan, A., Al Hussaini, H., and Jazieh, A. R. (2012). The roles of epidermal growth factor receptor (EGFR) inhibitors in the management of lung cancer. J. Infect. public health 5, S50–S60. doi:10.1016/j.jiph.2012.09.004
Alam, M., Alam, S., Shamsi, A., Adnan, M., Elasbali, A. M., Al-Soud, W. A., et al. (2022). Bax/Bcl-2 cascade is regulated by the EGFR pathway: Therapeutic targeting of non-small cell lung cancer. Front. Oncol. 12, 869672. doi:10.3389/fonc.2022.869672
Arrieta, O., Catalan, R., Guzman-Vazquez, S., Barron, F., Lara-Mejia, L., Soto-Molina, H., et al. (2020). Cost-effectiveness analysis of first and second-generation EGFR tyrosine kinase inhibitors as first line of treatment for patients with NSCLC harboring EGFR mutations. BMC Cancer 20, 829. doi:10.1186/s12885-020-07329-8
Ates, M. P., Karaman, Y., Guntekin, S., and Ergun, M. (2016). Analysis of genetics and risk factors of Alzheimer’s Disease. Neuroscience 325, 124–131. doi:10.1016/j.neuroscience.2016.03.051
Babu, S. K., Prabhakar, V., Ravindranath, L., Latha, J., and Hari, K. (2015). Nagamaddaiah Quinazoline derivatives and its biological significance–a review. Int. J. Curr. Trends Pharm. Res. 3, 997–1010.
Barbuti, A. M., Zhang, G. N., Gupta, P., Narayanan, S., and Chen, Z. S. (2019). “EGFR and HER2 inhibitors as sensitizing agents for cancer chemotherapy,” in Protein kinase inhibitors as sensitizing agents for chemotherapy 4, 1–11.
Bethune, G., Bethune, D., Ridgway, N., and Xu, Z. (2010). Epidermal growth factor receptor (EGFR) in lung cancer: An overview and update. J. Thorac. Dis. 2, 48–51.
Birecree, E., Whetsell, W. O., Stoscheck, C., King, L. E., and Nanney, L. B. (1988). Immunoreactive epidermal growth factor receptors in neuritic plaques from patients with Alzheimer's disease. J. Neuropathol. Exp. Neurol. 47, 549–560. doi:10.1097/00005072-198809000-00006
Branigan, G. L., Torrandell-Haro, G., and Brinton, R. D. (2021). Breast cancer therapies reduce risk of Alzheimer’s disease and dementia: A claims-based retrospective study with clinic to bench implications. Alzheimer's Dementia 17, e055602. doi:10.1002/alz.055602
Cai, Y., Sheng, Z., Dong, Z., and Wang, J. (2023). EGFR inhibitor CL-387785 suppresses the progression of lung adenocarcinoma. Curr. Mol. Pharmacol. 16, 211–216. doi:10.2174/1874467215666220329212300
Cannata, D., Fierz, Y., Vijayakumar, A., and Leroith, D. (2010). Type 2 diabetes and cancer: What is the connection? Mt. Sinai J. Med. A J. Transl. Personalized Med. A J. Transl. Personalized Med. 77, 197–213. doi:10.1002/msj.20167
Cataldo, J. K., Prochaska, J. J., and Glantz, S. A. (2010). Cigarette smoking is a risk factor for alzheimer's disease: An analysis controlling for tobacco industry affiliation. J. Alzheimer's Dis. 19, 465–480. doi:10.3233/JAD-2010-1240
Chen, K., Jiang, X., Wu, M., Cao, X., Bao, W., and Zhu, L.-Q. (2021). Ferroptosis, a potential therapeutic target in Alzheimer’s disease. Front. Cell Dev. Biol. 9, 704298. doi:10.3389/fcell.2021.704298
Chen, Y., Chen, X., Ding, X., and Wang, Y. (2019a). Afatinib, an EGFR inhibitor, decreases EMT and tumorigenesis of Huh-7 cells by regulating the ERK-VEGF/MMP9 signaling pathway. Mol. Med. Rep. 20, 3317–3325. doi:10.3892/mmr.2019.10562
Chen, Y. J., Hsu, C. C., Shiao, Y. J., Wang, H. T., Lo, Y. L., and Lin, A. M. Y. (2019b). Anti-inflammatory effect of afatinib (an EGFR-TKI) on OGD-induced neuroinflammation. Sci. Rep. 9, 2516. doi:10.1038/s41598-019-38676-7
Chi, A., Remick, S., and Tse, W. (2013). EGFR inhibition in non-small cell lung cancer: Current evidence and future directions. Biomark. Res. 1, 2–10. doi:10.1186/2050-7771-1-2
Chiang, H. C., Wang, L., Xie, Z., Yau, A., and Zhong, Y. (2010). PI3 kinase signaling is involved in Abeta-induced memory loss in Drosophila. Proc. Natl. Acad. Sci. U. S. A. 107, 7060–7065. doi:10.1073/pnas.0909314107
Cihan, Y. B. (2019). Lapatinib? Or radiotherapy? In cranial metastasis of breast cancer. Eur. J. Breast Health 15, 205–206. doi:10.5152/ejbh.2019.4874
Cohen, M. H., Johnson, J. R., Chen, Y.-F., Sridhara, R., and Pazdur, R. (2005). FDA drug approval summary: Erlotinib (Tarceva®) tablets. Oncol. 10, 461–466. doi:10.1634/theoncologist.10-7-461
Colclough, N., Chen, K., Johnstrom, P., Strittmatter, N., Yan, Y., Wrigley, G. L., et al. (2021). Preclinical comparison of the blood-brain barrier permeability of osimertinib with other EGFR TKIs. Clin. Cancer Res. 27, 189–201. doi:10.1158/1078-0432.CCR-19-1871
Cramer, P. E., Cirrito, J. R., Wesson, D. W., Lee, C. Y., Karlo, J. C., Zinn, A. E., et al. (2012). ApoE-directed therapeutics rapidly clear beta-amyloid and reverse deficits in AD mouse models. Science 335, 1503–1506. doi:10.1126/science.1217697
De, S., Zhou, H., Desantis, D., Croniger, C. M., Li, X., and Stark, G. R. (2015). Erlotinib protects against LPS-induced endotoxicity because TLR4 needs EGFR to signal. Proc. Natl. Acad. Sci. U. S. A. 112, 9680–9685. doi:10.1073/pnas.1511794112
Dhamodharan, J., Sekhar, G., and Muthuraman, A. (2022). Epidermal growth factor receptor kinase inhibitor ameliorates beta-amyloid oligomer-induced alzheimer disease in Swiss albino mice. Molecules 27, 5182. doi:10.3390/molecules27165182
Discafani, C. M., Carroll, M. L., Floyd, M. B., Hollander, I. J., Husain, Z., Johnson, B. D., et al. (1999). Irreversible inhibition of epidermal growth factor receptor tyrosine kinase with in vivo activity by N-[4-[(3-bromophenyl) amino]-6-quinazolinyl]-2-butynamide (CL-387,785). Biochem. Pharmacol. 57, 917–925. doi:10.1016/s0006-2952(98)00356-6
Dokduang, H., Jamnongkarn, W., Promraksa, B., Suksawat, M., Padthaisong, S., Thanee, M., et al. (2020). In vitro and in vivo anti-tumor effects of pan-HER inhibitor varlitinib on cholangiocarcinoma cell lines. Drug Des. Devel Ther. 14, 2319–2334. doi:10.2147/DDDT.S250061
Emmerzaal, T. L., Kiliaan, A. J., and Gustafson, D. R. (2015). 2003-2013: A decade of body mass index, alzheimer's disease, and dementia. J. Alzheimer's Dis. 43, 739–755. doi:10.3233/JAD-141086
Feng, Y.-C. A., Cho, K., Lindstrom, S., Kraft, P., Cormack, J., Liang, L., et al. (2017). Investigating the genetic relationship between Alzheimer’s disease and cancer using GWAS summary statistics. Hum. Genet. 136, 1341–1351. doi:10.1007/s00439-017-1831-6
Fischer, M., Grossmann, P., Padi, M., and Decaprio, J. A. (2016). Integration of TP53, DREAM, MMB-FOXM1 and RB-E2F target gene analyses identifies cell cycle gene regulatory networks. Nucleic acids Res. 44, 6070–6086. doi:10.1093/nar/gkw523
Fumarola, C., Bonelli, M. A., Petronini, P. G., and Alfieri, R. R. (2014). Targeting PI3K/AKT/mTOR pathway in non small cell lung cancer. Biochem. Pharmacol. 90, 197–207. doi:10.1016/j.bcp.2014.05.011
Ge, M., Zhuang, Y., Zhou, X., Huang, R., Liang, X., and Zhan, Q. (2017). High probability and frequency of EGFR mutations in non-small cell lung cancer with brain metastases. J. neuro-oncology 135, 413–418. doi:10.1007/s11060-017-2590-x
Gen, S., Tanaka, I., Morise, M., Koyama, J., Kodama, Y., Matsui, A., et al. (2022). Clinical efficacy of osimertinib in EGFR-mutant non-small cell lung cancer with distant metastasis. BMC Cancer 22, 654. doi:10.1186/s12885-022-09741-8
Greig, S. L. (2016). Brodalumab: First global approval. Drugs 76, 1403–1412. doi:10.1007/s40265-016-0634-8
Greulich, H., Chen, T.-H., Feng, W., Jänne, P. A., Alvarez, J. V., Zappaterra, M., et al. (2005). Oncogenic transformation by inhibitor-sensitive and-resistant EGFR mutants. PLoS Med. 2, e313. doi:10.1371/journal.pmed.0020313
Guerreiro, R., and Bras, J. (2015). The age factor in Alzheimer's disease. Genome Med. 7, 106. doi:10.1186/s13073-015-0232-5
Gupta, R., Dastane, A. M., Forozan, F., Riley-Portuguez, A., Chung, F., Lopategui, J., et al. (2009). Evaluation of EGFR abnormalities in patients with pulmonary adenocarcinoma: The need to test neoplasms with more than one method. Mod. Pathol. 22, 128–133. doi:10.1038/modpathol.2008.182
Hanahan, D., and Weinberg, R. A. (2011). Hallmarks of cancer: The next generation. Cell 144, 646–674. doi:10.1016/j.cell.2011.02.013
Hotte, S. J., Hirte, H. W., Turner, S., Jonker, D., Fortin, C., Barrett, E., et al. (2009). Abstract B54: A phase 1 study to assess the safety, tolerability and pharmacokinetics of the ErbB-family inhibitor ARRY-334543 in patients with advanced solid tumors. Mol. Cancer Ther. 8, B54. doi:10.1158/1535-7163.targ-09-b54
Hsu, P.-C., Jablons, D. M., Yang, C.-T., and You, L. (2019). Epidermal growth factor receptor (EGFR) pathway, yes-associated protein (YAP) and the regulation of programmed death-ligand 1 (PD-L1) in non-small cell lung cancer (NSCLC). Int. J. Mol. Sci. 20, 3821. doi:10.3390/ijms20153821
Ingelheim, B. (2016). Gilotrif [prescribing information](2014). Ridgefield, CT: Boehringer Ingelheim Pharmaceuticals, Inc.
Jang, H. Y., Oh, J. M., and Kim, I. W. (2022). Drug repurposing using meta-analysis of gene expression in Alzheimer's disease. Front. Neurosci. 16, 989174. doi:10.3389/fnins.2022.989174
Janjigian, Y. Y., Smit, E. F., Groen, H. J., Horn, L., Gettinger, S., Camidge, D. R., et al. (2014). Dual inhibition of EGFR with afatinib and cetuximab in kinase inhibitor-resistant EGFR-mutant lung cancer with and without T790M mutations. Cancer Discov. 4, 1036–1045. doi:10.1158/2159-8290.CD-14-0326
Jia, J.-N., Yin, X.-X., Li, Q., Guan, Q.-W., Yang, N., Chen, K.-N., et al. (2020). Neuroprotective effects of the anti-cancer drug lapatinib against epileptic seizures via suppressing glutathione peroxidase 4-dependent ferroptosis. Front. Pharmacol. 11, 601572. doi:10.3389/fphar.2020.601572
Jiang, J., Greulich, H., Janne, P. A., Sellers, W. R., Meyerson, M., and Griffin, J. D. (2005). Epidermal growth factor-independent transformation of Ba/F3 cells with cancer-derived epidermal growth factor receptor mutants induces gefitinib-sensitive cell cycle progression. Cancer Res. 65, 8968–8974. doi:10.1158/0008-5472.CAN-05-1829
Jiang, T., and Zhou, C. (2014). Clinical activity of the mutant-selective EGFR inhibitor AZD9291 in patients with EGFR inhibitor-resistant non-small cell lung cancer. Transl. Lung Cancer Res. 3, 370–372. doi:10.3978/j.issn.2218-6751.2014.08.02
Jolly, A. A., Nannoni, S., Edwards, H., Morris, R. G., and Markus, H. S. (2022). Prevalence and predictors of vascular cognitive impairment in patients with CADASIL. Neurology 99, e453–e461. doi:10.1212/WNL.0000000000200607
Khaddour, K., Jonna, S., Deneka, A., Patel, J. D., Abazeed, M. E., Golemis, E., et al. (2021). Targeting the epidermal growth factor receptor in EGFR-mutated lung cancer: Current and emerging therapies. Cancers 13, 3164. doi:10.3390/cancers13133164
Kim, J., Kim, S. J., Jeong, H. R., Park, J. H., Moon, M., and Hoe, H. S. (2022). Inhibiting EGFR/HER-2 ameliorates neuroinflammatory responses and the early stage of tau pathology through DYRK1A. Front. Immunol. 13, 903309. doi:10.3389/fimmu.2022.903309
Kiyohara, Y., Yamazaki, N., and Kishi, A. (2013). Erlotinib-related skin toxicities: Treatment strategies in patients with metastatic non-small cell lung cancer. J. Am. Acad. Dermatol 69, 463–472. doi:10.1016/j.jaad.2013.02.025
Kobayashi, S., Boggon, T. J., Dayaram, T., Jänne, P. A., Kocher, O., Meyerson, M., et al. (2005). EGFR mutation and resistance of non–small-cell lung cancer to gefitinib. N. Engl. J. Med. 352, 786–792. doi:10.1056/NEJMoa044238
Krstic, D., and Knuesel, I. (2013). Deciphering the mechanism underlying late-onset Alzheimer disease. Nat. Rev. Neurol. 9, 25–34. doi:10.1038/nrneurol.2012.236
Kuijper, M., Van Hoften, G., Janssen, B., Geurink, R., De Carlo, S., Vos, M., et al. (2015). FEI’s direct electron detector developments: Embarking on a revolution in cryo-TEM. J. Struct. Biol. 192, 179–187. doi:10.1016/j.jsb.2015.09.014
Lai, W.-C. V., Lebas, L., Milia, J., Barnes, T. A., Gautschi, O., Peters, S., et al. (2017). Afatinib in patients with metastatic HER2-mutant lung cancers: An international multicenter study. United States: American Society of Clinical Oncology.
Lee, H.-K., Noh, M. H., Hong, S.-W., Kim, S.-M., Kim, S. H., Kim, Y. S., et al. (2021a). Erlotinib activates different cell death pathways in EGFR-mutant lung cancer cells grown in 3D versus 2D culture systems. Anticancer Res. 41, 1261–1269. doi:10.21873/anticanres.14883
Lee, H. J., Jeon, S. G., Kim, J., Kang, R. J., Kim, S. M., Han, K. M., et al. (2021b). Ibrutinib modulates Aβ/tau pathology, neuroinflammation, and cognitive function in mouse models of Alzheimer's disease. Aging Cell 20, e13332. doi:10.1111/acel.13332
Lemmon, M. A., and Schlessinger, J. (2010). Cell signaling by receptor tyrosine kinases. Cell 141, 1117–1134. doi:10.1016/j.cell.2010.06.011
Liam, C. K. (2019). Central nervous system activity of first-line osimertinib in epidermal growth factor receptor-mutant advanced non-small cell lung cancer. Ann. Transl. Med. 7, 61. doi:10.21037/atm.2018.12.68
Lin, N. U., Carey, L. A., Liu, M. C., Younger, J., Come, S. E., Ewend, M., et al. (2008). Phase II trial of lapatinib for brain metastases in patients with human epidermal growth factor receptor 2–positive breast cancer. J. Clin. Oncol. 26, 1993–1999. doi:10.1200/JCO.2007.12.3588
Liu, B., Diaz Arguello, O. A., Chen, D., Chen, S., Saber, A., and Haisma, H. J. (2020). CRISPR-mediated ablation of overexpressed EGFR in combination with sunitinib significantly suppresses renal cell carcinoma proliferation. PLoS One 15, e0232985. doi:10.1371/journal.pone.0232985
Liu, C. Y., Chu, P. Y., Huang, C. T., Chen, J. L., Yang, H. P., Wang, W. L., et al. (2019). Varlitinib downregulates HER/ERK signaling and induces apoptosis in triple negative breast cancer cells. Cancers (Basel) 11, 105. doi:10.3390/cancers11010105
Lu, Z., Jiang, G., Blume-Jensen, P., and Hunter, T. (2001). Epidermal growth factor-induced tumor cell invasion and metastasis initiated by dephosphorylation and downregulation of focal adhesion kinase. Mol. Cell. Biol. 21, 4016–4031. doi:10.1128/MCB.21.12.4016-4031.2001
Lynch, T. J., Bell, D. W., Sordella, R., Gurubhagavatula, S., Okimoto, R. A., Brannigan, B. W., et al. (2004). Activating mutations in the epidermal growth factor receptor underlying responsiveness of non–small-cell lung cancer to gefitinib. N. Engl. J. Med. 350, 2129–2139. doi:10.1056/NEJMoa040938
Majd, S., Power, J., and Majd, Z. (2019). Alzheimer's disease and cancer: When two monsters cannot Be together. Front. Neurosci. 13, 155. doi:10.3389/fnins.2019.00155
Makhlin, I., Salinas, R. D., Zhang, D., Jacob, F., Ming, G. L., Song, H., et al. (2019). Clinical activity of the EGFR tyrosine kinase inhibitor osimertinib in EGFR-mutant glioblastoma. CNS Oncol. 8, CNS43. doi:10.2217/cns-2019-0014
Mansour, H. M., Fawzy, H. M., El-Khatib, A. S., and Khattab, M. M. (2021a). Inhibition of mitochondrial pyruvate carrier 1 by lapatinib ditosylate mitigates Alzheimer's-like disease in D-galactose/ovariectomized rats. Neurochem. Int. 150, 105178. doi:10.1016/j.neuint.2021.105178
Mansour, H. M., Fawzy, H. M., El-Khatib, A. S., and Khattab, M. M. (2021b). Lapatinib ditosylate rescues memory impairment in D-galactose/ovariectomized rats: Potential repositioning of an anti-cancer drug for the treatment of Alzheimer's disease. Exp. Neurol. 341, 113697. doi:10.1016/j.expneurol.2021.113697
Mansour, H. M., Fawzy, H. M., El-Khatib, A. S., and Khattab, M. M. (2021c). Potential repositioning of anti-cancer EGFR inhibitors in alzheimer's disease: Current perspectives and challenging prospects. Neuroscience 469, 191–196. doi:10.1016/j.neuroscience.2021.06.013
Mansour, H. M., Fawzy, H. M., El-Khatib, A. S., and Khattab, M. M. (2022). Repurposed anti-cancer epidermal growth factor receptor inhibitors: Mechanisms of neuroprotective effects in alzheimer's disease. Neural Regen. Res. 17, 1913–1918. doi:10.4103/1673-5374.332132
Matsumoto, A., Hayashida, T., Takahashi, M., Jinno, H., and Kitagawa, Y. (2018). Antitumor effect of lapatinib and cytotoxic agents by suppression of E2F1 in HER2-positive breast cancer. Mol. Med. Rep. 18, 958–964. doi:10.3892/mmr.2018.9068
Mayeux, R., and Stern, Y. (2012). Epidemiology of alzheimer disease. Cold Spring Harb. Perspect. Med. 2, a006239. doi:10.1101/cshperspect.a006239
Merrick, D. T., Kittelson, J., Winterhalder, R., Kotantoulas, G., Ingeberg, S., Keith, R. L., et al. (2006). Analysis of c-ErbB1/epidermal growth factor receptor and c-ErbB2/HER-2 expression in bronchial dysplasia: Evaluation of potential targets for chemoprevention of lung cancer. Clin. Cancer Res. 12, 2281–2288. doi:10.1158/1078-0432.CCR-05-2291
Mitsudomi, T., and Yatabe, Y. (2010). Epidermal growth factor receptor in relation to tumor development: EGFR gene and cancer. FEBS J. 277, 301–308. doi:10.1111/j.1742-4658.2009.07448.x
Mok, T. S., Wu, Y.-L., Thongprasert, S., Yang, C.-H., Chu, D.-T., Saijo, N., et al. (2009). Gefitinib or carboplatin–paclitaxel in pulmonary adenocarcinoma. N. Engl. J. Med. 361, 947–957. doi:10.1056/NEJMoa0810699
Moosavi, L., and Polineni, R. (2023). “Afatinib,” in StatPearls. (Treasure Island (FL) ineligible companies. Disclosure: Rahul Polineni declares no relevant financial relationships with ineligible companies (United States: StatPearls Publishing).
Morgan, T. M., Koreckij, T. D., and Corey, E. (2009). Targeted therapy for advanced prostate cancer: Inhibition of the PI3K/Akt/mTOR pathway. Curr. cancer drug targets 9, 237–249. doi:10.2174/156800909787580999
Morikawa, A., De Stanchina, E., Pentsova, E., Kemeny, M. M., Li, B. T., Tang, K., et al. (2019). Phase I study of intermittent high-dose lapatinib alternating with capecitabine for HER2-positive breast cancer patients with central nervous system metastases. Clin. Cancer Res. 25, 3784–3792. doi:10.1158/1078-0432.CCR-18-3502
Moy, B., and Goss, P. E. (2007). Lapatinib-associated toxicity and practical management recommendations. Oncologist 12, 756–765. doi:10.1634/theoncologist.12-7-756
Munoz, U., Bartolomé, F., Bermejo, F., and Martín-Requero, Ã. (2008). Enhanced proteasome-dependent degradation of the CDK inhibitor p27kip1 in immortalized lymphocytes from Alzheimer's dementia patients. Neurobiol. aging 29, 1474–1484. doi:10.1016/j.neurobiolaging.2007.03.013
Nishimura, T., Nakamura, K., Yamashita, S., Ikeda, S., Kigure, K., and Minegishi, T. (2015). Effect of the molecular targeted drug, erlotinib, against endometrial cancer expressing high levels of epidermal growth factor receptor. BMC Cancer 15, 957. doi:10.1186/s12885-015-1975-5
Niu, F.-Y., and Wu, Y.-L. (2014). Novel agents and strategies for overcoming EGFR TKIs resistance. Exp. Hematol. Oncol. 3, 2–5. doi:10.1186/2162-3619-3-2
Niu, M., Hu, J., Wu, S., Xiaoe, Z., Xu, H., Zhang, Y., et al. (2014). Structural bioinformatics-based identification of EGFR inhibitor gefitinib as a putative lead compound for BACE. Chem. Biol. Drug Des. 83, 81–88. doi:10.1111/cbdd.12200
Noble, S., and Faulds, D. (1999). Management of advanced non-small cell lung cancer: The potential role of gemcitabine. Dis. Manag. Health Outcomes 5, 167–179. doi:10.2165/00115677-199905030-00005
Nudelman, K. N., Mcdonald, B. C., Lahiri, D. K., and Saykin, A. J. (2019). Biological hallmarks of cancer in Alzheimer’s disease. Mol. Neurobiol. 56, 7173–7187. doi:10.1007/s12035-019-1591-5
Oakman, C., Pestrin, M., Zafarana, E., Cantisani, E., and Di Leo, A. (2010). Role of lapatinib in the first-line treatment of patients with metastatic breast cancer. Cancer Manag. Res. 2, 13–25. doi:10.2147/CMAR.S8951
Ogawa, O., Lee, H. G., Zhu, X., Raina, A., Harris, P. L., Castellani, R. J., et al. (2003). Increased p27, an essential component of cell cycle control, in Alzheimer's disease. Aging Cell 2, 105–110. doi:10.1046/j.1474-9728.2003.00042.x
Ohsaki, Y., Tanno, S., Fujita, Y., Toyoshima, E., Fujiuchi, S., Nishigaki, Y., et al. (2000). Epidermal growth factor receptor expression correlates with poor prognosis in non-small cell lung cancer patients with p53 overexpression. Oncol. Rep. 7, 603–607. doi:10.3892/or.7.3.603
Ozbeyli, D., Sari, G., Ozkan, N., Karademir, B., Yuksel, M., Cilingir Kaya, O. T., et al. (2017). Protective effects of different exercise modalities in an Alzheimer's disease-like model. Behav. Brain Res. 328, 159–177. doi:10.1016/j.bbr.2017.03.044
Porta, C., Paglino, C., and Mosca, A. (2014). Targeting PI3K/Akt/mTOR signaling in cancer. Front. Oncol. 4, 64. doi:10.3389/fonc.2014.00064
Pei, J. J., and Hugon, J. (2008). mTOR-dependent signalling in Alzheimer's disease. J. Cell. Mol. Med. 12, 2525–2532. doi:10.1111/j.1582-4934.2008.00509.x
Prüßing, K., Voigt, A., and Schulz, J. B. (2013). Drosophila melanogaster as a model organism for Alzheimer’s disease. Mol. Neurodegener. 8, 35–12. doi:10.1186/1750-1326-8-35
Purba, E. R., Saita, E.-I., and Maruyama, I. N. (2017). Activation of the EGF receptor by ligand binding and oncogenic mutations: The “rotation model”. Cells 6, 13. doi:10.3390/cells6020013
Robinson, D. R., Wu, Y.-M., Lonigro, R. J., Vats, P., Cobain, E., Everett, J., et al. (2017). Integrative clinical genomics of metastatic cancer. Nature 548, 297–303. doi:10.1038/nature23306
Romano, R., and Bucci, C. (2020). Role of EGFR in the nervous system. Cells 9, 1887. doi:10.3390/cells9081887
Rosell, R., Carcereny, E., Gervais, R., Vergnenegre, A., Massuti, B., Felip, E., et al. (2012). Erlotinib versus standard chemotherapy as first-line treatment for European patients with advanced EGFR mutation-positive non-small-cell lung cancer (EURTAC): A multicentre, open-label, randomised phase 3 trial. lancet Oncol. 13, 239–246. doi:10.1016/S1470-2045(11)70393-X
Roskoski, R. (2019). Small molecule inhibitors targeting the EGFR/ErbB family of protein-tyrosine kinases in human cancers. Pharmacol. Res. 139, 395–411. doi:10.1016/j.phrs.2018.11.014
Ryu, J. K., and Mclarnon, J. G. (2008). Thalidomide inhibition of perturbed vasculature and glial-derived tumor necrosis factor-alpha in an animal model of inflamed Alzheimer's disease brain. Neurobiol. Dis. 29, 254–266. doi:10.1016/j.nbd.2007.08.019
Sabbah, D. A., Hajjo, R., and Sweidan, K. (2020). Review on epidermal growth factor receptor (EGFR) structure, signaling pathways, interactions, and recent updates of EGFR inhibitors. Curr. Top. Med. Chem. 20, 815–834. doi:10.2174/1568026620666200303123102
Schlessinger, J. (2002). Ligand-induced, receptor-mediated dimerization and activation of EGF receptor. Cell 110, 669–672. doi:10.1016/s0092-8674(02)00966-2
Selvaggi, G., Novello, S., Torri, V., Leonardo, E., De Giuli, P., Borasio, P., et al. (2004). Epidermal growth factor receptor overexpression correlates with a poor prognosis in completely resected non-small-cell lung cancer. Ann. Oncol. 15, 28–32. doi:10.1093/annonc/mdh011
Sequist, L. V., Yang, J. C.-H., Yamamoto, N., O'byrne, K., Hirsh, V., Mok, T., et al. (2013). Phase III study of afatinib or cisplatin plus pemetrexed in patients with metastatic lung adenocarcinoma with EGFR mutations. J. Clin. Oncol. 31, 3327–3334. doi:10.1200/JCO.2012.44.2806
Serrano-Pozo, A., Frosch, M. P., Masliah, E., and Hyman, B. T. (2011). Neuropathological alterations in Alzheimer disease. Cold Spring Harb. Perspect. Med. 1, a006189. doi:10.1101/cshperspect.a006189
Shao, F., Gao, Y., Wang, W., He, H., Xiao, L., Geng, X., et al. (2022). Silencing EGFR-upregulated expression of CD55 and CD59 activates the complement system and sensitizes lung cancer to checkpoint blockade. Nat. Cancer 3, 1192–1210. doi:10.1038/s43018-022-00444-4
Shien, K., Ueno, T., Tsukuda, K., Soh, J., Suda, K., Kubo, T., et al. (2012). Knockdown of the epidermal growth factor receptor gene to investigate its therapeutic potential for the treatment of non-small-cell lung cancers. Clin. Lung Cancer 13, 488–493. doi:10.1016/j.cllc.2012.02.003
Sigismund, S., Avanzato, D., and Lanzetti, L. (2018). Emerging functions of the EGFR in cancer. Mol. Oncol. 12, 3–20. doi:10.1002/1878-0261.12155
Smith, J. (2005). Erlotinib: Small-molecule targeted therapy in the treatmentof non-small-cell lung cancer. Clin. Ther. 27, 1513–1534. doi:10.1016/j.clinthera.2005.10.014
Song, X., Liu, Z., and Yu, Z. (2020). EGFR promotes the development of triple negative breast cancer through JAK/STAT3 signaling. Cancer Manag. Res. 12, 703–717. doi:10.2147/CMAR.S225376
Srivatsa, S., Paul, M. C., Cardone, C., Holcmann, M., Amberg, N., Pathria, P., et al. (2017). EGFR in tumor-associated myeloid cells promotes development of colorectal cancer in mice and associates with outcomes of patients. Gastroenterology 153, 178–190.e10. doi:10.1053/j.gastro.2017.03.053
Talbot, K., Wang, H.-Y., Kazi, H., Han, L.-Y., Bakshi, K. P., Stucky, A., et al. (2012). Demonstrated brain insulin resistance in Alzheimer’s disease patients is associated with IGF-1 resistance, IRS-1 dysregulation, and cognitive decline. J. Clin. investigation 122, 1316–1338. doi:10.1172/JCI59903
Tanaka, H., Taima, K., Itoga, M., Ishioka, Y., Baba, K., Shiratori, T., et al. (2019). Real-world study of afatinib in first-line or re-challenge settings for patients with EGFR mutant non-small cell lung cancer. Med. Oncol. 36, 57. doi:10.1007/s12032-019-1278-9
Thomas, R., Zuchowska, P., Morris, A. W., Marottoli, F. M., Sunny, S., Deaton, R., et al. (2016). Epidermal growth factor prevents APOE4 and amyloid-beta-induced cognitive and cerebrovascular deficits in female mice. Acta Neuropathol. Commun. 4, 111. doi:10.1186/s40478-016-0387-3
Van Zandwijk, N. (2003). Tolerability of gefitinib in patients receiving treatment in everyday clinical practice. Br. J. Cancer 89, S9–S14. doi:10.1038/sj.bjc.6601477
Vengoji, R., Macha, M. A., Nimmakayala, R. K., Rachagani, S., Siddiqui, J. A., Mallya, K., et al. (2019). Afatinib and Temozolomide combination inhibits tumorigenesis by targeting EGFRvIII-cMet signaling in glioblastoma cells. J. Exp. Clin. Cancer Res. 38, 266. doi:10.1186/s13046-019-1264-2
Wang, B.-J., Her, G. M., Hu, M.-K., Chen, Y.-W., Tung, Y.-T., Wu, P.-Y., et al. (2017). ErbB2 regulates autophagic flux to modulate the proteostasis of APP-CTFs in Alzheimer’s disease. Proc. Natl. Acad. Sci. 114, E3129–E3138. doi:10.1073/pnas.1618804114
Wang, L., Chiang, H.-C., Wu, W., Liang, B., Xie, Z., Yao, X., et al. (2012). Epidermal growth factor receptor is a preferred target for treating Amyloid-β–induced memory loss. Proc. Natl. Acad. Sci. 109, 16743–16748. doi:10.1073/pnas.1208011109
Wang, L., Liang, B., and Zhong, Y. (2013). Reduced EGFR level potentially mediates the Aβ42-induced neuronal loss in transgenic fruit fly and mouse. Protein Cell 4, 647–649. doi:10.1007/s13238-013-3043-8
White, M. C., Holman, D. M., Boehm, J. E., Peipins, L. A., Grossman, M., and Henley, S. J. (2014). Age and cancer risk: A potentially modifiable relationship. Am. J. Prev. Med. 46, S7–S15. doi:10.1016/j.amepre.2013.10.029
Wong, R. W., and Guillaud, L. (2004). The role of epidermal growth factor and its receptors in mammalian CNS. Cytokine Growth Factor Rev. 15, 147–156. doi:10.1016/j.cytogfr.2004.01.004
Yang, C. H., Chou, H. C., Fu, Y. N., Yeh, C. L., Cheng, H. W., Chang, I. C., et al. (2015). EGFR over-expression in non-small cell lung cancers harboring EGFR mutations is associated with marked down-regulation of CD82. Biochim. Biophys. Acta 1852, 1540–1549. doi:10.1016/j.bbadis.2015.04.020
Yewale, C., Baradia, D., Vhora, I., Patil, S., and Misra, A. (2013). Epidermal growth factor receptor targeting in cancer: A review of trends and strategies. Biomaterials 34, 8690–8707. doi:10.1016/j.biomaterials.2013.07.100
Yu, X., Sharma, K. D., Takahashi, T., Iwamoto, R., and Mekada, E. (2002). Ligand-independent dimer formation of epidermal growth factor receptor (EGFR) is a step separable from ligand-induced EGFR signaling. Mol. Biol. Cell 13, 2547–2557. doi:10.1091/mbc.01-08-0411
Yun, C.-H., Mengwasser, K. E., Toms, A. V., Woo, M. S., Greulich, H., Wong, K.-K., et al. (2008). The T790M mutation in EGFR kinase causes drug resistance by increasing the affinity for ATP. Proc. Natl. Acad. Sci. 105, 2070–2075. doi:10.1073/pnas.0709662105
Zhang, D.-D., Ou, Y.-N., Yang, L., Ma, Y.-H., Tan, L., Feng, J.-F., et al. (2022). Investigating the association between cancer and dementia risk: A longitudinal cohort study. Alzheimer's Res. Ther. 14, 146–212. doi:10.1186/s13195-022-01090-9
Zhang, W., Wei, Y., Yu, D., Xu, J., and Peng, J. (2018). Gefitinib provides similar effectiveness and improved safety than erlotinib for east asian populations with advanced non–small cell lung cancer: A meta-analysis. BMC cancer 18, 780–814. doi:10.1186/s12885-018-4685-y
Zhang, Y., Miao, S., Wang, F., Fang, W., Chen, G., Chen, X., et al. (2017). The efficacy and toxicity of afatinib in advanced EGFR-positive non-small-cell lung cancer patients after failure of first-generation tyrosine kinase inhibitors: A systematic review and meta-analysis. J. Thorac. Dis. 9, 1980–1987. doi:10.21037/jtd.2017.06.08
Zhang, Z., Lee, J. C., Lin, L., Olivas, V., Au, V., Laframboise, T., et al. (2012). Activation of the AXL kinase causes resistance to EGFR-targeted therapy in lung cancer. Nat. Genet. 44, 852–860. doi:10.1038/ng.2330
Keywords: Alzheimer’s disease, EGFR, EGFR inhibitor, cancer, Aβ, learning and memory
Citation: Choi H-J, Jeong YJ, Kim J and Hoe H-S (2023) EGFR is a potential dual molecular target for cancer and Alzheimer’s disease. Front. Pharmacol. 14:1238639. doi: 10.3389/fphar.2023.1238639
Received: 13 June 2023; Accepted: 18 July 2023;
Published: 02 August 2023.
Edited by:
Guangrong Zheng, University of Florida, United StatesReviewed by:
Heba Mansour, Egyptian Drug Authority (EDA), EgyptZhixing Wu, University of Florida, United States
Copyright © 2023 Choi, Jeong, Kim and Hoe. This is an open-access article distributed under the terms of the Creative Commons Attribution License (CC BY). The use, distribution or reproduction in other forums is permitted, provided the original author(s) and the copyright owner(s) are credited and that the original publication in this journal is cited, in accordance with accepted academic practice. No use, distribution or reproduction is permitted which does not comply with these terms.
*Correspondence: Jieun Kim, jieunkim@kangwon.ac.kr; Hyang-Sook Hoe, sookhoe72@kbri.re.kr
†These authors have contributed equally to this work